- 1B lymphocytes, Autoimmunity and Immunotherapies (LBAI), Mixed Research Unit (UMR)1227 INSERM, University of Brest, Brest, France
- 2Service d’Odontologie, University Hospital (CHU) de Brest, Brest, France
Periodontal diseases are common inflammatory conditions characterized by bone loss in response to simultaneous bacterial aggression and host defenses. The etiology of such diseases is still not completely understood, however. It has been shown that specific pathogens involved in the build-up of dysbiotic biofilms participate actively in the establishment of periodontitis. This multifactorial pathology also depends on environmental factors and host characteristics, especially defenses. The immune response to the pathogens seems to be critical in preventing the disease from starting but also contributes to tissue damage. It is known that small molecules known as antimicrobial peptides (AMPs) are key actors in the innate immune response. They not only target microbes, but also act as immuno-modulators. They can help to recruit or activate cells such as neutrophils, monocytes, dendritic cells, or lymphocytes. AMPs have already been described in the periodontium, and their expression seems to be connected to disease activity. Alpha and beta defensins and LL37 are the AMPs most frequently linked to periodontitis. Additionally, leukocyte infiltrates, especially B-cells, have also been linked to the severity of periodontitis. Indeed, the particular subpopulations of B-cells in these infiltrates have been linked to inflammation and bone resorption. A link between B-cells and AMP could be relevant to understanding B-cells’ action. Some AMP receptors, such as chemokines receptors, toll-like receptors, or purinergic receptors, have been shown to be expressed by B-cells. Consequently, the action of AMPs on B—cell subpopulations could participate to B-cell recruitment, their differentiation, and their implication in both periodontal defense and destruction.
Introduction
Periodontal diseases are inflammatory conditions with an infectious etiology that can involve various pathogens such as Aggregatibacter actinomycetemcomitans, Fusobacterium nucleatum, Porphyromonas gingivalis, Prevotella intermedia, Treponema denticola, and Treponema forsythia (1). Periodontal diseases are the most common oral diseases in the world, with the moderate form affecting 45–50% of adults and the severe form 9–11% (2, 3). They can be divided into two main types: gingivitis and periodontitis. Periodontitis are chronic, multifactorial, immuno-inflammatory diseases associated with dysbiotic bacterial biofilms. They are characterized by the progressive destruction of the supporting apparatus of the teeth, often leading to tooth loss and finally to bone resorption (4, 5). The appearance and evolution of these diseases are dependent not only on the pathogenicity of periodonto-pathogenic bacteria, but also on genetics and environmental and behavioral risk factors that affect host susceptibility (1). In addition to being a worldwide public health concern, these diseases provide a strategic model to study inflammatory processes. The oral localization of the periodontium provides access for both clinical assessment and tissue biopsy, facilitating longitudinal studies. Furthermore, the mouth is the first place for most interactions between the self and non-self and is involved in immune system training from birth with probable heritability of microbiota (6). Any advances in the understanding of periodontal disease etiopathogenesis could improve the overall knowledge of chronic inflammation and some systemic conditions such as cardio-metabolic, neurodegenerative, and autoimmune diseases and cancer (1).
In the oral cavity, when facing periodontal pathogens, the innate and adaptive immune systems cooperate to combat this bacterial attack (7). One mechanism of the innate immune system to fight oral infections is the release of antimicrobial peptides (AMPs) (8–10). This appellation gathers small molecules (between 10 and 60 amino acids) expressed in animals with, most of the time, cationic properties (11). Produced by many cells, including neutrophils, macrophages, dendritic cells, and even lymphocytes, AMPs play an important role in innate immunity due to their rapid and broad-spectrum antimicrobial activity (12–15). These molecules are capable of neutralizing a large number of pathogenic microorganisms, including bacteria, fungi, parasites, and viruses (16–18). They have also been shown to perform various biological activities within the innate and adaptive immune systems (18–23). They can induce both chemoattraction and/or cell activation, and they also play a role in inflammation resolution (20). Their known targets are mainly neutrophils, monocytes, dendritic cells, and, to a lesser extent, T cells (24). They can also work in synergy with the cell mediator’s cytokine and chemokine, as has been shown for HNP1and RANTES (25) or IL1β (26).
AMPs have also been suggested to potentiate the innate immune response and function as a bridge between innate and adaptive immunity by regulating B and T lymphocytes and natural killer cells (27–29). Indeed, an increasing number of studies have been conducted on the action of AMPs in the regulation of T-cells (30, 31) mainly with TH17 response linked to cathelicidins, but few have explored B-cells, more precisely plasma cells (32). Until recently, the immunomodulatory functions of AMPs have been poorly studied for periodontitis, as most of the approaches were focused on their antimicrobial roles. The first therapeutical approach to these diseases was to target pathogenic bacteria, and AMPs are promising candidates for this purpose (33). However, managing the host reaction seems to be crucial to reach a potential curative strategy, as the appearance of the disease cannot be reduced to only a bacterial presence (34). Furthermore, AMPs are in the frontier between these two strategies, as they can act on both bacteria and leucocytes. Recent research on the immune response during periodontitis has underlined the role of B-cells in both defense mechanisms and bone resorption (35). Few connections, however, have been reported between B-cells and AMPs. This blind spot could be a critical handicap in the understanding of periodontal disease etiology and, more widely, chronic inflammatory diseases. The objective of this review is to answer to the pertinence of studying AMP action of B-cells. In this purpose, it is first needed to sum up which peptides are relevant in periodontal disease, what impact B-cells have on periodontitis, and if they can be a target for AMP. Peptides sharing both a role in periodontitis and a potential receptor on B-cells would be promising candidates for further analysis.
Functions of antimicrobial peptides in periodontal diseases
Oral infections are first managed by the innate immune system, especially with AMPs. Salivary gland epithelial cells, neutrophils, monocytes, and potentially other cells secrete these AMPs in the oral cavity. Their antimicrobial activity against oral pathogenic bacteria and their biofilms plays a central role in oral microbiota homeostasis (33). Three major families of AMP are found in saliva: defensins, cathelicidins, and histatins (34, 36). AMPs are primarily known for their antibacterial activity (elimination or inhibition of the growth of these microorganisms). In addition, they show antifungal and/or antiviral effects against a large number of other microorganisms (37, 38) and even exhibit antitumoral properties (39). Theses lytic activities are linked to AMPs’ capacity to create pores in their targets.
Independently of their antimicrobial activities, AMPs may play a role in the inflammatory response and the immune response, even serving as a link between the innate and adaptive immune responses (35). Their antimicrobial effect and their role in the regulation of immune responses are two aspects of particular interest in relation to periodontal disease. Natural AMPs have shown antibacterial effects against periodontal pathogens. Healthy subjects show distinct levels of natural AMPs compared to those with periodontitis (40), with most studies reporting higher levels of LL37, beta defensins, or HNP1, although the result remains heterogeneous between studies. The most studied AMPs in relation to the periodontal state are LL-37 and alpha and beta defensins (41).
In the literature, several roles have been reported for AMPs in periodontal disease, such as inhibiting the growth of pathogenic bacteria (LL37, HBD-1, HBD-2, HBD-3, histatin-2, HNP1, HNP2, HNP3), promoting periodontal tissue healing (LL37, HBD-1, HBD-2, HBD-3, histatin-2), promoting bone healing (HBD-2, histatin-1), and serving as a potential indicator of the severity of periodontal disease (HBD-2, HNP1, HNP2, HNP3). Additionally, AMPs have been reported to promote osteogenic differentiation and reduce bone loss (42). Histatin-5 has been shown to be an inhibitor of host and bacterial enzymes involved in periodontal destruction (43). Among natural AMPs, HBD are expressed in the buccal bone (44). HBD have been shown to promote the proliferation of human mesenchymal stem cells, osteoblasts, and keratinocytes in cultures (45). In addition, HBD and histatin-1 promote bone regeneration and prevent infection (46–48).
All these findings suggest that the AMPs relevant to the management of periodontal diseases could be LL-37, alpha and beta defensins, and histatins. In contexts other than the oral cavity immunity, these AMPs have all been described as immune modulators. Alpha defensins have been shown to inhibit monocyte/macrophage-produced pro-inflammatory cytokines such as IL6 and IL1β and to increase leucocyte recruitment directly or indirectly. Beta defensins can either be decreased or increased (49). Similar chemoattraction has been reported for beta defensins and LL37 with additional impact on immune cells’ signaling pathways (49). LL37 is strongly connected to neutrophil extracellular traps (NETs): DNA strands, released by dying neutrophils, that are covered by multiple proteins and peptides that play critical roles during both inflammation and microbial neutralization (50). These NETs activate BAFF production, which is a major B-cell activator (50). This activation could be critical, as neutrophils are strongly connected to periodontal diseases, and B-cells have been shown to be effectors of a part of periodontitis pathogenesis.
Functions of B-cells in periodontal diseases
The ontogenesis of B-cells takes place in the bone marrow from pluripotent hematopoietic stem cells (51). The latter form lymphocyte progenitors, some of which migrate to the thymus for the formation of T cells, while the rest remain in the bone marrow to form B-cells until the immature B stage. During antigen-independent maturation in the marrow, there are four distinct stages: the early pro-B cell, the late pro-B cell, the pre-B cell (precursor B), and the immature B cell. Immature B-cells then migrate to secondary lymphocyte organs (spleen, lymph nodes, tonsils, and lymphoid tissues associated with mucous membranes) in the form of transitional B-cells (52). These transitional B-cells transform into either marginal zone cells or follicular cells. All of these cells undergo a succession of T-independent or T-dependent differentiation, leading to the formation of memory cells or plasma cells (52).
Few studies have highlighted the roles of B-cells in periodontitis. Certain roles of B-cells in periodontitis have been recently discovered, however. A review of the literature showed the role of B-cells in periodontitis and the potential interest in using B-cells as a target for new treatments for severe periodontitis (53). Additionally, a study carried out by Demoersman highlighted the crucial role of B-cells in periodontal disease with an increase of memory B-cells and a reduction of their regulatory counterpart in severe forms (54). It has also been shown that anti-B lymphocyte therapy could be beneficial in improving periodontitis, suggesting a major role of B-cells in this disease (55). The 2015 study by Abe et al. 2015 suggests that B-cells have a more important role than T cells in bone resorption (56). It has been reported that, in periodontitis, the presence of B-cells specific to periodontal pathogenic bacteria is essential for the establishment of the bone resorption characteristic of periodontitis (57). Some authors have also suggested an involvement of B-cells in bone remodeling (58–60).
Bacterial infection remains important in the etiopathogenesis of periodontal disease. An immuno-inflammatory response is set up by the body against this bacterial infection. This reaction is the origin of the destruction of supporting tissues of the tooth, loss of attachment, and alveolar bone lysis. Inflammation-induced osteoclast genesis in periodontal disease involves several pathways of mechanisms that involve several biological molecules and their products. Cells of the B lymphoid lineage can contribute to the physiopathology of bone disorders by regulating osteoclast genesis in the context of periodontal infection through several pathways of mechanisms. The molecular mechanism pathways by which cells of the B lymphoid lineage regulate osteoclast genesis in periodontal disease have become better understood in recent years. As well as their antibody secretion, B-cells contribute to the destruction of the alveolar bone in RANKL-dependent periodontitis (61). B-cells express RANKL, a protein involved in osteoclast differentiation, activation, and survival. RANKL then binds to its RANK receptor expressed by osteoclast precursor cells and preosteoclasts to stimulate their differentiation into osteoclasts resorbing alveolar bone (59, 62–65). In periodontitis, the main source of RANKL are T-cells and B-cells (66), either of which themselves serve as progenitors of osteoclasts. Normal pro-B-cells may serve as osteoclast progenitor cells (67).
B-cells express SOFAT, an osteoclastogenic cytokine independent of RANKL. By stimulating osteoblasts, SOFAT modulates the production of osteoclastogenic cytokines and contributes to osteoclast formation and bone destruction in periodontitis. Human B-cells, plasma cells, and T-cells express SOFAT, which is a bone-destroying factor in periodontal disease lesions (68–70).
Pathogens that breach periodontal tissue express molecular patterns associated with pathogenic agents (PAMPs) such as lipopolysaccharides, lipoteichoic acid, peptidoglycan, bacterial DNA, and double-stranded RNA (71, 72). These PAMPs can be recognized by receptors such as Toll-like receptors (TLR) expressed on immune cells: macrophages, Langerhans cells, dendritic cells, and polymorphonuclear neutrophils. They can also be recognized by epithelial cells, gingival fibroblasts, fibroblasts of the periodontal ligament, osteoblasts, osteoclasts endothelial cells, and even lymphocytes (73, 74). The interaction between macrophages, dendritic cells, neutrophils, and PAMPs via TLRs leads to the production of pro-inflammatory cytokines and chemokines such as TNF-α, IL-1, IL-6 (CXCL-8: IL-8), IL-12, and IL-18. It should be noted, however, that dendritic cells act as antigen-presenting cells for B and T cells (19, 75–77). Neutrophils are one of the first inflammatory cells to arrive at the site of periodontal inflammation by chemotaxis (78), following chemoattractant such as IL-8 secreted by oral epithelial cells, connective fibroblasts, and immune cells (79) and growth-related gene product-α (80). Neutrophils contain cytoplasmic granules that in turn contain lytic enzymes and molecules with antimicrobial properties such as cathepsins, lactoferrin, lysozyme, and defensins, which destroy microorganisms (81).
Defensins are AMPs known for their antibacterial activity (elimination or total inhibition of the growth of these microorganisms), and several roles have been attributed to them in relation to periodontal diseases, such as inhibiting the growth of pathogenic bacteria, promoting the healing of periodontal tissues, promoting bone healing, and serving as a potential indicator of the severity of periodontal disease (42). TNF-α, IL-1, and IL-6 are osteotropic cytokines that stimulate osteoclast resorption in periodontitis (82) and are found in higher concentrations in patients with periodontal disease than in healthy individuals (83).
Cells of the B lymphoid lineage can participate in osteoclast genesis through two main pathways (Figure 1):
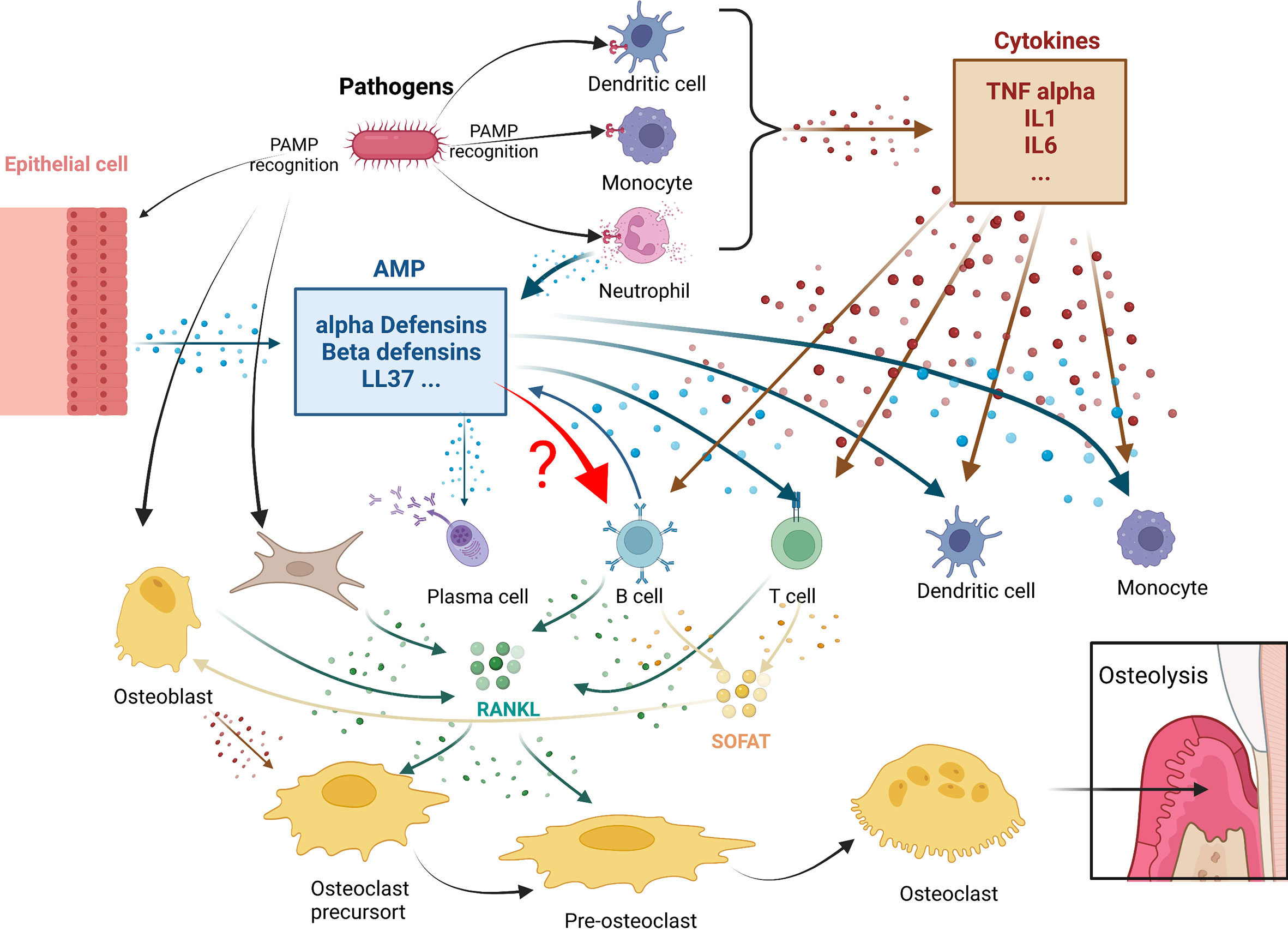
Figure 1 Antimicrobial peptides immunomodulation in periodontitis osteolysis: potential connections with leukocytes. In periodontitis, pathogens triggers both cytokines (in brown) or antimicrobial peptides (AMPS) (in blue) production. Both mediator’s categories are known to play roles in leukocytes actions. A crucial step in these diseases is the expression of RANKL and SOFAT. B and T cells, which are the main source of RANKL and SOFAT, are central in this orchestration of osteoclast differentiation and activity leading to osteolysis. The effect of AMPs in B in this environment still remain to be determined.
1. The B-cells express RANKL, the main factor involved in the differentiation, activation, and survival of osteoclasts. TNF-α secreted by macrophages and dendritic cells can stimulate osteoblasts, T cells, and B-cells to produce RANKL. In addition, periodontal ligament fibroblasts and gingival fibroblasts can regulate osteoclast activity by secreting RANKL (58, 84).
2. The B-cells express SOFAT, which is a bone-destroying factor in periodontal disease lesions (70), independent of RANKL. By co-opting osteoblasts to increase production of osteoclastogenic cytokines, SOFAT can exacerbate inflammation and promote osteoclast formation and bone destruction (68).
In all these interactions that occur during periodontal disease, however, it is not known whether there is a direct relation between AMPs and B-cells.
Can antimicrobial peptides affect the function of B-cells in periodontal diseases?
Most of the effects of AMPs on host cells are mediated via the specific activation of various cell surface receptors, membrane channels, or specific intracellular targets and pathways (85, 86). Relations have been established between the LL-37 peptide and at least nine receptors of different classes, including four G-protein-coupled receptors, three receptor tyrosine kinases, a ligand-gated ion channel, and TLRs (87, 88) (Figure 2). Several receptors and pathways involved in the immune functions of human beta defensins have been studied, such as TLRs, receptors of the purinoceptor family, and chemokine receptors (85) (Figure 2). Some receptors have been described for beta defensin, such as CCR6, CCR4, CCR2, TLR1/2/9, and P2X7 (85). However, specific alpha-defensin (HNP) receptors have not yet been clearly identified, only a G-protein-coupled receptor response has been confirmed so far (85). B-cell expression of some of these receptors conditioned their responsiveness to AMPs. Many chemokine receptors regarded as AMP receptors are expressed on T cells but can also be found on human B-cells, such as CXCR4 (89), CXCR5, CXCR3, CCR7, CCR1 (90, 91), CCR2 (92, 93), and CCR6 (94, 95). CCR6 probably plays an important role in B-cell trafficking in humans and is established as an efficient receptor on human B-cells (92). B-cells express several TLRs. TLR1, TLR2, TLR4, TLR5, TLR6, and TLR10 receptors are expressed on the cell membrane, while TLR3, TLR7, TLR8, and TLR9 are expressed in the endosomes. Some of them can identify AMPs such as TLR1, TLR2, and TLR9 (95–100). Their signaling in B-cells is related to the stage of activation and the tissue situation of the B-cells (101). It has also been reported that certain receptors of the purinoceptor family, such as P2X7 and P2Y11, are expressed on B-cells (102, 103).
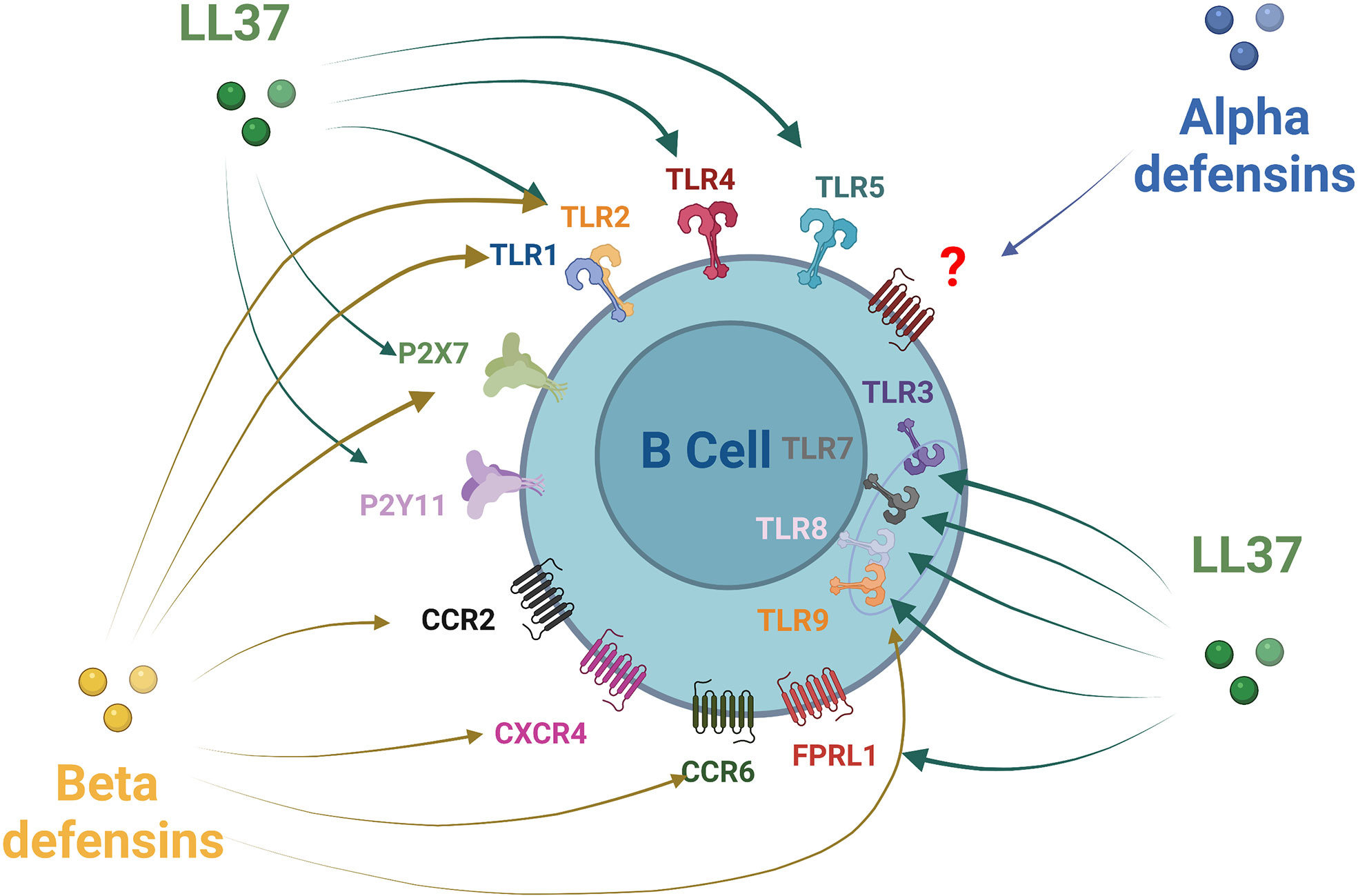
Figure 2 Antimicrobial peptides receptors expression on B-cells. Alpha defensin has been shown to recruit B-cells through a non-identified Protein G Coupled Receptor. Beta defensin family receptors are more documented with an action of HBD1 and HBD2 on CCR6 and HBD3 via CCR6, CCR2, CXCR4, P2X7, TLR1, TLR2, and TLR9. LL37 act on a larger number of receptors that can be expressed by B-cells: TLRs1-5, TLRs7-9, FPRL1 P2X7, and P2Y11.
Furthermore, the literature also mentions that B-cells themselves express AMPs such as alpha defensins (HNP-1–3), HBD-2, and cathelicidin LL-37 in the presence of pathogens such as Aggregatibacter actinomycetemcomitans (104, 105). Thus, B-cells can produce some of the AMPs found in periodontal tissue and potentially react to them. For now, however, the only clear link between B-cell lineage and their response to AMPs is the IgG production induced in plasma cells by LL-37 associated with NETs during systemic lupus erythematosus (32). If this IgG production is useful to predict the installation of the disease and participate in host defense. initially, no direct connection to osteolysis, the major concern in periodontitis, was described (106). More recently, anti-carbamylated LL37 autoantibodies have been linked to an increase in bone resorption in RA (107). Auto-immunity and periodontitis are connected in many ways. Patients with auto-immune conditions such as Lupus or RA are known to be more prone to developing periodontitis (55, 108). Bone destruction in periodontitis shares similarities with tissue damage observed during RA and presents a TH17 cytokines overexpression that can be also found in RA, Lupus psoriasis, and many other auto-immune conditions (109, 110). In addition, AMPs’ participation in autoimmunity has been investigated in the past few years. High concentrations of AMPs have been found in sera of Lupus patients or the joints of RA patients (107, 111, 112). For now, AMPs’ immunomodulatory role in autoimmunity is not fully understood, probably due to the difficulty in overcoming AMPs/cytokines/chemokines’ roles redundancy combined with dual detrimental and protective effects (113, 114). Regardless, the role of autoantibodies against AMPs can be critical in autoimmunity. LL37/DNA complexes during NETs release can be carbamylated or citrullinated during autoimmune disease, which can be the result of oral cavities pathogens such as PG or AA (115, 116). Modified LL37s can then be recognized by specific autoantibodies leading to the formation of immune complexes that have been described in Lupus, auto-immunes vasculitis, psoriasis, and RA (117–119). The fact that in RA such autoantibodies participate in osteolysis strengthens the idea of the implication of AMP in autoimmunity but also in inflammatory contexts such as periodontitis.
All these elements point to an implication of B-cells by their production of autoantibodies against AMPs in autoimmunity with, in parallel, a potential immunomodulatory action that remains to be investigated. For now, however, no causal relationship has been established between AMPs’ immunomodulation and B-cells participation in osteolysis. Presence of autoantibodies against AMPs could be of interest as an actor of osteolysis and potentially as a biomarker in diseases associated with bone self-destruction. As previously mentioned, a large number of AMPs have been identified in the oral cavity, and several exhibit antimicrobial effects against periodontal pathogens. They offer a broad spectrum of roles (antibacterial, antiviral, and/or antifungal or immunomodulation activities) that are critical in periodontal diseases. These characteristics make these natural molecules promising candidates for anti-infection strategies. Moreover, it has been proposed that the differential regulation of AMPs in periodontal disease makes them relevant biomarkers for the disease in saliva and gingival fluid (41). Promising strategies in the treatment of periodontitis can be derived from AMPs in periodontal diseases. They could be considered as a potential alternative to traditional antimicrobial therapy (antibiotics) in periodontal infections (38). Nevertheless, their immunomodulatory action must be better understood to avoid any unwanted effect. In this regard, B-cells’ involvement in AMP immunomodulation also needs to be known.
Conclusion
As only sporadic information is available on the effect of AMPs on B-cells, it may be tempting to think that they have no or few connections. However, the B lymphocyte family consists of various subtypes with specific patterns of receptors and functions. Global analysis of lymphocytes tends to be disconnected from functional reality, and the results are unclear due to the heterogeneity and low number of each category of cell. The only way to overcome this limitation is to study each lymphocyte subpopulation. This, however, has not been done for the immunomodulatory action of AMPs on B-cells. Only plasma cells and LL37 have been functionally connected. An in vivo or ex vivo tissue analysis would be convenient to assess this link due to the small B-cell subpopulation. In addition, AMPs have been shown to be critical in both the initiation and the chronicity of inflammation. As AMPs are already known to influence and participate actively in both the initiation and the persistence of inflammation (120), it would be unlikely that they are not involved in periodontitis-related immune system dysregulation. Recent studies on pathogenesis tend to involve more and more B-cells in osteolysis processes. An interaction between B-cells and AMPs could explain the pathogenic action of B-cells. As they can carry some identified AMP receptors, they should react to their presence.
A parallel is frequently made between periodontitis and auto-immune diseases.
In addition, periodontal diseases are chronic inflammatory conditions with changing inflammatory states (1). Longitudinal studies are still rare but may be needed to truly understand the molecular mechanisms. This time-dependent disease activity could explain why studies on AMP presence in periodontitis are frequently contradictory, with AMPs such as LL37 or defensins linked to both protective and pathogenic patterns of the disease. In a similar way, the action of B-cells depends on the result of each subpopulation action found at the same time in tissue. Regulatory B-cells seem decreased or inefficient in situations involving chronic inflammation, which has been confirmed in periodontitis (54). The variability of B-cell subpopulations seems to share some patterns with AMP presence. Elucidation of the relationship between AMPs and B-cells could clarify why the immune system participates in periodontal tissue destruction during its struggle against pathogens, and may clarified whether or not periodontitis can be considered as an autoimmune-like condition. Furthermore, this understanding could open new possibilities for treatments involving the targeting of B-cells and/or AMPs to optimize the immune response and reduce self-damage.
Data availability statement
The original contributions presented in the study are included in the article/supplementary material. Further inquiries can be directed to the corresponding author.
Author contributions
All authors contributed to the article and approved the submitted version.
Conflict of interest
The authors declare that the research was conducted in the absence of any commercial or financial relationships that could be construed as a potential conflict of interest.
Publisher’s note
All claims expressed in this article are solely those of the authors and do not necessarily represent those of their affiliated organizations, or those of the publisher, the editors and the reviewers. Any product that may be evaluated in this article, or claim that may be made by its manufacturer, is not guaranteed or endorsed by the publisher.
References
1. Hajishengallis G, Chavakis T. Local and systemic mechanisms linking periodontal disease and inflammatory comorbidities. Nat Rev Immunol (2021) 21(7):426–40. doi: 10.1038/s41577-020-00488-6
2. Nazir MA. Prevalence of periodontal disease, its association with systemic diseases and prevention. Int J Health Sci (2017) 11(2):72–80.
3. Chapple IL, Bouchard P, Cagetti MG, Campus G, Carra MC, Cocco F, et al. Interaction of lifestyle, behaviour or systemic diseases with dental caries and periodontal diseases: consensus report of group 2 of the joint EFP/ORCA workshop on the boundaries between caries and periodontal diseases. J Clin Periodontol (2017) 44 Suppl 18:S39–51. doi: 10.1111/jcpe.12685
4. Kinane DF, Stathopoulou PG, Papapanou PN. Periodontal diseases. Nat Rev Dis Primers (2017) 3(1):1–14. doi: 10.1038/nrdp.2017.38
5. Papapanou PN, Sanz M, Buduneli N, Dietrich T, Feres M, Fine DH, et al. Periodontitis: Consensus report of workgroup 2 of the 2017 world workshop on the classification of periodontal and peri-implant diseases and conditions. J Periodontology (2018) 89(S1):S173–S182. doi: 10.1111/jcpe.12946
6. Kaan AMM, Kahharova D, Zaura E. Acquisition and establishment of the oral microbiota. Periodontol 2000 (2021) 86(1):123–41. doi: 10.1111/prd.12366
7. Wiesner J, Vilcinskas A. Antimicrobial peptides: The ancient arm of the human immune system. Virulence (2010) 1(5):440–464. doi: 10.4161/viru.1.5.12983
8. Boman HG. Peptide antibiotics and their role in innate immunity. Annu Rev Immunol (1995) 13(1):61–92. doi: 10.1146/annurev.iy.13.040195.000425
9. Pasupuleti M, Schmidtchen A, Malmsten M. Antimicrobial peptides: Key components of the innate immune system. Crit Rev Biotechnol (2012) 32(2):143–171. doi: 10.3109/07388551.2011.594423
10. Izadi N, Keikha M, Ghazvini K, Karbalaei M. Oral antimicrobial peptides and new therapeutic strategies for plaque-mediated diseases. Gene Rep (2020), 21:100811. doi: 10.1016/j.genrep.2020.100811
11. Lazzaro BP, Zasloff M, Rolff J. Antimicrobial peptides: Application informed by evolution. Science. (2020) 368(6490):5480. doi: 10.1126/science.aau5480
12. De la Fuente-Núñez C, Silva ON, Lu TK, Franco OL. Antimicrobial peptides: Role in human disease and potential as immunotherapies. Pharmacol Ther (2017) 178:132–140. doi: 10.1016/j.pharmthera.2017.04.002
13. Ganz T. Defensins: Antimicrobial peptides of innate immunity. Nat Rev Immunol (2003) 3(9):710–720. doi: 10.1038/nri1180
14. Hoffmann JA, Kafatos FC, Janeway CA, Ezekowitz RAB. Phylogenetic perspectives in innate immunity. Science (1999) 284(5418):1313–1318. doi: 10.1126/science.284.5418.1313
15. Wang YJ, Traum D, Schug J, Gao L, Liu C, HPAP Consortium, et al. Multiplexed In situ imaging mass cytometry analysis of the human endocrine pancreas and immune system in type 1 diabetes. Cell Metab (2019) 29:769–783.e4. doi: 10.1016/j.cmet.2019.01.003
16. Mueller SN, Gebhardt T, Carbone FR, Heath WR. Memory T cell subsets, migration patterns, and tissue residence. Annu Rev Immunol (2013) 31(1):1377–161. doi: 10.1146/annurev-immunol-032712-095954
17. Rowley DA, Fitch FW. The road to the discovery of dendritic cells, a tribute to Ralph Steinman.Cell Immunol. (2012) 273(2): 95–8. doi: 10.1016/j.cellimm.2012.01.002
18. Ohlrich E, Cullinan M, Seymour G. The immunopathogenesis of periodontal disease. Aust Dental J (2009) 54:S2–S10. doi: 10.1111/j.1834-7819.2009.01139.x
19. Silva ON, de la Fuente-Núñez C, Haney EF, Fensterseifer ICM, Ribeiro SM, Porto WF, et al. An anti-infective synthetic peptide with dual antimicrobial and immunomodulatory activities. Sci Rep (2016) 6(1):17–11. doi: 10.1038/srep35465
20. Choi K-Y, Chow LNY, Mookherjee N. Cationic host defence peptides: Multifaceted role in immune modulation and inflammation. J Innate Immun (2012) 4:361–70. doi: 10.1159/000336630
21. Lai Y, Gallo RL. AMPed up immunity: How antimicrobial peptides have multiple roles in immune defense. Trends Immunol (2009) 30(3):131–141. doi: 10.1016/j.it.2008.12.003
22. Shin D-M, & Jo E-K. Antimicrobial peptides in innate immunity against mycobacteria. Immune Network (2011) 11(5):245. doi: 10.4110/in.2011.11.5.245
23. Steinstraesser L, Kraneburg U, Jacobsen F, & Al-Benna S. Host defense peptides and their antimicrobial-immunomodulatory duality. Immunobiology (2011) 216(3):322–333. doi: 10.1016/j.imbio.2010.07.003
24. Zhang QY, Yan ZB, Meng YM, Hong XY, Shao G, Ma JJ, et al. Antimicrobial peptides: mechanism of action, activity and clinical potential. Mil Med Res (2021) 8(1):48. doi: 10.1186/s40779-021-00343-2
25. Alard JE, Ortega-Gomez A, Wichapong K, Bongiovanni D, Horckmans M, Megens RT, et al. Recruitment of classical monocytes can be inhibited by disturbing heteromers of neutrophil HNP1 and platelet CCL5. Sci Transl Med (2015) 7(317):317ra196. doi: 10.1126/scitranslmed.aad5330
26. Yu J, Mookherjee N, Wee K, Bowdish DM, Pistolic J, Li Y, et al. Host defense peptide LL-37, in synergy with inflammatory mediator IL-1beta, augments immune responses by multiple pathways. J Immunol (2007) 179(11):7684–91. doi: 10.4049/jimmunol.179.11.7684
27. Allaker RP. Host defence peptides-a bridge between the innate and adaptive immune responses. Trans R Soc Trop Med Hygiene (2008) 102(1):3–4. doi: 10.1016/j.trstmh.2007.07.005
28. Kin NW, Chen Y, Stefanov EK, Gallo RL, & Kearney JF. Cathelin-related antimicrobial peptide differentially regulates T-and b-cell function. Eur J Immunol (2011) 41(10):3006–3016. doi: 10.1002/eji.201141606
29. Yang D, Chertov O, Bykovskaia SN, Chen Q, Buffo MJ, Shogan J, et al. Beta-defensins: Linking innate and adaptive immunity through dendritic and T cell CCR6. Science (1999) 286(5439):525–528. doi: 10.1126/science.286.5439.525
30. Xie GH, Chen QX, Cheng BL, Fang XM. Defensins and sepsis. BioMed Res Int (2014) 2014:180109. doi: 10.1155/2014/180109
31. Minns D, Smith KJ, Alessandrini V, Hardisty G, Melrose L, Jackson-Jones L, et al. The neutrophil antimicrobial peptide cathelicidin promotes Th17 differentiation. Nat Commun (2021) 12(1):1285. doi: 10.1038/s41467-021-21533-5
32. Gestermann N, Di Domizio J, Lande R, Demaria O, Frasca L, Feldmeyer L, et al. Netting neutrophils activate autoreactive b cells in lupus. J Immunol (2018) 200(10):3364–71. doi: 10.4049/jimmunol.1700778
33. da Silva BR, de Freitas VAA, Nascimento-Neto LG, Carneiro VA, Arruda FVS, de Aguiar ASW, et al. Antimicrobial peptide control of pathogenic microorganisms of the oral cavity: A review of the literature. Peptides (2012) 36(2):315–321. doi: 10.1016/j.peptides.2012.05.015
34. De Smet K, Contreras R. Human antimicrobial peptides: Defensins, cathelicidins and histatins. Biotechnol Lett (2005) 27(18):1337–1347. doi: 10.1007/s10529-005-0936-5
35. Patrzykat A, Douglas SE. Antimicrobial peptides: Cooperative approaches to protection. Protein Pept Lett (2005) 12(1):19–25. doi: 10.2174/0929866053406057
36. Gorr S-U. Antimicrobial peptides of the oral cavity. Periodontology 2000 (2009) 51(1):152–180. doi: 10.1111/j.1600-0757.2009.00310.x
37. Ageitos JM, Sánchez-Pérez A, Calo-Mata P, Villa TG. Antimicrobial peptides (AMPs): Ancient compounds that represent novel weapons in the fight against bacteria. Biochem Pharmacol (2017) 133:117–138. doi: 10.1016/j.bcp.2016.09.018
38. Seo M-D, Won H-S, Kim J-H, Mishig-Ochir T, Lee B-J. Antimicrobial peptides for therapeutic applications: A review. Molecules (2012) 17(10):12276–12286. doi: 10.3390/molecules171012276
39. Zhang B, Shi W, Li J, Liao C, Li M, Huang W, et al. Design, synthesis and biological evaluation of novel peptides as potential agents with anti-tumor and multidrug resistance-reversing activities. Amino Acids (2017) 49(8):1355–1364. doi: 10.1007/s00726-017-2434-1
40. Gorr S-U. Antimicrobial peptides in periodontal innate defense. Periodontal Dis (2012) 15:84–98. doi: 10.1159/000329673
41. Jourdain M-L, Velard F, Pierrard L, Sergheraert J, Gangloff SC, Braux J. Cationic antimicrobial peptides and periodontal physiopathology: A systematic review. J periodontal Res (2019) 54(6):589–600. doi: 10.1111/jre.12676
42. Niu JY, Yin IX, Mei ML, Wu WKK, Li Q-L, Chu CH. The multifaceted roles of antimicrobial peptides in oral diseases. Mol Oral Microbiol (2021) 36(3):159–71. doi: 10.1111/omi.12333
43. Gusman H, Travis J, Helmerhorst EJ, Potempa J, Troxler RF, Oppenheim FG. Salivary histatin 5 is an inhibitor of both host and bacterial enzymes implicated in periodontal disease. Infection Immun (2001) 69(3):1402–1408. doi: 10.1128/IAI.69.3.1402-1408.2001
44. Warnke PH, Springer IN, Russo PAJ, Wiltfang J, Essig H, Kosmahl M, et al. Innate immunity in human bone. Bone (2006) 38(3):400–408. doi: 10.1016/j.bone.2005.09.003
45. Warnke PH, Voss E, Russo PAJ, Stephens S, Kleine M, Terheyden H, et al. Antimicrobial peptide coating of dental implants : Biocompatibility assessment of recombinant human beta defensin-2 for human cells. Int J Oral Maxillofac Implants (2013) 28(4):982–988. doi: 10.11607/jomi.2594
46. Lee P-H, Chen M-Y, Lai Y-L, Lee S-Y, Chen H-L. Human beta-defensin-2 and-3 mitigate the negative effects of bacterial contamination on bone healing in rat calvarial defect. Tissue Eng Part A (2018) 24(7–8):653–661. doi: 10.1089/ten.tea.2017.0219
47. Peng Y, Li L, Yuan Q, Gu P, You Z, Zhuang A, et al. Effect of bifunctional β defensin 2-modified scaffold on bone defect reconstruction. ACS omega (2020) 5(8):4302–4312. doi: 10.1021/acsomega.9b04249
48. Sun P, Shi A, Shen C, Liu Y, Wu G, Feng J. Human salivary histatin-1 (Hst1) promotes bone morphogenetic protein 2 (BMP2)-induced osteogenesis and angiogenesis. FEBS Open Bio (2020) 10(8):1503–1515. doi: 10.1002/2211-5463.12906
49. Cai J, Li X, Du H, Jiang C, Xu S, Cao Y. Immunomodulatory significance of natural peptides in mammalians: Promising agents for medical application. Immunobiology. (2020) 225(3):151936. doi: 10.1016/j.imbio.2020.151936
50. Dömer D, Walther T, Möller S, Behnen M, Laskay T. Neutrophil extracellular traps activate proinflammatory functions of human neutrophils. Front Immunol (2021) 12:636954. doi: 10.3389/fimmu.2021.636954
51. Melchers F. Checkpoints that control B cell development. J Clin Invest. (2015) 125(6):2203–10. doi: 10.1172/JCI78083
52. Le Pottier L, Devauchelle V, Pers J-O, Jamin C, Youinou P. The mosaic of b-cell subsets (with special emphasis on primary sjögren’s syndrome). Autoimmun Rev (2007) 6(3):149–154. doi: 10.1016/j.autrev.2006.09.011
53. Zouali M. The emerging roles of b cells as partners and targets in periodontitis. Autoimmunity (2017) 50(1):61–70. doi: 10.1080/08916934.2016.1261841
54. Demoersman J, Pochard P, Framery C, Simon Q, Boisramé S, Soueidan A, et al. B cell subset distribution is altered in patients with severe periodontitis. PloS One (2018) 13(2):e0192986. doi: 10.1371/journal.pone.0192986
55. Coat J, Demoersman J, Beuzit S, Cornec D, Devauchelle-Pensec V, Saraux A, et al. Anti-b lymphocyte immunotherapy is associated with improvement of periodontal status in subjects with rheumatoid arthritis. J Clin Periodontology (2015) 42(9):817–823. doi: 10.1111/jcpe.12433
56. Abe T, AlSarhan M, Benakanakere MR, Maekawa T, Kinane DF, Cancro MP, et al. The b cell–stimulatory cytokines BLyS and APRIL are elevated in human periodontitis and are required for b cell–dependent bone loss in experimental murine periodontitis. J Immunol (2015) 195(4):1427–1435. doi: 10.4049/jimmunol.1500496
57. Harada Y, Han X, Yamashita K, Kawai T, Eastcott JW, Smith DJ, et al. Effect of adoptive transfer of antigen-specific b cells on periodontal bone resorption. J Periodontal Res (2006) 41(2):101–107. doi: 10.1111/j.1600-0765.2005.00839.x
58. Algate K, Haynes DR, Bartold PM, Crotti TN, Cantley MD. The effects of tumour necrosis factor-α on bone cells involved in periodontal alveolar bone loss; osteoclasts, osteoblasts and osteocytes. J periodontal Res (2016) 51(5):549–566. doi: 10.1111/jre.12339
59. Nagasawa T, Kiji M, Yashiro R, Hormdee D, Lu H, Kunze M, et al. Roles of receptor activator of nuclear factor-κB ligand (RANKL) and osteoprotegerin in periodontal health and disease. Periodontology 2000 (2007) 43(1):65–84. doi: 10.1159/000232942
60. Taubman MA, Valverde P, Han X, Kawai T. Immune response: The key to bone resorption in periodontal disease. J Periodontology (2005) 76(11S):2033–2041. doi: 10.1902/jop.2005.76.11-S.2033
61. Han YK, Jin Y, Miao YB, Shi T, Lin XP. Improved RANKL production by memory b cells: A way for b cells promote alveolar bone destruction during periodontitis. Int Immunopharmacol (2018) 64:232–7. doi: 10.1016/j.intimp.2018.08.033
62. Doucet P, Lowenstein M. Activation de l'ostéoclasie par les endotoxines bactériennes au cours des maladies parodontales [Osteoclasts activation by bacterial endotoxins during periodontal diseases]. Med Sci (Paris) (2006) 22(6-7):614–20. doi: 10.1051/medsci/20062267614
63. Hofbauer LC, Heufelder AE. Role of receptor activator of nuclear factor-kappaB ligand and osteoprotegerin in bone cell biology. J Mol Med (Berl) (2001) 79(5-6):243–53. doi: 10.1007/s001090100226
64. Walsh MC, Choi Y. Biology of the RANKL-RANK-OPG system in immunity, bone, and beyond. Front Immunol (2014) 5:511. doi: 10.3389/fimmu.2014.00511
65. Nagasawa T, Kobayashi H, Kiji M, Aramaki M, Mahanonda R, Kojima T, et al. LPS-stimulated human gingival fibroblasts inhibit the differentiation of monocytes into osteoclasts through the production of osteoprotegerin. Clin Exp Immunol (2002) 130(2):338–44. doi: 10.1046/j.1365-2249.2002.01990.x
66. Belibasakis GN, Bostanci N. The RANKL-OPG system in clinical periodontology. J Clin Periodontol (2012) 39(3):239–48. doi: 10.1111/j.1600-051X.2011.01810.x
67. Manabe N, Kawaguchi H, Chikuda H, Miyaura C, Inada M, Nagai R, et al. Connection between b lymphocyte and osteoclast differentiation pathways. J Immunol (2001) 167(5):2625–31. doi: 10.4049/jimmunol.167.5.2625
68. Napimoga MH, Demasi AP, Jarry CR, Ortega MC, de Araújo VC, Martinez EF. In vitro evaluation of the biological effect of SOFAT on osteoblasts. Int Immunopharmacol (2015) 26(2):378–83. doi: 10.1016/j.intimp.2015.04.033
69. Jarry CR, Duarte PM, Freitas FF, de Macedo CG, Clemente-Napimoga JT, Saba-Chujfi E, et al. Secreted osteoclastogenic factor of activated T cells (SOFAT), a novel osteoclast activator, in chronic periodontitis. Hum Immunol (2013) 74(7):861–6. doi: 10.1016/j.humimm.2013.04.013
70. Jarry CR, Martinez EF, Peruzzo DC, Carregaro V, Sacramento LA, Araújo VC, et al. Expression of SOFAT by T- and b-lineage cells may contribute to bone loss. Mol Med Rep (2016) 13(5):4252–8. doi: 10.3892/mmr.2016.5045
71. Cekici A, Kantarci A, Hasturk H, Van Dyke TE. Inflammatory and immune pathways in the pathogenesis of periodontal disease. Periodontol 2000 (2014) 64(1):57–80. doi: 10.1111/prd.12002
72. Han MX, Ding C, Kyung HM. Genetic polymorphisms in pattern recognition receptors and risk of periodontitis: Evidence based on 12,793 subjects. Hum Immunol (2015) 76(7):496–504. doi: 10.1016/j.humimm.2015.06.006
73. AlQranei MS, Chellaiah MA. Osteoclastogenesis in periodontal diseases: Possible mediators and mechanisms. J Oral Biosci (2020) 62(2):123–30. doi: 10.1016/j.job.2020.02.002
74. Beutler B. Inferences, questions and possibilities in toll-like receptor signalling. Nature. (2004) 430(6996):257–63. doi: 10.1038/nature02761
75. Di Benedetto A, Gigante I, Colucci S, Grano M. Periodontal disease: linking the primary inflammation to bone loss. Clin Dev Immunol (2013) 2013:503754. doi: 10.1155/2013/503754
76. Tew JG, El Shikh ME, El Sayed RM, Schenkein HA. Dendritic cells, antibodies reactive with oxLDL, and inflammation. J Dent Res (2012) 91(1):8–16. doi: 10.1177/0022034511407338
77. Watanabe K, Iizuka T, Adeleke A, Pham L, Shlimon AE, Yasin M, et al. Involvement of toll-like receptor 4 in alveolar bone loss and glucose homeostasis in experimental periodontitis. J Periodontal Res (2011) 46(1):21–30. doi: 10.1111/j.1600-0765.2010.01304.x
78. Freire MO, Van Dyke TE. Natural resolution of inflammation. Periodontol 2000 (2013) 63(1):149–64. doi: 10.1111/prd.12034
79. Han YW, Shi W, Huang GT, Kinder Haake S, Park NH, Kuramitsu H, et al. Interactions between periodontal bacteria and human oral epithelial cells: Fusobacterium nucleatum adheres to and invades epithelial cells. Infect Immun (2000) 68(6):3140–6. doi: 10.1128/IAI.68.6.3140-3146.2000
80. Chakravarti A, Allaeys I, Poubelle PE. Neutrophile et immunité: est-ce inné ou acquis? [Neutrophils and immunity: is it innate or acquired?]. Med Sci (Paris) (2007) 23(10):862–7. doi: 10.1051/medsci/20072310862
81. Borregaard N. Neutrophils, from marrow to microbes. Immunity. (2010) 33(5):657–70. doi: 10.1016/j.immuni.2010.11.011
82. Hienz SA, Paliwal S, Ivanovski S. Mechanisms of bone resorption in periodontitis. J Immunol Res (2015) 2015:615486. doi: 10.1155/2015/615486
83. Laurent F, Romagna C, Laurent Y, Chaux-Bodard AG, Veyre S, Hemar J, et al. Relations entre les pathologies cardiovasculaires et buccodentaires. ce que le cardiologue doit connaître [Relationship between coronary artery disease and periodontal disease. what the cardiologist must know]. Ann Cardiol Angeiol (Paris) (2007) 56(6):297–302.
84. Kawai T, Matsuyama T, Hosokawa Y, Makihira S, Seki M, Karimbux NY, et al. B and T lymphocytes are the primary sources of RANKL in the bone resorptive lesion of periodontal disease. Am J Pathol (2006) 169(3):987–98. doi: 10.2353/ajpath.2006.060180
85. Fruitwala S, El-Naccache DW, Chang TL. Multifaceted immune functions of human defensins and underlying mechanisms. Semin Cell Dev Biol (2019) 88:163–172. doi: 10.1016/j.semcdb.2018.02.023
86. Verjans E-T, Zels S, Luyten W, Landuyt B, Schoofs L. Molecular mechanisms of LL-37-induced receptor activation : An overview. Peptides (2016) 85:16–26. doi: 10.1016/j.peptides.2016.09.002
87. Vandamme D, Landuyt B, Luyten W, Schoofs L. A comprehensive summary of LL-37, the factotum human cathelicidin peptide. Cell Immunol (2012) 280(1):22–35. doi: 10.1016/j.cellimm.2012.11.009
88. Xhindoli D, Pacor S, Benincasa M, Scocchi M, Gennaro R, Tossi A. The human cathelicidin LL-37–a pore-forming antibacterial peptide and host-cell modulator. Biochim Biophys Acta (BBA)-Biomembranes (2016) 1858(3):546–566. doi: 10.1016/j.bbamem.2015.11.003
89. Burteau C, Willems L, Kettmann R. Les Chémokines et leurs récepteurs : Rôle dans les infections virales et dans les pathologies cancéreuses. biotechnol. Agron Soc Environ (2007) 11(2):141–50.
90. Liao F, Shirakawa A-K, Foley JF, Rabin RL, Farber JM. Human b cells become highly responsive to macrophage-inflammatory protein-3α/CC chemokine ligand-20 after cellular activation without changes in CCR6 expression or ligand binding. J Immunol (2002) 168(10):4871–4880. doi: 10.4049/jimmunol.168.10.4871
91. Okada T, Ngo VN, Ekland EH, Förster R, Lipp M, Littman DR, et al. Chemokine requirements for b cell entry to lymph nodes and peyer’s patches. J Exp Med (2002) 196(1):65–75. doi: 10.1084/jem.20020201
92. Liao F, Rabin RL, Smith CS, Sharma G, Nutman TB, Farber JM. CC-chemokine receptor 6 is expressed on diverse memory subsets of T cells and determines responsiveness to macrophage inflammatory protein 3α. J Immunol (1999) 162(1):186–194.
93. Frade JM, Mellado M, del Real G, Gutierrez-Ramos JC, Lind P, Martinez-A C. Characterization of the CCR2 chemokine receptor : Functional CCR2 receptor expression in b cells. J Immunol (1997) 159(11):5576–5584.
94. Avila EE. Functions of antimicrobial peptides in vertebrates. Curr Protein Pept Sci (2017) 18(11):1098–119. doi: 10.2174/1389203717666160813162629
95. Murdoch C, Finn A. Chemokine receptors and their role in inflammation and infectious diseases. Blood (2000) 95:3032–3043. doi: 10.1182/blood.v95.10.3032
96. Underhill DM, Gantner B. Integration of toll-like receptor and phagocytic signaling for tailored immunity. Microbes Infect (2004) 6(15):1368–73. doi: 10.1016/j.micinf.2004.08.016
97. Dasari P, Nicholson IC, Hodge G, Dandie GW, Zola H. Expression of toll-like receptors on b cells. Cell Immunol (2005) 236(1-2):140–5. doi: 10.1016/j.cellimm.2005.08.020
98. Gururajan M, Jacob J, Pulendran B. Toll-like receptor expression and responsiveness of distinct murine splenic and mucosal b-cell subsets. PloS One (2007) 2(9):e863. doi: 10.1371/journal.pone.0000863
99. Kang JY, Lee JO. Structural biology of the toll-like receptor family. Annu Rev Biochem (2011) 80:917–41. doi: 10.1146/annurev-biochem-052909-141507
100. Ghosh D, Stumhofer JS. Do you see what I see: Recognition of protozoan parasites by toll-like receptors. Curr Immunol Rev (2013) 9(3):129–40. doi: 10.2174/1573395509666131203225929
101. Singh K, Bayrak B, Riesbeck K. A role for TLRs in moraxella-superantigen induced polyclonal b cell activation. Front Biosci (Schol Ed) (2012) 4(3):1031–43. doi: 10.2741/s316
102. Castañeda-Sánchez JI, Duarte ARM, Domínguez-López M, de la Cruz-López JJ, Julieta Luna-Herrera J. B lymphocyte as a target of bacterial infections. In: Isvoranu G, editor. Lymphocyte updates - cancer, autoimmunity and infection [Internet]. London: IntechOpen (2017).
103. Conigrave AD, Fernando KC, Gu B, Tasevski V, Zhang W, Luttrell BM, et al. P2Y(11) receptor expression by human lymphocytes: evidence for two cAMP-linked purinoceptors. Eur J Pharmacol (2001) 426(3):157–63. doi: 10.1016/S0014-2999(01)01222-5
104. Pippel A, Beßler B, Klapperstück M, Markwardt F. Inhibition of antigen receptor-dependent Ca(2+) signals and NF-AT activation by P2X7 receptors in human b cells. Cell Calcium (2015) 57(4):275–89. doi: 10.1016/j.ceca.2015.01.010
105. Agerberth B, Charo J, Werr J, Olsson B, Idali F, Lindbom L, et al. The human antimicrobial and chemotactic peptides LL-37 and alpha-defensins are expressed by specific lymphocyte and monocyte populations. Blood (2000) 96(9):3086–93. doi: 10.1182/blood.V96.9.3086.
106. Han X, Lin X, Seliger AR, Eastcott J, Kawai T, Taubman MA. Expression of receptor activator of nuclear factor-kappaB ligand by b cells in response to oral bacteria. Oral Microbiol Immunol (2009) 24(3):190–6. doi: 10.1111/j.1399-302X.2008.00494.x
107. Ebersole JL, Hamzeh R, Nguyen L, Al-Sabbagh M, Dawson D 3rd. Variations in IgG antibody subclass responses to oral bacteria: Effects of periodontal disease and modifying factors. J Periodontal Res (2021) 56(5):863–76. doi: 10.1111/jre.12882
108. O'Neil LJ, Oliveira CB, Sandoval-Heglund D, Barrera-Vargas A, Merayo-Chalico J, Aguirre-Aguilar E, et al. Anti-carbamylated LL37 antibodies promote pathogenic bone resorption in rheumatoid arthritis. Front Immunol (2021) 12:715997. doi: 10.3389/fimmu.2021.715997
109. Bae SC, Lee YH. Causal association between periodontitis and risk of rheumatoid arthritis and systemic lupus erythematosus: a mendelian randomization. kausalzusammenhang zwischen periodontitis und dem risiko für rheumatoide arthritis und systemischen lupus erythematodes: eine Mendel-randomisierung. Z Rheumatol (2020) 79(9):929–36. doi: 10.1007/s00393-019-00742-w
110. de Molon RS, Rossa C Jr, Thurlings RM, Cirelli JA, Koenders MI. Linkage of periodontitis and rheumatoid arthritis: Current evidence and potential biological interactions. Int J Mol Sci (2019) 20(18):4541. doi: 10.3390/ijms20184541
111. Knochelmann HM, Dwyer CJ, Bailey SR, Amaya SM, Elston DM, Mazza-McCrann JM, et al. When worlds collide: Th17 and treg cells in cancer and autoimmunity. Cell Mol Immunol (2018) 15(5):458–69. doi: 10.1038/s41423-018-0004-4
112. Vordenbäumen S, Fischer-Betz R, Timm D, Sander O, Chehab G, Richter J, et al. Elevated levels of human beta-defensin 2 and human neutrophil peptides in systemic lupus erythematosus. Lupus. (2010) 19(14):1648–53. doi: 10.1177/0961203310377089
113. Bokarewa MI, Jin T, Tarkowski A. Intraarticular release and accumulation of defensins and bactericidal/permeability-increasing protein in patients with rheumatoid arthritis. J Rheumatol (2003) 30(8):1719–24.
114. Kienhöfer D, Hahn J, Schubert I, Reinwald C, Ipseiz N, Lang SC, et al. No evidence of pathogenic involvement of cathelicidins in patient cohorts and mouse models of lupus and arthritis. PloS One (2014) 9(12):e115474. doi: 10.1371/journal.pone.0115474
115. Liang W, Diana J. The dual role of antimicrobial peptides in autoimmunity. Front Immunol (2020) 11:2077. doi: 10.3389/fimmu.2020.02077
116. Konig MF, Abusleme L, Reinholdt J, Palmer RJ, Teles RP, Sampson K, et al. Aggregatibacter actinomycetemcomitans-induced hypercitrullination links periodontal infection to autoimmunity in rheumatoid arthritis. Sci Transl Med (2016) 8:369ra176. doi: 10.1126/scitranslmed.aaj1921
117. Moen K, Brun JG, Valen M, Skartveit L, Eribe EK, Olsen I, et al. Synovial inflammation in active rheumatoid arthritis and psoriatic arthritis facilitates trapping of a variety of oral bacterial DNAs. Clin Exp Rheumatol (2006) 24:656–63.
118. Lande R, Pietraforte I, Mennella A, Palazzo R, Spinelli FR, Giannakakis K, et al. Complementary effects of carbamylated and citrullinated LL37 in autoimmunity and inflammation in systemic lupus erythematosus. Int J Mol Sci (2021) 22(4):1650. doi: 10.3390/ijms22041650
119. Frasca L, Palazzo R, Chimenti MS, Alivernini S, Tolusso B, Bui L, et al. Anti-LL37 antibodies are present in psoriatic arthritis (PsA) patients: New biomarkers in PsA. Front Immunol (2018) 9:1936. doi: 10.3389/fimmu.2018.01936
120. Lande R, Palazzo R, Hammel P, Pietraforte I, Surbeck I, Gilliet M, et al. Generation of monoclonal antibodies specific for native LL37 and citrullinated LL37 that discriminate the two LL37 forms in the skin and circulation of Cutaneous/Systemic lupus erythematosus and rheumatoid arthritis patients. Antibodies (Basel) (2020) 9(2):14. doi: 10.3390/antib9020014
Keywords: B cell, anti-microbial peptides, periodontal diseases, periodontitis, immunomodulation
Citation: Lobognon VD and Alard J-E (2022) Could AMPs and B-cells be the missing link in understanding periodontitis? Front. Immunol. 13:887147. doi: 10.3389/fimmu.2022.887147
Received: 02 March 2022; Accepted: 01 August 2022;
Published: 21 September 2022.
Edited by:
Rizgar A Mageed, Queen Mary University of London, United KingdomReviewed by:
Loredana Frasca, National Institute of Health (ISS), ItalyDiptiman Choudhury, Thapar Institute of Engineering and Technology, India
Copyright © 2022 Lobognon and Alard. This is an open-access article distributed under the terms of the Creative Commons Attribution License (CC BY). The use, distribution or reproduction in other forums is permitted, provided the original author(s) and the copyright owner(s) are credited and that the original publication in this journal is cited, in accordance with accepted academic practice. No use, distribution or reproduction is permitted which does not comply with these terms.
*Correspondence: Jean-Eric Alard, amVhbmVyaWMuYWxhcmRAdW5pdi1icmVzdC5mcg==