- 1Duke Human Vaccine Institute, Duke University School of Medicine, Durham, NC, United States
- 2Department of Pediatrics, Duke University School of Medicine, Durham, NC, United States
- 3Department of Microbiology and Immunology, Emory Vaccine Center, Yerkes National Primate Research Center, Emory University, Atlanta, GA, United States
- 4Department of Pediatrics, Weill Cornell Medicine, New York, NY, United States
- 5Department of Pediatrics, Emory University School of Medicine, Atlanta, GA, United States
- 6Center for Childhood Infections and Vaccines of Children’s Healthcare of Atlanta and Emory University, Atlanta, GA, United States
Early initiation of antiretroviral therapy (ART) significantly improves clinical outcomes and reduces mortality of infants/children living with HIV. However, the ability of infected cells to establish latent viral reservoirs shortly after infection and to persist during long-term ART remains a major barrier to cure. In addition, while early ART treatment of infants living with HIV can limit the size of the virus reservoir, it can also blunt HIV-specific immune responses and does not mediate clearance of latently infected viral reservoirs. Thus, adjunctive immune-based therapies that are geared towards limiting the establishment of the virus reservoir and/or mediating the clearance of persistent reservoirs are of interest for their potential to achieve viral remission in the setting of pediatric HIV. Because of the differences between the early life and adult immune systems, these interventions may need to be tailored to the pediatric settings. Understanding the attributes and specificities of the early life immune milieu that are likely to impact the virus reservoir is important to guide the development of pediatric-specific immune-based interventions towards viral remission and cure. In this review, we compare the immune profiles of pediatric and adult HIV elite controllers, discuss the characteristics of cellular and anatomic HIV reservoirs in pediatric populations, and highlight the potential values of current cure strategies using immune-based therapies for long-term viral remission in the absence of ART in children living with HIV.
Introduction
In 2020, approximately 150,000 children were newly infected with HIV and 1.7 million children (<15 years of age) were living with HIV worldwide (1). Most of these children (˜90%) live in sub-Saharan Africa and were infected perinatally or during the breastfeeding period. Although antiretroviral therapy (ART) has significantly reduced the rate of vertical transmission, infant infections continue to occur due to incident of maternal HIV infections during pregnancy or breastfeeding, poor maternal access to ART treatment, challenges in maternal ART adherence, and lack of viral suppression in mothers receiving ART (2). Early ART treatment reduces mortality and improves clinical outcomes in infants with HIV. Thus, the current treatment guidelines recommend initiation of ART to infected infants as early as possible, regardless of clinical or immunological outcomes (3). Studies have shown that early ART initiation within the first months of life in infants with HIV suppresses viral replication and can result in a significantly smaller latent viral reservoir, preservation of CD4+ T cell counts, and decreased immune dysregulation (4–7). However, ART is not curative and does not eliminate the establishment of persistent latent viral reservoirs, which contribute to viral rebound when treatment is interrupted (8).
Previous studies have also reported that early ART initiation in infants with HIV is associated with a lack of circulating HIV-specific antibodies and T cell responses, likely due to low levels of circulating viral antigen and suppression of viral replication (6, 9–11). In these studies, approximately 36-46% of infants with perinatal HIV infection who were treated with ART within the first year of life became seronegative (9, 11–13). In a more recent study, Cotugno et al. reported that while these early ART-treated perinatally infected infants are seronegative, in vitro stimulation of the memory B cell population derived from peripheral blood mononuclear cells (PBMCs) with HIV peptides induced HIV-specific antibody responses, suggesting persistence of HIV-specific humoral memory despite the seronegative status (13). Interestingly, in vitro stimulation induced higher expression of transcriptional signature profiles of genes related to antibody production (PRDM1) and T-B cells cognate stimulation (CXCR4, IL21R) in early ART-treated infants who were seropositive compared to their seronegative counterparts, suggesting a truncated process of HIV-specific B cell maturation in seronegative children (13). Taken together these results suggest that while early ART initiation is beneficial for controlling viral replication, rapid elimination of viral antigen results in qualitatively distinct HIV-specific memory responses. Additional studies are needed to further define the impact of the timing of ART initiation on persistence of HIV-specific cellular and humoral immune responses in children and to define whether the distinct profiles of HIV-specific memory T and B cells in children who become seronegative has an impact on the potential of immunotherapies to promote cure.
The overall goal of this review is to discuss current knowledge of early life immunity that can be harnessed to develop future strategies aimed to achieve HIV remission in pediatric settings. Herein, we compare the characteristics of innate and adaptive immune cells in pediatric versus adult elite controllers, discuss the characteristics of cellular and anatomic HIV reservoirs in pediatric populations, and highlight the potential of novel immune-based interventions that have been studied in adults with HIV or in preclinical models to eliminate persistent latently infected viral reservoirs in children living with HIV.
Immune Profiles of Pediatric and Adult HIV Elite Controllers
HIV elite controllers (ECs) are a rare group of individuals with HIV that have the capacity to spontaneously control viral load without treatment (14). Specifically, these individuals maintain a viral load of <50 copies/ml at least three times over the course of a year (15) and do not demonstrate CD4+ T cell depletion (16). These individuals are distinguished from long-term non progressors (LTNPs) who have low-to-moderate viremia (<5000 RNA copies/ml) but preserved CD4+ T cell numbers (15–17). ECs make up ~0.5% of all adults with HIV and the number is at least six times lower in children with HIV (~0.08%) (18, 19). While most ECs eventually lose control of viremia, they provide a model for natural suppression of HIV replication that can guide immunotherapeutic interventions towards long-term ART-free virus control. Herein, we examine and compare the adaptive and innate immune cell profiles of pediatric and adult ECs to guide development of immune-based strategies for latent reservoir clearance and HIV remission tailored to the pediatric settings.
Cellular Immunity
CD8+ T Cells
The CD8+ T cell response has been identified as a distinct and crucial feature in HIV ECs (20). Both pediatric and adult ECs, compared to their progressor counterparts, possess a higher frequency of HIV Gag-specific, polyfunctional CD8+ T cells capable of cytokine release (IFN-g, TNF-a and IL-2), degranulation, and release of perforin and granzyme B (19, 21). Notably, in pediatric elite controllers (PECs), this polyfunctional population of HIV-specific CD8+ T cells is higher than in non-progressors, even though the overall production of cytokines is similar between these groups (19). Additionally, in line with previous observations in adults, pediatric progressors (PPs) had higher magnitude Nef-specific CD8+ T-cell responses compared to ECs (19). Additionally, Viera et al. reported that only two of seven adolescent ECs (median age 14.6 years, range 12.7–16.6 years) shared the protective HLA Class I type (HLA-B∗27/57/58 : 01/81 : 01) previously described in adult ECs, suggestive of potential distinct, age-relevant immune control mechanisms in pediatric age groups. Studies using nonhuman primate (NHP) models of HIV/SHIV/SIV in adult macaques showed differential impact of antiviral CD8+ T cell depletion between ECs and progressors in chronic and acute infections (22–24). CD8+ T cell depletion resulted in a more prominent increase in viral replication in ECs (22) with a subsequent recovery of viral control upon reconstitution of CD8+ T cells (23), and more profound CD4+ T effector memory cells activation and expansion (24). This finding further highlights the contribution of CD8+ T cells to the suppression of viremia, suggesting that elicitation of effective, polyfunctional antiviral HIV-specific CD8+ T cell responses will be a key for achieving long term viral control in pediatric HIV. NHP model of SIV infection in adult macaques showed viral suppression by CD8+ T cells that recapitulate HIV suppression in ECs (25, 26). These adult macaques were inoculated intravenously with SIVagm.sab92018 and demonstrated viral suppression below the levels of detection of conventional and single copy assays despite persistent reservoirs of replication competent virus. However, it is unclear whether similar characteristics could be achieved in a pediatric NHP model due to differences in early life immune milieus and different route of infection. Therefore, there is a critical need to develop NHP model that can be utilized to understand the mechanisms of viral control in pediatric HIV ECs.
CD4+ T Cells
HIV primarily targets CD4+ T cells expressing CXCR4 and CCR5 co-receptors, which undergo dramatic depletion during the acute phase of infection. Even after ART initiation, CD4+ T cell populations do not fully recover (27, 28). Unsurprisingly, PECs were observed to have higher proportion of naïve CD4+ T cells compared to PPs who had an increased effector memory CD4+ T cell population (19). Additionally, immune activation and exhaustion makers on central memory and transitional memory CD4+ T cells were lower in PECs compared to PPs (19). Functionally, HIV-specific CD4+ T cells in PECs are superior to those in PPs, with increased polyfunctionality despite lower activation (19, 29). This phenotype of lower immune activation and exhaustion markers is also strongly established in adult ECs (29, 30). In adult ECs, low HIV DNA viral reservoir is associated with lower activation of both CD4+ and CD8+ T cells (31, 32). However, while the lower activation phenotype is shared between adult and pediatric ECs, they are likely influenced by factors unique to their distinct immune milieus. The lower HIV reservoir and lower activation in CD4+ T cells in adults is attributed to the aggressive CD8+ T cell response in the acute phase of infection (33), while this phenotype in pediatric ECs is preceded by the tolerogenic environment (18). Taken together, these findings demonstrate that the CD4+ T cells in pediatric and adult ECs share similar phenotypes, in which they are less susceptible to infection and exhaustion. However, understanding of mechanisms and pathways that contribute to these phenotypes in the context of tolerogenic early life immunity could potentially benefit immunotherapeutic designs against pediatric HIV.
Regulatory T Cells
Tregs are crucial in dampening inflammatory responses, and hence, it is reasonable to hypothesize that Tregs, could be important in slowing progression of HIV pathogenesis. However, there could also be a conflicting dual role of these cells in HIV control. Adult ECs have normal Treg frequencies, with low HIV-specific immune activation while maintaining strong polyfunctional T cell responses (29). A study on pediatric slow progressors (PSP), who are characterized by high CD4+ T-cell counts and low immune activation despite having high viral load, indicated that these PSPs had a higher absolute number of Tregs expressing a suppressive phenotype compared to PPs (34). Additionally, PSPs had higher secretion of the immunosuppressive cytokine IL-10 and a higher frequency of central memory Tregs compared to PPs. Moreover, PSPs that progressed later had lower frequency of suppressive Tregs, lower Treg proliferation, and IL-10 production (34). Together, these findings suggest an active role of suppressive Tregs and anti-inflammatory responses in controlling immune activation thereby slowing progression to disease in children with HIV. Given that the function of Tregs may plausibly limit or promote HIV pathogenesis, more pediatric studies are required to clarify their roles, distributions, and functions in the context of HIV control. Some therapeutic strategies to target Tregs have in fact been studied in adults with HIV. As CTLA-4 on Tregs plays a role in suppressing HIV-specific T cells, blocking CTLA-4 has been proposed as a strategy to rescue an effective HIV-specific T cell response (35, 36). In a study of SIV-infected adult rhesus macaques CTLA-4 blockade resulted in reduced viral RNA in tissues (37). However, another study showed conflicting results when blockade was performed in the early stage of infection (38). Dual monoclonal antibody treatment using anti-PD-1 and anti-CTLA-4 in SIV-infected adult NHPs with long term ART enhanced both memory CD4+ and CD8+ T cells proliferation and effectors functions but was insufficient to control or delay viral rebound after ART interruption (39). Thus, dual CTLA-4/PD-1 blockade is likely insufficient to induce HIV remission and would likely require combination with enhanced killing strategies, such as therapeutic vaccination (40) or coadministration of neutralizing antibodies (41). The potential contribution of Tregs to HIV cure was further demonstrated in NHP model of SIV infection in adult macaques (42, 43). Tregs depletion in SIV infected adult macaques resulted in increased in reactivation of latent reservoirs as well as significant boost of SIV-specific cytotoxic T lymphocytes (CTLs) responses (42). However, Tregs depletion alone is insufficient as a strategy for HIV cure and would require combination with other viral reactivation therapies or vaccination strategies (44). Therefore, future research should focus on refining promising Treg treatments as a strategy for cure in pediatric HIV NHP model.
Th17
Th17 cell populations are crucial in maintaining the integrity of the gut mucosa by promoting epithelial cell regeneration as well as coordinating immune responses in this region (45). The absence of these cells results in microbial translocation due to reduced gut integrity, leading to the immune activation which promotes HIV-driven CD4+ T cell depletion, especially in the gut. Consistent with this pathogenic mechanism, studies have shown a higher frequency of Th17 cells in circulation among adult ECs compared to long-term non progressors and even HIV-negative individuals, suggesting a protective role for this cell subset (46). Unfortunately, studies on the distribution and expansion of Th17 cells in HIV infection in children are limited, let alone in the rare group of elite controllers. Dzanibe et al. recently reported that maternal HIV exposure alters the proportions of Th17 and Tregs CD4+ T cells in the periphery of HIV-exposed uninfected infants (HEUs) compared to HIV-unexposed uninfected (HUUs) control infants (47). Additionally, while peripheral Tregs showed inverse correlations with known damage-associated markers in the gut (CCL17, IL-7, CCL20) in HEUs, Th17 cell population was positively correlated with these markers. Taken together, these data suggest that there is an association between markers of gut damage and in utero HIV/ART exposure on the HEU infants Th17/Treg balance at birth. However, whether these findings extend to children who acquired HIV from their mothers is unclear.
T Follicular Helper Cells
Tfh cells are found in secondary lymphoid organs such as tonsils, spleen, and lymph nodes where ART penetration is not optimal, rendering them sites for ongoing HIV replication (48). Tfh cells have been shown to support HIV persistence, and more interestingly have been demonstrated to be the major HIV reservoirs within central memory CD4+ T cells in adults with chronic HIV infection and on ART (49–51). In adult ECs, however, Garcia et al. demonstrated that peripheral Tfh (pTfh) cells have smaller HIV reservoirs compared to individuals treated with combination ART (52). They postulate a possible link between the low infection of pTfh cells in ECs and their ability to suppress HIV (52). Functionally, pTfh cells in EC’s have been shown to have better B-cell helper activity than those in HIV progressors (53, 54). In children with HIV, the proportion of circulating Tfh cells are shown to be reduced and correlated with memory B cells as well as advanced disease (55, 56). Further research is required to fill the gap in knowledge of the role of Tfh cells in pediatric ECs as this could provide insight on the role of these cells in HIV control and in providing help for improved B cell functions for vaccine design.
Humoral Immunity
HIV disease progression is associated with phenotypic and functional abnormalities of B cells, several of which are driven by the chronic immune activation associated with HIV replication. However, few studies have evaluated the role of the humoral immune response in spontaneous control of viral replication. In adults, the median frequency of HIV-specific memory B cells in ECs was significantly higher compared to that found in individuals receiving ART (57). While this has not been evaluated in pediatric ECs, a recent study has highlighted B cell dysfunction as a hallmark of HIV disease progression in children with HIV. In this study, a cohort of pediatric long-term non-progressors (LTNPs; n=20) had lower naïve and resting memory B cell frequencies compared to uninfected children (58), while tissue-like memory B cells were lower in LTNPs compared to pediatric progressors. Interestingly, plasma levels of B lymphocyte stimulator (BLyS), a known regulator of B cell function and survival, were lower in pediatric LTNPs compared to progressors. In adults, BLyS expression levels are unaltered, and less B cell dysregulation was observed in non-progressors (59).
Recent work has highlighted a potential role for non-neutralizing HIV-specific antibodies in protection from disease progression and viral control, implicating features that could be exploited in attempts to achieve HIV cure. Muenchhoff et al. described that a subset of ART-naïve children with HIV who maintained normal CD4+ T cells also known as pediatric non progressors (PNPs) had higher p24-specific IgG levels than children under ART, and these anti-p24 IgG were mostly of the IgG1 subclass (60). Meanwhile in PPs, there was a greater contribution to IgG3 subclass to the p24-specific IgG responses. IgG1 mediates antibody responses to viral infection while IgG3 regulates proinflammatory effector functions (61). This suggests that in PNPs viral replication is under control without chronic inflammation, while PPs have more inflammatory responses. Interestingly, when compared to uninfected children, PNPs had no changes in bulk IgG galactosylation, which corresponds to low immune activation and inflammation (60). In PPs, agalactosylated IgG glycans were expanded, while digalactosylated glycans were decreased compared to HIV-uninfected children. Moreover, PNPs demonstrated increased HIV-specific Fc-mediated IgG effector functions, antibody-dependent cellular cytotoxicity (ADCC) and phagocytosis (ADCP), compared to progressors (60). Interestingly, robust ADCC (62, 63) and overall superior polyfunctional Fc dependent activity (64, 65) have been consistently observed in adult ECs. Broadly neutralizing antibodies (bNAbs) arise early with higher breadth and potency in children than in adults with HIV (66–68). While bNAbs in children mostly target similar epitopes to adult bNAbs (69), they also showed remarkable polyclonalities with some bNAbs targeting up to 4 distinct epitopes (69, 70). Altogether, these data suggest that successful monoclonal antibody-based therapeutics for viral remission in pediatric HIV will likely need to exploit neutralizing and non-neutralizing properties of HIV-specific bNAbs to control and mediate clearance of infected cells.
Innate Immunity
Natural Killer Cells
Natural killer (NK) cells are innate lymphocytes that directly kill cells infected by intracellular pathogens, usually viruses. The functionality of NK cells is governed by the interaction of both inhibitory and activating receptors with the MHC-I (71). NK cells are inhibited from killing uninfected cells that have normal levels of MHC-I. The absence of MHC-I molecules causes the loss of inhibitory signals on NK cells, permitting them to directly kill infected cells (71). Genovese et al. demonstrated an association between delayed progression to AIDS and the interaction of protective HLA-B alleles on infected cells and killer immunoglobulin receptors (KIR) on NK cells (72). The interactions between NK cell receptors (KIR3DL1 and KIR3DS1) and the protective HLA-B Bw4-80Ile on target cells are associated with delayed progression to AIDS has been documented (73–75). Interestingly, Tomescu et al. have demonstrated that adult ECs expressing KIR3DL1 on NK cells and HLA-B Bw4-80Ile on target cells had stronger NK cell mediated cytotoxicity compared to CD8+ T cell cytotoxicity from the same individuals (76). These findings suggest an association of NK cell phenotype with development of elite control.
There is a paucity of research on the distribution and function of NK cells in pediatric ECs. Given the immature adaptive immune system in children, NK cell response to infection is an important initial defense. Interestingly, NK cell populations in pediatric long-term non progressors (LTNP) are lower than healthy uninfected individuals but similar to ART-treated children (77). Research on the NK cell population in pediatric ECs is required to identify the distributional and functional correlates of NK cells to HIV control in order to better inform strategies for HIV cure.
Dendritic Cells
Dendritic cells (DCs) are innate immune cells are that are specialized in presenting foreign antigens to the adaptive immune system to mount an effective immune response and also release cytokines and chemokines to orchestrate adaptive immune responses. These cells are therefore important in HIV control as they present HIV antigen to T cells to mount effective immune responses. A study showed exposure of conventional DCs to HIV-1 resulted in rapid and sustained production of type I interferon and importantly stronger capacity to stimulate and expand HIV-1-specific CD8+ T cell responses in ECs (78). Furthermore, it was shown that plasmacytoid DCs (pDCs) from ECs had a higher capacity to reduce HIV production compared to those from viremic individuals (79). In adult rhesus chronic SIV infection model, DCs and pDCs influenced ongoing inflammation and T cell exhaustion in the mucosal tissues and there by contributed to persistence of viral reservoirs during ART (80). There is a lack of studies on the role of and functionality of dendritic cell subpopulations in pediatric ECs. During HIV infection, dendritic cells in children undergo numerical and functional deficits. Interestingly, with the administration of ART, the recovery of myeloid dendritic cells was observed whereas that of plasmacytoid dendritic cells was only partial (81).
Other Innate Immune Cells
Monocytes are an innate effector cell population which seem to be associated with suppression of HIV viremia. Adult ECs, have overall lower levels of monocyte activation (based on HLA-DR expression) with a reduced frequency of inflammatory monocyte (CD14++CD16+) subset compared to viremic subjects (30). In addition, elite controllers tend to exhibit significantly higher proportion of CD14+CD16+ monocytes, compared with HIV-negative controls (82).
Innate lymphoid cells (ILCs) are lymphoid-lineage cells that are further classified as ILC1, ILC2, and ILC3 based on the transcription factor they express, and their functions overlap with that of CD4+ T helper (Th)1, Th2, and Th17 lymphocytes, respectively. ILCs are severely depleted in the circulation of HIV-infected children however, initiation of ART at birth preserves ILC development and function (83). While a role for ILCs has not been established in elite controllers, the aforementioned study establishes a connection between the maintenance of ILCs in population and control of HIV replication in pediatric populations.
Gamma delta T cells (γδT) are a subset of innate-like T-cells with a transcriptional phenotype that blends characteristics of both NK and CD8+ T cells, thus exhibit highly cytotoxic activity (84, 85). Gamma delta T cells represent a small fraction (1-5%) of the overall T cell population but are more abundant in barrier sites like the skin, lung, digestive tract, and reproductive organ mucosa (86). Recent work demonstrated that interleukin (IL)-17-production by γδ T cells was better preserved in PBMCs from adult ECs than in untreated HIV-infected patients and was negatively associated with immune activation (87). Moreover, overall alterations in γδ T-cells were less prominent in ECs compared to untreated HIV- infected adults. The extent to which γδT cells contribute to natural control of HIV infection in children and adults remains elusive, however a few studies have highlighted the ability of γδT cells to inhibit HIV replication in vitro (88, 89).
Summary
There are several opportunities in the early life immune system that could be exploited towards an HIV cure. The immunotolerant and naive immune environment can allow low levels of activation and immune system maturation is highly adaptable. A better understanding of the early life immune ontogeny and mechanism of viral control in pediatric ECs could provide insight into novel immune-based strategies towards clearance of latently infected cells and viral remission in children with HIV.
HIV Reservoir in Pediatrics
Composition of the Latent Reservoir in Adults and Children
The latent HIV reservoirs are heterogenous in both cell types and tissue localizations within the body. Various CD4+ T cell types are susceptible to HIV infection including naïve cells, long-lived memory cells (stem cell and central memory cells), and terminally differentiated cells (90), but central memory cells CD4+ T cells are the most vital to the maintenance of the latent reservoir (91). HIV-infected CD4+ T cells exist broadly throughout the body and have been found in the gut-associated lymphoid tissue, genital tract, and lymph nodes (92). In addition to CD4+ T cells, myeloid immune cells have been implicated as HIV reservoirs. Macrophages are known to be infected by HIV (91, 93) and in vitro infectivity studies showed that they are not killed by HIV, suggesting that they are likely HIV reservoirs (93). Tissue resident macrophages are highly specialized cells and varied in susceptibility to HIV infection. Alveolar macrophages and microglial cells are susceptible to HIV infection and contribute to the formation of cell reservoirs in the lung and brain, respectively (94, 95).
The majority of studies that focused on kinetics and origin of persistent latent reservoirs that eventually contribute to viral rebound when ART is interrupted were conducted in adults with HIV or adult NHPs. Infant NHPs are highly relevant and translatable models for the study of pediatric HIV infection and persistent latent reservoirs. Several studies using oral infant NHP Simian-Human Immunodeficiency Virus (SHIV) infection model that mimic breastmilk transmission of HIV, the gastrointestinal (GI) tract was identified as major source of viral RNA during ART and upon viral rebound (96–98). Following ART discontinuation, while the GI tract showed the most rapid increase in viral RNA, virus was also detected in the nasal-associated lymphoid tissue (NALT), axillary lymph node, and spleen (97). An earlier study described that while the oral mucosa and tonsils are generally the anatomical location for initial establishment of a small founder population of infected cells, the anatomical location of persistent latent reservoirs in SHIV-infected infant NHPs continues to spread into distal lymph nodes and other organs including the brain, lungs, intestines, pancreas, and spleen (99).
In addition, due to differences in age-related immune landscapes, the latent HIV reservoirs in adults and children are defined by distinct characteristics. In perinatally-acquired HIV in children, the latent reservoir consists of transitional memory CD4+ T cells as opposed to the mostly central memory CD4+ T cells observed in adults (100, 101). Additionally, perinatally-infected children have the unique opportunity of initiating ART early, which has been reported to limit the establishment of the initial latent HIV reservoir (12, 102, 103). A recent study demonstrated that reactivation of latent HIV reservoirs with PMA/ionomycin from adolescent who were perinatally-infected and treated within the first 24-months of life, resulted in similar rate of cell activation but slower and lower magnitude of proviral load when compared to infected adults (104), suggesting that latent viral reservoirs in children were more resistant to ex vivo reactivation. This study highlights differences in inducibility of latent viral reservoirs resulting from perinatal versus adult HIV infections, which could impact strategies toward latent reservoirs clearance and viral remission in children.
Immune Signatures of the Viral Reservoir
CD4+ T cell immune signatures associated with the size of viral reservoir in adolescents and children who were vertically infected with HIV were recently described (105). Rinaldi et al., reported that while co-expression of PD-1 and TIGIT was associated with CD4+ T cell viral reservoir in both children and adult, the frequencies of PD-1 and TIGIT expressing CD4+ T cells along with HIV-specific CD4 T cells were able to discriminate between lower and higher viral reservoirs in perinatally-infected children (105). This finding suggests that while some immune signatures that could be targeted by interventions or therapies towards clearance of latent viral reservoirs are similar in children and adults, there are also unique signatures that immune-based strategies aimed at permanent HIV remission in children could target.
Current Immune-Based Therapies Potential for Pediatric HIV Viral Remission and Cure
Elimination of latently infected cells that are established early during infection remains as a major challenge to cure in children and adults living with HIV (8, 106, 107). While ART treatment can achieve sustained viral suppression, it does not eliminate latently infected cells, which lack active expression of viral proteins on their cell surface and therefore are invisible to the immune system. ART discontinuation reactivates latently infected cells harboring intact replication competent HIV provirus to produce HIV virions, resulting in sustained viral rebound in nearly all individuals with HIV (106–108). This indicates that the host immune system of most individuals with HIV is unable to eliminate virus producing and/or infected cells in persistent reservoirs. Therefore, novel strategies with combinatory effect to induce viral production by latently infected cells while on ART and to augment the ability of host immune responses in clearing infected cells are paramount to HIV cure. Additionally, as the majority of these novel interventions are currently developed in adults with HIV or preclinical models for adults, it is imperative to design novel combination strategies that are tailored to the developing immune system in children for eradication of pediatric HIV.
Therapeutic HIV Vaccines (T and B Cells)
The goal of therapeutic vaccination is to improve the functional capacity of the host immune system to kill infected CD4+ T cells and/or neutralize circulating viruses (109). Several therapeutic HIV vaccines have been assessed preclinically and clinically for their safety, immunogenicity, and effectiveness but these studies have largely focused on adult HIV population or SIV/adult nonhuman primate (NHP) models (107, 109). It is well established that perinatally-infected infants and children who are treated early with ART tend to have a small pool of latent viral reservoir, normal development of B and T cell compartments, and a high capacity to regenerate an immune repertoire (110, 111). While lessons from the “Mississippi baby” have indicated that early ART treatment is not enough to achieve life-long HIV remission, such characteristics do render perinatally-infected children as an ideal group to test therapeutic vaccines for functional HIV cure (110, 112). However, to date, only two clinical and one preclinical NHP therapeutic HIV/SIV vaccine studies have been conducted with a focus of HIV cure in infants and children (Table 1).
The Pediatric AIDS Clinical Trials Group 218 (ACTG 218 or NCT00000762) was the first clinical study conducted to evaluate the safety and immunogenicity of viral envelope proteins as therapeutic vaccine in infants and children with HIV. The rationale behind this study was that immunization with HIV envelope (Env) protein-based vaccines could alter the landscape of naïve immune responses to HIV and thereby delaying viral rebound. Three HIV Env-based vaccines were administered to infants and children with HIV aged 1 month to 18 years at the time of entry and then at 1, 2, 3, 4 and 6 months later. The vaccines were well tolerated with no adverse effects, secondary infections, or change in viral replication status. Around 65% of vaccinees demonstrated moderate to strong antigen-specific antibody responses and up to 56% of vaccinees exhibited vaccine antigen-specific lymphoproliferative responses, indicative of immunogenicity of the therapeutic vaccine (113).
The First Pediatric Randomized Trial (PEDVAC or NCT04301154) evaluated safety and immunogenicity of the multiclade, multigene HIV DNA therapeutic vaccine (HIVIS) in 10 HIV infected and ART treated children of 6 to 16 years of age. Administration of an HIV DNA vaccine construct expressing HIV-1 subtypes A, B, and C, Env, Rev, Gag, and reverse transcriptase (RT) was well tolerated and exhibited a safe profile except for local irritation, erythema with or without swelling or usual itching at the site of injection. Unlike, non-vaccinated children, vaccinees exhibited increased percentage of HIV-specific perforin-producing CD8+ T cells, compared to baseline, highlighting the immunogenicity of therapeutic HIV-DNA vaccines (114–116). However, there was no evidence that the vaccine had an impact on latent viral reservoir and viral rebound.
Preclinical studies demonstrated the promise of therapeutic vaccination with Ad26/MVA (recombinant adenovirus serotype 26 (Ad26) prime, modified vaccinia Ankara (MVA) boost) encoding SIV gag, pol, env in combination with a toll-like receptor 7 (TLR-7) agonist as an adjuvant against Simian Immunodeficiency Virus (SIV) using an adult NHP model (117). This vaccine regimen was recently translated to an infant SIV NHP model that simulated postnatal HIV infection through breastfeeding with ART-mediated suppression of viremia (118). In this study, a total of 8 infant NHPs were orally infected with SIVmac251 and treated with ART at 4 weeks post-infection followed by multiple immunization with Ad48/MVA encoding SIV gag, pol env plus TLR7 while remining on ART. The vaccinated group showed greater magnitude and breadth of polyfunctional CD4+ and CD8+ T cells when compared to control group and, as anticipated, the administration of the TLR-7 agonist (GS-986) led to activation of monocytes and T cells. This finding was similar to the observations found in adult NHPs (117). Additionally, the levels of SIV gp120-specific antibodies were significantly increased following the MVA boost. However, despite these robust vaccine-induced cellular and humoral immune responses, the size of viral reservoirs, time to viral rebound, and viral set point following analytical ART interruption (ATI) were similar in the vaccine and control groups. These findings suggest that while therapeutic vaccine-induced immunity may induce antiviral T and B cell responses, these responses may be insufficient to modulate viral rebound dynamics during ATI. Thus, there is a need to identify T and B cell responses that could potentially impact the latently infected HIV reservoir and to combine therapeutic vaccines with other immune therapies such as potent latency reversing agents (LRAs), passive transfer of broadly neutralizing antibodies (bNAbs), and check point blockade if this approach is pursued as strategy for HIV remission in children.
Passive Immunization Strategies
Broadly Neutralizing Antibodies
Recent development in the use of antibodies for HIV therapy showed promise in the ability of antibody-based strategies to reduce plasma viremia and to mediate clearance of infected cells to further delay viral rebound following ATI (119–123). Emerging evidence supports the effect of antibody-based therapies on persistent latent HIV reservoirs, which could potentially induce viral remission when used alone or in combination with ART and other novel therapeutic modalities (121).
Passive infusion with highly potent broadly neutralizing antibodies (bNAbs) against distinct HIV Env regions may represent an advantageous strategy due to the ability of bNAbs to neutralize free virions via the Fab domain and to engage cognate receptors on host innate and adaptive via the Fc domain. Passive infusion with single or combination bNAbs is actively evaluated as therapeutic strategy for HIV remission in the clinics by measuring the effects of antiviral activities of bNAbs on plasma viremia and viral suppression following ATI as well as impact on viral reservoir by delaying viral rebound (121). Most of these studies were conducted in adults with acute or chronic infections while on ART and similar studies in pediatric HIV population are lacking. To date, only two ongoing clinical trials of bNAb therapy as part of an HIV treatment strategy have been conducted in infants and children (Table 2). Passive infusion of the unmodified version and the long acting (LS) version of the CD4 binding site (CD4bs), VRC01, was shown to be safe and well tolerated in HIV-exposed infants (124, 125). Similarly, passive infusion of the modified and extended half-life version of the VRC01 with the LS mutation in combination with the V3-glycan bNAb, 10-1074, was also safe and well tolerated in the pediatric population (125). The ability of monotherapy and dual therapy using bNAbs to maintain viral suppression and delay viral rebound following ATI in the pediatric HIV population is still under investigation.
Preclinical data from SHIV infection in adult NHP models demonstrated that administration of single or combination bNAb therapy could potentially target, control, and eliminate viral reservoir, suggesting that ART free remission is attainable following ATI (126–132). These mono- or dual-bNAb therapies were administered either alone or in combination with other interventions strategies, including therapeutic vaccines (126, 127) and immune modulatory agents (130–133). Depletion of CD8+ T cells resulted in transient increase of plasma viremia in animals with controlled viral suppression, suggesting that mechanism of viral control is likely co-dependent on CD8+ T cell immune responses (126, 127). The potential immunomodulatory effect of bNAb therapy on CD8+ T cells was recently demonstrated in adults in the clinic, in which administration of combination bNAb therapy (3BNC117 and 10-1074) was associated with an increase in Gag-specific CD8+ T cells in the blood (134). However, whether the increase in HIV-specific CD8+ T cell immune responses contribute to viral control and suppression in the absence of ART remains to be seen. Taken together, these studies highlight the potential of bNAb therapy as an alternative or adjuvant to ART in mediating viral control and/or augmenting the host endogenous immune responses for viral control. Given the unique characteristics of early life immunity as discussed above, it is imperative that effort to advance the use of bNAb therapy in pediatric HIV be tailored to the developing immune system cell composition, activation state, and milieu. Indeed, the highly tolerogenic and anti-inflammatory immune environment during early life as well as differences in pathogenesis between adults and children with HIV may influence efficacy of bNAb therapy towards viral control and remission the pediatric HIV population (135). Thus, the endogenous immune responses in pediatric HIV could potentially be harnessed towards viral elimination and clearance of persistent reservoir to achieve viral remission in the absence of ART. Future studies should aim to investigate the effect of bNAb therapy alone or in combination with therapeutic vaccines and other immunomodulatory agents in augmenting endogenous immune responses as a potential strategy for HIV cure in the in the context of early life immunity.
Numerous efforts are currently being developed to ensure consistent high levels of bNAbs in the circulation including various modifications to extend their half-lives (120, 136–139) and expression of bNAbs using viral vectors (122, 140, 141). Additional strategies are currently being developed to enhance the ability of Fc effector functions, including antibody-mediated cell cytotoxicity (ADCC) and antibody-dependent cell phagocytosis (ADCP), to clear HIV-infected cells (121, 122, 142–144). The Fc region enhancement can be accomplished by (i) altering the antibody isotype (142, 143), (ii) modifying the antibody Fc domain amino acid sequences (139, 144), and (iii) modifying the antibody Fc glycans (122, 145). These approaches modulate antiviral breadth and potency of bNAb therapy by increasing bNAbs affinity to cognate Fc receptors on host immune cells and host complement proteins for elimination of HIV-infected cells. These current novel modalities represent tremendous opportunities for pediatric HIV cure. Passively acquired and endogenous ADCC-mediating antibodies contributed to better disease outcome in infants with HIV (146–149). This suggests that passive immunization with bNAbs exhibiting enhanced antiviral functions could be especially attractive for pediatric HIV treatment.
Engineered Antibody-Like Molecules
One major challenge in bNAb therapy is the emergence of viral escape to one or more bNAbs due to selection or mutation once the plasma levels of passively administered bNAbs start to decline (150). Antibody engineering through generation of bi- (151, 152) and tri-specific (153–155) bNAbs against HIV have been demonstrated to increase potency and breadth of combination bNAb therapy. An attractive option to improve bNAb performance and overcome their intrinsic limitations is the development of antibody-like molecules, in which antibodies are engineered to (i) improve affinity and recognition of viral epitopes, (ii) combine bi- or tri-specificities, and/or (iii) modify Fc domains to improve half-life and increase engagement of effector cells (156). Overall, there are two major classes of bi- or tri-specific antibody-like molecules: those with or without an antibody Fc region (157).
Asokan and colleagues constructed four different bi-specific immunoglobulins (IgGs) composed of independent antigen-binding fragments with a common Fc region: VRC07×10E8, VRC07×PGT121, VRC07×PG9-16, and 10E8×PG9-16. All four bi-specific IgGs neutralized over 94% of antigenically diverse viruses in a panel of 206 HIV-1 strains, with VRC07xPG9-16 showing the most positive neutralization profile (158). In a step further in the development of these molecules, a tri-specific antibody-like molecule was generated to include bNAbs with 3 HIV Env specificities, N6 (targeting the CD4bs), PGDM1400 (targeting the V2 glycan), and 10E8v4 (targeting the MPER). This tri-specific antibody-like molecule neutralized clade A SHIV BG505 more potently than any of the parental bNAbs (154), showing comparable potency but greater breadth than a previously developed tri-specific molecule including VRC01/PGDM1400/10E8v4 specificities (153). The newly improved tri-specific antibody-like molecule (N6-CD4bs/PGDM1400-V2 glycan/10E8v4-MPER) was shown to reduce viremia from 100- to 1000-fold in viremic SHIV-infected adult NHPs (154) although transient viral rebound was observed when ART was interrupted, likely due to decline of antibody-like molecule level. However, similar to prior observations with mono- and dual-bNAb therapy, rebound viremia was returned to low levels via CD8+ T cell mediated viral control (154).
Another interesting approach to engineer antibody-like molecule against HIV involves combining a domain to target the virus protein and another domain to target host cell receptors. Mainly, two different constructs have been developed following this strategy: Bi-specific T/NK cell engagers (BiTEs/BiKEs) and Dual Affinity Re-targeting Molecules (DARTs). BiTEs are composed of antibody domain targeting the HIV-1 Env protein gp120 through B12 or VRC01 and an anti-human CD3 single chain variable fragment (scFv) was shown to redirect lysis of HIV gp120-transfected CHO cells in vitro, as well as inhibited HIV replication in both HIV-infected PBMCs and macrophages cocultured with autologous CD8+T cells (159). Similarly, BiKEs are composed of antibody domains D6 and E11, which bind to human CD16 present on NK cells, and a soluble CD4 domain, which binds to HIV gp120. BiKEs activate NK cells in the presence of target cells in and mediate infected target cell lysis (160). DARTs are novel bi-specific-based constructs that seem to offer improved stability, manufacturability, and potency compared to the BiTEs (161). The variable domains of the two antigen-binding moieties in DART molecules are incorporated into a disulfide-linked heterodimer, stabilizing the structure. DART molecules with HIV-1 Env and CD3 specificities were evaluated for their capacity to recruit and redirect cytotoxic T effector cells to primary HIV infected CD4+ T cells.
Unfortunately, all studies with bi/tri-specific molecules so far focused on cells from adult participants, leaving unknown the potential as therapy for pediatric infection. Importantly, immune system development during early life will probably affect the therapeutic potential of bispecific molecules, and thus children’s effector cells might have improved activity profiles.
Latency Reversing Agents
Latency reversing agents (LRAs) are small-chemical compounds employed to activate and expose latently HIV-infected cells to the host immune system (92). LRAs target different mechanisms of HIV transcription and replication. The current classes of LRAs induce epigenetic modification, sequester transcription factors, and target the HIV Tat protein (162). Among the epigenetic LRAs, there are: histone deacetylase inhibitors, histone methyltransferase inhibitors, and bro- and extra terminal domain inhibitors. These drugs act at the HIV-1 LTR promoter and induce histone modifications at the chromatin levels, which prevent RNA polymerase from initiating transcription. There are also single-agonists LRAs like protein kinase C (PKC), that induce HIV latency reversal in model systems, which has broad impact activity and could potentially have dangerous side effects in vivo. The potential broad side effects of LRAs are one of the major challenges in employment of LRAs in the clinic for pediatric HIV treatment.
Many anti-tumor drugs approved for use in adults and children are currently under evaluation for their potential to act as LRAs in adults with HIV (Table 3). Existing anti-tumor drugs are easily translatable to LRA discovery because of the shared strategy to induce epigenetic changes that promote cell cycle arrest, apoptosis, autophagy, and cell death of cancer-infected cells (163). Several LRAs have undergone testing in adult clinical trials including the histone deacetylase inhibitors, such as vorinostat, panobinostat, belinostat, and romidepsin, as well as immunomodulatory compound such as IL-15 receptor superagonist complex (N-803). While some drugs have induced HIV-1 transcription and T-cell activation, no candidates have effectively reduced the size of the virus reservoir (162). Clinical trials in adults with HIV and treated with ART in combination with romidepsin and vorinostat showed increased levels of plasma HIV RNA and cell-associated unspliced RNA in CD4+ T cells respectively. However, no significant changes in virus reservoir size were observed (164, 165). Recently, a regimen of vorinostat along with hydroxychloroquine and maraviroc was reported to increase the levels of plasma HIV RNA in a cohort of adults on ART but no change in the virus reservoir size was detected (166). In addition, upon ART interruption, no difference in time to viral rebound between the vorinostat and control groups was observed (166). Miller et al. reported that escalating doses of the investigational drug N-803, an IL-15 receptor superagonist complex (IL-15/IL-15α-Fc) was safe and well-tolerated in adult living with HIV in Phase 1 clinical trial (167). However, future investigation is still needed to determine the impact of N-803 on HIV reservoirs.
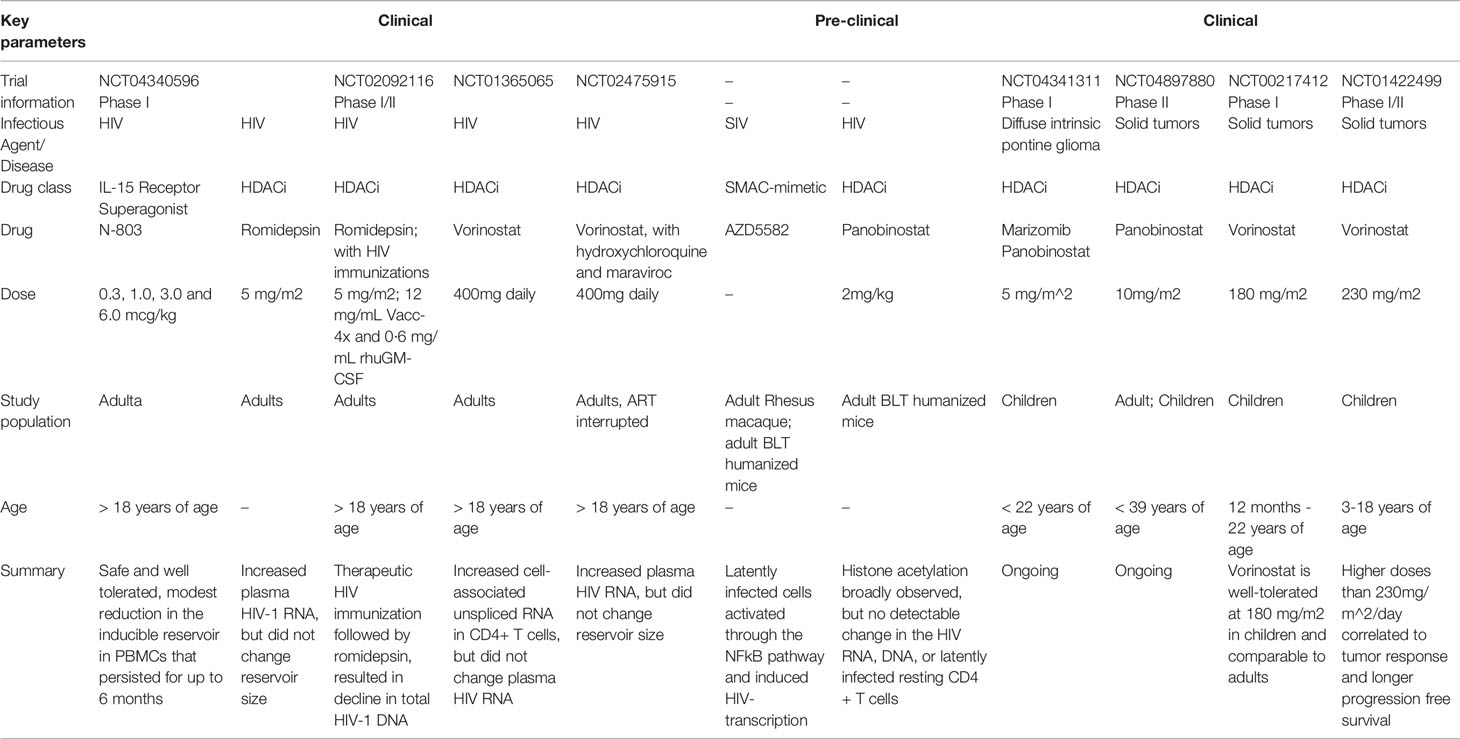
Table 3 Latency reversing agents in development for adults and for potential use in pediatric HIV treatment.
Currently, there are no clinical trials for evaluation of LRAs in pediatric HIV populations. However, several of the LRAs in clinical trials for adults have also been evaluated as anti-tumor drugs for pediatric cancer treatment (Table 3). The clinical trials of vorinostat and panobinostat in pediatric cancer populations showed that the drugs were well-tolerated, suggesting the potential opportunity to safely translate these drugs into LRAs to the pediatric HIV population (166, 168). Preclinical studies in adult rhesus and humanized mouse models showed promising results in using the mimetics of the second mitochondrial-derived activator caspases (SMAC) to activate latently HIV-infected cells via the non-canonical NF-Kb pathway, in which increased levels of SIV-RNAs were observed in resting CD4+ T cells derived from tissues following SMAC mimetic (AZD5582) treatment (169). However, a more recent study by Bricker et al, reported that while the pharmacokinetics and pharmacodynamics (PKPD) study of repeated AZD5582 dosing was safe in preclinical infant rhesus macaques, the investigational drug showed weaker latency reversing activity in infant than in adult rhesus macaques, likely due to altered pharmacokinetics and less inducibility of infant CD4+ T cells (170). Therefore, additional studies in both clinical and preclinical models to investigate the impact of novel latency reversing strategies, in the context of developing immune system, on latent viral reservoir reactivation and their combination with anti-viral immunotherapeutic strategies would highly benefit the quest for pediatric HIV cure.
Summary
The eradication of persistent latently infected HIV reservoirs will likely require combinations of multiple immune-based interventions, whether alone or in combination with ART. In addition to the treatment regimen composition, dosing and timing will likely be key elements in efforts to limit initial establishment of a founder population of infected cells or to purge latently infected cells harboring replication competent HIV provirus in children with HIV.
Conclusions
The unique context of early life immunity and the distinct nature of persistent viral reservoirs in pediatric settings suggest that pediatric-specific strategies will likely be required to achieve ART-free remission in children living with HIV. ART alone is insufficient and a combination of immune-based interventions that can directly target and/or augment host immune effector functions will probably be needed to eliminate latently HIV-infected cells. However, many of the recent advances in understanding HIV persistence and strategies to eliminate latent viral reservoirs for HIV cure using immune-based strategies are conducted in adults, and there is a paucity in studies involving children living with HIV. Current HIV cure strategies cannot be directly extrapolated to children due to (1) unique differences in early life and adult immune systems, (2) the distinct kinetics of latent virus reactivation in adult and children, and (3) differences in route of infection or transmission of HIV in adult and children. Immune-based intervention strategies such as therapeutic vaccines that target T and/or B cells or passive immunization using bNAbs or engineered Ab-like molecules in combination with LRAs will likely need to be adapted to the pediatric setting. Additionally, these intervention strategies will likely need to be tailored to both perinatal and postnatal HIV infections in children. ART interruption poses the risk of viral rebound, emergence of resistance strains, and other unknown risks (171). However, a study in small subset of perinatally infected infants (7 infants, age <12 weeks) treated early with ART, planned treatment interruption resulted in prolonged viral control (median of 57 weeks off treatment) and that restarting of treatment led to improvement in CD4+ T cell counts (171).
One of the major limitations of HIV cure research in the pediatric population is the ethical and risk-to-benefit considerations around the optimum time to interrupt treatment in order to assess successful eradication or prevention of long-term HIV reservoirs establishment (172, 173). The unique immune profiles of pediatric ECs could provide better understanding of the immune mechanisms involved in long term viral control in the absence of treatment and may guide the development of immune-based strategies targeted towards augmentation of infant early life immune responses for eradication of latent HIV reservoirs.
Future Directions
The use of infant NHP models for both perinatal and postnatal pediatric HIV infections in the context of ART is crucial in advancing our understanding of the persistent latent HIV reservoir and how it can be targeted in the unique setting of early life immune development. Additionally, development of infant NHP models that recapitulate the slower disease progression in pediatric ECs in the absence of treatment would be highly valuable in investigating the immune mechanisms involved in viral control and development of immune-based strategies for pediatric HIV cure.
Author Contributions
SB, AN, BY, RG, TS, and CG conceived, designed, and wrote the article. CP, SD, MN, and AB contributed to writing part of the article. GF, AC, SP, and RA contributed to the conception, design, review and editing of the manuscript.
Funding
This work was supported by grant P01 AI131276 from the (National Institute of Allergy and Infectious Diseases (NIAID) to AC, GF, and SP. AC, GF, SP are also supported by UM1 AI164566. SB is supported by the Interdisciplinary Research and Training Program in AIDS (5T32AI007392-31), Duke University.
Conflict of Interest
Author SP serves as a consultant for Moderna, Merck, Dynavax, Pfizer, and Hoopika CMV vaccine programs and leads a sponsored research program on CMV vaccines with Moderna and Merck.
The remaining authors declare that the research was conducted in the absence of any commercial or financial relationships that could be construed as a potential conflict of interest.
Publisher’s Note
All claims expressed in this article are solely those of the authors and do not necessarily represent those of their affiliated organizations, or those of the publisher, the editors and the reviewers. Any product that may be evaluated in this article, or claim that may be made by its manufacturer, is not guaranteed or endorsed by the publisher.
References
1. UNAIDS. Global HIV & AIDS Statistics — Fact Sheet (2021). Available at: https://www.unaids.org/en/resources/fact-sheet.
2. UNAIDS. UNAIDS Epidemiological Estimates (2021). Available at: https://aidsinfo.unaids.org/.
3. World Health Organization. Consolidated guidelines on HIV prevention, testing, treatment, service delivery and monitoring: recommendations for a public health approach, 2021 update. World Health Organization (2021). Available at: https://apps.who.int/iris/handle/10665/342899.
4. Shiau S, Abrams EJ, Arpadi SM, Kuhn L. Early Antiretroviral Therapy in HIV-Infected Infants: Can it Lead to HIV Remission? Lancet HIV (2018) 5(5):e250–e8. doi: 10.1016/S2352-3018(18)30012-2
5. Cotton MF, Violari A, Otwombe K, Panchia R, Dobbels E, Rabie H, et al. Early Time-Limited Antiretroviral Therapy Versus Deferred Therapy in South African Infants Infected With HIV: Results From the Children With HIV Early Antiretroviral (CHER) Randomised Trial. Lancet (2013) 382(9904):1555–63. doi: 10.1016/S0140-6736(13)61409-9
6. Luzuriaga K. Early Combination Antiretroviral Therapy Limits HIV-1 Persistence in Children. Annu Rev Med (2016) 67:201–13. doi: 10.1146/annurev-med-091114-111159
7. Garcia-Broncano P, Maddali S, Einkauf KB, Jiang C, Gao C, Chevalier J, et al. Early Antiretroviral Therapy in Neonates With HIV-1 Infection Restricts Viral Reservoir Size and Induces a Distinct Innate Immune Profile. Sci Transl Med (2019) 11(520):eaax7350. doi: 10.1126/scitranslmed.aax7350
8. Shiau S, Kuhn L. Antiretroviral Treatment in HIV-Infected Infants and Young Children: Novel Issues Raised by the Mississippi Baby. Expert Rev Anti Infect Ther (2014) 12(3):307–18. doi: 10.1586/14787210.2014.888311
9. Ananworanich J, Puthanakit T, Suntarattiwong P, Chokephaibulkit K, Kerr SJ, Fromentin R, et al. Reduced Markers of HIV Persistence and Restricted HIV-Specific Immune Responses After Early Antiretroviral Therapy in Children. AIDS (2014) 28(7):1015–20. doi: 10.1097/QAD.0000000000000178
10. Luzuriaga K, McManus M, Catalina M, Mayack S, Sharkey M, Stevenson M, et al. Early Therapy of Vertical Human Immunodeficiency Virus Type 1 (HIV-1) Infection: Control of Viral Replication and Absence of Persistent HIV-1-Specific Immune Responses. J Virol (2000) 74(15):6984–91. doi: 10.1128/JVI.74.15.6984-6991.2000
11. Persaud D, Patel K, Karalius B, Rainwater-Lovett K, Ziemniak C, Ellis A, et al. Influence of Age at Virologic Control on Peripheral Blood Human Immunodeficiency Virus Reservoir Size and Serostatus in Perinatally Infected Adolescents. JAMA Pediatr (2014) 168(12):1138–46. doi: 10.1001/jamapediatrics.2014.1560
12. Kuhn L, Paximadis M, Da Costa Dias B, Loubser S, Strehlau R, Patel F, et al. Age at Antiretroviral Therapy Initiation and Cell-Associated HIV-1 DNA Levels in HIV-1-Infected Children. PloS One (2018) 13(4):e0195514. doi: 10.1371/journal.pone.0195514
13. Cotugno N, Morrocchi E, Rinaldi S, Rocca S, Pepponi I, di Cesare S, et al. Early Antiretroviral Therapy-Treated Perinatally HIV-Infected Seronegative Children Demonstrate Distinct Long-Term Persistence of HIV-Specific T-Cell and B-Cell Memory. AIDS (2020) 34(5):669–80. doi: 10.1097/QAD.0000000000002485
14. Gebara NY, El Kamari V, Rizk N. HIV-1 Elite Controllers: An Immunovirological Review and Clinical Perspectives. J Virus Erad (2019) 5(3):163–6. doi: 10.1016/S2055-6640(20)30046-7
15. Deeks SG, Walker BD. Human Immunodeficiency Virus Controllers: Mechanisms of Durable Virus Control in the Absence of Antiretroviral Therapy. Immunity (2007) 27(3):406–16. doi: 10.1016/j.immuni.2007.08.010
16. Okulicz JF, Grandits GA, Weintrob AC, Landrum ML, Ganesan A, Crum-Cianflone NF, et al. CD4 T Cell Count Reconstitution in HIV Controllers After Highly Active Antiretroviral Therapy. Clin Infect Dis (2010) 50(8):1187–91. doi: 10.1086/651421
18. Vieira VA, Zuidewind P, Muenchhoff M, Roider J, Millar J, Clapson M, et al. Strong Sex Bias in Elite Control of Paediatric HIV Infection. Aids (2019) 33(1):67–75. doi: 10.1097/QAD.0000000000002043
19. Vieira VA, Millar J, Adland E, Muenchhoff M, Roider J, Guash CF, et al. Robust HIV-Specific CD4+ and CD8+ T-Cell Responses Distinguish Elite Control in Adolescents Living With HIV From Viremic Nonprogressors. Aids (2022) 36(1):95–105. doi: 10.1097/QAD.0000000000003078
20. Collins DR, Gaiha GD, Walker BD. CD8(+) T Cells in HIV Control, Cure and Prevention. Nat Rev Immunol (2020) 20(8):471–82. doi: 10.1038/s41577-020-0274-9
21. Yan J, Sabbaj S, Bansal A, Amatya N, Shacka JJ, Goepfert PA, et al. HIV-Specific CD8+ T Cells From Elite Controllers are Primed for Survival. J Virol (2013) 87(9):5170–81. doi: 10.1128/JVI.02379-12
22. Chowdhury A, Hayes TL, Bosinger SE, Lawson BO, Vanderford T, Schmitz JE, et al. Differential Impact of In Vivo CD8+ T Lymphocyte Depletion in Controller Versus Progressor Simian Immunodeficiency Virus-Infected Macaques. J Virol (2015) 89(17):8677–86. doi: 10.1128/JVI.00869-15
23. Mueller YM, Do DH, Boyer JD, Kader M, Mattapallil JJ, Lewis MG, et al. CD8+ Cell Depletion of SHIV89.6P-Infected Macaques Induces CD4+ T Cell Proliferation That Contributes to Increased Viral Loads. J Immunol (2009) 183(8):5006–12. doi: 10.4049/jimmunol.0900141
24. Okoye A, Park H, Rohankhedkar M, Coyne-Johnson L, Lum R, Walker JM, et al. Profound CD4+/CCR5+ T Cell Expansion is Induced by CD8+ Lymphocyte Depletion But Does Not Account for Accelerated SIV Pathogenesis. J Exp Med (2009) 206(7):1575–88. doi: 10.1084/jem.20090356
25. Pandrea I, Gaufin T, Gautam R, Kristoff J, Mandell D, Montefiori D, et al. Functional Cure of SIVagm Infection in Rhesus Macaques Results in Complete Recovery of CD4+ T Cells and is Reverted by CD8+ Cell Depletion. PloS Pathog (2011) 7(8):e1002170. doi: 10.1371/journal.ppat.1002170
26. Ma D, Xu C, Cillo AR, Policicchio B, Kristoff J, Haret-Richter G, et al. Simian Immunodeficiency Virus SIVsab Infection of Rhesus Macaques as a Model of Complete Immunological Suppression With Persistent Reservoirs of Replication-Competent Virus: Implications for Cure Research. J Virol (2015) 89(11):6155–60. doi: 10.1128/JVI.00256-15
27. Kelley CF, Kitchen CM, Hunt PW, Rodriguez B, Hecht FM, Kitahata M, et al. Incomplete Peripheral CD4+ Cell Count Restoration in HIV-Infected Patients Receiving Long-Term Antiretroviral Treatment. Clin Infect Dis (2009) 48(6):787–94. doi: 10.1086/597093
28. Battegay M, Nuesch R, Hirschel B, Kaufmann GR. Immunological Recovery and Antiretroviral Therapy in HIV-1 Infection. Lancet Infect Dis (2006) 6(5):280–7. doi: 10.1016/S1473-3099(06)70463-7
29. Owen RE, Heitman JW, Hirschkorn DF, Lanteri MC, Biswas HH, Martin JN, et al. HIV+ Elite Controllers Have Low HIV-Specific T-Cell Activation Yet Maintain Strong, Polyfunctional T-Cell Responses. Aids (2010) 24(8):1095–105. doi: 10.1097/QAD.0b013e3283377a1e
30. Bansal A, Sterrett S, Erdmann N, Westfall AO, Dionne-Odom J, Overton ET, et al. Normal T-Cell Activation in Elite Controllers With Preserved CD4+ T-Cell Counts. Aids (2015) 29(17):2245–54. doi: 10.1097/QAD.0000000000000860
31. Khoury G, Fromentin R, Solomon A, Hartogensis W, Killian M, Hoh R, et al. Human Immunodeficiency Virus Persistence and T-Cell Activation in Blood, Rectal, and Lymph Node Tissue in Human Immunodeficiency Virus-Infected Individuals Receiving Suppressive Antiretroviral Therapy. J Infect Dis (2017) 215(6):911–9. doi: 10.1093/infdis/jix039
32. Cockerham LR, Siliciano JD, Sinclair E, O'Doherty U, Palmer S, Yukl SA, et al. CD4+ and CD8+ T Cell Activation are Associated With HIV DNA in Resting CD4+ T Cells. PloS One (2014) 9(10):e110731. doi: 10.1371/journal.pone.0110731
33. Ndhlovu ZM, Kamya P, Mewalal N, Kloverpris HN, Nkosi T, Pretorius K, et al. Magnitude and Kinetics of CD8+ T Cell Activation During Hyperacute HIV Infection Impact Viral Set Point. Immunity (2015) 43(3):591–604. doi: 10.1016/j.immuni.2015.08.012
34. Roider J, Ngoepe A, Muenchhoff M, Adland E, Groll A, Ndung'u T, et al. Increased Regulatory T-Cell Activity and Enhanced T-Cell Homeostatic Signaling in Slow Progressing HIV-Infected Children. Front Immunol (2019) 10. doi: 10.3389/fimmu.2019.00213
35. Kaufmann DE, Kavanagh DG, Pereyra F, Zaunders JJ, Mackey EW, Miura T, et al. Upregulation of CTLA-4 by HIV-Specific CD4+ T Cells Correlates With Disease Progression and Defines a Reversible Immune Dysfunction. Nat Immunol (2007) 8(11):1246–54. doi: 10.1038/ni1515
36. Elrefaei M, Burke CM, Baker CA, Jones NG, Bousheri S, Bangsberg DR, et al. HIV-Specific TGF-Beta-Positive CD4+ T Cells do Not Express Regulatory Surface Markers and are Regulated by CTLA-4. AIDS Res Hum Retroviruses (2010) 26(3):329–37. doi: 10.1089/aid.2009.0149
37. Hryniewicz A, Boasso A, Edghill-Smith Y, Vaccari M, Fuchs D, Venzon D, et al. CTLA-4 Blockade Decreases TGF-Beta, IDO, and Viral RNA Expression in Tissues of SIVmac251-Infected Macaques. Blood (2006) 108(12):3834–42. doi: 10.1182/blood-2006-04-010637
38. Cecchinato V, Tryniszewska E, Ma ZM, Vaccari M, Boasso A, Tsai WP, et al. Immune Activation Driven by CTLA-4 Blockade Augments Viral Replication at Mucosal Sites in Simian Immunodeficiency Virus Infection. J Immunol (2008) 180(8):5439–47. doi: 10.4049/jimmunol.180.8.5439
39. Harper J, Gordon S, Chan CN, Wang H, Lindemuth E, Galardi C, et al. CTLA-4 and PD-1 Dual Blockade Induces SIV Reactivation Without Control of Rebound After Antiretroviral Therapy Interruption. Nat Med (2020) 26(4):519–28. doi: 10.1038/s41591-020-0782-y
40. Hansen SG, Ford JC, Lewis MS, Ventura AB, Hughes CM, Coyne-Johnson L, et al. Profound Early Control of Highly Pathogenic SIV by an Effector Memory T-Cell Vaccine. Nature (2011) 473(7348):523–7. doi: 10.1038/nature10003
41. Bar KJ, Sneller MC, Harrison LJ, Justement JS, Overton ET, Petrone ME, et al. Effect of HIV Antibody VRC01 on Viral Rebound After Treatment Interruption. N Engl J Med (2016) 375(21):2037–50. doi: 10.1056/NEJMoa1608243
42. He T, Brocca-Cofano E, Policicchio BB, Sivanandham R, Gautam R, Raehtz KD, et al. Cutting Edge: T Regulatory Cell Depletion Reactivates Latent Simian Immunodeficiency Virus (SIV) in Controller Macaques While Boosting SIV-Specific T Lymphocytes. J Immunol (2016) 197(12):4535–9. doi: 10.4049/jimmunol.1601539
43. Sivanandham R, Kleinman AJ, Sette P, Brocca-Cofano E, Kilapandal Venkatraman SM, Policicchio BB, et al. Nonhuman Primate Testing of the Impact of Different Regulatory T Cell Depletion Strategies on Reactivation and Clearance of Latent Simian Immunodeficiency Virus. J Virol (2020) 94(19):e00533-20. doi: 10.1128/JVI.00533-20
44. Kleinman AJ, Sivanandham R, Pandrea I, Chougnet CA, Apetrei C. Regulatory T Cells As Potential Targets for HIV Cure Research. Front Immunol (2018) 9. doi: 10.3389/fimmu.2018.00734
45. Falivene J, Ghiglione Y, Laufer N, Socias ME, Holgado MP, Ruiz MJ, et al. Th17 and Th17/Treg Ratio at Early HIV Infection Associate With Protective HIV-Specific CD8(+) T-Cell Responses and Disease Progression. Sci Rep (2015) 5:11511. doi: 10.1038/srep11511
46. Caetano DG, de Paula HHS, Bello G, Hoagland B, Villela LM, Grinsztejn B, et al. HIV-1 Elite Controllers Present a High Frequency of Activated Regulatory T and Th17 Cells. PloS One (2020) 15(2):e0228745. doi: 10.1371/journal.pone.0228745
47. Dzanibe S, Lennard K, Kiravu A, Seabrook MSS, Alinde B, Holmes SP, et al. Stereotypic Expansion of T Regulatory and Th17 Cells During Infancy Is Disrupted by HIV Exposure and Gut Epithelial Damage. J Immunol (2022) 208(1):27–37. doi: 10.4049/jimmunol.2100503
48. Fletcher CV, Staskus K, Wietgrefe SW, Rothenberger M, Reilly C, Chipman JG, et al. Persistent HIV-1 Replication is Associated With Lower Antiretroviral Drug Concentrations in Lymphatic Tissues. Proc Natl Acad Sci U S A (2014) 111(6):2307–12. doi: 10.1073/pnas.1318249111
49. Petrovas C, Yamamoto T, Gerner MY, Boswell KL, Wloka K, Smith EC, et al. CD4 T Follicular Helper Cell Dynamics During SIV Infection. J Clin Invest (2012) 122(9):3281–94. doi: 10.1172/JCI63039
50. Fukazawa Y, Lum R, Okoye AA, Park H, Matsuda K, Bae JY, et al. B Cell Follicle Sanctuary Permits Persistent Productive Simian Immunodeficiency Virus Infection in Elite Controllers. Nat Med (2015) 21(2):132–9. doi: 10.1038/nm.3781
51. Pallikkuth S, Sharkey M, Babic DZ, Gupta S, Stone GW, Fischl MA, et al. Peripheral T Follicular Helper Cells Are the Major HIV Reservoir Within Central Memory CD4 T Cells in Peripheral Blood From Chronically HIV-Infected Individuals on Combination Antiretroviral Therapy. J Virol (2015) 90(6):2718–28. doi: 10.1128/JVI.02883-15
52. Garcia M, Gorgolas M, Cabello A, Estrada V, Ligos JM, Fernandez-Guerrero M, et al. Peripheral T Follicular Helper Cells Make a Difference in HIV Reservoir Size Between Elite Controllers and Patients on Successful cART. Sci Rep (2017) 7(1):16799. doi: 10.1038/s41598-017-17057-y
53. Buranapraditkun S, Pissani F, Teigler JE, Schultz BT, Alter G, Marovich M, et al. Preservation of Peripheral T Follicular Helper Cell Function in HIV Controllers. J Virol (2017) 91(14):e00497-17. doi: 10.1128/JVI.00497-17
54. Claireaux M, Galperin M, Benati D, Nouel A, Mukhopadhyay M, Klingler J, et al. A High Frequency of HIV-Specific Circulating Follicular Helper T Cells Is Associated With Preserved Memory B Cell Responses in HIV Controllers. mBio (2018) 9(3):e00317-18. doi: 10.1128/mBio.00317-18
55. Muema DM, Macharia GN, Olusola BA, Hassan AS, Fegan GW, Berkley JA, et al. Proportions of Circulating Follicular Helper T Cells are Reduced and Correlate With Memory B Cells in HIV-Infected Children. PloS One (2017) 12(4):e0175570. doi: 10.1371/journal.pone.0175570
56. McCarty B, Mwamzuka M, Marshed F, Generoso M, Alvarez P, Ilmet T, et al. Low Peripheral T Follicular Helper Cells in Perinatally HIV-Infected Children Correlate With Advancing HIV Disease. Front Immunol (2018) 9. doi: 10.3389/fimmu.2018.01901
57. Buckner CM, Kardava L, Zhang X, Gittens K, Justement JS, Kovacs C, et al. Maintenance of HIV-Specific Memory B-Cell Responses in Elite Controllers Despite Low Viral Burdens. J Infect Dis (2016) 214(3):390–8. doi: 10.1093/infdis/jiw163
58. Aggarwal H, Khan L, Chaudhary O, Kumar S, Makhdoomi MA, Singh R, et al. Alterations in B Cell Compartment Correlate With Poor Neutralization Response and Disease Progression in HIV-1 Infected Children. Front Immunol (2017) 8. doi: 10.3389/fimmu.2017.01697
59. Fontaine J, Chagnon-Choquet J, Valcke HS, Poudrier J, Roger M, Montreal Primary HIVI, et al. High Expression Levels of B Lymphocyte Stimulator (BLyS) by Dendritic Cells Correlate With HIV-Related B-Cell Disease Progression in Humans. Blood (2011) 117(1):145–55. doi: 10.1182/blood-2010-08-301887
60. Muenchhoff M, Chung AW, Roider J, Dugast AS, Richardson S, Kloverpris H, et al. Distinct Immunoglobulin Fc Glycosylation Patterns Are Associated With Disease Nonprogression and Broadly Neutralizing Antibody Responses in Children With HIV Infection. mSphere (2020) 5(6):e00880-20. doi: 10.1128/mSphere.00880-20
61. Vidarsson G, Dekkers G, Rispens T. IgG Subclasses and Allotypes: From Structure to Effector Functions. Front Immunol (2014) 5. doi: 10.3389/fimmu.2014.00520
62. Lambotte O, Pollara J, Boufassa F, Moog C, Venet A, Haynes BF, et al. High Antibody-Dependent Cellular Cytotoxicity Responses are Correlated With Strong CD8 T Cell Viral Suppressive Activity But Not With B57 Status in HIV-1 Elite Controllers. PloS One (2013) 8(9):e74855. doi: 10.1371/journal.pone.0074855
63. Madhavi V, Wines BD, Amin J, Emery S, Group ES, Lopez E, et al. HIV-1 Env- and Vpu-Specific Antibody-Dependent Cellular Cytotoxicity Responses Associated With Elite Control of HIV. J Virol (2017) 91(18):e00700-17. doi: 10.1128/JVI.00700-17
64. Ackerman ME, Mikhailova A, Brown EP, Dowell KG, Walker BD, Bailey-Kellogg C, et al. Polyfunctional HIV-Specific Antibody Responses Are Associated With Spontaneous HIV Control. PloS Pathog (2016) 12(1):e1005315. doi: 10.1371/journal.ppat.1005315
65. Kant S, Zhang N, Barbe A, Routy JP, Tremblay C, Thomas R, et al. Polyfunctional Fc Dependent Activity of Antibodies to Native Trimeric Envelope in HIV Elite Controllers. Front Immunol (2020) 11. doi: 10.3389/fimmu.2020.583820
66. Muenchhoff M, Adland E, Karimanzira O, Crowther C, Pace M, Csala A, et al. Nonprogressing HIV-Infected Children Share Fundamental Immunological Features of Nonpathogenic SIV Infection. Sci Transl Med (2016) 8(358):358ra125. doi: 10.1126/scitranslmed.aag1048
67. Simonich CA, Williams KL, Verkerke HP, Williams JA, Nduati R, Lee KK, et al. HIV-1 Neutralizing Antibodies With Limited Hypermutation From an Infant. Cell (2016) 166(1):77–87. doi: 10.1016/j.cell.2016.05.055
68. Kumar S, Panda H, Makhdoomi MA, Mishra N, Safdari HA, Chawla H, et al. An HIV-1 Broadly Neutralizing Antibody From a Clade C-Infected Pediatric Elite Neutralizer Potently Neutralizes the Contemporaneous and Autologous Evolving Viruses. J Virol (2019) 93(4):e01495-18. doi: 10.1128/JVI.01495-18
69. Ditse Z, Muenchhoff M, Adland E, Jooste P, Goulder P, Moore PL, et al. HIV-1 Subtype C-Infected Children With Exceptional Neutralization Breadth Exhibit Polyclonal Responses Targeting Known Epitopes. J Virol (2018) 92(17):e00878-18. doi: 10.1128/JVI.00878-18
70. Landais E, Moore PL. Development of Broadly Neutralizing Antibodies in HIV-1 Infected Elite Neutralizers. Retrovirology (2018) 15(1):61. doi: 10.1186/s12977-018-0443-0
71. Paul S, Lal G. The Molecular Mechanism of Natural Killer Cells Function and Its Importance in Cancer Immunotherapy. Front Immunol (2017) 8. doi: 10.3389/fimmu.2017.01124
72. Genovese L, Nebuloni M, Alfano M. Cell-Mediated Immunity in Elite Controllers Naturally Controlling HIV Viral Load. Front Immunol (2013) 4. doi: 10.3389/fimmu.2013.00086
73. Martin MP, Qi Y, Gao X, Yamada E, Martin JN, Pereyra F, et al. Innate Partnership of HLA-B and KIR3DL1 Subtypes Against HIV-1. Nat Genet (2007) 39(6):733–40. doi: 10.1038/ng2035
74. Alter G, Martin MP, Teigen N, Carr WH, Suscovich TJ, Schneidewind A, et al. Differential Natural Killer Cell-Mediated Inhibition of HIV-1 Replication Based on Distinct KIR/HLA Subtypes. J Exp Med (2007) 204(12):3027–36. doi: 10.1084/jem.20070695
75. Kamya P, Boulet S, Tsoukas CM, Routy JP, Thomas R, Cote P, et al. Receptor-Ligand Requirements for Increased NK Cell Polyfunctional Potential in Slow Progressors Infected With HIV-1 Coexpressing KIR3DL1*h/*y and HLA-B*57. J Virol (2011) 85(12):5949–60. doi: 10.1128/JVI.02652-10
76. Tomescu C, Duh FM, Hoh R, Viviani A, Harvill K, Martin MP, et al. Impact of Protective Killer Inhibitory Receptor/Human Leukocyte Antigen Genotypes on Natural Killer Cell and T-Cell Function in HIV-1-Infected Controllers. Aids (2012) 26(15):1869–78. doi: 10.1097/QAD.0b013e32835861b0
77. Bhukkar R, Sachdeva RK, Suri D, Shandilya J, Rawat A, Saikia B, et al. Reduced Natural Killer Cell Subsets in Perinatally Acquired Long-Term Non-Progressor Human Immunodeficiency Virus-Infected Children. AIDS Res Hum Retroviruses (2019) 35(5):437–43. doi: 10.1089/aid.2018.0243
78. Martin-Gayo E, Buzon MJ, Ouyang Z, Hickman T, Cronin J, Pimenova D, et al. Potent Cell-Intrinsic Immune Responses in Dendritic Cells Facilitate HIV-1-Specific T Cell Immunity in HIV-1 Elite Controllers. PloS Pathog (2015) 11(6):e1004930. doi: 10.1371/journal.ppat.1004930
79. Machmach K, Leal M, Gras C, Viciana P, Genebat M, Franco E, et al. Plasmacytoid Dendritic Cells Reduce HIV Production in Elite Controllers. J Virol (2012) 86(8):4245–52. doi: 10.1128/JVI.07114-11
80. Kwa S, Kannanganat S, Nigam P, Siddiqui M, Shetty RD, Armstrong W, et al. Plasmacytoid Dendritic Cells are Recruited to the Colorectum and Contribute to Immune Activation During Pathogenic SIV Infection in Rhesus Macaques. Blood (2011) 118(10):2763–73. doi: 10.1182/blood-2011-02-339515
81. Zhang Z, Fu J, Zhao Q, He Y, Jin L, Zhang H, et al. Differential Restoration of Myeloid and Plasmacytoid Dendritic Cells in HIV-1-Infected Children After Treatment With Highly Active Antiretroviral Therapy. J Immunol (2006) 176(9):5644–51. doi: 10.4049/jimmunol.176.9.5644
82. Krishnan S, Wilson EM, Sheikh V, Rupert A, Mendoza D, Yang J, et al. Evidence for Innate Immune System Activation in HIV Type 1-Infected Elite Controllers. J Infect Dis (2014) 209(6):931–9. doi: 10.1093/infdis/jit581
83. Singh A, Kazer SW, Roider J, Krista KC, Millar J, Asowata OE, et al. Innate Lymphoid Cell Activation and Sustained Depletion in Blood and Tissue of Children Infected With HIV From Birth Despite Antiretroviral Therapy. Cell Rep (2020) 32(11):108153. doi: 10.1016/j.celrep.2020.108153
84. Pizzolato G, Kaminski H, Tosolini M, Franchini DM, Pont F, Martins F, et al. Single-Cell RNA Sequencing Unveils the Shared and the Distinct Cytotoxic Hallmarks of Human TCRVdelta1 and TCRVdelta2 Gammadelta T Lymphocytes. Proc Natl Acad Sci U S A (2019) 116(24):11906–15. doi: 10.1073/pnas.1818488116
85. Gutierrez-Arcelus M, Teslovich N, Mola AR, Polidoro RB, Nathan A, Kim H, et al. Lymphocyte Innateness Defined by Transcriptional States Reflects a Balance Between Proliferation and Effector Functions. Nat Commun (2019) 10(1):687. doi: 10.1038/s41467-019-08604-4
86. Mayassi T, Jabri B. Human Intraepithelial Lymphocytes. Mucosal Immunol (2018) 11(5):1281–9. doi: 10.1038/s41385-018-0016-5
87. Chevalier MF, Bhatnagar N, Didier C, Lopez-Gonzalez M, Pavie J, Bollens D, et al. Gammadelta T-Cell Subsets in HIV Controllers: Potential Role of Tgammadelta17 Cells in the Regulation of Chronic Immune Activation. Aids (2019) 33(8):1283–92. doi: 10.1097/QAD.0000000000002196
88. Poccia F, Battistini L, Cipriani B, Mancino G, Martini F, Gougeon ML, et al. Phosphoantigen-Reactive Vgamma9Vdelta2 T Lymphocytes Suppress In Vitro Human Immunodeficiency Virus Type 1 Replication by Cell-Released Antiviral Factors Including CC Chemokines. J Infect Dis (1999) 180(3):858–61. doi: 10.1086/314925
89. Garrido C, Clohosey ML, Whitworth CP, Hudgens M, Margolis DM, Soriano-Sarabia N. Gammadelta T Cells: An Immunotherapeutic Approach for HIV Cure Strategies. JCI Insight (2018) 3(12):e120121. doi: 10.1172/jci.insight.120121
90. Grau-Exposito J, Luque-Ballesteros L, Navarro J, Curran A, Burgos J, Ribera E, et al. Latency Reversal Agents Affect Differently the Latent Reservoir Present in Distinct CD4+ T Subpopulations. PloS Pathog (2019) 15(8):e1007991. doi: 10.1371/journal.ppat.1007991
91. Vanhamel J, Bruggemans A, Debyser Z. Establishment of Latent HIV-1 Reservoirs: What do We Really Know? J Virus Erad (2019) 5(1):3–9. doi: 10.1016/S2055-6640(20)30275-2
92. Ait-Ammar A, Kula A, Darcis G, Verdikt R, De Wit S, Gautier V, et al. Current Status of Latency Reversing Agents Facing the Heterogeneity of HIV-1 Cellular and Tissue Reservoirs. Front Microbiol (2019) 10. doi: 10.3389/fmicb.2019.03060
93. Stevenson M. Role of Myeloid Cells in HIV-1-Host Interplay. J Neurovirol (2015) 21(3):242–8. doi: 10.1007/s13365-014-0281-3
94. Jambo KC, Banda DH, Kankwatira AM, Sukumar N, Allain TJ, Heyderman RS, et al. Small Alveolar Macrophages are Infected Preferentially by HIV and Exhibit Impaired Phagocytic Function. Mucosal Immunol (2014) 7(5):1116–26. doi: 10.1038/mi.2013.127
95. Wallet C, De Rovere M, Van Assche J, Daouad F, De Wit S, Gautier V, et al. Microglial Cells: The Main HIV-1 Reservoir in the Brain. Front Cell Infect Microbiol (2019) 9. doi: 10.3389/fcimb.2019.00362
96. Obregon-Perko V, Bricker KM, Mensah G, Uddin F, Rotolo L, Vanover D, et al. Dynamics and Origin of Rebound Viremia in SHIV-Infected Infant Macaques Following Interruption of Long-Term ART. JCI Insight (2021) 6(23):e152526. doi: 10.1172/jci.insight.152526
97. Obregon-Perko V, Bricker KM, Mensah G, Uddin F, Kumar MR, Fray EJ, et al. Simian-Human Immunodeficiency Virus SHIV.C.CH505 Persistence in ART-Suppressed Infant Macaques Is Characterized by Elevated SHIV RNA in the Gut and a High Abundance of Intact SHIV DNA in Naive CD4(+) T Cells. J Virol (2020) 95(2):e01669-20. doi: 10.1128/JVI.01669-20
98. Mavigner M, Habib J, Deleage C, Rosen E, Mattingly C, Bricker K, et al. Simian Immunodeficiency Virus Persistence in Cellular and Anatomic Reservoirs in Antiretroviral Therapy-Suppressed Infant Rhesus Macaques. J Virol (2018) 92(18):e00562-18. doi: 10.1128/JVI.00562-18
99. Hessell AJ, Jaworski JP, Epson E, Matsuda K, Pandey S, Kahl C, et al. Early Short-Term Treatment With Neutralizing Human Monoclonal Antibodies Halts SHIV Infection in Infant Macaques. Nat Med (2016) 22(4):362–8. doi: 10.1038/nm.4063
100. Luzuriaga K, Tabak B, Garber M, Chen YH, Ziemniak C, McManus MM, et al. HIV Type 1 (HIV-1) Proviral Reservoirs Decay Continuously Under Sustained Virologic Control in HIV-1-Infected Children Who Received Early Treatment. J Infect Dis (2014) 210(10):1529–38. doi: 10.1093/infdis/jiu297
101. Chomont N, El-Far M, Ancuta P, Trautmann L, Procopio FA, Yassine-Diab B, et al. HIV Reservoir Size and Persistence are Driven by T Cell Survival and Homeostatic Proliferation. Nat Med (2009) 15(8):893–900. doi: 10.1038/nm.1972
102. Martinez-Bonet M, Puertas MC, Fortuny C, Ouchi D, Mellado MJ, Rojo P, et al. Establishment and Replenishment of the Viral Reservoir in Perinatally HIV-1-Infected Children Initiating Very Early Antiretroviral Therapy. Clin Infect Dis (2015) 61(7):1169–78. doi: 10.1093/cid/civ456
103. Foster C, Dominguez-Rodriguez S, Tagarro A, Gkouleli T, Heaney J, Watters S, et al. The CARMA Study: Early Infant Antiretroviral Therapy-Timing Impacts on Total HIV-1 DNA Quantitation 12 Years Later. J Pediatr Infect Dis Soc (2021) 10(3):295–301. doi: 10.1093/jpids/piaa071
104. Dhummakupt A, Rubens JH, Anderson T, Powell L, Nonyane BA, Siems LV, et al. Differences in Inducibility of the Latent HIV Reservoir in Perinatal and Adult Infection. JCI Insight (2020) 5(4):e134105. doi: 10.1172/jci.insight.134105
105. Rinaldi S, de Armas L, Dominguez-Rodriguez S, Pallikkuth S, Dinh V, Pan L, et al. T Cell Immune Discriminants of HIV Reservoir Size in a Pediatric Cohort of Perinatally Infected Individuals. PloS Pathog (2021) 17(4):e1009533. doi: 10.1371/journal.ppat.1009533
106. Siliciano JD, Kajdas J, Finzi D, Quinn TC, Chadwick K, Margolick JB, et al. Long-Term Follow-Up Studies Confirm the Stability of the Latent Reservoir for HIV-1 in Resting CD4+ T Cells. Nat Med (2003) 9(6):727–8. doi: 10.1038/nm880
107. Stephenson KE. Therapeutic Vaccination for HIV: Hopes and Challenges. Curr Opin HIV AIDS (2018) 13(5):408–15. doi: 10.1097/COH.0000000000000491
108. Crooks AM, Bateson R, Cope AB, Dahl NP, Griggs MK, Kuruc JD, et al. Precise Quantitation of the Latent HIV-1 Reservoir: Implications for Eradication Strategies. J Infect Dis (2015) 212(9):1361–5. doi: 10.1093/infdis/jiv218
109. Chen Z, Julg B. Therapeutic Vaccines for the Treatment of HIV. Transl Res (2020) 223:61–75. doi: 10.1016/j.trsl.2020.04.008
110. Zangari P, Palma P, Cotugno N, Rojo P, Tagarro A, Pepponi I, et al. Report From the First EPIICAL (Early-Treated Perinatally HIV-Infected Individuals: Improving Children's Actual Life With Novel Immunotherapeutic Strategies) General Assembly Meeting, 9-11 November 2017, Rome, Italy. J Virus Erad (2018) 4(1):51–4. doi: 10.1016/S2055-6640(20)30240-5
111. Tagarro A, Chan M, Zangari P, Ferns B, Foster C, De Rossi A, et al. Early and Highly Suppressive Antiretroviral Therapy Are Main Factors Associated With Low Viral Reservoir in European Perinatally HIV-Infected Children. J Acquir Immune Defic Syndr (2018) 79(2):269–76. doi: 10.1097/QAI.0000000000001789
112. Klein N, Palma P, Luzuriaga K, Pahwa S, Nastouli E, Gibb DM, et al. Early Antiretroviral Therapy in Children Perinatally Infected With HIV: A Unique Opportunity to Implement Immunotherapeutic Approaches to Prolong Viral Remission. Lancet Infect Dis (2015) 15(9):1108–14. doi: 10.1016/S1473-3099(15)00052-3
113. Lambert JS, McNamara J, Katz SL, Fenton T, Kang M, VanCott TC, et al. Safety and Immunogenicity of HIV Recombinant Envelope Vaccines in HIV-Infected Infants and Children. National Institutes of Health-Sponsored Pediatric AIDS Clinical Trials Group (ACTG-218). J Acquir Immune Defic Syndr Hum Retrovirol (1998) 19(5):451–61. doi: 10.1097/00042560-199812150-00003
114. Palma P, Romiti ML, Montesano C, Santilli V, Mora N, Aquilani A, et al. Therapeutic DNA Vaccination of Vertically HIV-Infected Children: Report of the First Pediatric Randomised Trial (PEDVAC). PloS One (2013) 8(11):e79957. doi: 10.1371/journal.pone.0079957
115. Palma P, Romiti ML, Li Pira G, Montesano C, Mora N, Aquilani A, et al. The PEDVAC Trial: Preliminary Data From the First Therapeutic DNA Vaccination in HIV-Infected Children. Vaccine (2011) 29(39):6810–6. doi: 10.1016/j.vaccine.2010.12.058
116. Palma P, Gudmundsdotter L, Finocchi A, Eriksson LE, Mora N, Santilli V, et al. Immunotherapy With an HIV-DNA Vaccine in Children and Adults. Vaccines (Basel) (2014) 2(3):563–80. doi: 10.3390/vaccines2030563
117. Borducchi EN, Cabral C, Stephenson KE, Liu J, Abbink P, Ng'ang'a D, et al. Ad26/MVA Therapeutic Vaccination With TLR7 Stimulation in SIV-Infected Rhesus Monkeys. Nature (2016) 540(7632):284–7. doi: 10.1038/nature20583
118. Bricker KM, Obregon-Perko V, Uddin F, Williams B, Uffman EA, Garrido C, et al. Therapeutic Vaccination of SIV-Infected, ART-Treated Infant Rhesus Macaques Using Ad48/MVA in Combination With TLR-7 Stimulation. PloS Pathog (2020) 16(10):e1008954. doi: 10.1371/journal.ppat.1008954
119. Rossignol E, Alter G, Julg B. Antibodies for Human Immunodeficiency Virus-1 Cure Strategies. J Infect Dis (2021) 223(12 Suppl 2):22–31. doi: 10.1093/infdis/jiaa165
120. Spencer DA, Shapiro MB, Haigwood NL, Hessell AJ. Advancing HIV Broadly Neutralizing Antibodies: From Discovery to the Clinic. Front Public Health (2021) 9. doi: 10.3389/fpubh.2021.690017
121. Hsu DC, Mellors JW, Vasan S. Can Broadly Neutralizing HIV-1 Antibodies Help Achieve an ART-Free Remission? Front Immunol (2021) 12. doi: 10.3389/fimmu.2021.710044
122. Phelps M, Balazs AB. Contribution to HIV Prevention and Treatment by Antibody-Mediated Effector Function and Advances in Broadly Neutralizing Antibody Delivery by Vectored Immunoprophylaxis. Front Immunol (2021) 12. doi: 10.3389/fimmu.2021.734304
123. Hvilsom CT, Sogaard OS. TLR-Agonist Mediated Enhancement of Antibody-Dependent Effector Functions as Strategy For an HIV-1 Cure. Front Immunol (2021) 12. doi: 10.3389/fimmu.2021.704617
124. Cunningham CK, McFarland EJ, Morrison RL, Capparelli EV, Safrit JT, Mofenson LM, et al. Safety, Tolerability, and Pharmacokinetics of the Broadly Neutralizing Human Immunodeficiency Virus (HIV)-1 Monoclonal Antibody VRC01 in HIV-Exposed Newborn Infants. J Infect Dis (2020) 222(4):628–36. doi: 10.1093/infdis/jiz532
125. McFarland EJ, Cunningham CK, Muresan P, Capparelli EV, Perlowski C, Morgan P, et al. Safety, Tolerability, and Pharmacokinetics of a Long-Acting Broadly Neutralizing Human Immunodeficiency Virus Type 1 (HIV-1) Monoclonal Antibody VRC01LS in HIV-1-Exposed Newborn Infants. J Infect Dis (2021) 224(11):1916–24. doi: 10.1093/infdis/jiab229
126. Nishimura Y, Gautam R, Chun TW, Sadjadpour R, Foulds KE, Shingai M, et al. Early Antibody Therapy can Induce Long-Lasting Immunity to SHIV. Nature (2017) 543(7646):559–63. doi: 10.1038/nature21435
127. Nishimura Y, Donau OK, Dias J, Ferrando-Martinez S, Jesteadt E, Sadjadpour R, et al. Immunotherapy During the Acute SHIV Infection of Macaques Confers Long-Term Suppression of Viremia. J Exp Med (2021) 218(1):e20201214. doi: 10.1084/jem.20201214
128. Borducchi EN, Liu J, Nkolola JP, Cadena AM, Yu WH, Fischinger S, et al. Antibody and TLR7 Agonist Delay Viral Rebound in SHIV-Infected Monkeys. Nature (2018) 563(7731):360–4. doi: 10.1038/s41586-018-0600-6
129. Hsu DC, Schuetz A, Imerbsin R, Silsorn D, Pegu A, Inthawong D, et al. TLR7 Agonist, N6-LS and PGT121 Delayed Viral Rebound in SHIV-Infected Macaques After Antiretroviral Therapy Interruption. PloS Pathog (2021) 17(2):e1009339. doi: 10.1371/journal.ppat.1009339
130. Barouch D MN, Chandrashekar A, Borducchi E, Nkolola J, Carr BA, Thomsen N, et al. (2020). PGT121 and Vesatolimod in Chronically Treated SHIV-Infected Rhesus Monkeys, in: Conference on Retroviruses and Opportunistic Infections Available at: https://www.croiconference.org
131. Lim SY OC, Lee J, Silva-Ayala D, Vikhe P, Chen E, Lundgren S, et al. (2020). Combination IL-15 Therapy in a SHIV NHP Model, in: Conference on Retroviruses and Opportunistic Infections Available at: https://www.croiconference.org
132. Barouch D MN, Chandrashekar A, Borducchi E, Nkolola J, Pau M, Schuitemaker H, et al. (2020). Combined Active and Passive Immunization in SHIV-Infected Rhesus Monkeys, in: Conference on Retroviruses and Opportunistic Infections Available at: https://www.croiconference.org
133. Gunst JD, Hojen JF, Sogaard OS. Broadly Neutralizing Antibodies Combined With Latency-Reversing Agents or Immune Modulators as Strategy for HIV-1 Remission. Curr Opin HIV AIDS (2020) 15(5):309–15. doi: 10.1097/COH.0000000000000641
134. Niessl J, Baxter AE, Mendoza P, Jankovic M, Cohen YZ, Butler AL, et al. Combination Anti-HIV-1 Antibody Therapy is Associated With Increased Virus-Specific T Cell Immunity. Nat Med (2020) 26(2):222–7. doi: 10.1038/s41591-019-0747-1
135. Berendam SJ, Nelson AN, Goswami R, Persaud D, Haigwood NL, Chahroudi A, et al. Pediatric HIV: The Potential of Immune Therapeutics to Achieve Viral Remission and Functional Cure. Curr HIV/AIDS Rep (2020) 17(3):237–48. doi: 10.1007/s11904-020-00495-1
136. Mackness BC, Jaworski JA, Boudanova E, Park A, Valente D, Mauriac C, et al. Antibody Fc Engineering for Enhanced Neonatal Fc Receptor Binding and Prolonged Circulation Half-Life. MAbs (2019) 11(7):1276–88. doi: 10.1080/19420862.2019.1633883
137. Zalevsky J, Chamberlain AK, Horton HM, Karki S, Leung IW, Sproule TJ, et al. Enhanced Antibody Half-Life Improves In Vivo Activity. Nat Biotechnol (2010) 28(2):157–9. doi: 10.1038/nbt.1601
138. Ko SY, Pegu A, Rudicell RS, Yang ZY, Joyce MG, Chen X, et al. Enhanced Neonatal Fc Receptor Function Improves Protection Against Primate SHIV Infection. Nature (2014) 514(7524):642–5. doi: 10.1038/nature13612
139. Saunders KO. Conceptual Approaches to Modulating Antibody Effector Functions and Circulation Half-Life. Front Immunol (2019) 10. doi: 10.3389/fimmu.2019.01296
140. Sherpa C, Le Grice SFJ. Adeno-Associated Viral Vector Mediated Expression of Broadly- Neutralizing Antibodies Against HIV-Hitting a Fast-Moving Target. Curr HIV Res (2020) 18(2):114–31. doi: 10.2174/1570162X18666200210121339
141. Lin A, Balazs AB. Adeno-Associated Virus Gene Delivery of Broadly Neutralizing Antibodies as Prevention and Therapy Against HIV-1. Retrovirology (2018) 15(1):66. doi: 10.1186/s12977-018-0449-7
142. Richardson SI, Ayres F, Manamela NP, Oosthuysen B, Makhado Z, Lambson BE, et al. HIV Broadly Neutralizing Antibodies Expressed as IgG3 Preserve Neutralization Potency and Show Improved Fc Effector Function. Front Immunol (2021) 12. doi: 10.3389/fimmu.2021.733958
143. Richardson SI, Lambson BE, Crowley AR, Bashirova A, Scheepers C, Garrett N, et al. IgG3 Enhances Neutralization Potency and Fc Effector Function of an HIV V2-Specific Broadly Neutralizing Antibody. PloS Pathog (2019) 15(12):e1008064. doi: 10.1371/journal.ppat.1008064
144. Edwards JM, Heydarchi B, Khoury G, Salazar-Quiroz NA, Gonelli CA, Wines B, et al. Enhancement of Antibody-Dependent Cellular Cytotoxicity and Phagocytosis in Anti-HIV-1 Human-Bovine Chimeric Broadly Neutralizing Antibodies. J Virol (2021) 95(13):e0021921. doi: 10.1128/JVI.00219-21
145. Lu J, Chu J, Zou Z, Hamacher NB, Rixon MW, Sun PD. Structure of FcgammaRI in Complex With Fc Reveals the Importance of Glycan Recognition for High-Affinity IgG Binding. Proc Natl Acad Sci USA (2015) 112(3):833–8. doi: 10.1073/pnas.1418812112
146. Yaffe ZA, Naiman NE, Slyker J, Wines BD, Richardson BA, Hogarth PM, et al. Improved HIV-Positive Infant Survival is Correlated With High Levels of HIV-Specific ADCC Activity in Multiple Cohorts. Cell Rep Med (2021) 2(4):100254. doi: 10.1016/j.xcrm.2021.100254
147. Naiman NE, Slyker J, Richardson BA, John-Stewart G, Nduati R, Overbaugh JM. Antibody-Dependent Cellular Cytotoxicity Targeting CD4-Inducible Epitopes Predicts Mortality in HIV-Infected Infants. EBioMedicine (2019) 47:257–68. doi: 10.1016/j.ebiom.2019.08.072
148. Milligan C, Richardson BA, John-Stewart G, Nduati R, Overbaugh J. Passively Acquired Antibody-Dependent Cellular Cytotoxicity (ADCC) Activity in HIV-Infected Infants is Associated With Reduced Mortality. Cell Host Microbe (2015) 17(4):500–6. doi: 10.1016/j.chom.2015.03.002
149. Himes JE, Goswami R, Mangan RJ, Kumar A, Jeffries TL Jr., Eudailey JA, et al. Polyclonal HIV Envelope-Specific Breast Milk Antibodies Limit Founder SHIV Acquisition and Cell-Associated Virus Loads in Infant Rhesus Monkeys. Mucosal Immunol (2018) 11(6):1716–26. doi: 10.1038/s41385-018-0067-7
150. Martinez DR, Tu JJ, Kumar A, Mangold JF, Mangan RJ, Goswami R, et al. Maternal Broadly Neutralizing Antibodies Can Select for Neutralization-Resistant, Infant-Transmitted/Founder HIV Variants. mBio (2020) 11(2):e00176-20. doi: 10.1128/mBio.00176-20
151. Khan SN, Sok D, Tran K, Movsesyan A, Dubrovskaya V, Burton DR, et al. Targeting the HIV-1 Spike and Coreceptor With Bi- and Trispecific Antibodies for Single-Component Broad Inhibition of Entry. J Virol (2018) 92(18):e00384-18. doi: 10.1128/JVI.00384-18
152. Davis-Gardner ME, Alfant B, Weber JA, Gardner MR, Farzan M. A Bispecific Antibody That Simultaneously Recognizes the V2- and V3-Glycan Epitopes of the HIV-1 Envelope Glycoprotein Is Broader and More Potent Than Its Parental Antibodies. mBio (2020) 11(1):e03080-19. doi: 10.1128/mBio.03080-19
153. Xu L, Pegu A, Rao E, Doria-Rose N, Beninga J, McKee K, et al. Trispecific Broadly Neutralizing HIV Antibodies Mediate Potent SHIV Protection in Macaques. Science (2017) 358(6359):85–90. doi: 10.1126/science.aan8630
154. Pegu A, Xu L, DeMouth ME, Fabozzi G, March K, Almasri CG, et al. Potent Anti-Viral Activity of a Trispecific HIV Neutralizing Antibody in SHIV-Infected Monkeys. Cell Rep (2022) 38(1):110199. doi: 10.1016/j.celrep.2021.110199
155. Steinhardt JJ, Guenaga J, Turner HL, McKee K, Louder MK, O'Dell S, et al. Rational Design of a Trispecific Antibody Targeting the HIV-1 Env With Elevated Anti-Viral Activity. Nat Commun (2018) 9(1):877. doi: 10.1038/s41467-018-03335-4
156. Grobben M, Stuart RA, van Gils MJ. The Potential of Engineered Antibodies for HIV-1 Therapy and Cure. Curr Opin Virol (2019) 38:70–80. doi: 10.1016/j.coviro.2019.07.007
157. Ferrari G, Haynes BF, Koenig S, Nordstrom JL, Margolis DM, Tomaras GD. Envelope-Specific Antibodies and Antibody-Derived Molecules for Treating and Curing HIV Infection. Nat Rev Drug Discovery (2016) 15(12):823–34. doi: 10.1038/nrd.2016.173
158. Asokan M, Rudicell RS, Louder M, McKee K, O'Dell S, Stewart-Jones G, et al. Bispecific Antibodies Targeting Different Epitopes on the HIV-1 Envelope Exhibit Broad and Potent Neutralization. J Virol (2015) 89(24):12501–12. doi: 10.1128/JVI.02097-15
159. Brozy J, Schlaepfer E, Mueller CKS, Rochat MA, Rampini SK, Myburgh R, et al. Antiviral Activity of HIV Gp120-Targeting Bispecific T Cell Engager Antibody Constructs. J Virol (2018) 92(14):e00491-18. doi: 10.1128/JVI.00491-18
160. Li W, Wu Y, Kong D, Yang H, Wang Y, Shao J, et al. One-Domain CD4 Fused to Human Anti-CD16 Antibody Domain Mediates Effective Killing of HIV-1-Infected Cells. Sci Rep (2017) 7(1):9130. doi: 10.1038/s41598-017-07966-3
161. Moore PA, Zhang W, Rainey GJ, Burke S, Li H, Huang L, et al. Application of Dual Affinity Retargeting Molecules to Achieve Optimal Redirected T-Cell Killing of B-Cell Lymphoma. Blood (2011) 117(17):4542–51. doi: 10.1182/blood-2010-09-306449
162. Margolis DM, Archin NM, Cohen MS, Eron JJ, Ferrari G, Garcia JV, et al. Curing HIV: Seeking to Target and Clear Persistent Infection. Cell (2020) 181(1):189–206. doi: 10.1016/j.cell.2020.03.005
163. van Tilburg CM, Milde T, Witt R, Ecker J, Hielscher T, Seitz A, et al. Phase I/II Intra-Patient Dose Escalation Study of Vorinostat in Children With Relapsed Solid Tumor, Lymphoma, or Leukemia. Clin Epigenetics (2019) 11(1):188. doi: 10.1186/s13148-019-0775-1
164. Elliott JH, Wightman F, Solomon A, Ghneim K, Ahlers J, Cameron MJ, et al. Activation of HIV Transcription With Short-Course Vorinostat in HIV-Infected Patients on Suppressive Antiretroviral Therapy. PloS Pathog (2014) 10(10):e1004473. doi: 10.1371/journal.ppat.1004473
165. Sogaard OS, Graversen ME, Leth S, Olesen R, Brinkmann CR, Nissen SK, et al. The Depsipeptide Romidepsin Reverses HIV-1 Latency In Vivo. PloS Pathog (2015) 11(9):e1005142. doi: 10.1371/journal.ppat.1005142
166. Kroon E, Ananworanich J, Pagliuzza A, Rhodes A, Phanuphak N, Trautmann L, et al. A Randomized Trial of Vorinostat With Treatment Interruption After Initiating Antiretroviral Therapy During Acute HIV-1 Infection. J Virus Erad (2020) 6(3):100004. doi: 10.1016/j.jve.2020.100004
167. Miller JS, Davis ZB, Helgeson E, Reilly C, Thorkelson A, Anderson J, et al. Safety and Virologic Impact of the IL-15 Superagonist N-803 in People Living With HIV: A Phase 1 Trial. Nat Med (2022) 28(2):392–400. doi: 10.1038/s41591-021-01651-9
168. Fouladi M, Park JR, Stewart CF, Gilbertson RJ, Schaiquevich P, Sun J, et al. Pediatric Phase I Trial and Pharmacokinetic Study of Vorinostat: A Children's Oncology Group Phase I Consortium Report. J Clin Oncol (2010) 28(22):3623–9. doi: 10.1200/JCO.2009.25.9119
169. Nixon CC, Mavigner M, Sampey GC, Brooks AD, Spagnuolo RA, Irlbeck DM, et al. Systemic HIV and SIV Latency Reversal via non-Canonical NF-kappaB Signalling In Vivo. Nature (2020) 578(7793):160–5. doi: 10.1038/s41586-020-1951-3
170. Bricker KM, Obregon-Perko V, Williams B, Oliver D, Uddin F, Neja M, et al. Altered Response Pattern Following AZD5582 Treatment of SIVInfected, ART-Suppressed Rhesus Macaque Infants. J Virol (2022) 96(7):e0169921. doi: 10.1128/jvi.01699-21
171. Fortuny C, Noguera-Julian A, Alsina L, Bellido R, Sanchez E, Munoz-Almagro C, et al. Impact of CD4 T Cell Count on the Outcome of Planned Treatment Interruptions in Early-Treated Human Immunodeficiency Virus-Infected Children. Pediatr Infect Dis J (2011) 30(5):435–8. doi: 10.1097/INF.0b013e3181ff8661
172. Shah SK, Persaud D, Wendler DS, Taylor HA, Gay H, Kruger M, et al. Research Into a Functional Cure for HIV in Neonates: The Need for Ethical Foresight. Lancet Infect Dis (2014) 14(9):893–8. doi: 10.1016/S1473-3099(14)70766-2
Keywords: pediatric HIV, cure, early life immunity, immune-based therapies, HIV cure strategies
Citation: Berendam SJ, Nelson AN, Yagnik B, Goswami R, Styles TM, Neja MA, Phan CT, Dankwa S, Byrd AU, Garrido C, Amara RR, Chahroudi A, Permar SR and Fouda GG (2022) Challenges and Opportunities of Therapies Targeting Early Life Immunity for Pediatric HIV Cure. Front. Immunol. 13:885272. doi: 10.3389/fimmu.2022.885272
Received: 27 February 2022; Accepted: 16 June 2022;
Published: 13 July 2022.
Edited by:
Savita Pahwa, University of Miami, United StatesReviewed by:
Ivona Pandrea, University of Pittsburgh, United StatesSuresh Pallikkuth, University of Miami, United States
Copyright © 2022 Berendam, Nelson, Yagnik, Goswami, Styles, Neja, Phan, Dankwa, Byrd, Garrido, Amara, Chahroudi, Permar and Fouda. This is an open-access article distributed under the terms of the Creative Commons Attribution License (CC BY). The use, distribution or reproduction in other forums is permitted, provided the original author(s) and the copyright owner(s) are credited and that the original publication in this journal is cited, in accordance with accepted academic practice. No use, distribution or reproduction is permitted which does not comply with these terms.
*Correspondence: Stella J. Berendam, c3RlbGxhLmJlcmVuZGFtQGR1a2UuZWR1; Genevieve G. Fouda, Z2VuZXZpZXZlLmZvdWRhQGR1a2UuZWR1
†Present address: Stella J. Berendam, GSK Center for Vaccines Research, Rockville, MD, United States