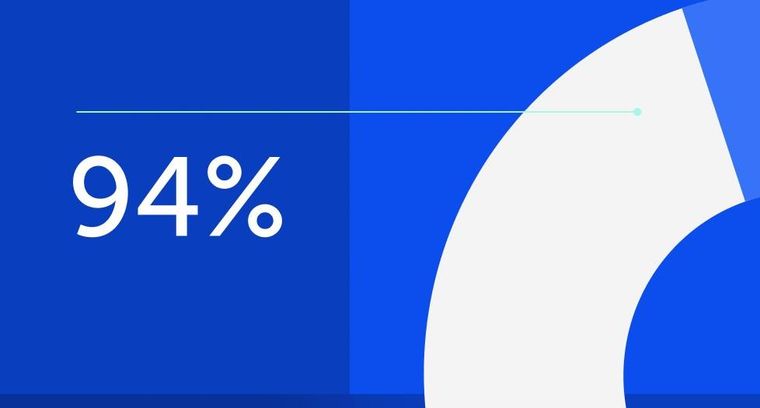
94% of researchers rate our articles as excellent or good
Learn more about the work of our research integrity team to safeguard the quality of each article we publish.
Find out more
REVIEW article
Front. Immunol., 28 April 2022
Sec. Inflammation
Volume 13 - 2022 | https://doi.org/10.3389/fimmu.2022.883116
This article is part of the Research TopicThe Role of Innate Immune and Resident Cells in Allergic Diseases and their Potential in Therapeutic InterventionView all 9 articles
Thioredoxin-1 (Trx1) is an important regulator of cellular redox homeostasis that comprises a redox-active dithiol. Trx1 is induced in response to various stress conditions, such as oxidative damage, infection or inflammation, metabolic dysfunction, irradiation, and chemical exposure. It has shown excellent anti-inflammatory and immunomodulatory effects in the treatment of various human inflammatory disorders in animal models. This review focused on the protective roles and mechanisms of Trx1 in allergic diseases, such as allergic asthma, contact dermatitis, food allergies, allergic rhinitis, and drug allergies. Trx1 plays an important role in allergic diseases through processes, such as antioxidation, inhibiting macrophage migration inhibitory factor (MIF), regulating Th1/Th2 immune balance, modulating allergic inflammatory cells, and suppressing complement activation. The regulatory mechanism of Trx1 differs from that of glucocorticoids that regulates the inflammatory reactions associated with immune response suppression. Furthermore, Trx1 exerts a beneficial effect on glucocorticoid resistance of allergic inflammation by inhibiting the production and internalization of MIF. Our results suggest that Trx1 has the potential for future success in translational research.
Thioredoxin (Trx) is a ubiquitously expressed protein with a low molecular weight of 12 kDa. It is a part of the Trx system that includes NADPH and Trx reductase (TrxR) (1). Trx shows thiol–disulphide reductase activity that is influenced by a highly conserved active site (-Cys32-Gly-Pro-Cys35-) (2). The reduced form of Trx transfers its reducing equivalents to disulphides within the target molecule and catalyzes their reduction. TrxR uses NADPH in this process to reduce the active site disulphide in the Trx substrates to dithiol (3) (Figure 1A). Overall, the Trx system plays a critical role in regulating the cellular redox balance through the reversible thiol–disulphide exchange reaction.
Figure 1 (A) Mechanism of redox regulation by the thioredoxin (Trx) system. The reduced form of Trx catalyzes the reduction of the disulphide bonds in the target protein. Oxidized thioredoxin is restored to its reduced state by the NADPH-dependent flavoenzyme thioredoxin reductase. (B) Trx1 inhibition of eosinophil activation and chemotaxis. Trx1 can eliminate reactive oxygen species (ROS) produced by eosinophils and directly inhibit the activation of the mitogen-activated protein kinase signal pathway when entering cells. Trx1 regulates Th2 response by inhibiting IL-13 production, which prevents IL-13 from stimulating epithelial cells or fibroblasts to produce eotaxin. In addition, Trx1 blocks the pro-inflammatory effect of the upstream chemokine macrophage migration inhibitory factor, which can directly induce the chemotaxis of eosinophils or promote the production of eotaxin by epithelial cells or fibroblasts to promote eosinophil recruitment. (C) Potential mechanisms of the effects of Trx1 on mast cell degranulation. Crosslinking of the allergen and IgE complex with FcϵRI activates the mast cell degranulation pathway, which then activates Lyn, Syk, Btk, and phospholipase Cγ (PLCγ). Activation of PLCγ eventually activates Ca2+ and protein kinase c (PKC), which contributes to degranulation. ROS induced in FcϵRI-stimulated mast cells activate mast cells by activating PLCγ, Ca2+ influx, and PKC. Accordingly, Trx1 prevents mast cell degranulation by scavenging ROS. The effective catalytic function of βII tryptase secreted by mast cells depends on the existence of normal disulphide bonds in molecules. The Trx1 system selectively reduces the number of disulphide bonds, which then reduces the catalytic activity of βII-tryptase.
Indeed, there are three distinct forms of human Trx, encoded by separate genes: cytosolic Trx (Trx1) and mitochondrial Trx (Trx2), and a spermatid-specific isoform of Trx (SpTrx/Trx3). Trx1 is a major isoform of Trx that is located in the cytoplasm but is also translocated to the nucleus. Trx1 can directly scavenge reactive oxygen species (ROS) and thereby protect against oxidative stress (4). It is also involved in various redox-dependent cellular processes, such as gene expression, signal transduction, and cell growth and apoptosis, and interacts with various target molecules (5). Under stress conditions, Trx1 is released into the extracellular space where it exerts a cytoprotective effect and shows cytokine-like activities (6). Trx2 is a mitochondrial redox protein sharing 35% sequence homology and similar catalytic properties with Trx1 in vitro, and possesses the Trx1 active-site but lacking additional structural cysteines (7). It plays a critical role for scavenging ROS to maintain a reducing status in the mitochondrial matrix (8).
Allergic diseases include immune-mediated disorders mainly characterized by a Th2 immune response phenotype. In patients with asthma, plasma Trx1 levels were significantly higher in the attack stage than those in the remission stage, and these levels of Trx1 substantially increased with the severity of the asthma attack (9). This implies that Trx1 can be a useful clinical parameter in asthma progression prediction.
The protective effects of Trx1 are associated with the pathogenesis of several human disorders, including metabolic syndromes and neurodegenerative, cardiovascular, and inflammatory diseases (10–13). In this review, we focused on recent studies on the underlying intercellular and intracellular mechanisms through which Trx1 regulates immune cells in response to allergic inflammatory diseases, such as allergic asthma, food and drug allergies, contact dermatitis, and allergic rhinitis (AR), as well as identifying the potential Trx-based therapeutic strategies for treating allergic diseases.
Airway inflammation in allergic asthma is a complex process, and Th2-type inflammation and excessive accumulation of eosinophils are the important features (14). At the site of airway inflammation, Th2 cells secrete large amounts of interleukin (IL)-3, -4, -5, -9 and -13 and recruit/activate eosinophils, mast cells, and basophils (15). IL-13 is crucial to the pathogenesis of asthma; overexpression of IL-13 significantly induces the occurrence of allergic asthma in a mouse model (16). Additionally, IL-13 induces not only the proliferation of goblet cells (the main effector cells for mucus production in the respiratory tract) but also subepithelial fibrosis which leads to airway remodeling (16, 17). Activated eosinophils migrate to the bronchial epithelium and release ROS and eosinophil granulocyte protein, resulting in airway hyper responsiveness (AHR) and epithelial damage that exacerbates the respiratory symptoms (18). The growth and survival signaling induced by ligand/receptor interactions in the airway smooth muscle cells are mediated through ROS (19). In addition, macrophage migration inhibitory factor (MIF), an important upstream regulator of airway inflammation, promotes eosinophil differentiation, survival, activation, and migration by binding to CD74 and CXCR4 on the surface of eosinophils (20). In the pathogenesis of allergic asthma, reduced expression of antioxidant genes is also observed, such as Nrf2. Clinical studies have shown that with the reduction of Nrf2 expression in the body, asthma becomes more serious (21, 22), and the application of Nrf2 agonists significantly relaxes the bronchi and improves the symptoms (23), suggesting that the occurrence of allergic asthma is not only related to Th2 inflammatory reaction but also has a close relationship with oxidative stress.
Clinical treatment of asthma mainly involves β2-receptor agonists, corticosteroids, and aminophylline. Although β2 agonists are currently the largest class of treatment agents for asthma, their use is controversial because of poor clinical reactions and possible life-threatening adverse events. For moderate and severe asthma, combination therapy with inhaled corticosteroids and long-acting β2-agonists is used; however, this combination cannot prevent, reverse, or treat the underlying causes of the disease. Moreover, this treatment requires continuous monitoring for side effects and resistance (24). For instance, aminophylline can often cause adverse reactions, such as palpitations, headache, and vomiting (25).
Trx1 is closely associated with asthma. Serum Trx1 levels in patients with acute asthma exacerbation are significantly high and there is a significant correlation between these levels and eosinophil cationic protein (9, 26). Exogenous Trx1 treatment can significantly improve AHR and airway inflammation in ovalbumin-sensitized mice (27). Similarly, in a mouse model of chronic asthma, the systemic use of Trx1 significantly inhibited airway remodeling, eosinophil infiltration, and AHR while reducing the expressions of eotaxin (an eosinophil chemokine), macrophage inflammatory protein-1 and IL-13 in the lungs; thus, Trx1 improves pathological changes in the airway to prevent remodeling and asthma development (28), in agreement with a recent report which indicated that Trx1 displayed pronounced protective effects on the manifestation of allergic airway inflammation (29). Trx1 also inhibits Th2 cytokine production by directly downregulating MIF production and indirectly inhibiting eosinophil chemotaxis. Notably, the realization of this process does not depend on the regulation of systemic Th1/Th2 immunity (30). The proliferation of goblet cells that secrete excessive mucus increases the morbidity and mortality of asthma patients; however, Trx1 prevents this proliferation or improves established goblet cell proliferation (31). Trx1 also regulates ARH and airway remodeling by directly reducing the production of intracellular ROS. Additionally, the clinical drug ephedrine may produce anti-asthma effects in vivo through inducing Trx1 production (32). The regulation of allergic asthma by Trx1 also involves the Trx1/Txnip system. Trx1 and Txnip are normally combined into a dimer, and the Trx1/Txnip dimer is separated in the presence of irritant factors such as inflammation. Txnip can activate such signaling pathways as ASK1, Bax, p38, and Caspase3, and induce apoptosis in the lung tissue (33). Moreover, Txnip can also activate inflammasomes to trigger inflammatory reactions (33), all of which aggravate the symptoms of allergic asthma. Trx1, on the other hand, inhibits these reactions, thereby maintaining the balance between Trx and Txnip. Overall, Trx1 may be useful for the treatment of asthma and may represent a therapeutic target for asthma control.
The inflammatory process of AR is similar to that of asthma. Many Th2 cells infiltrate the nasal mucosa and release cytokines (e.g., IL-4, IL-5 and IL-13) that promote IgE production by plasma cells. A lot of medical treatment modalities used as a treatment of AR, such as antihistamines, steroids, montelukast, and immunotherapy. However, these therapeutic modalities can fail on some occasions (34).
Generally, large-scale production and release of inflammatory cells, including eosinophils and ROS and their metabolites, plays a vital role in the pathogenesis of allergic inflammatory airway diseases (35, 36). As an endogenous antioxidant protein, Trx1 has strong antioxidative stress effects. Thus, administration of exogenous Trx1 can inhibit AHR induced by specific allergens via the inhibition of eosinophil accumulation in the airway of mouse models with asthma (27, 28). Additionally, a recent study indicated that the concentration of Trx1 in nasal tissues is significantly decreased in patients with chronic rhinosinusitis with nasal polyps and a connection between elevated ROS levels and decreased levels of Trx1 has also been observed (37). Quercetin has been suggested as a dietary supplement for improving the clinical symptoms of allergic diseases, such as AR, but its precise mechanisms of action remain unclear. Nevertheless, Trx1 levels in the nasal mucosa significantly increase after oral administration of quercetin; moreover, the frequency of nasal allergy-like symptoms, such as sneezing and nasal rubbing, are significantly reduced (38). These changes provide insights into the possible mechanism underlying the favorable effects of quercetin on AR.
Food allergies are caused by aberrant immune responses towards food antigens; these responses are skewed towards Th2 responses associated with IL-4, IL-5, and IL-13. Current treatments for IgE-mediated food allergies are largely confined to the avoidance of the suspected allergens, antihistamine treatments, and corticosteroid therapies with low efficacy and several side effects. Food allergen immunotherapy induces desensitization and promotes permanent immune tolerance to food allergens by gradually increasing exposure to the allergens (39); however, the incidence of adverse reactions is high and is a long-term treatment process (40).
Trx1 treatment has been effective against food allergies in previous studies. For example, the application of Trx1 significantly reduced allergic reactions in a wheat allergy dog model subjected to skin test. Therefore, Trx1 potentially reduces wheat sensitization by reducing the number of disulphide bonds in the major protein allergens of wheat (41). Similarly, Trx1 reduces the number of disulphide bonds in β-lactoglobulin, an allergen in bovine milk. The disulphide-reduced protein shows increased sensitivity to pepsin digestion and decreased hypersensitivity in vivo (42). Additionally, a Trx1-treated salt-soluble wheat allergen was shown to reduce IgE binding in children with asthma (43). Consistent with these results, active systemic and passive cutaneous anaphylaxis testing on guinea pigs showed that yeast extract rich in Trx1 significantly reduced egg mucin-induced anaphylaxis. It was hypothesized that the anti-allergic activity of Trx1 itself plays a role in these effects (44). Accordingly, Trx1-rich yeast extract can potentially be used to ferment foods, such as alcoholic beverages and bread. Recently, recombinant Trx1 rice has been shown to improve β-lactoglobulin digestion and decrease its allergenicity, thereby improving the feasibility and practicality of its large-scale application; a plant Trx system would be more cost-effective than those of Escherichia coli or animals (45).
Drug allergies (DAs) can be IgE- or non-IgE mediated. Some drugs, such as anesthetics, antibiotics, nonsteroidal anti-inflammatory drugs and codeine, are associated with a carrier protein through a prototype or its metabolite (46). Binding of cell-bound IgE molecules activates mast cells and releases various factors, such as histamines, leukotrienes, prostaglandins, and cytokines, which can cause extensive tissue damage. Trx1 is a stress-induced redox regulatory protein in vivo; thus, it inhibits histamine release by eliminating ROS in mast cells (47). The mechanisms of DAs may often be associated with non-IgE-mediated complement activation. Indeed, Trx1 is known to inhibit the activation of the complement cascade at different stages, e.g., suppressing C3 cleavage and C5 convertase activation (48, 49). The functions of Trx1 in mast cells and the complement system are described in section 3.5 “Suppression of Complement Activation”.
Contact dermatitis is a common inflammatory skin disorder that is usually characterized by alternating relief from and deterioration of symptoms, but it can be persistent at times (50). Contact dermatitis can be categorized as irritant contact dermatitis (ICD), a non-immunologically driven inflammatory reaction to an irritating substance, and allergic contact dermatitis, a type-IV delayed-type hypersensitivity reaction resulting from the activation of allergen-specific T cells, i.e., a second exposure to the allergen resulting in circulating memory T cells homing to the skin and eliciting an immunologic reaction that causes skin inflammation (51). Topical corticosteroid treatment is typically the first-choice treatment for contact dermatitis. However, corticosteroids are not suitable for long-term use because of multiple side effects, such as skin atrophy, telangiectasia, dermatoglyphics, and pigmentation. Oxidative stress is known to play a key role in contact dermatitis inflammation. In particular, ROS participate in dendritic cell activation (52). In addition to being an endogenous redox regulatory protein, Trx is an effective ROS scavenger (53). Because ROS regulate the function of dendritic cells that function in the sensitization phase of contact hypersensitivity, transgenic overexpression and systemic administration of exogenous Trx1 can suppress skin inflammation by inhibiting neutrophil recruitment during the elicitation phase, but not during the induction phase, in mice treated with 2,4-dinitrofluorobenzene (54). Transgenic overexpression of Trx1 and systemic administration of exogenous Trx1 can prevent the cutaneous inflammation caused by UV radiation through regulating the cellular redox status and ROS scavenging (55). We previously demonstrated that Trx1 ameliorates ICD by inhibiting epithelial production and releasing inflammatory cytokines and chemokines (56). Existing research suggests that Trx1 can be used to treat contact dermatitis; however, its exact therapeutic mechanism requires further clarification.
Trx1 can directly remove ROS produced in inflamed tissues and help maintain redox balance. Mitsui et al. showed that Trx1 transgenic mice had strong resistance to oxidative stress and a longer life span compared with wild-type (WT) animals (57). Compared with the Trx1 system, the intracellular redox system has similar antioxidant mechanisms, such as the glutathione and peroxidase systems, which defend against oxidative stress. The Trx1 and glutathione systems act as backup systems to provide electrons for each other, i.e., the two systems protect cells from oxidative damage synergistically (58, 59). In addition, Trx1 is required to provide electrons when peroxidase is used to reduce ROS in organisms (60). Thus, Trx1 plays a key role in the balance of multiple redox systems in the body, and it coordinates the normal operation and function of these systems. Moreover, Trx1 is also a downstream target molecule for the activation of many redox signals. For example, in the case of mitochondrial redox imbalance, Nrf2 signal that is important for redox is activated (61). Nrf2 signaling activates some specific targets, including Trx1 and glutathione (62).
In the allergic state, expression of Trx1 can be induced to reduce the damage caused by excessive ROS. Simultaneously, the Trx1 system restores and refolds oxidized and damaged proteins. Consequently, Trx1 likely plays an important protective role against allergic inflammation.
Human MIF, a member of the Trx1 family of proteins that displays thiol reductase activity, was first cloned from T cells in 1989 (63). It shows inhibitory properties against the migration of macrophages and plays an essential role in cellular immunity, particularly in delayed-type hypersensitivity (64). MIF is largely considered a pleiotropic inflammatory medium with a wide range of immunoregulatory and pro-inflammatory activities, including the induction of inflammatory cytokines, regulation of macrophage and lymphocyte proliferation, and functions similar to those of chemokines (64, 65). Furthermore, MIF is directly involved in eosinophil differentiation, survival, activation, and migration (20).
MIF shares the redox-active motif -Cys-Xxx-Xxx-Cys- with Trx1 (66). It has sulfhydryl reductase activity and direct redox reactions with Trx1 (67). Several preclinical studies using animal models have shown that Trx has beneficial MIF-related functions against various inflammatory diseases. For example, the serum MIF level of Trx1 transgenic mice was significantly lower than that of WT mice in a dextran sodium sulphate-induced colitis mouse model (68). In mice with systemic inflammatory reactions from smoking, MIF gene expression in the spleens of Trx1 transgenic mice was inhibited compared with the expression levels in control mice (69). Using a mouse model of asthma, Torii et al. determined that MIF production in the lungs of Trx1 transgenic mice was significantly reduced despite similar systemic Th2 responses and IgE concentrations, indicating that Trx1 can suppress airway inflammation by directly inhibiting MIF independent of systemic Th1/Th2 immune modulation (30).
In vitro studies have provided evidence on the strong anti-MIF effect of Trx1. For instance, the production of MIF in macrophages cultured with LPS and IFN-γ was significantly inhibited by Trx1 (68). MIF expression is also suppressed in Trx1-transfected cells (70), and topically applied exogenous Trx1 suppresses the expression of MIF in ICD skin tissues (56). Additionally, MIF can enter cells to induce a series of inflammatory reactions, and cell surface Trx1 is one of the target proteins for MIF internalization. Specifically, Trx1 on the cell surface binds to extracellular MIF with high affinity and blocks MIF internalization. Exogenous and intracellular Trx1 can also directly bind to MIF, thereby forming a complex that blocks MIF-induced inflammatory response (71).
In the cell microenvironment, proliferation and differentiation of Th1/Th2 cells are affected by various factors, such as cytokines, antigen properties, T cell receptor signal intensity, antigen-presenting cell types, and costimulatory molecules (72–74). In addition to these external factors, cell redox status is considered to play a role Th cell differentiation. T cells have limitations in terms of cystine uptake and require exogenous mercaptan for their activation to play a role in this process. During antigen presentation, after dendritic cells interact with T cells, the former generate and release Trx1, which reduces extracellular cystine to cysteine used by T cells; thus, the normal proliferative ability of T cells as well as an effective immune response are maintained (75, 76). Trx1 also controls the redox state of cell surface receptors, such as CD4 and CD30, and thereby affects the behavior of T cells (77, 78). When Th2 cytokine responses increase, Trx1 induces the expression of Th1-like cytokines, such as IL-1α, IL-1β, IL-1Ra, and IL-18, which in turn suppresses Th2-like cytokine expression (27). In recent studies, Trx1 has been confirmed as a specific target gene induced by the cytokine IFN-γ that directly drives the Th1 immune response (79, 80). Indeed, IFN-γ promotes Th1 differentiation and downregulates the Th2 response (81). Exogenous Trx1 can induce the expression and release of IFN-γ in Th1 cells, and the increased IFN-γ level in turn increases the Trx1 level. The intracellular Trx1 of IFN-γ-activated macrophages increases the secretion of the Th1 cytokine IL-12 by regulating the thiol redox state. Given the mutual induction and promotion of Trx1 and IFN-γ by immune cells during oxidative stress, a positive feedback mechanism could exist between Trx1 and IFN-γ as they participate in stimulating Th1 immunity (80). In addition, hTrx1 can bind to Dectin-1 and/or Dectin-2 on antigen presenting cells to secret IL-1β and IL-23, which influence Th2/Th17-polarizing milieu during the allergic sensitization in the skin (82). Recently, IL-4 has been identified as a new target of Trx1; specifically, its activity can be selectively suppressed by Trx1 (83) (Figure 2); thus, the production of IgE by B cells may also be effectively blocked. However, Trx1 does not directly affect the proliferation and differentiation of Th1/Th2 cells; instead, it suppresses inflammation by regulating the production and release of Th1/Th2 cytokines because lymphocytes isolated from Trx1-transgenic (Trx1-Tg) mice are similar to those from WT mice in terms of their ability to produce Th2 cytokines, such as IL-4, IL-5 and IL-13, once they leave an in vivo environment with high Trx1 (30). The recent report showed that regulatory T cells (Tregs) play an important role in maintaining immune tolerance to allergens by inhibiting the type 2 immune cells, inducing tolerogenic dendritic cells, regulatory B cells and IgG4-producing B cells in allergic disease (84). Increased Trx1 in Tregs enhances tolerance to oxidative stress (85). Thus, Trx1 may prevent the occurrence and progression of Th2-driven allergic inflammatory conditions by adjusting the Th1/Th2 balance. In response to various cytokines released by Th2 cells, such as IL-4, IL-5, IL-5 and IL-13, many inflammatory cells are activated, which in turn elicit an inflammatory response that leads to the clinical symptoms of allergic disease. The main effector cells are eosinophils, mast cells, and neutrophils in this complex immune response. A number of studies have shown that Trx1 directly modulate these cells through various mechanisms. Next, we focus on the role and the mechanisms of Trx1 on these inflammatory cells in allergic reactions.
Figure 2 Thioredoxin-1 (Trx1) regulation of Th1/Th2 balance. Most extracellular cysteine (cys-SH) equivalents exist in the oxidized form of cystine (cys-S-S-cys). T cells cannot ingest cystine and rely on antigen-presenting cells (e.g., dendritic cells) to provide cysteine for them. Dendritic cells convert extracellular cystine into cysteine through Trx secretion, thereby promoting the proliferation of activated T cells. A positive feedback mechanism exists between Trx and IFN-γ wherein Trx1 induces the expression and release of IFN-γ in Th1 cells and the increased IFN-γ level increases Trx1 levels in turn. IFN-γ-activated intracellular Trx1 of macrophages increases Th1 cytokine IL-12 secretion by regulating the thiol redox state. Furthermore, Trx1 selectively inactivates the cytokine activity of IL-4 and inhibits the Th2 immune response.
Excessive proliferation and infiltration of eosinophils is generally considered a marker of allergic inflammation. Trx1 inhibits the migration and activation of eosinophils by regulating the extracellular Th1/Th2 balance, cellular signaling pathway, and molecules that interact with eosinophil-produced cytokines. In allergic asthma, Trx1 inhibits eosinophil accumulation by inducing Th1 cytokine production and suppressing Th2 cytokine production (27). Low expression of MIF in the airways of Trx1-Tg mice significantly inhibits eosinophil aggregation and mucus metaplasia (30). Additionally, MIF can directly induce the production of eotaxin to promote eosinophil chemotaxis (86); however, as described previously in the text, Trx1 can bind to MIF inside and outside the cells to block its internalization and pro-inflammatory activity. Eotaxin, an eosinophil chemotactic chemokine, is mediated by the C–C chemokine receptor type 3 (CCR3) on the surface of eosinophils (87). Eotaxin-stimulated eosinophils incubated with Trx1 significantly decreased the activation of eotaxin-stimulated ERK1/2 and p38MAPK pathways (88–90); however, Trx1 does not affect CCR3 expression in eosinophils. Thus, chemokine-induced eosinophil migration is apparently attenuated by regulating the downstream signaling of CCR3. In addition, intraperitoneal Trx1 injection significantly reduces the overproduction of MIP-1α and IL-13, which are closely related to eosinophil chemotaxis in the lungs (28). In vitro studies have confirmed that Trx1-overexpressing human bronchial epithelial cells can be protected from eosinophil-induced damage (91). Furthermore, Trx1 directly suppresses the production of ROS in eosinophils (92). Overall, Trx1 exerts anti-allergic effects by regulating eosinophil activation and migration (Figure 1B).
Mast cell activation plays an important role in various immediate allergic diseases. ROS functions in FcϵRI-mediated degranulation of mast cells (93, 94), and several ROS are generated during FcϵRI-mediated activation of mast cells. Thus, blocking the production of intracellular ROS can prevent the release of FcϵRI-mediated allergic mediators from rat mast cells (95). Son et al. stimulated mast cells from WT and Trx1-Tg mice with Ag, DNP-bovine serum albumin. The levels of histamine secreted by mast cells from Trx1-Tg mice were significantly reduced compared with that in WT mice, and the levels of intracellular ROS suggested that Trx1 inhibits mast cell degranulation by blocking ROS production (47). As the underlying mechanism, ROS mainly activates phospholipase Cγ (PLCγ), protein kinase C (PKC) and Ca2+ influx to cause medium release (94, 96), whereas Trx1 effectively inhibits PLCγ, PKC, and Ca2+ influx in the signal transmission of ROS-activated FcϵRI-dependent mast cells (Figure 1C).
βII-tryptase is one of the most abundant proteins stored and released in mast cells, and it participates in various acute and chronic allergic processes. It is commonly noted in patients with asthma and AR (97, 98). The redox activity of the allosteric disulphide bond (Cys220-Cys248 disulphide bond) in βII-tryptase plays an essential role in exerting enzyme activity, and Trx1 is a related βII-tryptase reducing agent in vivo; it can selectively reduce the disulphide bonds and potently reduce the catalytic activity of βII-tryptase in the reduced state (99) (Figure 1C).
Neutrophil recruitment is an important step in the pathogenesis of allergic sensitization and inflammation (100). Trx1 has an obvious inhibitory effect on the binding of neutrophils to vascular endothelial cells. Nakamura et al. found that Trx1 can inhibit the adhesion of neutrophils to endothelial cells in a mouse air sac chemotactic model (101). CD62L is an important adhesion molecule that is expressed and released by neutrophils, and it plays a key chemotactic role in neutrophil adherence to vascular endothelium and blood vessel penetration (102). Specifically, exogenous Trx1 acts directly on neutrophils, inhibiting the activation of the p38 mitogen-activated protein kinase (MAPK) signaling pathway, which causes the downregulation of CD62L in neutrophils, and ultimately reduces the adhesion of CD62L to endothelial cells. We previously explained its specific mechanism of action (103). Additionally, C32S/C35S mutant Trx1, showing a mutation at the redox function site, cannot inhibit the adhesion of neutrophils to human umbilical vein endothelial cells, indicating that the redox site of Trx1 is necessary for the inhibition of neutrophil adhesion (101). Moreover, in an LPS-induced bronchial inflammation rat model intravenously injected with 8 mg/kg of Trx1 every day, neutrophil infiltration into the bronchial and lung tissues had significantly reduced (104). Although adhesion molecules, such as ICAM-1, expressed by endothelial cells play important roles in neutrophil extravasation, Trx1 does not alter the expression of such adhesion molecules in these cells (101, 104). Therefore, Trx1 can inhibit the neutrophil recruitment by other chemokines, and it may play a unique role in neutrophil exudation of allergic inflammation.
Excessive complement activation has been implicated in the pathogenesis of allergic inflammatory disorders, such as IgE-independent DAs, and the increased production of the anaphylactic toxins C3a and C5a contributes to the activation of mast cells or basophils, vasodilation, and smooth muscle contraction. Transgenic overexpression of Trx1 in vivo or exogenous Trx1 injection can reduce choroidal neovascularization formation in laser-injured mouse models, which is closely associated with the complement activation of the Trx1 inhibition alternative pathway (48). Complement factor H, a multidomain and multifunctional protein, functions within the negative feedback that occurs during complement alternative pathway activation. It competes with factor B for C3b binding and accelerates the degradation of C3 convertase into its component (105). Trx1 inhibits C3 cleavage into C3a and C3b in a dose-dependent manner and prevents the deposition of C3b, and it inhibits the activation of C3b and reduces the generation of C3 convertase by binding to complement factor H; thus, it enhances the inhibition of C3 cleavage by complement factor H (48).
Moreover, Trx1 inhibits the activation of C5 convertase through its active site, thereby preventing the production of C5a and the formation of the membrane attack complex (49) (Figure 3). The deposition of C5b and C9 is also inhibited by Trx1 in a concentration-dependent manner in all three pathways during their early stages; however, Trx1 does not inhibit the deposition of non-allergic toxin C3b, which has a conditioning effect on bacteria and promotes phagocyte phagocytosis (106). C5a shows strong chemotactic activity in neutrophils and stimulates them to produce a large amount of oxygen free radicals, prostaglandins, and arachidonic acid. When Trx1 is intravenously injected into mice, complement-mediated neutrophil recruitment is significantly inhibited (48, 49). Therefore, blockage of complement activation by Trx1 may represent a therapeutic target for relieving IgE-independent allergic inflammation.
Figure 3 Potential mechanism of thioredoxin-1 (Trx1) inhibition of complement activation. Serum Trx1 inhibits C3 cleavage in the alternative pathway alone and enhances factor H (FH)-induced inhibition of C3 cleavage by combining with FH, which reduces C3a levels and C3b deposition. In contrast, Trx1 on the surface of endothelial cells or serum Trx1 blocks the production and deposition of C5b by inhibiting C5 convertase activity in the three complement terminal pathways; moreover, C9 deposition is inhibited. At the same time, Trx1 inhibits the production of anaphylaxis toxin C5a, which reduces the chemotaxis of neutrophils.
Glucocorticoids (GCs), which stabilize mast cells to prevent degranulation and exert broad anti-inflammatory effects by binding to glucocorticoid receptors (GRs), are recognized as an effective first-line therapy for allergic diseases. Notably, GCs interfere with the division and proliferation of systemic lymphoid tissues under the action of antigens, affect the metabolism of lymphocytes, and induce lymphocyte apoptosis. Therefore, long-term administration of GCs attenuates host immunity to specific antigens and leads to the inhibition of the immune response to pathogenic microorganisms.
In previous studies, we have shown that the anti-inflammatory and anti-allergic effects of Trx1 may inhibit host immunity, which is in contrast to the effects of corticosteroids (47, 54). Long-term use of GCs can cause GC resistance or insensitivity, which is a major obstacle in the treatment of allergic diseases. MIF can be induced by GCs and it enhances GC resistance (107); specifically, it impairs GC sensitivity via MAP kinase phosphatase-1 (MKP-1) inhibition (108). MKP-1 is an important MAPK signal inhibitor that is induced by GCs and mediates GC inhibition of ERK, JNK, and p38 MAPK activities as well as cytokine production induced by pro-inflammatory stimuli, such as LPS or IL-1 (109–111). MIF has been shown to downregulate GC-induced leucine zipper (GILZ) expression through a unique set of effects on transcription factor expression and phosphorylation. Notably, MIF-induced regulation of MKP-1 and MAPK activation is mediated through GILZ (112). Furthermore, MIF affects the NF-κB/IκB signal cascade, leading to accentuated inflammation and GC resistance (113). Trx1 can bind to GR and enhance the response of the cells to glucocorticoids (114). In addition, it can bind directly to MIF inside and outside the cell (71). Thus, Trx1 represents a potential intervention target between GC and MIF balance (Figure 4A).
Figure 4 (A) Thioredoxin-1 (Trx1) improves glucocorticoid (GC) resistance through macrophage migration inhibitory factor (MIF). MIF impairs GC sensitivity via the inhibition of MAP kinase phosphatase-1 (MKP-1). MKP-1 is induced by GC to mediate GC inhibition of ERK, JNK, and p38MAPK activities as well as cytokine production. MIF inhibits GC-induced leucine zipper (GILZ) expression through unique effects on the expression of transcription factor and phosphorylation. MKP-1 and MAPK activation are regulated by MIF via GILZ. MIF also affects the NF-κB/IκB signal cascade. Trx1 may directly bind to GC receptor and enhance the response of cells to GCs. Both intracellular and extracellular Trx1 bind to MIF and form a heterodimer to prevent MIF entry into cells and MIF-induced GC resistance. (B) Potential clinical applications of Trx1 in allergic diseases. Administration of Trx1 suppresses the excessive allergic inflammatory response. Future clinical applications of Trx1 could include treatment of asthma or allergic rhinitis with a Trx1 inhaler, topical application for patients with contact dermatitis, and/or oral delivery for those with food allergies. It may also be promising to combine Trx1 with corticosteroids. Finally, Trx1 could potentially be administered as an intravenous injection.
Trx1 induction is considered an effective compensatory protective mechanism through which damaged tissue proteins are reduced or repaired. Trx1 exerts anti-inflammatory effects on a wide variety of inflammatory disorders. In this review, we summarized the available data on Trx1 and highlighted a variety of mechanisms underlying its beneficial effects against allergic inflammation. Trx1 improved GC resistance, thereby acting as a promising therapeutic target both as a supplement to existing treatments for allergic diseases and for patients with hormone intolerance. In addition, it has been reported that increased levels of Trx1 in plasma or serum are correlated with the progression of diseases, especially allergic asthma. Thus, Trx1 may also serve as a potential diagnostic marker and be useful in prognostic assessments.
A variety of protein expression systems, including yeasts, lactobacillus, algae and plants cells, have been developed with anti-allergic and anti-inflammatory activities that are comparable to those found for purified recombinant human thioredoxin (rhTrx); thus, feasible sources for production of thioredoxin protein currently exist. In future translational research focused on Trx1, it will be essential to conduct human studies. Importantly, clinical trials are now ongoing in which rhTrx1 is being administered to patients with atopic dermatitis and trans-tracheal inhalation experiment with rhTrx1 are being performed; Trx1 is showing good efficacy with no major side effects (unpublished data). Finally, we suggest that Trx1 will be an important potential target for anti-allergic and anti-inflammatory drug development in the future (Figure 4B).
JW, JY, and HT were involved in the conception and writing of the manuscript, JZ, CW, AF, and SL contributed to literature searches and extensive discussions, and all authors agreed to publish the paper.
Author HT was employed by Jiaozhimei Biotechnology (Shaoxing) Co., Ltd.
The remaining authors declare that the research was conducted in the absence of any commercial or financial relationships that could be construed as a potential conflict of interest.
All claims expressed in this article are solely those of the authors and do not necessarily represent those of their affiliated organizations, or those of the publisher, the editors and the reviewers. Any product that may be evaluated in this article, or claim that may be made by its manufacturer, is not guaranteed or endorsed by the publisher.
We deeply appreciate Pro. Takashi Inamoto for pointed advice and discussion when writing this paper.
1. Holmgren A. Thioredoxin. Annu Rev Biochem (1985) 54:237–71. doi: 10.1146/annurev.bi.54.070185.001321
2. Eklund H, Gleason FK, Holmgren A. Structural and Functional Relations Among Thioredoxins of Different Species. Proteins (1991) 11(1):13–28. doi: 10.1002/prot.340110103
3. Arner ES. Focus on Mammalian Thioredoxin Reductases–Important Selenoproteins With Versatile Functions. Biochim Biophys Acta (2009) 1790(6):495–526. doi: 10.1016/j.bbagen.2009.01.014
4. Rhee SG, Chae HZ, Kim K. Peroxiredoxins: A Historical Overview and Speculative Preview of Novel Mechanisms and Emerging Concepts in Cell Signaling. Free Radic Biol Med (2005) 38(12):1543–52. doi: 10.1016/j.freeradbiomed.2005.02.026
5. Lee S, Kim SM, Lee RT. Thioredoxin and Thioredoxin Target Proteins: From Molecular Mechanisms to Functional Significance. Antioxid Redox Signal (2013) 18(10):1165–207. doi: 10.1089/ars.2011.4322
6. Lillig CH, Holmgren A. Thioredoxin and Related Molecules–from Biology to Health and Disease. Antioxid Redox Signal (2007) 9(1):25–47. doi: 10.1089/ars.2007.9.25
7. Hanschmann EM, Godoy JR, Berndt C, Hudemann C, Lillig CH. Thioredoxins, Glutaredoxins, and Peroxiredoxins–Molecular Mechanisms and Health Significance: From Cofactors to Antioxidants to Redox Signaling. Antioxid Redox Signal (2013) 19(13):1539–605. doi: 10.1089/ars.2012.4599
8. Lu J, Holmgren A. Thioredoxin System in Cell Death Progression. Antioxid Redox Signal (2012) 17(12):1738–47. doi: 10.1089/ars.2012.4650
9. Yamada Y, Nakamura H, Adachi T, Sannohe S, Oyamada H, Kayaba H, et al. Elevated Serum Levels of Thioredoxin in Patients With Acute Exacerbation of Asthma. Immunol Lett (2003) 86(2):199–205. doi: 10.1016/s0165-2478(03)00006-3
10. Tinkov AA, Bjorklund G, Skalny AV, Holmgren A, Skalnaya MG, Chirumbolo S, et al. The Role of the Thioredoxin/Thioredoxin Reductase System in the Metabolic Syndrome: Towards a Possible Prognostic Marker? Cell Mol Life Sci (2018) 75(9):1567–86. doi: 10.1007/s00018-018-2745-8
11. Lu J, Holmgren A. The Thioredoxin Superfamily in Oxidative Protein Folding. Antioxid Redox Signal (2014) 21(3):457–70. doi: 10.1089/ars.2014.5849
12. Awan MUN, Yan F, Mahmood F, Bai L, Liu J, Bai J. The Functions of Thioredoxin 1 in Neurodegeneration. Antioxid Redox Signal (2021). doi: 10.1089/ars.2021.0186
13. Nakamura H, Hoshino Y, Okuyama H, Matsuo Y, Yodoi J. Thioredoxin 1 Delivery as New Therapeutics. Adv Drug Delivery Rev (2009) 61(4):303–9. doi: 10.1016/j.addr.2009.01.003
14. Barnes PJ. Pathophysiology of Asthma. Br J Clin Pharmacol (1996) 42(1):3–10. doi: 10.1046/j.1365-2125.1996.03721.x
15. Hammad H, Lambrecht BN. The Basic Immunology of Asthma. Cell (2021) 184(6):1469–85. doi: 10.1016/j.cell.2021.02.016
16. Wang X, Xu C, Ji J, Cai Y, Shu Y, Chao Y, et al. Il-4/Il-13 Upregulates Sonic Hedgehog Expression to Induce Allergic Airway Epithelial Remodeling. Am J Physiol Lung Cell Mol Physiol (2020) 318(5):L888–L99. doi: 10.1152/ajplung.00186.2019
17. Ingram JL, Kraft M. Il-13 in Asthma and Allergic Disease: Asthma Phenotypes and Targeted Therapies. J Allergy Clin Immunol (2012) 130(4):829–42. doi: 10.1016/j.jaci.2012.06.034
18. Papi A, Brightling C, Pedersen SE, Reddel HK. Asthma. Lancet (2018) 391(10122):783–800. doi: 10.1016/S0140-6736(17)33311-1
19. Das D, Wang YH, Hsieh CY, Suzuki YJ. Major Vault Protein Regulates Cell Growth/Survival Signaling Through Oxidative Modifications. Cell Signal (2016) 28(1):12–8. doi: 10.1016/j.cellsig.2015.10.007
20. Bozza MT, Lintomen L, Kitoko JZ, Paiva CN, Olsen PC. The Role of Mif on Eosinophil Biology and Eosinophilic Inflammation. Clin Rev Allergy Immunol (2020) 58(1):15–24. doi: 10.1007/s12016-019-08726-z
21. Qian Y, Sun Y, Chen Y, Mao Z, Shi Y, Wu D, et al. Nrf2 Regulates Downstream Genes by Targeting Mir-29b in Severe Asthma and the Role of Grape Seed Proanthocyanidin Extract in a Murine Model of Steroid-Insensitive Asthma. Pharm Biol (2022) 60(1):347–58. doi: 10.1080/13880209.2022.2032205
22. Hirai K, Shirai T, Rachi Y, Uehara S, Ueda M, Nakatani E, et al. Impact of Gene Expression Associated With Glucocorticoid-Induced Transcript 1 (Glcci1) on Severe Asthma and Future Exacerbation. Biol Pharm Bull (2019) 42(10):1746–52. doi: 10.1248/bpb.b19-00476
23. Brown RH, Reynolds C, Brooker A, Talalay P, Fahey JW. Sulforaphane Improves the Bronchoprotective Response in Asthmatics Through Nrf2-Mediated Gene Pathways. Respir Res (2015) 16:106. doi: 10.1186/s12931-015-0253-z
24. Weiss ST. Emerging Mechanisms and Novel Targets in Allergic Inflammation and Asthma. Genome Med (2017) 9(1):107. doi: 10.1186/s13073-017-0501-6
25. Mokra D, Tonhajzerova I, Mokry J, Petraskova M, Hutko M, Calkovska A. Cardiovascular Side Effects of Aminophylline in Meconium-Induced Acute Lung Injury. Adv Exp Med Biol (2013) 756:341–7. doi: 10.1007/978-94-007-4549-0_41
26. Ito W, Kobayashi N, Takeda M, Ueki S, Kayaba H, Nakamura H, et al. Thioredoxin in Allergic Inflammation. Int Arch Allergy Immunol (2011) 155 Suppl 1:142–6. doi: 10.1159/000327501
27. Ichiki H, Hoshino T, Kinoshita T, Imaoka H, Kato S, Inoue H, et al. Thioredoxin Suppresses Airway Hyperresponsiveness and Airway Inflammation in Asthma. Biochem Biophys Res Commun (2005) 334(4):1141–8. doi: 10.1016/j.bbrc.2005.07.007
28. Imaoka H, Hoshino T, Takei S, Sakazaki Y, Kinoshita T, Okamoto M, et al. Effects of Thioredoxin on Established Airway Remodeling in a Chronic Antigen Exposure Asthma Model. Biochem Biophys Res Commun (2007) 360(3):525–30. doi: 10.1016/j.bbrc.2007.06.019
29. Hanschmann EM, Berndt C, Hecker C, Garn H, Bertrams W, Lillig CH, et al. Glutaredoxin 2 Reduces Asthma-Like Acute Airway Inflammation in Mice. Front Immunol (2020) 11:561724. doi: 10.3389/fimmu.2020.561724
30. Torii M, Wang L, Ma N, Saito K, Hori T, Sato-Ueshima M, et al. Thioredoxin Suppresses Airway Inflammation Independently of Systemic Th1/Th2 Immune Modulation. Eur J Immunol (2010) 40(3):787–96. doi: 10.1002/eji.200939724
31. Imaoka H, Hoshino T, Okamoto M, Sakazaki Y, Sawada M, Takei S, et al. Endogenous and Exogenous Thioredoxin 1 Prevents Goblet Cell Hyperplasia in a Chronic Antigen Exposure Asthma Model. Allergol Int (2009) 58(3):403–10. doi: 10.2332/allergolint.09-OA-0086
32. Jia JJ, Zeng XS, Li Y, Ma S, Bai J. Ephedrine Induced Thioredoxin-1 Expression Through Beta-Adrenergic Receptor/Cyclic Amp/Protein Kinase a/Dopamine- and Cyclic Amp-Regulated Phosphoprotein Signaling Pathway. Cell Signal (2013) 25(5):1194–201. doi: 10.1016/j.cellsig.2013.02.007
33. Lim JO, Lee SJ, Kim WI, Pak SW, Moon C, Shin IS, et al. Titanium Dioxide Nanoparticles Exacerbate Allergic Airway Inflammation Via Txnip Upregulation in a Mouse Model of Asthma. Int J Mol Sci (2021) 22(18):9924. doi: 10.3390/ijms22189924
34. Kulka M. The Potential of Natural Products as Effective Treatments for Allergic Inflammation: Implications for Allergic Rhinitis. Curr Top Med Chem (2009) 9(17):1611–24. doi: 10.2174/156802609789941898
35. Kramer MF, Jordan TR, Klemens C, Hilgert E, Hempel JM, Pfrogner E, et al. Factors Contributing to Nasal Allergic Late Phase Eosinophilia. Am J Otolaryngol (2006) 27(3):190–9. doi: 10.1016/j.amjoto.2005.09.013
36. Shi Q, Lei Z, Cheng G, Li D, Wang Q, Luo S, et al. Mitochondrial Ros Activate Interleukin-1beta Expression in Allergic Rhinitis. Oncol Lett (2018) 16(3):3193–200. doi: 10.3892/ol.2018.8984
37. Lin H, Ba G, Tang R, Li M, Li Z, Li D, et al. Increased Expression of Txnip Facilitates Oxidative Stress in Nasal Epithelial Cells of Patients With Chronic Rhinosinusitis With Nasal Polyps. Am J Rhinol Allergy (2021) 35(5):607–14. doi: 10.1177/1945892420982411
38. Edo Y, Otaki A, Asano K. Quercetin Enhances the Thioredoxin Production of Nasal Epithelial Cells In Vitro and In Vivo. Medicines (Basel) (2018) 5(4):124. doi: 10.3390/medicines5040124
39. Vickery BP, Scurlock AM, Kulis M, Steele PH, Kamilaris J, Berglund JP, et al. Sustained Unresponsiveness to Peanut in Subjects Who Have Completed Peanut Oral Immunotherapy. J Allergy Clin Immunol (2014) 133(2):468–75. doi: 10.1016/j.jaci.2013.11.007
40. Sampson HA. Peanut Oral Immunotherapy: Is It Ready for Clinical Practice? J Allergy Clin Immunol Pract (2013) 1(1):15–21. doi: 10.1016/j.jaip.2012.10.009
41. Buchanan BB, Adamidi C, Lozano RM, Yee BC, Momma M, Kobrehel K, et al. Thioredoxin-Linked Mitigation of Allergic Responses to Wheat. Proc Natl Acad Sci USA (1997) 94(10):5372–7. doi: 10.1073/pnas.94.10.5372
42. del Val G, Yee BC, Lozano RM, Buchanan BB, Ermel RW, Lee YM, et al. Thioredoxin Treatment Increases Digestibility and Lowers Allergenicity of Milk. J Allergy Clin Immunol (1999) 103(4):690–7. doi: 10.1016/s0091-6749(99)70244-7
43. Matsumoto T, Shimada Y, Hirai S. Mitigated Binding of Ige to Thioredoxin-Treated Salt-Soluble Wheat Allergens in A Child With Baker’s Asthma. Ann Allergy Asthma Immunol (2007) 98(6):599–600. doi: 10.1016/S1081-1206(10)60746-6
44. Taketani Y, Kinugasa K, Furukawa S, Nakamura H, Otsuki R, Yasuda H, et al. Yeast Thioredoxin-Enriched Extracts for Mitigating the Allergenicity of Foods. Biosci Biotechnol Biochem (2011) 75(10):1872–9. doi: 10.1271/bbb.100734
45. Shahriari-Farfani T, Shahpiri A, Taheri-Kafrani A. Enhancement of Tryptic Digestibility of Milk Beta-Lactoglobulin Through Treatment With Recombinant Rice Glutathione/Thioredoxin and Nadph Thioredoxin Reductase/Thioredoxin Systems. Appl Biochem Biotechnol (2019) 187(2):649–61. doi: 10.1007/s12010-018-2793-4
46. Pichler WJ, Yerly D. Drug Hypersensitivity: We Need to Do More. J Allergy Clin Immunol (2018) 141(1):89–91. doi: 10.1016/j.jaci.2017.11.002
47. Son A, Nakamura H, Kondo N, Matsuo Y, Liu W, Oka S, et al. Redox Regulation of Mast Cell Histamine Release in Thioredoxin-1 (Trx) Transgenic Mice. Cell Res (2006) 16(2):230–9. doi: 10.1038/sj.cr.7310031
48. Inomata Y, Tanihara H, Tanito M, Okuyama H, Hoshino Y, Kinumi T, et al. Suppression of Choroidal Neovascularization by Thioredoxin-1 Via Interaction With Complement Factor H. Invest Ophthalmol Vis Sci (2008) 49(11):5118–25. doi: 10.1167/iovs.07-1659
49. King BC, Nowakowska J, Karsten CM, Kohl J, Renstrom E, Blom AM. Truncated and Full-Length Thioredoxin-1 Have Opposing Activating and Inhibitory Properties for Human Complement With Relevance to Endothelial Surfaces. J Immunol (2012) 188(8):4103–12. doi: 10.4049/jimmunol.1101295
50. Galli E, Neri I, Ricci G, Baldo E, Barone M, Belloni Fortina A, et al. Consensus Conference on Clinical Management of Pediatric Atopic Dermatitis. Ital J Pediatr (2016) 42:26. doi: 10.1186/s13052-016-0229-8
51. Leonard A, Guttman-Yassky E. The Unique Molecular Signatures of Contact Dermatitis and Implications for Treatment. Clin Rev Allergy Immunol (2019) 56(1):1–8. doi: 10.1007/s12016-018-8685-0
52. Miyachi Y, Uchida K, Komura J, Asada Y, Niwa Y. Auto-Oxidative Damage in Cement Dermatitis. Arch Dermatol Res (1985) 277(4):288–92. doi: 10.1007/BF00509082
53. Nakamura H, Masutani H, Yodoi J. Extracellular Thioredoxin and Thioredoxin-Binding Protein 2 in Control of Cancer. Semin Cancer Biol (2006) 16(6):444–51. doi: 10.1016/j.semcancer.2006.09.001
54. Fukunaga A, Horikawa T, Ogura K, Taguchi K, Yu X, Funasaka Y, et al. Thioredoxin Suppresses the Contact Hypersensitivity Response by Inhibiting Leukocyte Recruitment During the Elicitation Phase. Antioxid Redox Signal (2009) 11(6):1227–35. doi: 10.1089/ARS.2008.2340
55. Ono R, Masaki T, Dien S, Yu X, Fukunaga A, Yodoi J, et al. Suppressive Effect of Recombinant Human Thioredoxin on Ultraviolet Light-Induced Inflammation and Apoptosis in Murine Skin. J Dermatol (2012) 39(10):843–51. doi: 10.1111/j.1346-8138.2012.01566.x
56. Tian H, Matsuo Y, Fukunaga A, Ono R, Nishigori C, Yodoi J. Thioredoxin Ameliorates Cutaneous Inflammation by Regulating the Epithelial Production and Release of Pro-Inflammatory Cytokines. Front Immunol (2013) 4:269. doi: 10.3389/fimmu.2013.00269
57. Mitsui A, Hamuro J, Nakamura H, Kondo N, Hirabayashi Y, Ishizaki-Koizumi S, et al. Overexpression of Human Thioredoxin in Transgenic Mice Controls Oxidative Stress and Life Span. Antioxid Redox Signal (2002) 4(4):693–6. doi: 10.1089/15230860260220201
58. Du Y, Zhang H, Lu J, Holmgren A. Glutathione and Glutaredoxin Act as a Backup of Human Thioredoxin Reductase 1 to Reduce Thioredoxin 1 Preventing Cell Death by Aurothioglucose. J Biol Chem (2012) 287(45):38210–9. doi: 10.1074/jbc.M112.392225
59. Tan SX, Greetham D, Raeth S, Grant CM, Dawes IW, Perrone GG. The Thioredoxin-Thioredoxin Reductase System Can Function in Vivo as an Alternative System to Reduce Oxidized Glutathione in Saccharomyces Cerevisiae. J Biol Chem (2010) 285(9):6118–26. doi: 10.1074/jbc.M109.062844
60. Kang SW, Chae HZ, Seo MS, Kim K, Baines IC, Rhee SG. Mammalian Peroxiredoxin Isoforms Can Reduce Hydrogen Peroxide Generated in Response to Growth Factors and Tumor Necrosis Factor-Alpha. J Biol Chem (1998) 273(11):6297–302. doi: 10.1074/jbc.273.11.6297
61. Cvetko F, Caldwell ST, Higgins M, Suzuki T, Yamamoto M, Prag HA, et al. Nrf2 Is Activated by Disruption of Mitochondrial Thiol Homeostasis But Not by Enhanced Mitochondrial Superoxide Production. J Biol Chem (2021) 296:100169. doi: 10.1074/jbc.RA120.016551
62. Jaganjac M, Milkovic L, Sunjic SB, Zarkovic N. The Nrf2, Thioredoxin, and Glutathione System in Tumorigenesis and Anticancer Therapies Antioxidants (Basel). (2020) 9(11):1151. doi: 10.3390/antiox9111151
63. Weiser WY, Temple PA, Witek-Giannotti JS, Remold HG, Clark SC, David JR. Molecular Cloning of a Cdna Encoding a Human Macrophage Migration Inhibitory Factor. Proc Natl Acad Sci USA (1989) 86(19):7522–6. doi: 10.1073/pnas.86.19.7522
64. Lang T, Foote A, Lee JP, Morand EF, Harris J. Mif: Implications in the Pathoetiology of Systemic Lupus Erythematosus. Front Immunol (2015) 6:577. doi: 10.3389/fimmu.2015.00577
65. Das R, Moss JE, Robinson E, Roberts S, Levy R, Mizue Y, et al. Role of Macrophage Migration Inhibitory Factor in the Th2 Immune Response to Epicutaneous Sensitization. J Clin Immunol (2011) 31(4):666–80. doi: 10.1007/s10875-011-9541-7
66. Kleemann R, Kapurniotu A, Frank RW, Gessner A, Mischke R, Flieger O, et al. Disulfide Analysis Reveals a Role for Macrophage Migration Inhibitory Factor (Mif) as Thiol-Protein Oxidoreductase. J Mol Biol (1998) 280(1):85–102. doi: 10.1006/jmbi.1998.1864
67. Thiele M, Bernhagen J. Link Between Macrophage Migration Inhibitory Factor and Cellular Redox Regulation. Antioxid Redox Signal (2005) 7(9-10):1234–48. doi: 10.1089/ars.2005.7.1234
68. Tamaki H, Nakamura H, Nishio A, Nakase H, Ueno S, Uza N, et al. Human Thioredoxin-1 Ameliorates Experimental Murine Colitis in Association With Suppressed Macrophage Inhibitory Factor Production. Gastroenterology (2006) 131(4):1110–21. doi: 10.1053/j.gastro.2006.08.023
69. Sato A, Hara T, Nakamura H, Kato N, Hoshino Y, Kondo N, et al. Thioredoxin-1 Suppresses Systemic Inflammatory Responses Against Cigarette Smoking. Antioxid Redox Signal (2006) 8(9-10):1891–6. doi: 10.1089/ars.2006.8.1891
70. Kondo N, Ishii Y, Son A, Sakakura-Nishiyama J, Kwon YW, Tanito M, et al. Cysteine-Dependent Immune Regulation by Trx and Mif/Gif Family Proteins. Immunol Lett (2004) 92(1-2):143–7. doi: 10.1016/j.imlet.2003.11.030
71. Son A, Kato N, Horibe T, Matsuo Y, Mochizuki M, Mitsui A, et al. Direct Association of Thioredoxin-1 (Trx) With Macrophage Migration Inhibitory Factor (Mif): Regulatory Role of Trx on Mif Internalization and Signaling. Antioxid Redox Signal (2009) 11(10):2595–605. doi: 10.1089/ARS.2009.2522
72. Agnello D, Lankford CS, Bream J, Morinobu A, Gadina M, O’Shea JJ, et al. Cytokines and Transcription Factors That Regulate T Helper Cell Differentiation: New Players and New Insights. J Clin Immunol (2003) 23(3):147–61. doi: 10.1023/a:1023381027062
73. Nembrini C, Abel B, Kopf M, Marsland BJ. Strong Tcr Signaling, Tlr Ligands, and Cytokine Redundancies Ensure Robust Development of Type 1 Effector T Cells. J Immunol (2006) 176(12):7180–8. doi: 10.4049/jimmunol.176.12.7180
74. Yagi J, Arimura Y, Takatori H, Nakajima H, Iwamoto I, Uchiyama T. Genetic Background Influences Th Cell Differentiation by Controlling the Capacity for Il-2-Induced Il-4 Production by Naive Cd4+ T Cells. Int Immunol (2006) 18(12):1681–90. doi: 10.1093/intimm/dxl102
75. Angelini G, Gardella S, Ardy M, Ciriolo MR, Filomeni G, Di Trapani G, et al. Antigen-Presenting Dendritic Cells Provide the Reducing Extracellular Microenvironment Required for T Lymphocyte Activation. Proc Natl Acad Sci USA (2002) 99(3):1491–6. doi: 10.1073/pnas.022630299
76. Edinger AL, Thompson CB. Antigen-Presenting Cells Control T Cell Proliferation by Regulating Amino Acid Availability. Proc Natl Acad Sci USA (2002) 99(3):1107–9. doi: 10.1073/pnas.042707999
77. Matthias LJ, Yam PT, Jiang XM, Vandegraaff N, Li P, Poumbourios P, et al. Disulfide Exchange in Domain 2 of Cd4 Is Required for Entry of Hiv-1. Nat Immunol (2002) 3(8):727–32. doi: 10.1038/ni815
78. Schwertassek U, Balmer Y, Gutscher M, Weingarten L, Preuss M, Engelhard J, et al. Selective Redox Regulation of Cytokine Receptor Signaling by Extracellular Thioredoxin-1. EMBO J (2007) 26(13):3086–97. doi: 10.1038/sj.emboj.7601746
79. Kang MW, Jang JY, Choi JY, Kim SH, Oh J, Cho BS, et al. Induction of Ifn-Gamma Gene Expression by Thioredoxin: Positive Feed-Back Regulation of Th1 Response by Thioredoxin and Ifn-Gamma. Cell Physiol Biochem (2008) 21(1-3):215–24. doi: 10.1159/000113763
80. Kim SH, Oh J, Choi JY, Jang JY, Kang MW, Lee CE. Identification of Human Thioredoxin as a Novel Ifn-Gamma-Induced Factor: Mechanism of Induction and Its Role in Cytokine Production. BMC Immunol (2008) 9:64. doi: 10.1186/1471-2172-9-64
81. Schroder K, Hertzog PJ, Ravasi T, Hume DA. Interferon-Gamma: An Overview of Signals, Mechanisms and Functions. J Leukoc Biol (2004) 75(2):163–89. doi: 10.1189/jlb.0603252
82. Roesner LM, Ernst M, Chen W, Begemann G, Kienlin P, Raulf MK, et al. Human Thioredoxin, a Damage-Associated Molecular Pattern and Malassezia-Crossreactive Autoallergen, Modulates Immune Responses Via the C-Type Lectin Receptors Dectin-1 and Dectin-2. Sci Rep (2019) 9(1):11210. doi: 10.1038/s41598-019-47769-2
83. Plugis NM, Weng N, Zhao Q, Palanski BA, Maecker HT, Habtezion A, et al. Interleukin 4 Is Inactivated Via Selective Disulfide-Bond Reduction by Extracellular Thioredoxin. Proc Natl Acad Sci USA (2018) 115(35):8781–6. doi: 10.1073/pnas.1805288115
84. Boonpiyathad T, Sozener ZC, Akdis M, Akdis CA. The Role of Treg Cell Subsets in Allergic Disease. Asian Pac J Allergy Immunol (2020) 38(3):139–49. doi: 10.12932/AP-030220-0754
85. Mougiakakos D, Johansson CC, Jitschin R, Bottcher M, Kiessling R. Increased Thioredoxin-1 Production in Human Naturally Occurring Regulatory T Cells Confers Enhanced Tolerance to Oxidative Stress. Blood (2011) 117(3):857–61. doi: 10.1182/blood-2010-09-307041
86. Baugh JA, Bucala R. Macrophage Migration Inhibitory Factor. Crit Care Med (2002) 30(1 Supp):S27–35. doi: 10.1097/00003246-200201001-00004
87. Heath H, Qin S, Rao P, Wu L, LaRosa G, Kassam N, et al. Chemokine Receptor Usage by Human EosinophilsThe Importance of Ccr3 Demonstrated Using an Antagonistic Monoclonal Antibody. J Clin Invest (1997) 99(2):178–84. doi: 10.1172/JCI119145
88. Kobayashi N, Yamada Y, Ito W, Ueki S, Kayaba H, Nakamura H, et al. Thioredoxin Reduces C-C Chemokine-Induced Chemotaxis of Human Eosinophils. Allergy (2009) 64(8):1130–5. doi: 10.1111/j.1398-9995.2009.01969.x
89. Boehme SA, Sullivan SK, Crowe PD, Santos M, Conlon PJ, Sriramarao P, et al. Activation of Mitogen-Activated Protein Kinase Regulates Eotaxin-Induced Eosinophil Migration. J Immunol (1999) 163(3):1611–8.
90. Kampen GT, Stafford S, Adachi T, Jinquan T, Quan S, Grant JA, et al. Eotaxin Induces Degranulation and Chemotaxis of Eosinophils Through the Activation of Erk2 and P38 Mitogen-Activated Protein Kinases. Blood (2000) 95(6):1911–7. doi: 10.1182/blood.V95.6.1911
91. Chuang CY, Chang CH, Huang YL. Thioredoxin Mediates Remodeling Factors of Human Bronchial Epithelial Cells Upon Interaction With House Dust Mite-Stimulated Eosinophils. Inhal Toxicol (2009) 21(2):153–67. doi: 10.1080/08958370802368730
92. Kondo N, Nakamura H, Masutani H, Yodoi J. Redox Regulation of Human Thioredoxin Network. Antioxid Redox Signal (2006) 8(9-10):1881–90. doi: 10.1089/ars.2006.8.1881
93. Wolfreys K, Oliveira DB. Alterations in Intracellular Reactive Oxygen Species Generation and Redox Potential Modulate Mast Cell Function. Eur J Immunol (1997) 27(1):297–306. doi: 10.1002/eji.1830270143
94. Suzuki Y, Yoshimaru T, Inoue T, Niide O, Ra C. Role of Oxidants in Mast Cell Activation. Chem Immunol Allergy (2005) 87:32–42. doi: 10.1159/000087569
95. Yoshimaru T, Suzuki Y, Matsui T, Yamashita K, Ochiai T, Yamaki M, et al. Blockade of Superoxide Generation Prevents High-Affinity Immunoglobulin E Receptor-Mediated Release of Allergic Mediators by Rat Mast Cell Line and Human Basophils. Clin Exp Allergy (2002) 32(4):612–8. doi: 10.1046/j.0954-7894.2002.01263.x
96. Suzuki Y, Yoshimaru T, Matsui T, Inoue T, Niide O, Nunomura S, et al. Fc Epsilon Ri Signaling of Mast Cells Activates Intracellular Production of Hydrogen Peroxide: Role in the Regulation of Calcium Signals. J Immunol (2003) 171(11):6119–27. doi: 10.4049/jimmunol.171.11.6119
97. Cairns JA. Inhibitors of Mast Cell Tryptase Beta as Therapeutics for the Treatment of Asthma and Inflammatory Disorders. Pulm Pharmacol Ther (2005) 18(1):55–66. doi: 10.1016/j.pupt.2004.09.032
98. Castells M, Schwartz LB. Tryptase Levels in Nasal-Lavage Fluid as an Indicator of the Immediate Allergic Response. J Allergy Clin Immunol (1988) 82(3 Pt 1):348–55. doi: 10.1016/0091-6749(88)90005-x
99. Cook KM, McNeil HP, Hogg PJ. Allosteric Control of Betaii-Tryptase by a Redox Active Disulfide Bond. J Biol Chem (2013) 288(48):34920–9. doi: 10.1074/jbc.M113.523506
100. Hosoki K, Itazawa T, Boldogh I, Sur S. Neutrophil Recruitment by Allergens Contribute to Allergic Sensitization and Allergic Inflammation. Curr Opin Allergy Clin Immunol (2016) 16(1):45–50. doi: 10.1097/ACI.0000000000000231
101. Nakamura H, Herzenberg LA, Bai J, Araya S, Kondo N, Nishinaka Y, et al. Circulating Thioredoxin Suppresses Lipopolysaccharide-Induced Neutrophil Chemotaxis. Proc Natl Acad Sci USA (2001) 98(26):15143–8. doi: 10.1073/pnas.191498798
102. Rizoli SB, Rotstein OD, Kapus A. Cell Volume-Dependent Regulation of L-Selectin Shedding in NeutrophilsA Role for P38 Mitogen-Activated Protein Kinase. J Biol Chem (1999) 274(31):22072–80. doi: 10.1074/jbc.274.31.22072
103. Zhou J, Wang C, Wu J, Fukunaga A, Cheng Z, Wang J, et al. Anti-Allergic and Anti-Inflammatory Effects and Molecular Mechanisms of Thioredoxin on Respiratory System Diseases. Antioxid Redox Signal (2020) 32(11):785–801. doi: 10.1089/ars.2019.7807
104. Ueda S, Nakamura T, Yamada A, Teratani A, Matsui N, Furukawa S, et al. Recombinant Human Thioredoxin Suppresses Lipopolysaccharide-Induced Bronchoalveolar Neutrophil Infiltration in Rat. Life Sci (2006) 79(12):1170–7. doi: 10.1016/j.lfs.2006.03.026
105. Richards A, Kavanagh D, Atkinson JP. Inherited Complement Regulatory Protein Deficiency Predisposes to Human Disease in Acute Injury and Chronic Inflammatory Statesthe Examples of Vascular Damage in Atypical Hemolytic Uremic Syndrome and Debris Accumulation in Age-Related Macular Degeneration. Adv Immunol (2007) 96:141–77. doi: 10.1016/S0065-2776(07)96004-6
107. Aeberli D, Leech M, Morand EF. Macrophage Migration Inhibitory Factor and Glucocorticoid Sensitivity. Rheumatol (Oxford) (2006) 45(8):937–43. doi: 10.1093/rheumatology/kel142
108. Roger T, Chanson AL, Knaup-Reymond M, Calandra T. Macrophage Migration Inhibitory Factor Promotes Innate Immune Responses by Suppressing Glucocorticoid-Induced Expression of Mitogen-Activated Protein Kinase Phosphatase-1. Eur J Immunol (2005) 35(12):3405–13. doi: 10.1002/eji.200535413
109. Chen P, Li J, Barnes J, Kokkonen GC, Lee JC, Liu Y. Restraint of Proinflammatory Cytokine Biosynthesis by Mitogen-Activated Protein Kinase Phosphatase-1 in Lipopolysaccharide-Stimulated Macrophages. J Immunol (2002) 169(11):6408–16. doi: 10.4049/jimmunol.169.11.6408
110. Kassel O, Sancono A, Kratzschmar J, Kreft B, Stassen M, Cato AC. Glucocorticoids Inhibit Map Kinase Via Increased Expression and Decreased Degradation of Mkp-1. EMBO J (2001) 20(24):7108–16. doi: 10.1093/emboj/20.24.7108
111. Lasa M, Abraham SM, Boucheron C, Saklatvala J, Clark AR. Dexamethasone Causes Sustained Expression of Mitogen-Activated Protein Kinase (Mapk) Phosphatase 1 and Phosphatase-Mediated Inhibition of Mapk P38. Mol Cell Biol (2002) 22(22):7802–11. doi: 10.1128/MCB.22.22.7802-7811.2002
112. Fan H, Kao W, Yang YH, Gu R, Harris J, Fingerle-Rowson G, et al. Macrophage Migration Inhibitory Factor Inhibits the Antiinflammatory Effects of Glucocorticoids Via Glucocorticoid-Induced Leucine Zipper. Arthritis Rheumatol (2014) 66(8):2059–70. doi: 10.1002/art.38689
113. Wang FF, Zhu LA, Zou YQ, Zheng H, Wilson A, Yang CD, et al. New Insights Into the Role and Mechanism of Macrophage Migration Inhibitory Factor in Steroid-Resistant Patients With Systemic Lupus Erythematosus. Arthritis Res Ther (2012) 14(3):R103. doi: 10.1186/ar3828
114. Makino Y, Okamoto K, Yoshikawa N, Aoshima M, Hirota K, Yodoi J, et al. Thioredoxin: A Redox-Regulating Cellular Cofactor for Glucocorticoid Hormone ActionCross Talk Between Endocrine Control of Stress Response and Cellular Antioxidant Defense System. J Clin Invest (1996) 98(11):2469–77. doi: 10.1172/JCI119065
Keywords: allergic disease, anti-inflammatory, glucocorticoid resistance, migration inhibitory factor, thioredoxin-1
Citation: Wang J, Zhou J, Wang C, Fukunaga A, Li S, Yodoi J and Tian H (2022) Thioredoxin-1: A Promising Target for the Treatment of Allergic Diseases. Front. Immunol. 13:883116. doi: 10.3389/fimmu.2022.883116
Received: 24 February 2022; Accepted: 01 April 2022;
Published: 28 April 2022.
Edited by:
Heiko Mühl, Goethe University Frankfurt, GermanyReviewed by:
Christoph Hudemann, Philipps-University Marburg, GermanyCopyright © 2022 Wang, Zhou, Wang, Fukunaga, Li, Yodoi and Tian. This is an open-access article distributed under the terms of the Creative Commons Attribution License (CC BY). The use, distribution or reproduction in other forums is permitted, provided the original author(s) and the copyright owner(s) are credited and that the original publication in this journal is cited, in accordance with accepted academic practice. No use, distribution or reproduction is permitted which does not comply with these terms.
*Correspondence: Hai Tian, dGlhbmhhaTI4MjAwNDRAeWFob28uY28uanA=
Disclaimer: All claims expressed in this article are solely those of the authors and do not necessarily represent those of their affiliated organizations, or those of the publisher, the editors and the reviewers. Any product that may be evaluated in this article or claim that may be made by its manufacturer is not guaranteed or endorsed by the publisher.
Research integrity at Frontiers
Learn more about the work of our research integrity team to safeguard the quality of each article we publish.