- 1Aoyang Institute of Cancer, Affiliated Aoyang Hospital of Jiangsu University, Suzhou, China
- 2Zhenjiang Key Laboratory of High Technology Research on Exosome Foundation and Transformation Application, School of Medicine, Jiangsu University, Zhenjiang, China
Mesenchymal stem cell small extracellular vesicles (MSC-sEVs) are a priority for researchers because of their role in tissue regeneration. sEVs act as paracrine factors and carry various cargos, revealing the state of the parent cells and contributing to cell–cell communication during both physiological and pathological circumstances. Hepatic diseases are mainly characterized by inflammatory cell infiltration and hepatocyte necrosis and fibrosis, bringing the focus onto immune regulation and other regulatory mechanisms of MSCs/MSC-sEVs. Increasing evidence suggests that MSCs and their sEVs protect against acute and chronic liver injury by inducing macrophages (MΦ) to transform into the M2 subtype, accelerating regulatory T/B (Treg/Breg) cell activation and promoting immunosuppression. MSCs/MSC-sEVs also prevent the proliferation and differentiation of T cells, B cells, dendritic cells (DCs), and natural killer (NK) cells. This review summarizes the potential roles for MSCs/MSC-sEVs, including immunomodulation and tissue regeneration, in various liver diseases. There is also a specific focus on the use of MSC-sEVs for targeted drug delivery to treat hepatitis.
Introduction
Mesenchymal stem cells (MSCs) have gained attention for their potential in treating various diseases, advancing from animal experiments to clinical trials (1), and MSC biosafety has been widely indicated. We defined the renovation of MSCs in hepatic diseases over a decade ago using an in-house isolation culture system (2–7). MSCs differentiate into hepatocyte-like cells (8), informing the transition from liver to hepatocyte transplantation to improve liver function. The capacity of MSCs to reverse mouse hepatic injury (2) and Schistosoma japonicum-induced mouse liver injury was also shown (7). ERK1/2 phosphorylation was defined as an essential factor for differentiation (3), and concurrent overexpression of miR-106a, miR-574-3p, and miR-451 was shown to induce human umbilical cord-derived MSC (hucMSC) differentiation into functionally mature hepatocytes (9).
MSCs repair liver damage through both differentiation and exocrine regulation (10). Exosomes are nanosized (30–150 nm) membrane vesicles with a lipid bilayer that contains protein, DNA, RNA, and lipid molecules. The International Society for Extracellular Vesicles proposed membrane vesicles of 30-200 nm collectively referred to as small extracellular vesicles (sEVs) (11). These vesicles are classified as an exocrine form of MSCs that regenerate damaged tissues and organs, being considered as a novel type of intercellular communication. Disease occurrence and progression can be mapped by the transmission of sEVs. This paves the way for the use of sEVs as diagnostic markers and therapeutic targets, especially given that they offer more flexible modes of administration than MSCs (Figure 1). In this context, we have shown that MSC-sEVs can suppress hepatic oxidant injury and liver fibrosis by mediating immune responses (4–6).
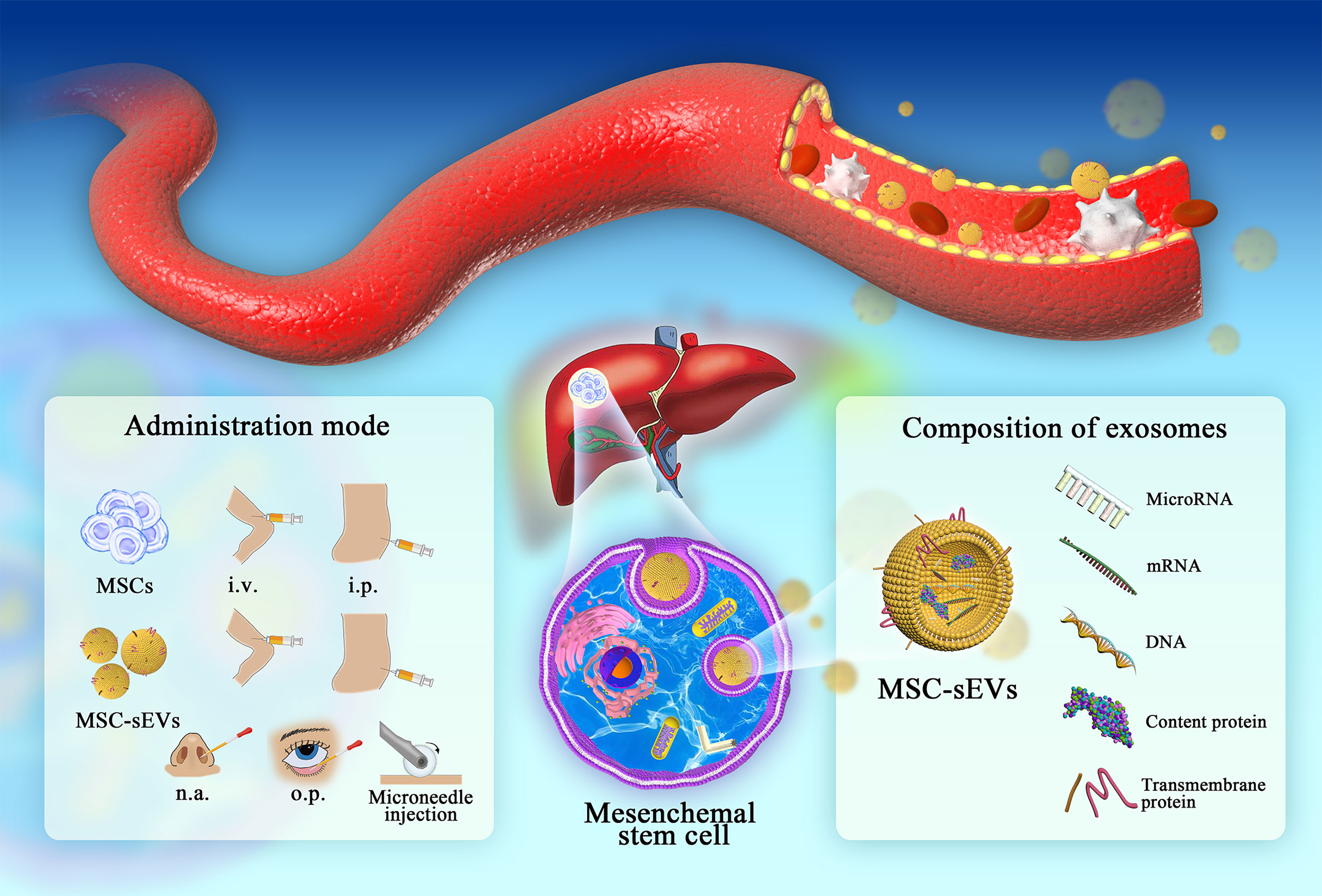
Figure 1 The administration of MSCs/MEC-sEVs and detailed composition of MSC-sEVs (i.v., intravenous injection; i.p., intraperitoneal injection; n.a., nasal drip; o.p., eye drop.). In liver diseases, MSC colonization and sEV circulation play important roles in damage repair. Research on the composition and administration of sEVs provides a novel chance for follow-up clinical promotion.
Although MSCs/MSC-sEVs play an important role in regulating inflammatory diseases, the potential mechanisms by which they regulate immune cells and reduce immune-mediated injury have not been fully clarified. In this review, we will discuss the prominent immunomodulatory mechanisms involved in MSCs/MSC-sEVs’ therapeutic potential, and we will unveil a reasonable modification to MSCs/MSC-sEVs for treating diverse hepatic injury, including acute and chronic hepatitis, metabolic liver diseases, self-development and complications of liver cirrhosis, and hepatocellular carcinoma (Table 1).
MSCs/MSC-sEVs as Neotype Immunosuppressants
To combat liver injury, tissues initiate innate and adaptive immune responses by inducing immune cell activation. An imbalance between liver injury and the immune response results in inflammation and further damage; thus, immunosuppression is necessary for hepatocytic regeneration and damage repair (60). MSC/MSC-sEV treatment reduces the secretion of the pro-inflammatory cytokines, IL-6, IL-1β, IL-12, IFN-α, IL-2, and IL-4 and increases the production of the anti-inflammatory cytokines, TGF-β, and IL-10 (61–63), leading to immunosuppression (Figure 2).
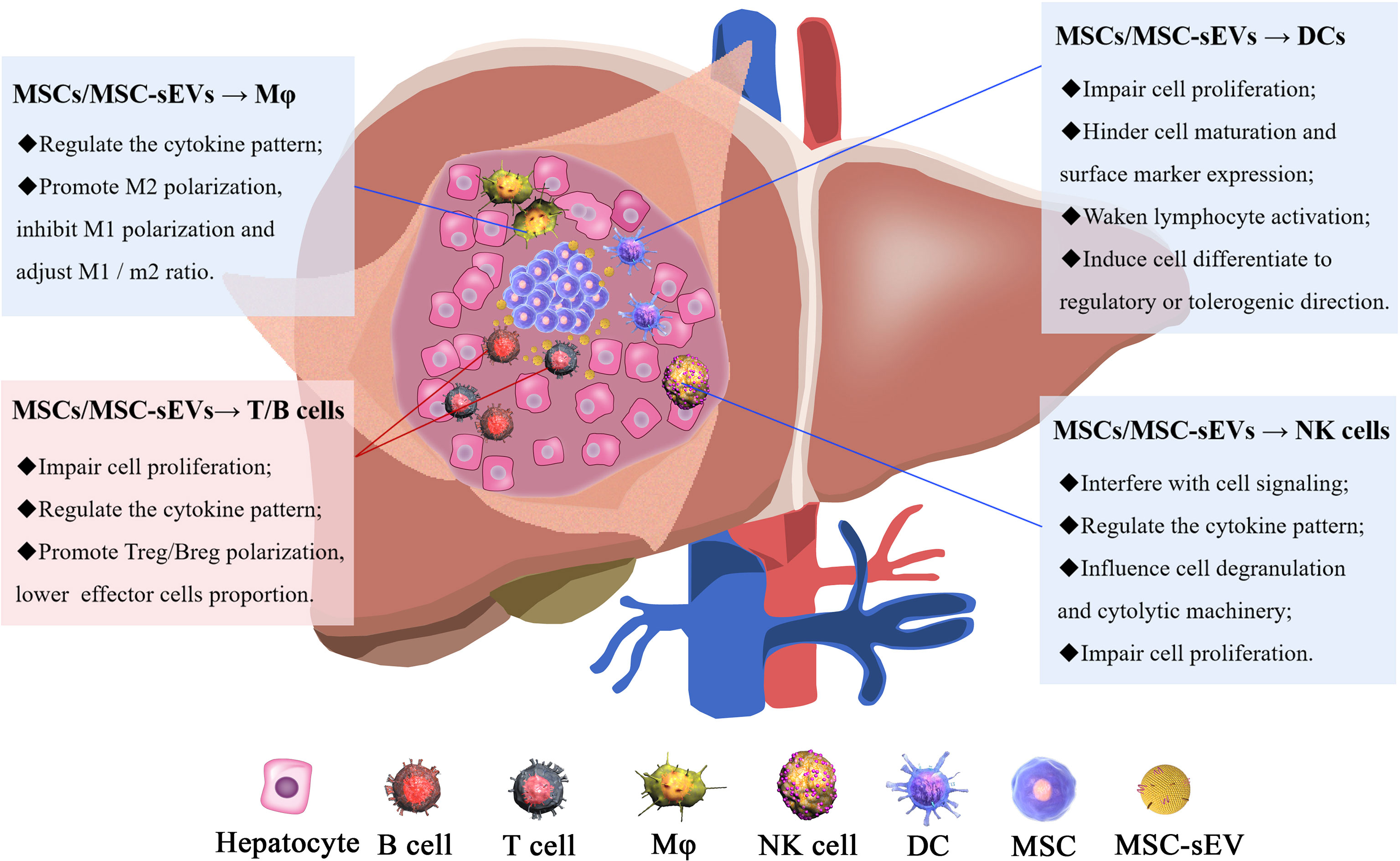
Figure 2 The mechanisms of MSC/MSC-sEV modulation of immune cells. The immunomodulatory effect of MSCs/MSC-sEVs is reflected in their ability to inhibit immune cell proliferation and function and induce anti-inflammatory cell activation.
Innate Immune Responses
MSCs and their sEVs effectively regulate innate immune cells such as DCs, NK cells, and MΦ. DC maturation and proliferation can be suppressed by MSCs/MSC-sEVs (64). Zhao et al. (65) discovered that DCs suppress inflammation through the CD200/CD200R signaling pathway. MiR-21-5p is enriched in MSC-sEVs, targeting degradation of CCR7 on DCs and modulating cell function (63). Yuan et al. (64) demonstrated that the IFN-γ/FLT3L-FLT3/CD1c+ DC axis plays a role in upregulating tolerogenic CD1c+ DCs and suppressing inflammation in response to MSC treatment. Regulatory DCs are characterized by low costimulatory molecule expression and may be synergistically stimulated by MSCs through Notch (66) and TGF-β signaling (67). In brief, insufficient maturation and reduced expression of DC maturation and activation markers (61) may impair antigen uptake and decrease lymphocyte proliferation (63).
MSCs/MSC-sEVs exert an immunomodulatory effect on NK cells by interfering with cell signal transmission, regulating cytokine secretion, preventing degranulation and cytolysis, and reducing proliferation (68). MSCs strongly decrease NK cell-induced IFN-γ production by secreting activin-A (69). While both MSC-derived conditioned medium (CM) and NK-MSC-cocultured CM prevent NK cell degranulation, the effect is more dramatic for cocultured CM. This finding suggests that the suppressing factor is increased when MSCs contact NK cells (70). In addition, MSCs that have been pre-activated by inflammatory cytokines secrete prostaglandin E2 to promote NK-cell inhibition and reduce the expression of the activation marker, CD69, the cytotoxic ligand, TRAIL, and the chemokine receptor, CXCR3, on the NK cell surface (71).
MSCs/MSC-sEVs promote MΦ polarization toward the M2-like phenotype and regulate the release of inflammatory factors. MSCs also inhibit MΦ polarization toward the CD86+ M1 phenotype through reduced production of NO and regulated production of pro-inflammatory factors like TNF-α, IL-1β, IL-6, IL-8, and NOS-2 (72). A significant decline in the M1/M2 MΦ ratio is observed following MSC or MSC-sEV treatment (73). Mechanistically, TGF-β secreted by MSCs facilitates the polarization of CD163+ M2 MΦ through the Akt/FoxO1 pathway (74), while MSC-sEVs deliver IL-10 to Kupffer cells and induce the expression of PTPN22, shifting Kupffer cells to an anti-inflammatory phenotype and mitigating liver inflammation (75). Enrichment of miRNA in MSC-sEVs negatively regulates the inflammatory environment by miR-223-3p targeting stathmin 1 (76), miR-21 targeting programmed cell death 4 (77), and miR-124-3p targeting nucleus signaling 1 (78).
Adaptive Immune Responses
T and B cells are directly modulated by MSCs/MSC-sEVs and are also affected by MSC-induced immunosuppression of innate immune cells (79). MSCs/MSC-sEVs limit the proliferation and activation of CD4+ T (80), CD8+ T (81), γδ T (82), and B cells (83). MSC-induced chitinase-3-like protein 1 inhibits STAT1/3 signaling in T cells by upregulating peroxisome proliferator-activated receptor δ (84). Naïve MSCs have a greater impact on T cells (79), while IFN-γ-primed MSCs strongly inhibit B cells (79, 85). Lee et al. (86) showed that phorbol myristate acetate primes MSCs to attract B cells in a CXCL10-dependent manner and induces apoptosis and concomitantly inhibits IgG production in a PD-L1-dependent manner. IgM also significantly decreases because of altered bio-function genes involved in cell-to-cell signaling and interaction and cellular movement (83).
MSCs/MSC-sEVs also induce Treg/Breg-cell differentiation. Promoting Treg differentiation (87) reverses the imbalance of T effector and Treg cells (88). MSC-sEVs induce differentiation through the miR-1246/Nfat5 axis (89) and the mTOR-mediated axis (90) and the secretion of hepatocyte growth factor by MSCs (91). The transfer of mitochondria from MSCs to T cells induces Treg-cell differentiation through an increase in Treg cell differentiation-related gene FoxP3 expression (92, 93). The anti-inflammatory factors, TGF-β, PGE2, and IL-10, secreted by MSCs contribute to Breg-cell generation and prevent T effector-cell proliferation and inflammatory factor secretion (94, 95). The ability of MSCs/MSC-sEVs to modulate the phenotype, function, and homing of immune cells could inform new therapies for inflammatory and autoimmune diseases.
Insights From Hepatic Diseases
The liver is an essential organ to maintain life activities, breaking down and creating nutrients and aiding energy metabolism (96). Hepatitis is divided into acute and chronic forms that can be caused by viruses, bacteria, parasites, chemical poisons, drugs, alcohol, or autoimmune factors. During chronic liver injury, collagen accumulation can lead to cirrhosis and eventually develop into hepatocellular cancer (97). Although vaccination and drug development have reduced the occurrence and progression of particular liver diseases, the prevalence and burden of these illnesses remain significant (97).
Acute Liver Failure
Acute liver failure (ALF) is a life-threatening clinical syndrome characterized by sudden massive hepatocellular necrosis and coagulopathy (98). Both human adipose-derived MSCs (hADSCs) (13) and hucMSCs (14) are effective against ALF, relieving hepatotoxicity, promoting hepatocyte regeneration (15), and alleviating inflammation. PGE2 secreted by BMSCs blocks MΦ activation by inhibiting TGF-β-activated kinase 1 (TAK1) signaling and the NLRP3 inflammasome and induces M2 MΦ by activating STAT6 and rapamycin (mTOR) signaling (19). Lipid-conjugated heparin was designed to enhance the delivery and retention of MSCs for better engraftment in the injured liver and improve repair of damaged tissues (13). Importantly, pro-inflammatory factor pre-stimulation may improve the therapeutic capacity of MSCs. IL-1β pretreatment (16) and VEGF165 gene modification (17) enhance MSC multipotency and improve their effectiveness, homing, and colonization. The therapeutic factor, miR-455-3p, targets PI3K signaling, ameliorating ALF as an sEV cargo (12).
MSC-CM, which contains a high number of cytokines and paracrine factors secreted by MSCs, including MSC-sEVs, has been used as a therapeutic agent in some studies (18, 20). After demonstrating the reversal of ALF in mice that received MSC-CM treatment, Chen et al. (18) investigated the effects of CM derived from MSCs that were cocultured with hepatocytes and showed that it prevented the release of liver injury biomarkers and promoted the recovery of liver structure. The introduction of silica magnetic graphene oxide increased the ability of MSC-CM to reduce necrosis, inflammation, and liver biochemical levels (20, 21).
Viral Hepatitis
Hepatitis pathogens entering the human body through routes like the skin and blood result in an infectious disease primarily caused by liver lesions. sEV-mediated infection is an essential route of viral transmission by carrying the structural protein and nucleic acid of the virus that is produced by infected cells (99–101). The release of sEVs provides pathogens with a mechanism for escaping the host immune system by impairing immune cell function (102). Blocking sEV release from infected cells prevents virus replication (101). Based on these findings, sEVs have been used as vectors for vaccine delivery (103). Masalova et al. (26) revealed that MSCs have adjuvant properties during DNA vaccination against hepatitis C. MSCs modified with five non-structural hepatitis C virus proteins resulted in a pronounced innate and adaptive immune response than DNA immunization, suggesting that MSCs may serve as an effective antiviral vaccine (28).
A recent study (22) assessed the interaction between NK cells and BMSCs during hepatitis B virus (HBV) infection. In vivo results showed that MSCs accumulate in the injured liver and minimize liver damage. However, limiting immune-mediated liver injury was at the cost of suppressing NK-cell activity and restricting innate immunity, which could promote HBV gene expression and replication. In a randomized controlled trial (23), patients with HBV-related acute-on-chronic liver failure received an allogeneic hBMSC infusion. While fever was more common for patients in the MSC group, serum total bilirubin levels were improved and end-stage liver disease scores were associated with less severe infection and a lower incidence of multiple-organ failure. Using the same injection method, Li et al. (24) showed significant improvement in liver function following hBMSC treatment while Xu et al. (25) found no short-term improvements. These differences may be explained by the different numbers of patients and MSC doses used in the two studies. A safe and effective case for autologous MSC infusion was reported by Salama et al. (27). The tolerability and beneficial effects on liver function and hepatic fibrosis are encouraging.
Liver Injury Complicated by COVID-19
COVID-19, which continues to sweep the globe, is commonly associated with complicated liver injury (104) which is severe in some cases (105). Fang et al. (106, 107) found that prognosis is particularly poor for COVID-19 patients with chronic HBV-infection. Moon et al. (108) and Iavarone et al. (109) both found higher mortality among patients with cirrhosis who were subsequently infected with COVID-19. Considering the multiple-organ failure associated with this disease, MSC/MSC-sEVs may be a potential treatment (110). The liver injury caused by COVID-19 may not result from direct viral invasion, but rather the cytokine storm caused by infection (104, 111). MSC/MSC-sEV administration results in MΦ polarization from the M1 to the M2 reparative phenotype (73) and immune suppression that can attenuate the cytokine storm (112).
In clinical remission of critically ill COVID-19 patients, the triple administration of allogenic hucMSCs with a 3-day interval, together with daily thymosin α1 and antibiotic injection, alleviated inflammation after the regular antiviral therapy was found to be ineffective (113). A clinical trial conducted in China showed the safety and efficacy of intravenous MSC transplantation among patients with COVID-19 pneumonia, especially those in critically severe conditions (114). HucMSC treatment effectively reduces serum IL-6 levels in patients, which is considered a biologically relevant biomarker for COVID-19 disease progression (115, 116). Emerging clinical evidence indicates that MSC treatment is safe and has fewer side effects, suggesting that it could be an effective therapy for patients with severe COVID-19 (Table 2).
Alcoholic Hepatitis and Non-Alcoholic Steatohepatitis
Total sEV numbers are increased in the plasma of patients with both alcoholic hepatitis (AH) and non-alcoholic steatohepatitis (NASH) (117). In a study of the protein expression profiles of sEVs enriched from the serum samples of 24 patients diagnosed with various fatty liver diseases, 61 proteins provided a confident differentiation, of which the most significantly upregulated proteins were α-2-macroglobulin for AH and apolipoprotein C3 for NASH (118). Alcohol-treated hepatocytes release sEVs with liver-specific miR-122 (119) and CD40L (120), both of which contribute to MΦ activation. Curtis et al. (121) assessed the capacity of BMSCs to relieve liver and lung inflammation in intoxicated and burned mice, suggesting that MSCs could be a novel therapy for restoring the homeostasis of multiple-organ systems in intoxicated burn patients.
NASH is closely related to obesity, insulin resistance, type 2 diabetes, and hyperlipidemia. HucMSC-sEVs alleviate type 2 diabetes by reversing peripheral insulin resistance and relieving β−cell destruction in both the liver and muscle (122), which is consistent with findings by Yang et al. (29) and Domingues et al. (30). In a streptozotocin and high-fat diet (HFD)-induced T2DM mouse model, hucMSC-CM was reported to reverse liver dysfunction, enhance liver total antioxidant capacity and mitochondrial function, and decrease inflammation (29). ADSC-sEVs facilitate metabolic homeostasis in obese mice by polarizing MΦ to M2 and beiging in white adipose tissue, promoting ADSC proliferation and establishing a cross-talk that facilitates immune and metabolic homeostasis (123). In a preestablished Mc4r-KO NASH mouse model, hADSCs and their sEVs are both able to adjust the inflammatory environment and enhance liver function (31). BMSCs reproduce the corresponding therapeutic effect in a rat model, protecting hepatocytes from lipotoxicity by regulating endoplasmic reticulum stress and calcium homeostasis (32).
Autoimmune Hepatitis
Autoimmune hepatitis (AIH) is a chronic liver disease characterized by positive autoantibodies (124) and featured with lymphocyte infiltration. MSC treatment can partially alleviate AIH (33). A recent study transfected IL-35, a cytokine involved in regulating Treg cells and essential in autoimmune diseases, into ADSCs to strengthen the protective effect against AIH (33). IL-35-ADSCs prevented hepatocyte apoptosis by reducing FASL expression and IFN-γ secretion by liver mononuclear cells, significantly reducing the necrotic area in injured liver. Both BMSC-sEVs and BMSC-sEVsmiR-223(+) significantly reversed AIH as shown by serum testing, liver histology, inflammation, and cell death, while BMSC-sEVsmiR-223(-) counteracted the therapeutic effect of sEVs, indicating a possible mechanism related to miR-223 enrichment in sEVs (35). A follow-up study (34) specific to miR-223-3p showed a reduction in the release of inflammatory cytokines by MΦ.
Liver Fibrosis and Cirrhosis
Liver repair involves the process of fibrosis to protect tissue integrity when the injury exceeds the regeneration ability of parenchymal cells, resulting in excessive deposition of the extracellular matrix, activation of hepatic stellate cells (HSCs), and a decrease in parenchymal cells (125, 126). HucMSCs ameliorate HSC activation and liver fibrosis by upregulating miR-455-3p through suppression of p21-activated kinase-2 (38). Reversal of liver function and histological damage was observed after BMSC injection (41). ADSC-CM reduced hepatic enzyme levels and collagen deposition (36), and ADSC-sEVs inhibited HSC proliferation by transferring miR-150-5p to reduce CXCL1 expression (39). BMSC-sEVs regulate Wnt/β-catenin signaling (40) while tonsil-derived MSC-sEVs suppress hedgehog signaling (42), renewing hepatocyte and liver function and controlling inflammation. circDIDO1 originates from BMSC-sEVs sponge for miR-141-3p and further suppresses HSC proliferation by blocking PTEN/AKT signaling (127). Erythropoietin was used as a target factor in a study by Wang et al. (37), promoting MSC cell viability and migration by activating PI3K/AKT and ERK1/2 signaling pathways and boosting anti-fibrotic efficacy.
When there is persistent liver injury, fibrosis develops into cirrhosis. Effective cirrhosis treatment suppresses HSC activation and fibrogenesis and induces fibrinolysis and the production of anti-inflammatory factors (128). MSCs strongly induce anti-inflammatory MΦ and, in combination with the medicine Juzentaihoto, activate NK cells and Treg cells to further attenuate liver damage and reverse liver fibrosis (43). Smad7-expressing MSCs treated liver fibrosis using the TGF-β1/Smad signaling (45). In clinical trials, hucMSCs can promote long-term survival with favorable liver function (44) while autologous hBMSCs suppress histologic fibrosis in patients with alcoholic cirrhosis in a dose-dependent manner for fibrosis quantification and Child–Pugh scores (46).
Complications After Liver Transplantation
Liver transplantation is typically the last resort for end-stage liver disease (129). Ischemia–reperfusion injury (IRI) and biliary complications are postoperative responses to liver transplantation (130). HucMSC-sEVs modulate CD154 expression on intrahepatic CD4+ T cells and interfere with the activation of subsequent effector cells (131), protecting against IRI-induced hepatic apoptosis both in vivo and in vitro (132). In a recent study, 12 patients with biliary tract lesions were recruited into the MSC group and after six doses of hucMSCs, there was a reduction in the biochemical indexes of their liver function with no short- or long-term adverse events, except only one self-limiting fever (50).
While the incidence of liver graft versus host disease (GvHD) is relatively rare following liver transplantation, the conflict between the abundance of graft liver lymphocytes and the recipient’s main histocompatibility antigen means that GvHD is often lethal. The use of MSCs to inhibit GvHD has been moved into the preclinical phase (133). In vivo fluorescence tracked the biodistribution of hucMSCs labeled with semiconducting nanocrystals after tail-vein injection and confirmed their regeneration potential (134). After a single injection of hucMSCs to liver allograft recipients that had experienced acute rejection, ALT decreased markedly and remained low through the 12-week follow-up period alongside a significant increase in the Treg/Th17 cell ratio (47). A 5-year follow-up (48) verified the long-term feasibility, safety, and tolerability of MSC infusion. There is likely more potential for MSC infusion as a prophylactic before transplantation rather than a regulator after transplantation (133). In a randomized open-label phase Ib/IIa clinical trial, a single pre-transplant intravenous infusion of third-party BMSCs induced mild positive changes in Treg and NK cells in the peripheral blood with no complications indicated at the 1-year follow-up (49). As a result of the low sample size, this experiment did not obtain statistical significance. However, the slight increase in the proportion of Treg cells along with the anti-donor CD8+ T-cell hypo-responsiveness suggests that a pro-tolerogenic environment developed in MSC transplant recipients could eventually result in immune tolerance. The ex vivo delivery of active MSCs to liver grafts was proposed and expected for regeneration and immune regulation following transplantation (135).
To address the limitation of ABO-compatible liver transplantation, clinicians began the use of ABO-incompatible grafts as an alternative option, which may result in aggravation of the postoperative immune response (136). To overcome this, an anti-CD20 monoclonal antibody, rituximab, was introduced; however, severe adverse effects threaten the survival of transplant recipients (137). A randomized, open-label clinical trial replaced rituximab with hucMSCs for immunosuppression following ABO-incompatible liver transplantation (51). There were no significant differences in 2-year graft and recipient survival, and there was an obvious decline in biliary complications and infection in the MSC group.
Hepatocellular Carcinoma
Liver cancer is usually the final sequelae of chronic liver disease given the high incidence of genetic alterations that accompany it (138). Hepatocellular carcinoma (HCC) accounts for a large proportion of liver cancer and is a leading cause of cancer-related death (139). Bioluminescence imaging has been applied to track the efficacy of tumor suppression following hucMSC treatment, and a significant variation in bioluminescence signal intensity indicates a positive role for MSCs in tumorigenesis (56). MSC-sEVs regulate the proliferation, migration, invasion, angiogenesis-stimulating, and self-renewal abilities of HCC cancer stem cells (53). miR-199a enriches MSC-sEV-sensitized HCC cells to doxorubicin by blocking the mTOR pathway (55). Wang et al. (57) proposed that tumors may have a specific impact on MSCs. Indeed, studies show a modest and largely reversible impact on MSC phenotype and glucose metabolism and a permanent impact on their secretory phenotype and tumor-promoting properties (57).
Hajighasemlou et al. (138) combined sorafenib, one of the standard treatments for advanced hepatocellular carcinoma, with MSCs to address drug resistance and side effects (58). MSCs, possessing well-established tumor-homing properties, serve as oncolytic adenovirus delivery tools. Under normoxic and hypoxic conditions, MSC infected with a low viral dose can effectively lyse HCC cells without significant hepatocellular toxicity (54). The anticancer drug, norcantharidin, was loaded into BMSC-sEVs to promote a continuous and slow release of the drug (59). MSCs pretreated with melatonin accepted the domestication of the tumor microenvironment and maximized the therapeutic outcome (52). MSCs were more attracted to heat-treated tumors both in vitro and in vivo, and MSC therapy with regional hyperthermia significantly enhanced the therapeutic efficacy (57). Numerous experiments have assessed the utility of MSC therapy to promote injury regeneration and immune regulation around the injury.
Advances and Perspectives
MSCs and their sEVs play an important role in tissue damage repair and tumor inhibition (140, 141). Their use in clinical practice is associated with improved prognoses (Figure 3) (44, 49, 142). Systemic regulation that relies on body fluid circulation and specific targeting of injured tissues make MSCs/MSC-sEVs relevant for the treatment of COVID-19 (143); a single administration is equivalent to a multidrug combination. While most research teams inject MSCs/MSC-sEVs in combination with antiviral therapy, this does not limit their importance (113–116, 144). However, a series of issues resulting in the slow progress of clinical treatment and development of MSCs/MSC-sEVs as an ideal treatment strategy remains to be addressed.
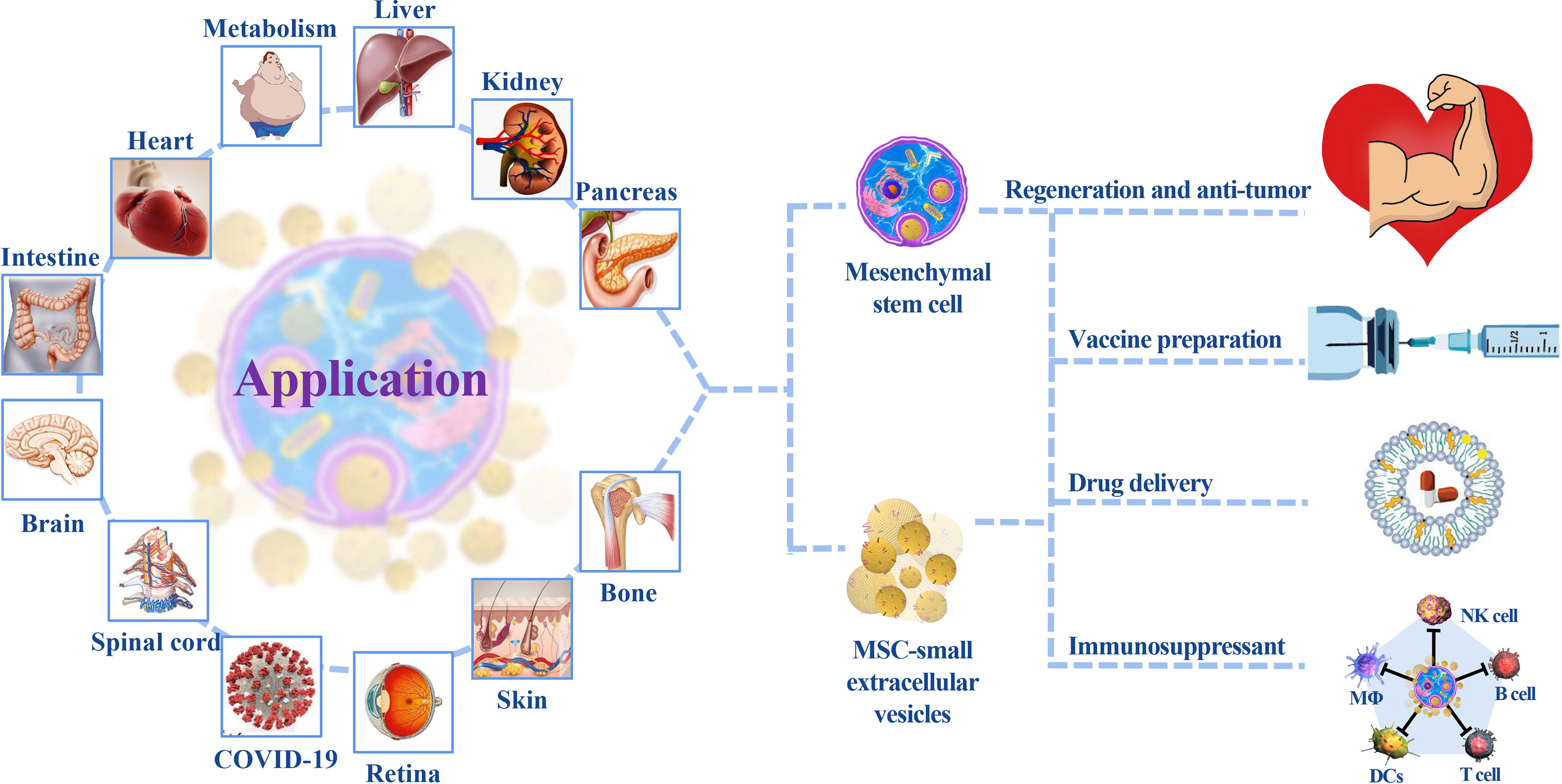
Figure 3 The application of MSCs/MSC-sEVs. MSC treatment primarily focuses on regenerative repair and autoimmune disease treatment. sEVs play a key role in cardiovascular, tumor, and nervous system diseases because of their diversified administration modes and ability to cross the blood–brain barrier. Their participation in multiple-organ repair provides insight into new medical technologies.
It is worth noting that the anticancer capability of MSCs was not unified across laboratories, because some experimental studies have indicated that MSCs/MSC-sEVs can promote cancer development (145, 146). This may be related to the methods used for cell extraction or sEV separation and purification. In addition, there are discrepancies in the therapeutic efficacy of MSCs/MSC-sEVs from different sources, species, and individuals (147). Thus, the donor selection of MSCs requires strict screening. For example, a pregnant woman’s physical status, age, history of metabolic, infectious, and familial genetic diseases, and fetal status need to be taken into account prior to hucMSC treatment.
In addition to the administered dose of MSCs/MSC-sEVs, a unified industry standard has not yet been established. In most experiments summarized in this paper, the MSC dose varies from 105 to 108 while the sEV dose ranges from μg to mg, indicating that most research teams agree that a particular concentration is essential, and increasing this could be as problematic as using too little. Partial experiments were conducted using dosage differences, and there was a certain correlation between the therapeutic effect and dosage (46). It was shown that hucMSC-sEVs accumulate in the liver after tail vein injection in normal mice. However, in mouse models of injury to other organs, sEVs target the injury site while still accumulating in the liver. Due to metabolic differences resulting from the route of administration (148) and phagocytosis (149) by mononuclear/MΦ, there are differences in the tendency of MSC-sEVs to migrate to different organs. The optimal dosage of MSCs/MSC-sEVs for different species and diseases remains a challenge for their use in clinical practice.
Research comparing outcomes to treatment with MSCs and their sEVs indicates that sEVs are superior (40). MSC and sEV treatment have their own merits. Cell therapy based on MSCs originated earlier than the sEV. Currently, clinical trials are mostly focused on MSC-based cell therapies. MSC treatment of liver disease contributes to both hepatocyte transformation and cytokine secretion, while sEV therapy is more one-sided. MSC infusion is often only required once, and MSC colonization at the injured site maintains a long-term effect, including the secretion of sEVs, as compared with the multiple infusions of sEVs required for metabolism. However, these benefits make it difficult to standardize the mechanism. Additionally, the safety of MSCs for aging and long-term tracking has not been confirmed. He et al. (150) proposed that dead MSCs may also play a role in immune regulation that is comparable to living cells, which impacts the original view of living cell therapy.
sEVs inherit the regenerative capacity of MSCs in the damaged environment and avoid the prognostic concerns caused by cell therapy. There is no unified standard for an optimal dose of sEV therapy. Cryopreservation (151) ensures long-term preservation, and convenient transportation avoids the wastage of biological activities, providing a strong guarantee for large-scale clinical promotion. The compilation of standards (T/CRHA 001-2021) in human MSC-sEVs by the Chinese Extracellular Vesicle Association has specified the preparation process of sEVs in detail, including storage and transportation, and is expected to standardize related scientific experiments. Naive sEVs can naturally be used as the targeted carriers of drugs or therapeutic molecules (152–154). Materials are applied externally to achieve a slow-release effect and consequently prolong the cycle time. Hydrogel, frequently used in medical materials, wrap up the sEVs and maintain their bioavailability so that they are not rapidly cleared under biodegradation. The sustained release is recorded for up to 1 month (155). The combination of modern drug therapy and material science greatly increases sEV acceptability and effectiveness.
Although the road from bench to bedside may be complex, MSCs/MSC-sEVs are a promising therapeutic agent for the treatment of tissue damage and tumors.
Author Contributions
YT searched the literature and wrote the first draft of the manuscript. PW and LL critically revised the work. Corresponding authors WX and JJ are responsible for the guidance, revision, and fund support. All authors contributed to the article and approved the submitted version.
Funding
This study was supported by grants from the National Natural Science Foundation of China (No. 81971757), the Jiangsu Province’s Major Project in Research and Development (BE2020680), Zhenjiang Key Laboratory of Exosome Foundation, and Transformation Application High-tech Research, China (SS2018003), the Clinical Major Disease Project of Suzhou (LCZX202019), the Technology Development Project of Suzhou (SKY2021018), and the Technology Project of Zhangjiagang (ZKCXY2106).
Conflict of Interest
The authors declare that the research was conducted in the absence of any commercial or financial relationships that could be construed as a potential conflict of interest.
Publisher’s Note
All claims expressed in this article are solely those of the authors and do not necessarily represent those of their affiliated organizations, or those of the publisher, the editors and the reviewers. Any product that may be evaluated in this article, or claim that may be made by its manufacturer, is not guaranteed or endorsed by the publisher.
References
1. Uccelli A, Laroni A, Ali R, Battaglia MA, Blinkenberg M, Brundin L, et al. Safety, Tolerability, and Activity of Mesenchymal Stem Cells Versus Placebo in Multiple Sclerosis (Mesems): A Phase 2, Randomised, Double-Blind Crossover Trial. Lancet Neurol (2021) 20(11):917–29. doi: 10.1016/s1474-4422(21)00301-x
2. Yan Y, Xu W, Qian H, Si Y, Zhu W, Cao H, et al. Mesenchymal Stem Cells From Human Umbilical Cords Ameliorate Mouse Hepatic Injury In Vivo. Liver Int (2009) 29(3):356–65. doi: 10.1111/j.1478-3231.2008.01855.x
3. Yan Y, Zhu Y, Sun F, Zhang B, Li L, Sun Z, et al. Extracellular Regulated Protein Kinases 1/2 Phosphorylation Is Required for Hepatic Differentiation of Human Umbilical Cord-Derived Mesenchymal Stem Cells. Exp Biol Med (Maywood NJ) (2015) 240(4):534–45. doi: 10.1177/1535370214548996
4. Li T, Yan Y, Wang B, Qian H, Zhang X, Shen L, et al. Exosomes Derived From Human Umbilical Cord Mesenchymal Stem Cells Alleviate Liver Fibrosis. Stem Cells Dev (2013) 22(6):845–54. doi: 10.1089/scd.2012.0395
5. Yan Y, Jiang W, Tan Y, Zou S, Zhang H, Mao F, et al. Hucmsc Exosome-Derived Gpx1 Is Required for the Recovery of Hepatic Oxidant Injury. Mol Ther J Am Soc Gene Ther (2017) 25(2):465–79. doi: 10.1016/j.ymthe.2016.11.019
6. Jiang W, Tan Y, Cai M, Zhao T, Mao F, Zhang X, et al. Human Umbilical Cord Msc-Derived Exosomes Suppress the Development of Ccl(4)-Induced Liver Injury Through Antioxidant Effect. Stem Cells Int (2018) 2018:6079642. doi: 10.1155/2018/6079642
7. Xu H, Qian H, Zhu W, Zhang X, Yan Y, Mao F, et al. Mesenchymal Stem Cells Relieve Fibrosis of Schistosoma Japonicum-Induced Mouse Liver Injury. Exp Biol Med (Maywood NJ) (2012) 237(5):585–92. doi: 10.1258/ebm.2012.011362
8. Afshari A, Shamdani S, Uzan G, Naserian S, Azarpira N. Different Approaches for Transformation of Mesenchymal Stem Cells Into Hepatocyte-Like Cells. Stem Cell Res Ther (2020) 11(1):54. doi: 10.1186/s13287-020-1555-8
9. Khosravi M, Azarpira N, Shamdani S, Hojjat-Assari S, Naserian S, Karimi MH. Differentiation of Umbilical Cord Derived Mesenchymal Stem Cells to Hepatocyte Cells by Transfection of Mir-106a, Mir-574-3p, and Mir-451. Gene (2018) 667:1–9. doi: 10.1016/j.gene.2018.05.028
10. Psaraki A, Ntari L, Karakostas C, Korrou-Karava D, Roubelakis MG. Extracellular Vesicles Derived From Mesenchymal Stem/Stromal Cells: The Regenerative Impact in Liver Diseases. Hepatol (Baltimore Md) (2021). doi: 10.1002/hep.32129
11. Théry C, Witwer KW, Aikawa E, Alcaraz MJ, Anderson JD, Andriantsitohaina R, et al Minimal Information for Studies of Extracellular Vesicles 2018 (misev2018): A Position Statement of the International Society for Extracellular Vesicles and Update of the Misev2014 Guidelines. J Extracell Vesicles (2018) 7(1):1535750
12. Shao M, Xu Q, Wu Z, Chen Y, Shu Y, Cao X, et al. Exosomes Derived From Human Umbilical Cord Mesenchymal Stem Cells Ameliorate Il-6-Induced Acute Liver Injury Through Mir-455-3p. Stem Cell Res Ther (2020) 11(1):37. doi: 10.1186/s13287-020-1550-0
13. Hwang Y, Kim JC, Tae G. Significantly Enhanced Recovery of Acute Liver Failure by Liver Targeted Delivery of Stem Cells via Heparin Functionalization. Biomaterials (2019) 209:67–78. doi: 10.1016/j.biomaterials.2019.04.019
14. Cai W, Sun J, Sun Y, Zhao X, Guo C, Dong J, et al. Nir-Ii Fl/Pa Dual-Modal Imaging Long-Term Tracking of Human Umbilical Cord-Derived Mesenchymal Stem Cells Labeled With Melanin Nanoparticles and Visible Humsc-Based Liver Regeneration for Acute Liver Failure. Biomater Sci (2020) 8(23):6592–602. doi: 10.1039/d0bm01221a
15. Liu M, He J, Zheng S, Zhang K, Ouyang Y, Zhang Y, et al. Human Umbilical Cord Mesenchymal Stem Cells Ameliorate Acute Liver Failure by Inhibiting Apoptosis, Inflammation and Pyroptosis. Ann Trans Med (2021) 9(21):1615. doi: 10.21037/atm-21-2885
16. Nie H, An F, Mei J, Yang C, Zhan Q, Zhang Q. Il-1β Pretreatment Improves the Efficacy of Mesenchymal Stem Cells on Acute Liver Failure by Enhancing Cxcr4 Expression. Stem Cells Int (2020) 2020:1498315. doi: 10.1155/2020/1498315
17. Chen H, Tang S, Liao J, Liu M, Lin Y. Vegf(165) Gene-Modified Human Umbilical Cord Blood Mesenchymal Stem Cells Protect Against Acute Liver Failure in Rats. J Gene Med (2021) 23(10):e3369. doi: 10.1002/jgm.3369
18. Chen L, Zhang J, Yang L, Zhang G, Wang Y, Zhang S. The Effects of Conditioned Medium Derived From Mesenchymal Stem Cells Cocultured With Hepatocytes on Damaged Hepatocytes and Acute Liver Failure in Rats. Stem Cells Int (2018) 2018:9156560. doi: 10.1155/2018/9156560
19. Wang J, Liu Y, Ding H, Shi X, Ren H. Mesenchymal Stem Cell-Secreted Prostaglandin E(2) Ameliorates Acute Liver Failure via Attenuation of Cell Death and Regulation of Macrophage Polarization. Stem Cell Res Ther (2021) 12(1):15. doi: 10.1186/s13287-020-02070-2
20. Foroutan T, Kabiri F, Motamedi E. Silica Magnetic Graphene Oxide Improves the Effects of Stem Cell-Conditioned Medium on Acute Liver Failure. ACS Omega (2021) 6(33):21194–206. doi: 10.1021/acsomega.0c05395
21. Foroutan T, Kassaee MZ, Salari M, Ahmady F, Molavi F, Moayer F. Magnetic Fe(3) O(4) @Graphene Oxide Improves the Therapeutic Effects of Embryonic Stem Cells on Acute Liver Damage. Cell Proliferation (2021) 54(11):e13126. doi: 10.1111/cpr.13126
22. Qu M, Yuan X, Liu D, Ma Y, Zhu J, Cui J, et al. Bone Marrow-Derived Mesenchymal Stem Cells Attenuate Immune-Mediated Liver Injury and Compromise Virus Control During Acute Hepatitis B Virus Infection in Mice. Stem Cells Dev (2017) 26(11):818–27. doi: 10.1089/scd.2016.0348
23. Lin BL, Chen JF, Qiu WH, Wang KW, Xie DY, Chen XY, et al. Allogeneic Bone Marrow-Derived Mesenchymal Stromal Cells for Hepatitis B Virus-Related Acute-On-Chronic Liver Failure: A Randomized Controlled Trial. Hepatol (Baltimore Md) (2017) 66(1):209–19. doi: 10.1002/hep.29189
24. Li YH, Xu Y, Wu HM, Yang J, Yang LH, Yue-Meng W. Umbilical Cord-Derived Mesenchymal Stem Cell Transplantation in Hepatitis B Virus Related Acute-On-Chronic Liver Failure Treated With Plasma Exchange and Entecavir: A 24-Month Prospective Study. Stem Cell Rev Rep (2016) 12(6):645–53. doi: 10.1007/s12015-016-9683-3
25. Xu WX, He HL, Pan SW, Chen YL, Zhang ML, Zhu S, et al. Combination Treatments of Plasma Exchange and Umbilical Cord-Derived Mesenchymal Stem Cell Transplantation for Patients With Hepatitis B Virus-Related Acute-On-Chronic Liver Failure: A Clinical Trial in China. Stem Cells Int (2019) 2019:4130757. doi: 10.1155/2019/4130757
26. Masalova OV, Lesnova EI, Klimova RR, Ivanov AV, Kushch AA. Mesenchymal Stem Cells Can Both Enhance and Inhibit the Cellular Response to Dna Immunization by Genes of Nonstructural Proteins of the Hepatitis C Virus. Int J Mol Sci (2021) 22(15):8121. doi: 10.3390/ijms22158121
27. Salama H, Zekri AR, Medhat E, Al Alim SA, Ahmed OS, Bahnassy AA, et al. Peripheral Vein Infusion of Autologous Mesenchymal Stem Cells in Egyptian Hcv-Positive Patients With End-Stage Liver Disease. Stem Cell Res Ther (2014) 5(3):70. doi: 10.1186/scrt459
28. Masalova OV, Lesnova EI, Klimova RR, Momotyuk ED, Kozlov VV, Ivanova AM, et al. Genetically Modified Mouse Mesenchymal Stem Cells Expressing Non-Structural Proteins of Hepatitis C Virus Induce Effective Immune Response. Vaccines (2020) 8(1):62. doi: 10.3390/vaccines8010062
29. Yang M, Cui Y, Song J, Cui C, Wang L, Liang K, et al. Mesenchymal Stem Cell-Conditioned Medium Improved Mitochondrial Function and Alleviated Inflammation and Apoptosis in Non-Alcoholic Fatty Liver Disease by Regulating Sirt1. Biochem Biophys Res Commun (2021) 546:74–82. doi: 10.1016/j.bbrc.2021.01.098
30. Domingues CC, Kundu N, Kropotova Y, Ahmadi N, Sen S. Antioxidant-Upregulated Mesenchymal Stem Cells Reduce Inflammation and Improve Fatty Liver Disease in Diet-Induced Obesity. Stem Cell Res Ther (2019) 10(1):280. doi: 10.1186/s13287-019-1393-8
31. Watanabe T, Tsuchiya A, Takeuchi S, Nojiri S, Yoshida T, Ogawa M, et al. Development of a non-Alcoholic Steatohepatitis Model With Rapid Accumulation of Fibrosis, and Its Treatment Using Mesenchymal Stem Cells and Their Small Extracellular Vesicles. Regenerative Ther (2020) 14:252–61. doi: 10.1016/j.reth.2020.03.012
32. Li L, Zeng X, Liu Z, Chen X, Li L, Luo R, et al. Mesenchymal Stromal Cells Protect Hepatocytes From Lipotoxicity Through Alleviation of Endoplasmic Reticulum Stress by Restoring Serca Activity. J Cell Mol Med (2021) 25(6):2976–93. doi: 10.1111/jcmm.16338
33. Wang W, Guo H, Li H, Yan Y, Wu C, Wang X, et al. Interleukin-35 Gene-Modified Mesenchymal Stem Cells Protect Concanavalin a-Induced Fulminant Hepatitis by Decreasing the Interferon Gamma Level. Hum Gene Ther (2018) 29(2):234–41. doi: 10.1089/hum.2017.171
34. Lu FB, Chen DZ, Chen L, Hu ED, Wu JL, Li H, et al. Attenuation of Experimental Autoimmune Hepatitis in Mice With Bone Mesenchymal Stem Cell-Derived Exosomes Carrying Microrna-223-3p. Molecules Cells (2019) 42(12):906–18. doi: 10.14348/molcells.2019.2283
35. Chen L, Lu FB, Chen DZ, Wu JL, Hu ED, Xu LM, et al. Bmscs-Derived Mir-223-Containing Exosomes Contribute to Liver Protection in Experimental Autoimmune Hepatitis. Mol Immunol (2018) 93:38–46. doi: 10.1016/j.molimm.2017.11.008
36. Pinheiro D, Dias I, Freire T, Thole AA, Stumbo AC, Cortez EAC, et al. Effects of Mesenchymal Stem Cells Conditioned Medium Treatment in Mice With Cholestatic Liver Fibrosis. Life Sci (2021) 281:119768. doi: 10.1016/j.lfs.2021.119768
37. Wang X, Wang H, Lu J, Feng Z, Liu Z, Song H, et al. Erythropoietin-Modified Mesenchymal Stem Cells Enhance Anti-Fibrosis Efficacy in Mouse Liver Fibrosis Model. Tissue Eng Regenerative Med (2020) 17(5):683–93. doi: 10.1007/s13770-020-00276-2
38. Zhou Q, Gu T, Zhang Y, Li H, Zhuansun X, Xu S, et al. Human Umbilical Cord Mesenchymal Stem Cells Ameliorate Hepatic Stellate Cell Activation and Liver Fibrosis by Upregulating Microrna-455-3p Through Suppression of P21-Activated Kinase-2. BioMed Res Int (2021) 2021:6685605. doi: 10.1155/2021/6685605
39. Du Z, Wu T, Liu L, Luo B, Wei C. Extracellular Vesicles-Derived Mir-150-5p Secreted by Adipose-Derived Mesenchymal Stem Cells Inhibits Cxcl1 Expression to Attenuate Hepatic Fibrosis. J Cell Mol Med (2021) 25(2):701–15. doi: 10.1111/jcmm.16119
40. Rong X, Liu J, Yao X, Jiang T, Wang Y, Xie F. Human Bone Marrow Mesenchymal Stem Cells-Derived Exosomes Alleviate Liver Fibrosis Through the Wnt/β-Catenin Pathway. Stem Cell Res Ther (2019) 10(1):98. doi: 10.1186/s13287-019-1204-2
41. Raafat N, Abdel Aal SM, Abdo FK, El Ghonaimy NM. Mesenchymal Stem Cells: In Vivo Therapeutic Application Ameliorates Carbon Tetrachloride Induced Liver Fibrosis in Rats. Int J Biochem Cell Biol (2015) 68:109–18. doi: 10.1016/j.biocel.2015.09.003
42. Kim J, Lee C, Shin Y, Wang S, Han J, Kim M, et al. Sevs From Tonsil-Derived Mesenchymal Stromal Cells Alleviate Activation of Hepatic Stellate Cells and Liver Fibrosis Through Mir-486-5p. Mol Ther J Am Soc Gene Ther (2021) 29(4):1471–86. doi: 10.1016/j.ymthe.2020.12.025
43. Iwasawa T, Nojiri S, Tsuchiya A, Takeuchi S, Watanabe T, Ogawa M, et al. Combination Therapy of Juzentaihoto and Mesenchymal Stem Cells Attenuates Liver Damage and Regresses Fibrosis in Mice. Regenerative Ther (2021) 18:231–41. doi: 10.1016/j.reth.2021.07.002
44. Shi M, Li YY, Xu RN, Meng FP, Yu SJ, Fu JL, et al. Mesenchymal Stem Cell Therapy in Decompensated Liver Cirrhosis: A Long-Term Follow-Up Analysis of the Randomized Controlled Clinical Trial. Hepatol Int (2021) 15(6):1431–41. doi: 10.1007/s12072-021-10199-2
45. Su DN, Wu SP, Xu SZ. Mesenchymal Stem Cell-Based Smad7 Gene Therapy for Experimental Liver Cirrhosis. Stem Cell Res Ther (2020) 11(1):395. doi: 10.1186/s13287-020-01911-4
46. Suk KT, Yoon JH, Kim MY, Kim CW, Kim JK, Park H, et al. Transplantation With Autologous Bone Marrow-Derived Mesenchymal Stem Cells for Alcoholic Cirrhosis: Phase 2 Trial. Hepatol (Baltimore Md) (2016) 64(6):2185–97. doi: 10.1002/hep.28693
47. Shi M, Liu Z, Wang Y, Xu R, Sun Y, Zhang M, et al. A Pilot Study of Mesenchymal Stem Cell Therapy for Acute Liver Allograft Rejection. Stem Cells Trans Med (2017) 6(12):2053–61. doi: 10.1002/sctm.17-0134
48. Vandermeulen M, Mohamed-Wais M, Erpicum P, Delbouille MH, Lechanteur C, Briquet A, et al. Infusion of Allogeneic Mesenchymal Stromal Cells After Liver Transplantation: A 5-Year Follow-Up. Liver Transplant (2022) 28(4):636–46. doi: 10.1002/lt.26323
49. Casiraghi F, Perico N, Podestà MA, Todeschini M, Zambelli M, Colledan M, et al. Third-Party Bone Marrow-Derived Mesenchymal Stromal Cell Infusion Before Liver Transplantation: A Randomized Controlled Trial. Am J Transplant (2021) 21(8):2795–809. doi: 10.1111/ajt.16468
50. Zhang YC, Liu W, Fu BS, Wang GY, Li HB, Yi HM, et al. Therapeutic Potentials of Umbilical Cord-Derived Mesenchymal Stromal Cells for Ischemic-Type Biliary Lesions Following Liver Transplantation. Cytotherapy (2017) 19(2):194–9. doi: 10.1016/j.jcyt.2016.11.005
51. Zhang Y, Zhang J, Yi H, Zheng J, Cai J, Chen W, et al. A Novel Msc-Based Immune Induction Strategy for Abo-Incompatible Liver Transplantation: A Phase I/Ii Randomized, Open-Label, Controlled Trial. Stem Cell Res Ther (2021) 12(1):244. doi: 10.1186/s13287-021-02246-4
52. El-Magd MA, Mohamed Y, El-Shetry ES, Elsayed SA, Abo Gazia M, Abdel-Aleem GA, et al. Melatonin Maximizes the Therapeutic Potential of Non-Preconditioned Mscs in a Den-Induced Rat Model of Hcc. Biomed Pharmacother = Biomed Pharmacotherapie (2019) 114:108732. doi: 10.1016/j.biopha.2019.108732
53. Gu H, Yan C, Wan H, Wu L, Liu J, Zhu Z, et al. Mesenchymal Stem Cell-Derived Exosomes Block Malignant Behaviors of Hepatocellular Carcinoma Stem Cells Through a Lncrna C5orf66-As1/Microrna-127-3p/Dusp1/Erk Axis. Hum Cell (2021) 34(6):1812–29. doi: 10.1007/s13577-021-00599-9
54. Yoon AR, Hong J, Li Y, Shin HC, Lee H, Kim HS, et al. Mesenchymal Stem Cell-Mediated Delivery of an Oncolytic Adenovirus Enhances Antitumor Efficacy in Hepatocellular Carcinoma. Cancer Res (2019) 79(17):4503–14. doi: 10.1158/0008-5472.Can-18-3900
55. Lou G, Chen L, Xia C, Wang W, Qi J, Li A, et al. Mir-199a-Modified Exosomes From Adipose Tissue-Derived Mesenchymal Stem Cells Improve Hepatocellular Carcinoma Chemosensitivity Through Mtor Pathway. J Exp Clin Cancer Res CR (2020) 39(1):4. doi: 10.1186/s13046-019-1512-5
56. Liu J, Shi Y, Han J, Zhang Y, Cao Z, Cheng J. Quantitative Tracking Tumor Suppression Efficiency of Human Umbilical Cord-Derived Mesenchymal Stem Cells by Bioluminescence Imaging in Mice Hepatoma Model. Int J Stem Cells (2020) 13(1):104–15. doi: 10.15283/ijsc19098
57. Tutter M, Schug C, Schmohl KA, Urnauer S, Kitzberger C, Schwenk N, et al. Regional Hyperthermia Enhances Mesenchymal Stem Cell Recruitment to Tumor Stroma: Implications for Mesenchymal Stem Cell-Based Tumor Therapy. Mol Ther J Am Soc Gene Ther (2021) 29(2):788–803. doi: 10.1016/j.ymthe.2020.10.009
58. Hajighasemlou S, Nikbakht M, Pakzad S, Muhammadnejad S, Gharibzadeh S, Mirmoghtadaei M, et al. Sorafenib and Mesenchymal Stem Cell Therapy: A Promising Approach for Treatment of Hcc. Evidence-Based Complementary Altern Med eCAM (2020) 2020:9602728. doi: 10.1155/2020/9602728
59. Liang L, Zhao L, Wang Y, Wang Y. Treatment for Hepatocellular Carcinoma Is Enhanced When Norcantharidin Is Encapsulated in Exosomes Derived From Bone Marrow Mesenchymal Stem Cells. Mol Pharmaceutics (2021) 18(3):1003–13. doi: 10.1021/acs.molpharmaceut.0c00976
60. Hu C, Wu Z, Li L. Mesenchymal Stromal Cells Promote Liver Regeneration Through Regulation of Immune Cells. Int J Biol Sci (2020) 16(5):893–903. doi: 10.7150/ijbs.39725
61. He JG, Li BB, Zhou L, Yan D, Xie QL, Zhao W. Indoleamine 2,3-Dioxgenase-Transfected Mesenchymal Stem Cells Suppress Heart Allograft Rejection by Increasing the Production and Activity of Dendritic Cells and Regulatory T Cells. J Invest Med (2020) 68(3):728–37. doi: 10.1136/jim-2019-001160
62. Shi B, Qi J, Yao G, Feng R, Zhang Z, Wang D, et al. Mesenchymal Stem Cell Transplantation Ameliorates Sjögren's Syndrome via Suppressing Il-12 Production by Dendritic Cells. Stem Cell Res Ther (2018) 9(1):308. doi: 10.1186/s13287-018-1023-x
63. Reis M, Mavin E, Nicholson L, Green K, Dickinson AM, Wang XN. Mesenchymal Stromal Cell-Derived Extracellular Vesicles Attenuate Dendritic Cell Maturation and Function. Front Immunol (2018) 9:2538. doi: 10.3389/fimmu.2018.02538
64. Shahir M, Mahmoud Hashemi S, Asadirad A, Varahram M, Kazempour-Dizaji M, Folkerts G, et al. Effect of Mesenchymal Stem Cell-Derived Exosomes on the Induction of Mouse Tolerogenic Dendritic Cells. J Cell Physiol (2020) 235(10):7043–55. doi: 10.1002/jcp.29601
65. Zhao Y, Su G, Wang Q, Wang R, Zhang M. The Cd200/Cd200r Mechanism in Mesenchymal Stem Cells' Regulation of Dendritic Cells. Am J Trans Res (2021) 13(8):9607–13.
66. Lu Z, Meng S, Chang W, Fan S, Xie J, Guo F, et al. Mesenchymal Stem Cells Activate Notch Signaling to Induce Regulatory Dendritic Cells in Lps-Induced Acute Lung Injury. J Trans Med (2020) 18(1):241. doi: 10.1186/s12967-020-02410-z
67. Li X, Dong Y, Yin H, Qi Z, Wang D, Ren S. Mesenchymal Stem Cells Induced Regulatory Dendritic Cells From Hemopoietic Progenitor Cells Through Notch Pathway and Tgf-β Synergistically. Immunol Lett (2020) 222:49–57. doi: 10.1016/j.imlet.2020.03.005
68. Najar M, Fayyad-Kazan M, Merimi M, Burny A, Bron D, Fayyad-Kazan H, et al. Mesenchymal Stromal Cells and Natural Killer Cells: A Complex Story of Love and Hate. Curr Stem Cell Res Ther (2019) 14(1):14–21. doi: 10.2174/1574888x13666180912125736
69. Chatterjee D, Marquardt N, Tufa DM, Hatlapatka T, Hass R, Kasper C, et al. Human Umbilical Cord-Derived Mesenchymal Stem Cells Utilize Activin-A to Suppress Interferon-γ Production by Natural Killer Cells. Front Immunol (2014) 5:662. doi: 10.3389/fimmu.2014.00662
70. Chatterjee D, Marquardt N, Tufa DM, Beauclair G, Low HZ, Hatlapatka T, et al. Role of Gamma-Secretase in Human Umbilical-Cord Derived Mesenchymal Stem Cell Mediated Suppression of Nk Cell Cytotoxicity. Cell Communication Signaling CCS (2014) 12:63. doi: 10.1186/s12964-014-0063-9
71. Ishida N, Ishiyama K, Saeki Y, Tanaka Y, Ohdan H. Cotransplantation of Preactivated Mesenchymal Stem Cells Improves Intraportal Engraftment of Islets by Inhibiting Liver Natural Killer Cells in Mice. Am J Transplant (2019) 19(10):2732–45. doi: 10.1111/ajt.15347
72. Huang Y, He B, Wang L, Yuan B, Shu H, Zhang F, et al. Bone Marrow Mesenchymal Stem Cell-Derived Exosomes Promote Rotator Cuff Tendon-Bone Healing by Promoting Angiogenesis and Regulating M1 Macrophages in Rats. Stem Cell Res Ther (2020) 11(1):496. doi: 10.1186/s13287-020-02005-x
73. Nakazaki M, Morita T, Lankford KL, Askenase PW, Kocsis JD. Small Extracellular Vesicles Released by Infused Mesenchymal Stromal Cells Target M2 Macrophages and Promote Tgf-β Upregulation, Microvascular Stabilization and Functional Recovery in a Rodent Model of Severe Spinal Cord Injury. J Extracellular Vesicles (2021) 10(11):e12137. doi: 10.1002/jev2.12137
74. Liu F, Qiu H, Xue M, Zhang S, Zhang X, Xu J, et al. Msc-Secreted Tgf-β Regulates Lipopolysaccharide-Stimulated Macrophage M2-Like Polarization via the Akt/Foxo1 Pathway. Stem Cell Res Ther (2019) 10(1):345. doi: 10.1186/s13287-019-1447-y
75. Zhang Y, Zhang X, Zhang H, Song P, Pan W, Xu P, et al. Mesenchymal Stem Cells Derived Extracellular Vesicles Alleviate Traumatic Hemorrhagic Shock Induced Hepatic Injury via Il-10/Ptpn22-Mediated M2 Kupffer Cell Polarization. Front Immunol (2021) 12:811164. doi: 10.3389/fimmu.2021.811164
76. Xin L, Lin X, Zhou F, Li C, Wang X, Yu H, et al. A Scaffold Laden With Mesenchymal Stem Cell-Derived Exosomes for Promoting Endometrium Regeneration and Fertility Restoration Through Macrophage Immunomodulation. Acta Biomaterialia (2020) 113:252–66. doi: 10.1016/j.actbio.2020.06.029
77. Yao M, Cui B, Zhang W, Ma W, Zhao G, Xing L. Exosomal Mir-21 Secreted by Il-1β-Primed-Mesenchymal Stem Cells Induces Macrophage M2 Polarization and Ameliorates Sepsis. Life Sci (2021) 264:118658. doi: 10.1016/j.lfs.2020.118658
78. Li R, Zhao K, Ruan Q, Meng C, Yin F. Bone Marrow Mesenchymal Stem Cell-Derived Exosomal Microrna-124-3p Attenuates Neurological Damage in Spinal Cord Ischemia-Reperfusion Injury by Downregulating Ern1 and Promoting M2 Macrophage Polarization. Arthritis Res Ther (2020) 22(1):75. doi: 10.1186/s13075-020-2146-x
79. Lee HK, Kim EY, Kim HS, Park EJ, Lee HJ, Lee TY, et al. Effect of Human Mesenchymal Stem Cells on Xenogeneic T and B Cells Isolated From Lupus-Prone Mrl.Fas (Lpr) Mice. Stem Cells Int (2020) 2020:5617192. doi: 10.1155/2020/5617192
80. Kuca-Warnawin E, Plebańczyk M, Bonek K, Kontny E. Inhibition of Allogeneic and Autologous T Cell Proliferation by Adipose-Derived Mesenchymal Stem Cells of Ankylosing Spondylitis Patients. Stem Cells Int (2021) 2021:6637328. doi: 10.1155/2021/6637328
81. Xu Y, Zhu J, Feng B, Lin F, Zhou J, Liu J, et al. Immunosuppressive Effect of Mesenchymal Stem Cells on Lung and Gut Cd8(+) T Cells in Lipopolysaccharide-Induced Acute Lung Injury in Mice. Cell Proliferation (2021) 54(5):e13028. doi: 10.1111/cpr.13028
82. Luque-Campos N, Contreras-López RA, Jose Paredes-Martínez M, Torres MJ, Bahraoui S, Wei M, et al. Mesenchymal Stem Cells Improve Rheumatoid Arthritis Progression by Controlling Memory T Cell Response. Front Immunol (2019) 10:798. doi: 10.3389/fimmu.2019.00798
83. Khare D, Or R, Resnick I, Barkatz C, Almogi-Hazan O, Avni B. Mesenchymal Stromal Cell-Derived Exosomes Affect Mrna Expression and Function of B-Lymphocytes. Front Immunol (2018) 9:3053. doi: 10.3389/fimmu.2018.03053
84. Liu Q, Chen X, Liu C, Pan L, Kang X, Li Y, et al. Mesenchymal Stem Cells Alleviate Experimental Immune-Mediated Liver Injury via Chitinase 3-Like Protein 1-Mediated T Cell Suppression. Cell Death Dis (2021) 12(3):240. doi: 10.1038/s41419-021-03524-y
85. Palomares Cabeza V, Hoogduijn MJ, Kraaijeveld R, Franquesa M, Witte-Bouma J, Wolvius EB, et al. Pediatric Mesenchymal Stem Cells Exhibit Immunomodulatory Properties Toward Allogeneic T and B Cells Under Inflammatory Conditions. Front Bioeng Biotechnol (2019) 7:142. doi: 10.3389/fbioe.2019.00142
86. Lee HK, Kim HS, Pyo M, Park EJ, Jang S, Jun HW, et al. Phorbol Ester Activates Human Mesenchymal Stem Cells to Inhibit B Cells and Ameliorate Lupus Symptoms in Mrl.Fas (Lpr) Mice. Theranostics (2020) 10(22):10186–99. doi: 10.7150/thno.46835
87. Zhang Q, Fu L, Liang Y, Guo Z, Wang L, Ma C, et al. Exosomes Originating From Mscs Stimulated With Tgf-β and Ifn-γ Promote Treg Differentiation. J Cell Physiol (2018) 233(9):6832–40. doi: 10.1002/jcp.26436
88. Gao Y, Li W, Bu X, Xu Y, Cai S, Zhong J, et al. Human Amniotic Mesenchymal Stem Cells Inhibit Agvhd by Regulating Balance of Treg and T Effector Cells. J Inflammation Res (2021) 14:3985–99. doi: 10.2147/jir.S323054
89. Zhang Y, Chen J, Fu H, Kuang S, He F, Zhang M, et al. Exosomes Derived From 3d-Cultured Mscs Improve Therapeutic Effects in Periodontitis and Experimental Colitis and Restore the Th17 Cell/Treg Balance in Inflamed Periodontium. Int J Oral Sci (2021) 13(1):43. doi: 10.1038/s41368-021-00150-4
90. Luo Y, Guo J, Zhang P, Cheuk YC, Jiang Y, Wang J, et al. Mesenchymal Stem Cell Protects Injured Renal Tubular Epithelial Cells by Regulating Mtor-Mediated Th17/Treg Axis. Front Immunol (2021) 12:684197. doi: 10.3389/fimmu.2021.684197
91. Chen QH, Wu F, Liu L, Chen HB, Zheng RQ, Wang HL, et al. Mesenchymal Stem Cells Regulate the Th17/Treg Cell Balance Partly Through Hepatocyte Growth Factor In Vitro. Stem Cell Res Ther (2020) 11(1):91. doi: 10.1186/s13287-020-01612-y
92. Agrawal M, Rasiah PK, Bajwa A, Rajasingh J, Gangaraju R. Mesenchymal Stem Cell Induced Foxp3(+) Tregs Suppress Effector T Cells and Protect Against Retinal Ischemic Injury. Cells (2021) 10(11):3006. doi: 10.3390/cells10113006
93. Court AC, Le-Gatt A, Luz-Crawford P, Parra E, Aliaga-Tobar V, Bátiz LF, et al. Mitochondrial Transfer From Mscs to T Cells Induces Treg Differentiation and Restricts Inflammatory Response. EMBO Rep (2020) 21(2):e48052. doi: 10.15252/embr.201948052
94. Chen X, Cai C, Xu D, Liu Q, Zheng S, Liu L, et al. Human Mesenchymal Stem Cell-Treated Regulatory Cd23(+)Cd43(+) B Cells Alleviate Intestinal Inflammation. Theranostics (2019) 9(16):4633–47. doi: 10.7150/thno.32260
95. Liu J, Liu Q, Chen X. The Immunomodulatory Effects of Mesenchymal Stem Cells on Regulatory B Cells. Front Immunol (2020) 11:1843. doi: 10.3389/fimmu.2020.01843
96. Michalopoulos GK, Bhushan B. Liver Regeneration: Biological and Pathological Mechanisms and Implications. Nat Rev Gastroenterol Hepatol (2021) 18(1):40–55. doi: 10.1038/s41575-020-0342-4
97. Xiao J, Wang F, Wong NK, He J, Zhang R, Sun R, et al. Global Liver Disease Burdens and Research Trends: Analysis From a Chinese Perspective. J Hepatol (2019) 71(1):212–21. doi: 10.1016/j.jhep.2019.03.004
98. Stravitz RT, Lee WM. Acute Liver Failure. Lancet (London England) (2019) 394(10201):869–81. doi: 10.1016/s0140-6736(19)31894-x
99. Shi Y, Du L, Lv D, Li Y, Zhang Z, Huang X, et al. Emerging Role and Therapeutic Application of Exosome in Hepatitis Virus Infection and Associated Diseases. J Gastroenterol (2021) 56(4):336–49. doi: 10.1007/s00535-021-01765-4
100. Jiang W, Ma P, Deng L, Liu Z, Wang X, Liu X, et al. Hepatitis a Virus Structural Protein Px Interacts With Alix and Promotes the Secretion of Virions and Foreign Proteins Through Exosome-Like Vesicles. J Extracellular Vesicles (2020) 9(1):1716513. doi: 10.1080/20013078.2020.1716513
101. Grünvogel O, Colasanti O, Lee JY, Klöss V, Belouzard S, Reustle A, et al. Secretion of Hepatitis C Virus Replication Intermediates Reduces Activation of Toll-Like Receptor 3 in Hepatocytes. Gastroenterology (2018) 154(8):2237–51.e16. doi: 10.1053/j.gastro.2018.03.020
102. Shi Y, Du L, Lv D, Li H, Shang J, Lu J, et al. Exosomal Interferon-Induced Transmembrane Protein 2 Transmitted to Dendritic Cells Inhibits Interferon Alpha Pathway Activation and Blocks Anti-Hepatitis B Virus Efficacy of Exogenous Interferon Alpha. Hepatol (Baltimore Md) (2019) 69(6):2396–413. doi: 10.1002/hep.30548
103. Zhang TY, Guo XR, Wu YT, Kang XZ, Zheng QB, Qi RY, et al. A Unique B Cell Epitope-Based Particulate Vaccine Shows Effective Suppression of Hepatitis B Surface Antigen in Mice. Gut (2020) 69(2):343–54. doi: 10.1136/gutjnl-2018-317725
104. Saviano A, Wrensch F, Ghany MG, Baumert TF. Liver Disease and Coronavirus Disease 2019: From Pathogenesis to Clinical Care. Hepatol (Baltimore Md) (2021) 74(2):1088–100. doi: 10.1002/hep.31684
105. Wander P, Epstein M, Bernstein D. Covid-19 Presenting as Acute Hepatitis. Am J Gastroenterol (2020) 115(6):941–2. doi: 10.14309/ajg.0000000000000660
106. Fan Z, Chen L, Li J, Cheng X, Yang J, Tian C, et al. Clinical Features of Covid-19-Related Liver Functional Abnormality. Clin Gastroenterol Hepatol (2020) 18(7):1561–6. doi: 10.1016/j.cgh.2020.04.002
107. Zou X, Fang M, Huang J. Liver Function Should be Monitored When Treating Covid-19 in Chronic Hbv-Infected Patients. Clin Gastroenterol Hepatol (2020) 18(13):3056–7. doi: 10.1016/j.cgh.2020.07.062
108. Moon AM, Webb GJ, Aloman C, Armstrong MJ, Cargill T, Dhanasekaran R, et al. High Mortality Rates for SARS-CoV-2 Infection in Patients With Pre-Existing Chronic Liver Disease and Cirrhosis: Preliminary Results From an International Registry. J Hepatol (2020) 73(3):705–8. doi: 10.1016/j.jhep.2020.05.013
109. Iavarone M, D'Ambrosio R, Soria A, Triolo M, Pugliese N, Del Poggio P, et al. High Rates of 30-Day Mortality in Patients With Cirrhosis and Covid-19. J Hepatol (2020) 73(5):1063–71. doi: 10.1016/j.jhep.2020.06.001
110. Khalaj K, Figueira RL, Antounians L, Lauriti G, Zani A. Systematic Review of Extracellular Vesicle-Based Treatments for Lung Injury: Are Evs a Potential Therapy for Covid-19? J Extracellular Vesicles (2020) 9(1):1795365. doi: 10.1080/20013078.2020.1795365
111. Efe C, Dhanasekaran R, Lammert C, Ebik B, Higuera-de la Tijera F, Aloman C, et al. Outcome of Covid-19 in Patients With Autoimmune Hepatitis: An International Multicenter Study. Hepatol (Baltimore Md) (2021) 73(6):2099–109. doi: 10.1002/hep.31797
112. Song N, Wakimoto H, Rossignoli F, Bhere D, Ciccocioppo R, Chen KS, et al. Mesenchymal Stem Cell Immunomodulation: In Pursuit of Controlling Covid-19 Related Cytokine Storm. Stem Cells (Dayton Ohio) (2021) 39(6):707–22. doi: 10.1002/stem.3354
113. Liang B, Chen J, Li T, Wu H, Yang W, Li Y, et al. Clinical Remission of a Critically Ill Covid-19 Patient Treated by Human Umbilical Cord Mesenchymal Stem Cells: A Case Report. Medicine (2020) 99(31):e21429. doi: 10.1097/md.0000000000021429
114. Leng Z, Zhu R, Hou W, Feng Y, Yang Y, Han Q, et al. Transplantation of Ace2(-) Mesenchymal Stem Cells Improves the Outcome of Patients With Covid-19 Pneumonia. Aging Dis (2020) 11(2):216–28. doi: 10.14336/ad.2020.0228
115. Dienz O, Rud JG, Eaton SM, Lanthier PA, Burg E, Drew A, et al. Essential Role of Il-6 in Protection Against H1n1 Influenza Virus by Promoting Neutrophil Survival in the Lung. Mucosal Immunol (2012) 5(3):258–66. doi: 10.1038/mi.2012.2
116. Zhang C, Wu Z, Li JW, Zhao H, Wang GQ. Cytokine Release Syndrome in Severe Covid-19: Interleukin-6 Receptor Antagonist Tocilizumab may be the Key to Reduce Mortality. Int J Antimicrobial Agents (2020) 55(5):105954. doi: 10.1016/j.ijantimicag.2020.105954
117. Devhare PB, Ray RB. Extracellular Vesicles: Novel Mediator for Cell to Cell Communications in Liver Pathogenesis. Mol Aspects Med (2018) 60:115–22. doi: 10.1016/j.mam.2017.11.001
118. Nguyen HQ, Lee D, Kim Y, Bang G, Cho K, Lee YS, et al. Label-Free Quantitative Proteomic Analysis of Serum Extracellular Vesicles Differentiating Patients of Alcoholic and Nonalcoholic Fatty Liver Diseases. J Proteomics (2021) 245:104278. doi: 10.1016/j.jprot.2021.104278
119. Momen-Heravi F, Bala S, Kodys K, Szabo G. Exosomes Derived From Alcohol-Treated Hepatocytes Horizontally Transfer Liver Specific Mirna-122 and Sensitize Monocytes to Lps. Sci Rep (2015) 5:9991. doi: 10.1038/srep09991
120. Verma VK, Li H, Wang R, Hirsova P, Mushref M, Liu Y, et al. Alcohol Stimulates Macrophage Activation Through Caspase-Dependent Hepatocyte Derived Release of Cd40l Containing Extracellular Vesicles. J Hepatol (2016) 64(3):651–60. doi: 10.1016/j.jhep.2015.11.020
121. Curtis BJ, Shults JA, Boe DM, Ramirez L, Kovacs EJ. Mesenchymal Stem Cell Treatment Attenuates Liver and Lung Inflammation After Ethanol Intoxication and Burn Injury. Alcohol (Fayetteville NY) (2019) 80:139–48. doi: 10.1016/j.alcohol.2018.09.001
122. Sun Y, Shi H, Yin S, Ji C, Zhang X, Zhang B, et al. Human Mesenchymal Stem Cell Derived Exosomes Alleviate Type 2 Diabetes Mellitus by Reversing Peripheral Insulin Resistance and Relieving β-Cell Destruction. ACS Nano (2018) 12(8):7613–28. doi: 10.1021/acsnano.7b07643
123. Zhao H, Shang Q, Pan Z, Bai Y, Li Z, Zhang H, et al. Exosomes From Adipose-Derived Stem Cells Attenuate Adipose Inflammation and Obesity Through Polarizing M2 Macrophages and Beiging in White Adipose Tissue. Diabetes (2018) 67(2):235–47. doi: 10.2337/db17-0356
124. Lleo A, de Boer YS, Liberal R, Colombo M. The Risk of Liver Cancer in Autoimmune Liver Diseases. Ther Adv Med Oncol (2019) 11:1758835919861914. doi: 10.1177/1758835919861914
125. Khatun M, Ray RB. Mechanisms Underlying Hepatitis C Virus-Associated Hepatic Fibrosis. Cells (2019) 8(10):1249. doi: 10.3390/cells8101249
126. Roehlen N, Crouchet E, Baumert TF. Liver Fibrosis: Mechanistic Concepts and Therapeutic Perspectives. Cells (2020) 9(4):875. doi: 10.3390/cells9040875
127. Ma L, Wei J, Zeng Y, Liu J, Xiao E, Kang Y, et al. Mesenchymal Stem Cell-Originated Exosomal Circdido1 Suppresses Hepatic Stellate Cell Activation by Mir-141-3p/Pten/Akt Pathway in Human Liver Fibrosis. Drug Delivery (2022) 29(1):440–53. doi: 10.1080/10717544.2022.2030428
128. An SY, Jang YJ, Lim HJ, Han J, Lee J, Lee G, et al. Milk Fat Globule-Egf Factor 8, Secreted by Mesenchymal Stem Cells, Protects Against Liver Fibrosis in Mice. Gastroenterology (2017) 152(5):1174–86. doi: 10.1053/j.gastro.2016.12.003
129. Sarin SK, Choudhury A. Acute-On-Chronic Liver Failure: Terminology, Mechanisms and Management. Nat Rev Gastroenterol Hepatol (2016) 13(3):131–49. doi: 10.1038/nrgastro.2015.219
130. Owen A, Newsome PN. Mesenchymal Stromal Cells, a New Player in Reducing Complications From Liver Transplantation? Front Immunol (2020) 11:1306. doi: 10.3389/fimmu.2020.01306
131. Zheng J, Lu T, Zhou C, Cai J, Zhang X, Liang J, et al. Extracellular Vesicles Derived From Human Umbilical Cord Mesenchymal Stem Cells Protect Liver Ischemia/Reperfusion Injury by Reducing Cd154 Expression on Cd4+ T Cells via Cct2. Advanced Sci (Weinheim Baden-Wurttemberg Germany) (2020) 7(18):1903746. doi: 10.1002/advs.201903746
132. Grange C, Bellucci L, Bussolati B, Ranghino A. Potential Applications of Extracellular Vesicles in Solid Organ Transplantation. Cells (2020) 9(2):369. doi: 10.3390/cells9020369
133. Podestà MA, Remuzzi G, Casiraghi F. Mesenchymal Stromal Cell Therapy in Solid Organ Transplantation. Front Immunol (2020) 11:618243. doi: 10.3389/fimmu.2020.618243
134. Chetty SS, Praneetha S, Govarthanan K, Verma RS, Vadivel Murugan A. Noninvasive Tracking and Regenerative Capabilities of Transplanted Human Umbilical Cord-Derived Mesenchymal Stem Cells Labeled With I-Iii-Iv Semiconducting Nanocrystals in Liver-Injured Living Mice. ACS Appl Materials Interfaces (2019) 11(9):8763–78. doi: 10.1021/acsami.8b19953
135. Verstegen MMA, Mezzanotte L, Ridwan RY, Wang K, de Haan J, Schurink IJ, et al. First Report on Ex Vivo Delivery of Paracrine Active Human Mesenchymal Stromal Cells to Liver Grafts During Machine Perfusion. Transplantation (2020) 104(1):e5–7. doi: 10.1097/tp.0000000000002986
136. Hann A, Osei-Bordom DC, Neil DAH, Ronca V, Warner S, Perera M. The Human Immune Response to Cadaveric and Living Donor Liver Allografts. Front Immunol (2020) 11:1227. doi: 10.3389/fimmu.2020.01227
137. Oh J, Kim JM. Immunologic Strategies and Outcomes in Abo-Incompatible Living Donor Liver Transplantation. Clin Mol Hepatol (2020) 26(1):1–6. doi: 10.3350/cmh.2019.0023
138. Forner A, Reig M, Bruix J. Hepatocellular Carcinoma. Lancet (London England) (2018) 391(10127):1301–14. doi: 10.1016/s0140-6736(18)30010-2
139. Yang JD, Hainaut P, Gores GJ, Amadou A, Plymoth A, Roberts LR. A Global View of Hepatocellular Carcinoma: Trends, Risk, Prevention and Management. Nat Rev Gastroenterol Hepatol (2019) 16(10):589–604. doi: 10.1038/s41575-019-0186-y
140. Wang LT, Liu KJ, Sytwu HK, Yen ML, Yen BL. Advances in Mesenchymal Stem Cell Therapy for Immune and Inflammatory Diseases: Use of Cell-Free Products and Human Pluripotent Stem Cell-Derived Mesenchymal Stem Cells. Stem Cells Trans Med (2021) 10(9):1288–303. doi: 10.1002/sctm.21-0021
141. Claridge B, Lozano J, Poh QH, Greening DW. Development of Extracellular Vesicle Therapeutics: Challenges, Considerations, and Opportunities. Front Cell Dev Biol (2021) 9:734720. doi: 10.3389/fcell.2021.734720
142. Schacher FC, Martins Pezzi da Silva A, Silla L, Álvares-da-Silva MR. Bone Marrow Mesenchymal Stem Cells in Acute-On-Chronic Liver Failure Grades 2 and 3: A Phase I-Ii Randomized Clinical Trial. Can J Gastroenterol Hepatol (2021) 2021:3662776. doi: 10.1155/2021/3662776
143. Rezakhani L, Kelishadrokhi AF, Soleimanizadeh A, Rahmati S. Mesenchymal Stem Cell (Msc)-Derived Exosomes as a Cell-Free Therapy for Patients Infected With Covid-19: Real Opportunities and Range of Promises. Chem Phys Lipids (2021) 234:105009. doi: 10.1016/j.chemphyslip.2020.105009
144. Meng F, Xu R, Wang S, Xu Z, Zhang C, Li Y, et al. Human Umbilical Cord-Derived Mesenchymal Stem Cell Therapy in Patients With Covid-19: A Phase 1 Clinical Trial. Signal Transduction Targeted Ther (2020) 5(1):172. doi: 10.1038/s41392-020-00286-5
145. Liu Y, Ren H, Zhou Y, Shang L, Zhang Y, Yang F, et al. The Hypoxia Conditioned Mesenchymal Stem Cells Promote Hepatocellular Carcinoma Progression Through Yap Mediated Lipogenesis Reprogramming. J Exp Clin Cancer Res CR (2019) 38(1):228. doi: 10.1186/s13046-019-1219-7
146. Zhang X, Sai B, Wang F, Wang L, Wang Y, Zheng L, et al. Hypoxic Bmsc-Derived Exosomal Mirnas Promote Metastasis of Lung Cancer Cells via Stat3-Induced Emt. Mol Cancer (2019) 18(1):40. doi: 10.1186/s12943-019-0959-5
147. Xie Y, Liu S, Wang L, Yang H, Tai C, Ling L, et al. Individual Heterogeneity Screened Umbilical Cord-Derived Mesenchymal Stromal Cells With High Treg Promotion Demonstrate Improved Recovery of Mouse Liver Fibrosis. Stem Cell Res Ther (2021) 12(1):359. doi: 10.1186/s13287-021-02430-6
148. Betzer O, Perets N, Angel A, Motiei M, Sadan T, Yadid G, et al. In Vivo Neuroimaging of Exosomes Using Gold Nanoparticles. ACS Nano (2017) 11(11):10883–93. doi: 10.1021/acsnano.7b04495
149. Wan Z, Zhao L, Lu F, Gao X, Dong Y, Zhao Y, et al. Mononuclear Phagocyte System Blockade Improves Therapeutic Exosome Delivery to the Myocardium. Theranostics (2020) 10(1):218–30. doi: 10.7150/thno.38198
150. He X, Hong W, Yang J, Lei H, Lu T, He C, et al. Spontaneous Apoptosis of Cells in Therapeutic Stem Cell Preparation Exert Immunomodulatory Effects Through Release of Phosphatidylserine. Signal Transduction Targeted Ther (2021) 6(1):270. doi: 10.1038/s41392-021-00688-z
151. Wu JY, Li YJ, Hu XB, Huang S, Xiang DX. Preservation of Small Extracellular Vesicles for Functional Analysis and Therapeutic Applications: A Comparative Evaluation of Storage Conditions. Drug Delivery (2021) 28(1):162–70. doi: 10.1080/10717544.2020.1869866
152. Wu P, Zhang B, Ocansey DKW, Xu W, Qian H. Extracellular Vesicles: A Bright Star of Nanomedicine. Biomaterials (2021) 269:120467. doi: 10.1016/j.biomaterials.2020.120467
153. Fu P, Zhang J, Li H, Mak M, Xu W, Tao Z. Extracellular Vesicles as Delivery Systems at Nano-/Micro-Scale. Advanced Drug Delivery Rev (2021) 179:113910. doi: 10.1016/j.addr.2021.113910
154. Cheng L, Hill AF. Therapeutically Harnessing Extracellular Vesicles. Nat Rev Drug Discovery (2022). doi: 10.1038/s41573-022-00410-w
155. Mardpour S, Ghanian MH, Sadeghi-Abandansari H, Mardpour S, Nazari A, Shekari F, et al. Hydrogel-Mediated Sustained Systemic Delivery of Mesenchymal Stem Cell-Derived Extracellular Vesicles Improves Hepatic Regeneration in Chronic Liver Failure. ACS Appl Materials Interfaces (2019) 11(41):37421–33. doi: 10.1021/acsami.9b10126
Keywords: hepatic diseases, mesenchymal stem cells, small extracellular vesicles, immunomodulatory effects, tissue regeneration
Citation: Tang Y, Wu P, Li L, Xu W and Jiang J (2022) Mesenchymal Stem Cells and Their Small Extracellular Vesicles as Crucial Immunological Efficacy for Hepatic Diseases. Front. Immunol. 13:880523. doi: 10.3389/fimmu.2022.880523
Received: 21 February 2022; Accepted: 11 April 2022;
Published: 06 May 2022.
Edited by:
Robert Chunhua Zhao, Chinese Academy of Medical Sciences and Peking Union Medical College, ChinaReviewed by:
Sina Naserian, Hôpital Paul Brousse, FrancePhilippe Lewalle, Jules Bordet Institute, Belgium
Copyright © 2022 Tang, Wu, Li, Xu and Jiang. This is an open-access article distributed under the terms of the Creative Commons Attribution License (CC BY). The use, distribution or reproduction in other forums is permitted, provided the original author(s) and the copyright owner(s) are credited and that the original publication in this journal is cited, in accordance with accepted academic practice. No use, distribution or reproduction is permitted which does not comply with these terms.
*Correspondence: Wenrong Xu, icls@ujs.edu.cn; Jiajia Jiang, jiangjiajia_2001@163.com
†These authors have contributed equally to this work