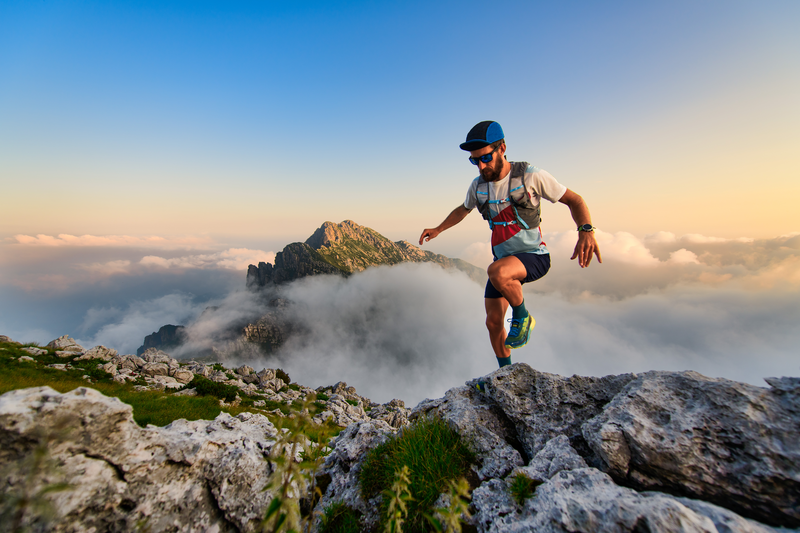
94% of researchers rate our articles as excellent or good
Learn more about the work of our research integrity team to safeguard the quality of each article we publish.
Find out more
MINI REVIEW article
Front. Immunol. , 11 May 2022
Sec. Molecular Innate Immunity
Volume 13 - 2022 | https://doi.org/10.3389/fimmu.2022.879552
This article is part of the Research Topic The Role of Immune Cells in Hepatic Ischemia Reperfusion View all 13 articles
Inflammation is crucial to tumorigenesis and the development of metastasis. Hepatic ischemia/reperfusion injury (IRI) is an unresolved problem in liver resection and transplantation which often establishes and remodels the inflammatory microenvironment in liver. More and more experimental and clinical evidence unmasks the role of hepatic IRI and associated inflammation in promoting the recurrence of hepatocellular carcinoma (HCC). Meanwhile, approaches aimed at alleviating hepatic IRI, such as machine perfusion, regulating the gut-liver axis, and targeting key inflammatory components, have been proved to prevent HCC recurrence. This review article highlights the underlying mechanisms and promising therapeutic strategies to reduce tumor recurrence through alleviating inflammation induced by hepatic IRI.
Hepatocellular carcinoma (HCC) is one of the most prevalent malignancies and the third leading cause of cancer-related mortality in the world (1). Surgical therapies, including hepatectomy and liver transplantation (LT), are the most efficient treatments for patients with HCC (2). However, the prognosis of HCC patients remains dismal due to the high incidence of metastasis and recurrence after surgery, with 5-year recurrence rates reaching up to 70% after liver resection (LR) and 40% after LT (3, 4). Ischemia/reperfusion injury (IRI) refers to the pathophysiological process in which ischemic tissue injury is accentuated following the restoration of blood flow after a period of ischemia (5). Hepatic IRI is an inevitable consequence of LR and LT, which is usually accompanied by intense inflammatory cascade and subsequent damage repair (5). In the mid-19th century, the link between inflammation and cancer was firstly suggested by Rudolf Virchow, based on discovering leukocyte infiltration in neoplastic tissues (6). Nowadays, inflammation has been demonstrated to be strongly associated with tumorigenesis and metastasis of most types of cancer. Targeting inflammation associated with tumor progress has gradually become a critical anti-cancer treatment (7). Currently, multiple preclinical and clinical studies suggest that inflammation induced by hepatic IRI promoted tumor relapse and metastasis after LR or LT (8–12). Meanwhile, alleviating inflammation induced by hepatic IRI is emerging as a promising therapeutic strategy for reducing liver damage and simultaneously suppressing tumor recurrence for HCC patients (13). In this review, we summarize the clinical evidence that hepatic IRI promotes tumor relapse and metastasis. Furthermore, we review recent advances in therapeutic strategies which suppress tumor recurrence through alleviating inflammation induced by hepatic IRI. These avenues of killing two birds with one stone may provide new insights into preventing HCC relapse.
LT, which removes the tumor and the diseased liver at the same time, is a radical treatment modality for HCC. However, tumor relapse is still the most severe threat to the survival of HCC patients after LT (14). Hepatic IRI is a common but thorny problem in different clinical settings such as LT, LR, trauma surgery, and shock. As essential procedures during LT, cold preservation of liver graft and subsequent warm reperfusion when implanted into the recipients result in hepatic IRI, which can be stratified into warm IRI and cold IRI (15). The severity of IRI and subsequent inflammation is positively correlated with ischemia time, which can also be partitioned into warm ischemia time (WIT) and cold ischemia time (CIT) (16).
Clinically, HCC patients with prolonged WIT and CIT are more likely to relapse after transplantation, revealing the links between hepatic IRI and HCC recurrence (8–12). The details of relevant clinical studies are listed in Table 1. A retrospective study that enrolled 391 patients found that CIT >10 hours and WIT >50 minutes were associated with significantly increased recurrence (P=0.015 and 0.036, respectively) (8). Another single-center study from Germany also showed that WIT>50 min was an independent risk factor for HCC recurrence (OR=15.5; P <0.001) (9). Orci et al. analyzed the data of 9724 HCC patients from the American Scientific Registry of Transplant Recipients (SRTR). They found that WIT >19 minutes was associated with an increased HCC recurrence risk (P = 0.025) (11). In 2018, our team reached a similar conclusion in HCC patients of China, where hepatitis-B constitutes the main cause of HCC. We recognized CIT >12 hours as the independent donor prognostic factor for predicting HCC recurrence (HR=2.23; P=0.007) (10). In addition to ischemia time, post-reperfusion serum aspartate transaminase (AST) and lactate dehydrogenase (LDH), important indicators reflecting the degree of hepatic inflammatory injury, have also been shown to correlate with the risk of tumor recurrence in patients within the Milan criteria (12).
These findings provide the clinical evidence that inflammation induced by hepatic IRI promoted HCC recurrence and indicate that minimizing ischemia time may be beneficial to alleviating inflammatory injury and reducing the risk of HCC recurrence. However, reducing ischemia time might be difficult in clinical practice, which depends on many factors such as organ allocation system, organ transport, surgery complexity, and experience of transplant teams. Therefore, repair of liver injury or targeting inflammatory mechanisms of hepatic IRI may be more practical solutions in the existing clinical context.
Currently, cold static storage (CS) is still the most commonly utilized method for liver preservation in LT. Meanwhile, due to the shortage of donor livers, extended criteria donor (ECD) liver grafts such as steatotic grafts, livers from older donors and donors after cardiac death (DCD) are utilized to expand the scarce donor pool (17). These ECD liver grafts are more susceptible to hepatic IRI and are linked to inferior post-transplant outcomes (18). Besides, the use of organs from DCD donors with prolonged WIT and severe steatotic donor livers was proved to increase the risk of HCC recurrence post LT (11, 19). In such a context, the limitations of CS become more obvious. As a future direction of graft preservation, there is increasing attention to machine perfusion (MP) due to its ability to preserve, evaluate and recondition such donor livers prior to transplantation (20).
Oldani et al. found that the use of ischemic rat liver graft accompanied by an increased serum pro-inflammatory cytokine profile increased the risk of cancer recurrence (21). Investigators approximated an in vivo normothermic perfusion by reperfusing the liver in vivo (animal alive) for two hours before flushing and retrieving. This method attenuated liver injury, measured by the release of liver enzymes (AST and ALT). Besides, prior-to-retrieval reperfusion decreased the recurrence and growth of HCC after transplantation. These effects were partly attributed to the improvement of serum inflammatory cytokine profile. In clinical practice, normothermic extracorporeal membrane oxygenation (NECMO) or NECMO-based normothermic regional perfusion (NRP) is the closest procedure to reproduce the studied model (22). As a technique that allows the in-situ perfusion of organs with oxygenated blood in DCD donors, NRP can reduce warm ischemia time and improve graft function (23). This investigation further revealed the possible potential of NRP in preventing hepatic IRI-associated HCC recurrence.
Hypothermic oxygenated perfusion (HOPE) is an emerging organ preservation strategy for marginal grafts (20). The use of artificial, cooled perfusion solutions with oxygenation applied in HOPE has been demonstrated to decrease oxidative stress and inflammatory damage in LT (24). To validate the protective effect of HOPE in HCC recurrence, Mueller et al. compared tumor recurrence rate between HOPE-treated DCD and unperfused DBD liver recipients after liver LT for HCC (25). The results showed that HOPE alleviated general inflammation (measured through plasma CRP of the recipient) and recovered liver functions. He also observed a significantly lower recurrence rate in patients who received end-ischemic HOPE treatment (5.7%, n = 4/70) compared to an unperfused DBD cohort (25.7%, 18/70), despite the use of high-risk DCD grafts (P=0.002). Besides, HOPE treatment improved recurrence-free survival and reduced tumor load significantly compared to an external, nonperfused DCD and DBD liver recipient cohort. HOPE before implantation protected the liver from reperfusion injury and subsequently prevented ongoing tissue inflammation and hypoxia, making the environment less favorable for tumor recurrence and metastasis.
Despite the ability to decrease damage and improve outcomes, hepatic IRI cannot be completely avoided using the techniques above. With the innovations of surgical techniques and NMP, the technique named ischemia-free liver transplantation (IFLT) has been created. Its application is able to procure, preserve, and implant liver grafts from DBD donors without stopping the blood and oxygen supply, thus entirely preventing hepatic IRI (26). A recent propensity-matched cohort study showed that IFLT recipients had a higher RFS rate at 1 and 3 years (92.2% and 86.7%) than conventional LT recipients (88.1% and 53.6%), with much lower liver damage (27). Though the oncological benefit of IFLT needs further observation and validation in a larger sample, this technique shows enormous potential not only for completely avoiding IRI but also for preventing associated HCC recurrence. However, this technique is not applicable to LDLT and DCD LT, which occupy the major types of LT.
The close interplay between the intestine and liver in anatomy and function is known as the gut-liver axis. In the liver, two-thirds of total blood flow originates from the portal circulation, which contains gut-derived bacterial products (28). As a “microbial organ”, gut microbiota is involved in the development of many liver disorders through the gut-liver axis (29, 30). During LT and LR, portal vein clamping usually results in venous congestion and hypoperfusion of the intestines, followed by intestinal mucosa damage and increased permeability (31). In these cases, endotoxin/lipopolysaccharide (LPS), a cell wall component of Gram-negative bacteria, could translocate to the portal blood through the impaired intestinal mucosal barrier and intensify hepatic IRI (32).
A recent study has revealed the connection between hepatic IRI and HCC recurrence from a brand-new perspective of the gut-liver axis. The study found that pedicle clamping induced the congestive injury of the bowel wall and subsequent increase of LPS in portal circulation. Upon re-establishment of portal blood flow, bacterial LPS activated the Toll-like receptor 4 (TLR4) signaling pathway in the liver, leading to aggravated IRI, exacerbated inflammatory response, and increased tumor burden (33). In this process, hyperactivation of TLR4 by LPS results in the activation and over-expression of pro-inflammatory transcription factors (TFs) such as nuclear factor-kappaB (NF-κB), activator protein 1 (AP-1) and interferon regulatory factor 3 (IRF3) (34). Increased production of pro-inflammatory cytokines (IL-6, TNF-α, etc.) and chemokines (CXCL1, CXCL10, etc.) intensify hepatic IRI and upregulate the components involved in the progression and metastasis of HCC including angiogenesis cytokines and immunosuppressive cells such as Tregs (35).
The researchers also explored several therapeutic approaches to suppress tumor growth by targeting the gut–liver axis (33). They found that remote ischemic preconditioning (RIPC) induced by brief and repeated sequences of femoral vascular bundle clamping and declamping could prevent liver injury and associated HCC recurrence through dampening small bowel venous ischemia and preventing bacterial translocation. Despite promising animal evidence supporting the protective effect of RIPC on hepatic IRI, the results of several clinical trials are inconsistent, indicating that RIPC’s clinical role remains to be further confirmed (36–39). Besides RIPC, the study also showed that gut decontamination (antibiotics) and pharmacological TLR4 inhibition confer systemic protection against inflammation-mediated accelerated HCC growth. Their results indicated that modulating the gut–liver axis during liver surgery could be a potential target in combating HCC recurrence. Predictably, gut microecological therapy will gradually become an emerging treatment modality in preventing inflammation-induced HCC recurrence. Figure 1 displays the schematic diagram of the gut-liver axis as a target in inflammation-associated cancer recurrence induced by hepatic IRI.
Figure 1 The gut-liver axis as a target in inflammation-associated cancer recurrence induced by hepatic IRI. Portal vein clamping during LT and LR leads to venous congestion and hypoperfusion of the intestines. Bacterial products released through damaged intestinal mucosa activate TLR4 signaling pathway in the liver, resulting in the exacerbated inflammatory response and increased tumor burden. RIPC, antibiotics, and TLR4 inhibition are able to act on the gut-liver axis to reduce inflammation-associated HCC recurrence.
Hepatic IRI is a dynamic process including two related stages: ischemic injury and inflammation-mediated reperfusion injury (40). In the phase of ischemia, hepatocytes are exposed to oxygen deprivation, ATP depletion, and pH decreasement (41). These changes result in the accumulation of reactive oxygen species (ROS), increasement of intracellular calcium concentrations which lead to hepatocyte damage and different cell death programs such as apoptosis, necrosis, ferroptosis and pyroptosis (13, 42). The following reperfusion triggers inflammatory cascade that aggravates hepatocyte injury through multiple mechanisms. In addition to cell death programs and metabolic disorders, innate immune activation plays a major role in this process (5). After sensing damage-associated molecular patterns (DAMPs) released from damaged or dead cells, Kupffer cells become activated through pattern-recognition receptors (PRRs) and release chemokines and cytokines to initiate a pro-inflammatory response (43).
The inflammatory cascade after reperfusion involves multiple inflammatory components and their interactions. Activated Kupffer cells release cytokines like IL-1β and chemokines such as CXCL1 and CXCL2 to activate and recruit neutrophils in damaged site (44). Platelets activated by DAMPs also serve as immune mediators which express CXCL4 and P-selectin and promote the recruitment of monocytes and neutrophils to the inflammation site (45–47). The expression of NKG2D (an activating receptor for NK cells) is significantly upregulated in the reperfused liver (48). Increased NK cells recruited to the liver can not only exacerbate IRI by producing IFNγ but also increase the production of IL-17, which promotes the recruitment of neutrophils (49, 50). Hepatic IRI also elicits a robust adaptive immune response in which CD4+ T cells mediate aggravated inflammatory damage (40).
The balance of pro-inflammatory and anti-inflammatory components determines the outcome of inflammation during IRI (44). Multiple clinical and experimental studies have suggested that intense and continuous inflammation induced by hepatic IRI promoted tumor recurrence via activating cellular signaling for tumor cell proliferation, adhesion, invasion and angiogenesis (8, 51). Hypoxia-inducible factor 1α (HIF1α), matrix metalloproteinase 9 (MMP9), vascular endothelial growth factor (VEGF) and other key molecules play important roles in this process (52).
After the initiation of inflammation, some anti-inflammatory components and repair factors will be recruited into the inflammatory microenvironment to inhibit the progress of inflammation and promote injury repair. Anti-inflammatory cytokines, such as IL-4 or IL-13, and apoptotic cells promote in situ macrophage polarization and reprogramming and then initiate the resolution phase of inflammation (53–55). Some anti-inflammatory neutrophil subtypes exert anti-inflammatory and repair functions through inhibiting cytotoxic T cells (CTLs), promoting macrophage polarization and angiogenesis (44, 56). Besides, regulatory T cells (Tregs), myeloid-derived suppressor cells (MDSCs), endothelial progenitor cells (EPCs), platelets and many other factors also play critical roles in regulating inflammation and its resolution (44, 57, 58).
However, in the setting of oncology, these beneficial components may also result in worsened oncologic outcomes. Some components responding to inflammation have been proved to promote HCC recurrence through different mechanisms, including mediating the immune escape and angiogenesis of tumor as well as causing tumor cells to be more aggressive by triggering tumor cell adhesion, migration, and invasion (13). Targeting key inflammatory components in the tumor microenvironment may suppress tumor recurrence. Compared to machine perfusion, this method provides more targeted treatment for HCC patients and can be applied to both LR and LT. Figure 2 displays the key inflammatory components in the tumor microenvironment and relevant therapies.
Figure 2 Targeting key inflammatory components in the tumor microenvironment. Hepatic IRI results in the recruitment of EPC, Treg, and MDSC as well as the release of pro-inflammatory cytokines. These changes in the tumor microenvironment induce tumor angiogenesis, immune evasion and promote tumor invasion and metastasis. The use of agents such as FTY720, YQ23, PGE1, Rosiglitazone and targeting key inflammatory pathways are able to attenuate hepatic IRI and prevent tumor recurrence.
Immune escape is a crucial characteristic during tumor growth and metastasis. MDSCs and Tregs have been identified as key immune cell subsets that mediate tumor immune escape (59). Of them, MDSCs are a heterogeneous population of immunosuppressive cells derived from myeloid progenitors, which can be upregulated by inflammatory mediators such as IL-2, GCSF, and so on (57). MDSCs suppress the immune system by various mechanisms including (i) inhibiting CTLs proliferation and activation by increased nitric oxide (NO), nitrotyrosine and ROS secretion, and decreased l-arginine production (ii) inducing immunosuppressive cells like T helper (Th) 17 cells and Tregs (60); Tregs are crucial suppressors of immune responses and are essential for maintaining immunological tolerance and controlling inflammatory diseases (61, 62). In the process of tumor immune escape, Tregs can suppress antigen presentation by DCs, CD4+ Th cell function and promote intratumoral T cell exhaustion through secreting TGF-β, IL-10 and IL-35 (63, 64). Recent findings also demonstrated the crosstalk between Tregs and MDSCs, which found that Tregs regulate MDSCs differentiation and function through TGF-β and the programmed death ligand 1 (B7-H1) (65, 66). In HCC patients, MDSCs and Tregs were found to be significantly elevated in the peripheral blood and tumor (67, 68). Besides, emerging tumor immunotherapy targeting MDSC and Tregs has effectively inhibited the progression of HCC (63, 69).
In addition to immune escape, tumor angiogenesis also plays a key role in tumor recurrence. EPCs are a kind of vascular progenitor with high proliferative potential that are derived from bone marrow (70). Inflammatory endothelial injury can trigger mobilization, homing, and transdifferentiation of EPCs, thereby contributing to the repair of injured endothelium (71). Recent studies have found that EPCs participate in neovascularization during acute ischemic injury (58). However, mobilization of EPCs also occurs in response to low oxygenation during tumor growth (72). They are able to facilitate the release of proangiogenic cytokines and promote vessel incorporation and stabilization (73). Multiple studies have shown that mobilization of circulating EPC results in tumor neovascularization and accelerates tumor growth and metastasis (74, 75). Besides, the inhibition of EPCs mobilization restrained angiogenesis and tumor progression (76).
In the inflammatory cascade triggered by hepatic IRI, chemokines and chemokine receptors are involved in the recruitment of inflammation-responsive components discussed above (77). Selectively targeting these pathways has been heralded as a promising avenue for suppressing these cell subsets. Through a series of clinical analyses, in vitro and in vivo experiments, Liu et al. found that MDSCs recruited by CXCL10/TLR4 during acute phase inflammation played a critical role in tumor recurrence after LT. Targeting MDSC mobilization via CXCL10/TLR4 signaling could not only protect the liver graft from IRI but also reduce tumor recurrence after transplantation (78). Ling et al. identified that post-transplant enhanced CXCL10/CXCR3 signaling in small-for-size liver grafts directly induced EPC mobilization, differentiation, and neovessel formation, which further promotes tumor growth (79). Besides, CXCL10/CXCR3 signaling upregulated at liver graft injury directly induced the mobilization and intragraft recruitment of Tregs, which further promoted HCC recurrence after transplantation (80). Targeting CXCL10/CXCR3 signaling inhibited the mobilization of Treg and EPC, attenuated early-phase liver graft injury, and prevented late-phase tumor recurrence/metastasis after transplantation (79, 80).
In addition to genetic approaches and pharmacological inhibitors, some drugs can also act through the above pathways. FTY720 (fingolimod) is a sphingosine-1-phosphate (S1P) receptor agonist that has been approved by FDA as a treatment for multiple sclerosis (MS) (81). In models of lung, kidney, and liver IRI, FTY720 unfolds a demonstrated anti-inflammatory effect (82–84). Furthermore, FTY720 has exhibited a strong anti-tumor activity in liver cancer, breast cancer, bladder cancer, and so on (85–88). Li et al. found that FTY720 significantly attenuated hepatic IRI and tumor metastasis after LR through the downregulating CXCL10/CXCR3 signaling pathway. In their study, FTY720 treatment reduced the population of circulating EPCs and Tregs and thereby limited tumor angiogenesis and enhanced antitumor immune response (89, 90). A novel oxygen carrier called YQ23 played a similar role in suppressing liver tumor metastasis after major hepatectomy and partial hepatic I/R injury through increasing liver oxygenation and reducing the number of circulating EPCs and Tregs (91).
A number of studies have indicated that proinflammatory cytokines such as E-selectin, VEGF and MMP activated by hepatic IRI promoted tumor invasion and metastasis (92–94). Ligands of PPARγ, such as rosiglitazone can downregulate the expression of proinflammatory cytokines that are associated with tumor metastases through inhibiting the NF-κB signaling pathway (95–97). In an experimental mouse model of hepatic IRI-induced HCC metastasis, rosiglitazone exerts a protective effect on hepatic IRI and significantly inhibits tumor metastasis following that. As reported in this article, the dual action may be attributed to inhibited NF-κB signaling and reduced expression of proinflammatory cytokines in liver (98).
Prostaglandins (PGs) are products of arachidonic acid metabolism via the cyclooxygenase pathway, which are produced primarily by activated Kupfer cells during hepatic IRI (99). PGs exert anti-inflammatory effects by prevention of leucocyte migration and down-regulation of pro-inflammatory cytokines (100). The administration of alprostadil, a synthetic stable form of prostaglandin E1(PGE1), was shown to attenuate hepatic IRI and improve liver graft function (101). In a retrospective clinical study, Kornberg et al. found that treating hepatic IRI with alprostadil reduced systemic inflammation levels and the risk of early HCC recurrence following LT (102).
Besides these mentioned strategies above, glutathione peroxidase 3, thymoquinone, and zinc finger protein A20 also show the potential to be “one stone for two birds” strategies that attenuate hepatic IR injury and prevent tumor recurrence after liver surgery (103–105). However, their inhibition of hepatic IRI-associated recurrence needs further experimental research and clinical verification.
Collectively, the inflammation induced by hepatic IRI plays a crucial role in the development and progression of HCC, which contributes to several hallmarks of HCC, such as promoting proliferative and survival signaling, inducing angiogenesis, evading immune surveillance, and activating invasion and metastasis. Besides, alleviating inflammation such as machine perfusion, regulating the gut-liver axis and targeting key inflammatory components or inflammatory pathways is believed to be an attractive therapeutic strategy. However, current evidence mainly comes from animal models. More clinical studies with larger numbers of patients will be required. Moreover, not all measures are applicable to LT or LR due to the differences in surgical procedures, use of immunosuppressive agents and postoperative management. Additional validation is needed in different models and patient populations. In the future, we advocate using advanced techniques such as single-cell multi-omics and spatial omics to explore the mechanism and targets for therapeutic intervention comprehensively. Meanwhile, we believe that combining different approaches at different time points should yield better outcomes for patients.
XX and DL designed and supervised the study. HC and XY performed the article searching and wrote the manuscript. ZH, CH, HL, ZL and MY revised the manuscript. All authors contributed to the article and approved the submitted version.
This work was supported by grants from The Major Research Plan of the National Natural Science Foundation of China (92159202), Key Program, National Natural Science Foundation of China (81930016), National Key Research and Development Program of China (2021YFA1100500), Key Research & Development Plan of Zhejiang Province (No. 2019C03050), and Young Program of National Natural Science Funds (No. 82000617).
The authors declare that the research was conducted in the absence of any commercial or financial relationships that could be construed as a potential conflict of interest.
All claims expressed in this article are solely those of the authors and do not necessarily represent those of their affiliated organizations, or those of the publisher, the editors and the reviewers. Any product that may be evaluated in this article, or claim that may be made by its manufacturer, is not guaranteed or endorsed by the publisher.
We thank all members of Key Laboratory of Integrated Oncology and Intelligent Medicine of Zhejiang Province for helpful suggestions regarding the manuscript. Figures were created with BioRender.
1. Torre LA, Bray F, Siegel RL, Ferlay J, Lortet-Tieulent J, Jemal A. Global Cancer Statistics, 2012. CA Cancer J Clin (2015) 65(2):87–108. doi: 10.3322/caac.21262
2. Kawaguchi Y, Honda G, Endo I, Cherqui D, Kokudo N. Current Technical Issues for Surgery of Primary Liver Cancer. Liver Cancer (2016) 6(1):51–8. doi: 10.1159/000449345
3. El-Serag HB. Hepatocellular Carcinoma. N Engl J Med (2011) 365(12):1118–27. doi: 10.1056/NEJMra1001683
4. Xia YX, Zhang F, Li XC, Kong LB, Zhang H, Li DH, et al. Surgical Treatment of Primary Liver Cancer: A Report of 10 966 Cases. Zhonghua Wai Ke Za Zhi (2021) 59(1):6–17. doi: 10.3760/cma.j.cn112139-20201110-00791
5. Zhou J, Chen J, Wei Q, Saeb-Parsy K, Xu X. The Role of Ischemia/Reperfusion Injury in Early Hepatic Allograft Dysfunction. Liver Transpl (2020) 26(8):1034–48. doi: 10.1002/lt.25779
6. Mollinedo F. Neutrophil Degranulation, Plasticity, and Cancer Metastasis. Trends Immunol (2019) 40(3):228–42. doi: 10.1016/j.it.2019.01.006
7. Lazennec G, Richmond A. Chemokines and Chemokine Receptors: New Insights Into Cancer-Related Inflammation. Trends Mol Med (2010) 16(3):133–44. doi: 10.1016/j.molmed.2010.01.003
8. Nagai S, Yoshida A, Facciuto M, Moonka D, Abouljoud MS, Schwartz ME, et al. Ischemia Time Impacts Recurrence of Hepatocellular Carcinoma After Liver Transplantation. Hepatology (2015) 61(3):895–904. doi: 10.1002/hep.27358
9. Kornberg A, Witt U, Kornberg J, Friess H, Thrum K. Extended Ischemia Times Promote Risk of Hcc Recurrence in Liver Transplant Patients. Dig Dis Sci (2015) 60(9):2832–9. doi: 10.1007/s10620-015-3541-z
10. Ling Q, Liu J, Zhuo J, Zhuang R, Huang H, He X, et al. Development of Models to Predict Early Post-Transplant Recurrence of Hepatocellular Carcinoma That Also Integrate the Quality and Characteristics of the Liver Graft: A National Registry Study in China. Surgery (2018) 164(1):155–164. doi: 10.1016/j.surg.2018.01.022
11. Orci LA, Berney T, Majno PE, Lacotte S, Oldani G, Morel P, et al. Donor Characteristics and Risk of Hepatocellular Carcinoma Recurrence After Liver Transplantation. Br J Surg (2015) 102(10):1250–7. doi: 10.1002/bjs.9868
12. Grąt M, Krawczyk M, Wronka KM, Stypułkowski J, Lewandowski Z, Wasilewicz M, et al. Ischemia-Reperfusion Injury and the Risk of Hepatocellular Carcinoma Recurrence After Deceased Donor Liver Transplantation. Sci Rep (2018) 8(1):8935. doi: 10.1038/s41598-018-27319-y
13. Liu H, Man K. New Insights in Mechanisms and Therapeutics for Short- and Long-Term Impacts of Hepatic Ischemia Reperfusion Injury Post Liver Transplantation. Int J Mol Sci (2021) 22(15):8210. doi: 10.3390/ijms22158210
14. Bruix J, Sherman M. Management of Hepatocellular Carcinoma. Hepatology (2005) 42(5):1208–36. doi: 10.1002/hep.20933
15. Heylen L, Pirenne J, Naesens M, Sprangers B, Jochmans I. "Time Is Tissue"-A Minireview on the Importance of Donor Nephrectomy, Donor Hepatectomy, and Implantation Times in Kidney and Liver Transplantation. Am J Transplant (2021) 21(8):2653–61. doi: 10.1111/ajt.16580
16. Ito T, Naini BV, Markovic D, Aziz A, Younan S, Lu M, et al. Ischemia-Reperfusion Injury and Its Relationship With Early Allograft Dysfunction in Liver Transplant Patients. Am J Transplant (2021) 21(2):614–25. doi: 10.1111/ajt.16219
17. Ceresa CDL, Nasralla D, Pollok JM, Friend PJ. Machine Perfusion of the Liver: Applications in Transplantation and Beyond. Nat Rev Gastroenterol Hepatol (2022) 19(3):199–209. doi: 10.1038/s41575-021-00557-8
18. Vodkin I, Kuo A. Extended Criteria Donors in Liver Transplantation. Clin Liver Dis (2017) 21(2):289–301. doi: 10.1016/j.cld.2016.12.004
19. Orci LA, Lacotte S, Oldani G, Slits F, De Vito C, Crowe LA, et al. Effect of Ischaemic Preconditioning on Recurrence of Hepatocellular Carcinoma in an Experimental Model of Liver Steatosis. Br J Surg (2016) 103(4):417–26. doi: 10.1002/bjs.10080
20. van Rijn R, Schurink IJ, de Vries Y, van den Berg AP, Cortes Cerisuelo M, Darwish Murad S, et al. Hypothermic Machine Perfusion in Liver Transplantation - A Randomized Trial. N Engl J Med (2021) 384(15):1391–401. doi: 10.1056/NEJMoa2031532
21. Oldani G, Crowe LA, Orci LA, Slits F, Rubbia-Brandt L, de Vito C, et al. Pre-Retrieval Reperfusion Decreases Cancer Recurrence After Rat Ischemic Liver Graft Transplantation. J Hepatol (2014) 61(2):278–85. doi: 10.1016/j.jhep.2014.03.036
22. Oldani G, Lacotte S, Orci L, Toso C. Reply To: Pre-Retrieval Reperfusion Decreases Cancer Recurrence After Rat Ischemic Liver Graft Transplantation. J Hepatol (2014) 61(4):962–3. doi: 10.1016/j.jhep.2014.06.011
23. Watson CJE, Hunt F, Messer S, Currie I, Large S, Sutherland A, et al. In Situ Normothermic Perfusion of Livers in Controlled Circulatory Death Donation May Prevent Ischemic Cholangiopathy and Improve Graft Survival. Am J Transplant (2019) 19(6):1745–58. doi: 10.1111/ajt.15241
24. Czigany Z, Lurje I, Schmelzle M, Schöning W, Öllinger R, Raschzok N, et al. Ischemia-Reperfusion Injury in Marginal Liver Grafts and the Role of Hypothermic Machine Perfusion: Molecular Mechanisms and Clinical Implications. J Clin Med (2020) 9(3):846. doi: 10.3390/jcm9030846
25. Mueller M, Kalisvaart M, O'Rourke J, Shetty S, Parente A, Muller X, et al. Hypothermic Oxygenated Liver Perfusion (Hope) Prevents Tumor Recurrence in Liver Transplantation From Donation After Circulatory Death. Ann Surg (2020) 272(5):759–65. doi: 10.1097/sla.0000000000004258
26. Guo Z, Zhao Q, Huang S, Huang C, Wang D, Yang L, et al. Ischaemia-Free Liver Transplantation in Humans: A First-In-Human Trial. Lancet Reg Health West Pac (2021) 16:100260. doi: 10.1016/j.lanwpc.2021.100260
27. Tang Y, Wang T, Ju W, Li F, Zhang Q, Chen Z, et al. Ischemic-Free Liver Transplantation Reduces the Recurrence of Hepatocellular Carcinoma After Liver Transplantation. Front Oncol (2021) 11:773535. doi: 10.3389/fonc.2021.773535
28. Tripathi A, Debelius J, Brenner DA, Karin M, Loomba R, Schnabl B, et al. The Gut-Liver Axis and the Intersection With the Microbiome. Nat Rev Gastroenterol Hepatol (2018) 15(7):397–411. doi: 10.1038/s41575-018-0011-z
29. Yang X, Lu D, Zhuo J, Lin Z, Yang M, Xu X. The Gut-Liver Axis in Immune Remodeling: New Insight Into Liver Diseases. Int J Biol Sci (2020) 16(13):2357–66. doi: 10.7150/ijbs.46405
30. Dapito DH, Mencin A, Gwak GY, Pradere JP, Jang MK, Mederacke I, et al. Promotion of Hepatocellular Carcinoma by the Intestinal Microbiota and Tlr4. Cancer Cell (2012) 21(4):504–16. doi: 10.1016/j.ccr.2012.02.007
31. Dello SA, Reisinger KW, van Dam RM, Bemelmans MH, van Kuppevelt TH, van den Broek MA, et al. Total Intermittent Pringle Maneuver During Liver Resection Can Induce Intestinal Epithelial Cell Damage and Endotoxemia. PloS One (2012) 7(1):e30539. doi: 10.1371/journal.pone.0030539
32. Colletti LM, Green M. Lung and Liver Injury Following Hepatic Ischemia/Reperfusion in the Rat Is Increased by Exogenous Lipopolysaccharide Which Also Increases Hepatic Tnf Production in Vivo and in Vitro. Shock (2001) 16(4):312–9. doi: 10.1097/00024382-200116040-00014
33. Orci LA, Lacotte S, Delaune V, Slits F, Oldani G, Lazarevic V, et al. Effects of the Gut-Liver Axis on Ischaemia-Mediated Hepatocellular Carcinoma Recurrence in the Mouse Liver. J Hepatol (2018) 68(5):978–85. doi: 10.1016/j.jhep.2017.12.025
34. Yamamoto M, Sato S, Hemmi H, Hoshino K, Kaisho T, Sanjo H, et al. Role of Adaptor Trif in the Myd88-Independent Toll-Like Receptor Signaling Pathway. Science (2003) 301(5633):640–3. doi: 10.1126/science.1087262
35. Sepehri Z, Kiani Z, Kohan F, Alavian SM, Ghavami S. Toll Like Receptor 4 and Hepatocellular Carcinoma; a Systematic Review. Life Sci (2017) 179:80–7. doi: 10.1016/j.lfs.2017.04.025
36. Kanoria S, Robertson FP, Mehta NN, Fusai G, Sharma D, Davidson BR. Effect of Remote Ischaemic Preconditioning on Liver Injury in Patients Undergoing Major Hepatectomy for Colorectal Liver Metastasis: A Pilot Randomised Controlled Feasibility Trial. World J Surg (2017) 41(5):1322–30. doi: 10.1007/s00268-016-3823-4
37. Rakić M, Patrlj L, Amić F, Aralica G, Grgurević I. Comparison of Hepatoprotective Effect From Ischemia-Reperfusion Injury of Remote Ischemic Preconditioning of the Liver Vs Local Ischemic Preconditioning of the Liver During Human Liver Resections. Int J Surg (2018) 54(Pt A):248–53. doi: 10.1016/j.ijsu.2018.05.001
38. Robertson FP, Goswami R, Wright GP, Imber C, Sharma D, Malago M, et al. Remote Ischaemic Preconditioning in Orthotopic Liver Transplantation (Ripcolt Trial): A Pilot Randomized Controlled Feasibility Study. HPB (Oxford) (2017) 19(9):757–67. doi: 10.1016/j.hpb.2017.05.005
39. Teo JY, Ho AFW, Bulluck H, Gao F, Chong J, Koh YX, et al. Effect of Remote Ischemic Preconditioning on Liver Injury in Patients Undergoing Liver Resection: The Eric-Liver Trial. HPB (Oxford) (2020) 22(9):1250–7. doi: 10.1016/j.hpb.2019.12.002
40. Zhai Y, Petrowsky H, Hong JC, Busuttil RW, Kupiec-Weglinski JW. Ischaemia-Reperfusion Injury in Liver Transplantation–from Bench to Bedside. Nat Rev Gastroenterol Hepatol (2013) 10(2):79–89. doi: 10.1038/nrgastro.2012.225
41. Selzner M, Selzner N, Jochum W, Graf R, Clavien PA. Increased Ischemic Injury in Old Mouse Liver: An Atp-Dependent Mechanism. Liver Transpl (2007) 13(3):382–90. doi: 10.1002/lt.21100
42. Lentsch AB, Kato A, Yoshidome H, McMasters KM, Edwards MJ. Inflammatory Mechanisms and Therapeutic Strategies for Warm Hepatic Ischemia/Reperfusion Injury. Hepatology (2000) 32(2):169–73. doi: 10.1053/jhep.2000.9323
43. Li P, He K, Li J, Liu Z, Gong J. The Role of Kupffer Cells in Hepatic Diseases. Mol Immunol (2017) 85:222–9. doi: 10.1016/j.molimm.2017.02.018
44. Hirao H, Nakamura K, Kupiec-Weglinski JW. Liver Ischaemia-Reperfusion Injury: A New Understanding of the Role of Innate Immunity. Nat Rev Gastroenterol Hepatol (2021) 19(4):239–56. doi: 10.1038/s41575-021-00549-8
45. Suzuki J, Hamada E, Shodai T, Kamoshida G, Kudo S, Itoh S, et al. Cytokine Secretion From Human Monocytes Potentiated by P-Selectin-Mediated Cell Adhesion. Int Arch Allergy Immunol (2013) 160(2):152–60. doi: 10.1159/000339857
46. Singh MV, Davidson DC, Jackson JW, Singh VB, Silva J, Ramirez SH, et al. Characterization of Platelet-Monocyte Complexes in Hiv-1-Infected Individuals: Possible Role in Hiv-Associated Neuroinflammation. J Immunol (2014) 192(10):4674–84. doi: 10.4049/jimmunol.1302318
47. Hwaiz R, Rahman M, Zhang E, Thorlacius H. Platelet Secretion of Cxcl4 Is Rac1-Dependent and Regulates Neutrophil Infiltration and Tissue Damage in Septic Lung Damage. Br J Pharmacol (2015) 172(22):5347–59. doi: 10.1111/bph.13325
48. Kimura S, Ozaki KS, Ueki S, Zhang M, Yokota S, Stolz DB, et al. Contribution of Alloantigens to Hepatic Ischemia/Reperfusion Injury: Roles of Natural Killer Cells and Innate Immune Recognition of Nonself. Liver Transpl (2016) 22(1):80–90. doi: 10.1002/lt.24330
49. Nakajima H, Mizuta N, Fujiwara I, Sakaguchi K, Ogata H, Magae J, et al. Blockade of the Fas/Fas Ligand Interaction Suppresses Hepatocyte Apoptosis in Ischemia-Reperfusion Rat Liver. Apoptosis (2008) 13(8):1013–21. doi: 10.1007/s10495-008-0234-5
50. Feng M, Li G, Qian X, Fan Y, Huang X, Zhang F, et al. Il-17a-Producing Nk Cells Were Implicated in Liver Injury Induced by Ischemia and Reperfusion. Int Immunopharmacol (2012) 13(2):135–40. doi: 10.1016/j.intimp.2012.03.007
51. Man K, Ng KT, Lo CM, Ho JW, Sun BS, Sun CK, et al. Ischemia-Reperfusion of Small Liver Remnant Promotes Liver Tumor Growth and Metastases–Activation of Cell Invasion and Migration Pathways. Liver Transpl (2007) 13(12):1669–77. doi: 10.1002/lt.21193
52. Orci LA, Lacotte S, Oldani G, Morel P, Mentha G, Toso C. The Role of Hepatic Ischemia-Reperfusion Injury and Liver Parenchymal Quality on Cancer Recurrence. Dig Dis Sci (2014) 59(9):2058–68. doi: 10.1007/s10620-014-3182-7
53. Blander JM. The Many Ways Tissue Phagocytes Respond to Dying Cells. Immunol Rev (2017) 277(1):158–73. doi: 10.1111/imr.12537
54. Bosurgi L, Cao YG, Cabeza-Cabrerizo M, Tucci A, Hughes LD, Kong Y, et al. Macrophage Function in Tissue Repair and Remodeling Requires Il-4 or Il-13 With Apoptotic Cells. Science (2017) 356(6342):1072–6. doi: 10.1126/science.aai8132
55. Soehnlein O, Lindbom L. Phagocyte Partnership During the Onset and Resolution of Inflammation. Nat Rev Immunol (2010) 10(6):427–39. doi: 10.1038/nri2779
56. Wang J. Neutrophils in Tissue Injury and Repair. Cell Tissue Res (2018) 371(3):531–9. doi: 10.1007/s00441-017-2785-7
57. Scalea JR, Lee YS, Davila E, Bromberg JS. Myeloid-Derived Suppressor Cells and Their Potential Application in Transplantation. Transplantation (2018) 102(3):359–67. doi: 10.1097/tp.0000000000002022
58. Minami E, Laflamme MA, Saffitz JE, Murry CE. Extracardiac Progenitor Cells Repopulate Most Major Cell Types in the Transplanted Human Heart. Circulation (2005) 112(19):2951–8. doi: 10.1161/circulationaha.105.576017
59. Kerkar SP, Restifo NP. Cellular Constituents of Immune Escape Within the Tumor Microenvironment. Cancer Res (2012) 72(13):3125–30. doi: 10.1158/0008-5472.Can-11-4094
60. De Cicco P, Ercolano G, Ianaro A. The New Era of Cancer Immunotherapy: Targeting Myeloid-Derived Suppressor Cells to Overcome Immune Evasion. Front Immunol (2020) 11:1680. doi: 10.3389/fimmu.2020.01680
61. Kwon HK, Chen HM, Mathis D, Benoist C. Different Molecular Complexes That Mediate Transcriptional Induction and Repression by Foxp3. Nat Immunol (2017) 18(11):1238–48. doi: 10.1038/ni.3835
62. Faustino LD, Griffith JW, Rahimi RA, Nepal K, Hamilos DL, Cho JL, et al. Interleukin-33 Activates Regulatory T Cells to Suppress Innate Γδ T Cell Responses in the Lung. Nat Immunol (2020) 21(11):1371–83. doi: 10.1038/s41590-020-0785-3
63. Li C, Jiang P, Wei S, Xu X, Wang J. Regulatory T Cells in Tumor Microenvironment: New Mechanisms, Potential Therapeutic Strategies and Future Prospects. Mol Cancer (2020) 19(1):116. doi: 10.1186/s12943-020-01234-1
64. Sullivan JA, Tomita Y, Jankowska-Gan E, Lema DA, Arvedson MP, Nair A, et al. Treg-Cell-Derived Il-35-Coated Extracellular Vesicles Promote Infectious Tolerance. Cell Rep (2020) 30(4):1039–51.e5. doi: 10.1016/j.celrep.2019.12.081
65. Fujimura T, Ring S, Umansky V, Mahnke K, Enk AH. Regulatory T Cells Stimulate B7-H1 Expression in Myeloid-Derived Suppressor Cells in Ret Melanomas. J Invest Dermatol (2012) 132(4):1239–46. doi: 10.1038/jid.2011.416
66. Lee CR, Kwak Y, Yang T, Han JH, Park SH, Ye MB, et al. Myeloid-Derived Suppressor Cells Are Controlled by Regulatory T Cells Via Tgf-B During Murine Colitis. Cell Rep (2016) 17(12):3219–32. doi: 10.1016/j.celrep.2016.11.062
67. Hoechst B, Ormandy LA, Ballmaier M, Lehner F, Krüger C, Manns MP, et al. A New Population of Myeloid-Derived Suppressor Cells in Hepatocellular Carcinoma Patients Induces Cd4(+)Cd25(+)Foxp3(+) T Cells. Gastroenterology (2008) 135(1):234–43. doi: 10.1053/j.gastro.2008.03.020
68. Viguier M, Lemaître F, Verola O, Cho MS, Gorochov G, Dubertret L, et al. Foxp3 Expressing Cd4+Cd25(High) Regulatory T Cells Are Overrepresented in Human Metastatic Melanoma Lymph Nodes and Inhibit the Function of Infiltrating T Cells. J Immunol (2004) 173(2):1444–53. doi: 10.4049/jimmunol.173.2.1444
69. Shimizu J, Yamazaki S, Sakaguchi S. Induction of Tumor Immunity by Removing Cd25+Cd4+ T Cells: A Common Basis Between Tumor Immunity and Autoimmunity. J Immunol (1999) 163(10):5211–8.
70. Reinisch A, Hofmann NA, Obenauf AC, Kashofer K, Rohde E, Schallmoser K, et al. Humanized Large-Scale Expanded Endothelial Colony-Forming Cells Function in Vitro and in Vivo. Blood (2009) 113(26):6716–25. doi: 10.1182/blood-2008-09-181362
71. Inoue T, Sata M, Hikichi Y, Sohma R, Fukuda D, Uchida T, et al. Mobilization of Cd34-Positive Bone Marrow-Derived Cells After Coronary Stent Implantation: Impact on Restenosis. Circulation (2007) 115(5):553–61. doi: 10.1161/circulationaha.106.621714
72. de la Puente P, Muz B, Azab F, Azab AK. Cell Trafficking of Endothelial Progenitor Cells in Tumor Progression. Clin Cancer Res (2013) 19(13):3360–8. doi: 10.1158/1078-0432.Ccr-13-0462
73. Gao D, Nolan D, McDonnell K, Vahdat L, Benezra R, Altorki N, et al. Bone Marrow-Derived Endothelial Progenitor Cells Contribute to the Angiogenic Switch in Tumor Growth and Metastatic Progression. Biochim Biophys Acta (2009) 1796(1):33–40. doi: 10.1016/j.bbcan.2009.05.001
74. Ruzinova MB, Schoer RA, Gerald W, Egan JE, Pandolfi PP, Rafii S, et al. Effect of Angiogenesis Inhibition by Id Loss and the Contribution of Bone-Marrow-Derived Endothelial Cells in Spontaneous Murine Tumors. Cancer Cell (2003) 4(4):277–89. doi: 10.1016/s1535-6108(03)00240-x
75. Peters BA, Diaz LA, Polyak K, Meszler L, Romans K, Guinan EC, et al. Contribution of Bone Marrow-Derived Endothelial Cells to Human Tumor Vasculature. Nat Med (2005) 11(3):261–2. doi: 10.1038/nm1200
76. Lyden D, Hattori K, Dias S, Costa C, Blaikie P, Butros L, et al. Impaired Recruitment of Bone-Marrow-Derived Endothelial and Hematopoietic Precursor Cells Blocks Tumor Angiogenesis and Growth. Nat Med (2001) 7(11):1194–201. doi: 10.1038/nm1101-1194
77. Colletti LM, Kunkel SL, Walz A, Burdick MD, Kunkel RG, Wilke CA, et al. The Role of Cytokine Networks in the Local Liver Injury Following Hepatic Ischemia/Reperfusion in the Rat. Hepatology (1996) 23(3):506–14. doi: 10.1002/hep.510230315
78. Liu H, Ling CC, Yeung WHO, Pang L, Liu J, Zhou J, et al. Monocytic Mdsc Mobilization Promotes Tumor Recurrence After Liver Transplantation Via Cxcl10/Tlr4/Mmp14 Signaling. Cell Death Dis (2021) 12(5):489. doi: 10.1038/s41419-021-03788-4
79. Ling CC, Ng KT, Shao Y, Geng W, Xiao JW, Liu H, et al. Post-Transplant Endothelial Progenitor Cell Mobilization Via Cxcl10/Cxcr3 Signaling Promotes Liver Tumor Growth. J Hepatol (2014) 60(1):103–9. doi: 10.1016/j.jhep.2013.08.017
80. Li CX, Ling CC, Shao Y, Xu A, Li XC, Ng KT, et al. Cxcl10/Cxcr3 Signaling Mobilized-Regulatory T Cells Promote Liver Tumor Recurrence After Transplantation. J Hepatol (2016) 65(5):944–52. doi: 10.1016/j.jhep.2016.05.032
81. Strader CR, Pearce CJ, Oberlies NH. Fingolimod (Fty720): A Recently Approved Multiple Sclerosis Drug Based on a Fungal Secondary Metabolite. J Nat Prod (2011) 74(4):900–7. doi: 10.1021/np2000528
82. Stone ML, Sharma AK, Zhao Y, Charles EJ, Huerter ME, Johnston WF, et al. Sphingosine-1-Phosphate Receptor 1 Agonism Attenuates Lung Ischemia-Reperfusion Injury. Am J Physiol Lung Cell Mol Physiol (2015) 308(12):L1245–52. doi: 10.1152/ajplung.00302.2014
83. Foster AD, Vicente D, Clark N, Leonhardt C, Elster EA, Davis TA, et al. Fty720 Effects on Inflammation and Liver Damage in a Rat Model of Renal Ischemia-Reperfusion Injury. Mediators Inflammation (2019) 2019:3496836. doi: 10.1155/2019/3496836
84. Man K, Ng KT, Lee TK, Lo CM, Sun CK, Li XL, et al. Fty720 Attenuates Hepatic Ischemia-Reperfusion Injury in Normal and Cirrhotic Livers. Am J Transplant (2005) 5(1):40–9. doi: 10.1111/j.1600-6143.2004.00642.x
85. Lee TK, Man K, Ho JW, Wang XH, Poon RT, Xu Y, et al. Fty720: A Promising Agent for Treatment of Metastatic Hepatocellular Carcinoma. Clin Cancer Res (2005) 11(23):8458–66. doi: 10.1158/1078-0432.Ccr-05-0447
86. Azuma H, Takahara S, Horie S, Muto S, Otsuki Y, Katsuoka Y. Induction of Apoptosis in Human Bladder Cancer Cells in Vitro and in Vivo Caused by Fty720 Treatment. J Urol (2003) 169(6):2372–7. doi: 10.1097/01.ju.0000064938.32318.91
87. Azuma H, Takahara S, Ichimaru N, Wang JD, Itoh Y, Otsuki Y, et al. Marked Prevention of Tumor Growth and Metastasis by a Novel Immunosuppressive Agent, Fty720, in Mouse Breast Cancer Models. Cancer Res (2002) 62(5):1410–9.
88. Zhou C, Ling MT, Kin-Wah Lee T, Man K, Wang X, Wong YC. Fty720, a Fungus Metabolite, Inhibits Invasion Ability of Androgen-Independent Prostate Cancer Cells Through Inactivation of Rhoa-Gtpase. Cancer Lett (2006) 233(1):36–47. doi: 10.1016/j.canlet.2005.02.039
89. Li CX, Shao Y, Ng KT, Liu XB, Ling CC, Ma YY, et al. Fty720 Suppresses Liver Tumor Metastasis by Reducing the Population of Circulating Endothelial Progenitor Cells. PloS One (2012) 7(2):e32380. doi: 10.1371/journal.pone.0032380
90. Li CX, Yang XX, Wang HW, Li XC, Ng KT, Lo CM, et al. Fty720 Suppresses Liver Tumor Growth and Metastasis by Reducing Circulating Regulating T Cells and Enhancing the Anti-Tumor Effect of Rapamycin. Onco Targets Ther (2020) 13:4743–54. doi: 10.2147/ott.S234394
91. Li CX, Wong BL, Ling CC, Ma YY, Shao Y, Geng W, et al. A Novel Oxygen Carrier "Yq23" Suppresses the Liver Tumor Metastasis by Decreasing Circulating Endothelial Progenitor Cells and Regulatory T Cells. BMC Cancer (2014) 14:293. doi: 10.1186/1471-2407-14-293
92. Uotani H, Yamashita I, Nagata T, Kishimoto H, Kashii Y, Tsukada K. Induction of E-Selectin After Partial Hepatectomy Promotes Metastases to Liver in Mice. J Surg Res (2001) 96(2):197–203. doi: 10.1006/jsre.2001.6095
93. Tamagawa K, Horiuchi T, Uchinami M, Doi K, Yoshida M, Nakamura T, et al. Hepatic Ischemia-Reperfusion Increases Vascular Endothelial Growth Factor and Cancer Growth in Rats. J Surg Res (2008) 148(2):158–63. doi: 10.1016/j.jss.2007.12.787
94. Cuzzocrea S. Peroxisome Proliferator-Activated Receptors Gamma Ligands and Ischemia and Reperfusion Injury. Vascul Pharmacol (2004) 41(6):187–95. doi: 10.1016/j.vph.2004.10.004
95. Wu QD, Wang JH, Condron C, Bouchier-Hayes D, Redmond HP. Human Neutrophils Facilitate Tumor Cell Transendothelial Migration. Am J Physiol Cell Physiol (2001) 280(4):C814–22. doi: 10.1152/ajpcell.2001.280.4.C814
96. Baud V, Karin M. Is Nf-Kappab a Good Target for Cancer Therapy? Hopes and Pitfalls. Nat Rev Drug Discovery (2009) 8(1):33–40. doi: 10.1038/nrd2781
97. Rogers AB, Fox JG. Inflammation and Cancer. I. Rodent Models of Infectious Gastrointestinal and Liver Cancer. Am J Physiol Gastrointest Liver Physiol (2004) 286(3):G361–6. doi: 10.1152/ajpgi.00499.2003
98. Liu YI, Liu Z, Chen Y, Xu K, Dong J. Pparγ Activation Reduces Ischemia/Reperfusion-Induced Metastasis in a Murine Model of Hepatocellular Carcinoma. Exp Ther Med (2016) 11(2):387–96. doi: 10.3892/etm.2015.2934
99. Nishizawa N, Ito Y, Eshima K, Ohkubo H, Kojo K, Inoue T, et al. Inhibition of Microsomal Prostaglandin E Synthase-1 Facilitates Liver Repair After Hepatic Injury in Mice. J Hepatol (2018) 69(1):110–20. doi: 10.1016/j.jhep.2018.02.009
100. Hossain MA, Wakabayashi H, Izuishi K, Okano K, Yachida S, Maeta H. The Role of Prostaglandins in Liver Ischemia-Reperfusion Injury. Curr Pharm Des (2006) 12(23):2935–51. doi: 10.2174/138161206777947678
101. Hsieh CC, Hsieh SC, Chiu JH, Wu YL. Protective Effects of N-Acetylcysteine and a Prostaglandin E1 Analog, Alprostadil, Against Hepatic Ischemia: Reperfusion Injury in Rats. J Tradit Complement Med (2014) 4(1):64–71. doi: 10.4103/2225-4110.124351
102. Kornberg A, Witt U, Kornberg J, Friess H, Thrum K. Treating Ischaemia-Reperfusion Injury With Prostaglandin E1 Reduces the Risk of Early Hepatocellular Carcinoma Recurrence Following Liver Transplantation. Aliment Pharmacol Ther (2015) 42(9):1101–10. doi: 10.1111/apt.13380
103. Qi X, Ng KT, Shao Y, Li CX, Geng W, Ling CC, et al. The Clinical Significance and Potential Therapeutic Role of Gpx3 in Tumor Recurrence After Liver Transplantation. Theranostics (2016) 6(11):1934–46. doi: 10.7150/thno.16023
104. Bimonte S, Albino V, Barbieri A, Tamma ML, Nasto A, Palaia R, et al. Dissecting the Roles of Thymoquinone on the Prevention and the Treatment of Hepatocellular Carcinoma: An Overview on the Current State of Knowledge. Infect Agent Cancer (2019) 14:10. doi: 10.1186/s13027-019-0226-9
Keywords: hepatic, ischemia/reperfusion injury, inflammation, hepatocellular carcinoma, liver transplantation, liver resection
Citation: Chen H, Lu D, Yang X, Hu Z, He C, Li H, Lin Z, Yang M and Xu X (2022) One Shoot, Two Birds: Alleviating Inflammation Caused by Ischemia/Reperfusion Injury to Reduce the Recurrence of Hepatocellular Carcinoma. Front. Immunol. 13:879552. doi: 10.3389/fimmu.2022.879552
Received: 19 February 2022; Accepted: 15 April 2022;
Published: 11 May 2022.
Edited by:
Tao Qiu, Renmin Hospital of Wuhan University, ChinaReviewed by:
Arshi Khanam, University of Maryland, United StatesCopyright © 2022 Chen, Lu, Yang, Hu, He, Li, Lin, Yang and Xu. This is an open-access article distributed under the terms of the Creative Commons Attribution License (CC BY). The use, distribution or reproduction in other forums is permitted, provided the original author(s) and the copyright owner(s) are credited and that the original publication in this journal is cited, in accordance with accepted academic practice. No use, distribution or reproduction is permitted which does not comply with these terms.
*Correspondence: Xiao Xu, emp4dUB6anUuZWR1LmNu
†These authors have contributed equally to this work
Disclaimer: All claims expressed in this article are solely those of the authors and do not necessarily represent those of their affiliated organizations, or those of the publisher, the editors and the reviewers. Any product that may be evaluated in this article or claim that may be made by its manufacturer is not guaranteed or endorsed by the publisher.
Research integrity at Frontiers
Learn more about the work of our research integrity team to safeguard the quality of each article we publish.