- 1Shiseido Global Innovation Center, Yokohama, Japan
- 2Center for Cancer Immunology and Cutaneous Biology Research Center, Department of Dermatology and Center for Cancer Research, Massachusetts General Hospital and Harvard Medical School, Boston, MA, United States
Skin acts as the primary interface between the body and the environment. The skin immune system is composed of a complex network of immune cells and factors that provide the first line of defense against microbial pathogens and environmental insults. Alarmin cytokines mediate an intricate intercellular communication between keratinocytes and immune cells to regulate cutaneous immune responses. Proper functions of the type 2 alarmin cytokines, thymic stromal lymphopoietin (TSLP), interleukin (IL)-25, and IL-33, are paramount to the maintenance of skin homeostasis, and their dysregulation is commonly associated with allergic inflammation. In this review, we discuss recent findings on the complex regulatory network of type 2 alarmin cytokines that control skin immunity and highlight the mechanisms by which these cytokines regulate skin immune responses in host defense, chronic inflammation, and cancer.
Introduction
Skin is the largest organ and acts as a protective barrier separating the body from the outside environment (1). Epidermis, dermis, and subcutaneous fat together with skin appendages, sweat glands, sebaceous glands, and hair follicles, form an integrated structure that enables proper skin function (2). The foremost physical barrier of the skin consists of the epidermis, which in its outermost layer is composed of brick and mortar-like stratum corneum and tight junctions that regulate the inward and outward passage of fluid and electrolytes in and out of the skin (1, 3). The skin has developed complex protective functions against constant exposure to various environmental insults, such as solar radiation, pollutants, a broad range of microbial pathogens, and allergens (4, 5). The immune system contributes to this first line of defense against microbial pathogens and chemical insults (2).
In the epidermis, Langerhans cells (LCs) are epidermal-resident antigen-presenting cells (APCs), which play a sentinel role as the first professional immune cells confronting the environmental insults (6, 7). Activated LCs capture foreign antigens by extending their dendrites through epidermal tight junctions. Next, they migrate to the lymph nodes to initiate cutaneous adaptive immunity (8, 9). In addition, tissue-resident memory T cells (TRM cells), which are noncirculating lymphocytes in peripheral tissues, persist in the epidermis to provide long-lasting protective defense against future immunological challenges at the most probable sites of invasion (10–12). The dermis is the stromal layer below the epidermis that encompasses an active immunological microenvironment (2). The superficial papillary dermis is composed of a relatively loose connective tissue and contains vessels and sensory nerves (13). A diverse range of immune cell types, including several T cell subsets, macrophages, dendritic cells (DCs), innate lymphoid cells (ILCs), and mast cells, is localized around vasculature and extracellular matrix proteins in the dermis (14–16). This complex network of dermal immune cells helps in many aspects of host physiology, including protection against pathogens, wound healing (17), sebum production (18), and hair follicle homeostasis (19).
Cytokine-mediated communication allows immune cells to achieve a context-appropriate response, as the homeostasis of a multi-cellular organism is made possible by the proper cell-cell communication across different cell types (20). The cytokine superfamily consists of many ligands and receptors that mediate key interactions between immune cells and non-immune cells, including keratinocytes, fibroblasts, and endothelial cells, in the skin microenvironment (21–23). As the primary barrier, which is constantly exposed to environmental insults, the epidermis functions as a key sensor and integrator of environmental cues to regulate immunity in the skin (24, 25). As such, epidermis-derived alarmin cytokines mediate an intricate intercellular communication between epidermal keratinocytes and immune cells to regulate cutaneous immune surveillance. Alarmins are endogenous molecules that function as danger signals and are rapidly released to the extracellular milieu in response to tissue damage to trigger defensive immune responses (26). Among them, the proper functions of type 2 alarmin cytokines, thymic stromal lymphopoietin (TSLP), interleukin (IL)-25 and IL-33, as central orchestrators of T helper 2 (Th2) immunity, are paramount to the skin homeostasis and their dysregulation is commonly associated with chronic allergic inflammation (27–29).
In this review, we discuss recent findings on the complex functions of type 2 alarmin cytokines in regulating epithelium-immune cell communication that governs host defense, chronic inflammation, and cancer.
TSLP
TSLP is a member of the IL-2 family of cytokines, and its receptor is a heterodimer that consists of the IL-7 receptor α chain (IL-7Rα), which is shared with IL-7, and the TSLP receptor (TSLPR) (30–32). TSLP is mainly expressed by the epithelial cells of the gut, lung, and skin. Other cell types including DCs, basophils, and mast cells can express TSLP (33, 34). At the organ level, TSLP is widely distributed in several organs including the heart, liver, testis, spleen, prostate, skin, lung, kidney, ovary, small intestine, and colon (35). A variety of endogenous and environmental factors, such as pro-inflammatory cytokines, tryptase, invading pathogens, allergens, irritants, pollutants, and cigarette smoke, stimulate epithelial cells to release TSLP at barrier surfaces (36–45). Activation of protease-activated receptor 2 (PAR2), Toll-like receptor 4 (TLR4), and a member of transient receptor potential vanilloid (TRPV) channel family, including TRPV1, on the cell membrane mediate the production of TSLP through transcription factors, nuclear factor of activated T cells (NFAT), nuclear factor-kappa B (NF-κB), and interferon regulatory factor 3 (IRF-3) (46–48). TSLP is post-translationally modified by endogenous proteases, and cleaved TSLP has an increased biological activity (49). Despite poor sequence homology of TSLP (43% amino acid identity) and TSLPR (39% amino acid identity) between humans and mice, TSLP has been shown to exhibit similar biological functions in humans and mice (50).
Hematopoietic cell populations and sensory neurons express TSLPR. TSLP first interacts with the cognate TSLPR, then IL-7Rα can be recruited to the TSLP/TSLPR assembly to form the extracellular ternary complex. This leads to the activation of an intricate network of signaling pathways, including Janus kinase/signal transducer activator of transcription (JAK/STAT) and phosphatidylinositol-3 kinase (PI3K) pathways (51). Similar to IL-7, this signaling plays a critical role in the activation and differentiation of immune cells, such as B cells and T cells (52). In contrast, TSLP also mediates Th2 immunity associated with protection from helminth parasites and the pathogenesis of allergic inflammation at barrier surfaces. TSLP strongly induces the expression of major histocompatibility complex (MHC) class I and II molecules and costimulatory molecules on DCs. TSLP-activated DCs produce Th2-attracting chemokines, such as CCL17 and CCL22 (53), and induce Th2 differentiation through OX40 ligand upregulation on these cells (54, 55). Furthermore, TSLP can directly activate naïve CD4+ T cells to differentiate and promote Th2 effector function in a TCR-dependent manner (56–58), indicating that TSLP is a key driver of Th2 immunity. In addition, TSLP acts on CD4+ T cells to promote T helper 9 (Th9) differentiation and function through STAT5 activation in airway inflammation (59). Th9 cells, IL-9 producing CD4+ T cells, are closely related to Th2 cells and contribute to allergic inflammation and anti-tumor immunity (60, 61). It remains unclear whether these cells represent a truly unique Th cell subset. TSLP also acts on basophils and group 2 ILCs (ILC2s) to induce Th2 responses (62, 63). Thus, TSLP orchestrates type 2 immune responses by innate and adaptive immune cells at barrier sites.
TSLP in Skin
Keratinocytes are a powerful source of TSLP in the skin under chronic and severe barrier disruption (64, 65). TSLP is released from keratinocytes in response to cutaneous pathogens, such as Staphylococcus aureus (66) and Malassezia yeasts (67), and environmental stimuli, such as ultraviolet radiation (68), mechanical injury (41), and air pollutants (69). The thermosensitive transient receptor potential channels TRPV3 and TRPV4 (70, 71), pattern recognition receptor TLR3 (72, 73), and PAR2 (38, 74) are among the receptors that can sense the external stimuli and induce the production of TSLP in keratinocytes. Vitamin D3 also induces the expression of TSLP in keratinocytes, which leads to the development of an atopic dermatitis-like phenotype (75). In contrast, TSLP is negatively regulated by retinoid X receptor αβ (RXRαβ) (76), aryl hydrocarbon receptor (AhR) (77), and Notch signaling (64), which function as a gatekeeper in keratinocytes. Chronic TSLP release by keratinocytes responding to cellular and tissue damage instigates type 2 immune response that leads to atopic dermatitis. High TSLP expression is observed in a broad spectrum of skin lesions of atopic dermatitis (53), psoriasis (78), Netherton syndrome (79), and keloid (80). Continuous skin barrier disruption, characterized by atopic dermatitis, facilitates epicutaneous sensitization, which accelerates TSLP expression in keratinocytes. Furthermore, TSLP promoter demethylation is detected in skin lesions from patients with atopic dermatitis (81).
TSLPR is broadly expressed by immune cells and sensory neurons in the skin. In particular, DCs are important TSLP-responsive immune cell populations (27, 53–55, 82). Several DC subsets, including epidermal LCs and dermal type 1 and 2 conventional DCs, respond to keratinocytes-derived TSLP signals to initiate cutaneous adaptive immunity and provide multiple soluble and surface-bound signals that help to guide T cell differentiation, in particular Th2 cells (83–86). In atopic dermatitis lesions, TSLP may contribute to the activation of LCs, which then migrate to the draining lymph nodes and prime allergen-specific Th2 responses (53). In addition, TSLP-mediated LC activation can promote the differentiation of CD4+ T cells into Th2 and follicular helper T cells (Tfh cells), which are important regulators of humoral responses (87). Moreover, TSLP and transforming growth factor-β1 (TGF-β1) synergistically contribute to the pool of LCs during inflammation via the promotion of LC differentiation from human blood BDCA-1+ DCs (88). Dermal DCs act as critical responders in TSLP-mediated type 2 allergic inflammatory responses. TSLP activates CCL17-producing CD11b+ dermal DCs to migrate to draining lymph nodes and attract naïve CD4+ T cells to differentiate into Th2 cells during contact hypersensitivity in mice (89). Furthermore, TSLP-stimulated DCs act not only on the priming of Th2 cells but also on the maintenance and further polarization of Th2 central memory cells in allergic inflammation (90). Besides Th2 priming, TSLP-mediated DC activation conducts multiple CD4+ T cell fate specifications in the skin, depending on the surrounding inflammatory microenvironment. TSLP drives the differentiation of IL-21-producing human Tfh cells through OX40 ligand in CD1c+ DCs and helps memory B cells to produce immunoglobulin G (IgG) and IgE in a Th2 cell-dominated environment (91). Furthermore, TSLP is highly produced by keratinocytes in patients with psoriasis, where it synergizes with CD40 ligand in skin DCs to promote the expression of the Th17-polarizing cytokine IL-23 (78).
TSLP can directly activate CD4+ T cells and induce the differentiation of a distinct population of effector Th2 cells in lymph nodes (58). TSLP/TSLPR signaling amplifies IL-4 production from CD4+ T cells, which results in driving a positive feedback loop between TSLP and IL-4 to exacerbate Th2 cell-mediated allergic inflammation (92). In fact, atopic dermatitis patients possess circulating CD4+ T cells expressing high TSLPR levels, and the frequency of this subset correlates with the severity of atopic dermatitis (93). In addition, Th2 cells express more TSLPR than Th1 or Th17 cells (94). ILC2s are also important targets of TSLP in allergic inflammation (95). TSLP-elicited ILC2 promotes allergic inflammation, whereas IL-25 and IL-33 are dispensable for this ILC2 response in an atopic dermatitis-like mouse model (95). Although IL-25 and IL-33 are critical mediators to elicit ILC2s in the gut and lung for anti-helminth immunity and allergic inflammation (96), skin-specific ILCs may have distinct properties. Accordingly, transcriptomic heterogeneity of ILC2s is apparent across tissues, and skin-resident ILC2s express relatively low levels of receptors of type 2 cytokines, such as TSLP, IL-25, and IL-33, and instead dominantly express IL-18 receptor (IL-18R1), compared with other tissues (97, 98). Indeed, IL-18 can activate skin ILC2s and synergize with type 2 cytokines in the development of atopic dermatitis-like disease (98). Furthermore, two distinct populations of ILC2s, consisting of skin-resident and circulating ILC2s, exist in the murine skin, and they exhibit distinctive phenotypes and functions (99). The response of ILC2s may ultimately depend on the nature of the inflammatory stimulus in the microenvironment.
Keratinocytes-released TSLP signals directly reach out to sensory neurons in the skin. PAR2-triggered release of TSLP can stimulate sensory neurons to evoke the itch response in allergic diseases such as atopic dermatitis, in a TSLPR- and TRPA1-dependent manner (38). In addition, TRPV4 triggers TSLP release, which activates sensory neurons through TSLPR and TRPV4 in a dry skin-induced pruritus model (71). TSLP is found to be involved in the later phase of itch progression in allergic inflammation while neutrophil-derived CXCL10 drives itch in the acute phase, which is mediated through CXCR3 on sensory neurons (100). TSLP widely impacts a broad array of dermal immune cells in the skin. TSLP elicits skin basophils, and TSLP-dependent basophil-derived IL-4 promotes ILC2 responses during atopic dermatitis-like inflammation (101). Furthermore, TSLP induces mast cell development through the activation of mouse double minute 2 (MDM2) and STAT6, which results in skin allergic inflammation (45, 102).
Excessive TSLP that is secreted by barrier-defective skin into the systemic circulation leads to sensitization of the lung airways to inhaled allergens characterized by allergic asthma-like phenotype in mice (103, 104). Thus, high systemic levels of skin-derived TSLP instigate the atopic march whereas IL-25 does not (105). Meanwhile, regulatory T cell (Treg)-mediated immunosuppression directly by TSLP from keratinocytes protects against progression from a local skin inflammatory response to a lethal systemic condition (106). TSLP has a dual function as a pro-inflammatory and pro-homeostatic modulator, and this may depend at least in part on the nature of surrounding immune signals and the type of cells responding to TSLP in the tissue microenvironment.
IL-25
IL-25 (also known as IL-17E) belongs to the IL-17 cytokine family, which consists of six members, and shares relatively low sequence similarity to the prototype member, IL-17 (alternative name IL-17A) (107–109). IL-25 receptor (IL-25R) is a heterodimer of the IL-17RA chain, which is shared with other IL-17 family members, and the IL-25-specific IL-17RB chain (108). Therefore, IL-25 exhibits a distinct function from other members of the IL-17 cytokine family and has been implicated as a type 2 cytokine that induces the production of IL-4, IL-5, and IL-13, which in turn inhibit the IL-17-dependent autoimmune diseases (110). Furthermore, IL-25 enhances Th9 cell response to prevent parasitic helminths infection (111, 112). IL-25 is produced by epithelial and immune cells including Th2 cells, macrophages, ILC2s, mast cells, basophils, and eosinophils (109, 113). In the extracellular space, IL-25 has been reported to be a substrate for proteolytic cleavage by matrix metalloproteinase-7 (MMP-7) from airway epithelial cells during inflammation, and cleaved IL-25 increases its activity to induce type 2 cytokines (114). Expression of IL-25R has been reported on non-immune cells, including fibroblasts and endothelial cells, and immune cells, such as Th2 cells, natural killer T cells (NKT cells), DCs, macrophages, ILC2s, mast cells, basophils, and eosinophils in the inflammatory state (115, 116).
IL-25 in Skin
IL-25 has been reported to be highly expressed in several skin inflammatory diseases, including atopic dermatitis (117), psoriasis (118), pyoderma gangrenosum (119), acute generalized exanthematous pustulosis (119), and cutaneous T-cell lymphoma (CTCL) (120). IL-4, IL-13, IL-22, endothelin-1, and periostin enhance the production of IL-25 from keratinocytes (120–122). IL-25 induces allergic skin inflammation, characterized by elevated expression of IL-4 and IL-5, dermal infiltration of immune cells, epidermal hyperplasia, and impairment of skin barrier function in mice (118). Epidermal keratinocytes-derived IL-25 is a central regulator of a broad array of allergic inflammatory responses, and the major targets of IL-25 are dermal ILC2s and macrophages in the skin (123). IL-25 activates ILC2s to promote IL-13 production, which in turn helps keratinocytes proliferate and produce immune cell-attracting chemokines (124, 125). IL-25 also promotes the recruitment of neutrophils via activation of macrophages in a p38-dependent manner (119). On the other hand, IL-25 responds to tissue injury and participates in cutaneous would healing through an amelioration of angiogenesis and collagen deposition in diabetic mice model (126).
The autocrine function of IL-25 in keratinocytes promotes proliferation and inflammatory responses via STAT3 transcriptional factor, which results in amplification of psoriatic skin inflammation (118). Unlike IL-17, IL-25 is not capable of inducing antimicrobial peptides, β-defensin 2 and LL-37, in keratinocytes (121). IL-25 acts synergistically with Th2 cytokines, IL-4 and IL-13, to down-regulate filaggrin expression in keratinocytes exacerbating skin barrier defects (127). Down-regulation of filaggrin expression by IL-25 is mediated at least in part through the activation of NF-κB (107), whereas Th2 cytokines activate STAT6 (128, 129). In the fluorescein isothiocyanate-induced contact hypersensitivity model, IL-25 induces hapten-specific Th17 immunity, rather than Th2 immunity, in the elicitation phase of contact hypersensitivity (130). Following the hapten challenge, dermal DCs release IL-1β in response to IL-25, and IL-1β directly activates Th17 cells. This contrasts with the observed role of TSLP in DC activation and hapten-specific Th2 cell differentiation in the sensitization phase of contact hypersensitivity (131). These findings indicate that type 2 alarmin cytokines have distinct mechanisms for the regulation of T cell responses during inflammation.
IL-33
IL-33 is the most recently discovered member of type 2 alarmin cytokines (132). IL-33 was first described in 2005. It belongs to the IL-1 family of cytokines, which includes IL-1α, IL-1β, IL-18, IL-36α, IL-36β, IL-36γ, and IL-37, and the receptor antagonists IL-1Ra, IL-36Ra, and IL-38 (133). In contrast to its other family members, IL-1 and IL-18, IL-33 has been shown to promote Th2 cytokine responses in helminth infection and allergic inflammation (132). IL-33 is mainly expressed by non-immune cells, including epithelial cells, endothelial cells, and fibroblasts. It can also be expressed by immune cells, including macrophages and mast cells, at the barrier sites, where it functions as an alarmin following tissue damage (134, 135). IL-33 is localized in the cell nucleus, and its N-terminal domain, which includes a chromatin-binding motif, is required for its nuclear localization (136). Unlike IL-1β and IL-18, the N-terminal portion of IL-33 does not require inflammasome-mediated cleavage by caspase-1 for extracellular release of the active form. Apoptosis-associated caspase-3 and caspase-7 cleave and inactivate IL-33 at a conserved residue, Asp178 (Asp175 in mouse), within the IL-1-like cytokine domain (137). On the other hand, N-terminal processing of extracellular full-length IL-33 can occur in the central domain between the nuclear domain and the IL-1-like cytokine domain. These residues are targeted by extracellular proteases in the inflammatory microenvironment, including neutrophil cathepsin G, neutrophil elastase, and mast cell serine proteases, and the resulting 18-21 kDa cytokine forms of IL-33 exhibit higher biological activity (138, 139). Full-length IL-33 can be rapidly cleaved in its central sensor domain by extracellular environmental allergens-derived proteases within 10-20 minutes (140). In contrast, cysteine oxidation of extracellular IL-33 diminishes its biological activity (141). Thus, following IL-33 release, the impact of IL-33 is tightly regulated by post-translational modifications in the extracellular milieu. Although IL-33 lacks a secretion sequence and is sequestered in the nucleus via chromatin binding, IL-33 seems to be released into extracellular space through an unconventional secretion pathway following various stimuli. Environmental allergens, including fungi and mites, and mechanical stress trigger the release of IL-33 (142–145). Two primary scenarios for IL-33 release have been proposed: passive release as alarmin from necrotic cells during tissue damage and unconventional secretion from living cells. A recent study suggests that IL-33 is secreted through the extracellular vesicles pathway, commonly referred to as exosomes, as surface-bound cargo, from living airway epithelial cells (146). However, the molecular mechanisms and pathways of IL-33 secretion in living cells remain unclear.
IL-33 binds to its receptor, suppressor of tumorigenesis 2 (ST2), on target cells (147, 148). ST2 is classified as a member of the IL-1 receptor superfamily, which has a common intracellular domain, known as the Toll/Interleukin-1 receptor (TIR) domain (147, 148). IL-33 signals via its cognate receptor ST2, which is highly expressed on Th2 cells, ILC2s, and mast cells, and induces Th2-skewed immunity to help with the removal of invading pathogens and helminths (29, 149). However, the detrimental effects of its chronic expression in response to environmental insults cause allergic inflammation. In addition, inappropriate activation of the IL-33/ST2 axis after tissue injury can lead to impaired wound healing and tissue remodeling (150–152). In contrast, IL-33 can also support tissue homeostasis and repair mediated by Tregs, which express ST2 predominantly in nonlymphoid tissue (153, 154).
IL-33 in Skin
Epidermal keratinocytes are the predominant producer of IL-33 while also expressing ST2 on their surface (132, 155). Dermal fibroblasts and macrophages can also produce IL-33 (104). IL-33 has been reported to be highly expressed in several skin diseases, including atopic dermatitis (155), psoriasis (156), and vitiligo (157). Serum IL-33 levels are higher in atopic dermatitis patients compared with healthy individuals and it correlates with excoriation and xerosis scores in atopic dermatitis (158). On the other hand, an increase in IL-33 is observed in skin lesions of psoriasis while no increase is observed in the serum (158). ST2 is distributed widely on dermal immune cells, including Th2 cells, Tregs, ILC2s, and mast cells. IL-33 is released from keratinocytes exposed to the invading pathogens, such as Staphylococcus aureus and house dust mite allergens, to instigate cutaneous immunity (159, 160). Environmental insults, such as ultraviolet B radiation and hypo-osmotic stress, and mechanical injury, also trigger the induction of IL-33 in keratinocytes (161–163). Interferon (IFN)-γ and tumor necrosis factor (TNF)-α are other known inducers of IL-33 in keratinocytes (164, 165). Nuclear IL-33 is elevated in human keratinocytes stimulated by TSLP and is required for TSLP-mediated suppression of epidermal barrier integrity components, indicating that nuclear IL-33 is a key mediator for chronic TSLP-induced skin barrier dysfunction (166).
IL-33 is implicated in type 2 immune response and the pathogenesis of allergic inflammatory diseases, such as atopic dermatitis (167). Excess IL-33 release from keratinocytes activates ILC2s to produce IL-5 and IL-13, which induce the accumulation of eosinophils in the dermis (168). Basophils induced by IL-33 also boost the ILC2 function via IL-4 signaling (169). IL-33 increases histamine generation in mast cells through p38 activation (170). Furthermore, IL-33 is involved in the induction of systemic allergic inflammation as keratinocyte-derived IL-33 can mediate skin-gut crosstalk culminating in the expansion of intestinal mast cells through ILC2 activation and food anaphylaxis (163, 171).
IL-33/ST2 signaling activates sensory neurons to mediate itch and pain responses (172–174). Excessive release of IL-33 from keratinocytes irradiated with poison ivy-derived allergen urushiol enhances the calcium influx in dermal peripheral dorsal root ganglia neurons through its receptor, ST2, to evoke itch and inflammatory responses (172). Neuronal ST2 signaling is a critical regulator of the development of the dry skin itch, but not an itch associated with atopic dermatitis (173). On the other hand, pathogen-derived lipopeptides, such as fibroblast-stimulating lipopeptide-1, can activate TLR2 in dorsal root ganglia, which, in turn, leads to infiltration of macrophages and release of IL-33 from keratinocytes. IL-33 activates the nociceptive sensory neurons at the superficial layers of the skin to instigate and prime the inflammatory pain responses (174). IL-33-mediated molecular mechanisms responsible for itch and pain are an active area of investigation.
IL-33 also plays a regulatory role in the inflamed tissue to restrain inflammation and promote remodeling in the skin, at least in part through the regulation of Tregs and M2 macrophages (175, 176). IL-33/ST2 signaling induces the expansion of Tregs, which have potent anti-inflammatory activity (177). IL-33 release from keratinocytes following skin barrier disruption induces antigen-specific Tregs to suppress excessive skin inflammation in a model of contact hypersensitivity (175). The diabetic mice model shows that IL-33 enhances extracellular matrix deposition and angiogenesis through the polarization of M2 macrophages to promote wound healing (176). Dysregulation of IL-33-mediated Treg induction causes aberrant chronic inflammation and fibrosis in the skin (151, 178). Furthermore, skin-resident Tregs from patients with systemic sclerosis are differentiated into Th2-like Tregs, which produce a higher amount of IL-4 and IL-13, by high expression of skin-localized IL-33, suggesting that IL-33 might be an important factor that contributes to fibrosis due to loss of normal skin-localized Tregs function (98). Single-cell RNA sequencing analysis of skin murine Tregs reveals a predominance of Th2-like Tregs, which preferentially express high levels of the master Th2 transcription factor, GATA3, and are more differentiated into cells, which have tissue reparative capacity (179). GATA3+ Tregs in skin express ST2, which enables them to enact reparative functions in response to alarmin IL-33 (180). Thus, IL-33/ST2 signaling has diverse impacts on skin-resident Tregs and their function in the steady-state and fibrosis development.
Beyond the Role of Type 2 Cytokines as Alarmins in Skin Health
TSLP, IL-25, and IL-33 alert the immune system in the skin to respond to environmental insults, and their chronic overexpression triggers allergic inflammation at barrier sites (Figure 1). Importantly, the function of type 2 alarmin cytokines extends beyond their physiological function in host defense and pathological function in allergic inflammation and involves other critical roles including skin cancer regulation and sebum secretion (Figure 2). Keratinocyte-derived TSLP protects the skin from carcinogenesis (181, 182). TSLP exerts its dominant anti-tumor effects through the induction of CD4+ Th2 cell immunity in the early stages of keratinocyte cancer development (181). In contrast, TSLP induces proliferation of the malignant CD4+ T cells in CTCL lesions, which are marked by a Th2 cell-dominant phenotype in advanced stages (183). It has also been shown that TSLP can recruit IgE-bearing basophils into inflamed skin, and IgE promotes inflammation-driven tumor growth during chronic tissue inflammation in a cutaneous squamous cell carcinoma model (184). Baseline TSLP expression in breast and pancreatic cancer has been linked to a pro-tumorigenic function (185–188). A tumor-myeloid cell axis independent of T cell response may mediate this tumor-promoting function of TSLP (189). However, systemic TSLP induction from the skin causes an effective CD4+ T cell-mediated anti-tumor immune response at the site of developing cancer in the breast (185). Topical treatment of calcipotriol, a TSLP inducer (75), blocks skin cancer development in mice in a TSLP-dependent manner, and synergistically with 5-fluorouracil (5-FU) induces an effective CD4+ T cell-mediated immunity against actinic keratosis, which is a precursor to cutaneous squamous cell carcinoma in humans (190). IL-25 also has potent anti-tumor effects against several tumor types, including melanoma, through an increase in eosinophils recruitment into the tumor (191, 192). In other models, IL-25 itself exhibits anti-tumor activity through the induction of apoptosis in cancer cells without affecting nonmalignant cells (193). In contrast, epidermal IL-33 contributes to a microenvironment that supports tumor growth and progression in murine skin. Nuclear IL-33 mediates focal adhesion kinase (FAK)-dependent secretion of soluble ST2, a decoy receptor, and CCL5 from squamous cell carcinoma cells, which stimulates immunosuppressive Tregs leading to cancer immune evasion (194). Continuous IL-33-driven stimulation of Tregs shapes a tumor-promoting immune environment associated with chronic inflammation in the murine skin, and an increase in IL-33 expression and Treg accumulation are observed in the perilesional skin of patients with cancer-prone chronic inflammation (178). Furthermore, IL-33 induces the recruitment of a subset of tumor-associated macrophages, which express ST2 and high-affinity IgE receptor, FcϵRIα, and produce TGF-β, in the tumor microenvironment, which results in tumor progression in a mouse model of squamous cell carcinoma (195). Nuclear IL-33 in keratinocytes also promotes intrinsic TGF-β signaling through the SMAD signaling pathway, which constitutes a cell-autonomous tumor promotion mechanism in chronic inflammation (196). IL-33-stimulated macrophages highly produce MMP-9, which proteolytically trims activating receptor natural killer group 2, member D (NKG2D) and its ligands MHC class I polypeptide-related sequence A/B (MICA/B) on the surface of tumor-infiltrating lymphocytes and melanoma cells, and thus impedes the immune surveillance of tumor-infiltrating lymphocytes (197). These findings indicate that type 2 alarmin cytokines have distinct mechanisms for the regulation of cutaneous malignancies.
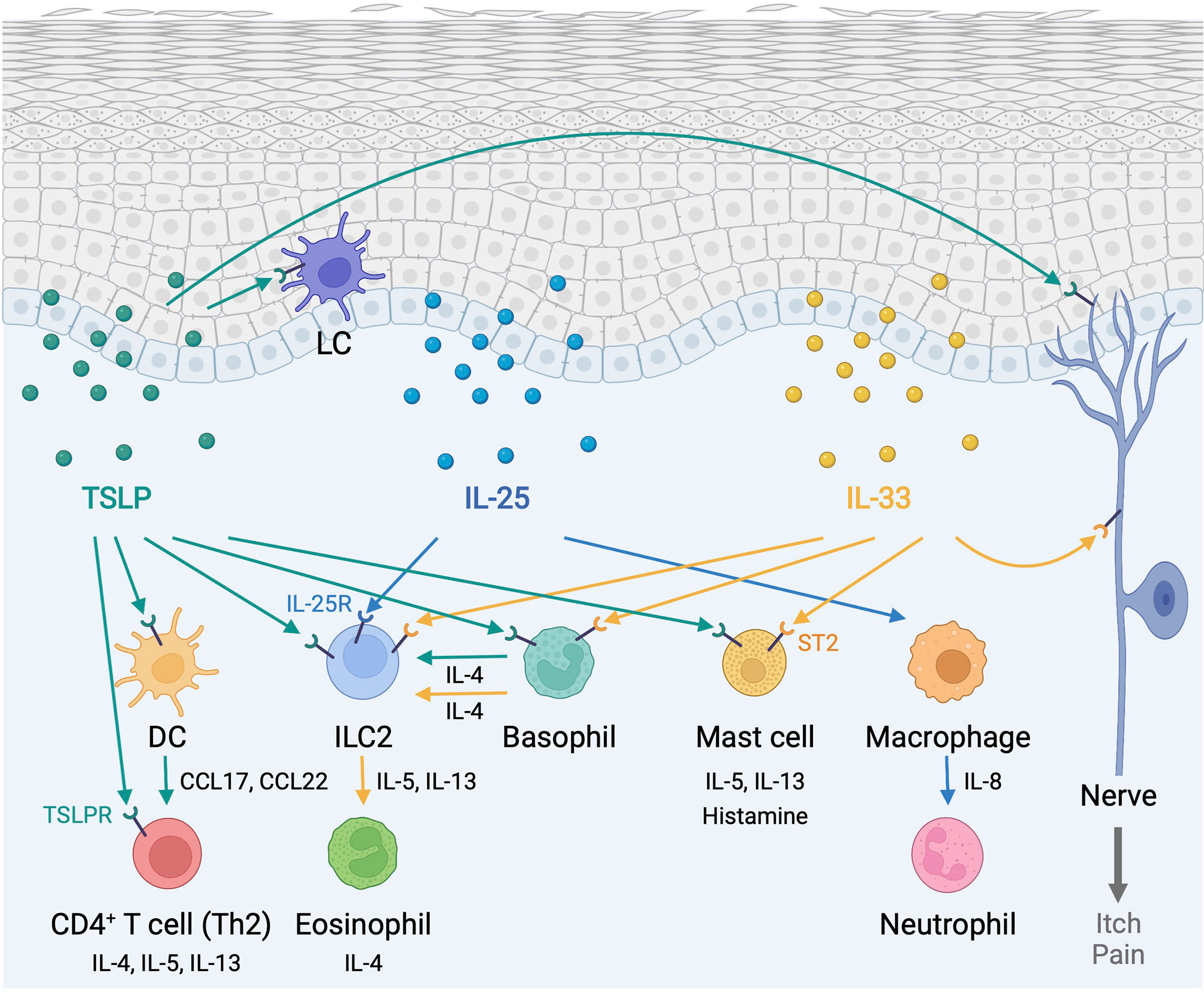
Figure 1 Type 2 alarmin cytokines orchestrate immune responses in the skin. Epidermis-derived TSLP, IL-25, and IL-33 act as alarmins to instigate cutaneous type 2 immunity through a complex and pleiotropic network of innate and adaptive immune cells. In addition, TSLP and IL-33 provoke sensory neurons to mediate itch and pain responses. DC, dendritic cell; ILC2, group2 innate lymphoid cell; LC, Langerhans cell; TSLP, thymic stromal lymphopoietin; TSLPR, thymic stromal lymphopoietin receptor. Created with BioRender.com.
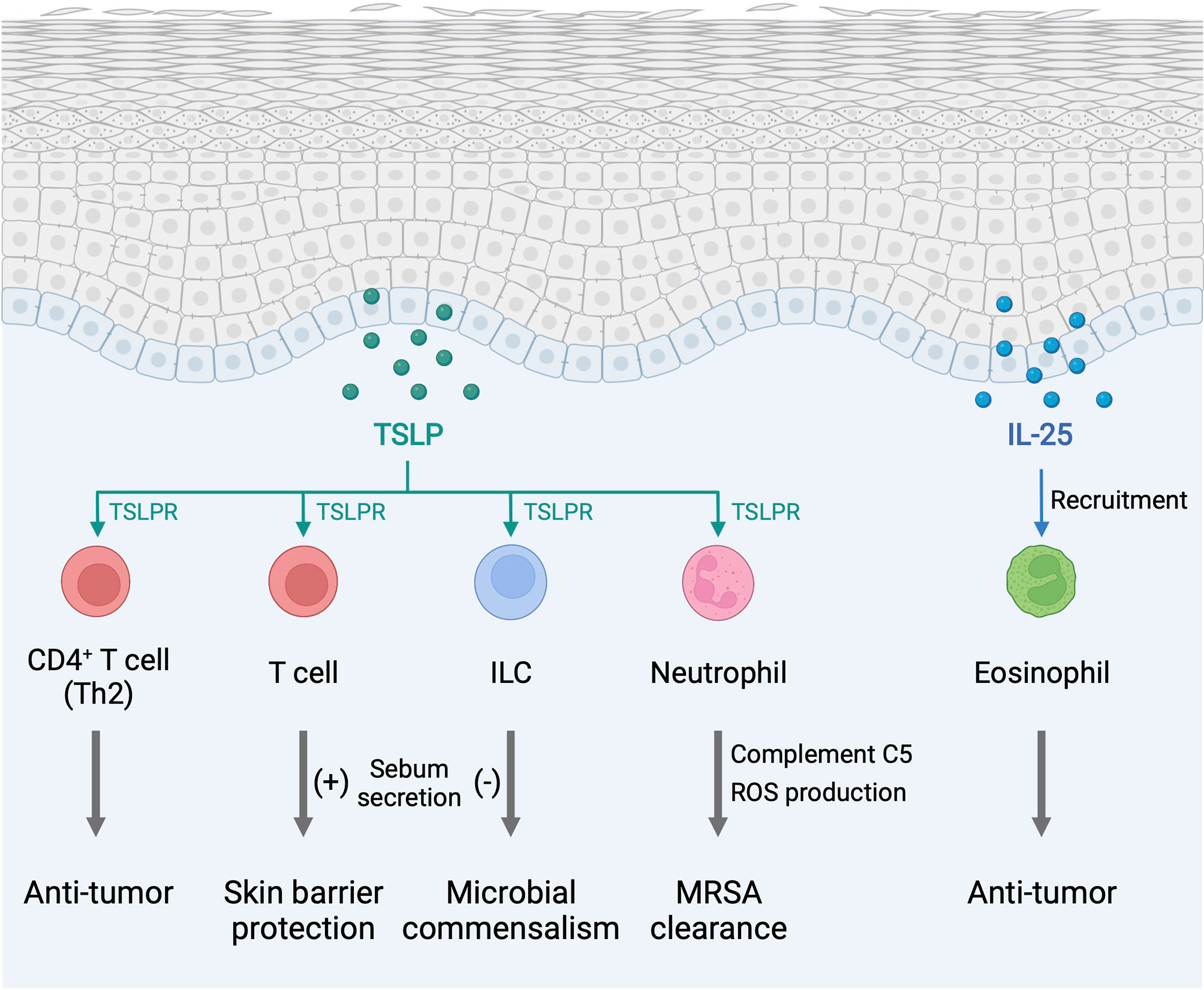
Figure 2 Type 2 alarmin cytokines exhibit pro-homeostatic properties in the skin. The function of type 2 alarmin cytokines extends beyond their conventional Th2-associated function in host defense and allergy. In the skin, these cytokines play critical roles in cancer regulation and sebum secretion. ILC, innate lymphoid cell; MRSA, methicillin-resistant Staphylococcus aureus; ROS, reactive oxygen species; TSLP, thymic stromal lymphopoietin; TSLPR, thymic stromal lymphopoietin receptor. Created with BioRender.com.
Endogenous TSLP controls the steady-state level of sebum secretion and sebum-associated antimicrobial peptide expression through the activation of T cells in murine skin. TSLP overexpression results in loss of white adipose tissue in conjunction with sebum hypersecretion (18). TSLP helps to maintain skin-resident RORγt+ ILCs within hair follicles, and, by the virtue of their location, ILCs negatively regulate surrounding sebaceous gland size and lipid content to regulate commensal bacteria equilibrium and fine-tune the skin barrier surface in mice (198). These findings suggest that TSLP-elicited ILCs and T cells play opposing functions in sebum secretion. Finally, TSLP is found to activate neutrophils to protect the skin from infection by methicillin-resistant Staphylococcus aureus (MRSA) (199).
Conclusion
Epidermis-derived TSLP, IL-25, and IL-33 act as alarmins to instigate cutaneous type 2 immunity through a complex and pleiotropic network of innate and adaptive immune cells. Accumulating evidence indicates that these type 2 alarmin cytokines not only function in concert but also have distinct physiological functions. Thus, the panoramic view of the communications between keratinocytes and immune cells through type 2 alarmin cytokines is required to fully understand how these cytokines regulate cutaneous immunity. The function of type 2 alarmin cytokines partly depends on the nature of the inflammatory stimulus, the presence of supporting cytokines and chemokines, and the surrounding microenvironment. Recent studies indicate that type 2 alarmin cytokines exhibit not only conventional type 2 inflammatory properties but also pro-homeostatic properties in the skin. Therefore, further understanding of the spectrum of biologic processes regulated by type 2 alarmin cytokines will provide new insights for the development of effective therapeutic approaches to combat allergic inflammation while utilizing the beneficial effects of these cytokines in the skin.
Author Contributions
All authors contributed to the writing of the manuscript and approved the submitted version.
Conflict of Interest
TH is an employee of Shiseido Co. Ltd.
The remaining author declares that the research was conducted in the absence of any commercial or financial relationships that could be construed as a potential conflict of interest.
Publisher’s Note
All claims expressed in this article are solely those of the authors and do not necessarily represent those of their affiliated organizations, or those of the publisher, the editors and the reviewers. Any product that may be evaluated in this article, or claim that may be made by its manufacturer, is not guaranteed or endorsed by the publisher.
Acknowledgments
Shadmehr Demehri, M.D., Ph.D., holds a Career Award for Medical Scientists award from the Burroughs Wellcome Fund.
References
1. Elias PM. Stratum Corneum Defensive Functions: An Integrated View. J Invest Dermatol (2005) 125(2):183–200. doi: 10.1111/j.0022-202X.2005.23668.x
2. Kabashima K, Honda T, Ginhoux F, Egawa G. The Immunological Anatomy of the Skin. Nat Rev Immunol (2019) 19(1):19–30. doi: 10.1038/s41577-018-0084-5
3. Simpson CL, Patel DM, Green KJ. Deconstructing the Skin: Cytoarchitectural Determinants of Epidermal Morphogenesis. Nat Rev Mol Cell Biol (2011) 12(9):565–80. doi: 10.1038/nrm3175
4. Suwanpradid J, Holcomb ZE, MacLeod AS. Emerging Skin T-Cell Functions in Response to Environmental Insults. J Invest Dermatol (2017) 137(2):288–94. doi: 10.1016/j.jid.2016.08.013
5. Suzuki T, Hidaka T, Kumagai Y, Yamamoto M. Environmental Pollutants and the Immune Response. Nat Immunol (2020) 21(12):1486–95. doi: 10.1038/s41590-020-0802-6
6. Doebel T, Voisin B, Nagao K. Langerhans Cells - The Macrophage in Dendritic Cell Clothing. Trends Immunol (2017) 38(11):817–28. doi: 10.1016/j.it.2017.06.008
7. Deckers J, Hammad H, Hoste E. Langerhans Cells: Sensing the Environment in Health and Disease. Front Immunol (2018) 9:93. doi: 10.3389/fimmu.2018.00093
8. Kubo A, Nagao K, Yokouchi M, Sasaki H, Amagai M. External Antigen Uptake by Langerhans Cells With Reorganization of Epidermal Tight Junction Barriers. J Exp Med (2009) 206(13):2937–46. doi: 10.1084/jem.20091527
9. Ouchi T, Kubo A, Yokouchi M, Adachi T, Kobayashi T, Kitashima DY, et al. Langerhans Cell Antigen Capture Through Tight Junctions Confers Preemptive Immunity in Experimental Staphylococcal Scalded Skin Syndrome. J Exp Med (2011) 208(13):2607–13. doi: 10.1084/jem.20111718
10. Mueller SN, Zaid A, Carbone FR. Tissue-Resident T Cells: Dynamic Players in Skin Immunity. Front Immunol (2014) 5:332. doi: 10.3389/fimmu.2014.00332
11. Watanabe R, Gehad A, Yang C, Scott LL, Teague JE, Schlapbach C, et al. Human Skin is Protected by Four Functionally and Phenotypically Discrete Populations of Resident and Recirculating Memory T Cells. Sci Transl Med (2015) 7(279):279ra39. doi: 10.1126/scitranslmed.3010302
12. Szabo PA, Miron M, Farber DL. Location, Location, Location: Tissue Resident Memory T Cells in Mice and Humans. Sci Immunol (2019) 4(34). doi: 10.1126/sciimmunol.aas9673
13. Watt FM, Fujiwara H. Cell-Extracellular Matrix Interactions in Normal and Diseased Skin. Cold Spring Harb Perspect Biol (2011) 3(4). doi: 10.1101/cshperspect.a005124
14. Nestle FO, Di Meglio P, Qin JZ, Nickoloff BJ. Skin Immune Sentinels in Health and Disease. Nat Rev Immunol (2009) 9(10):679–91. doi: 10.1038/nri2622
15. Eyerich S, Eyerich K, Traidl-Hoffmann C, Biedermann T. Cutaneous Barriers and Skin Immunity: Differentiating A Connected Network. Trends Immunol (2018) 39(4):315–27. doi: 10.1016/j.it.2018.02.004
16. Bhattacharjee O, Ayyangar U, Kurbet AS, Ashok D, Raghavan S. Unraveling the ECM-Immune Cell Crosstalk in Skin Diseases. Front Cell Dev Biol (2019) 7:68. doi: 10.3389/fcell.2019.00068
17. Brazil JC, Quiros M, Nusrat A, Parkos CA. Innate Immune Cell-Epithelial Crosstalk During Wound Repair. J Clin Invest (2019) 129(8):2983–93. doi: 10.1172/JCI124618
18. Choa R, Tohyama J, Wada S, Meng H, Hu J, Okumura M, et al. Thymic Stromal Lymphopoietin Induces Adipose Loss Through Sebum Hypersecretion. Science (2021) 373(6554). doi: 10.1126/science.abd2893
19. Rahmani W, Sinha S, Biernaskie J. Immune Modulation of Hair Follicle Regeneration. NPJ Regener Med (2020) 5:9. doi: 10.1038/s41536-020-0095-2
20. Altan-Bonnet G, Mukherjee R. Cytokine-Mediated Communication: A Quantitative Appraisal of Immune Complexity. Nat Rev Immunol (2019) 19(4):205–17. doi: 10.1038/s41577-019-0131-x
21. Jiang Y, Tsoi LC, Billi AC, Ward NL, Harms PW, Zeng C, et al. Cytokinocytes: The Diverse Contribution of Keratinocytes to Immune Responses in Skin. JCI Insight (2020) 5(20). doi: 10.1172/jci.insight.142067
22. Davidson S, Coles M, Thomas T, Kollias G, Ludewig B, Turley S, et al. Fibroblasts as Immune Regulators in Infection, Inflammation and Cancer. Nat Rev Immunol (2021) 21(11):704–17. doi: 10.1038/s41577-021-00540-z
23. Pober JS, Sessa WC. Evolving Functions of Endothelial Cells in Inflammation. Nat Rev Immunol (2007) 7(10):803–15. doi: 10.1038/nri2171
24. Kupper TS, Fuhlbrigge RC. Immune Surveillance in the Skin: Mechanisms and Clinical Consequences. Nat Rev Immunol (2004) 4(3):211–22. doi: 10.1038/nri1310
25. Denda M, Nakanishi S. Do Epidermal Keratinocytes Have Sensory and Information Processing Systems? Exp Dermatol (2021). doi: 10.1111/exd.14494
26. Rider P, Voronov E, Dinarello CA, Apte RN, Cohen I. Alarmins: Feel the Stress. J Immunol (2017) 198(4):1395–402. doi: 10.4049/jimmunol.1601342
27. Wang SH, Zuo YG. Thymic Stromal Lymphopoietin in Cutaneous Immune-Mediated Diseases. Front Immunol (2021) 12:698522. doi: 10.3389/fimmu.2021.698522
28. Borowczyk J, Shutova M, Brembilla NC, Boehncke WH. IL-25 (IL-17E) in Epithelial Immunology and Pathophysiology. J Allergy Clin Immunol (2021) 148(1):40–52. doi: 10.1016/j.jaci.2020.12.628
29. Martin NT, Martin MU. Interleukin 33 is a Guardian of Barriers and a Local Alarmin. Nat Immunol (2016) 17(2):122–31. doi: 10.1038/ni.3370
30. Pandey A, Ozaki K, Baumann H, Levin SD, Puel A, Farr AG, et al. Cloning of a Receptor Subunit Required for Signaling by Thymic Stromal Lymphopoietin. Nat Immunol (2000) 1(1):59–64. doi: 10.1038/76923
31. Park LS, Martin U, Garka K, Gliniak B, Di Santo JP, Muller W, et al. Cloning of the Murine Thymic Stromal Lymphopoietin (TSLP) Receptor: Formation of a Functional Heteromeric Complex Requires Interleukin 7 Receptor. J Exp Med (2000) 192(5):659–70. doi: 10.1084/jem.192.5.659
32. Tonozuka Y, Fujio K, Sugiyama T, Nosaka T, Hirai M, Kitamura T. Molecular Cloning of a Human Novel Type I Cytokine Receptor Related to Delta1/TSLPR. Cytogenet Cell Genet (2001) 93(1-2):23–5. doi: 10.1159/000056941
33. He R, Geha RS. Thymic Stromal Lymphopoietin. Ann N Y Acad Sci (2010) 1183:13–24. doi: 10.1111/j.1749-6632.2009.05128.x
34. Takai T. TSLP Expression: Cellular Sources, Triggers, and Regulatory Mechanisms. Allergol Int (2012) 61(1):3–17. doi: 10.2332/allergolint.11-RAI-0395
35. Quentmeier H, Drexler HG, Fleckenstein D, Zaborski M, Armstrong A, Sims JE, et al. Cloning of Human Thymic Stromal Lymphopoietin (TSLP) and Signaling Mechanisms Leading to Proliferation. Leukemia (2001) 15(8):1286–92. doi: 10.1038/sj.leu.2402175
36. Bernard M, Carrasco C, Laoubi L, Guiraud B, Rozieres A, Goujon C, et al. IL-1beta Induces Thymic Stromal Lymphopoietin and an Atopic Dermatitis-Like Phenotype in Reconstructed Healthy Human Epidermis. J Pathol (2017) 242(2):234–45. doi: 10.1002/path.4887
37. Segawa R, Shigeeda K, Hatayama T, Dong J, Mizuno N, Moriya T, et al. EGFR Transactivation is Involved in TNF-Alpha-Induced Expression of Thymic Stromal Lymphopoietin in Human Keratinocyte Cell Line. J Dermatol Sci (2018) 89(3):290–8. doi: 10.1016/j.jdermsci.2017.12.008
38. Wilson SR, The L, Batia LM, Beattie K, Katibah GE, McClain SP, et al. The Epithelial Cell-Derived Atopic Dermatitis Cytokine TSLP Activates Neurons to Induce Itch. Cell (2013) 155(2):285–95. doi: 10.1016/j.cell.2013.08.057
39. Wells JM, Rossi O, Meijerink M, van Baarlen P. Epithelial Crosstalk at the Microbiota-Mucosal Interface. Proc Natl Acad Sci USA (2011) 108 Suppl 1:4607–14. doi: 10.1073/pnas.1000092107
40. Cui X, Gao N, Me R, Xu J, Yu FX. TSLP Protects Corneas From Pseudomonas Aeruginosa Infection by Regulating Dendritic Cells and IL-23-IL-17 Pathway. Invest Ophthalmol Vis Sci (2018) 59(10):4228–37. doi: 10.1167/iovs.18-24672
41. Leyva-Castillo JM, Hener P, Jiang H, Li M. TSLP Produced by Keratinocytes Promotes Allergen Sensitization Through Skin and Thereby Triggers Atopic March in Mice. J Invest Dermatol (2013) 133(1):154–63. doi: 10.1038/jid.2012.239
42. Kumari V, Babina M, Hazzan T, Worm M. Thymic Stromal Lymphopoietin Induction by Skin Irritation is Independent of Tumour Necrosis Factor-Alpha, But Supported by Interleukin-1. Br J Dermatol (2015) 172(4):951–60. doi: 10.1111/bjd.13465
43. Bleck B, Grunig G, Chiu A, Liu M, Gordon T, Kazeros A, et al. MicroRNA-375 Regulation of Thymic Stromal Lymphopoietin by Diesel Exhaust Particles and Ambient Particulate Matter in Human Bronchial Epithelial Cells. J Immunol (2013) 190(7):3757–63. doi: 10.4049/jimmunol.1201165
44. Smelter DF, Sathish V, Thompson MA, Pabelick CM, Vassallo R, Prakash YS. Thymic Stromal Lymphopoietin in Cigarette Smoke-Exposed Human Airway Smooth Muscle. J Immunol (2010) 185(5):3035–40. doi: 10.4049/jimmunol.1000252
45. Allakhverdi Z, Comeau MR, Jessup HK, Yoon BR, Brewer A, Chartier S, et al. Thymic Stromal Lymphopoietin is Released by Human Epithelial Cells in Response to Microbes, Trauma, or Inflammation and Potently Activates Mast Cells. J Exp Med (2007) 204(2):253–8. doi: 10.1084/jem.20062211
46. Kouzaki H, O’Grady SM, Lawrence CB, Kita H. Proteases Induce Production of Thymic Stromal Lymphopoietin by Airway Epithelial Cells Through Protease-Activated Receptor-2. J Immunol (2009) 183(2):1427–34. doi: 10.4049/jimmunol.0900904
47. Jia X, Zhang H, Cao X, Yin Y, Zhang B. Activation of TRPV1 Mediates Thymic Stromal Lymphopoietin Release via the Ca2+/NFAT Pathway in Airway Epithelial Cells. FEBS Lett (2014) 588(17):3047–54. doi: 10.1016/j.febslet.2014.06.018
48. Kang JH, Yang HW, Park JH, Shin JM, Kim TH, Lee SH, et al. Lipopolysaccharide Regulates Thymic Stromal Lymphopoietin Expression via TLR4/MAPK/Akt/NF-kappaB-Signaling Pathways in Nasal Fibroblasts: Differential Inhibitory Effects of Macrolide and Corticosteroid. Int Forum Allergy Rhinol (2021) 11(2):144–52. doi: 10.1002/alr.22641
49. Poposki JA, Klingler AI, Stevens WW, Peters AT, Hulse KE, Grammer LC, et al. Proprotein Convertases Generate a Highly Functional Heterodimeric Form of Thymic Stromal Lymphopoietin in Humans. J Allergy Clin Immunol (2017) 139(5):1559–67 e8. doi: 10.1016/j.jaci.2016.08.040
50. Liu YJ, Soumelis V, Watanabe N, Ito T, Wang YH, Malefyt Rde W, et al. TSLP: An Epithelial Cell Cytokine That Regulates T Cell Differentiation by Conditioning Dendritic Cell Maturation. Annu Rev Immunol (2007) 25:193–219. doi: 10.1146/annurev.immunol.25.022106.141718
51. Verstraete K, van Schie L, Vyncke L, Bloch Y, Tavernier J, Pauwels E, et al. Structural Basis of the Proinflammatory Signaling Complex Mediated by TSLP. Nat Struct Mol Biol (2014) 21(4):375–82. doi: 10.1038/nsmb.2794
52. Ziegler SF, Artis D. Sensing the Outside World: TSLP Regulates Barrier Immunity. Nat Immunol (2010) 11(4):289–93. doi: 10.1038/ni.1852
53. Soumelis V, Reche PA, Kanzler H, Yuan W, Edward G, Homey B, et al. Human Epithelial Cells Trigger Dendritic Cell Mediated Allergic Inflammation by Producing TSLP. Nat Immunol (2002) 3(7):673–80. doi: 10.1038/ni805
54. Gilliet M, Soumelis V, Watanabe N, Hanabuchi S, Antonenko S, de Waal-Malefyt R, et al. Human Dendritic Cells Activated by TSLP and CD40L Induce Proallergic Cytotoxic T Cells. J Exp Med (2003) 197(8):1059–63. doi: 10.1084/jem.20030240
55. Ito T, Wang YH, Duramad O, Hori T, Delespesse GJ, Watanabe N, et al. TSLP-Activated Dendritic Cells Induce an Inflammatory T Helper Type 2 Cell Response Through OX40 Ligand. J Exp Med (2005) 202(9):1213–23. doi: 10.1084/jem.20051135
56. Omori M, Ziegler S. Induction of IL-4 Expression in CD4(+) T Cells by Thymic Stromal Lymphopoietin. J Immunol (2007) 178(3):1396–404. doi: 10.4049/jimmunol.178.3.1396
57. Rochman I, Watanabe N, Arima K, Liu YJ, Leonard WJ. Cutting Edge: Direct Action of Thymic Stromal Lymphopoietin on Activated Human CD4+ T Cells. J Immunol (2007) 178(11):6720–4. doi: 10.4049/jimmunol.178.11.6720
58. Ochiai S, Jagot F, Kyle RL, Hyde E, White RF, Prout M, et al. Thymic Stromal Lymphopoietin Drives the Development of IL-13(+) Th2 Cells. Proc Natl Acad Sci USA (2018) 115(5):1033–8. doi: 10.1073/pnas.1714348115
59. Yao W, Zhang Y, Jabeen R, Nguyen ET, Wilkes DS, Tepper RS, et al. Interleukin-9 is Required for Allergic Airway Inflammation Mediated by the Cytokine TSLP. Immunity (2013) 38(2):360–72. doi: 10.1016/j.immuni.2013.01.007
60. Angkasekwinai P, Dong C. IL-9-Producing T Cells: Potential Players in Allergy and Cancer. Nat Rev Immunol (2021) 21(1):37–48. doi: 10.1038/s41577-020-0396-0
61. Rivera Vargas T, Humblin E, Vegran F, Ghiringhelli F, Apetoh L. TH9 Cells in Anti-Tumor Immunity. Semin Immunopathol (2017) 39(1):39–46. doi: 10.1007/s00281-016-0599-4
62. Siracusa MC, Saenz SA, Hill DA, Kim BS, Headley MB, Doering TA, et al. TSLP Promotes Interleukin-3-Independent Basophil Haematopoiesis and Type 2 Inflammation. Nature (2011) 477(7363):229–33. doi: 10.1038/nature10329
63. Stier MT, Bloodworth MH, Toki S, Newcomb DC, Goleniewska K, Boyd KL, et al. Respiratory Syncytial Virus Infection Activates IL-13-Producing Group 2 Innate Lymphoid Cells Through Thymic Stromal Lymphopoietin. J Allergy Clin Immunol (2016) 138(3):814–24 e11. doi: 10.1016/j.jaci.2016.01.050
64. Demehri S, Liu Z, Lee J, Lin MH, Crosby SD, Roberts CJ, et al. Notch-Deficient Skin Induces a Lethal Systemic B-Lymphoproliferative Disorder by Secreting TSLP, a Sentinel for Epidermal Integrity. PloS Biol (2008) 6(5):e123. doi: 10.1371/journal.pbio.0060123
65. Zhang Z, Hener P, Frossard N, Kato S, Metzger D, Li M, et al. Thymic Stromal Lymphopoietin Overproduced by Keratinocytes in Mouse Skin Aggravates Experimental Asthma. Proc Natl Acad Sci USA (2009) 106(5):1536–41. doi: 10.1073/pnas.0812668106
66. Vu AT, Baba T, Chen X, Le TA, Kinoshita H, Xie Y, et al. Staphylococcus Aureus Membrane and Diacylated Lipopeptide Induce Thymic Stromal Lymphopoietin in Keratinocytes Through the Toll-Like Receptor 2-Toll-Like Receptor 6 Pathway. J Allergy Clin Immunol (2010) 126(5):985–93. doi: 10.1016/j.jaci.2010.09.002
67. Ishibashi Y, Sugawara K, Sugita T, Nishikawa A. Secretion of Thymic Stromal Lymphopoietin From Human Keratinocytes in Response to Malassezia Yeasts. J Dermatol Sci (2011) 62(2):134–8. doi: 10.1016/j.jdermsci.2011.02.012
68. Jang Y, Jeong SH, Park YH, Bae HC, Lee H, Ryu WI, et al. UVB Induces HIF-1alpha-Dependent TSLP Expression via the JNK and ERK Pathways. J Invest Dermatol (2013) 133(11):2601–8. doi: 10.1038/jid.2013.203
69. Hidaka T, Ogawa E, Kobayashi EH, Suzuki T, Funayama R, Nagashima T, et al. The Aryl Hydrocarbon Receptor AhR Links Atopic Dermatitis and Air Pollution via Induction of the Neurotrophic Factor Artemin. Nat Immunol (2017) 18(1):64–73. doi: 10.1038/ni.3614
70. Seo SH, Kim S, Kim SE, Chung S, Lee SE. Enhanced Thermal Sensitivity of TRPV3 in Keratinocytes Underlies Heat-Induced Pruritogen Release and Pruritus in Atopic Dermatitis. J Invest Dermatol (2020) 140(11):2199–209 e6. doi: 10.1016/j.jid.2020.02.028
71. Lee WJ, Shim WS. Cutaneous Neuroimmune Interactions of TSLP and TRPV4 Play Pivotal Roles in Dry Skin-Induced Pruritus. Front Immunol (2021) 12:772941. doi: 10.3389/fimmu.2021.772941
72. Vu AT, Chen X, Xie Y, Kamijo S, Ushio H, Kawasaki J, et al. Extracellular Double-Stranded RNA Induces TSLP via an Endosomal Acidification- and NF-kappaB-Dependent Pathway in Human Keratinocytes. J Invest Dermatol (2011) 131(11):2205–12. doi: 10.1038/jid.2011.185
73. Lee KH, Cho KA, Kim JY, Kim JY, Baek JH, Woo SY, et al. Filaggrin Knockdown and Toll-Like Receptor 3 (TLR3) Stimulation Enhanced the Production of Thymic Stromal Lymphopoietin (TSLP) From Epidermal Layers. Exp Dermatol (2011) 20(2):149–51. doi: 10.1111/j.1600-0625.2010.01203.x
74. Moniaga CS, Jeong SK, Egawa G, Nakajima S, Hara-Chikuma M, Jeon JE, et al. Protease Activity Enhances Production of Thymic Stromal Lymphopoietin and Basophil Accumulation in Flaky Tail Mice. Am J Pathol (2013) 182(3):841–51. doi: 10.1016/j.ajpath.2012.11.039
75. Li M, Hener P, Zhang Z, Kato S, Metzger D, Chambon P. Topical Vitamin D3 and Low-Calcemic Analogs Induce Thymic Stromal Lymphopoietin in Mouse Keratinocytes and Trigger an Atopic Dermatitis. Proc Natl Acad Sci USA (2006) 103(31):11736–41. doi: 10.1073/pnas.0604575103
76. Li M, Messaddeq N, Teletin M, Pasquali JL, Metzger D, Chambon P. Retinoid X Receptor Ablation in Adult Mouse Keratinocytes Generates an Atopic Dermatitis Triggered by Thymic Stromal Lymphopoietin. Proc Natl Acad Sci USA (2005) 102(41):14795–800. doi: 10.1073/pnas.0507385102
77. Jeong H, Shin JY, Kim MJ, Na J, Ju BG. Activation of Aryl Hydrocarbon Receptor Negatively Regulates Thymic Stromal Lymphopoietin Gene Expression via Protein Kinase Cdelta-P300-NF-kappaB Pathway in Keratinocytes Under Inflammatory Conditions. J Invest Dermatol (2019) 139(5):1098–109. doi: 10.1016/j.jid.2018.11.012
78. Volpe E, Pattarini L, Martinez-Cingolani C, Meller S, Donnadieu MH, Bogiatzi SI, et al. Thymic Stromal Lymphopoietin Links Keratinocytes and Dendritic Cell-Derived IL-23 in Patients With Psoriasis. J Allergy Clin Immunol (2014) 134(2):373–81. doi: 10.1016/j.jaci.2014.04.022
79. Hovnanian A. Netherton Syndrome: Skin Inflammation and Allergy by Loss of Protease Inhibition. Cell Tissue Res (2013) 351(2):289–300. doi: 10.1007/s00441-013-1558-1
80. Shin JU, Kim SH, Kim H, Noh JY, Jin S, Park CO, et al. TSLP Is a Potential Initiator of Collagen Synthesis and an Activator of CXCR4/SDF-1 Axis in Keloid Pathogenesis. J Invest Dermatol (2016) 136(2):507–15. doi: 10.1016/j.jid.2015.11.008
81. Luo Y, Zhou B, Zhao M, Tang J, Lu Q. Promoter Demethylation Contributes to TSLP Overexpression in Skin Lesions of Patients With Atopic Dermatitis. Clin Exp Dermatol (2014) 39(1):48–53. doi: 10.1111/ced.12206
82. Nakajima S, Igyarto BZ, Honda T, Egawa G, Otsuka A, Hara-Chikuma M, et al. Langerhans Cells Are Critical in Epicutaneous Sensitization With Protein Antigen via Thymic Stromal Lymphopoietin Receptor Signaling. J Allergy Clin Immunol (2012) 129(4):1048–55 e6. doi: 10.1016/j.jaci.2012.01.063
83. Malissen B, Tamoutounour S, Henri S. The Origins and Functions of Dendritic Cells and Macrophages in the Skin. Nat Rev Immunol (2014) 14(6):417–28. doi: 10.1038/nri3683
84. Boltjes A, van Wijk F. Human Dendritic Cell Functional Specialization in Steady-State and Inflammation. Front Immunol (2014) 5:131. doi: 10.3389/fimmu.2014.00131
85. Eisenbarth SC. Dendritic Cell Subsets in T Cell Programming: Location Dictates Function. Nat Rev Immunol (2019) 19(2):89–103. doi: 10.1038/s41577-018-0088-1
86. Oyoshi MK, Larson RP, Ziegler SF, Geha RS. Mechanical Injury Polarizes Skin Dendritic Cells to Elicit a T(H)2 Response by Inducing Cutaneous Thymic Stromal Lymphopoietin Expression. J Allergy Clin Immunol (2010) 126(5):976–84. doi: 10.1016/j.jaci.2010.08.041
87. Marschall P, Wei R, Segaud J, Yao W, Hener P, German BF, et al. Dual Function of Langerhans Cells in Skin TSLP-Promoted TFH Differentiation in Mouse Atopic Dermatitis. J Allergy Clin Immunol (2021) 147(5):1778–94. doi: 10.1016/j.jaci.2020.10.006
88. Martinez-Cingolani C, Grandclaudon M, Jeanmougin M, Jouve M, Zollinger R, Soumelis V. Human Blood BDCA-1 Dendritic Cells Differentiate Into Langerhans-Like Cells With Thymic Stromal Lymphopoietin and TGF-Beta. Blood (2014) 124(15):2411–20. doi: 10.1182/blood-2014-04-568311
89. Kitajima M, Ziegler SF. Cutting Edge: Identification of the Thymic Stromal Lymphopoietin-Responsive Dendritic Cell Subset Critical for Initiation of Type 2 Contact Hypersensitivity. J Immunol (2013) 191(10):4903–7. doi: 10.4049/jimmunol.1302175
90. Wang YH, Ito T, Wang YH, Homey B, Watanabe N, Martin R, et al. Maintenance and Polarization of Human TH2 Central Memory T Cells by Thymic Stromal Lymphopoietin-Activated Dendritic Cells. Immunity (2006) 24(6):827–38. doi: 10.1016/j.immuni.2006.03.019
91. Pattarini L, Trichot C, Bogiatzi S, Grandclaudon M, Meller S, Keuylian Z, et al. TSLP-Activated Dendritic Cells Induce Human T Follicular Helper Cell Differentiation Through OX40-Ligand. J Exp Med (2017) 214(5):1529–46. doi: 10.1084/jem.20150402
92. Kitajima M, Kubo M, Ziegler SF, Suzuki H. Critical Role of TSLP Receptor on CD4 T Cells for Exacerbation of Skin Inflammation. J Immunol (2020) 205(1):27–35. doi: 10.4049/jimmunol.1900758
93. Tatsuno K, Fujiyama T, Yamaguchi H, Waki M, Tokura Y. TSLP Directly Interacts With Skin-Homing Th2 Cells Highly Expressing Its Receptor to Enhance IL-4 Production in Atopic Dermatitis. J Invest Dermatol (2015) 135(12):3017–24. doi: 10.1038/jid.2015.318
94. Kitajima M, Lee HC, Nakayama T, Ziegler SF. TSLP Enhances the Function of Helper Type 2 Cells. Eur J Immunol (2011) 41(7):1862–71. doi: 10.1002/eji.201041195
95. Kim BS, Siracusa MC, Saenz SA, Noti M, Monticelli LA, Sonnenberg GF, et al. TSLP Elicits IL-33-Independent Innate Lymphoid Cell Responses to Promote Skin Inflammation. Sci Transl Med (2013) 5(170):170ra16. doi: 10.1126/scitranslmed.3005374
96. Halim TY. Group 2 Innate Lymphoid Cells in Disease. Int Immunol (2016) 28(1):13–22. doi: 10.1093/intimm/dxv050
97. Simoni Y, Fehlings M, Kloverpris HN, McGovern N, Koo SL, Loh CY, et al. Human Innate Lymphoid Cell Subsets Possess Tissue-Type Based Heterogeneity in Phenotype and Frequency. Immunity (2017) 46(1):148–61. doi: 10.1016/j.immuni.2016.11.005
98. Ricardo-Gonzalez RR, Van Dyken SJ, Schneider C, Lee J, Nussbaum JC, Liang HE, et al. Tissue Signals Imprint ILC2 Identity With Anticipatory Function. Nat Immunol (2018) 19(10):1093–9. doi: 10.1038/s41590-018-0201-4
99. Nakatani-Kusakabe M, Yasuda K, Tomura M, Nagai M, Yamanishi K, Kuroda E, et al. Monitoring Cellular Movement With Photoconvertible Fluorescent Protein and Single-Cell RNA Sequencing Reveals Cutaneous Group 2 Innate Lymphoid Cell Subtypes, Circulating ILC2 and Skin-Resident Ilc2. JID Innov (2021) 1(3):100035. doi: 10.1016/j.xjidi.2021.100035
100. Walsh CM, Hill RZ, Schwendinger-Schreck J, Deguine J, Brock EC, Kucirek N, et al. Neutrophils Promote CXCR3-Dependent Itch in the Development of Atopic Dermatitis. Elife (2019) 8. doi: 10.7554/eLife.48448
101. Kim BS, Wang K, Siracusa MC, Saenz SA, Brestoff JR, Monticelli LA, et al. Basophils Promote Innate Lymphoid Cell Responses in Inflamed Skin. J Immunol (2014) 193(7):3717–25. doi: 10.4049/jimmunol.1401307
102. Han NR, Oh HA, Nam SY, Moon PD, Kim DW, Kim HM, et al. TSLP Induces Mast Cell Development and Aggravates Allergic Reactions Through the Activation of MDM2 and STAT6. J Invest Dermatol (2014) 134(10):2521–30. doi: 10.1038/jid.2014.198
103. Demehri S, Morimoto M, Holtzman MJ, Kopan R. Skin-Derived TSLP Triggers Progression From Epidermal-Barrier Defects to Asthma. PloS Biol (2009) 7(5):e1000067. doi: 10.1371/journal.pbio.1000067
104. Li C, Li H, Jiang Z, Zhang T, Wang Y, Li Z, et al. Interleukin-33 Increases Antibacterial Defense by Activation of Inducible Nitric Oxide Synthase in Skin. PloS Pathog (2014) 10(2):e1003918. doi: 10.1371/journal.ppat.1003918
105. Han H, Xu W, Headley MB, Jessup HK, Lee KS, Omori M, et al. Thymic Stromal Lymphopoietin (TSLP)-Mediated Dermal Inflammation Aggravates Experimental Asthma. Mucosal Immunol (2012) 5(3):342–51. doi: 10.1038/mi.2012.14
106. Kashiwagi M, Hosoi J, Lai JF, Brissette J, Ziegler SF, Morgan BA, et al. Direct Control of Regulatory T Cells by Keratinocytes. Nat Immunol (2017) 18(3):334–43. doi: 10.1038/ni.3661
107. Lee J, Ho WH, Maruoka M, Corpuz RT, Baldwin DT, Foster JS, et al. IL-17E, a Novel Proinflammatory Ligand for the IL-17 Receptor Homolog IL-17rh1. J Biol Chem (2001) 276(2):1660–4. doi: 10.1074/jbc.M008289200
108. Moseley TA, Haudenschild DR, Rose L, Reddi AH. Interleukin-17 Family and IL-17 Receptors. Cytokine Growth Factor Rev (2003) 14(2):155–74. doi: 10.1016/s1359-6101(03)00002-9
109. Deng C, Peng N, Tang Y, Yu N, Wang C, Cai X, et al. Roles of IL-25 in Type 2 Inflammation and Autoimmune Pathogenesis. Front Immunol (2021) 12:691559. doi: 10.3389/fimmu.2021.691559
110. Kleinschek MA, Owyang AM, Joyce-Shaikh B, Langrish CL, Chen Y, Gorman DM, et al. IL-25 Regulates Th17 Function in Autoimmune Inflammation. J Exp Med (2007) 204(1):161–70. doi: 10.1084/jem.20061738
111. Angkasekwinai P, Chang SH, Thapa M, Watarai H, Dong C. Regulation of IL-9 Expression by IL-25 Signaling. Nat Immunol (2010) 11(3):250–6. doi: 10.1038/ni.1846
112. Angkasekwinai P, Srimanote P, Wang YH, Pootong A, Sakolvaree Y, Pattanapanyasat K, et al. Interleukin-25 (IL-25) Promotes Efficient Protective Immunity Against Trichinella Spiralis Infection by Enhancing the Antigen-Specific IL-9 Response. Infect Immun (2013) 81(10):3731–41. doi: 10.1128/IAI.00646-13
113. Paul WE, Zhu J. How Are T(H)2-Type Immune Responses Initiated and Amplified? Nat Rev Immunol (2010) 10(4):225–35. doi: 10.1038/nri2735
114. Goswami S, Angkasekwinai P, Shan M, Greenlee KJ, Barranco WT, Polikepahad S, et al. Divergent Functions for Airway Epithelial Matrix Metalloproteinase 7 and Retinoic Acid in Experimental Asthma. Nat Immunol (2009) 10(5):496–503. doi: 10.1038/ni.1719
115. Gaffen SL. Structure and Signalling in the IL-17 Receptor Family. Nat Rev Immunol (2009) 9(8):556–67. doi: 10.1038/nri2586
116. Yao X, Sun Y, Wang W, Sun Y. Interleukin (IL)-25: Pleiotropic Roles in Asthma. Respirology (2016) 21(4):638–47. doi: 10.1111/resp.12707
117. Hvid M, Vestergaard C, Kemp K, Christensen GB, Deleuran B, Deleuran M. IL-25 in Atopic Dermatitis: A Possible Link Between Inflammation and Skin Barrier Dysfunction? J Invest Dermatol (2011) 131(1):150–7. doi: 10.1038/jid.2010.277
118. Xu M, Lu H, Lee YH, Wu Y, Liu K, Shi Y, et al. An Interleukin-25-Mediated Autoregulatory Circuit in Keratinocytes Plays a Pivotal Role in Psoriatic Skin Inflammation. Immunity (2018) 48(4):787–98.e4. doi: 10.1016/j.immuni.2018.03.019
119. Senra L, Mylonas A, Kavanagh RD, Fallon PG, Conrad C, Borowczyk-Michalowska J, et al. IL-17e (IL-25) Enhances Innate Immune Responses During Skin Inflammation. J Invest Dermatol (2019) 139(8):1732–42 e17. doi: 10.1016/j.jid.2019.01.021
120. Nakajima R, Miyagaki T, Hirakawa M, Oka T, Takahashi N, Suga H, et al. Interleukin-25 is Involved in Cutaneous T-Cell Lymphoma Progression by Establishing a T Helper 2-Dominant Microenvironment. Br J Dermatol (2018) 178(6):1373–82. doi: 10.1111/bjd.16237
121. Borowczyk J, Buerger C, Tadjrischi N, Drukala J, Wolnicki M, Wnuk D, et al. IL-17e (IL-25) and IL-17a Differentially Affect the Functions of Human Keratinocytes. J Invest Dermatol (2020) 140(7):1379–89.e2. doi: 10.1016/j.jid.2019.12.013
122. Aktar MK, Kido-Nakahara M, Furue M, Nakahara T. Mutual Upregulation of Endothelin-1 and IL-25 in Atopic Dermatitis. Allergy (2015) 70(7):846–54. doi: 10.1111/all.12633
123. Roan F, Obata-Ninomiya K, Ziegler SF. Epithelial Cell-Derived Cytokines: More Than Just Signaling the Alarm. J Clin Invest (2019) 129(4):1441–51. doi: 10.1172/JCI124606
124. Salimi M, Barlow JL, Saunders SP, Xue L, Gutowska-Owsiak D, Wang X, et al. A Role for IL-25 and IL-33-Driven Type-2 Innate Lymphoid Cells in Atopic Dermatitis. J Exp Med (2013) 210(13):2939–50. doi: 10.1084/jem.20130351
125. Leyva-Castillo JM, Galand C, Mashiko S, Bissonnette R, McGurk A, Ziegler SF, et al. ILC2 Activation by Keratinocyte-Derived IL-25 Drives IL-13 Production at Sites of Allergic Skin Inflammation. J Allergy Clin Immunol (2020) 145(6):1606–14 e4. doi: 10.1016/j.jaci.2020.02.026
126. Zhang F, Liu Y, Wang S, Yan X, Lin Y, Chen D, et al. Interleukin-25-Mediated-IL-17rb Upregulation Promotes Cutaneous Wound Healing in Diabetic Mice by Improving Endothelial Cell Functions. Front Immunol (2022) 13:809755. doi: 10.3389/fimmu.2022.809755
127. Kim BE, Bin L, Ye YM, Ramamoorthy P, Leung DYM. IL-25 Enhances HSV-1 Replication by Inhibiting Filaggrin Expression, and Acts Synergistically With Th2 Cytokines to Enhance HSV-1 Replication. J Invest Dermatol (2013) 133(12):2678–85. doi: 10.1038/jid.2013.223
128. Howell MD, Kim BE, Gao P, Grant AV, Boguniewicz M, Debenedetto A, et al. Cytokine Modulation of Atopic Dermatitis Filaggrin Skin Expression. J Allergy Clin Immunol (2007) 120(1):150–5. doi: 10.1016/j.jaci.2007.04.031
129. Sehra S, Yao Y, Howell MD, Nguyen ET, Kansas GS, Leung DY, et al. IL-4 Regulates Skin Homeostasis and the Predisposition Toward Allergic Skin Inflammation. J Immunol (2010) 184(6):3186–90. doi: 10.4049/jimmunol.0901860
130. Suto H, Nambu A, Morita H, Yamaguchi S, Numata T, Yoshizaki T, et al. IL-25 Enhances TH17 Cell-Mediated Contact Dermatitis by Promoting IL-1beta Production by Dermal Dendritic Cells. J Allergy Clin Immunol (2018) 142(5):1500–9.e10. doi: 10.1016/j.jaci.2017.12.1007
131. Larson RP, Zimmerli SC, Comeau MR, Itano A, Omori M, Iseki M, et al. Dibutyl Phthalate-Induced Thymic Stromal Lymphopoietin is Required for Th2 Contact Hypersensitivity Responses. J Immunol (2010) 184(6):2974–84. doi: 10.4049/jimmunol.0803478
132. Schmitz J, Owyang A, Oldham E, Song Y, Murphy E, McClanahan TK, et al. IL-33, an Interleukin-1-Like Cytokine That Signals via the IL-1 Receptor-Related Protein ST2 and Induces T Helper Type 2-Associated Cytokines. Immunity (2005) 23(5):479–90. doi: 10.1016/j.immuni.2005.09.015
133. Garlanda C, Dinarello CA, Mantovani A. The Interleukin-1 Family: Back to the Future. Immunity (2013) 39(6):1003–18. doi: 10.1016/j.immuni.2013.11.010
134. Moussion C, Ortega N, Girard JP. The IL-1-Like Cytokine IL-33 is Constitutively Expressed in the Nucleus of Endothelial Cells and Epithelial Cells In Vivo: A Novel A’larmin’? PloS One (2008) 3(10):e3331. doi: 10.1371/journal.pone.0003331
135. Pichery M, Mirey E, Mercier P, Lefrancais E, Dujardin A, Ortega N, et al. Endogenous IL-33 is Highly Expressed in Mouse Epithelial Barrier Tissues, Lymphoid Organs, Brain, Embryos, and Inflamed Tissues: In Situ Analysis Using a Novel Il-33-LacZ Gene Trap Reporter Strain. J Immunol (2012) 188(7):3488–95. doi: 10.4049/jimmunol.1101977
136. Carriere V, Roussel L, Ortega N, Lacorre DA, Americh L, Aguilar L, et al. IL-33, the IL-1-Like Cytokine Ligand for ST2 Receptor, is a Chromatin-Associated Nuclear Factor In Vivo. Proc Natl Acad Sci USA (2007) 104(1):282–7. doi: 10.1073/pnas.0606854104
137. Luthi AU, Cullen SP, McNeela EA, Duriez PJ, Afonina IS, Sheridan C, et al. Suppression of Interleukin-33 Bioactivity Through Proteolysis by Apoptotic Caspases. Immunity (2009) 31(1):84–98. doi: 10.1016/j.immuni.2009.05.007
138. Lefrancais E, Roga S, Gautier V, Gonzalez-de-Peredo A, Monsarrat B, Girard JP, et al. IL-33 is Processed Into Mature Bioactive Forms by Neutrophil Elastase and Cathepsin G. Proc Natl Acad Sci USA (2012) 109(5):1673–8. doi: 10.1073/pnas.1115884109
139. Lefrancais E, Duval A, Mirey E, Roga S, Espinosa E, Cayrol C, et al. Central Domain of IL-33 is Cleaved by Mast Cell Proteases for Potent Activation of Group-2 Innate Lymphoid Cells. Proc Natl Acad Sci USA (2014) 111(43):15502–7. doi: 10.1073/pnas.1410700111
140. Cayrol C, Duval A, Schmitt P, Roga S, Camus M, Stella A, et al. Environmental Allergens Induce Allergic Inflammation Through Proteolytic Maturation of IL-33. Nat Immunol (2018) 19(4):375–85. doi: 10.1038/s41590-018-0067-5
141. Cohen ES, Scott IC, Majithiya JB, Rapley L, Kemp BP, England E, et al. Oxidation of the Alarmin IL-33 Regulates ST2-Dependent Inflammation. Nat Commun (2015) 6:8327. doi: 10.1038/ncomms9327
142. Kouzaki H, Iijima K, Kobayashi T, O’Grady SM, Kita H. The Danger Signal, Extracellular ATP, is a Sensor for an Airborne Allergen and Triggers IL-33 Release and Innate Th2-Type Responses. J Immunol (2011) 186(7):4375–87. doi: 10.4049/jimmunol.1003020
143. Hiraishi Y, Yamaguchi S, Yoshizaki T, Nambu A, Shimura E, Takamori A, et al. IL-33, IL-25 and TSLP Contribute to Development of Fungal-Associated Protease-Induced Innate-Type Airway Inflammation. Sci Rep (2018) 8(1):18052. doi: 10.1038/s41598-018-36440-x
144. de Kleer IM, Kool M, de Bruijn MJ, Willart M, van Moorleghem J, Schuijs MJ, et al. Perinatal Activation of the Interleukin-33 Pathway Promotes Type 2 Immunity in the Developing Lung. Immunity (2016) 45(6):1285–98. doi: 10.1016/j.immuni.2016.10.031
145. Sanada S, Hakuno D, Higgins LJ, Schreiter ER, McKenzie AN, Lee RT. IL-33 and ST2 Comprise a Critical Biomechanically Induced and Cardioprotective Signaling System. J Clin Invest (2007) 117(6):1538–49. doi: 10.1172/JCI30634
146. Katz-Kiriakos E, Steinberg DF, Kluender CE, Osorio OA, Newsom-Stewart C, Baronia A, et al. Epithelial IL-33 Appropriates Exosome Trafficking for Secretion in Chronic Airway Disease. JCI Insight (2021) 6(4). doi: 10.1172/jci.insight.136166
147. Yanagisawa K, Takagi T, Tsukamoto T, Tetsuka T, Tominaga S. Presence of a Novel Primary Response Gene ST2L, Encoding a Product Highly Similar to the Interleukin 1 Receptor Type 1. FEBS Lett (1993) 318(1):83–7. doi: 10.1016/0014-5793(93)81333-u
148. Xu Y, Tao X, Shen B, Horng T, Medzhitov R, Manley JL, et al. Structural Basis for Signal Transduction by the Toll/interleukin-1 Receptor Domains. Nature (2000) 408(6808):111–5. doi: 10.1038/35040600
149. Griesenauer B, Paczesny S. The ST2/IL-33 Axis in Immune Cells During Inflammatory Diseases. Front Immunol (2017) 8:475. doi: 10.3389/fimmu.2017.00475
150. Rankin AL, Mumm JB, Murphy E, Turner S, Yu N, McClanahan TK, et al. IL-33 Induces IL-13-Dependent Cutaneous Fibrosis. J Immunol (2010) 184(3):1526–35. doi: 10.4049/jimmunol.0903306
151. MacDonald KG, Dawson NAJ, Huang Q, Dunne JV, Levings MK, Broady R. Regulatory T Cells Produce Profibrotic Cytokines in the Skin of Patients With Systemic Sclerosis. J Allergy Clin Immunol (2015) 135(4):946–55.e9. doi: 10.1016/j.jaci.2014.12.1932
152. Molofsky AB, Savage AK, Locksley RM. Interleukin-33 in Tissue Homeostasis, Injury, and Inflammation. Immunity (2015) 42(6):1005–19. doi: 10.1016/j.immuni.2015.06.006
153. Schiering C, Krausgruber T, Chomka A, Frohlich A, Adelmann K, Wohlfert EA, et al. The Alarmin IL-33 Promotes Regulatory T-Cell Function in the Intestine. Nature (2014) 513(7519):564–8. doi: 10.1038/nature13577
154. Faustino LD, Griffith JW, Rahimi RA, Nepal K, Hamilos DL, Cho JL, et al. Interleukin-33 Activates Regulatory T Cells to Suppress Innate Gammadelta T Cell Responses in the Lung. Nat Immunol (2020) 21(11):1371–83. doi: 10.1038/s41590-020-0785-3
155. Savinko T, Matikainen S, Saarialho-Kere U, Lehto M, Wang G, Lehtimaki S, et al. IL-33 and ST2 in Atopic Dermatitis: Expression Profiles and Modulation by Triggering Factors. J Invest Dermatol (2012) 132(5):1392–400. doi: 10.1038/jid.2011.446
156. Theoharides TC, Zhang B, Kempuraj D, Tagen M, Vasiadi M, Angelidou A, et al. IL-33 Augments Substance P-Induced VEGF Secretion From Human Mast Cells and is Increased in Psoriatic Skin. Proc Natl Acad Sci USA (2010) 107(9):4448–53. doi: 10.1073/pnas.1000803107
157. Li P, Ma H, Han D, Mou K. Interleukin-33 Affects Cytokine Production by Keratinocytes in Vitiligo. Clin Exp Dermatol (2015) 40(2):163–70. doi: 10.1111/ced.12464
158. Tamagawa-Mineoka R, Okuzawa Y, Masuda K, Katoh N. Increased Serum Levels of Interleukin 33 in Patients With Atopic Dermatitis. J Am Acad Dermatol (2014) 70(5):882–8. doi: 10.1016/j.jaad.2014.01.867
159. Al Kindi A, Williams H, Matsuda K, Alkahtani AM, Saville C, Bennett H, et al. Staphylococcus Aureus Second Immunoglobulin-Binding Protein Drives Atopic Dermatitis via IL-33. J Allergy Clin Immunol (2021) 147(4):1354–68 e3. doi: 10.1016/j.jaci.2020.09.023
160. Dai X, Tohyama M, Murakami M, Shiraishi K, Liu S, Mori H, et al. House Dust Mite Allergens Induce Interleukin 33 (IL-33) Synthesis and Release From Keratinocytes via ATP-Mediated Extracellular Signaling. Biochim Biophys Acta Mol Basis Dis (2020) 1866(5):165719. doi: 10.1016/j.bbadis.2020.165719
161. Meephansan J, Komine M, Tsuda H, Tominaga S, Ohtsuki M. Ultraviolet B Irradiation Induces the Expression of IL-33 mRNA and Protein in Normal Human Epidermal Keratinocytes. J Dermatol Sci (2012) 65(1):72–4. doi: 10.1016/j.jdermsci.2011.10.004
162. Pietka W, Khnykin D, Bertelsen V, Lossius AH, Stav-Noraas TE, Hol Fosse J, et al. Hypo-Osmotic Stress Drives IL-33 Production in Human Keratinocytes-An Epidermal Homeostatic Response. J Invest Dermatol (2019) 139(1):81–90. doi: 10.1016/j.jid.2018.07.023
163. Leyva-Castillo JM, Galand C, Kam C, Burton O, Gurish M, Musser MA, et al. Mechanical Skin Injury Promotes Food Anaphylaxis by Driving Intestinal Mast Cell Expansion. Immunity (2019) 50(5):1262–75.e4. doi: 10.1016/j.immuni.2019.03.023
164. Meephansan J, Tsuda H, Komine M, Tominaga S, Ohtsuki M. Regulation of IL-33 Expression by IFN-Gamma and Tumor Necrosis Factor-Alpha in Normal Human Epidermal Keratinocytes. J Invest Dermatol (2012) 132(11):2593–600. doi: 10.1038/jid.2012.185
165. Sundnes O, Pietka W, Loos T, Sponheim J, Rankin AL, Pflanz S, et al. Epidermal Expression and Regulation of Interleukin-33 During Homeostasis and Inflammation: Strong Species Differences. J Invest Dermatol (2015) 135(7):1771–80. doi: 10.1038/jid.2015.85
166. Dai X, Muto J, Shiraishi K, Utsunomiya R, Mori H, Murakami M, et al. TSLP Impairs Epidermal Barrier Integrity by Stimulating the Formation of Nuclear IL-33/Phosphorylated STAT3 Complex in Human Keratinocytes. J Invest Dermatol (2022). doi: 10.1016/j.jid.2022.01.005
167. Imai Y. Interleukin-33 in Atopic Dermatitis. J Dermatol Sci (2019) 96(1):2–7. doi: 10.1016/j.jdermsci.2019.08.006
168. Imai Y, Yasuda K, Sakaguchi Y, Haneda T, Mizutani H, Yoshimoto T, et al. Skin-Specific Expression of IL-33 Activates Group 2 Innate Lymphoid Cells and Elicits Atopic Dermatitis-Like Inflammation in Mice. Proc Natl Acad Sci USA (2013) 110(34):13921–6. doi: 10.1073/pnas.1307321110
169. Imai Y, Yasuda K, Nagai M, Kusakabe M, Kubo M, Nakanishi K, et al. IL-33-Induced Atopic Dermatitis-Like Inflammation in Mice Is Mediated by Group 2 Innate Lymphoid Cells in Concert With Basophils. J Invest Dermatol (2019) 139(10):2185–94.e3. doi: 10.1016/j.jid.2019.04.016
170. Babina M, Wang Z, Franke K, Guhl S, Artuc M, Zuberbier T. Yin-Yang of IL-33 in Human Skin Mast Cells: Reduced Degranulation, But Augmented Histamine Synthesis Through P38 Activation. J Invest Dermatol (2019) 139(7):1516–25.e3. doi: 10.1016/j.jid.2019.01.013
171. Galand C, Leyva-Castillo JM, Yoon J, Han A, Lee MS, McKenzie ANJ, et al. IL-33 Promotes Food Anaphylaxis in Epicutaneously Sensitized Mice by Targeting Mast Cells. J Allergy Clin Immunol (2016) 138(5):1356–66. doi: 10.1016/j.jaci.2016.03.056
172. Liu B, Tai Y, Achanta S, Kaelberer MM, Caceres AI, Shao X, et al. IL-33/ST2 Signaling Excites Sensory Neurons and Mediates Itch Response in a Mouse Model of Poison Ivy Contact Allergy. Proc Natl Acad Sci USA (2016) 113(47):E7572–E9. doi: 10.1073/pnas.1606608113
173. Trier AM, Mack MR, Fredman A, Tamari M, Ver Heul AM, Zhao Y, et al. IL-33 Signaling in Sensory Neurons Promotes Dry Skin Itch. J Allergy Clin Immunol (2021). doi: 10.1016/j.jaci.2021.09.014
174. Huang J, Gandini MA, Chen L, M’Dahoma S, Stemkowski PL, Chung H, et al. Hyperactivity of Innate Immunity Triggers Pain via TLR2-IL-33-Mediated Neuroimmune Crosstalk. Cell Rep (2020) 33(1):108233. doi: 10.1016/j.celrep.2020.108233
175. Bruhs A, Proksch E, Schwarz T, Schwarz A. Disruption of the Epidermal Barrier Induces Regulatory T Cells via IL-33 in Mice. J Invest Dermatol (2018) 138(3):570–9. doi: 10.1016/j.jid.2017.09.032
176. He R, Yin H, Yuan B, Liu T, Luo L, Huang P, et al. IL-33 Improves Wound Healing Through Enhanced M2 Macrophage Polarization in Diabetic Mice. Mol Immunol (2017) 90:42–9. doi: 10.1016/j.molimm.2017.06.249
177. Kawai K, Uchiyama M, Hester J, Issa F. IL-33 Drives the Production of Mouse Regulatory T Cells With Enhanced In Vivo Suppressive Activity in Skin Transplantation. Am J Transplant (2021) 21(3):978–92. doi: 10.1111/ajt.16266
178. Ameri AH, Moradi Tuchayi S, Zaalberg A, Park JH, Ngo KH, Li T, et al. IL-33/Regulatory T Cell Axis Triggers the Development of a Tumor-Promoting Immune Environment in Chronic Inflammation. Proc Natl Acad Sci USA (2019) 116(7):2646–51. doi: 10.1073/pnas.1815016116
179. Kalekar LA, Cohen JN, Prevel N, Sandoval PM, Mathur AN, Moreau JM, et al. Regulatory T Cells in Skin Are Uniquely Poised to Suppress Profibrotic Immune Responses. Sci Immunol (2019) 4(39). doi: 10.1126/sciimmunol.aaw2910
180. Delacher M, Imbusch CD, Weichenhan D, Breiling A, Hotz-Wagenblatt A, Trager U, et al. Genome-Wide DNA-Methylation Landscape Defines Specialization of Regulatory T Cells in Tissues. Nat Immunol (2017) 18(10):1160–72. doi: 10.1038/ni.3799
181. Demehri S, Turkoz A, Manivasagam S, Yockey LJ, Turkoz M, Kopan R. Elevated Epidermal Thymic Stromal Lymphopoietin Levels Establish an Antitumor Environment in the Skin. Cancer Cell (2012) 22(4):494–505. doi: 10.1016/j.ccr.2012.08.017
182. Di Piazza M, Nowell CS, Koch U, Durham AD, Radtke F. Loss of Cutaneous TSLP-Dependent Immune Responses Skews the Balance of Inflammation From Tumor Protective to Tumor Promoting. Cancer Cell (2012) 22(4):479–93. doi: 10.1016/j.ccr.2012.08.016
183. Takahashi N, Sugaya M, Suga H, Oka T, Kawaguchi M, Miyagaki T, et al. Thymic Stromal Chemokine TSLP Acts Through Th2 Cytokine Production to Induce Cutaneous T-Cell Lymphoma. Cancer Res (2016) 76(21):6241–52. doi: 10.1158/0008-5472.CAN-16-0992
184. Hayes MD, Ward S, Crawford G, Seoane RC, Jackson WD, Kipling D, et al. Inflammation-Induced IgE Promotes Epithelial Hyperplasia and Tumour Growth. Elife (2020) 9. doi: 10.7554/eLife.51862
185. Demehri S, Cunningham TJ, Manivasagam S, Ngo KH, Moradi Tuchayi S, Reddy R, et al. Thymic Stromal Lymphopoietin Blocks Early Stages of Breast Carcinogenesis. J Clin Invest (2016) 126(4):1458–70. doi: 10.1172/JCI83724
186. Pedroza-Gonzalez A, Xu K, Wu TC, Aspord C, Tindle S, Marches F, et al. Thymic Stromal Lymphopoietin Fosters Human Breast Tumor Growth by Promoting Type 2 Inflammation. J Exp Med (2011). doi: 10.1084/jem.20102131
187. Olkhanud PB, Rochman Y, Bodogai M, Malchinkhuu E, Wejksza K, Xu M, et al. Thymic Stromal Lymphopoietin Is a Key Mediator of Breast Cancer Progression. J Immunol (2011). doi: 10.4049/jimmunol.1100463
188. De Monte L, Reni M, Tassi E, Clavenna D, Papa I, Recalde H, et al. Intratumor T Helper Type 2 Cell Infiltrate Correlates With Cancer-Associated Fibroblast Thymic Stromal Lymphopoietin Production and Reduced Survival in Pancreatic Cancer. J Exp Med (2011) 208(3):469–78. doi: 10.1084/jem.20101876
189. Kuan EL, Ziegler SF. A Tumor-Myeloid Cell Axis, Mediated via the Cytokines IL-1alpha and TSLP, Promotes the Progression of Breast Cancer. Nat Immunol (2018) 19(4):366–74. doi: 10.1038/s41590-018-0066-6
190. Cunningham TJ, Tabacchi M, Eliane JP, Tuchayi SM, Manivasagam S, Mirzaalian H, et al. Randomized Trial of Calcipotriol Combined With 5-Fluorouracil for Skin Cancer Precursor Immunotherapy. J Clin Invest (2017) 127(1):106–16. doi: 10.1172/JCI89820
191. Benatar T, Cao MY, Lee Y, Li H, Feng N, Gu X, et al. Virulizin Induces Production of IL-17E to Enhance Antitumor Activity by Recruitment of Eosinophils Into Tumors. Cancer Immunol Immunother (2008) 57(12):1757–69. doi: 10.1007/s00262-008-0502-9
192. Benatar T, Cao MY, Lee Y, Lightfoot J, Feng N, Gu X, et al. IL-17E, a Proinflammatory Cytokine, has Antitumor Efficacy Against Several Tumor Types In Vivo. Cancer Immunol Immunother (2010) 59(6):805–17. doi: 10.1007/s00262-009-0802-8
193. Furuta S, Jeng YM, Zhou L, Huang L, Kuhn I, Bissell MJ, et al. IL-25 Causes Apoptosis of IL-25R-Expressing Breast Cancer Cells Without Toxicity to Nonmalignant Cells. Sci Transl Med (2011) 3(78):78ra31. doi: 10.1126/scitranslmed.3001374
194. Serrels B, McGivern N, Canel M, Byron A, Johnson SC, McSorley HJ, et al. IL-33 and ST2 Mediate FAK-Dependent Antitumor Immune Evasion Through Transcriptional Networks. Sci Signal (2017) 10(508). doi: 10.1126/scisignal.aan8355
195. Taniguchi S, Elhance A, Van Duzer A, Kumar S, Leitenberger JJ, Oshimori N. Tumor-Initiating Cells Establish an IL-33-TGF-Beta Niche Signaling Loop to Promote Cancer Progression. Science (2020) 369(6501). doi: 10.1126/science.aay1813
196. Park JH, Ameri AH, Dempsey KE, Conrad DN, Kem M, Mino-Kenudson M, et al. Nuclear IL-33/SMAD Signaling Axis Promotes Cancer Development in Chronic Inflammation. EMBO J (2021) 40(7):e106151. doi: 10.15252/embj.2020106151
197. Wu J, Chen Z, Wickstrom SL, Gao J, He X, Jing X, et al. Interleukin-33 is a Novel Immunosuppressor That Protects Cancer Cells From TIL Killing by a Macrophage-Mediated Shedding Mechanism. Adv Sci (Weinh) (2021) 8(21):e2101029. doi: 10.1002/advs.202101029
198. Kobayashi T, Voisin B, Kim DY, Kennedy EA, Jo JH, Shih HY, et al. Homeostatic Control of Sebaceous Glands by Innate Lymphoid Cells Regulates Commensal Bacteria Equilibrium. Cell (2019) 176(5):982–97 e16. doi: 10.1016/j.cell.2018.12.031
Keywords: TSLP, IL-33, IL-25, skin immunlogy, allergic inflammation, cancer, host defense, skin homeostasis
Citation: Hasegawa T, Oka T and Demehri S (2022) Alarmin Cytokines as Central Regulators of Cutaneous Immunity. Front. Immunol. 13:876515. doi: 10.3389/fimmu.2022.876515
Received: 15 February 2022; Accepted: 08 March 2022;
Published: 30 March 2022.
Edited by:
Alicia R. Mathers, University of Pittsburgh, United StatesReviewed by:
Yongyao Fu, Indiana University, United StatesCopyright © 2022 Hasegawa, Oka and Demehri. This is an open-access article distributed under the terms of the Creative Commons Attribution License (CC BY). The use, distribution or reproduction in other forums is permitted, provided the original author(s) and the copyright owner(s) are credited and that the original publication in this journal is cited, in accordance with accepted academic practice. No use, distribution or reproduction is permitted which does not comply with these terms.
*Correspondence: Shadmehr Demehri, c2RlbWVocmkxQG1naC5oYXJ2YXJkLmVkdQ==