- 1Shenzhen Institute of Advanced Technology (SIAT), Chinese Academy of Science (CAS), Shenzhen, China
- 2Institute of Biochemistry and Cell Biology (IBBC), National Research Council (CNR), Napoli, Italy
- 3Stazione Zoologica Anton Dohrn, Napoli, Italy
Interleukin-1 is a cytokine with potent inflammatory and immune-amplifying effects, mainly produced by macrophages during defensive reactions. In mammals, IL-1 is a superfamily of eleven structurally similar proteins, all involved in inflammation or its control, which mainly act through binding to specific receptors on the plasma membrane of target cells. IL-1 receptors are also a family of ten structurally similar transmembrane proteins that assemble in heterocomplexes. In addition to their innate immune/inflammatory effects, the physiological role of IL-1 family cytokines seems to be linked to the development of adaptive immunity in vertebrates. We will discuss why IL-1 developed in vertebrates and what is its physiological role, as a basis for understanding when and how it can be involved in the initiation and establishment of pathologies.
Introduction
After many studies that described soluble endogenous factors responsible for inflammation, fever and lymphocyte activation, human IL-1β was molecularly identified in 1984 as a single factor mainly produced by leukocytes (in particular monocytes and macrophages) responsible for many inflammation-related functions that occur during the host reaction to infections and other stressful events (1, 2). IL-1β is responsible for the induction of fever (originally described as “endogenous pyrogen”) (3–6), thereby contributing to create an unfavorable environment for infectious microorganisms and facilitate lymphocyte functions (which in mammals are more pronounced at temperatures above 37°C). Also, it amplifies B and T lymphocyte proliferation to antigens/mitogens (7–13), stimulates neutrophilia and acute phase proteins (14, 15), induces the production of proteolytic enzymes and prostaglandins by fibroblasts, chondrocytes and other cells (16–19), upregulates the expression and production of chemokines (IL-8, MCP-1) and inflammatory cytokines (TNFα, IL-6, IL-1 itself), induces the production of ROS and NO, causes redistribution of zinc and iron in the tissues and regulates corticosteroids and glucose homoeostasis (20–22), and is a potent adjuvant of antigen-specific antibody responses in vivo (23–29).
Thus, IL-1β appears to contribute to the defensive response by acting at different levels, i.e., by directly participating to the inflammatory response (production of degrading enzymes and toxic molecules), by amplifying it (increase of body temperature, production of inflammatory factors, recruitment of effector cells, redistribution of nutrients and hormones) and eventually by non-specifically but directly activating/amplifying lymphocyte responses. The substantial disruption of homeostasis caused by IL-1β activation requires a complex and coordinated control system, which can inhibit IL-1 effects at various levels and dampen its activity once the defensive inflammatory reaction comes to an end. In addition, we should also consider that IL-1 is not a single molecule but rather a superfamily of structurally and functionally related cross-regulating cytokines.
A number of questions arise, when observing the plethora of IL-1 effects.
● Is IL-1 a typically innate/inflammatory molecule or is it involved in other functions beyond innate immunity? Why is it associated to vertebrates and to adaptive immunity? Does it have a physiological or homeostatic role? Does it have non-immune functions?
● Why do we have so many IL-1-like molecules that have overlapping effects? Is there a reason for such duplication of molecules, or is it just redundancy?
● Does IL-1 only act by binding to its receptors or does it have receptor-independent functions? Do the IL-1R have IL-1-independent functions?
In order to answer these questions, we need to know something more about the IL-1 superfamily.
The IL-1 Superfamily
The cytokines of the IL-1 superfamily are proteins of about 17-18 kDa, with a typical β-trefoil pyramidal barrel structure composed by six two-stranded β hairpins, a structure common to many proteins with different functions throughout evolution, from bacteria to mammals, in particular for carbohydrate recognition (ricin-type lectins) and as toxins (30–34). In humans (and most mammals), eleven IL-1-like cytokines have been described, some with inflammatory activity and some with anti-inflammatory functions. From evolutionary analysis (see below), the ancestral IL-1 family encompasses IL-1β (the prototypical IL-1 cytokine) and other eight cytokines derived from it (IL-1α, IL-1Ra, IL-36Ra, IL-36α, IL-36β, IL-36γ, IL-37 and IL-38) (35, 36). IL-1β, IL-1α and the three IL-36 isoforms have largely overlapping inflammatory activities, with IL-1β and IL-1α binding to the same receptor (35, 36). However, IL-1α is a moonlighting protein, with a number of diverse functions attributed to its long intracellular form, which differ from the inflammatory functions of the extracellular shorter cytokine (37, 38). The three IL-36 isoforms (which all bind to the same receptor) have a range of tissue-specific inflammatory activities and are differentially present in different tissues (39, 40). Other members of the family have antagonistic or inhibitory activity: IL-1Ra is a receptor antagonist that binds in an inactive fashion to the same receptor as IL-1β and IL-1α, thereby antagonizing them (41); IL-36Ra is a receptor antagonist for the three IL-36 isoforms (34); IL-37 is an anti-inflammatory cytokine with a wide range of targets, possibly binding to the IL-18 receptor and to the inhibitory receptor IL-1R8 (35, 42–44); IL-38 was also reported to have anti-inflammatory activity (although its functions are not fully clarified) (45–47).
In addition to the ancestral family, the broader IL-1 superfamily also includes IL-18 and IL-33, which are not evolutionarily related, but have substantial structural and functional similarities with IL-1 (48). Both cytokines have multiple activities that are not strictly inflammatory and that are largely involved in immune metabolism. IL-18 is present in circulation at measurable levels (implying that it is not directly inflammatory), it induces the production of IFN-γ in T and NK cells in concert with IL-12, and it contributes to the differentiation of Th1 cells (49, 50). In its extracellular form, IL-33 is a potent activator of Th2 cells, as well as mast cells, basophils and eosinophils, thereby contributing to type 2 inflammation (51–53). In addition, intracellular IL-33 localises to the nucleus and presumably binds to DNA, although with unknown effects on gene expression, and it was reported able to interact with NFκB to decrease NFκB-dependent inflammatory gene transcription (51–53).
The IL-1R Family
The receptors that bind the IL-1 superfamily cytokines are also structurally very similar, and are ten transmembrane proteins, which display an extracellular portion encompassing three Ig-like domains and a cytoplasmic portion that contains a Toll-IL-1R (TIR) signaling domain. The IL-1R complex that allows for cell activation is typically composed by a ligand-binding chain, which engages with the cytokine, and an accessory chain that associates with the dimeric ligand/receptor complex. The approximation of the two homologous intracellular TIR domains is the event that initiates signaling and eventual cell activation (54). The prototypical IL-1R is IL-1R1, the ligand-binding receptor for IL-1β and IL-1α. The accessory chain for IL-1R1 is IL-1R3, a promiscuous accessory chain that participates to several IL-1R complexes. Another ligand binding chain for IL-1β and IL-1α is the IL-1R2, which acts as a decoy receptor because it lacks the intracellular TIR domain and therefore can sequester ligands and IL-1R3 into an inactive receptor complex. The receptor antagonist IL-1Ra can inhibit binding of the active ligands IL-1β and IL-1α to IL-1R1. Other receptor complexes are similarly composed. The receptor for IL-36 encompasses the ligand binding chain IL-1R6 and the accessory chain IL-1R3, while the IL-33 receptor complex is composed by IL-1R4 and IL-1R3. The receptor complex for IL-18 includes the ligand binding chain IL-1R5 and a unique accessory protein, IL-1R7.
Other receptor-like chains included in the family have peculiar characteristics. The orphan receptors IL-1R9 and IL-1R10 are mainly expressed in the brain and do not have known ligands (54), although it has been proposed that IL-38 may bind IL-1R9 (46). The unique receptor IL-1R8 (also known as SIGIRR) is characterized by the presence of a single Ig-like domain in its extracellular portion and has the role of inhibiting receptor-mediated activation. Proposed mechanisms include interference with receptor complexes both at the extracellular and intracellular level (in this case active also in inhibiting TLR signaling), and acting as co-receptor for inhibitory ligands (hypothesized in the case of IL-37 bound to IL-1R5) (43). In teleost fish, another truncated receptor has been described, DIGIRR, which displays two Ig-like extracellular domains. DIGIRR is alternative to SIGIRR (same chromosomal location) and has similar anti-inflammatory capacity as SIGIRR, leading to hypothesize that it is a transition from between the classical receptors with 3 Ig domains and the single Ig domain SIGIRR (55).
A list of the human IL-1 and IL-1R molecules and genes, with some of the different names they are known with, is reported in Table 1, while Table 2 summarizes the main known ligand-receptor interactions.
Evolution of IL-1 and IL-1R
Evolution of the IL-1 Superfamily
The evolutionary history of IL-1 and IL-1R offers very interesting implications and can help us understanding more about IL-1 functions. As mentioned above, the structure of IL-1 superfamily cytokines is quite common (β-trefoil barrel), as it can be found in molecules with different recognition and defensive functions across evolution, from bacteria and fungi (e.g., pore-forming toxins and carbohydrate binding proteins) to plants (many lectins and toxins, Kunitz protease inhibitors), invertebrates (chitin-binding proteins and other lectins) and vertebrates (R-type lectins and domains in mannose and other receptors) (30–34). IL-1-like activities were extensively described in invertebrates (echinoderms, tunicates, insects) (56–66). However, no molecular characterization of invertebrate IL-1-like molecule(s) is at present available, and no gene orthologues were found in any clade besides vertebrates, based on the currently available genomes. This implies that IL-1 cytokines in vertebrates have taken functions that in invertebrates are performed by different molecules (58, 59, 63, 66). From evolutionary and chromosomal analysis, the ancestral proto-IL-1β gene appeared about 420 million years ago, i.e., coincidental with the emergence of the vertebrate subphylum (48), encoding for a protein that adopted the β-trefoil barrel structure common to many invertebrate defensive molecules.
From chromosomal location and surrounding gene positioning, three separate IL-1 families can be identified, i.e., IL-1, IL-18 and IL-33 (48). While the IL-1 and IL-18 families are present in all vertebrate species, IL-33 only appeared in mammals. From the proto-IL-1β gene are derived all other cytokines of the ancestral IL-1 family, which are orthologues generated by gene duplication. Of them, only IL-1Ra is present in all vertebrates (independently evolved in fish, derived from proto-IL-1β gene duplication in other vertebrates), whereas IL-1α, the IL-36 group, IL-37 and IL-38 appeared much more recently (between 320 and 160 million years ago), as they are only present in mammals (48), similar to IL-33 (Figure 1). Why all the cytokines of the ancestral IL-1 family only appeared in mammals is a matter of speculation, although they have likely developed for performing specific defensive/immunostimulating functions linked to the peculiar characteristics of mammalian physiology and anatomy (large brain, fetal development, lactation, hair-covered skin, complex anatomy).
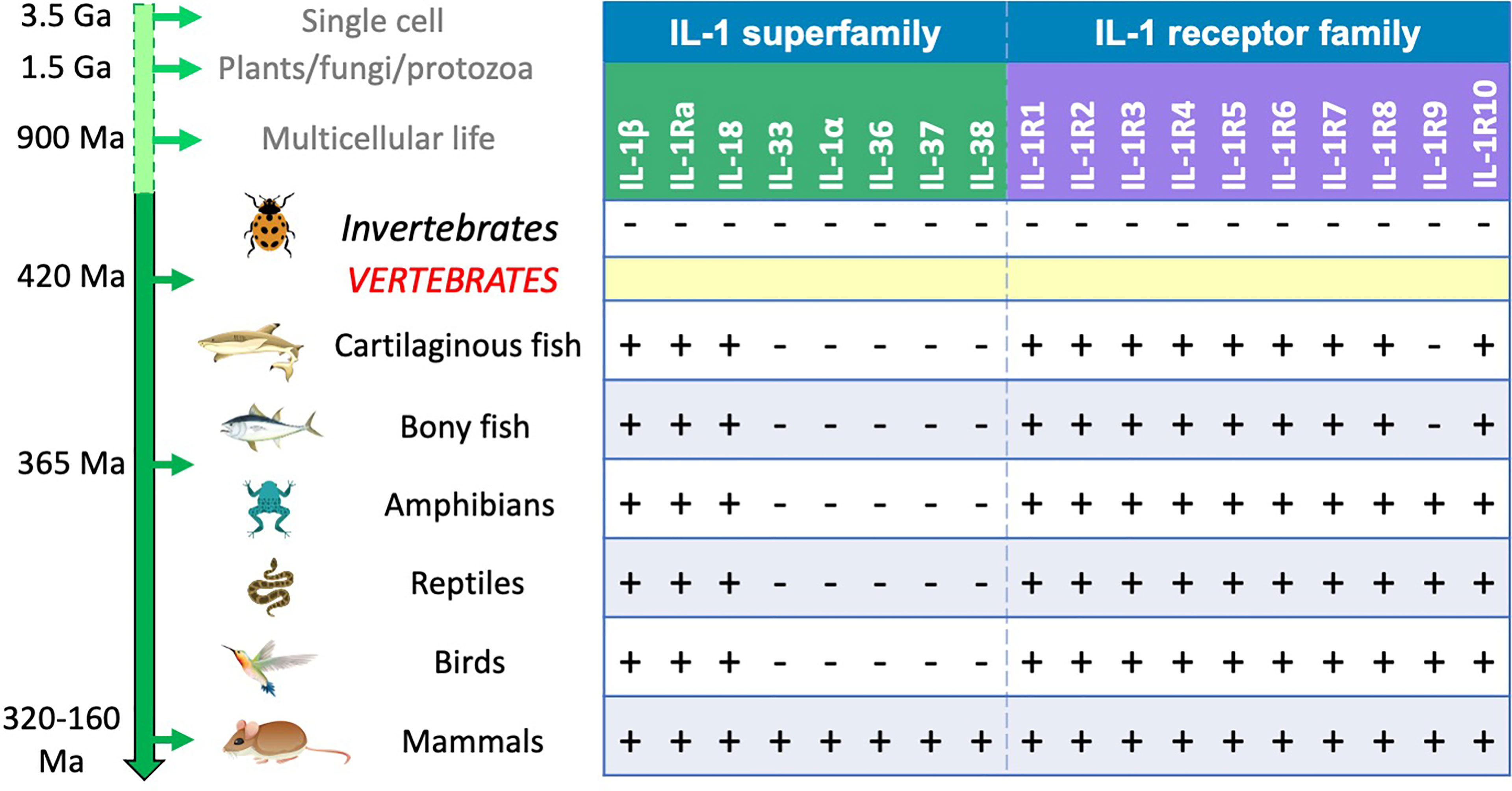
Figure 1 IL-1 and IL-1R family molecules in vertebrates. No IL-1-like homologous sequences have been identified in invertebrates, whereas numerous orthologues of IL-1R family genes were identified. This places the appearance of proto-IL-1 genes at the same time as the appearance of the vertebrate subphylum around 420 million years ago (48). The various IL-1R genes are present in all vertebrates, from cartilaginous fish to mammals, except for IL-1RAPL1, which only appeared after separation of bony fish and Tetrapoda clades about 365 million years ago, most likely from duplication of the IL1RAPL2 gene. All vertebrates possess the genes for IL-1β, IL-1Ra and IL-18, whereas all the other IL-1 genes only appeared in mammals; genes encoding IL-1α, the IL-36 group, IL-37 and IL-38 are likely derived from IL-1β gene duplication, while the gene for IL-33 has independently evolved. Please note that the receptor genes were present in evolution long before the appearance of their ligands. Minus and plus signs indicate gene absence or presence.
Evolution of the IL-1R Family
IL-1R-like genes are present in invertebrates and are predicted to encode proteins with a typical organisation encompassing an extracellular Ig-like domain, a transmembrane region and an intracellular signalling domain. Several IL-1R-like orthologous genes, with homologies to the vertebrate genes, have been identified in Cnidaria, Protostomia and Deuterostomia, implying important yet unidentified functions (67–69). In addition, a significant homology can be observed between the intracellular portion of IL-1R and the Toll/Toll-like receptors (TLR), the most represented innate/developmental receptors in invertebrates, in particular in the signalling domain that has been therefore dubbed TIR (from Toll-IL-1R) (70, 71). Thus, IL-1R molecules are hybrid transmembrane receptors with the same signalling domain as TLR but with a distinct extracellular portion displaying Ig-like domains. While Ig domains are present in many invertebrate proteins, their inclusion into Ig-like receptors has expanded from pre-vertebrate chordata (68, 69, 72) to vertebrates giving rise to a plethora of receptors with high interaction capacity and specificity [including antigen-recognising T and B cell receptors; (73-75)]. In this perspective, the presence of Ig-like domains in the binding portion of IL-1R molecules is predictive of high binding specificity, at variance with the broad pattern-recognition capacity of TLR and other innate receptors.
The sequence similarity and the chromosomal anatomy of IL-1R genes in vertebrates suggest that most IL-1R molecules belong to a single family derived from duplication of an ancestral IL-1R gene. These are the two IL-1 receptor genes IL1R1 and IL1R2, the IL-33 receptor gene IL1RL1, the IL-18 binding and accessory chain genes IL18R1 and IL18AP, the IL-36 receptor gene IL1RL2 and, by further duplication from one of the IL-1R genes, IL1RAPL2 and (from it) IL1RAPL1, which is only present in birds and mammals (48). The only two IL-1R genes that do not seem to belong to the IL-1R family are the orphan receptor gene SIGIRR and the IL-1R3 gene IL1RAP, possibly derived from IL18RAP (48). SIGIRR shares low sequence homology and no chromosomal anatomy with the other genes, suggesting no common ancestry. As mentioned above, SIGIRR is likely the result of a gradual loss of extracellular Ig domains from a distinct IL-1R-like ancestral gene. IL-1R molecules are present in all vertebrates, except IL1RAPL1, which is absent in fish and only appeared in Tetrapoda about 365 million years ago (Figure 1). Given the presence of the intracellular TIR domain, the signalling pathways of IL-1R and TLR are similar, involving an overlapping range of downstream signalling and regulatory molecules, and leading to activation of overlapping immune defensive responses.
Notably, the appearance of receptors preceded that of ligands. This is obvious in invertebrates, in which IL-1R are present in the absence of IL-1-like molecules, but also in vertebrates, as in the case of IL-1R4 for IL-33 and IL-1R6 for the IL-36 group. The notion that receptors were present long before their cytokine ligands underlines their different original functions and their re-use in mammals for additional, IL-1-mediated effects. That IL-1R have appeared for functions that do not require IL-1 ligand binding is supported by the case of the orphan receptors IL-1R10 and IL-1R9, which do not have known ligands and that have non-immune functions in the brain (54).
The Physiological Role of IL-1
IL-1 Cytokines in Innate and Adaptive Immunity
Despite the common notion that IL-1 is an innate/inflammatory cytokine, the observation that IL-1 appeared in vertebrates, coincidental with the development of adaptive immunity, leads to hypothesize that its functions are not exclusively linked to innate immunity. The co-existence of innate and adaptive immunity in vertebrates likely led to a progressive “simplification” of the innate immune defensive mechanisms, which are particularly complex in several invertebrates (67). For instance, TLR and NLR genes decreased from over 200 in sea urchin to 10-20 in human beings. In this context, IL-1 family cytokines may have developed for taking a double role, to maintain a functional innate immunity that could also amplify the adaptive immune responses.
IL-1 family cytokines are considered innate immune factors because their production does not depend on cognate signalling and their effects are likewise non-specific. In the case of the prototypical cytokine IL-1β, its production is inducible, i.e., the cytokine is produced upon stimulation, and there is no constitutive production in quiescent conditions, implying that its main role is in the reaction to triggering agents, typically infective microorganisms. Since the IL-1 receptors are receptors that signal through a TLR-like pathway, being TLR the main innate defensive receptors in invertebrate immunity, it is reasonable to say that IL-1β is a factor involved in immune defense, and we can therefore suggest that its main physiological role is inducing/participating to the innate immune protection from invasion and damage. However, the fact that IL-1 family cytokines are only present in vertebrates further suggests that their defensive activity goes beyond innate immunity and that they have a role in helping/amplifying adaptive immune responses.
IL-1β can non-specifically amplify T and B lymphocyte responses that, conversely, are triggered by specific antigenic signals. Thus, IL-1β can induce IL-2 secretion and expression of IL-2 receptors on T cells, induce expansion and commitment of naïve and memory CD4+ T cells (in particular Th17) in response to antigen and enhance their functions (76–87), thereby indirectly increasing B cell activation, and it can also increase CD8+ T cell activation and cytocidal capacity (88–90).
Likewise, the IL-1 superfamily member IL-18 is a potent activator of Th1 responses and participates to Th1 differentiation and activation, typically by inducing the production of IFN-γ in concert with IL-12 (49, 80, 91). IL-18 is constitutively produced by many cell types, and mainly released by monocytes and macrophages, and is present at measurable level in the circulation of healthy people, suggesting a homeostatic or surveillance role (91).
The other, most recent IL-1 superfamily member IL-33, present only in mammals, is an inducer of Th2 differentiation and amplification, thereby contributing to the specific defensive reaction against extracellular parasites (e.g., helmints), bacteria and toxins (and to the overall type 2 inflammatory reaction) (51, 80), as well as to the successful establishment of pregnancy (92, 93).
The Moonlighting IL-1 Superfamily Proteins
IL-1α is very similar in its effects as IL-1β in its mature extracellular form, including effects on T cell activation. This raises the question why, after duplication from IL-1β, IL-1α has divergently evolved and has been conserved in mammals. The most likely hypothesis is that IL-1α possesses different functions, non-redundant with those of IL-1β. Indeed, IL-1α is not a typical inflammatory cytokine, as its precursor protein (pro-IL-1α) is constitutively expressed in macrophages and many non-immune stromal cells (epithelial cells, endothelial cells, fibroblasts) in resting conditions, and it localises in the nucleus where it acts as transcription factor thereby regulating cellular functions, proliferation, senescence and apoptosis (37, 38, 94, 95). Upon inflammatory stimulation, pro-IL-1α can be cleaved and, while the C-terminal portion is exported outside the cell and acts as a cytokine (binding to the same receptor as IL-1β), the pro-piece can still act as transcription factor and upregulate the expression of inflammation-related genes (38, 95–98). Thus, the moonlighting nature of IL-1α, which has different functions in different conditions and in different compartments, is probably among the reasons why it has been conserved throughout mammalian evolution.
IL-33 shares with IL-1α its dual functions, both as a soluble IL-1-like cytokine active on Th2 cells and as an intracellular transcription factor constitutively present in several stromal cell types (37, 51). At variance with IL-1α, however, the nuclear IL-33 represses gene expression, as it does facilitate chromatin compaction (99), suggesting that IL-33 can act as a strong down-regulator of inflammatory responses and contribute to the re-establishment of tissue homeostasis during the resolution of a defensive reaction. Also, as mentioned above, the fact that IL-33 is only present in mammals suggests its major role in controlling inflammatory reactions during pregnancy, thereby facilitating embryo implantation and development (92, 93).
Agonists, Antagonists and Inverse Agonists in the IL-1 Cytokine and Receptor Families
Based on the above notions, we may consider the IL-1 superfamily as a hybrid cytokine family, which has developed for amplifying and controlling adaptive immune responses in vertebrates but that is non-specific in its induction and effects, as it simultaneously displays typical innate/inflammation-related activities. The IL-1R characteristics contribute to the mixed activities of the IL-1 family cytokines, since the receptors display specific ligand recognition and binding (with their Ig-like extracellular domains) and are differentially expressed on the surface of distinct lymphocyte populations (thereby specifically targeting the IL-1 effects to them), but are typical innate receptors in their downstream TIR-dependent effects.
However, the evolutionary data suggest a more complex scenario. Many of the IL-1R molecules appeared long before their IL-1 ligands, suggesting that these molecules had/have an original constitutive activity, independent of ligands. In mammals, this hypothesis is supported by some data showing ligand-independent receptor functions, as for instance in the case of IL-1R7 (100) and IL-1R9 (101, 102) in the brain. In the case of the IL-33 receptor IL-1R4, based on structural data, it has been proposed that its activation is brought about by interaction with the accessory chain IL-1R3 in a ligand-independent way, and that the function of IL-33 is merely that of stabilizing IL-1R4 in a conformation that promotes interaction with IL-1R3 (103, 104). We can hypothesize that the IL-1 ligands developed for downregulating and controlling the constitutive functions of such receptors, a strategy adopted by pathogenic microorganisms for inhibiting host receptors with antimicrobial capacity. These ligands would be inverse agonists, i.e., agonists that dampen the activity of constitutively active receptors. Thus, the inverse agonist molecules may have appeared in evolution before the agonist ligands and may be derived from microbial genes/molecules (which, as already mentioned, share the same β-trefoil fold structure as IL-1 family molecules). In the case of microbial inverse agonists, the agonist ligands are the countermeasures created by the host organism to antagonise the microbial inverse agonists, thereby neutralising the pathogen’s escape strategy. Similarly, the IL-1 family agonists may have developed from the inverse agonists, based on modifications of the same structure, to re-activate, control and fine-tune biological functions that are down-regulated by an inverse agonist (Figure 2). IL-1Ra and IL-1β appeared around the same time, i.e., about 420 million years ago with the emergence of vertebrates, underlining their mutual relationship, although we cannot know whether IL-1 emerged to counteract IL-1Ra or vice-versa. In mammals, IL-1R1 does not seem to have ligand-independent functions (which may have been lost during evolution), and IL-1β acts as agonist ligand, whereas IL-1Ra has mainly developed as antagonist ligand. However, it is notable that binding of IL-1β to IL-1R1 does not induce receptor activation, which can only be achieved after the engagement of a second non-ligand binding accessory chain. Thus, IL-1Ra does not counteract IL-1β by dumping IL-1β-induced receptor activation but rather by preventing interaction of the receptor with the accessory chain (Figure 2).
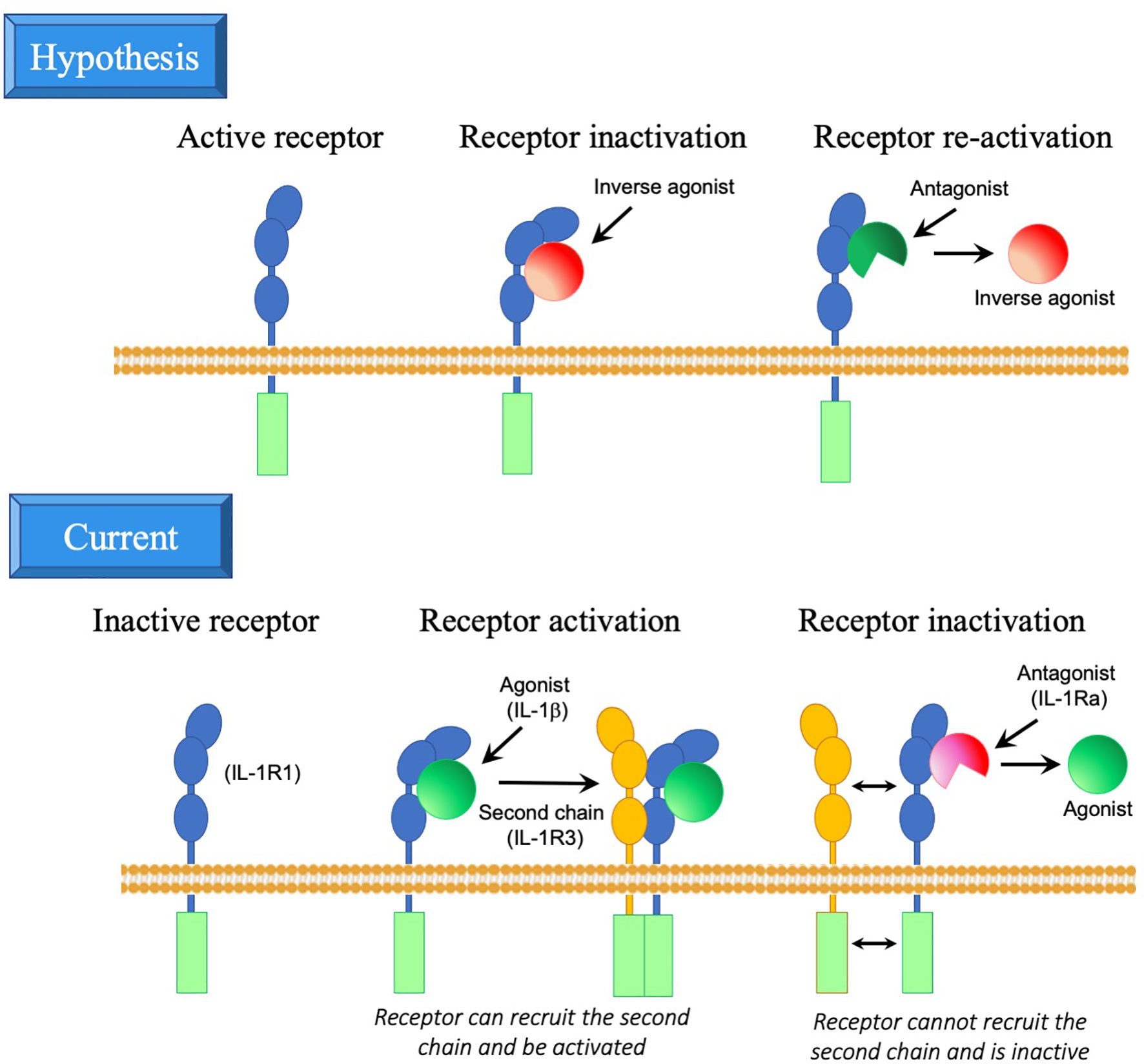
Figure 2 Inverse agonist hypothesis in the development of IL-1R ligands. Upper panel. Hypothesis on the possible development of IL-1R family molecules and their ligands. The receptor may have developed as a molecule with intrinsic activity, in the absence of interaction with ligands (this is still the case for instance for the orphan receptor IL-1R9). Ligands may have developed as a tool for inhibiting and modulating the receptor activity (inverse agonists, which inhibit rather than activate the receptor), likely by changing the receptor conformation. Consequently, antagonists may have developed as partial ligands, based on modifications of the inverse agonist structure, that bind to the receptor and displace the inverse agonists, while unable to modify the receptor conformation and its activity. Lower panel. Current model of IL-1R and ligand interaction. The receptor is intrinsically inactive (for instance, in the case of IL-1R1). Binding of an agonist (such as IL-1β) changes the receptor conformation thereby enabling the interaction with a second chain (such as IL-1R3) and consequent activation (by approximation of the intracellular domains of the two receptors). Antagonists (such as IL-1Ra) have a similar structure as agonists and are able to partially bind to the receptor, thereby displacing the agonist, but unable to modify the receptor conformation and thus preventing interaction with the second chain and consequent activation. Artwork by W.J. Yang.
Do IL-1 Family Cytokines Need Receptors?
IL-1 family molecules can exert a number of activities that are apparently independent of receptor engagement, as it has been reported in particular for the most ancient cytokines IL-1β and IL-1Ra. In ischemic brain damage, IL-1β can exacerbate damage also in the absence of IL-1R1, whereas IL-1Ra could only antagonise IL-1β in the presence of IL-1R1 (105). Likewise, IL-1β could modulate expression of a number of genes in primary microglial cells independently of IL-1R1, while other genes were only modulated depending on the receptor’s presence (106). In the same system, IL-1α only showed receptor-dependent effects (106). Most interestingly, IL-1Ra was observed having the same agonist activity as IL-1β in the hippocampus, with the IL-1Ra effects being IL-1R1-independent and those of IL-1β IL-1R1-dependent (107). The lack of correlation between receptor binding and activity in IL-1β is further supported by data on the IL-1R1-independent immunostimulatory capacity of the β-bulge surface loop between the 4th and the 5th β-strand in the IL-1β structure, and by the use of monoclonal antibodies and IL-1β mutants that showed a dissociation between IL-1R1 binding and different functional activities (108–112).
Notably, reminiscent of the lectin-like activity of β-trefoil proteins, IL-1β displays a lectin-like domain, distinct from the IL-1R1 binding sites, which can enable binding to specific carbohydrates (e.g., heparin, hyaluronic acid, GM4), thereby promoting interaction with glycoproteins on cell membrane and in the extracellular matrix (113–118). This capacity of interacting with glycoproteins not only can promote the tissue-specific localisation of the cytokine, as initially proposed, but it can actually underlie a more complex and more controlled activation mode, entailing the concomitant or differential engagement of receptor and glycoproteins for activation/inhibition of immune functions (114, 115, 119). IL-1α also has a carbohydrate-binding domain, which however is different from that of IL-1β, implying a distinct interaction mode and distinct activity regulation (114, 115, 118, 120), while IL-1Ra does not have such domain (118).
All these observations support the hypothesis that IL-1β may have developed at first as a receptor-independent functional molecule, and that it has been subsequently “re-used” to counteract IL-1Ra effects, but still maintaining both receptor-dependent and independent functions within a complex interplay of regulatory cross-interactions.
IL-1 Superfamily Cytokines in Pathology
The IL-1 family cytokine effects on immunity and inflammation imply that their dysregulation can cause pathological derangements. Excessive IL-1β is involved in a range of inflammation-related diseases, from chronic inflammatory diseases and autoinflammatory diseases to autoimmune syndromes, neurodegenerative diseases and cancer (2, 15, 35, 36, 41, 121). IL-1α released from necrotic cells is considered a major inducer of sterile inflammation in several ischemic conditions, by inducing neutrophil and macrophage recruitment and IL-1β expression and maturation (37, 121–123). The importance of IL-1β and IL-1α in sterile inflammation has been underlined by the clinical use of the recombinant IL-1Ra (Anakinra), which has allowed us to appreciate the involvement of these cytokines in many unexpected pathologies (41, 123), and by a rare genetic defect in IL-1Ra production that leads to a strong inflammatory pathology (124, 125). IL-1α in particular has also been linked to cancer development (95, 126, 127). Excessive IL-18 is associated to the development of autoimmune diseases, and the increased levels of IL-18 and of its inhibitor IL-18BP are a common marker of ongoing inflammation and ageing (49, 128–134). Increase in IL-36 is strongly linked to skin inflammatory diseases (39, 40, 134–136), while IL-33 can be involved in pathological type 2 inflammatory diseases, such as exacerbation of pulmonary inflammation in COPD and asthma/allergies (51, 134, 137, 138). Notably, the circulating levels of the soluble form of the IL-33 receptor IL-1R4 are also substantially increased in patients with inflammatory diseases, and high IL-1R4 levels are one of the predictive markers of heart failure (139).
Thus, all IL-1 cytokines, when dysregulated, can cause or participate to pathological outcomes, a notion that supports their role in maintaining immune functionality, i.e., preparedness to mounting a defensive reaction and capacity to control it.
Conclusions: Why Do We Have IL-1?
IL-1 family cytokines are a recent acquisition in the animal kingdom, suggesting that they are specifically needed for the optimal development and survival of vertebrates. IL-1 family cytokines have most likely emerged for improving the efficacy of the newly evolved adaptive immunity, which is highly specific (and therefore more efficient than innate immunity in reacting to individual dangers) but possibly too slow and too weak for an effective protection of the anatomically complex, multi-organ vertebrate organisms. Two different IL-1-like molecules independently appeared essentially at the same time as adaptive immunity, IL-1β and IL-18, implying distinct needs, most likely the amplification of strong anti-infective responses differentially initiated by Th17 and Th1 cells. IL-33 only appeared in mammals, although being involved in the activation of the more ancient type 2 responses. These responses are brought about by innate cells but also by Th2 cells (which are present in birds besides mammals). The need of IL-33 in mammals is therefore hypothetically linked to mammal-specific needs, such as pregnancy and embryonal implantation and survival, a process that implies the downregulation of type 1 responses (which would kill the embryo) and the enhancement of anti-inflammatory type 2 responses.
Thus, we can say that IL-1 family molecules are important for the adequate amplification of adaptive immune responses, acting by enhancing Th1, Th2, Th17 and CD8 functions. They act on adaptive immunity by using innate immune tools, through receptors that trigger innate immune responses and by using receptor-independent pathways that are still not fully known. Notably, the functions of IL-1 and IL-1R family molecules seem to be related to their organ localisation, with different molecules and different pathways and regulatory networks engaged in achieving tissue-specific immune protection. In addition, many questions are still open on the putative non-immune role of both the IL-1 family ligands and, importantly, their receptors, since the latter have evolved well before their ligands or do not have known ligands.
To the initial questions, we may try to give some answers, although still hypothetical.
● IL-1 family molecules indeed seem to have both homeostatic and inflammation-related defensive functions. Their presence in vertebrates underlines the association with adaptive immunity, and it seems that IL-1/IL-1R family molecules are important tools linking innate immune functions (lectin-like recognition domains in the ligands, TIR signalling domains in the receptors) with the amplification and regulation of adaptive immunity.
● The redundancy of IL-1 family cytokines is likely due to organ/tissue-specific activities and regulation, which may also explain why they have appeared in vertebrates (which have a more complex anatomy with specialised organs and organ-specific homoeostatic and defensive needs).
● That both IL-1 and IL-1R family molecules may retain ancestral non-immune functions or have developed them in specific organs (as the brain) is a realistic possibility, as it is also realistically possible that both IL-1 and IL-1R family molecules can have functions that do not depend on canonical ligand-receptor binding. The lectin-like interaction of some IL-1 cytokines with glycoproteins enlarges in a still unexplored fashion the range of their possible functions.
On these grounds, how IL-1 and IL-1R family molecules are involved in pathologies is still largely obscure, from a mechanistic point of view, and associations with diseases in most cases are made with circulating cytokine levels that may not reflect the relevant organ-specific alterations and may not imply a cause-effect relationship. Thus, our understanding of IL-1 family cytokines in health and disease is still at the beginning, and it will take a much deeper knowledge of their role in homeostasis and defence in different tissues and organs within the mammalian body before being able to harness their activity towards health promotion.
Author Contributions
DB devised and wrote the paper. The author confirms being the sole contributor of this work and has approved it for publication.
Funding
The author was supported by the EU Horizon 2020 projects PANDORA (GA 671881) and ENDONANO (GA 812661), the Italian MIUR InterOmics Flagship projects MEMORAT and MAME, and the Presidential International Fellowship Programme (PIFI) of the Chinese Academy of Science (2020VBA0028).
Conflict of Interest
The author declares that the study was conducted in the absence of any commercial or financial relationships that could be construed as a potential conflict of interest.
Publisher’s Note
All claims expressed in this article are solely those of the authors and do not necessarily represent those of their affiliated organizations, or those of the publisher, the editors and the reviewers. Any product that may be evaluated in this article, or claim that may be made by its manufacturer, is not guaranteed or endorsed by the publisher.
Acknowledgments
The author wishes to acknowledge the many colleagues with whom she has shared her life-long interest in IL-1, first of all Aldo Tagliabue. Special thanks to W. Jason Yang, who has critically revised the manuscript with an unbiased and curious mind.
References
1. Auron PE, Webb AC, Rosenwasser LJ, Mucci SF, Rich A, Wolff SM, et al. Nucleotide Sequence of Human Monocyte Interleukin 1 Precursor cDNA. Proc Natl Acad Sci USA (1984) 81:7907–11. doi: 10.1073/pnas.81.24.7907
2. Dinarello CA. IL-1: Discoveries, Controversies and Future Directions. Eur J Immunol (2010) 40:599–606. doi: 10.1002/eji.201040319
3. Bennett IL Jr, Beeson PB. Studies on the Pathogenesis of Fever. I. The Effect of the Injection of Extracts and Suspensions of Uninfected Rabbit Tissues Upon the Body Temperature of Normal Rabbits. J Exp Med (1953) 98:477–92. doi: 10.1084/jem.98.5.477
4. Bodel P. Studies on the Mechanism of Endogenous Pyrogen Production. III. Human Blood Monocytes. J Exp Med (1974) 140:954–64. doi: 10.1084/jem.140.4.954
5. Atkins E. Fever: The Old and the New. J Infect Dis (1984) 149:339–48. doi: 10.1093/infdis/149.3.339
6. Dinarello CA. Infection, Fever, and Exogenous and Endogenous Pyrogens: Some Concepts Have Changed. J Endotoxin Res (2004) 10:201–22. doi: 10.1179/096805104225006129
7. Gery I, Waksman BH. Potentiation of the T-Lymphocyte Response to Mitogens. II. The Cellular Source of Potentiating Mediator(s). J Exp Med (1972) 136:143–55. doi: 10.1084/jem.136.1.143
8. Rosenwasser LJ, Dinarello CA, Rosenthal AS. Adherent Cell Function in Murine T-Lymphocyte Antigen Recognition. IV. Enhancement of Murine T-Cell Antigen Recognition by Human Leukocytic Pyrogen. J Exp Med (1979) 150:709–14. doi: 10.1084/jem.150.3.709
9. Rosenwasser LJ, Dinarello CA. Ability of Human Leukocytic Pyrogen to Enhance Phytohemagglutinin Induced Murine Thymocyte Proliferation. Cell Immunol (1981) 63:134–42. doi: 10.1016/0008-8749(81)90034-4
10. Lipsky PE. Role of Interleukin-1 in Human B-Cell Activation. Contemp Top Mol Immunol (1985) 10:195–217. doi: 10.1007/978-1-4684-4838-2_8
11. Pike BL, Nossal GJV. Interleukin 1 can Act as a B-Cell Growth and Differentiation Factor. Proc Natl Acad Sci USA (1985) 82:8153–7. doi: 10.1073/pnas.82.23.8153
12. Lowenthal JW, Cerottini J-C, MacDonald HR. Interleukin 1-Dependent Induction of Both Interleukin 2 Secretion and Interleukin 2 Receptor Expression by Thymoma Cells. J Immunol (1986) 137:1226–31.
13. Lichtman AH, Chin J, Schmidt JA, Abbas AK. Role of Interleukin 1 in the Activation of T Lymphocytes. Proc Natl Acad Sci USA (1988) 85:9699–703. doi: 10.1073/pnas.85.24.9699
14. Cybulsky MI, Colditz IG, Movat HZ. The Role of Interleukin-1 in Neutrophil Leukocyte Emigration Induced by Endotoxin. Am J Pathol (1986) 124:367–72.
15. Dinarello CA. Interleukin-1 and the Pathogenesis of the Acute-Phase Response. N Engl J Med (1984) 311:1413–8. doi: 10.1056/NEJM198411293112205
16. Dayer JM, Bréard J, Chess L, Krane SM. Participation of Monocyte-Macrophages and Lymphocytes in the Production of a Factor That Stimulates Collagenase and Prostaglandin Release by Rheumatoid Synovial Cells. J Clin Invest (1979) 64:1386–92. doi: 10.1172/JCI109596
17. Postlethwaite AE, Lachman LB, Mainardi CL, Kang AH. Interleukin 1 Stimulation of Collagenase Production by Cultured Fibroblasts. J Exp Med (1983) 157:801–6. doi: 10.1084/jem.157.2.801
18. Matsushima K, Durum SK, Kimball ES, Oppenheim JJ. Purification of Human Interleukin 1 From Human Monocyte Culture Supernatants and Identity of Thymocyte Comitogenic Factor, Fibroblast-Proliferation Factor, Acute-Phase Protein-Inducing Factor, and Endogenous Pyrogen. Cell Immunol (1985) 92:290–301. doi: 10.1016/0008-8749(85)90010-3
19. Saklatvala J, Pilsworth LM, Sarsfield SJ, Gavrilovic J, Heath JK. Pig Catabolin is a Form of Interleukin 1. Cartilage and Bone Resorb, Fibroblasts Make Prostaglandin and Collagenase, and Thymocyte Proliferation is Augmented in Response to One Protein. Biochem J (1984) 224:461–6. doi: 10.1042/bj2240461
20. Powanda MC, Beisel WR. Leukocyte Endogenous Mediator/Endogenous Pyrogen/Lymphocyte-Activating Factor Modulates the Development of Nonspecific and Specific Immunity and Affects Nutritional Status. Am J Clin Nutr (1982) 35:762–8. doi: 10.1093/ajcn/35.4.762
21. Besedovsky H, del Rey A, Sorkin E, Dinarello CA. Immunoregulatory Feedback Between Interleukin-1 and Glucocorticoid Hormones. Science (1986) . 233:652–4. doi: 10.1126/science.3014662
22. del Rey A, Besedovsky H. Interleukin 1 Affects Glucose Homeostasis. Am J Physiol (1987) 22:R794–8. doi: 10.1152/ajpregu.1987.253.5.R794
24. Durum SK, Higuchi C, Ron Y. Accessory Cells and T Cell Activation. The Relationship Between Two Components of Macrophage Accessory Cell Function: I-A and IL-1. Immunobiology (1984) 168:213–31. doi: 10.1016/S0171-2985(84)80112-6
25. Nencioni L, Villa L, Tagliabue A, Antoni G, Presentini R, Perin F, et al. In Vivo Immunostimulating Activity of the 163-171 Peptide of Human IL-1β. J Immunol (1987) 139:800–4.
26. Boraschi D, Nencioni L, Villa L, Censini S, Bossù P, Ghiara P, et al. In Vivo Stimulation and Restoration of the Immune Response by the Noninflammatory Fragment 163-171 of Human Interleukin 1β. J Exp Med (1988) 168:675–86. doi: 10.1084/jem.168.2.675
27. Boraschi D, Villa L, Volpini G, Bossù P, Censini S, Ghiara P, et al. Differential Activity of Interleukin 1α and Interleukin 1β in the Stimulation of the Immune Response In Vivo. Eur J Immunol (1990) 20:317–21. doi: 10.1002/eji.1830200213
28. Ichinohe T, Lee HK, Ogura Y, Flavell R, Iwasaki A. Inflammasome Recognition of Influenza Virus is Essential for Adaptive Immune Responses. J Exp Med (2009) 206:79–87. doi: 10.1084/jem.20081667
29. Allen IC, Scull MA, Moore CB, Holl EK, McElvania-TeKippe E, Taxman DJ, et al. The NLRP3 Inflammasome Mediates In Vivo Innate Immunity to Influenza A Virus Through Recognition of Viral RNA. Immunity (2009) 30:556–65. doi: 10.1016/j.immuni.2009.02.005
30. Murzin AG, Lesk AM, Chothia C. β-Trefoil Fold. Patterns of Structure and Sequence in the Kunitz Inhibitors Interleukin-1β and 1α and Fibroblast Growth Factors. J Mol Biol (1992) 223:531–43. doi: 10.1016/0022-2836(92)90668-a
31. Heuck AP, Tweten RK, Johnson AE. β-Barrel Pore-Forming Toxins: Intriguing Dimorphic Proteins. Biochemistry (2001) 40:9063–5. doi: 10.1021/bi0155394
32. Kaus K, Olson R. Structure and Glycan-Binding Properties of the Vibrio vulnificus Hemolysin B-Trefoil Lectin. Biophys J (2014) 106:87A. doi: 10.1016/j.bpj.2013.11.555
33. Hatakeyama T, Unno H, Kouzuma Y, Uchida T, Eto S, Hidemura H, et al. C-Type Lectin-Like Carbohydrate Recognition of the Hemolytic Lectin CEL-III Containing Ricin-Type B-Trefoil Folds. J Biol Chem (2007) 282:37826–35. doi: 10.1074/jbc.M705604200
34. Cummings RD, Schnaar RL. R-Type Lectins. Chapter 31. In: Varki A, Cummings RD, Esko JD, Stanley P, Hart GW, Aebi M, et al, editors. Essential of Glycobiology, 3rd Edition. Cold Spring Harbor, NY: CSH Laboratory Press (2017).
35. Dinarello CA. The IL-1 Cytokine and Receptor Family. Immunol Rev 281. Oxford, UK: John Wiley & Sons Ltd (2018). 247 p.
36. Dinarello CA. Overview of the IL-1 Family in Innate Inflammation and Acquired Immunity. Immunol Rev (2018) 281:8–27. doi: 10.1111/imr.12621
37. Bertheloot D, Latz E. HMGB1, IL-1α, IL-33 and S100 Proteins: Dual Function Alarmins. Cell Mol Immunol (2017) 14:43–64. doi: 10.1038/cmi.2016.34
38. Werman A, Werman-Venkert R, White R, Lee J-K, Werman B, Krelin Y, et al. The Precursor Form of IL-1α is an Intracrine Proinflammatory Activator of Transcription. Proc Natl Acad Sci USA (2004) 101:2434–9. doi: 10.1073/pnas.0308705101
39. Gresnigt MS, van de Veerdonk FL. Biology of IL-36 Cytokines and Their Role in Disease. Semin Immunol (2013) 25:458–65. doi: 10.1016/j.smim.2013.11.003
40. Bassoy EY, Towne JE, Gabay C. Regulation and Function of Interleukin-36 Cytokines. Immunol Rev (2018) 281:169–78. doi: 10.1111/imr.12610
41. Dinarello CA, Thompson RC. Blocking IL-1: Interleukin 1 Receptor Antagonist In Vivo and In Vitro. Immunol Today (1991) 12:404–10. doi: 10.1016/0167-5699(91)90142-G
42. Boraschi D, Lucchesi D, Hainzl S, Leitner M, Maier E, Mangelberger D, et al. IL-37: A New Anti-Inflammatory Cytokine of the IL-1 Family. Eur Cytokine Netw (2011) 22:127–47. doi: 10.1684/ecn.2011.0288
43. Nold-Petry C, Lo CY, Rudloff I, Elgass KD, Li S, Gantier MP, et al. IL-37 Requires the Receptors IL-18rα and IL-1r8 (SIGIRR) to Carry Out its Multifaceted Anti-Inflammatory Program Upon Innate Signal Transduction. Nat Immunol (2015) 16:354–65. doi: 10.1038/ni.3103
44. Dinarello CA, Nold-Petry C, Nold M, Fujita M, Li S, Kim S, et al. Suppression of Innate Inflammation and Immunity by Interleukin-1 Family Member Interleukin-37. Eur J Immunol (2016) 46:1067–81. doi: 10.1002/eji.201545828
45. van de Veerdonk FL, Stoeckman AK, Wu G, Boeckermann AN, Azam T, Netea MG, et al. IL-38 Binds to the IL-36 Receptor and has Biological Effects on Immune Cells Similar to IL-36 Receptor Antagonist. Proc Natl Acad Sci USA (2012) 109:3001–5. doi: 10.1073/pnas.1121534109
46. Mora K, Schlemmer A, Wittig I, Richter F, Putyrski M, Frank A-C, et al. Interleukin-38 is Released from Apoptotic Cells to Limit Inflammatory Macrophage Responses. J Mol Cell Biol (2016) 8:426–38. doi: 10.1093/jmcb/mjw006
47. Garraud T, Harel M, Boutet M-A, Le Goff B, Blanchard F. The Enigmatic Role of IL-38 in Inflammatory Diseases. Cytokine Growth Fact Rev (2018) 39:26–35. doi: 10.1016/j.cytogfr.2018.01.001
48. Rivers-Auty J, Daniels MJD, Colliver I, Robertson DL, Brough D. Redefining the Ancestral Origins of the Interleukin-1 Superfamily. Nat Commun (2018) 9:1156. doi: 10.1038/s1467-018-03362-1
49. Kaplanski G. Interleukin-18: Biological Properties and Role in Disease Pathogenesis. Immunol Rev (2018) 281:138–53. doi: 10.1111/imr.12616
50. Nakanishi K. Unique Action of Interleukin-18 on T Cells and Other Immune Cells. Front Immunol (2018) 9:763. doi: 10.3389/fimmu.2018.00763
51. Martin NT, Martin MU. Interleukin 33 is a Guardian of Barriers and a Local Alarmin. Nat Immunol (2016) 17:122–31. doi: 10.1038/ni.3370
52. Baekkevold ES, Roussigné M, Yamanaka T, Johansen F-E, Jahnsen FL, Amalric F, et al. Molecular Characterization of NF-HEV, a Nuclear Factor Preferentially Expressed in Human High Endothelial Venules. Am J Pathol (2003) 163:69–79. doi: 10.1016/S0002-9440(10)63631-0
53. Ali S, Mohs A, Thomas M, Klare J, Ross R, Schmitz ML, et al. The Dual Function Cytokine IL-33 Interacts With the Transcription Factor NF- κB to Dampen NF-κB-Stimulated Gene Transcription. J Immunol (2011) 187:1609–16. doi: 10.4049/jimmunol.1003080
54. Boraschi D, Italiani P, Weil S, Martin MU. The Family of Interleukin-1 Receptors. Immunol Rev (2018) 281:197–232. doi: 10.1111/imr.12606
55. Gu Y-F, Fang Y, Jin Y, Dong W-R, Xiang L-X, Shao J-Z. Discovery of the DIGIRR Gene From Teleost Fish: A Novel Toll-IL-1 Receptor Family Member Serving as a Negative Regulator of IL-1 Signaling. J Immunol (2011) 187:2514–30. doi: 10.4049/jimmunol.1003457
56. Beck G, Habicht GS. Isolation and Characterization of a Primitive Interleukin-1-Like Protein From an Invertebrate, Asteria forbesi. Proc Natl Acad Sci USA (1986) 83:7429–33. doi: 10.1073/pnas.83.19.7429
57. Beck G, Vasta G, Marchalonis J, Habicht G. Characterization of an Interleukin 1 Activity in Tunicates. Comp Biochem Physiol (1989) 92B:93–8. doi: 10.1016/0305-0491(89)90318-0
58. Beck G, Habicht G, Stillman D, Cooper E, Raftos D. Invertebrate Cytokines III: Interleukin 1-Like Molecules Stimulate Phagocytosis by Tunicate and Echinoderm Cells. Cell Immunol (1993) 146:284–99. doi: 10.1006/cimm.1993.1027
59. Ottaviani E, Franchini A, Franceschi C. Presence of Several Cytokine-Like Molecules in Molluscan Hemocytes. Biochem Biophys Res Commun (1993) 195:984–8. doi: 10.1006/bbrc.1993.2141
60. Raftos D. Interactions of Tunicate Immunomodulatory Proteins With Mammalians Cells. Immun Cell Biol (1996) 74:26–31. doi: 10.1038/icb.1996.3
61. Pestarino M, De Anna E, Masini MA, Sturla M. Localization of Interleukin-1β mRNA in the Cerebral Ganglion of the Protochordate, Styela Plicata. Neurosci Lett (1997) 222:151–4. doi: 10.1016/S0304-3940(97)13362-6
62. Ottaviani E, Caselgrandi E, Franceschi C. Cytokines and Evolution: In Vitro Effects of IL-1α, IL-1β, TNF-α and TNF-β on an Ancestral Type of Stress Response. Biochem Biophys Res Commun (1995) 207:288–92. doi: 10.1006/bbrc.1995.1185
63. Beck G. Macrokines: Invertebrate Cytokine-Like Molecules? Front Biosci (1998) 3:d559–69. doi: 10.2741/a303
64. Beschin A, Bilej M, Brys L, Torreele E, Lucas R, Magez S, et al. Convergent Evolution of Cytokines. Nature (1999) 400:627–8. doi: 10.1038/23164
65. Wittwer D, Franchini A, Ottaviani E, Wiesner A. Presence of IL-1- and TNF-Like Molecules in Galleria mellonella (Lepidoptera) Haemocytes and in an Insect Cell Line Fromestigmene acraea (Lepidoptera). Cytokine (1999) 11:637–42. doi: 10.1006/cyto.1998.0481
66. Beschin A, Bilej M, Torreele E, De Batselier P. On the Existence of Cytokines in Invertebrates. Cell Mol Life Sci (2001) 58:801–14. doi: 10.1007/PL00000901
67. Rast JP, Messier-Solek C. Marine Invertebrate Genome Sequences and Our Evolving Understanding of Animal Immunity. Biol Bull (2008) 214:274–83. doi: 10.2307/25470669
68. Beck G, Ellis TW, Truong N. Characterization of an IL-1 Receptor From Asterias forbesi Coelomocytes. Cell Immunol (2000) (2000) 203:66–73. doi: 10.1006/cimm.2000.1674
69. Hibino T, Loza-Coll M, Messier C, Majeske AJ, Cohen AH, Terwilliger DP, et al. The Immune Gene Repertoire Encoded in the Purple Sea Urchin Genome. Dev Biol (2006) 300:349–65. doi: 10.1016/j.ydbio.2006.08.065
70. Gay NJ, Keith FJ. Drosophila Toll and IL-1 Receptor. Nature (1991) 351:355–6. doi: 10.1038/351355b0
71. Heguy A, Baldari CT, Macchia G, Telford JL, Melli M. Amino Acids Conserved in Interleukin-1 Receptors (IL-1Rs) and the Drosophila Toll Protein are Essential for IL-1R Signal Transduction. J Biol Chem (1992) 267:2605–9. doi: 10.1016/S0021-9258(18)45924-8
72. Chen R, Zhang L, Qi J, Zhang N, Zhang L, Yao S, et al. Insight Into the Evolution of the Ig-C2 Structure From Amphioxus Provides Discovery and Analysis of Invertebrate IgVJ Superfamily. J Immunol (2018) 200:2869–81. doi: 10.4049/jimmunol.1700906
73. Barclay AN. Ig-Like Domains: Evolution From Simple Interaction Molecules to Sophisticated Antigen Recognition. Proc Natl Acad Sci USA (1999) 96:14672–4. doi: 10.1073/pns.96.26.14672
74. Litman GW, Anderson MK, Rast JP. Evolution of Antigen Binding Receptors. Annu Rev Immunol (1999) 17:109–47. doi: 10.1146/annurev.immunol.17.1.109
75. Du Pasquier L. Speculations on the Origin of the Vertebrate Immune System. Immunol Lett (2004) 92:3–9. doi: 10.1016/j.imlet.2003.10.012
76. Chambers CA, Allison JP. Costimulatory Regulation of T Cell Function. Curr Opin Cell Biol (1999) 11:203–10. doi: 10.1016/S0955-0674(99)80027-1
77. Acosta-Rodriguez EV, Napolitani G, Lanzavecchia A, Sallusto F. Interleukins 1beta and 6 But Not Transforming Growth Factor-Beta are Essential for the Differentiation of Interleukin 17-Producing Human T Helper Cells. Nat Immunol (2007) 8:942–9. doi: 10.1038/ni1496
78. Chung Y, Chang SH, Martinez GJ, Yang XO, Nurieva R, Kang HS, et al. Critical Regulation of Early Th17 Cell Differentiation by IL-1 Signaling. Immunity (2009) 30:576–87. doi: 10.1016/j.immuni.2009.02.007
79. Ben-Sasson SZ, Hu-Li J, Quiel J, Cauchetaux S, Ratner M, Shapira I, et al. IL-1 Acts Directly on CD4 T Cells to Enhance Their Antigen-Driven Expansion and Differentiation. Proc Natl Acad Sci USA (2009) 106:7119–24. doi: 10.1073/pnas.0902745106
80. Guo L, Wei G, Zhu J, Liao W, Leonard WJ, Zhao K, et al. IL-1 Family Members and STAT Activators Induce Cytokine Production by Th2, Th17, and Th1 Cells. Proc Natl Acad Sci USA (2009) 106:13463–8. doi: 10.1073/pnas.0906988106
81. Nakae S, Naruse-Nakajima C, Sudo K, Horai R, Asano M, Iwakura Y. IL-1 Alpha, But Not IL-1 Beta, is Required for Contact-Allergen-Specific T Cell Activation During the Sensitization Phase in Contact Hypersensitivity. Int Immunol (2001) 13:1471–8. doi: 10.1093/intimm/13.12.1471
82. Lasigliè D, Traggiai E, Federici S, Alessio M, Buoncompagni A, Accogli A, et al. Role of IL-1 Beta in the Development of Human TH17 Cells: Lesson FromMutated Patients. PloS One (2011) 6:e20014. doi: 10.1371/journal.pone.0020014
83. Wuthrich M, LeBert V, Galles K, Hu-Li J, Ben-Sasson SZ, Paul WE, et al. Interleukin 1 Enhances Vaccine-Induced Antifungal T-Helper 17 Cells and Resistance Against Blastomyces Dermatitidis Infection. J Infect Dis (2013) 208:1175–82. doi: 10.1093/infdis/jit283
84. Santarlasci V, Cosmi L, Maggi L, Liotta F, Annunziato F. IL-1 and T Helper Immune Responses. Front Immunol (2013) 4:182. doi: 10.3389/fimmu.2013.00182
85. Jain A, Song R, Wakeland EK, Pasare C. T Cell-Intrinsic IL-1R Signaling Licenses Effector Cytokine Production by Memory CD4 T Cells. Nat Commun (2018) 9:3185. doi: 10.1038/s41467-018-05489-7
86. Paul-Gydéon Ritvo1 P-G, Klatzmann D. Interleukin-1 in the Response of Follicular Helper and Follicular Regulatory T Cells. Front Immunol (2019) 10:250. doi: 10.3389/fimmu.2019.00250
87. Van Den Eeckhout B, Tavernier J, Gerlo S. Interleukin-1 as Innate Mediator of T Cell Immunity. Front Immunol (2021) 11:621931. doi: 10.3389/fimmu.2020.621931
88. Ben-Sasson SZ, Hogg A, Hu-Li J, Wingfield P, Chen X, Crank M, et al. IL-1 Enhances Expansion, Effector Function, Tissue Localization, and Memory Response of Antigen-Specific CD8 T Cells. J Exp Med (2013) 210:491–502. doi: 10.1084/jem.20122006
89. Pang IK, Ichinohe T, Iwasaki A. IL-1R Signaling in Dendritic Cells Replaces Pattern-Recognition Receptors in Promoting CD8(+) T Cell Responses to Influenza A Virus. Nat Immunol (2013) 14:246–53. doi: 10.1038/ni.2514
90. Sarkar S, Yuzefpolskiy Y, Xiao H, Baumann FM, Yim S, Lee DL, et al. Programming of CD8 T Cell Quantity and Polyfunctionality by Direct IL-1 Signals. J Immunol (2018) 201:3641–50. doi: 10.4049/jimmunol.1800906
91. Dinarello CA, Novick D, Kim S, Kaplanski G. Interleukin-18 and IL-18 Binding Protein. Front Immuno (2013) 4:289. doi: 10.3389/fimmu.2013.00289
92. Wegmann TG, Lin H, Guilbert L, Mosmann TR. Bidirectional Cytokine Interactions in the Maternal-Fetal Relationship: Is Successful Pregnancy a TH2 Phenomenon? Immunol Today (1993) 14:353–6. doi: 10.1016/0167-5699(93)90235-D
93. Reinhard G, Noll A, Schlebusch H, Mallmann P, Ruecker AV. Shifts in the TH1/TH2 Balance During Human Pregnancy Correlate With Apoptotic Changes. Biochem Biophys Res Commun (1998) 245:933–8. doi: 10.1006/bbrc.1998.8549
94. Maier JAM, Statuto M, Ragnotti G. Endogenous Interleukin 1 Alpha Must be Transported to the Nucleus to Exert its Activity in Human Endothelial Cells. Mol Cell Biol (1994) 14:1845–51. doi: 10.1128/mcb.14.3.1845-1851.1994
95. Voronov E, Dotan S, Krelin Y, Song X, Elkabets M, Carmi Y, et al. Unique Versus Redundant Functions of IL-1α and IL-1β in the Tumor Microenvironment. Front Immunol (2013) 4:177. doi: 10.3389/fimmu.2013.00177
96. Lonnemann G, Shapiro L, Engler-Blum G, Müller GA, Koch KM, Dinarello CA. Cytokines in Human Renal Interstitial Fibrosis. I. Interleukin-1 is a Paracrine Growth Factor for Cultured Fibrosis-Derived Kidney Fibroblasts. Kidney Int (1995) 47:837–44. doi: 10.1038/ki.1995.126
97. Pollock AS, Turck J, Lovett DH. The Prodomain of Interleukin 1alpha Interacts With Elements of the RNA Processing Apparatus and Induces Apoptosis in Malignant Cells. FASEB J (2003) 17:203–13. doi: 10.1096/fj.02-0602com
98. Buryskova M, Pospisek M, Grothey A, Simmet T, Burysek L. Intracellular Interleukin-1alpha Functionally Interacts With Histone Acetyltransferase Complexes. J Biol Chem (2006) 279:4017–26. doi: 10.1074/jbc.M306342200
99. Roussel L, Erard M, Cayrol C, Girard J-P. Molecular Mimicry Between IL-33 and KSHV for Attachment to Chromatin Through the H2A-H2B Acidic Pocket. EMBO Rep (2008) 9:1006–12. doi: 10.1038/embor.2008.145
100. Gutcher I, Urich E, Wolter K, Prinz M, Becher B. Interleukin 18–Independent Engagement of Interleukin 18 Receptor-α is Required for Autoimmune Inflammation. Nat Immunol (2006) 7:946–53. doi: 10.1038/ni1377
101. Khan JA, Brint EK, O’Neill LAJ, Tong L. Crystal Structure of the Toll/Interleukin-1 Receptor Domain of Human IL-1rapl. J Biol Chem (2004) 279:31664–70. doi: 10.1074/jbc.M403434200
102. Hayashi T, Yoshida T, Ra M, Taguchi R, Mishina M. IL1RAPL1 Associated With Mental Retardation and Autism Regulates the Formation and Stabilization of Glutamatergic Synapses of Cortical Neurons Through RhoA Signaling Pathway. PloS One (2013) 8:e66254. doi: 10.1371/journal.pone.0066254
103. Günther S, Deredge D, Bowers AL, Luchini A, Bonsor DA, Beadenkopf R, et al. IL-1 Family Cytokines Use Distinct Molecular Mechanisms to Signal Through Their Shared Co-Receptor. Immunity (2017) 47:510–23. doi: 10.1016/j.immuni.2017.08.004
104. Fields JK, Günther S, Sundberg EJ. Structural Basis of IL-1 Family Cytokine Signaling. Front Immunol (2019) 10:1412. doi: 10.3389/fimmu.2019.01412
105. Touzani O, Boutin H, LeFeuvre R, Parker L, Miller A, Luheshi G, et al. Interleukin-1 Influences Ischemic Brain Damage in the Mouse Independently of the Interleukin-1 Type I Receptor. J Neurosci (2002) 22:38–43. doi: 10.1523/JNEUROSCI.22-01-00038.2002
106. Andre R, Moggs JG, Kimber I, Rothwell NJ, Pinteaux E. Gene Regulation by IL-1β Independent of IL-1R1 in the Mouse Brain. Glia (2006) 53:477–83. doi: 10.1002/glia.20302
107. Loscher CE, Mills KHG, Lynch MA. Interleukin-1 Receptor Antagonist Exerts Agonist Activity in the Hippocampus Independent of the Interleukin-1 Type I Receptor. J Neuroimmunol (2003) 137:117–24. doi: 10.1016/S0165-5728(03)00072-9x
108. Boraschi D, Volpini G, Villa L, Nencioni L, Scapigliati G, Nucci D, et al. A Monoclonal Antibody to the IL-1β Peptide 163-171 Blocks Adjuvanticity But Not Pyrogenicity of IL-1β In Vivo. J Immunol (1989) 143:131–4.
109. Boraschi D, Villa L, Ghiara P, Presentini R, Bossù P, Censini S, et al. Differential Inhibition of IL-1β Activities and Receptor Binding by Monoclonal Antibodies Mapping Within a Discrete Region of the Protein. Lymphokine Cytokine Res (1991) 10:377–84.
110. Boraschi D, Bossù P, Ruggiero P, Tagliabue A, Bertini R, Macchia G, et al. Mapping of Receptor Binding Sites on IL-1β by Reconstruction of IL-1ra-Like Domains. J Immunol (1995) 155:4719–25.
111. D’Ettorre C, De Chiara G, Casadei R, Boraschi D, Tagliabue A. Functional Epitope Mapping of Human Interleukin 1β by Surface Plasmon Resonance. Eur Cytokine Netw (1997) 8:161–71.
112. Casadio R, Frigimelica E, Bossù P, Neumann D, Martin MU, Tagliabue A, et al. Model of Interaction of the IL-1 Receptor Accessory Protein IL-1racp With the IL-1β/IL-1RI Complex. FEBS Lett (2001) 499:65–8. doi: 10.1016/s0014-5793(01)02515-7
113. Hession C, Decker JM, Sherblom AP, Kumar S, Yue CC, Mattaliano RJ, et al. Uromodulin (Tamm-Horsfall Glycoprotein): A Renal Ligand for Lymphokines. Science (1987) 237:1479–84. doi: 10.1126/science.3498215
114. Ramsden L, Rider CC. Selective and Differential Binding of Interleukin (IL)-1α, IL-1β, IL-2 and IL-6 to Glycosaminoglycans. Eur J Immunol (1992) 22:3027–31. doi: 10.1002/eji.1830221139
115. Cebo C, Dambrouck T, Maes E, Laden C, Strecker G, Michalski J-C, et al. Recombinant Human Interleukins IL-1α, IL-1β, IL-4, IL-6, and IL-7 Show Different and Specific Calcium-Independent Carbohydrate-Binding Properties. J Biol Chem (2001) 8:5685–91. doi: 10.1074/jbc.M008662200
116. Fukushima K, Hara-Kuge S, Ohkura T, Seko A, Ideo H, Inazu T, et al. Lectin-Like Characteristics of Recombinant Human Interleukin-1beta Recognizing Glycans of the Glycosylphosphatidylinositol Anchor. J Biol Chem (1997) 272:10579–84. doi: 10.1074/jbc.272.16.10579
117. Tandai-Hiruma M, Endo T, Kobata A. Detection of Novel Carbohydrate Binding Activity of Interleukin-1. J Biol Chem (1999) 274:4459–66. doi: 10.1074/jbc.274.7.4459
118. Vergoten G, Zanetta JP. Structural Differences Between the Putative Carbohydrate-Recognition Domains of Human IL-1 Alpha, IL-1 Beta and IL-1 Receptor Antagonist Obtained by in Silico Modelling. Glycoconj J (2007) 24:183–93. doi: 10.1007/s10719-006-9021-0
119. Dziarski R. Enhancement of Mixed Leukocyte Reaction and Cytotoxic Antitumor Responses by Heparin. J Immunol (1989) 143:356–65.
120. Muchmore AV, Decker JM. Evidence That Recombinant IL 1 Alpha Exhibits Lectin-Like Specificity and Binds to Homogeneous Uromodulin via N-Linked Oligosaccharides. J Immunol (1987) 138:2541–6.
121. Dinarello CA. The IL-1 Family of Cytokines and Receptors in Rheumatic Diseases. Nat Rev Rheumatol (2019) 15:612–32. doi: 10.1038/s41584-019-0277-8
122. Dinarello CA, Ikejima T, Warner SJ, Orencole SF, Lonnemann G, Cannon JG, et al. Interleukin 1 Induces Interleukin 1. I. Induction of Circulating Interleukin 1 in Rabbits In Vivo and in Human Mononuclear Cells In Vitro. J Immunol (1987) 139:1902–10.
123. Dinarello CA, Simon A, van der Meer JWM. Treating Inflammation by Blocking Interleukin-1 in a Broad Spectrum of Diseases. Nat Rev Drug Discovery (2012) 11:633–52. doi: 10.1038/nrd3800
124. Aksentijevich I, Masters SL, Ferguson PJ, Dancey P, Frenkel J, van Royen-Kerkhoff A, et al. An Autoinflammatory Disease With Deficiency of the Interleukin-1-Receptor Antagonist. N Engl J Med (2009) 360:2426–37. doi: 10.1056/NEJMoa0807865
125. Reddy S, Jia S, Geoffrey R, Lorier R, Suchi M, Broeckel U, et al. An Autoinflammatory Disease Due to Homozygous Deletion of the IL1RN Locus. N Engl J Med (2009) 360:2438–44. doi: 10.1056/NEJMoa0809568
126. Voronov E, Apte RN. Targeting the Tumor Microenvironment by Intervention in Interleukin-1 Biology. Curr Pharm Des (2017) 23:4893–905. doi: 10.2174/1381612823666170613080919
127. Voronov E, Dinarello CA, Apte RN. Interleukin-1alpha as an Intracellular Alarmin in Cancer Biology. Semin Immunol (2018) 38:3–14. doi: 10.1016/j.smim.2018.10.006
128. Bossù P, Neumann D, Del Giudice E, Ciaramella A, Gloaguen I, Fantuzzi G, et al. IL-18 cDNA Vaccination Protects Mice From Spontaneous Lupus-Like Autoimmune Disease. Proc Natl Acad Sci USA (2003) 100:14181–6. doi: 10.1073/pnas.2336094100
129. Gangemi S, Basile G, Merendino RA, Minciullo PL, Novick D, Rubinstein M, et al. Increased Circulating Interleukin-18 Levels in Centenarians With No Signs of Vascular Disease: Another Paradox of Longevity? Exp Gerontol (2003) 38:669–72. doi: 10.1016/s0531-5565(03)00061-5
131. Migliorini P, Anzilotti C, Pratesi F, Quattroni P, Bargagna M, Dinarello CA, et al. Serum and Urinary Levels of IL-18 and its Inhibitor IL-18BP in Systemic Lupus Erythematosus. Eur Cytokine Netw (2010) 21:264–71. doi: 10.1684/ecn.2010.0210
132. Italiani P, Puxeddu I, Napoletano S, Scala E, Melillo D, Manocchio S, et al. Circulating Levels of IL-1 Family Cytokines and Receptors in Alzheimer’s Disease: New Markers of Disease Progression? J Neuroinflamm (2018) 15:342. doi: 10.1186/s12974-018-1376-1
133. Migliorini P, Italiani P, Pratesi F, Puxeddu I, Boraschi D. Cytokines and Soluble Receptors of the IL-1 Family in Schnitzler Syndrome. Scand J Rheumatol (2019) 48:235–8. doi: 10.1080/03009742.2018.1550210
134. Migliorini P, Italiani P, Pratesi F, Puxeddu I, Boraschi D. The IL-1 Family Cytokines and Receptors in Autoimmune Diseases. Autoimmun Rev (2020) 11:102617. doi: 10.1016/j.autrev.2020.102617
135. Buhl A-L, Wenzel J. Interleukin-36 in Infectious and Inflammatory Skin Diseases. Front Immunol (2019) 10:1162. doi: 10.3389/fimmu.2019.01162
136. D’Erme AM, Wilsmann-Theis D, Wagenpfeil J, Hölzel M, Ferring-Schmitt S, Sternberg S, et al. IL-36γ (IL-1F9) is a Biomarker for Psoriasis Skin Lesions. J Invest Dermatol (2015) 135:1025–32. doi: 10.1038/jid.2014.532
137. Jackson DJ, Makrinioti H, Rana BM, Shamji BW, Trujillo-Torralbo MB, Footitt J, et al. IL-33-Dependent Type 2 Inflammation During Rhinovirus-Induced Asthma Exacerbations In Vivo. Am J Respir Crit Care Med (2014) 190:1373–82. doi: 10.1164/rccm.201406-1039OC
138. Kim RY, Oliver BG, Wark PAB, Hansbro PM, Donovan C. COPD Exacerbation: Targeting IL-33 as a New Therapy. Lancet Respir Med (2021) 9:1213–4. doi: 10.1016/S2213-2600(21)00182-X
Keywords: interleukin-1, inflammation, innate immunity, adaptive immunity, evolution
Citation: Boraschi D (2022) What Is IL-1 for? The Functions of Interleukin-1 Across Evolution. Front. Immunol. 13:872155. doi: 10.3389/fimmu.2022.872155
Received: 09 February 2022; Accepted: 11 March 2022;
Published: 06 April 2022.
Edited by:
Silvano Sozzani, Sapienza University of Rome, ItalyReviewed by:
Eric J Sundberg, Emory University, United StatesDetlef Neumann, Hannover Medical School, Germany
Copyright © 2022 Boraschi. This is an open-access article distributed under the terms of the Creative Commons Attribution License (CC BY). The use, distribution or reproduction in other forums is permitted, provided the original author(s) and the copyright owner(s) are credited and that the original publication in this journal is cited, in accordance with accepted academic practice. No use, distribution or reproduction is permitted which does not comply with these terms.
*Correspondence: Diana Boraschi, ZGlhbmEuYm9yYXNjaGlAaWJiYy5jbnIuaXQ=