- 1Department of Paediatrics and Adolescent Medicine, Li Ka Shing Faculty of Medicine, The University of Hong Kong, Hong Kong, Hong Kong SAR, China
- 2Bioland Laboratory (Guangzhou Regenerative Medicine and Health Guangdong Laboratory), Guangzhou, China
- 3Computational and Systems Biology Interdepartmental Program, University of California, Los Angeles, Los Angeles, CA, United States
Although γδ-T cell-based tumor immunotherapy using phosphoantigens to boost γδ-T cell immunity has shown success in some cancer patients, the clinical application is limited due to the rapid exhaustion of Vγ9Vδ2-T cells caused by repetitive stimulation from phosphoantigens and the profoundly immunosuppressive tumor microenvironment (TME). In this study, using a cell culture medium containing human and viral interleukin-10 (hIL-10 and vIL-10) secreted from EBV-transformed lymphoblastoid B cell lines (EBV-LCL) to mimic the immunosuppressive TEM, we found that the antitumor activity of Vγ9Vδ2-T cells was highly suppressed by endogenous hIL-10 and vIL-10 within the TME. CD137 costimulation could provide an anti-exhaustion signal to mitigate the suppressive effects of IL-10 in TME by suppressing IL-10R1 expression on Vγ9Vδ2-T cells. CD137 costimulation also improved the compromised antitumor activity of Vγ9Vδ2-T cells in TME with high levels of IL-10 in Rag2-/- γc-/- mice. In humanized mice, CD137 costimulation boosted the therapeutic effects of aminobisphosphonate pamidronate against EBV-induced lymphoma. Our study offers a novel approach to overcoming the obstacle of the hIL-10 and vIL-10-mediated immunosuppressive microenvironment by costimulating CD137 and enhancing the efficacy of γδ-T cell-based tumor therapy.
Introduction
Epstein-Barr virus (EBV) is a predominant type of human herpesviruses. It infects over 95% of the population by adulthood (1, 2). EBV infection is highly correlated with several human malignancies (1–3). As the first known human tumor virus, the carcinogenesis of EBV has been identified in various hematopoietic and epithelial cell cancers, including EBV-associated tumors and lymphoproliferative disorder (1, 2, 4). Current therapeutic approaches for EBV-associated tumors are restricted by undesirable side effects and ineffectiveness for refractory or relapsed diseases (5, 6). It was reported that EBV-specific CTL-based therapy is effective in the control of EBV-associated malignancy (5, 6). However, its clinical application is hampered due to insufficient quantity of EBV-specific CTL generated ex vivo (7).
As a major subset of human γδ-T cells, Vγ9Vδ2-T cells have been extensively demonstrated to have promising anti-tumor effects (8–13). Vγ9Vδ2-T cells can be activated specifically by phosphoantigens from isoprenoid biosynthesis in an MHC-unrestricted manner. Aminobisphosphonates pamidronate (PAM) and zoledronate (ZOL) are commonly used pharmacological phosphoantigens for osteoporosis and Paget’s disease treatment (11, 14). Previously, we demonstrated that direct administration of PAM could expand Vγ9Vδ2-T cells in vivo and thus control EBV-induced lymphoma in humanized mice, suggesting that Vγ9Vδ2-T cell-based immunotherapy is promising for treating EBV-associated tumors (15). A recent meta-analysis of about 18,000 human cancers revealed that tumor-infiltrating γδ T cells are the most favorable cancer-wide prognostic marker (16). However, the clinical application was limited by the rapid exhaustion of Vγ9Vδ2-T cells caused by the repetitive stimulation from phosphoantigens in vivo (17) and the profoundly immunosuppressive tumor microenvironment (TME) (18–20).
Interleukin (IL)-10, as a major immunosuppressive cytokine in TME secreted by tumor cells, can help tumor cells escape immunological recognition and destruction (21–24). Current evidence indicates that EBV codes a homologue of human IL-10 (vIL-10) with immunosuppressive properties to evade immunity and establish persistent/latent infections (25–28). EBV-LCL also express and release various amounts of human IL-10 (hIL-10) (29, 30). hIL-10 and vIL-10 are crucial for B cell transformation of B cell (31, 32) and oncogenesis of EBV-associated tumors (33). However, whether the antitumor activity of Vγ9Vδ2-T cells was suppressed by IL-10 in TME remained largely unknown.
CD137 (4-1BB), a membrane-bound receptor, is a costimulatory molecule expressed in many lymphocytes (34–36). Recently, we demonstrated that CD137 costimulation enhanced the activation and cytolytic activity of Vγ9Vδ2-T cells against virus-infected cells (37). Importantly, boosting cancer immunotherapy with agonistic CD137 antibodies has been demonstrated to be a promising therapeutic strategy for different tumors (38, 39). However, the roles of CD137 signaling for human Vγ9Vδ2-T cells in the immunosuppressive TME remained to be determined.
In this study, we aim to clarify whether IL-10 in the TME is responsible for the exhaustion of Vγ9Vδ2-T cells and determine whether targeting CD137 can enhance the antitumor activity of Vγ9Vδ2-T cells compromised by the immunosuppressive TME.
Materials and Methods
Vγ9Vδ2-T Cell Cultures
hPBMC were isolated from buffy coats by Ficoll-Hypaque gradient centrifugation of EBV+ healthy donors after informed consents were obtained. PAM-expanded Vγ9Vδ2-T cells were prepared according to the protocol we established before (40). Briefly, hPBMC were cultured in RPMI1640 medium with 10% fetal bovine serum (FBS) in the presence of PAM from day 0 to day 3 at a concentration of 9μg/ml. Recombinant human IL-2 was added to medium from day 3 to day 14 at a concentration of 500 IU/ml. After 2 weeks, the γδ-T cells were purified by positive selection with α-TCRγ/δ MicroBead (Miltenyi Biotec).
Cytotoxic Assay
Purified Vγ9Vδ2-T cells were cultured with IL-10low or IL-10high conditioned medium for 24h, RPMI 1640 with 10% FBS medium (plain medium, PM) as a control. The pretreated Vγ9Vδ2-T cells (effector cells, E) were cocultured with autologous EBV-LCL (target cells, T) at an E: T ratio of 10:1 for 4 to 6 h in the IL-10low/high CM or PM, and then the death of target cells was analyzed with flow cytometry. Cells were stained with anti-CD3 to identify Vγ9Vδ2-T cells and propidium iodide (PI) was used to identify dead cells. The death of EBV-LCL was shown as the percentage of PI+ cells in the CD3- population (40). In some experiments, neutralizing antibody against IL-10 (abcam) was added to block IL-10 mediated pathways. To confirm the suppressive role of IL-10 in the CM, recombinant hIL-10 (Peprotech) or recombinant vIL-10 (R&D systems) was added to culture medium at the indicated concentration.
Establishment of EBV-LCL
EBV-secreting cell lines B95-8 and B95.8EBfaV-GFP were cultured and EBV-containing supernatants were collected for the following infection. hPBMC were incubated with EBV-containing supernatants, and then cultured in the RPMI 1640 medium containing 15% FBS with the addition of cyclosporine-A (1μg/ml) as we describe before (15).
Collection of EBV-LCL Conditioned Medium
EBV-LCL were cultured in RPMI1640 medium for 24 h. The conditioned medium (CM) was collected, centrifuged at 5000 rpm at 4°C for 10 min to remove cell debris and then frozen at −80°C in aliquots. Stored CM was passed through a 0.22-μm syringe filter (Millipore) before use. Plain medium (PM) collected from complete medium without cell incubation under the same experimental conditions served as the control for CM.
Determination of hIL-10 and vIL-10 Levels
For hIL-10, the concentrations in conditioned medium were measured by ELISA. The procedures for human IL-10 ELISA kits (Biolegend, San Diego, CA, USA) were performed based on the manufacturer’s instructions. For vIL-10, the concentrations in conditioned medium were measured according to the method described before (41). The conditioned medium was concentrated by Amicon-Ultra centrifugation filters (Millipore) following the manufacturer’s instructions. Then, the concentrated conditioned medium was used for performing Western blot assay. Mouse monoclonal antibody against vIL-10 (R&D) was used as primary antibody for incubating transferred membranes at 4°C overnight. Horseradish peroxide conjugated goat anti-mouse secondary antibody (R&D) was used as secondary antibody for detecting vIL-10 levels. The bands of Western blot were quantified by “Gels” analysis tool of ImageJ. Recombinant vIL-10 was used as a standard to quantify the vIL-10 level in conditioned medium.
Establishment and Treatment of EBV-Associated Lymphoma in Mice
All animal studies were approved and performed in compliance with the guidelines for the use of experimental animals by the Committee on the Use of Live Animals in the Teaching and Research, the University of Hong Kong. Rag2−/−γc−/− mice were bred in Centre for Comparative Medicine Research of the University of Hong Kong. Humanized mice were generated according to the protocol we established before (14, 15). Rag2−/−γc−/− or humanized mice were inoculated with EBV-LCL expressing high or low level of IL-10 (0.1×106/mouse) by subcutaneous injection to establish the EBV-associated lymphoma model. For Rag2−/−γc−/− mice, PAM-expanded Vγ9Vδ2-T cells (5×106/mouse) with or without the addition of SA-hCD137L (5μg/mouse) were adoptively transferred intravenously into EBV-associated lymphoma murine model at indicated time. For humanized mice, PAM (5mg/kg body weight) and SA-hCD137L (15μg/mouse) were injected intraperitoneally at the indicated time. The mice treated with an equivalent volume of PBS or SA were used as controls. The tumor volume and mice survival were monitored every day and calculated at the indicated time. Mice were counted as dying when their subcutaneous tumor diameter was larger than 17 mm and thus sacrificed according to the regulation of Centre for Comparative Medicine Research of the University of Hong Kong. Otherwise, mice were monitored for 100 days before being sacrificed. The tumor tissues were reserved for immunohistochemical evaluation.
Preparation of the Recombinant SA-hCD137L Protein
Recombinant SA-hCD137L proteins were generated as described before (37). Briefly, the DNA sequences were synthesized encoding the extracellular domain of human CD137L (a.a. 58-254) and the core streptavidin (SA; a.a. 16-133) with an N-terminal 6×His tag. The recombinant SA-hCD137L protein was expressed in E. coli by inserting the SA-hCD137L DNA fragments into the pETH expression vector and transforming into competent cells. After purifying with Ni-nitrilotriacetic acid affinity chromatography (QIAGEN, Germany), the recombinant SA-hCD137L protein was filtered a and quantitated by BCA Protein Assay Kit (Pierce, USA).
Flow Cytometric Analysis
Cells were stained for surface molecules with the following antibodies: αIL10R (Miltenyi Biotec, clone REA239), αCD3 (Biolegend, clone HIT3a), αTCRγ9 (Biolegend, clone B3), αTCRVδ2 (Biolegend, clone B6), and αCD137 (Biolegend, clone 4B4-1). All samples were performed with a FACS LSR II (BD). The results were analyzed with FlowJo software.
Histological Staining and Immunohistochemical Assays
The tumor tissues were fixed with 10% formalin for 24 h and maintained in 70% ethanol. Fixed tumor tissues were embedded in paraffin and sectioned. The tumor sections were performed immunohistochemistry staining with αIL-10 antibody (abcam) (42).
Statistics
Data are shown in the form of mean ± standard error of the mean (SEM). All data were tested by Shapiro-Wilk test to verify the normality. For data that did not meet normal distribution, Mann-Whitney U test was used for analysis. For data that met normal distribution, one-way analysis of variance (ANOVA) with Bonferroni correction was used for analysis. For multiple variables, two-way ANOVA was used. Kaplan-Meier log-rank test was used for comparing survival among different groups. Two-tailed test was used for all analyses. P < 0.05 was regarded as significant.
Results
Antitumor Activity of Vγ9Vδ2-T Cells Was Inhibited by IL-10 Secreted From EBV-LCL In Vitro
To investigate the effects of IL-10 in TME on the antitumor activity of Vγ9Vδ2-T cells, conditioned medium (CM) was obtained by collecting the supernatant of EBV-LCL culture for modeling TME in vitro. As shown in Figure 1A, CM from EBV-LCL culture established from different donors contained distinct levels of IL-10, and vIL-10 accounted for about 9.56 ± 5.74% of total IL-10. CM collected from EBV-LCL1 and EBV-LCL6, which contained the lowest and highest concentrations of IL-10, was used as IL-10low CM and IL-10high CM, respectively, in the following experiments. Importantly, the cytotoxic activity of IL-10high CM-treated Vγ9Vδ2-T cells against EBV-LCL was significantly lower than IL-10low CM- or PM-treated Vγ9Vδ2-T cells (Figure 1B). To verify the immunosuppressive role of IL-10 in the CM, an IL-10 neutralizing mAb was applied to block IL-10 signaling during Vγ9Vδ2-T cells exposed to IL-10high/low CM. The reduced cytotoxicity of Vγ9Vδ2-T cells against EBV-LCL was significantly abrogated when blocked with the IL-10 neutralizing mAb (Figure 1C). Furthermore, both hIL-10 and vIL-10 recombinant proteins showed dose-dependent inhibitions in the cytotoxicity of Vγ9Vδ2-T cells against EBV-LCL in the PM (Figures 1D, E). Taken together, our data indicate that the antitumor activity of Vγ9Vδ2-T cells against EBV-LCL was suppressed by both the hIL-10 and vIL-10 in the CM from EBV-LCL in vitro.
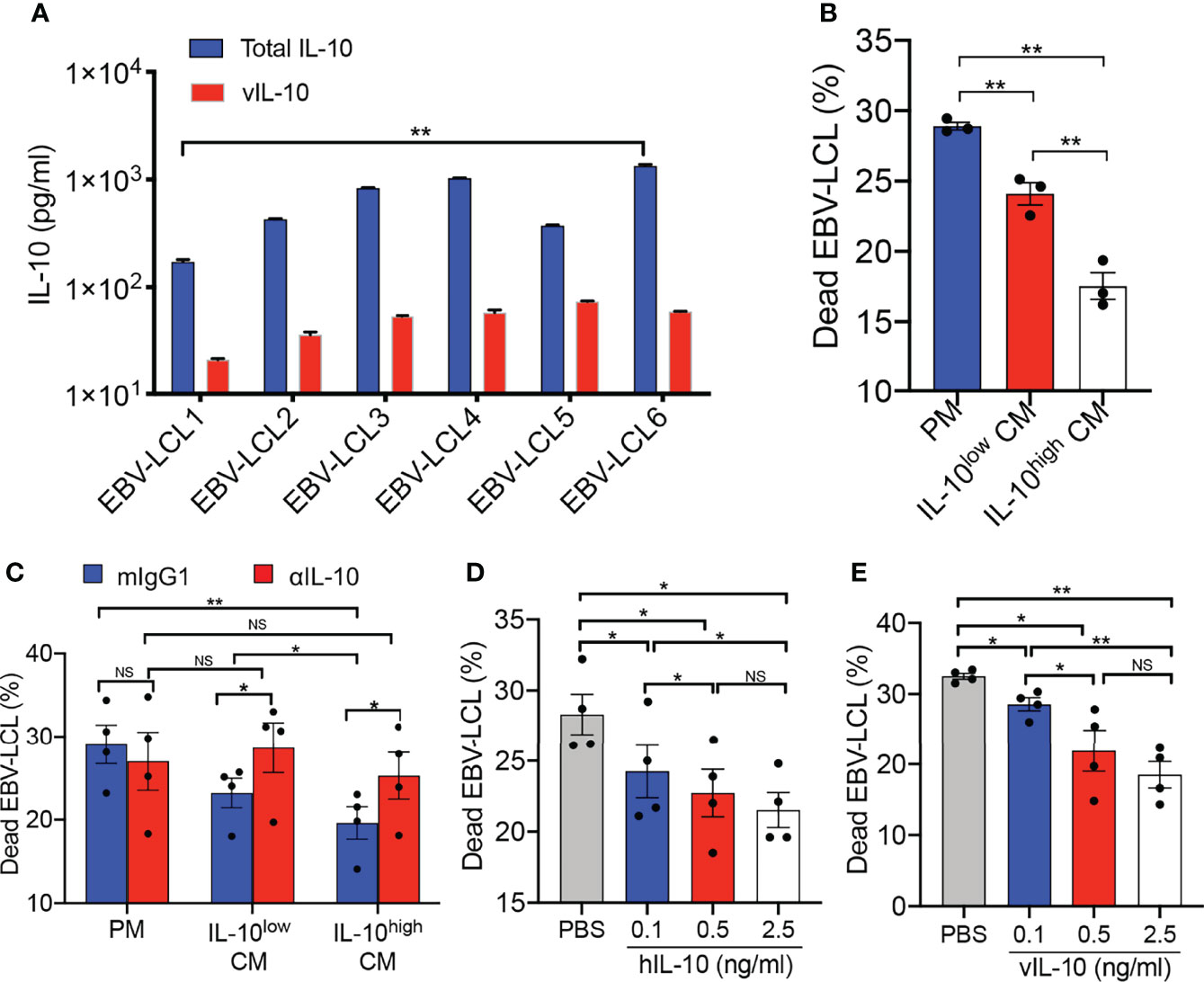
Figure 1 Antitumor activity of Vγ9Vδ2-T cells was inhibited by IL-10 secreted from EBV-LCL in vitro. (A) The concentration of vIL-10 and total IL-10 in conditioned medium (CM) collected from EBV-LCL established from different donors were detected. (B) Purified Vγ9Vδ2-T cells were pretreated in the IL-10low CM and IL-10high CM separately for 24 h, RPMI 1640 with 10% FBS medium (plain medium, PM) as a control. Pretreated Vγ9Vδ2-T cells then cocultured with autologous EBV-LCL at an effector: target (E:T) ratio of 10:1 for 4–6 h in the IL-10low/high CM and PM, respectively. Cytotoxicity was calculated as the proportion of dead EBV-LCL (CD3-PI+). (C) Purified Vγ9Vδ2-T cells were pretreated with IL-10low CM, IL-10high CM or PM in the presence of a neutralizing anti-IL-10 mAb (αIL-10, 5μg/ml) or isotype control (mIgG1, 5μg/ml), then cocultured with autologous EBV-LCL at an E:T ratio of 10:1 for 4–6 h in the IL-10low/high CM and PM respectively. Cytotoxicity was calculated as the proportion of dead EBV-LCL (CD3- PI+). (D, E) Purified Vγ9Vδ2-T cells were pretreated with recombinant hIL-10 (D) or vIL-10 (E) at different concentration, then cocultured with autologous EBV-LCL at an E:T ratio of 10:1 for 4–6h. The proportion of dead EBV-LCL (CD3- PI+) were detected by flow cytometry. All data are shown as mean ± SEM and representative of three independent experiments. *p < 0.05; **p < 0.01; ns, no significant difference.
Antitumor Activity of Vγ9Vδ2-T Cells Against EBV-Induced Lymphoma Was Decreased Under IL-10high TME In Vivo
To determine whether the therapeutic effects of Vγ9Vδ2-T cells on EBV-induced B cell lymphoma were inhibited by IL-10 within the TME, EBV-LCL1 expressing low levels of IL-10 (IL-10low LCL) and EBV-LCL6 expressing high levels of IL-10 (IL-10high LCL) were inoculated into Rag2-/- γc-/- mice, respectively (Figure 2A). After 21 days, large subcutaneous tumors developed in all the mice as detected by in vivo imaging (Figures 2B, C). The expressions of IL-10 in the tumor tissues generated from IL-10low LCL and IL-10high LCL were detected by immunohistochemistry (Figure 2D). Consistent with our previous results (15), Vγ9Vδ2-T cell treatment constrained tumor growth and prolonged the survival of tumor-bearing mice in contrast with the mice treated with PBS as the control (Figures 2E, F). Importantly, Vγ9Vδ2-T cells showed less efficacy in controlling EBV-induced lymphoma developed from IL-10high LCL compared with that developed from IL-10low LCL, along with larger tumor volume and lower survival rates (Figures 2E, F). These results suggest that the decreased antitumor activity of Vγ9Vδ2-T cells against EBV-induced lymphoma may be associated with IL-10high TME in vivo.
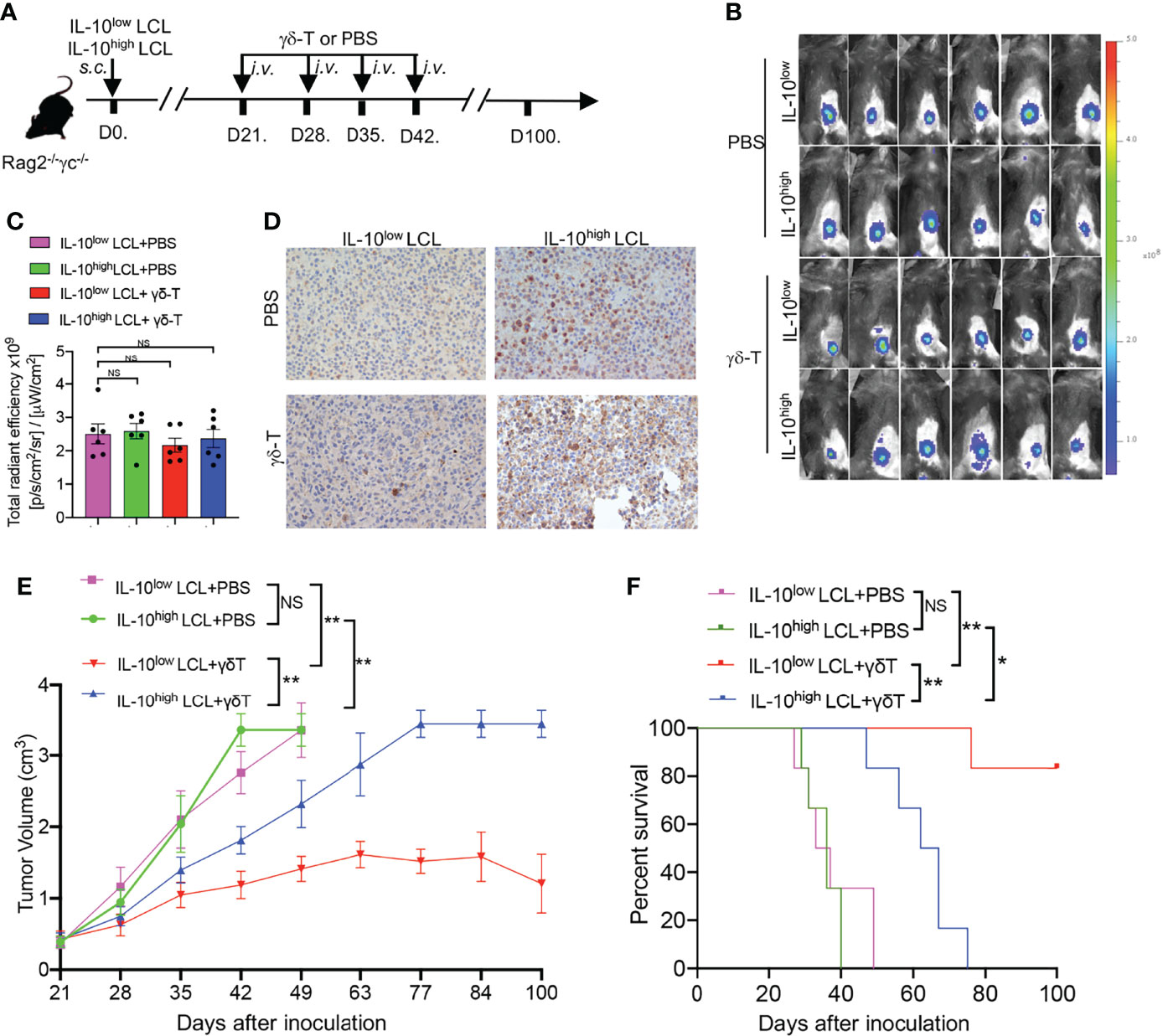
Figure 2 Antitumor activity of Vγ9Vδ2-T cells against EBV-induced lymphoma was decreased under IL-10high TME in vivo. (A) IL-10low LCL and IL-10high LCL were injected s.c. in Rag2−/−γc−/− mice separately. After 21 days, mice that had developed subcutaneous tumor were randomly divided into two groups respectively followed by the treatment with allogeneic Vγ9Vδ2-T cells or PBS at indicated time (six mice per group). (B, C) Whole-body fluorescence images (B) and total radiant efficiency (C) of mice before treatment with Vγ9Vδ2-T cells or PBS. (D) Representative histology of IL-10 in tumor sections that developed from IL-10low LCL and IL-10high LCL. (E, F) The tumor volume (E) and mouse survival (F) were determined at the indicated time. The tumor volume was compared using two-way ANOVA analysis, and mice survival was compared using Kaplan-Meier log-rank test. Data are representative for three independent experiments. *p < 0.05; **p < 0.01; ns, no significant difference.
CD137 Costimulation Suppressed IL-10R1 Expression and Restored the Antitumor Activity of Vγ9Vδ2-T Cells
IL-10 mediates its biological effects mainly through a heterodimeric membrane receptor composed of IL-10R1 and IL-10R2 (43). Since IL-10R2 is shared by more than five IL-10 family cytokines (44), we investigated the expression of IL-10R1 on Vγ9Vδ2-T cells exposed to the IL-10high CM upon γδ-TCR activation in vitro. Importantly, we found that following activation, IL-10R1+ Vγ9Vδ2-T cell subset expressed high levels of CD137 compared with IL-10R1-/lo Vγ9Vδ2-T cell subset in the IL-10high CM, indicating that CD137 could be an effective costimulatory signaling to restore the antitumor activity of Vγ9Vδ2-T cells compromised by the IL-10 in TME (Figures 3A, B).
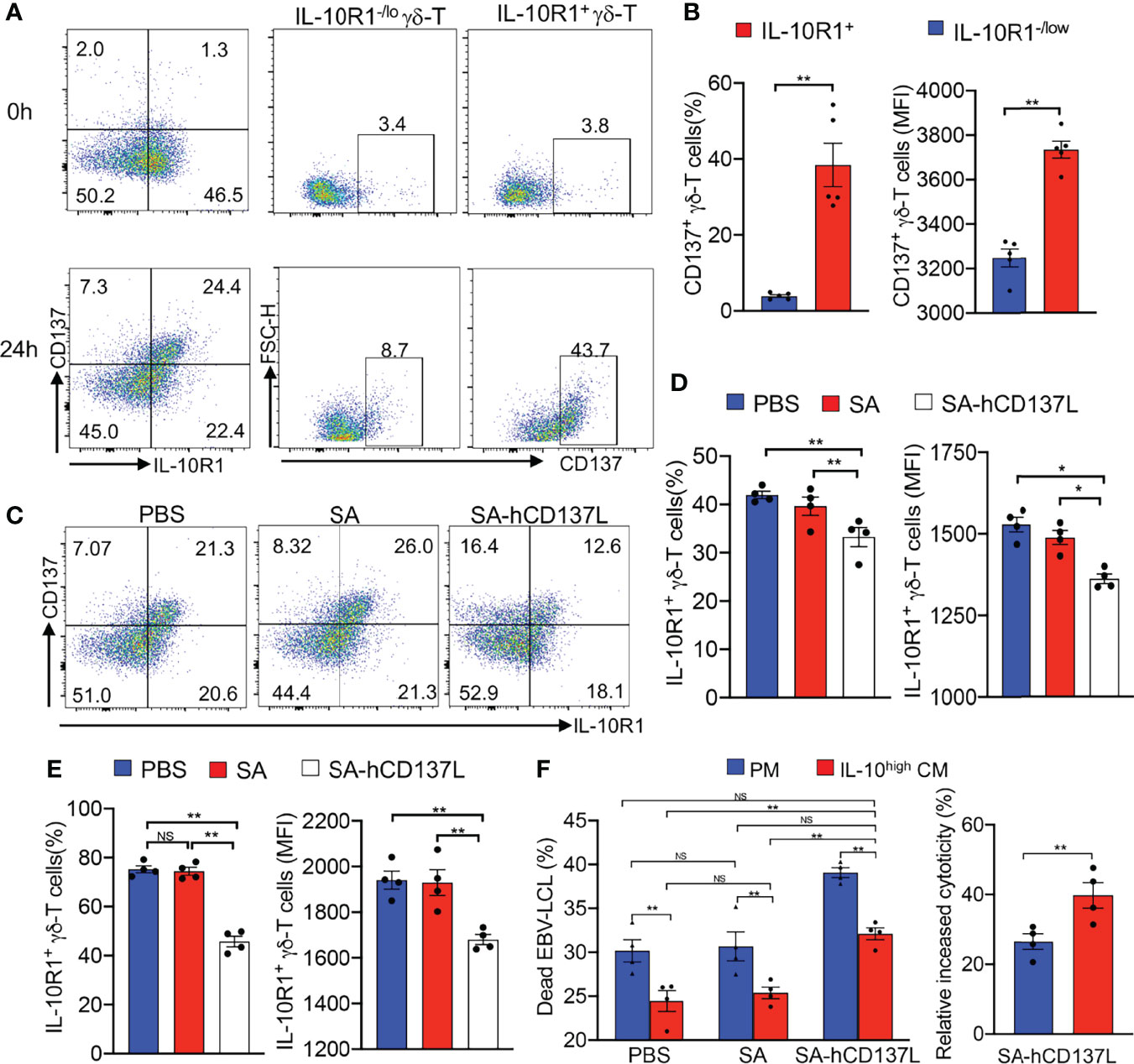
Figure 3 CD137 costimulation suppressed IL-10R1 expression and restored the antitumor activity of Vγ9Vδ2-T cells. (A) The expression of CD137 on IL-10R1-/lo and IL-10R1+ Vγ9Vδ2-T cells before (0h) and after stimulation with anti-γδ-TCR mAb for 24h (24h) in IL-10high CM. (B) The percentages and expression levels (mean fluorescence intensities, MFI) of CD137 on IL-10R1-/lo and IL-10R1+ Vγ9Vδ2-T cells upon stimulation for 24h in IL-10high CM. (C, E) The FACS patterns of CD137 and IL-10R1 expressions (C), surface expression of IL-10R1 in total Vγ9Vδ2-T cells (D) and in CD137+ Vγ9Vδ2-T cells (E) upon stimulation by anti-γδ-TCR mAb supplemented with SA-CD137L (500ng/ml), PBS and SA in IL-10high CM for 24h were detected by flow cytometry after surface staining of IL-10R1. (F) Purified Vγ9Vδ2-T cells were pretreated with SA-hCD137L (500ng/ml), PBS or SA for 24 h in the PM and IL-10high CM, and then cocultured with autologous EBV-LCL at an E: T ratio of 10:1 for 4-6h. The proportions of dead EBV-LCL (CD3- PI+, left), and the relative increase of cytotoxicity after being treated with SA-hCD137L (right) are shown. All data are shown as mean ± SEM and representative of three independent experiments. *p < 0.05; **p < 0.01; ns, no significant difference.
To determine whether CD137 costimulation could provide an anti-exhaustion signal to mitigate the inhibiting effects mediated by IL-10 in TME, a recombinant SA-hCD137L protein containing a core streptavidin (SA) molecule with the extracellular domains of human CD137L (hCD137L) was generated as we reported previously (37). We found that the addition of the recombinant SA-hCD137L protein significantly inhibited the surface expression of IL-10R1 in total and CD137+ Vγ9Vδ2-T cells in IL-10high CM in terms of both percentage and expression level (MFI) changes (Figures 3C–E). These results indicate that CD137 costimulation suppressed IL-10R1 expression in CD137+ Vγ9Vδ2-T cells, and thereby was able to reduce their sensitivity to endogenous IL-10 in the immunosuppressive TME.
To determine whether CD137 costimulation could rescue the impaired antitumor efficacy of Vγ9Vδ2-T cells in suppressive TME, the recombinant SA-hCD137L protein was added to the coculture of Vγ9Vδ2-T cells with EBV-LCL in IL-10high CM for mimicking the tumor milieu. As shown in Figure 3F, the SA-hCD137L protein significantly increased the cytotoxicity of Vγ9Vδ2-T cells against EBV-LCL under both the immunosuppressive and normal microenvironments mimicked by the IL-10high CM and the PM. Importantly, CD137 costimulation not only completely restored the reduced cytotoxicity of Vγ9Vδ2-T cells in the IL-10high CM to normal levels, but also had a better effect to enhance the cytotoxic activity of Vγ9Vδ2-T cells in IL-10high CM than that in PM (Figure 3F). These data demonstrate that CD137 engagement enables Vγ9Vδ2-T cells to withstand the hostile environment mediated by endogenous IL-10, resulting in the increase of the antitumor activity of Vγ9Vδ2-T cells in vitro.
CD137 Costimulation Enhanced the Compromised Antitumor Activity of Vγ9Vδ2-T Cells With IL-10high TME in Rag2-/- γc-/- Mice
Previously we have demonstrated that Vγ9Vδ2-T cells could control EBV-inducing lymphoma (15), and their antitumor activity in controlling EBV-induced lymphoma developed from IL-10high LCL was lower than that developed from IL-10low LCL (Figures 2E, F). To further elucidate the roles of CD137 costimulation in the compromised antitumor activity of Vγ9Vδ2-T cells in IL-10high TME in vivo, EGFP-expressing IL-10high LCL was inoculated in Rag2-/- γc-/- mice, s.c. (Figure 4A). Twenty-one days later, mice bearing subcutaneous tumors were randomly divided into three groups as detected by in vivo imaging (Figure 4B). No significant differences were found in fluorescent density from tumor cells among the three groups after 21 days of tumor cell inoculation (Figure 4C). PAM-expanded Vγ9Vδ2-T cells were adoptively transferred to one group of the tumor-bearing mice with the recombinant SA-hCD137L protein weekly from day 21 to day 42. The other two groups of mice were adoptively transferred with Vγ9Vδ2-T cells in the presence of PBS or SA as the controls. Importantly, Vγ9Vδ2-T cells in combination with SA-hCD137L treatment significantly limited tumor growth (Figure 4D) and improved mouse survival (Figure 4E) compared to treatments of Vγ9Vδ2-T cells with PBS or SA. These data indicate that the costimulation of CD137 efficiently enhanced the antitumor activity of Vγ9Vδ2-T cells in the highly immunosuppressive microenvironment mediated by IL-10 in vivo.
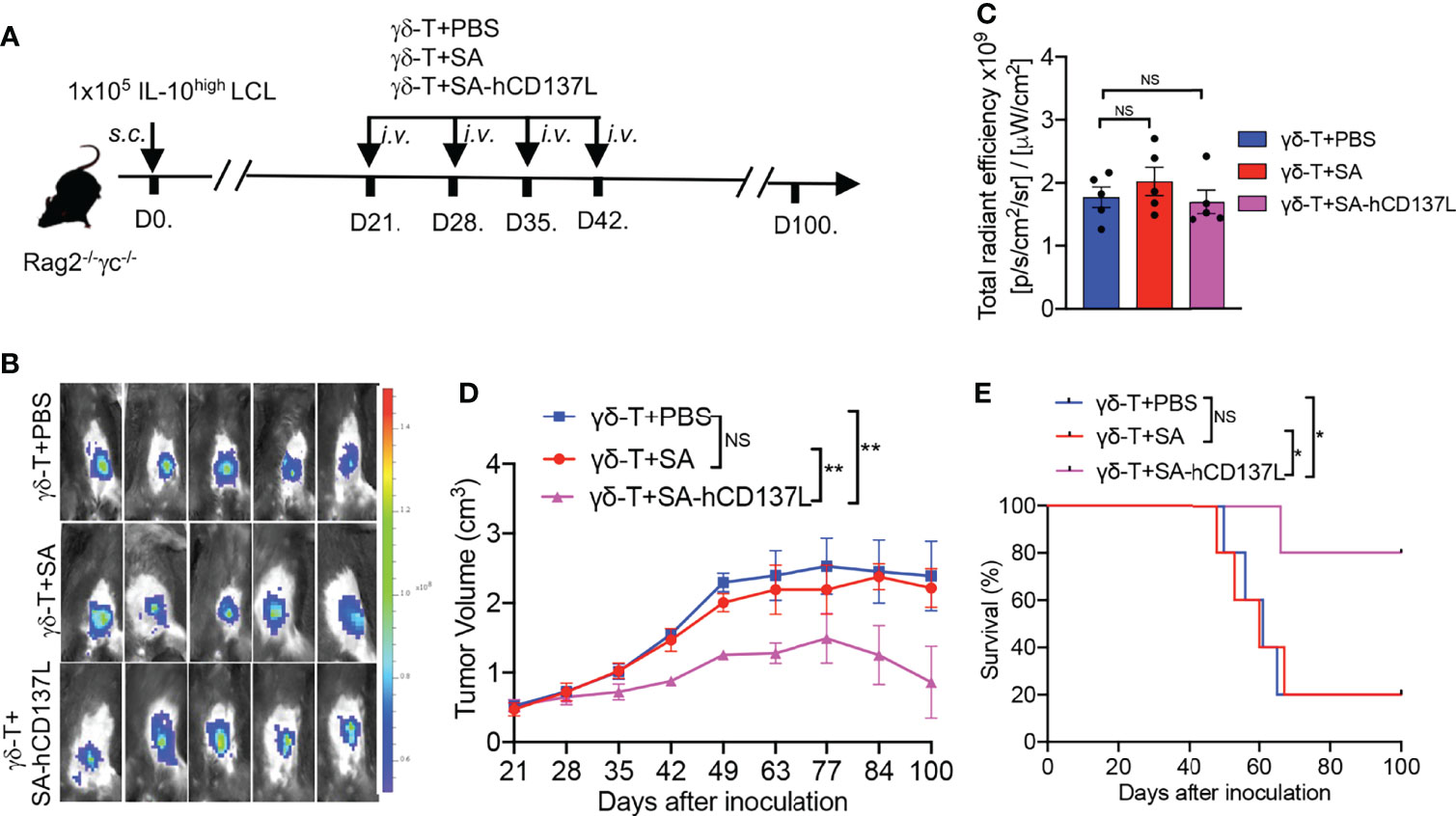
Figure 4 CD137 costimulation enhanced the compromised antitumor activity of Vγ9Vδ2-T cells with IL-10high TME in Rag2-/- γc-/- mice. (A) Protocol for evaluation of the synergistic therapeutic effect of Vγ9Vδ2-T cells and SA-hCD137L on EBV-induced lymphoma in Rag2-/- γc-/- mice (five mice per group). (B, C) Whole-body fluorescence images (B) and total radiant efficiency (C) of mice before treatment with PAM, SA-hCD137, SA, and PBS. (D, E) The tumor volume (D) and mouse survival (E) were determined at the indicated time. The tumor volume was analyzed by two-way ANOVA test, and mice survival was analyzed by Kaplan-Meier log-rank test. Data are representative for three independent experiments. *p < 0.05; **p < 0.01; ns, no significant difference.
CD137 Costimulation Improved the Therapeutic Effect of PAM in Controlling EBV-Induced Lymphoma With IL-10high TME in Humanized Mice
Previously we had demonstrated that PAM could expand Vγ9Vδ2-T cells in vivo to control EBV-induced lymphoma in humanized mice with functional hPBMC (15). We then investigated the role of CD137 costimulation on the therapeutic effect of PAM in controlling EBV-induced lymphoma with IL-10high TME in humanized mice. EBV-induced lymphoma with IL-10high TME model was generated by inoculation s.c. of IL-10high EBV-LCL in humanized mice (Figure 5A) (15). All humanized mice developed subcutaneous tumors after IL-10high EBV-LCL inoculation for 28 days with similar fluorescent density from tumor cells as detected by in vivo imaging (Figures 5B, C). PAM, SA-hCD137L, or the combination of these two agents were injected intraperitoneally (i.p.) at days 28, 35, 42, and 49 after IL-10high EBV-LCL inoculation (Figure 5A). PBS- and SA-treated mice were controls. As a result, PAM administration alone decreased the tumor volume significantly and extended the survival of the tumor-bearing humanized mice compared with the treatment with PBS, SA, or SA-hCD137L protein alone, respectively (Figures 5D, E). Importantly, the combination treatment of PAM with SA-hCD137L was more potent than PAM alone to control the development of EBV-induced lymphoma with IL-10high TME in humanized mice, in terms of tumor growth and survival (Figures 5D, E).
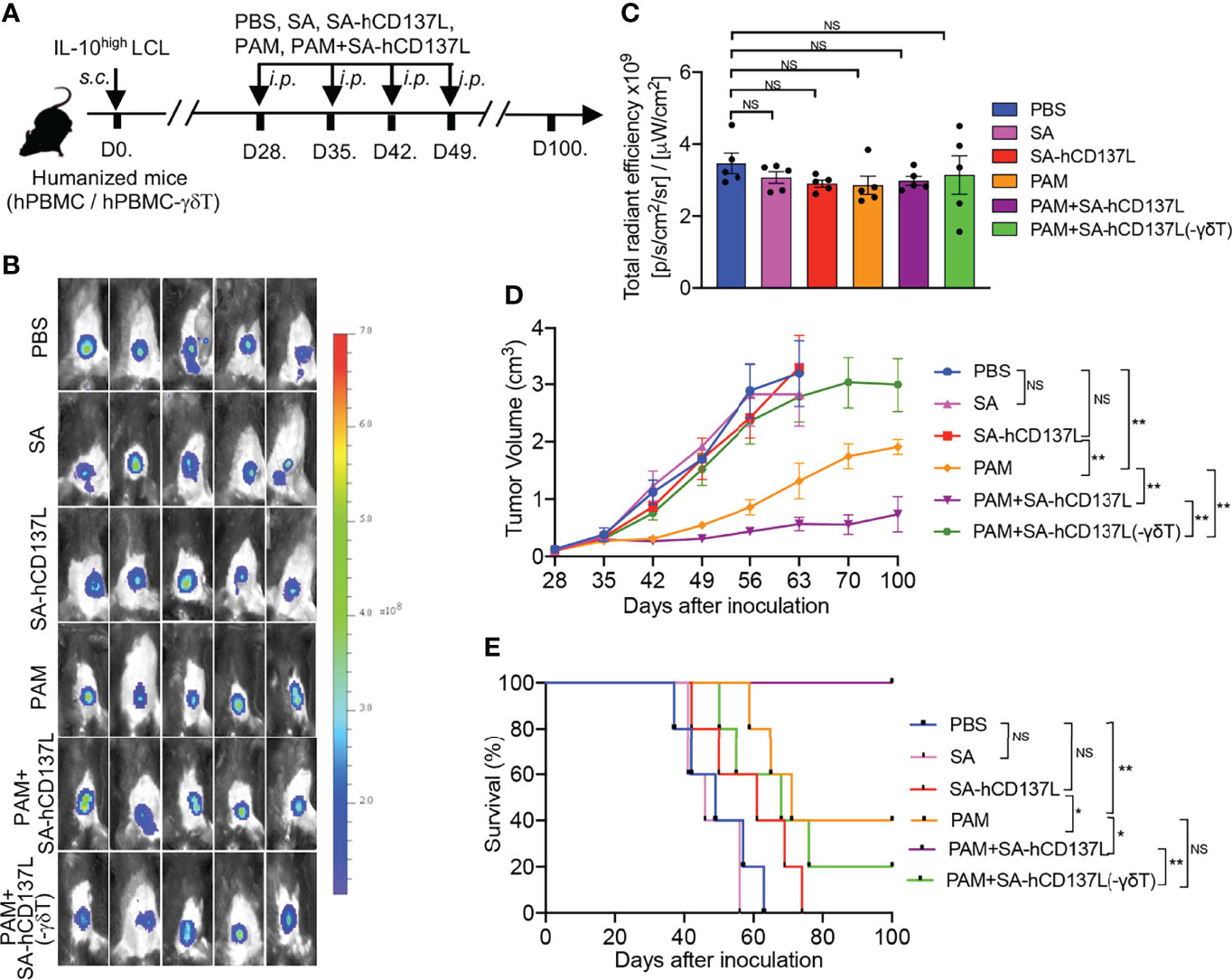
Figure 5 CD137 costimulation improved the therapeutic effect of PAM in controlling EBV-induced lymphoma with IL-10high TME in humanized mice. (A) The evaluation protocol of the synergistic therapeutic effect of PAM and SA-hCD137L on EBV-induced lymphoma in humanized mice (five mice per group). (B, C) Whole-body fluorescence images (B) and total radiant efficiency (C) of mice before treatment with PAM, SA-hCD137, SA and PBS. (D, E) The tumor volume (D) and mouse survival (E) were determined at the indicated time. The tumor volume was analyzed by two-way ANOVA test, and mice survival was analyzed by Kaplan-Meier log-rank test. Data are representative for three independent experiments. *p < 0.05; **p < 0.01; ns, no significant difference.
Humanized mice reconstituted with Vγ9Vδ2-T-cell-depleted hPBMC were also used to confirm whether the effect of SA-hCD137L costimulation on the control of EBV-induced lymphoma with IL-10high TME was mediated by Vγ9Vδ2-T cells (Figure 5A). As shown in Figures 5D, E, there were no therapeutic effects by the combination treatment of PAM with SA-hCD137L in humanized mice reconstituted with Vγ9Vδ2-T-cell-depleted hPBMC. These data demonstrate that the recombinant SA-hCD137L protein had a synergistic effect with PAM to overcome the barriers of IL-10high TME in vivo and this synergistic effect was mainly mediated by Vγ9Vδ2-T cells.
Discussion
In this study, we demonstrated that the antitumor activities of Vγ9Vδ2-T cells against EBV-LCL were inhibited by both hIL-10 and vIL-10 in vitro and in vivo. Importantly, we found that IL-10R1 was highly expressed on CD137+ Vγ9Vδ2-T cells compared to CD137-/lo Vγ9Vδ2-T cells following activation. CD137 engagement significantly suppressed IL-10R1 expression in Vγ9Vδ2-T cells, therefore reducing the Vγ9Vδ2-T cells’ sensitivity to IL-10 in the TME. We further demonstrated that SA-hCD137L in a tetrameric form of human CD137L protein obviously enhanced the therapeutic effects of adoptive transfer of ex vivo expanded Vγ9Vδ2-T cell in Rag2-/-γc-/- mice and direct administration of PAM in humanized mice for the treatment of EBV-induced lymphoma with IL-10high TME.
IL-10 is important as an immunoregulatory cytokine to suppress inflammatory responses. However, its effects on tumorigenesis and development are controversial (45). IL-10 can inhibit the process of antigen presentation by downregulating the expression of MHC-II in APCs (46) and MHC-I in tumor cells (47). Thus, IL-10 can facilitate tumor escape by contributing to an immunosuppressive environment. A meta-analysis of 1788 cancer patients also revealed that the elevated serum IL-10 can predict poor prognosis (48). Paradoxically, it was reported that IL-10 can also induce immune-dependent antitumor effects (15, 49–51). Therefore, the roles of IL-10 on tumor development are dependent on the local environment and physiopathological states. The inhibitory role of IL-10 on APCs, CD8+ T cells, and CD4+ T cells has been clearly defined, but its impact on Vγ9Vδ2-T cells remains unclear. In this study, our data supported that hIL-10 and vIL-10 derived from tumor cells and EBV significantly inhibited the cytotoxicity of Vγ9Vδ2-T cells, which substantially limits the antitumor efficacy of Vγ9Vδ2-T cells.
EBV has evolved to express vIL-10, thereby providing a suitable microenvironment for itself to evade immunity and cause tumorigenesis (52, 53). The structurally homologous viral and human IL-10 perform similarly in several biological properties, including inhibition of IFN-γ production, suppression of T cell proliferation in response to antigens and mitogens, and stimulation of B cell growth (54). This similarity has raised the possibility that EBV might have captured the IL-10 gene during evolution. Furthermore, IL-10 has been shown to be involved in the pathogenesis of lymphoid disorders (55, 56). Elevated IL-10 levels are correlated with shorter survival and adverse disease features in patients with EBV-associated tumors (33, 57). Thus, we reasoned that hIL-10 and vIL-10 may be associated with the suppression of Vγ9Vδ2-T cells’ antitumor activity. Such an interaction would provide a suitable microenvironment for viruses to evade immunity and cause tumorigenesis. Here, our in vitro data revealed that vIL-10 derived from EBV and hIL-10 derived from EBV-LCL were the dominant factors for inhibiting the antitumor activity of Vγ9Vδ2-T cells in TME. Our in vivo data also suggested that the reduced antitumor activity of Vγ9Vδ2-T cells against EBV-induced lymphoma may be associated with IL-10high TME. Further study using IL-10 neutralizing mAb or IL-10 knockout mice is required to determine the exact role of IL-10 in antitumor activity of Vγ9Vδ2-T cells in vivo. Of note, additional factors in the CM might also contribute to suppressing Vγ9Vδ2-T cells activity because a smaller extent of cytotoxicity reduction after treatment with recombinant hIL-10 and vIL-10 proteins was observed when compared with IL-10high CM (Figure 1).
Recently, clinical trials utilizing bisphosphonates, such as PAM and ZOL, to expand γδ-T cell in vivo in combination with IL-2 therapy or adoptive transfer of ex vivo cultured γδ-T cells were performed in patients with tumors and virus infections (11, 15, 58, 59). Administration of bisphosphonates with IL-2 and the transfer of expanded autologous Vγ9Vδ2 T-cells have been demonstrated to be safe with limited adverse events (60). However, there is only a modest efficacy in the treatment of some tumors (61, 62). One drawback of γδ-T cell-based immunotherapy is the rapid exhaustion of proliferation and effector responses due to repeated phosphoantigen treatments (17). Another drawback of this therapy is the impaired antitumor activity of γδ-T cells caused by the tumor immunosuppressive microenvironment (18, 63).
CD137 is a promising costimulatory immunologic target for enhancing antitumor immune responses (39). CD137 costimulation, known as “stepping on the accelerator,” is believed to be a compelling complement for “removing the brakes” via blocking inhibitory signaling. Importantly, it is now appreciated that CD137 signaling not only works as an “accelerator” to provide costimulation, but also breaks and reverses the established anergy in cytotoxic T lymphocytes (CTLs) (64, 65). However, the role of CD137 signaling in Vγ9Vδ2-T cells within the context of IL-10-mediated TME is not clear. Here, we revealed that IL-10R1+ Vγ9Vδ2 T-cell subset expressed high levels of CD137. Moreover, CD137 costimulation suppressed IL-10R1 in Vγ9Vδ2-T cells, suggesting that CD137 engagement possessed the potential to ameliorate the exhaustion and dysfunction of Vγ9Vδ2-T cells.
Ligation of CD137 is correlated with effective antitumor responses; however, the application of anti-CD137 agonistic antibodies in patients is limited by a variety of side effects (66). The natural CD137 ligand is an alternative to the CD137-specific antibodies to stimulate antitumor T cell responses. Shirwan lab reported that a streptavidin-conjugated murine CD137L (SA-mCD137L) complex could induce effective antitumor immune responses (67, 68). SA-mCD137L induces less pathological side effects than anti-CD137 agonistic antibody therapy, suggesting a higher therapeutic index of SA-mCD137L. Previously, we demonstrated that recombinant SA-hCD137L enhanced the cytotoxic effect of Vγ9Vδ2- T cells against influenza virus infection (37). Here, we further found that SA-hCD137L restored the antitumor activity of Vγ9Vδ2-T cells compromised by the IL-10-mediated TME. These data indicate that SA-hCD137L can provide an alternative to anti-CD137 agonistic for anti-tumor therapy.
There are no Vγ9Vδ2-T cells in mice, thus it is impossible to study these cells in mouse models (69). Previously, we successfully established humanized mice with a similar proportion of Vγ9Vδ2-T cells in murine peripheral blood to that in humans (12, 14, 70, 71). Importantly, here the synergistic effect of PAM and recombinant SA-hCD137L Vγ9Vδ2-T cells was verified in humanized mice.
In conclusion, our study further elucidates the role of CD137 in the antitumor activity of human Vγ9Vδ2-T cells in the IL-10-mediated immunosuppressive TME. The combination of a phosphoantigen and CD137 agonist also provides a novel strategy for treating EBV-induced tumors by avoiding Vγ9Vδ2-T cell exhaustion and enhancing the efficacy of Vγ9Vδ2-T cell-based therapy.
Author Contributors
YP, WT, and YL conceived and designed the study, interpreted the results, wrote and edited the manuscript; YP, KW, ZX, CT, XW, YZ, and XM designed and performed the experiments, analyzed the results. WT supervised this study. All authors contributed to the article and approved the submitted version.
Data Availability Statement
The original contributions presented in the study are included in the article/supplementary material. Further inquiries can be directed to the corresponding author.
Ethics Statement
The studies involving human participants were reviewed and approved by the institutional review board of The University of Hong Kong/Hospital Authority Hong Kong West Cluster. The patients/participants provided their written informed consent to participate in this study. The animal study was reviewed and approved by the Committee on the Use of Live Animals in Teaching and Research, The University of Hong Kong.
Funding
This study was supported partially by GRF, RGC (17122519, 17126317); Health and Medical Research Fund, Food and Health Bureau, Hong Kong SAR Government (18192021); Seed Funding for Strategic Interdisciplinary Research Scheme, the University of Hong Kong; Hong Kong SAR, China.
Conflict of Interest
The authors declare that the research was conducted in the absence of any commercial or financial relationships that could be construed as a potential conflict of interest.
Publisher’s Note
All claims expressed in this article are solely those of the authors and do not necessarily represent those of their affiliated organizations, or those of the publisher, the editors and the reviewers. Any product that may be evaluated in this article, or claim that may be made by its manufacturer, is not guaranteed or endorsed by the publisher.
References
1. Cohen JI, Fauci AS, Varmus H, Nabel GJ. Epstein-Barr Virus: An Important Vaccine Target for Cancer Prevention. Sci Transl Med (2011) 3(107):107fs7. doi: 10.1126/scitranslmed.3002878
2. Young LS, Yap LF, Murray PG. Epstein-Barr Virus: More Than 50 Years Old and Still Providing Surprises. Nat Rev Cancer (2016) 16(12):789–802. doi: 10.1038/nrc.2016.92
3. Zhu QY, Zhao GX, Li Y, Talakatta G, Mai HQ, Le QT, et al. Advances in Pathogenesis and Precision Medicine for Nasopharyngeal Carcinoma. MedComm (2020) (2021) 2(2):175–206. doi: 10.1002/mco2.32
4. Taylor GS, Long HM, Brooks JM, Rickinson AB, Hislop AD. The Immunology of Epstein-Barr Virus-Induced Disease. Annu Rev Immunol (2015) 33:787–821. doi: 10.1146/annurev-immunol-032414-112326
5. Israel BF, Kenney SC. Virally Targeted Therapies for EBV-Associated Malignancies. Oncogene (2003) 22(33):5122–30. doi: 10.1038/sj.onc.1206548
6. Dharnidharka VR, Mohanakumar T. New Approaches to Treating B-Cell Cancers Induced by Epstein-Barr Virus. N Engl J Med (2015) 372(6):569–71. doi: 10.1056/NEJMcibr1415117
7. Bollard CM, Rooney CM, Heslop HE. T-Cell Therapy in the Treatment of Post-Transplant Lymphoproliferative Disease. Nat Rev Clin Oncol (2012) 9(9):510–9. doi: 10.1038/nrclinonc.2012.111
8. Hayday AC. Gammadelta T Cell Update: Adaptate Orchestrators of Immune Surveillance. J Immunol (2019) 203(2):311–20. doi: 10.4049/jimmunol.1800934
9. Silva-Santos B, Mensurado S, Coffelt SB. Gammadelta T Cells: Pleiotropic Immune Effectors With Therapeutic Potential in Cancer. Nat Rev Cancer (2019) 19(7):392–404. doi: 10.1038/s41568-019-0153-5
10. Xiang Z, Tu W. Dual Face of Vgamma9Vdelta2-T Cells in Tumor Immunology: Anti- Versus Pro-Tumoral Activities. Front Immunol (2017) 8:1041. doi: 10.3389/fimmu.2017.01041
11. Tanaka Y, Murata-Hirai K, Iwasaki M, Matsumoto K, Hayashi K, Kumagai A, et al. Expansion of Human Gammadelta T Cells for Adoptive Immunotherapy Using a Bisphosphonate Prodrug. Cancer Sci (2018) 109(3):587–99. doi: 10.1111/cas.13491
12. Wang X, Xiang Z, Liu Y, Huang C, Pei Y, Wang X, et al. Exosomes Derived From Vδ2-T Cells Control Epstein-Barr Virus-Associated Tumors and Induce T Cell Antitumor Immunity. Sci Transl Med (2020) 12(563):eaaz3426. doi: 10.1126/scitranslmed.aaz3426
13. Wang X, Zhang Y, Mu X, Tu CR, Chung Y, Tsao SW, et al. Exosomes Derived From Gammadelta-T Cells Synergize With Radiotherapy and Preserve Antitumor Activities Against Nasopharyngeal Carcinoma in Immunosuppressive Microenvironment. J Immunother Cancer (2022) 10(2):e003832. doi: 10.1136/jitc-2021-003832
14. Tu W, Zheng J, Liu Y, Sia SF, Liu M, Qin G, et al. The Aminobisphosphonate Pamidronate Controls Influenza Pathogenesis by Expanding a Gammadelta T Cell Population in Humanized Mice. J Exp Med (2011) 208(7):1511–22. doi: 10.1084/jem.20110226
15. Xiang Z, Liu Y, Zheng J, Liu M, Lv A, Gao Y, et al. Targeted Activation of Human Vgamma9Vdelta2-T Cells Controls Epstein-Barr Virus-Induced B Cell Lymphoproliferative Disease. Cancer Cell (2014) 26(4):565–76. doi: 10.1016/j.ccr.2014.07.026
16. Gentles AJ, Newman AM, Liu CL, Bratman SV, Feng W, Kim D, et al. The Prognostic Landscape of Genes and Infiltrating Immune Cells Across Human Cancers. Nat Med (2015) 21(8):938–45. doi: 10.1038/nm.3909
17. Sicard H, Ingoure S, Luciani B, Serraz C, Fournié JJ, Bonneville M, et al. In Vivo Immunomanipulation of V Gamma 9V Delta 2 T Cells With a Synthetic Phosphoantigen in a Preclinical Nonhuman Primate Model. J Immunol (2005) 175(8):5471–80. doi: 10.4049/jimmunol.175.8.5471
18. Yi Y, He HW, Wang JX, Cai XY, Li YW, Zhou J, et al. The Functional Impairment of HCC-Infiltrating γδ T Cells, Partially Mediated by Regulatory T Cells in a Tgfβ- and IL-10-Dependent Manner. J Hepatol (2013) 58(5):977–83. doi: 10.1016/j.jhep.2012.12.015
19. Park JH, Lee HK. Function of γδ T Cells in Tumor Immunology and Their Application to Cancer Therapy. Exp Mol Med (2021) 53(3):318–27. doi: 10.1038/s12276-021-00576-0
20. Pitt JM, Marabelle A, Eggermont A, Soria JC, Kroemer G, Zitvogel L. Targeting the Tumor Microenvironment: Removing Obstruction to Anticancer Immune Responses and Immunotherapy. Ann Oncol (2016) 27(8):1482–92. doi: 10.1093/annonc/mdw168
21. Dennis KL, Blatner NR, Gounari F, Khazaie K. Current Status of Interleukin-10 and Regulatory T-Cells in Cancer. Curr Opin Oncol (2013) 25(6):637–45. doi: 10.1097/CCO.0000000000000006
22. Kajino K, Nakamura I, Bamba H, Sawai T, Ogasawara K. Involvement of IL-10 in Exhaustion of Myeloid Dendritic Cells and Rescue by CD40 Stimulation. Immunology (2007) 120(1):28–37. doi: 10.1111/j.1365-2567.2006.02474.x
23. Gassa A, Jian F, Kalkavan H, Duhan V, Honke N, Shaabani N, et al. IL-10 Induces T Cell Exhaustion During Transplantation of Virus Infected Hearts. Cell Physiol Biochem (2016) 38(3):1171–81. doi: 10.1159/000443067
24. Shi R, Tang YQ, Miao H. Metabolism in Tumor Microenvironment: Implications for Cancer Immunotherapy. MedComm (2020) 1(1):47–68. doi: 10.1002/mco2.6
25. Stuart AD, Stewart JP, Arrand JR, Mackett M. The Epstein-Barr Virus Encoded Cytokine Viral Interleukin-10 Enhances Transformation of Human B Lymphocytes. Oncogene (1995) 11(9):1711–9.
26. Brooks DG, Trifilo MJ, Edelmann KH, Teyton L, McGavern DB, Oldstone MB. Interleukin-10 Determines Viral Clearance or Persistence In Vivo. Nat Med (2006) 12(11):1301–9. doi: 10.1038/nm1492
27. Ejrnaes M, Filippi CM, Martinic MM, Ling EM, Togher LM, Crotty S, et al. Resolution of a Chronic Viral Infection After Interleukin-10 Receptor Blockade. J Exp Med (2006) 203(11):2461–72. doi: 10.1084/jem.20061462
28. Jochum S, Moosmann A, Lang S, Hammerschmidt W, Zeidler R. The EBV Immunoevasins vIL-10 and BNLF2a Protect Newly Infected B Cells From Immune Recognition and Elimination. PLos Pathog (2012) 8(5):e1002704. doi: 10.1371/journal.ppat.1002704
29. Wilson AD, Hopkins JC, Morgan AJ. In Vitro Cytokine Production and Growth Inhibition of Lymphoblastoid Cell Lines by CD4+ T Cells From Epstein-Barr Virus (EBV) Seropositive Donors. Clin Exp Immunol (2001) 126(1):101–10. doi: 10.1046/j.1365-2249.2001.01641.x
30. Miyauchi K, Urano E, Yoshiyama H, Komano J. Cytokine Signatures of Transformed B Cells With Distinct Epstein-Barr Virus Latencies as a Potential Diagnostic Tool for B Cell Lymphoma. Cancer Sci (2011) 102(6):1236–41. doi: 10.1111/j.1349-7006.2011.01924.x
31. Lambert SL, Martinez OM. Latent Membrane Protein 1 of EBV Activates Phosphatidylinositol 3-Kinase to Induce Production of IL-10. J Immunol (2007) 179(12):8225–34. doi: 10.4049/jimmunol.179.12.8225
32. Miyazaki I, Cheung RK, Dosch HM. Viral Interleukin 10 is Critical for the Induction of B Cell Growth Transformation by Epstein-Barr Virus. J Exp Med (1993) 178(2):439–47. doi: 10.1084/jem.178.2.439
33. Lech-Maranda E, Bienvenu J, Michallet AS, Houot R, Robak T, Coiffier B, et al. Elevated IL-10 Plasma Levels Correlate With Poor Prognosis in Diffuse Large B-Cell Lymphoma. Eur Cytokine Netw (2006) 17(1):60–6.
34. Wang C, Lin GH, McPherson AJ, Watts TH. Immune Regulation by 4-1BB and 4-1BBL: Complexities and Challenges. Immunol Rev (2009) 229(1):192–215. doi: 10.1111/j.1600-065X.2009.00765.x
35. Stoll A, Bruns H, Fuchs M, Völkl S, Nimmerjahn F, Kunz M, et al. CD137 (4-1BB) Stimulation Leads to Metabolic and Functional Reprogramming of Human Monocytes/Macrophages Enhancing Their Tumoricidal Activity. Leukemia (2021) 35(12):3482–96. doi: 10.1038/s41375-021-01287-1
36. Shuford WW, Klussman K, Tritchler DD, Loo DT, Chalupny J, Siadak AW, et al. 4-1BB Costimulatory Signals Preferentially Induce CD8+ T Cell Proliferation and Lead to the Amplification In Vivo of Cytotoxic T Cell Responses. J Exp Med (1997) 186(1):47–55. doi: 10.1084/jem.186.1.47
37. Pei Y, Wen K, Xiang Z, Huang C, Wang X, Mu X, et al. CD137 Costimulation Enhances the Antiviral Activity of Vγ9vδ2-T Cells Against Influenza Virus. Signal Transduct Target Ther (2020) 5(1):74. doi: 10.1038/s41392-020-0174-2
38. Chester C, Sanmamed MF, Wang J, Melero I. Immunotherapy Targeting 4-1BB: Mechanistic Rationale, Clinical Results, and Future Strategies. Blood (2018) 131(1):49–57. doi: 10.1182/blood-2017-06-741041
39. Hashimoto K. CD137 as an Attractive T Cell Co-Stimulatory Target in the TNFRSF for Immuno-Oncology Drug Development. Cancers (Basel) (2021) 13(10):2288–303. doi: 10.3390/cancers13102288
40. Qin G, Mao H, Zheng J, Sia SF, Liu Y, Chan PL, et al. Phosphoantigen-Expanded Human Gammadelta T Cells Display Potent Cytotoxicity Against Monocyte-Derived Macrophages Infected With Human and Avian Influenza Viruses. J Infect Dis (2009) 200(6):858–65. doi: 10.1086/605413
41. Jog NR, Chakravarty EF, Guthridge JM, James JA. Epstein Barr Virus Interleukin 10 Suppresses Anti-Inflammatory Phenotype in Human Monocytes. Front Immunol (2018) 9:2198. doi: 10.3389/fimmu.2018.02198
42. Vahl JM, Friedrich J, Mittler S, Trump S, Heim L, Kachler K, et al. Interleukin-10-Regulated Tumour Tolerance in Non-Small Cell Lung Cancer. Br J Cancer (2017) 117(11):1644–55. doi: 10.1038/bjc.2017.336
43. Moore KW, de Waal Malefyt R, Coffman RL, O'Garra A. Interleukin-10 and the Interleukin-10 Receptor. Annu Rev Immunol (2001) 19:683–765. doi: 10.1146/annurev.immunol.19.1.683
44. Donnelly RP, Sheikh F, Kotenko SV, Dickensheets H. The Expanded Family of Class II Cytokines That Share the IL-10 Receptor-2 (IL-10R2) Chain. J Leukoc Biol (2004) 76(2):314–21. doi: 10.1189/jlb.0204117
45. Mannino MH, Zhu Z, Xiao H, Bai Q, Wakefield MR, Fang Y. The Paradoxical Role of IL-10 in Immunity and Cancer. Cancer Lett (2015) 367(2):103–7. doi: 10.1016/j.canlet.2015.07.009
46. Steinbrink K, Jonuleit H, Müller G, Schuler G, Knop J, Enk AH. Interleukin-10-Treated Human Dendritic Cells Induce a Melanoma-Antigen-Specific Anergy in CD8(+) T Cells Resulting in a Failure to Lyse Tumor Cells. Blood (1999) 93(5):1634–42. doi: 10.1182/blood.V93.5.1634
47. Adris S, Klein S, Jasnis M, Chuluyan E, Ledda M, Bravo A, et al. IL-10 Expression by CT26 Colon Carcinoma Cells Inhibits Their Malignant Phenotype and Induces a T Cell-Mediated Tumor Rejection in the Context of a Systemic Th2 Response. Gene Ther (1999) 6(10):1705–12. doi: 10.1038/sj.gt.3301012
48. Zhao S, Wu D, Wu P, Wang Z, Huang J. Serum IL-10 Predicts Worse Outcome in Cancer Patients: A Meta-Analysis. PLos One (2015) 10(10):e0139598. doi: 10.1371/journal.pone.0139598
49. Wang Y, Sun SN, Liu Q, Yu YY, Guo J, Wang K, et al. Autocrine Complement Inhibits IL10-Dependent T-Cell-Mediated Antitumor Immunity to Promote Tumor Progression. Cancer Discov (2016) 6(9):1022–35. doi: 10.1158/2159-8290.CD-15-1412
50. Fujii S, Shimizu K, Shimizu T, Lotze MT. Interleukin-10 Promotes the Maintenance of Antitumor CD8(+) T-Cell Effector Function in Situ. Blood (2001) 98(7):2143–51. doi: 10.1182/blood.V98.7.2143
51. Emmerich J, Mumm JB, Chan IH, LaFace D, Truong H, McClanahan T, et al. IL-10 Directly Activates and Expands Tumor-Resident CD8(+) T Cells Without De Novo Infiltration From Secondary Lymphoid Organs. Cancer Res (2012) 72(14):3570–81. doi: 10.1158/0008-5472.CAN-12-0721
52. Salek-Ardakani S, Arrand JR, Mackett M. Epstein-Barr Virus Encoded Interleukin-10 Inhibits HLA-Class I, ICAM-1, and B7 Expression on Human Monocytes: Implications for Immune Evasion by EBV. Virology (2002) 304(2):342–51. doi: 10.1006/viro.2002.1716
53. Yoon SI, Jones BC, Logsdon NJ, Harris BD, Kuruganti S, Walter MR. Epstein-Barr Virus IL-10 Engages IL-10R1 by a Two-Step Mechanism Leading to Altered Signaling Properties. J Biol Chem (2012) 287(32):26586–95. doi: 10.1074/jbc.M112.376707
54. Slobedman B, Barry PA, Spencer JV, Avdic S, Abendroth A. Virus-Encoded Homologs of Cellular Interleukin-10 and Their Control of Host Immune Function. J Virol (2009) 83(19):9618–29. doi: 10.1128/JVI.01098-09
55. Mocellin S, Marincola F, Rossi CR, Nitti D, Lise M. The Multifaceted Relationship Between IL-10 and Adaptive Immunity: Putting Together the Pieces of a Puzzle. Cytokine Growth Factor Rev (2004) 15(1):61–76. doi: 10.1016/j.cytogfr.2003.11.001
56. Benjamin D, Knobloch TJ, Dayton MA. Human B-Cell Interleukin-10: B-Cell Lines Derived From Patients With Acquired Immunodeficiency Syndrome and Burkitt's Lymphoma Constitutively Secrete Large Quantities of Interleukin-10. Blood (1992) 80(5):1289–98. doi: 10.1182/blood.V80.5.1289.1289
57. Nacinović-Duletić A, Stifter S, Dvornik S, Skunca Z, Jonjić N. Correlation of Serum IL-6, IL-8 and IL-10 Levels With Clinicopathological Features and Prognosis in Patients With Diffuse Large B-Cell Lymphoma. Int J Lab Hematol (2008) 30(3):230–9. doi: 10.1111/j.1751-553X.2007.00951.x
58. Kabelitz D, Serrano R, Kouakanou L, Peters C, Kalyan S. Cancer Immunotherapy With Gammadelta T Cells: Many Paths Ahead of Us. Cell Mol Immunol (2020) 17(9):925–39. doi: 10.1038/s41423-020-0504-x
59. Zheng J, Liu Y, Lau YL, Tu W. Gammadelta-T Cells: An Unpolished Sword in Human Anti-Infection Immunity. Cell Mol Immunol (2013) 10(1):50–7. doi: 10.1038/cmi.2012.43
60. Xu Y, Xiang Z, Alnaggar M, Kouakanou L, Li J, He J, et al. Allogeneic Vgamma9Vdelta2 T-Cell Immunotherapy Exhibits Promising Clinical Safety and Prolongs the Survival of Patients With Late-Stage Lung or Liver Cancer. Cell Mol Immunol (2021) 18(2):427–39. doi: 10.1038/s41423-020-0515-7
61. Kakimi K, Matsushita H, Masuzawa K, Karasaki T, Kobayashi Y, Nagaoka K, et al. Adoptive Transfer of Zoledronate-Expanded Autologous Vγ9vδ2 T-Cells in Patients With Treatment-Refractory Non-Small-Cell Lung Cancer: A Multicenter, Open-Label, Single-Arm, Phase 2 Study. J Immunother Cancer (2020) 8(2):e001185. doi: 10.1136/jitc-2020-001185
62. Sakamoto M, Nakajima J, Murakawa T, Fukami T, Yoshida Y, Murayama T, et al. Adoptive Immunotherapy for Advanced Non-Small Cell Lung Cancer Using Zoledronate-Expanded γδtcells: A Phase I Clinical Study. J Immunother (2011) 34(2):202–11. doi: 10.1097/CJI.0b013e318207ecfb
63. Lafont V, Sanchez F, Laprevotte E, Michaud HA, Gros L, Eliaou JF, et al. Plasticity of γδ T Cells: Impact on the Anti-Tumor Response. Front Immunol (2014) 5:622. doi: 10.3389/fimmu.2014.00622
64. Wilcox RA, Tamada K, Flies DB, Zhu G, Chapoval AI, Blazar BR, et al. Ligation of CD137 Receptor Prevents and Reverses Established Anergy of CD8+ Cytolytic T Lymphocytes In Vivo. Blood (2004) 103(1):177–84. doi: 10.1182/blood-2003-06-2184
65. Long AH, Haso WM, Shern JF, Wanhainen KM, Murgai M, Ingaramo M, et al. 4-1BB Costimulation Ameliorates T Cell Exhaustion Induced by Tonic Signaling of Chimeric Antigen Receptors. Nat Med (2015) 21(6):581–90. doi: 10.1038/nm.3838
66. Ascierto PA, Simeone E, Sznol M, Fu YX, Melero I. Clinical Experiences With Anti-CD137 and Anti-PD1 Therapeutic Antibodies. Semin Oncol (2010) 37(5):508–16. doi: 10.1053/j.seminoncol.2010.09.008
67. Barsoumian HB, Batra L, Shrestha P, Bowen WS, Zhao H, Egilmez NK, et al. A Novel Form of 4-1BBL Prevents Cancer Development via Nonspecific Activation of CD4(+) T and Natural Killer Cells. Cancer Res (2019) 79(4):783–94. doi: 10.1158/0008-5472.CAN-18-2401
68. Srivastava AK, Dinc G, Sharma RK, Yolcu ES, Zhao H, Shirwan H. SA-4-1BBL and Monophosphoryl Lipid A Constitute an Efficacious Combination Adjuvant for Cancer Vaccines. Cancer Res (2014) 74(22):6441–51. doi: 10.1158/0008-5472.CAN-14-1768-A
69. Born WK, Reardon CL, O'Brien RL. The Function of Gammadelta T Cells in Innate Immunity. Curr Opin Immunol (2006) 18(1):31–8. doi: 10.1016/j.coi.2005.11.007
70. Chen Q, Wen K, Lv A, Liu M, Ni K, Xiang Z, et al. Human Vgamma9Vdelta2-T Cells Synergize CD4(+) T Follicular Helper Cells to Produce Influenza Virus-Specific Antibody. Front Immunol (2018) 9:599. doi: 10.3389/fimmu.2018.00599
Keywords: CD137, γδ-T cells, antitumor acitivity, IL-10, immunotherapy
Citation: Pei Y, Xiang Z, Wen K, Tu CR, Wang X, Zhang Y, Mu X, Liu Y and Tu W (2022) CD137 Costimulation Enhances the Antitumor Activity of Vγ9Vδ2-T Cells in IL-10-Mediated Immunosuppressive Tumor Microenvironment. Front. Immunol. 13:872122. doi: 10.3389/fimmu.2022.872122
Received: 09 February 2022; Accepted: 17 May 2022;
Published: 17 June 2022.
Edited by:
Andy Hee-Meng Tan, Bioprocessing Technology Institute (A*STAR), SingaporeReviewed by:
Graham Robert Leggatt, The University of Queensland, AustraliaAlice Cheung, Singapore General Hospital, Singapore
Copyright © 2022 Pei, Xiang, Wen, Tu, Wang, Zhang, Mu, Liu and Tu. This is an open-access article distributed under the terms of the Creative Commons Attribution License (CC BY). The use, distribution or reproduction in other forums is permitted, provided the original author(s) and the copyright owner(s) are credited and that the original publication in this journal is cited, in accordance with accepted academic practice. No use, distribution or reproduction is permitted which does not comply with these terms.
*Correspondence: Wenwei Tu, wwtu@hku.hk