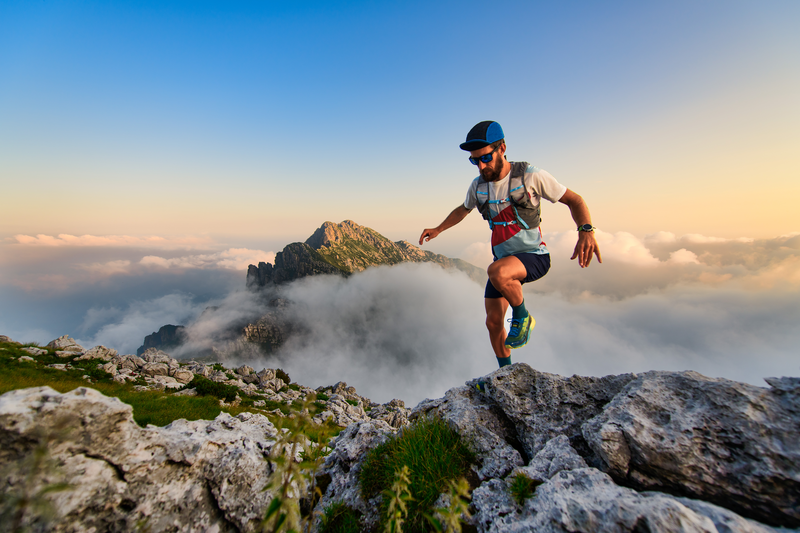
95% of researchers rate our articles as excellent or good
Learn more about the work of our research integrity team to safeguard the quality of each article we publish.
Find out more
REVIEW article
Front. Immunol. , 22 April 2022
Sec. T Cell Biology
Volume 13 - 2022 | https://doi.org/10.3389/fimmu.2022.867181
This article is part of the Research Topic Thymus Function and Aging: A Focus on Thymic Epithelial Cells View all 10 articles
Thymic Epithelial Tumors (TETs) arise from epithelial cells of the thymus and are very rare neoplasms comprising Thymoma, Thymic carcinoma, and Thymic Neuroendocrine tumors that still require in-depth molecular characterization. Long non-coding RNAs (lncRNAs) are emerging as relevant gene expression modulators involved in the deregulation of several networks in almost all types of human cancer, including TETs. LncRNAs act at different control levels in the regulation of gene expression, from transcription to translation, and modulate several pathways relevant to cell fate determination under normal and pathological conditions. The activity of lncRNAs is strongly dependent on their expression, localization, and post-transcriptional modifications. Starting from our recently published studies, this review focuses on the involvement of lncRNAs in the acquisition of malignant traits by neoplastic thymic epithelial cells, and describes the possible use of these molecules as targets for the design of novel therapeutic approaches specific for TET. Furthermore, the involvement of lncRNAs in myasthenia gravis (MG)-related thymoma, which is still under investigation, is discussed.
The thymus is the primary lymphoid organ located at the level of the anterior mediastinum. The thymus plays an essential role in educating and enabling the maturation of prelymphocytes into mature T lymphocytes, which are involved in the adaptive immune response. Thymic epithelial-reticular cells (TECs) play a major role in the maturation of T lymphocytes. However, TECs may undergo neoplastic transformation, resulting in certain types of tumors, such as thymic epithelial tumors (TETs) (1).
TETs are relatively rare neoplasms in middle-aged or elderly adults that represent 0.2-1.5% of all cancers and comprise thymoma, thymic carcinoma (TC), and thymic neuroendocrine tumors (2). TETs are characterized by wide variability and heterogeneity in their malignant behavior. In 30% of cases, TETs are asymptomatic; however, in 40% of cases, local symptoms, such as chest pain, cough, dyspnea, and hoarseness, are displayed while in the remaining 30% of cases, systemic symptoms, with superior vena cava syndrome (SVC), and in the most aggressive forms, weight loss, ensue. To date, the etiology of TETs has not been established and the risk factors remain unclear (3).
TETs classification has remained controversial subject for many years. After different classification approaches [Bernatz et al. in 1961, Rosai and Levine in 1976, Marino and Muller- Hermelink, in 1985 (4)], Rosai and Sobin published a new classification in the World Health Organization (WHO) series in 1999, dividing thymic tumors into three major subgroups based on the morphology of epithelial cells and the percentage of epithelial and lymphocyte populations: type A, type B, and type C (thymic carcinoma). Type A thymomas are tumors with a component of spindle-oval EC but lack lymphocytes, whereas type B thymomas are characterized by large EC with dendritic or plump (epithelioid) morphology, forming networks where lymphocytes are attracted. Notably, the combination of these two morphologies has been designated as type AB (5). Thymic carcinoma (type C) is a rare malignancy, representing less than 1% of thymic tumors, and is characterized by cytological atypia, more aggressive behavior, and local and distant metastases (liver, lymph nodes, or bones) (6).
Recently, a new WHO classification of thoracic cancers was established, which includes new diagnostic criteria and rare entities, such as hyalinizing clear cell carcinoma (7).
Interestingly, thymoma is strongly associated with various paraneoplastic syndromes (PNS), such as myasthenia gravis (MG), red cell aplasia, polymyositis, systemic lupus erythematosus, Cushing syndrome, and syndrome of inappropriate antidiuretic hormone secretion (8).
Thymomas are associated with MG in 30-50% of cases, and thymoma occurs in 10-15% of cases of MG (9). MG (myos = muscle, asthenos = weakness, gravis = severe) is an autoimmune disease that affects the neuromuscular junction (NMJ) of the skeletal muscle, causing muscle weakness of different severity, several complications such as myasthenia crisis, and in some cases, acute respiratory paralysis (10–13). MG can develop at any age, particularly in young women (>30 years) and older men (>60 years) (14).
Thymoma-associated myasthenia gravis (TAMG) is frequently reported in adults and is characterized by alterations in thymus function (14). The association between MG and thymoma is due to the dysregulation of positive and negative selection of T cells in the thymus (15). For example, in cortical thymoma, the lack of medullary epithelial cell function and defects in the autoimmune regulator complex (AIRE), which is responsible for negative selection, leads to the production of autoreactive T cells specific for acetylcholine receptors (AChR) that are exported to the periphery, where autoreactive T cells stimulate and activate B cells to produce antibodies against AChR (14, 16). Although the required treatment for thymoma-associated MG is tumor removal, remission is not inevitable. Moreover, removal of thymoma in non-myasthenic patients does not prevent the subsequent onset of MG. Patients with thymoma have been observed to develop antibodies against AChR and symptoms of myasthenia after the resection of the tumor (17).
Although the etiology of TETs is still poorly understood, advanced next-generation sequencing (NGS) techniques have recently allowed the mapping of gene mutations and epigenetic alterations occurring in thymic tumors.
One of the most frequently mutated genes in TETs is GTF2I, whose mutation is specifically associated with types A and AB (78%), but is less frequent in more aggressive histological types, such as thymic carcinomas (8%) (18). The overexpression of EGFR and HER2 and mutations in KIT, IGR-1, and neurotrophin receptors have been recently demonstrated in some cases, as reviewed by Scorsetti et al. (6). Gain-of-function mutations in HRAS and NRAS and loss-of-function mutations in TP53 are less common, but are considered founder mutations (19).
Thymic carcinoma is characterized by loss of chromosome 16q, mutations in epigenetic regulatory genes (BAP1, ASXL1, SETD2, SMARCA4, TET2, DNMT3A, and WT1), and anti-apoptotic genes (BCL2 copy number gains) (8, 20).
Notably, gene expression profiling in TGCA study revealed four molecular subtypes, represented respectively by type B (subtype 1), TC (subtype 2), AB (subtype 3), and a mix of types A and AB (subtype 4). TGCA study also revealed four distinct molecular clusters using PARADIGM analysis. In particular, the upregulation of TP53 and downregulation of oncogenes, such as MYC/Max, MYB, and FOXM, characterize the A-like cluster, while the downregulation of TP53 and upregulation of MYC/Max, MYB, FOXM1, and E2F1 in AB-, B-, and C-like clusters are consistent with the high aggressiveness of B3 and TC tumors. Furthermore, this study highlighted that types A, AB, B, and TC are not a continuum of diseases, but are instead distinct biological entities (5, 19), Recently, metaplastic thymomas were reported to harbor the YAP1-MAML2 translocation, whereas 6% of pretreated types B2 and B3 and a combined TC and B3 thymoma (but not in thymoma and “pure” TCs) may be associated with KMT2A-MAML2 translocation (7).
Alterations in inflammatory and thymus function, which occur in thymic neoplasms, and mutations in the AIRE gene locus promote the development of autoimmune diseases, such as MG (16). EOMG is associated with HLA-DR3, HLA-B8, and other autoimmune risk genes, whereas LOMG is weakly associated with HLA-DR2, HLA-B7, and mLA-DR-B1*15:0 (14). Aneuploidy and intratumoral overexpression of genes that have a similar sequence to autoimmune targets (CHRNA1, RYR3, and NEFM) are common in patients (19).
In addition to mutations in protein-coding genes, alterations in ncRNA molecules have been reported to significantly impact the initiation, progression, and response of TETs and MG to therapy, as described in the next section.
Despite being considered “junk” for a long time, ncRNAs have emerged as functionally relevant in nearly all physiological and pathological cellular processes (21, 22). These new discoveries have been aided by powerful high-throughput approaches, such as next-generation sequencing (NGS), transcriptome studies, molecular network analyses, and artificial intelligence-guided prediction of ncRNA function (23).
ncRNAs play a role in many biological, physiological, and developmental processes, including several diseases and tumors. ncRNAs are produced by transcription from different genomic regions and post-transcriptional maturation and modification. ncRNAs can be divided into two classes according to their length (24): small non-coding RNAs (ncRNAs) and lncRNAs (usually > 200 nt).
In recent years, ncRNAs have been found to play an important role in gene regulation at different levels. Several studies have demonstrated the involvement of ncRNAs in transcriptional regulation, RNA maturation, chromatin remodeling, post-transcriptional RNA regulation, and modification (25). Dysregulation of ncRNAs is involved in many human diseases and in tumor initiation and progression (26). Regulatory ncRNAs can be divided into two classes: circular and linear.
Circular RNAs (circRNAs) are a class of covalently closed RNA molecules and are thus more stable than linear RNAs. The expression patterns of circRNAs are cell type-, tissue-, and developmental stage-specific (27, 28). Depending on their localization, circRNAs can exert different functions, including acting as microRNA (miRNA) sponges, modulating the activity of RNA-binding proteins (RBPs), or acting as protein scaffolds, which can be translated into polypeptides owing to the presence of internal ribosome entry sites (IRES) or m6A modifications (27, 29). Many circRNAs are differentially expressed in several cancer types compared with their untransformed counterparts and are related to tumor growth, metastasis, and therapy resistance (30, 31). Interestingly, several studies have demonstrated that circRNAs are differentially expressed in thymoma and MG (32, 33), and have highlighted their important role as biomarkers for the diagnosis of this disease (34).
Another major group of regulatory ncRNAs is represented by linear ncRNAs, comprising small ncRNA (18-200 nt) and lncRNAs (>200 nt) (35).
Among small ncRNAs, miRNAs play a predominant role in post-transcriptional regulation, binding to specific mRNA targets and causing their degradation or translation inhibition. miRNAs are single-stranded RNAs with an average length of 22 nucleotides that are derived from hairpin-structured precursors (36). Several miRNAs are altered in different human diseases, including cancer. The roles of miRNAs in thymus differentiation, development, and involution have been extensively described by Cron et al. (37). Moreover, their relevance in the treatment, diagnosis, and prognosis of TETs and MG is emerging (38).
Several research groups have identified miRNAs that are differentially expressed between thymic tumors and normal samples, as well as between thymic carcinoma and thymoma and histotype classes (39–41). In particular, Bellissimo et al. found that miR-145-5p was epigenetically downregulated in thymic carcinoma cells (42), confirming its well-known tumor suppressor role (43). Similar to circRNAs, miRNAs are excellent biomarkers for cancer diagnosis found in the serum of patients with TETs and MG (44–49).
Although miRNAs represent one of the most studied biomarkers involved in thymic tumorigenesis among all ncRNA classes, the study of lncRNAs is becoming more relevant. Based on their localization, lncRNAs can bind to genes, transcripts, miRNAs, and proteins that regulate different cellular processes, such as gene expression, transcription, and post-transcriptional regulation, through different mechanisms of action. Further, lncRNAs can act as guides of chromatin modification complexes, including DNA methyltransferase and histone-modifying enzymes, on specific genomic loci that induce activation or inhibition of target genes in cis or trans (50); function as decoys for transcription factors and other effectors, impairing their regulatory activity (50); serve as modular scaffolds, and bind and drive two or more physically distant proteins into specific genomic regions to regulate gene expression (51); act as sponges of miRNAs, sequestering them and preventing their ability to promote degradation or repression of target genes (52, 53); and influence splicing (54) and the stability of mRNAs, regulating their post-transcriptional expression (55). Therefore, according to their mechanisms of action, lncRNAs are involved in the regulation of various biological processes, including cellular survival, proliferation, differentiation, apoptosis, invasion, and metastasis. Consequently, aberrant lncRNA expression is tightly correlated with cancer development (56).
Similar to many types of human tumors, such as breast cancer (57), lung cancer (58), colorectal carcinoma (59), ovarian cancer (60) and prostate cancer (61), dysregulated lncRNAs in TETs may contribute to tumor onset and progression. Different studies have identified a large spectrum of lncRNAs in thymic epithelial tumors using high-throughput sequencing technologies. In this context, most of the identified lncRNAs act as miRNA sponges, playing oncogenic or tumor suppressor roles. An example of this type of regulation is represented by lncRNA LOXL1-AS1, miR‐525-5p, and the HSPA9 gene network. Data from TCGA study showed that high expression of LOXL1-AS1 and downregulation of miR-525-5p correlated with poor prognosis in TET (62). Similar to other cancer types (63, 64) in thymic tumors, miR-525-5p acts as a tumor suppressor, inhibiting cell growth and invasion, and inducing apoptosis by repressing the target gene, HSPA9. HSPA9 is upregulated in thymoma and thymic carcinoma and correlated with poor patient survival. The positive association between LOXL1-AS1 and HSPA9, which is consistent with the downregulation of miR-525-5p, was confirmed by in vitro experiments. The silencing of LOXL1-AS1 promotes thymic tumor progression by acting as a sponge of miR-525-5p and increasing the expression of HSPA9 (62). Similar to LOXL1-AS1, another network is represented by the interaction between lncRNA LINC00174, miR-145-5p, and miR-145-5p predicted target genes involved in thymic tumorigenesis (65). In this study, the upregulation of lncRNA LINC00174 in frozen tissue samples of thymoma compared to its normal counterparts was identified. LINC00174 is negatively associated with miR-145-5p, a well-known tumor suppressor of miRNA downregulation in TETs (39, 42), and positively correlated with miR-145-5p predicted targets (Figure 1). The inhibition or overexpression of miR-145-5p modulates LINC00174 expression and its associated genes (65). Notably, the poor prognosis of TET patients, characterized by high expression of LINC00174 and its associated genes and low expression of miR-145-5p, suggests an oncogenic role of LINC00174 in TETs. According to these data, LINC00174 silencing impairs cell growth and proliferation, cell migration, and lipid metabolism. Similar to LINC00174, MALAT1 can act as a sponge for miR-145-5p in TET. MALAT1 is a well-known oncogenic lncRNA that regulates different biological processes, such as cell proliferation, apoptosis, angiogenesis, invasion, and metastasis, and contributes to cancer development (66–68). Using a luciferase assay, the interaction between miR-145-5p and MALAT1 was demonstrated in the thymic cancer cell line, IU-TAB1. In this thymic tumor context, the downregulation of MALAT1 increased miR-145-5p expression and led to a reduction in cell proliferation and an increase in the apoptosis rate compared to that observed in the control. Additionally, the combination of MALAT1 silencing and miR-145-5p overexpression induces a synergistic effect, suggesting that MALAT1 may regulate the thymic cancer phenotype by inhibiting miR-145-5p (69). Recently, the expression of MALAT1 has been a focus in our studies where the relationship between lncRNA MALAT1 and METTL3, a methyltransferase enzyme that catalyzes the N6-methyladenosine (m6A) modification, were described in the thymic carcinoma cell line, TC1889. Of note, the expression of lncRNAs can be regulated by m6A modifications (70), and the downregulation of METTL3 leads to increased localization of MALAT1 in nuclear speckles and decreased m6A modification of MALAT1 lncRNA (71), which probably impinges on its functional activity. In the past, we observed a similar delocalization of MALAT1 in nuclear speckles with consequent altered splicing (72) due to the presence of mutant p53 protein in breast cancer cells. Another interesting lncRNA-miRNA-target network in the control of thymoma progression was reported by Yang et al. (73). The lncRNA RP11-424C20.2 regulates the expression of the UHRF1 gene (ubiquitin-like containing PHD ring finger 1) by sponging miR-378a-3p; the RP11-424C20.2/UHRF1 axis is strongly associated with a better outcome in thymoma patients, which is related to the different types of infiltrating immune cells, such as B cells, macrophages, CD8+ and CD4+ cells, neutrophils, and dendritic cells. The role of UHRF1 is well established; it is an epigenetic modifier that regulates immune infiltration and the tumor immune microenvironment through its interaction with DNA histone deacetylase genes (73, 74). Therefore, RP11-424C20.2 expression can influence the prognosis of patients with thymoma by regulating the expression of UHRF1 via miR-378a-3p sponging (73).
Figure 1 Contribution of lncRNAs in TETs and MG. LINC00174 and LOXL1-AS1 are up-regulated in thymoma and thymic carcinoma, where they act as sponges of miR-145-5p and miR-525-5p, respectively, promoting cell growth, metastasis, migration, and lipid metabolism and inhibiting cell death. LncRNAs are deregulated in MG, an autoimmune disease strongly associated with thymoma. Oebiotech_1193 and XLOC_003810 are overexpressed in MG patients and are involved in inflammatory pathways.
Owing to this recent evidence, the identification of altered lncRNAs in TETs and the characterization of their role in the promotion of tumorigenesis could provide new potential therapeutic targets relevant for the treatment of TETs.
Studies on the profiles of lncRNAs expressed in thymoma tissue samples have revealed that altered expression of specific lncRNAs may correlate with overall or disease-free survival. For example, Su et al. (75) identified a panel of lncRNAs that predicts the recurrence of thymic epithelial tumors. They analyzed a cohort of 114 TET patients from TCGA study and identified four lncRNAs, ADAMTS9-AS1, HSD52, LINC00968, and LINC01697, which are significantly related to recurrence-free survival (RFS). These lncRNAs can be used to divide TET patients into high-risk and low-risk groups, respectively, with shorter and longer RFS. Based on ROC analysis, these lncRNAs represent a better prognostic model for the RFS of patients than the WHO classification and Masaoka stage. Although these lncRNAs constitute a good factor of discrimination between different TETs subtypes and their associated stages, the trial had some limitations: few samples from TCGA, absence of evidence of their predictive power in other types of cancer, and biological characterization of their role in TETs (75). Furthermore, the altered expression of ADAMTS9-AS1 (one of the four RFS-related lncRNAs) with five other lncRNAs, namely AFAP1-AS1, LINC00324, VLDLR-AS1, LINC00968, and NEAT1, was detected in another study by RNA-seq and profiling expression analysis in 25 thymoma patients and 25 healthy individuals (76). These lncRNAs are involved in the development of different types of cancers and regulate several biological processes and molecular pathways. For example, ADAMTS9-AS1 induces cell migration and proliferation in colorectal carcinoma, affecting β-catenin expression (77); LINC00968 reduces drug resistance and invasion of tumor cells in breast cancer (78); AFAP1-AS1 increases epithelial-mesenchymal transition by impairing RhoC, ROCK1, p38MAPK, and Twist1 signaling pathways in osteosarcoma (79); LINC00324 inhibits the NOTCH pathway, regulating apoptosis and cell proliferation in papillary thyroid cancer (80); VLDLR- AS1 modulates the expression of genes involved in fat loss in cancer cachexia, acting as a sponge of hsa-miR-1224-3p (81) and finally, NEAT1, by sponging miR-193b-3p, activates cyclin D, promoting cell proliferation in cervical cancer (82). Notably, the differential expression of these lncRNAs in TETs affects the disease-free survival of patients. In particular, the high expression of ADAMTS9-AS1 and low expression of LINC00324 are correlated with the worst prognosis of patients. Similar to other types of cancer, the expression of these lncRNAs was found to be correlated with the deregulation of miRNA clusters and target genes involved in the regulation of tumorigenic signaling pathways, including PI3K/Akt, FoxO, HIF-1, and Notch, supporting their oncogenic role in the tumorigenesis process (76). Moreover, Gong et al. found that AFAP1-AS1, LINC00324, and VLDLR-AS1 were associated with the RFS of patients with TETs (83).
According to these data, bioinformatic analysis performed for different types of TETs (A, B, AB, and TC) revealed that different competitive endogenous RNA (ceRNA) networks were significantly associated with the overall survival of individuals. The two most important lncRNAs in this ceRNA network were LINC00665 and NR2F1-AS1. The association between their expression and patient prognosis aligns with their biological function (84). LINC00665 binds to mRNAs MYO10 and WASF3 through the miRNAs, hsa-miR-140 and hsa-miR-3199. LINC00665 is upregulated in lung cancer, regulates cellular proliferation and invasive ability in lung adenocarcinoma, and is a predictive factor of this tumor (85). NR2F1-AS1 can indirectly interact with FBN1, GALNT16, HAND2, and MCAM through miR-140, miR-139, and miR-141. NR2F1-AS1 leads to the impairment of osteosarcoma, acting as a sponge of miR-483-3p and increasing FOXA1 gene expression (86).
Based on these recent studies, profiling analysis of lncRNA expression can be used as a potential and innovative strategy for the detection and follow-up of thymic epithelial tumors (Table 1).
The alteration of lncRNA expression could play a prominent role in distinguishing thymomatous and non-thymomatous MG and clarifying the molecular mechanisms underlying its pathogenesis. In this context, by using lncRNA and mRNAs microarray analyses, Luo et al. (87) identified an aberrant expression of different lncRNAs between MG patients with thymoma and healthy controls, and MG patients without thymoma and normal individuals. In the first case, lncRNAs upregulated in MG patients with thymoma were associated with different regulatory pathways that contribute to thymic cancer progression and immune cell proliferation, such as cell response to interferon-γ, positive regulation of cytokine production, chemokine receptor binding, and regulation of smooth muscle cell proliferation. In particular, the most upregulated lncRNA in MG patients with thymoma is Oebiotech_11933, an lncRNA related to the MAPK, chemokine, and Toll-like receptor signaling pathways (87–89). In the second case, although altered lncRNAs in MG patients without thymoma revealed their association with the same cellular pathways in MG patients with thymoma (i.e., positive regulation of cytokine production and chemokine receptor binding), they showed a lower association with cell response to interferon-γ. These data highlight that the discrimination between MG patients with or without thymoma may depend on the presence of altered lncRNAs involved in the regulation of IFN-γ expression (90). Additionally, these lncRNAs have been observed to function by regulating the transcription of genes in cis or trans (87). Consistent with this study, Ke et al. found another lncRNA, XLOC_003810, which is highly expressed in MG-associated thymoma patients, and revealed an increase in activated CD4+ T cells compared to that in control samples. In vitro experiments using thymic mononuclear cells demonstrated that the overexpression of XLOC_003810 leads to an increase in CD4+ T cells and production of the inflammatory cytokines IFN-γ, TNF-α, and IL-1β. In contrast, the downregulation of XLOC_003810 caused the opposite results. Consequently, as the activation of CD4+ T cells and inflammatory cytokines plays an important role in the development of thymoma-associated MG, XLOC_003810 lncRNA could contribute to the pathogenesis of these cellular pathways (91). The study by Niu et al. supports the role of XLOC_003810 in MG with thymoma. XLOC_003810 affects the balance between T helper 17 (Th17) and T regulatory cells (Tregs) (92). T helper cells are active in the adaptive immune response against antigens and pathogens, and Tregs have suppressive potential, preventing autoimmune diseases (93). The overexpression of XLOC_003810 leads to higher levels of Th17 cells than Tregs, which, on the contrary, increases upon silencing of this lncRNA. This association is also evident in MG-T patients and is characterized by an increase in CD4+ T cells and Th17 cells and a decrease in Treg cells (92). As the number of Tregs increases in MG-T patients upon immunosuppressive treatment (94) and the number of Th17 cells correlates with the severity of the disease (95), the alteration of XLOC_003810 expression could enhance the imbalance in the Th17/Treg ratio, favoring the pathogenetic mechanism. Moreover, the discrimination between patients with MG with or without thymoma is also determined by the different hypomethylation and hypermethylation levels associated with the aberrant expression of lncRNAs. The presence of DNA methylation sites has been observed in three immune-related lncRNAs, namely AC004943.1, FOXG1-AS1, and WT1-AS, in (MG-T) patients. DNA methylation is an epigenetic modification catalyzed by DNA methyltransferase enzymes that promote the silencing of gene expression. In this context, tissue samples of thymoma patients with MG are characterized by lower methylation levels of these lncRNAs than those without MG. Consequently, MG-T patients showed a higher expression of these immune-related lncRNAs that correlate with their involvement in pathogenesis, regulating different biological processes, such as transmission at the neuromuscular junctions, cell cycle, actin and Ras GTPase binding, and herpes simplex virus 1 infection associated with MG development (96). Although the lncRNA, MALAT1, is a known oncogene that promotes thymic cancer development, as described previously, it plays a protective role in MG (Figure 2). Compared to healthy individuals, lower expression of MALAT1 has been observed in MG patients, together with higher expression of miR-338-3p, an oncogenic miRNA that directly targets MSL2, a gene involved in chromatin organization and DNA damage response. A previous study revealed the interaction between MALAT1, miR-338-3p, and MSL2 by luciferase assay, demonstrating that the silencing of MALAT1 leads to an increase in miR-338-3p expression, reducing MSL2 protein levels (97). The downregulation of MALAT1 in MG patients suggests its involvement in the inhibition of T lymphocytes, suggesting that it could be a specific target for MG treatment. Finally, different lncRNAs are involved in the regulation of hydrolase, phosphorylase, and dephosphorylase enzyme activities, which affect the activation of T cells during selection in the thymus, promoting MG development (98).
Figure 2 Different roles of lncRNA MALAT1 in TETs and MG. MALAT1 has an oncogenic role in TETs. It promotes cell proliferation by acting as a sponge of miR-145-5p. MALAT1 is m6A-modified due to METTL3 overexpression in TET and induces c-MYC protein, further contributing to proliferation. In MG, MALAT1 plays a protective role by binding mir-338-3p and avoiding T lymphocyte activation.
Based on the evidence, a large spectrum of lncRNAs can regulate different signaling pathways that contribute to the development of associated MG-thymoma. As a result, they could be used as biomarkers to distinguish between two types of MG, namely MG with or without thymoma. Moreover, the data suggest the possible use of these pathogenesis-related molecules as therapeutic targets (Table 2).
Recent advances in next-generation sequencing technologies have enabled the study of the role of ncRNAs in the development and progression of cancer. Particularly, the aberrant expression of the most studied groups of ncRNAs, such as miRNAs, circRNAs, and lncRNAs, is associated with tumorigenesis, highlighting the role of ncRNAs as oncogenes or tumor suppressors. In this review, we sought to provide an overview of lncRNA regulation in the initiation and progression of TET and MG. Many lncRNAs identified in these diseases play an oncogenic role, acting as sponges of tumor suppressor miRNAs and consequently regulating many cellular pathways that contribute to the cancer phenotype. The identification of aberrant expression of lncRNAs and studies on their inhibition or overexpression allow us to understand their contribution to the thymic cancer phenotype and suggest specific targeted therapies. Various lncRNAs differentially expressed in tumor vs. normal tissues in patients with TET are potential powerful biomarkers for the detection and follow-up of diseases.
The role of the lncRNA, MALAT1, which has several opposing functions, is particularly intriguing. In thymoma and thymic carcinoma, MALAT1 regulates cell proliferation by acting as an miR-145-5p sponge and contributing to c-MYC induction, following its change in subnuclear localization due to METTL3 methylation. In contrast, MALAT1 has a protective role in MG, acting as an miRNA sponge and inhibiting T lymphocyte activation. Although several lncRNAs have been identified to date, the function and expression of many lncRNAs in TETs and MG pathogenesis and progression remain unclear. Therefore, further studies on these ncRNAs are necessary. Moreover, the development of novel lncRNA-directed therapeutic strategies could represent a promising and powerful approach for the management of TET and MG.
AI and CT designed and wrote the manuscript. SM, GF, MM, and FF reviewed and edited the manuscript. FG, AS, EG, AF, EM, VP, FV, and GB conceptualized the study and provided feedback regarding the content of the manuscript. All authors approved the final version of the manuscript.
AIRC IG 2018 - ID. 21406 project, ‘Progetti Ateneo’ Sapienza University of Rome and PRIN 2017-Prot. 2017TATYMP_003 to FF.; AIRC IG 2018 - ID. 21434 Project to GF; and ‘Progetti Ateneo,’ Sapienza University of Rome to VP.
The authors declare that the research was conducted in the absence of any commercial or financial relationships that could be construed as a potential conflict of interest.
All claims expressed in this article are solely those of the authors and do not necessarily represent those of their affiliated organizations, or those of the publisher, the editors and the reviewers. Any product that may be evaluated in this article, or claim that may be made by its manufacturer, is not guaranteed or endorsed by the publisher.
The authors thank the Biobank of the IRCCS Regina Elena National Cancer Institute (BBIRE), Rome, Italy, for providing study samples and for preserving the data.
1. Thapa P, Farber DL. The Role of the Thymus in the Immune Response. Thorac Surg Clin (2019) 29(2):123. doi: 10.1016/j.thorsurg.2018.12.001
2. Benjamin DJ, Klapheke A, Lara PN, Cress RD, Riess JW. A Population-Based Study of Incidence and Survival of 1588 Thymic Malignancies: Results From the California Cancer Registry. Clin Lung Cancer (2019) 20(6):477–83. doi: 10.1016/j.cllc.2019.06.005
3. Venuta F, Rendina EA, Anile M, de Giacomo T, Vitolo D, Coloni GF. Thymoma and Thymic Carcinoma. Gen Thorac Cardiovasc Surg (2012) 60(1):1–12. doi: 10.1007/s11748-011-0814-0
4. Kashima J, Okuma Y. New Histological Classification and Staging of Thymic Malignancies: ITMIG Consensus Statements and the 8th TNM Staging System. J Thorac Dis (2017) 9(10):3656. doi: 10.21037/jtd.2017.09.126
5. Marino M, Marx A, Anemona L, Lauriola L, Ströbel P, Müller-Hermelink HK. Juan Rosai as Master of Our Comprehensive Understanding of Thymus and Thymoma. Pathologica (2021) 113(5):360–70. doi: 10.32074/1591-951X-539
6. Scorsetti M, Leo F, Trama A, D’Angelillo R, Serpico D, Macerelli M, et al. Thymoma and Thymic Carcinomas. Crit Rev Oncol Hematol (2016) 99:332–50. doi: 10.1016/j.critrevonc.2016.01.012
7. Marx A, Chan JKC, Chalabreysse L, Dacic S, Detterbeck F, French CA, et al. The 2021 WHO Classification of Tumors of the Thymus and Mediastinum: What Is New in Thymic Epithelial, Germ Cell, and Mesenchymal Tumors? J Thorac Oncol (2022) 17(2):200–13. doi: 10.1016/j.jtho.2021.10.010
8. Conforti F, Pala L, Giaccone G, De Pas T. Thymic Epithelial Tumors: From Biology to Treatment. Cancer Treat Rev (2020) 86:102014. doi: 10.1016/j.ctrv.2020.102014
9. Zhang Z, Cui Y, Jia R, Xue L, Liang H. Myasthenia Gravis in Patients With Thymoma Affects Survival Rate Following Extended Thymectomy. Oncol Lett (2016) 11(6):4177–82. doi: 10.3892/ol.2016.4528
10. Beloor Suresh A, Asuncion RMD. Myasthenia Gravis. StatPearls. StatPearls Publishing, Treasure Island (FL) (2022).
11. Koneczny I, Herbst R. Myasthenia Gravis: Pathogenic Effects of Autoantibodies on Neuromuscular Architecture. Cells (2019) 8(7):671. doi: 10.3390/cells8070671
12. Wang S, Breskovska I, Gandhy S, Punga AR, Guptill JT, Kaminski HJ. Advances in Autoimmune Myasthenia Gravis Management. Expert Rev Neurother (2018) 18(7):573–88. doi: 10.1080/14737175.2018.1491310
13. Lazaridis K, Tzartos SJ. Autoantibody Specificities in Myasthenia Gravis; Implications for Improved Diagnostics and Therapeutics. Front Immunol (2020) 11:212. doi: 10.3389/fimmu.2020.00212
14. Gilhus NE, Verschuuren JJ. Myasthenia Gravis: Subgroup Classification and Therapeutic Strategies. Lancet Neurol (2015) 14(10):1023–36. doi: 10.1016/S1474-4422(15)00145-3
15. Romi F, Hong Y, Gilhus NE. Pathophysiology and Immunological Profile of Myasthenia Gravis and its Subgroups. Curr Opin Immunol (2017) 49:9–13. doi: 10.1016/j.coi.2017.07.006
16. Marx A, Yamada Y, Simon-Keller K, Schalke B, Willcox N, Ströbel P, et al. Thymus and Autoimmunity. Semin Immunopathol (2021) 43(1):45–64. doi: 10.1007/s00281-021-00842-3
17. Fujii Y. The Thymus, Thymoma and Myasthenia Gravis. Surg Today (2013) 43(5):461–6. doi: 10.1007/s00595-012-0318-2
18. Petrini I, Meltzer PS, Kim I-K, Lucchi M, Park K-S, Fontanini G, et al. A Specific Missense Mutation in GTF2I Occurs at High Frequency in Thymic Epithelial Tumors. Nat Genet (2014) 46(8):844–9. doi: 10.1038/ng.3016
19. Radovich M, Pickering CR, Felau I, Ha G, Zhang H, Jo H, et al. The Integrated Genomic Landscape of Thymic Epithelial Tumors. Cancer Cell (2018) 33(2):244–258.e10. doi: 10.1016/j.ccell.2018.01.003
20. Wang Y, Thomas A, Lau C, Rajan A, Zhu Y, Killian JK, et al. Mutations of Epigenetic Regulatory Genes Are Common in Thymic Carcinomas. Sci Rep (2015) 4(1):7336. doi: 10.1038/srep07336
21. Anastasiadou E, Jacob LS, Slack FJ. Non-Coding RNA Networks in Cancer. Nat Rev Cancer (2017) 18(1):5–18. doi: 10.1038/nrc.2017.99
22. Palazzo AF, Lee ES. Non-Coding RNA: What Is Functional and What Is Junk? Front Genet (2015) 6:2. doi: 10.3389/fgene.2015.00002
23. Wolfien M, Brauer DL, Bagnacani A, Wolkenhauer O. Workflow Development for the Functional Characterization of ncRNAs. In: Methods in Molecular Biology. Clifton, NJ: Methods Mol Biol (2019). p. 111–32.
24. Zhang P, Wu W, Chen Q, Chen M. Non-Coding RNAs and Their Integrated Networks. J Integr Bioinform (2019) 16(3). doi: 10.1515/jib-2019-0027
25. Grillone K, Riillo C, Scionti F, Rocca R, Tradigo G, Guzzi PH, et al. Non-Coding RNAs in Cancer: Platforms and Strategies for Investigating the Genomic “Dark Matter”. J Exp Clin Cancer Res (2020) 39(1):117. doi: 10.1186/s13046-020-01622-x
26. López-Jiménez E, Andrés-León E. The Implications of ncRNAs in the Development of Human Diseases. Non-Coding RNA (2021) 7(1):17. doi: 10.3390/ncrna7010017
27. Kristensen LS, Andersen MS, Stagsted LVW, Ebbesen KK, Hansen TB, Kjems J. The Biogenesis, Biology and Characterization of Circular RNAs. Nat Rev Genet (2019) 20(11):675–91. doi: 10.1038/s41576-019-0158-7
28. Qu S, Liu Z, Yang X, Zhou J, Yu H, Zhang R, et al. The Emerging Functions and Roles of Circular RNAs in Cancer. Cancer Lett (2018) 414:301–9. doi: 10.1016/j.canlet.2017.11.022
29. Shi Y, Jia X, Xu J. The New Function of circRNA: Translation. Clin Transl Oncol (2020) 22(12):2162–9. doi: 10.1007/s12094-020-02371-1
30. Patop IL, Kadener S. circRNAs in Cancer. Curr Opin Genet Dev (2018) 48:121–7. doi: 10.1016/j.gde.2017.11.007
31. Guarnerio J, Bezzi M, Jeong JC, Paffenholz SV, Berry K, Naldini MM, et al. Oncogenic Role of Fusion-circRNAs Derived From Cancer-Associated Chromosomal Translocations. Cell (2016) 165(2):289–302. doi: 10.1016/j.cell.2016.03.020
32. Wu Q, Luo X, Li H, Zhang L, Su F, Hou S, et al. Identification of Differentially Expressed Circular RNAs Associated With Thymoma. Thorac cancer (2021) 12(9):1312–9. doi: 10.1111/1759-7714.13873
33. Lai X, Bi Z, Yang X, Hu R, Wang L, Jin M, et al. Upregulation of Circ-FBL Promotes Myogenic Proliferation in Myasthenia Gravis by Regulation of miR-133/Pax7. Cell Biol Int (2021) 45(11):2287–93. doi: 10.1002/cbin.11676
34. Lv J, Ren L, Han S, Zhang J, Zhao X, Zhang Y, et al. Peripheral Blood Hsa-Circrna5333-4: A Novel Biomarker for Myasthenia Gravis. Clin Immunol (2021) 224:108676. doi: 10.1016/j.clim.2021.108676
35. Romano G, Veneziano D, Acunzo M, Croce CM. Small Non-Coding RNA and Cancer. Carcinogenesis (2017) 38(5):485. doi: 10.1093/carcin/bgx026
36. Lu TX, Rothenberg ME. MicroRNA. J Allergy Clin Immunol (2018) 141(4):1202. doi: 10.1016/j.jaci.2017.08.034
37. Cron MA, Guillochon É, Kusner L, Le Panse R. Role of miRNAs in Normal and Myasthenia Gravis Thymus. Front Immunol (2020) 11:1074. doi: 10.3389/fimmu.2020.01074
38. Blandino G, Fazi F, Donzelli S, Kedmi M, Sas-Chen A, Muti P, et al. Tumor Suppressor microRNAs: A Novel Non-Coding Alliance Against Cancer. FEBS Lett (2014) 588(16):2639–52. doi: 10.1016/j.febslet.2014.03.033
39. Ganci F, Vico C, Korita E, Sacconi A, Gallo E, Mori F, et al. MicroRNA Expression Profiling of Thymic Epithelial Tumors. Lung Cancer (2014) 85(2):197–204. doi: 10.1016/j.lungcan.2014.04.008
40. Enkner F, Pichlhöfer B, Zaharie AT, Krunic M, Holper TM, Janik S, et al. Molecular Profiling of Thymoma and Thymic Carcinoma: Genetic Differences and Potential Novel Therapeutic Targets. Pathol Oncol Res (2017) 23(3):551. doi: 10.1007/s12253-016-0144-8
41. Radovich M, Solzak JP, Hancock BA, Conces ML, Atale R, Porter RF, et al. A Large microRNA Cluster on Chromosome 19 Is a Transcriptional Hallmark of WHO Type A and AB Thymomas. Br J Cancer (2016) 114(4):477. doi: 10.1038/bjc.2015.425
42. Bellissimo T, Ganci F, Gallo E, Sacconi A, Tito C, De Angelis L, et al. Thymic Epithelial Tumors Phenotype Relies on miR-145-5p Epigenetic Regulation. Mol Cancer (2017) 16(1):88. doi: 10.1186/s12943-017-0655-2
43. Ye D, Shen Z, Zhou S. Function of microRNA-145 and Mechanisms Underlying its Role in Malignant Tumor Diagnosis and Treatment. Cancer Manag Res (2019) 11:969–79. doi: 10.2147/CMAR.S191696
44. Sohel MMH. Circulating microRNAs as Biomarkers in Cancer Diagnosis. Life Sci (2020) 248:117473. doi: 10.1016/j.lfs.2020.117473
45. Nogales-Gadea G, Ramos-Fransi A, Suarez-Calvet X, Navas M, Rojas-García R, Mosquera JL, et al. Analysis of Serum miRNA Profiles of Myasthenia Gravis Patients. PloS One (2014) 9(3):e91927. doi: 10.1371/journal.pone.0091927
46. Bellissimo T, Russo E, Ganci F, Vico C, Sacconi A, Longo F, et al. Circulating miR-21-5p and miR-148a-3p as Emerging Non-Invasive Biomarkers in Thymic Epithelial Tumors. Cancer Biol Ther (2016) 17(1):79. doi: 10.1080/15384047.2015.1108493
47. Lu J, Yan M, Wang Y, Zhang J, Yang H, Tian F, et al. Altered Expression of miR-146a in Myasthenia Gravis. Neurosci Lett (2013) 555:85–90. doi: 10.1016/j.neulet.2013.09.014
48. Bortone F, Scandiffio L, Marcuzzo S, Bonanno S, Frangiamore R, Motta T, et al. miR-146a in Myasthenia Gravis Thymus Bridges Innate Immunity With Autoimmunity and Is Linked to Therapeutic Effects of Corticosteroids. Front Immunol (2020) 11. doi: 10.3389/fimmu.2020.00142
49. Punga T, Panse R, Andersson M, Truffault F, Berrih-Aknin S, Punga AR. Circulating miRNAs in Myasthenia Gravis: miR-150-5p as a New Potential Biomarker. Ann Clin Transl Neurol (2014) 1(1):49–58. doi: 10.1002/acn3.24
50. Gao N, Li Y, Li J, Gao Z, Yang Z, Li Y, et al. Long Non-Coding RNAs: The Regulatory Mechanisms, Research Strategies, and Future Directions in Cancers. Front Oncol (2020) 10. doi: 10.3389/fonc.2020.598817
51. Sun T-T, He J, Liang Q, Ren L-L, Yan T-T, Yu T-C, et al. LncRNA GClnc1 Promotes Gastric Carcinogenesis and May Act as a Modular Scaffold of WDR5 and KAT2A Complexes to Specify the Histone Modification Pattern. Cancer Discovery (2016) 6(7):784–801. doi: 10.1158/2159-8290.CD-15-0921
52. Yu F, Zheng J, Mao Y, Dong P, Lu Z, Li G, et al. Long Non-Coding RNA Growth Arrest-Specific Transcript 5 (GAS5) Inhibits Liver Fibrogenesis Through a Mechanism of Competing Endogenous RNA. J Biol Chem (2015) 290(47):28286–98. doi: 10.1074/jbc.M115.683813
53. Huang W, Tian Y, Dong S, Cha Y, Li J, Guo X, et al. The Long Non-Coding RNA SNHG3 Functions as a Competing Endogenous RNA to Promote Malignant Development of Colorectal Cancer. Oncol Rep (2017) 38(3):1402–10. doi: 10.3892/or.2017.5837
54. Yuan J, Liu X, Wang T, Pan W, Tao Q, Zhou W, et al. The MBNL3 Splicing Factor Promotes Hepatocellular Carcinoma by Increasing PXN Expression Through the Alternative Splicing of lncRNA-PXN-As1. Nat Cell Biol (2017) 19(7):820–32. doi: 10.1038/ncb3538
55. He R-Z, Luo D-X, Mo Y-Y. Emerging Roles of lncRNAs in the Post-Transcriptional Regulation in Cancer. Genes Dis (2019) 6(1):6–15. doi: 10.1016/j.gendis.2019.01.003
56. Bhat SA, Ahmad SM, Mumtaz PT, Malik AA, Dar MA, Urwat U, et al. Long Non-Coding RNAs: Mechanism of Action and Functional Utility. Non-coding RNA Res (2016) 1(1):43–50. doi: 10.1016/j.ncrna.2016.11.002
57. Zhao W, Geng D, Li S, Chen Z, Sun M. LncRNA HOTAIR Influences Cell Growth, Migration, Invasion, and Apoptosis via the miR-20a-5p/ HMGA2 Axis in Breast Cancer. Cancer Med (2018) 7(3):842–55. doi: 10.1002/cam4.1353
58. Wang M, Sun X, Wang H, Xin Y, Jiao W. Long Non-Coding RNAs In Non-Small Cell Lung Cancer: Functions and Distinctions From Other Malignancies. Transl Cancer Res (2019) 8(7):2636–53. doi: 10.21037/tcr.2019.10.22
59. Zhang H, Lu Y, Wu J, Feng J. LINC00460 Hypomethylation Promotes Metastasis in Colorectal Carcinoma. Front Genet (2019) 10. doi: 10.3389/fgene.2019.00880
60. Wang J, Lu A, Chen L. LncRNAs in Ovarian Cancer. Clin Chim Acta (2019) 490:17–27. doi: 10.1016/j.cca.2018.12.013
61. Wen S, Wei Y, Zen C, Xiong W, Niu Y, Zhao Y. Long Non-Coding RNA NEAT1 Promotes Bone Metastasis of Prostate Cancer Through N6-Methyladenosine. Mol Cancer (2020) 19(1):171. doi: 10.1186/s12943-020-01293-4
62. Wang J, Huang H, Zhang X, Ma H. LOXL1−AS1 Promotes Thymoma and Thymic Carcinoma Progression by Regulating Mir−525−5p− HSPA9. Oncol Rep (2021) 45(6):117. doi: 10.3892/or.2021.8068
63. Chen M, Liu L. MiR-525-5p Repressed Metastasis and Anoikis Resistance in Cervical Cancer via Blocking UBE2C/ZEB1/2 Signal Axis. Dig Dis Sci (2020) 65(8):2442–51. doi: 10.1007/s10620-019-05916-9
64. Jiang H-Y, Wang Z-J. ADPGK-AS1 Promotes the Progression of Colorectal Cancer via Sponging miR-525 to Upregulate FUT1. Eur Rev Med Pharmacol Sci (2020) 24(5):2380–6. doi: 10.26355/eurrev_202003_20505
65. Tito C, Ganci F, Sacconi A, Masciarelli S, Fontemaggi G, Pulito C, et al. LINC00174 Is a Novel Prognostic Factor in Thymic Epithelial Tumors Involved in Cell Migration and Lipid Metabolism. Cell Death Dis (2020) 11(11):959. doi: 10.1038/s41419-020-03171-9
66. Li Z-X, Zhu Q-N, Zhang H-B, Hu Y, Wang G, Zhu Y-S. MALAT1: A Potential Biomarker in Cancer. Cancer Manag Res (2018) 10:6757–68. doi: 10.2147/CMAR.S169406
67. Hu L, Wu Y, Tan D, Meng H, Wang K, Bai Y, et al. Up-Regulation of Long Noncoding RNA MALAT1 Contributes to Proliferation and Metastasis in Esophageal Squamous Cell Carcinoma. J Exp Clin Cancer Res (2015) 34(1):7. doi: 10.1186/s13046-015-0123-z
68. Han Y, Wu Z, Wu T, Huang Y, Cheng Z, Li X, et al. Tumor-Suppressive Function of Long Noncoding RNA MALAT1 in Glioma Cells by Downregulation of MMP2 and Inactivation of ERK/MAPK Signaling. Cell Death Dis (2016) 7(3):e2123–3. doi: 10.3892/ol.2021.12846
69. Tan S, Chen J. Si−MALAT1 Attenuates Thymic Cancer Cell Proliferation and Promotes Apoptosis via the Mir−145−5p/HMGA2 Pathway. Oncol Lett (2021) 22(2):585.
70. Fazi F, Fatica A. Interplay Between N6-Methyladenosine (M6a) and Non-Coding RNAs in Cell Development and Cancer. Front Cell Dev Biol (2019) 7:116. doi: 10.3389/fcell.2019.00116
71. Iaiza A, Tito C, Ianniello Z, Ganci F, Laquintana V, Gallo E, et al. METTL3-Dependent MALAT1 Delocalization Drives C-Myc Induction in Thymic Epithelial Tumors. Clin Epigenetics (2021) 13(1):173. doi: 10.1186/s13148-021-01159-6
72. Pruszko M, Milano E, Forcato M, Donzelli S, Ganci F, Di Agostino S, et al. The Mutant P53-ID4 Complex Controls VEGFA Isoforms by Recruiting lncRNA Malat1. EMBO Rep (2017) 18(8):1331–51. doi: 10.18632/aging.102197
73. Yang J, Zhang Y, Song H. A Disparate Role of RP11-424c20.2/UHRF1 Axis Through Control of Tumor Immune Escape in Liver Hepatocellular Carcinoma and Thymoma. Aging (Albany NY) (2019) 11(16):6422–39.
74. Obata Y, Furusawa Y, Endo TA, Sharif J, Takahashi D, Atarashi K, et al. The Epigenetic Regulator Uhrf1 Facilitates the Proliferation and Maturation of Colonic Regulatory T Cells. Nat Immunol (2014) 15(6):571–9. doi: 10.1038/ni.2886
75. Su Y, Chen Y, Tian Z, Lu C, Chen L, Ma X. lncRNAs Classifier to Accurately Predict the Recurrence of Thymic Epithelial Tumors. Thorac Cancer (2020) 11(7):1773–83. doi: 10.1111/1759-7714.13439
76. Ji G, Ren R, Fang X. Identification and Characterization of Non-Coding RNAs in Thymoma. Med Sci Monit (2021) 27:e929727. doi: 10.12659/MSM.929727
77. Li N, Li J, Mi Q, Xie Y, Li P, Wang L, et al. Long Non-Coding RNA ADAMTS9-AS1 Suppresses Colorectal Cancer by Inhibiting the Wnt/β-Catenin Signalling Pathway and Is a Potential Diagnostic Biomarker. J Cell Mol Med (2020) 24(19):11318–29. doi: 10.1111/jcmm.15713
78. Xiu D-H, Liu G-F, Yu S-N, Li L-Y, Zhao G-Q, Liu L, et al. RETRACTED ARTICLE: Long Non-Coding RNA LINC00968 Attenuates Drug Resistance of Breast Cancer Cells Through Inhibiting the Wnt2/β-Catenin Signaling Pathway by Regulating WNT2. J Exp Clin Cancer Res (2019) 38(1):94. doi: 10.1186/s13046-019-1100-8
79. Shi D, Wu F, Mu S, Hu B, Zhong B, Gao F, et al. LncRNA AFAP1-AS1 Promotes Tumorigenesis and Epithelial-Mesenchymal Transition of Osteosarcoma Through RhoC/ROCK1/p38MAPK/Twist1 Signaling Pathway. J Exp Clin Cancer Res (2019) 38(1):375. doi: 10.26355/eurrev_202006_21671
80. Wan JF, Wan JY, Dong C, Li L. Linc00324 Promotes the Progression of Papillary Thyroid Cancer via Regulating Notch Signaling Pathway. Eur Rev Med Pharmacol Sci (2020) 24(12):6818–24.
81. Liu H, Zhou T, Wang B, Li L, Ye D, Yu S. Identification and Functional Analysis of a Potential Key lncRNA Involved in Fat Loss of Cancer Cachexia. J Cell Biochem (2018) 119(2):1679–88. doi: 10.1002/jcb.26328
82. Han D, Wang J, Cheng G. LncRNA NEAT1 Enhances the Radio-Resistance of Cervical Cancer via miR-193b-3p/CCND1 Axis. Oncotarget (2018) 9(2):2395–409. doi: 10.18632/oncotarget.23416
83. Gong J, Jin S, Pan X, Wang G, Ye L, Tao H, et al. Identification of Long Non-Coding RNAs for Predicting Prognosis Among Patients With Thymoma. Clin Lab (2018) 64(07+08/2018):1193–8. doi: 10.7754/Clin.Lab.2018.180136
84. Chen K, Bai L, Ji L, Wu L, Li G. Bioinformatics Analysis of the Key Potential ceRNA Biomarkers in Human Thymic Epithelial Tumors. Med (Baltimore) (2021) 100(24):e26271. doi: 10.1097/MD.0000000000026271
85. Cong Z, Diao Y, Xu Y, Li X, Jiang Z, Shao C, et al. Long non-Coding RNA Linc00665 Promotes Lung Adenocarcinoma Progression and Functions as ceRNA to Regulate AKR1B10-ERK Signaling by Sponging miR-98. Cell Death Dis (2019) 10(2):84. doi: 10.1038/s41419-019-1361-3
86. Li S, Zheng K, Pei Y, Wang W, Zhang X. Long Noncoding RNA NR2F1-AS1 Enhances the Malignant Properties of Osteosarcoma by Increasing Forkhead Box A1 Expression via Sponging of microRNA-483-3p. Aging (Albany NY) (2019) 11(23):11609–23. doi: 10.18632/aging.102563
87. Luo Z, Li Y, Liu X, Luo M, Xu L, Luo Y, et al. Systems Biology of Myasthenia Gravis, Integration of Aberrant lncRNA and mRNA Expression Changes. BMC Med Genomics (2015) 8(1):13. doi: 10.1186/s12920-015-0087-z
88. Uzawa A, Kawaguchi N, Himuro K, Kanai T, Kuwabara S. Serum Cytokine and Chemokine Profiles in Patients With Myasthenia Gravis. Clin Exp Immunol (2014) 176(2):232–7. doi: 10.1111/cei.12272
89. Cordiglieri C, Marolda R, Franzi S, Cappelletti C, Giardina C, Motta T, et al. Innate Immunity in Myasthenia Gravis Thymus: Pathogenic Effects of Toll-Like Receptor 4 Signaling on Autoimmunity. J Autoimmun (2014) 52:74–89. doi: 10.1016/j.jaut.2013.12.013
90. Yilmaz V, Tütüncü Y, Barış Hasbal N, Parman Y, Serdaroglu P, Deymeer F, et al. Polymorphisms of Interferon-γ, Interleukin-10, and Interleukin-12 Genes in Myasthenia Gravis. Hum Immunol (2007) 68(6):544–9. doi: 10.1016/j.humimm.2007.02.003
91. Hu B, Niu L, Jiang Z, Xu S, Hu Y, Cao K. LncRNA XLOC_003810 Promotes T Cell Activation and Inhibits PD-1/PD-L1 Expression in Patients With Myasthenia Gravis-Related Thymoma. Scand J Immunol (2020) 92(1):e12886. doi: 10.1111/sji.12886
92. Niu L, Jiang J, Yin Y, Hu B. LncRNA XLOC_003810 Modulates Thymic Th17/Treg Balance in Myasthenia Gravis With Thymoma. Clin Exp Pharmacol Physiol (2020) 47(6):989–96. doi: 10.1111/1440-1681.13280
93. Corthay A. How do Regulatory T Cells Work? Scand J Immunol (2009) 70(4):326–36. doi: 10.1111/j.1365-3083.2009.02308.x
94. Wu Y, Luo J, Garden OA. Immunoregulatory Cells in Myasthenia Gravis. Front Neurol. (2020) 11:. doi: 10.3389/fneur.2020.593431
95. Wang Z, Wang W, Chen Y, Wei D. T Helper Type 17 Cells Expand in Patients With Myasthenia-Associated Thymoma. Scand J Immunol (2012) 76(1):54–61. doi: 10.3389/fgene.2021.756493
96. Zhuang J, Guan M, Liu M, Liu Y, Yang S, Hu Z, et al. Immune-Related Molecular Profiling of Thymoma With Myasthenia Gravis. Front Genet (2021) 12.
97. Kong X, Wang J, Cao Y, Zhang H, Lu X, Wang Y, et al. The Long Noncoding RNA MALAT-1 Functions as a Competing Endogenous RNA to Regulate MSL2 Expression by Sponging miR-338-3p in Myasthenia Gravis. J Cell Biochem (2019) 120(4):5542–50. doi: 10.1002/jcb.27838
Keywords: thymoma, thymic carcinoma, thymic epithelial tumors (TETs), ncRNAs (non coding RNAs), miRNA - microRNA, lncRNA - long noncoding RNA, MALAT1, myasthenia gravis
Citation: Iaiza A, Tito C, Ganci F, Sacconi A, Gallo E, Masciarelli S, Fontemaggi G, Fatica A, Melis E, Petrozza V, Venuta F, Marino M, Blandino G and Fazi F (2022) Long Non-Coding RNAs in the Cell Fate Determination of Neoplastic Thymic Epithelial Cells. Front. Immunol. 13:867181. doi: 10.3389/fimmu.2022.867181
Received: 31 January 2022; Accepted: 21 March 2022;
Published: 22 April 2022.
Edited by:
Valentin Shichkin, OmniFarma, UkraineReviewed by:
Prerna Guleria, Southern Command Hospital, IndiaCopyright © 2022 Iaiza, Tito, Ganci, Sacconi, Gallo, Masciarelli, Fontemaggi, Fatica, Melis, Petrozza, Venuta, Marino, Blandino and Fazi. This is an open-access article distributed under the terms of the Creative Commons Attribution License (CC BY). The use, distribution or reproduction in other forums is permitted, provided the original author(s) and the copyright owner(s) are credited and that the original publication in this journal is cited, in accordance with accepted academic practice. No use, distribution or reproduction is permitted which does not comply with these terms.
*Correspondence: Francesco Fazi, ZnJhbmNlc2NvLmZhemlAdW5pcm9tYTEuaXQ=; Giovanni Blandino, Z2lvdmFubmkuYmxhbmRpbm9AaWZvLml0; Mirella Marino, bWlyZWxsYS5tYXJpbm9AaWZvLmdvdi5pdA==
†These authors share first authorship
Disclaimer: All claims expressed in this article are solely those of the authors and do not necessarily represent those of their affiliated organizations, or those of the publisher, the editors and the reviewers. Any product that may be evaluated in this article or claim that may be made by its manufacturer is not guaranteed or endorsed by the publisher.
Research integrity at Frontiers
Learn more about the work of our research integrity team to safeguard the quality of each article we publish.