Erratum: Virus-like particles are efficient tools for boosting mRNA-induced antibodies
- 1Department of Rheumatology and Immunology, University Hospital, Bern, Switzerland
- 2Department of BioMedical Research, University of Bern, Bern, Switzerland
- 3Division of Allergology and Clinical Immunology, Department of Pneumology, Inselspital, Bern University Hospital, University of Bern, Bern, Switzerland
- 4Allergy Unit, Department of Dermatology, University Hospital of Zurich, Zurich, Switzerland
- 5Erasmus Medical Center, Department of Viroscience, Rotterdam, Netherlands
- 6Artemis Bio-Support, Delft, Netherlands
- 7Latvian Biomedical Research & Study Centre, Riga, Latvia
- 8Nuffield Department of Medicine, Centre for Cellular and Molecular Physiology (CCMP), The Jenner Institute, University of Oxford, Oxford, United Kingdom
mRNA based vaccines against COVID-19 have proven most successful at keeping SARS-CoV-2 pandemic at bay in many countries. Recently, there is an increased interest in heterologous prime-boost vaccination strategies for COVID-19 to maintain antibody responses for the control of continuously emerging SARS-CoV-2 variants of concern (VoCs) and to overcome other obstacles such as supply shortage, costs and reduced safety issues or inadequatly induced immune-responses. In this study, we investigated the antibody responses induced by heterologous prime-boost with vaccines based on mRNA and virus-like particles (VLPs). The VLP-based mCuMVTT-RBM vaccine candidate and the approved mRNA-1273 vaccine were used for this purpose. We find that homologous prime boost regimens with either mRNA or VLP induced high levels of high avidity antibodies. Optimal antibody responses were, however, induced by heterologous regimens both for priming with mRNA and boosting with VLP and vice versa, priming with VLP and boosting with mRNA. Thus, heterologous prime boost strategies may be able to optimize efficacy and economics of novel vaccine strategies.
Introduction
Multiple immunizations are usually required for most vaccines to be successful in protecting against pathogens. For instance, five-dose series of Tetanus, Pertussis and Diphtheria (DTaP) vaccine is required during childhood and an adolescent booster dose to elucidate the aimed protection (1). It is not entirely clear why some vaccines require more additional boosters than others; however, it is well accepted that multiple immunizations are essentials for better responses, in particular for non-replicating vaccines (2). An annual dose of influenza vaccine is also recommended for persons who may be at increased risk for complications from influenza infections.
Additionally, heterologous prime-boost regimens in particular when using vectored vaccines have previously shown better immunogenicity; for example, priming with DNA and boosting with viral vectors (3, 4). The need to change the vector may be explained by the induction of neutralizing antibodies against the vector, which compromises further boosting with the same vector. Vaccine-specific antibodies have also been reported to suppress the cytotoxic (CTL) response when using the non-infectious virus-like particles (VLPs) derived from human papilloma virus (HPV) or Qβ-p33 for immunization (5, 6).
COVID-19 pandemic has overwhelmed the healthcare systems worldwide and several countries have prioritized the development of SARS-CoV-2 vaccine to contain the virus. Currently, 30 vaccines are approved in various countries and around 80 vaccines are in clinical development, of which 19 are in phase 3 (7). Several leading vaccines have been shown to confer protection and induction of neutralizing antibodies against the wild type SARS-CoV-2. However, with continuous emergence of SARS-CoV-2 variants and the relatively rapid drop in antibody titers induced in vaccinated individuals, concerns are raised by public health organizations regarding the duration and efficacy of antibody responses by current vaccines (8).
Next-generation COVID-19 vaccines which have been approved for emergency use, namely mRNA vaccines such as BNT162b2, mRNA-1273 and adenovirus-based vaccines, have their own advantages and disadvantages. Obviously, the main advantage is their remarkable efficacy of 95% for Pfizer® mRNA vaccine, 94.1% for Moderna® and around 80% for Ad26.COV2.S (vector based vaccine) respectively (9–11). On the other hand, their adverse reactions has raised concerns with respect to their safety. The incidence of local and systemic adverse reactions (AR) has been shown to be relatively high for mRNA and adenovirus-based vaccines with Local AR reaching 40%-88.9% and systemic AR between 44% and 86%, respectively (12).
Virus-like particles (VLPs) are considered traditional vaccine platforms. They are virus-derived structures and have the ability to self-assemble and mimic the parental virus in size and shape. One important aspect is that VLPs lack any genetic materials; accordingly, they are not capable of infecting the host cell or replication (13). Such traditional VLP-based vaccines have been approved for human use against different viruses decades ago. For example, vaccines against Hepatitis B and E viruses (HBV and HEV), Human Papilloma Virus (HPV) and the newly developed malaria vaccine (14). There are currently three VLP-based vaccines which have also been tested for COVID-19 in preclinical and clinical trials (15). The traditional vaccine platforms have shown lower incidence of adverse side effects (16); however they typically also showed inferior immunogenicity in comparison to the next-generation mRNA vaccines (17–19).
In line with the above, heterologous prime-boost vaccination strategies using different vectors should be tested to elicit broader and more efficient protective immune-responses with better and improved safety profiles. This strategy may meet the emergency needs in the current pandemic. In the current study, we evaluated different prime and boost regimens combining mRNA-1273 with a VLP-based vaccine based, namely mCuMVTT-RBM. We have previously designed and developed the scalable and immunogenic VLP-based COVID-19 vaccine mCuMVTT-RBM, which has been shown to induce RBD-specific IgG and IgA antibodies with strong neutralizing capability (17). We show that priming and boosting with mRNA-1273 or mCuMVTT-RBM induced high levels of high avidity antibodies. Interestingly, highest antibody responses were measured, when vaccination was performed by heterologous administration of mRNA for prime and VLP as boost and vice versa, priming with VLP and boosting with mRNA. Thus, these findings, heterologous prime boost strategies may be able to improve efficacy and economics of current vaccine protocols.
Results
Heterologous Prime-Boost Vaccine Administration Induces High Levels of RBD- Specific Antibodies Following the Booster Dose
We have designed different heterologous vaccination regimens using mRNA-1273 vaccine (Moderna®) and our newly developed mosaic COVID-19 VLPs-based vaccine mCuMVTT-RBM (17) as illustrated in Figure 1A. For SARS-CoV-2 specific genetic vaccine, the mRNA provides expression of the spike protein of COVID-19 (20). The mRNA of the spike protein is then translated by the host, thus allowing the host to mount an antibody response against the expressed protein (21, 22). mRNA-1273 has shown >90% effectiveness in preventing SARS-CoV-2 infection, at least for original Wuhan strain (10). mCuMVTT-RBM is a plant-derived VLPs which incorporates the receptor-binding motif (RBM) of SARS-CoV-2 using genetic fusion techniques. mCuMVTT-RBM also incorporates in its interior surface a tetanus toxin (TT) epitope which is believed to enhance the immune response in elderly people. Specifically, the TT-epitope is expected to augment interaction between TT-specific T helper (TH) cells and RMB-specific B cells. This is supported by the fact that pre-existing immunity to the chosen TT epitope is very broad in humans (and animals) as the peptide binds essentially to all HLA-DR molecules and most people have been immunized many times against TT. Additionally, mCuMVTT-RBM is packaged with ssRNA, a TLR7/8 ligand which serves as a natural adjuvant (23, 24).
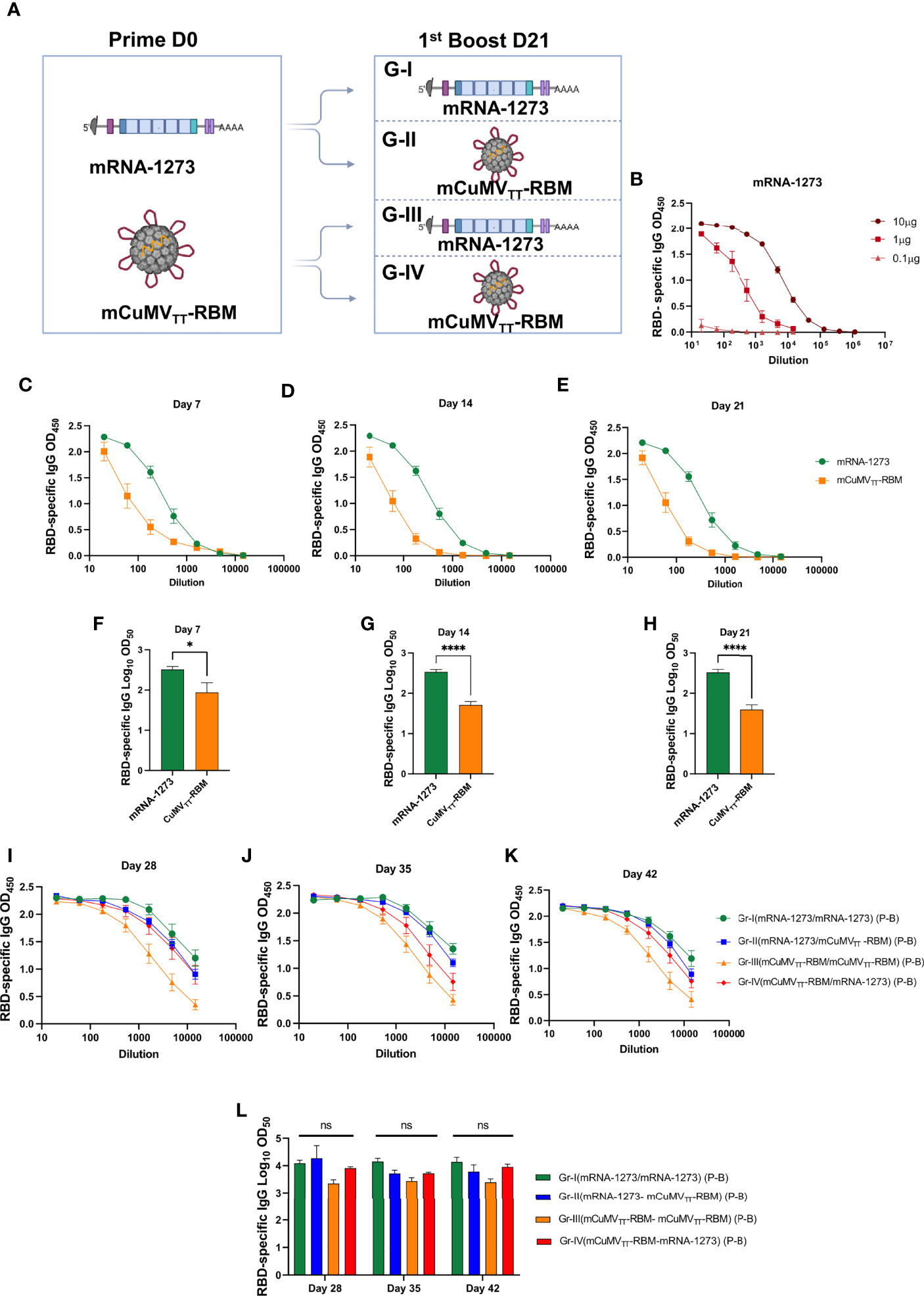
Figure 1 Heterologous prime boost vaccination induces high levels of RBD-specific antibodies. (A) Vaccination regimen used for Prime and Boost at day D0/D21 and the corresponding groups: Group I (mRNA-1273 → mRNA-1273), Group II (mRNA-1273 → mCuMVTT-RBM), Group III (mCuMVTT-RBM → mCuMVTT-RBM) and Group IV (mCuMVTT-RBM → mRNA-1273). Illustration was created using BioRender.com. (B) RBD-specific IgG for mice vaccinated with different concentrations of mRNA-1273 (mRNA titration) (C–E) OD450 of RBD-specific IgG for the groups vaccinated with mRNA-1273 or mCuMVTT-RBM on days 7, 14 and 21. (F–H) Log10OD50 of RBD-specific IgG titers for the groups vaccinated with mRNA-1273 or mCuMVTT-RBM on days 7, 14 and 21. (I–K) OD450 of RBD-specific IgG for the groups boosted with mRNA-1273 or mCuMVTT-RBM on days 28, 35 and 42. (L) Log10OD50 of RBD-specific IgG titers for the groups boosted with mRNA-1273 or mCuMVTT-RBM on days 28, 35 and 42 using D0/D21 regimen. Statistical analysis (mean ± SEM) using one- way ANOVA in K or Student’s t test in E-G, n=10 or 5. One representative of 3 similar experiments is shown. The value of *p < 0.05 was considered statistically significant (****p < 0.0001). ns, statistically not significant.
10μg/dose of mRNA-1273 vaccine and 100μg/dose of mCuMVTT-RBM were used in this experiment for priming and boosting (Figure 1A). The mRNA dose of 10µg was chosen, as the 1μg/dose induced a lower and the 0.1μg/dose failed to induce a response (Figure 1B). Due to this unexpected steep dose-response, 10μg of mRNA was used for the experiments throughout. Balb/c mice were divided into two groups for subcutaneous (s.c.) priming with mRNA-1273 or mCuMVTT-RBM vaccines (Figure 1A). Blood samples were collected on a weekly basis to measure the induced RBD-specific antibodies by ELISA. Our results showed successful induction of RBD-specific antibodies 7 days after s.c injection of mRNA-1273 or mCuMVTT-RBM (Figure 1C). mRNA-1273 was superior to mCuMVTT-RBM in inducing RBD-specific antibodies measured on days 7, 14 and 21 at the 10μg dose (Figures 1C–H) but the VLPs also was effective at inducing rapid IgG responses.
The primed mice were divided next into four groups (Figure 1A) and received a booster dose on day 21 using mRNA-1273 or mCuMVTT-RBM. Therefore, mice are grouped and immunized as follows: Group I (mRNA-1273 → mRNA-1273), Group II (mRNA-1273 → mCuMVTT-RBM), Group III (mCuMVTT-RBM → mCuMVTT-RBM) and Group IV (mCuMVTT-RBM → mRNA-1273). Blood samples were collected on days 28 and 35 to measure the induced RBD-specific antibodies by ELISA. Interestingly, heterologous boost with mRNA-1273 or mCuMVTT-RBM showed a similar induction of RBD-specific antibodies with no statistical difference for sera collected on days 28, 35 and 42 (Figures 1I–L). This demonstrates that mCuMVTT-RBM can significantly boost previously primed B-cells to a similar titer when using a homologous mRNA vaccine.
A Second Booster Dose Further Enhances the Induced Immune Response
Taking into account the slightly inferior immunogenicity of mCuMVTT-RBM vaccine to mRNA-1273, and our previous data showing that a 2nd booster dose of mCuMVTT-RBM would enhance the quality of the induced antibodies (17), a 2nd booster dose was performed on day 56 in Groups II, III and IV as illustrated in Figure 2A. As Group I represents the standard immunization strategy followed in almost all countries, no booster dose was applied. ELISA data for OD450 and OD50 for days 63 and 70 did not show a further increase in RBD-specific antibodies (Figures 2B–E).
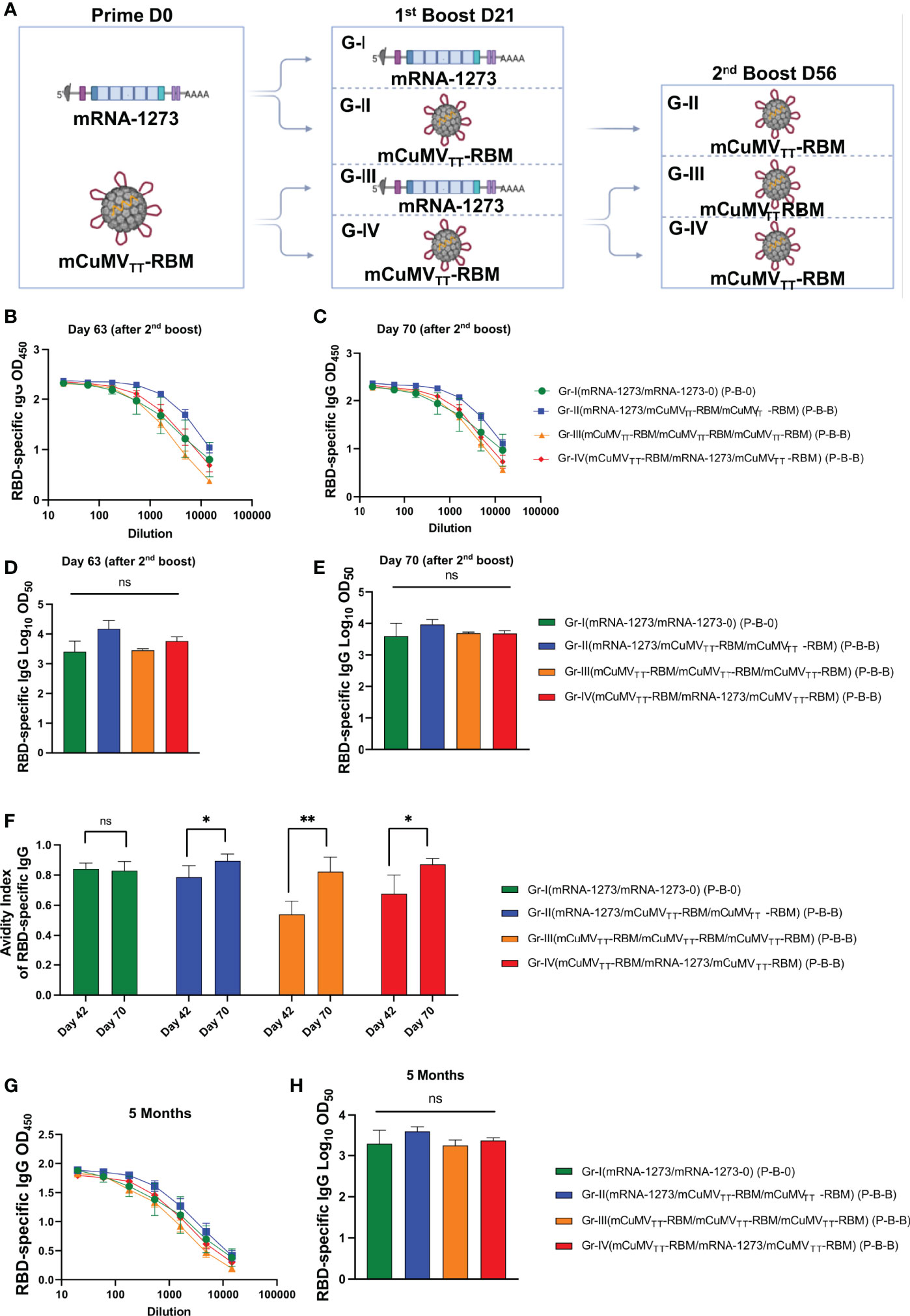
Figure 2 Enhanced immune response with second booster dose. (A) Vaccination regimen (Prime-Boost-Boost) D0/D21/D56 and groups. Illustration was created using BioRender.com (B, C) OD450 of RBD-specific IgG for the groups vaccinated with mRNA-1273 or mCuMVTT-RBM on days 63 and 70 using D0/D21/D56 regimen. (D, E) Log10OD50 of RBD-specific IgG titers for the groups vaccinated with mRNA-1273 or mCuMVTT-RBM on 63 and 70 using D0/D21/D56 regimen. (F) Avidity Index of RBD- specific IgG in mice vaccinated with mRNA-1273 or mCuMVTT- RBM using D0/D21 or D0/D21/D56 regimens, sera were treated with PBST or 7 M Urea. (G) OD450 of RBD-specific longevity IgG for the groups vaccinated with mRNA-1273 or mCuMVTT-RBM 5 months after priming using D0/D21/D56 regimen. (H) Log10OD50 of RBD-specific IgG titers for the groups vaccinated with mRNA-1273 or mCuMVTT-RBM 5 month after priming, using D0/D21/D56 regimen. Statistical analysis (mean ± SEM) using one- way ANOVA in (D, E, H) or Student’s t test in (F), n=10 or 5. One representative of 3 similar experiments is shown. The value of *p < 0.05 was considered statistically significant (**p < 0.01). ns, statistically not significant.
The avidity of the induced antibodies was assessed using a modified immunoassay containing 7M urea which facilitates the detachment of the low avidity antibodies. More specifically, we compared the avidity of RBD-specific antibodies on days 42 and 70, i.e. after 2 or 3 injections. For group I, which was primed and boosted with mRNA-1273, the avidity of RBD-specific antibodies did not differ on days 42 and 70 (p. 0.7460). In Group II (which received a prime with mRNA-1273 and 2 booster doses of mCuMVTT-RBM) ~80% of RBD-specific antibodies were of high avidity after the 1st boost in comparison to ~90% following the 2nd booster dose (p. 0.0436). This finding was similar in group IV (which received a prime with mCuMVTT-RBM, followed by a 1st boost with mRNA-1273 and 2nd boost with mCuMVTT-RBM), again with statistically significant difference in avidity between day 42 and 70 (p. 0.0243) (Figure 2F). The induction of higher avidity antibodies following 3 vaccinations with mCuMVTT-RBM is consistent with our previous findings (17).
It was also of interest to study the longevity of the induced antibodies after vaccination with the different heterologous strategies. Accordingly, we have tested RBD-specific antibodies up to 5 months after the priming dose. The results revealed a slight drop in the antibody titer in group I (received 2 doses of mRNA-1273), which however was not statistically different when compared to the other groups. Groups II, III and IV showed a stable antibody titer comparable to the titers seen on days 42 and 70 (Figures 2G, H).
The Induced Antibodies Recognize SARS-CoV-2 Variants of Concern (VoCs) Efficiently
To test the capacity of the induced antibodies following the heterologous prime-boost regimen to neutralize SARS-CoV-2 wild-type and its mutated VoCs, we used a reduction of cytopathic effect (CPE) assay. 100 TCID50 of SARS-CoV-2/ABS/NL20, delta strain, south African, Brazilian and the most recent Omicron strain have been used and titers have been expressed as the highest dilution that inhibits 50% CPE formation. No significant difference was detected between the four groups when measuring the neutralization titer against SARS-CoV-2 wild-type (Figure 3A). When comparing the induced neutralization titer against the delta VoC (Figure 3B), our results show a significant difference between Group I (2xmRNA) and III (3xVLP) (p. 0.039) confirming the slight superiority of mRNA only over VLP-only based vaccines. However, no statistical differences have been detected between Group I and II (p. 0.2558) or between Group I and IV (p >0.999) (Figure 3B). VoCs haven been use to test the neutralization capacity (Figures 3B–E). While neutralization of the Brazilian variant and the Omicron variant was reduced, the different vaccine regimens did not result in different neutralization capacity. This indicates that mRNA may be used to boost VLP-induced antibody responses; or vice versa, and probably more importantly, VLPs may be used to boost RNA-induced responses. Hence, classical VLP-based vaccines may be used to boost mRNA induced antibody responses.
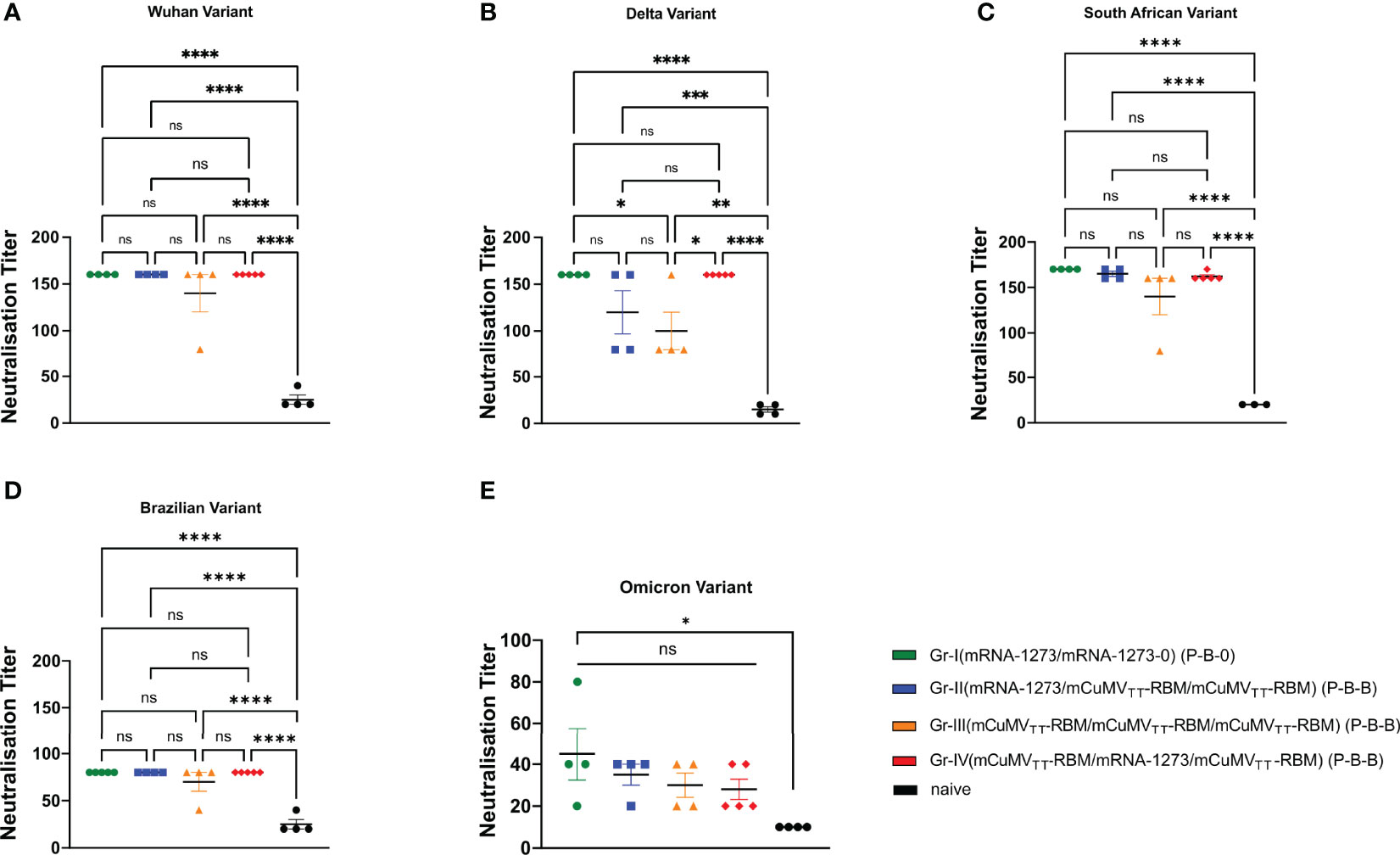
Figure 3 Recognition of SARS-CoV-2 variants of concern. (A–E) Neutralization titer (CPE) for sera from mice vaccinated with mRNA-1273 and mice vaccinated with mCuMVTT-RBM, sera from day 63 (after 2nd boost). Statistical analysis (mean ± SEM) using ANOVA, n=5. One representative of 2 similar experiments is shown. The value of *p < 0.05 was considered statistically significant (**p < 0.01, ***p < 0.001, ****p < 0.0001) ns, statistically not significant.
Discussion
The highly contagious SARS-CoV-2 virus has put a heavy toll on the worlds public health systems and caused a disruption of the world economy (25). The use of masks, physical distancing, contact tracing as well as isolation of sick or infected people are important but insufficient measures to reduce the spread of the corona virus disease (26). Accordingly, vaccine campaigns are needed to curb the spread of SARS-CoV-2 and thereby reducing the mortality and morbidity associated with the disease.
The commercially available mRNA vaccines require stringent storage conditions during transport and at vaccination sites as they have a short half-life (27). According to manufactures information, mRNA-1273 is stable up to 6 month when kept at -20°C, whereas to store BNT162b2 for the same time-period, temperatures between -60°C to -80°C are needed (9, 10). With regard to stability at room temperature, mRNA-1273 is stable for up to 12h; in contrast BNT162b2 has to be administered within 6h after thawing (28). These strict storage conditions represent a major obstacle for efficient use in less affluent parts of the world. Beside the challenging storage conditions, a further obstacle represents the production-costs of RNA-based vaccines, limiting their global access. BNT162b2 has a production cost of $19.50 and mRNA-1273 with even higher costs at $32-37 (12, 29). This can cause a major problem in low-income countries, to afford these types of vaccines. Therefore, cost effective and efficient vaccine candidates are of high importance considering the continuous persistence of the virus and the obstacles in eradicating it worldwide. We have previously shown high stability of our newly developed VLP-based vaccine-candidate mCuMVTT-RBM which was stable for at least 14 months at 4°C without signs of degradation (17). This has also been confirmed with other preclinical studies showing the stability of mCuMVTT -based vaccines for up to 12 months when kept at 4°C. Thus indicating a significant advantage for such VLP-based vaccines (30). Additionally, the production of mCuMVTT-RBM is highly scalable allowing the production of millions of the doses in a single 1000L fermenter run (15). The rapid pace of mRNA vaccine development accompanied with fears of potential long-term adverse effects have raised some concerns in global community. VLP-based vaccines are therefore of high interest as they display a safe platform, due to the lack of replicating genetic material which is also been confirmed in the approved and marketed vaccines (HBV-HEV-HPV and recently Mosquirix™) (13). In addition, the here employed mCuMVTT technology does not need use of an adjuvant.
Our data show that both vaccine-types, mRNA-1273 and mCuMVTT-RBM are capable of inducing high RBD-specific antibody titers 7 days following the priming dose. Interestingly, mRNA-1273 shows a superior induction of antibody response in comparison to the VLP-based vaccine, at least at the high dose chosen. However, after the administration of the 1st homologous boosting, this difference was not statistically significant anymore (Group I and III). The same was true for the heterologous boosts (Group II and IV), indicating the ability of VLPs to efficiently boost the B cells primed by mRNA. We have previously hypothesized that natural infection with SARS-CoV-2 virus induces short-lived neutralizing antibodies due to the unusually large distance between RBD-epitopes embedded in the membrane surface (31, 32). This obstacle may be overcome by grafting RBM or RBD onto highly repetitive nanoparticles such as the here used mCuMVTT VLPs, resulting in successful display of the virus-epitopes at optimal distances of 5-10nm. This pathogen-associated structural pattern (PASP) may also play a role in the induction of higher avidity antibodies as shown after the 2nd booster dose.
With the continuous emergence of SARS-CoV-2 VoCs, it may be essential to administer repetitive booster doses, as higher antibody levels may need to be maintained for sustained protection. Indeed, our data demonstrate that a 2nd boost can maintain antibody titers. Furthermore, the 2nd boost in the different heterologous regimens in groups II, III and IV have significantly enhanced the avidity of the antibodies, likely a key attribute for neutralization of emerging new variants.
The neutralizing capacity of the induced antibodies following the homologous or heterologous vaccination strategies showed that both approaches are efficient against the wild-type SARS-CoV-2. No statistical difference in the ability to neutralize different VoCs has been detected between groups I, II and IV. Furthermore, heterologous boosting of mice primed with mRNA-1273 or mCuMVTT-RBM revealed similar outcome. These data confirm the ability of mCuMVTT-RBM to efficiently boost antibodies primed with mRNA-1273 vaccine in a similar way to the standard followed vaccination strategy (mRNA-1273 prime/boost).
Collectively, our data support premise of using a heterologous prime/boost vaccination regimen against COVID-19. CuMVTT-RBM VLPs may therefore constitute an efficient platform for boosting previously primed B cell responses; here we demonstrate this for mRNA primed responses, but it is likely that this may extend to B cell responses primed by other modalities, including vector- or virus induced immune reactions.
Materials and Methods
Mice
In vivo experiments were performed using 8-12 weeks-old female, BALB/cOlaHsd mice purchased from Envigo (Amsterdam, Netherlands). All mice were maintained in microisolater cages with free access to water and food. Mice were kept on standard chow diet (diet Catalog # 3430); Granovit AG-Kliba Nafag, Switzerland). All animals could acclimatize to the facility for one week before experiments were performed. All animal procedures were conducted in accordance with the Swiss Animal Act (455.109.1 – September 2008, 5th) of University of Bern. All animals were treated for experimentation according to protocols approved by the Swiss Federal Veterinary Office.
Vaccines
mRNA-1273 (Moderna®) was kindly provided the Inselspital, Bern University Hospital. Mosaic (mCuMVTT-RBM) vaccine was prepared as previously described by Mohsen et al. (15).
Vaccination Regimen/Dose/Sera Collection
Wild type BALB/cOlaHsd mice were vaccinated s.c. using different regimens as summarized in Table 1. 10μg (Prime on day 0) and 10μg for a booster dose on day 21 were used. mCuMVTT-RBM vaccine and mRNA-1273 were diluted in 1xPBS in a final volume of 100μl for final injection. Serum was collected on a weekly basis. The three doses regimen (prime- 1st boost- 2nd boost) was either 10μg prime and 100μg 1st and 2nd boost or 100μg prime and 10μg 1st boost and 100mg 2nd boost given at days 0, 21 and 56.
Expression and Purification of RBD
SARS-CoV-2 RBDwildtype, was cloned as a synthetic gene into pTWIST-CMV-BetaGlobin-WPRE-Neo vector (Twist Biosciences, CA, USA) and expressed in HEK293F cells through the Expi293 system (ThermoFisher Scientific, MA, USA). Purification was performed by IMAC using a HiTrap TALON crude column (Cytiva, Uppsala, Sweden).
Enzyme-Linked Immunosorbant Assay (ELISA)
To determine the total IgG antibodies against the vaccine mCuMVTT-RBM as well as mRNA-1273 in sera of vaccinated mice, ELISA plates (96 well half-area ELISA plates; Costar, Corning, Catalog # 3690) were coated with SARS-CoV-2 RBD (wildtype) at a concentration of 1μg/ml overnight at 4°C. ELISA plates were washed with PBS-0.01% Tween and blocked using 100μl PBS-Casein 0.15% for 2h in RT. Sera from vaccinated mice was serially diluted 1:3 starting with a dilution of 1:20 and incubated for 2h at RT. After washing with PBS-0.01%Tween, goat anti-mouse IgG conjugated to Horseradish Peroxidase (HRP) (Jackson ImmunoResearch, West Grove, Pennsylvania) was added at 1/1000 and incubated for 1h at RT ELISA was developed with tetramethylbenzidine (TMB), stopped by adding equal 1 M H2SO4 solution. OD450 was measured using the SpectraMax M5 ELISA reader (Molecular Devices, Catalog # M5) and read at OD450 nm or expressed as Log OD50. Detecting RBD-specific IgGs against mutated RBDs was carried out in a similar way. Detecting antibody response from mice injected with different doses of mRNA-1273 (10μg, 1μg and 0.1μg) was done in a similar set up.
Avidity (ELISA)
To test IgG antibody avidity against RBD protein, threefold serial dilutions of 1/20 diluted mice sera, were added to ELISA plates (96 well half-area ELISA plates; Costar, Corning, Catalog # 3690) coated over night at 4°C with 1μg/ml RBD. After incubation at RT for 1h, the plates were washed once in PBS-0.01% Tween, and then washed 3x with 7M urea in PBS-0.05%Tween or with PBS-0.05% Tween for 5min every time. After washing with PBS-0.05%Tween, goat anti-mouse IgG conjugated to Horseradish Peroxidase (HRP) (Jackson ImmunoResearch, West Grove, Pennsylvania) was added 1/1000 and incubated for 1h at RT. Plates were developed and read at OD450 nm.
Cytopathic Effect-Based Neutralization Assay (CPE)
To determine neutralization ability and capacity of vaccine induced antibodies a CPE was performed using wild-type SARS-CoV-2 (SARS-CoV-2/ABS/NL20), delta strain, South African strain, Brazilian strain and Omicron strain. Serum samples were heat-inactivated for 30min at 56°C. Two-fold serial dilutions were prepared starting at 1:20 up to 1:160. 100 TCID50 of the virus was added to each well and incubated for 37°C for 1h. The mixture has been added on a monolayer of Vero cells and incubated again for 37°C for 4 days. Four days later the cells were inspected for cytopathic effect. The titer was expressed as the highest dilution that fully inhibits formation of CPE.
Statistical Analysis
All data are presented as mean ± SEM. Data were analyzed using Ordinary One-way ANOVA for multiple comparisons and Students’ t-test when comparing two groups. At least two independent experiments were performed. Statistical significance was set at p ≤ 0.05. *P < 0.05, **P < 0.01, ***P < 0.001, ****P < 0.0001. Analyses were performed using GraphPad PRISM 9.0 (Graph-Pad Software, Inc., La Jolla, CA, USA).
Data Availability Statement
The datasets generated during and/or analysed during the current study are available from the corresponding author on reasonable request.
Ethics Statement
The animal study was reviewed and approved by Swiss Federal Veterinary Office.
Author Contributions
A-CV, LJ, PK, XC, AZ, MM, and MB: Design of experiments, acquisition of data, interpretation, and analysis of data. A-CV, MM, MV, and MB: Writing, revision, and editing of manuscript. LJ, MV, and AZ: Technical, material, and tool support. MM and MFB: Study supervision. All authors read and approved the final manuscript.
Funding
This work was supported by Saiba AG and Inselspital Bern. The funder was not involved in the study design, collection, analysis, interpretation of data, the writing of this article or the decision to submit it for publication. Open access funding was provided by the University Of Bern.
Conflict of Interest
Author BM was employed by company Artemis Bio-Support. MB is a board member of Saiba AG and holds the patent of CuMVTT-VLPs. MM received payments by Saiba AG to work on the development of vaccines. MB and MM are shareholder of Saiba AG.
The remaining authors declare that the research was conducted in the absence of any commercial or financial relationships that could be construed as a potential conflict of interest.
The reviewer SL declared a shared affiliation with the author MB to the handling editor at the time of review.
Publisher’s Note
All claims expressed in this article are solely those of the authors and do not necessarily represent those of their affiliated organizations, or those of the publisher, the editors and the reviewers. Any product that may be evaluated in this article, or claim that may be made by its manufacturer, is not guaranteed or endorsed by the publisher.
References
1. Liang JL, Tiwari T, Moro P, Messonnier NE, Reingold A, Sawyer M, et al. Prevention of Pertussis, Tetanus, and Diphtheria With Vaccines in the United States: Recommendations of the Advisory Committee on Immunization Practices (ACIP). MMWR Recomm Rep (2018) 67(2):1–44. doi: 10.15585/mmwr.rr6702a1
2. Lu S. Heterologous Prime-Boost Vaccination. Curr Opin Immunol (2009) 21(3):346–51. doi: 10.1016/j.coi.2009.05.016
3. Kent SJ, Zhao A, Best SJ, Chandler JD, Boyle DB, Ramshaw IA. Enhanced T-Cell Immunogenicity and Protective Efficacy of a Human Immunodeficiency Virus Type 1 Vaccine Regimen Consisting of Consecutive Priming With DNA and Boosting With Recombinant Fowlpox Virus. J Virol (1998) 72(12):10180–8. doi: 10.1128/JVI.72.12.10180-10188.1998
4. Schneider J, Gilbert SC, Blanchard TJ, Hanke T, Robson KJ, Hannan CM, et al. Enhanced Immunogenicity for CD8+ T Cell Induction and Complete Protective Efficacy of Malaria DNA Vaccination by Boosting With Modified Vaccinia Virus Ankara. Nat Med (1998) 4(4):397–402. doi: 10.1038/nm0498-397
5. Da Silva DM, Pastrana DV, Schiller JT, Kast WM. Effect of Preexisting Neutralizing Antibodies on the Anti-Tumor Immune Response Induced by Chimeric Human Papillomavirus Virus-Like Particle Vaccines. Virology (2001) 290(2):350–60. doi: 10.1006/viro.2001.1179
6. Keller SA, Schwarz K, Manolova V, von Allmen CE, Kinzler MG, Bauer M, et al. Innate Signaling Regulates Cross-Priming at the Level of DC Licensing and Not Antigen Presentation. Eur J Immunol (2010) 40(1):103–12. doi: 10.1002/eji.200939559
7. Skowronski DM, De Serres G. Safety and Efficacy of the BNT162b2 mRNA Covid-19 Vaccine. N Engl J Med (2021) 384(16):1576–7. doi: 10.1056/NEJMc2036242
8. He Q, Mao Q, An C, Zhang J, Gao F, Bian L, et al. Heterologous Prime-Boost: Breaking the Protective Immune Response Bottleneck of COVID-19 Vaccine Candidates. Emerg Microbes Infect (2021) 10(1):629–37. doi: 10.1080/22221751.2021.1902245
9. Baden LR, El Sahly HM, Essink B, Kotloff K, Frey S, Novak R, et al. Efficacy and Safety of the mRNA-1273 SARS-CoV-2 Vaccine. N Engl J Med (2021) 384(5):403–16. doi: 10.1056/NEJMoa2035389
10. Polack FP, Thomas SJ, Kitchin N, Absalon J, Gurtman A, Lockhart S, et al. Safety and Efficacy of the BNT162b2 mRNA Covid-19 Vaccine. N Engl J Med (2020) 383(27):2603–15. doi: 10.1056/NEJMoa2034577
11. Corchado-Garcia J, Zemmour D, Hughes T, Bandi H, Cristea-Platon T, Lenehan P, et al. Analysis of the Effectiveness of the Ad26.COV2.S Adenoviral Vector Vaccine for Preventing COVID-19. JAMA Netw Open (2021) 4(11):e2132540. doi: 10.1001/jamanetworkopen.2021.32540
12. He Q, Mao Q, Zhang J, Bian L, Gao F, Wang J, et al. COVID-19 Vaccines: Current Understanding on Immunogenicity, Safety, and Further Considerations. Front Immunol (2021) 12:669339. doi: 10.3389/fimmu.2021.669339
13. Nooraei S, Bahrulolum H, Hoseini ZS, Katalani C, Hajizade A, Easton AJ, et al. Virus-Like Particles: Preparation, Immunogenicity and Their Roles as Nanovaccines and Drug Nanocarriers. J Nanobiotech (2021) 19(1):59. doi: 10.1186/s12951-021-00806-7
14. Mohsen MO, Zha L, Cabral-Miranda G, Bachmann MF. Major Findings and Recent Advances in Virus-Like Particle (VLP)-Based Vaccines. Semin Immunol (2017) 34:123–32. doi: 10.1016/j.smim.2017.08.014
15. Raman R, Patel KJ, Ranjan K. COVID-19: Unmasking Emerging SARS-CoV-2 Variants, Vaccines and Therapeutic Strategies. Biomolecules (2021) 11(7):1–30. doi: 10.3390/biom11070993
16. Karandikar SM,A, Waybhase V, Patravale V, Patankar S. Micro and Nano Technologies. Ecaterina Andronescu AMG, editor. Amsterdam, The Netherlands: Elsevier (2017).
17. Mohsen MO, Balke I, Zinkhan S, Zeltina V, Liu XL, Chang XY, et al. A Scalable and Highly Immunogenic Virus-Like Particle-Based Vaccine Against SARS-CoV-2. Allergy (2021) 77:243–257. doi: 10.22541/au.162659077.72476255/v1
18. Chang X, Zeltins A, Mohsen MO, Gharailoo Z, Zha L, Liu X, et al. A Novel Double Mosaic Virus-Like Particle-Based Vaccine Against SARS-CoV-2 Incorporates Both Receptor Binding Motif (RBM) and Fusion Domain. Vaccines (Basel) (2021) 9(11):1–13 . doi: 10.3390/vaccines9111287
19. Rothen DA, Krenger PS, Nonic A, Balke I, Vogt AS, Chang X, et al. Intranasal Administration of a VLP-Based Vaccine Induces Neutralizing Antibodies Against SARS-CoV-2 and Variants of Concern. Allergy (2022):1–13. doi: 10.1111/all.15311
20. Zhang NN, Li XF, Deng YQ, Zhao H, Huang YJ, Yang G, et al. A Thermostable mRNA Vaccine Against COVID-19. Cell (2020) 182(5):1271–83.e16. doi: 10.1016/j.cell.2020.07.024
21. Anand P, Stahel VP. Review the Safety of Covid-19 mRNA Vaccines: A Review. Patient Saf Surg (2021) 15(1):20. doi: 10.1186/s13037-021-00291-9
22. Van Lint S, Heirman C, Thielemans K, Breckpot K. mRNA: From a Chemical Blueprint for Protein Production to an Off-The-Shelf Therapeutic. Hum Vaccin Immunother. (2013) 9(2):265–74. doi: 10.4161/hv.22661
23. Mohsen MO, Rothen D, Balke I, Martina B, Zeltina V, Inchakalody V, et al. Neutralization of MERS Coronavirus Through a Scalable Nanoparticle Vaccine. NPJ Vaccines (2021) 6(1):2–9. doi: 10.1038/s41541-021-00365-w
24. Zeltins A, West J, Zabel F, El Turabi A, Balke I, Haas S, et al. Incorporation of Tetanus-Epitope Into Virus-Like Particles Achieves Vaccine Responses Even in Older Recipients in Models of Psoriasis, Alzheimer's and Cat Allergy. NPJ Vaccines (2017) 2:30. doi: 10.1038/s41541-017-0030-8
25. Augusto G, Mohsen MO, Zinkhan S, Liu XL, Vogel M, Bachmann MF. In Vitro Data Suggest That Indian Delta Variant B.1.617 of SARS-CoV-2 Escapes Neutralization by Both Receptor Affinity and Immune Evasion. Allergy (2021)77:111–117. doi: 10.1111/all.15065
26. Puranik A, Lenehan PJ, Silvert E, Niesen MJM, Corchado-Garcia J, O'Horo JC, et al. Comparison of Two Highly-Effective mRNA Vaccines for COVID-19 During Periods of Alpha and Delta Variant Prevalence. medRxiv (2021). doi: 10.1101/2021.08.06.21261707
27. Crommelin DJA, Anchordoquy TJ, Volkin DB, Jiskoot W, Mastrobattista E. Addressing the Cold Reality of mRNA Vaccine Stability. J Pharm Sci (2021) 110(3):997–1001. doi: 10.1016/j.xphs.2020.12.006
28. Information for Healthcare Professionals on Pfizer BioNTech COVID-19 Vaccine. In: UK Department of Health and Social Care. Health and Social Care UK, Health and Security Agency. Available at: https://www.gov.uk/government/publications/regulatory-approval-of-pfizer-biontech-vaccine-for-covid-19/information-for-healthcare-professionals-on-pfizerbiontech-covid-19-vaccine; accessed on February 2022.
29. Meo SA, Bukhari IA, Akram J, Meo AS, Klonoff DC. COVID-19 Vaccines: Comparison of Biological, Pharmacological Characteristics and Adverse Effects of Pfizer/BioNTech and Moderna Vaccines. Eur Rev Med Pharmacol Sci (2021) 25(3):1663–9. doi: 10.26355/eurrev_202102_24877
30. Lynch A, Meyers AE, Williamson AL, Rybicki EP. Stability Studies of HIV-1 Pr55(gag) Virus-Like Particles Made in Insect Cells After Storage in Various Formulation Media. Virol J (2012) 9:1–5. doi: 10.1186/1743-422X-9-210
31. Bachmann MF, Mohsen MO, Zha LS, Vogel M, Speiser DE. SARS-CoV-2 Structural Features may Explain Limited Neutralizing-Antibody Responses. NPJ Vaccines (2021) 6(1):1–5. doi: 10.1038/s41541-020-00264-6
Keywords: SARS-CoV-2, vaccine, virus-like particles, mRNA, COVID-19
Citation: Vogt A-CS, Jörg L, Martina B, Krenger PS, Chang X, Zeltins A, Vogel M, Mohsen MO and Bachmann MF (2022) Virus-Like Particles Are Efficient Tools for Boosting mRNA-Induced Antibodies. Front. Immunol. 13:864718. doi: 10.3389/fimmu.2022.864718
Received: 28 January 2022; Accepted: 05 May 2022;
Published: 16 June 2022.
Edited by:
Allan Randrup Thomsen, University of Copenhagen, DenmarkReviewed by:
Stephanie Longet, University of Oxford, United KingdomKishu Ranjan, Yale University, United States
Copyright © 2022 Vogt, Jörg, Martina, Krenger, Chang, Zeltins, Vogel, Mohsen and Bachmann. This is an open-access article distributed under the terms of the Creative Commons Attribution License (CC BY). The use, distribution or reproduction in other forums is permitted, provided the original author(s) and the copyright owner(s) are credited and that the original publication in this journal is cited, in accordance with accepted academic practice. No use, distribution or reproduction is permitted which does not comply with these terms.
*Correspondence: Anne-Cathrine S. Vogt, YW5uZS1jYXRocmluZS52b2d0QHN0dWRlbnRzLnVuaWJlLmNo; Mona O. Mohsen, bW9uYS5tb2hzZW5AZGJtci51bmliZS5jaA==
†These authors have contributed equally to this work