- 1State Key Laboratory of Cellular Stress Biology, Innovation Center for Cell Signaling Network, School of Life Sciences, Xiamen University, Xiamen, China
- 2Department of Biochemistry, Universidad Autónoma de Madrid (UAM), Instituto de Investigaciones Biomédicas Alberto Sols (CSIC-UAM), Madrid, Spain
- 3Institute of Laboratory Animals, Graduate School of Medicine, Kyoto University, Kyoto, Japan
- 4Department of Immunology and Microbiology, The Scripps Research Institute, La Jolla, CA, United States
A microRNA (miRNA) often regulates the expression of hundreds of target genes. A fundamental question in the field of miRNA research is whether a miRNA exerts its biological function through regulating a small number of key targets or through small changes in the expression of hundreds of target genes. We addressed this issue by performing functional analysis of target genes regulated by miR-148a. We previously identified miR-148a as a critical regulator of B cell central tolerance and found 119 target genes that may mediate its function. We selected 4 of them for validation and demonstrated a regulatory role for Bim, Pten, and Gadd45a in this process. In this study, we performed functional analysis of the other miR-148a target genes in in vitro and in vivo models of B cell central tolerance. Our results show that those additional target genes play a minimal role, if any, in miR-148a-mediated control of B cell central tolerance, suggesting that the function of miRNAs is mediated by a few key target genes. These findings have advanced our understanding of molecular mechanisms underlying miRNA regulation of gene expression and B cell central tolerance.
Introduction
B cells mount humoral responses against pathogens following their recognition of a vast variety of foreign antigens through their B cell receptors (BCRs). The large diversity of BCR specificities required for this is generated through V(D)J recombination of the heavy and light chains of the immunoglobulin (Ig) M during the early pro-B and pre-B stages of B cell development in the bone marrow. The largely random and combinatorial nature of these rearrangements also generates many autoreactive B cells that recognize self-tissues and, thus, can lead to autoimmunity. To test their autoreactivity, IgM-expressing immature B cells are scrutinized by the process of central tolerance. If a B cell is autoreactive, it continues to rearrange its light chain to eliminate self-reactivity through receptor editing. If this process is not successful, the autoreactive cell is deleted from the repertoire by apoptosis. This is known as clonal deletion. If the cell is non-autoreactive or eliminated its autoreactivity through receptor editing, it exits to the periphery to become a mature B cell (1–3). B cell central tolerance is a tightly regulated process, but the molecular mechanisms governing this checkpoint remain largely unexplored. Expanding this knowledge is important because defects in B cell tolerance have been shown in many patients with autoimmune diseases such as systemic lupus erythematosus (SLE), type 1 diabetes, rheumatoid arthritis, and multiple sclerosis (4, 5).
MiRNAs are small non-coding RNAs of approximately 22 nucleotides in length that regulate the expression of protein-coding genes at the post-transcriptional level, resulting in a fast and robust control of virtually all cellular processes, including development, activation, proliferation, differentiation, and apoptosis (6–12). MiRNA expression levels are tightly regulated and highly conserved throughout evolution, which supports their functional importance (13–15). Hundreds of genes can be targeted in any given cell by an individual miRNA through its cognate binding sites on their mRNAs (16, 17). MiRNA studies frequently validate a limited number of predicted or experimentally identified target genes, without examining all potential target genes in a systematic manner. Hence, a fundamental question in the field that remains to be answered is whether miRNAs exert their function in a given cellular context through simultaneous regulation of a large number of target genes or, alternatively, through a few critical ones (11, 16, 18, 19).
In a recent study, we identified miR-148a as the first miRNA that functions as a critical regulator of B cell tolerance using the IgMb-macroself mouse model of B cell tolerance (20). The IgMb-macroself mice ubiquitously express an engineered membrane-bound superantigen reactive to the constant region of IgM heavy chain. In these mice, early B cell development occurs normally, resulting in an immature B cell pool with a large variety of antigen specificities in their bone marrow. However, once immature B cells express IgM molecules on the cell surface, they are recognized by the engineered superantigen. This mimics the recognition of self-antigens by BCR. These “autoreactive” B cells first attempt to eliminate their autoreactivity through receptor editing and then die by apoptosis (clonal deletion), resulting in the absence of B cells in the peripheral lymphoid organs. This murine model provides a robust and clean system to identify novel regulators of B cell tolerance through a hematopoietic and precursor stem cell (HPSC) transduction and reconstitution approach or reconstitution with bone marrow cells from transgenic or knockout mice, with the presence of B cells in the spleens of reconstituted IgMb-macroself mice indicating a break of B cell tolerance. MiR-148a expression is tightly regulated during B cell development and differentiation, and its expression levels frequently increased in lymphocytes of patients with lupus erythematosus and lupus-prone mice even before disease onset (13, 20–22). Increased expression of miR-148a in B cells compromised B cell tolerance in IgMb-macroself mice and facilitated lethal autoimmunity in MRL-lpr mice, a classical mouse model of lupus. Importantly, this effect was recapitulated in WEHI-231 cells, an in vitro model of clonal deletion. Moreover, 119 protein coding genes were identified as potential miR-148a target genes. Four of them were further investigated and 3 of them (Bcl2l11, Pten and Gadd45a) were demonstrated to be bona fide miR-148a target genes and regulate B cell central tolerance in IgMb-macroself mice (20).
However, this study did not investigate the potential contribution of the other 115 target genes to miR-148a regulation of B cell tolerance. This provided the opportunity to perform a systematic and unbiased functional analysis of a miRNA targetome in B cell tolerance to determine whether this miRNA exerts its biological function through a large number or only a few key target genes in this cellular context. No study addressing this question has been previously conducted to our knowledge. By performing in vitro and in vivo functional analysis of miR-148a target genes in B cell tolerance, this work aims to advance our current understanding of miRNA biology in B cell tolerance, with potential implications for the therapy of autoimmune diseases.
Materials and Methods
Mice
B4galt5fl/+ mice were obtained from Masahide Asano (23). B4galt5fl/+ mice were bred with Mb1Cre mice (The Jackson Laboratory, Stock 020505) to obtain Mb1Cre;B4galt5fl/fl mice. Nsd1fl/+ mice were purchased from Gempharmatech (T018412), bred with Mb1Cre mice to obtain Mb1Cre;Nsd1fl/fl mice. IgMb-macroself mice were generated by David Nemazee´s laboratory (The Scripps Research Institute, La Jolla, CA) as previously described (20, 24). All mouse strains were housed and bred in pathogen-free conditions.
Cell Culture
The immature B cell line WEHI-231 was originally obtained from David Nemazee (The Scripps Research Institute, La Jolla, CA) and cultured in DMEM (Gibco, Cat:10569-044, containing GlutaMAX and Sodium Pyruvate) supplemented with 10% FBS, 1% Pen/Strep, and 50 μM 2-mercaptoethanol. The HEK293T cell line was originally obtained from Jun Lu (Yale University, New Haven, CT) and cultured in DMEM (Gibco, Cat:10569-044, containing GlutaMAX and Sodium Pyruvate) supplemented with 10% FBS and 1% Pen/Strep.
Retroviral TransOMIC-shRNA Library Generation
The TransOMIC-shRNA sublibrary was obtained from the RNAi Core at La Jolla Institute for Allergy and Immunology (La Jolla, CA), amplified from a glycerol stock, and DNA prepared using an endotoxin-free DNA purification kit (K210001, ThermoFisher). Retrovirus preparations were obtained as previously described (20). Briefly, packaging vectors GAG-POL, VSV-G and individual shRNA-encoding or empty plasmids were co-transfected into HEK293T cells using TransIT-LT1 (Mirus) following the manufacturer´s instructions. Supernatants containing retrovirus were collected after 48 and 72 hours, filtered through a 0.45μm low protein retention filter, aliquoted and stored at −80°C until use.
Flow Cytometry Analysis
For WEHI-231 apoptosis analysis, the active Caspase 3 kit (C92-605, BD Pharmingen) was used following the manufacturer’s instructions. Briefly, cells were fixed and permeabilized with Fix/Perm Buffer for 30 min at 4°C, stained with an antibody for active Caspase 3 labelled with APC for 30 min at 4°C, and analyzed by flow cytometry. For splenic B cell analysis of IgMb-macroself mice reconstituted with retrovirus-transduced HPSCs or bone marrow cells from donor mice, single-cell suspensions were obtained by disaggregating spleens through a 0.70μm cell strainer, depleted of red blood cells by incubation for 5 min RT with ACK lysis buffer, stained with CD45.1-PerCP/Cy5.5 (A20; Biolegend), CD45.2-PE (104; Biolegend), IgM-APC (RMM-1; Biolegend) and CD19 PE/Cy7 (1D3, eBioscience) for 20 min at 4°C, and analyzed by flow cytometry. Data were acquired on FACS Calibur, Novocyte or Fortessa X-20 flow cytometers, and analyzed with Flowjo Version10.7.1.
In Vitro Screen of Stable shRNA WEHI- 231 Cell Lines
To generate stable WEHI-231 cell lines, 0.5x106 cells were transduced with retroviruses encoding individual shRNAs in 24-well plates overnight. Media was replaced with fresh one the next morning. After 24 hours of culture, 10µg/ml puromycin was added to medium to select transduced cells for 5 days. After selection, stable cell lines were maintained in the presence of 2µg/ml puromycin in culture medium. Stable WEHI-231 cells were stimulated with 2µg/ml anti-IgM (115-006-020, µ chain specific, Jackson) for 72 hours, stained for intracellular active caspase-3, and analyzed by flow cytometry as described above.
In Vivo Validation of Positive Hits in IgMb-Macroself Mice
For in vivo validation, plasmids encoding positive shRNA hits were modified to delete the puromycin resistance gene, amplified using an endotoxin-free DNA purification kit (12362, Qiagen), and packaged to generate retroviral supernatants as described above. For HPSC enrichment, wild type C57BL/6J mice were treated with 4 mg/mouse 5-fluorouracil (5-FU) (Sigma) by intravenous (iv) injection, followed by bone marrow cell collection 5 days later. HPSCs were stimulated with SCF (100ng/ml, Novoprotein), IL-3 (20ng/ml, Novoprotein) and IL-6 (25ng/ml, Novoprotein) for 36 hours before transduction. Retrovirus transduction of HPSCs was performed by spinoculation (2000rpm, 2 hours without brake) and media was replaced 4 hours later. Transduced cells were harvested after 20 hours for intravenous transfer into IgMb-macroself mice. Alternatively, freshly isolated BM cells from mutant mice were depleted of red blood cells using ACK lysis buffer, counted, and resuspended in PBS. 1-10x106 retrovirally transduced HPSCs or freshly isolated BM cells were iv injected into recipient mice. IgMb-macroself mice were irradiated with 6 Gy by X-Ray before reconstitution. Recipient mice were euthanized and their splenocytes analyzed by flow cytometry 8 weeks after reconstitution.
Statistical Analysis
Data were analyzed with an unpaired two-tailed Student’s t test or one-way ANOVA using the Prism software Version9 as indicated in the figure legends. Statistical significance was set at P<0.05.
Results
In Vitro Functional Screen Platform Development
WEHI-231 is a commonly used immature B cell line that undergoes cell cycle arrest at G0/G1 phase and apoptosis upon anti-IgM-induced BCR crosslinking (25). This mimics the recognition of self-antigens by and subsequent clonal deletion (apoptosis) of autoreactive B cells that occur in vivo under homeostatic conditions. We previously found that miR-148a protects WEHI-231 cells from BCR engagement-induced apoptosis triggered by anti-IgM stimulation. This recapitulated the role of miR-148a in B cell central tolerance, where it prevented clonal deletion of autoreactive B cells following self-antigen encounter (20). We further identified 119 protein-coding genes that contained predicted miR-148a binding sites in their mRNAs and showed at least 1.5-fold reduction in their mRNA levels upon miR-148a overexpression. Four of them were chosen for experimental validation. Among them, Bcl2l11 (encoding Bim), Pten and Gadd45a were validated as regulators of B cell tolerance, while Tnfrsf1b did not play any significant role in this process (20). In this study, we investigated the function of the other miR-148a target genes in B cell tolerance, first in the WEHI-231 system, followed by validation in animal models (Figure 1A). To this end, a retroviral TransOMIC shRNA library containing 288 shRNAs (Supplementary Table 1) covering 106 miR-148a target genes, with one to nine shRNAs for each gene, was generated. An empty vector (EV) and a vector encoding an shRNA against Bcl2l11 (shRNA-Bcl2l11) were included as negative and positive controls. The backbone vector used to generate this library contains a shRNA cassette followed by a puromycin resistance gene that enables puromycin selection of transduced cells (Figure 1B). Retroviruses were produced from individual constructs to generate the shRNA retroviral library as previously described (20).
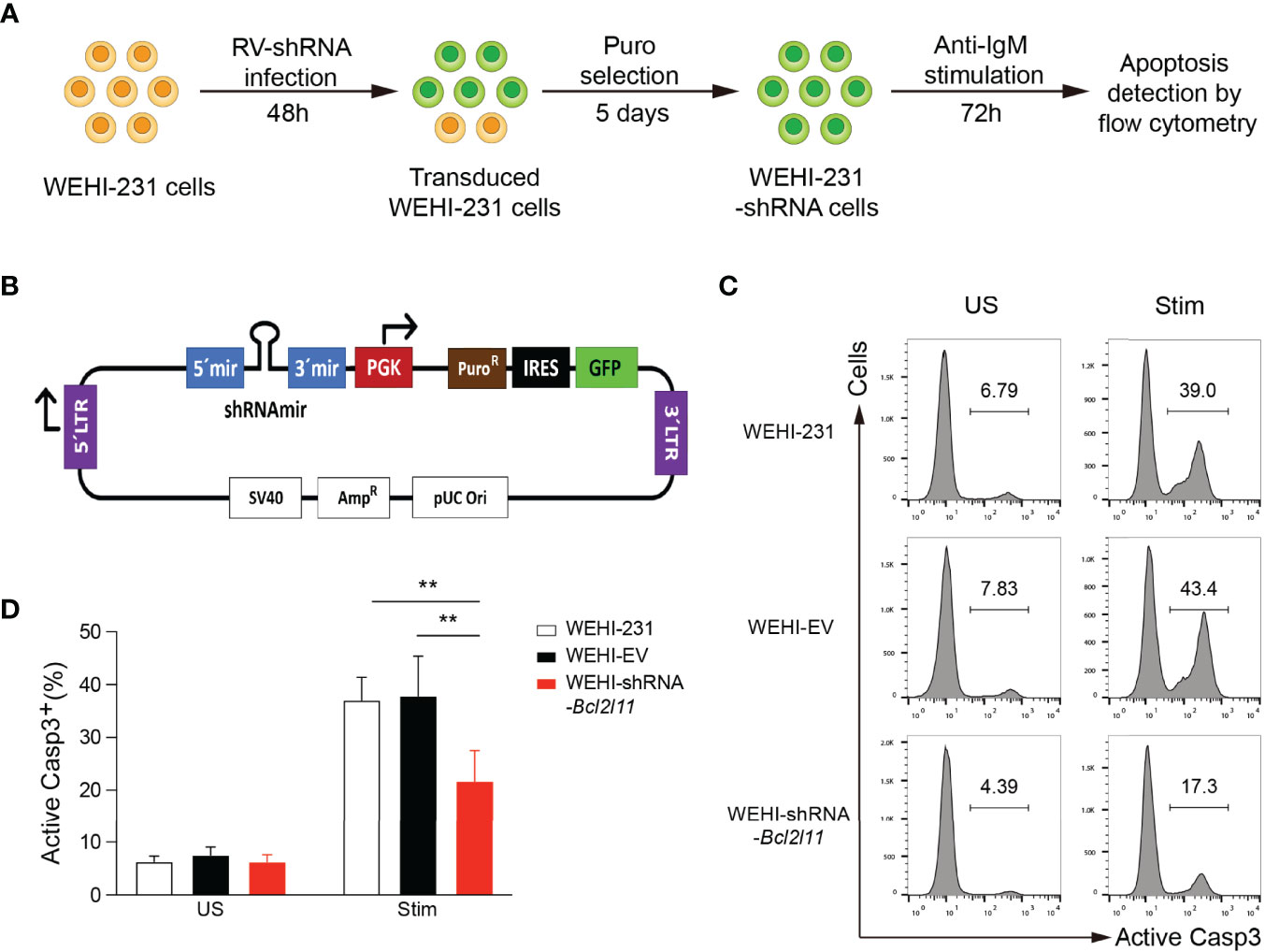
Figure 1 In vitro functional screen platform. (A) Screen strategy outline. WEHI-231 cells were transduced with retroviruses encoding shRNAs. After 48 hours, transduced cells were selected with puromycin for 5 days, stimulated with anti-IgM for 72 hours, stained for active caspase 3 (Casp3), and analyzed by flow cytometry. (B) Scheme of the TransOMIC library pMLP vector including the shRNA and puromycin selection cassettes. (C) Flow cytometry histograms showing apoptosis of control non-transduced WEHI-231, WEHI-EV (empty vector) and WEHI–shRNA-Bcl2l11 cells stimulated for 72 hours with 2µg/ml anti-IgM (Stim) or left unstimulated (US), assessed by staining of active caspase 3. (D) Frequency of active caspase-3-positive (Casp3+) cells among total cells as in (C). The results are representative of 4 independent experiments. Data are represented as mean + SD. Statistical analysis was performed with a one-way ANOVA test with **P < 0.01.
The strategy for our in vitro functional screen is outlined in Figure 1A. WEHI-231 cells were transduced with retroviruses expressing individual shRNAs. After 48 hours, transduced cells were selected with puromycin for 5 days. Half of the cells were then stimulated with anti-IgM (2μg/ml) for 72 hours to induce BCR-engagement induced apoptosis, while the other half were left without stimulation and served as control. After 72 hours of stimulation, cells were harvested, stained for active Caspase 3, and analyzed by flow cytometry to quantify the percentage of apoptotic cells as a readout for clonal deletion. WEHI-231 cells transduced with empty retroviruses (WEHI-EV) and with retroviruses encoding shRNA-Bcl2l11 (WEHI-shRNA-Bcl2l11) were included in every independent experiment as controls.
The feasibility of this approach was first validated with WEHI-EV and WEHI-shRNA-Bcl2l11 controls. As expected, a significant fraction of non-transduced and WEHI-EV-transduced WEHI-231 cells died by apoptosis when stimulated with anti-IgM, and cell death was largely abrogated in shRNA-Bcl2l11-transduced cells (Figures 1C, D). This protection from clonal deletion by decreasing the expression levels of Bim recapitulated our previous results obtained in the IgMb-macroself B cell tolerance mouse model (20).
Functional Screen of the miR-148a Targetome shRNA Library
Once the in vitro screen platform had been validated, 288 WEHI-231 stable cell lines expressing individual shRNAs for miR-148a target genes were generated. WEHI-231 cells were transduced with retroviruses encoding individual shRNAs, selected with puromycin, stimulated with anti-IgM, and analyzed for apoptosis as described above (Figure 1A). The effect of shRNAs that significantly impaired BCR engagement-induced apoptosis were confirmed in three independent experiments.
Among the 106 miR-148a target genes tested, nearly half of them showed an effect in protecting WEHI-231 cells from BCR-induced apoptosis when knocked down by shRNAs, with protection ratios ranging from 5% to 65% (Figure 2A). Among those, 22 shRNAs, which correspond to 13 genes, showed an average protection effect greater than 30% (Figure 2B and Figure 3). Of note, knockdown of B4galt5, Phip and Nsd1 showed the most robust protection against BCR engagement-induced apoptosis, with all shRNAs showing consistent effect (Figures 2C, D).
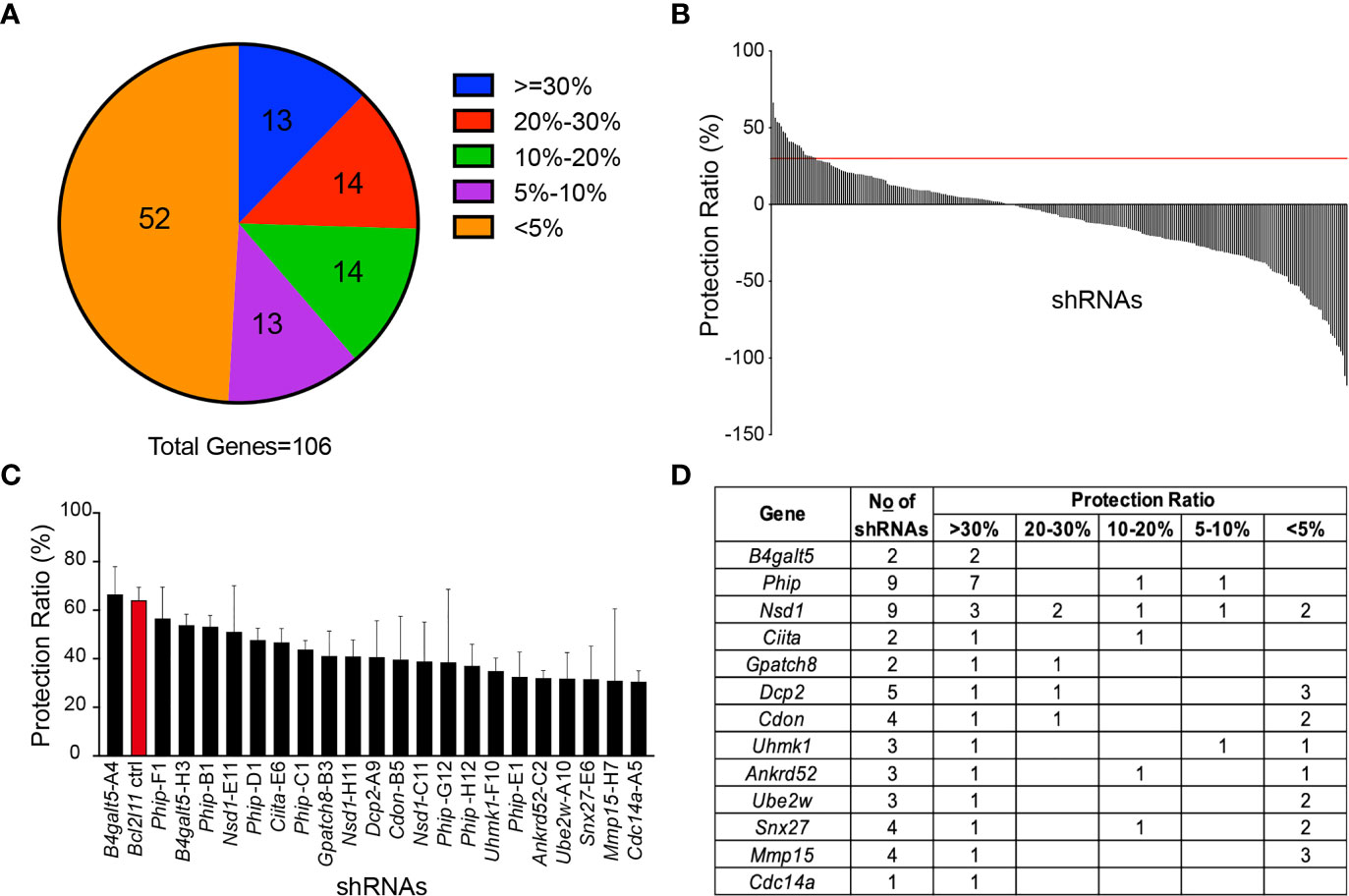
Figure 2 Functional screen of the TransOMIC shRNA library. (A) Pie chart summarizing the screen results. Numbers inside the chart indicate the number of genes with shRNAs protecting WEHI-231 cells from BCR engagement-induced apoptosis at various ratios. The protection ratio = 1 – (active caspase 3+ % of WEHI-shRNA)/(active caspase 3+ % of WEHI-EV) under stimulated conditions. (B) Bar graph showing protection ratios of individual shRNAs. The red line indicates the 30% protection threshold. (C) Bar graph detailing individual shRNAs with >30% protection ratios. (D) List of genes with shRNAs exhibiting >30% protection ratios. Data are representative of 2 independent experiments for B4galt5-H3 and Phip-E1, 3 for B4galt5-A4, Phip-F1, Phip-B1, Nsd1-E11, Phip-D1, Ciita-E6, Phip-C1, Nsd1-H11, Dcp2-A9, Cdon-B5, Phip-G12 and Cdc14a-A5, 4 for Bcl2l11, BGpatch8-B3, Nsd1-C11, Phip-H12, Uhmk1-F10, Ankrd52-C2, Ube2w-A10 and Snx27-E6, and 5 for Mmp15-H7. Graph bars indicate mean + SD.
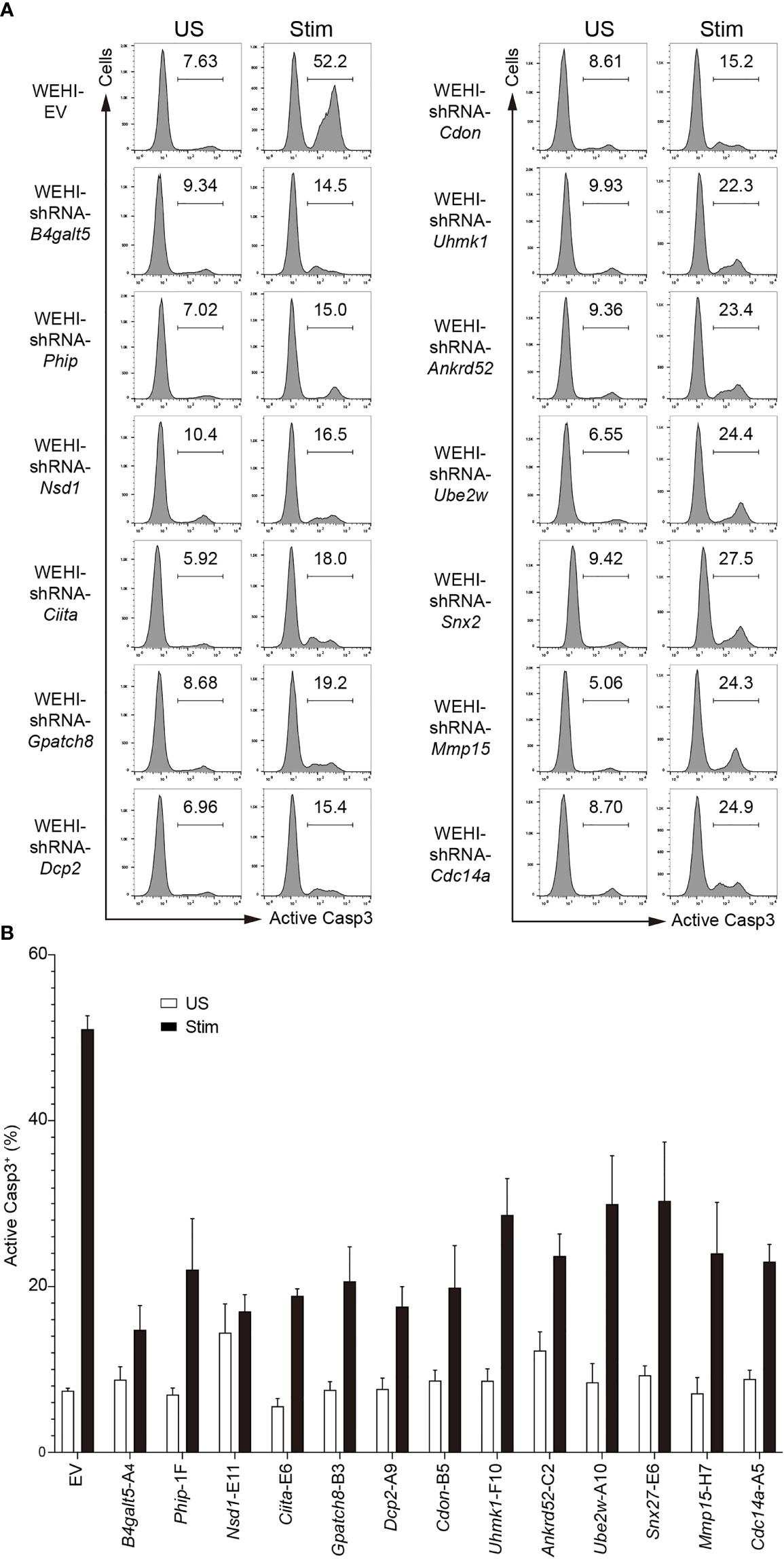
Figure 3 Effect of the top hit shRNAs in the in vitro functional screen. (A) Representative flow cytometry histograms showing apoptosis of WEHI-231 transduced with retroviruses encoding shRNAs or empty vector (EV), stimulated for 72 hours with 2µg/ml anti-IgM (Stim) or left unstimulated (US), followed by flow cytometry analysis of active caspase 3 (Casp3). (B) Frequency of active caspase-3-positive (Casp3+) cells among total cells in (A). Every independent experiment included an internal WEHI-EV control. A representative WEHI-EV control experiment is shown. Results are pooled from 3 independent experiments for EV, Phip-F1, Nsd1-E11, Ciita-E6, Dcp2-A9, Cdon-B5 and Cdc14a-A5, 4 for Gpatch8-B3, Uhmk1-F10, Ankrd52-C2, Ube2w-A10 and Snx27-E6, and 5 for B4galt5-A4 and Mmp15-H7. Graph bars indicate mean + SD.
In Vivo Analysis of Positive Hits in IgMb-Macroself Mouse Model
We next investigated the function of those positive hits in regulating B cell tolerance in vivo, by retroviral transduction of HSPCs and reconstitution of IgMb-macroself mice. Briefly, C57BL/6J mice were treated with 5-fluorouracil (5-FU) for 5 days. HSPCs from treated mice were enriched, transduced with shRNA-encoding retroviruses, and transferred to sublethally irradiated IgMb-macroself mice. After 8 weeks, the presence of peripheral B cells was analyzed in the spleen of these reconstituted mice by flow cytometry (Figure 4A). We set the presence of 5% splenic B cells as the threshold to consider an effect of breaking B cell tolerance because irradiated IgMb-macroself mice reconstituted with wild-type C57BL/6J bone marrow cells show some background of splenic B cells after 8 weeks, up to this percentage in some experiments.
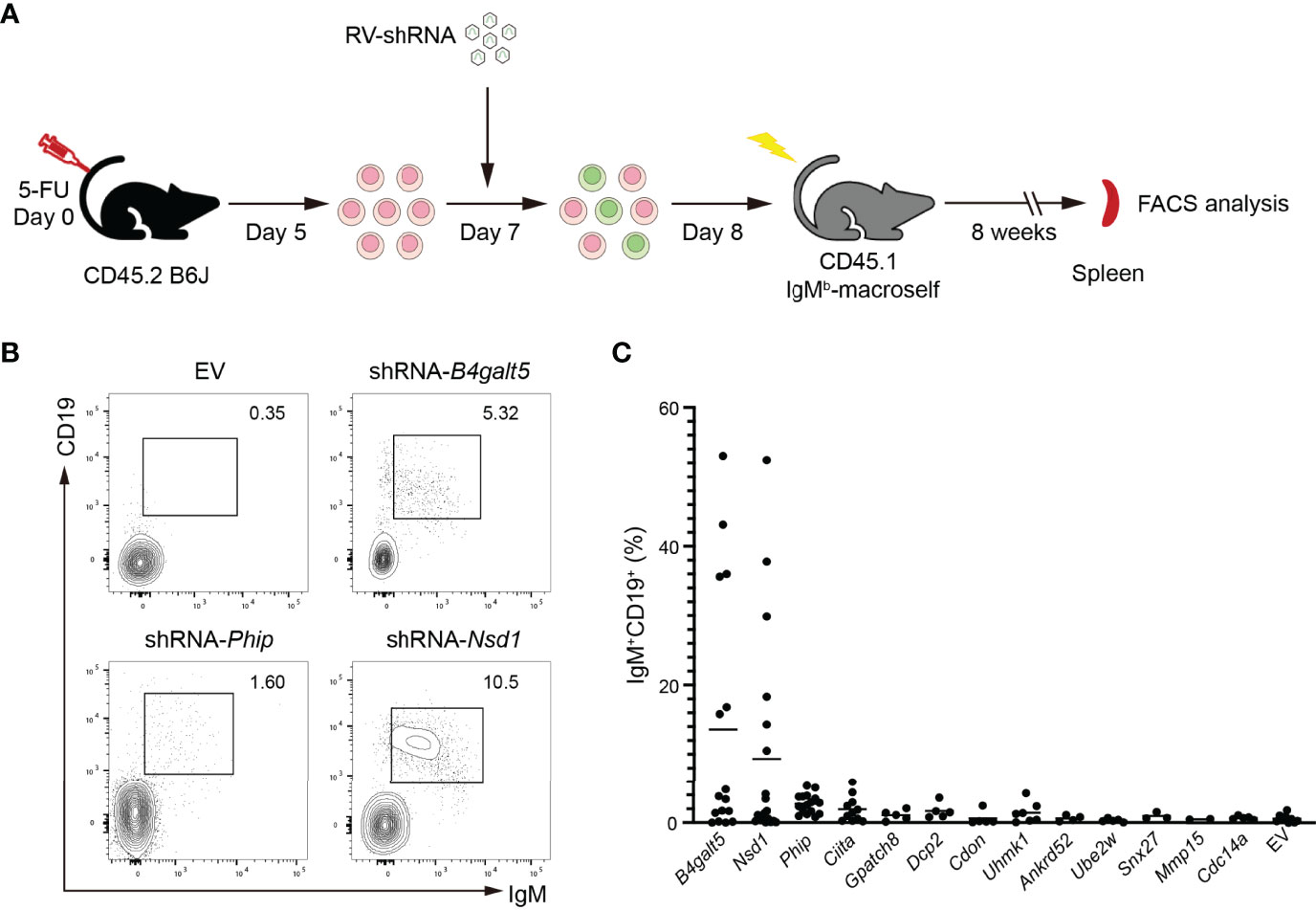
Figure 4 In vivo validation of positive hits in IgMb-macroself mice. (A) Experimental outline of the in vivo validation strategy. HPSCs were enriched from 5-FU-treated C57BL/6J, transduced with retroviruses encoding individual shRNAs or empty vector (EV), and used to reconstitute sublethally irradiated IgMb-macroself mice. After 8 weeks, the presence of splenic B cells was analyzed in these mice by flow cytometry to determine a potential break of B cell tolerance. (B) Representative flow cytometry plots of splenocytes from IgMb-macroself recipient mice reconstituted with donor HSPCs transduced with retrovirus encoding the ‘top hit’ shRNAs (B4galt5 and Nsd1) or no shRNA (EV, empty vector) at terminal analysis. Numbers adjacent to outlined areas indicate percentage of IgM+CD19+ cells (B cells) among splenocytes. (C) Graph summarizing percentages of IgM+CD19+ splenic B cells in IgMb-macroself mouse model reconstituted with HPSCs transduced with top hit shRNAs. Each dot indicates an individual mouse, and the horizontal lines indicate the mean value of mice analyzed for each group with n=16 for B4galt5, n=19 for Nsd1, n=16 for Phip, n=11 for Ciita, n=7 for Uhmk1, n=5 for Gpatch8, Dcp2, Cdon,Ube2w and Cdc14a, n=4 for Ankrd52, n=3 for Snx27, n=2 for Mmp15, and n=9 for EV. No statistically significant differences were found.
We observed a significant number of splenic B cells in 6 out of 16 (37%) and 6 out of 19 (32%) IgMb-macroself mice reconstituted with HPSCs transduced with shRNA-B4galt5 and shRNA-Nsd1, respectively. Of note, a combination of two different shRNAs for B4galt5 was required for this effect, as transduction of HSPCs with individual shRNAs failed to break B cell tolerance (Supplementary Figure 1). The remaining mice did not show compromised B cell tolerance (Figures 4B, C). Although shRNA-Phip showed a significant protection against BCR engagement-induced apoptosis in WEHI-231 cells, those shRNAs did not show much effect in IgMb-macroself mice, suggesting that Phip does not play any significant role in regulating B cell tolerance (Figures 4B, C). All other hits from the screen failed to break B cell tolerance in vivo.
In Vivo Validation by a Genetic Approach
To confirm that B4galt5 and Nsd1 indeed regulate B cell tolerance, we obtained conditional knockout (KO) mice with specific deletion of these genes in B cells. B4galt5fl/+ and Nsd1fl/+ mice were bred with Mb1Cre mice to drive specific deletion in the B cell lineage at the early pro-B cell stage. Deletion of these genes was validated in the resulting Mb1Cre;B4galt5fl/fl and Mb1Cre;Nsd1fl/fl mice by qRT-PCR analysis of splenic B cells (Figures 5A, B). Bone marrow cells from Mb1Cre;B4galt5fl/fl and Mb1Cre;Nsd1fl/fl mice were used to reconstitute irradiated IgMb-macroself mice. Splenic B cells of recipient mice were analyzed 8 weeks after reconstitution. No accumulation of splenic B cells was observed in IgMb-macroself mice reconstituted with bone marrow cells from Mb1Cre;B4galt5fl/fl, Mb1Cre;Nsd1fl/fl or their littermate controls B4galt5fl/fl and Nsd1fl/fl at terminal analysis (Figures 5C, G). Concurrently, bone marrow cells from heterozygous Mb1Cre;B4galt5fl/+ also failed to break B cell tolerance in IgMb-macroself mice (Supplementary Figure 2). This indicates that B4galt5 and Nsd1 do not play significant roles in the regulation of B cell tolerance. Taken together, our previous and this studies show that Bcl2l11, Pten and Gadd45a play key roles in mediating miR-148a-regulation of B cell central tolerance, with minimal, if any, contribution from the other target genes (20).
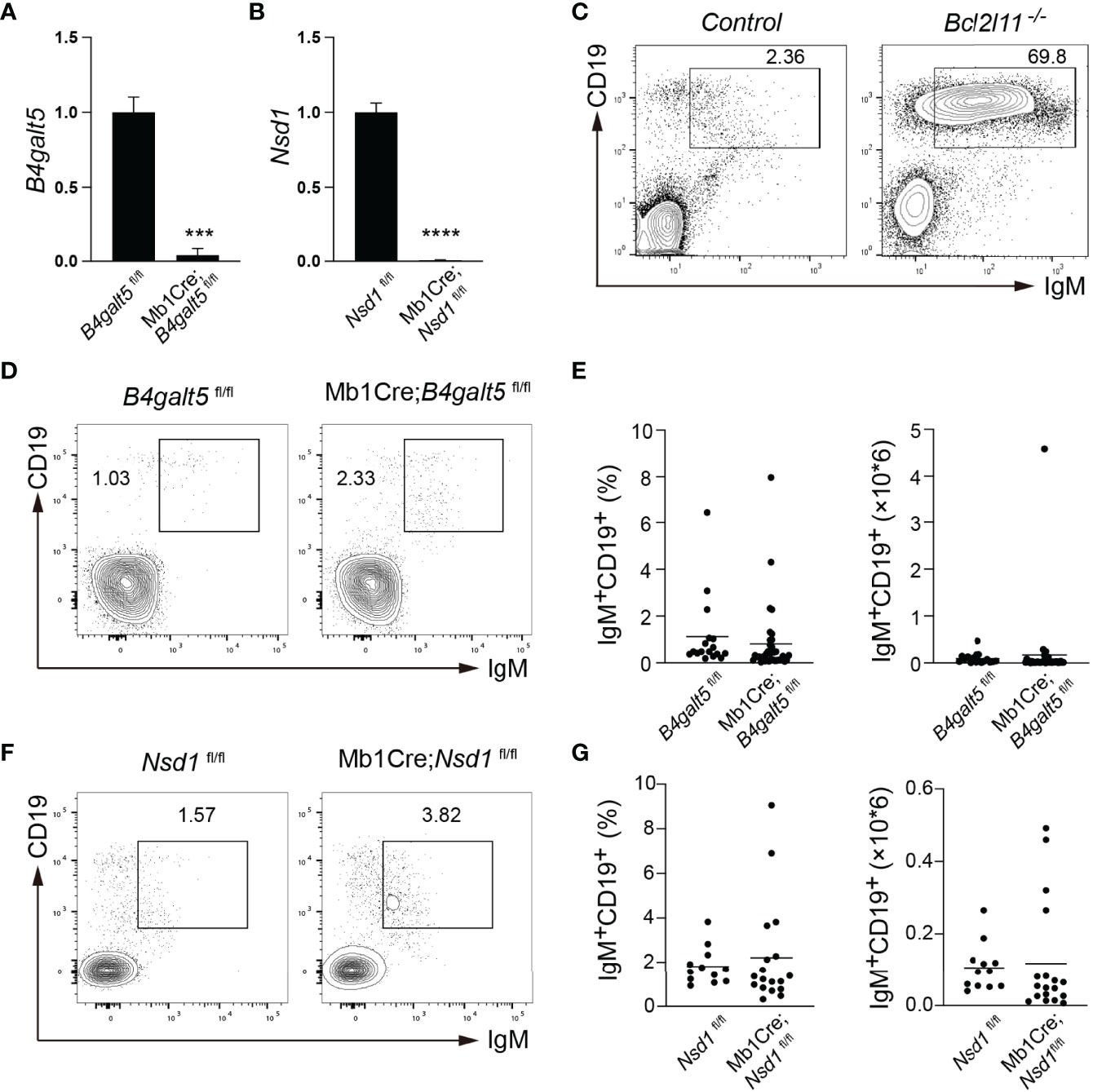
Figure 5 In vivo validation in IgMb-macroself mice with bone marrow cells from conditional knockout mice. (A, B) Expression levels of B4galt5 (A) and Nsd1 (B) in splenic B cells from Mb1Cre;B4galt5fl/fl and Mb1Cre;Nsd1fl/fl mice analyzed by qRT-PCR. Bar graph shows mean + SD. Data are representative of 2 independent experiments using technical triplicates. (C) Representative flow cytometry plots analyzing splenocytes from IgMb-macroself mice reconstituted with bone marrow cells from C57BL/6J mice and Bcl2l11-/- mice at terminal analysis. Numbers adjacent to outlined areas indicate percentage of IgM+CD19+ B cells among splenocytes. (D) Representative flow cytometry plots analyzing splenocytes from IgMb-macroself mice reconstituted with bone marrow cells from B4galt5fl/fl and Mb1Cre;B4galt5fl/fl mice at terminal analysis. Numbers adjacent to outlined areas indicate percentage of IgM+CD19+ B cells among splenocytes. (E) Graphs summarizing percentages and numbers of IgM+CD19+ splenic B cells of mice analyzed in (D). (F) Representative flow cytometry analysis of splenocytes from IgMb-macroself mice reconstituted with bone marrow cells from Nsd1fl/fl and Mb1Cre;Nsdfl/fl mice at terminal analysis, with numbers adjacent to outlined areas indicating percentage of IgM+CD19+ B cells among splenocytes. (G) Graphs summarizing percentages and numbers of IgM+CD19+ splenic B cells of mice analyzed in (D). Data in (E) and (G) are pooled from 3 and 2 independent experiments, respectively with n=17 for B4galt5fl/fl, n=41 for Mb1Cre;B4galt5fl/fl, n=12 for Nsd1fl/fl, and n=18 for Mb1Cre;Nsd1fl/fl mice. Data in (C) are adapted from (20). Statistical analysis was performed with an unpaired two-tailed Student’s T-test with ***P < 0.001 and ****P < 0.0001. No statistically significant differences were found in (D, F).
Discussion
B cell tolerance mechanisms are essential for the generation of a vast pool of B cells that enable the defense against a large variety of pathogens without attacking self-tissues. When B cell tolerance checkpoints are compromised, the development of autoimmune diseases ensue (1, 6, 20, 26–33). Aiming to advance our knowledge on B cell tolerance, we investigated the function of more than one hundred miR-148a target genes in this process.
Our previous study showed that elevated expression of miR-148a impaired BCR engagement-induced apoptosis in WEHI-231 cells, and that this was recapitulated in IgMb-macroself mice, suggesting that WEHI-231 cells are a good in vitro model for studying B cell central tolerance. In this study, we performed initial screen of miR-148a target genes in WEHI-231 cells and identified 13 genes whose knockdown protected BCR engagement-induced apoptosis by more than 30%. While this murine immature B cell line have proven useful to advance our understanding of B cell tolerance, it does not fully recapitulate the behavior of primary immature B cells in mice, in which cells interact with other cell subsets from their environment such as bone marrow stromal cells. Further validation of the screen hits by retroviral transduction and bone marrow reconstitution of IgMb-macroself mice showed that shRNA-mediated knockdown of two of those 13 genes, B4galt5 or Nsd1, led to break of B cell tolerance in a fraction of IgMb-macroself mice. When irradiated IgMb-macroself mice were reconstituted with bone marrow cells from mutant mice with B cell-specific deletion of B4galt5 or Nsd1, very few B cells escaped B cell central tolerance. Therefore, none of those 13 genes whose knockdown protected BCR engagement-induced apoptosis in WEHI-231 cells plays important roles in regulating B cell central tolerance. We speculate that the escape of B cell tolerance in some of the IgMb-macroself mice reconstituted with HPSCs transduced with retroviruses encoding shRNAs for B4galt5 or Nsd1 was due to off-target effect of these shRNAs.
MiRNAs bind target gene mRNAs mainly through pairing with their seed regions, allowing for the regulation of hundreds of target genes by one miRNA. Exactly how many targets mediate the function of a miRNA has been hotly debated. Cellular context-dependence of miRNA functions adds another layer of complexity to this issue (16, 18, 34, 35). For example, miR-148a regulates antigen presentation of TLR-triggered dendritic cells (36), osteoclastogenesis of CD14+ peripheral blood mononuclear cells (37), LDL receptor activity in human hepatic cells (38), and functions as a tumor suppressor miRNA in various types of cancers including gastric, colorectal, hepatocellular and pancreatic cancers (39–42). How the same miRNA exerts such diverse functions in different cell types remains elusive. It is possible that these differences rely on the differential gene expression programs of each individual cell type and that miRNA-mediated regulation of target genes is only efficient when target genes are expressed at appropriate levels in the cell type of interest. Another possibility is that only a small number of the hundreds of target genes regulated by a given miRNA are both functionally important and sensitive to changes in their cellular concentrations in any given cell type. Those genes would be the key target genes mediating the function of this miRNA in this particular cellular context. In the context of B cell central tolerance, three key target genes (i.e. Bcl2l11, Pten and Gadd45a) drive the function of miR-148a upon engagement of their BCRs with anti-IgM, with a minor contribution, if any, from the other one hundred or so target genes. Further exploring if miR-148a functions similarly or not in B cells receiving different stimuli such as TLR agonist or anti-CD40 and anti-IgM would be of interest (21, 43–45).
Our results support the concept that miRNAs exert their regulatory functions through a limited number of key target genes. In future studies, it would be interesting to perform functional analysis of miR-148a target genes in other cellular contexts to find out whether the same or different sets of key target genes mediate the function of this miRNA in different types of cells.
Data Availability Statement
The raw data supporting the conclusions of this article will be made available by the authors, without undue reservation.
Ethics Statement
The animal study was reviewed and approved by Animal Care and Use Committees of Xiamen University and Autonoma University of Madrid.
Author Contributions
AG-M and CX designed and supervised the project. FM performed most of the experiments, analyzed the data and prepared the figures. YZ, RB-C, WY, AG-M, and MA performed some experiments. ZH and RB-C helped with data analysis and manuscript writing. AG-M, FM, ZH, and CX wrote the manuscript. All authors contributed to the article and approved the submitted version.
Funding
This work was supported by the grants RTI2018-100008-A-I00 funded by Spanish Ministry of Science and Innovation MCIN/AEI/10.13039/501100011033/and FEDER, SI1-PJI-2019-00241 from Community of Madrid (Spain), merit award RyC-21155 funded by Spanish Ministry of Science and Innovation MCIN/AEI/10.13039/501100011033 and FSE to AG-M, and by the National Natural Science Foundation of China (81830047 to CX).
Conflict of Interest
The authors declare that the research was conducted in the absence of any commercial or financial relationships that could be construed as a potential conflict of interest.
Publisher’s Note
All claims expressed in this article are solely those of the authors and do not necessarily represent those of their affiliated organizations, or those of the publisher, the editors and the reviewers. Any product that may be evaluated in this article, or claim that may be made by its manufacturer, is not guaranteed or endorsed by the publisher.
Supplementary Material
The Supplementary Material for this article can be found online at: https://www.frontiersin.org/articles/10.3389/fimmu.2022.861655/full#supplementary-material
Supplementary Table 1 | List of the shRNA sequences encoded by the TransOMIC-shRNA library.
Supplementary Figure 1 | Retroviral transduction of HSPCs with individual shRNAs for B4galt5 does not induce a break of B cell tolerance. (A) Representative flow cytometry plots showing IgM+CD19+ splenic B cells of IgMb-macroself mice reconstituted with HSPCs transduced with an empty retrovirus, retroviruses encoding shRNA-B4galt5-H3, shRNA-B4galt5-A4 or a combination of both shRNAs (H3+A4), as indicated, 8 weeks after reconstitution. The total amount of viral particles was kept constant in all conditions. (B) Graph summarizing percentage of IgM+CD19+ splenic B cells among total cells of mice analyzed in (A). Data in (B) are pooled from 4 independent experiments with n=16 for shRNA-B4galt5-(H3+A4), n=3 for shRNA-B4galt5-(H3+A4), n=4 for shRNA-B4Galt5-A4, and n=9 for EV. No statistical differences were found.
Supplementary Figure 2 | Heterozigous deletion of B4Galt5 does not compromise B cell tolerance. (A) Representative flow cytometry plots showing IgM+CD19+ splenic B cells of IgMb-macroself mice reconstituted with bone marrow cells from B4galt5fl/fl and Mb1Cre;B4galt5fl/+ mice, as indicated, 8 weeks after reconstitution. (B) Graph showing percentage of IgM+CD19+ splenic B cells among total cells in mice analyzed in (A). n=4 for B4galt5fl/fl and n=6 for Mb1Cre;B4galt5fl/+ mice. No statistical differences were found.
References
1. Nemazee D. Mechanisms of Central Tolerance for B Cells. Nat Rev Immunol (2017) 17(5):281–94. doi: 10.1038/nri.2017.19
2. Melchers F. Checkpoints That Control B Cell Development. J Clin Invest (2015) 125(6):2203–10. doi: 10.1172/JCI78083
3. Pieper K, Grimbacher B, Eibel H. B-Cell Biology and Development. J Allergy Clin Immunol (2013) 131(4):959–71. doi: 10.1016/j.jaci.2013.01.046
4. Yap DYH, Chan TM. B Cell Abnormalities in Systemic Lupus Erythematosus and Lupus Nephritis-Role in Pathogenesis and Effect of Immunosuppressive Treatments. Int J Mol Sci (2019) 20(24):6231. doi: 10.3390/ijms20246231
5. Dörner T, Giesecke C, Lipsky PE. Mechanisms of B Cell Autoimmunity in SLE. (2011) 13(5):1–12. doi: 10.1186/ar3433
6. Coffre M, Koralov SB. miRNAs in B Cell Development and Lymphomagenesis. Trends Mol Med (2017) 23(8):721–36. doi: 10.1016/j.molmed.2017.06.001
7. Zheng B, Xi Z, Liu R, Yin W, Sui Z, Ren B, et al. The Function of MicroRNAs in B-Cell Development, Lymphoma, and Their Potential in Clinical Practice. Front Immunol (2018) 9:936. doi: 10.3389/fimmu.2018.00936
8. Adams BD, Guo S, Bai H, Guo Y, Megyola CM, Cheng J, et al. An In Vivo Functional Screen Uncovers miR-150-Mediated Regulation of Hematopoietic Injury Response. Cell Rep (2012) 2(4):1048–60. doi: 10.1016/j.celrep.2012.09.014
9. Shaffi SK, Galas D, Etheridge A, Argyropoulos C. Role of MicroRNAs in Renal Parenchymal Diseases-A New Dimension. Int J Mol Sci (2018) 19(6):1797. doi: 10.3390/ijms19061797
10. Yan S, Yim LY, Lu L, Lau CS, Chan VSF. MicroRNA Regulation in Systemic Lupus Erythematosus Pathogenesis. Immune Netw (2014) 14(3):138–48. doi: 10.4110/in.2014.14.3.138
11. Ebert MS, Sharp PA. Roles for microRNAs in Conferring Robustness to Biological Processes. Cell (2012) 149(3):515–24. doi: 10.1016/j.cell.2012.04.005
12. Friedman RC, Farh KKH, Burge CB, Bartel DP. Most Mammalian mRNAs are Conserved Targets of microRNAs. Genome Res (2009) 19(1):92–105. doi: 10.1101/gr.082701.108
13. Basso K, Sumazin P, Morozov P, Schneider C, Maute RL, Kitagawa Y, et al. Identification of the Human Mature B Cell Mirnome. Immunity (2009) 30(5):744–52. doi: 10.1016/j.immuni.2009.03.017
14. Zhang J, Jima DD, Jacobs C, Fischer R, Gottwein E, Huang G, et al. Patterns of microRNA Expression Characterize Stages of Human B-Cell Differentiation. Blood (2009) 113(19):4586–94. doi: 10.1182/blood-2008-09-178186
15. Jensen K, Brusletto BS, Aass HCD, Olstad OK, Kierulf P, Gautvik KM. Transcriptional Profiling of mRNAs and microRNAs in Human Bone Marrow Precursor B Cells Identifies Subset- and Age-Specific Variations. PloS One (2013) 8(7):e70721. doi: 10.1371/journal.pone.0070721
16. Bartel DP. MicroRNAs: Target Recognition and Regulatory Functions. Cell (2009) 136(2):215–33. doi: 10.1016/j.cell.2009.01.002
17. Bushati N, Cohen SM. microRNA Functions. Annu Rev Cell Dev Biol (2007) 23:175–205. doi: 10.1146/annurev.cellbio.23.090506.123406
18. Xiao C, Rajewsky K. MicroRNA Control in the Immune System: Basic Principles. Cell (2009) 136(1):26–36. doi: 10.1016/j.cell.2008.12.027
19. Vigorito E, Kohlhaas S, Lu D, Leyland R. miR-155: An Ancient Regulator of the Immune System. Immunol Rev (2013) 253(1):146–57. doi: 10.1111/imr.12057
20. Gonzalez-Martin A, Adams BD, Lai M, Shepherd J, Salvador-Bernaldez M, Salvador JM, et al, et al. The microRNA miR-148a Functions as a Critical Regulator of B Cell Tolerance and Autoimmunity. Nat Immunol (2016) 17(4):433–40. doi: 10.1038/ni.3385
21. Meffre E. The Establishment of Early B Cell Tolerance in Humans: Lessons From Primary Immunodeficiency Diseases. Ann N Y Acad Sci 2011 (1246) 1–10. doi: 10.1111/j.1749-6632.2011.06347.x.
22. Tsubata T. B-Cell Tolerance and Autoimmunity. F1000Res (2017) 6:391. doi: 10.12688/f1000research.10583.1
23. Yoshihara T, Satake H, Nishie T, Okino N, Hatta T, Otani H, et al. Lactosylceramide Synthases Encoded by B4galt5 and 6 Genes are Pivotal for Neuronal Generation and Myelin Formation in Mice. PloS Genet (2018) 14(8):e1007545. doi: 10.1371/journal.pgen.1007545
24. Duong BH, Ota T, Aoki-Ota M, Cooper AB, Ait-Azzouzene D, Vela JL, et al. Negative Selection by IgM Superantigen Defines a B Cell Central Tolerance Compartment and Reveals Mutations Allowing Escape. J Immunol (2011) 187(11):5596–605. doi: 10.4049/jimmunol.1102479
26. Yurasov S, Wardemann H, Hammersen J, Tsuiji M, Meffre E, Pascual V, et al. Defective B Cell Tolerance Checkpoints in Systemic Lupus Erythematosus. J Exp Med (2005) 201(5):703–11. doi: 10.1084/jem.20042251
27. Tobon GJ, Izquierdo JH, Canas CA. B Lymphocytes: Development, Tolerance, and Their Role in Autoimmunity-Focus on Systemic Lupus Erythematosus. Autoimmune Dis 2013 (2013) p:827254. doi: 10.1155/2013/827254
28. Sanz I. Rationale for B Cell Targeting in SLE. Semin Immunopathology (2014) 36(3):365–75. doi: 10.1007/s00281-014-0430-z
29. Zhao L, Ye Y, Zhang X. B Cells Biology in Systemic Lupus Erythematosus-From Bench to Bedside. Sci China Life Sci (2015) 58(11):1111–25. doi: 10.1007/s11427-015-4953-x
30. Nguyen TT, Baumgarth N. Natural IgM and the Development of B Cell-Mediated Autoimmune Diseases. Crit Rev Immunol (2016) 36(2):163–77. doi: 10.1615/CritRevImmunol.2016018175
31. Rawlings DJ, Metzler G, Wray-Dutra M, Jackson SW. Altered B Cell Signalling in Autoimmunity. Nat Rev Immunol (2017) 17(7):421–36. doi: 10.1038/nri.2017.24
32. Tsubata T. Inhibitory B Cell Co-Receptors and Autoimmune Diseases. Immunol Med (2019) 42(3):108–16. doi: 10.1080/25785826.2019.1660038
33. Lai M, Gonzalez-Martin A, Cooper AB, Oda H, Jin HY, Shepherd J, et al. Regulation of B-Cell Development and Tolerance by Different Members of the miR-17 Approximately 92 Family microRNAs. Nat Commun (2016) 7:12207. doi: 10.1038/ncomms12207
35. Jin HY, Xiao C. MicroRNA Mechanisms of Action: What Have We Learned From Mice? Front Genet (2015) 6:328. doi: 10.3389/fgene.2015.00328
36. Liu X, Zhan Z, Xu L, Ma F, Li D, Guo Z, et al. MicroRNA-148/152 Impair Innate Response and Antigen Presentation of TLR-Triggered Dendritic Cells by Targeting Camkiiα. J Immunol (2010) 185(12):7244–51. doi: 10.4049/jimmunol.1001573
37. Cheng P, Chen C, He HB, Hu R, Zhou HD, Xie H, et al. miR-148a Regulates Osteoclastogenesis by Targeting V-Maf Musculoaponeurotic Fibrosarcoma Oncogene Homolog B. J Bone Mineral Res (2013) 28(5):1180–90. doi: 10.1002/jbmr.1845
38. Goedeke L, Rotllan N, Canfrán-Duque A, Aranda JF, Ramírez CM, Araldi E, et al. MicroRNA-148a Regulates LDL Receptor and ABCA1 Expression to Control Circulating Lipoprotein Levels. Nat Med (2015) 21(11):1280–9. doi: 10.1038/nm.3949
39. Zheng B, Liang L, Wang C, Huang S, Cao X, Zha R, et al. MicroRNA-148a Suppresses Tumor Cell Invasion and Metastasis by Downregulating ROCK1 in Gastric Cancer. Clin Cancer Res (2011) 17(24):7574–83. doi: 10.1158/1078-0432.CCR-11-1714
40. Hibino Y, Sakamoto N, Naito Y, Goto K, Oo HZ, Sentani K, et al. Significance of miR-148a in Colorectal Neoplasia: Downregulation of miR-148a Contributes to the Carcinogenesis and Cell Invasion of Colorectal Cancer. Pathobiology (2015) 82(5):233–41. doi: 10.1159/000438826
41. Pan L, Huang S, He R, Rong M, Dang Y, Chen G. Decreased Expression and Clinical Significance of miR-148a in Hepatocellular Carcinoma Tissues. Eur J Med Res (2014) 19(1):1–6. doi: 10.1186/s40001-014-0068-2
42. Liffers S-T, Munding JB, Vogt M, Kuhlmann JD, Verdoodt B, Nambiar S, et al. MicroRNA-148a is Down-Regulated in Human Pancreatic Ductal Adenocarcinomas and Regulates Cell Survival by Targeting CDC25B. Lab Invest (2011) 91(10):1472–9. doi: 10.1038/labinvest.2011.99
43. Hua Z, Hou B. TLR Signaling in B-Cell Development and Activation. Cell Mol Immunol (2013) 10(2):103–6. doi: 10.1038/cmi.2012.61
44. Malapati S, Pierce SK. The Influence of CD40 on the Association of the B Cell Antigen Receptor With Lipid Rafts in Mature and Immature Cells. Eur J Immunol (2001) 31(12):3789–97. doi: 10.1002/1521-4141(200112)31:12<3789::AID-IMMU3789>3.0.CO;2-V
Keywords: B cell tolerance, microRNA, clonal deletion, miR-148a, target gene
Citation: Ma F, Zhan Y, Bartolomé-Cabrero R, Ying W, Asano M, Huang Z, Xiao C and González-Martín A (2022) Analysis of a miR-148a Targetome in B Cell Central Tolerance. Front. Immunol. 13:861655. doi: 10.3389/fimmu.2022.861655
Received: 24 January 2022; Accepted: 15 April 2022;
Published: 12 May 2022.
Edited by:
Paolo Casali, The University of Texas Health Science Center at San Antonio, United StatesReviewed by:
Egest James Pone, University of California, Irvine, United StatesHong Zan, The University of Texas Health Science Center at San Antonio, United States
Virginia G. De Yebenes, Universidad Complutense de Madrid, Spain
Copyright © 2022 Ma, Zhan, Bartolomé-Cabrero, Ying, Asano, Huang, Xiao and González-Martín. This is an open-access article distributed under the terms of the Creative Commons Attribution License (CC BY). The use, distribution or reproduction in other forums is permitted, provided the original author(s) and the copyright owner(s) are credited and that the original publication in this journal is cited, in accordance with accepted academic practice. No use, distribution or reproduction is permitted which does not comply with these terms.
*Correspondence: Alicia González-Martín, agonzalez@iib.uam.es; Changchun Xiao, cxiao@xmu.edu.cn
†Present addresses: Zhe Huang, Sanofi Institute for Biomedical Research, Suzhou, China
Changchun Xiao, Sanofi Institute for Biomedical Research, Suzhou, China