- Department of Obstetrics and Gynecology, The First Affiliated Hospital of University of Science and Technology of China, Hefei, China
The cervicovaginal microbiota plays a key role in the health and reproductive outcomes of women. In reality epidemiological studies have demonstrated that there is an association between the structure of cervicovaginal microbiota and reproductive health, although key mechanistic questions regarding these effects remain unanswered and understanding the interplay between the immune system and the structure of the cervicovaginal microbiota. Here, we review existing literature relating to the potential mechanisms underlying the interaction between vaginal microbes and the immune system; we also describe the composition and function of the microbiome and explain the mechanisms underlying the interactions between these microbial communities and various aspects of the immune system. Finally, we also discuss the diseases that are caused by disorders of the reproductive tract and how the immune system is involved. Finally, based on the data presented in this review, the future perspectives in research directions and therapeutic opportunities are explored.
Introduction
Microbial communities are hypothesized to play an important role in promoting homeostasis. It is known that certain types of cervicovaginal (CV) communities are associated with multitude of adverse outcomes and some CV communities are associated with lower than expected risk of these outcomes. Again, it is important to recognize the difference between causation and association. Compared to other parts of the body, the vagina appears to have a particularly simple and low-diversity microbial community (1). The microbial community in women at childbearing age can be divided into five different categories, referred to as community-state types (CSTs). Four of these CST species are dominated by Lactobacillus, namely L. crispatus (CST-I), L. iners (CST-III), L. gasseri (CST-II) and L. jensenii (CST-V). The CST-IV category does not feature many Lactobacillus species; rather, this category consists of multiple microbial mixtures of strict and facultative anaerobes, including Gardnerella, Atopobium, Mobiluncus, and Putelltella (2). There are many kinds of cervicovaginal microbiota, which symbiotic and antagonize each other, and participate in the formation of a complex micro-ecosystem. There is always a dynamic balance between the microbiota and the host, the microbiota and the microbiota, and the microbiota and the environment. This coordinated dynamic balance plays a decisive role in resisting the invasion of pathogenic microorganisms. However, it is hypothesized that the stability of vaginal micro-ecosystems depends on its function and not simply their composition.
Previously, it was assumed that the vaginal microbiota of healthy women was dominated by Lactobacillus. However, it is now recognized that the stability of vaginal micro-ecosystems is based on their true function and not simply their composition (3). Evidence shows that the vaginal bacterial community is maintained in a state of dynamic equilibrium and that the vaginal microbiota is affected by personal hygiene, menstruation, hormone levels, and disease states (4). furthermore, Pawel Gajer et al. found that there were five longitudinal patterns of change in vaginal microbial community composition. Moreover, in some women, the vaginal microbial community composition changed markedly and rapidly over time, whereas in others it was relatively stable (5). Disruption of the vaginal ecosystem contributes to the overgrowth of pathogens, thus leading to complex vaginal infections such as bacterial vaginosis (6), sexually transmitted infections (7), and vulvar vaginal candidiasis (8). Interestingly, vaginal microbes can be also used to predict the success of in vitro fertilization (9).
The Uterine Microbiota
Compared to the vaginal microbiota, the upper reproductive tract remains largely unexplored. Previously, the endometrial cavity in healthy women was considered sterile because of the cervical mucus plug. However, the application of next-generation sequencing technologies has increased our perception of the microbiota of the human mucosal surface. Many recent studies have found that certain changes in the uterine microbiota may be related to diseases, such as pelvic inflammation and endometrial cancer (10), and the failure of embryos to undergo implantation (11). For example, Oleer et al. (12)reported the presence of uterine colonies that mainly consisted of Gardenella, Enterobacter bacteria, and Streptococcus lactose. Using 16S rRNA gene sequencing and Lactobacillus-specific (L. iners & L. crispatus) qPCR, Andrew et al. reported that Lactobacillus was rarely found in the endometrium, while the distribution of bacteria in the endometrium and cervix was dominated by Gardnerella vaginalis, Enterobacter and Streptococcus agalactiae (13).
Innate Immunity of the Genital Tract (The Mucosal Immune System)
An Epithelial Barrier in the Mucosa of the Female Genital Tract
The female reproductive tract includes the fallopian tubes, uterus, cervix and vagina. The mucosa of the female reproductive tract varies between the upper and lower reproductive channels. The upper reproductive tract includes the fallopian tube, uterus, and inner cervix, and is covered by a monolayer of columnar epithelium. The lower reproductive tract includes the cervix and vagina; these are covered by a stratified squamous epithelium that forms a more protective barrier than the columnar epithelium. This is a unique system that can balance mucosal immunity to microorganisms and immune tolerance to the sperm, embryo, and fetus (14).
The mucosal immune system is the first line of defense against viral, bacterial, fungal, and parasitic pathogens (15). In the vagina, the main mucosal cells are the epithelial cells, stromal fibroblasts, and leukocytes; these line the surface of the vaginal mucosa and provide a barrier that controls epithelial cell barrier function (Figure 1). Estradiol (E2) increases the proliferation of vaginal epithelium cells, and high levels of progesterone (P4) are associated with vaginal epithelial thinning in animal models, although this has not been observed in humans (16).
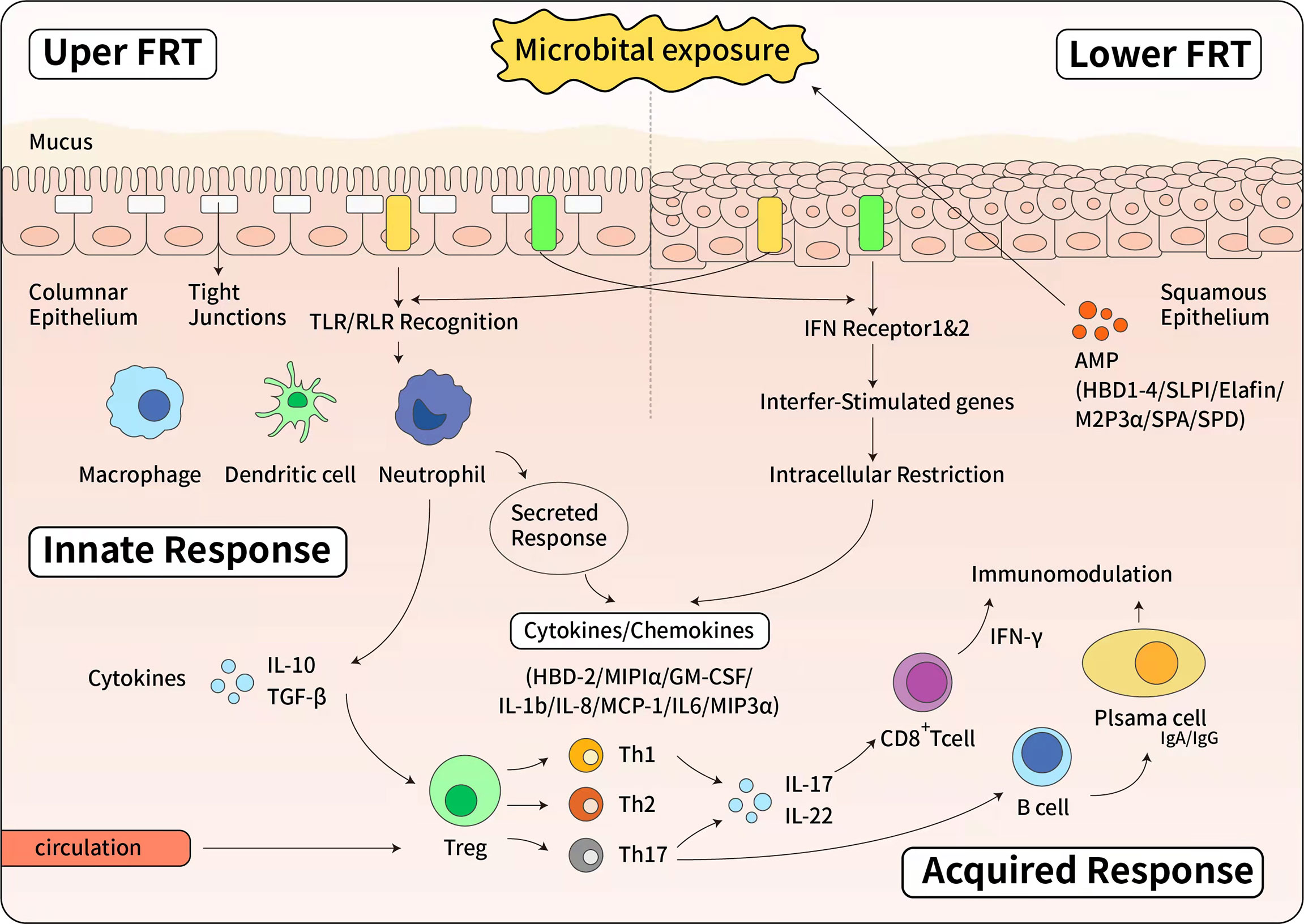
Figure 1 The immune response of female reproductive tract. Upside: The innate immune response of upper female reproductive tract. The upper female reproductive tract, consisting of the Fallopian tubes, uterine endometrium and endocervix is lined by a single layer of columnar epithelial cells linked by tight junctions. The lower female reproductive tract, consisting of the ectocervix and vagina, is covered by a layer of stratified squamous epithelial cells. Below the epithelial layer are innate and adaptive immune cells, as well as some AMPs. When pathogen invades to the epithelial cells, epithelial cells express a panel of Toll-like receptors (TLRs) and RIG-like receptors (RLRs) that can recognize and respond to bacteria or viruses. The Type I interferon (IFN) response is a potent defense system in female reproductive tract cells. Additionally, in response to pathogens, antimicrobials and cytokines/chemokines are secreted to confer broad spectrum protection. Below: The effector of adaptive immune response. Pathogen specific adaptive responses are driven by mucosal macrophages, dendritic cells, and epithelial cells that directly present antigens to T and B cells. Once activated by cytokine stimulation, T and B cells proliferate and differentiate. The cell-mediated response is characterized by the production of IFN and cytotoxic CD8+ T cells that cause apoptosis of infected cells. IFN also stimulates the expression of intracellular antiviral genes that block viral replication. The humoral response is mediated by B cells differentiation into plasma cells that secrete antibodies. Both IgG and IgA are produced in the female reproductive tract and secreted into the mucous.
Epithelial cells are connected by tight junctions that regulate the movement of molecules through the epithelium. Tight junctions predominate between the basal epithelial cells of the stratified squamous epithelium of the lower female reproductive tract. In contrast, the columnar epithelium in the upper female reproductive tract has a more tightly connected and powerful network (17). A recent study demonstrated that destruction of the epithelium in the female reproductive tract increases the risk of human immunodeficiency virus (HIV) infection by interfering with barrier protection and by promoting the recruitment of HIV target cells. It was shown that the vaginal use of tenofovir (TFV) and tenofovir alafamide (TAF) (a modified TFV prodrug) in HIV prophylaxis trials caused a significant delay in wound closure in the endometrium (EM), endocervix (CX) and ectocervix (ECX). Reconstitution of the tight junctions in epithelial cells of the EM and CX is compromised even after wound closure (18).
A recent study of the mucosal barrier in the reproductive tract showed that the treatment of bovine endometrial epithelial cell lines with astaxanthin (AST, a natural antioxidant carotenoid) reduced the production of lipopolysaccharide-induced interleukin-6 and tumor necrosis factor, increased the activity of cell superoxide dismutase and catalase, and promoted the production of insulin-like growth factor and epithelial growth factor. Furthermore, AST significantly increased the expression of claudin, a tight junction protein that may play an important role in maintaining the host endometrial defense barrier against pathogenic infection. Collectively, these results suggest that AST is a promising agent for endometritis (19).
Innate Immune Cells
Uterine Natural Killer (NK) Cells
Human natural killer (NK) cells are a class of innate immune cells that play an important role against pathogenic immunity; this is due to their ability to recognize and lyse infected cells. NK cells are also the dominant form of immune cells at the maternal and fetal interface (20). During the proliferative phase of the endometrium, only a few NK cells are scattered in the matrix of the functional layer. However, during the proliferative phase of the menstrual cycle, only a few NK cells are scattered throughout the stroma of the functional layer. In contrast, there is a dramatic increase in the number of NK after ovulation (Figure 2). During the late secretory phase, the number of NK cells surges up (by up to 30-40% of cells) in the stromal compartment and the number of endometrial leukocytes increases up to 70% (17, 23) but numbers of uterine NK cells are thought to reduce in the second half of pregnancy but the mechanism for this reduction is unclear (24).
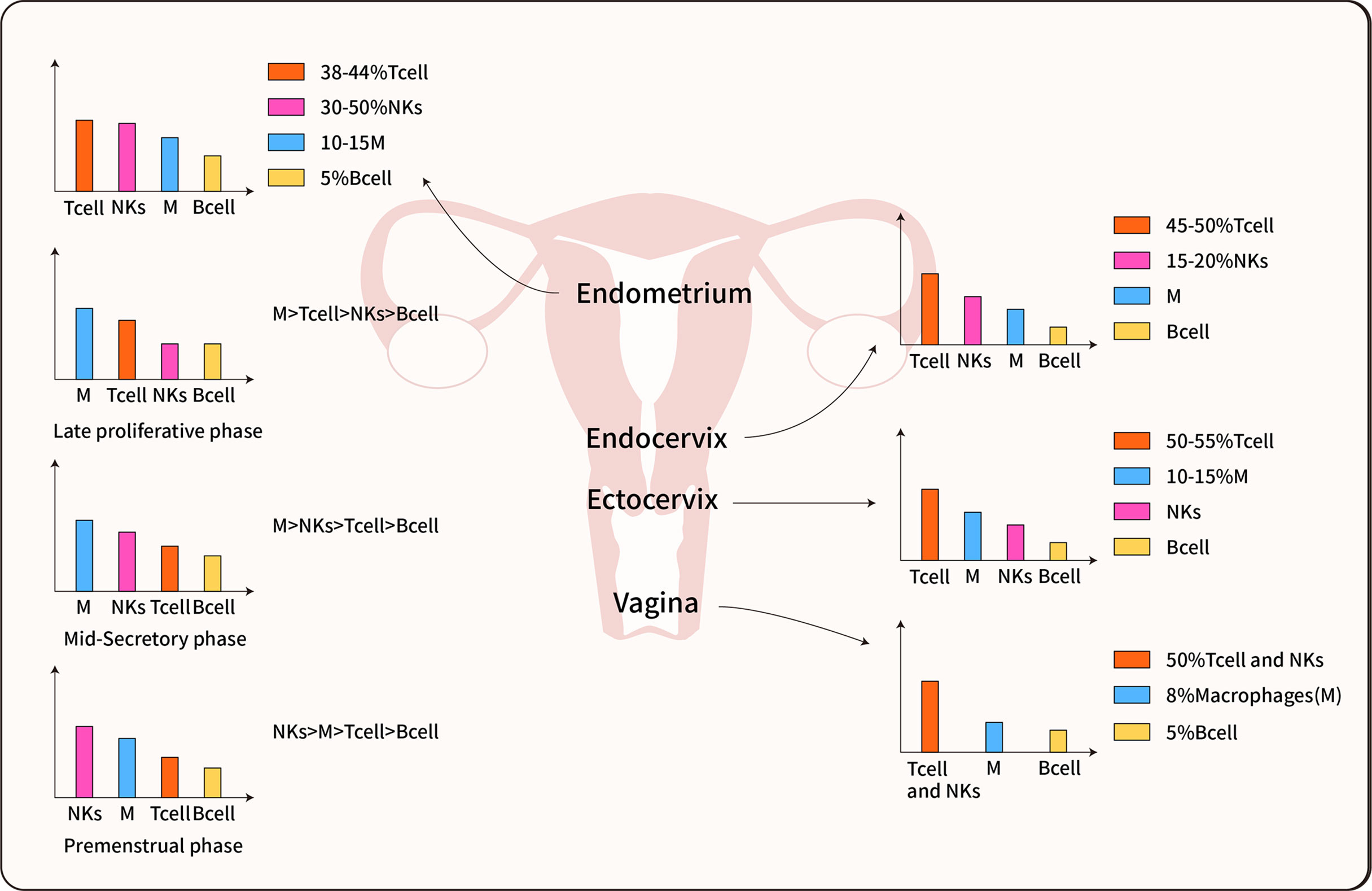
Figure 2 Immune cell distribution in the female reproductive tract. The predominant immune cells are T cells, Macrophages, NK cells, and B cells. The immune cells inconsistent distributed in each organ of the female reproductive tract, furthermore, most data indicate the immune cells also differentially populations in all phases of the menstrual cycle (14, 21, 22).
Three major subpopulations of NK cells have been identified in the decidua based on mRNA expression profiles, cell surface antigens, and metabolic behavior; these subpopulations are referred to as dNK1, dNK2, and dNK3 (25). Recently, Lamond et al. reported that NK cells protect the placenta to avoid invasion by Listeria, an intracellular bacterial pathogen (26). NK cells can also prevent infection-induced abortion via the injection of granulysin into the placental trophoblast; this removes intracellular pathogens without damaging placental cells, thus reflecting a mechanism that maintains the tolerance of the maternal-fetal interface to external abnormal factors (20).
Macrophages
Macrophage are the second most abundant subset of immune cells in the endometrium after uterine NK cells (24). The population of macrophages increases significantly during the secretion phase of the menstrual cycle and accounts for 10–20% of the population of decidual leukocytes (24). Macrophages bind to molecules that are specific to the cell wall of pathogens by specific pattern recognition receptors that participate in the recognition, phagocytosis, and degradation of microbial cells or ‘self’ cells (27). The phage function of macrophages in the female reproductive tract is controlled by dendritic cell-specific regulators that are locally synthesized by cells (e.g., uterine epithelial cells) and regulated by estrogen (28). Recent research suggest that dysregulation of the functions and estrogen responsiveness of female reproductive tract macrophages may be associated with infertility, estrogen- and macrophage-dependent gynecological diseases (29).
Dendritic Cells
Dendritic cells are a heterogeneous and dynamic population of leukocytes. These are the most potent antigen capture cells (immature dendritic cells) and antigen presentation cells (mature dendritic cells) (30). Recent research demonstrated that uterine dendritic cells exhibit a tolerant phenotype and both uterine dendritic cells and uterine macrophages produce IL-10, TGF-β, and indoleamine 2,3 -dioxygenase, thus helping to maintain a steady state in the microenvironment (31). Dendritic cells in the cervical mucosa can effectively promote the replication of human immunodeficiency virus 1 (HIV-1) and systemic viral dissemination in the cervical mucosa through siglec-1 antibodies (32). A previous study demonstrated that decidualization is a process that involves phenotypic and functional changes of the endometrial stromal cells to sustain immune homeostasis.
Adaptive Immunity of the Reproductive Tract (Specific Immunity)
T Cells and B Cells
Adaptive immune cells in the reproductive tract include both B and T lymphocytes. Although B cells are relatively rare in the female reproductive tract, a recent study showed that circulating memory B cells clustered together in a chemokine receptor 3-dependent manner in the vaginal mucosa after secondary infection with herpes simplex virus and then secreted virus-specific IgG2b, IgG2c and IgA into the lumen. This data indicated that circulating memory B cells act as a rapid induction source of mucosal antibodies in the female genital tract (33).
Tissue-resident memory T cells (TRM cells) are composed of both CD4 and CD8 T cell subsets. We found that the distribution of TRM subsets was uneven in the female genital tract with significantly higher levels of CD69+CD103+CD4 TRM in the vaginal tissue than in cervical tissue (34). Compared to B cells, T cells are always present in the vagina and uterus. Tissue-resident memory T cells in the mucosa of the reproductive tract respond rapidly to reproductive pathogens via the innate and adaptive immune systems. In cervical tissues, CD103+CD8TRM cells are preferentially localized to the cervical epithelial cells, whereas CD69+CD8TRM cells are evenly distributed in the epithelial cells and stroma (35). The production of TRM cells in the vaginal mucosa can provide advanced levels of defense against pathogens. Therefore, T cell-induced vaccines can persistently prevent infections in the mucosa of the reproductive tract, such as HIV (36). Hormone levels are also involved in the regulation of tissue T cells in the female reproductive tract. For example, estradiol treatment for herpes simplex virus 2 in mice led to increased levels of Th1 and Th17 TRM cells in the vagina (37). Estradiol has also been shown to prevent Herpes simplex virus type 2 (38), although the underlying mechanisms remain unknown. Recent studies show that compared with pre-menopausal women, the intra-and extra-cervical CD8+ T cells can increase cytotoxic activity in post-menopausal women (39).
The Secretion of Antimicrobial Peptides (AMP) by the Mucosa of the Reproductive Tract
The mucosal surface of the female reproductive tract represents the frontline with regards to defense against microbial challenges from the external environment. Antimicrobial peptides are a class of peptides with both antimicrobial and immunomodulatory properties; these are located at the host barrier. Antimicrobial peptides are effective against bacteria, fungi, enveloped viruses, and protozoa (40) and can even kill tumor cells (Figure 3). Importantly, most antimicrobial peptides are non-toxic or less toxic to normal eukaryotic cells and have little pharmacogenetic resistance. Because of this actions, antimicrobial peptides are also known as “endogenous antibiotics”. Antimicrobial peptides are known to protect the reproductive tract and regulate the vaginal microbiome in the lower female reproductive tract to prevent the entry of microbes into the upper female reproductive tract. In addition, antimicrobial peptides can evolve simultaneously with pathogenic lesions (40). Like other tissues, the female reproductive tract has a unique set of antimicrobial peptides that are mainly secreted by epithelial cells (ECs) and neutrophils in the female reproductive tract after exposure to inflammation or microbial stimulation (40). The female reproductive tract expresses a series of AMP, including human beta defensin (HBD), LL -37, SLPI, Elafifin, S100 protein, C-Type lectins, Lysozyme, Iron metabolism proteins, and Kinocidins (Table 1). Recent findings indicate the presence of additional antimicrobial peptides in the female reproductive tract (including histone, thrombospondin, lipophilic protein, cystatin A, and ubiquitin) (88), although the complete antimicrobial profile in secretions from the female reproductive tract has yet to be elucidated.
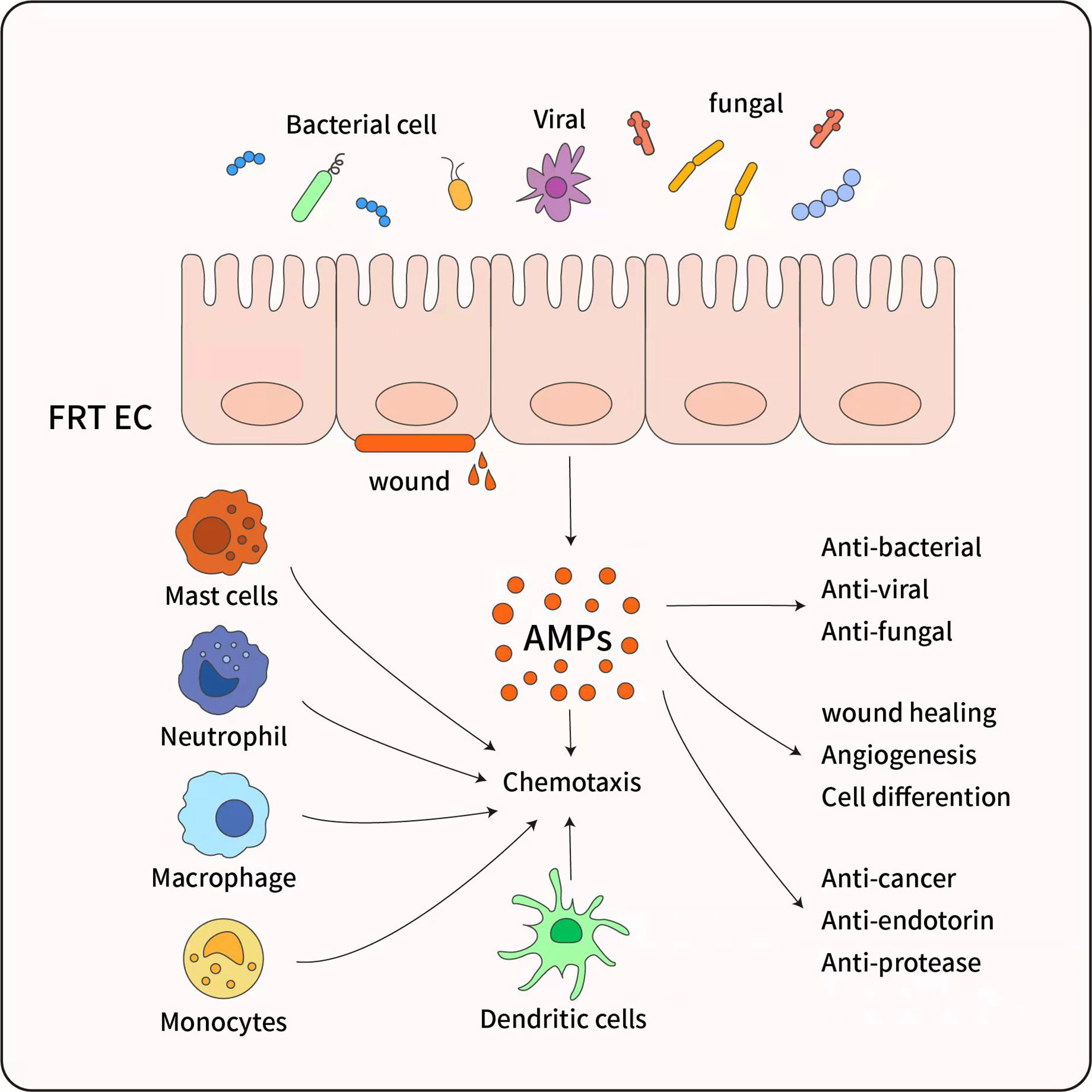
Figure 3 The pleiotropic functions of antimicrobial peptides. AMP have diverse biological effects, which are mainly secreted by female reproductive tract epithelial cells (FRT EC) following exposure to inflammatory or microbial stimuli. AMP have a broad spectrum of activity against bacteria and exhibit anti-fungal and antiviral activity, And AMP promote wound healing and angiogenesis through triggering cell differentiation, ultimate tissue homeostasis is maintained. Although AMP are most recognized for their microbicidal and anti-inflammatory function, AMP also possess immunomodulatory properties through activation of Mast cells, Monocytes/Macrophages, Neutrophils, and Dendritic cells, and inducing chemotaxis to infection sites.
Cytokines
Inflammatory cytokines can also exert effect on microbes, as confirmed by the presence of specific receptors (89). Cytokines play a unique role in microbial inflammation and can inhibit the growth of Lactobacillus and increase the resistance of this genus of microbes to adverse factors (90). Epithelial cells and potential antigen-presenting cells exert inflammatory responses to Prevotella, Mobiluncus, and Sneathia via the production of proinflammatory cytokines. For example, IL-1α, IL-1β, and tumor necrosis factor-α (91), along with bacterial vaginosis, may be associated with genetic polymorphisms in the innate immune Toll-like-receptor (TLR1, TLR 2, TLR 4 and TLR 9) and proinflammatory cytokines (IL-1β, IL-1ra, IL-6, IL-6, CXCL8, and IL-10) (92). IL-1 stimulates the resistance of Lactobacillus vaginalis to adverse factors, whereas IL-8 and tumor necrosis factor-α primarily increase resistance to peptidoglycans (89).
Protective Effects of Microbiota in the Reproductive Tract on Hosts
Symbiotic microorganisms are known to interact with the human immune system (93). Lactobacillus maintains homeostasis in the reproductive tract to prevent the invasion of pathogens (94). Lactobacillus exerts functionality through several mechanisms: (i) by competing for nutrients; (ii) by degrading the glycogen released from vaginal cells to produce organic acids (especially lactic acid) which lowers the vaginal pH, thereby exerting selective antibacterial activity on abnormal microbes; (iii) by producing antimicrobial substances such as bacteriocins and hydrogen peroxide (H2O2); and (iv) by helping to regulate the local immune system (95). It is important to note that not all Lactobacillus species have the same protective capacity; women with a predominant population of inert Lactobacillus are known to exhibit higher levels of viral infection (95).
Lactoferrin is a cationic multifunctional glycoprotein that binds iron and plays an important role in immune regulation by exerting antibacterial, antifungal, antiviral, and antiparasitic effects; it can also promote cell growth. When the female genital tract is infected by Neisseria gonorrhoeae, Chlamydia trachomatis, vaginal trichomas, or vaginal dysregulation, the increased abundance of lactoferrin in the sub-genital mucosa can promote both innate and adaptive immune responses (96). In cases of vaginal dysregulation that are characterized by a small number of vaginal Lactobacillus bacteria and an increased number of endogenous anaerobes, the increased abundance of lactoferrin can act as an immunomodulator to maintain the normal healthy microbiota of the vaginal mucosa. Thus, Lactobacillus and lactoferrin can be considered as biomarkers of altered microbial homeostasis at the vaginal level. Furthermore, Lactobacillus and lactoferrin can be influenced by paracrine activity induced by female hormones and a variety of cytokines. A recent study showed that 17β-estradiol increased adhesion to the vaginal mucosal epithelial cells by altering the morphology of Lactobacillus crispatus and inducing the production of biosurfactants (97). Therefore, hormones can be assumed to act as potential mediators to protect or restore vaginal homeostasis.
Disorders of the Microbiota in the Reproductive Tract Exerts Effect on the Immune System
The ability of a host to resist pathogenic microorganisms depends on a bidirectional relationship between the immune system and the microbiota (98). Changes in the composition of the vaginal microbiota, even in a small number of microbiota, can induce local immune responses (Figure 4). Bacteria related to vaginal dysregulation often produce mucin degradation enzymes (7, 99), induce a pro-inflammatory response (99), damage the mucosal barrier, and promote invasion by sexually transmitted pathogens (7). Disorders of the microbiota in the reproductive tract can also cause inflammatory and non-inflammatory infections in the reproductive tract, especially bacterial vaginitis caused by opportunistic microorganisms, vulvar vaginal candidiasis, and cervical intraepithelial neoplasia (100–102).
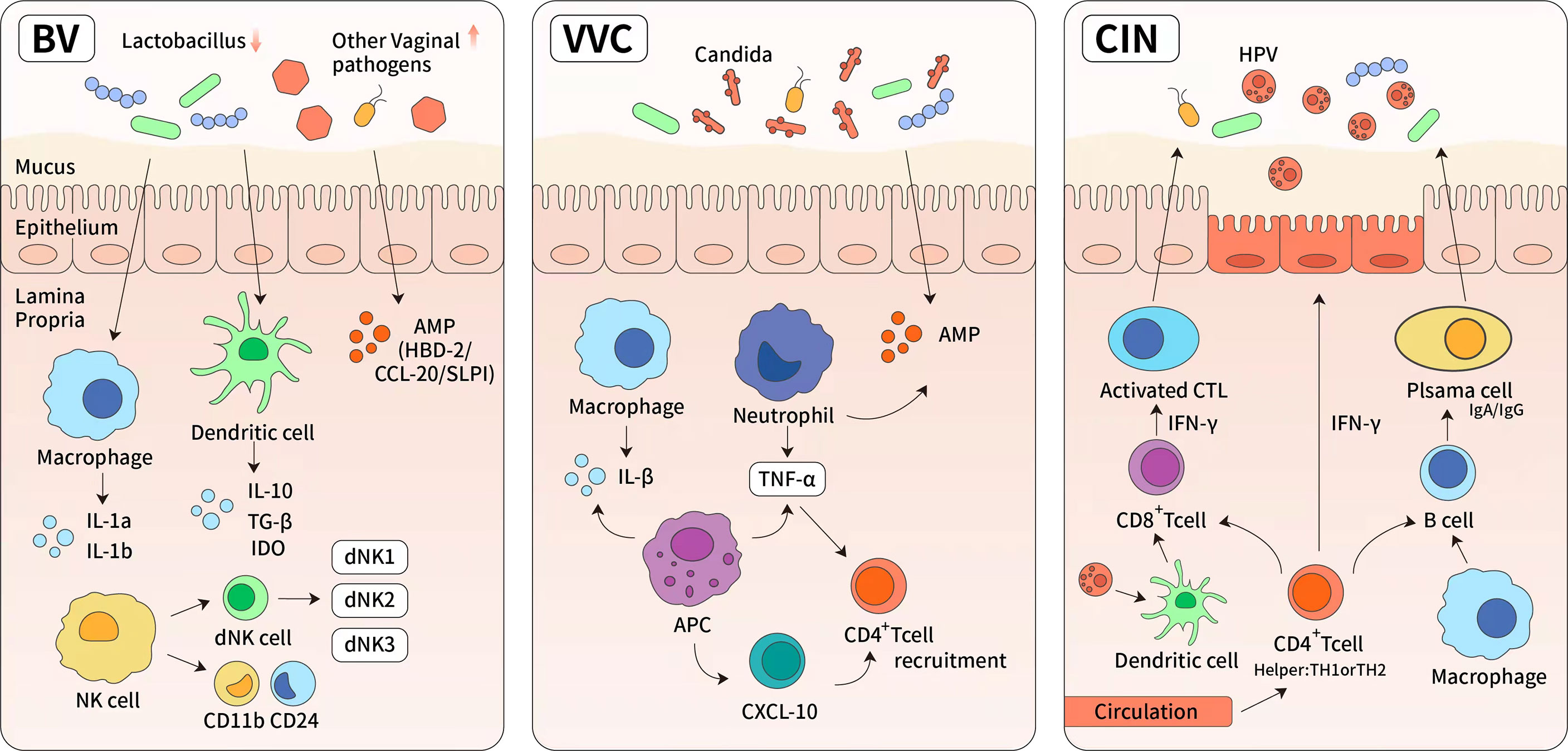
Figure 4 The immune response of reproductive tract diseases. (1) Bacterial vaginosis (BV) is characterized by significant reduction of normal Lactobacillus-dominated microbiota and the overgrowth of anaerobic organisms. These changes lead to higher vaginal pH and increased cytokines, such as IL-8, IL-1a, IL1b, interferon and tumor necrosis factor. (2) Vulvar vaginal candida is predominantly caused by albicans. It induces the upregulation of light chain 3, lysosome-associated membrane protein 1, and cytokines (tumor necrosis factor-α and IL-1), then leading to the activation of cellular autophagy. The specific bacterial species were found in highly diverse and Lactobacillus-deficient cervicovaginal bacteria communities. Antigen presentation cells have also been shown to produce chemokine (C-X-C motif) ligand 10 (CXCL10) which can lead to an increase in the number of activated CD4+ T cells. (3) Antivirus-specific immune responses are essential for the eradication of HPV infection, which requires the cooperation of CD4 + Th cells (TH) and cytotoxic CD8+ T cells. It has also been demonstrated that there are numerous CD4+ Th cells and activated TH-1 and TH-2 cells in persistent high-risk human papillomavirus infection. The high levels of interferon-γ secreted by Th-1 cells are known to mediate cytotoxic T cells and directly block viral cytotoxic activity.
Bacterial Vaginosis
Bacterial vaginosis is characterized by significant reduction of normal Lactobacillus-dominated microbiota and the overgrowth of anaerobic organisms (including Gardnerella, Prevotella, and vaginal fungi); collectively, these changes lead to an increase in vaginal pH and foul-smelling secretions. The diversity of microbial communities dominated by bacteria associated with bacterial vaginosis leads to an increase in the levels of cytokines, such as IL-8, IL-1a, IL1b, interferon and tumor necrosis factor (103). In a previous study, antimicrobial peptides of human beta-defensin-2, C-C (cervical cancer) chemokine ligand 20 (CCL20), and secretory leucocyte peptidase inhibitor, were found to be upregulated in cases of bacterial vaginosis, although there was no significant change in CCL20 expression following colonization with Lactobacillus species (63). The production of CCL20 is known to be regulated by tumor necrosis factor-α and IL-1β (104). Notably, both CCL20 and HBD-2 encode antimicrobial peptides and ligands for C-C chemokine receptor 6 (CCR6); this is a receptor that is specifically expressed on CD4+ T cells, leukocytes, and dendritic cell populations, and regulates the migration of these cells during inflammation. A recent study, based on a three-dimensional human cervical epithelial cell model, found that the expression levels of IL36G were significantly increased in bacterial vaginosis-positive cervical epithelial cells, thus proving that IL-36G is a key regulator of mucosal inflammation, neutrophil transport, and low immunity in the female reproductive tract (105).
Vulvar Vaginal Candidiasis
Vulvar vaginal candidiasis is the second most common cause of vaginal inflammation and is predominantly causes by Candida albicans as the main pathogen. When Candida albicans invades the vaginal mucosa, it activates the host innate immune system; this induces the upregulation of light chain 3, lysosome-associated membrane protein 1, and cytokines (tumor necrosis factor-αand IL-1), thus leading to the activation of cellular autophagy (106). The specific bacterial species that were found in highly diverse and Lactobacillus-deficient cervicovaginal bacteria communities, were not only associated with sharply elevated levels of genital pro-inflammatory cytokines but also associated with increased genital antigen presentation cell activation through the lipopolysaccharide sensing pathway. Alternatively, antigen presentation cells have also been shown to produce chemokine (C-X-C motif) ligand 10 (CXCL10) which can lead to an increase in the number of activated CD4+ T cells (91). Therefore, a small fraction of the vaginal microbiota regulates the local immune system and inflammatory response, thus affecting the susceptibility of infection.
Cervical Intraepithelial Neoplasia
Disorders of the vaginal microbiota are risk factors for the development of cervical intraepithelial neoplasia. Human papillomavirus infection plays an important role in the etiology and pathogenesis of cervical cancer lesions. Vaginal Lactobacillus maintains a low pH environment and produces bacteriocin, thereby maintaining the barrier function of the cervical epithelium to inhibit human papilloma virus (HPV) from entering the basal cells (42). When pathogenic bacteria colonize the epithelium of the reproductive tract, they produce enzymes and metabolites that may impair the barrier and promote the entry of HPV. Recent studies have shown that the risk of developing cervical intraepithelial neoplasia in patients with an abnormal genital microbiota was twice than that in the healthy population (107). The immune response to acute HPV infection was shown to be initially mediated by mucosal NK cells and the production of epithelial antiviral antimicrobial peptides (108). Antivirus-specific immune responses are essential for the eradication of HPV infection; this requires cooperation between CD4 + Th cells (TH) and cytotoxic CD8+ T cells (109). It has also been demonstrated in persistent high-risk human papillomavirus infection, that there are numerous CD4+ Th cells, CD25+ regulatory T cells, and activated TH-1 and TH-2 cells (110). The high levels of interferon-γsecreted by Th-1 cells are known to mediate cytotoxic T cells and directly block viral cytotoxic activity (111). A previous clinical study confirmed the strong correlation between the Th-1 pattern and the clearance of high risk human papilloma virus (HR-HPV) (112). In contrast, IL-17 has been shown to inhibit immune response effectors in HPV-related diseases (113). The high-risk human papillomavirus has evolved different mechanisms to evade host adaptive responses, including reduced protein secretion or the manipulation of antigen processing machinery (114). The clearance of infection is not a rare event and is often associated with the specific composition of the vaginal microbiota (7).
Microbiota with a reduced content of Lactobacillus may contribute to HPV persistence. For example, the prevalence of bacterial vaginosis in women with persistent HR-HPV was reported to be 11%, while the ratio of bacterial vaginosis in women clearing HR-HPV was only 5% (103). Persistent infection with HR-HPV is the leading cause of cervical cancer worldwide (115). Further studies found that HPV infection and/or subsequent clearance was not associated with inflammation or alterations in the subpopulation of cervical T cells but was associated with an increased number of Langerhans cells (116).
Conclusion
The most important function of the microbiota in the reproductive tract is to maintain immune homeostasis to prevent infection by harmful pathogens. Clinically, external auxiliary factors are used to treat gynecological diseases caused by disorders of the bacterial microbiota, including vaginal acidity, probiotics, hormone therapy and antibiotics. Antibacterial peptides, located on the frontline of the host barrier defense and widely considered as “endogenous antibiotics”, not only prevent host infection by pathogens but can also evolve with pathogens. Most antimicrobial peptides are not toxic or only minimally toxic to normal eukaryotic cells. Therefore, the activation of antibacterial peptides is a strategy to inhibit the pathogenic bacteria to maintain homeostasis in the reproductive tract. Finally, the regulation of cervicovaginal microbiota dysbiosis and immunity may also have important clinical significance and provide new challenges for treating gynecological disease.
Author Contributions
WZ and ZC planned the project and supervised the overall work. YW and XW wrote the manuscript. LG, MZ, XL, and KS was involved in the article modification. All authors approved the final version to be published.
Funding
This work was supported by the National Natural Science Foundation of China (82172774), the Fundamental Research Funds for the Central Universities (WK9110000150), and the University Synergy Innovation Program of Anhui Province (GXXT-2019-044).
Conflict of Interest
The authors declare that the research was conducted in the absence of any commercial or financial relationships that could be construed as a potential conflict of interest.
Publisher’s Note
All claims expressed in this article are solely those of the authors and do not necessarily represent those of their affiliated organizations, or those of the publisher, the editors and the reviewers. Any product that may be evaluated in this article, or claim that may be made by its manufacturer, is not guaranteed or endorsed by the publisher.
References
1. Human Microbiome Project Consortium. Structure, Function and Diversity of the Healthy Human Microbiome. Nature (2012) 486(7402):207–14. doi: 10.1038/nature11234
2. Ravel J, Gajer P, Abdo Z, Schneider GM, Koenig SS, McCulle SL, et al. Vaginal Microbiome of Reproductive-Age Women. Proc Natl Acad Sci USA (2011) 108 Suppl 1:4680–7. doi: 10.1073/pnas.1002611107
3. Ma B, Forney LJ, Ravel J. Vaginal Microbiome: Rethinking Health and Disease. Annu Rev Microbiol (2012) 66:371–89. doi: 10.1146/annurev-micro-092611-150157
4. Greenbaum S, Greenbaum G, Moran-Gilad J, Weintraub AY. Ecological Dynamics of the Vaginal Microbiome in Relation to Health and Disease. Am J Obstetrics Gynecology (2019) 220(4):324–35. doi: 10.1016/j.ajog.2018.11.1089
5. Gajer P, Brotman RM, Bai G, Sakamoto J, Schutte UM, Zhong X, et al. Temporal Dynamics of the Human Vaginal Microbiota. Sci Transl Med (2012) 4(132):132ra52. doi: 10.1126/scitranslmed.3003605
6. Lev-Sagie A, Goldman-Wohl D, Cohen Y, Dori-Bachash M, Leshem A, Mor U, et al. Vaginal Microbiome Transplantation in Women With Intractable Bacterial Vaginosis. Nat Med (2019) 25(10):1500–4. doi: 10.1038/s41591-019-0600-6
7. Torcia MG. Interplay Among Vaginal Microbiome, Immune Response and Sexually Transmitted Viral Infections. Int J Mol Sci (2019) 20(2):266. doi: 10.3390/ijms20020266
8. d’Enfert C, Kaune AK, Alaban LR, Chakraborty S, Cole N, Delavy M, et al. The Impact of the Fungus-Host-Microbiota Interplay Upon Candida Albicans Infections: Current Knowledge and New Perspectives. FEMS Microbiol Rev (2021) 45(3):fuaa060. doi: 10.1093/femsre/fuaa060
9. Schoenmakers S, Laven J. The Vaginal Microbiome as a Tool to Predict IVF Success. Curr Opin Obstet Gynecol (2020) 32(3):169–78. doi: 10.1097/GCO.0000000000000626
10. Walther-Antonio MR, Chen J, Multinu F, Hokenstad A, Distad TJ, Cheek EH, et al. Potential Contribution of the Uterine Microbiome in the Development of Endometrial Cancer. Genome Med (2016) 8(1):122. doi: 10.1186/s13073-016-0368-y
11. Ichiyama T, Kuroda K, Nagai Y, Urushiyama D, Ohno M, Yamaguchi T, et al. Analysis of Vaginal and Endometrial Microbiota Communities in Infertile Women With a History of Repeated Implantation Failure. Reprod Med Biol (2021) 20(3):334–44. doi: 10.1002/rmb2.12389
12. Birger R, Mdller F, Poul T, Lars F, S0ren C. Mogensen. Sterility of the Uterine Cavity. Acta Obstet Gynecol Scand (1995) 74(3):216–9. doi: 10.3109/00016349509008942
13. Winters AD, Romero R, Gervasi MT, Gomez-Lopez N, Tran MR, Garcia-Flores V, et al. Does the Endometrial Cavity Have a Molecular Microbial Signature? Sci Rep (2019) 9(1):9905. doi: 10.1038/s41598-019-46173-0
14. Trifonova RT, Lieberman J, van Baarle D. Distribution of Immune Cells in the Human Cervix and Implications for HIV Transmission. Am J Reprod Immunol (2014) 71(3):252–64. doi: 10.1111/aji.12198
15. Wira CR, Rodriguez-Garcia M, Patel MV. The Role of Sex Hormones in Immune Protection of the Female Reproductive Tract. Nat Rev Immunol (2015) 15(4):217–30. doi: 10.1038/nri3819
16. Galand P, Leroy F, Chretien J. Effect of Oestradiol on Cell Proliferation and Histological Changes in the Uterus and Vagina of Mice. J Endocrinol (1971) 49(2):243–52. doi: 10.1677/joe.0.0490243
17. Lee SK, Kim CJ, Kim DJ, Kang JH. Immune Cells in the Female Reproductive Tract. Immune Netw (2015) 15(1):16–26. doi: 10.4110/in.2015.15.1.16
18. Rodriguez-Garcia M, Patel MV, Shen Z, Bodwell J, Rossoll RM, Wira CR. Tenofovir Inhibits Wound Healing of Epithelial Cells and Fibroblasts From the Upper and Lower Human Female Reproductive Tract. Sci Rep (2017) 8:45725. doi: 10.1038/srep45725
19. Wan FC, Zhang C, Jin Q, Wei C, Zhao HB, Zhang XL, et al. Protective Effects of Astaxanthin on Lipopolysaccharide-Induced Inflammation in Bovine Endometrial Epithelial Cellsdagger. Biol Reprod (2020) 102(2):339–47. doi: 10.1093/biolre/ioz187
20. Dogra P, Farber DL. Stealth Killing by Uterine NK Cells for Tolerance and Tissue Homeostasis. Cell (2020) 182(5):1074–6. doi: 10.1016/j.cell.2020.08.018
21. Givan AL, White HD, Stern JE, Colby E, Gosselin EJ, Guyre PM, et al. Flow Cytometric Analysis of Leukocytes in the Human Female Reproductive Tract: Comparison of Fallopian Tube, Uterus, Cervix, and Vagina. Am J Reprod Immunol (1997) 38(5):350–9. doi: 10.1111/j.1600-0897.1997.tb00311.x
22. Salamonsen LA, Woolley DE. Menstruation: Induction by Matrix Metalloproteinases and Inflammatory Cells. J Reprod Immunol (1999) 44(1-2):1–27. doi: 10.1016/S0165-0378(99)00002-9
23. A K. Uterine Leukocytes and Decidualization. Hum Reprod Update (2000) 6(1):28–36. doi: 10.1093/humupd/6.1.28
24. Bulmer JN, Williams PJ, Lash GE. Immune Cells in the Placental Bed. Int J Dev Biol (2010) 54(2-3):281–94. doi: 10.1387/ijdb.082763jb
25. Vento-Tormo R, Efremova M, Botting RA, Turco MY, Vento-Tormo M, Meyer KB, et al. Single-Cell Reconstruction of the Early Maternal-Fetal Interface in Humans. Nature (2018) 563(7731):347–53. doi: 10.1038/s41586-018-0698-6
26. Lamond NM, Freitag NE. Vertical Transmission of Listeria Monocytogenes: Probing the Balance Between Protection From Pathogens and Fetal Tolerance. Pathogens (2018) 7(2):52. doi: 10.3390/pathogens7020052
27. Weiss G, Schaible UE. Macrophage Defense Mechanisms Against Intracellular Bacteria. Immunol Rev (2015) 264(1):182–203. doi: 10.1111/imr.12266
28. Moldenhauer LM, Keenihan SN, Hayball JD, Robertson SA. GM-CSF is an Essential Regulator of T Cell Activation Competence in Uterine Dendritic Cells During Early Pregnancy in Mice. J Immunol (2010) 185(11):7085–96. doi: 10.4049/jimmunol.1001374
29. Pepe G, Locati M, Della Torre S, Mornata F, Cignarella A, Maggi A, et al. The Estrogen-Macrophage Interplay in the Homeostasis of the Female Reproductive Tract. Hum Reprod Update (2018) 24(6):652–72. doi: 10.1093/humupd/dmy026
30. Agostinis C, Mangogna A, Bossi F, Ricci G, Kishore U, Bulla R. Uterine Immunity and Microbiota: A Shifting Paradigm. Front Immunol (2019) 10:2387. doi: 10.3389/fimmu.2019.02387
31. Racicot K, Kwon JY, Aldo P, Silasi M, Mor G. Understanding the Complexity of the Immune System During Pregnancy. Am J Reprod Immunol (2014) 72(2):107–16. doi: 10.1111/aji.12289
32. Perez-Zsolt D, Cantero-Perez J, Erkizia I, Benet S, Pino M, Serra-Peinado C, et al. Dendritic Cells From the Cervical Mucosa Capture and Transfer HIV-1 via Siglec-1. Front Immunol (2019) 10:825. doi: 10.3389/fimmu.2019.00825
33. Oh JE, Iijima N, Song E, Lu P, Klein J, Jiang R, et al. Migrant Memory B Cells Secrete Luminal Antibody in the Vagina. Nature (2019) 571(7763):122–6. doi: 10.1038/s41586-019-1285-1
34. Woodward Davis AS, Vick SC, Pattacini L, Voillet V, Hughes SM, Lentz GM, et al. The Human Memory T Cell Compartment Changes Across Tissues of the Female Reproductive Tract. Mucosal Immunol (2021) 14(4):862–72. doi: 10.1038/s41385-021-00406-6
35. Peng T, Phasouk K, Bossard E, Klock A, Jin L, Laing KJ, et al. Distinct Populations of Antigen-Specific Tissue-Resident CD8+ T Cells in Human Cervix Mucosa. JCI Insight (2021) 6(15):e149950. doi: 10.1172/jci.insight.149950
36. Arunachalam PS, Charles TP, Joag V, Bollimpelli VS, Scott MKD, Wimmers F, et al. T Cell-Inducing Vaccine Durably Prevents Mucosal SHIV Infection Even With Lower Neutralizing Antibody Titers. Nat Med (2020) 26(6):932–40. doi: 10.1038/s41591-020-0858-8
37. Bagri P, Ghasemi R, McGrath JJC, Thayaparan D, Yu E, Brooks AG, et al. Estradiol Enhances Antiviral CD4(+) Tissue-Resident Memory T Cell Responses Following Mucosal Herpes Simplex Virus 2 Vaccination Through an IL-17-Mediated Pathway. J Virol (2020) 95(1):e01206–20. doi: 10.1128/JVI.01206-20
38. Bhavanam S, Snider DP, Kaushic C. Intranasal and Subcutaneous Immunization Under the Effect of Estradiol Leads to Better Protection Against Genital HSV-2 Challenge Compared to Progesterone. Vaccine (2008) 26(48):6165–72. doi: 10.1016/j.vaccine.2008.08.045
39. Rodriguez-Garcia M, Shen Z, Fortier JM, Wira CR. Differential Cytotoxic Function of Resident and Non-Resident CD8+ T Cells in the Human Female Reproductive Tract Before and After Menopause. Front Immunol (2020) 11:1096. doi: 10.3389/fimmu.2020.01096
40. Yarbrough VL, Winkle S, Herbst-Kralovetz MM. Antimicrobial Peptides in the Female Reproductive Tract: A Critical Component of the Mucosal Immune Barrier With Physiological and Clinical Implications. Hum Reprod Update (2015) 21(3):353–77. doi: 10.1093/humupd/dmu065
41. G€unter Weiss UES. Macrophage Defense Mechanisms Against Intracellular Bacteria. Immunol Rev (2015) 204(1):182–203. doi: 10.1111/imr.12266
42. Borgdorff H, Gautam R, Armstrong SD, Xia D, Ndayisaba GF, van Teijlingen NH, et al. Cervicovaginal Microbiome Dysbiosis is Associated With Proteome Changes Related to Alterations of the Cervicovaginal Mucosal Barrier. Mucosal Immunol (2016) 9(3):621–33. doi: 10.1038/mi.2015.86
43. Canny GO, Trifonova RT, Kindelberger DW, Colgan SP, Fichorova RN. Expression and Function of Bactericidal/PermeabilityIncreasing Protein in Human Genital Tract Epithelial Cells. J Infect Dis (2006) 194(4):498–502. doi: 10.1086/505712
44. Menzies FM, Oldham RS, Waddell C, Nelson SM, Nibbs RJB. A Comprehensive Profile of Chemokine Gene Expression in the Tissues of the Female Reproductive Tract in Mice. Immunol Invest (2020) 49(3):264–86. doi: 10.1080/08820139.2019.1655573
45. Mukura LR, Hickey DK, Rodriguez-Garcia M, Fahey JV, Wira CR. Chlamydia Trachomatis Regulates Innate Immune Barrier Integrity and Mediates Cytokine and Antimicrobial Responses in Human Uterine ECC-1 Epithelial Cells. Am J Reprod Immunol (2017) 78(6):264–86. doi: 10.1111/aji.12764
46. Scagnolari C, Cannella F, Pierangeli A, Mellinger Pilgrim R, Antonelli G, Rowley D, et al. Insights Into the Role of Innate Immunity in Cervicovaginal Papillomavirus Infection From Studies Using Gene-Deficient Mice. J Virol (2020) 94(12):e00087–20. doi: 10.1128/JVI.00087-20
47. Patel MV, Ghosh M, Fahey JV, Ochsenbauer C, Rossoll RM, Wira CR. Innate Immunity in the Vagina (Part II): Anti-HIV Activity and Antiviral Content of Human Vaginal Secretions. Am J Reprod Immunol (2014) 72(1):22–33. doi: 10.1111/aji.12218
48. Longatto-Filho A, Fregnani JH, Mafra da Costa A, de Araujo-Souza PS, Scapulatempo-Neto C, Herbster S, et al. Evaluation of Elafin Immunohistochemical Expression as Marker of Cervical Cancer Severity. Acta Cytol (2021) 65(2):165–74. doi: 10.1159/000512010
49. Kadomoto S, Izumi K, Mizokami A. The CCL20-CCR6 Axis in Cancer Progression. Int J Mol Sci (2020) 21(15):5186. doi: 10.3390/ijms21155186
50. Stock SJ, Duthie L, Tremaine T, Calder AA, Kelly RW, Riley SC. Elafin (SKALP/Trappin-2/Proteinase Inhibitor-3) Is Produced by the Cervix in Pregnancy and Cervicovaginal Levels are Diminished in Bacterial Vaginosis. Reprod Sci (2009) 16(12):1125–34. doi: 10.1177/1933719109341998
51. Alvarez AH, Martinez Velazquez M, Prado Montes de Oca E. Human Beta-Defensin 1 Update: Potential Clinical Applications of the Restless Warrior. Int J Biochem Cell Biol (2018) 104:133–7. doi: 10.1016/j.biocel.2018.09.007
52. Varrey A, Romero R, Panaitescu B, Miller D, Chaiworapongsa T, Patwardhan M, et al. Human Beta-Defensin-1: A Natural Antimicrobial Peptide Present in Amniotic Fluid That is Increased in Spontaneous Preterm Labor With Intra-Amniotic Infection. Am J Reprod Immunol (2018) 80(4):e13031. doi: 10.1111/aji.13031
53. Patel MV, Fahey JV, Rossoll RM, Wira CR. Innate Immunity in the Vagina (Part I): Estradiol Inhibits HBD2 and Elafin Secretion by Human Vaginal Epithelial Cells. Am J Reprod Immunol (2013) 69(5):463–74. doi: 10.1111/aji.12078
54. Schaefer TM FJ, Wright JA, Wira CR. Innate Immunity in the Human Female Reproductive Tract: Antiviral Response of Uterine Epithelial Cells to the TLR3 Agonist Poly(I:C). J Immunol (2005) 174(2):992–1002. doi: 10.4049/jimmunol.174.2.992
55. Nittayananta W, Kemapunmanus M, Amornthatree K, Talungchit S, Sriplung H. Oral Human β-Defensin 2 in HIV-Infected Subjects With Long-Term Use of Antiretroviral Therapy. J Oral Pathol Med (2013) 42(1):53–60. doi: 10.1111/j.1600-0714.2012.01183.x
56. Jakubowska J, Maciejewska A, Pawlowski R, Bielawski KP. mRNA Profiling for Vaginal Fluid and Menstrual Blood Identification. Forensic Sci Int Genet (2013) 7(2):272–8. doi: 10.1016/j.fsigen.2012.11.005
57. Saha D, Koli S, Patgaonkar M, Reddy KV. Expression of Hemoglobin-Alpha and Beta Subunits in Human Vaginal Epithelial Cells and Their Functional Significance. PloS One (2017) 12(2):e0171084. doi: 10.1371/journal.pone.0171084
58. Chen SQ, Li JB, Jiang HY, Yuan L, Niu G, Yao SZ. Expression of Human Beta-Defensin-2 in the Eutopic and Ectopic Endometrial Tissues in Patients With Endometriosis. Arch Gynecol Obstet (2013) 287(6):1151–7. doi: 10.1007/s00404-012-2686-7
59. Galgano MT, Hampton GM, Frierson HF Jr. Comprehensive Analysis of HE4 Expression in Normal and Malignant Human Tissues. Mod Pathol (2006) 19(6):847–53. doi: 10.1038/modpathol.3800612
60. Twishasri DasGupta EIN, Hong Y, Liming W, Jin J, Ghosh SK, Kawsar HI, et al. Human Papillomavirus Oncogenic E6 Protein Regulates Human β-Defensin 3 (Hbd3) Expression via the Tumor Suppressor Protein P53. Oncotarget (2016) 7(19):27430–44. doi: 10.18632/oncotarget.8443
61. Wei Meng M, Rong DU, Wang Y, Chen Z, Ding Y. Human β-Defensin Messenger RNA is Overexpressed in the Cervical Epithelia of Patients With Nongonococcal Cervicitis. J Low Genit Tract Dis (2013) 17(4):440–5. doi: 10.1097/LGT.0b013e318281f1a0
62. Casalicchio G, Freato N, Maestri I, Comar M, Crovella S, Segat L. Beta Defensin-1 Gene Polymorphisms and Susceptibility to Atypical Squamous Cells of Undetermined Significance Lesions in Italian Gynecological Patients. J Med Virol (2014) 86(12):1999–2004. doi: 10.1002/jmv.23878
63. Doerflinger SY, Throop AL, Herbst-Kralovetz MM. Bacteria in the Vaginal Microbiome Alter the Innate Immune Response and Barrier Properties of the Human Vaginal Epithelia in a Species-Specific Manner. J Infect Dis (2014) 209(12):1989–99. doi: 10.1093/infdis/jiu004
64. Kotani H, Koshizuka T, Matsubara K, Nishiyama K, Sugiyama T, Suzutani T. Relationship Between Human Beta-Defensin 2 and the Vaginal Environment. Jpn J Infect Dis (2020) 73(3):214–20. doi: 10.7883/yoken.JJID.2019.190
65. King AE, Fleming DC, Critchley HOD, Kelly RW. Differential Expression of the Natural Antimicrobials, Beta-Defensins 3 and 4, in Human Endometrium. J Reprod Immunol (2003) 59(1):1–16. doi: 10.1016/S0165-0378(02)00083-9
66. Polettini J, Takitane J, Peracoli JC, Silva MG. Expression of Beta Defensins 1, 3 and 4 in Chorioamniotic Membranes of Preterm Pregnancies Complicated by Chorioamnionitis. Eur J Obstet Gynecol Reprod Biol (2011) 157(2):150–5. doi: 10.1016/j.ejogrb.2011.03.014
67. Aguilar-Jimenez W, Zapata W, Caruz A, Rugeles MT. High Transcript Levels of Vitamin D Receptor are Correlated With Higher mRNA Expression of Human Beta Defensins and IL-10 in Mucosa of HIV-1-Exposed Seronegative Individuals. PloS One (2013) 8(12):e82717. doi: 10.1371/journal.pone.0082717
68. Drapkin R, von Horsten HH, Lin Y, Mok SC, Crum CP, Welch WR, et al. Human Epididymis Protein 4 (HE4) is a Secreted Glycoprotein That is Overexpressed by Serous and Endometrioid Ovarian Carcinomas. Cancer Res (2005) 65(6):2162–9. doi: 10.1158/0008-5472.CAN-04-3924
69. Wei J, S KG, Flyckt R, Kalinowska M, Starks D, Jurevic R, et al. Bacterial Colonization and Beta Defensins in the Female Genital Tract in HIV Infection. Curr HIV Res (2012) 10(6):504–12. doi: 10.2174/157016212802429848
70. Han JH, Kim MS, Lee MY, Kim TH, Lee MK, Kim HR, et al. Modulation of Human Beta-Defensin-2 Expression by 17beta-Estradiol and Progesterone in Vaginal Epithelial Cells. Cytokine (2010) 49(2):209–14. doi: 10.1016/j.cyto.2009.09.005
71. Kell DB, Heyden EL, Pretorius E. The Biology of Lactoferrin, an Iron-Binding Protein That Can Help Defend Against Viruses and Bacteria. Front Immunol (2020) 11:1221. doi: 10.3389/fimmu.2020.01221
72. Alvendal C, Kamolvit W, Wagner S, Brauner A, Bohm-Starke N. Expression of Psoriasin in Human Papillomavirus-Induced Cervical High-Grade Squamous Intraepithelial Lesions. J Low Genit Tract Dis (2019) 23(1):33–8. doi: 10.1097/LGT.0000000000000438
73. Mary E, Klotman AR, Teleshova N, Micsenyi A, Jarvis GA, Lu W, et al. Neisseria Gonorrhoeae -Induced Human Role in Enhanced Transmission Defensins 5 and 6 Increase HIV Infectivity. J Immunol (2008) 180(9):6176–85. doi: 10.4049/jimmunol.180.9.6176
74. Dalgetty DM, Sallenave JM, Critchley HO, Williams AR, Tham WY, King AE, et al. Altered Secretory Leukocyte Protease Inhibitor Expression in the Uterine Decidua of Tubal Compared With Intrauterine Pregnancy. Hum Reprod (2008) 23(7):1485–90. doi: 10.1093/humrep/den130
75. Suff N, Karda R, Diaz JA, Ng J, Baruteau J, Perocheau D, et al. Cervical Gene Delivery of the Antimicrobial Peptide, Human Beta-Defensin (HBD)-3, in a Mouse Model of Ascending Infection-Related Preterm Birth. Front Immunol (2020) 11:106. doi: 10.3389/fimmu.2020.00106
76. Tarhini M, Pizzoccaro A, Benlyamani I, Rebaud C, Greige-Gerges H, Fessi H, et al. Human Serum Albumin Nanoparticles as Nanovector Carriers for Proteins: Application to the Antibacterial Proteins "Neutrophil Elastase" and "Secretory Leukocyte Protease Inhibitor". Int J Pharm (2020) 579:119150. doi: 10.1016/j.ijpharm.2020.119150
77. Radtke AL, Quayle AJ, Herbst-Kralovetz MM. Microbial Products Alter the Expression of Membrane-Associated Mucin and Antimicrobial Peptides in a Three-Dimensional Human Endocervical Epithelial Cell Model. Biol Reprod (2012) 87(6):132. doi: 10.1095/biolreprod.112.103366
78. Orfanelli T, Jayaram A, Doulaveris G, Forney LJ, Ledger WJ, Witkin SS. Human Epididymis Protein 4 and Secretory Leukocyte Protease Inhibitor in Vaginal Fluid: Relation to Vaginal Components and Bacterial Composition. Reprod Sci (2014) 21(4):538–42. doi: 10.1177/1933719113503416
79. Sotiriadis G, Dodagatta-Marri E, Kouser L, Alhamlan FS, Kishore U, Karteris E. Surfactant Proteins SP-A and SP-D Modulate Uterine Contractile Events in ULTR Myometrial Cell Line. PloS One (2015) 10(12):e0143379. doi: 10.1371/journal.pone.0143379
80. Kay S, Metkari SM, Madan T. Ovarian Hormones Regulate SP-D Expression in the Mouse Uterus During Estrous Cycle and Early Pregnancy. Am J Reprod Immunol (2015) 74(1):77–88. doi: 10.1111/aji.12369
81. Erhart W, Alkasi O, Brunke G, Wegener F, Maass N, Arnold N, et al. Induction of Human Beta-Defensins and Psoriasin in Vulvovaginal Human Papillomavirus-Associated Lesions. J Infect Dis (2011) 204(3):391–9. doi: 10.1093/infdis/jir079
82. Kumar R, Vicari M, Gori I, Achtari C, Fiche M, Surbeck I, et al. Compartmentalized Secretory Leukocyte Protease Inhibitor Expression and Hormone Responses Along the Reproductive Tract of Postmenopausal Women. J Reprod Immunol (2011) 92(1-2):88–96. doi: 10.1016/j.jri.2011.06.103
83. Valere K, Rapista A, Eugenin E, Lu W, Chang TL. Human Alpha-Defensin HNP1 Increases HIV Traversal of the Epithelial Barrier: A Potential Role in STI-Mediated Enhancement of HIV Transmission. Viral Immunol (2015) 28(10):609–15. doi: 10.1089/vim.2014.0137
84. MacNeill C, Umstead TM, Phelps DS, Lin Z, Floros J, Shearer DA, et al. An Innate Immune Factor, is Expressed in the Vaginal Mucosa and is Present in Vaginal Lavage Fluid. Immunology (2004) 111(1):91–9. doi: 10.1111/j.1365-2567.2004.01782.x
85. Pandit H, Kale K, Yamamoto H, Thakur G, Rokade S, Chakraborty P, et al. Surfactant Protein D Reverses the Gene Signature of Transepithelial HIV-1 Passage and Restricts the Viral Transfer Across the Vaginal Barrier. Front Immunol (2019) 10. doi: 10.3389/fimmu.2019.00264
86. Itaoka N, Nagamatsu T, Schust DJ, Ichikawa M, Sayama S, Iwasawa-Kawai Y, et al. Cervical Expression of Elafin and SLPI in Pregnancy and Their Association With Preterm Labor. Am J Reprod Immunol (2015) 73(6):536–44. doi: 10.1111/aji.12354
87. Oberley RE, Goss KL, Hoffmann DS, Ault KA, Neff TL, Ramsey KH, et al. Regulation of Surfactant Protein D in the Mouse Female Reproductive Tract In Vivo. Mol Hum Reprod (2007) 13(12):863–8. doi: 10.1093/molehr/gam074
88. Wira CR, Patel MV, Ghosh M, Mukura L, Fahey JV. Innate Immunity in the Human Female Reproductive Tract: Endocrine Regulation of Endogenous Antimicrobial Protection Against HIV and Other Sexually Transmitted Infections. Am J Reprod Immunol (2011) 65(3):196–211. doi: 10.1111/j.1600-0897.2011.00970.x
89. Hogbom M, Ihalin R. Functional and Structural Characteristics of Bacterial Proteins That Bind Host Cytokines. Virulence (2017) 8(8):1592–601. doi: 10.1080/21505594.2017.1363140
90. Sgibnev AV, Kremleva EA. Inflammation Mediators Regulate the Microbiota Resistance to Adverse Factors. Bull Exp Biol Med (2020) 170(1):49–52. doi: 10.1007/s10517-020-05002-5
91. Anahtar MN, Gootenberg DB, Mitchell CM, Kwon DS. Cervicovaginal Microbiota and Reproductive Health: The Virtue of Simplicity. Cell Host Microbe (2018) 23(2):159–68. doi: 10.1016/j.chom.2018.01.013
92. Murphy K, Mitchell CM. The Interplay of Host Immunity, Environment and the Risk of Bacterial Vaginosis and Associated Reproductive Health Outcomes. J Infect Dis (2016) 214 Suppl 1:S29–35. doi: 10.1093/infdis/jiw140
93. Taddei CR, Cortez RV, Mattar R, Torloni MR, Daher S. Microbiome in Normal and Pathological Pregnancies: A Literature Overview. Am J Reprod Immunol (2018) 80(2):e12993. doi: 10.1111/aji.12993
94. O’Callaghan JL, Turner R, Dekker Nitert M, Barrett HL, Clifton V, Pelzer ES. Re-Assessing Microbiomes in the Low-Biomass Reproductive Niche. BJOG (2020) 127(2):147–58. doi: 10.1111/1471-0528.15974
95. Aroutcheva A, Gariti D, Simon M, Shott S, Faro J, Simoes JA, et al. Defense Factors of Vaginal Lactobacilli. Am J Obstet Gynecol (2001) 185(2):375–9. doi: 10.1067/mob.2001.115867
96. Spear GT, Kendrick SR, Chen HY, Thomas TT, Bahk M, Balderas R, et al. Multiplex Immunoassay of Lower Genital Tract Mucosal Fluid From Women Attending an Urban STD Clinic Shows Broadly Increased IL1ss and Lactoferrin. PloS One (2011) 6(5):e19560. doi: 10.1371/journal.pone.0019560
97. Clabaut M, Suet A, Racine PJ, Tahrioui A, Verdon J, Barreau M, et al. Effect of 17beta-Estradiol on a Human Vaginal Lactobacillus Crispatus Strain. Sci Rep (2021) 11(1):7133. doi: 10.1038/s41598-021-86628-x
98. Sansonetti PJ. To be or Not to be a Pathogen: That is the Mucosally Relevant Question. Mucosal Immunol (2011) 4(1):8–14. doi: 10.1038/mi.2010.77
99. Amabebe E, Anumba DOC. The Vaginal Microenvironment: The Physiologic Role of Lactobacilli. Front Med (Lausanne) (2018) 5:181. doi: 10.3389/fmed.2018.00181
100. Peebles K, Velloza J, Balkus JE, McClelland RS, Barnabas RV. High Global Burden and Costs of Bacterial Vaginosis: A Systematic Review and Meta-Analysis. Sex Transm Dis (2019) 46(5):304–11. doi: 10.1097/OLQ.0000000000000972
101. Goncalves B, Ferreira C, Alves CT, Henriques M, Azeredo J, Silva S. Vulvovaginal Candidiasis: Epidemiology, Microbiology and Risk Factors. Crit Rev Microbiol (2016) 42(6):905–27. doi: 10.3109/1040841X.2015.1091805
102. Escobar N, Plugge E. Prevalence of Human Papillomavirus Infection, Cervical Intraepithelial Neoplasia and Cervical Cancer in Imprisoned Women Worldwide: A Systematic Review and Meta-Analysis. J Epidemiol Community Health (2020) 74(1):95–102. doi: 10.1136/jech-2019-212557
103. Kyrgiou M, Mitra A, Moscicki AB. Does the Vaginal Microbiota Play a Role in the Development of Cervical Cancer? Transl Res (2017) 179:168–82. doi: 10.1016/j.trsl.2016.07.004
104. Reibman J, Hsu Y, Chen LC, Bleck B, Gordon T. Airway Epithelial Cells Release MIP-3alpha/CCL20 in Response to Cytokines and Ambient Particulate Matter. Am J Respir Cell Mol Biol (2003) 28(6):648–54. doi: 10.1165/rcmb.2002-0095OC
105. Gardner JK, Laniewski P, Knight A, Haddad LB, Swaims-Kohlmeier A, Herbst-Kralovetz MM. Interleukin-36gamma Is Elevated in Cervicovaginal Epithelial Cells in Women With Bacterial Vaginosis and In Vitro After Infection With Microbes Associated With Bacterial Vaginosis. J Infect Dis (2020) 221(6):983–8. doi: 10.1093/infdis/jiz514
106. Shroff A, Sequeira R, Reddy KVR. Human Vaginal Epithelial Cells Augment Autophagy Marker Genes in Response to Candida Albicans Infection. Am J Reprod Immunol (2017) 77(4):10.1111/aji.12639. doi: 10.1111/aji.12639
107. Mitra A, MacIntyre DA, Lee YS, Smith A, Marchesi JR, Lehne B, et al. Cervical Intraepithelial Neoplasia Disease Progression is Associated With Increased Vaginal Microbiome Diversity. Sci Rep (2015) 5:16865. doi: 10.1038/srep16865
108. Mitra A, MacIntyre DA, Marchesi JR, Lee YS, Bennett PR, Kyrgiou M. The Vaginal Microbiota, Human Papillomavirus Infection and Cervical Intraepithelial Neoplasia: What do We Know and Where are We Going Next? Microbiome (2016) 4(1):58. doi: 10.1186/s40168-016-0203-0
109. Stanley M. Immune Responses to Human Papillomavirus. Vaccine (2006) 24 Suppl 1:S16–22. doi: 10.1016/j.vaccine.2005.09.002
110. Bonin CM, Padovani CTJ, da Costa IP, Avila LS, Ferreira AMT, Fernandes CES, et al. Detection of Regulatory T Cell Phenotypic Markers and Cytokines in Patients With Human Papillomavirus Infection. J Med Virol (2019) 91(2):317–25. doi: 10.1002/jmv.25312
111. Iijima N, Linehan MM, Zamora M, Butkus D, Dunn R, Kehry MR, et al. Dendritic Cells and B Cells Maximize Mucosal Th1 Memory Response to Herpes Simplex Virus. J Exp Med (2008) 205(13):3041–52. doi: 10.1084/jem.20082039
112. Scott M, Stites DP, Moscicki AB. Th1 Cytokine Patterns in Cervical Human Papillomavirus Infection. Clin Diagn Lab Immunol (1999) 6(5):751–5. doi: 10.1128/CDLI.6.5.751-755.1999
113. Gosmann C, Mattarollo SR, Bridge JA, Frazer IH, Blumenthal A. IL-17 Suppresses Immune Effector Functions in Human Papillomavirus-Associated Epithelial Hyperplasia. J Immunol (2014) 193(5):2248–57. doi: 10.4049/jimmunol.1400216
114. Jee B, Yadav R, Pankaj S, Shahi SK. Immunology of HPV-Mediated Cervical Cancer: Current Understanding. Int Rev Immunol (2021) 40(5):359–78. doi: 10.1080/08830185.2020.1811859
115. Ferlay J, Colombet M, Soerjomataram I, Mathers C, Parkin DM, Pineros M, et al. Estimating the Global Cancer Incidence and Mortality in 2018: GLOBOCAN Sources and Methods. Int J Cancer (2019) 144(8):1941–53. doi: 10.1002/ijc.31937
Keywords: vaginal, endometrial, female reproductive tract, immunology, microbiota
Citation: Wang Y, Wang X, Zhu M, Ge L, Liu X, Su K, Chen Z and Zhao W (2022) The Interplay Between Cervicovaginal Microbial Dysbiosis and Cervicovaginal Immunity. Front. Immunol. 13:857299. doi: 10.3389/fimmu.2022.857299
Received: 18 January 2022; Accepted: 21 February 2022;
Published: 10 March 2022.
Edited by:
Paola Massari, Tufts University School of Medicine, United StatesReviewed by:
Ana Paula Lourenco, Tufts University School of Medicine, United StatesPawel Gajer, University of Maryland, United States
Copyright © 2022 Wang, Wang, Zhu, Ge, Liu, Su, Chen and Zhao. This is an open-access article distributed under the terms of the Creative Commons Attribution License (CC BY). The use, distribution or reproduction in other forums is permitted, provided the original author(s) and the copyright owner(s) are credited and that the original publication in this journal is cited, in accordance with accepted academic practice. No use, distribution or reproduction is permitted which does not comply with these terms.
*Correspondence: Weidong Zhao, dmN0b3J6aGFvQHVzdGMuZWR1LmNu; Zhengzheng Chen, emhlbmctMDkxNkAxNjMuY29t