- 1Department of Hepatopancreatobiliary Surgery, Shenzhen Institute of Translational Medicine, Health Science Center, The First Affiliated Hospital of Shenzhen University, Shenzhen Second People’s Hospital, Shenzhen, China
- 2Shenzhen Xenotransplantation Medical Engineering Research and Development Center, Shenzhen Institute of Translational Medicine, Health Science Center, The First Affiliated Hospital of Shenzhen University, Shenzhen Second People’s Hospital, Shenzhen, China
- 3Faculty of Arts and Science, University of Toronto, Toronto, ON, Canada
- 4Center for Transplantation Sciences, Department of Surgery, Massachusetts General Hospital, Boston, MA, United States
- 5Department of Life Science, Bellevue College, Bellevue, WA, United States
- 6Imaging Department, Shenzhen Institute of Translational Medicine, The First Affiliated Hospital of Shenzhen University, Shenzhen Second People’s Hospital, Shenzhen, China
Pig islet xenotransplantation is a potential treatment for patients with type 1 diabetes. Current efforts are focused on identifying the optimal pig islet source and overcoming the immunological barrier. The optimal age of the pig donors remains controversial since both adult and neonatal pig islets have advantages. Isolation of adult islets using GMP grade collagenase has significantly improved the quantity and quality of adult islets, but neonatal islets can be isolated at a much lower cost. Certain culture media and coculture with mesenchymal stromal cells facilitate neonatal islet maturation and function. Genetic modification in pigs affords a promising strategy to prevent rejection. Deletion of expression of the three known carbohydrate xenoantigens (Gal, Neu5Gc, Sda) will certainly be beneficial in pig organ transplantation in humans, but this is not yet proven in islet transplantation, though the challenge of the ‘4th xenoantigen’ may prove problematic in nonhuman primate models. Blockade of the CD40/CD154 costimulation pathway leads to long-term islet graft survival (of up to 965 days). Anti-CD40mAbs have already been applied in phase II clinical trials of islet allotransplantation. Fc region-modified anti-CD154mAbs successfully prevent the thrombotic complications reported previously. In this review, we discuss (I) the optimal age of the islet-source pig, (ii) progress in genetic modification of pigs, (iii) the immunosuppressive regimen for pig islet xenotransplantation, and (iv) the reduction in the instant blood-mediated inflammatory reaction.
Introduction
Type 1 diabetes (T1D) is a chronic autoimmune disease characterized by pancreatic islet cell destruction by CD4+ and CD8+ T cells and autoantibodies, resulting in insulin deficiency and hyperglycemia (1). Conventional treatment of T1D includes exogenous insulin therapy, which reduces, but may not prevent, the development of the long-term complications of hyperglycemia. In late-stage T1D patients, especially those with ‘brittle’ diabetes, it is difficult to prevent complications such as cardiovascular disease, retinopathy, nephropathy, and life-threatening hypoglycemic episodes (1).
Islet allotransplantation has been identified as an efficient therapy for T1D, but, faced with the shortage of pancreases from deceased human donors, pig-to-human islet xenotransplantation has emerged as a potential alternative (2). Although pig-to-nonhuman primate (NHP) islet xenotransplantation has resulted in insulin independence, several problems remain.
The age of the islet-source pig may be important to islet quality. Adult pigs have a mature islet structure, lower galactose-α1,3-galactose (Gal) expression on islets, and a higher islet yield (3). Neonatal pig islets are easier to isolate and at a lower cost (3). To overcome immunological rejection of pig-to-NHP islet transplants, genetic modification of the source pig plays an important role by deleting xenoantigen expression and introducing human ‘protective’ proteins (4). New alternative modifications, e.g., expression of programmed cell death ligand 1 (PD-L1), are being explored. A consensus has been reached that, in regard to the transplantation of pig organs into humans, the expression of the three known carbohydrate xenoantigens (Gal, Neu5Gc, Sda) should be deleted (resulting in triple-knockout [TKO] pigs) (4, 5), but this remains uncertain after pig islet transplantation. However, there is a limitation in the TKO pig-to-NHP model because of the problem of the ‘4th xenoantigen’.
The selection of the immunosuppressive regimen plays a critical role in preventing the adaptive immune response (6). Although conventional immunosuppressive regimens are inefficient in preventing the adaptive response to pig cells, blockade of the CD40/CD154 costimulation pathway is successful, and has resulted in insulin-independence for a maximum of 965 days) (7). Emerging Fc region-modified anti-CD154mAbs successfully prevent the thrombotic complications seen previously (8, 9). Although anti-CD154 agents may be preferable, anti-CD40mAbs have already been applied in phase II clinical trials of human kidney allotransplantation (10).
In this review, we consider (i) the optimal age of the islet-source pig, (ii) the potential of genetic modification of the pig, (iii) the selection of the immunosuppressive regimen for pig-to-primate islet xenotransplantation, and (iv) potential steps to reduce the instant blood-mediated inflammatory reaction (IBMIR). We also briefly discuss the possible directions for future research.
Donor Age
Based on previous studies of pig-to-NHP islet xenotransplantation, pigs can be divided into three age groups: adult (>12 weeks), neonatal (~first 14 days after birth), and fetal. Their characteristics are summarized in Table 1. As fetal pig islets are not currently considered ideal sources for xenotransplantation due to defects in β-cell yield and immunogenicity, we will focus on adult and neonatal pigs.
Adult Pig Islets
To date, adult pig islets transplanted into NHPs have displayed the longest survival time (965 days) and have always been considered the primary source for islet xenotransplantation due to their superior islet yield, immediate insulin response, lower Gal expression, and higher β-cell percentage compared with neonatal pigs (Table 1). Female adult pigs that have produced >2 litters (retired breeders, usually >2 years old and > 200 kg) are preferred over young adult pigs because they consistently provide a higher yield of high-quality islets (3, 11). We add the ref: Bottino R, 2007 Our previous review summarized the above advantages (3). Using GMP-grade collagenase (collagenase AF-1 and liberase MTF C/T), one adult pig can yield up to 720,000IEQ (12), which is enough for islet xenotransplantation in a diabetic patient of approximately 60kg in weight. However, the limitations of adult pig islets include difficulty in isolation, higher costs for pig maintenance and islet isolation, and poor proliferative capacity (3) (Table 1).
Neonatal Islet Cell Clusters (NICC)
There have been only a few reports using NICC for transplantation into NHPs, with the longest survival being 260 days (13–16). The advantages of NICCs include (I) the need for only a short period of pig maintenance after birth, thus reducing the costs, (ii) easier isolation, thus increasing success and reducing isolation cost ($0.02/IEQ) compared to adult pig islets ($0.09/IEQ) (17), and (iii) greater proliferative capacity (3). However, there are some limitations. First, NICCs must be cultured to reaggregate the islet cluster before transplantation, although various modified culture media, the addition of growth factors, and coculture with mesenchymal stromal cells facilitate NICC islet maturation and function (18–21). Second, there is a delay in the in vivo response to glucose after transplantation (that may be >4 weeks in NHPs), and so measuring islet loss is difficult (3).
The difference in the glucose-stimulated insulin secretion index between adult pig islets and NICC remains controversial. Some research has indicated that NICC has a significantly higher stimulation index (4.7± 0.58) than adult pig islets (1.75 ± 0.60) (17), but other studies show the opposite (summarized in Table 2) (12, 17, 21–23). Therefore, the glucose-stimulated insulin secretion of adult pig islets and NICC may be equivalent.
In summary, a consensus on the optimal age for pig islet xenotransplantation has not been reached. Adult pig islets should be the primary option as better results have been achieved following transplantation into NHPs, but NICCs are regarded as a promising alternative islet source with several significant superiorities.
Gene Modification
The development of CRISPR/Cas9, an efficient genome editing technique, provides the capacity to produce pigs with multiple genetic modifications for xenotransplantation (Table 3) (24–41). We will here mainly focus on gene modification targets for carbohydrate xenoantigens and cellular immune response-related genes.
Carbohydrate Xenoantigen Genes
A consensus has been reached that the three known carbohydrate xenoantigen genes (Gal, Neu5Gc, Sda) should be knocked-out for pig-to-human organ transplantation (Table 4), but this is not ideal for pig-to-NHP organ transplantation because of the problems associated with the ‘4th xenoantigen’ (discussed in 42–46). It is well-known that pig organ grafts from CMAHKO pigs are associated with increased NHP IgM and IgG binding and serum complement-mediated cytotoxicity, resulting in acute xenograft rejection (42–46).
To our knowledge, the transplantation of TKO pig islets into NHPs has not been reported, and it remains unknown whether the ‘4th xenoantigen’ is exposed in TKO pig islets as it is in vascular endothelial cells. Whether TKO islets would provide an advantage in this regard remains uncertain.
Of relevance to this point, there were no statistically significant differences in human IgM and IgG binding to isolated islet cells from GTKO/hCD46 and GTKO/hCD46/NeuGcKO pigs (47). Knockout of CMAH may therefore possibly have a different effect in islets than in solid organs. In one report, GTKO/CMAHKO pigs developed pathological features that are similar to those seen in anemia, possibly associated with variations in glycosylation on the red blood cell membranes of these pigs (48). Obukhova et al. have reviewed CMAH comprehensively (49).
If neonatal pigs are the source of islets (i.e., NICCs), in which expression of Gal is considerable, the deletion of expression of Gal (and possibly of Neu5Gc and Sda) will be advantageous.
Differences in N- and O-glycan profiles between human and porcine islets might prove to be the next gene modification sites. Novel xenoantigens include complex-type N-glycans with terminal neuraminic acid residues and high-mannose-type N-glycans with core fucosylation (50). Carbohydrate antigen microarrays in pigs and cynomolgus monkeys have revealed natural non-αGal antigens (e.g., Tn antigen, T antigen, GM2 glycolipid) and novel carbohydrate structures (e.g., Galβ1-4GlcNAcβ1-3Galβ1 and N-linked glycans with Manα1-6 (GlcNAcβ1-2Manα1-3)Manβ1-4GlcNAcβ) that are responsible for the IgM and IgG anti-carbohydrate antibody responses (51, 52). These findings suggest future gene modification sites to eliminate anti-carbohydrate antibody responses in pig-to-primate islet xenotransplantation.
For future studies of the 4th xenoantigen(s), several sources might be helpful, e.g., the database of Glycomics (http://www.functionalglycomics.org/). The National Center for Functional Glycomics (NCFG) (https://ncfg.hms.harvard.edu/) offers a CFG mammalian-type glycan microarray, with 600 glycans present, that might be helpful in studying xenoantigens in the future.
Cellular Immune Response-Related Genes
Progress in gene modification aimed at protecting xenografts from the adaptive immune response has been made recently. For example, knock-in of CTLA4-Ig or the high-affinity variant LEA29Y (36, 53), knockout or knockdown swine leukocyte antigen (SLA) class I and class II (37, 54), and in vitro tests on SLA class I and class II-silenced cells have reported significantly reduced xenogeneic T cell and natural killer cell responses, and antibody-mediated cell-dependent responses to islet cell clusters (55). However, CTLA4-Ig or LEA29Y transgenic pigs face the problems of hypoimmunity (36, 56).
Immune checkpoint blockade is a promising approach to control pathogenic immune responses. Immunomodulation with PD-L1 improves islet allotransplantation outcomes (57–63), and may facilitate successful xenotransplantation. PD-L1 is a ligand that reduces the proliferation and activation of T cells, B cells, and monocytes through interaction with PD-L1 receptors on these cells, and prevents cell-mediated lysis from CD8+ T cells by reducing their proliferation and cytokine secretion (40). Programmed cell death protein 1 blockade has successfully achieved clinical objectives in the treatment of cancer (64–66). In xenotransplantation, pigs transgenic for PD-L1 have been successfully generated, and cells from these pigs prevent human T cell cytotoxicity and B cell activation in vitro (57, 58), with similar results in a pig-to-rat xenotransplantation model (67). In contrast, islet PD-L1 deficiency has been associated with increased allograft rejection and increased inflammatory cell infiltration (68). Testing of the transplantation of pig islets expressing PD-L1 in NHPs should be a future research direction.
In summary, whether the 4th xenoantigen is exposed in islets after CMAHKO remains uncertain, and more research on the cellular response (that will be the next obstacle to explore) is required (69).
Immunosuppressive Regimen
The main objective of the immunosuppressive regimen is to inhibit T cell activation and prevent subsequent T cell-dependent dendritic cell activation and activation of B cells and macrophages. Immunosuppressive regimens based on conventional (FDA-approved) therapy have proved inadequate, although islet graft survival of 222 days has been reported (70). In contrast, blockade of the CD40/CD154 costimulation pathway has resulted in maximal islet graft survival of 965 days (Table 5) (7, 13, 70–76). The major mechanistic effects, advantages, and side-effects of the key immunosuppressive agents of relevance to xenotransplantation have been reviewed by Bikhet and his colleagues (77). Samy et al. have reviewed the role of costimulation pathway blockade in xenotransplantation (78). Here we will focus on novel immunosuppressive regimens based on blockade of the CD40/CD154 costimulation pathway.
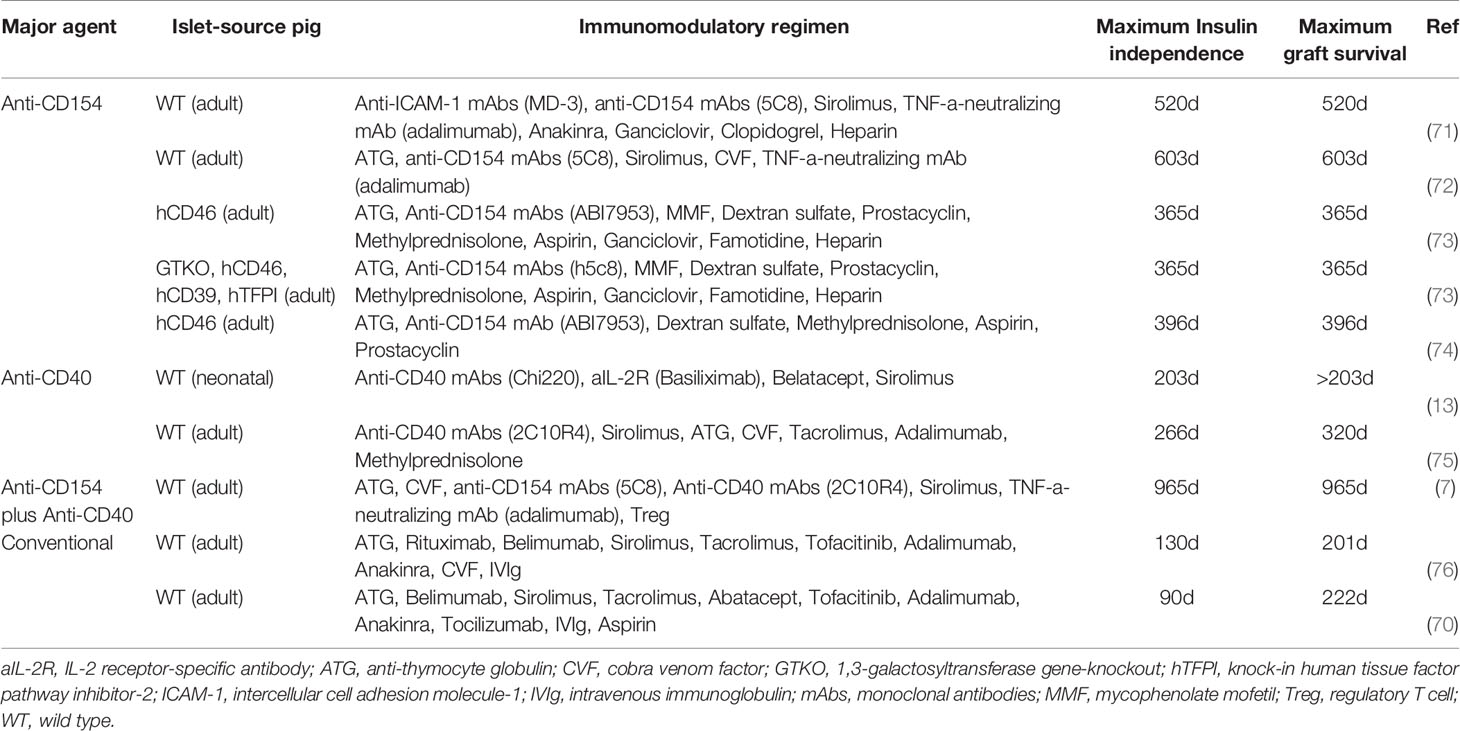
Table 5 Immunosuppressive protocols associated with prolonged periods of insulin-independence and islet graft survival.
Immunosuppressive Regimens Based on Anti-CD40mAbs
Anti-CD40mAbs are a chimeric form of Fab combined with IgG Fc fragments to prevent the stimulation of B and T cells through blockade of the CD40/CD154 pathway, which also participates in regulating thrombosis, tissue inflammation, and hematopoiesis (79). Unlike anti-CD154mAbs, no significant thrombogenic complications have been observed in anti-CD40mAb studies (10). Islet graft survivals are summarized in Table 5.
To date, some anti-CD40mAbs have completed phase II clinical trials of allotransplantation (but not in islet transplantation). These included bleselumab (ASKP1240), iscalimab (CFZ533), and BI 655064 (10, 80, 81). Among them, ASKP1240 demonstrated good results with a favorable benefit-risk ratio and no thromboembolic events in a phase II clinical kidney transplantation trial (10). Treatment with 2C10R4 was associated with the longest pig islet graft survival in NHPs to date (maximum insulin-independence 950 days, maximum graft survival 965 days) (7). However, anti-CD40mAbs may be associated with adverse effects, e.g., a temporary increase in liver enzymes (ASKP1240) (82, 83), significant depletion of peripheral blood B cells (Chi220) (13), and inhibition of T regulatory cell (Treg) expansion (2C10R4) (84).
Immunosuppressive Regimens Based on Anti-CD154 Agents
Anti-CD154 agents also provide efficient CD40/CD154 pathway blockade (85), but were originally associated with thromboembolic complications (BG9588, hu5c8, IDEC-131, ABI793) (86–88), although the situation with IDEC-131 remains controversial (89). They were demonstrated to be preferable to anti-C40mAbs in pig islet transplantation in NHPs (Table 5) (72, 75). Modifications to the Fc region on CD154 agents, the binding site for the Fc receptor (FcgRIIA) on platelets (85), appear to have eliminated thromboembolic events (e.g., CDP7657 and BMS-986004 in rhesus macaques, and MEDI4920 in cynomolgus monkeys) (77). To date, CDP7657, BMS-986004, and MEDI4920 have completed phase I or II clinical trials (not in islet transplantation) without obvious complications (8, 9, 90–92).
Overall, although anti-CD40mAbs have proved successful in pig-to-NHP islet xenotransplantation, the new anti-CD154 agents may prove preferable for clinical trials (Table 6) (9, 10, 75, 80–83, 90, 92–102). Of importance, ongoing studies at the Massachusetts General Hospital indicate that monotherapy with an anti-CD154mAb (with no additional immunosuppressive therapy) prevents rejection of heterotopic heart and life-supporting kidney allografts in monkeys (Robin Pierson and Tatsuo Kawai, personal communications). This regimen, or a modification of it, has not yet been tested in xenograft models
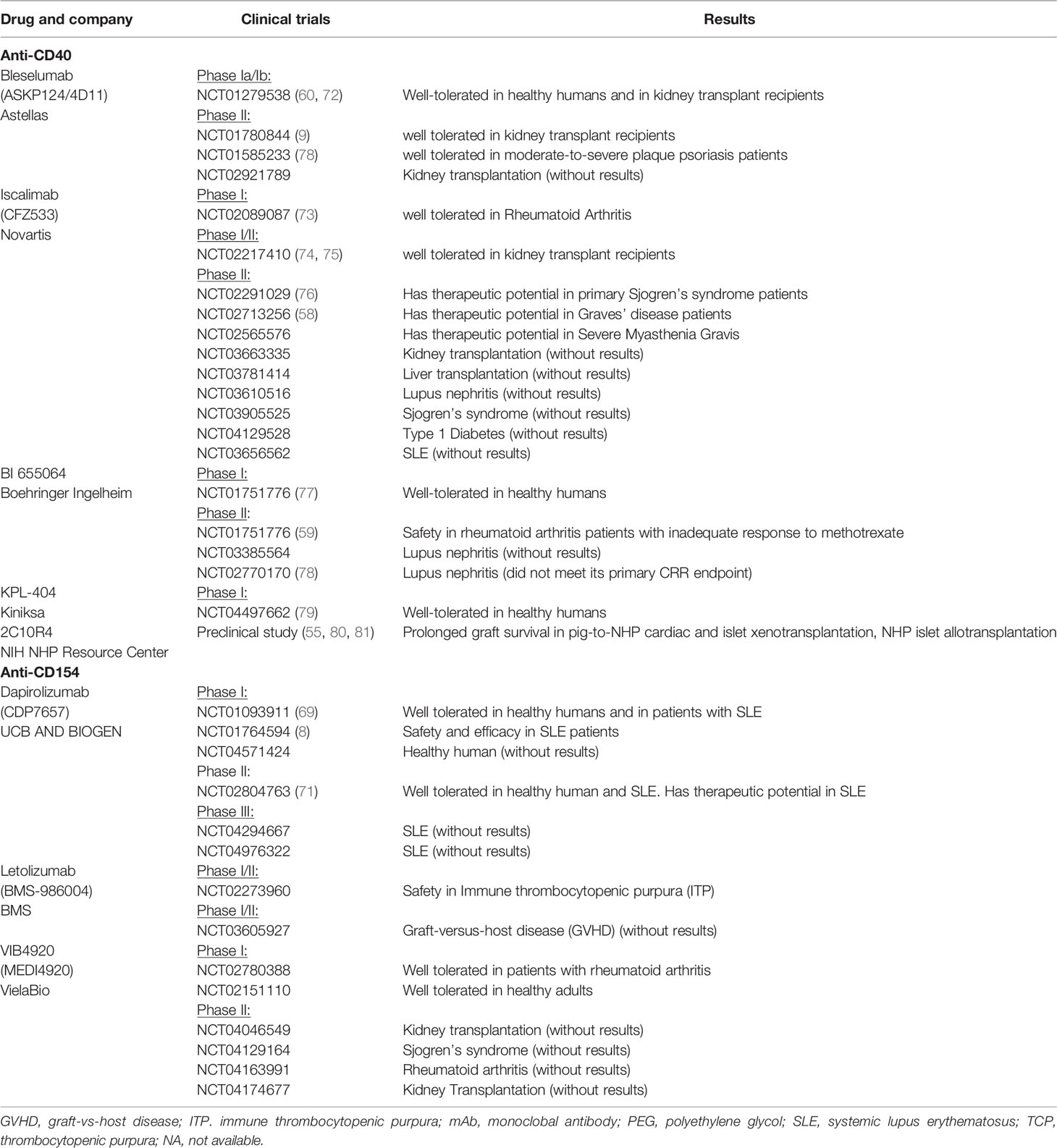
Table 6 Agents that block the CD40/CD154 costimulation pathway that are currently in clinical trials and preclinical studies, an update of Bikhet 2021 (58).
Bikhet et al. published an immunosuppressive regimen that has proved moderately successful in pig solid organ transplantation in NHPs (77), but such a regimen may be too intensive to warrant use in patients with islet xenografts.
The Instant Blood-Mediated Inflammatory Reaction (IBMIR)
After infusion of islets into the portal vein (the preferred site at present), a substantial percentage of islets are lost in the immediate post-transplant period through an inflammatory response termed IBMIR. The loss is significantly greater if the islets are xeno-islets, e.g., pig islets into NHPs and pig islets to human blood in vitro (103–107). Coagulation, platelet aggregation, complement activation, and neutrophil and monocyte infiltration play roles in this reaction (108). Several approaches to reduce the loss of islets have been explored, e.g., anticoagulation, complement depletion (109), and modified islet culture medium (110), but none has been entirely successful yet. The transplantation of islets from pigs with one or multiple genetic modifications may help protect the islets from early injury and loss (14, 74, 111–115). Moreover, alternative transplantation sites in intrapleural space greatly reduced IBMIR (116).
It is beneficial to add heparin or dextran sulfate to the peri-transplant regimen for their anticoagulant and complement-modulating properties that reduce islet loss from IBMIR (109, 117–120). Low molecular dextran sulfate at low doses demonstrated good results in the prevention of IBMIR in phase II clinical islet allotransplantation study (NCT00789308) (119). Nanoparticle-based techniques improve the therapeutic efficacy of heparin. For example, polymeric nanocoating islets with heparin-polyethylene glycol (PEG) or chondroitin sulfate-PEG in an NHP islet allotransplant model was associated with significantly longer islet survival with reduced loss to IBMIR compared with PEG and naked islets (121, 122). Conjugated nanoparticles (heparin-immobilized superparamagnetic iron oxide) conjugated onto the surface of the islets attenuated IBMIR in a rat-to-mouse islet xenotransplantation model (123). Islet-surface modifications with streptavidin-CD47 protein, a chimeric construct expressing CD47 on the extracellular domain, efficiently prevent islet loss from IBMIR (124).
Cibinetide (Araim Pharmaceuticals Inc., Tarrytown, NY, USA) (a non-hematopoietic erythropoietin analogue) also showed islet-protective effects by reducing IBMIR-induced platelet consumption (125). Based on these studies, agents that reduce IBMIR, combined with the transplantation of islets from genetically-engineered pigs (e.g., pigs not expressing the known carbohydrate xenoantigens, but expressing human complement- and coagulation-regulatory proteins), and an optimal immunosuppressive regimen may increase graft survival and the therapeutic efficacy of islet xenotransplantation.
Comment
Key factors in successfully developing pig islet xenotransplantation include determination of the optimal age of the islet-source pig (adult or neonatal), the optimal genetic modifications that should be made to the pig, and the optimal immunosuppressive regimen that should be administered to the recipient. Whether the ‘4th’ xenoantigen is problematic in the pig-to-NHP islet transplantation model needs to be clarified. More attention needs to be directed to genetic modifications that might reduce the instant blood-mediated inflammatory reaction and/or the adaptive immune response to pig islets. The advantages and disadvantages of immunosuppressive regimens based on anti-CD40 and anti-CD154 agents require clarification. Since the first case of successful pig-to-human kidney and heart transplantation had been reported recently (126, 127), we anticipate that pig islet xenotransplantation will become clinically successful when these remaining questions have been resolved.
Author Contributions
LM, DC, and ZP initiated the review. LM and GS wrote the manuscript. DC, YL, JC, SZ, JD, YH, YN, YZ, and ZC revised the manuscript. All authors contributed to the article and approved the submitted version.
Funding
This work was supported by grants from the Shenzhen Foundation of Science and Technology (grant numbers GJHZ20200731095207021), the National Key R&D Program of China (2017YFC1103704) and from the Special Funds for the Construction of High Level Hospitals in Guangdong Province (2019).
Conflict of Interest
The authors declare that the research was conducted in the absence of any commercial or financial relationships that could be construed as a potential conflict of interest.
Publisher’s Note
All claims expressed in this article are solely those of the authors and do not necessarily represent those of their affiliated organizations, or those of the publisher, the editors and the reviewers. Any product that may be evaluated in this article, or claim that may be made by its manufacturer, is not guaranteed or endorsed by the publisher.
Abbreviations
CMAH, cytidine monophospho-N-acetylneuraminic acid hydroxylase; Gal, galactose-α1,3-galactose; GTKO, 1,3-galactosyltransferase gene-knockout; IBMIR, instant blood-mediated inflammatory reaction; mAbs, monoclonal antibodies; Neu5Gc, N-glycolylneuraminic acid; NHP, nonhuman primate; NICC, neonatal islet cell clusters; PD-L1, programmed cell death ligand 1.
References
1. DiMeglio LA, Evans-Molina C, Oram RA. Type 1 Diabetes. Lancet (2018) 391:2449–62. doi: 10.1016/S0140-6736(18)31320-5
2. Liu Z, Hu W, He T, Dai Y, Hara H, Bottino R, et al. Pig-to-Primate Islet Xenotransplantation: Past, Present, and Future. Cell Transplant (2017) 26:925–47. doi: 10.3727/096368917X694859
3. Nagaraju S, Bottino R, Wijkstrom M, Trucco M, Cooper DKC. Islet Xenotransplantation: What Is the Optimal Age of the Islet-Source Pig? Xenotransplantation (2015) 22:7–19. doi: 10.1111/xen.12130
4. Cooper DKC, Hara H, Iwase H, Yamamoto T, Li Q, Ezzelarab M, et al. Justification of Specific Genetic Modifications in Pigs for Clinical Organ Xenotransplantation. Xenotransplantation (2019) 26:e12516. doi: 10.1111/xen.12516
5. Estrada JL, Martens G, Li P, Adams A, Newell KA, Ford ML, et al. Evaluation of Human and Non-Human Primate Antibody Binding to Pig Cells Lacking GGTA1/CMAH/β4galnt2 Genes. Xenotransplantation (2015) 22:194–202. doi: 10.1111/xen.12161
6. Hong S-H, Kim H-J, Kang S-J, Park C-G. Novel Immunomodulatory Approaches for Porcine Islet Xenotransplantation. Curr Diabetes Rep (2021) 21:3. doi: 10.1007/s11892-020-01368-y
7. Shin J-S, Min B-H, Kim J-M, Kim J-S, Yoon IH, Kim HJ, et al. Failure of Transplantation Tolerance Induction by Autologous Regulatory T Cells in the Pig-to-Non-Human Primate Islet Xenotransplantation Model. Xenotransplantation (2016) 23:300–9. doi: 10.1111/xen.12246
8. Karnell JL, Albulescu M, Drabic S, Wang L, Moate R, Baca M, et al. A CD40L-Targeting Protein Reduces Autoantibodies and Improves Disease Activity in Patients With Autoimmunity. Sci Transl Med (2019) 11:eaar6584. doi: 10.1126/scitranslmed.aar6584
9. Chamberlain C, Colman PJ, Ranger AM, Burkly LC, Johnston GI, Otoul C, et al. Repeated Administration of Dapirolizumab Pegol in a Randomised Phase I Study Is Well Tolerated and Accompanied by Improvements in Several Composite Measures of Systemic Lupus Erythematosus Disease Activity and Changes in Whole Blood Transcriptomic Profiles. Ann Rheum Dis (2017) 76:1837–44. doi: 10.1136/annrheumdis-2017-211388
10. Harland RC, Klintmalm G, Jensik S, Yang H, Bromberg J, Holman J, et al. Efficacy and Safety of Bleselumab in Kidney Transplant Recipients: A Phase 2, Randomized, Open-Label, Noninferiority Study. Am J Transplant (2020) 20:159–71. doi: 10.1111/ajt.15591
11. Bottino R, Balamurugan AN, Smetanka C, Bertera S, He J, Rood PPM, et al. Isolation Outcome and Functional Characteristics of Young and Adult Pig Pancreatic Islets for Transplantation Studies. Xenotransplantation (2007) 14:74–82. doi: 10.1111/j.1399-3089.2006.00374.x
12. Kwak K, Park J-K, Shim J, Ko N, Kim H-J, Lee Y, et al. Comparison of Islet Isolation Result and Clinical Applicability According to GMP-Grade Collagenase Enzyme Blend in Adult Porcine Islet Isolation and Culture. Xenotransplantation (2021) 28:e12703. doi: 10.1111/xen.12703
13. Thompson P, Cardona K, Russell M, Badell IR, Shaffer V, Korbutt G, et al. CD40-Specific Costimulation Blockade Enhances Neonatal Porcine Islet Survival in Nonhuman Primates. Am J Transplant (2011) 11:947–57. doi: 10.1111/j.1600-6143.2011.03509.x
14. Thompson P, Badell IR, Lowe M, Cano J, Song M, Leopardi F, et al. Islet Xenotransplantation Using Gal-Deficient Neonatal Donors Improves Engraftment and Function. Am J Transplant (2011) 11:2593–602. doi: 10.1111/j.1600-6143.2011.03720.x
15. Thompson P, Badell IR, Lowe M, Turner A, Cano J, Avila J, et al. Alternative Immunomodulatory Strategies for Xenotransplantation: CD40/154 Pathway-Sparing Regimens Promote Xenograft Survival. Am J Transplant (2012) 12:1765–75. doi: 10.1111/j.1600-6143.2012.04031.x
16. Cardona K, Korbutt GS, Milas Z, Lyon J, Cano J, Jiang W, et al. Long-Term Survival of Neonatal Porcine Islets in Nonhuman Primates by Targeting Costimulation Pathways. Nat Med (2006) 12:304–6. doi: 10.1038/nm1375
17. Vanderschelden R, Sathialingam M, Alexander M, Lakey JRT. Cost and Scalability Analysis of Porcine Islet Isolation for Islet Transplantation: Comparison of Juvenile, Neonatal and Adult Pigs. Cell Transplant (2019) 28:967–72. doi: 10.1177/0963689719847460
18. Lau H, Corrales N, Rodriguez S, Luong C, Zaldivar F, Alexander M, et al. An Islet Maturation Media to Improve the Development of Young Porcine Islets During In Vitro Culture. Islets (2020) 12:41–58. doi: 10.1080/19382014.2020.1750933
19. He S, Wang C, Du X, Chen Y, Zhao J, Tian B, et al. MSCs Promote the Development and Improve the Function of Neonatal Porcine Islet Grafts. FASEB J (2018) 32:3242–53. doi: 10.1096/fj.201700991R
20. Montanari E, Szabó L, Balaphas A, Meyer J, Perriraz-Mayer N, Pimenta J, et al. Multipotent Mesenchymal Stromal Cells Derived From Porcine Exocrine Pancreas Improve Insulin Secretion From Juvenile Porcine Islet Cell Clusters. Xenotransplantation (2021) 28:e12666. doi: 10.1111/xen.12666
21. Hassouna T, Seeberger KL, Salama B, Korbutt GS. Functional Maturation and In Vitro Differentiation of Neonatal Porcine Islet Grafts. Transplantation (2018) 102:e413–23. doi: 10.1097/TP.0000000000002354
22. Smith KE, Purvis WG, Davis MA, Min CG, Cooksey AM, Weber CS, et al. In Vitro Characterization of Neonatal, Juvenile, and Adult Porcine Islet Oxygen Demand, β-Cell Function, and Transcriptomes. Xenotransplantation (2018) 25:e12432. doi: 10.1111/xen.12432
23. Emamaullee JA, Shapiro AMJ, Rajotte RV, Korbutt G, Elliott JF. Neonatal Porcine Islets Exhibit Natural Resistance to Hypoxia-Induced Apoptosis. Transplantation (2006) 82:945–52. doi: 10.1097/01.tp.0000238677.00750.32
24. Dai Y, Vaught TD, Boone J, Chen S-H, Phelps CJ, Ball S, et al. Targeted Disruption of the Alpha1,3-Galactosyltransferase Gene in Cloned Pigs. Nat Biotechnol (2002) 20:251–5. doi: 10.1038/nbt0302-251
25. Phelps CJ, Koike C, Vaught TD, Boone J, Wells KD, Chen S-H, et al. Production of Alpha 1,3-Galactosyltransferase-Deficient Pigs. Science (2003) 299:411–4. doi: 10.1126/science.1078942
26. Kim GA, Lee EM, Jin J-X, Lee S, Taweechaipaisankul A, Hwang JI, et al. Generation of CMAHKO/GTKO/shTNFRI-Fc/HO-1 Quadruple Gene Modified Pigs. Transgenic Res (2017) 26:435–45. doi: 10.1007/s11248-017-0021-6
27. Wang R-G, Ruan M, Zhang R-J, Chen L, Li X-X, Fang B, et al. Antigenicity of Tissues and Organs From GGTA1/CMAH/β4galnt2 Triple Gene Knockout Pigs. J BioMed Res (2018) 33:194–202. doi: 10.7555/JBR.32.20180018
28. Kim GA, Lee EM, Cho B, Alam Z, Kim SJ, Lee S, et al. Generation by Somatic Cell Nuclear Transfer of GGTA1 Knockout Pigs Expressing Soluble Human TNFRI-Fc and Human HO-1. Transgenic Res (2019) 28:91–102. doi: 10.1007/s11248-018-0103-0
29. Diamond LE, Quinn CM, Martin MJ, Lawson J, Platt JL, Logan JS. A Human CD46 Transgenic Pig Model System for the Study of Discordant Xenotransplantation. Transplantation (2001) 71:132–42. doi: 10.1097/00007890-200101150-00021
30. Murakami H, Nagashima H, Takahagi Y, Fujimura T, Miyagawa S, Okabe M, et al. Production of Transgenic Pigs Expressing Human DAF (CD55) Regulated by the Porcine MCP Gene Promoter. Transplant Proc (2000) 32:2505–6. doi: 10.1016/s0041-1345(00)01769-3
31. Niemann H, Verhoeyen E, Wonigeit K, Lorenz R, Hecker J, Schwinzer R, et al. Cytomegalovirus Early Promoter Induced Expression of Hcd59 in Porcine Organs Provides Protection Against Hyperacute Rejection. Transplantation (2001) 72:1898–906. doi: 10.1097/00007890-200112270-00006
32. Petersen B, Ramackers W, Tiede A, Lucas-Hahn A, Herrmann D, Barg-Kues B, et al. Pigs Transgenic for Human Thrombomodulin Have Elevated Production of Activated Protein C. Xenotransplantation (2009) 16:486–95. doi: 10.1111/j.1399-3089.2009.00537.x
33. Cho J, Kim G, Qamar AY, Fang X, Roy PK, Tanga BM, et al. Improved Efficiencies in the Generation of Multigene-Modified Pigs by Recloning and Using Sows as the Recipient. Zygote (2021) 30:1–8. doi: 10.1017/S0967199421000423
34. Ahrens H, Petersen B, Cunha A, Queisser A-L, Herrmann D, Kues W, et al. Production and Characterization of TF Knock-Down Pigs and hTFPI Transgenic Pigs. Xenotransplantation (2011) 18:296–6. doi: 10.1111/j.1399-3089.2011.00661.x
35. Choi K, Shim J, Ko N, Eom H, Kim J, Lee J-W, et al. Production of Heterozygous Alpha 1,3-Galactosyltransferase (GGTA1) Knock-Out Transgenic Miniature Pigs Expressing Human CD39. Transgenic Res (2017) 26:209–24. doi: 10.1007/s11248-016-9996-7
36. Bähr A, Käser T, Kemter E, Gerner W, Kurome M, Baars W, et al. Ubiquitous Lea29y Expression Blocks T Cell Co-Stimulation But Permits Sexual Reproduction in Genetically Modified Pigs. PloS One (2016) 11:e0155676. doi: 10.1371/journal.pone.0155676
37. Hara H, Witt W, Crossley T, Long C, Isse K, Fan L, et al. Human Dominant-Negative Class II Transactivator Transgenic Pigs - Effect on the Human Anti-Pig T-Cell Immune Response and Immune Status. Immunology (2013) 140:39–46. doi: 10.1111/imm.12107
38. Wang Y, Du Y, Zhou X, Wang L, Li J, Wang F, et al. Efficient Generation of B2m-Null Pigs via Injection of Zygote With TALENs. Sci Rep (2016) 6:38854. doi: 10.1038/srep38854
39. Tena A, Germana S, Turcotte N, Leto Barone AA, Arn S, Terlouw SL, et al. Miniature Swine Expressing Human Cd47 to Enhance Bone Marrow Engraftment in Non-Human Primates: 1651. Transplantation (2012) 94:776. doi: 10.1097/00007890-201211271-01520
40. Buermann A, Petkov S, Petersen B, Hein R, Lucas-Hahn A, Baars W, et al. Pigs Expressing the Human Inhibitory Ligand PD-L1 (CD 274) Provide a New Source of Xenogeneic Cells and Tissues With Low Immunogenic Properties. Xenotransplantation (2018) 25:e12387. doi: 10.1111/xen.12387
41. Yue Y, Xu W, Kan Y, Zhao H-Y, Zhou Y, Song X, et al. Extensive Germline Genome Engineering in Pigs. Nat BioMed Eng (2021) 5:134–43. doi: 10.1038/s41551-020-00613-9
42. Foote JB, Jagdale A, Yamamoto T, Hara H, Bikhet MH, Schuurman H-J, et al. Histopathology of Pig Kidney Grafts With/Without Expression of the Carbohydrate Neu5Gc in Immunosuppressed Baboons. Xenotransplantation (2021) 28:e12715. doi: 10.1111/xen.12715
43. Ariyoshi Y, Takeuchi K, Pomposelli T, Ekanayake-Alper DK, Shimizu A, Boyd L, et al. Antibody Reactivity With New Antigens Revealed in Multi-Transgenic Triple Knockout Pigs may Cause Early Loss of Pig Kidneys in Baboons. Xenotransplantation (2021) 28:e12642. doi: 10.1111/xen.12642
44. Yamamoto T, Hara H, Iwase H, Jagdale A, Bikhet MH, Morsi MA, et al. The Final Obstacle to Successful Pre-Clinical Xenotransplantation? Xenotransplantation (2020) 27:e12596. doi: 10.1111/xen.12596
45. Yamamoto T, Iwase H, Patel D, Jagdale A, Ayares D, Anderson D, et al. Old World Monkeys Are Less Than Ideal Transplantation Models for Testing Pig Organs Lacking Three Carbohydrate Antigens (Triple-Knockout). Sci Rep (2020) 10:9771. doi: 10.1038/s41598-020-66311-3
46. Yamamoto T, Hara H, Ayares D, Cooper DKC. The Problem of the “4th Xenoantigen” After Pig Organ Transplantation in Non-Human Primates may be Overcome by Expression of Human “Protective” Proteins. Xenotransplantation (2021) 28:e12658. doi: 10.1111/xen.12658
47. Lee W, Hara H, Ezzelarab MB, Iwase H, Bottino R, Long C, et al. Initial In Vitro Studies on Tissues and Cells From GTKO/CD46/NeuGcKO Pigs. Xenotransplantation (2016) 23:137–50. doi: 10.1111/xen.12229
48. Choe HM, Luo Z-B, Kang J-D, Oh MJ, An HJ, Yin X-J. Pathological Features in “Humanized” Neonatal Pig. Anim Biotechnol (2021), 1–9. doi: 10.1080/10495398.2021.1962896
49. Obukhova P, Tsygankova S, Chinarev A, Shilova N, Nokel A, Kosma P, et al. Are There Specific Antibodies Against Neu5Gc Epitopes in the Blood of Healthy Individuals? Glycobiology (2020) 30:395–406. doi: 10.1093/glycob/cwz107
50. Nanno Y, Shajahan A, Sonon RN, Azadi P, Hering BJ, Burlak C. High-Mannose Type N-Glycans With Core Fucosylation and Complex-Type N-Glycans With Terminal Neuraminic Acid Residues Are Unique to Porcine Islets. PloS One (2020) 15:e0241249. doi: 10.1371/journal.pone.0241249
51. Nanno Y, Sterner E, Gildersleeve JC, Hering BJ, Burlak C. Carbohydrate Antigen Microarray Analysis of Serum IgG and IgM Antibodies Before and After Adult Porcine Islet Xenotransplantation in Cynomolgus Macaques. PloS One (2021) 16:e0253029. doi: 10.1371/journal.pone.0253029
52. Nanno Y, Sterner E, Gildersleeve JC, Hering BJ, Burlak C. Profiling Natural Serum Antibodies of Non-Human Primates With a Carbohydrate Antigen Microarray. Xenotransplantation (2020) 27:e12567. doi: 10.1111/xen.12567
53. Buerck LW, Schuster M, Oduncu FS, Baehr A, Mayr T, Guethoff S, et al. LEA29Y Expression in Transgenic Neonatal Porcine Islet-Like Cluster Promotes Long-Lasting Xenograft Survival in Humanized Mice Without Immunosuppressive Therapy. Sci Rep (2017) 7:3572. doi: 10.1038/s41598-017-03913-4
54. Fischer K, Rieblinger B, Hein R, Sfriso R, Zuber J, Fischer A, et al. Viable Pigs After Simultaneous Inactivation of Porcine MHC Class I and Three Xenoreactive Antigen Genes GGTA1, CMAH and B4GALNT2. Xenotransplantation (2020) 27:e12560. doi: 10.1111/xen.12560
55. Carvalho Oliveira M, Valdivia E, Verboom M, Yuzefovych Y, Sake HJ, Pogozhykh O, et al. Generating Low Immunogenic Pig Pancreatic Islet Cell Clusters for Xenotransplantation. J Cell Mol Med (2020) 24:5070–81. doi: 10.1111/jcmm.15136
56. Phelps CJ, Ball SF, Vaught TD, Vance AM, Mendicino M, Monahan JA, et al. Production and Characterization of Transgenic Pigs Expressing Porcine CTLA4-Ig. Xenotransplantation (2009) 16:477–85. doi: 10.1111/j.1399-3089.2009.00533.x
57. Ding Q, Lu L, Zhou X, Zhou Y, Chou K-Y. Human PD-L1-Overexpressing Porcine Vascular Endothelial Cells Induce Functionally Suppressive Human CD4+CD25hiFoxp3+ Treg Cells. J Leukoc Biol (2011) 90:77–86. doi: 10.1189/jlb.1210691
58. Buermann A, Römermann D, Baars W, Hundrieser J, Klempnauer J, Schwinzer R. Inhibition of B-Cell Activation and Antibody Production by Triggering Inhibitory Signals via the PD-1/PD-Ligand Pathway. Xenotransplantation (2016) 23:347–56. doi: 10.1111/xen.12261
59. Li R, Lee J, Kim M, Liu V, Moulik M, Li H, et al. PD-L1-Driven Tolerance Protects Neurogenin3-Induced Islet Neogenesis to Reverse Established Type 1 Diabetes in NOD Mice. Diabetes (2015) 64:529–40. doi: 10.2337/db13-1737
60. Batra L, Shrestha P, Zhao H, Woodward KB, Togay A, Tan M, et al. Localized Immunomodulation With PD-L1 Results in Sustained Survival and Function of Allogeneic Islets Without Chronic Immunosuppression. J Immunol (2020) 204:2840–51. doi: 10.4049/jimmunol.2000055
61. Coronel MM, Martin KE, Hunckler MD, Barber G, O’Neill EB, Medina JD, et al. Immunotherapy via PD-L1-Presenting Biomaterials Leads to Long-Term Islet Graft Survival. Sci Adv (2020) 6:eaba5573. doi: 10.1126/sciadv.aba5573
62. Li T, Ma R, Zhu JY, Wang FS, Huang L, Leng XS. PD-1/PD-L1 Costimulatory Pathway-Induced Mouse Islet Transplantation Immune Tolerance. Transplant Proc (2015) 47:165–70. doi: 10.1016/j.transproceed.2014.10.043
63. Li T, Ma R, Zhu J, Wang F, Huang L, Leng X. Blockade of the OX40/OX40L Pathway and Induction of PD-L1 Synergistically Protects Mouse Islet Allografts From Rejection. Chin Med J (Engl) (2014) 127:2686–92. doi: 10.3760/cma.j.issn.0366-6999.20140740
64. Kraehenbuehl L, Weng C-H, Eghbali S, Wolchok JD, Merghoub T. Enhancing Immunotherapy in Cancer by Targeting Emerging Immunomodulatory Pathways. Nat Rev Clin Oncol (2022) 19:37–50. doi: 10.1038/s41571-021-00552-7
65. Lemaire V, Shemesh CS, Rotte A. Pharmacology-Based Ranking of Anti-Cancer Drugs to Guide Clinical Development of Cancer Immunotherapy Combinations. J Exp Clin Cancer Res (2021) 40:311. doi: 10.1186/s13046-021-02111-5
66. Tan AC, Bagley SJ, Wen PY, Lim M, Platten M, Colman H, et al. Systematic Review of Combinations of Targeted or Immunotherapy in Advanced Solid Tumors. J Immunother Cancer (2021) 9:e002459. doi: 10.1136/jitc-2021-002459
67. Plege-Fleck A, Lieke T, Römermann D, Düvel H, Hundrieser J, Buermann A, et al. Pig to Rat Cell Transplantation: Reduced Cellular and Antibody Responses to Xenografts Overexpressing PD-L1. Xenotransplantation (2014) 21:533–42. doi: 10.1111/xen.12121
68. Ma D, Duan W, Li Y, Wang Z, Li S, Gong N, et al. PD-L1 Deficiency Within Islets Reduces Allograft Survival in Mice. PloS One (2016) 11:e0152087. doi: 10.1371/journal.pone.0152087
69. Ladowski J, Martens G, Estrada J, Tector M, Tector J. The Desirable Donor Pig to Eliminate All Xenoreactive Antigens. Xenotransplantation (2019) 26:e12504. doi: 10.1111/xen.12504
70. Kim J-M, Hong S-H, Chung H, Shin J-S, Min B-H, Kim HJ, et al. Long-Term Porcine Islet Graft Survival in Diabetic Non-Human Primates Treated With Clinically Available Immunosuppressants. Xenotransplantation (2021) 28:e12659. doi: 10.1111/xen.12659
71. Lee J-I, Kim J, Choi Y-J, Park H-J, Park H-J, Wi HJ, et al. The Effect of Epitope-Based Ligation of ICAM-1 on Survival and Retransplantation of Pig Islets in Nonhuman Primates. Xenotransplantation (2018) 25:e12362. doi: 10.1111/xen.12362
72. Shin JS, Kim JM, Kim JS, Min BH, Kim YH, Kim HJ, et al. Long-Term Control of Diabetes in Immunosuppressed Nonhuman Primates (NHP) by the Transplantation of Adult Porcine Islets. Am J Transplant (2015) 15:2837–50. doi: 10.1111/ajt.13345
73. Bottino R, Knoll MF, Graeme-Wilson J, Klein EC, Ayares D, Trucco M, et al. Safe Use of Anti-CD154 Monoclonal Antibody in Pig Islet Xenotransplantation in Monkeys. Xenotransplantation (2017) 24:e12283. doi: 10.1111/xen.12283
74. van der Windt DJ, Bottino R, Casu A, Campanile N, Smetanka C, He J, et al. Long-Term Controlled Normoglycemia in Diabetic Non-Human Primates After Transplantation With Hcd46 Transgenic Porcine Islets. Am J Transplant (2009) 9:2716–26. doi: 10.1111/j.1600-6143.2009.02850.x
75. Shin J-S, Kim J-M, Min B-H, Yoon IH, Kim HJ, Kim J-S, et al. Pre-Clinical Results in Pig-to-Non-Human Primate Islet Xenotransplantation Using Anti-CD40 Antibody (2C10R4)-Based Immunosuppression. Xenotransplantation (2018) 25:e12356. doi: 10.1111/xen.12356
76. Kim J-M, Hong S-H, Shin J-S, Min B-H, Kim HJ, Chung H, et al. Long-Term Control of Diabetes in a Nonhuman Primate by Two Separate Transplantations of Porcine Adult Islets Under Immunosuppression. Am J Transplant (2021) 21:3561–72. doi: 10.1111/ajt.16704
77. Bikhet M, Iwase H, Yamamoto T, Jagdale A, Foote JB, Ezzelarab M, et al. What Therapeutic Regimen Will be Optimal for Initial Clinical Trials of Pig Organ Transplantation? Transplantation (2021) 105:1143–55. doi: 10.1097/TP.0000000000003622
78. Samy KP, Butler JR, Li P, Cooper DKC, Ekser B. The Role of Costimulation Blockade in Solid Organ and Islet Xenotransplantation. J Immunol Res (2017) 2017:8415205. doi: 10.1155/2017/8415205
79. Tang T, Cheng X, Truong B, Sun L, Yang X, Wang H. Molecular Basis and Therapeutic Implications of CD40/CD40L Immune Checkpoint. Pharmacol Ther (2021) 219:107709. doi: 10.1016/j.pharmthera.2020.107709
80. Kahaly GJ, Stan MN, Frommer L, Gergely P, Colin L, Amer A, et al. A Novel Anti-CD40 Monoclonal Antibody, Iscalimab, for Control of Graves Hyperthyroidism-a Proof-of-Concept Trial. J Clin Endocrinol Metab (2020) 105:dgz013. doi: 10.1210/clinem/dgz013
81. Visvanathan S, Daniluk S, Ptaszyński R, Müller-Ladner U, Ramanujam M, Rosenstock B, et al. Effects of BI 655064, an Antagonistic Anti-CD40 Antibody, on Clinical and Biomarker Variables in Patients With Active Rheumatoid Arthritis: A Randomised, Double-Blind, Placebo-Controlled, Phase IIa Study. Ann Rheum Dis (2019) 78:754–60. doi: 10.1136/annrheumdis-2018-214729
82. Vincenti F, Klintmalm G, Yang H, Ram Peddi V, Blahunka P, Conkle A, et al. A Randomized, Phase 1b Study of the Pharmacokinetics, Pharmacodynamics, Safety, and Tolerability of Bleselumab, a Fully Human, Anti-CD40 Monoclonal Antibody, in Kidney Transplantation. Am J Transplant (2020) 20:172–80. doi: 10.1111/ajt.15560
83. Anil Kumar MS, Papp K, Tainaka R, Valluri U, Wang X, Zhu T, et al. Randomized, Controlled Study of Bleselumab (ASKP1240) Pharmacokinetics and Safety in Patients With Moderate-to-Severe Plaque Psoriasis. Biopharm Drug Dispos (2018) 39:245–55. doi: 10.1002/bdd.2130
84. Oura T, Hotta K, Rosales I, Dehnadi A, Kawai K, Lee H, et al. Addition of Anti-CD40 Monoclonal Antibody to Nonmyeloablative Conditioning With Belatacept Abrogated Allograft Tolerance Despite Induction of Mixed Chimerism. Transplantation (2019) 103:168–76. doi: 10.1097/TP.0000000000002417
85. Lee J-I, Choi Y-J, Park H-J, Jung KC, Park SH. RD-05, a Novel Anti-CD154 Antibody, Efficiently Inhibits Generation of Anti-Drug Antibody Without the Risk of Thrombus Formation in Non-Human Primates. Biochem Biophys Res Commun (2018) 498:996–1001. doi: 10.1016/j.bbrc.2018.03.099
86. Boumpas DT, Furie R, Manzi S, Illei GG, Wallace DJ, Balow JE, et al. A Short Course of BG9588 (Anti-CD40 Ligand Antibody) Improves Serologic Activity and Decreases Hematuria in Patients With Proliferative Lupus Glomerulonephritis. Arthritis Rheum (2003) 48:719–27. doi: 10.1002/art.10856
87. Ferrant JL, Benjamin CD, Cutler AH, Kalled SL, Hsu Y-M, Garber EA, et al. The Contribution of Fc Effector Mechanisms in the Efficacy of Anti-CD154 Immunotherapy Depends on the Nature of the Immune Challenge. Int Immunol (2004) 16:1583–94. doi: 10.1093/intimm/dxh162
88. Sifuentes Giraldo WA, García Villanueva MJ, Boteanu AL, Lois Iglesias A, Zea Mendoza AC. New Targets in Systemic Lupus (Part 2/2). Reumatol Clin (2012) 8:263–9. doi: 10.1016/j.reuma.2012.01.013
89. Fadul CE, Mao-Draayer Y, Ryan KA, Noelle RJ, Wishart HA, Channon JY, et al. Safety and Immune Effects of Blocking Cd40 Ligand in Multiple Sclerosis. Neurol Neuroimmunol Neuroinflamm (2021) 8:e1096. doi: 10.1212/NXI.0000000000001096
90. Tocoian A, Buchan P, Kirby H, Soranson J, Zamacona M, Walley R, et al. First-In-Human Trial of the Safety, Pharmacokinetics and Immunogenicity of a PEGylated Anti-CD40L Antibody Fragment (CDP7657) in Healthy Individuals and Patients With Systemic Lupus Erythematosus. Lupus (2015) 24:1045–56. doi: 10.1177/0961203315574558
91. Kim SC, Wakwe W, Higginbotham LB, Mathews DV, Breeden CP, Stephenson AC, et al. Fc-Silent Anti-Cd154 Domain Antibody Effectively Prevents Nonhuman Primate Renal Allograft Rejection. Am J Transplant (2017) 17:1182–92. doi: 10.1111/ajt.14197
92. Furie RA, Bruce IN, Dörner T, Leon MG, Leszczyński P, Urowitz M, et al. Phase 2, Randomized, Placebo-Controlled Trial of Dapirolizumab Pegol in Patients With Moderate-to-Severe Active Systemic Lupus Erythematosus. Rheumatol (Oxford) (2021) 60:keab381. doi: 10.1093/rheumatology/keab381
93. Goldwater R, Keirns J, Blahunka P, First R, Sawamoto T, Zhang W, et al. A Phase 1, Randomized Ascending Single-Dose Study of Antagonist Anti-Human CD40 ASKP1240 in Healthy Subjects. Am J Transplant (2013) 13:1040–6. doi: 10.1111/ajt.12082
94. Espié P, He Y, Koo P, Sickert D, Dupuy C, Chokoté E, et al. First-In-Human Clinical Trial to Assess Pharmacokinetics, Pharmacodynamics, Safety, and Tolerability of Iscalimab, an Anti-CD40 Monoclonal Antibody. Am J Transplant (2020) 20:463–73. doi: 10.1111/ajt.15661
95. CNI-Free Therapy With Iscalimab (Anti-Cd40 Mab) Preserves Allograft Histology Compared to Standard of Care After Kidney Transplantation, in: ATC Abstracts. Available at: https://atcmeetingabstracts.com/abstract/cni-free-therapy-with-iscalimab-anti-cd40-mab-preserves-allograft-histology-compared-to-standard-of-care-after-kidney-transplantation/ (Accessed December 17, 2021).
96. CFZ533, A New Anti-Cd40 Mab Demonstrates Comparable Efficacy and Better Renal Function Versus Tacrolimus in De-Novo Cni-Free Kidney Transplantation, in: ATC Abstracts. Available at: https://atcmeetingabstracts.com/abstract/cfz533-a-new-anti-cd40-mab-demonstrates-comparable-efficacy-and-better-renal-function-versus-tacrolimus-in-de-novo-cni-free-kidney-transplantation/ (Accessed December 17, 2021).
97. Fisher BA, Szanto A, Ng W-F, Bombardieri M, Posch MG, Papas AS, et al. Assessment of the Anti-CD40 Antibody Iscalimab in Patients With Primary Sjögren’s Syndrome: A Multicentre, Randomised, Double-Blind, Placebo-Controlled, Proof-of-Concept Study. Lancet Rheumatol (2020) 2:e142–52. doi: 10.1016/S2665-9913(19)30135-3
98. Schwabe C, Rosenstock B, Doan T, Hamilton P, Dunbar PR, Eleftheraki AG, et al. Safety, Pharmacokinetics, and Pharmacodynamics of Multiple Rising Doses of Bi 655064, an Antagonistic Anti-CD40 Antibody, in Healthy Subjects: A Potential Novel Treatment for Autoimmune Diseases. J Clin Pharmacol (2018) 58:1566–77. doi: 10.1002/jcph.1278
99. Jayne D, Steffgen J, Romero-Diaz J, Amano H, Noppakun K, Gomez HM, et al. Pos0687 a Randomised Dose Ranging, Placebo-Controlled, Phase Ii Study Assessing the Efficacy and Safety of Bi 655064, an Antagonistic Anti-CD40 Antibody, in Patients With Lupus Nephritis. Ann Rheumatic Dis (2021) 80:589–90. doi: 10.1136/annrheumdis-2021-eular.1401
100. Kiniksa Announces Positive Final Data From Phase 1 Trial of KPL-404, in: Kiniksa Pharmaceuticals. Available at: https://investors.kiniksa.com/news-releases/news-release-details/kiniksa-announces-positive-final-data-phase-1-trial-kpl-404/ (Accessed October 29, 2021).
101. Mohiuddin MM, Singh AK, Corcoran PC, Thomas Iii ML, Clark T, Lewis BG, et al. Chimeric 2C10R4 Anti-CD40 Antibody Therapy Is Critical for Long-Term Survival of GTKO.hCD46.hTBM Pig-to-Primate Cardiac Xenograft. Nat Commun (2016) 7:11138. doi: 10.1038/ncomms11138
102. Singh A, Ramachandran S, Graham ML, Daneshmandi S, Heller D, Suarez-Pinzon WL, et al. Long-Term Tolerance of Islet Allografts in Nonhuman Primates Induced by Apoptotic Donor Leukocytes. Nat Commun (2019) 10:3495. doi: 10.1038/s41467-019-11338-y
103. Kim JH, Oh BJ, Lee HN, Park HS, Park SG, Park KS. Endothelial Colony-Forming Cell Coating of Pig Islets Prevents Xenogeneic Instant Blood-Mediated Inflammatory Reaction. Cell Transplant (2011) 20:1805–15. doi: 10.3727/096368911X566154
104. van der Windt DJ, Bottino R, Casu A, Campanile N, Cooper DKC. Rapid Loss of Intraportally Transplanted Islets: An Overview of Pathophysiology and Preventive Strategies. Xenotransplantation (2007) 14:288–97. doi: 10.1111/j.1399-3089.2007.00419.x
105. Nagaraju S, Bertera S, Tanaka T, Hara H, Rayat GR, Wijkstrom M, et al. In Vitro Exposure of Pig Neonatal Isletlike Cell Clusters to Human Blood. Xenotransplantation (2015) 22:317–24. doi: 10.1111/xen.12178
106. Samy KP, Davis RP, Gao Q, Martin BM, Song M, Cano J, et al. Early Barriers to Neonatal Porcine Islet Engraftment in a Dual Transplant Model. Am J Transplant (2018) 18:998–1006. doi: 10.1111/ajt.14601
107. van der Windt DJ, Marigliano M, He J, Votyakova TV, Echeverri GJ, Ekser B, et al. Early Islet Damage After Direct Exposure of Pig Islets to Blood: Has Humoral Immunity Been Underestimated? Cell Transplant (2012) 21:1791–802. doi: 10.3727/096368912X653011
108. Martin BM, Samy KP, Lowe MC, Thompson PW, Cano J, Farris AB, et al. Dual Islet Transplantation Modeling of the Instant Blood-Mediated Inflammatory Reaction. Am J Transplant (2015) 15:1241–52. doi: 10.1111/ajt.13098
109. Rood PPM, Bottino R, Balamurugan AN, Smetanka C, Ayares D, Groth C-G, et al. Reduction of Early Graft Loss After Intraportal Porcine Islet Transplantation in Monkeys. Transplantation (2007) 83:202–10. doi: 10.1097/01.tp.0000250680.36942.c6
110. Ma X, Yang C, Zhang J, Wang J, Li W, Xu C, et al. Culturing With Modified EGM2 Medium Enhances Porcine Neonatal Islet-Like Cell Clusters Resistance to Apoptosis in Islet Xenotransplantation. Xenotransplantation (2018) 25:e12358. doi: 10.1111/xen.12358
111. Casu A, Echeverri GJ, Bottino R, van der Windt DJ, He J, Ekser B, et al. Insulin Secretion and Glucose Metabolism in Alpha 1,3-Galactosyltransferase Knock-Out Pigs Compared to Wild-Type Pigs. Xenotransplantation (2010) 17:131–9. doi: 10.1111/j.1399-3089.2010.00572.x
112. Bottino R, Wijkstrom M, van der Windt DJ, Hara H, Ezzelarab M, Murase N, et al. Pig-To-Monkey Islet Xenotransplantation Using Multi-Transgenic Pigs. Am J Transplant (2014) 14:2275–87. doi: 10.1111/ajt.12868
113. Wijkstrom M, Bottino R, Iwase H, Hara H, Ekser B, van der Windt D, et al. Glucose Metabolism in Pigs Expressing Human Genes Under an Insulin Promoter. Xenotransplantation (2015) 22:70–9. doi: 10.1111/xen.12145
114. Samy KP, Gao Q, Davis RP, Song M, Fitch ZW, Mulvihill MS, et al. The Role of Human CD46 in Early Xenoislet Engraftment in a Dual Transplant Model. Xenotransplantation (2019) 26:e12540. doi: 10.1111/xen.12540
115. Song M, Fitch ZW, Samy KP, Martin BM, Gao Q, Patrick Davis R, et al. Coagulation, Inflammation, and CD46 Transgene Expression in Neonatal Porcine Islet Xenotransplantation. Xenotransplantation (2021) 28:e12680. doi: 10.1111/xen.12680
116. Lei J, Zhang A, Deng H, Yang Z, Peters CW, Lee KM, et al. Intrapleural Transplantation of Allogeneic Pancreatic Islets Achieves Glycemic Control in a Diabetic Non-Human Primate. Am J Transplant (2021) 22:966–72. doi: 10.1111/ajt.16875
117. Gustafson E, Asif S, Kozarcanin H, Elgue G, Meurling S, Ekdahl KN, et al. Control of Ibmir Induced by Fresh and Cryopreserved Hepatocytes by Low Molecular Weight Dextran Sulfate Versus Heparin. Cell Transplant (2017) 26:71–81. doi: 10.3727/096368916X692609
118. Johansson H, Goto M, Dufrane D, Siegbahn A, Elgue G, Gianello P, et al. Low Molecular Weight Dextran Sulfate: A Strong Candidate Drug to Block IBMIR in Clinical Islet Transplantation. Am J Transplant (2006) 6:305–12. doi: 10.1111/j.1600-6143.2005.01186.x
119. von Zur-Mühlen B, Lundgren T, Bayman L, Berne C, Bridges N, Eggerman T, et al. Open Randomized Multicenter Study to Evaluate Safety and Efficacy of Low Molecular Weight Sulfated Dextran in Islet Transplantation. Transplantation (2019) 103:630–7. doi: 10.1097/TP.0000000000002425
120. Cabric S, Sanchez J, Lundgren T, Foss A, Felldin M, Källen R, et al. Islet Surface Heparinization Prevents the Instant Blood-Mediated Inflammatory Reaction in Islet Transplantation. Diabetes (2007) 56:2008–15. doi: 10.2337/db07-0358
121. Park H, Haque MR, Park JB, Lee KW, Lee S, Kwon Y, et al. Polymeric Nano-Shielded Islets With Heparin-Polyethylene Glycol in a Non-Human Primate Model. Biomaterials (2018) 171:164–77. doi: 10.1016/j.biomaterials.2018.04.028
122. Yang J, Jiang S, Guan Y, Deng J, Lou S, Feng D, et al. Pancreatic Islet Surface Engineering With a starPEG-Chondroitin Sulfate Nanocoating. Biomater Sci (2019) 7:2308–16. doi: 10.1039/c9bm00061e
123. Hwang YH, Kim MJ, Lee DY. MRI-Sensitive Contrast Agent With Anticoagulant Activity for Surface Camouflage of Transplanted Pancreatic Islets. Biomaterials (2017) 138:121–30. doi: 10.1016/j.biomaterials.2017.05.038
124. Shrestha P, Batra L, Tariq Malik M, Tan M, Yolcu ES, Shirwan H. Immune Checkpoint CD47 Molecule Engineered Islets Mitigate Instant Blood-Mediated Inflammatory Reaction and Show Improved Engraftment Following Intraportal Transplantation. Am J Transplant (2020) 20:2703–14. doi: 10.1111/ajt.15958
125. Yao M, Domogatskaya A, Ågren N, Watanabe M, Tokodai K, Brines M, et al. Cibinetide Protects Isolated Human Islets in a Stressful Environment and Improves Engraftment in the Perspective of Intra Portal Islet Transplantation. Cell Transplant (2021) 30:9636897211039740. doi: 10.1177/09636897211039739
126. Cooper DKC. Genetically Engineered Pig Kidney Transplantation in a Brain-Dead Human Subject. Xenotransplantation (2021) 28:e12718. doi: 10.1111/xen.12718
127. Man Gets Genetically-Modified Pig Heart in World-First Transplant, in: BBC News (2022). Available at: https://www.bbc.com/news/world-us-canada-59944889 (Accessed January 11, 2022).
Keywords: immunosuppression, islets, nonhuman primate, pig, genetically-engineered, type 1 diabetes, islet transplantation, xenotransplantation
Citation: Mou L, Shi G, Cooper DKC, Lu Y, Chen J, Zhu S, Deng J, Huang Y, Ni Y, Zhan Y, Cai Z and Pu Z (2022) Current Topics of Relevance to the Xenotransplantation of Free Pig Islets. Front. Immunol. 13:854883. doi: 10.3389/fimmu.2022.854883
Received: 14 January 2022; Accepted: 14 March 2022;
Published: 01 April 2022.
Edited by:
Mohammad Hossein Karimi, Shiraz University of Medical Sciences, IranReviewed by:
Nalu Navarro-Alvarez, Instituto Nacional de Ciencias Médicas y Nutrición Salvador Zubirán (INCMNSZ), MexicoHidetaka Hara, University of Alabama at Birmingham, United States
Haitao Zhu, Xi’an Jiaotong University, China
Copyright © 2022 Mou, Shi, Cooper, Lu, Chen, Zhu, Deng, Huang, Ni, Zhan, Cai and Pu. This is an open-access article distributed under the terms of the Creative Commons Attribution License (CC BY). The use, distribution or reproduction in other forums is permitted, provided the original author(s) and the copyright owner(s) are credited and that the original publication in this journal is cited, in accordance with accepted academic practice. No use, distribution or reproduction is permitted which does not comply with these terms.
*Correspondence: Zuhui Pu, cHVwZXRlcjE5MEAxNjMuY29t; Lisha Mou, bGlzaGFtb3VAZ21haWwuY29t
†These authors have contributed equally to this work
‡These authors share senior authorship