- 1State Key Laboratory of Animal Nutrition, College of Animal Science and Technology, China Agricultural University, Beijing, China
- 2Poultry Mineral Nutrition Laboratory, College of Animal Science and Technology, Yangzhou University, Yangzhou, China
Enterococcus faecium (E. faecium) is a protective role that has crucial beneficial functions on intestinal homeostasis. This study aimed to investigate the effects of E. faecium on the laying performance, egg quality, host metabolism, intestinal mucosal immunity, and gut microbiota of laying hens under the Salmonella Enteritidis (S. Enteritidis) challenge. A total of 400 45-week-old laying hens were randomly divided into four treatments (CON, EF, SCON, and SEF groups) with five replicates for each group and 20 hens per replicate and fed with a basal diet or a basal diet supplemented with E. faecium (2.5 × 108 cfu/g feed). The experiment comprised two phases, consisting of the pre-salmonella challenged phase (from day 14 to day 21) and the post-salmonella challenged phase (from day 21 to day 42). At day 21 and day 22, the hens in SCON and SEF groups were orally challenged with 1.0 ml suspension of 109 cfu/ml S. Enteritidis (CVCC3377) daily, whereas the hens in CON and EF groups received the same volume of sterile PBS. Herein, our results showed that E. faecium administration significantly improved egg production and shell thickness during salmonella infection. Also, E. faecium affected host lipid metabolism parameters via downregulating the concentration of serum triglycerides, inhibited oxidative stress, and enhanced immune functions by downregulating the level of serum malondialdehyde and upregulating the level of serum immunoglobulin G. Of note, E. faecium supplementation dramatically alleviated intestinal villi structure injury and crypt atrophy, and improved intestinal mucosal barrier injuries caused by S. Enteritidis challenge. Moreover, our data revealed that E. faecium supplementation ameliorated S. Enteritidis infection-induced gut microbial dysbiosis by altering the gut microbial composition (reducing Bacteroides, Desulfovibrio, Synergistes, and Sutterella, and increasing Barnesiella, Butyricimonas, Bilophila, and Candidatus_Soleaferrea), and modulating the gut microbial function, such as cysteine and methionine metabolism, pyruvate metabolism, fatty acid metabolism, tryptophan metabolism, salmonella infection, and the PI3K-Akt signaling pathway. Taken together, E. faecium has a strong capacity to inhibit the S. Enteritidis colonization of hens. The results highlight the potential of E. faecium supplementation as a dietary supplement to combat S. Enteritidis infection in animal production and to promote food safety.
Introduction
Salmonella enterica serovar Enteritidis (S. enterica) infection is an important public health problem and also associated with significant morbidity and mortality in those infected with the pathogen (1, 2). Intestinal damage and diarrhea caused by S. enterica infection are a severe gastrointestinal disease responsible for the annual economic loss in the global poultry industry (3). Salmonella infection is associated with intestinal damage, impaired absorption of nutrients, and poor overall performance in poultry. Also, salmonella is one of the most common reasons of foodborne disease (4–6). Salmonella, as a common foodborne pathogen that affects humans, poses a serious threat to human health via contaminated poultry products, including laying eggs and meat (6). It was well known that the risks of acquiring this disease are greatly influenced by the prevalence of salmonella in poultry (7). Also, infection with salmonella significantly increases chick mortality and disrupts egg formation to reduce laying performance and egg quality in hens (8, 9). Very importantly, the stages of the laying period of laying hens are generally 27–50 weeks old. Egg production and egg quality by the hens in the peak egg production stage are affected by manifold factors, especially salmonella infection (10, 11). Besides, salmonella infection in hens also disrupts host metabolism and intestinal barrier functions and promotes host inflammatory responses and oxidative stress. Hence, to prevent the salmonella contamination of poultry products worldwide, growing studies demonstrated that feed additives are also considered as effective measures to minimize salmonella infection in poultry production (12, 13). In recent years, antibiotic use is the main approach to confront pathogenic infection; however, due to the emergence of antibiotic-resistant bacteria in animal husbandry (14, 15), there is an urgent need to find suitable alternatives to improve the intestinal health and maintain animal and human health in the post-antibiotics era (2, 16). Many studies have demonstrated that probiotic supplementation might be an effective strategy to solve this public problem in poultry (17, 18).
The current definition formulated by the Food and Agriculture Organization of the United Nations and World Health Organization working group experts states that probiotics are “live strains of strictly selected microorganisms which, when administered in adequate amounts, confer a health benefit on the host” (19, 20). Therefore, the use of probiotics is a promising measure for the prevention and treatment of intestinal disorders or diseases, such as inflammatory bowel disease (IBD), diarrhea, and intestinal homeostasis dysfunction (21–23). Numerous studies have also shown that some microbes, including Bacillus, Lactobacilli, and Enterococci, could significantly alleviate the severity and damage of intestinal ecosystems in poultry (24–26). Enterococcus faecium (E. faecium) is a ubiquitous bacterium that has been observed in the microbiota of many animals and humans (27). Also, the commensal strains of E. faecium have been reported to protect animals from enteric pathogenic infection and potentially improve host metabolism, immune responses, and intestinal homeostasis (27, 28). Studies have shown that the chicken supplement E. faecium could significantly improve the growth performance of broiler chickens and promote the digestion and absorption of nutrients (29). Also, another study revealed that E. faecium NCIMB 10415 has inhibited the pathogenic infection in piglets (30). For example, E. faecium has a considerable therapeutic effect on S. typhimurium infection in pigs (31). Moreover, current studies have demonstrated that the supplementation of E. faecium NCIMB11181 can decrease salmonella colonization in the intestine of broiler chickens infected with Escherichia coli O78 (32). While some previous studies have begun to reveal the functions of E. faecium in animal husbandry, the underlying mechanisms of action and specific protective factors for E. faecium that are related with salmonella susceptibility in laying hens are still unknown.
Therefore, our study mainly explored the detailed protective mechanism of E. faecium against S. enterica infection in laying hens, from the sides of crucial phenotypes, host metabolism and immune responses, intestinal barrier function, gut microbial community and structure, and salmonella colonization.
Materials and Methods
The experiment was carried out following the guidelines of China Agricultural Animal Care and Use Ethics Committee and conducted according to the relevant guidelines and regulations (AW02211202-1-1, Beijing, China). All experimental protocols were in accordance with the recommendations of the Guide for Guidelines for Experimental Animals of the Ministry of Science and Technology (Beijing, China).
Animals, Housing, and Experimental Design
A total of 400 45-week-old laying hens (Yukou Poultry Co., Ltd., Beijing, China) were assigned according to the body weight (1.80 ± 0.10 kg) and egg production (88 ± 2.10%) and then randomly allocated to four groups with five replicates for each group and 20 hens per replicate. Each of the groups consisted of five replicates in ten different cages (two birds per cage). The cages (Height 45 cm × Width 45 cm × Depth 45 cm) were equipped with one nipple drinker and an exterior feed trough that expanded the length of the cage. The whole experiment comprised 6 weeks, consisting of a 2-week adaptation period and 4- week experimental period. As shown in Figure 1, the experiment comprised two phases, consisting of the pre-salmonella challenged phase (from day 14 to day 21) and the post-salmonella challenged phase (from day 21 to day 42). The E. faecium powder (2.5 × 108 cfu/g feed) used in this study was provided by a commercial company (Smistyle Sci. & Tech. Development Co., Ltd, Beijing, China). The laying hens were fed with a basal diet (powder) or a basal diet supplemented with E. faecium (2.5 × 108 cfu/g feed). The four treatment groups were as follows: CON group (basal diet), EF group (basal diet + 2.5 × 108 cfu E. faecium per g feed), SCON group (basal diet + S. Enteritidis), and SEF group (basal diet + 2.5 × 108 cfu E. faecium per g feed + S. Enteritidis). In our study, the required quality of probiotics was added to the commercial powdered feed for homogenous mixing. Also, the basal diet was formulated to meet the recommended nutrient content by the National Research Council (1994) and was shown in Table S1. Room temperature was thermostatically controlled at 22 ± 3°C, with 16 h of light/d. The laying hens were provided with ad libitum feed and water throughout the experiment. In our study, challenged and non-challenged hens were fed in two independent, mechanically ventilated hen houses under the same environmental conditions to avoid cross-infection. Before and during the experiment, the salmonella- free status of diets, water, and environment samples was determined by using RT-qPCR analysis as we have discussed previously (33).
S. Enteritidis Inoculum and Challenge
S. enterica spp. enterica serovar Enteritidis (S. Enteritidis, CVCC3377) was obtained from the China Institute of Veterinary Drug Control (Beijing, China). The frozen culture was recovered by using 10 ml of sterile tryptone soy broth and incubated at 37°C with orbital shaking for 24 h. Subsequently, 5 ml of S. Enteritidis pre-culture were transferred to 100 ml of tryptone soy broth and incubated with orbital shaking overnight at 37°C. To determine the concentration of viable S. Enteritidis in the culture, the inoculum was diluted with sterile phosphate buffer saline (PBS) (pH = 7.2), then plated on xylose lysine doxycholate (XLD) and incubated at 37°C for 24 h. The stock culture was prepared in sterile PBS and adjusted to 1 ×109 cfu/ml of S. Enteritidis to be used as an inoculum. At day 8 and day 9, the hens in SCON and SEF groups were orally challenged with 1.0 ml suspension of 109 cfu/ml S. Enteritidis daily, whereas the hens in CON and EF groups received the same volume of sterile PBS. A syringe with an attached flexible tube was used for the administration of the suspension and the physiological saline solution.
Laying Performance and Egg Quality
During the study, feed intake was recorded weekly by calculating the difference between full bucket weights and the remaining feed. The hen-day egg production and egg weight were recorded daily, and the body weight was recorded weekly on a replication basis. The egg mass was calculated. The feed conversion ratio (FCR) was calculated as the grams of feed intake per gram of egg mass produced. At the end of the experiment, 30 eggs from each treatment were randomly collected to assess egg quality parameters. The eggshell strength and eggshell thickness were measured with a digital egg tester (ESTG-01; Orka Food Technology Ltd., Ramat Hasharon, Israel). The Haugh unit, yolk color, and egg weight were measured by a multifunctional egg quality tester (EA-01; Orka Food Technology Ltd., Ramat Hasharon, Israel). The eggshell was weighed, and yolks were separated using a separator and were then weighed to determine the relative yolk and albumen proportion as previously described (34).
Blood Sampling and Biochemical Analysis
At the end of the experiment, blood samples were collected from birds via the wing vein on sampling days as previously described. The serum was centrifuged at 3,000 rpm for 15 min at room temperature. Serum samples were aspirated by a pipette and stored in 1.5 ml tubes at -20°C until analyzed. The serum concentrations of total protein (TP), albumin (ALB), globulin (GLB), alanine aminotransferase (ALT), aspartate aminotransferase (AST), alkaline phosphatase (ALP), high-density lipoprotein cholesterol (HDL-C), low-density lipoprotein cholesterol (LDL-C), total cholesterol (TC), triglycerides (TGs), calcium (Ca), phosphate (P), immunoglobulin A (IgA), immunoglobulin G (IgG), and immunoglobulin M (IgM) were measured by an automatic biochemical analyzer (7600; Hitachi, Tokyo, Japan), following the manufacturer’s instructions. Also, the levels of total superoxide dismutase (T-SOD), total antioxidant capacity (T-AOC), malondialdehyde (MDA), interleukin-1β (IL-1β), interleukin-6 (IL-6), interferon-γ (IFN-γ), and tumor necrosis factor-α (TNF-α) were evaluated with enzyme-linked immunosorbent assay kits (Nanjing Jiancheng Biology Engineering Institute, Nanjing, China). All operations are in accordance with the instructions. Hens were humanely euthanized using an injection of pentobarbital sodium (0.4 ml kg/body weight; Sile Biological Technology Co. Ltd., Guangzhou City, China).
Tissue Collection, Fixation, and Histochemistry
At the end of the experiment, duodenum, jejunum, and ileum tissues were fixed in 4% paraformaldehyde, embedded in paraffin, cut into 5 μm thick sections, and subsequently stained with hematoxylin and eosin (H&E). Images were collected and analyzed using the CaseViewer 2.4 software (3DHISTECH Ltd., Budapest, Hungary). The villus height (VH) was measured from the top of the villus to the crypt mouth, and the crypt depth (CD) was defined as the depth of the invagination between adjacent crypt mouths. The villus width was measured at the bottom of the villus. Intestinal tissue damage was scored as previously described (35, 36), and the epithelial loss of intestinal villi and the infiltration of inflammatory cells were evaluated.
Immunofluorescence Staining and Analysis
Paraffin-embedded 5 μm thick sections were deparaffinized by heating to 60°C for 15 min and cleared with xylene, followed by an ethanol gradient (75%, 95%, and 100%) and water and steamed for 30 min in a citrate buffer for antigen retrieval. The levels of apoptosis in the colon tissues were detected by terminal deoxynucleotidyl transferase (TdT) dUTP nick-end labeling (TUNEL) staining according to the instructions provided as previously described (Roche, Basel, Switzerland). The 4’,6-diamidino-2-phenylindole, dilactate (DAPI) blue nuclei with the same label were selected as the total cells, and the TUNEL-positive cell number per field of intestinal epithelial cells was analyzed. Cell apoptosis was observed by green fluorescence microscopy (×200 magnification).
The intestinal tissues, including duodenum, jejunum, and ileum, harvested from hens were fixed in 4% PFA in PBS and incubated in 50% ethanol overnight. After fixation, the tissues were embedded in paraffin, sectioned, and subjected. For immunofluorescence analysis, samples were incubated with a ZO-1 rabbit polyclonal antibody (Cat: #A0659, ABclonal Technology; 1:200) and occludin (OCLN) rabbit polyclonal antibody (Cat: # A2601, ABclonal Technology; 1:200) for 30 min at 37°C. After three washes, the samples were stained with a Cy3-conjugated goat anti-rabbit secondary antibody (Beyotime, Beijing, China) and DAPI (Cat: #D21490, Invitrogen Life Technologies, Carlsbad, CA, USA). Images were obtained using a fluorescence microscope (Carl Zeiss, Oberkochen, Germany).
Cecal DNA Extraction, Amplification, and Sequencing of 16s RNA Gene
All the fresh cecal contents were collected and immediately frozen in liquid nitrogen and instantly sent to the laboratory for DNA extraction. Microbial DNA was isolated by the Omega Bio-Tek stool DNA kit (Omega, Norcross, GA, United States) and quantified by a NanoDrop 2000 spectrophotometer (Thermo Fisher Scientific, Waltham, MA, USA). Then, the V3–V4 region of the 16S rRNA gene was amplified with 338F and 806R primers with the sequence of 5’-ACTCCTACGGGAGCAGCA-3’ and 5’-GGACTACHVGGGTWTCTAAT-3’. DNA samples were quantified, followed by the amplification of the V3–V4 hypervariable region of the 16S rDNA. The final amplicon pool was evaluated by the AxyPrep DNA gel extraction kit. The purified PCR products were sequenced on the Illumina MiSeq PE300 platform at Major Biomedical Technology Co., Ltd. (Shanghai, China).
After demultiplexing, the resulting sequences were merged with FLASH (v1.2.11) and quality-filtered with fastp (0.19.6). Then, the high-quality sequences were de-noised using the DADA2 plugin in the QIIME2 (version 2020.2) [81] pipeline with the recommended parameters, which obtains single-nucleotide resolution based on the error profiles within samples. DADA2-denoised sequences are usually called amplicon sequence variants (ASVs). To minimize the effects of sequencing depth on the alpha and beta diversity measure, the number of sequences from each sample was rarefied to 4,000, which still yielded an average Good’s coverage of 97.90%. The taxonomic assignment of ASVs was performed using the naive Bayes consensus taxonomy classifier implemented in QIIME2 and the SILVA 16S rRNA database (v138). The analyses of the 16S rRNA microbiome sequencing data were performed using the free online platform Majorbio Cloud Platform.
Determination Gene Copy Numbers of S. Enteritidis in Cecal Contents
Five randomly chosen hens from SCON and SEF groups were euthanized, respectively. The samples of fresh cecal contents were aseptically collected and immediately frozen. The genomic DNA from samples was isolated with the QIAamp DNA Stool Mini Kit (Qiagen GmbH, Hilden, Germany) according to the manufacturer’s protocols. Finally, the genomic DNA of S. Enteritidis in these samples was determined in 0.1 μl aliquot of the DNA template for RT-qPCR detection.
The sequences of the primer pairs used for RT-qPCR detection were designed according to the S. Enteritidis special Prot6E nucleotide sequence (NO. U66901). The production size was 175 bp. The sequences of the primer pairs were as follows: Prot6E-F: 5’-ACAGGGGCACAATAACCGTA-3’ and Prot6E-R: 5’-TGCATCCCTGTCACAACATT-3’. The PCR and data acquisition and analysis were performed using the iCycler iQ Optical system software (version 3.1; Bio-Rad, California, USA). The number of target copies in the reaction was deduced from the threshold cycle (Ct) values. The Ct value corresponds to the fractional cycle number at which the fluorescence emission exceeds the standard deviation of the mean baseline emission by 15-fold. The plasmid DNA containing the target amplicon was diluted to contain 101–108 copies of the target DNA per test tube and used as the plasmid standard series. All samples were analyzed three times by the RT-qPCR assay, and the concentrations of the target DNA detected were expressed as the mean log10 of the bacterial genome copy number per milligram of cecal material.
Statistical Analysis
The data of the laying performance, egg quality, histomorphological parameters, and cecum content of salmonella copies were analyzed by means of one-way ANOVA using the SPSS 22.0 software (SPSS Inc., Chicago, IL, United States). Statistical significance was declared at P < 0.05 and trended at P < 0.1. The data were expressed as mean ± standard error of mean (SEM), and graphs were generated by the GraphPad Prism software v 8.0 (GraphPad Software, Inc., San Diego, USA).
All statistical analyses were performed in the R environment (version: V3.6.0, http://www.r-project.org/). The alpha diversity indices, including the Sobs index, Ace index, Chao index, and Shannon index, were determined by sampling-based ASV analysis and presented by the observed ASV, which was calculated using the MOTHUR program (version v.1.30.1). Principal coordinates analysis (PCoA) was conducted by the R package (http://www.R-project.org/) to display microbiome beta diversity. The Bray–Curtis metric distances, unweighted Unifrac distances, and weighted Unifrac distances were calculated with the phyloseq package. Adonis (999 permutations) was used to evaluate the effect of E. faecium supplementation on the bacterial community structure using the vegan package in R. The differences in the abundance and enrichment of bacterial genera were examined using Kruskal–Wallis tests, and ternary plots were created using the “vcd” package and visualized by the “ggplot2” package in R. The predominance of bacterial communities between groups was analyzed by the linear discriminant analysis (LDA; LDA score > 2.0) effect size method. Based on the normalized relative abundance matrix, the features with significantly different abundances between assigned taxa were determined by the linear discriminant analysis effect size (LEfSe) with the Kruskal–Wallis rank-sum test (P < 0.05), and LDA was used to assess the effect size of each feature. The PICRUSt (version 2.0) software was used to predict the Kyoto Encyclopedia of Genes and Genomes (KEGG) ortholog functional profiles of bacterial communities in rhizospheres and roots using the 16S rRNA sequences.
Results
E. faecium Alleviated the Laying Performance and Egg Quality Damage of S. Enteritidis-Challenged Laying Hens
During the post-salmonella challenge period (from day 21 to day 42), compared with the CON group, the egg production of SCON hens had a decreasing trend (0.05 < P < 0.1, Table 1). Importantly, the level of egg production was significantly higher (P < 0.05) from SEF hens than in SCON hens (Table 1). Moreover, compared to the CON group, the yolk color was significantly decreased (P < 0.05) in the EF group and the shell thickness was decreased (P < 0.05) in the SCON group (Table 2). Further, the level of shell thickness in the SEF hens was significantly higher (P < 0.05) than in SCON hens (Table 2). Also, there was no significant difference (P > 0.05) in the laying performance, including the egg weight, feed intake, egg mass, and feed efficiency, and in the egg quality, including the yolk weight, albumen weight, eggshell weight, eggshell strength, and Haugh unit of these hens among the treatment groups (Tables 1, 2).

Table 1 Effect of dietary supplementation with E. faecium on laying performance of hens challenged with S. Enteritidis.
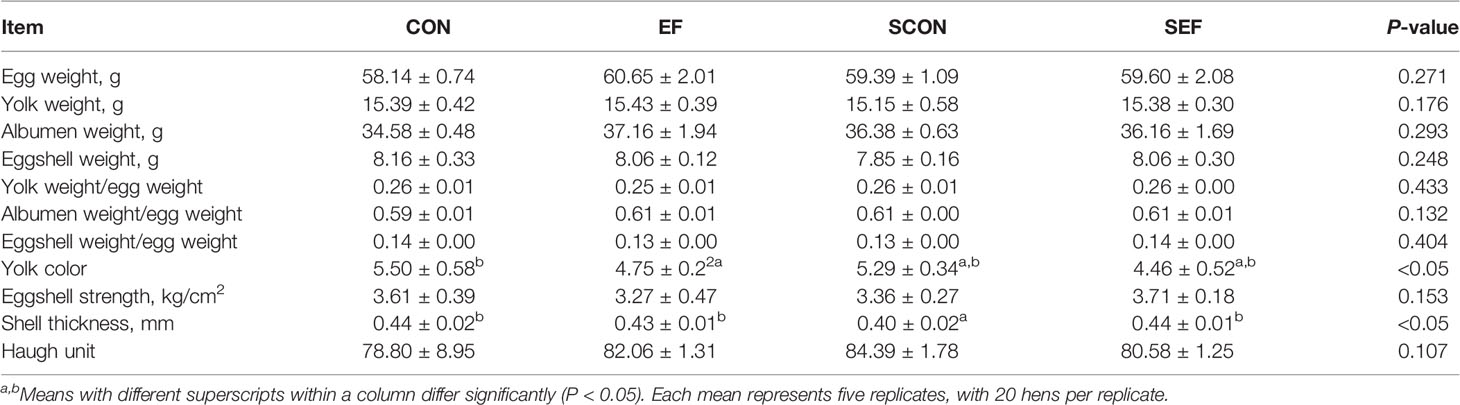
Table 2 Effect of dietary supplement with E. faecium on egg quality of layers challenged with S. Enteritidis.
E. faecium Affected Host Metabolism, Oxidative Stress Parameters, Inflammatory Cytokines, and Immunoglobulin Levels of S. Enteritidis-challenged laying hens
To evaluate the effects of dietary E. faecium supplementation on the host health of laying hens, the protein metabolism, lipid metabolism, oxidative stress, and inflammatory immune responses were recorded and calculated at different phases (the pre-salmonella challenge period and post-salmonella challenge period). As shown in Figure 2, during the post-salmonella challenge period (from day 21 to day 42), S. Enteritidis infection increased (P < 0.05) the concentration of serum ALP and decreased (P < 0.05) the levels of serum LDL-C, TC, TG, P, and MDA (Figures 2H, J–M, Q), while E. faecium treatment significantly decreased (P < 0.05) the levels of ALP, T-SOD, IL-1β, IL-6, and TNF-α (Figures 2H, O, R, S, U). Also, on day 42, compared to the CON hens, E. faecium supplementation downregulated the concentrations of serum TG and MDA and upregulated the level of IgG in the EF group (Figure 2W).
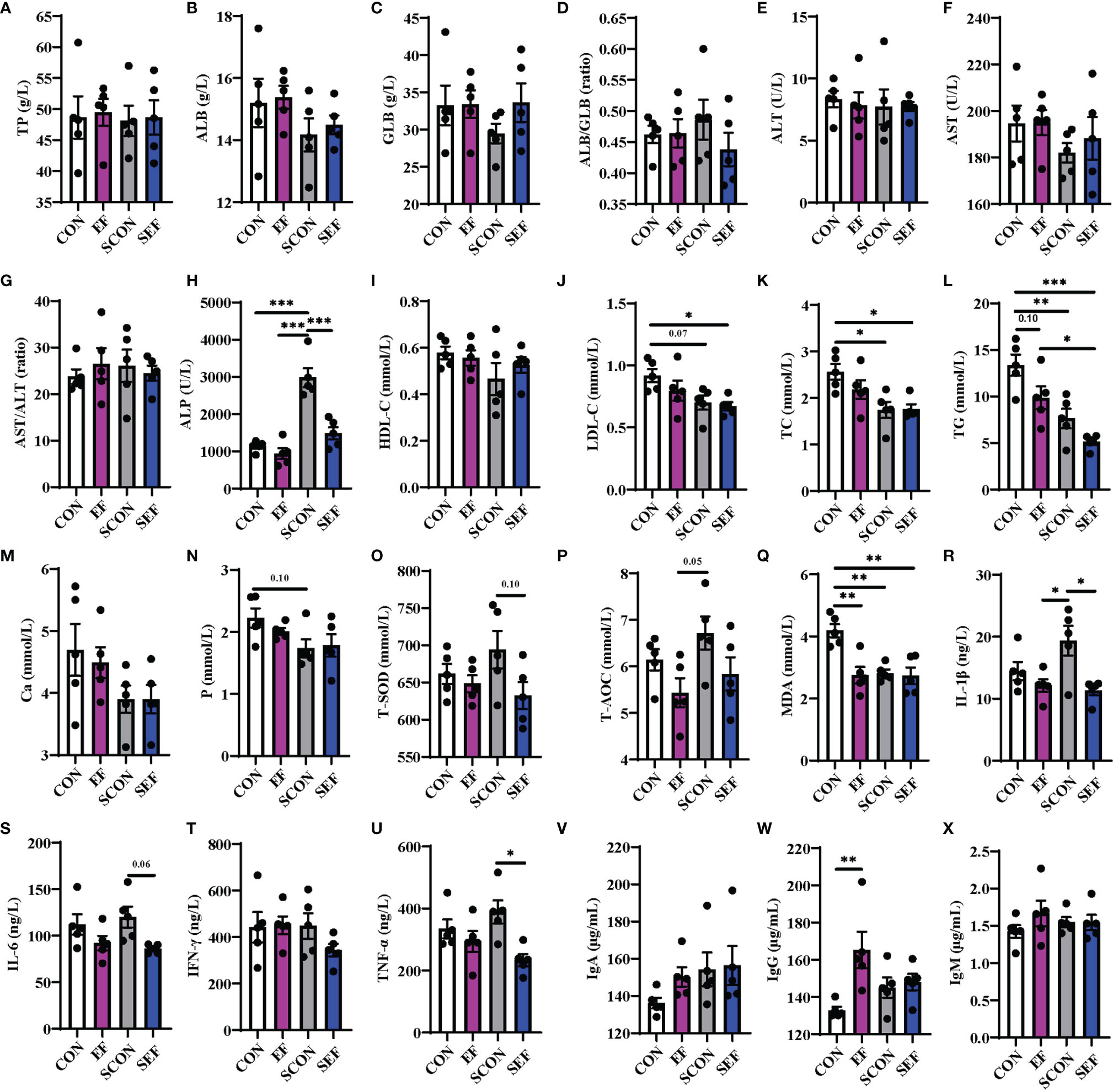
Figure 2 Effect of dietary supplementation with E. faecium on serum parameters of hens challenged with S. Enteritidis. (A–H) TP, ALB, GLB, ALB/GLB, ALT, AST, AST/ALT, and ALP levels in serum samples were measured in different treatments. (I–N) HDL-C, LDL-C, TC, TG, Ca, and P levels in serum samples were measured. (O–X) T-SOD, T-AOC, MDA, IL-1β, IL-6, IFN-γ, TNF-α, IgA, and IgM levels in serum samples were measured among these groups. Asterisks denote significant differences (*p ≤ 0.05, **p ≤ 0.01, ***p ≤ 0.001), n = five per group; data are represented as mean ± SEM.
E. faecium Improved Intestinal Histopathologic Changes, Alleviated Intestinal Barrier, and Reduced Intestinal Apoptosis of S. Enteritidis-Challenged Laying Hens
The intestinal morphology of the duodenum, jejunum, and ileum was displayed in Figure 2. In our study, obvious hemorrhagic spots were observed (P < 0.05) in both jejunum and ileum parts after S. Enteritidis challenge. Hematoxylin–eosin (H&E) staining revealed that the intestinal epithelial villi and crypt in the segments of duodenum, jejunum, and ileum were severely damaged (P < 0.05) in response to S. Enteritidis, as evidenced by the broken villi structure and crypt atrophy (Figure 3). However, E. faecium administration decreased (P < 0.05) the degree of intestinal injury, and reduced the CD, and increased the villus-to-crypt ratio (VCR) in the duodenum, jejunum, and ileum (Figures 3B–J). Besides, the salmonella infection significantly decreased (P < 0.05) the expression of intestinal barrier-related proteins, such as ZO-1 (TJP1) and OCLN-1 in the duodenum, jejunum, and ileum. However, the expression of TJP1 and OCLN in the SEF group was significantly higher (P < 0.05) compared with the SCON group (Figure 4). Meanwhile, TUNEL assay results demonstrated that S. Enteritidis infection significantly increased the number of positive cells in the duodenum, jejunum, and ileum, while there was a decline following E. faecium administration (Figure 5).
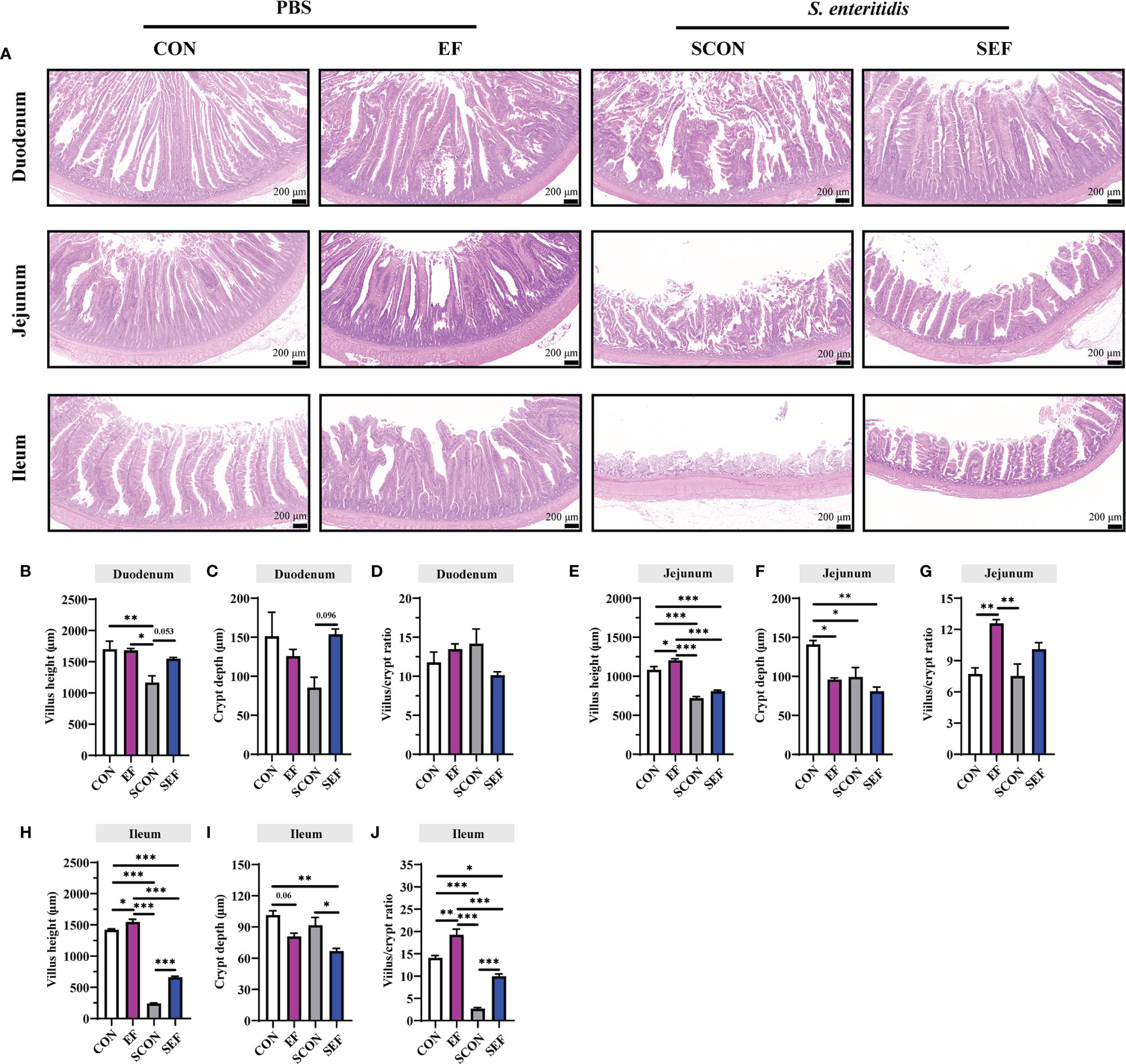
Figure 3 Effect of dietary supplement with E. faecium on histomorphological parameters of jejunum and ileum of layers challenged with S. Enteritidis. (A) Histomorphometric analysis of the Duodenum, Jejunum, and Ileum by Hematoxylin & Eosin (H&E) staining. (B–J) The villus height, crypt depth, and villus/crypt ratio shown in the pictures were randomly measured in each sample from each group. Scale bar: 200 μm. Asterisks denote significant differences (*p ≤ 0.05, **p ≤ 0.01, ***p ≤ 0.001), n = five per group; data are represented as mean ± SEM.
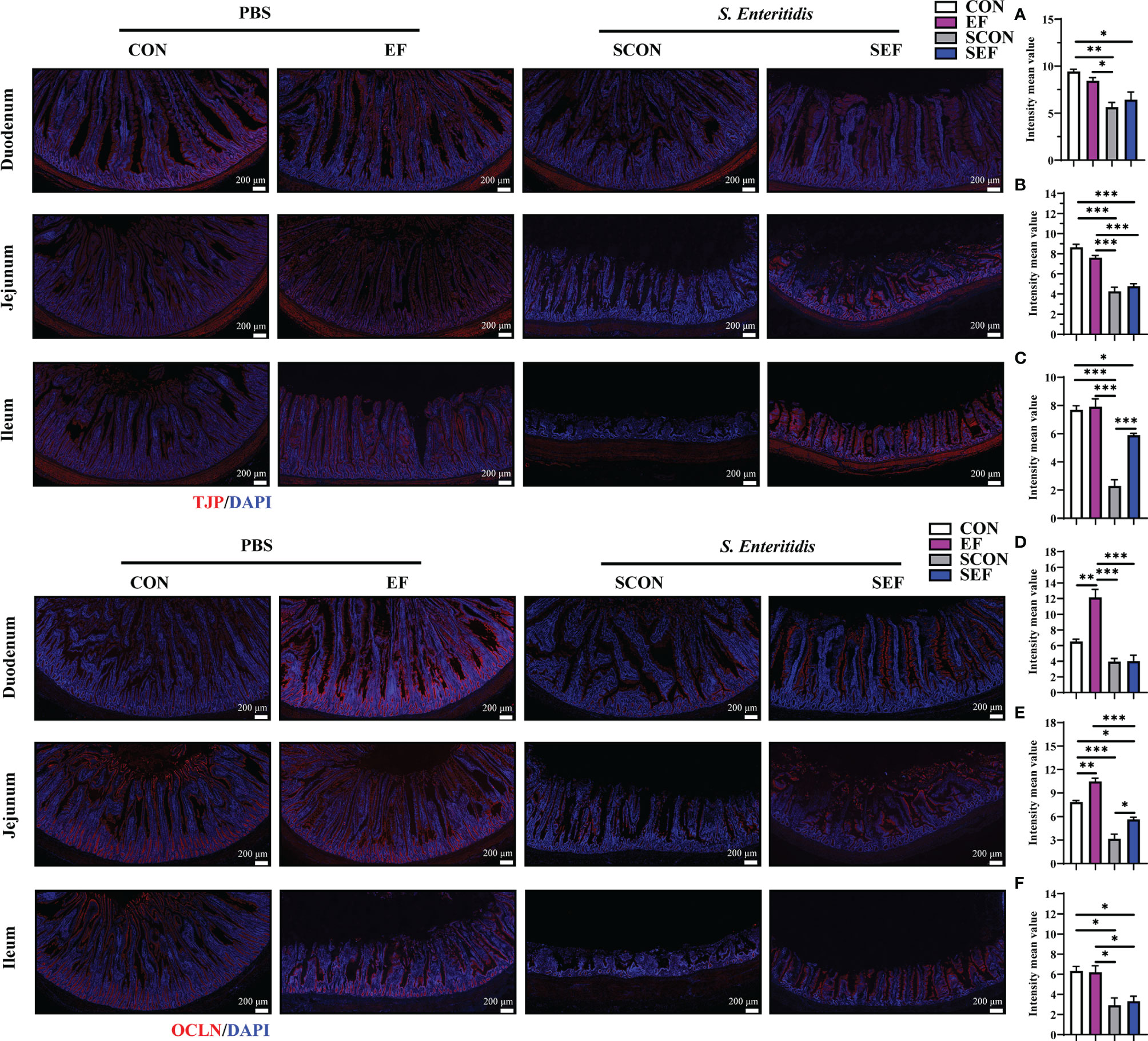
Figure 4 Effect of dietary supplement with E. faecium on intestinal epithelial barrier function of layers challenged with S. Enteritidis. Representative images of the immunohistochemical staining of TJP in the duodenum (A), Jejunum (B), and ileum (C) mucosa. The positive cells are stained red. Representative images of the immunohistochemical staining of OCLN in the duodenum (D), Jejunum (E), and ileum (F) mucosa. The positive cells are stained red. Scale bar: 200 μm. Asterisks denote significant differences (*p ≤ 0.05, **p ≤ 0.01, ***p ≤ 0.001), n = five per group; data are represented as mean ± SEM.
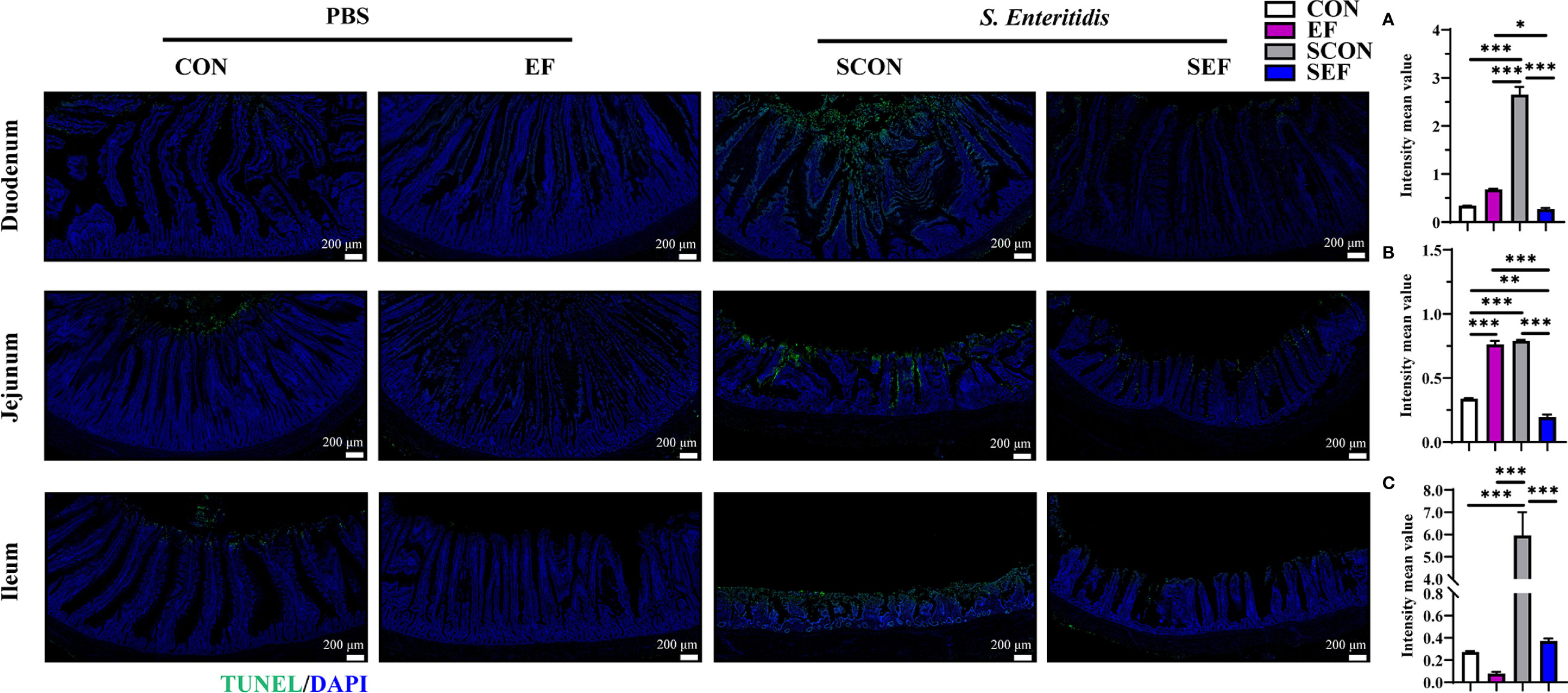
Figure 5 Effect of dietary supplement with E. faecium on intestinal epithelial cell apoptosis of layers challenged with S. Enteritidis. Representative images of the immunohistochemical staining of TUNEL in the duodenum (A), jejunum (B), and ileum (C) mucosa. Scale bar: 200 μm. Asterisks denote significant differences (*p ≤ 0.05, **p ≤ 0.01, ***p ≤ 0.001), n = five per group; data are represented as mean ± SEM.
E. faecium Reshifted the Gut Microbial Community and Structure of S. Enteritidis-Challenged Laying Hens
We next used the high-throughput 16S rRNA gene sequencing to determine whether E. faecium supplementation affected the gut microbial composition in S. Enteritidis-infected hens. V3-V4 16S rRNA gene sequencing was performed on the cecal samples collected from CON, EF, SCON, and SEF hens (n = 5 per group). As shown in Figures 6A–C, no significant difference was observed (P > 0.05) in the alpha diversity, including the Sobs, Ace, and Chao indices of these hens. A principal coordinate analysis (PCoA) was performed to assess the similarities and differences among groups (Figures 6D–H). Our results indicated distinct clusters of the gut microbial composition among these groups. The Adonis analysis based on the Bray–Curtis distance was performed to quantify the differences in species diversity. As shown in Figures 6D–H, the data revealed that E. faecium administration significantly altered (P < 0.05) the β diversity index compared to the control group (EF vs. CON, SEF vs. SCON, respectively). However, these results demonstrated that S. Enteritidis infection did not alter the β diversity index of the gut microbiome (Figures 6G, H).
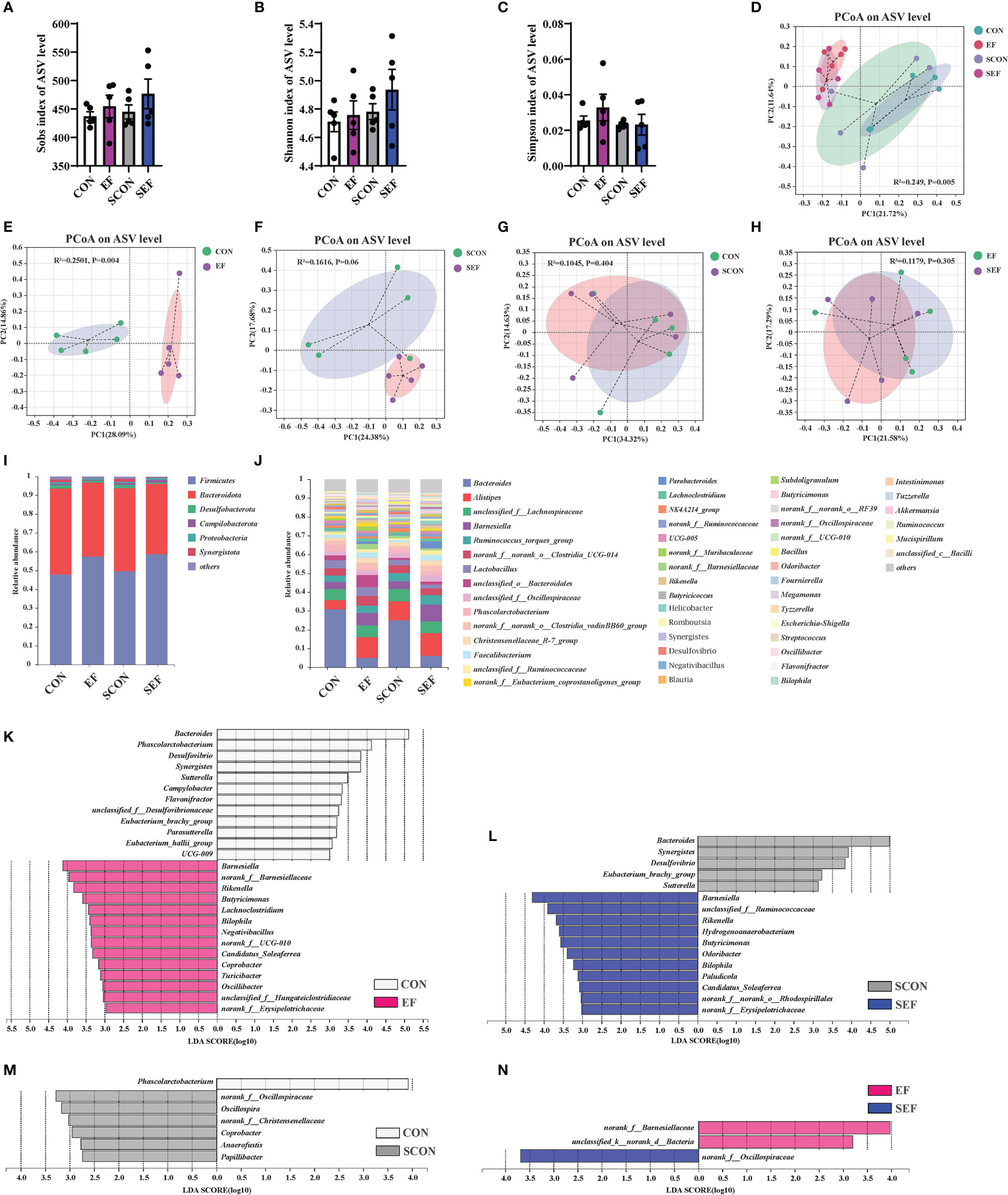
Figure 6 Effect of dietary supplement with E. faecium on the gut microbial community and structure of cecum in hens. (A) Sobs index of ASV level. (B) Shannon index of ASV level. (C) Simpson index of ASV level. (D–H) PCoA plots assessed by Adonis analysis among these treatments. (I–J) Shows the relative abundance of phylum and genus in different groups. Linear discriminate analysis effect size (LEfSe) was performed to determine the difference in abundance among these treatments (K–N).
As shown in Figures 6I, J, phylogenetic analysis revealed the most abundant composition of cecal microbiota at phylum and genera levels among all treatments. At the phylum level, Firmicutes, Bacteroidota, Desulfobacterota, Campilobacterota, Proteobacteria, and Synergistota were dominant (Figure 6I). The composition of the gut microbiome at the genera level is shown in Figure 6J. The predominant genera were Bacteroides, Alistipes, unclassified_f:Lachnospiraceae, Barnesiella, Ruminococcus_torques_group, norank_f:norank_o:Clostridia_UCG-014, Lactobacillus, unclassified_o:Bacteroidales, Phascolarctobacterium, Faecalibacterium, Parabacteroides, and so on (Figrure 6J). The specific bacterial taxa associated with E. faecium and S. Enteritidis treatments was identified through the linear discriminant analysis effect size (LEfSe, LDA score > 2.0) analysis. As shown in Figure 6K (CON group vs. EF group), there was a significant increase in the relative abundance of Barnesiella, norank_f:Barnesiellaceae, Rikenella, Butyricimonas, Lachnoclostridium, Bilophila, Negativibacillus, Candidatus_Soleaferrea, Coprobacter, Turicibacter, and Oscillibacter and reduction in the relative abundance of Bacteroides, Phascolarctobacterium, Desulfovibrio, Synergistes, Sutterella, Campylobacter, Flavonifractor, and Parasutterella in the hens fed with an E. faecium-supplemented diet compared to the control hens. As shown in Figure 6L (SCON group vs. SEF group), the predominant bacterial strains of SEF were Barnesiella, Rikenella, Hydrogenoanaerobacterium, Butyricimonas, Odoribacter, Bilophila, Paludicola, and Candidatus_Soleaferrea, while the predominant bacterial strains in the control group (SCON) were Bacteroides, Synergistes, Desulfovibrio, Eubacterium_brachy_group, and Sutterella. Also, the predominant bacterial strain in the CON group was Phascolarctobacterium, while the predominant bacterial strains in the SCON group were Oscillospira, Coprobacter, Anaerofustis, and Papillibacter (Figure 6M). As shown in Figure 6N, the predominant bacterial strains in the EF group were norank_f:Barnesiellaceae and unclassified_k:norank_d:Bacteria, while the predominant bacterial strain in the SEF group was norank_f:Oscillospiraceae.
In the present study, Phylogenetic Investigation of Communities by Reconstruction of Unobserved States 2.0 (PICRUSt2) was based on the ASV tree in the Greengenes database to achieve the prediction of the metabolic function of the gut microbiota. As shown in Figure 7A, compared to the CON group, the relative abundance of cysteine and methionine metabolism, tryptophan metabolism, secondary bile acid biosynthesis, and the NOD-like receptor signaling pathway were significantly increased in the EF group, while nitrogen metabolism, pyruvate metabolism, ferroptosis, and apoptosis were markedly decreased. Also, our results showed that the abundance of cysteine and methionine metabolism, pyruvate metabolism, fatty acid metabolism, tryptophan metabolism, salmonella infection, and the PI3K-Akt signaling pathway were dramatically increased in the SEF group, while the levels of purine metabolism and apoptosis were decreased compared with the SCON group (Figure 7B).
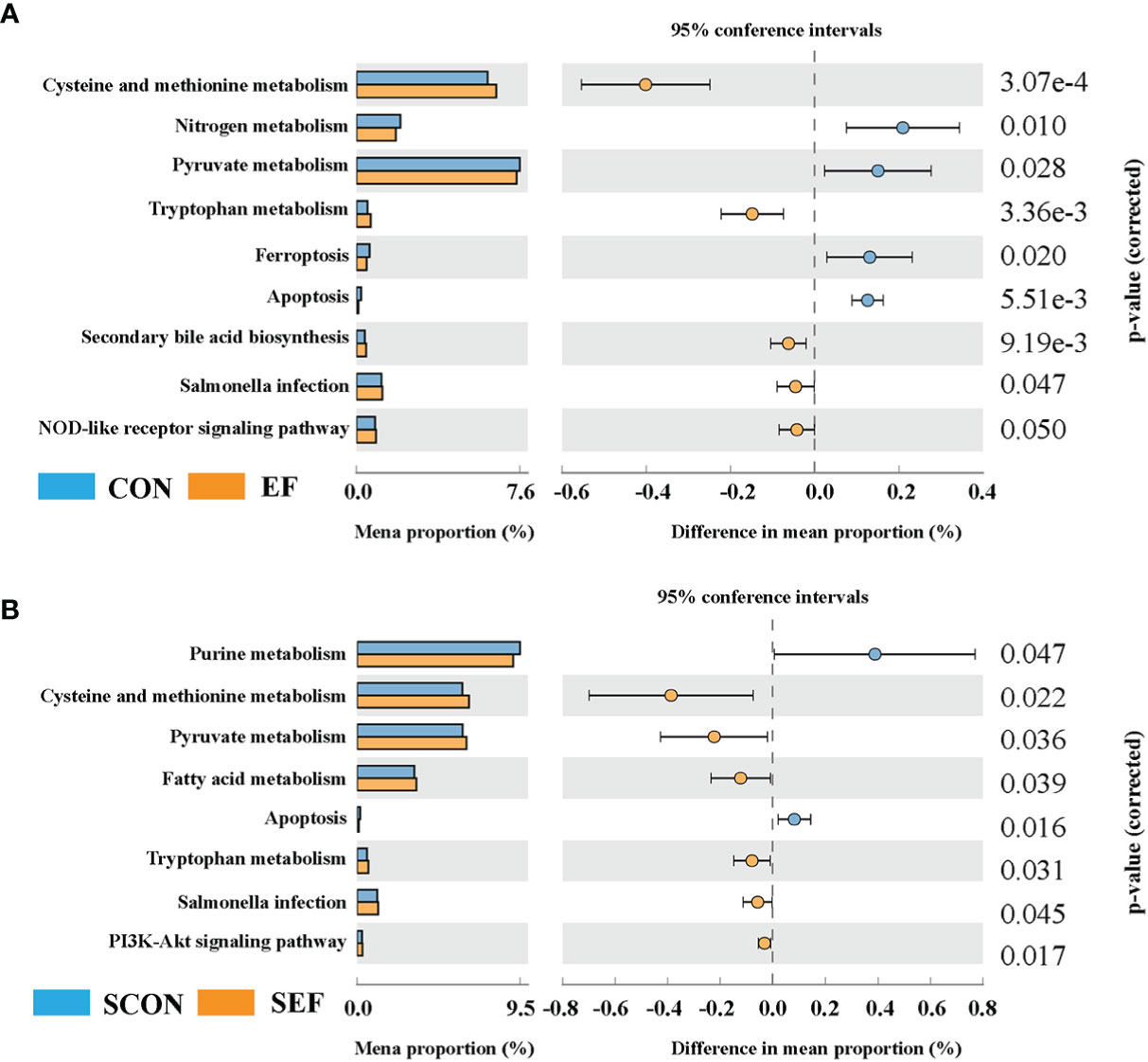
Figure 7 Effect of dietary supplement with E. faecium on the gut microbial function of cecum in hens. (A) The functional profile of cecum contents between CON and EF groups. (B) The functional profile of cecum contents between SCON and SEF groups.
E. faecium Inhibited S. Enteritidis Colonization of Laying Hens
S. Enteritidis copies were detected using the RT-qPCR from cecal samples collected at different time points. Samples were collected from SCON and SEF groups at days 1, 7, 14, and 21 post-infection (1, 7, 14, and 21 dpi). Also, the samples collected from the negative control groups (CON and EF) were negative for S. Enteritidis throughout the experiments (data not shown). As shown in Figures 8A, B, hens developed infection with S. Enteritidis observed in the cecal samples at 1 dpi, while no significant difference was observed between the SCON and SEF groups (P > 0.05). Importantly, compared to the SCON, the supplementation of E. faecium in the SEF group dramatically decreased (P < 0.05) salmonella copies in cecal samples at 7, 14, and 21 dpi (Figures 8C–E). These results indicate that E. faecium has a strong capacity to inhibit the S. Enteritidis colonization of laying hens (Figure 8F).
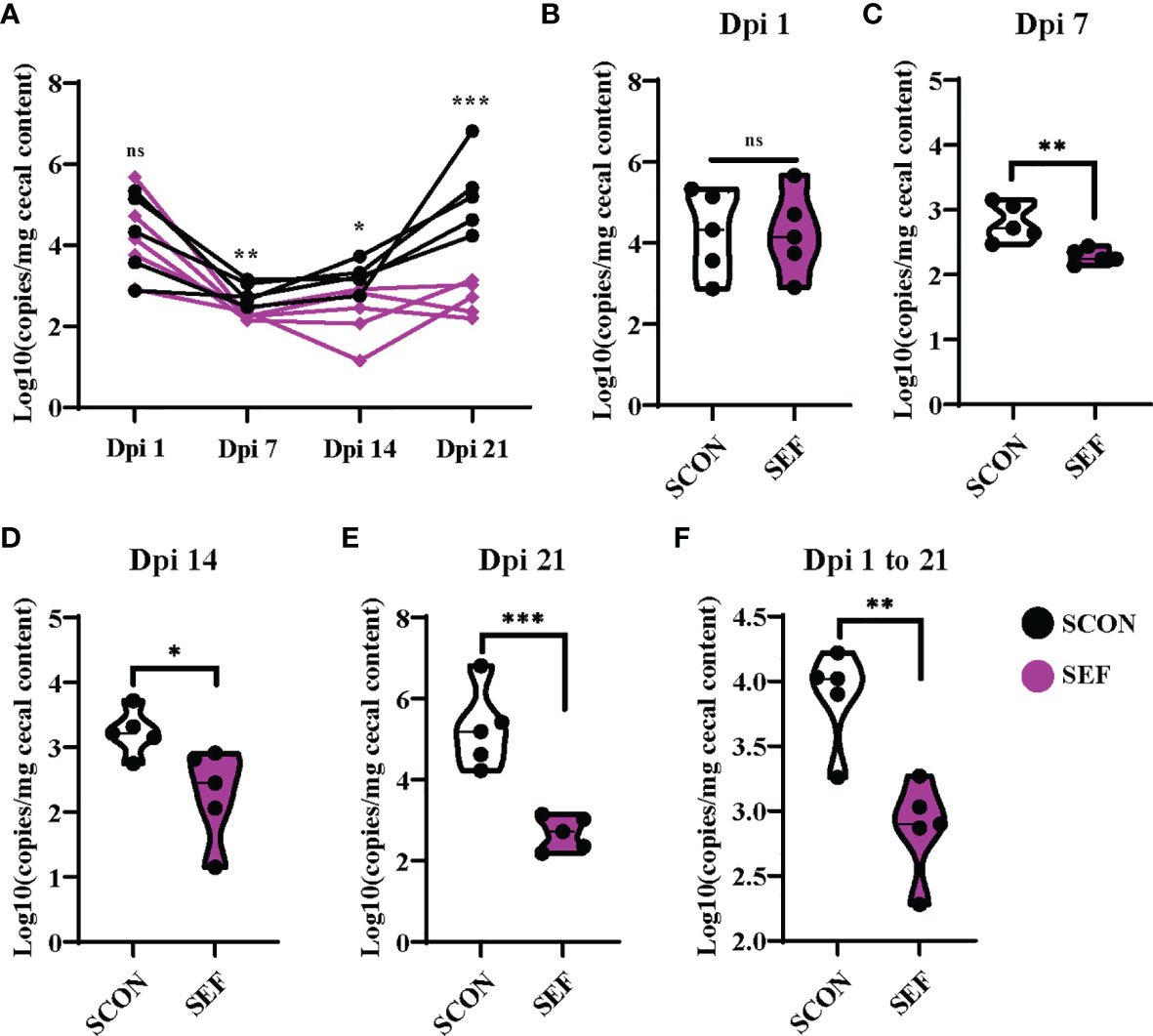
Figure 8 Effect of dietary supplementation with E. faecium on salmonella copies in cecum of hens challenged with S. Enteritidis. (A–F) salmonella copies in the cecum contents determined by RT-qPCR at day 1, 7, 14, 21 post infection (1 Dpi, 7 Dpi, 14 Dpi and 21 Dpi). Asterisks denote significant differences (ns, not significant; *p ≤ 0.05, **p ≤ 0.01, ***p ≤ 0.001), n = five per group; data are represented as mean ± SEM.
Discussion
S. Enteritidis infection is a serious problem in animal production (e.g., egg products and poultry meat) that ultimately affects the quality and safety of animal-derived foods and human health (37). Numerous previous studies have shown that the S. Enteritidis challenge led to a compromised growth performance of poultry. In this study, we found that salmonella exposure resulted in a significant laying performance and egg quality loss, whereas E. faecium supplementation reversed these changes. As we have known, the intestine is believed to be the main target organ for salmonella infection. It can destroy the intestinal mucosal barrier, increase gut permeability, and induce intestinal inflammation and bacterial translocation (4). Herein, the S. Enteritidis challenge significantly caused host metabolic dysfunction, intestinal histopathologic damage, and intestinal mucosal injuries, whereas E. faecium supplementation attenuated these detrimental effects, suggesting the capacity of E. faecium to improve the laying performance and support the intestinal homeostasis. In the present study, we have recently identified a crucial role for E. faecium in regulating hen cecal commensal microbes that improve beneficial bacteria and inhibit S. Enteritidis colonization. These results also revealed that the supplementation of E. faecium markedly enriched the cysteine and methionine metabolism, pyruvate metabolism, fatty acid metabolism, tryptophan metabolism, and the PI3K-Akt signaling pathway of hens. Therefore, the supplementation with E. faecium has the potential to improve laying hen outcomes.
Previous studies revealed that host cells and organs undergo metabolic reprogramming and activate inflammatory pathways in response to salmonella infection (5, 38). In the present study, S. Enteritidis infection significantly increased the serum ALP, whereas E. faecium supplementation reversed these alterations. According to a previous study, the abnormalities in liver enzyme levels are frequent during severe intestinal injuries due to S. Enteritidis infection (39), which is in line with these findings. However, the level of ALP was decreased in the serum of broilers with fungal infection (40). As reported, oral supplementation with intestinal alkaline phosphatase (IAP) protects mice from infections with S. Typhimurium as well as with Clostridium Difficile (41). Also, the animals given IAP maintained their weight, had reduced clinical severity and gut inflammation, and showed improved survival (41). Therefore, it remains unclear which dietary supplementation with E. faecium directly influences the serum ALP of hens infected with S. Enteritidis, the underlying mechanism by which the beneficial functions in E. faecium leads to host health needs further study. In laying hens, S. Enteritidis has been found in the internal tissues of infected chickens, including the intestine, liver, spleen, lung, ovary, and oviduct (42). Gast et al. reported that salmonella can persist in the liver as a chronic infection for up to 5 weeks after the oral inoculation of hens (43). It is well known that the liver is an important organ for lipid metabolism and the de novo fatty acid synthesis (44). Importantly, lipid metabolism in the liver has also been regarded as having an important role in egg maturation and production (44). The induction and maintenance of egg productivity are dependent upon the expression of genes related to lipid metabolism and the generation of egg yolk lipid that is mostly composed of low-density lipoproteins (LDLs) (45). Therefore, S. Enteritidis infection could elevate lipid synthesis and reduce lipid transportation in the chicken hepatocytes. It was well known that the concentrations of serum lipid indices are indicative of the host metabolic regulations in a steady state. Of note, as shown in our results, the concentrations of serum TG and LDL-C in the S. Enteritidis infection group were decreased compared with the CON group, while no significant differences were observed in the lipid metabolism, including the HDL-C, LDL-C, TC, and TG of these hens (SCON vs. SEF groups). In line with these results, a study revealed that the supplementation of E. faecium had no effect on serum lipid parameters, including HDL-C, LDL-C, TG, TC, and other lipid indices of broilers (46). Also, a previous study reported that the administration of E. faecium did not affect the serum TG and TC of broilers (47). In brief, it appeared that the serum protein and lipid metabolic parameter response observed in the current study was similar to those of poultry models in previous studies. Therefore, the administration of E. faecium did not affect the lipid metabolism of hens, which is likely due to the unchanged lipogenic and lipolytic enzyme activities in the tissues.
The host immune system also activates inflammatory pathways in response to infection with salmonella (48). It was well known that salmonella infection increases susceptibility to intestinal inflammation and contributes to intestinal or systemic inflammation (35, 49). Also, the dysfunction of the host immune system caused by infection is the key factor for salmonella-induced inflammatory injuries (49). Therefore, when the intestinal barrier function is destroyed upon salmonella infection, these pro-inflammatory cytokines, including IL-1β, IL-6, IL-8, IFN, and TNF-α, are activated (5, 50). A previous study reported that a number of cytokines, including IL-1β and TNF-α, are produced during the initial salmonella infection (51). In the current study, no significant difference was observed in the systemic inflammation, including the IL-1β, IL-6, IFN-γ, and TNF-α of these hens (CON and SCON groups), while the pro-inflammatory cytokines IL-1β, IL-6, and TNF-α in the SEF hens were markedly decreased and the IgG was increased than those in the SCON hens. According to previous studies, these cytokines have all been demonstrated to be important for salmonella infection and clearance (52). In line with the outcome, previous studies have demonstrated that some lactic acid bacteria are capable of activating immune responses against pathogen infection and then alleviating host inflammation (53). Importantly, E. faecium is one of the first batch of probiotics approved by the European Union and Food and Drug Administration for animal feed (54). As reported that the treatment of intestinal porcine enterocyte cell line (IPEC) cell lines with E. faecium HDRsEf1 was found to be effective against pathogen infection (E. coli, ETEC) (55). Similarly, numerous studies reported that E. faecium showed many beneficial functions, including inhibiting pathogen adhesion and infection, enhancing the anti-inflammatory effect, as well as promoting immune system development (30, 47, 56, 57). These results suggested that E. faecium might suppress salmonella-induced systemic inflammation by reducing pro-inflammatory cytokines.
It is well known that gut integrity is a prerequisite for maintaining the host and intestinal homeostasis (58, 59). According to previous studies, the intestinal mucosal barrier comprises connecting epithelial cells that are overlaid by a host-secreted mucous layer and serve as the first line of defense against pathogens and potentially harmful commensal bacteria (60–63). However, the impaired intestinal barrier functions caused by pathogen infection compromises the immune tolerance of the intestines and causes systemic inflammatory responses, which aggravate the systemic immune response and host body damage (64). As we have known, salmonella invades and destroys the intestinal epithelial cells and then crosses the intestinal epithelial barrier to cause intestinal and even systemic inflammation (65, 66). In the current study, significant hemorrhagic spots were observed in the duodenum, jejunum, and ileum on the dpi 42. Also, during the post-salmonella challenge period, the intestinal epithelial villi and crypt in the segments of duodenum, jejunum, and ileum were significantly damaged. For example, the broken villi structure and crypt atrophy were observed, which are consistent with the results from previous reports. Interestingly, the supplementation of E. faecium significantly increased the VH and VCR and decreased CD in the duodenum, jejunum, and ileum of hens, and ultimately effectively alleviated the damages of S. Enteritidis infection. As previous reported, high VCR is widely regarded as a good biomarker of intestinal mucosal turnover and is related to strong digestion and absorption capacity (67). Similarly, Zhang et al., reported that E. faecium YQH2 effectively reduced the colonization of S. Typhimurium, which may be attributed to the alleviation of intestinal barrier function damage (66). Meanwhile, according to previous studies, salmonella infection has been shown to regulate certain tight junction proteins, which ultimately promotes the translocation of the bacteria through the intestinal epithelial cell monolayer (68). Also, the manipulation of tight junction proteins serves to damage the intestinal epithelial barrier by increasing its permeability, thereby allowing salmonella to more effectively invade the basolateral side of the epithelial cell monolayer (48). Upon infection with salmonella, OCLN becomes dephosporylated and subsequently removed from epithelial tight junctions (68). In addition, ZO-1 (TJP) is recruited from the cytosol to the membrane, suggesting that salmonella changes the intracellular distribution of this tight junction protein (68). In line with previous studies, S. Enteritidis infection significantly downregulated TJP and OCLN protein expression in the small intestinal tissues, exacerbating systemic inflammation in hens. Interestingly, after E. faecium supplementation was given to the hens, the improvement expression levels of TJP and OCLN suggested that E. faecium restored the intestinal mucosal barrier functions and intestinal health. A previous study reported that E. faecium YQH2 improved the intestinal mucosal damage caused by salmonella in chicken (66), which was consistent with the outcome. Also, E. faecium YQH2 significantly stimulated the Wnt/β-catenin pathway to promote the repair of intestinal epithelial cells and reduced the intestinal inflammation level (66). Therefore, through the present study, these results indicated that the integrity of intestinal barrier functions in hens infected with S. Enteritidis was dramatically destroyed, while E. faecium supplementation resulted in the improvement of intestinal or systemic inflammation.
Accumulating evidence has shown that the gut microbiome is consistent with host physiological states, and intestinal physiological structure and function (69–72), and has been regarded as a potential nutritional intervention for the improvement of several intestinal diseases, especially pathogen infection (59, 64, 73). The gut microbiota is increasingly recognized for playing a critical role in human or animal health and disease, especially pathogenic infections, such as salmonella (74). In the present study, both salmonella infection and E. faecium supplementation affected the gut microbial alpha- and beta-diversity parameters. Similarly, previous studies indicated that the exposure of chickens to salmonella influences and shapes their gut microbial community and structure (75). As shown in a previous study, salmonella can multiply rapidly and destroy the gut microbiome of young chicks (76). In addition, the salmonella-associated alteration of the gut microbiota could be a result of either pathogen-commensal microbiota interaction or host intestinal mucosal immune responses to the pathogen or even a combination of both (75, 77). As previously reported, the supplementation of E. faecium significantly altered the gut microbiota composition of broilers and enriched the relative abundance of short-chain fatty acid-producing microbes (78). Also, E. faecium is a natural commensal bacteria of the poultry gastrointestinal tract and is commercially used as a probiotic in poultry diets (47), previous studies reported that numerous types of probiotic (e.g., Bacillus subtilis, Lactobacillus, and E. faecium) supplementation can alter the community and structure of the gut microbiota and improve its diversity (11, 79–81). Based on the PCoA and LEfSe analyses, the gut microbial composition and function are altered in hens, followed with E. faecium supplementation. In our study, for both non-infected and infected hens, the relative abundance of Barnesiella, Butyricimonas, and Bilophila were markedly enriched in the supplementation of E. faecium, while the abundance of Bacteroides was increased in the control hens (CON and SCON groups). As we have known, even though some strains of Bacteroides have an anti-inflammatory property, toxigenic Bacteroides fragilis induces intestinal inflammation and can cause intestinal diseases and colon cancer (82). For example Bacteroides, especially Gallibacterium, is an indigenous bacterial pathogen in chicken and one of the major pathogens causing reproductive tract disorders in laying hens (83). As this study reported, these results revealed that orally administered E. faecium significantly decreased the relative abundance of Bacteroides and then substantially inhibited the intestinal injuries in hens challenged with S. Enteritidis, which was similar to the previous result to some extent (84). Earlier studies found that certain E. faecium spp. can produce folate, an essential vitamin, which is needed by the body for cell metabolism, cell division, and the synthesis of vitamins and amino acids (54, 85). Also, animal research results indicated that E. faecium spp. may boost immune cell function, improve the regulation of cell proliferation, and elevate fat-burning capacity (30, 77, 86). Therefore, E. faecium has been confirmed to use some functional compounds as nutritional and immunological substrates to metabolize and regulate beneficial compounds for effectively affecting host and gut microbial metabolism (85, 87, 88). Based on the PICRUSt2 analysis, dietary E. faecium may modulate the cysteine and methionine metabolism, Tryptophan metabolism, the NOD-like receptor signaling pathway, and PI3K-Akt signaling pathway and ultimately inhibit salmonella infection by improving the intestinal homeostasis, which was similar to a previous result to some extent. However, the underlying mechanism of effect of E. faecium on the bacterial function and host metabolism during salmonella infection needs further research.
Conclusion
In summary, we found that E. faecium supplementation significantly improved the laying performance and egg quality to combat the S. Enteritidis challenge. Also, these results demonstrated that E. faecium administration dramatically alleviated the intestinal histopathologic damage and improved the intestinal mucosal barrier function injuries caused by S. Enteritidis infection. Moreover, the data of 16S rRNA high- throughput sequencing of cecal microflora revealed that Barnesiella, Butyricimonas, Bilophila, and Candidatus_Soleaferrea dominated the cecal microflora of hens with E. faecium supplementation, which have a higher relative abundance in the known functional genes for cysteine and methionine metabolism, pyruvate metabolism, fatty acid metabolism, tryptophan metabolism, and the PI3K-Akt signaling pathway in E. faecium-treated hens from the PICRUSt2 analysis. Very importantly, these results indicate that E. faecium has a strong capacity to inhibit the S. Enteritidis colonization of laying hens. Therefore, the maintenance of the gut microbial composition protects the intestinal barrier from injury under S. Enteritidis infection, as demonstrated by decreased intestinal permeability, enhanced the inhibition of the translocation of bacteria and toxins, and suppressed intestinal inflammation. Our findings provide a scientific foundation for E. faecium application in poultry feed in the future.
Data Availability Statement
The datasets presented in this study can be found in online repositories. The names of the repository/repositories and accession number(s) can be found below: https://www.ncbi.nlm.nih.gov/, PRJNA796704.
Ethics Statement
The animal study was reviewed and approved by the institutional Animal Care and Use Committee of China Agricultural University.
Author Contributions
The authors’ responsibilities were as follows: SH, CJ, JZ, QM, and LZ designed the study. SH, XR, ML, ZL, YG, XW, CJ, and QM conducted the experiments and draft the manuscript. SH and QM polished the manuscript and finished the submission. SH, YG, JZ, CJ, and QM guided to analyze the experimental data. SH, CJ, and QM helped with revisiting and reviewing the manuscript. All authors read and approved the final manuscript.
Funding
This study was supported by the National Science Foundation of China (grant No.31772621), a Special Fund for China Agricultural Research System program (CARS-40-K08), and the Special Fund from Chinese Universities Scientific Fund (2018TC043).
Conflict of Interest
The authors declare that the research was conducted in the absence of any commercial or financial relationships that could be construed as a potential conflict of interest.
Publisher’s Note
All claims expressed in this article are solely those of the authors and do not necessarily represent those of their affiliated organizations, or those of the publisher, the editors and the reviewers. Any product that may be evaluated in this article, or claim that may be made by its manufacturer, is not guaranteed or endorsed by the publisher.
Acknowledgments
We thank all technicians in the experimental animal facility of China Agricultural University for providing daily care of laying hens. We also thank Prof. Rainer Mosenthin for support in the manuscript preparation.
Supplementary Material
The Supplementary Material for this article can be found online at: https://www.frontiersin.org/articles/10.3389/fimmu.2022.853954/full#supplementary-material
References
1. Foley SL, Lynne AM, Nayak R. Salmonella Challenges: Prevalence in Swine and Poultry and Potential Pathogenicity of Such Isolates. J Anim Sci (2008) 86:E149–162. doi: 10.2527/jas.2007-0464
2. Uddin TM, Chakraborty AJ, Khusro A, Zidan BMRM, Mitra S, Emran TB, et al. Antibiotic Resistance in Microbes: History, Mechanisms, Therapeutic Strategies and Future Prospects. J Infection Public Health (2021) 14:1750–66. doi: 10.1016/j.jiph.2021.10.020
3. Batz MB, Hoffmann S, Morris JG Jr. Ranking the Disease Burden of 14 Pathogens in Food Sources in the United States Using Attribution Data From Outbreak Investigations and Expert Elicitation. J Food Prot (2012) 75:1278–91. doi: 10.4315/0362-028X.JFP-11-418
4. Larock DL, Chaudhary A, Miller SI. Salmonellae Interactions With Host Processes. Nat Rev Microbiol (2015) 13:191–205. doi: 10.1038/nrmicro3420
5. Galán JE. Salmonella Typhimurium and Inflammation: A Pathogen-Centric Affair. Nat Rev Microbiol (2021) 19:716–25. doi: 10.1038/s41579-021-00561-4
6. Sobotik EB, Ramirez S, Roth N, Tacconi A, Pender C, Murugesan R, et al. Evaluating the Effects of a Dietary Synbiotic or Synbiotic Plus Enhanced Organic Acid on Broiler Performance and Cecal and Carcass Salmonella Load. Poult Sci (2021) 100:101508. doi: 10.1016/j.psj.2021.101508
7. Wideman N, Bailey M, Bilgili SF, Thippareddi H, Wang L, Bratcher C, et al. Evaluating Best Practices for Campylobacter and Salmonella Reduction in Poultry Processing Plants. Poultry Sci (2016) 95:306–15. doi: 10.3382/ps/pev328
8. Shivaprasad HL, Timoney JF, Morales S, Lucio B, Baker RC. Pathogenesis of Salmonella Enteritidis Infection in Laying Chickens. I. Studies on Egg Transmission, Clinical Signs, Fecal Shedding, and Serologic Responses. Avian Dis (1990) 34:548–57. doi: 10.2307/1591243
9. Wang CL, Fan YC, Chun-Hsien T, Chiu CH, Tsai HJ, Chou CH. Salmonella Enteritidis Infection Slows Steroidogenesis and Impedes Cell Growth in Hen Granulosa Cells. Avian Dis (2014) 58:511–7. doi: 10.1637/10846-041414-Reg.1
10. Park JW, Jeong JS, Lee SI, Kim IH. Effect of Dietary Supplementation With a Probiotic (Enterococcus Faecium) on Production Performance, Excreta Microflora, Ammonia Emission, and Nutrient Utilization in ISA Brown Laying Hens. Poult Sci (2016) 95:2829–35. doi: 10.3382/ps/pew241
11. Wang J, Wan C, Shuju Z, Yang Z, Celi P, Ding X, et al. Differential Analysis of Gut Microbiota and the Effect of Dietary Enterococcus Faecium Supplementation in Broiler Breeders With High or Low Laying Performance. Poult Sci (2021) 100:1109–19. doi: 10.1016/j.psj.2020.10.024
12. Berge AC, Wierup M. Nutritional Strategies to Combat Salmonella in Mono-Gastric Food Animal Production. Animal (2012) 6:557–64. doi: 10.1017/S1751731111002217
13. Alali WQ, Hofacre CL. Preharvest Food Safety in Broiler Chicken Production. Microbiol Spectr (2016) 4:1–13. doi: 10.1128/microbiolspec.PFS-0002-2014
14. Ventola CL. The Antibiotic Resistance Crisis: Part 1: Causes and Threats. P T: peer-reviewed J formulary Manage (2015) 40:277–83.
15. Aslam B, Wang W, Arshad MI, Khurshid M, Muzammil S, Rasool MH, et al. Antibiotic Resistance: A Rundown of a Global Crisis. Infect Drug Resist (2018) 11:1645–58. doi: 10.2147/IDR.S173867
16. Landers TF, Cohen B, Wittum TE, Larson EL. A Review of Antibiotic Use in Food Animals: Perspective, Policy, and Potential. Public Health Rep (2012) 127(1):4–22. doi: 10.1177/003335491212700103
17. Gueimonde M, Sánchez B, DeLos Reyes-Gavilan C,G, Margolles A. Antibiotic Resistance in Probiotic Bacteria. Front Microbiol (2013) 4:202. doi: 10.3389/fmicb.2013.00202
18. Selvin J, Maity D, Sajayan A, Kiran GS. Revealing Antibiotic Resistance in Therapeutic and Dietary Probiotic Supplements. J Glob Antimicrob Resist (2020) 22:202–5. doi: 10.1016/j.jgar.2020.02.007
19. Fijan S. Microorganisms With Claimed Probiotic Properties: An Overview of Recent Literature. Int J Environ Res Public Health (2014) 11:4745–67. doi: 10.3390/ijerph110504745
20. Markowiak P, Slizewska K. The Role of Probiotics, Prebiotics and Synbiotics in Animal Nutrition. Gut Pathog (2018) 10:21. doi: 10.1186/s13099-018-0250-0
21. Gareau MG, Sherman PM, Walker WA. Probiotics and the Gut Microbiota in Intestinal Health and Disease. Nat Rev Gastroenterol Hepatol (2010) 7:503–14. doi: 10.1038/nrgastro.2010.117
22. Cai J, Zhao C, Du Y, Zhang Y, Zhao M, Zhao Q. Comparative Efficacy and Tolerability of Probiotics for Antibiotic-Associated Diarrhea: Systematic Review With Network Meta-Analysis. United Eur Gastroenterol J (2018) 6:169–80. doi: 10.1177/2050640617736987
23. Jakubczyk D, Leszczyńska K, Górska S. The Effectiveness of Probiotics in the Treatment of Inflammatory Bowel Disease (IBD)-A Critical Review. Nutrients (2020) 12:1–25. doi: 10.3390/nu12071973
24. Jayaraman S, Thangavel G, Kurian H, Mani R, Mukkalil R, Chirakkal H. Bacillus Subtilis PB6 Improves Intestinal Health of Broiler Chickens Challenged With Clostridium Perfringens-Induced Necrotic Enteritis. Poult Sci (2013) 92:370–4. doi: 10.3382/ps.2012-02528
25. Caly DL, D’inca R, Auclair E, Drider D. Alternatives to Antibiotics to Prevent Necrotic Enteritis in Broiler Chickens: A Microbiologist’s Perspective. Front Microbiol (2015) 6:1336. doi: 10.3389/fmicb.2015.01336
26. Qing X, Zeng D, Wang H, Ni X, Liu L, Lai J, et al. Preventing Subclinical Necrotic Enteritis Through Lactobacillus Johnsonii BS15 by Ameliorating Lipid Metabolism and Intestinal Microflora in Broiler Chickens. AMB Express (2017) 7:139. doi: 10.1186/s13568-017-0439-5
27. Van Tyne D, Gilmore MS. Friend Turned Foe: Evolution of Enterococcal Virulence and Antibiotic Resistance. Annu Rev Microbiol (2014) 68:337–56. doi: 10.1146/annurev-micro-091213-113003
28. Zheng A, Luo J, Meng K, Li J, Bryden WL, Chang W, et al. Probiotic (Enterococcus Faecium) Induced Responses of the Hepatic Proteome Improves Metabolic Efficiency of Broiler Chickens (Gallus Gallus). BMC Genomics (2016) 17:89. doi: 10.1186/s12864-016-2371-5
29. Cao GT, Zeng XF, Chen AG, Zhou L, Zhang L, Xiao YP, et al. Effects of a Probiotic, Enterococcus Faecium, on Growth Performance, Intestinal Morphology, Immune Response, and Cecal Microflora in Broiler Chickens Challenged With Escherichia Coli K88. Poult Sci (2013) 92:2949–55. doi: 10.3382/ps.2013-03366
30. Lodemann U, Hübener K, Jansen N, Martens H. Effects of Enterococcus Faecium NCIMB 10415 as Probiotic Supplement on Intestinal Transport and Barrier Function of Piglets. Arch Anim Nutr (2006) 60:35–48. doi: 10.1080/17450390500468099
31. Szabó I, Wieler LH, Tedin K, Scharek-Tedin L, Taras D, Hensel A, et al. Influence of a Probiotic Strain of Enterococcus Faecium on Salmonella Enterica Serovar Typhimurium DT104 Infection in a Porcine Animal Infection Model. Appl Environ Microbiol (2009) 75:2621–8. doi: 10.1128/AEM.01515-08
32. Huang L, Luo L, Zhang Y, Wang Z, Xia Z. Effects of the Dietary Probiotic, Enterococcus Faecium NCIMB11181, on the Intestinal Barrier and System Immune Status in Escherichia Coli O78-Challenged Broiler Chickens. Probiotics Antimicrob Proteins (2019) 11:946–56. doi: 10.1007/s12602-018-9434-7
33. Liu Y, Mosenthin R, Zhao L, Zhang J, Ji C, Ma Q. Vitamin K Alleviates Bone Calcium Loss Caused by Salmonella Enteritidis Through Carboxylation of Osteocalcin. J Anim Sci Biotechnol (2021) 12:80. doi: 10.1186/s40104-021-00604-z
34. Geng S, Huang S, Ma Q, Li F, Gao Y, Zhao L, et al. Alterations and Correlations of the Gut Microbiome, Performance, Egg Quality, and Serum Biochemical Indexes in Laying Hens With Low-Protein Amino Acid-Deficient Diets. ACS Omega (2021) 6:13094–104. doi: 10.1021/acsomega.1c00739
35. Schultz BM, Salazar GA, Paduro CA, Pardo-Roa C, Pizarro DP, Salazar-Echegarai FJ, et al. Persistent Salmonella Enterica Serovar Typhimurium Infection Increases the Susceptibility of Mice to Develop Intestinal Inflammation. Front Immunol (2018) 9:1166. doi: 10.3389/fimmu.2018.01166
36. Huang S, Wu Z, Liu C, Han D, Feng C, Wang S, et al. Milk Fat Globule Membrane Supplementation Promotes Neonatal Growth and Alleviates Inflammation in Low-Birth-Weight Mice Treated With Lipopolysaccharide. BioMed Res Int (2019) 2019:4876078. doi: 10.1155/2019/4876078
37. Pires SM, Vieira AR, Hald T, Cole D. Source Attribution of Human Salmonellosis: An Overview of Methods and Estimates. Foodborne Pathog Dis (2014) 11:667–76. doi: 10.1089/fpd.2014.1744
38. Thompson A, Fulde M, Tedin K. The Metabolic Pathways Utilized by Salmonella Typhimurium During Infection of Host Cells. Environ Microbiol Rep (2018) 10:140–54. doi: 10.1111/1758-2229.12628
39. González-Quintela A, Campos J, Alende R, López-Soto A, Tomé S, Otero E, et al. Abnormalities in Liver Enzyme Levels During Salmonella Enteritidis Enterocolitis. Rev Esp Enferm Dig (2004) 96:559–562; 563–556. doi: 10.4321/s1130-01082004000800005
40. Ali Rajput S, Sun L, Zhang N, Mohamed Khalil M, Gao X, Ling Z, et al. Ameliorative Effects of Grape Seed Proanthocyanidin Extract on Growth Performance, Immune Function, Antioxidant Capacity, Biochemical Constituents, Liver Histopathology and Aflatoxin Residues in Broilers Exposed to Aflatoxin B₁. Toxins (Basel) (2017) 9:1–16. doi: 10.3390/toxins9110371
41. Alam SN, Yammine H, Moaven O, Ahmed R, Moss AK, Biswas B, et al. Intestinal Alkaline Phosphatase Prevents Antibiotic-Induced Susceptibility to Enteric Pathogens. Ann Surg (2014) 259:715–22. doi: 10.1097/SLA.0b013e31828fae14
42. Hoop RK, Pospischil A. Bacteriological, Serological, Histological and Immunohistochemical Findings in Laying Hens With Naturally Acquired Salmonella Enteritidis Phage Type 4 Infection. Vet Rec (1993) 133:391–3. doi: 10.1136/vr.133.16.391
43. Gast RK, Holt PS. Deposition of Phage Type 4 and 13a Salmonella Enteritidis Strains in the Yolk and Albumen of Eggs Laid by Experimentally Infected Hens. Avian Dis (2000) 44:706–10. doi: 10.2307/1593116
44. Wang CL, Fan YC, Wang C, Tsai HJ, Chou CH. The Impact of Salmonella Enteritidis on Lipid Accumulation in Chicken Hepatocytes. Avian Pathol (2016) 45:450–7. doi: 10.1080/03079457.2016.1162280
45. Yen CF, Lin EC, Wang YH, Wang PH, Lin HW, Hsu JC, et al. Abundantly Expressed Hepatic Genes and Their Differential Expression in Liver of Prelaying and Laying Geese. Poult Sci (2009) 88:1955–62. doi: 10.3382/ps.2008-00473
46. Zhao X, Guo Y, Guo S, Tan J. Effects of Clostridium Butyricum and Enterococcus Faecium on Growth Performance, Lipid Metabolism, and Cecal Microbiota of Broiler Chickens. Appl Microbiol Biotechnol (2013) 97:6477–88. doi: 10.1007/s00253-013-4970-2
47. Capcarova M, Weiss J, Hrncar C, Kolesarova A, Pal G. Effect of Lactobacillus Fermentum and Enterococcus Faecium Strains on Internal Milieu, Antioxidant Status and Body Weight of Broiler Chickens. J Anim Physiol Anim Nutr (Berl) (2010) 94:e215–224. doi: 10.1111/j.1439-0396.2010.01010.x
48. Patel S, Mccormick BA. Mucosal Inflammatory Response to Salmonella Typhimurium Infection. Front Immunol (2014) 5:311. doi: 10.3389/fimmu.2014.00311
49. Zha L, Garrett S, Sun J. Salmonella Infection in Chronic Inflammation and Gastrointestinal Cancer. Diseases (2019) 7:1–14. doi: 10.3390/diseases7010028
50. Kurtz JR, Goggins JA, Mclachlan JB. Salmonella Infection: Interplay Between the Bacteria and Host Immune System. Immunol Lett (2017) 190:42–50. doi: 10.1016/j.imlet.2017.07.006
51. Rydström A, Wick MJ. Monocyte Recruitment, Activation, and Function in the Gut-Associated Lymphoid Tissue During Oral Salmonella Infection. J Immunol (2007) 178:5789–801. doi: 10.4049/jimmunol.178.9.5789
52. Nauciel C, Espinasse-Maes F. Role of Gamma Interferon and Tumor Necrosis Factor Alpha in Resistance to Salmonella Typhimurium Infection. Infect Immun (1992) 60:450–4. doi: 10.1128/iai.60.2.450-454.1992
53. Dowdell P, Chankhamhaengdecha S, Panbangred W, Janvilisri T, Aroonnual A. Probiotic Activity of Enterococcus Faecium and Lactococcus Lactis Isolated From Thai Fermented Sausages and Their Protective Effect Against Clostridium Difficile. Probiotics Antimicrob Proteins (2020) 12:641–8. doi: 10.1007/s12602-019-09536-7
54. Franz CM, Huch M, Abriouel H, Holzapfel W, Galvez A. Enterococci as Probiotics and Their Implications in Food Safety. Int J Food Microbiol (2011) 151:125–40. doi: 10.1016/j.ijfoodmicro.2011.08.014
55. Tian Z, Liu X, Dai R, Xiao Y, Wang X, Bi D, et al. Enterococcus Faecium HDRsEf1 Protects the Intestinal Epithelium and Attenuates ETEC-Induced IL-8 Secretion in Enterocytes. Mediators Inflammation (2016) 2016:7474306. doi: 10.1155/2016/7474306
56. Bednorz C, Guenther S, Oelgeschläger K, Kinnemann B, Pieper R, Hartmann S, et al. Feeding the Probiotic Enterococcus Faecium Strain NCIMB 10415 to Piglets Specifically Reduces the Number of Escherichia Coli Pathotypes That Adhere to the Gut Mucosa. Appl Environ Microbiol (2013) 79:7896–904. doi: 10.1128/AEM.03138-13
57. Klingspor S, Martens H, Caushi D, Twardziok S, Aschenbach JR, Lodemann U. Characterization of the Effects of Enterococcus Faecium on Intestinal Epithelial Transport Properties in Piglets. J Anim Sci (2013) 91:1707–18. doi: 10.2527/jas.2012-5648
58. Citi S. Intestinal Barriers Protect Against Disease. Science (2018) 359:1097–8. doi: 10.1126/science.aat0835
59. Martens EC, Neumann M, Desai MS. Interactions of Commensal and Pathogenic Microorganisms With the Intestinal Mucosal Barrier. Nat Rev Microbiol (2018) 16:457–70. doi: 10.1038/s41579-018-0036-x
60. Macdonald TT, Monteleone G. Immunity, Inflammation, and Allergy in the Gut. Science (2005) 307:1920–5. doi: 10.1126/science.1106442
61. Odenwald MA, Turner JR. The Intestinal Epithelial Barrier: A Therapeutic Target? Nat Rev Gastroenterol Hepatol (2017) 14:9–21. doi: 10.1038/nrgastro.2016.169
62. Vancamelbeke M, Vermeire S. The Intestinal Barrier: A Fundamental Role in Health and Disease. Expert Rev Gastroenterol Hepatol (2017) 11:821–34. doi: 10.1080/17474124.2017.1343143
63. Thoo L, Noti M, Krebs P. Keep Calm: The Intestinal Barrier at the Interface of Peace and War. Cell Death Dis (2019) 10:849. doi: 10.1038/s41419-019-2086-z
64. Kamada N, Seo SU, Chen GY, Núñez G. Role of the Gut Microbiota in Immunity and Inflammatory Disease. Nat Rev Immunol (2013) 13:321–35. doi: 10.1038/nri3430
65. Chong A, Cooper KG, Kari L, Nilsson OR, Hillman C, Fleming BA, et al. Cytosolic Replication in Epithelial Cells Fuels Intestinal Expansion and Chronic Fecal Shedding of Salmonella Typhimurium. Cell Host Microbe (2021) 29:1177–1185 e1176. doi: 10.1016/j.chom.2021.04.017
66. Zhang H, Wang M, Jia J, Zhao J, Radebe SM, Yu Q. The Protective Effect of E. Faecium on S. Typhimurium Infection Induced Damage to Intestinal Mucosa. Front Vet Sci (2021) 8:740424. doi: 10.3389/fvets.2021.740424
67. Wells JM, Brummer RJ, Derrien M, Macdonald TT, Troost F, Cani PD, et al. Homeostasis of the Gut Barrier and Potential Biomarkers. Am J Physiol Gastrointest Liver Physiol (2017) 312:G171–93. doi: 10.1152/ajpgi.00048.2015
68. Kohler H, Sakaguchi T, Hurley BP, Kase BA, Reinecker HC, Mccormick BA. Salmonella Enterica Serovar Typhimurium Regulates Intercellular Junction Proteins and Facilitates Transepithelial Neutrophil and Bacterial Passage. Am J Physiol Gastrointest Liver Physiol (2007) 293:G178–187. doi: 10.1152/ajpgi.00535.2006
69. Round JL, Mazmanian SK. The Gut Microbiota Shapes Intestinal Immune Responses During Health and Disease. Nat Rev Immunol (2009) 9:313–23. doi: 10.1038/nri2515
70. Shreiner AB, Kao JY, Young VB. The Gut Microbiome in Health and in Disease. Curr Opin Gastroenterol (2015) 31:69–75. doi: 10.1097/MOG.0000000000000139
71. Garrett WS. Immune Recognition of Microbial Metabolites. Nat Rev Immunol (2019) 20(2):91–92. doi: 10.1038/s41577-019-0252-2
72. Neurath MF. Host-Microbiota Interactions in Inflammatory Bowel Disease. Nat Rev Gastroenterol Hepatol (2019) 17:76–77. doi: 10.1038/s41575-019-0248-1
73. Ruff WE, Greiling TM, Kriegel MA. Host-Microbiota Interactions in Immune-Mediated Diseases. Nat Rev Microbiol (2020) 18(9):521–38. doi: 10.1038/s41579-020-0367-2
74. Bratburd JR, Keller C, Vivas E, Gemperline E, Li L, Rey FE, et al. Gut Microbial and Metabolic Responses to Salmonella Enterica Serovar Typhimurium and Candida Albicans. mBio (2018) 9:1–14. doi: 10.1128/mBio.02032-18
75. Mon KK, Saelao P, Halstead MM, Chanthavixay G, Chang HC, Garas L, et al. Salmonella Enterica Serovars Enteritidis Infection Alters the Indigenous Microbiota Diversity in Young Layer Chicks. Front Vet Sci (2015) 2:61. doi: 10.3389/fvets.2015.00061
76. Barrow PA, Freitas Neto OC. Pullorum Disease and Fowl Typhoid–New Thoughts on Old Diseases: A Review. Avian Pathol (2011) 40:1–13. doi: 10.1080/03079457.2010.542575
77. Barman M, Unold D, Shifley K, Amir E, Hung K, Bos N, et al. Enteric Salmonellosis Disrupts the Microbial Ecology of the Murine Gastrointestinal Tract. Infect Immun (2008) 76:907–15. doi: 10.1128/IAI.01432-07
78. Wang W, Cai H, Zhang A, Chen Z, Chang W, Liu G, et al. Enterococcus Faecium Modulates the Gut Microbiota of Broilers and Enhances Phosphorus Absorption and Utilization. Anim (Basel) (2020) 10:1–13. doi: 10.3390/ani10071232
79. Hosoi T, Ametani A, Kiuchi K, Kaminogawa S. Changes in Fecal Microflora Induced by Intubation of Mice With Bacillus Subtilis (Natto) Spores are Dependent Upon Dietary Components. Can J Microbiol (1999) 45:59–66. doi: 10.1139/w98-206
80. Jin LZ, Ho YW, Abdullah N, Jalaludin S. Digestive and Bacterial Enzyme Activities in Broilers Fed Diets Supplemented With Lactobacillus Cultures. Poult Sci (2000) 79:886–91. doi: 10.1093/ps/79.6.886
81. Luo J, Zheng A, Meng K, Chang W, Bai Y, Li K, et al. Proteome Changes in the Intestinal Mucosa of Broiler (Gallus Gallus) Activated by Probiotic Enterococcus Faecium. J Proteomics (2013) 91:226–41. doi: 10.1016/j.jprot.2013.07.017
82. Zakharzhevskaya NB, Vanyushkina AA, Altukhov IA, Shavarda AL, Butenko IO, Rakitina DV, et al. Outer Membrane Vesicles Secreted by Pathogenic and Nonpathogenic Bacteroides Fragilis Represent Different Metabolic Activities. Sci Rep (2017) 7:5008. doi: 10.1038/s41598-017-05264-6
83. Wang C, Pors SE, Olsen RH, Bojesen AM. Transmission and Pathogenicity of Gallibacterium Anatis and Escherichia Coli in Embryonated Eggs. Vet Microbiol (2018) 217:76–81. doi: 10.1016/j.vetmic.2018.03.005
84. Zhou X, Willems RJL, Friedrich AW, Rossen JWA, Bathoorn E. Enterococcus Faecium: From Microbiological Insights to Practical Recommendations for Infection Control and Diagnostics. Antimicrob Resist Infect Control (2020) 9:130. doi: 10.1186/s13756-020-00770-1
85. Kim B, Wang YC, Hespen CW, Espinosa J, Salje J, Rangan KJ, et al. Enterococcus Faecium Secreted Antigen A Generates Muropeptides to Enhance Host Immunity and Limit Bacterial Pathogenesis. Elife (2019) 8:1–35. doi: 10.7554/eLife.45343
86. Steck N, Hoffmann M, Sava IG, Kim SC, Hahne H, Tonkonogy SL, et al. Enterococcus Faecalis Metalloprotease Compromises Epithelial Barrier and Contributes to Intestinal Inflammation. Gastroenterology (2011) 141:959–71. doi: 10.1053/j.gastro.2011.05.035
87. Wagner T, Joshi B, Janice J, Askarian F, Škalko-Basnet N, Hagestad OC, et al. Enterococcus Faecium Produces Membrane Vesicles Containing Virulence Factors and Antimicrobial Resistance Related Proteins. J Proteomics (2018) 187:28–38. doi: 10.1016/j.jprot.2018.05.017
88. Kim MH, Kim SY, Son JH, Kim SI, Lee H, Kim S, et al. Production of Membrane Vesicles by Enterococcus Faecium Cultured With or Without Subinhibitory Concentrations of Antibiotics and Their Pathological Effects on Epithelial Cells. Front Cell Infect Microbiol (2019) 9:295. doi: 10.3389/fcimb.2019.00295
Keywords: E. faecium, hens, S. Enteritidis, intestinal health, gut microbiota, performance
Citation: Huang S, Rong X, Liu M, Liang Z, Geng Y, Wang X, Zhang J, Ji C, Zhao L and Ma Q (2022) Intestinal Mucosal Immunity-Mediated Modulation of the Gut Microbiome by Oral Delivery of Enterococcus faecium Against Salmonella Enteritidis Pathogenesis in a Laying Hen Model. Front. Immunol. 13:853954. doi: 10.3389/fimmu.2022.853954
Received: 13 January 2022; Accepted: 15 February 2022;
Published: 15 March 2022.
Edited by:
Wentao Yang, Jilin Agriculture University, ChinaReviewed by:
Ji Ping Li, Northeast Agricultural University, ChinaHaijun Zhang, Key Laboratory for Feed Biotechnology, Feed Research Institute (CAAS), China
Copyright © 2022 Huang, Rong, Liu, Liang, Geng, Wang, Zhang, Ji, Zhao and Ma. This is an open-access article distributed under the terms of the Creative Commons Attribution License (CC BY). The use, distribution or reproduction in other forums is permitted, provided the original author(s) and the copyright owner(s) are credited and that the original publication in this journal is cited, in accordance with accepted academic practice. No use, distribution or reproduction is permitted which does not comply with these terms.
*Correspondence: Qiugang Ma, maqiugang@cau.edu.cn