- 1Cheeloo College of Medicine, Shandong University, Jinan, China
- 2Department of Radiation Oncology, Shandong Cancer Hospital and Institute, Shandong First Medical University and Shandong Academy of Medical Sciences, Jinan, China
- 3Department of Nuclear Medicine, Shandong Cancer Hospital and Institute, Shandong First Medical University and Shandong Academy of Medical Sciences, Jinan, China
Brain metastases (BMs) in non-small-cell lung cancer (NSCLC) patients are associated with significant morbidity and poor prognosis. Immune checkpoint inhibitors (ICIs) have resulted in a paradigm shift in the management of advanced NSCLC. However, the value of ICIs in NSCLC patients with BMs remains unclear because patients with BMs are routinely excluded in numerous prospective trials on ICIs. Here, starting from the mechanisms of ICIs for BMs, we will reveal the value of ICIs by reviewing the efficacy and adverse effects of ICIs monotherapy as well as promising combination strategies, such as combinations with chemotherapy, radiotherapy, and anti-angiogenic drugs, etc. In addition, the methods of patient selection and response assessment will be summarized to assist clinical practice and further studies.
Introduction
Brain metastases (BMs) are frequent complications in patients with non-small cell lung cancer (NSCLC), present in 10% ~ 20% of patients at diagnosis, and approximately 20% ~ 40% eventually (1, 2). The incidence of NSCLC BMs is increasing, partly due to the improvements in testing techniques and the popularity of screening, as well as the improvements in therapies that extend patient survival (3).
NSCLC-BMs are associated with poor prognosis (4). In patients with driver-gene positive NSCLC-BMs, such as those harboring epidermal growth factor receptor (EGFR) mutation or anaplastic lymphoma kinase (ALK) rearrangement, new-generation targeting reagents have a favorable intracranial response rate (66%–78%) (5, 6). However, treatments for those driver-gene negative patients are extremely limited. Radiotherapy is the mainstream treatment for symptomatic BMs, such as whole brain radiotherapy (WBRT) and stereotactic radiation therapy (SRT). Surgical resection is only appropriate for a limited number of carefully selected patients. Management of multiple asymptomatic BMs often involves systemic therapy only. However, conventional systemic therapies could not achieve desired intracranial efficacy and survival improvements (7). Overall, platinum-based chemotherapy has obtained 30%~40% intracranial response, while the benefit is short-lasting and the toxicity is enormous (8). Hence, optimization of the treatment of NSCLC-BMs is urgently needed.
Blocking the programmed death protein-1 (PD-1)/its ligand (PD-L1) axis with immune checkpoint inhibitors (ICIs) has revolutionized the treatment landscape for advanced NSCLC, covering from first-line treatment to post-line treatment (9). In addition, ICIs in combination with chemotherapy or radiotherapy were approved for metastatic NSCLC. Cytotoxic T lymphocyte-associated antigen-4 (CTLA-4) inhibitors, such as ipilimumab, have a good performance in combination treatment, despite poor performance as monotherapy for NSCLC. Unfortunately, the value of ICIs for NSCLC-BMs is indeterminate because those patients have generally been excluded or underrepresented in over half of the clinical trials (10). There are several reasons for this issue: (1) The poor survival of patients with BMs may increase the probability of trial failure (11); (2) Drugs are difficult to penetrate through the blood-brain barrier (BBB) to intracranial lesions (12); (3) The tumor microenvironment of BMs is immunologically “cold” (13); (4) Patients with symptomatic BMs often need steroids, which may conflict with immunotherapy (14). Although some clinical trials enrolled asymptomatic BMs, the outcomes of the BMs subgroup were rarely reported (10). Until recently, several clinical trials of ICI treatment for advanced NSCLC, especially combination therapy, have published the results containing BMs subgroup analysis. Although the evidence is still limited, NSCLC-BMs are navigating towards the era of immunotherapy.
The main aim of this review is to reveal the value of ICIs for NSCLC-BMs. Based on the possible mechanisms through which BMs can benefit from ICIs, we summarize clinical evidence, including pivotal prospective trials and representative studies. Future challenges and perspectives will also be sketched out in order to better understand and optimize ICI-containing treatments in patients with BMs.
Mechanisms
Historically, the physiological brain was regarded as an immune-privileged organ, mainly due to the BBB and genetically special immune spectrum (15). However, the mechanism of ICIs, unlike targeted-tumor cell drugs, theoretically relates to modified immune cell activity rather than a direct action of tumor cells in the brain. Moreover, changes in the neuroimmunology background have rekindled interests in immunotherapy for BMs.
It was generally believed that molecules with large molecular weight and low liposolubility, as well as peripheral immune cells, cannot penetrate the BBB (16). Actually, the existence of BMs and the management of anti-tumor treatments can lead BBB to become “not too dense” (17, 18). The BBB is induced to structural changes and dysfunction by BMs, and possible mechanisms include the increase in vascular endothelial growth factor (VEGF)-mediated angiogenesis and multiple adhesion molecules (such as VCAM-1 and ICAM-1) and chemokines (such as CXCL12-CSCR4 axis)-mediated trans-endothelial migration (18). Indeed recently, nivolumab has been measured in cerebrospinal fluid (CSF) of five patients with suspected leptomeningeal metastases, with CSF/plasma ratios ranging from 1/52 to 1/299 (19). Furthermore, radiotherapy can loosen the BBB. After brain radiotherapy, the CSF/plasma ratio of trastuzumab could increase by six times (20). Unfortunately, pharmacokinetic studies of ICIs after radiotherapy are absent. Collectively, during the development and treatment of BMs, the tight fences of BBB may be opened.
A deeper understanding of the tumor microenvironment (TME) is necessary to develop immunotherapy (21). Tumor infiltrating lymphocytes (TILs) are essential for the efficacy of immunotherapy. Other immune cells in the brain TME, such as tumor-associated macrophages (TAMs), microglia, and astrocytes that surround brain tumors are involved in tumor progression and immune evasion (22). Whether lymphocytes can cross the BBB had remained controversial for decades. Recent studies have refuted the notion of immune isolation in the brain. In the 1980s, an antigen exit route from the brain to the deep cervical lymph nodes was discovered (23). In 2015, functional lymph-vessel found in the meninges provided a direct drainage route for immune cells from the brain to cervical lymph nodes (24). Despite the discovery of T cell infiltration in primary brain tumors (25), outcomes from PD-1/PD-L1 ICB trials in gliomas are disappointing to date (26). It is partially attributed to insufficiency of TILs infiltration, low expression of PD-1/PD-L1, and low tumor mutation burden (TMB) in gliomas (26), which are not conducive to the ICIs to revive the anti-tumor immune response. Compared with gliomas, however, BMs are more abundant and diverse with TILs and neutrophils (27). Relatively high infiltration of TILs has been found in BMs from melanoma, renal cell carcinoma, and NSCLC (28). Comparing PD-L1 expression and TILs densities between primary tumor and matched BMs revealed a lower burden of TILs but a higher PD-L1 expression in NSCLC BMs (29–31). Given the discordance between BMs and primary tumors, these differences may contribute to the differential activity of immunotherapy for NSCLC-BMs. The intracranial efficacy of pembrolizumab was confirmed despite the relative scarcity of intracranial TILs (32). Hence, it is necessary to determine whether the intracranial efficacy of ICIs depends on activation of intracranial immune cells in situ, or migrating from the peripheral environment, or both. Using a high-dimensional single-cell approach, Friebel et al. revealed that BMs were characterized by high infiltration of peripherally-derived leukocytes, especially CD8+ T cells (33). Moreover, CD8+ T cells in BMs appear to be more exhausted than those in peripheral and normal intracranial environments, partially because immune suppressive signals, such as PD-1 and CTLA-4, are upregulated, a mechanism that ICIs can potentially improve. Studies have shown that blocking PD-1 could induce the migration of immune cells to the brain, in which IFN-γ played a key role (34). Up-regulation of IFN-γ can modulate multiple adhesion molecules (such as VCAM-1 and ICAM-1) and chemokines (such as CXCL10)-mediated T cell migration and can also induce the turning on of BBB (35, 36). Notably, activated CD4+ T cells in the brain can loosen the BBB through local IFN-γ production (37), which may produce positive feedback.
In conclusion, these findings indicated that NSCLC patients with BMs might benefit from ICIs treatment by activated intracranial and extracranial immune (Figure 1).
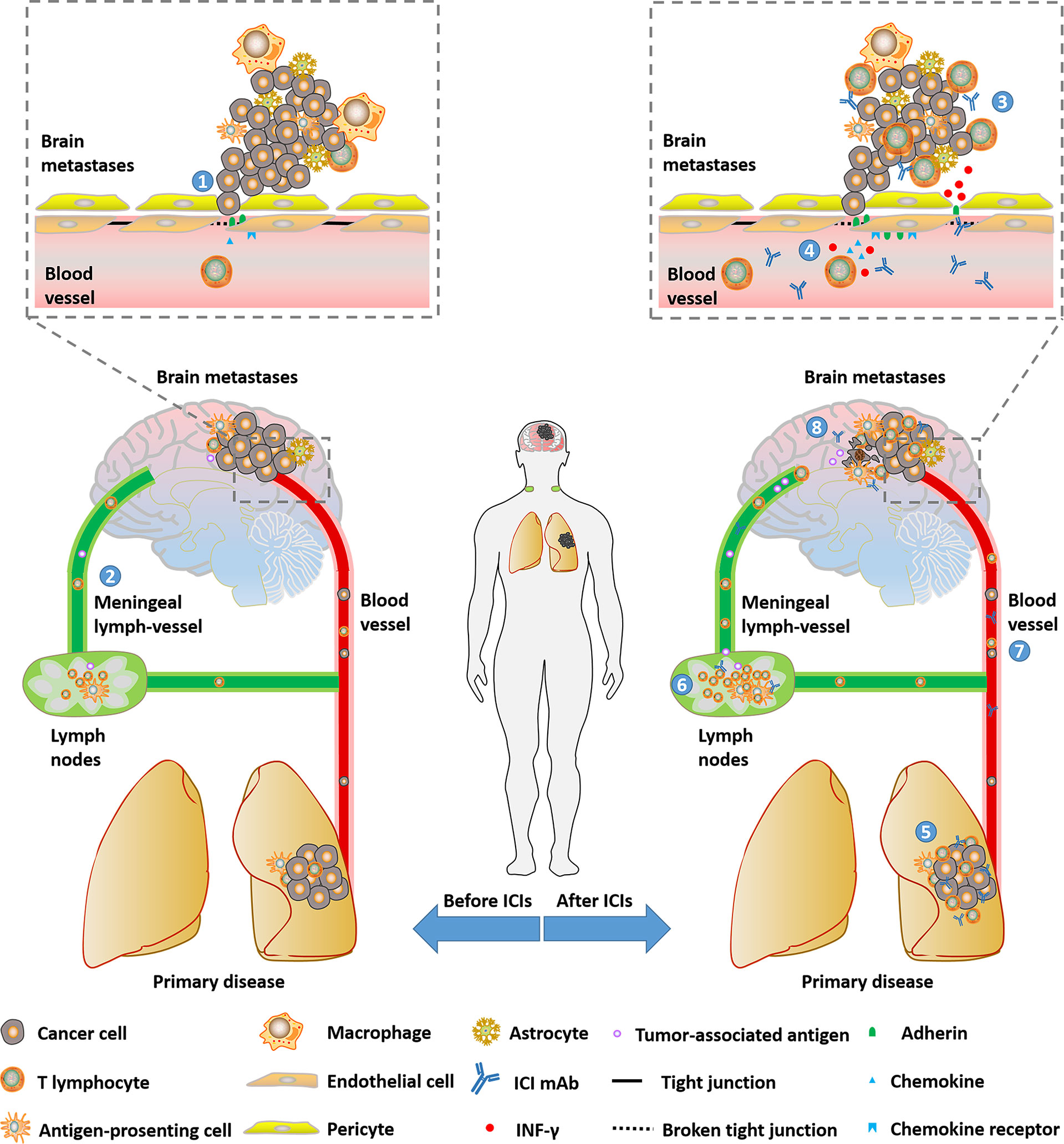
Figure 1 Potential mechanisms that NSCLC patients with brain metastases could benefit from ICIs. (1) BBB will be loosened with the progression of BMs. (2) Immune cells and tumor-associated antigen could be transported between intracranial and peripheral environments by meningeal lymph-vessels. (3) Part of ICI mAbs can enter the intracranial environment and be detected. (4) BBB can get loose due to broken tight conjunction and enhanced trans-endothelial migration through the up-regulation of adhesins and chemokines mediated by INF-γ inducing T-cell- secreted INF-γ. (6)(7)(8) ICIs revive anti-tumor immune in lymph nodes, primary disease and peripheral circulation. ICI, immune checkpoint inhibitor; RT, radiotherapy; CT, chemotherapy; IFN, interferon; BBB, blood-brain barrier; mAb, monoclonal antibody; IFN, interferon.
ICI Monotherapy for NSCLC-BMs
Response
For a long time, it has been controversial whether ICI monotherapy induces an intracranial response in NSCLC-BMs. Intracranial examinations were routinely not been performed in the follow-up when observing the response of ICIs for advanced NSCLC. Several large randomized trials on ICIs treatment for gliomas (Checkmate-143, Checkmate-498, Checkmate-548) failed to prolong PFS and OS (26), casting a shadow over research on metastatic brain tumors. This nebulous status continued until Goldberg and his colleagues firstly reported the outcome of pembrolizumab for patients with untreated BMs (32). In their phase 2 trial, patients with at least one 5~20mm untreated asymptomatic BMs were divided into two cohorts. Cohort 1 was patients with PD-L1 positive (PD-L1≥1%) and cohort 2 PD-L1 negative (PD-L1<1%) or unknown. The results showed that 11 of 37 patients (29.7%) in cohort 1 had an intracranial response. However, there was no response in cohort 2 (5 patients). Furthermore, 29.7% of patients in cohort 1 had a systemic response, and 7 of the intracranial responders had a systemic response simultaneously. Several retrospective studies also provided valuable evaluations of intracranial response (38–43). In short, intracranial objective response rate (ORR) ranges from 16.4% to 36.6% regardless of PD-L1 expression. For patients with PD-L1≥50%, intracranial ORR may exceed 50%.
Compared with chemotherapy, a higher systemic response has been observed in ICI monotherapy for patients with BMs. Recently, a pooled analysis based on KEYNOTE-001, 010, 024, and 042 (44) showed that systemic ORR with pembrolizumab was superior to chemotherapy in PD-L1≥1% NSCLC patients with BMs (26.1% vs. 18.1%). The single-arm FIR trial (45) enrolled 13 advanced NSCLC patients treated with atezolizumab into its cohort 3 (second-line with treated BMs). All eligible patients were PD-L1 positive. Investigator-assessed systemic ORR was 23%. Of particular interest, the outcome of FIR trial as well as other retrospective data suggested that there was no significant difference between patients with asymptomatic BMs and patients without BMs (45) (Table 1).
Survival
Benefits in survival are relatively clear-cut for NSCLC patients with BMs. A pooled analysis of KEYNOTE-001, 010, 024, and 042 (44) showed that pembrolizumab improved overall survival (OS) versus chemotherapy (13.4 months vs. 10.3 months, HR=0.83). In the subgroup of NSCLC patients with high PD-L1 expression (PD-L1≥50%), the magnitude of benefit with pembrolizumab compared with chemotherapy increased to 19.7 months vs. 9.7 months (HR=0.67). Importantly, both the magnitude of benefit and the toxicity profile with pembrolizumab were similar to those in patients without BMs (38, 40). A pooled analysis of CheckMate-017 and 057 (46) compared the long-term outcomes of nivolumab with docetaxel in the BMs subgroup. Nivolumab improved OS (7.6 months vs. 6.2 months, HR=0.81) and 5-year OS rate (8% vs. 0%) versus docetaxel. Clinical evidence that NSCLC patients with BMs can benefit from nivolumab mainly came from several real-world studies in European regions (41, 47–51). Several retrospective studies that did not distinguish the types of anti-PD-1/PD-L1 monotherapy were also shown in Table 1. Cemiplimab is a newly approved anti-PD-1 ICI for advanced NSCLC with high PD-L1 expression(≥50%) based on a phase 3 EMPOWER-Lung 1 study (53). Results have demonstrated that cemiplimab monotherapy present significantly superior progression-free survival (PFS) (HR=0.45) and OS (HR=0.17) compared with platinum-double chemotherapy in the BMs subgroup. A similar benefit could be observed in OAK trials comparing atezolizumab with docetaxel (52). Notably, the development of new BMs was delayed by atezolizumab. In patients with baseline BMs, the median time to the development of new BMs was not reached in the atezolizumab arm, and was 9.3 months in the docetaxel arm (HR=0.38). Interestingly, in PACIFIC study for inoperable stage 3 NSCLC patients (55), the maintenance treatment of durvalumab after chemoradiotherapy was associated with a halved incidence of developing new BMs. These findings indicated PD-L1 inhibitors might prevent or at least delay the occurrence of BMs.
Safety
According to the published data, it was generally accepted that ICI monotherapy had a better tolerance than standard chemotherapy. The prospective trial focusing on BMs from Goldberg et al. (32) reported that no intracranial Grade≥3 adverse events (AEs) occurred, and the incidence of systemic Grade≥3 AEs was 14%. In the pooled analysis of KEYNOTE-001, 010, 024, and 042 (44), comparing pembrolizumab and chemotherapy, the incidence of intracranial Grade≥3 AEs was 9.7% vs. 26.7%, while the incidence of systemic Grade≥3 AEs was 14.8% vs. 45.6%. The Expanded Access Program from Italy included 466 NSCLC patients with BMs who were asymptomatic after radiotherapy. 7% nonsquamous NSCLC patients and 8% squamous NSCLC patients suffered Grade≥3 systemic AEs (50, 51). Another real-world study reported similar incidence, regardless of neither histological type nor condition of BMs (56). The BMs subgroup analysis from OAK trial (52) indicated the better systemic safety of atezolizumab compared with docetaxel. Notably, Grade≥3 neurologic treatment-related AEs (trAEs) were higher in the atezolizumab arm, although the rate of neurocognitive AEs was quite low in both arms (5.0% vs. 1.8%), and no Grade 4~5 neurologic trAEs occurred. Collectively, the incidence of Grade≥3 trAEs ranges from 14%~25%, as well as a low incidence of intracranial AEs.
To sum up, anti-PD-1/PD-L1 ICI monotherapy is beneficial for NSCLC patients with BMs, which can diminish intracranial disease, reduce adverse events and improve survival, particularly for those with high PD-L1 expression (Table 1). However, we still need more large-scale clinical data to support this view and precisely pre-stratify patients who may benefit.
Combination Strategy for NSCLC-BMs
Although ICI monotherapy could yield benefits to NSCLC patients with BMs, the response of ICI monotherapy is generally less than 30%. Moreover, due to the slow onset of the anti-tumor immune response, there is often a cross point on the Kaplan-Meier survival curves between ICI monotherapy group and the control group when curves are priming, suggesting that the early efficacy of ICI treatment was inferior to chemotherapy or radiotherapy for some patients. The outcomes of the Keynote-001 study showed that about 75% of patients who produced early response on immunotherapy achieved long-term survival (57). Hence, improving ICI efficacy and expanding the pool of beneficiaries are urgently needed. Actually, immunotherapy can be reciprocally beneficial to other treatments (Figure 2), such as radiotherapy and chemotherapy.
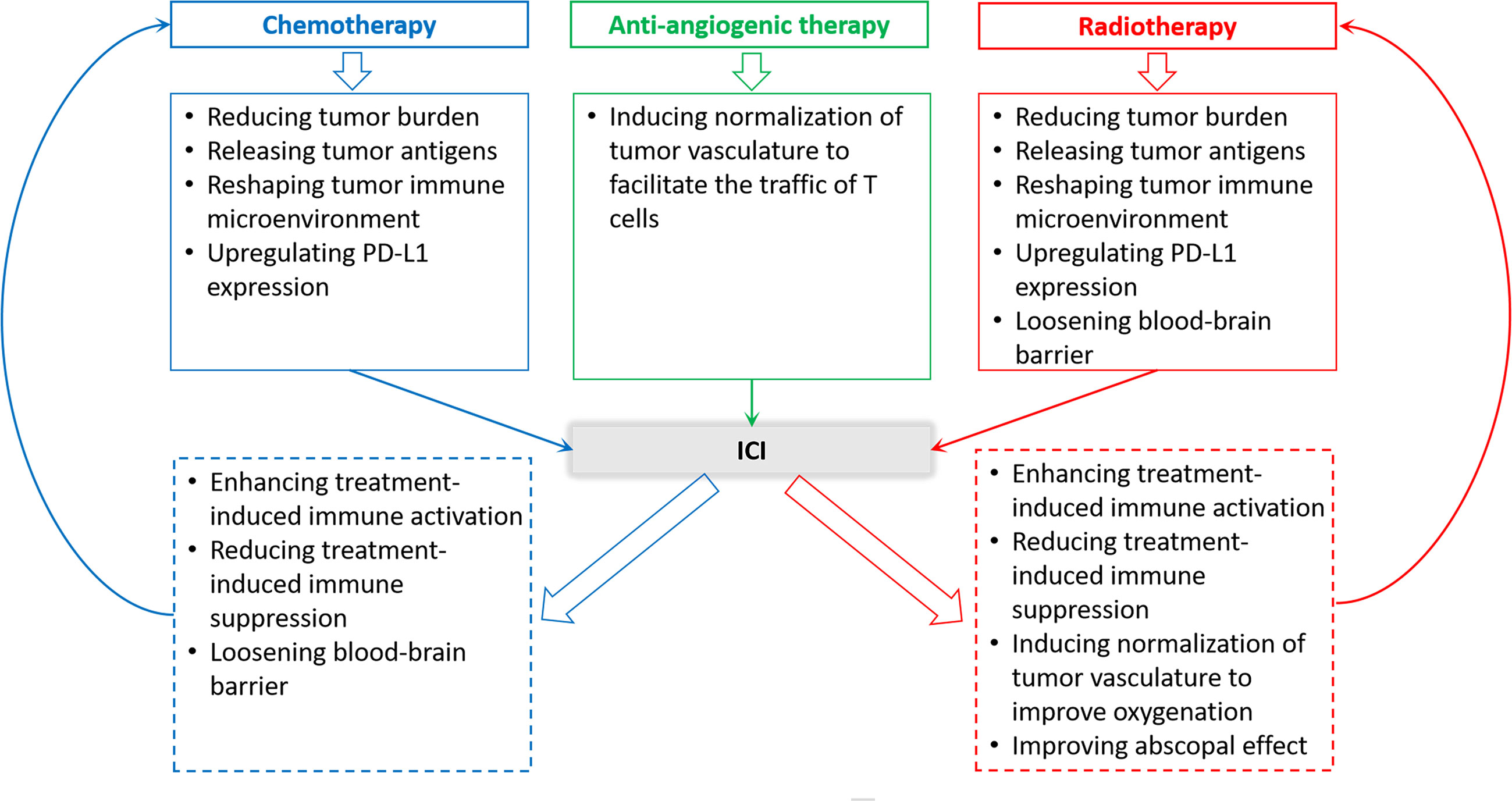
Figure 2 Rationality of ICI therapy and other therapies combination in the treatment of NSCLC patients with BMs.
ICI Combined With Radiotherapy
Given almost all patients with metastatic NSCLC require radiotherapy, especially those with BMs, radiotherapy and immunotherapy possibly form the best alliance in clinical practice. On the one hand, a growing body of evidence supports synergistic mechanisms between radiotherapy and immunotherapy (58–60), which are present in Figure 2. On the other hand, from a clinical point of view, the response of immunotherapy is slow but persistent, whereas radiotherapy can quickly relieve nervous symptoms in BMs patients, although the response is not long-lasting (60). Therefore, combination treatment can bring effective and long-term benefits to BMs patients from NSCLC.
Some clinical studies have provided support for the value of this alliance. ICIs combined with radiotherapy can convey benefits to NSCLC patients with BMs by elevation in intracranial disease control, prolongation survival as well as improvement in neurocognitive function. A multicenter phase II trial (61) examined the effect of combining nivolumab with SRT. It included 26 patients (22 NSCLC), having ≤10 cc of BMs with no prior irradiation or immunotherapy. Of the 21 NSCLC patients with assessable PD-L1 status, 12 patients had PD-L1≥50%. High intracranial control was present: median intracranial PFS was 5.0 months, the 1-year cumulative incidence of intracranial relapse was 20% accounting for death as a competing risk. Median OS was 14 months. In the sixth month, neurocognitive function showed potential improvements. Compared with radiotherapy alone, radiotherapy plus ICI significantly improved local disease control and survival. A representative example is an investigation with a large sample size from National Cancer Databank (NCDB), showing that the median OS of NSCLC-BMs patients treated with ICI+RT increased 1.5 times (13.1 months vs. 9.7 months) than those treated with RT alone (62). Moreover, combination therapy can decrease neurological death (63). More data are listed in Table 2.
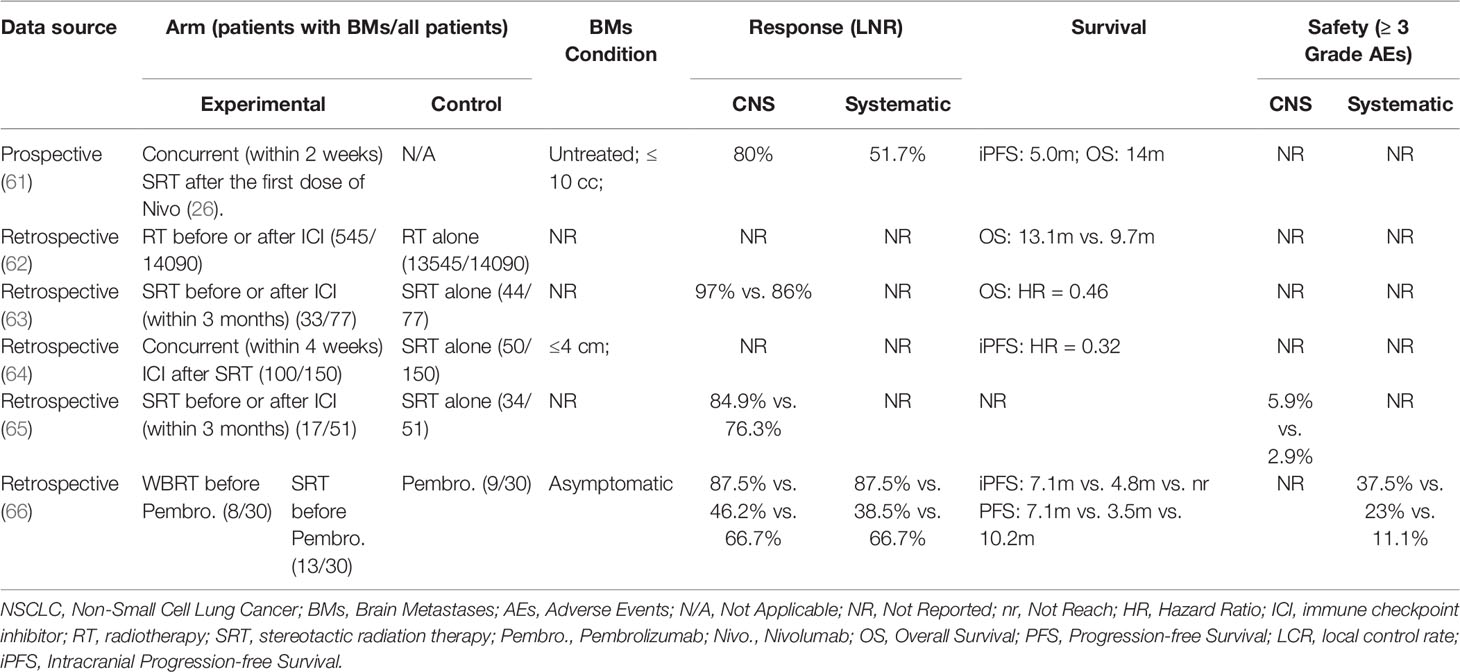
Table 2 Clinical investigations of ICI and radiotherapy combination in the treatment of NSCLC patients with BMs.
While the advantages of radiotherapy combined with immunotherapy are promising, three concerns remain for clinicians. The primary concern is safety. In recent years, an increased incidence of radionecrosis was reported when applying ICIs in patients undergoing SRT for BMs. Radionecrosis significantly impacts the quality of life, leading to focal neurologic deficits, headaches, nausea, and seizures. A retrospective research has shown that receipt of immunotherapy was associated with symptomatic radionecrosis after adjustment for tumor histology (HR=2.56); this association was stronger in BMs patients from melanoma(n=145) (HR=4.02) (67). The outcome of NSCLC with BMs was not published. There were also more recent studies suggesting that ICIs were not associated with the increasing incidence of radionecrosis in NSCLC-BMs (64). This discordance may be attributed to histology heterogeneity and difficulty to distinguish radionecrosis from tumor progression in radiology. Hence, more studies are needed to clarify the association between immunotherapy and radionecrosis. Another major concern is the optimal timing of combining radiation and immunotherapy. Based on the aforementioned reciprocal mechanisms, some investigators have proposed that immunotherapy should be administered after radiotherapy so that the reciprocal effect could be maximized while activated immune cells avoid being damaged by radiation (68). Many retrospective investigations observed better outcomes in RT+ICI. However, they did not limit the order of between RT and ICI management, and the intervals between these two managements varied widely, ranging from two weeks to three months (64, 65, 69). A meta-analysis showed that concurrent SRT with ICI performed better OS than sequential therapy in the treatment of NSCLC patients with BMs (HR=0.39), but there were only two studies involved (70). In addition, the radiation dose is also attracting attention. Some investigations considered that hypofraction radiotherapy (HFRT) or SRT might better activate anti-tumor immune responses and preserve lymphocytes than traditional radiotherapy (71–73). However, there were also retrospective data showing that WBRT plus ICI performed better than SRT plus ICI in the treatment of NSCLC patients with BMs (66). Therefore, prospective trials are needed to determine the optimal dose-fraction scheme.
ICI Combined With Chemotherapy
Like radiotherapy, there are also synergy effects between immunotherapy and chemotherapy, which is the theoretical basis of combination (74, 75). Recently, numerous prospective clinical evidence has confirmed the advantages of this combination (Table 3).
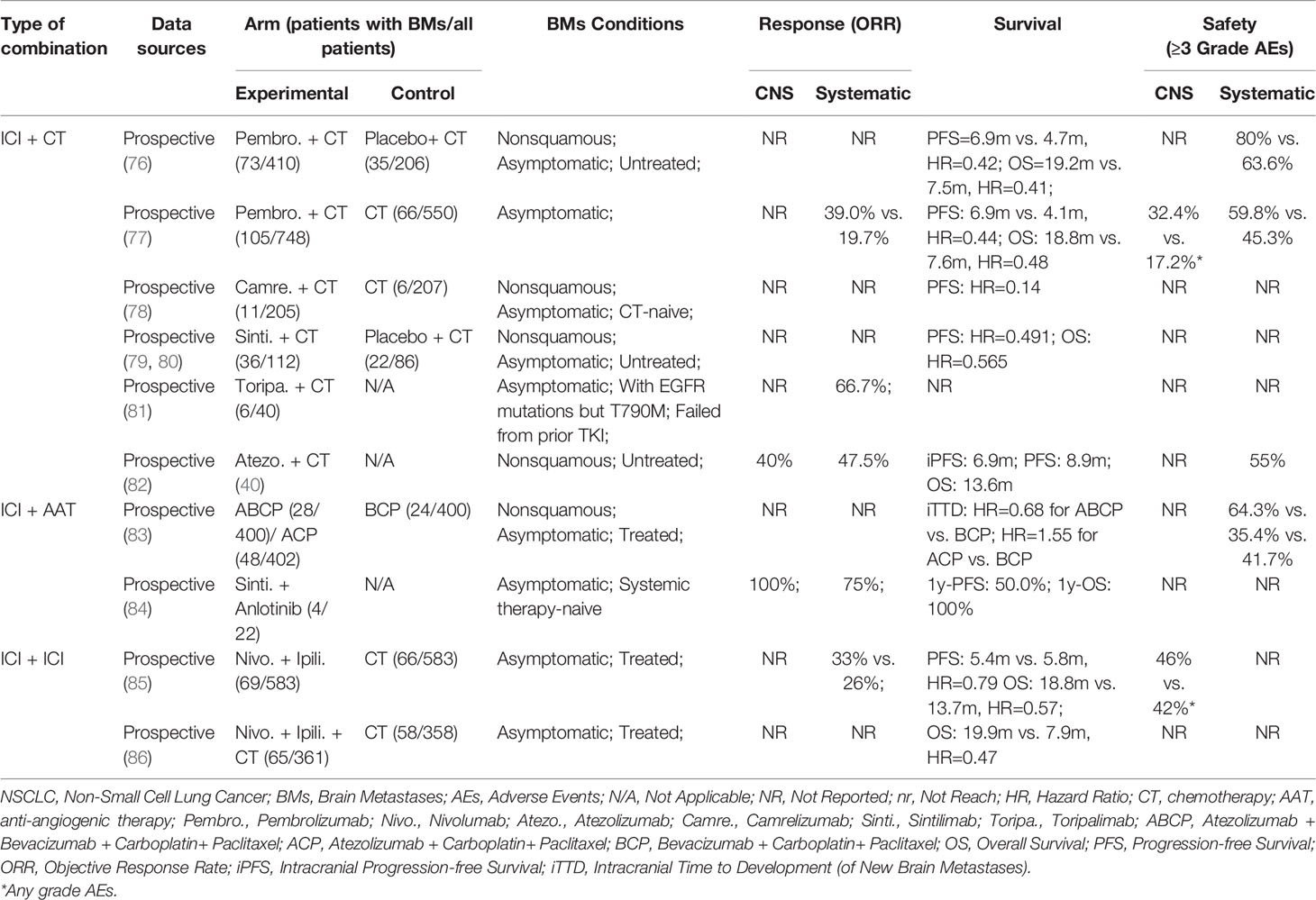
Table 3 Clinical investigations of ICI and other systematic therapies combination in the treatment of NSCLC patients with BMs.
Synergies between chemotherapy and ICIs yield to NSCLC patients with BMs in prolonged survival, including PFS and OS. This benefit has been observed in the KEYNOTE-189 study of pembrolizumab and chemotherapy vs. chemotherapy alone (76). The HR for PFS was 0.48 in the overall population, 0.48 in patients without metastases, and 0.42 in patients with BMs, and the HR for OS was 0.56, 0.59, 0.41 in the three groups above, respectively. Particularly, compared with chemotherapy alone, OS was observed a remarkable prolongation in pembrolizumab combined with chemotherapy (19.2 months vs. 7.5 months). Similar conclusions were supported in a pooled analysis of KEYNOTE-021, 189, 407 (77), including 171 NSCLC patients with asymptomatic BMs in 1298 advanced NSCLC. Systematic ORR in the combination arm was also significantly enhanced as compared with the chemotherapy alone arm (39.0% vs. 19.7%). Notably, for NSCLC patients with BMs, the magnitude of benefit from ICIs combined with chemotherapy seems to be realized regardless of PD-L1 expression. In addition, for those advanced NSCLC patients with EGFR mutation who failed from prior first-line targeting therapy, ICIs and chemotherapy combination may work well, though observed only in the trial with a small sample (81). Collectively, adding ICIs into chemotherapy can significantly improve survival for NSCLC patients with BMs. Relevant prospective clinical studies are listed in Table 3.
Like radiation therapy, the sequence and dose of chemotherapy combined with ICI also make a difference in the efficacy of treatment (87). Zhu et al. found docetaxel delivery before anti-PD-1 ICI with an interval of two days could initiate a more powerful anti-tumor response than simultaneous delivery and post delivery in multiple tumor models (88). However, patients in large randomized clinical trials are almost administered with chemotherapy drugs and ICI on the same day, followed by ICI maintenance (with or without chemotherapy), probably due to patient compliance. To date, no consensus has been reached regarding the dose and sequence strategies used in combinational cancer immunotherapies. How to optimize the scheme of administration relative to each kind of drug remains to be studied.
ICI Combined With Anti-Angiogenic Agents
It is commonly believed that anti-angiogenic agents limit tumors growth by inhibiting the tumor vasculature. Nevertheless, a low dose of anti-angiogenic agent may instead induce the normalization of abnormal tumor vessels, decreasing tumor-promoting hypoxia and increasing accessibility for immune cells and other therapeutic agents to reach the TME, which facilitate the efficacy of immunotherapy (89).
In IMpower150 trial (83), patients were randomized 1:1:1 to receive atezolizumab + bevacizumab + carboplatin/paclitaxel (ABCP), atezolizumab + carboplatin/paclitaxel(ACP), or bevacizumab + carboplatin/paclitaxel (BCP). With a good tolerance, significantly improved PFS and OS were observed in the ABCP group compared with the BCP group for metastatic nonsquamous NSCLC, regardless of PD-L1 expression and EGFR or ALK genetic alteration status. Outcomes from the latest IMpower150 exploratory analyses in the subgroup with BMs indicated that the ABCP regimen could delay the time to development of new BMs (HR=0.68) for ABCP versus BCP and 1.55 for ACP versus BCP). A phase 1b trial assessed sintilimab (a PD-1 inhibitor) combined with anlotinib (a multi-target tyrosine kinase inhibitor with anti-angiogenic action) in the frontline setting for advanced NSCLC (84). This chemotherapy-free regimen presented encouraging efficacy, durability, and safety profile regardless of PD-L1 expression. Notably, all four involved patients with asymptomatic BMs at baseline achieved intracranial complete response (CR), and three of them achieved overall partial response (PR), indicating that sintilimab plus anlotinib had synergistic effects in the brain. The outcomes of a further trial are worth expecting.
The primary concern for this combination is still safety. In the BMs subgroup of Impower150 trial (83), the ABCP group had the highest incidence of Grade 3~4 trAEs among the three groups. Besides, treatment withdrawal due to AEs occurred in 42.9% of patients in the ABCP arm. On the plus side, there were no Grade 5 AEs with ABCP. Additionally, an understandable concern for AEs is intracranial hemorrhage, which should be highly regarded, although the management of anti-VEGF regents may make no contribution to an increased risk of intracerebral hemorrhage in NSCLC patients (90). Due to the lack of higher-level evidence, it is necessary to closely monitor the risk of intracerebral hemorrhage in patients with BMs when using ICIs plus anti-angiogenic agent regimens. Another imperative concern in clinical practice is the cost. After all, the promising regimen in Impower150 trial involves four drugs. A cost-effectiveness analysis from the United States showed that the incremental cost-effectiveness ratio for ABCP was $568,967 per quality-adjusted life-year (QALY) compared with BCP and $516,114 per QALY compared with CP (91). The issue of cost should be taken seriously by all parties.
Multiple ICIs Combination
Blocking multiple immune checkpoints seems natural to activate anti-tumor immunity to a greater extent. Nivolumab plus ipilimumab have been approved for metastatic melanoma and NSCLC by FDA (92), but the failure of durvalumab plus ipilimumab gave us a warning that not all double-ICIs regimens worked well. Despite the fact that all ICIs play roles in releasing brakes that limit the immune system, the specific mechanisms for reviving anti-tumor immunity are peculiar. For instance, circulatory and resident T cells are subject to blocking the PD-1/PD-L1 axis, whereas lymphocytes in lymph nodes are subject to be activating by CTLA-4 inhibitors (93). Furthermore, different ICIs may function on their preferred subsets of T cells (94). Therefore, it is rational that the combination of dual ICIs may produce spatio-temporal synergies.
Checkmate-227 part 1 evaluated nivolumab plus ipilimumab versus chemotherapy in the treatment of advanced NSCLC. A post-hoc analysis (85) demonstrated that the double-ICIs arm presented higher ORR (33% vs. 26%), with a longer duration of response (24.9 months vs. 8.4 months). Longer OS was observed in the double-ICIs arm (18.8 months vs. 13.7 months). The rate of any-grade CNS AEs was 46% in BMs patients treated with double-ICIs, most of which were Grade 1~2, while it was 42% for those treated with chemotherapy. Chemotherapy or radiotherapy can be added into a double-ICIs regimen. Nivolumab + ipilimumab + two-cycle chemotherapy has been proved to have an advantage over four-cycle chemotherapy in the BMs subgroup of Checkmate-9L trial (86). Of note, double-ICIs combined with short-course chemotherapy eliminated the cross point of OS curve in Checkmate-027, which demonstrated the advantage of the combination strategy. Concurrent/sequential SBRT combined with nivolumab and ipilimumab was well tolerated (95). Multimodality therapy is valued to achieve durable metastases control and survival (Table 3).
Challenges and Perspectives
Based on the basic researches and clinical data presented in this review, sufficient evidence exists to support the continued exploration of the novel value of immunotherapy for NSCLC BMs. An ICI-containing algorithm in the management of NSCLC-BMs is presented in Figure 3. However, it must be noted that until more robust clinical trials are conducted, NSCLC-BMs patients should be individually evaluated by multidisciplinary tumor boards in highly experienced centers. Also, several considerations need to be adequately addressed before the development of a clinical trial designed to widely test the setting.
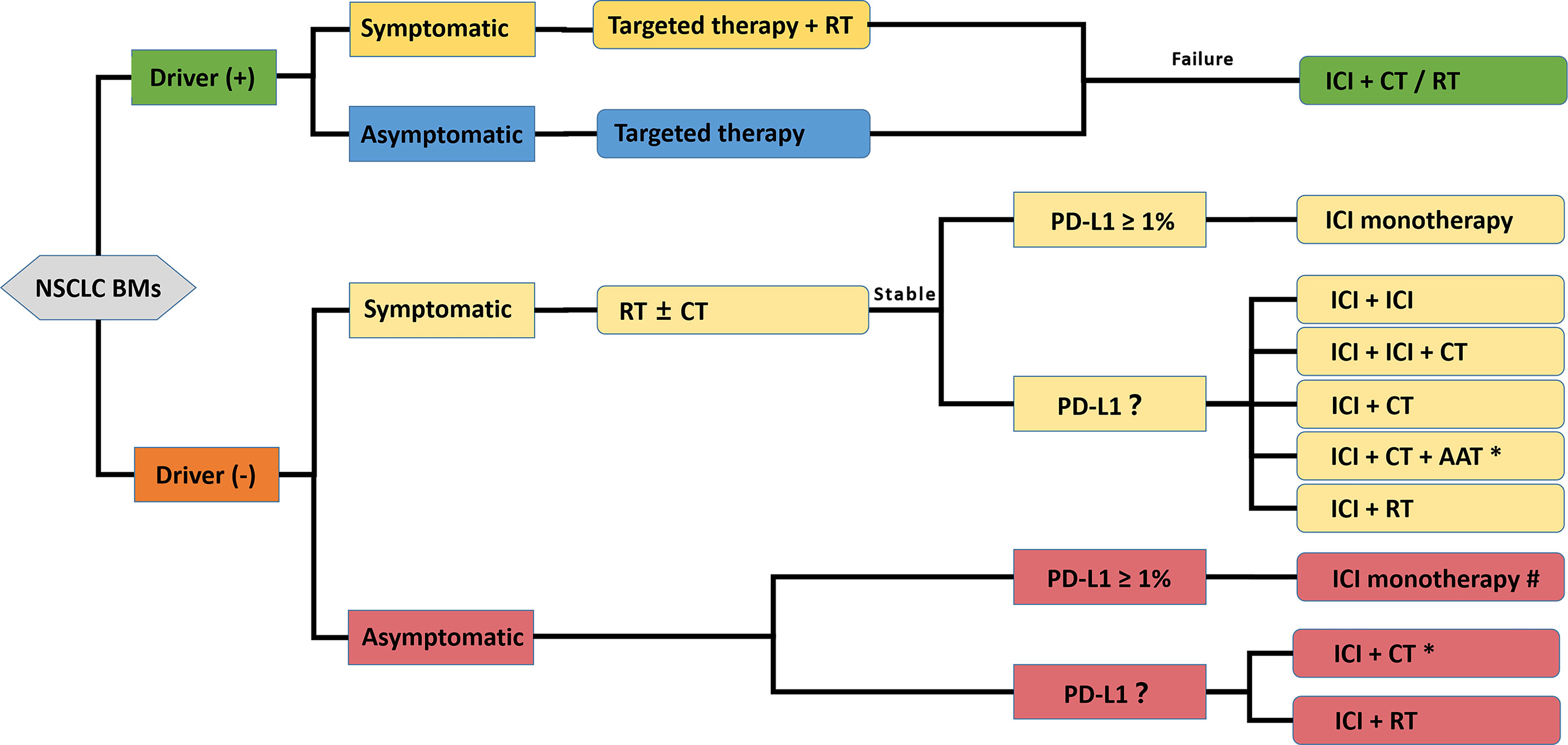
Figure 3 A propositional management algorithm for NSCLC patients with BMs who are candidates for ICI-containing comprehensive treatment. This algorithm considers patients who are candidates for an ICI-containing comprehensive therapy after the standard patient examination and tumor specimen evaluation (driver-gene status and PD-L1 expression evaluation). Targeted reagent-centered therapy should be recommended first for driver-positive patients owing to a favorable intracranial response rate. Considering the poor prognosis of BMs, ICI monotherapy is recommended with more caution, and ICI-containing combination therapy is encouraged. ICI Patients with symptomatic or multiple BMs could be treated with RT strategies. The final therapeutic decision should be made by a multidisciplinary tumor board. * only appropriate for nonsquamous NSCLC; # optimal when PD-L1 ≥ 50%; ICI, Immune Checkpoint Inhibitor; CT, Chemotherapy; RT, Radiotherapy; TT, Targeted therapy; AAT, Anti-angiogenic therapy.
Patient Selection
In the era of immunotherapy, the appropriate patient selection remains of paramount importance. In NSCLC BMs, prognosis significantly depends on several factors, including age, Karnofsky performance status, extracranial metastases, number of BMs, and the presence of driver-gene mutations. Together, these clinical parameters constitute lung-graded prognostic assessment (lung-GPA), the most established tool to estimate survival in lung cancer. GPA of 4.0 and 0.0 correlate with the best and worst prognosis, respectively, with OS varying widely from 7 months to 47 months (96). Some studies have shown that some clinical parameters, including but not limited to those involved in GPA, are associated with intracranial outcomes and survival in ICIs treatment of NSCLC BMs (11, 97). As noted previously, the benefit acquired from ICIs in NSCLC patients with asymptomatic BMs is likely to have no different from that in patients without BMs. Hence, we recommend that NSCLC patients with asymptomatic BMs are not supposed to be excluded routinely from clinical trials on ICIs. Symptomatic BMs often have poor efficacy and outcome. Besides active BMs per se, the decline in the efficacy of immunotherapy is attributed to steroids, which are considered immune suppressors and routinely used to control intracranial symptoms and modify side effects of other therapies in patients with BMs (14, 98). Moreover, harboring driver-gene mutation, indicating a favorable prognosis, is associated with low benefits from ICIs (99). Hence, GPA in the setting of immunotherapy may need to be updated.
Developing reliable biomarkers is an important approach to accurately select patients. Currently, for anti-PD-1/PD-L1 treatment, PD-L1 remains the most commonly used stratified biomarker in both clinical practice and trials. A study demonstrated that PD-L1 expression might predict OS in NSCLC BMs patients receiving immunotherapy. Importantly, it was independent of lung-GPA (100). It also noted that intracranial PFS did not show an association with PD-L1 expression (100). However, the specimens for testing PD-L1 expression in almost all studies are from primary lesions. As mentioned earlier, PD-L1 expression is at variance between BMs and primary lesions (30, 31). Therefore, whether PD-L1 can be a robust marker for ICIs intracranial response remains to require further investigations. Besides PD-L1, tumor mutational burden (TMB) is also approved as a biomarker of ICIs therapy, such as pembrolizumab-based therapy and nivolumab + ipilimumab combination therapy, in pan-cancer (101–103). Remarkably, TMB is site-specific in NSCLC and is highest in lung adenocarcinoma BMs (104). Beyond PD-L1 and TMB, emerging genomic biomarkers for immunotherapy are under development (105, 106).
A major restriction of studying the tumor immune microenvironment of BMs is that it is extremely challenging to obtain intracranial specimens. Therefore, simple substitutions are required. Liquid biopsy technique based on cerebrospinal fluid provides the opportunity to precisely acquire and monitor BMs in real-time and guide immunotherapy. Cell-free DNA (ct-DNA) and immune cell RNA profiling of CFS enable to characterize genomic information and immune cells infiltration of BMs and predict prognosis eventually (107–110). The presence of circulating tumor cells (CTCs) has been reported to be associated not only with NSCLC recurrence and metastasis but also with worse tumor response to ICI (111). Methods have been developed to characterize CTCs of NSCLC-BMs in CSF (112). Further work is needed to confirm the potential value of CTCs in predicting the efficacy of ICI for NSCLC-BMs. Besides liquid biopsy, advanced imaging techniques and artificial intelligence in radiomics will bring about a revolutionary shift in predicting cancer outcomes (113). For instance, the deep learning models from CT (114) or PET-CT (115) provide a noninvasive method to predict high PD-L1 expression of NSCLC and infer clinical outcomes in response to immunotherapy.
With the digitalization of radiology, histopathology, genomics and clinical information, it is necessary to integrate and analyze these data, because none of them can fully characterize tumors alone. In other words, they are complementary. For example, radiological scans and pathology specimens describe tumors spatially at different dimensions. However, at present, even when these data are available, they are rarely integrated. Artificial intelligence and deep learning provide an opportunity for multimodal data integration (116). One example is that, by integrating PET-CT imaging, RNA-sequencing, and histology, differential immuno-metabolic crosstalk in lung squamous cell carcinoma and adenocarcinoma was observed (117). Another study found that the combination of features from histological imaging and MRI outperformed unimodal classifiers for the stratification of brain tumor subtypes (118). BMs possess the intricate characteristics of both primary solid tumors and neurological tumors. Thus, the integration of information may be a promising direction for developing reliable biomarkers for BMs.
Response Assessment
The response assessment of BMs depends not only on changes in the size of the targeted lesions but also on changes in neurological status and steroid dosage. Previous measures, such as tumor shrinkage rate and survival time, to evaluate the efficacy of immunotherapy are not comprehensive and are not able to imply early efficacy because some lesions have a temporary pseudoprogression and then respond well. Published studies have reported a wide range of incidences of pseudoprogression (from less than 1% to more than 20%) (119–121). Regardless, consequences with failure to identify pseudoprogression are substantive and undesired, including premature discontinuation of an effective therapy and overestimating the efficacy of a subsequent therapy. Therefore, with the shift of the management of BMs in the era of immunotherapy, understanding of the response assessment of BMs needs renovation.
Firstly, the criteria of response assessment to BMs are constantly evolving (Figure 4). Most solid tumors were evaluated by Response Evaluation Criteria in Solid Tumors (RECIST) 1.1. Given the use of steroids and changes in patients’ neurological status, MacDonald Criteria was established, primarily applied to gliomas (122). Subsequently, to cope with the challenges posed by the pseudoprogression after radiotherapy as well as the pseudoremission after anti-angiogenic therapy, the Response Assessment in Neuro-Oncology (RANO) working group published the Response Assessment in Neuro-Oncology for High-grade Gliomas (RANO-HGG) (123). Although MacDonald Criteria and RANO-HGG could be extended to BMs, BMs possess the characteristics of both primary solid tumors and neurological tumors. Hence, for high-quality assessment of BMs, the RANO working group established RANO for BMs (RANO-BM) (124). The RANO-BM uses a one-dimensional method to measure tumor size, requiring measurable lesions to be at least 10 mm in diameter, allowing up to 5 lesions to be targeted, and incorporates the patient’s performance status and steroid use as a basis for evaluation when determining disease remission or progression. Qian et al. proposed modified RECIST (mRECIST) 1.1 criteria to adapt to the application of immunotherapy in the treatment of BMs (125). The standard eased the restriction of measurable lesion length to ≥5 mm, allowing more patients to be included in clinical studies. Immune-related Response Criteria (irRC) pointed out that if there was no significant decrease in the patient’s clinical performance status, progression could not be determined by an increase in the volume of an early lesion or the appearance of a new lesion unless subsequent imaging tests could confirm (126). Based on irRC, the RANO working group developed Immunotherapy RANO (iRANO) for patients with neurologic tumors who received immunotherapy, including BMs (127). It recommended that immunotherapy for six months was required in patients without clinical response. Patients with radiological progression should undertake a radiological follow-up after three months and clinicians should compare the two images to review post-treatment outcomes. iRANO is proposed to address the potential pseudoprogression after immunotherapy, with a 6-month window to assess true response, avoiding premature discontinuation of treatment in patients who are likely to benefit from immunotherapy potentially. However, iRANO has not yet been widely manipulated in clinical trials and practice because of its complicated implementation, which limits the popularity of iRANO to a certain extent.
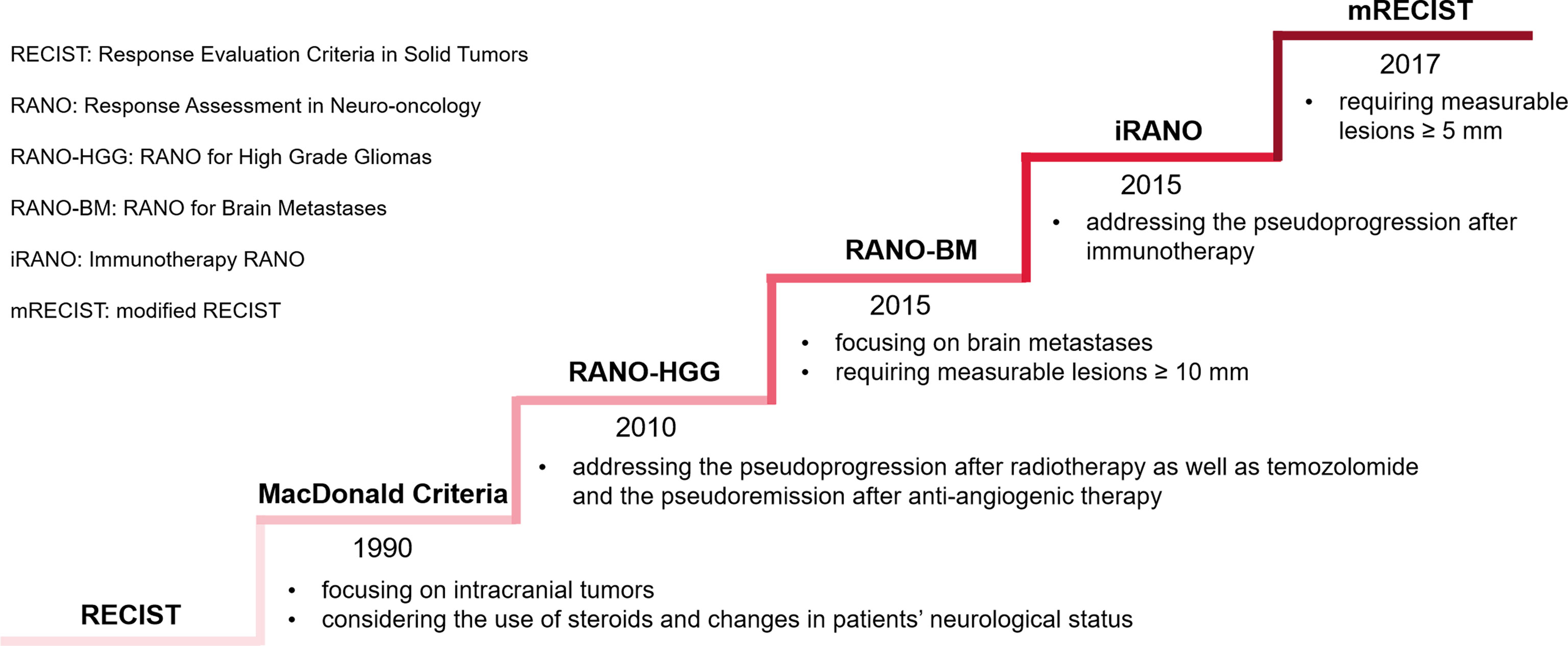
Figure 4 Evolving criteria of response assessment to BMs. The ladder rises by the time a standard is first released rather than its popularity.
Similarly, advanced tools are emerging to facilitate response assessment. An example is that magnetic resonance (MR) spectroscopy and perfusion might increase the accuracy of differentiating recurrent tumors from radionecrosis in patients with gliomas or BMs (128). Of particular interest are radiolabeled amino acids for brain tumor imaging using positron emission tomography (PET) because of their increased uptake in neoplastic tissue but low uptake in the normal brain parenchyma (129), which allows the accurate depiction of BMs to delineate BMs extent, assess treatment response, and differentiate treatment-related changes from tumor progression (130). Recently, this imaging technique was strongly recommended by the RANO working group (131, 132). Other tracers, such as radiolabeled analog to the nucleoside thymidine, were developed to assess cellular proliferation and may be of great value in the differentiation of BMs pseudoprogression after immunotherapy (133).
Importantly, on the basis of more investigations, more consensus needs to be reached in the context of the existence of various criteria and assessment tools.
Conclusion
Advances in ICIs have resulted in the management of BMs patients from NSCLC navigating toward the immunotherapy era. ICI monotherapy and combination have embodied novel value in enhancing intracranial response, prolonging survival, delaying BMs, and improving quality of life. In summary, the activity of ICIs for the treatment of NSCLC BMs should not be drastically underestimated, especially for selected patients. Considering the poor prognosis of BMs as well as the reciprocity between immunotherapy and other therapies, the synergistic combination treatment is promising. Critically, before the extensive application of this combination protocol in clinical practice, more preclinical and clinical trials are urgently needed to provide definite evidence and resolve the challenges discussed above.
Author Contributions
GY contributed to the original draft, investigation, and resources. LX contributed to review, and funding acquisition. XS contributed to study design, review, and funding acquisition. All authors read and approved the submitted manuscript.
Funding
The study was funded by the National Natural Science Foundation of China (No. 82172866).
Conflict of Interest
The authors declare that the research was conducted in the absence of any commercial or financial relationships that could be construed as a potential conflict of interest.
Publisher’s Note
All claims expressed in this article are solely those of the authors and do not necessarily represent those of their affiliated organizations, or those of the publisher, the editors and the reviewers. Any product that may be evaluated in this article, or claim that may be made by its manufacturer, is not guaranteed or endorsed by the publisher.
Abbreviations
NSCLC, Non-small cell lung cancer; BMs, Brain metastases; EGFR, Epidermal growth factor receptor; ALK, Anaplastic lymphoma kinase; PD-1, Programmed cell death-1; PD-L1, Programmed cell death ligand-1; CTLA-4, Cytotoxic T-lymphocyte antigen-4; ICI, Immune checkpoint inhibitor; mAb, Monoclonal antibody; RT, Radiotherapy; SRT, Stereotactic radiotherapy; WBRT, Whole-brain radiotherapy; HFRT, Hypofraction radiotherapy; CT, Chemotherapy; Computed tomography; AAT, Anti-angiogenic therapy; BBB, Blood-brain barrier; CSF, Cerebrospinal fluid; TME, Tumor microenvironment; TILs, Tumor-infiltrating lymphocytes; TAMs, Tumor-associated macrophages; Tregs, Regulatory T cells; MDSCs, Myeloid-derived suppressor cells; VEGF, Vascular endothelial growth factor; VEGFR, Vascular endothelial growth factor receptor; VCAM-1, Vascular cell adhesion molecule-1; ICAM-1, Intercellular adhesion molecule 1; INF, Interferon; CNS, Central never system; HR, Hazard ratio; CI, Confidence interval; CR, Complete response; PR, Partial response; ORR, Objective response rate; OS, Overall survival; PFS, Progression free survival; iPFS, Intracranial progression-free survival; iTTD, Intracranial time to development; AEs, Adverse events; trAEs, Treatment-related adverse events; QALY, Quality-adjusted life-year; FDA, Food and Drug Administration; lung-GPA, Lung-graded prognostic assessment; Ct-DNA, Circulating tumor DNA; CTCs,irculating tumor cells; RECIST, Response Evaluation Criteria in Solid Tumors; RANO, Response Assessment in Neuro-oncology; RANO-HGG, RANO for High Grade Gliomas; RANO-BM, RANO for Brain Metastases; iRANO, immunotherapy RANO; mRECIST, modified RECIST; PET, Positron emission tomography; MR, Magnetic resonance.
Glossary
References
1. Lah TT, Novak M, Breznik B. Brain Malignancies: Glioblastoma and Brain Metastases. Semin Cancer Biol (2020) 60:262–73. doi: 10.1016/j.semcancer.2019.10.010
2. Waqar SN, Samson PP, Robinson CG, Bradley J, Devarakonda S, Du L, et al. Non-Small-Cell Lung Cancer With Brain Metastasis at Presentation. Clin Lung Cancer (2018) 19(4):e373–e9. doi: 10.1016/j.cllc.2018.01.007
3. Poon MTC, Brennan PM, Jin K, Sudlow CLM, Figueroa JD. Might Changes in Diagnostic Practice Explain Increasing Incidence of Brain and Central Nervous System Tumors? A Population-Based Study in Wales (United Kingdom) and the United States. Neuro-oncology (2021) 23(6):979–89. doi: 10.1093/neuonc/noaa282
4. Cagney DN, Martin AM, Catalano PJ, Redig AJ, Lin NU, Lee EQ, et al. Incidence and Prognosis of Patients With Brain Metastases at Diagnosis of Systemic Malignancy: A Population-Based Study. Neuro-Oncology (2017) 19(11):1511–21. doi: 10.1093/neuonc/nox077
5. O'Kane GM, Leighl NB. Systemic Therapy of Lung Cancer CNS Metastases Using Molecularly Targeted Agents and Immune Checkpoint Inhibitors. CNS Drugs (2018) 32(6):527–42. doi: 10.1007/s40263-018-0526-4
6. Reungwetwattana T, Nakagawa K, Cho BC, Cobo M, Cho EK, Bertolini A, et al. CNS Response to Osimertinib Versus Standard Epidermal Growth Factor Receptor Tyrosine Kinase Inhibitors in Patients With Untreated EGFR-Mutated Advanced Non-Small-Cell Lung Cancer. J Clin Oncol Off J Am Soc Clin Oncol (2018) 36(33):3290–7. doi: 10.1200/JCO.2018.78.3118
7. Barlesi F, Tomasini P. Non-Small-Cell Lung Cancer Brain Metastases and PD-(L)1 Immune Checkpoint Inhibitors. Lancet Oncol (2020) 21(5):607–8. doi: 10.1016/S1470-2045(20)30207-2
8. Page S, Milner-Watts C, Perna M, Janzic U, Vidal N, Kaudeer N, et al. Systemic Treatment of Brain Metastases in Non-Small Cell Lung Cancer. Eur J Cancer (2020) 132:187–98. doi: 10.1016/j.ejca.2020.03.006
9. Remon J, Hendriks LEL, Besse B. Paving the Way for Long-Term Survival in Non-Small-Cell Lung Cancer. J Clin Oncol Off J Am Soc Clin Oncol (2021) 39(21):2321–3. doi: 10.1200/JCO.21.00760
10. McCoach CE, Berge EM, Lu X, Barón AE, Camidge DR. A Brief Report of the Status of Central Nervous System Metastasis Enrollment Criteria for Advanced Non-Small Cell Lung Cancer Clinical Trials: A Review of the ClinicalTrials.gov Trial Registry. J Thorac Oncol Off Publ Int Assoc Study Lung Cancer (2016) 11(3):407–13. doi: 10.1016/j.jtho.2015.10.024
11. Tozuka T, Kitazono S, Sakamoto H, Yoshida H, Amino Y, Uematsu S, et al. Poor Efficacy of Anti-Programmed Cell Death-1/Ligand 1 Monotherapy for Non-Small Cell Lung Cancer Patients With Active Brain Metastases. Thorac Cancer (2020) 11(9):2465–72. doi: 10.1111/1759-7714.13557
12. Pardridge WM. Drug Transport Across the Blood-Brain Barrier. J Cereb Blood Flow Metab Off J Int Soc Cereb Blood Flow Metab (2012) 32(11):1959–72. doi: 10.1038/jcbfm.2012.126
13. Kudo Y, Haymaker C, Zhang J, Reuben A, Duose DY, Fujimoto J, et al. Suppressed Immune Microenvironment and Repertoire in Brain Metastases From Patients With Resected Non-Small-Cell Lung Cancer. Ann Oncol Off J Eur Soc Med Oncol (2019) 30(9):1521–30. doi: 10.1093/annonc/mdz207
14. AK C, Laura M, Niamh L, Hira R, Edouard A, Andy N, et al. Impact of Baseline Steroids on Efficacy of Programmed Cell Death-1 and Programmed Death-Ligand 1 Blockade in Patients With Non-Small-Cell Lung Cancer. J Clin Oncol Off J Am Soc Clin Oncol (2018) 36(28):2872–8. doi: 10.1200/JCO.2018.79.0006.
15. Engelhardt B, Vajkoczy P, Weller RO. The Movers and Shapers in Immune Privilege of the CNS. Nat Immunol (2017) 18(2):123–31. doi: 10.1038/ni.3666
16. Chen Y, Liu L. Modern Methods for Delivery of Drugs Across the Blood-Brain Barrier. Advanced Drug Deliv Rev (2012) 64(7):640–65. doi: 10.1016/j.addr.2011.11.010
17. Fidler IJ, Yano S, Zhang RD, Fujimaki T, Bucana CD. The Seed and Soil Hypothesis: Vascularisation and Brain Metastases. Lancet Oncol (2002) 3(1):53–7. doi: 10.1016/S1470-2045(01)00622-2
18. Weiss N, Miller F, Cazaubon S, Couraud PO. The Blood-Brain Barrier in Brain Homeostasis and Neurological Diseases. Biochim Biophys Acta (2009) 1788(4):842–57. doi: 10.1016/j.bbamem.2008.10.022
19. Pluim D, Ros W, van Bussel MTJ, Brandsma D, Beijnen JH, Schellens JHM. Enzyme Linked Immunosorbent Assay for the Quantification of Nivolumab and Pembrolizumab in Human Serum and Cerebrospinal Fluid. J Pharm Biomed Anal (2019) 164:128–34. doi: 10.1016/j.jpba.2018.10.025
20. Stemmler HJ, Schmitt M, Willems A, Bernhard H, Harbeck N, Heinemann V. Ratio of Trastuzumab Levels in Serum and Cerebrospinal Fluid is Altered in HER2-Positive Breast Cancer Patients With Brain Metastases and Impairment of Blood-Brain Barrier. Anti-cancer Drugs (2007) 18(1):23–8. doi: 10.1097/01.cad.0000236313.50833.ee
21. Sadeghi Rad H, Monkman J, Warkiani ME, Ladwa R, O'Byrne K, Rezaei N, et al. Understanding the Tumor Microenvironment for Effective Immunotherapy. Med Res Rev (2021) 41(3):1474–98. doi: 10.1002/med.21765
22. Luo L, Liu P, Zhao K, Zhao W and Zhang X. The Immune Microenvironment in Brain Metastases of Non-Small Cell Lung Cancer. Front Oncol (2021) 11:698844. doi: 10.3389/fonc.2021.698844.
23. Cserr HF, Harling-Berg CJ, Knopf PM. Drainage of Brain Extracellular Fluid Into Blood and Deep Cervical Lymph and Its Immunological Significance. Brain Pathol (Zurich Switzerland) (1992) 2(4):269–76. doi: 10.1111/j.1750-3639.1992.tb00703.x
24. Louveau A, Smirnov I, Keyes TJ, Eccles JD, Rouhani SJ, Peske JD, et al. Structural and Functional Features of Central Nervous System Lymphatic Vessels. Nature (2015) 523(7560):337–41. doi: 10.1038/nature14432
25. Lohr J, Ratliff T, Huppertz A, Ge Y, Dictus C, Ahmadi R, et al. Effector T-Cell Infiltration Positively Impacts Survival of Glioblastoma Patients and is Impaired by Tumor-Derived TGF-β. Clin Cancer Res Off J Am Assoc Cancer Res (2011) 17(13):4296–308. doi: 10.1158/1078-0432.CCR-10-2557
26. Fakhoury KR, Ney DE, Ormond DR, Rusthoven CG. Immunotherapy and Radiation for High-Grade Glioma: A Narrative Review. Transl Cancer Res (2021) 10(5):2537–70. doi: 10.21037/tcr-20-1933
27. Klemm F, Maas RR, Bowman RL, Kornete M, Soukup K, Nassiri S, et al. Interrogation of the Microenvironmental Landscape in Brain Tumors Reveals Disease-Specific Alterations of Immune Cells. Cell (2020) 181(7):1643–60.e17. doi: 10.1016/j.cell.2020.05.007
28. Berghoff AS, Fuchs E, Ricken G, Mlecnik B, Bindea G, Spanberger T, et al. Density of Tumor-Infiltrating Lymphocytes Correlates With Extent of Brain Edema and Overall Survival Time in Patients With Brain Metastases. Oncoimmunology (2016) 5(1):e1057388. doi: 10.1080/2162402X.2015.1057388
29. Berghoff AS, Inan C, Ricken G, Widhalm G, Dieckmann K, Birner P, et al. 1324p - Tumor-Infiltrating Lymphocytes (Tils) and Pd-L1 Expression in Non- Small Cell Lung Cancer Brain Metastases (Bm) and Matched Primary Tumors (Pt). Ann Oncol (2014) 25:iv465. doi: 10.1093/annonc/mdu349.103
30. Mansfield AS, Aubry MC, Moser JC, Harrington SM, Dronca RS, Park SS, et al. Temporal and Spatial Discordance of Programmed Cell Death-Ligand 1 Expression and Lymphocyte Tumor Infiltration Between Paired Primary Lesions and Brain Metastases in Lung Cancer. Ann Oncol Off J Eur Soc Med Oncol (2016) 27(10):1953–8. doi: 10.1093/annonc/mdw289
31. Camy F, Karpathiou G, Dumollard JM, Magne N, Perrot JL, Vassal F, et al. Brain Metastasis PD-L1 and CD8 Expression is Dependent on Primary Tumor Type and Its PD-L1 and CD8 Status. J Immunother Cancer (2020) 8(2):e000597. doi: 10.1136/jitc-2020-000597
32. Goldberg SB, Schalper KA, Gettinger SN, Mahajan A, Herbst RS, Chiang AC, et al. Pembrolizumab for Management of Patients With NSCLC and Brain Metastases: Long-Term Results and Biomarker Analysis From a Non-Randomised, Open-Label, Phase 2 Trial. Lancet Oncol (2020) 21(5):655–63. doi: 10.1016/S1470-2045(20)30111-X
33. Friebel E, Kapolou K, Unger S, Núñez NG, Utz S, Rushing EJ, et al. Single-Cell Mapping of Human Brain Cancer Reveals Tumor-Specific Instruction of Tissue-Invading Leukocytes. Cell (2020) 181(7):1626–42.e20. doi: 10.1016/j.cell.2020.04.055
34. Peng W, Liu C, Xu C, Lou Y, Chen J, Yang Y, et al. PD-1 Blockade Enhances T-Cell Migration to Tumors by Elevating IFN-γ Inducible Chemokines. Cancer Res (2012) 72(20):5209–18. doi: 10.1158/0008-5472.CAN-12-1187
35. Bonney S, Seitz S, Ryan CA, Jones KL, Clarke P, Tyler KL, et al. Gamma Interferon Alters Junctional Integrity via Rho Kinase, Resulting in Blood-Brain Barrier Leakage in Experimental Viral Encephalitis. mBio (2019) 10(4):e01675–19. doi: 10.1128/mBio.01675-19
36. Sonar SA, Shaikh S, Joshi N, Atre AN, Lal G. IFN-γ Promotes Transendothelial Migration of CD4(+) T Cells Across the Blood-Brain Barrier. Immunol Cell Biol (2017) 95(9):843–53. doi: 10.1038/icb.2017.56
37. Iwasaki A. Immune Regulation of Antibody Access to Neuronal Tissues. Trends Mol Med (2017) 23(3):227–45.doi: 10.1016/j.molmed.2017.01.004
38. Sun L, Davis CW, Hwang WT, Jeffries S, Sulyok LF, Marmarelis ME, et al. Outcomes in Patients With Non-Small-Cell Lung Cancer With Brain Metastases Treated With Pembrolizumab-Based Therapy. Clin Lung Cancer (2021) 22(1):58–66 e3. doi: 10.1016/j.cllc.2020.10.017
39. Nikolaj F, Jens K, Daniel M, Claudia V, Christian G, Burkhard M, et al. Pembrolizumab as First-Line Palliative Therapy in PD-L1 Overexpressing (≥ 50%) NSCLC: Real-World Results With Special Focus on PS ≥ 2, Brain Metastases, and Steroids. Clin Lung Cancer (2021) 22(5):411–22. doi: 10.1016/j.cllc.2021.02.001.
40. Kazushige W, Michitoshi Y, Hiroaki K, Naoya N, Taichi M, Eriko M, et al. Efficacy of Pembrolizumab in Patients With Brain Metastasis Caused by Previously Untreated Non-Small Cell Lung Cancer With High Tumor PD-L1 Expression. Lung Cancer (Amsterdam Netherlands) (2020) 151:60–8. doi: 10.1016/j.lungcan.2020.11.009.
41. Zhang G, Cheng R, Wang H, Zhang Y, Yan X, Li P, et al. Comparable Outcomes of Nivolumab in Patients With Advanced NSCLC Presenting With or Without Brain Metastases: A Retrospective Cohort Study. Cancer Immunol Immunother (2020) 69(25):399–405. doi: 10.1007/s00262-019-02462-1
42. Teixeira Loiola de Alencar V, Guedes Camandaroba MP, Pirolli R, Fogassa CAZ, Cordeiro de Lima VC. Immunotherapy as Single Treatment for Patients With NSCLC With Brain Metastases: A Systematic Review and Meta-Analysis-The META-L-BRAIN Study. J Thorac Oncol Off Publ Int Assoc Study Lung Cancer (2021) 16(8):1379–91. doi: 10.1016/j.jtho.2021.04.014
43. Jiamin S, Hui L, Xiaoqing Y, Sizhe Y, Kaiyan C, Guoqiang P, et al. Efficacy of PD-1/PD-L1 Inhibitors in Patients With Non-Small Cell Lung Cancer and Brain Metastases: A Real-World Retrospective Study in China. Thorac Cancer (2021) 12(22):3019–31. doi: 10.1111/1759-7714.14171.
44. Mansfield AS, Herbst RS, de Castro G Jr., Hui R, Peled N, Kim DW, et al. Outcomes With Pembrolizumab Monotherapy in Patients With Programmed Death-Ligand 1-Positive NSCLC With Brain Metastases: Pooled Analysis of KEYNOTE-001, 010, 024, and 042. JTO Clin Res Rep (2021) 2(8):100205. doi: 10.1016/j.jtocrr.2021.100205
45. Spigel DR, Chaft JE, Gettinger S, Chao BH, Dirix L, Schmid P, et al. FIR: Efficacy, Safety, and Biomarker Analysis of a Phase II Open-Label Study of Atezolizumab in PD-L1-Selected Patients With NSCLC. J Thorac Oncol Off Publ Int Assoc Study Lung Cancer (2018) 13(11):1733–42. doi: 10.1016/j.jtho.2018.05.004
46. Borghaei H, Gettinger S, Vokes EE, Chow LQM, Burgio MA, Carpeno J, et al. Five-Year Outcomes From the Randomized, Phase III Trials CheckMate 017 and 057: Nivolumab Versus Docetaxel in Previously Treated Non–Small-Cell Lung Cancer. J Clin Oncol (2021) 39(7):723–33. doi: 10.1200/JCO.20.01605
47. Assié JB, Corre R, Levra MG, Calvet CY, Gaudin AF, Grumberg V, et al. Nivolumab Treatment in Advanced Non-Small Cell Lung Cancer: Real-World Long-Term Outcomes Within Overall and Special Populations (the UNIVOC Study). Ther Adv Med Oncol (2020) 12:1758835920967237. doi: 10.1177/1758835920967237
48. Debieuvre D, Juergens RA, Asselain B, Audigier-Valette C, Auliac J-B, Barlesi F, et al. Two-Year Survival With Nivolumab in Previously Treated Advanced Non–Small-Cell Lung Cancer: A Real-World Pooled Analysis of Patients From France, Germany, and Canada. Lung Cancer (Amsterdam Netherlands) (2021) 157:40–7. doi: 10.1016/j.lungcan.2021.04.022
49. Grossi F, Genova C, Crinò L, Delmonte A, Turci D, Signorelli D, et al. Real-Life Results From the Overall Population and Key Subgroups Within the Italian Cohort of Nivolumab Expanded Access Program in Non-Squamous Non-Small Cell Lung Cancer. Eur J Cancer (Oxford Engl 1990) (2019) 123:72–80. doi: 10.1016/j.ejca.2019.09.011
50. Crino L, Bronte G, Bidoli P, Cravero P, Minenza E, Cortesi E, et al. Nivolumab and Brain Metastases in Patients With Advanced Non-Squamous Non-Small Cell Lung Cancer. Lung Cancer (Amsterdam Netherlands) (2019) 129:35–40. doi: 10.1016/j.lungcan.2018.12.025
51. Cortinovis D, Chiari R, Catino A, Grossi F, DEM F, Sperandi F, et al. Italian Cohort of the Nivolumab EAP in Squamous NSCLC: Efficacy and Safety in Patients With CNS Metastases. Anticancer Res (2019) 39(8):4265–71. doi: 10.21873/anticanres.13590
52. Gadgeel SM, Lukas RV, Goldschmidt J, Conkling P, Park K, Cortinovis D, et al. Atezolizumab in Patients With Advanced Non-Small Cell Lung Cancer and History of Asymptomatic, Treated Brain Metastases: Exploratory Analyses of the Phase III OAK Study. Lung Cancer (Amsterdam Netherlands) (2019) 128:105–12. doi: 10.1016/j.lungcan.2018.12.017
53. Sezer A, Kilickap S, Gümüş M, Bondarenko I, Özgüroğlu M, Gogishvili M, et al. Cemiplimab Monotherapy for First-Line Treatment of Advanced Non-Small-Cell Lung Cancer With PD-L1 of at Least 50%: A Multicentre, Open-Label, Global, Phase 3, Randomised, Controlled Trial. Lancet (2021) 397(10274):592–604. doi: 10.1016/s0140-6736(21)00228-2
54. Shinkichi T, Takefumi K, Emily P. Survival Benefit From Immunocheckpoint Inhibitors in Stage IV Non-Small Cell Lung Cancer Patients With Brain Metastases: A National Cancer Database Propensity-Matched Analysis. Cancer Med (2020) 10(3):923–32. doi: 10.1002/cam4.3675.
55. Antonia SJ, Villegas A, Daniel D, Vicente D, Murakami S, Hui R, et al. Overall Survival With Durvalumab After Chemoradiotherapy in Stage III NSCLC. N Engl J Med (2018) 379(24):2342–50. doi: 10.1056/NEJMoa1809697
56. Barlesi F, Dixmier A, Debieuvre D, Raspaud C, Auliac JB, Benoit N, et al. Effectiveness and Safety of Nivolumab in the Treatment of Lung Cancer Patients in France: Preliminary Results From the Real-World EVIDENS Study. Oncoimmunology (2020) 9(1):1744898. doi: 10.1080/2162402X.2020.1744898
57. Garon EB, Hellmann MD, Rizvi NA, Carcereny E, Leighl NB, Ahn MJ, et al. Five-Year Overall Survival for Patients With Advanced Non‒Small-Cell Lung Cancer Treated With Pembrolizumab: Results From the Phase I KEYNOTE-001 Study. J Clin Oncol Off J Am Soc Clin Oncol (2019) 37(28):2518–27. doi: 10.1200/JCO.19.00934
58. Bernstein MB, Krishnan S, Hodge JW, Chang JY. Immunotherapy and Stereotactic Ablative Radiotherapy (ISABR): A Curative Approach? Nat Rev Clin Oncol (2016) 13(8):516–24. doi: 10.1038/nrclinonc.2016.30
59. Wang Y, Liu ZG, Yuan H, Deng W, Li J, Huang Y, et al. The Reciprocity Between Radiotherapy and Cancer Immunotherapy. Clin Cancer Res Off J Am Assoc Cancer Res (2019) 25(6):1709–17. doi: 10.1158/1078-0432.CCR-18-2581
60. Weichselbaum RR, Liang H, Deng L, Fu YX. Radiotherapy and Immunotherapy: A Beneficial Liaison? Nat Rev Clin Oncol (2017) 14(6):365–79. doi: 10.1038/nrclinonc.2016.211
61. Masucci GL, Boucher O, Marie F, Plourde ME, Panet-Raymond V, Pavic M, et al. Prospective Neurocognitive Functions of Patients Treated With Concurrent Nivolumab and Stereotactic Brain Radiosurgery for NSCLC and RCC Brain Metastases. Int J Radiat Oncol Biol Phys (2021) 111(3S):e574. doi: 10.1016/j.ijrobp.2021.07.1547
62. Patruni S, Khattab A, Abel S, Hasan S, Rizwan S, Finley GG, et al. A Comparative Analysis of Survival in Patients With Non-Small Cell Lung Cancer With Brain Metastases Receiving Intracranial Radiation With and Without Immunotherapy. J Clin Oncol (2019) 37(15):2. doi: 10.1200/JCO.2019.37.15_suppl.9025
63. Enright TL, Witt JS, Burr AR, Yadav P, Leal T, Baschnagel AM. Combined Immunotherapy and Stereotactic Radiotherapy Improves Neurologic Outcomes in Patients With Non-Small-Cell Lung Cancer Brain Metastases. Clin Lung Cancer (2021) 22(2):110–9. doi: 10.1016/j.cllc.2020.10.014
64. Silvia S, Emanuela O, Valentina P, Falchetto OM, Rossella DF, Saverio C, et al. Immunotherapy in Association With Stereotactic Radiotherapy for Non-Small Cell Lung Cancer Brain Metastases: Results From a Multicentric Retrospective Study on Behalf of AIRO. Neuro-Oncology (2021) 23(10):1750–64. doi: 10.1093/neuonc/noab129.
65. Shepard M, Xu ZY, Donahue J, Muttikkal TE, Codeiro D, Hansen L, et al. Stereotactic Radiosurgery With and Without Checkpoint Inhibition for Patients With Metastatic Non-Small Cell Lung Cancer to the Brain: A Matched Cohort Study. Neurosurg Off J Congress Neurol Surgeons (2019) 66:68–9. doi: 10.1093/neuros/nyz310_217
66. Metro G, Gili A, Signorelli D, De Toma A, Garaffa M, Galetta D, et al. Upfront Pembrolizumab as an Effective Treatment Start in Patients With PD-L1 >/= 50% non-Oncogene Addicted non-Small Cell Lung Cancer and Asymptomatic Brain Metastases: An Exploratory Analysis. Clin Trans Oncol (2021) 23(9):1818–26. doi: 10.1007/s12094-021-02588-8
67. Martin AM, Cagney DN, Catalano PJ, Alexander BM, Redig AJ, Schoenfeld JD, et al. Immunotherapy and Symptomatic Radiation Necrosis in Patients With Brain Metastases Treated With Stereotactic Radiation. JAMA Oncol (2018) 4(8):1123–4. doi: 10.1001/jamaoncol.2017.3993
68. Young KH, Baird JR, Savage T, Cottam B, Friedman D, Bambina S, et al. Optimizing Timing of Immunotherapy Improves Control of Tumors by Hypofractionated Radiation Therapy. PloS One (2016) 11(6):e0157164. doi: 10.1371/journal.pone.0157164
69. Qian JM, Martin AM, Martin K, Hammoudeh L, Catalano PJ, Hodi FS, et al. Response Rate and Local Recurrence After Concurrent Immune Checkpoint Therapy and Radiotherapy for non-Small Cell Lung Cancer and Melanoma Brain Metastases. Cancer (2020) 126(24):5274–82. doi: 10.1002/cncr.33196.
70. Yin Y, Lei D, Yufan Y, Tao Z, Yuqi W, Luhua W, et al. Efficacy and Safety of Combined Brain Radiotherapy and Immunotherapy in Non-Small-Cell Lung Cancer With Brain Metastases: A Systematic Review and Meta-Analysis. Clin Lung Cancer (2021) 23(2):95–107. doi: 10.1016/j.cllc.2021.06.009.
71. Filatenkov A, Baker J, Mueller AM, Kenkel J, Ahn GO, Dutt S, et al. Ablative Tumor Radiation Can Change the Tumor Immune Cell Microenvironment to Induce Durable Complete Remissions. Clin Cancer Res Off J Am Assoc Cancer Res (2015) 21(16):3727–39. doi: 10.1158/1078-0432.CCR-14-2824
72. Lee Y, Auh SL, Wang Y, Burnette B, Wang Y, Meng Y, et al. Therapeutic Effects of Ablative Radiation on Local Tumor Require CD8+ T Cells: Changing Strategies for Cancer Treatment. Blood (2009) 114(3):589–95. doi: 10.1182/blood-2009-02-206870
73. Chen D, Patel RR, Verma V, Ramapriyan R, Barsoumian HB, Cortez MA, et al. Interaction Between Lymphopenia, Radiotherapy Technique, Dosimetry, and Survival Outcomes in Lung Cancer Patients Receiving Combined Immunotherapy and Radiotherapy. Radiother Oncol (2020) 150:114–20. doi: 10.1016/j.radonc.2020.05.051
74. Leonetti A, Wever B, Mazzaschi G, Assaraf YG, Rolfo C, Quaini F, et al. Molecular Basis and Rationale for Combining Immune Checkpoint Inhibitors With Chemotherapy in non-Small Cell Lung Cancer. Drug Resist Update (2019) 46:12. doi: 10.1016/j.drup.2019.100644
75. Zitvogel L, Apetoh L, Ghiringhelli F, Kroemer G. Immunological Aspects of Cancer Chemotherapy. Nat Rev Immunol (2008) 8(1):59–73. doi: 10.1038/nri2216
76. Shirish G, Delvys R-A, Giovanna S, Emilio E, Enriqueta F, Manuel D, et al. Updated Analysis From KEYNOTE-189: Pembrolizumab or Placebo Plus Pemetrexed and Platinum for Previously Untreated Metastatic Nonsquamous Non-Small-Cell Lung Cancer. J Clin Oncol Off J Am Soc Clin Oncol (2020) 38(14):1505–17. doi: 10.1200/JCO.19.03136
77. Powell SF, Rodriguez-Abreu D, Langer CJ, Tafreshi A, Paz-Ares L, Kopp HG, et al. Outcomes With Pembrolizumab Plus Platinum-Based Chemotherapy for Patients With Non-Small-Cell Lung Cancer and Stable Brain Metastases: Pooled Analysis of KEYNOTE-021, 189, and 407. J Thorac Oncol Off Publ Int Assoc Study Lung Cancer (2021) 16(11):1883–92. doi: 10.1016/j.jtho.2021.06.020
78. Zhou C, Chen G, Huang Y, Zhou J, Lin L, Feng J, et al. Camrelizumab Plus Carboplatin and Pemetrexed Versus Chemotherapy Alone in Chemotherapy-Naive Patients With Advanced non-Squamous non-Small-Cell Lung Cancer (CameL): A Randomised, Open-Label, Multicentre, Phase 3 Trial. Lancet Respir Med (2021) 9(3):305–14. doi: 10.1016/S2213-2600(20)30365-9
79. Yang Y, Wang Z, Fang J, Yu Q, Han B, Cang S, et al. Efficacy and Safety of Sintilimab Plus Pemetrexed and Platinum as First-Line Treatment for Locally Advanced or Metastatic Nonsquamous NSCLC: A Randomized, Double-Blind, Phase 3 Study (Oncology Program by InnovENT Anti-PD-1-11). J Thorac Oncol Off Publ Int Assoc Study Lung Cancer (2020) 15(10):1636–46. doi: 10.1016/j.jtho.2020.07.014
80. Yang Y, Sun J, Wang Z, Fang J, Yu Q, Han B, et al. Updated Overall Survival Data and Predictive Biomarkers of Sintilimab Plus Pemetrexed and Platinum as First-Line Treatment for Locally Advanced or Metastatic Nonsquamous NSCLC in the Phase 3 ORIENT-11 Study. J Thorac Oncol (2021) 16(12):2109–20. doi: 10.1016/j.jtho.2021.07.015
81. Jiang T, Wang P, Zhang J, Zhao Y, Zhou J, Fan Y, et al. Toripalimab Plus Chemotherapy as Second-Line Treatment in Previously EGFR-TKI Treated Patients With EGFR-Mutant-Advanced NSCLC: A Multicenter Phase-II Trial. Signal Transduction Targeted Ther (2021) 6(1):355. doi: 10.1038/s41392-021-00751-9
82. Nadal E, Massuti B, Huidobro G, Castro RL, Estival A, Mosquera J, et al. OA09.02 Atezo-Brain: Single Arm Phase II Study of Atezolizumab Plus Chemotherapy in Stage IV NSCLC With Untreated Brain Metastases. J Thorac Oncol (2021) 16(10):S863. doi: 10.1016/j.jtho.2021.08.062
83. Nogami N, Barlesi F, Socinski MA, Reck M, Thomas CA, Cappuzzo F, et al. IMpower150 Final Exploratory Analyses for Atezolizumab Plus Bevacizumab and Chemotherapy in Key NSCLC Patient Subgroups With EGFR Mutations or Metastases in the Liver or Brain. J Thorac Oncol Off Publ Int Assoc Study Lung Cancer (2021) 17(2):309–23. doi: 10.1016/j.jtho.2021.09.014.
84. Chu T, Zhong R, Zhong H, Zhang B, Zhang W, Shi C, et al. Phase 1b Study of Sintilimab Plus Anlotinib as First-Line Therapy in Patients With Advanced NSCLC. J Thorac Oncol Off Publ Int Assoc Study Lung Cancer (2021) 16(4):643–52. doi: 10.1016/j.jtho.2020.11.026
85. Borghaei H, Pluzanski A, Caro RB, Provencio M, Burgers S, Carcereny E, et al. Abstract CT221: Nivolumab (NIVO) + Ipilimumab (IPI) as First-Line (1L) Treatment for Patients With Advanced non-Small Cell Lung Cancer (NSCLC) With Brain Metastases: Results From CheckMate 227. Cancer Res (2020) 80:CT221–CT. doi: 10.1158/1538-7445.AM2020-CT221
86. Reck M, Ciuleanu TE, Cobo M, Schenker M, Zurawski B, Menezes J, et al. First-Line Nivolumab Plus Ipilimumab With Two Cycles of Chemotherapy Versus Chemotherapy Alone (Four Cycles) in Advanced non-Small-Cell Lung Cancer: CheckMate 9LA 2-Year Update. ESMO Open (2021) 6(5):100273. doi: 10.1016/j.esmoop.2021.100273
87. Kwon M, Jung H, Nam GH, Kim IS. The Right Timing, Right Combination, Right Sequence, and Right Delivery for Cancer Immunotherapy. J Controlled Release Off J Controlled Release Soc (2021) 331:321–34. doi: 10.1016/j.jconrel.2021.01.009
88. Zhu C, Shi Y, Li Q, Luo L, Li X, Luo Z, et al. Rational Administration Sequencing of Immunochemotherapy Elicits Powerful Anti-Tumor Effect. J Controlled Release Off J Controlled Release Soc (2022) 341:769–81. doi: 10.1016/j.jconrel.2021.12.022
89. Huang Y, Yuan J, Righi E, Kamoun WS, Ancukiewicz M, Nezivar J, et al. Vascular Normalizing Doses of Antiangiogenic Treatment Reprogram the Immunosuppressive Tumor Microenvironment and Enhance Immunotherapy. Proc Natl Acad Sci USA (2012) 109(43):17561–6. doi: 10.1073/pnas.1215397109
90. Sandler A, Hirsh V, Reck M, von Pawel J, Akerley W, Johnson DH. An Evidence-Based Review of the Incidence of CNS Bleeding With Anti-VEGF Therapy in non-Small Cell Lung Cancer Patients With Brain Metastases. Lung Cancer (Amsterdam Netherlands) (2012) 78(1):1–7. doi: 10.1016/j.lungcan.2012.07.004
91. Wan X, Luo X, Tan C, Zeng X, Zhang Y, Peng L. First-Line Atezolizumab in Addition to Bevacizumab Plus Chemotherapy for Metastatic, Nonsquamous non-Small Cell Lung Cancer: A United States-Based Cost-Effectiveness Analysis. Cancer (2019) 125(20):3526–34. doi: 10.1002/cncr.32368
92. Vellanki PJ, Mulkey F, Jaigirdar AA, Rodriguez L, Wang Y, Xu Y, et al. FDA Approval Summary: Nivolumab With Ipilimumab and Chemotherapy for Metastatic Non-Small Cell Lung Cancer, A Collaborative Project Orbis Review. Clin Cancer Res Off J Am Assoc Cancer Res (2021) 27(13):3522–7. doi: 10.1158/1078-0432.CCR-20-4338
93. Robert L, Tsoi J, Wang X, Emerson R, Homet B, Chodon T, et al. CTLA4 Blockade Broadens the Peripheral T-Cell Receptor Repertoire. Clin Cancer Res Off J Am Assoc Cancer Res (2014) 20(9):2424–32. doi: 10.1158/1078-0432.CCR-13-2648
94. Wei SC, Levine JH, Cogdill AP, Zhao Y, Anang NAS, Andrews MC, et al. Distinct Cellular Mechanisms Underlie Anti-CTLA-4 and Anti-PD-1 Checkpoint Blockade. Cell (2017) 170(6):1120–33.e17. doi: 10.1016/j.cell.2017.07.024
95. Bestvina CM, Pointer KB, Karrison T, Al-Hallaq H, Hoffman PC, Jelinek MJ, et al. A Phase I Trial of Concurrent Or Sequential Ipilimumab, Nivolumab, and Stereotactic Body Radiotherapy in Patients With Stage IV Non-Small Cell Lung Cancer (COSINR Study). Int J Radiat Oncol Biol Phys (2020) 108(3):S72. doi: 10.1016/j.ijrobp.2020.07.2214.
96. SP W, Shane M, Jing L, Daniel C, Ayal A, LN U, et al. Survival in Patients With Brain Metastases: Summary Report on the Updated Diagnosis-Specific Graded Prognostic Assessment and Definition of the Eligibility Quotient. J Clin Oncol (2020) 38(22):3773–84. doi: 10.1200/JCO.20.01255.
97. Konstantinos R, Marcus S, Dimitrios M, Luigi DP, Sofia A, Simon E, et al. Correlation of Clinical Parameters With Intracranial Outcome in Non-Small Cell Lung Cancer Patients With Brain Metastases Treated With Pd-1/Pd-L1 Inhibitors as Monotherapy. Cancers (2021) 13(7):1562. doi: 10.3390/cancers13071562
98. Jessurun CAC, Hulsbergen AFC, de Wit AE, Tewarie IA, Snijders TJ, Verhoeff JJC, et al. The Combined Use of Steroids and Immune Checkpoint Inhibitors in Brain Metastasis Patients: A Systematic Review and Meta-Analysis. Neuro-oncology (2021) 23(8):1261–72. doi: 10.1093/neuonc/noab046
99. Huang Q, Zhang H, Hai J, Socinski MA, Lim E, Chen H, et al. Impact of PD-L1 Expression, Driver Mutations and Clinical Characteristics on Survival After Anti-PD-1/PD-L1 Immunotherapy Versus Chemotherapy in non-Small-Cell Lung Cancer: A Meta-Analysis of Randomized Trials. Oncoimmunology (2018) 7(12):e1396403. doi: 10.1080/2162402X.2017.1396403
100. Hulsbergen AFC, Mammi M, Nagtegaal SHJ, Lak AM, Kavouridis V, Smith TR, et al. Programmed Death Receptor Ligand One Expression May Independently Predict Survival In Non-Small Cell Lung Carcinoma Brain Metastases Patients Receiving Immunotherapy. Int J Radiat Oncol Biol Phys (2020) 108(3S):258–67. doi: 10.1016/j.ijrobp.2020.04.018.
101. Hellmann MD, Ciuleanu TE, Pluzanski A, Lee JS, Otterson GA, Audigier-Valette C, et al. Nivolumab plus Ipilimumab in Lung Cancer with a High Tumor Mutational Burden. N Engl J Med (2018) 378(22):2093–104. doi: 10.1056/NEJMoa1801946
102. Waqar SN, Govindan R. The Mystic Role of Tumor Mutational Burden in Selecting Patients With Lung Cancer for First-Line Immunotherapy. JAMA Oncol (2020) 6(5):674–5. doi: 10.1001/jamaoncol.2020.0264
103. Hellmann MD, Nathanson T, Rizvi H, Creelan BC, Sanchez-Vega F, Ahuja A, et al. Genomic Features of Response to Combination Immunotherapy in Patients With Advanced Non-Small-Cell Lung Cancer. Cancer Cell (2018) 33(5):843–52 e4. doi: 10.1016/j.ccell.2018.03.018
104. Stein MK, Pandey M, Xiu J, Tae H, Swensen J, Mittal S, et al. Tumor Mutational Burden Is Site Specific in Non-Small-Cell Lung Cancer and Is Highest in Lung Adenocarcinoma Brain Metastases. JCO Precis Oncol (2019) 3:1–13. doi: 10.1200/PO.18.00376
105. Lagos GG, Izar B, Rizvi NA. Beyond Tumor PD-L1: Emerging Genomic Biomarkers for Checkpoint Inhibitor Immunotherapy. Am Soc Clin Oncol Educ Book Am Soc Clin Oncol Annu Meeting (2020) 40:1–11. doi: 10.1200/EDBK_289967
106. Morrison C, Pabla S, Conroy JM, Nesline MK, Glenn ST, Dressman D, et al. Predicting Response to Checkpoint Inhibitors in Melanoma Beyond PD-L1 and Mutational Burden. J Immunother Cancer (2018) 6(1):32. doi: 10.1186/s40425-018-0344-8
107. Seoane J, De Mattos-Arruda L, Le Rhun E, Bardelli A, Weller M. Cerebrospinal Fluid Cell-Free Tumour DNA as a Liquid Biopsy for Primary Brain Tumours and Central Nervous System Metastases. Ann Oncol Off J Eur Soc Med Oncol (2019) 30(2):211–8. doi: 10.1093/annonc/mdy544
108. Rubio-Perez C, Planas-Rigol E, Trincado JL, Bonfill-Teixidor E, Arias A, Marchese D, et al. Immune Cell Profiling of the Cerebrospinal Fluid Enables the Characterization of the Brain Metastasis Microenvironment. Nat Commun (2021) 12(1):1503. doi: 10.1038/s41467-021-21789-x
109. Wei Zou P, Stephanie J, Yaung P, Frederike Fuhlbr¨uck M, Marcus Ballinger P, Eric Peters P, et al. ctDNA Predicts Overall Survival in Patients With NSCLC Treated With PD-L1 Blockade or With Chemotherapy. Jco Precis Oncol (2021) 5:827–38. doi: 10.1200/PO.21.00057
110. Kulasinghe A, O'Leary C, Monkman J, Bharti V, Irwin D, Dutta S, et al. The Identification of Circulating Tumour DNA Using MassARRAY Technology in non-Small-Cell Lung Cancer (NSCLC). Lung Cancer (Amsterdam Netherlands) (2021) 160:73–7. doi: 10.1016/j.lungcan.2021.08.005
111. Tamminga M, de Wit S, Hiltermann TJN, Timens W, Schuuring E, Terstappen L, et al. Circulating Tumor Cells in Advanced non-Small Cell Lung Cancer Patients are Associated With Worse Tumor Response to Checkpoint Inhibitors. J Immunother Cancer (2019) 7(1):173. doi: 10.1186/s40425-019-0649-2
112. Ruan H, Zhou Y, Shen J, Zhai Y, Xu Y, Pi L, et al. Circulating Tumor Cell Characterization of Lung Cancer Brain Metastases in the Cerebrospinal Fluid Through Single-Cell Transcriptome Analysis. Clin Trans Med (2020) 10(8):e246. doi: 10.1002/ctm2.246
113. Bera K, Braman N, Gupta A, Velcheti V, Madabhushi A. Predicting Cancer Outcomes With Radiomics and Artificial Intelligence in Radiology. Nat Rev Clin Oncol (2021) 19(2):132–46. doi: 10.1038/s41571-021-00560-7
114. Panwen T, Bingxi H, Wei M, Kunqin L, Li L, Hao Z, et al. Assessing PD-L1 Expression in non-Small Cell Lung Cancer and Predicting Responses to Immune Checkpoint Inhibitors Using Deep Learning on Computed Tomography Images. Theranostics (2021) 11(5):2098–107. doi: 10.7150/thno.48027
115. Wei M, Lei J, Yu S, Ilke T, GJ E, Evangelia K, et al. Non-Invasive Measurement of PD-L1 Status and Prediction of Immunotherapy Response Using Deep Learning of PET/CT Images. J Immunother Cancer (2021) 9(6):e002118. doi: 10.1136/jitc-2020-002118
116. Boehm KM, Khosravi P, Vanguri R, Gao J, Shah SP. Harnessing Multimodal Data Integration to Advance Precision Oncology. Nat Rev Cancer (2021) 22(2):114–26. doi: 10.1038/s41568-021-00408-3
117. Leitner BP, Givechian KB, Ospanova S, Beisenbayeva A, Politi K, Perry RJ. Multimodal Analysis Suggests Differential Immuno-Metabolic Crosstalk in Lung Squamous Cell Carcinoma and Adenocarcinoma. NPJ Precis Oncol (2022) 6(1):8. doi: 10.1038/s41698-021-00248-2
118. Rathore S, Akbari H, Rozycki M, Abdullah KG, Nasrallah MP, Binder ZA, et al. Radiomic MRI Signature Reveals Three Distinct Subtypes of Glioblastoma With Different Clinical and Molecular Characteristics, Offering Prognostic Value Beyond IDH1. Sci Rep (2018) 8(1):5087. doi: 10.1038/s41598-018-22739-2
119. Akhoundova D, Hiltbrunner S, Mader C, Förster R, Kraft J, Schwanhäusser B, et al. 18f-FET PET for Diagnosis of Pseudoprogression of Brain Metastases in Patients With Non-Small Cell Lung Cancer. Clin Nucl Med (2020) 45(2):113–7. doi: 10.1097/RLU.0000000000002890
120. Urban H, Steidl E, Hattingen E, Filipski K, Meissner M, Sebastian M, et al. Immune Checkpoint Inhibitor-Induced Cerebral Pseudoprogression: Patterns and Categorization. Front Immunol (2021) 12:798811. doi: 10.3389/fimmu.2021.798811
121. Hendriks LEL, Henon C, Auclin E, Mezquita L, Ferrara R, Audigier-Valette C, et al. Outcome of Patients With Non-Small Cell Lung Cancer and Brain Metastases Treated With Checkpoint Inhibitors. J Thorac Oncol Off Publ Int Assoc Study Lung Cancer (2019) 14(7):1244–54. doi: 10.1016/j.jtho.2019.02.009
122. Macdonald DR, Cascino TL, Schold SC Jr., Cairncross JG. Response Criteria for Phase II Studies of Supratentorial Malignant Glioma. J Clin Oncol Off J Am Soc Clin Oncol (1990) 8(7):1277–80. doi: 10.1200/JCO.1990.8.7.1277
123. Wen PY, Macdonald DR, Reardon DA, Cloughesy TF, Sorensen AG, Galanis E, et al. Updated Response Assessment Criteria for High-Grade Gliomas: Response Assessment in Neuro-Oncology Working Group. J Clin Oncol Off J Am Soc Clin Oncol (2010) 28(11):1963–72. doi: 10.1200/JCO.2009.26.3541
124. Lin NU, Lee EQ, Aoyama H, Barani IJ, Barboriak DP, Baumert BG, et al. Response Assessment Criteria for Brain Metastases: Proposal From the RANO Group. Lancet Oncol (2015) 16(6):e270–8. doi: 10.1016/S1470-2045(15)70057-4
125. Qian JM, Mahajan A, Yu JB, Tsiouris AJ, Goldberg SB, Kluger HM, et al. Comparing Available Criteria for Measuring Brain Metastasis Response to Immunotherapy. J Neurooncol (2017) 132(3):479–85. doi: 10.1007/s11060-017-2398-8
126. Wolchok JD, Hoos A, O'Day S, Weber JS, Hamid O, Lebbé C, et al. Guidelines for the Evaluation of Immune Therapy Activity in Solid Tumors: Immune-Related Response Criteria. Clin Cancer Res Off J Am Assoc Cancer Res (2009) 15(23):7412–20. doi: 10.1158/1078-0432.CCR-09-1624
127. Okada H, Weller M, Huang R, Finocchiaro G, Gilbert MR, Wick W, et al. Immunotherapy Response Assessment in Neuro-Oncology: A Report of the RANO Working Group. Lancet Oncol (2015) 16(15):e534–e42. doi: 10.1016/S1470-2045(15)00088-1
128. Chuang MT, Liu YS, Tsai YS, Chen YC, Wang CK. Differentiating Radiation-Induced Necrosis From Recurrent Brain Tumor Using MR Perfusion and Spectroscopy: A Meta-Analysis. PloS One (2016) 11(1):e0141438. doi: 10.1371/journal.pone.0141438
129. Langen KJ, Galldiks N, Hattingen E, Shah NJ. Advances in Neuro-Oncology Imaging. Nat Rev Neurol (2017) 13(5):279–89. doi: 10.1038/nrneurol.2017.44
130. Langen KJ, Watts C. Neuro-Oncology: Amino Acid PET for Brain Tumours - Ready for the Clinic? Nat Rev Neurol (2016) 12(7):375–6. doi: 10.1038/nrneurol.2016.80
131. Galldiks N, Langen KJ, Albert NL, Chamberlain M, Soffietti R, Kim MM, et al. PET Imaging in Patients With Brain Metastasis-Report of the RANO/PET Group. Neuro-Oncology (2019) 21(5):585–95. doi: 10.1093/neuonc/noz003
132. Law I, Albert NL, Arbizu J, Boellaard R, Drzezga A, Galldiks N, et al. Joint EANM/EANO/RANO Practice Guidelines/SNMMI Procedure Standards for Imaging of Gliomas Using PET With Radiolabelled Amino Acids and [(18)F]FDG: Version 1.0. Eur J Nucl Med Mol Imaging (2019) 46(3):540–57. doi: 10.1007/s00259-018-4207-9
133. Nguyen NC, Yee MK, Tuchayi AM, Kirkwood JM, Tawbi H, Mountz JM. Targeted Therapy and Immunotherapy Response Assessment With F-18 Fluorothymidine Positron-Emission Tomography/Magnetic Resonance Imaging in Melanoma Brain Metastasis: A Pilot Study. Front Oncol (2018) 8:18. doi: 10.3389/fonc.2018.00018
Keywords: brain metastases (BMs), non-small cell lung cancer (NSCLC), immune checkpoint inhibitors (ICI), combination strategies, patient selection, response assessment
Citation: Yang G, Xing L and Sun X (2022) Navigate Towards the Immunotherapy Era: Value of Immune Checkpoint Inhibitors in Non-Small Cell Lung Cancer Patients With Brain Metastases. Front. Immunol. 13:852811. doi: 10.3389/fimmu.2022.852811
Received: 11 January 2022; Accepted: 28 February 2022;
Published: 29 March 2022.
Edited by:
Tao Jiang, Shanghai Pulmonary Hospital, ChinaReviewed by:
Theresa Bunse, German Cancer Research Center (DKFZ), GermanyArutha Kulasinghe, The University of Queensland, Australia
Copyright © 2022 Yang, Xing and Sun. This is an open-access article distributed under the terms of the Creative Commons Attribution License (CC BY). The use, distribution or reproduction in other forums is permitted, provided the original author(s) and the copyright owner(s) are credited and that the original publication in this journal is cited, in accordance with accepted academic practice. No use, distribution or reproduction is permitted which does not comply with these terms.
*Correspondence: Xiaorong Sun, MjUxNDAwMDY3QHFxLmNvbQ==