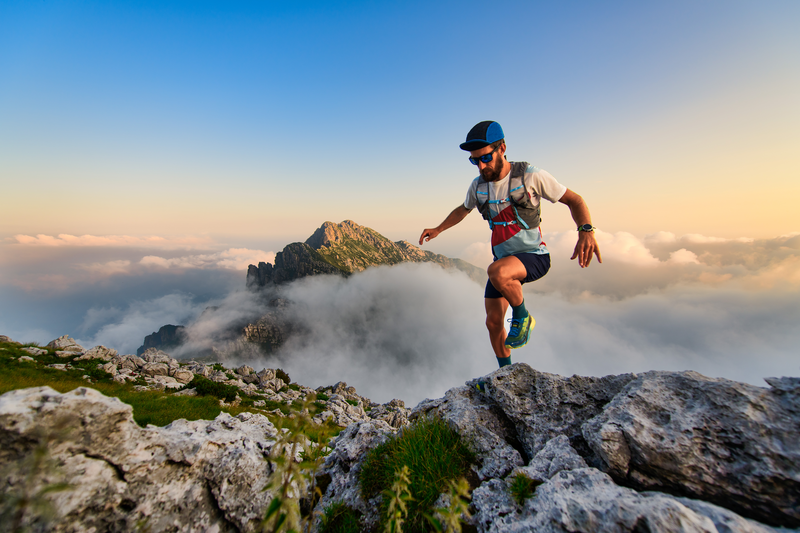
95% of researchers rate our articles as excellent or good
Learn more about the work of our research integrity team to safeguard the quality of each article we publish.
Find out more
ORIGINAL RESEARCH article
Front. Immunol. , 31 March 2022
Sec. Vaccines and Molecular Therapeutics
Volume 13 - 2022 | https://doi.org/10.3389/fimmu.2022.848835
This article is part of the Research Topic Insights in Vaccines and Molecular Therapeutics: 2021 View all 11 articles
Background: Antibiotic-driven dysbiosis may impair immune function and reduce vaccine-induced antibody titers.
Objectives: This study aims to investigate the impacts of early-life antibiotic exposure on subsequent varicella and breakthrough infections.
Methods: This is a nationwide matched cohort study. From Taiwan’s National Health Insurance Research Database, we initially enrolled 187,921 children born from 1997 to 2010. Since 2003, the Taiwan government has implemented a one-dose universal varicella vaccination program for children aged 1 year. We identified 82,716 children born during the period 1997 to 2003 (pre-vaccination era) and 48,254 children born from July 1, 2004, to 2009 (vaccination era). In the pre-vaccination era, 4,246 children exposed to antibiotics for at least 7 days within the first 2 years of life (Unvaccinated A-cohort) were compared with reference children not exposed to antibiotics (Unvaccinated R-cohort), with 1:1 matching for gender, propensity score, and non-antibiotic microbiota-altering medications. Using the same process, 9,531 children in the Vaccinated A-cohort and Vaccinated R-cohort were enrolled from the vaccination era and compared. The primary outcome was varicella. In each era, demographic characteristics were compared, and cumulative incidences of varicella were calculated. Cox proportional hazards model was used to examine associations.
Results: In the pre-vaccination era, the 5-year cumulative incidence of varicella in the Unvaccinated A-cohort (23.45%, 95% CI 22.20% to 24.70%) was significantly higher than in the Unvaccinated R-cohort (16.72%, 95% CI 15.62% to 17.82%) (p<.001). In the vaccination era, a significantly higher 5-year cumulative incidence of varicella was observed in the Vaccinated A-cohort (1.63%, 95% 1.32% to 1.93%) than in the Vaccinated R-cohort (1.19%, 95% CI 0.90% to 0.45%) (p=0.006). On multivariate analyses, early-life antibiotic exposure was an independent risk factor for varicella occurrence in the pre-vaccination (adjusted hazard ratio [aHR] 1.92, 95% CI 1.74 to 2.12) and vaccination eras (aHR 1.66, 95% CI 1.24 to 2.23). The use of penicillins, cephalosporins, macrolides, or sulfonamides in infancy was all positively associated with childhood varicella regardless of vaccine administration.
Conclusions: Antibiotic exposure in early life is associated with varicella occurrence and breakthrough infections.
The early-life microbiome has a fundamental role in human immunity. Indigenous microbiota provides crucial signals for maturation and modulation of the immune system (1, 2). In contrast, dysbiosis in infancy might cause stunting and dysregulation of immunity (3, 4). The composition of gut microbiota also correlates with vaccine immunogenicity (5). Evidence has suggested the association between early-life microbial colonization and sustainable vaccine-specific memory T-cells and antibody responses (6).
Exposure to medications, particularly antibiotics, is a common cause of dysbiosis (7, 8). Even short-term or low-dose antibiotics can disturb the delicate ecosystem of the infant microbiome (9, 10). Early-life antibiotic exposure has been linked to a higher risk of various conditions, including inflammatory bowel disease, type 2 diabetes, and atopic disorders (11–13). However, little is known about the effect of infantile antibiotic exposure on susceptibility to later-life infections. In addition, although antibiotic-driven dysbiosis has been found to impair vaccine responses (10, 14, 15), limitations are that most studies were conducted with a small sample size and in animal models or adults. Whether early-life antibiotic exposures decrease vaccine efficacy or increase the risk of breakthrough infections in the pediatric population remains to be elucidated.
Varicella was once associated with a significant impact on public health in Taiwan (16). Since the implementation of universal varicella vaccination (UVV) in 2003, disease transmission has been successfully controlled (17). However, varicella outbreaks among schoolchildren still occurred occasionally (18), and breakthrough infections continue to be reported despite high rates of national vaccination coverage (19). The present study was aimed to investigate the effect of early-life antibiotic exposure on childhood varicella risk and breakthrough infections.
We conducted a nationwide cohort study using Taiwan’s National Health Insurance Research Database (NHIRD) from 1997 to 2013. The NHIRD contains detailed healthcare information from more than 99% of Taiwan’s population of 25 million people. Diagnoses are documented in the NHIRD using codes based on the International Classification of Diseases, Revision 9, Clinical Modification (ICD-9-CM). The accuracy of diagnosis in the NHIRD has been validated (20, 21), and the data have been used extensively in clinical epidemiology and health service research (22, 23). Personal information, including body weight, height, lifestyle, occupation, and cluster history, is unavailable from the database. This study has been approved by the ethical review board of Taichung Veterans General Hospital (No. CE20224B).
The live attenuated varicella vaccine was approved for use in Taiwan in 1997. Two brands of OKA-strain varicella vaccines, Varivax (Merck) and Varilrix (GlaxoSmithKline) are available in Taiwan. The vaccines have been first provided free to children aged 1 year in Taipei City and Taichung City since 1998 and 1999, respectively. In 2003, the Taiwan government implemented the UVV program, targeting 1-year-old children. The self-paid second-dose booster has been recommended for children aged 4 to 6 years. Despite unavailable 2-dose vaccination rates, the cumulative coverage rate of at least one dose of varicella vaccine among children born after July 1, 2004, has reached more than 94% to date (24).
From the NHIRD, children born from 1997 to 2010 were eligible for enrollment. Children born from 1997 to 2003 and living in regions other than Taipei City and Taichung City were considered unvaccinated (pre-vaccination era). Children born during July 1, 2004, and 2009 were deemed vaccinated (vaccination era). We excluded children with a follow-up period of less than one year, death registration, malignancy, immunodeficiency disorders, white blood cell disorders, transplantation, chemotherapy, or immunotherapy before varicella development. The diagnostic codes for these comorbidities are presented in Supplemental Table 1.
Early life, especially from conception to 2 years of age, is a critical window for microbiota development and immune maturation (25, 26). In the vaccination era, children who received antibiotics for at least 7 days within the first 2 years of life were included in the antibiotic cohort (Vaccinated A-cohort). The reference cohort (Vaccinated R-cohort) comprised children who had not received antibiotics. We identified the Unvaccinated A-cohort and the Unvaccinated R-cohort in the pre-vaccination era using the same process.
The index date was defined as the first day of the third year of life. All sampled children were followed up from age 2 years to the development of outcome of interest or death. Each child was followed up for a maximum of 5 years.
In each era, 1:1 matching of children in both cohorts was carried out for gender, propensity score, and non-antibiotic microbiota-altering medications. The propensity score was calculated via logistic regression model (27) that included infectious diseases, non-bacterial gastroenteritis, and constipation (Supplemental Table 1). These have been common pediatric comorbidities that promote intestinal dysbiosis. Histamine type-2 receptor antagonists (H2RAs), proton pump inhibitors (PPIs), and laxatives have been found to cause perturbation of gut microbiota (28). Non-antibiotic microbiota-altering medication exposure was defined as using any of these drugs for at least 7 days within the first 2 years of life.
The primary outcome was varicella with diagnostic code (ICD-9-CM code 052) in the NHIRD. Children with varicella before the index date were still censored during the follow-up. However, to evaluate the association between early-life antibiotic exposure and subsequent varicella, only varicella that occurred in children after 2 years of age was identified.
Breakthrough varicella has been defined as varicella occurring over 6 weeks after at least one dose of vaccination (17, 19). Since the age at varicella vaccination in Taiwan was previously reported to be 1 to 1.97 years (17), the identified varicella cases in the vaccination era were considered breakthrough events.
Demographic factors such as gender, comorbidities, and medication were considered potential confounders. Comorbidities were defined as diseases based on diagnostic codes (Supplemental Table 1) after the index date. Exposure to drugs related to dysbiosis, including H2RAs, PPIs, or laxatives, was defined as the use of such medications for at least 7 days within the first 2 years of life. Exposure to immunomodulatory drugs, such as systemic corticosteroids and disease-modifying antirheumatic drugs, was defined as using these drugs for more than 30 days per year on average. The aforementioned medication is listed in Supplemental Table 2.
We first analyzed the demographic data, comorbidities, and medications. The categorical variables and prevalence rates of varicella in the study cohorts of each era were compared using the chi-square test. The cumulative incidences of varicella were calculated using the Kaplan-Meier method. The differences in the full time-to-event distributions between the two cohorts of each era were tested via the 2-tailed log-rank test.
We next performed multivariate analyses with modified Cox proportional hazard models to determine whether antibiotic exposure is an independent risk factor for subsequent varicella. The adjusted variables were gender, hospital visit number during the follow-up period, and well-known factors for dysbiosis, including antibiotic exposure, use of non-antibiotic microbiota-altering medications, infectious diseases, non-bacterial gastroenteritis, and constipation. We also conducted sub-analyses to examine the risk of exposure to different antibiotics in early life on varicella development.
All data were managed via SAS 9.4 software (SAS Institute Inc., Cary, NC, USA) and the “cmprsk” package of R. The results are expressed as an estimated number with 95% confidence interval (CI).
We initially enrolled 187,921 children born from 1997 to 2010 from Taiwan’s NHIRD. Among them, 82,716 children not living in Taipei City or Taichung City were born from 1997 to 2003, and 48,254 children were born from July 1, 2004, to 2009. A total of 8,293 children with a follow-up period of less than 1 year or with comorbidities or therapy that may increase the risk of infections before the occurrence of varicella were excluded. Finally, 81,596 children were included in the pre-vaccination era group and 47,533 were included in the vaccination era group (Figure 1). The baseline characteristics of the children in both groups are presented in Supplemental Table 3.
Figure 1 Flow chart of the patient selection process. NHIRD, National Health Insurance Research Database; A-cohort, antibiotic cohort; R-cohort, reference cohort.
In the pre-vaccination era, 69,430 children exposed to antibiotics for at least 7 days within the first 2 years were included in the Unvaccinated A-cohort, and 4,975 children in the reference group not exposed to antibiotics within the first 2 years of life were included in the Unvaccinated R-cohort. The baseline characteristics of children in both cohorts are shown in Supplemental Table 4. After matching for gender, propensity score, and non-antibiotic microbiota-altering medications at a ratio of 1:1, there were 4,246 children in each cohort (Figure 1). Using the same process, we selected subjects from the vaccination era, with 9,531 children each in the Vaccinated A-cohort and the Vaccinated R-cohort (Figure 1).
Demographic characteristics and comorbidities were comparable between the cohorts in each era, except higher numbers of hospital visits in both A-cohorts compared to the respective R-cohorts (median 72 vs. 59 in pre-vaccination era, and 77 vs.71 in vaccination era) (Table 1). In the Unvaccinated A-cohort, penicillins (59.6%) were most common, followed by cephalosporins (33.4%), macrolides (32.0%), and sulfonamides (22.7%). In the Vaccinated A-cohort, penicillins (61.1%) and cephalosporins (15.4%) were most common (Supplemental Table 5). Ages at varicella occurrence and hospitalization for varicella were comparable between the cohorts in each era.
Table 1 Demographic characteristics and outcomes of matched study subjects in antibiotic and reference cohorts in the pre-vaccination and vaccination eras.
A significantly higher 5-year cumulative incidence of varicella was observed in the pre-vaccination era group (22.22%, 95% confidence interval [CI] 21.94-22.51%) than in the vaccination era group (1.40%, 95% CI 1.27-1.53%) (p<.001) (Supplemental Figure 1).
In the pre-vaccination era, the 5-year cumulative incidence of varicella in the Unvaccinated A-cohort (23.45%, 95% CI 22.20-24.70%) was significantly higher than in the Unvaccinated R-cohort (16.72%, 95% CI 15.62-17.82%) (p<.001) (Figure 2A). In the vaccination era, a significantly higher 5-year cumulative incidence of varicella was observed in the Vaccinated A-cohort (1.63%, 95% 1.32-1.93%) than in the Vaccinated R-cohort (1.19%, 95% CI 0.90-1.45%) (p=0.006) (Figure 2B).
Figure 2 Cumulative incidences of varicella in patients exposed to antibiotics within the first 2 years of life and matched controls. The differences between the two study cohorts in the (A) pre-vaccination era and (B) vaccination era were determined by log-rank test.
In the pre-vaccination era, antibiotic exposure for at least 7 days within the first 2 years of life was independently associated with varicella occurrence (adjusted hazard ratio [aHR] 1.92, 95% CI 1.74-2.12). This risk was weaker but still significant among children born in the vaccination era (aHR 1.66, 95% CI 1.24-2.23) (Table 2).
Table 2 Multivariate analyses of antibiotic effects for varicella in the pre- vaccination and vaccination eras.
Further analyses demonstrated that exposure to a specific type of the commonly-used antibiotics, including penicillins (aHR 1.47, 95% CI 1.31-1.66), cephalosporins (aHR 1.19, 95% CI 1.04-1.36), macrolides (aHR 1.46, 95% CI 1.28-1.67), and sulfonamides (aHR 1.27, 95% CI 1.09-1.48), were also independent risk factors for varicella occurrence in the pre-vaccination era. However, exposure to these antibiotics in the vaccination era was positively associated with subsequent varicella but without statistical significance (Table 2).
This nationwide cohort study suggests that antibiotic exposure early in life is an independent risk factor for childhood varicella. Even though herd immunity has been reached in the vaccination era, a significantly higher incidence of breakthrough varicella is observed in children exposed to antibiotics in early life. The present study adds to the mounting evidence that antibiotic-driven dysbiosis during infancy may cause sequelae linked with immune dysfunction, including increased susceptibility to infections.
Commensal-pathogen interactions involve the direct microbiota-related colonization resistance and the indirect microbiome-mediated immune modulation (29). Commensal microbiota can limit colonization of the invading pathogen through upregulating epithelial barrier function, competition for specific resources, and bactericidal or bacteriostatic effects (29, 30). Eubiotic microbiota also supports healthy immune development, shaping optimal innate and acquired immune responses against infective challenges (1, 2).
Evidence has demonstrated that a decrease in bacterial taxa, vacant nutrient niches, and metabolic environment changes after antibiotic administration predispose individuals to certain infections (31, 32), whereas the commensals may progressively return to baseline following antibiotic cessation (33). On the other hand, antibiotic-driven dysbiosis, especially in early life, might result in enduring immune alterations and long-lasting health impacts (3, 4). Animal studies have demonstrated that infant mice exposed to antibiotics had reduced and dysfunctional interferon-γ-producing CD8 T cells, resulting in subsequent increased mortality from vaccinia virus infection (34). In humans, children exposed to early-life antibiotics have been found to exhibit lower infection-induced cytokines, including interleukin 1β, interferon α, interferon γ, tumor necrosis factor α, and IP10 protein (35). Our results align with these immunological findings and support the microbiome-immune-infection axis theory. Early-life antibiotic exposure is associated with dysbiosis and impaired anti-infectious immunity and increases susceptibility to future varicella infections.
The role of the microbiome in the modulation of vaccine immunogenicity has recently been addressed (5). Several observational studies have documented the correlation between microbiota composition, such as the abundance of Bifidobacterium and Bacteroides species, and vaccine responses (6, 36, 37). Immunomodulatory molecules derived from microbiota, such as flagellin, peptidoglycan, and lipopolysaccharides, regulate T cell priming and immunoglobulin production in response to antigenic stimulation (36, 38, 39). Increasing data also suggests that epitopes encoded by the microbiota can cross-reactive with pathogen-encoded epitopes, presumably with vaccine-encoded epitopes (40, 41).
Despite the association between microbiome and vaccine responses, controversy exists over the influence of microbial perturbation on immunization. Antibiotic-driven dysbiosis impairs vaccine immunogenicity in infant mice but not in adult mice (14). From human research, adults with low pre-existing immunity have been found to present markedly reduced post-vaccination antibody titers after experiencing antibiotic treatment (10). Nevertheless, antibiotic exposure in early life has not significantly affected immunogenicity induced by routine infant vaccines, while sample sizes of these studies were modest (42, 43). Additionally, effects of prebiotics or probiotics on vaccine response are variable, depending on the antigens, probiotic strains, and population (44–46). To date, none of these microbiota-targeted interventions have been transferred from research into clinical practice. Our study assessed incidence of breakthrough varicella among children exposed to early-life antibiotics. Although the UVV program has provided robust protection, infantile antibiotic exposure was still an independent risk factor for childhood breakthrough varicella. Such risk might result from increased varicella pathogenicity following antibiotic exposure overwhelming the vaccine protective efficacy or alteration of vaccine responses induced by antibiotic-driven dysbiosis. Further studies are needed to clarify the effects of early-life antibiotic exposure on immunization and vaccine efficacy.
The microbiota changes related to antibiotics depend on the type of antibiotic used. Previous studies have suggested that almost all types of antibiotics affect gut microbiota. The penicillin family of antibiotics, such as amoxicillin, piperacillin, and ticarcillin, may increase the abundance of Enterococcus spp. and decrease the abundance of anaerobes (47). Cephalosporins, quinolones, and sulfonamides have been associated with abundant Enterobacteriaceae except for Escherichia coli (47). Macrolide treatment has been linked to long-term gut microbiota perturbations among pre-school children, including depletion of Actinobacteria and increases in Bacteroidetes and Proteobacteria (48). The antimicrobial spectrum also influences the impact of antibiotics on the immune response to vaccination. An adult study has demonstrated that the proportion of vaccinees with a more than 2-fold anti-rotavirus antibody titer by 7 days post-vaccination was significantly higher among subjects treated with vancomycin only than those treated with broad-spectrum antibiotics (15). In the present study, early-life exposure to penicillins, cephalosporins, macrolides, or sulfonamides were all independent risk factors for childhood varicella in the pre-vaccination era. The risk of breakthrough varicella due to exposure to these antibiotics in the vaccination era was also observed, although without statistical significance owing to the small number of cases. Relationship between the risk and antimicrobial spectrum of the administered antibiotic remains to be elucidated, since we only examined the effects of using different types of antibiotics rather than the specific antibiotic on varicella occurrence. Overall, caution is warranted in prescribing any type of antibiotic to infants despite their benefits. It should also be taken into account the effects on the human microbiome when administering antibiotic therapy.
Our study has several strengths. The population-based cohort study design enabled us to assess the association between antibiotic exposure and varicella infections. By utilizing the nationwide NHIRD, we enrolled a large sample size, which prevented selection bias, allowing us to identify relatively rare conditions such as post-vaccination infection, and provide reliability in terms of statistics with a smaller margin of error.
Despite these strengths, there are several limitations. First, as this was an observational study, we could only report an association between antibiotic exposure and subsequent varicella but could not infer causality. Second, patient-specific information such as lifestyle, contact history, seeking healthcare in private practice, and over-the-counter medication use was unavailable from the NHIRD. To minimize biases, cohorts possessed comparable characteristics after matching gender, propensity score, and non-antibiotic microbiota-altering medications. We also performed multivariable analyses to adjust for potential confounders. Third, the specific date of vaccination, the total number of varicella vaccines administered, whether concomitant vaccinations were used or not, and the interval from antibiotic exposure to vaccination were not recorded in the dataset. Therefore, it is difficult to assess the effects of antibiotic exposure on immunization. Instead, we reported the association between antibiotic therapy in infancy and varicella during childhood regardless of herd immunity. Finally, as our study focused on varicella, the generalizability of our results may be limited. Nevertheless, it provided valuable information on the microbiome-immune-infection axis theory.
In conclusion, children exposed to antibiotics in infancy are associated with varicella later in life. Antibiotic exposure is an independent risk factor for varicella occurrence, even though herd immunity has been reached. These findings suggest caution when administering antibiotics in early life to prevent increased infection susceptibility and poor vaccine efficacy.
The data analyzed in this study is subject to the following licenses/restrictions: All researchers who wish to use the NHIRD and its data subsets are required to sign a written agreement declaring that they have no intention of attempting to obtain information that could potentially violate the privacy of patients or care providers. Requests to access these datasets should be directed to Center for Biomedical Resources of NHRI, https://nhird.nhri.org.tw/en/Data_Protection.html.
The studies involving human participants were reviewed and approved by the ethical review board of Taichung Veterans General Hospital (No. CE20224B). Written informed consent from the participants’ legal guardian/next of kin was not required to participate in this study in accordance with the national legislation and the institutional requirements.
Study concept and design: T-LL, Y-HF, Y-JC, C-YW. Statistical analysis: Y-LC, HH. Analysis and interpretation of data: T-LL, L-LL, Y-JC. Drafting of the manuscript: T-LL, Y-JC. Critical revision of the manuscript for important intellectual content: T-LL, C-YW, Y-JC. All authors read and approved the final manuscript.
This work is supported in part by the Ministry of Science and Technology, Taiwan (MOST 109-2327-B-010-005; 109-2410-H-110-016-MY2).
The authors declare that the research was conducted in the absence of any commercial or financial relationships that could be construed as a potential conflict of interest.
All claims expressed in this article are solely those of the authors and do not necessarily represent those of their affiliated organizations, or those of the publisher, the editors and the reviewers. Any product that may be evaluated in this article, or claim that may be made by its manufacturer, is not guaranteed or endorsed by the publisher.
The Supplementary Material for this article can be found online at: https://www.frontiersin.org/articles/10.3389/fimmu.2022.848835/full#supplementary-material
1. Gensollen T, Iyer SS, Kasper DL, Blumberg RS. How Colonization by Microbiota in Early Life Shapes the Immune System. Science (2016) 352:539–44. doi: 10.1126/science.aad9378
2. Rooks MG, Garrett WS. Gut Microbiota, Metabolites and Host Immunity. Nat Rev Immunol (2016) 16:341–52. doi: 10.1038/nri.2016.42
3. Tamburini S, Shen N, Wu HC, Clemente JC. The Microbiome in Early Life: Implications for Health Outcomes. Nat Med (2016) 22:713–22. doi: 10.1038/nm.4142
4. Ruff WE, Greiling TM, Kriegel MA. Host-Microbiota Interactions in Immune-Mediated Diseases. Nat Rev Microbiol (2020) 18:521–38. doi: 10.1038/s41579-020-0367-2
5. Lynn DJ, Benson SC, Lynn MA, Pulendran B. Modulation of Immune Responses to Vaccination by the Microbiota: Implications and Potential Mechanisms. Nat Rev Immunol (2022) 22:33–46. doi: 10.1038/s41577-021-00554-7
6. Huda MN, Ahmad SM, Alam MJ, Khanam A, Kalanetra KM, Taft DH, et al. Bifidobacterium Abundance in Early Infancy and Vaccine Response at 2 Years of Age. Pediatrics. (2019) 143:e20181489. doi: 10.1542/peds.2018-1489
7. Vangay P, Ward T, Gerber JS, Knights D. Antibiotics, Pediatric Dysbiosis, and Disease. Cell Host Microbe (2015) 175:553–64. doi: 10.1016/j.chom.2015.04.006
8. Ianiro G, Tilg H, Gasbarrini A. Antibiotics as Deep Modulators of Gut Microbiota: Between Good and Evil. Gut. (2016) 65:1906–15. doi: 10.1136/gutjnl-2016-312297
9. Rodriguez JG, Rogers AB, Robine N, Loke P, Blaser MJ. Altering the Intestinal Microbiota During a Critical Developmental Window has Lasting Metabolic Consequences. Cell. (2014) 158:705–21. doi: 10.1016/j.cell.2014.05.052
10. Hagan T, Cortese M, Rouphael N, Boudreau C, Linde C, Maddur MS, et al. Antibiotics-Driven Gut Microbiome Perturbation Alters Immunity to Vaccines in Humans. Cell. (2019) 178:1313–28.e1313. doi: 10.1016/j.cell.2019.08.010
11. Kronman MP, Zaoutis TE, Haynes K, Feng R, Coffin SE. Antibiotic Exposure and IBD Development Among Children: A Population-Based Cohort Study. Pediatrics. (2012) 130:e794–803. doi: 10.1542/peds.2011-3886
12. Mikkelsen KH, Knop FK, Frost M, Hallas J, Pottegård A. Use of Antibiotics and Risk of Type 2 Diabetes: A Population-Based Case-Control Study. J Clin Endocrinol Metab (2015) 100:3633–40. doi: 10.1210/jc.2015-2696
13. Mitre E, Susi A, Kropp LE, Schwartz DJ, Gorman GH, Nylund CM. Association Between Use of Acid-Suppressive Medications and Antibiotics During Infancy and Allergic Diseases in Early Childhood. JAMA Pediatr (2018) 172:e180315. doi: 10.1001/jamapediatrics.2018.0315
14. Lynn MA, Tumes DJ, Choo JM, Sribnaia A, Blake SJ, Leong LEX, et al. Early-Life Antibiotic-Driven Dysbiosis Leads to Dysregulated Vaccine Immune Responses in Mice. Cell Host Microbe (2018) 23:653–60.e655. doi: 10.1016/j.chom.2018.04.009
15. Harris VC, Haak BW, Handley SA, Jiang B, Velasquez DE, Hykes BL Jr, et al. Effect of Antibiotic-Mediated Microbiome Modulation on Rotavirus Vaccine Immunogenicity: A Human, Randomized-Control Proof-Of-Concept Trial. Cell Host Microbe (2018) 24:197–207.e194. doi: 10.1016/j.chom.2018.07.005
16. Lin YH, Huang LM, Chang IS, Tsai FY, Chang LY. Disease Burden and Epidemiological Characteristics of Varicella in Taiwan From 2000 to 2005. J Microbiol Immunol Infect (2009) 42:5–12.
17. Cheng HY, Chang LY, Lu CY, Huang LM. Epidemiology of Breakthrough Varicella After the Implementation of a Universal Varicella Vaccination Program in Taiwan, 2004-2014. Sci Rep (2018) 8:17192. doi: 10.1038/s41598-018-35451-y
18. Lai CC, Chen SC, Jiang DD. An Outbreak of Varicella Among Schoolchildren in Taipei. BMC Public Health (2011) 11:226. doi: 10.1186/1471-2458-11-226
19. Huang WC, Huang LM, Chang IS, Tsai FY, Chang LY. Varicella Breakthrough Infection and Vaccine Effectiveness in Taiwan. Vaccine. (2011) 29:2756–60. doi: 10.1016/j.vaccine.2011.01.092
20. Lin CC, Lai MS, Syu CY, Chang SC, Tseng FY. Accuracy of Diabetes Diagnosis in Health Insurance Claims Data in Taiwan. J Formos Med Assoc (2005) 104:157–63.
21. Cheng CL, Kao YH, Lin SJ, Lee CH, Lai ML. Validation of the National Health Insurance Research Database With Ischemic Stroke Cases in Taiwan. Pharmacoepidemiol Drug Saf (2011) 20:236–42. doi: 10.1002/pds.2087
22. Lin TL, Fan YH, Chang YL, Ho HJ, Wu CY, Chen YJ. Early-Life Infections in Association With the Development of Atopic Dermatitis in Infancy and Early Childhood: A Nationwide Nested Case-Control Study. J Eur Acad Dermatol Venereol (2022) 36:615–22. doi: 10.1111/jdv.17908
23. Lin TL, Wu CY, Fan YH, Chang YL, Ho HJ, Chen YJ. Association Between Early Life Laxative Exposure and Risk of Allergic Diseases. Ann Allergy Asthma Immunol (2022) 128:291–98. doi: 10.1016/j.anai.2021.12.016
24. Taiwan Centers for Disease Control. Statistics of Communicable Diseases and Surveillance Report. Available at: https://www.cdc.gov.tw/InfectionReport/List/DRiONFTwYxu8T162Hm6yFw (Accessed 30 December 2021).
25. Wopereis H, Oozeer R, Knipping K, Belzer C, Knol J. The First Thousand Days - Intestinal Microbiology of Early Life: Establishing a Symbiosis. Pediatr Allergy Immunol (2014) 25:428–38. doi: 10.1111/pai.12232
26. Robertson RC, Manges AR, Finlay BB, Prendergast AJ. The Human Microbiome and Child Growth - First 1000 Days and Beyond. Trends Microbiol (2019) 27:131–47. doi: 10.1016/j.tim.2018.09.008
27. Rosenbaum PR, Rubin DB. Reducing Bias in Observational Studies Using Subclassification on the Propensity Score. Am Stat Assoc (1984) 79:516–24. doi: 10.1080/01621459.1984.10478078
28. Weersma RK, Zhernakova A, Fu J. Interaction Between Drugs and the Gut Microbiome. Gut. (2020) 69:1510–9. doi: 10.1136/gutjnl-2019-320204
29. Libertucci J, Young VB. The Role of the Microbiota in Infectious Diseases. Nat Microbiol (2019) 4:35–45. doi: 10.1038/s41564-018-0278-4
30. George S, Aguilera X, Gallardo P, Farfán M, Lucero Y, Torres JP, et al. Bacterial Gut Microbiota and Infections During Early Childhood. Front Microbiol (2022) 12:793050. doi: 10.3389/fmicb.2021.793050
31. Ng KM, Ferreyra JA, Higginbottom SK, Lynch JB, Kashyap PC, Gopinath S, et al. Microbiota-Liberated Host Sugars Facilitate Post-Antibiotic Expansion of Enteric Pathogens. Nature. (2013) 502:96–9. doi: 10.1038/nature12503
32. Theriot CM, Koenigsknecht MJ, Carlson PE Jr, Hatton GE, Nelson AM, Li B, et al. Antibiotic-Induced Shifts in the Mouse Gut Microbiome and Metabolome Increase Susceptibility to Clostridium Difficile Infection. Nat Commun (2014) 5:3114. doi: 10.1038/ncomms4114
33. Ng KM, Aranda-Díaz A, Tropini C, Frankel MR, Van Treuren W, O’Loughlin CT, et al. Recovery of the Gut Microbiota After Antibiotics Depends on Host Diet, Community Context, and Environmental Reservoirs. Cell Host Microbe (2019) 26:650–65.e654. doi: 10.1016/j.chom.2019.10.011
34. Gonzalez-Perez G, Hicks AL, Tekieli TM, Radens CM, Williams BL, Lamousé-Smith ES. Maternal Antibiotic Treatment Impacts Development of the Neonatal Intestinal Microbiome and Antiviral Immunity. J Immunol (2016) 196:3768–79. doi: 10.4049/jimmunol.1502322
35. Semic-Jusufagic A, Belgrave D, Pickles A, Telcian AG, Bakhsoliani E, Sykes A, et al. Assessing the Association of Early Life Antibiotic Prescription With Asthma Exacerbations, Impaired Antiviral Immunity, and Genetic Variants in 17q21: A Population-Based Birth Cohort Study. Lancet Respir Med (2014) 2:621–30. doi: 10.1016/S2213-2600(14)70096-7
36. Harris VC, Armah G, Fuentes S, Korpela KE, Parashar U, Victor JC, et al. Significant Correlation Between the Infant Gut Microbiome and Rotavirus Vaccine Response in Rural Ghana. J Infect Dis (2017) 215:34–41. doi: 10.1093/infdis/jiw518
37. Harris V, Ali A, Fuentes S, Korpela K, Kazi M, Tate J, et al. Rotavirus Vaccine Response Correlates With the Infant Gut Microbiota Composition in Pakistan. Gut Microbes (2018) 9:93–101. doi: 10.1080/19490976.2017.1376162
38. Oh JZ, Ravindran R, Chassaing B, Carvalho FA, Maddur MS, Bower M, et al. TLR5-Mediated Sensing of Gut Microbiota Is Necessary for Antibody Responses to Seasonal Influenza Vaccination. Immunity. (2014) 41:478–92. doi: 10.1016/j.immuni.2014.08.009
39. Kim D, Kim YG, Seo SU, Kim DJ, Kamada N, Prescott D, et al. Nod2-Mediated Recognition of the Microbiota Is Critical for Mucosal Adjuvant Activity of Cholera Toxin. Nat Med (2016) 22:524–30. doi: 10.1038/nm.4075
40. Bremel RD, Homan EJ. Extensive T-Cell Epitope Repertoire Sharing Among Human Proteome, Gastrointestinal Microbiome, and Pathogenic Bacteria: Implications for the Definition of Self. Front Immunol (2015) 6:538. doi: 10.3389/fimmu.2015.00538
41. Carrasco Pro S, Lindestam Arlehamn CS, Dhanda SK, Carpenter C, Lindvall M, Faruqi AA, et al. Microbiota Epitope Similarity Either Dampens or Enhances the Immunogenicity of Disease-Associated Antigenic Epitopes. PloS One (2018) 13:e0196551. doi: 10.1371/journal.pone.0196551
42. Grassly NC, Praharaj I, Babji S, Kaliappan SP, Giri S, Venugopal S, et al. The Effect of Azithromycin on the Immunogenicity of Oral Poliovirus Vaccine: A Double-Blind Randomised Placebo-Controlled Trial in Seronegative Indian Infants. Lancet Infect Dis (2016) 16:905–14. doi: 10.1016/S1473-3099(16)30023-8
43. Zimmermann P, Perrett KP, Ritz N, Flanagan KL, Robins-Browne R, van der Klis FRM, et al. Biological Sex Influences Antibody Responses to Routine Vaccinations in the First Year of Life. Acta Paediatr (2020) 109:147–57. doi: 10.1111/apa.14932
44. Soh SE, Ong DQ, Gerez I, Zhang X, Chollate P, Shek LP, et al. Effect of Probiotic Supplementation in the First 6 Months of Life on Specific Antibody Responses to Infant Hepatitis B Vaccination. Vaccine. (2010) 28:2577–9. doi: 10.1016/j.vaccine.2010.01.020
45. Matsuda F, Chowdhury MI, Saha A, Asahara T, Nomoto K, Tarique AA, et al. Evaluation of a Probiotics, Bifidobacterium Breve BBG-01, for Enhancement of Immunogenicity of an Oral Inactivated Cholera Vaccine and Safety: A Randomized, Double-Blind, Placebo-Controlled Trial in Bangladeshi Children Under 5 Years of Age. Vaccine. (2011) 29:1855–8. doi: 10.1016/j.vaccine.2010.12.133
46. Maruyama M, Abe R, Shimono T, Iwabuchi N, Abe F, Xiao JZ. The Effects of Non-Viable Lactobacillus on Immune Function in the Elderly: A Randomised, Double-Blind, Placebo-Controlled Study. Int J Food Sci Nutr (2016) 67:67–73. doi: 10.3109/09637486.2015.1126564
47. Zimmermann P, Curtis N. The Effect of Antibiotics on the Composition of the Intestinal Microbiota - a Systematic Review. J Infect (2019) 796:471–89. doi: 10.1016/j.jinf.2019.10.008
Keywords: varicella, breakthrough infection, vaccine, antibiotic, microbiota, dysbiosis, pediatric population
Citation: Lin T-L, Fan Y-H, Chang Y-L, Ho HJ, Liang L-L, Chen Y-J and Wu C-Y (2022) Early-Life Antibiotic Exposure Associated With Varicella Occurrence and Breakthrough Infections: Evidence From Nationwide Pre-Vaccination and Post-Vaccination Cohorts. Front. Immunol. 13:848835. doi: 10.3389/fimmu.2022.848835
Received: 05 January 2022; Accepted: 10 March 2022;
Published: 31 March 2022.
Edited by:
Lee Mark Wetzler, Boston University, United StatesReviewed by:
Anastasia N. Vlasova, The Ohio State University, United StatesCopyright © 2022 Lin, Fan, Chang, Ho, Liang, Chen and Wu. This is an open-access article distributed under the terms of the Creative Commons Attribution License (CC BY). The use, distribution or reproduction in other forums is permitted, provided the original author(s) and the copyright owner(s) are credited and that the original publication in this journal is cited, in accordance with accepted academic practice. No use, distribution or reproduction is permitted which does not comply with these terms.
*Correspondence: Yi-Ju Chen, eWpjaGVubWRAdmdodGMuZ292LnR3; Chun-Ying Wu, ZHIud3UudGFpd2FuQGdtYWlsLmNvbQ==
Disclaimer: All claims expressed in this article are solely those of the authors and do not necessarily represent those of their affiliated organizations, or those of the publisher, the editors and the reviewers. Any product that may be evaluated in this article or claim that may be made by its manufacturer is not guaranteed or endorsed by the publisher.
Research integrity at Frontiers
Learn more about the work of our research integrity team to safeguard the quality of each article we publish.