- 1Department of Organ Transplantation, The Second Affiliated Hospital of Hainan Medical University, The Transplantation Institute of Hainan Medical University, Haikou, China
- 2Institute of Organ Transplantation, Tongji Hospital, Tongji Medical College, Huazhong University of Science and Technology, Wuhan, China
- 3Key Laboratory of Organ Transplantation, Ministry of Education and National Health Commission (NHC), Chinese Academy of Medical Sciences, Wuhan, China
- 4Genetic Engineering Department, Chengdu Clonorgan Biotechnology Co., Ltd., Chengdu, China
- 5Center for Transplantation Sciences, Department of Surgery, Massachusetts General Hospital/Harvard Medical School, Boston, MA, United States
- 6Clinical Immunology Translational Medicine Key Laboratory of Sichuan Province, Sichuan Academy of Medical Sciences & Sichuan Provincial People’s Hospital, Chengdu, China
- 7Department of Urology, Second Affiliated Hospital of University of South China, Hengyang, China
Kidney xenotransplantation is expected to contribute to resolving the shortage of kidneys from deceased human donors. Although progress in experimental life-supporting pig renal xenotransplantation has been encouraging, there are still issues to be considered before a clinical trial can be initiated. We attempted to clarify some of these by an in vitro study. Blood was drawn from healthy volunteers (Volunteers, n=20), patients with end-stage renal disease (ESRD, n=20) pre-operation (Pre), and on Day 1 (POD 1) and Day 14 (POD 14) after renal allotransplantation, brain-dead organ donors (DBD, n=20), and renal allotransplant recipients who were currently experiencing T cell-mediated rejection (Allo-TCMR, n=20). Serum IgM/IgG binding to, and complement-dependent cytotoxicity (CDC) of, PBMCs and RBCs from (a) wild-type (WT), (b) α1,3-galactosyltransferase gene-knockout (GTKO), (c) GTKO/beta-1,4-N-acety1 galactosaminyltransferase 2-knockout (GTKO/β4GalNT2KO), (d) GTKO/cytidine monophosphate-N-acetylneuraminic acid hydroxylase-knockout (GTKO/CMAHKO), and (e) GTKO/β4GalNT2KO/CMAHKO/hCD55 (TKO/hCD55) pigs were measured by flow cytometry. We obtained the following results: (i) Serum IgM/IgG binding and CDC in Volunteers were significantly greater to WT, GTKO, and GTKO/β4GalNT2KO PBMCs or RBCs than to GTKO/CMAHKO and TKO/hCD55 cells; (ii) ESRD, DBD, and Allo-TCMR serum antibody binding and CDC to WT pig PBMCs were significantly greater than to GTKO, GTKO/β4GalNT2KO, GTKO/CMAHKO, and TKO/hCD55 cells; (iii) antibody binding to GTKO/CMAHKO pig cells was significantly lower in hemodialysis than peritoneal dialysis patients. (iv) Two of twenty allotransplantation recipients’ serum IgG binding to GTKO pig PBMCs increased on POD14 compared with Pre, but IgG binding to GTKO pig RBCs did not; (v) In all sera, the lowest antibody binding and CDC were to GTKO/CMAHKO and TKO/CD55 pig cells. We conclude (i) CMAHKO in the pig may be critical to the success of clinical pig kidney xenotransplantation, and may be the most important after GTKO, at least in Chinese patients; (ii) subjects with ESRD, or who are immunosuppressed after kidney allotransplantation, and DBD, have lower levels of antibody binding and CDC to genetically-engineered pig cells than do volunteers; (iii) TKO pigs with selected human ‘protective’ transgenes, e.g., CD55, are likely to prove to be the optimal sources of kidneys for clinical xenotransplantation.
Introduction
There is a critical shortage of deceased human donor organs for transplantation in patients with end-stage renal disease (ESRD) (1). Genetically-engineered pigs are a potential alternative source of kidneys for these patients. Pig-to-nonhuman primate kidney transplantation Is now associated with encouraging results with recipient and graft survival extending to >1 year in several cases (2–4). However, there are still some major issues that must be resolved before a clinical trial can be initiated, e.g., (i) what genetically-modified pigs should be the sources of kidneys for clinical renal xenotransplantation; and (ii) whether a new xenotransplantation model needs to be identified because of differences in antibody binding and complement-dependent cytotoxicity (CDC) to pig cells between humans and Old World monkeys (OWMs) (5).
Pigs that do not express Gal or Sda (GTKO/β4GalNT2KO pigs), with or without added human transgenes, may be the optimal source of organs for OWMs (Table 1), whereas pigs in which expression of all 3 known carbohydrate xenoantigens has been deleted [triple-knockout (TKO) pigs], with or without added human transgenes, are likely to be optimal for human recipients (5–8). Humans have low (or no) antibody levels and CDC to cells from TKO pigs.
Whether or not swine leukocyte antigen (SLA) expression needs to be deleted remains uncertain. SLA is the homolog of human leukocyte antigen (HLA), a protein complex expressed on human tissue capable of stimulating the development of new antibodies in allotransplantation. Some in vitro studies have indicated that HLA-sensitized patients will not be at greater risk of rejecting a pig organ than HLA-non-sensitized patients (9–14), but other studies indicate that HLA-sensitized patients have a greater risk of rejecting a pig organ (15–17), and so it would be prudent not to select HLA-sensitized patients for the first clinical trials of pig kidney transplantation (18).
Subjects with brain-death (DBD subjects) are a frequent source of organs for transplantation, and transplantation of a pig organ into a brain-dead human recipient has recently been carried out (19). However, brain death is associated with dysfunction of the cardiovascular, pulmonary, endocrine, thermoregulation, renal, hematologic and inflammatory systems (20–23). If DBD subjects are used as recipients in preclinical models of pig renal xenotransplantation, there is concern that these pathophysiological consequences may affect the xenograft, e.g., by activation of T and B lymphocytes, release of cytokines, etc. (19).
The aims of the present study were (i) to measure serum anti-pig antibodies in healthy human volunteers, patients with ESRD pre- and post-renal allotransplantation, DBD subjects, and patients with renal allografts who were currently experiencing acute T cell-mediated rejection (Allo-TCMR), and (ii) to provide further data to help select pigs with the optimal genotype for clinical renal xenotransplantation.
Materials and Methods
Human Sera
Blood was drawn from (i) healthy volunteers (Volunteers, n=20; ABO blood types A n=6; B n=6; AB n=3; O n=5), (ii) patients with ESRD (n=20), pre-renal transplantation (Pre) and on Day 1 (POD 1) and Day 14 (POD 14) after renal allotransplantation, (iii) brain-dead organ donors (DBD, n=20) and (iv) patients with renal allografts who were currently experiencing episodes of acute T cell-mediated rejection (Allo-TCMR, n=20) (Table 1). Sera were obtained from de-identified remnant/discarded clinical laboratory samples.
Sera from Volunteers were obtained from the Second Affiliated Hospital of Hainan Medical University, and all experimental protocols were approved by the ethics committee of the Second Affiliated Hospital of Hainan Medical University. All procedures involving humans were performed in accordance with the relevant guidelines and regulations, and had no adverse effects on the subjects.
Pigs
Blood was obtained from wild-type (WT, i.e., genetically-unmodified) pigs (n=4) and from different genetically-modified pigs (n=4) (Table 1) (Chengdu Clonorgan Biotechnology, Chengdu, Sichuan, China).
Detection of Expression of Xenoantigens on Selected Pig Cells by Flow Cytometry
Pig RBCs and PBMCs were stained for expression of Gal (by isolectin BSI-B4), Sda (Dolichos biflorus agglutinin,DBA), Neu5Gc (chicken anti-Neu5Gc mAb), and SLA (anti-human β2-microglobulin antibody, β2M).
Binding of Human Serum IgM and IgG to pRBCs and pPBMCs by Flow Cytometry
Binding of human antibodies to pig cells was measured by flow cytometry using the relative geometric mean (rGM), as previously described (11). Briefly, pRBCs were separated from whole blood, washed x3 with phosphate-buffered saline (PBS), and centrifuged at 700g for 5min at 4°C. The washed RBCs were suspended in fluorescence-activated cell sorting (FACS) buffer (PBS containing 1% bovine serum albumin). pPBMCs were isolated using Ficoll (HaoYang, Tianjin, China) and suspended in FACS buffer for IgM/IgG binding assays. The isolated pRBCs (5x105/tube) and pPBMCs (5x105/tube) were incubated with heat-inactivated human serum at 4°C for 30min, respectively, and the final serum concentration was 20%. After incubation, cells were washed with PBS to remove unbound antibodies and were blocked with 10% goat serum for 15min at 4°C. After further washing with PBS, anti-human IgM or anti-human IgG (Jackson ImmunoResearch Laboratories, West Grove, PA, USA) (IgG: concentration 1:1000 for pRBCs and pPBMCs; IgM: concentration 1:1600 for pRBCs and pPBMCs) was added, and the cells were incubated for 30min at 4°C. After washing with PBS, 100μL PBS buffer was added. Flow cytometry was carried out using BD FACSCelesta (Becton Dickinson, San Jose, CA, USA).
Human Serum CDC of Pig PBMCs by Flow Cytometry
Briefly, PBMCs (5×105 cells in 250µL FACS buffer) were incubated with 50µL heat-inactivated human serum at 4°C for 1h. After washing with PBS, FACS buffer (200µL) and rabbit complement (50µL, Cedarlane, Hornby, CA, USA) were added (final concentration 20%), and incubation was carried out at 37°C for 30min. After washing with PBS, the cells were incubated in the dark at 4°C for 15min with propidium iodide, and finally 200µL FACS buffer was added. Flow cytometry was carried out using BD FACSCelesta.
Cytotoxicity was calculated, as follows (11):
where A represented the percentage of dead cells, B was the maximal percentage of dead cells (PBMCs fixed with 70% ethanol), and C was the minimal percentage of dead cells (PBMCs incubated with medium only).
Statistical Analysis
Significance of the difference between two groups was determined by student t-test or Wilcoxon test. Continuous variables were expressed as mean ± SD. Comparisons among multiple groups were performed using a One-way ANOVA test (Tukey test) or nonparametric test (Dunn’s test). A p value of <0.05 was considered statistically significant. All statistical analyses were performed using social sciences software GraphPad Prism 8 (GraphPad Software, San Diego, CA, USA).
Results
Expression of Gal, Sda, Neu5Gc, and SLA on Pig PBMCs and/or RBCs
PBMCs
PBMCs from WT pigs expressed Gal, Sda, Neu5Gc, and SLA (Figure 1A). As anticipated, PBMCs from a GTKO pig did not express the Gal antigen, but expressed Sda and Neu5Gc. Those from a GTKO/β4GalNT2KO pig did not express Gal or Sda, but expressed Neu5Gc. Those from a GTKO/CMAHKO pig did not express Gal or Neu5Gc, but expressed Sda. And those from a TKO/hCD55 pig did not express any of the 3 carbohydrate xenoantigens, but expressed hCD55. Neu5Gc that was still expressed a small amount in GKTO/CMAHKO and TKO/hCD55 pig PBMCs was a false positive which was caused by Rabbit anti-Chicken IgY/Alexa Fluor 555 antibody (Supplemental Material).
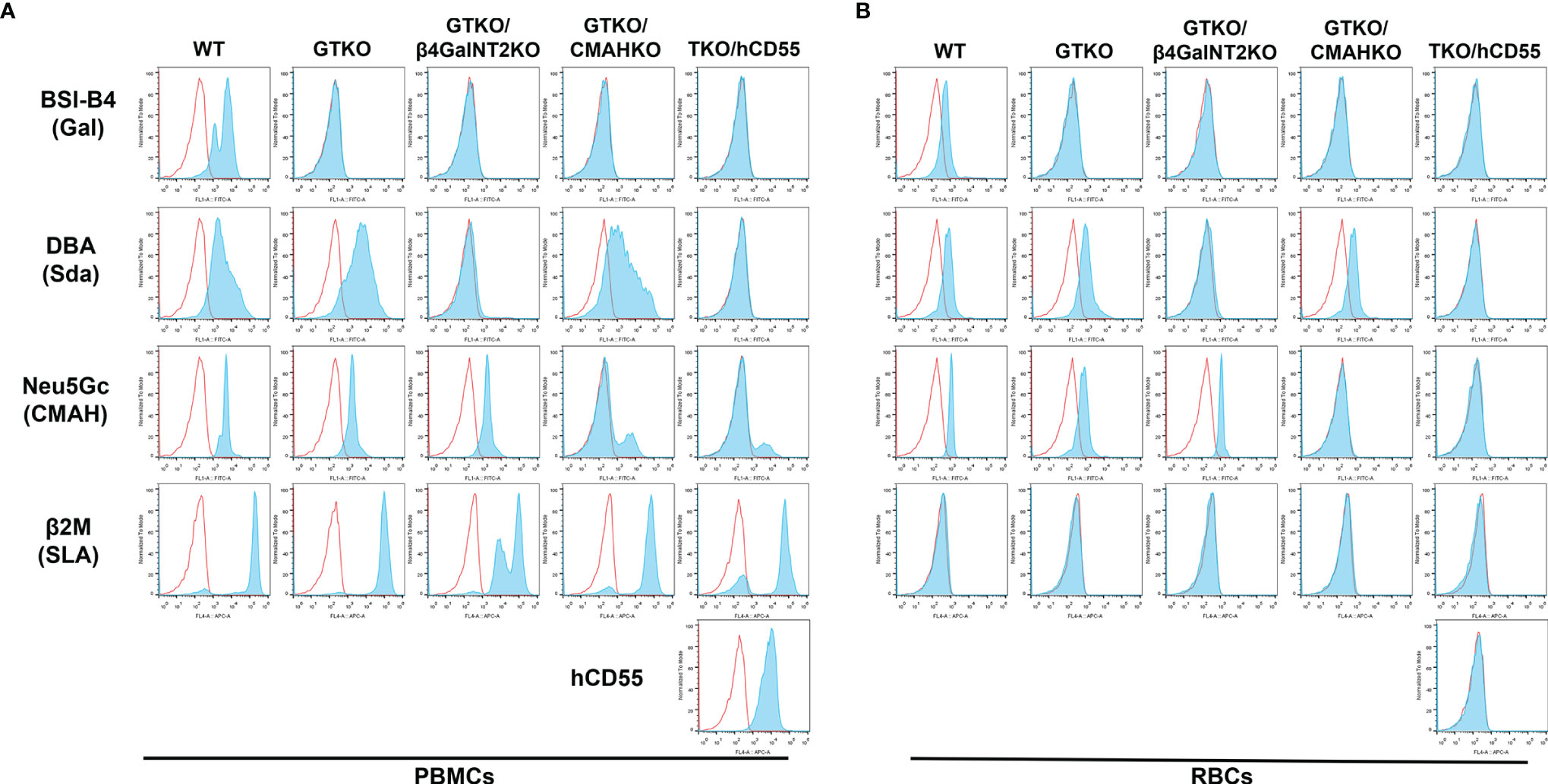
Figure 1 Expression of Gal, Sda, Neu5Gc, SLA, and hCD55 on WT, GTKO, GTKO/β4GalNT2KO, GTKO/CMAHKO and TKO/hCD55 pig PBMCs and RBCs by flow cytometry. (A) PBMCs and (B) RBCs from WT pigs expressed Gal, Sda, and Neu5Gc. GTKO expressed Sda and Neu5Gc. GTKO/β4GalNT2KO expressed Neu5Gc. GTKO/CMAHKO expressed Sda. TKO/hCD55 PBMCs (but not RBCs) expressed hCD55. All PBMCs expressed SLA, but RBCs did not express SLA.
There was a positive expression The false positive expression of Neu5Gc on GTKO/CMAHKO and TKO/hCD55 pig PBMCs were caused by Rabbit anti-Chicken IgY/Alexa Fluor 555 antibody (Supplemental Material).
RBCs
Expression of xenoantigens on RBCs from all of the above pigs followed the same pattern as that to PBMCs except that they did not express SLA or hCD55 (Figure 1B).
Effect of Different Human Sera on IgM and IgG Binding and CDC to WT and Various Genetically-Modified Pig PBMCs by Flow Cytometry
The aim was to compare the binding of different human sera to various pig PBMCs.
IgM Binding
Mean IgM binding to WT, GTKO and GTKO/β4GalNT2KO PBMCs was significantly greater in Volunteers than in the other three sera. Mean IgM binding to GTKO/CMAHKO and TKO/hCD55 PBMCs was minimal and not significantly different between all four groups of sera (Volunteers, ESRD, DBD, and Allo-TCMR) (Figure 2A).
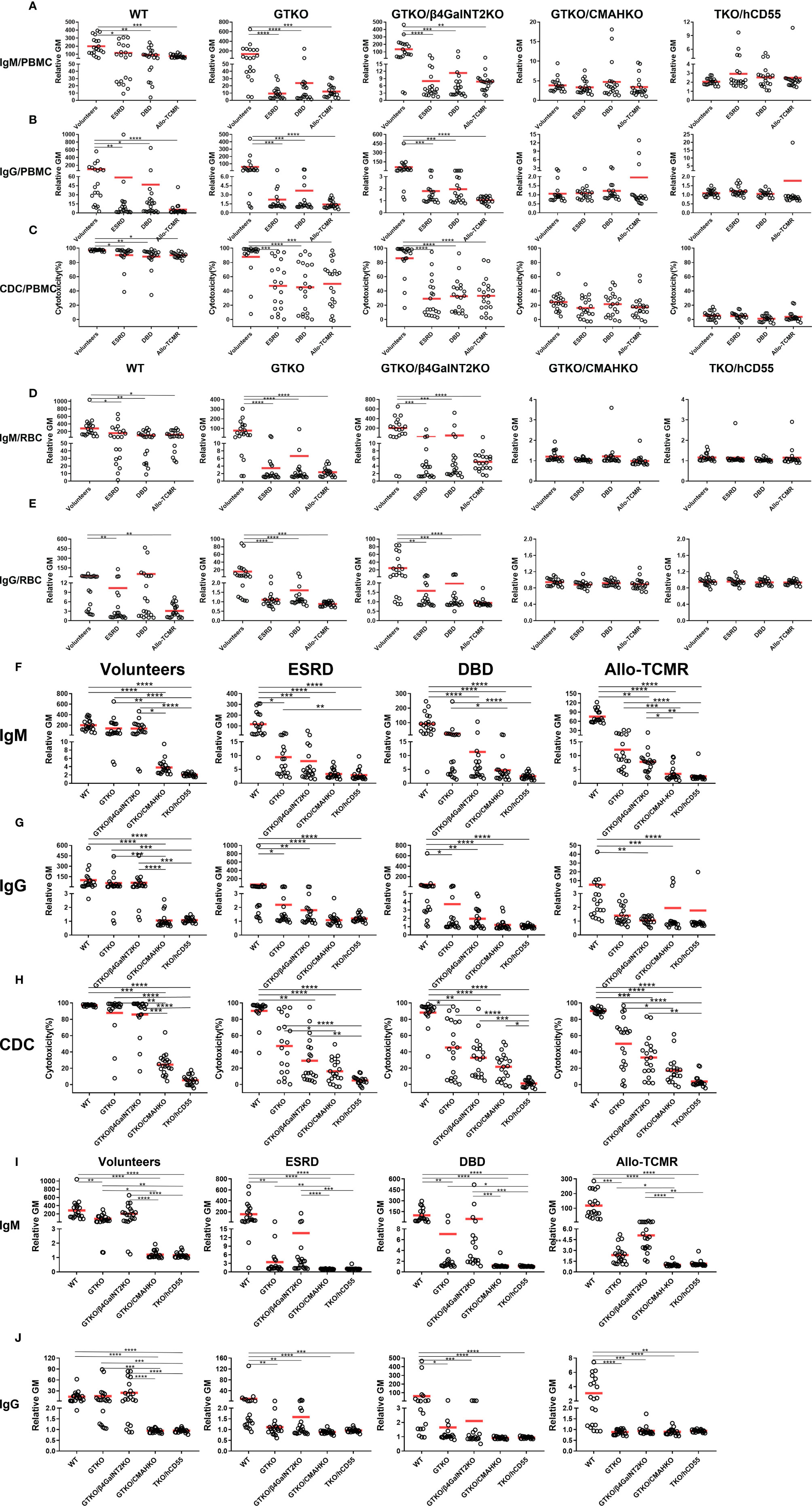
Figure 2 Effect of different human sera on IgM and IgG binding and CDC to various pig PBMCs and RBCs and effect of genetic-engineering of pig PBMCs and RBCs on human IgM and IgG binding and CDC by flow cytometry. Comparison of mean (A) IgM/(B) IgG binding and (C) CDC of sera from Volunteers, ESRD, DBD and Allo-TCMR to WT, GTKO, GTKO/β4GalNT2KO, GTKO/CMAHKO, and TKO/hCD55 pig PBMCs. Mean of (D) IgM and (E) IgG comparing binding of sera from Volunteers, ESRD, DBD, and Allo-TCMR to WT, GTKO, GTKO/β4GalNT2KO, GTKO/CMAHKO, and TKO/hCD55 pig RBCs. (*p <0.05, **p<0.01, ***p<0.005, ****p<0.001). Volunteers had very low levels of IgM/IgG binding and CDC to GTKO/CMAHKO and TKO/hCD55 pig PBMCs (F-H) or RBCs (I, J). IgM and IgG binding and CDC to GTKO, GTKO/β4GalNT2KO, GTKO/CMAHKO, and TKO/hCD55 PBMCs (F–H) or RBCs (I, J) were low in sera from ESRD, DBD, and Allo-TCMR. (*p<0.05, **p<0.01, ***p<0.005, ****p<0.001).
IgG Binding
Mean IgG binding to WT, GTKO and GTKO/β4GalNT2KO PBMCs was significantly greater in Volunteers than in the other three sera. No sera showed more than minimal binding to GTKO/CMAHKO and TKO/CD55 PBMCs (Figure 2B).
CDC
Mean serum CDC to GTKO/CMAHKO and TKO/hCD55 pig PBMCs was not significantly different between Volunteers, ESRD, DBD, and Allo-TCMR (Figure 2C). However, mean CDC to WT, GTKO and GTKO/β4GalNT2KO pig PBMCs was significantly greater in Volunteers than in ESRD, DBD, and Allo-TCMR. CDC of GTKO/CMAHKO PBMCS was low or negative in all sera, and almost no sera caused any killing of TKO/CD55 PBMCs.
Effect of Different Human Sera on IgM and IgG Binding to WT and Various Genetically-Modified Pig RBCs by Flow Cytometry
The aim was to compare the binding of different human sera to various pig RBCs.
IgM Binding
Mean IgM binding to WT, GTKO and GTKO/β4GalNT2KO RBCs was significantly greater in Volunteers compared with ESRD, DBD, and Allo-TCMR. Mean IgM binding to GTKO/CMAHKO and TKO/hCD55 RBCs was minimal in all sera and was not significantly different between all four groups of sera (Figure 2D).
IgG Binding
Mean IgG binding to WT was significantly greater in Volunteers compared with ESRD an Allo-TCMR. Mean IgG binding to GTKO and GTKO/β4GalNT2KO RBCs was significantly greater in Volunteers than in ESRD, DBD, and Allo-TCMR. Mean IgG binding was absent or minimal to GTKO/CMAHKO and TKO/CD55 RBCs (Figure 2E).
The Effect of Genetic-Engineering of Pig PBMCs on Human IgM and IgG Binding and CDC by Flow Cytometry
The data presented in relation to differences in binding of various human sera to pig cells (Figures 2A–E) were re-presented to more clearly illustrate the effect of different pig genotypes (Figures 2F–J).
IgM Binding
Mean IgM binding in serum from Volunteers to WT, GTKO, and GTKO/β4GalNT2KO PBMCs was significantly greater than to GTKO/CMAHKO and TKO/hCD55 PBMCs. Mean IgM binding in serum from ESRD to WT PBMCs was significantly greater than to GTKO, GTKO/β4GalNT2KO, GTKO/CMAHKO and TKO/hCD55 PBMCs, and mean IgM binding to GTKO PBMCs was significantly greater than to TKO/hCD55 PBMCs. Mean IgM binding in serum from DBD and Allo-TCMR to WT PBMCs was significantly greater than to GTKO/β4GalNT2KO, GTKO/CMAHKO and TKO/hCD55 PBMCs, and the mean IgM binding to GTKO PBMCs was significantly greater than to GTKO/CMAHKO and TKO/hCD55 PBMCs, what’s more, Mean IgM binding in serum from Allo-TCMR to GTKO/β4GalNT2KO PBMCs was significantly greater than to GTKO/CMAHKO and TKO/hCD55 PBMCs (Figure 2F).
In healthy human sera (Volunteers), mean IgM binding was reduced by approximately 20% by GTKO (compared to WT), but by approximately 80% by CMAHKO (WT vs GTKO: 199 vs 138, p=ns; WT vs CMAHKO: 199 vs 4, p<0.001) (Figure 2F).
IgG Binding
Mean IgG binding was almost lower than mean IgM binding in all sera (Figure 3A). Mean IgG binding in Volunteers to WT, GTKO and GTKO/β4GalNT2KO PBMCs was significantly greater than to GTKO/CMAHKO and TKO/hCD55 PBMCs. Mean IgG binding in ESRD and DBD to WT PBMCs was significantly greater than to GTKO, GTKO/β4GalNT2KO, GTKO/CMAHKO and TKO/hCD55 PBMCs. Mean IgG binding in serum from Allo-TCMR to WT PBMCs was significantly greater than to GTKO/β4GalNT2KO, GTKO/CMAHKO and TKO/hCD55 PBMCs (Figure 2G). In all sera, binding to GTKO/CMAHKO and TKO/CD55 PBMCs was minimal or absent.
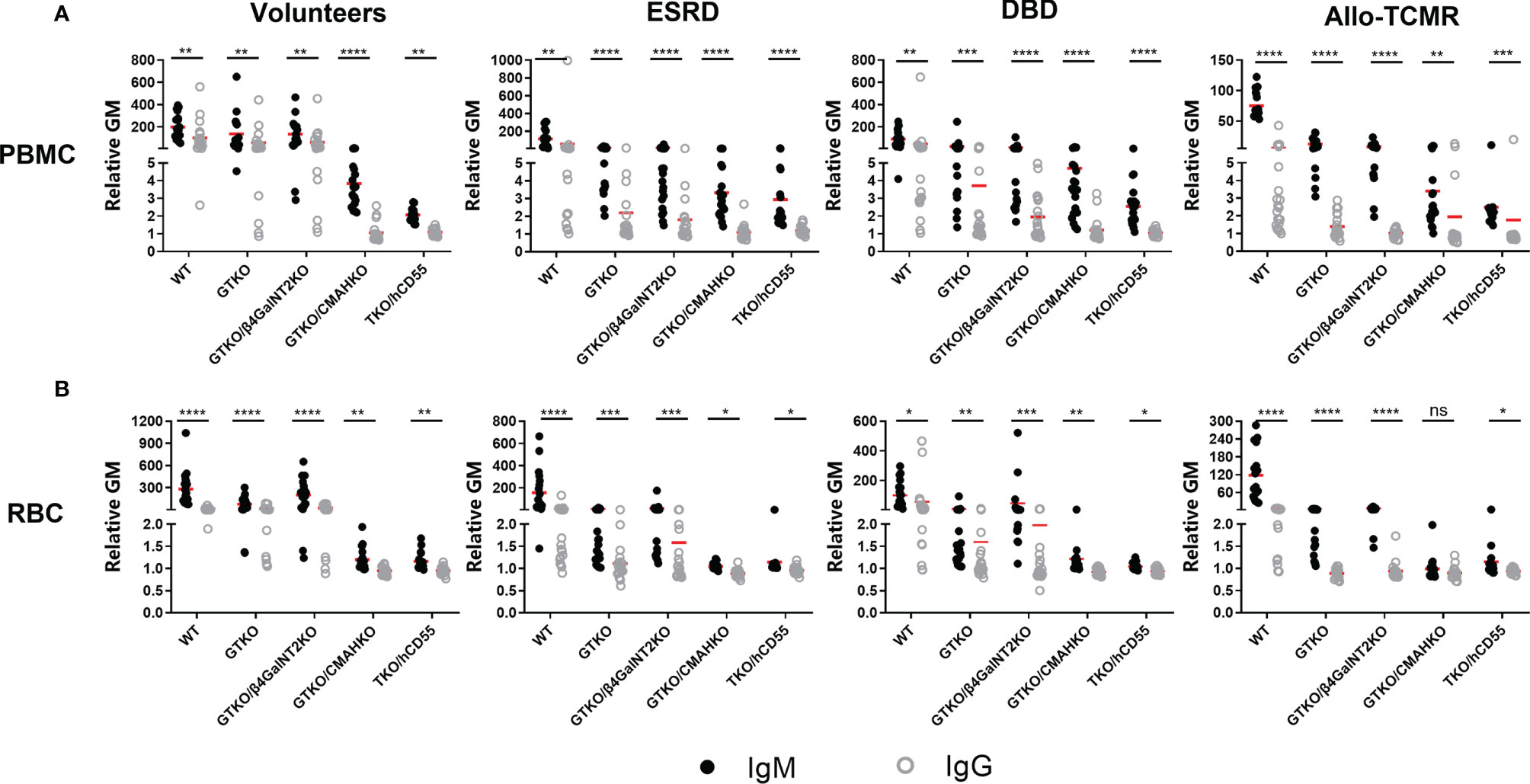
Figure 3 Comparison of serum IgM and IgG binding to PBMCs and RBCs. All human subjects had lower levels of IgG binding (compared to IgM) to WT, GTKO, GTKO/β4GalNT2KO, GTKO/CMAHKO, and TKO/hCD55 PBMCs (A) and RBCS (B). (*p<0.05, **p<0.01, ***p<0.005, ****p<0.001).
In healthy human sera (Volunteers), mean IgG binding was reduced by approximately 5% by GTKO (compared to WT) but by approximately 90% by CMAHKO (WT vs GTKO: 100 vs 58, p=ns; WT vs CMAHKO: 100 vs 1, p<0.01)
CDC
Although there were many variations in CDC depending on the serum and the source of PBMCs (Figure 2H), the most obvious finding was that CDC to GTKO/CMAHKO and, particularly, TKO/CD55 PBMCs was generally low or absent.
In healthy human sera (Volunteers), mean CDC was reduced by approximately 10% by GTKO (compared to WT) but by approximately 80% by CMAHKO (WT vs GTKO: 97 vs 88, p=ns; WT vs CMAHKO: 97 vs 25, p<0.001).
The Effect of Genetic-Engineering of Pig RBCs on Human IgM and IgG Binding by Flow Cytometry
IgM Binding
Mean serum IgM binding followed the same pattern as for IgM binding to PBMCs, with minimal binding to GTKO/CMAHKO and TKO/CD55 RBCs in all sera (Figure 2I).
IgG Binding
Mean serum IgG binding was lower in all sera than IgM binding (Figure 3B). Binding was greatest in the sera from Volunteers, but again was minimal to GTKO/CMAHKO and TKO/CD55 RBCs (Figure 2J).
Comparison of IgM/IgG Antibody Binding and CDC to Pig PBMCs or RBCs Between Hemodialysis Patients and Peritoneal Dialysis Patients
Patients with ESRD were divided into 2 groups based on whether they were undergoing hemodialysis (n=15) or peritoneal dialysis (n=5).
IgM/IgG Binding
There were no significant differences in IgM/IgG binding to WT, GTKO, GTKO/β4GalNT2KO, and TKO/hCD55 PBMCs and RBCs between hemodialysis and peritoneal dialysis patients (Figure 4), but serum IgM binding to GTKO/CMAHKO PBMCs and RBCs was significantly lower in hemodialysis patients than in peritoneal dialysis patients.
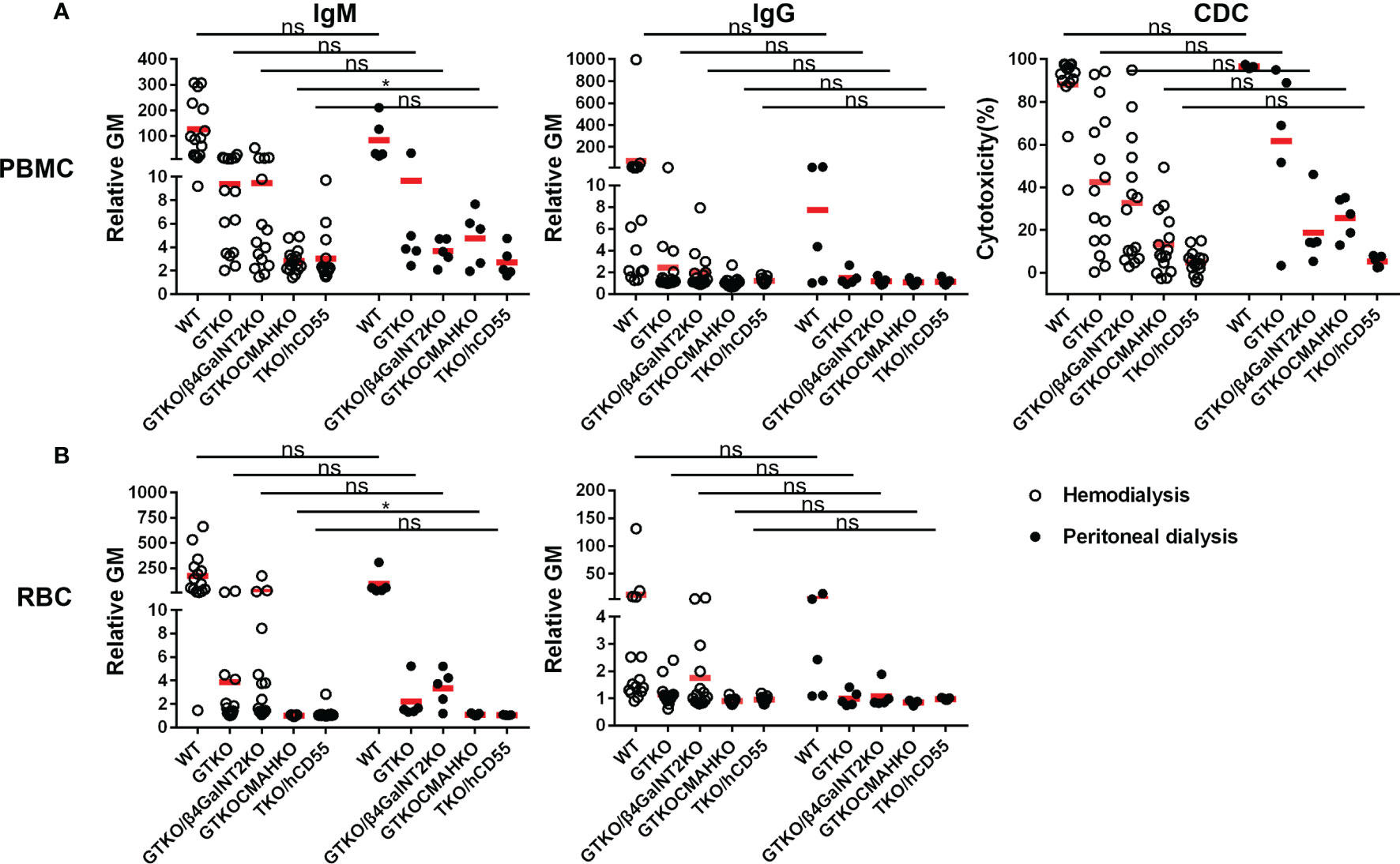
Figure 4 Comparison of serum IgM/IgG binding and CDC to PBMCs or RBCs from WT, GTKO, GTKO/β4GalNT2KO, GTKO/CMAHKO and TKO/hCD55 pig in patients receiving hemodialysis or peritoneal dialysis. (A) There were no differences in IgM/IgG binding or CDC to WT, GTKO, GTKO/β4GalNT2KO, and TKO/hCD55 PBMCs, but IgM binding to GTKO/CMAHKO to PBMCs was significantly lower in hemodialysis patients. (B) There were no differences in IgM/IgG binding to WT, GTKO, GTKO/β4GalNT2KO, GTKO/CMAHKO, and TKO/hCD55 RBCs, but IgM binding to GTKO/CMAHKO to RBCs was again significantly lower in hemodialysis patients. (*p<0.05; ns=not significant).
CDC
There was no significant difference in CDC to any cell type between hemodialysis and peritoneal dialysis patients (Figure 4).
Changes in the Levels of IgM and IgG Binding in Patients With ESRD Who Underwent Kidney Allotransplantation
Serum samples from renal allotransplant recipients were collected pretransplant (Pre), and on POD1 and POD14, and the levels of anti-pig IgM and IgG antibodies were measured (Figure 5). In the majority of patients (n=18), there was no change in IgM/IgG binding to PBMCs or RBCs between Pre, POD 1, and POD 14, although in some patients anti-pig IgM/IgG decreased transiently on POD1, but recovered to Pre levels by POD14. (This may possibly be related to hemodilution by perioperative fluid infusion.) However, in 2 of the recipients (red dot), serum IgG binding to GTKO PBMCs increased by POD14, compared with Pre and POD1. There was no change in IgG binding to GTKO RBCs (that do not express SLA), nor in binding to PBMCs and RBCs from the other pig genotypes.
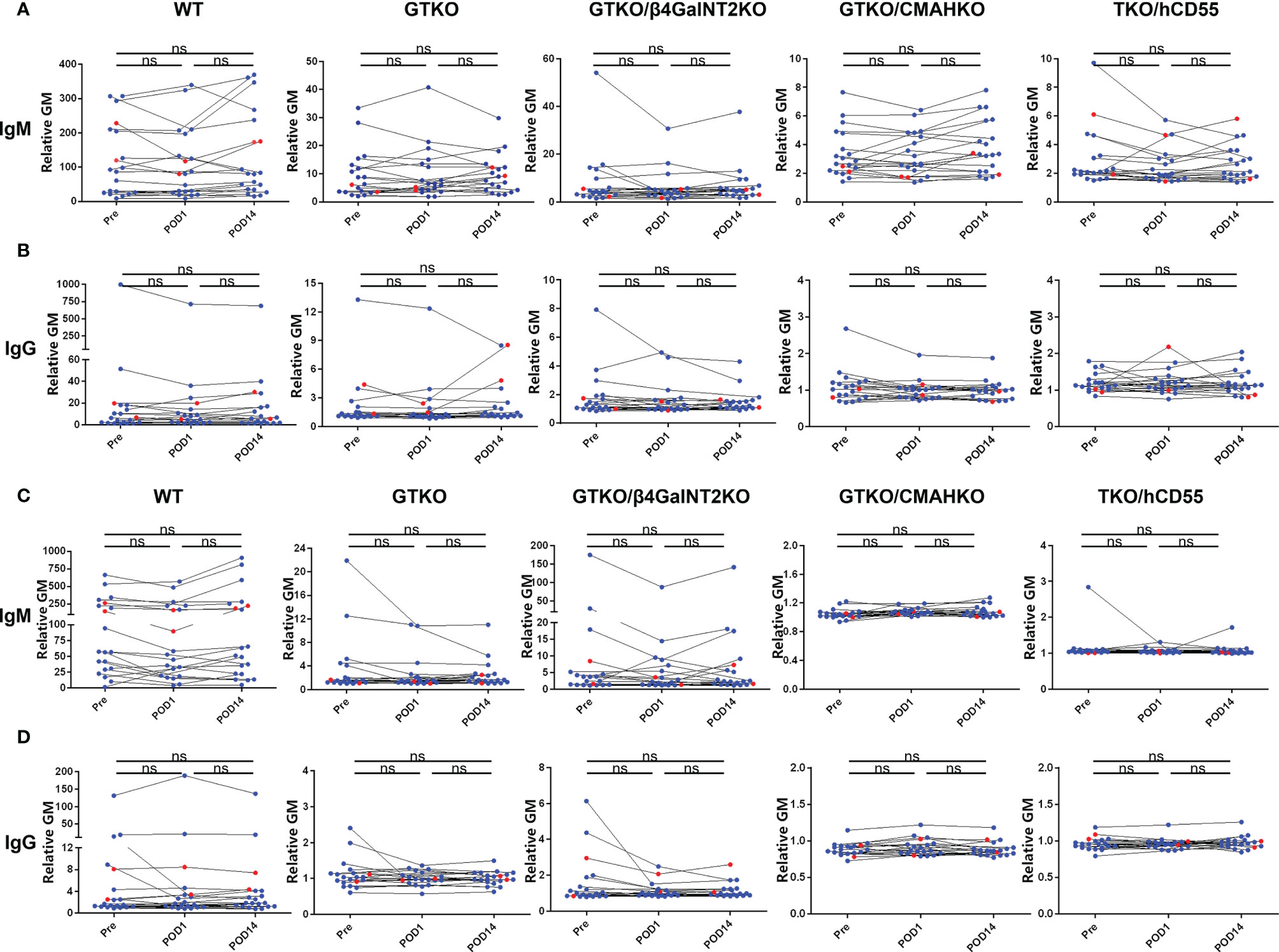
Figure 5 Changes in IgM and IgG binding to pig cells in patients with ESRD pre and early post-renal allotransplantation. Serum samples from renal allotransplant recipients were collected Pre, and on POD1 and POD14 to measure IgM (A) and IgG (B) binding to pig PBMCs or to pig RBCs (C, D). In the majority of cases, there were no significant changes in binding between the time-intervals, although in some there was a transient reduction in IgM and IgG binding on POD1 (possibly associated with hemodilution or binding to the pig cells) that had recovered to Pre levels by POD14. In two sera, IgG binding to GTKO PBMCs increased on POD14 compared with Pre and POD1, but there was no change in binding to GTKO RBCs, nor to pig cells of other genotypes. (ns, not significant).
Influence of ABO Blood Type of Healthy Human Volunteers on Serum IgM and IgG Binding and CDC to WT and Various Genetically-Modified Pig PBMCs or RBCs by Flow Cytometry
Healthy volunteers were divided into 4 groups based on ABO blood type.
IgM/IgG Binding
There were no significant differences in IgM/IgG binding to WT, GTKO, GTKO/β4GalNT2KO, and TKO/hCD55 PBMCs and RBCs in relation to ABO blood type, but IgM binding to GTKO/CMAHKO RBCs was significantly lower in sera from subjects with A blood type compared to those with O blood type (Figure 6).
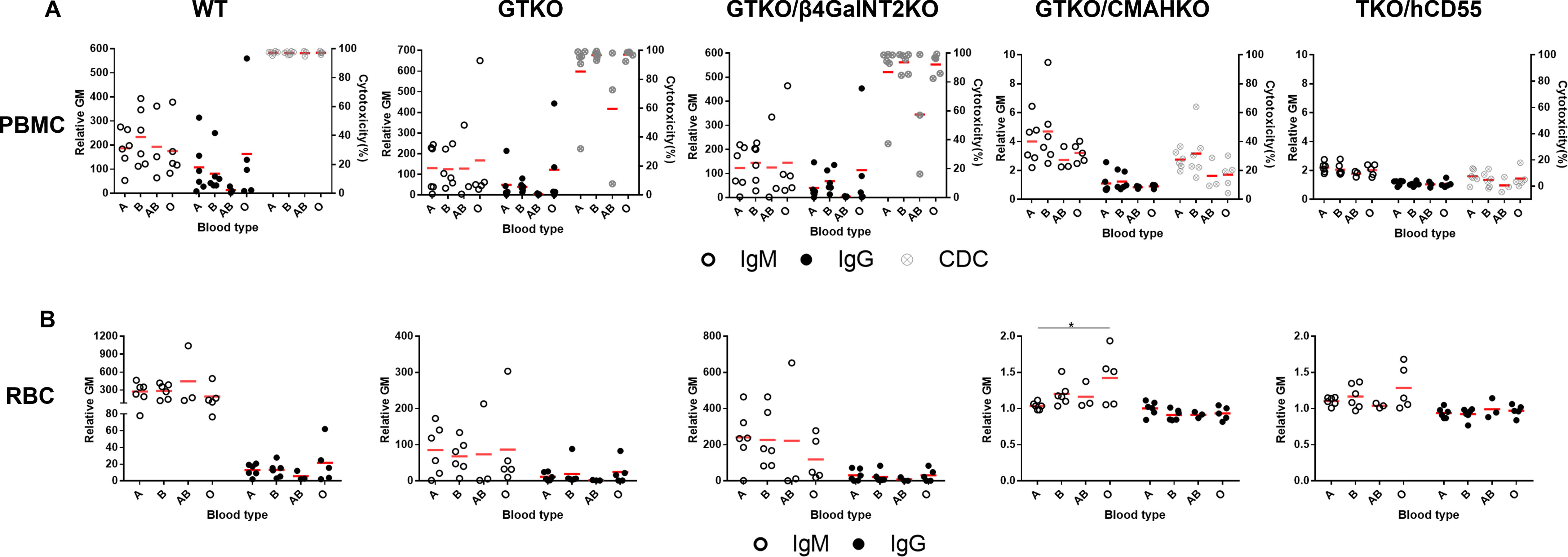
Figure 6 Comparison of IgM/IgG binding and CDC to PBMCs or RBCs from WT, GTKO, GTKO/β4GalNT2KO, GTKO/CMAHKO and TKO/hCD55 pigs in sera from healthy human volunteers of different ABO blood types. (A) There were no differences in IgM (left) or IgG (middle) binding or CDC (right) to WT, GTKO, GTKO/β4GalNT2KO, GTKO/CMAHKO, and TKO/hCD55 PBMCs in sera from humans of various ABO blood types. (B) There were no differences in IgM (left) or IgG (right) binding to WT, GTKO, GTKO/β4GalNT2KO, and TKO/hCD55 RBCs, but IgM binding to GTKO/CMAHKO RBCs was significantly lower in sera from A blood type subjects than in those of O blood type. (*p<0.05).
CDC
There was no significant difference in serum CDC between subjects of the four blood types (Figure 6).
Discussion
Effect of CMAHKO
The first important observation in this study was that our data showed that healthy volunteer serum IgM binding to WT, GTKO, GTKO/β4GalNT2KO PBMCs was significantly greater than to CMAHKO and TKO/hCD55 PBMCs, strongly suggesting that CMAHKO will be important for clinical renal xenotransplantation, as others have also reported (24, 25). However, deletion of expression of Neu5Gc appeared to play a more important role in reducing human antibody binding to the pig cells than deletion of expression of Gal, which is in contrast to some previous studies in which GTKO had a much greater impact than CMAHKO on IgM binding (14, 26). The reduction in IgM and IgG binding to GTKO pig cells when compared to WT cells was less than reported in most previous studies (25), but, in contrast, the reduction in binding and CDC was significantly greater after deletion of expression of Neu5Gc. This is most likely explained by differences in the antibody profiles of the Chinese participants in this study compared with those of some other ethnic groups.
Neu5Gc is expressed in pigs, apes and OWMs, but not in humans (27–29), and therefore only humans develop anti-Neu5Gc antibodies (30). Gao and her colleagues drew attention to the fact that natural antibodies are largely associated with exposure to glycans expressed on flora in the gastrointestinal tract (26), as suggested by others previously (31). In humans, anti-Neu5Gc antibodies develop during the first 6 months of life, and reach adult levels by the end of the first year (32). Both anti-Neu5Gc IgM and IgG increase soon after the infant is exposed to cow’s milk and baby foods containing red meat, which express Neu5Gc (32).
All of the subjects from whom blood was drawn in the present study were Chinese and had been resident in China throughout most of their lives, whereas those in other reported studies were from a variety of ethnic and geographic backgrounds. This suggests that maybe in Chinese patients (or in patients who have been exposed to Chinese environmental factors, e.g., diet, for a prolonged period of time), an absence of expression of Neu5Gc in the pig organ may be as important, if not more important, than absence of expression of Gal.
We therefore suggest that the Chinese subjects included in the present study expressed different gastrointestinal flora (perhaps based on differences in diet), than other groups that have been studied in other geographic regions (26, 33), thus rendering Neu5Gc a more important stimulus to natural antibody production. However, of interest, no correlation between diet and anti-pig antibody levels was found in a previous study in Taipei (34). There may other ‘ethnic’ or ‘environmental’ differences in other population groups that have not yet been investigated.
There are increasing in vitro data indicating that TKO pig organs will prove to be a major advance over GTKO organs for transplantation into humans (5, 7, 35) which is consistent with our conclusions from the present study.
Healthy Human Volunteers vs Other Groups
A second major observation made in this study was that (i) patients with ESRD, brain-dead donors, and immunosuppressed patients with kidney allografts generally had significantly lower levels of anti-pig antibodies than healthy human volunteers, except in regard to WT pig cells. The trends in CDC were similar to those of IgM and IgG. In addition, the ABO blood type of the donor of the serum appeared to play no part in influencing the results.
The clinical impact of ESRD on anti-pig immunity remains to some extent uncertain because immune dysfunction in ESRD includes both immunoactivation and immunosuppression. Heparin-induced antibodies (HIA) (36, 37) and anticardiolipin antibodies (IgG-ACA) (38) are elevated in ESRD and the complement system can be activated (39, 40). However, It is well-recognized that ESRD patients are to some extent immunocompromised (41, 42), e.g., reduced number of NK cells, reduced phagocytic activity of neutrophils (43). Anti-pig antibodies are low in infants (44) and in patients with ESRD (14), the last of which observations is consistent with our present results.
Patients with kidney allografts are receiving chronic immunosuppressive therapy, and presumably this has prevented an increase in antibody levels even though T cell activation had taken place in the patients we studied. The explanation in brain-dead donors is not so obvious, but may be associated with the infusion of fluids to maintain an adequate hemodynamic state, resulting in hemodilution.
However, there was no difference in serum IgM/IgG binding to GTKO/CMAHKO and TKO/hCD55 PBMCs or RBCs in the 4 groups, indicating that the effect of deletion of Neu5Gc expression on the pig cell was sufficient to reduce antibody binding and CDC to negligible levels whatever the source of the serum. Expression of CD55 on TKO pig PBMCs further reduced CDC of the cells.
Hemodialysis vs Peritoneal Dialysis
Our data showed that there was no significant difference in serum IgM/IgG binding to any of the pig cell types between hemodialysis and peritoneal dialysis patients, with the exception of lower IgM binding to GTKO/CMAHKO cells in patients on hemodialysis. This suggests that hemodialysis might remove anti-β4GalNT2 antibodies. However, it is unlikely that hemodialysis directly removes antibodies since standard hemodialysis filters typically have a cut-off size of between 10–20 kDa, whereas IgM/IgG molecules are >150 kDa, and are thus not removed by hemodialysis (45).
Experimental Models of Pig Organ Xenotransplantation
As is well-known, the most widely used xenotransplantation model is the genetically-modified pig-to-OWM. A major difference between humans and OWMs is that OWMs express Neu5Gc on the vascular endothelium, whereas humans do not. OWMs, therefore, are far from ideal models for xenotransplantation, as there is markedly increased OWM serum antibody binding and CDC to TKO pig cells. Although New World monkeys have some advantage in this respect (5), their small size and the ineffectiveness of some immunosuppressive drugs in them negates their suitability as a surrogate recipient for pig organ transplantation (46–50). Is there an alternative recipient as a surrogate for living humans?
Could DBD subjects be used as recipients in preclinical studies of xenotransplantation? Our data indicate that their levels of anti-pig antibodies are significantly lower than healthy volunteers (possibly associated with hemodilution through the need for fluid administration to maintain hemodynamic stability), and comparable to those in patients with ESRD. However, activation of innate immunity and inflammation can occur in brain-dead subjects (21). This observation, and because of their hemodynamic instability that may limit follow-up to days rather than months, reduces their suitability as potential surrogates for living recipients (51, 52).
Our observation that 2 of 20 patients undergoing kidney allotransplantation developed increased anti-pig antibody binding on POD14 may suggest that, at least in some patients, there may be cross-reactivity between anti-HLA antibodies and SLA epitopes, but the data are too few to draw any definite conclusions. (Unfortunately, anti-HLA antibodies were not investigated.)
Conclusions
In summary, on the basis of the present study, (i) CMAHKO in the pig may be critical to the success of clinical pig kidney xenotransplantation, and may be the most important after α1,3-galactosyltransferase gene be knockout, at least in Chinese patients; (ii) subjects with ESRD, or who are immunosuppressed after kidney allotransplantation, and brain-dead organ donors, all have lower levels of antibody binding and CDC to genetically-engineered pig cells than do healthy human volunteers; (iii) brain-dead subjects may mimic ESRD patients in that they both have low levels of anti-pig antibody levels, but experimental pig organ transplants in this group are unlikely to provide significant information of real value; (iv) TKO pigs with selected human ‘protective’ transgenes, e.g., CD55, are likely to prove to be optimal sources of kidneys for clinical xenotransplantation. (v) The role of SLAKO or SLA knockdown remains uncertain.
Data Availability Statement
The raw data supporting the conclusions of this article will be made available by the authors, without undue reservation.
Ethics Statement
The studies involving human participants were reviewed and approved by Ethics Committee of the Second Affiliated Hospital of Hainan Medical University. Written informed consent for participation was not required for this study in accordance with the national legislation and the institutional requirements. The animal study was reviewed and approved by ethics committee of the Hainan Medical University.
Author Contributions
YW and GC designed the experiments. TL, HF, JD, QX, and SH participated in the performance of the research and data analysis. DP provided transgenic pigs for experiments. TL, DC, and HJ prepared the figures and wrote the article. DC, YW, and GC critically revised the article. All authors contributed to the article and approved the submitted version.
Funding
This study was supported in part by the Major Scientific and Technological Project of Hainan province (ZDKJ2019009) and Hainan Provincial Natural Science Foundation of China (821QN413). The funder was not involved in the study design, collection, analysis, interpretation of data, the writing of this article or the decision to submit it for publication. Work on xenotransplantation at the Second Affiliated Hospital of Hainan Medical University, is supported in part by the Major Scientific and Technological Project of Hainan province (ZDKJ2019009) and Hainan Provincial Natural Science Foundation of China (821QN413).
Conflict of Interest
Author JD is employed by Chengdu Clonorgan Biotechnology Co., LTD.
The remaining authors declare that the research was conducted in the absence of any commercial or financial relationships that could be construed as a potential conflict of interest.
Publisher’s Note
All claims expressed in this article are solely those of the authors and do not necessarily represent those of their affiliated organizations, or those of the publisher, the editors and the reviewers. Any product that may be evaluated in this article, or claim that may be made by its manufacturer, is not guaranteed or endorsed by the publisher.
Supplementary Material
The Supplementary Material for this article can be found online at: https://www.frontiersin.org/articles/10.3389/fimmu.2022.844632/full#supplementary-material
Abbreviations
Allo-TCMR, allograft recipients who were currently experiencing T cell-mediated rejection; β4GalNT2KO, beta-1,4-N-acety1 galactosaminyltransferase 2-knockout; CMAHKO, cytidine monophosphate-N-acetylneuraminic acid hydroxylase-knockout; CDC, complement-dependent cytotoxicity; DBD, brain-dead donors; ESRD, end-stage renal disease; GTKO, α1,3-galactosyltransferase-knockout; HLA, human leukocyte antigens; hCD55, human complement-regulatory protein, CD55; OWMs, Old World monkeys; PBMCs, peripheral blood mononuclear cells; RBCs, red blood cells; SLA, swine leukocyte antigens; WT, wild-type.
References
1. Jagdale A, Cooper DKC, Iwase H, Gaston RS. Chronic Dialysis in Patients With End-Stage Renal Disease: Relevance to Kidney Xenotransplantation. Xenotransplantation (2019) 26:e12471. doi: 10.1111/xen.12471
2. Adams AB, Lovasik BP, Faber DA, Burlak C, Breeden C, Estrada JL, et al. Anti-C5 Antibody Tesidolumab Reduces Early Antibody-Mediated Rejection and Prolongs Survival in Renal Xenotransplantation. Ann Surg (2021) 274:473–80. doi: 10.1097/SLA.0000000000004996
3. Higginbotham L, Mathews D, Breeden CA, Song M, Farris AB 3rd, Larsen CP, et al. Pre-Transplant Antibody Screening and Anti-CD154 Costimulation Blockade Promote Long-Term Xenograft Survival in a Pig-to-Primate Kidney Transplant Model. Xenotransplantation (2015) 22:221–30. doi: 10.1111/xen.12166
4. Kim SC, Mathews DV, Breeden CP, Higginbotham LB, Ladowski J, Martens G, et al. Long-Term Survival of Pig-to-Rhesus Macaque Renal Xenografts is Dependent on CD4 T Cell Depletion. Am J Transplant (2019) 19:2174–85. doi: 10.1111/ajt.15329
5. Yamamoto T, Iwase H, Patel D, Jagdale A, Ayares D, Anderson D, et al. Old World Monkeys are Less Than Ideal Transplantation Models for Testing Pig Organs Lacking Three Carbohydrate Antigens (Triple-Knockout). Sci Rep (2020) 10:9771. doi: 10.1038/s41598-020-66311-3
6. Adams AB, Kim SC, Martens GR, Ladowski JM, Estrada JL, Reyes LM, et al. Xenoantigen Deletion and Chemical Immunosuppression can Prolong Renal Xenograft Survival. Ann Surg (2018) 268:564–73. doi: 10.1097/SLA.0000000000002977
7. Cui Y, Yamamoto T, Raza SS, Morsi M, Nguyen HQ, Ayares D, et al. Evidence for GTKO/β4galnt2ko Pigs as the Preferred Organ-Source for Old World Nonhuman Primates as a Preclinical Model of Xenotransplantation. Transplant Direct (2020) 6:e590. doi: 10.1097/TXD.0000000000001038
8. Estrada JL, Martens G, Li P, Adams A, Newell KA, Ford ML, et al. Evaluation of Human and non-Human Primate Antibody Binding to Pig Cells Lacking GGTA1/CMAH/β4galnt2 Genes. Xenotransplantation (2015) 22:194–202. doi: 10.1111/xen.12161
9. Albritton A, Leonard DA, Leto Barone A, Keegan J, Mallard C, Sachs DH, et al. Lack of Cross-Sensitization Between Alpha-1,3-Galactosyltransferase Knockout Porcine and Allogeneic Skin Grafts Permits Serial Grafting. Transplantation (2014) 97:1209–15. doi: 10.1097/TP.0000000000000093
10. Cooper DK, Tseng YL, Saidman SL. Alloantibody and Xenoantibody Cross-Reactivity in Transplantation. Transplantation (2004) 77:1–5. doi: 10.1097/01.TP.0000105116.74032.63
11. Hara H, Ezzelarab M, Rood PP, Lin YJ, Busch J, Ibrahim Z, et al. Allosensitized Humans are at No Greater Risk of Humoral Rejection of GT-KO Pig Organs Than Other Humans. Xenotransplantation (2006) 13:357–65. doi: 10.1111/j.1399-3089.2006.00319.x
12. Li Q, Hara H, Zhang Z, Breimer ME, Wang Y, Cooper DKC. Is Sensitization to Pig Antigens Detrimental to Subsequent Allotransplantation? Xenotransplantation (2018) 25:e12393. doi: 10.1111/xen.12393
13. Wong BS, Yamada K, Okumi M, Weiner J, O'Malley PE, Tseng YL, et al. Allosensitization Does Not Increase the Risk of Xenoreactivity to Alpha1,3-Galactosyltransferase Gene-Knockout Miniature Swine in Patients on Transplantation Waiting Lists. Transplantation (2006) 82:314–9. doi: 10.1097/01.tp.0000228907.12073.0b
14. Zhang Z, Hara H, Long C, Iwase H, Qi H, Macedo C, et al. Immune Responses of HLA Highly Sensitized and Nonsensitized Patients to Genetically Engineered Pig Cells. Transplantation (2018) 102:e195–204. doi: 10.1097/TP.0000000000002060
15. Fischer K, Rieblinger B, Hein R, Sfriso R, Zuber J, Fischer A, et al. Viable Pigs After Simultaneous Inactivation of Porcine MHC Class I and Three Xenoreactive Antigen Genes GGTA1, CMAH and B4GALNT2. Xenotransplantation (2020) 27:e12560. doi: 10.1111/xen.12560
16. Ladowski JM, Reyes LM, Martens GR, Butler JR, Wang ZY, Eckhoff DE, et al. Swine Leukocyte Antigen Class II Is a Xenoantigen. Transplantation (2018) 102:249–54. doi: 10.1097/TP.0000000000001924
17. Martens GR, Reyes LM, Li P, Butler JR, Ladowski JM, Estrada JL, et al. Humoral Reactivity of Renal Transplant-Waitlisted Patients to Cells From GGTA1/CMAH/B4GalNT2, and SLA Class I Knockout Pigs. Transplantation (2017) 101:e86–92. doi: 10.1097/TP.0000000000001646
18. Jagdale A, Kumar V, Anderson DJ, Locke JE, Hanaway MJ, Eckhoff DE, et al. Suggested Patient Selection Criteria for Initial Clinical Trials of Pig Kidney Xenotransplantation in the United States. Transplantation (2021) 105:1904–8. doi: 10.1097/TP.0000000000003632
19. Cooper DKC. Genetically Engineered Pig Kidney Transplantation in a Brain-Dead Human Subject. Xenotransplantation (2021) 28(6):e12718. doi: 10.1111/xen.12718
20. McKeown DW, Bonser RS, Kellum JA. Management of the Heartbeating Brain-Dead Organ Donor. Br J Anaesth (2012) 108 Suppl 1:i96–107. doi: 10.1093/bja/aer351
21. Barklin A. Systemic Inflammation in the Brain-Dead Organ Donor. Acta Anaesthesiol Scand (2009) 53:425–435w. doi: 10.1111/j.1399-6576.2008.01879.x
22. Iwase H, Ekser B, Zhou H, Liu H, Satyananda V, Humar R, et al. Further Evidence for Sustained Systemic Inflammation in Xenograft Recipients (SIXR). Xenotransplantation (2015) 22:399–405. doi: 10.1111/xen.12182
23. Li T, Lee W, Hara H, Long C, Ezzelarab M, Ayares D, et al. An Investigation of Extracellular Histones in Pig-to-Baboon Organ Xenotransplantation. Transplantation (2017) 101:2330–9. doi: 10.1097/TP.0000000000001676
24. Burlak C, Paris LL, Lutz AJ, Sidner RA, Estrada J, Li P, et al. Reduced Binding of Human Antibodies to Cells From GGTA1/CMAH KO Pigs. Am J Transplant (2014) 14:1895–900. doi: 10.1111/ajt.12744
25. Yamamoto T, Hara H, Iwase H, Jagdale A, Bikhet MH, Morsi MA, et al. The Final Obstacle to Successful Pre-Clinical Xenotransplantation? Xenotransplantation (2020) 27:e12596. doi: 10.1111/xen.12596
26. Gao B, Long C, Lee W, Zhang Z, Gao X, Landsittel D, et al. Anti-Neu5Gc and Anti-non-Neu5Gc Antibodies in Healthy Humans. PloS One (2017) 12:e0180768. doi: 10.1371/journal.pone.0180768
27. Bouhours D, Pourcel C, Bouhours JE. Simultaneous Expression by Porcine Aorta Endothelial Cells of Glycosphingolipids Bearing the Major Epitope for Human Xenoreactive Antibodies (Gal Alpha 1-3Gal), Blood Group H Determinant and N-Glycolylneuraminic Acid. Glycoconj J (1996) 13:947–53. doi: 10.1007/BF01053190
28. Miwa Y, Kobayashi T, Nagasaka T, Liu D, Yu M, Yokoyama I, et al. Are N-Glycolylneuraminic Acid (Hanganutziu-Deicher) Antigens Important in Pig-to-Human Xenotransplantation? Xenotransplantation (2004) 11:247–53. doi: 10.1111/j.1399-3089.2004.00126.x
29. Padler-Karavani V, Varki A. Potential Impact of the non-Human Sialic Acid N-Glycolylneuraminic Acid on Transplant Rejection Risk. Xenotransplantation (2011) 18:1–5. doi: 10.1111/j.1399-3089.2011.00622.x
30. Zhu A, Hurst R. Anti-N-Glycolylneuraminic Acid Antibodies Identified in Healthy Human Serum. Xenotransplantation (2002) 9:376–81. doi: 10.1034/j.1399-3089.2002.02138.x
31. Galili U, Mandrell RE, Hamadeh RM, Shohet SB, Griffiss JM. Interaction Between Human Natural Anti-Alpha-Galactosyl Immunoglobulin G and Bacteria of the Human Flora. Infect Immun (1988) 56:1730–7. doi: 10.1128/iai.56.7.1730-1737.1988
32. Taylor RE, Gregg CJ, Padler-Karavani V, Ghaderi D, Yu H, Huang S, et al. Novel Mechanism for the Generation of Human Xeno-Autoantibodies Against the Nonhuman Sialic Acid N-Glycolylneuraminic Acid. J Exp Med (2010) 207:1637–46. doi: 10.1084/jem.20100575
33. Kumar G, Satyananda V, Fang J, Zhou H, Fujita M, Ekser B, et al. Is There a Correlation Between Anti-Pig Antibody Levels in Humans and Geographic Location During Childhood? Transplantation (2013) 96:387–93. doi: 10.1097/TP.0b013e3182992a84
34. Chao SH, Chu SH, Lin CY, Hsieh RP, Chen KM. Epidemiologic Profile of Anti-Pig Natural Antibodies in the Taiwan Population. Transplant Proc (1994) 26:1369–71.
35. Cooper DKC, Hara H, Iwase H, Yamamoto T, Li Q, Ezzelarab M, et al. Justification of Specific Genetic Modifications in Pigs for Clinical Organ Xenotransplantation. Xenotransplantation (2019) 26:e12516. doi: 10.1111/xen.12516
36. Asmis LM, Segal JB, Plantinga LC, Fink NE, Kerman JS, Kickler TS, et al. Heparin-Induced Antibodies and Cardiovascular Risk in Patients on Dialysis. Thromb Haemost (2008) 100:498–504. doi: 10.1160/TH08-03-0144
37. Zhao D, Sun X, Yao L, Lin H, Li J, Zhao J, et al. The Clinical Significance and Risk Factors of Anti-Platelet Factor 4/Heparin Antibody on Maintenance Hemodialysis Patients: A Two-Year Prospective Follow-Up. PloS One (2013) 8:e62239. doi: 10.1371/journal.pone.0062239
38. Prakash R, Miller CC 3rd, Suki WN. Anticardiolipin Antibody in Patients on Maintenance Hemodialysis and its Association With Recurrent Arteriovenous Graft Thrombosis. Am J Kidney Dis (1995) 26:347–52. doi: 10.1016/0272-6386(95)90656-8
39. Craddock PR, Fehr J, Brigham KL, Kronenberg RS, Jacob HS. Complement and Leukocyte-Mediated Pulmonary Dysfunction in Hemodialysis. N Engl J Med (1977) 296:769–74. doi: 10.1056/NEJM197704072961401
40. Tang S, Leung JC, Chan LY, Tsang AW, Chen CX, Zhou W, et al. Regulation of Complement C3 and C4 Synthesis in Human Peritoneal Mesothelial Cells by Peritoneal Dialysis Fluid. Clin Exp Immunol (2004) 136:85–94. doi: 10.1111/j.1365-2249.2004.02407.x
41. Litjens NH, Boer K, Zuijderwijk JM, Klepper M, Peeters AM, Verschoor W, et al. Natural Regulatory T Cells From Patients With End-Stage Renal Disease can be Used for Large-Scale Generation of Highly Suppressive Alloantigen-Specific Tregs. Kidney Int (2017) 91:1203–13. doi: 10.1016/j.kint.2016.09.043
42. Vaziri ND, Pahl MV, Crum A, Norris K. Effect of Uremia on Structure and Function of Immune System. J Ren Nutr (2012) 22:149–56. doi: 10.1053/j.jrn.2011.10.020
43. Pappas EM, Mpournaka S, Katopodis P, Chardalias A, Tsakas S, Theodoros T, et al. The Effect of Dialysis Modality and Membrane Performance on Native Immunity in Dialysis Patients. Pril (Makedon Akad Nauk Umet Odd Med Nauki) (2019) 40:25–32. doi: 10.2478/prilozi-2019-0011
44. Li Q, Hara H, Banks CA, Yamamoto T, Ayares D, Mauchley DC, et al. Anti-Pig Antibody in Infants: Can a Genetically Engineered Pig Heart Bridge to Allotransplantation? Ann Thorac Surg (2020) 109:1268–73. doi: 10.1016/j.athoracsur.2019.08.061
45. Gondouin B, Hutchison CA. High Cut-Off Dialysis Membranes: Current Uses and Future Potential. Adv Chronic Kidney Dis (2011) 18:180–7. doi: 10.1053/j.ackd.2011.02.006
46. Iwase H, Hara H, Ezzelarab M, Li T, Zhang Z, Gao B, et al. Immunological and Physiological Observations in Baboons With Life-Supporting Genetically Engineered Pig Kidney Grafts. Xenotransplantation (2017) 24:10.1111/xen.12293. doi: 10.1111/xen.12293
47. Iwase H, Klein EC, Cooper DK. Physiologic Aspects of Pig Kidney Transplantation in Nonhuman Primates. Comp Med (2018) 68:332–40. doi: 10.30802/AALAS-CM-17-000117
48. Iwase H, Liu H, Wijkstrom M, Zhou H, Singh J, Hara H, et al. Pig Kidney Graft Survival in a Baboon for 136 Days: Longest Life-Supporting Organ Graft Survival to Date. Xenotransplantation (2015) 22:302–9. doi: 10.1111/xen.12174
49. Tanabe T, Watanabe H, Shah JA, Sahara H, Shimizu A, Nomura S, et al. Role of Intrinsic (Graft) Versus Extrinsic (Host) Factors in the Growth of Transplanted Organs Following Allogeneic and Xenogeneic Transplantation. Am J Transplant (2017) 17:1778–90. doi: 10.1111/ajt.14210
50. Yamamoto T, Ladowski JM, Bikhet M, Cooper DKC, Hara H. Efficacy of ATG and Rituximab in Capuchin Monkeys (a New World Monkey)-An In Vitro Study Relevant to Xenotransplantation. Xenotransplantation (2020) 27:e12627. doi: 10.1111/xen.12627
51. Carpenter DJ, Granot T, Matsuoka N, Senda T, Kumar BV, Thome JJC, et al. Human Immunology Studies Using Organ Donors: Impact of Clinical Variations on Immune Parameters in Tissues and Circulation. Am J Transplant (2018) 18:74–88. doi: 10.1111/ajt.14434
Keywords: brain-dead organ donors, complement-mediated cytotoxicity, end-stage renal disease, kidney, pig, xenotransplantation
Citation: Li T, Feng H, Du J, Xia Q, Cooper DKC, Jiang H, He S, Pan D, Chen G and Wang Y (2022) Serum Antibody Binding and Cytotoxicity to Pig Cells in Chinese Subjects: Relevance to Clinical Renal Xenotransplantation. Front. Immunol. 13:844632. doi: 10.3389/fimmu.2022.844632
Received: 28 December 2021; Accepted: 18 February 2022;
Published: 10 March 2022.
Edited by:
Hanchao Gao, Shenzhen Longhua District Central Hospital, ChinaReviewed by:
Yifan Dai, Nanjing Medical University, ChinaYuanzheng Peng, Shenzhen Third People’s Hospital, China
Copyright © 2022 Li, Feng, Du, Xia, Cooper, Jiang, He, Pan, Chen and Wang. This is an open-access article distributed under the terms of the Creative Commons Attribution License (CC BY). The use, distribution or reproduction in other forums is permitted, provided the original author(s) and the copyright owner(s) are credited and that the original publication in this journal is cited, in accordance with accepted academic practice. No use, distribution or reproduction is permitted which does not comply with these terms.
*Correspondence: Yi Wang, d2F5bmUwMTA4QDEyNi5jb20=; Gang Chen, Z2NoZW5AdGpoLnRqbXUuZWR1LmNu; Dengke Pan, cGFuZGVuZ2tlMjAwMkAxNjMuY29t
†These authors have contributed equally to this work