- Department of Nephrology, The Second Hospital of Jilin University, Changchun, China
Lupus nephritis (LN) is a significant cause of various acute and chronic renal diseases, which can eventually lead to end-stage renal disease. The pathogenic mechanisms of LN are characterized by abnormal activation of the immune responses, increased cytokine production, and dysregulation of inflammatory signaling pathways. LN treatment is an important issue in the prevention and treatment of systemic lupus erythematosus. Mesenchymal stem cells (MSCs) have the advantages of immunomodulation, anti-inflammation, and anti-proliferation. These unique properties make MSCs a strong candidate for cell therapy of autoimmune diseases. MSCs can suppress the proliferation of innate and adaptive immune cells, such as natural killer cells (NKs), dendritic cells (DCs), T cells, and B cells. Furthermore, MSCs suppress the functions of various immune cells, such as the cytotoxicity of T cells and NKs, maturation and antibody secretion of B cells, maturation and antigen presentation of DCs, and inhibition of cytokine secretion, such as interleukins (ILs), tumor necrosis factor (TNF), and interferons (IFNs) by a variety of immune cells. MSCs can exert immunomodulatory effects in LN through these immune functions to suppress autoimmunity, improve renal pathology, and restore kidney function in lupus mice and LN patients. Herein, we review the role of immune cells and cytokines in the pathogenesis of LN and the mechanisms involved, as well as the progress of research on the immunomodulatory role of MSCs in LN.
Introduction
Systemic lupus erythematosus (SLE) is a chronic autoimmune inflammatory disease with manifestations of multi-organ damage due to extensive deposition of immune complexes (IC). Kidney involvement in SLE is lupus nephritis (LN), and approximately 20–60% of SLE cases eventually develop LN (1) and is the main predictor of poor prognosis in SLE. The development of LN is based on the loss of immune tolerance to self-nuclear antigens and inflammation induced by the IC. Deposition of antigen-antibody complexes in the kidney induces LN, which triggers an inflammatory cascade that includes complement activation, activation of Fc receptors, activation of renal lamina propria cells and aggregation of inflammatory cells, and further activation of the immune system by mediators released by tissue injury, T cells, B cells, dendritic cells (DCs), macrophages, and the cytokines they produce. This can ultimately lead to LN by disrupting immune tolerance and inducing the onset of inflammation (2, 3). Despite significant advances in the diagnosis and treatment of SLE, its prevalence has increased over time (4) In addition, the treatment of refractory LN has become a subject of great interest as some refractory patients do not achieve the expected efficacy with standard therapies (hormones and immunosuppressants) and experience considerable side effects (infections and secondary malignancies).
Mesenchymal stem cells (MSCs) are pluripotent stem cells with abundant sources, such as bone marrow, umbilical cord, umbilical blood, adipose tissue, and embryonic tissue (5). MSCs have been shown to have immunomodulatory abilities and reduce inflammatory responses (6). Mesenchymal stem cell transplantation (MSCT) has been used to treat a variety of autoimmune diseases, including SLE, and has benefited patients who are resistant to conventional therapies (7, 8). In recent years, research related to stem cell therapy for LN has been rapidly developing. In this review, we focus on the role of various immune cells and cytokines in LN and how MSCs exert immunomodulatory activity in LN by regulating the corresponding cells or cytokines, which provides a reference for MSCs to target and regulate specific cells and cytokines.
Defective MSCs in SLE
One research study found that there was no treatment effect within 14 days after autologous MSCT in two SLE patients (9). Other numerous reports have suggested that MSCs in SLE are defective and may be involved in the pathogenesis of SLE, and therefore, SLE is assumed to be a “stem cell disease”. MSCs of SLE patients grow more slowly than those of healthy individuals and have a lower proliferative capacity; normal MSCs are uniformly shuttle-shaped, but MSCs of SLE patients become bulky and flattened from the third generation onwards (10). MSCs from SLE patients show signs of senescence such as deep staining of nuclei, disrupted F-actin cytoskeleton, increased reactive oxygen species production, increased senescence associated β-galactosidase staining, increased telomerase activity, and increased DNA damage and repair (10–12). The endoplasmic reticulum stress response induces G1 cell cycle arrest in bone marrow MSCs of SLE patients and is involved in the senescence of MSCs in SLE patients (13). The mitochondrial antiviral signaling protein (MAVS) is an articulatory protein that induces IFN-β, and the MAVS-IFNβ-positive feedback loop mediates the senescence of MSCs in SLE (12). Studies have shown that aberrant activation of several signaling pathways, such as Wnt/β-catenin (14), p53/p21 (14), PI3K/Akt (15), PTEN/Akt-p27 (16), and JAK-STAT (11), is involved in the aging process of MSCs in SLE. At the same time, MSCs in SLE exhibit impaired migration, differentiation, and immunomodulation (17, 18). Expression levels of mRNA for IL-6 and IL-17 are downregulated in MSCs from SLE patients (10). Indoleamine 2,3 dioxygenase (IDO) is a tryptophan metabolizing enzyme. The mRNA level of IDO secreted by bone marrow MSCs from patients with active lupus was significantly reduced, affecting its suppressive effect on T cells (19). Decreased CCL2 expression in bone marrow MSCs of SLE affects their inhibitory effect on B cells (17). These findings suggest that MSCs from SLE are defective.
Immunomodulatory Activity of MSCs on Immune Cell Subsets in LN
SLE is characterized by T-cell dysfunction and polyclonal B-cell activation, and various immune cells and cytokines are involved in the pathogenesis of LN (20). Owing to their unique immunomodulatory properties (21), MSCs are strong candidates for cell therapy and can be used in a wide range of immune-related diseases. MSCs can polarize various immune cells to an inactive or anti-inflammatory state (22), while inhibiting their proliferation and attenuating cytotoxicity (Figure 1).
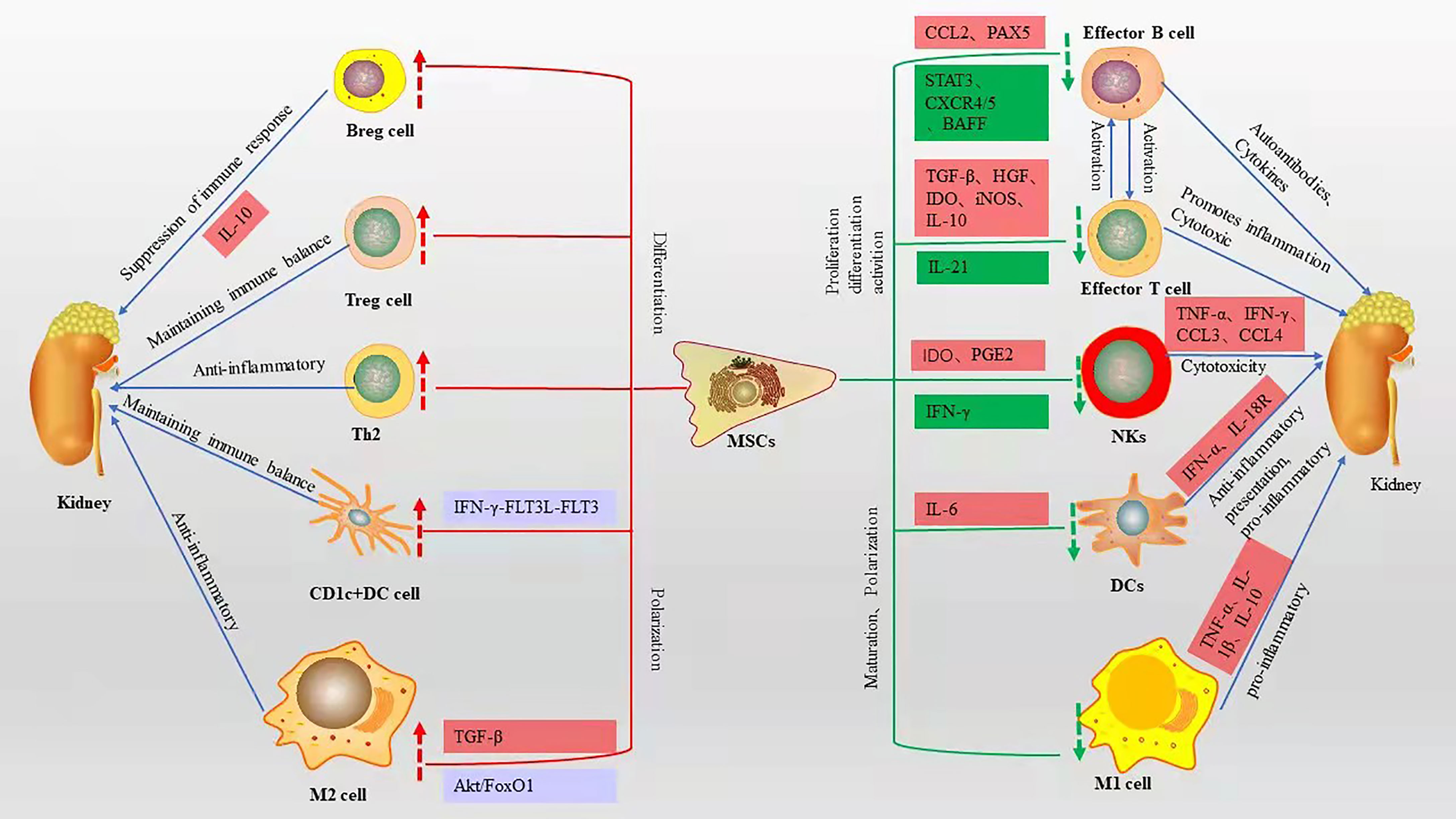
Figure 1 Immunomodulatory effects of MSCs on various immune cells and related mechanisms, red boxes represent elevated, green boxes represent decreased, blue boxes represent pathways or signaling axes. The red up arrow represents an upward adjustment and the green down arrow a downward adjustment.
Immunomodulatory Activity of MSCs on B Cells
B cells are a hallmark of the adaptive immune system. Researchers have shown lupus-prone mice lacking B cells do not develop nephritis (23). In contrast, lupus-like renal disease occurs in wild-type mice injected with lupus-derived autoantibodies (24). B cells can produce autoantibodies against nuclear proteins and DNA, especially anti-double-stranded DNA (anti-dsDNA) (25). Meanwhile, B cells can act as antigen-presenting cells and promote the activation of T cells in MRL-lpr/lpr mice (26), and different subpopulations of B cells produce large amounts of cytokines involved in immune regulation (27). Regulatory B cells (Breg) are a class of B cells with immunomodulatory functions that play an important role in maintaining immune tolerance and suppressing harmful immune responses (28). Plasma cells are effector B cells and are present in large numbers in the tubulointerstitium of SLE-prone NZB/W mice, and the degree of infiltration correlates positively with ds-DNA-IgG titer and the severity of LN pathology (29). B cell activating factor (BAFF) is a TNF family cytokine secreted mainly by DCs, macrophages, and neutrophils, and the development and survival of B cells depend on BAFF stimulation (30). CD4+ and CD8+ T cells from SLE patients express BAFF mRNA, but not in normal subjects (31). Severe proteinuria and mortality were shown to be significantly reduced in BAFF-deficient 6–7-month-old NEM mice (32), in addition, BAFF transgenic (Tg) mice showed a large increase in the number of mature B cells and effector T cells, similar to human SLE, anti-dsDNA antibodies, and immunoglobulin deposits in the kidney (33).
MSCs can affect the proliferation and differentiation of B cells, reduce plasma cell production, decrease the secretion of immunoglobulins, and inhibit B cell chemotaxis (34). The MSC-derived chemokine ligand 2 (CCL2) inhibits plasma cell production of immunoglobulins by suppressing activation of transcription factor 3 (STAT3) and inducing transcription factor PAX5 (17, 35). MSCs can downregulate chemokine receptor 4/5(CXCR4/CXCR5) chemotactic receptors, thereby inhibiting B cell chemotaxis (36). Ma et al. (37) showed that bone marrow MSCs derived from BALB/c mice injected intravenously into MRL/lpr mice inhibited B cell maturation and differentiation by suppressing BAFF production, resulting in a significant reduction in proteinuria and glomerular inflammation. Meanwhile, in vitro experiments showed that bone marrow MSCs from BALB/c mice may inhibit the decrease in BAFF levels secreted by DCs through downregulation of IL-10 and upregulation of transforming growth factor (TGF-β), leading to B cell activation and immunoglobulin production. Transplantation of human-derived adipose MSCs into Roquin san/san C57BL/6 mice via tail vein increased IL-10-producing Breg cells and significantly improved kidney tract proliferation and interstitial inflammatory cell infiltration, as well as increased IL-10-producing Breg cells in vitro (38). Human gingival-derived MSCs inhibit B cell proliferation, activation, plasma cell differentiation, and improve LN symptoms such as reduced proteinuria and glomerulonephritis, which through the CD39-CD73 signaling axis in vitro and in vivo (39). These studies suggest that MSCs can improve LN symptoms by inhibiting the proliferation, differentiation, and chemotaxis of B cells (Figure 2).
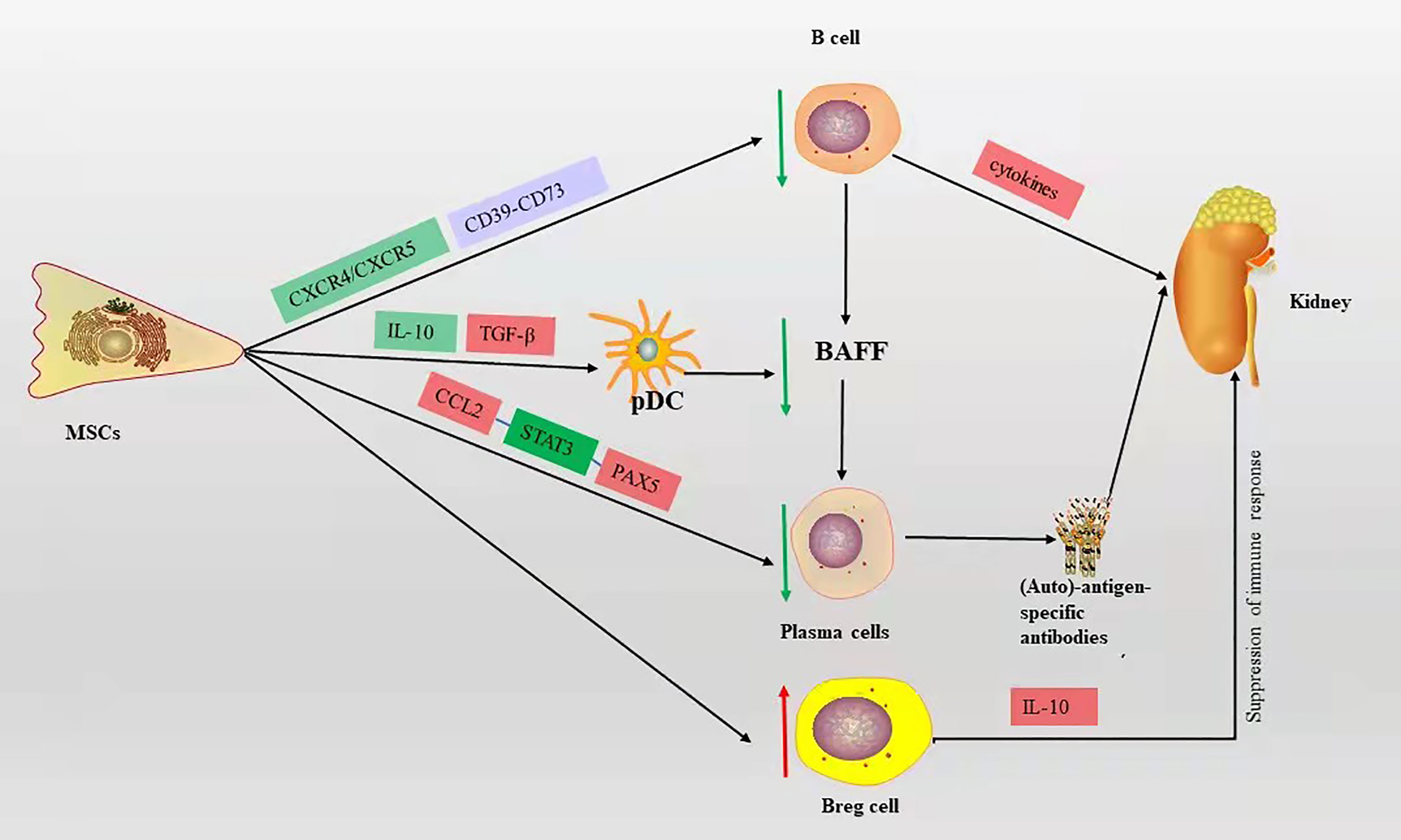
Figure 2 The mechanism of B cell action on LN and the immunomodulatory mechanism of MSCs on B cells in LN, red box represents elevation, green box represents decrease, blue box represents pathway or signaling axis. The red up arrow represents an upward adjustment and the green down arrow a downward adjustment.
Immunomodulatory Activity of MSCs on T Cells
T cells play a central and multiple role in LN and are another type of adaptive immune cell. T cells amplify the inflammatory response by secreting various proinflammatory cytokine cascades and assist B cells to produce autoantibodies. Imbalance of T cell subsets can affect the course of LN. Large numbers of T cells infiltrate the LN kidney tissue through cytotoxicity or by promoting the activation and recruitment of macrophages and NKs, which directly or indirectly damage renal parenchymal cells (40). Many functional defects of CD4+ T cells in SLE patients (41, 42). Activated CD4+ T cells can differentiate into various T helper (Th) subpopulations with different functions, such as Th1, Th2, Th17, follicular helper T (Tfh) cells, and regulatory T (Treg) cells (43, 44). TGF-β and hepatocyte growth factor (HGF) mediate the inhibition of T cell proliferation by MSCs, leading to a decrease in cyclin D2 and an increase in p27 kip1z at the molecular level, resulting in stagnation of T cell proliferation in G1 phase (45, 46). Plumas et al. (47) showed that the conversion of tryptophan to kynurenine by MSC-derived IDO in the presence of IFN-γ induces apoptosis in activated T cells. Bone marrow MSCs mediate T cell apoptosis via the FAS ligand/FAS pathway in the systemic sclerosis disease model (48). However, the lack of functional Fas in B6.lpr mice leads to a defect in the T cell apoptotic process, and it has been shown that umbilical cord MSCs promote apoptosis of CD4+ T cells in B6.lpr mice and have a significant effect in an in vitro setting, the mechanism of which is not yet clear (49). MSCs induce a shift from a proinflammatory to an anti-inflammatory state in T cells (50). Collectively, these reports show that MSCs improve LN by suppressing T cell proliferation, promoting apoptosis, and improving inflammatory status (Figure 3).
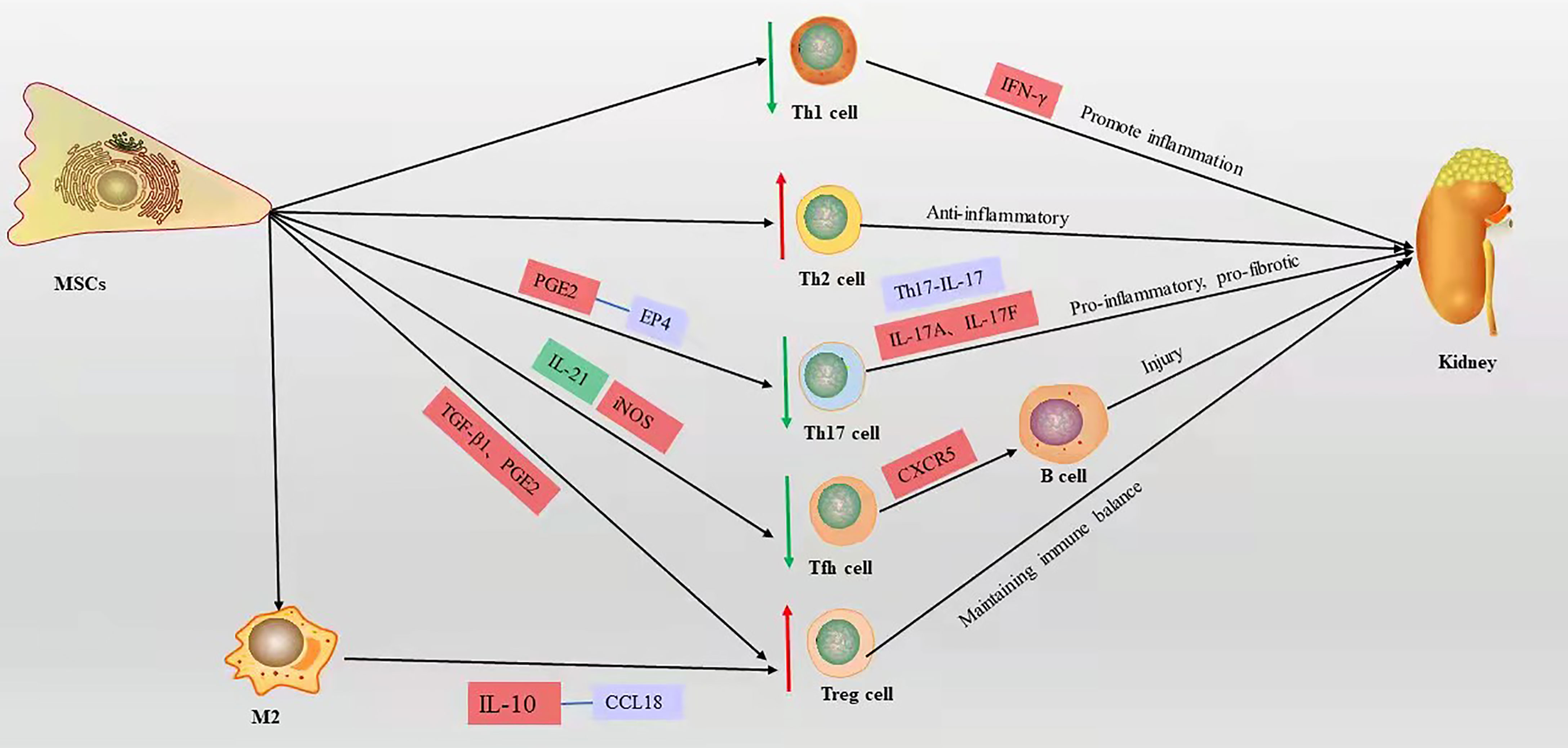
Figure 3 The mechanism of T cell action on LN and the immunomodulatory mechanism of MSCs on T cells in LN, red box represents elevation, green box represents decrease, blue box represents pathway or signaling axis. The red up arrow represents an upward adjustment and the green down arrow a downward adjustment.
Immunomodulatory Activity of MSCs on Th1/Th2
An imbalance in T cell subsets severely affects the disease process in LN. Elevated Th1/Th2 (IFN-γ/IL-4) ratios in peripheral blood is a characteristic feature of LN, and the balance of Th1/Th2 in peripheral cells of LN patients shows a polarization toward the Th1 phenotype, and the expression level of IFN-γ produced by Th1 cells parallels the severity of renal injury (51). Sigdel et al. (52) showed that Th1 phenotype cells predominate in peripheral blood in SLE with diffuse proliferative LN. As in human diffuse proliferative LN, MRL/lpr mouse nephritis was associated with a polarized Th1 cell phenotype (53). Allogeneic chondrogenic MSCs suppress the differentiation and proliferation of pro-inflammatory Th1 cells and promote the differentiation of T cells into the anti-inflammatory Th2 subpopulation (54). Choi et al. (55) demonstrated that long-term continuous administration of human adipose tissue-derived MSCs downregulated the Th1/Th2 ratio in the spleen of C3.MRL-Fas lpr/J mice, significantly reduced anti-dsDNA levels, and decreased renal inflammatory cell infiltration and glomerular C3 deposition. Human umbilical cord-derived MSCs reduced the Th1/Th2 ratio in the spleen of B6.lpr mice, improved renal function, and reduced infiltration of renal lesions and inflammatory cells (49). Thus, MSCs improve LN by balancing the Th1/Th2 ratio.
Immunomodulatory Activity of MSCs on Th17/Treg
Sakaguchi et al. (56) isolated a unique type of CD4+ CD25+ T cells capable of suppressing immune responses and maintaining immune tolerance, which were later named Treg cells. The presence of a limited number and/or impaired function of Treg cells in patients with SLE is associated with increased disease activity (57, 58). The defective immune response of Treg cells in SLE is characterized by reduced expression of CD4, CD25, and Forkhead box P3 (Foxp3), known as inducible Treg (iTreg) (59). Foxp3 is a transcription factor that is important for Treg cell development, function, and maintenance of immune homeostasis (60, 61). IL-17A and IL-17F are the major cytokines secreted by Th17 cells (62), and their receptors are expressed in most renal cells and are involved in activating pro-inflammatory and pro-fibrotic pathways. Th17 is involved in the pathogenesis of LN (63, 64); Th17/IL-17 axially recruits Th17 cells in the kidney and is involved in pro-inflammatory responses and pro-fibrotic actions in the kidney, leading to renal fibrosis and loss of renal function (65). The increase in peripheral Th17 cells in LN patients is accompanied by a decrease in Tregs, suggesting that Th17/Treg imbalance is involved in LN disease progression (64). In vitro, unstimulated bone marrow MSCs from BALB/c mice secrete TGF-β to induce Foxp3 expression to promote Treg cell development (66). As with TGF-β1, PGE2 secreted by human MSCs derived from bone marrow induces Foxp3+ Treg cell differentiation in vitro (67). Darlan et al. (59) showed that TGF-β1 released from human umbilical cord MSCs promotes the production of iTreg cells in peripheral blood mononuclear cells from lupus patients, characterized by Foxp3 expression. In vitro experiments showed that inhibition of CD4+ T cell differentiation to Th17 by MSCs derived from mouse bone marrow is triggered by cell-cell contacts and mediated by prostaglandin E2 (PGE2) via receptor 4 (EP4) (68). Transplantation of human umbilical cord MSCs into SLE patients decreased peripheral blood Th17 cells and elevated Treg cells, which were confirmed to be mediated by PGE2 and TGF-β secreted by MSCs in in vitro experiments (69). Makino et al. (70) showed that human supernumerary tooth-derived stem cells upregulate Tregs and downregulate Th17 activity and IL-17 in the kidney in MRL/lpr mice, while improving renal function, reducing collagen and IgG deposition in the glomerulus, and thinning the basement membrane. These studies indicate that MSCs directly upregulate Tregs or downregulate Th17 cells, thereby improving SLE or LN via the regulation of the Th17/Treg ratio.
Immunomodulatory Activity of MSCs on Tfh Cells
Tfh cells are a specialized subset of CD4+ T cells that are located in B cell follicles. Studies have demonstrated that dysregulated Tfh cells are present in both SLE patients and animal models (71–74). Tfh cells can migrate into B cell follicles via CXCR5, leading to germinal center formation, promote the differentiation of B cells in the germinal centers into memory B cells or plasma cells (75). Studies showed that upon the existence of a defective checkpoint in the maintenance of peripheral B cell tolerance, which appears to be specific to patients with SLE, Tfh cells provide their cognate response B cells with the essential help needed to survive and pass the tolerance checkpoint (76, 77). Moreover, before B cells in the germinal center differentiate into memory B cells or plasma cells, Tfh cells signal B cells to undergo Ab class switching and affinity maturation (76). Studies have shown that in MRL/lpr mice, large numbers of Tfh cells are closely associated with excessive germinal center formation, high autoantibody titers, and damage from LN (78). IL-21 is a key cytokine produced by Tfh cells, which promotes the differentiation of Tfh. Bone marrow MSCs from C57BL/6 inhibit Tfh cell proliferation in vivo and in vitro, suppress Tfh differentiation by inhibiting IL-21, inhibit Tfh differentiation in the spleen of MRL/lpr mice, attenuate proteinuria and renal pathology, and prolong the survival of mice (79). Human umbilical cord MSCs inhibit Tfh proliferation by activating inducible NOS (iNOS) in lupus-prone B6.MRL-Faslpr mice, and improve the reduction of interstitial and peritubular inflammatory cell infiltration and reducing glomerular IgG deposition in mice (80). Human bone marrow MSCs can improve renal function and pathological alterations by inhibiting the emergence of Tfh in NZB/W mice, such as reduced proteinuria, decreased immune complex deposition, glomerular proliferation, and plasma cell infiltration, and in vitro human bone marrow MSCs directly inhibit the differentiation of nascent CD4+ T cells to Tfh cells in a contact-dependent manner (81). In summary, MSCs improve LN by inhibiting the proliferation and differentiation of Tfh cells.
Immunomodulatory Activity of MSCs on DCs
DCs are the most functional and powerful antigen-presenting cells in the body, linking innate and adaptive immunity (82). Depending on the origin of DCs, they can be classified as plasmacytoid dendritic cells (pDCs), myeloid dendritic cells (mDCs), and peripheral blood monocyte-derived dendritic cells (PBMDCs), which accumulate in the kidney of LN patients and participate in the immune inflammatory response by expressing multiple receptors, co-stimulatory molecules, and inflammatory factors. pDCs are decreased in the peripheral blood and increased in the renal tissue of patients with active LN (83). pDCs secrete a large amount of type I interferons to trigger antiviral immune responses, and they have been identified as an important source of IFN-α production in SLE (84). Studies have shown that pDCs highly express IL-18R, which interacts with the cytokine IL-18 secreted by monocytes/macrophages to induce Th homeostasis to Th1 cell polarization (84). The main role of pDCs in SLE is to recognize nuclear autoantigens (85).pDCs promote the proliferation and cytotoxic effects of NKs and promote the renal inflammatory response (84). IFN-α is a type I IFN, and type I IFNs plays a key role in the pathogenesis of LN. IFN-α induces B cell differentiation and activation (86), upregulates BAFF (87, 88), promotes survival and maturation of DC cells (89), decreases Treg cells, and enhances Th cell functions (90). Elevated serum IFN-α levels in SLE patients correlate with disease activity and severity (91). Injection of IFN-α adenovirus rapidly induces T cell activation and extensive GC formation and produces large numbers of short-lived plasma cells producing IgG2a and IgG3 autoantibodies, leading to glomerulonephritis in lupus-prone NZB/W mice (87). mDCs are activated by IC and upregulate the expression of co-stimulatory molecules (CD86 and CD40), MHC molecules, and inflammatory factors (IL-6, TNF-α, etc.) to present antigens to T lymphocytes. Monocytes induced to differentiate into DCs can effectively present nuclear antigens, leading to cell proliferation, increasing secretion of inflammatory factors, and upregulated expression of co-stimulatory molecules, which play a vital part in maintaining a high level of an inflammatory environment. They have enhanced chemotactic properties that allow them to accumulate in secondary lymph nodes and inflammatory sites (92). CD1c+ DCs may be a key DC subtype for improving immune dysfunction and maintaining immune homeostasis. CD1c+ DCs were markedly reduced in SLE patients, especially LN patients, compared to that in healthy individuals (93).
MSCs can impair the antigen-presenting function of DCs by inhibiting their differentiation, maturation, and migration. MSCs have been shown to inhibit the differentiation of monocytes to DCs by reducing the expression of CD1-α, CD80, CD83, CD86, and HLA-DR on the surface of DCs (94, 95). MSCs inhibit DC cell maturation by secreting IL-6 (96). MSCs can also induce the production of regulatory DCs and evade apoptosis, further enhancing phagocytosis and inhibiting T cell activation and proliferation (97, 98). Bone marrow MSCs derived from C57BL/6 mice abolished the generation of functional IFN-α-producing pDCs, while they interfered with the maturation of mDCs (99). Significantly increased CD1c+ DCs and decreased proteinuria levels in SLE patients after human umbilical cord MSCT demonstrated that proliferation of tolerogenic CD1c+ DCs and inhibition of their apoptosis were promoted through IFNγ-FLT3L-FLT3 interactions (93). Human bone marrow-derived MSCs inhibit inflammatory factor IFN- α expression in Adriamycin (ADR)-induced nephropathy mice to suppress renal inflammation. In addition, MSCT prevents podocyte injury and renal fibrosis (100). In conclusion, MSCs can improve LN by inhibiting the differentiation and maturation of DCs and by suppressing the production of their cytokine, IFN-α.
Immunomodulatory Activity of MSCs on Macrophages
Macrophages are a prevalent infiltrative population in the kidney of LN patients, and studies have shown that renal macrophage infiltration is related to poor prognosis, and that macrophage depletion improves LN, suggesting a key role for macrophages in LN (101). Simultaneously, activated macrophages may be involved in LN through TNF-α and IL-1β-mediated podocyte injury (102, 103). Recently, new concepts of macrophage subsets have emerged, including the classically activated pro-inflammatory M1 type and the alternative activated anti-inflammatory M2 type (104). CD206 is an important marker of M2 type macrophages, and macrophage CD206 is expressed at low levels in B6.MRL-Faslpr mice and SLE patients (105). The balance between M1 and M2 macrophages is associated with the pathogenesis of nephritis, and dysregulated M2 macrophages play a pro-inflammatory part in LN (106). Iwata et al. (107) showed that in lupus-susceptible MRL/lpr mice, abnormal macrophages fail to shift from a “destructive” inflammatory phenotype to a “healing” anti-inflammatory phenotype, which triggers LN. Furthermore, certain cytokines produced by macrophages such as IL-10 (108, 109), IL-12 (51), and TNF-α (110, 111) play important roles in LN. Human placental MSCs shift macrophage differentiation from M1 into M2 macrophages, helping to aid in the regression of inflammation and further tissue regeneration (112). Murine MSCs induce macrophage M2 polarization through secreted TGF-β to exert anti-inflammatory effects and enhance phagocytosis via the Akt/FoxO1 pathway (113). Human umbilical cord MSCs increase CD206 expression in lupus-prone mice and SLE patients to promote M2 type macrophages and their phagocytosis, and improve inflammatory response and renal injury (105). Zhang et al. (103) demonstrated that human umbilical cord MSCT into B6.lpr mice polarize macrophages to the anti-inflammatory M2 type while reducing macrophage infiltration in the kidney of B6.lpr mice to prevent podocyte injury and improve LN; examples include reduction of proteinuria and glomerular thylakoid cell proliferation, and thylakoid matrix deposition. Thus, MSCs improve LN by promoting the M2-type polarization of macrophages.
Immunomodulatory Activity of MSCs on NKs
NKs are large granular lymphocytes which act as a bridge between the innate and adaptive immune systems. NKs also produce a variety of cytokines and chemokines, for instance TNF-α, CCL3, and CCL4, which amplify and recruit inflammatory responses through various mechanisms involved in the LN disease process (114, 115). Several studies have shown that the proportion of NKs and the total number of NKs in the blood of SLE patients are significantly lower, especially in LN patients; also, defective killing activity of NKs has been shown in the peripheral blood of first-degree relatives of SLE patients (116–118). Studies have shown that renal NKs from mice with active disease produced more cytotoxic granules (perforin and granzyme B) and IFN-γ when stimulated with PMA+ ionomycin, which contributed in part to the renal damage in SLE (119, 120). Human bone marrow MSCs inhibit IL-12-induced proliferation of NKs through the derived immunomodulatory factors IDO and PGE2, and MSCs inhibit cytotoxic activity and their cytokine IFN-γ production (121, 122). When MSCs are exposed to exogenous IFN-γ, the expression of MHC-I molecules is upregulated and NKs are insensitive to the killing ability of MSCs (123). NKs can kill autologous or allogeneic MSCs when activated by IL-12 and IL-15 (124, 125). In short, MSCs may improve LN by inhibiting the proliferation and activity of NKs; however, in an inflammatory environment, NKs may have the potential to lyse MSCs and affect their immuno-modulatory function.
Immunomodulatory Activity of MSCs on Cytokines in LN
Large amounts of inflammatory mediators are produced in the LN kidney, and as the disease progresses, the response spreads (126). It is widely believed that ICs trigger the production of key mediators of inflammation, including cytokines, chemokines, and adhesion molecules, leading to glomerular infiltration of individual nucleated cells, tissue damage, and renal failure in mouse models of LN, and their absence or inhibition greatly diminishes disease activity. MSCs can exert immune activity by regulating the secretion of certain cytokines, for example IL-10, IL-17, TGF-β, TNF-α, GM-CSF, and HMGB-1 (Table 1).
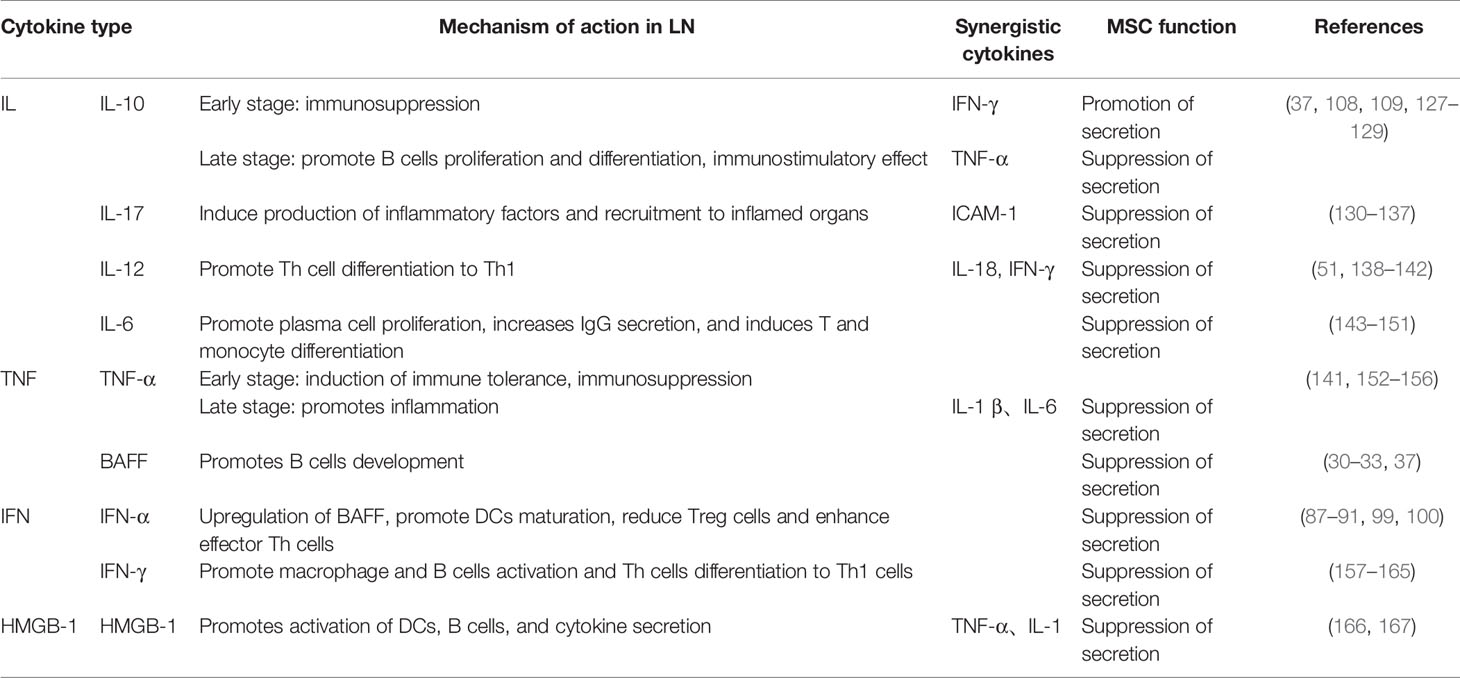
Table 1 The role of cytokines in the pathogenesis of LN and the role of MSCs in the treatment of LN discussed in the text.
Immunomodulatory Activity of MSCs on IL-10
IL-10 is a regulatory cytokine produced by B cells or certain CD+ 4 T cells and may have multiple roles in lupus, playing a major part in the stability of cellular and humoral immunity, with immunostimulatory and suppressive effects. Studies have indicated high levels of IL-10 in SLE patients and mouse models of lupus (108). IL-10 promotes B cell proliferation and differentiation (109), and continuous administration of anti-interleukin 10 antibody delays autoimmunity in NZB/W F1 mice by upregulating TNF-α (167). In vitro experiments show that bone marrow MSCs from BALB/c mice can reduce IL-10 secretion (37). However, genetic defects in IL-10 lead to more severe glomerulonephritis in mice with lupus in the MRL background, and IL-10 downregulates IFN-γ in the early stages of lupus to inhibit the pathogenicity of Th1 cells and delay the progression of lupus (127). Choi et al. (128) demonstrated that human adipose tissue-derived MSCs significantly increased the expression levels of IL-10 and IL-4 cytokines in the serum of (NZB × NZW) F1 mice and that they had decreased serum urea nitrogen levels and increased survival. In summary, MSCs improve LN by regulating IL-10 expression at different stages of LN.
Immunomodulatory Activity of MSCs on IL-17
IL-17 is mainly produced by Th17 cells and other T cell subsets and plays a central part in the pathogenesis of LN. IL-17 induces the production of more inflammatory cytokines and chemokines, and promotes the recruitment of inflammatory cells (monocytes and neutrophils) to inflammatory organs (129, 130). IL-17 promotes T cell infiltration in tissues by stimulating the expression of intercellular adhesion molecule (ICAM)-1 (131) and promotes autoantibody production and renal inflammation in SLE (132). IL-17 plays a vital part in the pathogenesis of SLE and presents at high levels in the serum of SLE patients and lupus mice (133). IL-17 was detected in damaged renal tissues of SLE patients, and IL-17 in urine sediment was associated with LN activity (134). Sun et al. (135) showed that mouse bone marrow MSCs inhibited IL-17 in the bone marrow and spleen of MRL/lpr mice, improved kidney function, reduced circulating immunoglobulins, IgG and IgM, and restored glomerular structure. Adipose MSCs derived from C57BL/6 mice reduced IL-17 expression in the serum of MRL/lpr mice and IL-17 mRNA expression in renal tissues by inhibiting the mTORC1 pathway, improving renal function, reducing glomerular tract proliferation, inflammatory cell infiltration, and C3 deposition (136). Therefore, MSCs prevent and ameliorate LN by downregulating the expression of IL-17.
Immunomodulatory Activity of MSCs on IL-12
IL-12 is a 70 kDa heterodimer (IL-12p70) produced by macrophages and DCs, and together with IL-18, it promotes the production of IFN-γ and is involved in the differentiation and activation of various Th cell subpopulations (51, 137). Tucci et al. (51) demonstrated that serum IL-12 levels are higher in SLE patients; IL-12 triggers an inflammatory response in the kidney and promotes the conversion of Th cells to Th1, and that IL-12 responds to the severity of LN. IL-12 is upregulated in the kidney and serum of MRL-Faslpr mice with LN and its expression further increases with disease progression (138, 139). Human umbilical cord blood MSCT reduces NZB/W F1 mouse IL-12 to inhibit the inflammatory response, reduces the severity of proteinuria, the progression of renal function deterioration, and reduces glomerular tract proliferation and sclerosis (140). Human adipose-derived MSCs reduce serum IL-12 levels in MRL/lpr mice and decrease inflammatory cell infiltration, IgG and C3 deposition in the kidney (141).
Immunomodulatory Activity of MSCs on IL-6
IL-6 is a multipotent cytokine. IL-6 promotes plasma cell survival and proliferation (142), enhances IgG secretion (143), induces differentiation of T cells into effector cells (144), and induces differentiation of monocytes into macrophages (145, 146). IL-6 is significantly elevated in the urine of LN patients, may be associated with LN activity (147, 148), and has emerged as a potential biomarker, with IL-6 deficiency reducing macrophages, CD4+ and CD8+ lymphocytes, along with reduced renal IgG and C3 deposition and delayed LN in MRL-Faslpr mice (149). Thiel et al. (150) showed that human embryonic stem cell-derived MSCs reduce serum IL-6 levels in BWF1 mice and have the ability to prevent or slow down lupus-associated glomerular disease.
Immunomodulatory Activity of MSCs on TNF-α
TNF-α is a multipotent cytokine, mainly produced by activated macrophages and lymphocytes, that exerts pro-inflammatory and immunomodulatory effects in LN. TNF-α has an immunosuppressive effect at the onset of LN, and administration of TNF-α to pre-onset NZB/W mice delayed the onset of LN; TNF-α may exert a protective effect by inducing tolerance (151). However, TNF-α causes end-organ damage in late disease (151). Studies have demonstrated a major involvement in the inflammatory cascade response in kidney injury, such as the promotion of IL-1 β and IL-6 secretion mediated by dsDNA antibodies (152), elevated TNF-α levels in the kidney in LN, and correlation with disease activity (153, 154). Chang et al. (140) showed experimentally that human umbilical cord blood MSCT could inhibit the production of the pro-inflammatory factor TNF-α and improve LN. Liu et al. (155) showed that human placenta-derived MSCT downregulated the expression of the inflammatory marker TNF-α in the kidney and improved renal function and pathology, such as reducing proteinuria, glomerular inflammatory infiltrates, and immune complex deposition.
Immunomodulatory Activity of MSCs on IFN-γ
IFN-γ is a type II IFN, mainly produced by Th1 and NKs. IFN-γ promotes B cell activation and production of IgG2a, IgA, and IgM (156, 157). IFN-γ mediates the activation of macrophages (158), induces differentiation of Th cells to Th1 cells, and inhibits the proliferation of Th2 cells (159, 160). Glomerulonephritis is severely reduced in IFN-gamma-/- mice and overproduction of IFN-γ is required for the development of lupus (161). Jacob et al. (162) showed that treatment with IFN-γ accelerated the development of nephritis in (NZB × NZW) F1 mice and that application of a specific IFN-γ monoclonal antibody delayed the development of LN. Ozmen et al. (163) showed that treatment of NZB/W mice with soluble IFN-γ receptors delayed the development of glomerulonephritis. Significantly lower levels of IFN-γ were found in T lymphocytes from SLE patients co-cultured with human umbilical cord-derived MSCs (164).
Immunomodulatory Activity of MSCs on HMGB-1
HMGB-1 is an immunostimulatory cytokine mediator that mediates IC to stimulate DC and B cell activation and production of cytokines, such as TNF-α and IL-1, and plays a main part in the development of LN (165). Gu et al. (166) found that mRNA expression of HMGB-1 was reduced in the umbilical cord MSC group compared with the control group by transplanting human umbilical cord MSCs in MRL/lpr mice; the rate of positive immunohistochemical staining for HMGB-1 was lower than that of the control group, also improved kidney function and crescent reduction was demonstrated.
Failure of MSC Therapy and Analysis of Causes
Table 2 demonstrates several preclinical studies of MSCs in lupus-susceptible mice, which have been extensively conducted and yielded preliminary results, and these results have facilitated current clinical studies. Many in vivo studies have shown differences in the immunomodulatory activity of MSCs in LN, and the previous section of this review described the immunomodulatory activity and potential mechanisms of MSCs in LN, which support the preclinical and clinical applications of MSCs; however, the applications of MSCs in regenerative medicine are still challenging; hence, this section focuses on elucidating the contradictory findings of preclinical and clinical studies and analyzing the reasons for the conflicting results.
Most MSCTs have been shown to improve LN, including improving renal function such as reducing proteinuria, serum urea nitrogen, and creatinine levels, and improving renal pathology such as reducing glomerular tract proliferation, inflammatory cell infiltration, and immune complex deposition. However, there are contradictory results, as Youd et al. showed in a pilot study that bone marrow MSCs from BALB/c mice injected intraperitoneally into NZB/W mice showed that MSCT-treated mice exacerbated the disease instead of benefiting from it (168), probably due to the intraperitoneal injection method, in which MSCs injected intraperitoneally into mice could form aggregates with B20 aggregates, lymphocytes, and macrophages, which then attach to the peritoneal wall, thus limiting the entry of MSCs into the body circulation (170). Che et al. (17) also found that transplantation of bone marrow MSCs derived from MRL/lpr mice into MRL/lpr mice did not improve renal immune complex deposition and had no significant inhibitory effect on B lymphocytes, due to the defective immune function of bone marrow MSCs derived from lupus mice. Tani et al. (169) showed that transplantation of bone marrow MSCs derived from C57BL/6J-derived bone marrow MSCs transplanted into NZB/Wf1 mice showed only delayed onset of proteinuria, no improvement in anti-ds-DNA titers and renal function scores, more inflammatory cell deposition, B cell overexpression and fewer Treg cells. This may be due to different murine models of lupus and the multiplicity and heterogeneity of MSCs, among other reasons.
Clinical cases of MSC-treated LN have accumulated through a large number of preclinical trials. Most clinical trials used an intravenous injection of 1 × 106 MSCs/kg, with good results in LN patients. Wang et al. (8) observed the clinical performance of 40 SLE patients treated with human Umbilical Cord-MSCs. After 12 months of follow-up, the overall survival rate was 92.5%. In the UC-MSC group, SLEDAI scores decreased, as also the levels of serum creatinine, urea nitrogen, and proteinuria. Gu et al. (171) showed that 81 patients with refractory or active LN treated who had undergone allogeneic bone marrow MSCT for LN had an overall survival rate of 95% at 12-month follow-up. Patient survival had improved, and SLEDAI scores, serum creatinine, and urine protein levels had decreased. The observation of 87 patients with refractory SLE and receiving intravenous human bone marrow/umbilical cord MSCs demonstrated an overall survival rate of 94%, an improved renal function, and the recovery of serum albumin and complement C3 levels during 4 years of follow-up, suggesting that allogeneic MSCT can lead to clinical remission and improvement of renal dysfunction in patients with drug resistant SLE (172). However, there are still a few reports of adverse events associated with MSC transplantation. The disease activity of two SLE patients failed to improve after autologous MSCT, and the observed increase in Treg cells was not associated with clinical benefits, mainly due to the possible presence of defective MSCs in lupus patients (9). Deng et al. (173) used a randomized two-way blinded approach to divide patients into a human umbilical Cord-MSC group and a placebo group. There were two adverse events in each group: stroke and ascites in the placebo group, and leukopenia and pneumonia in the human umbilical Cord-MSC group, with one patient dying of pneumonia. Allogeneic normal MSCT is more effective than autologous MSCT in treating SLE (9, 174).
In SLE, the body is in a chronic inflammatory state in which MSCs can act to promote or suppress the immune system. High levels of TGF-β production by umbilical cord MSCs, upregulation of Treg cells and PGE2, and downregulation of Th17 cells are responses to stimulation of the lupus microenvironment to induce immune tolerance (69). Overexpression of IL-37 may enhance the immunosuppressive effect of MSCs by suppressing the inflammatory microenvironment, while transplanted MSCs may improve the damaged microenvironment in SLE (175). However, high levels of TNF-α in SLE patients significantly inhibit the migration and homing ability of bone marrow MSCs (18). The inflammatory environment promotes the secretion of inflammatory cytokines such as IL-1β and TNF-α by MSCs, and in response to the co-stimulation of IL-1β and TNF-α, MSCs promote inflammatory responses and Th17 differentiation and participate in the progression of SLE disease (176). MSCs with SLE are more susceptible to the effects of the matrix proteins of the bone marrow microenvironment and cytokines and chemokines secreted by stromal cells during culture, which accelerate senescence (10). The immunomodulatory effects of MSCs are influenced by the tissue microenvironment, and modification of MSCs with cytokines or drugs to enhance their therapeutic potential requires further exploration.
Conclusion and Outlook
With the development and application of stem cell technology, the immunomodulatory activity of stem cells can be utilized to improve LN, which has greatly contributed to the development of LN treatment. We illustrate the defective MSCs in SLE and describe the pathogenesis of LN in detail in terms of immune cells and cytokines and discussed the immunomodulatory activity and potential mechanisms of MSCs in LN, all of which support the preclinical and clinical applications of MSCs. Although MSCs are promising in the treatment of LN, the application of MSCs in regenerative medicine remains challenging, as there are many differences in the immunomodulatory activity of MSCs due to multiple factors, for example, differences in the source of MSCs, injection routes, and the amount of injected dose. Also, the immunomodulatory effect of MSCs may be influenced by the layout microenvironment, so there is a need to find standardized ways or pretreatment of MSCs to avoid these situations. In the future, we will fully exploit the immunomodulatory activity of MSCs and use it to optimize their therapeutic effects. We are optimistic that MSCs will be valued as immunomodulators of autoimmune responses, as there is ample proof of principle for conducting preclinical and clinical experiments.
Author Contributions
JL: conceptualization and writing – original draft. ML: writing – review and editing. BL: visualization. YL: writing – review and editing. YZ: writing – review and editing. XB: writing – review and editing. BS: visualization. XL: review and project administration. PL: funding acquisition, and supervision. All authors contributed to the article and approved the submitted version.
Funding
This work was supported by the National Natural Science Foundation of China [grant number 81970628(PL)] and the Science and Technology Development Plan Project of Jilin province [grant numbers 20200201488JC(BL) and 20190304042YY(PL)].
Conflict of Interest
The authors declare that the research was conducted in the absence of any commercial or financial relationships that could be construed as a potential conflict of interest.
Publisher’s Note
All claims expressed in this article are solely those of the authors and do not necessarily represent those of their affiliated organizations, or those of the publisher, the editors and the reviewers. Any product that may be evaluated in this article, or claim that may be made by its manufacturer, is not guaranteed or endorsed by the publisher.
Abbreviations
ADR, Adriamycin; Anti-dsDNA, anti-double-stranded DNA; Breg, Regulatory B cells; BAFF, B cell activating factor; CCL2, Chemokine ligand 2; CXCR4/CXCR5, chemokine receptor 4/5; DCs, Dendritic cells; ERS, Endoplasmic reticulum stress response; EP4, receptor 4; FOXP3, Forkhead box P3; HGF, Hepatocyte growth factor; IC, Immune complexes; ICAM, Intercellular adhesion molecule; IDO, Indoleamine 2,3dioxygenase; IL, Interleukin; INF, Interferon; LN, Lupus nephritis; iTreg, inducible Treg; iNOS, inducible NOS; mDCs, Myeloid dendritic cells; MSCs, Mesenchymal stem cells; MSCT, Mesenchymal stem cell transplantation; MAVS, Mtochondrial antiviral signaling; NKs, Natural killer cells; PBMDCs, Peripheral blood monocyte-derived dendritic cells; pDCs, Plasmacytoid dendritic cells; PGE2, prostaglandin E2; ROS, Reactive oxygen species; SA-b-gal, Senescence associated b-galactosidase; SLE, Systemic lupus erythematosus; STAT3, Signal transducer and activator of transcription 3; TGF- b, Transforming growth factor b; Th, T helper cells; Tfh, Follicular helper T cells; TNF, Tumor necrosis factor; Treg, Regulatory T cells.
References
1. Rovin BH, Adler SG, Barratt J, Bridoux F, Burdge KA, Chan TM, et al. KDIGO 2021 Clinical Practice Guideline for the Management of Glomerular Diseases. Kidney Int (2021) 100(4s):S1–276. doi: 10.1016/j.kint.2021.05.021
2. Lech M, Anders HJ. The Pathogenesis of Lupus Nephritis. J Am Soc Nephrol (2013) 24(9):1357–66. doi: 10.1681/asn.2013010026
3. Parikh SV, Almaani S, Brodsky S, Rovin BH. Update on Lupus Nephritis: Core Curriculum 2020. Am J Kidney Dis (2020) 76(2):265–81. doi: 10.1053/j.ajkd.2019.10.017
4. Rees F, Doherty M, Grainge MJ, Lanyon P, Zhang W. The Worldwide Incidence and Prevalence of Systemic Lupus Erythematosus: A Systematic Review of Epidemiological Studies. Rheumatology (Oxf Engl) (2017) 56(11):1945–61. doi: 10.1093/rheumatology/kex260
5. Ding DC, Shyu WC, Lin SZ. Mesenchymal Stem Cells. Cell Transplant (2011) 20(1):5–14. doi: 10.3727/096368910x
6. Uccelli A, Moretta L, Pistoia V. Mesenchymal Stem Cells in Health and Disease. Nat Rev Immunol (2008) 8(9):726–36. doi: 10.1038/nri2395
7. Ben-Ami E, Berrih-Aknin S, Miller A. Mesenchymal Stem Cells as an Immunomodulatory Therapeutic Strategy for Autoimmune Diseases. Autoimmun Rev (2011) 10(7):410–5. doi: 10.1016/j.autrev.2011.01.005
8. Wang D, Li J, Zhang Y, Zhang M, Chen J, Li X, et al. Umbilical Cord Mesenchymal Stem Cell Transplantation in Active and Refractory Systemic Lupus Erythematosus: A Multicenter Clinical Study. Arthritis Res Ther (2014) 16(2):R79. doi: 10.1186/ar4520
9. Carrion F, Nova E, Ruiz C, Diaz F, Inostroza C, Rojo D, et al. Autologous Mesenchymal Stem Cell Treatment Increased T Regulatory Cells With No Effect on Disease Activity in Two Systemic Lupus Erythematosus Patients. Lupus (2010) 19(3):317–22. doi: 10.1177/0961203309348983
10. Nie Y, Lau CS, Lie AK, Chan GC, Mok MY. Defective Phenotype of Mesenchymal Stem Cells in Patients With Systemic Lupus Erythematosus. Lupus (2010) 19(7):850–9. doi: 10.1177/0961203310361482
11. Ji J, Wu Y, Meng Y, Zhang L, Feng G, Xia Y, et al. JAK-STAT Signaling Mediates the Senescence of Bone Marrow-Mesenchymal Stem Cells From Systemic Lupus Erythematosus Patients. Acta Biochim Biophys Sin (2017) 49(3):208–15. doi: 10.1093/abbs/gmw134
12. Gao L, Bird AK, Meednu N, Dauenhauer K, Liesveld J, Anolik J, et al. Bone Marrow-Derived Mesenchymal Stem Cells From Patients With Systemic Lupus Erythematosus Have a Senescence-Associated Secretory Phenotype Mediated by a Mitochondrial Antiviral Signaling Protein-Interferon-β Feedback Loop. Arthritis Rheumatol (Hoboken NJ) (2017) 69(8):1623–35. doi: 10.1002/art.40142
13. Gu Z, Meng Y, Tao T, Guo G, Tan W, Xia Y, et al. Endoplasmic Reticulum Stress Participates in the Progress of Senescence of Bone Marrow-Derived Mesenchymal Stem Cells in Patients With Systemic Lupus Erythematosus. Cell Tissue Res (2015) 361(2):497–508. doi: 10.1007/s00441-015-2131-x
14. Gu Z, Tan W, Feng G, Meng Y, Shen B, Liu H, et al. Wnt/β-Catenin Signaling Mediates the Senescence of Bone Marrow-Mesenchymal Stem Cells From Systemic Lupus Erythematosus Patients Through the P53/P21 Pathway. Mol Cell Biochem (2014) 387(1-2):27–37. doi: 10.1007/s11010-013-1866-5
15. Chen H, Shi B, Feng X, Kong W, Chen W, Geng L, et al. Leptin and Neutrophil-Activating Peptide 2 Promote Mesenchymal Stem Cell Senescence Through Activation of the Phosphatidylinositol 3-Kinase/Akt Pathway in Patients With Systemic Lupus Erythematosus. Arthritis Rheumatol (Hoboken NJ) (2015) 67(9):2383–93. doi: 10.1002/art.39196
16. Tan W, Gu Z, Shen B, Jiang J, Meng Y, Da Z, et al. PTEN/Akt-P27(Kip1) Signaling Promote the BM-MSCs Senescence and Apoptosis in SLE Patients. J Cell Biochem (2015) 116(8):1583–94. doi: 10.1002/jcb.25112
17. Che N, Li X, Zhang L, Liu R, Chen H, Gao X, et al. Impaired B Cell Inhibition by Lupus Bone Marrow Mesenchymal Stem Cells Is Caused by Reduced CCL2 Expression. J Immunol (Baltimore Md 1950) (2014) 193(10):5306–14. doi: 10.4049/jimmunol.1400036
18. Geng L, Li X, Feng X, Zhang J, Wang D, Chen J, et al. Association of TNF-α With Impaired Migration Capacity of Mesenchymal Stem Cells in Patients With Systemic Lupus Erythematosus. J Immunol Res (2014) 2014:169082. doi: 10.1155/2014/169082
19. Wang D, Feng X, Lu L, Konkel JE, Zhang H, Chen Z, et al. A CD8 T Cell/Indoleamine 2,3-Dioxygenase Axis Is Required for Mesenchymal Stem Cell Suppression of Human Systemic Lupus Erythematosus. Arthritis Rheumatol (Hoboken NJ) (2014) 66(8):2234–45. doi: 10.1002/art.38674
20. Frangou E, Georgakis S, Bertsias G. Update on the Cellular and Molecular Aspects of Lupus Nephritis. Clin Immunol (Orlando Fla) (2020) 216:108445. doi: 10.1016/j.clim.2020.108445
21. Song N, Scholtemeijer M, Shah K. Mesenchymal Stem Cell Immunomodulation: Mechanisms and Therapeutic Potential. Trends Pharmacol Sci (2020) 41(9):653–64. doi: 10.1016/j.tips.2020.06.009
22. Wang M, Yuan Q, Xie L. Mesenchymal Stem Cell-Based Immunomodulation: Properties and Clinical Application. Stem Cells Int (2018) 2018:3057624. doi: 10.1155/2018/3057624
23. Shlomchik MJ, Madaio MP, Ni D, Trounstein M, Huszar D. The Role of B Cells in Lpr/Lpr-Induced Autoimmunity. J Exp Med (1994) 80(4):1295–306. doi: 10.1084/jem.180.4.1295
24. Vlahakos DV, Foster MH, Adams S, Katz M, Ucci AA, Barrett KJ, et al. Anti-DNA Antibodies Form Immune Deposits at Distinct Glomerular and Vascular Sites. Kidney Int (1992) 41(6):1690–700. doi: 10.1038/ki.1992.242
25. Madaio MP, Shlomchik MJ. Emerging Concepts Regarding B Cells and Autoantibodies in Murine Lupus Nephritis. B Cells Have Multiple Roles; All Autoantibodies Are Not Equal. J Am Soc Nephrol (1996) 7(3):387–96. doi: 10.1681/asn.V73387
26. Chan O, Shlomchik MJ. A New Role for B Cells in Systemic Autoimmunity: B Cells Promote Spontaneous T Cell Activation in MRL-Lpr/Lpr Mice. J Immunol (Baltimore Md 1950) (1998) 160(1):51–9.
27. Harris DP, Haynes L, Sayles PC, Duso DK, Eaton SM, Lepak NM, et al. Reciprocal Regulation of Polarized Cytokine Production by Effector B and T Cells. Nat Immunol (2000) 1(6):475–82. doi: 10.1038/82717
28. Rosser EC, Mauri C. Regulatory B Cells: Origin, Phenotype, and Function. Immunity (2015) 42(4):607–12. doi: 10.1016/j.immuni.2015.04.005
29. Espeli M, Bökers S, Giannico G, Dickinson HA, Bardsley V, Fogo AB, et al. Local Renal Autoantibody Production in Lupus Nephritis. J Am Soc Nephrol (2011) 22(2):296–305. doi: 10.1681/asn.2010050515
30. Mackay F, Schneider P, Rennert P, Browning J. BAFF AND APRIL: A Tutorial on B Cell Survival. Annu Rev Immunol (2003) 21:231–64. doi: 10.1146/annurev.immunol.21.120601.141152
31. Morimoto S, Nakano S, Watanabe T, Tamayama Y, Mitsuo A, Nakiri Y, et al. Expression of B-Cell Activating Factor of the Tumour Necrosis Factor Family (BAFF) in T Cells in Active Systemic Lupus Erythematosus: The Role of BAFF in T Cell-Dependent B Cell Pathogenic Autoantibody Production. Rheumatology (Oxf Engl) (2007) 46(7):1083–6. doi: 10.1093/rheumatology/kem097
32. Jacob CO, Pricop L, Putterman C, Koss MN, Liu Y, Kollaros M, et al. Paucity of Clinical Disease Despite Serological Autoimmunity and Kidney Pathology in Lupus-Prone New Zealand Mixed 2328 Mice Deficient in BAFF. J Immunol (Baltimore Md 1950) (2006) 177(4):2671–80. doi: 10.4049/jimmunol.177.4.2671
33. Mackay F, Woodcock SA, Lawton P, Ambrose C, Baetscher M, Schneider P, et al. Mice Transgenic for BAFF Develop Lymphocytic Disorders Along With Autoimmune Manifestations. J Exp Med (1999) 190(11):1697–710. doi: 10.1084/jem.190.11.1697
34. Franquesa M, Hoogduijn MJ, Bestard O, Grinyó JM. Immunomodulatory Effect of Mesenchymal Stem Cells on B Cells. Front Immunol (2012) 3:212:212. doi: 10.3389/fimmu.2012.00212
35. Rafei M, Hsieh J, Fortier S, Li M, Yuan S, Birman E, et al. Mesenchymal Stromal Cell-Derived CCL2 Suppresses Plasma Cell Immunoglobulin Production via STAT3 Inactivation and PAX5 Induction. Blood (2008) 112(13):4991–8. doi: 10.1182/blood-2008-07-166892
36. Corcione A, Benvenuto F, Ferretti E, Giunti D, Cappiello V, Cazzanti F, et al. Human Mesenchymal Stem Cells Modulate B-Cell Functions. Blood (2006) 107(1):367–72. doi: 10.1182/blood-2005-07-2657
37. Ma X, Che N, Gu Z, Huang J, Wang D, Liang J, et al. Allogenic Mesenchymal Stem Cell Transplantation Ameliorates Nephritis in Lupus Mice via Inhibition of B-Cell Activation. Cell Transplant (2013) 22(12):2279–90. doi: 10.3727/096368912x658692
38. Park MJ, Kwok SK, Lee SH, Kim EK, Park SH, Cho ML. Adipose Tissue-Derived Mesenchymal Stem Cells Induce Expansion of Interleukin-10-Producing Regulatory B Cells and Ameliorate Autoimmunity in a Murine Model of Systemic Lupus Erythematosus. Cell Transplant (2015) 24(11):2367–77. doi: 10.3727/096368914x685645
39. Dang J, Xu Z, Xu A, Liu Y, Fu Q, Wang J, et al. Human Gingiva-Derived Mesenchymal Stem Cells Are Therapeutic in Lupus Nephritis Through Targeting of CD39(-)CD73 Signaling Pathway. J Autoimmun (2020) 113:102491. doi: 10.1016/j.jaut.2020.102491
40. Tilstra JS, Avery L, Menk AV, Gordon RA, Smita S, Kane LP, et al. Kidneyinfiltrating T Cells in Murine Lupus Nephritis Are Metabolically and Functionally Exhausted. J Clin Invest (2018) 128(11):4884–97. doi: 10.1172/jci120859
41. Messner RP, Lindström FD, Williams RC Jr. Peripheral Blood Lymphocyte Cell Surface Markers During the Course of Systemic Lupus Erythematosus. J Clin Invest (1973) 52(12):3046–56. doi: 10.1172/jci107503
42. Glinski W, Gershwin ME, Steinberg AD. Fractionation of Cells on a Discontinuous Ficoll Gradient. Study of Subpopulations of Human T Cells Using Anti-T-Cell Antibodies From Patients With Systemic Lupus Erythematosus. J Clin Invest (1976) 57(3):604–14. doi: 10.1172/jci108316
43. Takeuchi A, Saito T. CD4 CTL, a Cytotoxic Subset of CD4(+) T Cells, Their Differentiation and Function. Front Immunol (2017) 8:194. doi: 10.3389/fimmu.2017.00194
44. O'Garra A. Cytokines Induce the Development of Functionally Heterogeneous T Helper Cell Subsets. Immunity (1998) 8(3):275–83. doi: 10.1016/s1074-7613(00)80533-6
45. Di Nicola M, Carlo-Stella C, Magni M, Milanesi M, Longoni PD, Matteucci P, et al. Human Bone Marrow Stromal Cells Suppress T-Lymphocyte Proliferation Induced by Cellular or Nonspecific Mitogenic Stimuli. Blood (2002) 99(10):3838–43. doi: 10.1182/blood.v99.10.3838
46. Glennie S, Soeiro I, Dyson PJ, Lam EW, Dazzi F. Bone Marrow Mesenchymal Stem Cells Induce Division Arrest Anergy of Activated T Cells. Blood (2005) 105(7):2821–7. doi: 10.1182/blood-2004-09-3696
47. Plumas J, Chaperot L, Richard MJ, Molens JP, Bensa JC, Favrot MC. Mesenchymal Stem Cells Induce Apoptosis of Activated T Cells. Leukemia (2005) 19(9):1597–604. doi: 10.1038/sj.leu.2403871
48. Akiyama K, Chen C, Wang D, Xu X, Qu C, Yamaza T, et al. Mesenchymal-Stemcell-Induced Immunoregulation Involves FAS-Ligand-/FAS-Mediated T Cell Apoptosis. Cell Stem Cell (2012) 10(5):544–55. doi: 10.1016/j.stem.2012.03.007
49. Huang S, Wu S, Zhang Z, Deng W, Fan J, Feng R, et al. Mesenchymal Stem Cells Induced CD4+ T Cell Apoptosis in Treatment of Lupus Mice. Biochem Biophys Res Commun (2018) 507(1-4):30–5. doi: 10.1016/j.bbrc.2018.10.133
50. Castro-Manrreza ME, Montesinos JJ. Immunoregulation by Mesenchymal Stem Cells: Biological Aspects and Clinical Applications. J Immunol Res (2015) 2015:394917. doi: 10.1155/2015/394917
51. Tucci M, Lombardi L, Richards HB, Dammacco F, Silvestris F. Overexpression of Interleukin-12 and T Helper 1 Predominance in Lupus Nephritis. Clin Exp Immunol (2008) 154(2):247–54. doi: 10.1111/j.1365-2249.2008.03758.x
52. Sigdel KR, Duan L, Wang Y, Hu W, Wang N, Sun Q, et al. Serum Cytokines Th1, Th2, and Th17 Expression Profiling in Active Lupus Nephritis-IV: From a Southern Chinese Han Population. Mediators Inflamm (2016) 2016:4927530. doi: 10.1155/2016/4927530
53. Takahashi S, Fossati L, Iwamoto M, Merino R, Motta R, Kobayakawa T, et al. Imbalance Towards Th1 Predominance Is Associated With Acceleration of Lupus-Like Autoimmune Syndrome in MRL Mice. J Clin Invest (1996) 97(7):1597–604. doi: 10.1172/jci118584
54. Kiernan CH, Asmawidjaja PS, Fahy N, Witte-Bouma J, Wolvius EB, Brama PAJ, et al. Allogeneic Chondrogenic Mesenchymal Stromal Cells Alter Helper T Cell Subsets in CD4+ Memory T Cells. Tissue Eng Part A (2020) 26(9-10):490–502. doi: 10.1089/ten.TEA.2019.0177
55. Choi EW, Lee M, Song JW, Shin IS, Kim SJ. Mesenchymal Stem Cell Transplantation can Restore Lupus Disease-Associated miRNA Expression and Th1/Th2 Ratios in a Murine Model of SLE. Sci Rep (2016) 6:38237. doi: 10.1038/srep38237
56. Sakaguchi S, Sakaguchi N, Asano M, Itoh M, Toda M. Immunologic Self-Tolerance Maintained by Activated T Cells Expressing IL-2 Receptor Alpha-Chains (CD25). Breakdown of a Single Mechanism of Self-Tolerance Causes Various Autoimmune Diseases. J Immunol (Baltimore Md 1950) (1995) 155(3):1151–64.
57. Barath S, Aleksza M, Tarr T, Sipka S, Szegedi G, Kiss E. Measurement of Natural (CD4+CD25high) and Inducible (CD4+IL-10+) Regulatory T Cells in Patients With Systemic Lupus Erythematosus. Lupus (2007) 16(7):489–96. doi: 10.1177/0961203307080226
58. Bonelli M, Savitskaya A, von Dalwigk K, Steiner CW, Aletaha D, Smolen JS, et al. Quantitative and Qualitative Deficiencies of Regulatory T Cells in Patients With Systemic Lupus Erythematosus (SLE). Int Immunol (2008) 20(7):861–8. doi: 10.1093/intimm/dxn044
59. Darlan DM, Munir D, Putra A, Jusuf NK. MSCs-Released Tgfβ1 Generate CD4(+)CD25(+)Foxp3(+) in T-Reg Cells of Human SLE PBMC. J Formos Med Assoc (2021) 120(1 Pt 3):602–8. doi: 10.1016/j.jfma.2020.06.028
60. Lin W, Haribhai D, Relland LM, Truong N, Carlson MR, Williams CB, et al. Regulatory T Cell Development in the Absence of Functional Foxp3. Nat Immunol (2007) 8(4):359–68. doi: 10.1038/ni1445
61. Sakaguchi S, Yamaguchi T, Nomura T, Ono M. Regulatory T Cells and Immune Tolerance. Cell (2008) 133(5):775–87. doi: 10.1016/j.cell.2008.05.009
62. Iwakura Y, Ishigame H, Saijo S, Nakae S. Functional Specialization of Interleukin-17 Family Members. Immunity (2011) 34(2):149–62. doi: 10.1016/j.immuni.2011.02.012
63. Paust HJ, Turner JE, Steinmetz OM, Peters A, Heymann F, Hölscher C, et al. The IL-23/Th17 Axis Contributes to Renal Injury in Experimental Glomerulonephritis. J Am Soc Nephrol (2009) 20(5):969–79. doi: 10.1681/asn.2008050556
64. Talaat RM, Mohamed SF, Bassyouni IH, Raouf AA. Th1/Th2/Th17/Treg Cytokine Imbalance in Systemic Lupus Erythematosus (SLE) Patients: Correlation With Disease Activity. Cytokine (2015) 72(2):146–53. doi: 10.1016/j.cyto.2014.12.027
65. Paquissi FC, Abensur H. The Th17/IL-17 Axis and Kidney Diseases, With Focus on Lupus Nephritis. Front Med (2021) 8:654912. doi: 10.3389/fmed.2021.654912
66. Svobodova E, Krulova M, Zajicova A, Pokorna K, Prochazkova J, Trosan P, et al. The Role of Mouse Mesenchymal Stem Cells in Differentiation of Naive T-Cells Into Anti-Inflammatory Regulatory T-Cell or Proinflammatory Helper T-Cell 17 Population. Stem Cells Dev (2012) 21(6):901–10. doi: 10.1089/scd.2011.0157
67. English K, Ryan JM, Tobin L, Murphy MJ, Barry FP, Mahon BP. Cell Contact, Prostaglandin E(2) and Transforming Growth Factor Beta 1 Play Non-Redundant Roles in Human Mesenchymal Stem Cell Induction of CD4+CD25(High) Forkhead Box P3+ Regulatory T Cells. Clin Exp Immunol (2009) 156(1):149–60. doi: 10.1111/j.1365-2249.2009.03874.x
68. Duffy MM, Pindjakova J, Hanley SA, McCarthy C, Weidhofer GA, Sweeney EM, et al. Mesenchymal Stem Cell Inhibition of T-Helper 17 Cell- Differentiation Is Triggered by Cell-Cell Contact and Mediated by Prostaglandin E2 via the EP4 Receptor. Eur J Immunol (2011) 41(10):2840–51. doi: 10.1002/eji.201141499
69. Wang D, Huang S, Yuan X, Liang J, Xu R, Yao G, et al. The Regulation of the Treg/Th17 Balance by Mesenchymal Stem Cells in Human Systemic Lupus Erythematosus. Cell Mol Immunol (2017) 14(5):423–31. doi: 10.1038/cmi.2015.89
70. Makino Y, Yamaza H, Akiyama K, Ma L, Hoshino Y, Nonaka K, et al. Immune Therapeutic Potential of Stem Cells From Human Supernumerary Teeth. J Dental Res (2013) 92(7):609–15. doi: 10.1177/0022034513490732
71. Dong W, Zhu P, Wang Y, Wang Z. Follicular Helper T Cells in Systemic Lupus Erythematosus: A Potential Therapeutic Target. Autoimmun Rev (2011) 10(6):299–304. doi: 10.1016/j.autrev.2010.11.004
72. Le Coz C, Joublin A, Pasquali JL, Korganow AS, Dumortier H, Monneaux F. Circulating TFH Subset Distribution is Strongly Affected in Lupus Patients With an Active Disease. PloS One (2013) 8(9):e75319. doi: 10.1371/journal.pone.0075319
73. Kim SJ, Lee K, Diamond B. Follicular Helper T Cells in Systemic Lupus Erythematosus. Front Immunol (2018) 9:1793. doi: 10.3389/fimmu.2018.01793
74. Linterman MA, Rigby RJ, Wong RK, Yu D, Brink R, Cannons JL, et al. Follicular Helper T Cells Are Required for Systemic Autoimmunity. J Exp Med (2009) 206(3):561–76. doi: 10.1084/jem.20081886
75. Crotty S. Follicular Helper CD4 T Cells (TFH). Annu Rev Immunol (2011) 29:621–63. doi: 10.1146/annurev-immunol-031210-101400
76. Cappione A 3rd, Anolik JH, Pugh-Bernard A, Barnard J, Dutcher P, Silverman G, et al. Germinal Center Exclusion of Autoreactive B Cells Is Defective in Human Systemic Lupus Erythematosus. J Clin Invest (2005) 115(11):3205–16. doi: 10.1172/jci24179
77. Vinuesa CG, Sanz I, Cook MC. Dysregulation of Germinal Centres in Autoimmune Disease. Nat Rev Immunol (2009) 9(12):845–57. doi: 10.1038/nri2637
78. Yang X, Yang J, Chu Y, Wang J, Guan M, Zhu X, et al. T Follicular Helper Cells Mediate Expansion of Regulatory B Cells via IL-21 in Lupus-Prone MRL/lpr Mice. PloS One (2013) 8(4):e62855. doi: 10.1371/journal.pone.0062855
79. Yang X, Yang J, Li X, Ma W, Zou H. Bone Marrow-Derived Mesenchymal Stem Cells Inhibit T Follicular Helper Cell in Lupus-Prone Mice. Lupus (2018) 27(1):49–59. doi: 10.1177/0961203317711013
80. Zhang Z, Feng R, Niu L, Huang S, Deng W, Shi B, et al. Human Umbilical Cord Mesenchymal Stem Cells Inhibit T Follicular Helper Cell Expansion Through the Activation of iNOS in Lupus-Prone B6.MRL-Fas(lpr) Mice. Cell Transplant (2017) 26(6):1031–42. doi: 10.3727/096368917x694660
81. Jang E, Jeong M, Kim S, Jang K, Kang BK, Lee DY, et al. Infusion of Human Bone Marrow-Derived Mesenchymal Stem Cells Alleviates Autoimmune Nephritis in a Lupus Model by Suppressing Follicular Helper T-Cell Development. Cell Transplant (2016) 25(1):1–15. doi: 10.3727/096368915x688173
82. Wculek SK, Cueto FJ, Mujal AM, Melero I, Krummel MF, Sancho D. Dendritic Cells in Cancer Immunology and Immunotherapy. Nat Rev Immunol (2020) 20(1):7–24. doi: 10.1038/s41577-019-0210-z
83. Tucci M, Calvani N, Richards HB, Quatraro C, Silvestris F. The Interplay of Chemokines and Dendritic Cells in the Pathogenesis of Lupus Nephritis. Ann N Y Acad Sci (2005) 1051:421–32. doi: 10.1196/annals.1361.084
84. Rönnblom L, Alm GV. A Pivotal Role for the Natural Interferon Alpha-Producing Cells (Plasmacytoid Dendritic Cells) in the Pathogenesis of Lupus. J Exp Med (2001) 194(12):F59–63. doi: 10.1084/jem.194.12.f59
85. Lorenz G, Anders HJ. Neutrophils, Dendritic Cells, Toll-Like Receptors, and Interferon-α in Lupus Nephritis. Semin Nephrol (2015) 35(5):410–26. doi: 10.1016/j.semnephrol.2015.08.003
86. De Groof A, Ducreux J, Aleva F, Long AJ, Ferster A, van der Ven A, et al. STAT3 Phosphorylation Mediates the Stimulatory Effects of Interferon Alpha on B Cell Differentiation and Activation in SLE. Rheumatol (Oxf Engl) (2020) 59(3):668–77. doi: 10.1093/rheumatology/kez354
87. Mathian A, Weinberg A, Gallegos M, Banchereau J, Koutouzov S. IFN-Alpha Induces Early Lethal Lupus in Preautoimmune (New Zealand Black X New Zealand White) F1 But Not in BALB/c Mice. J Immunol (Baltimore Md 1950) (2005) 174(5):2499–506. doi: 10.4049/jimmunol.174.5.2499
88. Jacob N, Guo S, Mathian A, Koss MN, Gindea S, Putterman C, et al. B Cell and BAFF Dependence of IFN-α-Exaggerated Disease in Systemic Lupus Erythematosusprone NZM 2328 Mice. J Immunol (Baltimore Md 1950) (2011) 186(8):4984–93. doi: 10.4049/jimmunol.1000466
89. Blanco P, Palucka AK, Gill M, Pascual V, Banchereau J. Induction of Dendritic Cell Differentiation by IFN-Alpha in Systemic Lupus Erythematosus. Science (New York NY) (2001) 294(5546):1540–3. doi: 10.1126/science.1064890
90. Pace L, Vitale S, Dettori B, Palombi C, La Sorsa V, Belardelli F, et al. APC Activation by IFN-Alpha Decreases Regulatory T Cell and Enhances Th Cell Functions. J Immunol (Baltimore Md 1950) (2010) 184(11):5969–79. doi: 10.4049/jimmunol.0900526
91. Hooks JJ, Moutsopoulos HM, Geis SA, Stahl NI, Decker JL, Notkins AL. Immune Interferon in the Circulation of Patients With Autoimmune Disease. N Engl J Med (1979) 301(1):5–8. doi: 10.1056/nejm197907053010102
92. Vermi W, Riboldi E, Wittamer V, Gentili F, Luini W, Marrelli S, et al. Role of ChemR23 in Directing the Migration of Myeloid and Plasmacytoid Dendritic Cells to Lymphoid Organs and Inflamed Skin. J Exp Med (2005) 201(4):509–15. doi: 10.1084/jem.20041310
93. Yuan X, Qin X, Wang D, Zhang Z, Tang X, Gao X, et al. Mesenchymal Stem Cell Therapy Induces FLT3L and CD1c(+) Dendritic Cells in Systemic Lupus Erythematosus Patients. Nat Commun (2019) 10(1):2498. doi: 10.1038/s41467-019-10491-8
94. Jiang XX, Zhang Y, Liu B, Zhang SX, Wu Y, Yu XD, et al. Human Mesenchymal Stem Cells Inhibit Differentiation and Function of Monocyte-Derived Dendritic Cells. Blood (2005) 105(10):4120–6. doi: 10.1182/blood-2004-02-0586
95. Abomaray FM, Al Jumah MA, Kalionis B, AlAskar AS, Al Harthy S, Jawdat D, et al. Human Chorionic Villous Mesenchymal Stem Cells Modify the Functions of Human Dendritic Cells, and Induce an Anti-Inflammatory Phenotype in CD1+ Dendritic Cells. Stem Cell Rev Rep (2015) 11(3):423–41. doi: 10.1007/s12015-014-9562-8
96. Djouad F, Charbonnier LM, Bouffi C, Louis-Plence P, Bony C, Apparailly F, et al. Mesenchymal Stem Cells Inhibit the Differentiation of Dendritic Cells Through an Interleukin-6-Dependent Mechanism. Stem Cells (Dayton Ohio) (2007) 25(8):2025–32. doi: 10.1634/stemcells.2006-0548
97. Zhang B, Liu R, Shi D, Liu X, Chen Y, Dou X, et al. Mesenchymal Stem Cells Induce Mature Dendritic Cells Into a Novel Jagged-2-Dependent Regulatory Dendritic Cell Population. Blood (2009) 113(1):46–57. doi: 10.1182/blood-2008-04-154138
98. Lu Z, Chang W, Meng S, Xu X, Xie J, Guo F, et al. Mesenchymal Stem Cells Induce Dendritic Cell Immune Tolerance via Paracrine Hepatocyte Growth Factor to Alleviate Acute Lung Injury. Stem Cell Res Ther (2019) 10(1):372. doi: 10.1186/s13287-019-1488-2
99. Hackstein H, Tschipakow I, Bein G, Nold P, Brendel C, Baal N. Contact-Dependent Abrogation of Bone Marrow-Derived Plasmacytoid Dendritic Cell Differentiation by Murine Mesenchymal Stem Cells. Biochem Biophys Res Commun (2016) 476(1):15–20. doi: 10.1016/j.bbrc.2016.05.108
100. Kim HS, Lee JS, Lee HK, Park EJ, Jeon HW, Kang YJ, et al. Mesenchymal Stem Cells Ameliorate Renal Inflammation in Adriamycin-Induced Nephropathy. Immune Netw (2019) 19(5):e36. doi: 10.4110/in.2019.19.e36
101. Chalmers SA, Chitu V, Ramanujam M, Putterman C. Therapeutic Targeting of Macrophages in Lupus Nephritis. Discov Med (2015) 20(108):43–9.
102. Ikezumi Y, Suzuki T, Karasawa T, Kawachi H, Nikolic-Paterson DJ, Uchiyama M. Activated Macrophages Down-Regulate Podocyte Nephrin and Podocin Expression via Stress-Activated Protein Kinases. Biochem Biophys Res Commun (2008) 376(4):706–11. doi: 10.1016/j.bbrc.2008.09.049
103. Zhang Z, Niu L, Tang X, Feng R, Yao G, Chen W, et al. Mesenchymal Stem Cells Prevent Podocyte Injury in Lupus-Prone B6.MRL-Faslpr Mice via Polarizing Macrophage Into an Anti-Inflammatory Phenotype. Nephrol Dial Transplant (2019) 34(4):597–605. doi: 10.1093/ndt/gfy195
104. Mosser DM, Edwards JP. Exploring the Full Spectrum of Macrophage Activation. Nat Rev Immunol (2008) 8(12):958–69. doi: 10.1038/nri2448
105. Deng W, Chen W, Zhang Z, Huang S, Kong W, Sun Y, et al. Mesenchymal Stem Cells Promote CD206 Expression and Phagocytic Activity of Macrophages Through IL-6 in Systemic Lupus Erythematosus. Clin Immunol (Orlando Fla) (2015) 161(2):209–16. doi: 10.1016/j.clim.2015.07.011
106. Kishimoto D, Kirino Y, Tamura M, Takeno M, Kunishita Y, Takase-Minegishi K, et al. Dysregulated Heme Oxygenase-1(Low) M2-Like Macrophages Augment Lupus Nephritis via Bach1 Induced by Type I Interferons. Arthritis Res Ther (2018) 20(1):64. doi: 10.1186/s13075-018-1568-1
107. Iwata Y, Boström EA, Menke J, Rabacal WA, Morel L, Wada T, et al. Aberrant Macrophages Mediate Defective Kidney Repair That Triggers Nephritis in Lupus-Susceptible Mice. J Immunol (Baltimore Md 1950) (2012) 188(9):4568–80. doi: 10.4049/jimmunol.1102154
108. Blenman KR, Duan B, Xu Z, Wan S, Atkinson MA, Flotte TR, et al. IL-10 Regulation of Lupus in the NZM2410 Murine Model. Lab Invest J Tech Methods Pathol (2006) 86(11):1136–48. doi: 10.1038/labinvest.3700468
109. Caielli S, Veiga DT, Balasubramanian P, Athale S, Domic B, Murat E, et al. A CD4(+) T Cell Population Expanded in Lupus Blood Provides B Cell Help Through Interleukin-10 and Succinate. Nat Med (2019) 25(1):75–81. doi: 10.1038/s41591-018-0254-9
110. McCarthy EM, Smith S, Lee RZ, Cunnane G, Doran MF, Donnelly S, et al. The Association of Cytokines With Disease Activity and Damage Scores in Systemic Lupus Erythematosus Patients. Rheumatol (Oxf Engl) (2014) 53(9):1586–94. doi: 10.1093/rheumatology/ket428
111. Mahto H, Tripathy R, Meher BR, Prusty BK, Sharma M, Deogharia D, et al. TNF-α Promoter Polymorphisms (G-238A and G-308A) Are Associated With Susceptibility to Systemic Lupus Erythematosus (SLE) and P. Falciparum Malaria: A Study in Malaria Endemic Area. Sci Rep (2019) 9(1):11752. doi: 10.1038/s41598-019-48182-5
112. Abumaree MH, Al Jumah MA, Kalionis B, Jawdat D, Al Khaldi A, Abomaray FM, et al. Human Placental Mesenchymal Stem Cells (pMSCs) Play a Role as Immune Suppressive Cells by Shifting Macrophage Differentiation From Inflammatory M1 to Anti-Inflammatory M2 Macrophages. Stem Cell Rev Rep (2013) 9(5):620–41. doi: 10.1007/s12015-013-9455-2
113. Liu F, Qiu H, Xue M, Zhang S, Zhang X, Xu J, et al. MSC-Secreted TGF-β Regulates Lipopolysaccharide-Stimulated Macrophage M2-Like Polarization via the Akt/FoxO1 Pathway. Stem Cell Res Ther (2019) 10(1):345. doi: 10.1186/s13287-019-1447-y
114. Cooper MA, Fehniger TA, Caligiuri MA. The Biology of Human Natural Killer-Cell Subsets. Trends Immunol (2001) 22(11):633–40. doi: 10.1016/s1471-4906(01)02060-9
115. Maghazachi AA. Role of Chemokines in the Biology of Natural Killer Cells. Curr Top Microbiol Immunol (2010) 341:37–58. doi: 10.1007/82_2010_20
116. Riccieri V, Spadaro A, Parisi G, Taccari E, Moretti T, Bernardini G, et al. Down-Regulation of Natural Killer Cells and of Gamma/Delta T Cells in Systemic Lupus Erythematosus. Does it Correlate to Autoimmunity and to Laboratory Indices of Disease Activity? Lupus (2000) 9(5):333–7. doi: 10.1191/096120300678828460
117. Erkeller-Yuksel FM, Lydyard PM, Isenberg DA. Lack of NK Cells in Lupus Patients With Renal Involvement. Lupus (1997) 6(9):708–12. doi: 10.1177/096120339700600905
118. Green MR, Kennell AS, Larche MJ, Seifert MH, Isenberg DA, Salaman MR. Natural Killer Cell Activity in Families of Patients With Systemic Lupus Erythematosus: Demonstration of a Killing Defect in Patients. Clin Exp Immunol (2005) 141(1):165–73. doi: 10.1111/j.1365-2249.2005.02822.x
119. Huang Z, Fu B, Zheng SG, Li X, Sun R, Tian Z, et al. Involvement of CD226+ NK Cells in Immunopathogenesis of Systemic Lupus Erythematosus. J Immunol (Baltimore Md 1950) (2011) 186(6):3421–31. doi: 10.4049/jimmunol.1000569
120. Spada R, Rojas JM, Pérez-Yagüe S, Mulens V, Cannata-Ortiz P, Bragado R, et al. NKG2D Ligand Overexpression in Lupus Nephritis Correlates With Increased NK Cell Activity and Differentiation in Kidneys But Not in the Periphery. J Leukoc Biol (2015) 97(3):583–98. doi: 10.1189/jlb.4A0714-326R
121. Spaggiari GM, Capobianco A, Abdelrazik H, Becchetti F, Mingari MC, Moretta L. Mesenchymal Stem Cells Inhibit Natural Killer-Cell Proliferation, Cytotoxicity, and Cytokine Production: Role of Indoleamine 2,3-Dioxygenase and Prostaglandin E2. Blood (2008) 111(3):1327–33. doi: 10.1182/blood-2007-02-074997
122. Aggarwal S, Pittenger MF. Human Mesenchymal Stem Cells Modulate Allogeneic Immune Cell Responses. Blood (2005) 105(4):1815–22. doi: 10.1182/blood-2004-04-1559
123. Crop MJ, Korevaar SS, de Kuiper R, IJzermans JN, van Besouw NM, Baan CC, et al. Human Mesenchymal Stem Cells Are Susceptible to Lysis by CD8(+) T Cells and NK Cells. Cell Transplant (2011) 20(10):1547–59. doi: 10.3727/096368910x564076
124. Spaggiari GM, Capobianco A, Becchetti S, Mingari MC, Moretta L. Mesenchymal Stem Cell-Natural Killer Cell Interactions: Evidence That Activated NK Cells Are Capable of Killing MSCs, Whereas MSCs can Inhibit IL-2-Induced NK-Cell Proliferation. Blood (2006) 107(4):1484–90. doi: 10.1182/blood-2005-07-2775
125. Sotiropoulou PA, Perez SA, Gritzapis AD, Baxevanis CN, Papamichail M. Interactions Between Human Mesenchymal Stem Cells and Natural Killer Cells. Stem Cells (Dayton Ohio) (2006) 24(1):74–85. doi: 10.1634/stemcells.2004-0359
126. Lema GP, Maier H, Nieto E, Vielhauer V, Luckow B, Mampaso F, et al. Chemokine Expression Precedes Inflammatory Cell Infiltration and Chemokine Receptor and Cytokine Expression During the Initiation of Murine Lupus Nephritis. J Am Soc Nephrol (2001) 12(7):1369–82. doi: 10.1681/asn.V1271369
127. Yin Z, Bahtiyar G, Zhang N, Liu L, Zhu P, Robert ME, et al. IL-10 Regulates Murine Lupus. J Immunol (Baltimore Md 1950) (2002) 169(4):2148–55. doi: 10.4049/jimmunol.169.4.2148
128. Choi EW, Lee HW, Shin IS, Park JH, Yun TW, Youn HY, et al. Comparative Efficacies of Long-Term Serial Transplantation of Syngeneic, Allogeneic, Xenogeneic, or CTLA4Ig-Overproducing Xenogeneic Adipose Tissue-Derived Mesenchymal Stem Cells on Murine Systemic Lupus Erythematosus. Cell Transplant (2016) 25(6):1193–206. doi: 10.3727/096368915x689442
129. Burkett PR, Meyer zu Horste G, Kuchroo VK. Pouring Fuel on the Fire: Th17 Cells, the Environment, and Autoimmunity. J Clin Invest (2015) 125(6):2211–9. doi: 10.1172/jci78085
130. Ruddy MJ, Shen F, Smith JB, Sharma A, Gaffen SL. Interleukin-17 Regulates Expression of the CXC Chemokine LIX/CXCL5 in Osteoblasts: Implications for Inflammation and Neutrophil Recruitment. J Leukoc Biol (2004) 76(1):135–44. doi: 10.1189/jlb.0204065
131. Albanesi C, Cavani A, Girolomoni G. IL-17 Is Produced by Nickel-Specific T Lymphocytes and Regulates ICAM-1 Expression and Chemokine Production in Human Keratinocytes: Synergistic or Antagonist Effects With IFN-Gamma and TNF-Alpha. J Immunol (Baltimore Md 1950) (1999) 162(1):494–502.
132. Pisitkun P, Ha HL, Wang H, Claudio E, Tivy CC, Zhou H, et al. Interleukin-17 Cytokines Are Critical in Development of Fatal Lupus Glomerulonephritis. Immunity (2012) 37(6):1104–15. doi: 10.1016/j.immuni.2012.08.014
133. Amarilyo G, Lourenço EV, Shi FD, La Cava A. IL-17 Promotes Murine Lupus. J Immunol (Baltimore Md 1950) (2014) 193(2):540–3. doi: 10.4049/jimmunol.1400931
134. Kwan BC, Tam LS, Lai KB, Lai FM, Li EK, Wang G, et al. The Gene Expression of Type 17 T-Helper Cell-Related Cytokines in the Urinary Sediment of Patients With Systemic Lupus Erythematosus. Rheumatology (Oxf Engl) (2009) 48(12):14917. doi: 10.1093/ / rheumatology kep255
135. Sun L, Akiyama K, Zhang H, Yamaza T, Hou Y, Zhao S, et al. Mesenchymal Stem Cell Transplantation Reverses Multiorgan Dysfunction in Systemic Lupus Erythematosus Mice and Humans. Stem Cells (Dayton Ohio) (2009) 27(6):1421–32. doi: 10.1002/stem.68
136. Wei S, Xie S, Yang Z, Peng X, Gong L, Zhao K, et al. Allogeneic Adipose-Derived Stem Cells Suppress Mtorc1 Pathway in a Murine Model of Systemic Lupus Erythematosus. Lupus (2019) 28(2):199–209. doi: 10.1177/0961203318819131
137. Morimoto S, Tokano Y, Nakano S, Watanabe T, Tamayama Y, Mitsuo A, et al. Chemoattractant Mechanism of Th1 Cells in Class III and IV Lupus Nephritis. Autoimmunity (2009) 42(2):143–9. doi: 10.1080/08916930802438790
138. Schwarting A, Tesch G, Kinoshita K, Maron R, Weiner HL, Kelley VR. IL-12 Drives IFN-Gamma-Dependent Autoimmune Kidney Disease in MRL-Fas(lpr) Mice. J Immunol (Baltimore Md 1950) (1999) 163(12):6884–91.
139. Fan X, Oertli B, Wüthrich RP. Up-Regulation of Tubular Epithelial Interleukin-12 in Autoimmune MRL-Fas(lpr) Mice With Renal Injury. Kidney Int (1997) 51(1):79–86. doi: 10.1038/ki.1997.10
140. Chang JW, Hung SP, Wu HH, Wu WM, Yang AH, Tsai HL, et al. Therapeutic Effects of Umbilical Cord Blood-Derived Mesenchymal Stem Cell Transplantation in Experimental Lupus Nephritis. Cell Transplant (2011) 20(2):245–57. doi: 10.3727/096368910x520056
141. Choi EW, Shin IS, Song JW, Yun TW, Yang J, Choi KS, et al. Transplantation of Adipose Tissue-Derived Mesenchymal Stem Cells Prevents the Development of Lupus Dermatitis. Stem Cells Dev (2015) 24(17):2041–51. doi: 10.1089/scd.2015.0021
142. Jego G, Bataille R, Pellat-Deceunynck C. Interleukin-6 is a Growth Factor for Nonmalignant Human Plasmablasts. Blood (2001) 97(6):1817–22. doi: 10.1182/blood.v97.6.1817
143. Bertolini JN, Benson EM. The Role of Human Interleukin-6 in B-Cell Isotype Regulation and Differentiation. Cell Immunol (1990) 125(1):197–209-. doi: 10.1016/0008-8749(90)90074-2
144. Okada M, Kitahara M, Kishimoto S, Matsuda T, Hirano T, Kishimoto T. IL-6/BSF2 Functions as a Killer Helper Factor in the In Vitro Induction of Cytotoxic T Cells. J Immunol (Baltimore Md 1950) (1988) 141(5):1543–9.
145. Tanigawa T, Nicola N, McArthur GA, Strasser A, Begley CG. Differential Regulation of Macrophage Differentiation in Response to Leukemia Inhibitory Factor/Oncostatin-M/interleukin-6: The Effect of Enforced Expression of the SCL Transcription Factor. Blood (1995) 85(2):379–90.
146. Chomarat P, Banchereau J, Davoust J, Palucka AK. IL-6 Switches the Differentiation of Monocytes From Dendritic Cells to Macrophages. Nat Immunol (2000) 1(6):510–4. doi: 10.1038/82763
147. Reyes-Thomas J, Blanco I, Putterman C. Urinary Biomarkers in Lupus Nephritis. Clin Rev Allergy Immunol (2011) 40(3):138–50. doi: 10.1007/s12016010-8197-z
148. Tsai CY, Wu TH, Yu CL, Lu JY, Tsai YY. Increased Excretions of Beta2microglobulin, IL-6, and IL-8 and Decreased Excretion of Tamm-Horsfall Glycoprotein in Urine of Patients With Active Lupus Nephritis. Nephron (2000) 85(3):207–14. doi: 10.1159/000045663
149. Cash H, Relle M, Menke J, Brochhausen C, Jones SA, Topley N, et al. Interleukin 6 (IL-6) Deficiency Delays Lupus Nephritis in MRL-Faslpr Mice: The IL-6 Pathway as a New Therapeutic Target in Treatment of Autoimmune Kidney Disease in Systemic Lupus Erythematosus. J Rheumatol (2010) 37(1):60–70. doi: 10.3899/jrheum.090194
150. Thiel A, Yavanian G, Nastke MD, Morales P, Kouris NA, Kimbrel EA, et al. Human Embryonic Stem Cell-Derived Mesenchymal Cells Preserve Kidney Function and Extend Lifespan in NZB/W F1 Mouse Model of Lupus Nephritis. Sci Rep (2015) 5:17685. doi: 10.1038/srep17685
151. Brennan DC, Yui MA, Wuthrich RP, Kelley VE. Tumor Necrosis Factor and IL-1 in New Zealand Black/White Mice. Enhanced Gene Expression and Acceleration of Renal Injury. J Immunol (Baltimore Md 1950) (1989) 143(11):3470–5.
152. Yung S, Tsang RC, Sun Y, Leung JK, Chan TM. Effect of Human Anti-DNA Antibodies on Proximal Renal Tubular Epithelial Cell Cytokine Expression: Implications on Tubulointerstitial Inflammation in Lupus Nephritis. J Am Soc Nephrol (2005) 16(11):3281–94. doi: 10.1681/asn.2004110917
153. Malide D, Russo P, Bendayan M. Presence of Tumor Necrosis Factor Alpha and Interleukin-6 in Renal Mesangial Cells of Lupus Nephritis Patients. Hum Pathol (1995) 26(5):558–64. doi: 10.1016/0046-8177(95)90253-8
154. Aringer M, Feierl E, Steiner G, Stummvoll GH, Höfler E, Steiner CW, et al. Increased Bioactive TNF in Human Systemic Lupus Erythematosus: Associations With Cell Death. Lupus (2002) 11(2):102–8. doi: 10.1191/0961203302lu160oa
155. Liu J, Lu X, Lou Y, Cai Y, Cui W, Wang J, et al. Xenogeneic Transplantation of Human Placenta-Derived Mesenchymal Stem Cells Alleviates Renal Injury and Reduces Inflammation in a Mouse Model of Lupus Nephritis. BioMed Res Int (2019) 2019:9370919. doi: 10.1155/2019/9370919
156. Snapper CM, Paul WE. Interferon-Gamma and B Cell Stimulatory Factor-1 Reciprocally Regulate Ig Isotype Production. Science (New York NY) (1987) 236(4804):944–7. doi: 10.1126/science.3107127
157. Snapper CM, Rosas F, Moorman MA, Jin L, Shanebeck K, Klinman DM, et al. IFN-Gamma Is a Potent Inducer of Ig Secretion by Sort-Purified Murine B Cells Activated Through the mIg, But Not the CD40, Signaling Pathway. Int Immunol (1996) 8(6):877–85. doi: 10.1093/intimm/8.6.877
158. Hu X, Chakravarty SD, Ivashkiv LB. Regulation of Interferon and Toll-Like Receptor Signaling During Macrophage Activation by Opposing Feedforward and Feedback Inhibition Mechanisms. Immunol Rev (2008) 226:41–56. doi: 10.1111/j.1600-065X.2008.00707.x
159. Oriss TB, McCarthy SA, Morel BF, Campana MA, Morel PA. Crossregulation Between T Helper Cell (Th)1 and Th2: Inhibition of Th2 Proliferation by IFN-Gamma Involves Interference With IL-1. J Immunol (Baltimore Md 1950) (1997) 158(8):3666–72.
160. Szabo SJ, Sullivan BM, Peng SL, Glimcher LH. Molecular Mechanisms Regulating Th1 Immune Responses. Annu Rev Immunol (2003) 21:713–58. doi: 10.1146/annurev.immunol.21.120601.140942
161. Balomenos D, Rumold R, Theofilopoulos AN. Interferon-Gamma is Required for Lupus-Like Disease and Lymphoaccumulation in MRL-Lpr Mice. J Clin Invest (1998) 101(2):364–71. doi: 10.1172/jci750
162. Jacob CO, van der Meide PH, McDevitt HO. In Vivo Treatment of (NZB X NZW)F1 Lupus-Like Nephritis With Monoclonal Antibody to Gamma Interferon. J Exp Med (1987) 166(3):798–803. doi: 10.1084/jem.166.3.798
163. Ozmen L, Roman D, Fountoulakis M, Schmid G, Ryffel B, Garotta G. Experimental Therapy of Systemic Lupus Erythematosus: The Treatment of NZB/W Mice With Mouse Soluble Interferon-Gamma Receptor Inhibits the Onset of Glomerulonephritis. Eur J Immunol (1995) 25(1):6–12. doi: 10.1002/eji.1830250103
164. Zheng B, Zhang P, Yuan L, Chhetri RK, Guo Y, Deng D. Effects of Human Umbilical Cord Mesenchymal Stem Cells on Inflammatory Factors and miR-181a in T Lymphocytes From Patients With Systemic Lupus Erythematosus. Lupus (2020) 29(2):126–35. doi: 10.1177/0961203319896417
165. Wang H, Yang H, Czura CJ, Sama AE, Tracey KJ. HMGB1 as a Late Mediator of Lethal Systemic Inflammation. Am J Respir Crit Care Med (2001) 164(10 Pt 1):1768–73. doi: 10.1164/ajrccm.164.10.2106117
166. Gu Z, Akiyama K, Ma X, Zhang H, Feng X, Yao G, et al. Transplantation of Umbilical Cord Mesenchymal Stem Cells Alleviates Lupus Nephritis in MRL/lpr Mice. Lupus (2010) 19(13):1502–14. doi: 10.1177/0961203310373782
167. Ishida H, Muchamuel T, Sakaguchi S, Andrade S, Menon S, Howard M. Continuous Administration of Anti-Interleukin 10 Antibodies Delays Onset of Autoimmunity in NZB/W F1 Mice. J Exp Med (1994) 179(1):305–10. doi: 10.1084/jem.179.1.305
168. Youd M, Blickarz C, Woodworth L, Touzjian T, Edling A, Tedstone J, et al. Allogeneic Mesenchymal Stem Cells do Not Protect NZBxNZW F1 Mice From Developing Lupus Disease. Clin Exp Immunol (2010) 161(1):176–86. doi: 10.1111/j.1365-2249.2010.04158.x
169. Tani C, Vagnani S, Carli L, Querci F, Kühl AA, Spieckermann S, et al. Treatment With Allogenic Mesenchymal Stromal Cells in a Murine Model of Systemic Lupus Erythematosus. Int J Stem Cells (2017) 10(2):160–8. doi: 10.15283/ijsc17014
170. Bazhanov N, Ylostalo JH, Bartosh TJ, Tiblow A, Mohammadipoor A, Foskett A, et al. Intraperitoneally Infused Human Mesenchymal Stem Cells Form Aggregates With Mouse Immune Cells and Attach to Peritoneal Organs. Stem Cell Res Ther (2016) 7:27. doi: 10.1186/s13287-016-0284-5
171. Gu F, Wang D, Zhang H, Feng X, Gilkeson GS, Shi S, et al. Allogeneic Mesenchymal Stem Cell Transplantation for Lupus Nephritis Patients Refractory to Conventional Therapy. Clin Rheumatol (2014) 33(11):1611–9. doi: 10.1007/s10067-014-2754-4
172. Wang D, Zhang H, Liang J, Li X, Feng X, Wang H, et al. Allogeneic Mesenchymal Stem Cell Transplantation in Severe and Refractory Systemic Lupus Erythematosus: 4 Years of Experience. Cell Transplant (2013) 22(12):2267–77. doi: 10.3727/096368911X582769c
173. Deng D, Zhang P, Guo Y, Lim TO. A Randomised Double-Blind, Placebo-Controlled Trial of Allogeneic Umbilical Cord-Derived Mesenchymal Stem Cell for Lupus Nephritis. Ann Rheum Dis (2017) 76(8):1436–9. doi: 10.1136/annrheumdis-2017-211073
174. Li X, Wang D, Liang J, Zhang H, Sun L. Mesenchymal SCT Ameliorates Refractory Cytopenia in Patients With Systemic Lupus Erythematosus. Bone Marrow Transplant (2013) 48(4):544–50. doi: 10.1038/bmt.2012.184
175. Xu J, Chen J, Li W, Lian W, Huang J, Lai B, et al. Additive Therapeutic Effects of Mesenchymal Stem Cells and IL-37 for Systemic Lupus Erythematosus. J Am Soc Nephrol (2020) 31(1):54–65. doi: 10.1681/asn.2019050545
Keywords: mesenchymal stem cells, lupus nephritis, immunomodulation, immune cells, cytokines, autoimmune
Citation: Li J, Luo M, Li B, Lou Y, Zhu Y, Bai X, Sun B, Lu X and Luo P (2022) Immunomodulatory Activity of Mesenchymal Stem Cells in Lupus Nephritis: Advances and Applications. Front. Immunol. 13:843192. doi: 10.3389/fimmu.2022.843192
Received: 25 December 2021; Accepted: 17 February 2022;
Published: 10 March 2022.
Edited by:
Philippe Saas, INSERM U1098 Interactions Hôte-Greffon-Tumeur & Ingénierie Cellulaire et Génique, FranceReviewed by:
Helen Michelle McGettrick, University of Birmingham, United KingdomJehan J. El-Jawhari, Nottingham Trent University, United Kingdom
Copyright © 2022 Li, Luo, Li, Lou, Zhu, Bai, Sun, Lu and Luo. This is an open-access article distributed under the terms of the Creative Commons Attribution License (CC BY). The use, distribution or reproduction in other forums is permitted, provided the original author(s) and the copyright owner(s) are credited and that the original publication in this journal is cited, in accordance with accepted academic practice. No use, distribution or reproduction is permitted which does not comply with these terms.
*Correspondence: Xuehong Lu, changchunlxh@foxmail.com; Ping Luo, luopingjen@163.com