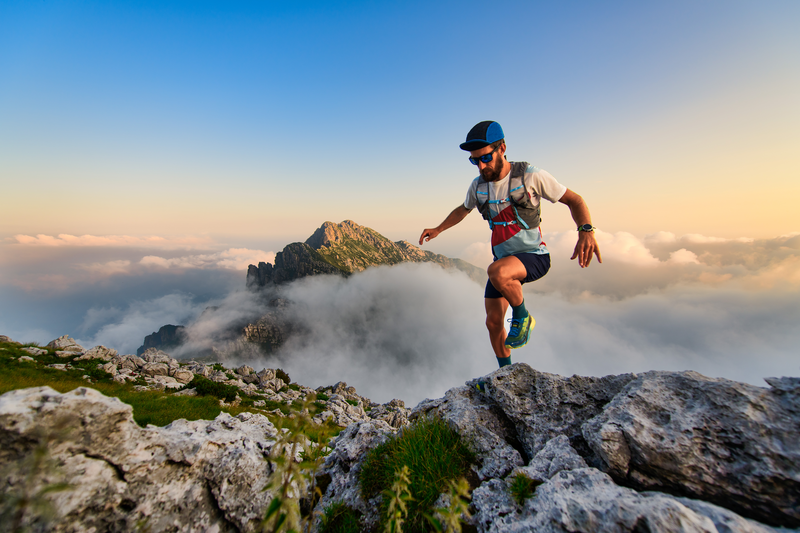
95% of researchers rate our articles as excellent or good
Learn more about the work of our research integrity team to safeguard the quality of each article we publish.
Find out more
ORIGINAL RESEARCH article
Front. Immunol. , 10 March 2022
Sec. Viral Immunology
Volume 13 - 2022 | https://doi.org/10.3389/fimmu.2022.835333
Human coronavirus OC43 (HCoV-OC43) is the coronavirus most associated with “common colds”, infections of the upper respiratory tract. Previously, we reported that direct interactions of nucleocapsid (N) protein and C-terminal domain of Spike protein (Spike CD) are essential for replication of SARS-CoV-2 and MERS-CoV. Thus, we developed a novel ELISA-based strategy targeting these specific interactions to detect SARS-CoV-2 and MERS-CoV. Here, we investigated whether the same principles apply to HCoV-OC43. We discovered that the S protein of HCoV-OC43 interacts with N protein and that cell penetrating Spike CD peptide inhibits virus protein expression and replication of HCoV-OC43. The interaction between HCoV-OC43 S and N proteins were recapitulated with a recombinant HCoV-OC43 Spike CD fusion protein and a recombinant HCoV-OC43 N fusion protein in vitro. By producing an anti-HCoV-OC43 N protein-specific monoclonal antibody, we established a virus detection system based on the interaction between recombinant Spike CD and N protein of HCoV-OC43. We suggest that the interaction between Spike CD and N protein is conserved in coronaviruses and therefore could be a target for therapeutics against both novel coronavirus and its variants.
Coronaviruses (CoVs) cause infections of the upper respiratory tract that range in severity from mild to lethal. CoVs did not attract much scientific attention until the outbreak of severe acute respiratory syndrome (SARS) in 2002 (1). CoVs are enveloped, non-segmented positive-sense RNA viruses belonging to the Coronaviridae family. CoVs are divided into four genera including Alphacoronaviruses, Betacoronaviruses, Gammacoronaviruses, and Deltacoronaviruses by International Committee for Taxonomy of Viruses (2, 3). CoVs infect many different hosts, including bats, cattle, avians, swine, and humans. Currently, there are seven reported human coronaviruses (HCoVs). Two HCoVs, HCoV-229E and HCoV-NL63, are classified in the Alphacoronavirus genera, whereas the other five HCoVs are in the Betacoronaviruses genera. Within the Betacoronaviruses genera, HCoVs are divided by lineage, as HCoV-HKU1 and HCoV-OC43 belong to lineage A, severe acute respiratory syndrome coronavirus (SARS-CoV) and SARS-CoV-2 belong to lineage B, and Middle East respiratory syndrome coronavirus (MERS-CoV) belongs to lineage C (4, 5). Highly pathogenic coronaviruses belonging to Betacoronaviruses emerged in 2002 (SARS-CoV), in 2012 (MERS-CoV), and in 2019 (SARS-CoV-2). SARS-CoV-2 is now the causative agent of the ongoing COVID-19 pandemic, highlighting the importance of studying HCoVs (6).
All HCoVs contain four major structural protein complexes: spike glycoprotein (S protein), nucleocapsid phosphoprotein (N protein), Membrane glycoprotein (M protein) and small envelope glycoprotein (E protein) (7). Many studies have explored the mechanism of receptor-mediated host cell entry by S protein binding of Betacoronaviruses. SARS-CoV and SARS-CoV-2 S protein binds to angiotensin-converting enzyme 2 (ACE2) (8, 9), MERS-CoV S protein binds to dipeptidyl peptidase 4 (DPP4) (10), and HCoV-OC43 S protein binds to 9-O-acetylated sialic acid (11). Taken together, these results suggest that S proteins are ideal targets for therapeutic agents against pathogenic coronaviruses.
In addition to S protein, N protein is considered another potential therapeutic target against coronavirus infection (12). N protein dimers bind to viral RNA and are essential for CoV replication (13). N protein consists of two major domains: the N-terminal domain (NTD) and C-terminal domain (CTD). Many investigators have characterized the viral RNA binding of N proteins in SARS-CoV (14, 15), SARS-CoV-2 (16, 17), MERS-CoV (18, 19), and HCoV-OC43 (20) through structural analysis. Interactions between M and N proteins play an important role in the assembly of SARS-CoV (21), SARS-CoV-2 (22), MERS-CoV (23), and HCoV-OC43 (24). Recently, we suggested that a direct interaction between the S protein and N protein contributes to the replication of MERS-CoV and SARS-CoV-2 (25). However, interactions between S and N proteins have yet to be identified in other coronaviruses.
Here, we identified a direct interaction between the S and N proteins in HCoV-OC43-infected cells. We found that inhibition of this interaction by synthetic cell-penetrating peptide corresponding to the C-terminal domain of S protein (Spike CD) of HCoV-OC43 reduced the production of HCoV-OC43 viruses in infected cells. Thus, therapeutic agents targeting the interaction of S and N proteins could be effective against HCoV-OC43.
Vero cells originating from African green monkey kidneys were obtained from the Korean Cell Line Bank (Seoul, Korea). The cells were cultured in Dulbecco’s modified Eagle’s medium (DMEM, ATCC, Manassas, VA, USA) containing 10% fetal bovine serum (FBS, Thermo Fisher Scientific, Waltham, MA, USA), penicillin (100 U/ml), and streptomycin (100 µg/ml). Cells were maintained in 95% atmospheric air and 5% CO2 at 37°C.
HCoV-OC43 (KBPV-VR-8) was provided by the Korea Bank for Pathogenic Viruses (College of Medicine, Korea University, Seoul, Korea). The virus amplification was carried out as described previously [26]. Briefly, Vero cells cultured in six-well plates (3 × 105 cells/well) were infected with HCoV-OC43 suspended in phosphate-buffered saline (PBS) at a multiplicity of infection (MOI) of 0.03. After incubation for 1 h at 37°C with shaking every 10 min, the cells were cultured in DMEM containing 2% FBS at 37°C in a 5% CO2 incubator. At 4 days post-infection, the cell culture supernatants were harvested and centrifuged for 10 min at 3,000 rpm. Supernatants were collected and stored at -70°C. HCoV-OC43 amplification was performed under biosafety level 2 (BSL-2) conditions in the Translational Research Center of Hallym University. Both MERS-CoV (MERS-CoV/KOR/KNIH/002_05_2015) and SARS-CoV-2 S clade (hCoV-19/South Korea/KCDC03/2020, NCCP43326) were provided by the National Culture Collection for Pathogens (Osong, Korea). MERS-CoV and SARS-CoV-2 amplification and cell culture procedures were performed according to biosafety level 3 (BSL-3) conditions in the Hallym Clinical and Translational Science Institute in accordance with the recommendations of the Institutional Biosafety Committee of Hallym University (Permit no. Hallym2020-12).
HCoV-OC43 titers were quantified by plaque assay as described previously (26, 27). Briefly, Vero cells (7 × 105 cells/well) were cultured in 6-well plates (Corning, NY, USA) for 18 h. The cells were washed with PBS and inoculated with tenfold serial dilutions of the virus-infected culture supernatants in PBS at 37°C. After 1 h of infection, the medium was aspirated from the wells and 3 ml DMEM/F12 (Thermo Fisher Scientific) mixed with 2% Oxoid agar was added to the wells. At 5 days post-infection, the overlay medium was removed, and plaque formation was visualized by staining with 0.1% crystal violet.
To analyze the interaction between the S and N proteins, Spike protein C-terminal domain (Spike CD HCoV-OC43) peptide was synthesized based on the HCoV-OC43 S protein sequence (YP_009555241.1: Spike CD HCoV-OC43, 1320CCTGCGTSCFKKCGGCCDDYTGYQELVIKTSHDD1353. The peptide was conjugated with nine D-arginine residues at the N-terminus (R-Spike CD HCoV-OC43) to penetrate host cells. A negative control cell-penetrating peptide (R-CP-1) was synthesized by Anygen Co., Ltd. (Gwang Ju, South Korea) to contain nine D-arginine residues (NH2-d-RRRRRRRRR-AQARRKNYGQLDIFP-COOH) (28).
Antibodies against HCoV-OC43 N (Mouse anti-HCoV-OC43 N monoclonal Ab, Catalog No. LS-C79764) and S protein (Rabbit anti-HCoV-OC43 S Ab, Catalog No. LS-C371066) were purchased from LifeSpan BioSciences (Seattle, WA, USA). Both the peroxidase-conjugated streptavidin (Catalog No. S5512) and antibody for β-actin were obtained from Sigma-Aldrich (St. Louis, MO, USA). The antibody against the Fc domain of human IgG (Catalog No. 790-035-098) was obtained from Jackson ImmunoResearch Laboratories (West Grove, PA, USA).
Vero cells were grown in six-well plates at a density of 3 × 105 cells/well. The cells were cultured for 18 h and infected with HCoV-OC43 (MOI of 0.1) in PBS for 1 h in a 5% CO2 incubator at 37°C. After infection, the supernatants were removed, and the culture medium was changed with DMEM containing 2% FBS. After 3 days of infection, HCoV-OC43-infected Vero cells were lysed in cell lysis buffer (10 mM HEPES, 150 mM NaCl, 5 mM EDTA, 100 mM NaF, 2 mM Na3VO4, protease inhibitor cocktail, and 1% NP-40) for 30 min at 4°C. Cell lysates were collected by centrifugation at 14,000 rpm at 4°C for 20 min. Equal amounts of proteins from each cell lysate were resolved on 4–12% Bis-Tris gradient gels (Bolt™ 4–12% Bis-Tris Plus gel, Thermo Fisher Scientific) and transferred onto nitrocellulose membranes. The membranes were blocked with 3% BSA, incubated with either anti-HCoV-OC43 N monoclonal Ab or anti-HCoV-OC43 S Ab overnight at 4°C, then subsequently incubated with horseradish peroxidase (HRP)-conjugated secondary antibodies. Protein bands were developed using enhanced chemiluminescence reagent (Thermo Fisher Scientific) and analyzed with ChemiDoc (Bio-Rad, Hercules, CA, USA).
The HCoV-OC43-infected cell lysates were incubated with anti-HCoV-OC43 S Ab for 3 h at 4°C. Protein A beads (CaptivA™ PriMAB 52% (v/v) slurry, Repligen, Waltham, MA, USA) were added into the cell lysates. After 1 h incubation at 4°C, the immunocomplexes were collected by centrifugation, then separated by 4–12% gradient SDS-PAGE (Thermo Fisher Scientific) and subjected to western blotting analysis using either anti-HCoV-OC43 N monoclonal Ab or anti-HCoV-OC43 S Ab.
To analyze the inhibitory effect of the HCoV-OC43 Spike CD peptide on HCoV-OC43 S protein and N protein expression, confocal images were analyzed as described previously (29). Vero cells (5 × 104 cells/well) were cultivated on coverslips on 12-well plates for 18 h. The cells were infected with HCoV-OC43 (MOI of 0.1) in PBS for 1 h in a 5% CO2 incubator at 37°C. After infection, the culture medium was changed to DMEM containing 2% FBS. The cells were then treated 6 h post-infection with R-Spike CD HCoV-OC43 peptide or Spike CD HCoV-OC43 peptide. After 48 h incubation in a 5% CO2 incubator at 37°C, the cells were fixed with 4% paraformaldehyde then permeabilized with Tween-20 in PBS (PBST) containing 1% bovine serum albumin (BSA). The permeabilized cells were incubated with anti-HCoV-OC43 N monoclonal Ab (1:5,000 dilution) or anti-HCoV-OC43 S Ab (1:200 dilution) for 2 h, washed with PBST containing 1% BSA, and incubated for 1 h with Alexa Fluor 488-conjugated secondary antibody (Thermo Fisher Scientific). Hoechst 33258 was used for nuclei staining. The cells were observed using a Carl Zeiss LSM710 microscope.
To investigate the effect of HCoV-OC43 Spike CD peptide on HCoV-OC43 replication, we also performed plaque reduction assay as described previously (29, 30). Vero cells (7 × 105 cells/well) were cultured on six-well plates for 18 h. HCoV-OC43 (200 pfu) was mixed with two-fold serially diluted R-Spike CD HCoV-OC43 peptide, Spike CD HCoV-OC43 peptide or R-CP-1 peptide in PBS. Vero cells on six-well plates were washed with PBS and then the diluted R-Spike CD HCoV-OC43 peptide, Spike CD HCoV-OC43 peptide, or R-CP-1 peptide mixtures was added to the Vero cells. The cells were incubated for 1 h in a 5% CO2 incubator at 37°C, shaking at 15–20-min intervals. After incubation, the supernatants were removed, and the virus replication was quantified using the previously described plaque assay.
To examine the cytotoxic effect of the cell-penetrating peptides in cells, Vero cells (5 × 103 cells/well) were cultured on 96-well plates in DMEM containing 10% FBS. After cultivation for 18 h, the culture media was changed to DMEM containing 2% FBS and cultured for 6 h. The cells were then incubated with two-fold serially diluted R-Spike CD HCoV-OC43 peptide, Spike CD HCoV-OC43 peptide or R-CP-1 peptide for 48 h. Lastly, the cells were incubated with Cell Counting Kit-8 (CCK-8) solution (Dojindo Molecular Technologies, Rockville, MD, USA) for 2 h at 37°C. Soluble formazan was measured by absorbance at 450 nm using a microplate reader (Thermo Fisher Scientific, Ratastie, Finland).
A fusion gene encoding a biotin peptide-6xHis-tagged (NSGSLHHILDAQKMVWNHR-DRNLPPLAPLGPHHHHHH) HCoV-OC43 N protein (GenBank ID: NC006213.1, nucleotide number 29079-30425, protein YP_009555245.1) was synthesized (Bioneer, Daejeon, Korea) with restriction enzyme sites (Not I and Kpn I) included at 5′ and 3′ ends, respectively. The biotin peptide sequence was recognized by the Escherichia coli biotin holoenzyme synthetase, BirA (31–33). The synthesized fusion gene was inserted into the modified pcDNA 3.4 expression vector (Invitrogen, Waltham, MA, USA) containing IL-2 signal sequences (pcDNA3.4-HCoV-OC43 N protein-Biotin-His6) for mammalian cell expression. The HCoV-OC43 N protein-Biotin-His6 was expressed in ExpiCHO cells (catalog No. A29133, Thermo Fisher Scientific) with modified expression vector containing BirA (pTT3-secreted BirA-8xHis, Catalog No. #32408; Addgene, Watertown, MA, USA). The HCoV-OC43 N protein-Biotin-His6 was purified from ExpiCHO culture supernatants after 14 days of cell culture at 32°C using Ni-NTA-agarose (Qiagen) chromatography. The expression and purification of HCoV-OC43 N protein-Biotin-His6 was confirmed by SDS-PAGE and western blot analysis with the anti-His-tag antibody.
Fusion gene encoding HCoV-OC43 Spike CD (GenBank ID: NC_006213, nucleotide number 27600-27701, protein YP_009555241.1) and human IgG1 Fc domain (GenBank ID: AK123800.1. protein) was synthesized (Bioneer, Daejeon, Korea), including restriction enzyme sites (Not I and Kpn I) at 5′ and 3′ ends, respectively. This fusion gene was inserted into the modified pcDNA 3.4 expression vector containing IL-2 signal sequences (pcDNA3.4-HCoV-OC43 Spike CD-Fc) for mammalian cell expression. The HCoV-OC43 Spike CD-Fc fusion protein was expressed using the in Gibco™ ExpiCHO™ Expression System Kit according to the manufacturer’s instructions. At 14 days after ExpiCHO culturing at 32°C, the HCoV-OC43 Spike CD-Fc fusion protein was purified using Protein A affinity chromatography. The purity of the HCoV-OC43 Spike CD-Fc fusion protein was evaluated using SDS-PAGE analysis.
Monoclonal antibodies against HCoV-OC43 N protein were produced using 4-week-old BALB/c mice (female, H-2b) provided by Nara-Biotec (Seoul, Korea). A mixture of recombinant HCoV-OC43 N-Bio-His6 protein (50 μg) and MB-ODN 4531(O) (CpG-DNA, (50 μg) was encapsulated in the DOPE : CHEMS complex (molar ratio of 1:1) as reported previously (34). BALB/c mice were intraperitoneally immunized with the HCoV-OC43 N protein complex three times at 14-day intervals. Animal care and experimental protocols were approved by the Hallym University Institutional Animal Care and Use Committee (Hallym2021-12).
Mouse SP2/0 myeloma cells were fused with splenocytes derived from HCoV-OC43 N protein-immunized mice using polyethylene glycol solution (PEG, Sigma-Aldrich) to generate hybridoma cells. Hybridoma cells were obtained and cloned in HAT medium (Sigma-Aldrich) and HT medium (Sigma-Aldrich) according to the standard hybridoma production method (34). Hybridoma cells producing mouse monoclonal antibody against HCoV-OC43 N protein were injected into the peritoneal cavity of BALB/c mice. Then, ascites was collected from the peritoneal cavity of BALB/c mice, and monoclonal antibody against HCoV-OC43 N protein was purified using Protein A column chromatography.
Streptavidin (2 μg/well) in ELISA coating buffer (0.1 M carbonate buffer, pH 9.6) was coated in 96-we1l immunoplates (Thermo Fisher Scientific) overnight at 4°C. The plates were blocked with PBS supplemented with PBST containing 3% BSA. Recombinant HCoV-OC43 N-Bio-His6 protein (2 μg/well) was added to each well for 2 h and then mouse sera, hybridoma culture supernatants, ascites, or purified monoclonal antibody solution were added to each well to measure HCoV-OC43 N protein-specific antibody levels by standard ELISA as described previously (34). The subclass of the monoclonal antibody was identified with HRP-conjugated anti-mouse IgG (each subclass) antibodies (Southern Biotech, Birmingham, AL, USA).
The binding affinity of the purified HCoV-OC43 N protein-specific monoclonal antibody was measured by ELISA as described previously (35). Briefly, streptavidin (2 μg/well) was coated in 96-well immunoplates (Thermo Fisher Scientific), and then recombinant HCoV-OC43 N-Bio-His6 protein (3 μg/well) was added into each well along with serially diluted (1:5) monoclonal antibody (clone 1C7D7 mAb) in PBST. The monoclonal antibody binding to HCoV-OC43 N protein was detected with HRP-conjugated anti-mouse IgG antibody (1:5,000 dilution, Catalog No. 715-035-150, Jackson ImmunoResearch Laboratories) and tetramethylbenzidine (TMB) peroxidase substrate (Kirkegaard and Perry Laboratories, Gaithersburg, MD, USA). The absorbance of each well was measured at 450 nm using the Spectra Max 250 microplate reader (Molecular Devices, San Jose, CA, USA). SigmaPlot was used to determine the EC50 value (27). To evaluate the specificity of the monoclonal antibody, recombinant MERS-CoV N protein-Bio-His6 and SARS-CoV-2 N protein-Bio-His6 (35) were analyzed as a control.
To investigate the specificity of monoclonal antibody (clone 1C7D7 mAb) obtained against HCoV-OC43 N protein, cell lysates were prepared from Vero cells infected with SARS-CoV-2, MERS-CoV, or HCoV-OC43 as described previously (26). Vero cells (3 × 105 cells) were cultured in six-well plates for 18 h. After washing the cells with PBS, each virus (0.1 MOI) was inoculated into each well in PBS and then incubated for 1 h in a 5% CO2 incubator at 37°C with shaking every 15 min. Cells were washed with PBS then cultured for 72 h at 37°C in a CO2 incubator in either 2 ml of DMEM/F12 for MERS-CoV or DMEM medium containing 2% FBS for SARS-CoV-2 and HCoV-OC43. After culturing, the cells were washed with PBS and lysed for 30 min with cell lysis buffer (10 mM HEPES, 150 mM NaCl, 5 mM EDTA, 100 mM NaF, 2 mM Na3VO4, protease inhibitor cocktail, 1% NP-40). The cell lysates were centrifuged at 13,000 rpm at 4°C for 10 min, and supernatants were collected and stored at -80°C.
The interaction of HCoV-OC43 Spike CD and HCoV-OC43 N protein was measured by ELISA as described previously (35). Briefly, HCoV-OC43 N protein-specific monoclonal antibody (clone 1C7D7 mAb, 3 μg/well) was coated in 96-well immunoplates overnight at 4°C, then blocked with PBST containing 1% BSA. HCoV-OC43 viral particles in cell culture supernatants were lysed with cell lysis buffer, serially diluted (1:3) in PBST, and added to the wells of the plate. After incubation for 2 h at room temperature, recombinant Fc domain or HCoV-OC43 Spike CD-Fc fusion protein were added along with goat anti-human IgG Fc antibody conjugated with HRP (1:5000 dilution, Catalog No.109-035-008, Jackson ImmunoResearch Laboratories). After developing with TMB peroxidase substrate, the amount of HCoV-OC43 N protein in each well was quantified using a Spectra Max 250 microplate reader (absorbance at 450 nm).
To analyze the interaction of HCoV-OC43 Spike CD and HCoV-OC43 N protein, we designed a “bait and prey” ELISA, described previously (35). Briefly, streptavidin (2 μg/well) was coated in 96-well immunoplates then blocked with PBST containing 1% BSA. HCoV-OC43 N protein-Biotin-His6 (3 μg/well) was added to each well and incubated for 2 h at room temperature. Recombinant MERS-CoV N protein-Bio-His6 and SARS-CoV-2 N protein-Bio-His6 (35) were used as a bait control to confirm the specificity of the interaction. After washing with PBST, HCoV-OC43 Spike CD-Fc fusion protein serially diluted to 1:3 was added to each well in PBST, then incubated for 2 h at room temperature. After washing with PBST, goat anti-human IgG Fc antibody conjugated with HRP (1:5000 dilution) was added to each well. Interactions between HCoV-OC43 Spike CD-human Fc fusion protein and HCoV-OC43 N protein were measured by developing with TMB peroxidase substrate. To analyze the interaction of HCoV-OC43 Spike CD-human Fc fusion protein and HCoV-OC43 N protein in the viral particles, cell culture supernatants containing HCoV-OC43 were lysed with cell lysis buffer and then added to the recombinant Fc domain or HCoV-OC43 Spike CD-Fc protein. After incubation for 2 h at 4°C, Protein A beads were added, and the immunocomplexes were collected and then subjected to western blotting analysis using anti-HCoV-OC43 N monoclonal Ab (clone 1C7D7 mAb).
Results are shown as the mean ± standard deviation. Differences between the samples were analyzed using an unpaired, two-tailed nonparametric t-test of significance (Instat; GraphPad Inc., San Diego, CA, USA). p-values < 0.05 were considered statistically significant.
In our previous work, we reported that Spike CD and N proteins directly interact during virus assembly of MERS-CoV and SARS-CoV-2 (25). Therefore, we hypothesized that the interaction between S protein and N protein might be conserved across coronaviruses. Here, we identified an interaction between S and N proteins of HCoV-OC43 through the immunocomplex analysis after immunoprecipitation using lysates of HCoV-OC43-infected Vero cells and a polyclonal antibody against the HCoV-OC43 S protein (anti-HCoV-OC43 S Ab). HCoV-OC43 S and N proteins were expressed in infected cells and N protein was coimmunoprecipitated with S protein (Figures 1A, B).
Figure 1 HCoV-OC43 S protein and N protein interact. (A) Expression of S and N proteins in HCoV-OC43-infected cells. Lysates of uninfected and HCoV-OC43 (0.1 MOI)-infected Vero cells were prepared and analyzed by western blotting with rabbit anti-HCoV-OC43 S antibody or mouse anti-HCoV-OC43 N monoclonal antibody. Anti-β-actin antibody was used as a loading control. S, spike protein. N, nucleocapsid protein. (B) Co-immunoprecipitation of N and S proteins. Equal amounts of the lysates (150 μg protein) were immunoprecipitated with anti-HCoV-OC43 S antibody. The immunocomplexes were analyzed by western blotting with rabbit anti-HCoV-OC43 S antibody or mouse anti-HCoV-OC43 N monoclonal antibody. HC, heavy chain. Ab, antibody. (C) HCoV-OC43 S and N protein expression is reduced by R-Spike CD HCoV-OC43 peptide. Vero cells were infected with HCoV-OC43 (0.1 MOI). After 6 h, the cells were treated with 2 μM of R-Spike CD HCoV-OC43 peptide or Spike CD HCoV-OC43 peptide. Cell lysates were prepared at 48 h after infection and analyzed by western blotting with the indicated antibodies.
Previously, we found that interaction between S and N proteins of MERS-CoV and SARS-CoV-2 was reduced by each cell-penetrating Spike CD peptide because the cell-penetrating Spike CD peptides interfered with the intracellular production of MERS-CoV and SARS-CoV-2 S and N proteins (25). Therefore, we investigated the effect of the cell-penetrating Spike CD HCoV-OC43 peptide (R-Spike CD HCoV-OC43) on the production of HCoV-OC43 S and N proteins. We found that S and N protein levels in HCoV-OC43-infected cells were consistently reduced by R-Spike CD HCoV-OC43 peptide compared to PBS control or cell-impermeable Spike CD HCoV-OC43 peptide (Figure 1C). We confirmed the inhibitory effect of R-Spike CD HCoV-OC43 peptide on S and N protein production by confocal image analysis. Confocal images showed remarkably reduced S and N protein production by R-Spike CD peptide in a concentration-dependent manner compared to PBS or Spike CD peptide controls (Figure 2).
Figure 2 R-Spike CD HCoV-OC43 peptide reduces N and S protein production. Vero cells were infected with HCoV-OC43 (0.1 MOI). After 6 h, the cells were treated with PBS control or R-Spike CD HCoV-OC43 peptide (1 or 2 μM) or Spike CD HCoV-OC43 peptide (2 μM). The cells were cultured for 48 h and then stained with rabbit anti-HCoV-OC43 S antibody (A) or mouse anti-HCoV-OC43 N monoclonal antibody and then (B) Alexa Fluor 488-conjugated secondary antibodies. The expression of HCoV-OC43 S protein and N protein were analyzed by confocal microscopy. Expression of S and N proteins measured by the fluorescence was decreased in the presence of R-Spike CD HCoV-OC43 peptide. Scale bar, 20 μm.
To investigate the effect of cell penetration by Spike CD HCoV-OC43 peptide on the replication of HCoV-OC43, Vero cells were infected with HCoV-OC43 in combination with R-Spike CD HCoV-OC43, Spike CD HCoV-OC43 or R-CP-1. Plaque formation assays revealed that HCoV-OC43 replication was significantly reduced by the treatment of R-Spike HCoV-OC43 peptide compared to Spike HCoV-OC43 peptide treatment (Figure 3A). Treatment with control R-CP-1 had some inhibitory effect at 2.5 μM and significant inhibitory effects at 5 or 10 μM on plaque formation, suggesting nonspecific effects of the cell-permeable peptides especially at high concentrations. To investigate potential side effects of cell-penetrating Spike CD peptides, we measured the cytotoxicity of R-Spike CD HCoV-OC43, Spike CD HCoV-OC43 or R-CP-1 in Vero cells. Cytotoxic effects of the cell-penetrating peptides were not observed at concentrations below 10 μM in Vero cells (Figure 3B), suggesting that there are no side effects of cell-penetrating Spike CD peptides at the concentration we used for functional assays in Figures 1, 2 (2 μM). Our results showed that inhibition of the interaction between the Spike CD and the N protein using a cell-permeable Spike CD HCoV-OC43 peptide reduces HCoV-OC43 replication.
Figure 3 R-Spike CD HCoV-OC43 peptide reduces HCoV-OC43 replication without cytotoxicity. (A) Vero cells in 6-well plates were infected with HCoV-OC43 (200 pfu/well) with indicated concentration of R-Spike CD HCoV-OC43 peptide, Spike CD HCoV-OC43 peptide, or R-CP-1 peptide (n=3). Plaques formed for 5 days were counted and compared between the peptide-treated samples and control samples treated with virus only. The plaque formation was reduced by R-Spike CD HCoV-OC43 peptide. (B) Vero cells were cultured with the indicated concentrations of cell-penetrating peptides for 48 h and subjected to viability assay. There was no cytotoxic effect of the peptides at concentrations below 10 μM. *p < 0.05, **p < 0.01, ***p < 0.001.
Previously, we developed an ELISA-based “bait and prey” system to confirm direct interaction between SARS-CoV-2 Spike CD and N protein using recombinant fusion proteins (35). Here, we used this ELISA-based “bait and prey” system to confirm the interaction between HCoV-OC43 Spike CD and N protein in vitro. To do this, we expressed and purified recombinant HCoV-OC43 N protein-Bio-His6 fusion protein (Figure 4A) and HCoV-OC43 Spike CD-Fc fusion protein (Figure 4B). Expected molecular mass of the recombinant Fc proteins are 25.5 kDa and 29.5 kDa, respectively, and most of the purified recombinant proteins showed major single bands with similar mass. However, there were minor protein bands with about two-fold higher molecular mass suggesting partial formation of dimers (Figure 4B). We designed a bait and prey assay system employing streptavidin, HCoV-OC43 N protein-Bio-His6, HCoV-OC43 Spike CD-Fc, and anti-human IgG Fc antibody conjugated with HRP (Figure 4C). The ELISA results showed that HCoV-OC43 Spike CD-Fc binds to HCoV-OC43 N protein-Bio-His6 in a concentration-dependent manner. There was weak binding of N protein-Bio-His6 to the Fc control at high concentrations and no binding in PBS control (Figure 4D). To further confirm the specificity of the interaction, we performed the same experiments with MERS-CoV N protein-Bio-His6 and SARS-CoV-2 N protein-Bio-His6 as a bait and found only weak binding of HCoV-OC43 Spike CD-Fc to other virus recombinant N proteins (Figure 4E). Taken together, our results support the idea that the Spike CD and the N protein of HCoV-OC43 interact specifically and directly with one another and suggest that our bait and prey system can be used to quantitatively assess this interaction in vitro.
Figure 4 The Spike CD-Fc fusion protein interacts with N protein-Bio-His6 recombinant protein in HCoV-OC43-infected cells. (A) Expression of biotin peptide-6×His-tagged HCoV-OC43 N protein (HCoV-OC43 N protein-Bio-His6). The purified recombinant protein was analyzed by SDS-PAGE (left) and western blotting with peroxidase-conjugated streptavidin (right). R, reducing condition; NR, non-reducing condition. (B) Expression of HCoV-OC43 Spike CD-Fc. The purified recombinant Fc domain protein and the HCoV-OC43 Spike CD-Fc fusion protein were analyzed by SDS-PAGE and Coomassie blue staining. (C) Schematic of the bait and prey assay system. (D, E) The amount of HCoV-OC43 Spike CD-Fc bound to HCoV-OC43 N protein-Bio-His6 recombinant protein in the wells was determined by ELISA. PBS, Fc domain, MERS-CoV N protein-Bio-His6 and SARS-CoV-2 N protein-Bio-His6 were used as a bait control. HCoV-OC43 Spike CD-Fc specifically interacted with HCoV-OC43 N protein-Bio-His6.
To detect the N protein of HCoV-OC43 particles, we produced a monoclonal antibody against N protein of HCoV-OC43 using recombinant HCoV-OC43 N protein-Bio-His6 and CpG-DNA co-encapsulated in a liposome (DOPE : CHEMS) as described previously (34). First, we confirmed production of antibody against recombinant HCoV-OC43 N protein from immunized mice (Figure 5A). Second, one hybridoma clone (1C7D7) producing HCoV-OC43 N protein-specific monoclonal antibody was selected from several clones and the HCoV-OC43 N protein-specific monoclonal antibody (1C7D7 mAb) was purified from the ascitic fluids of mice injected with hybridoma cells (Figures 5B, C). Third, we characterized the purified monoclonal antibody. The IgG subclass of the purified monoclonal antibody was IgG1 (Figure 5D). Binding of the monoclonal antibody to recombinant HCoV-OC43 N protein-Bio-His6 was measured by ELISA and the EC50 value was ~ 1.2 nM (Figure 5E). The purified monoclonal antibody recognized N protein in cell lysates of HCoV-OC43-infected Vero cells but not in MERS-CoV- or SARS-CoV-2-infected Vero cells (Figure 5F). Through ELISA analysis, we proved that the antibody specifically recognized HCoV-OC43 N protein-Bio-His6 but not MERS-CoV N protein-Bio-His6 or SARS-CoV-2 N protein-Bio-His6 (Figure 5G). Next, we confirmed a direct interaction between Spike CD and N protein in HCoV-OC43-infected cells. We incubated the purified HCoV-OC43 Spike CD-Fc or Fc control with lysates of HCoV-OC43 virus, pulled down complexes with Protein A agarose beads, and detected HCoV-OC43 N protein using the HCoV-OC43 N protein-specific monoclonal antibody (1C7D7 mAb). The results revealed that N protein of HCoV-OC43 specifically interacts with HCoV-OC43 Spike CD-Fc but not with the Fc control (Figure 6). The Fc control and HCoV-OC43 Spike CD-Fc recombinant proteins showed two protein bands composed of major monomers and minor dimers as we have seen in Figure 4B (Figure 6B).
Figure 5 Production and characterization of the anti-HCoV-OC43 N protein-specific monoclonal antibody. (A) ELISA was performed to determine the production of recombinant HCoV-OC43 N-Bio-His6 protein-specific antibody in the immunized mice. (B) Ascites was collected from mice injected with cloned hybridoma cells (1C7D7). Production of recombinant HCoV-OC43 N-Bio-His6 protein-specific antibody in the ascites was determined by ELISA. (C) The purified monoclonal antibody in the ascitic fluid was analyzed by SDS-PAGE. HC, heavy chain. LC, light chain. (D) Subclasses of the purified monoclonal antibody were determined by ELISA. (E) Binding of the purified monoclonal antibody (clone 1C7D7 mAb) to recombinant HCoV-OC43 N-Bio-His6 protein. (F) Mock-infected or HCoV-OC43-, MERS-CoV- or SARS-CoV-2-infected cell lysates were analyzed by western blotting with the purified monoclonal antibody. Anti-β-actin antibody was used as a control. The purified monoclonal antibody specifically recognizes N protein of HCoV-OC43. (G) Recombinant HCoV-OC43 N-Bio-His6, SARS-CoV-2 N-Bio-His6 or MERS-CoV N-Bio-His6 proteins were captured on streptavidin-coated 96-well immunoplates and then incubated with the purified monoclonal antibody for ELISA. Th antibody was specific to recombinant HCoV-OC43 N-Bio-His6.
Figure 6 N protein in HCoV-OC43 particles interacts with HCoV-OC43 Spike CD. Cell culture supernatants containing HCoV-OC43 were lysed with cell lysis buffer and incubated with Fc or HCoV-OC43 Spike CD-Fc. Fc-bound proteins were pulled down with Protein A beads and subjected to western blotting using anti-HCoV-OC43 N monoclonal antibody (clone 1C7D7 mAb) (A) or anti-hIgG Fc antibody (B). Virus lysates were analyzed using anti-HCoV-OC43 N monoclonal antibody (clone 1C7D7 mAb) as a loading control (C).
Similar to our previously reported “bait and prey” system (35), we designed an ELISA-based detection system for HCoV-OC43 using the anti-HCoV-OC43 N protein antibody and HCoV-OC43 Spike CD-Fc (Figure 7A). The HCoV-OC43 N protein-specific monoclonal antibody (1C7D7 mAb) was used to capture N proteins of HCoV-OC43 particles in lysates, and the N protein was allowed to interact with HCoV-OC43 Spike CD-Fc. This ELISA system successfully detected HCoV-OC43 in a concentration-dependent manner (Figure 7B), suggesting that the interaction between N protein and Spike CD Fc fusion protein can be applied as a novel method for the detection of HCoV-OC43
Figure 7 Detection of HCoV-OC43 in cell culture media with HCoV-OC43 N protein-specific monoclonal antibody and recombinant HCoV-OC43 Spike CD-Fc protein. (A) Schematic of the ELISA system. (B) HCoV-OC43 in cell culture supernatants were lysed with cell lysis buffer and serially diluted in PBST. The virus lysates were added into 96-well immunoplates coated with HCoV-OC43 N protein-specific monoclonal antibody (clone 1C7D7 mAb) and incubated for 2 h at room temperature. After washing with PBST, recombinant Spike CD-Fc protein was added, and then HRP-conjugated anti-human IgG Fc antibody was added to each well. The amount of HCoV-OC43 N protein in each well was determined by ELISA.
Since the emergence of SARS-CoV in 2002, research on highly pathogenic coronaviruses has heavily focused on identifying pathophysiological mechanisms as targets for novel therapeutics. For example, vaccines that suppress the infection mechanism of SARS-CoV-2 are now being administered worldwide, and many studies are being conducted to develop therapeutic agents for SARS-CoV-2. However, studies on other HCoVs, like HCoV-229E, HCoV-NL63, HCoV-HKU1, and HCoV-OC43, have yet to identify many therapeutic targets. Identifying targets for these and other coronaviruses is important because they not only cause most human common colds, but they also have the potential to cross species barriers with risky variants. Previously, we reported interaction of S and N proteins in MERS-CoV- and SARS-CoV-2-infected cells for the assembly of the viruses. Furthermore, we verified that the Spike CDs of MERS-CoV and SARS-CoV-2 are involved in the interaction with the N protein of each corona virus. In addition, we suggested that interactions of S and N protein are required for packaging of the viral genome as reduction of interactions between S and N protein by cell-penetrating Spike CD of MERS-CoV and SARS-CoV-2 treatment resulted in reduced viral protein expression and virus replication (25). Here, we investigated the interaction of HCoV-OC43 Spike CD and N protein with the hope of identifying a new therapeutic target for HCoV-OC43. We confirmed that Spike CD and N protein interact in HCoV-OC43 and found that the cell-penetrating Spike CD HCoV-OC43 peptide inhibited the production of HCoV-OC43 in infected cells. Taken together, our results indicate that the interaction of Spike CD and N protein is conserved across coronavirus and these interactions play an important role in coronavirus replication. Therefore, this would be a fantastic therapeutic target for future coronavirus treatments.
To detect interaction between SARS-CoV-2 Spike CD and N protein, we recently developed a novel assay based on the bait and prey ELISA system using the recombinant fusion proteins SARS-CoV-2 Spike CD-Fc and SARS-CoV-2 N-Bio-His6 (35). Here, we applied the same strategy to HCoV-OC43. We expressed the HCoV-OC43 Spike CD-Fc and HCoV-OC43 N-Bio-His6 recombinant proteins and confirmed specific and direct interaction between these proteins in vitro. Furthermore, we confirmed that the system can be modified to detect HCoV-OC43 using anti-HCoV-OC43 N protein-specific monoclonal antibody that we produced in this study. Taken together, our data suggest that the Spike CD-N protein interaction may be conserved among coronaviruses and this interaction can be used as a quantitative measurement of respective virus particles using our bait and prey ELISA system. Moreover, we believe that our ELISA system can be used to screen for potential drugs that inhibit the Spike CD-N protein interaction and thereby block coronavirus replication.
The raw data supporting the conclusions of this article will be made available by the authors, without undue reservation.
The animal study was reviewed and approved by Hallym University Institutional Animal Care and Use Committee.
H-JK and YL conceived of the project. H-JK and YL designed the experiments and wrote the manuscript. JK, MYK, DK, SP, MJK, KB, J-KC, SM, and MA carried out the experiments. H-JK, JK, MYK, DK, and YL analyzed the data. All authors contributed to the article and approved the submitted version.
This research was supported by grants from the National Research Foundation (NRF-2019M3A9E4032628, NRF-2020M3A9I2107294) funded by the Ministry of Science and ICT in the Republic of Korea.
The authors declare that the research was conducted in the absence of any commercial or financial relationships that could be construed as a potential conflict of interest.
All claims expressed in this article are solely those of the authors and do not necessarily represent those of their affiliated organizations, or those of the publisher, the editors and the reviewers. Any product that may be evaluated in this article, or claim that may be made by its manufacturer, is not guaranteed or endorsed by the publisher.
We thank the Korea Bank for Pathogenic Viruses (College of Medicine, Korea University, Seoul, Korea) for supplying HCoV-OC43 (KBPV-VR-8). We also thank the National Culture Collection for Pathogens (Osong, Korea) for supplying MERS-CoV/KOR/KNIH/002_05_2015 and SARS-CoV-2 S clade (hCoV-19/South Korea/KCDC03/2020, NCCP43326).
1. Chan-Yeung M, Ooi GC, Hui DS, Ho PL, Tsang KW. Severe Acute Respiratory Syndrome. Int J Tuberc Lung Dis (2003) 7:1117–30.
2. Gorbalenya AE, Snijder EJ, Spaan WJM. Severe Acute Respiratory Syndrome Coronavirus Phylogeny: Toward Consensus. J Virol (2004) 78:7863–6. doi: 10.1128/JVI.78.15.7863-7866.2004
3. McBride R, Fielding BC. The Role of Severe Acute Respiratory Syndrome (SARS)-Coronavirus Accessory Proteins in Virus Pathogenesis. Viruses (2012) 4:2902–23. doi: 10.3390/v4112902
4. Fung TS, Liu DX. Human Coronavirus: Host-Pathogen Interaction. Annu Rev Microbiol (2019) 73:529–57. doi: 10.1146/annurev-micro-020518-115759
5. Ye ZW, Yuan S, Yuen KS, Fung SY, Chan CP, Jin DY. Zoonotic Origins of Human Coronaviruses. Int J Biol Sci (2020) 16:1686–97. doi: 10.7150/ijbs.45472
6. Awadasseid A, Wu Y, Tanaka Y, Zhang W. Initial Success in the Identification and Management of the Coronavirus Disease 2019 (COVID-19) Indicates Human-to-Human Transmission in Wuhan, China. Int J Biol Sci (2020) 16:1846–60. doi: 10.7150/ijbs.45018
7. Fehr AR, Perlman S. Coronaviruses: An Overview of Their Replication and Pathogenesis. Methods Mol Biol (2015) 1282:1–23. doi: 10.1007/978-1-4939-2438-7_1
8. Li W, Moore MJ, Vasilieva N, Sui J, Wong SK, Berne MA, et al. Angiotensin-Converting Enzyme 2 Is a Functional Receptor for the SARS Coronavirus. Nature (2003) 426:450–4. doi: 10.1038/nature02145
9. Shang J, Wan Y, Luo C, Ye G, Geng Q, Auerbach A, et al. Cell Entry Mechanisms of SARS-CoV-2. Proc Natl Acad Sci U S A (2020) 117:11727–34. doi: 10.1073/pnas.2003138117
10. Raj VS, Mou H, Smits SL, Dekkers DH, Müller MA, Dijkman R, et al. Dipeptidyl Peptidase 4 is a Functional Receptor for the Emerging Human Coronavirus-EMC. Nature (2013) 495:251–4. doi: 10.1038/nature12005
11. Hulswit RJG, Lang Y, Bakkers MJG, Li W, Li Z, Schouten A, et al. Human Coronaviruses OC43 and HKU1 Bind to 9-O-Acetylated Sialic Acids via a Conserved Receptor-Binding Site in Spike Protein Domain A. Proc Natl Acad Sci U S A (2019) 116:2681–90. doi: 10.1073/pnas.1809667116
12. Chang CK, Hou MH, Chang CF, Hsiao CD, Huang TH. The SARS Coronavirus Nucleocapsid Protein–Forms and Functions. Antiviral Res (2014) 103:39–50. doi: 10.1016/j.antiviral.2013.12.009
13. Zeng Z, Deng F, Shi K, Ye G, Wang G, Fang L, et al. Dimerization of Coronavirus Nsp9 With Diverse Modes Enhances Its Nucleic Acid Binding Affinity. J Virol (2018) 92:e00692–18. doi: 10.1128/JVI.00692-18
14. Chen CY, Chang CK, Chang YW, Sue SC, Bai HI, Riang L, et al. Structure of the SARS Coronavirus Nucleocapsid Protein RNA-Binding Dimerization Domain Suggests a Mechanism for Helical Packaging of Viral RNA. J Mol Biol (2007) 368:1075–86. doi: 10.1016/j.jmb.2007.02.069
15. Takeda M, Chang CK, Ikeya T, Güntert P, Chang YH, Hsu YL, et al. Solution Structure of the C-Terminal Dimerization Domain of SARS Coronavirus Nucleocapsid Protein Solved by the SAIL-NMR Method. J Mol Biol (2008) 380:608–22. doi: 10.1016/j.jmb.2007.11.093
16. Kang S, Yang M, Hong Z, Zhang L, Huang Z, Chen X, et al. Crystal Structure of SARS-CoV-2 Nucleocapsid Protein RNA Binding Domain Reveals Potential Unique Drug Targeting Sites. Acta Pharm Sin B (2020) 10:1228–38. doi: 10.1016/j.apsb.2020.04.009
17. Zhou R, Zeng R, von Brunn A, Lei J. Structural Characterization of the C-Terminal Domain of SARS-CoV-2 Nucleocapsid Protein. Mol Biomed (2020) 1:2. doi: 10.1186/s43556-020-00001-4
18. Nguyen THV, Lichière J, Canard B, Papageorgiou N, Attoumani S, Ferron F, et al. Structure and Oligomerization State of the C-Terminal Region of the Middle East Respiratory Syndrome Coronavirus Nucleoprotein. Acta Crystallogr D Struct Biol (2019) 75(Pt1):8–15. doi: 10.1107/S2059798318014948
19. Papageorgiou N, Lichière J, Baklouti A, Ferron F, Sévajol M, Canard B, et al. Structural Characterization of the N-Terminal Part of the MERS-CoV Nucleocapsid by X-Ray Diffraction and Small-Angle X-Ray Scattering. Acta Crystallogr D Struct Biol (2016) 72(Pt 2):192–202. doi: 10.1107/S2059798315024328
20. Chen IJ, Yuann JM, Chang YM, Lin SY, Zhao J, Perlman S, et al. Crystal Structure-Based Exploration of the Important Role of Arg106 in the RNA-Binding Domain of Human Coronavirus OC43 Nucleocapsid Protein. Biochim Biophys Acta (2013) 1834:1054–62. doi: 10.1016/j.bbapap.2013.03.003
21. Tseng YT, Wang SM, Huang KJ, Lee AI, Chiang CC, Wang CT. Self-Assembly of Severe Acute Respiratory Syndrome Coronavirus Membrane Protein. J Biol Chem (2010) 285:12862–72. doi: 10.1074/jbc.M109.030270
22. Lu S, Ye Q, Singh D, Cao Y, Diedrich JK, Yates JR 3rd, et al. The SARS-CoV-2 Nucleocapsid Phosphoprotein Forms Mutually Exclusive Condensates With RNA and the Membrane-Associated M Protein. Nat Commun (2021) 12:502. doi: 10.1038/s41467-020-20768-y
23. Hsin WC, Chang CH, Chang CY, Peng WH, Chien CL, Chang MF, et al. Nucleocapsid Protein-Dependent Assembly of the RNA Packaging Signal of Middle East Respiratory Syndrome Coronavirus. J Biomed Sci (2018) 25:47. doi: 10.1186/s12929-018-0449-x
24. de Haan CA, Rottier PJ. Molecular Interactions in the Assembly of Coronaviruses. Adv Virus Res (2005) 64:165–230. doi: 10.1016/S0065-3527(06)66005-3
25. Park BK, Kim J, Park S, Kim D, Kim M, Baek K, et al. MERS-CoV and SARS-CoV-2 Replication can be Inhibited by Targeting the Interaction Between the Viral Spike Protein and the Nucleocapsid Protein. Theranostics (2021) 11:3853–67. doi: 10.7150/thno.55647
26. Maharjan S, Kang M, Kim J, Kim D, Park S, Kim M, et al. Apoptosis Enhances the Replication of Human Coronavirus Oc43. Viruses (2021) 13:2199. doi: 10.3390/v13112199
27. Park BK, Kim D, Park S, Maharjan S, Kim J, Choi JK, et al. Differential Signaling and Virus Production in Calu-3 Cells and Vero Cells Upon SARS-CoV-2 Infection. Biomol Ther (Seoul) (2021) 29:273–81. doi: 10.4062/biomolther.2020.226
28. Kim D, Maharjan S, Kim J, Park S, Park JA, Park BK, et al. MUC1-C Influences Cell Survival in Lung Adenocarcinoma Calu-3 Cells After SARS-CoV-2 Infection. BMB Rep (2021) 54:425–30. doi: 10.5483/BMBRep.2021.54.8.018
29. Park BK, Maharjan S, Lee SI, Kim J, Bae JY, Park MS, et al. Generation and Characterization of a Monoclonal Antibody Against MERS-CoV Targeting the Spike Protein Using a Synthetic Peptide Epitope-CpG-DNA-Liposome Complex. BMB Rep (2019) 52:397–402. doi: 10.5483/BMBRep.2019.52.6.185
30. Kandeel M, Yamamoto M, Park BK, Al-Taher A, Watanabe A, Gohda J, et al. Discovery of New Potent Anti-MERS CoV Fusion Inhibitors. Front Pharmacol (2021) 12:685161. doi: 10.3389/fphar.2021.685161
31. Altman JD, Moss PA, Goulder PJ, Barouch DH, McHeyzer-Williams MG, Bell JI, et al. Phenotypic Analysis of Antigen-Specific T Lymphocytes. Science (1996) 274:94–6. doi: 10.1126/science.274.5284.94
32. Brown MH, Boles K, van der Merwe PA, Kumar V, Mathew PA, Barclay AN. 2B4, the Natural Killer and T Cell Immunoglobulin Superfamily Surface Protein, is a Ligand for CD48. J Exp Med (1998) 188:2083–90. doi: 10.1084/jem.188.11.2083
33. Schatz PJ. Use of Peptide Libraries to Map the Substrate Specificity of a Peptide-Modifying Enzyme: A 13 Residue Consensus Peptide Specifies Biotinylation in. Escherichia coli Biotechnol (1993) 11:1138–43. doi: 10.1038/nbt1093-1138
34. Kim D, Kwon S, Rhee JW, Kim KD, Kim YE, Park CS, et al. Production of Antibodies With Peptide-CpG-DNA-Liposome Complex Without Carriers. BMC Immunol (2011) 12:29. doi: 10.1186/1471-2172-12-29
35. Kim D, Kim J, Park S, Kim M, Baek K, Kang M, et al. Production of SARS-CoV-2 N Protein-Specific Monoclonal Antibody and its Application in an ELISA-Based Detection System and Targeting the Interaction Between the Spike C-Terminal Domain and N Protein. Front Microbiol (2021) 12:726231. doi: 10.3389/fmicb.2021.726231
Keywords: HCoV-OC43, spike protein, nucleocapsid protein, anti-HCoV-OC43 N antibody, bait and prey, virus detection, ELISA
Citation: Kim J, Kim M, Kim D, Park S, Kang M, Baek K, Choi J-K, Maharjan S, Akauliya M, Lee Y and Kwon H-J (2022) Targeting the Interaction Between Spike Protein and Nucleocapsid Protein for Suppression and Detection of Human Coronavirus OC43. Front. Immunol. 13:835333. doi: 10.3389/fimmu.2022.835333
Received: 15 December 2021; Accepted: 18 February 2022;
Published: 10 March 2022.
Edited by:
Rong Hai, University of California, Riverside, United StatesReviewed by:
Rohit K. Jangra, LSU Health Sciences Center-Shreveport, United StatesCopyright © 2022 Kim, Kim, Kim, Park, Kang, Baek, Choi, Maharjan, Akauliya, Lee and Kwon. This is an open-access article distributed under the terms of the Creative Commons Attribution License (CC BY). The use, distribution or reproduction in other forums is permitted, provided the original author(s) and the copyright owner(s) are credited and that the original publication in this journal is cited, in accordance with accepted academic practice. No use, distribution or reproduction is permitted which does not comply with these terms.
*Correspondence: Younghee Lee, eWhsNDE3N0BjYm51LmFjLmty; Hyung-Joo Kwon, aGpvb2t3b25AaGFsbHltLmFjLmty
†These authors have contributed equally to this work
Disclaimer: All claims expressed in this article are solely those of the authors and do not necessarily represent those of their affiliated organizations, or those of the publisher, the editors and the reviewers. Any product that may be evaluated in this article or claim that may be made by its manufacturer is not guaranteed or endorsed by the publisher.
Research integrity at Frontiers
Learn more about the work of our research integrity team to safeguard the quality of each article we publish.