- Department of Neurology, The First Bethune Hospital of Jilin University, Changchun, China
Depressive disorder is the most prevalent affective disorder today. Depressive disorder has been linked to changes in the white matter. White matter changes in depressive disorder could be a result of impaired cerebral blood flow (CBF) and CBF self-regulation, impaired blood-brain barrier function, inflammatory factors, genes and environmental factors. Additionally, white matter changes in patients with depression are associated with clinical variables such as differential diagnosis, severity, treatment effect, and efficacy assessment. This review discusses the characteristics, possible mechanisms, clinical relevance, and potential treatment of white matter alterations caused by depressive disorders.
Introduction
A depressive disorder, an affective disorder, is defined by significant and persistent depression. It is frequently accompanied by a variety of cognitive impairments and somatic symptoms. Depressive disorders are classified as disruptive mood disorder, major depressive disorder (MDD), persistent mood disorder (PSD), transitory depressive disorder (TSD), persistent mood disorder, premenstrual dysphoric disorder, substance/medication-induced depressive disorder, other disease-induced depressive disorder, other specified depressive disorder, and unspecified depressive disorder in the Diagnostic and Statistical Manual of Mental Disorders-5 (DMS-5). International studies revealed a significantly higher prevalence of depressive disorders in people with white matter lesions (WMLs) than in health (1–3). Additionally, the correlation between white matter alterations in various parts of the brain and depressive disorder is different (4). Therefore, this review will focus on the white matter changes associated with MDD and their research progress.
Possible Pathogenesis of White Matter Changes Caused by MDD
The white matter is made up of nerve fibers that have aggregated. Most of the nerve fibers are myelinated. Thus, white matter injury primarily affects the myelin sheath of nerve fibers. It is easily influenced by a variety of factors, including ischemia, hypoxia, and inflammatory stimulation. The pathogenesis of white matter changes in MDD is still unclear. According to current research, it may be related to cerebral blood flow regulation and cerebral blood flow self-regulation, blood-brain barrier damage, inflammatory factors, genetic and environmental factors.
Cerebral Blood Flow and Cerebral Blood Flow Self-Regulation
Appropriate cerebral blood flow (CBF) is required to maintain the brain’s structural and functional integrity. In health, self-regulation of CBF can help maintain a constant CBF (5). Reduced CBF, on the other hand, does not have to result in ischemia to influence brain function. Mild CBF insufficiency may impair cognitive and emotional function (6). White matter in the terminal area of the deep perforating artery blood supply has a lower capillary density than the cortex between adjacent small arteries (7). Hence, white matter is more susceptible to insufficient CBF. In comparison to the control group, those with a depressive disorder had less white matter CBF in the bilateral anterior cerebral artery regions and the left middle cerebral artery region (8). Increased white matter hyperintensity (WMH) was associated with decreased CBF in the rostral anterior cingulate cortex volumes in late-life depression, according to an MRI study (9). Colloby et al. discovered that when participants’ depression was in remission, white matter CBF was significantly higher than in the control group, but not in patients with current depression (10). It demonstrates the point in the opposite direction.
CBF self-regulation occurs via three primary mechanisms: cerebral autoregulation (CA), cerebrovascular reactivity (CVR), and neurovascular coupling (NVC). CA is a mechanism that rapidly stabilizes CBF by adjusting cerebrovascular resistance in response to changes in cerebral perfusion pressure. There are two types of cerebral autoregulation: static cerebral autoregulation (sCA) and dynamic cerebral autoregulation (dCA). CA was initially believed to be a static, stable process that contributed to slow, gradual blood pressure changes. Static cerebral autoregulation is primarily quantified using Single-Photon Emission Computed Tomography (SPECT) or Computed Tomography Perfusion imaging (CTPI). With the development of monitoring measurements, the term “dynamic autoregulation” was coined (11). Numerous methods for inducing blood pressure changes have been proposed to investigate dCA, including pharmacological means, thigh-cuff release, body tilt, handgrip, lower-body negative pressure, valsalva or sit-to-stand maneuvers, and transient carotid artery compression (12). However, this method is not appropriate for all people. With many mathematical methods proposed, it is possible that induced changes in ABP are not completely necessary (13). Transcranial Doppler (TCD) measures cerebral blood flow velocity (CBFV), which is a proxy for cerebral blood flow, while vascular unloading techniques in the fingers monitor changes in the finger’s blood pressure. Combining the two measurements is a widely used technique for determining the dCA because it is non-invasive, simple to implement, and cooperative. Luo et al. (14) also applied the same method to investigate the dCA’s role in depression. The study demonstrated that depression significantly impairs cerebral autoregulation, and that the higher the depression score, the more compromised CBF self-regulation is. Purkayastha et al. (15) then demonstrated a link between compromised cerebrovascular hemodynamics (pulsatility index and dCA) and white matter structural integrity. arteriosclerosis is one factor that contributes to decreased cerebrovascular reactivity. According to a cross-sectional study by van Sloten et al. (16), the degree of arteriosclerosis is directly proportional to the severity of depression, and a portion of the arteriosclerosis is associated with white matter lesions. These findings suggest that there may be a link between self-regulation of CBF and white matter changes in depressive disorder.
Inflammatory Factors
Inflammatory factors play a critical role in the onset and progression of MDD. In comparison to healthy controls, MDD patients have higher levels of IL-6, tumor necrosis factor (TNF)-, IL-10, soluble IL-2 receptor, IL-13, C-C chemokine ligand-2, IL-13, IL-18, IL-12, IL-1 receptor antagonist, and soluble TNF receptor 2 in their peripheral blood (17). Increased inflammation is also associated with white matter damage. C-reactive protein, a marker of inflammation, is elevated in middle-aged people. The level is associated with a decrease in white matter changes related to MDD (18). Sugimoto et al. demonstrated that the fractional anisotropy (FA) values of the bilateral inferior fronto-occipital fasciculus (IFOF) and corpus callosum genu were significantly lower in MDD patients than in healthy controls and were negatively correlated with IL-1β levels (19). IL-1β can cause inflammation, resulting in cell death and damage in the central nervous system. Not only can IL-1β reduce myelination (20), but injection of IL-1β into the brain of newborn rats can decrease the number of developing oligodendrocytes, thereby causing direct myelination damage (21).
As a result, the increased level of inflammatory factors in the central nervous system of patients with MDD can result in inflammation, impairing the formation and integrity of the myelin sheath, ultimately resulting in white matter damage.
Blood-Brain Barrier Damage
The blood-brain barrier (BBB) regulates material exchange and maintains the nervous system’s homeostasis due to its unique structure. An investigation carried out by Lesniak et al. (22) reveals that trauma destroys the BBB in mice. The material exchange function was out of control, resulting in nerve growth factor leakage. It exacerbated the mice’s depression-like behavior. Above all, MDD may be associated with the breakdown of the BBB structure. Chronic social stress, according to a study (23) using a mouse model of depression, has been shown to decrease the expression of claudin-5 in mice. It increases the BBB’s permeability and facilitates the entry of blood inflammatory factors such as interleukin (IL)-6 into the central nervous system. These factors contribute to the development of depression-like behavior in mice. Similarly, patients with MDD have decreased claudin-5 expression (24). MDD patients were found to have increased permeability because of an abnormal BBB structure. The research of Pearlman et al. (25) corroborated the preceding assertion. Normally, astrocytes S100β was found in the brain parenchyma. The study did discover, however, that S100β can be detected in the serum of MDD patients. Its level is significantly correlated with acute depressive symptoms fluctuations. Moreover, the breakdown of the BBB is corelated with white matter alterations. Kerkho et al. (26) used dynamic enhanced MRI to investigate patients with cerebral small vessel disease. They discovered that every 2 mm closer to WMLs, the change in parenchymal diffusivity increased by 0.01%. Additionally, the change in parenchymal diffusion over two years is positively correlated with the baseline permeability of the BBB. Indeed, it corroborates the hypothesis that the BBB is the cause of WMLs. The results of Topakian et al. (27) demonstrated that the normal white matter area exhibited increased permeability of the BBB. This demonstrates that the BBB is damaged prior to the white matter changes. Although there is no direct evidence that BBB disruption is associated with white matter changes in MDD patients. However, as previously stated, a destroyed BBB results in an excessive leakage of toxins such as inflammatory factors into the central nervous system, which can destroy the integrity of the white matter structure.
Genes and Environmental Factors
A growing number of studies have established an association between certain psychiatric disorders and genetic and environmental factors (28, 29). MDD is one of them. The meta-analysis results of Sullivan et al. suggested that MDD has a heritability of 31% to 42% (CI=95%) (30). Methylation of the brain-derived neurotrophic factor (BDNF) promoter region has been shown to be negatively correlated with the FA value of the right radiated crown in patients with MDD (31). White matter structure is associated with genes, which are also found in MDD. A study of untreated patients with MDD showed damage to the brain white matter fiber bundles in the emotional control circuit. And it was associated with the TPH2-rs4570625 GG polymorphism (32). Furthermore, the serotonin transporter is involved in neuronal serotonin reuptake, which is the primary target of selective serotonin reuptake inhibitors. It is critical in MDD. Lesch et al. (33) demonstrated that the S allele of the 5HTTLPR polymorphism slows the serotonin transporter’s transcription in neurons. According to one default Diffusion Tensor Imaging (DTI) study, depressed elderly S-allele carriers have a lower FA than L homozygotes in frontolimbic brain areas such as the dorsal and rostral anterior cingulate, posterior cingulate, dorsolateral and medial prefrontal regions, and thalamus, among others (34). Moreover, won et al. (35) discovered a significant correlation between the white matter integrity of the corpus callosum and increased DNA methylation of the serotonin transporter SLC6A4 gene in MDD patients. It suggests a possible regulatory mechanism for gene-environmental interactions affecting white matter integrity in MDD. Although the well-known behaviorist psychologist John Broadus Watson’s “environmental determinism” overemphasizes the environment’s influence, the influence of environment on human growth and development cannot be ignored. A study on primates established that a poor feeding environment influences the integrity of white matter fiber bundles in the frontal lobes of Bonnet macaques (36). Witnessing domestic violence as a child is a traumatic experience that increases one’s risk of developing depression (37), post-traumatic stress disorder (38), and other affective disorders. The FA value of the left suboccipital longitudinal tract was significantly lower in adolescents who witnessed domestic violence than in the control group, and was negatively correlated with the depression and anxiety scale scores (39). However, Meinert et al. argued that damage to the white matter tracts in MDD was caused by childhood abuse, not by the MDD diagnosis itself (40).
Not only can the pathogenesis exist independently, but they can also interact. For instance, insufficient CBF results in white matter ischemia. Then oxidative stress can occur, resulting in an increase in reactive oxygen species. Furthermore, reactive oxygen species can result in the breakdown of the blood-brain barrier, promote white blood cell migration, and enhance myelin phagocytosis. Additionally, it harmed the fragile central nervous system’s essential macromolecular substances by mediating cell damage (41). Finally, those factors caused continuous damage to the white matter. Simultaneously, ischemia and hypoxia of the white matter can activate astrocytes while activating the central protection mechanism. It induces an inflammatory response, aggravating the response to white matter injury
White Matter Alterations in MDD
The advancement of imaging technology has made it possible to conduct non-invasive research on affective disorders, with Diffusion Weighted Imaging (DWI) playing a critical role in identifying brain abnormalities correlated with mental illness (42). In comparison, the majority of imaging examinations focus on macroscopic changes or functions, whereas the advancement and development of DTI, has shifted researchers’ attention to changes in the microstructure of the brain. It quantifies water molecule movement in the brain and is increasingly used to assess changes in the white matter microstructure. FA, which is the proportion of water molecules in the total dispersion tensor, can be used to determine the thickness of the myelin sheath and the degree of white matter damage. The Mean Diffusivity (MD) is a measure of the diffusion coefficient and serves as an indicator of the membrane’s density. The Axial Diffusivity (AD) is used to determine the number, diameter, and organization of axons; while the Radial Diffusivity (RD) provides additional information about the myelin sheath (43). Low FA and high MD values are both common manifestations of white matter changes associated with depression and other affective disorders (44). Many subsequent studies confirm the correlation between MDD and alterations in white matter.
White Matter Fundamental Changes in MDD
White matter changes are also common in aging healthy individuals. Age-related white matter changes typically manifest as fuzzy linear, cap-shaped, or ring-shaped changes surrounding the ventricle or as multi-point and patch-shaped abnormal signals under the cortex (45). Rabins et al. (46) examined MDD patients aged 60 years or older. In comparison to the control group, MDD patients’ subcortical WMLs were more severe and contained more basal ganglia lesions. Nonetheless, the periventricular hyperintensity levels were comparable. As a result, periventricular hyperintensity may be unrelated to depression.
Neuroimaging techniques were used to map changes in white matter microstructure in MDD. According to the largest multicenter study to date, the DTI results for the MDD adult group revealed that the FA was lower in 16 of 25 interested white matter tracts than in the control group (47). It may reveal extensive structural connection defects in depression patients. The study of Cole et al. also supports this result (48). Among the damaged white matter, the corpus callosum and radiation crown were the most dissimilar parts of FA. Another meta-analysis of 641 depression patients and 581 normal controls revealed that depression patients had lower FA in the genu of the corpus callosum and the anterior limb of the internal capsule than the health (49). The callosum, as the largest inter-hemisphere association, connects the anterior cingulate cortex and orbitofrontal cortex of the two hemispheres and is critical for emotional regulation. Working memory and attention persistence are associated with the anterior cingulate cortex (50). The superior longitudinal fasciculus, inferior longitudinal fasciculus, fronto-occipital fasciculus, and posterior thalamic radiation have all been reported to have decreased FA of white matter (32, 51). As mentioned previously, the findings of several studies are inconsistent. The reason for the inconsistent findings could be the type and clinical phenotype of depression. Some scholars questioned the association between white matter FA and depressive symptoms. Dillon et al. found that anhedonia is negatively correlated with the FA in the genu, cingulum, and uncinate fasciculus and positively correlated with radial diffusivity (52). Affected white matter pathways connect the critical regions for value coding (53). In depression, Coloigner et al. discovered a positive correlation between anxiety and the FA of the genu and splenium of the corpus callosum, the anterior corona radiata, and the posterior thalamic radiation (54). As a result, the inconsistent findings may be explained by the interference of various clinical symptoms. The characteristics of white matter changes vary between different MDD groups. Late-onset depression primarily impairs the integrity of fiber tracts in the frontal lobe system (55). Compared with other types of depression, executive function is significantly impaired, white matter microstructure is significantly altered, and the incidence is higher (56, 57). Postpartum depression primarily affects the left anterior limb of the internal capsule. This change is related to the severity of MDD (58). Furthermore, Zhang et al. (59) discovered that functional connectivity within white matter bundles and between white matter and gray matter was decreased in MDD patients. The internal capsule’s functional connectivity with the cingulate cortex is the most severely damaged. To summarize, patients with MDD not only have structural damage to their white matter, but also have some functional impairment (Table 1 and Figure 1 provide the summary of white matter alterations in MDD in this review).
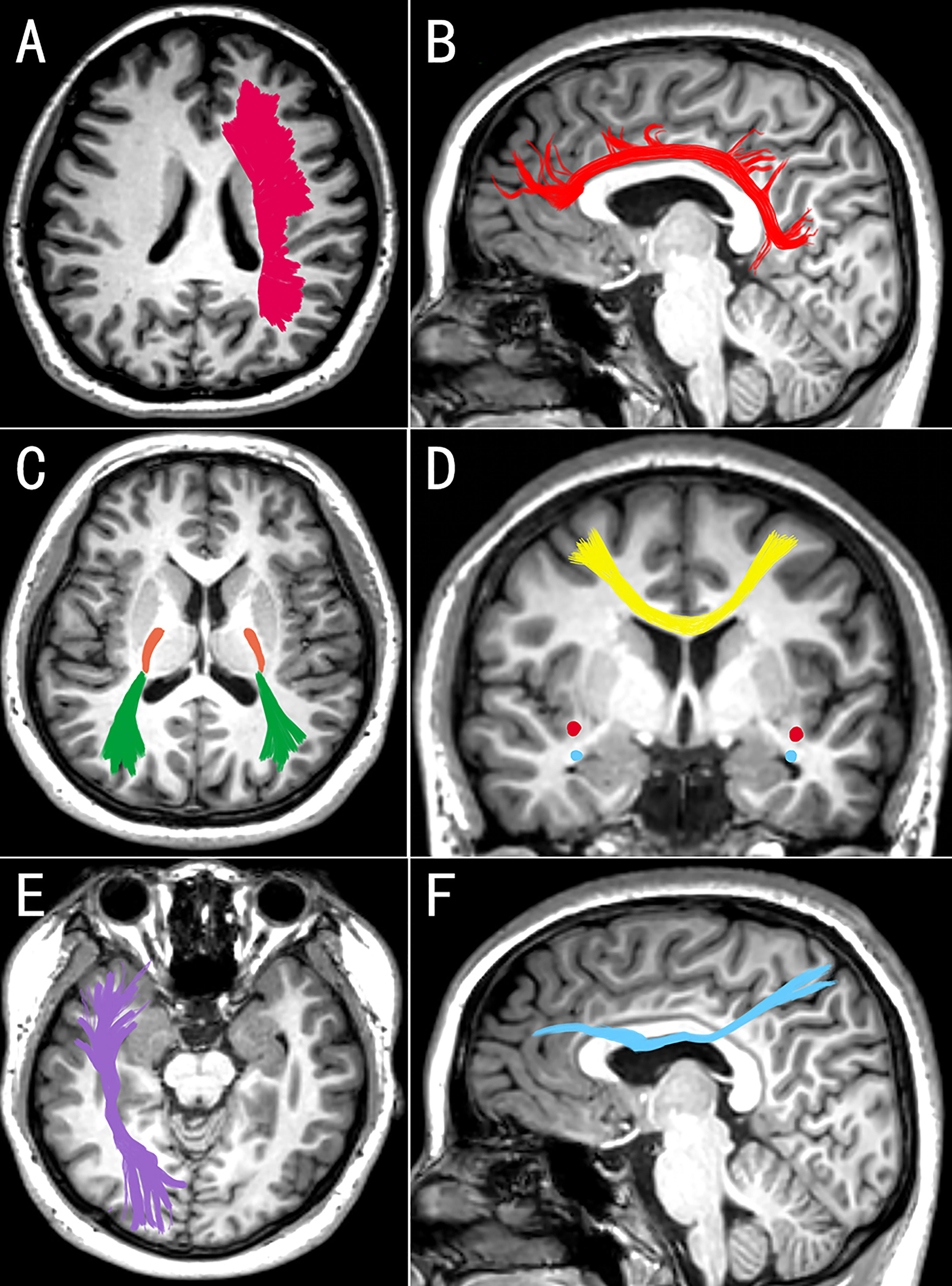
Figure 1 Images (A, C, E) are seen from above, medial image (D) is seen from behind, and images (B, F) are seen from the right. The white matter changes in corona radiata (A), cingulum (B), posterior thalamic radiation (C, green), posterior limb of the internal capsule (C, orange), corpus callosum (D, yellow), inferior fronto-occipital fasciculus (C, red), uncinate fasciculus (D, blue), inferior longitudinal fasciculus (E), and superior longitudinal fasciculus (F) may be associated with MDD. These changes in white matter may be bilateral or unilateral (left or right) in different studies (Edited by Photoshop).
The Effect of MDD on Cognition and Its Relationship With White Matter Abnormalities
Previous Studies have shown that cognitive dysfunction is common in MDD patients and may persist in the period of remission (60, 61). Residual cognitive impairment may adversely affect occupational and social function (62). A variety of cognitions are impaired in MDD, such as set-shifting, processing speed, memory, learning, and verbal fluency (63, 64). And decreased executive function is the predominant cognitive manifestation. WMH is also correlated with cognitive impairment. Particularly, periventricular WMH associated with executive function and processing speed (65). Herrmann et al. illustrated that late-onset depression mainly impaired executive function and processing speed (56). There may be a potential link between white matter alterations and cognitive impairment in patients with depression (66). Compared with the control group, MDD patients showed significantly reduced FA in the right posterior cingulate cluster (PCC) which is positively correlated with performance in a verbal naming task (67). Moreover, Sheline et al. (68) reported that depression patients had greater WMH in the tracts of superior longitudinal fasciculus, fronto-occipital fasciculus, uncinate fasciculus, extreme capsule, and inferior longitudinal fasciculus. These white matter fibers connect the cortex associated with cognition and emotion functions. Hence, structural dysconnectivity between regions of gray matter may be the source of cognitive changes in depressed patients. Superior longitudinal fasciculus, fronto-occipital fasciculus and extreme capsule are the part of dorsolateral Prefrontal circuit that connects the dorsolateral prefrontal cortex and the basal ganglia. Impaired dorsolateral prefrontal circuits in MDD patients present the executive dysfunction, such as working memory, set switching, and inhibitory control (54, 69). Additionally, the response to happy faces is reduced in right fusiform activation (70), which may result from white matter disruption in this region (51). A study on late life depression demonstrated that the poorer attentional set-shifting, slower processing speed, and dysexecutive behavior is associated with the structural dysconnectivity of cognitive-control, sensorimotor, and attentional networks caused by WMH (71). Therefore, we consider that cognitive impairment in MDD may be the result of the structural dysconnectivity of multiple functional networks. The relationship between depression and cognitive dysfunction is still not fully understood, and whether it is cause, effect or interaction is still not fully unified (72). Hence, the above content only discussed the impact of depression on cognition.
The Relationship Between White Matter Changes and MDD Clinical Relevance
The changes in the white matter of patients with MDD are highly correlated with clinical practice. It provides new concepts for diagnosing psychiatric disorders and relates to a variety of factors, including differential diagnosis, severity evaluation, quantification of treatment effects, and prognosis assessment.
Bipolar Depression (BD) and Schizophrenia (SZ) are both well-known mental illnesses. Depression is frequently the only symptom in the early stages of both diseases (73). Therefore, effective methods for identifying them are required. Significant differences in the limbic system were discovered between schizophrenia/bipolar disorder and major depressive disorder in large-scale meta-analyses (74, 75). Additionally, the callosal, limbic-paralimbic-hetermodal, cortico-cortical, thalamocortical, and cerebellar white matter integrity is significantly altered in the bipolar disorder and schizophrenia groups. In these areas, the MDD group, in contrast to the bipolar disorder and schizophrenia groups, exhibits no significant changes in white matter integrity. According to the preceding contents, MDD can be distinguished from bipolar disorder and schizophrenia based on the severity and extent of white matter changes.
MDD severity is associated to the location of white matter changes. Zhu et al. (76) demonstrated a negative correlation between the FA in the left anterior limb of the internal capsule and the severity of depression. However, in patients with late-onset depression, the severity of the condition is significantly associated with the microstructural changes in the left superior longitudinal fasciculus and the right uncinate fasciculus (77). Therefore, different types of depression may have distinct white matter regions that can be used to assess depression severity. Additional detailed analysis is required prior to clinical application.
Many studies have also been conducted on white matter changes in the treatment effect and prognosis evaluation of MDD. Emotional fluctuations have an effect on the integrity of white matter (78). A study comparing elderly depression patients before and after a year using DTI indicated that patients who did not achieve remission had a lower FA value for white matter in the anterior cingulate cortex than patients who did achieve remission or the control group (79). Then, some researchers examined changes in the white matter of MDD patients before and after treatment. Pillai et al. reported that the FA values between the raphe and left amygdala of patients who were not cured were significantly higher than those of cured patients (80). Accordingly, the integrity of the white matter connections within the emotional regulation network may be a critical characteristic of effective antidepressant treatment (81). Prefrontal and amygdala/hippocampal white matter connectivity has been demonstrated to be associated with antidepressant treatment response (82). Moreover, alterations in white matter are associated with the persistence and recurrence of depression (83).
Potential Therapies
As previously stated, white matter changes in MDD are associated with depressive symptoms, and some are reversible. They provide us with a novel method of treating depression. It is known that antidepressant medications work by increasing the expression of neurotrophic factors such as BDNF, increasing the availability of serotonin and norepinephrine, and exerting anti-inflammatory effects. Antidepressant medications have been shown to improve white matter volume (84). Additionally, Wang et al. demonstrated that desvenlafaxine protects white matter from injury caused by stress (85). Cilostazol, phosphodiesterase-3, is an antiplatelet agent inhibitor. recently, phosphodiesterase is being used in the treatment of affective disorders. Phosphodiesterase regulate the intracellular levels of cyclic adenosine monophosphate (cAMP) and cyclic guanosine monophosphate (cGMP) via hydrolyzing cyclic nucleotides (86). Increased cAMP may stimulate transcription of the cAMP response element-binding protein (87, 88), thereby increasing BDNF expression (88). BDNF is involved in the regulation of neuronal pathways and synaptic plasticity. According to the research of Khadivi et al., the HAMD score decreased significantly more in MDD patients treated with cilostazol and sertraline for six weeks than in those treated with sertraline alone, and the safety was no difference (89). When Cilostazol combined with escitalopram, similar results were obtained (90). A case report found that cilostazol was used intensively to treat a patient with intractable depression complicated by WMH. The comparison of before and after treatment data revealed an improvement in the patient’s depressive symptoms and CBF to WMH regions (91). Due to the small sample size, however, the potential role of cilostazol in MDD patients with WMH requires further investigation.
Exercise also has a similar molecular mechanism with antidepressant drugs to improve depression symptoms (92). A study examined the effects of exercise training (stationary bicycle) on healthy and schizophrenic patients over a six-month period. They discovered that regardless of the psychiatric diagnosis, exercise significantly improves white matter integrity (93). Therefore, it is reasonable to consider whether exercise also influence white matter integrity in MDD.
Antidepressant medications indirectly repair white matter via a variety of molecular mechanisms. Repetitive transcranial electrical stimulation (rTMS) is a relatively new treatment modality that is primarily used in the treatment of major depressive disorder (MDD) and treatment-resistant depression. Even though the underlying mechanism for treating MDD remains unknown, one proposed mechanism involves directly altering abnormal brain functional and structural connectivity (94). An abnormal frontier-limbic network is the root of emotion regulation disorder (49). Studies have established that rTMS significantly alters the neural activity in fronto-limbic brain regions in MDD patients (95, 96). Additionally, a meta-analysis found that rTMS was more likely to induce remission (97).
Conclusion
Many studies have established that white matter changes are characteristic of MDD. Moreover, those changes in MDD patients are related to a variety of factors, including differential diagnosis, the severity of MDD, treatment effect evaluation, and prognosis assessment. Hence, we can use and develop imaging methods to provide patients with a more objective diagnosis and comprehensive evaluation, as well as an individualized treatment plan to enhance the treatment effect and prognosis
Author Contributions
EH conceived the review, collected the data, wrote the paper. ML helped analyze the data. SG, XF, and YH revise the manuscript. FD supervised the review and approved the final version of the manuscript. All authors contributed to the article and approved the submitted version.
Funding
This work was supported by a grant from the National Natural Science Foundation of China (82071293).
Conflict of Interest
The authors declare that the research was conducted in the absence of any commercial or financial relationships that could be construed as a potential conflict of interest.
Publisher’s Note
All claims expressed in this article are solely those of the authors and do not necessarily represent those of their affiliated organizations, or those of the publisher, the editors and the reviewers. Any product that may be evaluated in this article, or claim that may be made by its manufacturer, is not guaranteed or endorsed by the publisher.
References
1. Heiden A, Kettenbach J, Fischer P, Schein A, Ba-Ssalamah A, Frey R, et al. White Matter Hyperintensities and Chronicity of Depression. J Psychiatr Res (2005) 39(3):285–93. doi: 10.1016/j.jpsychires.2004.07.004
2. Taylor WD, Steffens DC, MacFall JR, McQuoid DR, Payne ME, Provenzale JM, et al. White Matter Hyperintensity Progression and Late-Life Depression Outcomes. Arch Gen Psychiatry (2003) 60(11):1090–6. doi: 10.1001/archpsyc.60.11.1090
3. Hickie I, Scott E, Wilhelm K, Brodaty H. Subcortical Hyperintensities on Magnetic Resonance Imaging in Patients With Severe Depression–A Longitudinal Evaluation. Biol Psychiatry (1997) 42(5):367–74. doi: 10.1016/S0006-3223(96)00363-0
4. Wang L, Leonards CO, Sterzer P, Ebinger L. White Matter Lesions and Depression: A Systematic Review and Meta-Analysis. J Psychiatr Res (2014) 56:56–64. doi: 10.1016/j.jpsychires.2014.05.005
5. Donnelly J, Budohoski KP, Smielewski P, Czosnyka M. Regulation of the Cerebral Circulation: Bedside Assessment and Clinical Implications. Crit Care (2016) 20(1):129. doi: 10.1186/s13054-016-1293-6
6. Taylor WD, Aizenstein HJ, Alexopoulos GS. The Vascular Depression Hypothesis: Mechanisms Linking Vascular Disease With Depression. Mol Psychiatry (2013) 18(9):963–74. doi: 10.1038/mp.2013.20
7. Anstrom JA, Brown WR, Moody DM, Thore CR, Challa VR, Block SM. Anatomical Analysis of the Developing Cerebral Vasculature in Premature Neonates: Absence of Precapillary Arteriole-to-Venous Shunts. Pediatr Res (2002) 52(4):554–60. doi: 10.1203/00006450-200210000-00015
8. Wang Y, Zhang H, Tang S, Liu X, O'Neil A, Turner A, et al. Assessing Regional Cerebral Blood Flow in Depression Using 320-Slice Computed Tomography. PloS One (2014) 9(9):e107735. doi: 10.1371/journal.pone.0107735
9. Abi Zeid Daou M, Boyd BD, Donahue MJ, Albert K, Taylor WD. Anterior-Posterior Gradient Differences in Lobar and Cingulate Cortex Cerebral Blood Flow in Late-Life Depression. J Psychiatr Res (2018) 97:1–7. doi: 10.1016/j.jpsychires.2017.11.005
10. Colloby SJ, Firbank MJ, He J, Thomas AJ, Vasudev A, Parry SW, et al. Regional Cerebral Blood Flow in Late-Life Depression: Arterial Spin Labelling Magnetic Resonance Study. Br J Psychiatry (2012) 200(2):150–5. doi: 10.1192/bjp.bp.111.092387
11. Tiecks FP, Lam AM, Aaslid R, Newell DW. Comparison of Static and Dynamic Cerebral Autoregulation Measurements. Stroke (1995) 26(6):1014–9. doi: 10.1161/01.STR.26.6.1014
12. Claassen JA, Meel-van den Abeelen AS, Simpson DM, Panerai RB. Transfer Function Analysis of Dynamic Cerebral Autoregulation: A White Paper From the International Cerebral Autoregulation Research Network. J Cereb Blood Flow Metab (2016) 36(4):665–80. doi: 10.1177/0271678X15626425
13. Xiong L, Liu X, Shang T, Smielewski P, Donnelly J, Guo ZN, et al. Impaired Cerebral Autoregulation: Measurement and Application to Stroke. J Neurol Neurosurg Psychiatry (2017) 88(6):520–31. doi: 10.1136/jnnp-2016-314385
14. Luo MY, Guo ZN, Qu Y, Zhang P, Wang Z, Jin H, et al. Compromised Dynamic Cerebral Autoregulation in Patients With Depression. Front Psychiatry (2019) 10:373. doi: 10.3389/fpsyt.2019.00373
15. Purkayastha S, Fadar O, Mehregan A, Salat DH, Moscufo N, Meier DS, et al. Impaired Cerebrovascular Hemodynamics are Associated With Cerebral White Matter Damage. J Cereb Blood Flow Metab (2014) 34(2):228–34. doi: 10.1038/jcbfm.2013.180
16. van Sloten TT, Mitchell GF, Sigurdsson S, van Buchem MA, Jonsson PV, Garcia ME, et al. Associations Between Arterial Stiffness, Depressive Symptoms and Cerebral Small Vessel Disease: Cross-Sectional Findings From the AGES-Reykjavik Study. J Psychiatry Neurosci (2016) 41(3):162–8. doi: 10.1503/jpn.140334
17. Köhler CA, Freitas TH, Maes M, de Andrade NQ, Liu CS, Fernandes BS, et al. Peripheral Cytokine and Chemokine Alterations in Depression: A Meta-Analysis of 82 Studies. Acta Psychiatr Scand (2017) 135(5):373–87. doi: 10.1111/acps.12698
18. O'Donovan A, Bahorik A, Sidney S, Launer LJ, Yaffe K. Relationships of Inflammation Trajectories With White Matter Volume and Integrity in Midlife. Brain Behav Immun (2021) 91:81–8. doi: 10.1016/j.bbi.2020.09.006
19. Sugimoto S, Watanabe K, Katsuki A, Ueda I, Igata N, et al. Relationship Between White Matter Integrity and Serum Inflammatory Cytokine Levels in Drug-Naive Patients With Major Depressive Disorder: Diffusion Tensor Imaging Study Using Tract-Based Spatial Statistics. Transl Psychiatry (2018) 8(1):141. doi: 10.1038/s41398-018-0174-y
20. Favrais G, van de Looij Y, Fleiss B, Ramanantsoa N, Bonnin P, Stoltenburg-Didinger G, et al. Systemic Inflammation Disrupts the Developmental Program of White Matter. Ann Neurol (2011) 70(4):550–65. doi: 10.1002/ana.22489
21. Cai Z, Lin S, Pang Y, Rhodes PG. Brain Injury Induced by Intracerebral Injection of Interleukin-1beta and Tumor Necrosis Factor-Alpha in the Neonatal Rat. Pediatr Res (2004) 56(3):377–84. doi: 10.1203/01.PDR.0000134249.92944.14
22. Lesniak A, Poznański P, Religa P, Nawrocka A, Bujalska-Zadrozny M, Sacharczuk M. Loss of Brain-Derived Neurotrophic Factor (BDNF) Resulting From Congenital- Or Mild Traumatic Brain Injury-Induced Blood-Brain Barrier Disruption Correlates With Depressive-Like Behaviour. Neuroscience (2021) 458:1–10. doi: 10.1016/j.neuroscience.2021.01.013
23. Menard C, Pfau ML, Hodes GE, Kana V, Wang VX, Bouchard S, et al. Social Stress Induces Neurovascular Pathology Promoting Depression. Nat Neurosci (2017) 20(12):1752–60. doi: 10.1038/s41593-017-0010-3
24. Greene C, Hanley N, Campbell M. Blood-Brain Barrier Associated Tight Junction Disruption is a Hallmark Feature of Major Psychiatric Disorders. Transl Psychiatry (2020) 10(1):373. doi: 10.1038/s41398-020-01054-3
25. Pearlman DM, Brown JR, MacKenzie TA, Hernandez F Jr, Najjar S. Blood Levels of S-100 Calcium-Binding Protein B, High-Sensitivity C-Reactive Protein, and Interleukin-6 for Changes in Depressive Symptom Severity After Coronary Artery Bypass Grafting: Prospective Cohort Nested Within a Randomized, Controlled Trial. PloS One (2014) 9(10):e111110. doi: 10.1371/journal.pone.0111110
26. Kerkhofs D, Wong SM, Zhang E, Staals J, Jansen JFA, van Oostenbrugge RJ, et al. Baseline Blood-Brain Barrier Leakage and Longitudinal Microstructural Tissue Damage in the Periphery of White Matter Hyperintensities. Neurology (2021) 96(17):e2192–200. doi: 10.1212/WNL.0000000000011783
27. Topakian R, Barrick TR, Howe FA, Markus HS. Blood-Brain Barrier Permeability is Increased in Normal-Appearing White Matter in Patients With Lacunar Stroke and Leucoaraiosis. J Neurol Neurosurg Psychiatry (2010) 81(2):192–7. doi: 10.1136/jnnp.2009.172072
28. Kendler KS, Walters EE, Neale MC, Kessler RC, Heath AC, Eaves KS. The Structure of the Genetic and Environmental Risk Factors for Six Major Psychiatric Disorders in Women. Phobia, Generalized Anxiety Disorder, Panic Disorder, Bulimia, Major Depression, and Alcoholism. Arch Gen Psychiatry (1995) 52(5):374–83. doi: 10.1001/archpsyc.1995.03950170048007
29. Cross-Disorder Group of the Psychiatric Genomics Consortium. Identification of Risk Loci With Shared Effects on Five Major Psychiatric Disorders: A Genome-Wide Analysis. Lancet (2013) 381(9875):1371–9. doi: 10.1016/S0140-6736(12)62129-1
30. Sullivan PF, Neale MC, Kendler KS. Genetic Epidemiology of Major Depression: Review and Meta-Analysis. Am J Psychiatry (2000) 157(10):1552–62. doi: 10.1176/appi.ajp.157.10.1552
31. Choi S, Han KM, Won E, Yoon BJ, Lee MS, Ham BJ. Association of Brain-Derived Neurotrophic Factor DNA Methylation and Reduced White Matter Integrity in the Anterior Corona Radiata in Major Depression. J Affect Disord (2015) 172:74–80. doi: 10.1016/j.jad.2014.09.042
32. Ping L, Xu J, Zhou C, Lu J, Lu Y, Shen Z, et al. Tryptophan Hydroxylase-2 Polymorphism is Associated With White Matter Integrity in First-Episode, Medication-Naïve Major Depressive Disorder Patients. Psychiatry Res Neuroimaging (2019) 286:4–10. doi: 10.1016/j.pscychresns.2019.02.002
33. Lesch KP, Bengel D, Heils A, Sabol SZ, Greenberg BD, Petri S, et al. Association of Anxiety-Related Traits With a Polymorphism in the Serotonin Transporter Gene Regulatory Region. Science (1996) 274(5292):1527–31. doi: 10.1126/science.274.5292.1527
34. Alexopoulos GS, Murphy CF, Gunning-Dixon CF, Glatt CE, Latoussakis V, Kelly RE Jr, et al. Serotonin Transporter Polymorphisms, Microstructural White Matter Abnormalities and Remission of Geriatric Depression. J Affect Disord (2009) 119(1-3):132–41. doi: 10.1016/j.jad.2009.03.004
35. Won E, Choi S, Kang J, Kim A, Han KM, Chang HS, et al. Association Between Reduced White Matter Integrity in the Corpus Callosum and Serotonin Transporter Gene DNA Methylation in Medication-Naive Patients With Major Depressive Disorder. Transl Psychiatry (2016) 6(8):e866. doi: 10.1038/tp.2016.137
36. Coplan JD, Abdallah CG, Tang CY, Mathew SJ, Martinez J, Hof PR, et al. The Role of Early Life Stress in Development of the Anterior Limb of the Internal Capsule in Nonhuman Primates. Neurosci Lett (2010) 480(2):93–6. doi: 10.1016/j.neulet.2010.06.012
37. Nicodimos S, Gelaye BS, Williams MA, Berhane Y. Associations Between Witnessing Parental Violence and Experiencing Symptoms of Depression Among College Students. East Afr J Public Health (2009) 6(2):184–90. doi: 10.4314/eajph.v6i2.51764
38. Luthra R, Abramovitz R, Greenberg R, Schoor A, Newcorn J, Schmeidler J, et al. Relationship Between Type of Trauma Exposure and Posttraumatic Stress Disorder Among Urban Children and Adolescents. J Interpers Violence (2009) 24(11):1919–27. doi: 10.1177/0886260508325494
39. Choi J, Jeong B, Polcari A, Rohan ML, Teicher MH. Reduced Fractional Anisotropy in the Visual Limbic Pathway of Young Adults Witnessing Domestic Violence in Childhood. Neuroimage (2012) 59(2):1071–9. doi: 10.1016/j.neuroimage.2011.09.033
40. Meinert S, Repple J, Nenadic I, Krug A, Jansen A, Grotegerd D, et al. Reduced Fractional Anisotropy in Depressed Patients Due to Childhood Maltreatment Rather Than Diagnosis. Neuropsychopharmacology (2019) 44(12):2065–72. doi: 10.1038/s41386-019-0472-y
41. van Horssen J, Schreibelt G, Drexhage J, Hazes T, Dijkstra CD, van der Valk P, et al. Severe Oxidative Damage in Multiple Sclerosis Lesions Coincides With Enhanced Antioxidant Enzyme Expression. Free Radic Biol Med (2008) 45(12):1729–37. doi: 10.1016/j.freeradbiomed.2008.09.023
42. Kubicki M, Shenton ME. Diffusion Tensor Imaging Findings and Their Implications in Schizophrenia. Curr Opin Psychiatry (2014) 27(3):179–84. doi: 10.1097/YCO.0000000000000053
43. Alexander AL, Hurley SA, Samsonov AA, Adluru N, Hosseinbor AP, Mossahebi P, et al. Characterization of Cerebral White Matter Properties Using Quantitative Magnetic Resonance Imaging Stains. Brain Connect (2011) 1(6):423–46. doi: 10.1089/brain.2011.0071
44. Shizukuishi T, Abe O, Aoki S. Diffusion Tensor Imaging Analysis for Psychiatric Disorders. Magn Reson Med Sci (2013) 12(3):153–9. doi: 10.2463/mrms.2012-0082
45. Woodworth DC, Scambray KA, Corrada MM, Kawas CH, Sajjadi SA. Neuroimaging in the Oldest-Old: A Review of the Literature. J Alzheimers Dis (2021) 82(1):129–47. doi: 10.3233/JAD-201578
46. Rabins PV, Pearlson GD, Aylward E, Kumar AJ, Dowell K. Cortical Magnetic Resonance Imaging Changes in Elderly Inpatients With Major Depression. Am J Psychiatry (1991) 148(5):617–20. doi: 10.1176/ajp.148.5.617
47. van Velzen LS, Kelly S, Isaev D, Aleman A, Aftanas LI, Bauer J, et al. White Matter Disturbances in Major Depressive Disorder: A Coordinated Analysis Across 20 International Cohorts in the ENIGMA MDD Working Group. Mol Psychiatry (2020) 25(7):1511–25. doi: 10.1038/s41380-019-0477-2
48. Cole J, Chaddock CA, Farmer AE, Aitchison KJ, Simmons A, McGuffin P, et al. White Matter Abnormalities and Illness Severity in Major Depressive Disorder. Br J Psychiatry (2012) 201(1):33–9. doi: 10.1192/bjp.bp.111.100594
49. Chen G, Hu X, Li L, Huang X, Lui S, Kuang W, et al. Disorganization of White Matter Architecture in Major Depressive Disorder: A Meta-Analysis of Diffusion Tensor Imaging With Tract-Based Spatial Statistics. Sci Rep (2016) 6:21825. doi: 10.1038/srep21825
50. Yamada S, Takahashi S, Ukai T, Tsuji T, Iwatani J, Tsuda K, et al. Microstructural Abnormalities in Anterior Callosal Fibers and Their Relationship With Cognitive Function in Major Depressive Disorder and Bipolar Disorder: A Tract-Specific Analysis Study. J Affect Disord (2015) 174:542–8. doi: 10.1016/j.jad.2014.12.022
51. Liao Y, Huang X, Wu Q, Yang C, Kuang W, Du M, et al. Is Depression a Disconnection Syndrome? Meta-Analysis of Diffusion Tensor Imaging Studies in Patients With MDD. J Psychiatry Neurosci (2013) 38(1):49–56. doi: 10.1503/jpn.110180
52. Dillon DG, Gonenc A, Belleau E, Pizzagalli DA. Depression is Associated With Dimensional and Categorical Effects on White Matter Pathways. Depress Anxiety (2018) 35(5):440–7. doi: 10.1002/da.22734
53. Haber SN, Behrens TE. The Neural Network Underlying Incentive-Based Learning: Implications for Interpreting Circuit Disruptions in Psychiatric Disorders. Neuron (2014) 83(5):1019–39. doi: 10.1016/j.neuron.2014.08.031
54. Coloigner J, Batail JM, Commowick O, Corouge I, Robert G, Barillot C, et al. White Matter Abnormalities in Depression: A Categorical and Phenotypic Diffusion MRI Study. NeuroImage Clin (2019) 22:101710. doi: 10.1016/j.nicl.2019.101710
55. Alexopoulos GS. 'Vascular Depression' Hypothesis. Arch Gen Psychiatry (1997) 54(10):915. doi: 10.1001/archpsyc.1997.01830220033006
56. Herrmann LL, Goodwin GM, Ebmeier KP. The Cognitive Neuropsychology of Depression in the Elderly. Psychol Med (2007) 37(12):1693–702. doi: 10.1017/S0033291707001134
57. Herrmann LL, Le Masurier M, Ebmeier KP. White Matter Hyperintensities in Late Life Depression: A Systematic Review. J Neurol Neurosurg Psychiatry (2008) 79(6):619–24. doi: 10.1136/jnnp.2007.124651
58. Silver M, Moore CM, Villamarin V, Jaitly N, Hall JE, Rothschild AJ, et al. White Matter Integrity in Medication-Free Women With Peripartum Depression: A Tract-Based Spatial Statistics Study. Neuropsychopharmacology (2018) 43(7):1573–80. doi: 10.1038/s41386-018-0023-y
59. Zhang Y, Kong Y, Liu X, Gao H, Yin Y, Hou Z, et al. Desynchronized Functional Activities Between Brain White and Gray Matter in Major Depression Disorder. J Magn Reson Imaging (2021) 53(5):1375–86. doi: 10.1002/jmri.27466
60. McIntyre RS, Xiao HX, Syeda K, Vinberg M, Carvalho AF, Mansur RB, et al. The Prevalence, Measurement, and Treatment of the Cognitive Dimension/Domain in Major Depressive Disorder. CNS Drugs (2015) 29(7):577–89. doi: 10.1007/s40263-015-0263-x
61. Knight MJ, Baune BT. Cognitive Dysfunction in Major Depressive Disorder. Curr Opin Psychiatry (2018) 31(1):26–31. doi: 10.1097/YCO.0000000000000378
62. Fried EI, Nesse RM. The Impact of Individual Depressive Symptoms on Impairment of Psychosocial Functioning. PloS One (2014) 9(2):e90311. doi: 10.1371/journal.pone.0090311
63. Baune BT, Air T. Clinical, Functional, and Biological Correlates of Cognitive Dimensions in Major Depressive Disorder - Rationale, Design, and Characteristics of the Cognitive Function and Mood Study (CoFaM-Study). Front Psychiatry (2016) 7:150. doi: 10.3389/fpsyt.2016.00150
64. Motter JN, Pimontel PA, Rindskopf D, Devanand DP, Doraiswamy PM, Sneed JR, et al. Computerized Cognitive Training and Functional Recovery in Major Depressive Disorder: A Meta-Analysis. J Affect Disord (2016) 189:184–91. doi: 10.1016/j.jad.2015.09.022
65. Bolandzadeh N, Davis JC, Tam R, Handy TC, Liu-Ambrose T. The Association Between Cognitive Function and White Matter Lesion Location in Older Adults: A Systematic Review. BMC Neurol (2012) 12:126. doi: 10.1186/1471-2377-12-126
66. Ye Q, Su F, Gong L, Shu H, Liao W, Xie C, et al. Divergent Roles of Vascular Burden and Neurodegeneration in the Cognitive Decline of Geriatric Depression Patients and Mild Cognitive Impairment Patients. Front Aging Neurosci (2017) 9:288. doi: 10.3389/fnagi.2017.00288
67. Alves GS, Karakaya T, Fußer F, Kordulla M, O'Dwyer DM, Christl J, et al. Association of Microstructural White Matter Abnormalities With Cognitive Dysfunction in Geriatric Patients With Major Depression. Psychiatry Res (2012) 203(2-3):194–200. doi: 10.1016/j.pscychresns.2011.12.006
68. Sheline YI, Price JL, Vaishnavi SN, Mintun MA, Barch DM, Alves GS, et al. Regional White Matter Hyperintensity Burden in Automated Segmentation Distinguishes Late-Life Depressed Subjects From Comparison Subjects Matched for Vascular Risk Factors. Am J Psychiatry (2008) 165(4):524–32. doi: 10.1176/appi.ajp.2007.07010175
69. Adólfsdóttir S, Wehling HJ, Ystad M, Lundervold A, Lundervold AJ. Salient Measures of Inhibition and Switching are Associated With Frontal Lobe Gray Matter Volume in Healthy Middle-Aged and Older Adults. Neuropsychology (2014) 28(6):859–69. doi: 10.1037/neu0000082
70. Surguladze S, Brammer MJ, Keedwell P, Giampietro V, Young AW, Travis MJ, et al. A Differential Pattern of Neural Response Toward Sad Versus Happy Facial Expressions in Major Depressive Disorder. Biol Psychiatry (2005) 57(3):201–9. doi: 10.1016/j.biopsych.2004.10.028
71. Respino M, Jaywant A, Kuceyeski A, Victoria LW, Hoptman MJ, Scult MA, et al. The Impact of White Matter Hyperintensities on the Structural Connectome in Late-Life Depression: Relationship to Executive Functions. NeuroImage Clin (2019) 23:101852. doi: 10.1016/j.nicl.2019.101852
72. Bennett S, Thomas AJ. Depression and Dementia: Cause, Consequence or Coincidence? Maturitas (2014) 79(2):184–90. doi: 10.1016/j.maturitas.2014.05.009
73. Häfner H, Maurer K, Trendler G, van der Heiden W, Schmidt M, Könnecke R. Schizophrenia and Depression: Challenging the Paradigm of Two Separate Diseases–a Controlled Study of Schizophrenia, Depression and Healthy Controls. Schizophr Res (2005) 77(1):11–24. doi: 10.1016/j.schres.2005.01.004
74. Koshiyama D, Fukunaga M, Okada N, Morita K, Nemoto K, Usui K, et al. White Matter Microstructural Alterations Across Four Major Psychiatric Disorders: Mega-Analysis Study in 2937 Individuals. Mol Psychiatry (2020) 25(4):883–95. doi: 10.1038/s41380-019-0553-7
75. Chang M, Womer FY, Edmiston EK, Bai C, Zhou Q, Jiang X, et al. Neurobiological Commonalities and Distinctions Among Three Major Psychiatric Diagnostic Categories: A Structural MRI Study. Schizophr Bull (2018) 44(1):65–74. doi: 10.1093/schbul/sbx028
76. Zhu X, Wang X, Xiao J, Zhong M, Liao J, Yao S. Altered White Matter Integrity in First-Episode, Treatment-Naive Young Adults With Major Depressive Disorder: A Tract-Based Spatial Statistics Study. Brain Res (2011) 1369:223–9. doi: 10.1016/j.brainres.2010.10.104
77. Dalby RB, Frandsen J, Chakravarty MM, Ahdidan J, Sørensen L, Rosenberg R, et al. Depression Severity is Correlated to the Integrity of White Matter Fiber Tracts in Late-Onset Major Depression. Psychiatry Res (2010) 184(1):38–48. doi: 10.1016/j.pscychresns.2010.06.008
78. Magioncalda P, Martino M, Conio B, Piaggio N, Teodorescu R, Escelsior A, et al. Patterns of Microstructural White Matter Abnormalities and Their Impact on Cognitive Dysfunction in the Various Phases of Type I Bipolar Disorder. J Affect Disord (2016) 193:39–50. doi: 10.1016/j.jad.2015.12.050
79. Taylor WD, Macfall JR, Boyd B, Payne ME, Sheline YI, Krishnan RR, et al. One-Year Change in Anterior Cingulate Cortex White Matter Microstructure: Relationship With Late-Life Depression Outcomes. Am J Geriatr Psychiatry (2011) 19(1):43–52. doi: 10.1097/JGP.0b013e3181e70cec
80. Pillai RLI, Huang C, LaBella A, Zhang M, Yang Y, Trivedi M, et al. Examining Raphe-Amygdala Structural Connectivity as a Biological Predictor of SSRI Response. J Affect Disord (2019) 256:8–16. doi: 10.1016/j.jad.2019.05.055
81. Riva-Posse P, Choi KS, Holtzheimer PE, McIntyre CC, Gross RE, Chaturvedi A, et al. Defining Critical White Matter Pathways Mediating Successful Subcallosal Cingulate Deep Brain Stimulation for Treatment-Resistant Depression. Biol Psychiatry (2014) 76(12):963–9. doi: 10.1016/j.biopsych.2014.03.029
82. Tatham EL, Hall GB, Clark D, Foster J, Ramasubbu R. The 5-HTTLPR and BDNF Polymorphisms Moderate the Association Between Uncinate Fasciculus Connectivity and Antidepressants Treatment Response in Major Depression. Eur Arch Psychiatry Clin Neurosci (2017) 267(2):135–47. doi: 10.1007/s00406-016-0702-9
83. Vilgis V, Vance A, Cunnington R, TJ. White Matter Microstructure in Boys With Persistent Depressive Disorder. J Affect Disord (2017) 221:11–6. doi: 10.1016/j.jad.2017.06.020
84. Zeng LL, Liu L, Liu Y, Shen H, Li Y, Hu D. Antidepressant Treatment Normalizes White Matter Volume in Patients With Major Depression. PloS One (2012) 7(8):e44248. doi: 10.1371/journal.pone.0044248
85. Wang J, Qiao J, Zhang Y, Wang H, Zhu S, Zhang H, et al. Desvenlafaxine Prevents White Matter Injury and Improves the Decreased Phosphorylation of the Rate-Limiting Enzyme of Cholesterol Synthesis in a Chronic Mouse Model of Depression. J Neurochem (2014) 131(2):229–38. doi: 10.1111/jnc.12792
86. Delhaye S, Bardoni B. Role of Phosphodiesterases in the Pathophysiology of Neurodevelopmental Disorders. Mol Psychiatry (2021) 26(9):4570–82. doi: 10.1038/s41380-020-00997-9
87. Lee JH, Kim KY, Lee YK, Park SY, Kim CD, Lee WS, et al. Cilostazol Prevents Focal Cerebral Ischemic Injury by Enhancing Casein Kinase 2 Phosphorylation and Suppression of Phosphatase and Tensin Homolog Deleted From Chromosome 10 Phosphorylation in Rats. J Pharmacol Exp Ther (2004) 308(3):896–903. doi: 10.1124/jpet.103.061853
88. Li YJ, Xu M, Gao ZH, Wang YQ, Yue Z, Zhang YX, et al. Alterations of Serum Levels of BDNF-Related miRNAs in Patients With Depression. PloS One (2013) 8(5):e63648. doi: 10.1371/journal.pone.0063648
89. Khadivi A, Shobeiri P, Momtazmaneh S, Samsami FS, Shalbafan M, Shirazi E, et al. Cilostazol as an Adjunctive Treatment in Major Depressive Disorder: A Pilot Randomized, Double-Blind, and Placebo-Controlled Clinical Trial. Psychopharmacol (Berl) (2022) 239(2):551–9. doi: 10.1007/s00213-021-06041-0
90. Abdallah MS, Ramadan AN, Omara-Reda H, Mansour NO, Elsokary MA, Elsawah HK, et al. Double-Blind, Randomized, Placebo-Controlled Pilot Study of the Phosphodiesterase-3 Inhibitor Cilostazol as an Adjunctive to Antidepressants in Patients With Major Depressive Disorder. CNS Neurosci Ther (2021) 27(12):1540–8. doi: 10.1111/cns.13731
91. Takahashi K, Oshima A, Inoue K, Takeyoshi H, Fukuda M, Mikuni M. Novel Augmentation Therapy With Cilostazol for the Geriatric Major Depressive Disorder Patient With Deep White Matter Hyperintensities on T2-Weighted Brain MRI: A Case Report. Pharmacopsychiatry (2008) 41(1):37–9. doi: 10.1055/s-2007-993208
92. Russo-Neustadt AA, Beard RC, Huang YM, Cotman CW. Physical Activity and Antidepressant Treatment Potentiate the Expression of Specific Brain-Derived Neurotrophic Factor Transcripts in the Rat Hippocampus. Neuroscience (2000) 101(2):305–12. doi: 10.1016/S0306-4522(00)00349-3
93. Svatkova A, Mandl RC, Scheewe TW, Cahn W, Kahn RS, HE. Physical Exercise Keeps the Brain Connected: Biking Increases White Matter Integrity in Patients With Schizophrenia and Healthy Controls. Schizophr Bull (2015) 41(4):869–78. doi: 10.1093/schbul/sbv033
94. Anderson RJ, Hoy KE, Daskalakis ZJ, Fitzgerald PB. Repetitive Transcranial Magnetic Stimulation for Treatment Resistant Depression: Re-Establishing Connections. Clin Neurophysiol (2016) 127(11):3394–405. doi: 10.1016/j.clinph.2016.08.015
95. Kito S, Fujita K, Koga Y. Changes in Regional Cerebral Blood Flow After Repetitive Transcranial Magnetic Stimulation of the Left Dorsolateral Prefrontal Cortex in Treatment-Resistant Depression. J Neuropsychiatry Clin Neurosci (2008) 20(1):74–80. doi: 10.1176/jnp.2008.20.1.74
96. Kozel FA, Johnson KA, Nahas Z, Nakonezny PA, Morgan PS, Anderson BS, et al. Fractional Anisotropy Changes After Several Weeks of Daily Left High-Frequency Repetitive Transcranial Magnetic Stimulation of the Prefrontal Cortex to Treat Major Depression. J ECT (2011) 27(1):5–10. doi: 10.1097/YCT.0b013e3181e6317d
Keywords: major depressive disorder, pathogenesis, white matter alterations, clinical relevance, depression, diffusion tenor imaging
Citation: He E, Liu M, Gong S, Fu X, Han Y and Deng F (2022) White Matter Alterations in Depressive Disorder. Front. Immunol. 13:826812. doi: 10.3389/fimmu.2022.826812
Received: 01 December 2021; Accepted: 22 March 2022;
Published: 12 May 2022.
Edited by:
Norbert Müller, Ludwig Maximilian University of Munich, GermanyReviewed by:
Ermelinda De Meo, San Raffaele Hospital (IRCCS), ItalyLu-sha Tong, Zhejiang University, China
Copyright © 2022 He, Liu, Gong, Fu, Han and Deng. This is an open-access article distributed under the terms of the Creative Commons Attribution License (CC BY). The use, distribution or reproduction in other forums is permitted, provided the original author(s) and the copyright owner(s) are credited and that the original publication in this journal is cited, in accordance with accepted academic practice. No use, distribution or reproduction is permitted which does not comply with these terms.
*Correspondence: Fang Deng, ZGVuZ19mYW5nQGpsdS5lZHUuY24=