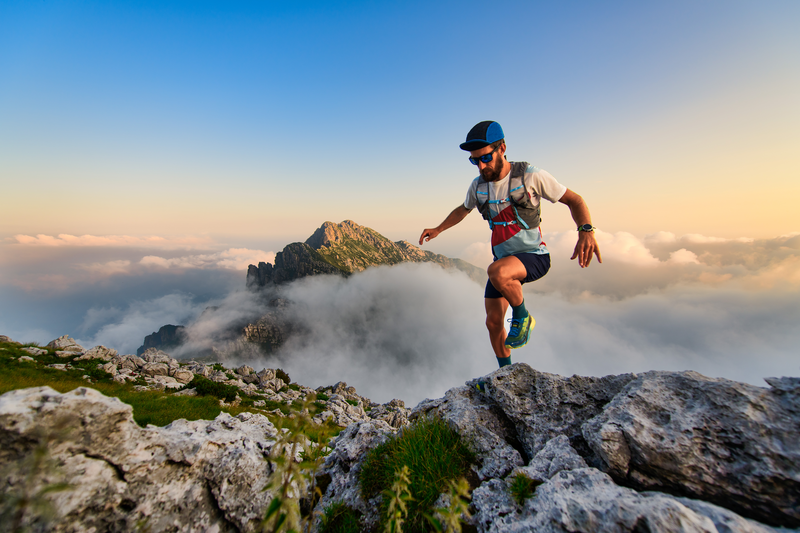
95% of researchers rate our articles as excellent or good
Learn more about the work of our research integrity team to safeguard the quality of each article we publish.
Find out more
SYSTEMATIC REVIEW article
Front. Immunol. , 22 March 2022
Sec. Autoimmune and Autoinflammatory Disorders
Volume 13 - 2022 | https://doi.org/10.3389/fimmu.2022.825103
The coronavirus disease 2019 (COVID-19) pandemic has had an enormous impact on the world, affecting people’s lifestyle, economy, and livelihood. Recently, with the development of vaccines, the number of infected cases has decreased. Many case reports have revealed that COVID-19 may induce other serious comorbidities such as anti-N-methyl-d-aspartate (anti-NMDA) receptor encephalitis. Anti-NMDA receptor encephalitis is an acute autoimmune disease that occurs more commonly in women than in men. To explore the association between COVID-19 and anti-NMDA receptor encephalitis, the microRNA (miRNA) biomarkers of COVID-19, anti-NMDA receptor encephalitis, and other related diseases from the literature are reviewed; then on the basis of these miRNA biomarkers, the relationship between COVID-19 and anti-NMDA receptor encephalitis is discussed. miRNAs are small non-coding RNAs that play important roles in cell differentiation, development, cell-cycle regulation, and apoptosis. miRNAs have been used as biological biomarkers for many diseases. The results in this study reveal that the relationship between anti-NMDA receptor encephalitis and COVID-19 infection or COVID-19 vaccination cannot be excluded; however, the risk that COVID-19 triggers the anti-NMDA receptor encephalitis is not high.
Coronavirus disease 2019 (COVID-19), caused by severe acute respiratory syndrome coronavirus 2 (SARS-CoV-2), has infected more than 173 million people and has had a huge impact all over the world, affecting people’s lifestyle, economy, and livelihood (1). The outbreak of COVID-19 is similar to the prior infectious disease severe acute respiratory syndrome (SARS) that was reported in the early 2000s. SARS was caused by SARS-CoV or SARS-CoV-1 (2). COVID-19 appears to be far more infectious than SARS-CoV (3). Recently, due to the development of vaccines, the number of identified cases has decreased in countries with high vaccination rates. The relationships between daily increasing cases or daily increasing deaths due to COVID-19 and vaccination in 187 countries and regions were studied (4). The results revealed that vaccination can effectively reduce the number of new cases and deaths due to COVID-19. Nevertheless, the vaccines may not be very useful in preventing new variants of SARS-CoV-2; however, they may prevent the risk of developing severe illness.
The origin of SARS-CoV-2 has been widely discussed, and it is more controversial in this sense compared with SARS-CoV-1. It was first identified in Wuhan, China, in December 2019, before rapidly spreading to other countries. Phylogenetic analysis showed that the nucleotide sequences of SARS-CoV-2 samples in China had the lowest diversity, followed by those in Europe and the USA. This result coincided with the transmission order of this virus, whereby SARS-CoV-2 started in China, then broke out in Europe, and finally arrived in the USA (5). SARS-CoV-2 might have been transmitted from bats to humans via an intermediary animal such as the pangolin. Bats are the most likely host of SARS-CoV-2 because bat coronaviruses are 96% identical to SARS-CoV-2 at the whole-genome level (6).
COVID-19 has been reported to trigger some other diseases such as autoimmune or autoinflammatory conditions (7). It might trigger a severe inflammatory state known as the “cytokine storm” and the development of thrombotic phenomena, both of which are associated with high mortality. In this paper, the association between COVID-19 and the anti-N-methyl-d-aspartate (anti-NMDA) receptor encephalitis is explored. Anti-NMDA receptor encephalitis is an acute autoimmune disease with a multistage illness progressing from initial psychiatric symptoms to memory disturbances, dyskinesia, seizures, and catatonia (8). It occurs more often in females than in males. The cause of this disease is often unknown. Tumors might induce anti-NMDA receptor encephalitis (9). In addition, virus infection and vaccination might also induce anti-NMDA receptor encephalitis. Vaccination against H1N1 influenza or tetanus, diphtheria, pertussis, and poliomyelitis, or Japanese encephalitis (JE) might induce anti-NMDA receptor encephalitis (10). The association of viruses and various tumors with anti-NMDA receptor encephalitis may account for the comorbidity of these conditions. Anti-NMDA receptor encephalitis may not be diagnosed at an early stage, which may lead to treatment delays and unsatisfactory outcomes.
Several COVID-19 cases associated with anti-NMDA receptor encephalitis have been reported in the literature. A 30-year-old female patient was admitted to the psychiatry department due to paranoid ideation, psychomotor agitation, dysarthria with dysprosody, and visual hallucinations (11). Later, she presented fever, and polymerase chain reaction (PCR) assay results were positive for SARS-CoV-2. In this case, SARS-CoV-2 infection was suspected to trigger the onset of anti-NMDA receptor encephalitis. A 7-year-old boy was diagnosed with anti-NMDA receptor encephalitis associated with COVID-19 without pulmonary involvement or fever (12). A 23-year-old SARS-CoV-2-infected Ecuadorian male patient was hospitalized for psychotic symptoms, and a cerebrospinal fluid (CSF) examination was performed with evidence of anti-NMDA receptor antibodies (13). A 50-year-old man presented an acute onset of psychiatric symptoms, and the test for NMDA receptor antibodies in CSF was positive, while it was negative in serum (14). PCR confirmed SARS-CoV-2 infection. A 23-month-old girl developed fever and movement disorder symptoms, and her PCR test screening for SARS-CoV-2 was positive (15). Her autoantibody testing demonstrated NMDA receptor IgG positivity in the serum and CSF. A 53-year-old woman, during the onset of anti-NMDA receptor encephalitis, had palilalia as a prominent clinical manifestation, accompanied by SARS-CoV-2 infection (16). An 18-year-old girl was admitted to the hospital with generalized tonic-clonic seizures (17). Her CSF PCR was positive for COVID-19, and she also had NMDA receptor antibodies present in the CSF. In addition to these reported cases of COVID-19 infection associated with anti-NMDA receptor encephalitis, a case of anti-NMDA receptor encephalitis triggered by COVID-19 vaccination was reported. A young female presented with acute psychosis, motor dysfunction and a transient bout of aphasia after receiving her first dose of the Pfizer-BioNTech COVID-19 vaccine (18). Later, this patient was diagnosed with anti-NMDA receptor encephalitis by a CSF test. Due to prompt therapies targeting anti-NMDAR antibodies, this patient achieved an excellent clinical outcome.
The virus attaches to a host cell by binding to specific receptor proteins on the cell membrane of the target cell. Different viruses have different binding receptors. SARS-CoV-2 binds the same cell entry receptor, angiotensin-converting enzyme II (ACE2) as SARS-CoV. In addition to these two well-known coronaviruses, SARS-CoV and SARS-CoV-2, another notable coronavirus is the Middle East respiratory syndrome (MERS) coronavirus that binds another cellular receptor, dipeptidyl peptidase 4 (DPP4) (19, 20). The interaction with cell receptors is a key initial step in the life cycle of infectious viruses, and it plays an important regulatory role in viral pathogenesis. Therefore, understanding the molecular interactions of viruses and their receptors can help develop new antiviral therapies and vaccine technologies (21). As a result, it can be concluded that viruses that bind the same receptor may have a more similar pathogenesis than those that bind other receptors. This assumption may apply to other biological mechanisms such as microRNA (miRNA) regulation. In this study, miRNA biomarkers of SARS-CoV-2 and other viruses are reviewed, and the results are used to explore the association between COVID-19 and anti-NMDA receptor encephalitis.
miRNAs are small single-stranded non-coding RNAs that play important roles in cell differentiation, development, apoptosis, and modulating gene expression. There are over 2000 miRNAs that have been discovered in humans. miRNAs can be used as genetic biomarkers for various diseases. miRNAs can regulate the protein-coding genes in the human genome (22), and they are well known to be involved in the initiation and progression of many diseases including cancers, neurological diseases, gastroesophageal reflux, and diabetes (23–30). In addition, miRNAs might be important modulators of viral infections and play a role in the treatment of viral infections (31). COVID-19 might use the interplay between miRNAs and other molecules to avoid being recognized and attacked by the host immune protection system, as well as to inactivate related genes that are vital to the immune system (32). SARS-CoV-2 alters self-gene regulation and seizes host miRNAs to provide a protective environment maintaining its latency (33).
miRNA therapy and miRNA biomarkers for COVID-19 infection have been studied (Table 1). Honeysuckle is one of the commonly used herbs in traditional Chinese medicine. The absorption of an miRNA in honeysuckle decoction (HD), HD-miR-2911, was demonstrated to inhibit SARS-CoV-2 replication (34). The peripheral blood miRNA levels of 10 patients with COVID-19 and 4 healthy individuals were studied (34). miR‐16‐2‐3p, miR-501‐5p, and miR‐618 were upregulated, while miR‐183‐5p, miR‐627‐5p, and miR‐144‐3p were downregulated in COVID-19 patients. COVID-19 targeted human miRNAs involved in various diseases, with miR-15a, miR-15b, miR-548c, miR-548d, miR-409, miR-30b, and miR-505 exhibiting higher target scores (35). Four miRNAs, miR‐146a‐5p, miR‐21‐5p, miR‐142‐3p, and miR‐15b‐5p, were identified as potential contributors to COVID-19 pathogenesis and might be candidate therapeutic targets (38). The levels of circulating miRNAs of mechanically-ventilated COVID-19 patients and healthy controls were compared (40). Compared with healthy controls, the serum concentration levels of miR-499, miR-21, miR-155, and miR-208a were significantly increased in COVID-19 patients. The circulating expression levels of miR-146a-5p, miR-21-5p, and miR-126-3p were significantly lower in COVID-19 patients compared with gender-matched healthy controls (39). Among the miRNAs in Table 1, several were identified by at least two studies. miR‐16‐2, miR-15b, miR-126, miR-146a, and miR-548d were identified by two studies, while miR-21 was identified by three studies (Table 2). This reveals that miR‐16‐2, miR-15b, miR-548d, miR-126, miR-146a, and miR-21 could be further investigated as useful miRNA COVID-19 biomarkers. Whereas HD-miR-2911 was also discussed in two studies, it is not a human miRNA.
miRNA biomarkers for anti-NMDA receptor encephalitis and other diseases related to anti-NMDA receptor encephalitis have been discussed in the literature. First, the miRNA biomarkers for anti-NMDA receptor encephalitis were reviewed. So far, only a few studies have investigated miRNA expression for anti-NMDA receptor encephalitis. Zhang et al. recruited anti-NMDA receptor encephalitis patients at the prodromal phase and psychotic phase before immunotherapy, and they studied their blood samples in comparison with a control group (41). The blood samples of five anti-NMDA receptor encephalitis patients and five controls were used for microarray analyses. The miRNAs, let-7a, let-7b, let-7d, and let-7f were found to be significantly downregulated in anti-NMDA receptor encephalitis cases compared to the controls. Next, additional patients and controls were recruited for PCR analysis. The miRNAs let-7a, let-7b, let-7d, and let-7f of the anti-NMDA receptor encephalitis patients were significantly downregulated compared with the controls (see Table 3). Finally, they concluded that only let-7b could be used as a biomarker for anti-NMDA receptor encephalitis because the other three miRNAs, let-7a, let-7d, and let-7f, were significantly downregulated in other nervous system diseases compared to the normal controls.
Table 3 miRNA biomarkers related to anti-NMDA receptor encephalitis (41).
Anti-NMDA receptor encephalitis was found to be related to tumors including ovarian teratoma, testis teratoma, neuroendocrine tumor, small-cell lung cancer, dura mater lesions, and mediastinal teratoma (42–47). The miRNA biomarkers for these tumors were discussed in Wang (2019) (9) (see Table 4). In addition, the miRNAs related to viruses and vaccines associated with anti-NMDA receptor encephalitis are listed in Table 5. These viruses include the herpes simplex virus (HSV) and JE virus, while the vaccines include those against H1N1 influenza, pertussis, and poliomyelitis.
Table 4 miRNA biomarkers for tumors related to anti-NMDA receptor encephalitis (9).
Table 5 miRNAs biomarkers for vaccines or viruses related to anti-NMDA receptor encephalitis (10).
To explore the association between COVID-19 and anti-NMDA receptor encephalitis, the common miRNA biomarkers for COVID-19, anti-NMDA receptor encephalitis, or tumors or vaccines related to anti-NMDA receptor encephalitis were first checked by comparing Table 1 with Tables 3–5. Seven common miRNA biomarkers were identified: miR-107, miR-29b, let-7a, let-7f, miR-26b, miR-21 and miR-155 (Figure 1).
Figure 1 Seven common miRNA biomarkers for COVID-19, anti-NMDA receptor encephalitis, tumors or vaccines related to anti-NMDA receptor encephalitis.
Among these miRNAs, let-7a and let-7f were directly related to anti-NMDA receptor encephalitis (Table 3). As mentioned above, although Zhang et al. found that let-7a, let-7b, let-7d, and let-7f were significantly downregulated in patients with anti-NMDA receptor encephalitis compared with the controls, they concluded that only let-7b could be used as the main biomarker for anti-NMDA receptor encephalitis, because let-7a, let-7d, and let-7f were also related to other neurological diseases (41). Several studies reported that miRNA let-7a and let-7f were detected in certain neurological diseases, probably explaining the COVID-19 comorbidity with these conditions. So far, although several cases of anti-NMDA receptor encephalitis triggered by COVID-19 have been reported in the literature, there are not as many as other complications. This may coincide with the fact that the main anti-NMDA receptor encephalitis biomarker, let-7b, was not reported to be a miRNA biomarker of COVID-19. The flowchart of this analysis is provided in Figure 2. In addition, two miRNA biomarkers of COVID-19, let-7a and let-7f, were associated with testis teratoma and the H1N1 vaccine, respectively. A 30-year-old male patient with a testicular teratoma and seminoma developed anti-NMDA receptor encephalitis (46). A patient was confirmed to have positive anti-NMDA receptor antibodies after vaccination against H1N1 influenza (48). This may provide indirect evidence showing a relationship between COVID-19 and anti-NMDA receptor encephalitis.
The other five common biomarkers of COVID-19—miR-29b, miR-107, miR-21, miR-155, and miR-26b—were also related to tumors or vaccines associated with anti-NMDA receptor encephalitis. miR-29b was related to both the JE virus and neuroendocrine tumor. miR-107 and miR-21 were related to neuroendocrine tumor. miR-155 was related to JE virus, while miR-26b was associated with ovarian teratoma. This may also provide indirect evidence showing a relationship between COVID-19 and anti-NMDA receptor encephalitis. The relationship between neuroendocrine tumor and anti-NMDA receptor encephalitis has been discussed (44, 49–53). JE vaccination or JE infection has been associated with anti-NMDA receptor encephalitis. A 7-year-old boy was diagnosed with JE, as well as with anti-NMDA receptor encephalitis symptoms (54). Three JE patients who developed relapsing movement or behavioral disorders were examined for anti-NMDA receptor immunoglobulin G (IgG), and positive results were obtained for all the samples (55). Further cases for JE triggering anti-NMDA receptor encephalitis have been discussed (10, 56). Ovarian teratoma has been detected in some female anti-NMDA receptor encephalitis patients (57–61). Patients with ovarian teratomas presented more severe neurological conditions; thus early operative treatment might lead to complete recovery and reduce the risk of relapse.
Only a few cases of COVID-19 triggering anti-NMDA receptor encephalitis have been reported in the literature. This indicates that the risk is not high. Table 1 presents more than 50 COVID-19 miRNA biomarkers. Among these biomarkers, only seven miRNAs are related to tumors or vaccines associated with anti-NMDA receptor encephalitis, yielding a ratio less than 0.14. This may explain the low risk of developing anti-NMDA receptor encephalitis triggered by COVID-19 infection or COVID-19 vaccination (Figure 3).
Figure 3 COVID-19 infection or COVID-19 vaccination has a risk of triggering anti-NMDA receptor encephalitis, but the risk is not high.
In spite of the low risk, we may still be interested in investigating the causality of COVID-19 triggering anti-NMDA receptor encephalitis. This can be discussed in several aspects. A molecular mimicry mechanism might contribute to the development of COVID-19-associated neuropsychiatric symptoms (62). Thus, understanding the homology between SARS CoV-2 and human proteins can help explore the mimicry mechanisms during infection. SARS CoV-2 proteins may simulate human proteins, mislead the immune system, and slow down its response. SARS-CoV-2 proteins could interact directly with GABA receptors and NMDA glutamate receptors because of the structural similarities between the NMDA receptor GluN1 and GluN2a subunits with the SARS-CoV-2 nonstructural proteins 8 and 9, respectively (63). As a result, SARS-CoV-2 may induce immune-mediated cross-reactivity to the NMDA receptor.
As, also mentioned above, SARS-CoV-2 can seize host miRNAs to provide a protective environment. It may hijack the miRNA pathway, either by depleting host miRNAs or by producing its own miRNAs. Pawlica et al. discovered a small viral noncoding RNA, named CoV2-miR-O7a, expressed by SARS-CoV-2 (64). CoV2-miR-O7a has the potential to regulate host transcripts by associating with the cellular RNA interference machinery. This finding revealed that SARS-CoV-2 itself could produce miR-like molecules that can influence host gene transcription. These miR-like molecules might resemble miRNAs involved in the function of the NMDA receptor, thereby leading to its dysfunction. In addition, CoV2-miR-O7a was derived from the ORF7a sequence that resembled viral accessory protein ORF7a. Several SARS-CoV-2 proteins including ORF7a, ORF7b, ORF8, and ORF9b are involved in provoking an autoimmune response (65).
These pathological mechanisms might explain the causality of COVID-19 inducing anti-NMDA receptor encephalitis. As these seven miRNAs are common miRNA biomarkers, COVID-19 patients with abnormal expression of at least one of these seven miRNAs might be at a higher risk for anti-NMDA receptor encephalitis.
The seven common miRNA biomarkers in Figure 1 were used to discuss the risk of COVID-19 infection or COVID-19 vaccination triggering anti-NMDA receptor encephalitis. To examine whether these seven miRNAs can be used as main miRNA biomarkers for COVID-19, a phylogenetic tree for the human COVID-19 miRNA biomarkers in Table 1 (Figure 4) was plotted to study the relationship between these seven biomarkers and other COVID-19 biomarkers. The phylogenetic tree was plotted by Matlab software using the Jukes-Cantor substitution model.
Figure 4 Phylogenetic tree of human COVID-19 miRNA biomarkers. The seven common miRNAs are marked with asterisks.
Figure 4 shows that these seven miRNA biomarkers were all located on the inner branches of the phylogenetic tree. As they are close to most other COVID-19 miRNA biomarkers in Table 1, these seven miRNAs can be used to study the association between COVID-19 and anti-NMDA receptor encephalitis.
In addition, most of these miRNA biomarkers of COVID-19 are involved in the inflammatory pathways in COVID-19. Coronaviruses enter the human body through respiratory droplets, fecal-oral transmission, etc. The virus enters the host cell and then releases its RNA genome inside the nucleus before initiating the virus replication. At this stage, the body produces an immune response. However, SARS-CoV-2 infection becomes severe as the inflammatory pathways get activated. The inflammatory pathways involved in COVID-19 include the interleukin-6/Janus kinase/STAT (IL-6/JAK/STAT), the interferon (IFN) cell signaling pathway, the TNFα-NF-κB inflammatory signaling pathway, the JAK/STAT pathway, the toll-like receptor (TLR) pathway, the antibody-mediated pathway, the Bruton tyrosine kinase (BTK) pathway, and the renin-angiotensin system (RAS) pathway (66).
Two of these inflammatory pathways, the JAK/STAT pathway and the RAS pathway, are discussed in this study. The miRTarbase database was used to determine the miRNA biomarkers of COVID-19 involved in these two pathways (67). Table 6 provides the miRNA biomarkers involved in the JAK/STAT pathway and the RAS pathway. Among the COVID-19 human miRNA biomarkers, only a small number of them were not involved in these two pathways. This reveals that most COVID-19 miRNA biomarkers may play an important role in activating the inflammatory response to SARS-CoV-2 infection. In addition, six of the seven common miRNA biomarkers were involved in both the JAK/STAT and the RAS pathways; the exception was let-7f that was only involved in the JAK/STAT pathway. These inflammatory pathways might contribute to the pathological mechanisms of both COVID-19 and anti-NMDA receptor encephalitis.
In this paper, the association between COVID-19 and anti-NMDA receptor encephalitis was discussed. COVID-19 causes many short-term or long-term complications including autoimmune reactions and neurological symptoms. Anti-NMDA receptor encephalitis is not the only complication of COVID-19. As the two COVID-19 miRNA biomarkers let-7a and let-7f are related to other neurological diseases, this may explain why neurological complications other than anti-NMDA receptor encephalitis could be caused by COVID-19.
Many other neurological complications have been reported to be associated with COVID-19 including acute cerebrovascular disease, Guillain-Barré syndrome, and hemophagocytic lymphohistiocytosis (68). A retrospective study investigated 841 patients hospitalized with COVID-19 and found that 57% of patients had neurological symptoms (69). The Spanish Society of Neurology ran a national registry of neurological complications in patients with COVID-19 infection; on the basis of this dataset, a retrospective study investigated patients with encephalopathy or encephalitis (70). A total of 232 patients were recorded in this dataset, including 51 cases of encephalitis or encephalopathy. They concluded that patients with encephalitis were younger than those with other neurological syndromes, but older encephalitis patients had severe encephalopathy and seizures. A child was reported to have encephalitis associated with COVID-19 (71). An 11-year-old boy presented with status epilepticus, and encephalitis was confirmed from the CSF test. His nasopharyngeal swab was shown to be positive for COVID-19. He achieved complete recovery without treatment in 6 days. A Wuhan male had a fever, shortness of breath, and myalgia, with a positive test for SARS-CoV-2 (72). Later, he was diagnosed to have encephalitis. A 79-year-old female was admitted to hospital following a seizure (73). Her second nasopharyngeal sample PCR was positive for COVID-19. The computed tomography (CT) scan and repeat brain MRI suggested limbic encephalitis. A 39-year-old-man presenting with acute encephalitis was diagnosed as having a COVID-19 infection (74). This patient achieved full recovery, suggesting that this condition could be amenable to therapeutic modulation. The effect of plasmapheresis treatment in COVID-19-related autoimmune encephalitis was investigated (75). A 40-year-old woman with type 2 diabetes was admitted to hospital with encephalitis (76). On admission, her nasopharyngeal swab was positive for SARS-CoV-2.
Moreover, many chronic diseases or treatments can increase the risk of COVID-19 infection or increase the risk of developing serious illness from COVID-19. Gastroesophageal reflux (GERD) is a digestive disorder that occurs when acidic stomach fluids back up from the stomach into the esophagus. A GERD drug, proton pump inhibitor (PPI), was shown to increase the risk of COVID-19 infection (77, 78). Collagen could be used as an alternative to treat GERD, as it did not increase the risk of COVID-19 infection (79). Patients with hypertension, cardiac diseases, or diabetes, who receive ACE2-increasing drugs, were found to be at a higher risk of severe COVID-19 infection (80). Obesity was associated with a variety of comorbidities related to the increased morbidity and mortality of COVID-19 (81).
In addition to COVID-19 infection, COVID-19 vaccines have been reported to cause neurological complications. Recently, with the development of vaccines, cases of infection have decreased. Although the vaccines may provide efficient protection against COVID-19, encephalomyelitis, neurological complications, or autoimmune reactions after receiving COVID-19 vaccines have been reported. A 56-year-old female with a history of post-infectious rhombencephalitis developed an acute disseminated encephalomyelitis (ADEM)-like disorder shortly after COVID-19 vaccination (82). Autoimmune hepatitis caused by any vaccination is very rare. Two autoimmune hepatitis cases, a 38-year-old female and a 62-year-old diabetic male, were reported to be triggered by COVID-19 vaccination (83). Two patients with no psychiatric or neurological history presented with encephalopathy and seizures after receiving Moderna COVID-19 vaccines (4). No associations between COVID‐19 vaccination and Bell’s palsy (or Guillain‐Barré syndrome) were found; however, there are special considerations around COVID‐19 vaccines for patients who take immunosuppressive medications (84). On the basis of the results discussed in this paper and the fact that anti-NMDA receptor encephalitis is an autoimmune and neurological disorder, the risk of COVID-19 infection or COVID-19 vaccination triggering anti-NMDA receptor encephalitis cannot be excluded; however, the risk is not high.
Anti-NMDA receptor encephalitis is an acute disorder. Several cases have been reported to be related to COVID-19 infection. This study investigated miRNA biomarkers to explore the relationship between anti-NMDA receptor encephalitis and COVID-19 infection or COVID-19 vaccination. The results revealed that there were not many common miRNA biomarkers of COVID-19 and anti-NMDA receptor encephalitis-related tumors or vaccines. This may explain why the risk of anti-NMDA receptor encephalitis triggered by COVID-19 infection or COVID-19 vaccines is not high. Nevertheless, there remains a small risk that COVID-19 or COVID-19 vaccination could trigger anti-NMDA receptor encephalitis. Therefore, for COVID-19 patients or people receiving COVID-19 vaccination who develop psychiatric or neurological symptoms, a diagnosis of anti-NMDA receptor encephalitis should be considered if other complications are excluded.
The original contributions presented in the study are included in the article/supplementary material. Further inquiries can be directed to the corresponding author.
HW conceived the presented idea, analyzed the data and wrote the paper.
This work was supported by the Ministry of Science and Technology 109-2118-M-009-005-MY2, Taiwan.
The author declares that the research was conducted in the absence of any commercial or financial relationships that could be construed as a potential conflict of interest.
All claims expressed in this article are solely those of the authors and do not necessarily represent those of their affiliated organizations, or those of the publisher, the editors and the reviewers. Any product that may be evaluated in this article, or claim that may be made by its manufacturer, is not guaranteed or endorsed by the publisher.
1. Wei M, Yang N, Wang F, Zhao G, Gao H, Li Y. Epidemiology of Coronavirus Disease 2019 (COVID-19) Caused by Severe Acute Respiratory Syndrome Coronavirus 2 (SARS-CoV-2). Disaster Med Public Health Prep (2020) 14(6):796–804. doi: 10.1017/dmp.2020.155
2. Kirtipal N, Bharadwaj S, Kang SG. From SARS to SARS-CoV-2, Insights on Structure, Pathogenicity and Immunity Aspects of Pandemic Human Coronaviruses. Infect Genet Evol (2020) 85:104502. doi: 10.1016/j.meegid.2020.104502
3. Shu Y, He H, Shi X, Lei Y, Li J. Coronavirus Disease−2019. (2021) 3(2):1–1. doi: 10.3892/wasj.2021.83
4. Liu BD, Ugolini C, Jha P. Two Cases of Post-Moderna COVID-19 Vaccine Encephalopathy Associated With Nonconvulsive Status Epilepticus. Cureus (2021) 13(7):e16172. doi: 10.7759/cureus.16172
5. Chen YH, Wang H. Exploring Diversity of COVID19 Based on Substitution Distance. Infect Drug Resist (2020) 13:3887–94. doi: 10.2147/IDR.S277620
6. Perlman S. Another Decade, Another Coronavirus. N Engl J Med (2020) 382(8):760–2. doi: 10.1056/NEJMe2001126
7. Canas CA. The Triggering of Post-COVID-19 Autoimmunity Phenomena Could be Associated With Both Transient Immunosuppression and an Inappropriate Form of Immune Reconstitution in Susceptible Individuals. Med Hypotheses (2020) 145:110345. doi: 10.1016/j.mehy.2020.110345
8. Wang H. Efficacies of Treatments for Anti-NMDA Receptor Encephalitis. Front Biosci (Landmark Ed) (2016) 21:651–63. doi: 10.2741/4412
9. Wang H. Phylogenetic Analysis to Explore the Association Between Anti-NMDA Receptor Encephalitis and Tumors Based on microRNA Biomarkers. Biomolecules (2019) 9(10):572. doi: 10.3390/biom9100572
10. Wang H. Anti-NMDA Receptor Encephalitis and Vaccination. Int J Mol Sci (2017) 18(1):193. doi: 10.3390/ijms18010193
11. Bravo GA, Torrenta LRI. Anti-NMDA Receptor Encephalitis Secondary to SARS-CoV-2 Infection. Neurologia (2020) 35(9):699–700. doi: 10.1016/j.nrl.2020.07.013
12. Sarigecili E, Arslan I, Ucar HK, Celik U. Pediatric Anti-NMDA Receptor Encephalitis Associated With COVID-19. Childs Nerv Syst (2021) (37):3919–22. doi: 10.1007/s00381-021-05155-2
13. Panariello A, Bassetti R, Radice A, Rossotti R, Puoti M, Corradin M, et al. Anti-NMDA Receptor Encephalitis in a Psychiatric Covid-19 Patient: A Case Report. Brain Behav Immun (2020) 87:179–81. doi: 10.1016/j.bbi.2020.05.054
14. Monti G, Giovannini G, Marudi A, Bedin R, Melegari A, Simone AM, et al. Anti-NMDA Receptor Encephalitis Presenting as New Onset Refractory Status Epilepticus in COVID-19. Seizure (2020) 81:18. doi: 10.1016/j.seizure.2020.07.006
15. Burr T, Barton C, Doll E, Lakhotia A, Sweeney M. N-Methyl-D-Aspartate Receptor Encephalitis Associated With COVID-19 Infection in a Toddler. Pediatr Neurol (2021) 114:75–6. doi: 10.1016/j.pediatrneurol.2020.10.002
16. McHattie AW, Coebergh J, Khan F, Morgante F. Palilalia as a Prominent Feature of Anti-NMDA Receptor Encephalitis in a Woman With COVID-19. J Neurol (2021) 268(11):3995–7. doi: 10.1007/s00415-021-10542-5
17. Allahyari F, Hosseinzadeh R, Nejad JH, Heiat M, Ranjbar R. A Case Report of Simultaneous Autoimmune and COVID-19 Encephalitis. J Neurovirol (2021). doi: 10.1007/s13365-021-00978-w
18. Flannery P, Yang I, Keyvani M, Sakoulas GJ. Acute Psychosis Due to Anti-N-Methyl D-Aspartate Receptor Encephalitis Following COVID-19 Vaccination: A Case Report. J Front Neurol (2021) 12. doi: 10.3389/fneur.2021.764197
19. Wang NS, Shi XL, Jiang LW, Zhang SY, Wang DL, Tong P, et al. Structure of MERS-CoV Spike Receptor-Binding Domain Complexed With Human Receptor DPP4. Cell Res (2013) 23(8):986–93. doi: 10.1038/cr.2013.92
20. Lu L, Liu Q, Zhu Y, Chan KH, Qin LL, Li Y, et al. Structure-Based Discovery of Middle East Respiratory Syndrome Coronavirus Fusion Inhibitor. Nat Commun (2014) 5:3067. doi: 10.1038/ncomms4067
21. Maginnis MS. Virus-Receptor Interactions: The Key to Cellular Invasion. J Mol Biol (2018) 430(17):2590–611. doi: 10.1016/j.jmb.2018.06.024
22. Lewis BP, Burge CB, Bartel DP. Conserved Seed Pairing, Often Flanked by Adenosines, Indicates That Thousands of Human Genes are microRNA Targets. Cell (2005) 120(1):15–20. doi: 10.1016/j.cell.2004.12.035
23. Wang H. MicroRNAs, Parkinson’s Disease, and Diabetes Mellitus. Int J Mol Sci (2021) 22(6):2953. doi: 10.3390/ijms22062953
24. Galka-Marciniak P, Urbanek-Trzeciak MO, Nawrocka PM, Dutkiewicz A, Giefing M, Lewandowska MA, et al. Somatic Mutations in miRNA Genes in Lung Cancer-Potential Functional Consequences of Non-Coding Sequence Variants. Cancers (2019) 11(6):793. doi: 10.3390/cancers11060793
25. Wang H. Predicting MicroRNA Biomarkers for Cancer Using Phylogenetic Tree and Microarray Analysis. Int J Mol Sci (2016) 17(5):773. doi: 10.3390/ijms17050773
26. Kou XJ, Chen DD, Chen N. The Regulation of microRNAs in Alzheimer’s Disease. Front Neurol (2020) 11. doi: 10.3389/fneur.2020.00288
27. Fan BY, Chopp M, Zhang ZG, Liu XS. Emerging Roles of microRNAs as Biomarkers and Therapeutic Targets for Diabetic Neuropathy. Front Neurol (2020) 11. doi: 10.3389/fneur.2020.558758
28. Chen YH, Wang H. The Association Between Depression and Gastroesophageal Reflux Based on Phylogenetic Analysis of miRNA Biomarkers. Curr Medicinal Chem (2020) 27(38):6536–47. doi: 10.2174/0929867327666200425214906
29. Chen YH, Wang HY. The Association Between Migraine and Depression Based on miRNA Biomarkers and Cohort Studies. Curr Medicinal Chem (2021) 28(27):5648–56. doi: 10.2174/0929867327666201117100026
30. Wang H. MicroRNA, Diabetes Mellitus and Colorectal Cancer. Biomedicines (2020) 8(12). doi: 10.3390/biomedicines8120530
31. Canatan D, De Sanctis V. The Impact of MicroRNAs (miRNAs) on the Genotype of Coronaviruses. Acta BioMed (2020) 91(2):195–8. doi: 10.23750/abm.v91i2.9534
32. Zhang S, Amahong K, Sun X, Lian X, Liu J, Sun H, et al. The miRNA: A Small But Powerful RNA for COVID-19. Brief Bioinform (2021) 22(2):1137–49. doi: 10.1093/bib/bbab062
33. Farshbaf A, Mohtasham N, Zare R, Mohajertehran F, Rezaee SA. Potential Therapeutic Approaches of microRNAs for COVID-19: Challenges and Opportunities. J Oral Biol Craniofac Res (2021) 11(2):132–7. doi: 10.1016/j.jobcr.2020.12.006
34. Zhou Z, Zhou Y, Jiang XM, Wang Y, Chen X, Xiao G, et al. Decreased HD-MIR2911 Absorption in Human Subjects With the SIDT1 Polymorphism Fails to Inhibit SARS-CoV-2 Replication. Cell Discovery (2020) 6:63. doi: 10.1038/s41421-020-00206-5
35. Fulzele S, Sahay B, Yusufu I, Lee TJ, Sharma A, Kolhe R, et al. COVID-19 Virulence in Aged Patients Might Be Impacted by the Host Cellular MicroRNAs Abundance/Profile. Aging Dis (2020) 11(3):509–22. doi: 10.14336/AD.2020.0428
36. Zhou LK, Zhou Z, Jiang XM, Zheng Y, Chen X, Fu Z, et al. Absorbed Plant MIR2911 in Honeysuckle Decoction Inhibits SARS-CoV-2 Replication and Accelerates the Negative Conversion of Infected Patients. Cell Discovery (2020) 6:54. doi: 10.1038/s41421-020-00197-3
37. Chow JT, Salmena L. Prediction and Analysis of SARS-CoV-2-Targeting MicroRNA in Human Lung Epithelium. Genes (Basel) (2020) 11(9). doi: 10.3390/genes11091002
38. Tang H, Gao YA, Li ZH, Miao YS, Huang ZH, Liu XX, et al. The Noncoding and Coding Transcriptional Landscape of the Peripheral Immune Response in Patients With COVID-19. Clin Trans Med (2020) 10(6). doi: 10.1002/ctm2.200
39. Sabbatinelli J, Giuliani A, Matacchione G, Latini S, Laprovitera N, Pomponio G, et al. Decreased Serum Levels of the Inflammaging Marker miR-146a are Associated With Clinical non-Response to Tocilizumab in COVID-19 Patients. Mech Ageing Dev (2021) 193:111413. doi: 10.1016/j.mad.2020.111413
40. Garg A, Seeliger B, Derda AA, Xiao K, Gietz A, Scherf K, et al. Circulating Cardiovascular microRNAs in Critically Ill COVID-19 Patients. Eur J Heart Failure (2021) 23(3):468–75. doi: 10.1002/ejhf.2096
41. Zhang J, Xu X, Zhao S, Gong Z, Liu P, Guan W, et al. The Expression and Significance of the Plasma Let-7 Family in Anti-N-Methyl-D-Aspartate Receptor Encephalitis. J Mol Neurosci (2015) 56(3):531–9. doi: 10.1007/s12031-015-0489-6
42. Tanyi JL, Marsh EB, Dalmau J, Chu CS. Reversible Paraneoplastic Encephalitis in Three Patients With Ovarian Neoplasms. Acta Obstet Gynecol Scand (2012) 91(5):630–4. doi: 10.1111/j.1600-0412.2011.01365.x
43. Suzuki H, Kitada M, Ueno S, Tanaka K, Kusunoki S. Anti-NMDAR Encephalitis Preceded by Dura Mater Lesions. Neurol Sci (2013) 34(6):1021–2. doi: 10.1007/s10072-012-1169-8
44. Hara M, Morita A, Kamei S. [Associated Tumors in Patients With Anti-N-Methyl-D-Aspartate Receptor (NMDAR) Encephalitis]. Rinsho Shinkeigaku (2012) 52(11):979–81. doi: 10.5692/clinicalneurol.52.979
45. Stover DG, Eisenberg R, Johnson DH. Anti-N-Methyl-D-Aspartate Receptor Encephalitis in a Young Woman With a Mature Mediastinal Teratoma. J Thorac Oncol (2010) 5(11):1872–3. doi: 10.1097/JTO.0b013e3181eba81d
46. Eker A, Saka E, Dalmau J, Kurne A, Kurne C, Ozen H, et al. Testicular Teratoma and Anti-N-Methyl-D-Aspartate Receptor-Associated Encephalitis. J Neurol Neurosurg Psychiatry (2008) 79(9):1082–3. doi: 10.1136/jnnp.2008.147611
47. Jeraiby M, Depince-Berger A, Bossy V, Antoine JC, Paul S. A Case of Anti-NMDA Receptor Encephalitis in a Woman With a NMDA-R(+) Small Cell Lung Carcinoma (SCLC). Clin Immunol (2016) 166-167:96–9. doi: 10.1016/j.clim.2016.03.011
48. Dalmau J, Lancaster E, Martinez-Hernandez E, Rosenfeld MR, Balice-Gordon R. Clinical Experience and Laboratory Investigations in Patients With Anti-NMDAR Encephalitis. Lancet Neurol (2011) 10(1):63–74. doi: 10.1016/S1474-4422(10)70253-2
49. Afanasiev V, Brechemier ML, Boisseau W, Ducoudray R, Mayeur ME, Meyronet D, et al. Anti-NMDA Receptor Antibody Encephalitis and Neuroendocrine Pancreatic Tumor: Causal Link? Neurology (2016) 87(1):112–3. doi: 10.1212/WNL.0000000000002809
50. Lim EW, Yip CW. Anti-N-Methyl-D-Aspartate Receptor Encephalitis Associated With Hepatic Neuroendocrine Carcinoma: A Case Report. J Clin Neurosci (2017) 41:70–2. doi: 10.1016/j.jocn.2017.02.038
51. Kobayashi M, Nishioka K, Takanashi M, Hattori A, Shojima Y, Hayashida A, et al. Anti-NMDA Receptor Encephalitis Due to Large-Cell Neuroendocrine Carcinoma of the Uterus. J Neurological Sci (2017) 383:72–4. doi: 10.1016/j.jns.2017.10.024
52. Kumpfel T, Gerdes LA, Heck C, Pruss H. Delayed Diagnosis of Extraovarian Teratoma in Relapsing Anti-NMDA Receptor Encephalitis. Neurol Neuroimmunol Neuroinflamm (2016) 3(4):e250. doi: 10.1212/NXI.0000000000000250
53. Coban A, Gundogdu G, Poyraz M, Yegen G, Demirtas-Tatlidede A, Bilgic B, et al. NMDA Receptor Encephalitis With Cancer of Unknown Primary Origin. Tumori (2016) 102(Suppl. 2):S3–4. doi: 10.5301/tj.5000447
54. Tian M, Li J, Lei W, Shu XJN. Japanese Encephalitis Virus-Induced Anti-N-Methyl-D-Aspartate Receptor Encephalitis: A Case Report and Review of Literature. (2019) 50(02):111–5. doi: 10.1055/s-0038-1675607
55. Pastel H, Chakrabarty B, Saini L, Kumar A, Gulati SJNI, et al. A Case of Anti-N-Methyl-D-Aspartate (NMDA) Receptor Encephalitis Possibly Triggered by an Episode of Japanese B Encephalitis. (2017) 65(4):895. doi: 10.4103/neuroindia.NI_340_16
56. Shaik RS, Netravathi M, Nitish L, Mani R, Shah P, Damodar T, et al. A Rare Case of Japanese Encephalitis-Induced Anti-N-Methyl-D-Aspartate Receptor Encephalitis. (2018) 66(5):1495. doi: 10.4103/0028-3886.241335
57. Lwin S, San Yi M, Mardiana K, Woon SY, Nwe TM. Ovarian Teratoma-Associated Anti-NMDAR Encephalitis in a 12-Year-Old Girl. Med J Malaysia (2020) 75(6):731–3. doi: 10.1590/S1516-44462007000200017
58. Yu M, Li S, Cheng J, Zhou L, Jiang Z, Di W. Ovarian Teratoma-Associated Anti-NMDAR Encephalitis: A Single-Institute Series of Six Patients From China. Arch Gynecol Obstet (2021) 303(5):1283–94. doi: 10.1007/s00404-020-05861-3
59. Chefdeville A, Treilleux I, Mayeur ME, Couillault C, Picard G, Bost C, et al. Immunopathological Characterization of Ovarian Teratomas Associated With Anti-N-Methyl-D-Aspartate Receptor Encephalitis. Acta Neuropathol Commun (2019) 7(1):38. doi: 10.1186/s40478-019-0693-7
60. Sato M, Yasumoto H, Arai T. General Anesthesia With Propofol for Ovarian Teratoma Excision Associated With Anti-N-Methyl-D-Aspartate Receptor Encephalitis. JA Clin Rep (2018) 4(1):14. doi: 10.1186/s40981-018-0153-6
61. Nolan A, Buza N, Margeta M, Rabban JT. Ovarian Teratomas in Women With Anti-N-Methyl-D-Aspartate Receptor Encephalitis Topography and Composition of Immune Cell and Neuroglial Populations Is Compatible With an Autoimmune Mechanism of Disease. Am J Surg Pathol (2019) 43(7):949–64. doi: 10.1097/PAS.0000000000001249
62. Yapici-Eser H, Koroglu YE, Oztop-Cakmak O, Keskin O, Gursoy A, Gursoy-Ozdemir YJF, et al. Neuropsychiatric Symptoms of COVID-19 Explained by SARS-CoV-2 Proteins’ Mimicry of Human Protein Interactions. Front Hum Neurosci (2021) 15:126. doi: 10.3389/fnhum.2021.656313
63. Vasilevska V, Guest PC, Bernstein HG, Schroeter ML, Geis C, Steiner J, et al. Molecular Mimicry of NMDA Receptors may Contribute to Neuropsychiatric Symptoms in Severe COVID-19 Cases. J Neuroinflamm (2021) 18(1):245. doi: 10.1186/s12974-021-02293-x
64. Pawlica P, Yario TA, White S, Wang J, Moss WN, Hui P, et al. SARS-CoV-2 Expresses a microRNA-Like Small RNA Able to Selectively Repress Host Genes. Proc Natl Acad Sci USA (2021) 118(52). doi: 10.1073/pnas.2116668118
65. Khavinson V, Terekhov A, Kormilets D, Maryanovich A. Homology Between SARS CoV-2 and Human Proteins. Sci Rep (2021) 11(1):17199. doi: 10.1038/s41598-021-96233-7
66. Choudhary S, Sharma K, Silakari O. The Interplay Between Inflammatory Pathways and COVID-19: A Critical Review on Pathogenesis and Therapeutic Options. Microbial Pathogenesis (2021) 150:104673. doi: 10.1016/j.micpath.2020.104673
67. Huang HY, Lin YC, Li J, Huang KY, Shrestha S, Hong HC, et al. Mirtarbase 2020: Updates to the Experimentally Validated microRNA-Target Interaction Database. Nucleic Acids Res (2020) 48(D1):D148–54. doi: 10.1093/nar/gkz896
68. Bridwell R, Long B, Gottlieb M. Neurologic Complications of COVID-19. Am J Emerg Med (2020) 38(7):1549.e3–1549.e7. doi: 10.1016/j.ajem.2020.05.024
69. Romero-Sanchez CM, Diaz-Maroto I, Fernandez-Diaz E, Sanchez-Larsen A, Layos-Romero A, Garcia-Garcia J, et al. Neurologic Manifestations in Hospitalized Patients With COVID-19: The ALBACOVID Registry. Neurology (2020) 95(8):e1060–70. doi: 10.1212/WNL.0000000000009937
70. Abenza Abildua MJ, Atienza S, Carvalho Monteiro G, Erro Aguirre ME, Imaz Aguayo L, Freire Alvarez E, et al. Encephalopathy and Encephalitis During Acute SARS-CoV-2 Infection. Spanish Society of Neurology COVID-19 Registry. Neurologia (Engl Ed) (2021) 36(2):127–34. doi: 10.1016/j.nrleng.2020.11.003
71. McAbee GN, Brosgol Y, Pavlakis S, Agha R, Gaffoor M. Encephalitis Associated With COVID-19 Infection in an 11-Year-Old Child. Pediatr Neurol (2020) 109:94. doi: 10.1016/j.pediatrneurol.2020.04.013
72. Ye MX, Ren Y, Lv TF. Encephalitis as a Clinical Manifestation of COVID-19. Brain Behav Immun (2020) 88:945–6. doi: 10.1016/j.bbi.2020.04.017
73. Hosseini AA, Shetty AK, Sprigg N, Auer DP, Constantinescu CS. Delirium as a Presenting Feature in COVID-19: Neuroinvasive Infection or Autoimmune Encephalopathy? Brain Behav Immun (2020) 88:68–70. doi: 10.1016/j.bbi.2020.06.012
74. Freire-Alvarez E, Guillen L, Lambert K, Baidez A, Garcia-Quesada M, Andreo N, et al. COVID-19-Associated Encephalitis Successfully Treated With Combination Therapy. Clin Infect Pract (2020) 7:100053. doi: 10.1016/j.clinpr.2020.100053
75. Dogan L, Kaya D, Sarikaya T, Zengin R, Dincer A, Akinci IO, et al. Plasmapheresis Treatment in COVID-19–Related Autoimmune Meningoencephalitis: Case Series. (2020) 87:155–8. doi: 10.1016/j.bbi.2020.05.022
76. Huang YH, Jiang D, Huang JT. SARS-CoV-2 Detected in Cerebrospinal Fluid by PCR in a Case of COVID-19 Encephalitis. Brain Behav Immun (2020) 87:149. doi: 10.1016/j.bbi.2020.05.012
77. Lee SW, Ha EK, Yeniova AO, Moon SY, Kim SY, Koh HY, et al. Severe Clinical Outcomes of COVID-19 Associated With Proton Pump Inhibitors: A Nationwide Cohort Study With Propensity Score Matching. Gut (2021) 70(1):76–84. doi: 10.1136/gutjnl-2020-322248
78. Almario CV, Chey WD, Spiegel BMR. Response to Hajifathalian and Katz Regarding Increased Risk of COVID-19 Among Users of Proton Pump Inhibitors. Am J Gastroenterol (2020) 115(11):1922–3. doi: 10.14309/ajg.0000000000000993
79. Wang HJP. A Review of the Effects of Collagen Treatment in Clinical Studies. Polymers (2021) 13(22):3868. doi: 10.3390/polym13223868
80. Fang L, Karakiulakis G, Roth M. Are Patients With Hypertension and Diabetes Mellitus at Increased Risk for COVID-19 Infection? Lancet Respir Med (2020) 8(4):E21–1. doi: 10.1016/S2213-2600(20)30116-8
81. Albashir AAD. The Potential Impacts of Obesity on COVID-19. Clin Med (2020) 20(4):E109–13. doi: 10.7861/clinmed.2020-0239
82. Vogrig A, Janes F, Gigli GL, Curcio F, Negro ID, D'Agostini S, et al. Acute Disseminated Encephalomyelitis After SARS-CoV-2 Vaccination. Clin Neurol Neurosurg (2021) 208:106839. doi: 10.1016/j.clineuro.2021.106839
83. Rela M, Jothimani D, Vij M, Rajakumar A, Rammohan A. Auto-Immune Hepatitis Following COVID Vaccination. J Autoimmun (2021) 123:102688. doi: 10.1016/j.jaut.2021.102688
Keywords: anti-NMDA receptor encephalitis, biomarkers, COVID−19, microRNA, vaccine
Citation: Wang H (2022) COVID−19, Anti-NMDA Receptor Encephalitis and MicroRNA. Front. Immunol. 13:825103. doi: 10.3389/fimmu.2022.825103
Received: 30 November 2021; Accepted: 28 February 2022;
Published: 22 March 2022.
Edited by:
Zhichao Fan, UCONN Health, United StatesReviewed by:
Honghao Wang, Southern Medical University, ChinaCopyright © 2022 Wang. This is an open-access article distributed under the terms of the Creative Commons Attribution License (CC BY). The use, distribution or reproduction in other forums is permitted, provided the original author(s) and the copyright owner(s) are credited and that the original publication in this journal is cited, in accordance with accepted academic practice. No use, distribution or reproduction is permitted which does not comply with these terms.
*Correspondence: Hsiuying Wang, d2FuZ0BzdGF0Lm55Y3UuZWR1LnR3
Disclaimer: All claims expressed in this article are solely those of the authors and do not necessarily represent those of their affiliated organizations, or those of the publisher, the editors and the reviewers. Any product that may be evaluated in this article or claim that may be made by its manufacturer is not guaranteed or endorsed by the publisher.
Research integrity at Frontiers
Learn more about the work of our research integrity team to safeguard the quality of each article we publish.