- 1Department of Pulmonary and Critical Care Medicine, Tongji Hospital, Tongji Medical College, Huazhong University of Science and Technology, Wuhan, China
- 2Key Laboratory of Pulmonary Diseases, National Ministry of Health of The People’s Republic of China, Wuhan, China
Exosomes are small extracellular vesicles that are secreted by almost all types of cells and exist in almost all extracellular spaces. As an important mediator of intercellular communication, exosomes encapsulate the miRNA, lncRNA, cirRNA, mRNA, cytokine, enzyme, lipid, and other components from the cytoplasm into its closed single membrane structure and transfer them to recipient units in an autocrine, paracrine, or endocrine manner. Hypoxia is a state of low oxygen tension and is involved in many pathological processes. Hypoxia influences the size, quantity, and expression of exosome cargos. Exosomes derived from hypoxic tumor cells transfer genetics, proteins, and lipids to the recipient units to exert pleiotropic effects. Different donor cells produce different cargo contents, target different recipient units and lead to different biological effects. Hypoxic exosomes derived from tumor cells uptaken by normoxic tumor cells lead to promoted proliferation, migration, and invasion; uptaken by extracellular space or liver lead to promoted metastasis; uptaken by endothelial cells lead to promoted angiogenesis; uptaken by immune cells lead to promoted macrophage polarization and changed tumor immune microenvironment. In addition to various types of tumors, hypoxic exosomes also participate in the development of diseases in the cardiovascular system, neuron system, respiratory system, hematology system, endocrine system, urinary system, reproduction system, and skeletomuscular system. Understanding the special characteristics of hypoxic exosomes provide new insight into elaborating the pathogenesis of hypoxia related disease. This review summarizes hypoxia induced cargo changes and the biological effects of hypoxic exosomes in tumors and non-malignant diseases in different systems.
Introduction
Hypoxia, a state of inadequate oxygen supply, is a common feature of many different diseases, like solid tumor (1), hypoxia induced pulmonary arterial hypertension (2), sleep-disordered breathing (3), hypoxic kidney injury (4), placental hypoxia (5) and so on. The main molecular mechanism to sense oxygen stress is Hypoxia Induced Factors (HIFs), which functions as a master regulator of oxygen homeostasis in all metazoan species (6). HIFs are dimeric proteins composed of an oxygen-sensitive -subunit (HIF-1α, HIF-2α, or HIF-3α) and a β-subunit (HIF-1β) (7). HIF-1α is ubiquitously expressed whereas HIF-2α and HIF-3α are selectively expressed in certain tissues (8). Under normoxia, HIF-α subunits are hydroxylated by prolyl hydroxylases (PHD1-3) and recognized and targeted for proteasomal degradation. The activity of PHD1-3 is oxygen dependent, so under hypoxia, the rate of HIF-α hydroxylation is suppressed (9), accumulated HIF-1α dimerizes with HIF-1β and binds to hypoxia-responsive elements(HREs) in promoters of target genes to promote a concerted transcriptional response (10).
Hundreds of genes are now known to respond directly or indirectly to hypoxia via HIFs (11). Human large-scale genomic sequencing projects have revealed that less than 2% of transcriptional output encodes for proteins, while the remaining genome encrypts different classes of non-coding RNAs, including miRNA, lncRNA, cirRNA, and so on (12, 13). Hypoxia upregulates or downregulates the expression of some key factors, including the non-coding RNAs, as well as mRNAs, proteins, and lipids in hypoxic cells and the hypoxic exosomes secreted by these cells. The upregulated or downregulated key factors were transferred to recipient cells or tissues through exosomes, leading to various biological effects involving angiogenesis, invasion, metastasis, and immune escape in tumor development (14), and other hypoxia involved disease mentioned above. Recently, myriads of research exploring hypoxia induced changes in exosomes loads and subsequent effects have emerged rapidly. This review tries to focus on the impact of hypoxia on exosomes secretion and cargo changes, and summarizing various biological results in various diseases.
Biogenesis and Recipience of Exosomes
The exosome is one kind of extracellular vesicle. Extracellular vesicles are mainly classified into three types according to biogenesis. Namely, apoptotic bodies range from 500-2000 nm, microvesicles range from 200-2000 nm and exosomes range from 40-200 nm (15). The nomenclature of extracellular vesicles has not reached a broad agreement today (16–18), and in this review, we only discuss those studies clearly stated “exosome” in their elaboration. The formation of exosomes is the budding process of membrane organizations. Firstly, the inward budding of the cell membrane leads to the formation of the endosome (19). Endosome goes through different stages: early endosome, recycling endosome, and end endosome (20), during the maturation from early endosome to end endosome, further inward budding inside an intracellular endosome occurs and leads to the formation of multivesicle body, characterized by the presence of intraluminal vesicles (21–23). During the process of endosome inward budding, cytosolic contents, transmembrane, and peripheral proteins are incorporated into the invaginating membrane (24). The membrane of the multivesicle body fuses either with lysosome leading to the degradation of vesicles contents (25) or fuses with cell membrane leading to the release of vesicles contents, namely exosomes (26, 27). In contrast to inward budding, the outward budding process leads to the formation of microvesicles or apoptotic bodies (15).
Extracellular vesicles were first found during the maturation of sheep reticulocytes in 1983 (28). In the beginning, people thought it was just a kind of cell debris for the disposal of cell waste (22, 29). Later, researchers found that exosomes encapsulate RNAs, DNA, proteins, and lipids, and play a significant role both in cell to cell and cell to its milieu communication by transferring its encapsulated contents, both locally and distally (30, 31) and these contents are not randomized distributed but a specific subset from endosomes, the plasm membrane and the cytosol (32), suggesting an endosomal sorting complex required for transport (ESCRT) during exosome formation. The ESCRT dependent and independent manner of sorting mechanism are described in detail by Guillaume van Niel (33). As for the uptake mechanisms of exosomes by recipient cells, at least five mechanisms are involved, including clathrin-dependent, micropinocytosis, lipid-raft, membrane fusion, and caveolin-dependent endocytosis (34). In another word, exosomes sort specific contents to load and uptaken by specific recipient cells to exert their function (Figure 1). In addition, exosomes are secreted by almost all kinds of cells (35, 36) in biological and pathological conditions. And exists in almost all body fluids like blood, urine, saliva, synovial fluid, bile, cerebrospinal fluid, bronchoalveolar fluid, nasal fluid, uterine fluid, amniotic fluid, breast milk, feces, seminal plasma (30). It has been reported that many factors, including hypoxia, the change in pH, temperature, oxidative stress, radiation, and shear stress can affect the secretion level and composition of exosomes (37). Here, we focus on the impact of hypoxia on the production and cargo changes of exosomes and subsequent biological effects, and we made a brief summary in Table 1.
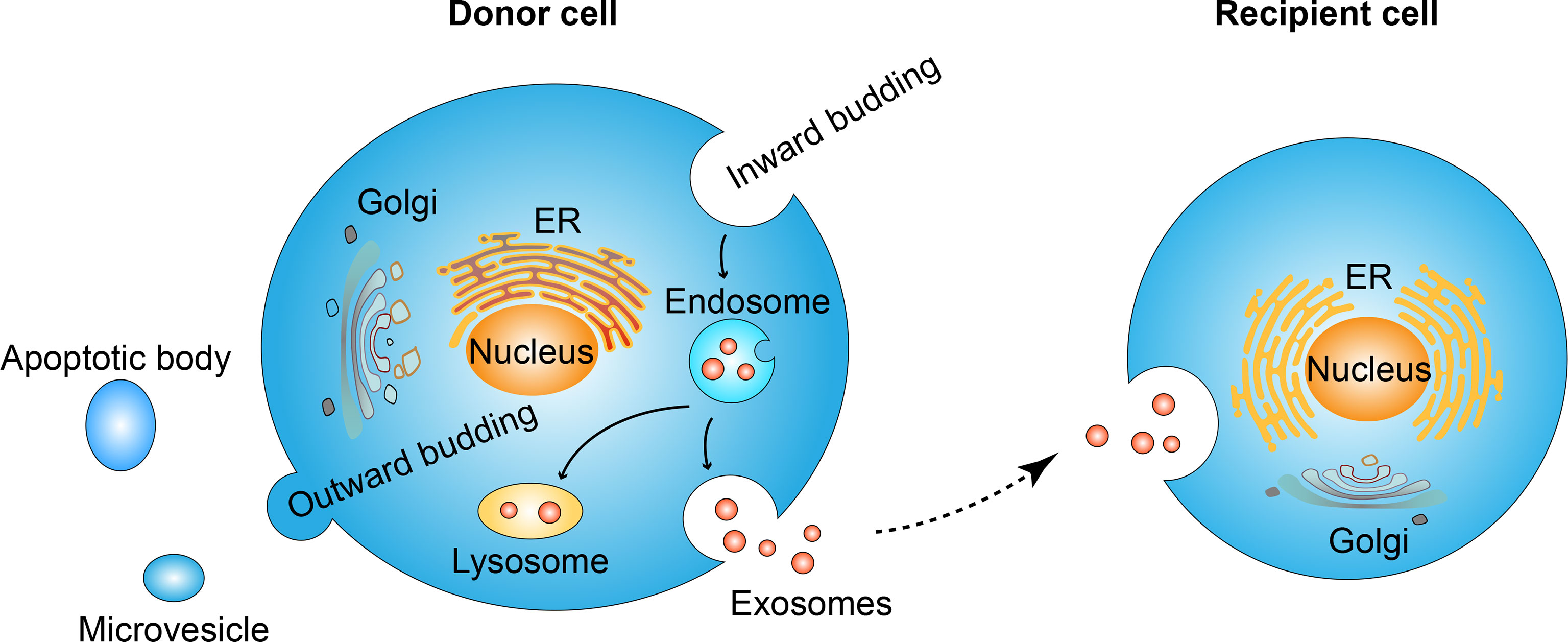
Figure 1 Exosome formation and uptaking process. Inward budding of cell membrane leads to the formation of the endosome. Further inward budding which encapsulates cell components leads to the formation of vesicles inside the endosome. The membrane of endosome fuse either with lysosome and result in the degradation of endosome contents, or fuse with cell membrane and lead to the release of its vesicles, namely exosomes. Secreted exosomes will be uptaken by recipient cells and the internalized contents are thus transferred in an autocrine, paracrine, or endocrine manner. In contrast to the inward budding process, the out ward budding of the membrane leads to the formation of microvesicles or apoptotic bodies.
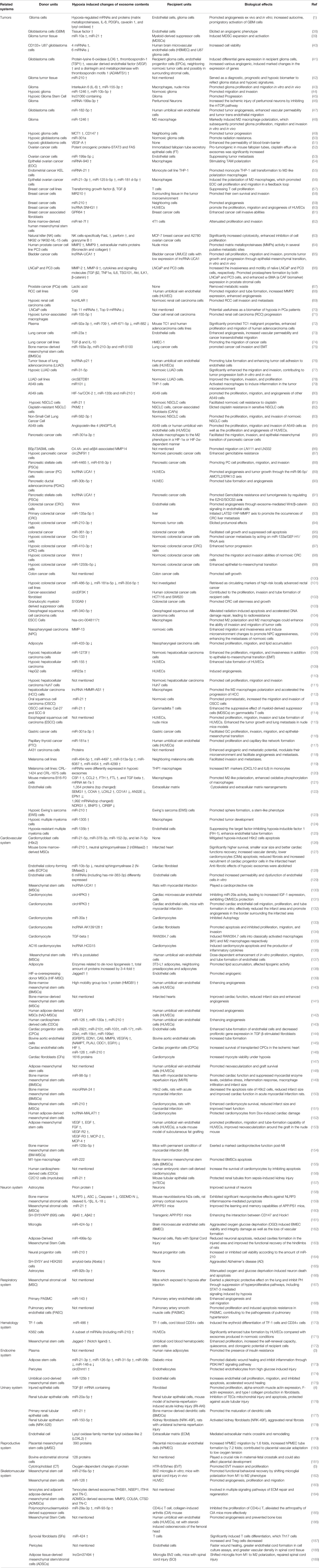
Table 1 Summary of hypoxia induced changes of exosome cargos from different donor units to their corresponding target recipient units, and leading to different biological effects.
Hypoxic Exosomes Promote the Development of Different Types of Tumors
Hypoxia is a common phenomenon in the solid tumor microenvironment. The release of exosomes and the contents of exosomes change dramatically under a short supply of oxygen, resulting in altered biological effects. Here, we make a summary of hypoxia induced changes in the cargos and subsequent biological effects of tumor cell derived exosomes in various types of tumor, including glioma, ovarian cancer, breast cancer, prostate cancer, lung cancer, pancreatic cancer, colorectal cancer, liver cancer, oesophageal squamous cell carcinoma, and other types of cancer we retrieved (Figure 2).
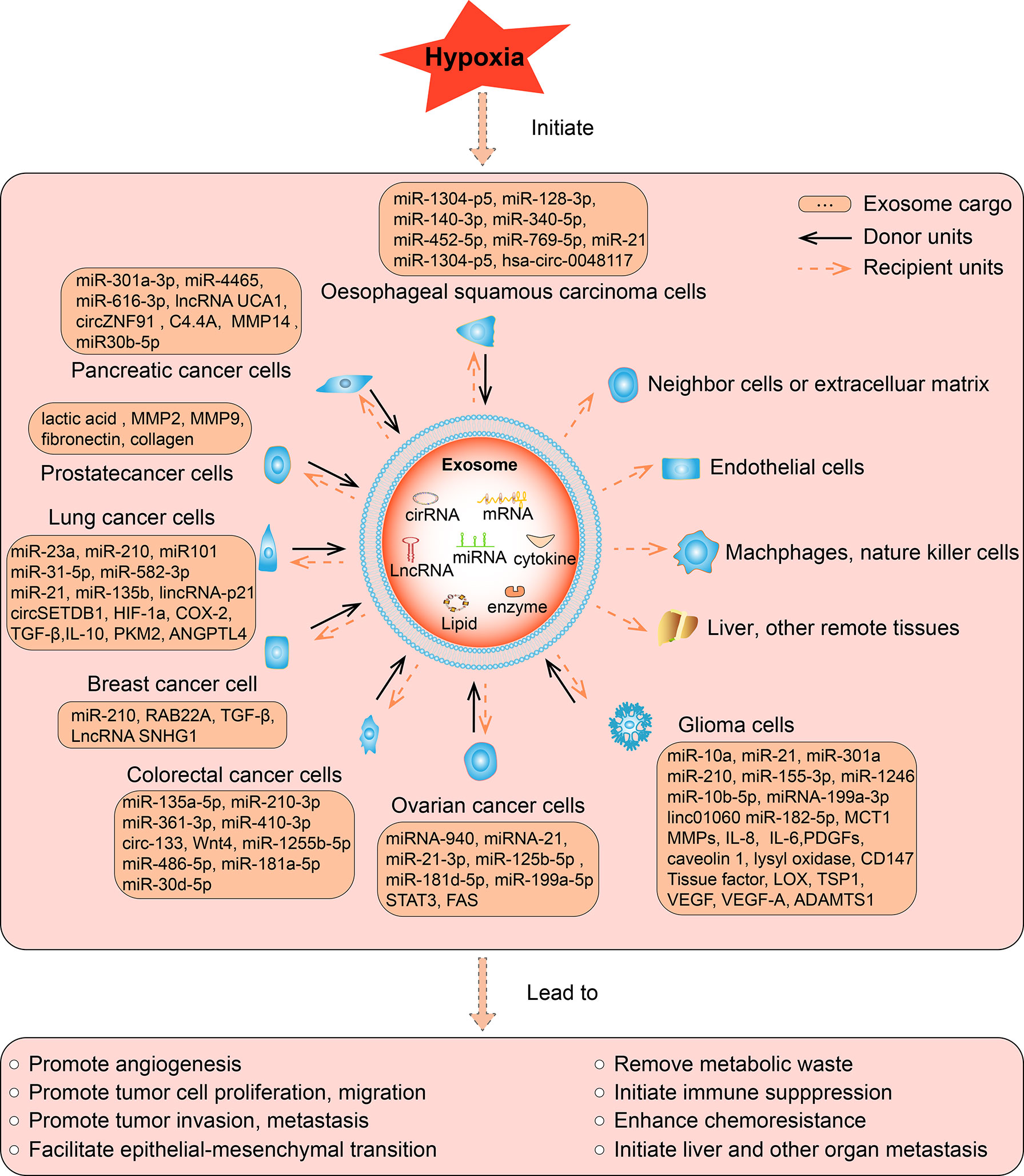
Figure 2 The function of hypoxic exosomes in tumors. In different tumors, hypoxia increased the secretion of exosomes from cancer cells, accompanied by altered expression of its contents, which can be miRNA, lncRNA, cirRNA, mRNA, protein, or lipid. The contents can be upregulated or downregulated in hypoxic exosomes than normoxic exosomes, such contents are briefly listed in the square box. As most studies reported, hypoxia induce the upregulation of its contents, such hypoxic exosomes transmit the key messages to recipient units, result in various biological effects, eventually promote the development of tumors. The solid arrows indicate the donor cells that secret the exosomes, the dashed arrows indicate the recipient cells, organs, and tissues that receive the exosomes. Exosomes are secreted by various hypoxic tumor cells in different types of tumors, and they can be uptaken by normoxic tumor cells, neighboring cells or extracellular matrix, endothelial cells, remote organs or tissues in an autocrine, paracrine, or endocrine manner to exert its function.
In glioma, hypoxia promoted the secretion of glioma-derived exosomes and hypoxic exosomes which contain higher expression of miR-10a and miR-21 have a stronger ability than normoxic exosomes to induce myeloid-derived suppressor cells (MDSCs) expansion and potentiate their immunosuppressive functions (39). Hypoxic exosomes isolated from glioblastoma multiforme (GBM) cells have different miRNA and protein quantitative profiles and they were potent inducers of proliferation and angiogenesis in vivo and in vitro. Moreover, exosomes derived from the hypoxia condition show increased autocrine, promigratory activation of GBM cells (1, 38, 40). Several miRNAs were reported to be upregulated in hypoxic exosomes compared to normoxic exosomes and resulted in further deterioration of glioma. MiR-1246 and miR-10b-5p were enriched in exosomes that produced in the hypoxic microenvironment, and such exosomes were transferred to normoxic glioma cells, promoting their migration and invasion in vitro and in vivo (44). Plus, miR-1246 containing exosomes markedly induced M2 macrophage polarization, facilitating the formation of the immunosuppressive microenvironment, thus contributing to promoted glioma proliferation, migration, and invasion in vitro and in vivo (48). MiR-155-3p and interleukin 6 (IL-6) were highly expressed in hypoxic glioma-derived exosomes, and such exosomes also induced M2-like macrophage polarization and eventually promotes glioma progression (43). MiR-182-5p expression was significantly upregulated in glioblastoma secreted exosomes in hypoxic conditions. Exosomal miR-182-5p was uptaken by human umbilical vein endothelial cells, leading to KLF2 and KLF4 suppression and VEGFR accumulation, thus promoting tumor angiogenesis (47). miR-301a was also reported to be encapsulated in the hypoxic exosomes dervied from glioblastoma cells, and was transferred to normoxic glioblastoma cells to promote radiation resistance by targeting the tumor suppressor gene TCEAL7 (50). In addition to miRNAs, some proteins were also reported to be increased in the hypoxic exosomes. Hypoxia upregulated the expression of lysyl oxidase homolog 2 and VEGF-F in the glioblastoma derived exosomes, the latter was able to enhance the blood-brain-barrier by interrupting the expression of claudin-5 and occludin (51, 190). Hypoxia also upregulated the monocarboxylate transporter 1(MCT1) and the cluster of differentiation 147 (CD147) in glioma cells and its secreted exosomes, transporting these pro-oncogenic molecules to recipient neighboring cells led to promoted tumor progression (49).
Besides contributing to glioma progression, miRNA containing exosomes also contributed to ischemic injury of peritumoral neurons. MiR-199a-3p was upregulated in a HIF-α dependent way in hypoxic culturing glioma cells derived exosomes. Exosomal miR-199a-3p mediated the oxygen-glucose deprivation (OGD)/reperfusion neuronal injury process by suppressing the mTOR signaling pathway (46). In patients with glioma, serum exosome miR-210 was significantly increased compared to healthy controls. The increased expression level reflected the hypoxic state of glioma patients by positively associated with HIF-α, and it also reflected the changes in malignant glioma loads (42). Apart from miRNA, lncRNA and protein were also encapsulated and transferred by exosomes in hypoxic microenvironment. It was reported that hypoxic glioma stem cells transferred lnc01060 containing exosomes to glioma cells, activating prooncogenic signaling and promoting disease progression. In addition, lnc01060 was upregulated in glioma patients and was significantly correlated with tumor grade and poor clinical prognosis (45). The protein content of secreted exosomes from GBM cells was able to induce differential gene expression in recipient glioma cells. Thus, intercellular communication was facilitated via exosomes secreted from hypoxic GBM cells. And prominent changes of genes expression were induced in neighboring normoxic tumor cells and possibly in surrounding stromal cells, many of which are involved in cancer progression and treatment resistance mechanisms (41).
In ovarian cancer, hypoxia also promoted the release of exosomes from cancer cells, and miRNA containing exosomes played a vital role in facilitating tumor progression. MiRNA-940 was upregulated in hypoxic exosomes derived from epithelial ovarian cancer (EOC) (54). EOC cell-derived exosomes delivered miRNAs to induce M2 macrophage polarization, which promoted EOC cell proliferation and migration (56). A similar situation occured in endometrial cancer, the expression of miRNA-21 was increased in exosomes derived from endometrial cancer KLE cells cultured in hypoxia. Exosomal transfer of miRNA-21 to monocyte THP-1 cell promoted its transformation to M2-like polarization macrophages, forming the immune microenvironment in cancer progression (55). In some cases, miRNA was downregulated to facilitate tumor progression. MiR‐199a‐5p was reported to be downregulated in cancer tissue. Hypoxic culturing further decreased the miR‐199a‐5p level in both ovarian cancer cells and their secreted exosomes. The downregulation of miR‐199a‐5p promoted tumor metastasis through targeting HIF-2α to regulate Wnt/β-catenin path-way (53). Besides miRNAs, protein containing exosomes were also involved in ovarian cancer development. It was found that exosomes from hypoxic culturing ovarian cancer cell lines carried more potent oncogenic proteins-STAT3 and FAS, and significantly increased cell migration, invasion, and chemo-resistance in vitro and promoted tumor progression, metastasis in vivo. In addition, cisplatin efflux via exosomes was significantly increased in ovarian cancer cells under hypoxic conditions, contributing to chemo-resistance (52).
In breast cancer, activation of hypoxic signaling also resulted in a significant increase in exosome release. Exposing three different breast cancer cell lines to moderate (1% O2) and severe (0.1% O2) hypoxia led to a significant increase in the number of exosomes and an increase of miR-210 in exosomes (58). MiR-210 was involved in the expression of vascular remodeling related genes, such as Ephrin A3 and PTP1B, which function to promote angiogenesis (59). In addition to miR-210, lncRNA SNHG1, which was enriched in the hypoxic breast cancer cells secreted exosomes, also promoted the angiogenesis, as well as the proliferation and migration of HUVECs (60). And some research indicated that hypoxic exosomes were preferentially taken up by hypoxic cancer cells (191). The autophagy-associated GPR64 was reported to be upregulated in the hypoxic exosomes derived from the breast cancer associated fibroblast, it stimulated the NF-KB pathway to enhance the invasiveness in recipient breast cancer cells (61). microRNA let-7f was reported to be upregulated both in bone marrow-derived human mesenchymal stem cells (hMSCs) and its secreted exosomes under hypoxic culture, and miR-let-7f attenuated the proliferation and invasion in recipient 4T1 cells (62). In addition to autocrine, hypoxic exosomes also function in a paracrine manner in tumor development. It is reported that under hypoxia, breast cancer cells encapsulate TGF-β into exosomes, such exosomes were able to be taken up by T cells and mediated the suppression of T cell proliferation. Such immunosuppression microenvironment contributes to tumor progression (57). In contrast to cancer cell derived exosomes, natural killer (NK) cells, which play an important role in the tumor immune microenvironment, can also produce exosomes in the tumor microenvironment. Hypoxia enhanced the release of exosomes and increased the expression of three functional proteins of NK cells-specifically FasL, perforin, and granzyme B in hypoxic exosomes. And such hypoxic exosomes exhibited significantly increased cytotoxicity, enhanced inhibition of cell proliferation on breast and pancreatic cancer cells than normoxic exosomes (63).
In prostate cancer, cancer cells secreted more exosomes in a hypoxic microenvironment as a survival mechanism to remove metabolic waste like lactic acid (67). Hypoxia changed not only the secretion amount but also the average size of exosomes secreted by prostate cancer cells. Hypoxic exosomes had a smaller average size and a higher level of exosome biomarkers compared to normoxic exosomes. Co-culturing of hypoxic exosomes with normoxic prostate cancer cells increased the stemness, motility, and invasiveness, promoted prostasphere formation, and enhanced α-SMA expression. Compared to normoxia, hypoxic exosomes showed higher metalloproteinases activity and a higher number of proteins, primarily associated with the remodeling of the epithelial adherens junction pathway (66, 70). Hypoxic exosomes were also involved in cancer metastasis progress. Exosomes derived from hypoxic culturing prostate cancer cells enhanced the level of MMP2, MMP9, and extracellular matrix proteins (fibronectin and collagen) at selective pre-metastatic niches sites, contributing to cancer metastasis (64). Hypoxic exosomes also prompted the development of bladder tumors and renal cell carcinoma (RCC). In bladder tumors, hypoxic exosomes derived from tumor cells showed higher expression levels of lncRNA-UCA1 which could promote tumor growth and progression through epithelial-mesenchymal transition, in vitro and in vivo (65). In RCC, hypoxia and treatment with CoCl2, a hypoxia mimic agent, increased the CA9 level in exosomes in all RCC cell lines. CA9 exosomes released from hypoxic RCC were postulated to enhance angiogenesis in the microenvironment, thereby contributing to cancer progression (68). MiR-155-5p was found to be upregulated in RCC specimens and hypoxia promoted its selective enrichment in exosomes secreted by hypoxic tumor-associated macrophages (TAM). The exosomes transferred miR-155-5p to RCC and promoted the tumor progression partially through activating IGF1R/PI3K/AKT cascades (71). lncHILAR was also reported to be secreted by hypoxic cancer cells and transferred to normoxic cancer cells through exosomes to activate the miR-613/206/1-1-3p/Jagged-1/Notch/CXCR4 axis, thereby promoting cell invasion and metastasis (69).
In lung cancer, cancer cells produced more exosomes under hypoxic conditions than normoxic conditions. MiRNA, cirRNA, lncRNA, and protein were both reported to be encapsulated in cancer cell derived exosomes and contributed to tumor progression. MiR-23a was significantly upregulated in hypoxic exosomes, resulting in enhanced angiogenesis through regulation of endothelial cells (73). Besides immunosuppressive function, TGF-β, as well as IL-10 which were increased in hypoxic lung cancer cell derived exosomes, also played a positive role in regulating cancer cell migration (74). MiR-31-5p and miR-582-3p were largely internalized within hypoxic exosomes. Exosomal transfer of miRNAs from hypoxic cancer cells to normoxic cancer cells significantly enhanced the proliferation, migration, and invasion of receptive normoxic lung cancer cells in vitro, and promoted lung adenocarcinoma metastasis (77, 83). Similarly, hypoxia induced the upregulation of angiopoietin-like 4 (ANGPTL4), HIF-1α/COX-2 and miR-135b and miR-210 in the exosome cargo of A549 cells, exosome transfer of these factors to other A549 cells led to the enhanced proliferation, migration, angiogenesis, and tumor progression (80, 84). In hypoxic tumor microenvironment, bone marrow-derived mesenchymal stem cells (BMSCs) secreted exosomes that contained miR-193a-3p, miR-210-3p and miR-5100, these exosomes were taken up by neighboring epithelial lung cancer cells, led to STAT3 signaling activation and increased expression of mesenchymal related molecules (75). In addition to metastasis and tumor progression, chemoresistance is also involved. Hypoxia upregulated the miR-21 expression in non-small cell lung cancer (NSCLC) cells and cell-derived exosomes, while exosomal miR-21 contributed to cisplatin resistance by downregulating phosphatase and tensin homolog (PTEN) in its recipient normoxic NSCLC cells (81). In some cases, hypoxia decreased the expression of some key genes. It was reported that hypoxic stress suppressed the expression of miR101 in A549 cell secreted exosomes, the exosomes with suppressed expression of miR101 were transferred to THP-1 cells and upregulating the expression of IL-1 and IL-6, contributing to the promoted inflammation of macrophages in the tumor microenvironment (79). Besides miRNA and protein, cirRNA and lncRNA also play a role in tumor progression. circSETDB1 was found to be significantly upregulated in hypoxia-induced exosomes from lung adenocarcinoma (LUAD) cell lines in comparison to normoxic exosomes. And such exosomes improved the migration, invasion, and proliferation capacity of normoxic LUAD cells (78). LncRNA-p21 was reported to be upregulated by hypoxia in non-small cell lung cancer tumor tissue and a higher level of lncRNA-p21 encapsulated exosomes in blood indicates a shorter time to relapse and shorter overall survival. In addition, lncRNA-p21 enriched exosomes promoted tube formation of endothelial cells and enhanced tumor cell adhesion to endothelial cells (76). Besides continuous hypoxia, intermittent hypoxia also has a stronger prosurvival effect than normoxia. Circulating exosomes released under intermittent hypoxia conditions significantly promoted lung carcinoma cells’ malignant properties (72). Hypoxic exosomes also play a role in the chemoresistance of lung cancer therapy. In NSCLC, normoxic and hypoxic exosomes derived from cisplatin-resistant cancer cells were analyzed. As a result, the expression of PKM2 was elevated in hypoxic exosomes. PKM2 promoted glycolysis and finally may neutralize reactive oxygen species (ROS) induced by cisplatin, eventually promoting cisplatin resistance in sensitive NSCLC (82).
In the digestive system malignant disease, including pancreatic cancer, colorectal cancer (CRC), hepatocellular cancer, oesophageal squamous cell carcinoma (OSCC), and other relatively less frequently diagnosed malignant disease like oral squamous cell carcinoma, nasopharyngeal carcinoma, and gastric cancer, hypoxia also exerted a great impact on exosomes and thus contributed to tumor development.
In pancreatic cancer, hypoxic pancreatic cancer cells secreted exosomes that contained miRNA, lncRNA, cirRNA or protein to promote tumor progression. Hypoxia was reported to upregulate a series of miRAN including hsa-miR-29b-3p and hsa-miR-216a-5p,hsa-miR-148a-3p and islet cell damage marker hsa-miR-375 in the exosomes derived from human islets (192). MiR-301a-3p-rich exosomes were induced in the hypoxic microenvironment from pancreatic cancer cells, such exosomes then polarized macrophages to promote malignant behaviors of pancreatic cancer cells (85). miR-30b-5p enriched hypoxic exosomes that derived from pancreatic ductal adenocarcinoma (PDAC) cells, was transferred to HUVEC, leading to enhanced tube formation and angiogenesis (90). LncRNA UCA1 was also reported to be highly expressed in exosomes derived from hypoxic pancreatic cancer cells. Exosomal transfer of lncRNA UCA1 promoted cell migration and tube formation of human umbilical vein endothelial cells (HUVECs), contributing to angiogenesis and tumor growth (89). Interestingly, lncRNA UCA1 was also enriched in exosomes derived from pancreatic stellate cells (PSCs), and such exosome was able to promote the Gemcitabine resistance of pancreatic cancer cells through SOCS3/EZH2 Axis (91). CircZNF91 was encapsulated and transmitted to normoxic pancreatic cancer cells through hypoxic exosomes, eventually enhancing the stability of HIF-1α and leading to glycolysis and chemoresistance of normoxic pancreatic cancer cells (87). C4.4A, a molecular which was upregulated in several tumor types, was upregulated in the pancreatic cancer cells and its secreted exosomes, promoting wound healing and tumor metastasis (86). Pancreatic stellate cells (PSCs), the important components of the tumor microenvironment in pancreatic cancer (PC), also contributed to its development and metastasis through exosome delivery. In tumor hypoxic microenvironment, miR-4465 and miR-616-3p were encapsulated in PSCs secreted exosomes and transmitted to pancreatic cancer cells, promoting PC cell proliferation, migration, and invasion, contributing to PC progression and metastasis (88).
In colorectal cancer (CRC), the hypoxic microenvironment boosted exosome release. Hypoxia promoted colon cancer cells to release more exosomes and thereby promoting self-proliferation in a time-and dose-dependent manner through shortening mitosis duration and upregulating phosphorylated STAT3 (100). Many proteins, miRNAs, cirRNAs were indicated to be upregulated in hypoxic conditions and internalized into exosomes and transferred from hypoxic CRC cancer cells to normoxic cancer cells to promote tumor deterioration. Wnt4, S100A9, MiR-210-3p, miR-361-3p, miR-410-3p, circ-133 were reported to be remarkably elevated in hypoxic CRC exosomes, and can be transferred to normoxic CRC cells, leading to facilitated cell growth and suppressed cell apoptosis, eliciting a protumoral effect, promoting cancer metastasis and enhancing tumor progression. In addition, some miRNA or cirRNA were also enriched in the plasma exosomes of CRC patients and positively associated with a poor prognosis of colorectal cancer (94–98, 103). Some hypoxic colorectal cancer derived exosomes, like exosomes enriched with miR-135a-5p, were phagocytosed by Kupffer cells and transferred from blood circulation into the liver, initiating the large tumor suppressor kinase 2-yes-associated protein-matrix metalloproteinase 7 axis to promote liver metastasis (93). It is worth noting that CRC cells not only secreted exosomes to promote tumor development, but also uptaken exosomes in some case. For example, hypoxic exosomes that was enrich with circEIF3K and derived from cancer-associated fibroblasts (CAF), could be uptaken by colorectal cancer cells (CRC) HCT116 and SW620, and contributed the proliferation, invasion, and tube formation. Knocking down circEIF3K in CAF could mitigate hypoxia induced CRC progression (102). Hypoxic exosomes derived from colorectal cancer cells also enhanced angiogenesis in tumor progression by promoting the proliferation and migration of endothelial cells. Suppression of exosome secretion inhibited these effects (92). In some cases, hypoxia suppressed specific gene expression to adapt to oxygen stress. It was reported that hypoxia downregulated the exosomal miR-1255b-5p that was secreted by colorectal cancer cells and eventually enhanced the epithelial-to-mesenchymal transition as a response to hypoxia (99). Hypoxia also downregulated miR-486-5p and miR-181a-5p while upregulated miR-30d-5p in the cancer cell derived exosomes, both of which were associated with organ-invasiveness and lymph node metastases (101).
In liver cancer, hypoxia promoted tumor self-growth through exosome transfer. Hypoxic exosomes derived from hypoxic hepatocellular carcinoma cancer cells promoted cell proliferation, migration and invasion of normoxic cancer cells through a paracrine manner (111). miR-1273f was reported to be upregulated by hypoxia in the exosomes derived from hepatocellular carcinoma cells, and it was transferred to normoxic cancer cells to promote their proliferation, migration, and invasiveness by targeting LHX6 and subsequently activating Wnt/beta-catenin signaling pathway (108). LncRNA HMMR-AS1 was also increased in the hypoxic exosomes from hepatocellular carcinoma cells, and could be transferred to promote the M2 macrophages polarization and promote the progression of HCC (112). In addition to hepatocellular carcinoma cells, hypoxic tumor exosomes can also be uptaken by HUVECs. It was reported that hypoxia upregulated the miR-155 and miR23a in the hepatocellular carcinoma secreted exosomes. Such exosomes remarkably enhanced tube formation of HUVECs, indicating its facilitating role in promoting angiogenesis in hepatocellular carcinoma (109, 110).
Hypoxia induced significant upregulation of a series of miRNAs in exosomes isolated from oesophageal squamous cell carcinoma (OSCC) cell lines, among which miR-340-5p was reported to be transferred to normoxic OSCC cells through hypoxic exosomes. Such hypoxic exosomes alleviated radiation-induced apoptosis and accelerated DNA damage repair, leading to radioresistance (104). Besides miRNA, hsa-circ-0048117 rich exosomes were also generated in the hypoxic microenvironment in OSCC. Exosomal hsa-circ-0048117 could be transmitted to macrophages to promote M2 polarization and M2 macrophages could enhance the ability of invasion and migration of tumor cells (105). It was reported that hypoxic exosomes derived from esophageal squamous cell carcinoma cells had stronger effects than normoxic exosomes both in vitro and in vivo. Hypoxic exosomes which contains altered gene information had better effects in promoting proliferation, migration, invasion and tube formation of HUVECs than normoxic exosomes. And hypoxic exosomes also significantly promoted the tumor growth and lung metastasis in nude mice (115). Hypoxic tumor derived exosomes were also reported to influence tumor immune microenvironment. It was reported that the OSCC cell lines: Cal-27 and SCC-9 secreted hypoxic exosomes which contain miR-21 and such exosomes were transferred to myeloid-derived suppressor cells (MDSCs), promoting its suprressive effect on gammadelta T cells, eventually affecting the anti-and pro-tumoral equilibrium (114).
In oral squamous cell carcinoma cells, a hypoxic microenvironment promoted the enrichment of miR-21 in its secreted exosomes. These exosomes were delivered to normoxic cells and promoted metastatic behaviors (113). In nasopharyngeal carcinoma, hypoxic adipocyte-derived exosomes transferred low expression of miR-433-3p into cancer cells, promoting proliferation, migration, and lipid accumulation in cancer cells (107). In many cases, HIF was involved in the pathological process of tumor malignant behaviors. Hypoxic cancer cell derived exosomes can enhance the metastases in a HIF-1α dependent manner. HIF-1α stimulated MMPs expression to affect cell migration and invasion (106). In gastric cancer, hypoxia promoted miR-301a-3p expression in a HIF-α dependent manner and exosomes released from cancer cells were also increased in the hypoxic tumor microenvironment. The miR-301a-3p enriched exosomes were transmitted among gastric cancer cells, eventually leading to cancer cell proliferation, invasion, migration, and epithelial-mesenchymal transition (116).
In many cell types, hypoxia induced the change of RNA and proteins components in cells and such changes were also reflected in hypoxic exosomes (122). In papillary thyroid cancer, hypoxia promoted both the expression of miR-181a in cancer cells and the secretion of miR-181a enriched exosomes from cancer cells. Human umbilical vein endothelial cells (HUVECs) which uptake such exosomes exhibited enhanced proliferation and capillary-like network formation, contributing to tumor angiogenesis (117). In skin carcinoma A431 cells, hypoxia induced the expression of proteins involved in angiogenesis, focal adhesion, extracellular matrix-receptor interaction, and immune cell recruitment. These proteins were encapsulated into exosomes and facilitated angiogenesis and metastasis in the microenvironment (118). In melanoma cells, hypoxia induced the upregulation of 15 miRNAs and downregulation of 3 miRNAs in exosomes derived from cancer cells through miRNA profile analysis (119). Co-culturing of hypoxic exosomes derived from melanoma cells which contained alternated miRNA profiles with THP1 macrophages led to increased M1 markers (CXCL10 and IL6) in macrophages (120). Hypoxia also upregulated the immunomodulatory proteins and chemokines including CSF-1, CCL2, FTH, FTL, and TGFbeta in exosomes from mouse melanoma B16-F0 cells, and such exosomes promoted M2-like polarization of macrophages. And the upregulated miRNA let-7a in hypoxic exosomes enhanced oxidative phosphorylation in macrophages (121). In Ewing’s sarcoma (EWS), hypoxic cancer cells secreted miR-210 enriched exosomes to normoxic cancer cells, leading to promoted sphere formation by targeting the proapoptotic protein CASP8AP2 (123). In multiple myeloma, hypoxia upregulated the expression of miR-1305 in cancer cell secreted exosomes, thus the cellular miR-1305 decreased and its target genes increased, eventually promoting the oncogenic activity of multiple myeloma cells. On the other hand, macrophages uptaken the miR-1305 containing exosomes and exhibited tumor-promoting, M2-macrophage phenotypes (124). miR-135b was also reported to be increased by hypoxia in the exosomes derived from multiple myeloma cells. Such exosomes enhanced endothelial tube formation under hypoxia (125). To conclude, hypoxia increase the release of exosomes from malignant cells and the content of exosomes changed under low oxygen tension, which induce recipient cells to alter gene expression and eventually promote tumor progression.
Hypoxic Exosomes in Cardiology
Cardioprotective Effect of Hypoxic Exosomes
Under hypoxia, a series of miRNA were encapsulated into the exosomes and transferred to recipient cells to exhibit cardioprotective effects. A series of cardioprotective miRNAs, including miR-21-5p, miR-378-3p, miR-152-3p, and let-7i-5p were identified in hypoxic exosomes derived from cardiomyocyte H9c2 cells. These anti-apoptotic miRNAs mitigate hypoxia-induced H9c2 cells apoptosis (126). In contrast to these miRNAs, lncRNA HCG15 in the exosomes derived from hypoxic cardiomyocytes led to increased apoptosis, reduced proliferation, and release of inflammatory factors (136). Hypoxia also promoted the accumulation of TGF-beta in exosomes from cardiomyocyte H9c2 cells, and such exosomes could be taken up by RAW264.7 cells and induce the polarization of macrophages and reduce the apoptosis of cardiomyocytes (135). There were large amounts of studies that investigated exosomes derived from mesenchymal stem cells, such exosomes exhibited protective effects in cardiac injury and many miRNAs were reported to be encapsulated in exosome cargo. Especially, exosomes derived from hypoxia pretreated bone marrow-derived mesenchymal stem cells (BMSCs) were able to improve the cardiac function, reduce the infarct size and enhance the angiogenesis of the infarcted hearts (141). miR-98-5p was upregulated by hypoxia in exosomes derived from BMSCs and injection of such exosomes to rats with myocardial ischemia-reperfusion injury improved the cardiac function by targeting TRL4 and activating PI3K/AKT signaling pathway (149). miR-24 in exosomes derived from BMSCs was also upregulated by hypoxia, and such exosomes decreased the apoptosis rate of H9c2 cells, reduced infarct sized and improved cardiac function in acute myocardial infarction rats (150). miR-210 in exosomes derived from mesenchymal stem cells was increased under hypoxic culture, administration of such exosomes enhanced cardiomyocyte survival to hypoxia in vitro, reduced infarct size and improved heart function in vivo (151). Injecting such hypoxic exosomes into the infarcted heart of C57BL/6 mouse resulted in significantly higher survival, smaller scar size, and better cardiac functions recovery. Hypoxic exosomes conferred increased vascular density, lower cardiomyocytes apoptosis, reduced fibrosis, and increased recruitment of cardiac progenitor cells in the infarcted heart relative to exosomes isolated from the same cell line cultured under normoxia (127). miR-125b-5p in the exosomes from mesenchymal stem cells was also upregulated by hypoxia, adminstration of such exosomes to mice with myocardial infarction suppressed the expression of the proapoptotic genes p53 and BAK1 in cardiomyocytes and exerted a marked cardioprotective function post-infarction (154). Hypoxic exosomes derived from human cardiosphere-derived cells also exhibited protective effects in cardiomyocytes by inhibiting apoptosis (156).
Although most miRNAs were upregulated in hypoxic exosomes, some miRNAs were enriched in normoxic exosomes rather than hypoxic exosomes. miR-10b-5p was reported to be downregulated in hypoxic exosomes derived from endothelial colony forming cells compared to normoxic exosomes. Due to a reduction of miR-10b-5p, which targets the fibrotic genes Smurf1 and HDAC4, the anti-fibrotic effects of exosomes were abolished (128). Besides continuous hypoxia, intermittent hypoxia also alters endothelial cell derived exosome cargo, including has-mir-383-3p, and such exosomes promote increased permeability and dysfunction of endothelial cells, contributing to cardiovascular dysfunction (129). Apart from miRNAs, lncRNA and cirRNA are also important non-coding RNAs that are induced and transferred through hypoxic exosomes. LncRNA-UCA1 is elevated both in myocardial infarction patients and exosomes derived from hypoxic culturing mesenchymal stem cells (hMSCs). Intramyocardial injection of lncRNA-UCA1 containing exosomes to rats with myocardial infarction demonstrated that hypoxic exosomes had a better cardioprotection effect than normoxic exosomes (130). lncRNA-MALAT1 was increased by hypoxia in exosomes from human adipose-derived mesenchymal stem cells, such exosomes functioned as competing endogenous RNAs (ceRNAs) binding to miR-92a-3p to protect cardiomyocyte from doxorubicin (Dox) induced cardiac damage (152). CirRNA is also involved in cardioprotective effects. circHIPK3 expression was found to be significantly upregulated in hypoxic exosomes compared to normoxic exosomes. Such circHIPK3 encapsulated exosomes were transferred from cardiomyocytes to cardiac microvascular endothelial cells (CMVECs). Leading to promoted endothelial cell migration, proliferation, and tube formation in vitro, and can effectively reduce the infarct area and promote angiogenesis in the border surrounding the infarcted area in myocardial infarction mice (132). Hypoxia also upregulated a series of proteins, like vascular endothelial growth factor (VEGF), epidermal growth factor (EGF), fibroblast growth factor (FGF) and their receptors (VEGF-R2, VEGF-R3), and monocyte chemoattractant protein 2 (MCP-2), monocyte chemoattractant protein 4 (MCP-4) in the exosomes derived from adipose mesenchymal stem cells, administration of such hypoxic exosomes promoted proliferation, migration, and tube-formation capability of HUVECs, and also improved neovascularization around the graft in the nude mice model of fat grafting (153).
In addition to protective effects, the hypoxia augmented miRNA in exosomes can also be harmful in some cases. MiR-30a was highly enriched in exosomes either from the culture medium of cardiomyocytes after hypoxic stimulation in vitro or the serum of acute myocardial infarction (AMI) patients in vivo. The miR-30a enriched exosomes were regulated by HIF-α and were efficiently transferred between cardiomyocytes in an autocrine manner after hypoxia. But miR-30a inhibits the expression of core autophagy regulators, which are beneficial in ischemic heart disease. Inhibition of such exosomes release or inhibition of miR-30a was considered a promising treatment strategy in AMI (133). miR-222 was also reported to be transferred under hypoxic culture from M1-type macrophages to bone marrow mesenchymal stem cells, and co-culturing with such exosomes led to decreased cell viability, migration and increased apoptosis in the recipient cells, such effects were partly abolished by the exosome secretion inhibitor GM4869. Similarly, lncRNA AK139128 was also increased in hypoxia and played a negative role. Cardiomyocyte derived exosomal which contained lncRNA AK139128 promoted apoptosis and inhibited proliferation, migration, and invasion in cardiac fibroblasts. Such exosomes could also exacerbate myocardial infarction in the rat model (134).
Hypoxic Exosomes Promote Angiogenesis in Cardiology
Upon hypoxia, exosomes and microvesicles released by bone marrow mesenchymal stem cells (MSCs) were easily taken up by human umbilical vein endothelial cells (HUVECs), which led to promoted angiogenesis and improved cardiac function in infarction attack (137). The total amount of proteins secreted from exosomes increased by 3-4 folds under hypoxic conditions (138). Overexpressing HIF-1α in MSCs led to an increased angiogenic capacity mediated by its secreted exosomes (139). High mobility group box 1 protein (HMGB1) was induced by hypoxia in MSCs derived exosomes. Exosomal HMGB1 transferred to HUVECs activated JNK signaling and induced HIF-1α dependent VEGF expression, eventually led to enhanced angiogenesis (140). Compared with normoxia, hypoxic exosomes were more easily to be taken up by HUVECs and their angiogenesis stimulatory activity was also significantly enhanced. The expression of vascular endothelial growth factor (VEGF) and activation of the protein kinase A (PKA) signaling pathway in HUVECs was significantly increased by hypoxia-exposed exosomes (142). Besides, HUVECs also uptaken exosomes derived from hypoxic culturing human cardiosphere derived cells and exhibited enhanced tube formation. Pro-angiogenic exosomal miRNAs including miR-126, miR-130a, and miR-210 showed a substantial increase in the hypoxic exosomes compared to normoxic exosomes (143).
Besides bone marrow meschmenal stem cells, cardiac progenitor cells (CPCs), and other stem cell types were also attractive candidates for the treatment of myocardial infarction, however, the role of exosomes in the treatment remains unclear. Recent research shows that upon hypoxia, exosomes secreted by cardiac progenitor cells enhanced tube formation of endothelial cells and decreased profibrotic gene expression in TGF-β-stimulated fibroblasts. Microarray analysis identified 11 miRNAs that were upregulated in hypoxic exosomes compared with normoxic exosomes. Hypoxic exosomes improved cardiac function and reduced fibrosis (144). In research involving different degrees of hypoxia, human CPCs were cultured under normoxia (21% O2), physoxia (5% O2), and hypoxia (1% O2) conditions. As a result, the release of exosomes under physoxia increased 1.6 folds and significantly increased tube formation compared to normoxia and hypoxia (145). Interestingly, exosomes secreted by endothelial cells cultured under hypoxia can be taken up by CPCs, giving them increased tolerance when subjected to in vitro hypoxic stress. These exosomes overexpress HIF1 and have higher contents of miR-126 and miR-210 which activated prosurvival kinases and induced a glycolytic switch in recipient cells (146). These studies revealed close crosstalk between cardiac progenitor cells and endothelial cells via exosomes. For stem cell derived exosomes, the stemness of the cell is crucial for the treatment effect. In research evaluating the effect of donor’s age and hypoxia to exosomes, cardiac progenitor cells secreted exosomes from older children were reparative only when subjected to hypoxic conditions (193).
Hypoxic Exosomes in Non-Malignant Disease From Different Systems
Besides cardiovascular system, hypoxic exosomes also play a comprehensive role in diseases from other systems. A wide range of donor units and recipient units are involved, and various biological effects are induced (Figure 3).
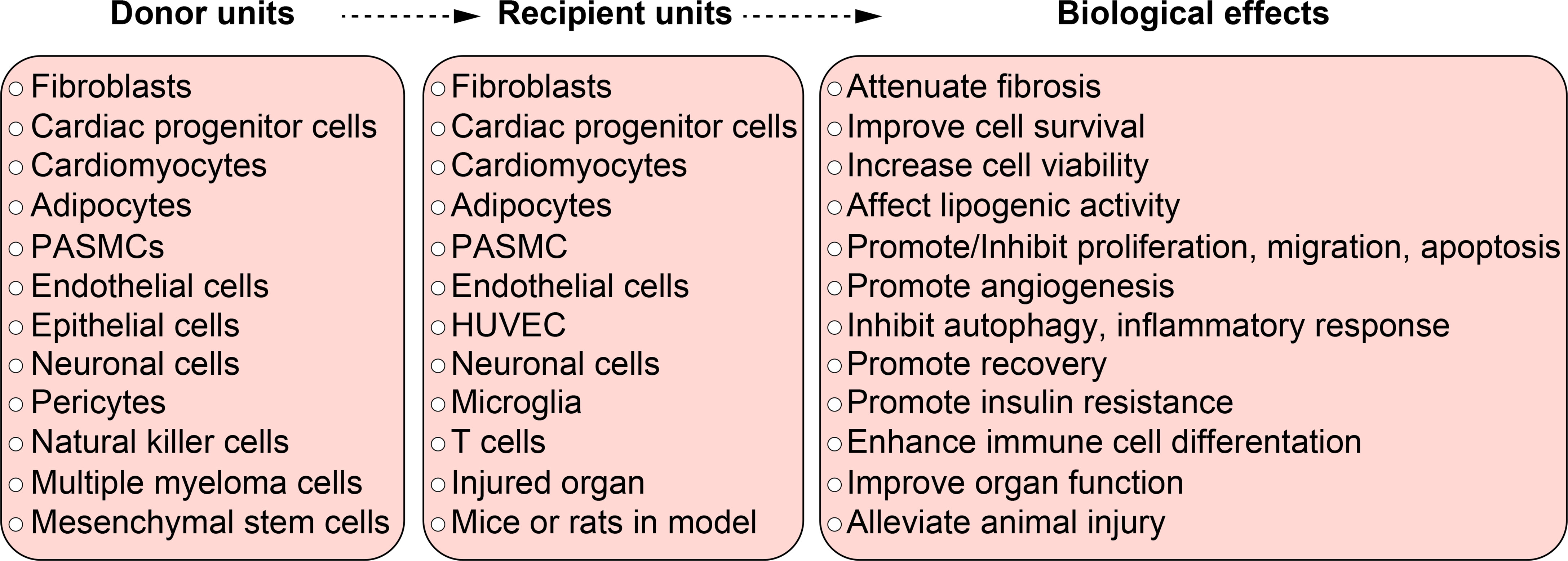
Figure 3 The function of hypoxic exosomes in non-malignant diseases. A summary of donor units, recipient units, and biological effects in different systems. Each box briefly summarizes the corresponding contents from different systems, they don’t relate to each other line to line. Specific relationship among the donor units, recipient units, and biological effects can be found in Table 1 and in the body text.
Neuron System
In the neuron system, different hypoxic exosomal content has been reported and they play a neuron protective role from different perspectives. Amyloid-β (Aβ), which is related to the pathogenesis of Alzheimer’s disease, was accumulated during a hypoxic environment. Hypoxia increased the level of Aβ40 and Aβ42 in human neuroblastoma SH-SY5Y cells derived exosomes and such exosomes promote amyloids propagation (161). Hypoxia also increased Amyloid-beta (Aβ) both in the extracellular and exosomes of HEK293, which aggravated Alzheimer’s disease (AD) (165). Prion protein in astrocytes is a sensor for oxidative stress and mediates beneficial cellular responses. Under hypoxic and ischemic conditions, the release of exosomes carrying enriched prion protein and other molecules led to improved survival of neurons (158). The expression of NLRP3, ASC, Caspase-1, GSDMD-N, cleaved IL-1β and IL-18 were decreased in neuron cells after taking up bone marrow mesenchymal stromal cells-derived exosomes. Hypoxic exosomes had more significant effects to decrease these molecules, and exhibited a more pronounced neuroprotective effect against oxygen-glucose deprivation injury (159). MiR-21 was also reported to be increased by hypoxia in exosomes derived from mesenchymal stromal cells and mediated the improvement of neurologic conditions. And hypoxic exosomes were demonstrated to have better effects than normoxic exosomes to ameliorate cognitive decline in APP/PS1 mice by rescuing synaptic dysfunction and regulating inflammatory responses (160). miR-92b-3p was reported to be upregulated in exosomes derived from oxygen-glucose deprivated (OGD) astrocytes, such exosomes could be uptaken by neurons and attenuated OGD-induced neuron death and apoptosis (166). miR-499a-5p that was encapsulated into exosomes from hypoxic adipose tissue-derived stromal cells (ADSCs) reduced neuronal apoptosis after oxygen-glucose deprivation and reperfusion (OGD/R) in vitro, and relieved the damage of rats with spinal cord injury (SCI) in vivo (163).
Apart from the protective role, hypoxic exosomal content can be harmful as well. MiR-424-5p was upregulated in the exosomes derived from oxygen-glucose deprivation activated microglia. Such exosomes induced significant cell damage and permeability of microvascular endothelial cells. This interpreted how microglia secreted exosomes participate in neurovascular unit injury under the ischemic and hypoxic state (162). miR-210 was upregulated by hypoxia in exosomes from neural stem/progenitor cells, but high levels of miR-210 inhibited the cell viability of recipient neural stem/progenitor cells (164).
Respiratory System
Hypoxia is a broad accepted factor to induce vascular remodeling and the development of pulmonary hypertension. Hypoxic exosomes from different donor cells play a different role in this process. Hypoxia and inflammation could induce pulmonary artery endothelial cells (PAECs) to release exosomes. These exosomes were involved in overproliferation and apoptosis resistance in pulmonary arterial smooth muscle cells (PASMCs), by which they may contribute to the pathogenesis of pulmonary hypertension (169). In contrast, hypoxia can also induce PASMCs to release exosomes and the exosomes were transferred to PAECs in a paracrine manner, affecting the migration and apoptosis of PAECs, then promoting the development of pulmonary arterial hypertension (168). Exosomes transferred between PAECs and PASMCs promoted the development of pulmonary hypertension while exosomes derived from mesenchymal stromal cells prevented the development of pulmonary hypertension. Hypoxic mesenchymal stromal cells derived exosomes exerted a pleiotropic protective effect on the lung and inhibit hypoxic pulmonary hypertension through suppression of hyperproliferative pathways, including STAT-3 mediated signaling induced by hypoxia (2).
Hematology System
Hypoxia enhanced the package of miRNA and protein in exosomes derived from hematology system and such exosomes could be taken up by endothelial cells and leading to enhanced tube formation, or they could be taken up by other hematology cells and promoting further differentiation. Compared to normoxia, hypoxia significantly upregulated a subset of miRNAs, including miR-210, in exosomes derived from human leukemia cells. Coculturing such exosomes with HUVECs remarkably enhanced tube formation by HUVECs compared with exosomes produced in normoxic conditions (171). MiR-486 was also upregulated by hypoxia both in intracellular space and in the secreted exosomes of erythroleukemia TF-1 cells. Exosomal miR-486 played critical roles in regulating the hypoxia-induced erythroid differentiation of hematopoietic cells (170). Hypoxia also upregulated the the Jagged-1 (Notch ligand) in the exosomes from mesenchymal stem cells, and co-culture such exosomes with hematopoietic stem cells (HSCs) enhanced the proliferation, increased the self-renewal capacity, quiescence, and clonogenic potential of HSCs (172).
Endocrine System
Hypoxic exosomes play a bilateral role in diabetic disease. On one hand, hypoxic exosomes led to insulin resistance in some cases. Exosomes isolated from sleep fragmentation and intermittent hypoxia exposed mice induced attenuated p-AKT/total AKT responses to exogenous insulin, leading to insulin resistance in adipocytes (3). On the other hand, hypoxic exosomes internalized miRNA or lncRNA to exert a protective role in diabetic disease. Compared to normoxia, miR-21-3p, miR-126-5p, miR-31-5p were significantly upregulated while miR-99b and miR-146-a were significantly downregulated in hypoxic exosomes derived from adipose stem cells. Hypoxic exosomes exhibited significantly better effects in promoting wound healing than normoxic exosomes when they were injected into diabetic mice (173). miR-125b was also upregulated by hypoxia in the exosomes from umbilical cord-derived mesenchymal stem cells (ucMSCs), such exosomes can be taken up by endothelial cells and promoted cell proliferation, migration, inhibited apoptosis, and accelerated wound healing by targeting and suppressing the tumor protein p53 inducible nuclear protein 1 (TP53INP1) (175). In addition, it was reported that hypoxia upregulated circEhmt1 both in pericytes and its secreted exosomes. CircEhmt1 that were encapsulated into exosomes were transmitted to endotheliocytes, protecting endotheliocytes against high glucose induced injury (174). In another word, hypoxic exosomes mediated impaired insulin sensitivity in the intermittent hypoxia process.
Urinary System
In the urinary system, hypoxia did not change the average size of exosomes secreted by rat renal proximal tubular cells, but significantly increased exosome production in a time-dependent manner. HIF-1 induction also promoted exosome secretion, pharmacological and genetic suppression of HIF-1 abrogated the increase of exosome secretion under hypoxia (194). Many miRNAs were reported to be encapsulated in the exosomes from tubular epithelial cells and exhibited renal protective effects both in vivo and in vitro. Hypoxia induced the enrichment of miR-20a-5p in exosomes from renal tubular epithelial cells. Such exosomes were protective in acute tubular injury by promoting tubular epithelial cells proliferation and improving mitochondrial functions (176). Hypoxia increased the miR-21 both in tubular epithelial cells and in the exosomes of bone marrow-derived dendritic cells. And it was postulated that the upregulated miR-21 of tubular epithelial cells promoted dentritic cell maturation (177). Hypoxia upregulated the miR-150-5p in the exosomes from rat renal tubular epithelium cells (NRK-52E), such exosomes could activate rat kidney fibroblasts (NRK-49F), and injection of such exosomes alleviated renal fibrosis in rats with unilateral ischemia reperfusion injury (178). Hypoxia also upregulated the miR-21 in the exosomes from myotubes C2C12 cells, and such exosomes protected renal tubes from sepsis-induced kidney injury (157). In addition to tubular epithelial cells, exosomes from epithelial cells were also more active under hypoxia. Hypoxia promoted epithelial cells to produce an increased number of exosomes containing TGF-β1 mRNA, to activate fibroblasts, contributing to fibrosis that involved in the pathology of hypoxia induced kidney injury (4). Hypoxia upregulated the lysyl oxidase family member lysyl oxidase-like 2 (LOXL2) in the exosomes from endothelial cells, and such exosomes mediated extracellular matrix crosslink and remodelling (179).
Reproductive System
Different degree of hypoxia has different impacts on exosome contents. Compared to the controls (8% O2), exosomes released from placental mesenchymal stem cells increased by 3.3 folds in 1% O2 and 6.7 folds in 3% O2 respectively. Such changes may contribute to placental vascular adaptation to low oxygen tension under both physiological and pathological conditions (180). Similarly, in endometrial stromal cells, the secretion of exosomes cultured at 1% O2 was increased by about 3.6 folds compared with 8% O2. Mass spectrometry analysis identified 128 proteins unique to exosomes produced at 1% O2 compared with only 46 proteins unique to those produced at 8% O2. Differential production of proteins was associated with different specific biological processes (181). It was reported that hypoxic cytotrophoblast derived exosomes promoted extravillous trophoblasts’ invasion and proliferation (5). Implying the mechanism of hypoxic placenta contributing to the pathophysiology of preeclampsia. Besides proteins encapsulated in hypoxic exosomes, hypoxic exosomal miRNA may also contribute to the pathogenesis of preeclampsia. The level of circulating exosomal total-miRNA and the hypoxia sensitive hsa-miR-210 was elevated in preeclampsia and it was higher in the severe form. Hsa-miR-210 was secreted via exosomes, which may play a role in the pathomechanism of the disease (195).
Skeletomuscular System
In the skeletomuscular system, hypoxic exosomes play an important role to promote angiogenesis and injury recovery in different joint damage. In the spinal cord injury model, hypoxia resulted in an enrichment of miR-216a-5p in the exosomes from mesenchymal stem cells (MSCs). Hypoxic exosomes promoted functional behavioral recovery by shifting microglial polarization from M1 to M2 phenotype in vivo and in vitro (182). LncGm37494 was also reported to be upregulated by hypoxia in the exosomes from adipose tissue-derived mesenchymal stem/stromal cells, and such hypoxic exosomes were more effective than normoxic exosomes in repairing spinal cord injury (189). In spinal cord angiogenesis, preconditioning pericyte with hypoxia mimic and coculture it with endothelial cells resulted in faster wound healing, greater endothelial cord formation, and greater vascular density in the spinal cord tissue. Exosome secretion and the physical presence of stimulated pericytes were necessary for the promotion of angiogenic outcomes (188). In a research of bone fracture healing, hypoxia enhanced the production of exosomal miR-126 through the activation of HIF-1α. Such exosomes were transferred to HUVECs and promoted angiogenesis, proliferation, and migration in vitro. In vivo experiments demonstrated that hypoxic exosome administration promoted bone fracture healing through exosomal miR-126 transfer (183). In rotator cuff tendon injuries (RCTI), the hypoxic environment triggered tenocytes and adjacent adipose-derived mesenchymal stem cells (ADMSCs) to release exosomes to the extracellular matrix (ECM). Tenocytes secreted exosomes encapsulated THSB1, NSEP1, ITIH4, and TN-C. ADMSCs secreted exosomes encapsulated MMP2, COL6A, CTSD, and TN-C. These proteins were involved in multiple signaling pathways of ECM repair and regeneration, protecting the tendon matrix from injury (184). In the arthropathy of collagen-induced arthritis (CIA), hypoxia upregulated the expression of miR-29a-3p and miR-93-5p in exosomes derived from polymorphonuclear myeloid-derived suppressor cells. Compared to normoxic exosomes, hypoxic exosomes could inhibit the proliferation of CD4(+) T cells more effectively. And the administration of hypoxic exosomes alleviated mice with CIA more effectively (185). In steroid-induced osteonecrosis of the femoral head (ONFH) model, hypoxic exosomes derived from bone marrow mesenchymal stem cells (BMMSCs) exhibited better therapeutic effects in promoting angiogenesis and preventing bone loss (186). In rheumatoid arthritis, hypoxia induced the upregulation of miR-424 in exosomes derived from synovial fibroblasts. Exosomal miR-424 could significantly induce T cells differentiation and contribute to the aggravation of rheumatoid arthritis (187).
Conclusion
In many hypoxia related diseases, miRNAs have been reported most to be internalized into exosomes under hypoxia. The master regulator of oxygen homeostasis and a hypoxia sensitive transcription factor HIF, has been reported to have crosstalk with miRNA by many studies. HIF-1α/HIF-1β dimmer can bind to the promoters of target miRNAs and regulate extensive cellular processes, including proliferation, metastasis, apoptosis, and drug resistance, etc. Also, tumor-related miRNAs can modulate the activity of HIF-1α, and play a positive or negative role in tumor development (196). Whether HIF regulated miRNAs are more easily to be encapsulated into exosomes and if there is crosstalk between HIF and other lncRNAs, cirRNAs, proteins, and Lipids that internalized into hypoxic exosomes still awaits further investigation.
As mentioned above, in various types of tumors and other diseases, hypoxia not only increases the quantities of exosome release but also alters the contents of exosomes. Since exosomes are a crucial mediator of genetic, protein, and lipid information carrier and transmitter, manipulating the release, degradation, cargo sorting, and receiving of exosomes becomes a promising strategy to interfere with disease development. In tumor development, hypoxic tumor cells secret more exosomes, such hypoxic exosomes disseminate the malignant information to recipient cells, tissue, and organs, contributing to tumor progression. Reducing exosomes release, promoting lysosome degradation, inhibiting key factors encapsulating, or disrupting exosomes uptaking could be a possible way to prevent the invasion and metastasis of tumors. In fact, through high-throughput screening, a series of inhibitors and activators of exosomes biogenesis have been identified in prostate cancer cells (197). And the mechanisms of how inhibitors function have been well reviewed (198, 199). But targeting exosome donor cells exploration, a broader range of cell line study and the in vivo effects investigation is warranted. Vice versa, when exosomes are beneficial, for example, exosomes derived from mesenchymal stem cells play a cardioprotective role and promote angiogenesis in acute myocardial infarction, and prevent the development of pulmonary hypertension. Under such circumstances, facilitating the secretion and function of exosomes is profitable for improving health conditions. In fact, there are already attempt to applying exosomes in cancer therapy. Reprocessing exosomes that target immune cells to induce an immune response against the tumor, as well as other infectious diseases has been investigated by researchers (200). Such strategies were also explored in several tumor types, including colorectal cancer, metastatic melanoma, and non-small cell lung cancer (201). More research is needed to extend such kind of engineer for disease control.
In addition, although the release of exosomes is increased under hypoxia, there is also baseline release of exosomes under a physiological state. In another word, almost all cell types within the body produce exosomes and the mixed exosomes exist in almost all intercellular space and body fluids. The increased amount of exosome secretion and some specific encapsulated markers make exosomes a potential biomarker for detecting tumors. The closed and naturally biocompatible membrane structure, stable circulation in the blood, and ability to cross the blood-brain-barrier make exosomes ideal candidates to deliver drugs or genes to target spots (202, 203). But how to classify the exosomes, distinguish their parent cells, identify their target cells remains less elucidated. More research is needed to investigate the specific molecular markers of exosomes to categorize them. Elucidating the specific mechanism of exosomes network and function has great potential for deep understanding and accurate interferer with disease progression.
To conclude, hypoxia increased the release of exosomes in tumor development and other diseases. Donor cells grown in hypoxic microenvironment release exosomes that encapsulate miRNA, lncRNA, circRNA, mRNA, cytokines, and enzymes and transfer them to recipient cells, tissue or, organs in an autocrine, paracrine, or endocrine manner. Such exosomal transfer eventually leads to promoted angiogenesis, tumor progression and metastasis, epithelial meschemenal transition, metabolic waste disposal, immune suppression, and chemoresistance in tumor development. In non-malignant disease, hypoxic exosomes can lead to attenuated fibrosis, altered cell proliferation/migration/apoptosis, autography and inflammation inhibition, immune cell differentiation, insulin resistance, injury recovery, and organ function improvement. Understanding the biogenesis, sorting, and receiving of exosomes provide new perspectives to interfere with the disease.
Author Contributions
HJ, writing—original draft. HZ and MZ, writing—discussing about the ideas in the draft. YH and XCL, writing—review and editing. YX and XSL, resources, funding acquisition, project administration, and writing—review and editing. All authors contributed to the article and approved the submitted version.
Funding
This study was funded by grants from the National Natural Science Foundation of China (No. 81973987, 81700051 and 81700052).
Conflict of Interest
The authors declare that the research was conducted in the absence of any commercial or financial relationships that could be construed as a potential conflict of interest.
Publisher’s Note
All claims expressed in this article are solely those of the authors and do not necessarily represent those of their affiliated organizations, or those of the publisher, the editors and the reviewers. Any product that may be evaluated in this article, or claim that may be made by its manufacturer, is not guaranteed or endorsed by the publisher.
Acknowledgments
Adobe Illustrator (v2020) and ScienceSlides (v2016) were used to generate the figures.
Glossary
References
1. Kucharzewska P, Christianson HC, Welch JE, Svensson KJ, Fredlund E, Ringner M, et al. Exosomes Reflect the Hypoxic Status of Glioma Cells and Mediate Hypoxia-Dependent Activation of Vascular Cells During Tumor Development. Proc Natl Acad Sci USA (2013) 110:7312–7. doi: 10.1073/pnas.1220998110
2. Lee C, Mitsialis SA, Aslam M, Vitali SH, Vergadi E, Konstantinou G, et al. Exosomes Mediate the Cytoprotective Action of Mesenchymal Stromal Cells on Hypoxia-Induced Pulmonary Hypertension. Circulation (2012) 126:2601–11. doi: 10.1161/CIRCULATIONAHA.112.114173
3. Khalyfa A, Gozal D, Masa JF, Marin JM, Qiao Z, Corral J, et al. Sleep-Disordered Breathing, Circulating Exosomes, and Insulin Sensitivity in Adipocytes. Int J Obes (Lond) (2018) 42:1127–39. doi: 10.1038/s41366-018-0099-9
4. Borges FT, Melo SA, Ozdemir BC, Kato N, Revuelta I, Miller CA, et al. TGF-Beta1-Containing Exosomes From Injured Epithelial Cells Activate Fibroblasts to Initiate Tissue Regenerative Responses and Fibrosis. J Am Soc Nephrol (2013) 24:385–92. doi: 10.1681/ASN.2012101031
5. Salomon C, Kobayashi M, Ashman K, Sobrevia L, Mitchell MD, Rice GE. Hypoxia-Induced Changes in the Bioactivity of Cytotrophoblast-Derived Exosomes. PloS One (2013) 8:e79636. doi: 10.1371/journal.pone.0079636
6. Semenza GL. Hypoxia-Inducible Factors in Physiology and Medicine. Cell (2012) 148:399–408. doi: 10.1016/j.cell.2012.01.021
7. Noman MZ, Hasmim M, Messai Y, Terry S, Kieda C, Janji B, et al. Hypoxia: A Key Player in Antitumor Immune Response. A Review in the Theme: Cellular Responses to Hypoxia. Am J Physiol Cell Physiol (2015) 309:C569–79. doi: 10.1152/ajpcell.00207.2015
8. Majmundar AJ, Wong WJ, Simon MC. Hypoxia-Inducible Factors and the Response to Hypoxic Stress. Mol Cell (2010) 40:294–309. doi: 10.1016/j.molcel.2010.09.022
9. Schönenberger MJ. Hypoxia Signaling Pathways: Modulators of Oxygen-Related Organelles. Front Cell Dev Biol (2015) 3. doi: 10.3389/fcell.2015.00042
10. Keith B, Johnson RS, Simon MC. HIF1α and HIF2α: Sibling Rivalry in Hypoxic Tumour Growth and Progression. Nat Rev Cancer (2011) 12:9–22. doi: 10.1038/nrc3183
11. Pugh CW, Ratcliffe PJ. New Horizons in Hypoxia Signaling Pathways. Exp Cell Res (2017) 356:116–21. doi: 10.1016/j.yexcr.2017.03.008
12. Choudhry H, Harris AL. Advances in Hypoxia-Inducible Factor Biology. Cell Metab (2018) 27:281–98. doi: 10.1016/j.cmet.2017.10.005
13. Goodall GJ, Wickramasinghe VO. RNA in Cancer. Nat Rev Cancer (2021) 21:22–36. doi: 10.1038/s41568-020-00306-0
14. Shao C, Yang F, Miao S, Liu W, Wang C, Shu Y, et al. Role of Hypoxia-Induced Exosomes in Tumor Biology. Mol Cancer (2018) 17:120. doi: 10.1186/s12943-018-0869-y
15. Shao H, Im H, Castro CM, Breakefield X, Weissleder R, Lee H. New Technologies for Analysis of Extracellular Vesicles. Chem Rev (2018) 118:1917–50. doi: 10.1021/acs.chemrev.7b00534
16. Couch Y, Buzas EI, Vizio DD, Gho YS, Harrison P, Hill AF, et al. A Brief History of Nearly EV-Erything - The Rise and Rise of Extracellular Vesicles. J Extracell Vesicles (2021) 10:e12144. doi: 10.1002/jev2.12144
17. Witwer KW, Thery C. Extracellular Vesicles or Exosomes? On Primacy, Precision, and Popularity Influencing a Choice of Nomenclature. J Extracell Vesicles (2019) 8:1648167. doi: 10.1080/20013078.2019.1648167
18. Gould SJ, Raposo G. As We Wait: Coping With an Imperfect Nomenclature for Extracellular Vesicles. J Extracell Vesicles (2013) 2:10.3402. doi: 10.3402/jev.v2i0.20389
19. Klumperman J, Raposo G. The Complex Ultrastructure of the Endolysosomal System. Cold Spring Harb Perspect Biol (2014) 6:a16857. doi: 10.1101/cshperspect.a016857
20. Thebaud B, Stewart DJ. Exosomes: Cell Garbage can, Therapeutic Carrier, or Trojan Horse? Circulation (2012) 126:2553–5. doi: 10.1161/CIRCULATIONAHA.112.146738
21. Pan BT, Teng K, Wu C, Adam M, Johnstone RM. Electron Microscopic Evidence for Externalization of the Transferrin Receptor in Vesicular Form in Sheep Reticulocytes. J Cell Biol (1985) 101:942–8. doi: 10.1083/jcb.101.3.942
22. Harding C, Heuser J, Stahl P. Receptor-Mediated Endocytosis of Transferrin and Recycling of the Transferrin Receptor in Rat Reticulocytes. J Cell Biol (1983) 97:329–39. doi: 10.1083/jcb.97.2.329
23. Huotari J, Helenius A. Endosome Maturation. EMBO J (2011) 30:3481–500. doi: 10.1038/emboj.2011.286
24. Hurley JH, Hanson PI. Membrane Budding and Scission by the ESCRT Machinery: It’s All in the Neck. Nat Rev Mol Cell Biol (2010) 11:556–66. doi: 10.1038/nrm2937
25. Luzio JP, Gray SR, Bright NA. Endosome-Lysosome Fusion. Biochem Soc Trans (2010) 38:1413–6. doi: 10.1042/BST0381413
26. Colombo M, Raposo G, Thery C. Biogenesis, Secretion, and Intercellular Interactions of Exosomes and Other Extracellular Vesicles. Annu Rev Cell Dev Biol (2014) 30:255–89. doi: 10.1146/annurev-cellbio-101512-122326
27. Thery C. Exosomes: Secreted Vesicles and Intercellular Communications. F1000 Biol Rep (2011) 3:15. doi: 10.3410/B3-15
28. Pan BT, Johnstone RM. Fate of the Transferrin Receptor During Maturation of Sheep Reticulocytes In Vitro: Selective Externalization of the Receptor. Cell (1983) 33:967–78. doi: 10.1016/0092-8674(83)90040-5
29. Nagarajah S. Exosome Secretion - More Than Simple Waste Disposal? Implications for Physiology, Diagnostics and Therapeutics. J Circ Biomark (2016) 5:7. doi: 10.5772/62975
30. Yanez-Mo M, Siljander PR, Andreu Z, Zavec AB, Borras FE, Buzas EI, et al. Biological Properties of Extracellular Vesicles and Their Physiological Functions. J Extracell Vesicles (2015) 4:27066. doi: 10.3402/jev.v4.27066
31. Milane L, Singh A, Mattheolabakis G, Suresh M, Amiji MM. Exosome Mediated Communication Within the Tumor Microenvironment. J Control Release (2015) 219:278–94. doi: 10.1016/j.jconrel.2015.06.029
32. Thery C, Boussac M, Veron P, Ricciardi-Castagnoli P, Raposo G, Garin J, et al. Proteomic Analysis of Dendritic Cell-Derived Exosomes: A Secreted Subcellular Compartment Distinct From Apoptotic Vesicles. J Immunol (2001) 166:7309–18. doi: 10.4049/jimmunol.166.12.7309
33. van Niel G, D’Angelo G, Raposo G. Shedding Light on the Cell Biology of Extracellular Vesicles. Nat Rev Mol Cell Biol (2018) 19:213–28. doi: 10.1038/nrm.2017.125
34. Li Q, Huang Q, Huyan T, Wang Y, Huang Q, Shi J. Bifacial Effects of Engineering Tumour Cell-Derived Exosomes on Human Natural Killer Cells. Exp Cell Res (2018) 363:141–50. doi: 10.1016/j.yexcr.2017.12.005
35. Banizs AB, Huang T, Nakamoto RK, Shi W, He J. Endocytosis Pathways of Endothelial Cell Derived Exosomes. Mol Pharm (2018) 15:5585–90. doi: 10.1021/acs.molpharmaceut.8b00765
36. Mulcahy LA, Pink RC, Carter DR. Routes and Mechanisms of Extracellular Vesicle Uptake. J Extracell Vesicles (2014) 3:10.3402. doi: 10.3402/jev.v3.24641
37. Yaghoubi S, Najminejad H, Dabaghian M, Karimi MH, Abdollahpour-Alitappeh M, Rad F, et al. How Hypoxia Regulate Exosomes in Ischemic Diseases and Cancer Microenvironment? IUBMB Life (2020) 72:1286–305. doi: 10.1002/iub.2275
38. Svensson KJ, Kucharzewska P, Christianson HC, Skold S, Lofstedt T, Johansson MC, et al. Hypoxia Triggers a Proangiogenic Pathway Involving Cancer Cell Microvesicles and PAR-2-Mediated Heparin-Binding EGF Signaling in Endothelial Cells. Proc Natl Acad Sci USA (2011) 108:13147–52. doi: 10.1073/pnas.1104261108
39. Guo X, Qiu W, Liu Q, Qian M, Wang S, Zhang Z, et al. Immunosuppressive Effects of Hypoxia-Induced Glioma Exosomes Through Myeloid-Derived Suppressor Cells via the miR-10a/Rora and miR-21/Pten Pathways. Oncogene (2018) 37:4239–59. doi: 10.1038/s41388-018-0261-9
40. Zhang G, Zhang Y, Cheng S, Wu Z, Liu F, Zhang J. CD133 Positive U87 Glioblastoma Cells-Derived Exosomal microRNAs in Hypoxia- Versus Normoxia-Microenviroment. J Neurooncol (2017) 135:37–46. doi: 10.1007/s11060-017-2566-x
41. Kore RA, Edmondson JL, Jenkins SV, Jamshidi-Parsian A, Dings R, Reyna NS, et al. Hypoxia-Derived Exosomes Induce Putative Altered Pathways in Biosynthesis and Ion Regulatory Channels in Glioblastoma Cells. Biochem Biophys Rep (2018) 14:104–13. doi: 10.1016/j.bbrep.2018.03.008
42. Lan F, Yue X, Xia T. Exosomal microRNA-210 is a Potentially Non-Invasive Biomarker for the Diagnosis and Prognosis of Glioma. Oncol Lett (2020) 19:1967–74. doi: 10.3892/ol.2020.11249
43. Xu J, Zhang J, Zhang Z, Gao Z, Qi Y, Qiu W, et al. Hypoxic Glioma-Derived Exosomes Promote M2-Like Macrophage Polarization by Enhancing Autophagy Induction. Cell Death Dis (2021) 12:373. doi: 10.1038/s41419-021-03664-1
44. Qian M, Chen Z, Guo X, Wang S, Zhang Z, Qiu W, et al. Exosomes Derived From Hypoxic Glioma Deliver miR-1246 and miR-10b-5p to Normoxic Glioma Cells to Promote Migration and Invasion. Lab Invest (2021) 101:612–24. doi: 10.1038/s41374-020-00522-0
45. Li J, Liao T, Liu H, Yuan H, Ouyang T, Wang J, et al. Hypoxic Glioma Stem Cell-Derived Exosomes Containing Linc01060 Promote Progression of Glioma by Regulating the MZF1/c-Myc/HIF1α Axis. Cancer Res (2021) 81:114–28. doi: 10.1158/0008-5472.CAN-20-2270
46. Zhao JL, Tan B, Chen G, Che XM, Du ZY, Yuan Q, et al. Hypoxia-Induced Glioma-Derived Exosomal miRNA-199a-3p Promotes Ischemic Injury of Peritumoral Neurons by Inhibiting the mTOR Pathway. Oxid Med Cell Longev (2020) 2020:5609637. doi: 10.1155/2020/5609637
47. Li J, Yuan H, Xu H, Zhao H, Xiong N. Hypoxic Cancer-Secreted Exosomal miR-182-5p Promotes Glioblastoma Angiogenesis by Targeting Kruppel-Like Factor 2 and 4. Mol Cancer Res (2020) 18:1218–31. doi: 10.1158/1541-7786.MCR-19-0725
48. Qian M, Wang S, Guo X, Wang J, Zhang Z, Qiu W, et al. Hypoxic Glioma-Derived Exosomes Deliver microRNA-1246 to Induce M2 Macrophage Polarization by Targeting TERF2IP via the STAT3 and NF-kappaB Pathways. Oncogene (2020) 39:428–42. doi: 10.1038/s41388-019-0996-y
49. Thakur A, Qiu G, Xu C, Han X, Yang T, Ng SP, et al. Label-Free Sensing of Exosomal MCT1 and CD147 for Tracking Metabolic Reprogramming and Malignant Progression in Glioma. Sci Adv (2020) 6:z6119. doi: 10.1126/sciadv.aaz6119
50. Yue X, Lan F, Xia T. Hypoxic Glioma Cell-Secreted Exosomal miR-301a Activates Wnt/beta-Catenin Signaling and Promotes Radiation Resistance by Targeting Tceal7. Mol Ther (2019) 27:1939–49. doi: 10.1016/j.ymthe.2019.07.011
51. Zhao C, Wang H, Xiong C, Liu Y. Hypoxic Glioblastoma Release Exosomal VEGF-A Induce the Permeability of Blood-Brain Barrier. Biochem Biophys Res Commun (2018) 502:324–31. doi: 10.1016/j.bbrc.2018.05.140
52. Dorayappan K, Wanner R, Wallbillich JJ, Saini U, Zingarelli R, Suarez AA, et al. Hypoxia-Induced Exosomes Contribute to a More Aggressive and Chemoresistant Ovarian Cancer Phenotype: A Novel Mechanism Linking STAT3/Rab Proteins. Oncogene (2018) 37:3806–21. doi: 10.1038/s41388-018-0189-0
53. Lian XY, Zhang H, Liu Q, Lu X, Zhou P, He SQ, et al. Ovarian Cancer-Excreted Exosomal miR-199a-5p Suppresses Tumor Metastasis by Targeting Hypoxia-Inducible Factor-2alpha in Hypoxia Microenvironment. Cancer Commun (Lond) (2020) 40:380–5. doi: 10.1002/cac2.12034
54. Chen X, Ying X, Wang X, Wu X, Zhu Q, Wang X. Exosomes Derived From Hypoxic Epithelial Ovarian Cancer Deliver microRNA-940 to Induce Macrophage M2 Polarization. Oncol Rep (2017) 38:522–8. doi: 10.3892/or.2017.5697
55. Xiao L, He Y, Peng F, Yang J, Yuan C. Endometrial Cancer Cells Promote M2-Like Macrophage Polarization by Delivering Exosomal miRNA-21 Under Hypoxia Condition. J Immunol Res (2020) 2020:9731049. doi: 10.1155/2020/9731049
56. Chen X, Zhou J, Li X, Wang X, Lin Y, Wang X. Exosomes Derived From Hypoxic Epithelial Ovarian Cancer Cells Deliver microRNAs to Macrophages and Elicit a Tumor-Promoted Phenotype. Cancer Lett (2018) 435:80–91. doi: 10.1016/j.canlet.2018.08.001
57. Rong L, Li R, Li S, Luo R. Immunosuppression of Breast Cancer Cells Mediated by Transforming Growth Factor-Beta in Exosomes From Cancer Cells. Oncol Lett (2016) 11:500–4. doi: 10.3892/ol.2015.3841
58. King HW, Michael MZ, Gleadle JM. Hypoxic Enhancement of Exosome Release by Breast Cancer Cells. BMC Cancer (2012) 12:421. doi: 10.1186/1471-2407-12-421
59. Jung KO, Youn H, Lee CH, Kang KW, Chung JK. Visualization of Exosome-Mediated miR-210 Transfer From Hypoxic Tumor Cells. Oncotarget (2017) 8:9899–910. doi: 10.18632/oncotarget.14247
60. Dai G, Yang Y, Liu S, Liu H. Hypoxic Breast Cancer Cell-Derived Exosomal SNHG1 Promotes Breast Cancer Growth and Angiogenesis via Regulating miR-216b-5p/JAK2 Axis. Cancer Manag Res (2022) 14:123–33. doi: 10.2147/CMAR.S327621
61. Xi L, Peng M, Liu S, Liu Y, Wan X, Hou Y, et al. Hypoxia-Stimulated ATM Activation Regulates Autophagy-Associated Exosome Release From Cancer-Associated Fibroblasts to Promote Cancer Cell Invasion. J Extracell Vesicles (2021) 10:e12146. doi: 10.1002/jev2.12146
62. Egea V, Kessenbrock K, Lawson D, Bartelt A, Weber C, Ries C. Let-7f miRNA Regulates SDF-1alpha- and Hypoxia-Promoted Migration of Mesenchymal Stem Cells and Attenuates Mammary Tumor Growth Upon Exosomal Release. Cell Death Dis (2021) 12:516. doi: 10.1038/s41419-021-03789-3
63. Jiang Y, Jiang H, Wang K, Liu C, Man X, Fu Q. Hypoxia Enhances the Production and Antitumor Effect of Exosomes Derived From Natural Killer Cells. Ann Transl Med (2021) 9:473. doi: 10.21037/atm-21-347
64. Deep G, Jain A, Kumar A, Agarwal C, Kim S, Leevy WM, et al. Exosomes Secreted by Prostate Cancer Cells Under Hypoxia Promote Matrix Metalloproteinases Activity at Pre-Metastatic Niches. Mol Carcinog (2020) 59:323–32. doi: 10.1002/mc.23157
65. Xue M, Chen W, Xiang A, Wang R, Chen H, Pan J, et al. Hypoxic Exosomes Facilitate Bladder Tumor Growth and Development Through Transferring Long Non-Coding RNA-Uca1. Mol Cancer (2017) 16:143. doi: 10.1186/s12943-017-0714-8
66. Ramteke A, Ting H, Agarwal C, Mateen S, Somasagara R, Hussain A, et al. Exosomes Secreted Under Hypoxia Enhance Invasiveness and Stemness of Prostate Cancer Cells by Targeting Adherens Junction Molecules. Mol Carcinog (2015) 54:554–65. doi: 10.1002/mc.22124
67. Panigrahi GK, Praharaj PP, Peak TC, Long J, Singh R, Rhim JS, et al. Author Correction: Hypoxia-Induced Exosome Secretion Promotes Survival of African-American and Caucasian Prostate Cancer Cells. Sci Rep (2018) 8:6645. doi: 10.1038/s41598-018-24997-6
68. Horie K, Kawakami K, Fujita Y, Sugaya M, Kameyama K, Mizutani K, et al. Exosomes Expressing Carbonic Anhydrase 9 Promote Angiogenesis. Biochem Biophys Res Commun (2017) 492:356–61. doi: 10.1016/j.bbrc.2017.08.107
69. Hu G, Ma J, Zhang J, Chen Y, Liu H, Huang Y, et al. Hypoxia-Induced lncHILAR Promotes Renal Cancer Metastasis via ceRNA for the miR-613/206/1-1-3p/Jagged-1/Notch/CXCR4 Signaling Pathway. Mol Ther (2021) 29:2979–94. doi: 10.1016/j.ymthe.2021.05.020
70. Panigrahi GK, Ramteke A, Birks D, Abouzeid AH, Venkataraman S, Agarwal C, et al. Exosomal microRNA Profiling to Identify Hypoxia-Related Biomarkers in Prostate Cancer. Oncotarget (2018) 9:13894–910. doi: 10.18632/oncotarget.24532
71. Gu W, Gong L, Wu X, Yao X. Hypoxic TAM-Derived Exosomal miR-155-5p Promotes RCC Progression Through HuR-Dependent IGF1R/AKT/PI3K Pathway. Cell Death Discov (2021) 7:147. doi: 10.1038/s41420-021-00525-w
72. Almendros I, Khalyfa A, Trzepizur W, Gileles-Hillel A, Huang L, Akbarpour M, et al. Tumor Cell Malignant Properties Are Enhanced by Circulating Exosomes in Sleep Apnea. Chest (2016) 150:1030–41. doi: 10.1016/j.chest.2016.08.1438
73. Hsu YL, Hung JY, Chang WA, Lin YS, Pan YC, Tsai PH, et al. Hypoxic Lung Cancer-Secreted Exosomal miR-23a Increased Angiogenesis and Vascular Permeability by Targeting Prolyl Hydroxylase and Tight Junction Protein ZO-1. Oncogene (2017) 36:4929–42. doi: 10.1038/onc.2017.105
74. Wang Y, Yi J, Chen X, Zhang Y, Xu M, Yang Z. The Regulation of Cancer Cell Migration by Lung Cancer Cell-Derived Exosomes Through TGF-Beta and IL-10. Oncol Lett (2016) 11:1527–30. doi: 10.3892/ol.2015.4044
75. Zhang X, Sai B, Wang F, Wang L, Wang Y, Zheng L, et al. Hypoxic BMSC-Derived Exosomal miRNAs Promote Metastasis of Lung Cancer Cells via STAT3-Induced EMT. Mol Cancer (2019) 18:40. doi: 10.1186/s12943-019-0959-5
76. Castellano JJ, Marrades RM, Molins L, Vinolas N, Moises J, Canals J, et al. Extracellular Vesicle lincRNA-P21 Expression in Tumor-Draining Pulmonary Vein Defines Prognosis in NSCLC and Modulates Endothelial Cell Behavior. Cancers (Basel) (2020) 12:734. doi: 10.3390/cancers12030734
77. Yu F, Liang M, Huang Y, Wu W, Zheng B, Chen C. Hypoxic Tumor-Derived Exosomal miR-31-5p Promotes Lung Adenocarcinoma Metastasis by Negatively Regulating SATB2-Reversed EMT and Activating MEK/ERK Signaling. J Exp Clin Cancer Res (2021) 40:179. doi: 10.1186/s13046-021-01979-7
78. Xu L, Liao WL, Lu QJ, Zhang P, Zhu J, Jiang GN. Hypoxic Tumor-Derived Exosomal Circular RNA SETDB1 Promotes Invasive Growth and EMT via the miR-7/Sp1 Axis in Lung Adenocarcinoma. Mol Ther Nucleic Acids (2021) 23:1078–92. doi: 10.1016/j.omtn.2021.01.019
79. Li J, Xu P, Wu D, Guan M, Weng X, Lu Y, et al. Hypoxic Stress Suppresses Lung Tumor-Secreted Exosomal Mir101 to Activate Macrophages and Induce Inflammation. Cell Death Dis (2021) 12:776. doi: 10.1038/s41419-021-04030-x
80. Chen J, Xu R, Xia J, Huang J, Su B, Wang S. Aspirin Inhibits Hypoxia-Mediated Lung Cancer Cell Stemness and Exosome Function. Pathol Res Pract (2019) 215:152379. doi: 10.1016/j.prp.2019.03.008
81. Dong C, Liu X, Wang H, Li J, Dai L, Li J, et al. Hypoxic Non-Small-Cell Lung Cancer Cell-Derived Exosomal miR-21 Promotes Resistance of Normoxic Cell to Cisplatin. Onco Targets Ther (2019) 12:1947–56. doi: 10.2147/OTT.S186922
82. Wang D, Zhao C, Xu F, Zhang A, Jin M, Zhang K, et al. Cisplatin-Resistant NSCLC Cells Induced by Hypoxia Transmit Resistance to Sensitive Cells Through Exosomal PKM2. Theranostics (2021) 11:2860–75. doi: 10.7150/thno.51797
83. Wang J, Zhao J, Zhu J, Zhang S. Hypoxic Non-Small-Cell Lung Cancer Cell-Secreted Exosomal microRNA-582-3p Drives Cancer Cell Malignant Phenotypes by Targeting Secreted Frizzled-Related Protein 1. Cancer Manag Res (2020) 12:10151–61. doi: 10.2147/CMAR.S263768
84. Mo F, Xu Y, Zhang J, Zhu L, Wang C, Chu X, et al. Effects of Hypoxia and Radiation-Induced Exosomes on Migration of Lung Cancer Cells and Angiogenesis of Umbilical Vein Endothelial Cells. Radiat Res (2020) 194:71–80. doi: 10.1667/RR15555.1
85. Wang X, Luo G, Zhang K, Cao J, Huang C, Jiang T, et al. Hypoxic Tumor-Derived Exosomal miR-301a Mediates M2 Macrophage Polarization via PTEN/PI3Kgamma to Promote Pancreatic Cancer Metastasis. Cancer Res (2018) 78:4586–98. doi: 10.1158/0008-5472.CAN-17-3841
86. Ngora H, Galli UM, Miyazaki K, Zoller M. Membrane-Bound and Exosomal Metastasis-Associated C4.4A Promotes Migration by Associating With the Alpha(6)Beta(4) Integrin and MT1-MMP. Neoplasia (2012) 14:95–107. doi: 10.1593/neo.111450
87. Zeng Z, Zhao Y, Chen Q, Zhu S, Niu Y, Ye Z, et al. Hypoxic Exosomal HIF-1alpha-Stabilizing CircZNF91 Promotes Chemoresistance of Normoxic Pancreatic Cancer Cells via Enhancing Glycolysis. Oncogene (2021) 40:5505–17. doi: 10.1038/s41388-021-01960-w
88. Cao W, Zeng Z, He Z, Lei S. Hypoxic Pancreatic Stellate Cell-Derived Exosomal Mirnas Promote Proliferation and Invasion of Pancreatic Cancer Through the PTEN/AKT Pathway. Aging (Albany NY) (2021) 13:7120–32. doi: 10.18632/aging.202569
89. Guo Z, Wang X, Yang Y, Chen W, Zhang K, Teng B, et al. Hypoxic Tumor-Derived Exosomal Long Noncoding RNA UCA1 Promotes Angiogenesis via miR-96-5p/AMOTL2 in Pancreatic Cancer. Mol Ther Nucleic Acids (2020) 22:179–95. doi: 10.1016/j.omtn.2020.08.021
90. Chen K, Wang Q, Liu X, Wang F, Yang Y, Tian X. Hypoxic Pancreatic Cancer Derived Exosomal miR-30b-5p Promotes Tumor Angiogenesis by Inhibiting GJA1 Expression. Int J Biol Sci (2022) 18:1220–37. doi: 10.7150/ijbs.67675
91. Chi Y, Xin H, Liu Z. Exosomal lncRNA UCA1 Derived From Pancreatic Stellate Cells Promotes Gemcitabine Resistance in Pancreatic Cancer via the SOCS3/EZH2 Axis. Front Oncol (2021) 11:671082. doi: 10.3389/fonc.2021.671082
92. Huang Z, Feng Y. Exosomes Derived From Hypoxic Colorectal Cancer Cells Promote Angiogenesis Through Wnt4-Induced Beta-Catenin Signaling in Endothelial Cells. Oncol Res (2017) 25:651–61. doi: 10.3727/096504016X14752792816791
93. Sun H, Meng Q, Shi C, Yang H, Li X, Wu S, et al. Hypoxia-Inducible Exosomes Facilitate Liver-Tropic Premetastatic Niche in Colorectal Cancer. Hepatology (2021) 74:2633–51. doi: 10.1002/hep.32009
94. Ge L, Zhou F, Nie J, Wang X, Zhao Q. Hypoxic Colorectal Cancer-Secreted Exosomes Deliver miR-210-3p to Normoxic Tumor Cells to Elicit a Protumoral Effect. Exp Biol Med (Maywood) (2021) 246:1895–906. doi: 10.1177/15353702211011576
95. Li J, Yang P, Chen F, Tan Y, Huang C, Shen H, et al. Hypoxic Colorectal Cancer-Derived Extracellular Vesicles Deliver microRNA-361-3p to Facilitate Cell Proliferation by Targeting TRAF3 via the Noncanonical NF-kappaB Pathways. Clin Transl Med (2021) 11:e349. doi: 10.1002/ctm2.349
96. Yang H, Zhang H, Yang Y, Wang X, Deng T, Liu R, et al. Hypoxia Induced Exosomal circRNA Promotes Metastasis of Colorectal Cancer via Targeting GEF-H1/RhoA Axis. Theranostics (2020) 10:8211–26. doi: 10.7150/thno.44419
97. Hu X, Mu Y, Liu J, Mu X, Gao F, Chen L, et al. Exosomes Derived From Hypoxic Colorectal Cancer Cells Transfer miR-410-3p to Regulate Tumor Progression. J Cancer (2020) 11:4724–35. doi: 10.7150/jca.33232
98. Huang Z, Yang M, Li Y, Yang F, Feng Y. Exosomes Derived From Hypoxic Colorectal Cancer Cells Transfer Wnt4 to Normoxic Cells to Elicit a Prometastatic Phenotype. Int J Biol Sci (2018) 14:2094–102. doi: 10.7150/ijbs.28288
99. Zhang X, Bai J, Yin H, Long L, Zheng Z, Wang Q, et al. Exosomal miR-1255b-5p Targets Human Telomerase Reverse Transcriptase in Colorectal Cancer Cells to Suppress Epithelial-to-Mesenchymal Transition. Mol Oncol (2020) 14:2589–608. doi: 10.1002/1878-0261.12765
100. Ren R, Sun H, Ma C, Liu J, Wang H. Colon Cancer Cells Secrete Exosomes to Promote Self-Proliferation by Shortening Mitosis Duration and Activation of STAT3 in a Hypoxic Environment. Cell Biosci (2019) 9:62. doi: 10.1186/s13578-019-0325-8
101. Bjornetro T, Redalen KR, Meltzer S, Thusyanthan NS, Samiappan R, Jegerschold C, et al. An Experimental Strategy Unveiling Exosomal microRNAs 486-5p, 181a-5p and 30d-5p From Hypoxic Tumour Cells as Circulating Indicators of High-Risk Rectal Cancer. J Extracell Vesicles (2019) 8:1567219. doi: 10.1080/20013078.2019.1567219
102. Yang K, Zhang J, Bao C. Exosomal Circeif3k From Cancer-Associated Fibroblast Promotes Colorectal Cancer (CRC) Progression via miR-214/PD-L1 axis. BMC Cancer (2021) 21:933. doi: 10.1186/s12885-021-08669-9
103. Wang Y, Yin K, Tian J, Xia X, Ma J, Tang X, et al. Granulocytic Myeloid-Derived Suppressor Cells Promote the Stemness of Colorectal Cancer Cells Through Exosomal S100a9. Adv Sci (Weinh) (2019) 6:1901278. doi: 10.1002/advs.201901278
104. Chen F, Xu B, Li J, Yang X, Gu J, Yao X, et al. Hypoxic Tumour Cell-Derived Exosomal miR-340-5p Promotes Radioresistance of Oesophageal Squamous Cell Carcinoma via KLF10. J Exp Clin Cancer Res (2021) 40:38. doi: 10.1186/s13046-021-01834-9
105. Lu Q, Wang X, Zhu J, Fei X, Chen H, Li C. Hypoxic Tumor-Derived Exosomal Circ0048117 Facilitates M2 Macrophage Polarization Acting as miR-140 Sponge in Esophageal Squamous Cell Carcinoma. Onco Targets Ther (2020) 13:11883–97. doi: 10.2147/OTT.S284192
106. Shan Y, You B, Shi S, Shi W, Zhang Z, Zhang Q, et al. Hypoxia-Induced Matrix Metalloproteinase-13 Expression in Exosomes From Nasopharyngeal Carcinoma Enhances Metastases. Cell Death Dis (2018) 9:382. doi: 10.1038/s41419-018-0425-0
107. Yin H, Qiu X, Shan Y, You B, Xie L, Zhang P, et al. HIF-1alpha Downregulation of miR-433-3p in Adipocyte-Derived Exosomes Contributes to NPC Progression via Targeting SCD1. Cancer Sci (2021) 112:1457–70. doi: 10.1111/cas.14829
108. Yu Y, Min Z, Zhou Z, Linhong M, Tao R, Yan L, et al. Hypoxia-Induced Exosomes Promote Hepatocellular Carcinoma Proliferation and Metastasis via miR-1273f Transfer. Exp Cell Res (2019) 385:111649. doi: 10.1016/j.yexcr.2019.111649
109. Matsuura Y, Wada H, Eguchi H, Gotoh K, Kobayashi S, Kinoshita M, et al. Exosomal miR-155 Derived From Hepatocellular Carcinoma Cells Under Hypoxia Promotes Angiogenesis in Endothelial Cells. Dig Dis Sci (2019) 64:792–802. doi: 10.1007/s10620-018-5380-1
110. Sruthi TV, Edatt L, Raji GR, Kunhiraman H, Shankar SS, Shankar V, et al. Horizontal Transfer of miR-23a From Hypoxic Tumor Cell Colonies can Induce Angiogenesis. J Cell Physiol (2018) 233:3498–514. doi: 10.1002/jcp.26202
111. Zou M, You Y, He S, Wu XL. Effects of Hypoxic Exosomes on the Proliferation, Migration and Invasion of Hepatocellular Carcinoma Huh7 Cells. Zhonghua Gan Zang Bing Za Zhi (2019) 27:363–8. doi: 10.3760/cma.j.issn.1007-3418.2019.05.008
112. Wang X, Zhou Y, Dong K, Zhang H, Gong J, Wang S. Exosomal lncRNA HMMR-AS1 Mediates Macrophage Polarization Through miR-147a/ARID3A Axis Under Hypoxia and Affects the Progression of Hepatocellular Carcinoma. Environ Toxicol (2022). doi: 10.1002/tox.23489
113. Li L, Li C, Wang S, Wang Z, Jiang J, Wang W, et al. Exosomes Derived From Hypoxic Oral Squamous Cell Carcinoma Cells Deliver miR-21 to Normoxic Cells to Elicit a Prometastatic Phenotype. Cancer Res (2016) 76:1770–80. doi: 10.1158/0008-5472.CAN-15-1625
114. Li L, Cao B, Liang X, Lu S, Luo H, Wang Z, et al. Microenvironmental Oxygen Pressure Orchestrates an Anti- and Pro-Tumoral Gammadelta T Cell Equilibrium via Tumor-Derived Exosomes. Oncogene (2019) 38:2830–43. doi: 10.1038/s41388-018-0627-z
115. Mao Y, Wang Y, Dong L, Zhang Y, Zhang Y, Wang C, et al. Hypoxic Exosomes Facilitate Angiogenesis and Metastasis in Esophageal Squamous Cell Carcinoma Through Altering the Phenotype and Transcriptome of Endothelial Cells. J Exp Clin Cancer Res (2019) 38:389. doi: 10.1186/s13046-019-1384-8
116. Xia X, Wang S, Ni B, Xing S, Cao H, Zhang Z, et al. Hypoxic Gastric Cancer-Derived Exosomes Promote Progression and Metastasis via MiR-301a-3p/PHD3/HIF-1alpha Positive Feedback Loop. Oncogene (2020) 39:6231–44. doi: 10.1038/s41388-020-01425-6
117. Wang Y, Cen A, Yang Y, Ye H, Li J, Liu S, et al. miR-181a, Delivered by Hypoxic PTC-Secreted Exosomes, Inhibits DACT2 by Downregulating MLL3, Leading to YAP-VEGF-Mediated Angiogenesis. Mol Ther Nucleic Acids (2021) 24:610–21. doi: 10.1016/j.omtn.2021.02.027
118. Park JE, Tan HS, Datta A, Lai RC, Zhang H, Meng W, et al. Hypoxic Tumor Cell Modulates its Microenvironment to Enhance Angiogenic and Metastatic Potential by Secretion of Proteins and Exosomes. Mol Cell Proteomics (2010) 9:1085–99. doi: 10.1074/mcp.M900381-MCP200
119. Wozniak M, Peczek L, Czernek L, Duchler M. Analysis of the miRNA Profiles of Melanoma Exosomes Derived Under Normoxic and Hypoxic Culture Conditions. Anticancer Res (2017) 37:6779–89. doi: 10.21873/anticanres.12138
120. Khalyfa A, Trzepizur W, Gileles-Hillel A, Qiao Z, Sanz-Rubio D, Marin JM, et al. Heterogeneity of Melanoma Cell Responses to Sleep Apnea-Derived Plasma Exosomes and to Intermittent Hypoxia. Cancers (Basel) (2021) 13:4781. doi: 10.3390/cancers13194781
121. Park JE, Dutta B, Tse SW, Gupta N, Tan CF, Low JK, et al. Hypoxia-Induced Tumor Exosomes Promote M2-Like Macrophage Polarization of Infiltrating Myeloid Cells and microRNA-Mediated Metabolic Shift. Oncogene (2019) 38:5158–73. doi: 10.1038/s41388-019-0782-x
122. de Jong OG, Verhaar MC, Chen Y, Vader P, Gremmels H, Posthuma G, et al. Cellular Stress Conditions are Reflected in the Protein and RNA Content of Endothelial Cell-Derived Exosomes. J Extracell Vesicles (2012) 1:10.3402. doi: 10.3402/jev.v1i0.18396
123. Kling MJ, Chaturvedi NK, Kesherwani V, Coulter DW, McGuire TR, Sharp JG, et al. Exosomes Secreted Under Hypoxia Enhance Stemness in Ewing’s Sarcoma Through miR-210 Delivery. Oncotarget (2020) 11:3633–45. doi: 10.18632/oncotarget.27702
124. Lee JY, Ryu D, Lim SW, Ryu KJ, Choi ME, Yoon SE, et al. Exosomal miR-1305 in the Oncogenic Activity of Hypoxic Multiple Myeloma Cells: A Biomarker for Predicting Prognosis. J Cancer (2021) 12:2825–34. doi: 10.7150/jca.55553
125. Umezu T, Tadokoro H, Azuma K, Yoshizawa S, Ohyashiki K, Ohyashiki JH. Exosomal miR-135b Shed From Hypoxic Multiple Myeloma Cells Enhances Angiogenesis by Targeting Factor-Inhibiting HIF-1. Blood (2014) 124:3748–57. doi: 10.1182/blood-2014-05-576116
126. Zhang J, Ma J, Long K, Qiu W, Wang Y, Hu Z, et al. Overexpression of Exosomal Cardioprotective miRNAs Mitigates Hypoxia-Induced H9c2 Cells Apoptosis. Int J Mol Sci (2017) 18:711. doi: 10.3390/ijms18040711
127. Zhu J, Lu K, Zhang N, Zhao Y, Ma Q, Shen J, et al. Myocardial Reparative Functions of Exosomes From Mesenchymal Stem Cells are Enhanced by Hypoxia Treatment of the Cells via Transferring microRNA-210 in an Nsmase2-Dependent Way. Artif Cells Nanomed Biotechnol (2018) 46:1659–70. doi: 10.1080/21691401.2017.1388249
128. Liu W, Zhang H, Mai J, Chen Z, Huang T, Wang S, et al. Distinct Anti-Fibrotic Effects of Exosomes Derived From Endothelial Colony-Forming Cells Cultured Under Normoxia and Hypoxia. Med Sci Monit (2018) 24:6187–99. doi: 10.12659/MSM.911306
129. Khalyfa A, Zhang C, Khalyfa AA, Foster GE, Beaudin AE, Andrade J, et al. Effect on Intermittent Hypoxia on Plasma Exosomal Micro RNA Signature and Endothelial Function in Healthy Adults. Sleep (2016) 39:2077–90. doi: 10.5665/sleep.6302
130. Sun L, Zhu W, Zhao P, Wang Q, Fan B, Zhu Y, et al. Long Noncoding RNA UCA1 From Hypoxia-Conditioned hMSC-Derived Exosomes: A Novel Molecular Target for Cardioprotection Through miR-873-5p/XIAP Axis. Cell Death Dis (2020) 11:696. doi: 10.1038/s41419-020-02783-5
131. Wang Y, Zhao R, Liu W, Wang Z, Rong J, Long X, et al. Exosomal CircHIPK3 Released From Hypoxia-Pretreated Cardiomyocytes Regulates Oxidative Damage in Cardiac Microvascular Endothelial Cells via the miR-29a/IGF-1 Pathway. Oxid Med Cell Longev (2019) 2019:7954657. doi: 10.1155/2019/7954657
132. Wang Y, Zhao R, Shen C, Liu W, Yuan J, Li C, et al. Exosomal CircHIPK3 Released From Hypoxia-Induced Cardiomyocytes Regulates Cardiac Angiogenesis After Myocardial Infarction. Oxid Med Cell Longev (2020) 2020:8418407. doi: 10.1155/2020/8418407
133. Yang Y, Li Y, Chen X, Cheng X, Liao Y, Yu X. Exosomal Transfer of miR-30a Between Cardiomyocytes Regulates Autophagy After Hypoxia. J Mol Med (Berl) (2016) 94:711–24. doi: 10.1007/s00109-016-1387-2
134. Wang L, Zhang J. Exosomal lncRNA AK139128 Derived From Hypoxic Cardiomyocytes Promotes Apoptosis and Inhibits Cell Proliferation in Cardiac Fibroblasts. Int J Nanomedicine (2020) 15:3363–76. doi: 10.2147/IJN.S240660
135. Zhang Z, Xu Y, Cao C, Wang B, Guo J, Qin Z, et al. Exosomes as a Messager to Regulate the Crosstalk Between Macrophages and Cardiomyocytes Under Hypoxia Conditions. J Cell Mol Med (2022) 26:1486–500. doi: 10.1111/jcmm.17162
136. Lin B, Chen X, Lu C, Xu J, Qiu Y, Liu X, et al. Loss of Exosomal lncRNA HCG15 Prevents Acute Myocardial Ischemic Injury Through the NF-Kappab/P65 and P38 Pathways. Cell Death Dis (2021) 12:1007. doi: 10.1038/s41419-021-04281-8
137. Bian S, Zhang L, Duan L, Wang X, Min Y, Yu H. Extracellular Vesicles Derived From Human Bone Marrow Mesenchymal Stem Cells Promote Angiogenesis in a Rat Myocardial Infarction Model. J Mol Med (Berl) (2014) 92:387–97. doi: 10.1007/s00109-013-1110-5
138. Sano S, Izumi Y, Yamaguchi T, Yamazaki T, Tanaka M, Shiota M, et al. Lipid Synthesis is Promoted by Hypoxic Adipocyte-Derived Exosomes in 3T3-L1 Cells. Biochem Biophys Res Commun (2014) 445:327–33. doi: 10.1016/j.bbrc.2014.01.183
139. Gonzalez-King H, Garcia NA, Ontoria-Oviedo I, Ciria M, Montero JA, Sepulveda P. Hypoxia Inducible Factor-1alpha Potentiates Jagged 1-Mediated Angiogenesis by Mesenchymal Stem Cell-Derived Exosomes. Stem Cells (2017) 35:1747–59. doi: 10.1002/stem.2618
140. Gao W, He R, Ren J, Zhang W, Wang K, Zhu L, et al. Exosomal HMGB1 Derived From Hypoxia-Conditioned Bone Marrow Mesenchymal Stem Cells Increases Angiogenesis via the JNK/HIF-1alpha Pathway. FEBS Open Bio (2021) 11:1364–73. doi: 10.1002/2211-5463.13142
141. Xiong Y, Tang R, Xu J, Jiang W, Gong Z, Zhang L, et al. Sequential Transplantation of Exosomes and Mesenchymal Stem Cells Pretreated With a Combination of Hypoxia and Tongxinluo Efficiently Facilitates Cardiac Repair. Stem Cell Res Ther (2022) 13:63. doi: 10.1186/s13287-022-02736-z
142. Xue C, Shen Y, Li X, Li B, Zhao S, Gu J, et al. Exosomes Derived From Hypoxia-Treated Human Adipose Mesenchymal Stem Cells Enhance Angiogenesis Through the PKA Signaling Pathway. Stem Cells Dev (2018) 27:456–65. doi: 10.1089/scd.2017.0296
143. Namazi H, Mohit E, Namazi I, Rajabi S, Samadian A, Hajizadeh-Saffar E, et al. Exosomes Secreted by Hypoxic Cardiosphere-Derived Cells Enhance Tube Formation and Increase Pro-Angiogenic miRNA. J Cell Biochem (2018) 119:4150–60. doi: 10.1002/jcb.26621
144. Gray WD, French KM, Ghosh-Choudhary S, Maxwell JT, Brown ME, Platt MO, et al. Identification of Therapeutic Covariant microRNA Clusters in Hypoxia-Treated Cardiac Progenitor Cell Exosomes Using Systems Biology. Circ Res (2015) 116:255–63. doi: 10.1161/CIRCRESAHA.116.304360
145. Dougherty JA, Patel N, Kumar N, Rao SG, Angelos MG, Singh H, et al. Human Cardiac Progenitor Cells Enhance Exosome Release and Promote Angiogenesis Under Physoxia. Front Cell Dev Biol (2020) 8:130. doi: 10.3389/fcell.2020.00130
146. Ong SG, Lee WH, Huang M, Dey D, Kodo K, Sanchez-Freire V, et al. Cross Talk of Combined Gene and Cell Therapy in Ischemic Heart Disease: Role of Exosomal microRNA Transfer. Circulation (2014) 130:S60–9. doi: 10.1161/CIRCULATIONAHA.113.007917
147. Cosme J, Guo H, Hadipour-Lakmehsari S, Emili A, Gramolini AO. Hypoxia-Induced Changes in the Fibroblast Secretome, Exosome, and Whole-Cell Proteome Using Cultured, Cardiac-Derived Cells Isolated From Neonatal Mice. J Proteome Res (2017) 16:2836–47. doi: 10.1021/acs.jproteome.7b00144
148. Han YD, Bai Y, Yan XL, Ren J, Zeng Q, Li XD, et al. Co-Transplantation of Exosomes Derived From Hypoxia-Preconditioned Adipose Mesenchymal Stem Cells Promotes Neovascularization and Graft Survival in Fat Grafting. Biochem Biophys Res Commun (2018) 497:305–12. doi: 10.1016/j.bbrc.2018.02.076
149. Zhang L, Wei Q, Liu X, Zhang T, Wang S, Zhou L, et al. Exosomal microRNA-98-5p From Hypoxic Bone Marrow Mesenchymal Stem Cells Inhibits Myocardial Ischemia-Reperfusion Injury by Reducing TLR4 and Activating the PI3K/Akt Signaling Pathway. Int Immunopharmacol (2021) 101:107592. doi: 10.1016/j.intimp.2021.107592
150. Zhang CS, Shao K, Liu CW, Li CJ, Yu BT. Hypoxic Preconditioning BMSCs-Exosomes Inhibit Cardiomyocyte Apoptosis After Acute Myocardial Infarction by Upregulating microRNA-24. Eur Rev Med Pharmacol Sci (2019) 23:6691–9. doi: 10.26355/eurrev_201908_18560
151. Cheng H, Chang S, Xu R, Chen L, Song X, Wu J, et al. Hypoxia-Challenged MSC-Derived Exosomes Deliver miR-210 to Attenuate Post-Infarction Cardiac Apoptosis. Stem Cell Res Ther (2020) 11:224. doi: 10.1186/s13287-020-01737-0
152. Xia W, Chen H, Xie C, Hou M. Long-Noncoding RNA MALAT1 Sponges microRNA-92a-3p to Inhibit Doxorubicin-Induced Cardiac Senescence by Targeting ATG4a. Aging (Albany NY) (2020) 12:8241–60. doi: 10.18632/aging.103136
153. Han Y, Ren J, Bai Y, Pei X, Han Y. Exosomes From Hypoxia-Treated Human Adipose-Derived Mesenchymal Stem Cells Enhance Angiogenesis Through VEGF/VEGF-R. Int J Biochem Cell Biol (2019) 109:59–68. doi: 10.1016/j.biocel.2019.01.017
154. Zhu LP, Tian T, Wang JY, He JN, Chen T, Pan M, et al. Hypoxia-Elicited Mesenchymal Stem Cell-Derived Exosomes Facilitates Cardiac Repair Through miR-125b-Mediated Prevention of Cell Death in Myocardial Infarction. Theranostics (2018) 8:6163–77. doi: 10.7150/thno.28021
155. Qi Y, Zhu T, Zhang T, Wang X, Li W, Chen D, et al. M1 Macrophage-Derived Exosomes Transfer miR-222 to Induce Bone Marrow Mesenchymal Stem Cell Apoptosis. Lab Invest (2021) 101:1318–26. doi: 10.1038/s41374-021-00622-5
156. Namazi H, Namazi I, Ghiasi P, Ansari H, Rajabi S, Hajizadeh-Saffar E, et al. Exosomes Secreted by Normoxic and Hypoxic Cardiosphere-Derived Cells Have Anti-Apoptotic Effect. Iran J Pharm Res (2018) 17:377–85.
157. Pan T, Jia P, Chen N, Fang Y, Liang Y, Guo M, et al. Delayed Remote Ischemic Preconditioning ConfersRenoprotection Against Septic Acute Kidney Injury via Exosomal miR-21. Theranostics (2019) 9:405–23. doi: 10.7150/thno.29832
158. Guitart K, Loers G, Buck F, Bork U, Schachner M, Kleene R. Improvement of Neuronal Cell Survival by Astrocyte-Derived Exosomes Under Hypoxic and Ischemic Conditions Depends on Prion Protein. Glia (2016) 64:896–910. doi: 10.1002/glia.22963
159. Kang X, Jiang L, Chen X, Wang X, Gu S, Wang J, et al. Exosomes Derived From Hypoxic Bone Marrow Mesenchymal Stem Cells Rescue OGD-Induced Injury in Neural Cells by Suppressing NLRP3 Inflammasome-Mediated Pyroptosis. Exp Cell Res (2021) 405:112635. doi: 10.1016/j.yexcr.2021.112635
160. Cui GH, Wu J, Mou FF, Xie WH, Wang FB, Wang QL, et al. Exosomes Derived From Hypoxia-Preconditioned Mesenchymal Stromal Cells Ameliorate Cognitive Decline by Rescuing Synaptic Dysfunction and Regulating Inflammatory Responses in APP/PS1 Mice. FASEB J (2018) 32:654–68. doi: 10.1096/fj.201700600R
161. Xie JC, Ma XY, Liu XH, Yu J, Zhao YC, Tan Y, et al. Hypoxia Increases Amyloid-Beta Level in Exosomes by Enhancing the Interaction Between CD147 and Hook1. Am J Transl Res (2018) 10:150–63.
162. Xie L, Zhao H, Wang Y, Chen Z. Exosomal Shuttled miR-424-5p From Ischemic Preconditioned Microglia Mediates Cerebral Endothelial Cell Injury Through Negatively Regulation of FGF2/STAT3 Pathway. Exp Neurol (2020) 333:113411. doi: 10.1016/j.expneurol.2020.113411
163. Liang Y, Wu JH, Zhu JH, Yang H. Exosomes Secreted by Hypoxia-Pre-Conditioned Adipose-Derived Mesenchymal Stem Cells Reduce Neuronal Apoptosis in Rats With Spinal Cord Injury. J Neurotrauma (2022). doi: 10.1089/neu.2021.0290
164. Zhao M, Gao Y, Wang F, Cheng X, Zhao T, Zhao Y, et al. Neural Progenitor Cells-Secreted Exosomal miR-210 Induced by Hypoxia Influences Cell Viability. Neuroreport (2020) 31:798–805. doi: 10.1097/WNR.0000000000001490
165. Xie J, Li X, Zhou Y, Wu J, Tan Y, Ma X, et al. Resveratrol Abrogates Hypoxia-Induced Up-Regulation of Exosomal Amyloid-Beta Partially by Inhibiting Cd147. Neurochem Res (2019) 44:1113–26. doi: 10.1007/s11064-019-02742-3
166. Xu L, Cao H, Xie Y, Zhang Y, Du M, Xu X, et al. Exosome-Shuttled miR-92b-3p From Ischemic Preconditioned Astrocytes Protects Neurons Against Oxygen and Glucose Deprivation. Brain Res (2019) 1717:66–73. doi: 10.1016/j.brainres.2019.04.009
167. Lee C, Mitsialis SA, Aslam M, Vitali SH, Vergadi E, Konstantinou G, et al. Exosomes Mediate the Cytoprotective Action of Mesenchymal Stromal Cells on Hypoxia-Induced Pulmonary Hypertension. Circulation (2012) 126:2601–11. doi: 10.1161/CIRCULATIONAHA.112.114173
168. Deng L, Blanco FJ, Stevens H, Lu R, Caudrillier A, McBride M, et al. MicroRNA-143 Activation Regulates Smooth Muscle and Endothelial Cell Crosstalk in Pulmonary Arterial Hypertension. Circ Res (2015) 117:870–83. doi: 10.1161/CIRCRESAHA.115.306806
169. Zhao L, Luo H, Li X, Li T, He J, Qi Q, et al. Exosomes Derived From Human Pulmonary Artery Endothelial Cells Shift the Balance Between Proliferation and Apoptosis of Smooth Muscle Cells. Cardiology (2017) 137:43–53. doi: 10.1159/000453544
170. Shi XF, Wang H, Kong FX, Xu QQ, Xiao FJ, Yang YF, et al. Exosomal miR-486 Regulates Hypoxia-Induced Erythroid Differentiation of Erythroleukemia Cells Through Targeting Sirt1. Exp Cell Res (2017) 351:74–81. doi: 10.1016/j.yexcr.2016.12.023
171. Tadokoro H, Umezu T, Ohyashiki K, Hirano T, Ohyashiki JH. Exosomes Derived From Hypoxic Leukemia Cells Enhance Tube Formation in Endothelial Cells. J Biol Chem (2013) 288:34343–51. doi: 10.1074/jbc.M113.480822
172. Niazi V, Ghafouri-Fard S, Verdi J, Jeibouei S, Karami F, Pourhadi M, et al. Hypoxia Preconditioned Mesenchymal Stem Cell-Derived Exosomes Induce Ex Vivo Expansion of Umbilical Cord Blood Hematopoietic Stem Cells CD133+ by Stimulation of Notch Signaling Pathway. Biotechnol Prog (2022) 38:e3222. doi:10.1002/btpr.322
173. Wang J, Wu H, Peng Y, Zhao Y, Qin Y, Zhang Y, et al. Hypoxia Adipose Stem Cell-Derived Exosomes Promote High-Quality Healing of Diabetic Wound Involves Activation of PI3K/Akt Pathways. J Nanobiotechnol (2021) 19:202. doi: 10.1186/s12951-021-00942-0
174. Ye L, Guo H, Wang Y, Peng Y, Zhang Y, Li S, et al. Exosomal Circehmt1 Released From Hypoxia-Pretreated Pericytes Regulates High Glucose-Induced Microvascular Dysfunction via the NFIA/NLRP3 Pathway. Oxid Med Cell Longev (2021) 2021:8833098. doi: 10.1155/2021/8833098
175. Zhang XF, Wang T, Wang ZX, Huang KP, Zhang YW, Wang GL, et al. Hypoxic ucMSC-Secreted Exosomal miR-125b Promotes Endothelial Cell Survival and Migration During Wound Healing by Targeting TP53INP1. Mol Ther Nucleic Acids (2021) 26:347–59. doi: 10.1016/j.omtn.2021.07.014
176. Yu W, Zeng H, Chen J, Fu S, Huang Q, Xu Y, et al. miR-20a-5p is Enriched in Hypoxia-Derived Tubular Exosomes and Protects Against Acute Tubular Injury. Clin Sci (Lond) (2020) 134:2223–34. doi: 10.1042/CS20200288
177. Liang YR, Zhang T, Jia P, Xu XL, Fang Y, Ding XQ. Interaction Between Bone Marrow-Derived Dendritic Cells and miR-21 of Tubular Renal Epithelial Cells Under Hypoxia. Eur Rev Med Pharmacol Sci (2019) 23:1641–51. doi: 10.26355/eurrev_201902_17124
178. Zhou X, Zhao S, Li W, Ruan Y, Yuan R, Ning J, et al. Tubular Cell-Derived Exosomal miR-150-5p Contributes to Renal Fibrosis Following Unilateral Ischemia-Reperfusion Injury by Activating Fibroblast In Vitro and In Vivo. Int J Biol Sci (2021) 17:4021–33. doi: 10.7150/ijbs.62478
179. de Jong OG, van Balkom BW, Gremmels H, Verhaar MC. Exosomes From Hypoxic Endothelial Cells Have Increased Collagen Crosslinking Activity Through Up-Regulation of Lysyl Oxidase-Like 2. J Cell Mol Med (2016) 20:342–50. doi: 10.1111/jcmm.12730
180. Salomon C, Ryan J, Sobrevia L, Kobayashi M, Ashman K, Mitchell M, et al. Exosomal Signaling During Hypoxia Mediates Microvascular Endothelial Cell Migration and Vasculogenesis. PloS One (2013) 8:e68451. doi: 10.1371/journal.pone.0068451
181. Koh YQ, Peiris HN, Vaswani K, Reed S, Rice GE, Salomon C, et al. Characterization of Exosomal Release in Bovine Endometrial Intercaruncular Stromal Cells. Reprod Biol Endocrinol (2016) 14:78. doi: 10.1186/s12958-016-0207-4
182. Liu W, Rong Y, Wang J, Zhou Z, Ge X, Ji C, et al. Exosome-Shuttled miR-216a-5p From Hypoxic Preconditioned Mesenchymal Stem Cells Repair Traumatic Spinal Cord Injury by Shifting Microglial M1/M2 Polarization. J Neuroinflamm (2020) 17:47. doi: 10.1186/s12974-020-1726-7
183. Liu W, Li L, Rong Y, Qian D, Chen J, Zhou Z, et al. Hypoxic Mesenchymal Stem Cell-Derived Exosomes Promote Bone Fracture Healing by the Transfer of miR-126. Acta Biomater (2020) 103:196–212. doi: 10.1016/j.actbio.2019.12.020
184. Thankam FG, Chandra I, Diaz C, Dilisio MF, Fleegel J, Gross RM, et al. Matrix Regeneration Proteins in the Hypoxia-Triggered Exosomes of Shoulder Tenocytes and Adipose-Derived Mesenchymal Stem Cells. Mol Cell Biochem (2020) 465:75–87. doi: 10.1007/s11010-019-03669-7
185. Chu X, Chen L, Zhang Y. Exosomes Derived From PMN-MDSCs Preconditioned by Hypoxia Attenuate Arthropathy of Collagen-Induced Arthritis Mice. Xi Bao Yu Fen Zi Mian Yi Xue Za Zhi (2021) 37:728–35.
186. Yuan N, Ge Z, Ji W, Li J. Exosomes Secreted From Hypoxia-Preconditioned Mesenchymal Stem Cells Prevent Steroid-Induced Osteonecrosis of the Femoral Head by Promoting Angiogenesis in Rats. BioMed Res Int (2021) 2021:6655225. doi: 10.1155/2021/6655225
187. Ding Y, Wang L, Wu H, Zhao Q, Wu S. Exosomes Derived From Synovial Fibroblasts Under Hypoxia Aggravate Rheumatoid Arthritis by Regulating Treg/Th17 Balance. Exp Biol Med (Maywood) (2020) 245:1177–86. doi: 10.1177/1535370220934736
188. Mayo JN, Bearden SE. Driving the Hypoxia-Inducible Pathway in Human Pericytes Promotes Vascular Density in an Exosome-Dependent Manner. Microcirculation (2015) 22:711–23. doi: 10.1111/micc.12227
189. Shao M, Jin M, Xu S, Zheng C, Zhu W, Ma X, et al. Exosomes From Long Noncoding RNA-Gm37494-ADSCs Repair Spinal Cord Injury via Shifting Microglial M1/M2 Polarization. Inflammation (2020) 43:1536–47. doi: 10.1007/s10753-020-01230-z
190. Yoon JH, Kim J, Kim KL, Kim DH, Jung SJ, Lee H, et al. Proteomic Analysis of Hypoxia-Induced U373MG Glioma Secretome Reveals Novel Hypoxia-Dependent Migration Factors. Proteomics (2014) 14:1494–502. doi: 10.1002/pmic.201300554
191. Jung KO, Jo H, Yu JH, Gambhir SS, Pratx G. Development and MPI Tracking of Novel Hypoxia-Targeted Theranostic Exosomes. Biomaterials (2018) 177:139–48. doi: 10.1016/j.biomaterials.2018.05.048
192. Saravanan PB, Vasu S, Yoshimatsu G, Darden CM, Wang X, Gu J, et al. Differential Expression and Release of Exosomal miRNAs by Human Islets Under Inflammatory and Hypoxic Stress. Diabetologia (2019) 62:1901–14. doi: 10.1007/s00125-019-4950-x
193. Agarwal U, George A, Bhutani S, Ghosh-Choudhary S, Maxwell JT, Brown ME, et al. Experimental, Systems, and Computational Approaches to Understanding the MicroRNA-Mediated Reparative Potential of Cardiac Progenitor Cell-Derived Exosomes From Pediatric Patients. Circ Res (2017) 120:701–12. doi: 10.1161/CIRCRESAHA.116.309935
194. Zhang W, Zhou X, Yao Q, Liu Y, Zhang H, Dong Z. HIF-1-Mediated Production of Exosomes During Hypoxia is Protective in Renal Tubular Cells. Am J Physiol Renal Physiol (2017) 313:F906–13. doi: 10.1152/ajprenal.00178.2017
195. Biro O, Alasztics B, Molvarec A, Joo J, Nagy B, Rigo JJ. Various Levels of Circulating Exosomal Total-miRNA and miR-210 hypoxamiR in Different Forms of Pregnancy Hypertension. Pregnancy Hypertens (2017) 10:207–12. doi: 10.1016/j.preghy.2017.09.002
196. Yang W, Ma J, Zhou W, Cao B, Zhou X, Zhang H, et al. Reciprocal Regulations Between miRNAs and HIF-1alpha in Human Cancers. Cell Mol Life Sci (2019) 76:453–71. doi: 10.1007/s00018-018-2941-6
197. Datta A, Kim H, McGee L, Johnson AE, Talwar S, Marugan J, et al. High-Throughput Screening Identified Selective Inhibitors of Exosome Biogenesis and Secretion: A Drug Repurposing Strategy for Advanced Cancer. Sci Rep (2018) 8:8161. doi: 10.1038/s41598-018-26411-7
198. Catalano M, O’Driscoll L. Inhibiting Extracellular Vesicles Formation and Release: A Review of EV Inhibitors. J Extracell Vesicles (2020) 9:1703244. doi: 10.1080/20013078.2019.1703244
199. Zhang H, Lu J, Liu J, Zhang G, Lu A. Advances in the Discovery of Exosome Inhibitors in Cancer. J Enzyme Inhib Med Chem (2020) 35:1322–30. doi: 10.1080/14756366.2020.1754814
200. Hao S, Liu Y, Yuan J, Zhang X, He T, Wu X, et al. Novel Exosome-Targeted CD4+ T Cell Vaccine Counteracting CD4+25+ Regulatory T Cell-Mediated Immune Suppression and Stimulating Efficient Central Memory CD8+ CTL Responses. J Immunol (2007) 179:2731–40. doi: 10.4049/jimmunol.179.5.2731
201. Dai S, Wei D, Wu Z, Zhou X, Wei X, Huang H, et al. Phase I Clinical Trial of Autologous Ascites-Derived Exosomes Combined With GM-CSF for Colorectal Cancer. Mol Ther (2008) 16:782–90. doi: 10.1038/mt.2008.1
202. Brinton LT, Sloane HS, Kester M, Kelly KA. Formation and Role of Exosomes in Cancer. Cell Mol Life Sci (2015) 72:659–71. doi: 10.1007/s00018-014-1764-3
Keywords: hypoxia, exosome, cargo, tumor, changes
Citation: Jiang H, Zhao H, Zhang M, He Y, Li X, Xu Y and Liu X (2022) Hypoxia Induced Changes of Exosome Cargo and Subsequent Biological Effects. Front. Immunol. 13:824188. doi: 10.3389/fimmu.2022.824188
Received: 29 November 2021; Accepted: 25 February 2022;
Published: 04 April 2022.
Edited by:
Russel J. Reiter, The University of Texas Health Science Center at San Antonio, United StatesReviewed by:
Veronika Lukacs-Kornek, University of Bonn, GermanyAiqun Xu, The Second People’s Hospital of Hefei, China
Daxiong Zeng, Soochow University, China
Copyright © 2022 Jiang, Zhao, Zhang, He, Li, Xu and Liu. This is an open-access article distributed under the terms of the Creative Commons Attribution License (CC BY). The use, distribution or reproduction in other forums is permitted, provided the original author(s) and the copyright owner(s) are credited and that the original publication in this journal is cited, in accordance with accepted academic practice. No use, distribution or reproduction is permitted which does not comply with these terms.
*Correspondence: Xiansheng Liu, ZG9jdG9ybGl1NjlAMTI2LmNvbQ==