- 1Centro de Investigaciones, Diagnóstico y Referencia, Instituto “Pedro Kourí” de Medicina Tropical, La Habana, Cuba
- 2IHPE, Univ Montpellier, CNRS, IFREMER, Univ Perpignan Via Domitia, Perpignan, France
One of the most interesting biological models is that of snail–trematode interactions, many of which ultimately result in the transmission of several important diseases, particularly in the tropics. Herein, we review the scientific advances on a trematode–snail system in which certain populations of Pseudosuccinea columella (a common host species for trematodes) have been demonstrated naturally-resistant to Fasciola hepatica, in association with an effective encapsulation of the parasite by innate immune cells of the host, the hemocytes. Emphasis is made on the molecular and immunological features characterizing each P. columella phenotype in relation to their anti-parasitic competence, their distinctive ecological patterns and the existence of a significant cost of resistance. An integrative overview of the resistance to F. hepatica through comparative immunobiology, genetics and ecology is presented to hypothesize on the possible origins and evolution of this phenomenon and to postulate significant roles for parasite mediated-selection and environmental factors in shaping and maintaining the resistant phenotype in the field. Lastly, clues into future experimental perspectives to deeply characterize the interplay between P. columella and F. hepatica and the immunobiology of the resistance are also included. The advances revised in the present paper are only beginning to unravel mechanisms of anti-parasite innate defense responses and their evolutionary bases, and can facilitate the development of prospective approaches towards practical applications of P. columella resistance.
Introduction
The effects of parasitism on populations of the host are significant: (i) from exerting density-dependent regulation, (ii) actively contributing to the diversity patterns of populations of the host, (iii) affecting their genetic structure through selection, and possibly, (iv) the maintenance of sexual reproduction, to (v) leading in many cases, to co-speciation through host–parasite interactions (1). Concomitantly, the study of host–parasite interactions is particularly essential for the comprehension of the epidemiology and transmission of infectious diseases as there is a reciprocal natural selection between host resistance and parasite infectivity that impacts not only the spatio-temporal dynamics of parasite circulation but also the genetic structure of both entities (2). In this sense, such interplay also contributes to shaping the defense system of the host, with the latter evolving under the influence of two different but equally important selective pressures: to fight back, in a regulated and effective manner, the plethora of infective or harmful agents while remaining tolerant to host self (namely, microbiota).
As parasites continuously evolve to improve their fitness for infecting, surviving, and to be transmitted while thriving at the expense of host resources, a reduction in fitness is expected to occur in parasitized hosts compared to non-parasitized, due to the inherent mechanical, energetic and/or physiological damage exerted by the parasite. This could result in a significant selective pressure as, in turn, hosts must adapt to parasite changes to resist or to tolerate the aggression in an evolutionary “arms race” (3). Nonetheless, examples of host resistance in nature are not plentiful, as these very rare genotypes are usually less abundant due to a fitness cost of such resistance in the absence of parasitism (4). Notwithstanding, a few different types of selection of resistance have been suggested from theoretical studies on the evolution of resistance to parasites [see (5) for review]. Briefly, there is the well-known: i) frequency-dependent selection related to co-evolution of hosts and parasite under the Red Queen principle in which the parasite “tracks” the common hosts genotypes, ultimately reducing their fitness and favoring rare genotypes in a time-lagged parasite-driven genetic selection in host populations. Other theories include: ii) the directional selection for increased resistance or iii) susceptibility, and iv) the stabilizing or v) disruptive selections, all of which are tightly related to the extent of the selective pressure exerted by the parasite and/or to the degree of the associated cost of resistance. The type of selection that occurs varies between particular localities or years and it is postulated that such variation is strongly related to the nature, strength, and shape of the trade-offs associated with resistance, which are highly influenced by ecological factors (5).
The acknowledgment of the impact of environmental variables (e.g., climate, seasonality, other infections, etc.) on host–parasite interactions has been on the rise with several studies accounting for their direct influence on host susceptibility and pathogen dynamics, as environmental changes can alter the encounter and compatibility filters, the immune competence of the host and the immunobiological interplay of the system, and the final outcome of infection [see (6–8) for review]. Furthermore, the indelible link between ecological interactions and evolutionary changes through space and time in host and parasite populations (and their interplay) determines the need for integrative approaches across multiple scales of biological organization (9). This would help to comprehend evolutionary dynamics of disease transmission, to identify general processes and principles within host–parasites interactions that transcend taxonomic boundaries (2), and to attempt the understanding of spatio-temporal variation in ecological factors and their influence on parasite-mediated selection through associated trade-offs for resistance (5).
Within host–parasite systems, snail–trematode models are significant for comparative immunology, evolutionary biology, and health sciences (10). The class Trematoda (Platyhelminthes) groups many parasites highly relevant to public and veterinary health and they present an almost strict dependence upon molluscs, particularly snails acting as intermediate hosts. As a result, snails need to cope with the deleterious effects of infection in an ever-changing environment. As a very interesting model, the interaction of the snail Pseudosuccinea columella with the trematode Fasciola hepatica has aroused a multi-approach interest integrating disciplines, from classical malacology and parasitology to combined immunological, ecological, and evolutionary perspectives. This snail–trematode system stands out by presenting two different phenotypes within the same species P. columella when infection by F. hepatica occurs: some populations have susceptible individuals (with a varying degree of compatibility among populations) while others are completely resistant to the parasite. There are studies that show the worldwide distribution of susceptible populations (11) usually displaying variations in compatibility traits [see (12, 13) for discussion]. Such variation, dependant on the genotypes of the infecting parasites, reveals a co-evolution towards a certain degree of genetic matching between the host and the parasite known as polymorphism of compatibility (14, 15). However, resistant populations have been only reported until now in specific natural field sites within the Caribbean island of Cuba and whenever tested, always succeed in fighting back the infection regardless of the F. hepatica isolate, the parasitic dose, or even the infection scheme used (e.g., (12, 13, 16). Immunological and molecular studies reflect a constitutively enhanced immune competence of resistant compared to susceptible populations (17, 18), although such selection for resistance is thought to carry a cost in fitness in the absence of parasites; reproductive constraints have been associated to resistant P. columella (18–20). In this sense, distinctive ecological patterns have been related to each phenotype; in particular, resistant P. columella always occurs in relatively soft/acid waters with low malacological diversity (19).
Herein, we will briefly present this host–parasite system by reviewing the current knowledge and advances made on the topic of P. columella resistance from different biological perspectives, namely, phenotypic, molecular, immunological, and ecological standpoints. Afterwards, we will discuss the possible origins of the resistant phenotype based on the preliminary evidences and on theoretical understanding of parasite and environmental-driven selection. Lastly, as much remains to be studied for a proper understanding of the mechanistic and evolutionary bases featuring the resistant/susceptible phenotypes, clues into some future experimental perspectives will be briefly stated. The advances revised herein are beginning to unravel mechanisms of anti-parasite innate defense responses and their evolutionary bases, and can facilitate the development of prospective approaches towards practical applications of P. columella resistance.
Resistance of P. columella to F. hepatica in Cuba: What We Know
Cuba, the biggest archipelago of the Caribbean basin, is mainly plains and floodable lowlands under a tropical regime of warm temperatures and frequent rainfall throughout the year, all of which favor the occurrence of the two lymnaeid snails Galba cubensis and P. columella (21). Both species act as intermediate hosts of a number of parasites, namely, the medically and veterinary relevant trematode F. hepatica (19, 22, 23), the main etiological agent of the (re-)emergent significant zoonosis known as fasciolosis [see (24)]. In this sense, elevated rates of transmission of F. hepatica in nature (mainly related to human activities such as cattle husbandry) are believed to occur all year-round (22, 25) supported by high prevalence of infection in both lymnaeid snail species reported from field (19, 22, 26) and laboratory studies [e.g., (12, 13)]. Although ecological and parasitological studies point at G. cubensis as the main intermediate host of F. hepatica in Cuba and in the Caribbean (13, 21, 22, 27), P. columella is also a worldwide-recognized host of the parasite (11). In Cuba, P. columella presents a wide distribution in the western and central regions of the country (19). Moreover, high infection rates of P. columella by F. hepatica from 1 to 10% (19, 23), and even up to 100% prevalence (13) have been recorded respectively from the field and from experimental studies in Cuba.
However, one of the most interesting facts related to P. columella in Cuba is the occurrence of, at least six field populations that are naturally resistant to F. hepatica infection. The six populations occur in the localities of La Playita, Loma Candelaria, La Palma, El Azufre, La Coca, and Babiney, with the latter being the only one found in central Cuba [see (19) for details on their distribution]. A distinctive morphological feature consisting of a belt-like shape of small, highly abundant sharp-whitish spots in the central region of the mantle with wider and sparser spots in the upper and lowers sides has been associated to these populations [see (18, 28), and Figure 1]. All P. columella snails displaying such phenotypic markers have never been found infected with F. hepatica, either in nature or after experimental challenges, regardless of the isolates of the parasite used, the parasite dose or the infection scheme (12, 13, 16, 20, 28). In this sense, histological sections of exposed resistant P. columella showed that the penetration of F. hepatica miracidium into the snail is rapidly followed by an effective encapsulation of the parasite larvae by the hemocytes of the host (circulating immune cells of snails), observed at 24 h post-exposure (28). This powerful immunological reaction is believed to be at the base of the resistance of P. columella to F. hepatica (17, 28).
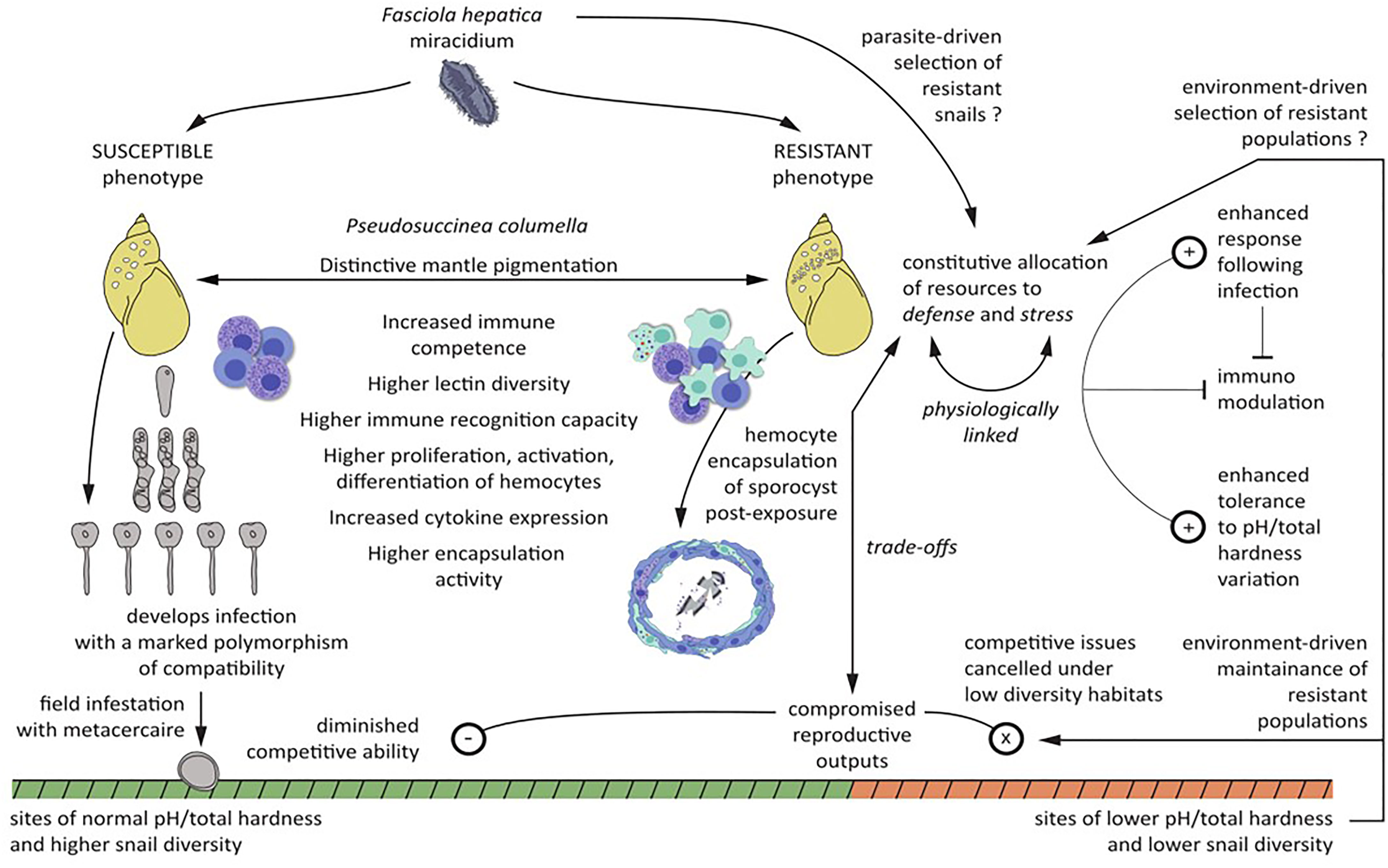
Figure 1 Comprehensive view of the main phenotypic features of resistant and susceptible Pseudosuccinea columella to Fasciola hepatica and the main results and hypothesis derived from comparative molecular and mechanistic immunobiological, ecological, and evolutionary approaches applied to this system. Symbols in circles refers to enhanced (+), diminished (−), and canceled (x) pathways.
Although resistant and susceptible P. columella belongs to the same species accordingly to significant anatomical, conchiological and genetic data (18, 29), nuclear polymorphic genetic markers and mitochondrial haplotypes cluster resistant populations in a different clade from susceptible snails, with little within-group polymorphism (11, 16, 19). This segregation implies a certain genetic determinism of resistant P. columella and opens to the possibility that such phenomenon was selected from or has derived in a different genetic pool of the majority of susceptible P. columella populations worldwide (11, 19). Furthermore, resistant populations, particularly those from La Coca and Babiney, showed an overall higher allelic richness and higher genotypic diversity than susceptible populations, and they not only significantly differed from susceptible populations but also between themselves, possibly as a result of different introductions between western and central regions of Cuba and to their in situ evolution (19).
Comparative Molecular and Immunobiological Studies Between P. columella Phenotypes: Evidences of Increased Immune Competence Towards F. hepatica Associated With the Resistant Phenotype
For two decades, several efforts have been made to challenge the resistant phenotype by increasing the odds for F. hepatica success. In this sense, resistant P. columella snails have been exposed to different sympatric and allopatric F. hepatica from Cuba [e.g., (13, 16)], which are usually genetically diversified [see (25) for details]. In addition, they have been also confronted against isolates of F. hepatica from different countries (Dominican Republic—narrow geographic scale and France—large geographic scale), trickle infections (3 exposures every 3 days) and single infections with increasing parasite loads (12). Although these attempts aimed at increasing the probability of encounter of compatible genotypic host–parasite individual combinations and at potentially decreasing the effectivity of the immune response of the host (such effects have been observed in susceptible populations used as control), they have all failed in rendering phenotypically resistant snails infected. Such stability and universality (at least at the above mentioned scale) of this phenotype paired to the potent immune response associated to it (17, 28), point at the occurrence of an immunological resistance that is not restricted by specific interactions of matching genotypes between the host and the parasite. It is, therefore, rather a phenomenon of immunological resistance than one of incompatibility and that, noteworthy, has been selected in nature (18, 28). We have postulated that a major factor/function associated to a single locus or a few loci is present or highly abundant in the genetic clade of resistant P. columella snails. Such feature is believed to be involved proximately or ultimately, in the immunobiological interaction with F. hepatica, interfering with biologically relevant functions of the parasite coded by a single locus or a few loci highly conserved (17). Contrastingly, given the polymorphism of compatibility resulted from exposing different susceptible snails to different parasite isolates [see (12, 13) for discussion], it was speculated that the major factor highly present in resistant snails is absent/scarce in susceptible P. columella and, consequently, it is the interplay among several other factors/functions between the susceptible host and the parasite that dictates the final outcome and the intensity of the infection, possibly preferentially following a matching allele model (17).
In this sense, the comprehension of the molecular and mechanistic bases that mediate P. columella resistance to F. hepatica is pivotal for comparative immunology and evolutionary biology. The rapid orchestration of the protective cellular immune response against F. hepatica in the resistant phenotype during the first 24 h post-infection (28) supports the notion of the occurrence of at least some constitutive elements participating in the resistance (18). In fact, hemocytes from naive (non-exposed) resistant snails significantly associate to higher encapsulation activity of F. hepatica sporocysts in vitro, compared to immune cells from naive susceptible snails (17). With all this in mind, the whole-snail transcriptome of P. columella and the differential proteome of the albumen gland (organ involved in both reproduction and immune response) prior infection (naïve conditions) were analyzed to compare the constitutive “molecular base line” between resistant and susceptible phenotypes (18). From this study, significant enrichments of five functions/biological processes in the resistant phenotype were recorded, among which the immune/stress response was particularly noted. Such enrichment in these biological functions was supported by the significant abundance of different transcripts identified as orthologues of recognized immune molecules involved in the recognition of pathogens, in immune regulation, and defense activation/response in association to resistant P. columella. In this sense, several types of lectins and pattern recognition receptors, CD109, cytokines and cytokine related molecules [e.g., macrophage migration inhibitory factor (MIF), granulocyte colony stimulatory factor receptor (G-CSFR), transforming growth factor 1-beta (TGF1β)], and also of signaling/regulatory transcripts (e.g., Toll-like receptors (TLR), protein C kinase (PKC), members of the superfamily of tumoral necrosis factor (TNF) receptors, interferon regulatory factors (IRF) and molecules with leucine rich repeats), and enzymatic antioxidants and molecular effectors such as ferritine, nitrite oxide synthase, catalase and superoxide dismutase where found overexpressed in resistant versus susceptible snails (18). Orthologues of these transcripts have been recognized as relevant for the immune response in other mollusk species such as Lymnaea stagnalis and Biomphalaria glabrata, and particularly, in their defense against trematodes [e.g., (30–35)]. In addition, other significant immune effectors, i.e., a G-type lysozyme and a lipopolysaccharide-binding protein/bactericidal permeability-increasing protein (LBP/BPI) (36, 37), were also revealed from the analysis of the albumen gland to be only present in the proteome of resistant snails (18). The latter made patent, at the molecular level, the significant and constitutive allocation of resources towards self-maintenance, which possibly occur at the expenses of other competing biological functions [e.g., reproduction; (38)] and thus, implying possible trade-offs associated to resistance.
In terms of P. columella–F. hepatica interaction, apparently, mannosylated residues in the surface of F. hepatica sporocysts and mannose recognition factors have central roles in the molecular interplay between the parasitic larvae and the snail hemocytes. Indeed, a marked inhibition of parasite encapsulation by P. columella hemocytes was observed when the cells were pre-incubated with mannose, irrespective of the phenotype (17). Among the previously mentioned overrepresented molecules associated with resistant P. columella, the high abundance of different types of lectins and in particular, those involved in mannose recognition, e.g., mannose-binding protein C, C-type mannose receptor 2, mannose binding protein, paired with the overexpression of immune-related signaling molecules (18) might be fundamental in driving a protective response in the resistant phenotype that begins by the proper recognition of F. hepatica. At the functional level, qualitative and quantitative differences in the surface of hemocytes between phenotypes can be presumed from the different degrees of inhibition of the encapsulation of F. hepatica by P. columella hemocytes recorded when the cells were pre-incubated with a variety of carbohydrates [i.e., glucose, galactose, fructose; (17)].
Other functional differences were observed when different aspects of the immune response were studied and compared between P. columella phenotypes following experimental infection. A significant increase of the number of circulating hemocytes, of the proliferative activity of blast-like hemocytes and in the adherence and spreading capacity of large hemocytes were verified to occur in resistant snails, at 24 h post-exposure to F. hepatica. Notably, the highest in vitro encapsulation activity, resulting in the total formation of a hemocyte capsule around F. hepatica sporocyst was recorded only in exposed resistant snails, at 24 h post-exposure (17). Additionally, a rapid increase of the expression of the cytokine granuline was recorded at 6 h post-exposure only in resistant snails (17). This cytokine participates in the proliferation, activation, and differentiation of hemocytes in trematode-challenged snails and increased levels have been associated to a decrease of the susceptibility to the infection in B. glabrata–Schistosoma mansoni system (39). Therefore, not only do resistant snails present a more competent molecular and functional base line prior infection, but they also respond more rapidly and efficiently to the parasite following infection (17, 18). These features endorse the potent immune phenotype that develops in these snails towards F. hepatica and indicate that constitutive and inducible elements share roles in the orchestration and amplification of the protective immune response that occurs in resistant P. columella (17).
Contrastingly, exposure to F. hepatica of susceptible snails rendered a less competent immune phenotype not only compared to resistant snails but also to their naïve conditions. No significant increase, neither of the total hemocytes counts nor of blast-like cells proliferation, were observed in exposed-susceptible snails. In addition, large hemocytes displaying less and/or shorter pseudopods without clear tendency to cohesion or aggregation at 24 h post-exposure compared to naïve conditions were noted, which possibly relates to the diminishment of the encapsulation capacity of F. hepatica larvae also recorded at that time point. Furthermore, a significant decrease of granulin expression at 12 h and 24 h time points, below constitutive levels, observed in the susceptible phenotype accounted for an almost 3-fold differential increased expression in resistant compared to susceptible snails at 24 h post-exposure (17). From these results, we suggested that infection by F. hepatica involves immunomodulatory/immunosuppressive parasite-driven effects that are rapidly put in place and that only affect the susceptible phenotype, possibly resulting in infection when compatible combinations take place. It has been hypothesized that the constitutive allocation of resources to defence observed in resistant snails contributes to speedily impair such parasite-mediated immunomodulation or that F. hepatica immunosuppressive factors are inefficient against resistant snails and, concomitantly, trigger the powerful protective response that develops following F. hepatica penetration [see (17) for discussion].
Selection for resistance is often associated with fitness costs, thus understanding how resistant phenotype could maintained in the field is an intricate question. To answer this question it is important to consider the environmental conditions in which host and parasite species evolved. This is particularly true for resistant P. columella that always occurs in relatively soft/acid waters with low malacological diversity. The links between resistance, fitness cost and environmental factors need thus to be investigated in the P. columella/F. hepatica interaction.
Ecological Patterns Associated With the Resistant Phenotype: Evidences of a Cost for Resistance and Environmental Abiotic Factor Selective Pressures
Taking into account the heavy investment on immune or stress response described in P. columella (see section Comparative Molecular and Immunobiological Studies Between P. columella Phenotypes: Evidences of Increased Immune Competence Towards F. hepatica Associated With the Resistant Phenotype), particularly in naïve conditions, one could ask if there is an ecological cost of P. columella resistance to F. hepatica in the absence of parasitism?
Results from Alba et al. (19) indicate that from all the malacological records of P. columella in Cuba, the majority (more than 90% of all field records) belongs to the susceptible phenotype. This contrasts with the supposition that resistant populations are the result of more ancient introductions in the island given its high genetic diversity in relation to the mostly monomorphic nature of susceptible P. columella snails from Cuba. This is particularly interesting knowing the diminished competitive potential of resistant snails compared to susceptible snails, resistance seems to impair their apparent capacity to invade and successfully colonize new habitats. In this sense, the analysis of the ecological factors occurring in the different sites where each P. columella phenotype exists has rendered a peculiar association: resistant P. columella populations were always present in sites of slightly acidic (pH = 6.2 ± 0.12), softer water (total hardness, TH = 6.3 ± 1.03°d) and lower malacological diversity [3.2 ± 1.02 freshwater snail richness (19)]. Extensive ecological studies performed state that most freshwater sites in Cuba are featured by higher pH/TH values [ranging from 7–8.5 to 12–18°d; (40)], and in fact, resistant populations represent only the 1.2% of all the registered accounts of freshwater snail populations in Cuba (19).
To clarify the significance of pH/TH on the occurrence of resistance and susceptible P. columella, life-history traits experiments were performed in the laboratory simulating the differential values recorded in the field in association with each phenotype [i.e., 5.9/4°d or 7.8/14°d; (19)]. According to this study, survival and fertility traits of P. columella snails are negatively affected by low pH/TH irrespective of the phenotype whereas, overall, all populations performed better at common pH/TH values (similar to those corresponding to the finding of susceptible populations in the field). Nonetheless, resistant populations showed a diminished reproductive potential featured by a lower fecundity rate and late reproductive peaks (La Coca population) or by delayed egg hatching (La Palma population) when compared to susceptible P. columella, regardless of the experimental setting (19). Previous studies also reported lower fecundity rates for resistant (La Palma population) compared to susceptible P. columella (20). All these results suggest a possible fitness and physiological cost associated with the resistant phenotype of P. columella (19, 20).
Interestingly, resistant snails showed higher tolerance to the low pH/TH setting than susceptible snails. Resistant snails presented higher survival, life expectancy at birth and proportion of viable eggs compared to susceptible snails (19). These results suggest that the restricted distribution of the resistant phenotype in low pH/TH water is due to the conjunction of their higher tolerance to these stringent environmental conditions and the compensation of their diminished reproductive and competitive abilities in sites of low malacological diversity (19). It has been reported, under experimental conditions, that the presence of susceptible P. columella when rearing resistant snails results in a decrease of the net reproductive rates of the latter. Contrastingly, susceptible snails increase both growth and net reproductive rates when paired with resistant snails (41), which could be related to an unbalanced competition in favor of susceptible snails. In nature, a temporal invasion of a traditionally susceptible P. columella-occurring site by a nearby resistant population coincide with the decrease of pH/TH values from 7.5 to 6.5 (19). Furthermore, ecological follow-up studies of temporal dynamics on El Azufre and La Coca (sites of low pH/TH) have shown that resistant P. columella occurs always as the more stable population compared to the other mollusk species, even compared to the acknowledged highly invasive gastropods Tarebia granifera and Physa acuta [see (19, 42)]. Both species are widely distributed in Cuba, and particularly T. granifera, has been pointed as strong competitor for pulmonates including lymnaeids.
It is significant to point out that results from the molecular studies are in harmony with what have been observed from the ecological approaches. The molecular base line of resistant P. columella is characterized by a significant allocation of resources to the constitutive production of molecules involved in immune defense and stress responses, which could not only endorse the enhanced capacity to react to F. hepatica infection but also to abiotic stressors such as particular environmental conditions (18). In this sense, and concordantly with the higher tolerance displayed for pH/TH variations, several transcripts, particularly involved in the regulation of osmotic and pH variations of the internal milieu (43, 44), e.g., several carbonic anhydrase isoforms, and major ion transporters such as Na+/K+-ATPase, H+-ATPase, Na+/H+-exchanger, are constitutively overexpressed in resistant compared to susceptible snails [see (18)]. This constitutive over-expression of genes involved in adaptation to acidic water in the resistant phenotype may specifically trade-off against reproduction. Concomitantly, resistant snails trigger a powerful protective immune cellular response involving the production of immune effectors following F. hepatica penetration (17), resulting also in a potential reproduction trade-off. This is particularly evidenced by the comparative proteomic approach conducted on the albumen gland. Albumen gland guarantees the nourishment of embryos through the production of different elements of the perivitelline fluid (45, 46), but is also involved in production of immune effectors. Thus, the metabolic rearrangements favoring the production of particular immune effectors, regulatory and catabolic enzymes in detriment of overall protein synthesis in albumen gland could be at the base of the reproductive constraints associated to the resistant phenotype (18).
Possible Influence of Parasite vs. Environmental Factors: Discussing the Selection of Resistance
As previously mentioned, resistant P. columella populations could tolerate slightly acid and soft waters better than susceptible snails and they experienced certain reproduction constraints compared to susceptible populations (19, 20). Interestingly, both, a protective immune response towards F. hepatica and an enhanced tolerance to pH/TH variations, involved higher molecular and phenotypical fitness to respond to stressors (biotic or abiotic), whereas the impairment of reproductive traits is likely a consequence of energetic trade‐offs among potentially competing physiological processes [i.e., self-maintenance/survival vs. reproduction; (38)]. All these could explain the limited distribution of resistant P. columella in the field to localities where being more tolerant to variations of water acidity/hardness or more immunologically resistant could be considered as an advantage (i.e., sites with low pH/TH or high F. hepatica pressure) thus, compensating for their low reproductive or competitive capacities.
However, the question of the origins of the primary feature of resistant snails (i.e., the immunological resistance to F. hepatica) and its association with the higher tolerance to pH/TH variations remain. Noteworthy, both the immune response and acid–base/osmotic regulation, are physiologically linked through several molecular functions, effectors and signaling pathways (47, 48). Changes on environmental and internal pH could lead to an improved immunological condition through an increase of different defense-related traits as observed in other invertebrates, e.g., increase haematopoiesis (49), phagocytic capacities of the hemocytes (48, 49), and acid-balance and anti-oxidative regulatory potential (43). The boosting of such features with pivotal roles in processes such as immune surveillance and immune response [see (50–53) for some examples], could make the snails more prone to efficiently respond to infectious challenges by being “better prepared” to recognize, phagocyte/encapsulate and kill intruders. On the other hand, an enhanced immune responsiveness may involve a metabolic reconfiguration towards a relative increase of pathways related to energy and metabolite production; e.g., respiratory rate, glycolysis, proteolysis and/or lipolysis (54). This could lead to a concomitant increase of protons, reactive oxygen species and CO2 levels in the internal milieu, a biochemical setting that it is, then, coped through an enrichment of other related functions, such as antioxidant enzymes, carbonic anhydrase, and certain ion transporters (55, 56). Therefore, the enhanced tolerance to pH/TH variations associated to resistant snails could be a result of such a metabolic reconfiguration as, in aquatic species, the acid–base and osmotic balances are closely linked through the coupled movement of H+ and HCO3− with that of Na+ via ion transporters (44). In this sense, an overexpression of immune-related transcripts/proteins with pro-activating and pro-inflammatory roles, an enrichment of energetic metabolic process, and of carbonic anhydrase and major ion regulators featured the constitutive molecular base line of the resistant phenotype (18).
Unfortunately, all these experimental and theoretical outlines remain inconclusive and from them, the hypothesis on the selection of the primary feature associated to resistance could go either way: is it the higher pH/TH tolerance a “by-product” resulting from parasite-driven selection of resistance, or is it the resistant phenotype a merely “side effect” of an environmental-driven selection for an increased fitness for compensating osmotic and acid–base variations? An environmental-driven selection could, perhaps, easily explain the broad resistance towards F. hepatica, irrespective of the infective isolate [see (12, 13, 16)], through an overall enhancement of multiple and “universal” immune functions in the snails. However, from such an environmentally-linked immune reactiveness not selected from specific parasitic pressures, it might be also expected for it to remain fairly “constant” and not to strongly develop into a highly competent induced defence following a particular infection, and possibly, for it to be extended to other infectious challenges. Contrastingly, evidences of a potent and significantly different induced immune response following exposure to F. hepatica in terms of hemocyte morphology, proliferation and encapsulation activities, and of granulin expression are reported from resistant but not from susceptible P. columella snails. Contrastingly, resistant and susceptible P. columella were equally sensitive to experimental infection by Trichobilharzia sp. from which high infection rates and cercarial outputs were attained [see (57)]. Moreover, it is noteworthy that resistant snails from La Coca have been found naturally infected with rediae, cercariae and metacercariae of Echinostomatidae (author’s unpublished data). These results suggest that there is a certain specificity of such “enhanced immune competence” towards F. hepatica that allows to uniquely recognize and to “better” respond to this particular parasitic challenge and thus, possibly fitting better into a parasite-driven selection hypothesis.
However, we have to be careful to not oversimplify the situation, considering exclusively a simple dichotomy, without considering that both selection favoring resistance and selection favoring pH tolerance could be involved in the observed natural resistant phenotype. To present an integrative view of all these results and of the possible influence of both the parasite and the environment, we hypothesized that a parasite-driven selection took place resulting in a phenotype featured by an immunological resistance to F. hepatica and a high tolerance to pH/TH changes that traded-off against reproduction. Frequency dependent selection and/or disruptive selection on resistance associate evolution of resistance through parasite mediated-selection with the occurrence of a fitness cost and of an increase of the genetic diversity of host populations [see (58, 59) for examples], could have occurred in the case of resistant P. columella [see (11, 19, 20) for genetic diversity and cost of resistance in P. columella)]. While it should be kept in mind that evolutionary processes are time-lagged, it might be argued that impaired competitive capacities due to the trade-off against reproduction could have probably already determined a negative selection for the resistant but less ecologically-competitive phenotype with the diminishment of the parasitic pressure that followed the primary selection for resistance. However, it should be considered that resistant P. columella occurred mostly in western Cuba (19), a region that has been recognized for decades to be one of the most heavily impacted by fasciolosis (19, 22, 25, 60, 61). In particular, four resistant populations are even located within or in close proximity to localities where specific transmission foci of F. hepatica have been described [i.e., La Palma, El Azufre, La Coca, Loma de Candelaria; see (20, 42, 62, 63)]. Furthermore, high prevalence of infection in lymnaeid snails (19, 22) and in livestock (25, 64), and even human cases and outbreaks (61, 65) have been historically reported in central and western Cuba, all of which presume high odds for host–parasite encounters all year-round. Snail–trematode encountering rate has proved to be significant in the field, even in sites showing low prevalence of patient infections, by the use for example of sentinel snails for Bulinus globosus/Schistosoma haematobium interaction (66). Concomitantly, experimental and observational evidences endorse a significant role for the environment, maybe in the primary selection but, in any case, in the maintenance of the phenotype in the field, as the trade-offs associated with P. columella resistance are compensated by their higher tolerance to the more restrained environmental conditions for other competitor snails that comes with lower pH/TH values. This could be particularly significant if a disruptive or even a directional selection of resistance took place, as the fitness cost associated with P. columella resistance is unlikely to be relatively weak given the limited distribution of these populations in nature in spite of their ancient presence in the island.
In any case, while resistant populations have been found so far in Cuba, it might be considered that the primary features of these populations could have been selected somewhere in North America from where P. columella is suggested to have originated. However, no exhaustive sampling towards tracking this resistant phenotype has ever been carried out in this region.
Figure 1 aimed at providing an integrative synthesis of the main phenotypic features of resistant and susceptible P. columella to F. hepatica and the main results and hypothesis derived from comparative molecular and mechanistic immunobiological, ecological, and evolutionary approaches applied to this system.
Future Experimental Perspectives: Where Do We Go From Here
There is a growing interest for understanding immunobiological interactions within vector snails as they can increase our comprehension of innate defenses from mechanistic and evolutionary points of view, of the dynamic of parasite transmission and of the multiple factors influencing parasite circulation. In the case of P. columella–F. hepatica, future studies aiming at deciphering the particular immune pathways and molecules involved during resistant/susceptible host–parasite interactions are essential to shed light on the differential determinants mediating each phenotype and to propose global mechanisms of resistance/infection for this system. Multiple “omics” approaches coupled with functional validation (RNA interference: siRNA, dsRNA) of the identified pathways following parasite exposure need to be applied to decipher the molecular and metabolic scenario in susceptible and resistant snails. In this sense, it will be also interesting to establish the inheritance pattern of the identified specific markers through mating experiments, for theoretical and practical uses. However, as self-crossing constitutes the almost exclusive reproductive strategy of P. columella (11, 19), it is likely that directed castration should be put in place to resolve this issue.
Due to the association of the resistant phenotype with an increase molecular and phenotypical competence to cope with stress (18, 19), experimental infections on stressed-snails could be worth testing in this model. Environmental factors such as an increase of temperature and starvation are known to affect snail–parasite interaction by decreasing immune capabilities of the host (67, 68), increasing their susceptibility to digenean infections (69) and, even reverting certain resistant phenotypes (70, 71). In this resistant P. columella model, the exposure to heat waves, low pH/TH, and low food income prior infection could negatively affect the energetic budget necessary for the sustainability of its enhanced immune competence following F. hepatica exposure. Meanwhile, it would be worth exploring if housing susceptible snails in low pH/TH conditions could decrease their sensitivity to F. hepatica as it could strengthen the argument towards an environmental-mediated resistance to the parasite. Furthermore, experimental studies assessing life traits and competing capacities of resistant populations reared in sympatry with other freshwater snail species at common pH/TH conditions could gauge the cost of resistance against the most frequently found gastropods in Cuba.
An interesting interaction to approach in the future in this model would be the possibility of P. columella of actively avoiding F. hepatica infection. Recent studies depicting a scenario referred to as ‘a landscape of disgust’ explain how parasite avoidance shapes the ecology and evolution of host-parasite interactions (72). Heavily miracidia-infested areas in portions of ponds, rivers or other freshwater bodies may prevent P. columella of settling, and remain in distal regions with slightly different physical conditions. This, however, would be an interesting fact to explore given that snails usually become infected passively and have little to none chance of avoidance by a direct change in behavior.
Furthermore, field and experimental measures to characterize the encounter filter of resistant P. columella (e.g., encounter rates with F. hepatica, attractiveness of F. hepatica miracidia) could be also a motivating research with implications not only for biological sciences but also for epidemiology.
In addition, experimental evolution over few generations of parasite resistance on susceptible P. columella by selectively pressing with F. hepatica or with low pH/TH housing conditions might shed light on the mechanistic bases behind the phenotypic features recorded in resistant populations. It could be also interesting to evaluate the sensibility of resistant and susceptible P. columella populations to other trematode parasites, namely, those phylogenetically close to F. hepatica and compatible with P. columella (e.g., Fasciola gigantica and Fascioloides magna) to deeply characterize the specificity of the resistance. All of these approaches deserve further investigation.
Author Contributions
Investigation, AA, AV, and BG. Writing—Original Draft Preparation, AA, AV, and BG. Writing—Review & Editing, AA, AV, JS, and BG. Project Administration, AA and BG. Funding Acquisition, AA, AV, and BG. All authors contributed to the article and approved the submitted version.
Funding
Partial financial support for this investigation was provided by the subventions granted to AA by the French Embassy in Cuba and to AV by the Institut de Recherche pour le Développement (BEST grant). This study is set within the framework of the “Laboratoires d’Excellences (LABEX)” TULIP (ANR‐10‐LABX‐41). BG was supported by the ANR JCJC INVIMORY (number ANR 13-JSV7-0009) from the French National Research Agency (ANR).
Conflict of Interest
The authors declare that the research was conducted in the absence of any commercial or financial relationships that could be construed as a potential conflict of interest.
Publisher’s Note
All claims expressed in this article are solely those of the authors and do not necessarily represent those of their affiliated organizations, or those of the publisher, the editors and the reviewers. Any product that may be evaluated in this article, or claim that may be made by its manufacturer, is not guaranteed or endorsed by the publisher.
Acknowledgments
The authors would like to thank the editors Dr. Joanna Bridger and Dr. Mathilde Knight for the kind invitation to contribute to the special issue.
References
1. Joachim H, Little T, Ebert D. Genetic Variation in a Host-Parasite Association: Potential for Coevolution and Frequency-Dependent Selection. Evolution (2001) 55:1136–45. doi: 10.1111/j.0014-3820.2001.tb00633.x
2. Hawley D, Altizer S. Disease Ecology Meets Ecological Immunology: Understanding the Links Between Organismal Immunity and Infection Dynamics in Natural Populations. Funct Ecol (2011) 25:48–60. doi: 10.1111/j.1365-2435.2010.01753.x
3. Hurtrez-Boussès S, Meunier C, Durand P, Renaud F. Dynamics of Host–Parasite Interactions: The Example of Population Biology of the Liver Fluke (Fasciola Hepatica). Microbes Infect (2001) 3:841–9. doi: 10.1016/S1286-4579(01)01442-3
4. Lively CM, Dybdahl MF. Parasite Adaptation to Locally Common Host Genotypes. Nature (2000) 405:679–81. doi: 10.1038/35015069
5. Duffy MA, Forde SE. Ecological Feedbacks and the Evolution of Resistance. J Anim Ecol (2009) 78:1106–12. doi: 10.1111/j.1365-2656.2009.01568.x
6. Budria A, Candolin U. How Does Human-Induced Environmental Change Influence Host-Parasite Interactions? Parasitology (2014) 141:462–74. doi: 10.1017/S0031182013001881
7. Lafferty KD, Kuris AM. How Environmental Stress Affects the Impacts of Parasites. Limnol Oceanogr (1999) 44:925–31. doi: 10.4319/lo.1999.44.3_part_2.0925
8. Wolinska J, King KC. Environment can Alter Selection in Host-Parasite Interactions. Trends Parasitol (2009) 25:236–44. doi: 10.1016/j.pt.2009.02.004
9. Schmid-Hempel P. Evolutionary Parasitology: The Integrated Study of Infections, Immunology, Ecology and Genetics. Oxford: Oxford University Press (2011).
10. Loker ES. Gastropod Immunobiology. Adv Exp Med Biol (2010) 708:17–43. doi: 10.1007/978-1-4419-8059-5_2
11. Lounnas M, Correa AC, Vázquez AA, Dia A, Escobar JS, Nicot A, et al. Self-Fertilization, Long-Distance Flash Invasion and Biogeography Shape the Population Structure of Pseudosuccinea Columella at the Worldwide Scale. Mol Ecol (2017) 26:887–903. doi: 10.1111/mec.13984
12. Alba A, Vázquez AA, Sánchez J, Duval D, Hernández H, SABOURIN E, et al. Fasciola Hepatica - Pseudosuccinea Columella Interaction: Effect of Increasing Parasite Doses, Successive Exposures and Geographic Origin on the Infection Outcome of Naturally-Resistant and Susceptible Snails From Cuba. Parasit Vectors (2018) 11:559. doi: 10.1186/s13071-018-3155-3
13. Vázquez AA, Sánchez J, Pointier JP, Théron A, Hurtrez-Boussès S. Fasciola Hepatica in Cuba: Compatibility of Different Isolates With Two Intermediate Intermediate Hosts, Galba Cubensis and Pseudosuccinea Columella. J Helminthol (2014) 88:434–40. doi: 10.1017/S0022149X13000382
14. Thèron A, Coustau C. Are Biomphalaria Snails Resistant to Schistosoma Mansoni? J Helminthol (2005) 79:189–91. doi: 10.1079/JOH2005299
15. Thèron A, Rognon A, Gourbal B, Mitta G. Multi-Parasite Host Susceptibility and Multi-Host Parasite Infectivity: A New Approach of the Biomphalaria Glabrata/Schistosoma Mansoni Compatibility Polymorphism. Infect Genet Evol (2014) 26:80–8. doi: 10.1016/j.meegid.2014.04.025
16. Calienes AF, Fraga J, Pointier JP, Yong M, Sánchez J, Coustau C, et al. Detection and Genetic Distance of Resistant Populations of Pseudosuccinea Columella (Mollusca: Lymnaeidae) to Fasciola Hepatica (Trematoda: Digenea) Using RAPD Markers. Acta Trop (2004) 92:83–7. doi: 10.1016/j.actatropica.2004.05.014
17. Alba A, Duval D, Sanchez J, Perez AB, Pinaud S, Galiner R, et al. The Immunobiological Interplay Between Pseudosuccinea Columella Resistant/Susceptible Snails With Fasciola Hepatica: Hemocytes in the Spotlight. Dev Comp Immunol (2020) 102:103485. doi: 10.1016/j.dci.2019.103485
18. Alba A, Tetrau G, Chaparro C, Sánchez J, Vázquez AA, Gourbal B. Field-Occurring Resistance to Fasciola Hepatica (Trematoda) in Pseudosuccinea Columella Snails: A Review From Literature and Insights From Comparative “Omic” Analyses. Dev Comp Immunol (2019) 101:103463. doi: 10.1016/j.dci.2019.103463
19. Alba A, Vázquez AA, Sánchez J, Lounnas M, Pointier JP, Hurtrez-Boussès S, et al. Patterns of Distribution, Population Genetics and Ecological Requirements of Field-Occurring Resistant and SusceptiblePseudosuccinea Columella Snails to Fasciola Hepatica in Cuba. Sci Rep (2019) 9(1):14359. doi: 10.1038/s41598-019-50894-7
20. Gutiérrez A, Perera G, Yong M, Lin W. Fasciola Hepatica (Trematoda: Digenea): Its Effect on the Life History Traits of Pseudosuccinea Columella (Gastropoda: Lymnaeidae), an Uncommon Interaction. Parasitol Res (2002) 88:535–9. doi: 10.1007/s00436-002-0625-4
21. Vázquez AA, Hevia Y, Sánchez J. Distribución Y Preferencia De Hábitats De Moluscos Hospederos Intermediarios De Fasciola Hepatica En Cuba. Rev Cubana Med Trop (2009) 61:248–53.
22. Alba A, Vázquez AA, Sanchez J, Fraga J, Martínez E, Hernández H, et al. Assessment of the FasciMol-ELISA in the Detection of the Trematode Fasciola Hepatica in Field-Collected Galba Cubensis: A Novel Tool for the Malacological Survey of Fasciolosis Transmission. Parasit Vectors (2016) 9:22. doi: 10.1186/s13071-016-1303-1
23. Gutiérrez A, Vázquez AA, Hevia Y, Sánchez J, Correa AC, Hurtrez-Bouseès S, et al. First Report of Larval Stages of Fasciola Hepatica in a Wild Population of Pseudosuccinea Columella From Cuba and the Caribbean. J Helminthol (2011) 85:109–11. doi: 10.1017/S0022149X10000350
24. Alba A, Vázquez AA, Hurtrez-Boussés S. Towards the Comprehension of Fasciolosis Re-Emergence: An Integrative Overview. Parasitology (2021) 148:385–407. doi: 10.1017/S0031182020002255
25. Vázquez AA, Lounnas M, Sánchez J, Alba A, Milesi A, Hurtrez-Boussés S. Genetic and Infective Diversity of the Common Liver Fluke Fasciola Hepatica (Trematoda: Digenea) From Cuba. J Helminthol (2016) 14:1–7. doi: 10.1017/S0022149X15001029
26. Vázquez AA, Sánchez J, Alba A, Pointier JP, Hurtrez-Boussés S. Natural Prevalence in Cuban Populations of the Lymnaeid Snail Galba Cubensis Infected With the Liver Fluke Fasciola Hepatica: Small Values do Matter. Parasitol Res (2015) 114(11):4205–10. doi: 10.1007/s00436-015-4653-2
27. Vázquez AA, Vargas M, Alba A, Sánchez J, Alda P, Sabourin E, et al. Reviewing Fasciola Hepatica Transmission in the West Indies and Novel Perceptions From Experimental Infections of Sympatric vs. Allopatric Snail/Fluke Combinations. Vet Parasitol (2019) 275:108955. doi: 10.1016/j.vetpar.2019.108955
28. Gutiérrez A, Pointier JP, Yong M, Sánchez J, Théron A. Evidence of Phenotypic Differences Between Resistant and Susceptible Isolates of Pseudosuccinea Columella (Gastropoda: Lymnaeidae) to Fasciola Hepatica (Trematoda: Digenea) in Cuba. Parasitol Res (2003) 90:129–34. doi: 10.1007/s00436-002-0824-z
29. Gutiérrez A, Pointier JP, Fraga J, Jobet E, Modat S, Pérez RT, et al. Fasciola Hepatica: Identification of Molecular Markers for Resistant and Susceptible Pseudosuccinea Columella Snail Hosts. Exp Parasitol (2003) 105:211–8. doi: 10.1016/j.exppara.2003.12.006
30. Baeza-Garcia A, Pierce RJ, Gourbal B, Werkmeister E, Colinet D, Reichhart JM, et al. Involvement of the Cytokine MIF in the Snail Host Immune Response to the Parasite Schistosoma Mansoni. PLoS Pathog (2010) 6:e1001115. doi: 10.1371/journal.ppat.1001115
31. Hahn UK, Bender RC, Bayne CJ. Involvement of Nitric Oxide in Killing of Schistosoma Mansoni Sporocysts by Hemocytes From Resistant Biomphalaria Glabrata. J Parasitol (2001) 87:778–85. doi: 10.1645/0022-3395(2001)087[0778:IONOIK]2.0.CO;2
32. Lacchini AH, Davies AJ, Mackintosh D, Walker AJ. Beta-1, 3-Glucan Modulates PKC Signalling in Lymnaea Stagnalis Defence Cells: A Role for PKC in H2O2 Production and Downstream ERK Activation. J Exp Biol (2006) 209:4829–40. doi: 10.1242/jeb.02561
33. Lockyer AE, Emery AM, Kane RA, Walker AJ, Mayer CD, Mitta G, et al. Early Differential Gene Expression in Haemocytes From Resistant and Susceptible Biomphalaria Glabrata Strains in Response to Schistosoma Mansoni. PLoS One (2012) 7:e51102. doi: 10.1371/journal.pone.0051102
34. Pila EA, Tarrabain M, Kabore AL, Hanington PC. A Novel Toll-Like Receptor (TLR) Influences Compatibility Between the Gastropod Biomphalaria Glabrata, and the Digenean Trematode Schistosoma Mansoni. PLoS Pathog (2016) 12:e1005513. doi: 10.1371/journal.ppat.1005513
35. Wu XJ, Dinguirard N, Sabat G, Lui HD, Gonzalez L, Gehring M, et al. Proteomic Analysis of Biomphalaria Glabrata Plasma Proteins With Binding Affinity to Those Expressed by Early Developing Larval Schistosoma Mansoni. PLoS Pathog (2017) 13:e1006081. doi: 10.1371/journal.ppat.1006081
36. Baron OL, Van West P, Industri B, Ponchet M, Dubreuil G, Gourbal B, et al. Parental Transfer of the Antimicrobial Protein LBP/BPI Protects Biomphalaria Glabrata Eggs Against Oomycete Infections. PLoS Pathog (2013) 9:e1003792. doi: 10.1371/journal.ppat.1003792
37. Zhang SH, Zhu DD, Chang MX, Zhao QP, Jiao R, Huang B, et al. Three Goose-Type Lysozymes in the Gastropod Oncomelania Hupensis: cDNA Sequences and Lytic Activity of Recombinant Proteins. Dev Comp Immunol (2012) 36:241–6. doi: 10.1016/j.dci.2011.06.014
39. Pila EA, Gordy MA, Phillips VK, Kabore AL, Rudko SP, Hanington PC. Endogenous Growth Factor Stimulation of Hemocyte Proliferation Induces Resistance to Schistosoma Mansoni Challenge in the Snail Host. Proc Natl Acad Sci (2016) 113:5305–10. doi: 10.1073/pnas.1521239113
40. Perera G. Écologie Des Mollusques D’eau Douce D’intérêt Médical Et Vétérinaire a Cuba. Thèse de Doctorat, Université de Perpignan (1996). 185 p.
41. Gutiérrez A, Yong M, Sanchez J, Wong M, Pointier JP. Competition Between Fossaria Cubensis and Two Isolates (Susceptible and Resistant to Fasciola Hepatica) of Pseudosuccinea Columella Under Laboratory Conditions. Haliotis (2005) 35:1–11.
42. Gutiérrez A, Hernández DF, Sánchez J. Variation of Snail’s Abundance in Two Water Bodies Harboring Strains of Pseudosuccinea Columella Resistant and Susceptible to Fasciola Hepatica Miracidial Infection, in Pinar Del Río Province, Cuba. Mem Inst Oswaldo Cruz (2005) 100:725–7. doi: 10.1590/S0074-02762005000700007
43. Freitas R, Pires A, Moreira A, Wrona FJ, Figueira E, Soares AM. Biochemical Alterations Induced in Hediste Diversicolor Under Seawater Acidification Conditions. Mar Environ Res (2006) 117:75–84. doi: 10.1016/j.marenvres.2016.04.003
44. Mattews PGD. Acid-Base Regulation in Insect Hemolymph. In: Weihrauch D, O’donnell M, editors. Acid-Base Balance and Nitrogen Excretion in Invertebrates: Mechanisms and Strategies in Various Invertebrate Groups With Considerations of Challenges Caused by Ocean Acidification. Cham, Switzerland: Springer International Publishing AG (2017).
46. Geraerts WPM, Joosse J. Freshwater Snails (Basommatophora). In: Tompa AS, Verdonk NH, Van den Biggelaar JAM, editors. The Mollusca. Orlando: Academic Press (1984).
47. Demas GE, Adamo SA, French SS. Neuroendocrine-Immune Crosstalk in Vertebrates and Invertebrates: Implications for Host Defence. Funct Ecol (2011) 25:29–39. doi: 10.1111/j.1365-2435.2010.01738.x
48. Matozzo V, Chinellato A, Munari M, Finos L, Bressan M, Marin MG. First Evidence of Immunomodulation in Bivalves Under Seawater Acidification and Increased Temperature. PLoS One (2012) 7:e33820. doi: 10.1371/journal.pone.0033820
49. Meseck SL, Alix JH, Swiney KM, Long WC, Wikfors GH, Foy RJ. Ocean Acidification Affects Hemocyte Physiology in the Tanner Crab (Chionoecetes Bairdi). PLoS One (2016) 11:e0148477. doi: 10.1371/journal.pone.0148477
50. Goodall CP, Bender RC, Broderick EJ, Bayne CJ. Constitutive Differences in Cu/Zn Superoxide Dismutase mRNA Levels and Activity in Hemocytes of Biomphalaria Glabrata (Mollusca) That Are Either Susceptible or Resistant to Schistosoma Mansoni (Trematoda). Mol Biochem Parasitol (2004) 137:321–8. doi: 10.1016/j.molbiopara.2004.06.011
51. Larson MK, Bender RC, Bayne CJ. Resistance of Biomphalaria Glabrata 13-16-R1 Snails to Schistosoma Mansoni PR1 Is a Function of Haemocyte Abundance and Constitutive Levels of Specific Transcripts in Haemocytes. Int J Parasitol (2014) 44(6):343–53. doi: 10.1016/j.ijpara.2013.11.004
52. Connors VA, De Buron I, Granath WOJ. Schistosoma Mansoni: Interleukin-1 Increases Phagocytosis and Superoxide Production by Hemocytes and Decreases Output of Cercariae in Schistosome-Susceptible Biomphalaria Glabrata. Exp Parasitol (1995) 80:139–48. doi: 10.1006/expr.1995.1016
53. Nikapitiya C, De Zoysa M, Whang I, Kim SJ, Choi CY, Lee JS, et al. Characterization and Expression Analysis of EF Hand Domain-Containing Calcium-Regulatory Gene From Disk Abalone: Calcium Homeostasis and its Role in Immunity. Fish Shellfish Immunol (2010) 29:334–42. doi: 10.1016/j.fsi.2010.04.004
54. Lochmiller RL, Deerenberg C. Trade-Offs in Evolutionary Immunology: Just What is the Cost of Immunity? OIKOS (2000) 88:87–98. doi: 10.1034/j.1600-0706.2000.880110.x
55. Epstein T, Xu L, Gillies RJ, Gatenby RA. Separation of Metabolic Supply and Demand: Aerobic Glycolysis as a Normal Physiological Response to Fluctuating Energetic Demands in the Membrane. Cancer Metab (2014) 2:7–16. doi: 10.1186/2049-3002-2-7
56. Biller-Takahashi JD, Takahashi LS, Mingatto FE, Urbinati EC. The Immune System is Limited by Oxidative Stress: Dietary Selenium Promotes Optimal Antioxidative Status and Greatest Immune Defense in Pacu Piaractus Mesopotamicus. Fish Shellfish Immunol (2015) 47:360–7. doi: 10.1016/j.fsi.2015.09.022
57. Alba A. Comparative Biology of Susceptible and Naturally-Resistant Pseudosuccinea Columella Snails to Fasciola Hepatica Infection in Cuba: Ecological, Molecular and Phenotypical Aspects. PhD Thesis in Biology Sciences, Université de Perpignan (2018). 197 p.
58. Duffy MA, Brassil CE, Hall SR, Tessier AJ, Cáceres CE, Conner JK. Parasite-Mediated Disruptive Selection in a Natural Daphnia Population. BMC Evol Biol (2008) 8:80. doi: 10.1186/1471-2148-8-80
59. Dybdahl MF, Lively CM. Host-Parasite Coevolution: Evidence for Rare Advantage and Time-Lagged Selection in a Natural Population. Evolution (1998) 52:1057–66. doi: 10.1111/j.1558-5646.1998.tb01833.x
61. Rojas L, Vázquez AA, Domenech I, Robertson L. Fascioliasis: Can Cuba Conquer This Emerging Parasitosis? Trends Parasitol (2010) 26:26–34. doi: 10.1016/j.pt.2009.10.005
62. Alba A. Desarrollo De Ensayos Inmunoenzimático Y Molecular Para La Detección De Fasciola Hepatica (Trematoda: Digenea) En Moluscos Hospederos: Evaluación Del Sistema FasciMol-ELISA Como Herramienta Para La Vigilancia Epidemiológica. PhD Thesis in Health Sciences, from Instituto Pedro Kouri de Medicina Tropical de la Habana, Cuba 152 p (2016). doi: 10.13140/RG.2.2.12459.08485
63. Wong L, Vázquez AA, Quesada M, Sánchez J, Hevia Y, Fuentes J, et al. Estudios Ecológicos En Moluscos De Importancia Médico-Veterinaria En La Granja De Desarrollo La Coca. Rev Cubana Med Trop (2010) 62:16–20.
64. Brito E, Hernández MA, de la Fé Rodríguez P, Silveira EA. Prevalencia, Decomisos De Hígado Y Pérdidas Económicas Por Fasciola Hepatica En Mataderos Bovinos De Tres Provincias De La Región Central De Cuba. REDVET (2010) 11:n040410.
65. Díaz R, Garcés M, Millán LM, Pérez J, Millán JC. Comportamiento Clínico-Terapéutico De Fasciola Hepatica En Una Serie De 87 Pacientes. Rev Cubana Med Trop (2011) 63:268–74.
66. Allan F, Dunn AM, Emery AM, Stothard JR, Johnston DA, Kane RA, et al. Use of Sentinel Snails for the Detection of Schistosoma Haematobium Transmission on Zanzibar and Observations on Transmission Patterns. Acta Trop (2013) 128:234–40. doi: 10.1016/j.actatropica.2013.01.003
67. Leicht K, Jokela J, Seppälä O. An Experimental Heat Wave Changes Immune Defense and Life History Traits in a Freshwater Snail. Ecol Evol (2013) 3:4861–71. doi: 10.1002/ece3.874
68. Seppälä O, Jokela J. Maintenance of Genetic Variation in Immune Defense of a Freshwater Snail: Role of Environmental Heterogeneity. Evolution (2010) 64:2397–407. doi: 10.1111/j.1558-5646.2010.00995.x
69. Leicht K, Seppälä O. Infection Success of Echinoparyphium Aconiatum (Trematoda) in Its Snail Host Under High Temperature: Role of Host Resistance. Parasit Vectors (2014) 7:192. doi: 10.1186/1756-3305-7-192
70. Ittiprasert W, Knight M. Reversing the Resistance Phenotype of the Biomphalaria Glabrata Snail Host Schistosoma Mansoni Infection by Temperature Modulation. PLoS Pathog (2012) 8:e1002677. doi: 10.1371/journal.ppat.1002677
71. Knight M, Ittiprasert W, Arican-Goktas HD, Bridger JM. Epigenetic Modulation, Stress and Plasticity in Susceptibility of the Snail Host, Biomphalaria Glabrata, to Schistosoma Mansoni Infection. Int J Parasitol (2016) 46:389–94. doi: 10.1016/j.ijpara.2016.03.003
Keywords: host–parasite interaction, snail immunity, cost of resistance, selection of resistance, immuno-ecology
Citation: Alba A, Vázquez AA, Sánchez J and Gourbal B (2022) Immunological Resistance of Pseudosuccinea columella Snails From Cuba to Fasciola hepatica (Trematoda) Infection: What We Know and Where We Go on Comparative Molecular and Mechanistic Immunobiology, Ecology and Evolution. Front. Immunol. 13:794186. doi: 10.3389/fimmu.2022.794186
Received: 13 October 2021; Accepted: 03 January 2022;
Published: 24 January 2022.
Edited by:
Mathilde Knight, George Washington University, United StatesReviewed by:
Xiaocui He, La Jolla Institute for Immunology (LJI), United StatesMichael Zimmermann, Shenandoah University, United States
Maurine Neiman, The University of Iowa, United States
Copyright © 2022 Alba, Vázquez, Sánchez and Gourbal. This is an open-access article distributed under the terms of the Creative Commons Attribution License (CC BY). The use, distribution or reproduction in other forums is permitted, provided the original author(s) and the copyright owner(s) are credited and that the original publication in this journal is cited, in accordance with accepted academic practice. No use, distribution or reproduction is permitted which does not comply with these terms.
*Correspondence: Benjamin Gourbal, benjamin.gourbal@univ-perp.fr; Annia Alba, annia.alba@gmail.com