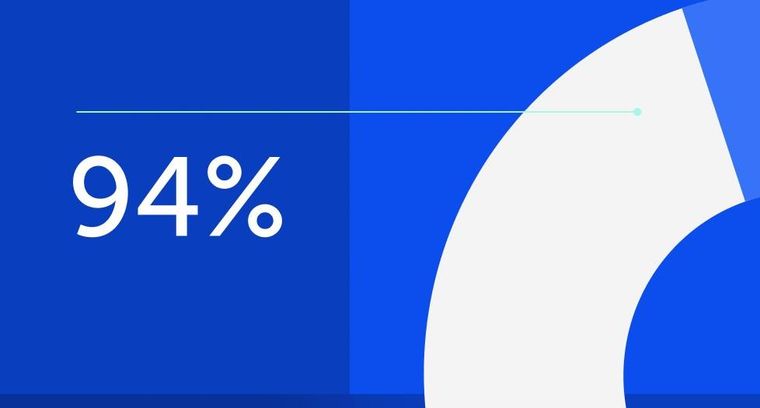
94% of researchers rate our articles as excellent or good
Learn more about the work of our research integrity team to safeguard the quality of each article we publish.
Find out more
REVIEW article
Front. Immunol., 22 August 2022
Sec. Cancer Immunity and Immunotherapy
Volume 13 - 2022 | https://doi.org/10.3389/fimmu.2022.757480
CD47 is ubiquitously expressed on the surface of cells and plays a critical role in self-recognition. By interacting with SIRPα, TSP-1 and integrins, CD47 modulates cellular phagocytosis by macrophages, determines life span of individual erythrocytes, regulates activation of immune cells, and manipulates synaptic pruning during neuronal development. As such, CD47 has recently be regarded as one of novel innate checkpoint receptor targets for cancer immunotherapy. In this review, we will discuss increasing awareness about the diverse functions of CD47 and its role in immune system homeostasis. Then, we will discuss its potential therapeutic roles against cancer and outlines, the possible future research directions of CD47- based therapeutics against cancer.
CD47 is a membrane receptor glycoprotein composed of a heavily glycosylated N-terminal IgV domain and five transmembrane helices domain with a short cytoplasmic tail (1–3). It was originally identified as a tumor antigen on human ovarian cancer specimens (4). This transmembrane protein is well recognized for its role in “don’t eat me” anti-phagocytic signals by binding to SIRPα (signal regulatory protein alpha) (5). Additionally, TSP-1 (thrombospondin-1) and integrin α2β1 and αvβ3 have also been shown to be CD47 ligands (1, 6) (Figure 1).
Figure 1 CD47 binding partners and downstream signaling mediators of CD47-SIRPα interaction.The type of signals triggered by CD47 relies on many factors, of which the foremost is the type of ligand that it binds. Three major groups of ligands are identified to exhibit capacity of binding to CD47, namely, SIRPα, TSP-1, and integrins. The interaction between CD47 and SIRPα causes cytoplasmic ITIM phosphorylation and subsequently recruits SHP-1/2. Both of SHP-1 and SHP-2 can inhibit accumulation of myosin-IIA at the phagocytic synapse, which finally inhibit the process of phagocytosis.
CD47 is involved in multiple fundamental cellular functions, including cell migration, apoptosis, and axon development (7–9). It is widely expressed on the surfaces of normal cells, especially hematopoietic cells and is best known for its interaction with SIRPα (10). SIRPα is a myeloid-specific immune checkpoint and has been classically described as counterbalanced by a variety of activating membrane receptors (11).The interaction between CD47 and SIRPα emerges as a key regulatory component of innate immune checkpoint by regulating signal transduction and cell clearance. In addition, CD47 is recognized as pivotal for erythrocyte homeostasis, during which both SIRPα and TSP ligands have been shown to be involved (12).
In this article, we will discuss basic functions of CD47 and its associated ligands, review the current knowledge about the involvement of CD47 in host cell phagocytosis, and survey current strategies for developing CD47-based immunotherapy for tumors. In addition, side effects and future research directions for CD47-based immunotherapy will be evaluated.
The most investigated binding partner of CD47 is SIRPα, which is highly expressed in neurons and a subset of myeloid hematopoietic cells such as dendritic cells (DCs) and macrophages (13). SIRPα is a transmembrane protein comprised of cytoplasmic region with four immunoreceptor tyrosine-based inhibition motifs (ITIMs) and extracellular region with three immunoglobulin (Ig)-like domains (14). The NH2-terminal V-like domain of SIRPα is responsible for the binding the extracellular Ig-domain of CD47, whereas ITIMs provide the binding sites for the src homology-2 (SH2)-domain-containing protein tyrosine phosphatases SHP-1 and SHP-2 (15). Recruitment of SHP-1 and SHP-2 phosphatases prevents myosin-IIA accumulation at the phagocytic synapse (16).
By combining different ligands, different signaling pathways can be triggered: When CD47 interacts with SIRPα on the surface of phagocytes, it promotes phosphorylation of the intracellular ITIMs and activates the inhibitory phosphatases SHP-1 and SHP-2, which will inhibit the activation of immune cells by dephosphorylation of proteins containing immunoreceptor tyrosine-based activation motifs (10) (Figure 1). Meanwhile, the dephosphorylation cascade initiated by CD47 inside phagocytes lead to deactivation of myosin-II and thereby preventing contractile engulfment (16). Molecular modeling revealed that a VMM motif in C-terminus of TSP-1 is an optimal locus to bind to CD47 (17). TSP-1-CD47 interaction regulates multiple biological functions such as cellular migration, angiogenesis, adhesion, cell aging (18). 4N1K, a peptide corresponding to the CD47 binding region of TSP on IL-12 and TNF-α production, was reported to mediate significant biological effects that were CD47‐independent (19–21). PKHB1, the first‐described serum‐stable soluble CD47‐agonist peptide, directly induced T‐leukemic cell death by engaging the CD47 receptor (20).
CD47 was originally also known as integrin-associated protein (IAP) in earlier studies because of its interaction with aIIbβ3 and a2β1 and αvβ3 integrins (22). In this manner, CD47 functions as a key regulator of migration of smooth muscle cell and platelet activation, etc. (6, 23).
There are other SIRP-family members sharing significantly conserved amino acid sequences within the extracellular domains but different signaling potentials, which are recognized as “paired receptors” (10, 24). In addition to aforementioned SIRPα, the SIRP family have the other members, SIRPβ1, SIRPβ2, SIRPγ, and SIRPδ (13, 25). Among these members, only SIRPγ exhibit a lower affinity with CD47 than that for SIRPα (Figure 2).
Figure 2 Schematics of structure of SIRP family members. Besides SIRPα, SIRPβ1, and SIRPγ have also been identified in humans. Both of SIRPβ1 and SIRPγ consist of three Ig-like loops in their extracellular domains. SIRPβ1 is characterized by a basic amino acid side chain in its transmembrane domain with a very short cytoplasmic region. This transmembrane region is indispensable for binding of DAP12 (DNAX activation protein 12). It has been established that SIRPβ1 can mobilize the tyrosine kinase Syk, which was followed by MAPK (mitogen-activated protein kinase) activation and microglial phagocytosis enhancement (26). However, it remains unknown what the extracellular ligand for SIRPβ1 and how it might regulate cellular function. There is also a short cytoplasmic region in SIRPγ, but it is quite different from SIRPβ1. The former lacks a charged amino acid residue in its transmembrane region. Nevertheless, SIRPγ can still interact with CD47 by the way of protein-protein binding studies (27). One study has demonstrated that endothelial cell CD47 interacting with SIRPγ plays an important role in T-cell trans-endothelial migration (28). SIRPβ2 is expressed by cells of the monocyte-macrophage lineage and presumably has an association with DAP12 or a similar adaptor (14). SIRPδ has only one domain and has not yet been found any obvious means of membrane attachment (10).
In addition, several cytoplasmic binding partners of CD47 have been identified, including protein linking IAP and cytoskeleton-1 (PLIC-1), ubiquilin-2 (PLIC-2), vascular endothelial growth factor receptor (VEGFR2), and exportin-1 (18, 29–31). These binding receptors are being exploited in the design of new immune-therapeutic approaches and more robust data are yet to follow on clinical significance of them.
In earlier times, CD47-SIRPα interaction was best known for its role as a negative regulator during erythrocytes clearance (32, 33). The original evidence for this came from compelling research in which CD47 knockdown red blood cells, which were quickly cleared by spleen macrophage (33). In contrast, normal erythrocytes can survive for more than 40 days in mice by the reason of CD47 expression (33). Subsequently, it was found that aging erythrocytes have some conformational changes (possibly induced by oxidative modification) in CD47, which change from a “don’t-eat me” configuration into an “eat-me” signal (12). By this token, CD47 not only serves as a pro-phagocytic signal but also behaves as a molecular switch regulating erythrocyte homeostasis (Figure 3). This process is thought to be highly associated with Gaucher disease, which is a genetic disorders with glucocerebroside accumulations in cells (40). Comparative analyses of membranous CD47 expression showed that erythrocytes from untreated Gaucher patients have week CD47 expression but can be overturned upon enzyme-replacement remedy (41).
Figure 3 Signals involved in the interaction between macrophages and erythrocytes regulating clearance.Macrophages exhibit some receptors for phosphatidylserine, such as Tim- 1, CD300, Stabilin-2, and Tim-4, which are presumed to deliver a pro-phagocytic signal (34–37). Band 3 is the major target of Nabs. Band 3 clustering and opsonization with Nabs and complements on the erythrocytes facilitates binding to the macrophage via Fc receptors and CR-1 and thereby promotes phagocytosis (38, 39). CD47 is a “don’t eat me” signal that plays a crucial role in erythrocytes homeostasis. Binding of CD47 to SIRPα generates an erythrocyte phagocytosis-associated deactivation of myosin IIA, which is a primary contributor to phagocytosis because of its participation in macrophage actinomyosin contraction. Tim, T cell immunoglobulin; Nabs, natural occurring antibodies; CR-1, complement receptor.
Kusakari and colleagues’ study revealed that CD47-SIRPα interaction lead to the internalization of partial membrane by contiguous cells (42). Then it is proved that CD47 did not inhibit, but rather facilitated both phosphatidylserine-independent and phosphatidylserine-dependent uptake of apoptotic cells in the murine system (43). These results confirm prior evidence that CD47 is responsible for removal of some cells rather than just one negative regulator of phagocytosis.
CD47 can be found expressed on virtually all immune cells, but the level of expression varies markedly in severity depending on cell types and pathologic conditions (2). For T cells, CD47 signaling is associated with a wide array of cellular processes, from activation to induction of deaths (44). For example, intracellular signaling induced by CD4 stimulates their proliferation, thereby improving the immunological response (45–47). Additionally, CD47 is preferentially expressed on long-lived memory T cell progenitors, which may increase their survival time by preventing clearance by macrophages (48).
CD4+ T cells differentiation can be regulated not only by CD47 on themselves but also on antigen presenting cells (APCs). Avice et al. demonstrated that CD47 ligation can selectively inhibit the development of naive T cells into Th1 effectors, which are characterized by production of high levels of IFN-γ, lymphotoxin-a (LT-α), and TNF-α (49, 50). Specifically, inhibiting the expression of IL-12R via T cells, impairing the responsiveness of T cells to IL-12, and decreasing IFN-γ production by dendritic cells are among different options how CD47 influence Th1 response (49, 51). Moreover, application of F(ab’)2 fragments from CD47-binding peptides or an anti-CD47 mAb has been shown to elicit similar effects (49, 52). The research from Cham recently indicated that CD47 blockade enhances T cell responses and speeds lymphocytic choriomeningitis virus clearance (53). These evidences suggest that targeting CD47 holds much promise to treat a wide range of immunity function disturbance with undesirable immune responses.
Liu et al. reveal data suggesting that T cells are required for tumor regression and mediate most of the anti-tumor effects of the CD47 blockade (54). Using syngeneic mouse models of cancer, rather than transplanted xenografts, they first demonstrated that the therapeutic effect mediated by the CD47 blockade is specifically CD8+ cytotoxic T cell–dependent. Phagocytosis of tumor cells by DCs and cross-present antigens to activate downstream CD8+ T initiate adaptive immunity, thereby effectively bridge innate immunity and adaptive immunity. Mechanistically, these anti-tumor effects rely on the cytosolic DNA sensor STING expressed by CD11c+ DCs instead of signaling through MyD88, for example by Toll-like receptors.
CD47 promotes the differentiation of Treg cells and regulates homeostasis of activated CD103+ Treg cells (18, 55); however, a deficiency of CD47 does not alter the inhibitory function of Treg cells (56). Interestingly, naive T cells exhibit an elevated expression of FoxP3 after treatment with anti-CD47/anti-TSP-1, which is akin to the effects of TGF-β and IL-10 (55). CD47 is found highly expressed in the Treg cells in atopic dermatitis patients, suggesting it may contribute to the increased population of Treg cells in this type of eczema (57). In addition, Tregs can protect dopaminergic neurons against MPP+ neurotoxicity underlying CD47-SIRPα interaction (58). This finding provides a novel perspective into how to effect neurodegenerative disease progression by sustaining neuroprotective immunity.
Macrophages are involved in recruitment of immune cells to eliminate foreign materials, aid in tissue repair, and eventually return the tissue to homeostasis (59, 60). CD47 is the dominant macrophage checkpoint, which acts as a “don’t eat me signal” (33). Through its interaction with SIRPα, aged erythrocytes and other ineffective normal cells are quickly cleared by macrophage in the spleen along with accelerated phagocytosis. Further evidence suggest that CD47-SIRPα axis contributes to macrophage activity regulation and alters the polarization state of macrophages (61).
DCs are professional antigen presenting cells with the unique ability to induce naïve T cells activation. They also represent an abundant and stable source of TSP, which can function as an autocrine factor suppressing IL-12 and IFN-γ production by means of interaction with CD47 (62, 63). Meanwhile, the contact between CD47 on T cells and SIRPα on DCs may also participate in the maintenance of immune hemostasis (52). Compared with macrophages, DCs appeared to be the major APCs that cross-prime cytotoxic T cells following CD47 blockade since the therapeutic effect was severely impaired after DC depletion rather than macrophages (54). The authors attributed this different efficiency to higher expression of Ifna mRNA on DCs (54).
CD47 plays negative roles in NK cells activation and proliferations upon binding its ligand TSP-1, while lack of CD47 increase NK cells activation and cytotoxicity (64, 65). As a SIRPα counter-receptor, CD47 is involved in NK precursors engraftment in humanized mice (66, 67). In tumor microenvironment, CD47 impairs the recruitment of NK cells, whereas treatment with anti-CD47 antibody increases NK cells killing against tumor cells by enhancing expression of granzyme B and IFN-γ (65). Though future studies are still needed to better understand the intricate mechanisms recruiting NK cells, targeting CD47 has a therapeutic potential as a NK cell checkpoint in tumor micro-environment (68).
Emerging evidences indicate that CD47 plays an important role in trans-endothelial migration of neutrophil and other leukocytes (69, 70). Diverse anti-CD47 mAbs have been illustrated to suppress neutrophil movements across cell mono-layers in vitro (7, 71, 72). Additionally, neutrophil mobilization was shown to be retarded in vivo in CD47-deficient mice by means of intraperitoneal inoculation of Escherichia coli (70). Also, recent studies demonstrated that CD47 molecule expressed on myeloid DCs is a critical factor in controlling efficiently traffic across lymphatic and endothelial vessels, seeding in secondary lymphoid organs and participating in T-cell priming (54, 73).
In the current era in oncology, checkpoints immunotherapy of hematopoietic and solid malignancies are becoming a promising mode of treatment for cancer patients (74, 75). But the fact that not all cancer patients benefit from adaptive checkpoints immunotherapy catalyzed enormous interests in the targeting of novel immune checkpoint receptors. Considering innate immune system is the first line of defense directly target cancer cells, harnessing innate immunity represents another potential therapeutic avenue for cancer treatments. Innate checkpoints have recently received some attention regarding their possible roles in tumor-mediated immune escape. A list of innate checkpoints of interest are under investigation, including CD47-SIPα axis, TAM family (Tyro3, Axl, and MerTK), Siglec-9, CD24-Siglec-10 axis (76–79).
The theoretical basis for CD47 functioning as a promising checkpoint in cancer therapy is due to its pivotal role in balancing both inhibitory and stimulating activities of myeloid cells (Figure 4). Firstly, CD47 ligation induce apoptosis of tumor cell through a caspase-independent mechanism (Figure 5) (84, 85). Secondly, anti-CD47 leads to tumor cell phagocytic uptake by antigen presenting cells and subsequent antigen presentation to T cells (76, 86). Thirdly, anti-CD47 abrogates the TSP-1-mediated inhibitory effect against human NK cells but increase NK cells activation and cytotoxicity (65). CD47 blockades have shown initial success in early- phase clinical trials for many human cancers, either alone or in combination with other agents (Table 1) (87, 88). Fourthly, preclinical study by Xiaojuan L et al. demonstrated that therapeutic effect of CD47 blockade depends on STING (stimulator of interferon genes), which induces a type I/II IFN response mediated by dendritic cells and CD8+ T-cells (54). Fifthly, some literatures revealed that CD47/TSP-1 pathway also has pleiotropic effects on immunity system and may present a new target for potential cancer therapeutics (89, 90).
Figure 4 Targeting CD47 immune checkpoint for cancer immunotherapy.Cancer cells rely on the expression of “don’t eat me” signals such as CD47 to inhibit their phagocytotic clearance by macrophages, while blocking CD47 reduces tumor growth by enabling macrophages to phagocytose the cancer cells [reviewed in refs (22)].
Figure 5 Mechanisms of CD47-induced caspase-independent cell death.CD47 induces a caspase-independent cell death, characterized by intact or slightly modified nuclei, reduced cell viability (80). The process of cell apoptosis includes PS (phosphatidylserine) exposure, disruption of mitochondrial function, and cytoskeleton rearrangement (1): K+ is a critical component of volume regulatory response, and leakage of K+ is a simple way to accommodate a rapid decrease in cell volume; CD47-induced cell death was dependent on K+ efflux (81); (2) Mechanisms involved in PS exposure remain poorly understood. Veronique M et al. proposed that CD47 ligation initially induce triggering of actin polymerization, perhaps via Cdc42/WASP pathway. This event then leads to mitochondrial changes including matrix swelling and ΔΨm (mitochondrial transmembrane potential) loss, followed by PS exposure or bypass of mitochondria and direct induction of PS externalization (81); (3) In addition, Bcl-2 homology 3 (BH3)-only protein 19 kDa interacting protein-3 (BNIP3) is indispensable for the pro-apoptotic effect of CD47 (82). A simple model-based on the translocation of BNIP3 from the inner surface of the cell membrane to the mitochondria is proposed since BNIP3 can only exert its pro-apoptotic effect at the mitochondria membrane (83).
Whether blocking CD47 alone is sufficient to induce phagocytosis is a matter of dispute (91, 92). Some evidence supports that targeting CD47 would be sufficient for elimination of tumor cells by macrophage (93, 94). It should be noted that most of the antibodies tested before include an Fc region, which recognizes Fc receptor γ-chain (FcRγ) on macrophage, neutrophils, and NK cells (95, 96). On one hand, activating FcRs associated with FcRγ of immunoreceptor tyrosine-based activation motif (ITAM) and, thereby, trigger phagocytosis of macrophage (96). On the other hand, the interaction between Fc region and FcRs simultaneously opsonize the target cells for antibody-dependent cell-mediated cytotoxicity (ADCC) since NK cells are the crucial mediators of this reaction in vivo (87, 97). Moreover, FcR-activated NK cells can also secrete IFN-γ, TNF-α, and lead to increased expression of interleukin-21 receptor that suppress tumor growth (98, 99). Therefore, insights raise intriguing questions as to whether anti-tumor effects induced by CD47-antibodies occur through Fc-dependent mechanisms? Which acts as the predominant signal responsible for anti-tumor effects, modulation of phagocytosis or ADCC?
It is of great significance to the development of therapeutic agents because of balance between their direct killing effect against cancer cells and their potentially hazardous side effects on normal CD47-positive cells. If ADCC generally dominates during the stage of immune response, tolerable toxicity can be achieved with an impaired therapeutic efficacy (100).
To minimize the effect of Fc-dependent effector functions, investigators designed a chimeric antibody (Hu5F9-G4) containing the Fc portion that binds to FcRs with a lower affinity compared to other human IgG subclasses (101). ADCC and complement- dependent cytotoxicity (CDC) activities were tested, and Hu5F9-G4 was unable to induce ADCC and CDC at any of the concentration tested (101). But it still triggered potent macrophage-mediated phagocytosis against human hematological cells, suggesting that CD47-SIRPα blockade alone may be sufficient to induce tumor regression (101). However, the application of the agent having lower affinity with FcRs did not completely exclude the direct ADCC since even minimal engagement of FcRs could be enough to activate signaling cascade of phagocytosis.
The best way to completely exclude direct ADCC is to use F(ab’)2 fragment antibodies. F(ab’)2 fragment is characterized with removal of most of the Fc fragment, while leaving the hinge region intact. Research data showed that anti-CD47 F(ab’)2 fragment was able to promote engulfment of Raji and other lymphoma cells in vitro (88, 102). Intriguingly, this phenomenon of engulfment activation was not applicable to Jurkat and various colon carcinoma cell lines (91, 102). Studies later confirmed that the first group of tumor cells expressed other phagocytic receptors that can bypass the need for FcR engagement (88).
CD47 antibodies with devoid of Fc portion are theoretically an ideal candidate for the development of therapy approaches to modulate CD47-SIRPα interaction. This can be achieved via the form of nanobody or single-chain variable fragment (103). For example, HuNb1-IgG4, an innovative anti-CD47 nanobody, has been developed to reduce adverse effects of blocking CD47-SIRPα interaction (103). It was shown that HuNb1-IgG4 presented potent anti-tumor activities in vivo, and more importantly, it exhibited high safety for hematopoietic system in cynomolgus monkeys (103).
As is discussed before, CD47-SIRPα blockade alone, in most cases, is not sufficient for the induction of significant phagocytosis and subsequent antitumor activity. A secondary stimulus, such as other prophagocytic signal (i.e., calreticulin, SLAMF7, and macrophage-1 antigen) or an opsonizing antibody (i.e., rituximab) is indispensable (104). Calreticulin is proposed to be a pro-phagocytic signal recognized by lipoprotein receptor-related protein-1 (LRP-1) on macrophage (105, 106). SLAMF7 (signaling lymphocytic activation molecule family 7, also known as CRACC, CS1, and CD319) remarkably facilitates engulfment of a few hematopoietic tumor cells expressing SLAMF7 such as Raji during CD47-SIRPα blockade (45, 102). This explains why anti-CD47 F(ab’)2 fragment was able to promote engulfment of Raji (107). In addition, SLAMF7 interact with macrophage-1 antigen (MAC-1) to form a protein complex on the macrophage surface (102). The complex consists of two ITAM-containing receptors, FcRγ and DAP12, which trigger the phagocytic machinery through SRC kinase, spleen tyrosine kinase, and Bruton’s tyrosine kinase (102). All the three prophagocytic receptors can be exploited to enhance anti-tumor response during CD47-SIRPα blockade.
In some aspects, small-molecule agents are superior to therapeutic antibodies since they can increase active time period and absorbability in the body (108). Research on small-molecule inhibitors targeting CD47 has also been one of the focuses for cancer immunotherapy. These inhibitors can modulate CD47 at the transcriptional, translational, and post-translational modification levels. In a concentration-dependent manner, both NCGC00138783 and pep-20 bind to CD47 and exert their blocking functions (109, 110). Some small-molecule agents can suppress CD47 expression at transcriptional or translational levels, including RRx-001, metformin, 4-methylumbelliferone, JQ1, and gefitinib (111). Excitedly, RRx-001 has showed potent anti-cancer activities via different pathways and has been proceeded into Phase III clinical trial (NCT03699956) (112, 113). At the post-translational modification level, QPCTL (glutaminyl-peptide cyclo-transferase-like protein) disrupts CD47 pyroglutamate formation and is regarded a novel target to augment antibody therapy of cancer (114, 115). To sum up, there have been big progress in this endeavor recently and this field deserves increased attention in future.
Given the ubiquitous expression of CD47 on normal cells, potential side effects such as anemia will need to be considered though several clinical trials are underway and have produced impressive clinical results in recent years (Figure 6).
Figure 6 Proposed solutions to the side effects of anti-CD47 treatments. (A) antibody fusion proteins, e.g., TTI-621 (116) or ALX-148 (26); (B) “initiation doses” followed by “therapeutic doses”, e.g., Hu5F9-G4 (117); (C) pro-drug vesicle-induced immunogenic cell death combined with CD47 blockade (54); or (D) tumor-targeting nanoparticles loaded with anti-CD47 antibody (27).
In several preclinical solid tumor models, tumor-specific delivery of CD47 blockade demonstrated superior accumulation and fewer side effects at tumor sites compared with systemic administration (118) (119). Novel drug delivery system has emerged as a critical modulator in the development of anti-CD47 therapeutics, such as nanomedicine and gel matrix (117, 120–122).
Bispecific antibodies are helpful for the purpose to limit bystander effects due to widespread CD47 expression. Heretofore, some bispecific antibodies have been developed with one arm binding and blocking CD47 to prohibit its interaction with SIRPα, and the other arm binding tumor-associated antigens such as CD19, CD20, and PD-L1 (123). In comparison to other CD47 antibodies, bispecific antibody was engineered to have a lower affinity towards CD47, limiting binding activity with normal cells expressing CD47. Here, proof was provided by an anti-CD47/CD19 bispecific antibody that selectively block the CD47-SIRPα on malignant cells expressing CD19 and interact weakly with normal cells expressing CD47 (124). Such a bispecific design could be applied to limit the negatively impacts caused by “antigen sink”.
In addition to drug delivery system and bispecific antibody, there are many other strategies to prevent anemia caused by anti-CD47 therapeutics: gradually increase therapeutic dose (125); differentiate erythrocytes CD47 and tumor cell-expressing CD47 (114, 126–130); sacrifice antibodies mediated ADCP (antibody-dependent cellular phagocytosis) (131–133); pro-body technology (134) (120). Apart from anemia, issues with thrombocytopenia, hyperbilirubinemia, and neutropenia have also limited the use of anti-CD47. For further reading on this field, we recommend to the curious reader one excellent review by Yuchen W et al. (135). The review also high-light recent advances in tumor therapy targeted on the CD47-SIRPα axis and provides ideas for further clinical transformation.
Although early data with agents targeting either CD47 or SIRPα have highlighted the therapeutic potential by sending a potent “don’t eat me” signal to prevent phagocytosis, no Phase III study convincingly demonstrated the efficacy of these novel therapeutic targets for cancer treatment so far. ENHANCE (NCT04313881), a randomized, double-blind, placebo-controlled multicenter Phase III study from Forty-Seven (Gilead Sciences) aimed to compare the effects of treatment with magrolimab plus azacitidine and placebo plus azacitidine in untreated patients with myelodysplastic syndrome, has recently resumed after FDA lifted the partial clinical hold placed on the item. This is the only Phase III clinical trial related to CD47 blocking therapy. Inhibitors and antibodies against CD47 in immunotherapy have several limitations to emerge, and some features of the therapy must be considered.
The first limitation is to search for safe potent antibodies that are not highly bound to erythrocytes at early step in terms of safety. Earlier detection of the potential toxicity during antibody development can help cut costs and improve success rate. Dual targeting bi-specific antibodies of both TAA (tumor-associated antigens) and CD47 can also be designed to resolve this issue, which can direct CD47 blockade selectively to cancer target cells, thereby improving the safety of CD47 blocking therapy (136) (137).
Secondly, immunotherapy with CD47 blockade has achieved impressive successes in treatment of hematological malignancies. But the clinical efficacy of CD47 blockades in solid tumors has been much less rewarding due to the tumor heterogeneity and intricacies of the tumor microenvironment. Compared with macromolecule agents, small molecule inhibitors are more penetrative to the targets and have broad prospects for development and application in solid tumors (138). For example, a humanized CD47/GPC3 (Glypican-3) BsAb enhances the Fc-mediated effector function and has significantly better efficacy than the combined treatment using CD47 and GPC3 monoclonal antibodies in the hepatocellular carcinoma model of xeno-transplantation (139). Apart from bispecific antibodies, nanobodies have also received widespread attention due to their deeper penetration in solid tumors. HuNb1-IgG4, a new type of CD47 nano-antibody that has a low affinity for human erythrocytes, enhances tumor phagocytosis mediated by macrophages in vitro, and shows strong anti-tumor activity in ovarian cancer and lymphoma (103). Thirdly, CD47 can also be used in other therapeutic areas, including atherosclerosis, neurological disorders, and autoimmune diseases. By administration of CD47-blocking antibodies, the atheroprone mice would develop significantly smaller atherosclerotic plaques compared to IgG controls (140). A potential role for CD47 in Alzheimer’s disease has been identified with studies showing CD47 facilitates Aβ oligomers internalization by microglia (141, 142). Even in the infancy of understanding its diverse function and potentiality, CD47 will be further revealed as an important mediator in these therapeutic areas.
Inspiring progress has been made with respect to the understanding of the role of CD47 in normal cell growth cycle as well as in the molecular pathogenesis of diverse diseases. CD47 serves as a receptor for TSP, integrins, and SIRP family members and has been shown to trigger a wide variety of cellular functions. For example, several lines of evidence suggests CD47 ligation appears to co-stimulate T cell proliferation and induce their arrest (54).
Utilizing anti-CD47 antibodies open up exciting avenues in cancer, but there are still unsolved questions in this field. First, the underlying mechanisms need to be elucidated. What is the importance of FcR engagement during CD47 blockade? How to avoid an uncontrolled immune response since the ubiquitous expression of CD47? The cell specific function of CD47 is not fully understood so far and its downstream signaling is still unclear. Secondly, there exists more than one binding receptor of CD47. It, therefore, would be challenging to decide what are the specific contribution of each partner in this intricate network? Thirdly, the question remains whether NK cells or other immune cells contribute to the effect of CD47 blockade rather than macrophage. Finally, some researchers asserted that anti-CD47 antibody did not induce apparent side effects in animal models, but whether if it is equally safe in human body still needs further elucidation. In conclusion, though there is still long way to go about mechanistic issue of CD47 blockade, immunological and clinical studies are yielding impressive results in this field. An exciting new era of innate checkpoint strategy targeting CD47 is likely to come especially when we fully understand underlying mechanisms.
All authors listed have made a substantial, direct, and intellectual contribution to the work, and approved it for publication.
The authors declare that the research was conducted in the absence of any commercial or financial relationships that could be construed as a potential conflict of interest.
All claims expressed in this article are solely those of the authors and do not necessarily represent those of their affiliated organizations, or those of the publisher, the editors and the reviewers. Any product that may be evaluated in this article, or claim that may be made by its manufacturer, is not guaranteed or endorsed by the publisher.
1. Wang X, Wang Y, Hu J, Xu H. An antitumor peptide RS17-targeted CD47, design, synthesis, and antitumor activity. Cancer Med (2021) 10:2125–36. doi: 10.1002/cam4.3768
2. Lindberg FP, Gresham HD, Schwarz E, Brown EJ. Molecular cloning of integrin-associated protein: An immunoglobulin family member with multiple membrane-spanning domains implicated in α(v)β3- dependent ligand binding. J Cell Biol (1993) 123:485–96. doi: 10.1083/jcb.123.2.485
3. Fenalti G, Villanueva N, Griffith M, Pagarigan B, Lakkaraju SK, Huang RYC, et al. Structure of the human marker of self 5-transmembrane receptor CD47. Nat Commun (2021) 12:5218. doi: 10.1038/s41467-021-25475-w
4. Poels LG, Peters D, van Megen Y, Vooijs GP, Verheyen RN, Willemen A, et al. Monoclonal antibody against human ovarian tumor-associated antigens. J Natl Cancer Inst (1986) 76:781–91.
5. Cheng L, Li Y, Zhang SB, Teng XD. Molecular pathology of lung cancer: Key to personalized medicine. Chin J Pathol (2012) 41:715–20. doi: 10.3760/cma.i.issn.0529-5807.2012.10.019
6. Brown EJ, Frazier WA. Integrin-associated protein (CD47) and its ligands. Trends Cell Biol (2001) 11:130–5. doi: 10.1016/S0962-8924(00)01906-1
7. Liu Y, Merlin D, Burst SL, Pochet M, Madara JL, Parkos CA. The role of CD47 in neutrophil transmigration. J Biol Chem (2001) 276:40156–66. doi: 10.1074/jbc.M104138200
8. Jaiswal S, Jamieson CHM, Pang WW, Park CY, Chao MP, Majeti R, et al. CD47 is upregulated on circulating hematopoietic stem cells and leukemia cells to avoid phagocytosis. Cell (2009) 138:271–85. doi: 10.1016/j.cell.2009.05.046
9. Sarfati M, Fortin G, Raymond M, Susin S. CD47 in the immune response: Role of thrombospondin and SIRP-α reverse signaling. Curr Drug Targets (2008) 9:842–50. doi: 10.2174/138945008785909310
10. Barclay AN, van den Berg TK. The interaction between signal regulatory protein alpha (SIRP α) and CD47: Structure, function, and therapeutic target. Annu Rev Immunol (2014) 32:25–50. doi: 10.1146/annurev-immunol-032713-120142
11. Liu J, Xavy S, Mihardja S, Chen S, Sompalli K, Feng D, et al. Targeting macrophage checkpoint inhibitor SIRPα for anticancer therapy. JCI Insight (2020). doi: 10.1172/jci.insight.134728
12. Burger P, Hilarius-Stokman P, de Korte D, van den Berg TK, van Bruggen R. CD47 functions as a molecular switch for erythrocyte phagocytosis. Blood (2012) 119:5512–21. doi: 10.1182/blood-2011-10-386805
13. Barclay AN, Brown MH. The SIRP family of receptors and immune regulation. Nat Rev Immunol (2006) 6:457–64. doi: 10.1038/nri1859
14. van Beek EM, Cochrane F, Barclay AN, van den Berg TK. Signal regulatory proteins in the immune system. J Immunol (2005) 175:7781–7. doi: 10.4049/jimmunol.175.12.7781
15. Vernon-Wilson EF, Kee WJ, Willis AC, Barclay AN, Simmons DL, Brown MH. CD47 is a ligand for rat macrophage membrane signal regulatory protein SIRP (OX41) and human SIRPalpha 1. Eur J Immunol (2000) 30:2130–7. doi: 10.1002/1521-4141(2000)30:8<2130::AID-IMMU2130>3.0.CO;2-8
16. Tsai RK, Discher DE. Inhibition of “self” engulfment through deactivation of myosin-II at the phagocytic synapse between human cells. J Cell Biol (2008) 180:989–1003. doi: 10.1083/jcb.200708043
17. Isenberg JS, Annis DS, Pendrak ML, Ptaszynska M, Frazier WA, Mosher DF, et al. Differential interactions of thrombospondin-1, -2, and -4 with CD47 and effects on cGMP signaling and ischemic injury responses. J Biol Chem (2009) 284:1116–25. doi: 10.1074/jbc.M804860200
18. Hayat SMG, Bianconi V, Pirro M, Jaafari MR, Hatamipour M, Sahebkar A. CD47: Role in the immune system and application to cancer therapy. Cell Oncol (2020) 43:19–30. doi: 10.1007/s13402-019-00469-5
19. Tulasne D, Judd BA, Johansen M, Asazuma N, Best D, Brown EJ, et al. C-terminal peptide of thrombospondin-1 induces platelet aggregation through the fc receptor γ-chain-associated signaling pathway and by agglutination. Blood (2001) 98:3346–52. doi: 10.1182/blood.V98.12.3346
20. Leclair P, Kim MJ, Lim CJ. Peptide analogues PKHB1 and 4N1K induce cell death through CD47-independent mechanisms. Cancer Sci (2020) 111:1028–30. doi: 10.1111/cas.14310
21. Leclair P, Lim CJ. CD47-independent effects mediated by the TSP-derived 4N1K peptide. PloS One (2014) 9:e98358. doi: 10.1371/journal.pone.0098358
22. Feng R, Zhao H, Xu J, Shen C CD47: The next checkpoint target for cancer immunotherapy. Crit Rev Oncol Hematol (2020) 152:103014. doi: 10.1016/j.critrevonc.2020.103014
23. Engelbertsen D, Autio A, Verwilligen RAF, Depuydt MAC, Newton G, Rattik S, et al. Increased lymphocyte activation and atherosclerosis in CD47-deficient mice. Sci Rep (2019) 9:10608. doi: 10.1038/s41598-019-46942-x
24. Hatherley D, Graham SC, Turner J, Harlos K, Stuart DI, Barclay AN. Paired receptor specificity explained by structures of signal regulatory proteins alone and complexed with CD47. Mol Cell (2008) 31:266–77. doi: 10.1016/j.molcel.2008.05.026
25. Brooke G, Holbrook JD, Brown MH, Barclay AN. Human lymphocytes interact directly with CD47 through a novel member of the signal regulatory protein (SIRP) family. J Immunol (2004) 173:2562–70. doi: 10.4049/jimmunol.173.4.2562
26. Hanisch UK, Kettenmann H. Microglia: Active sensor and versatile effector cells in the normal and pathologic brain. Nat Neurosci (2007) 10:1387–94. doi: 10.1038/nn1997
27. Piccio L, Vermi W, Boles KS, Fuchs A, Strader CA, Facchetti F, et al. Adhesion of human T cells to antigen-presenting cells through SIRPβ2-CD47 interaction costimulates T-cell proliferation. Blood (2005) 105:2421–7. doi: 10.1182/blood-2004-07-2823
28. Stefanidakis M, Newton G, Lee WY, Parkos CA, Luscinskas FW. Endothelial CD47 interaction with SIRPγ is required for human T-cell transendothelial migration under shear flow conditions in vitro. Blood (2008) 112:1280–9. doi: 10.1182/blood-2008-01-134429
29. Kaur S, Saldana AC, Elkahloun AG, Petersen JD, Arakelyan A, Singh SP, et al. CD47 interactions with exportin-1 limit the targeting of m7G-modified RNAs to extracellular vesicles. J Cell Commun Signal (2021). doi: 10.1007/s12079-021-00646-y
30. Nguyen K, Puthenveetil R, Vinogradova O. Investigation of the adaptor protein PLIC-2 in multiple pathways. Biochem Biophys Rep (2017) 9:341–8. doi: 10.1016/j.bbrep.2017.01.013
31. Kaur S, Roberts DD. CD47 applies the brakes to angiogenesis via vascular endothelial growth factor receptor-2. Cell Cycle (2011) 10:10–2. doi: 10.4161/cc.10.1.14324
32. Olsson M, Oldenborg PA. CD47 on experimentally senescent murine RBCs inhibits phagocytosis following fcγ receptor-mediated but not scavenger receptor-mediated recognition by macrophages. Blood (2008) 112:4259–67. doi: 10.1182/blood-2008-03-143008
33. Oldenborg P-A. Role of CD47 as a marker of self on red blood cells. Science (80-) (2000) 288:2051–4. doi: 10.1126/science.288.5473.2051
34. Kobayashi N, Karisola P, Peña-Cruz V, Dorfman DM, Jinushi M, Umetsu SE, et al. TIM-1 and TIM-4 glycoproteins bind phosphatidylserine and mediate uptake of apoptotic cells. Immunity (2007) 27:927–40. doi: 10.1016/j.immuni.2007.11.011
35. Park S-Y, Jung M-Y, Kim H-J, Lee S-J, Kim S-Y, Lee B-H, et al. Rapid cell corpse clearance by stabilin-2, a membrane phosphatidylserine receptor. Cell Death Differ (2008) 15:192–201. doi: 10.1038/sj.cdd.4402242
36. Murakami Y, Tian L, Voss OH, Margulies DH, Krzewski K, Coligan JE. CD300b regulates the phagocytosis of apoptotic cells via phosphatidylserine recognition. Cell Death Differ (2014) 21:1746–57. doi: 10.1038/cdd.2014.86
37. Simhadri VR, Andersen JF, Calvo E, Choi S-C, Coligan JE, Borrego F. Human CD300a binds to phosphatidylethanolamine and phosphatidylserine, and modulates the phagocytosis of dead cells. Blood (2012) 119:2799–809. doi: 10.1182/blood-2011-08-372425
38. Velliquette RW, Aeschlimann J, Kirkegaard J, Shakarian G, Lomas-Francis C, Westhoff CM. Monoclonal anti-CD47 interference in red cell and platelet testing. Transfusion (2019) 59:730–7. doi: 10.1111/trf.15033
39. Low P, Waugh S, Zinke K, Drenckhahn D. The role of hemoglobin denaturation and band 3 clustering in red blood cell aging. Science (80-) (1985) 227:531–3. doi: 10.1126/science.2578228
40. Stirnemann J, Belmatoug N, Camou F, Serratrice C, Froissart R, Caillaud C, et al. A review of gaucher disease pathophysiology, clinical presentation and treatments. Int J Mol Sci (2017) 18:441. doi: 10.3390/ijms18020441
41. Bratosin D, Tissier J-P, Lapillonne H, Hermine O, de Villemeur TB, Cotoraci C, et al. A cytometric study of the red blood cells in gaucher disease reveals their abnormal shape that may be involved in increased erythrophagocytosis. Cytom Part B Clin Cytom (2011) 80B:28–37. doi: 10.1002/cyto.b.20539
42. Kusakari S, Ohnishi H, Jin F-J, Kaneko Y, Murata T, Murata Y, et al. Trans-endocytosis of CD47 and SHPS-1 and its role in regulation of the CD47-SHPS-1 system. J Cell Sci (2008) 121:1213–23. doi: 10.1242/jcs.025015
43. Nilsson A, Oldenborg P-A. CD47 promotes both phosphatidylserine-independent and phosphatidylserine-dependent phagocytosis of apoptotic murine thymocytes by non-activated macrophages. Biochem Biophys Res Commun (2009) 387:58–63. doi: 10.1016/j.bbrc.2009.06.121
44. Azcutia V, Routledge M, Williams MR, Newton G, Frazier WA, Manica A, et al. CD47 plays a critical role in T-cell recruitment by regulation of LFA-1 and VLA-4 integrin adhesive functions. Mol Biol Cell (2013) 24:3358–68. doi: 10.1091/mbc.e13-01-0063
45. Feng M, Jiang W, Kim BYS, Zhang CC, Fu Y-X, Weissman IL. Phagocytosis checkpoints as new targets for cancer immunotherapy. Nat Rev Cancer (2019) 19:568–86. doi: 10.1038/s41568-019-0183-z
46. Russ A, Hua AB, Montfort WR, Rahman B, Riaz IB, Khalid MU, et al. Blocking “don’t eat me” signal of CD47-SIRPα in hematological malignancies, an in-depth review. Blood Rev (2018) 32:480–89. doi: 10.1016/j.blre.2018.04.005
47. Ticchioni M, Deckert M, Mary F, Bernard G, Brown EJ, Bernard A. Integrin-associated protein (CD47) is a comitogenic molecule on CD3-activated human T cells. J Immunol (1997) 158:677–84.
48. Van VQ, Raymond M, Baba N, Rubio M, Wakahara K, Susin SA, et al. CD47 high expression on CD4 effectors identifies functional long-lived memory T cell progenitors. J Immunol (2012) 188:4249–255. doi: 10.4049/jimmunol.1102702
49. Avice M-N, Rubio M, Sergerie M, Delespesse G, Sarfati M. CD47 ligation selectively inhibits the development of human naive T cells into Th1 effectors. J Immunol (2000) 165:4624–31. doi: 10.4049/jimmunol.165.8.4624
50. Bouguermouh S, Van VQ, Martel J, Gautier P, Rubio M, Sarfati M. CD47 expression on T cell is a self-control negative regulator of type 1 immune response. J Immunol (2008) 180:8073–82. doi: 10.4049/jimmunol.180.12.8073
51. Demeure CE, Tanaka H, Mateo V, Rubio M, Delespesse G, Sarfati M. CD47 engagement inhibits cytokine production and maturation of human dendritic cells. J Immunol (2000) 164:2193–199. doi: 10.4049/jimmunol.164.4.2193
52. Latour S, Tanaka H, Demeure C, Mateo V, Rubio M, Brown EJ, et al. Bidirectional negative regulation of human T and dendritic cells by CD47 and its cognate receptor signal-regulator protein-α: Down-regulation of IL-12 responsiveness and inhibition of dendritic cell activation. J Immunol (2001) 167:2547–54. doi: 10.4049/jimmunol.167.5.2547
53. Cham LB, Torrez Dulgeroff LB, Tal MC, Adomati T, Li F, Bhat H, et al. Immunotherapeutic blockade of CD47 inhibitory signaling enhances innate and adaptive immune responses to viral infection. Cell Rep (2020) 31:107494. doi: 10.1016/j.celrep.2020.03.058
54. Liu X, Pu Y, Cron K, Deng L, Kline J, Frazier WA, et al. CD47 blockade triggers T cell–mediated destruction of immunogenic tumors. Nat Med (2015) 21:1209–15. doi: 10.1038/nm.3931
55. Rodríguez-Jiménez P, Chicharro P, Llamas-Velasco M, Cibrian D, Trigo-Torres L, Vara A, et al. Thrombospondin-1/CD47 interaction regulates Th17 and treg differentiation in psoriasis. Front Immunol (2019) 10:1268. doi: 10.3389/fimmu.2019.01268
56. Van VQ, Darwiche J, Raymond M, Lesage S, Bouguermouh S, Rubio M, et al. Cutting edge: CD47 controls the In vivo proliferation and homeostasis of peripheral CD4 + CD25 + Foxp3 + regulatory T cells that express CD103. J Immunol (2008) 181:5204–208. doi: 10.4049/jimmunol.181.8.5204
57. Lee N, Shin JU, Jin S, Yun KN, Kim JY, Park CO, et al. Upregulation of CD47 in regulatory T cells in atopic dermatitis. Yonsei Med J (2016) 57:1435. doi: 10.3349/ymj.2016.57.6.1435
58. Huang Y, Liu Z, Cao BB, Qiu YH, Peng YP. Treg cells protect dopaminergic neurons against MPP + neurotoxicity via CD47-SIRPA interaction. Cell Physiol Biochem (2017) 41:1240–54. doi: 10.1159/000464388
59. Crayne CB, Albeituni S, Nichols KE, Cron RQ. The immunology of macrophage activation syndrome. Front Immunol (2019) 10:119. doi: 10.3389/fimmu.2019.00119
60. Zhao H, Bo C, Kang Y, Li H. What else can CD39 tell us? Front Immunol (2017) 8:727. doi: 10.3389/fimmu.2017.00727
61. Weiskopf K. Cancer immunotherapy targeting the CD47/SIRPα axis. Eur J Cancer (2017) 76:100–109. doi: 10.1016/j.ejca.2017.02.013
62. Ferrari D, Gorini S, Callegari G, la Sala A. Shaping immune responses through the activation of dendritic cells’ P2 receptors. Purinergic Signal (2007) 3:99–107. doi: 10.1007/s11302-006-9024-0
63. Bell BM, Kirk ID, Hiltbrunner S, Gabrielsson S, Bultema JJ. Designer exosomes as next-generation cancer immunotherapy. Nanomed Nanotechnol Biol Med (2016) 12:163–9. doi: 10.1016/j.nano.2015.09.011
64. Nath PR, Gangaplara A, Pal-Nath D, Mandal A, Maric D, Sipes JM, et al. CD47 expression in natural killer cells regulates homeostasis and modulates immune response to lymphocytic choriomeningitis virus. Front Immunol (2018) 9:2985. doi: 10.3389/fimmu.2018.02985
65. Nath PR, Pal-Nath D, Mandal A, Cam MC, Schwartz AL, Roberts DD. Natural killer cell recruitment and activation are regulated by cd47 expression in the tumor microenvironment. Cancer Immunol Res (2019) 7:1547–61. doi: 10.1158/2326-6066.CIR-18-0367
66. Pierson B, Gupta K, Hu W, Miller J. Human natural killer cell expansion is regulated by thrombospondin- mediated activation of transforming growth factor-beta 1 and independent accessory cell-derived contact and soluble factors. Blood (1996) 87:180–9. doi: 10.1182/blood.V87.1.180.bloodjournal871180
67. Przewoznik M, Hömberg N, Naujoks M, Pötzl J, Münchmeier N, Brenner CD, et al. Recruitment of natural killer cells in advanced stages of endogenously arising b-cell lymphoma. J Immunother (2012) 35:217–22. doi: 10.1097/CJI.0b013e318247440a
68. Bald T, Krummel MF, Smyth MJ, Barry KC. The NK cell–cancer cycle: advances and new challenges in NK cell–based immunotherapies. Nat Immunol (2020) 21:835–47. doi: 10.1038/s41590-020-0728-z
69. Lee Y-T, Ko E-J, Lee Y, Lee Y-N, Bian Z, Liu Y, et al. CD47 plays a role as a negative regulator in inducing protective immune responses to vaccination against influenza virus. J Virol (2016) 90:6746–58. doi: 10.1128/JVI.00605-16
70. Liu Y, O’Connor MB, Mandell KJ, Zen K, Ullrich A, Bühring H-J, et al. Peptide-mediated inhibition of neutrophil transmigration by blocking CD47 interactions with signal regulatory protein α. J Immunol (2004) 172:2578–85. doi: 10.4049/jimmunol.172.4.2578
71. Parkos CA, Colgan SP, Liang TW, Nusrat A, Bacarra AE, Carnes DK, et al. CD47 mediates post-adhesive events required for neutrophil migration across polarized intestinal epithelia. J Cell Biol (1996) 132:437–50. doi: 10.1083/jcb.132.3.437
72. Cooper D, Lindberg FP, Gamble JR, Brown EJ, Vadas MA. Transendothelial migration of neutrophils involves integrin-associated protein (CD47). Proc Natl Acad Sci (1995) 92:3978–82. doi: 10.1073/pnas.92.9.3978
73. Van VQ, Lesage S, Bouguermouh S, Gautier P, Rubio M, Levesque M, et al. Expression of the self-marker CD47 on dendritic cells governs their trafficking to secondary lymphoid organs. EMBO J (2006) 25:5560–8. doi: 10.1038/sj.emboj.7601415
74. Topalian SL, Taube JM, Pardoll DM. Neoadjuvant checkpoint blockade for cancer immunotherapy. Science (80-) (2020) 367:eaax0182. doi: 10.1126/science.aax0182
75. Ribas A, Wolchok JD. Cancer immunotherapy using checkpoint blockade. Science (80-) (2018) 359:1350–5. doi: 10.1126/science.aar4060
76. Advani R, Flinn I, Popplewell L, Forero A, Bartlett NL, Ghosh N, et al. CD47 blockade by Hu5F9-G4 and rituximab in non-hodgkin’s lymphoma. N Engl J Med (2018) 379:1711–21. doi: 10.1056/NEJMoa1807315
77. Demaria O, Cornen S, Daëron M, Morel Y, Medzhitov R, Vivier E. Harnessing innate immunity in cancer therapy. Nature (2019) 574:45–56. doi: 10.1038/s41586-019-1593-5
78. Barkal AA, Brewer RE, Markovic M, Kowarsky M, Barkal SA, Zaro BW, et al. CD24 signalling through macrophage siglec-10 is a target for cancer immunotherapy. Nature (2019) 572:392–96. doi: 10.1038/s41586-019-1456-0
79. Jalil AR, Andrechak JC, Discher DE. Macrophage checkpoint blockade: results from initial clinical trials, binding analyses, and CD47-SIRPα structure–function. Antib Ther (2020) 3:80–94. doi: 10.1093/abt/tbaa006
80. Mateo V, Lagneaux L, Bron D, Biron G, Armant M, Delespesse G, et al. CD47 ligation induces caspase-independent cell death in chronic lymphocytic leukemia. Nat Med (1999) 5:1277–84. doi: 10.1038/15233
81. Mateo V, Brown EJ, Biron G, Rubio M, Fischer A, Le Deist F, et al. Mechanisms of CD47-induced caspase-independent cell death in normal and leukemic cells: Link between phosphatidylserine exposure and cytoskeleton organization. Blood (2002) 100:2882–90. doi: 10.1182/blood-2001-12-0217
82. Lamy L, Ticchioni M, Rouquette-Jazdanian AK, Samson M, Deckert M, Greenberg AH, et al. CD47 and the 19 kDa interacting protein-3 (BNIP3) in T cell apoptosis. J Biol Chem (2003) 278:23915–21. doi: 10.1074/jbc.M301869200
83. Burton TR, Gibson SB. The role of bcl-2 family member BNIP3 in cell death and disease: NIPping at the heels of cell death. Cell Death Differ (2009) 16:515–23. doi: 10.1038/cdd.2008.185
84. Zhao XW, van Beek EM, Schornagel K, van der Maaden H, Van Houdt M, Otten MA, et al. CD47-signal regulatory protein- (SIRP) interactions form a barrier for antibody-mediated tumor cell destruction. Proc Natl Acad Sci (2011) 108:18342–7. doi: 10.1073/pnas.1106550108
85. Barkal AA, Weiskopf K, Kao KS, Gordon SR, Rosental B, Yiu YY, et al. Engagement of MHC class I by the inhibitory receptor LILRB1 suppresses macrophages and is a target of cancer immunotherapy. Nat Immunol (2018) 19:76–84. doi: 10.1038/s41590-017-0004-z
86. Feng R, Zhao H, Xu J, Shen C. CD47: the next checkpoint target for cancer immunotherapy. Crit Rev Oncol Hematol (2020) 152:103014. doi: 10.1016/j.critrevonc.2020.103014
87. Willingham SB, Volkmer J-P, Gentles AJ, Sahoo D, Dalerba P, Mitra SS, et al. The CD47-signal regulatory protein alpha (SIRPa) interaction is a therapeutic target for human solid tumors. Proc Natl Acad Sci (2012) 109:6662–7. doi: 10.1073/pnas.1121623109
88. Chao MP, Alizadeh AA, Tang C, Myklebust JH, Varghese B, Gill S, et al. Anti-CD47 antibody synergizes with rituximab to promote phagocytosis and eradicate non-Hodgkin lymphoma. Cell (2010) 142:699–713. doi: 10.1016/j.cell.2010.07.044
89. Gao L, Chen K, Gao Q, Wang X, Sun J, Yang Y-G. CD47 deficiency in tumor stroma promotes tumor progression by enhancing angiogenesis. Oncotarget (2017) 8:22406–13. doi: 10.18632/oncotarget.9899
90. Jeanne A, Sarazin T, Charlé M, Moali C, Fichel C, Boulagnon-Rombi C, et al. Targeting ovarian carcinoma with TSP-1:CD47 antagonist TAX2 activates anti-tumor immunity. Cancers (Basel) (2021) 13:5019. doi: 10.3390/cancers13195019
91. Zhao XW, Kuijpers TW, van den Berg TK. Is targeting of CD47-SIRPα enough for treating hematopoietic malignancy? Blood (2012) 119:4333–4. doi: 10.1182/blood-2011-11-391367
92. Zhao XW, Matlung HL, Kuijpers TW, van den Berg TK. On the mechanism of CD47 targeting in cancer. Proc Natl Acad Sci (2012) 109:E2843–3. doi: 10.1073/pnas.1209265109
93. Chao MP, Tang C, Pachynski RK, Chin R, Majeti R, Weissman IL. Extranodal dissemination of non-Hodgkin lymphoma requires CD47 and is inhibited by anti-CD47 antibody therapy. Blood (2011) 118:4890–901. doi: 10.1182/blood-2011-02-338020
94. Jaiswal S, Chao MP, Majeti R, Weissman IL. Macrophages as mediators of tumor immunosurveillance. Trends Immunol (2010) 31:212–9. doi: 10.1016/j.it.2010.04.001
95. Ben Mkaddem S, Benhamou M, Monteiro RC. Understanding fc receptor involvement in inflammatory diseases: From mechanisms to new therapeutic tools. Front Immunol (2019) 10:811. doi: 10.3389/fimmu.2019.00811
96. Németh T, Futosi K, Szabó M, Aradi P, Saito T, Mócsai A, et al. Importance of fc receptor γ-chain ITAM tyrosines in neutrophil activation and in vivo autommune arthritis. Front Immunol (2019) 10. doi: 10.3389/fimmu.2019.00252
97. Mentlik James A, Cohen AD, Campbell KS. Combination immune therapies to enhance anti-tumor responses by NK cells. Front Immunol (2013) 4:481. doi: 10.3389/fimmu.2013.00481
98. Cooper MA, Fehniger TA, Turner SC, Chen KS, Ghaheri BA, Ghayur T, et al. Human natural killer cells: A unique innate immunoregulatory role for the CD56bright subset. Blood (2001) 97:3146–51. doi: 10.1182/blood.V97.10.3146
99. McMichael EL, Courtney NB, Duggan MC, Wesolowski R, Quiroga D, Kondadasula SV, et al. Activation of the FcgammaReceptorIIIa on human natural killer cells leads to increased expression of functional interleukin-21 receptor. Oncoimmunology (2017) 6:e1312045. doi: 10.1080/2162402X.2017.1312045
100. Kaur S, Cicalese KV, Banerjee R, Roberts DD. Preclinical and clinical development of therapeutic antibodies targeting functions of CD47 in the tumor microenvironment. Antib Ther (2020) 3:179–92. doi: 10.1093/abt/tbaa017
101. Liu J, Wang L, Zhao F, Tseng S, Narayanan C, Shura L, et al. Pre-clinical development of a humanized anti-CD47 antibody with anti-cancer therapeutic potential. PloS One (2015) 10:e0137345. doi: 10.1371/journal.pone.0137345
102. Chen J, Zhong M-C, Guo H, Davidson D, Mishel S, Lu Y, et al. SLAMF7 is critical for phagocytosis of haematopoietic tumour cells via mac-1 integrin. Nature (2017) 544:493–7. doi: 10.1038/nature22076
103. Ma L, Zhu M, Gai J, Li G, Chang Q, Qiao P, et al. Preclinical development of a novel CD47 nanobody with less toxicity and enhanced anti-cancer therapeutic potential. J Nanobiotechnol (2020) 18:12. doi: 10.1186/s12951-020-0571-2
104. Chao MP, Jaiswal S, Weissman-Tsukamoto R, Alizadeh AA, Gentles AJ, Volkmer J, et al. Calreticulin is the dominant pro-phagocytic signal on multiple human cancers and is counterbalanced by CD47. Sci Transl Med (2010) 2:63ra94–63ra94. doi: 10.1126/scitranslmed.3001375
105. Yuan H, Jiang W, Von Roemeling CA, Qie Y, Liu X, Chen Y, et al. Multivalent bi-specific nanobioconjugate engager for targeted cancer immunotherapy. Nat Nanotechnol (2017) 12:763–9. doi: 10.1038/nnano.2017.69
106. von Roemeling CA, Wang Y, Qie Y, Yuan H, Zhao H, Liu X, et al. Therapeutic modulation of phagocytosis in glioblastoma can activate both innate and adaptive antitumour immunity. Nat Commun (2020) 11:1–12. doi: 10.1038/s41467-020-15129-8
107. Veillette A, Chen J. SIRPα–CD47 immune checkpoint blockade in anticancer therapy. Trends Immunol (2018) 39:173–84. doi: 10.1016/j.it.2017.12.005
108. Kim BYS, Rutka JT, Chan WCW. Nanomedicine. N Engl J Med (2010) 363:2434–43. doi: 10.1056/NEJMra0912273
109. Miller TW, Amason JD, Garcin ED, Lamy L, Dranchak PK, Macarthur R, et al. Quantitative high-throughput screening assays for the discovery and development of SIRPα-CD47 interaction inhibitors. (2019) 14:e0218897. doi: 10.1371/journal.pone.0218897
110. Wang H, Sun Y, Zhou X, Chen C, Jiao L, Li W, et al. CD47/SIRPα blocking peptide identification and synergistic effect with irradiation for cancer immunotherapy. J Immunother Cancer (2020) 8:1–13. doi: 10.1136/jitc-2020-000905
111. Yu W, Ye Z, Chen X, Shi J, Lu J. The development of small-molecule inhibitors targeting CD47. Drug Discov Today (2020) 00. doi: 10.1016/j.drudis.2020.11.003
112. Oronsky B, Scicinski J, Cabrales P, Minchinton A. RRx-001, an epigenetic-based radio- and chemosensitizer, has vascular normalizing effects on SCCVII and U87 tumors. Clin Epigenet (2016) 8:53. doi: 10.1186/s13148-016-0220-7
113. Zhao H, Ning S, Nolley R, Scicinski J, Oronsky B, Knox SJ, et al. The immunomodulatory anticancer agent, RRx-001, induces an interferon response through epigenetic induction of viral mimicry. Clin Epigenet (2017) 9:4. doi: 10.1186/s13148-017-0312-z
114. Logtenberg MEW, Jansen JHM, Raaben M, Toebes M, Franke K, Brandsma AM, et al. Glutaminyl cyclase is an enzymatic modifier of the CD47- SIRPα axis and a target for cancer immunotherapy. Nat Med (2019) 25:612–19. doi: 10.1038/s41591-019-0356-z
115. Bresser K, Logtenberg MEW, Toebes M, Proost N, Sprengers J, Siteur B, et al. QPCTL regulates macrophage and monocyte abundance and inflammatory signatures in the tumor microenvironment. Oncoimmunology (2022) 11. doi: 10.1080/2162402X.2022.2049486
116. Petrova PS, Viller NN, Wong M, Pang X, Lin GHY, Dodge K, et al. TTI-621 (SIRPαFc): A CD47-blocking innate immune checkpoint inhibitor with broad antitumor activity and minimal erythrocyte binding. Clin Cancer Res (2017) 23:1068–79. doi: 10.1158/1078-0432.CCR-16-1700
117. Fisher B. Biological research in the evolution of cancer surgery: A personal perspective. Cancer Res (2008) 68:10007–20. doi: 10.1158/0008-5472.CAN-08-0186
118. Chen H, Yang Y, Deng Y, Wei F, Zhao Q, Liu Y, et al. Delivery of CD47 blocker SIRPα-fc by CAR-T cells enhances antitumor efficacy. J Immunother Cancer (2022) 10:1–12. doi: 10.1136/jitc-2021-003737
119. Ingram JR, Blomberg OS, Sockolosky JT, Ali L, Schmidt FI, Pishesha N, et al. Localized CD47 blockade enhances immunotherapy for murine melanoma. Proc Natl Acad Sci (2017) 114:201710776. doi: 10.1073/pnas.1710776114
120. Zhang R, Drobenaire H, Li H, Pizzarella V, Lin H. Abstract 1611: A novel anti-human CD47 antibody prodrug as cancer therapeutics to lower on-target side effects. Cancer Res (2021) 81:1611–1. doi: 10.1158/1538-7445.AM2021-1611
121. Ni K, Luo T, Culbert A, Kaufmann M, Jiang X, Lin W. Nanoscale metal–organic framework Co-delivers TLR-7 agonists and anti-CD47 antibodies to modulate macrophages and orchestrate cancer immunotherapy. J Am Chem Soc (2020) 142:12579–84. doi: 10.1021/jacs.0c05039
122. Huang L, Zhang Y, Li Y, Meng F, Li H, Zhang H, et al. Time-programmed delivery of sorafenib and anti-CD47 antibody via a double-Layer-Gel matrix for postsurgical treatment of breast cancer. Nano-Micro Lett (2021) 13:141. doi: 10.1007/s40820-021-00647-x
123. Shi R, Chai Y, Duan X, Bi X, Huang Q, Wang Q, et al. The identification of a CD47-blocking “hotspot” and design of a CD47/PD-L1 dual-specific antibody with limited hemagglutination. Signal Transduct Target Ther (2020) 5:16. doi: 10.1038/s41392-020-0121-2
124. Dheilly E, Moine V, Broyer L, Salgado-Pires S, Johnson Z, Papaioannou A, et al. Selective blockade of the ubiquitous checkpoint receptor CD47 is enabled by dual-targeting bispecific antibodies. Mol Ther (2017) 25:523–33. doi: 10.1016/j.ymthe.2016.11.006
125. Sallman DA, Asch AS, Al Malki MM, Lee DJ, Donnellan WB, Marcucci G, et al. The first-in-Class anti-CD47 antibody magrolimab (5F9) in combination with azacitidine is effective in MDS and AML patients: Ongoing phase 1b results. Blood (2019) 134:569–9. doi: 10.1182/blood-2019-126271
126. Wilson WC, Richards JO, Andrejeva G, Capoccia BJ, Puro RJ, Donio MJ, et al. AO-176, a differentiated clinical-stage anti-CD47 antibody, demonstrates potent anti-tumor activity across multiple preclinical models of b cell neoplasms. Blood (2021) 138:2277–7. doi: 10.1182/blood-2021-152896
127. Meng Z, Wang Z, Guo B, Cao W, Shen H. TJC4, a differentiated anti-CD47 antibody with novel epitope and RBC sparing properties. Blood (2019) 134:4063–3. doi: 10.1182/blood-2019-122793
128. Mehta A, Harb W, Xu C, Meng Y, Lee L, Yuan V, et al. Lemzoparlimab, a differentiated anti-CD47 antibody in combination with rituximab in relapsed and refractory non-hodgkin’s lymphoma: Initial clinical results. Blood (2021) 138:3542–2. doi: 10.1182/blood-2021-150606
129. Qi J, Li J, Jiang B, Jiang B, Liu H, Cao X, et al. A phase I/IIa study of lemzoparlimab, a monoclonal antibody targeting CD47, in patients with relapsed and/or refractory acute myeloid leukemia (AML) and myelodysplastic syndrome (MDS): Initial phase I results. Blood (2020) 136:30–1. doi: 10.1182/blood-2020-134391
130. Maute R, Xu J, Weissman IL. CD47–SIRPα-targeted therapeutics: Status and prospects. Immuno-Oncology Technol (2022) 13:100070. doi: 10.1016/j.iotech.2022.1000705
131. Kim TM, Lakhani N, Gainor J, Kamdar M, Fanning P, Squifflet P, et al. ALX148, a CD47 blocker, in combination with rituximab in patients with non-Hodgkin lymphoma. Blood (2020) 136:13–4. doi: 10.1182/blood-2020-135941
132. Chow LQM, Gainor JF, Lakhani NJ, Lee KW, Chung HC, Lee J, et al. A phase I study of ALX148, a CD47 blocker, in combination with standard anticancer antibodies and chemotherapy regimens in patients with advanced malignancy. J Clin Oncol (2020) 38:3056–6. doi: 10.1200/JCO.2020.38.15_suppl.3056
133. Foppoli M, Ferreri AJM. Gamma-delta t-cell lymphomas. Eur J Haematol (2015) 94:206–18. doi: 10.1111/ejh.12439
134. Autio KA, Boni V, Humphrey RW, Naing A. Probody therapeutics: An emerging class of therapies designed to enhance on-target effects with reduced off-tumor toxicity for use in immuno-oncology. Clin Cancer Res (2020) 26:984–9. doi: 10.1158/1078-0432.CCR-19-1457
135. Wang Y, Zhao C, Liu Y, Wang C, Jiang H, Hu Y, et al. Recent advances of tumor therapy based on the CD47-SIRPα axis. Mol Pharm (2022) 19:1273–93. doi: 10.1021/acs.molpharmaceut.2c00073
136. Ferlin W, Masternak K, Shang L. Selective CD47 targeting with a bispecific antibody. Cancer Immunol Immunother (2021) 70:1161–2. doi: 10.1007/s00262-020-02812-4
137. Chen RP, Shinoda K, Rampuria P, Jin F, Zhao C, Yang F, et al. Bispecific antibodies for immune cell retargeting against cancer. Expert Opin Biol Ther (2022) 00:1–18. doi: 10.1080/14712598.2022.2072209
138. Zhong S, Jeong J-H, Chen Z, Chen Z, Luo J-L. Targeting tumor microenvironment by small-molecule inhibitors. Transl Oncol (2020) 13:57–69. doi: 10.1016/j.tranon.2019.10.001
139. Du K, Li Y, Liu J, Chen W, Wei Z, Luo Y, et al. A bispecific antibody targeting GPC3 and CD47 induced enhanced antitumor efficacy against dual antigen-expressing HCC. Mol Ther (2021) 29:1572–84. doi: 10.1016/j.ymthe.2021.01.006
140. Kojima Y, Volkmer JP, McKenna K, Civelek M, Lusis AJ, Miller CL, et al. CD47-blocking antibodies restore phagocytosis and prevent atherosclerosis. Nature (2016) 536:86–90. doi: 10.1038/nature18935
141. Bamberger ME, Harris ME, McDonald DR, Husemann J, Landreth GE. A cell surface receptor complex for fibrillar β-amyloid mediates microglial activation. J Neurosci (2003) 23:2665–74. doi: 10.1523/jneurosci.23-07-02665.2003
Keywords: CD47, SIRPα, immune modulation, immunotherapies, cancer immunotherapy, atherosclerosis, neurological disorders
Citation: Zhao H, Song S, Ma J, Yan Z, Xie H, Feng Y and Che S (2022) CD47 as a promising therapeutic target in oncology. Front. Immunol. 13:757480. doi: 10.3389/fimmu.2022.757480
Received: 12 August 2021; Accepted: 28 July 2022;
Published: 22 August 2022.
Edited by:
Alessandro Poggi, San Martino Hospital (IRCCS), ItalyReviewed by:
Sukhbir Kaur, National Institutes of Health (NIH), United StatesCopyright © 2022 Zhao, Song, Ma, Yan, Xie, Feng and Che. This is an open-access article distributed under the terms of the Creative Commons Attribution License (CC BY). The use, distribution or reproduction in other forums is permitted, provided the original author(s) and the copyright owner(s) are credited and that the original publication in this journal is cited, in accordance with accepted academic practice. No use, distribution or reproduction is permitted which does not comply with these terms.
*Correspondence: Shusheng Che, c2h1c2hlbmc2MTAwQHFkdS5lZHUuY24=
Disclaimer: All claims expressed in this article are solely those of the authors and do not necessarily represent those of their affiliated organizations, or those of the publisher, the editors and the reviewers. Any product that may be evaluated in this article or claim that may be made by its manufacturer is not guaranteed or endorsed by the publisher.
Research integrity at Frontiers
Learn more about the work of our research integrity team to safeguard the quality of each article we publish.