- 1Biodesign Center for Immunotherapy, Vaccines, and Virotherapy, Arizona State University, Tempe, AZ, United States
- 2School of Mathematical and Natural Sciences, Arizona State University West Campus, Glendale, AZ, United States
Altering T cell trafficking to mucosal regions can enhance immune responses towards pathogenic infections and cancers at these sites, leading to better outcomes. All-trans-retinoic acid (ATRA) promotes T cell migration to mucosal surfaces by inducing transcription of the mucosal-homing receptors CCR9 and α4β7 via binding to retinoic acid receptors (RARs), which heterodimerize with retinoid X receptors (RXRs) to function. However, the unstable nature and toxicity of ATRA limit its use as a widespread treatment modality for mucosal diseases. Therefore, identifying alternatives that could reduce or eliminate the use of ATRA are needed. Rexinoids are synthetically derived compounds structurally similar to ATRA. Originally named for their ability to bind RXRs, rexinoids can enhance RAR-mediated gene transcription. Furthermore, rexinoids are more stable than ATRA and possess an improved safety profile, making them attractive candidates for use in clinical settings. Here we show that select novel rexinoids act as ATRA mimics, as they cause increased CCR9 and α4β7 expression and enhanced migration to the CCR9 ligand, CCL25 in vitro, even in the absence of ATRA. Conversely, other rexinoids act synergistically with ATRA, as culturing cells with suboptimal doses of both compounds resulted in CCR9 expression and migration to CCL25. Overall, our findings show that rexinoids can be used independently or synergistically with ATRA to promote mucosal homing of T cells in vitro, and lends support for the prospective clinical use of these compounds in immunotherapeutic approaches for pathogenic infections or cancers at mucosal surfaces.
Introduction
Mucosal surfaces represent a main entryway for pathogens to anatomic access and are common sites for cancer development. Enhancing immunity at these regions can provide better protection and improve strategies for treating these diseases. Our previous work in mouse models has shown that increasing the migration of vaccinia virus (VACV)-specific memory T cells to mucosal regions during vaccination boosts protection at these sites during VACV challenge (1). Correlative evidence also exists in non-human primate models; in rhesus macaques, the use of an attenuated cytomegalovirus (CMV) vaccine vector for simian immunodeficiency virus (SIV) increases effector T cell numbers at mucosal regions, resulting in vastly improved control and clearance of SIV following viral challenge (2, 3). In humans, clinical evidence further suggests that enhancing immune presence at mucosal sites corresponds positively with protection (4–9). Individuals with vitamin A deficiencies exhibit severely impaired mucosal immunity, resulting in increased susceptibility to infections (10–13). As the heightened immune protection seen is predominantly a result of increased effector T cell presence in the mucosal regions, identifying ways to promote T cell migration to these areas is likely to improve resistance to diseases affecting these areas (1, 14, 15).
Effector T cell trafficking to and entry into mucosal regions is governed by their expression of receptors that instigate mucosal homing, including C-C chemokine receptor type 9 (CCR9) and α4β7 integrin (α4β7) (1, 15–17). Upregulation of these mucosal homing receptors during T cell activation is dependent on signaling via retinoic acid receptor, a type II nuclear receptor that heterodimerizes with another nuclear receptor, the retinoid X receptor, to mediate transcription (12, 15, 17). Both the RAR and RXR possess α, β, and γ isotypes, with activation of the RARα/RXRα heterodimer implicated in transcription of CCR9 and the α4 subunit of α4β7, via cooperation NFATc2 (12, 15, 16, 18–21). Binding of all-trans retinoic acid (ATRA), a biologically active vitamin A metabolite and the most abundant naturally occurring pan-RAR ligand, to the ligand-binding pocket (LBP) of the RAR triggers activation of the heterodimer, ultimately resulting in RAR-mediated transcription (22–24).
Like the RAR, the RXR also possesses an LBP, and ligand bound to both the RAR and RXR has been shown to enhance transcription of retinoid-dependent genes (18, 23, 25, 26). However, identification of endogenously occurring RXR ligands has remained limited. 9-cis-retinoic acid (9cRA), a naturally occurring stereoisomer of ATRA, has been reported as a high affinity RXR ligand, however its detection in vivo remains elusive (27–29). Fatty acids such as docosahexaenoic acid and phytanic acid are also capable of binding the RXR, however endogenously occurring levels are likely too low to activate the receptor under most physiologic scenarios (28, 29). The challenge to conclusively identify naturally occurring RXR ligands has led many groups to utilize synthetic agonists, which have since been coined ‘rexinoids’.
Despite studies showing that ATRA can promote the expression of mucosal T cell homing proteins and subsequent migration to mucosal sites in vivo, resulting in better protection against mucosal infection, little is known about the effect of rexinoids on effector T cells. The functional similarity seen between rexinoids and ATRA in experimental models indicates that these synthetic small molecules may be able to exert similar effects as ATRA on effector T cells, by influencing their migration to mucosal-associated regions (30, 31). The ability of rexinoids to bind the RXR suggests that they may improve the impact of endogenous ATRA on T cell mucosal-related function. Additionally, reports that some rexinoids bind to the RAR indicates they may be able to mimic the effect ATRA has on T cell activity (30, 32).
Here we assessed the ability of a panel of rexinoids (Figure 1) to induce expression of CCR9 and α4β7 and to promote T cell migration in vitro. These rexinoids include a fluorobexarotene analog, A18, halogenated bexarotene analogs A20-A22, rexinoids A30-A41 which are described in our previous work and references therein, and rexinoids A52-A63 which are again described in our prior work and citations therein (33–36). A subset of rexinoids (A18, A20, and A41) were capable of exerting this effect independently of ATRA, suggesting these compounds can act as ATRA mimics while retaining lower toxicity and enhanced stability. Conversely, other rexinoids (A55, A56, and A57) displayed synergy with suboptimal doses of ATRA to enhance CCR9 expression. Moreover, treatment with ATRA mimics induced T cell migration in vitro towards the CCR9 ligand CCL25, while treatment with the ATRA cooperating rexinoids also resulted in improved migration. Furthermore, preliminary in vivo data suggest rexinoid treatment is accompanied by reduced toxicity compared to ATRA. Together, these data suggest that rexinoids may have potential to be used as a novel immunotherapeutic treatment modality for mucosal diseases by either replacing ATRA-based strategies or by being used in conjunction with non-toxic ATRA levels to bolster efficacy.
Materials and Methods
Rexinoid and ATRA Preparation
A panel of novel rexinoids and bexarotene (BEX) were generously donated by the Wagner, Marshall, and Jurutka labs at 1mM and diluted in 95% ethanol (Koptec) or DMSO (Sigma-Aldrich) to 2x105 nM. Powdered all-trans retinoic acid (Sigma-Aldrich) was dissolved in DMSO and stored in the dark at -20C.
Lymphocyte Isolation and Culture
Spleens were harvested from B6.Cg-Tcratm1MomTg(TcrLCMV)327Sdz/TacMmjax (P14), B6.Cg-PtprcaPepcbTg(TcrLCMV)1Aox/PpmJ (SMARTA), or C57BL/6-Tg(TcraTcrb)1100Mjb/J (OT-1) transgenic mice (Jackson Labs) and mechanically dissociated into a single cell suspension using a 70μm nylon mesh strainer (Fisherbrand). All mouse experiments were conducted under institutional care and use committee approval. T cells were stimulated with 1μg/mL of appropriate viral peptide (LCMV gp33-41, LCMV gp61-80, or OVA257-264, respectively) (GenScript; Anaspec). Cells were cultured in a 96-well plate for 8 days using RPMI complete medium (10% FBS, 1% PSG 100X) supplemented with 2.5x10-5 μg/μL IL-2 (Gibco) and 100nM of indicated rexinoid treatment or ATRA in a final volume of 200μL. 8 day treatment timeframe was determined using a time course assay (Supplementary Figure 1). Fresh culture medium with IL-2 and rexinoid or ATRA was replaced every 48 hours. Vitamin A deficient media was made using charcoal-stripped FBS (ThermoFisher).
Flow Cytometry
Expression of mucosal homing receptors was determined using flow cytometry. Cells were stained with a 1:100 dilution of the following fluorochrome-conjugated anti-mouse monoclonal antibodies: CCR9 (CW-1.2) and α4β7 (DATK32). P14 cells were further stained with Thy1.1 (HIS51) and CD8α (53-6.7); SMARTA cells were further stained with CD4 (GK1.5) and Vα2 (B20.1); OT-1 cells were further stained with CD8α (53-6.7)and Vα2 (B20.1). All antibodies were purchased from ThermoFisher. Flow cytometry data were collected using a BD LSR Fortessa flow cytometer (BD Biosciences) and analyzed using FlowJo 8.8.7 software. Graphs were created using Prism 8 software (GraphPad). Error bars indicate SD from the mean. Data from SMARTA mice included in Supporting Information.
In Vitro Migration Assay
P14 or SMARTA splenocytes, processed and cultured as described above for 7 days, were plated into the upper chamber of a 96 well HTS Transwell plate insert with 3.0um pore size (Corning) at a concentration of 5x105 cells in 75 μL of chemotaxis buffer (RPMI medium containing 0.1% FBS). Recombinant mouse CCL25/TECK protein (R&D Systems) was reconstituted to 10 μg/mL in 1X PBS (GenClone) containing 0.1% FBS, resuspended in 235 μL chemotaxis buffer at a concentration of 250nM, and plated into the lower chamber. Control wells received no chemokine. Cells were incubated for 6 hours at 37C in 5% CO2. Live cells that migrated into the lower chamber were subjected to a 1:2 trypan blue stain and manually quantified using a Neubauer improved C-Chip hemocytometer (INCYTO). Assays using P14 splenocytes were performed in triplicate, while those using SMARTA splenocytes were performed in duplicate. Graphs were created using Prism 8 software. Statistical significance calculated using 2-way ANOVA. Data from SMARTA mice included in Supporting Information.
In Vivo Toxicity
6-12 week old female Balb/cJ mice (Jackson Labs) were inoculated via the tail vein with 1x106 K7M2 cells (ATCC; cells not tested for mycoplasma) at day 0, and treated daily for 14 days with either 40mg/kg of vehicle control (n=4), ATRA (n=5), or rexinoid A55 (n=5) delivered via intraperitoneal (i.p.) injection, or 100mg/kg vehicle control (n=5), ATRA (n=5), or rexinoid A41 (n=6) delivered via oral gavage. Treatment timeframe was determined using previous unpublished data showing lung tumor establishment by Day 14 (data not shown). K7M2 cells were cultured using DMEM complete medium (10% FBS, 1% PSG 100X) under sterile conditions. Vehicle control, ATRA, and rexinoids were dissolved using DMSO and diluted to working concentrations using soybean oil (Sigma-Aldrich). Mouse weights were taken every 24 hours during the course of treatment. For liver toxicity, serum used to measure alanine transaminase (ALT) levels was obtained following cardiac puncture at Day 14, and analyzed using liquid ALT reagent kits (Pointe Scientific). Graphs created using Prism 8 software.
Statistical Analyses
One-way and two-way Analysis of Variance (ANOVA) were used for data analysis to establish the impacts of rexinoid and/or ATRA on the percentage of CCR9 and α4β7 expression. Follow-up tests for pairwise comparisons among groups were also performed post-ANOVA using Fisher’s Least Significant Difference (LSD) test. All tests were performed at the α = 0.05 significance level in JMP Pro 16, a statistical software package.
Results
Effector CD8+ T Cells Increase Expression of CCR9 and α4β7In Vitro Following Rexinoid Treatment
ATRA is capable of modifying T cell expression of the mucosal homing markers CCR9 and α4β7. As rexinoids have displayed functional similarity to ATRA in other studies, we sought to determine if our panel of novel rexinoids could also modulate T cell expression of CCR9 and α4β7. To do this, splenocytes isolated from naïve P14 mice, expressing a transgenic TCR specific for the H-2Db restricted GP33-41 peptide of LCMV, were activated in vitro and cultured with a panel of 40 rexinoids for 8 days. Many rexinoids administered at 100nM were able to significantly enhance CD8+ T cell expression of CCR9, compared to negative controls (Figures 2A–C). Culture with the FDA approved rexinoid bexarotene (BEX) also significantly enhanced CCR9 expression on responding T cells compared to negative controls (Figure 2C). Interestingly, rexinoid A41 improved T cell expression of CCR9 better than BEX, identifying a candidate that may possess improved functional efficacy compared to a current existing treatment. Rexinoid treatment also significantly enhanced α4β7 expression at day 8 of activation compared to negative controls, with A41 again outperforming BEX (Figure 2D).
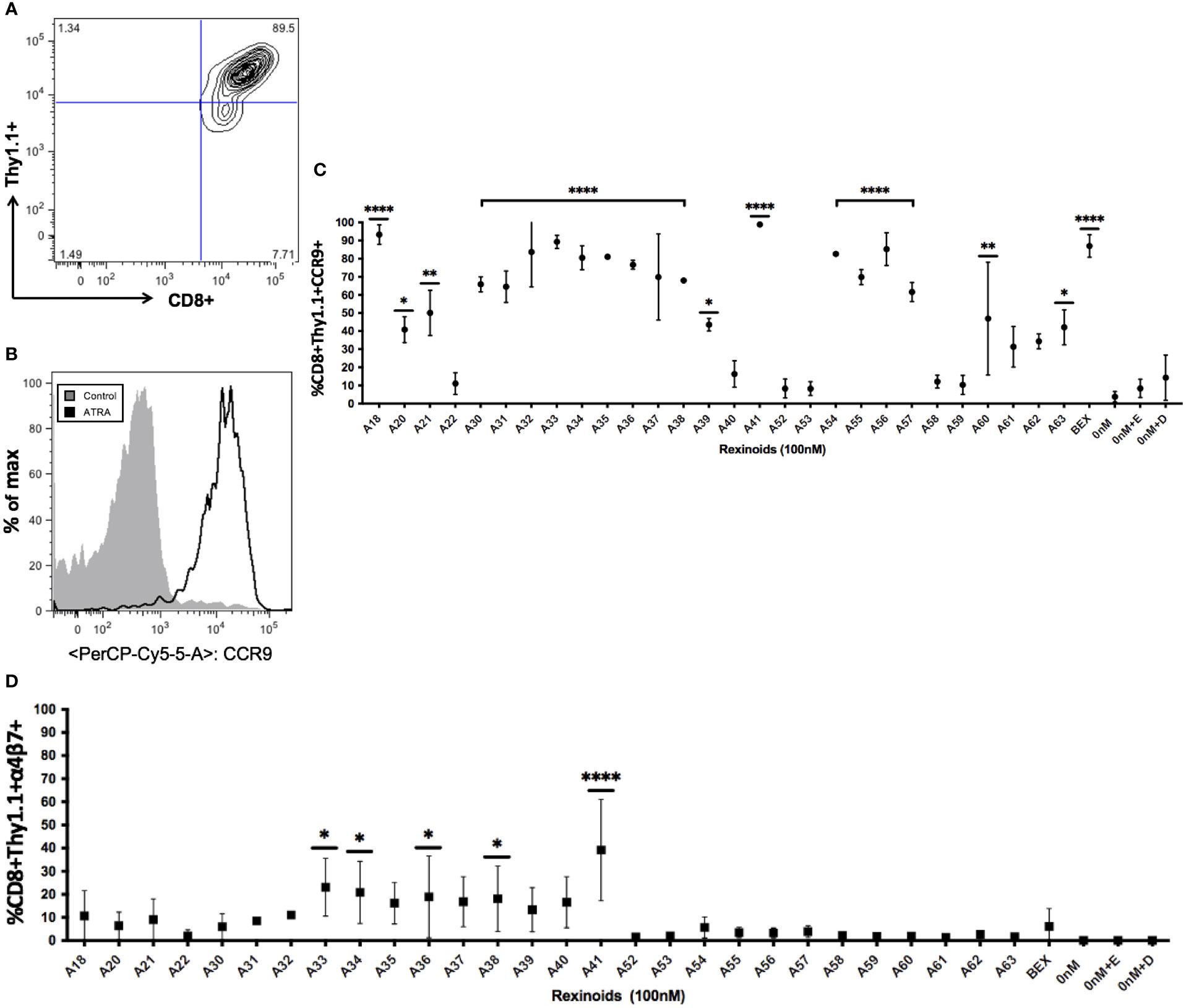
Figure 2 Rexinoid treatment enhances CCR9 and α4β7 expression on effector CD8+ T cells in vitro. Splenocytes obtained from a naïve P14 mouse were stimulated with GP33-41 peptide and cultured for 8 days with a large panel of novel rexinoids delivered at a 100nM concentration (treatment every 48 hours). Cells were then analyzed for changes in expression of CCR9 and α4β7. Analysis was performed using flow cytometry. (A) Antigen-specific effector CD8+ T cells gated using appropriate markers. (B) CCR9 expression is upregulated on antigen-specific effector CD8+ T cells following ATRA treatment given over an 8 day time course. (C) shows % positive CCR9 expression on antigen-specific effector CD8+ T cells following 8 day rexinoid treatment. Experiment performed in triplicate. (D) α4β7 expression on antigen-specific effector CD8+ T cells following 8 day rexinoid treatment. Experiment performed in duplicate. Connecting letters report used to determine statistical significance, with ordered differences report used to compare p-values between groups (* = p < 0.05, ** = p < 0.005, **** = p < 0.0001). All error bars represent SD from the mean.
The Ability of Rexinoids to Enhance CCR9 Expression on Effector T Cells Is Independent of Antigen and MHC Specificity
As rexinoids had a pronounced effect on CCR9 expression, our subsequent experiments focused primarily on the expression of this chemokine receptor as an indicator of mucosal homing protein expression. To determine if the change in T cell expression of CCR9 was antigen or MHC specific, we cultured T cells from either SMARTA and OT-1 mice, TCR transgenic mice expressing TCR specific for different peptide (LCMV GP61-80 and OVA257-264, respectively) presented in the context of a different MHC (H2-IAb and H-2Kb, respectively). Rexinoid treatment of T cells from these other TCR transgenic mouse strains also resulted in increased CCR9 expression (Figures 3A, B). Moreover, the patterns of increased expression were similar to that obtained for T cells from P14 mice, with no significant differences seen between CD8 and CD4 T cells (p= >0.05). These data suggest that the ability of rexinoids to modulate T cell expression of CCR9 is not limited by antigen specificity or MHC. Moreover, both CD4 and CD8 T cells are able to increase expression of mucosal homing proteins.
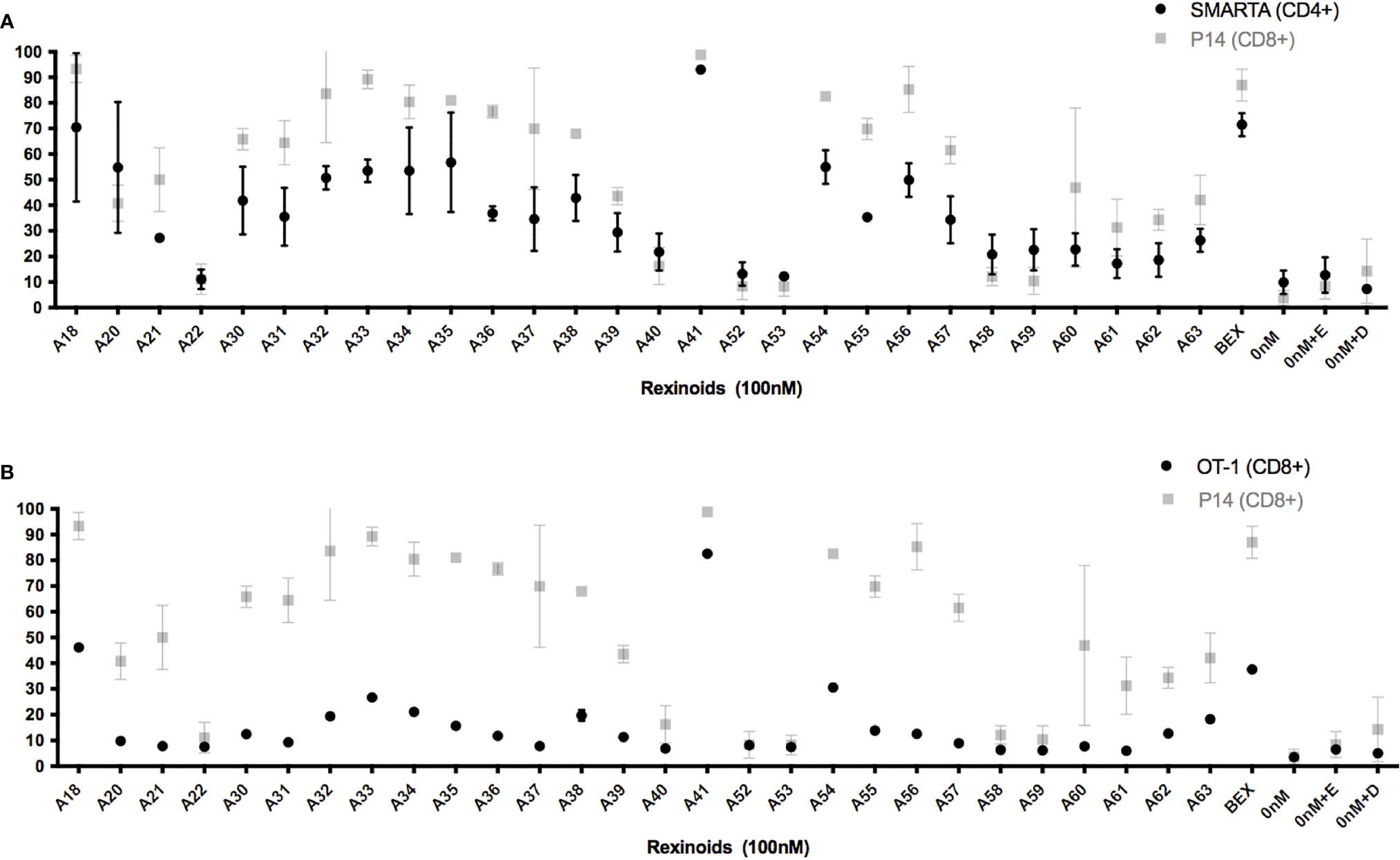
Figure 3 Rexinoid treatment enhances CCR9 expression on effector T cells of different antigen specificity in vitro. Splenocytes obtained from naïve SMARTA and OT-1 mice were stimulated with GP61-80 peptide and OVA257-264, respectively, and cultured for 8 days with the same panel of novel rexinoids delivered at a 100nM concentration (treatment every 48 hours). Cells were then analyzed for changes in expression of CCR9. Analysis was performed using flow cytometry. (A) CCR9 expression on antigen-specific effector CD4+ T cells from SMARTA mice following rexinoid treatment (black circles) superimposed onto results from Figure 2C (gray squares). SMARTA experiment performed in duplicate. (B) CCR9 expression on antigen-specific effector CD8+ T cells from OT-1 mice following rexinoid treatment (black circles) superimposed onto results from Figure 2C (gray squares). OT-1 experiment performed in duplicate. All error bars represent SD from the mean.
Some Rexinoids Act Independently of ATRA to Enhance T Cell Expression of CCR9
We next sought to determine which rexinoids were capable of altering CCR9 expression independently of ATRA. As charcoal stripping FBS removes lipophilic substances from the serum, including ATRA and other vitamin A derivatives, we supplemented RPMI medium with charcoal stripped FBS in place of standard FBS to create appropriate ATRA deficient culture conditions. P14 T cells were cultured as described above with the indicated rexinoids but without ATRA. The ability of a majority of the rexinoids to alter T cell expression of CCR9 declined to background levels when vitamin A/ATRA was removed from the medium, indicating their dependence on ATRA for increased expression of mucosal homing proteins (Figure 4A and Supplementary Figure 2). However, some rexinoids (A18, A20, A41) retained their ability to enhance CCR9 expression, despite the lack of vitamin A/ATRA in the culture medium. Overall, these findings demonstrate that select rexinoids can mimic the effects of ATRA in enhancing T cell expression of CCR9, while retaining enhanced safety and stability profiles.
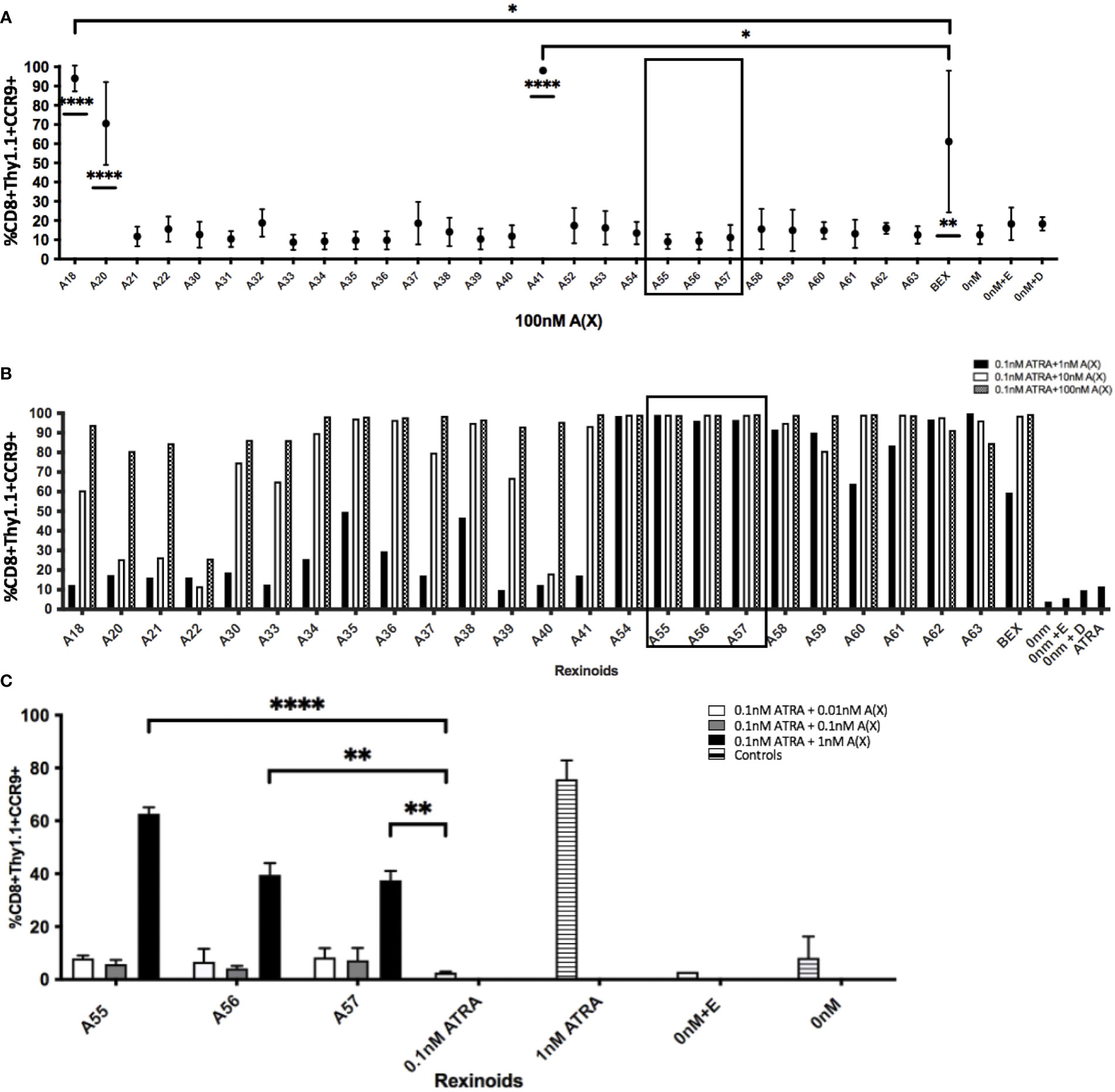
Figure 4 Rexinoids can enhance effector CD8+ T cell expression of CCR9 independently or in combination with ATRA. Splenocytes obtained from P14 mice were stimulated with GP33-41 peptide and cultured either with 100nM rexinoids in vitamin A deficient media (top), or in vitamin A deficient media supplemented with suboptimal doses of rexinoids and ATRA. After 8 day culture, effector CD8+ T cells were analyzed for expression of CCR9 using flow cytometry. (A) Rexinoids A18, A20, A41 and BEX are able to significantly enhance CCR9 expression independent of ATRA presence, compared to no treatment. Rexinoids A18 and A41 also significantly enhance CCR9 expression, compared to BEX. Experiment performed in triplicate. Connecting letters report used to determine statistical significance, with ordered differences report used to compare p-values between groups (* = p < 0.05, ** = p < 0.005, **** = p < 0.0001). (B) Suboptimal doses of several rexinoids cooperate with suboptimal doses of ATRA to enhance CCR9 expression. Boxed region identifies rexinoids that had minimal effect on CCR9 expression when previously delivered at 100nM. (boxed region 3A; graph representative of one experiment). (C) Replicate data obtained from culturing cells with suboptimal doses of rexinoid and ATRA. Rexinoids selected were those that showed high cooperativity with ATRA from 3B (boxed region). Suboptimal doses of selected rexinoid combined with suboptimal ATRA significantly improved CCR9 expression, compared to suboptimal ATRA alone (** = p < 0.005, **** = p < 0.0001). Rexinoid dosages lower than 1nM did not result in enhanced CCR9 expression. Experiment performed in triplicate. All error bars represent SD from the mean.
Some Rexinoids Act Synergistically With ATRA to Enhance T Cell Expression of CCR9
In order to test whether other rexinoids may act synergistically with ATRA, we cultured T cells from P14 mice as described above in charcoal-stripped media that had been supplemented with suboptimal amounts of ATRA (0.1nM) and rexinoids (1nM); neither ATRA nor rexinoids at these concentrations caused expression of CCR9 above background levels (rexinoids only Figure 4A, ATRA only Figure 4B). T cells cultured with selected rexinoids and ATRA at suboptimal concentrations showed significantly improved expression of CCR9 (boxed region Figures 4B, C) compared to treatment with an equivalent dose of ATRA alone (Figure 4B) or rexinoid alone (Figure 4A). These data suggest that select rexinoids act synergistically with ATRA to promote CCR9 expression. This is supported by previously published in vitro data that shows low activation of RAR (Table 1). The ability of these rexinoids to cooperate with lower concentrations of ATRA in vitro suggests a potential strategy to use these compounds in cooperation with physiological levels of vitamin A levels in vivo to promote T cell migration to mucosal regions.
Rexinoid Treatment Improves Chemokine-Mediated Migration of Effector T Cells
To evaluate the ability of rexinoid-treated T cells to migrate towards chemokine, we performed an in vitro transwell migration assay using the CCR9 ligand CCL25. P14 or SMARTA splenocytes were cultured as described above with selected rexinoids that showed the potential to act as an ATRA mimic (A18, A20, A41), or rexinoids that showed synergistic activity with ATRA (A55, A56, A57). Following culture, cells were seeded into the upper well of a transwell plate and incubated to allow for migration through the cell-permeable membrane towards CCL25 in the lower chamber. CD8+ T cells treated with the ATRA mimicking rexinoids A18 and A41 displayed significant migration towards CCL25 (Figure 5A). Notably, A41 treatment significantly increased CD8+ T cell migration compared to ATRA treatment (Figure 5A). CD8+ T cells treated with the ATRA cooperating rexinoids A55, A56, or A57 also showed significantly better migration towards CCL25 (Figure 5B). These results indicate that treatment with ATRA mimicking or ATRA cooperating rexinoids induces effector T cell migration, with some rexinoids outperforming ATRA and BEX. Rexinoid-treated CD4+ T cells displayed increased migration when treated with A41 and A56 (Supplementary Figure 3).
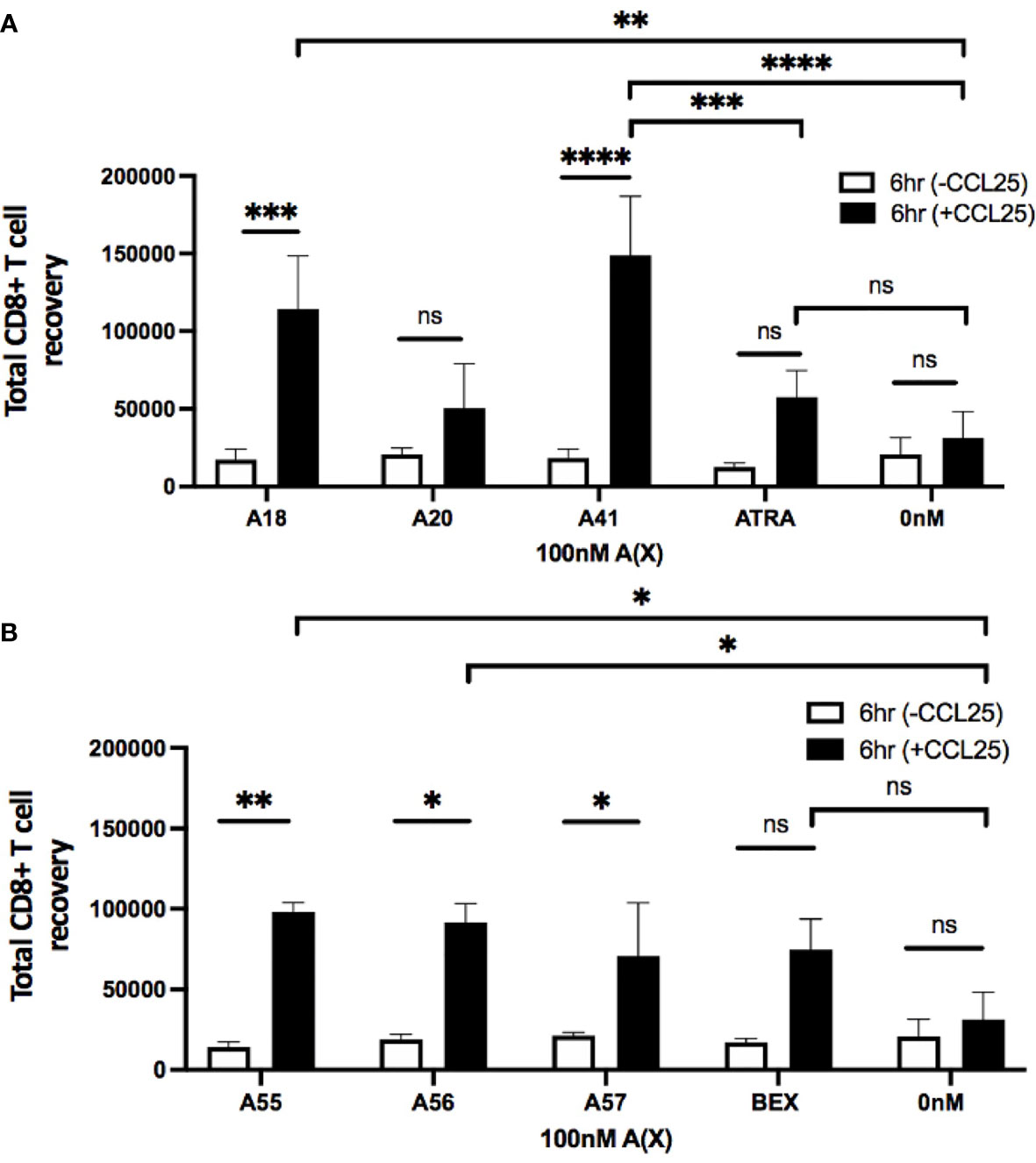
Figure 5 Treatment with ATRA independent and ATRA cooperating rexinoids enhances effector CD8+ T cell migration towards the mucosally expressed chemokine CCL25 in vitro. Splenocytes obtained from P14 mice were stimulated with GP33-41 peptide and cultured for 7 days with 100nM selected rexinoids or 10nM ATRA. Cells were then subjected to a Boyden chamber assay. 5x105 splenocytes resuspended in chemotaxis buffer were seeded into the top insert of a 96 well HTS Transwell plate and allowed 6 hours to migrate through a membrane (3.0um pore size) towards CCL25 (250nM concentration) plated in the lower chamber. Cells were then isolated from the bottom chamber and manually counted using a hemocytometer. (A) Migration following cell culture with ATRA independent rexinoids or ATRA. T cell migration towards CCL25 was significantly improved when cells were cultured with A18 and A41 (adjusted p values = 0.0009 and < 0.0001, respectively). Treatment with A18 or A41 also significantly improved migration towards CCL25 compared to no treatment given (adjusted p values = 0.004 and 0.0001, respectively). Treatment with A41 also significantly improved migration compared to treatment with ATRA (adjusted p value = 0.0008). All ATRA independent rexinoids and ATRA tested in triplicate. (B) Migration following cell culture with ATRA cooperating rexinoids or BEX. Migration towards CCL25 was significantly improved when cells were cultured with A55, A56, and A57 (adjusted p values = 0.001, 0.01, and 0.02, respectively). Treatment with A55 or A56 significantly improved migration towards CCL25 compared to no treatment given (adjusted p values = 0.01 and 0.02, respectively). A55, A56, and BEX rexinoids tested in duplicate, A57 rexinoid tested in triplicate. Statistics were calculated using a two-way ANOVA (* = p < 0.05, ** = p < 0.005, *** = p < 0.0005, **** = p < 0.0001). All error bars represent SD from the mean. ns, not significant.
Rexinoid Treatment Displays Lower Toxicity Potential In Vivo Compared to ATRA
To measure the in vivo toxicity of rexinoid treatment, we used an established metastatic osteosarcoma (mOS) mouse model for which ATRA has previously been used. Briefly, Balb/cJ mice were inoculated with K7M2 cells via tail vein injection prior to daily i.p. treatment with vehicle control or a previously established effective dose of 40mg/kg ATRA or 40mg/kg rexinoid A55. As a measure of toxicity, mouse weights were taken every 24 hours over the course of treatment. Mice that were treated with vehicle control or rexinoid A55 displayed minimal weight changes during the course of treatment, while mice treated with ATRA had significantly higher weight loss (Figure 6A), skin erythema, and fur loss (images not shown). In vivo toxicity was further examined using a high concentration of treatment delivered orally. Balb/cJ mice were similarly inoculated with K7M2 cells, and treated daily with a predetermined dose of 100mg/kg vehicle control, ATRA, or rexinoid A41, delivered via oral gavage. Mice treated with vehicle control or rexinoid A41 displayed minimal weight changes, while ATRA-treated mice displayed significant losses following treatment onset (Figure 6B). ATRA-treated mice were removed from study after 5 days treatment, due to rapid physical decompensation. Balb/cJ mice treated with an oral dose of 40mg/kg ATRA also showed greater elevation of the liver enzyme ALT at day 14 compared to mice treated with 40mg/kg vehicle control, A55, or A41 (Supplementary Figure 4). Together, these findings suggest that rexinoids are better tolerated and less toxic than ATRA when delivered as a therapeutic modality.
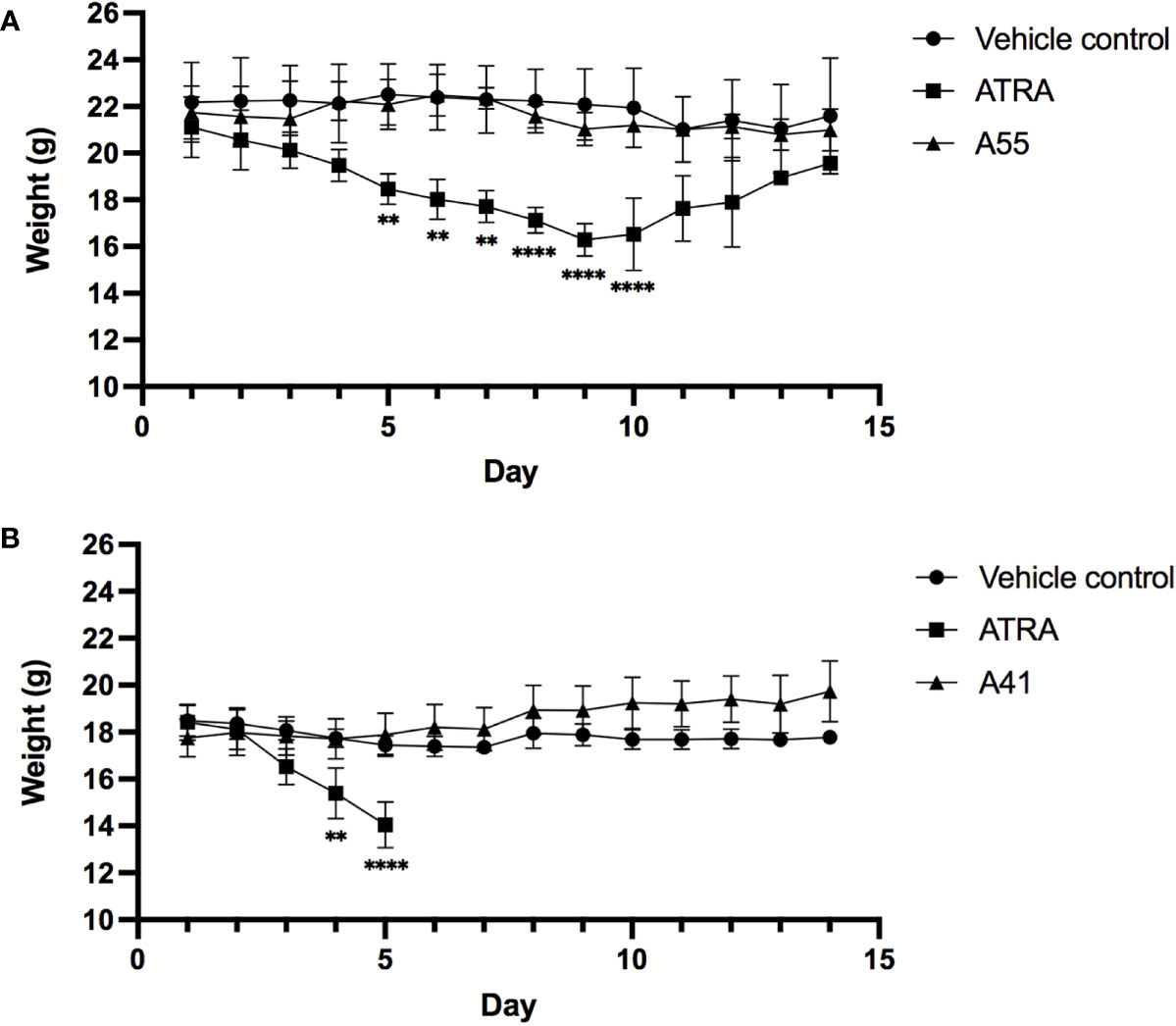
Figure 6 Mice inoculated with K7M2 cells were treated daily with either vehicle control (n=4), rexinoid A55 (n=5), or ATRA (n=4), delivered i.p. at a previously determined concentration of 40mg/kg for 14 days, or with vehicle control (n=5), rexinoid A41 (n=6), or ATRA (n=5) delivered orally at 100mg/kg for 14 days. (A) Mice that received A55 treatment i.p. had weight loss similar to negative control mice, while mice that received ATRA treatment displayed significantly larger weight losses during treatment (** = p < 0.005, **** = p < 0.0001). (B) Mice that received high dose oral A41 treatment showed slight weight gain, while ATRA-treated mice displayed significant weight loss that necessitated removal from study (** = p < 0.005, **** = p < 0.001). All error bars represent SD from the mean.
Discussion
Identifying compounds that can favorably alter T cell migration to mucosal surfaces has the potential to improve immune responses towards diseases at these surfaces. Here we tested a panel of novel rexinoids for their ability to both influence effector T cell expression of mucosal homing markers CCR9 and α4β7 and to affect their migration towards a mucosally expressed chemokine in vitro. Our results show that many rexinoids are capable of enhancing CCR9 and α4β7 expression on responding T cells. Several rexinoids induced T cell expression of CCR9 independently, mimicking the naturally occurring biologic ATRA, while others worked synergistically with subtoxic doses of ATRA to enhance expression, indicating a potential to cooperate with vitamin A present in vivo. Furthermore, both ATRA mimicking and ATRA cooperating rexinoids were seen to improve T cell migration towards the CCR9 ligand CCL25, with some outperforming bexarotene and ATRA. These findings introduce several rexinoids that can imprint T cells with a mucosal homing phenotype and influence their migration, and may have clinical relevance in treating mucosal diseases.
In addition to CCR9 and α4β7, the expression of a myriad of other genes have also been shown to be under the control of RAR signaling, including those that inhibit cell cycle progression and promote apoptosis (37–42). These discoveries have led to ATRA being used clinically as an anti-cancer drug; combination treatments that include ATRA have been successful in inducing cancer remission, most notably with acute promyelocytic leukemia (APL), a disease marked by an RARα translocation (43–45). Unfortunately, these favorable results are dampened by adverse side effects attributed to ATRA usage. Various toxicities, including hepatotoxicity due to retinyl ester buildup in hepatic stellate cells (HSCs), and mucocutaneous toxicity, have been reported in cancer patients receiving ATRA treatment (46–49). Here we confirmed that mice treated with ATRA fare poorly, as evidenced by their severe weight loss, physical appearance, and higher ALT levels. An additional complication seen with ATRA use is differentiation syndrome (DS), which can be life-threatening (49–52). Surprisingly, similar adverse health effects have also been reported following the use of synthetic vitamin A derivatives such as isotretinoin and acitretin, which has led us to postulate that the toxicities seen may be due to aberrant activation of the other RAR isoforms (53–55). This is supported by the finding that RARγ deficient mice show resistance to ATRA-mediated toxicity (56). Additionally, activation of all three RAR isoforms have been shown to display teratogenic potential (57). As the ATRA cooperating rexinoids demonstrate high selectivity for the RXR, their use could avoid such toxicity. Furthermore, the widespread use of ATRA is limited due to its instability when exposed to ubiquitous elements such as ultraviolet (UV) light, ambient temperatures, and oxygen (49, 58). The improved stability of rexinoids compared to ATRA is another attractive characteristic; their long shelf life and resistance to fluctuations in temperature, UV light, or oxygen presence makes them more durable treatment options.
In animal models of lung cancer, rexinoid use has been seen to mediate similar antiproliferative and proapoptotic effects on cancer cells as is observed with ATRA (59, 60). Importantly, rexinoid treatment has been shown to be better tolerated than ATRA in both animal and human models. Clinical trial results show that bexarotene, which is currently used as a treatment modality for patients with cutaneous T cell lymphoma (CTCL), can be safely administered at dosages of 300mg/m2/day, while side effects are seen with ATRA dosages higher than 45mg/m2/day (49, 61–67). However, it is currently unknown for most rexinoids whether they are behaving as ATRA mimics or acting in synergy with ATRA. Here we have not only identified RXR ligands that act similarly to ATRA in altering mucosal homing capabilities, but we have further determined if this effect is dependent on ATRA or not. The rexinoids capable of exerting their effect independently of ATRA have the potential to replace ATRA in therapeutic settings, as they could provide a similar efficacy with a considerably reduced ability to induce toxicity. ATRA cooperating rexinoids also have potential for use in treatment settings; combining these compounds with a much lower dose of ATRA may enhance ATRA mediated effects while minimizing toxicity side effects.
It is well-established that effector T cell infiltration into affected tissues positively correlates with protection from viral infection and tumor regression, therefore identifying methods that can specifically impact their migratory ability may improve immune responses in these microenvironments (1, 14, 68, 69). Our discovery of several rexinoids that favorably modulate T cell mucosal homing abilities in vitro indicates that they may be useful as an adjuvant during vaccination towards viruses that infect mucosal surfaces, and in immunotherapies targeting tumors that form at mucosal sites. We have previously shown in mouse models that ATRA has the potential to function as an adjuvant; i.p. injection of ATRA during vaccination increases the number of virus specific T cells to mucosal regions and boosts protection during viral challenge (1). However, this treatment is physically taxing to the mice, resulting in weight loss and inflammation at the injection site. Changing the route of delivery may improve tolerability, however the tradeoff is a reduction in ATRA bioavailability. Our preliminary in vivo work has shown that mice are not subject to the same physical discomforts following rexinoid treatment delivered via i.p. injection, as observed by their minimal weight loss during treatment (Figure 6A). Furthermore, high dose rexinoid delivered orally was well-tolerated, which could compensate for reduced bioavailability when delivered a more preferable route, unlike high dose ATRA (Figure 6B). Thus, administering either the ATRA mimicking or ATRA cooperating rexinoids via the same route as ATRA may result in similar immune modulating activity, without the associated pathology.
Adoptive cell transfer (ACT) and immune checkpoint blockade (ICB) are immunotherapies currently showing great promise as cancer treatment modalities (70–73). The ability of our rexinoids to modulate T cell migration suggests that their use in tandem with either ACT or ICB therapy may enhance the efficacy of these treatments by directing more effector T cells to tumors at mucosal sites. With ACT, the treatment of ex vivo expanded tumor-specific T cells with rexinoids prior to re-infusion can result in more T cells effectively homing to the mucosal tumor, which would result in tumor reduction and possible elimination while avoiding the majority of toxicity issues associated with ATRA use in vivo. ICB therapy using a combination of PD-L1 and CTLA-4 blocking antibodies has been shown to reverse tumor-specific effector T cell exhaustion and increase the number of tumor-infiltrating lymphocytes (TILs) present, resulting in improved anti-tumor immune responses (68, 72, 74). Inhibitory interactions between TILs and tumor cells are blocked by anti-PD-L1, while the use of anti-CTLA-4 likely both promotes the activation of new tumor-specific T cells and overcomes regulatory T cell inhibitory pathways. Although promising, this approach currently displays limited efficacy in a subset of patients (69, 75, 76). This may be due to the newly activated T cells ineffectively migrating to the tumor site, resulting in the current TILs becoming overwhelmed, and subsequent re-loss of function. Coupling this ICB approach with our identified rexinoids may ameliorate treatment efficacy towards mucosal cancers by better directing the migration of newly activated tumor-specific T cells to these sites. This would result in larger numbers of functional effector T cells present in the mucosal tumors, resulting in improved cancer control and patient survival.
While this work focuses on immune function resulting from interactions between the RXR and RAR, it should be noted that the RXR is promiscuous. It is an essential partner for a multitude of other receptors, all of which require heterodimeric formation with the RXR to exert their function (26, 77). Rexinoids that did not affect RAR/RXR mediated transcription in terms of CCR9 and α4β7 expression may play a role in mediating expression of non-immune RAR/RXR dependent genes, or may influence the expression of genes under the control of other RXR heterodimers. The potential of rexinoid treatment to beneficially regulate a variety of biological processes is an exciting and growing research area.
Data Availability Statement
The authors acknowledge that the data presented in this study must be deposited and made publicly available in an acceptable repository, prior to publication.
Ethics Statement
All animal studies were reviewed and approved by Arizona State University Institutional Animal Care and Use Committee (IACUC #19-1676R).
Author Contributions
KM performed experiments, statistical analyses, and wrote the first draft of the manuscript. PM, CW, and PJ contributed to study design and rexinoid synthesis. CW and PJ wrote sections of the manuscript. MM wrote sections of the manuscript and performed statistical analyses. HD performed experiments. JB designed and implemented the study, and wrote sections of the manuscript. All authors read, revised, and approved the manuscript prior to submission.
Funding
This work was supported in part by funds received from the NIH (NIH SBIR AI089290-01 to JB, NIH R01 AI110720-03 to JB, NIH 1R15CA139364-01A2 to CW, co-Is: PM and PJ, and NIH 1R15CA249617-01 to CW and PJ, co-I: PM) and Arizona State University (ASU GPSA and SOLS RTI funds to KM, Barrett, The Honors College Honors Thesis Reimbursement funds to HD). Patent applications covering the technologies described in this work have been applied for on behalf of the Arizona Board of Regents. This content is solely the responsibility of the authors and does not necessarily represent the official views of the National Institutes of Health.
Conflict of Interest
The authors declare that the research was conducted in the absence of any commercial or financial relationships that could be construed as a potential conflict of interest.
Publisher’s Note
All claims expressed in this article are solely those of the authors and do not necessarily represent those of their affiliated organizations, or those of the publisher, the editors and the reviewers. Any product that may be evaluated in this article, or claim that may be made by its manufacturer, is not guaranteed or endorsed by the publisher.
Acknowledgments
The first author wholeheartedly thanks Evelyn Luna, Nicole Appel, Juliane Daggett-Vondras, Jacquelyn Kilbourne, and Kenneth Lowe for their assistance provided in obtaining the in vivo data.
Supplementary Material
The Supplementary Material for this article can be found online at: https://www.frontiersin.org/articles/10.3389/fimmu.2022.746484/full#supplementary-material
Glossary
References
1. Tan X, Sande JL, Pufnock JS, Blattman JN, Greenberg PD. Retinoic Acid as a Vaccine Adjuvant Enhances CD8+ T Cell Response and Mucosal Protection From Viral Challenge. J Virol (2011) 85:8316–27. doi: 10.1128/JVI.00781-11
2. Hansen SG, Ford JC, Lewis MS, Ventura AB, Hughes CM, Coyne-Johnson L, et al. Profound Early Control of Highly Pathogenic SIV by an Effector Memory T-Cell Vaccine. Nature (2011) 473:523–7. doi: 10.1038/nature10003
3. Hansen SG, Vieville C, Whizin N, Coyne-Johnson L, Siess DC, Drummond DD, et al. Effector Memory T Cell Responses Are Associated With Protection of Rhesus Monkeys From Mucosal Simian Immunodeficiency Virus Challenge. Nat Med (2009) 15:293–9. doi: 10.1038/nm.1935
4. Burnett E, Parashar UD, Tate JE. Real-World Effectiveness of Rotavirus Vaccines, 2006-19: A Literature Review and Meta-Analysis. Lancet Glob Health (2020) 8:e1195–202. doi: 10.1016/S2214-109X(20)30262-X
5. Saluja T, Mogasale VV, Excler JL, Kim JH, Mogasale V. An Overview of Vaxchora(TM), a Live Attenuated Oral Cholera Vaccine. Hum Vaccin Immunother (2020) 16:42–50. doi: 10.1080/21645515.2019.1644882
6. Desai SN, Clemens JD. An Overview of Cholera Vaccines and Their Public Health Implications. Curr Opin Pediatr (2012) 24:85–91. doi: 10.1097/MOP.0b013e32834eb625
7. Anh DD, Lopez AL, Thiem VD, Grahek SL, Duong TN, Park JK, et al. Use of Oral Cholera Vaccines in an Outbreak in Vietnam: A Case Control Study. PloS Negl Trop Dis (2011) 5:e1006. doi: 10.1371/journal.pntd.0001006
8. Onorato IM, Modlin JF, McBean AM, Thoms ML, Losonsky GA, Bernier RH. Mucosal Immunity Induced by Enhance-Potency Inactivated and Oral Polio Vaccines. J Infect Dis (1991) 163:1–6. doi: 10.1093/infdis/163.1.1
9. Mallory RM, Bandell A, Ambrose CS, Yu J. A Systematic Review and Meta-Analysis of the Effectiveness of LAIV4 and IIV in Children Aged 6 Months to 17 Years During the 2016-2017 Season. Vaccine (2020) 38:3405–10. doi: 10.1016/j.vaccine.2019.12.015
10. Stephensen CB. Vitamin A, Infection, and Immune Function. Annu Rev Nutr (2001) 21:167–92. doi: 10.1146/annurev.nutr.21.1.167
11. Huang Z, Liu Y, Qi G, Brand D, Zheng SG. Role of Vitamin A in the Immune System. J Clin Med (2018) 7:258. doi: 10.3390/jcm7090258
12. Pino-Lagos K, Guo Y, Noelle RJ. Retinoic Acid: A Key Player in Immunity. Biofactors (2010) 36:430–6. doi: 10.1002/biof.117
13. Yang Y, Yuan Y, Tao Y, Wang W. Effects of Vitamin A Deficiency on Mucosal Immunity and Response to Intestinal Infection in Rats. Nutrition (2011) 27:227–32. doi: 10.1016/j.nut.2009.11.024
14. Holechek SA, McAfee MS, Nieves LM, Guzman VP, Manhas K, Fouts T, et al. Retinaldehyde Dehydrogenase 2 as a Molecular Adjuvant for Enhancement of Mucosal Immunity During DNA Vaccination. Vaccine (2016) 34:5629–35. doi: 10.1016/j.vaccine.2016.09.013
15. Iwata M, Hirakiyama A, Eshima Y, Kagechika H, Kato C, Song SY. Retinoic Acid Imprints Gut-Homing Specificity on T Cells. Immunity (2004) 21:527–38. doi: 10.1016/j.immuni.2004.08.011
16. Bono MR, Tejon G, Flores-Santibanez F, Fernandez D, Rosemblatt M, Sauma D. Retinoic Acid as a Modulator of T Cell Immunity. Nutrients (2016) 8:349. doi: 10.3390/nu8060349
17. Habtezion A, Nguyen LP, Hadeiba H, Butcher EC. Leukocyte Trafficking to the Small Intestine and Colon. Gastroenterology (2016) 150:340–54. doi: 10.1053/j.gastro.2015.10.046
18. Takeuchi H, Yokota A, Ohoka Y, Kagechika H, Kato C, Song SY, et al. Efficient Induction of CCR9 on T Cells Requires Coactivation of Retinoic Acid Receptors and Retinoid X Receptors (RXRs): Exaggerated T Cell Homing to the Intestine by RXR Activation With Organotins. J Immunol (2010) 185:5289–99. doi: 10.4049/jimmunol.1000101
19. Ohoka Y, Yokota A, Takeuchi H, Maeda N, Iwata M. Retinoic Acid-Induced CCR9 Expression Requires Transient TCR Stimulation and Cooperativity Between NFATc2 and the Retinoic Acid Receptor/Retinoid X Receptor Complex. J Immunol (2011) 186:733–44. doi: 10.4049/jimmunol.1000913
20. Leal AS, Reich LA, Moerland JA, Zhang D, Liby KT. Potential Therapeutic Uses of Rexinoids. Adv Pharmacol (2021) 91:141–83. doi: 10.1016/bs.apha.2021.01.004
21. DeNucci CC, Pagan AJ, Mitchell JS, Shimizu Y. Control of Alpha4beta7 Integrin Expression and CD4 T Cell Homing by the Beta1 Integrin Subunit. J Immunol (2010) 184:2458–67. doi: 10.4049/jimmunol.0902407
22. Huang P, Chandra V, Rastinejad F. Retinoic Acid Actions Through Mammalian Nuclear Receptors. Chem Rev (2014) 114:233–54. doi: 10.1021/cr400161b
23. Aranda A, Pascual A. Nuclear Hormone Receptors and Gene Expression. Physiol Rev (2001) 81:1269–304. doi: 10.1152/physrev.2001.81.3.1269
24. Goverse G, Olivier BJ, Molenaar R, Knippenberg M, Greuter M, Konijn T, et al. Vitamin A Metabolism and Mucosal Immune Function are Distinct Between BALB/c and C57BL/6 Mice. Eur J Immunol (2015) 45:89–100. doi: 10.1002/eji.201343340
25. Minucci S, Leid M, Toyama R, Saint-Jeannet JP, Peterson VJ, Horn V, et al. Retinoid X Receptor (RXR) Within the RXR-Retinoic Acid Receptor Heterodimer Binds its Ligand and Enhances Retinoid-Dependent Gene Expression. Mol Cell Biol (1997) 17:644–55. doi: 10.1128/MCB.17.2.644
26. Kliewer SA, Umesono K, Mangelsdorf DJ, Evans RM. Retinoid X Receptor Interacts With Nuclear Receptors in Retinoic Acid, Thyroid Hormone and Vitamin D3 Signalling. Nature (1992) 355:446–9. doi: 10.1038/355446a0
27. Heyman RA, Mangelsdorf DJ, Dyck JA, Stein RB, Eichele G, Evans RM, et al. 9-Cis Retinoic Acid is a High Affinity Ligand for the Retinoid X Receptor. Cell (1992) 68:397–406. doi: 10.1016/0092-8674(92)90479-V
28. Wolf G. Is 9-Cis-Retinoic Acid the Endogenous Ligand for the Retinoic Acid-X Receptor? Nutr Rev (2006) 64:532–8. doi: 10.1111/j.1753-4887.2006.tb00186.x
29. Ruhl R, Krezel W, de Lera AR. 9-Cis-13,14-Dihydroretinoic Acid, a New Endogenous Mammalian Ligand of Retinoid X Receptor and the Active Ligand of a Potential New Vitamin A Category: Vitamin A5. Nutr Rev (2018) 76:929–41. doi: 10.1093/nutrit/nuy057
30. Gorgun G, Foss F. Immunomodulatory Effects of RXR Rexinoids: Modulation of High-Affinity IL-2R Expression Enhances Susceptibility to Denileukin Diftitox. Blood (2002) 100:1399–403. doi: 10.1182/blood-2002-01-0300
31. Qiu JJ, Zeisig BB, Li S, Liu W, Chu H, Song Y, et al. Critical Role of Retinoid/Rexinoid Signaling in Mediating Transformation and Therapeutic Response of NUP98-RARG Leukemia. Leukemia (2015) 29:1153–62. doi: 10.1038/leu.2014.334
32. Sato Y, Ramalanjaona N, Huet T, Potier N, Osz J, Antony P, et al. The “Phantom Effect” of the Rexinoid LG100754: Structural and Functional Insights. PloS One (2010) 5:e15119. doi: 10.1371/journal.pone.0015119
33. Wagner CE, Jurutka PW, Marshall PA, Groy TL, van der Vaart A, Ziller JW, et al. Modeling, Synthesis and Biological Evaluation of Potential Retinoid X Receptor (RXR) Selective Agonists: Novel Analogues of 4-[1-(3,5,5,8,8-Pentamethyl-5,6,7,8-Tetrahydro-2-Naphthyl)Ethynyl]Benzoic Acid (Bexarotene). J Med Chem (2009) 52:5950–66. doi: 10.1021/jm900496b
34. Furmick JK, Kaneko I, Walsh AN, Yang J, Bhogal JS, Gray GM, et al. Modeling, Synthesis and Biological Evaluation of Potential Retinoid X Receptor-Selective Agonists: Novel Halogenated Analogues of 4-[1-(3,5,5,8,8-Pentamethyl-5,6,7,8-Tetrahydro-2-Naphthyl)Ethynyl]Benzoic Acid (Bexarotene). ChemMedChem (2012) 7:1551–66. doi: 10.1002/cmdc.201290042
35. Jurutka PW, Kaneko I, Yang J, Bhogal JS, Swierski JC, Tabacaru CR, et al. Modeling, Synthesis, and Biological Evaluation of Potential Retinoid X Receptor (RXR) Selective Agonists: Novel Analogues of 4-[1-(3,5,5,8,8-Pentamethyl-5,6,7,8-Tetrahydro-2-Naphthyl)Ethynyl]Benzoic Acid (Bexarotene) and (E)-3-(3-(1,2,3,4-Tetrahydro-1,1,4,4,6-Pentamethylnaphthalen-7-Yl)-4-Hydroxypheny L)Acrylic Acid (CD3254). J Med Chem (2013) 56:8432–54. doi: 10.1021/jm4008517
36. Heck MC, Wagner CE, Shahani PH, MacNeill M, Grozic A, Darwaiz T, et al. Modeling, Synthesis, and Biological Evaluation of Potential Retinoid X Receptor (RXR)-Selective Agonists: Analogues of 4-[1-(3,5,5,8,8-Pentamethyl-5,6,7,8-Tetrahydro-2-Naphthyl)Ethynyl]Benzoic Acid (Bexarotene) and 6-(Ethyl(5,5,8,8-Tetrahydronaphthalen-2-Yl)Amino)Nicotinic Acid (NEt-TMN). J Med Chem (2016) 59:8924–40. doi: 10.1021/acs.jmedchem.6b00812
37. Zhu WY, Jones CS, Kiss A, Matsukuma K, Amin S, De Luca LM. Retinoic Acid Inhibition of Cell Cycle Progression in MCF-7 Human Breast Cancer Cells. Exp Cell Res (1997) 234:293–9. doi: 10.1006/excr.1997.3589
38. Chen Q, Ross AC. Retinoic Acid Regulates Cell Cycle Progression and Cell Differentiation in Human Monocytic THP-1 Cells. Exp Cell Res (2004) 297:68–81. doi: 10.1016/j.yexcr.2004.02.017
39. Hu XT, Zuckerman KS. Role of Cell Cycle Regulatory Molecules in Retinoic Acid- and Vitamin D3-Induced Differentiation of Acute Myeloid Leukaemia Cells. Cell Prolif (2014) 47:200–10. doi: 10.1111/cpr.12100
40. Hatoum A, El-Sabban ME, Khoury J, Yuspa SH, Darwiche N. Overexpression of Retinoic Acid Receptors Alpha and Gamma Into Neoplastic Epidermal Cells Causes Retinoic Acid-Induced Growth Arrest and Apoptosis. Carcinogenesis (2001) 22:1955–63. doi: 10.1093/carcin/22.12.1955
41. Zhang H, Satyamoorthy K, Herlyn M, Rosdahl I. All-Trans Retinoic Acid (atRA) Differentially Induces Apoptosis in Matched Primary and Metastatic Melanoma Cells – a Speculation on Damage Effect of atRA via Mitochondrial Dysfunction and Cell Cycle Redistribution. Carcinogenesis (2003) 24:185–91. doi: 10.1093/carcin/24.2.185
42. Nagy L, Thomazy VA, Heyman RA, Davies PJ. Retinoid-Induced Apoptosis in Normal and Neoplastic Tissues. Cell Death Differ (1998) 5:11–9. doi: 10.1038/sj.cdd.4400337
43. Huang ME, Ye YC, Chen SR, Chai JR, Lu JX, Zhoa L, et al. Use of All-Trans Retinoic Acid in the Treatment of Acute Promyelocytic Leukemia. Blood (1988) 72:567–72. doi: 10.1182/blood.V72.2.567.567
44. Thomas X. Acute Promyelocytic Leukemia: A History Over 60 Years-From the Most Malignant to the Most Curable Form of Acute Leukemia. Oncol Ther (2019) 7:33–65. doi: 10.1007/s40487-018-0091-5
45. Liquori A, Ibanez M, Sargas C, Sanz MA, Barragan E, Cervera J. Acute Promyelocytic Leukemia: A Constellation of Molecular Events Around a Single PML-RARA Fusion Gene. Cancers (Basel) (2020) 12:624. doi: 10.3390/cancers12030624
46. Sindermann J, Foerster E, Kienast J. Severe Hepatobiliary Complication in a Patient With Acute Promyelocytic Leukemia Treated With All-Trans Retinoic Acid. Acta Oncol (1996) 35:499–501. doi: 10.3109/02841869609109933
47. de-Medeiros BC, Strapasson E, Pasquini R, de-Medeiros CR. Effect of All-Trans Retinoic Acid on Newly Diagnosed Acute Promyelocytic Leukemia Patients: Results of a Brazilian Center. Braz J Med Biol Res (1998) 31:1537–43. doi: 10.1590/S0100-879X1998001200005
48. Park SH, Gray WC, Hernandez I, Jacobs M, Ord RA, Sutharalingam M, et al. Phase I Trial of All-Trans Retinoic Acid in Patients With Treated Head and Neck Squamous Carcinoma. Clin Cancer Res (2000) 6:847–54.
49. Cancer, I. A. f. R. o. IARC Handbooks of Cancer Prevention. In: Retinoids. Lyon, France: World Health Organization (1999).
50. Frankel SR, Eardley A, Lauwers G, Weiss M, Warrell RP Jr. The “Retinoic Acid Syndrome” in Acute Promyelocytic Leukemia. Ann Intern Med (1992) 117:292–6. doi: 10.7326/0003-4819-117-4-292
51. De Botton S, Dombret H, Sanz M, Miguel JS, Caillot D, Zittoun R, et al. Incidence, Clinical Features, and Outcome of All Trans-Retinoic Acid Syndrome in 413 Cases of Newly Diagnosed Acute Promyelocytic Leukemia. Eur APL Group Blood (1998) 92:2712–8. doi: 10.1182/blood.V92.8.2712
52. Montesinos P, Sanz MA. The Differentiation Syndrome in Patients With Acute Promyelocytic Leukemia: Experience of the Pethema Group and Review of the Literature. Mediterr J Hematol Infect Dis (2011) 3:e2011059. doi: 10.4084/mjhid.2011.059
53. Ellis CN, Krach KJ. Uses and Complications of Isotretinoin Therapy. J Am Acad Dermatol (2001) 45:S150–157. doi: 10.1067/mjd.2001.113717
54. Khan S, Guddati AK, Marak C. Acitretin Induced Lung Injury by Differentiation Syndrome. Respir Med Case Rep (2020) 31:101138. doi: 10.1016/j.rmcr.2020.101138
55. Pilkington T, Brogden RN. Acitretin: A Review of its Pharmacology and Therapeutic Use. Drugs (1992) 43:597–627. doi: 10.2165/00003495-199243040-00010
56. Look J, Landwehr J, Bauer F, Hoffmann AS, Bluethmann H, LeMotte P. Marked Resistance of RAR Gamma-Deficient Mice to the Toxic Effects of Retinoic Acid. Am J Physiol (1995) 269:E91–8. doi: 10.1152/ajpendo.1995.269.1.E91
57. Elmazar MM, Reichert U, Shroot B, Nau H. Pattern of Retinoid-Induced Teratogenic Effects: Possible Relationship With Relative Selectivity for Nuclear Retinoid Receptors RAR Alpha, RAR Beta, and RAR Gamma. Teratology (1996) 53:158–67. doi: 10.1002/(SICI)1096-9926(199603)53:3<158::AID-TERA3>3.0.CO;2-0
58. Morgenstern J, Fleming T, Kliemank E, Brune M, Nawroth P, Fischer A. Quantification of All-Trans Retinoic Acid by Liquid Chromatography-Tandem Mass Spectrometry and Association With Lipid Profile in Patients With Type 2 Diabetes. Metabolites (2021) 11:60. doi: 10.3390/metabo11010060
59. Moerland JA, Zhang D, Reich LA, Carapellucci S, Lockwood B, Leal AS, et al. The Novel Rexinoid MSU-42011 is Effective for the Treatment of Preclinical Kras-Driven Lung Cancer. Sci Rep (2020) 10:22244. doi: 10.1038/s41598-020-79260-8
60. Liby K, Royce DB, Risingsong R, Williams CR, Wood MD, Chandraratna RA, et al. A New Rexinoid, NRX194204, Prevents Carcinogenesis in Both the Lung and Mammary Gland. Clin Cancer Res (2007) 13:6237–43. doi: 10.1158/1078-0432.CCR-07-1342
61. Duvic M, Martin AG, Kim Y, Olsen E, Wood GS, Crowley CA, et al. Phase 2 and 3 Clinical Trial of Oral Bexarotene (Targretin Capsules) for the Treatment of Refractory or Persistent Early-Stage Cutaneous T-Cell Lymphoma. Arch Dermatol (2001) 137:581–93. doi: 10.1200/JCO.2001.19.9.2456
62. Duvic M, Hymes K, Heald P, Breneman D, Martin AG, Myskowski P, et al. Bexarotene is Effective and Safe for Treatment of Refractory Advanced-Stage Cutaneous T-Cell Lymphoma: Multinational Phase II-III Trial Results. J Clin Oncol (2001) 19:2456–71. doi: 10.1200/JCO.2001.19.9.2456
63. Conley BA, Egorin MJ, Sridhara R, Finley R, Hemady R, Wu S, et al. Phase I Clinical Trial of All-Trans-Retinoic Acid With Correlation of its Pharmacokinetics and Pharmacodynamics. Cancer Chemother Pharmacol (1997) 39:291–9. doi: 10.1007/s002800050575
64. Ferla V, SciumË M, Gianelli U, Baldini L, Fracchiolla N. Multiple Adverse Drug Reactions During All-Trans Retinoic Acid Treatment for Acute Promyelocytic Leukemia: Differentiation Syndrome, Bradycardia, Intestinal Necrosis. Explor Target Antitumor Ther (2020) 1:109–16. doi: 10.37349/etat.2020.00007
65. Paydas S, Sahin B, Zorludemir S, Hazar B. All Trans Retinoic Acid as the Possible Cause of Necrotizing Vasculitis. Leuk Res (1998) 22:655–7. doi: 10.1016/S0145-2126(98)00062-9
66. Ipek Y, Hulya D, Melih A. Disseminated Exfoliative Dermatitis Associated With All-Transretinoic Acid in the Treatment of Acute Promyelocytic Leukemia. Case Rep Med (2012) 2012:236174. doi: 10.1155/2012/236174
67. Kurzrock R, Estey E, Talpaz M. All-Trans Retinoic Acid: Tolerance and Biologic Effects in Myelodysplastic Syndrome. J Clin Oncol (1993) 11:1489–95. doi: 10.1200/JCO.1993.11.8.1489
68. Lussier DM, Johnson JL, Hingorani P, Blattman JN. Combination Immunotherapy With Alpha-CTLA-4 and Alpha-PD-L1 Antibody Blockade Prevents Immune Escape and Leads to Complete Control of Metastatic Osteosarcoma. J Immunother Cancer (2015) 3:21. doi: 10.1186/s40425-015-0067-z
69. Melero I, Rouzaut A, Motz GT, Coukos G. T-Cell and NK-Cell Infiltration Into Solid Tumors: A Key Limiting Factor for Efficacious Cancer Immunotherapy. Cancer Discov (2014) 4:522–6. doi: 10.1158/2159-8290.CD-13-0985
70. Dudley ME, Wunderlich JR, Robbins PF, Yang JC, Hwu P, Schwartzentruber DJ, et al. Cancer Regression and Autoimmunity in Patients After Clonal Repopulation With Antitumor Lymphocytes. Science (2002) 298:850–4. doi: 10.1126/science.1076514
71. Rosenberg SA, Restifo NP, Yang JC, Morgan RA, Dudley ME. Adoptive Cell Transfer: A Clinical Path to Effective Cancer Immunotherapy. Nat Rev Cancer (2008) 8:299–308. doi: 10.1038/nrc2355
72. Rotte A. Combination of CTLA-4 and PD-1 Blockers for Treatment of Cancer. J Exp Clin Cancer Res (2019) 38:255. doi: 10.1186/s13046-019-1259-z
73. Blattman JN, Greenberg PD. Cancer Immunotherapy: A Treatment for the Masses. Science (2004) 305:200–5. doi: 10.1126/science.1100369
74. Plesca I, Tunger A, Muller L, Wehner R, Lai X, Grimm MO, et al. Characteristics of Tumor-Infiltrating Lymphocytes Prior to and During Immune Checkpoint Inhibitor Therapy. Front Immunol (2020) 11:364. doi: 10.3389/fimmu.2020.00364
75. Fares CM, Van Allen EM, Drake CG, Allison JP, Hu-Lieskovan S. Mechanisms of Resistance to Immune Checkpoint Blockade: Why Does Checkpoint Inhibitor Immunotherapy Not Work for All Patients? Am Soc Clin Oncol Educ Book (2019) 39:147–64. doi: 10.1200/EDBK_240837
76. Jenkins RW, Barbie DA, Flaherty KT. Mechanisms of Resistance to Immune Checkpoint Inhibitors. Br J Cancer (2018) 118:9–16. doi: 10.1038/bjc.2017.434
Keywords: migration, mucosal, rexinoids, retinoic acid, retinoid, T-cell, toxicity, vitamin A
Citation: Manhas KR, Marshall PA, Wagner CE, Jurutka PW, Mancenido MV, Debray HZ and Blattman JN (2022) Rexinoids Modulate Effector T Cell Expression of Mucosal Homing Markers CCR9 and α4β7 Integrin and Direct Their Migration In Vitro. Front. Immunol. 13:746484. doi: 10.3389/fimmu.2022.746484
Received: 23 July 2021; Accepted: 03 January 2022;
Published: 27 January 2022.
Edited by:
Christoph Siegfried Niki Klose, Charité University Medicine Berlin, GermanyReviewed by:
Guoxun Chen, The University of Tennessee, United StatesNavin Kumar Verma, Nanyang Technological University, Singapore
Copyright © 2022 Manhas, Marshall, Wagner, Jurutka, Mancenido, Debray and Blattman. This is an open-access article distributed under the terms of the Creative Commons Attribution License (CC BY). The use, distribution or reproduction in other forums is permitted, provided the original author(s) and the copyright owner(s) are credited and that the original publication in this journal is cited, in accordance with accepted academic practice. No use, distribution or reproduction is permitted which does not comply with these terms.
*Correspondence: Joseph N. Blattman, joseph.blattman@asu.edu