- 1School of Systems Biology, George Mason University, Fairfax, VA, United States
- 2Research Centre for Medical Genetics, Moscow, Russia
- 3Institute of Neuropsychiatry, The Affiliated Brain Hospital of Nanjing Medical University, Nanjing, China
- 4Department of Psychiatry, The Affiliated Brain Hospital of Nanjing Medical University, Nanjing, China
Objectives: Recent studies suggest that asthma may have a protective effect on COVID-19.We aimed to investigate the causality between asthma and two COVID-19 outcomes and explore the mechanisms underlining this connection.
Methods: Summary results of GWAS were used for the analyses, including asthma (88,486 cases and 447,859 controls), COVID-19 hospitalization (6,406 hospitalized COVID-19 cases and 902,088 controls), and COVID-19 infection (14,134 COVID-19 cases and 1,284,876 controls). The Mendelian randomization (MR) analysis was performed to evaluate the causal effects of asthma on the two COVID-19 outcomes. A cross-trait meta-analysis was conducted to analyze genetic variants within two loci shared by COVID-19 hospitalization and asthma.
Results: Asthma is associated with decreased risk both for COVID-19 hospitalization (odds ratio (OR): 0.70, 95% confidence interval (CI): 0.70-0.99) and for COVID-19 infection (OR: 0.83, 95%CI: 0.51-0.95). Asthma and COVID-19 share two genome-wide significant genes, including ABO at the 9q34.2 region and OAS2 at the 12q24.13 region. The meta-analysis revealed that ABO and ATXN2 contain variants with pleiotropic effects on both COVID-19 and asthma.
Conclusion: In conclusion, our results suggest that genetic liability to asthma is associated with decreased susceptibility to SARS-CoV-2 and to severe COVID-19 disease, which may be due to the protective effects of ongoing inflammation and, possibly, related compensatory responses against COVID-19 in its early stage.
Introduction
The COVID-19 pandemic due to the severe acute respiratory syndrome coronavirus 2 (SARS-CoV-2) infections caused a public health crisis worldwide. By the end of March 2021, the COVID-19 pandemic has incurred 128 million infections worldwide, including close to three million deaths, with a mortality rate of 2.2%, according to Johns Hopkins Coronavirus Resource Center (https://coronavirus.jhu.edu/map.html). Although the majority of infected persons experience mild no obvious symptoms, approximately 10-20% of people with COVID-19 infection need hospitalization (1, 2). In hospitalized patients, comorbid hypertension, obesity, and diabetes are common (1, 2).
There is substantial variability in terms of symptoms, severity, and prognosis of the disease. Infected individuals with older age or medical complications are more likely to develop severe symptoms, with some young and seemingly healthy individuals also having serious outcomes. Host genetics is considered to play an essential role in an individual’s propensity to contract infectious diseases (3). Other medical conditions may exert an influence on an individual’s susceptibility to COVID-19 both by disease-driven conditioning of the immune system, and by shared genetic variations, which may either predispose to comorbid conditions or aid in resisting disease phenotype.
As COVID-19 disease is known to affect both upper and lower airways, it is not surprising that the links between SARS-CoV-2 severity and pre-existing lung inflammation were soon uncovered. In particular, in lung epithelium cell datasets from SARS-CoV-2 infection and idiopathic pulmonary fibrosis, transcriptomic analyses pinpointed a set of shared pathways and hub genes (4). Similarly made observations genetically connected SARS-CoV-2 withpulmonary arterial hypertension (5).
It is commonly accepted that asthmatics and patients with respiratory allergies have increased susceptibility and severity for viral infections (6). Therefore, asthma was initially considered as a risk factor for COVID-19, and a higher prevalence of asthma in COVID-19 hospitalized patients has been reported (7). Asthma was reported to be associated with a higher risk of morbidity in COVID-19 patients (8). However, some studies reported that the prevalence of asthma in patients with COVID-19 is lower than expected (9, 10), suggesting that having asthma may exert a protective effect (11). Some evidence indicates that asthma is not associated with outcomes of COVID-19 (12–14). Until now, the relationship between COVID-19 and asthma remains controversial and is under active debate (15–18). The associations reported by observational studies may suffer from limited support for causality. Therefore, there is an urgent need to determine their association using more fundamental evidence and to elucidate the mechanisms underlying the association between COVID-19 and asthma.
Mendelian randomization (MR) is an analytic framework that utilizes genetic variants as instrumental variables to test the causative association between an exposure and an outcome (19), which has been widely used in recent studies (20–22). In particular, previous MR analyses have reported causal risk factors for a severe course of COVID-19, including body mass index and smoking intensity (23–25). In this study, summary-level Genome-Wide Association Studies (GWAS) data were utilized to test for putative causal associations between asthma and two COVID-19 outcomes. Furthermore, we annotated the COVID-19 GWAS results by performing functional analyses for the discovered genes. These asthma-related findings may shed more insight into the COVID-19 pathophysiology.
Methods
Study Design and Participants
Two-sample MR was employed to investigate causal relationships between asthma and COVID-19 outcomes in the summary-level GWAS datasets. The asthma GWAS dataset included 88,486 cases and 447,859 controls (97.2% of the participants were of European origins) (26). Two datasets were obtained from the COVID-19 Host Genetic Initiative GWAS meta-analyses round 4 (Release Date: October 20, 2020) (27), with outcomes including either COVID-19 hospitalization (6,406 hospitalized COVID-19 cases and 902,088 controls), or COVID-19 infection (14,134 COVID-19 cases and 1,284,876 controls). COVID-19 infection reflects the overall susceptibility to the disease, whereas COVID-19 hospitalization cases represent the relative severity of the disease. In both the COVID-19 datasets, all the participants were of European origins.
MR Analysis and Genetic Correlation Estimation
Causality was tested using inverse variance-weighted (IVW) analysis (28). To evaluate the sensitivity, we further test the causal effect using the MR-Egger regression (29) and the weighted median method (30). The intercept from the MR-Egger model was used as a measure of directional pleiotropy. All the above analyses, and the heterogeneity analysis, were conducted using TwoSampleMR v0.5.5 (31). Single-nucleotide polymorphisms (SNPs) associated with asthma at genome-wide significance (P < 5.0E-8) were selected as instrumental variants and further pruned using a clumping r2 cutoff of 0.01. The genetic correlations of asthma with COVID-19 outcomes were calculated using linkage disequilibrium (LD) score regression (32). Statistical significance of the analyses was accepted when P values were < 0.05.
Annotation of the COVID-19 and Asthma GWAS Results
Functional mapping and annotation (FUMA) software was used to map SNPs to genes and identify LD-independent genomic regions (33). All genes located within 10 kb vicinity of each variant were mapped. Independent significant SNPs (IndSigSNPs) were extracted when their P-value were genome-wide significant (P ≤ 5.0E-08) and independent of each other (r2 < 0.6). Lead SNPs were identified as a subset of the independent significant SNPs that were in LD with each other at r2 < 0.1 within a 500 Kb window. Genomic risk loci were identified by merging lead SNPs located at a distance of less than 500 kb from each other. Clumping procedures were carried out in accordance with the European 1000 Genomes Project phase 3 reference panel. Due to extensive LD, the entire major histocompatibility complex (MHC) locus was merged into one region (chr6:25-35Mb). Regional association results of the loci were plotted using LocusZoom (34).
Cross-Trait Meta-Analysis of COVID-19 Hospitalization and Asthma
We conducted a cross-trait meta-analysis to identified pleiotropic genetic variants shared by asthma and COVID-19 hospitalization. ASSET is an agnostic approach that performs cross-trait meta-analysis by allowing a subset of the input GWASs to have no effect on a given SNP (35). This technique identifies the strongest association signal by exhaustive exploration of all possible subsets of GWAS and their inputs within a fixed-effect framework.
Tissue Specificity and Pathway Enrichment Analyses
Tissue specificity of the genome-wide genes was measured against each of the differentially expressed gene (DEG) sets from GTEx v8 (36) using the hypergeometric test (33). For each genome-wide gene, enrichment in canonical pathways was evaluated using FUMA (33). All analyses were done using R v4.0.3 or Python v3.7. A detailed description of the methods is provided in the Supplementary File.
Gene Overlap Analysis for COVID-19 and Asthma
To identify overlapped risk genes between COVID-19 and asthma, we retrieved genome-wide risk genes for two traits from GWAS-catalog (https://www.ebi.ac.uk/gwas/). For COVID-19, we combined the results from GWAS-catalog and the genes identified in our present study.
Results
MR Analysis and Genetic Correlation Estimation
As shown in Table 1 and Figure 1, our MR analysis unequivocally indicated that asthma is associated with decreased risk for either COVID-19 infection (OR: 0.83, 95%CI: 0.70-0.99, P = 0.037) or hospitalization (OR: 0.70, 95%CI: 0.51-0.95, P = 0.023). The sensitivity analyses suggested that the directions of causal effect estimates across the methods were the same. Tests of MR-Egger regression did not support the directional pleiotropy of the genetic instrumental variables for both the causal associations (MR-Egger intercept < 0.001, P > 0.05). The heterogeneity test did not support the existence of heterogeneity in the MR analysis (all P > 0.05). There were no genetic correlations between asthma and COVID-19 hospitalization (r = -0.03, P = 0.631) or COVID-19 infection (r = 0.11, P = 0.120).
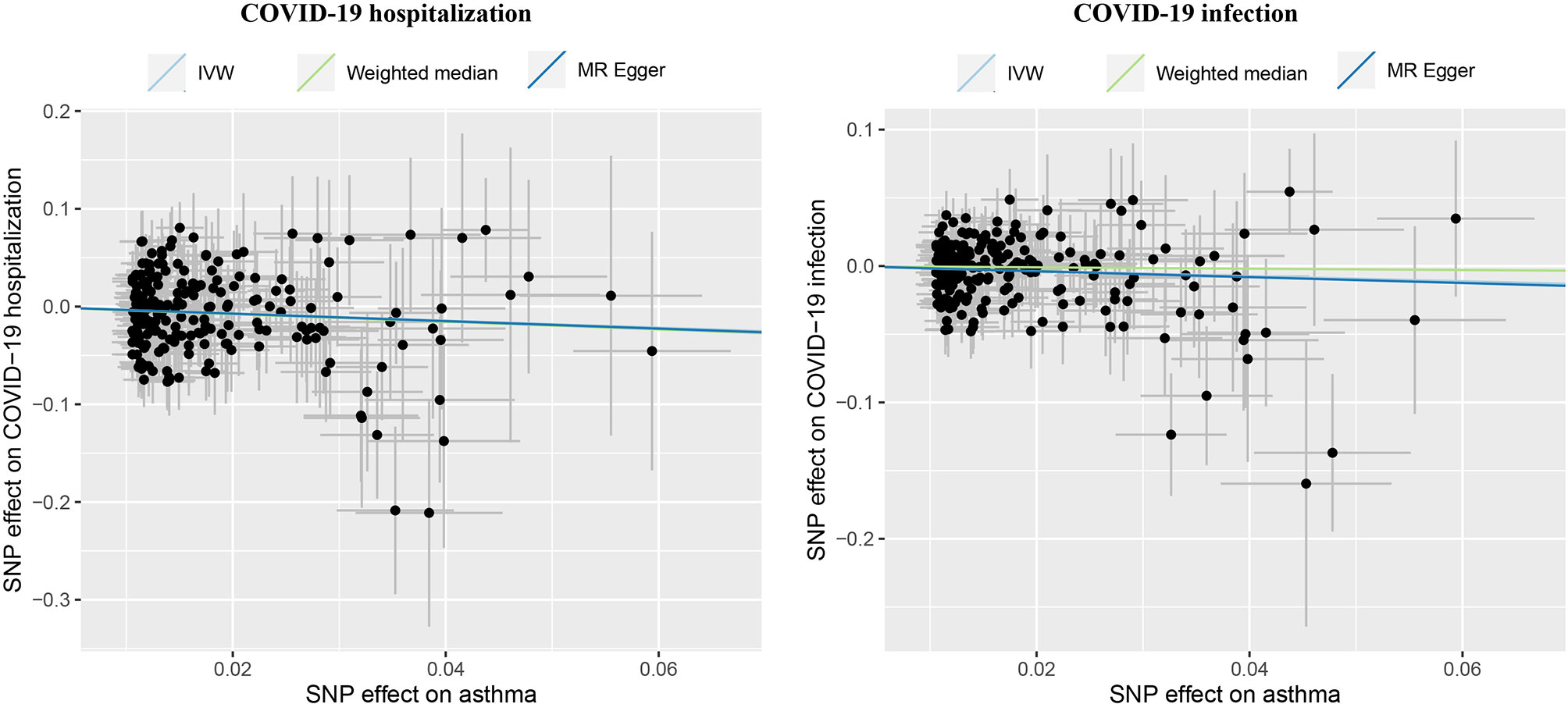
Figure 1 Causal effects of asthma on COVID-19 outcomes, including hospitalization and infection. IVW, inverse variance weighted; MR, Mendelian randomization. The lines denote effect sizes (b).
Annotation of the COVID-19 and Asthma GWAS Results
A total of six and four genomic loci were associated with COVID-19 hospitalization and with infection, respectively (Table 2 and Figure 2). All the four loci implicated in COVID-19 infection overlapped with the six loci associated with COVID-19 hospitalization. For both datasets, the 3p21.31 locus had the largest amount of signals within protein-coding genes.
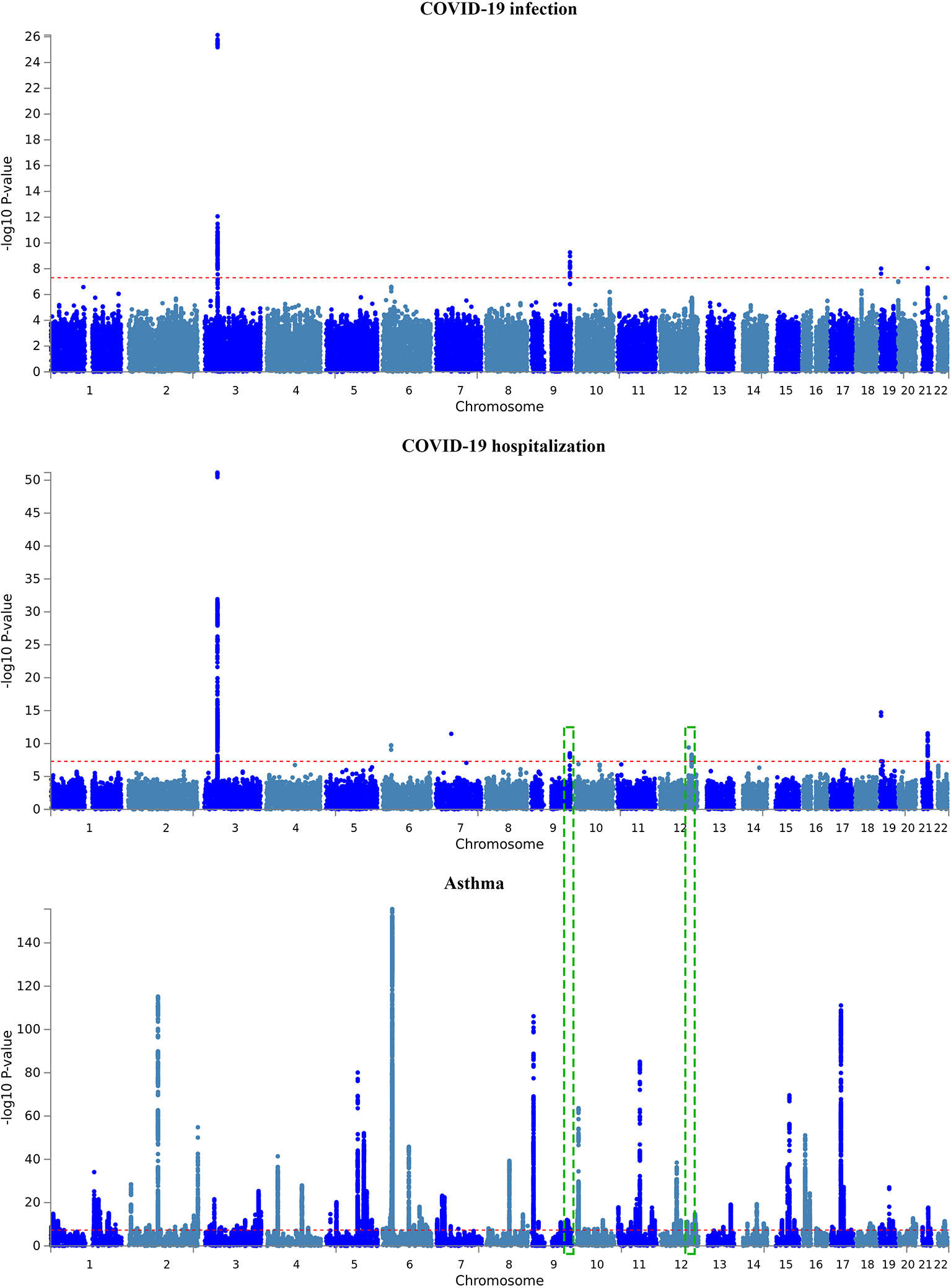
Figure 2 Manhattan plot of GWAS results of the COVID-19 outcomes and asthma. The x-axis is chromosomal position of SNPs and the y-axis is the significance of the SNPs (-log10P). Each horizontal dashed line denotes genome-wide significance level of 5E-8. Dashed green rectangles indicate the two overlapped genomic loci between COVID-19 hospitalization and asthma.
A total of 19 and 10 protein-coding genes were detected for COVID-19 hospitalization and infection, respectively (Table 2). All the 10 coding genes implicated in COVID-19 infection overlapped with the gene set for the COVID-19 hospitalization. Therefore, the present study revealed a total of 19 genome-wide risk genes for COVID-19, including ABO, CCR1, CCR2, CCR3, CCR9, CCRL2, CXCR6, DPP9, FYCO1, IFNAR2, LIMD1, LTF, LZTFL1, OAS1, OAS2, OAS3, SLC6A20, VSTM2A, and XCR1.
For asthma, a total of 169 genomic loci were associated with the illness. Interestingly, two loci were overlapped with those of COVID-19 hospitalization, including the 9q34.2 locus and the 12q24.13 locus (Table 2 and Figure 2). The ABO gene within the 9q34.2 locus was implicated in both asthma and COVID-19.
Cross-Trait Meta-Analysis of COVID-19 Hospitalization and Asthma
The cross-trait meta-analysis identified 63 significant associations (P < 5E-8), including two SNPs shared by COVID-19 and asthma (Figure 3 and Supplementary Table 1). The rs1381383189 within ABO was implicated in both the traits in the same direction (3.00E-08), while rs35350651 within ATXN2 was implicated in both the traits in the opposite directions (2.33E-09).
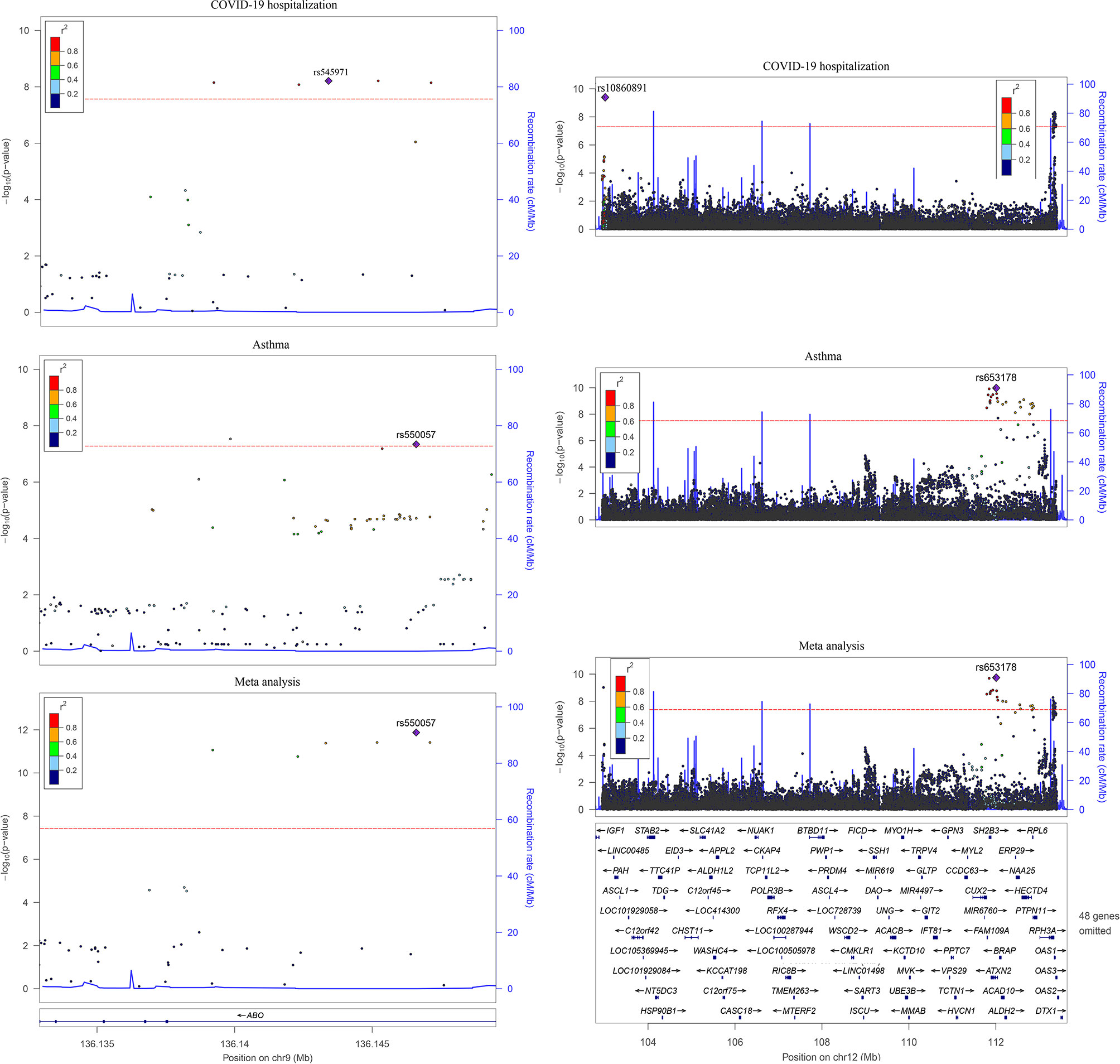
Figure 3 Two overlapped loci between COVID-19 hospitalization and asthma. Left is the 9q34.2 locus and right is the 12q24.13 locus in hg19. The linkage disequilibrium information is from phase 3 of the 1000 Genomes Project. The dashed line represents the threshold for genome-wide significance (P < 5.0E-08).
Tissue Specificity and Pathway Enrichment Analyses
Gene-based tissue enrichment analysis showed that the set of 19 genes of COVID-19 was upregulated in the spleen, lung, and blood (Supplementary Figure 1A). SNP-based tissue enrichment analysis of asthma showed that the GWAS hits of asthma were over-represented in blood, spleen, lung, and small intestine (Supplementary Figure 1B). The pathway enrichment analysis highlighted multiple pathways, including cytokine interaction with their receptors, chemokine and interferon signaling, human oligoadenylate synthetase (OAS) antiviral response, G protein-coupled receptor signaling, and natural killer T pathway (Figure 4). These pathways are predominantly involved in the inflammatory function.
Overlapped Genome-Wide Risk Genes Between COVID-19 and Asthma
In the GWAS catalog, there were 19 and 1,293 genes for COVID-19 and asthma, respectively. After merging with the 27 genes extracted in this study, a set of 34 risk genes for COVID-19 was formed. Overlap analysis revealed that two protein-coding genes were shared between the two conditions, namely, ABO and OAS2.
Discussion
Our study shows that asthma has a protective effect on the risk of COVID-19 infection and hospitalization, representing a surprising departure from other common respiratory viral outbreaks. Notably, COVID-19 progression relies on the over-activation of innate immunity and ‘cytokine storms’. Predominantly allergic immune responses, which are characteristic of asthma, may mediate the protective effect of asthma against COVID-19. The receptor-binding domain of SARS-CoV-2 spike protein docks to Angiotensin-Converting Enzyme 2 (ACE2), which is encoded by the gene reported as less active in asthma patients, thus, possibly limiting the entry of the virus into the epithelium of the asthmatic’ airways (37). The expression levels of ACE2 negatively correlate with the levels of Th2 cytokines in airway epithelial cells (IL-4, IL-5, and IL-13) and with total amounts of IgE (37, 38). In asthma, the predominance of Th2 response may alleviate the viral-induced release of interferons, and downregulate the cytokine storm which is typical for advanced COVID-19, thus, preventing hospitalization.
On the other hand, both the asthma treatments, namely, inhaled corticosteroids (39), and the cross-reactivity to T cell epitopes of common airborne allergens (40) may directly decrease the risk of contracting SARS-CoV-2 infection either by alleviating inflammation or by providing pre-existing immunity.
In this paper, we identified a set of 19 protein-coding risk genes associated with COVID-19 susceptibility and severity. These genes are located within six genomic loci, with chromosome 3p21.31 displaying the peak association across the two COVID-19 datasets and encompassing a cluster of chemokine receptor genes. Thus, our study supports the 3p21.31 locus as the most critical among COVID-19-related regions, which has been identified and highlighted by previous GWASs and functional analyses (41–43).
Our study revealed loci within the 9q34.2 and the 12q24.13 region as influencing both asthma and COVID-19 hospitalization. The ABO gene is the single gene within the 9q34.2 locus. Previously GWASs have identified it as a risk gene for critical illness of COVID-19 (41, 42) and asthma (26), while the present study suggests its involvement of COVID-19 infection and hospitalization as well. In previous studies, blood group A was associated with an increased risk for severe COVID-19 (OR = 1.45), while blood group O was shown to confer a protective effect (OR = 0.65) (42). Epidemiological studies reported a similar risk pattern for contracting COVID-19 (44, 45). Interestingly, ABO was also highlighted as a genome-wide gene for asthma by Han et al. (26), with blood group O being specifically reported as a risk factor for asthma in a recent review (46). Therefore, the effects incurred by the blood types on both diseases seem genuine.
Chromosome 12q24.13 contains a cluster of genes for the oligoadenylate synthase family (OAS1, OAS2, and OAS3). These enzymes synthesize 2’,5’-oligoadenylates (2-5As), which aid in degrading viral RNAs and inhibiting viral replication by activating latent RNase L (47). The association of OAS2 with asthma was reported in an earlier GWAS (48). Moreover, one study showed that expression levels of OAS2 correlate with reticular basement membrane thickness (49). Notably, OAS2 was recently suggested as one of the hub genes for coordinating innate immune responses in COVID-19 and a potential to-be-augmented target for the treatments of this illness (50). In particular, inhibitors of endogenous phosphodiesterase 12 (PDE-12) enzyme, which cleaves the host 2-5As, were proposed for this purpose (51).
Our meta-analysis supports that the effects of variation within the ABO and ATXN2 genes are shared between COVID-19 and asthma. Since ATXN2 has been associated with asthma at the genome-wide level, our meta-analysis suggests ATXN2 may be a novel risk gene for COVID-19. Ataxin-2, which is encoded by the ATXN2 gene, is a multifunctional protein of the rough endoplasmic reticulum and plasma membrane (52), where it modulates mTOR signals by participating in its translational regulation by associating with polyribosomes (53). In stressed cells, ataxin-2 also is involved in the formation of stress granules, where untranslated mRNAs are translationally inhibited (54, 55). Notably, stress granules attract certain viral proteins, including that of positive-strand RNA viruses SARS-CoV-2 (56) and Zika (57). In fact, induced disassembly of the stress granules is required for the production of viral particles (58). While the role of ataxin-2 in supporting the replication of SARS-CoV-2 is yet to be investigated, Zika (ZIKV) decreases its viral production in response to ataxin-2 depletion (57). Moreover, the N protein of SARS-CoV-2 (56, 59) and ataxin-2 (60) both aid in the formation of high-density protein/RNA condensates through their intrinsically disordered regions, possibly competing with each other.
The role of ataxin-2 in immunity is less clear. Previous studies have connected the genetic variation in the SH2B3/ATXN2 region with CD4+ T cells counts (61), and a variety of autoimmune conditions, including alopecia areata (62) and sarcoidosis (63).
Functional analyses showed that the set of 19 genome-wide risk genes for COVID-19 is expressed at a high level in the spleen, lung, and blood, supporting the involvement of the local immune responses in course of the COVID-19. Interestingly, GWAS hits of asthma were also enriched in three tissues mentioned above, and in the small intestine. Pathway analysis supports that the severity-related set of 19 genes predominately participates in cytokine and chemokine signaling, consistent with their enrichment in gene sets associated with several immune-related conditions. Our results strengthen the proposed viewpoint that COVID-19 progression depends on over-activated innate immunity and resultant ‘cytokine storm’.
The strengths of this study include the MR design, which is known to help avoid the causality pitfalls of traditional observational epidemiological studies. All or the vast majority of the participants were of European ancestry, reducing the potential population heterogeneity. Several limitations should be acknowledged, including pleiotropy as a potential source of bias capable of undermining the validity of an MR study. In the present study, both COVID-19 and asthma datasets contained samples from the UK biobank; this sample overlap may contribute to pleiotropy. However, the pleiotropy test revealed no indication of directional pleiotropy in the MR analysis.
In conclusion, our results suggest that genetic liability to asthma is associated with decreased risk for COVID-19 infection and hospitalization. This phenomenon may be due to the protective effects of ongoing inflammatory responses against the early stages of COVID-19.
Data Availability Statement
Publicly available datasets were analyzed in this study. This data can be found here: COVID-19 Host Genetic Initiative (https://www.covid19hg.org/results/r4/) and The NHGRI-EBI Catalog (https://www.ebi.ac.uk/gwas/).
Author Contributions
FZ contributed to the study design and data analysis. FZ and AB wrote the manuscript. All authors contributed to revising the work and approved the final manuscript.
Funding
This work was supported by the National Natural Science Foundation of China (81471364).
Conflict of Interest
The authors declare that the research was conducted in the absence of any commercial or financial relationships that could be construed as a potential conflict of interest.
Publisher’s Note
All claims expressed in this article are solely those of the authors and do not necessarily represent those of their affiliated organizations, or those of the publisher, the editors and the reviewers. Any product that may be evaluated in this article, or claim that may be made by its manufacturer, is not guaranteed or endorsed by the publisher.
Supplementary Material
The Supplementary Material for this article can be found online at: https://www.frontiersin.org/articles/10.3389/fimmu.2022.705379/full#supplementary-material
References
1. Richardson S, Hirsch JS, Narasimhan M, Crawford JM, McGinn T, Davidson KW, et al. Clarification of Mortality Rate and Data in Abstract, Results, and Table 2. JAMA (2020) 323(20):2098. doi: 10.1001/jama.2020.7681
2. Richardson S, Hirsch JS, Narasimhan M, Crawford JM, McGinn T, Davidson KW, et al. Presenting Characteristics, Comorbidities, and Outcomes Among 5700 Patients Hospitalized With COVID-19 in the New York City Area. JAMA (2020) 323(20):2052–9. doi: 10.1001/jama.2020.6775
3. Chapman SJ, Hill AV. Human Genetic Susceptibility to Infectious Disease. Nat Rev Genet (2012) 13(3):175–88. doi: 10.1038/nrg3114
4. Taz TA, Ahmed K, Paul BK, Kawsar M, Aktar N, Mahmud SMH, et al. Network-Based Identification Genetic Effect of SARS-CoV-2 Infections to Idiopathic Pulmonary Fibrosis (IPF) Patients. Brief Bioinform (2021) 22(2):1254–66. doi: 10.1093/bib/bbaa235
5. Taz TA, Ahmed K, Paul BK, Al-Zahrani FA, Mahmud SMH, Moni MA. Identification of Biomarkers and Pathways for the SARS-CoV-2 Infections That Make Complexities in Pulmonary Arterial Hypertension Patients. Brief Bioinform (2021) 22(2):1451–65. doi: 10.1093/bib/bbab026
6. Corne JM, Marshall C, Smith S, Schreiber J, Sanderson G, Holgate ST, et al. Frequency, Severity, and Duration of Rhinovirus Infections in Asthmatic and non-Asthmatic Individuals: A Longitudinal Cohort Study. Lancet (2002) 359(9309):831–4. doi: 10.1016/S0140-6736(02)07953-9
7. Khawaja AP, Warwick AN, Hysi PG, Kastner A, Dick A, Khaw PT, et al. Associations With Covid-19 Hospitalisation Amongst 406,793 Adults: The UK Biobank Prospective Cohort Study. medRxiv (2020). doi: 10.1101/2020.05.06.20092957
8. Choi HG, Wee JH, Kim SY, Kim JH, Il Kim H, Park JY, et al. Association Between Asthma and Clinical Mortality/Morbidity in COVID-19 Patients Using Clinical Epidemiologic Data From Korean Disease Control and Prevention. Allergy (2021) 76(3):921–4. doi: 10.1111/all.14675
9. Wang JY, Pawankar R, Tsai HJ, Wu LS, Kuo WS. COVID-19 and Asthma, the Good or the Bad? Allergy (2021) 76(2):565–7. doi: 10.1111/all.14480
10. Avdeev S, Moiseev S, Brovko M, Yavorovskiy A, Umbetova K, Akulkina L, et al. Low Prevalence of Bronchial Asthma and Chronic Obstructive Lung Disease Among Intensive Care Unit Patients With COVID-19. Allergy (2020) 75(10):2703–4. doi: 10.1111/all.14420
11. Carli G, Cecchi L, Stebbing J, Parronchi P, Farsi A. Is Asthma Protective Against COVID-19? Allergy (2020) 76(3):866–8. doi: 10.1111/all.14426
12. Beken B, Ozturk GK, Aygun FD, Aydogmus C, Akar HH. Asthma and Allergic Diseases are Not Risk Factors for Hospitalization in Children With Coronavirus Disease 2019. Ann Allergy Asthma Immunol (2021) 126(5):569–75. doi: 10.1016/j.anai.2021.01.018
13. Timberlake DT, Strothman K, Grayson MH. Asthma, Severe Acute Respiratory Syndrome Coronavirus-2 and Coronavirus Disease 2019. Curr Opin Allergy Clin Immunol (2021) 21(2):182–7. doi: 10.1097/ACI.0000000000000720
14. Kim S, Jung CG, Lee JY, Kim G, Choi SW, Jin HJ, et al. Characterization of Asthma and Risk Factors for Delayed SARS-CoV-2 Clearance in Adult COVID-19 Inpatients in Daegu. Allergy (2021) 76(3):918–21. doi: 10.1111/all.14609
15. Johnston SL. Asthma and COVID-19: Is Asthma a Risk Factor for Severe Outcomes? Allergy (2020) 75(7):1543–5. doi: 10.1111/all.14348
16. Morais-Almeida M, Barbosa MT, Sousa CS, Aguiar R, Bousquet J. Update on Asthma Prevalence in Severe COVID-19 Patients. Allergy (2021) 76(3):953–4. doi: 10.1111/all.14482
17. Song J, Zeng M, Wang H, Qin C, Hou HY, Sun ZY, et al. Distinct Effects of Asthma and COPD Comorbidity on Disease Expression and Outcome in Patients With COVID-19. Allergy (2021) 76(2):483–96. doi: 10.1111/all.14517
18. Kow CS, Capstick T, Hasan SS. Are Severe Asthma Patients at Higher Risk of Developing Severe Outcomes From COVID-19? Allergy (2021) 76(3):959–60. doi: 10.1111/all.14589
19. Lawlor DA, Harbord RM, Sterne JA, Timpson N, Davey Smith G. Mendelian Randomization: Using Genes as Instruments for Making Causal Inferences in Epidemiology. Stat Med (2008) 27(8):1133–63. doi: 10.1002/sim.3034
20. Zhang F, Rao S, Cao H, Zhang X, Wang Q, Xu Y, et al. Genetic Evidence Suggests Posttraumatic Stress Disorder as a Subtype of Major Depressive Disorder. J Clin Invest (2022) 132(3):e145942. doi: 10.1172/JCI145942
21. Baranova A, Cao H, Zhang F. Shared Genetic Liability and Causal Effects Between Major Depressive Disorder and Insomnia. Hum Mol Genet (2021). doi: 10.1093/hmg/ddab328
22. Zhang F, Cao H, Baranova A. Shared Genetic Liability and Causal Associations Between Major Depressive Disorder and Cardiovascular Diseases. Front Cardiovasc Med (2021) 8:735136. doi: 10.3389/fcvm.2021.735136
23. Initiative C-HG. Mapping the Human Genetic Architecture of COVID-19. Nature (2021) 600(7889):472–7. doi: 10.1038/s41586-021-03767-x
24. Rao S, Baranova A, Cao H, Chen J, Zhang X, Zhang F. Genetic Mechanisms of COVID-19 and its Association With Smoking and Alcohol Consumption. Brief Bioinform (2021) 22(6):bbab284. doi: 10.1093/bib/bbab284
25. Zhang F, Baranova A. Smoking Quantitatively Increases Risk for COVID-19. Eur Respir J (2021). doi: 10.1183/13993003.01273-2021
26. Han Y, Jia Q, Jahani PS, Hurrell BP, Pan C, Huang P, et al. Genome-Wide Analysis Highlights Contribution of Immune System Pathways to the Genetic Architecture of Asthma. Nat Commun (2020) 11(1):1776. doi: 10.1038/s41467-020-15649-3
27. Initiative C-HG. The COVID-19 Host Genetics Initiative, a Global Initiative to Elucidate the Role of Host Genetic Factors in Susceptibility and Severity of the SARS-CoV-2 Virus Pandemic. Eur J Hum Genet (2020) 28(6):715–8. doi: 10.1038/s41431-020-0636-6
28. Burgess S, Butterworth A, Thompson SG. Mendelian Randomization Analysis With Multiple Genetic Variants Using Summarized Data. Genet Epidemiol (2013) 37(7):658–65. doi: 10.1002/gepi.21758
29. Bowden J, Davey Smith G, Burgess S. Mendelian Randomization With Invalid Instruments: Effect Estimation and Bias Detection Through Egger Regression. Int J Epidemiol (2015) 44(2):512–25. doi: 10.1093/ije/dyv080
30. Bowden J, Davey Smith G, Haycock PC, Burgess S. Consistent Estimation in Mendelian Randomization With Some Invalid Instruments Using a Weighted Median Estimator. Genet Epidemiol (2016) 40(4):304–14. doi: 10.1002/gepi.21965
31. Hemani G, Zheng J, Elsworth B, Wade KH, Haberland V, Baird D, et al. The MR-Base Platform Supports Systematic Causal Inference Across the Human Phenome. Elife (2018) 7:e34408. doi: 10.7554/eLife.34408
32. Bulik-Sullivan BK, Loh PR, Finucane HK, Ripke S, Yang J, Schizophrenia Working Group of the Psychiatric Genomics C, et al. LD Score Regression Distinguishes Confounding From Polygenicity in Genome-Wide Association Studies. Nat Genet (2015) 47(3):291–5. doi: 10.1038/ng.3211
33. Watanabe K, Taskesen E, van Bochoven A, Posthuma D. Functional Mapping and Annotation of Genetic Associations With FUMA. Nat Commun (2017) 8(1):1826. doi: 10.1038/s41467-017-01261-5
34. Pruim RJ, Welch RP, Sanna S, Teslovich TM, Chines PS, Gliedt TP, et al. LocusZoom: Regional Visualization of Genome-Wide Association Scan Results. Bioinformatics (2010) 26(18):2336–7. doi: 10.1093/bioinformatics/btq419
35. Bhattacharjee S, Rajaraman P, Jacobs KB, Wheeler WA, Melin BS, Hartge P, et al. A Subset-Based Approach Improves Power and Interpretation for the Combined Analysis of Genetic Association Studies of Heterogeneous Traits. Am J Hum Genet (2012) 90(5):821–35. doi: 10.1016/j.ajhg.2012.03.015
36. Consortium GT. The GTEx Consortium Atlas of Genetic Regulatory Effects Across Human Tissues. Science (2020) 369(6509):1318–30. doi: 10.1126/science.aaz1776
37. Jackson DJ, Busse WW, Bacharier LB, Kattan M, O'Connor GT, Wood RA, et al. Association of Respiratory Allergy, Asthma, and Expression of the SARS-CoV-2 Receptor ACE2. J Allergy Clin Immunol (2020) 146(1):203–206 e203. doi: 10.1016/j.jaci.2020.04.009
38. Kimura H, Francisco D, Conway M, Martinez FD, Vercelli D, Polverino F, et al. Type 2 Inflammation Modulates ACE2 and TMPRSS2 in Airway Epithelial Cells. J Allergy Clin Immunol (2020) 146(1):80–88 e88. doi: 10.1016/j.jaci.2020.05.004
39. Kumar K, Hinks TSC, Singanayagam A. Treatment of COVID-19-Exacerbated Asthma: Should Systemic Corticosteroids be Used? Am J Physiol Lung Cell Mol Physiol (2020) 318(6):L1244–7. doi: 10.1152/ajplung.00144.2020
40. Balz K, Chen M, Kaushik A, Cemic F, Heger V, Renz H, et al. Homologies Between SARS-CoV-2 and Allergen Proteins may Direct T Cell-Mediated Heterologous Immune Responses. Res Sq (2020). doi: 10.21203/rs.3.rs-86873/v1
41. Severe Covid GG, Ellinghaus D, Degenhardt F, Bujanda L, Buti M, Albillos A, et al. Genomewide Association Study of Severe Covid-19 With Respiratory Failure. N Engl J Med (2020) 383(16):1522–34. doi: 10.1056/NEJMoa2020283
42. Pairo-Castineira E, Clohisey S, Klaric L, Bretherick AD, Rawlik K, Pasko D, et al. Genetic Mechanisms of Critical Illness in COVID-19. Nature (2021) 591(7848):92–8. doi: 10.1038/s41586-020-03065-y
43. Baranova A, Cao H, Zhang F. Unraveling Risk Genes of COVID-19 by Multi-Omics Integrative Analyses. Front Med (Lausanne) (2021) 8:738687. doi: 10.3389/fmed.2021.738687
44. Zhao J, Yang Y, Huang H, Li D, Gu D, Lu X, et al. Relationship Between the ABO Blood Group and the COVID-19 Susceptibility. Clin Infect Dis (2020) 73(2):328–31. doi: 10.1093/cid/ciaa1150
45. Muniz-Diaz E, Llopis J, Parra R, Roig I, Ferrer G, Grifols J, et al. Relationship Between the ABO Blood Group and COVID-19 Susceptibility, Severity and Mortality in Two Cohorts of Patients. Blood Transfus (2021) 19(1):54–63. doi: 10.2450/2020.0256-20
46. Dahalan NH, Tuan Din SA, Mohamad SMB. Association of ABO Blood Groups With Allergic Diseases: A Scoping Review. BMJ Open (2020) 10(2):e029559. doi: 10.1136/bmjopen-2019-029559
47. Choi UY, Kang JS, Hwang YS, Kim YJ. Oligoadenylate Synthase-Like (OASL) Proteins: Dual Functions and Associations With Diseases. Exp Mol Med (2015) 47:e144. doi: 10.1038/emm.2014.110
48. Almoguera B, Vazquez L, Mentch F, Connolly J, Pacheco JA, Sundaresan AS, et al. Identification of Four Novel Loci in Asthma in European American and African American Populations. Am J Respir Crit Care Med (2017) 195(4):456–63. doi: 10.1164/rccm.201604-0861OC
49. Bazan-Socha S, Buregwa-Czuma S, Jakiela B, Zareba L, Zawlik I, Myszka A, et al. Reticular Basement Membrane Thickness Is Associated With Growth- and Fibrosis-Promoting Airway Transcriptome Profile-Study in Asthma Patients. Int J Mol Sci (2021) 22(3):998. doi: 10.3390/ijms22030998
50. Prasad K, Khatoon F, Rashid S, Ali N, AlAsmari AF, Ahmed MZ, et al. Targeting Hub Genes and Pathways of Innate Immune Response in COVID-19: A Network Biology Perspective. Int J Biol Macromol (2020) 163:1–8. doi: 10.1016/j.ijbiomac.2020.06.228
51. Wood ER, Bledsoe R, Chai J, Daka P, Deng H, Ding Y, et al. The Role of Phosphodiesterase 12 (PDE12) as a Negative Regulator of the Innate Immune Response and the Discovery of Antiviral Inhibitors. J Biol Chem (2015) 290(32):19681–96. doi: 10.1074/jbc.M115.653113
52. van de Loo S, Eich F, Nonis D, Auburger G, Nowock J. Ataxin-2 Associates With Rough Endoplasmic Reticulum. Exp Neurol (2009) 215(1):110–8. doi: 10.1016/j.expneurol.2008.09.020
53. Satterfield TF, Pallanck LJ. Ataxin-2 and its Drosophila Homolog, ATX2, Physically Assemble With Polyribosomes. Hum Mol Genet (2006) 15(16):2523–32. doi: 10.1093/hmg/ddl173
54. Nonhoff U, Ralser M, Welzel F, Piccini I, Balzereit D, Yaspo ML, et al. Ataxin-2 Interacts With the DEAD/H-Box RNA Helicase DDX6 and Interferes With P-Bodies and Stress Granules. Mol Biol Cell (2007) 18(4):1385–96. doi: 10.1091/mbc.E06-12-1120
55. Ralser M, Albrecht M, Nonhoff U, Lengauer T, Lehrach H, Krobitsch S. An Integrative Approach to Gain Insights Into the Cellular Function of Human Ataxin-2. J Mol Biol (2005) 346(1):203–14. doi: 10.1016/j.jmb.2004.11.024
56. Savastano A, Ibanez de Opakua A, Rankovic M, Zweckstetter M. Nucleocapsid Protein of SARS-CoV-2 Phase Separates Into RNA-Rich Polymerase-Containing Condensates. Nat Commun (2020) 11(1):6041. doi: 10.1038/s41467-020-19843-1
57. Bonenfant G, Williams N, Netzband R, Schwarz MC, Evans MJ, Pager CT. Zika Virus Subverts Stress Granules To Promote and Restrict Viral Gene Expression. J Virol (2019) 93(12):e00520–19. doi: 10.1128/JVI.00520-19
58. Luo L, Li Z, Zhao T, Ju X, Ma P, Jin B, et al. SARS-CoV-2 Nucleocapsid Protein Phase Separates With G3BPs to Disassemble Stress Granules and Facilitate Viral Production. Sci Bull (Beijing) (2021) 66(12):1194–204. doi: 10.1016/j.scib.2021.01.013
59. Perdikari TM, Murthy AC, Ryan VH, Watters S, Naik MT, Fawzi NL. SARS-CoV-2 Nucleocapsid Protein Phase-Separates With RNA and With Human hnRNPs. EMBO J (2020) 39(24):e106478. doi: 10.15252/embj.2020106478
60. Bakthavachalu B, Huelsmeier J, Sudhakaran IP, Hillebrand J, Singh A, Petrauskas A, et al. RNP-Granule Assembly via Ataxin-2 Disordered Domains Is Required for Long-Term Memory and Neurodegeneration. Neuron (2018) 98(4):754–766 e754. doi: 10.1016/j.neuron.2018.04.032
61. Orru V, Steri M, Sole G, Sidore C, Virdis F, Dei M, et al. Genetic Variants Regulating Immune Cell Levels in Health and Disease. Cell (2013) 155(1):242–56. doi: 10.1016/j.cell.2013.08.041
62. Betz RC, Petukhova L, Ripke S, Huang H, Menelaou A, Redler S, et al. Genome-Wide Meta-Analysis in Alopecia Areata Resolves HLA Associations and Reveals Two New Susceptibility Loci. Nat Commun (2015) 6:5966. doi: 10.1038/ncomms6966
Keywords: asthma, COVID-19, Mendelian randomization, inflammation, OAS2, ABO, ATXN2
Citation: Baranova A, Cao H, Chen J and Zhang F (2022) Causal Association and Shared Genetics Between Asthma and COVID-19. Front. Immunol. 13:705379. doi: 10.3389/fimmu.2022.705379
Received: 05 May 2021; Accepted: 25 February 2022;
Published: 21 March 2022.
Edited by:
Ming Li, Kunming Institute of Zoology, Chinese Academy of Sciences (CAS), ChinaCopyright © 2022 Baranova, Cao, Chen and Zhang. This is an open-access article distributed under the terms of the Creative Commons Attribution License (CC BY). The use, distribution or reproduction in other forums is permitted, provided the original author(s) and the copyright owner(s) are credited and that the original publication in this journal is cited, in accordance with accepted academic practice. No use, distribution or reproduction is permitted which does not comply with these terms.
*Correspondence: Fuquan Zhang, emhhbmdmcUBuam11LmVkdS5jbg==