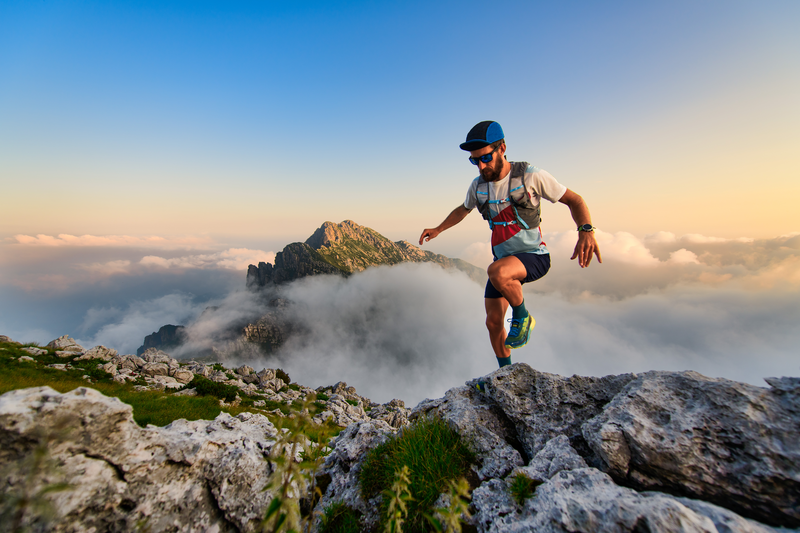
94% of researchers rate our articles as excellent or good
Learn more about the work of our research integrity team to safeguard the quality of each article we publish.
Find out more
REVIEW article
Front. Immunol. , 01 April 2022
Sec. Multiple Sclerosis and Neuroimmunology
Volume 13 - 2022 | https://doi.org/10.3389/fimmu.2022.688619
As a vital pivot for the human circulatory system, the brain-gut axis is now being considered as an important channel for many of the small immune molecules’ transductions, including interleukins, interferons, neurotransmitters, peptides, and the chemokines penetrating the mesentery and blood brain barrier (BBB) during the development of an ischemic stroke (IS). Hypoxia-ischemia contributes to pituitary and neurofunctional disorders by interfering with the molecular signal release and communication then providing feedback to the gut. Suffering from such a disease on a long-term basis may cause the peripheral system’s homeostasis to become imbalanced, and it can also lead to multiple intestinal complications such as gut microbiota dysbiosis (GMD), inflammatory bowel disease (IBD), necrotizing enterocolitis (NEC), and even the tumorigenesis of colorectal carcinoma (CRC). Correspondingly, these complications will deteriorate the cerebral infarctions and, in patients suffering with IS, it can even ruin the brain’s immune system. This review summarized recent studies on abnormal immunological signal exchange mediated polarization subtype changes, in both macrophages and microglial cells as well as T-lymphocytes. How gut complications modulate the immune signal transduction from the brain are also elucidated and analyzed. The conclusions drawn in this review could provide guidance and novel strategies to benefit remedies for both IS and relative gut lesions from immune-prophylaxis and immunotherapy aspects.
As one of the most high-risk cerebrum lesions in the world, ischemic stroke (IS) exhibits cerebral artery stenosis, occlusion or acute blood circulation barricade and takes possession of 75% to 85% of all-type strokes, with the specific characters of burstiness, rapidity, disability and high mortality (1–3). The pathogenesis of IS originates from multiple systematic symptoms, such as thrombus-induced atherosclerosis, cardiovascular inflammation, arrhythmia, and supplementary blood deficiency in the cerebrum (4, 5). Clinical manifestations include anterior or intracranial artery damages such as uncontrolled behavior, alogia, hypersomnia, and neurodegenerative disease, especially the onset of tumors and the growth of glioblastoma multiforme which may thoroughly destroy the brain’s central nervous system (CNS) (6–8). When a cerebral artery embolism grows, the intracellular environment experiences a conspicuous deficiency of blood, then promotes multiple immune signals such as cytokines, chemokines and matrix metalloproteinases (MMP) 1, 2 and 9 which discharges from the brain and flows into the gut (9, 10). The consequent blood reperfusion facilitates the infiltration of blood cells, including allowing natural killers (NKs), monocytes, lymphocytes or neutrophils to enter into the damaged brain parenchyma (11). During such a procedure, those signals or immune factors are transmitted into other organs, like the spleen, to separately trigger the inflammations (12). Many cases have been elucidated, but among all of the human organs the gut is the most versatile one in continually acquiring the immune signals that are released from the IS-triggered injury regions in the brain, and then conversely inducing the creation of different intestinal lesions (13–15) (Figure 1).
Figure 1 Graphical abstract. To better understand the rationale of IS initiation-induced immune signal exchange to multiple intestinal lesions or complications through the brain-gut axis, such as gut microbiota dysbiosis (GMD), inflammatory bowel disease (IBD), necrotizing enterocolitis (NEC), colorectal carcinoma (CRC), we have reviewed and summarized recent articles and drawn reasonable guidance on how to take precautions and alleviate each creation.
A recent study illustrated that the development of IS was corresponding to some gut abnormalities such as constipation, microbiota diversity dysbiosis, intestinal motility disorder and tract bleeding. More seriously, such abnormalities could turn into complications such as gut microbiota dysbiosis (GMD), inflammatory bowel disease (IBD), necrotizing enterocolitis (NEC) and colorectal carcinoma (CRC) (16–19). Of these, IS-mediated GMD has a relatively higher frequency of occurrence. For example, patients with IS may be diagnosed with post-stroke cognitive impairment, which reflects obvious GMD symptoms. The abundance of Firmicutes, including the same species of Clostridia and Lachnospiraceae in the guts is experiencing an obvious reduction (20). Following such phenomena, the degradation of mucus proteins and goblet T-lymphocytes apoptosis are also augmented in the intra-intestinal epithelium (21, 22). In addition, from those middle cerebral artery occlusion (MCAO) rat models, the fecal flora transplantation recreates the ecological balance of gut microbiota, alleviates the cerebrovascular embolism generation speed, then attenuates the infarct size, finally eliminates the brain edema (23). Moreover, the peripheral adaptive immunity-mediated neuroinflammatory response, which is a common stressor in IS, has a strong connection with GMD through the bidirectional hypothalamic–pituitary–adrenal axis mediated signal transmission (24). Further investigations must still be carried out to demonstrate whether microbiota dysbiosis is the prerequisite for other gut complications triggered by IS however.
T-lymphocyte colony subtypes, including helper T-cells such as Th1, Th2, Th17 and regulatory T-cells such as Treg, have different functions and performances in the beginning and during the development of IS (25). Th2 and Treg cells are antagonists in preventing inflammation in brain injuries (26), while Th1 and Th17, which are the agonists regulated by Th2 and Treg, controversially perform the neuroprotective role in the post-stroke neurogenesis process (27). Th1 secretes IL-2, IL-12, tumor necrosis factor-α (TNF-α) and interferon-γ (IFN-γ) to activate the immune response (28). Relatively, cytokines IL-6, IL-21, IL-22 and IL-17 can be secreted from Th17 (29). However, this T-lymphocyte subtype may tear down the neurovascular unit after penetrating the BBB and then work against the recovery from IS (30). Differently, Th2 and Treg, which are correlative to secreted cytokines, such as IL-4, IL-35, IL-10, transform growth factor-β (TGF-β) and exhibit anti-inflammation and protective cerebral effects (31–33). Accordingly, regulating T-lymphocyte polarization direction to Th2 and Treg contributes to IS attenuation.
The initial M2 subtype polarization of macrophage can be switched to M1. Locating on the M1 subtype of the macrophage surface membrane, CD16/32, CD86, CD40, and the major histocompatibility complex (MHC-II) could induce the discharge of proinflammatory factors including IL-1β, IL-6, IL-12, IL-23, TNFα. Some chemokines are also involved in for example like C-C motif chemokine ligand (CCL) 8, CCL15, C-X-C motif chemokine ligand (CXCL)10 and CXCL19 (34–36). Meanwhile, some transcription factors are also activated, such as STAT1, IRF3, NFκB and AP-1 (36). The M1 macrophages subtype can participate in Th1 creation, which is differentiated from CD4+ and CD8+ T-lymphocytes and plays a vital role against the formation of pathogens, and tumorigenesis (37). Correspondingly, the M2 macrophages subtype has surface membrane markers such as Arg-1, CD163, Fizz-1, scavenger receptor (SR) and mannose receptor (MR) to facilitate the releases of the chemokines like CCL13, CCL14, CCL17, CCL18, or cytokines like TGFβ, IL-10 (38, 39). Such signals will drive the decrease of inducible nitric oxide synthase (iNOS) and the increase of arginase-1 (Arg-1), which could break the Arginine metabolism’s balance, or lead to nitric oxide (NO) creation (40, 41). Differently to the M1 subtype, M2 macrophages benefit revascularization, tissue remodeling and wound healing (42–44). Interestingly, the IS-mediated polarization of microglial cells is also stimulated and reversibly differentiated from M2 subtype polarization to M1 (45). Unlike M1 and M2 macrophage polarization, the M1 microglial cells subtype could deteriorate the IS-mediated area of the brain injury, and even damage the central nervous system (46). Those hints are all manifested that the polarization in either macrophage or microglial cells should play completely opposite roles to the IS triggered intestinal lesions.
This review summarizes the involvement of IS in the immune signal exchange from the axis of the gut to the brain, as well as from the brain to the gut, when under multiple intestinal lesions. We screened all the potential independent or overlapping targets for IS, as well as each of the intestinal complications, through a target interaction network based on the STRING, TCMSP, Swiss Target Prediction, SEA, GeneCards, DrugBank and DisGeNet databases (Figure 2 and Supplementary Material). After that, we selected all the cellular immune signal targets with significant differences from the patients suffered with IS, including chemokines, cytokines, interleukins, interferons, neuro-immune and hormone-immune factors. It is worth to mention that those of them are also correlated to the events of T-lymphocytes differentiation, macrophage or microglial cells polarization. Following this, we then established four cluster panels to indicate their inner connections through the Software Cytoscape Version 3.6.0 (Figure 3). This data and the recent reports were gathered together to highlight the rationales behind such immunotherapeutic regulations. Such of that may provide cure strategies for how to prevent IS-induced gut complications, as well as facilitate medicinal developments for these diseases.
Figure 2 The Venn diagram for all the potential targets correlated to IS and GMD, IBD, NEC, CRC respectively. The numbers in each diagram represent the quantification statistics of the potential target numbers for IS and other lesions. All the data has come from the multiple databases of STRING, TCMSP, Swiss Target Prediction, SEA, GeneCards, DrugBank and DisGeNet and has been reproduced using Microsoft Software.
Figure 3 Interaction networks for the selected cellular immune signals from overlapping targets. These are those that have been identified from the overlapping results of the Venn diagram statistics in (A) IS-GMD, (B) IS-IBD, (C) IS-NEC, (D) IS-CRC group to correlate with T-lymphocytes differentiation or macrophage and microglial cells polarization. The node from each pane represents a selected target and the gray line reflects the interaction with each group. All the data has been reproduced through Cytoscape Version 3.6.0. software.
The presence of gut microbiota dysbiosis (GMD) will further break the immune system’s balance and stability by leading to a declining biosynthesis of γδT lymphocytes, which directly impairs the regulatory T-lymphocyte differentiation even the neurological functions and infarct size in IS (47, 48). It is worth mentioning that IL-17 secreted from γδT lymphocytes plays a crucial role in such an intervention procedure (49). Recent reports demonstrated that IS-induced GMD gave rise to the migration of T-lymphocytes from the Peyer patches, or intestinal lamina propria, to the brain leptomeninges, and then stimulated the augmentation of γδT lymphocytes in these regions of the brain (47). However, the accumulated γδT could discharge more IL-17 secretions and deteriorate brain injuries (50). Besides, Singh and colleagues transplanted the feces from the mice suffering with IS into the germ-free mice with the same disease. Then, they realized either IFN-γ or IL-17 surged in both of Th1 and Th17 cells during mice brain injuries (48). Also, they found that bacterial colonization reduced the stroke volumes in the colonized ex-germfree and specific pathogen-free (SPF) mice, when compared to germfree ones, meanwhile it increased the cerebral cytokines expression, such as IL-1β, TNF-α, IL-10, IL-17, as well as the microglia or macrophage numbers (51). Reversely, removing IL-17 leads to a provision of blood and oxygen for the brain’s recovery, IS symptom alleviation and GMD rebalance, also it activates the body’s defense function (52, 53). For example, constraining the T-lymphocyte differentiation to an excessive release of γδT and IL-17 in the mesenteric dendritic cells would restore GMD and begin to promote the accumulation of CD4+ T lymphocytes (Th17), thereby holding back the immigration of γδT lymphocytes from the gut to the region of the brain’s injuries (54, 55).
Except from the specific interleukin, some exogenous compounds metabolized by gut microbiota also correlate to γδT boosting under IS. For example, lactulose alters the composition of gut microbiota and contributes to the number of Foxp3+ Treg cells that markedly drop off. Those phenotypes are also responsible for the proliferation of T-lymphocytes through secreting IL-6, IL-17, IL10 and TNF-α cytokines and then up-regulating the short-chain fatty acids (SCFAs), which contain acetate, propionate and butyrate, in the intestine or serum (56). Some scientists also noticed that gut microbiota-mediated SCFAs synthesis can perform an immune-modulating effect on the microglia cells-induced synaptic plasticity recovery after the generation of IS, as well as evoke the secretion of IL-10 from T-lymphocytes-differentiated Th1 cells in the gut (57, 58). The abnormal synthesis of such fatty acids driven by IS promotes the creation of GMD and γδT and then revitalizes inflammation spreading (59). Besides, administering a single-course of amoxicillin to the mice may severely disrupt the gut microbiota’s components, enrich the pathobionts of Klebsiella and Escherichia-Shigella but dramatically delete the prebiotics of Bifidobacterium and Lactobacillus (60). Moreover, amoxicillin retards the accumulation of Treg, but facilitates the differentiation of T-lymphocytes with helper Th1 in the gut (61). Further study has also revealed that amoxicillin can be used to antagonize the Varicella Zoster or Syphilis virus, following with recovering the homeostasis of cerebrospinal fluid circulation in the brain (62, 63). All the evidence implies that the compromising of the GMD by amoxicillin is related to the immune signal circulations from the gut to the brain.
Some growth and neurotrophic factors such as the nerve growth factor (NGF), fibroblast growth factor (FGF), glial cell-derived neurotrophic factor (GDNF) or brain-derived neurotrophic factor (BDNF), can also take part in IS-induced immune signal exchanges and migration (64–66). By coordinating with other interleukins, interferons, chemokines or cytokines, BDNF can participate in the microglial cells’ polarization-induced immune signal exchange through the brain-gut axis, while also breaking the gut microbiota’s balance and setting up a responsibility for the creation of GMD (67). Downregulation of FGF, GDNF and their receptors during the initiation of IS connects with the M1 microglial cells subtype. This is followed by high volume IL-23 secretions to reverse the outgrowth of phosphatidylinositol 3-kinase (PI3K) and cAMP-response element binding protein (CREB) pathways. It is done while GMD is facilitated through augmentations of NF-κB, GSK-3β, TGF-β-mediated Smad2/3 and p38 MAPK signals (68, 69). In reverse, as an interplay communication, some pro-inflammatory cytokines such as IL-6 and TNF-α have high levels in the spinal cord, which when under GMD conditions, can accelerate the release of growth-associated protein 43 (GAP-43), neurotrophin-3 (NT-3) and BDNF from the brain neurocytes, and then modulate the axonal plasticity to spontaneously attenuate the IS-derived brain injuries (70). Similarly, the lipopolysaccharide (LPS) induced inflammatory formation impacts the constitution of the gut microbiota in aggravating GMD. This thus contributes to the discharging of BDNF from the gut’s microglial cells, followed by an increase in multiple IS inactivation cytokines excreted from astrocytes, such as CCL2, IL-15, IL-17, IL-10 and TGF-β (71–76). Interestingly, GMD-mediated abnormal synthesis of SCFAs can ameliorate microglia cells transition, intervene in the immune signal transmission induced by IS and has been confirmed to activate the pathway of BDNF-TrkB and exert neurogenesis (77, 78). This implies that interleukins, endogenous compounds and neurotrophic factors all influence the immune responses in either the brain or the gut.
As a severe gut disorder, inflammatory bowel disease (IBD) is characterized by a recurrent and chronic gastrointestinal inflammation and it is divided into three subtypes including ulcerative colitis, Crohn’s disease and indeterminate colitis (79). The majority of clues lead to the fact that IBD initiation can be found in multiple variations for the gut and relative symptoms, such as gut permeability, microbiota colonization enlargement, bacteria translocation and hypothalamic-pituitary-adrenal axis response elimination (80). Out of all the IBD-induced gut variations, the immune response activation in gut mucosal cells is the most versatile one in triggering T-lymphocyte differentiation and transformation into helper Th1, Th2, Th17 or regulatory Tregs (81). However, only the other stress-mediated Th2 and Treg from M2 macrophages could be responsible for IBD alleviation (82). Proinflammatory Th1 and Th17 polarization, stimulation and macrophage infiltration in the gut antagonize the immunopathology of IBD (83). Similarly, T-cells’ differentiations between Th1 and Th17 expedite IS development (84), which implies that restricting such a procedure may be beneficial to either IS or IBD patients. Moreover, the migration of CX3CR1+ CD28- CD4+ T-lymphocytes reflecting as a Th1 phenotype localized in the brain leptomeninges enhances IS-mediated IBD by increasing the production of multiple chemokines and the local infiltrations of cytotoxic immune cells (85). All of this elucidates the T-lymphocytes’ activities including migration, differentiation, and in particular, the polarization-triggered immune signal transmission that can influence the IS-derived onset of IBD.
Some pathogen-associated molecular patterns (PAMPs), which are associated to the pattern recognition receptors (PRRs) that mediate immune signal release and communication, are also responsible for GMD-induced IBD, and are verified to have intersections with an IS-origin (86). Current reports show that Toll-like receptors (TLRs) from PAMPs modulated the susceptibility and permeability of IBD were further able to participate in the GMD and immune signal exchange (87). The distributions of the TLR subtypes are found in multiple types of human cellular surface membranes, such as intestinal epithelial cells (IECs), macrophages, dendritic cells (DCs), mast cells, lymphocyte, neutrophils and CNS enteric glial cells (EGCs) (88–91). Therefore, studies demonstrated that microbial component variation in the gut can regulate TLR5, trigger an increase in the T-lymphocytes transformation and even cut-off the interferons and inflammation factors, including those of IL-1β, IL-6, IL-8 and TNF-α (92). One of the subtypes, TLR2, is now confirmed to be concerned with GMD-mediated aberrant immune responses, and taking that out of animals can assuage the dextran sulphate sodium salt (DSS)-induced colitis (93). Similarly, when comparing the mice, scientists found that relative to the IBD-mediated GMD group, TLR4 knockout mice displayed more neuroinflammation and gastrointestinal disturbances (93, 94). In addition, some other vital genes also involved in the aberrant immune response to GMD in IBD patients, such as nucleotide-binding oligomerization domain-containing-2 (NOD2), Caspase-recruitment domain 15 (CARD15), immunity-related GTPase M (IRGM), autophagy-related 16-like 1 (ATG16L1) (95). These independently take part in the processing of innate human immune responses and coordinate with vital pathways to modulate the extent of IS through proinflammatory Th1/Th17 polarization or macrophage infiltration (96–98). These perform a central role in immune signal amplification and transmission during the pathogenesis of IBD.
As one of the most devastating intestinal lesions, necrotizing enterocolitis (NEC) affects 5–10% infants, or 2-5% of all preterm neonates, however its clinical treatment in neonatology still remains unclear (99). The overall mortality rate approaches 100% in infants suffering from NEC, along with other congenital fundamental diseases such as intestinal perforation, peritonitis and so on (100). It was once considered to primarily originate from ischemic mucosal injury from the immature gut of a preterm, but now theories on its cause have turned to some major antenatal or postnatal risk factors. These primarily include chorioamnionitis and maternal antibiotics abuse (101, 102). Correspondingly, postnatal risk factors include gut motility and enzymatic function reverse, hemostasis of immune system disorder, mucus production and composition alteration, defense stress, intestinal hypoxia-ischemia-reperfusion activation, and normal neonatal gut colonization intervention (103). NEC-induced GMD is a common feature in these patients (104). For example, insufficient colonization by Firmicutes and Proteobacteria in preterm babies is attributed to immune tolerance inactivation and provoking an overwhelming intestinal inflammation reaction followed by NEC (105). Successive studies have also shown that during the development of NEC, a significant alteration existed in each fecal microbiota colonization of Klebsiella, Tatumella, Citrobacter and Sphingomonas spp. Under such conditions, the enterococcal microbe of the depletion of the gut’s microbiota will also be determined (106, 107).
Manifestations have demonstrated that the initiation of IS is tightly connected to NEC, and then it is noticeably responsible for its development. Some tools such as remote ischemic conditioning (RIC), can be used to prevent single intestinal injuries (18). Under such conditions, the polarization and anti-inflammation of the macrophage have been activated (108). Currently, the counting number ratio of M1/M2 subtypes of polarized macrophage has been unanimously approved as an important determination index and is used for measuring and verifying a patient’s degree of NEC (109, 110). During such a procedure, multiple immune signals are stimulated to play the repairing role for NEC. For instance, through intraperitoneal injections of Gr-1 antibodies combined with carrageenan with significantly depleted polymorphonuclear leukocytes (PMNs) and macrophage in the NEC mice model induced by cronobacter sakazakii (CS), led scientists to realize that the cytokines including IL-1β, IL-12, IL-6, TNF-α, iNOS were all up-regulated, the apoptosis of vascular epithelial cells significantly surged, and those with variations could exacerbate NEC-mediated intestinal lesions (111). Anand and colleagues explored how extra IFN-γ can activate the function performance of macrophages by inhibiting the phosphorylation of connexin, while Cx43 in enterocytes can then turn down the intercellular junctions and migration ability. Therefore, such interferon will alleviate the risks for macrophage M1 subtype transformation and prevent the pathogenesis of NEC (112, 113). Halpern and colleagues elucidated how rat milk substitute (RMS), asphyxia and cold stress can induce the creation of NEC in newborn rats. They also investigated how IFN-γ secretion-boosting triggered the aggregation of cytokine IL-18 and IL-12-positive monocytes or macrophages (IL-12 is the marker of M1 macrophage subtype) (114). Taken together, these findings imply that IFN-γ could be vital for macrophage polarization and M1 subtype creation during the early onset of NEC.
Colorectal carcinoma (CRC) is one of the most common malignant carcinomas in a human being’s digestive tract and has major repercussions on the efficacy of immunotherapy. Ranking third in terms of incidence and second in its worldwide mortality rate, the surging number of CRC patients is now counted at 1.8 million new cases and 881,000 deaths in 2018 alone, and it is predicted to increase to 2.2 million new cases and 1.1 million deaths worldwide by 2030 (115, 116). Patients with IS may have a higher risk of suffering from CRC (117). Both IS and CRC share the same complications, including atrial fibrillation (AF), arterial thromboembolism, hypercoagulability induced by elevated carotid endarterectomy (CEA) and cardiovascular disease (CVD), which fully illustrates the tight physiological connection between each of them (19, 118, 119). On the other hand, GMD, which broke the immune hemostasis, is also considered responsible for the initiation and development of CRC (120). Some species such as Enterococcus faecalis, Clostridium septicum, Bacteroides fragilis, Helicobacter pylori, Streptococcus bovis, Escherichia coli and Fusobacterium spp. are supposedly vital in the pathogenesis of colorectal tumor growth and metastasis (121). Recent report referred, 13 out of the 18 genera microbiota, such as Streptococcus, experienced a significant boost in the normal colorectal tissues when compared to the carcinoma ones (122). Furthermore, mutating the adenomatous polyposis coli (APC) tumor-suppressor gene in the C57BL/6J mice destroyed the diversity of the gut microbiota and spontaneously developed intestinal tumorigenesis (123). Further studies also demonstrated that as the key metabolites, overcharging of the SCFAs modulated T-cell differentiation, and in addition, not only did it deteriorate the creation of IS-induced dysbacteriosis but it was also responsible for the tumorigenesis of CRC (124–126). By suppressing the creation and release of inflammatory factors, such an aberrant phenotype will barricade the proliferation of the effector CD4+ T-lymphocytes, while also breaking the intestinal immune homeostasis (127). Among all the ingredients of SCFAs, butyrate and propionate are correlated to the extrathymic generation of colitis and functional differentiation and proliferation elimination of regulatory T-lymphocytes (128–130). Moreover, both of them can influence the modulation of the gut’s macrophages polarization by suppressing the histone deacetylase which thereby drives the tolerance increase for intestinal dysbacteriosis (131). Accordingly, GMD-induced CRC impairs the gut-brain signal circulation and mainly dominates through endogenous substances, metabolites and substitutions.
During the commencement of IS, the majority of immunosuppressive molecules such as the immune checkpoint receptor of programmed cell death 1 (PD-1) or programmed cell death ligand 1 (PD-L1) are stimulated and activated to prevent inflammatory generations such as the arterial wall damage by vasculitis or the mediated multiple immune responses (132). Reversely, PD-L1 deficiency amplifies the infarct sizes and deteriorates neurological deficits in the MACO C57BL/6 mice, and then promotes the polarization of macrophages or microglial cells (133). High volumes of IFN-γ conversely stimulate the up-regulation of PD-L1 and then mediate the multiple immune suppression for IS correlative cytokines or signal amplification pathways, such as PI3K/AKT and JAK/STAT3, to attenuate the anti-tumor immune positive responses (134, 135). Accordingly, PD-1 or the ligand PD-L1 is crucial for either IS or the tumorigenesis procedure of CRC (136, 137). In the early stages of CRC, characterized mismatch repair-deficient (dMMR) and microsatellite instability (MSI) have a relatively high level of CD8+ cytotoxic T-cells as well as PD-1/PD-L1 expression (138, 139). Further reports demonstrated that PD-1/PD-L1 can regulate the Th9 tumor-infiltrating lymphocytes (TILs) in CRC and drive the CD8+ T-cell expansion but not CD4+ T-cell (140). Interestingly, CD163+ M2 macrophages accumulation significantly increased in the cerebral infarction area of those patients suffering with IS (141). To strengthen the augment, PD-L1 was found to be expressed on tumor cells or CD68+/CD163+ M2 tumor-associated macrophages in MSI CRC patients and was attributed to tumor invasion extension and immune escape (142). Since polarized macrophages can be infiltrated from the brain to the gut with CD4+ T-lymphocyte (143), it may be possible that PD-L1 is also involved with such a macrophage transformation. Further investigations are necessary in order to research whether the activation of PD-1 and PD-L1 under CRC induces the polarizations of macrophages or whether microglial cells can transmit the immune signals back to the brain. Once this is elucidated, we may be able to completely understand the integral picture on how to intervene with CRC or IS through PD-1 or PD-L1 blockers.
As one of the major lethal diseases in the world, scientists are primarily focusing on IS’ pathogenesis mechanisms but overlooking the potential complications, especially potential lesions in the gut, such as GMD, IBD, NEC and CRC. Through the brain-gut circulation, IS-induced signal exchanges coordinate with events including the activation of the neurohormone secretion, immunosignal release, lymphocyte differentiation, and even the oncogene resuscitation. It is worth mentioning that these major intestinal lesions accompanied with IS should be independent and mutually reflected in one or more complications. Recent reports reflected that the mice accepted the fecal transplants from higher stroke-associated dysbiosis patterns and that patients could suffer from severe brain injuries when accelerating the IL-17+ γδ T-cell creation in their guts (144). Relatively, the increase in expression of IL-17+ γδ T-lymphocytes are certified as the main elements that induce IBD, NEC and CRC (145–147). Accordingly, analyzing the functional alterations for specific gut microbiota colonization would be helpful to understand which species of flora are the most probably related to such phenomena.
The secreted interleukins from the aberrant differentiations or polarizations of T-lymphocytes, as well as the macrophages or microglial cells, form up the major communication signals for IS-derived intestinal complications. For example, the majority of reports implied that IL-6 was the most vital biomarker in IBD or NEC initiation (148, 149). Coincidentally, this interleukin is also the predictive biomarker for infections associated with strokes, and those situations could significantly increase its secretion (150). Moreover, IFN-γ could be another predominant transmissive immune signal related to gut complications and IS. Following IS deterioration, there is a boost in IFN-γ secretions (151). More IFN-γ triggers the generation of Th1 and Th17, which in parallel will stimulate PD-L1 recruitment and the JAK/STAT pathway, that in turn is relative and conducive to a CRC prognosis (152, 153). Further study still needs to be carried out in order to verify whether eliminating IFN-γ production could alleviate IS or CRC, or even reverse T-lymphocyte polarization.
In summary, this review illustrates cellular immunity-induced signal exchanges from IS-derived brain-to-gut complications including GMD, IBD, NEC and CRC. Some important cytokines and interleukins could become the primary therapeutic targets used to intervene in these complications, though basic scientific studies and clinical trials still need to be performed to verify the inner rationales of their roles in IS. The synergistic interaction between IS and intestinal complications would be particularly valuable for the efforts to modulate immunity for therapeutic purposes, as well as to advance stroke therapy to the next level.
ZY has drafted this manuscript. FW, BZ, and XX have provided the editing and writing assistance and suggestions. YL, MW, and RC have critically revised it for important intellectual content. GS and XS have approved the final version for publication. All authors contributed to the article and approved the submitted version.
This work was supported by the Natural Sciences Foundation for Young Scientists of China (82104494), key project grant from the National Natural Science Foundation of China (U20A20405), National Natural Science Foundation of China (81891012) and CAMS Innovation Fund for Medical Sciences (CIFMS) (2021-12M-1-031).
The authors declare that the research was conducted in the absence of any commercial or financial relationships that could be construed as a potential conflict of interest.
All claims expressed in this article are solely those of the authors and do not necessarily represent those of their affiliated organizations, or those of the publisher, the editors and the reviewers. Any product that may be evaluated in this article, or claim that may be made by its manufacturer, is not guaranteed or endorsed by the publisher.
The Supplementary Material for this article can be found online at: https://www.frontiersin.org/articles/10.3389/fimmu.2022.688619/full#supplementary-material
Supplementary Data Sheet | The statistics of all the potential varied targets corelated to IS combing with each complication of GMD, IBD, NEC, CRC, respectively. All the data has come from the multiple databases of STRING, TCMSP, Swiss Target Prediction, SEA, GeneCards, DrugBank and DisGeNet and has been reproduced using Microsoft Software.
1. Chen WH, Chui C, Yin HL. Zoster Sine Herpete, Vertebral Artery Stenosis, and Ischemic Stroke. J Stroke Cerebrovasc Dis (2013) 22:e234–7. doi: 10.1016/j.jstrokecerebrovasdis.2012.07.017
2. Roy-O'Reilly M, McCullough LD. Age and Sex Are Critical Factors in Ischemic Stroke Pathology. Endocrinology (2018) 159:3120–31. doi: 10.1210/en.2018-00465
3. Edwards DN, Bix GJ. Roles of Blood-Brain Barrier Integrins and Extracellular Matrix in Stroke. Am J Physiol Cell Physiol (2019) 316:C252–63. doi: 10.1152/ajpcell.00151.2018
4. Lien LM, Lin KH, Huang LT, Tseng MF, Chiu HC, Chen RJ, et al. Licochalcone A Prevents Platelet Activation and Thrombus Formation Through the Inhibition of Plcγ2-PKC, Akt, and MAPK Pathways. Int J Mol Sci (2017) 18:1500. doi: 10.3390/ijms18071500
5. Choi SW, Kim BB, Choi DH, Park G, Shin BC, Song H, et al. Stroke or Left Atrial Thrombus Prediction Using Antithrombin III and Mean Platelet Volume in Patients With Nonvalvular Atrial Fibrillation. Clin Cardiol (2017) 40:1013–9. doi: 10.1002/clc.22759
6. Hsu WH, Shen YC, Shiao YJ, Kuo CH, Lu CK, Lin TY, et al. Combined Proteomic and Metabolomic Analyses of Cerebrospinal Fluid From Mice With Ischemic Stroke Reveals the Effects of a Buyang Huanwu Decoction in Neurodegenerative Disease. PloS One (2019) 14:e0209184. doi: 10.1371/journal.pone.0209184
7. Pajediene E, Pajeda A, Urnieziute G, Paulekas E, Liesiene V, Bileviciute-Ljungar I, et al. Subjective and Objective Features of Sleep Disorders in Patients With Acute Ischemic or Haemorrhagic Stroke: It is Not Only Sleep Apnoea Which is Important. Med Hypotheses (2020) 136:109512. doi: 10.1016/j.mehy.2019.109512
8. Kasapas K, Malli A, Kassioti E, Valkimadi PE. Posterior Circulation Ischemic Stroke Secondary to High-Grade Glioma: A Rare Case Report and Review of the Literature. Cureus (2020) 12:e9824. doi: 10.7759/cureus.9824
9. Victoria ECG, Toscano ECB, Oliveira FMS, Carvalho BA, Caliari MV, Teixeira AL, et al. Up-Regulation of Brain Cytokines and Metalloproteinases 1 and 2 Contributes to Neurological Deficit and Brain Damage in Transient Ischemic Stroke. Microvasc Res (2020) 129:103973. doi: 10.1016/j.mvr.2019.103973
10. Yang CJ, Yang Y, DeMars KM, Rosenberg GA, Candelario-Jalil E. Genetic Deletion or Pharmacological Inhibition of Cyclooxygenase-2 Reduces Blood-Brain Barrier Damage in Experimental Ischemic Stroke. Front Neurol (2020) 11:887. doi: 10.3389/fneur.2020.00887
11. O'Connell GC, Treadway MB, Tennant CS, Lucke-Wold N, Chantler PD, Barr TL. Shifts in Leukocyte Counts Drive the Differential Expression of Transcriptional Stroke Biomarkers in Whole Blood. Transl Stroke Res (2019) 10:26–35. doi: 10.1007/s12975-018-0623-1
12. Liu QK, Johnson EM, Lam RK, Wang Q, Ye HB, Wilson EN, et al. Peripheral TREM1 Responses to Brain and Intestinal Immunogens Amplify Stroke Severity. Nat Immunol (2019) 20:1023–34. doi: 10.1038/s41590-019-0421-2
13. Crapser J, Ritzel R, Verma R, Venna VR, Liu FD, Chauhan A, et al. Ischemic Stroke Induces Gut Permeability and Enhances Bacterial Translocation Leading to Sepsis in Aged Mice. Aging (Albany NY) (2016) 8:1049–63. doi: 10.18632/aging.100952
14. Ahnstedt H, Patrizz A, Chauhan A, Roy-O'Reilly M, Furr JW, Spychala MS, et al. Sex Differences in T Cell Immune Responses, Gut Permeability and Outcome After Ischemic Stroke in Aged Mice. Brain Behav Immun (2020) 87:556–67. doi: 10.1016/j.bbi.2020.02.001
15. Liu YN, Luo SJ, Kou L, Tang CG, Huang RX, Pei Z, et al. Ischemic Stroke Damages the Intestinal Mucosa and Induces Alteration of the Intestinal Lymphocytes and CCL19 mRNA in Rats. Neurosci Lett (2017) 658:165–70. doi: 10.1016/j.neulet.2017.08.061
16. Yin J, Liao SX, He Y, Wang S, Xia GH, Liu FT, et al. Dysbiosis of Gut Microbiota With Reduced Trimethylamine-N-Oxide Level in Patients With Large-Artery Atherosclerotic Stroke or Transient Ischemic Attack. J Am Heart Assoc (2015) 4:e002699. doi: 10.1161/JAHA.115.002699
17. Zhao L, Xiong QT, Stary CM, Mahgoub OK, Ye YZ, Gu LJ, et al. Bidirectional Gut-Brain-Microbiota Axis as a Potential Link Between Inflammatory Bowel Disease and Ischemic Stroke. J Neuroinflamm (2018) 15:339. doi: 10.1186/s12974-018-1382-3
18. Koike Y, Li B, Ganji N, Zhu HT, Muyake H, Chen Y, et al. Remote Ischemic Conditioning Counteracts the Intestinal Damage of Necrotizing Enterocolitis by Improving Intestinal Microcirculation. Nat Commun (2020) 11:4950. doi: 10.1038/s41467-020-18750-9
19. Qin QX, Cheng XM, Lu LZ, Wei YF, Wang DC, Li HH, et al. Biomarkers and Potential Pathogenesis of Colorectal Cancer-Related Ischemic Stroke. World J Gastroenterol (2018) 24:4950–8. doi: 10.3748/wjg.v24.i43.4950
20. Ling Y, Gong TY, Zhang JM, Gu QL, Gao XX, Weng XP, et al. Gut Microbiome Signatures Are Biomarkers for Cognitive Impairment in Patients With Ischemic Stroke. Front Aging Neurosci (2020) 12:511562. doi: 10.3389/fnagi.2020.511562
21. Wehrwein EA, Orer HS, Barman SM. Overview of the Anatomy, Physiology, and Pharmacology of the Autonomic Nervous System. Compr Physiol (2016) 6:1239–78. doi: 10.1002/cphy.c150037
22. Houlden A, Goldrick M, Brough D, Vizi ES, Lénárt N, Martinecz B, et al. Brain Injury Induces Specific Changes in the Caecal Microbiota of Mice via Altered Autonomic Activity and Mucoprotein Production. Brain Behav Immun (2016) 57:10–20. doi: 10.1016/j.bbi.2016.04.003
23. Zhang F, Zhai MT, Wu Q, Jia XY, Wang Y, Wang N. Protective Effect of Tong-Qiao-Huo-Xue Decoction on Inflammatory Injury Caused by Intestinal Microbial Disorders in Stroke Rats. Biol Pharm Bull (2020) 43:788–800. doi: 10.1248/bpb.b19-00847
24. Battaglini D, Pimentel-Coelho PM, Robba C, Santos CCD, Cruz FF, Pelosi P, et al. Gut Microbiota in Acute Ischemic Stroke: From Pathophysiology to Therapeutic Implications. Front Neurol (2020) 11:598. doi: 10.3389/fneur.2020.00598
25. Zhen Y, Yao L, Zhong SX, Song Y, Yan C, Li SS. Enhanced Th1 and Th17 Responses in Peripheral Blood in Active Non-Segmental Vitiligo. Arch Dermatol Res (2016) 308:703–10. doi: 10.1007/s00403-016-1690-3
26. Kubick N, Flournoy PCH, Enciu A, Manda G, Mickael ME. Drugs Modulating CD4+ T Lymphocytes Blood-Brain Barrier Interaction in Alzheimer's Disease. Pharmaceutics (2020) 12:880. doi: 10.3390/pharmaceutics12090880
27. Kim SH, Oh K, Jin HK, Bae JS. Immune Inflammatory Modulation as a Potential Therapeutic Strategy of Stem Cell Therapy for ALS and Neurodegenerative Diseases. BMB Rep (2018) 51:545–6. doi: 10.5483/BMBRep.2018.51.11.255
28. Seyed N, Rafati S. Th1 Concomitant Immune Response Mediated by IFN-γ Protects Against Sand Fly Delivered Leishmania Infection: Implications for Vaccine Design. Cytokine (2020) 29:155247. doi: 10.1016/j.cyto.2020.155247
29. Halwani R, Sultana A, Vazquez-Tello A, Jamhawi A, Al-Masri AA, Al-Muhsen S. Th-17 Regulatory Cytokines IL-21, IL-23, and IL-6 Enhance Neutrophil Production of IL-17 Cytokines During Asthma. J Asthma (2017) 54:893–904. doi: 10.1080/02770903.2017.1283696
30. Shekhar S, Cunningham MW, Pabbidi MR, Wang SX, Booz GW, Fan F. Targeting Vascular Inflammation in Ischemic Stroke: Recent Developments on Novel Immunomodulatory Approaches. Eur J Pharmacol (2018) 833:531–44. doi: 10.1016/j.ejphar.2018.06.028
31. Xu LF, Huang LN, Lian CY, Xue HL, Lu YF, Chen XJ. Vaginal Microbiota Diversity of Patients With Embryonic Miscarriage by Using 16s rDNA High-Throughput Sequencing. Int J Genomics (2020) 2020:1764959. doi: 10.1155/2020/1764959
32. Mangalam A, Shahi SK, Luckey D, Karau M, Marietta E, Luo N, et al. Human Gut-Derived Commensal Bacteria Suppress CNS Inflammatory and Demyelinating Disease. Cell Rep (2017) 20:1269–77. doi: 10.1016/j.celrep.2017.07.031
33. Liblau R. Glatiramer Acetate for the Treatment of Multiple Sclerosis: Evidence for a Dual Anti-Inflammatory and Neuroprotective Role. J Neurol Sci (2009) 287:S17–23. doi: 10.1016/S0022-510X(09)71296-1
34. Trujillo JA, Fleming EL, Perlman S. Transgenic CCL2 Expression in the Central Nervous System Results in a Dysregulated Immune Response and Enhanced Lethality After Coronavirus Infection. J Virol (2013) 87:2376–89. doi: 10.1128/JVI.03089-12
35. Ivashkiv LB. Epigenetic Regulation of Macrophage Polarization and Function. Trends Immunol (2013) 34:216–23. doi: 10.1016/j.it.2012.11.001
36. Sica A, Mantovani A. Macrophage Plasticity and Polarization: In Vivo Veritas. J Clin Invest (2012) 122(3):787–95. doi: 10.1172/JCI59643
37. Pushalkar S, Hundeyin M, Daley D, Zambirinis CP, Kurz E, Mishra A, et al. The Pancreatic Cancer Microbiome Promotes Oncogenesis by Induction of Innate and Adaptive Immune Suppression. Cancer Discov (2018) 8:403–16. doi: 10.1158/2159-8290.CD-17-1134
38. Kim Y, Udayanga KGS, Totsuka N, Weinberg JB, Nunez G, Shibuya A. Gut Dysbiosis Promotes M2 Macrophage Polarization and Allergic Airway Inflammation via Fungi-Induced PGE2. Cell Host Microbe (2014) 15:95–102. doi: 10.1016/j.chom.2013.12.010
39. Yue YP, Huang W, Liang JJ, Guo J, Ji J, Yao YL, et al. IL4I1 Is a Novel Regulator of M2 Macrophage Polarization That Can Inhibit T Cell Activation via L-Tryptophan and Arginine Depletion and IL-10 Production. PloS One (2015) 10:e0142979. doi: 10.1371/journal.pone.0142979
40. Recalcati S, Locati M, Marini A, Santambrogio P, Zaninotto F, Pizzol MD, et al. Differential Regulation of Iron Homeostasis During Human Macrophage Polarized Activation. Eur J Immunol (2010) 40:824–35. doi: 10.1002/eji.200939889
41. Corna G, Campana L, Pignatti E, Castiglioni A, Tagliafico E, Bosurgi L, et al. Polarization Dictates Iron Handling by Inflammatory and Alternatively Activated Macrophages. Haematologica (2010) 95:1814–22. doi: 10.3324/haematol.2010.023879
42. Barbay V, Houssari M, Banquet MS, Edwards-Lévy F, Henry J, Dumsenil A, et al. Role of M2-Like Macrophage Recruitment During Angiogenic Growth Factor Therapy. Angiogenesis (2015) 18:191–200. doi: 10.1007/s10456-014-9456-z
43. Zhang Y, Böse T, Unger RE, Jansen JA, Kirkpatrick CJ, van den Beucken JJJP. Macrophage Type Modulates Osteogenic Differentiation of Adipose Tissue MSCs. Cell Tissue Res (2017) 369:273–86. doi: 10.1007/s00441-017-2598-8
44. Krzyszczyk P, Kang HJ, Kumar S, Meng YX, O'Reggio MD, Patel K, et al. Anti-Inflammatory Effects of Haptoglobin on LPS-Stimulated Macrophages: Role of HMGB1 Signaling and Implications in Chronic Wound Healing. Wound Repair Regener (2020) 28:493–505. doi: 10.1111/wrr.12814
45. Liu R, Liao XY, Pan MX, JC T, Chen SF, Zhang Y, et al. Glycine Exhibits Neuroprotective Effects in Ischemic Stroke in Rats Through the Inhibition of M1 Microglial Polarization via the NF-κb P65/Hif-1α Signaling Pathway. J Immunol (2019) 202:1704–14. doi: 10.4049/jimmunol.1801166
46. Kwon YW, Cheon SY, Park SY, Song J, Lee JH. Tryptanthrin Suppresses the Activation of the LPS-Treated BV2 Microglial Cell Line via Nrf2/HO-1 Antioxidant Signaling. Front Cell Neurosci (2017) 11:18. doi: 10.3389/fncel.2017.00018
47. Benakis C, Brea D, Caballero S, Faraco G, Moore J, Murphy M, et al. Commensal Microbiota Affects Ischemic Stroke Outcome by Regulating Intestinal γδ T Cells. Nat Med (2016) 22:516–23. doi: 10.1038/nm.4068
48. Singh V, Roth S, Llovera G, Sadler R, Garzetti D, Stecher B, et al. Microbiota Dysbiosis Controls the Neuroinflammatory Response After Stroke. J Neurosci (2016) 36:7428–40. doi: 10.1523/JNEUROSCI.1114-16.2016
49. Gelderblom M, Arunachalam P, Magnus T. γδ T Lymphocytesas Early Sensors of Tissue Damage and Mediators of Secondary Neurodegeneration. Front Cell Neurosci (2014) 8:368. doi: 10.3389/fncel.2014.00368
50. Gelderblom M, Weymar A, Bernreuther C, Velden J, Arunachalam P, Steinbach K, et al. Neutralization of the iL-17 Axis Diminishes Neutrophil Invasion and Protects From Ischemic Stroke. Blood (2012) 120:3793–802. doi: 10.1182/blood-2012-02-412726
51. Singh V, Sadler R, Heindl S, Llovera G, Roth S, Benakis C, et al. The Gut Microbiome Primes a Cerebroprotective Immune Response After Stroke. J Cereb Blood Flow Metab (2018) 38:1293–8. doi: 10.1177/0271678X18780130
52. Dai QQ, Li SJ, Liu T, Zheng JY, Han S, Qu AJ. Interleukin-17A-Mediated Alleviation of Cortical Astrocyte Ischemic Injuries Affected the Neurological Outcome of Mice With Ischemic Stroke. J Cell Biochem (2019) 120:11498–509. doi: 10.1002/jcb.28429
53. Cheng HY, Ning MX, Chen DK, Ma WT. Interactions Between the Gut Microbiota and the Host Innate Immune Response Against Pathogens. Front Immunol (2019) 10:607. doi: 10.3389/fimmu.2019.00607
54. Wang X, Darcy J, Cai C, Jin JF, Bartke A, Cao DL. Intestinal Immunity in Hypopituitary Dwarf Mice: Effects of Age. Aging (Albany NY) (2018) 10:358–70. doi: 10.18632/aging.101393
55. Ekmekciu I, von Klitzing E, Fiebiger U, Escher U, Neumann C, Bacher P, et al. Immune Responses to Broad-Spectrum Antibiotic Treatment and Fecal Microbiota Transplantation in Mice. Front Immunol (2017) 8:397. doi: 10.3389/fimmu.2017.00397
56. Chen X, Zhang Z, Hu Y, Cui J, Zhi X, Li XQ, et al. Lactulose Suppresses Osteoclastogenesis and Ameliorates Estrogen Deficiency-Induced Bone Loss in Mice. Aging Dis (2020) 11:629–41. doi: 10.14336/AD.2019.0613
57. Sadler R, Cramer JV, Heindl S, Kostidis S, Betz D, Zuurbier KR, et al. Short-Chain Fatty Acids Improve Poststroke Recovery via Immunological Mechanisms. J Neurosci (2020) 40:1162–73. doi: 10.1523/JNEUROSCI.1359-19.2019
58. Sun MM, Wu W, Chen L, Yang WJ, Huang XS, Ma CY, et al. Microbiota-Derived Short-Chain Fatty Acids Promote Th1 Cell IL-10 Production to Maintain Intestinal Homeostasis. Nat Commun (2018) 9:3555. doi: 10.1038/s41467-018-05901-2
59. Lee JY, d'Aigle J, Atadja L, Quaicoe V, Honarpisheh P, Ganesh BP, et al. Gut Microbiota-Derived Short-Chain Fatty Acids Promote Poststroke Recovery in Aged Mice. Circ Res (2020) 127:453–65. doi: 10.1161/CIRCRESAHA.119.316448
60. Lin H, Wang Q, Yuan M, Liu L, Chen ZY, Zhao YH, et al. The Prolonged Disruption of a Single-Course Amoxicillin on Mice Gut Microbiota and Resistome, and Recovery by Inulin, Bifidobacterium Longum and Fecal Microbiota Transplantation. Environ Pollut (2020) 265:114651. doi: 10.1016/j.envpol.2020.114651
61. Lin H, Wang Q, Liu L, Chen ZY, Das R, Zhao YH, et al. Colonization of Mice With Amoxicillin-Associated Klebsiella Variicola Drives Inflammation via Th1 Induction and Treg Inhibition. Front Microbiol (2020) 11:1256. doi: 10.3389/fmicb.2020.01256
62. Guedes M, Filipe R, Costa A, Soares C, Sarmento A, Tavares M. Central Nervous System Varicella Zoster Vasculopathy in an Immunocompromised Patient. ID cases (2018) 15:e00483. doi: 10.1016/j.idcr.2018.e00483
63. Imoto W, Arima H, Yamada K, Kanzaki T, Nakagawa C, Kuwabara G, et al. Incidental Finding of Neurosyphilis With Intracranial Hemorrhage and Cerebral Infarction: A Case Report. J Infect Chemother (2020) 20:30360–3. doi: 10.1016/j.jiac.2020.10.001
64. Gao Y, Deng K, Hou J, Bryson JB, Barco A, Nikulina E, et al. Activated CREB is Sufficient to Overcome Inhibitors in Myelin and Promote Spinal Axon Regeneration In Vivo. Neuron (2004) 44:609–21. doi: 10.1016/j.neuron.2004.10.030
65. Caracciolo L, Marosi M, Mazzitelli J, Latifi S, Sano Y, Galvan L, et al. CREB Controls Cortical Circuit Plasticity and Functional Recovery After Stroke. Nat Commun (2018) 9:2250. doi: 10.1038/s41467-018-04445-9
66. Ravina K, Briggs DI, Kislal S, Warraich Z, Nguyen T, Lam RK, et al. Intracerebral Delivery of Brain-Derived Neurotrophic Factor Using HyStem®-C Hydrogel Implants Improves Functional Recovery and Reduces Neuroinflammation in a Rat Model of Ischemic Stroke. Int J Mol Sci (2018) 19:3782. doi: 10.3390/ijms19123782
67. Heyck M, Ibarra A. Microbiota and Memory: A Symbiotic Therapy to Counter Cognitive Decline? Brain Circ (2019) 5:124–9. doi: 10.4103/bc.bc_34_19
68. Xia CY, Zhang S, Gao Y, Wang ZZ, Chen NH. Selective Modulation of Microglia Polarization to M2 Phenotype for Stroke Treatment. Int Immunopharmacol. (2015) 25:377–82. doi: 10.1016/j.intimp.2015.02.019
69. Ohta M, Chosa N, Kyakumoto S, Yokota S, Okubo N, Nemoto A, et al. IL-1β and TNF-α Suppress TGF-β-Promoted NGF Expression in Periodontal Ligament-Derived Fibroblasts Through Inactivation of TGF-β-Induced Smad2/3- and P38 MAPK-Mediated Signals. Int J Mol Med (2018) 42:1484–94. doi: 10.3892/ijmm.2018.3714
70. Sist B, Fouad K, Winship IR. Plasticity Beyond Peri-Infarct Cortex: Spinal Up Regulation of Structural Plasticity, Neurotrophins, and Inflammatory Cytokines During Recovery From Cortical Stroke. Exp Neurol (2014) 252:47–56. doi: 10.1016/j.expneurol.2013.11.019
71. Li Y, Chen N, Wu C, Lu Y, Gao G, Duan C, et al. Galectin-1 Attenuates Neurodegeneration in Parkinson's Disease Model by Modulating Microglial MAPK/Iκb/Nfκb Axis Through its Carbohydrate-Recognition Domain. Brain Behav Immun (2020) 83:214–25. doi: 10.1016/j.bbi.2019.10.015
72. Lai S-W, Chen J-H, Lin H-Y, Liu Y-S, Tsai C-F, Chang P-C, et al. Regulatory Effects of Neuroinflammatory Responses Through Brain-Derived Neurotrophic Factor Signaling in Microglial Cells. Mol Neurobiol (2018) 55:7487–99. doi: 10.1007/s12035-018-0933-z
73. Strack A, Asensio VC, Campbell IL, Schlüter D, Deckert M. Chemokines are Differentially Expressed by Astrocytes, Microglia and Inflammatory Leukocytes in Toxoplasma Encephalitis and Critically Regulated by Interferon-Gamma. Acta Neuropathol (2002) 103:458–68. doi: 10.1007/s00401-001-0491-7
74. Cekanaviciute E, Fathali N, Doyle KP, Williams AM, Han J, Buckwalter MS. Astrocytic Transforming Growth Factor-Beta Signaling Reduces Subacute Neuroinflammation After Stroke in Mice. Glia (2014) 62:1227–40. doi: 10.1002/glia.22675
75. Lee GA, Lin TN, Chen CY, Mau SY, Huang WZ, Kao YC, et al. Interleukin 15 Blockade Protects the Brain From Cerebral Ischemia-Reperfusion Injury. Brain Behav Immun (2018) 73:562–70. doi: 10.1016/j.bbi.2018.06.021
76. Lin Y, Zhang JC, Yao CY, Wu Y, Abdelgawad AF, Yao SL, et al. Critical Role of Astrocytic Interleukin-17 A in Post-Stroke Survival and Neuronal Differentiation of Neural Precursor Cells in Adult Mice. Cell Death Dis (2016) 7:e2273. doi: 10.1038/cddis.2015.284
77. Hasan MR, Kim JH, Kim YJ, Kwon KJ, Shin CY, Kim HY, et al. Effect of HDAC Inhibitors on Neuroprotection and Neurite Outgrowth in Primary Rat Cortical Neurons Following Ischemic Insult. Neurochem Res (2013) 38:1921–34. doi: 10.1007/s11064-013-1098-9
78. Jaworska J, Zalewska T, Sypecka J, Ziemka-Nalecz M. Effect of the HDAC Inhibitor, Sodium Butyrate, on Neurogenesis in a Rat Model of Neonatal Hypoxia-Ischemia: Potential Mechanism of Action. Mol Neurobiol (2019) 56:6341–70. doi: 10.1007/s12035-019-1518-1
79. Haboubi N. Reporting Colonic Biopsies in Patients With Inflammatory Bowel Disease; a Practical Approach. Inflammation Bowel Dis (2019) 25:679–84. doi: 10.1093/ibd/izy288
80. Abautret-Daly A, Dempsey E, Parra-Blanco A, Medina C, Harkin A. Gut-Brain Actions Underlying Comorbid Anxiety and Depression Associated With Inflammatory Bowel Disease. Acta Neuropsychiatr (2018) 30:275–96. doi: 10.1017/neu.2017.3
81. Yang Y, Liu L, Liu XL, Zhang YY, Shi HN, Jia WZ, et al. Extracellular Vesicles Derived From Trichinella Spiralis Muscle Larvae Ameliorate TNBS-Induced Colitis in Mice. Front Immunol (2020) 11:1174. doi: 10.3389/fimmu.2020.01174
82. Kim DH, Kim S, Lee JH, Kim JH, Che XM, Ma HW, et al. Lactobacillus Acidophilus Suppresses Intestinal Inflammation by Inhibiting Endoplasmic Reticulum Stress. J Gastroenterol Hepatol (2019) 34:178–85. doi: 10.1111/jgh.14362
83. Sanctuary MR, Huang RH, Jones AA, Luck ME, Aherne CM, Jedlicka P, et al. miR-106a Deficiency Attenuates Inflammation in Murine IBD Models. Mucosal Immunol (2019) 12:200–11. doi: 10.1038/s41385-018-0091-7
84. Wang SW, Zhang H, Xu Y. Crosstalk Between Microglia and T Lymphocytes Contributes to Brain Damage and Recovery After Ischemic Stroke. Neurol Res (2016) 38:495–503. doi: 10.1080/01616412.2016.1188473
85. Kobayashi T, Okamoto S, Iwakami Y, Nakazawa A, Hisamatsu T, Chinen H, et al. Exclusive Increase of CX3CR1+CD28-CD4+ T Lymphocytesin Inflammatory Bowel Disease and Their Recruitment as Intraepithelial Lymphocytes. Inflammation Bowel Dis (2007) 13:837–46. doi: 10.1002/ibd.20113
86. Hyland NP, Cryan JF. Microbe-Host Interactions: Influence of the Gut Microbiota on the Enteric Nervous System. Dev Biol (2016) 417:182–7. doi: 10.1016/j.ydbio.2016.06.027
87. Lu Y, Li XR, Liu SS, Zhang YF, Zhang D. Toll-Like Receptors and Inflammatory Bowel Disease. Front Immunol (2018) 9:72. doi: 10.3389/fimmu.2018.00072
88. McKernan DP, Finn DP. An Appealing New Therapeutic for Ulcerative Colitis? Gut (2014) 63:1207–8. doi: 10.1136/gutjnl-2013-305929
89. Inoue R, Yajima T, Tsukahara T. Expression of TLR2 and TLR4 in Murine Small Intestine During Postnatal Development. Biosci Biotechnol Biochem (2017) 81:350–8. doi: 10.1080/09168451.2016.1254534
90. Niedzwiedzka-Rystwej P, Ratajczak W, Tokarz-Deptula B, Deptula W. Mechanisms of Type I Interferon Action and its Role in Infections and Diseases Transmission in Mammals. Acta Biochim Pol (2017) 64:199–205. doi: 10.18388/abp.2016_1403
91. Berger M, Hsieh CY, Bakele M, Marcos V, Rieber N, Kormann M, et al. Neutrophils Express Distinct RNA Receptors in a Non-Canonical Way. J Biol Chem (2012) 287:19409–17. doi: 10.1074/jbc.M112.353557
92. Man AL, Bertelli E, Rentini S, Regoli M, Briars G, Marini M, et al. Age-Associated Modifications of Intestinal Permeability and Innate Immunity in Human Small Intestine. Clin Sci (Lond) (2015) 129:515–27. doi: 10.1042/CS20150046
93. Chang YC, Ching YH, Chiu CC, Liu JY, Hung SW, Huang WC, et al. TLR2 and Interleukin-10 are Involved in Bacteroides Fragilis-Mediated Prevention of DSS-Induced Colitis in Gnotobiotic Mice. PloS One (2017) 12:e0180025. doi: 10.1371/journal.pone.0180025
94. Alhasson F, Das S, Seth R, Dattaroy D, Chandrashekaran V, Ryan CN, et al. Altered Gut Microbiome in a Mouse Model of Gulf War Illness Causes Neuroinflammation and Intestinal Injury via Leaky Gut and TLR4 Activation. PloS One (2017) 12:e0172914. doi: 10.1371/journal.pone.0172914
95. Friswell M, Campbell B, Rhodes J. The Role of Bacteria in the Pathogenesis of Inflammatory Bowel Disease. Gut Liver (2010) 4:295–306. doi: 10.5009/gnl.2010.4.3.295
96. Costa FR, Françozo MCS, de Oliveira GG, Ignacio A, Castoldi A, Zamboni DS, et al. Gut microbiota translocation to the pancreatic lymph nodes triggers NOD2 activation and contributes to T1D onset. J Exp Med (2016) 213:1223–39. doi: 10.1084/jem.20150744
97. Lei QS, Li L, Cai J, Huang WX, Qin B, Zhang SJ. ORF3 of Hepatitis E Virus Inhibits the Expression of Proinflammatory Cytokines and Chemotactic Factors in LPS-Stimulated Human PMA-THP1 Cells by Inhibiting NF-κb Pathway. Viral Immunol (2016) 29:105–11. doi: 10.1089/vim.2015.0107
98. Halme L, Turunen U, Paavola-Sakki P, Heliö T, Lappalainen M, Färkkilä M, et al. CARD15 Frameshift Mutation in Patients With CROHN Disease is Associated With Immune Dysregulation. Scand J Gastroenterol (2004) 39(12):1243–9. doi: 10.1080/00365520410003489
99. Lin PW. Stoll BJ.Necrotising Enterocolitis. Lancet (2006) 368:1271–83. doi: 10.1016/S0140-6736(06)69525-1
100. Garzoni L, Faure C, Frasch MG. Fetal Cholinergic Anti-Inflammatory Pathway and Necrotizing Enterocolitis: The Brain-Gut Connection Begins. Utero Front Integr Neurosci (2013) 7:57. doi: 10.3389/fnint.2013.00057
101. Been JV, Lievense S, Zimmermann LJI, Kramer BW, Wolfs TGAM. Chorioamnionitis as a Risk Factor for Necrotizing Enterocolitis: A Systematic Review and Meta-Analysis. J Pediatr (2013) 162:236–42. doi: 10.1016/j.jpeds.2012.07.012
102. Santana DS, Cecatti JG, Surita FG, Tedesco RP, Passini R, Souza RT, et al. Maternal and Perinatal Outcomes and Factors Associated With Twin Pregnancies Among Preterm Births: Evidence From the Brazilian Multicenter Study on Preterm Birth (EMIP). Int J Gynaecol Obstet (2020) 149:184–91. doi: 10.1002/ijgo.13107
103. Morgan JA, Young L, McGuire W. Pathogenesis and Prevention of Necrotizing Enterocolitis. Curr Opin Infect Dis (2011) 24:183–9. doi: 10.1097/QCO.0b013e328345d5b5
104. Levy EI, Hoang DM, Vandenplas Y. The Effects of Proton Pump Inhibitors on the Microbiome in Young Children. Acta Paediatr (2020) 109:1531–8. doi: 10.1111/apa.15213
105. Lee JKF, Tan LTH, Ramadas A, Mutalib NSA, Lee LH. Exploring the Role of Gut Bacteria in Health and Disease in Preterm Neonates. Int J Environ Res Public Health (2020) 17:6963. doi: 10.3390/ijerph17196963
106. Carlisle EM, Poroyko V, Caplan MS, Alverdy JA, Liu D. Gram Negative Bacteria are Associated With the Early Stages of Necrotizing Enterocolitis. PloS One (2011) 6(3):e18084. doi: 10.1371/journal.pone.0018084
107. Stewart CJ, Nelson A, Scribbins D, Marrs ECL, Lanyon C, Perry JD. Bacterial and Fungal Viability in the Preterm Gut: NEC and Sepsis. Arch Dis Child Fetal Neonatal Ed (2013) 98:F298–303. doi: 10.1136/archdischild-2012-302119
108. Vaibhav K, Braun M, Khan MB, Fatima S, Saad N, Shankar A, et al. Remote Ischemic Post-Conditioning Promotes Hematoma Resolution via AMPK-Dependent Immune Regulation. J Exp Med (2018) 215:2636–54. doi: 10.1084/jem.20171905
109. Wei J, Tang DX, Lu CJ, Yang J, Lu YL, Wang YD, et al. Irf5 Deficiency in Myeloid Cells Prevents Necrotizing Enterocolitis by Inhibiting M1 Macrophage Polarization. Mucosal Immunol (2019) 12:888–96. doi: 10.1038/s41385-019-0169-x
110. Wei J, Besner GE. M1 to M2 Macrophage Polarization in Heparin-Binding Epidermal Growth Factor-Like Growth Factor Therapy for Necrotizing Enterocolitis. J Surg Res (2015) 197:126–38. doi: 10.1016/j.jss.2015.03.023
111. Emami CN, Mittal R, Wang L, Ford HR, Prasadarao NV. Role of Neutrophils and Macrophages in the Pathogenesis of Necrotizing Enterocolitis Caused by Cronobacter Sakazakii. J Surg Res (2012) 172:18–28. doi: 10.1016/j.jss.2011.04.019
112. Leaphart CL, Qureshi F, Cetin S, Li J, Dubowski T, Baty C, et al. Interferon-Gamma Inhibits Intestinal Restitution by Preventing Gap Junction Communication Between Enterocytes. Gastroenterology (2007) 132:2395–411. doi: 10.1053/j.gastro.2007.03.029
113. Anand RJ, Dai SP, Rippel C, Leaphart C, Qureshi F, Gribar SC, et al. Activated Macrophages Inhibit Enterocyte Gap Junctions via the Release of Nitric Oxide. Am J Physiol Gastrointest Liver Physiol (2008) 294:G109–119. doi: 10.1152/ajpgi.00331.2007
114. Halpern MD, Holubec H, Dominguez JA, Williams CS, Meza YG, McWilliam DL, et al. Up-Regulation of IL-18 and IL-12 in the Ileum of Neonatal Rats With Necrotizing Enterocolitis. Pediatr Res (2002) 51:733–9. doi: 10.1203/00006450-200206000-00012
115. Bray F, Ferlay J, Soerjomataram I, Siegel RL, Torre LA, Jemal A. Global Cancer Statistics 2018: GLOBOCAN Estimates of Incidence and Mortality Worldwide for 36 Cancers in 185 Countries. CA Cancer J Clin (2018) 68:394–424. doi: 10.3322/caac.21492
116. Arnold M, Sierra MS, Laversanne M, Soerjomataram I, Jemal A, Bray F. Global Patterns and Trends in Colorectal Cancer Incidence and Mortality. Gut (2017) 66:683–91. doi: 10.1136/gutjnl-2015-310912
117. Selvik HA, Thomassen L, Logallo N, Nass H. Prior Cancer in Patients With Ischemic Stroke: The Bergen NORSTROKE Study. J Stroke Cerebrovasc Dis (2014) 23:919–25. doi: 10.1016/j.jstrokecerebrovasdis.2013.07.041
118. Aspberg S, Yu LY, Gigante B, Smedby KE, Singer DE. Risk of Ischemic Stroke and Major Bleeding in Patients With Atrial Fibrillation and Cancer. J Stroke Cerebrovasc Dis (2020) 29:104560. doi: 10.1016/j.jstrokecerebrovasdis.2019.104560
119. Yuan S, Carter P, Bruzelius M, Vithayathil M, Kar S, Mason AM, et al. Effects of Tumour Necrosis Factor on Cardiovascular Disease and Cancer: A Two-Sample Mendelian Randomization Study. EBioMedicine (2020) 59:102956. doi: 10.1016/j.ebiom.2020.102956
120. Song MY, Chan AT. Diet, Gut Microbiota, and Colorectal Cancer Prevention: A Review of Potential Mechanisms and Promising Targets for Future Research. Curr Colorectal Cancer Rep (2017) 13:429–39. doi: 10.1007/s11888-017-0389-y
121. Kostic AD, Chun E, Robertson L, Glickman JN, Gallini CA, Michaud M, et al. Fusobacterium Nucleatum Potentiates Intestinal Tumorigenesis and Modulates the Tumor-Immune Microenvironment. Cell Host Microbe (2013) 14:207–15. doi: 10.1016/j.chom.2013.07.007
122. Sobhani I, Tap J, Roudot-Thoraval F, Roperch JP, Letulle S, Langella P, et al. Microbial Dysbiosis in Colorectal Cancer (CRC) Patients. PloS One (2011) 6:e16393. doi: 10.1371/journal.pone.0016393
123. Martin OCB, Bergonzini A, D'Amico F, Chen P, Shay JW, Dupuy J, et al. Infection With Genotoxin-Producing Salmonella Enterica Synergises With Loss of the Tumour Suppressor APC in Promoting Genomic Instability via the PI3K Pathway in Colonic Epithelial Cells. Cell Microbiol (2019) 21:e13099. doi: 10.1111/cmi.13099
124. O'Keefe SJD, Li JV, Lahti L, Ou JH, Carbonero F, Mohammed K, et al. Fat, Fibre and Cancer Risk in African Americans and Rural Africans. Nat Commun (2015) 6:6342. doi: 10.1038/ncomms7342
125. Chen HM, Yu YN, Wang JL, Lin YW, Kong X, Yang CQ, et al. Decreased Dietary Fiber Intake and Structural Alteration of Gut Microbiota in Patients With Advanced Colorectal Adenoma. Am J Clin Nutr (2013) 97:1044–52. doi: 10.3945/ajcn.112.046607
126. Hu Y, Leu RKL, Christophersen CT, Somashekar R, Conlon MA, Meng XQ, et al. Manipulation of the Gut Microbiota Using Resistant Starch is Associated With Protection Against Colitis-Associated Colorectal Cancer in Rats. Carcinogenesis (2016) 37:366–75. doi: 10.1093/carcin/bgw019
127. Furusawa Y, Obata Y, Fukuda S, Enda TA, Nakato G, Takahashi D, et al. Commensal Microbe-Derived Butyrate Induces the Differentiation of Colonic Regulatory T Cells. Nature (2013) 504:446–50. doi: 10.1038/nature12721
128. Singh N, Gurav A, Sivaprakasam S, Brady E, Padia R, Shi HD, et al. Activation of Gpr109a, Receptor for Niacin and the Commensal Metabolite Butyrate, Suppresses Colonic Inflammation and Carcinogenesis. Immunity (2014) 40:128–39. doi: 10.1016/j.immuni.2013.12.007
129. Arpaia N, Campbell C, Fan XY, Dikiy S, van der Veeken J, deRoos P, et al. Metabolites Produced by Commensal Bacteria Promote Peripheral Regulatory T-Cell Generation. Nature (2013) 504:451–5. doi: 10.1038/nature12726
130. Smith PM, Howitt MR, Panikov N, Michaud M, Gallini CA, Bohlooly-y M, et al. The Microbial Metabolites, Short-Chain Fatty Acids, Regulate Colonic Treg Cell Homeostasis. Science (2013) 341:569–73. doi: 10.1126/science.1241165
131. Sanchez HN, Moroney JB, Gan HQ, Shen T, Im JL, Li TB, et al. B Cell-Intrinsic Epigenetic Modulation of Antibody Responses by Dietary Fiber-Derived Short-Chain Fatty Acids. Nat Commun (2020) 11:60. doi: 10.1038/s41467-019-13603-6
132. Zhang H, Watanable R, Berry GJ, Vaglio A, Liao YPJ, Warrington KJ, et al. Immunoinhibitory Checkpoint Deficiency in Medium and Large Vessel Vasculitis. Proc Natl Acad Sci USA (2017) 114:E970–9. doi: 10.1073/pnas.1616848114
133. Ren XF, Akiyoshi K, Vandenbark AA, Hurn PD, Offner H. Programmed Death-1 Pathway Limits Central Nervous System Inflammation and Neurologic Deficits in Murine Experimental Stroke. Stroke (2011) 42:2578–83. doi: 10.1161/STROKEAHA.111.613182
134. Gao Y, Yang JJ, Cai YX, Fu SL, Zhang N, Fu XN, et al. IFN-γ-Mediated Inhibition of Lung Cancer Correlates With PD-L1 Expression and is Regulated by PI3K-AKT Signaling. Int J Cancer (2018) 143:931–43. doi: 10.1002/ijc.31357
135. Mimura K, Teh JL, Okayama H, Shiraishi K, Kua LF, Koh V, et al. PD-L1 Expression is Mainly Regulated by Interferon Gamma Associated With JAK-STAT Pathway in Gastric Cancer. Cancer Sci (2018) 109:43–53. doi: 10.1111/cas.13424
136. Bodhankar S, Chen YX, Vandenbark AA, Murphy SJ, Offner H. PD-L1 Enhances CNS Inflammation and Infarct Volume Following Experimental Stroke in Mice in Opposition to PD-1. J Neuroinflamm (2013) 10:111. doi: 10.1186/1742-2094-10-111
137. Yaghoubi N, Soltani A, Ghazvini K, Hassanian SM, Hashemy SI. PD-1/ PD-L1 Blockade as a Novel Treatment for Colorectal Cancer. BioMed Pharmacother (2019) 110:312–8. doi: 10.1016/j.biopha.2018.11.105
138. Le DT, Uram JN, Wang H, Bartlett BR, Kemberling H, Eyring AD, et al. PD-1 Blockade in Tumors With Mismatch-Repair Deficiency. N Engl J Med (2015) 372:2509–20. doi: 10.1056/NEJMoa1500596
139. Llosa NJ, Cruise M, Tam A, Wicks EC, Hechenbleikner EM, Taube JM, et al. The Vigorous Immune Microenvironment of Microsatellite Instable Colon Cancer is Balanced by Multiple Counter-Inhibitory Checkpoints. Cancer Discov (2015) 5:43–51. doi: 10.1158/2159-8290.CD-14-0863
140. Wang CF, Lu YY, Chen L, Gao T, Yang Q, Zhu CQ, et al. Th9 Cells are Subjected to PD-1/PD-L1-Mediated Inhibition and are Capable of Promoting CD8 T Cell Expansion Through IL-9R in Colorectal Cancer. Int Immunopharmacol. (2020) 78:106019. doi: 10.1016/j.intimp.2019.106019
141. Tatebayashi K, Takagi T, Fujita M, Doe N, Nakagomi T, Matsuyama T, et al. Adipose-Derived Stem Cell Therapy Inhibits the Deterioration of Cerebral Infarction by Altering Macrophage Kinetics. Brain Res (2019) 1712:139–50. doi: 10.1016/j.brainres.2019.01.037
142. Korehisa S, Oki E, Iimori M, Nakaji Y, Shimokawa M, Saeki H, et al. Clinical Significance of Programmed Cell Death-Ligand 1 Expression and the Immune Microenvironment at the Invasive Front of Colorectal Cancers With High Microsatellite Instability. Int J Cancer (2018) 142:822–32. doi: 10.1002/ijc.31107
143. Feng YK, XF H, Luo SJ, Chen XF, Long SM, Liang FY, et al. Chronic Colitis Induces Meninges Traffic of Gut-Derived T Cells, Unbalances M1 and M2 Microglia/Macrophage and Increases Ischemic Brain Injury in Mice. Brain Res (2019) 1707:8–17. doi: 10.1016/j.brainres.2018.11.019
144. Xia GH, You C, Gao XX, Zeng XL, Zhu JJ, Xu KY, et al. Stroke Dysbiosis Index (SDI) in Gut Microbiome Are Associated With Brain Injury and Prognosis of Stroke. Front Neurol (2019) 10:397. doi: 10.3389/fneur.2019.00397
145. Li M, Gao JL, Tang Y, Liu MS, Wu SJ, Qu KL, et al. Traditional Herbal Medicine-Derived Sulforaphene LFS-01 Reverses Colitis in Mice by Selectively Altering the Gut Microbiota and Promoting Intestinal Gamma-Delta T Cells. Front Pharmacol (2018) 8:959. doi: 10.3389/fphar.2017.00959
146. Weitkamp JH, Rosen MJ, Zhao ZG, Koyama T, Geem D, Denning TL, et al. Small Intestinal Intraepithelial Tcrγδ+ T Lymphocytes are Present in the Premature Intestine But Selectively Reduced in Surgical Necrotizing Enterocolitis. PloS One (2014) 9:e99042. doi: 10.1371/journal.pone.0099042
147. Ma RL, Yuan DW, Guo YZ, Yan R, Li K. Immune Effects of γδ T Cells in Colorectal Cancer: A Review. Front Immunol (2020) 11:1600. doi: 10.3389/fimmu.2020.01600
148. Desreumaux P. Specific Targeting of IL-6 Signaling Pathway: A New Way to Treat IBD? Gut (2000) 47:465–6. doi: 10.1136/gut.47.4.465
149. Li ZH, Sheng L. Significance of Dynamic Evolution of TNF-α, IL-6 and Intestinal Fatty Acid-Binding Protein Levels in Neonatal Necrotizing Enterocolitis. Exp Ther Med (2018) 15:1289–92. doi: 10.3892/etm.2017.5532
150. Kwan J, Horsfield G, Bryant T, Gawne-Cain M, Durward G, Byrne CD, et al. IL-6 is a Predictive Biomarker for Stroke Associated Infection and Future Mortality in the Elderly After an Ischemic Stroke. Exp Gerontol (2013) 48:960–5. doi: 10.1016/j.exger.2013.07.003
151. Liesz A, Suri-Payer E, Veltkamp C, Doerr H, Sommer C, Rivest S, et al. Regulatory T Cells are Key Cerebroprotective Immunomodulators in Acute Experimental Stroke. Nat Med (2009) 15:192–9. doi: 10.1038/nm.1927
152. Xu WH, Li RM, Sun YC. Increased IFN-γ-Producing Th17/Th1 Cells and Their Association With Lung Function and Current Smoking Status in Patients With Chronic Obstructive Pulmonary Disease. BMC Pulm Med (2019) 19:137. doi: 10.1186/s12890-019-0899-2
Keywords: ischemic stroke, brain-gut axis, intestinal complications, cellular immunity, necrotizing enterocolitis, gut microbiota dysbiosis, inflammatory bowel disease, colorectal carcinoma
Citation: Yang Z, Wei F, Zhang B, Luo Y, Xing X, Wang M, Chen R, Sun G and Sun X (2022) Cellular Immune Signal Exchange From Ischemic Stroke to Intestinal Lesions Through Brain-Gut Axis. Front. Immunol. 13:688619. doi: 10.3389/fimmu.2022.688619
Received: 31 March 2021; Accepted: 16 March 2022;
Published: 01 April 2022.
Edited by:
Peng Wu, Southern Medical University, ChinaReviewed by:
Xianli Meng, Chengdu University of Traditional Chinese Medicine, ChinaCopyright © 2022 Yang, Wei, Zhang, Luo, Xing, Wang, Chen, Sun and Sun. This is an open-access article distributed under the terms of the Creative Commons Attribution License (CC BY). The use, distribution or reproduction in other forums is permitted, provided the original author(s) and the copyright owner(s) are credited and that the original publication in this journal is cited, in accordance with accepted academic practice. No use, distribution or reproduction is permitted which does not comply with these terms.
*Correspondence: Guibo Sun, Z2JzdW5AaW1wbGFkLmFjLmNu; Xiaobo Sun, c3VuLXhpYW9ib0AxNjMuY29t
Disclaimer: All claims expressed in this article are solely those of the authors and do not necessarily represent those of their affiliated organizations, or those of the publisher, the editors and the reviewers. Any product that may be evaluated in this article or claim that may be made by its manufacturer is not guaranteed or endorsed by the publisher.
Research integrity at Frontiers
Learn more about the work of our research integrity team to safeguard the quality of each article we publish.