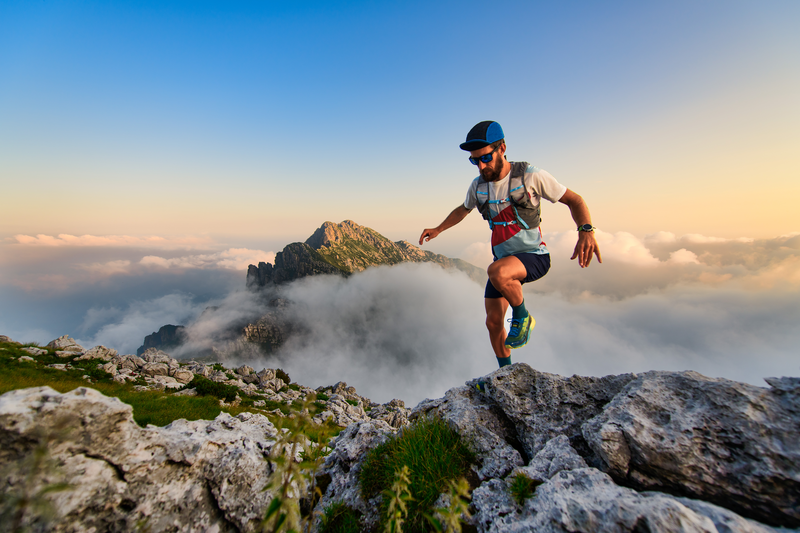
95% of researchers rate our articles as excellent or good
Learn more about the work of our research integrity team to safeguard the quality of each article we publish.
Find out more
REVIEW article
Front. Immunol. , 04 January 2023
Sec. Viral Immunology
Volume 13 - 2022 | https://doi.org/10.3389/fimmu.2022.1112234
This article is part of the Research Topic Focus on HBV: Antiviral, Pregnancy, and Immunization View all 24 articles
In order to develop immune tolerant to the fetal, maternal immune system will have some modification comparing to the time before pregnancy. Immune tolerance starts and develops at the maternal placental interface. In innate immunity, decidual natural killer (dNK) cells, macrophages and dendritic cells play a key role in immue tolerance. In adaptive immunity, a moderate increase of number and immune inhibition function of regulatory T cells (Treg) are essential for immune tolerance. The trophoblast cells and immune cells expressing indoleamine 2,3-dioxygenase (IDO), the trophoblast cells expressing HLA-G, and Th1/Th2 shifting to Th2 dominant and Th17/Treg shifting to Treg domiant are in favor of maternal fetal immune tolerance. Steroids (estrogen and progesterone) and human chorionic gonadotropin (HCG) also participate in immune tolerance by inducing Treg cells or upregulating immunosuppressive cytokines. Most of the patients with chronic HBV infection are in the “HBV immune tolerance period” before pregnancy, and the liver disease is relatively stable during pregnancy. In chronic HBV infection women, after delivery, the relative immunosuppression in vivo is reversed, and Th1 is dominant in Th1/Th2 and Th17 is dominant in Th17/Treg balance. After delivery, the number of Treg decrease and NK cells increase in quantity and cytotoxicity in peripheral blood. Liver NK cells may cause liver inflammation through a non-antigen specific mechanism. After delivery, the number of CD8+ T cells will increase and HBV specific T cell response recovers from the disfunction in pregnancy. Under the background of postpartum inflammation, the rapid decrease of cortisol after delivery, and especially the enhancement of HBV specific T cell response induced by HBV DNA and cytokines, are the main reasons for postpartum hepatitis. HBeAg positive, especially HBeAg<700 S/CO, and HBV DNA>3-5Log10IU/ml are risk factors for postpartum hepatitis. Antiviral treatment in late pregnancy can reduce the incidence of mother to child transmission (MTCT) in chronic HBV infection women. Chronic HBV infection women have hepatitis both during pregnancy and more often in 12 weeks postpartum. It is generally agreed that postpartum hepatitis is mild symptoms and self-limited. Delaying drug withdrawal to 48 weeks can increase the seroconversion rate of HBeAg in delivery women with elevated alanine aminotransferase (ALT) in pregnancy.
In pregnant women with chronic HBV infection, a certain proportion of postpartum hepatitis may occur after delivery. The mechanisms of postpartum hepatitis are complex, involving the changes in the immune system and pregnancy-associated hormone of women in pregnancy and after delivery, the natural stage of chronic HBV infection period, whether preventive antiviral therapy (AVT) and the time of withdrawal AVT after delivery.
Postpartum hepatitis refers to the occurrence of hepatitis after delivery, which is characterized by the elevated ALT with or without fatigue and corresponding digestive tract symptoms. The standards of ALT elevation vary from>1 upper limit of normal range (ULN) or >2ULN to >5ULN. Different countries have different ULN standards, most of which are >40U/L, but >25U/L or >19U/L for female in the United States (1). At present, research on postpartum hepatitis has focused on women with chronic HBV infection.
Postpartum hepatitis mostly occurs within 12 weeks after delivery, but a few also occur in 12-24 weeks. The two peak periods of postpartum hepatitis are 3-4 weeks and 9-12 weeks respectively (2). If postpartum follow-up is only 12 weeks, postpartum hepatitis occurring after 12 weeks is often not monitored. The degree of postpartum hepatitis is mostly asymptomatic or mild to moderate. There is little chance of elevated bilirubin level or clinical decompensation of liver function. Postpartum hepatitis, either without oral AVT in late pregnancy or withdrawal AVT after delivery, is usually self-limited and does not require medical intervention (2–4). It is occasionally reported that a few patients have severe postpartum hepatitis flare (5). In chronic HBV infection women, the incidence of hepatitis during pregnancy can reach 6-14%, and it is more common after delivery (5). In chronic HBV infection women, percentage of postpartum hepatitis is 3.5%-50% (2, 4). The use of oral AVT to prevent MTCT in late pregnancy can also reduce the incidence of postpartum hepatitis. Postpartum hepatitis associated with MTCT is usually accompanied by a mild flare, usually without the need for AVT again (3).
The maternal-fetal interface mainly consists of trophoblast cells from the embryo and decidua from the pregnant women. In order to adapt to those process, the uterus innate immune system is activated in the early pregnancy, then gradually weakens in most of the pregnancy, and reactivates in the late pregnancy (6). The enhancement of innate immunity at the early pregnancy plays an important action in trophoblast growth, angiogenesis and placentation. The maternal placenta is rich in white blood cells, including about 70% decidual natural killer cells (dNK), 20% mononuclear macrophages, and roughly 10% T cells (7).
NK cells come from bone marrow and circulate to decidual tissue of uterus. During pregnancy, the quantity, phenotype and function of dNK cells at the maternal-fetal interface are different from those before pregnancy. The percentage of dNK cells increased in the first trimester of pregnancy accounting for 70-90% of decidual mononuclear cells, then decreased gradually in the second and third trimesters of pregnancy accounting for 35-40% (8, 9). The function of dNK cells may be influenced by the hormone level during pregnancy (10). High levels of adrenocortical hormone (ACH) during pregnancy directly inhibit NK cell activity (10). The phenotype of dNK cells is different from that of peripheral blood (11, 12). It is CD56brightCD16-NK, which can recognize non classical MHC-I molecules, and has weak cytotoxicity (8, 10). dNK cells can produce generous TNF-α and IFN-α which promote the invasion of extravillous trophoblast (EVT) cells and the formation of decidual vessels and spiral arteries (8, 9, 11). dNK cell function is regulated by the balance of inhibitory and active receptor signals (6). During pregnancy, the expression of dNK activated receptor NKG2D decreased, and the expression of inhibitory receptor CD94/NKG2A increased.The basic feature of inhibitory receptor is the structure of immunoreceptor tyrosine inhibitory motif (ITIM) in the cytoplasm. KIR can transmit inhibition signal through ITIM, thus inhibiting NK cells’ killing activity against target cells. The dysfunction of dNK is related to abnormal pregnancy, such as recurrent abortion and fetal growth retardation.
In pregnancy, the number of circulating monocytes and granulocytes increased and they were activated by up-regulating activation markers of CD11b and CD14, but the phagocytosis of monocytes decreased (13, 14). The decidual MФs mainly remodel the uterine blood vessels, participating in the regulation of immune tolerance and antigen presentation (13, 15). In early pregnancy, MФs actively remove apoptotic cells, produce proteases that degrade extracellular matrix, and participate in uterine vascular remodeling (16). MФ has high plasticity and can polarize into different phenotypes under the stimulation of different microenvironments. Decidual MФs are classified into classically activated macrophages (M1) and alternatively activated macrophages (M2) (16). M1 is dominant during both embryo implantation and during labor. MФs in decidua can induce Foxp3+Treg proliferation in vitro. Most decidual MФs produce indoleamine 2,3-dioxygenase (IDO), which is conducive to maternal immune tolerance to the half allogeneic fetus. In early pregnancy, most decidual T cells show strong expression of PD-1. Decidual MФs express B7 family costimulatory molecules and then inhibit T cells from producing IFN-γ through B7-H1/PD-1 signal pathway (17).
Dendritic cells (DC), derived from bone marrow hematopoietic stem cells, are the most important professional antigen presenting cells in the body. DC also acts as an important immune cell for immune tolerance in pregnancy. DC is divided into mature DC and immature DC. IDC is the main type of DC, and only a few mature CD83+DC in endometrium during pregnancy. IDC has strong antigen capture function, but weak antigen presentation function (18). Without the costimulatory signal on the surface, iDC can induce the disability of effector T lymphocytes (Teff), induce the production of Tregs, and promote immune tolerance in maternal fetal interface. IDC induces antigen specific T cell tolerance by secretes IL-10 and TGF-β. In the mouse model, DC at the maternal and fetal interface is difficult to migrate to the lymphatic vessels of the uterus and lymph nodes even if stimulated by LPS, which can minimize the exposure of fetal or placental antigens to immunogenic T cells and is beneficial to maternal fetal immune tolerance (19).
Other immune cells, such as mast cells. Mast cells were stimulated by cell surface-expressed pattern recognition receptors (PRR) from bacteria, released IL-6, leukotrienes, TNF and histamine. Two receptors of H2R and H4R in mast cells regulate the production of Treg (20, 21). The number of neutrophils from maternal fetal interfaces remained relatively constant during pregnancy and began to increase rapidly before delivery. In the first-trimester, the maternal leukocytes of decidua have significant function in the implantation and early development of embryo and placenta. Neutrophils produce reactive oxygen and lytic enzymes by phagocytosis of bacteria, and promote inflammatory cascade reaction (22).
The absolute counts of T, B lymphocytes and CD4+, CD8+ T cell subsets in early pregnancy decreased, and the proportion of lymphocytes remained unchanged (9). T cells account for 10%~15% of white blood cells in decidual tissue in early pregnancy, and this proportion increases to 70% in late pregnancy (7).
Treg cells are an important CD4+ T lymphocyte subset of immune tolerance in pregnancy (23–25). Treg cells are distributed in iliac and inguinal lymph nodes, spleen and peripheral blood, and later found in uterus (26). The exhaustion of Tregs will lead to pathological pregnancy. At present, the research mainly focuses on the inducing factors of amplification and the molecular action mechanism of Tregs. During early pregnancy, the number of Tregs in the pregnant woman increased and migrated to the surface of the mother and fetus (27). During the third trimester of pregnancy and after delivery, the number of Treg cells decreased significantly (28). CCL22/CCL17 at the maternal fetal interface can chemotactic Treg cells. Treg surface molecules include Foxp3, GITR (glucocorticoid induced TNF receptor), CTLA-4, and TGF-β. Tregs are usually represented by CD4+CD25+FoxP3+Tregs (24, 26). TGF-β can regulate the differentiation of Th17 cells and Treg cell lineage. The interaction between GITR and its ligand can eliminate the inhibition of CD25+CD4+Tregs and enhance the activation, proliferation and cytokine production of T cells. Treg exerts its immune regulation function mainly through two ways: 1. Direct contact between cells. Treg can inhibit the proliferation of immune cells by directly binding with corresponding receptors of CD4+ T, CD8+ T and DCs through surface molecules (25, 29). For example, the interaction between the Treg inhibitory receptor CTLA-4 and its ligand CD80/CD86 induces macrophages or DCs to express indolamine 2,3-dioxygenase, which inhibits T cell activity and promotes maternal tolerance to the fetus (30, 31). Treg can inhibit the activation and amplification of immune cells such as CD4+ T and CD8+ T cells. 2. Secretion of inhibitory cytokines. For example, Tregs secretes IL-10 and TGF-β which are also important for self reproduction. In normal pregnancy, Tregs promote Th2 immune tolerance, but do not reduce Th1 cytokines, promoting maternal fetal immune tolerance (28, 32). The interaction between dNK and monocytes can also activate Treg (33). Many cytokines and hormones can regulate Treg production. During the first six months of pregnancy, semen contains TGF-β, IL-10 and prostaglandin E2 (PGE2), paternal antigen, can significantly increase Treg cells in peripheral blood and decidual tissue (34). The number of Treg cells increased significantly with the dramatic increase of human chorionic gonadotropin (HCG) (35).
The most abundant lymphocytes in decidual tissue are CD8+ T cells. Exhausted and senescent CD8+ T cells exist at the maternal fetal interface (36). In the maternal fetal interface, the inhibition receptors of CD8+ T cells such as CTLA4, PD1 and LAG3, suggest a phenotype of T cell depletion or dysfunction. The function of CD8+ T cells is temporarily inhibited and can still proliferate and produce IFN-γ, TNF-α, perforin and granzyme when stimulated (37, 38). During early pregnancy, the regulatory CD8+T cell population co-expressing PD-1 and T cell immunoglobulin and mucin domain containing protein 3 (Tim-3) which are enriched in decidua, and can proliferate and produce Th2 type cytokines (39). In the first trimester of pregnancy, apoptosis of T cells which express Fas on the surfaces of cells were induced by FasL from maternal decidua and placental trophoblast cells (40). Antigen presenting cells (APC) which express PD-L1 combined with T cells which express PD1 to induce T cell apoptosis (41). αβT cells are the most important T cells in the body. Due to the lack of effective stimulus of MHC molecules, the harm of αβT cells to the fetus are almost inhibited in pregnant women. TCRγδT cells involved in the immune tolerance of pregnancy (25, 42). By recognizing HLA G and HLA E antigens of trophoblasts, r&T cells are activated and release inhibitory cytokines of IL-10, TGF-β, and inhibit their cytotoxicity in pregnancy (6).
B lymphocyte cells have the ability to produce specific antibodies, in addition, they have the function of secondary antigen presenting cells, and can produce various immune regulatory cytokines. Adaptive cellular immunity was down regulated and humoral immunity was up regulated during pregnancy (43). Regulatory B cells (Bregs) are B lymphocyte subsets with strong immunosuppressive function. Studies have shown that under physiological conditions, the number of Breg is very small. However, in animal experiments, it is found that mature B cells of pregnant rats gather in the abdominal cavity and uterine lymph nodes (44), which may increase the number of serum immunoglobulins (43). Breg inhibits the toxic activity of Th1, Th17, and CD8+ T cells, and they can also induce CD4+ T cells to differentiate into FoxP3+Tregs (45, 46). Breg also inhibited TNF-α production by monocytes and IFN-α production by plasmacytoid dendritic cells (PDCs). Bregs are negative regulators of the body’s immune system through the anti-inflammatory cytokines IL-10, TGF-β. Breg inhibits the immunity through direct contact between cells or the indirect action of cytokines such as IL-35 (45).
Myeloid-derived suppressor cells (MDSCs) are a group of heterogeneous immature cells from bone marrow. MDSCs have immunosuppression function, and become new immune regulating cells at the maternal-fetal interface. MDSCs play an inhibitory role in normal pregnancy. MDSCs are characterized by myeloid origin and immature state, which can inhibit T cells. MDSCs suppress immunity through two ways. One way is through arginase 1 (Arg1), iDO, NO, ROS, and PGE2. Arg1, causing hunger environment by consuming essential amino acids such as arginine and tryptophan, leads to T cell inhibition (47). Another way is through TGF-β which can induce Treg production (48). MDSCs can inhibit NK through NO, ROS and inhibitory receptor TIGIT. MDSCs can also inhibit the activation of T cells by DCs (49).
Natural killer T (NKT) cell is a special T cell subgroup both with T cell receptor and NK cell receptor on the cell. NKT cells can produce a large number of cytokines, and play a similar cytotoxic role to NK cells. The NKT activation process requires the mutual activation of APC and NKT. The decidual NKT has obvious Th2 tendency, which is very important at the maternal fetal interface (50). Under normal conditions, decidual NKT inhibits immune rejection against paternal antigens, which is beneficial to the growth of trophoblast.
There are a large number of immune cells infiltrating in the uterus which construct the endometrial immune microenvironment and enable the embryo to obtain “ immune privilege” (51, 52). At present, it is generally believed that in addition to the placental barrier, maternal fetal immune tolerance maintains mainly by increasing the expression of human leukocyte antigen (HLA) G molecule in the placenta, Th1/Th2 and T17/Treg balance shifting to Th2 and Treg dominant, and trophoblast and immune cells expressing iDO and so on (Figure 1).
Figure 1 Causes of maternal fetal immune tolerance. There are five main reasons: IDO at the maternal-fetal interface increases which induces maternal fetal immune tolerance; EVTs express HLA-G to induce immune tolerance; Changes of Th1, Th2, Th17 and Treg balance; hormone change during pregnancy; maternal-fetal barrier. Abbreviation: Extraxtravillous Trophoblast (EVT).
(1) Immune cells and IDO mechanism in maternal fetus immune tolerance
Cellular immunity is the central link of maternal immune tolerance to fetus. IDO is involved in maternal immune tolerance to fetus by cellular immunity. The expression level of IDO is low under normal conditions, and lipopolysaccharide and IFN-γ can induce the expression of IDO. From the early pregnancy, trophoblasts, NK, macrophages, DC and T cells at the maternal infant interface began to express IDO, and the amount of IDO gradually increased (53). IDO is a monomer enzyme of heme and the only limiting rate enzyme outside the liver that can catalyze tryptophan metabolism. IDO decomposes tryptophan along the kynurenine (KYN) pathway and produce a series of metabolites including quinolinic acid. Tryptophan is an essential amino acid to maintain cell activation and proliferation. IDO mainly suppresses cellular immunity through depletion of tryptophan (53). Firstly, iDO degrades L-tryptophan in local microenvironment, and limits T lymphocyte proliferation. Secondly, iDO produces KYN by metabolizing L-tryptophan (54). KYN is an endogenous ligand of Aromatic hydrocarbon receptor (AhR). KYN combines with AhR to cause immature CD4+ T cells to differentiate into inhibitory T cells. In addition, the combination of KYN and AhR can also induce the expression of IDO and further inhibit the immune response of T cells. Macrophages expressing IDO inhibit T cell function and promote the polarization of macrophages to M2 phenotype. IDO also inhibits NK cell activation by down regulating the surface receptors of NKp46 and NKG2D.
(2) HLA-G mainly expressing by trophoblast cells in maternal fetal immune tolerance (55)
Human trophoblast cells do not express MHC II molecule, HLA-A and HLA-B molecules of classical MHC I molecule, but express classical HLA-C and non classical HLA-E, HLA-F, and HLA-G to protect from cellular immune damage. HLA Ib molecules are the key to the generation and maintenance of maternal fetal immune tolerance. The research mainly focuses on the mechanism of immune escape induced by HLA-G. At present, HLA-C, E is also considered important. In the maternal-fetal interface, HLA class Ib molecules expressed in trophoblast cells were binded to inhibitory receptors of decidual immune cells (DICs), and then shifted Th1/Th2 balance toward Th2 bias. HLA-G as immune tolerance molecules is expressed in EVT of placenta and immune cells in vivo. There are two main mechanisms for HLA-G to induce immune tolerance. The first mechanism is that membrane-bound or soluble type of HLA-G can bind to the inhibitory receptors of immune cells (NK, T, B, monocytes and DC), which is a direct inhibition of immune cells. The second mechanism includes inducing tolerance of DC (56). HLA-G specifically inhibits T cell amplification and function in a dose related form (57, 58). The HLA-G antigen specifically combines to the ILT-4 receptor on the surface of DC, activates the downstream IL-6/STAT3 signal pathway, downregulates the expression of MHC-II and costimulatory molecules CD80/CD86, inhibits the maturation and differentiation of DCs, and induces the formation of tolerant DCs (56, 59). HLA-G also mediates the function of MDSC by ILT-4 receptor (58). HLA-C expressed by trophoblast cells can bind with KIRs of dNK cells and take part in trophoblast invasion into the uterine (60). HLA-G and HLA-E molecule expressed by EVTs can combine with the inhibitory receptor CD94/NKG2A of dNK cells, thereby inhibit dNK cell activation (61, 62). Other researchers found that HLA-G molecules could also promote the expression of HLA-E molecules, thereby indirectly reducing the toxicity of dNK cells (63).
(3) Th1/Th2/Th17 and Treg cell models in maternal fetal immune tolerance (64–66) (Figure 2).
Figure 2 Naive CD4+ T cells differentiation in the pregnancy. In different microenvironment, naive CD4+T cells can differentiate into Th1, Th2, Th17, and Treg under the antigen stimulation which is presented by dendritic cells (DC). For example, IL12 and IFN-γ can induce the differentiation of Th1 cells, which secrete cytokines IFN-γ and TNF-ɑ. In the Th1/Th2 balance during pregnancy, Th2 is dominant. In the Th17/Treg balance, Treg was dominant.
The differentiation of CD4+ T cells is affected by many factors and cytokines. It is generally believed that Th cell precursor can differentiate into Th0 cells under the stimulation of antigen. In different microenvironments, Th0 cells can selectively differentiate into Th1, Th2, Th17 and Treg (Figure 2). It is traditionally believed that the Th1/Th2 balance at the maternal fetal interface during pregnancy tends to Th2 dominant (67–69). Th1 cells can release cytokines that cause local inflammation and activate MФS, NK cells and T lymphocytes. Th2 cells can release cytokines and promote humoral immune response. Th2 cells was once considered as the main T cell subgroup that assisted B cells to produce humoral immune response. The latest research suggests that follicular helper T cell (Tfh) belonging to CD4+ T is the main subgroup of helper B cells (70), and plays a regulatory role in B cell activation and Ig class conversion.
Treg/Th17 maybe play a much more important immune regulation role than Th1/Th2. At present, it has developed to Th1/Th2/Th17 and Treg cell models (71). Th17 cells have strong proinflammatory effect and can secrete IL-17A, IL-17F, IL-22, IL-21 and other cytokines. Th17 cells are differentiated by TGF-β, which can also induce regulatory T cells (iTreg) (72). IL-17 is the main effector of Th17 cells, which has proinflammatory effect which is in favor of the production of proinflammatory cytokines and chemokines (70, 73). The symptoms of RA usually improve during pregnancy, indicating that Th17 cells may decrease during pregnancy (74). The shift of Th17/Treg balance to Treg dominant may be favor to the outcome of pregnancy (75).
(4) Changes of pregnancy-associated hormone level in the maternal fetal immune tolerance
Hormonal factors are relative to maternal fetal immune tolerance including estrogen and progesterone and HCG. HCG can be detected from maternal serum on the first day after implantation of the fertilized eggs, reaching a peak at 8-10 weeks of gestation, and then dropping rapidly. Estrogen is produced by corpus luteum in early pregnancy, and is mainly synthesized by placental unit after 10 weeks of pregnancy. The concentration of estrogen gradually increases until the third trimester of pregnancy. Progesterone is produced by the corpus luteum of ovarian in the early pregnancy. After 8-10 weeks of pregnancy, placental syncytiotrophoblast cells begin to produce progesterone. The local concentration of the above hormones in the placenta is much higher than that in the whole body, which is mainly a regional effect and has little impact on all systems in the whole body.
During pregnancy, the hypothalamic neuropeptide corticotropin releasing hormone (CRH) mainly comes from endometrium and placenta. CRH induces the expression of Fas ligand (FasL) in DCs at the fetal maternal interface and increases apoptosis of activated T lymphocytes through Fas-FasL induction (76). High levels of adrenocortical hormone during pregnancy inhibit the immune system and directly inhibit NK cell activity. Cortisol secretion during pregnancy increased several times, and the active free cortisol was only 10% (77). Glucocorticoid (GC) can inhibit APC and Th1 cells which produce IFN-α, IFN-γ, TNF-α and IL-12, and promote Th2 cells which produce IL-4, IL-10 and IL-13 (78). The increase of GC level may lead to the maternal fetal local Th1/T2 to Th2 type, but it is not systemic inhibition (78).
Estrogen and progesterone levels increase during pregnancy. Research proved that progesterone not only increased the proportion of CD4+ CD25+ Treg cells and the expression of IL-10, but also enhanced its inhibitory function in mice (79). 17β-Estradiol (E2) can start immunosuppression through CD4+ CD25+ Treg cells in early pregnancy, reduce the production of Th1/Th17 inflammatory cytokines and up regulate the production of anti-inflammatory cell factors (80). Progesterone induces Tregs amplification, which is mediated by glucocorticoid receptors (81). Progesterone promotes Th2 cytokine production during normal pregnancy (82). In mice, progesterone directly or indirectly induced DC immune tolerance (83). Progesterone inhibits the activation and proliferation of lymphocytes (84). Progesterone can also inhibit the production of proinflammatory cytokine IL-17 (80). HCG can not only increase the number and activity of Treg, but also promote the migration of Tregs from peripheral blood to the maternal fetal interface (35, 85). In animal experiments, estradiol triggers expansion and activation of regulatory B cells. High level of HCG in pregnant women can induce Breg cells to expand, promote Breg to produce IL-10, and inhibit the proliferation of CD4+ T cells (86). During pregnancy, high levels of progesterone and estrogen promote humoral immunity by activating the T follicular helper cells (TFH)/B cell axis (87).
There are three hypotheses about the formation of immune tolerance in pregnancy as follows: Self-non-self models of immunity, danger model and Evolutionary non-self model. Self-non-self models of immunity, that is, if “non self” is recognized, antigen specific cytotoxic T lymphocytes (CTL) will be activated through a series of intermediate links. If it is “self”, antigen specific CTL will not be activated. Maternal fetal immune tolerance during pregnancy is achieved by limiting antigen specific T cell activity (88, 89). For the danger model, if there is no danger signal, the antigen expression from the father will not activate T cells (90). Dangerous signals are related to the activation of DCs or their costimulatory signals in the process of activating T cells. The danger model was validated in adverse pregnancy outcomes. “Evolutionary non-self” model: The presence of fetal antigen on the maternal fetal interface may not activate the immune system, but when infection occurs, the immune response mediated by PRR occurs to protect the maternal fuction (91). In short, the “Evolutionary non-self” model is more accurate in distinguishing between self and non self, and can accurately distinguish “microbial non self”, “changed self”, etc.
4) Most chronic HBV infected pregnant women in the “HBV immune tolerance period” before pregnancy
In China, most chronic HBV infections are transmitted by MTCT. After chronic infection, there are four periods: immune tolerance period, immune clearance period, immune control period and reactivation period. After acute HBV infection, CD4+ T cells secrete cytokines, activate CD8+ T cells, and kill HBV from infected hepatocytes through cell lysis and non lysis cell mode. HBV specific CD4+ T cells also stimulate B cells to produce antibodies and neutralize free viruses. This antiviral response is often unsuccessful in the immune tolerance period of chronic HBV infected patients, mainly manifested by the dysfunction or exhaustion of HBV specific CD8+ T cells (92). In chronic HBV infection, HBV specific CD8+ T mediated liver injury is caused firstly by antigen non-specific cells (such as NK cells). Age is an important factor in determining the immune tolerance period (93). It is generally believed that once age exceeds 30 or 40 years old, the immune tolerance is gradually broken (94). Most women of childbearing age (25-35 years old) are in the HBV immune tolerance period, with high HBsAg, HBeAg positive, and HBV DNA>108IU/ml. During immune tolerance period, the immune cells will not directly or indirectly attack the liver cells, and the liver inflammation is very slight. The condition of chronic HBV infection women during pregnancy is relatively stable, with few HBV related progress or liver failure (95). In 2008, it was reported that in 35 pregnant women without antiviral treatment, abnormal liver function were 34.6% during pregnancy and 50% after delivery (4). There was no statistical difference in the rate of postpartum hepatitis in receiving AVT group in late pregnancy compared with not receiving AVT group (39.4% vs 38.7%, P=0.942) in chronic HBV infection pregnant women (96). During pregnancy, high levels of progesterone and estrogen and Glucocorticoid are favour to the Th2 and Treg dominant mode accompanied by the increase of corresponding cytokines. To sum up, chronic HBV infection pregnant women in immune tolerance period before pregnancy remain keep “immune tolerance to HBV” during pregnancy (97).
There are conflicting results about the quantitative changes of HBV DNA during pregnancy. This may be caused by the mix factors such as the natural stage of chronic HBV infection, HBeAg status and the level of HBV DNA before pregnancy. In the Liu J’study, HBeAg positive pregnant women group was enrolled with baseline HBV DNA >7.0 log10IU/mL, HBsAg >4.0 log10IU/mL and HBeAg >3.0 log10S/CO in the pregnancy (98). It has been found that in pregnant women without AVT in late pregnancy, no matter whether the ALT is normal or abnormal during pregnancy and postpartum, HBV DNA, HBsAg and HBeAg quantification have almost remained stable (98). However, some studies have found that HBV DNA had elevated in some patients during pregnancy. Chang CY found that about 9% of chronic HBV infection pregnant women with low baseline virus load had elevated HBV DNA levels during pregnancy (99). It was also found that there was an increasing trend on HBV DNA level during pregnancy, with average value of 0.4-1log10IU/ml (100). Analysis of causes: On the one hand, high levels of adrenocortical hormone during pregnancy may increase HBV replication (101). The increase of corticosteroid during pregnancy can activate glucocorticoid response elements in HBV gene, further enhance HBV replication and HBV gene expression. But the increase of estrogen level during pregnancy has been proved to reduce HBV replication in animal experiments (102). On the other hand, the predominance of Th2 in chronic HBV infection women during pregnancy is conducive to maintain immune tolerance to HBV in vivo (103).
After the fetus is delivered, maternal fetal immune tolerance is no longer required and maternal cell immunity gradually recovers (Figure 3). In the first trimester pregnancy, moderate inflammatory reaction is conducive to placenta implantation, and inflammation is gradually reduced in the second trimester pregnancy. In the third trimester of pregnancy, the uterine is again in an inflammatory state, so as to prepare for the delivery of the fetus and the face of birth canal infection.
Figure 3 Maternal Postpartum changes in immune system and hormone. It mainly includes four aspects: Fetal delivery; changes of immune cells; changes in maternal hormone levels; changes of Th1, Th2, Th17 and Treg balance in vivo. Abbreviation: MCP-1: monocyte chemoattractant protein-1, MDC:macrophage derived chemokine.
Both innate and adaptive immunity were activated after delivery. NK cells gathered at the maternal fetal interface during pregnancy returns to the peripheral blood after delivery. The increase of NK cell activity in postpartum mothers was mainly caused by the change of single NK cell activity (104). The percentage and absolute count of NK cells after delivery increased temporarily (9). It was found that the frequency of NK cells increased from 6 weeks after delivery and held plateau at 6-24 weeks (105). Postpartum neutrophils increased. Postpartum monocyte chemoattractant protein-1 (MCP-1), also known as CCL2 and macrophage derived chemokine (MDC) levels increased after delivery (106). As CC subclass chemokines, MCP-1 and DC can cause chemotactic migration of dendritic cells, monocytes and T cells, especially Th1 cells. During labor, macrophages in the cervix rapidly aggregate, showing M1 proinflammatory phenotype, and participate in regulating cervical maturation and the beginning of labor by producing proinflammatory cytokines (15). In the mouse model, it was found that postpartum cervical macrophages obtained M2 phenotype, which may be related to postpartum tissue repair (107).
Postpartum adaptive immune adjusts accordingly. In not receiving AVT patients with chronic HBV infection, the increase of Th1 cytokines during perinatal period can be slight. Due to the lack of IDO and HLA-G, their inhibition on immune cells is relieved. Th and CTL increased from 1 to 4 months postpartum, while inhibitory T cells increased at 7 months postpartum (108). As an important component of adaptive immunity, B cells can produce specific antibodies, present antigens and regulate immunity. CD5- and CD5+ B cells further decreased at 1 month postpartum, but CD5+ B cells significantly increased at 7-10 months postpartum (108). Plasma IL-6 and hsC-reactive protein (hsCRP) levels were higher in the early postpartum period. After delivery, IFN-γ, IL-2 and TNF-α were detected to be low level, and recovered 3-4 months later (109).
In a word, after the delivery of the fetus, maternal immunity presents Th1 and T17 dominant. The specific cellular immunity gradually increases in 3-4 months (109). Postpartum humoral immunity also increased after 6 months. The change of immune system lasts for 1 year after delivery.
HCG reached its peak at 8-10 weeks of gestation, then decreased rapidly, and disappeared within 2 weeks after delivery. Estrogen reached its peak at the end of pregnancy and decreased rapidly after delivery. Progesterone decreased rapidly after delivery. Postpartum progesterone rebound to baseline levels before pregnancy and no longer promotes the production of Th2 cytokine (110). Maternal HCG, estrogen and progesterone levels will recover in one month and the decrease of estrogen, progesterone and HCG promote the transformation from Th2 and Treg dominant in pregnancy to Th1 and Th17 dominant after delivery.
In conclusion, there is no need for maternal fetal immune tolerance after delivery. In the background of uterine inflammation and disappearance of immune tolerance after delivery, the immunosuppression in innate and adaptive immune is reversed. Under the influence of various postpartum factors, there are shifts of Th1/Th2 to Th1 dominant and Th17/Treg to Th17 dominant type. The rapid recovery of hormones of estrogen, progesterone, HCG and cortisol levels aggravate the inflammatory process after delivery. (Figure 3)
The causes of postpartum hepatitis are complex. Although immune tolerance to antigens during pregnancy mainly occurs at the maternal-fetal interface, it also affects the systemic immune system. With postpartum uterine inflammation, the body immunity is mainly Th1 and Th17 type. The change of postpartum hormone level does not seem to directly cause postpartum hepatitis, but it can make Th1 cells predominate. The rapid decrease of postpartum cortisol level is similar to the effect of stopping cortisol, which reduces the inhibition of liver inflammation and may aggravate the liver inflammatory reaction (111, 112). In the above condition, if stimulated by HBV DNA, postpartum hepatitis will flare (Figure 4).
Figure 4 Causes of postpartum hepatitis. It mainly includes: fetal delivery (maternal fetal immune tolerance disappears);local inflammation of uterus after delivery; recovery of immune system after delivery; changes of Th1, Th2, Th17 and Treg balance after delivery; after delivery, GC decreases which is similar to “hormone withdrawal” reaction; HBV stimulation. Abbreviation: GC, Corticosteroid.
The changes of innate immunity and adaptive immunity after delivery mainly lead to postpartum hepatitis flare. The immune effect of pregnancy lasts about 1 year after delivery (108). After delivery, the immune tolerance to the fetus disappears rapidly. The number and function inhibition of innate immune cells often recovery earlier after delivery. There are many reasons for postpartum hepatitis, including proinflammatory factor release, the withdrawal of corticosteroids, especially the enhancement of HBV specific T cell response induced by viral HBV DNA, cytokines, etc. Postpartum hepatitis should also exclude various common causes of abnormal liver function, such as non-alcoholic fatty liver disease and drug-induced liver damage.
The largest number of innate immune cells in the liver is NK, followed by macrophages. After delivery, the delivery woman is in an inflammatory state caused by bacteria or other reasons. Postpartum inflammatory cytokines and chemokines may also activate innate immunity or adaptive immunity, causing hepatitis. The number and activity of NK cells in postpartum peripheral blood increased. Liver NK cells may cause liver inflammation through a non-antigen specific mechanism through (TRAIL/TRAIL R) mediated apoptosis, which can be initiated by cytokines such as IFN-α (113). Under the stimulation of inflammation, macrophages derived from Kupffer cells or monocytes in the liver are activated. Macrophages play the role of double swords. On the one hand, they are conducive to controlling infection, on the other hand, they can release chemokines, recruit more monocytes and lymphocytes to the liver, damage liver cells, and promote the occurrence of fibrosis.
In cellular immunity, CD8+ T plays a major role in immune cells proliferation and activation. It has been reported that the postpartum ALT and Th1 cytokines in untreated CHB patients increased slightly, and the serum HBsAg and HBV DNA content did not change (95). It is considered that in this study the increase of Th1 cytokines is not strong enough to cause immune suppression to HBV after postpartum (95). Compared with the group without postpartum hepatitis, the number of Treg in delivery woman with postpartum hepatitis was low, the ratio of CD4+ T cells expressing activated marker CD69 was significantly higher. In postpartum hepatitis mother, CD4+ T cells secreted more IFN-γ, IL-21, IL-2, TNF-α, and secreted more pro-inflammatory/anti-inflammatory cytokines (114). After delivery, CD8+ T and its subgroup effector memory T cell (Tem) and terminally differentiated effector memory T cells (TEMRA) in postpartum hepatitis flares group were significantly activated, and the expression levels of perforin and granzyme B increased (108, 115). In postpartum hepatitis flare group, activation of CD8+ T cells can produce TNF-α and IFN-α and induce postpartum hepatitis (114, 115). Finally, postpartum hepatitis may be accompanied by the decrease of HBV DNA, and HBeAg or HBsAg.
For the first pregnancy, most HBeAg-positive women of childbearing age with chronic HBV infection are in the HBV immune tolerance period, and a small part are in the immune control period. ALT and HBeAg status can help us distinguish immune tolerance status from immune clearance phase (116). Most pregnant woman are in the period of immune tolerance or immune control before pregnancy. At the early stage, it is believed that pregnancy and childbirth have little impact on the natural process of chronic HBV infection. Women with chronic HBV infection rarely develop severe HBV related liver disease during pregnancy, which may be due to the predominance of Th2 cells in women during pregnancy. Early research supported that the virology and serology of chronic HBV infection were no significant change in pregnancy and postpartum (98). The quantification of HBV DNA, HBsAg/HBeAg had no significant change from pregnancy to 12 months after delivery in patients with e antigen positive or e antigen negative chronic HBV infection. But now it seems that serological conversion of HBeAg can occur after delivery. As an immune tolerance factor, HBeAg is also a risk factor for postpartum hepatitis. Postpartum hepatitis in HBeAg positive women was 2.56 times higher than that in HBeAg negative delivery women (117). Postpartum immune reactivation can lead to increased ALT, and women with HBeAg positive with low titer can transit to the immune clearance period accompanied by the decline of HBsAg and HBeAg, and even HBeAg serological conversion after delivery (117). Postpartum HBeAg serum conversion rate ranged from 7-17% (117–119). Compared with non pregnant women, the HBeAg seroconversion rate of pregnant women with an average age of 30.7 ± 3.6 years increased from 2.2% to 14.3% (4). The clearance rate of HBeAg in HBeAg positive and NAVT delivery women was closely related to decrease in both HBeAg titer and/or HBV-DNA concentration in 1-2 months after delivery (118). Forty HBeAg positive pregnant women were studied in Taiwan, with an average age of 29.9 ± 5.3 years. During the follow-up period from the beginning of labor to 1 year, it was found that prepartum HBeAg titer<1:650 was associated with high HBeAg seroconversion rate, and there was no significant correlation with genotype B and genotype C (119). Observing the data of 214 HBeAg positive and NAVT delivery woman, HBsAg<1.0×104IU/mL, and HBeAg<7.36×102S/CO, HBV DNA level<1.0 ×107 IU/mL at delivery were independent predictors of HBeAg seroconversion within 12 months postpartum (120). The presence of PC, BCP, and PC and BCP mutations during labor was associated with an increased likelihood of spontaneous postnatal HBeAg seroconversion (all P<0.05) (120). Other studies have similar findings. The NAVT HBeAg positive delivery woman were observed for 6.4 years. The baseline HBV DNA<3x107IU/mL or HBeAg<770 S/CO in pregnancy was the predictive factor of HBeAg serological conversion (121).
Because of the interaction between HBV and immune response, postpartum hepatitis is more common in chronic HBV infection women. HBV DNA during delivery is also a predictor of postpartum hepatitis. There is 30% probability for postpartum hepatitis in pregnant women who can detect HBV DNA during delivery, and 20% probability in pregnant women who do not detect viremia in chronic HBV infected patients (2). The study found that hepatitis during pregnancy was 4.6% (2/44) and 7.1% (5/68) respectively for HBV DNA<2000 IU/mL and HBV DNA>2000 IU/mL, and hepatitis after delivery was 7.4% (2/28) and 13.0% (3/23) respectively (99). Some studies believe that the cutoff value of postpartum hepatitis is HBV DNA ≥ 5 log10IU/mL at the delivery of untreated chronic HBV infected pregnant women with positive predictive value is 14.4% and the negative predictive value is 98.2% (2). It is speculated that the postpartum hepatitis flare can only be triggered when the HBV DNA load reaches a certain level. Among HBeAg negative pregnant women, about 30% of pregnant women have postpartum hepatitis, which may be related to reactivation in the immune control period. Age is related to HBV immune tolerance. It is believed that some 30 years old HBeAg positive women are in the transition stage from immune tolerance to immune clearance. The study found that once postpartum hepatitis occured, the quantitative level of HBsAg in delivery women would decrease significantly at 6-8 weeks or 15-18 weeks (114). HBsAg<100 IU/ml was the predictive factor of HBsAg seroconversion after delivery (121).
Early articles suggested that HBV DNA, ALT level, HBeAg status or any other characteristics of pregnant women could not predict postpartum hepatitis during pregnancy (51). However, in recent studies, the pregnant women were further differentiated according to the natural period of chronic HBV infection and HBeAg positive, and it was found that there were characteristic indicators to predict the onset of postpartum hepatitis (Table 1). In addition to the mentioned HBeAg positive and <700S/CO, HBV DNA>5Log10IU/ml at delivery is a predictor of postpartum hepatitis. Postpartum hepatitis can occur in chronic HBV infected people receiving AVT or NAVT (120–123). The increase rate of hepatitis during pregnancy and after delivery was 14% and 16%, respectively (5). ALT at delivery is elevated, which is also a high-risk predictor of postpartum hepatitis (2). In 241 pregnant women, 33.6% of the women had elevated serum ALT during pregnancy. Multivariate analysis showed that elevated ALT during pregnancy was associated with postpartum hepatitis after telbivudine withdrawal (124). The cutoff value of predicting postpartum hepatitis in pregnant women was 29 years old, the prenatal ALT was greater than 14.8 U/L, and the postnatal HBeAg level was less than 3.1 log10 S/CO (125). Studies on pregnant women showed that ALT at 32 weeks of gestation and HBcAb were risk factors of postpartum hepatitis (126). HBeAg positivity and gestational diabetes were associated with postpartum hepatitis (123). When oral AVT is used in late pregnancy and stopped after delivery, the prediction model of postpartum hepatitis includes the decrease of HBsAg and HBV DNA from baseline to gestation 32 weeks or delivery, respectively (127).
At first, scholars questioned the correctness of AVT in late pregnancy. According to previous research reports, 62% of women will have postpartum hepatitis after stopping antiviral treatment after delivery, which is significantly higher than that of women who do not receive antiviral treatment (36% vs 62%) (51). Ayres et al. reported that although short-term lamivudine treatment during pregnancy moderately reduced maternal viremia, HBV rtM204I/V and rtA181T mutations were detected (128). With the emergence of powerful and highly resistant barrier drugs, guidelines recommend antiviral treatment for pregnant women in late pregnancy with high viral load of hepatitis B virus infection.
Whether stopping AVT after delivery is a risk factor for postpartum hepatitis in chronic HBV infected women who have received oral nucleoside/nucleotides analogs in late pregnancy? It is believed that even if prophylaxis MTCT treatment continues until 12 weeks postpartum, postnatal hepatitis will still occur during this period. It is believed that postpartum hepatitis is generally not serious and reversible if preventive AVT is stopped after delivery (124). Postpartum hepatitis occurred in 25% of women with elevated ALT during pregnancy after discontinuation of telbivudine (124). In immune related studies, lower IFN-α during pregnancy and higher IFN-α after delivery may be related to postpartum hepatitis (125). In the population receiving antiviral treatment, the number of Treg is relatively reduced in chronic HBV infected women with postpartum hepatitis, the production of pro-inflammatory factors IFN-γ, IL-2 and TNF-α is more, and the production of anti-inflammatory cytokines (IL-10) is less (114).
(4) Postpartum hepatitis and treatment to prevent MTCT
AVT in the third trimester of pregnancy can reduce the incidence of MTCT (129–132). After delivery, the immune reactivation in chronic HBV infection women is the basis of hepatitis flare (106). In addition, many factors are involved in the occurrence of postpartum hepatitis. During AVT, there is a negative correlation between the enhancement of Th1/Th2 immunity and the decrease of HBV DNA load (133). Oral AVT reduces HBV DNA load which may further promote to the recovery of HBV specific T cell function. Treatment of prevention MTCT can obviously increase the frequency of postpartum CD83+ pDCs and CD86+ pDCs (134). The increase in the frequency of differentiated mature DC means that the immune presentation function is enhanced, which is conducive to activating specific cellular immunity. HBV specific immune enhancement participates the damage of HBV infected liver cells, on the other hand, it is conducive to the HBeAg seroconversion. The incidence of postpartum hepatitis after withdrawal of AVT after delivery ranged from 5% to 62%, which may be related to hepatitis postpartum criteria, the frequency of ALT monitoring, and the withdrawal time of AVT. Postpartum immune effect was maintained for 1 year (108). Professor Xie Yao’s team conducted a prospective study on pregnant women with HBeAg positive chronic HBV infection, including 96 cases of withdrawal the drug immediately postpartum, and 37 cases of were delayed withdrawal to six weeks postpartum (135). The conclusion of the study suggests that withdrawal of antiviral drugs immediately or six weeks postpartum does not affect the rate of postpartum hepatitis, but may delay postpartum hepatitis until twelve weeks postpartum (135). AVT in late pregnancy prolonged to 12 weeks postpartum did not decrease the occurance rate of hepatitis after delivery compared with immediate withdrawal postpartum. In pregnant women who with elevated ALT prolonged AVT to 52 weeks postpartum, HBeAg serum conversion rate increased significantly, and HBsAg titer decreased significantly (124).
Postpartum anti-HBV treatment is often a good time for achieving the seroclearance of HBeAg or HBsAg. In the chronic HBV infected women who were in immune tolerance period before pregnancy, some people would enter the immune clearance period after delivery. Previous studies have shown that in the pursuit of HBeAg seroconversion or clinical cure, a more effective treatment is the interferon-based treatment. If there is no contraindication of interferon, oral nucleoside/nucleotides analogs combined with pegylated-interferon can often achieve higher HBeAg seroclearance or HBsAg seroclearance. A research was conducted in pregnant women with ALT <1ULN, HBeAg positive and HBV DNA>6log10IU/ml who had received oral AVT in the late pregnancy. If the postpartum women showed the characteristics of ALT>2ULN with HBeAg decreased by more than 20% or HBV DNA decreased by more than 2log10IU/ml compared with baseline of late pregnancy, they were included in the study group which were given pegylated-interferon combined with Adefovir, while the control group stopped taking AVT orally in 12 weeks after delivery. In the study group, 56.7% (17/30) patients achieved HBeAg seroclearance and 26.7% (8/30) patients achieved HBsAg seroclearance. No one in the control group achieved the seroclearance of HBeAg or HBsAg (136).
In conclusion, during pregnancy, maternal fetal immune tolerance makes immunosuppression in specific immunity and non-specific immunity. The elevation of pregnancy related hormones will also affect immunity. The condition of pregnant women with chronic HBV infection seems to be relatively stable during pregnancy. Postpartum human immune system reverses the trend of maternal fetal immune tolerance and were dominated by uterine inflammation. NK’s non-specific immunity is enhanced, Th1 cells and corresponding cytokines are increased, DC’ antigen presenting function is enhanced. The content of serum cortisol dropped rapidly after delivery, which was equivalent to hormone withdrawal reaction. In delivery women with chronic HBV infection, when HBV DNA can be detected, it can often induce or aggravate the hepatitis flare. There are several indicators to predict postpartum hepatitis, including HBeAg positive and<700 S/CO, childbearing age and<29 years old, and HBV DNA>3-5Log10 IU/ml. When delaying the time for withdrawal AVT to 6-12 weeks postpartum, postpartum hepatitis rate cannot decrease. For pregnant women with elevated ALT during pregnancy, the HBeAg serum conversion rate can be increased by prolonging the AVT to more than 48 weeks if HBeAg tends to decline from the baseline in the pregnant or delivery. If the postpartum biochemical, virological or other indicators indicate that the delivery women have entered the immune clearance period, it is recommended to consider anti-HBV treatment. If there is no interferon contraindication, the interferon-based treatmment is more conducive to HBeAg or HBsAg seroclearance after delivery. In rare cases postpartum hepatitis flare would be severe, and oral AVT is recommended.
ML, YLu, YJ and YX contributed to study concept and design. TJ, YY, WD, HL, SW, RL, MC, SWu, YG, HH, GS, XC, LH, LY, XB and YLin collected and sorted out literatures. LZ wrote the fifirst draft and drew pictures. TJ, YY, WD, HL and SW edited the English version. ML, YLu, YJ and YX modify the version to be submitted. All authors contributed to the article and approved the submitted version.
National Key R&D Program of China (2022YFC2603505); Beijing Hospitals Authority Clinical medicine Development of special funding support (XMLX 202127); The Digestive Medical Coordinated Development Center of Beijing Hospitals Authority (XXZ0302 and XXT28); High-level Public Health Technical Personnel Training Program of Beijing Municipal Health Commission (2022-3-050); National Science and Technology Major Project of China (2017ZX10201201-001-006, 2017ZX10201201-002-006, 2018ZX10715-005-003-005); Project supported by Beijing science and technology commission (Z211100002921059); Special Public Health Project for Health Development in Capital (2022-1-2172).
The authors declare that the research was conducted in the absence of any commercial or financial relationships that could be construed as a potential conflict of interest. The reviewer XC declared a shared affiliation with the authors LZ, TJ, WD, HL, SW, RL, MC, WS, YG, HH, GS, XM, XC, LH, LY, XB, YL, YJ, ML, YX to the handling editor at the time of review.
All claims expressed in this article are solely those of the authors and do not necessarily represent those of their affiliated organizations, or those of the publisher, the editors and the reviewers. Any product that may be evaluated in this article, or claim that may be made by its manufacturer, is not guaranteed or endorsed by the publisher.
1. Terrault NA, Lok A, McMahon BJ, Chang KM, Hwang JP, Jonas MM, et al. Update on prevention, diagnosis, and treatment of chronic hepatitis b: AASLD 2018 hepatitis b guidance. Hepatology (2018) 67(4):1560–99. doi: 10.1002/hep.29800
2. Yi W, Pan CQ, Li MH, Wan G, Lv YW, Liu M, et al. The characteristics and predictors of postpartum hepatitis flares in women with chronic hepatitis b. Am J Gastroenterol (2018) 113(5):686–93. doi: 10.1038/s41395-018-0010-2
3. Bzowej NH, Tran TT, Li R, Belle SH, Smith CI, Khalili M, et al. Total alanine aminotransferase (ALT) flares in pregnant north American women with chronic hepatitis b infection: Results from a prospective observational study. Am J Gastroenterol (2019) 114(8):1283–91. doi: 10.14309/ajg.0000000000000221
4. Tan HH, Lui HF, Chow WC. Chronic hepatitis b virus (HBV) infection in pregnancy. Hepatol Int (2008) 2(3):370–5. doi: 10.1007/s12072-008-9063-4
5. Kushner T, Shaw PA, Kalra A, Magaldi L, Monpara P, Bedi G, et al. Incidence, determinants and outcomes of pregnancy-associated hepatitis b flares: A regional hospital-based cohort study. Liver Int (2018) 38(5):813–20. doi: 10.1111/liv.13594
6. Bonney EA. Immune regulation in pregnancy: A matter of perspective. Obstet Gynecol Clin North Am (2016) 43(4):679–98. doi: 10.1016/j.ogc.2016.07.004
7. Erlebacher A. Immunology of the maternal-fetal interface. Annu Rev Immunol (2013) 31:387–411. doi: 10.1146/annurev-immunol-032712-100003
8. Mavoungou E. Interactions between natural killer cells, cortisol and prolactin in malaria during pregnancy. Clin Med Res (2006) 4(1):33–41. doi: 10.3121/cmr.4.1.33
9. Iwatani Y, Amino N, Tachi J, Kimura M, Ura I, Mori M, et al. Changes of lymphocyte subsets in normal pregnant and postpartum women: postpartum increase in NK/K (Leu 7) cells. Am J Reprod Immunol Microbiol (1988) 18(2):52–5. doi: 10.1111/j.1600-0897.1988.tb00235.x
10. Matera L, Cardoso E, Veglia F, Cesano A, Bellone G, Vuolo A, et al. Effect of cortisol on the native and in vitro induced non-MHC restricted cytotoxicity of large granular lymphocytes. J Clin Lab Immunol (1988) 27(2):77–81.
11. Slukvin II, Breburda EE, Golos TG. Dynamic changes in primate endometrial leukocyte populations: differential distribution of macrophages and natural killer cells at the rhesus monkey implantation site and in early pregnancy. Placenta (2004) 25(4):297–307. doi: 10.1016/j.placenta.2003.08.019
12. Vacca P, Chiossone L, Mingari MC, Moretta L. Heterogeneity of NK cells and other innate lymphoid cells in human and murine decidua. Front Immunol (2019) 10:170. doi: 10.3389/fimmu.2019.00170
13. Tang MX, Hu XH, Liu ZZ, Kwak-Kim J, Liao AH. What are the roles of macrophages and monocytes in human pregnancy. J Reprod Immunol (2015) 112:73–80. doi: 10.1016/j.jri.2015.08.001
14. Jakubzick CV, Randolph GJ, Henson PM. Monocyte differentiation and antigen-presenting functions. Nat Rev Immunol (2017) 17(6):349–62. doi: 10.1038/nri.2017.28
15. Nagamatsu T, Schust DJ. The immunomodulatory roles of macrophages at the maternal-fetal interface. Reprod Sci (2010) 17(3):209–18. doi: 10.1177/1933719109349962
16. Mosser DM, Edwards JP. Exploring the full spectrum of macrophage activation. Nat Rev Immunol (2008) 8(12):958–69. doi: 10.1038/nri2448
17. Sayama S, Nagamatsu T, Schust DJ, Itaoka N, Ichikawa M, Kawana K, et al. Human decidual macrophages suppress IFN-γ production by T cells through costimulatory B7-H1:PD-1 signaling in early pregnancy. J Reprod Immunol (2013) 100(2):109–17. doi: 10.1016/j.jri.2013.08.001
18. Kim MK, Kim J. Properties of immature and mature dendritic cells: phenotype, morphology, phagocytosis, and migration. RSC Adv (2019) 9(20):11230–8. doi: 10.1039/c9ra00818g
19. Collins MK, Tay CS, Erlebacher A. Dendritic cell entrapment within the pregnant uterus inhibits immune surveillance of the maternal/fetal interface in mice. J Clin Invest (2009) 119(7):2062–73. doi: 10.1172/JCI38714
20. Emerson MR, Orentas DM, Lynch SG, LeVine SM. Activation of histamine H2 receptors ameliorates experimental allergic encephalomyelitis. Neuroreport (2002) 13(11):1407–10. doi: 10.1097/00001756-200208070-00012
21. Dijkstra D, Leurs R, Chazot P, Shenton FC, Stark H, Werfel T, et al. Histamine downregulates monocyte CCL2 production through the histamine H4 receptor. J Allergy Clin Immunol (2007) 120(2):300–7. doi: 10.1016/j.jaci.2007.03.024
22. Bert S, Ward EJ, Nadkarni S. Neutrophils in pregnancy: New insights into innate and adaptive immune regulation. Immunology (2021) 164(4):665–76. doi: 10.1111/imm.13392
23. Rowe JH, Ertelt JM, Xin L, Way SS. Pregnancy imprints regulatory memory that sustains anergy to fetal antigen. Nature (2012) 490(7418):102–6. doi: 10.1038/nature11462
24. Mold JE, Michaëlsson J, Burt TD, Muench MO, Beckerman KP, Busch MP, et al. Maternal alloantigens promote the development of tolerogenic fetal regulatory T cells in utero. Science (2008) 322(5907):1562–5. doi: 10.1126/science.1164511
25. Ghaebi M, Nouri M, Ghasemzadeh A, Farzadi L, Jadidi-Niaragh F, Ahmadi M, et al. Immune regulatory network in successful pregnancy and reproductive failures. BioMed Pharmacother (2017) 88:61–73. doi: 10.1016/j.biopha.2017.01.016
26. Aluvihare VR, Kallikourdis M, Betz AG. Regulatory T cells mediate maternal tolerance to the fetus. Nat Immunol (2004) 5(3):266–71. doi: 10.1038/ni1037
27. Tilburgs T, Roelen DL, van der Mast BJ, de Groot-Swings GM, Kleijburg C, Scherjon SA, et al. Evidence for a selective migration of fetus-specific CD4+CD25bright regulatory T cells from the peripheral blood to the decidua in human pregnancy. J Immunol (2008) 180(8):5737–45. doi: 10.4049/jimmunol.180.8.5737
28. Alijotas-Reig J, Llurba E, Gris JM. Potentiating maternal immune tolerance in pregnancy: a new challenging role for regulatory T cells. Placenta (2014) 35(4):241–8. doi: 10.1016/j.placenta.2014.02.004
29. Schlößer HA, Theurich S, Shimabukuro-Vornhagen A, Holtick U, Stippel DL, von Bergwelt-Baildon M. Overcoming tumor-mediated immunosuppression. Immunotherapy (2014) 6(9):973–88. doi: 10.2217/imt.14.58
30. Munn DH, Zhou M, Attwood JT, Bondarev I, Conway SJ, Marshall B, et al. Prevention of allogeneic fetal rejection by tryptophan catabolism. Science (1998) 281(5380):1191–3. doi: 10.1126/science.281.5380.1191
31. Fallarino F, Grohmann U, Hwang KW, Orabona C, Vacca C, Bianchi R, et al. Modulation of tryptophan catabolism by regulatory T cells. Nat Immunol (2003) 4(12):1206–12. doi: 10.1038/ni1003
32. Collison LW, Workman CJ, Kuo TT, Boyd K, Wang Y, Vignali KM, et al. The inhibitory cytokine IL-35 contributes to regulatory T-cell function. Nature (2007) 450(7169):566–9. doi: 10.1038/nature06306
33. Vacca P, Cantoni C, Vitale M, Prato C, Canegallo F, Fenoglio D, et al. Crosstalk between decidual NK and CD14+ myelomonocytic cells results in induction of tregs and immunosuppression. Proc Natl Acad Sci U.S.A. (2010) 107(26):11918–23. doi: 10.1073/pnas.1001749107
34. Erlebacher A. Mechanisms of T cell tolerance towards the allogeneic fetus. Nat Rev Immunol (2013) 13(1):23–33. doi: 10.1038/nri3361
35. Schumacher A, Heinze K, Witte J, Poloski E, Linzke N, Woidacki K, et al. Human chorionic gonadotropin as a central regulator of pregnancy immune tolerance. J Immunol (2013) 190(6):2650–8. doi: 10.4049/jimmunol.1202698
36. van Egmond A, van der Keur C, Swings GM, Scherjon SA, Claas FH. The possible role of virus-specific CD8(+) memory T cells in decidual tissue. J Reprod Immunol (2016) 113:1–8. doi: 10.1016/j.jri.2015.09.073
37. van der Zwan A, Bi K, Norwitz ER, Crespo ÂC, Claas F, Strominger JL, et al. Mixed signature of activation and dysfunction allows human decidual CD8(+) T cells to provide both tolerance and immunity. Proc Natl Acad Sci U.S.A. (2018) 115(2):385–90. doi: 10.1073/pnas.1713957115
38. Slutsky R, Romero R, Xu Y, Galaz J, Miller D, Done B, et al. Exhausted and senescent T cells at the maternal-fetal interface in preterm and term labor. J Immunol Res (2019) 2019:3128010. doi: 10.1155/2019/3128010
39. Wang SC, Li YH, Piao HL, Hong XW, Zhang D, Xu YY, et al. PD-1 and Tim-3 pathways are associated with regulatory CD8+ T-cell function in decidua and maintenance of normal pregnancy. Cell Death Dis (2015) 6(5):e1738. doi: 10.1038/cddis.2015.112
40. Jerzak M, Kasprzycka M, Wierbicki P, Kotarski J, Górski A. Apoptosis of T cells in the first trimester human decidua. Am J Reprod Immunol (1998) 40(3):130–5. doi: 10.1111/j.1600-0897.1998.tb00404.x
41. Wang LL, Li ZH, Hu XH, Muyayalo KP, Zhang YH, Liao AH. The roles of the PD-1/PD-L1 pathway at immunologically privileged sites. Am J Reprod Immunol (2017) 78(2):e12710. doi: 10.1111/aji.12710
42. Kang S, Wu Q, Huang J, Yang B, Liang C, Chi P, et al. Tissue resident memory γδT cells in murine uterus expressed high levels of IL-17 promoting the invasion of trophocytes. Front Immunol (2020) 11:588227. doi: 10.3389/fimmu.2020.588227
43. Li L, Wang L, Huang C, Diao L, Zhang Y, Zhang X, et al. Chronic hepatitis b infection alters peripheral immune response in women with reproductive failure. Am J Reprod Immunol (2019) 81(3):e13083. doi: 10.1111/aji.13083
44. Muzzio DO, Soldati R, Ehrhardt J, Utpatel K, Evert M, Zenclussen AC, et al. B cell development undergoes profound modifications and adaptations during pregnancy in mice. Biol Reprod (2014) 91(5):115. doi: 10.1095/biolreprod.114.122366
45. Rosser EC, Mauri C. Regulatory b cells: origin, phenotype, and function. Immunity (2015) 42(4):607–12. doi: 10.1016/j.immuni.2015.04.005
46. Wang L, Fu Y, Chu Y. Regulatory b cells. Adv Exp Med Biol (2020) 1254:87–103. doi: 10.1007/978-981-15-3532-1_8
47. Petroff MG. Immune interactions at the maternal-fetal interface. J Reprod Immunol (2005) 68(1-2):1–13. doi: 10.1016/j.jri.2005.08.003
48. Gomez-Lopez N, StLouis D, Lehr MA, Sanchez-Rodriguez EN, Arenas-Hernandez M. Immune cells in term and preterm labor. Cell Mol Immunol (2014) 11(6):571–81. doi: 10.1038/cmi.2014.46
49. Zhang Y, Wang X, Zhang R, Wang X, Fu H, Yang W. MDSCs interactions with other immune cells and their role in maternal-fetal tolerance. Int Rev Immunol (2022) 41(5):534–51. doi: 10.1080/08830185.2021.1938566
50. Meggyes M, Nagy DU, Saad Al Deen I, Parkanyi B, Szereday L. CD8+ and CD8- NKT cells exhibit phenotypic changes during pregnancy. Immunol Invest (2022), 1–17. doi: 10.1080/08820139.2022.2119863
51. ter Borg MJ, Leemans WF, de Man RA, Janssen HL. Exacerbation of chronic hepatitis b infection after delivery. J Viral Hepat (2008) 15(1):37–41. doi: 10.1111/j.1365-2893.2007.00894.x
52. Valencia-Ortega J, Saucedo R, Peña-Cano MI, Hernández-Valencia M, Cruz-Durán JG. Immune tolerance at the maternal-placental interface in healthy pregnancy and pre-eclampsia. J Obstet Gynaecol Res (2020) 46(7):1067–76. doi: 10.1111/jog.14309
53. Chang RQ, Li DJ, Li MQ. The role of indoleamine-2,3-dioxygenase in normal and pathological pregnancies. Am J Reprod Immunol (2018) 79(4):e12786. doi: 10.1111/aji.12786
54. Ploder M, Spittler A, Kurz K, Neurauter G, Pelinka LE, Roth E, et al. Accelerated tryptophan degradation predicts poor survival in trauma and sepsis patients. Int J Tryptophan Res (2010) 3:61–7. doi: 10.4137/ijtr.s3983
55. Rao JS, Hosny N, Kumbha R, Naqvi RA, Singh A, Swanson Z, et al. HLA-G1(+) expression in GGTA1KO pigs suppresses human and monkey anti-pig T, b and NK cell responses. Front Immunol (2021) 12:730545. doi: 10.3389/fimmu.2021.730545
56. Carosella ED, Rouas-Freiss N, Tronik-Le Roux D, Moreau P, LeMaoult J. HLA-G: An immune checkpoint molecule. Adv Immunol (2015) 127:33–144. doi: 10.1016/bs.ai.2015.04.001
57. Le Gal FA, Riteau B, Sedlik C, Khalil-Daher I, Menier C, Dausset J, et al. HLA-g-mediated inhibition of antigen-specific cytotoxic T lymphocytes. Int Immunol (1999) 11(8):1351–6. doi: 10.1093/intimm/11.8.1351
58. LeMaoult J, Krawice-Radanne I, Dausset J, Carosella ED. HLA-G1-expressing antigen-presenting cells induce immunosuppressive CD4+ T cells. Proc Natl Acad Sci U.S.A. (2004) 101(18):7064–9. doi: 10.1073/pnas.0401922101
59. Köstlin N, Ostermeir AL, Spring B, Schwarz J, Marmé A, Walter CB, et al. HLA-G promotes myeloid-derived suppressor cell accumulation and suppressive activity during human pregnancy through engagement of the receptor ILT4. Eur J Immunol (2017) 47(2):374–84. doi: 10.1002/eji.201646564
60. Hiby SE, Apps R, Sharkey AM, Farrell LE, Gardner L, Mulder A, et al. Maternal activating KIRs protect against human reproductive failure mediated by fetal HLA-C2. J Clin Invest (2010) 120(11):4102–10. doi: 10.1172/JCI43998
61. Söderström K, Corliss B, Lanier LL, Phillips JH. CD94/NKG2 is the predominant inhibitory receptor involved in recognition of HLA-G by decidual and peripheral blood NK cells. J Immunol (1997) 159(3):1072–5.
62. Cristiani CM, Palella E, Sottile R, Tallerico R, Garofalo C, Carbone E. Human NK cell subsets in pregnancy and disease: Toward a new biological complexity. Front Immunol (2016) 7:656. doi: 10.3389/fimmu.2016.00656
63. Gong H, Chen Y, Xu J, Xie X, Yu D, Yang B, et al. The regulation of ovary and conceptus on the uterine natural killer cells during early pregnancy. Reprod Biol Endocrinol (2017) 15(1):73. doi: 10.1186/s12958-017-0290-1
64. Saito S, Nakashima A, Shima T, Ito M. Th1/Th2/Th17 and regulatory T-cell paradigm in pregnancy. Am J Reprod Immunol (2010) 63(6):601–10. doi: 10.1111/j.1600-0897.2010.00852.x
65. Maeda Y, Ohtsuka H, Tomioka M, Oikawa M. Effect of progesterone on Th1/Th2/Th17 and regulatory T cell-related genes in peripheral blood mononuclear cells during pregnancy in cows. Vet Res Commun (2013) 37(1):43–9. doi: 10.1007/s11259-012-9545-7
66. Li X, Wang B, Li Y, Wang L, Zhao X, Zhou X, et al. The Th1/Th2/Th17/Treg paradigm induced by stachydrine hydrochloride reduces uterine bleeding in RU486-induced abortion mice. J Ethnopharmacol (2013) 145(1):241–53. doi: 10.1016/j.jep.2012.10.059
67. Marzi M, Vigano A, Trabattoni D, Villa ML, Salvaggio A, Clerici E, et al. Characterization of type 1 and type 2 cytokine production profile in physiologic and pathologic human pregnancy. Clin Exp Immunol (1996) 106(1):127–33. doi: 10.1046/j.1365-2249.1996.d01-809.x
68. Wegmann TG, Lin H, Guilbert L, Mosmann TR. Bidirectional cytokine interactions in the maternal-fetal relationship: is successful pregnancy a TH2 phenomenon. Immunol Today (1993) 14(7):353–6. doi: 10.1016/0167-5699(93)90235-D
69. Lin H, Mosmann TR, Guilbert L, Tuntipopipat S, Wegmann TG. Synthesis of T helper 2-type cytokines at the maternal-fetal interface. J Immunol (1993) 151(9):4562–73.
70. Wang W, Sung N, Gilman-Sachs A, Kwak-Kim J. T Helper (Th) cell profiles in pregnancy and recurrent pregnancy losses: Th1/Th2/Th9/Th17/Th22/Tfh cells. Front Immunol (2020) 11:2025. doi: 10.3389/fimmu.2020.02025
71. Lee YK, Mukasa R, Hatton RD, Weaver CT. Developmental plasticity of Th17 and treg cells. Curr Opin Immunol (2009) 21(3):274–80. doi: 10.1016/j.coi.2009.05.021
72. Basu R, Hatton RD, Weaver CT. The Th17 family: flexibility follows function. Immunol Rev (2013) 252(1):89–103. doi: 10.1111/imr.12035
73. Logiodice F, Lombardelli L, Kullolli O, Haller H, Maggi E, Rukavina D, et al. Decidual interleukin-22-Producing CD4+ T cells (Th17/Th0/IL-22+ and Th17/Th2/IL-22+, Th2/IL-22+, Th0/IL-22+), which also produce IL-4, are involved in the success of pregnancy. Int J Mol Sci (2019) 20(2):428. doi: 10.3390/ijms20020428
74. Taams LS. Interleukin-17 in rheumatoid arthritis: Trials and tribulations. J Exp Med (2020) 217(3):e20192048. doi: 10.1084/jem.20192048
75. Lee SK, Kim JY, Lee M, Gilman-Sachs A, Kwak-Kim J. Th17 and regulatory T cells in women with recurrent pregnancy loss. Am J Reprod Immunol (2012) 67(4):311–8. doi: 10.1111/j.1600-0897.2012.01116.x
76. Makrigiannakis A, Zoumakis E, Kalantaridou S, Mitsiades N, Margioris A, Chrousos GP, et al. Corticotropin-releasing hormone (CRH) and immunotolerance of the fetus. Biochem Pharmacol (2003) 65(6):917–21. doi: 10.1016/s0006-2952(02)01547-2
77. Carr BR, Parker CR Jr, Madden JD, MacDonald PC, Porter JC. Maternal plasma adrenocorticotropin and cortisol relationships throughout human pregnancy. Am J Obstet Gynecol (1981) 139(4):416–22. doi: 10.1016/0002-9378(81)90318-5
78. Elenkov IJ. Glucocorticoids and the Th1/Th2 balance. Ann N Y Acad Sci (2004) 1024:138–46. doi: 10.1196/annals.1321.010
79. Mao G, Wang J, Kang Y, Tai P, Wen J, Zou Q, et al. Progesterone increases systemic and local uterine proportions of CD4+CD25+ treg cells during midterm pregnancy in mice. Endocrinology (2010) 151(11):5477–88. doi: 10.1210/en.2010-0426
80. AbdulHussain G, Azizieh F, Makhseed M, Raghupathy R. Effects of progesterone, dydrogesterone and estrogen on the production of Th1/Th2/Th17 cytokines by lymphocytes from women with recurrent spontaneous miscarriage. J Reprod Immunol (2020) 140:103132. doi: 10.1016/j.jri.2020.103132
81. Solano ME, Arck PC. Steroids, pregnancy and fetal development. Front Immunol (2019) 10:3017. doi: 10.3389/fimmu.2019.03017
82. Piccinni MP, Giudizi MG, Biagiotti R, Beloni L, Giannarini L, Sampognaro S, et al. Progesterone favors the development of human T helper cells producing Th2-type cytokines and promotes both IL-4 production and membrane CD30 expression in established Th1 cell clones. J Immunol (1995) 155(1):128–33.
83. Thiele K, Hierweger AM, Riquelme J, Solano ME, Lydon JP, Arck PC. Impaired progesterone-responsiveness of CD11c+ dendritic cells affects the generation of CD4+ regulatory T cells and is associated with intrauterine growth restriction in mice. Front Endocrinol (Lausanne) (2019) 10:96. doi: 10.3389/fendo.2019.00096
84. Shah NM, Imami N, Johnson MR. Progesterone modulation of pregnancy-related immune responses. Front Immunol (2018) 9:1293. doi: 10.3389/fimmu.2018.01293
85. Schumacher A, Brachwitz N, Sohr S, Engeland K, Langwisch S, Dolaptchieva M, et al. Human chorionic gonadotropin attracts regulatory T cells into the fetal-maternal interface during early human pregnancy. J Immunol (2009) 182(9):5488–97. doi: 10.4049/jimmunol.0803177
86. Liu J, Chen X, Hao S, Zhao H, Pang L, Wang L, et al. Human chorionic gonadotropin and IL-35 contribute to the maintenance of peripheral immune tolerance during pregnancy through mediating the generation of IL-10(+) or IL-35(+) breg cells. Exp Cell Res (2019) 383(2):111513. doi: 10.1016/j.yexcr.2019.111513
87. Monteiro C, Kasahara T, Sacramento PM, Dias A, Leite S, Silva VG, et al. Human pregnancy levels of estrogen and progesterone contribute to humoral immunity by activating T(FH) /B cell axis. Eur J Immunol (2021) 51(1):167–79. doi: 10.1002/eji.202048658
88. Bretscher P, Cohn M. A theory of self-nonself discrimination. Science (1970) 169(3950):1042–9. doi: 10.1126/science.169.3950.1042
89. Cohn M. The evolutionary context for a self-nonself discrimination. Cell Mol Life Sci (2010) 67(17):2851–62. doi: 10.1007/s00018-010-0438-z
90. Anderson CC, Matzinger P. Danger: the view from the bottom of the cliff. Semin Immunol (2000) 12(3):231–8; discussion 257-344. doi: 10.1006/smim.2000.0236
91. Janeway CA Jr. The immune system evolved to discriminate infectious nonself from noninfectious self. Immunol Today (1992) 13(1):11–6. doi: 10.1016/0167-5699(92)90198-G
92. Fisicaro P, Barili V, Rossi M, Montali I, Vecchi A, Acerbi G, et al. Pathogenetic mechanisms of T cell dysfunction in chronic HBV infection and related therapeutic approaches. Front Immunol (2020) 11:849. doi: 10.3389/fimmu.2020.00849
93. Yi X, Yuan Y, Li N, Yi L, Wang C, Qi Y, et al. A mouse model with age-dependent immune response and immune-tolerance for HBV infection. Vaccine (2018) 36(6):794–801. doi: 10.1016/j.vaccine.2017.12.071
94. Vlachogiannakos J, Papatheodoridis GV. HBV: Do I treat my immunotolerant patients. Liver Int (2016) 36 Suppl 1:93–9. doi: 10.1111/liv.12996
95. Joshi SS, Wong D, Castillo E, Swain MG, Coffin CS. Peripartum cytokine flares in a multiethnic cohort of chronic hepatitis b carriers does not correlate with hepatitis b virus suppression or increased risk of liver disease. Am J Reprod Immunol (2017) 78(4):e12707. doi: 10.1111/aji.12707
96. Wang XX, Lu JF, Wu YL, Ma LN, Jin Y, Cao ZH, et al. [Clinical study on liver function, virology, serological changes and the safety of drug withdrawal in pregnant women who are chronic HBV carriers during pregnancy and postpartum]. Zhonghua Gan Zang Bing Za Zhi (2019) 27(4):261–6. doi: 10.3760/cma.j.issn.1007-3418.2019.04.005
97. Stoop JN, van der Molen RG, Baan CC, van der Laan LJ, Kuipers EJ, Kusters JG, et al. Regulatory T cells contribute to the impaired immune response in patients with chronic hepatitis b virus infection. Hepatology (2005) 41(4):771–8. doi: 10.1002/hep.20649
98. Liu J, Bi Y, Xu C, Liu L, Xu B, Chen T, et al. Kinetic changes of viremia and viral antigens of hepatitis b virus during and after pregnancy. Med (Baltimore) (2015) 94(45):e2001. doi: 10.1097/MD.0000000000002001
99. Chang CY, Aziz N, Poongkunran M, Javaid A, Trinh HN, Lau D, et al. Serum alanine aminotransferase and hepatitis b DNA flares in pregnant and postpartum women with chronic hepatitis b. Am J Gastroenterol (2016) 111(10):1410–5. doi: 10.1038/ajg.2016.296
100. Söderström A, Norkrans G, Lindh M. Hepatitis b virus DNA during pregnancy and post partum: aspects on vertical transmission. Scand J Infect Dis (2003) 35(11-12):814–9. doi: 10.1080/00365540310016547
101. Zhong Z, Liao W, Dai L, Feng X, Su G, Gao Y, et al. Average corticosteroid dose and risk for HBV reactivation and hepatitis flare in patients with resolved hepatitis b infection. Ann Rheum Dis (2022) 81(4):584–91. doi: 10.1136/annrheumdis-2021-221650
102. Almog Y, Klein A, Adler R, Laub O, Tur-Kaspa R. Estrogen suppresses hepatitis b virus expression in male athymic mice transplanted with HBV transfected hep G-2 cells. Antiviral Res (1992) 19(4):285–93. doi: 10.1016/0166-3542(92)90010-3
103. Li MH, Zhang D, Zhang L, Qu XJ, Lu Y, Shen G, et al. Ratios of T-helper 2 cells to T-helper 1 cells and cytokine levels in patients with hepatitis b. Chin Med J (Engl) (2017) 130(15):1810–5. doi: 10.4103/0366-6999.211541
104. Hidaka Y, Amino N, Iwatani Y, Kaneda T, Mitsuda N, Morimoto Y, et al. Changes in natural killer cell activity in normal pregnant and postpartum women: increases in the first trimester and postpartum period and decrease in late pregnancy. J Reprod Immunol (1991) 20(1):73–83. doi: 10.1016/0165-0378(91)90024-k
105. Wang F, Xie S, Ran C, Hao H, Jiang T, Deng W, et al. Effect of antiviral therapy during pregnancy on natural killer cells in pregnant women with chronic HBV infection. Front Immunol (2022) 13:893628. doi: 10.3389/fimmu.2022.893628
106. Joshi SS, Coffin CS. Hepatitis b and pregnancy: Virologic and immunologic characteristics. Hepatol Commun (2020) 4(2):157–71. doi: 10.1002/hep4.1460
107. Timmons BC, Fairhurst AM, Mahendroo MS. Temporal changes in myeloid cells in the cervix during pregnancy and parturition. J Immunol (2009) 182(5):2700–7. doi: 10.4049/jimmunol.0803138
108. Watanabe M, Iwatani Y, Kaneda T, Hidaka Y, Mitsuda N, Morimoto Y, et al. Changes in T, b, and NK lymphocyte subsets during and after normal pregnancy. Am J Reprod Immunol (1997) 37(5):368–77. doi: 10.1111/j.1600-0897.1997.tb00246.x
109. Groer ME, Jevitt C, Ji M. Immune changes and dysphoric moods across the postpartum. Am J Reprod Immunol (2015) 73(3):193–8. doi: 10.1111/aji.12322
110. Elenkov IJ, Wilder RL, Bakalov VK, Link AA, Dimitrov MA, Fisher S, et al. IL-12, TNF-alpha, and hormonal changes during late pregnancy and early postpartum: implications for autoimmune disease activity during these times. J Clin Endocrinol Metab (2001) 86(10):4933–8. doi: 10.1210/jcem.86.10.7905
111. Harrison L, Gleeson D. Stopping immunosuppressive treatment in autoimmune hepatitis (AIH): Is it justified (and in whom and when). Liver Int (2019) 39(4):610–20. doi: 10.1111/liv.14051
112. Czaja AJ. Late relapse of type 1 autoimmune hepatitis after corticosteroid withdrawal. Dig Dis Sci (2010) 55(6):1761–9. doi: 10.1007/s10620-010-1243-0
113. Dunn C, Brunetto M, Reynolds G, Christophides T, Kennedy PT, Lampertico P, et al. Cytokines induced during chronic hepatitis b virus infection promote a pathway for NK cell-mediated liver damage. J Exp Med (2007) 204(3):667–80. doi: 10.1084/jem.20061287
114. Huang M, Gao Y, Yin X, Zhang X, Hao Y, Hu J, et al. Characterization of T cell immunity in chronic hepatitis b virus-infected mothers with postpartum alanine transaminase flare. BMC Infect Dis (2021) 21(1):922. doi: 10.1186/s12879-021-06634-2
115. Song A, Liu Y, Cao Z, Lu J, Ren S, Zheng S, et al. Clinical features and T cell immune characteristics of postpartum hepatitis flare in pregnant women with HBeAg-positive chronic HBV infection. Front Immunol (2022) 13:881321. doi: 10.3389/fimmu.2022.881321
116. Zhang L, Li MH, Cao WH, Qi TL, Lu Y, Wu SL, et al. Negative correlation of serum hepatitis b surface antigen and hepatitis b e antigen levels with the severity of liver inflammation in treatment-naïve patients with chronic hepatitis b virus infection. Chin Med J (Engl) (2017) 130(22):2697–702. doi: 10.4103/0366-6999.218000
117. Giles M, Visvanathan K, Lewin S, Bowden S, Locarnini S, Spelman T, et al. Clinical and virological predictors of hepatic flares in pregnant women with chronic hepatitis b. Gut (2015) 64(11):1810–5. doi: 10.1136/gutjnl-2014-308211
118. Lin HH, Chen PJ, Chen DS, Sung JL, Yang KH, Young YC, et al. Postpartum subsidence of hepatitis b viral replication in HBeAg-positive carrier mothers. J Med Virol (1989) 29(1):1–6. doi: 10.1002/jmv.1890290102
119. Lin HH, Wu WY, Kao JH, Chen DS. Hepatitis b post-partum e antigen clearance in hepatitis b carrier mothers: Correlation with viral characteristics. J Gastroenterol Hepatol (2006) 21(3):605–9. doi: 10.1111/j.1440-1746.2006.04198.x
120. Luo Y, Pan M, Ning M, Chenyu X, Liu L, Chen L, et al. High mutation prevalence of precore and basal core promoter in pregnant women who underwent spontaneous HBeAg seroconversion within one year postpartum. Dig Liver Dis (2020) 52(2):199–204. doi: 10.1016/j.dld.2019.06.021
121. Hu Y, Feng Z, Liu J, Chen J, Zhang S, Zhou YH. Virological determinants of spontaneous postpartum e antigen seroconversion and surface antigen seroclearance in pregnant women infected with hepatitis b virus. Arch Med Res (2016) 47(3):207–13. doi: 10.1016/j.arcmed.2016.06.008
122. Samadi Kochaksaraei G, Shaheen AA, Seow CH, Barkema HW, Coffin CS. Tenofovir disoproxil fumarate therapy to prevent hepatitis b virus vertical transmission-a review of maternal and infant outcomes. Liver Int (2022) 42(8):1712–30. doi: 10.1111/liv.15249
123. Li L, Zou H, Xu M, Li S, Zhu Y, Zheng S, et al. Risk factors related to postpartum hepatic inflammation in pregnant women with chronic hepatitis b. J Int Med Res (2020) 48(11):300060520966439. doi: 10.1177/0300060520966439
124. Liu J, Wang J, Jin D, Qi C, Yan T, Cao F, et al. Hepatic flare after telbivudine withdrawal and efficacy of postpartum antiviral therapy for pregnancies with chronic hepatitis b virus. J Gastroenterol Hepatol (2017) 32(1):177–83. doi: 10.1111/jgh.13436
125. Lu J, Wang X, Zhu Y, Ma L, Zheng S, Hu Z, et al. Clinical and immunological factors associated with postpartum hepatic flares in immune-tolerant pregnant women with hepatitis b virus infection treated with telbivudine. Gut Liver (2021) 15(6):887–94. doi: 10.5009/gnl21001
126. Quan M, Liu XM, Liu C, Li W, Xing HC. Antiviral therapy for prevention of perinatal hepatitis b virus transmission reduces the incidence of postpartum hepatitis flare. BioMed Res Int (2022) 2022:7046955. doi: 10.1155/2022/7046955
127. Wang CR, Zhong GC, Chen ZW, Hu P. A nomogram for predicting non-rebound in HBV-infected pregnant women with mother-to-Child transmission prevention. Front Med (Lausanne) (2021) 8:746759. doi: 10.3389/fmed.2021.746759
128. Ayres A, Yuen L, Jackson KM, Manoharan S, Glass A, Maley M, et al. Short duration of lamivudine for the prevention of hepatitis b virus transmission in pregnancy: lack of potency and selection of resistance mutations. J Viral Hepat (2014) 21(11):809–17. doi: 10.1111/jvh.12212
129. He T, Bai Y, Cai H, Ou X, Liu M, Yi W, et al. Safety and efficacy of lamivudine or telbivudine started in early pregnancy for mothers with active chronic hepatitis b. Hepatol Int (2018) 12(2):118–25. doi: 10.1007/s12072-017-9839-5
130. Yi W, Li MH, Xie Y, Wu J, Hu YH, Zhang D, et al. Prospective cohort study on the efficacy and safety of telbivudine used throughout pregnancy in blocking mother-to-child transmission of hepatitis b virus. J Viral Hepat (2017) 24 Suppl 1:49–56. doi: 10.1111/jvh.12788
131. Yi W, Liu M, Cai HD. Safety of lamivudine treatment for chronic hepatitis b in early pregnancy. World J Gastroenterol (2012) 18(45):6645–50. doi: 10.3748/wjg.v18.i45.6645
132. Liu M, Cai H, Yi W. Safety of telbivudine treatment for chronic hepatitis b for the entire pregnancy. J Viral Hepat (2013) 20 Suppl 1:65–70. doi: 10.1111/jvh.12066
133. Jiang Y, Ma Z, Xin G, Yan H, Li W, Xu H, et al. Th1 and Th2 immune response in chronic hepatitis b patients during a long-term treatment with adefovir dipivoxil. Mediators Inflammation (2010) 2010:143026. doi: 10.1155/2010/143026
134. Wang F, Song M, Hu Y, Yang L, Bi X, Lin Y, et al. The relation of the frequency and functional molecules expression on plasmacytoid dendritic cells to postpartum hepatitis in women with HBeAg-positive chronic hepatitis b virus infection. Front Immunol (2022) 13:1062123. doi: 10.3389/fimmu.2022.1062123
135. Li M, Sun F, Bi X, Lin Y, Yang L, Jiang T, et al. Effects of antiviral therapy and drug withdrawal on postpartum hepatitis in pregnant women with chronic HBV infection. Hepatol Int (2022) 1–10. doi: 10.1007/s12072-022-10412-w
Keywords: pregnant, HBV, immune tolerant, treg, postpartum hepatitis, antiviral therapy (AVT)
Citation: Zhang L, Jiang T, Yang Y, Deng W, Lu H, Wang S, Liu R, Chang M, Wu S, Gao Y, Hao H, Shen G, Xu M, Chen X, Hu L, Yang L, Bi X, Lin Y, Lu Y, Jiang Y, Li M and Xie Y (2023) Postpartum hepatitis and host immunity in pregnant women with chronic HBV infection. Front. Immunol. 13:1112234. doi: 10.3389/fimmu.2022.1112234
Received: 30 November 2022; Accepted: 16 December 2022;
Published: 04 January 2023.
Edited by:
Siqing Fu, University of Texas MD Anderson Cancer Center, United StatesReviewed by:
Xinyue Chen, Beijing Youan Hospital, Capital Medical University, ChinaCopyright © 2023 Zhang, Jiang, Yang, Deng, Lu, Wang, Liu, Chang, Wu, Gao, Hao, Shen, Xu, Chen, Hu, Yang, Bi, Lin, Lu, Jiang, Li and Xie. This is an open-access article distributed under the terms of the Creative Commons Attribution License (CC BY). The use, distribution or reproduction in other forums is permitted, provided the original author(s) and the copyright owner(s) are credited and that the original publication in this journal is cited, in accordance with accepted academic practice. No use, distribution or reproduction is permitted which does not comply with these terms.
*Correspondence: Yao Lu, MTM5MTEwODkxMTZAMTYzLmNvbQ==; Yuyong Jiang, anl1eTExQDEyNi5jb20=; Minghui Li, d3VobTIwMDBAc2luYS5jb20=; Yao Xie, eGlleWFvMDAxMjAxODRAc2luYS5jb20=
†These authors share first authorship
‡ORCID: Minghui Li, orcid.org/0000-0003-3233-5473
Yao Xie, orcid.org/0000-0003-4108-7037
Disclaimer: All claims expressed in this article are solely those of the authors and do not necessarily represent those of their affiliated organizations, or those of the publisher, the editors and the reviewers. Any product that may be evaluated in this article or claim that may be made by its manufacturer is not guaranteed or endorsed by the publisher.
Research integrity at Frontiers
Learn more about the work of our research integrity team to safeguard the quality of each article we publish.