- 1Department of Metabolism, Digestion and Reproduction, Institute of Developmental Reproductive and Developmental Biology, Imperial College London, London, United Kingdom
- 2The Fertility Centre, Chelsea and Westminster Hospital, London, United Kingdom
- 3Department of Cellular Pathology, Imperial College Healthcare NHS Trust, Charing Cross Hospital, London, United Kingdom
A significant proportion of recurrent miscarriage, recurrent implantation failure and infertility are unexplained, and these conditions have been proposed to have an etiology of immunological dysfunction at the maternal-fetal interface. Uterine Natural Killer cells (uNK) comprise three subsets and are the most numerous immune cells found in the uterine mucosa at the time of implantation. They are thought to play an important role in successful pregnancy by regulation of extravillous trophoblast (EVT) invasion and spiral artery remodelling. Here, we examine the frequency, phenotype and function of uNK1-3 from the uterine mucosa of 16 women with unexplained reproductive failure compared to 11 controls with no reproductive problems, during the window of implantation. We report that KIR2DL1/S1 and LILRB1 expression is lower in the reproductive failure group for both uNK (total uNK, uNK 2 and 3) and pNK. We also show that degranulation activity is significantly reduced in total uNK, and that TNF-α production is lower in all uNK subsets in the reproductive failure group. Taken together, our findings suggest that reproductive failure is associated with global reduction in expression of uNK receptors important for interaction with HLA-C and HLA-G on EVT during early pregnancy, leading to reduced uNK activation. This is the first study to examine uNK subsets during the window of implantation in women with reproductive failure and will serve as a platform to focus on particular aspects of phenotype and function of uNK subsets in future studies. Further understanding of uNK dysregulation is important to establish potential diagnostic and therapeutic targets in the population of women with unexplained reproductive failure.
Introduction
Subfertility affects up to 1 in 7 couples and is commonly defined as failure to conceive after regular unprotected sexual intercourse for 12 months (1, 2). Recurrent miscarriage (RM) can be defined as loss of two (3, 4), three (5) or more clinical pregnancies and affects 1-2% of couples trying to conceive. Recurrent implantation failure (RIF) is a term used to describe failure to conceive after several attempts of in vitro fertilization (IVF). Similar to recurrent miscarriage, it has variable definitions which involve failure to achieve pregnancy after two (6) or three (7–9) fresh or frozen cycles with transfer of good quality embryos in women under the age of 40. Although these three conditions represent different clinical entities, a significant proportion of the cases in each group remain unexplained. Indeed, 1 in every 4 cases of subfertility do not have a cause identified after routine investigations (unexplained infertility: UI) (2, 10) and up to 50% cases of recurrent miscarriage (11).
It has been suggested that these conditions may have an immune etiology, resulting from dysfunctional molecular mechanisms involving the immune system at the maternal fetal interface. Indeed, immune dysregulation has been proposed to cause defective implantation and deep placentation resulting in a spectrum of pregnancy disorders such as recurrent miscarriage, pre-eclampsia, intra-uterine growth restriction and preterm labour, collectively termed “Great Obstetrical Syndromes” (12, 13). In line with this, the term “reproductive failure” was coined based on the theory that RM and RIF have a common pathogenic pathway involving a complex interplay of hormonal and immunological factors to ensure optimum endometrial receptivity (14). Here, we use the term “reproductive failure” to refer to women with UI, RM and RIF.
Uterine natural killer (uNK) cells are the most abundant immune cells found in placental bed of first trimester pregnancy, accounting for up to 70% of immune cells (15, 16). It is widely recognized that uNK are important for the development of the placenta by facilitating trophoblast invasion and vascular remodelling (17, 18). However, there is still uncertainty about how NK cells are associated with reproductive failure. Initially, it was thought that uNK, similar to peripheral blood NK cells (pNK), exert cytotoxicity and have the potential to kill invading trophoblasts (19, 20). However, this was quickly shown to be unlikely because uNK are less able to kill classical NK cell targets than pNK and have almost no ability to kill trophoblasts (21).
Immunogenetic studies have revealed interesting discoveries about interactions between uNK receptors KIR, LILRB1 and CD94/NKG2 heterodimer with their ligands HLA-C, HLA-G and HLA-E, respectively, on extravillous trophoblast cells (EVT). The most compelling evidence comes from studies on KIR/HLA-C interaction. KIR expressed by uNK can confer either an activating or inhibitory signal, which in turn is dependent on the genes inherited by each individual. The genetic polymorphism of KIR can be simplified by considering two main KIR haplotypes, KIR A and KIR B. The genetic polymorphism of KIR can be simplified by considering two main KIR haplotypes, KIR A and KIR B. KIR A is comprised of mostly inhibitory genes such as KIR2DL1 and KIR2DL3. KIR B contains the inhibitory genes KIR2DL1 and KIR2DL2, but in addition contains activating genes such as KIR2DS1 and KIR2DS2. The ligand for 2-domain KIR are HLA-C molecules, which are expressed by all EVT and carry either a C1 or C2 epitope. The combination of maternal KIR and paternally derived HLA-C in fetus can be widely diverse, resulting in net activation or inhibition of NK cells (22). In a study of women with RM and RIF undergoing assisted reproduction using their own or donor oocytes, women with a KIR AA genotype had lower livebirth rates compared to those with KIR AB or KIR BB genotypes, suggesting that reduced uNK activation is associated with negative reproductive outcomes (23). The livebirth rate was also decreased as fetal HLA-C2 load is increased which has implications for future clinical utility in selection of fetus with HLA-C that is “compatible” with maternal KIR. (23). However, this is still considered to be at the pre-clinical stage owing to several limitations of present evidence and practical considerations in the clinical setting (22).
On the other hand, it has been proposed that increased uNK number or cytokine production could cause excessive premature angiogenesis which in turn cause harmful oxidative stress to the feto-placental unit (24). Research on uNK cytokine production has been inconclusive, with conflicting reports on which cytokine is excessive or deficient in reproductive failure (25). Potential reasons for this are the use of different assays to measure cytokine level, addition of other cytokines (such as IL-2 or IL-15) into culture to recover immune cells which alters their cytokine production profile, and use of decidual samples collected after miscarriage potentially introducing the confounding factor of inflammatory processes that occur after a miscarriage (13).
Since the landmark discovery of three uNK subsets by single-cell RNA sequencing (scRNAseq) (26), it has become apparent that these subsets exhibit different phenotypes and function and thus are likely to have different roles in pregnancy (18). A better characterization of the three uNK subsets was identified to be essential to the field (18). We have recently characterized these uNK subsets throughout the normal human reproductive cycle (27) and have shown that all three subsets upregulate KIR and LILRB1 expression during first trimester. uNK also have the ability to increase cytokine production during the mid-secretory phase of the menstrual cycle, which aligns with period of embryo implantation. Further scRNAseq data have demonstrated that uNK1 frequency is lower and uNK3 higher in women with RM compared to pregnant controls (28, 29). However, these studies were performed in decidua collected after miscarriage which may have been affected by inflammatory processes thus not reflecting the immune environment before the miscarriage. Therefore, the question remains, how do uNK subsets behave in the endometrium of women with reproductive failure? Is one subset more affected than the others? Elucidating the answer to this may help us to focus research on a particular uNK subset to understand the pathophysiology behind reproductive failure. Therefore, we aim to evaluate differences in receptor expression, activation/cytokine production and proportion of matched pNK and uNK subsets in women with reproductive failure compared to controls and in women with ongoing compared to no ongoing pregnancy after in vitro fertilization (IVF).
Materials and methods
Primary tissue
Collection of human tissue was approved by Solihull Research Ethics Committee (study number: 22/WM/0041). Inclusion criteria for patient groups were as follows: Infertility defined as women below 40 years old with failure to conceive after regular unprotected intercourse for more than one year, RM defined as loss of two or more clinical pregnancies and RIF defined as absence of pregnancy after two or more fresh or frozen transfer of good quality embryos. Exclusion criteria were any other causes identified for the reproductive failure after routine investigations including uterine, tubal, ovarian, male factor, endocrinological, parental chromosomal abnormalities and haematological (e.g. thrombophilia and antiphospholipid syndrome) factors. Inclusion criteria for the control group were women below 40 years old undergoing coil insertion for contraception with a regular menstrual cycle between 25 to 35 days and no history of reproductive problems. Exclusion criterion was usage of any coil or hormonal contraception within one month prior to sampling. The research participants’ characteristics are summarised in Table 1.
Matched peripheral blood and endometrial samples were obtained for 16 reproductive failure patients (9 UI, 4 RM, 3 RIF) and 11 controls during mid-luteal phase. Endometrial biopsies were obtained by Pipelle sampler, immediately suspended in RPMI and processed within 2 hours. For the control group, endometrial samples were obtained before coil insertion for contraception between day 18-23 and mid-luteal phase was confirmed by histological dating and serum progesterone level. For the patient group, samples were obtained seven to nine days after ovulation as determined by serial urine luteinizing hormone (LH) tests.
For extraction of peripheral blood lymphocytes (PBL), whole blood was layered onto Histopaque (Sigma Aldrich), centrifuged (700 xg 21°C, 20 minutes) and the enriched PBL were washed twice (500 xg, 10 minutes, 4°C) with Dulbecco’s Phosphate-Buffered Saline (Life Tech). For endometrial lymphocytes extraction, endometrial tissue was pressed through 100 µm cell strainer, pelleted (700 xg, 10 minutes, 4°C), resuspended in Dulbecco’s Phosphate-Buffered Saline supplemented by 10% Fetal Calf Serum (Sigma Aldrich), passed through 70µm strainer, and layered on Histopaque to isolate immune cells as above. 0.2 to 1 x106 endometrial lymphocytes and 1 x106 PBL were allocated per condition of either fresh stain or culture.
Short-term culture for measurement of cytokine production
Both PBL and endometrial lymphocytes were suspended in RPMI supplemented with antibiotics, non-essential amino acids 10% fetal calf serum, 1 µM sodium pyruvate, 2.5 mM HEPES and 50 µM β-mercaptoethanol (all from Gibco) and divided into unstimulated and stimulated wells. Anti-CD107a BV605 (100 µl/ml; clone H4A3, Biolegend), Brefeldin (10µg/ml) and Monensin (2µM/ml) were added to all wells and Phorbol 12-myristate 13-acetate (PMA) (50 ng/ml) and ionomycin (1µg/ml) into the stimulated wells only. Cells were incubated for 4 hours at 37°C then harvested and stained.
Flow cytometry
The following anti-human antibodies were used for surface staining: Anti-CD56 Brilliant Violet (BV) 650 (clone NCAM 16.2, BD Bioscience), anti-CD39 BV421 (clone A1, Biolegend), anti-CD3 BV711 (clone SK7, Biolegend), anti-CD103 BV785 (clone Ber-ACT8, Biolegend), anti-CD16 Alexa Fluor(AF)700 (clone 3G8, Biolegend), anti-CD9 phycoerythrin(PE)/Dazzle 594 (clone HI9a, Biolegend), anti-CD49a PE/Cy7 (clone TS2/7, Biolegend), anti-CD45 allophycocyanin (APC) (clone HI30, Biolegend), anti-CD94 PE (Clone HP-3D9, BD Bioscience), anti-CD94 PECy7 (Clone DX22, Biolegend), anti-CD158a/h (KIR2DL1/DS1) VioBright 515 (clone REA1010, Miltenyi Biotec), anti-CD158a (KIR2DL1) PE (clone HP-DM1, Biolegend) anti-CD158b (KIR2DL2/DL3) APC vio 770 (clone REA 1006, Miltenyi Biotec), CD85j (ILT2 or LILRB1) Peridinin chlorophyll protein (PerCP)-eFluor 710 (clone HP-F1, Thermo Fisher Scientific). The following anti-human antibodies were used for intracellular cytokine staining: anti-IL-8 PE (clone G265-8, BD Bioscience), anti-IFN-γ APCvio770 (clone REA600, Miltenyi Biotec), anti GM-CSF PERCP/Cyanine 5.5 (clone BVD2-21C11, Biolegend), anti-TNFα FITC (clone MAb11, Biolegend).
Cells were incubated with fixable viability dye (Live/Dead Fixable Aqua Dead Cell stain kit, LifeTech) and surface antibodies (15 minutes, 4°C). For intracellular staining, human FoxP3 buffer (BD Biosciences) was used according to manufacturer’s instructions for fixation and permeabilization before staining with intracellular antibodies (30 minutes, 4°C). Excess antibodies were washed off (5 minutes, 500 xg, 4°C) between each incubation and twice after the final incubation with intracellular antibodies.
Statistical analysis
Data were acquired on an BD Fortessa and analysed using FlowJo (Tree Star, Ashland, OR). In order to ensure reproducibility of results, CS&T and application settings were used. Statistical analysis was performed using PRISM (GraphPad Software Inc.). Data were assessed for normality using Shapiro-Wilk tests to determine whether a parametric or a non-parametric statistical test was appropriate. The appropriate statistical test was used to compare patient and control groups as specified in figure legends. The Holm-Bonferroni correction for multiple hypothesis testing was applied to reduce family-wise error rate and both uncorrected (p) and corrected (p’) values are given in figures and legends. Fisher’s exact test was used to test for significant differences in proportions of patients and controls expressing KIR2DS1.
Results
No difference in uNK or pNK frequencies in women with reproductive failure compared to controls
In women with recurrent miscarriage, assessment by scRNAseq on decidual samples after miscarriage found that uNK1 level is lower but uNK3 level is higher compared to controls, although these findings may represent inflammatory changes subsequent to fetal demise (28, 29). Therefore, we evaluated proportion of uNK and its subsets from mid-luteal phase endometrial samples as total uNK, uNK1, 2 and 3 as proportion of CD45+ lymphocytes and the latter three as proportion of total uNK. NK cells were identified as live CD3-CD56+ lymphocytes, and CD49a was used to distinguish uterine from circulating NK cells. The uNK subsets were subsequently identified by CD103 and CD39 expression (26) (Figure 1A). pNK were identified by conventional gating strategy; CD3-CD56+ cells with gating by CD56 expression level and on CD16 to distinguish CD56bright and CD56dim.
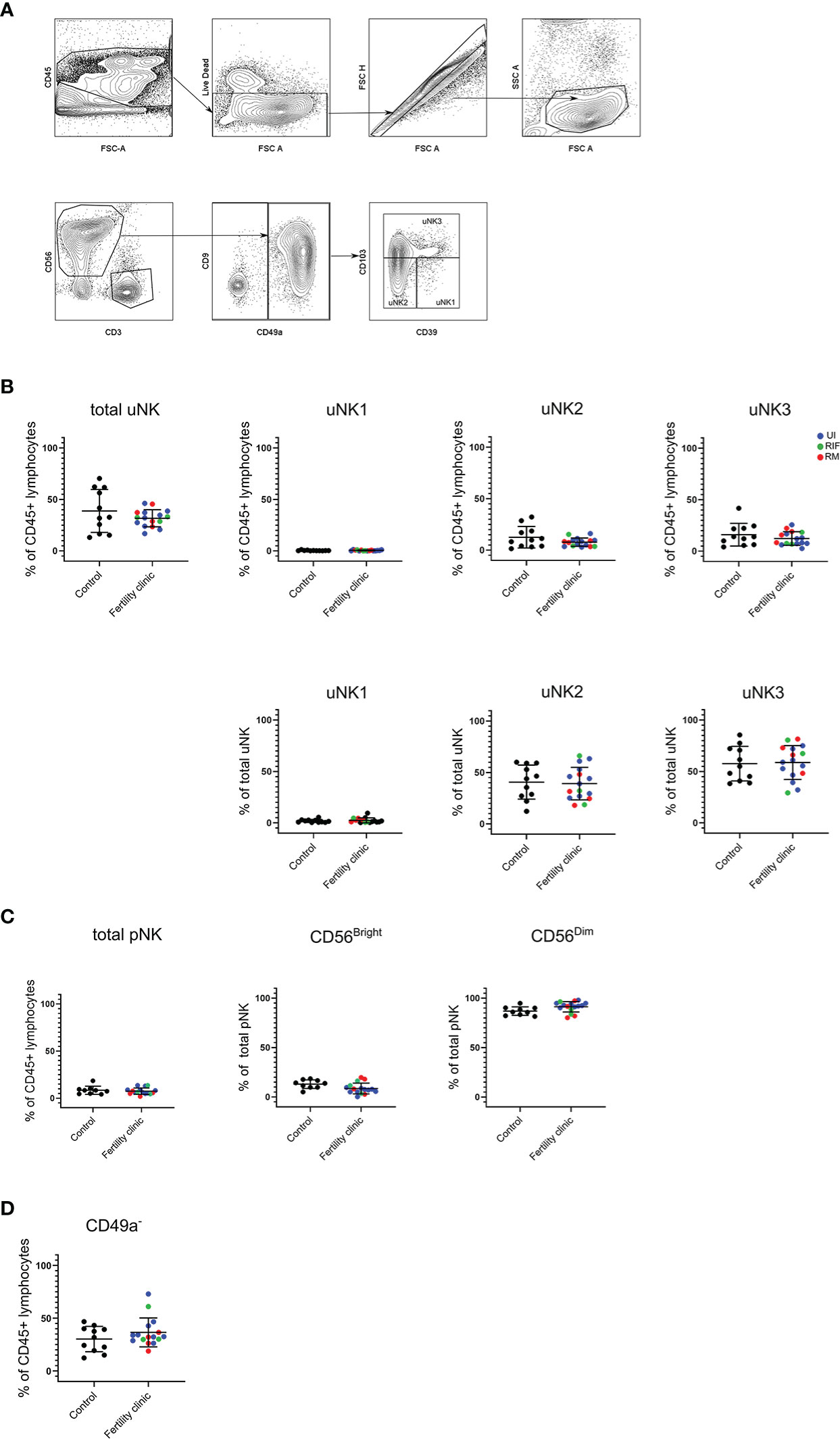
Figure 1 uNK and pNK proportion in unexplained subfertility, recurrent miscarriage and implantation failure compared to controls (A) FACs gating strategy used to identify three uNK subsets (representative example shown) (B) Graphs of total uNK from CD45+ lymphocytes then frequency of each uNK subset (uNK1, -2, -3) as proportion of CD45+ lymphocytes and proportion of total uNK. (C) Graphs of total pNK from CD45+ lymphocytes then frequency of CD56Bright and CD56Dim as proportion of total pNK. (D) Graph of CD3-CD56+CD49a- lymphocytes as proportion of total uNK. Means and standard deviations are shown for controls (n=11) shown in black and patients from fertility clinic including unexplained infertility (UI; n=9) showed in blue, recurrent miscarriage (RM; n=34) shown in red and recurrent implantation failure (RIF; n=3) shown in green. Statistical testing was done using unpaired t-test for normal or Mann-Whitney U test for non-normal distribution and showed no significant difference in any of the comparisons.
In keeping with our previous finding at this stage in the menstrual cycle (27) uNK1 frequency was lower than that of uNK 2 and 3 in the endometrium in both control and UI/RM/RIF groups (Figure 1B). However, there was no significant difference in frequency of total uNK or uNK subsets in reproductive failure compared to control group (Figure 1B). Similarly, there was no significant difference in pNK (Figure 1C). Additionally, no significant difference was detected even when each subgroup of reproductive failure was considered separately (data not shown).
In our previous meta-analysis, it was found that CD16+ cells, which are likely to represent CD56dim pNK, are more frequency in the endometrium of women with recurrent miscarriage compared to controls (25). Therefore, we examined the frequency of CD3-CD56+CD49a- NK cells in the endometrium, since these more accurately represent pNK. Overall, no significant difference was detected in reproductive failure compared to control group (Figure 1D).
KIR2DL1/S1 and LILRB1 expression are lower in women with reproductive failure
KIR, LILRB1 and CD94/NKG2 heterodimer are uNK receptors that interact with HLA-C, HLA-G and HLA-E on EVT respectively (30). These receptors are present in all uNK subsets throughout the menstrual cycle and in all trimesters of pregnancy, albeit at different frequencies. Previously, we have shown that KIR2DL1/S1 and LILRB1 were upregulated in all three uNK subsets during first trimester, suggesting that these receptors are important for cross-talk with EVT at the time of placentation (27).
Here, we assessed KIR2DL1/S1, KIR2DL2/S2/L3, LILRB1 and CD94 expression on total uNK, uNK1, uNK2, uNK3 from mid-luteal phase endometrial samples and CD56bright and CD56dim pNK from matched peripheral blood samples in women with reproductive failure (RM, RIF or UI) compared to controls attending the clinic for contraceptive coil fitting. Representative histograms of staining from each receptor are shown in Figure 2A.
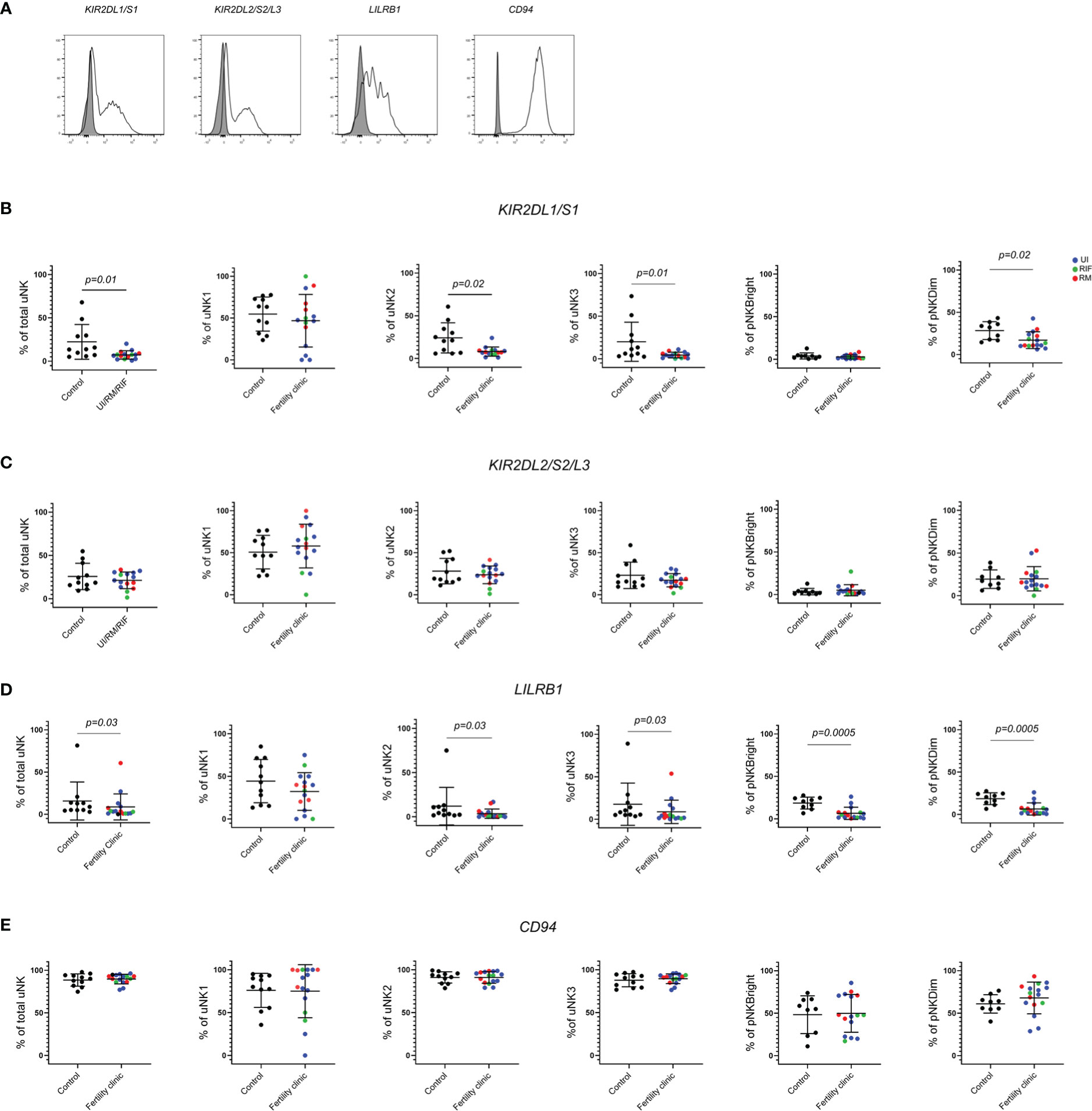
Figure 2 KIR2DL1/S1 and LILRB1 expression is lower in women with reproductive failure. (A) Uterine and peripheral NK cells taken from control and UI, RM and RIF groups were freshly stained for phenotypic markers. Representative staining of two samples from total uNK are shown in clear alongside fluorescence minus one control in grey. Graphs showing frequencies of KIR2DL1/S1 (B), KIR2DL2/3 (C), LILRB1 (D) and CD94 (E) on uNK and pNK. Means and standard deviations are shown for controls (n=11) shown in black and patients from fertility clinic including unexplained infertility (UI; n=9) showed in blue, recurrent miscarriage (RM; n=4) shown in red and recurrent implantation failure (RIF; n=3) shown in green. Statistical testing was done using unpaired t-test for normal or Mann-Whitney U test for non-normal distribution and p-values are shown on the graphs. Holm-Bonferroni correction for multiple hypothesis testing showed no significance except for LILRB1 on pNKBright and pNK Dim which remained significant at p’=0.003.
KIR2DL1/S1 expression was significantly lower in total uNK in the reproductive failure group, with further investigation of expression in subsets revealing lower expression in all three uNK subsets, although this only reached statistical significance for uNK2 and 3 (Figure 2B) In peripheral blood, KIR2DL1/S1 was also observed to be significantly lower in the reproductive failure group among CD56dim but not CD56bright cells (Figure 2B). Immunogenetic studies have found that maternal KIR haplotypes lacking KIR2DS1 are associated with failures of early pregnancy (31, 32), so one possibility is that this finding reflects a reduction in the proportion of individuals who have the KIR2DS1 gene in the patient group, compared to controls. To investigate this, we accessed cryopreserved PBL from the patient group to determine if the patient expresses KIR2DS1 or not (Supplementary Figure 4 and Supplementary Table 1). 56% (9/16) of the patient group expressed any KIR2DS1, similar to the 47% (33) or 44% (31) KIR2DS1 positive previously reported in UK cohorts. Cryopreserved PBL were not available from the original control group, but in recognition of the fact that the population at our hospital in London may differ from that elsewhere in the UK, we compared the frequency of KIR2DS1 positivity in our patient cohort with a group of women with no history of reproductive problems recruited from our hospital: these were 65% (13/20) KIR2DS1 positive. Therefore a lower proportion of patients was positive for KIR2DS1 than controls, although this finding was not significant (p=0.73).
LILRB1 was also significantly lower in total uNK and all three uNK subsets in the reproductive failure group, although this only reached statistical significance for uNK2 and 3 (Figure 2D). In the blood, LILRB1 expression was lower in for the reproductive failure group for both CD56dim and CD56bright pNK (Figure 2D). However, no trend was noted for KIR2DL2/S2/L3 (Figure 2C) or CD94 (Figure 2E) in either uNK or pNK.
uNK are less activated in women with reproductive failure
To examine the ability of uNK to degranulate and produce cytokines, they were cultured for 4 hours in the presence of the protein transport inhibitors Brefeldin and Monensin. The ability of cells to respond directly ex vivo was determined by culturing them without stimulation, whereas their potential to respond was determined by adding PMA/I as a strong non-specific stimulus. Degranulation, as measured by the internalisation of anti-CD107a is a good measure of uNK activation (34, 35) and using this measurement we have previously found that uNK are most active at the time of implantation during secretory phase and first trimester (27). CD107a trended upwards in secretory phase and was significantly higher in first trimester compared to third trimester in uNK2 and 3 after stimulation. We also found that TNFα, IFNγ and IL-8 production across all uNK subsets is highest after stimulation with PMA and ionomycin during secretory phase (27).
Here, we replicated the assessment for functional responses with and without stimulation on uNK and pNK from women with reproductive failure compared to controls (Figure 3). Strikingly, we observed significantly lower degranulation by total uNK in women with reproductive failure, compared to controls, without stimulation (Figure 3B). When uNK subsets were considered separately, the reduction was most pronounced on uNK3 although this did not reach statistical significance. However, no discernible difference was seen for degranulation by total uNK or uNK subsets with stimulation (Figure 3C). A paired comparison of CD107a expression with and without stimulation in controls and patients revealed that CD107a was generally increased with stimulation, as expected, but it was significantly reduced in uNK1 of fertility patients only (Supplementary Figure 3).
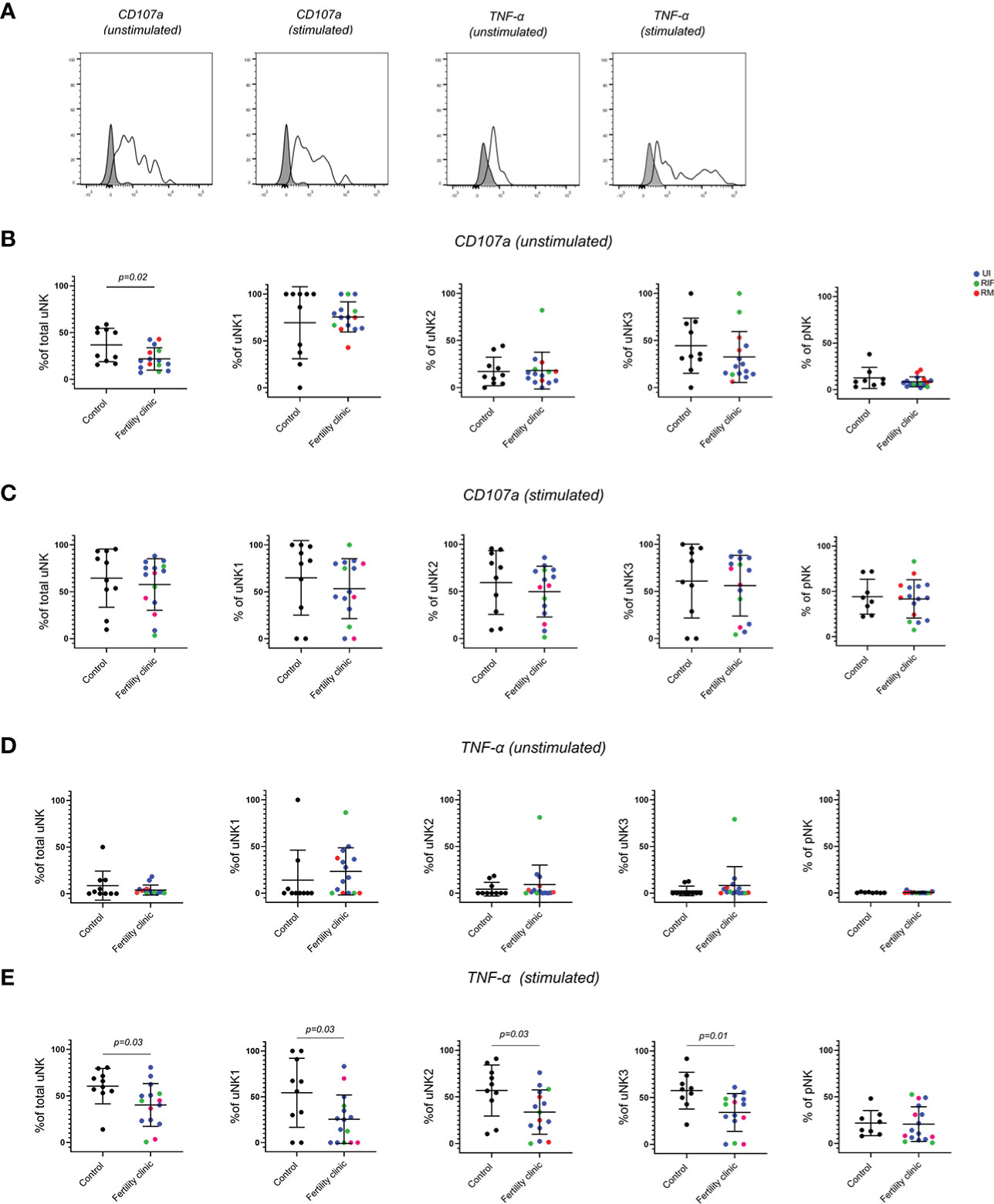
Figure 3 uNK are less active in women with reproductive failure. (A) Uterine and peripheral NK cells taken from control and UI, RM and RIF groups were cultured with or without PMA and ionomycin stimulation. Representative staining of one sample from total uNK is shown in clear alongside fluorescence minus one control in grey. Graphs showing frequencies of CD107a (B, C) and TNF-α (D, E) on unstimulated and stimulated uNK and pNK. Means and standard deviations are shown for controls (n=11) shown in black and patients from fertility clinic including unexplained infertility (UI; n=9) showed in blue, recurrent miscarriage (RM; n=3) shown in red and recurrent implantation failure (RIF; n=3) shown in green. Statistical testing was done using unpaired t-test for normal or Mann-Whitney U test for non-normal distribution and p-values are shown on the graphs. Holm-Bonferroni correction for multiple hypothesis testing showed no significance for all the comparisons.
Among all the cytokines (TNFα, IFNγ, IL-8 and GM-CSF) that were assessed, TNFα emerged as the only one that was significantly lower in all three uNK subsets in the reproductive failure group with stimulation (Figures 3D, E) Notably, none of the functional changes in uNK were mirrored by pNK. (Figure 3 and Supplementary Figure 1)
No difference in phenotype or function of uNK in women with ongoing versus no ongoing pregnancy after IVF
Next, we prospectively followed up women from the reproductive failure group to obtain data on their pregnancy outcome after IVF. We compared women who had an ongoing pregnancy (OP) with women with no ongoing pregnancy (NOP). The latter group consisted of women who did not fall pregnant as well as women who fell pregnant but miscarried. Due to the small numbers from each subgroup, they were pooled into the NOP group. The same phenotypic and functional parameters as above were assessed.
No significant difference was seen in any of the KIR (Figures 4A, B), LILRB1 (Figure 4C) or CD94 (Figure 4D) expression in either uNK or pNK. However, a trend of lower KIR expression was seen in total uNK and uNK subsets for the NOP group. On removal of the two datapoints of women who fell pregnant but miscarried from the NOP group, KIR2DL2/S2/L3 was significantly lower specifically in the uNK3 subgroup (data not shown). No other significant difference emerged from similar sensitivity analysis on the other receptors. In terms of functional activity, no significant difference was observed in CD107a (Figures 4E, F), TNF-α (Figures 4G, H), or any of the cytokines examined (Supplementary Figure 2).
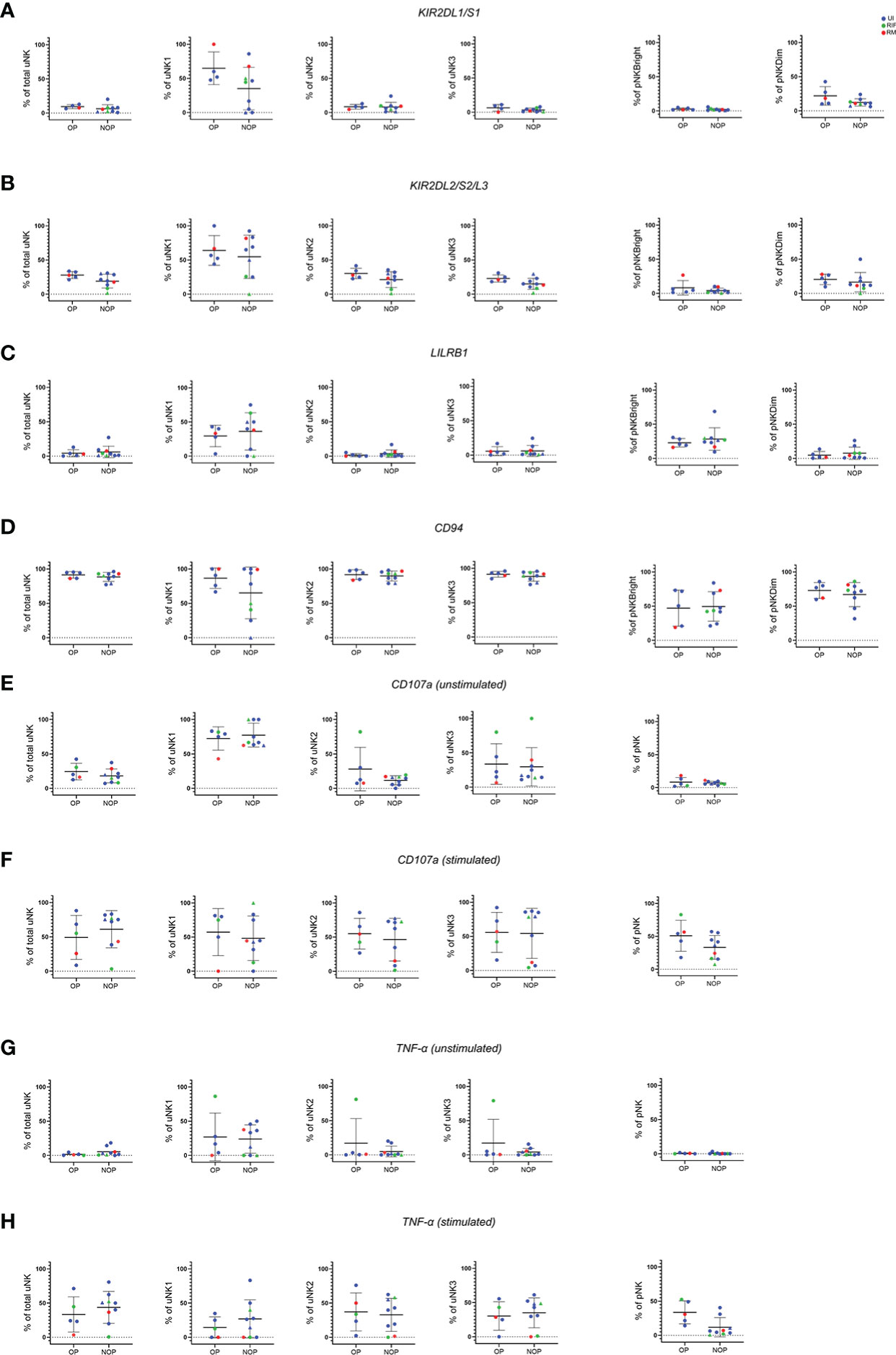
Figure 4 No difference in phenotype or degranulation in patients who had ongoing pregnancy compared to no ongoing pregnancy after IVF. Means and standard deviations are shown for KIR2DL1/S1 (A), KIR2DL2/S2/L3 (B), LILRB1 (C), CD94 (D), CD107a (E, F), and TNF-α (G, H) with and without stimulation. NOP group consist of n=7 who did not become (represented as dots) and n=2 who became pregnant but miscarried (represented as triangles). Unexplained infertility (UI) is showed in blue, recurrent miscarriage (RM) is shown in red and recurrent implantation failure (RIF) is shown in green. Statistical testing was done using unpaired t-test for normal or Mann-Whitney U test for non-normal distribution and showed no significance in all of the comparisons.
Discussion
In this study, we have examined the frequency, phenotype and function of pNK and uNK subsets from timed endometrial samples obtained from mid-luteal phase in women with reproductive failure compared to controls. In contrast to previous studies of endometrial samples from UI, RM or RIF patients, which analyzed total CD3-CD56+ cells, here we also present data on the three uNK subsets individually.
Our previous systematic review showed significantly raised uNK in women with RM and RIF when considering total CD56+ NK cells in the endometrium during mid-luteal phase, but not in decidua collected after miscarriage (25). In women with RM, two recent studies using scRNAseq and mass cytometry demonstrated reduced uNK1 and raised uNK3 level in RM group (28, 29), albeit the studies compared termination samples to those collected after miscarriage, so the results could be confounded by inflammatory changes occurring subsequent to fetal demise (13). In comparison, we did not observe any difference in the frequency of either CD56+ uNK or uNK subsets. One possibility could be that our cohort included patients with a mixture of types of reproductive failure, although each subgroup did not show any difference when considered separately. Another possibility is that this small study was not sufficiently powered to detect a difference, although no emerging trend was apparent from our results. A third possibility is that the comparison is not perfect because the timing of samples for control group was done by serum progesterone and histological dating instead of urine LH tracking, which increases the variance in the control group, thus reducing the power to detect differences. However, it should also not be discounted that the lack of difference is partially due to significantly lower level of uNK1 in the endometrium compared to decidua in first trimester, therefore no inherent difference in the frequency of endometrial NK cells may be present (27).
We found significantly lower expression of KIR2DL1/S1 in uNK subsets in the reproductive failure group compared to controls, and these findings were mirrored in pNK. Our finding in pNK is consistent with previous work which has reported a reduction in the expression of KIR2DL1 expression by pNK associated with reproductive failure (36–39), although we did not find a reduction in KIR2DL2 expression, which has also been reported (36). Studies on uNK KIR expression have thus far included samples retrieved after termination of pregnancy stratified to high and low risk of resistance index on uterine artery doppler (40) or post-surgical management of miscarriage (41), which reported reduction in KIR2DL1/S1 but no change in KIR2DL2/L3/S2 associated with worse pregnancy outcomes. Therefore our findings that uNK and pNK from UI, RM and RIF patients express lower levels of KIR2DL1/S1 are in line with previous work looking at other disorders of pregnancy.
Our finding that KIRD2L1/S1 expression was reduced on both uNK and pNK suggests that the deficiency in KIR expression is not tissue-specific. Early immunogenetic approaches demonstrated that women with KIR AA genotype in combination with fetus carrying HLA-C2 epitope are at higher risk of disorders of placentation associated with pre-eclampsia, and identified KIR2DS1, specifically, as protective (33, 42). Similarly, in IVF patients maternal KIR AA genotype is associated with higher miscarriage rate compared to KIR AB and BB, and this risk is augmented by presence of HLA-C2 on fetus (23, 32). Furthermore, in vitro studies found that stimulation of the activating KIR2DS1 and KIR2DS4 on uNK release GM-CSF that promoted trophoblast invasion (34, 35), although there is some evidence that this production of GM-CSF is not occurring in response to KIR2DS1 ligation by EVT (43).
A key question is whether the underexpression of KIRD2L1/S1 we observed is due to individuals who lack the KIR2DS1 gene being overrepresented among patients compared to controls: our finding that the proportion of patients who do not express KIR2DS1 is similar to that in the general population suggests that this is not the case but recruitment of larger cohorts is needed to confirm this. Studies in larger cohorts should also examine the expression of KIR2DL1 and -S1 separately, to determine which of these KIRs, specifically, is reduced in reproductive failure. It is also important to consider factors other than simple presence of absence of genes which could impact KIR expression. These could include NK developmental processes that are influenced by genetic factors outside the KIR locus, such as the influence of HLA on NK cell education (44) or HCMV seropositivity, which is known to increase KIR expression on peripheral NK cells (45). HCMV seropositivity could also be relevant because uNK can respond to HCMV-infected stromal and trophoblast cells in a manner that is at least partially mediated by KIR2DS1 (46), highlighting a potential for KIR2DS1 expression to protect against HCMV-mediated early pregnancy failures. A limitation of our study was that HCMV serostatus for the donors was not available.
We also observed reduced LILRB1 expression in both uNK and pNK from reproductive failure patients. Although LILRB1 ligation inhibits pNK, recent research has found that ligation of LILRB1on uNK by HLA-G promotes growth factor secretion by uNK which in turn promotes development of the fetus through the placenta (47). A subset of uNK which express NKG2C and LILRB1 has been reported in multiparous women (48). It has been suggested that these cells could account for the greater likelihood of success in second and subsequent pregnancies (49) and if so, this would be consistent with a role for LILRB1 in optimizing placental development. For women with reproductive failure, studies on LILRB1 have mostly concentrated on pNK in RM patients (39, 50) except for one study on uNK using termination samples stratified by uterine artery resistance (51). On balance, and in line with our findings, these studies reported reduced expression of LILRB1 in women with high risk of reproductive problems. KIR2DL4 has also been proposed to recognize HLA-G although this line of evidence is controversial (13). Future studies are warranted to investigate this relationship in view of the lower expression of LILRB1 in our cohort of reproductive failure patients.
We found significantly lower degranulation by total uNK in the reproductive failure group, although no one subset accounted for this reduction, and this finding lends weight to the hypothesis that underactivation of uNK is associated with reproductive failure. Unexpectedly, degranulation was decreased by stimulation in uNK1 of fertility patients: uNK2, uNK3 and pNK in fertility patients, as well as all NK subsets in controls, increased degranulation in response to stimulation, as expected. This observation could suggest that reduced ability of uNK1 to be activated despite stimulation contributes to the pathophysiology of reproductive failure. Notably, reduced activation was specific to uNK as there was no evidence of reduced degranulation in pNK from reproductive failure patients. This is a clinically important finding and complements our previous report that pNK and uNK numbers were not correlated (27), challenging the growing tendency among IVF clinics to test pNK numbers and function.
Similarly, the only cytokine that was altered between controls and reproductive failure patients was TNFα, whose production after stimulation was reduced specifically in all uNK subsets, but not in pNK, from reproductive failure patients. This finding was in line with an earlier report that uNK from mid-luteal biopsies display reduced production of TNFα in women with RM Fukui et al. (52). On the other hand, there are reports that RM is associated with increased uNK production of TNFα and IFN-γ, although a major limitation is that all of these studies were performed on decidua collected after miscarriage hence associated with the confounding factor of inflammatory processes occurring after fetal demise (53–57). There is some evidence that TNFα inhibits EVT invasion by mechanisms such as trophoblast apoptosis, inhibition of proliferation and reduced matrix metalloprotease production (58, 59). However, more recently it has been suggested that implantation is a pro-inflammatory process, in which case TNFα production might be expected to be beneficial (60). In support of this theory, our previous results demonstrated that TNFα and IL-8 production by uNK peak in secretory phase, potentially indicating a role for these cytokines during the window of implantation (27). Here, we have shown deficiencies of uNK activation and TNFα production during mid-luteal phase in the reproductive failure group which supports the hypothesis that pro-inflammatory cytokines are important during the implantation window.
Taken together, our findings suggest global reduction of NK cell receptors important for cross-talk with HLA molecules on EVT leads to reduced activation specifically in uNK, which in turn impairs implantation and placental development, although some other studies have suggested that RM is associated with excess of NK cell activation (61–63). However, our finding that expression of KIR and LILRB1, and uNK degranulation, is not predictive of successful pregnancy outcome sounds a note of caution about this interpretation, since it could suggest that the phenotypic and functional differences we observe are an effect, rather than a cause, of repeated failures of implantation. The insight gained from our findings will aid the direction of future research on uNK subsets in women with reproductive problems. Further larger cohorts should be recruited for studies on phenotype and function of uNK subsets, with a focus on KIR genotype and phenotype as well as uNK activation.
Data availability statement
The original contributions presented in the study are included in the article/Supplementary Material. Further inquiries can be directed to the corresponding author. Raw data and analysis files have been deposited at the Open Science Framework, doi: 10.17605/OSF.IO/Y2PCD.
Ethics statement
The studies involving human participants were reviewed and approved by Solihull Research Ethics Committee. The patients/participants provided their written informed consent to participate in this study.
Author contributions
EW, MJ and VM designed the study. EW, DN, KM and JN-T participated in recruitment of patients and collection of clinical samples. PB performed histological dating. EW and AC carried out laboratory experiments. EW and VM analysed results and wrote the manuscript. All authors contributed to the article and approved the submitted version.
Funding
This study was funded by the preterm birth charity Borne (grant number: P84654).
Acknowledgments
We would like to thank all the people from Fertility Centre, Chelsea and Westminster Hospital and John Hunter Clinic (London, UK) who contributed samples to this study, as well as the medical, nursing and embryology staff who helped in the collection of samples. We would also like to thank Emily Whettlock for her contribution in optimizing the original flow cytometry panel.
Conflict of interest
The authors declare that the research was conducted in the absence of any commercial or financial relationships that could be construed as a potential conflict of interest.
Publisher’s note
All claims expressed in this article are solely those of the authors and do not necessarily represent those of their affiliated organizations, or those of the publisher, the editors and the reviewers. Any product that may be evaluated in this article, or claim that may be made by its manufacturer, is not guaranteed or endorsed by the publisher.
Supplementary material
The Supplementary Material for this article can be found online at: https://www.frontiersin.org/articles/10.3389/fimmu.2022.1108163/full#supplementary-material
References
1. World Health Organization. ICD-11: International classification of diseases (11th revision) (2019). Available at: https://icd.who.int/.
2. Carson SA, Kallen AN. Diagnosis and management of infertility: A review. JAMA (2021) 326(1):65–76. doi: 10.1001/jama.2021.4788
3. Practice Committee of the American Society for Reproductive Medicine, 2012. Evaluation and treatment of recurrent pregnancy loss: A committee opinion. Fertil Steril (2012) 98(5):1103–11. doi: 10.1016/j.fertnstert.2012.06.048
4. Bender Atik R, Christiansen OB, Elson J, Kolte AM, Lewis S, Middeldorp S, et al. ESHRE guideline: recurrent pregnancy loss. Hum Reprod Open (2018) 2018(2). doi: 10.1093/HROPEN/HOY004
5. RCOG. Investigation and treatment of couples with recurrent miscarriage (Green-top guideline no. 17). London: RCOG (2011).
6. Polanski LT, Baumgarten MN, Quenby S, Brosens J, Campbell BK, Raine-Fenning NJ. What exactly do we mean by `recurrent implantation failure’? a systematic review and opinion. Reprod Biomed Online (2014) 28(4):409–23. doi: 10.1016/j.rbmo.2013.12.006
7. Margalioth EJ, Ben-Chetrit A, Gal M, Eldar-Geva T. Investigation and treatment of repeated implantation failure following IVF-ET. Hum Reprod (2006) 21(12):3036–43. doi: 10.1093/humrep/del305
8. Coughlan C, Ledger W, Wang Q, Liu F, Demirol A, Gurgan T, et al. Recurrent implantation failure: Definition and management. Reprod BioMed Online (2014) 28(1):14–38. doi: 10.1016/j.rbmo.2013.08.011
9. Mascarenhas M, Jeve Y, Polanski L, Sharpe A, Yasmin E, Bhandari HM. Management of recurrent implantation failure: British fertility society policy and practice guideline. Hum Fertil (2021) 25(5):813–37. doi: 10.1080/14647273.2021.1905886
10. National Institute for Health and Care Excellence (NICE). Fertility problems: assessment and treatment. (2013).
11. Rai R, Regan L. Recurrent miscarriage. Lancet (2006) 368(9535):601–11. doi: 10.1016/S0140-6736(06)69204-0
12. Brosens I, Pijnenborg R, Vercruysse L, Romero R. The “great obstetrical syndromes” are associated with disorders of deep placentation. Am J Obstet Gynecol (2011) 204(3):193–201. doi: 10.1016/j.ajog.2010.08.009
13. Moffett A, Shreeve N. ‘Local immune recognition of trophoblast in early human pregnancy: controversies and questions.’ nature reviews. Immunology (2022) pp:1–14. doi: 10.1038/s41577-022-00777-2
14. Makrigiannakis A, Petsas G, Toth B, Relakis K, Jeschke U. Recent advances in understanding immunology of reproductive failure. J Reprod Immunol (2011) 90(1):96–104. doi: 10.1016/j.jri.2011.03.006
15. Bulmer JN, Morrison L, Longfellow M, Ritson A, Pace D. Granulated lymphocytes in human endometrium: Histochemical and immunohistochemical studies. Hum Reprod (1991) 6(6):791–8. doi: 10.1093/oxfordjournals.humrep.a137430
16. King A, Balendran N, Wooding P, Loke YW. Cd3– leukocytes present in the human uterus during early placentation: Phenotypic and morphologic characterization of the Cd56++ population. Dev Immunol (1991) 1(3):169–90. doi: 10.1155/1991/83493
17. Moffett-King A. Natural killer cells and pregnancy. Nat Rev Immunol (2002) 2(9):656–63. doi: 10.1038/nri886
18. Huhn O, Zhao X, Esposito L, Moffett A, Colucci F, Sharkey AM. How do uterine natural killer and innate lymphoid cells contribute to successful pregnancy? Front Immunol (2021) 12:607669. doi: 10.3389/FIMMU.2021.607669
19. Chao KH, Yang YS, Ho HN, Chen SU, Chen HF, Dai HJ, et al. Decidual natural killer cytotoxicity decreased in normal pregnancy but not in anembryonic pregnancy and recurrent spontaneous abortion. Am J Reprod Immunol (New York N.Y.: 1989) (1995) 34(5):274–80. doi: 10.1111/j.1600-0897.1995.tb00953.x
20. Ruiz JE, Kwak JY, Baum L, Gilman-Sachs A, Beaman KD, Kim YB, et al. Effect of intravenous immunoglobulin G on natural killer cell cytotoxicity in vitro in women with recurrent spontaneous abortion. J Reprod Immunol (1996) 31(1–2):125–41. doi: 10.1016/0165-0378(96)00969-2
21. King A, Birkby C, Loke YW. Early human decidual cells exhibit NK activity against the K562 cell line but not against first trimester trophoblast. Cell Immunol (1989) 118(2):337–44. doi: 10.1016/0008-8749(89)90382-1
22. Moffett A, Chazara O, Colucci F, Johnson MH. Variation of maternal KIR and fetal HLA-c genes in reproductive failure: too early for clinical intervention. Reprod Biomed Online (2016) 33(6):763–9. doi: 10.1016/j.rbmo.2016.08.019
23. Alecsandru D, Barrio A, Garrido NN, Aparicio P, Pellicer A, Moffett A, et al. Parental human leukocyte antigen-c allotypes are predictive of live birth rate and risk of poor placentation in assisted reproductive treatment. Fertil Steril (2020) 114(4):809–17. doi: 10.1016/j.fertnstert.2020.05.008
24. Chen X, Mariee N, Jiang L, Liu Y, Wang CC, Li TC, et al. Measurement of uterine natural killer cell percentage in the periimplantation endometrium from fertile women and women with recurrent reproductive failure: establishment of a reference range. Am J Obstet Gynecol (2017) 217(6):680. doi: 10.1016/j.ajog.2017.09.010
25. Woon EV, Greer O, Shah N, Nikolaou D, Johnson M, Male V, et al. Number and function of uterine natural killer cells in recurrent miscarriage and implantation failure: A systematic review and meta-analysis. Hum Reprod Update (2022) 28(4):548–82. doi: 10.1093/humupd/dmac006
26. Vento-Tormo R, Efremova M, Botting RA, Turco MY. Single-cell reconstruction of the early maternal–fetal interface in humans. Nature (2018) 563(7731):347–53. doi: 10.1038/s41586-018-0698-6
27. Whettlock EM, Woon EV, Cuff AO, Browne B, Johnson MR, Male V. Dynamic changes in uterine NK cell subset frequency and function over the menstrual cycle and pregnancy. Front Immunol (2022) 13:880438. doi: 10.3389/fimmu.2022.880438
28. Guo C, Cai P, Jin L, Sha Q, Yu Q, Zhang W, et al. Single-cell profiling of the human decidual immune microenvironment in patients with recurrent pregnancy loss. Cell Discov (2021) 7(1):1. doi: 10.1038/s41421-020-00236-z
29. Wu Z, Wang M, Liang G, Jin P, Wang P, Xu Y, et al. Pro-inflammatory signature in decidua of recurrent pregnancy loss regardless of embryonic chromosomal abnormalities. Front Immunol (2021) 12:772729. doi: 10.3389/fimmu.2021.772729
30. Parham P, Moffett A. Variable NK cell receptors and their MHC class i ligands in immunity, reproduction and human evolution. Nat Rev Immunol (2013) 13(2):133–44. doi: 10.1038/nri3370
31. Hiby SE, Regan L, Lo W, Farrell L, Carrington M, Moffett A. Association of maternal killer-cell immunoglobulin-like receptors and parental HLA-c genotypes with recurrent miscarriage. Hum Reprod (Oxford England) (2008) 23(4):972–6. doi: 10.1093/humrep/den011
32. Alecsandru D, Garrido N, Vicario JL, Barrio A, Aparicio P, Requena A, et al. Maternal KIR haplotype influences live birth rate after double embryo transfer in IVF cycles in patients with recurrent miscarriages and implantation failure. Hum Reprod (2014) 29(12):2637–43. doi: 10.1093/humrep/deu251
33. Hiby SE, Walker JJ, O’shaughnessy KM, Redman CWG, Carrington M, Trowsdale J, et al. Combinations of maternal KIR and fetal HLA-c genes influence the risk of preeclampsia and reproductive success. J Exp Med (2004) 200(8):957–65. doi: 10.1084/jem.20041214
34. Xiong S, Sharkey AM, Kennedy PR, Gardner L, Farrell LE, Chazara O, et al. Maternal uterine NK cell-activating receptor KIR2DS1 enhances placentation. J Clin Invest (2013) 123(10):4264–72. doi: 10.1172/JCI68991
35. Kennedy PR, Chazara O, Gardner L, Ivarsson MA, Farrell LE, Xiong S, et al. Activating KIR2DS4 is expressed by uterine NK cells and contributes to successful pregnancy. J Immunol (2016) 197(11):4292–300. doi: 10.4049/jimmunol.1601279
36. Ntrivalas E, Paterakis G, Papasaranti C, Mantouvalou E, Mantouvalos H, Stavropoulos-Giokas C. Phenotypic analysis of natural killer (NK) cells in women with recurrent spontaneous abortions of immune etiology. Arch Hellenic Med (2002) 19(1):64–70.
37. Yamada H, Shimada S, Kato EH, Morikawa M, Iwabuchi K, Kishi R, et al. Decrease in a specific killer cell immunoglobulin-like receptor on peripheral natural killer cells in women with recurrent spontaneous abortion of unexplained etiology. Am J Reprod Immunol (New York N.Y.: 1989) (2004) 51(3):241–7. doi: 10.1111/j.1600-0897.2004.00139.x
38. Faridi RM, Agrawal S. Killer immunoglobulin-like receptors (KIRs) and HLA-c allorecognition patterns implicative of dominant activation of natural killer cells contribute to recurrent miscarriages. Hum Reprod (2011) 26(2):491–7. doi: 10.1093/humrep/deq341
39. Kniotek M, Roszczyk A, Zych M, Wrzosek M, Szafarowska M, Zagożdżon R, et al. Sildenafil citrate downregulates PDE5A mRNA expression in women with recurrent pregnancy loss without altering angiogenic factors-a preliminary study. J Clin Med (2021) 10(21):5086. doi: 10.3390/jcm10215086
40. Wallace AE, Whitley GS, Thilaganathan B, Cartwright JE. Decidual natural killer cell receptor expression is altered in pregnancies with impaired vascular remodeling and a higher risk of pre-eclampsia. J Leukocyte Biol (2015) 97(1):79–86. doi: 10.1189/jlb.2a0614-282r
41. Wang S, Li Y-P, Ding B, Zhao Y-R, Chen Z-J, Xu C-Y, et al. Recurrent miscarriage is associated with a decline of decidual natural killer cells expressing killer cell immunoglobulin-like receptors specific for human leukocyte antigen c. J Obstet Gynaecol Res (2014) 40(5):1288–95. doi: 10.1111/jog.12329
42. Hiby SE, Apps R, Sharkey AM, Farrell LE, Gardner L, Mulder A, et al. Maternal activating KIRs protect against human reproductive failure mediated by fetal HLA-C2. J Clin Invest (2010) 120(11):4102–10. doi: 10.1172/JCI43998
43. Tilburgs T, Evans JH, Crespo ÂC, Strominger JL. The HLA-G cycle provides for both NK tolerance and immunity at the maternal-fetal interface. Proc Natl Acad Sci United States America (2015) 112(43):13312–7. doi: 10.1073/pnas.1517724112
44. Höglund P, Brodin P. Current perspectives of natural killer cell education by MHC class I molecules. Nat Rev Immunol (2010) 10(10):724–34. doi: 10.1038/nri2835
45. Forrest C, Gomes A, Reeves M, Male V. NK cell memory to cytomegalovirus: implications for vaccine development. Vaccines (2020) 8(3):394. doi: 10.3390/vaccines8030394
46. Crespo ÂC, Strominger JL, Tilburgs T. Expression of KIR2DS1 by decidual natural killer cells increases their ability to control placental HCMV infection. Proc Natl Acad Sci USA (2016) 113(52):15072–7. doi: 10.1073/pnas.1617927114
47. Fu B, Zhou Y, Ni X, Tong X, Xu X, Dong Z, et al. Natural killer cells promote fetal development through the secretion of growth-promoting factors. Immunity (2017) 47(6):1100. doi: 10.1016/j.immuni.2017.11.018
48. Gamliel M, Goldman-Wohl D, Isaacson B, Gur C, Stein N, Yamin R, et al. Trained memory of human uterine NK cells enhances their function in subsequent pregnancies. Immunity (2018) 48(5):951–962.e5. doi: 10.1016/j.immuni.2018.03.030
49. Goldman-Wohl D, Gamliel M, Mandelboim O, Yagel S. Learning from experience: cellular and molecular bases for improved outcome in subsequent pregnancies. Am J Obstet Gynecol (2019) 221(3):183–93. doi: 10.1016/j.ajog.2019.02.037
50. Habets DHJ, Schlütter A, van Kuijk SMJ, Spaanderman MEA, Al-Nasiry S, Wieten L. Natural killer cell profiles in recurrent pregnancy loss: Increased expression and positive associations with TACTILE and LILRB1. Am J Reprod Immunol (New York N.Y.: 1989) (2022) 88(5):e13612. doi: 10.1111/aji.13612
51. Wallace AE, Fraser R, Gurung S, Goulwara SS, Whitley GS, Johnstone AP, et al. Increased angiogenic factor secretion by decidual natural killer cells from pregnancies with high uterine artery resistance alters trophoblast function. Hum Reprod (2014) 29(4):652–60. doi: 10.1093/humrep/deu017
52. Fukui A, Funamizu A, Fukuhara R, Shibahara H. Expression of natural cytotoxicity receptors and cytokine production on endometrial natural killer cells in women with recurrent pregnancy loss or implantation failure, and the expression of natural cytotoxicity receptors on peripheral blood natural kille. J Obstet Gynaecol Res (2017) 43(11):1678–86. doi: 10.1111/jog.13448
53. Dong P, Wen X, Liu J, Yan C-Y, Yuan J, Luo L-R, et al. Simultaneous detection of decidual Th1/Th2 and NK1/NK2 immunophenotyping in unknown recurrent miscarriage using 8-color flow cytometry with FSC/Vt extended strategy. Biosci Rep (2017) 37(3). doi: 10.1042/BSR20170150
54. Liu J, Dong P, Wang S, Li J. Natural killer, natural killer T, helper and cytotoxic T cells in the decidua from recurrent spontaneous abortion with normal and abnormal chromosome karyotypes. Biochem Biophys Res Commun (2019) 508(2):354–60. doi: 10.1016/j.bbrc.2018.11.156
55. Liu J, Dong P, Jia N, Wen X, Luo L, Wang S, et al. The expression of intracellular cytokines of decidual natural killer cells in unexplained recurrent pregnancy loss. J Matern Fetal Neonat Med (2020) 35(16):3209–15. doi: 10.1080/14767058.2020.1817369
56. Fonseca BM, Cunha SC, Gonçalves D, Mendes A, Braga J, Correia-da-Silva G, et al. Decidual NK cell-derived conditioned medium from miscarriages affects endometrial stromal cell decidualisation: endocannabinoid anandamide and tumour necrosis factor-α crosstalk. Hum Reprod (Oxford England) (2020) 35(2):265–74. doi: 10.1093/humrep/dez260
57. Takeyama R, Fukui A, Mai C, Yamamoto M, Saeki S, Yamaya A, et al. Co-Expression of NKp46 with activating or inhibitory receptors on, and cytokine production by, uterine endometrial NK cells in recurrent pregnancy loss. J Reprod Immunol (2021) 145:103324. doi: 10.1016/j.jri.2021.103324
58. Lash GE, Otun HA, Innes BA, Kirkley M, De Oliveira L, Searle RF, et al. Interferon-γ inhibits extravillous trophoblast cell invasion by a mechanism that involves both changes in apoptosis and protease levels. FASEB J (2006) 20(14):2512–8. doi: 10.1096/fj.06-6616com
59. Otun HA, Lash GE, Innes BA, Bulmer JN, Naruse K, Hannon T, et al. Effect of tumour necrosis factor-α in combination with interferon-γ on first trimester extravillous trophoblast invasion. J Reprod Immunol (2011) 88(1):1–11. doi: 10.1016/j.jri.2010.10.003
60. Wang W, Sung N, Gilman-Sachs A, Kwak-Kim J. T Helper (Th) cell profiles in pregnancy and recurrent pregnancy losses: Th1/Th2/Th9/Th17/Th22/Tfh cells. Front Immunol (2020) 11:2025. doi: 10.3389/fimmu.2020.02025
61. Flores AC, Marcos CY, Paladino N, Arruvito L, Williams F, Middleton D, et al. KIR receptors and HLA-c in the maintenance of pregnancy. Tissue Antigens (2007) 69:112–3. doi: 10.1111/j.1399-0039.2006.762_8.x
62. Faridi RM, Das V, Tripthi G, Talwar S, Parveen F, Agrawal S, et al. Influence of activating and inhibitory killer immunoglobulin-like receptors on predisposition to recurrent miscarriages. Hum Reprod (Oxford England) (2009) 24(7):1758–64. doi: 10.1093/humrep/dep047
63. Morin SJ, Franasiak JM, Juneau CR, Treff NR, Scott RT, Han M. Uterine natural killer cell immunoglobulin receptor (KIR) genotypes and trophoblastic human leukocyte antigen c (HLA-c) ligands influence risk of pregnancy loss: Data from euploid transfers. Reprod BioMed Online (2016) 33:e.1. doi: 10.1016/j.rbmo.2016.06.011
Keywords: NK cells, endometrium, pregnancy, ILC, recurrent miscarriage, infertility, recurrent implantation failure, reproductive failure
Citation: Woon EV, Nikolaou D, MacLaran K, Norman-Taylor J, Bhagwat P, Cuff AO, Johnson MR and Male V (2023) Uterine NK cells underexpress KIR2DL1/S1 and LILRB1 in reproductive failure. Front. Immunol. 13:1108163. doi: 10.3389/fimmu.2022.1108163
Received: 25 November 2022; Accepted: 28 December 2022;
Published: 13 January 2023.
Edited by:
Gendie Lash, Guangzhou Medical University, ChinaReviewed by:
Andrew Sharkey, University of Cambridge, United KingdomSvetlana Dambaeva, Rosalind Franklin University of Medicine and Science, United States
Copyright © 2023 Woon, Nikolaou, MacLaran, Norman-Taylor, Bhagwat, Cuff, Johnson and Male. This is an open-access article distributed under the terms of the Creative Commons Attribution License (CC BY). The use, distribution or reproduction in other forums is permitted, provided the original author(s) and the copyright owner(s) are credited and that the original publication in this journal is cited, in accordance with accepted academic practice. No use, distribution or reproduction is permitted which does not comply with these terms.
*Correspondence: Ee Von Woon, ZWUudm9uQG5ocy5uZXQ=