- 1Center for Regenerative and Reconstructive Medicine, Med-X Institute, First Affiliated Hospital of Xi’an Jiaotong University, Xi’an, Shaanxi, China
- 2Department of Hepatobiliary Surgery, First Affiliated Hospital of Xi’an Jiaotong University, Xi’an, Shaanxi, China
- 3Department of Radiotherapy, Xi’an Medical University, Xi’an, Shaanxi, China
- 4Department of Surgical Oncology, Shaanxi Provincial People’s Hospital, Xi’an, Shaanxi, China
Liver fibrosis is a fibrogenic and inflammatory process that results from hepatocyte injury and is characterized by hepatic architectural distortion and resultant loss of liver function. There is no effective treatment for advanced fibrosis other than liver transplantation, but it is limited by expensive costs, immune rejection, and postoperative complications. With the development of regenerative medicine in recent years, mesenchymal stem cell (MSCs) transplantation has become the most promising treatment for liver fibrosis. The underlying mechanisms of MSC anti-fibrotic effects include hepatocyte differentiation, paracrine, and immunomodulation, with immunomodulation playing a central role. This review discusses the immune cells involved in liver fibrosis, the immunomodulatory properties of MSCs, and the immunomodulation mechanisms of MSC-based strategies to attenuate liver fibrosis. Meanwhile, we discuss the current challenges and future directions as well.
1. Introduction
Liver fibrosis is a complex fibrogenic and inflammatory process that results from chronic liver injury. The primary pathophysiology of liver fibrosis is increased collagen deposition of types I and III in the extracellular matrix (ECM) (1, 2). Major etiological agents of liver fibrosis include alcohol, viruses, metabolic, and congenital disorders, all of which can lead to hepatocyte injury and hepatic stellate cell (HSC) activation, resulting in excessive ECM deposition and structural disorders in the liver (3, 4). Despite distinct pathogenesis, their common outcome is the development of liver cirrhosis. In recent years, the incidence and mortality of hepatic fibrosis have increased steadily and become a substantial global health burden. Other than liver transplantation, there is no curative treatment for end-stage cirrhosis. However, expensive costs, immune rejection, and postoperative complications limit liver transplantation (5, 6).
Stem cell transplantation has emerged as the most promising treatment for liver fibrosis since the advent of regenerative medicine (7–9). Stem cells are a population of cells with self-renewal, proliferation, and pluripotent differentiation potential that can differentiate into multiple cell types under defined conditions (10, 11). Embryonic stem cells (ESCs) (12, 13), induced pluripotent stem cells (iPSCs) (14–17), and mesenchymal stem cells (MSCs) (18–22) have been investigated the most in the treatment of liver fibrosis. ESCs, the prototype of pluripotent stem cells, have nearly unlimited self-renewal capacity and differentiation potential (23). iPSCs display similar surface antigens expression, proliferation capacity, morphology, and gene expression characteristics as embryonic stem cells (24). ESCs and iPSCs achieve therapeutic effects mainly by differentiating into mature hepatocytes in vitro or in vivo (8). MSCs, on the other hand, not only have the potential for self-renewal and differentiation (25) and can regulate the immune response (7). Moreover, since abnormal immune responses are the primary cause of liver fibrosis, MSCs are the most promising stem cells for treating liver fibrosis, as they can slow or even reverse the progression of liver fibrosis (26–28).
This review focuses on the immune cells involved in liver fibrosis, the immunomodulatory properties of MSCs, and the immunomodulation mechanisms of MSC-based strategies to attenuate liver fibrosis. The challenges and future directions were also discussed.
2. Immune cells participate in the process of liver fibrosis
According to common knowledge, the liver is composed of primary hepatocytes, cholangiocytes, Kupffer cells (KCs), liver sinusoidal endothelial cells (LSECs), HSCs, fibroblasts, lymphocytes, oval cells, lymphocytes, and other immune cells (29). The liver’s blood supply originates from the hepatic artery and portal vein and passes through infections or toxins of systemic and intestinal origin. Hence, the liver is highly susceptible to pathogens that cause acute or chronic liver injury (30). Injury to intrahepatic parenchymal cells, such as hepatocytes or cholangiocytes, causes liver fibrosis. Varied etiologies could contribute to liver damage, including inflammation, chronic viral hepatitis, alcohol consumption, chronic cholestasis, and non-alcoholic steatohepatitis (NASH). Although the etiologies are different, the initial phase often includes hepatocyte injury, which subsequently causes the release of oxygen radicals and inflammatory molecules. These pro-inflammatory mediators stimulate KCs and hepatic sinusoidal endothelial cells, leading to the transdifferentiation of HSCs from a quiescent to an activated phenotype (1–3). In addition, other liver-specific immune cells, including natural killer (NK) cells, natural killer T cells (NKT) cells, dendritic cells (DCs), and neutrophils, react to injured hepatocytes by producing cytokines and initiating inflammatory responses. These inflammatory cytokines (such as transforming growth factor-β (TGF-β) and platelet-derived growth factor (PDGF)) could stimulate and transdifferentiate HSCs into myofibroblasts (3, 4). HSCs are the most critical non-parenchymal cells of the liver, located in the Disse space, and play a crucial role in developing liver fibrosis. Fibroblasts in the bone marrow or circulating blood, as well as hepatocytes and cholangiocytes, can also be transdifferentiated into myofibroblasts by epithelial-mesenchymal transition (EMT). However, those derived from activated HSCs are the most common (4).
Activation of HSCs results in the generation of a massive ECM and the expression of α-smooth muscle actin (α-SMA). TGF-β is the primary cytokine involved in the activation of HSC transdifferentiation and the EMT signal. TGF-β1 activation increases ECM synthesis and inhibits ECM degradation, thereby accelerating the liver fibrosis development. In addition, myofibroblasts are known to produce tissue inhibitors of matrix metalloproteinases (TIMPs) to prevent matrix metalloproteinases (MMPs) from degrading the ECM and maintaining ECM integrity (Figure 1) (31, 32).
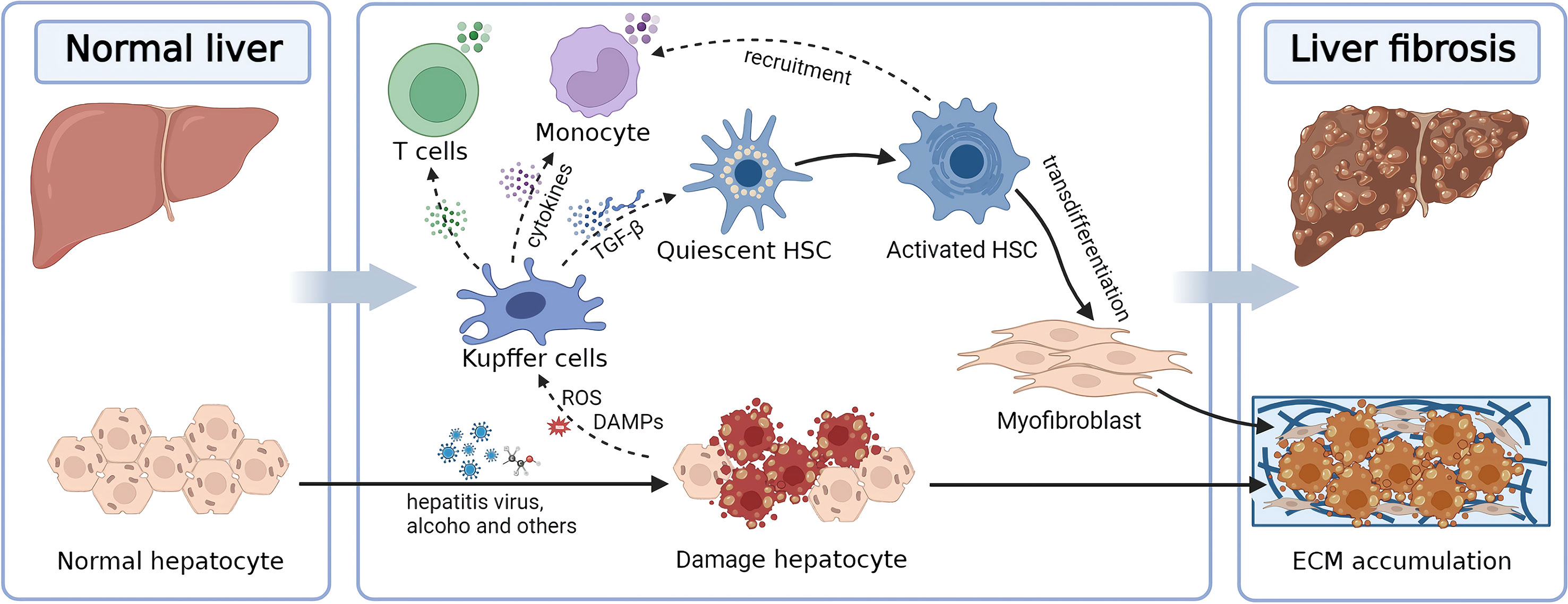
Figure 1 Diagram illustrating the pathological mechanisms underlying liver fibrosis. Hepatic fibrosis is induced by the imbalance between ECM production and degradation, driven by HSCs activated by hepatocyte injury. ECM, extracellular matrix; HSC, hepatic stellate cell; TGF-β, transforming growth factor-β.
3. The immunomodulatory properties of mesenchymal stem cells
MSCs were initially utilized primarily for tissue repair and regeneration. Subsequently, they have been increasingly used to treat graft-versus-host disease (GVHD) (33) and autoimmune diseases like lupus (34) and Crohn’s disease (35). Furthermore, the clinical potential of MSCs has been extended to treat myocardial infarction (36), stroke (37, 38), multiple sclerosis (39), liver cirrhosis (18, 40), diabetes (41), lung injuries (42, 43), and cancer (44). MSCs have been isolated and expanded from numerous adult and perinatal tissues, including bone marrow, adipose tissue, peripheral blood, fetal tissues, dental pulp, umbilical cord, and placental tissues (45).
Currently, it is believed that MSC must possess at least the following three characteristics (46): first, the cells must be able to grow on plates; second, CD90, CD73, and CD105 must be expressed, while HLA class II, CD19 or CD79a and CD14, CD34, CD45 or CD11b are negative; and third, the cells must be able to differentiate into osteoblasts, chondrocytes, and adipocytes.
MSCs are multipotent cells emerging as the most promising method of allogeneic cell treatment (45). MSCs have natural immunomodulatory properties, trophic capacities, and strong in vitro self-renewal capacity, and their immune-modulatory actions can be easily manipulated (44). MSCs influence most immune cell functions through direct contact and factors in the local microenvironment. Previous research has revealed that cytokines released by MSCs are primarily responsible for the immunomodulatory actions of MSCs (28). However, recent research has shown that apoptotic and metabolically inactive MSCs still possess the immunomodulatory capability, with regulatory T cells and monocytes playing a vital role (47).
MSCs regulate both innate and adaptive immunity (47), and their immunomodulatory functions are predominantly exerted through cell-to-cell contact and paracrine activity with macrophages, monocytes, neutrophils, T cells, B cells and natural killer (NK) cells. By secreting prostaglandin E2, MSCs convert pro-inflammatory M1 into anti-inflammatory M2 macrophages (PGE2) (48). MSCs can also modulate immune responses by activating the Notch 1 signaling pathway, releasing HLA-G5, PGE2, and TGF-1, and boosting the activation and proliferation of CD4+CD25+FoxP3+ regulatory T cells (Tregs) (49). MSCs decrease the proliferative potential of CD8+ T lymphocytes by producing indoleamine 2,3-dioxygenase (IDO) and heme oxygenase-1 (HO-1) and increase the rate of CD4+ T lymphocytes changing from type 1 T helper (TH1) to TH2 phenotype (50). In addition, hepatocyte growth factor (HGF) and IL-6 produced by MSCs, for instance, prevent the differentiation of monocytes into dendritic cells and reduce their propensity to cause inflammation, reduce the secretion of pro-inflammatory cytokines IL-12 and IFN-γ, and increase the production of anti-inflammatory cytokine IL-10, thereby inhibiting T-cell activation (51). MSCs reduce the generation of the pro-inflammatory cytokine TNF by inhibiting the activity of blast cells.
Successful immunomodulation and tissue regeneration depend on the interplay between MSCs and macrophages, especially juxtacrine mechanisms and cell–cell contact (52). Inflammatory factors released by M1 macrophages or activated T cells stimulate MSCs to secrete cytokines to differentiate monocytes toward the M2 type of the anti-inflammatory phenotype (53). It is known that MSCs may release anti-inflammatory or pro-inflammatory cytokines (such as IL1b, IL-6, IL-8, and IL-9) to mediate their immunomodulatory effects (54). As stated above, MSCs either inhibit or promote inflammation based on their exposure to pathological circumstances. The ultimate immunomodulatory effect may depend on the ratio of anti-inflammatory to pro-inflammatory factors in their surrounding environment (55).
4. Mesenchymal stem cell therapies for liver cirrhosis: Immune regulation plays a central role
4.1. Potential mechanisms of MSC-based treatment of liver fibrosis
Many studies have investigated the mechanisms of MSCs in the treatment of liver fibrosis from diverse perspectives, and they have been classified into three types (13, 22, 56). (1) MSCs can transdifferentiate into hepatocytes or merge with existing hepatocytes when introduced into injured liver tissue, making them a valuable resource for liver tissue regeneration and repair; (2) MSCs are capable of producing various cytokines, growth factors, and exosomes, which stimulate the regeneration of damaged liver tissue; (3) MSCs possess inhibitory effects on several other cell types, including NKs, B and T lymphocytes, allowing them to exercise immunomodulatory effects on liver diseases.
By migrating to damaged tissues, transplanted MSCs contribute to the regeneration of the damaged liver via hepatocyte differentiation mechanisms. Adding specific growth factors to in vitro culture can promote the differentiation of MSCs into hepatocyte-like cells with liver-specific morphology and functions, such as uptake of low-density lipoprotein and indocyanine green, secretion of albumin and urea, glycogen storage, and cytochrome P450 activity (25, 57). For instance, intrasplenic-grafted MSCs transplanted into liver tissue treated with carbon tetrachloride (CCl4) undergo hepatogenic differentiation into HLCs with typical hepatocyte morphology and form a three-dimensional structure (58). Transplantation of hepatocyte-differentiated MSCs further inhibited hepatocyte necrosis and stimulated liver regeneration, thereby enhancing the survival rate of the ALF model after transplantation into damaged liver tissue.
However, several studies have demonstrated that transplanted MSCs rarely undergo hepatic differentiation and vanish from the liver within one month (59). These studies indicate that MSCs stimulate liver regeneration via immune regulation and paracrine mechanisms (25, 56, 60). By regulating innate and adaptive immune cells, including T lymphocytes, regulatory T cells, helper T cells, B lymphocytes, and regulatory B cells, MSCs create a tolerant environment for maintaining immune homeostasis in vivo (61–64). As previously mentioned, the development of liver fibrosis is a wound-healing process involving key events, including hepatocyte injury, immune cell infiltration, HSCs activation, and excessive ECM deposition. Therefore, the immunomodulatory function of MSCs can be exploited to ameliorate liver fibrosis, and it has been an area of intense study interest (Figure 2).
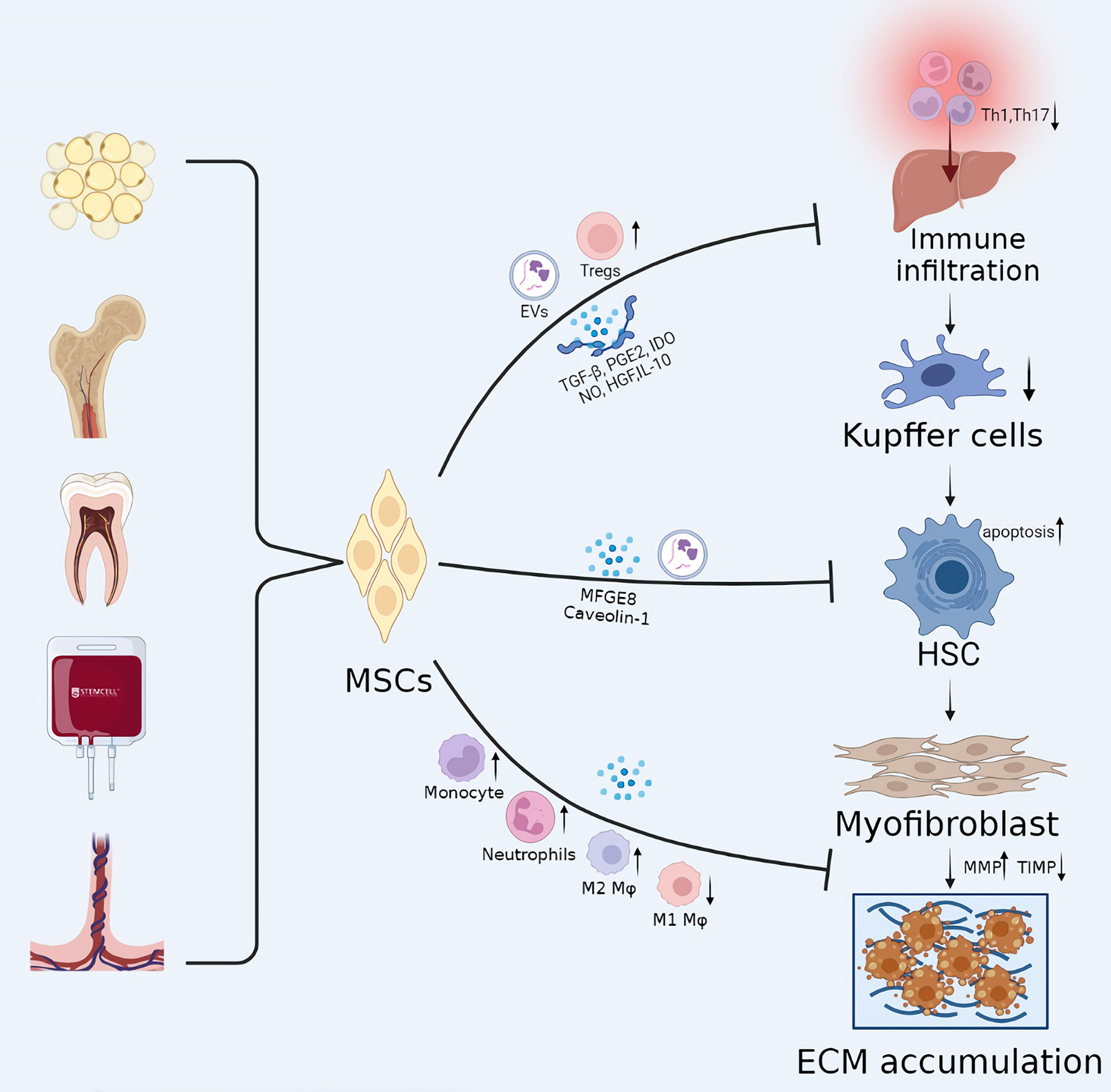
Figure 2 The potential mechanisms of MSCs in liver cirrhosis. The development of liver fibrosis is a wound-healing process involving key events, including hepatocyte injury, immune cell infiltration, HSC activation, and excessive ECM deposition. MSC transplantation can play a therapeutic role in every stage of liver fibrosis, with immunomodulatory effects playing a central role. EVs, extracellular vesicles; Mφ, Macrophages; IDO, indoleamine 2,3-dioxygenase.
4.2. MSC can inhibit immune cell infiltration
Infiltration of immune cells is a necessary stage in liver injury. MSCs create an immunological-tolerant milieu in liver tissue by reducing the infiltration of pro-inflammatory immune cells and promoting the recruitment of anti-inflammatory immune cells, preventing acute or chronic liver damage. Producing soluble factors, including TGF-β, PGE2, IDO, NO, and HGF, MSCs were able to inhibit the activation of T cells. MSCs can induce the transdifferentiation of CD4+ T cells into CD25+Foxp3+ regulatory T cells (Tregs) through the release of TGF-β (7, 49). Studies have demonstrated that MSCs dramatically decreased the number of CD4+ T cells invading the liver, the proportion of activated CD4+ T lymphocytes, and the total concentration of Th1 cells, and subsequently induced regulatory DCs and Tregs in the liver to ameliorate liver damage (65). MSCs significantly alleviated CCl4-mediated liver fibrosis by decreasing the proportion of Th17 cells and increasing the levels of CD4+IL-10+ T cells and immunosuppressive factors (including kynurenine, IDO, and IL-10). In addition, MSCs enhanced liver function and ameliorated clinical symptoms in patients with hepatitis B virus-mediated decompensated cirrhosis by significantly downregulating the expression levels of IL-6 and TNF-α, while upregulating the expression level of IL-10 (66).
4.3. Blocking HSC is a key target for MSC to attenuate liver fibrosis
Blocking the activation of HSCs is one of the most crucial intervention targets for liver fibrosis (8). Pro-inflammatory mediators, oxidative stress molecules, and inflammatory stimulants produced by apoptosis or necrosis of liver parenchymal cells initiate the activation of HSCs (4). Activated HSCs subsequently release a variety of inflammatory chemicals that enhance the liver’s inflammatory response. TGF-β is considered to be one of the most important signaling molecules for the activation of HSCs.
According to certain studies, milk fat globule-EGF factor 8 (MFGE8), one of the mediators secreted by MSC, is an anti-fibrotic protein that prevents the activation of HSCs by suppressing TGF-β type I receptor (TGFBR1) (67). Moreover, Caveolin-1, another possible target for MSC therapy, inhibited HSCs significantly (68). The expression of Wnt pathway-related proteins such as PPARγ, Wnt3a, Wnt10b, β-catenin, and WISP1 and Cyclin D1 is also known to be critical for HSC activation, and MSC has inhibitory effects on several molecules of this pathway (19). Ohara et al. (69) demonstrated that amniotic membrane-derived MSCs (AMSCs) could inhibit HSCs activation by downregulating the upstream steps of the LPS/TLR4 signaling pathway without inhibiting downstream NF-κB transcriptional activity. Qiao et al. (70) proved that hBM-MSCs significantly inhibited the proliferation of activated HSCs by inducing the apoptotic process of activated HSCs. Moreover, hBM-MSCs decreased the expression of peroxisome proliferator-activated receptor γ and α1(I) collagen and α-smooth muscle actin (α-SMA) in activated HSCs by decreasing the signalling pathway of NADPH oxidase, thereby delaying the progression of liver fibrosis.
4.4. MSC’s function in ECM degradation and remodeling
As mentioned above, liver fibrosis is associated with excessive ECM deposition and decreased ECM lytic activity. ECM degradation and remodelling are considered vital targets for reversing liver fibrosis and delaying the progression of liver fibrosis. Modulating TGF-β signalling is one of the important mechanisms of MSC-based modulation of liver fibrosis (8). MSCs participate in the regulation of TGF-β downstream pathways. MSCs were able to significantly down-regulate the mRNA expression of TGF-β1 and TGFBR1 downstream molecule SMAD3 and increase the mRNA expression of SMAD7 (71). It has been demonstrated that SMAD3 upregulates the expression of the pro-fibrotic factor α-SMA or Col1a1, whereas SMAD7 has an anti-fibrotic impact. ECM components laminin and hyaluronic acid were significantly decreased when BMSC overexpressed SMAD7.
In addition, it was demonstrated that by overexpressing SMAD7, MSC could enhance serum MMP-1 levels and reduce TIMP-1 levels. MMP-1 is a matrix metalloproteinase that degrades matrix collagen type I (Col1a1) and collagen type III (Col3a1). TIMPs, on the other hand, inhibit MMP activity by forming reversible covalent complexes with the corresponding MMPs. MSCs have been demonstrated to induce the infiltration of host monocytes and neutrophils into the liver and relieve fibrosis via MMP release (18). Luo et al. (72) demonstrate for the first time that BM-MSC transplantation promotes activation of MMP13-expressing M2 macrophages and suppresses M1 macrophages, which further inhibit HSCs, which play a synergistic role in attenuating liver fibrosis.
4.5. MSC can alleviate liver fibrosis via extracellular vehicles
In addition to direct cell-to-cell contact and paracrine cytokines, MSC can ameliorate liver fibrosis via extracellular vehicles (EVs). EVs produced by MSCs include exosomes (40-100 nm in diameter) and microvesicles (MVs, 0.1-1 mm in diameter). EVs contribute to the therapeutic potential of MSCs by enhancing intercellular contacts for the transport of paracrine substances during angiogenesis, tissue repair, and immunomodulation (73). Exosomes are nanoscale EVs derived from MVB, secreted into the extracellular microenvironment by the fusion of MVB with the plasma membrane. Exosomes can be taken up by target cells in the local milieu or transported to distant regions via biofluids. Exosomes contain numerous cytoplasmic and membrane proteins, such as nucleic acids (miRNA, mRNA, dsDNA, ssDNA, and mtDNA), ECM proteins, lipids, transcription factors, and receptors. Currently, the therapeutic mechanism of EVs is based on two main cargoes, RNA (especially miRNA) and proteins (74).
Exosomes have been shown to play an essential role in critical events in the development of liver fibrosis, including hepatocyte injury, immune cell infiltration, HSCs activation, and excessive ECM deposition (75–77). Several animal models of liver disease, including liver fibrosis and drug-induced acute liver injury, have been found to be alleviated by mesenchymal stem cell exosomes (MSCS-Ex) (78–81). For example, AMSC-Ex significantly decreased fiber accumulation, KCs number, and HSCs activation in rats with liver fibrosis. In vitro, AMSC-Ex significantly inhibited KC and HSC activation and suppressed the lipopolysaccharide (LPS)/toll-like receptor 4 (TLR4) signalling pathway. By decreasing collagen production and TGF-1 release, MSCs-Ex were also able to reduce the severity of liver fibrosis caused by ClC4 (79, 82). MSC-originated exosomes circDIDO1 sponged miR143-3p in HSCs, causing cell cycle arrest, suppression, and apoptosis through promoting PTEN and repressing the ratio of p-AKT/AKT. Furthermore, umbilical cord mesenchymal stem cell exosomes (UCMSC-Ex) boosted the expression of the epithelium-associated marker E-cadherin while decreasing the expression of N-cadherin and vimentin-positive cells, suppressing EMT and preventing hepatocyte apoptosis. Jiang et al. (83) demonstrated that UCMSCs-Ex reduced CCl4-mediated hepatocyte injury and liver fibrosis by inhibiting hepatocyte apoptosis and oxidative stress. In addition, MSCs can release immunopotent exosomes, allowing them to exert immunomodulatory effects on the differentiation, activation, and functionality of various subsets of lymphocytes. Tamura et al. (84) found that MSC-derived exosomes increased the production of anti-inflammatory cytokines and the number of T regulatory cells in mice with concanavalin A-induced liver injury, indicating immunosuppressive properties.
The ability of MSCs-Ex to execute drug transport tasks is another therapeutic use for these cells. Recent research has demonstrated that MSCs may package and distribute active medicines via their exosomes. These studies pave the way for researching and developing more robust and homing-capable novel medications employing MSCs. Cell-free treatment techniques avoid the potential for carcinogenesis, unneeded differentiation, embolization, cell injection, and infection dissemination associated with MSCs transplantation (21, 80). Furthermore, these therapies are safer, less costly, and more effective. Although MSCs-Ex shows considerable potential for treating liver disease, the absence of a consistent and efficient production process remains a key impediment. Thus, additional studies will be needed to address these important issues.
5. Challenges and future directions
Over the past two decades, there has been a rapid increase in cell therapy techniques for treating liver fibrosis. These technologies have accumulated knowledge on enhancing in vitro cell manipulation and cell transplantation processes to combat liver fibrosis and enhance liver repair. With the help of cutting-edge technologies such as bioreactors, microfluidics, and 3D bioprinting, these studies are now contributing to the development of new technologies aimed at producing in vitro systems that can yield liver-like tissue or whole bioengineered livers (85–87). However, it has been reported that MSCs therapy still has certain limitations for clinical application, including safety, limited cell survival, standardized production, ethical concerns, unavailability of trustworthy animal models, and lack of appropriate injection routes.
First, and perhaps most important, the safety of MSC-based therapy is still under discussion, especially in the context of long-term follow-up. A major concern is the undesired differentiation of transplanted MSCs and their propensity to impair antitumor immune responses and develop new blood vessels, which may contribute to tumor development and spread (88). In addition, neither the administration of MSCs nor their application in clinical studies has been standardized. Stem cells can readily differentiate into other cell types (except myofibroblasts), mediating communication between stem cells and HSCs, hepatocytes, or other intrahepatic cells associated with liver fibrosis, thereby triggering an immune response to promote fibrosis or prevent the reversal of liver fibrosis. Therefore, there is an urgent need for more sizeable prospective validation trials to verify the efficacy and safety of this treatment.
Second, low cell survival and poor integration with host tissue are well-recognized hurdles that confront the field of in vivo MSC delivery, and these may be improved by the pretreatment of stem cells (89, 90). Primary stem cells often fail to achieve the desired therapeutic effect due to poor homing ability, a low survival rate, and cellular senescence or decreased viability during in vitro culture. Pretreatments include transgenics, hypoxia, inflammatory factors, bioactive compounds, 3D cultures, disease-associated cells, or patient serum. For example, the proliferation of HSCs and collagen deposition in rats with liver fibrosis were more effectively inhibited by HGF gene-transduced MSC (MSCs/HGF) (91). In a separate study, miR122-modified ADMSCs (ADMSC-122) constructed by lentivirus-mediated transfer of miR-122 had a similar effect (92). HGF is a hepatocyte growth factor that promotes hepatocyte regeneration, whereas miR-122 is crucial for inhibiting the proliferation and activation of HSCs. In conjunction with MSCs, they act on intrahepatic cells to treat liver fibrosis and overcome the lack of physiological activity of MSCs cells following transplantation.
Third, the effectiveness and safety of MSCs in treating patients with liver fibrosis have been studied in numerous clinical trials over the last decade. According to National Institutes of Health ClinicalTrials.gov, since January 1, 2012, 44 relevant clinical studies have been registered, 9 of which have been completed. Of these, China (23 cases; 52.3%), Vietnam (4 cases; 9.1%), India (3 cases; 6.8%), Japan (3 cases; 6.8%), South Korea (2 cases; 4.5%), and Belgium (2 cases; 4.5%) are the top six countries. In terms of cell origin, MSCs can be derived from human umbilical cord (14 cases; 31.82%), bone marrow (10 cases; 22.7%), adipose tissue (3 case; 6.8%), teeth (1 case; 3.2%), and unknown (16 cases, 36.4%). Simultaneously, several studies describing the outcomes of clinical trials using MSCs have been published. For instance, Yuwei et al. (93) published a meta-analysis and systematic review of randomized controlled trials (RCTs) to assess the efficacy and safety of MSCs therapy for patients with chronic liver disease. They evaluated 12 RCTs, including 846 patients who met their selection criteria. The results indicated that MSCs improved liver function compared to conventional treatment, primarily in ALB, TBIL, MELD score, and coagulation levels, but there were no significant changes in ALT and AST levels. Furthermore, MSCs treatment could not significantly improve the overall survival rate, with only a slightly positive trend. Five of these studies reported that the only side effect of MSC treatment was fever, with no other serious side effects. In terms of cell source, these clinical trials also show a tendency toward shifting from autologous to allogeneic MSCs. Allogeneic MSCs do not require the painful and complicated process of autologous cells collection (94). In addition, some MSCs can be made into off-the-shelf products due to the absence of immune rejection and the potential for massive expansion. Among these, UCMSC is a promising stem cell that is expected to be produced commercially (95). Many studies have shown that UCMSCs are an ideal source of MSCs because of young cellular age, relative ease of collection, easy batch production, and low allogeneic reactivity (96, 97).
In addition, there are various routes of MSC transplantation, including trans-portal, hepatic artery, peripheral vein transplantation, intrasplenic transplantation, peripheral vein transplantation, intrahepatic and abdominal transplantation, and the respective characteristics of these routes remain unknown. Under identical conditions, MSCs from different transplantation routes have different biodistribution in vivo. They may exert their effects on liver fibrosis via mechanisms influenced by the microenvironment, resulting in different therapeutic effects on fibrosis. Selecting a suitable stem cell transplantation route to increase the amount of stem cell colonization in liver tissue, enhance cell activity, and maintain the survival time of stem cells in liver tissue may be beneficial to improve the anti-fibrotic effect of MSCs. The indications for different transplantation routes in clinical practice must be further investigated (98, 99).
In contrast to cell-based pretreatment, cell-free therapy is a hot topic for future research. Cell-free strategies have low tumorigenic potential, low preservation costs, and low risk of exogenous infection and thrombosis. Unfortunately, no clinical trial has involved the use of stem cell-derived EVs to treat liver fibrosis and cirrhosis. This phenomenon is primarily due to the lack of standardized methods for extracting large numbers of EVs and the unknown dose and half-life of EVs.
6. Conclusion
The immune response is crucial to the genesis and progression of liver fibrosis. Due to their immunomodulatory properties, hepatic differentiation potential, and capacity to produce trophic factors, MSCs and MSC-Ex have emerged as promising agents for treating liver fibrosis. However, many issues need to be addressed before MSCs can be used clinically, including sufficient cell numbers, higher integration efficiency, consistency of in vitro and in vivo studies, and optimal timing and route of cell transplantation. Therefore, large randomized controlled clinical trials with longer follow-up periods and experimental animal studies are needed to improve the safety and efficacy of MSCs for fibrosis treatment.
Author contributions
PL: Conceptualization, methodology, investigation, funding acquisition, writing – original draft. YQ: Investigation, writing-original draft. XL: Investigation, methodology. XFZ: Investigation. YL: Conceptualization, supervision, funding acquisition. JX: Conceptualization, supervision, writing-review & editing, funding acquisition. All authors contributed to the article and approved the submitted version.
Funding
This work was supported by the National Natural Science Foundation of China (82000624), the Fundamental Research Funds for the Central Universities (xjh012020035) and the Institution Foundation of the Shaanxi Province Natural Science Basic Research Program (2020JQ-528).
Conflict of interest
The authors declare that the research was conducted in the absence of any commercial or financial relationships that could be construed as a potential conflict of interest.
Publisher’s note
All claims expressed in this article are solely those of the authors and do not necessarily represent those of their affiliated organizations, or those of the publisher, the editors and the reviewers. Any product that may be evaluated in this article, or claim that may be made by its manufacturer, is not guaranteed or endorsed by the publisher.
References
1. Ginès P, Krag A, Abraldes JG, Solà E, Fabrellas N, Kamath PS. Liver cirrhosis. Lancet (2021) 398:1359–76. doi: 10.1016/S0140-6736(21)01374-X
2. Parola M, Pinzani M. Liver fibrosis: Pathophysiology, pathogenetic targets and clinical issues. Mol Aspects Med (2019) 65:37–55. doi: 10.1016/j.mam.2018.09.002
3. Kisseleva T, Brenner D. Molecular and cellular mechanisms of liver fibrosis and its regression. Nat Rev Gastroenterol Hepatol (2021) 18:151–66. doi: 10.1038/s41575-020-00372-7
4. Tsuchida T, Friedman SL. Mechanisms of hepatic stellate cell activation. Nat Rev Gastroenterol Hepatol (2017) 14:397–411. doi: 10.1038/nrgastro.2017.38
5. Hibi T, Wei CA, Chi-Yan CA, Bhangui P. Current status of liver transplantation in Asia. Int J Surg (2020) 82S:4–08. doi: 10.1016/j.ijsu.2020.05.071
6. Belli LS, Duvoux C, Artzner T, Bernal W, Conti S, Cortesi PA, et al. Liver transplantation for patients with acute-on-chronic liver failure (ACLF) in Europe: Results of the ELITA/EF-CLIF collaborative study (ECLIS). J Hepatol (2021) 75:610–22. doi: 10.1016/j.jhep.2021.03.030
7. Yao L, Hu X, Dai K, Yuan M, Liu P, Zhang Q, et al. Mesenchymal stromal cells: Promising treatment for liver cirrhosis. Stem Cell Res Ther (2022) 13:308. doi: 10.1186/s13287-022-03001-z
8. Liu P, Mao Y, Xie Y, Wei J, Yao J. Stem cells for treatment of liver fibrosis/cirrhosis: Clinical progress and therapeutic potential. Stem Cell Res Ther (2022) 13:356. doi: 10.1186/s13287-022-03041-5
9. Cao Y, Ji C, Lu L. Mesenchymal stem cell therapy for liver fibrosis/cirrhosis. Ann Transl Med (2020) 8:562. doi: 10.21037/atm.2020.02.119
11. Zakrzewski W, Dobrzyński M, Szymonowicz M, Rybak Z. Stem cells: Past, present, and future. Stem Cell Res Ther (2019) 10:68. doi: 10.1186/s13287-019-1165-5
12. Moriya K, Yoshikawa M, Ouji Y, Saito K, Nishiofuku M, Matsuda R, et al. Embryonic stem cells reduce liver fibrosis in CCl4-treated mice. Int J Exp Pathol (2008) 89:401–09. doi: 10.1111/j.1365-2613.2008.00607.x
13. Forbes SJ, Gupta S, Dhawan A. Cell therapy for liver disease: From liver transplantation to cell factory. J Hepatol (2015) 62:S157–69. doi: 10.1016/j.jhep.2015.02.040
14. Coll M, Perea L, Boon R, Leite SB, Vallverdú J, Mannaerts I, et al. Generation of hepatic stellate cells from human pluripotent stem cells enables in vitro modeling of liver fibrosis. Cell Stem Cell (2018) 23:101–13. doi: 10.1016/j.stem.2018.05.027
15. Vallverdú J, Martínez GDLT, Mannaerts I, Verhulst S, Smout A, Coll M, et al. Directed differentiation of human induced pluripotent stem cells to hepatic stellate cells. Nat Protoc (2021) 16:2542–63. doi: 10.1038/s41596-021-00509-1
16. Tasnim F, Xing J, Huang X, Mo S, Wei X, Tan MH, et al. Generation of mature kupffer cells from human induced pluripotent stem cells. Biomaterials (2019) 192:377–91. doi: 10.1016/j.biomaterials.2018.11.016
17. Povero D, Pinatel EM, Leszczynska A, Goyal NP, Nishio T, Kim J, et al. Human induced pluripotent stem cell-derived extracellular vesicles reduce hepatic stellate cell activation and liver fibrosis. JCI Insight (2019) 5:e125652. doi: 10.1172/jci.insight.125652
18. Watanabe Y, Tsuchiya A, Seino S, Kawata Y, Kojima Y, Ikarashi S, et al. Mesenchymal stem cells and induced bone marrow-derived macrophages synergistically improve liver fibrosis in mice. Stem Cells Transl Med (2019) 8:271–84. doi: 10.1002/sctm.18-0105
19. Rong X, Liu J, Yao X, Jiang T, Wang Y, Xie F. Human bone marrow mesenchymal stem cells-derived exosomes alleviate liver fibrosis through the wnt/β-catenin pathway. Stem Cell Res Ther (2019) 10:98. doi: 10.1186/s13287-019-1204-2
20. He Y, Guo X, Lan T, Xia J, Wang J, Li B, et al. Human umbilical cord-derived mesenchymal stem cells improve the function of liver in rats with acute-on-chronic liver failure via downregulating notch and Stat1/Stat3 signaling. Stem Cell Res Ther (2021) 12:396. doi: 10.1186/s13287-021-02468-6
21. Hu C, Wu Z, Li L. Mesenchymal stromal cells promote liver regeneration through regulation of immune cells. Int J Biol Sci (2020) 16:893–903. doi: 10.7150/ijbs.39725
22. Lou G, Chen Z, Zheng M, Liu Y. Mesenchymal stem cell-derived exosomes as a new therapeutic strategy for liver diseases. Exp Mol Med (2017) 49:e346. doi: 10.1038/emm.2017.63
23. Nishikawa S, Jakt LM, Era T. Embryonic stem-cell culture as a tool for developmental cell biology. Nat Rev Mol Cell Biol (2007) 8:502–07. doi: 10.1038/nrm2189
24. Yamanaka S. Induced pluripotent stem cells: past, present, and future. Cell Stem Cell (2012) 10:678–84. doi: 10.1016/j.stem.2012.05.005
25. Xiang JX, Peng L, Yang LF, Su JB, Zhang XF, Liu XM, et al. Hepatic differentiation of bone marrow mesenchymal stem cells induced by additional growth factors inhibits chronic liver fibrosis. Chin J Tissue Eng Res (2018), 22(33):5286–5291.
26. Pinheiro D, Dias I, Ribeiro Silva K, Stumbo AC, Thole A, Cortez E, et al. Mechanisms underlying cell therapy in liver fibrosis: An overview. Cells (2019) 8:1339. doi: 10.3390/cells8111339
27. Wu R, Fan X, Wang Y, Shen M, Zheng Y, Zhao S, et al. Mesenchymal stem cell-derived extracellular vesicles in liver immunity and therapy. Front Immunol (2022) 13:833878. doi: 10.3389/fimmu.2022.833878
28. Huang B, Cheng X, Wang H, Huang W, la Ga Hu Z, Wang D, et al. Mesenchymal stem cells and their secreted molecules predominantly ameliorate fulminant hepatic failure and chronic liver fibrosis in mice respectively. J Transl Med (2016) 14. doi: 10.1186/s12967-016-0792-1
29. Cheng ML, Nakib D, Perciani CT, MacParland SA. The immune niche of the liver. Clin Sci (Lond) (2021) 135:2445–66. doi: 10.1042/CS20190654
30. Piñeiro-Carrero VM, Piñeiro EO. Liver. Pediatrics (2004) 113:1097–106. doi: 10.1542/peds.113.S3.1097
31. Iannacone M, Guidotti LG. Immunobiology and pathogenesis of hepatitis b virus infection. Nat Rev Immunol (2022) 22:19–32. doi: 10.1038/s41577-021-00549-4
32. Peiseler M, Schwabe R, Hampe J, Kubes P, Heikenwälder M, Tacke F. Immune mechanisms linking metabolic injury to inflammation and fibrosis in fatty liver disease - novel insights into cellular communication circuits. J Hepatol (2022) 77:1136–60. doi: 10.1016/j.jhep.2022.06.012
33. Zhou T, He C, Lai P, Yang Z, Liu Y, Xu H, et al. miR-204-containing exosomes ameliorate GVHD-associated dry eye disease. Sci Adv (2022) 8:j9617. doi: 10.1126/sciadv.abj9617
34. Li A, Guo F, Pan Q, Chen S, Chen J, Liu HF, et al. Mesenchymal stem cell therapy: Hope for patients with systemic lupus erythematosus. Front Immunol (2021) 12:728190. doi: 10.3389/fimmu.2021.728190
35. Wang R, Yao Q, Chen W, Gao F, Li P, Wu J, et al. Stem cell therapy for crohn's disease: systematic review and meta-analysis of preclinical and clinical studies. Stem Cell Res Ther (2021) 12:463. doi: 10.1186/s13287-021-02533-0
36. Zhao J, Li X, Hu J, Chen F, Qiao S, Sun X, et al. Mesenchymal stromal cell-derived exosomes attenuate myocardial ischaemia-reperfusion injury through miR-182-regulated macrophage polarization. Cardiovasc Res (2019) 115:1205–16. doi: 10.1093/cvr/cvz040
37. Chrostek MR, Fellows EG, Crane AT, Grande AW, Low WC. Efficacy of stem cell-based therapies for stroke. Brain Res (2019) 1722:146362. doi: 10.1016/j.brainres.2019.146362
38. Chung JW, Chang WH, Bang OY, Moon GJ, Kim SJ, Kim SK, et al. Efficacy and safety of intravenous mesenchymal stem cells for ischemic stroke. Neurology (2021) 96:e1012–23. doi: 10.1212/WNL.0000000000011440
39. Uccelli A, Laroni A, Ali R, Battaglia MA, Blinkenberg M, Brundin L, et al. Safety, tolerability, and activity of mesenchymal stem cells versus placebo in multiple sclerosis (MESEMS): a phase 2, randomised, double-blind crossover trial. Lancet Neurol (2021) 20:917–29. doi: 10.1016/S1474-4422(21)00301-X
40. Qu Y, Zhang Q, Cai X, Li F, Ma Z, Xu M, et al. Exosomes derived from miR-181-5p-modified adipose-derived mesenchymal stem cells prevent liver fibrosis via autophagy activation. J Cell Mol Med (2017) 21:2491–502. doi: 10.1111/jcmm.13170
41. Pixley JS. Mesenchymal stem cells to treat type 1 diabetes. Biochim Biophys Acta Mol Basis Dis (2020) 1866:165315. doi: 10.1016/j.bbadis.2018.10.033
42. Yao Y, Zheng Z, Song Q. Mesenchymal stem cells: A double-edged sword in radiation-induced lung injury. Thorac Cancer (2018) 9:208–17. doi: 10.1111/1759-7714.12573
43. Chen J, Zhang X, Xie J, Xue M, Liu L, Yang Y, et al. Overexpression of TGFβ1 in murine mesenchymal stem cells improves lung inflammation by impacting the Th17/Treg balance in LPS-induced ARDS mice. Stem Cell Res Ther (2020) 11:311. doi: 10.1186/s13287-020-01826-0
44. Li P, Gong Z, Shultz LD, Ren G. Mesenchymal stem cells: From regeneration to cancer. Pharmacol Ther (2019) 200:42–54. doi: 10.1016/j.pharmthera.2019.04.005
45. Fu X, Liu G, Halim A, Ju Y, Luo Q, Song AG. Mesenchymal stem cell migration and tissue repair. Cells (2019) 8:784. doi: 10.3390/cells8080784
46. Kuntin D, Genever P. Mesenchymal stem cells from biology to therapy. Emerg Top Life Sci (2021) 5:539–48. doi: 10.1042/ETLS20200303
47. Jiang W, Xu J. Immune modulation by mesenchymal stem cells. Cell Prolif (2020) 53:e12712. doi: 10.1111/cpr.12712
48. Prockop DJ. Concise review: Two negative feedback loops place mesenchymal stem/stromal cells at the center of early regulators of inflammation. Stem Cells (2013) 31:2042–46. doi: 10.1002/stem.1400
49. Boumaza I, Srinivasan S, Witt WT, Feghali-Bostwick C, Dai Y, Garcia-Ocana A, et al. Autologous bone marrow-derived rat mesenchymal stem cells promote PDX-1 and insulin expression in the islets, alter T cell cytokine pattern and preserve regulatory T cells in the periphery and induce sustained normoglycemia. J Autoimmun (2009) 32:33–42. doi: 10.1016/j.jaut.2008.10.004
50. Ezquer F, Ezquer M, Contador D, Ricca M, Simon V, Conget P. The antidiabetic effect of mesenchymal stem cells is unrelated to their transdifferentiation potential but to their capability to restore Th1/Th2 balance and to modify the pancreatic microenvironment. Stem Cells (2012) 30:1664–74. doi: 10.1002/stem.1132
51. Deng Y, Zhang Y, Ye L, Zhang T, Cheng J, Chen G, et al. Umbilical cord-derived mesenchymal stem cells instruct monocytes towards an IL10-producing phenotype by secreting IL6 and HGF. Sci Rep (2016) 6:37566. doi: 10.1038/srep37566
52. Loi F, Córdova LA, Zhang R, Pajarinen J, Lin TH, Goodman SB, et al. The effects of immunomodulation by macrophage subsets on osteogenesis in vitro. Stem Cell Res Ther (2016) 7:15. doi: 10.1186/s13287-016-0276-5
53. Lee KC, Lin HC, Huang YH, Hung SC. Allo-transplantation of mesenchymal stem cells attenuates hepatic injury through IL1Ra dependent macrophage switch in a mouse model of liver disease. J Hepatol (2015) 63:1405–12. doi: 10.1016/j.jhep.2015.07.035
54. Vizoso FJ, Eiro N, Cid S, Schneider J, Perez-Fernandez R. Mesenchymal stem cell secretome: Toward cell-free therapeutic strategies in regenerative medicine. Int J Mol Sci (2017) 18:1852. doi: 10.3390/ijms18091852
55. Waterman RS, Tomchuck SL, Henkle SL, Betancourt AM. A new mesenchymal stem cell (MSC) paradigm: polarization into a pro-inflammatory MSC1 or an immunosuppressive MSC2 phenotype. PloS One (2010) 5:e10088. doi: 10.1371/journal.pone.0010088
56. Alfaifi M, Eom YW, Newsome PN, Baik SK. Mesenchymal stromal cell therapy for liver diseases. J Hepatol (2018) 68:1272–85. doi: 10.1016/j.jhep.2018.01.030
57. Zhou R, Li Z, He C, Li R, Xia H, Li C, et al. Human umbilical cord mesenchymal stem cells and derived hepatocyte-like cells exhibit similar therapeutic effects on an acute liver failure mouse model. PloS One (2014) 9:e104392. doi: 10.1371/journal.pone.0104392
58. Tao XR, Li WL, Su J, Jin CX, Wang XM, Li JX, et al. Clonal mesenchymal stem cells derived from human bone marrow can differentiate into hepatocyte-like cells in injured livers of SCID mice. J Cell Biochem (2009) 108:693–704. doi: 10.1002/jcb.22306
59. di Bonzo LV, Ferrero I, Cravanzola C, Mareschi K, Rustichell D, Novo E, et al. Human mesenchymal stem cells as a two-edged sword in hepatic regenerative medicine: Engraftment and hepatocyte differentiation versus profibrogenic potential. Gut (2008) 57:223–31. doi: 10.1136/gut.2006.111617
60. Eom YW, Shim KY, Baik SK. Mesenchymal stem cell therapy for liver fibrosis. Korean J Intern Med (2015) 30:580–89. doi: 10.3904/kjim.2015.30.5.580
61. Harrell CR, Jovicic N, Djonov V, Arsenijevic N, Volarevic V. Mesenchymal stem cell-derived exosomes and other extracellular vesicles as new remedies in the therapy of inflammatory diseases. Cells (2019) 8:1605. doi: 10.3390/cells8121605
62. Hu C, Zhao L, Zhang L, Bao Q, Li L. Mesenchymal stem cell-based cell-free strategies: Safe and effective treatments for liver injury. Stem Cell Res Ther (2020) 11:377. doi: 10.1186/s13287-020-01895-1
63. Liu H, Li R, Liu T, Yang L, Yin G, Xie Q. Immunomodulatory effects of mesenchymal stem cells and mesenchymal stem cell-derived extracellular vesicles in rheumatoid arthritis. Front Immunol (2020) 11:1912. doi: 10.3389/fimmu.2020.01912
64. Harrell CR, Markovic BS, Fellabaum C, Arsenijevic A, Volarevic V. Mesenchymal stem cell-based therapy of osteoarthritis: Current knowledge and future perspectives. BioMed Pharmacother (2019) 109:2318–26. doi: 10.1016/j.biopha.2018.11.099
65. Zhang Y, Cai W, Huang Q, Gu Y, Shi Y, Huang J, et al. Mesenchymal stem cells alleviate bacteria-induced liver injury in mice by inducing regulatory dendritic cells. Hepatology (2014) 59:671–82. doi: 10.1002/hep.26670
66. Fallowfield JA, Mizuno M, Kendall TJ, Constandinou CM, Benyon RC, Duffield JS, et al. Scar-associated macrophages are a major source of hepatic matrix metalloproteinase-13 and facilitate the resolution of murine hepatic fibrosis. J Immunol (2007) 178:5288–95. doi: 10.4049/jimmunol.178.8.5288
67. Yao Y, Xia Z, Cheng F, Jang Q, He J, Pan C, et al. Human placental mesenchymal stem cells ameliorate liver fibrosis in mice by upregulation of Caveolin1 in hepatic stellate cells. Stem Cell Res Ther (2021) 12:294. doi: 10.1186/s13287-021-02358-x
68. Tourkina E, Richard M, Oates J, Hofbauer A, Bonner M, Gööz P, et al. Caveolin-1 regulates leucocyte behaviour in fibrotic lung disease. Ann Rheum Dis (2010) 69:1220–26. doi: 10.1136/ard.2009.117580
69. Ohara M, Ohnishi S, Hosono H, Yamamoto K, Yuyama K, Nakamura H, et al. Extracellular vesicles from amnion-derived mesenchymal stem cells ameliorate hepatic inflammation and fibrosis in rats. Stem Cells Int (2018) 2018:3212643. doi: 10.1155/2018/3212643
70. Qiao H, Zhou Y, Qin X, Cheng J, He Y, Jiang Y. NADPH oxidase signaling pathway mediates mesenchymal stem cell-induced inhibition of hepatic stellate cell activation. Stem Cells Int (2018) 2018:1239143. doi: 10.1155/2018/1239143
71. Su DN, Wu SP, Xu SZ. Mesenchymal stem cell-based Smad7 gene therapy for experimental liver cirrhosis. Stem Cell Res Ther (2020) 11:395. doi: 10.1186/s13287-020-01911-4
72. Luo XY, Meng XJ, Cao DC, Wang W, Zhou K, Li L, et al. Transplantation of bone marrow mesenchymal stromal cells attenuates liver fibrosis in mice by regulating macrophage subtypes. Stem Cell Res Ther (2019) 10:16. doi: 10.1186/s13287-018-1122-8
73. Li T, Yan Y, Wang B, Qian H, Zhang X, Shen L, et al. Exosomes derived from human umbilical cord mesenchymal stem cells alleviate liver fibrosis. Stem Cells Dev (2013) 22:845–54. doi: 10.1089/scd.2012.0395
74. Lai CP, Mardini O, Ericsson M, Prabhakar S, Maguire C, Chen JW, et al. Dynamic biodistribution of extracellular vesicles in vivo using a multimodal imaging reporter. ACS Nano (2014) 8:483–94. doi: 10.1021/nn404945r
75. Ha DH, Kim HK, Lee J, Kwon HH, Park GH, Yang SH, et al. Mesenchymal Stem/Stromal cell-derived exosomes for immunomodulatory therapeutics and skin regeneration. Cells (2020) 9:1157. doi: 10.3390/cells9051157
76. Liu Y, Lin L, Zou R, Wen C, Wang Z, Lin F. MSC-derived exosomes promote proliferation and inhibit apoptosis of chondrocytes via lncRNA-KLF3-AS1/miR-206/GIT1 axis in osteoarthritis. Cell Cycle (2018) 17:2411–22. doi: 10.1080/15384101.2018.1526603
77. Zhang S, Chuah SJ, Lai RC, Hui J, Lim SK, Toh WS. MSC exosomes mediate cartilage repair by enhancing proliferation, attenuating apoptosis and modulating immune reactivity. Biomaterials (2018) 156:16–27. doi: 10.1016/j.biomaterials.2017.11.028
78. Zhang XW, Zhou JC, Peng D, Hua F, Li K, Yu JJ, et al. Disrupting the TRIB3-SQSTM1 interaction reduces liver fibrosis by restoring autophagy and suppressing exosome-mediated HSC activation. Autophagy (2020) 16:782–96. doi: 10.1080/15548627.2019.1635383
79. Gao J, Wei B, de Assuncao TM, Liu Z, Hu X, Ibrahim S, et al. Hepatic stellate cell autophagy inhibits extracellular vesicle release to attenuate liver fibrosis. J Hepatol (2020) 73:1144–54. doi: 10.1016/j.jhep.2020.04.044
80. Liu R, Li X, Zhu W, Wang Y, Zhao D, Wang X, et al. Cholangiocyte-derived exosomal long noncoding RNA H19 promotes hepatic stellate cell activation and cholestatic liver fibrosis. Hepatology (2019) 70:1317–35. doi: 10.1002/hep.30662
81. Chen L, Chen R, Kemper S, Cong M, You H, Brigstock DR. Therapeutic effects of serum extracellular vesicles in liver fibrosis. J Extracell Vesicles (2018) 7:1461505. doi: 10.1080/20013078.2018.1461505
82. Ono R, Yoshioka Y, Furukawa Y, Naruse M, Kuwagata M, Ochiya T, et al. Novel hepatotoxicity biomarkers of extracellular vesicle (EV)-associated miRNAs induced by CCl4. Toxicol Rep (2020) 7:685–92. doi: 10.1016/j.toxrep.2020.05.002
83. Jiang W, Tan Y, Cai M, Zhao T, Mao F, Zhang X, et al. Human umbilical cord MSC-derived exosomes suppress the development of CCl(4)-induced liver injury through antioxidant effect. Stem Cells Int (2018) 2018:6079642. doi: 10.1155/2018/6079642
84. Tamura R, Uemoto S, Tabata Y. Immunosuppressive effect of mesenchymal stem cell-derived exosomes on a concanavalin a-induced liver injury model. Inflammation Regener (2016) 36:26. doi: 10.1186/s41232-016-0030-5
85. Huang KC, Chuang MH, Lin ZS, Lin YC, Chen CH, Chang CL, et al. Transplantation with GXHPC1 for liver cirrhosis: Phase 1 trial. Cell Transplant (2019) 28:100S–11S. doi: 10.1177/0963689719884885
86. Zhang Z, Lin H, Shi M, Xu R, Fu J, Lv J, et al. Human umbilical cord mesenchymal stem cells improve liver function and ascites in decompensated liver cirrhosis patients. J Gastroenterol Hepatol (2012) 27(Suppl 2):112–20. doi: 10.1111/j.1440-1746.2011.07024.x
87. Mohamadnejad M, Alimoghaddam K, Mohyeddin-Bonab M, Bagheri M, Bashtar M, Ghanaati H, et al. Phase 1 trial of autologous bone marrow mesenchymal stem cell transplantation in patients with decompensated liver cirrhosis. Arch Iran Med (2007) 10:459–66. doi: 07104/aim.008
88. Deng Y, Xia B, Chen Z, Wang F, Lv Y, Chen G. Stem cell-based therapy strategy for hepatic fibrosis by targeting intrahepatic cells. Stem Cell Rev Rep (2022) 18:77–93. doi: 10.1007/s12015-021-10286-9
89. Li M, Jiang Y, Hou Q, Zhao Y, Zhong L, Fu X. Potential pre-activation strategies for improving therapeutic efficacy of mesenchymal stem cells: current status and future prospects. Stem Cell Res Ther (2022) 13:146. doi: 10.1186/s13287-022-02822-2
90. Yang X, Han ZP, Zhang SS, Zhu PX, Hao C, Fan TT, et al. Chronic restraint stress decreases the repair potential from mesenchymal stem cells on liver injury by inhibiting TGF-β1 generation. Cell Death Dis (2014) 5:e1308. doi: 10.1038/cddis.2014.257
91. Kim MD, Kim SS, Cha HY, Jang SH, Chang DY, Kim W, et al. Therapeutic effect of hepatocyte growth factor-secreting mesenchymal stem cells in a rat model of liver fibrosis. Exp Mol Med (2014) 46:e110. doi: 10.1038/emm.2014.49
92. Lou G, Yang Y, Liu F, Ye B, Chen Z, Zheng M, et al. MiR-122 modification enhances the therapeutic efficacy of adipose tissue-derived mesenchymal stem cells against liver fibrosis. J Cell Mol Med (2017) 21:2963–73. doi: 10.1111/jcmm.13208
93. Liu Y, Dong Y, Wu X, Xu X, Niu J. The assessment of mesenchymal stem cells therapy in acute on chronic liver failure and chronic liver disease: A systematic review and meta-analysis of randomized controlled clinical trials. Stem Cell Res Ther (2022) 13:204. doi: 10.1186/s13287-022-02882-4
94. Li C, Zhao H, Cheng L, Wang B. Allogeneic vs. autologous mesenchymal stem/stromal cells in their medication practice. Cell Biosci (2021) 11:187. doi: 10.1186/s13578-021-00698-y
95. Shi M, Li YY, Xu RN, Meng FP, Yu SJ, Fu JL, et al. Mesenchymal stem cell therapy in decompensated liver cirrhosis: A long-term follow-up analysis of the randomized controlled clinical trial. Hepatol Int (2021) 15:1431–41. doi: 10.1007/s12072-021-10199-2
96. Mebarki M, Abadie C, Larghero J, Cras A. Human umbilical cord-derived mesenchymal stem/stromal cells: A promising candidate for the development of advanced therapy medicinal products. Stem Cell Res Ther (2021) 12:152. doi: 10.1186/s13287-021-02222-y
97. Zhao J, Yu G, Cai M, Lei X, Yang Y, Wang Q, et al. Bibliometric analysis of global scientific activity on umbilical cord mesenchymal stem cells: A swiftly expanding and shifting focus. Stem Cell Res Ther (2018) 9:32. doi: 10.1186/s13287-018-0785-5
98. Truong NH, Nguyen NH, Le TV, Vu NB, Huynh N, Nguyen TV, et al. Comparison of the treatment efficiency of bone marrow-derived mesenchymal stem cell transplantation via tail and portal veins in CCl4-induced mouse liver fibrosis. Stem Cells Int (2016) 2016:5720413. doi: 10.1155/2016/5720413
Keywords: liver fibrosis, mesenchymal stem cell, immunomodulatory effects, exosome, antifibrosis
Citation: Liu P, Qian Y, Liu X, Zhu X, Zhang X, Lv Y and Xiang J (2023) Immunomodulatory role of mesenchymal stem cell therapy in liver fibrosis. Front. Immunol. 13:1096402. doi: 10.3389/fimmu.2022.1096402
Received: 12 November 2022; Accepted: 15 December 2022;
Published: 04 January 2023.
Edited by:
Jinhang Gao, Sichuan University, ChinaCopyright © 2023 Liu, Qian, Liu, Zhu, Zhang, Lv and Xiang. This is an open-access article distributed under the terms of the Creative Commons Attribution License (CC BY). The use, distribution or reproduction in other forums is permitted, provided the original author(s) and the copyright owner(s) are credited and that the original publication in this journal is cited, in accordance with accepted academic practice. No use, distribution or reproduction is permitted which does not comply with these terms.
*Correspondence: Junxi Xiang, eGp4NzIyQDE2My5jb20=; Yi Lv, bHV5aTE2OUAxMjYuY29t