- 1University of Bristol, Bristol, United Kingdom
- 2Dongfang Hospital, Beijing University of Chinese Medicine, Beijing, China
- 3Tianjin Nankai Hospital, Tianjin, China
Inflammatory bowel diseases, including Crohn’s disease and ulcerative colitis, is a chronic relapsing gastrointestinal inflammatory disease mediated by dysregulated immune responses to resident intestinal microbiota. Current conventional approaches including aminosalicylates, corticosteroids, immunosuppressive agents, and biological therapies are focused on reducing intestinal inflammation besides inducing and maintaining disease remission, and managing complications. However, these therapies are not curative and are associated with various limitations, such as drug resistance, low responsiveness and adverse events. Recent accumulated evidence has revealed the involvement of mucin-degrading bacterium Akkermansia muciniphila (A. muciniphila) in the regulation of host barrier function and immune response, and how reduced intestinal colonisation of probiotic A. muciniphila can contribute to the process and development of inflammatory bowel diseases, suggesting that it may be a potential target and promising strategy for the therapy of inflammatory bowel disease. In this review, we summarise the current knowledge of the role of A. muciniphila in IBD, especially focusing on the related mechanisms, as well as the strategies based on supplementation with A. muciniphila, probiotics and prebiotics, natural diets, drugs, and herbs to promote its colonisation in the gut, and holds promise for A. muciniphila-targeted and -based therapies in the treatment of inflammatory bowel disease.
1. Introduction
Inflammatory Bowel Disease (IBD), an immunological disorder of the intestinal tract which mainly consists of two clinical phenotypes: ulcerative colitis (UC) and Crohn’s disease (CD), has become a global health problem seriously affecting the quality of life of patients (1, 2). Classic strategies including aminosalicylates, corticosteroids, immunosuppressive agents, and biological therapies to treat IBD are focused on decreasing intestinal mucosal inflammation, inducing and extending disease remission, and managing complications (3, 4). However, these approaches are not curative and are associated with various limitations, such as drug resistance, low responsiveness and adverse events (5). It is, therefore, of fundamental importance to explore the pathogenesis of IBD and develop novel and safe therapeutic strategies.
The intestinal microbiota is a complex and dynamic ecosystem harbouring trillions of microbes that reside in the human intestine and plays a fundamental role in maintaining human health (6). This dynamic interaction between the microbiota and host contributes to the maintenance of the gut epithelial barrier and development and maturation of host immune system (7). Accumulated evidence suggests that intestinal dysbiosis may cause dysregulated mucosal immune responses leading to the onset of IBD in genetically susceptible (8). Therefore, targeted regulation of the composition and function of gut microbiota via direct supplement of some specific strains, drug intervention, or dietary structure adjustment plays an indispensable role in maintaining the homoeostatic immune responses and is now considered a valuable therapeutic approach to treat IBD patients. However, how to regulate gut microflora and which specific intestinal strains to regulate have not been clarified. Probiotics such as Lactobacillus or Bacillus cereus have been widely used in the prevention and treatment of IBD. However, a large number of studies show that these probiotics are only used as adjunctive therapy of first-line drugs, and their effectiveness needs to be further evaluated and optimised. Improper usage of probiotics might not only exacerbate existing conditions, but also take advantage of the weak host immunity and cause life-threatening pneumonia, sepsis or gut barrier impairments. A previous review of probiotics revealed that, despite its wide usage, lactobacilli infection poses a considerable threat, with a mortality rate of 48% within one year after infection onset (9). Some strains of lactobacilli such as L. rhamnousus have been associated with a high chance of translocation, potentially leading to fatal conditions including human sepsis (10). Thus, the extrapolations of the effects of probiotics need to be carefully scrutinised, and the search for safer, more effective probiotic treatments is urgently needed. Recent studies have revealed the involvement of mucin-degrading bacterium Akkermansia muciniphila (A.muciniphila) in the regulation of host barrier function and immune response (11), suggesting that it may act as a novel functional microbe with probiotic properties and play an important role in human health and diseases. A. muciniphila, a gram-negative bacterium and oval anaerobe which has been proposed as a novel functional microbe with probiotic properties, is initially isolated from human faeces in 2004 (12). However, recent studies have found that A. muciniphila is not absolutely anaerobic and can tolerate a small amount of oxygen, as evidenced by its yield and growth rate could increase under micro aerobic conditions (13). As a unique representative strain of the phylum Verrucomicrobia, A. muciniphila can be easily detected in phylogenetic and metagenome studies. The abundance of A. muciniphila in the human intestinal tract represents 1-4% of the total faecal microbiota, and will change with age, diet, body mass and immune state during a lifetime (14, 15). A. muciniphila is known to colonise the mucosal layer of the human intestine by utilising the glycoprotein mucin as its direct source of carbon and nitrogen (16), which means it has a unique survival advantage, that is, it can still survive during nutrient deficiency and under harsh conditions (17). With the characteristics of mucosal degradation, A. muciniphila has the function of regulating the intestinal barrier and immune response, and plays an important role in human health and diseases. Reduced levels of A. muciniphila have been observed in patients with IBD, indicating that it may contribute to the process and development of inflammatory bowel diseases, and maybe a potential target and promising strategy for the therapy of inflammatory bowel disease (18). However, the exact role and specific mechanisms of A. muciniphila in inflammatory bowel disease require further investigation.
Here, we reviewed the latest research and summarised the current knowledge of the role of A. muciniphila in IBD. This review reveals an interesting phenomenon that not only viable A. muciniphila, but also pasteurised A. muciniphila, and its ingredients such as AmEVs, Amuc_1100 and P9 also can alleviate intestinal inflammation, suggesting a therapeutic potential of A. muciniphila in the treatment of IBD. The protective role of A. muciniphila and its ingredients in IBD mainly involves immune regulation and enhanced barrier function, modulation of gut dysbiosis and colonisation against other pathogens. Moreover, we summarised the strategies based on supplementation with A. muciniphila, probiotics and prebiotics, natural diets, drugs, and herbs to promote its colonisation in the gut, and hold promise for A. muciniphila-targeted and -based therapies in the treatment of IBD.
2. Relationship between A. muciniphila and inflammatory bowel disease
2.1. Insights from clinical studies
Accumulated evidence has revealed a significant reduction in A. muciniphila in both faecal samples and mucosal biopsies of patients with IBD. The research from Png et al. showed a significant increase in the total abundance of mucosa-associated bacteria, while the numbers of A. muciniphila were reduced by mean 92-fold and 172-fold in noninflamed and inflamed UC, respectively, and 14.8-fold in inflamed CD, suggesting that A. muciniphila may have a potential protective or anti-inflammatory role in health (19). Earley et al. quantitatively analysed the amount of A. muciniphila in mucus brushings of the colonic mucus. They found a significant reduction in all four areas of the colon, caecum, transverse colon, left colon and rectum from patients with active UC compared to quiescent UC and healthy controls. In addition, the abundance in quiescent UC returns towards levels observed in the healthy colon, which strongly demonstrates an inverse relationship between A. muciniphila and intestinal inflammation (18). They further revealed a positive association between A. muciniphila abundance and the percentage of sulphated mucin in the mucus gel layer. James et al. collected the total faecal output of patients with UC in remission, and assessed faecal microbial abundances by quantitative PCR. The result showed that faeces from patients with UC had more diverse microbiota in their Clostridium cluster XIVa and a lower proportion of A. muciniphila (20). L.K. Vigsnæs et al. investigated the alterations in the composition of the gram-negative bacterial population in patients either with UC in remission or with active disease, and in healthy controls and found a significantly lower density of A. muciniphila in UC patients with active disease, but no significant difference between the healthy controls and the UC patients in remission, suggesting a possibility that reduction of A. muciniphila may play a role in the development of UC (21). Rajilić-Stojanović et al. also found that faeces of patients with UC in remission showed a significant reduction (approximately 5-fold) in the abundance of A. muciniphila (22). Wang L et al. comfirmed that A. muciniphila in stool samples was significantly reduced in patients with UC (23). Paediatric studies were mostly consistent with the above findings, and Dunn et al. reported that CD patients aged 10 to 16 had lower microbial diversity than the healthy controls, and the abundance of A. muciniphila was lower in patients who did not achieve sustained remission than those who did (24).
However, there are also a few studies that showed contradictory results. Lopez-Siles and colleagues found no difference in A. muciniphila across different disease states, whether it is UC, CD or colorectal cancer (CRC), although CD patients whose disease onset were below 16 years of age observed a marked depletion of this species (25). Moreover, the relative representation of A. muciniphila was found to increase in CD patients according to Danilova et al. (26). Thus, the role of A. muciniphila in IBD still requires further investigations. Table 1 demonstrates the overview of clinical studies of A. muciniphila related to inflammatory bowel disease.
2.2. Insights from animal studies
Similar to human clinical subjects, preclinical reports have also shown that the amount of A. muciniphila from animals with experimental colitis is negatively correlated with the severity of the disease. Previous studies showed that the abundance of A. muciniphila was significantly decreased in experimental colitis mice. Alrafas et al. investigated the gut microbial composition in BALB/c mice with 2,4,6-trinitrobenzene sulfonic acid (TNBS) -induced colitis by analysing the caecal flush from these mice (27). They reported that Bacteroides acidifaciens, a species that could lead to the aggravation and development of colitis, has significantly increased in colitis, whereas A. muciniphila has markedly decreased. The reduction in A. muciniphila suggested its possible role in maintaining gut homeostasis and that the restoration of this species may contribute to alleviating microbial dysbiosis due to IBD. Wang and colleagues agreed with the above conclusion, and they analysed the faecal samples of C57BL/6 mice with DSS-induced colitis, and although the animal model was different, they also found that the abundance of A. muciniphila experienced a marked decreased in colitis (24). Mice with chronic restraint stress and DSS collectively induced colitis also showed marked a decrease in A. muciniphila but an increase in the proportion of Parabacteroides, Erysipelatoclostridium and Enterococcus, suggesting a dysbiosis in gut microbiota after disease induction (28). Studies on epithelium-specific autophagy-related 5 (Atg5) knockout mice, a strain that is innately susceptible to colitis, were also consistent with the aforementioned animal or clinical studies: inflammation-controlling A. muciniphila was reduced, while pro-inflammatory bacteria (e.g., Candidatus Arthromitus) and potential pathogens observed an increase (29).
However, a small number of mice studies, especially those focusing on mice with genetic mutations, have shown inconsistent or even contradictory results. Mahoro et al. did not observe any significant difference in A. muciniphila abundance between SD rats with or without DSS treatment (30), while colonic mucosal samples from female C57BL/6 mice with DSS-induced colitis even showed higher A. muciniphila abundance than their counterparts in the control group according to Håkansson’s team (31). Furthermore, investigations on the faecal samples from two genetically colitis-prone mice, namely IL10-/- and Winnie-/- mice, both witnessed more abundant A. muciniphila than their wild-type littermates (32, 33). Cyp27b1-/- mice with 1,25(OH)2D3 deficiency is another colitis-prone strain that develops colonic inflammation at the age of 8-10 month. Zhu et al. confirmed that A. muciniphila was significantly enriched in Cyp27b1-/- mice, and supplementation with 1,25(OH)2D3 could reduce A. muciniphila abundance. Interestingly, while this results from experiment indicate that 1,25(OH)2D3 limits the colonisation of A. muciniphila, vitamin D deficiency has been shown to reduce the level of A. muciniphila in wild type BALB/c and C57BL/6 mice fed with high-fat diet (34, 35). The authors suggest that this discrepancy might be due to difference in mouse models (36), suggesting that genotypes have to be taken into consideration when studying the role of A. muciniphila in health and in disease states.
It should be noted that the strain-specific properties of A. muciniphila should also be taken into consideration. Zhai et al. investigated and compared the characteristics of A. mucinipihila type strain ATCC BAA-835 and murine A. muciniphiola strain 139 and reported interesting results. Both strains exhibited anti-inflammatory effects including inhibiting IL-8 production by TNF-α-stimulated, co-cultured HT-29 cells in in-vitro anti-inflammatory test. However, these two strains functioned differently in DSS-induced colitis mice models. While administrations of both strains alleviated colitis symptoms by downregulating pro-inflammatory cytokines, only the DSS+ATCC group observed a significant reduction of spleen weight and inflammation index in comparison to the DSS group. DSS+139 group. In addition, the DSS+ATCC group was the only group whose histological score recovered to the level of the healthy control group. On the other hand, while recovery from chronic colitis was also accelerated in the DSS+139 group, the results were not significant (37). Overview of animal studies of A. muciniphila related to inflammatory bowel disease is shown in Table 2.
3. The role of A. muciniphila in inflammatory bowel disease
3.1. Immunomodulatory properties
Gut microbial structure can regulate host metabolism and homeostasis by influencing the immune system, thereby regulating intestinal endocrine system and preventing pathogen overgrowth. Previous studies suggested that A. muciniphila can reduce the level of colon infiltrating macrophages and cytotoxic T lymphocytes (CTLs) to alleviate colitis (24), but increase the number of anti-inflammatory regulatory T (Treg) cells in high-fat diet-fed mice (38). The increase in Tregs may be due to the elevation in short-chain fatty acids (SCFAs; e.g., acetate, propionate, iso-buturate, iso-valeric and valeric) in the host gut following promoted A. muciniphila, which activates G protein-coupled receptor 43 (GPR43) and GPR41, thereby promoting Foxp3+Tregs in the host colon, relieving colitis and changing the composition of the gut microbiota (39). The conversion from CD4+T cells (CD3+CD4+) to Foxp3+ Treg was also promoted in mice treated with A. muciniphila (37). While the proportion of dendritic cells infiltrating mLNs did not significantly change, the mean fluorescence intensity of antigen-presenting molecule MHC-II on CD11b-CD103+ dendritic cell subpopulation was reduced in their study, indicating a decrease in activities of these subpopulations. Katiraei et al. further explored the immunomodulatory effects of A. muciniphila in mice. They revealed that A. muciniphila increased the total B cell population without changing the percentage of follicular or mucosal B cells in mesenteric lymph nodes (mLN), whereas total T cell and total neutrophil populations observed a marked decrease (40). Ansaldo et al. also studied the intestinal adaptive immune response induced by A. muciniphila and demonstrated that A. muciniphila triggers the production of IgG1 antibodies and induces antigen-specific T cell responses in mice, promoting the differentiation from naïve T cells to T follicular helper cells (41).
Interestingly, both pasteurised A. muciniphila and Amuc_1100, a specific outer membrane protein of the bacterium, relieved colitis symptoms, including colon shortening, colon injuries, inflammatory cell infiltration and impaired barrier functions. Flow cytometry analysis supported these findings, showing pronounced downregulation of pro-inflammatory cytokines such as TNF-α, IFN-γ, IL-1β, IL-6, IL-18 and IL-33. Specifically, CD16/32+ macrophages and CD8+ CTLs in the colon, as well as the level of CD16/32+ macrophages in the spleen and mesenteric lymph nodes were reduced in colitis mice (23). Hippala et al. reported that A. muciniphila increased the release of anti-inflammatory cytokine IL-10, although it also induces a low-level pro-inflammatory IL-8, which may be beneficial as it keeps the host immune system alerted (42). Ottman et al. backed their results by revealing that Amuc_1100 can activate Toll-like receptor2, which then leads to the production of IL-10 (43). Beyond improving colitis, Amuc_1100 also triggers immune responses that delay tumour formation and inhibit tumour growth. γH2AX or Ki67 expression were both reduced after pasteurised A. muciniphila or Amuc_1100 administration, indicating that these treatments may attenuate double-stranded DNA breaks and inhibit cell proliferation, respectively (23).
However, A. muciniphila has also been reported to induce colitis in germ-free IL10-deficient mice, indicating that A. muciniphila is not an elixir for IBD under all circumstances. A. muciniphila caused increased bacterial translocation, while both viable and heat-killed increased the production of cytokines (IL-6 and IL-12p40) of IL10-/- mice bone-marrow-derived macrophages. Further analysis suggests that the immunostimulatory effect of A. muciniphila in these genetically susceptible mice was not due to its proteins or nucleic acid, but its polysaccharide. Therefore, potential A. muciniphila-based therapy should only be taken after evaluating the genotypes and disease states of the subject (32).
3.2. Intestinal mucosal barrier function
Mucus layer thickness is an essential measure of intestinal permeability that is decreased in diet-induced obesity and IBD. Secreted by the goblet cells, intestinal mucus consists of about 20% protein and 80% carbohydrates, making it a substrate and source of nutrients for A. muciniphila. Gut mucus can be further divided into two sections, an inner layer with the absence of bacteria, and a thicker layer inhabited by commensal bacteria (44). The mucus forms a physical barrier between the gut and noxious agents and microorganisms, preventing them from directly contacting the epithelial cells and potentially entering the circulation. Thinner mucus layers and increased gut permeability lead to the translocation of lipopolysaccharide (LPS) from the intestine to circulation, consequently resulting inflammation and metabolic endotoxemia. A number of studies have shown that A. muciniphila can improve mucus thickness and thus gut barrier integrity. Our previous study revealed that A. muciniphila colonisation in mice promotes goblet cells, which were significantly reduced due to C. rodentium infection-induced colitis (45). Everard et al. showed that oral administration of viable or pasteurised A. muciniphila could significantly increase the population of mucin-secreting goblet cells, thereby restoring the 46% loss of mucus during obesity (46). Shin et al. proposed that increased goblet cells was resulted from A. muciniphila stimulating mucus turnover rate (38). As A. muciniphila degrades mucin into SCFAs, goblet cells’ preferable source of energy, mucin synthesis can be significantly boosted. Conversely, the amount of mucin can influence the abundance of A. muciniphila. Earley et al. hypothesised that the reduced abundance of A. muciniphila, as observed in UC patients, is resulted from a lack of sulphomucin, a substrate of A. muciniphila. Their hypothesis was supported by the observed association between inflamed mucosa and reduced sulphomucin in acute UC patients (18). However, the lack of sulphomucin is unlikely the sole contributor, as altered glycosylation and reduced MUC2 mucin may also have an impact on microbial survival (47). In line with these observations, the results of a previous study from our team also showed that colonisation of mice with A. muciniphila displayed markedly increased numbers of goblet cells in colons, resulted in upregulated expressions of gene encoding mucin, including muc1, muc5, and muc13. These results suggest a protective effect of A. muciniphila on colitis involved regulation of the mucus barrier in the gut (46).
Another study on A. muciniphila extracellular vesicles (AmEVs), a bilayer structure composed of lipid, protein, lipopolysaccharides as well as other molecules, showed the ability to regulate intestinal barrier permeability by altering tight junction protein expression. The three major tight junction proteins responsible for maintaining barrier integrity, namely occludin, zonal occludins and claudin-5, were promoted after AmEVs administration, thus alleviating the reduction of these proteins during high fat diet (HFD) treatment (48). This improvement is likely to result from Amuc_1100 activating TLR2, a receptor responsible for regulating a wide range of tight junction proteins including occludin (49). Their conclusion is evidenced by in vitro cell cultures: Caco-2 cell barrier integrity was improved by AmEVs via AMPK activation, which in turn may be promoted by increasing SCFA levels as suggested by previous studies (50). Li et al. also reported that occludin and ZO-1 were up-regulated in Apoe-/- mice as A. muciniphila prevented the Western diet- induced inflammation and endotoxemia (51).
3.3. Modulation of gut dysbiosis and colonisation against other pathogens
A number of studies have showed that administrations of A. muciniphila can influence the diversity and number of gut microbiota, which are altered in IBD. Suspected pathogenic bacterial agents for IBD include Escherichia coli and the Helicobacter species, and IBD is also accompanied by reductions of beneficial species, such as Lactobacillus, Bifidobacterium and A. muciniphila itself (52, 53). Bian et al. reported a positive correlation between A. muciniphila administration upregulated cytokine IL-10, which in turn and was positively correlated with a number of species that was promoted by A. muciniphila (e.g., Verrucomicrobia, Akkermansia and Ruminococcaceae) (54). On the other hand, Bacteroidetes, a harmful species associated with colitis progression was reduced by A. muciniphila administration.
In addition to promoting preferred bacteria, A. muciniphila may also inhibit the harmful effects of unwanted bacteria in the colon. Recent analysis of A. muciniphila genome suggested that A. muciniphila might produce cysteine by utilising hydrogen sulphide. Hydrogen sulphide is the end product of sulphate-reducing bacteria, and both are involved in the pathogenesis of chronic bowel diseases including UC (55). Hydrogen sulphide is also a potent genotoxin that may be associated with colorectal cancer (56). With A. muciniphila consuming hydrogen sulphide, it may partially limit the toxicity of sulphate-reducing bacteria to the host (42). Moreover, A. muciniphila augmented the expression of RegIIIγ, the primary anti-microbial peptides produced by Paneth cells. RegIIIγ, along with other anti-microbial peptides, participate in maintaining the innate immunity of the gut barrier as well as the gut microbiota composition (47). qRT-PCR analysis from our previous studies further evidenced the promoting effect of A. muciniphila on antimicrobial peptides: RegIIIγ and CRAMP were both upregulated, and both of which contributes to the protection against pathobionts infection. A. muciniphila also increased IL-22 expression, which has been suggested to augment mucus and antimicrobial proteins expression and subsequently facilitate the dissemination of pathogenic bacteria in the host gut (46). The role of A. muciniphila in IBD is shown in Figure 1.
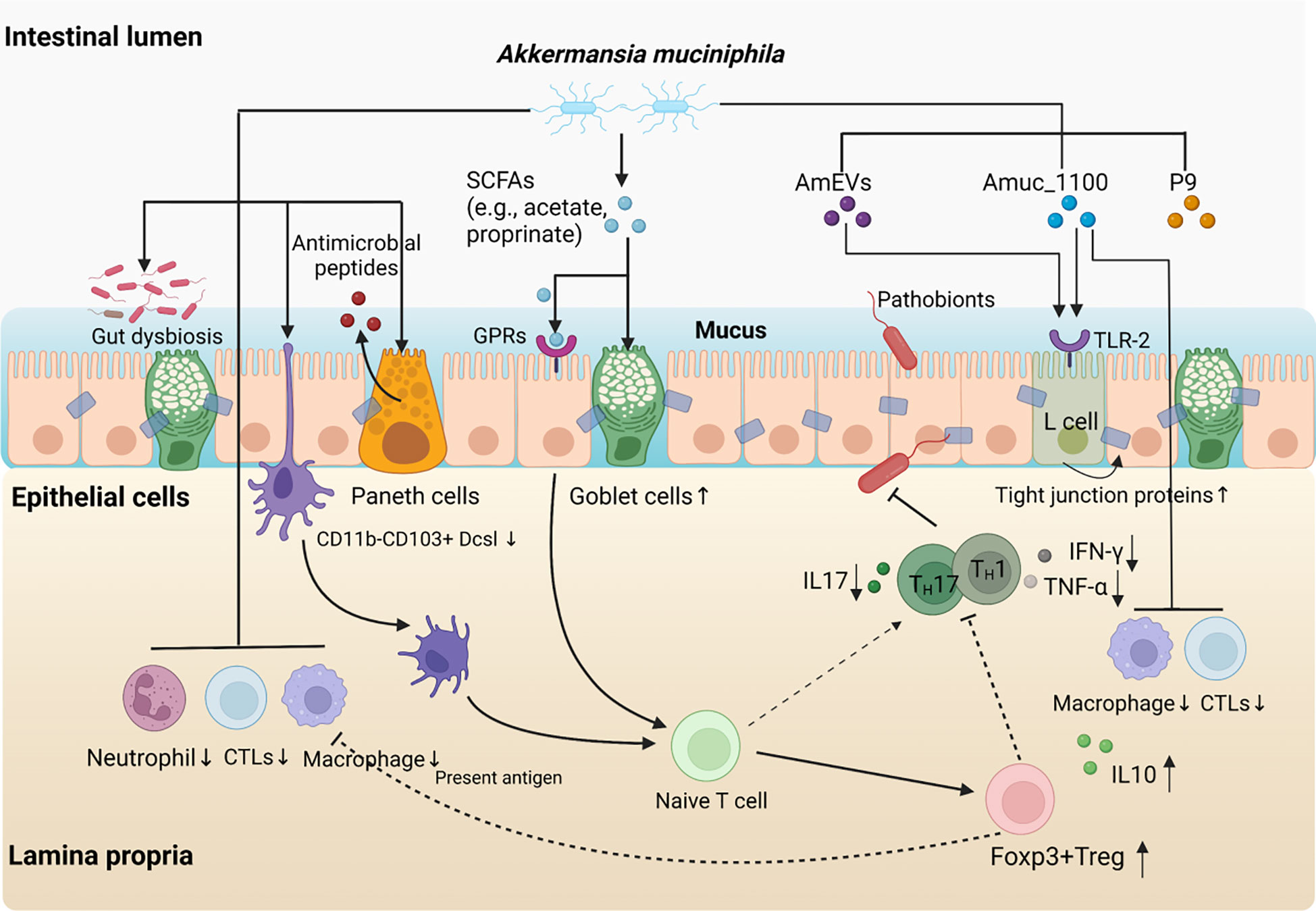
Figure 1 The role of A. muciniphila in inflammatory bowel disease. Supplement with A. muciniphila reduces the levels of colonic infiltrating macrophages and cytotoxic T lymphocytes (CTLs) and relieves intestinal inflammation. The protective effects of A. muciniphila on intestinal homeostasis are associated with increased numbers of goblet cells, enhanced mucus barrier and anti-microbial responses. A. muciniphila supplement partially attenuates gut dysbiosis and resist the colonisation against other pathogens. Moreover, A. muciniphila derived SCFAs promoted the conversion from Naïve T cells to Foxp3+Treg cells through GPRs signal pathway, which may be accompanied by reduced Th1/17 cell-mediated inflammatory response. Interestingly, both pasteurised A. muciniphila and Amuc_1100, a specific outer membrane protein of the bacterium also play a similar role as A. muciniphila itself in immune protection and inhibit the infiltration macrophages and CTLs. Furthermore, A. muciniphila-derived extracellular vesicles (AmEVs), a bilayer structure composed of lipid, protein, lipopolysaccharides as well as other molecules, and Amuc_1100 also regulate intestinal barrier permeability by altering tight junction protein expression through activating TLR2 pathway.
4. Strategies to promote abundance of Akkermansia muciniphila
Given that A. muciniphila is negatively correlated with IBD and plays an important role in maintaining homeostasis of intestinal barrier and mucosal immunity, we believe that the elevated abundance of A. muciniphila in the gut microenvironment via direct supplement of A. muciniphila and its components, indirect interventions such as derivatives, drugs, dietary or herbs may be a novel and promising strategies for the treatment of IBD. Here, we summarised these strategies, discussed their potential as therapeutic reagents, and holds promise for A. muciniphila-targeted and -based therapies in the treatment of IBD (Tables 3, 4).
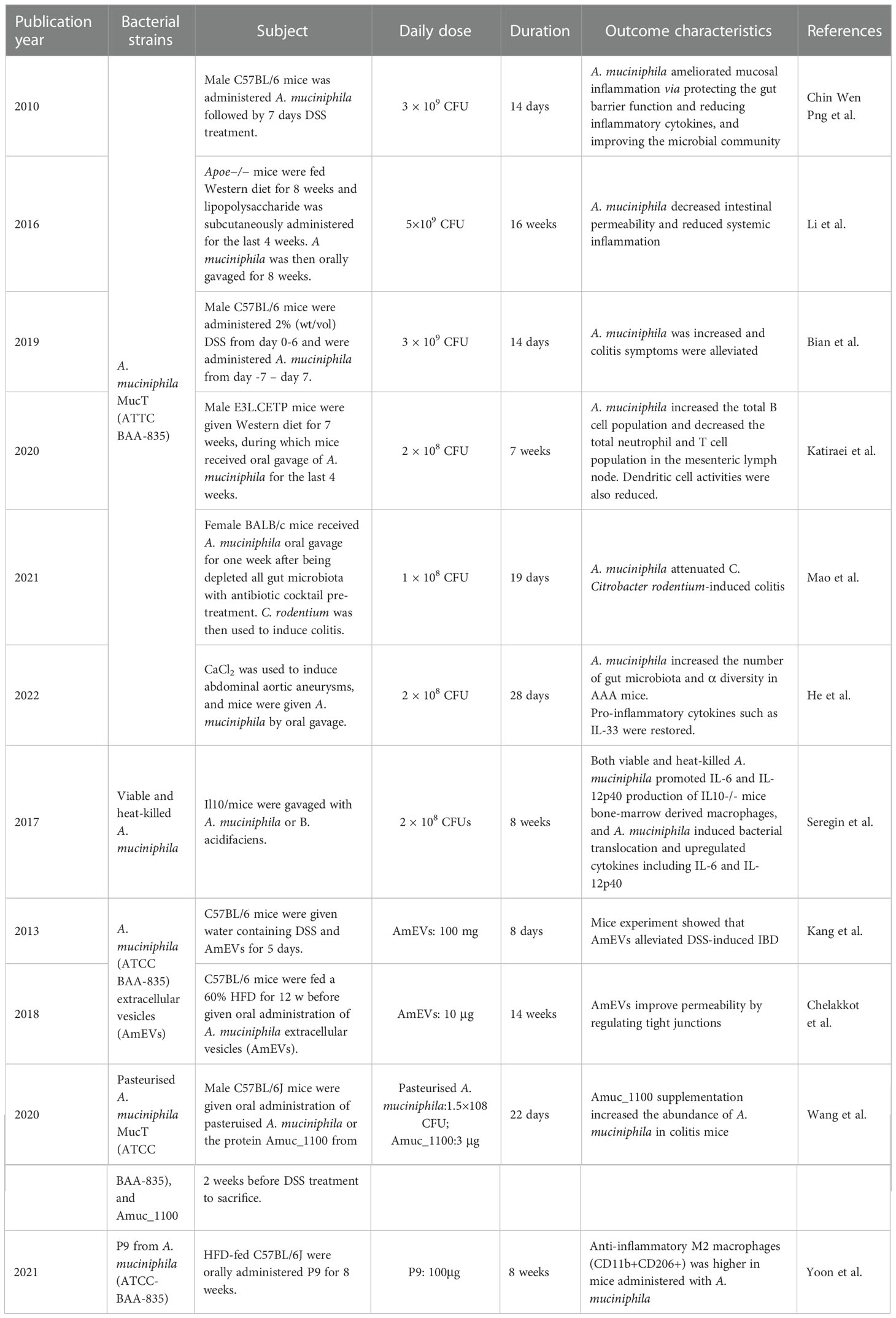
Table 3 Pre-clinical studies on the administration of the A. muciniphila and its components for protection against inflammatory bowel disease.
4.1. Supplementation with viable A. muciniphila
A. muciniphila has been isolated, identified and studied for almost 20 years, yet very few human studies on direct A. muciniphila supplementation are available at the current stage, especially for patients with IBD. However, several mice intervention studies have been reported and all of them showed a significant protection of A. muciniphila against intestinal inflammation. Bian et al. did a similar study focusing on viable A. muciniphila, separating their mice into three groups, namely the control group (CP), experimental group (DP) and A. muciniphila group (AKK). 2% (wt/vol) DSS was dissolved in the drinking water of DP and AKK group for 7 days (day 0-6) before changing back to normal water. A. muciniphila was administered solely to AKK group via oral gavage for 14 consecutive days (day -7 to day 7). Their results also support A. muciniphila’s modulative role on barrier function by showcasing the alleviation of DSS-induced colitis symptoms (e.g., weight loss, colon shortening) after A. muciniphila administration on mice (54). A. muciniphila improved gut microbial composition by promoting species such as Akkermansia and Rikenellaceae, although there are also some inconsistent results from other studies showing that A. muciniphila treatment did not change the gut microbiota profile (other than A. muciniphila itself) (53). Similar experimental design was adapted to test the effect of a range of A. muciniphila concentration and on different mice models: lower daily dose of A. muciniphila (1× 109 - 2× 109 CFU) was proved to relieve colitis in BALB/c and AAA mice, separately (45, 53).At the meantime, APOE-/- and E3L.CETP mice given Western diet witnessed a reduction in intestinal permeability and systemic inflammation after 8 weeks and 4 weeks of A. muciniphila supplementation, respectively, as indicated by the studies of Li et al. and Katiraei et al. (40, 51).
4.2. Supplementation with pasteurised or heat-killed A. muciniphila
In addition to viable A. muciniphila, pasteurised and heat-killed A. muciniphila as well as Amuc_1100 are now gaining increasing interests of research to test their abilities in regulating gut microflora and mucosal functions. In a study conducted by Everard et al., mice fed with control or high fat diet were treated with either A. muciniphila MucT (ATTC BAA-835 (2.108 cfu/0.2mL) or heat-killed A. muciniphila via oral gavage. Mice caecal and colonic content witnessed 100-fold more viable bacteria of interest in the A. muciniphila-treated group (9.5 ± 1.02 log10 cells/mg of content) than their counterparts from the high fat and heat-killed bacteria group (6.8 ± 0.51 log10 cells/mg of content). Heat-killed A. muciniphila also lost their ability to restore colonic mucus impairments or metabolic defects triggered by high-fat diet and obesity (46). While viable A. muciniphila counteracted high-fat diet-induced metabolic endotoxemia and reduced mucus layer thickness, heat-killed A. muciniphila failed to make any significant improvements. These findings further evidenced that A. muciniphila has to be alive to exert its regulatory effect on the mucosal barrier. Wang et al. investigated the effects of pasteurisation-inactivated A. muciniphila on blunting colitis development as well as the associated tumorigenesis. 1.5×108 CFU pasteurised A. muciniphila was administered to colitis or colorectal cancer mice models established using DSS and azoxymethane. qPCR, 16S rRNA sequencing, and flow cytometry results confirmed that pasteurised A. muciniphila positively influenced hosts’ immune system by reducing infiltrating macrophages, decreased proportions of CD8+ cytotoxic T lymphocytes in the colon and relieved ameliorated colitis in mice. These data indicated that pasteurised A. muciniphila provides potential prevention and treatment strategies for colitis as well as other IBDs (23).
4.3. The outer membrane protein Amuc_1100, A. muciniphila-derived extracellular vesicles and A. muciniphila-secreted protein P9
In addition to viable or heat-killed A. muciniphila, emerging evidence shows that the outer membrane protein Amuc_1100, bacteria-derived extracellular vesicle (AmEVs) and A. muciniphila-secreted protein (P9) also play important roles in preventing and/or treating IBD. To test the effects of Amuc_1100 on IBD, Wang et al. gave DSS-induced colitis model mice oral administration of 3μg Amuc_1100 from 2 weeks before DSS treatment to sacrifice. Analysis on stool and colon samples showed that significantly alleviated disease activity index and attenuated colonic histological injuries and inflammations. Interestingly, Amuc_1100 also downregulated pro-inflammatory cytokines including TNF-α and IFN-γ, suggesting an anti-inflammatory effect in the host gut (Wang et al., 2019). Extracellular vesicles are spherical structures consisting of lipid bilayers. They are released by bacteria and are capable of entering the host’s systemic circulation and even delivering their components into host cells. Thus, these structures can induce immunological and metabolic responses, and consequently interact with gut microbiota or the host (48, 57). Kang et al. reported that oral administration of AmEVs ameliorated symptoms of colitis including weight loss, colon shortening and inflammatory cell infiltration of gut barrier (57). In vitro experiments conducted on colon carcinoma cells showed that AmEVs application can reduce the secretion of pro-inflammatory cytokine IL-6 from colon epithelial cells, providing a possible explanation for AmEVs’ mechanism in regulating intestinal immunity and homeostasis. Their findings were supported by Chelakkot and colleagues, who reported that AmEVs improved gut barrier integrity and reduced intestinal epithelial layer damage from high fat diet (48). Despite the effects of AmEVs, it remains unclear whether AmEVs contain Amuc_1100 or other confounding factors that may exert an effect on gut immunity and metabolism. Therefore, more studies are required to investigate the properties of these bacterial structures. P9 is an 84kDa, glucagon-like peptide 1 (GLP-1)-inducing protein produced by A. muciniphila. Yoon et al. administered 100μg P9 daily to HFD-fed mice for 8 weeks and concluded that the administration of P9 prevented mice against obesity and improved glucose tolerance by regulating GLP-1 secretion (58). Several studies have shown that L cell-secreted GLP-1 has been associated with reduced levels of pro-inflammatory cytokine IL-1β, increased mucus-secreting goblet cells and improved gut epithelial architecture. Therefore, it is thus reasonable to postulate that P9 may alleviate the inflammation and intestinal injuries in IBD by modulating GLP-1 expression (59). However, a recent study also showed that P9 induced the expression of IL-6 in macrophages, suggesting its pro-inflammatory role in the occurrence and development of intestinal inflammation. On the whole, research for the properties and effects of this protein is very scarce, and further in vitro studies as well as mice experiments are needed for researchers to fully understand its mechanisms in regulating gut homeostasis and potentially alleviating IBD.
4.3.1. Probiotics
Administering several strains of probiotic treatments has been proved to have a promoting effect on A. muciniphila abundance. In addition to direct administration of A. muciniphila, a single probiotic treatment with Lactobacillus pentosus on mice with DSS-induced colitis was proved to increase the level of A.muciniphila abundance. Inflammatory cell infiltration and colonic injuries due to colitis were also alleviated post-treatment, thus inhibiting the development of the disease (60). Like Lactobacillu pentosus, other members of the Lactobacillu species have been widely researched as promising probiotic to regulate gut microbiota and promote intestinal health, although not all strains have been directly linked with promoting A. muciniphila or improving IBD. Lactobacillus plantarum, strain WCFS1, for example, had been proved to enhance TLR2 signalling activities, occluding expression, and subsequently benefit the mucosal barrier of the gut (61). It is thus reasonable to postulate that these modulations may associate with an increase in A. muciniphila level. A recent study supported this hypothesis by showing that, four weeks of 3 × 1010 CFU/day Lactobacillus plantarum administration to healthy triathletes significantly increased the abundance of A. muciniphila (62). Several mixtures of probiotics have also been found to be able to rebuild the gut microbiota structure and consequently improve IBD. Bifidobacterium infantis, Lactobacillus acidophilus, Enterococcus faecalis and Bacillus cereus collectively exert a promoting effect on A. muciniphila, almost restoring the gut microflora structure to normal state (63). Another study showed that administering Bifidobacterium animalis subsp. lactis LMG P-28149 or a mixture with Lactobacillus rhamnosus (Mix) for 14 weeks can restore the composition of host gut microbiota and significantly promote A. muciniphila abundance. Moreover, the Mix enhanced the production of SCFAs (e.g., butyrate and acetate), which could potentially promote mucin growth and consequently feedforward to A. muciniphila level increase, bringing beneficial changes to the host intestinal immunity. It is worth noticing that the protective effect of the Mix is likely resulted from Bifidobacterium animalis subsp. lactis, as administering Lactobacillus rhamnosus in its own decreased the abundance of A. muciniphila (64).
4.3.2. Prebiotics
Prebiotic are dietary substances that stimulates growth or activity of specific species in the gut microbiota that give rise to beneficial effects to the host (65). Bu et al. tested the effect of 7-day prebiotics administration on host mice gut microbiota. Interestingly, mice treated with the total flavone of Abelmoschus Manihot (TFA), a prebiotic of A. muciniphila, showed a direct promoting effect on A. muciniphila in a dose-dependent manner within certain range. The inhibiting effect on pro-inflammatory cytokines was also boosted with increased concentration of TFA, which subsequently contributed to improved gut barrier permeability and immunity. Notably, experimental colitis reduced the mRNA expression of KLF4, MUC2 and ZO-1in their experiment. KLF4 is a transcription factor associated with intestinal cell proliferation (especially goblet cells). Mucin MUC2 blcoks pathogens in the gut lumen, and deficiency in MUC2 leads to a destruction of symbiotic bacterial habitat. ZO-1 is involved in maintain the integrity of gut barrier and contributes to the recruitment of other component during the assembly of tight junctions. KLF4, MUC2 and ZO-1 expression were all improved after TFA treatment (66). Hyaluronan, a glycosaminoglycan polymer, has also shown prebiotic properties facilitating the maintenance of gut homeostasis and ameliorating IBD symptoms. Our previous studies showed that hyaluronan attenuated C. rodentium-induced colitis by boosting A. muciniphila abundance, promoting the expression of antimicrobial peptides, restoring the gut microbiota composition, and consecutively protecting the host against C. rodentium infection. What’s more, dietary supplements of prebiotic were also widely investigated for their effects on promoting A. muciniphila. For example, vitamin D3 supplementation promoted A. muciniphila (among other beneficial probiotics such as Bifidobacterium) in healthy human participants. Zhou and colleagues found that the level of vitamin D3 was significantly reduced in DSS- and Azoxymethane- induced CRC model mice and in CRC patients. While vitamin D deficiency is associated with aggravated enteritis deterioration, supplementation of this substance restores the number of A. muciniphila and boosts A. muciniphila-mediated colon barrier integrity (35). Intake of inulin, a fermentable fructo-oligosaccharide, has been associated with increased level of A. muciniphila in both healthy and obese participants (67), while giving mice inulin supplements in addition to a fat-enriched diet also increased the level of A. muciniphila in the host gut (68). Another recent study revealed that anthocyanin-containing purple potatoes in the diet alleviated DSS-induced colitis. Li et al. fed their colitis model mice a diet supplemented with 15% or 25% baked and freeze-dried purple potato powder and observed very intriguing outcomes: adding either proportion of purple potato powder suppressed colitis-induced body weight loss and inflammation. Pro-inflammatory interleukins IL-6 and IL-17 were downregulated and pathogenic bacteria such as Enterobacteriaceae abundance were reduced. 25% purple potato powder diet also elevated A. muciniphila abundance by four folds (69). Administrations of dietary polyphenols, natural antioxidants that sometimes possess antimicrobial properties, gave inconsistent results in mice studies. Concord grape polyphenols and epigallocatechin-3-gallate, a bioactive polyphenol constituent in green tea promoted A. muciniphila abundance in mice (70, 71). Wu et al. gave DSS-induced colitis mice 3 days of oral or rectal administration of epigallocatechin-3-gallate following disease induction, showing that this green tea-derived polyphenol attenuated colitis symptoms such as colon shortening. However, supplementing 4% green tea powder to HFD-fed mice did not induce any significant changes in A. muciniphila abundance (72). These results suggest that the properties of dietary polyphenols may be dependent on their sources and dose (73).
4.3.3. Diets
Difference in dietary habits may also influence microbiota and subsequently colonic mucosal integrity. Ketogenic diets (KD), characterised by high-fat and low-carbonhydrate, was initially used in treating epilepsy, but has now shown increasing potential in treating a wide range of other diseases. Recent studies suggest the possibility that the therapeutic potential of KD may attributed by its impact on the gut microbiota. Olson and colleagues gave healthy specific pathogen-free Swiss Webster mice a 6:1 fat: protein KD diet and found that these mice showed significant increase in the relative abundances of A. muciniphila (74). Kong et al. confirmed the effect of KD on alleviating IBD and found that KD (89% kcal fat, 10% kcal protein and <1% carbohydrate) enriched A. muciniphila abundance in mice gut and mesenteric lymph nodes, regardless of whether these mice have colitis. A. muciniphila dominated the gut microbiota in KD-treated colitis model mice, while colitis symptoms were also alleviated. In addition to changes in the gut microbiota composition, metabolites such as L-asparagine and glycine observed an increase, while the amount of ILC3 cell production was reduced. On the other hand, low carbohydrate diet (LCD, 70% kcal fat, 20% kcal protein, 10% kcal carbohydrate) decreased A. muciniphila level in the host gut and aggravated intestinal inflammation by promoting the production of pro-inflammatory IL-18 (75). High-fat diets had been previously associated with impairments of intestinal function, and it is yet unclear how KD exerts a protective effect on the intestinal barrier. However, it is postulated that increased L-asparagine resulting from KD could inhibit intestinal inflammation and protect the intestinal barrier from LPS-induced damages (75, 76). Mediterranean diet, characterised by high monosaturated fatty acids, fibre, whole grain, beans, nuts and low consumption of meats and sweets, is considered to have beneficial effects on immune and metabolic systems. In contrast, Western diet consumed by most industrialised countries is low in fruits and vegetable, but high in fat (especially animal fats) and sugar. Shankar et al. compared the distal gut microbiota composition in Egyptian (Mediterranean diet) and U.S. (Western diet) teenagers. They found that the abundance of A. muciniphila is significantly higher in Egyptian teenagers, while the gut of U.S. teenagers had relatively more Bacteroides, which might indicate an adaptation to elevated inflammatory levels in Western populations (77). The effects of dietary amino acid composition on treating IBD were also investigated in prior studies. Li et al. developed two recipes (Recipe 1 and 2, or R1 and R2) for amino acid-balanced diets based on grains (rice, millet, quinoa and mung bean), and beta-glucan and fructooligosaccharides were adopted for better functional and sensory qualities. Feeding DSS-induced colitis model mice with amino acid-balanced diets ameliorated IBD symptoms, and particularly, R1 (amino acid score: 86.64 ± 0.45, chemical scores: 104.36 ± 3, essential amino acid index: 61.02 ± 0.9) diet restored the decreased abundance of A. muciniphila (78).
4.3.4. Drugs
Given the close relationship of A. muciniphila with gut microbiota homeostasis, more and more researchers are now seeing this bacterium as a potential target for pharmaceutical intervention. Postulated treatments to modify the microbial environment of the host gut range from traditional antimicrobics to the relative novel nanomedicines (79–81). One of the most studied drugs known to promote A. muciniphila is metformin, a drug that has been used in type 2 diabetes treatment. While its therapeutic mechanisms are not yet fully understood, recent studies have revealed that this drug is involved in the modulation of gut microbiota, which consecutively contributes to reducing inflammation or obesity. De la Cuesta-Zuluaga et al. examined 28 type-2 diabetic patients- 14 taking metformin whilst others did not- to investigate the gut microbiota composition in their faecal samples (82). Their research has shown that A. muciniphila was more abundant in diabetic patients taking metformin than either diabetic participants who were not taking the drug, or their healthy counterparts. C57BL/6 mice experiments were consistent with the above clinical studies: metformin was given to mice with DSS-induced colitis via intraperitoneal injection (150ng/kg/day) either 3 days after metformin treatment, or MD group) or 1 day before (pre-treatment, or p group) disease induction. Both MD and p group significantly reduced inflammation, inhibited colon shortening and increased A. muciniphila (83). Metformin treatment via 4-10 weeks of oral gavage (100-300 mg/bw) showed a similar result in terms of promoting A. muciniphila (38). However, debate remains regarding whether the beneficial effects in these studies resulted from metformin or unknown confounding factors: an antibiotic combination (carbenicillin, metronidazole, neomycin, vancomycin) pre-treatment abolished the antidiabetic effect (38). The interaction between metformin and antibiotic drugs seem to suggest that the effect of metformin is subjected to gut microflora activities. Metformin also increases the number of goblet cells in the mice ileum, which may have a bidirectional boosting effect with A. muciniphila.
In addition to metformin, antibiotic treatments also often lead to alterations in microbial diversity. A recent study revealed that vancomycin treatment on mice promoted A. muciniphila as the most abundant species in the host gut (>85%), while the abundance of previously dominant phylum Firmicutes and Bacteroidetes significantly decreased (80). Dubourg et al. conducted a study on two patients receiving broad-spectrum antibiotic treatment, which supported the above findings: patient A was receiving a combination of doxycycline, hydroxychloroquine, piperacillin/tazobactam and teicoplanin at the time of stool collection, while patient B received impinem. Surprisingly, despite the fact that A. muciniphila is susceptible to imipenem and piperacillin, it was found to make up > 40% of the total gut microbiota (44.91% in patient A and 84.64% in patient B) without inducing any significant gastrointestinal disorders (84).
4.3.5. Herbs
Complementary and alternative medicines, especially herbs, have increasingly been used in the treatment of UC patients in recent years, and has been shown a fundamental role in regulating the gut microbiota during UC management (85). Zingiber officinale and Panax ginseng have been used together to clinically treat ulcerative colitis with synergistic effects for thousands of years, whose mechanism was related to regulating gut microbiota according to Wan et al.’s study showing that the relative abundance of beneficial bacteria (such as Akkermansia) was significantly increased and the abundance of pathogenic bacteria was markedly decreased after the intervention of Zingiber officinale-Panax ginseng herb pair (86). Some herbal prescriptions from traditional Asian medicines were also researched for their therapeutic effects on IBD. Baitouweng decoction is a prescription in traditional Chinese medicine that consists of four herbs (i.e., Radix pulsatilla, Cortex phellodendri, Rhizoma coptidis and Cortex fraxini) and it is used to treat UC. Results from a DSS-induced colitis model mice study showed that Baitouweng decoction reduced the production of several pro-inflammatory cytokines. Furthermore, Bacteroidetes was reduced after Baitouweng dedoction intragastrical administration, whereas A. muciniphila abundance was promoted (87). Similar to this study, the results from Li et al. showed that the main active components of Jiawei Gegen Qinlian decoction protected against ulcerative colitis under different dietary environments in a gut microbiota-dependent manner, especially regulating the gut microbiota by increasing the abundance of Akkermansia and decreasing the colonisation of Escherichia-Shigella (88). Similar to this study, a study from Cai et al. also showed a specific enrichment of genus Akkermansia in DSS-induced intestinal inflammation. All these results showed a significant role of herbs in the enrichment of A. muciniphila abundance (89).
5. Conclusions and perspectives
The current evidence has demonstrated its potential protective effect of A. muciniphila in the process and development of intestinal inflammation. Abnormal reduction in abundance of A. muciniphila maybe a hallmark of IBD, which is associated with gut dysbiosis, decreased mucosal barrier function and altered immune response. However, the role of commensal A. muciniphila in IBD is still controversial, and even plays a pro-inflammatory role in the occurrence and development of intestinal inflammation, which might be due to difference in genotypes of host, strain specificity of A. muciniphila and the coexistence of enteropathogens. Therefore, we should always hold a reasonable dose of expectation and skepticism in terms of the overwhelming “good effects” of A. muciniphila in health and disease states.
Nevertheless, A. muciniphila -based and -targeted therapies are now considered a valuable therapeutic approach to treat IBD patients. However, how to selectively enrich the growth and colonisation of A. muciniphila in the host intestine with direct or indirect interventions have not been clarified. This review reveals an interesting phenomenon that not only viable A. muciniphila, but also pasteurised A. muciniphila, and its ingredients such as AmEVs, Amuc_1100 and P9 also can alleviate intestinal inflammation, suggesting a therapeutic potential of A. muciniphila in the treatment of IBD. But there is still a long way to go to develop the clinically available products. Firstly, the strict anaerobic characteristics of A. muciniphila make it difficult to isolate, purify and preserve, so there is an urgent need for the development of novel strategies for the industrial production of A. muciniphila. Secondly, considering the controversial role of A. muciniphila, host genotypes and intestinal microenvironment, especially the coexistence of pathogenic bacteria in gut, have to be comprehensively and fully taken into consideration before its clinical application. Thirdly, safety issues of A. muciniphila needs to be further evaluated in the treatment of IBD. Up to now, there is still a lack of clinical studies in treating of IBD with A. muciniphila alone, which means that the effective dose, optimal dose, treatment course and adverse effects are not clarified and need to be further determined.
More importantly, because of the natural characteristics individual strains grow, we believe that it is more promising weapon for the treatment of IBD to improve the intestinal microenvironment of A. muciniphila than to simply increase the number of individual bacteria itself. Therefore, it has become a research hotspot in recent years to improve intestinal microenvironment and indirectly promote the colonisation of A. muciniphila through the supplementation of other probiotic or prebiotics, natural diets, drugs, and herbs, and have achieved good progress (Figure 2), which holds promise for A. muciniphila -based and -targeted therapies in the treatment of IBD.
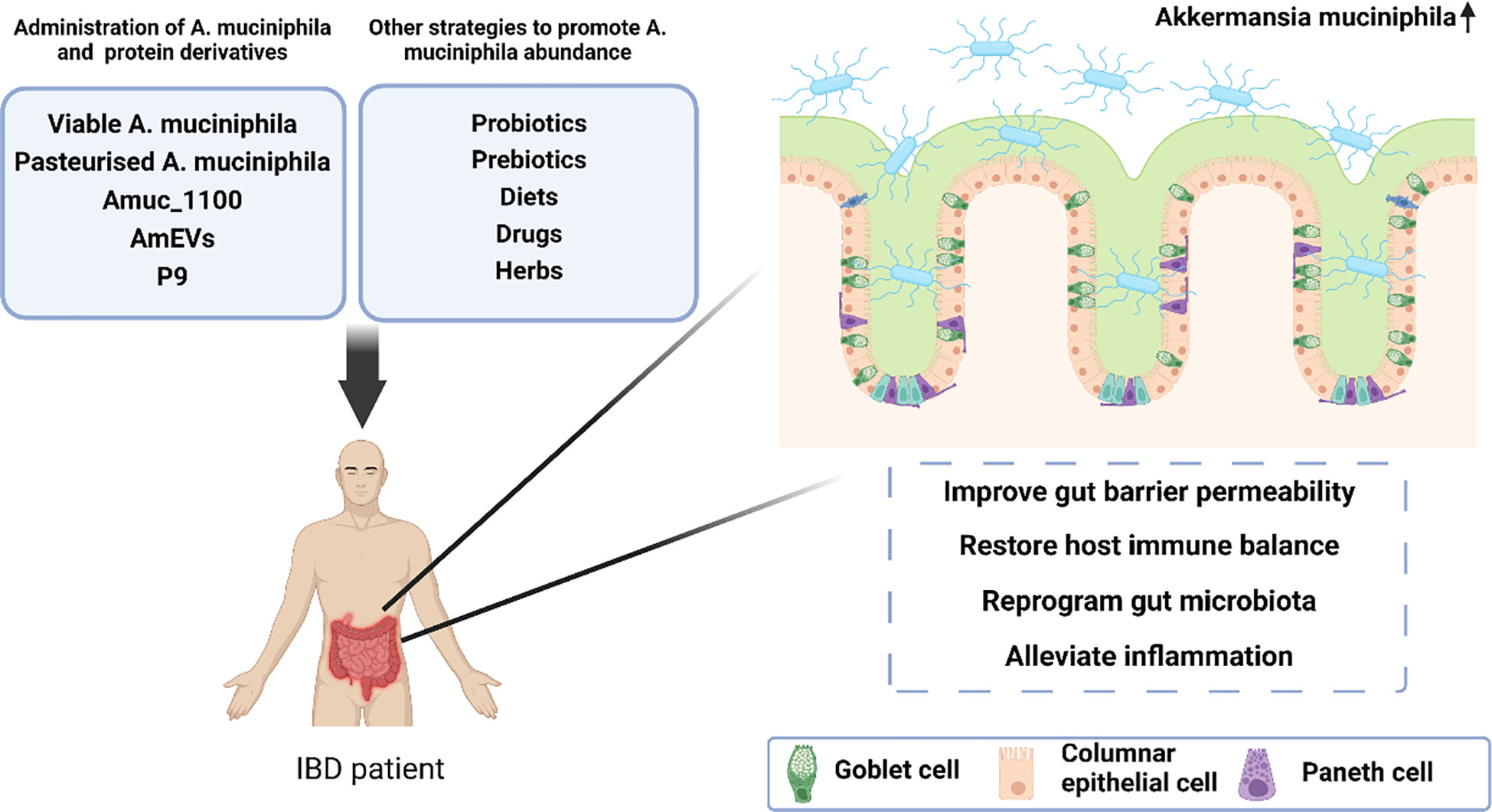
Figure 2 A proposed model illustrating the protective effect of A. muciniphila-targeted and -based therapies in the treatment of inflammatory bowel disease.
Author contributions
TM and JL conceived and designed the study. MZ, RH and YY collect literatures, organized documents, and wrote the first draft of the manuscript. YX, WZ, ZS and YL participated in editing of specific paragraphs and figures. TM supervised and critically revised the manuscript. All authors read and approved the manuscript. All authors contributed to the article and approved the submitted version.
Funding
This work was supported by National Science Foundation of China (No. 81903990), Capital’s Funds for Health Improvement and Research (shoufa 2022-4-4205).
Conflict of interest
The authors declare that the research was conducted in the absence of any commercial or financial relationships that could be construed as a potential conflict of interest.
Publisher’s note
All claims expressed in this article are solely those of the authors and do not necessarily represent those of their affiliated organizations, or those of the publisher, the editors and the reviewers. Any product that may be evaluated in this article, or claim that may be made by its manufacturer, is not guaranteed or endorsed by the publisher.
References
1. Lin L, Zhou G, Chen P, Wang Y, Han J, Chen M, et al. Which long noncoding RNAs and circular RNAs contribute to inflammatory bowel disease? Cell Death Dis (2020) 11(6):456. doi: 10.1038/s41419-020-2657-z
2. Couto MR, Gonçalves P, Magro F, Martel F. Microbiota-derived butyrate regulates intestinal inflammation: Focus on inflammatory bowel disease. Pharmacol Res (2020) 159:104947. doi: 10.1016/j.phrs.2020.104947
3. Sales-Campos H, Basso PJ, Alves VBF, Fonseca MTC, Bonfá G, Nardini V, et al. Classical and recent advances in the treatment of inflammatory bowel diseases. Braz J Med Biol Res (2015) 48:96–107. doi: 10.1590/1414-431X20143774
4. Jeong DY, Kim S, Son MJ, Son CY, Kim JY. Kronbichler a induction and maintenance treatment of inflammatory bowel disease: A comprehensive review. Autoimmun Rev (2019) 18:439–54. doi: 10.1016/j.autrev.2019.03.002
5. Oka A, Sartor RB. Microbial-based and microbial-targeted therapies for inflammatory bowel diseases. Dig Dis Sci (2020) 65:757–88. doi: 10.1007/s10620-020-06090-z
6. Rooks M, Garrett W. Gut microbiota, metabolites and host immunity. Nat Rev Immunol (2016) 16(6):341–52. doi: 10.1038/nri.2016.42
7. Belkaid Y, Harrison O. Homeostatic immunity and the microbiota. Immunity (2017) 46(4):562–76. doi: 10.1016/j.immuni.2017.04.008
8. Amoroso C, Perillo F, Strati F, Fantini M, Caprioli F, Facciotti F. The role of gut microbiota biomodulators on mucosal immunity and intestinal inflammation. Cells (2020) 9(5):1234. doi: 10.3390/cells9051234
9. Kothari D, Patel S, Kim S-K. Probiotic supplements might not be universally-effective and safe: A review. Biomed Pharmacother (2019) 111:537–47. doi: 10.1016/j.biopha.2018.12.104
10. Salminen MK, Tynkkynen S, Rautelin H, Saxelin M, Vaara M, Ruutu P, et al. Lactobacillusbacteremia during a rapid increase in probiotic use oflactobacillus rhamnosusGG in Finland. Clin Infect Dis (2002) 35(10):1155–60. doi: 10.1086/342912
11. Derrien M, Belzer C, de Vos W. Akkermansia muciniphila and its role in regulating host functions. Microb Pathogen (2017) 106:171–81. doi: 10.1016/j.micpath.2016.02.005
12. Derrien M, Vaughan E, Plugge C, de Vos W. Akkermansia muciniphila gen. nov., sp. nov., a human intestinal mucin-degrading bacterium. Int J Of Syst And Evol Microbiol (2004) 54(5):1469–76. doi: 10.1099/ijs.0.02873-0
13. Ouwerkerk JP, van der Ark K, Davids M, Claassens NJ, Finestra TR, de Vos WM, et al. Adaptation of akkermansia muciniphila to the oxic-anoxic interface of the mucus layer. Appl Environ Microbiol (2016) 82(23):6983–93. doi: 10.1128/AEM.01641-16
14. Geerlings S, Kostopoulos I, de Vos W, Belzer C. Akkermansia muciniphila in the human gastrointestinal tract: When, where, and how? Microorganisms (2018) 6(3):75. doi: 10.3390/microorganisms6030075
15. Derrien M, Collado M, Ben-Amor K, Salminen S, de Vos W. The mucin degrader akkermansia muciniphila is an abundant resident of the human intestinal tract. Appl And Environ Microbiol (2008) 74(5):1646–8. doi: 10.1128/aem.01226-07t
16. Zhou JC, Zhang XW. Akkermansia muciniphila: A promising target for the therapy of metabolic syndrome and related diseases. Chin J Natural Medicines (2019) 17(11):835–41. doi: 10.1016/S1875-5364(19)30101-3
17. Gómez-Gallego C, Pohl S, Salminen S, De Vos WM, Kneifel W. Akkermansia muciniphila: A novel functional microbe with probiotic properties. Beneficial Microbes (2016) 7(4):571–84. doi: 10.3920/BM2016.0009
18. Earley H, Lennon G, Balfe Á, Coffey JC, Winter DC, O'Connell PR. The abundance of akkermansia muciniphila and its relationship with sulphated colonic mucins in health and ulcerative colitis. Sci Rep (2019) 9(1):15683. doi: 10.1038/s41598-019-51878-3
19. Png CW, Lindén SK, Gilshenan KS, Zoetendal EG, McSweeney CS, Sly LI, et al. Mucolytic bacteria with increased prevalence in IBD mucosa augment in vitro utilisation of mucin by other bacteria. Am J Gastroenterol (2010) 105(11):2420–8. doi: 10.1038/ajg.2010.281
20. James SL, Christophersen CT, Bird AR, et al. Abnormal fibre usage in UC in remission. Gut (2015) 64(4):562–70. doi: 10.1136/gutjnl-2014-307198
21. Vigsnæs LK, Brynskov J, Steenholdt C, Wilcks A, Licht TR. Gram-negative bacteria account for main differences between faecal microbiota from patients with ulcerative colitis and healthy controls. Beneficial Microbes (2012) 3(4):287–97. doi: 10.3920/BM2012.0018
22. Rajilić-Stojanović M, Shanahan F, Guarner F, de Vos WM. Phylogenetic analysis of dysbiosis in ulcerative colitis during remission. Inflammatory bowel Dis (2013) 19(3):481–8. doi: 10.1097/MIB.0b013e31827fec6d
23. Wang L, Tang L, Feng Y, Zhao S, Han M, Zhang C, et al. A purified membrane protein from akkermansia muciniphila or the pasteurised bacterium blunts colitis associated tumourigenesis by modulation of CD8+ T cells in mice. Gut (2020) 69(11):1988–97. doi: 10.1136/gutjnl-2019-320105
24. Dunn KA, Moore-Connors J, MacIntyre B, Stadnyk AW, Thomas NA, Noble A, et al. Early changes in microbial community structure are associated with sustained remission after nutritional treatment of pediatric crohn's disease. Inflammatory bowel Dis (2016) 22(12):2853–62. doi: 10.1097/MIB.0000000000000956
25. Lopez-Siles M, Enrich-Capó N, Aldeguer X, Sabat-Mir M, Duncan SH, Garcia-Gil LJ, et al. Alterations in the abundance and Co-occurrence of akkermansia muciniphila and faecalibacterium prausnitzii in the colonic mucosa of inflammatory bowel disease subjects. Front Cell infect Microbiol (2018) 8:281. doi: 10.3389/fcimb.2018.00281
26. Danilova NA, Abdulkhakov SR, Grigoryeva TV, Markelova MI, Vasilyev IY, Boulygina EA, et al. Markers of dysbiosis in patients with ulcerative colitis and crohn's disease. Terapevticheskii arkhiv (2019) 91(4):17–24. doi: 10.26442/00403660.2019.04.000211
27. Alrafas HR, Busbee PB, Nagarkatti M, Nagarkatti PS. Resveratrol modulates the gut microbiota to prevent murine colitis development through induction of tregs and suppression of Th17 cells. J leukoc Biol (2019) 106(2):467–80. doi: 10.1002/JLB.3A1218-476RR
28. Chen T, Wang R, Duan Z, Yuan X, Ding Y, Feng Z, et al. Akkermansia muciniphila protects against psychological disorder-induced gut microbiota-mediated colonic mucosal barrier damage and aggravation of colitis. Front Cell infect Microbiol (2021) 11:723856. doi: 10.3389/fcimb.2021.723856
29. Yang L, Liu C, Zhao W, He C, Ding J, Dai R, et al. Impaired autophagy in intestinal epithelial cells alters gut microbiota and host immune responses. Appl Environ Microbiol (2018) 84(18):e00880-18. doi: 10.1128/AEM.00880-18
30. Mahoro P, Moon HJ, Yang HJ, Kim KA, Cha YS. Protective effect of gochujang on inflammation in a DSS-induced colitis rat model. Foods (Basel Switzerland) (2021) 10(5):1072. doi: 10.3390/foods10051072
31. Håkansson Å, Tormo-Badia N, Baridi A, Xu J, Molin G, Hagslätt M-L, et al. Immunological alteration and changes of gut microbiota after dextran sulfate sodium (DSS) administration in mice. Clin Exp Med (2015) 15(1):107–20. doi: 10.1007/s10238-013-0270-5
32. Seregin SS, Golovchenko N, Schaf B, Chen J, Pudlo NA, Mitchell J, et al. NLRP6 protects Il10-/- mice from colitis by limiting colonisation of akkermansia muciniphila. Cell Rep (2017) 19(4):733–45. doi: 10.1016/j.celrep.2017.03.080
33. Liso M, De Santis S, Verna G, Dicarlo M, Calasso M, Santino A, et al. A specific mutation in Muc2 determines early dysbiosis in colitis-prone winnie mice. Inflammatory bowel Dis (2020) 26(4):546–56. doi: 10.1093/ibd/izz279
34. Su D, Nie Y, Zhu A, Chen Z, Wu P, Zhang L, et al. Vitamin d signaling through induction of paneth cell defensins maintains gut microbiota and improves metabolic disorders and hepatic steatosis in animal models. Front Physiol (2016) 7. doi: 10.3389/fphys.2016.00498
35. Zhou X, Chen C, Zhong YN, Zhao F, Hao Z, Xu Y, et al. Effect and mechanism of vitamin d on the development of colorectal cancer based on intestinal flora disorder. J Gastroenterol Hepatol (2020) 35:1023–1031. doi: 10.1111/jgh.14949
36. Zhu W, Yan J, Zhi C, Zhou Q, Yuan X. 1,25(OH)2d3 deficiency-induced gut microbial dysbiosis degrades the colonic mucus barrier in CYP27B1 knockout mouse model. Gut Pathog (2019) 11(1):2–6. doi: 10.1186/s13099-019-0291-z
37. Zhai R, Xue X, Zhang L, Yang X, Zhao L, Zhang C. Strain-specific anti-inflammatory properties of two akkermansia muciniphila strains on chronic colitis in mice. Front Cell infect Microbiol (2019) 9:239. doi: 10.3389/fcimb.2019.00239
38. Shin NR, Lee JC, Lee HY, Kim M-S, Whon TW, Lee M-S, et al. An increase in the akkermansia spp. population induced by metformin treatment improves glucose homeostasis in diet-induced obese mice. Gut (2014) 63(5):727–35. doi: 10.1136/gutjnl-2012-303839
39. Smith P, Howitt M, Panikov N, Michaud M, Gallini CA, Bohlooly-Y M, et al. The microbial metabolites, short-chain fatty acids, regulate colonic T reg cell homeostasis. Science (2013) 341(6145):569–73. doi: 10.1126/science.1241165
40. Katiraei S, de Vries MR, Costain AH, Thiem K, Hoving LR, Diepen JA, et al. Akkermansia muciniphila exerts lipid-lowering and immunomodulatory effects without affecting neointima formation in hyperlipidemic APOE*3-Leiden.CETP mice. Mol Nutr Food Res (2020) 64(15):e1900732. doi: 10.1002/mnfr.201900732
41. Ansaldo E, Slayden LC, Ching KL, Koch MA, Wolf NK, Plichta DR, et al. Akkermansia muciniphila induces intestinal adaptive immune responses during homeostasis. Sci (New York N.Y.) (2019) 364(6446):1179–84. doi: 10.1126/science.aaw7479
42. Hiippala K, Jouhten H, Ronkainen A, Hartikainen A, Kainulainen V, Jalanka J, et al. The potential of gut commensals in reinforcing intestinal barrier function and alleviating inflammation. Nutrients (2018) 10(8):988. doi: 10.3390/nu10080988
43. Ottman N, Reunanen J, Meijerink M, Pietilä TE, Kainulainen V, Klievink J, et al. Pili-like proteins of akkermansia muciniphila modulate host immune responses and gut barrier function. PLoS One (2017) 12(3):e0173004. doi: 10.1371/journal.pone.0173004
44. Johansson ME, Phillipson M, Petersson J, Velcich A, Holm L, Hansson GC. The inner of the two Muc2 mucin-dependent mucus layers in colon is devoid of bacteria. Proc Natl Acad Sci United States America (2008) 105(39):15064–9. doi: 10.1073/pnas.0803124105
45. Mao T, Su CW, Ji Q, Chen C-Y, Wang R, Vijaya Kumar D, et al. Hyaluronan-induced alterations of the gut microbiome protects mice against citrobacter rodentium infection and intestinal inflammation. Gut Microbes (2021) 13(1):1972757. doi: 10.1080/19490976.2021.1972757
46. Everard A, Belzer C, Geurts L, Ouwerkerk JP, Druart C, Bindels LB, et al. Cross-talk between akkermansia muciniphila and intestinal epithelium controls diet-induced obesity. Proc Natl Acad Sci United States America (2013) 110(22):9066–71. doi: 10.1073/pnas.1219451110
47. Larsson JM, Karlsson H, Crespo JG, Johansson MEV, Eklund L, Sjövall H, et al. Altered O-glycosylation profile of MUC2 mucin occurs in active ulcerative colitis and is associated with increased inflammation. Inflammatory bowel Dis (2011) 17(11):2299–307. doi: 10.1002/ibd.21625
48. Chelakkot C, Choi Y, Kim DK, Park HT, Ghim J, Kwon Y, et al. Akkermansia muciniphila-derived extracellular vesicles influence gut permeability through the regulation of tight junctions. Exp Mol Med (2018) 50(2):e450. doi: 10.1038/emm.2017.282
49. Plovier H, Everard A, Druart C, Depommier C, Van Hul M, Geurts L, et al. A purified membrane protein from akkermansia muciniphila or the pasteurised bacterium improves metabolism in obese and diabetic mice. Nat Med (2017) 23(1):107–13. doi: 10.1038/nm.4236
50. Gonzalez A, Krieg R, Massey HD, Carl D, Ghosh S, Gehr TW, et al. Sodium butyrate ameliorates insulin resistance and renal failure in CKD rats by modulating intestinal permeability and mucin expression. Nephrol dialysis Transplant (2019) 34(5):783–94. doi: 10.1093/ndt/gfy238
51. Li J, Lin S, Vanhoutte PM, Woo CW, Xu A. Akkermansia muciniphila protects against atherosclerosis by preventing metabolic endotoxemia-induced inflammation in apoe-/- mice. Circulation (2016) 133(24):2434–46. doi: 10.1161/CIRCULATIONAHA.115.019645
52. Gu ZY, Pei WL, Zhang Y, Zhu J, Li L, Zhang Z. Akkermansia muciniphila in inflammatory bowel disease and colorectal cancer. Chin Med J (2021) 134(23):2841–3. doi: 10.1097/CM9.0000000000001829
53. He X, Bai Y, Zhou H, Wu K. Akkermansia muciniphila alters gut microbiota and immune system to improve cardiovascular diseases in murine model. Front Microbiol (2022) 13:906920. doi: 10.3389/fmicb.2022.906920
54. Bian X, Wu W, Yang L, Lv L, Wang Q, Li Y, et al. Administration of akkermansia muciniphila ameliorates dextran sulfate sodium-induced ulcerative colitis in mice. Front Microbiol (2019) 10:2259. doi: 10.3389/fmicb.2019.02259
55. Roediger WE, Moore J, Babidge W. Colonic sulfide in pathogenesis and treatment of ulcerative colitis. Digestive Dis Sci (1997) 42(8):1571–9. doi: 10.1023/a:1018851723920
56. Attene-Ramos MS, Wagner ED, Plewa MJ, Gaskins HR. Evidence that hydrogen sulfide is a genotoxic agent. Mol Cancer Res MCR (2006) 4(1):9–14. doi: 10.1158/1541-7786.MCR-05-0126
57. Kang CS, Ban M, Choi EJ, Moon H-G, Jeon J-S, Kim D-K, et al. Extracellular vesicles derived from gut microbiota, especially akkermansia muciniphila, protect the progression of dextran sulfate sodium-induced colitis. PLoS One (2013) 8(10):e76520. doi: 10.1371/journal.pone.0076520
58. Yoon HS, Cho CH, Yun MS, Jang SJ, You HJ, Kim J-H, et al. Akkermansia muciniphila secretes a glucagon-like peptide-1-inducing protein that improves glucose homeostasis and ameliorates metabolic disease in mice. Nat Microbiol (2021) 6(5):563–73. doi: 10.1038/s41564-021-00880-5
59. Anbazhagan AN, Thaqi M, Priyamvada S, Jayawardena D, Kumar A, Gujral T, et al. GLP-1 nanomedicine alleviates gut inflammation. Nanomed: Nanotechnol Biol Med (2017) 13(2):659–65. doi: 10.1016/j.nano.2016.08.004
60. Ma Y, Hu C, Yan W, Jiang H, Liu G. Lactobacillus pentosus increases the abundance of akkermansia and affects the serum metabolome to alleviate DSS-induced colitis in a murine model. Front Cell Dev Biol (2020) 8:591408. doi: 10.3389/fcell.2020.591408
61. Karczewski J, Troost FJ, Konings I, Dekker J, Kleerebezem M, Brummer R-JM, et al. Regulation of human epithelial tight junction proteins by lactobacillus plantarum in vivo and protective effects on the epithelial barrier. Am J Physiol-Gastrointest Liver Physiol (2010) 298(6):G856–7. doi: 10.1152/ajpgi.00327.2009
62. Huang W-C, Pan C-H, Wei C-C, Huang H-Y. Lactobacillus plantarum PS128 improves physiological adaptation and performance in triathletes through gut microbiota modulation. Nutrients (2020) 12(8):2315. doi: 10.3390/nu12082315
63. Chen Y, Zhang L, Hong G, Huang C, Qian W, Bai T, et al. Probiotic mixtures with aerobic constituent promoted the recovery of multi-barriers in DSS-induced chronic colitis. Life Sci (2020) 240:117089. doi: 10.1016/j.lfs.2019.117089
64. Alard J, Lehrter V, Rhimi M, Mangin I, Peucelle V, Abraham A-L, et al. Beneficial metabolic effects of selected probiotics on diet-induced obesity and insulin resistance in mice are associated with improvement of dysbiotic gut microbiota. Environ Microbiol (2016) 18(5):1484–97. doi: 10.1111/1462-2920.13181
65. Roberfroid M, Gibson GR, Hoyles L, McCartney AL, Rastall R, Rowland I, et al. Prebiotic effects: Metabolic and health benefits. Br J Nutr (2010) 104:S2. doi: 10.1017/S0007114510003363
66. Bu F, Ding Y, Chen T, Wang Q, Wang R, Zhou J-Y, et al. Total flavone of abelmoschus manihot improves colitis by promoting the growth of akkermansia in mice. Sci Rep (2021) 11(1):20787. doi: 10.1038/s41598-021-00070-7
67. Verhoog S, Taneri PE, Roa Díaz ZM, Marques-Vidal P, Troup JP, Bally L, et al. Dietary factors and modulation of bacteria strains of akkermansia muciniphila and faecalibacterium prausnitzii: A systematic review. Nutrients (2019) 11(7):1565. doi: 10.3390/nu11071565
68. Pérez-Monter C, Álvarez-Arce A, Nuño-Lambarri N, Escalona-Nández I, Juárez-Hernández E, Chávez-Tapia NC, et al. Inulin improves diet-induced hepatic steatosis and increases intestinal akkermansia genus level. Int J Mol Sci (2022) 23(2):991. doi: 10.3390/ijms23020991
69. Li S, Wang T, Wu B, Fu W, Xu B, Pamuru RR, et al. Anthocyanin-containing purple potatoes ameliorate DSS-induced colitis in mice. J Nutr Biochem (2021) 93:108616. doi: 10.1016/j.jnutbio.2021.108616
70. Wu Z, Huang S, Li T, Li N, Han D, Zhang B, et al. Gut microbiota from green tea polyphenol-dosed mice improves intestinal epithelial homeostasis and ameliorates experimental colitis. Microbiome (2021) 9(1):184. doi: 10.1186/s40168-021-01115-9
71. Roopchand DE, Carmody RN, Kuhn P, Moskal K, Rojas-Silva P, Turnbaugh PJ, et al. Dietary polyphenols promote growth of the gut bacterium akkermansia muciniphila and attenuate high-fat diet-induced metabolic syndrome. Diabetes (2015) 64(8):2847–58. doi: 10.2337/db14-1916
72. Axling U, Olsson C, Xu J, Fernandez C, Larsson S, Ström K, et al. Green tea powder and lactobacillus plantarum affect gut microbiota, lipid metabolism and inflammation in high-fat fed C57BL/6J mice. Nutr Metab (2012) 9(1):105. doi: 10.1186/1743-7075-9-105
73. Zhou K. Strategies to promote abundance of akkermansia muciniphila, an emerging probiotics in the gut, evidence from dietary intervention studies. J Funct foods (2017) 33:194–201. doi: 10.1016/j.jff.2017.03.045
74. Olson CA, Vuong HE, Yano JM, Liang QY, Nusbaum DJ, Hsiao EY. The gut microbiota mediates the anti-seizure effects of the ketogenic diet. Cell (2018) 173:3. doi: 10.1016/j.cell.2018.04.027
75. Kong C, Yan X, Liu Y, Huang L, Zhu Y, He J, et al. Ketogenic diet alleviates colitis by reduction of colonic group 3 innate lymphoid cells through altering gut microbiome. Signal Transduct Target Ther (2021) 6:1–8. doi: 10.1038/s41392-021-00549-9
76. Chen S, Liu Y, Wang X, Wang H, Li S, Shi H, et al. Asparagine improves intestinal integrity, inhibits TLR4 and nod signaling, and differently regulates p38 and ERK1/2 signaling in weanling piglets after LPS challenge. Innate Immun (2016) 22(8):577–87. doi: 10.1177/1753425916664124
77. Shankar V, Gouda M, Moncivaiz J, Gordon A, Reo NV, Hussein L, et al. Differences in gut metabolites and microbial composition and functions between Egyptian and U.S. children are consistent with their diets. mSystems (2017) 2:1–5. doi: 10.1128/mSystems.00169-16
78. Li S, Yang S, Zhang Y, Huang K, Liang T, Chen Y, et al. Amino acid-balanced diets improved DSS-induced colitis by alleviating inflammation and regulating gut microbiota. Eur J Nutr (2022) 61(7):3531–43. doi: 10.1007/s00394-022-02906-y
79. Zhang Z, Wu X, Cao S, Cromie M, Shen Y, Feng Y, et al. Chlorogenic acid ameliorates experimental colitis by promoting growth of akkermansia in mice. Nutrients (2017) 9(7):677. doi: 10.3390/nu9070677
80. Hansen CH, Krych L, Nielsen DS, Vogensen FK, Hansen LH, Sørensen SJ, et al. Early life treatment with vancomycin propagates akkermansia muciniphila and reduces diabetes incidence in the NOD mouse. Diabetologia (2012) 55(8):2285–94. doi: 10.1007/s00125-012-2564-7
81. Lee Y, Sugihara K, Gillilland MG, Jon S, Kamada N, Moon JJ. Hyaluronic acid-bilirubin nanomedicine for targeted modulation of dysregulated intestinal barrier, microbiome and immune responses in colitis. Nat mater (2020) 19(1):118–26. doi: 10.1038/s41563-019-0462-9
82. de la Cuesta-Zuluaga J, Mueller NT, Corrales-Agudelo V, Velásquez-Mejía EP, Carmona JA, Abad JM, et al. Metformin is associated with higher relative abundance of mucin-degrading akkermansia muciniphila and several short-chain fatty acid-producing microbiota in the gut. Diabetes Care (2017) 40(1):54–62. doi: 10.2337/dc16-1324
83. Liu Z, Liao W, Zhang Z, Sun R, Luo Y, Chen Q, et al. Metformin affects gut microbiota composition and diversity associated with amelioration of dextran sulfate sodium-induced colitis in mice. Front Pharmacol (2021) 12:640347. doi: 10.3389/fphar.2021.640347
84. Dubourg G, Lagier JC, Armougom F, Robert C, Audoly G, Papazian L, et al. High-level colonisation of the human gut by verrucomicrobia following broad-spectrum antibiotic treatment. Int J antimicrob Agents (2013) 41(2):149–55. doi: 10.1016/j.ijantimicag.2012.10.012
85. Feng W, Zhu L, Shen H. Traditional Chinese medicine alleviates ulcerative colitis via modulating gut microbiota. Evidence-Based complementary Altern Med eCAM (2022) 2022:8075344. doi: 10.1155/2022/8075344
86. Wan Y, Yang L, Li H, Ren H, Zhu K, Dong Z, et al. Zingiber officinale and panax ginseng ameliorate ulcerative colitis in mice via modulating gut microbiota and its metabolites. J chromatogr B Anal Technol Biomed Life Sci (2022) 1203:123313. doi: 10.1016/j.jchromb.2022.123313
87. Xuan-Qing C, Xiang-Yu LV, Shi-Jia L. Baitouweng decoction alleviates dextran sulfate sodium-induced ulcerative colitis by regulating intestinal microbiota and the IL-6/STAT3 signaling pathway. J ethnopharmacol (2021) 265:113357. doi: 10.1016/j.jep.2020.113357
88. Li Q, Cui Y, Xu B, Wang Y, Lv F, Li Z, et al. Main active components of jiawei gegen qinlian decoction protects against ulcerative colitis under different dietary environments in a gut microbiota-dependent manner. Pharmacol Res (2021) 170:105694. doi: 10.1016/j.phrs.2021.105694
Keywords: Akkermansia muciniphila, inflammatory bowel disease, barrier function, mucosal immunity, gut microbiota
Citation: Zheng M, Han R, Yuan Y, Xing Y, Zhang W, Sun Z, Liu Y, Li J and Mao T (2023) The role of Akkermansia muciniphila in inflammatory bowel disease: Current knowledge and perspectives. Front. Immunol. 13:1089600. doi: 10.3389/fimmu.2022.1089600
Received: 04 November 2022; Accepted: 20 December 2022;
Published: 06 January 2023.
Edited by:
Qinglong Wu, Baylor College of Medicine, United StatesReviewed by:
Bo Zhu, University of Texas MD Anderson Cancer Center, United StatesZhangran Chen, Xiamen University, China
Yina Huang, Anhui Polytechnic University, China
Copyright © 2023 Zheng, Han, Yuan, Xing, Zhang, Sun, Liu, Li and Mao. This is an open-access article distributed under the terms of the Creative Commons Attribution License (CC BY). The use, distribution or reproduction in other forums is permitted, provided the original author(s) and the copyright owner(s) are credited and that the original publication in this journal is cited, in accordance with accepted academic practice. No use, distribution or reproduction is permitted which does not comply with these terms.
*Correspondence: Junxiang Li, lijunxiang1226@163.com; Tangyou Mao, maotangyouqun@126.com
†These authors have contributed equally to this work