- 1College of Animal Science and Technology, Yangzhou University, Yangzhou, China
- 2Joint International Research Laboratory of Agricultural & Agri-Product Safety, The Ministry of Education of China, Yangzhou University, Yangzhou, China
Berberine (BBR), an isoquinoline alkaloid extracted from Coptidis Rhizoma, has a long history of treating dysentery in the clinic. Over the past two decades, the polytrophic, pharmacological, and biochemical properties of BBR have been intensively studied. The key functions of BBR, including anti-inflammation, antibacterial, antioxidant, anti-obesity, and even antitumor, have been discovered. However, the underlying mechanisms of BBR-mediated regulation still need to be explored. Given that BBR is also a natural nutrition supplement, the modulatory effects of BBR on nutritional immune responses have attracted more attention from investigators. In this mini-review, we summarized the latest achievements of BBR on inflammation, gut microbes, macrophage polarization, and immune responses associated with their possible tools in the pathogenesis and therapy of ulcerative colitis and cancer in recent 5 years. We also discuss the therapeutic efficacy and anti-inflammatory actions of BBR to benefit future clinical applications.
Introduction
In modern medicine, natural products are closely linked to numerous health complications treatment and great therapeutic approaches. In particular, the functional metabolites derived from plants are suggested to perform various biological activities involving anti-obesity, anti-inflammation, antibacterial, anti-fatty liver, and anti-cancer (1, 2). Alkaloids act as the chemical defense in plants when producing secondary metabolites with beneficial pharmacological roles and account for 3/5 of plant-derived medicals. The bioactive components of alkaloids have been used for immunomodulatory therapeutic potentials and anti-inflammation (3). Among them, berberine (BBR) is an isoquinoline alkaloid purified from Chinese herbs and a naturally occurring compound extracted from Coptidis Rhizoma (4). In recent years, a series of actions of BBR-mediated anti-intestinal diseases, anti-cancer, antioxidative stress, and anti-inflammatory has been reported in vivo and in vitro (5–7). Indeed, intestinal disorders and cancer are tightly associated and always characterized by inflammation, oxidative stress, and a couple of immune outputs (8). Inflammation response of the body would benefit the recovery when exposed to infection or invasion events. In this process, immune cells are stimulated by BBR to fight the inflammatory responses in these diseases (9). It has been reported that the potential mechanisms of the anti-inflammation of alkaloids would be attributed to the inhibition of several pro-inflammatory enzyme complexes enrolled in inflammatory signaling processes (10). Moreover, evidence indicates that BBR could ameliorate intestinal lesions and tumor development by reducing macrophage and oxidative stress inflammatory responses (11). The possible underlying mechanisms, in particular, of signaling pathways, have been documented (12). It is plausible that at least part of the observed anti-inflammation roles is due to the activations of classic inflammatory signaling factors, including adenosine monophosphate-activated protein kinase (AMPK) and Wnt/β-catenin (13). Additionally, the extracellular signal-regulated protein kinase 1/2 (ERK1/2) (14), signal transducer and activator of transcription 1 (STAT1), protein kinase B (AKT), nuclear factor kappa-light-chain-enhancer of activated B cells (NF-kB), and nitric oxide (NO), prostaglandin E2 (PEG2), along with chemokines and cytokines (15–18) have also been illustrated. Again, the modulation of gut microbes and microphage polarization has previously been registered in BBR-mediated inflammatory regulation (19). This mini-review aims to collect updated information on BBR in the fields of inflammation and immune responses for a better understanding of the potential mechanisms in the pathogenesis process of various human and animal ulcerative colitis (UC) and cancer research. This will encourage researchers to explore further addressing all aspects of the utilization of BBR for new treatments and therapeutic strategies.
BBR in ulcerative colitis-the anti-inflammatory, immunomodulatory effects and potential mechanisms
Ulcerative colitis (UC) is a chronic inflammatory disease of the bowel with unclear etiology. It is characterized by mucous purulent, abdominal pain, and recurrent diarrhea and is a modern refractory disease with an extremely high risk of colorectal cancer (CRC) (20). Currently, immunosuppressants, anti-inflammatory drugs, and biological agents are the main therapeutic approaches for UC. However, it is still difficult to cure because none of the approaches can reverse the colon injury, and a proportion of patients will have recurrent attacks once ceasing the treatments (21). Emerging evidence suggests that traditional Chinese medicine has positive clinical outputs for UC, including reducing recurrent diarrhea, ameliorating intestinal inflammatory responses, and improving the patient’s life quality (22, 23). Given that BBR has a long history in Chinese medicine used as an antibacterial agent to treat dysentery, it is promising to repurpose BBR for UC and other inflammatory-associated diseases. Tang and coworkers have recently demonstrated in a rodent model that oral administration of BBR effectively alleviates animals’ colitis symptoms when combined with another Chinese herb Atractylodes macrocephala Koidz (24). The underlying mechanisms involve local- and systemic regulations of the immune system, including the reduced pro-inflammatory cytokines IL-4, IL-6, IL-1β, TNF-α, and myeloperoxidase (MPO), and IgA, IgG levels. Indeed, large-scale genome-wide association studies (GWAS) revealed hundreds of loci associated with UC and implicated genes and core cytokines pathways underlying inflammatory pathology. Such as IFN-γ, IL-17, and IL-13, etc., by which immune cells coordinate their functions and intercellular communications (25). In the dextran sulfate sodium (DSS)-induced colitis mice model, treatment of BBR attenuated all pathologic alterations, especially the suppression of the IFN-γ signaling pathway. BBR treatment consistently down-regulated the IFN-γ targeted genes (e.g., IRF8, IRF1, Ifit1 and Ifit3) in UC mice. In addition, BBR markedly decreased serum pro-inflammatory cytokines/chemokines IL-17, TNF-α, CXCL1, and CXCL9 levels (26). In contrast, studies demonstrate that BBR can block the excessive pro-inflammatory cytokine production in UC rodents via the IL-6/STAT3/NF-κB signaling pathway (27, 28). Following this signaling, BBR exerts antisepsis and antioxidative stress activities by affecting mucosal immunity while improving gut barrier function (27–29). Moreover, the Wnt/β-catenin signaling is pivotal for intestinal epithelial homeostasis and tissue regeneration and is dysregulated during inflammatory responses (30). In line with this, Dong et al. demonstrated that BBR acts as an effective drug for UC treatment in a Wnt/β-catenin signaling-dependent manner (31) where BBR administration maintained intestinal mucosal barrier homeostasis and modulated the colonic T cell response, including the transcription and populations of Th17 and regulatory T cells (Treg).
Notably, in the phase I clinical trial, BBR is shown to lower the Geboes Score (GS, a histological score as a UC indicator) in UC patients from a Chinese cohort (32). Accordingly, it suggests that the GS lowering, inflammation suppression, and tissue-repairing effects of BBR in UC may be mediated via the chemosensory Tuft cells-controlled IL-25/C2/13 immune pathway in the colon tissues (33). Meanwhile, Li et al. reported that BBR reduces the colonic infiltration of neutrophils, macrophage and dendritic cells, and innate lymphoid cells (ILCs) and decreases NK cell activation in UC (34). It impedes the colitis from further advancing via the JAK-STAT, ERK, and AKT signaling in intestinal stromal cells. Moreover, a protective effect is observed where BBR preserves the colonic mucosal tight junction and modifies the Th17/Treg dynamic equilibrium in DSS-induced colitis mice (35). In addition, the crosstalk of enteric glial-intestinal epithelium-immune cells has been suggested in the BBR regulation of colitis, where Th17 inhibition is a key component (36, 37). With a similar pathway, in another intestinal lesion model induced by cecal ligation and puncture, BBR is demonstrated to exert a protective effect on cecal ligation and puncture (CLP)-induced intestinal injury by reducing the pro-inflammatory response (38). The mechanisms of BBR’s mediation should result from the accumulated proportion of Treg cells and CTLA-4 linked cell-cell contact pathway. Shaping of intestinal macrophage function is a key element of infection resistance and tissue reparation. Therefore, it plays a dominant role in UC pathogenesis and regulation. In this regard, maintaining macrophage polarization homeostasis is critical for UC treatment (22). It is worth mentioning that BBR has been validated to target macrophage polarization and its downstream regulation in health and inflammation; therefore could be a potential therapeutic approach for UC (20, 39, 40).
Microbiology studies in human and animal models have shown that UC stems from skewed immune responses toward one’s commensal microflora or microbiota dysbiosis. In contrast, numerous studies indicate that maintaining gut microbiota homeostasis or providing beneficial microbes/probiotics can substantially improve mucosal barrier function and ameliorate UC (20, 41). Intriguingly, BBR regulates intestinal microbiota, possibly via boosting Blautia sp., Lactobacillus sp., Bacteroides sp., Bifidobacterium sp., and Akkermansia sp. growth while inhibiting the pathogenic bacteria Enterococci sp. and Escherichia coli in mice with inflammation (42). It is worth noting that BBR has been shown to improve gut tight junction (TJ) protein expression and reduce the Th17/Treg ratio in DSS-induced colitis by promoting intestinal Bacteroides fragilis and the associated IL-6 inhibition (35). Again, BBR could modulate intestinal microecology by boosting specific microflora (e.g., bifidobacteria), and enriching bacterial fermentation. Therefore, BBR-promoted gut microbiota balance facilitates its protection of intestinal mucosa and barrier integrity in UC (33). Because gut microbiota is vulnerable to high-fat diets, BBR effectively ameliorates the expression of genes involved in short-chain fatty acids synthesis, improves mucosal immunity, and enhances the host inflammatory response against gut lesions induced by the high-fat challenge (43). In contrast, BBR-mediated actions are sensitized to the gut microbiome. For example, BBR weakens the generation of trimethylamine by microbiota to lessen choline-induced atherosclerosis in mice (44). With the enrichment of quote-generating gut microbiome, BBR attenuates ovariectomy-triggered anxiety-like illness. In a human study, BBR exhibits an antidiabetic function in type 2 diabetes by reducing secondary bile acid by repressing Ruminococcus bromii growth (45). These studies provide clues of BBR-derived regulations via gut microbiome in inflammatory-associated diseases. However, the deep mechanism of BBR on the interaction between gut microbiota and colitis still needs to be explored.
The anti-tumor activity of BBR and its potential roles in cancer therapy
The antitumor actions of BBR mainly include inducing tumor cell apoptosis, suppressing cancer cell proliferation via cell cycle arrest, autophagy, scavenging free radicals, and inhibiting the metastasis of tumor cells without causing overt side effects on normal cells (46, 47). In comparison, a number of pathways of these actions have been studied such as inhibition of the PI3K/AKT/mTOR, Wnt/β-catenin, MAPK/ERK, EGF receptor, Her2/neu, and the VEGF receptor signaling along with induction of Cip1/p21, Rb expression, p53, and Kip1/p27. These are associated with BBR’s anti-inflammation and antioxidant properties (46, 48). It is well-known that chronic inflammation is one of the main factors to cause human cancers (49). And cancer-linked inflammation indicates the seventh hallmark of cancer development and progress (49). In this process, tumor-infiltrating immune cells produce inflammatory mediators involving cytokines, reactive oxygen species (ROS), and free radicals, resulting in a pre-malignant state (50). Subsequently, the released pro-inflammatory cytokines and growth factors stimulate signaling pathways like PI3K/AKT/mTOR, MAPK/ERK, STAT3, and NF-κB. By inhibiting these cascades, medicinal plants or their bioactive extracts, including BBR, can have a preventative effect on tumor onsets (51).
Cancer development is observed when AKT/PI3K/mTOR pathway is activated. At the same time, BBR performs a vital function in tumor management by strongly suppressing the PI3K/AKT/mTOR signaling (46). In a study of gastric cancer, BBR is validated to increase cellular apoptosis, blocks PI3K/AKT/mTOR, and causes the dephosphorylation of the AKT and p38 pathways (52). Inflammation-linked cancer could produce several chemokines and cytokines via NF-κB, which directly binds to the specific gene promoters (53). BBR administration efficiently decreases the NF-κB signaling accompanied by pro-inflammatory cytokines IL-1, IL-6, and TNF-α productions (54). It has been noted that BBR drastically suppresses lung cancer cell proliferation via NF-κB/COX-2 (55). Furthermore, BBR reduces the activation of the NF-κB pathway via enhancing IκBα and inhibits the elevated phosphorylation of c-Fos/Jun in the scratched cancer cells MDA-MB-231 (56). Again, the pro-inflammatory cytokines, interleukins and TNF-α are all suppressed in response to BBR treatment in TNBC cells, this would further inhibit the tumor metastasis (57). Moreover, BBR blunts cancer metastasis of melanoma cells by the reduction of ERK signaling and the activation of the AMPK pathway (58). In agreement, BBR-activated AMPK is a dominant reason to inhibit colorectal carcinogenesis, which could suppress the growth of a colon xenograft tumor when AMPK is activated via phosphating AMPK signaling at Thr172 (59). Interestingly, p53 and p38 AMPK are also reported functioning in antitumor processes with BBR treatment (60, 61). In contrast, when BBR inhibits the MAPK/mTOR/p70-S6K pathway, gastric cancer cell growth is markedly suppressed due to cytostatic autophagy (62). It is noteworthy that Wnt/β-catenin signaling activation is strongly associated with CRC initiation (63). BBR presents a strong cytostatic efficiency against human CRC via blocking the Wnt/β-catenin pathway to stimulate the caspase‐dependent apoptosis and diminish cancer cell survival (64). This further inhibits the metastasis of CRC because of the cell cycle arrest at G1/S and G2/M phases, DNA damage, and topoisomerase poisoning in colon tumor cells (65).
It suggests that macrophages play complicated roles in cancer depending on cytokines derived from the microenvironment (20). Notably, it has been reported that BBR manipulates the macrophage polarization, reducing the IL-10 and TGF-β pathways in a mouse melanoma model to reinstall their anti-tumor immune responses (66). By increasing the MHC-II and CD40 expression on macrophages, BBR also activates the cytotoxic T lymphocytes (CTL) activity and stimulates the CD4+ T-cells derived IFN-γ production (66). Furthermore, BBR performs anti-tumor roles in diffuse large B cell lymphoma (DLBCL) related to rituximab-based immunochemotherapy and CD47-targeted immunotherapy (67). BBA exerts a remarkable synergistic action to enhance the CD47 inhibition resultant-tumor repression by c-myc and promote the phagocytosis of macrophages (67). Finally, BBR could prevent lung cancer by modulating the peptidylarginine deiminase 4 (PADI4)-related macrophage inflammatory responses by up-regulating CD86 and decreasing CD163 and CD206 in the PADI4 overexpressed macrophages (68).
Gut bacteria are tightly linked to cancer oncogenesis and progression, while BBR has exhibited therapeutic potential in bacteria-induced cancer (69). BBR maintains Fusobacterium nucleatum-induced intracellular signaling pathways and reduces the secretion of mucosal immune factors, including IL-21, IL-22, IL-31, and CD40 (70). Therefore, BBR facilitates intestinal microbiota homeostasis by increasing Tenericutes and Verrucomicrobia populations and reduces F. nucleatum colonization. BBR modulates the intestinal microbiome by regulating sodium butyrate production and inhibits colon cancer (71). BBR boosts the α and β diversity of microbiota, and the abundance of Bacteroidetes and Proteobacteria, whereas alters the biomarkers and metabolic outputs of the intestinal microbe and decreases the abundance of Ruminococcus (71). Given that immunotherapy is a critical part of cancer treatment, BBR has been validated to function on the immune system, showing great potential in cancer immunotherapy (72). For instance, BBR serves as a dopamine D1- and D2-like receptor antagonist to diminish IL-6, IL-1β, IFN-γ, and TNF-α production in the LPS-stimulated lymphocytes (73). It is also addressed that BBR boosts autoimmune neuropathy via decreasing IL-1 and TNF-α concentrations together with suppressing CD4+ T cell proliferation (74). Again, IFN-γ-induced indoleamine-2,3-dioxygenase 1 (IDO1) expression is reduced when BBR causes the inhibition of STAT1 phosphorylation (75). Moreover, BBR inhibits the PD-1/PD-L1 pathway by inactivating CSN5 deubiquitination in non-small-cell lung carcinoma (NSCLC) and improves anti-cancer T-cell immunity (9). It suggests a rationale for the therapeutic potential of BBR, which can be used as an efficient antagonist of PD-L1 in cancer immunotherapy.
Conclusions and perspectives
In the past several decades, we have witnessed a tremendous advance in exploring the potential mechanisms behind the pathogenesis of ulcerative colitis and cancer therapy. Nevertheless, the therapeutic approaches are still waiting for the findings of more reliably targetable players and available administrators. BBR is a multi-functional herbal medicine. The characteristics of BBR offer it a pivotal candidate for inflammation-associated UC and cancer treatment and attract more attention to study its targets and modes. In this mini-review, we summarized the latest advances in the main actions of BBR on inflammation and immune responses in UC and cancer research. As shown in the schematic diagram (Figure 1), inflammatory and immune factors include the signaling pathways of MAPK, NF-κB, Akt, AMPK, and Wnt/β-catenin interleukins, TNF-α, CD4+, CD40, and gut microbes, as well as macrophage polarization, are addressed. Although BBR exerts the marked repression of various targets as aforementioned in basic research, the preventive and therapeutic use of BBR against UC and cancer must be explored and validated in clinical studies.
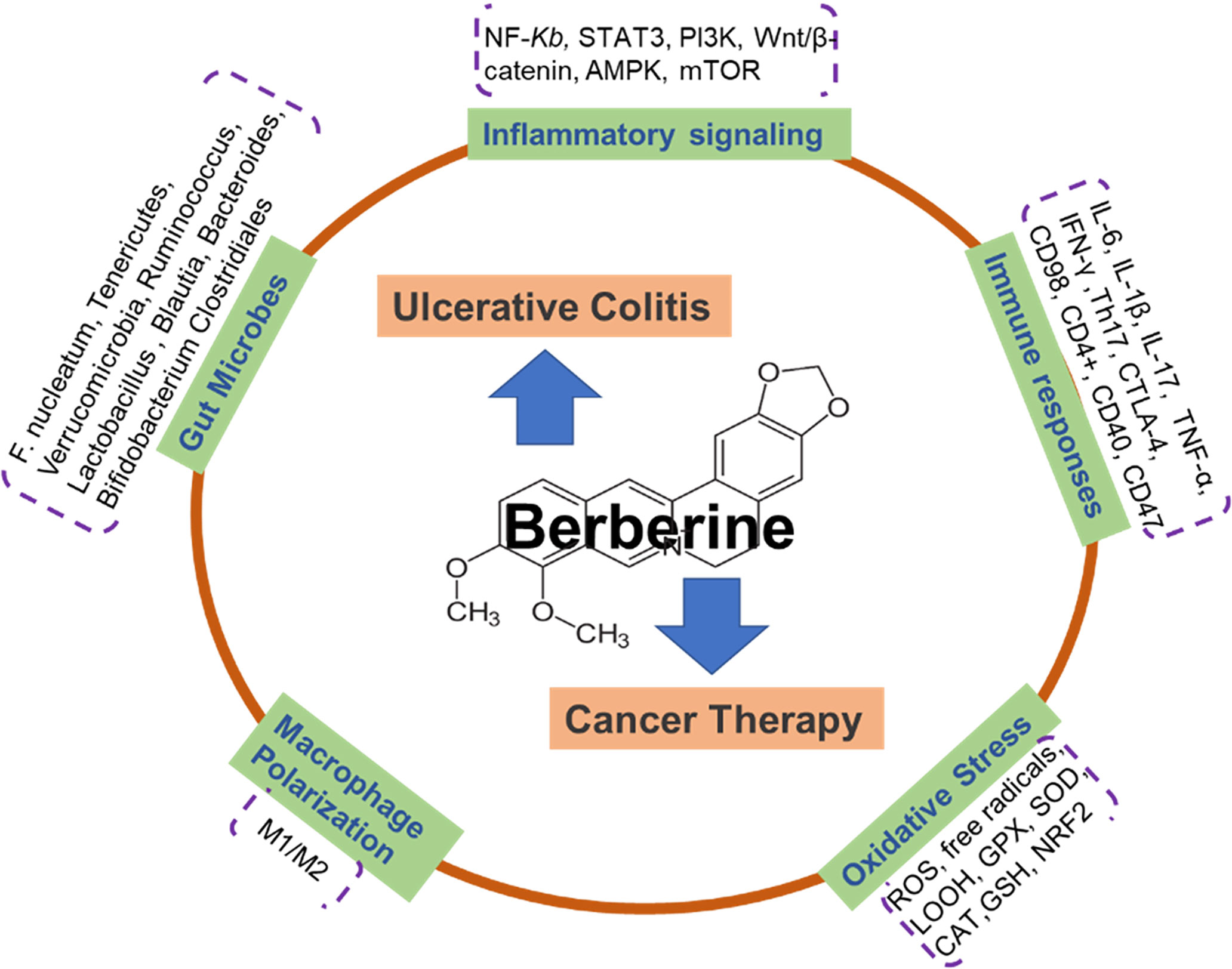
Figure 1 Schematic illustration depicting that berberine actions on ulcerative colitis and cancer therapy via multiple mechanisms. Berberine is an isoquinoline alkaloid purified from Chinese herbs and a naturally occurring compound extracted from Coptidis Rhizoma. There are 5 potential functions of BBR on the treatment of ulcerative colitis and cancer, including: inflammatory signaling pathways (NF-κB, STAT3, PI3K, Wnt/β-catenin, AMPK, mTOR); gut microbes (F. nucleatum, Tenericutes, Verrucomicrobia, Ruminococcus, Lactobacillus, Blautia, Bacteroides, Bifidobacterium, Clostridiales); immune responses (IL-6, IL-1β, IL-17, TNF-α, IFN-γ, Th17, CTLA-4, CD98, CD4+, CD40, CD47); macrophage polarization; Oxidative Stress (ROS, free radicals, LOOH, GPX, SOD, CAT, GSH, NRF2).
Author contributions
DC and H-YL: conceptualization. CZ, KL, PH, XP, ZW, and T-JY: writing the original draft. H-YL, and DC: review and editing. All authors contributed to the article and approved the submitted version.
Funding
This work was supported by the Postgraduate Research & Practice Innovation Program of Yangzhou University (X20211025), the Natural Science Foundation of Jiangsu Province (BK20200932, BK20220582), the Priority Academic Program Development of Jiangsu Higher Education Institutions (PAPD).
Conflict of interest
The authors declare that the research was conducted in the absence of any commercial or financial relationships that could be construed as a potential conflict of interest.
Publisher’s note
All claims expressed in this article are solely those of the authors and do not necessarily represent those of their affiliated organizations, or those of the publisher, the editors and the reviewers. Any product that may be evaluated in this article, or claim that may be made by its manufacturer, is not guaranteed or endorsed by the publisher.
References
1. Chen SR, Dai Y, Zhao J, Lin L, Wang Y, Wang Y. A mechanistic overview of triptolide and celastrol, natural products from tripterygium wilfordii hook f. Front Pharmacol (2018) 9:104. doi: 10.3389/fphar.2018.00104
2. Ma L, Sun Z, Zeng Y, Luo M, Yang J. Molecular mechanism and health role of functional ingredients in blueberry for chronic disease in human beings. Int J Mol Sci (2018) 19(9):2785. doi: 10.3390/ijms19092785
3. Song Z, Gao C, Jiang Q, Xu J, Xiong L, Liu K, et al. Diterpenoid alkaloids from delphinium forrestii var. viride and their anti-inflammation activity. Phytochemistry (2021) 192:112971. doi: 10.1016/j.phytochem.2021.112971
4. Xu Z, Feng W, Shen Q, Yu N, Yu K, Wang S, et al. Rhizoma coptidis and berberine as a natural drug to combat aging and aging-related diseases via anti-oxidation and AMPK activation. Aging Dis (2017) 8(6):760–77. doi: 10.14336/AD.2016.0620
5. Song D, Hao J, Fan D. Biological properties and clinical applications of berberine. Front Med (2020) 14(5):564–82. doi: 10.1007/s11684-019-0724-6
6. Zhang W, Xu JH, Yu T, Chen QK. Effects of berberine and metformin on intestinal inflammation and gut microbiome composition in db/db mice. BioMed Pharmacother (2019) 118:109131. doi: 10.1016/j.biopha.2019.109131
7. Zhang L, Wu X, Yang R, Chen F, Liao Y, Zhu Z, et al. Effects of berberine on the gastrointestinal microbiota. Front Cell Infect Microbiol (2020) 10:588517. doi: 10.3389/fcimb.2020.588517
8. Han M, Jung YS, Kim WH, Cheon JH, Park S. Cancer risk in patients with intestinal behcet's disease: A nationwide population-based study. Gut Liver (2018) 12(4):433–9. doi: 10.5009/gnl17324
9. Liu Y, Liu X, Zhang N, Yin M, Dong J, Zeng Q, et al. Berberine diminishes cancer cell PD-L1 expression and facilitates antitumor immunity via inhibiting the deubiquitination activity of CSN5. Acta Pharm Sin B (2020) 10(12):2299–312. doi: 10.1016/j.apsb.2020.06.014
10. Angelidis C, Kotsialou Z, Kossyvakis C, Vrettou AR, Zacharoulis A, Kolokathis F, et al. Colchicine pharmacokinetics and mechanism of action. Curr Pharm Des (2018) 24(6):659–63. doi: 10.2174/1381612824666180123110042
11. Yarmohammadi F, Hayes AW, Karimi G. The therapeutic effects of berberine against different diseases: A review on the involvement of the endoplasmic reticulum stress. Phytother Res (2022) 36(8):3215–31. doi: 10.1002/ptr.7539
12. Pang B, Zhao LH, Zhou Q, Zhao TY, Wang H, Gu CJ, et al. Application of berberine on treating type 2 diabetes mellitus. Int J Endocrinol (2015) 2015:905749. doi: 10.1155/2015/905749
13. Vishnoi K, Ke R, Saini KS, Viswakarma N, Nair RS, Das S, et al. Berberine represses beta-catenin translation involving 4E-BPs in hepatocellular carcinoma cells. Mol Pharmacol (2021) 99(1):1–16. doi: 10.1124/molpharm.120.000029
14. Ma CY, Shi XY, Wu YR, Zhang Y, Yao YH, Qu HL, et al. Berberine attenuates atherosclerotic lesions and hepatic steatosis in ApoE(-/-) mice by down-regulating PCSK9 via ERK1/2 pathway. Ann Transl Med (2021) 9(20):1517. doi: 10.21037/atm-20-8106
15. Li C, Xi Y, Li S, Zhao Q, Cheng W, Wang Z, et al. Berberine ameliorates TNBS induced colitis by inhibiting inflammatory responses and Th1/Th17 differentiation. Mol Immunol (2015) 67(2 Pt B):444–54. doi: 10.1016/j.molimm.2015.07.013
16. Zhu L, Han J, Yuan R, Xue L, Pang W. Berberine ameliorates diabetic nephropathy by inhibiting TLR4/NF-kappaB pathway. Biol Res (2018) 51(1):9. doi: 10.1186/s40659-018-0157-8
17. Zhou Y, Liu SQ, Yu L, He B, Wu SH, Zhao Q, et al. Berberine prevents nitric oxide-induced rat chondrocyte apoptosis and cartilage degeneration in a rat osteoarthritis model via AMPK and p38 MAPK signaling. Apoptosis (2015) 20(9):1187–99. doi: 10.1007/s10495-015-1152-y
18. Wang Y, Zhang S. Berberine suppresses growth and metastasis of endometrial cancer cells via miR-101/COX-2. BioMed Pharmacother (2018) 103:1287–93. doi: 10.1016/j.biopha.2018.04.161
19. Wang S, Ren H, Zhong H, Zhao X, Li C, Ma J, et al. Combined berberine and probiotic treatment as an effective regimen for improving postprandial hyperlipidemia in type 2 diabetes patients: a double blinded placebo controlled randomized study. Gut Microbes (2022) 14(1):2003176. doi: 10.1080/19490976.2021.2003176
20. Guo F, Cai D, Li Y, Gu H, Qu H, Zong Q, et al. How early-life gut microbiota alteration sets trajectories for health and inflammatory bowel disease? Front Nutr (2021) 8:690073. doi: 10.3389/fnut.2021.690073
21. Zhang T, Zhang B, Tian W, Wang F, Zhang J, Ma X, et al. Research trends in ulcerative colitis: A bibliometric and visualized study from 2011 to 2021. Front Pharmacol (2022) 13:951004. doi: 10.3389/fphar.2022.951004
22. Yang Z, Lin S, Feng W, Liu Y, Song Z, Pan G, et al. A potential therapeutic target in traditional Chinese medicine for ulcerative colitis: Macrophage polarization. Front Pharmacol (2022) 13:999179. doi: 10.3389/fphar.2022.999179
23. Yin J, Wei L, Wang N, Li X, Miao M. Efficacy and safety of adjuvant curcumin therapy in ulcerative colitis: A systematic review and meta-analysis. J Ethnopharmacol (2022) 289:115041. doi: 10.1016/j.jep.2022.115041
24. Tang X, Yang M, Gu Y, Jiang L, Du Y, Liu J. Orally deliverable dual-targeted pellets for the synergistic treatment of ulcerative colitis. Drug Des Devel Ther (2021) 15:4105–23. doi: 10.2147/DDDT.S322702
25. Graham DB, Xavier RJ. Pathway paradigms revealed from the genetics of inflammatory bowel disease. Nature (2020) 578(7796):527–39. doi: 10.1038/s41586-020-2025-2
26. Yang T, Ma X, Wang R, Liu H, Wei S, Jing M, et al. Berberine inhibits IFN-gamma signaling pathway in DSS-induced ulcerative colitis. Saudi Pharm J (2022) 30(6):764–78. doi: 10.1016/j.jsps.2022.03.015
27. Zhang LC, Wang Y, Tong LC, Sun S, Liu WY, Zhang S, et al. Berberine alleviates dextran sodium sulfate-induced colitis by improving intestinal barrier function and reducing inflammation and oxidative stress. Exp Ther Med (2017) 13(6):3374–82. doi: 10.3892/etm.2017.4402
28. Zhu L, Gu P, Shen H. Protective effects of berberine hydrochloride on DSS-induced ulcerative colitis in rats. Int Immunopharmacol (2019) 68:242–51. doi: 10.1016/j.intimp.2018.12.036
29. Wang Y, Du P, Jiang D. Berberine functions as a negative regulator in lipopolysaccharide -induced sepsis by suppressing NF-kappaB and IL-6 mediated STAT3 activation. Pathog Dis (2020) 78(7):ftaa047. doi: 10.1093/femspd/ftaa047
30. Moparthi L, Koch S. Wnt signaling in intestinal inflammation. Differentiation (2019) 108:24–32. doi: 10.1016/j.diff.2019.01.002
31. Dong Y, Fan H, Zhang Z, Jiang F, Li M, Zhou H, et al. Berberine ameliorates DSS-induced intestinal mucosal barrier dysfunction through microbiota-dependence and wnt/beta-catenin pathway. Int J Biol Sci (2022) 18(4):1381–97. doi: 10.7150/ijbs.65476
32. Xu L, Zhang Y, Xue X, Liu J, Li ZS, Yang GY, et al. A phase I trial of berberine in Chinese with ulcerative colitis. Cancer Prev Res (Phila) (2020) 13(1):117–26. doi: 10.1158/1940-6207.CAPR-19-0258
33. Xiong X, Cheng Z, Wu F, Hu M, Liu Z, Dong R, et al. Berberine in the treatment of ulcerative colitis: A possible pathway through tuft cells. BioMed Pharmacother (2021) 134:111129. doi: 10.1016/j.biopha.2020.111129
34. Li H, Feng C, Fan C, Yang Y, Yang X, Lu H, et al. Intervention of oncostatin m-driven mucosal inflammation by berberine exerts therapeutic property in chronic ulcerative colitis. Cell Death Dis (2020) 11(4):271. doi: 10.1038/s41419-020-2470-8
35. Zheng C, Wang Y, Xu Y, Zhou L, Hassan S, Xu G, et al. Berberine inhibits dendritic cells differentiation in DSS-induced colitis by promoting bacteroides fragilis. Int Immunopharmacol (2021) 101(Pt A):108329. doi: 10.1016/j.intimp.2021.108329
36. Li YH, Xiao HT, Hu DD, Fatima S, Lin CY, Mu HX, et al. Berberine ameliorates chronic relapsing dextran sulfate sodium-induced colitis in C57BL/6 mice by suppressing Th17 responses. Pharmacol Res (2016) 110:227–39. doi: 10.1016/j.phrs.2016.02.010
37. Li H, Fan C, Lu H, Feng C, He P, Yang X, et al. Protective role of berberine on ulcerative colitis through modulating enteric glial cells-intestinal epithelial cells-immune cells interactions. Acta Pharm Sin B (2020) 10(3):447–61. doi: 10.1016/j.apsb.2019.08.006
38. He Y, Yuan X, Zuo H, Sun Y, Feng A. Berberine exerts a protective effect on gut-vascular barrier via the modulation of the Wnt/Beta-catenin signaling pathway during sepsis. Cell Physiol Biochem (2018) 49(4):1342–51. doi: 10.1159/000493412
39. Noh JW, Jun MS, Yang HK, Lee BC. Cellular and molecular mechanisms and effects of berberine on obesity-induced inflammation. Biomedicines (2022) 10(7):1739. doi: 10.3390/biomedicines10071739
40. Liu YC, Hsiao YY, Ku KL, Liao HF, Chao WC. Mahonia oiwakensis extract and its bioactive compounds exert anti-inflammatory activities and VEGF production through M2-macrophagic polarization and STAT6 activation. J Med Food (2018) 21(7):654–64. doi: 10.1089/jmf.2017.4084
41. Shen ZH, Zhu CX, Quan YS, Yang ZY, Wu S, Luo WW, et al. Relationship between intestinal microbiota and ulcerative colitis: Mechanisms and clinical application of probiotics and fecal microbiota transplantation. World J Gastroenterol (2018) 24(1):5–14. doi: 10.3748/wjg.v24.i1.5
42. Neyrinck AM, Sanchez CR, Rodriguez J, Cani PD, Bindels LB, Delzenne NM. Prebiotic effect of berberine and curcumin is associated with the improvement of obesity in mice. Nutrients (2021) 13(5):1436. doi: 10.3390/nu13051436
43. Li J, Li J, Ni J, Zhang C, Jia J, Wu G, et al. Berberine relieves metabolic syndrome in mice by inhibiting liver inflammation caused by a high-fat diet and potential association with gut microbiota. Front Microbiol (2021) 12:752512. doi: 10.3389/fmicb.2021.752512
44. Li X, Su C, Jiang Z, Yang Y, Zhang Y, Yang M, et al. Berberine attenuates choline-induced atherosclerosis by inhibiting trimethylamine and trimethylamine-n-oxide production via manipulating the gut microbiome. NPJ Biofilms Microbiomes (2021) 7(1):36. doi: 10.1038/s41522-021-00205-8
45. Chou S, Zhang S, Guo H, Chang YF, Zhao W, Mou X. Targeted antimicrobial agents as potential tools for modulating the gut microbiome. Front Microbiol (2022) 13:879207. doi: 10.3389/fmicb.2022.879207
46. Li G, Zhang C, Liang W, Zhang Y, Shen Y, Tian X. Berberine regulates the Notch1/PTEN/PI3K/AKT/mTOR pathway and acts synergistically with 17-AAG and SAHA in SW480 colon cancer cells. Pharm Biol (2021) 59(1):21–30. doi: 10.1080/13880209.2020.1865407
47. Tai CJ, Jassey A, Liu CH, Tai CJ, Richardson CD, Wong SH, et al. Targeting autophagy augments BBR-mediated cell death in human hepatoma cells harboring hepatitis c virus RNA. Cells (2020) 9(4):908. doi: 10.3390/cells9040908
48. Hesari A, Ghasemi F, Cicero AFG, Mohajeri M, Rezaei O, Hayat SMG, et al. Berberine: A potential adjunct for the treatment of gastrointestinal cancers? J Cell Biochem (2018) 119(12):9655–63. doi: 10.1002/jcb.27392
49. Singh N, Baby D, Rajguru JP, Patil PB, Thakkannavar SS, Pujari VB. Inflammation and cancer. Ann Afr Med (2019) 18(3):121–6. doi: 10.4103/aam.aam_56_18
50. Kennel KB, Greten FR. Immune cell - produced ROS and their impact on tumor growth and metastasis. Redox Biol (2021) 42:101891. doi: 10.1016/j.redox.2021.101891
51. Xia Y, Chen S, Cui J, Wang Y, Liu X, Shen Y, et al. Berberine suppresses bladder cancer cell proliferation by inhibiting JAK1-STAT3 signaling via upregulation of miR-17-5p. Biochem Pharmacol (2021) 188:114575. doi: 10.1016/j.bcp.2021.114575
52. Kou Y, Tong B, Wu W, Liao X, Zhao M. Berberine improves chemo-sensitivity to cisplatin by enhancing cell apoptosis and repressing PI3K/AKT/mTOR signaling pathway in gastric cancer. Front Pharmacol (2020) 11:616251. doi: 10.3389/fphar.2020.616251
53. Filippi I, Carraro F, Naldini A. Interleukin-1beta affects MDAMB231 breast cancer cell migration under hypoxia: Role of HIF-1alpha and NFkappaB transcription factors. Mediators Inflammation (2015) 2015:789414. doi: 10.1155/2015/789414
54. Almatroodi SA, Alsahli MA, Rahmani AH. Berberine: An important emphasis on its anticancer effects through modulation of various cell signaling pathways. Molecules (2022) 27(18):5889. doi: 10.3390/molecules27185889
55. Fu L, Chen W, Guo W, Wang J, Tian Y, Shi D, et al. Berberine targets AP-2/hTERT, NF-kappaB/COX-2, HIF-1alpha/VEGF and cytochrome-c/Caspase signaling to suppress human cancer cell growth. PloS One (2013) 8(7):e69240. doi: 10.1371/journal.pone.0069240
56. Zhao L, Zhang C. Berberine inhibits MDA-MB-231 cells by attenuating their inflammatory responses. BioMed Res Int (2020) 2020:3617514. doi: 10.1155/2020/3617514
57. Yao M, Fan X, Yuan B, Takagi N, Liu S, Han X, et al. Berberine inhibits NLRP3 inflammasome pathway in human triple-negative breast cancer MDA-MB-231 cell. BMC Complement Altern Med (2019) 19(1):216. doi: 10.1186/s12906-019-2615-4
58. Liu JF, Lai KC, Peng SF, Maraming P, Huang YP, Huang AC, et al. Berberine inhibits human melanoma A375.S2 cell migration and invasion via affecting the FAK, uPA, and NF-kappaB signaling pathways and inhibits PLX4032 resistant A375.S2 cell migration in vitro. Molecules (2018) 23(8):2019. doi: 10.3390/molecules23082019
59. Li W, Hua B, Saud SM, Lin H, Hou W, Matter MS, et al. Berberine regulates AMP-activated protein kinase signaling pathways and inhibits colon tumorigenesis in mice. Mol Carcinog (2015) 54(10):1096–109. doi: 10.1002/mc.22179
60. Zhang X, Gu L, Li J, Shah N, He J, Yang L, et al. Degradation of MDM2 by the interaction between berberine and DAXX leads to potent apoptosis in MDM2-overexpressing cancer cells. Cancer Res (2010) 70(23):9895–904. doi: 10.1158/0008-5472.CAN-10-1546
61. Wang ZS, Lu FE, Xu LJ, Dong H. Berberine reduces endoplasmic reticulum stress and improves insulin signal transduction in hep G2 cells. Acta Pharmacol Sin (2010) 31(5):578–84. doi: 10.1038/aps.2010.30
62. Zhang Q, Wang X, Cao S, Sun Y, He X, Jiang B, et al. Berberine represses human gastric cancer cell growth in vitro and in vivo by inducing cytostatic autophagy via inhibition of MAPK/mTOR/p70S6K and akt signaling pathways. BioMed Pharmacother (2020) 128:110245. doi: 10.1016/j.biopha.2020.110245
63. Bian J, Dannappel M, Wan C, Firestein R. Transcriptional regulation of wnt/beta-catenin pathway in colorectal cancer. Cells (2020) 9(9):2125. doi: 10.3390/cells9092125
64. Jiang X, Jiang Z, Jiang M, Sun Y. Berberine as a potential agent for the treatment of colorectal cancer. Front Med (Lausanne) (2022) 9:886996. doi: 10.3389/fmed.2022.886996
65. Liu Y, Hua W, Li Y, Xian X, Zhao Z, Liu C, et al. Berberine suppresses colon cancer cell proliferation by inhibiting the SCAP/SREBP-1 signaling pathway-mediated lipogenesis. Biochem Pharmacol (2020) 174:113776. doi: 10.1016/j.bcp.2019.113776
66. Shah D, Challagundla N, Dave V, Patidar A, Saha B, Nivsarkar M, et al. Berberine mediates tumor cell death by skewing tumor-associated immunosuppressive macrophages to inflammatory macrophages. Phytomedicine (2021) 99:153904. doi: 10.1016/j.phymed.2021.153904
67. Ren S, Cai Y, Hu S, Liu J, Zhao Y, Ding M, et al. Berberine exerts antitumor activity in diffuse large b-cell lymphoma by modulating c-myc/CD47 axis. Biochem Pharmacol (2021) 188:114576. doi: 10.1016/j.bcp.2021.114576
68. Gu W, Zhang M, Gao F, Niu Y, Sun L, Xia H, et al. Berberine regulates PADI4-related macrophage function to prevent lung cancer. Int Immunopharmacol (2022) 110:108965. doi: 10.1016/j.intimp.2022.108965
69. Chen H, Ye C, Cai B, Zhang F, Wang X, Zhang J, et al. Berberine inhibits intestinal carcinogenesis by suppressing intestinal pro-inflammatory genes and oncogenic factors through modulating gut microbiota. BMC Cancer (2022) 22(1):566. doi: 10.1186/s12885-022-09635-9
70. Li S, Liu J, Zheng X, Ren L, Yang Y, Li W, et al. Tumorigenic bacteria in colorectal cancer: mechanisms and treatments. Cancer Biol Med (2021) 19(2):147–162. doi: 10.20892/j.issn.2095-3941.2020.0651
71. Huang C, Sun Y, Liao SR, Chen ZX, Lin HF, Shen WZ. Suppression of berberine and probiotics (in vitro and in vivo) on the growth of colon cancer with modulation of gut microbiota and butyrate production. Front Microbiol (2022) 13:869931. doi: 10.3389/fmicb.2022.869931
72. Wang YX, Pang WQ, Zeng QX, Deng ZS, Fan TY, Jiang JD, et al. Synthesis and biological evaluation of new berberine derivatives as cancer immunotherapy agents through targeting IDO1. Eur J Med Chem (2018) 143:1858–68. doi: 10.1016/j.ejmech.2017.10.078
73. Kawano M, Takagi R, Kaneko A, Matsushita S. Berberine is a dopamine D1- and D2-like receptor antagonist and ameliorates experimentally induced colitis by suppressing innate and adaptive immune responses. J Neuroimmunol (2015) 289:43–55. doi: 10.1016/j.jneuroim.2015.10.001
74. Li H, Li XL, Zhang M, Xu H, Wang CC, Wang S, et al. Berberine ameliorates experimental autoimmune neuritis by suppressing both cellular and humoral immunity. Scand J Immunol (2014) 79(1):12–9. doi: 10.1111/sji.12123
75. Sharma A, Tirpude NV, Bhardwaj N, Kumar D, Padwad Y. Berberis lycium fruit extract and its phytoconstituents berberine and rutin mitigate collagen-CFA-induced arthritis (CIA) via improving GSK3beta/STAT/Akt/MAPKs/NF-kappaB signaling axis mediated oxi-inflammation and joint articular damage in murine model. Inflammopharmacology (2022) 30(2):655–66. doi: 10.1007/s10787-022-00941-z
Keywords: berberine, ulcerative colitis, inflammation, microbes, cancer
Citation: Zhu C, Li K, Peng X-X, Yao T-J, Wang Z-Y, Hu P, Cai D and Liu H-Y (2022) Berberine a traditional Chinese drug repurposing: Its actions in inflammation-associated ulcerative colitis and cancer therapy. Front. Immunol. 13:1083788. doi: 10.3389/fimmu.2022.1083788
Received: 29 October 2022; Accepted: 21 November 2022;
Published: 06 December 2022.
Edited by:
Yi Wu, Nanjing Agricultural University, ChinaReviewed by:
Mingchao Liu, Agricultural University of Hebei, ChinaHelen Fu, Jiangsu Provincial Hospital of Traditional Chinese Medicine, China
Copyright © 2022 Zhu, Li, Peng, Yao, Wang, Hu, Cai and Liu. This is an open-access article distributed under the terms of the Creative Commons Attribution License (CC BY). The use, distribution or reproduction in other forums is permitted, provided the original author(s) and the copyright owner(s) are credited and that the original publication in this journal is cited, in accordance with accepted academic practice. No use, distribution or reproduction is permitted which does not comply with these terms.
*Correspondence: Hao-Yu Liu, aGFveXUubGl1QHl6dS5lZHUuY24=; Demin Cai, ZGVtaW5jYWlAeXp1LmVkdS5jbg==
†These authors have contributed equally to this work