- 1Department of Endosecretory Metabolic Diseases, Affiliated Hospital of Changchun University of Chinese Medicine, Changchun, Jilin, China
- 2School of Pharmacy, Changchun University of Chinese Medicine, Changchun, Jilin, China
- 3Department of Acupuncture and Massage, The Third Affiliated Hospital of Changchun University of Chinese Medicine, Changchun, Jilin, China
Metabolic syndrome mainly includes obesity, type 2 diabetes (T2DM), alcoholic fatty liver (NAFLD) and cardiovascular diseases. According to the ancient experience philosophy of Yin-Yang, monarch-minister compatibility of traditional Chinese medicine, prescription is given to treat diseases, which has the advantages of small toxic and side effects and quick effect. However, due to the diversity of traditional Chinese medicine ingredients and doubts about the treatment theory of traditional Chinese medicine, the mechanism of traditional Chinese medicine is still in doubt. Gastrointestinal tract is an important part of human environment, and participates in the occurrence and development of diseases. In recent years, more and more TCM researches have made intestinal microbiome a new frontier for understanding and treating diseases. Clinically, nonalcoholic fatty liver disease (NAFLD) and diabetes mellitus (DM) often co-occur. Our aim is to explain the mechanism of interaction between gastrointestinal microbiome and traditional Chinese medicine (TCM) or traditional Chinese medicine formula to treat DM and NAFLD. Traditional Chinese medicine may treat these two diseases by influencing the composition of intestinal microorganisms, regulating the metabolism of intestinal microorganisms and transforming Chinese medicinal compounds.
1. Introduction
Intestinal microecology is an important part of human environment. It is composed of intestinal microbiota, intestinal epithelial cells and immune system, forms intestinal mucosal barrier and plays an important role in energy metabolism. Both external and genetic factors affect the composition and function of intestinal microecology. The steady state of microbiota is closely related to human health. There is growing evidence that intestinal microbiota and their metabolites play an important role in the development of obesity, diabetes and nonalcoholic fatty liver disease (1). Diabetes and non-alcoholic fatty liver are two diseases closely related to intestinal microbial homeostasis. T2DM is the main type of diabetes, mainly manifested as metabolic disorders, such as hyperglycemia, hyperlipidemia and insulin resistance. Diabetes can lead to a variety of serious complications, such as retinopathy and diabetic nephropathy, gestational diabetes, atherosclerosis and other cardiovascular diseases, these complications affect the quality of life of a large number of people around the world. The rapid growth of diabetes has brought a great burden on the global society and economic society (2). Nonalcoholic fatty liver disease (NAFLD), as a common chronic liver disease, can be divided into fatty liver, steatohepatitis and liver fibrosis according to the degree of inflammation and fibrosis. The main manifestations of NAFLD are steatosis, lipotoxicity and inflammatory injury, which are associated with glucose homeostasis and persistent low-grade inflammation (1) (3). Studies have found that intestinal flora and metabolites can reverse some metabolic disorders, including high fat, tissue inflammation and low insulin sensitivity and secretion (4). This suggests that intestinal flora can be used in the treatment of diabetes and fatty liver (5).
As an important supplementary means of clinical medicine, traditional Chinese medicine has been widely adopted in some East Asian countries. In some western countries, such as the United States, Britain and Germany, the trend of using traditional Chinese medicine as a treatment for diseases is becoming more and more obvious. Different from chemical drugs and biological agents, traditional Chinese medicine and traditional Chinese medicine formulations under the guidance of traditional Chinese medicine theory are often difficult to determine specific bioactive components. Traditional Chinese medicine prescribes prescriptions to treat diseases under the guidance of ancient empirical philosophy, such as yin-yang, monarch-minister compatibility and so on. Traditional Chinese medicine advocates the concept of wholeness and regards organs such as internal organs as a whole. the destruction of intestinal microbial homeostasis promotes the development of metabolic syndrome such as diabetes, fatty liver and cardiovascular syndrome, which is in line with the “whole” concept of TCM theory. There has been a sharp increase in patients with diabetes and non-alcoholic fatty liver disease worldwide, which studies have shown may be associated with insulin resistance. Patients usually suffer from these two diseases at the same time, which is a difficult problem in clinical treatment (6). As insulin resistance plays an important role in the development of non-alcoholic fatty liver, diabetes drugs are often used as the treatment option for the treatment of non-alcoholic fatty liver (7) (8). On the one hand: there are no approved drugs for nonalcoholic fatty liver disease, and the only approved treatment option is to improve diet and lose weight. On the other hand: the drugs used to treat diabetes are still defective in the treatment of fatty liver. With the discovery of plant-derived natural products quercetin, resveratrol, polysaccharides, berberine and curcumin in the treatment of diseases, researchers have focused on “simple, convenient and low-toxic” herbs. Researchers have found that single herbs such as Coptis chinensis, Radix Astragali, Ginseng and herbal formulations such as SiMiao, Gegen Qinlian decoction, Huanglian jiedu decoction and LLKL have potential therapeutic effects on T2DM and NAFLD. These herbs exert pharmacological effects through intestinal microflora and mainly include two ways: changing the composition of intestinal microorganisms and affecting the metabolism of intestinal microflora. The main purpose of this review is to explain the therapeutic effect of intestinal microbiota on diabetes and non-alcoholic fatty liver.
2. Association between diabetes mellitus and non-alcoholic fatty liver disease
2.1. Diabetes mellitus and non-alcoholic fatty liver disease – two clinically associated diseases
Diabetes is a metabolic disorder characterized by hyperglycemia caused by deficiency of insulin secretion and/or deficiency of insulin action. There are two main types of diabetes, insulin-dependent type 1 diabetes (T1DM) and insulin-independent type 2 diabetes mellitus (T2DM), of which type 2 diabetes accounts for 90% of patients with diabetes (9). Nonalcoholic fatty liver disease (NAFLD) is considered to be the most common form of liver disease in the world, including fatty liver, steatohepatitis (NASH) and liver fibrosis (10). NASH is a progressive form of nonalcoholic fatty liver. NAFLD is a risk factor for metabolic disorders such as obesity, diabetes, especially T2DM and cardiovascular disease. Among obese people undergoing bariatric surgery, the prevalence of NAFLD is as high as 90%, and among diabetics, the prevalence of NAFLD can be as high as 71%. Insulin resistance (IR) exists in almost all patients with NAFLD and T2DM. Through the evaluation of the homeostasis model of insulin resistance, it was found that there was a significant correlation between IR and the prevalence of steatohepatitis in NAFLD. The relationship between diabetes and nonalcoholic fatty liver gradually evolved into the relationship with simple steatosis (SS), NASH and liver fibrosis (11) (12). Cross-sectional studies have shown that non-alcoholic fatty liver disease usually occurs in patients with type 2 diabetes (13). A systematic review and meta-analysis of 27 clinical trials confirmed the direct relationship between fatty liver and the incidence of diabetes (14). The probability of developing diabetes is also different in different steatosis states. Follow-up results showed that the incidence of diabetes in patients without steatosis, intermittent steatosis and persistent steatosis increased by 5.1%, 14.1% and 27.1%, respectively. It can be speculated that early intervention of steatosis has a resistant effect on the development of diabetes (15) A study based on patients with nonalcoholic fatty liver disease and first-degree relatives in the United States found that familial aggregation of insulin resistance syndrome has a genetic susceptibility to supporting nonalcoholic fatty liver disease (16). Family history of diabetes, especially in non-diabetic patients, is associated with nonalcoholic steatohepatitis (NASH) and fibrosis in NAFLD (17). In addition, the occurrence and development of cardiovascular diseases such as obesity, retinopathy, renal failure, peripheral neuropathy and atherosclerosis are also related to diabetes (9).
2.2. Beneficial effects of various anti-diabetic drugs on non-alcoholic fatty liver disease
In the above part, we have explained the clinical correlation between NAFLD and T2DM. However, NAFLD does not have an explicitly approved drug, and the only approved treatment option is to change diet and exercise. IR plays an important role in the development of NAFLD, and many hypoglycemic drugs have been evaluated for the treatment of NAFLD. These drugs mainly include biguanides, glucagon-like peptide 1 receptor (GLP-1) agonists, peroxisome proliferator-activated receptor (PPAR) agonists and farnesoid X receptor (FXR) agonists. Metformin is known to improve lipid metabolism by activating adenylate-activated protein kinase (AMPK), an important regulator of energy metabolism (18). Metformin exerts the preventive effect of NAFLD by increasing AMPK phosphorylation, inhibiting macrophage polarization, reducing macrophage infiltration and the expression of pro-inflammatory cytokines (TNF- α, IL-1 β and IL-6), relieving liver inflammation and fat accumulation (19, 20). In addition, metformin alleviates fatty liver degeneration in obese mice by affecting the protein levels of CYP7B1 and CH25H, a cholesterol hydroxylase, to regulate cholesterol secretion and metabolism (21). Glucagon-like peptide-1 (GLP-1) is an enterotropic insulin secreted by intestinal endocrine L cells that regulates glucose regulation by slowing gastric emptying and glucose-dependent inhibition of glucagon secretion. GLP-1 can improve liver insulin sensitivity (22, 23)and enhance the direct effect of lipid hydrolysis and oxidation on liver (24–26). Lilarutide is a kind of GLP-1 analogue. Studies have shown that liralutide can reduce liver enzymes, including alanine aminotransferase (ALT) and aspartate aminotransferase (AST) (27). Lipopeptide is associated with liver lipid metabolism, total cholesterol (TC) and triglyceride (TG) (28). Pioglitazone belongs to PPAR- γ agonist and has insulin sensitizing effect (29). Pioglitazone reduces the accumulation of lipids in the liver (30) by improving fatty acid uptake and transport. Farnesoid X receptor (FXR) is a kind of nuclear receptor activated by bile acid, which is highly expressed in the liver and intestines and is related to bile acid and lipid metabolism (31). FXR agonists can reduce insulin resistance, improve lipid metabolism disorders, and alleviate fatty liver degeneration (32).
2.3. Summary
In conclusion, there is a close relationship between diabetes and nonalcoholic fatty liver disease. Metformin, liralutide and pioglitazone are used in the treatment of diabetes and drugs can be developed for the treatment of non-alcoholic fatty liver.
3. How does the gut microbiota influence T2DM and NAFLD
3.1. Intestinal microbes
Intestinal microecology is an extremely complex ecosystem, which is composed of intestinal microflora, intestinal epithelial cells and intestinal immune system. Intestinal microecology is regarded as an important “organ”, which plays an important role in regulating human metabolism (33). Intestinal microflora, also known as intestinal bacteria, is a complex microbial community living in the gastrointestinal tract of the human body in a symbiotic way, which mainly includes two phyla, thick-walled bacteria and Bacteroides (34). Diabetes and nonalcoholic fatty liver disease are metabolic diseases related to obesity (35). Obesity increases the risk of diabetes and NAFLD in humans (36, 37). Intestinal flora disorders have been repeatedly observed in these metabolic diseases, which seem to be related to changes in the proportion of thick-walled bacteria and actinomycetes in the intestines (38, 39). In patients with T2DM, it was observed that the abundance of Streptococcus faecalis and Rosobacter increased, while the abundance of Shigella and Bifidobacterium decreased (40). The decrease of microbial diversity and the increase of Prevotella abundance were observed in the feces of children with NAFLD. Today, unhealthy Western diets are promoting and aggravating the course of T2DM and NAFLD, which may be reduced or reversed by intestinal flora treatment (41–43).
3.2. Intestinal microbial metabolites
3.2.1. SCFAs
Intestinal ecological disorders usually lead to changes in intestinal SCFAs levels. Short-chain fatty acids (such as acetate, propionate and butyrate) produced by intestinal microorganisms not only provide nutrition and energy for the host (44), but also participate in lipid metabolism and glucose metabolism through a variety of pathways (45), thus affecting the development of T2DM and NAFLD. It has been found that human cells respond to SCFAs mainly by activating G protein coupled receptor (GPR41,GPR43) and inhibiting histone deacetylase (HDAC) (46, 47). G protein coupled receptors are expressed in adipose tissue (48), liver (49) and pancreatic β cells (50). Acetate is an important substrate for fatty acid synthesis, and the increase of acetate will lead to the accumulation of triglycerides (51, 52). Propionate is an important precursor of gluconeogenesis, and an increase in propionate levels will promote gluconeogenesis in the liver (53). Acetate and propionate activate GPR43 receptors, inhibit insulin signal transduction in adipocytes, inhibit fat accumulation and promote lipid and glucose metabolism in other tissues (54, 55). Butyrate promotes the expression of gluconeogenesis-related genes in a cAMP-dependent manner. In addition, SCFAs stimulates intestinal endocrine cells to secrete glucagon-like peptide 1 (GLP-1) and YY peptide (PYY) through a GPR-dependent mechanism. These two hormones inhibit appetite, promote fat oxidation, promote insulin secretion and reduce glucagon, and inhibit hepatic steatosis and the development of diabetes (47, 56). In addition, propionate and butyrate can also act as HDAC inhibitors to induce increased PYY mRNA levels (57).
3.2.2. TMAO, BAs and BCAAs
Trimethylamine N-oxide (TMAO) is a metabolite associated with diabetes, liver steatosis and other chronic diseases (58). TMAO is derived from intestinal microflora that metabolizes choline. Choline is converted to trimethylamine (TMA) through Flavin-containing monooxygenase, and TMA is converted into TMAO in the liver (59). It was found that TMAO accumulated in the serum of patients with T2DM and NAFLD (60–62). TMAO can play a role in NAFLD by changing bile acid metabolism (63). In addition, TMAO may induce pancreatic β-cell dysfunction and promote the pathogenesis of T2D (64). Bile acids include primary bile acids and secondary bile acids. Primary bile acids are synthesized by cholesterol in the liver. Primary bile acids enter the intestine and are converted into secondary bile acids by intestinal flora. As an important mediator of intestinal-liver crosstalk, bile acid mainly acts on two key receptors, farnesoid X receptor (FXR) and Takeda G protein-coupled receptor 5 (TGR5), and regulates glucose homeostasis and lipid metabolism (65, 66). Bile acid metabolism is associated with the onset and progression of type 2 diabetes and NAFLD (67). Bile acid chelating agents can inhibit FXR activity in intestinal L cells, promote the production and secretion of GLP-1, and improve blood glucose (68). It can also reverse hepatic steatosis, inflammation and fibrosis by interrupting intestinal bile acid reabsorption (69). Branched chain amino acids (BCAAs) are essential amino acids, including leucine, isoleucine and valine (70). Intestinal microflora can produce and degrade branched chain amino acids. The increase of host branched chain amino acids is related to metabolic fatty liver disease and diabetes (71, 72). Amino acid-induced insulin signal transduction damage and G protein coupled receptor involvement lead to insulin resistance and type 2 diabetes mellitus (73). Leucine affects glucose metabolism by activating rapamycin complex 1mTORC1 (74). Host circulating branched chain amino acids were positively correlated with higher cholesterol level, liver fat content and insulin resistance (IR) (75). However, some studies have found that supplementation of branched chain amino acids can reduce the expression of adipogenesis-related genes FAS and ACC in the liver and reduce fat accumulation in the liver of rats fed with high-fat diet (72, 76).
3.3. Intestinal permeability and inflammation
The intestinal barrier consists of mucin layer and epithelial cells. The destruction of intestinal barrier makes it easier for bacterial metabolites and inflammatory cytokines to enter the circulatory system, which is related to the occurrence of metabolic syndrome (77). It is known that secondary bile acid pass inhibits the expression of intestinal tight junction protein and increases intestinal permeability (78). The production of LPS results from the overgrowth of Gram-negative bacteria in the intestinal tract. LPS circulates through the portal vein to the liver to induce liver injury and inflammation (79, 80). The increase of intestinal permeability and inflammation induced by LPS is mediated by TLR4-dependent activation of ganglion (81). By inducing the activation of TLR4/NF- κ B signal pathway, LPS upregulates the levels of inflammatory factors such as TNF- α, IL-1 and IL-10, and promotes oxidative stress, resulting in insulin resistance and NAFLD (82, 83). Similarly, SCFAs reduces intestinal inflammation by inhibiting the LPS/NF-kappa B/TLR4 pathway (84). SCFAs reduces inflammation by inhibiting the activity of histone deacetylase (HDAC) and promoting the production of regulatory T cells (Treg) (85).
3.4. Summary
From the above introduction, it can be known that intestinal microbiota disorder is the key to the occurrence and development of T2DM and NAFLD. It can induce local organ or systemic inflammation by changing the diversity of intestinal flora, affecting microbial metabolism and destroying intestinal barrier.
4. Intestinal flora– the “target organ” of traditional Chinese medicine in the treatment of diseases
Traditional Chinese medicine has a history of treating diseases in China for more than 2000 years, including single drug treatment and compound drug treatment. T2DM and NAFLD are metabolic diseases characterized by hyperglycemia and fat accumulation. Intestinal flora mediates the occurrence and development of metabolic diseases and is used as an important organ to participate in metabolic regulation. A series of experimental results also show that the hypoglycemic and lipid-lowering effect of traditional Chinese medicine is related to intestinal flora. Below, we will introduce the molecular mechanism of traditional Chinese medicine in the treatment of diabetes and fatty liver from the point of view of intestinal flora structure, intestinal barrier and intestinal metabolites. The mechanism of the therapeutic effect of traditional Chinese medicine (TCM) is shown in Figures 1, 2.
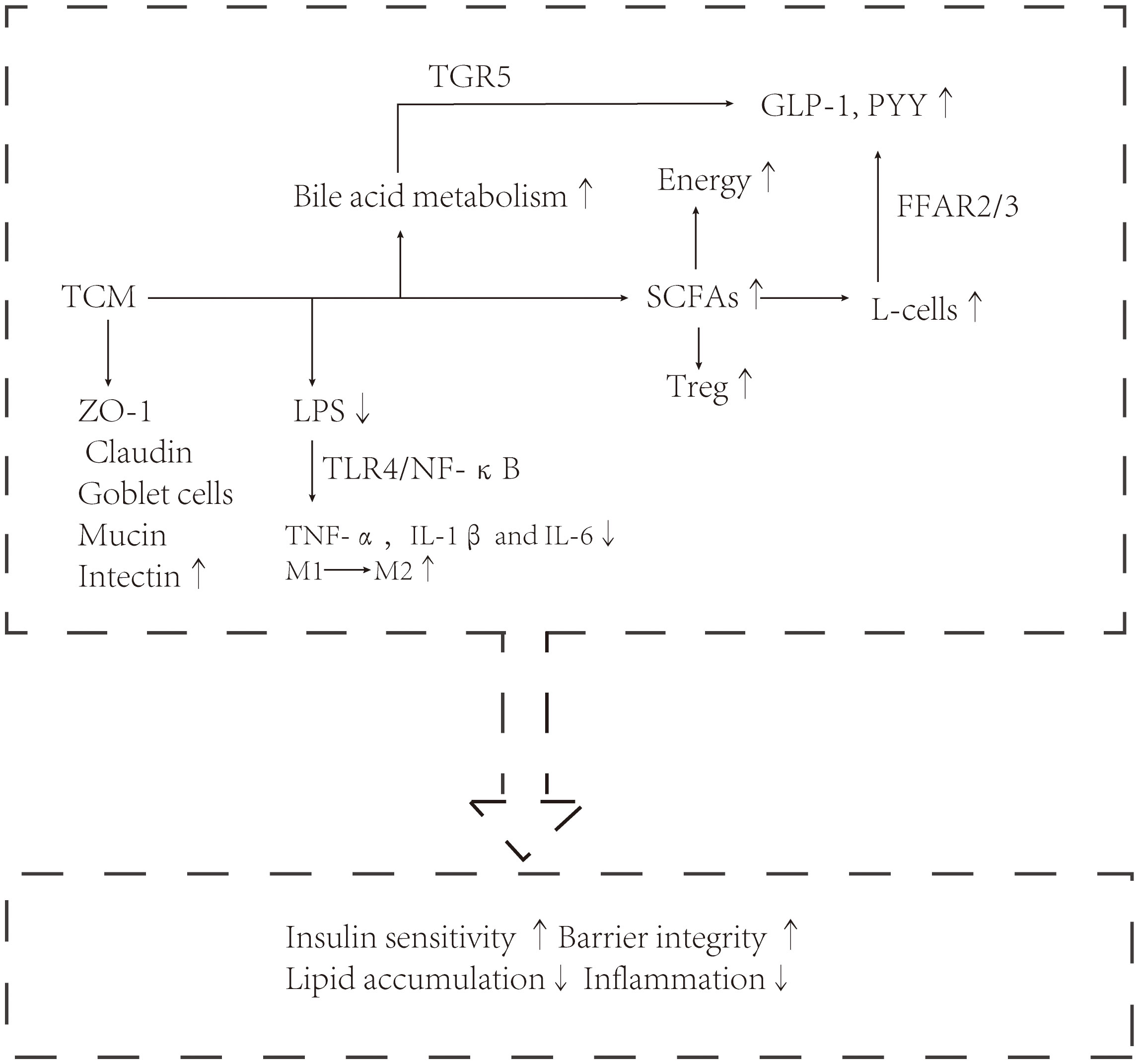
Figure 2 Traditional Chinese medicine exerts a therapeutic effect through intestinal flora, such as enterococcus, Akkermansia, and Vibrio desulfurization.
4.1. Individual herbs or herbal extracts
It is well known that traditional Chinese medicine extracts resveratrol, berberine, ginsenosides and curcumin play a beneficial regulatory role in lipid and glucose metabolism. Resveratrol is a natural polyphenol compound found in most herbs and has the potential to relieve diabetes and liver steatosis (86). It has been proved that the therapeutic effect of resveratrol is mediated by intestinal flora. For example, resveratrol alleviates the progression of diabetic nephropathy by reversing the low levels of Bacteroides, Alistipes, Rikenella, Odoribacter, Bacteroides and Alloprevotella in db/db mouse model. The therapeutic effect of resveratrol on db/db mice is related to resveratrol reversing the imbalance of intestinal flora, improving intestinal barrier, reducing intestinal permeability and inflammation (87). In addition, resveratrol can act as a potential NAFLD replacement therapy, and its therapeutic effect has been evaluated and confirmed in a number of trials. Previous experimental results have shown that resveratrol can improve lipid metabolism and reduce lipogenesis and inflammation in high-fat-fed mice, thus reducing hepatic steatosis (88). A new study found that high-fat diet (HFD)-induced NAFLD mice treated with resveratrol reduced the enrichment of lipid and glucose metabolism-related pathways, and this change was closely related to changes in intestinal flora. Resveratrol can reshape the diversity and composition of intestinal flora at different levels of the family. At the phylum level, the number of thick-walled bacteria increased significantly, while that of Bacteroides decreased significantly; at the family level, the erysipelaceae increased; at the genus level, the Olsenella content increased. Resveratrol reduces the invasion of harmful substances by up-regulating tight junction protein zo-1 and ameliorates liver inflammation by down-regulating inflammatory factors (IL-1, TNF- α, MyD88 and TLR-4) (89).
Berberine is a natural isoquinoline alkaloid extracted from herbal plants, which is the main activity of Coptis chinensis and Berberis (90, 91). The interaction between berberine and intestinal flora can alleviate metabolic disorders such as T2DM and NAFLD. Intestinal flora affects the absorption and transformation of berberine in gastrointestinal tract, and berberine also interferes with the structure and function of intestinal flora (92). In a study of Sprague-Dawley (SD) rats, the intestinal microflora diversity and richness of rats treated with berberine changed. At the gate level, there are higher abundance of Bacteroides and lower abundance of Proteus and verrucous microorganisms. At the family level, the family of Lactobacillus was significantly up-regulated. The concentrations of tyrosine, tryptophan and phenylalanine, the metabolites of intestinal flora, decreased in intestine and serum. Some studies have shown that high concentrations of aromatic amino acids are positively correlated with the risk of diabetes (93). Therefore, berberine treatment reduced the risk of diabetes in SD rats (94). Berberine is metabolized to berberine in the liver (95). It has been proved that berberine can regulate bile acid metabolism, activate intestinal farnesoid X receptor (FXR) and inhibit hepatic gluconeogenesis, and has significant lipid-lowering and hypoglycemic effects (95). Interestingly, by analyzing the composition of intestinal flora in high-fat (HFD)-fed mice, the researchers found that berberine increased intestinal beneficial bacteria, such as ileobacteria and myxobacteria. In addition, berberine reduced fat accumulation in the liver of HFD mice and decreased the levels of ALT and AST, which were beneficial to the treatment of NAFLD. Berberine can improve the imbalance of glucose homeostasis in HFD mice by affecting the expression of proteins related to glucose metabolism (PPAR γ, G6Pase, GLUT2,p-GSK) (96).
Ginsenosides are a kind of bioactive components extracted from plant medicine Ginseng. Ginsenosides can fight a variety of diseases through intestinal flora (97). Ginsenoside Rg1 can relieve T2D symptoms induced by HFD and streptozotocin (STZ) in rats, which may be related to the increase of the proportion of lactic acid bacteria and Lachnospiraceae and the decrease of the proportion of Lactobacillus by Rg1. Spearman correlation analysis showed that Lactobacillus was positively correlated with IL-1 β, IL-6, TNF- α and ROS levels (98). Lachnospiraceae is the main source of intestinal SCFAs, especially butyric acid (99, 100). Rg5 relieves inflammation by reducing plasma LPS levels and inhibiting the activation of TLR4-related signaling pathways in db/db mice. The hypoglycemic effect of Rg5 is related to its reducing the abundance of thick-walled bacteria and verrucous microorganisms and increasing the abundance of Bacteroides and Proteus in db/db mice (101). 25-hydroxy-protopanaxatriol (T19) is a new type of ginsenoside. Lachnospiraceae is a beneficial bacteria that regulates glucose and lipid metabolism. It was found that T19 significantly improved the abnormal glycolipid levels induced by HFD and STZ by significantly increasing the relative abundance of Lachnospiraceae family (102).
Curcumin is a polyphenol compound, mainly found in turmeric root (103). Curcumin attenuates dextran sulfate-induced T2MD symptoms in mice by reshaping the balance of Th17 and Treg in lymphoid cells. Th17 and Treg are related to the secretion of pro-inflammatory factor IL-17A and anti-inflammatory factor IL-10, respectively. Spearman analysis showed that curcumin mainly relieved chronic inflammation caused by T2MD by increasing the level of Roseburia and decreasing the levels of Erysipelatoclostridum and norank_f_Oscillospiraceae (104). Tetrahydrocurcumin (THC) is the main metabolite of curcumin. THC improves diabetes in db/db mice by reducing the relative abundance of Proteus and actinomycetes and promoting the expression of GLP-1 in the pancreas (105).
Herbs such as Polygala, licorice, Scutellaria baicalensis and Lycium barbarum also have the potential to treat diabetes and chronic liver disease. Polygala polygala extract (PTE) inhibits fat accumulation by promoting the expression of PPAR α. In addition, PTE regulates metabolism by enriching Proteus and reducing deferrifying bacteria (106). Licorice extract can reduce intestinal inflammation by reducing the levels of NF- κ B, Toll-like receptor 4 (TLR4) and tumor necrosis factor-α (TNF- α) in the colon of diabetic mice. The recovery of intestinal microbiology by licorice extract is related to the decrease of Lachnospiraceae _ NK4A136 content at genus level (107). The water extract of Scutellaria baicalensis Georgi can treat diabetes and complications by regulating the interaction between intestinal flora and bile acid metabolism. FXR is highly expressed in liver and intestine and is the key receptor of bile acid. Scutellaria baicalensis water extract can inhibit the expression of FXR in diabetic rats. Water extract of Scutellaria baicalensis Georgi can reverse the low levels of thephylaTenericutes and Patescibacteria and decrease the abundance of Lactobacillus and feacalibaculum in diabetic rats (108). Lycium barbarum polysaccharides can increase the proportion of probiotics, such as Ackermania, Lactobacillus and Prevaceae; Lycium barbarum polysaccharides can reduce intestinal pH and regulate the intestinal environment; Lycium barbarum polysaccharides can also stimulate innate immunity in the intestinal mucosa, such as macrophages or lymphocytes (109, 110). In addition, some Chinese herbal and natural plant extracts, such as cinnamon, Dendrobium, Radix Astragali, rhubarb, Aristolochia manshuriensis, cichoric acid, inulin, polyphenols, Ganoderma lucidum and mulberry polysaccharides are also effective in preventing and treating T2DM, NAFLD and related metabolic diseases. More details are shown in Table 1.
4.2. Chinese herbal formulae
The formula of traditional Chinese medicine is another important means for the treatment of diseases in traditional Chinese medicine, and it is often used in the diagnosis and treatment of clinical diseases as a supplement to western medicine. The compatibility of traditional Chinese medicine is not random. On the contrary, it is necessary to follow the principle of compatibility of traditional Chinese medicine and the principle of diagnosis and treatment of traditional Chinese medicine (140).
Pi-Dan-Jian-Qingdecoction (PDJQ) contains Radix Astragali, Radix Pseudostellariae, Coptis chinensis, Scutellaria baicalensis, Rhizoma Atractylodes, Salvia miltiorrhiza and Litchi. PDJQ has a good intervention effect on the clinical treatment of diabetes. In addition to regulating intestinal flora and inhibiting inflammation, the mechanism of PDJQ in treating diabetes is also related to the regulation of tryptophan metabolism, histamine metabolism and tricarboxylic acid (TCA) circulation. The specific results were as follows: at the genus level, PDJQ increased the relative abundance of Lactobacillus, Brucella, Bacteroides, Vibrio Desulfuricus and Ackermania, and decreased the relative abundance of Prevos. In addition, correlation analysis showed that the regulatory effects of PDJQ on tryptophan metabolism, histidine metabolism and TCA cycle pathway were related to the abundance changes of Lactobacillus, Bacteroides and Ackermann bacteria (141).
Gegen Qinlian Decoction (GQD) is composed of seven traditional Chinese medicines: Pueraria lobata, Coptis chinensis, Scutellaria baicalensis, Anemarrhena anemarrhena, American ginseng, red peony root and dried ginger. The mechanism of GQD in the treatment of diabetes is similar to that of berberine. GQD restores glucose homeostasis by increasing butyrate-producing bacteria, such as Faecalibacterium and Roseburia (142).
LingguiZhugan (LGZG) formula, a traditional Chinese medicine formula composed of Poria cocos, cassia twig, Atractylodes macrocephala and licorice, plays a useful role in the treatment of obesity-related diabetes. LGZG plays a role in controlling blood glucose and reducing insulin resistance, which may be mediated by intestinal microorganism OscillospiraandHelicobacte (143).
The effective components of inQiJiangtangTablet (JQJT) tablets are berberine, chlorogenic acid, astragalus polysaccharides and astragaloside IV mainly from Coptis chinensis, astragalus membranaceus and honeysuckle. Studies have shown that these active components are related to intestinal bacteria relieving insulin resistance and low-grade host inflammation. JQJT can increase the concentration of SCFAs in T2DM mice, especially butyric acid. JQJT treatment group showed lower desulphurization vibrio and higher Ackermania (144).
Xiexin T ang was first recorded in the synopsis of the Golden Chamber, an ancient Chinese medical book, and consists of rhubarb, Scutellaria baicalensis and Coptis chinensis. In traditional medicine, diabetes is called diabetes. Xiexin T ang has a long history in the treatment of diabetes and its effect is obvious. The new study found that Xiexin T ang improved diabetic symptoms in rats by changing the levels of bacteria that produce SCFAs and anti-inflammatory bacteria, such as Adlercreutzia, Barnesiella, and Prevotellaceae NK3B31 group (145).
In addition, other TCM formulations and TCM preparations derived from TCM formulations, such as Simiao Wan, Qijian mixturen, Naoxintong capsule and Herbal formula LLKL, have also been found to play a therapeutic role through intestinal flora. More details are shown in Table 2.
5. Discussion
Traditional Chinese medicine can affect the abundance of intestinal microbiota at different levels (Tables 1, 2). Therefore, we believe that the role of traditional Chinese medicine in the treatment of T2DM and NAFLD is probably related to its role in mediating intestinal microbial changes. There are differences in intestinal flora changes and therapeutic mechanisms mediated by different Chinese medicines.
5.1. Intestinal barrier
The damage of intestinal mucosa and the increase of inflammatory factors are related to T2DM and T2DM related metabolic diseases. Traditional Chinese medicine alleviates metabolic inflammation by increasing intestinal mucus and tight connection (152).Restoratol, ginsenoside Rg5, Curcumin, Nuciferinehe and traditional Chinese medicine formula Si Miao maintain the integrity of intestinal barrier by promoting the expression of tight junction protein ZO-1 and blocking protein. Nuciferine also enhances the intestinal barrier by increasing the expression of goblet cells and mucin2 (138). intestinal epithelium from damage by producing certain enzymes in the intestine. Resveratrol, Inulin, Rhubarb, Quercetin, and traditional Chinese medicine formulas such as Simiao Wan, JinQi Jiangtang T ablet, Huang Lian Jie Du Division can increase the abundance of Akkermansia. Escherichia coli is not conducive to maintaining the integrity of the intestinal barrier. The metabolic enzyme StcE produced will break down mucin, increase intestinal permeability and induce intestinal inflammation. Dendrobium can reduce the content of Escherichia coli in the intestine of db/db mice (116). Oscillibacter belonging to Ruminal Cocci family can also increase intestinal permeability. Astragaloside IV inhibits the increase of intestinal permeability by reducing the abundance of Oscillibacter (117).
5.2. Inflammation
LPS entering the intestinal tract will induce intestinal inflammation, and LPS mainly comes from vibrio desulfuricus (153). Inverterol, Pueraria lobata starch and Nuciferine can reduce the abundance of harmful bacteria, Vibrio desulfurization. LPS combines with TLR of intestinal epithelial cells to induce the release of proinflammatory factors and aggravate the host’s inflammatory response. Berberis kansuensis, Rhubarb, Quercetin, Morchella esculenta mushroom polysaccharide, Gynostemma pentaphyllum, Pueraria lobata start, as well as Chinese herbal formula Gegen Qinlian Reaction and JinQi Jiangtang T ablet can reduce TNF- α, IL-1 β And IL-6 levels, thereby relieving inflammation caused by bacterial endotoxin. Salviae polysaccharide, Laurolitsine, Inulin and Curcumin can increase the level of anti-inflammatory factor IL-10 (104, 122, 130, 136). In addition, Restoratol, Curcumin, Nuciferine and Chinese herbal formula LLKL can reduce TLR4/MyD88/NF- κ B pathway inhibits LPS induced inflammatory mediator production (89) (113, 138). In particular, Curcumin alleviates T2DM symptoms by maintaining the balance of immune cells Th17 and Treg, reducing intestinal mucosal damage and infiltration of inflammatory cells (104). Chiric Acid and laurolitsine regulate AMPK/NF- κ B signal pathway can reduce systemic inflammation caused by LPS (121) (130). Oxidative stress is another factor leading to inflammatory response. Astragaloside IV can reduce the level of oxidative stress through AMPK/SIRT1 and PI3K/AKT signaling pathways. Dendrobium, Mulberry fruit polysaccharide and Ganoderma lucidum extract have antioxidant capacity, which can reduce the level of malondialdehyde (MDA) and increase the content of superoxide dismutase (SOD), catalase (CA T) and glutathione (GSH) (116, 120, 127). The anti-inflammatory effect of traditional Chinese medicine may be mediated by increasing the abundance of anti-inflammatory bacteria Akkermania, Parabolides, Lactobacillus, Bacteroides and Blautia (141, 151).
5.3. SCFAs
TCM affects T2DM and NAFLD by affecting the abundance of SCFAs producing bacteria and the metabolism of SCFAs. SCFAs (acetate, propionate and butyrate) are produced by selective fermentation of intestinal microorganisms (154). Acetate participates in host energy metabolism by promoting the secretion of intestinal hormones (GLP-1 and PYY). Acetate is mainly produced by bifidobacteria and lactobacillus (155). Acetate can be converted to butyric acid by Firmicutes bacteria. Butyrate can protect the intestinal barrier and reduce inflammation (156). Clostridia, Bacteroides and Bifidobasteria are related to the production of butyric acid (157). Propionate is believed to reduce fat production, and serum cholesterol level has a beneficial effect on disorders of lipid metabolism (158). Green Tea Polyphenols increased the levels of acetic acid and butyric acid, which may be related to the increase of Clostridium populati, Blautia luti, Akkermania muciniphila and Thiothrix unzii (125). The increase of SCFAs content in Pueraria lobata star may be due to the increase of the content of Lactobacillus, Bifidobacterium and Turicibacte (135). Using Inulin in NAFLD treatment, it was found that SCFAs were positively correlated with Bacteroidetes, Akkermania and Bifidobasterium, and negatively correlated with Proteobasteria, Blautia and Ileiberium (122). In addition, the study also found that ginsenoside Rg1 can increase Lachnospiracea_ NK4A136_ The proportion of group, Roseburia and Romboutsia increases the content of SCFAs (98).
5.4. Bile acid metabolism
Bile acid metabolism, as an important part of the body’s regulation of glucose and lipid metabolism, is mainly mediated by G-protein coupled BA receptor (TGR5) and nuclear receptor Farni X receptor (FXR) (159). TGR5 is expressed in intestinal epithelial cells. The activation of TGR5 is conducive to the renewal of intestinal epithelial cells and the repair of intestinal barrier function (160). Cholesterol - 7 α- Hydroxylase (CYP7A1) is the rate limiting enzyme for converting cholesterol into BA (161). The changes of intestinal flora involved in bile acid metabolism mainly include bile salt hydrolase (BSH) and α- Dehydroxylated genera decreased and taurine metabolism related genera increased (137). Radix Scutellariae, Cinnamaldehyde, Astragaloside IV, Morchella esculenta mushroom polysaccharide, Nuciferine and Simiao Wan can all improve glycolipid disorder through bile acid metabolism. Detailed mechanisms are shown in Tables 1 and 2.
6. Conclusion and prospect
Traditional Chinese medicine has the potential to treat metabolic diseases such as diabetes and non-alcoholic fatty liver. Reshaping intestinal flora and regulating intestinal microbial metabolism is the key for traditional Chinese medicine to play a therapeutic role. at the same time, intestinal flora also provides a new opportunity to clarify the mechanism of traditional Chinese medicine in the treatment of diseases. The main mechanisms of traditional Chinese medicine include: improving the proportion of thick-walled bacteria and Bacteroides, increasing dominant flora and reducing harmful flora; regulating intestinal microbial metabolites such as short-chain fatty acids and bile acids; and restoring intestinal barrier. Increase the expression of tight junction proteins and reduce the level of inflammatory factors. It can be seen that maintaining the stability of intestinal microecology is of great significance to human health. The intestinal microecology is stable and healthy, and the destruction of intestinal microecology leads to the occurrence of disease. Lactobacillus acidophilus, Streptococcus thermophilus, Lactobacillus bulgaricus and/or Bifidobacterium can improve blood glucose levels in patients with diabetes. It can be inferred that dietary fiber, probiotics and probiotics are beneficial to the recovery of the disease. In addition, fecal microorganism transplantation has therapeutic potential in chronic inflammation, functional bowel disease, insulin resistance and morbid obesity. Herbs can be used as a treasure trove of potential probiotics for more in-depth research.
Author contributions
All authors contributed to the article and approved the submitted version.
Funding
This work was supported by the National Natural Science Foundation of China (Grant No. 82003985, 81973712), China Postdoctoral Science Foundation (Grant No. 2020M670825, 2020T130568), Jilin Province Science and Technology Development Project in China (Grant No. 20210101192JC, 20210204013YY, 20200504005YY), National College Students' innovation and entrepreneurship training program (Grant No. 202210199004, 202210199011X).
Conflict of interest
The authors declare that the research was conducted in the absence of any commercial or financial relationships that could be construed as a potential conflict of interest.
Publisher’s note
All claims expressed in this article are solely those of the authors and do not necessarily represent those of their affiliated organizations, or those of the publisher, the editors and the reviewers. Any product that may be evaluated in this article, or claim that may be made by its manufacturer, is not guaranteed or endorsed by the publisher.
References
1. Li HS, Hu YY. Intestinal microecology: An important target for Chinese medicine treatment of non-alcoholic fatty liver disease. Chin J Integr Med (2020) 26(10):723–8. doi: 10.1007/s11655-020-3268-3
2. Liu L, Zhang J, Cheng Y, Zhu M, Xiao Z, Ruan G, et al. Gut microbiota: A new target for T2DM prevention and treatment. Front Endocrinol (Lausanne) (2022) 13:958218. doi: 10.3389/fendo.2022.958218
3. Cobbina E, Akhlaghi F. Non-alcoholic fatty liver disease (NAFLD) - pathogenesis, classification, and effect on drug metabolizing enzymes and transporters. Drug Metab Rev (2017) 49(2):197–211. doi: 10.1080/03602532.2017.1293683
4. Upadhyaya S, Banerjee G. Type 2 diabetes and gut microbiome: at the intersection of known and unknown. Gut Microbes (2015) 6(2):85–92. doi: 10.1080/19490976.2015.1024918
5. Zhang B, Liu K, Yang H, Jin Z, Ding Q, Zhao L. Gut microbiota: The potential key target of TCM’s therapeutic effect of treating different diseases using the same method–UC and T2DM as examples. Front Cell Infection Microbiol (2022) 12:855075. doi: 10.3389/fcimb.2022.855075
6. Bril F, Cusi K. Management of nonalcoholic fatty liver disease in patients with type 2 diabetes: A call to action. Diabetes Care (2017) 40(3):419–30. doi: 10.2337/dc16-1787
7. Blazina I, Selph S. Diabetes drugs for nonalcoholic fatty liver disease: a systematic review. Syst Rev (2019) 8(1):295. doi: 10.1186/s13643-019-1200-8
8. Kim KS, Lee BW. Beneficial effect of anti-diabetic drugs for nonalcoholic fatty liver disease. Clin Mol Hepatol (2020) 26(4):430–43. doi: 10.3350/cmh.2020.0137
9. American Diabetes A. Diagnosis and classification of diabetes mellitus. Diabetes Care (2013) 36 Suppl 1(Suppl 1):S67–74. doi: 10.2337/dc13-S067
10. Han R, Qiu H, Zhong J, Zheng N, Li B, Hong Y, et al. Si Miao formula attenuates non-alcoholic fatty liver disease by modulating hepatic lipid metabolism and gut microbiota. Phytomedicine (2021) 85:153544. doi: 10.1016/j.phymed.2021.153544
11. Bril F, Lomonaco R, Orsak B, Ortiz-Lopez C, Webb A, Tio F, et al. Relationship between disease severity, hyperinsulinemia, and impaired insulin clearance in patients with nonalcoholic steatohepatitis. Hepatology (2014) 59(6):2178–87. doi: 10.1002/hep.26988
12. Parise ER. NONALCOHOLIC FATTY LIVER DISEASE (NAFLD), MORE THAN a LIVER DISEASE. Arq Gastroenterol (2019) 56(3):243–5. doi: 10.1590/s0004-2803.201900000-45
13. Chang Y, Jung HS, Yun KE, Cho J, Cho YK, Ryu S. Cohort study of non-alcoholic fatty liver disease, NAFLD fibrosis score, and the risk of incident diabetes in a Korean population. Am J Gastroenterol (2013) 108(12):1861–8. doi: 10.1038/ajg.2013.349
14. Movahedian M, Rahmani J, Hashemi Nazari SS, Mohamadi S, Naik G, Hekmatdoost A. Fatty liver index and risk of diabetes incidence: A systematic review and dose-response meta-analysis of cohort studies. Prim Care Diabetes (2020) 14(6):577–83. doi: 10.1016/j.pcd.2020.02.011
15. Chung SM, Kang MK, Jung J, Yoon JS, Won KC, Lee HW, et al. Long-term effects of the changes in hepatic steatosis status on the risk of incident type 2 diabetes mellitus: A 15-year community-based prospective cohort study. Diabetes Res Clin Pract (2022) 184:109208. doi: 10.1016/j.diabres.2022.109208
16. Abdelmalek MF, Liu C, Shuster J, Nelson DR, Asal NR. Familial aggregation of insulin resistance in first-degree relatives of patients with nonalcoholic fatty liver disease. Clin Gastroenterol Hepatol (2006) 4(9):1162–9. doi: 10.1016/j.cgh.2006.06.001
17. Loomba R, Abraham M, Unalp A, Wilson L, Lavine J, Doo E, et al. Association between diabetes, family history of diabetes, and risk of nonalcoholic steatohepatitis and fibrosis. Hepatology (2012) 56(3):943–51. doi: 10.1002/hep.25772
18. Hasanvand A. The role of AMPK-dependent pathways in cellular and molecular mechanisms of metformin: a new perspective for treatment and prevention of diseases. Inflammopharmacology (2022) 30(3):775–88. doi: 10.1007/s10787-022-00980-6
19. Woo SL, Xu H, Li H, Zhao Y, Hu X, Zhao J, et al. Metformin ameliorates hepatic steatosis and inflammation without altering adipose phenotype in diet-induced obesity. PloS One (2014) 9(3):e91111. doi: 10.1371/journal.pone.0091111
20. Zamani-Garmsiri F, Ghasempour G, Aliabadi M, Hashemnia SMR, Emamgholipour S, Meshkani R. Combination of metformin and chlorogenic acid attenuates hepatic steatosis and inflammation in high-fat diet fed mice. IUBMB Life (2021) 73(1):252–63. doi: 10.1002/iub.2424
21. Guo WR, Liu J, Cheng LD, Liu ZY, Zheng XB, Liang H, et al. Metformin alleviates steatohepatitis in diet-induced obese mice in a SIRT1-dependent way. Front Pharmacol (2021) 12:704112. doi: 10.3389/fphar.2021.704112
22. Gedulin BR, Nikoulina SE, Smith PA, Gedulin G, Nielsen LL, Baron AD, et al. Exenatide (exendin-4) improves insulin sensitivity and {beta}-cell mass in insulin-resistant obese fa/fa zucker rats independent of glycemia and body weight. Endocrinology (2005) 146(4):2069–76. doi: 10.1210/en.2004-1349
23. Svegliati-Baroni G, Saccomanno S, Rychlicki C, Agostinelli L, De Minicis S, Candelaresi C, et al. Glucagon-like peptide-1 receptor activation stimulates hepatic lipid oxidation and restores hepatic signalling alteration induced by a high-fat diet in nonalcoholic steatohepatitis. Liver Int (2011) 31(9):1285–97. doi: 10.1111/j.1478-3231.2011.02462.x
24. Ding X, Saxena NK, Lin S, Gupta NA, Anania FA. Exendin-4, a glucagon-like protein-1 (GLP-1) receptor agonist, reverses hepatic steatosis in ob/ob mice. Hepatology (2006) 43(1):173–81. doi: 10.1002/hep.21006
25. Lee J, Hong SW, Chae SW, Kim DH, Choi JH, Bae JC, et al. Exendin-4 improves steatohepatitis by increasing Sirt1 expression in high-fat diet-induced obese C57BL/6J mice. PloS One (2012) 7(2):e31394. doi: 10.1371/journal.pone.0031394
26. Mells JE, Fu PP, Sharma S, Olson D, Cheng L, Handy JA, et al. Glp-1 analog, liraglutide, ameliorates hepatic steatosis and cardiac hypertrophy in C57BL/6J mice fed a Western diet. Am J Physiol Gastrointest Liver Physiol (2012) 302(2):G225–235. doi: 10.1152/ajpgi.00274.2011
27. Tian F, Zheng Z, Zhang D, He S, Shen J. Efficacy of liraglutide in treating type 2 diabetes mellitus complicated with non-alcoholic fatty liver disease. Biosci Rep (2018) 38(6):1–9. doi: 10.1042/bsr20181304
28. Wu YR, Shi XY, Ma CY, Zhang Y, Xu RX, Li JJ. Liraglutide improves lipid metabolism by enhancing cholesterol efflux associated with ABCA1 and ERK1/2 pathway. Cardiovasc Diabetol (2019) 18(1):146. doi: 10.1186/s12933-019-0954-6
29. Liu L, Fan L, Chan M, Kraakman MJ, Yang J, Fan Y, et al. PPARγ deacetylation confers the antiatherogenic effect and improves endothelial function in diabetes treatment. Diabetes (2020) 69(8):1793–803. doi: 10.2337/db20-0217
30. Yang H, Suh DH, Kim DH, Jung ES, Liu KH, Lee CH, et al. Metabolomic and lipidomic analysis of the effect of pioglitazone on hepatic steatosis in a rat model of obese type 2 diabetes. Br J Pharmacol (2018) 175(17):3610–25. doi: 10.1111/bph.14434
31. Jiang L, Zhang H, Xiao D, Wei H, Chen Y. Farnesoid X receptor (FXR): Structures and ligands. Comput Struct Biotechnol J (2021) 19:2148–59. doi: 10.1016/j.csbj.2021.04.029
32. Han SY, Song HK, Cha JJ, Han JY, Kang YS, Cha DR. Farnesoid X receptor (FXR) agonist ameliorates systemic insulin resistance, dysregulation of lipid metabolism, and alterations of various organs in a type 2 diabetic kidney animal model. Acta Diabetol (2021) 58(4):495–503. doi: 10.1007/s00592-020-01652-z
33. Sun T, Xue M, Yang J, Pei Z, Zhang N, Qin K, et al. Metabolic regulation mechanism of fucoidan via intestinal microecology in diseases. J Sci Food Agric (2021) 101(11):4456–63. doi: 10.1002/jsfa.11202
34. Chen Y, Zhou J, Wang L. Role and mechanism of gut microbiota in human disease. Front Cell Infect Microbiol (2021) 11:625913. doi: 10.3389/fcimb.2021.625913
35. Wu J, Wang K, Wang X, Pang Y, Jiang C. The role of the gut microbiome and its metabolites in metabolic diseases. Protein Cell (2021) 12(5):360–73. doi: 10.1007/s13238-020-00814-7
36. Lembo E, Russo MF, Verrastro O, Anello D, Angelini G, Iaconelli A, et al. Prevalence and predictors of non-alcoholic steatohepatitis in subjects with morbid obesity and with or without type 2 diabetes. Diabetes Metab (2022) 48(5):101363. doi: 10.1016/j.diabet.2022.101363
37. Rohm TV, Meier DT, Olefsky JM, Donath MY. Inflammation in obesity, diabetes, and related disorders. Immunity (2022) 55(1):31–55. doi: 10.1016/j.immuni.2021.12.013
38. Tsai MC, Liu YY, Lin CC, Wang CC, Wu YJ, Yong CC, et al. Gut microbiota dysbiosis in patients with biopsy-proven nonalcoholic fatty liver disease: A cross-sectional study in Taiwan. Nutrients (2020) 12(3):820. doi: 10.3390/nu12030820
39. Cheng Z, Zhang L, Yang L, Chu H. The critical role of gut microbiota in obesity. Front Endocrinol (Lausanne) (2022) 13:1025706. doi: 10.3389/fendo.2022.1025706
40. Yang HT, Liu JK, Xiu WJ, Tian TT, Yang Y, Hou XG, et al. Gut microbiome-based diagnostic model to predict diabetes mellitus. Bioengineered (2021) 12(2):12521–34. doi: 10.1080/21655979.2021.2009752
41. Gulnaz A, Nadeem J, Han JH, Lew LC, Son JD, Park YH, et al. Lactobacillus sps in reducing the risk of diabetes in high-fat diet-induced diabetic mice by modulating the gut microbiome and inhibiting key digestive enzymes associated with diabetes. Biol (Basel) (2021) 10(4):348. doi: 10.3390/biology10040348
42. Kang H, You HJ, Lee G, Lee SH, Yoo T, Choi M, et al. Interaction effect between NAFLD severity and high carbohydrate diet on gut microbiome alteration and hepatic de novo lipogenesis. Gut Microbes (2022) 14(1):2078612. doi: 10.1080/19490976.2022.2078612
43. Sangineto M, Grander C, Grabherr F, Mayr L, Enrich B, Schwärzler J, et al. Recovery of bacteroides thetaiotaomicron ameliorates hepatic steatosis in experimental alcohol-related liver disease. Gut Microbes (2022) 14(1):2089006. doi: 10.1080/19490976.2022.2089006
44. Hu J, Lin S, Zheng B, Cheung PCK. Short-chain fatty acids in control of energy metabolism. Crit Rev Food Sci Nutr (2018) 58(8):1243–9. doi: 10.1080/10408398.2016.1245650
45. Chu H, Duan Y, Yang L, Schnabl B. Small metabolites, possible big changes: a microbiota-centered view of non-alcoholic fatty liver disease. Gut (2019) 68(2):359–70. doi: 10.1136/gutjnl-2018-316307
46. Kasubuchi M, Hasegawa S, Hiramatsu T, Ichimura A, Kimura I. Dietary gut microbial metabolites, short-chain fatty acids, and host metabolic regulation. Nutrients (2015) 7(4):2839–49. doi: 10.3390/nu7042839
47. Zhang S, Zhao J, Xie F, He H, Johnston LJ, Dai X, et al. Dietary fiber-derived short-chain fatty acids: A potential therapeutic target to alleviate obesity-related nonalcoholic fatty liver disease. Obes Rev (2021) 22(11):e13316. doi: 10.1111/obr.13316
48. Al Mahri S, Malik SS, Al Ibrahim M, Haji E, Dairi G, Mohammad S. Free fatty acid receptors (FFARs) in adipose: Physiological role and therapeutic outlook. Cells (2022) 11(4):750. doi: 10.3390/cells11040750
49. Secor JD, Fligor SC, Tsikis ST, Yu LJ, Puder M. Free fatty acid receptors as mediators and therapeutic targets in liver disease. Front Physiol (2021) 12:656441. doi: 10.3389/fphys.2021.656441
50. Priyadarshini M, Wicksteed B, Schiltz GE, Gilchrist A, Layden BT. SCFA receptors in pancreatic β cells: Novel diabetes targets? Trends Endocrinol Metab (2016) 27(9):653–64. doi: 10.1016/j.tem.2016.03.011
51. den Besten G, Lange K, Havinga R, van Dijk TH, Gerding A, van Eunen K, et al. Gut-derived short-chain fatty acids are vividly assimilated into host carbohydrates and lipids. Am J Physiol Gastrointest Liver Physiol (2013) 305(12):G900–910. doi: 10.1152/ajpgi.00265.2013
52. Alves-Bezerra M, Cohen DE. Triglyceride metabolism in the liver. Compr Physiol (2017) 8(1):1–8. doi: 10.1002/cphy.c170012
53. Yoshida H, Ishii M, Akagawa M. Propionate suppresses hepatic gluconeogenesis via GPR43/AMPK signaling pathway. Arch Biochem Biophys (2019) 672:108057. doi: 10.1016/j.abb.2019.07.022
54. Hong YH, Nishimura Y, Hishikawa D, Tsuzuki H, Miyahara H, Gotoh C, et al. Acetate and propionate short chain fatty acids stimulate adipogenesis via GPCR43. Endocrinology (2005) 146(12):5092–9. doi: 10.1210/en.2005-0545
55. Kimura I, Ozawa K, Inoue D, Imamura T, Kimura K, Maeda T, et al. The gut microbiota suppresses insulin-mediated fat accumulation via the short-chain fatty acid receptor GPR43. Nat Commun (2013) 4:1829. doi: 10.1038/ncomms2852
56. Zhang L, Chu J, Hao W, Zhang J, Li H, Yang C, et al. Gut microbiota and type 2 diabetes mellitus: Association, mechanism, and translational applications. Mediators Inflammation (2021) 2021:5110276. doi: 10.1155/2021/5110276
57. Larraufie P, Martin-Gallausiaux C, Lapaque N, Dore J, Gribble FM, Reimann F, et al. SCFAs strongly stimulate PYY production in human enteroendocrine cells. Sci Rep (2018) 8(1):74. doi: 10.1038/s41598-017-18259-0
58. Coutinho-Wolino KS, de Cardozo F.C.L.F.M., de Oliveira Leal V, Mafra D, Stockler-Pinto MB. Can diet modulate trimethylamine n-oxide (TMAO) production? what do we know so far? Eur J Nutr (2021) 60(7):3567–84. doi: 10.1007/s00394-021-02491-6
59. Arias N, Arboleya S, Allison J, Kaliszewska A, Higarza SG, Gueimonde M, et al. The relationship between choline bioavailability from diet, intestinal microbiota composition, and its modulation of human diseases. Nutrients (2020) 12(8):2340. doi: 10.3390/nu12082340
60. Li X, Hong J, Wang Y, Pei M, Wang L, Gong Z. Trimethylamine-N-Oxide pathway: A potential target for the treatment of MAFLD. Front Mol Biosci (2021) 8:733507. doi: 10.3389/fmolb.2021.733507
61. Kalagi NA, Thota RN, Stojanovski E, Alburikan KA, Garg ML. Association between plasma trimethylamine n-oxide levels and type 2 diabetes: A case control study. Nutrients (2022) 14(10):2093. doi: 10.3390/nu14102093
62. Li SY, Chen S, Lu XT, Fang AP, Chen YM, Huang RZ, et al. Serum trimethylamine-n-oxide is associated with incident type 2 diabetes in middle-aged and older adults: a prospective cohort study. J Transl Med (2022) 20(1):374. doi: 10.1186/s12967-022-03581-7
63. León-Mimila P, Villamil-Ramírez H, Li XS, Shih DM, Hui ST, Ocampo-Medina E, et al. Trimethylamine n-oxide levels are associated with NASH in obese subjects with type 2 diabetes. Diabetes Metab (2021) 47(2):101183. doi: 10.1016/j.diabet.2020.07.010
64. Krueger ES, Beales JL, Russon KB, Elison WS, Davis JR, Hansen JM, et al. Gut metabolite trimethylamine n-oxide protects INS-1 β-cell and rat islet function under diabetic glucolipotoxic conditions. Biomolecules (2021) 11(12):1892. doi: 10.3390/biom11121892
65. Shao JW, Ge TT, Chen SZ, Wang G, Yang Q, Huang CH, et al. Role of bile acids in liver diseases mediated by the gut microbiome. World J Gastroenterol (2021) 27(22):3010–21. doi: 10.3748/wjg.v27.i22.3010
66. Du L, Li Q, Yi H, Kuang T, Tang Y, Fan G. Gut microbiota-derived metabolites as key actors in type 2 diabetes mellitus. BioMed Pharmacother (2022) 149:112839. doi: 10.1016/j.biopha.2022.112839
67. Li R, Andreu-Sánchez S, Kuipers F, Fu J. Gut microbiome and bile acids in obesity-related diseases. Best Pract Res Clin Endocrinol Metab (2021) 35(3):101493. doi: 10.1016/j.beem.2021.101493
68. Trabelsi MS, Daoudi M, Prawitt J, Ducastel S, Touche V, Sayin SI, et al. Farnesoid X receptor inhibits glucagon-like peptide-1 production by enteroendocrine l cells. Nat Commun (2015) 6:7629. doi: 10.1038/ncomms8629
69. Takahashi S, Luo Y, Ranjit S, Xie C, Libby AE, Orlicky DJ, et al. Bile acid sequestration reverses liver injury and prevents progression of nonalcoholic steatohepatitis in Western diet-fed mice. J Biol Chem (2020) 295(14):4733–47. doi: 10.1074/jbc.RA119.011913
70. Du C, Liu WJ, Yang J, Zhao SS, Liu HX. The role of branched-chain amino acids and branched-chain α-keto acid dehydrogenase kinase in metabolic disorders. Front Nutr (2022) 9:932670. doi: 10.3389/fnut.2022.932670
71. Gojda J, Cahova M. Gut microbiota as the link between elevated BCAA serum levels and insulin resistance. Biomolecules (2021) 11(10):1414. doi: 10.3390/biom11101414
72. Zhang R, Mu H, Li Z, Zeng J, Zhou Q, Li H, et al. Oral administration of branched-chain amino acids ameliorates high-fat diet-induced metabolic-associated fatty liver disease via gut microbiota-associated mechanisms. Front Microbiol (2022) 13:920277. doi: 10.3389/fmicb.2022.920277
73. Zakaria NF, Hamid M, Khayat ME. Amino acid-induced impairment of insulin signaling and involvement of G-protein coupling receptor. Nutrients (2021) 13(7):2229. doi: 10.3390/nu13072229
74. Cuomo P, Capparelli R, Iannelli A, Iannelli D. Role of branched-chain amino acid metabolism in type 2 diabetes, obesity, cardiovascular disease and non-alcoholic fatty liver disease. Int J Mol Sci (2022) 23(8):4325. doi: 10.3390/ijms23084325
75. Lo EKK, Felicianna, Xu JH, Zhan Q, Zeng Z, El-Nezami H. The emerging role of branched-chain amino acids in liver diseases. Biomedicines (2022) 10(6):1444. doi: 10.3390/biomedicines10061444
76. Iwao M, Gotoh K, Arakawa M, Endo M, Honda K, Seike M, et al. Supplementation of branched-chain amino acids decreases fat accumulation in the liver through intestinal microbiota-mediated production of acetic acid. Sci Rep (2020) 10(1):18768. doi: 10.1038/s41598-020-75542-3
77. Portincasa P, Bonfrate L, Khalil M, Angelis M, Calabrese FM, D’Amato M, et al. Intestinal barrier and permeability in health, obesity and NAFLD. Biomedicines (2021) 10(1):83. doi: 10.3390/biomedicines10010083
78. Murakami Y, Tanabe S, Suzuki T. High-fat diet-induced intestinal hyperpermeability is associated with increased bile acids in the Large intestine of mice. J Food Sci (2016) 81(1):H216–222. doi: 10.1111/1750-3841.13166
79. Cornell RP. Gut-derived endotoxin elicits hepatotrophic factor secretion for liver regeneration. Am J Physiol (1985) 249(5 Pt 2):R551–562. doi: 10.1152/ajpregu.1985.249.5.R551
80. Zheng Z, Wang B. The gut-liver axis in health and disease: The role of gut microbiota-derived signals in liver injury and regeneration. Front Immunol (2021) 12:775526. doi: 10.3389/fimmu.2021.775526
81. Guo S, Nighot M, Al-Sadi R, Alhmoud T, Nighot P, Ma TY. Lipopolysaccharide regulation of intestinal tight junction permeability is mediated by TLR4 signal transduction pathway activation of FAK and MyD88. J Immunol (2015) 195(10):4999–5010. doi: 10.4049/jimmunol.1402598
82. Nolan JP. The role of intestinal endotoxin in liver injury: a long and evolving history. Hepatology (2010) 52(5):1829–35. doi: 10.1002/hep.23917
83. Ferro D, Baratta F, Pastori D, Cocomello N, Colantoni A, Angelico F, et al. New insights into the pathogenesis of non-alcoholic fatty liver disease: Gut-derived lipopolysaccharides and oxidative stress. Nutrients (2020) 12(9):2762. doi: 10.3390/nu12092762
84. Tian B, Zhao J, Zhang M, Chen Z, Ma Q, Liu H, et al. Lycium ruthenicum anthocyanins attenuate high-fat diet-induced colonic barrier dysfunction and inflammation in mice by modulating the gut microbiota. Mol Nutr Food Res (2021) 65(8):e2000745. doi: 10.1002/mnfr.202000745
85. Arpaia N, Campbell C, Fan X, Dikiy S, van der Veeken J, deRoos P, et al. Metabolites produced by commensal bacteria promote peripheral regulatory T-cell generation. Nature (2013) 504(7480):451–5. doi: 10.1038/nature12726
86. Hoca M, Becer E, Vatansever HS. The role of resveratrol in diabetes and obesity associated with insulin resistance. Arch Physiol Biochem (2021) 1-7:1–7. doi: 10.1080/13813455.2021.1893338
87. Cai TT, Ye XL, Li RR, Chen H, Wang YY, Yong HJ, et al. Resveratrol modulates the gut microbiota and inflammation to protect against diabetic nephropathy in mice. Front Pharmacol (2020) 11:1249. doi: 10.3389/fphar.2020.01249
88. Andrade JM, Paraíso AF, de Oliveira MV, Martins AM, Neto JF, Guimarães AL, et al. Resveratrol attenuates hepatic steatosis in high-fat fed mice by decreasing lipogenesis and inflammation. Nutrition (2014) 30(7-8):915–9. doi: 10.1016/j.nut.2013.11.016
89. Du F, Huang R, Lin D, Wang Y, Yang X, Huang X, et al. Resveratrol improves liver steatosis and insulin resistance in non-alcoholic fatty liver disease in association with the gut microbiota. Front Microbiol (2021) 12:611323. doi: 10.3389/fmicb.2021.611323
90. Khoshandam A, Imenshahidi M, Hosseinzadeh H. Pharmacokinetic of berberine, the main constituent of berberis vulgaris l.: A comprehensive review. Phytother Res (2022) 36(11):4063–79. doi: 10.1002/ptr.7589
91. Yang Y, Cao S, Xu W, Zang C, Zhang F, Xie Y, et al. Dual modulation of gut bacteria and fungi manifests the gut-based anti-hyperlipidemic effect of coptidis rhizoma. BioMed Pharmacother (2022) 153:113542. doi: 10.1016/j.biopha.2022.113542
92. Wang H, Zhang H, Gao Z, Zhang Q, Gu C. The mechanism of berberine alleviating metabolic disorder based on gut microbiome. Front Cell Infect Microbiol (2022) 12:854885. doi: 10.3389/fcimb.2022.854885
93. Ruiz-Canela M, Guasch-Ferré M, Toledo E, Clish CB, Razquin C, Liang L, et al. Plasma branched chain/aromatic amino acids, enriched Mediterranean diet and risk of type 2 diabetes: case-cohort study within the PREDIMED trial. Diabetologia (2018) 61(7):1560–71. doi: 10.1007/s00125-018-4611-5
94. Yao Y, Chen H, Yan L, Wang W, Wang D. Berberine alleviates type 2 diabetic symptoms by altering gut microbiota and reducing aromatic amino acids. BioMed Pharmacother (2020) 131:110669. doi: 10.1016/j.biopha.2020.110669
95. Sun R, Kong B, Yang N, Cao B, Feng D, Yu X, et al. The hypoglycemic effect of berberine and berberrubine involves modulation of intestinal farnesoid X receptor signaling pathway and inhibition of hepatic gluconeogenesis. Drug Metab Dispos (2021) 49(3):276–86. doi: 10.1124/dmd.120.000215
96. Yang S, Cao S, Li C, Zhang J, Liu C, Qiu F, et al. Berberrubine, a main metabolite of berberine, alleviates non-alcoholic fatty liver disease via modulating glucose and lipid metabolism and restoring gut microbiota. Front Pharmacol (2022) 13:913378. doi: 10.3389/fphar.2022.913378
97. Chen Z, Zhang Z, Liu J, Qi H, Li J, Chen J, et al. Gut microbiota: Therapeutic targets of ginseng against multiple disorders and ginsenoside transformation. Front Cell Infect Microbiol (2022) 12:853981. doi: 10.3389/fcimb.2022.853981
98. Peng M, Wang L, Su H, Zhang L, Yang Y, Sun L, et al. Ginsenoside Rg1 improved diabetes through regulating the intestinal microbiota in high-fat diet and streptozotocin-induced type 2 diabetes rats. J Food Biochem (2022) 46(10):e14321. doi: 10.1111/jfbc.14321
99. Vacca M, Celano G, Calabrese FM, Portincasa P, Gobbetti M, De Angelis M. The controversial role of human gut lachnospiraceae. Microorganisms (2020) 8(4):573. doi: 10.3390/microorganisms8040573
100. Berger K, Burleigh S, Lindahl M, Bhattacharya A, Patil P, Stålbrand H, et al. Xylooligosaccharides increase bifidobacteria and lachnospiraceae in mice on a high-fat diet, with a concomitant increase in short-chain fatty acids, especially butyric acid. J Agric Food Chem (2021) 69(12):3617–25. doi: 10.1021/acs.jafc.0c06279
101. Wei Y, Yang H, Zhu C, Deng J, Fan D. Hypoglycemic effect of ginsenoside Rg5 mediated partly by modulating gut microbiota dysbiosis in diabetic db/db mice. J Agric Food Chem (2020) 68(18):5107–17. doi: 10.1021/acs.jafc.0c00605
102. Xu J, Li T, Xia X, Fu C, Wang X, Zhao Y. Dietary ginsenoside T19 supplementation regulates glucose and lipid metabolism via AMPK and PI3K pathways and its effect on intestinal microbiota. J Agric Food Chem (2020) 68(49):14452–62. doi: 10.1021/acs.jafc.0c04429
103. Filardi T, Varì R, Ferretti E, Zicari A, Morano S, Santangelo C. Curcumin: Could this compound be useful in pregnancy and pregnancy-related complications? Nutrients (2020) 12(10):3179. doi: 10.3390/nu12103179
104. Xiao QP, Zhong YB, Kang ZP, Huang JQ, Fang WY, Wei SY, et al. Curcumin regulates the homeostasis of Th17/Treg and improves the composition of gut microbiota in type 2 diabetic mice with colitis. Phytother Res (2022) 36(4):1708–23. doi: 10.1002/ptr.7404
105. Yuan T, Yin Z, Yan Z, Hao Q, Zeng J, Li L, et al. Tetrahydrocurcumin ameliorates diabetes profiles of db/db mice by altering the composition of gut microbiota and up-regulating the expression of GLP-1 in the pancreas. Fitoterapia (2020) 146:104665. doi: 10.1016/j.fitote.2020.104665
106. Wang CC, Yen JH, Cheng YC, Lin CY, Hsieh CT, Gau RJ, et al. Polygala tenuifolia extract inhibits lipid accumulation in 3T3-L1 adipocytes and high-fat diet-induced obese mouse model and affects hepatic transcriptome and gut microbiota profiles. Food Nutr Res (2017) 61(1):1379861. doi: 10.1080/16546628.2017.1379861
107. Zhang Y, Xu Y, Zhang L, Chen Y, Wu T, Liu R, et al. Licorice extract ameliorates hyperglycemia through reshaping gut microbiota structure and inhibiting TLR4/NF-kappaB signaling pathway in type 2 diabetic mice. Food Res Int (2022) 153:110945. doi: 10.1016/j.foodres.2022.110945
108. Zhao L, Ma P, Peng Y, Wang M, Peng C, Zhang Y, et al. Amelioration of hyperglycaemia and hyperlipidaemia by adjusting the interplay between gut microbiota and bile acid metabolism: Radix scutellariae as a case. Phytomedicine (2021) 83:153477. doi: 10.1016/j.phymed.2021.153477
109. Zhu W, Zhou S, Liu J, McLean RJC, Chu W. Prebiotic, immuno-stimulating and gut microbiota-modulating effects of lycium barbarum polysaccharide. BioMed Pharmacother (2020) 121:109591. doi: 10.1016/j.biopha.2019.109591
110. Yang Y, Chang Y, Wu Y, Liu H, Liu Q, Kang Z, et al. A homogeneous polysaccharide from lycium barbarum: Structural characterizations, anti-obesity effects and impacts on gut microbiota. Int J Biol Macromol (2021) 183:2074–87. doi: 10.1016/j.ijbiomac.2021.05.209
111. Chen M, Hou P, Zhou M, Ren Q, Wang X, Huang L, et al. Resveratrol attenuates high-fat diet-induced non-alcoholic steatohepatitis by maintaining gut barrier integrity and inhibiting gut inflammation through regulation of the endocannabinoid system. Clin Nutr (2020) 39(4):1264–75. doi: 10.1016/j.clnu.2019.05.020
112. Xu T, Ge Y, Du H, Li Q, Xu X, Yi H, et al. Berberis kansuensis extract alleviates type 2 diabetes in rats by regulating gut microbiota composition. J Ethnopharmacol (2021) 273:113995. doi: 10.1016/j.jep.2021.113995
113. Huang J, Guan B, Lin L, Wang Y. Improvement of intestinal barrier function, gut microbiota, and metabolic endotoxemia in type 2 diabetes rats by curcumin. Bioengineered (2021) 12(2):11947–58. doi: 10.1080/21655979.2021.2009322
114. Zhao XQ, Guo S, Lu YY, Hua Y, Zhang F, Yan H, et al. Lycium barbarum l. leaves ameliorate type 2 diabetes in rats by modulating metabolic profiles and gut microbiota composition. BioMed Pharmacother (2020) 121:109559. doi: 10.1016/j.biopha.2019.109559
115. Zhao H, Wu H, Duan M, Liu R, Zhu Q, Zhang K, et al. Cinnamaldehyde improves metabolic functions in streptozotocin-induced diabetic mice by regulating gut microbiota. Drug Des Devel Ther (2021) 15:2339–55. doi: 10.2147/DDDT.S288011
116. Li XW, Chen HP, He YY, Chen WL, Chen JW, Gao L, et al. Effects of rich-polyphenols extract of dendrobium loddigesii on anti-diabetic, anti-inflammatory, anti-oxidant, and gut microbiota modulation in db/db mice. Molecules (2018) 23(12):3245. doi: 10.3390/molecules23123245
117. Gong P, Xiao X, Wang S, Shi F, Liu N, Chen X, et al. Hypoglycemic effect of astragaloside IV via modulating gut microbiota and regulating AMPK/SIRT1 and PI3K/AKT pathway. J Ethnopharmacol (2021) 281:114558. doi: 10.1016/j.jep.2021.114558
118. Zhai Y, Zhou W, Yan X, Qiao Y, Guan L, Zhang Z, et al. Astragaloside IV ameliorates diet-induced hepatic steatosis in obese mice by inhibiting intestinal FXR via intestinal flora remodeling. Phytomedicine (2022) 107:154444. doi: 10.1016/j.phymed.2022.154444
119. Li QM, Zha XQ, Zhang WN, Liu J, Pan LH, Luo JP. Laminaria japonica polysaccharide prevents high-fat-diet-induced insulin resistance in mice via regulating gut microbiota. Food Funct (2021) 12(12):5260–73. doi: 10.1039/d0fo02100h
120. Chen C, You LJ, Huang Q, Fu X, Zhang B, Liu RH, et al. Modulation of gut microbiota by mulberry fruit polysaccharide treatment of obese diabetic db/db mice. Food Funct (2018) 9(7):3732–42. doi: 10.1039/c7fo01346a
121. Ding X, Jian T, Li J, Lv H, Tong B, Li J, et al. Chicoric acid ameliorates nonalcoholic fatty liver disease via the AMPK/Nrf2/NFκB signaling pathway and restores gut microbiota in high-Fat-Diet-Fed mice. Oxid Med Cell Longev (2020) 2020:9734560. doi: 10.1155/2020/9734560
122. Bao T, He F, Zhang X, Zhu L, Wang Z, Lu H, et al. Inulin exerts beneficial effects on non-alcoholic fatty liver disease via modulating gut microbiome and suppressing the lipopolysaccharide-Toll-Like receptor 4-Mψ-Nuclear factor-κB-Nod-Like receptor protein 3 pathway via gut-liver axis in mice. Front Pharmacol (2020) 11:558525. doi: 10.3389/fphar.2020.558525
123. Regnier M, Rastelli M, Morissette A, Suriano F, Le Roy T, Pilon G, et al. Rhubarb supplementation prevents diet-induced obesity and diabetes in association with increased akkermansia muciniphila in mice. Nutrients (2020) 12(10):2932. doi: 10.3390/nu12102932
124. Yang S, Hu T, Liu H, Lv YL, Zhang W, Li H, et al. Akebia saponin d ameliorates metabolic syndrome (MetS) via remodeling gut microbiota and attenuating intestinal barrier injury. BioMed Pharmacother (2021) 138:111441. doi: 10.1016/j.biopha.2021.111441
125. Wang L, Zeng B, Liu Z, Liao Z, Zhong Q, Gu L, et al. Green tea polyphenols modulate colonic microbiota diversity and lipid metabolism in high-fat diet treated HFA mice. J Food Sci (2018) 83(3):864–73. doi: 10.1111/1750-3841.14058
126. Porras D, Nistal E, Martínez-Flórez S, Olcoz JL, Jover R, Jorquera F, et al. Functional interactions between gut microbiota transplantation, quercetin, and high-fat diet determine non-alcoholic fatty liver disease development in germ-free mice. Mol Nutr Food Res (2019) 63(8):e1800930. doi: 10.1002/mnfr.201800930
127. Lv XC, Wu Q, Cao YJ, Lin YC, Guo WL, Rao PF, et al. Ganoderic acid a from ganoderma lucidum protects against alcoholic liver injury through ameliorating the lipid metabolism and modulating the intestinal microbial composition. Food Funct (2022) 13(10):5820–37. doi: 10.1039/d1fo03219d
128. Chen M, Xiao D, Liu W, Song Y, Zou B, Li L, et al. Intake of ganoderma lucidum polysaccharides reverses the disturbed gut microbiota and metabolism in type 2 diabetic rats. Int J Biol Macromol (2020) 155:890–902. doi: 10.1016/j.ijbiomac.2019.11.047
129. Rehman AU, Siddiqui NZ, Farooqui NA, Alam G, Gul A, Ahmad B, et al. Morchella esculenta mushroom polysaccharide attenuates diabetes and modulates intestinal permeability and gut microbiota in a type 2 diabetic mice model. Front Nutr (2022) 9:984695. doi: 10.3389/fnut.2022.984695
130. Yong Z, Ruiqi W, Yanan Y, Ning M, Zhi Z, Yinfeng T, et al. Laurolitsine ameliorates type 2 diabetes by regulating the hepatic LKB1-AMPK pathway and gut microbiota. Phytomedicine (2022) 106:154423. doi: 10.1016/j.phymed.2022.154423
131. Shen SH, Zhong TY, Peng C, Fang J, Lv B. Structural modulation of gut microbiota during alleviation of non-alcoholic fatty liver disease with gynostemma pentaphyllum in rats. BMC Complement Med Ther (2020) 20(1):34. doi: 10.1186/s12906-020-2835-7
132. Yue SR, Tan YY, Zhang L, Zhang BJ, Jiang FY, Ji G, et al. Gynostemma pentaphyllum polysaccharides ameliorate non-alcoholic steatohepatitis in mice associated with gut microbiota and the TLR2/NLRP3 pathway. Front Endocrinol (Lausanne) (2022) 13:885039. doi: 10.3389/fendo.2022.885039
133. Tan YY, Yue SR, Lu AP, Zhang L, Ji G, Liu BC, et al. The improvement of nonalcoholic steatohepatitis by poria cocos polysaccharides associated with gut microbiota and NF-κB/CCL3/CCR1 axis. Phytomedicine (2022) 103:154208. doi: 10.1016/j.phymed.2022.154208
134. Zhong M, Yan Y, Yuan H, Rong A, Xu G, Cai F, et al. Astragalus mongholicus polysaccharides ameliorate hepatic lipid accumulation and inflammation as well as modulate gut microbiota in NAFLD rats. Food Funct (2022) 13(13):7287–301. doi: 10.1039/d2fo01009g
135. Yang Y, Li M, Wang Q, Huang H, Zhao Y, Du F, et al. Pueraria lobata starch regulates gut microbiota and alleviates high-fat high-cholesterol diet induced non-alcoholic fatty liver disease in mice. Food Res Int (2022) 157:111401. doi: 10.1016/j.foodres.2022.111401
136. Li L, Lan X, Peng X, Shi S, Zhao Y, Liu W, et al. Polysaccharide from salviae miltiorrhizae radix et rhizoma attenuates the progress of obesity-induced non-alcoholic fatty liver disease through modulating intestinal microbiota-related gut-liver axis. Int J Mol Sci (2022) 23(18):10620. doi: 10.3390/ijms231810620
137. Sun J, Fan J, Li T, Yan X, Jiang Y. Nuciferine protects against high-fat diet-induced hepatic steatosis via modulation of gut microbiota and bile acid metabolism in rats. J Agric Food Chem (2022) 70(38):12014–28. doi: 10.1021/acs.jafc.2c04817
138. Fan J, Sun J, Li T, Yan X, Jiang Y. Nuciferine prevents hepatic steatosis associated with improving intestinal mucosal integrity, mucus-related microbiota and inhibiting TLR4/MyD88/NF-κB pathway in high-fat induced rats. J Funct Foods (2022) 88:104859. doi: 10.1016/j.jff.2021.104859
139. Zhao W, Guo M, Feng J, Gu Z, Zhao J, Zhang H, et al. Myristica fragrans extract regulates gut microbes and metabolites to attenuate hepatic inflammation and lipid metabolism disorders via the AhR-FAS and NF-kappaB signaling pathways in mice with non-alcoholic fatty liver disease. Nutrients (2022) 14(9):1699. doi: 10.3390/nu14091699
140. Luan X, Zhang LJ, Li XQ, Rahman K, Zhang H, Chen HZ, et al. Compound-based Chinese medicine formula: From discovery to compatibility mechanism. J Ethnopharmacol (2020) 254:112687. doi: 10.1016/j.jep.2020.112687
141. Xie X, Liao J, Ai Y, Gao J, Zhao J, Qu F, et al. Pi-Dan-Jian-Qing decoction ameliorates type 2 diabetes mellitus through regulating the gut microbiota and serum metabolism. Front Cell Infect Microbiol (2021) 11:748872. doi: 10.3389/fcimb.2021.748872
142. Xu X, Gao Z, Yang F, Yang Y, Chen L, Han L, et al. Antidiabetic effects of gegen qinlian decoction via the gut microbiota are attributable to its key ingredient berberine. Genomics Proteomics Bioinf (2020) 18(6):721–36. doi: 10.1016/j.gpb.2019.09.007
143. Wu R, Zhao D, An R, Wang Z, Li Y, Shi B, et al. Linggui zhugan formula improves glucose and lipid levels and alters gut microbiota in high-fat diet-induced diabetic mice. Front Physiol (2019) 10:918. doi: 10.3389/fphys.2019.00918
144. Cao Y, Yao G, Sheng Y, Yang L, Wang Z, Yang Z, et al. JinQi jiangtang tablet regulates gut microbiota and improve insulin sensitivity in type 2 diabetes mice. J Diabetes Res (2019) 2019:1872134. doi: 10.1155/2019/1872134
145. Wei X, Tao J, Xiao S, Jiang S, Shang E, Zhu Z, et al. Xiexin tang improves the symptom of type 2 diabetic rats by modulation of the gut microbiota. Sci Rep (2018) 8(1):3685. doi: 10.1038/s41598-018-22094-2
146. Xiao S, Zhang Z, Chen M, Zou J, Jiang S, Qian D, et al. Xiexin tang ameliorates dyslipidemia in high-fat diet-induced obese rats via elevating gut microbiota-derived short chain fatty acids production and adjusting energy metabolism. J Ethnopharmacol (2019) 241:112032. doi: 10.1016/j.jep.2019.112032
147. Chen Y, Zhu L, Hu W, Wang Y, Wen X, Yang J. Simiao wan modulates the gut microbiota and bile acid metabolism during improving type 2 diabetes mellitus in mice. Phytomedicine (2022) 104:154264. doi: 10.1016/j.phymed.2022.154264
148. Gao K, Yang R, Zhang J, Wang Z, Jia C, Zhang F, et al. Effects of qijian mixture on type 2 diabetes assessed by metabonomics, gut microbiota and network pharmacology. Pharmacol Res (2018) 130:93–109. doi: 10.1016/j.phrs.2018.01.011
149. Yan Z, Wu H, Zhou H, Chen S, He Y, Zhang W, et al. Integrated metabolomics and gut microbiome to the effects and mechanisms of naoxintong capsule on type 2 diabetes in rats. Sci Rep (2020) 10(1):10829. doi: 10.1038/s41598-020-67362-2
150. Li M, Ding L, Hu YL, Qin LL, Wu Y, Liu W, et al. Herbal formula LLKL ameliorates hyperglycaemia, modulates the gut microbiota and regulates the gut-liver axis in zucker diabetic fatty rats. J Cell Mol Med (2021) 25(1):367–82. doi: 10.1111/jcmm.16084
151. Chen M, Liao Z, Lu B, Wang M, Lin L, Zhang S, et al. Huang-Lian-Jie-Du-Decoction ameliorates hyperglycemia and insulin resistant in association with gut microbiota modulation. Front Microbiol (2018) 9:2380. doi: 10.3389/fmicb.2018.02380
152. Gao Z, Li Q, Wu X, Zhao X, Zhao L, Tong X. New insights into the mechanisms of Chinese herbal products on diabetes: A focus on the “Bacteria-mucosal immunity-Inflammation-Diabetes” axis. J Immunol Res (2017) 2017:1813086. doi: 10.1155/2017/1813086
153. Cui HX, Zhang LS, Luo Y, Yuan K, Huang ZY, Guo Y. A purified anthraquinone-glycoside preparation from rhubarb ameliorates type 2 diabetes mellitus by modulating the gut microbiota and reducing inflammation. Front Microbiol (2019) 10:1423. doi: 10.3389/fmicb.2019.01423
154. Coppola S, Avagliano C, Calignano A, Berni Canani R. The protective role of butyrate against obesity and obesity-related diseases. Molecules (2021) 26(3):682. doi: 10.3390/molecules26030682
155. Hernández MAG, Canfora EE, Jocken JWE, Blaak EE. The short-chain fatty acid acetate in body weight control and insulin sensitivity. Nutrients (2019) 11(8):1943. doi: 10.3390/nu11081943
156. Bach Knudsen KE, Lærke HN, Hedemann MS, Nielsen TS, Ingerslev AK, Gundelund Nielsen DS, et al. Impact of diet-modulated butyrate production on intestinal barrier function and inflammation. Nutrients (2018) 10(10):1499. doi: 10.3390/nu10101499
157. Fu X, Liu Z, Zhu C, Mou H, Kong Q. Nondigestible carbohydrates, butyrate, and butyrate-producing bacteria. Crit Rev Food Sci Nutr (2019) 59(sup1):S130–s152. doi: 10.1080/10408398.2018.1542587
158. Hosseini E, Grootaert C, Verstraete W, Van de Wiele T. Propionate as a health-promoting microbial metabolite in the human gut. Nutr Rev (2011) 69(5):245–58. doi: 10.1111/j.1753-4887.2011.00388.x
159. Shapiro H, Kolodziejczyk AA, Halstuch D, Elinav E. Bile acids in glucose metabolism in health and disease. J Exp Med (2018) 215(2):383–96. doi: 10.1084/jem.20171965
160. Sorrentino G, Perino A, Yildiz E, El Alam G, Bou Sleiman M, Gioiello A, et al. Bile acids signal via TGR5 to activate intestinal stem cells and epithelial regeneration. Gastroenterology (2020) 159(3):956–968.e958. doi: 10.1053/j.gastro.2020.05.067
Keywords: gastrointestinal flora, diabetes mellitus, non-alcoholic fatty liver disease, traditional Chinese medicine, inflammation, short chain fatty acids
Citation: Bao Y, Han X, Liu D, Tan Z and Deng Y (2022) Gut microbiota: The key to the treatment of metabolic syndrome in traditional Chinese medicine – a case study of diabetes and nonalcoholic fatty liver disease. Front. Immunol. 13:1072376. doi: 10.3389/fimmu.2022.1072376
Received: 17 October 2022; Accepted: 09 December 2022;
Published: 23 December 2022.
Edited by:
Zhe-Sheng Chen, St. John’s University, United StatesReviewed by:
Leilei Du, Chengdu University of Traditional Chinese Medicine, ChinaYajuan Rui, Zhejiang University, China
Copyright © 2022 Bao, Han, Liu, Tan and Deng. This is an open-access article distributed under the terms of the Creative Commons Attribution License (CC BY). The use, distribution or reproduction in other forums is permitted, provided the original author(s) and the copyright owner(s) are credited and that the original publication in this journal is cited, in accordance with accepted academic practice. No use, distribution or reproduction is permitted which does not comply with these terms.
*Correspondence: Yongzhi Deng, NjI3NDczNTQ0QHFxLmNvbQ==; Zhaolin Tan, NDA4MDE4MjYwQHFxLmNvbQ==; Da Liu, bGl1ZGFfMTk4NkAxNjMuY29t