- 1Department of Interventional Oncology, Renji Hospital, Shanghai Jiao Tong University School of Medicine, Pudong, Shanghai, China
- 2School of Clinical Medicine, Jining Medical University, Jining, Shandong, China
- 3Department of Radiology, Shanghai Jiao Tong University School of Medicine, Pudong, Shanghai, China
- 4Department of Radiology, People’s Hospital of Qintong, Taizhou, Jiangsu, China
- 5Department of Ultrasound, Shanghai Jiao Tong University School of Medicine, Pudong, Shanghai, China
- 6Department of Interventional Radiology, The First Hospital of China Medical University, Shenyang, Liaoning, China
Introduction: To investigate immunogenic changes after percutaneous microwave ablation (MWA) in pulmonary malignancies.
Methods: Twenty-two consecutive patients with pulmonary malignancies who underwent percutaneous lung tumor MWA were prospectively enrolled in this study. Peripheral blood samples were collected on the day before (D0) and one month (M1) after MWA. Changes in immune cell subsets (CD3+, CD4+, and CD8+ T cells, and B, natural killer, regulatory T (Treg), and CD3-CD20+ cells) and cytokines (interleukin [IL]-2, 4, 6, 10, 17A, tumor necrosis factor [TNF]-α, and interferon-γ) were noted and compared. Progression-free survival (PFS) and potentially related factors were analyzed.
Results: The proportion of CD8+ T cells increased from 22.95 ± 7.38% (D0) to 25.95 ± 9.16% (M1) (p = 0.031). The proportion of Treg cells decreased from 10.82 ± 4.52% (D0) to 8.77 ± 2.05% (M1) (p = 0.049). The IL-2 concentration was also decreased from 1.58 ± 0.46 pg/mL (D0) to 1.26 ± 0.60 pg/mL (M1) (p = 0.028). The reduction in Treg cells predicted PFS independently of clinical prognostic features in multivariate analysis (hazard ratio = 4.97, 95% confidence interval: 1.32–18.66, p = 0.018). A reduction in the proportion of Treg cells was observed in 15 patients (68.2%) and the average of the reduction was 2.05 ± 4.60%. Those patients with a reduction in the proportion of Treg cells that was more than average showed a significantly longer median PFS time than those with a reduction that was less than average (16 months vs. 8.5 months, p = 0.025).
Discussion: Percutaneous MWA of pulmonary malignancies leads to immunogenic changes. The reduction in the proportion of Treg cells was independently associated with PFS.
Introduction
Thermal ablation has been widely used for the local ablation of solid tumors, with the advantages of high treatment efficacies and low complication risks (1–4). An increasing number of studies have demonstrated that thermal ablation not only destroys the tumor locally, but also leads to a change in systemic anti-tumor immunity (5–7). Such a change in immunity has been shown to predict the prognosis of patients (8, 9).
Although the mechanism of how thermal ablation can activate anti-tumor immunity has not been fully elucidated, recent studies have suggested that thermal ablation could modulate systemic anti-tumor immunity by activating various steps in the cancer immunity cycle, including: release of neoantigen and danger signals (10, 11); upregulation of immune cells in peripheral blood (12, 13); increased tumor-infiltrating lymphocytes (14, 15); elevation of interferon-gamma (IFN-γ) and other pro-inflammatory cytokines (16, 17), and a reduction in immunosuppressive regulatory T (Treg) cells (18).
In recent years, thermal ablation of primary and metastatic lung malignancies has gained in popularity for patients whose tumors are not suitable for surgical resection (19–22). However, previous studies regarding the immunity change caused by thermal ablation mainly focused on hepatocellular carcinoma (HCC), and breast, renal cell, and prostate cancers. Few to no studies have investigated immunity changes after thermal ablation of pulmonary malignancies (23). Thus, in the present study, immune cell subsets and cytokines were determined in the peripheral blood of patients, and compared before and after the percutaneous microwave ablation (MWA) of pulmonary malignancies. The aim of the study was to investigate immunogenic change after percutaneous lung MWA and its relationship with the prognosis of patients.
Methods
Study design
This monocentric prospective study was performed at our institution from January 2020 to December 2020. This study was approved by the local Institutional Review Board and informed consent was obtained from all eligible patients. After a multidisciplinary meeting that included surgeons, interventional radiologists, pathologists and oncologists, 104 consecutive patients who were recommended to receive MWA for pulmonary malignancies were assessed. Patients aged > 18 years, with a diagnosis of primary and metastatic lung malignancies by pathology, with less than five lesions, and with tumors that had a maximum diameter ≤5cm were included. Exclusion criteria included: 1) those with contraindications of MWA; 2) received chemotherapy, immunotherapy, radiotherapy or MWA within three months before enrollment in the study or within one month after MWA; 3) experienced severe complications from MWA such as infections or massive bleeding; 4) received glucocorticoid treatment or immunosuppressive therapy; 5) declined to participate in the study.
The day when MWA was performed was defined as D1. Patients’ peripheral blood samples were collected on the day before (D0) and one month (M1) after MWA, and were used to measure changes in immune cell subsets and cytokines. All patients received a clinical follow-up (Figure 1).
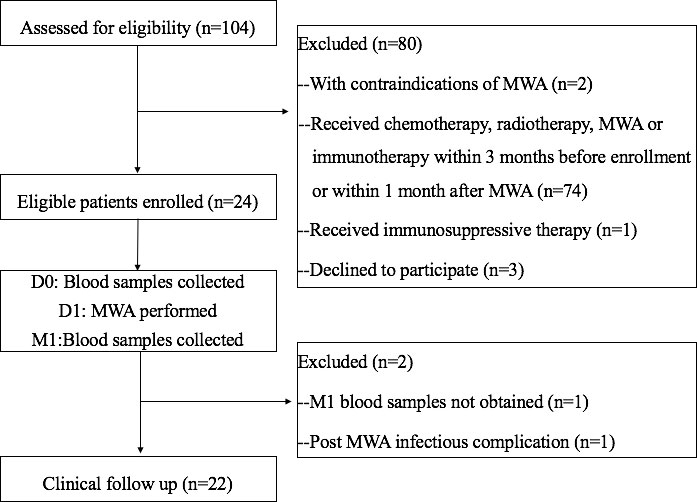
Figure 1 Flowchart of the study. D0, day before MWA; D1, day of MWA; M1, one month after MWA; MWA, percutaneous microwave ablation.
Percutaneous microwave ablation
Percutaneous MWA was performed by an experienced interventional radiologist under the guidance of a dual-source computed tomography (CT) system (SOMATOM Force, Siemens Healthcare, Forchheim, Germany) with local anesthesia (lidocaine). The MWA system (MTC-3C, Vison-China Medical Devices R&D Center, Nanjing, China) consisted of a 2450 ± 50MHz generator generating a maximum of 100 W and a water-cooled antenna (outer diameter was 16G or 18G and effective length was 150 mm or 180 mm).
Tumors with a maximal diameter less than 3 cm were treated with a single applicator that was placed in the center of the tumor. To ensure the ablation range completely covered the tumors and 0.5 cm around each tumor, tumors with a diameter larger than 3 cm were treated using two to three different sites based on tumor size and shape. A second or even third ablation was performed for those with a progression in ablation lesions.
A tumor treated according to the protocol and with a patient who was completely recovered as determined at the time of the procedure was deemed a “technical success”.
All patients received a chest CT scan the next day after MWA to assess for pneumothorax or hemorrhage. Analgesics and antipyretics were prescribed if necessary.
Measurement of immune cell subsets and cytokines
Peripheral blood samples were collected from patients on D0 and M1. T cells (CD3+), CD4+ T cells (CD3+CD4+), CD8+ T cells (CD3+CD8+), B cells (CD3-CD19+), natural killer (NK) cells (CD3-CD16+CD56+), CD20+ B cells (CD45+CD3-CD20+), and Treg cells (CD3+CD4+CD25+CD127-) were analyzed by flow cytometry (BD FACSCalibur, BD Biosciences, Sparks, MD, USA) using BD Multi-TEST IMK Kits (BD Biosciences, Shanghai, China). Levels of cytokines: interleukin-2 (IL-2), IL-4, IL-6, IL-10, IL-17A, tumor necrosis factor-alpha (TNF-α), and IFN-γ were determined by a Luminex 200 analyzer (Shanghai Tellgen Life Science Co. Ltd, Shanghai, China) using cytokine detection kits (Bio-Rad, Hercules, CA, USA).
Treatments after MWA
For primary lung malignancies, patients with sensitive mutations were treated with epidermal growth factor receptor-tyrosine kinase inhibitors (EGFR-TKIs) or anaplastic lymphoma (ALK) kinase inhibitors. For those without mutations, platinum-based chemotherapy was administered. For patients with metastatic lung malignancies, systematic chemotherapy was administered according to the pathology of the primary tumor. For all patients, if local therapy (such as radiotherapy, a second thermal ablation, transhepatic arterial chemotherapy, and embolization) or immunotherapy was necessary, such treatments were administered one month after MWA.
Complications and follow-up
Treatment-related complications were noted within 30 days after ablation, and were classified in accordance with the Common Terminology Criteria for Adverse Events version 4.0 (24). Patients with major complications were excluded from the study.
Patient follow-up was conducted at 1 month and every 3 months thereafter until death or the last follow-up. Follow-up evaluation included physical examination, laboratory testing, and contrast-enhanced imaging (ultrasound, CT, magnetic resonance imaging, or positron emission tomography).
Technique efficacy referred to a defined prospective time point when “complete ablation” of the macroscopic tumor was achieved as noted on follow-up imaging. Complete ablation was defined as lesion disappearance, complete cavitation formation, fibrotic progression or scar formation, solid nodule involution or no change, and/or atelectasis presenting as no contrast-enhanced signs on follow-up CT images. Incomplete ablation was indicated by incomplete cavernous formation with several remaining solid or liquid components; partial fibrosis or fibrotic lesions with solid residues; and/or solid nodules with unchanged or increased size displaying irregular peripheral or internal enhancement signs on follow-up CT images.
Treatment response was evaluated according to the Response Evaluation of Criteria in Solid Tumors version 4.0 (25). Progression-free survival (PFS), was defined as the interval between initial treatment and disease progression or death.
Statistical analysis
Statistical software (SPSS version 19.0; SPSS, Chicago, IL, USA) was used for analysis. Continuous variables were presented as mean ± standard deviation and compared by paired sample t test. Categorical variables were presented as frequencies and compared using the chi-square test. Univariate and multivariate analyses were used to identify factors associated with PFS. Progression-free survival was analyzed using Kaplan–Meier curves and a log-rank test. A Cox proportional hazards model was used to examine risk factors associated with PFS. Variables with a p value less than 0.1 were entered into the multivariate model. The relationship among those variables was analyzed by using spearman correlation coefficients. A p value less than 0.05 indicated a significant difference.
Results
Characteristics of study population
Between January 2020 and December 2020, 104 consecutive patients were assessed. A total of 24 eligible patients were enrolled in the study. Technical success was achieved in all 24 patients. One patient was excluded due to experiencing pneumonia after MWA. Another patient was excluded due to a lack of M1 blood sample. Finally, 22 patients finished the study. Table 1 summarizes the main characteristics of the studied population.
Variation in immune cell subsets and cytokines after MWA
The variations in immune cell subsets and cytokines after MWA are shown in Table 2 and Figures 2A, B. The proportion of CD8+ T cells increased from 22.95 ± 7.38% (D0) to 25.95 ± 9.16% (M1) (p = 0.031). An increase in the proportion of CD8+ T cells was observed in 15 (68.2%) patients. The proportion of Treg cells decreased from 10.82 ± 4.52% (D0) to 8.77 ± 2.05% (M1) (p = 0.049). A decrease in proportion of Treg cells was observed in 15 (68.2%) patients. The IL-2 concentration at M1 was significantly lower than that at D0 (1.26 ± 0.60 pg/mL vs. 1.58 ± 0.46 pg/mL, p = 0.028). A decrease in the IL-2 concentration was observed in 13 (59.1%) patients. Other immune cell subsets and cytokines showed no significant difference between D0 and M1.
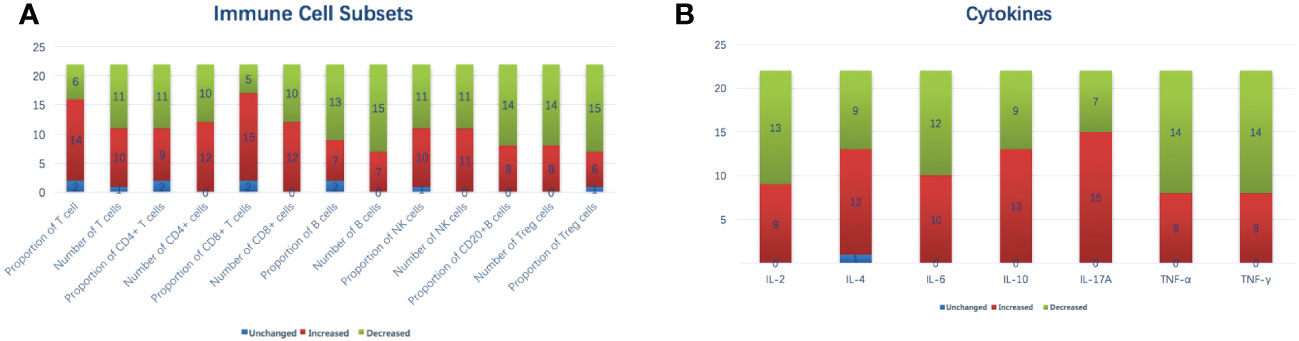
Figure 2 Variations in immune cell subsets (A) and cytokines (B) after MWA on a per-patient basis. IL, interleukin; MWA, percutaneous microwave ablation; NK, natural killer; TNF, tumor necrosis factor.
Follow-up analysis
No major post-treatment complications occurred in all patients after MWA. The average follow-up interval was 12.68 ± 7.56 (3 – 24) months. Technical efficacy was achieved in 16 patients (72.7%) during follow-up. One patient was lost to follow-up 15 months after MWA without disease progression. During the follow-up, a total of 16 patients (72.7%) showed disease progression.
In a Cox regression analysis of variables potentially associated with PFS (Table 3), gender (hazard ratio [HR] = 0.22, 95% confidence interval [CI]: 0.06–0.82), stage (HR = 4.26, 95% CI: 0.88–20.71), PS (HR = 1.98, 95% CI: 1.15–3.42), technical efficacy (HR = 0.31, 95% CI: 0.10–0.97), and a reduction in the proportion of Treg cells (HR = 3.30, 95% CI: 1.06–10.25) were significantly associated with PFS in univariate analysis. No linear correlation was found among the variables that entered into the multivariate model according to spearman correlation analysis (all p > 0.05). In multivariate analysis, a reduction in the proportion of Treg cells (HR = 4.97, 95% CI: 1.32–18.67, p = 0.018) was independently associated with PFS.
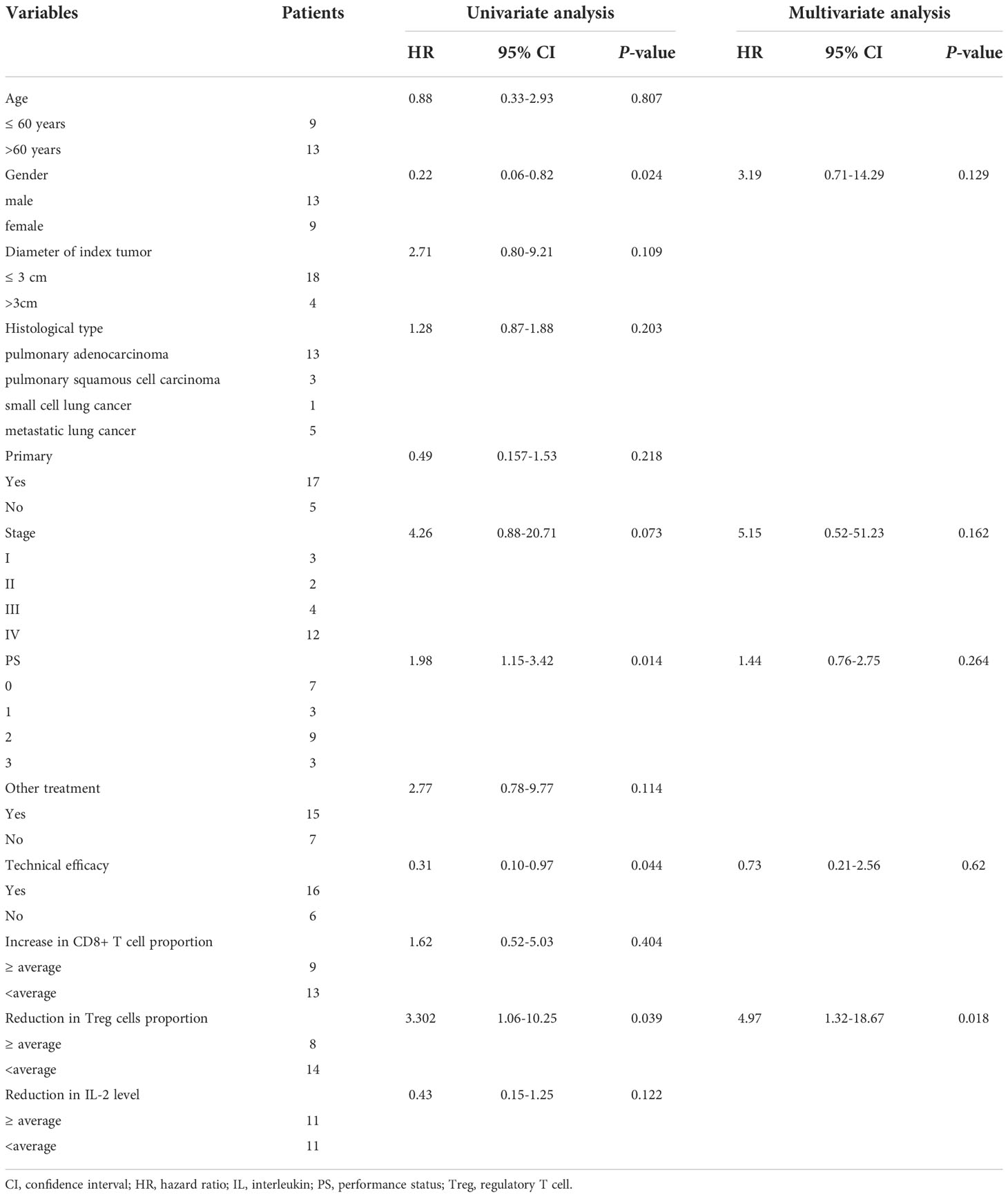
Table 3 Univariate and multivariate Cox regression analyses of variables potentially associated with PFS.
Patients with an absolute percentage decrease in the proportion of Treg cells over the average showed longer PFS (median PFS: 16.0 months) than patients with an absolute percentage decrease below the average (median PFS: 8.5 months) (Figure 3A, log-rank test: p = 0.025. Figure 3B, showed a case of a reduction in proportion of Treg cells predict PFS).
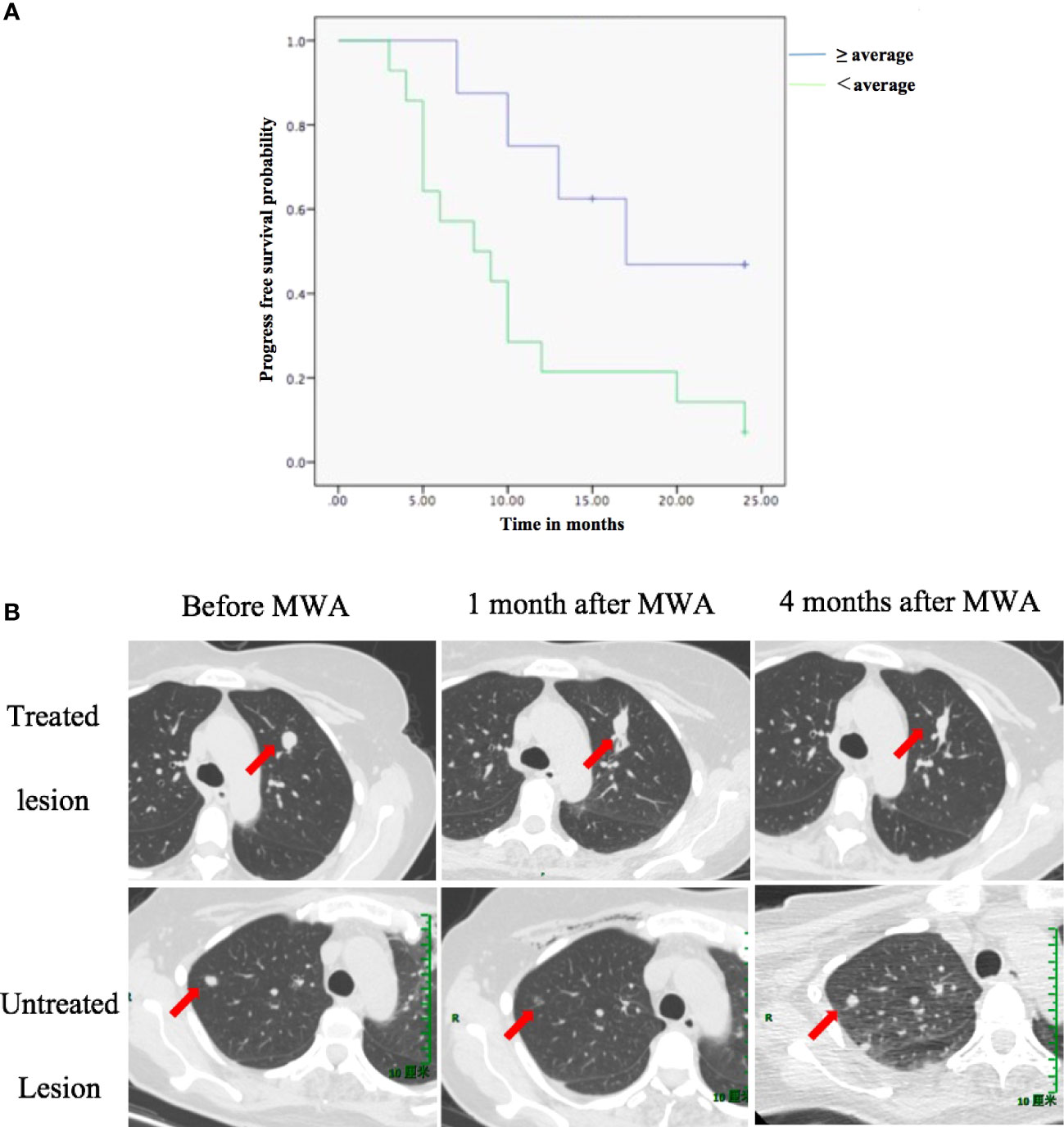
Figure 3 The reduction in the proportion of Treg cells is associated with PFS. (A) PFS according to a reduction in the proportion of Treg cells between D0 and M1. (B) A case showing that a reduction in proportion of Treg cells predict PFS. A 49-year-old female was diagnosed with pulmonary oligometastasis of urothelial carcinoma and underwent MWA (upper row: arrow indicated the change of treated lesion at different time point). One month after ablation of the index tumor, an abscopal effect was observed: untreated tumor regression (lower row: arrow indicated the change of untreated lesion at different time point). At M1, the proportion of CD8+ T cells increased from 39% (D0) to 53% (M1). However, the proportion of Treg cells also increased from 8.1% (D0) to 9.7% (M1). Finally, tumor progression was observed 4 months after MWA. The patient’s overall survival time was 10 months. D0, day before MWA; M1, one month after MWA; MWA, percutaneous microwave ablation; PFS, progression-free survival; Treg, regulatory T cell.
Discussion
The present prospective study investigated immunogenic changes after percutaneous lung MWA and analyzed potentially related factors associated with patients’ prognosis. Our data indicated that percutaneous MWA of pulmonary malignancies leads to an immunogenic change, including an increase in the proportion of CD8+ T cells and a decrease in the proportion of Treg cells and IL-2 concentration. The reduction in the proportion of Treg cells was independently associated with PFS.
Several studies have investigated the relationship between thermal ablation of lung tumors and systemic anti-tumor immunity. Fietta et al. (13) reported that 30 days after radiofrequency ablation (RFA) of lung tumor, a significant reduction in Treg cells count with an increase in CD4+ T cell proliferation and number of IFN-γ–secreting cells was observed. The reduction in Treg cells lasted up to 90 days after treatment. Schneider et al. (26) showed that treatment of patients with RFA led to an activated and highly T-cell–stimulatory phenotype in dendritic cells, and that the RFA-induced necrotic tumor debris can serve as an in-situ antigen source to induce an autologous anti-tumor immune response. Wang et al. (27) reported that after RFA, the level of Th1 and Th1/Th2 cells increased, whereas levels of Th2, Th17, and Treg cells declined, indicating an improvement in anti-tumor immunity. Our data reinforce current evidence that a thermal ablation of lung tumor can trigger systemic immunological effects.
Preclinical studies have shown that lymphocytes that infiltrate the tumor as a result of thermal ablation are predominantly CD4+ and CD8+ T cells (15, 28). However, an increase in T-cell subsets was not very marked in peripheral blood samples. Zhou et al. (29) reported that levels of circulating T-cell subsets, except for Th17 cells, were relatively stable after MWA in patients with HCC. In the present study, only the proportion of CD8+ T cells increased after MWA. The amount of CD8+ T cells was not increased significantly, indicating that the change in T-cell subsets induced by MWA may be mild or transient. Other studies (18, 30) also reported that NK cell and macrophage infiltration appears to increase with thermal ablation. However, in our study, a significant change in NK and B cells was not detected. A decline in Treg cells post-ablation has been reported in several studies (13, 27). Treg cells could facilitate tumor progression by suppressing the antitumor immune response, and was reported negatively correlated with survival (31, 32). A higher number of CD4+ and CD8+ T cells and a lower number of Treg cells post-thermal ablation have been shown to have a positive effect on tumor progression and survival (33, 34). In the present study, the reduction in Treg cells was the only feature that predicted PFS independently in multivariate analysis, indicating that the MWA-induced reduction of Treg cells plays an essential role in the enhancement of systemic anti-tumor immunity.
Changes in cytokines after thermal ablation have also been reported in several studies. Elevated IFN-γ levels post-ablation has been shown to be associated with tumor-infiltrating lymphocytes (35, 36). The pro-inflammatory cytokines, IL-1β, IL-6, and IL-8, have also been noted to be elevated post-thermal ablation (17, 37). These cytokines have been shown to assist with T-cell proliferation and trafficking (38). However, in the present study, only a decrease in IL-2 concentration was found. Interleukin-2 plays an important role in the immune system by binding to the IL-2 receptor (IL-2R) on the surface of lymphocytes. It has the ability to activate CD8+ T and NK cells, and promote their proliferation. However, IL-2 can also activate Treg cells, which inhibits the anti-tumor immune response of T cells (39). The IL-2R is another key point in the process; high affinity IL-2R (complex of α/β/γ) is expressed on Treg cells, while low affinity IL-2R (complex of β/γ) is expressed on CD8+T and NK cells. Therefore, the trimer high affinity complex on Treg cells consumes a large amount of IL-2, which requires a higher dose of IL-2 to achieve cytotoxic T lymphocyte (CTL)–mediated anti-tumor immunity (40, 41). Today, research on IL-2 therapy focuses on how to enhance the activation of IL-2 on CTLs and reduce or block the activation of Treg cells (42, 43). In the present study, an increase in proportion of CD8+ T cells and a decrease in proportion of Treg cells and IL-2 concentration were observed at the same. Thus, it is postulated that MWA may induce a change in IL-2 bias; that is, IL-2 may selectively bind IL-2R on effector T cells, and reduce the binding of IL-2R on Treg cells. This hypothesis is worthy of further investigation in order to explore the potential of the combination of MWA and IL-2 therapy. Previous studies have shown that the combination of radiotherapy or PD-L1 blockade and IL-2 therapy potently stimulates systemic anti-tumor immunity (44, 45).
Several limitations exist in the present study. First, the study population was strictly selected and quite small. It may give rise to bias in statistical analysis, and the reliability of study result needs to be confirmed by further research. Second, only changes in immune cells and cytokines on D0 and M1 were analyzed. Further time points, such as an early (Day 1, Day 7) change and long term (M3, M6) change of immunity should be further investigated. Third, the mechanism of how MWA regulated immune cells and cytokines was not investigated in the present study; however, it is worthwhile to further explore the regimen in order to amplify the anti-tumor immunity induced by thermal ablation. Fourth, as the only biological material studied was blood, it is not possible to know if the observed variations were due to tumor infiltration or solely due to blood dynamics. Finally, only PFS and potentially related factors were analyzed in this study. In a further study, long-term follow-up should be conducted and overall survival should also be analyzed.
In conclusion, our study demonstrated that percutaneous MWA of pulmonary malignancies leads to immunogenic changes. The reduction found in the proportion of Treg cells was independently associated with PFS.
Data availability statement
The raw data supporting the conclusions of this article will be made available by the authors, without undue reservation.
Ethics statement
The studies involving human participants were reviewed and approved by Ethic Committee of Renji Hospital, School of Medicine, Shanghai Jiao Tong University. The patients/participants provided their written informed consent to participate in this study.
Author contributions
LZ, BF and JS contributed to conception and design of the study. MZ performed statistical analysis and wrote sections of the manuscript. JW wrote the first draft of the manuscript. LZ, JS and JX performed MWA. TW and YL performed the follow-up. All authors contributed to manuscript revision, read, and approved the submitted version.
Funding
This study was funded by Shanghai Health Committee (Grant Number 20204Y0041) and Shanghai Jiao Tong University (Grant Number YG2022QN024).
Conflict of interest
The authors declare that the research was conducted in the absence of any commercial or financial relationships that could be construed as a potential conflict of interest.
Publisher’s note
All claims expressed in this article are solely those of the authors and do not necessarily represent those of their affiliated organizations, or those of the publisher, the editors and the reviewers. Any product that may be evaluated in this article, or claim that may be made by its manufacturer, is not guaranteed or endorsed by the publisher.
References
1. Ruers T, Van Coevorden F, Punt CJA, Pierie JPEN, Borel-Rinkes I, Ledermann JA, et al. Local treatment of unresectable colorectal liver metastases: Results of a randomized phase II trial. J Natl Cancer Institute (2017) 109:djx015. doi: 10.1093/jnci/djx015
2. Reig M, Forner A, Rimola J, Ferrer-Fàbrega J, Burrel M, Garcia-Criado Á, et al. BCLC strategy for prognosis prediction and treatment recommendation: The 2022 update. J Hepatol (2022) 76:681–93. doi: 10.1016/j.jhep.2021.11.018
3. Venturini M, Cariati M, Marra P, Masala S, Pereira PL, Carrafiello G. CIRSE standards of practice on thermal ablation of primary and secondary lung tumours. Cardiovasc Interventional Radiol (2020) 43:667–83. doi: 10.1007/s00270-020-02432-6
4. Yu J, Zhang X, Liu H, Zhang R, Yu X, Cheng Z, et al. Percutaneous microwave ablation versus laparoscopic partial nephrectomy for cT1a renal cell carcinoma: A propensity-matched cohort study of 1955 patients. Radiology (2020) 294:698–706. doi: 10.1148/radiol.2020190919
5. Haen SP, Pereira PL, Salih HR, Rammensee HG, Gouttefangeas C. More than just tumor destruction: Immunomodulation by thermal ablation of cancer. Clin Dev Immunol (2011) 2011:160250. doi: 10.1155/2011/160250
6. Chu KF, Dupuy DE. Thermal ablation of tumours: Biological mechanisms and advances in therapy. Nat Rev Cancer (2014) 14:199–208. doi: 10.1038/nrc3672
7. Dumolard L, Ghelfi J, Roth G, Decaens T, Jilkova ZM. Percutaneous ablation-induced immunomodulation in hepatocellular carcinoma. Int J Mol Sci (2020) 21:1–11. doi: 10.3390/ijms21124398
8. Leuchte K, Staib E, Thelen M, Gödel P, Lechner A, Zentis P, et al. Microwave ablation enhances tumor-specific immune response in patients with hepatocellular carcinoma. Cancer Immunology Immunotherapy (2021) 70:893–907. doi: 10.1007/s00262-020-02734-1
9. Lemdani K, Mignet N, Boudy V, Seguin J, Oujagir E, Bawa O, et al. Local immunomodulation combined to radiofrequency ablation results in a complete cure of local and distant colorectal carcinoma. OncoImmunology (2019) 8:1550342. doi: 10.1080/2162402X.2018.1550342
10. Ghanamah M, Berber E, Siperstein A. Pattern of carcinoembryonic antigen drop after laparoscopic radiofrequency ablation of liver metastasis from colorectal carcinoma. Cancer (2006) 107:149–53. doi: 10.1002/cncr.21959
11. Domingo-Musibay E, Heun JM, Nevala WK, Callstrom M, Atwell T, Galanis E, et al. Endogenous heat-shock protein induction with or without radiofrequency ablation or cryoablation in patients with stage IV melanoma. Oncologist (2017) 22:1026–e93. doi: 10.1634/theoncologist.2017-0060
12. Zerbini A, Pilli M, Penna A, Pelosi G, Schianchi C, Molinari A, et al. Radiofrequency thermal ablation of hepatocellular carcinoma liver nodules can activate and enhance tumor-specific T-cell responses. Cancer Res (2006) 66:1139–46. doi: 10.1158/0008-5472.CAN-05-2244
13. Fietta AM, Morosini M, Passadore I, Cascina A, Draghi P, Dore R, et al. Systemic inflammatory response and downmodulation of peripheral CD25+Foxp3+ T-regulatory cells in patients undergoing radiofrequency thermal ablation for lung cancer. Hum Immunol (2009) 70:477–86. doi: 10.1016/j.humimm.2009.03.012
14. Ito F, Vardam TD, Appenheimer MM, Eng KH, Gollnick SO, Muhitch JB, et al. In situ thermal ablation augments antitumor efficacy of adoptive T cell therapy. Int J Hyperthermia (2019) 36:22–36. doi: 10.1080/02656736.2019.1653500
15. Zhang L, Xu L, Wang Y, Liu X, Zhang M, Li W. Antitumor immunity augmented by combining radiofrequency ablation with anti–CTLA-4 therapy in a subcutaneous murine hepatoma model. J Vasc Interventional Radiol (2020) 31:1178–86. doi: 10.1016/j.jvir.2020.01.022
16. Zhang H, Hou X, Cai H, Zhuang X. Effects of microwave ablation on T-cell subsets and cytokines of patients with hepatocellular carcinoma. Minimally Invasive Ther Allied Technol (2017) 26:207–11. doi: 10.1080/13645706.2017.1286356
17. Erinjeri JP, Thomas CT, Samoilia A, Fleisher M, Gonen M, Sofocleous CT, et al. Image-guided thermal ablation of tumors increases the plasma level of interleukin-6 and interleukin-10. J Vasc Interventional Radiol (2013) 24:1105–12. doi: 10.1016/j.jvir.2013.02.015
18. Zhou L, Fu JL, Lu YY, Fu BY, Wang CP, An LJ, et al. Regulatory T cells are associated with post-cryoablation prognosis in patients with hepatitis b virus-related hepatocellular carcinoma. J Gastroenterol (2010) 45:968–78. doi: 10.1007/s00535-010-0243-3
19. Hasegawa T, Takaki H, Kodama H, Yamanaka T, Nakatsuka A, Sato Y, et al. Three-year survival rate after radiofrequency ablation for surgically resectable colorectal lung metastases: A prospective multicenter study. Radiology (2020) 294:686–95. doi: 10.1148/radiol.2020191272
20. Wei Z, Ye X, Yang X, Huang G, Li W, Han X, et al. Efficacy and safety of microwave ablation in the treatment of patients with oligometastatic non-small-cell lung cancer: a retrospective study. Int J Hyperthermia (2019) 36:827–34. doi: 10.1080/02656736.2019.1642522
21. Iezzi R, Cioni R, Basile D, Tosoratti N, Posa A, Busso M, et al. Standardizing percutaneous microwave ablation in the treatment of lung tumors: a prospective multicenter trial (MALT study). Eur Radiol (2021) 31:2173–82. doi: 10.1007/s00330-020-07299-2
22. Aufranc V, Farouil G, Abdel-Rehim M, Smadja P, Tardieu M, Aptel S, et al. Percutaneous thermal ablation of primary and secondary lung tumors: Comparison between microwave and radiofrequency ablation. Diagn Interventional Imaging (2019) 100:781–91. doi: 10.1016/j.diii.2019.07.008
23. Rangamuwa K, Leong T, Weeden C, Asselin-Labat ML, Bozinovski S, Christie M, et al. Thermal ablation in non-small cell lung cancer: A review of treatment modalities and the evidence for combination with immune checkpoint inhibitors. Trans Lung Cancer Res (2021) 10:2842–57. doi: 10.21037/tlcr-20-1075
24. National Cancer Institute. Common terminology criteria for adverse events0. (CTCAE) version 4.0 Bethesda, MD (2009).
25. Watanabe H, Okada M, Kaji Y, Satouchi M, Sato Y, Yamabe Y, et al. New response evaluation criteria in solid tumours - revised RECIST guideline (version 1.1). Japanese J Cancer Chemotherapy (2009) 36:2495–501.
26. Schneider T, Hoffmann H, Dienemann H, Herpel E, Heussel CP, Enk AH, et al. Immune response after radiofrequency ablation and surgical resection in nonsmall cell lung cancer. Semin Thorac Cardiovasc Surg (2016) 28:585–92. doi: 10.1053/j.semtcvs.2016.02.008
27. Shaobin W, Yu X, Jiatian L, Zaizhong C, Luping D, Junhui C. Changes of CD4+ T-cell subsets after radiofrequency ablation in lung cancer and its significance. J Cancer Res Ther (2016) 12:C166–70. doi: 10.4103/0973-1482.200609
28. Shi L, Chen L, Wu C, Zhu Y, Xu B, Zheng X, et al. PD-1 blockade boosts radiofrequency ablation-elicited adaptive immune responses against tumor. Clin Cancer Res (2016) 22:1173–84. doi: 10.1158/1078-0432.CCR-15-1352
29. Zhou Y, Xu X, Ding J, Jing X, Wang F, Wang Y, et al. Dynamic changes of T-cell subsets and their relation with tumor recurrence after microwave ablation in patients with hepatocellular carcinoma. J Cancer Res Ther (2018) 14:40–5. doi: 10.4103/jcrt.JCRT_775_17
30. Zhang J, Dong B, Liang P, Yu X, Su L, Yu D, et al. Significance of changes in local immunity in patients with hepatocellular carcinoma after percutaneous microwave coagulation therapy. Chin Med J (2002) 115:1367–71.
31. Wang H, Franco F, Ho PC. Metabolic regulation of tregs in cancer: Opportunities for immunotherapy. Trends Cancer (2017) 3:583–92. doi: 10.1016/j.trecan.2017.06.005
32. Shang B, Liu Y, Jiang SJ, Liu Y. Prognostic value of tumor-infiltrating FoxP3+ regulatory T cells in cancers: A systematic review and meta-analysis. Sci Rep (2015) 5:15179. doi: 10.1038/srep15179
33. Rochigneux P, Nault JC, Mallet F, Chretien AS, Barget N, Garcia AJ, et al. Dynamic of systemic immunity and its impact on tumor recurrence after radiofrequency ablation of hepatocellular carcinoma. OncoImmunology (2019) 8:1615818. doi: 10.1080/2162402X.2019.1615818
34. Mizukoshi E, Yamashita T, Arai K, Sunagozaka H, Ueda T, Arihara F, et al. Enhancement of tumor-associated antigen-specific T cell responses by radiofrequency ablation of hepatocellular carcinoma. Hepatology (2013) 57:1448–57. doi: 10.1002/hep.26153
35. Zhang L, Wang J, Jiang J, Zhang M, Shen J. CTLA-4 blockade suppresses progression of residual tumors and improves survival after insufficient radiofrequency ablation in a subcutaneous murine hepatoma model. Cardiovasc Interventional Radiol (2020) 43:1353–61. doi: 10.1007/s00270-020-02505-6
36. Yang X, Li X, Guo Z, Si T, Yu H, Xing W. Immunological response induced by cryoablation against murine H22 hepatoma cell line in vivo. Cryobiology (2018) 80:114–8. doi: 10.1016/j.cryobiol.2017.11.005
37. Ahmad F, Gravante G, Bhardwaj N, Strickland A, Basit R, West K, et al. Changes in interleukin-1β and 6 after hepatic microwave tissue ablation compared with radiofrequency, cryotherapy and surgical resections. Am J Surg (2010) 200:500–6. doi: 10.1016/j.amjsurg.2009.12.025
38. Fisher DT, Appenheimer MM, Evans SS. The two faces of IL-6 in the tumor microenvironment. Semin Immunol (2014) 26:38–47. doi: 10.1016/j.smim.2014.01.008
39. Sim GC, Martin-Orozco N, Jin L, Yang Y, Wu S, Washington E, et al. IL-2 therapy promotes suppressive ICOS+ treg expansion in melanoma patients. J Clin Invest (2014) 124:99–110. doi: 10.1172/JCI46266
40. Karakus U, Sahin D, Mittl PRE, Mooij P, Koopman G, Boyman O. Receptor-gated IL-2 delivery by an anti-human IL-2 antibody activates regulatory T cells in three different species. Sci Trans Med (2020) 12:eabb9283. doi: 10.1126/scitranslmed.abb9283
41. Spolski R, Li P, Leonard WJ. Biology and regulation of IL-2: from molecular mechanisms to human therapy. Nat Rev Immunol (2018) 18:648–59. doi: 10.1038/s41577-018-0046-y
42. Bentebibel SE, Hurwitz ME, Bernatchez C, Haymaker C, Hudgens CW, Kluger HM, et al. A first-in-human study and biomarker analysis of NKTR-214, a novel IL2Rβγ-biased cytokine, in patients with advanced or metastatic solid tumors. Cancer Discovery (2019) 9:711–21. doi: 10.1158/2159-8290.CD-18-1495
43. Mullard A. Restoring IL-2 to its cancer immunotherapy glory. Nat Rev Drug Discovery (2021) 20:163–5. doi: 10.1038/d41573-021-00034-6
44. Diab A, Tannir NM, Bentebibel SE, Hwu P, Papadimitrakopoulou V, Haymaker C, et al. Bempegaldesleukin (NKTR-214) plus nivolumab in patients with advanced solid tumors: Phase I dose-escalation study of safety, effi cacy, and immune activation (PIVOT-02). Cancer Discovery (2020) 10:1158–73. doi: 10.1158/2159-8290.CD-19-1510
Keywords: CD8+ T cell, IL-2, percutaneous microwave ablation, pulmonary malignancies, regulatory T cells, immune system
Citation: Zhang L, Zhang M, Wang J, Li Y, Wang T, Xia J, Feng B and Shen J (2022) Immunogenic change after percutaneous microwave ablation in pulmonary malignancies: Variation in immune cell subsets and cytokines in peripheral blood. Front. Immunol. 13:1069192. doi: 10.3389/fimmu.2022.1069192
Received: 13 October 2022; Accepted: 28 November 2022;
Published: 09 December 2022.
Edited by:
Yueyong Xiao, Chinese People’s Liberation Army General Hospital, ChinaReviewed by:
Xin Li, People’s Liberation Army General Hospital, ChinaShigenori Goto, Seta Clinic Tokyo, Japan
Copyright © 2022 Zhang, Zhang, Wang, Li, Wang, Xia, Feng and Shen. This is an open-access article distributed under the terms of the Creative Commons Attribution License (CC BY). The use, distribution or reproduction in other forums is permitted, provided the original author(s) and the copyright owner(s) are credited and that the original publication in this journal is cited, in accordance with accepted academic practice. No use, distribution or reproduction is permitted which does not comply with these terms.
*Correspondence: Jialin Shen, jialinshen123@163.com; Bo Feng, fb6772@163.com
†These authors share first authorship