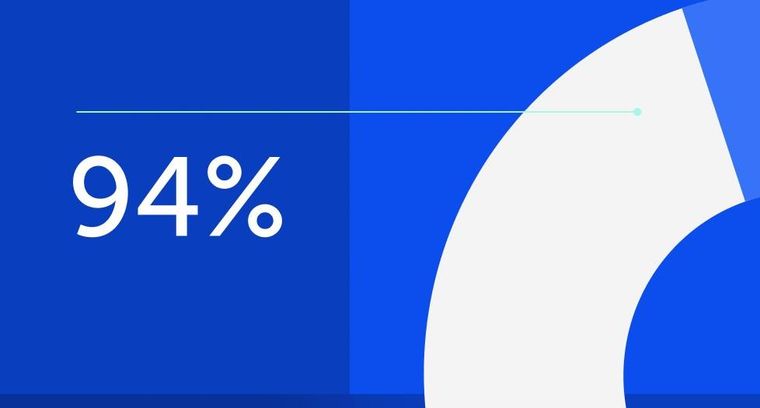
94% of researchers rate our articles as excellent or good
Learn more about the work of our research integrity team to safeguard the quality of each article we publish.
Find out more
REVIEW article
Front. Immunol., 24 November 2022
Sec. Cancer Immunity and Immunotherapy
Volume 13 - 2022 | https://doi.org/10.3389/fimmu.2022.1060497
Gastric cancer (GC) is one of the main causes of cancer incidence rate and mortality worldwide. As the main breakthrough direction, the application of immune checkpoint inhibitors makes patients with GC have better prognosis, where PD-L1/PD-1 inhibitors in immunotherapy have good anti-tumor immune efficacy. Further understanding of the regulatory mechanism of PD-L1 in GC may bring substantial progress to the immunotherapy. In this review, we provide information on the endogenous and exogenous regulatory mechanisms of PD-L1 and its biological functions combined with current clinical trials of PD-L1/PD-1 inhibitors in GC. The malignant biological phenotypes caused by PD-L1 and the corresponding clinical combined treatment scheme have been reported. Identifying the biomarkers of the potential efficacy of immunotherapy and specifying the clinical immunotherapy scheme in combination with molecular characteristics of patients may maximize clinical benefits and better prognosis.
Gastric cancer (GC) is one of the five most common cancers in the world and the fourth most common cause of cancer death, is responsible for over one million new cases in 2020 and an estimated 769,000 deaths (1). Helicobacter pylori infection, special age and high salt diet may induce the occurrence of GC (2). The Cancer Genome Atlas (TCGA) research network has published the latest genetic classification of gastric cancer, including Epstein Barr virus positive (EBV+), microsatellite install (MSI), genomically stable, and chromosomal unstable (CIN) (3). Surgical or endoscopic resection is still a mandatory backbone in treatment with curative intent. Continuous chemotherapy is used in the treatment of advanced GC, and platinum and fluoropyrimidine combined chemotherapy is the first-line treatment. Clinically, patients with GC, especially advanced GC, chemotherapy is a routine treatment, commonly include fluorouracil (5-FU)/capecitabine, paclitaxel (paclitaxel or docetaxel) and platinum, or a combination of these chemotherapeutic drugs (4). Targeted therapies for GC have been reported as follows: trastuzumab for the treatment of human epidermal growth factor (HER2) positive patients, Ramoximab for specific targeted angiogenesis, and anti-PD-L1(programmed cell death-Ligand 1)/PD-1(programmed death-1) inhibitors for patients with advanced GC, including nivolumab or pembrolizumab (5). Tumor immunotherapy is a sort of new tumor treatment which has developed rapidly compared with traditional treatment such as surgery, radiotherapy and chemotherapy and has great clinical application prospects. Immune checkpoint blockade is now established as a sort of combination treatment for chemorefractory gastric cancer (cancer which has progressed after two or more lines of chemotherapy) (6). However, such monitoring is not always perfect, tumor cells can escape immune regulation through a variety of ways under certain conditions. Compared with traditional treatment, tumor immunotherapy has multiple advantages of strong specificity and less side effects, which could provide a prospective view for treatment of solid tumors (7). At present, cancer immunotherapies include immune checkpoint inhibitor therapy, adoptive cell immunotherapy(ACT), cancer vaccine and some emerging immunotherapy (including LAG3、TIM3、VISTA and B7-H3). This article mainly discusses the regulation and biological consequences of intracellular PD-L1 expression level and the clinical application of PD-L1/PD-1 inhibitors in immunosuppressive checkpoints in GC.
In gastric adenocarcinoma (8, 9), there are tumor-infiltrating lymphocytes (TIL) in the tumor area or the surrounding matrix. The effectiveness of antitumor immune response may bring a better prognosis for patients with this type of GC. After curative surgery, patients with high TIL tumors had better disease-free survival (DFS) and overall survival (OS) than patients with low TIL tumors (8). Inhibiting the expression of PD-L1 on tumor cells can enhance immune surveillance and reduce the function of PD-L1 derived immune checkpoint (10). Agents against PD-L1/PD-1 have shown significant clinical significance in non-small-cell-lung-cancer(NSCLC) (11), melanoma (12) and GC (5) amongst others. Immune checkpoint receptor PD-1 is a co-inhibitory molecule, which physiologically expresses on the cell surface of immune cells, such as T and B lymphocytes or myeloid cells, and provides signal immune system activity to terminate immunity. PD-1 receptor has two natural ligands, PD-L1 (B7-H1) and PD-L2 (B7-DC). It can be expressed not only in immune cells, but also in tumor cells, and represents a potential mechanism of immune surveillance escape (13). In general, PD-L1/PD-1 axis is considered as a significant factor of poor prognosis across different types of GC patients. In this article we aim to describe the biological mechanism and function of PD-L1 regulation and clinical application of immune checkpoint inhibition as a therapeutic strategy in GC, and to discuss the progress and perspective on immune checkpoint inhibitors (ICIs).
The regulatory mechanism of PD-L1 in GC is mainly summarized in Figure 1.
Figure 1 The regulatory mechanism of PD-L1 expression in GC cells. Me, methylation; Ub, Ubiquitination.
The expression of PD-L1 can be simply induced by the change of gene copy number and chromosome rearrangement in the chromosome region (9p24.1) of PD-L1 and PD-L2 loci (14, 15). Such events mainly occur in hematological malignancies. Nevertheless, the destruction or mutation of the 3’ untranslated region of PD-L1 can directly affect its expression level in GC (16, 17). Some oncogenes have been identified, such as MYC, which regulates the anti-tumor immune response through CD47 and PD-L1, and ALK, which affect the expression of PD-L1 through STAT3 pathway. Concurrently, other molecules, including HIF1/2 α, hosting hypoxia-mediated escape from adaptive immunity, NF-κB, MAPK, mediating resistance to BRAF inhibition and upregulating PD-L1 expression, PTEN/PI3K, LKB1, loss of which could lead to elevated PD-L1 expression (18, 19). EGFR, whose activation closely relate to expression of PD-L1, contributes to immune escape in various tumors. PD-L1 has been reported to bind to EGFR and activate EGFR to promote the progression of GC (20). Expression level of PD-L1 was significantly inhibited when EGFR/HER2 signaling pathway was blocked in GC. Hypoxia-HIF-1α-VEGF/ANG signaling also weighs heavily in advanced GC (21). Overexpression of CXXC4 in GC leads to down-regulation of MAPK signaling pathway (22). For patients with MSI-H GC, who own better prognosis among diverse molecular types of GC patients, the expression of PD-L1 in ARID1A-deficient tumors was significantly higher (23). Hedgehog signal induces PD-L1 upregulation and tumor cell proliferation in GC (24). Nor is this all, the transcription effector Gli of hedgehog signal also can induce the expression of PD-L1 in GC through mTOR pathway (25).
The expression of PD-L1 in GC can also be regulated by miRNA and non-coding long chain RNA. For example, Mir-bart5-5p is expressed in EBV+ GC cells and specimens and promotes the expression of PD-L1 and the occurrence of GC subsequently through PIAS3/pSTAT3 pathway (26). Mir-105-5p, regulates PD-L1 expression and tumor immunogenicity in GC (27). LncRNA PROX1-AS1 promotes GC progression through mir-877-5p/PD-L1 axis (28). Tristetraprolin (TTP), a RNA-binding protein, binds to adenosine-uridine AU-rich elements in the 3’-untranslated region of messenger RNAs and facilitates rapid degradation of the target mRNAs. High TTP expression downregulates PD-L1 and proceed to affect GC cell survival and apoptosis, increasing peripheral blood mononuclear lymphocyte (PBML)-mediated cytotoxicity and slowing down tumor progression (29). One research in 2021 showed that PD-L1 was significantly hypermethylated during gastric carcinogenesis, and the DNA methylation of PD-L1 was negatively correlated with the expression of PD-L1 in GC samples (30). Meanwhile, some studies have also found that mir-105-5p is controlled by DNA methylation of Gabra3 promoter in GC, affecting the expression of PD-L1 and the progression of GC (27). In another study, after treating GC cells with compound oleanolic acid (OA), the expression specificity of DNA demethylase TET3 decreased and expression of downstream interleukin-1β (IL-1 β) was downregulated, so is PD-L1. In this process, DNA demethylase TET3, as an important mechanistic molecule, can largely restore the effect of OA on PD-L1 (31). Upregulation of MHC class I and MHC class II by DNA methyltransferases inhibitors (DNMTi) has appeared in many cancers (32). It is clearly reported that in gastric cancer, the association between PD-L1 positivity and histone N-methyltransferase 2 (KMT2) family member mutations remained significant in the proficient-MMR and microsatellite stable subgroup (33).
Ubiquitination ligases can greatly affect tumorigenesis in different ways. For example, it can regulate the stability of oncogenes and tumor suppressor genes. Ubiquitination labeled proteins are transported to proteasome or lysosome for degradation. Ubiquitin specific peptidase (USP7) is a member of ubiquitination related protein family, which can promote the stability of various proteins. Wang, Z et al. demonstrated that USP7 was highly expressed in GC, and poorly differentiated patients with high USP7 expression has worse overall survival. USP7 can directly interact with and de-ubiquitinate PD-L1, and then enhance the immune escape level in tumor immunity and promote the progression of GC (34). Ubiquitination is an important mechanism for PD-L1 to maintain protein stability in cancers.
As we know, except for tumor cells, PD-L1 is highly expressed on the surface of tumor infiltrating immune cell, such as neutrophils, macrophages, and mast cells. Since GC is a solid tumor with relatively weak immunogenicity, we found that there are many studies related to immunotherapy of GC target the immune cells infiltrating around the tumor. Figure 2 briefly describes the expression of PD-L1 on immune cells in the microenvironment of GC.
In GC, the expression of PD-L1 of neutrophils can be induced by tumor derived granulocyte-macrophage colony-stimulating factor (GM-CSF), which can be activated through Janus kinase (JAK) - signal transducer and activator of transcription 3 (STAT3) pathway, to a large extent, inhibiting tumor immunity and promote progression of GC (35). Similarly, while chemokine colony stimulating factor 2 (CSF-2) secreted by GC cells, macrophages are stimulated to secrete lots of chemokine CXCL8. In this autocrine form, macrophages continue to receive positive feedback, resulting PD-L1 highly expresses on the cell surface, to play the above tumor immunosuppression (36). In the tumor microenvironment, in some degree cytokines and chemokines are responsible for recruiting immune cells. Among them, GC cells can recruit mast cells through CXCR4-CXCL12 receptor-ligand binding. Then, under the condition that GC cells secrete a large amount of tumor necrosis factor a (TNF-a), while mast cells express TNF-a II ligand to receive such signals, and highly express PD-L1 through NF-kB pathway, to further promote the malignant progression of GC by cascade (37).
Induction and activation of JAK2/STAT3 signal by interferon γ (IFN- γ) can promote the upregulation of PD-L1 expression in GC and various kinds of solid tumors (38–40). In GC, in addition to the EGFR mentioned above, there are c-Met, mTOR, PI3K-Akt, JAK2/STAT3 and NF KB signaling pathways or key molecules (41, 42) that can make a difference to PD-L1. When it comes to STAT signal related genes, some researchers found that some DNA double strand breaks can activate STAT signal pathway and enhance the expression of PD-L1 (43). Besides, cytokine TNF- α and IL-6 also activate NF-KB and STAT3 signaling pathways in regulating the expression of PD-L1 (44). Other inflammatory cytokines, such as toll like receptor 3(TLR-3), transforming growth factor β (TGF-β), IFN- α/β and IL-4/6/17/27 have been shown to enhance the expression of PD-L1 mRNA on tumor cells or tumor associated stromal cells. In tissue samples from patients with GC, the expression of PD-L1 on tumor cell membrane was positively correlated with the presence of CD8 positive T cells and IFN- γ in matrix (45, 46). Overexpressing PD-L1 GC cell could recruit and enrich myeloid-Derived Suppressor Cells (MDSCs) by releasing lots of cytokines and promote the occurrence of gastric tumors by inhibiting tumor infiltrating CD8 + T cells (47). There are reports in the document that IFN- γ could restrain autophagy in the mechanism of promoting PD-L1 gene transcription (38, 40). Lately, Wang et al. found that autophagy, one specific mode of cell death, can directly down regulate PD-L1 through p62/SQSTM1-NF-κB pathway in GC (48).
Gastrointestinal cancer cells can up-regulate PD-L1 under the condition of 5-FU treatment. The improvement of prognosis of patients with gastrointestinal adenocarcinoma has been shown in various RCTs of the combination of 5-FU and PD-1/PD-L1 inhibitors (49). In another study, exogenous PD-L1 treatment could also significantly enhance the apoptosis of Jurkat T cells and inhibit T cell activation in PBMC. After combining nivolumab, a PD-L1 inhibitor, the immunosuppression of T cells was significantly alleviated (50). Vivien Koh et al. found that rapamycin blocked the transcriptional regulation of PD-L1 by Gli1 and Gli2 signals (25).
There are many different typing ways for GC cells, including molecular typing and histologic typing, such as EBV positive and MSI high cells. In addition, there are some special ones, such as GC cell lines with CSC characteristics (NCC-S1M), GC mesenchymal stem cell (GCMSC) and so on. NCC-S1M is the only widely recognized syngeneic GC transplantational model cell line. EBV+ GC cells not only promoted the amplification of CD274 gene (encoding PD-L1) by activating IRF3/CD274 axis, but also caused the up-regulation of PD-L1 constitutive expression (51). EBV infection stimulated elevated IFN- γ in GC, inducing the enhanced adaptive expression of PD-L1 (51–53). In another molecular type of GC, the expression of PD-L1 was also up-regulated, in which patients have the better clinical prognosis (54–58). For instance, the potential mechanism of high expression of PD-L1 in MSI-high GC was reported by Fang et al., which may be related to secretion of lots of new antigen in GC cells, therefore T lymphocytes infiltrate and secrete a large amount of IFN- γ, leading to GC progression (59). It should be noted that CD274 is highly expressed in NCC-S1M cells compared with normal gastric tissues. Treatment with PD-1 inhibitor can effectively retard the tumorigenesis of NCC-S1M cells (60). Mesenchymal stem cells get many biological functions, including secreting various chemokines, promoting angiogenesis, maintaining cell stemness and cellular immunity. GCMSC can secrete IL-8 and promote the high expression of PD-L1 in gastric tumor cells via classic STAT3/mTOR-c-MYC pathway (61). According to a recent report that GCMSC can further enhance the CSC-like characteristics of GC cells through PD-L1 associated with CTCF, to promote the invasion and drug resistance of GC cells (62).
The increased expression of PD-L1 in GC is related to the epithelial mesenchymal transformation phenotype (EMT), which can further increase the potential of tumor metastasis. The causal role of PD-L1 in promoting EMT has been confirmed in gastric carcinoma that IFN- γ upregulates the expression of PD-L1 in solid tumor cells through JAK signal transducer and transcriptional pathway activator, and weakens the cytotoxicity of tumor antigen specific CTL to tumor cells. After pretreatment with IFN- γ, cells were treated with anti-PD-L1 mAb, the decreased anti-tumor CTL activity by IFN- γ reached a level higher than that of non-therapeutic control target (45). Mir-940/Cbl-b/STAT5A axis up regulates the expression of PD-L1 and promotes the proliferation and EMT of GC cells (63). In another study, it is also confirmed that EMT can enhance the migration and invasion of GC cells through nuclear factor κB (NF-κB) pathway upregulates PD-L1 (64). Another study showed that the induction of EMT and PD-L1 expression was bidirectional. The induction of EMT upregulates the expression of PD-L1 mainly by activating PI3K-Akt pathway. These results demonstrate that the high expression level of PD-L1 is directly related to the phenotype of epithelial mesenchymal transformation.
The high expression of PD-L1 in GC can increase the potential of gastric CSCs and further promote the proliferation and progression of GC. Gastric CSCs also express PD-L1 (65). It is reported that GC cells were heterogeneous, and that PD-L1 in GC cells had different reactivity to GCMSCs and PD-L1 associated with CTCF to contribute to the stemness and self-renewal of GC cells, and GC mesenchymal cells (GCMSCS) enhance the CSC like characteristics of GC cells through PD-L1, resulting in the resistance of GC cells to chemotherapy (62). In vivo, PD-L1 positive GC cells have greater stem cell potential and tumorigenicity than PD-L1 negative GC cells (62). We find that the specific mechanism between PD-L1 and CSC in tumor cells in the latest study this year, that, the interaction between PD-L1 and frizled 6 receptor up-regulates β-Catenin targeted gene expression and promotes cancer progression through the maintenance and expansion of CSC (66).
The most famous biological function of PD-1/PD-L1 axis is to promote apoptosis of effector T cells, the basic principle behind which, is tumor immune escape. In 1992, Ishida et al. found PD-1 expressed transiently during inducing T cell death apoptosis in mice (67). In 2002, Minato et al. found that PD-L1 and PD-1 signaling pathways are involved in the regulation of tumor immunity for the first time (68). It’s reported that endogenous PD-L1 expression in gastric adenocarcinoma epithelial cells can promote T cell apoptosis (69). In another study, exogenous PD-L1 from GC can also induce Jurkat T cell apoptosis and inhibit T cell activation (50). PD-L1 can activate epidermal growth factor receptor (EGFR) through mir-429 to resist apoptosis induced by tumor necrosis factor-related apoptosis-inducing ligand (TRAIL) in GC (20). The most classic mechanism of PD-L1 promoting apoptosis and inhibiting T cell activation can be explained as follows: after PD-L1 binding to PD-1, tyrosine residues located in ITSM of PD-1 are phosphorylated and protein tyrosine phosphatases (PTPs), such as SHP2, are recruited. These PTPs can dephosphorylate various key signal kinases and resist the positive signal events caused by the co-stimulation of TCR-CD28 receptor during T cell activation. To some extent, they preferentially inhibit the pathways driven by TCR rather than the CD28 mediated pathway. We also find an important role of PD-L1 in another cell death, autophagy. In general, autophagy is negatively controlled by the PI3K/Akt/mTOR pathway, and PD-L1 induced activation of this pathway seems to lead to the down regulation of autophagy. It is recently reported that autophagy can inhibit the expression of PD-L1 in GC cells reversely (48). Another study has explored the mechanism that autophagy downregulates PD-L1 by reducing the expression of histone deacetylase (HDAC) (70).
A recurring observation among phase III gastric and esophageal trials combining chemotherapy and immunotherapy has been differential activity based on PD-L1 expression (71). Some studies have reported that PD-L1 can reduce the cytotoxicity sensitivity to cytokine induced killer cell (CIK) therapy in GC (72). The expression of PD-L1 can also enhance cisplatin resistance in GC (73). Low dose Diosbulbin-B (DB) (12.5 μ M) activates tumor-intrinsic PD-L1/NLRP3 signaling pathway to restore the sensitivity of the cisplatin resistant GC (CR-GC) cells to cisplatin, the level of PD-L1 downregulates simultaneously in GC cells (74). These results further confirm that PD-L1 can reduce the sensitivity of GC cells to cisplatin. However, one research reports that non-responders to XELOX chemotherapy, all of whom were PD-L1 negative, failed to upregulate PD-L1 expression on treatment or favorably remodel their TME and exhibited minimal change in T-cell infiltration but rather an increase in LAG3 expression and B-cell infiltration (75). The relationship between PD-L1 expression and cytotoxic chemotherapy response has not been examined in detail, but response is numerically lower (41% vs. 46%) in PD-L1–negative patients in the large (n = 1,581) phase III CheckMate-649 trial (76).
Systemic chemotherapy, radiotherapy, surgery, immunotherapy, and targeted therapy all have proven efficacy in gastric adenocarcinoma; therefore, multidisciplinary treatment is paramount to treatment selection. Triplet chemotherapy for resectable gastric cancer is now accepted and could represent a plateau of standard cytotoxic chemotherapy for localized disease (77). Classification of gastric cancer based on molecular subtypes is providing an opportunity for personalized therapy. The expression level of PD-L1 in GC patients with different molecular types differs. According to the PD-L1 score in gastric cancer, anti PD-L1/PD-1 inhibitor drugs are recommended respectively. The use of pembrolizumab as a third line treatment for patients with PD-L1 positive (CPS ≥ 1) gastric adenocarcinoma is based on many important clinical trials (77), which are described in Table 1. According to the Cancer Genome Atlas Project (TCGA), EBV positive tumors were not considered as a separate entity but they largely fell into the MSS/TP53 + and MSS/EMT subgroups. While they differ in terms of etiopathogenesis, molecular characteristics and determinants of immune-sensitivity, the MSI and the EBV subtypes (or EBV positive tumors in the ACGR classification) have been recognized as the gastric cancer subtypes that could benefit most from ICIs (78–80).
In different clinical trials of PD-1/PD-L1 inhibitors, most clinical trials have benefited from OS and PFS to varying degrees. ATTRACTION-4 and CHECKMATE-649 trials suggest that the combination of nivolumab with chemotherapy might be more effective than chemotherapy alone (76, 81). KEYNOTE-062, a phase 3 randomized clinical trial demonstrated that pembrolizumab was noninferior to chemotherapy for OS in patients with CPS of 1 or greater, but pembrolizumab monotherapy was not superior to chemotherapy in patients with CPS of 1 or greater (82). However, there have also been certain cases of failure. For example, the use of avelumab alone in the third-line treatment of GC patients can not significantly improve the overall survival (OS) of patients compared with the chemotherapy drugs selected by doctors. However, the results of Javelin gastric 300 (clinical trial NCT02625610) provide an important reference for us to continue to explore the role of avelumab in the treatment of GC. According to different drug regimens of PD-L1/PD-1 inhibitors, we divided them into first-line, second-line, third-line, and late treatment according to the combination of Europe, the USA, and Asia guideline for gastric cancer, as shown in Table 1.
Chromosomal events in patients with CIN GC include HER2 + or ERBB2 amplification, FGFR2b expression, FGFR amplification, EGFR amplification and MET amplification. Trastuzumab plus chemotherapy is the first-line drug for GC patients with HER-2 (+) and ERBB2 amplification in the current medical guidelines (Figure 3). Trastuzumab Deruxtecan DS-8201 (China) has been reported as a third-line drug for HER2 + GC (83). ADC targeting HER2 and immune checkpoint inhibitor are important potential biomarkers for clinical treatment, as well. Pembrolizumab currently be used in the second-line treatment of MSI GC. Tumor tissues were collected from patients participating in ITACA-S, and randomized adjuvant chemotherapy trials were carried out. According to MSI status, inflammatory response, and the expression of PD-L1, the prognosis and regressive analysis of MSI GC were analyzed. The results show that MSI status may be a meaningful prognostic biomarker in patients with radical resection stage II-III GC. The first-line regimens of PD-1/PD-L1 inhibitors currently used in GC patients expressing PD-L1 are: (1) nivolumab plus chemotherapy (CPS ≥ 5%); (2) Sintilimab plus chemotherapy (China). Third-line therapeutic schedule is pembrolizumab (CPS ≥ 1%). PD-1 monoclonal antibody may also be used as a potential first-line regimen for the treatment of MSS/TMB-high GC. At present, there is no suitable PD-1/PD-L1 agents for EBV positive GC, but PD-1 monoclonal antibody may be a potential first-line treatment for EBV cytotoxic T lymphocytes. Nivolumab (Asia) and Apatinib (China) can be used as a third-line regimen for some GC patients without clear molecular classification. At the same time, PD-1 monoclonal antibody combined with multiple kinase TKI (Stivarga/Lenvatinib) and PD-1 monoclonal antibody combined with PARP inhibitor can also be used as candidate immunotherapy schemes for this kind of groups.
As many of the patients with GC are PD-L1 negative, combination therapy is attractive. Furthermore, even some patients who are PD-L1 positive have quite limited benefit from anti-PD-1 therapy alone and relapse/recurrence frequently occurs due to various resistance mechanisms (84). The limitation of anti PD-L1/PD-1 monotherapy is due to the complex tumor microenvironment (TME) and multiple immunosuppressive factors in each step of the cancer immune cycle (85). In order to achieve effective tumor control and eradication, three levels of immunotherapy capabilities are required. First, CD4+T, CD8+T and B lymphocytes are activated to become antigen specific effector cells. Second, the inhibitory factors in the turbine microenvironment, represented by PD-L1 and others, are neutralized. Third, tumor cells that express related targets are recognized and killed (86). Therefore, increasing the benefit of checkpoint-immune blockade through combinations to achieve long-term efficacy may be helpful (87, 88). At present, PD-1/PD-L1 inhibitors combined with various chemotherapeutic drugs or targeted regimens have been reported in clinical trials.
Purpose of current immunotherapy is to change the immunogenicity of tumor cells, turn “cold” tumors into “hot” tumors, make more prominent T cells and cytotoxic T cells infiltrate around the tumors, reduce the density and number of corresponding immunosuppressive cells, and further increase the density of TILs and the concentration of chemokines in the tumor microenvironment. The results of such treatment strategies have also been well repeated under the treatment of many chemotherapeutic drugs (89). Some studies have shown that the potential mechanism of the synergistic effect of chemotherapeutic drugs and immune checkpoint inhibitors may include the following: (1) induced immunogenic tumor cell death, (2) anti-angiogenesis, (3) selective depletion of myeloid immunosuppressive cells, (4) loss of lymphopenia (90). The commonly used chemotherapy regimens for GC include Cisplatin, Oxaliplatin, Adriamycin, 5-Fluorouracil, Paclitaxel and Docetaxel. In-ho Kim et al. reported that PD-L1 positive GC patients owned down-regulated PD-L1 expression level after treated with cisplatin and had a better clinical prognosis (91). 5-FU, the most widely used chemotherapy drug for GC, can increase the expression of PD-L1 in GC cells and reduce bone marrow mesenchymal stem cells, and then weaken the CSC dryness of tumor cell. The latest clinical trial (NCT04065282) results show that as a neoadjuvant chemotherapy regimen, Sintilimab combined with oxaliplatin and capecitabine can significantly improve the postoperative remission rate of patients with advanced resettable GC (92).
However, not all chemotherapy plus immunotherapy will bring better prognosis to patients with GC. A randomized phase III trial demonstrated that patients with GC expressing PD-L1 had a worse prognosis after pembrolizumab combined chemotherapy, which includes cisplatin, fluorouracil and capecitabine (82). Therefore, specific problems need to be analyzed to determine the appropriate synergistic treatment through accurate clinical trials.
Radiotherapy (RT) is used to eliminate tumor cells by ionizing radiation site-specifically. The immune principle of radiotherapy for tumors also includes that radiation can induce immunogenic cell death (ICD), so that tumor antigen can be presented and promote T cells to kill tumor cells (93). However, radiotherapy does not always have an immune promoting effect on tumors. Due to immunosuppressive cytokines, like TGF- β and IL-10, and chemokines, in the process of radiation, tumor cells can also interact with immunosuppressive related cells (94). Here, surprisingly, we found that radiotherapy also had a certain correlation with PD-L1 expression. It has been revealed that after gastrointestinal cancer cells receive fractionated radiotherapy, T cells, referring to CD8 (+) T cells mostly, in the tumor microenvironment, have capacity to secrete IFN-γ to induce the up-regulation of PD-L1 in tumor cells (95). In these reports, most tumor patients with up-regulated PD-L1 after radiotherapy commonly have a worse clinical prognosis. As for the combination of radiotherapy and immunotherapy, in non-small cell lung cancer, the combination of RT and PD-1 inhibitor can significantly inhibit tumor growth and prolong survival (96). These results leave us enlightenment whether it is necessary to combine radiotherapy and PD-1/PD-L1 inhibitors in GC, and whether it may benefit patients.
GC is a typical heterogeneous tumor, whose high expression of HER2+ own similar targeting regimen scheme compared with HER2+ breast cancer. It has been reported that the combination of PD-1 inhibitor and anti-HER2 treatment can induce T cell activation and promote tumor inhibition (97). In several clinical trials of PD-1/PD-L1 inhibitors against HER2+ GC mentioned above, keynote-811, NCT02901301 and NCT02954536 have revealed a clinical result that such combinations can show a certain advantage in inhibiting progress of solid tumors. Currently, the combination of PD-1/PD-L1 inhibitors and small molecule inhibitors has been reported in many literatures and clinical trials, which can bring great benefits to different kinds of cancer patients, including nivolumab, pembrolizumab, PDR001, TSR-042, avelumab, atezolizumab, etc. Although no specific combination of PD-1/PD-L1 immune checkpoint inhibitors and small molecule inhibitors has been reported in GC, we still can learn the relevant details of drug combination in different malignant diseases in other studies (98). Among them, small molecule inhibitors used in combination with anti-PD-1/PD-L1 agents include but are not limited to EGFR inhibitors, vascular endothelial growth factor(VEGF/VEGFR) inhibitors, PI3K and MAPK pathway inhibitors, DNA methyltransferase (DNMT) inhibitors and histone deacetylase (HDAC) inhibitors, indolamine 2,3-dioxygenase 1(IDO1) inhibitors poly ADP ribose polymerase (PARP) inhibitor, Bruton’s tyrosine kinase inhibitor, TGF β Receptor I (TGF-β RI), MDM2 inhibitor, CHK1 inhibitor, etc. Such kind of combination ought to be one sort of great research trend in the future, because the basic research on the relationship between these targets and PD-L1 is in full swing. In addition to the combination of immunotherapy, there are reports on the novel treatment of nano new drugs carrying PD-L1 monoclonal antibody. Cell-uptake experiment further confirmed that NPs combined with PD-L1 monoclonal antibody can improve the uptake of the drug, suggesting the possibility of improving the drug regimen for clinical GC patients (99).
Immunosuppressive molecules like cytotoxic T lymphocyte antigen 4 (CTLA-4) and lymphocyte activation gene 3 (LAG3) are also popular and widely used besides PD-1. In GC, the combination of anti-PD-1 and anti-CTLA4 has been tested in clinical trial that checkmate-032 clinical trial revealed great clinical result of this combination (100). Meanwhile, the combination of anti-PD1 and anti-CTLA4 can significantly inhibit the proliferation, apoptosis, migration, invasion and EMT in MKN45 GC cells (101). Clinically established combination therapies have been approved for the treatment of metastatic melanoma, advanced renal cell carcinoma and metastatic colorectal cancer with MMR/MSI-H distortion, such as CTLA-4 inhibitor ipilimumab and PD-1/PD-L1 inhibitor nivolumab.
The synergy between LAG3 and PD-1 is still in clinical trials, although there is currently no such regimen as a clinical guideline for frontline medication for GC patients. However, the effectiveness and possibility of such combination therapy have been reported in the previous documents. In ovarian cancer (102), lymphoma (102) and melanoma (103), combination of anti-LAG3 and anti-PD1 can lead to significant tumor regression.
Aiming at PD-L1 expressed in tumor cell surface and PD-1 targets expressed in T cells of immune system, Innovent Biologics, Inc. has launched bis-specific combined drug, made solid fundamental research in terms of its pharmacokinetics and metabolomics, and is currently carrying out clinical trials (104). However, the disadvantages of this bispecific antibodies with anti-PD-1 or anti-PD-L1 arms may exist: (1) inaccurate drug targeting and easy to produce off target effect (2) the drug may have the same ADCC toxic effect on T cells induced by natural kill (NK) cells as tumor cells.
In terms of mentioned above dual ICIs treatment scheme, the application of immune checkpoint inhibitors can amplify the immune response, T lymphocytes were over-activated, leading to the immune-related adverse events (irAEs). Gastrointestinal tract, lung, joint and other organs could be injured, such as inflammatory arthritis, synovitis, synovitis and Sjogren’s syndrome, antinuclear antibody positive and other immune diseases would appear. Patients treated with anti-PD-1/PD-L1 were at greater risk of hypothyroidism, hepatotoxicity, and pneumonia.
The current application of PD-L1/PD-1 inhibitors in patients with advanced GC has greatly improved the prognosis and quality of life of patients (105). Moreover, we found that about 12.5% of the patients who received immunotherapy could benefit which based on the data of patients who met the qualification criteria of ICI treatment in 2018, The proportion of patients who benefit from this is less in GC. Therefore, the drug regimen of PD-L1/PD-1 inhibitor is recommended only for some patients with advanced GC with high expression of PD-L1. In these patients, acquired drug resistance also occurs in the later stage of treatment due to different reasons such as tumor heterogeneity, epitope change, PD-L1 blocking and so on (106).
In addition, the use of ICIs and the benefits of patients may also be related to the intestinal microbial environment. Recently, it has been reported that intestinal flora may affect the therapeutic effect of anti PD-1 inhibitors on patients through the level of tumor microenvironment (107), which has been further verified in patients with melanoma (108). Intestinal microbiome analysis showed a significant increase in bifidobacterial species in mice with slow tumor growth and the consequent increased benefit of PD-1 therapy (107, 109). The intestinal diversity of patients who responded to PD-1 treatment significantly increased their microbiota, some of which were relatively rich, such as Clostridium and Fecal bacilli (108). At present, it is not clear which specific composition of intestinal microbiota is most conducive to promoting anti-tumor immune response or promoting immunotherapy where the research on intestinal microbial ecology and tumor microenvironment is in full swing.
Immunotherapy such as anti-PD-1/PD-L1 further leads to the rapid progression of some tumors, which is called hyper progressive disease (HPD). The incidence rate of HPD in AGC is not clear, but the risk is certain. It was previously reported that when nivolumab was administered to AGC patients, liver metastasis and size of tumor at baseline were significantly associated with HPD. Some researchers explained that it may be necessary to further replace the concept of HPD with super HPD (naturally rapid growth of tumor after treatment) (110). At the same time, a separate case reported the occurrence of HPD in patients with advanced GC treated with nivolumab (111). However, it has been reported that there is no statistical correlation between PD-L1 expression in GC and hyper-progressive diseases (112).
In addition to the corresponding side effects, it has also been reported that new nanoparticles composed of platinum loaded complexes can produce positive synergistic effects in a large dose range, thereby achieving higher immunotherapeutic effects and lower side effects (113). MSI-H/dMMR phenotype, high tumor mutational burden and EBV etiopathogenesis appear to be more reliable indicators of tumor immunogenicity and sensitivity to PD-1L/PD-1 inhibitors. Indeed, PD-L1/PD-1 inhibition can substantially improve the outcome of these kinds of GC patients, the problem to be solved is to better identify the patients and the corresponding inclusion criteria for PD-L1/PD-1 therapy, while further clinical trials are needed to explore such specific biomarkers (114).
The immunogenicity of gastric cancer varies through molecular types. We classified it by different molecular types and corresponding clinical trial results, and listed several clinical trials of PD-L1 inhibitors and corresponding prognosis results. We believe that in the current clinical guidelines, patients with relatively high immunogenicity of gastric cancer types can be treated with PD-L1 inhibitors to achieve better efficacy under the premise of excluding some cases of acute side effects. With the exploration of various basic articles, the regulatory mechanism of PD-L1 cells has gradually been recognized more clearly. We provide the latest mechanistic regulatory pathway, which is described as PD-L1 can be regulated by various star signals inside and outside the cell, including IFN-r and some miRNAs. These regulation pathways are reflected in transcriptional, translational, and epigenetic levels, which provides a good thinking for the future development of clinical drugs.
First choice for operable GC patients should be surgery. At present, for patients with advanced GC, our treatment guidelines have clearly adopted the use of ICIs. In the case of combined chemotherapy and other drugs, obvious benefits have been brought to patients with AGC. On this basis, anti-PD-L1/anti-PD-1 treatment for patients with GC has been significantly beneficial in combination, and its application scope is not limited in the phase III guidelines mentioned above. There are many aspects to be considered such as irAEs and super progression events in the use of anti-PD-L1/anti-PD-1 regimes as systemic immunotherapy for GC patients, but it still has been highly valued, which may improve the survival time and quality of life of patients to varying degrees.
YZ contributed to the Conceptualization, Writing – original draft. YY, YC, WL, XC, JL, and YH contributed to the Writing – review & editing. HW and LT contributed to the Supervision, Validation, Writing – review & editing. All authors contributed to the article and approved the submitted version.
This work was supported by Science and Technology Program of Zhejiang Province [2021c03119] and Natural Science Foundation of Zhejiang Province [LQ18H160012].
Figures created with BioRender.com.
The authors declare that the research was conducted in the absence of any commercial or financial relationships that could be construed as a potential conflict of interest.
All claims expressed in this article are solely those of the authors and do not necessarily represent those of their affiliated organizations, or those of the publisher, the editors and the reviewers. Any product that may be evaluated in this article, or claim that may be made by its manufacturer, is not guaranteed or endorsed by the publisher.
1. Sung H, Ferlay J, Siegel RL, Laversanne M, Soerjomataram I, Jemal A, et al. Global cancer statistics 2020: GLOBOCAN estimates of incidence and mortality worldwide for 36 cancers in 185 countries. CA Cancer J Clin (2021) 71:209–49. doi: 10.3322/caac.21660
2. Bray F, Ferlay J, Soerjomataram I, Siegel R. L, Torre L. A., Jemal A. Global cancer statistics 2018: GLOBOCAN estimates of incidence and mortality worldwide for 36 cancers in 185 countries. CA Cancer J Clin (2018) 68:394–424. doi: 10.3322/caac.21492
3. Comprehensive molecular characterization of gastric adenocarcinoma. Nature (2014) 513:202–9. doi: 10.1038/nature13480
4. Smyth EC. Chemotherapy for resectable microsatellite instability-high gastric cancer? Lancet Oncol (2020) 21:204. doi: 10.1016/s1470-2045(20)30025-5
5. Fuchs CS, Doi T, Jang RW, Muro K, Satoh T, Machado M, et al. Safety and efficacy of pembrolizumab monotherapy in patients with previously treated advanced gastric and gastroesophageal junction cancer: Phase 2 clinical KEYNOTE-059 trial. JAMA Oncol (2018) 4:e180013. doi: 10.1001/jamaoncol.2018.0013
6. Smyth EC, Nilsson M, Grabsch HI, van Grieken NC, Lordick F. Gastric cancer. Lancet (2020) 396:635–48. doi: 10.1016/s0140-6736(20)31288-5
7. Bang YJ, Kang YK, Catenacci DV, Muro K, Fuchs CS, Geva R, et al. Pembrolizumab alone or in combination with chemotherapy as first-line therapy for patients with advanced gastric or gastroesophageal junction adenocarcinoma: results from the phase II nonrandomized KEYNOTE-059 study. Gastric Cancer (2019) 22:828–37. doi: 10.1007/s10120-018-00909-5
8. Zhang D, He W, Wu C, Tan Y, He Y, Xu B, et al. Scoring system for tumor-infiltrating lymphocytes and its prognostic value for gastric cancer. Front Immunol (2019) 10:71. doi: 10.3389/fimmu.2019.00071
9. Kang BW, Seo AN, Yoon S, Bae HI, Jeon SW, Kwon OK, et al. Prognostic value of tumor-infiltrating lymphocytes in Epstein-Barr virus-associated gastric cancer. Ann Oncol (2016) 27:494–501. doi: 10.1093/annonc/mdv610
11. Antonia SJ, Villegas A, Daniel D, Vicente D, Murakami S, Hui R, et al. Durvalumab after chemoradiotherapy in stage III non-Small-Cell lung cancer. N Engl J Med (2017) 377:1919–29. doi: 10.1056/NEJMoa1709937
12. Weber J, Mandala M, Del Vecchio M, Gogas HJ, Arance AM, Cowey CL, et al. Adjuvant nivolumab versus ipilimumab in resected stage III or IV melanoma. N Engl J Med (2017) 377:1824–35. doi: 10.1056/NEJMoa1709030
13. Sun C, Mezzadra R, Schumacher TN. Regulation and function of the PD-L1 checkpoint. Immunity (2018) 48:434–52. doi: 10.1016/j.immuni.2018.03.014
14. Chong LC, Twa DD, Mottok A, Ben-Neriah S, Woolcock BW, Zhao Y, et al. Comprehensive characterization of programmed death ligand structural rearrangements in b-cell non-Hodgkin lymphomas. Blood (2016) 128:1206–13. doi: 10.1182/blood-2015-11-683003
15. Budczies J, Bockmayr M, Denkert C, Klauschen F, Gröschel S, Darb-Esfahani S, et al. Pan-cancer analysis of copy number changes in programmed death-ligand 1 (PD-L1, CD274) - associations with gene expression, mutational load, and survival. Genes Chromosomes Cancer (2016) 55:626–39. doi: 10.1002/gcc.22365
16. Kataoka K, Shiraishi Y, Takeda Y, Sakata S, Matsumoto M, Nagano S, et al. Aberrant PD-L1 expression through 3'-UTR disruption in multiple cancers. Nature (2016) 534:402–6. doi: 10.1038/nature18294
17. Wang W, Sun J, Li F, Li R, Gu Y, Liu C, et al. A frequent somatic mutation in CD274 3'-UTR leads to protein over-expression in gastric cancer by disrupting miR-570 binding. Hum Mutat (2012) 33:480–4. doi: 10.1002/humu.22014
18. Parsa AT, Waldron JS, Panner A, Crane CA, Parney IF, Barry JJ, et al. Loss of tumor suppressor PTEN function increases B7-H1 expression and immunoresistance in glioma. Nat Med (2007) 13:84–8. doi: 10.1038/nm1517
19. Xu C, Fillmore CM, Koyama S, Wu H, Zhao Y, Chen Z, et al. Loss of Lkb1 and pten leads to lung squamous cell carcinoma with elevated PD-L1 expression. Cancer Cell (2014) 25:590–604. doi: 10.1016/j.ccr.2014.03.033
20. Lv J, Guo T, Qu X, Che X, Li C, Wang S, et al. PD-L1 under regulation of miR-429 influences the sensitivity of gastric cancer cells to TRAIL by binding of EGFR. Front Oncol (2020) 10:1067. doi: 10.3389/fonc.2020.01067
21. Saeed A, Park R, Sun W. The integration of immune checkpoint inhibitors with VEGF targeted agents in advanced gastric and gastroesophageal adenocarcinoma: a review on the rationale and results of early phase trials. J Hematol Oncol (2021) 14:13. doi: 10.1186/s13045-021-01034-0
22. Li P, Luo X, Xie Y, Li P, Hu F, Chu J, et al. GC-derived EVs enriched with MicroRNA-675-3p contribute to the MAPK/PD-L1-Mediated tumor immune escape by targeting CXXC4. Mol Ther Nucleic Acids (2020) 22:615–26. doi: 10.1016/j.omtn.2020.08.020
23. Kim YB, Ahn JM, Bae WJ, Sung CO, Lee D. Functional loss of ARID1A is tightly associated with high PD-L1 expression in gastric cancer. Int J Cancer (2019) 145:916–26. doi: 10.1002/ijc.32140
24. Chakrabarti J, Holokai L, Syu L, Steele NG, Chang J, Wang J, et al. Hedgehog signaling induces PD-L1 expression and tumor cell proliferation in gastric cancer. Oncotarget (2018) 9:37439–57. doi: 10.18632/oncotarget.26473
25. Koh V, Chakrabarti J, Torvund M, Steele N, Hawkins JA, Ito Y, et al. Hedgehog transcriptional effector GLI mediates mTOR-induced PD-L1 expression in gastric cancer organoids. Cancer Lett (2021) 518:59–71. doi: 10.1016/j.canlet.2021.06.007
26. Yoon CJ, Chang MS, Kim DH, Kim W, Koo BK, Yun SC, et al. Epstein-Barr Virus-encoded miR-BART5-5p upregulates PD-L1 through PIAS3/pSTAT3 modulation, worsening clinical outcomes of PD-L1-positive gastric carcinomas. Gastric Cancer (2020) 23:780–95. doi: 10.1007/s10120-020-01059-3
27. Miliotis C, Slack FJ. miR-105-5p regulates PD-L1 expression and tumor immunogenicity in gastric cancer. Cancer Lett (2021) 518:115–26. doi: 10.1016/j.canlet.2021.05.037
28. Guo T, Wang W, Ji Y, Zhang M, Xu G, Lin S. LncRNA PROX1-AS1 facilitates gastric cancer progression via miR-877-5p/PD-L1 axis. Cancer Manag Res (2021) 13:2669–80. doi: 10.2147/cmar.S275352
29. Guo J, Qu H, Shan T, Chen Y, Chen Y, Xia J, et al. Tristetraprolin overexpression in gastric cancer cells suppresses PD-L1 expression and inhibits tumor progression by enhancing antitumor immunity. Mol Cells (2018) 41:653–64. doi: 10.14348/molcells.2018.0040
30. Amini M, Hejazi M, Ghorban K, Mokhtarzadeh A, Baradaran B. Identification of functional methylated CpG loci in PD-L1 promoter as the novel epigenetic biomarkers for primary gastric cancer. Gene (2021) 772:145376. doi: 10.1016/j.gene.2020.145376
31. Lu X, Li Y, Yang W, Tao M, Dai Y, Xu J, et al. Inhibition of NF-κB is required for oleanolic acid to downregulate PD-L1 by promoting DNA demethylation in gastric cancer cells. J Biochem Mol Toxicol (2021) 35:e22621. doi: 10.1002/jbt.22621
32. Chen X, Pan X, Zhang W, Guo H, Cheng S, He Q, et al. Epigenetic strategies synergize with PD-L1/PD-1 targeted cancer immunotherapies to enhance antitumor responses. Acta Pharm Sin B (2020) 10:723–33. doi: 10.1016/j.apsb.2019.09.006
33. Wang J, Xiu J, Baca Y, Battaglin F, Arai H, Kawanishi N, et al. Large-Scale analysis of KMT2 mutations defines a distinctive molecular subset with treatment implication in gastric cancer. Oncogene (2021) 40:4894–905. doi: 10.1038/s41388-021-01840-3
34. Wang Z, Kang W, Li O, Qi F, Wang J, You Y, et al. Abrogation of USP7 is an alternative strategy to downregulate PD-L1 and sensitize gastric cancer cells to T cells killing. Acta Pharm Sin B (2021) 11:694–707. doi: 10.1016/j.apsb.2020.11.005
35. Wang TT, Zhao YL, Peng LS, Chen N, Chen W, Lv YP, et al. Tumour-activated neutrophils in gastric cancer foster immune suppression and disease progression through GM-CSF-PD-L1 pathway. Gut (2017) 66:1900–11. doi: 10.1136/gutjnl-2016-313075
36. Lin C, He H, Liu H, Li R, Chen Y, Qi Y, et al. Tumour-associated macrophages-derived CXCL8 determines immune evasion through autonomous PD-L1 expression in gastric cancer. Gut (2019) 68:1764–73. doi: 10.1136/gutjnl-2018-316324
37. Lv Y, Zhao Y, Wang X, Chen N, Mao F, Teng Y, et al. Increased intratumoral mast cells foster immune suppression and gastric cancer progression through TNF-α-PD-L1 pathway. J Immunother Cancer (2019) 7:54. doi: 10.1186/s40425-019-0530-3
38. Yan XL, Luo QY, Zhou SN, Pan WT, Zhang L, Yang DJ, et al. MicroRNA-375 reverses the expression of PD-L1 by inactivating the JAK2/STAT3 signaling pathways in gastric cancer. Clin Res Hepatol Gastroenterol (2021) 45:101574. doi: 10.1016/j.clinre.2020.10.015
39. Dong H, Strome SE, Salomao DR, Tamura H, Hirano F, Flies DB, et al. Tumor-associated B7-H1 promotes T-cell apoptosis: a potential mechanism of immune evasion. Nat Med (2002) 8:793–800. doi: 10.1038/nm730
40. Garcia-Diaz A, Shin DS, Moreno BH, Saco J, Escuin-Ordinas H, Rodriguez GA, et al. Interferon receptor signaling pathways regulating PD-L1 and PD-L2 expression. Cell Rep (2017) 19:1189–201. doi: 10.1016/j.celrep.2017.04.031
41. Green MR, Monti S, Rodig SJ, Juszczynski P, Currie T, O'Donnell E, et al. Integrative analysis reveals selective 9p24.1 amplification, increased PD-1 ligand expression, and further induction via JAK2 in nodular sclerosing Hodgkin lymphoma and primary mediastinal large b-cell lymphoma. Blood (2010) 116:3268–77. doi: 10.1182/blood-2010-05-282780
42. Prestipino A, Emhardt AJ, Aumann K, O'Sullivan D, Gorantla SP, Duquesne S, et al. Oncogenic JAK2(V617F) causes PD-L1 expression, mediating immune escape in myeloproliferative neoplasms. Sci Transl Med (2018) 10. doi: 10.1126/scitranslmed.aam7729
43. Sato H, Niimi A, Yasuhara T, Permata TBM, Hagiwara Y, Isono M, et al. DNA Double-strand break repair pathway regulates PD-L1 expression in cancer cells. Nat Commun (2017) 8:1751. doi: 10.1038/s41467-017-01883-9
44. Ju X, Zhang H, Zhou Z, Chen M, Wang Q. Tumor-associated macrophages induce PD-L1 expression in gastric cancer cells through IL-6 and TNF-ɑ signaling. Exp Cell Res (2020) 396:112315. doi: 10.1016/j.yexcr.2020.112315
45. Mimura K, Teh JL, Okayama H, Shiraishi K, Kua LF, Koh V, et al. PD-L1 expression is mainly regulated by interferon gamma associated with JAK-STAT pathway in gastric cancer. Cancer Sci (2018) 109:43–53. doi: 10.1111/cas.13424
46. Imai Y, Chiba T, Kondo T, Kanzaki H, Kanayama K, Ao J, et al. Interferon-γ induced PD-L1 expression and soluble PD-L1 production in gastric cancer. Oncol Lett (2020) 20:2161–8. doi: 10.3892/ol.2020.11757
47. Kim W, Chu TH, Nienhüser H, Jiang Z, Del Portillo A, Remotti HE, et al. PD-1 signaling promotes tumor-infiltrating myeloid-derived suppressor cells and gastric tumorigenesis in mice. Gastroenterology (2021) 160:781–96. doi: 10.1053/j.gastro.2020.10.036
48. Wang X, Wu WKK, Gao J, Li Z, Dong B, Lin X, et al. Autophagy inhibition enhances PD-L1 expression in gastric cancer. J Exp Clin Cancer Res (2019) 38:140. doi: 10.1186/s13046-019-1148-5
49. Van Der Kraak L, Goel G, Ramanan K, Kaltenmeier C, Zhang L, Normolle DP, et al. 5-fluorouracil upregulates cell surface B7-H1 (PD-L1) expression in gastrointestinal cancers. J Immunother Cancer (2016) 4:65. doi: 10.1186/s40425-016-0163-8
50. Zhang M, Fan Y, Che X, Hou K, Zhang C, Li C, et al. 5-FU-Induced upregulation of exosomal PD-L1 causes immunosuppression in advanced gastric cancer patients. Front Oncol (2020) 10:492. doi: 10.3389/fonc.2020.00492
51. Nakano H, Saito M, Nakajima S, Saito K, Nakayama Y, Kase K, et al. PD-L1 overexpression in EBV-positive gastric cancer is caused by unique genomic or epigenomic mechanisms. Sci Rep (2021) 11:1982. doi: 10.1038/s41598-021-81667-w
52. Shinozaki-Ushiku A, Kunita A, Fukayama M. Update on Epstein-Barr virus and gastric cancer (review). Int J Oncol (2015) 46:1421–34. doi: 10.3892/ijo.2015.2856
53. Casey SC, Tong L, Li Y, Do R, Walz S, Fitzgerald KN, et al. MYC regulates the antitumor immune response through CD47 and PD-L1. Science (2016) 352:227–31. doi: 10.1126/science.aac9935
54. Kim H, An JY, Noh SH, Shin SK, Lee YC, Kim H, et al. High microsatellite instability predicts good prognosis in intestinal-type gastric cancers. J Gastroenterol Hepatol (2011) 26:585–92. doi: 10.1111/j.1440-1746.2010.06487.x
55. Fang WL, Chang SC, Lan YT, Huang KH, Chen JH, Lo SS, et al. Microsatellite instability is associated with a better prognosis for gastric cancer patients after curative surgery. World J Surg (2012) 36:2131–8. doi: 10.1007/s00268-012-1652-7
56. Beghelli S, de Manzoni G, Barbi S, Tomezzoli A, Roviello F, Di Gregorio C, et al. Microsatellite instability in gastric cancer is associated with better prognosis in only stage II cancers. Surgery (2006) 139:347–56. doi: 10.1016/j.surg.2005.08.021
57. Kim SY, Choi YY, An JY, Shin HB, Jo A, Choi H, et al. The benefit of microsatellite instability is attenuated by chemotherapy in stage II and stage III gastric cancer: Results from a large cohort with subgroup analyses. Int J Cancer (2015) 137:819–25. doi: 10.1002/ijc.29449
58. An JY, Kim H, Cheong JH, Hyung WJ, Kim H, Noh SH, et al. Microsatellite instability in sporadic gastric cancer: its prognostic role and guidance for 5-FU based chemotherapy after R0 resection. Int J Cancer (2012) 131:505–11. doi: 10.1002/ijc.26399
59. Kim JH, Park HE, Cho NY, Lee HS, Kang GH. Characterisation of PD-L1-positive subsets of microsatellite-unstable colorectal cancers. Br J Cancer (2016) 115:490–6. doi: 10.1038/bjc.2016.211
60. Park JW, Um H, Yang H, Ko W, Kim DY, Kim HK, et al. Proteogenomic analysis of NCC-S1M, a gastric cancer stem cell-like cell line that responds to anti-PD-1. Biochem Biophys Res Commun (2017) 484:631–5. doi: 10.1016/j.bbrc.2017.01.153
61. Sun L, Wang Q, Chen B, Zhao Y, Shen B, Wang H, et al. Gastric cancer mesenchymal stem cells derived IL-8 induces PD-L1 expression in gastric cancer cells via STAT3/mTOR-c-Myc signal axis. Cell Death Dis (2018) 9:928. doi: 10.1038/s41419-018-0988-9
62. Sun L, Huang C, Zhu M, Guo S, Gao Q, Wang Q, et al. Gastric cancer mesenchymal stem cells regulate PD-L1-CTCF enhancing cancer stem cell-like properties and tumorigenesis. Theranostics (2020) 10:11950–62. doi: 10.7150/thno.49717
63. Fan Y, Che X, Hou K, Zhang M, Wen T, Qu X, et al. MiR-940 promotes the proliferation and migration of gastric cancer cells through up-regulation of programmed death ligand-1 expression. Exp Cell Res (2018) 373:180–7. doi: 10.1016/j.yexcr.2018.10.011
64. Xu D, Li J, Li RY, Lan T, Xiao C, Gong P, et al. PD-L1 expression is regulated by NF-κB during EMT signaling in gastric carcinoma. Onco Targets Ther (2019) 12:10099–105. doi: 10.2147/ott.S224053
65. Chen K, Cheng G, Zhang F, Zhang N, Li D, Jin J, et al. Prognostic significance of programmed death-1 and programmed death-ligand 1 expression in patients with esophageal squamous cell carcinoma. Oncotarget (2016) 7:30772–80. doi: 10.18632/oncotarget.8956
66. Fu L, Fan J, Maity S, McFadden G, Shi Y, Kong W, et al. PD-L1 interacts with frizzled 6 to activate β-catenin and form a positive feedback loop to promote cancer stem cell expansion. Oncogene (2022) 41:1100–13. doi: 10.1038/s41388-021-02144-2
67. Ishida Y, Agata Y, Shibahara K, Honjo T. Induced expression of PD-1, a novel member of the immunoglobulin gene superfamily, upon programmed cell death. EMBO J (1992) 11:3887–95. doi: 10.1002/j.1460-2075.1992.tb05481.x
68. Iwai Y, Ishida M, Tanaka Y, Okazaki T, Honjo T, Minato N, et al. Involvement of PD-L1 on tumor cells in the escape from host immune system and tumor immunotherapy by PD-L1 blockade. Proc Natl Acad Sci U.S.A. (2002) 99:12293–7. doi: 10.1073/pnas.192461099
69. Chiu YM, Tsai CL, Kao JT, Hsieh CT, Shieh DC, Lee YJ, et al. PD-1 and PD-L1 up-regulation promotes T-cell apoptosis in gastric adenocarcinoma. Anticancer Res (2018) 38:2069–78. doi: 10.21873/anticanres.12446
70. Booth L, Roberts JL, West C, Von Hoff D, Dent P. GZ17-6.02 initiates DNA damage causing autophagosome-dependent HDAC degradation resulting in enhanced anti-PD1 checkpoint inhibitory antibody efficacy. J Cell Physiol (2020) 235:8098–113. doi: 10.1002/jcp.29464
71. Reck M, Rodríguez-Abreu D, Robinson AG, Hui R, Csőszi T, Fülöp A, et al. Five-year outcomes with pembrolizumab versus chemotherapy for metastatic non-Small-Cell lung cancer with PD-L1 tumor proportion score ≥ 50. J Clin Oncol (2021) 39:2339–49. doi: 10.1200/jco.21.00174
72. Li J, Chen L, Xiong Y, Zheng X, Xie Q, Zhou Q, et al. Knockdown of PD-L1 in human gastric cancer cells inhibits tumor progression and improves the cytotoxic sensitivity to CIK therapy. Cell Physiol Biochem (2017) 41:907–20. doi: 10.1159/000460504
73. Wu L, Cai S, Deng Y, Zhang Z, Zhou X, Su Y, et al. PD-1/PD-L1 enhanced cisplatin resistance in gastric cancer through PI3K/AKT mediated p-gp expression. Int Immunopharmacol (2021) 94:107443. doi: 10.1016/j.intimp.2021.107443
74. Li C, Qiu J, Xue Y. Low-dose diosbulbin-b (DB) activates tumor-intrinsic PD-L1/NLRP3 signaling pathway mediated pyroptotic cell death to increase cisplatin-sensitivity in gastric cancer (GC). Cell Biosci (2021) 11:38. doi: 10.1186/s13578-021-00548-x
75. Kim R, An M, Lee H, Mehta A, Heo YJ, Kim KM, et al. Early tumor-immune microenvironmental remodeling and response to first-line fluoropyrimidine and platinum chemotherapy in advanced gastric cancer. Cancer Discovery (2022) 12:984–1001. doi: 10.1158/2159-8290.Cd-21-0888
76. Janjigian YY, Shitara K, Moehler M, Garrido M, Salman P, Shen L, et al. First-line nivolumab plus chemotherapy versus chemotherapy alone for advanced gastric, gastro-oesophageal junction, and oesophageal adenocarcinoma (CheckMate 649): a randomised, open-label, phase 3 trial. Lancet (2021) 398:27–40. doi: 10.1016/s0140-6736(21)00797-2
77. Joshi SS, Badgwell BD. Current treatment and recent progress in gastric cancer. CA Cancer J Clin (2021) 71:264–79. doi: 10.3322/caac.21657
78. Chiaravalli AM, Feltri M, Bertolini V, Bagnoli E, Furlan D, Cerutti R, et al. Intratumour T cells, their activation status and survival in gastric carcinomas characterised for microsatellite instability and Epstein-Barr virus infection. Virchows Arch (2006) 448:344–53. doi: 10.1007/s00428-005-0066-4
79. Grogg KL, Lohse CM, Pankratz VS, Halling KC, Smyrk TC. Lymphocyte-rich gastric cancer: associations with Epstein-Barr virus, microsatellite instability, histology, and survival. Mod Pathol (2003) 16:641–51. doi: 10.1097/01.Mp.0000076980.73826.C0
80. Kim KJ, Lee KS, Cho HJ, Kim YH, Yang HK, Kim WH, et al. Prognostic implications of tumor-infiltrating FoxP3+ regulatory T cells and CD8+ cytotoxic T cells in microsatellite-unstable gastric cancers. Hum Pathol (2014) 45:285–93. doi: 10.1016/j.humpath.2013.09.004
81. Kang YK, Chen LT, Ryu MH, Oh DY, Oh SC, Chung HC, et al. Nivolumab plus chemotherapy versus placebo plus chemotherapy in patients with HER2-negative, untreated, unresectable advanced or recurrent gastric or gastro-oesophageal junction cancer (ATTRACTION-4): a randomised, multicentre, double-blind, placebo-controlled, phase 3 trial. Lancet Oncol (2022) 23:234–47. doi: 10.1016/s1470-2045(21)00692-6
82. Shitara K, Van Cutsem E, Bang YJ, Fuchs C, Wyrwicz L, Lee KW, et al. Efficacy and safety of pembrolizumab or pembrolizumab plus chemotherapy vs chemotherapy alone for patients with first-line, advanced gastric cancer: The KEYNOTE-062 phase 3 randomized clinical trial. JAMA Oncol (2020) 6:1571–80. doi: 10.1001/jamaoncol.2020.3370
83. Shitara K, Bang YJ, Iwasa S, Sugimoto N, Ryu MH, Sakai D, et al. Trastuzumab deruxtecan in previously treated HER2-positive gastric cancer. N Engl J Med (2020) 382:2419–30. doi: 10.1056/NEJMoa2004413
84. Sharma P, Siddiqui BA, Anandhan S, Yadav SS, Subudhi SK, Gao J, et al. The next decade of immune checkpoint therapy. Cancer Discovery (2021) 11:838–57. doi: 10.1158/2159-8290.Cd-20-1680
85. Chen DS, Mellman I. Oncology meets immunology: the cancer-immunity cycle. Immunity (2013) 39:1–10. doi: 10.1016/j.immuni.2013.07.012
86. Lu L, Jiang J, Zhan M, Zhang H, Wang QT, Sun SN, et al. Targeting tumor-associated antigens in hepatocellular carcinoma for immunotherapy: Past pitfalls and future strategies. Hepatology (2021) 73:821–32. doi: 10.1002/hep.31502
87. Yap TA, Parkes EE, Peng W, Moyers JT, Curran MA, Tawbi HA, et al. Development of immunotherapy combination strategies in cancer. Cancer Discovery (2021) 11:1368–97. doi: 10.1158/2159-8290.Cd-20-1209
88. Meric-Bernstam F, Larkin J, Tabernero J, Bonini C. Enhancing anti-tumour efficacy with immunotherapy combinations. Lancet (2021) 397:1010–22. doi: 10.1016/s0140-6736(20)32598-8
89. Cristescu R, Mogg R, Ayers M, Albright A, Murphy E, Yearley J, et al. Pan-tumor genomic biomarkers for PD-1 checkpoint blockade-based immunotherapy. Science (2018) 362. doi: 10.1126/science.aar3593
90. Salas-Benito D, érez-Gracia JL, Ponz-Sarvisé M, Rodriguez-Ruiz ME, Martínez-Forero I, Castañón E, et al. Paradigms on immunotherapy combinations with chemotherapy. Cancer Discovery (2021) 11:1353–67. doi: 10.1158/2159-8290.Cd-20-1312
91. Yang JH, Kim H, Roh SY, Lee MA, Park JM, Lee HH, et al. Discordancy and changes in the pattern of programmed death ligand 1 expression before and after platinum-based chemotherapy in metastatic gastric cancer. Gastric Cancer (2019) 22:147–54. doi: 10.1007/s10120-018-0842-x
92. Jiang H, Yu X, Li N, Kong M, Ma Z, Zhou D, et al. Efficacy and safety of neoadjuvant sintilimab, oxaliplatin and capecitabine in patients with locally advanced, resectable gastric or gastroesophageal junction adenocarcinoma: early results of a phase 2 study. J Immunother Cancer (2022) 10. doi: 10.1136/jitc-2021-003635
93. Golden EB, Frances D, Pellicciotta I, Demaria S, Helen Barcellos-Hoff M, Formenti SC, et al. Radiation fosters dose-dependent and chemotherapy-induced immunogenic cell death. Oncoimmunology (2014) 3:e28518. doi: 10.4161/onci.28518
94. Shevtsov M, Sato H, Multhoff G, Shibata A. Novel approaches to improve the efficacy of immuno-radiotherapy. Front Oncol (2019) 9:156. doi: 10.3389/fonc.2019.00156
95. Dovedi SJ, Adlard AL, Lipowska-Bhalla G, McKenna C, Jones S, Cheadle EJ, et al. Acquired resistance to fractionated radiotherapy can be overcome by concurrent PD-L1 blockade. Cancer Res (2014) 74:5458–68. doi: 10.1158/0008-5472.Can-14-1258
96. Herter-Sprie GS, Koyama S, Korideck H, Hai J, Deng J, Li YY, et al. Synergy of radiotherapy and PD-1 blockade in kras-mutant lung cancer. JCI Insight (2016) 1:e87415. doi: 10.1172/jci.insight.87415
97. Taylor C, Hershman D, Shah N, Suciu-Foca N, Petrylak DP, Taub R, et al. Augmented HER-2 specific immunity during treatment with trastuzumab and chemotherapy. Clin Cancer Res (2007) 13:5133–43. doi: 10.1158/1078-0432.Ccr-07-0507
98. Wang M, Liu Y, Cheng Y, Wei Y, Wei X. Immune checkpoint blockade and its combination therapy with small-molecule inhibitors for cancer treatment. Biochim Biophys Acta Rev Cancer (2019) 1871:199–224. doi: 10.1016/j.bbcan.2018.12.002
99. Xu S, Cui F, Huang D, Zhang D, Zhu A, Sun X, et al. PD-L1 monoclonal antibody-conjugated nanoparticles enhance drug delivery level and chemotherapy efficacy in gastric cancer cells. Int J Nanomedicine (2019) 14:17–32. doi: 10.2147/ijn.S175340
100. Janjigian YY, Bendell J, Calvo E, Kim JW, Ascierto PA, Sharma P, et al. CheckMate-032 study: Efficacy and safety of nivolumab and nivolumab plus ipilimumab in patients with metastatic esophagogastric cancer. J Clin Oncol (2018) 36:2836–44. doi: 10.1200/jco.2017.76.6212
101. Wang B, Qin L, Ren M, Sun H. Effects of combination of anti-CTLA-4 and anti-PD-1 on gastric cancer cells proliferation, apoptosis and metastasis. Cell Physiol Biochem (2018) 49:260–70. doi: 10.1159/000492876
102. Huang RY, Eppolito C, Lele S, Shrikant P, Matsuzaki J, Odunsi K. LAG3 and PD1 co-inhibitory molecules collaborate to limit CD8+ T cell signaling and dampen antitumor immunity in a murine ovarian cancer model. Oncotarget (2015) 6:27359–77. doi: 10.18632/oncotarget.4751
103. Goding SR, Wilson KA, Xie Y, Harris KM, Baxi A, Akpinarli A, et al. Restoring immune function of tumor-specific CD4+ T cells during recurrence of melanoma. J Immunol (2013) 190:4899–909. doi: 10.4049/jimmunol.1300271
104. Kotanides H, Li Y, Malabunga M, Carpenito C, Eastman SW, Shen Y, et al. Bispecific targeting of PD-1 and PD-L1 enhances T-cell activation and antitumor immunity. Cancer Immunol Res (2020) 8:1300–10. doi: 10.1158/2326-6066.Cir-20-0304
105. Ott PA, Bang YJ, Piha-Paul SA, Razak ARA, Bennouna J, Soria JC, et al. T-Cell-Inflamed gene-expression profile, programmed death ligand 1 expression, and tumor mutational burden predict efficacy in patients treated with pembrolizumab across 20 cancers: KEYNOTE-028. J Clin Oncol (2019) 37:318–27. doi: 10.1200/jco.2018.78.2276
106. Haslam A, Prasad V. Estimation of the percentage of US patients with cancer who are eligible for and respond to checkpoint inhibitor immunotherapy drugs. JAMA Netw Open (2019) 2:e192535. doi: 10.1001/jamanetworkopen.2019.2535
107. Sivan A, Corrales L, Hubert N, Williams JB, Aquino-Michaels K, Earley ZM, et al. Commensal bifidobacterium promotes antitumor immunity and facilitates anti-PD-L1 efficacy. Science (2015) 350:1084–9. doi: 10.1126/science.aac4255
108. Gopalakrishnan V, Spencer CN, Nezi L, Reuben A, Andrews MC, Karpinets TV, et al. Gut microbiome modulates response to anti-PD-1 immunotherapy in melanoma patients. Science (2018) 359:97–103. doi: 10.1126/science.aan4236
109. Vétizou M, Pitt JM, Daillère R, Lepage P, Waldschmitt N, Flament C, et al. Anticancer immunotherapy by CTLA-4 blockade relies on the gut microbiota. Science (2015) 350:1079–84. doi: 10.1126/science.aad1329
110. Kubota Y, Yoshimura K, Hamada K, Hirasawa Y, Shida M, Taniguchi M, et al. Rare nivolumab-associated super hyper progressive disease in patients with advanced gastric cancer. In Vivo (2021) 35:1865–75. doi: 10.21873/invivo.12449
111. Huang LT, Ma JT, Zhang SL, Li XH, Sun L, Jing W, et al. Durable clinical response to pyrotinib after resistance to prior anti-HER2 therapy for HER2-positive advanced gastric cancer: A case report. Front Oncol (2019) 9:1453. doi: 10.3389/fonc.2019.01453
112. Liu J, Wu Q, Wu S, Xie X. Investigation on potential biomarkers of hyperprogressive disease (HPD) triggered by immune checkpoint inhibitors (ICIs). Clin Transl Oncol (2021) 23:1782–93. doi: 10.1007/s12094-021-02579-9
113. Xue Y, Gao S, Gou J, Yin T, He H, Wang Y, et al. Platinum-based chemotherapy in combination with PD-1/PD-L1 inhibitors: preclinical and clinical studies and mechanism of action. Expert Opin Drug Delivery (2021) 18:187–203. doi: 10.1080/17425247.2021.1825376
Keywords: gastric cancer, programmed death-ligand 1, immune checkpoint inhibitor (ICI), clinical trials, tumor microenvironment
Citation: Zhang Y, Yang Y, Chen Y, Lin W, Chen X, Liu J, Huang Y, Wang H and Teng L (2022) PD-L1: Biological mechanism, function, and immunotherapy in gastric cancer. Front. Immunol. 13:1060497. doi: 10.3389/fimmu.2022.1060497
Received: 03 October 2022; Accepted: 09 November 2022;
Published: 24 November 2022.
Edited by:
Shyamasree Ghosh, National Institute of Science Education and Research (NISER), IndiaReviewed by:
Amy De Haar-Holleman, University Hospital Brussels, BelgiumCopyright © 2022 Zhang, Yang, Chen, Lin, Chen, Liu, Huang, Wang and Teng. This is an open-access article distributed under the terms of the Creative Commons Attribution License (CC BY). The use, distribution or reproduction in other forums is permitted, provided the original author(s) and the copyright owner(s) are credited and that the original publication in this journal is cited, in accordance with accepted academic practice. No use, distribution or reproduction is permitted which does not comply with these terms.
*Correspondence: Lisong Teng, bHN0ZW5nQHpqdS5lZHUuY24=; Haiyong Wang, bGFuY2V0ZXIxQHpqdS5lZHUuY24=
Disclaimer: All claims expressed in this article are solely those of the authors and do not necessarily represent those of their affiliated organizations, or those of the publisher, the editors and the reviewers. Any product that may be evaluated in this article or claim that may be made by its manufacturer is not guaranteed or endorsed by the publisher.
Research integrity at Frontiers
Learn more about the work of our research integrity team to safeguard the quality of each article we publish.