- 1Department of Translational Medical Sciences, University of Naples Federico II, Naples, Italy
- 2Department of Oncology and Molecular Medicine, Istituto Superiore di Sanità, Rome, Italy
- 3World Allergy Organization (WAO), Center of Excellence (CoE), Naples, Italy
- 4Center for Basic and Clinical Immunology Research (CISI), University of Naples Federico II, Naples, Italy
- 5Institute of Experimental Endocrinology and Oncology “G. Salvatore”, National Research Council (CNR), Naples, Italy
- 6Department of Medicine, Division of Allergy and Clinical Immunology, Johns Hopkins Asthma and Allergy Center, Johns Hopkins University, Baltimore, MD, United States
Human basophils, first identified over 140 years ago, account for just 0.5-1% of circulating leukocytes. While this scarcity long hampered basophil studies, innovations during the past 30 years, beginning with their isolation and more recently in the development of mouse models, have markedly advanced our understanding of these cells. Although dissimilarities between human and mouse basophils persist, the overall findings highlight the growing importance of these cells in health and disease. Indeed, studies continue to support basophils as key participants in IgE-mediated reactions, where they infiltrate inflammatory lesions, release pro-inflammatory mediators (histamine, leukotriene C4: LTC4) and regulatory cytokines (IL-4, IL-13) central to the pathogenesis of allergic diseases. Studies now report basophils infiltrating various human cancers where they play diverse roles, either promoting or hampering tumorigenesis. Likewise, this activity bears remarkable similarity to the mounting evidence that basophils facilitate wound healing. In fact, both activities appear linked to the capacity of basophils to secrete IL-4/IL-13, with these cytokines polarizing macrophages toward the M2 phenotype. Basophils also secrete several angiogenic factors (vascular endothelial growth factor: VEGF-A, amphiregulin) consistent with these activities. In this review, we feature these newfound properties with the goal of unraveling the increasing importance of basophils in these diverse pathobiological processes.
Introduction
Paul Ehrlich discovered, over 140 years ago, peripheral blood basophils and tissue mast cells using novel hematological techniques that combined the use of alkaline dyes and conventional light microscopy (1, 2). Unlike mast cells, which are only found as mature cells in tissues, basophils represent just 0.5-1% of all leukocytes in the bone marrow and peripheral blood (3, 4). Basophils and mast cells are long recognized as being morphologically similar in appearance and for sharing several unique features (5, 6). For example, they are the only two cells that express the full tetrameric (αβγ2) form of the high-affinity receptor for IgE (FcϵRI). They both also uniquely store histamine in cytoplasmic granules (7), releasing it and other proinflammatory mediators (e.g., cysteinyl leukotrienes) when appropriately activated (5, 8). In fact, these shared characteristics continue to cause misperceptions, leading some to believe that basophils and mast cells are one and the same. However, compelling evidence over the last decades now supports that human basophils possess morphological, immunological, biochemical, and pharmacological characteristics quite different from those of human mast cells (5–7, 9).
Until recently, there was some dispute as to whether mice have basophils. However, the work of Ann M. Dvorak using electron microscopy, clearly identified basophils in mice as a rare population of bone marrow cells, with some ultrastructural characteristics like those observed in human basophils (7, 10, 11). As discussed below, there remains considerable debate as to whether mouse basophils are truly representative of human cells, particularly with regard to function (11–17). Of course, much of this debate often defaults to issues pertaining to the disparities expected with in vitro vs. in vivo experiments (18). Nonetheless, the one newest function perhaps most shared by basophils from both species is their capacity to secrete large quantities of IL-4, even though debate persists about the stimuli most responsible for this response.
Many fundamentals of basophil biology have been extensively reviewed elsewhere, especially regarding their role in allergic diseases (5, 19–24). In this review, we briefly touch on this research field but will additionally focus on the concept of basophils participating in tumorigenesis and wound-healing and how these processes are seemingly linked and driven by the capacity of these cells to secrete IL-4, IL-13, angiogenic factors and pro-fibrotic cytokines.
Basophil development
Basophils originate from stem cell progenitors in the bone marrow (25–27). Both in humans and mice, IL-3 is the most important growth factor for basophil development (12, 17, 28, 29). In fact, basophils from both species can be developed in vitro by simply culturing bone marrow cells (or CD34+ precursors in humans) in the presence of IL-3 for 10-14 days (12, 30–32).
While IL-3 is clearly most important for basophil development from precursors, other growth factors are reported to facilitate expansion/function. For example, the FMS-like tyrosine kinase 3 ligand (Flt3L) has been combined with IL-3 to expand the number of culture-derived basophils (33). Siracusa and co-workers reported that mouse basophils can be generated by thymic stromal lymphopoietin (TSLP) through the engagement of the heterodimeric TSLP receptor (TSLPR/IL-7Rα) (34). These authors demonstrated that IL-3 and TSLP induced the differentiation of two types of murine basophils displaying different gene expression and functions (35). In humans, it has been suggested that about 10% of basophils from asthmatics express the TSLP receptor and release histamine and cytokines in response to TSLP (15). In contrast, more recent studies have shown that human basophils do not express the IL-7Rα subunit of the heterodimeric TSLP receptor (14) and do not respond to in vitro TSLP stimulation (12, 14, 16). By contrast, TSLP induces the release of IL-4, IL-13, CXCL1, and CXCL2 from mouse basophils (12).
Heterogeneity of basophils: In species and tissue versus peripheral blood
Human and mouse basophils express FcεRI (36, 37) and will up-regulate the degranulation markers, CD63 (38–40) and CD203c when activated appropriately (9, 39, 41–43). Basophils from humans and mice express the IL-3 (IL-3Rα/CD123) (34, 44), GM-CSF (CD116) (45, 46), and IL-33 (ST2/IL1RL1) receptors (47–50). The heterodimeric TSLP receptor, TSLPR/IL-7Rα, is expressed by mouse basophils (12, 34), but the presence of this receptor on basophils from allergic donors and healthy subjects remains controversial (12, 14–16). Human basophils reportedly express receptors for IL-5 (CD125) (51) and for Nerve Growth Factor (NGF) (tropomyosin receptor kinase A: TrkA) (52–54). Both human and mouse basophils display a variety of chemokine receptors (5, 55–60). The IgG receptors FcγRIIA, FcγRIIB, and small amounts of FcγRIIIB are expressed by human basophils, whereas mouse basophils express FcγRIIB and FcγRIIIA (61, 62).
Preformed mediators, such as histamine (≃ 1 pg/cell), basogranulin (63, 64) and very low concentrations of tryptase (65) are present in human basophils. Human (66) and mouse basophils release granzyme B (67), that reportedly exerts cytotoxic effects on tumor cells (68, 69). Basophils from both species can synthesize cysteinyl leukotriene C4 (LTC4) through the 5-lipoxygenase pathway (70). Mouse basophils additionally produce prostaglandin D2 (PGD2) and prostaglandin E2 (PGE2) through the cyclooxygenase pathway (71, 72). Human basophils do not synthesize detectable levels of PGD2 or other mediators requiring cyclooxygenase activity (12, 73).
Substantial evidence now shows that human (12, 24, 74–82) and mouse (12, 47, 80) basophils secrete IL-4. Both human (12, 75, 76, 78, 81–85) and mouse basophils (12, 47) also generate and release IL-13, yet the evidence for this response is far more prevalent in the former species. Mouse basophils can release IL-6 (47, 86, 87) and TNF-α (47, 86). Two reports indicate that these cytokines are secreted from human basophils (88, 89), even though they do not appear to be products commonly released by these cells. Human and mouse basophils release granzyme B (66, 67) that exerts a cytotoxic effect on tumor cells.
Human basophils secrete several angiogenic factors such as vascular endothelial growth factor-A (VEGF-A) (64), angiopoietin-1 (ANGPT1) (90), hepatocyte growth factor (HGF) (47, 91), and amphiregulin (AREG) (92–94). Mouse (47) and human basophils (91) express Hgf and release, under certain conditions, AREG (94) and VEGF-A (Gambardella et al., unpublished).
The life-span of circulating basophils is relatively short (≃ 2.5 days in mice) (95) and therefore newly generated basophils are constantly supplied from the bone marrow to the blood (25). Basophils physiologically circulate in peripheral blood and migrate within tissues mainly during certain types of inflammation in mice (86, 95–98) and humans (99–104). Basophils, present during mouse lung development, exhibit a phenotype different from circulating blood basophils (47). In the lung, specific gene signature of lung-resident basophils is modulated by IL-33 and GM-CSF (47). These cells play a prominent role in the development and polarization toward the M2 state of alveolar macrophages, raising the possibility that in tumors associated with M2 macrophages (105–107), basophils contribute the polarization of tumor-associated macrophages.
Basophils derived from murine bone marrow cells are often used as a model system for studies of the immunological functions of these cells (86, 97, 108–111). It should be pointed out that these cells, developed by murine bone marrow cells in the presence of IL-3, have an activated phenotype (82, 112). Recently, Pellefigues et al. carefully demonstrated functional heterogeneity between naïve murine basophils obtained from spleen and bone marrow-derived basophils (108). In humans, functional heterogeneity of peripheral blood basophils has been demonstrated by applying mass cytometry (CyTOF) to simultaneously assess several proteins and functions of basophils (113).
Angiogenic factors released by basophils
Angiogenesis occurs physiologically during embryonic development, pathologically in inflammation and cancer (114, 115). Both cancer and immune cells (116, 117) produce several proangiogenic factors (118, 119). The vascular endothelial growth factor (VEGF) family includes VEGF-A, VEGF-B, VEGF-C, and VEGF-D. VEGFs activate specific receptors (VEGFR1, VEGFR2, and VEGFR3) on blood endothelial cells (BECs). VEGF/VEGFR axis plays pivotal roles in tumor and inflammatory angiogenesis (118). VEGF-A is released by human basophils (64). All members of the VEGF family are chemotactic for human basophils through the engagement of VEGFR2 on their surface (64, 120). Therefore, VEGFs released by cancer cells and immune cells in the tumor microenvironment (TME) (118, 120–123) can favor basophil infiltration in TME.
Angiopoietins (ANGPTs) are other players of inflammatory and tumor angiogenesis (124, 125). ANGPT1, released by perivascular mural cells, binds to the Tie2 receptor on endothelial cells and promotes endothelial stabilization (126). ANGPT2, secreted by activated endothelial cells, induces vascular permeability (127). ANGPT1 and ANGPT2 mRNAs are expressed by human basophils (90), and their activation induces ANGPT1 release. Mouse lung-resident basophils express mRNA for HGF, a potent angiogenic factor (47, 91, 128).
Cysteinyl leukotrienes (cys-LTs) are powerful proinflammatory mediators (129). The cys-LTs include leukotriene C4 (LTC4), the main lipid mediator synthesized by human and mouse basophils (54, 70). ɤ-glutamyl transpeptidases metabolize LTC4 to LTD4 and to LTE4 by the membrane-bound enzymes (129). Cys-LTs are potent agonists of three different receptors (CysLTRs) CysLT1R, CysLT2R, and CysLT3R (130–132). LTC4 and LTD4 induced the formation of angiogenesis (133). The angiogenic properties of LTC4 and LTD4 were mediated in vivo by the activation of CysLT2R on BECs. In mouse models, pharmacologic antagonism of CysLT2R inhibited tumor growth and metastasis formation (133). These results illustrate the relevance of cys-LTs as non-canonical angiogenic factors in cancer. Moreover, these findings suggest that CysLT2R might be a target in cancer (133). LTC4 is released by activated human (70, 134) and mouse (54) basophils and future studies should investigate whether basophil-derived LTC4 might contribute to angiogenesis in human cancer.
Formation of extracellular DNA traps by basophils
Activated neutrophils (135–137), eosinophils (138, 139), mast cells (140–143), macrophages (144–148), and basophils (149, 150) can release extracellular traps (ETs), which are DNA structures decorated with a variety of proteins [e.g., myeloperoxidase and elastase) (151), lactoferrin and pentraxin 3) (151, 152), and matrix metalloproteinase 9) (151)]. ETs released by human neutrophils (neutrophils extracellular traps: NETs) were initially characterized by their antibacterial activity (138, 151, 153, 154). Increasing evidences demonstrate that ETs, particularly NETs, play a role in asthma (137) and in fundamental aspects of tumorigenesis (155). NETs favor the formation of metastasis in mice and in humans (156–159) and awaken dormant cancer cells (160). An increase of NET release occurs when neutrophils from myeloproliferative neoplasms are associated with JAK2V617F mutations and mice with knock-in of JAK2V617F (161). We have provided evidence that anaplastic thyroid cancer cells can induce NET formation (162). Collectively, these findings demonstrate that NETs can promote tumor growth and metastasis formation. Basophils from humans and mouse can release extracellular DNA traps (BETs) in vitro and in vivo (149, 150, 163). The translational relevance of these findings should be explored in experimental models and human cancers.
Basophils in allergic disorders
Basophils play a major role in a variety of allergic disorders (8, 164–166). Anaphylaxis is a rapid-onset, potentially life-threatening allergic reaction caused by the release of vasoactive mediators from mast cells and basophils after allergen exposure (167). Mouse models of anaphylaxis suggest that basophils play a major role in the IgG-, but not IgE-mediated anaphylaxis (168). In these studies, the depletion of basophils by anti-CD200R3 mAb inhibited IgG-mediated anaphylaxis, whereas it had minor effect on IgE-mediated anaphylaxis. By contrast, mast cells are central for IgE-mediated mouse models of anaphylaxis (168, 169).
Several lines of indirect evidence suggest that basophils participate in human anaphylaxis (24). For example, the number of circulating basophils was significantly lower in subjects undergoing anaphylactic reactions compared to healthy controls (170). Peanut-induced allergic reactions also resulted in a significant decrease in circulating basophil counts and an increase in CCL2 levels compared with those in pre-challenge samples.
While there is a plethora of information from murine models regarding the role of basophils in allergic/asthma-like inflammation, the involvement of basophils in human asthma again derives mainly from indirect evidence (164). Most compelling, basophils have been found in the airways of asthmatics (171, 172), in post-mortem cases of fatal asthma (173) and after antigen challenge of airway mucosa (174). Basophil releasability (i.e., the ability of a basophil to release a given percentage of histamine in response to a given immunological stimulus) is long reported to be increased in asthma and more recently subject to circadian changes (175). Moreover, allergen-induced asthmatic responses are accompanied by infiltration of basophils expressing IL-4 mRNA (103). The in vitro secretion of both IL-4 and IL-13 has been shown to track with the basophil-enriched fractions of cells recovered after infiltrating the lung following segmental allergen challenge (176, 177). Moreover, these so-called basophil cytokine responses also correlated with the frequency of eosinophils recovered from the lung. Thus, basophils might represent an important source of Th2-like cytokines (IL-4 and IL-13) in the lung microenvironment, particularly that associated with human allergic disease.
Brooks and collaborators reported that basophils are increased in the sputum of patients with eosinophilic asthma compared to those with non-eosinophilic asthma (178). In asthmatics, basophils were positively correlated with sputum eosinophils and inversely with sputum neutrophils, but not with FEV1, FEV1/FVC or bronchodilator reversibility. Sputum basophils positively correlated with sputum eosinophils (179). In comparison with blood basophils, sputum basophils have a higher expression of activation markers (e.g., CD203c) (179). These findings indicate that basophils may be involved in eosinophilic asthma and that sputum basophil assessment could be a useful additional indicator of “Th2-high” asthma. Basophil counts in peripheral blood during childhood asthma are associated with exacerbations (180). The proportion of degranulated basophils can also be associated with recurrent exacerbations.
Hill et al. reported that omalizumab, a mAb that targets IgE and neutralizes it from binding to FcεRIα on basophils, reduces blood basophil frequencies in asthmatic children (181). Furthermore, treatment of severe asthma patients with benralizumab, a mAb against IL-5Rα, markedly decreased the number of both eosinophils and basophils (182–184). These findings suggest that benralizumab may have a positive effect on severe asthmatics by reducing not only eosinophils but also basophils.
A number of mouse studies indicate that basophils are involved in the development of asthma-like pathology. In an ovalbumin-induced asthma model, basophils recruited to the lungs, amplify the Th2 cell differentiation (185). In a papain-induced asthma model, basophil-derived IL-4 induces the IL-5 and CCL11 expression in ILC2 cells, causing eosinophil infiltration (68). Indeed, in a model of IgE-dependent dermatitis, the production of IL-4 from basophils was shown to directly condition endothelium for increased VCAM-1 expression, which facilitated the in vivo entry of eosinophils into lesion sites (186). This mechanistic observation may help elucidate the eosinophil/basophil IL-4 associations commonly seen in human disease.
Chronic spontaneous urticaria (CSU) is a common skin disease, characterized by spontaneous appearance of wheals, angioedema or both, for more than 6 weeks due to known or unknown causes (187, 188). A role for basophils in the pathophysiology of CSU is suggested by a number of findings (189, 190). CSU subjects have been shown to have significant increases in the numbers of intradermal basophils compared with non-atopic control subjects (191). Basopenia has long been reported in patients with CSU (192) and more recently postulated as the result of basophil migration from the circulation into the skin (104, 191, 193). The degree of basopenia often correlates with disease severity (194) and improves during times of remission (195). CSU subjects exhibit enhanced expression of the activation markers CD63 and CD69 on basophils compared to non-allergic subjects (196).
Rauber et al. identified three distinct immunologic phenotypes of CSU (197). One group of patients’ basophils reacted to FcϵRI stimulation, whereas the others had anti-FcϵRI nonreactive basophils. Among the latter, it was found a subgroup with basopenia. This subgroup had augmented serum-induced basophil activation, increased levels of autoantibodies against thyroid peroxidase, and worse quality of life. These phenotypes were associated with different clinical characteristics, pointing to basophils as important players in CSU pathophysiology (197). Oda et al. demonstrated that basophils from CSU patients had higher FcεRI expression compared to healthy controls. The proportion of CD203chigh basophils after anti-IgE or anti-FcεRI stimulation was lower in CSU patients compared to controls and characteristics of more severe patients (198).
Omalizumab is a mAb anti-IgE often used in treating severe allergic asthma (199, 200). More recently, it has also proved highly effective in patients with CSU (201). Surprisingly, this treatment, regardless of the disease being treated, is associated with increased expression of Syk, which is often also manifested by basophils showing greater histamine release in vitro when undergoing IgE/FcεRIα-dependent stimulation. This enhanced responsiveness is seen even through cell-surface FcεRIα/IgE levels are reduced with this treatment (196, 202). These observations have since prompted the same group of authors to suggest that Syk expression and IgE-mediated histamine release in basophils could function as biomarkers for predicting the clinical efficacy of omalizumab in patients receiving this therapy (203).
In following CSU subjects treated with omalizumab, MacGlashan and collaborators have also identified three basophil phenotypes in CSU patients: 1) subjects with basopenia, 2) normal basophil numbers with normal IgE-mediated histamine release, and 3) normal basophil numbers with poor histamine release. Basopenia was associated with the presence of autoantibodies to unoccupied FcϵRI and basophil numbers did not change during omalizumab treatment. Omalizumab resulted in similar kinetics for decreases in surface FcϵRI and IgE in all three groups of CSU patients (204).
Atopic dermatitis is a common inflammatory skin disorder characterized by chronic eczema and severe itching (205). Th2 cells mediate inflammation in atopic dermatitis with the release of IL-4 and IL-13, which contribute to clinical manifestations (206, 207). Keratinocyte-derived alarmins, such as IL-33, TSLP, and IL-25 (IL-17E) that elicit Th2 cytokines responses by activating group-2 innate lymphoid cells (ILC2s) play an upstream pathogenic role in atopic dermatitis (208, 209). Recent evidence indicates that LTC4 also plays a role in mouse models of atopic dermatitis (210). IgG autoantibodies against IgE from atopic dermatitis can induce the release of IL-4/IL-13 and LTC4 from human basophils (134, 211), indicating that these cells contribute to this allergic disorder.
Early studies reported that up to 80% of food-allergic children exhibit high spontaneous basophil histamine release (212). Moreover, food-allergic children release histamine in response to an IgE-dependent histamine-releasing factor (213). Schroeder and collaborators demonstrated that basophils from food-allergic children also spontaneously release IL-4 and overexpress CD203c (214). Interestingly, spontaneous basophil histamine release and IL-4 secretion decreased in children undergoing sublingual immunotherapy (215). In vitro studies show that this enhanced releasability of histamine and IL-4 from basophils of food-allergic children is transferred to basophils of normal subjects by sensitizing normal cells with plasma from the former group. However, the addition of omalizumab during this passive sensitization completely abated the responses, thus pointing to the involvement of IgE in transferring hyperresponsiveness (214).
Figure 1 schematically illustrates the versatile contribution of basophils and their mediators to the development of allergic disorders.
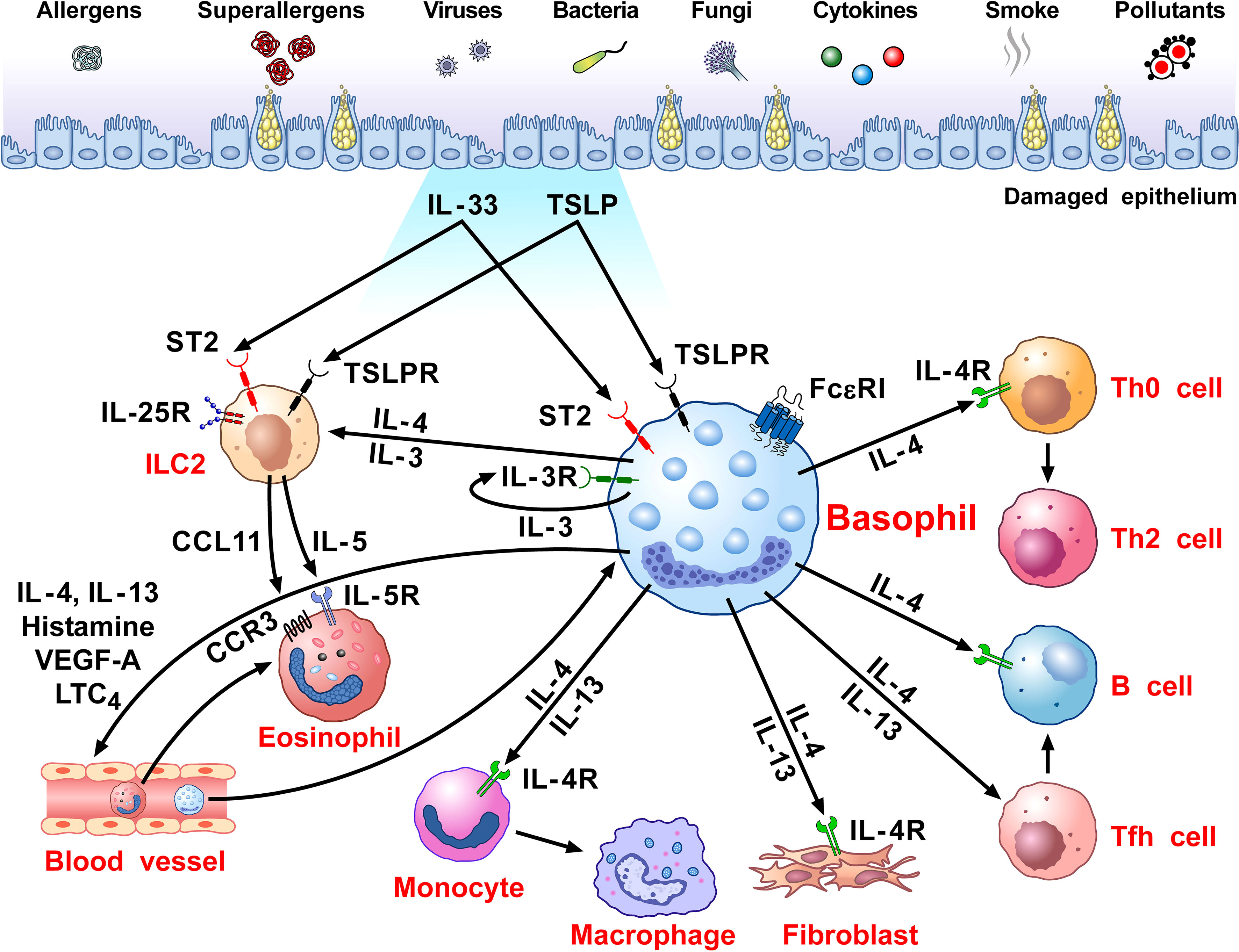
Figure 1 Schematic representation of the versatile role of basophils in the pathobiology of allergic disorders. Several immunological (i.e., allergens, superallergens, viral, bacterial and fungal proteins, cytokines) and non-immunological stimuli (e.g., pollutants, diesel exhaust particles) activate mucosal (i.e., lung and gut) and skin barriers to release different alarmins (i.e., TSLP, IL-33, IL-25) (130, 216, 217). Alarmins activate group 2 innate lymphoid cells (ILC2s) through the engagement of specific receptors (TSLPR, ST2, IL-25R, respectively) (218, 219) to release IL-5 and CCL11 that promote eosinophil infiltration into inflamed tissue (220, 221). Human and mouse basophils express the high-affinity receptor for IgE (FcεRI) (36, 37) and the receptors for IL-3 (IL-3Rα/CD123) (34, 44), GM-CSF (CD116) (45, 46), IL-33 (ST2/IL1RL1) (47–50), IL-5 (CD125) (51) and a variety of chemokine receptors (5, 55–60). The TSLP receptor (TSLPR/IL-7Rα) is expressed by mouse basophils (12, 34), but its presence on basophils from allergic and healthy donors remains controversial (12, 14–16). TSLP activates mouse but not human basophils (12, 17). IL-3 plays a key role in the development, survival and activation of human and mouse basophils (17). IL-3 activates human and mouse basophils to release cytokines and chemokines (12, 17, 46, 222). IgE-FcεRI crosslinking by antigens, superallergens and functional anti-IgE autoantibodies activates basophils to release a wide spectrum of inflammatory and immunomodulatory factors (24, 70, 75, 76, 78, 79, 134, 211, 223). IL-33 activates human and mouse basophils to release several cytokines and chemokines (12). Activated human (12, 24, 74–82) and mouse (12, 47, 80) basophils secrete large amounts of IL-4. Both human (12, 75, 76, 78, 81–85) and mouse basophils (12, 47) also release IL-13. Human basophils secrete several angiogenic factors such as vascular endothelial growth factor-A (VEGF-A) (64). Basophil-derived IL-4 activates ILC2s to enhance the release of IL-5 and CCL11, leading to eosinophil infiltration (68). IL-4 promotes Th2 cell differentiation and enhances humoral immune responses (224). IL-4, together with IL-13, induces T follicular helper cells (Tfh) to promote IgE responses (225, 226). Basophil-derived IL-4 and IL-13 act on inflammatory monocytes inducing their differentiation into M2 macrophages (227). IL-4 and IL-13 activate fibroblasts to promote the production of chemokines (CCL5 and CCL11) (228) and collagen (229). IL-4 and IL-13 and vasoactive mediators (histamine, LTC4, VEGF-A) act on blood endothelial cells (64, 230) to upregulate the expression of vascular cell adhesion molecule-1 (VCAM-1) (231), leading to enhanced trans-endothelial migration of eosinophils and basophils (186).
Peripheral blood basophils in human hematological tumors
Polycythemia vera (PV) is a clonal proliferation of erythroid, megakaryocytic, and myeloid cell lines (232, 233). More than 90% of patients with JAK2-STAT activating mutations (JAK2V617F or exon 12 mutations) are characterized by an overactive JAK-STAT pathway (234, 235). Pruritus and increased basophil-derived mediators (e.g., histamine) are common in PV patients (233, 236, 237). Peripheral blood basophils (238) and CD63 expression are increased in PV patients and are hyperresponsive to IL-3. Increased releasability of histamine from PV basophils can contribute to pruritus in these patients.
Basophilia can develop during the advanced phase of chronic myeloid leukemia (CML) (239) and the transcription factor IKAROS is reduced in the bone marrow from these patients (240). Basophils from CML patients express HGF, promoting CML cell expansion (91). In a mouse model of CML, basophil-derived CCL3 promotes CML development (241, 242). The presence of basophilia is considered an independent risk factor for the progression from myelodysplastic syndrome to acute myeloid leukemia (243, 244).
Basophils in solid cancers
Basophils are physiologically present in low numbers in peripheral blood. Under certain inflammatory circumstances, the number of circulating basophils can be altered, activated, or migrate from the bloodstream to the sites of inflammation (23, 245). Increased and decreased peripheral blood basophils can be associated with the progression of certain human solid cancers (Table 1) (256, 257). Basophilia positively correlates with improved outcomes in melanoma (246, 247), ovarian cancer (248), non-small cell lung cancer (NSCLC) (251), and glioblastoma (252), while basopenia is associated with a poor prognosis for colorectal cancer (245, 249, 250). Basophilia is also linked to improved outcomes in melanoma patients receiving immunotherapy (247). By contrast, in other solid tumors, such as prostate (253) and gastric cancers (250), a detrimental role of circulating or tissue-infiltrating basophils has been reported. Moreover, baseline basophil count predicts recurrence in bladder cancer patients receiving bacillus Calmette-Guérin (BCG) following resection (254). Interestingly, in a mouse model of breast cancer, basopenia correlated with an increased number of pulmonary metastasis (258). However, basophils are not associated with prognosis in breast cancer patients (259). Basophils may support humoral immunity by secreting several B-cell modulating molecules. Once activated, basophils may express CD40L, IL-4, and IL-6 to sustain B-cell proliferation and empower the production of IgM and IgG1. Gomez and colleagues demonstrated that, in vitro and in vivo, basophils sustain plasma cell survival (245, 260). Histamine is released from basophils and it has been suggested that it can be involved in colon carcinoma (CRC) (245).
Bax and coworkers have investigated the presence and functions of basophils from peripheral blood and in ovarian cancer (248). The same group reported that basophilia and basophils who possess greater ability for ex vivo stimulation are associated with improved outcomes (261). Additionally, a positive correlation between improved progression-free survival of patients and activated basophil markers (CD63+, CD203c+, CD123, CCR3, FcεRI) was observed in the TME of ovarian cancer (Bax, Chauhan et al., 2020). These results indicate that activated peripheral blood and intratumoral basophils correlate with a survival benefit in ovarian cancer patients (261). Nevertheless, these favorable effects that basophils might mediate in targeting the tumor for destruction can potentially result in unfavorable outcomes. For example, basophils have been found in ascitic fluid from ovarian cancer patients and it has been suggested that their release of vasoactive mediators (e.g., histamine) may exacerbate fluid accumulation in the peritoneal cavity (58).
It has been reported that the expression of cytokines by lung-resident basophils can be induced by local signals (e.g., IL-33, GM-CSF) (47, 102), emphasizing the plasticity of these cells. Hence, the lung microenvironment might influence the transcriptional and functional development of basophils. Likewise, these resident basophils seemingly play an important role in lung development and function by forming cellular networks and facilitating so-called macrophage imprinting. Low percentages of basophils (0.4%) were located in the immune infiltrate of human non-small cell lung cancer (NSCLC) tumors (262). Basophils have been identified in the immune landscape in early (stage I) lung adenocarcinoma and in non-involved lung tissue (nLung) (102). It remains unclear what the exact function basophils mediate in the TME, yet emerging evidence points to their capacity to secrete IL-4 and IL-13 as playing a potential role. For example, mouse and human studies have shown that basophils, by secreting these cytokines, facilitate the development/expansion of M2-like monocytes/macrophages (227, 263–265), which are often a prominent part of the immune cell landscape of the TME. However, in chronic inflammation, the exposure of basophils to certain cytokines, such as IL-33, may induce the polarization of lung macrophages to M2-like phenotype characterized by the expression of anti-inflammatory genes Clec7a, Arg1, Itgax. In this context, basophils are participants in the inflammatory entourage in lung cancer (261). It seems equally possible that basophil-derived IL-4/IL-13 also favor tumorigenesis by diminishing Th1-like immunity that is better suited to contest the cancer (80). Should these hypotheses prove correct, then another important question that arises pertains to the endogenous stimulus responsible for inducing these cytokines. In this regard, Schroeder et al. demonstrated that purified human basophils release histamine, IL-4 and IL-13 when co-cultured with the lung adenocarcinoma cell line A549 (16). Unexpectedly, these effects required IgE-expressing basophils and were suppressed by specific inhibitors of FcεRI signaling. A subsequent study revealed that the IgE-binding lectin, galectin-3, expressed on the A549 cells, was responsible for this model of basophil activation (223). In fact, galectin-3 is a biomarker and/or factor implicated in many kinds of cancer, chronic inflammation, cardiovascular disease, autoimmunity, and also beneficially in wound healing (266). These results thus reveal an innovative mechanism by which galectin-3 expressed by human lung carcinoma cells are able to activate basophils [and likely other cell types, namely dendritic cells (DCs) and monocytes] (267) to release cytokines and pro-inflammatory mediators. Further studies are necessary to understand the role of galectin-3 in activating basophils, and how IL-4/IL-13 and other mediators could contribute to human and experimental lung cancer.
Interestingly, many immune cells and markers that have a mounting prominence in cancer/tumorigenesis are also observed in experimental models of wound healing. For example, scaffolds that promote wound-healing often induce Th2 immune responses, whereby IL-4 and IL-13 are recognized as critical cytokines that help initiate the process (268). M2 cells, whose development is often dependent on the actions of IL-4/IL-13, are also widely implicated in wound healing. Not surprisingly, much emphasis is placed on the role of Th2 cells in being the source of IL-4/IL-13. However, in a recent publication that explored the mechanisms associated with wound healing following experimental myocardial infarction (MI), basophils were identified as a critical source of IL-4/IL-13 required for the healing process. Specifically targeting basophils using conditional knockouts or by antibody-mediated depletion, significantly impaired this wound healing (269). Moreover, the administration of IPSE-α1, an IgE-binding glycoprotein isolated from helminth eggs and well known for activating basophils for IL-4/IL-13, greatly augmented healing following the MI. While the endogenous ligand for stimulating IL-4/IL-13 from basophils in this model was not reported, it is intriguing to speculate that galectin-3 is involved. Indeed, galectin-3 is often a prominent marker in wound healing, both at the transcriptional and protein levels (266).
Investigation on the role of basophils in models of melanoma has provided interesting results in Foxp3DTR mice, in which these cells caused melanoma rejection (270). CCL3 and CCL4 produced by intratumoral basophils induced CD8+ lymphocyte recruitment in TME. The administration of FcϵRI (MAR-1) mAb in Foxp3DTR melanoma-bearing mice depleted basophils and abrogated the recruitment of CD8+ T cells thus preventing the rejection of melanoma. Furthermore, the IL-3/anti-IL-3 antibody complexes combined with adoptive T cell transfer induced basophilia and consequent T cell infiltration, which positively correlated with melanoma rejection. Unfortunately, the MAR-1 antibody can also deplete/activate other immune cells (e.g., mast cells, DCs, monocytes) which express FcϵRI (271, 272). Thus, studies in newer genetically engineered basophil-deficient mouse models (80, 97) appear necessary to establish the role of basophils in melanoma.
IL-33 is a cytokine that induces tumoricidal functions in eosinophils (273, 274) and upregulates granzyme B mRNA and the surface expression of CD63 (67), suggesting phenotypic and functional activation. Moreover, IL-33-activated basophils co-cultured with B16.F10 melanoma cells, inhibited tumor cell-growth compared to melanoma cells co-cultured with unstimulated basophils (67).
In a pioneering observation, Ann M. Dvorak first demonstrated piecemeal degranulation of basophils in human pancreatic cancer (PC) (7). Elegant studies evaluated the role of basophils in experimental and human ductal adenocarcinoma (PDAC) (80). In PDAC patients, they identified IL4 expressing basophils in tumor-draining lymph nodes (TDLNs). Basophils in TDLNs were an independent negative prognostic biomarker of patient survival. They also evaluated basophil role in PC using the Mcpt8-Cre basophil deficient (275) and wild-type (WT) mice. After PC implant, cancer was detected in 80% WT, but not in basophil-deficient mice. Basophils were found in TDLNs and cancer-associated fibroblasts (CAFs) released TSLP, which activated DCs to produce IL-3 from CD4+ T cells. CCL7, produced by DCs and CD14+ monocytes, induced basophil migration into TDLNs. Basophils activated by IL-3 played a pro-tumorigenic role through the production of IL-4, which favored Th2 and M2 polarization. These findings are consistent with our results indicating that basophil-derived IL-4 (and IL-13) promote M2-like cells (263).
Topical exposure of the skin of mice to an environmental DNA-damaging xenobiotic [i.e., 7,12-dimethylbenz [a] anthracene (DMBA)] caused the development of squamous-cell carcinomas (SCCs), high serum levels of IgE and tumor infiltration of IgE-bearing basophils (276). In this model, FcϵRI+ basophils mediated the DMBA-induced IgE protection against carcinogenesis. In contrast, topical exposure of the skin of mice to the proinflammatory agent 12-0-tetradecanoylphorbol-13-acetate (TPA) increased serum IgE and IgE-bearing basophils in the skin that promoted carcinogenesis (97). In a two-stage model of epithelial carcinogenesis (DMBA and subsequent exposure to TPA), Hayes and coworkers also discovered that mice lacking IgE (lgh7-/-) were less responsive to tumor development compared to WT mice (97). IgE-signaling was crucial for mediator release from basophils and infiltrating tissue basophils showed expression of Cxcr2, Cxcr4, and Ptgdr2 (CRTH2, the PGD2 receptor). Basophil infiltration into the inflamed skin was mediated by TSLP/IL-3-mediated upregulation of CXCR4 on basophils. The Mcpt8Cre/+ mice, presenting normal mast cell numbers but strongly reduced basophils (275), were less responsive to tumor growth. Table 2 summarizes the role of basophils in the TME of different solid cancers.
Colony-stimulating factor 1 (CSF1) is a primary regulator of monocytes/macrophage that sustains macrophage polarization towards an M2-like phenotype (278). Mouse basophils resident in the lung express high levels of Csf1 and contribute to M2 polarization of lung macrophage (47). The functional relevance of basophil-derived CSF1 was also underlined in vivo in a murine model of atopic dermatitis, where it promoted M2-like macrophage polarization (279). Interestingly, an inhibitor of CSF1/CSF1 receptor signaling reduced tumor-associated macrophage (TAM) infiltration in the TME of sarcoma models (278). These experimental findings may have translational relevance in cancer: there is the possibility that CSF1, in conjunction with basophil-derived IL-4/IL-13, might enhance the M2-like/TAM polarization of macrophage in TME (280).
A synopsis of the above findings signifies some conflicting views of the role that basophils potentially mediate in tumorigenesis. A more classical interpretation (from the ovarian, lung, colorectal, and melanoma data) suggests basophils mediate anti-tumor effects (248, 261, 270, 276). While the mechanisms underlying the beneficial outcomes are poorly defined, it has been proposed that some basophil-derived mediators (e.g., granzyme B and TNF-α) exert tumoricidal activity while others (e.g., CCL3 and CCL4) facilitate the recruitment of cytotoxic CD8+ T cells (Figure 2). In contrast, there is growing evidence that basophils, under certain circumstances, can promote tumorigenesis (Figure 3). In this instance, the tumor cell itself seemingly modulates basophil responses, causing a release of cytokines that favor the development of protumorigenic TME. Interestingly, this latter scenario shares many similarities with that seen in wound healing.
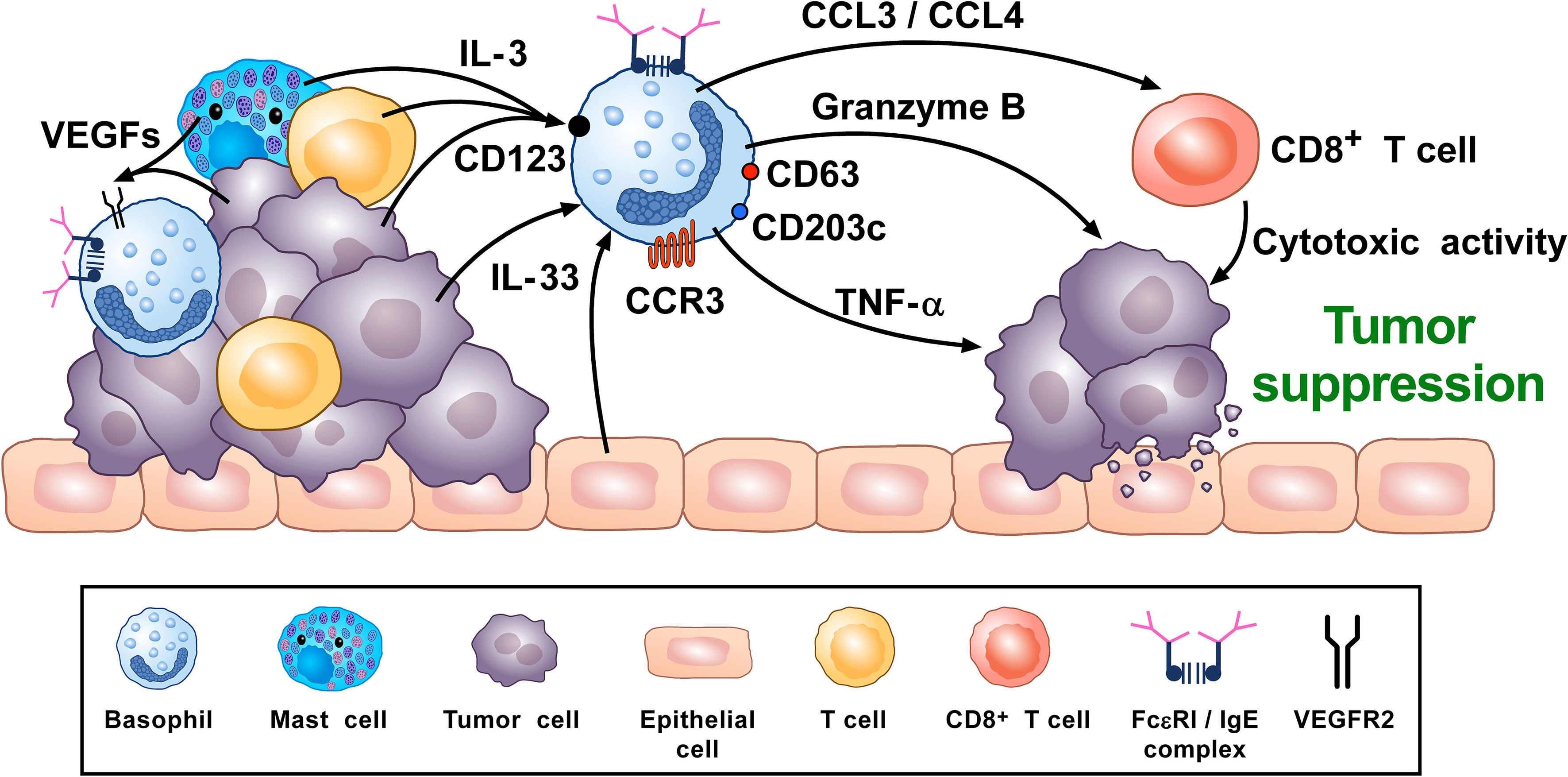
Figure 2 Theoretical representation of how basophils can promote tumor suppression. Basophils have been located in the immune infiltrate of several human (80, 102, 261, 262) and experimental tumors (80, 97, 102, 276). Vascular endothelial growth factors released by cancer cells and immune cells in tumor microenvironment (TME) (e.g., mast cells, macrophages) (118, 120–123) can favor basophil infiltration in TME through the engagement of VEGFR2 on these cells (120). IL-3, produced by intratumoral lymphocytes, mast cells and cancer cells (17, 82, 281), is the most important growth and activating factor for human and mouse basophils, through the engagement of the IL-3 receptor (IL-3Rα/CD123) (17). CCL3/CCL4 secreted by intratumoral basophils induces CD8+ T cell recruitment in TME, promoting melanoma rejection in mice (270). IL-33, a pleiotropic cytokine produced by epithelial and tumor cells (282), plays a central role in tumorigenesis (282). IL-33 upregulates granzyme B mRNA and the surface expression of CD63, suggesting functional and phenotypic basophil activation. IL-33-activated mouse basophils induce melanoma cell death in vitro (67). Mouse (47, 86) and, under specific circumstances, human basophils (88, 89) release TNF-α. Human and mouse basophils release granzyme B (66, 67). Both TNF-α and granzyme B exert cytotoxic effects on tumor cells (68, 69). Activated signature (CD123, CCR3, CD63, CD203c gene expression) in tumor resident basophils is associated with improved outcome in ovarian cancer patients (248, 261). Topical exposure to a DNA-damaging carcinogen promotes tumor-protective IgE response through skin infiltrating basophils (276). Taken together, these results suggest that, in certain experimental and clinical conditions, basophils and their mediators may play an anti-tumorigenic role.
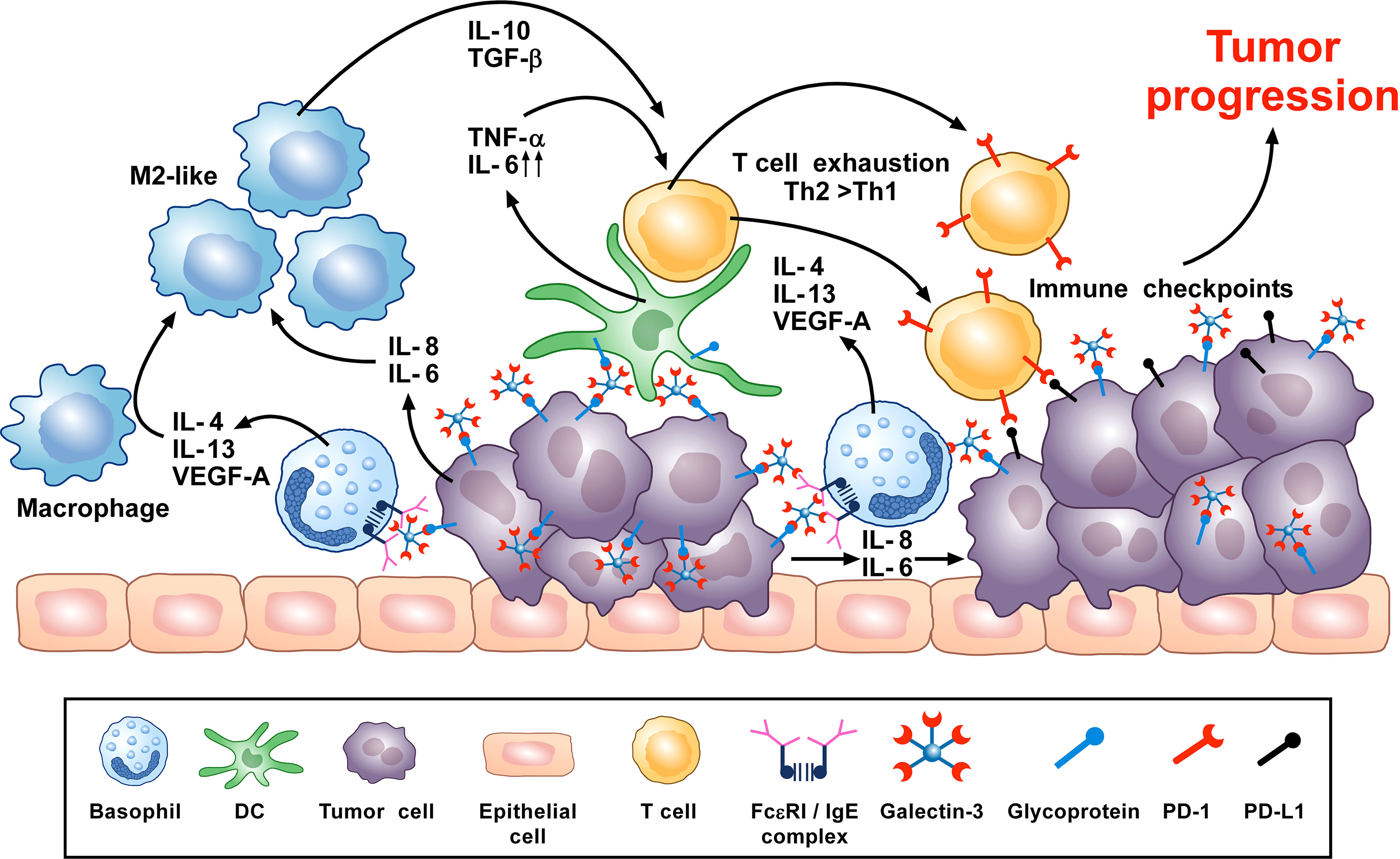
Figure 3 Theoretical representation of how basophils can promote tumor progression. Galectin-3 (Gal-3) is a lectin highly expressed by many types of cancer cells, frequently manifesting as a marker of poor prognosis with capacity to mediate immunosuppression within the tumor microenvironment (TME) (266). Recent in vitro studies show that Gal-3, expressed by the A549 adenocarcinoma cell line (or EC-Gal-3), has the capacity to activate basophils to secrete copious amounts of IL-4/IL-13 (16, 223). Both cytokines are known to promote M2-like macrophages, which are major players in the TME (227, 263–265). IL-4-producing basophils have been identified in the TME of human pancreatic cancer, with mouse models indicating that this IL-4 promotes a Th2>Th1 response that is more conducive to tumorigenesis (80). Additionally, basophils are long known to secrete VEGF-A (64) that promotes angiogenesis. Other studies show that basophils can induce IL-6/IL-8 secretion from cell lines through a mechanism requiring cell-to-cell contact (283) (JTS, unpublished). This tumor cell-derived IL-6/IL-8 is implicated in playing a critical role in metastasis formation (284). Likewise, dendritic cells and monocytes activated by EC-Gal-3 are shown to produce high levels of TNF-α/IL-6 in vitro (285). Chronic production of these cytokines, when combined with M2 cell-derived IL-10/TGF-β, are implicated in promoting T-cell exhaustion by up-regulating checkpoint inhibitors (e.g., PD-1) that interact with tumor cell-associated markers (PD-L1) to suppress cytotoxic T cell activity (286). V-domain immunoglobulin suppressor of T-cell activation (VISTA) is another immune checkpoint receptor which plays a role in cancer progression (287, 288) and regulates allergen-specific Th2-mediated immune responses (289). Overall, it is proposed that the combined actions of these dysregulated innate immune responses synergize to promote tumorigenesis.
Conclusions
Basophils were initially considered as effector cells of allergic diseases (166, 230). The discovery that murine (290) and human basophils produce immunomodulatory cytokines (e.g., IL-4, IL-3, and IL-13) (28, 76–78, 81, 85, 291) changed dramatically this erroneous concept. In addition, human and murine basophils release several canonical (24, 64, 90, 91) and non-canonical angiogenic factors (133) that play a pivotal role in inflammatory and tumor angiogenesis. Further in vitro and in vivo studies are needed to investigate the contribution of angiogenic factors released by mouse and human basophils in experimental and human tumors.
Basophils have been identified in human lung (102), gastric (99, 100), pancreatic (7, 80) and ovarian cancer (248). Lung-resident basophils (47) can provoke M2 polarization of lung macrophages, as occurs in several tumors (105, 106). The presence of basophils and their activation signatures appear to be linked with more favorable patient outcomes in certain tumors (melanoma, lung cancer, ovarian cancer) (248, 261, 270). Otherwise, with particular reference to gastric and pancreatic cancers, increased tumor-infiltrating basophils are negatively associated with less favorable overall survival (80, 255, 277).
Basophil functions in vivo have been evaluated through several models of basophil-deficient mice (275, 292–294). It should be remembered that, in some instances, studies using antibody-depleted basophils have produced erroneous findings due to lack of antibody specificity (271, 272) and even new mouse basophil-targeted mutants have some off-target hematological alterations (295). Therefore, the evaluation of basophil functions in complex and heterogeneous disorders, such as cancer and allergic diseases using multiple genetically engineered models of basophil deficiency, demands caution in data interpretation.
Collectively, recent findings highlight the critical contributions of basophils during homeostatic conditions and beyond their ability to promote allergic inflammation. Further studies are needed to understand the mechanisms and environmental factors driving basophils to play a pro- or anti-tumorigenic role in experimental and human cancers. A better knowledge of the involvement and functions of basophils in human immunity appears necessary considering the participation of these cells in immune and cancer cell crosstalk and in priming of several immune cell types. Single-cell RNA-seq of the immune landscape of tumor cells will be of paramount importance to characterize the role of basophils in different types of human and experimental cancer. Understanding of the molecular mechanisms orchestrated by basophils in the TME of several cancer types could allow to develop novel pharmacological/immunological strategies to modulate basophil functions and perhaps to prevent tumor progression.
Author contributions
RP and ARG are co-first authors of this manuscript. All authors contributed to the article and approved the submitted version. GS and GV are co-senior authors of this manuscript.
Funding
This work was supported in part by grants from the CISI-Lab Project (University of Naples Federico II), TIMING Project (Regione Campania) to GM, GV, from AIRC IG 21366 to GS and NIAID and by NIH grants AI115703 and AI141486 to JTS.
Acknowledgments
The authors apologize to the many researchers who have contributed importantly to this field and whose work was not cited due to space and citation limitations. The authors thank Dr. Gjada Criscuolo for her excellent managerial assistance in preparing this manuscript, scientists of CISI Laboratory and Schiavoni Laboratory not listed as authors for invaluable collaboration to the work reviewed, the administrative staff (Dr. Roberto Bifulco, Dr. Anna Ferraro and Dr. Maria Cristina Fucci), and the medical graphic artist Fabrizio Fiorbianco for the elaboration of figures.
Conflict of interest
The authors declare that the research was conducted in the absence of any commercial or financial relationships that could be construed as a potential conflict of interest.
Publisher’s note
All claims expressed in this article are solely those of the authors and do not necessarily represent those of their affiliated organizations, or those of the publisher, the editors and the reviewers. Any product that may be evaluated in this article, or claim that may be made by its manufacturer, is not guaranteed or endorsed by the publisher.
Abbreviations
ANGPT, angiopoietin; AREG: amphiregulin; BCG, bacillus Calmette-Guérin; BEC, blood endothelial cells; BET, basophil extracellular traps; CAF, cancer-associated fibroblast; CML, chronic myeloid leukemia; CRC, colon carcinoma; CSF1, colony-stimulating factor 1; CSU, chronic spontaneous urticaria; cys-LT, cysteinyl leukotriene; cys-LTR, cysteinyl leukotriene receptor; CyTOF, cytometry by Time-Of-Flight; DC, dendritic cell; DMBA, 7,12- dimethylbenz[a] amthracene; ET, extracellular trap; Flt3L, l, FMS-like tyrosine kinase 3 ligand; HGF, hepatocyte growth factor; ILC2, group-2 innate lymphoid cell; JAK2, janus kinase 2; LTC4, leukotriene C4; MI, myocardial infarction; NET, neutrophil extracellular trap; NGF, nerve growth factor; nLung, non-involved lung tissue; NSCLC, non-small cell lung cancer; PC, pancreatic cancer; PDAC, pancreatic ductal adenocarcinoma; PV, polycythemia vera; PGD2, prostaglandin D2; PGE2, prostaglandin E2; SCC, squamous-cell carcinoma; TAM, tumor-associated macrophage; TDLN, tumor-draining lymph nodes; Tfh, T follicular helper cell; TME, tumor microenvironment; TPA, 12-0-tetradecanoylphorbol-13-acetate; Treg cell, T regulatory cell; TrkA, tropomyosin receptor kinase A; TSLP, thymic stromal lymphopoietin; TSLPR, TSLP receptor; VEGF, vascular endothelial growth factor; VISTA, V-domain immunoglobulin suppressor of T-cell activation; WT, wild type.
References
1. Ehrlich P. Ueber die specifischen granulationen des blutes. Archiv fuer Anat Und Physiol: Physiol Abteilung (1879) 3:571–9.
2. Ehrlich P. Beitrage sur theorie und praxis der histologischen farbung. Leipzig, Germany: University of Leipzig (1878).
3. Varricchi G, de Paulis A, Marone G, Galli SJ. Future needs in mast cell biology. Int J Mol Sci (2019) 20:E4397. doi: 10.3390/ijms20184397
4. Varricchi G, Marone G. Mast cells: Fascinating but still elusive after 140 years from their discovery. Int J Mol Sci (2020) 21:464. doi: 10.3390/ijms21020464
5. Varricchi G, Raap U, Rivellese F, Marone G, Gibbs BF. Human mast cells and basophils-how are they similar how are they different? Immunol Rev (2018) 282:8–34. doi: 10.1111/imr.12627
6. Galli SJ, Tsai M, Marichal T, Tchougounova E, Reber LL, Pejler G. Approaches for analyzing the roles of mast cells and their proteases in vivo. Adv Immunol (2015) 126:45–127. doi: 10.1016/bs.ai.2014.11.002
7. Dvorak AM. Ultrastructural analysis of human mast cells and basophils. Chem Immunol (1995) 61:1–33. doi: 10.1159/000424579
8. Marone G, Borriello F, Varricchi G, Genovese A, Granata F. Basophils: historical reflections and perspectives. Chem Immunol Allergy (2014) 100:172–92. doi: 10.1159/000358734
9. Metcalfe DD, Pawankar R, Ackerman SJ, Akin C, Clayton F, Falcone FH, et al. Biomarkers of the involvement of mast cells, basophils and eosinophils in asthma and allergic diseases. World Allergy Organ J (2016) 9:7. doi: 10.1186/s40413-016-0094-3
10. Dvorak AM, Nabel G, Pyne K, Cantor H, Dvorak HF, Galli SJ. Ultrastructural identification of the mouse basophil. Blood (1982) 59:1279–85. doi: 10.1182/blood.V59.6.1279.1279
11. Dvorak AM. The mouse basophil, a rare and rarely recognized granulocyte. Blood (2000) 96:1616–7. doi: 10.1182/blood.V96.4.1616
12. Gambardella AR, Poto R, Tirelli V, Schroeder JT, Marone G, Mattei F, et al. Differential effects of alarmins on human and mouse basophils. Front Immunol (2022) 13:894163. doi: 10.3389/fimmu.2022.894163
13. Lee JJ, McGarry MP. When is a mouse basophil not a basophil? Blood (2007) 109:859–61. doi: 10.11820/blood-2006-06-027490
14. Salabert-Le Guen N, Hemont C, Delbove A, Poli C, Braudeau C, Fantou A, et al. Thymic stromal lymphopoietin does not activate human basophils. J Allergy Clin Immunol (2018) 141:1476–9.e6. doi: 10.1016/j.jaci.2017.11.012
15. Salter BM, Oliveria JP, Nusca G, Smith SG, Watson RM, Comeau M, et al. Thymic stromal lymphopoietin activation of basophils in patients with allergic asthma is IL-3 dependent. J Allergy Clin Immunol (2015) 136:1636–44. doi: 10.1016/j.jaci.2015.03.039
16. Schroeder JT, Bieneman AP. Activation of human basophils by A549 lung epithelial cells reveals a novel IgE-dependent response independent of allergen. J Immunol (2017) 199:855–65. doi: 10.4049/jimmunol.1700055
17. Varricchi G, Poto R, Marone G, Schroeder JT. IL-3 in the development and function of basophils. Semin Immunol (2021) 54:101510. doi: 10.1016/j.smim.2021.101510
18. Min B. Basophils: what they 'can do' versus what they 'actually do'. Nat Immunol (2008) 9:1333–9. doi: 10.1038/ni.f.217
19. Voehringer D. Protective and pathological roles of mast cells and basophils. Nat Rev Immunol (2013) 13:362–75. doi: 10.1038/nri3427
20. Nakashima C, Otsuka A, Kabashima K. Recent advancement in the mechanism of basophil activation. J Dermatol Sci (2018) 91:3–8. doi: 10.1016/j.jdermsci.2018.03.007
21. Siracusa MC, Kim BS, Spergel JM, Artis D. Basophils and allergic inflammation. J Allergy Clin Immunol (2013) 132:789–801. doi: 10.1016/j.jaci.2013.07.046
22. Kubo M. Mast cells and basophils in allergic inflammation. Curr Opin Immunol (2018) 54:74–9. doi: 10.1016/j.coi.2018.06.006
23. Yamanishi Y, Miyake K, Iki M, Tsutsui H, Karasuyama H. Recent advances in understanding basophil-mediated Th2 immune responses. Immunol Rev (2017) 278:237–45. doi: 10.1111/imr.12548
24. Schroeder JT. Basophils: emerging roles in the pathogenesis of allergic disease. Immunol Rev (2011) 242:144–60. doi: 10.1111/j.1600-065X.2011.01023.x
25. Arinobu Y, Iwasaki H, Gurish MF, Mizuno S, Shigematsu H, Ozawa H, et al. Developmental checkpoints of the basophil/mast cell lineages in adult murine hematopoiesis. Proc Natl Acad Sci U.S.A. (2005) 102:18105–10. doi: 10.1073/pnas.0509148102
26. Hamey FK, Lau WWY, Kucinski I, Wang X, Diamanti E, Wilson NK, et al. Single-cell molecular profiling provides a high-resolution map of basophil and mast cell development. Allergy (2021) 76:1731–42. doi: 10.1111/all.14633
27. Wanet A, Bassal MA, Patel SB, Marchi F, Mariani SA, Ahmed N, et al. E-cadherin is regulated by GATA-2 and marks the early commitment of mouse hematopoietic progenitors to the basophil and mast cell fates. Sci Immunol (2021) 6:eaba0178. doi: 10.1126/sciimmunol.aba0178
28. Schroeder JT, Chichester KL, Bieneman AP. Human basophils secrete IL-3: evidence of autocrine priming for phenotypic and functional responses in allergic disease. J Immunol (2009) 182:2432–8. doi: 10.4049/jimmunol.0801782
29. Kirshenbaum AS, Goff JP, Dreskin SC, Irani AM, Schwartz LB, Metcalfe DD. IL-3-dependent growth of basophil-like cells and mastlike cells from human bone marrow. J Immunol (1989) 142:2424–9.
30. Valent P, Besemer J, Muhm M, Majdic O, Lechner K, Bettelheim P. Interleukin 3 activates human blood basophils via high-affinity binding sites. Proc Natl Acad Sci U.S.A. (1989) 86:5542–6. doi: 10.1073/pnas.86.14.5542
31. Valent P, Schmidt G, Besemer J, Mayer P, Zenke G, Liehl E, et al. Interleukin-3 is a differentiation factor for human basophils. Blood (1989) 73:1763–9. doi: 10.1182/blood.V73.7.1763.1763
32. Yoshimoto T, Nakanishi K. Generation and characterization of mouse basophils from bone marrow and purification of basophils from spleen. Curr Protoc Immunol (2012). doi: 10.1002/0471142735.im0324s98. Chapter 3: Unit 3 24.
33. MacGlashan D Jr. Modulating the human basophil phenotype during its development and maturation: Basophils derived from In vitro cultures of CD34(+) progenitor cells. Methods Mol Biol (2020) 2163:69–83. doi: 10.1007/978-1-0716-0696-4_6
34. Siracusa MC, Saenz SA, Hill DA, Kim BS, Headley MB, Doering TA, et al. TSLP promotes interleukin-3-independent basophil haematopoiesis and type 2 inflammation. Nature (2011) 477:229–33. doi: 10.1038/nature10329
35. Siracusa MC, Wojno ED, Artis D. Functional heterogeneity in the basophil cell lineage. Adv Immunol (2012) 115:141–59. doi: 10.1016/B978-0-12-394299-9.00005-9
36. Daeron M. Innate myeloid cells under the control of adaptive immunity: the example of mast cells and basophils. Curr Opin Immunol (2016) 38:101–8. doi: 10.1016/j.coi.2015.12.004
37. Malveaux FJ, Conroy MC, Adkinson NF Jr., Lichtenstein LM. IgE receptors on human basophils. relationship to serum IgE concentration. J Clin Invest (1978) 62:176–81. doi: 10.1172/JCI109103
38. Pellefigues C, Mehta P, Prout MS, Naidoo K, Yumnam B, Chandler J, et al. The Basoph8 mice enable an unbiased detection and a conditional depletion of basophils. Front Immunol (2019) 10:2143. doi: 10.3389/fimmu.2019.02143
39. Mukai K, Gaudenzio N, Gupta S, Vivanco N, Bendall SC, Maecker HT, et al. Assessing basophil activation by using flow cytometry and mass cytometry in blood stored 24 hours before analysis. J Allergy Clin Immunol (2017) 139:889–99.e11. doi: 10.1016/j.jaci.2016.04.060
40. MacGlashan D Jr. Expression of CD203c and CD63 in human basophils: relationship to differential regulation of piecemeal and anaphylactic degranulation processes. Clin Exp Allergy (2010) 40:1365–77. doi: 10.1111/j.1365-2222.2010.03572.x
41. MacGlashan DW Jr. Basophil activation testing. J Allergy Clin Immunol (2013) 132:777–87. doi: 10.1016/j.jaci.2013.06.038
42. Tsai SH, Kinoshita M, Kusu T, Kayama H, Okumura R, Ikeda K, et al. The ectoenzyme e-NPP3 negatively regulates ATP-dependent chronic allergic responses by basophils and mast cells. Immunity (2015) 42:279–93. doi: 10.1016/j.immuni.2015.01.015
43. Salter BM, Nusca G, Tworek D, Oliveria JP, Smith SG, Watson RM, et al. Expression of activation markers in circulating basophils and the relationship to allergen-induced bronchoconstriction in subjects with mild allergic asthma. J Allergy Clin Immunol (2016) 137:936–8.e7. doi: 10.1016/j.jaci.2015.08.024
44. Lantz CS, Boesiger J, Song CH, Mach N, Kobayashi T, Mulligan RC, et al. Role for interleukin-3 in mast-cell and basophil development and in immunity to parasites. Nature (1998) 392:90–3. doi: 10.1038/32190
45. Kampfer SS, Odermatt A, Dahinden CA, Fux M. Late IL-3-induced phenotypic and functional alterations in human basophils require continuous IL-3 receptor signaling. J Leukoc Biol (2017) 101:227–38. doi: 10.1189/jlb.2A0715-292RR
46. Borriello F, Galdiero MR, Varricchi G, Loffredo S, Spadaro G, Marone G. Innate immune modulation by GM-CSF and IL-3 in health and disease. Int J Mol Sci (2019) 20:834. doi: 10.3390/ijms20040834
47. Cohen M, Giladi A, Gorki AD, Solodkin DG, Zada M, Hladik A, et al. Lung single-cell signaling interaction map reveals basophil role in macrophage imprinting. Cell (2018) 175:1031–1044.e18. doi: 10.1016/j.cell.2018.09.009
48. Pecaric-Petkovic T, Didichenko SA, Kaempfer S, Spiegl N, Dahinden CA. Human basophils and eosinophils are the direct target leukocytes of the novel IL-1 family member IL-33. Blood (2009) 113:1526–34. doi: 10.1182/blood-2008-05-157818
49. Smithgall MD, Comeau MR, Yoon BR, Kaufman D, Armitage R, Smith DE. IL-33 amplifies both Th1- and Th2-type responses through its activity on human basophils, allergen-reactive Th2 cells, iNKT and NK cells. Int Immunol (2008) 20:1019–30. doi: 10.1093/intimm/dxn060
50. Suzukawa M, Iikura M, Koketsu R, Nagase H, Tamura C, Komiya A, et al. An IL-1 cytokine member, IL-33, induces human basophil activation via its ST2 receptor. J Immunol (2008) 181:5981–9. doi: 10.4049/jimmunol.181.9.5981
51. Wright AKA, Weston C, Rana BMJ, Brightling CE, Cousins DJ. Human group 2 innate lymphoid cells do not express the IL-5 receptor. J Allergy Clin Immunol (2017) 140:1430–3.e4. doi: 10.1016/j.jaci.2017.04.025
52. Burgi B, Otten UH, Ochensberger B, Rihs S, Heese K, Ehrhard PB, et al. Basophil priming by neurotrophic factors. activation through the trk receptor. J Immunol (1996) 157:5582–8.
53. Sin AZ, Roche EM, Togias A, Lichtenstein LM, Schroeder JT. Nerve growth factor or IL-3 induces more IL-13 production from basophils of allergic subjects than from basophils of nonallergic subjects. J Allergy Clin Immunol (2001) 108:387–93. doi: 10.1067/mai.2001.117459
54. Wang F, Trier AM, Li F, Kim S, Chen Z, Chai JN, et al. A basophil-neuronal axis promotes itch. Cell (2021) 184:422–40.e17. doi: 10.1016/j.cell.2020.12.033
55. Pellefigues C, Dema B, Lamri Y, Saidoune F, Chavarot N, Loheac C, et al. Prostaglandin D2 amplifies lupus disease through basophil accumulation in lymphoid organs. Nat Commun (2018) 9:725. doi: 10.1038/s41467-018-03129-8
56. Dahinden CA, Geiser T, Brunner T, von Tscharner V, Caput D, Ferrara P, et al. Monocyte chemotactic protein 3 is a most effective basophil- and eosinophil-activating chemokine. J Exp Med (1994) 179:751–6. doi: 10.1084/jem.179.2.751
57. Mack M, Schneider MA, Moll C, Cihak J, Bruhl H, Ellwart JW, et al. Identification of antigen-capturing cells as basophils. J Immunol (2005) 174:735–41. doi: 10.4049/jimmunol.174.2.735
58. Khan ANH, Emmons TR, Wong JT, Alqassim E, Singel KL, Mark J, et al. Quantification of early-stage myeloid-derived suppressor cells in cancer requires excluding basophils. Cancer Immunol Res (2020) 8:819–28. doi: 10.1158/2326-6066.CIR-19-0556
59. Satoh T, Moroi R, Aritake K, Urade Y, Kanai Y, Sumi K, et al. Prostaglandin D2 plays an essential role in chronic allergic inflammation of the skin via CRTH2 receptor. J Immunol (2006) 177:2621–9. doi: 10.4049/jimmunol.177.4.2621
60. Shiratori I, Yamaguchi M, Suzukawa M, Yamamoto K, Lanier LL, Saito T, et al. Down-regulation of basophil function by human CD200 and human herpesvirus-8 CD200. J Immunol (2005) 175:4441–9. doi: 10.4049/jimmunol.175.7.4441
61. Cassard L, Jonsson F, Arnaud S, Daeron M. Fcgamma receptors inhibit mouse and human basophil activation. J Immunol (2012) 189:2995–3006. doi: 10.4049/jimmunol.1200968
62. Meknache N, Jonsson F, Laurent J, Guinnepain MT, Daeron M. Human basophils express the glycosylphosphatidylinositol-anchored low-affinity IgG receptor FcgammaRIIIB (CD16B). J Immunol (2009) 182:2542–50. doi: 10.4049/jimmunol.0801665
63. McEuen AR, Calafat J, Compton SJ, Easom NJ, Buckley MG, Knol EF, et al. Mass, charge, and subcellular localization of a unique secretory product identified by the basophil-specific antibody BB1. J Allergy Clin Immunol (2001) 107:842–8. doi: 10.1067/mai.2001.114650
64. de Paulis A, Prevete N, Fiorentino I, Rossi FW, Staibano S, Montuori N, et al. Expression and functions of the vascular endothelial growth factors and their receptors in human basophils. J Immunol (2006) 177:7322–31. doi: 10.4049/jimmunol.177.10.7322
65. Jogie-Brahim S, Min HK, Fukuoka Y, Xia HZ, Schwartz LB. Expression of alpha-tryptase and beta-tryptase by human basophils. J Allergy Clin Immunol (2004) 113:1086–92. doi: 10.1016/j.jaci.2004.02.032
66. Tschopp CM, Spiegl N, Didichenko S, Lutmann W, Julius P, Virchow JC, et al. A novel mediator of allergic inflammation: its induction and release in blood basophils and human asthma. Blood (2006) 108:2290–9. doi: 10.1182/blood-2006-03-010348
67. Marone G, Gambardella AR, Mattei F, Mancini J, Schiavoni G, Varricchi G. Basophils in tumor microenvironment and surroundings. Adv Exp Med Biol (2020) 1224:21–34. doi: 10.1007/978-3-030-35723-8_2
68. Motomura Y, Morita H, Moro K, Nakae S, Artis D, Endo TA, et al. Basophil-derived interleukin-4 controls the function of natural helper cells, a member of ILC2s, in lung inflammation. Immunity (2014) 40:758–71. doi: 10.1016/j.immuni.2014.04.013
69. Voskoboinik I, Whisstock JC, Trapani JA. Perforin and granzymes: function, dysfunction and human pathology. Nat Rev Immunol (2015) 15:388–400. doi: 10.1038/nri3839
70. MacGlashan DW Jr., Peters SP, Warner J, Lichtenstein LM. Characteristics of human basophil sulfidopeptide leukotriene release: releasability defined as the ability of the basophil to respond to dimeric cross-links. J Immunol (1986) 136:2231–9.
71. Ugajin T, Satoh T, Kanamori T, Aritake K, Urade Y, Yokozeki H. FcepsilonRI, but not FcgammaR, signals induce prostaglandin D2 and E2 production from basophils. Am J Pathol (2011) 179:775–82. doi: 10.1016/j.ajpath.2011.04.023
72. Bando T, Fujita S, Nagano N, Yoshikawa S, Yamanishi Y, Minami M, et al. Differential usage of COX-1 and COX-2 in prostaglandin production by mast cells and basophils. Biochem Biophys Rep (2017) 10:82–7. doi: 10.1016/j.bbrep.2017.03.004
73. Marone G, Galdiero MR, Pecoraro A, Pucino V, Criscuolo G, Triassi M, et al. Prostaglandin D2 receptor antagonists in allergic disorders: safety, efficacy, and future perspectives. Expert Opin Investig Drugs (2019) 28:73–84. doi: 10.1080/13543784.2019.1555237
74. Brunner T, Heusser CH, Dahinden CA. Human peripheral blood basophils primed by interleukin 3 (IL-3) produce IL-4 in response to immunoglobulin e receptor stimulation. J Exp Med (1993) 177:605–11. doi: 10.1084/jem.177.3.605
75. Genovese A, Borgia G, Bjorck L, Petraroli A, de Paulis A, Piazza M, et al. Immunoglobulin superantigen protein l induces IL-4 and IL-13 secretion from human fc epsilon RI+ cells through interaction with the kappa light chains of IgE. J Immunol (2003) 170:1854–61. doi: 10.4049/jimmunol.170.4.1854
76. Patella V, Florio G, Petraroli A, Marone G. HIV-1 gp120 induces IL-4 and IL-13 release from human fc epsilon RI+ cells through interaction with the VH3 region of IgE. J Immunol (2000) 164:589–95. doi: 10.4049/jimmunol.164.2.589
77. Patella V, Giuliano A, Bouvet JP, Marone G. Endogenous superallergen protein fv induces IL-4 secretion from human fc epsilon RI+ cells through interaction with the VH3 region of IgE. J Immunol (1998) 161:5647–55.
78. Redrup AC, Howard BP, MacGlashan DW Jr., Kagey-Sobotka A, Lichtenstein LM, Schroeder JT. Differential regulation of IL-4 and IL-13 secretion by human basophils: their relationship to histamine release in mixed leukocyte cultures. J Immunol (1998) 160:1957–64.
79. Schroeder JT, MacGlashan DW Jr., Kagey-Sobotka A, White JM, Lichtenstein LM. IgE-dependent IL-4 secretion by human basophils. the relationship between cytokine production and histamine release in mixed leukocyte cultures. J Immunol (1994) 153:1808–17.
80. De Monte L, Wormann S, Brunetto E, Heltai S, Magliacane G, Reni M, et al. Basophil recruitment into tumor-draining lymph nodes correlates with Th2 inflammation and reduced survival in pancreatic cancer patients. Cancer Res (2016) 76:1792–803. doi: 10.1158/0008-5472.CAN-15-1801-T
81. Galeotti C, Stephen-Victor E, Karnam A, Das M, Gilardin L, Maddur MS, et al. Intravenous immunoglobulin induces IL-4 in human basophils by signaling through surface-bound IgE. J Allergy Clin Immunol (2019) 144:524–535 e8. doi: 10.1016/j.jaci.2018.10.064
82. Sharma M, Das M, Stephen-Victor E, Galeotti C, Karnam A, Maddur MS, et al. Regulatory T cells induce activation rather than suppression of human basophils. Sci Immunol (2018) 3:eaan0829. doi: 10.1126/sciimmunol.aan0829
83. Gibbs BF, Haas H, Falcone FH, Albrecht C, Vollrath IB, Noll T, et al. Purified human peripheral blood basophils release interleukin-13 and preformed interleukin-4 following immunological activation. Eur J Immunol (1996) 26:2493–8. doi: 10.1002/eji.1830261033
84. Li H, Sim TC, Alam R. IL-13 released by and localized in human basophils. J Immunol (1996) 156:4833–8.
85. Ochensberger B, Daepp GC, Rihs S, Dahinden CA. Human blood basophils produce interleukin-13 in response to IgE-receptor-dependent and -independent activation. Blood (1996) 88:3028–37. doi: 10.1182/blood.V88.8.3028.bloodjournal8883028
86. Piliponsky AM, Shubin NJ, Lahiri AK, Truong P, Clauson M, Niino K, et al. Basophil-derived tumor necrosis factor can enhance survival in a sepsis model in mice. Nat Immunol (2019) 20:129–40. doi: 10.1038/s41590-018-0288-7
87. Yuk CM, Park HJ, Kwon BI, Lah SJ, Chang J, Kim JY, et al. Basophil-derived IL-6 regulates TH17 cell differentiation and CD4 T cell immunity. Sci Rep (2017) 7:41744. doi: 10.1038/srep41744
88. Falkencrone S, Poulsen LK, Bindslev-Jensen C, Woetmann A, Odum N, Poulsen BC, et al. IgE-mediated basophil tumour necrosis factor alpha induces matrix metalloproteinase-9 from monocytes. Allergy (2013) 68:614–20. doi: 10.1111/all.12143
89. Chen K, Xu W, Wilson M, He B, Miller NW, Bengten E, et al. Immunoglobulin d enhances immune surveillance by activating antimicrobial, proinflammatory and b cell-stimulating programs in basophils. Nat Immunol (2009) 10:889–98. doi: 10.1038/ni.1748
90. Prevete N, Staiano RI, Granata F, Detoraki A, Necchi V, Ricci V, et al. Expression and function of angiopoietins and their tie receptors in human basophils and mast cells. J Biol Regul Homeost Agents (2013) 27:827–39.
91. Cerny-Reiterer S, Ghanim V, Hoermann G, Aichberger KJ, Herrmann H, Muellauer L, et al. Identification of basophils as a major source of hepatocyte growth factor in chronic myeloid leukemia: a novel mechanism of BCR-ABL1-independent disease progression. Neoplasia (2012) 14:572–84. doi: 10.1593/neo.12724
92. Qi Y, Operario DJ, Oberholzer CM, Kobie JJ, Looney RJ, Georas SN, et al. Human basophils express amphiregulin in response to T cell-derived IL-3. J Allergy Clin Immunol (2010) 126:1260–6.e4. doi: 10.1016/j.jaci.2010.08.040
93. Zaiss DMW, Gause WC, Osborne LC, Artis D. Emerging functions of amphiregulin in orchestrating immunity, inflammation, and tissue repair. Immunity (2015) 42:216–26. doi: 10.1016/j.immuni.2015.01.020
94. Meulenbroeks C, van Weelden H, Schwartz C, Voehringer D, Redegeld FAM, Rutten V, et al. Basophil-derived amphiregulin is essential for UVB irradiation-induced immune suppression. J Invest Dermatol (2015) 135:222–8. doi: 10.1038/jid.2014.329
95. Ohnmacht C, Voehringer D. Basophil effector function and homeostasis during helminth infection. Blood (2009) 113:2816–25. doi: 10.1182/blood-2008-05-154773
96. Wada T, Ishiwata K, Koseki H, Ishikura T, Ugajin T, Ohnuma N, et al. Selective ablation of basophils in mice reveals their nonredundant role in acquired immunity against ticks. J Clin Invest (2010) 120:2867–75. doi: 10.1172/JCI42680
97. Hayes MD, Ward S, Crawford G, Seoane RC, Jackson WD, Kipling D, et al. Inflammation-induced IgE promotes epithelial hyperplasia and tumour growth. Elife (2020) 9:e51862. doi: 10.7554/eLife.51862
98. Webb LM, Oyesola OO, Fruh SP, Kamynina E, Still KM, Patel RK, et al. The notch signaling pathway promotes basophil responses during helminth-induced type 2 inflammation. J Exp Med (2019) 216:1268–79. doi: 10.1084/jem.20180131
99. de Paulis A, Prevete N, Fiorentino I, Walls AF, Curto M, Petraroli A, et al. Basophils infiltrate human gastric mucosa at sites of helicobacter pylori infection, and exhibit chemotaxis in response to h. pylori-derived peptide Hp(2-20). J Immunol (2004) 172:7734–43. doi: 10.4049/jimmunol.172.12.7734
100. de Paulis A, Prevete N, Rossi FW, Rivellese F, Salerno F, Delfino G, et al. Helicobacter pylori Hp(2-20) promotes migration and proliferation of gastric epithelial cells by interacting with formyl peptide receptors in vitro and accelerates gastric mucosal healing in vivo. J Immunol (2009) 183:3761–9. doi: 10.4049/jimmunol.0900863
101. Guo CB, Liu MC, Galli SJ, Bochner BS, Kagey-Sobotka A, Lichtenstein LM. Identification of IgE-bearing cells in the late-phase response to antigen in the lung as basophils. Am J Respir Cell Mol Biol (1994) 10:384–90. doi: 10.1165/ajrcmb.10.4.7510984
102. Lavin Y, Kobayashi S, Leader A, Amir ED, Elefant N, Bigenwald C, et al. Innate immune landscape in early lung adenocarcinoma by paired single-cell analyses. Cell (2017) 169:750–765.e17. doi: 10.1016/j.cell.2017.04.014
103. Nouri-Aria KT, Irani AM, Jacobson MR, O'Brien F, Varga EM, Till SJ, et al. Basophil recruitment and IL-4 production during human allergen-induced late asthma. J Allergy Clin Immunol (2001) 108:205–11. doi: 10.1067/mai.2001.117175
104. Ito Y, Satoh T, Takayama K, Miyagishi C, Walls AF, Yokozeki H. Basophil recruitment and activation in inflammatory skin diseases. Allergy (2011) 66:1107–13. doi: 10.1111/j.1398-9995.2011.02570.x
105. DeNardo DG, Ruffell B. Macrophages as regulators of tumour immunity and immunotherapy. Nat Rev Immunol (2019) 19:369–82. doi: 10.1038/s41577-019-0127-6
106. Mantovani A, Marchesi F, Malesci A, Laghi L, Allavena P. Tumour-associated macrophages as treatment targets in oncology. Nat Rev Clin Oncol (2017) 14:399–416. doi: 10.1038/nrclinonc.2016.217
107. Balestrieri B, Granata F, Loffredo S, Petraroli A, Scalia G, Morabito P, et al. Phenotypic and functional heterogeneity of low-density and high-density human lung macrophages. Biomedicines (2021) 9:505. doi: 10.3390/biomedicines9050505
108. Pellefigues C, Mehta P, Chappell S, Yumnam B, Old S, Camberis M, et al. Diverse innate stimuli activate basophils through pathways involving syk and IkappaB kinases. Proc Natl Acad Sci U.S.A. (2021) 118:e2019524118. doi: 10.1073/pnas.2019524118
109. Schneider E, Petit-Bertron AF, Bricard R, Levasseur M, Ramadan A, Girard JP, et al. IL-33 activates unprimed murine basophils directly in vitro and induces their in vivo expansion indirectly by promoting hematopoietic growth factor production. J Immunol (2009) 183:3591–7. doi: 10.4049/jimmunol.0900328
110. Hsu CL, Chhiba KD, Krier-Burris R, Hosakoppal S, Berdnikovs S, Miller ML, et al. Allergic inflammation is initiated by IL-33-dependent crosstalk between mast cells and basophils. PloS One (2020) 15:e0226701. doi: 10.1371/journal.pone.0226701
111. Rignault-Bricard R, Machavoine F, Mecheri S, Hermine O, Schneider E, Dy M, et al. IL-3-producing basophils are required to exacerbate airway hyperresponsiveness in a murine inflammatory model. Allergy (2018) 73:2342–51. doi: 10.1111/all.13480
112. Voehringer D. Basophil modulation by cytokine instruction. Eur J Immunol (2012) 42:2544–50. doi: 10.1002/eji.201142318
113. Vivanco Gonzalez N, Oliveria JP, Tebaykin D, Ivison GT, Mukai K, Tsai MM, et al. Mass cytometry phenotyping of human granulocytes reveals novel basophil functional heterogeneity. iScience (2020) 23:101724. doi: 10.1016/j.isci.2020.101724
114. Marone G, Varricchi G, Loffredo S, Granata F. Mast cells and basophils in inflammatory and tumor angiogenesis and lymphangiogenesis. Eur J Pharmacol (2016) 778:146–51. doi: 10.1016/j.ejphar.2015.03.088
115. Marcella S, Petraroli A, Braile M, Parente R, Ferrara AL, Galdiero MR, et al. Vascular endothelial growth factors and angiopoietins as new players in mastocytosis. Clin Exp Med (2021) 21:415–27. doi: 10.1007/s10238-021-00693-0
116. Sammarco G, Varricchi G, Ferraro V, Ammendola M, De Fazio M, Altomare DF, et al. Mast cells, angiogenesis and lymphangiogenesis in human gastric cancer. Int J Mol Sci (2019) 20:2106. doi: 10.3390/ijms20092106
117. Varricchi G, Loffredo S, Borriello F, Pecoraro A, Rivellese F, Genovese A, et al. Superantigenic activation of human cardiac mast cells. Int J Mol Sci (2019) 20:1828. doi: 10.3390/ijms20081828
118. Varricchi G, Loffredo S, Galdiero MR, Marone G, Cristinziano L, Granata F, et al. Innate effector cells in angiogenesis and lymphangiogenesis. Curr Opin Immunol (2018) 53:152–60. doi: 10.1016/j.coi.2018.05.002
119. Hanahan D, Weinberg RA. Hallmarks of cancer: the next generation. Cell (2011) 144:646–74. doi: 10.1016/j.cell.2011.02.013
120. Detoraki A, Staiano RI, Granata F, Giannattasio G, Prevete N, de Paulis A, et al. Vascular endothelial growth factors synthesized by human lung mast cells exert angiogenic effects. J Allergy Clin Immunol (2009) 123:1142–9. doi: 10.1016/j.jaci.2009.01.044
121. Granata F, Frattini A, Loffredo S, Staiano RI, Petraroli A, Ribatti D, et al. Production of vascular endothelial growth factors from human lung macrophages induced by group IIA and group X secreted phospholipases A2. J Immunol (2010) 184:5232–41. doi: 10.4049/jimmunol.0902501
122. Loffredo S, Borriello F, Iannone R, Ferrara AL, Galdiero MR, Gigantino V, et al. Group V secreted phospholipase A2 induces the release of proangiogenic and antiangiogenic factors by human neutrophils. Front Immunol (2017) 8:443. doi: 10.3389/fimmu.2017.00443
123. Braile M, Fiorelli A, Sorriento D, Di Crescenzo RM, Galdiero MR, Marone G, et al. Human lung-resident macrophages express and are targets of thymic stromal lymphopoietin in the tumor microenvironment. Cells (2021) 10:2012. doi: 10.3390/cells10082012
124. Thomas M, Augustin HG. The role of the angiopoietins in vascular morphogenesis. Angiogenesis (2009) 12:125–37. doi: 10.1007/s10456-009-9147-3
125. Bilimoria J, Singh H. The angiopoietin ligands and tie receptors: potential diagnostic biomarkers of vascular disease. J Recept Signal Transduct Res (2019) 39:187–93. doi: 10.1080/10799893.2019.1652650
126. Leligdowicz A, Richard-Greenblatt M, Wright J, Crowley VM, Kain KC. Endothelial activation: The Ang/Tie axis in sepsis. Front Immunol (2018) 9:838. doi: 10.3389/fimmu.2018.00838
127. Poto R, Cristinziano L, Modestino L, de Paulis A, Marone G, Loffredo S, et al. Neutrophil extracellular traps, angiogenesis and cancer. Biomedicines (2022) 10:431. doi: 10.3390/biomedicines10020431
128. Jiang WG, Martin TA, Parr C, Davies G, Matsumoto K, Nakamura T. Hepatocyte growth factor, its receptor, and their potential value in cancer therapies. Crit Rev Oncol Hematol (2005) 53:35–69. doi: 10.1016/j.critrevonc.2004.09.004
129. Kanaoka Y, Austen KF. Roles of cysteinyl leukotrienes and their receptors in immune cell-related functions. Adv Immunol (2019) 142:65–84. doi: 10.1016/bs.ai.2019.04.002
130. Bankova LG, Dwyer DF, Yoshimoto E, Ualiyeva S, McGinty JW, Raff H, et al. The cysteinyl leukotriene 3 receptor regulates expansion of IL-25-producing airway brush cells leading to type 2 inflammation. Sci Immunol (2018) 3:eaat9453. doi: 10.1126/sciimmunol.aat9453
131. Bankova LG, Lai J, Yoshimoto E, Boyce JA, Austen KF, Kanaoka Y, et al. Leukotriene E4 elicits respiratory epithelial cell mucin release through the G-protein-coupled receptor, GPR99. Proc Natl Acad Sci U.S.A. (2016) 113:6242–7. doi: 10.1073/pnas.1605957113
132. Barrett NA, Fernandez JM, Maekawa A, Xing W, Li L, Parsons MW, et al. Cysteinyl leukotriene 2 receptor on dendritic cells negatively regulates ligand-dependent allergic pulmonary inflammation. J Immunol (2012) 189:4556–65. doi: 10.4049/jimmunol.1201865
133. Duah E, Teegala LR, Kondeti V, Adapala RK, Keshamouni VG, Kanaoka Y, et al. Cysteinyl leukotriene 2 receptor promotes endothelial permeability, tumor angiogenesis, and metastasis. Proc Natl Acad Sci U.S.A. (2019) 116:199–204. doi: 10.1073/pnas.1817325115
134. Marone G, Casolaro V, Paganelli R, Quinti I. IgG anti-IgE from atopic dermatitis induces mediator release from basophils and mast cells. J Invest Dermatol (1989) 93:246–52. doi: 10.1111/1523-1747.ep12277582
135. Papayannopoulos V. Neutrophil extracellular traps in immunity and disease. Nat Rev Immunol (2018) 18:134–47. doi: 10.1038/nri.2017.105
136. Yousefi S, Simon D, Stojkov D, Karsonova A, Karaulov A, Simon HU. In vivo evidence for extracellular DNA trap formation. Cell Death Dis (2020) 11:300. doi: 10.1038/s41419-020-2497-x
137. Varricchi G, Modestino L, Poto R, Cristinziano L, Gentile L, Postiglione L, et al. Neutrophil extracellular traps and neutrophil-derived mediators as possible biomarkers in bronchial asthma. Clin Exp Med (2022) 22:285–300. doi: 10.1007/s10238-021-00750-8
138. Yousefi S, Gold JA, Andina N, Lee JJ, Kelly AM, Kozlowski E, et al. Catapult-like release of mitochondrial DNA by eosinophils contributes to antibacterial defense. Nat Med (2008) 14:949–53. doi: 10.1038/nm.1855
139. Gevaert E, Zhang N, Krysko O, Lan F, Holtappels G, De Ruyck N, et al. Extracellular eosinophilic traps in association with staphylococcus aureus at the site of epithelial barrier defects in patients with severe airway inflammation. J Allergy Clin Immunol (2017) 139:1849–1860.e6. doi: 10.1016/j.jaci.2017.01.019
140. von Kockritz-Blickwede M, Goldmann O, Thulin P, Heinemann K, Norrby-Teglund A, Rohde M, et al. Phagocytosis-independent antimicrobial activity of mast cells by means of extracellular trap formation. Blood (2008) 111:3070–80. doi: 10.1182/blood-2007-07-104018
141. Lopes JP, Stylianou M, Nilsson G, Urban CF. Opportunistic pathogen candida albicans elicits a temporal response in primary human mast cells. Sci Rep (2015) 5:12287. doi: 10.1038/srep12287
142. Campillo-Navarro M, Leyva-Paredes K, Donis-Maturano L, Rodriguez-Lopez GM, Soria-Castro R, Garcia-Perez BE, et al. Mycobacterium tuberculosis catalase inhibits the formation of mast cell extracellular traps. Front Immunol (2018) 9:1161. doi: 10.3389/fimmu.2018.01161
143. Clark M, Kim J, Etesami N, Shimamoto J, Whalen RV, Martin G, et al. Group a streptococcus prevents mast cell degranulation to promote extracellular trap formation. Front Immunol (2018) 9:327. doi: 10.3389/fimmu.2018.00327
144. Liu P, Wu X, Liao C, Liu X, Du J, Shi H, et al. Escherichia coli and candida albicans induced macrophage extracellular trap-like structures with limited microbicidal activity. PloS One (2014) 9:e90042. doi: 10.1371/journal.pone.0090042
145. Zhang X, Zhang L, Tan YM, Liu YP, Li JJ, Deng QM, et al. Hepcidin gene silencing ameliorated inflammation and insulin resistance in adipose tissue of db/db mice via inhibiting METs formation. Mol Immunol (2021) 133:110–21. doi: 10.1016/j.molimm.2021.02.015
146. Okubo K, Kurosawa M, Kamiya M, Urano Y, Suzuki A, Yamamoto K, et al. Macrophage extracellular trap formation promoted by platelet activation is a key mediator of rhabdomyolysis-induced acute kidney injury. Nat Med (2018) 24:232–8. doi: 10.1038/nm.4462
147. Conceicao-Silva F, Reis CSM, De Luca PM, Leite-Silva J, Santiago MA, Morrot A, et al. The immune system throws its traps: Cells and their extracellular traps in disease and protection. Cells (2021) 10:1891. doi: 10.3390/cells10081891
148. Kummarapurugu AB, Zheng S, Ma J, Ghosh S, Hawkridge A, Voynow JA. Neutrophil elastase triggers the release of macrophage extracellular traps: Relevance to cystic fibrosis. Am J Respir Cell Mol Biol (2022) 66:76–85. doi: 10.1165/rcmb.2020-0410OC
149. Morshed M, Hlushchuk R, Simon D, Walls AF, Obata-Ninomiya K, Karasuyama H, et al. NADPH oxidase-independent formation of extracellular DNA traps by basophils. J Immunol (2014) 192:5314–23. doi: 10.4049/jimmunol.1303418
150. Yousefi S, Morshed M, Amini P, Stojkov D, Simon D, von Gunten S, et al. Basophils exhibit antibacterial activity through extracellular trap formation. Allergy (2015) 70:1184–8. doi: 10.1111/all.12662
151. Brinkmann V, Reichard U, Goosmann C, Fauler B, Uhlemann Y, Weiss DS, et al. Neutrophil extracellular traps kill bacteria. Science (2004) 303:1532–5. doi: 10.1126/science.1092385
152. Jaillon S, Peri G, Delneste Y, Fremaux I, Doni A, Moalli F, et al. The humoral pattern recognition receptor PTX3 is stored in neutrophil granules and localizes in extracellular traps. J Exp Med (2007) 204:793–804. doi: 10.1084/jem.20061301
153. Pilsczek FH, Salina D, Poon KK, Fahey C, Yipp BG, Sibley CD, et al. A novel mechanism of rapid nuclear neutrophil extracellular trap formation in response to staphylococcus aureus. J Immunol (2010) 185:7413–25. doi: 10.4049/jimmunol.1000675
154. Yipp BG, Petri B, Salina D, Jenne CN, Scott BN, Zbytnuik LD, et al. Infection-induced NETosis is a dynamic process involving neutrophil multitasking in vivo. Nat Med (2012) 18:1386–93. doi: 10.1038/nm.2847
155. Jorch SK, Kubes P. An emerging role for neutrophil extracellular traps in noninfectious disease. Nat Med (2017) 23:279–87. doi: 10.1038/nm.4294
156. Cools-Lartigue J, Spicer J, McDonald B, Gowing S, Chow S, Giannias B, et al. Neutrophil extracellular traps sequester circulating tumor cells and promote metastasis. J Clin Invest (2013) 123:3446–58. doi: 10.1172/JCI67484
157. Park J, Wysocki RW, Amoozgar Z, Maiorino L, Fein MR, Jorns J, et al. Cancer cells induce metastasis-supporting neutrophil extracellular DNA traps. Sci Transl Med (2016) 8:361ra138. doi: 10.1126/scitranslmed.aag1711
158. Teijeira A, Garasa S, Gato M, Alfaro C, Migueliz I, Cirella A, et al. CXCR1 and CXCR2 chemokine receptor agonists produced by tumors induce neutrophil extracellular traps that interfere with immune cytotoxicity. Immunity (2020) 52:856–871.e8. doi: 10.1016/j.immuni.2020.03.001
159. Yang L, Liu Q, Zhang X, Liu X, Zhou B, Chen J, et al. DNA Of neutrophil extracellular traps promotes cancer metastasis via CCDC25. Nature (2020) 583:133–8. doi: 10.1038/s41586-020-2394-6
160. Albrengues J, Shields MA, Ng D, Park CG, Ambrico A, Poindexter ME, et al. Neutrophil extracellular traps produced during inflammation awaken dormant cancer cells in mice. Science (2018) 361:eaao4227. doi: 10.1126/science.aao4227
161. Wolach O, Sellar RS, Martinod K, Cherpokova D, McConkey M, Chappell RJ, et al. Increased neutrophil extracellular trap formation promotes thrombosis in myeloproliferative neoplasms. Sci Transl Med (2018) 10:eaan8292. doi: 10.1126/scitranslmed.aan8292
162. Cristinziano L, Modestino L, Loffredo S, Varricchi G, Braile M, Ferrara AL, et al. Anaplastic thyroid cancer cells induce the release of mitochondrial extracellular DNA traps by viable neutrophils. J Immunol (2020) 204:1362–72. doi: 10.4049/jimmunol.1900543
163. Schorn C, Janko C, Latzko M, Chaurio R, Schett G, Herrmann M. Monosodium urate crystals induce extracellular DNA traps in neutrophils, eosinophils, and basophils but not in mononuclear cells. Front Immunol (2012) 3:277. doi: 10.3389/fimmu.2012.00277
164. Marone G, Triggiani M, de Paulis A. Mast cells and basophils: friends as well as foes in bronchial asthma? Trends Immunol (2005) 26:25–31. doi: 10.1016/j.it.2004.10.010
165. Bochner BS, Lichtenstein LM. Anaphylaxis. N Engl J Med (1991) 324:1785–90. doi: 10.1056/NEJM199106203242506
166. Borriello F, Granata F, Varricchi G, Genovese A, Triggiani M, Marone G. Immunopharmacological modulation of mast cells. Curr Opin Pharmacol (2014) 17:45–57. doi: 10.1016/j.coph.2014.07.002
167. Reber LL, Hernandez JD, Galli SJ. The pathophysiology of anaphylaxis. J Allergy Clin Immunol (2017) 140:335–48. doi: 10.1016/j.jaci.2017.06.003
168. Tsujimura Y, Obata K, Mukai K, Shindou H, Yoshida M, Nishikado H, et al. Basophils play a pivotal role in immunoglobulin-g-mediated but not immunoglobulin-e-mediated systemic anaphylaxis. Immunity (2008) 28:581–9. doi: 10.1016/j.immuni.2008.02.008
169. Mukai K, Obata K, Tsujimura Y, Karasuyama H. New insights into the roles for basophils in acute and chronic allergy. Allergol Int (2009) 58:11–9. doi: 10.2332/allergolint.08-RAI-0059
170. Korosec P, Turner PJ, Silar M, Kopac P, Kosnik M, Gibbs BF, et al. Basophils, high-affinity IgE receptors, and CCL2 in human anaphylaxis. J Allergy Clin Immunol (2017) 140:750–758.e15. doi: 10.1016/j.jaci.2016.12.989
171. Liu MC, Hubbard WC, Proud D, Stealey BA, Galli SJ, Kagey-Sobotka A, et al. Immediate and late inflammatory responses to ragweed antigen challenge of the peripheral airways in allergic asthmatics. cellular, mediator, and permeability changes. Am Rev Respir Dis (1991) 144:51–8. doi: 10.1164/ajrccm/144.1.51
172. Gauvreau GM, Lee JM, Watson RM, Irani AM, Schwartz LB, O'Byrne PM. Increased numbers of both airway basophils and mast cells in sputum after allergen inhalation challenge of atopic asthmatics. Am J Respir Crit Care Med (2000) 161:1473–8. doi: 10.1164/ajrccm.161.5.9908090
173. Koshino T, Teshima S, Fukushima N, Takaishi T, Hirai K, Miyamoto Y, et al. Identification of basophils by immunohistochemistry in the airways of post-mortem cases of fatal asthma. Clin Exp Allergy (1993) 23:919–25. doi: 10.1111/j.1365-2222.1993.tb00276.x
174. KleinJan A, McEuen AR, Dijkstra MD, Buckley MG, Walls AF, Fokkens WJ. Basophil and eosinophil accumulation and mast cell degranulation in the nasal mucosa of patients with hay fever after local allergen provocation. J Allergy Clin Immunol (2000) 106:677–86. doi: 10.1067/mai.2000.109621
175. Casolaro V, Spadaro G, Marone G. Human basophil releasability. VI. changes in basophil releasability in patients with allergic rhinitis or bronchial asthma. Am Rev Respir Dis (1990) 142:1108–11. doi: 10.1164/ajrccm/142.5.1108
176. Schroeder JT, Bieneman AP, Chichester KL, Breslin L, Xiao H, Liu MC. Pulmonary allergic responses augment interleukin-13 secretion by circulating basophils yet suppress interferon-alpha from plasmacytoid dendritic cells. Clin Exp Allergy (2010) 40:745–54. doi: 10.1111/j.1365-2222.2010.03456.x
177. Schroeder JT, Lichtenstein LM, Roche EM, Xiao H, Liu MC. IL-4 production by human basophils found in the lung following segmental allergen challenge. J Allergy Clin Immunol (2001) 107:265–71. doi: 10.1067/mai.2001.112846
178. Brooks CR, van Dalen CJ, Hermans IF, Gibson PG, Simpson JL, Douwes J. Sputum basophils are increased in eosinophilic asthma compared with non-eosinophilic asthma phenotypes. Allergy (2017) 72:1583–6. doi: 10.1111/all.13185
179. Suzuki Y, Wakahara K, Nishio T, Ito S, Hasegawa Y. Airway basophils are increased and activated in eosinophilic asthma. Allergy (2017) 72:1532–9. doi: 10.1111/all.13197
180. Leffler J, Jones AC, Hollams EM, Prastanti F, Le Souef PN, Holt PG, et al. Basophil counts in PBMC populations during childhood acute wheeze/asthma are associated with future exacerbations. J Allergy Clin Immunol (2018) 142:1639–1641.e5. doi: 10.1016/j.jaci.2018.07.003
181. Hill DA, Siracusa MC, Ruymann KR, Tait Wojno ED, Artis D, Spergel JM. Omalizumab therapy is associated with reduced circulating basophil populations in asthmatic children. Allergy (2014) 69:674–7. doi: 10.1111/all.12375
182. Nair P, Wenzel S, Rabe KF, Bourdin A, Lugogo NL, Kuna P, et al. Oral glucocorticoid-sparing effect of benralizumab in severe asthma. N Engl J Med (2017) 376:2448–58. doi: 10.1056/NEJMoa1703501
183. Numata T, Miyagawa H, Nishioka S, Okuda K, Utsumi H, Hashimoto M, et al. Efficacy of benralizumab for patients with severe eosinophilic asthma: a retrospective, real-life study. BMC Pulm Med (2020) 20:207. doi: 10.1186/s12890-020-01248-x
184. Lommatzsch M, Marchewski H, Schwefel G, Stoll P, Virchow JC, Bratke K. Benralizumab strongly reduces blood basophils in severe eosinophilic asthma. Clin Exp Allergy (2020) 50:1267–9. doi: 10.1111/cea.13720
185. Wakahara K, Van VQ, Baba N, Begin P, Rubio M, Delespesse G, et al. Basophils are recruited to inflamed lungs and exacerbate memory Th2 responses in mice and humans. Allergy (2013) 68:180–9. doi: 10.1111/all.12072
186. Cheng LE, Sullivan BM, Retana LE, Allen CD, Liang HE, Locksley RM. IgE-activated basophils regulate eosinophil tissue entry by modulating endothelial function. J Exp Med (2015) 212:513–24. doi: 10.1084/jem.20141671
188. Zuberbier T, Abdul Latiff AH, Abuzakouk M, Aquilina S, Asero R, Baker D, et al. The international EAACI/GA(2)LEN/EuroGuiDerm/APAAACI guideline for the definition, classification, diagnosis, and management of urticaria. Allergy (2022) 77:734–66. doi: 10.1111/all.15090
189. Vonakis BM, Saini SS. New concepts in chronic urticaria. Curr Opin Immunol (2008) 20:709–16. doi: 10.1016/j.coi.2008.09.005
190. Saini SS, Omachi TA, Trzaskoma B, Hulter HN, Rosen K, Sterba PM, et al. Effect of omalizumab on blood basophil counts in patients with chronic Idiopathic/Spontaneous urticaria. J Invest Dermatol (2017) 137:958–61. doi: 10.1016/j.jid.2016.11.025
191. Ying S, Kikuchi Y, Meng Q, Kay AB, Kaplan AP. TH1/TH2 cytokines and inflammatory cells in skin biopsy specimens from patients with chronic idiopathic urticaria: comparison with the allergen-induced late-phase cutaneous reaction. J Allergy Clin Immunol (2002) 109:694–700. doi: 10.1067/mai.2002.123236
192. Rorsman H. Basopenia in urticaria. Acta Allergol (1961) 16:185–215. doi: 10.1111/j.1398-9995.1961.tb02894.x
193. Caproni M, Giomi B, Volpi W, Melani L, Schincaglia E, Macchia D, et al. Chronic idiopathic urticaria: infiltrating cells and related cytokines in autologous serum-induced wheals. Clin Immunol (2005) 114:284–92. doi: 10.1016/j.clim.2004.10.007
194. Grattan CE, Dawn G, Gibbs S, Francis DM. Blood basophil numbers in chronic ordinary urticaria and healthy controls: diurnal variation, influence of loratadine and prednisolone and relationship to disease activity. Clin Exp Allergy (2003) 33:337–41. doi: 10.1046/j.1365-2222.2003.01589.x
195. Kern F, Lichtenstein LM. Defective histamine release in chronic urticaria. J Clin Invest (1976) 57:1369–77. doi: 10.1172/JCI108405
196. Vasagar K, Vonakis BM, Gober LM, Viksman A, Gibbons SP Jr., Saini SS. Evidence of in vivo basophil activation in chronic idiopathic urticaria. Clin Exp Allergy (2006) 36:770–6. doi: 10.1111/j.1365-2222.2006.02494.x
197. Rauber MM, Pickert J, Holiangu L, Mobs C, Pfutzner W. Functional and phenotypic analysis of basophils allows determining distinct subtypes in patients with chronic urticaria. Allergy (2017) 72:1904–11. doi: 10.1111/all.13215
198. Oda Y, Fukunaga A, Washio K, Imamura S, Hatakeyama M, Ogura K, et al. Low responsiveness of basophils via FcepsilonRI reflects disease activity in chronic spontaneous urticaria. J Allergy Clin Immunol Pract (2019) 7:2835–2844 e7. doi: 10.1016/j.jaip.2019.05.020
199. Alhossan A, Lee CS, MacDonald K, Abraham I. "Real-life" effectiveness studies of omalizumab in adult patients with severe allergic asthma: Meta-analysis. J Allergy Clin Immunol Pract (2017) 5:1362–1370 e2. doi: 10.1016/j.jaip.2017.02.002
200. Esquivel A, Busse WW, Calatroni A, Togias AG, Grindle KG, Bochkov YA, et al. Effects of omalizumab on rhinovirus infections, illnesses, and exacerbations of asthma. Am J Respir Crit Care Med (2017) 196:985–92. doi: 10.1164/rccm.201701-0120OC
201. Maurer M, Rosen K, Hsieh HJ, Saini S, Grattan C, Gimenez-Arnau A, et al. Omalizumab for the treatment of chronic idiopathic or spontaneous urticaria. N Engl J Med (2013) 368:924–35. doi: 10.1056/NEJMoa1215372
202. Zaidi AK, Saini SS, Macglashan DW Jr. Regulation of syk kinase and FcRbeta expression in human basophils during treatment with omalizumab. J Allergy Clin Immunol (2010) 125:902–908.e7. doi: 10.1016/j.jaci.2009.12.996
203. MacGlashan DW Jr., Saini SS. Syk expression and IgE-mediated histamine release in basophils as biomarkers for predicting the clinical efficacy of omalizumab. J Allergy Clin Immunol (2017) 139:1680–1682.e10. doi: 10.1016/j.jaci.2016.12.965
204. MacGlashan D Jr., Saini S, Schroeder JT. Response of peripheral blood basophils in subjects with chronic spontaneous urticaria during treatment with omalizumab. J Allergy Clin Immunol (2021) 147:2295–2304.e12. doi: 10.1016/j.jaci.2021.02.039
205. Weidinger S, Beck LA, Bieber T, Kabashima K, Irvine AD. Atopic dermatitis. Nat Rev Dis Primers (2018) 4:1. doi: 10.1038/s41572-018-0001-z
206. Oetjen LK, Mack MR, Feng J, Whelan TM, Niu H, Guo CJ, et al. Sensory neurons Co-opt classical immune signaling pathways to mediate chronic itch. Cell (2017) 171:217–228.e13. doi: 10.1016/j.cell.2017.08.006
207. Eichenfield LF, Tom WL, Chamlin SL, Feldman SR, Hanifin JM, Simpson EL, et al. Guidelines of care for the management of atopic dermatitis: section 1. diagnosis and assessment of atopic dermatitis. J Am Acad Dermatol (2014) 70:338–51. doi: 10.1016/j.jaad.2013.10.010
208. Savinko T, Matikainen S, Saarialho-Kere U, Lehto M, Wang G, Lehtimaki S, et al. IL-33 and ST2 in atopic dermatitis: expression profiles and modulation by triggering factors. J Invest Dermatol (2012) 132:1392–400. doi: 10.1038/jid.2011.446
209. Imai Y, Yasuda K, Nagai M, Kusakabe M, Kubo M, Nakanishi K, et al. IL-33-Induced atopic dermatitis-like inflammation in mice is mediated by group 2 innate lymphoid cells in concert with basophils. J Invest Dermatol (2019) 139:2185–2194.e3. doi: 10.1016/j.jid.2019.04.016
210. Voisin T, Perner C, Messou MA, Shiers S, Ualiyeva S, Kanaoka Y, et al. The CysLT2R receptor mediates leukotriene C4-driven acute and chronic itch. Proc Natl Acad Sci U.S.A. (2021) 118:e2022087118. doi: 10.1073/pnas.2022087118
211. Poto R, Quinti I, Marone G, Taglialatela M, de Paulis A, Casolaro V, et al. IgG autoantibodies against IgE from atopic dermatitis can induce the release of cytokines and proinflammatory mediators from basophils and mast cells. Front Immunol (2022) 13:880412. doi: 10.3389/fimmu.2022.880412
212. May CD. High spontaneous release of histamine in vitro from leukocytes of persons hypersensitive to food. J Allergy Clin Immunol (1976) 58:432–7. doi: 10.1016/0091-6749(76)90124-x
213. Sampson HA, Broadbent KR, Bernhisel-Broadbent J. Spontaneous release of histamine from basophils and histamine-releasing factor in patients with atopic dermatitis and food hypersensitivity. N Engl J Med (1989) 321:228–32. doi: 10.1056/NEJM198907273210405
214. Schroeder JT, Bieneman AP, Chichester KL, Keet CA, Hamilton RG, MacGlashan DW Jr., et al. Spontaneous basophil responses in food-allergic children are transferable by plasma and are IgE-dependent. J Allergy Clin Immunol (2013) 132:1428–31. doi: 10.1016/j.jaci.2013.08.033
215. Keet CA, Frischmeyer-Guerrerio PA, Thyagarajan A, Schroeder JT, Hamilton RG, Boden S, et al. The safety and efficacy of sublingual and oral immunotherapy for milk allergy. J Allergy Clin Immunol (2012) 129:448–55, 455 e1-5. doi: 10.1016/j.jaci.2011.10.023
216. Brandt EB, Bolcas PE, Ruff BP, Khurana Hershey GK. TSLP contributes to allergic airway inflammation induced by diesel exhaust particle exposure in an experimental model of severe asthma. Clin Exp Allergy (2020) 50:121–4. doi: 10.1111/cea.13512
217. Kita H. Gasdermin d pores for IL-33 release. Nat Immunol (2022) 23:989–91. doi: 10.1038/s41590-022-01249-4
218. de Kleer IM, Kool M, de Bruijn MJ, Willart M, van Moorleghem J, Schuijs MJ, et al. Perinatal activation of the interleukin-33 pathway promotes type 2 immunity in the developing lung. Immunity (2016) 45:1285–98. doi: 10.1016/j.immuni.2016.10.031
219. Barlow JL, Peel S, Fox J, Panova V, Hardman CS, Camelo A, et al. IL-33 is more potent than IL-25 in provoking IL-13-producing nuocytes (type 2 innate lymphoid cells) and airway contraction. J Allergy Clin Immunol (2013) 132:933–41. doi: 10.1016/j.jaci.2013.05.012
220. Varricchi G, Bagnasco D, Borriello F, Heffler E, Canonica GW. Interleukin-5 pathway inhibition in the treatment of eosinophilic respiratory disorders: evidence and unmet needs. Curr Opin Allergy Clin Immunol (2016) 16:186–200. doi: 10.1097/ACI.0000000000000251
221. Varricchi G, Canonica GW. The role of interleukin 5 in asthma. Expert Rev Clin Immunol (2016) 12:903–5. doi: 10.1080/1744666X.2016.1208564
222. Hida S, Yamasaki S, Sakamoto Y, Takamoto M, Obata K, Takai T, et al. Fc receptor gamma-chain, a constitutive component of the IL-3 receptor, is required for IL-3-induced IL-4 production in basophils. Nat Immunol (2009) 10:214–22. doi: 10.1038/ni.1686
223. Schroeder JT, Adeosun AA, Do D, Bieneman AP. Galectin-3 is essential for IgE-dependent activation of human basophils by A549 lung epithelial cells. J Allergy Clin Immunol (2019) 144:312–315.e1. doi: 10.1016/j.jaci.2019.03.001
224. Miyake K, Shibata S, Yoshikawa S, Karasuyama H. Basophils and their effector molecules in allergic disorders. Allergy (2021) 76:1693–706. doi: 10.1111/all.14662
225. Varricchi G, Bencivenga L, Poto R, Pecoraro A, Shamji MH, Rengo G. The emerging role of T follicular helper (TFH) cells in aging: Influence on the immune frailty. Ageing Res Rev (2020) 61:101071. doi: 10.1016/j.arr.2020.101071
226. Gowthaman U, Chen JS, Zhang B, Flynn WF, Lu Y, Song W, et al. Identification of a T follicular helper cell subset that drives anaphylactic IgE. Science (2019) 365:eaaw6433. doi: 10.1126/science.aaw6433
227. Egawa M, Mukai K, Yoshikawa S, Iki M, Mukaida N, Kawano Y, et al. Inflammatory monocytes recruited to allergic skin acquire an anti-inflammatory M2 phenotype via basophil-derived interleukin-4. Immunity (2013) 38:570–80. doi: 10.1016/j.immuni.2012.11.014
228. Bhattacharjee A, Shukla M, Yakubenko VP, Mulya A, Kundu S, Cathcart MK. IL-4 and IL-13 employ discrete signaling pathways for target gene expression in alternatively activated monocytes/macrophages. Free Radic Biol Med (2013) 54:1–16. doi: 10.1016/j.freeradbiomed.2012.10.553
229. Marone G, Granata F, Pucino V, Pecoraro A, Heffler E, Loffredo S, et al. The intriguing role of interleukin 13 in the pathophysiology of asthma. Front Pharmacol (2019) 10:1387. doi: 10.3389/fphar.2019.01387
230. Marone G. Basophils, historical reflections and perspectives. In: Bergman, Ring, editors. History of allergy (Basel: Karger), vol. 214 (2014).
231. Bochner BS, Klunk DA, Sterbinsky SA, Coffman RL, Schleimer RP. IL-13 selectively induces vascular cell adhesion molecule-1 expression in human endothelial cells. J Immunol (1995) 154:799–803.
232. Spivak JL. How I treat polycythemia vera. Blood (2019) 134:341–52. doi: 10.1182/blood.2018834044
233. Vannucchi AM, Antonioli E, Guglielmelli P, Rambaldi A, Barosi G, Marchioli R, et al. Clinical profile of homozygous JAK2 617V>F mutation in patients with polycythemia vera or essential thrombocythemia. Blood (2007) 110:840–6. doi: 10.1182/blood-2006-12-064287
234. Tefferi A, Barbui T. Polycythemia vera and essential thrombocythemia: 2019 update on diagnosis, risk-stratification and management. Am J Hematol (2019) 94:133–43. doi: 10.1002/ajh.25303
235. Tefferi A, Lavu S, Mudireddy M, Lasho TL, Finke CM, Gangat N, et al. JAK2 exon 12 mutated polycythemia vera: Mayo-careggi MPN alliance study of 33 consecutive cases and comparison with JAK2V617F mutated disease. Am J Hematol (2018) 93:E93–6. doi: 10.1002/ajh.25017
236. Ishii T, Wang J, Zhang W, Mascarenhas J, Hoffman R, Dai Y, et al. Pivotal role of mast cells in pruritogenesis in patients with myeloproliferative disorders. Blood (2009) 113:5942–50. doi: 10.1182/blood-2008-09-179416
237. Hashimoto T, Rosen JD, Sanders KM, Yosipovitch G. Possible roles of basophils in chronic itch. Exp Dermatol (2019) 28:1373–9. doi: 10.1111/exd.13705
238. Pieri L, Bogani C, Guglielmelli P, Zingariello M, Rana RA, Bartalucci N, et al. The JAK2V617 mutation induces constitutive activation and agonist hypersensitivity in basophils from patients with polycythemia vera. Haematologica (2009) 94:1537–45. doi: 10.3324/haematol.2009.007047
239. Denburg JA, Browman G. Prognostic implications of basophil differentiation in chronic myeloid leukemia. Am J Hematol (1988) 27:110–4. doi: 10.1002/ajh.2830270208
240. Beer PA, Knapp DJ, Miller PH, Kannan N, Sloma I, Heel K, et al. Disruption of IKAROS activity in primitive chronic-phase CML cells mimics myeloid disease progression. Blood (2015) 125:504–15. doi: 10.1182/blood-2014-06-581173
241. Baba T, Naka K, Morishita S, Komatsu N, Hirao A, Mukaida N. MIP-1alpha/CCL3-mediated maintenance of leukemia-initiating cells in the initiation process of chronic myeloid leukemia. J Exp Med (2013) 210:2661–73. doi: 10.1084/jem.20130112
242. Baba T, Tanabe Y, Yoshikawa S, Yamanishi Y, Morishita S, Komatsu N, et al. MIP-1alpha/CCL3-expressing basophil-lineage cells drive the leukemic hematopoiesis of chronic myeloid leukemia in mice. Blood (2016) 127:2607–17. doi: 10.1182/blood-2015-10-673087
243. Matsushima T, Handa H, Yokohama A, Nagasaki J, Koiso H, Kin Y, et al. Prevalence and clinical characteristics of myelodysplastic syndrome with bone marrow eosinophilia or basophilia. Blood (2003) 101:3386–90. doi: 10.1182/blood-2002-03-0947
244. Wimazal F, Germing U, Kundi M, Noesslinger T, Blum S, Geissler P, et al. Evaluation of the prognostic significance of eosinophilia and basophilia in a larger cohort of patients with myelodysplastic syndromes. Cancer (2010) 116:2372–81. doi: 10.1002/cncr.25036
245. Liu Q, Luo D, Cai S, Li Q, Li X. Circulating basophil count as a prognostic marker of tumor aggressiveness and survival outcomes in colorectal cancer. Clin Transl Med (2020) 9:6. doi: 10.1186/s40169-019-0255-4
246. Wagner NB, Luttermann F, Gassenmaier M, Forschner A, Leiter U, Garbe C, et al. Absolute and relative differential blood count predicts survival of AJCC stage I-II melanoma patients scheduled for sentinel lymph node biopsy. Australas J Dermatol (2020) 61:e310–8. doi: 10.1111/ajd.13248
247. Rosner S, Kwong E, Shoushtari AN, Friedman CF, Betof AS, Brady MS, et al. Peripheral blood clinical laboratory variables associated with outcomes following combination nivolumab and ipilimumab immunotherapy in melanoma. Cancer Med (2018) 7:690–7. doi: 10.1002/cam4.1356
248. Bax HJ, Chauhan J, Stavraka C, Khiabany A, Nakamura M, Pellizzari G, et al. Basophils from cancer patients respond to immune stimuli and predict clinical outcome. Cells (2020) 9:1631. doi: 10.3390/cells9071631
249. Wei Y, Zhang X, Wang G, Zhou Y, Luo M, Wang S, et al. The impacts of pretreatment circulating eosinophils and basophils on prognosis of stage - colorectal cancer. Asia Pac J Clin Oncol (2018) 14:e243–51. doi: 10.1111/ajco.12871
250. Wu J, Ge XX, Zhu W, Zhi Q, Xu MD, Duan W, et al. Values of applying white blood cell counts in the prognostic evaluation of resectable colorectal cancer. Mol Med Rep (2019) 19:2330–40. doi: 10.3892/mmr.2019.9844
251. Hiltbrunner S, Spohn ML, Wechsler R, Akhoundova D, Bankel L, Kasser S, et al. Comprehensive statistical exploration of prognostic (Bio-)Markers for responses to immune checkpoint inhibitor in patients with non-small cell lung cancer. Cancers (Basel) (2021) 14:75. doi: 10.3390/cancers14010075
252. Zheng L, Yu M, Zhang S. Prognostic value of pretreatment circulating basophils in patients with glioblastoma. Neurosurg Rev (2021) 44:3471–8. doi: 10.1007/s10143-021-01524-2
253. Hadadi A, Smith KE, Wan L, Brown JR, Russler G, Yantorni L, et al. Baseline basophil and basophil-to-lymphocyte status is associated with clinical outcomes in metastatic hormone sensitive prostate cancer. Urol Oncol (2022) 40:271.e9–271.e18. doi: 10.1016/j.urolonc.2022.03.016
254. Ferro M, Di Lorenzo G, Vartolomei MD, Bruzzese D, Cantiello F, Lucarelli G, et al. Absolute basophil count is associated with time to recurrence in patients with high-grade T1 bladder cancer receiving bacillus calmette-guerin after transurethral resection of the bladder tumor. World J Urol (2020) 38:143–50. doi: 10.1007/s00345-019-02754-2
255. Wu C, Qiu Y, Zhang R, Li X, Liang H, Wang M, et al. Association of peripheral basophils with tumor M2 macrophage infiltration and outcomes of the anti-PD-1 inhibitor plus chemotherapy combination in advanced gastric cancer. J Transl Med (2022) 20:386. doi: 10.1186/s12967-022-03598-y
256. Varricchi G, Ameri P, Cadeddu C, Ghigo A, Madonna R, Marone G, et al. Antineoplastic drug-induced cardiotoxicity: A redox perspective. Front Physiol (2018) 9:167. doi: 10.3389/fphys.2018.00167
257. Marone G, Schroeder JT, Mattei F, Loffredo S, Gambardella AR, Poto R, et al. Is there a role for basophils in cancer? Front Immunol (2020) 11:2103. doi: 10.3389/fimmu.2020.02103
258. Wang C, Chen YG, Gao JL, Lyu GY, Su J, Zhang QI, et al. Low local blood perfusion, high white blood cell and high platelet count are associated with primary tumor growth and lung metastasis in a 4T1 mouse breast cancer metastasis model. Oncol Lett (2015) 10:754–60. doi: 10.3892/ol.2015.3304
259. Cihan YB, Arslan A, Cetindag MF, Mutlu H. Lack of prognostic value of blood parameters in patients receiving adjuvant radiotherapy for breast cancer. Asian Pac J Cancer Prev (2014) 15:4225–31. doi: 10.7314/apjcp.2014.15.10.4225
260. Rodriguez Gomez M, Talke Y, Goebel N, Hermann F, Reich B, Mack M. Basophils support the survival of plasma cells in mice. J Immunol (2010) 185:7180–5. doi: 10.4049/jimmunol.1002319
261. Chauhan J, Stavraka C, Grandits M, Palhares L, Josephs DH, Lacy KE, et al. Clinical and translational significance of basophils in patients with cancer. Cells (2022) 11:438. doi: 10.3390/cells11030438
262. Stankovic B, Bjorhovde HAK, Skarshaug R, Aamodt H, Frafjord A, Muller E, et al. Immune cell composition in human non-small cell lung cancer. Front Immunol (2018) 9:3101. doi: 10.3389/fimmu.2018.03101
263. Borriello F, Longo M, Spinelli R, Pecoraro A, Granata F, Staiano RI, et al. IL-3 synergises with basophil-derived IL-4 and IL-13 to promote the alternative activation of human monocytes. Eur J Immunol (2015) 45:2042–51. doi: 10.1002/eji.201445303
264. Kuroda E, Ho V, Ruschmann J, Antignano F, Hamilton M, Rauh MJ, et al. SHIP represses the generation of IL-3-induced M2 macrophages by inhibiting IL-4 production from basophils. J Immunol (2009) 183:3652–60. doi: 10.4049/jimmunol.0900864
265. Pernot S, Evrard S, Khatib AM. The give-and-Take interaction between the tumor microenvironment and immune cells regulating tumor progression and repression. Front Immunol (2022) 13:850856. doi: 10.3389/fimmu.2022.850856
266. Sciacchitano S, Lavra L, Morgante A, Ulivieri A, Magi F, De Francesco GP, et al. Galectin-3: One molecule for an alphabet of diseases, from a to z. Int J Mol Sci (2018) 19:379. doi: 10.3390/ijms19020379
267. Storti P, Marchica V, Giuliani N. Role of galectins in multiple myeloma. Int J Mol Sci (2017) 18:2740. doi: 10.3390/ijms18122740
268. Sadtler K, Wolf MT, Ganguly S, Moad CA, Chung L, Majumdar S, et al. Divergent immune responses to synthetic and biological scaffolds. Biomaterials (2019) 192:405–15. doi: 10.1016/j.biomaterials.2018.11.002
269. Sicklinger F, Meyer IS, Li X, Radtke D, Dicks S, Kornadt MP, et al. Basophils balance healing after myocardial infarction via IL-4/IL-13. J Clin Invest (2021) 131:e136778. doi: 10.1172/JCI136778
270. Sektioglu IM, Carretero R, Bulbuc N, Bald T, Tuting T, Rudensky AY, et al. Basophils promote tumor rejection via chemotaxis and infiltration of CD8+ T cells. Cancer Res (2017) 77:291–302. doi: 10.1158/0008-5472.CAN-16-0993
271. Hammad H, Plantinga M, Deswarte K, Pouliot P, Willart MA, Kool M, et al. Inflammatory dendritic cells–not basophils–are necessary and sufficient for induction of Th2 immunity to inhaled house dust mite allergen. J Exp Med (2010) 207:2097–111. doi: 10.1084/jem.20101563
272. Hubner MP, Larson D, Torrero MN, Mueller E, Shi Y, Killoran KE, et al. Anti-FcepsilonR1 antibody injections activate basophils and mast cells and delay type 1 diabetes onset in NOD mice. Clin Immunol (2011) 141:205–17. doi: 10.1016/j.clim.2011.08.004
273. Lucarini V, Ziccheddu G, Macchia I, La Sorsa V, Peschiaroli F, Buccione C, et al. IL-33 restricts tumor growth and inhibits pulmonary metastasis in melanoma-bearing mice through eosinophils. Oncoimmunology (2017) 6:e1317420. doi: 10.1080/2162402X.2017.1317420
274. Andreone S, Spadaro F, Buccione C, Mancini J, Tinari A, Sestili P, et al. IL-33 promotes CD11b/CD18-mediated adhesion of eosinophils to cancer cells and synapse-polarized degranulation leading to tumor cell killing. Cancers (Basel) (2019) 11:1664. doi: 10.3390/cancers11111664
275. Ohnmacht C, Schwartz C, Panzer M, Schiedewitz I, Naumann R, Voehringer D. Basophils orchestrate chronic allergic dermatitis and protective immunity against helminths. Immunity (2010) 33:364–74. doi: 10.1016/j.immuni.2010.08.011
276. Crawford G, Hayes MD, Seoane RC, Ward S, Dalessandri T, Lai C, et al. Epithelial damage and tissue gammadelta T cells promote a unique tumor-protective IgE response. Nat Immunol (2018) 19:859–70. doi: 10.1038/s41590-018-0161-8
277. He X, Cao Y, Gu Y, Fang H, Wang J, Liu X, et al. Clinical outcomes and immune metrics in intratumoral basophil-enriched gastric cancer patients. Ann Surg Oncol (2021) 28:6439–50. doi: 10.1245/s10434-021-09815-0
278. Fujiwara T, Yakoub MA, Chandler A, Christ AB, Yang G, Ouerfelli O, et al. CSF1/CSF1R signaling inhibitor pexidartinib (PLX3397) reprograms tumor-associated macrophages and stimulates T-cell infiltration in the sarcoma microenvironment. Mol Cancer Ther (2021) 20:1388–99. doi: 10.1158/1535-7163.MCT-20-0591
279. Pellefigues C, Naidoo K, Mehta P, Schmidt AJ, Jagot F, Roussel E, et al. Basophils promote barrier dysfunction and resolution in the atopic skin. J Allergy Clin Immunol (2021) 148:799–812 e10. doi: 10.1016/j.jaci.2021.02.018
280. Gieseck RL 3rd, Wilson MS, Wynn TA. Type 2 immunity in tissue repair and fibrosis. Nat Rev Immunol (2018) 18:62–76. doi: 10.1038/nri.2017.90
281. Plaut M, Pierce JH, Watson CJ, Hanley-Hyde J, Nordan RP, Paul WE. Mast cell lines produce lymphokines in response to cross-linkage of fc epsilon RI or to calcium ionophores. Nature (1989) 339:64–7. doi: 10.1038/339064a0
282. Afferni C, Buccione C, Andreone S, Galdiero MR, Varricchi G, Marone G, et al. The pleiotropic immunomodulatory functions of IL-33 and its implications in tumor immunity. Front Immunol (2018) 9:2601. doi: 10.3389/fimmu.2018.02601
283. Wong CK, Cao J, Yin YB, Lam CW. Interleukin-17A activation on bronchial epithelium and basophils: a novel inflammatory mechanism. Eur Respir J (2010) 35:883–93. doi: 10.1183/09031936.00088309
284. Jayatilaka H, Tyle P, Chen JJ, Kwak M, Ju J, Kim HJ, et al. Synergistic IL-6 and IL-8 paracrine signalling pathway infers a strategy to inhibit tumour cell migration. Nat Commun (2017) 8:15584. doi: 10.1038/ncomms15584
285. Schroeder JT, Adeosun AA, Bieneman AP. Epithelial cell-associated galectin-3 activates human dendritic cell subtypes for pro-inflammatory cytokines. Front Immunol (2020) 11:524826. doi: 10.3389/fimmu.2020.524826
286. Todoric J, Antonucci L, Karin M. Targeting inflammation in cancer prevention and therapy. Cancer Prev Res (Phila) (2016) 9:895–905. doi: 10.1158/1940-6207.CAPR-16-0209
287. Yasinska IM, Meyer NH, Schlichtner S, Hussain R, Siligardi G, Casely-Hayford M, et al. Ligand-receptor interactions of galectin-9 and VISTA suppress human T lymphocyte cytotoxic activity. Front Immunol (2020) 11:580557. doi: 10.3389/fimmu.2020.580557
288. Lines JL, Pantazi E, Mak J, Sempere LF, Wang L, O'Connell S, et al. VISTA is an immune checkpoint molecule for human T cells. Cancer Res (2014) 74:1924–32. doi: 10.1158/0008-5472.CAN-13-1504
289. Ohno T, Zhang C, Kondo Y, Kang S, Furusawa E, Tsuchiya K, et al. The immune checkpoint molecule VISTA regulates allergen-specific Th2-mediated immune responses. Int Immunol (2018) 30:3–11. doi: 10.1093/intimm/dxx070
290. Seder RA, Paul WE, Dvorak AM, Sharkis SJ, Kagey-Sobotka A, Niv Y, et al. Mouse splenic and bone marrow cell populations that express high-affinity fc epsilon receptors and produce interleukin 4 are highly enriched in basophils. Proc Natl Acad Sci U.S.A. (1991) 88:2835–9. doi: 10.1073/pnas.88.7.2835
291. MacGlashan D Jr., White JM, Huang SK, Ono SJ, Schroeder JT, Lichtenstein LM. Secretion of IL-4 from human basophils. the relationship between IL-4 mRNA and protein in resting and stimulated basophils. J Immunol (1994) 152:3006–16.
292. Mukai K, BenBarak MJ, Tachibana M, Nishida K, Karasuyama H, Taniuchi I, et al. Critical role of P1-Runx1 in mouse basophil development. Blood (2012) 120:76–85. doi: 10.1182/blood-2011-12-399113
293. Sullivan BM, Liang HE, Bando JK, Wu D, Cheng LE, McKerrow JK, et al. Genetic analysis of basophil function in vivo. Nat Immunol (2011) 12:527–35. doi: 10.1038/ni.2036
294. Shibata S, Miyake K, Tateishi T, Yoshikawa S, Yamanishi Y, Miyazaki Y, et al. Basophils trigger emphysema development in a murine model of COPD through IL-4-mediated generation of MMP-12-producing macrophages. Proc Natl Acad Sci U.S.A. (2018) 115:13057–62. doi: 10.1073/pnas.1813927115
Keywords: allergy, angiogenesis, angiopoietins, basophil, cancer, cysteinyl leukotrienes, cytokines, vascular endothelial growth factors
Citation: Poto R, Gambardella AR, Marone G, Schroeder JT, Mattei F, Schiavoni G and Varricchi G (2022) Basophils from allergy to cancer. Front. Immunol. 13:1056838. doi: 10.3389/fimmu.2022.1056838
Received: 29 September 2022; Accepted: 21 November 2022;
Published: 12 December 2022.
Edited by:
Christophe Pellefigues, CNRS EMR8252 Centre de Recherche sur l’Inflammation, FranceReviewed by:
Vadim V Sumbayev, University of Kent, United KingdomFrancesca Levi-Schaffer, Hebrew University of Jerusalem, Israel
Copyright © 2022 Poto, Gambardella, Marone, Schroeder, Mattei, Schiavoni and Varricchi. This is an open-access article distributed under the terms of the Creative Commons Attribution License (CC BY). The use, distribution or reproduction in other forums is permitted, provided the original author(s) and the copyright owner(s) are credited and that the original publication in this journal is cited, in accordance with accepted academic practice. No use, distribution or reproduction is permitted which does not comply with these terms.
*Correspondence: Gilda Varricchi, gildanet@gmail.com; Giovanna Schiavoni, giovanna.schiavoni@iss.it
†These authors share first authorship
‡These authors share senior authorship
§ORCID: Remo Poto, orcid.org/0000-0002-4723-0167
Adriana Rosa Gambardella, orcid.org/0000-0002-5335-8650
Gianni Marone, orcid.org/0000-0002-9849-4701
John T. Schroeder, orcid.org/0000-0002-5263-7850
Fabrizio Mattei, orcid.org/0000-0002-5357-7773
Giovanna Schiavoni, orcid.org/0000-0001-9135-0556
and Gilda Varricchi, orcid.org/0000-0002-9285-4657