- Department of Gastroenterology, Chongqing Key Laboratory of Digestive Malignancies, Daping Hospital, Army Medical University (Third Military Medical University), Chongqing, China
Th17 cells play an important role in the abnormal immune response in inflammatory bowel disease (IBD) and are involved in the development and progression of inflammation and fibrosis. An increasing amount of data has shown that gut microbes are important parts of intestinal immunity and regulators of Th17 cellular immunity. Th17 cell differentiation is regulated by intestinal bacteria and cytokines, and Th17 cells regulate the intestinal mucosal immune microenvironment by secreting cytokines, such as IL-17, IL-21, and IL-26. Solid evidence showed that, regarding the treatment of IBD by targeting Th17 cells, the therapeutic effect of different biological agents varies greatly. Fecal bacteria transplantation (FMT) in the treatment of IBD has been a popular research topic in recent years and is safe and effective with few side effects. To further understand the role of Th17 cells in the progression of IBD and associated therapeutic prospects, this review will discuss the progress of related research on Th17 cells in IBD by focusing on the interaction and immune regulation between Th17 cells and gut microbiota.
1 Introduction
Inflammatory bowel disease (IBD) is a chronic inflammatory gastrointestinal disease whose etiology and pathogenesis are not completely understood. IBD can be divided into three categories, Crohn’s disease (CD), ulcerative colitis (UC), and inflammatory bowel disease unclassified (IBDU). It is generally believed that IBD was induced by environmental factors acting on genetically susceptible populations, where the body initiates an abnormal immune response with the involvement of gut microbes, thereby leading to pathological processes such as intestinal mucosal barrier damage and inflammatory cell infiltration. Activated CD4+ T cells are the dominant effector cells mediating abnormal immune responses and subsequent inflammation in the intestinal mucosa. Previous studies have proved the relevance of CD and UC to T helper (Th)1- and Th2-mediated immune responses (1). Recent researches have affirmed that Th17 cells also play an essential role in the abnormal immune response in IBD pathophysiology (2, 3). Th17 cells were first described as a discrete subpopulation of Th0 cells in 2005 (4). They are differentiated from naive CD4+ T cells under the regulation of antigen-presenting cells (APCs) and their secreted cytokines, which specifically secrete interleukin (IL)-17 and cause tissue inflammation (4, 5).
The expression of chemokine receptor 6 (CCR6) on the surface of Th17 cells allows them to migrate to specific intestinal tissue targets in the presence of the CCR6 ligand chemokine (C-C motif) ligand 20 (CCL20) (6). Normally, Th17 cells and their secreted cytokines play essential roles in fighting infections and retaining intestinal immune homeostasis (7). When dysregulated, the hyperproliferation and activation of Th17 cells induce abnormal immune responses and lead to various autoimmune diseases, including IBD (8). As one of the essential members of the "gut microecosystems", the gut microbiota is involved in the regulation of Th17 cells and the progression of IBD. To further understand the role of Th17 cells in IBD progression and their therapeutic potential, this article will review the progress of Th17 cell research, their role in IBD, and their association with gut microbiota.
2 The role of Th17 cells and their secreted cytokines in the pathogenesis of IBD
Th17 cells are predominantly located on the lamina propria of intestinal lumen and defend the host from invasion by pathogenic microorganisms. Abundant mucosal Th17 cells are common in IBD patients and animal models. Compared with IBD remission stage, Th17 cells increased in peripheral blood and intestinal mucosa at the active stage. These cells are principally involved in disease progression by secreting cytokines such as IL-17A, IL-17F, IL-21, IL-22, and IL-26. Hereinafter, we will elaborate on the role of Th17 cells from the perspective of the disease occurrence and progression of IBD, as shown in Figure 1.
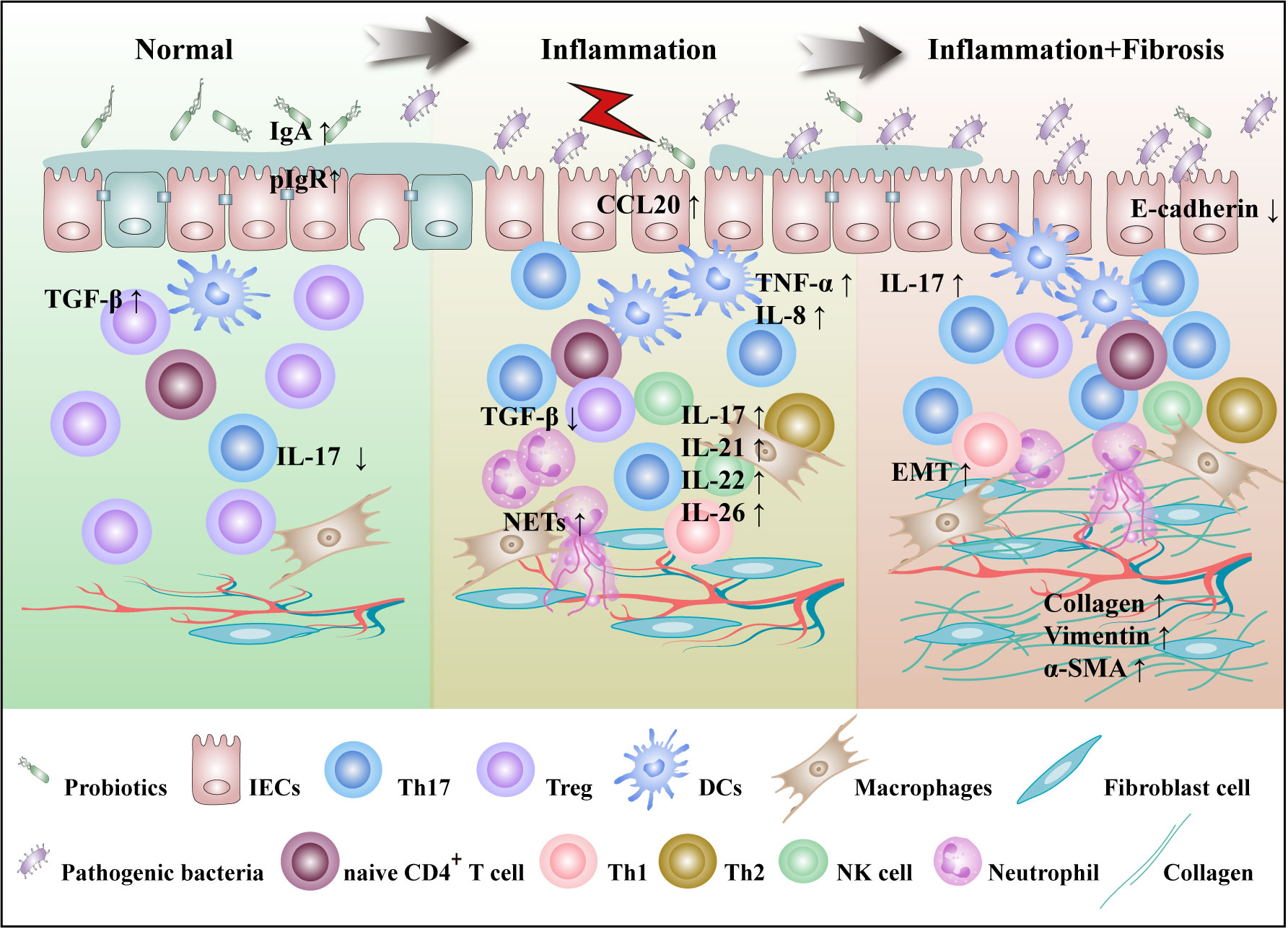
Figure 1 Th17 cells are involved in the pathogenesis of IBD. The pathogenesis of IBD is characterized by gut microbes imbalance, mucosal damage, inflammation and fibrosis. In the normal intestinal microecosystem, Th17 cells and Tregs are in dynamic balance. IL-17 secreted by Th17 cells inhibits the colonization of pathogenic bacteria by targeting IECs that enhance the secretion of IgA and antimicrobial peptides, while Tregs inhibit excessive immunity of T cells. Under the influence of external stimuli and susceptibility genes, imbalances in gut microbiota lead to a decrease in SCFA-producing bacteria, including R.intestinalis, and an increase in pathogenic bacteria, including SFB. DCs are activated to produce cytokines such as IL-6, IL-23, and TGF-β. Th17 cells migrate by CCL20 secreted by IECs to the site of inflammation, where they activate and secrete a large number of cytokines to participate in the progression of inflammation. At the same time, IL-17 induces IECs to express IL-8, which recruits a large number of neutrophils and NETs at sites of inflammation, positively regulating Th17 cell feedback. IL-21 is involved in the regulation of Th17 cell differentiation in an autocrine manner. IL-22 secretion by Th17 cells was shown to act on NK cells and goblet cells and promote goblet cell mucus production. With the continuous progression of inflammation, IL-17 can induce fibroblasts to secrete ECM and promote the progression of intestinal fibrosis. R.intestinalis, Roseburia intestinalis; NETs, neutrophil extracellular traps; ECM, extracellular matrix.
2.1 Th17 cells in the maintenance of intestinal homeostasis
Th17 cells are implicated in gastrointestinal immune responses. Under homeostasis conditions, "gut microecosystems" are in dynamic balance, under the joint action of intestinal microorganisms, cytokine signals, and costimulatory signals, Th17 cells and regulatory T cells (Tregs) are in dynamic balance, they collaboratively maintain the intestinal immune microenvironment homeostasis and intestinal health. Th17 cells express and secrete small quantities of IL-17 and IL-22 to promote epithelial cell proliferation and upregulate antimicrobial peptides and tight junction proteins expression, thus protecting the intestinal mucosa from infections by various pathogens.
IL-17 can promote intestinal epithelial cells (IECs) proliferation, increase the polymeric Ig receptor (pIgR) expression, promote intestinal IgA secretion, and secrete a variety of antimicrobial peptides to accelerate the healing of intestinal mucosal injury, thereby enhancing the intestinal barrier function (9). By binding to Th1 cell surface receptors, IL-17 also inhibits from secreting IL-23R, IFN-γ, IL-12Rp2, and other proinflammatory factors, thereby inhibiting their immune regulation (10). IL-17 inhibits intestinal inflammation by upregulating the number of atypical M2 macrophage subsets (11). IL-22 promotes signal transducer and activator of transcription 3 (STAT3) activation in colonic epithelial cells and induces mucus-related molecule expression and secretion of mucus goblet cell repair products (12). Upregulation of TGF-β expression in the gut microenvironment mediates Th17 cells transdifferentiation into Tregs. Tregs express the anti-inflammatory factor TGF-β, which can inhibit the excessive immune response of effector T cells and protect intestinal tissue from injury.
2.2 Th17 cells in inflammatory bowel diseases
2.2.1 Th17 cells with inflammation
Under the regulation of susceptibility genes and environmental hazards, the bacterial diversity, composition, and abundance of the gut microbiota changed. Pathogenic bacteria promote colonization and infection by breaking down mucins as nutrients, releasing toxic gases and secreting enzymes to disrupt mucosal barriers. The number of Th17 cells with upregulated IL-17 expression increased in inflammation foci in IBD, while the number of Tregs were decreased and the expression of TGF-β was downregulated (13).
Th17 cells promote inflammation primarily through the secretion of cytokines. IL-17 can exert proinflammatory effects through the following pathways: IL-17 alone or in conjunction with tumor necrosis factor (TNF)-α acts on IECs to promote the secretion of inflammatory mediators, chemokines, and proteases (e.g., IL-6, IL-8, inducible nitric oxide synthase (iNOS), CXCL8, TNF-α, matrix metalloproteinase (MMP) and granulocyte-macrophage colony-stimulating factor (GM-CSF)). These factors induce inflammation and promote the enlisting, activation and movement of neutrophil to target tissues, causing intestinal mucosal injury (14). Additionally, IL-17 can synergistically activate the NF-κB, ERK1/2, and p38 signaling pathways with TNF-α to induce IL-17C secretion from enteric neuroendocrine cells and goblet cells and promote the expression of the Th17 chemokine CCL20 in IECs. The upregulation of CCL20 expression and the occurrence of neutrophil production of neutrophil extracellular traps (NETs) further promotes the recruitment and activation of Th17 cells, leading to aggravation of the inflammatory response. IL-26 amplifies the aberrant inflammatory immune response; upregulates IL-6 and IL-8 expression through the STAT1, STAT3, PI3K/Akt, and MAPK signaling pathways; induces the adhesion molecules MMP-8 and MMP-9 expression; and activates macrophages (15, 16).
CD and UC are caused by Th1 and Th2 cells, respectively. Th17 cells have the ability to redifferentiate into Th1 or Th2 cells. IL-12 induces a rapid transformation of the Th1-like phenotype by restimulating the Th17 precursor (17). IFNγ secreted by progeny of Th17 precursors is pathogenic in some autoimmune models. In addition, IL-1β, IL-4, and IL-23 induced naive CD4+ T cells to differentiate into double-positive Th2-Th17 cells (18). Notably, IL-4 repolarizes Th17 precursors to a Th2 phenotype. CD161 is a specific marker for Th17 progenitor cells that are not expressed by traditional Th1 and Th2 cell populations, that can be used to distinguish whether Th1 or Th2 cells are transdifferentiated from Th17 cells.
2.2.2 Th17 cells with fibrosis
Intestinal fibrosis, which is usually the consequence of chronic inflammation, is a common outcome in IBD patients, resulting in internal strictures, progressive structural deformation and loss of function. More than 50% of CD patients develop penetrations or stenosis due to fibrous stenosis, which in most cases requires surgery. During UC progression, the frequency of stricture formation varies from 3.2% to 11.2%, with 68% of stenosis cases occurring in the rectum (19). Surgery is the only option available to improve intestinal stenosis and obstruction, but recurrence after surgery is extremely high. The pathogenesis of intestinal fibrosis points to a new concept of the central role of Th17 and IL-17 in the immune response, although their accurate roles in chronic inflammation and IBD are controversial (20).
Th17 cells have been demonstrated to promote a fibrotic phenotype in a variety of diseases, including oral submucosal lesions, vascular fibrosis, colon fibrosis and renal fibrosis (21–24). IL-17A promotes fibrosis by targeting different cells in different tissues, including hepatic stellate cells (HSCs) and Kupffer cells in the liver, and induces cardiac fibrosis by promoting cardiac fibroblast proliferation and HSC activation. In the murine model, inhibition of IL-23 and IL-17 attenuated 2,4,6-trinitrobenzene sulfonic acid (TNBS)-induced intestinal fibrosis. Following persistent intestinal inflammation, IL-17A promotes the production of extracellular matrix (ECM) components and reduces the migratory capacity of myofibroblasts (25). IL-17A overexpression is observed in CD stenotic surgical samples compared to nonstenotic regions from the same individuals and healthy intestinal samples from control individuals (19). IL-17 is overexpressed in stenosis in CD fibrosis, and IL-17A promotes fibroblast proliferation and survival through metabolic reprogramming (26), and induces epithelial-mesenchymal transition (EMT) in IECs, resulting in raised expression of vimentin, snail and α-SMA and lessened E-cadherin expression (27). Increasing evidence indicates that IL-22 is a key molecule in cell proliferation, mucosal healing and tissue repair in relieving fibrosis (20). It is worth noting that some murine CD models exhibit exacerbated midgut fibrosis when the IL-23/IL-22 signaling pathway is upregulated (28).
Th17/IL-17 is proposed to be the core of tissue fibrosis, which promotes myofibroblast proliferation and collagen deposition by connecting established profibrotic molecules and pathways such as TGF-β, various growth factors, and novel profibrotic mediators TL1A/DR3 and Ang-II, thus leading to intestinal fibrosis. Further research on the role of IL-22 and IL-26 in IBD fibrosis is needed.
3 Differentiation and regulation of Th17 cells
The differentiation of Th17 cells is influenced by gut microbes, metabolic pathways, cytokines, transcription factors, and signal transduction pathways. The specific mechanisms are illustrated in Figure 2 and Table 1. Gut microbes promote naive CD4+ T cells differentiation into Th17 cells by upregulating the expression of STAT3 and retinoic acid receptor-related orphan nuclear receptor γt (RORγt) by inducing APCs to secrete cytokines as IL-6 and IL-23 or by metabolites and direct induction (62).
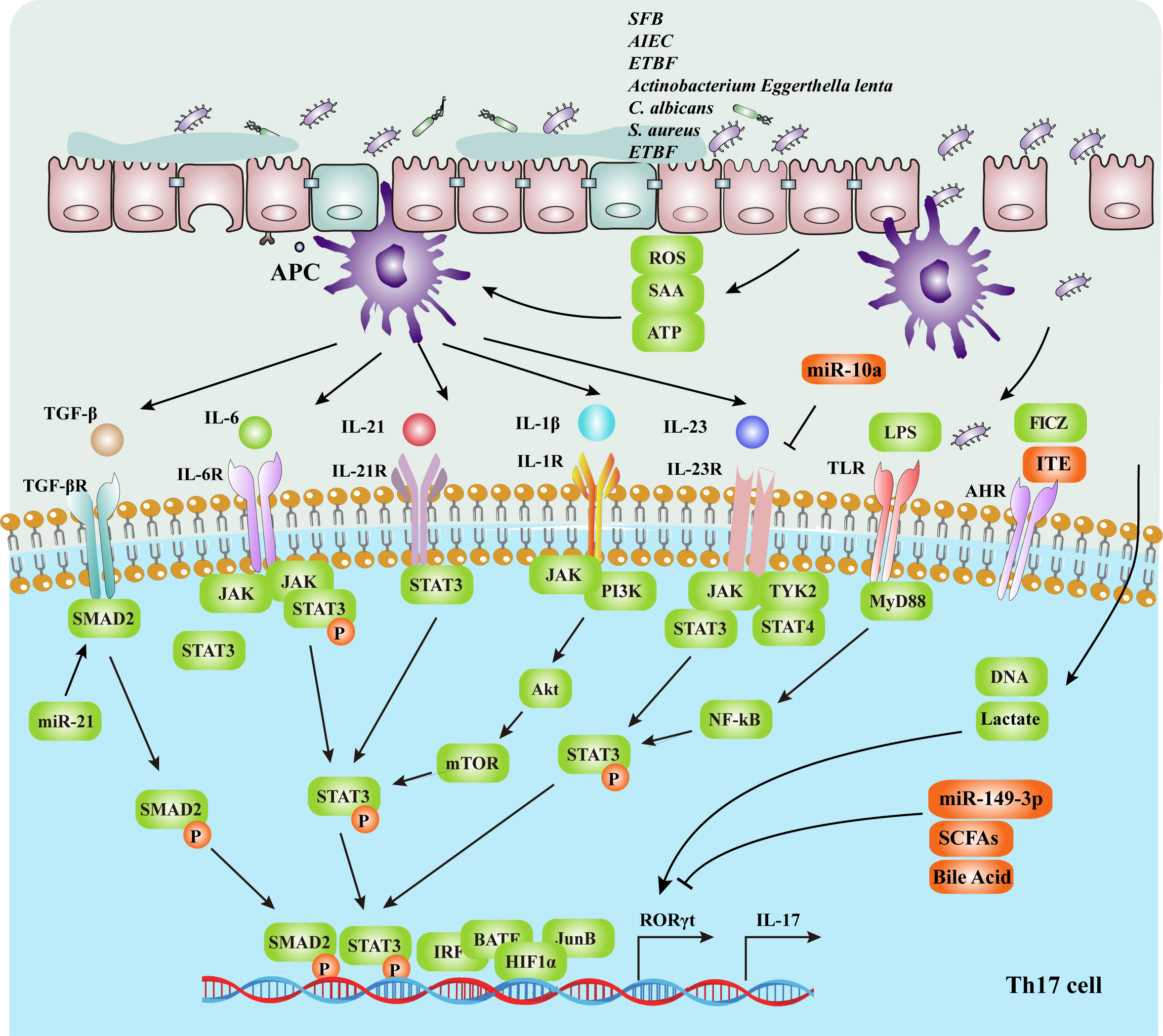
Figure 2 The intestinal mucosal microenvironment, composed of immune cells, cytokines, transcription factors and gut microbiota, regulates Th17 cell differentiation. The gut microbiota activates APCs and upregulates the expression of IL-6, IL-1β and IL-23. IL-6, IL-22, TGF-β, IL-1β and IL-23 can promote the differentiation of Th17 cells by upregulating the expression of the RORγt gene, which depends on the STAT3 signaling pathway. RORγt is a Th17 cell-specific transcription factor that directly affects the differentiation of Th17 cells. In addition, The gut microbiota regulates the differentiation of CD4+ T cells through metabolites and energy metabolism, such as LPS, SCFAs and secondary bile acid. Upregulation of the transcription factors IRF4, BATF, HIF-1 and JunB promotes the expression of the RORγt gene. Activation of the TLRs signaling pathways also promotes Th17 proliferation, thereby promoting naive T-cell differentiation into Th17 cells and IL-17 secretion. SFB, segmented filamentous bacteria; AIEC, adherent invasive Escherichia coli; ETBF, enterotoxigenic Bacteroides fragilis; SCFAs, short-chain fatty acids; SAA, serum amyloid A; APCs, antigen-presenting cells; Th17, T helper 17 cells; RORγt, retinoic acid receptor-related orphan nuclear receptor γt.
3.1 Regulation of Th17 cell differentiation by gut microbiota and its metabolites
The microbiota is thought to influence the development and progression of IBD. In previous studies, intestinal Th17 cells increased and mediated colonic inflammation in germ-free mice by transferring gut microbes from IBD mice (63). Bacterial activation of Th17 cells exacerbates autoimmune responses in IBD. Adherent invasive Escherichia coli (AIEC) induces an active Th17 response in mice, increasing transmural inflammation and fibrosis (30). Existing studies have suggested that the gut microbiota can induce Th17 cell differentiation either directly through contact with immune cells or through metabolites indirectly. A complex microenvironment of commensal flora drives IL-23 production by IECs or dendritic cells (DCs) in the ileum propria (64, 65). Microbial antigens are able to stimulate naive T cells to differentiate into Th17 cells when combined with TLR5 on the surface of CD11chiCD11bhi lamina propria DCs.
Segmented filamentous bacteria (SFB) can stimulate reactive oxygen species (ROS) production and use microbial adhesion-triggered endocytosis to transfer antigenic proteins into IECs, thus upregulating the secretion of IL-1β and IL-23 and inducing Th17 cell differentiation. SFB colonization induces the production of SAA in terminal ilea, and SAA may induce DCs to secrete IL-23 to maintain Th17 survival and activation as well as IL-17 expression, thereby leading to aggravation of IBD while maintaining chronic intestinal inflammation (29). Notably, SFB expansion is limited by Th17-derived IL-17A in turn through upregulating ROS and antimicrobial peptide production. The Actinobacterium Eggerthella lenta expresses the cardiac glycoside reductase 2 (Cgr2) enzyme. Cgr2 activates intestinal Th17 cells to upregulate IL-17A expression in a RORc-dependent manner (32). Candida albicans (C. albicans) is usually a beneficial species of the human gut microbiota, which can become pathogenic in immunocompromised hosts. C. albicans hyphae directly activate B cells to express IgG1 and IL-6, leading to the induction of Th17 cell differentiation and eventual bacterial clearance (33). Staphylococcus aureus (S. aureus)-specific Th17 cells express and secrete IL-17, along with IL-10 after restimulation (34).
Commensal bacteria affect Th17 cell differentiation by regulating miRNAs, such as miR-149-3p, miR21 and miR-10a. Enterotoxigenic Bacteroides fragilis (ETBF) downregulated miR-149-3p. MiR-149-3p can be released from exosomes and mediate enteritis by regulating the differentiation of Th17 cells (31). MiR-21 promotes Th17 cell development by targeting and inhibiting SMAD-7, which negatively regulates TGF-β signaling (35). MiR-10a is downregulated by microorganisms through a MyD88-dependent pathway that promotes Th17 cells in the gut via the IL-12/IL-23p40 signaling pathway (35). Gut microbes and miRNAs can form a regulatory network with the immune system.
Enterobacterial metabolites are important players in immune regulation. Lipopolysaccharide (LPS) is a bacterial surface glycolipid produced by gram-negative bacteria that induces Th17 cell differentiation and mediates chronic and acute inflammatory responses (36). Symbiotic bacteria provide high concentrations of adenosine triphosphate (ATP). Gut microbiota-derived ATP activates CD70highCD11clow lamina propria cells through P2X and P2Y receptors, thus enhancing the secretion of IL-1β, IL-6 and IL-23 and promoting a Th17-polarizing microenvironment (37). 2-(1’H-indole-3’carbonyl)-thiazole-4-carboxylic acid methyl ester (ITE) and 6-formylindolo (3-2b) carbazole (FICZ) are metabolically produced by tryptophanase-positive bacteria such as Lactobacillus reuteri and are involved in immune regulation through the activation of aryl hydrocarbon receptors (AHRs). FICZ upregulates IL-17 and IL-22 production in CD4+ T cells, and ITE induces Tregs (38).
In addition, Clostridium can inhibit the release of the proinflammatory factor IL-17 through short-chain fatty acids (SCFAs), thereby reducing the differentiation of Th1 and Th17 cells (39, 40). The mechanism by which SCFAs regulate T-cell differentiation includes the mTOR pathway, G-protein-coupled receptor signaling, and epigenome remodeling to promote Treg cell differentiation. For example, butyrate promotes Treg cell differentiation by acetylating histone H3 at the forkhead box protein P3 (Foxp3) locus, and inhibits Th17 cell differentiation by inhibiting C-myc and HDAC-related metabolism. Microorganisms regulate Th17 cell differentiation through bile acid metabolism (41, 66, 67). Saiyu Hang et al. discovered that two different lithocholic acids (LCA) derivatives, isoalloLCA and 3-oxoLCA, act as T-cell regulators in mice to regulate Th17-cell differentiation (41, 42). IsoalloLCA promotes Treg cell differentiation by producing mitochondrial ROS (mROS), and 3-OxoLCA inhibits Th17 cell differentiation by directly binding to RORγt. In a related study, Riping Xiao et al. studied the binding affinity of bile acid metabolites to the IL-17A transcription factor RORγt by synthesizing the sulfated product of LCA, LCA-3-sulfate (LCA-3-S). LCA-3-S specifically targets RORγt to inhibit Th17 cell differentiation, a process that does not affect Th1, Th2, and Treg cell differentiation (68).
The intestinal contents combined with the intestinal immune microenvironment constitute the "gut microecosystems". In the gut microecosystem, the gut microbes occupies a considerable proportion, participates in the regulation of the immune microenvironment and is a valued participant in the regulation of Th17 cell differentiation. Unfortunately, the gut microbiota is complicated, and little was known about the contribution of different strains to Th17 cell differentiation. In addition, for the known gut microbes and their metabolites involved in the regulation of cell differentiation, the molecular mechanisms of their involvement in regulation also need to be further explored.
3.2 Metabolic pathways affect Th17 cell differentiation
Polyamine metabolism, lipid oxidative metabolism, and glycolytic programs are involved in the mediation of naive T cell differentiation (43, 44, 69, 70). For example, the glycolytic pathway regulates Treg differentiation through the transcription factor HIF1α/mTOR signaling pathway, and blocking glycolysis promotes Treg differentiation while inhibiting Th17 development (45, 71). Targeting the glycolytic enzyme glucose phosphate isomerase (Gpi1) to suppress glycolysis is considered to be an effective therapeutic option for Th17-mediated autoimmune diseases with the advantages of minimal toxicity (72). Glutaminase deficiency affects the proliferation and activation of naive T cells and diminishes the differentiation of Th17 cells (73). Lactate has long been considered a waste byproduct of cellular metabolism that accumulates at inflammation sites. Recently, the role of lactate has been redefined as an active metabolite in cell signaling (74, 75). Lactate and the associated hydrion regulate Th17 cell differentiation and are generally considered negative regulators of immunosuppression (75, 76). Under the Warburg effect, tumor cells secrete a large amount of lactate, which enhances TLR ligand-mediated IL-23 expression, resulting in increased IL-17A expression (46). Sodium lactic acid induces the differentiation of CD4+ T cells to Th17 cells in rheumatoid arthritis patients; in contrast, lactic acid causes loss of cytolytic function in CD8+ T cells (77). These results indicate that the metabolic pathway plays a crucial role in Th17 cell differentiation. However, the possible targets and potential mechanisms of this regulation are not fully understood, and more experimental data are needed to support it.
3.3 Role of cytokines in Th17 cell differentiation
IL-6 is an essential molecule that upregulates the expression of the RORγt gene and promotes Th17 cell differentiation via activating the JAK2-STAT3 signaling pathway (47). TGF-β is also crucial in Th17 cell differentiation, as it upregulates the expression of the RORγt gene while inhibiting its ability to induce IL-17 expression (78). TGF-β induces Th17 cell differentiation and promotes the emergence of IL-22 production through Smad2 activation, a pathway that can be inhibited by TNF receptor-associated factor 6 (TRAF6) (48). Naive CD4+ T cells differentiate into Tregs at high concentration of TGF-β, while, differentiate into Th17 cells at low levels of TGF-β in conjunction with IL-6 (49). Notably, the presence of Th17 cells was not detected in the scarcity of IL-23. IL-23 promotes the stabilization and proliferation of Th17 cells without altering differentiation. In addition, IL-1β not only promotes Th17 polarization via the IL1R/PI3K-mTOR signaling pathway but also inhibits TGF-β-induced Foxp3 expression in CD4+ T cells (50), thereby inhibiting the differentiation of Tregs (79). Additionally, IL-21 is secreted by Th17 cells, upregulates the expression of IL-17 and RORγt and promotes Th17 cell differentiation through the STAT3 signaling pathway (51).
3.4 Regulation of Th17 cell differentiation by transcription factors
Under the induction of cytokines such as IL-6, phosphorylation-activated STAT3 binds to the IL-17 promoter and directly controls the expression of RORγt for Th17 cell differentiation (52). RORγt is a transcription factor that is specific to Th17 cells and directly influences their differentiation (47). Studies have shown that enhanced RORγt promotes Th17 cell differentiation, while RORγt-deficient T cells cannot differentiate into Th17 cells. In addition, IL-17 gene transcription was induced by RORγt (47). B-cell activating transcription factor (ATF-like factor, BATF), JunB, interferon regulatory factor 4 (IRF4), hypoxia inducible factor 1 (HIF1) and basic leucine zipper transcription factor can interfere with Th17 cell differentiation (54–56, 80). IRF4 binds directly to the IL-17 promoter and upregulates the expression of RORγt and IL-17 genes (53). The activator protein 1 (AP-1) transcription factors BATF and JunB are considered essential for Th17 cell differentiation and are considered to be primarily involved in RORγt transcription (54, 55). HIF1a enhances and activates the upregulation of RORγt receptor expression under the glycolytic pathway, thereby increasing the proportion of Th17 cells while inhibiting Foxp3 expression and weakening the differentiation of naive CD4+ cells to Tregs (45).
3.5 TLR-dependent T-cell differentiation
Activation of the TLR2, TLR3, TLR4, and TLR8 signaling pathways likewise promotes Th17 proliferation, while activation of the TLR7 signaling pathway downregulates STAT3, thereby inhibiting Th17 cell differentiation and IL-17 secretion (57–61). NETs and their histones are mediated downstream of TLR2, resulting in the phosphorylation of STAT3 (57). Activation of TLR2 in DCs mediates IL-23 production. TLR3 and TLR9 respond to pathogen-associated microbial patterns including unmethylated DNA and dsRNA. In the presence of anti-CD3 and anti-CD28, poly (I:C) induces IL-17A and IL-21 synthesis by costimulating naive CD4+ T cells through TLR3 (58). Activation of TLR2 in DCs mediates IL-23 production. These results indicated that Th17 cell differentiation and activation are regulated by transcription factor activation and TLR-like receptor signaling.
4 The therapeutic potential of IBD by targeting Th17 cells
Existing clinical treatments for IBD utilize monoclonal antibodies targeting TNF, and significant progress has been made in this regard, although some patients do not respond to this modality or lose their response over time. Accordingly, IBD requires additional effective and tolerable treatment options. Th17 cells are predominantly located on the surface of the intestinal mucosa and protect the host from the invasion of pathogenic microorganisms.Th17 cells and their secreted cytokines have been shown to play a vital role in IBD pathogenesis, and the imbalance of Treg/Th17 cells can cause IBD. Tregs suppress the excessive immune response of effector T cells and protect intestinal tissue from damage. Some of the existing Th17 cell-related treatment modalities for IBD will be discussed in this section (Table 2 and Figure 3). Above all, the regulation of T-cell differentiation and activation by gut microbiota suggests that a rationally designed bacterial consortium reduces inflammatory cytokines and Th1 and Th17 cells, treats chronic immune-mediated colitis, and restores intestinal homeostasis (100).
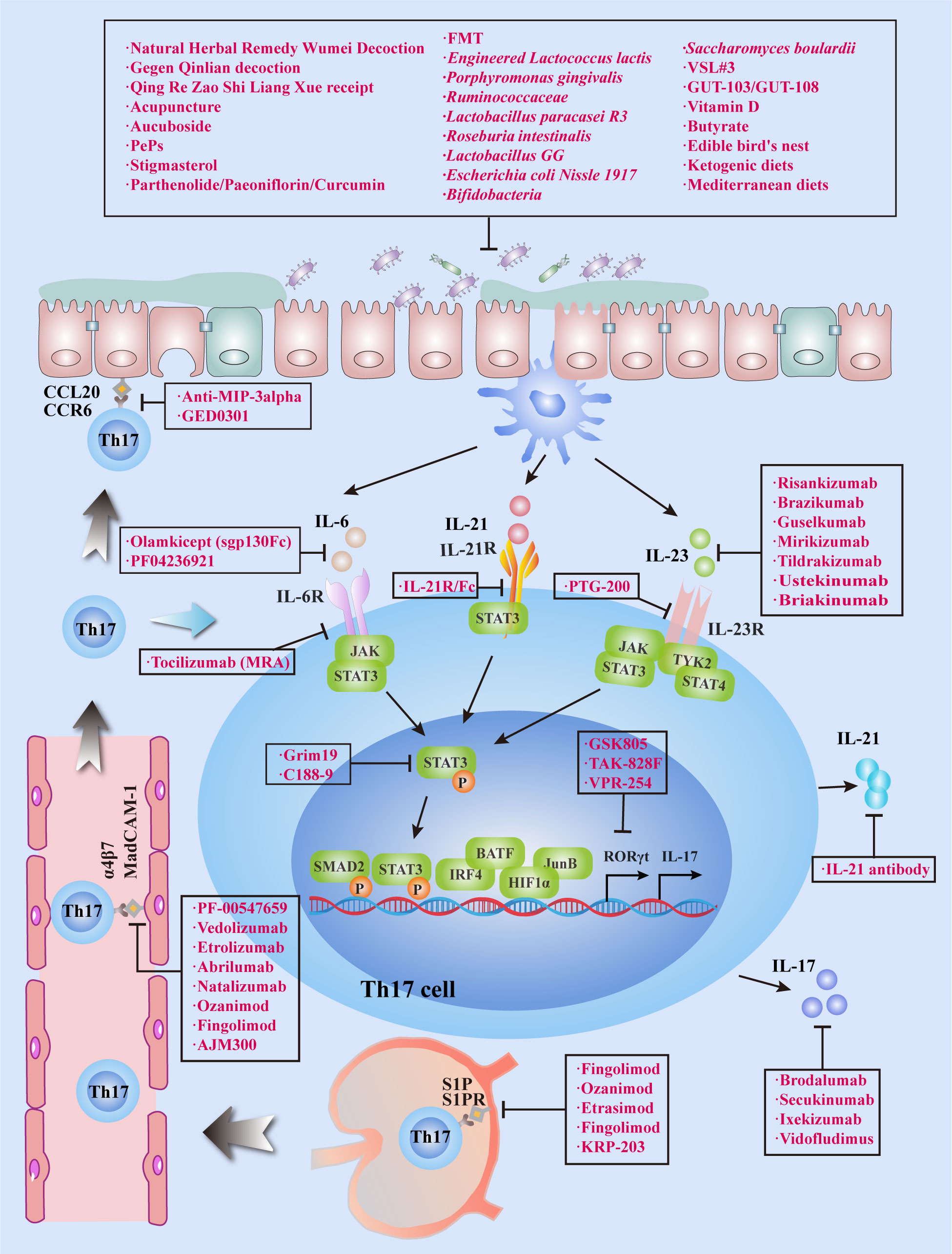
Figure 3 Targeting Th17 cells for treat IBD. IBD can be treated by inhibiting Th17 cell migration, infiltration, differentiation and secretion of cytokines. In addition to conventional treatment methods, medicine or phytochemicals, FMT and probiotics can also inhibit Th17 cell differentiation and IL-17 expression by regulating the bacterial flora, which eventually leads to clinical remission of IBD.
4.1 Regulating gut microbiota inhibits Th17 cell differentiation and activation
In vivo, a complex microenvironment of commensal flora drives IL-23 production by DCs in the ileum propria and CCL20 expression by IECs. SFB, Escherichia coli O157, and Citrobacter rodentium are examples of bacteria that attach to IECs and selectively induce Th17 responses in an IL-23-dependent manner. (64, 65). The treatment of IBD by regulating gut microbes is effective and has become a research hotspot in recent years; its main mode of action is to regulate the recruitment, differentiation and activation of Th17 cells in the intestinal mucosal microenvironment.
Traditional Chinese medicine therapy is usually characterized by safe and remarkable therapeutic effects. Chinese medicinal herbal decoctions, such as Natural Herbal Remedy Wumei decoction, Gegen Qinlian decoction and Qing Re Zao Shi Liang Xue receipt, ameliorate intestinal mucosal inflammation by maintaining microbial homeostasis and restoring Th17/Tregs balance. These decoctions change the diversity and community landscape of the gut microbiota and metabolic profiles and in particular significantly increased the abundance of SCFAs produced by the gut microbiota (81–83). Similarly, the relative abundance of Roseburia faecis and Faecalibacterium prausnitzii in gut microbiota was increased, and the expression of Th17 cell-related cytokines was decreased in CD patients treated with acupuncture (84).
Plant extract is an effective preparation for the treatment of IBD. Aucuboside is a compound extracted from traditional Chinese medicine that significantly inhibits the production of Th17 cells in colitis, accompanied by the inhibition of IL-17 expression (85). Periploca sepium periplosides (PePs) are cardiosinole-free progestane glycosides derived from the rhizocarp of rhizocarp, changes the gut microbes and plays a vital role in regulating intestinal Th17 cell immunity (86). Stigmasterol regulates the gut microbes of mice with enteritis and increases butyric acid-producing bacteria. Butyrate can promote Treg differentiation, inhibit Th17 differentiation, and reduce colonic inflammation in experimental mice (87). Parthenolide, paeoniflorin, curcumin, 6-gingerol, tripterygium wilfords polyglycoside, baicalein, baicalin and rhubarb peony decoction improve colonic inflammation by regulating the Treg/Th17 balance via the gut microbes (88).
In recent years, some researchers have also proposed that FMT can be used to reconstruct gut microbes and have clinically proven its effectiveness in the treatment of IBD (89, 90, 137, 138). The high cost of FMT led researchers to propose the concept of "precise capsules". In our previous study, Roseburia intestinalis was shown to reduce inflammation in animal models (95). After treating IBD mice with Porphyromonas gingivalis, Lactobacillus rhamnosus GG, Ruminococcaceae or Lactobacillus paracasei R3, intestinal inflammation was relieved, where Tregs increased significantly in the intestinal tissue, while Th17 cells decreased, and intestinal inflammation was relieved (92–94). Engineered Lactococcus lactis, Escherichia coli Nissle 1917, Bifidobacteria and Saccharomyces boulardii strains have achieved good effects in clinical research of IBD by regulating intestinal microecology (91, 97–99). GUT-103 and GUT-108, which are composed of 8, 17 and 11 intestinal bacteria, respectively, are used to treat IBD and mediate the reduction of inflammatory factors and Th17 cells (100). Unfortunately, the molecular mechanism of other functional strains in the treatment of IBD has not been further investigated. In recent years, although the interaction between the gut microbiota and the host has been gradually revealed in the progression of IBD, the mechanism needs to be further elucidated.
Vitamin D, SCFAs, and metabolites of gut microbes have anti-inflammatory properties that protect the intestinal epithelium, including promoting Treg cell differentiation and upregulating the expression of anti-inflammatory factors (101, 102). Nutrient intake is one of the most important factors affecting gut microbes and intestinal mucosal immunity. For example, in a pilot study in humans subjects, a moderate high-salt challenge increased Th17 cells by reducing intestinal survival of Lactobacillus spp. Excessive sugar intake mediates the increase in the cytokines IL-1β and Th17 cells, which induce inflammation (139). Edible bird's nest can recuperate dextran sulphate sodium (DSS)-induced UC in C57BL/6J mice by recovering the Th17/Treg cell balance (103). Ketogenic and Mediterranean diets decrease gut Th17 cells by altering the gut microbiome (104, 105). Therefore, diet control is of great significance in the adjuvant treatment of IBD.
In addition to the abovementioned treatments, OPS-2071 is a quinolone antibiotic used to treat intestinal infections with low-absorption properties, dose-dependent reduction of colitis histological score and colonic weight to length ratio in a mouse model (140). Mesenchymal stem cells may alleviate IBD by regulating Treg/Th17 cell differentiation (141), thus providing a new option for IBD treatment. Due to the unknown molecular mechanism of their regulation of Treg/Th17 cells, we will not go into detail here.
4.2 Inhibition of Th17 cell migration
In lymphatic endothelial cells, the sphingosine 1-phosphate receptor (S1PR) is highly expressed. Th17 cells migrate through the sphingosine 1-phosphate (S1P) -S1PR axis in lymphatic vessels. Therefore, targeting regulation of the S1P-S1PR axis to inhibit Th17 cell migration is a potential therapeutic approach for IBD. The S1P modulator fingolimod has been shown to be effective in animal models of enteritis (106). Modulators of S1PR, including ozanimod, etrasimod, ponvory (ponesimod), and KRP-203, have achieved good clinical efficacy (106–109).
Adhesion and infiltration of Th17 cells depend on the binding of α4β7 integrin to the adhesion molecule MAdCAM-1. Anti-α4β7 integrin antibodies relieve local inflammatory responses by blocking Th17 cell migration to inflammation site. Vedolizumab, etrolizumab, abrilumab, and natalizumab are some of the reported anti-α4β7 integrin antibodies that have shown good safety and efficacy in the treatment of IBD (110–113). Natalizumab and vedolizumab are FDA-approved for the therapy of moderate-to-severe IBD. Etrolizumab was shown to alleviate UC inflammation although the effectiveness is but was not better than adalimumab (111). Abrilumab is associated with extremely high remission, clinical response, and mucosal healing rates in patients with moderate-to-severe UC. In addition, other molecules with therapeutic potential are α4β7-specific ozanimod, fingolimod, and AJM300 (106, 111, 114). PF-00547659, an antibody target endothelial MAdCAM-1, has also shown therapeutic potential for UC but not for CD (115).
The homing and migration of immune cells depend on the expression of chemokine receptors. Macrophage inflammatory protein (MIP)-3α is elevated in IBD is considered to be a CD4 T-cell directed chemokine. Anti-MIP-3alpha significantly reduced T-cell recruitment and TNBS-mediated colonic injury (116). CCL20 is a CCR6 homologue ligand with strong affinity. Th17 cells have strong CCR6 expression, which promotes their migration to inflammatory tissues with high levels of CCL20. Mongersen-anti-sense oligonucleotide to Smad7 (GED0301) is a short oligonucleotide that indirectly targets CCL20 and alleviates inflammation in a TNBS-induced mouse model of IBD (6).
4.3 Inhibiting Th17 cell proliferation and differentiation
Th17 cell differentiation, proliferation, and activation are induced by a variety of cytokines, such as IL-6, IL-21, and IL-23. Neutralization of these cytokines or inhibition of their receptors by monoclonal antibodies or small molecule inhibitors can inhibit Th17 cell production and achieve IBD therapy. In animal models, recombinant anti-IL-6 antibodies can alleviate symptoms of colonic inflammation by hindering the secretion of inflammatory factors, reducing inflammatory cell aggregation, and promoting T-cell apoptosis (118, 119). PF04236921 is a human IL-6 monoclonal antibody. A clinical study involving 247 patients with IBD showed that the clinical response rate and remission rate of the treatment group taking the drug were better than those of the placebo group, and there was a certain dose-effect relationship (119). Tocilizumab (MRA), a humanized monoclonal antibody directed against the IL-6 receptor, showed promising efficacy in patients with active CD (120).
Studies found that after the administration of the recombinant protein IL-22 to mice with colitis, symptoms and intestinal inflammation were relieved, but there are no reports on the clinical application of IL-22 (28). Anti-TNFα preparations upregulate the ratio of Tregs versus effector T cells, mainly by reducing the expression of inflammatory factors (including Th17-cell-related IL-17, IL-23 and IL-6), increasing the number of Tregs, and promoting effector T-cell apoptosis. The specific binding of infliximab to TNFα can block the binding of the latter to cell surface receptors, thereby inhibiting the inflammatory response and rapidly inducing apoptosis of intestinal mucosa T cells. However, the drug is only effective for some patients; its efficacy may be related to the type of IBD and various inflammatory cytokines.
Ustekinumab, an antibody that blocks IL-12 and IL-23, has been greenlighted for the treatment of CD and UC (122). Briakinumab, another antibody that inhibits IL-23 and IL-12 in combination, is effective against IBD although it has been discontinued (123). Several anti-IL-23p19-specific antibodies have undergone or are presently undertaking clinical studies for IBD, such as brazikumab, risankizumab, mirikizumab, and guselkumab (124–127). PTG-200 is an IL-23R antagonists therefore also specifically interfere with IL-23 signaling (121).
In addition to targeting cytokines associated with mediating Th17 cell differentiation, the master transcriptional regulator RORγt itself is an intriguing therapeutic target that may have impacts not only on Th17 cells but also on type 17 cells generally. It targets cytokines linked to facilitating Th17 cell development. The RORγt antagonists VPR-254, GSK805, and TAK-828F, which have shown promise efficacy in preclinical models of IBD, are among those now being developed (128–130). The underlying mechanisms of action, important target cells (such as Th17 cells versus ILC3s), and impacts on the homeostatic balance between Th17 cells and Tregs are all unanswered problems involving RORγt antagonism. In addition, the transcription factor STAT3 can also be used as a target to inhibit Th17. STAT3-related inhibitors, such as gene associated with retinoid interferon-induced mortality (Grim) 19, metformin and C188-9, have shown good therapeutic effects in murine models of CD and UC (131, 132).
4.4 Neutralization or inhibition of cytokines produced by Th17 cells
Neutralizing antibodies and small molecule inhibitors targeting Th17 effector molecules can exhibit different therapeutic effects in different enteritis states. Although IL-17 is overexpressed in IBD patients, the IL-17A neutralizing antibody (secukinumab and ixekizumab) and the IL-17A receptor neutralizing antibody (brodalumab) are not effective in CD patients, and some patients have adverse reactions (133–135). Wedebye Schmidt et al. discovered that simultaneous inhibition of IL-17A and IL-17F expression can effectively alleviate T-cell transplantation-induced intestinal inflammation in mice (142). Vidofludimus is an oral immunosuppressant that can downregulate IL-17A and IL-17F levels by inhibiting STAT3 and NF-κB, demonstrating safety, efficacy and tolerability in hormone-dependent IBD patients (136). These results suggested that combined therapy and targets multiple cytokines is expected to become a new option for IBD treatment. Clinical trials have confirmed that secukinumab, ixekizumab, and brodalumab can effectively alleviate various diseases, such as multiple sclerosis (MS), active ankylosing spondylitis (AS), rheumatoid arthritis (RA) or psoriasis, and are ineffective in the treatment of CD and UC (133–135). IL-21 receptor blockers alleviate DSS-induced colitis in mice (117), indicating that IL-21 may also be a potential target for the treatment of IBD in the future.
5 Conclusion
Unlike other autoimmune diseases, the pathogenesis of IBD occurs in the gut, where the largest proportion of intestinal symbiotes and intestinal microbes are colonized. Through the review of Th17 cell immunity, we have gained more understanding of the pathogenesis and treatment of IBD. Th17 cells are potently affected by the gut microecosystem and are fully involved in the disease progression of IBD from intestinal homeostasis to inflammation to fibrosis. In recent years, a growing number of studies have shown that the gut microbes were involved in intestinal homeostasis and IBD by regulating Th17 cell differentiation and activation. Traditional Chinese medicine decoction, plant extract and gut microbes have attracted tremendous interest in the treatment of IBD through the gut microbes-Th17 cell axis, which is safe, effective and has few side effects, providing more possibilities for a better prognosis of IBD. These therapies complement the traditional Th17 cell-targeted immunobiologics for IBD. Unfortunately, the existing findings do not fully explain the immunomodulatory mechanisms of the gut microbiome. More evidence was needed to evaluate the contribution of gut microbes to mucosal immunity.
6 Perspective
Previous studies have shown that Th17 cells and their secreted cytokines play a crucial role in the occurrence and development of IBD and serve as a bridge between gut microbes and the gut immune microenvironment information. Theoretically, the superiority of Th17-targeted immunotherapy lies in alleviating IBD inflammation while improving intestinal fibrosis. The ineffectiveness of secukinumab, ixekizumab and brodalumab in the treatment of CD patients and the development of resistance after long-term biologic treatment suggest that the molecular mechanism of Th17 cells in IBD needs to be further investigated. We speculate that the diversity of intestinal microbes increases the complexity of the mucosal microenvironment and provides more possibilities for interaction between Th17 cells and other immune cells, making the situation in other tissues and organs not applicable to the intestinal microenvironment.
In the future, the in-depth study of gut microbes and Th17 cells in IBD, it will be of great help to the diagnosis, disease status assessment, drug efficacy and prognosis evaluation of IBD. Adjustment of intestinal microecology, selection of specific microorganisms and FMT combined with biological therapies may revolutionize the treatment of IBD and ultimately improve patient prognosis.
Author contributions
LC, DC, and YW wrote the manuscript. GR, YC, and AY conducted the literature survey. LC generated themes and ideas and edited the manuscript. This work was supervised by DC and YW. All authors contributed to the article and approved the submitted version.
Funding
This study was funded by the National Natural Science Foundation of China, Grant/Award Numbers: 82200614; the National Natural Science Foundation of China, Grant/Award Numbers: 82070571; and the key talent cultivation plan of the excellent talent pool of the Army Military Medical University, Grant/Award Number: No.
Conflict of interest
The authors declare that the research was conducted in the absence of any commercial or financial relationships that could be construed as a potential conflict of interest.
Publisher’s note
All claims expressed in this article are solely those of the authors and do not necessarily represent those of their affiliated organizations, or those of the publisher, the editors and the reviewers. Any product that may be evaluated in this article, or claim that may be made by its manufacturer, is not guaranteed or endorsed by the publisher.
References
1. Peluso I, Pallone F, Monteleone G. Interleukin-12 and Th1 immune response in crohn's disease: Pathogenetic relevance and therapeutic implication. World J Gastroenterol (2006) 12(35):5606–10. doi: 10.3748/wjg.v12.i35.5606
2. Yan JB, Luo MM, Chen ZY, He BH. The function and role of the Th17/Treg cell balance in inflammatory bowel disease. J Immunol Res (2020) p:8813558. doi: 10.1155/2020/8813558
3. Yang J, Sundrud MS, Skepner J, Yamagata T. Targeting Th17 cells in autoimmune diseases. Trends Pharmacol Sci (2014) 35(10):493–500. doi: 10.1016/j.tips.2014.07.006
4. Harrington LE, Hatton RD, Mangan PR, Turner H, Murphy TL, Murphy KM, et al. Interleukin 17-producing CD4+ effector T cells develop via a lineage distinct from the T helper type 1 and 2 lineages. Nat Immunol (2005) 6(11):1123–32. doi: 10.1038/ni1254
5. Park H, Li Z, Yang XO, Chang SH, Nurieva R, Wang YH, et al. A distinct lineage of CD4 T cells regulates tissue inflammation by producing interleukin 17. Nat Immunol (2005) 6(11):1133–41. doi: 10.1038/ni1261
6. Meitei HT, Jadhav N, Lal G. CCR6-CCL20 axis as a therapeutic target for autoimmune diseases. Autoimmun Rev (2021) 20(7):102846. doi: 10.1016/j.autrev.2021.102846
7. Abraham C, Medzhitov R. Interactions between the host innate immune system and microbes in inflammatory bowel disease. Gastroenterology (2011) 140(6):1729–37. doi: 10.1053/j.gastro.2011.02.012
8. Ueno A, Jeffery L, Kobayashi T, Hibi T, Ghosh S, Jijon H. Th17 plasticity and its relevance to inflammatory bowel disease. J Autoimmun (2018) 87:38–49. doi: 10.1016/j.jaut.2017.12.004
9. Matsunaga Y, Clark T, Wanek AG, Bitoun JP, Gong Q, Good M, et al. Intestinal IL-17R signaling controls secretory IgA and oxidase balance in citrobacter rodentium infection. J Immunol (2021) 206(4):766–75. doi: 10.4049/jimmunol.2000591
10. Chen J, Ye X, Pitmon E, Lu M, Wan J, Jellison ER, et al. IL-17 inhibits CXCL9/10-mediated recruitment of CD8(+) cytotoxic T cells and regulatory T cells to colorectal tumors. J Immunother Cancer (2019) 7(1):324. doi: 10.1186/s40425-019-0757-z
11. Nishikawa K, Seo N, Torii M, Ma N, Muraoka D, Tawara I, et al. Interleukin-17 induces an atypical M2-like macrophage subpopulation that regulates intestinal inflammation. PloS One (2014) 9(9):e108494. doi: 10.1371/journal.pone.0108494
12. Tawiah A, Moreau F, Kumar M, Tiwari S, Falguera J, Chadee K. High MUC2 mucin biosynthesis in goblet cells impedes restitution and wound healing by elevating endoplasmic reticulum stress and altered production of growth factors. Am J Pathol (2018) 188(9):2025–41. doi: 10.1016/j.ajpath.2018.05.013
13. Bsat M, Chapuy L, Rubio M, Wassef R, Richard C, Schwenter F, et al. Differential pathogenic Th17 profile in mesenteric lymph nodes of crohn's disease and ulcerative colitis patients. Front Immunol (2019) 10:1177. doi: 10.3389/fimmu.2019.01177
14. Huang FC. The interleukins orchestrate mucosal immune responses to salmonella infection in the intestine. Cells (2021) 10(12):3492. doi: 10.3390/cells10123492
15. Fujii M, Nishida A, Imaeda H, Ohno M, Nishino K, Sakai S, et al. Expression of interleukin-26 is upregulated in inflammatory bowel disease. World J Gastroenterol (2017) 23(30):5519–29. doi: 10.3748/wjg.v23.i30.5519
16. Song D, Lai L, Lu J, Tong J, Ran Z. Interleukin-26 expression in inflammatory bowel disease and its immunoregulatory effects on macrophages. Front Med (Lausanne) (2022) 9:797135. doi: 10.3389/fmed.2022.797135
17. Annunziato F, Cosmi L, Santarlasci V, Maggi L, Liotta F, Mazzinghi B, et al. Phenotypic and functional features of human Th17 cells. J Exp Med (2007) 204(8):1849–61. doi: 10.1084/jem.20070663
18. Cosmi L, Maggi L, Santarlasci V, Capone M, Cardilicchia E, Frosali F, et al. Identification of a novel subset of human circulating memory CD4(+) T cells that produce both IL-17A and IL-4. J Allergy Clin Immunol (2010) 125(1):222–30 e1-4. doi: 10.1016/j.jaci.2009.10.012
19. D'Alessio S, Ungaro F, Noviello D, Lovisa S, Peyrin-Biroulet L, Danese S. Revisiting fibrosis in inflammatory bowel disease: the gut thickens. Nat Rev Gastroenterol Hepatol (2022) 19(3):169–84. doi: 10.1038/s41575-021-00543-0
20. Latella G, Viscido A. Controversial contribution of Th17/IL-17 toward the immune response in intestinal fibrosis. Dig Dis Sci (2020) 65(5):1299–306. doi: 10.1007/s10620-020-06161-1
21. Liu S, Liu Z, Shan Z, Liu Y, Chen T, Fang L, et al. Skewed Th17/Treg balance during progression and malignant transformation of oral submucous fibrosis. Oral Dis (2021) 28(8):2119–30. doi: 10.1111/odi.13853
22. Shi X, Huang H, Zhou M, Liu Y, Wu H, Dai M. Paeonol attenuated vascular fibrosis through regulating Treg/Th17 balance in a gut microbiota-dependent manner. Front Pharmacol (2021) 12:765482. doi: 10.3389/fphar.2021.765482
23. Qiao L, Fang L, Zhu J, Xiang Y, Xu H, Sun X, et al. Total flavone of abelmoschus manihot ameliorates TNBS-induced colonic fibrosis by regulating Th17/Treg balance and reducing extracellular matrix. Front Pharmacol (2021) 12:769793.
24. Oshalim M, Johansson E, Rabe H, Gilljam M, Lindblad A, Jonsson B. Th17 associated cytokines in sputum samples from patients with cystic fibrosis. Pathog Dis (2020) 78(6):ftaa050. doi: 10.1093/femspd/ftaa050
25. Biancheri P, Pender SL, Ammoscato F, Giuffrida P, Sampietro G, Ardizzone S, et al. The role of interleukin 17 in crohn's disease-associated intestinal fibrosis. Fibrogenesis Tissue Repair (2013) 6(1):13. doi: 10.1186/1755-1536-6-13
26. Majumder S, Amatya N, Revu S, Jawale CV, Wu D, Rittenhouse N, et al. IL-17 metabolically reprograms activated fibroblastic reticular cells for proliferation and survival. Nat Immunol (2019) 20(5):534–45. doi: 10.1038/s41590-019-0367-4
27. Zhang HJ, Zhang YN, Zhou H, Guan L, Li Y, Sun MJ. IL-17A promotes initiation and development of intestinal fibrosis through EMT. Dig Dis Sci (2018) 63(11):2898–909. doi: 10.1007/s10620-018-5234-x
28. Mathur R, Alam MM, Zhao XF, Liao Y, Shen J, Morgan S, et al. Induction of autophagy in Cx3cr1(+) mononuclear cells limits IL-23/IL-22 axis-mediated intestinal fibrosis. Mucosal Immunol (2019) 12(3):612–23. doi: 10.1038/s41385-019-0146-4
29. Lee JY, Hall JA, Kroehling L, Wu L, Najar T, Nguyen HH, et al. Serum amyloid a proteins induce pathogenic Th17 cells and promote inflammatory disease. Cell (2020) 180(1):79–91 e16. doi: 10.1016/j.cell.2019.11.026
30. Viladomiu M, Kivolowitz C, Abdulhamid A, Dogan B, Victorio D, Castellanos JG, et al. IgA-coated e. coli enriched in crohn's disease spondyloarthritis promote TH17-dependent inflammation. Sci Transl Med (2017) 9(376):eaaf9655. doi: 10.1126/scitranslmed.aaf9655
31. Cao Y, Wang Z, Yan Y, Ji L, He J, Xuan B, et al. Enterotoxigenic bacteroidesfragilis promotes intestinal inflammation and malignancy by inhibiting exosome-packaged miR-149-3p. Gastroenterology (2021) 161(5):1552–1566 e12. doi: 10.1053/j.gastro.2021.08.003
32. Alexander M, Ang QY, Nayak RR, Bustion AE, Sandy M, Zhang B, et al. Human gut bacterial metabolism drives Th17 activation and colitis. Cell Host Microbe (2022) 30(1):17–30 e9. doi: 10.1016/j.chom.2021.11.001
33. Ferreira-Gomes M, Wich M, Bode S, Hube B, Jacobsen ID, Jungnickel B. B cell recognition of candida albicans hyphae via TLR 2 promotes IgG1 and IL-6 secretion for TH17 differentiation. Front Immunol (2021) 12:698849. doi: 10.3389/fimmu.2021.698849
34. Reiss-Mandel A, Rubin C, Zayoud M, Rahav G, Regev-Yochay G. Staphylococcus aureus colonization induces strain-specific suppression of interleukin-17. Infect Immun (2018) 86(3):e00834–17. doi: 10.1128/IAI.00834-17
35. Wu W, Chen F, Liu Z, Cong Y. Microbiota-specific Th17 cells: Yin and yang in regulation of inflammatory bowel disease. Inflammation Bowel Dis (2016) 22(6):1473–82. doi: 10.1097/MIB.0000000000000775
36. Candelli M, Franza L, Pignataro G, Ojetti V, Covino M, Piccioni A, et al. Interaction between lipopolysaccharide and gut microbiota in inflammatory bowel diseases. Int J Mol Sci (2021) 22(12):6242. doi: 10.3390/ijms22126242
37. Atarashi K, Nishimura J, Shima T, Umesaki Y, Yamamoto M, Onoue M, et al. ATP drives lamina propria T(H)17 cell differentiation. Nature (2008) 455(7214):808–12. doi: 10.1038/nature07240
38. Haase S, Haghikia A, Wilck N, Muller DN, Linker RA. Impacts of microbiome metabolites on immune regulation and autoimmunity. Immunology (2018) 154(2):230–8. doi: 10.1111/imm.12933
39. Zhao Q, Yang WR, Wang XH, Li GQ, Xu LQ, Cui X, et al. Clostridium butyricum alleviates intestinal low-grade inflammation in TNBS-induced irritable bowel syndrome in mice by regulating functional status of lamina propria dendritic cells. World J Gastroenterol (2019) 25(36):5469–82. doi: 10.3748/wjg.v25.i36.5469
40. Xiao Z, Liu L, Pei X, Sun W, Jin Y, Yang ST, et al. A potential probiotic for diarrhea: Clostridium tyrobutyricum protects against LPS-induced epithelial dysfunction via IL-22 produced by Th17 cells in the ileum. Front Immunol (2021) 12:758227. doi: 10.3389/fimmu.2021.758227
41. Hang S, Paik D, Yao L, Kim E, Trinath J, Lu J, et al. Bile acid metabolites control TH17 and treg cell differentiation. Nature (2019) 576(7785):143–8. doi: 10.1038/s41586-019-1785-z
42. Hang S, Paik D, Yao L, Kim E, Trinath J, Lu J, et al. Author correction: Bile acid metabolites control TH17 and treg cell differentiation. Nature (2020) 579(7798):E7. doi: 10.1038/s41586-020-2030-5
43. Puleston DJ, Baixauli F, Sanin DE, Edwards-Hicks J, Villa M, Kabat AM, et al. Polyamine metabolism is a central determinant of helper T cell lineage fidelity. Cell (2021) 184(16):4186–4202 e20. doi: 10.1016/j.cell.2021.06.007
44. Michalek RD, Gerriets VA, Jacobs SR, Macintyre AN, MacIver NJ, Mason EF, et al. Cutting edge: distinct glycolytic and lipid oxidative metabolic programs are essential for effector and regulatory CD4+ T cell subsets. J Immunol (2011) 186(6):3299–303. doi: 10.4049/jimmunol.1003613
45. Shi LZ, Wang R, Huang G, Vogel P, Neale G, Green DR, et al. HIF1alpha-dependent glycolytic pathway orchestrates a metabolic checkpoint for the differentiation of TH17 and treg cells. J Exp Med (2011) 208(7):1367–76. doi: 10.1084/jem.20110278
46. Yabu M, Shime H, Hara H, Saito T, Matsumoto M, Seya T, et al. IL-23-dependent and -independent enhancement pathways of IL-17A production by lactic acid. Int Immunol (2011) 23(1):29–41. doi: 10.1093/intimm/dxq455
47. Ivanov II, McKenzie BS, Zhou L, Tadokoro CE, Lepelley A, Lafaille JJ, et al. The orphan nuclear receptor RORgammat directs the differentiation program of proinflammatory IL-17+ T helper cells. Cell (2006) 126(6):1121–33. doi: 10.1016/j.cell.2006.07.035
48. Perez LG, Kempski J, McGee HM, Pelzcar P, Agalioti T, Giannou A, et al. TGF-beta signaling in Th17 cells promotes IL-22 production and colitis-associated colon cancer. Nat Commun (2020) 11(1):2608. doi: 10.1038/s41467-020-16363-w
49. Neurath MF. IL-23 in inflammatory bowel diseases and colon cancer. Cytokine Growth Factor Rev (2019) 45:1–8. doi: 10.1016/j.cytogfr.2018.12.002
50. Long D, Chen Y, Wu H, Zhao M, Lu Q. Clinical significance and immunobiology of IL-21 in autoimmunity. J Autoimmun (2019) 99:1–14. doi: 10.1016/j.jaut.2019.01.013
51. Acosta-Rodriguez EV, Napolitani G, Lanzavecchia A, Sallusto F. Interleukins 1beta and 6 but not transforming growth factor-beta are essential for the differentiation of interleukin 17-producing human T helper cells. Nat Immunol (2007) 8(9):942–9. doi: 10.1038/ni1496
52. Durant L, Watford WT, Ramos HL, Laurence A, Vahedi G, Wei L, et al. Diverse targets of the transcription factor STAT3 contribute to T cell pathogenicity and homeostasis. Immunity (2010) 32(5):605–15. doi: 10.1016/j.immuni.2010.05.003
53. Mudter J, Yu J, Zufferey C, Brustle A, Wirtz S, Weigmann B, et al. IRF4 regulates IL-17A promoter activity and controls RORgammat-dependent Th17 colitis in vivo. Inflammation Bowel Dis (2011) 17(6):1343–58. doi: 10.1002/ibd.21476
54. Schraml BU, Hildner K, Ise W, Lee WL, Smith WA, Solomon B, et al. The AP-1 transcription factor batf controls T(H)17 differentiation. Nature (2009) 460(7253):405–9. doi: 10.1038/nature08114
55. Yamazaki S, Tanaka Y, Araki H, Kohda A, Sanematsu F, Arasaki T, et al. The AP-1 transcription factor JunB is required for Th17 cell differentiation. Sci Rep (2017) 7(1):17402. doi: 10.1038/s41598-017-17597-3
56. Dang EV, Barbi J, Yang HY, Jinasena D, Yu H, Zheng Y, et al. Control of T(H)17/T(reg) balance by hypoxia-inducible factor 1. Cell (2011) 146(5):772–84. doi: 10.1016/j.cell.2011.07.033
57. Wilson AS, Randall KL, Pettitt JA, Ellyard JI, Blumenthal A, Enders A, et al. Neutrophil extracellular traps and their histones promote Th17 cell differentiation directly via TLR2. Nat Commun (2022) 13(1):528. doi: 10.1038/s41467-022-28172-4
58. Holm CK, Petersen CC, Hvid M, Petersen L, Paludan SR, Deleuran B, et al. TLR3 ligand polyinosinic:polycytidylic acid induces IL-17A and IL-21 synthesis in human Th cells. J Immunol (2009) 183(7):4422–31. doi: 10.4049/jimmunol.0804318
59. Qu X, Han J, Zhang Y, Wang X, Fan H, Hua F, et al. TLR4-RelA-miR-30a signal pathway regulates Th17 differentiation during experimental autoimmune encephalomyelitis development. J Neuroinflamm (2019) 16(1):183. doi: 10.1186/s12974-019-1579-0
60. Ye J, Wang Y, Liu X, Li L, Opejin A, Hsueh EC, et al. TLR7 signaling regulates Th17 cells and autoimmunity: Novel potential for autoimmune therapy. J Immunol (2017) 199(3):941–54. doi: 10.4049/jimmunol.1601890
61. Tamassia N, Arruda-Silva F, Wright HL, Moots RJ, Gardiman E, Bianchetto-Aguilera F, et al. Human neutrophils activated via TLR8 promote Th17 polarization through IL-23. J Leukoc Biol (2019) 105(6):1155–65. doi: 10.1002/JLB.MA0818-308R
62. Zhang M, Zhou L, Xu Y, Yang M, Xu Y, Komaniecki GP, et al. A STAT3 palmitoylation cycle promotes TH17 differentiation and colitis. Nature (2020) 586(7829):434–9. doi: 10.1038/s41586-020-2799-2
63. Britton GJ, Contijoch EJ, Mogno I, Vennaro OH, Llewellyn SR, Ng R, et al. Microbiotas from humans with inflammatory bowel disease alter the balance of gut Th17 and RORgammat(+) regulatory T cells and exacerbate colitis in mice. Immunity (2019) 50(1):212–224 e4. doi: 10.1016/j.immuni.2018.12.015
64. Atarashi K, Tanoue T, Ando M, Kamada N, Nagano Y, Narushima S, et al. Th17 cell induction by adhesion of microbes to intestinal epithelial cells. Cell (2015) 163(2):367–80. doi: 10.1016/j.cell.2015.08.058
65. Ivanov II, Atarashi K, Manel N, Brodie EL, Shima T, Karaoz U, et al. Induction of intestinal Th17 cells by segmented filamentous bacteria. Cell (2009) 139(3):485–98. doi: 10.1016/j.cell.2009.09.033
66. Paik D, Yao L, Zhang Y, Bae S, D'Agostino GD, Zhang M, et al. Human gut bacteria produce TauEta17-modulating bile acid metabolites. Nature (2022) 603(7903):907–12. doi: 10.1038/s41586-022-04480-z
67. Hindson J. Bile acid metabolites produced by gut bacteria suppress TH17 cells. Nat Rev Gastroenterol Hepatol (2022) 19(5):280. doi: 10.1038/s41575-022-00614-w
68. Xiao R, Lei K, Kuok H, Deng W, Zhuang Y, Tang Y, et al. Synthesis and identification of lithocholic acid 3-sulfate as RORgammat ligand to inhibit Th17 cell differentiation. J Leukoc Biol (2022) 112(4):835–43.
69. Hochrein SM, Wu H, Eckstein M, Arrigoni L, Herman JS, Schumacher F, et al. The glucose transporter GLUT3 controls T helper 17 cell responses through glycolytic-epigenetic reprogramming. Cell Metab (2022) 34(4):516–532 e11. doi: 10.1016/j.cmet.2022.02.015
70. Xu K, Yin N, Peng M, Stamatiades EG, Chhangawala S, Shyu A, et al. Glycolytic ATP fuels phosphoinositide 3-kinase signaling to support effector T helper 17 cell responses. Immunity (2021) 54(5):976–987 e7. doi: 10.1016/j.immuni.2021.04.008
71. Wagner A, Wang C, Fessler J, DeTomaso D, Avila-Pacheco J, Kaminski J, et al. Metabolic modeling of single Th17 cells reveals regulators of autoimmunity. Cell (2021) 184(16):4168–4185 e21. doi: 10.1016/j.cell.2021.05.045
72. Wu L, Hollinshead KER, Hao Y, Au C, Kroehling L, Ng C, et al. Niche-selective inhibition of pathogenic Th17 cells by targeting metabolic redundancy. Cell (2020) 182(3):641–654 e20. doi: 10.1016/j.cell.2020.06.014
73. Johnson MO, Wolf MM, Madden MZ, Andrejeva G, Sugiura A, Contreras DC, et al. Distinct regulation of Th17 and Th1 cell differentiation by glutaminase-dependent metabolism. Cell (2018) 175(7):1780–1795 e19. doi: 10.1016/j.cell.2018.10.001
74. de Roock S, van Elk M, Hoekstra MO, Prakken BJ, Rijkers GT, de Kleer IM. Gut derived lactic acid bacteria induce strain specific CD4(+) T cell responses in human PBMC. Clin Nutr (2011) 30(6):845–51. doi: 10.1016/j.clnu.2011.05.005
75. Caslin HL, Abebayehu D, Pinette JA, Ryan JJ. Lactate is a metabolic mediator that shapes immune cell fate and function. Front Physiol (2021) 12:688485. doi: 10.3389/fphys.2021.688485
76. Luo Y, Li L, Chen X, Gou H, Yan K, Xu Y. Effects of lactate in immunosuppression and inflammation: Progress and prospects. Int Rev Immunol (2022) 41(1):19–29. doi: 10.1080/08830185.2021.1974856
77. Haas R, Smith J, Rocher-Ros V, Nadkarni S, Montero-Melendez T, D'Acquisto F, et al. Lactate regulates metabolic and pro-inflammatory circuits in control of T cell migration and effector functions. PloS Biol (2015) 13(7):e1002202. doi: 10.1371/journal.pbio.1002202
78. Manel N, Unutmaz D, Littman DR. The differentiation of human T(H)-17 cells requires transforming growth factor-beta and induction of the nuclear receptor RORgammat. Nat Immunol (2008) 9(6):641–9. doi: 10.1038/ni.1610
79. Ikeda S, Saijo S, Murayama MA, Shimizu K, Akitsu A, Iwakura Y. Excess IL-1 signaling enhances the development of Th17 cells by downregulating TGF-beta-induced Foxp3 expression. J Immunol (2014) 192(4):1449–58. doi: 10.4049/jimmunol.1300387
80. Brustle A, Heink S, Huber M, Rosenplanter C, Stadelmann C, Yu P, et al. The development of inflammatory T(H)-17 cells requires interferon-regulatory factor 4. Nat Immunol (2007) 8(9):958–66. doi: 10.1038/ni1500
81. Wu X, Chen H, Gao X, Gao H, He Q, Li G, et al. Natural herbal remedy wumei decoction ameliorates intestinal mucosal inflammation by inhibiting Th1/Th17 cell differentiation and maintaining microbial homeostasis. Inflammation Bowel Dis (2022) 28(7):1061–71. doi: 10.1093/ibd/izab348
82. Wang Y, Zhang J, Xu L, Ma J, Lu M, Ma J, et al. Modified gegen qinlian decoction regulates Treg/Th17 balance to ameliorate DSS-induced acute experimental colitis in mice by altering the gut microbiota. Front Pharmacol (2021) 12:756978. doi: 10.3389/fphar.2021.756978
83. Zhang M, Fan H, Tan S, Tang Q, Liu X, Zuo D, et al. The Chinese medicinal herb decoction QRZSLXF enhances anti-inflammatory effect in TNBS-induced colitis via balancing Th17/Tregs differentiation. J Ethnopharmacol (2020) 251:112549. doi: 10.1016/j.jep.2020.112549
84. Bao C, Wu L, Wang D, Chen L, Jin X, Shi Y, et al. Acupuncture improves the symptoms, intestinal microbiota, and inflammation of patients with mild to moderate crohn's disease: A randomized controlled trial. EClinicalMedicine (2022) 45:101300. doi: 10.1016/j.eclinm.2022.101300
85. Mei C, Wang X, Meng F, Zhang X, Chen L, Yan S, et al. Aucuboside inhibits the generation of Th17 cells in mice colitis. Front Pharmacol (2021) 12:696599. doi: 10.3389/fphar.2021.696599
86. Lin Z, Chen L, Cheng M, Zhu F, Yang X, Zhao W, et al. Cortex periplocae modulates the gut microbiota to restrict colitis and colitis-associated colorectal cancer via suppression of pathogenic Th17 cells. BioMed Pharmacother (2022) 153:113399. doi: 10.1016/j.biopha.2022.113399
87. Wen S, He L, Zhong Z, Zhao R, Weng S, Mi H, et al. Stigmasterol restores the balance of Treg/Th17 cells by activating the butyrate-PPARgamma axis in colitis. Front Immunol (2021) 12:741934. doi: 10.3389/fimmu.2021.741934
88. Chang Y, Zhai L, Peng J, Wu H, Bian Z, Xiao H. Phytochemicals as regulators of Th17/Treg balance in inflammatory bowel diseases. BioMed Pharmacother (2021) 141:111931. doi: 10.1016/j.biopha.2021.111931
89. Sokol H, Landman C, Seksik P, Berard L, Montil M, Nion-Larmurier I, et al. Fecal microbiota transplantation to maintain remission in crohn's disease: a pilot randomized controlled study. Microbiome (2020) 8(1):12. doi: 10.1186/s40168-020-0792-5
90. Crothers JW, Chu ND, Nguyen LTT, Phillips M, Collins C, Fortner K, et al. Daily, oral FMT for long-term maintenance therapy in ulcerative colitis: results of a single-center, prospective, randomized pilot study. BMC Gastroenterol (2021) 21(1):281. doi: 10.1186/s12876-021-01856-9
91. Plavec TV, Kuchar M, Benko A, Liskova V, Cerny J, Berlec A, et al. Engineered lactococcus lactis secreting IL-23 receptor-targeted REX protein blockers for modulation of IL-23/Th17-Mediated inflammation. Microorganisms (2019) 7(5):152. doi: 10.3390/microorganisms7050152
92. Jia L, Wu R, Han N, Fu J, Luo Z, Guo L, et al. Porphyromonas gingivalis and lactobacillus rhamnosus GG regulate the Th17/Treg balance in colitis via TLR4 and TLR2. Clin Transl Immunol (2020) 9(11):e1213. doi: 10.1002/cti2.1213
93. Wang L, Liao Y, Yang R, Zhu Z, Zhang L, Wu Z, et al. An engineered probiotic secreting Sj16 ameliorates colitis via ruminococcaceae/butyrate/retinoic acid axis. Bioeng Transl Med (2021) 6(3):e10219. doi: 10.1002/btm2.10219
94. Huang J, Yang Z, Li Y, Chai X, Liang Y, Lin B, et al. Lactobacillus paracasei R3 protects against dextran sulfate sodium (DSS)-induced colitis in mice via regulating Th17/Treg cell balance. J Transl Med (2021) 19(1):356. doi: 10.1186/s12967-021-02943-x
95. Ruan G, Chen M, Chen L, Xu F, Xiao Z, Yi A, et al. Roseburia intestinalis and its metabolite butyrate inhibit colitis and upregulate TLR5 through the SP3 signaling pathway. Nutrients (2022) 14(15). doi: 10.3390/nu14153041
96. Oliva S, Di Nardo G, Ferrari F, Mallardo S, Rossi P, Patrizi G, et al. Randomised clinical trial: the effectiveness of lactobacillus reuteri ATCC 55730 rectal enema in children with active distal ulcerative colitis. Aliment Pharmacol Ther (2012) 35(3):327–34. doi: 10.1111/j.1365-2036.2011.04939.x
97. Henker J, Muller S, Laass MW, Schreiner A, Schulze J. Probiotic escherichia coli nissle 1917 (EcN) for successful remission maintenance of ulcerative colitis in children and adolescents: an open-label pilot study. Z Gastroenterol (2008) 46(9):874–5. doi: 10.1055/s-2008-1027463
98. Kato K, Mizuno S, Umesaki Y, Ishii Y, Sugitani M, Imaoka A, et al. Randomized placebo-controlled trial assessing the effect of bifidobacteria-fermented milk on active ulcerative colitis. Aliment Pharmacol Ther (2004) 20(10):1133–41. doi: 10.1111/j.1365-2036.2004.02268.x
99. Guslandi M. Saccharomyces boulardii plus rifaximin in mesalamine-intolerant ulcerative colitis. J Clin Gastroenterol (2010) 44(5):385. doi: 10.1097/MCG.0b013e3181cb4233
100. van der Lelie D, Oka A, Taghavi S, Umeno J, Fan TJ, Merrell KE, et al. Rationally designed bacterial consortia to treat chronic immune-mediated colitis and restore intestinal homeostasis. Nat Commun (2021) 12(1):3105. doi: 10.1038/s41467-021-23460-x
101. El Amrousy D, El Ashry H, Hodeib H, Hassan S. Vitamin d in children with inflammatory bowel disease: A randomized controlled clinical trial. J Clin Gastroenterol (2021) 55(9):815–20. doi: 10.1097/MCG.0000000000001443
102. Li G, Lin J, Zhang C, Gao H, Lu H, Gao X, et al. Microbiota metabolite butyrate constrains neutrophil functions and ameliorates mucosal inflammation in inflammatory bowel disease. Gut Microbes (2021) 13(1):1968257. doi: 10.1080/19490976.2021.1968257
103. Teng X, Qi Y, Li J, Wu J. Effect of exclusive enteral nutrition on Th17 cells in juvenile rats with inflammatory bowel disease. Inflammation (2021) 44(1):261–9. doi: 10.1007/s10753-020-01328-4
104. Ang QY, Alexander M, Newman JC, Tian Y, Cai J, Upadhyay V, et al. Ketogenic diets alter the gut microbiome resulting in decreased intestinal Th17 cells. Cell (2020) 181(6):1263–1275 e16. doi: 10.1016/j.cell.2020.04.027
105. Garcia-Montero C, Fraile-Martinez O, Gomez-Lahoz AM, Pekarek L, Castellanos AJ, Noguerales-Fraguas F, et al. Nutritional components in Western diet versus Mediterranean diet at the gut microbiota-immune system interplay. implications for health and disease. Nutrients (2021) 13(2). doi: 10.3390/nu13020699
106. Dal Buono A, Gabbiadini R, Alfarone L, Solitano V, Repici A, Vetrano S, et al. Sphingosine 1-phosphate modulation in inflammatory bowel diseases: Keeping lymphocytes out of the intestine. Biomedicines (2022) 10(7). doi: 10.3390/biomedicines10071735
107. Sandborn WJ, Peyrin-Biroulet L, Zhang J, Chiorean M, Vermeire S, Lee SD, et al. Efficacy and safety of etrasimod in a phase 2 randomized trial of patients with ulcerative colitis. Gastroenterology (2020) 158(3):550–61. doi: 10.1053/j.gastro.2019.10.035
108. D'Haens G, Danese S, Davies M, Watanabe M, Hibi T. A phase II, multicentre, randomised, double-blind, placebo-controlled study to evaluate safety, tolerability, and efficacy of amiselimod in patients with moderate to severe active crohn's disease. J Crohns Colitis (2022) 16(5):746–56. doi: 10.1093/ecco-jcc/jjab201
109. Radeke HH, Stein J, Van Assche G, Rogler G, Lakatos PL, Muellershausen F, et al. A multicentre, double-blind, placebo-controlled, parallel-group study to evaluate the efficacy, safety, and tolerability of the S1P receptor agonist KRP203 in patients with moderately active refractory ulcerative colitis. Inflamm Intest Dis (2020) 5(4):180–90. doi: 10.1159/000509393
110. Pudipeddi A, Ko Y, Paramsothy S, Leong RW. Vedolizumab has longer persistence than infliximab as a first-line biological agent but not as a second-line biological agent in moderate-to-severe ulcerative colitis: real-world registry data from the persistence Australian national IBD cohort (PANIC) study. Therap Adv Gastroenterol (2022) 15:17562848221080793. doi: 10.1177/17562848221080793
111. Rubin DT, Dotan I, DuVall A, Bouhnik Y, Radford-Smith G, Higgins PDR, et al. Etrolizumab versus adalimumab or placebo as induction therapy for moderately to severely active ulcerative colitis (HIBISCUS): two phase 3 randomised, controlled trials. Lancet Gastroenterol Hepatol (2022) 7(1):17–27. doi: 10.1016/S2468-1253(21)00338-1
112. Sandborn WJ, Cyrille M, Hansen MB, Feagan BG, Loftus EV Jr., Rogler G, et al. Efficacy and safety of abrilumab in a randomized, placebo-controlled trial for moderate-to-Severe ulcerative colitis. Gastroenterology (2019) 156(4):946–957 e18. doi: 10.1053/j.gastro.2018.11.035
113. Park SC, Jeen YT. Anti-integrin therapy for inflammatory bowel disease. World J Gastroenterol (2018) 24(17):1868–80. doi: 10.3748/wjg.v24.i17.1868
114. Matsuoka K, et al. AJM300 (carotegrast methyl), an oral antagonist of alpha4-integrin, as induction therapy for patients with moderately active ulcerative colitis: a multicentre, randomised, double-blind, placebo-controlled, phase 3 study. Lancet Gastroenterol Hepatol (2022) 7(7):648–57. doi: 10.1016/S2468-1253(22)00022-X
115. Vermeire S, Sandborn WJ, Danese S, Hebuterne X, Salzberg BA, Klopocka M, et al. Anti-MAdCAM antibody (PF-00547659) for ulcerative colitis (TURANDOT): a phase 2, randomised, double-blind, placebo-controlled trial. Lancet (2017) 390(10090):135–44. doi: 10.1016/S0140-6736(17)30930-3
116. Katchar K, Kelly CP, Keates S, O'Brien M J, Keates AC. MIP-3alpha neutralizing monoclonal antibody protects against TNBS-induced colonic injury and inflammation in mice. Am J Physiol Gastrointest Liver Physiol (2007) 292(5):G1263–71. doi: 10.1152/ajpgi.00409.2006
117. Fina D, Sarra M, Fantini MC, Rizzo A, Caruso R, Caprioli F, et al. Regulation of gut inflammation and th17 cell response by interleukin-21. Gastroenterology (2008) 134(4):1038–48. doi: 10.1053/j.gastro.2008.01.041
118. Schreiber S, Aden K, Bernardes JP, Conrad C, Tran F, Hoper H, et al. Therapeutic interleukin-6 trans-signaling inhibition by olamkicept (sgp130Fc) in patients with active inflammatory bowel disease. Gastroenterology (2021) 160(7):2354–2366 e11. doi: 10.1053/j.gastro.2021.02.062
119. Danese S, Vermeire S, Hellstern P, Panaccione R, Rogler G, Fraser G, et al. Randomised trial and open-label extension study of an anti-interleukin-6 antibody in crohn's disease (ANDANTE I and II). Gut (2019) 68(1):40–8. doi: 10.1136/gutjnl-2017-314562
120. Ito H, Takazoe M, Fukuda Y, Hibi T, Kusugami K, Andoh A, et al. A pilot randomized trial of a human anti-interleukin-6 receptor monoclonal antibody in active crohn's disease. Gastroenterology (2004) 126(4):989–96; discussion 947. doi: 10.1053/j.gastro.2004.01.012
121. Moschen AR, Tilg H, Raine T. IL-12, IL-23 and IL-17 in IBD: immunobiology and therapeutic targeting. Nat Rev Gastroenterol Hepatol (2019) 16(3):185–96. doi: 10.1038/s41575-018-0084-8
122. Biancone L, Ardizzone S, Armuzzi A, Castiglione F, D'Inca R, Danese S, et al. Ustekinumab for treating ulcerative colitis: an expert opinion. Expert Opin Biol Ther (2020) 20(11):1321–9. doi: 10.1080/14712598.2020.1792882
123. Davies SC, Nguyen TM, Parker CE, MacDonald JK, Jairath V, Khanna R. Anti-IL-12/23p40 antibodies for maintenance of remission in crohn's disease. Cochrane Database Syst Rev (2019) 12:CD012804. doi: 10.1002/14651858.CD012804.pub2
124. Feagan BG, Sandborn WJ, D'Haens G, Panes J, Kaser A, Ferrante M, et al. Induction therapy with the selective interleukin-23 inhibitor risankizumab in patients with moderate-to-severe crohn's disease: A randomised, double-blind, placebo-controlled phase 2 study. Lancet (2017) 389(10080):1699–709. doi: 10.1016/S0140-6736(17)30570-6
125. Sands BE, Chen J, Feagan BG, Penney M, Rees WA, Danese S, et al. Efficacy and safety of MEDI2070, an antibody against interleukin 23, in patients with moderate to severe crohn's disease: A phase 2a study. Gastroenterology (2017) 153(1):77–86 e6. doi: 10.1053/j.gastro.2017.03.049
126. Wong U, Cross RK. Expert opinion on interleukin-12/23 and interleukin-23 antagonists as potential therapeutic options for the treatment of inflammatory bowel disease. Expert Opin Investig Drugs (2019) 28(5):473–9. doi: 10.1080/13543784.2019.1597053
127. Sandborn WJ, Ferrante M, Bhandari BR, Berliba E, Feagan BG, Hibi T, et al. Efficacy and safety of mirikizumab in a randomized phase 2 study of patients with ulcerative colitis. Gastroenterology (2020) 158(3):537–549 e10. doi: 10.1053/j.gastro.2019.08.043
128. Withers DR, Hepworth MR, Wang X, Mackley EC, Halford EE, Dutton EE, et al. Transient inhibition of ROR-gammat therapeutically limits intestinal inflammation by reducing TH17 cells and preserving group 3 innate lymphoid cells. Nat Med (2016) 22(3):319–23. doi: 10.1038/nm.4046
129. Igaki K, Nakamura Y, Komoike Y, Uga K, Shibata A, Ishimura Y, et al. Pharmacological evaluation of TAK-828F, a novel orally available RORgammat inverse agonist, on murine colitis model. Inflammation (2019) 42(1):91–102. doi: 10.1007/s10753-018-0875-7
130. Fitzpatrick LR, Small J, O'Connell R, Talbott G, Alton G, Zapf J. VPR-254: an inhibitor of ROR-gamma T with potential utility for the treatment of inflammatory bowel disease. Inflammopharmacology (2020) 28(2):499–511. doi: 10.1007/s10787-019-00643-z
131. Kim JK, Lee SH, Lee SY, Kim EK, Kwon JE, Seo HB, et al. Grim19 attenuates DSS induced colitis in an animal model. PloS One (2016) 11(6):e0155853. doi: 10.1371/journal.pone.0155853
132. Kasembeli MM, Bharadwaj U, Robinson P, Tweardy DJ. Contribution of STAT3 to inflammatory and fibrotic diseases and prospects for its targeting for treatment. Int J Mol Sci (2018) 19(8). doi: 10.3390/ijms19082299
133. Targan SR, Feagan B, Vermeire S, Panaccione R, Melmed GY, Landers C, et al. A randomized, double-blind, placebo-controlled phase 2 study of brodalumab in patients with moderate-to-Severe crohn's disease. Am J Gastroenterol (2016) 111(11):1599–607. doi: 10.1038/ajg.2016.298
134. Hueber W, Sands BE, Lewitzky S, Vandemeulebroecke M, Reinisch W, Higgins PD, et al. Secukinumab, a human anti-IL-17A monoclonal antibody, for moderate to severe crohn's disease: unexpected results of a randomised, double-blind placebo-controlled trial. Gut (2012) 61(12):1693–700. doi: 10.1136/gutjnl-2011-301668
135. Philipose J, Ahmed M, Idiculla PS, Mulrooney SM, Gumaste VV. Severe de novo ulcerative colitis following ixekizumab therapy. Case Rep Gastroenterol (2018) 12(3):617–21. doi: 10.1159/000493922
136. Herrlinger KR, Diculescu M, Fellermann K, Hartmann H, Howaldt S, Nikolov R, et al. Efficacy, safety and tolerability of vidofludimus in patients with inflammatory bowel disease: the ENTRANCE study. J Crohns Colitis (2013) 7(8):636–43. doi: 10.1016/j.crohns.2012.09.016
137. Cui B, Feng Q, Wang H, Wang M, Peng Z, Li P, et al. Fecal microbiota transplantation through mid-gut for refractory crohn's disease: safety, feasibility, and efficacy trial results. J Gastroenterol Hepatol (2015) 30(1):51–8. doi: 10.1111/jgh.12727
138. Ianiro G, Bibbo S, Porcari S, Settanni CR, Giambo F, Curta AR, et al. Fecal microbiota transplantation for recurrent c. difficile infection in patients with inflammatory bowel disease: experience of a large-volume European FMT center. Gut Microbes (2021) 13(1):1994834. doi: 10.1080/19490976.2021.1994834
139. Ma X, Nan F, Liang H, Shu P, Fan X, Song X, et al. Excessive intake of sugar: An accomplice of inflammation. Front Immunol (2022) 13:988481. doi: 10.3389/fimmu.2022.988481
140. Sato M, Fujii K, Takagi H, Shibuya I, Oka D, Yamaya N, et al. Antibacterial and immunosuppressive effects of OPS-2071, a candidate therapy for inflammatory bowel disease. Dig Dis Sci (2022) 67(8):3993–4007. doi: 10.1007/s10620-021-07237-2
141. Tian J, Zhu Q, Zhang Y, Bian Q, Hong Y, Shen Z, et al. Olfactory ecto-mesenchymal stem cell-derived exosomes ameliorate experimental colitis via modulating Th1/Th17 and treg cell responses. Front Immunol (2020) 11:598322. doi: 10.3389/fimmu.2020.598322
Keywords: inflammatory bowel disease, T helper 17 cells, inflammatory, intestinal fibrosis, gut microbiota
Citation: Chen L, Ruan G, Cheng Y, Yi A, Chen D and Wei Y (2023) The role of Th17 cells in inflammatory bowel disease and the research progress. Front. Immunol. 13:1055914. doi: 10.3389/fimmu.2022.1055914
Received: 28 September 2022; Accepted: 28 November 2022;
Published: 09 January 2023.
Edited by:
Shenghong Zhang, The First Affiliated Hospital of Sun Yat-sen University, ChinaReviewed by:
Zikai Wang, People’s Liberation Army General Hospital, ChinaYubei Gu, Shanghai Jiao Tong University, China
Qiyi Chen, Tongji University, China
Copyright © 2023 Chen, Ruan, Cheng, Yi, Chen and Wei. This is an open-access article distributed under the terms of the Creative Commons Attribution License (CC BY). The use, distribution or reproduction in other forums is permitted, provided the original author(s) and the copyright owner(s) are credited and that the original publication in this journal is cited, in accordance with accepted academic practice. No use, distribution or reproduction is permitted which does not comply with these terms.
*Correspondence: Yanling Wei, bGluZ3ppMDE2QDEyNi5jb20=; Dongfeng Chen, Y2hlbmRmMTk4MUAxMjYuY29t