- 1Department of General Surgery, The First Affiliated Hospital of Xi’an Jiaotong University, Xi’an, Shaanxi, China
- 2Department of General Surgery, the First Affiliated Hospital of USTC, Division of Life Sciences and Medicine, University of Science and Technology of China, Hefei, Anhui, China
Gasdermins (GSDMs) protein family express in intestinal epithelial cells or lamina propria immune cells, and play a nonnegligible function during gut homeostasis. With the gradually in-depth investigation of GSDMs protein family, the proteases that cleave GSDMA-E have been identified. Intestinal GSDMs-induced pyroptosis is demonstrated to play a crucial role in the removal of self-danger molecules and clearance of pathogenic organism infection by mediating inflammatory reaction and collapsing the protective niche for pathogens. Simultaneously, excessive pyroptosis leading to the release of cellular contents including inflammatory mediators into the extracellular environment, enhancing the mucosal immune response. GSDMs-driver pyroptosis also participates in a novel inflammatory cell death, PANoptosis, which makes a significant sense to the initiation and progression of gut diseases. Moreover, GSDMs are expressed in healthy intestinal tissue without obvious pyroptosis and inflammation, indicating the potential intrinsic physiological functions of GSDMs that independent of pyroptotic cell death during maintenance of intestinal homeostasis. This review provides an overview of the latest advances in the physiological and pathological properties of GSDMs, including its mediated pyroptosis, related PANoptosis, and inherent functions independent of pyroptosis, with a focus on their roles involved in intestinal inflammation and tumorigenesis.
1. Introduction
The intestine represents one of the largest immune organs of the human body mostly because of its surface microscopic architecture (1–3). Majority of immunological processes occur in the mucosa, including the epithelium and the underlying lamina propria (4). In general, the commensal bacteria resident in gut lumen, intestinal epithelium and its secretions (such as mucin and antimicrobial peptides), as well as immune cells in the lamina propria, collaboratively maintain intestinal homeostasis (5–7). Remarkably, there is mounting evidence that the protein family of Gasdermins (GSDMs) play a nonnegligible function under such circumstance.
The GSDMs are a recently characterized family of conserved proteins with diverse functions (8). The nomenclature of Gasdermins is based on their expression pattern along the gastrointestinal tract (gas) and skin (dermis) (9). Human genome encodes six paralogous GSDMs genes: GSDMA, GSDMB, GSDMC, GSDMD, GSDME (also known as DFNA5) and DFNB59 (also known as PJVK) (9). All proteins of GSDMs family except DFNB59, consist of a conserved N-terminal (NT) and C-terminal (CT) domains that interconnected by a linker region (10). Proteolytic cleavage of GSDMs liberates the NT fragment, which assembles in membrane to form pores and executes lytic cell death, namely pyroptosis (11). However, full-length GSDMs are normally not able to induce such pyroptotic cell death due to the presence of the CT domain, which usually interacts with the NT domain by charge-charge and hydrophobic interfaces to auto-inhibit pore formation function (12). Intestinal homeostasis is frequently disrupted due to the stimulation of endogenous damage-associated molecular patterns (DAMPs) and microorganism-derived pathogen-associated molecular patterns (PAMPs), so as to provoke immune response and lead to tissue damage (13). Intestinal GSDMs-induced pyroptosis plays an essential role in the removal of self-danger molecules and clearance of pathogenic organism infection by mediating inflammatory reaction and collapsing the protective niche for pathogens (14). Simultaneously, excessive pyroptosis results in the release of cellular contents including inflammatory mediators into the extracellular environment (15), which will enhance the immune response and further aggravate intestinal injury. In recent years, the relationship between pyroptosis and cancer has become increasingly prominent, and pyroptosis plays a complex role in tumor progression (16). Furthermore, in addition to GSDMs-NT fragment-induced pyroptosis, emerging evidence indicates GSDMs possess intrinsic physiological functions that independent of pyroptotic cell death in gut. This review provides an overview of the latest advances in the physiological and pathological properties of GSDMs, with a focus on their roles involved in intestinal inflammation and tumorigenesis.
2. Expression pattern of Gasdermins in gut
Although GSDMs display unique tissue expression pattern in human, it has been found that GSDMA-E exist in the gastrointestinal tract except DFNB59. GSDMA is expressed in epithelial cells within the gastrointestinal tract while frequently silenced in primary gastric cancers and in gastric cancer cell lines (17, 18). GSDMB is expressed in the epithelium of the digestive tract that can be detected in esophagus, stomach, small intestine, colon and rectum (19). A recent study reveal differential distribution of GSDMB among colonic epithelial cell subtypes, predominantly in colonocytes, crypt top colonocytes, and goblet cells, through the supervised analysis of a large-scale scRNA-seq dataset from health controls and inflammatory bowel disease patients (20). GSDMC is prominently expressed in esophagus, stomach, small intestine, caecum and colon, especially in gut epithelial tissue including enterocytes and goblet cells (21–23). GSDME is also mainly expressed in epithelial cells in the intestine, and relative to normal tissue, GSDME expression in gastric and colorectal cancer is epigenetically suppressed by methylation (11, 24–26). Contrast to other members of the GSDMs family, GSDMD is relatively universally expressed in gastrointestinal tract, not only in epithelium, but also in lamina propria immune cells (especially macrophages and dendritic cells), suggesting that GSDMD may play an indispensable role in intestinal homeostasis (15, 27).
3. Molecular mechanisms of Gasdermins cleavage and activation
With the gradually in-depth investigation of GSDMs protein family, the proteases that activate GSDMA-E have been identified. Although the types and cleavage sites of the proteases vary considerably, they share a common mechanism to implement such inflammatory cell death. That is, the GSDMs-NT is unleashed and couples with acidic phospholipids in the inner leaflet of cell membrane to oligomerize into pores with an inner diameter of about 18 nm, which leads to water influx, cell swelling and rapid lytic cell death with the extravasation of cytoplasmic contents (12, 28).
Concretely, GSDMA is recently discovered to be cleaved at Gln246 site by cysteine protease streptococcal pyrogenic exotoxin B (SpeB) virulence factor, releasing the active NT fragment that triggers pyroptosis (29). GSDMA acts as not only the sensor and substrate of Streptococcus pyogenes SpeB, but also the effector to trigger pyroptosis, adding a novel mechanism for host immune recognition and response to microbial pathogen infection (29). Cytotoxic T lymphocytes (CTL) and natural killer (NK) cells-derived granzyme A (GrzA) cleaves the major Lys244 physiological site of GSDMB to induce pyroptotic cell death (30). Among numerous cytokines, IFN-γ is the one that exhibits broad effects of up-regulation for GSDMB expression in multiple cell lines and promotes GrzA-mediated pyroptosis (30). A previous study has showed that GSDMC is specifically cleaved at Asp365 site by active caspase 8 after TNF-mediated death receptor signaling to trigger pyroptosis (31). Intriguingly, another recently published study has demonstrated that the metabolite α-ketoglutarate (α-KG) induces pyroptosis through active caspase-8-mediated cleavage of GSDMC, but at different amino acid residue site (Asp240, mouse Asp233) (32). GSDMD is the best-characterized GSDMs family protein, and various proteases with capacity to cleave GSDMD have been discovered. Depending on the upstream stimulus signals, when the canonical inflammasomes, including AIM2 sensor by cytosolic double-stranded DNA (dsDNA) or NLRP3 sensor by cellular disturbances and plasma membrane integrity disruption, are activated, GSDMD is cleaved by downstream active caspase-1 at Asp275 site (mouse Asp276) to initiate pyroptosis, accompanied by IL-1 family cytokines IL-1β and IL-18 maturation (33, 34). By comparison, the noncanonical inflammasomes-mediated caspase-4, 5 (mouse caspase-11) activation, also leads to robust cleavage of GSDMD at Asp275 site, but without IL-1 family cytokines production (35). Moreover, in response to pathogenic Yersinia infection, where the transforming growth factor beta-activated kinase 1 (TAK1) and IκB kinases are blocked by the Yersinia effector protein YopJ, a receptor-interacting protein kinase 1 (RIPK1)-caspase-8 pathway can be activated to cleave GSDMD at this same amino acid site, which provides host defense and maintains homeostasis (36, 37). In addition to the caspases above, granule-associated proteases neutrophil elastase (ELANE) has been shown to cleave and activate GSDMD at Cys268 (mouse Val251) site and cathepsin G cleaves mouse GSDMD directly at Leu274 to provoke pyroptotic cell death (38, 39). GSDME has been shown to be cleaved by active caspase-3 at Asp270 (mouse Asp271) site when stimulated with apoptotic stimuli, which converts apoptosis into a rapid inflammatory pyroptotic death in GSDME-expressing cells (40). In addition, the serine protease granzyme B (GrzB) released from cytotoxic lymphocytes is found to cleave GSDME at the same site to induce pyroptosis (41). Molecular mechanisms of individual GSDMs that activated by specific proteases are summarized in Figure 1.
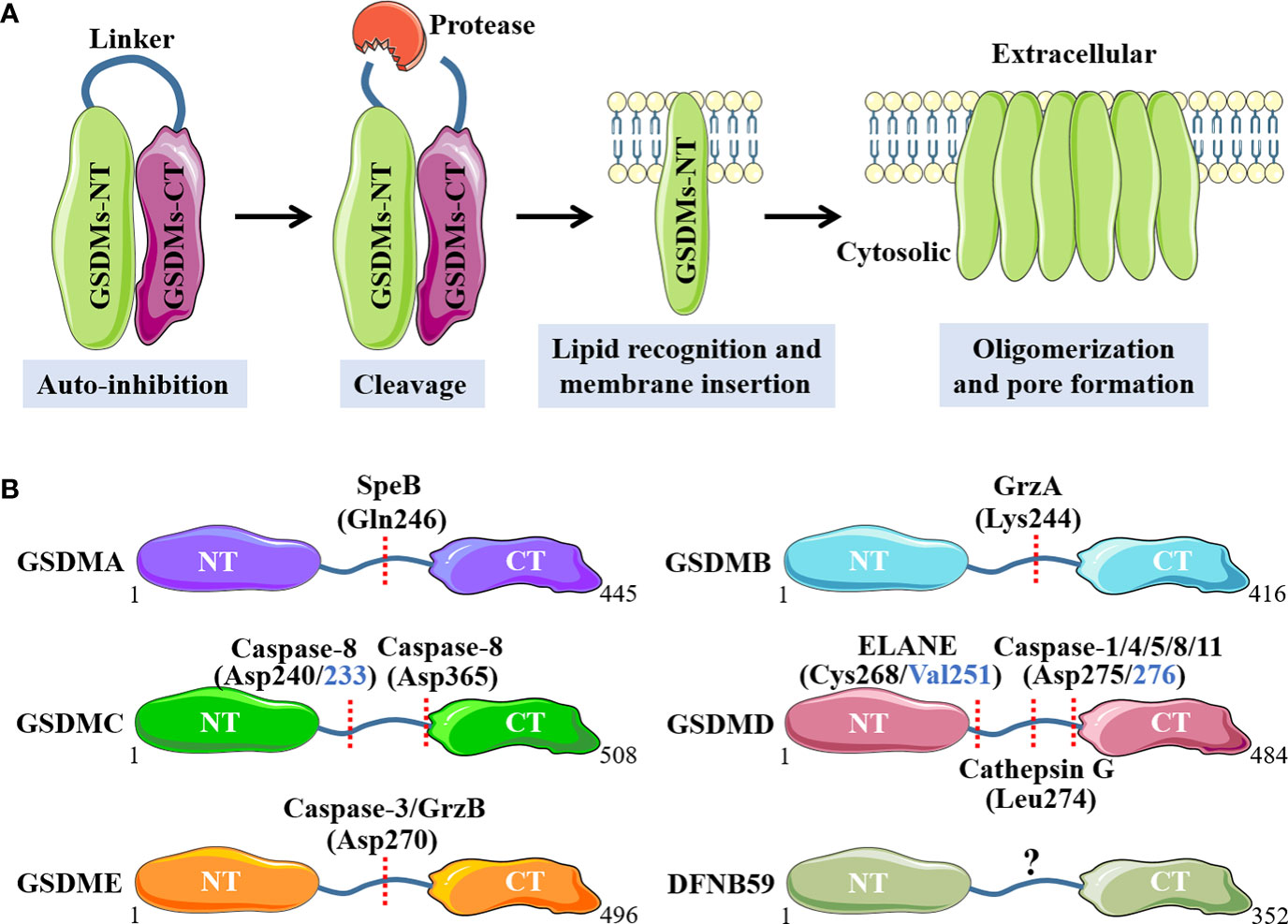
Figure 1 Molecular mechanisms of GSDMs that activated by specific proteases. (A). Main processes of GSDMs membrane pores formation. (B). Respective proteases and cleavage sites for individual GSDMs. NT, N-terminal; CT, C-terminal; SpeB, streptococcal pyrogenic exotoxin B; GrzA, granzyme A; GrzB, granzyme B; ELANE, neutrophil elastase.
4. Function of Gasdermins-mediated pyroptosis in gut homeostasis
When the intestine encounters the attack from self-DAMPs or microbial PAMPs, the mucosal immune system will be initiated, which triggers the immune inflammatory response and destroys the gut homeostasis. Due to the universal existence of GSDMs protein family in intestine, either in epithelium or lamina propria immune cells, its mediated pyroptosis plays a crucial role in the maintenance of abnormal inflammation and clearance of pathogens, including the beneficial and detrimental, depending on the pathological environment at that time. Increasingly emerging evidence indicates that GSDMs-mediated pyroptosis plays an important role in a variety of intestinal diseases, including inflammatory bowel diseases (IBD), sepsis‐induced intestinal injury, enteropathogenic microbial infection, intestinal ischemia/reperfusion (I/R) injury, radiation-induced enteropathy, gastrointestinal dysmotility, colorectal cancer (CRC) and so on.
4.1. Gasdermins-mediated pyroptosis in IBD-associated intestinal inflammation
IBD, including Crohn’s disease (CD) and ulcerative colitis (UC), represents a chronic and complex intestinal disorder characterized by uncontrolled and excessive mucosal pathogenic inflammation (42). It is wide recognized that DAMPs released from stressed or dead cells are important pathogenic stimuli for inducing and maintaining abnormal mucosal inflammation during IBD (43). Our recent studies demonstrated that nuclear DAMPs, spliceosome-associated protein 130 (SAP130), released from gut mucosa could induce GSDMD-mediated pyroptosis in lamina propria macrophages through sensing by macrophage-inducible C-type lectin/spleen tyrosine kinase (Mincle/Syk) signaling, which exacerbated mucosal inflammation and provoked intestinal tissue injury during CD (44, 45). We also uncovered that adaptor protein stimulator of interferon genes (STING) regulated intestinal inflammation dependent on GSDMD-related pyroptosis in acute dextran sulfate sodium (DSS)-induced colitis, while STING deficiency alleviated intestinal damage that associated with decreased expression of cleaved form of pyroptosis executive protein GSDMD (46).
A recent study demonstrated that the protein phosphatase, pleckstrin homology domain leucine-rich repeat protein phosphatase 2 (PHLPP2), was downregulated in UC and regulated GSDMD-induced intestinal epithelial cells (IECs) pyroptosis by modulating the NF-κB signaling, and PHLPP2 depletion increased the susceptibility to colitis by inducing dramatic activation of caspase-1/GSDMD in IECs (47). Wang et al. (48) observed that the expression of monocarboxylate transporter 4 (MCT4) was markedly increased in intestinal mucosal tissue of IBD, and overexpression of MCT4 triggered caspase-1/GSDMD-mediated canonical pyroptosis in IECs to aggravate intestinal inflammation through the ERK1/2-NF-kB pathway. CD147, a highly glycosylated transmembrane protein, was recently proved to induce pyroptosis in IECs by enhancing of phosphorylation of NF-κB, which was attributed to activation of caspase-1/GSDMD as well as GSDME, leading to aggravation of IBD (49). It is found that GSDMD in IECs, but not infiltrating immune cells, was activated by dysregulated commensal and in turn modulated microbiota-driven colitis by promoting IL-18 release form pyroptotic cell death (50). Caspase-8 and its adapter Fas associated with death domain (FADD) act on epithelial cells to maintain intestinal immune homeostasis, and FADD prevents intestinal inflammation by inhibiting caspase-8/GSDMD-dependent pyroptosis of IECs (51). In addition, Tan et al. (26) demonstrated that GSDME-mediated IECs pyroptosis induced intestinal inflammation through the release of proinflammatory intracellular contents and participated in the pathogenesis of CD. They also found that GSDME converted TNF-α-induced IECs shedding into a pyroptotic cell death process, and GSDME depletion mice presented an alleviative intestinal barrier dysfunction (52).
Apart from the pyroptosis of IECs, pyroptosis in mucosal immune cells especially macrophages can also regulate intestinal inflammation strikingly. A recent study identified a dominant gain-of-function missense variant of NLRP3, encoded by rs772009059, promoted NLRP3 inflammasome activation and GSDMD-mediated pyroptosis in macrophages, thereby contributing to very-early onset IBD development (53). Cai et al. (54) observed that human umbilical cord mesenchymal stem cell (hucMSC)-derived exosomes carrying miR-378a-5p inhibited NLRP3/caspase-1/GSDMD pathway and abrogated macrophage pyroptosis to protect against DSS-induced colitis. Roseburia intestinalis, a butyrate−producing bacterium, -derived flagellin was demonstrated to inhibit the activation of NLRP3/caspase-1/GSDMD signaling-triggered pyroptosis in macrophages by targeting miR−223−3p and ameliorate colitis (55). In contrast, Ma et al. (56) showed that GSDMD and its mediated pyroptosis in macrophages protected against colitis by negatively regulating cyclic GMP-AMP synthase (cGAS)-dependent inflammation, while GSDMD deficiency in macrophages exacerbated experimental colitis.
Taken together, almost all the above studies support that GSDMD or GSDME-mediated pyroptosis in IECs or macrophages provoked intestinal inflammation and exacerbated the disease progression in IBD (Figure 2). This has stimulated the search for some drug interventions with the anti-pyroptosis effects to ameliorate intestinal inflammation. It is recently found that 10-hydroxy-2-decenoic acid (10-HDA), the most abundant fatty acid and major lipid component in royal jelly, alleviated DSS-induced colitis and enhancing colonic barrier function by regulating the NLRP3/caspase-1/GSDMD-mediated pyroptotic pathway (57). Schisandrin B, a type of natural products, was confirmed to suppress NLRP3/caspase-1/GSDMD-related pyroptosis in IECs of experimental colitis through the activation of AMP-activated protein kinase/Nuclear factor erythroid 2-related factor 2 (AMPK/Nrf2)-dependent reactive oxygen species (ROS)-induced mitochondrial damage (58). Furthermore, apple polyphenols extract (APE) has been reported to significantly ameliorate DSS-induced acute colitis through inhibiting caspase-1/caspase-11-GSDMD-dependent pyroptosis pathway in IECs (59).
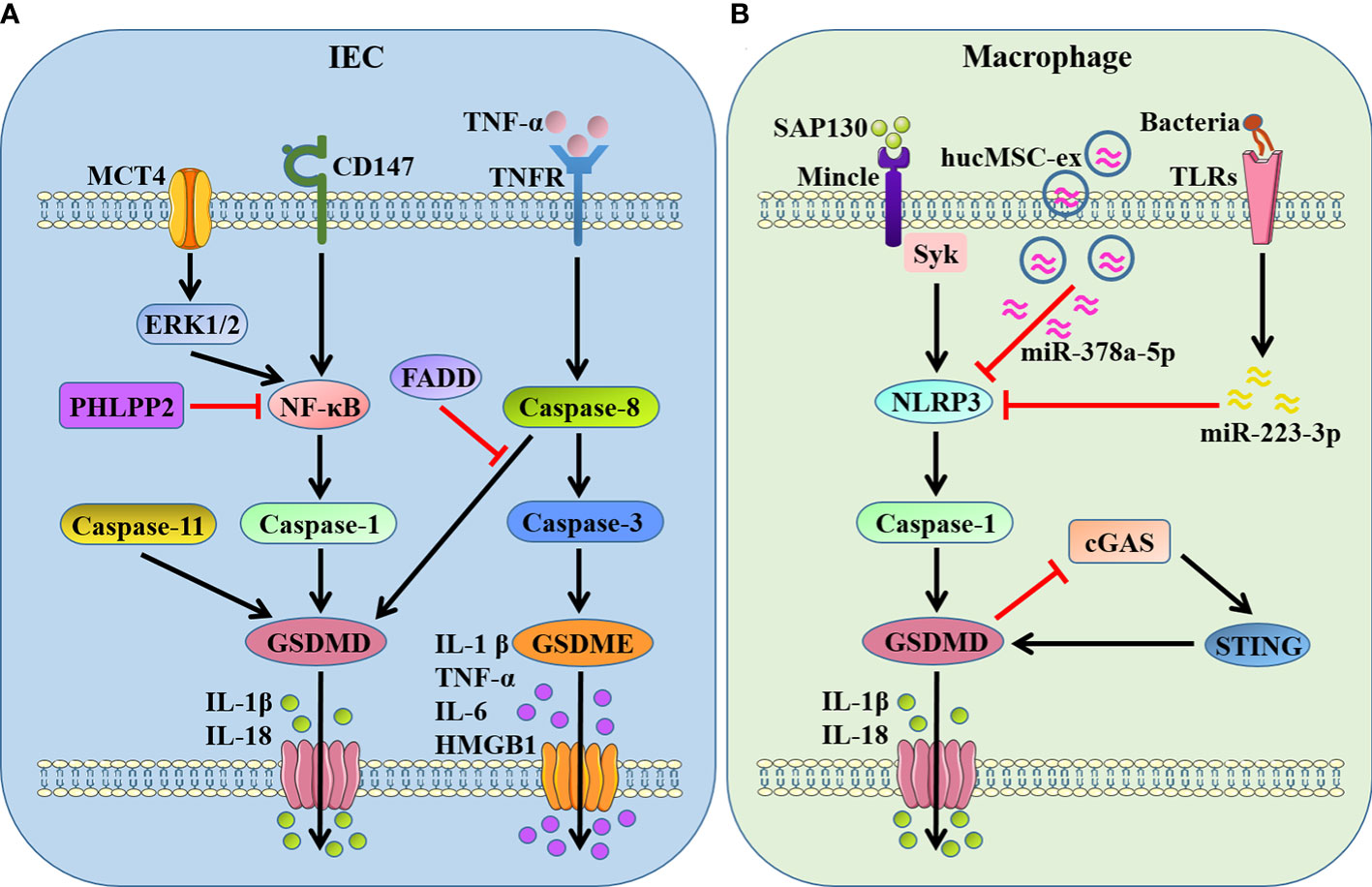
Figure 2 GSDMs-mediated pyroptosis in IBD-associated intestinal inflammation. (A) GSDMD- and GSDME-dependent pyroptosis in IECs. GSDMD is cleaved by active caspase-1/8/11 when NF-κB being activated by MCT4-ERK1/2 or CD147, promoting the release of IL-1β and IL-18. PHLPP2 and FADD inhibit GSDMD-dependent pyroptosis by suppressing NF-κB and caspase-8, respectively. GSDME is cleaved by active caspase-3 after caspase-8 activation in response to TNF-α stimulation, promoting the release of proinflammatory mediators IL-1β, TNF-α, IL-6 and HMGB1. (B) GSDMD-dependent pyroptosis in macrophages. GSDMD is cleaved by NLRP3/caspase-1 when Mincle/Syk pathway being activated in response to SAP130, and STING provokes GSDMD cleavage as well. In turn, GSDMD-mediated pyroptosis inhibits cGAS/STING signal. hucMSC-ex carrying miR-378a-5p and flagellin targeting miR−223−3p restrain NLRP3/caspase-1/GSDMD signaling-triggered pyroptosis. MCT4, monocarboxylate transporter 4; PHLPP2, pleckstrin homology domain leucine-rich repeat protein phosphatase 2; FADD, Fas associated with death domain; SAP130, spliceosome-associated protein 130; Mincle, macrophage-inducible C-type lectin; Syk, spleen tyrosine kinase; hucMSC-ex, human umbilical cord mesenchymal stem cell-derived exosomes; cGAS, cyclic GMP-AMP synthase; STING, stimulator of interferon genes.
4.2. Gasdermins are associated with endotoxemia- and sepsis‐related intestinal injury
Sepsis is defined as life-threatening organ dysfunction caused by a dysregulated host response to infection (60). The gut has long been considered to be the “motor” of sepsis, playing an essential role in the initiation and transmission of critical illness (61). A growing number of studies have confirmed GSDMs-mediated pyroptosis are involved in organ dysfunction of endotoxemia and sepsis, including in lung, kidney, liver and heart-related damage, but its role and specific mechanism in endotoxemia- or sepsis‐associated intestinal injury remains largely unknown.
Mandal et al. (62) investigated that neither caspase-11 nor pro-apoptotic caspase-8 was individually sufficient for endotoxic shock. They identified both caspase-11-GSDMD pyroptosis axis and caspase-8 apoptosis signaling collaborated to drive IECs shedding and amplify inflammatory signals associated with intestinal tissue damage during endotoxic shock (62). Intriguingly, another study indicated that both GSDMD-mediated pyroptosis and RIPK3-mediated necroptosis pathway contributed to inflammatory intestinal injury induced by bacterial sepsis (63). Sheng et al. (64) showed that intestinal colonization with commensal fungi inhibited GSDMD-mediated pyroptosis in response to lipopolysaccharide (LPS) challenge, while antifungal therapy aggravated endotoxin sepsis through promoting GSDMD cleavage in the distal small intestine. Moreover, CD4-positive T lymphocytes and IECs could secrete GSDMD-NT in high-fat diet (HFD)-induced systemic endotoxemia, and GSDMD-NT killed the Proteobacteria phylum via directly interacting with cardiolipin, thereby restraining gut barrier impairment and intestinal inflammation (65). Bromodomain-containing protein 4 (BRD4) was recently found to play a critical role in endotoxemia colon by regulating NLRP3/caspase-1/GSDMD-mediated pyroptosis, and BRD4 inhibition could prevent endotoxemia-induced colon damage through blocking inflammation-related pyroptosis (66).
Therefore, GSDMs-dependent pyroptosis is indeed involved in the progression of sepsis-related intestinal injury. Moderate pyroptosis in endotoxemia or sepsis can control pathogen infection, but excessive pyroptosis may lead to a dysregulated host immune response and even organ dysfunction. More studies are needed to elucidate the precise mechanism of activation and regulation of pyroptosis in intestinal injury associated with endotoxemia and sepsis.
4.3. Gasdermins-mediated lytic cell death in enteropathogenic microbial infections
The gastrointestinal tract not only has the functions of digestion and nutrient absorption, but also provides a physical barrier against amounts of commensal or pathogenic microbes in the gut lumen (67). The intestine employs multiple innate immune mechanisms to prevent and clear enteropathogenic microbial infections. Recent research upon GSDMs-dependent pyroptosis provided vital insights into host defense against pathogen.
Salmonella, a gram-negative facultative intracellular bacterium, is spread through polluted food or water and cause gastrointestinal disease that characterized by nausea, vomiting, abdominal pain and diarrhea after infection (68). Salmonella plasmid virulence C (SpvC) suppressed GSDMD-mediated pyroptosis in macrophages through negative modulation of both canonical and non-canonical inflammasome pathways, which related to the inhibition of intestinal inflammation while promotion of systemic bacterial dissemination (69). Ventayol et al. (70) demonstrated that Salmonella infection triggered NAIP/NLRC4/caspase-1/GSDMD-dependent focal epithelial contractions that preceded and uncoupled from IECs death and expulsion, thereby preventing subsequent tissue disintegration. Intestinal macrophages could also suffer NLRC4/caspase-1/GSDMD-dependent pyroptosis, which promoted inflammatory response and aggravated Salmonella-induced intestinal injury (71). Moreover, Salmonella was recently shown to induce pyroptosis of enteroendocrine L cells via NLRP3/caspase-1/GSDMD axis, which reduced the secretion of glucagon-like peptide 1, a significant intestinal hormone for regulating glucose homeostasis, and eventually resulted in hyperglycemia (72).
Cronobacter sakazakii, a gram-negative bacterial pathogen, was shown to upregulate NF-κB via TLR4/MyD88 to promote activation of the NLRP3 inflammasome and GSDMD-mediated pyroptosis, leading to the intestinal damage and development of necrotizing enterocolitis (73, 74). The translocated intimin receptor of enteropathogenic Escherichia coli triggered rapid Ca2+ influx in IECs, which induced LPS internalisation, resulting in the activation of caspase-4/GSDMD-driven pyroptosis of IECs (75). Melhem et al. (76) found that epithelial G protein-coupled receptor 35 protected against Citrobacter rodentium infection through guarding goblet cells from dysregulated caspase-11/GSDMD-mediated pyroptosis and maintaining mucosal barrier integrity. The canonical NLRP3/caspase-1/GSDMD pathway-dependent pyroptosis in macrophages contributed to the immunopathology and innate inflammatory responses upon gastrointestinal norovirus infection (77). Remarkably, Hansen et al. (78) recently elucidated that GSDMB, as a central executioner of intracellular bacterial killing, functioned lytic microbiocidal activity through recognition of phospholipids on gram-negative bacterial membranes. They also identified Shigella flexneri counteracted this host defense mechanism via ubiquitinated proteasome destruction pathway of GSDMB (78).
Intestinal parasite is another enteropathogenic microorganism that causes severe gastrointestinal inflammation and systemic infection. Enterocyte-intrinsic NLRP6/caspase-1/GSDMD-mediated pyroptosis played a critical role in enabling early control of Cryptosporidium replication by the local release of the proinflammatory cytokine IL-18 (79). Interestingly, Entamoeba histolytica-triggered caspase-1/4 activation in the cleavage of GSDMD to induce pore formation and sustain bioactive IL-1β secretion in the absence of pyroptotic cell death in macrophages (80). Type 2 cytokines, IL-4 or IL-13, triggered by worm infection, drastically up-regulated the expression of GSDMC in the gut, thereby enhancing the release of antiparasitic factors from enterocytes by GSDMC-mediated pyroptosis and facilitating the clearance of worms (81). Moreover, it was reported that epithelial O-linked N-Acetylglucosamine protein modification was initiated upon helminth infection, boosting GSDMC-mediated membrane pore formation and the unconventional secretion of IL-33, which contributed greatly to anti-helminth immunity and induced intestinal inflammation (23).
4.4. Gasdermins-regulated pyroptotic cell death during colorectal cancer
4.4.1. Anti‐tumorigenesis effect of Gasdermins
Accumulating evidence suggested that GSDMs-mediated pyroptosis might impact all stages of tumorigenesis and played a complex role in cancer biology depending on the different cellular context and genetic backgrounds (Figure 3). Obviously, as a type of cell death, pyroptosis inhibits the occurrence and progression of tumors. Our recent study clarified that STING-mediated Syk signaling attenuated the tumorigenesis of colitis-associated colorectal cancer (CAC) by enhancing GSDMD-driven pyroptosis of tumor cells (46). Thioredoxin reductase 3 (Txnrd3) as selenoprotein was recently proved to cause intracellular calcium outflow and increase oxidative stress in colonic epithelial cell line, thereby activating the canonical GSDMD-dependent pyroptosis to inhibit the growth and proliferation of colon cancer cells (82). Interestingly, it was reported that conjugated linolenic acid produced by the probiotic bacterium Lactobacillus exerted the anti-cancer effect through inducing GSDMD-mediated pyroptosis of colon cancer cells (83).
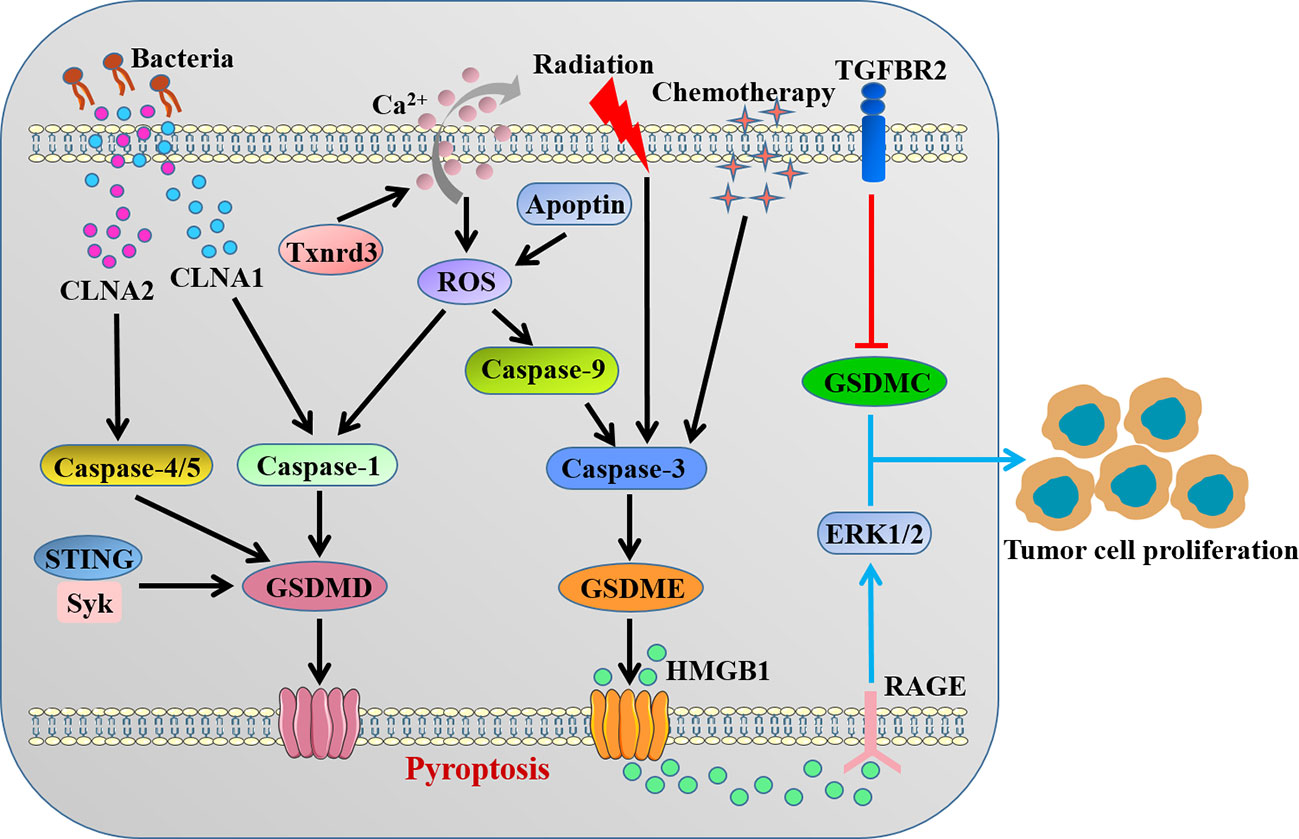
Figure 3 The contradictory role of GSDMs in colorectal cancer. CLNAs from probiotic bacterium and intracellular calcium outflow-caused ROS activate caspase-1/4/5 to cleave GSDMD. STING-mediated Syk signaling induces GSDMD-driven pyroptosis. Apoptin/ROS/caspase-9 axis, radiation and chemotherapy activate caspase-3 to cleave GSDME. The cleavage of GSDMD or GSDME inhibits the occurrence and progression of tumors by inducing pyroptosis. GSDMC and GSDME-related HMGB1 release promote tumor cell proliferation. CLNA, conjugated linolenic acid; Txnrd3, thioredoxin reductase 3; ROS, reactive oxygen species.
Previous studies indicated that GSDME gene methylation might play a vital role in the development of CRC, and GSDME as well as its methylation could be a promising molecular biomarker in CRC (84, 85). GSDME, rather than GSDMD, was demonstrated to be cleaved in lobaplatin-induced pyroptosis in colon cancer cells due to caspase-3 activation, which clarified the mechanism of lobaplatin eradicating neoplastic cells (86). It was reported that apoptin triggered caspase-9/caspase-3/GSDME-dependent pyroptosis of colon cancer cells through mitochondrial related ROS pathway and significantly inhibited tumor growth in vivo (87). Tan et al. (88) observed that radiation could induce caspase-3/GSDME-mediated pyroptosis in colon cancer cells, which recruited NK cells to provoke antitumor immunity and facilitated the radiosensitivity. The combination of oxaliplatin and farnesoid X receptor agonist GW4064 triggered caspase-3/GSDME-driven pyroptosis and slowed tumor growth, thereby enhancing the chemosensitivity of CRC (89). A recent study identified that small molecule inhibitors BI 2536 and (S)-(+)-camptothecin via organoid-based drug screen were able to induce GSDME-mediated pyroptosis concurrent with caspase-3-dependent apoptosis, which suppressed CRC tumor growth and enhanced the efficacy of immunotherapy (90). In contrast, Croes et al. (91) showed that there was no major differences for tumor features between GSDME-/- and wild-type mice in both chemically induced and genetic intestinal cancer models, in spite of less severe inflammation observed in GSDME deficiency mice.
4.4.2. The pro-tumor effect of Gasdermins
Beyond the anti-tumor function, the inflammatory microenvironment caused by pyroptosis may be suitable for tumor cell growth, which is closely related to the tumorigenesis. Recent evidence implicated that GSDMs also acted as oncogenes that participated in the initiation and progression of cancer. GSDMB was found to exert pro-tumor function by promoting proliferation, migration and invasion of tumor cells in both breast neoplasias and bladder cancer (92, 93). GSDMD could enhance tumor proliferation in non−small cell lung cancer and promote the invasion capacity of adenoid cystic carcinoma (94, 95).
For the colorectal cancer, Miguchi et al. (96) showed that GSDMC promoted tumor cell proliferation in colorectal carcinogenesis, and GSDMC facilitated xenograft tumor growth in vivo. In addition, GSDME-mediated pyroptosis promoted the development of CAC by releasing high-mobility group box protein 1 (HMGB1), which boosted tumor cell proliferation through the ERK1/2 pathway (97). Nevertheless, potential molecular mechanisms of pro-tumor effect of GSDMs in CRC remains largely unknown, further studies are warranted to unveil the relationship between GSDMs and tumor microenvironment.
4.5. Gasdermins-involved pyroptosis in other gut disorders
Intestinal I/R injury induced intestinal inflammation through activation of the NLRP3/caspase-1/GSDMD-driven pyroptosis, and metformin and dexmedetomidine were demonstrated to protect against intestine I/R injury by reducing expression of pyroptosis-related proteins (98, 99). Pyroptosis was recently observed to play an essential role in radiation-induced enteropathy, one of the most common and fatal complications of abdominal radiotherapy, and micheliolide significantly ameliorated radiation-induced intestinal tissue damage by lessening NLRP3/caspase-1/GSDMD pyroptosis pathway (100). Ye et al. (101) showed that Western diet induced increased myenteric neuronal pyroptosis via caspase-11/GSDMD pathway, leading to the myenteric nitrergic neuronal degeneration and colonic dysmotility. Caspase-11/GSDMD-triggered pyroptosis also contributed to acute graft-versus-host disease-related intestinal injury in allogeneic hematopoietic stem cell transplantation, while deletion of caspase-11 or GSDMD relieved intestinal inflammation (102). Circulating plasma exosomes derived from intestinal Behçet’s syndrome patients were proved to induce pyroptosis of intestinal epithelial cells via the activation of NLRP3/caspase-1/GSDMD pathway (103). Kerr et al. (104) revealed that GSDMD-mediated pyroptosis of intestinal macrophages resulted in increased gut permeability after thrombotic stroke, which provided a novel possible mechanism underlying gut complications after stroke. Further investigation was needed to clarify the pathogenic mechanism by which GSDMs facilitates gut homeostasis.
5. Pyroptosis-related PANoptosis in gut
It is widely known that GSDMs-dependent pyroptosis is a kind of inflammatory programmed cell death in response to pathogens or sterile insults. Emerging evidence states that multiple cell death pathways apart from pyroptosis, such as apoptosis and necroptosis, are induced during inflammation and immune response. Mechanistically, apoptosis is activated by the executioner caspase-3, caspase-6 or caspase-7, downstream of the initiator caspase-8, caspase-9 and caspase-10 (105). Necroptosis is initiated by phosphorylation of mixed lineage kinase domain-like pseudokinase (MLKL) downstream of the RIPK1 and RIPK3 signaling axis (105). Indeed, under such condition of homeostasis disbalance, a significant crosstalk among these three cell death pathways was observed, conceptualizing as a novel and united modality of death, namely PANoptosis (Pyroptosis-Apoptosis-Necroptosis) (106). PANoptosis is modulated by the multi-protein PANoptosome complex, which provides a molecular scaffold for simultaneous engagement of key molecules from pyroptosis, apoptosis and necroptosis (107). According to the biological functions, compositions of the PANoptosome consist of sensors, adaptors and catalytic effectors in general, such as Z-DNA binding protein 1 (ZBP1), FADD and caspase-1, respectively (108). Since PANoptosis has been triggered during various pathogenic infections, autoinflammatory diseases and cancer (107), indicating that it has significant pathophysiological relevance.
Recent studies suggested that interventions targeting PANoptosis-related molecules made a significant sense to the initiation and progression of gut diseases. Kanneganti’s group identified interferon regulatory factor 1 (IRF1) in both the myeloid and epithelial compartments could act as a master regulator of PANoptosis in the colon to induce cancer cell death, thus protecting against tumorigenesis in CAC (109). They confirmed synergism of cytokines TNF-α and IFN-γ was capable of inducing PANoptosis of colon cancer cells through activation of GSDMD, GSDME, caspase-8, caspase-3, caspase-7 and MLKL, thereby suppressing tumor growth (110). Their group also demonstrated that combining interferons and nuclear export inhibitors activated ZBP1-dependent PANoptosis and thus dramatically regressed colon tumor in vivo (111). By contrast, adenosine deaminase acting on RNA 1 (ADAR1), a RNA editor that critical for development and survival, interacted with ZBP1 and suppressed ZBP1-mediated PANoptosis, promoting tumorigenesis (111). Lin et al. (112) observed that cysteine desulfurase (NFS1), a rate-limiting enzyme in iron-sulfur cluster biogenesis, weakened oxaliplatin-based chemosensitivity of CRC by reducing the level of ROS to prevent PANoptosis.
Therefore, the existing research results unanimously suggested induction of PANoptosis in cancer cells would be a promising strategy for inhibiting tumorigenesis of CRC (Figure 4). Further studies may be warranted to develop novel agonists that can drive cancer cell PANoptosis. In addition, more studies are urgently needed to understand the specific functions and mechanisms of PANoptosis in other gut disorders.
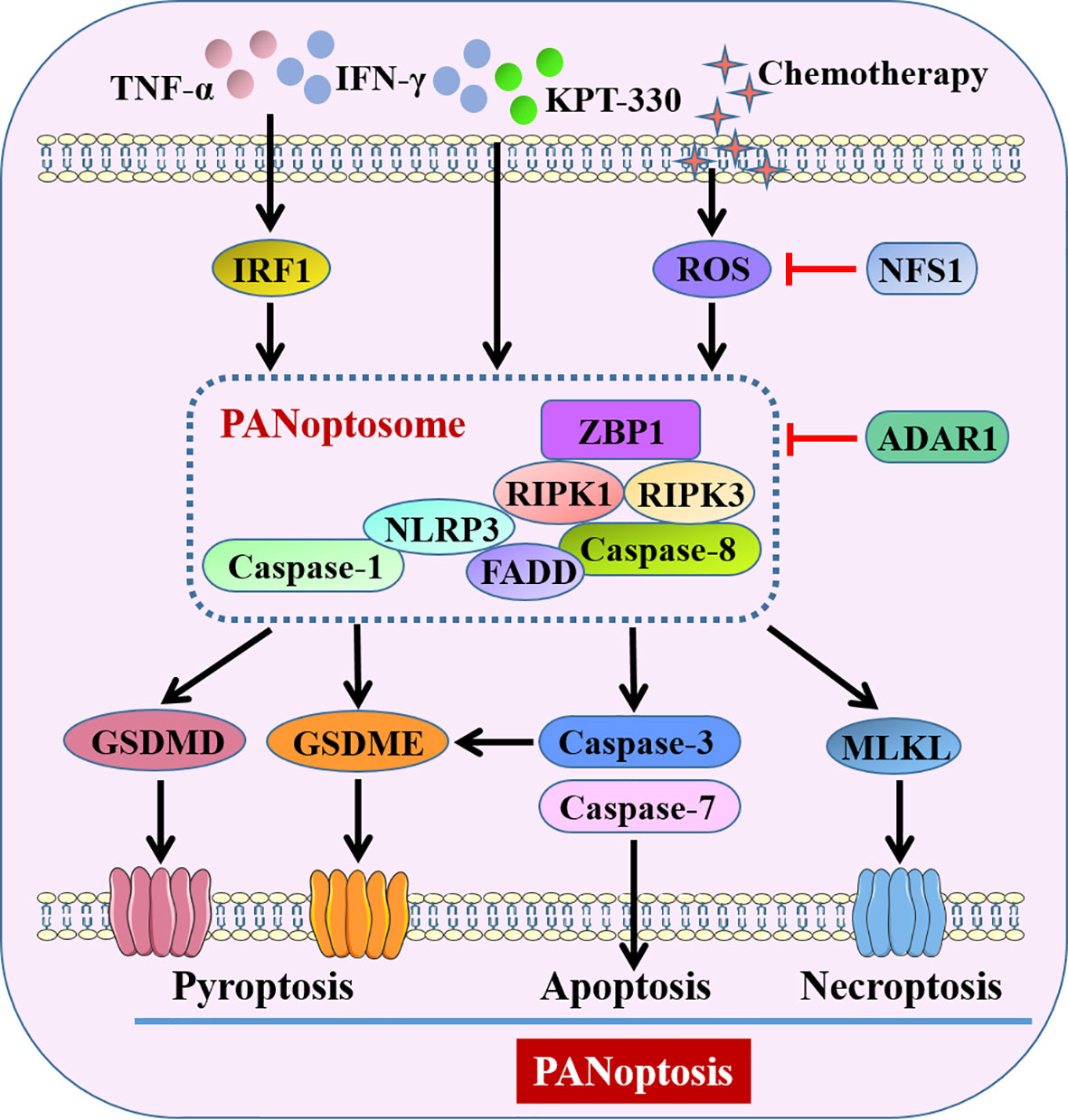
Figure 4 Pyroptosis-related PANoptosis in intestine. IFN-γ combined with TNF-α or KPT-330, and chemotherapy-induced ROS activate PANoptosome to trigger GSDMs-mediated pyroptosis, caspase-3/7-mediated apoptosis and MLKL-mediated necroptosis, conceptualizing as PANoptosis. ADAR1 and decreased ROS by NFS1 suppress PANoptosis through intervention of PANoptosome. KPT-330, a nuclear export inhibitor; NFS1, cysteine desulfurase; ADAR1, adenosine deaminase acting on RNA 1; ZBP1, Z-DNA binding protein 1; RIPK, receptor-interacting protein kinase; MLKL, mixed lineage kinase domain-like pseudokinase.
6. Intrinsic functions of Gasdermins in intestine independent of pyroptosis
The GSDMs have been primarily known for their role as pore-forming effector proteins upon cleavage, oligomerization, and translocation of the N-terminal domain to the plasma membrane to cause pyroptosis. Nonetheless, GSDMs are also expressed in many healthy tissues without obvious pyroptosis and inflammation especially the gastrointestinal tract, suggesting potential physiological functions associated with GSDMs that independent of pyroptosis may be modulated in the steady state. Recently, emerging studies have confirmed the nonpyroptotic role of GSDMs during maintenance of intestinal homeostasis (Figure 5).
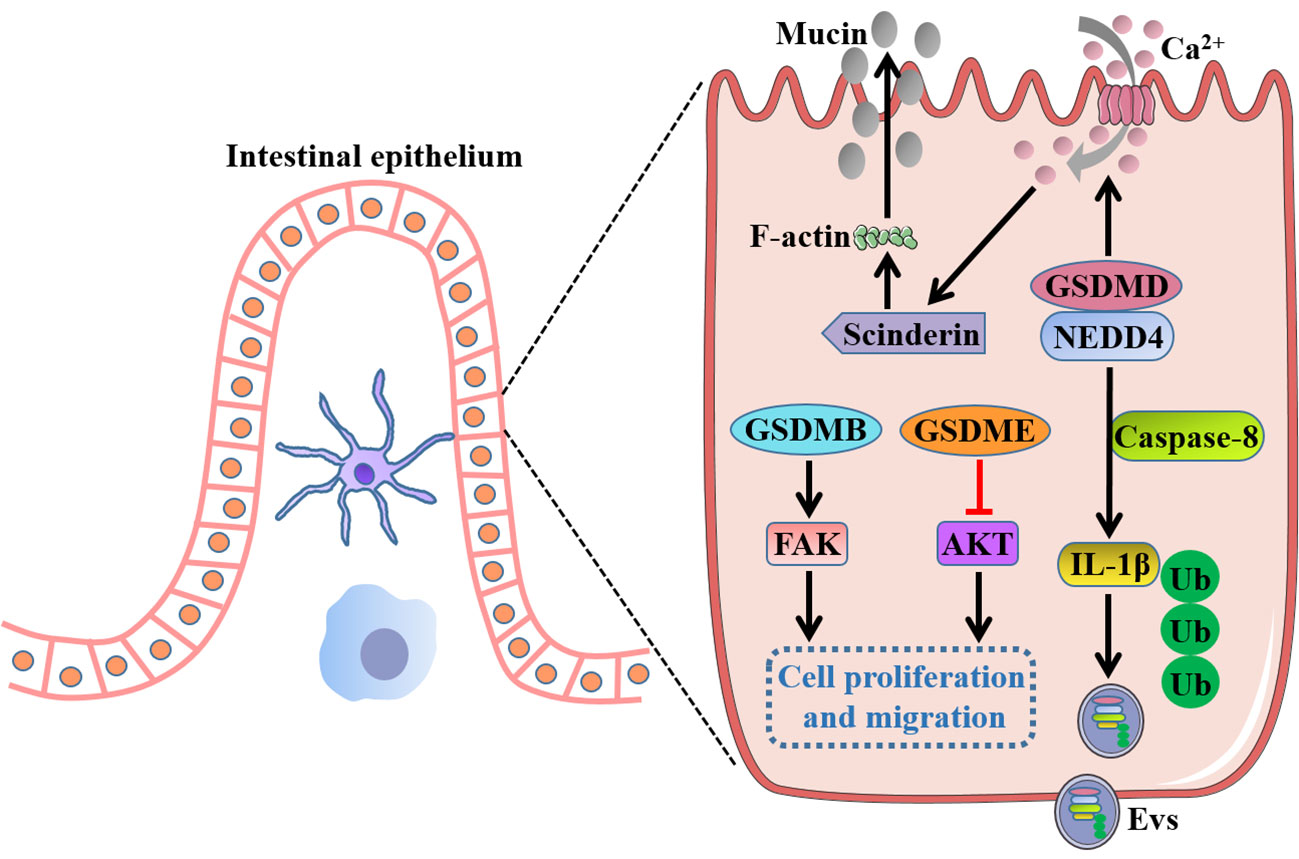
Figure 5 Intrinsic functions of GSDMs in gut independent of pyroptosis. Full-length GSDMB promotes cell proliferation and migration by regulation of FAK, whereas full-length GSDME suppresses cell growth by negative regulation of AKT pathway. GSDMD drives mucin secretion through calcium ion influx-dependent scinderin-mediated cortical F-actin disassembly, and recruits NEDD4 to mediate the release of polyubiquitinated IL-1β in the form of Evs via a caspase-8-dependent manner. FAK, focal adhesion kinase; NEDD4, an E3 ligase; Evs, extracellular vesicles.
Rana et al. (20) reported GSDMB was increased in inflamed colonocytes and crypt top colonocytes of IBD patients, and GSDMB enhanced IECs proliferation and migration by regulation of focal adhesion kinase (FAK) phosphorylation rather than its driven pyroptosis, thus promoting restoration of epithelial barrier function and the resolution of inflammation. GSDMD was recently found to recruit the E3 ligase NEDD4 to mediate the release of polyubiquitinated IL-1β via a caspase-8-dependent, nonpyroptotic pathway in IECs, which was crucial for the development of intestinal inflammation (113). Moreover, a recent study showed that GSDMD drove mucin secretion through calcium ion-dependent scinderin-mediated cortical F-actin disassembly, a key step in granule exocytosis, highlighting the distinctive function of GSDMD in intestinal goblet cell mucin secretion and mucus layer formation that uncoupled from pyroptotic cell death (114). Interestingly, a previous study showed that there were no gross histological abnormalities in the small intestinal or the colonic epithelium of GSDMD-/- mice, and GSDMD-/- mice appeared to exhibit comparable numbers of the differentiated cell lineages with wild-type mice such as goblet cells, Paneth cells and enteroendocrine cells, indicating an inessential role of GSDMD for intestinal epithelium development (115). These inconsistent results for GSDMD in gut homeostasis may attract researchers to further investigate the inherent functions of GSDMs. In addition, full-length GSDME was demonstrated to markedly decrease the cell growth and colony-forming ability of colon cancer cells by negative regulation of AKT pathway, whereas knockdown of GSDME enhanced cancer cell cycle progression and cell growth, implicating a potential tumor suppressor role for GSDME in CRC (116). However, the precise mechanism by which GSDMs exert the intrinsic functions independent of pyroptosis in intestinal homeostasis remains largely unknown and needs to be further elucidated.
7. Conclusions and perspectives
GSDMs are widely expressed in the intestine, while GSDMA, GSDMB, GSDMC and GSDME tend to appear prominently in intestinal epithelium, and GSDMD presents in both IECs and lamina propria immune cells. There is no doubt that GSDMs play a vital role in the intestinal mucosal immunity and homeostasis, due to its mediated pyroptosis, related PANoptosis, and inherent functions independent of pyroptotic cell death. The promotion or resistance effects of GSDMs on intestinal inflammation and tumorigenesis may depend on the biological context, in particular the interaction of GSDMs-expressing cells with the immune or tumor microenvironment. In these sense, the development of both agonists and blockers of GSDMs might be useful to govern therapy response and disease progression.
In fact, current research on GSDMs is just the tip of the iceberg, there are still multiple hurdles to resolve to fully unveil the role of GSDMs in gut homeostasis. Firstly, active GSDMs are important during cell death and inflammation, and GSDMs have been found to be cleaved and activated by caspases, SpeB virulence factor, neutrophil elastase, cathepsin G or granzymes. Whether GSDMs can be cleaved and activated by additional cellular proteases as well as pathogen-encoded proteases in gut remains to be explored. Secondly, as the key perpetrators of inflammation, the specific mechanisms of GSDMs being initiated and activated by which stimulating factors in intestinal homeostasis need to be further determined. Thirdly, it is notable that GSDMs are aberrantly expressed in CRC and are observed to be either promoters or suppressers of cancer. However, how GSDMs function to shape CRC cells or the tumor microenvironment in pyroptosis-dependent or -independent manners are largely unclear. Fourthly, several mutations of GSDMs have been demonstrated to increase the incidence risk of certain gut diseases, but whether it is caused by pyroptotic cell death or inherent function of GSDMs needs future investigation. In any case further insights into the roles of GSDMs in gut will not only strengthen our understanding of the daedal mechanisms underlying intestinal inflammation and tumorigenesis but will also help to exploit novel therapeutic strategies for gut homeostasis imbalance.
Author contributions
WG and KY drafted the manuscript and designed the figure. WZ and JZ revised the manuscript. JY, KG and XS conceived the topic. All authors contributed to the article and approved the submitted version.
Funding
This work was supported by the Institutional Foundation of The First Affiliated Hospital of Xi'an Jiaotong University (Grant serial numbers: 2022QN-07) and National Natural Science Foundation of China (Grant serial numbers: 82002547).
Conflict of interest
The authors declare that the research was conducted in the absence of any commercial or financial relationships that could be construed as a potential conflict of interest.
Publisher’s note
All claims expressed in this article are solely those of the authors and do not necessarily represent those of their affiliated organizations, or those of the publisher, the editors and the reviewers. Any product that may be evaluated in this article, or claim that may be made by its manufacturer, is not guaranteed or endorsed by the publisher.
References
1. Mowat AM, Agace WW. Regional specialization within the intestinal immune system. Nat Rev Immunol (2014) 14(10):667–85. doi: 10.1038/nri3738
2. Goll R, van Beelen Granlund A. Intestinal barrier homeostasis in inflammatory bowel disease. Scand J Gastroenterol (2015) 50(1):3–12. doi: 10.3109/00365521.2014.971425
3. Wolter M, Grant ET, Boudaud M, Steimle A, Pereira GV, Martens EC, et al. Leveraging diet to engineer the gut microbiome. Nat Rev Gastroenterol Hepatol (2021) 18(12):885–902. doi: 10.1038/s41575-021-00512-7
4. Kurashima Y, Goto Y, Kiyono H. Mucosal innate immune cells regulate both gut homeostasis and intestinal inflammation. Eur J Immunol (2013) 43(12):3108–15. doi: 10.1002/eji.201343782
5. Amoroso C, Perillo F, Strati F, Fantini MC, Caprioli F, Facciotti F. The role of gut microbiota biomodulators on mucosal immunity and intestinal inflammation. Cells (2020) 9(5):1234. doi: 10.3390/cells9051234
6. Okumura R, Takeda K. Roles of intestinal epithelial cells in the maintenance of gut homeostasis. Exp Mol Med (2017) 49(5):e338. doi: 10.1038/emm.2017.20
7. De Schepper S, Verheijden S, Aguilera-Lizarraga J, Viola MF, Boesmans W, Stakenborg N, et al. Self-maintaining gut macrophages are essential for intestinal homeostasis. Cell (2018) 175(2):400–415.e13. doi: 10.1016/j.cell.2018.07.048
8. Aglietti RA, Dueber EC. Recent insights into the molecular mechanisms underlying pyroptosis and gasdermin family functions. Trends Immunol (2017) 38(4):261–71. doi: 10.1016/j.it.2017.01.003
9. Tamura M, Tanaka S, Fujii T, Aoki A, Komiyama H, Ezawa K, et al. Members of a novel gene family, gsdm, are expressed exclusively in the epithelium of the skin and gastrointestinal tract in a highly tissue-specific manner. Genomics (2007) 89(5):618–29. doi: 10.1016/j.ygeno.2007.01.003
10. Schutter ED, Roelandt R, Riquet FB, Camp VN, Wullaert A, Vandenabeele P. Punching holes in cellular membranes: Biology and evolution of gasdermins. Trends Cell Biol (2021) 31(6):500–13. doi: 10.1016/j.tcb.2021.03.004
11. Liu X, Xia S, Zhang Z, Wu H, Lieberman J. Channelling inflammation: gasdermins in physiology and disease. Nat Rev Drug Discovery (2021) 20(5):384–405. doi: 10.1038/s41573-021-00154-z
12. Ding J, Wang K, Liu W, She Y, Sun Q, Shi J, et al. Pore-forming activity and structural autoinhibition of the gasdermin family. Nature (2016) 535(7610):111–6. doi: 10.1038/nature18590
13. Gong W, Liu P, Zheng T, Wu X, Zhao Y, Ren J. The ubiquitous role of spleen tyrosine kinase (Syk) in gut diseases: From mucosal immunity to targeted therapy. Int Rev Immunol (2021) 41(5):552–63. doi: 10.1080/08830185.2021.1962860
14. Wang M, Chen X, Zhang Y. Biological functions of gasdermins in cancer: From molecular mechanisms to therapeutic potential. Front Cell Dev Biol (2021) 9:638710. doi: 10.3389/fcell.2021.638710
15. Liu X, Lieberman J. Knocking 'em dead: Pore-forming proteins in immune defense. Annu Rev Immunol (2020) 38:455–85. doi: 10.1146/annurev-immunol-111319-023800
16. Xia X, Wang X, Cheng Z, Qin W, Lei L, Jiang J, et al. The role of pyroptosis in cancer: pro-cancer or pro-"host"? Cell Death Dis (2019) 10(9):650. doi: 10.1038/s41419-019-1883-8
17. Saeki N, Kuwahara Y, Sasaki H, Satoh H, Shiroishi T. Gasdermin (Gsdm) localizing to mouse chromosome 11 is predominantly expressed in upper gastrointestinal tract but significantly suppressed in human gastric cancer cells. Mamm Genome (2000) 11(9):718–24. doi: 10.1007/s003350010138
18. Laubhahn K, Böck A, Zeber K, Unterschemmann S, Kunze S, Schedel M, et al. 17q12-21 risk-variants influence cord blood immune regulation and multitrigger-wheeze. Pediatr Allergy Immunol (2022) 33(2):e13721. doi: 10.1111/pai.13721
19. Das S, Miller M, Beppu AK, Mueller J, McGeough MD, Vuong C, et al. GSDMB induces an asthma phenotype characterized by increased airway responsiveness and remodeling without lung inflammation. Proc Natl Acad Sci U S A (2016) 113(46):13132–7. doi: 10.1073/pnas.1610433113
20. Rana N, Privitera G, Kondolf HC, Bulek K, Lechuga S, Salvo CD, et al. GSDMB is increased in IBD and regulates epithelial restitution/repair independent of pyroptosis. Cell (2022) 185(2):283–298.e17. doi: 10.1016/j.cell.2021.12.024
21. Saeki N, Usui T, Aoyagi K, Kim DH, Sato M, Mabuchi T, et al. Distinctive expression and function of four GSDM family genes (GSDMA-d) in normal and malignant upper gastrointestinal epithelium. Genes Chromosomes Cancer (2009) 48(3):261–71. doi: 10.1002/gcc.20636
22. Kusumaningrum N, Lee DH, Yoon HS, Kim YK, Park CH, Chung JH, et al. Gasdermin c is induced by ultraviolet light and contributes to MMP-1 expression via activation of ERK and JNK pathways. J Dermatol Sci (2018) 90(2):180–9. doi: 10.1016/j.jdermsci.2018.01.015
23. Zhao M, Ren K, Xiong X, Xin Y, Zou Y, Maynard JC, et al. Epithelial STAT6 O-GlcNAcylation drives a concerted anti-helminth alarmin response dependent on tuft cell hyperplasia and gasdermin c. Immunity (2022) 55(4):623–638.e5. doi: 10.1016/j.immuni.2022.03.009
24. Van Laer L, Van Camp G, van Zuijlen D, Green ED, Verstreken M, Schatteman I, et al. Refined mapping of a gene for autosomal dominant progressive sensorineural hearing loss (DFNA5) to a 2-cM region, and exclusion of a candidate gene that is expressed in the cochlea. Eur J Hum Genet (1997) 5(6):397–405. doi: 10.1159/000484798
25. Delmaghani S, del Castillo FJ, Michel V, Leibovici M, Aghaie A, Ron U, et al. Mutations in the gene encoding pejvakin, a newly identified protein of the afferent auditory pathway, cause DFNB59 auditory neuropathy. Nat Genet (2006) 38(7):770–8. doi: 10.1038/ng1829
26. Tan G, Huang C, Chen J, Chen B, Zhi F. Gasdermin-e-mediated pyroptosis participates in the pathogenesis of crohn's disease by promoting intestinal inflammation. Cell Rep (2021) 35(11):109265. doi: 10.1016/j.celrep.2021.109265
27. Katoh M, Katoh M. Identification and characterization of human DFNA5L, mouse Dfna5l, and rat Dfna5l genes in silico. Int J Oncol (2004) 25(3):765–70. doi: 10.3892/ijo.25.3.765
28. Xia S, Zhang Z, Magupalli VG, Pablo JL, Dong Y, Vora SM, et al. Gasdermin d pore structure reveals preferential release of mature interleukin-1. Nature (2021) 593(7860):607–11. doi: 10.1038/s41586-021-03478-3
29. Deng W, Bai Y, Deng F, Pan Y, Mei S, Zheng Z, et al. Streptococcal pyrogenic exotoxin b cleaves GSDMA and triggers pyroptosis. Nature (2022) 602(7897):496–502. doi: 10.1038/s41586-021-04384-4
30. Zhou Z, He H, Wang K, Shi X, Wang Y, Su Y, et al. Granzyme a from cytotoxic lymphocytes cleaves GSDMB to trigger pyroptosis in target cells. Science (2020) 368(6494):eaaz7548. doi: 10.1126/science.aaz7548
31. Hou J, Zhao R, Xia W, Chang CW, You Y, Hsu JM, et al. PD-L1-mediated gasdermin c expression switches apoptosis to pyroptosis in cancer cells and facilitates tumour necrosis. Nat Cell Biol (2020) 22(10):1264–75. doi: 10.1038/s41556-020-0575-z
32. Zhang JY, Zhou B, Sun RY, Ai YL, Cheng K, Li FN, et al. The metabolite α-KG induces GSDMC-dependent pyroptosis through death receptor 6-activated caspase-8. Cell Res (2021) 31(9):980–97. doi: 10.1038/s41422-021-00506-9
33. Shi J, Zhao Y, Wang K, Shi X, Wang Y, Huang H, et al. Cleavage of GSDMD by inflammatory caspases determines pyroptotic cell death. Nature (2015) 526(7575):660–5. doi: 10.1038/nature15514
34. Sharma BR, Kanneganti TD. NLRP3 inflammasome in cancer and metabolic diseases. Nat Immunol (2021) 22(5):550–9. doi: 10.1038/s41590-021-00886-5
35. Kayagaki N, Stowe IB, Lee BL, O'Rourke K, Anderson K, Warming S, et al. Caspase-11 cleaves gasdermin d for non-canonical inflammasome signalling. Nature (2015) 526(7575):666–71. doi: 10.1038/nature15541
36. Orning P, Weng D, Starheim K, Ratner D, Best Z, Lee B, et al. Pathogen blockade of TAK1 triggers caspase-8-dependent cleavage of gasdermin d and cell death. Science (2018) 362(6418):1064–9. doi: 10.1126/science.aau2818
37. Sarhan J, Liu BC, Muendlein HI, Li P, Nilson R, Tang AY, et al. Caspase-8 induces cleavage of gasdermin d to elicit pyroptosis during yersinia infection. Proc Natl Acad Sci U S A (2018) 115(46):E10888–97. doi: 10.1073/pnas.1809548115
38. Sollberger G, Choidas A, Burn GL, Habenberger P, Lucrezia RD, Kordes S, et al. Gasdermin d plays a vital role in the generation of neutrophil extracellular traps. Sci Immunol (2018) 3(26):eaar6689. doi: 10.1126/sciimmunol.aar6689
39. Burgener SS, Leborgne NGF, Snipas SJ, Salvesen GS, Ian Bird P, Benarafa C, et al. Cathepsin G inhibition by Serpinb1 and Serpinb6 prevents programmed necrosis in neutrophils and monocytes and reduces GSDMD-driven inflammation. Cell Rep (2019) 27(12):3646–3656.e5. doi: 10.1016/j.celrep.2019.05.065
40. Rogers C, Fernandes-Alnemri T, Mayes L, Alnemri D, Cingolani G, Alnemri ES. Cleavage of DFNA5 by caspase-3 during apoptosis mediates progression to secondary necrotic/pyroptotic cell death. Nat Commun (2017) 8:14128. doi: 10.1038/ncomms14128
41. Zhang Z, Zhang Y, Xia S, Kong Q, Li S, Liu X, et al. Gasdermin e suppresses tumour growth by activating anti-tumour immunity. Nature (2020) 579(7799):415–20. doi: 10.1038/s41586-020-2071-9
42. Xavier RJ, Podolsky DK. Unravelling the pathogenesis of inflammatory bowel disease. Nature (2007) 448(7152):427–34. doi: 10.1038/nature06005
43. Boyapati RK, Rossi AG, Satsangi J, Ho GT. Gut mucosal DAMPs in IBD: from mechanisms to therapeutic implications. Mucosal Immunol (2016) 9(3):567–82. doi: 10.1038/mi.2016.14
44. Gong W, Guo K, Zheng T, Fang M, Xie H, Li W, et al. Preliminary exploration of the potential of spliceosome-associated protein 130 for predicting disease severity in crohn's disease. Ann N Y Acad Sci (2020) 1462(1):128–38. doi: 10.1111/nyas.14240
45. Gong W, Zheng T, Guo K, Ang M, Xie H, Li W, et al. Mincle/Syk signalling promotes intestinal mucosal inflammation through induction of macrophage pyroptosis in crohn's disease. J Crohns Colitis (2020) 14(12):1734–47. doi: 10.1093/ecco-jcc/jjaa088
46. Gong W, Liu P, Zhao F, Liu J, Hong Z, Ren H, et al. STING-mediated syk signaling attenuates tumorigenesis of colitis−associated colorectal cancer through enhancing intestinal epithelium pyroptosis. Inflammation Bowel Dis (2022) 28(4):572–85. doi: 10.1093/ibd/izab217
47. Li D, Chang X, Zhao J, Chen X, Xu Z, Zhang D, et al. Colonic epithelial PHLPP2 deficiency promotes colonic epithelial pyroptosis by activating the NF- κ b signaling pathway. Oxid Med Cell Longev (2021) 2021:5570731. doi: 10.1155/2021/5570731
48. Wang Y, Zhou X, Zou K, Chen G, Huang L, Yang F, et al. Monocarboxylate transporter 4 triggered cell pyroptosis to aggravate intestinal inflammation in inflammatory bowel disease. Front Immunol (2021) 12:644862. doi: 10.3389/fimmu.2021.644862
49. Xu Z, Liu R, Huang L, Xu Y, Su M, Chen J, et al. CD147 aggravated inflammatory bowel disease by triggering NF- κ b-mediated pyroptosis. BioMed Res Int (2020) 2020:5341247. doi: 10.1155/2020/5341247
50. Gao H, Cao M, Yao Y, Hu W, Sun H, Zhang Y, et al. Dysregulated microbiota-driven gasdermin d activation promotes colitis development by mediating IL-18 release. Front Immunol (2021) 12:750841. doi: 10.3389/fimmu.2021.750841
51. Schwarzer R, Jiao H, Wachsmuth L, Tresch A, Pasparakis M. FADD and caspase-8 regulate gut homeostasis and inflammation by controlling MLKL- and GSDMD-mediated death of intestinal epithelial cells. Immunity (2020) 52(6):978–993.e6. doi: 10.1016/j.immuni.2020.04.002
52. Tan G, Huang C, Chen J, Chen B, Shi Y, Zhi F. An IRF1-dependent pathway of TNFα-induced shedding in intestinal epithelial cells. J Crohns Colitis (2022) 16(1):133–42. doi: 10.1093/ecco-jcc/jjab134
53. Zhou L, Liu T, Huang B, Luo M, Chen Z, Zhao Z, et al. Excessive deubiquitination of NLRP3-R779C variant contributes to very-early-onset inflammatory bowel disease development. J Allergy Clin Immunol (2021) 147(1):267–79. doi: 10.1016/j.jaci.2020.09.003
54. Cai X, Zhang Z, Yuan J, Ocansey DKW, Tu Q, Zhang X, et al. hucMSC-derived exosomes attenuate colitis by regulating macrophage pyroptosis via the miR-378a-5p/NLRP3 axis. Stem Cell Res Ther (2021) 12(1):416. doi: 10.1186/s13287-021-02492-6
55. Wu X, Pan S, Luo W, Shen Z, Meng X, Xiao M, et al. Roseburia intestinalis−derived flagellin ameliorates colitis by targeting miR−223−3p−mediated activation of NLRP3 inflammasome and pyroptosis. Mol Med Rep (2020) 22(4):2695–704. doi: 10.3892/mmr.2020.11351
56. Ma C, Yang D, Wang B, Wu C, Wu Y, Li S, et al. Gasdermin d in macrophages restrains colitis by controlling cGAS-mediated inflammation. Sci Adv (2020) 6(21):eaaz6717. doi: 10.1126/sciadv.aaz6717
57. Huang S, Tao R, Zhou J, Qian L, Wu J. Trans-10-Hydroxy-2-Decenoic acid alleviates dextran sulfate sodium-induced colitis in mice via regulating the inflammasome-mediated pyroptotic pathway and enhancing colonic barrier function. Mol Nutr Food Res (2022) 66(12):e2100821. doi: 10.1002/mnfr.202100821
58. Zhang W, Wang W, Shen C, Wang X, Pu Z, Yin Q. Network pharmacology for systematic understanding of schisandrin b reduces the epithelial cells injury of colitis through regulating pyroptosis by AMPK/Nrf2/NLRP3 inflammasome. Aging (Albany NY) (2021) 13(19):23193–209. doi: 10.18632/aging.203611
59. Liu Y, Wang X, Cui Y, Yin Y, Qiu D, Li S, et al. Apple polyphenols extract (APE) alleviated dextran sulfate sodium induced acute ulcerative colitis and accompanying neuroinflammation via inhibition of apoptosis and pyroptosis. Foods (2021) 10(11):2711. doi: 10.3390/foods10112711
60. Evans L, Rhodes A, Alhazzani W, Antonelli M, Coopersmith CM, French C, et al. Surviving sepsis campaign: international guidelines for management of sepsis and septic shock 2021. Intensive Care Med (2021) 47(11):1181–247. doi: 10.1007/s00134-021-06506-y
61. Meng M, Klingensmith NJ, Coopersmith CM. New insights into the gut as the driver of critical illness and organ failure. Curr Opin Crit Care (2017) 23(2):143–8. doi: 10.1097/MCC.0000000000000386
62. Mandal P, Feng Y, Lyons JD, Berger SB, Otani S, DeLaney A, et al. Caspase-8 collaborates with caspase-11 to drive tissue damage and execution of endotoxic shock. Immunity (2018) 49(1):42–55.e6. doi: 10.1016/j.immuni.2018.06.011
63. Chen H, Li Y, Wu J, Li G, Tao X, Lai K, et al. RIPK3 collaborates with GSDMD to drive tissue injury in lethal polymicrobial sepsis. Cell Death Differ (2020) 27(9):2568–85. doi: 10.1038/s41418-020-0524-1
64. Sheng B, Chen Y, Sun L, Xu P, Han B, Li X, et al. Antifungal treatment aggravates sepsis through the elimination of intestinal fungi. Oxid Med Cell Longev (2021) 2021:2796700. doi: 10.1155/2021/2796700
65. Shi Y, Zou Y, Xiong Y, Zhang S, Song M, An X, et al. Host gasdermin d restrains systemic endotoxemia by capturing proteobacteria in the colon of high-fat diet-feeding mice. Gut Microbes (2021) 13(1):1946369. doi: 10.1080/19490976.2021.1946369
66. Chen L, Zhong X, Cao W, Mao M, Li W, Yang H, et al. JQ1 as a BRD4 inhibitor blocks inflammatory pyroptosis-related acute colon injury induced by LPS. Front Immunol (2021) 12:609319. doi: 10.3389/fimmu.2021.609319
67. Blyth GAD, Connors L, Fodor C, Cobo ER. The network of colonic host defense peptides as an innate immune defense against enteropathogenic bacteria. Front Immunol (2020) 11:965. doi: 10.3389/fimmu.2020.00965
68. Santos RL. Pathobiology of salmonella, intestinal microbiota, and the host innate immune response. Front Immunol (2014) 5:252. doi: 10.3389/fimmu.2014.00252
69. Zuo L, Zhou L, Wu C, Wang Y, Li Y, Huang R, et al. Salmonella spvC gene inhibits pyroptosis and intestinal inflammation to aggravate systemic infection in mice. Front Microbiol (2020) 11:562491. doi: 10.3389/fmicb.2020.562491
70. Ventayol PS, Geiser P, Martino MLD, Florbrant A, Fattinger SA, Walder N, et al. Bacterial detection by NAIP/NLRC4 elicits prompt contractions of intestinal epithelial cell layers. Proc Natl Acad Sci U S A (2021) 118(16):e2013963118. doi: 10.1073/pnas.2013963118
71. Cui C, Liu Q, Duan B, Liu X, Wei H, Peng J. Bioactive triple peptide inhibits inflammasome activation to alleviate salmonella-induced intestinal inflammation in mice via modulation of host defense and bacterial virulence. Food Funct (2022) 13(6):3512–25. doi: 10.1039/D1FO03891E
72. Zong Y, Chen W, Zhao Y, Suo X, Yang X. Salmonella infection causes hyperglycemia for decreased GLP-1 content by enteroendocrine l cells pyroptosis in pigs. Int J Mol Sci (2022) 23(3):1272. doi: 10.3390/ijms23031272
73. Chen Z, Zhang Y, Lin R, Meng X, Zhao W, Shen W, et al. Cronobacter sakazakii induces necrotizing enterocolitis by regulating NLRP3 inflammasome expression via TLR4. J Med Microbiol (2020) 69(5):748–58. doi: 10.1099/jmm.0.001181
74. Fan H, Chen Z, Lin R, Liu Y, Wu X, Puthiyakunnon S, et al. Bacteroides fragilis strain ZY-312 defense against cronobacter sakazakii-induced necrotizing enterocolitis In vitro and in a neonatal rat model. mSystems (2019) 4(4):e00305–19. doi: 10.1128/mSystems.00305-19
75. Zhong Q, Roumeliotis TI, Kozik Z, Cepeda-Molero M, Fernández LA, Shenoy AR, et al. Clustering of tir during enteropathogenic e. coli infection triggers calcium influx-dependent pyroptosis in intestinal epithelial cells. PloS Biol (2020) 18(12):e3000986. doi: 10.1371/journal.pbio.3000986
76. Melhem H, Kaya B, Kaymak T, Wuggenig P, Flint E, Roux J, et al. Epithelial GPR35 protects from citrobacter rodentium infection by preserving goblet cells and mucosal barrier integrity. Mucosal Immunol (2022) 15(3):443–58. doi: 10.1038/s41385-022-00494-y
77. Dubois H, Sorgeloos F, Sarvestani ST, Martens L, Saeys Y, Mackenzie JM, et al. Nlrp3 inflammasome activation and gasdermin d-driven pyroptosis are immunopathogenic upon gastrointestinal norovirus infection. PloS Pathog (2019) 15(4):e1007709. doi: 10.1371/journal.ppat.1007709
78. Hansen JM, de Jong MF, Wu Q, Zhang L, Heisler DB, Alto LT, et al. Pathogenic ubiquitination of GSDMB inhibits NK cell bactericidal functions. Cell (2021) 184(12):3178–3191.e18. doi: 10.1016/j.cell.2021.04.036
79. Sateriale A, Gullicksrud JA, Engiles JB, McLeod BI, Kugler EM, Henao-Mejia J, et al. The intestinal parasite cryptosporidium is controlled by an enterocyte intrinsic inflammasome that depends on NLRP6. Proc Natl Acad Sci U S A (2021) 118(2):e2007807118. doi: 10.1073/pnas.2007807118
80. Wang S, Moreau F, Chadee K. The colonic pathogen entamoeba histolytica activates caspase-4/1 that cleaves the pore-forming protein gasdermin d to regulate IL-1β secretion. PloS Pathog (2022) 18(3):e1010415. doi: 10.1371/journal.ppat.1010415
81. Xi R, Montague J, Lin X, Lu C, Lei W, Tanaka K, et al. Up-regulation of gasdermin c in mouse small intestine is associated with lytic cell death in enterocytes in worm-induced type 2 immunity. Proc Natl Acad Sci U S A (2021) 118(30):e2026307118. doi: 10.1073/pnas.2026307118
82. Liu Q, Du P, Zhu Y, Zhang X, Cai J, Zhang Z, et al. Thioredoxin reductase 3 suppression promotes colitis and carcinogenesis via activating pyroptosis and necrosis. Cell Mol Life Sci (2022) 79(2):106. doi: 10.1007/s00018-022-04155-y
83. Ren Q, Yang B, Zhu G, Wang S, Fu C, Zhang H, et al. Antiproliferation activity and mechanism of c9, t11, c15-CLNA and t9, t11, c15-CLNA from lactobacillus plantarum ZS2058 on colon cancer cells. Molecules (2020) 25(5):1225. doi: 10.3390/molecules25051225
84. Yokomizo K, Harada Y, Kijima K, Shinmura K, Sakata M, Sakuraba K, et al. Methylation of the DFNA5 gene is frequently detected in colorectal cancer. Anticancer Res (2012) 32(4):1319–22.
85. Ibrahim J, de Beeck KO, Fransen E, Croes L, Beyens M, Suls A, et al. Methylation analysis of gasdermin e shows great promise as a biomarker for colorectal cancer. Cancer Med (2019) 8(5):2133–45. doi: 10.1002/cam4.2103
86. Yu J, Li S, Qi J, Chen Z, Wu Y, Guo J, et al. Cleavage of GSDME by caspase-3 determines lobaplatin-induced pyroptosis in colon cancer cells. Cell Death Dis (2019) 10(3):193. doi: 10.1038/s41419-019-1441-4
87. Liu Z, Li Y, Zhu Y, Li N, Li W, Shang C, et al. Apoptin induces pyroptosis of colorectal cancer cells via the GSDME-dependent pathway. Int J Biol Sci (2022) 18(2):717–30. doi: 10.7150/ijbs.64350
88. Tan G, Lin C, Huang C, Chen B, Chen J, Shi Y, et al. Radiosensitivity of colorectal cancer and radiation-induced gut damages are regulated by gasdermin e. Cancer Lett (2022) 529:1–10. doi: 10.1016/j.canlet.2021.12.034
89. Guo J, Zheng J, Mu M, Chen Z, Xu Z, Zhao C, et al. GW4064 enhances the chemosensitivity of colorectal cancer to oxaliplatin by inducing pyroptosis. Biochem Biophys Res Commun (2021) 548:60–6. doi: 10.1016/j.bbrc.2021.02.043
90. Zhang Y, Xu Z, Feng W, Gao H, Xu Z, Miao Y, et al. Small molecule inhibitors from organoid-based drug screen induce concurrent apoptosis and gasdermin e-dependent pyroptosis in colorectal cancer. Clin Transl Med (2022) 12(4):e812. doi: 10.1002/ctm2.812
91. Croes L, Fransen E, Hylebos M, Buys K, Hermans C, Broeckx G, et al. Determination of the potential tumor-suppressive effects of gsdme in a chemically induced and in a genetically modified intestinal cancer mouse model. Cancers (Basel) (2019) 11(8):1214. doi: 10.3390/cancers11081214
92. Hergueta-Redondo M, Sarrió D, Molina-Crespo Á, Megias D, Mota A, Rojo-Sebastian A, et al. Gasdermin-b promotes invasion and metastasis in breast cancer cells. PloS One (2014) 9(3):e90099. doi: 10.1371/journal.pone.0090099
93. He H, Yi L, Zhang B, Yan B, Xiao M, Ren J, et al. USP24-GSDMB complex promotes bladder cancer proliferation via activation of the STAT3 pathway. Int J Biol Sci (2021) 17(10):2417–29. doi: 10.7150/ijbs.54442
94. Gao J, Qiu X, Xi G, Liu H, Zhang F, Lv T, et al. Downregulation of GSDMD attenuates tumor proliferation via the intrinsic mitochondrial apoptotic pathway and inhibition of EGFR/Akt signaling and predicts a good prognosis in non−small cell lung cancer. Oncol Rep (2018) 40(4):1971–84. doi: 10.3892/or.2018.6634
95. Shen X, Zhang Q, He Z, Xiao S, Li H, Huang Z, et al. Overexpression of gasdermin d promotes invasion of adenoid cystic carcinoma. Int J Clin Exp Pathol (2020) 13(7):1802–11.
96. Miguchi M, Hinoi T, Shimomura M, Adachi T, Saito Y, Niitsu H, et al. Gasdermin c is upregulated by inactivation of transforming growth factor β receptor type II in the presence of mutated apc, promoting colorectal cancer proliferation. PloS One (2016) 11(11):e0166422. doi: 10.1371/journal.pone.0166422
97. Tan G, Huang C, Chen J, Zhi F. HMGB1 released from GSDME-mediated pyroptotic epithelial cells participates in the tumorigenesis of colitis-associated colorectal cancer through the ERK1/2 pathway. J Hematol Oncol (2020) 13(1):149. doi: 10.1186/s13045-020-00985-0
98. Jia Y, Cui R, Wang C, Feng Y, Li Z, Tong Y, et al. Metformin protects against intestinal ischemia-reperfusion injury and cell pyroptosis via TXNIP-NLRP3-GSDMD pathway. Redox Biol (2020) 32:101534. doi: 10.1016/j.redox.2020.101534
99. Liu X, Leng Y, Lv X, Lv G, Zhang M, Shi Y, et al. Dexmedetomidine inhibits endoplasmic reticulum stress to suppress pyroptosis of hypoxia/reoxygenation-induced intestinal epithelial cells via activating the SIRT1 expression. J Bioenerg Biomembr (2021) 53(6):655–64. doi: 10.1007/s10863-021-09922-3
100. Wu D, Li J, Shen R, Li J, Yu Y, Li L, et al. Autophagy induced by micheliolide alleviates acute irradiation-induced intestinal injury via inhibition of the NLRP3 inflammasome. Front Pharmacol (2022) 12:773150. doi: 10.3389/fphar.2021.773150
101. Ye L, Li G, Goebel A, Raju AV, Kong F, Lv Y, et al. Caspase-11-mediated enteric neuronal pyroptosis underlies Western diet-induced colonic dysmotility. J Clin Invest (2020) 130(7):3621–36. doi: 10.1172/JCI130176
102. Lu Y, Meng R, Wang X, Xu Y, Tang Y, Wu J, et al. Caspase-11 signaling enhances graft-versus-host disease. Nat Commun (2019) 10(1):4044. doi: 10.1038/s41467-019-11895-2
103. Hou C, Ma H, Ye J, Luo D, Bao H, Guan J, et al. Plasma exosomes derived from patients with intestinal behçet's syndrome induce intestinal epithelial cell pyroptosis. Clin Rheumatol (2021) 40(10):4143–55. doi: 10.1007/s10067-021-05755-y
104. Kerr NA, Sanchez J, O'Connor G, Watson BD, Daunert S, Bramlett HM, et al. Inflammasome-regulated pyroptotic cell death in disruption of the gut-brain axis after stroke. Transl Stroke Res (2022) 13(6):898–912. doi: 10.1007/s12975-022-01005-8
105. Galluzzi L, Vitale I, Aaronson SA, Abrams JM, Adam D, Agostinis P, et al. Molecular mechanisms of cell death: recommendations of the nomenclature committee on cell death 2018. Cell Death Differ (2018) 25(3):486–541. doi: 10.1038/s41418-017-0012-4
106. Christgen S, Zheng M, Kesavardhana S, Karki R, Malireddi RKS, Banoth B, et al. Identification of the PANoptosome: A molecular platform triggering pyroptosis, apoptosis, and necroptosis (PANoptosis). Front Cell Infect Microbiol (2020) 10:237. doi: 10.3389/fcimb.2020.00237
107. Wang Y, Kanneganti TD. From pyroptosis, apoptosis and necroptosis to PANoptosis: A mechanistic compendium of programmed cell death pathways. Comput Struct Biotechnol J (2021) 19:4641–57. doi: 10.1016/j.csbj.2021.07.038
108. Jiang W, Deng Z, Dai X, Zhao W. PANoptosis: A new insight into oral infectious diseases. Front Immunol (2021) 12:789610. doi: 10.3389/fimmu.2021.789610
109. Karki R, Sharma BR, Lee E, Banoth B, Malireddi RKS, Samir P, et al. Interferon regulatory factor 1 regulates PANoptosis to prevent colorectal cancer. JCI Insight (2020) 5(12):e136720. doi: 10.1172/jci.insight.136720
110. Malireddi RKS, Karki R, Sundaram B, Kancharana B, Lee S, Samir P, et al. Inflammatory cell death, PANoptosis, mediated by cytokines in diverse cancer lineages inhibits tumor growth. Immunohorizons (2021) 5(7):568–80. doi: 10.4049/immunohorizons.2100059
111. Karki R, Sundaram B, Sharma BR, Lee S, Malireddi RKS, Nguyen LN, et al. ADAR1 restricts ZBP1-mediated immune response and PANoptosis to promote tumorigenesis. Cell Rep (2021) 37(3):109858. doi: 10.1016/j.celrep.2021.109858
112. Lin J, Hu P, Wang Y, Tan Y, Yu K, Liao K, et al. Phosphorylated NFS1 weakens oxaliplatin-based chemosensitivity of colorectal cancer by preventing PANoptosis. Signal Transduct Target Ther (2022) 7(1):54. doi: 10.1038/s41392-022-00889-0
113. Bulek K, Zhao J, Liao Y, Rana N, Corridoni D, Antanaviciute A, et al. Epithelial-derived gasdermin d mediates nonlytic IL-1β release during experimental colitis. J Clin Invest (2020) 130(8):4218–34. doi: 10.1172/JCI138103
114. Zhang J, Yu Q, Jiang D, Yu K, Yu W, Chi Z, et al. Epithelial gasdermin d shapes the host-microbial interface by driving mucus layer formation. Sci Immunol (2022) 7(68):eabk2092. doi: 10.1126/sciimmunol.abk2092
115. Fujii T, Tamura M, Tanaka S, Gasdermin D. (Gsdmd) is dispensable for mouse intestinal epithelium development. Genesis (2008) 46(8):418–23. doi: 10.1002/dvg.20412
Keywords: Gasdermins, pyroptosis, intestinal inflammation, tumorigenesis, intestinal homeostasis
Citation: Gong W, Yang K, Zhao W, Zheng J, Yu J, Guo K and Sun X (2022) Intestinal Gasdermins for regulation of inflammation and tumorigenesis. Front. Immunol. 13:1052111. doi: 10.3389/fimmu.2022.1052111
Received: 29 September 2022; Accepted: 14 November 2022;
Published: 25 November 2022.
Edited by:
Bhesh Raj Sharma, St. Jude Children’s Research Hospital, United StatesReviewed by:
Sarang Tartey, IGM Biosciences, United StatesKatarzyna Bulek, Cleveland Clinic, United States
Copyright © 2022 Gong, Yang, Zhao, Zheng, Yu, Guo and Sun. This is an open-access article distributed under the terms of the Creative Commons Attribution License (CC BY). The use, distribution or reproduction in other forums is permitted, provided the original author(s) and the copyright owner(s) are credited and that the original publication in this journal is cited, in accordance with accepted academic practice. No use, distribution or reproduction is permitted which does not comply with these terms.
*Correspondence: Junhui Yu, eXVqdW5odWlAbWFpbC54anR1LmVkdS5jbg==; Kun Guo, Z3Vva3VubWVkaWNhbEAxMjYuY29t; Xuejun Sun, c3VueHlAbWFpbC54anR1LmVkdS5jbg==
†These authors have contributed equally to this work