- Department of Pharmacology, School of Medicine, Southern University of Science and Technology, Shenzhen, China
Until May 2022, zoonotic infectious disease monkeypox (MPX) caused by the monkeypox virus (MPXV) was one of the forgotten viruses considered to be geographically limited in African countries even though few cases outside of Africa were identified. Central and West African countries are known to be endemic for MPXV. However, since the number of human MPX cases has rapidly increased outside of Africa the global interest in this virus has markedly grown. The majority of infected people with MPXV have never been vaccinated against smallpox virus. Noteworthily, the MPXV spreads fast in men who have sex with men (MSM). Preventive measures against MPXV are essential to be taken, indeed, vaccination is the key. Due to the antigenic similarities, the smallpox vaccine is efficient against MPXV. Nevertheless, there is no specific MPXV vaccine until now. Nucleic acid vaccines deserve special attention since the emergency approval of two messenger RNA (mRNA)-based coronavirus disease 2019 (COVID-19) vaccines in 2020. This milestone in vaccinology has opened a new platform for developing more mRNA- or DNA-based vaccines. Certainly, this type of vaccine has a number of advantages including time- and cost-effectiveness over conventional vaccines. The platform of nucleic acid-based vaccines gives humankind a huge opportunity. Ultimately, there is a strong need for developing a universal vaccine against MPXV. This review will shed the light on the strategies for developing nucleic acid vaccines against MPXV in a timely manner. Consequently, developing nucleic acid-based vaccines may alleviate the global threat against MPXV.
Introduction
Widely neglected disease– monkeypox (MPX) deserves remarkable attention since it crossed African borders and cases have increased fast. MPX is a zoonotic infectious disease characterized by smallpox-like symptoms but with less severity and a lower fatality rate. Monkeypox virus (MPXV) belongs to the Orthopoxvirus genus in the family of Poxviridae and has two clades– West and Central African clades. Remarkably, the Central African clade has more severe outcomes and a higher mortality rate, up to 11% (1, 2). Furthermore, it is more severe in younger children where the mortality rate can reach up to 15% (1, 3). MPXV that is spread outside of Africa represents the West African clade which has milder symptoms and a significantly lower mortality rate, ~1-3.6% (2–4). Besides, the recent outbreak demonstrated that the virus causes more mortality in developing endemic countries compared with developed countries probably because of the less capable health system (2). MPXV was first identified in macaque monkeys in a Denmark research facility (5). Since then, this infectious disease is called “MPX”. However, the natural hosts of this virus are considered to be rodents (6).
The first human case was reported in 1970 during the active smallpox surveillance in Bokenda, a village in the Democratic Republic of Congo (DRC) (also called Zaire). The infected patient was a 9-month-old child whose sample was sent to the World Health Organization (WHO) Smallpox Reference Centre in Moscow and eventually revealed MPXV by isolation. Interestingly, the patient’s family admitted that monkeys were eaten occasionally as a delicacy but they were not able to recall if it happened during the last month or whether the child had any contact with the monkey in recent times (7). Importantly, the boy was not vaccinated against smallpox unlike the other members of the family (8). After that, MPX outbreaks mainly occurred in West and Central Africa until 2003, following the import of infected prairie dogs from Ghana to the United States (US). Remarkably, those dogs were housed or transported together with African rodents from Ghana. In total, 47 MPX cases were reported with no death (9, 10). In 2021, two cases of MPXV were reported in the US, both imported from Nigeria (11, 12). Apart from the US, few single MPX cases were reported in different countries such as the United Kingdom (UK), Israel, and Singapore (3, 13–16). All were related to travel to Africa, particularly Nigeria. However, since May 2022, the confirmed cases of MPXV dramatically increased outside of Africa, and currently, it is a global concern. WHO declared the MPX outbreak a global health emergency on July 23, 2022 (17). The timeline of MPXV spread since 1958 is given in Figure 1.
MPXV represents a linear double-stranded DNA (dsDNA) virus and it has two forms like other poxviruses– intracellular mature virus (IMV) and extracellular enveloped virus (EEV). MPXV has a large DNA genome (18) and is quite stable in the environment as DNA viruses mutate less frequently than RNA viruses (5, 19). Until 2022, the spread of the virus via secondary transmission outside Africa was limited (20). The current non-endemic MPXV outbreak is the first and largest outbreak to date that is different from the previous waves as an intensive human-to-human transmission takes place (3). The MPXV can be transmitted via exposure sources such as scratches and bites from an infected animal, saliva, respiratory excretions, contact with the lesion exudate, or even feces (8, 21). Except for the similarities to the symptoms of smallpox, unlike it, the clinical manifestation of MPX also includes lymphadenopathy. The rash appears 1 to 3 days after the onset of fever and lymph node enlargement. It can be distributed all over the body but mainly concentrated on the extremities (8), genitals, and anus (3, 22–25).
Despite the availability of a smallpox vaccine that has high efficiency to MPXV, there is no specific vaccine for MPXV. Hence, it is urgent to develop a strategy for designing a universal MPXV vaccine for further unexpected epidemics/pandemics preparedness. Nucleic acid vaccines, particularly mRNA vaccines have elicited high efficiency against ongoing coronavirus disease 2019 (COVID-19) pandemic (26–31). Moreover, DNA vaccines also show great results (32–36), e.g., India has approved a DNA vaccine against severe acute respiratory syndrome coronavirus 2 (SARS-CoV-2) (37). Nucleic acid vaccines have a number of advantages compared with traditional vaccines. Current review addresses the MPXV classification, structure, pathogenesis, and the strategy for designing nucleic acid-based universal vaccine candidates against MPXV.
Monkeypox viruses– classification and structure
Along with MPXV, the genus Orthopoxvirus comprises three more human-pathogen species: variola virus (VARV)– the causative agent of smallpox, vaccinia virus (VACV), and cowpox. VARV and MPXV often cause life-threatening diseases, while VACV and cowpox are usually associated with local lesions. Out of two clades, the West African clade of MPXVs is characterized with less antigenic drift and virulence (38, 39). The Central African clade (clade 1) which is particularly endemic to the Congo Basin causes more severe symptoms of the disease as it is more virulent and transmissible (40, 41). The MPXVs isolated since 2017 are categorized as a clade 3 which along with clade 2 belongs to the West African clade (41, 42). MPXVs identified during the 2017/2019 outbreaks belong to lineages A.1, A.2, and A1.1, while MPXVs isolated during the current multi-country outbreak belong to lineage B.1 (41, 43). Importantly, clade 3 is characterized by the high number of mutations allowing increasing the adaptability to humans (42).
MPXV like other poxviruses is a large (~280 nm X ~220 nm) (13), brick- or oval-shaped enveloped virus. The viral core is dumbbell-shaped and contains the enzymes necessary for uncoating and replication as well as the large ~197 kb long viral genome that is a linear dsDNA comprising over 190 open reading frames (ORFs) (3, 18). The MPXV has a complex structure and its genome is not fully characterized. Although there are at least 90 essential ORFs, most of the ORFs still need to be identified and studied (3, 44) (Figure 2). Like other poxviruses, MPXV also has two forms–EEV and IMV. EEV has an additional outer membrane and is considered to play a major role in early dissemination while IMV is released during the cell lysis. Both forms induce the infection (45, 46).
Infection, pathogenesis, and clinical manifestation
After MPXV transmission through contact with an infected animal, human, or contaminated objects, the virus enters the body, disseminates systematically via monocytic cells, and can infect most mammalian cells (47). According to the clinical studies, lymphoid tissues in the neck and throat represent the primary replication areas for MPXV. After the primary lymphatic dissemination of the virus, liver and spleen are the major targets for the infection. The spread of the virus into small dermal blood vessels gives rise to the skin infection and lesions (48). The extracellular proteins of the poxviruses attach the glycans (laminin, heparin, and chondroitin sulfates) of host cells (49–51). H3L (heparan-binding surface IMV membrane protein), A29L (heparan-binding IMV surface membrane fusion protein), and E8L (chondroitin sulfate-binding IMV surface membrane adsorption protein) are among the proteins that are responsible for the attachment (49, 51). After the pH-dependent fusion and entry into the host cell, viral transcription takes place. Notably, transcription occurs via the viral DNA-dependent RNA polymerase. Hence, unlike other DNA viruses, MPXV does not need to be transported into the nucleus, instead, with its own machinery, the transcription takes place in the cytoplasm. Following transcription, translation occurs on the ribosomes of the host cell (52, 53). The majority of IMVs remain intracellularly and are released only upon the cell lysis while some of them become enveloped (intracellular enveloped virus (IEV)) by the additional outer membranes derived by the endoplasmic reticulum or Golgi apparatus. After the MPXV gains an additional membrane, it is either transported into the neighbor cell or outside the cell and becomes EEV (54). It is known that EEV infects the cells more efficiently compared with the IMV (55). Usually, the incubation period of MPXV lasts for approximately two weeks (56) and typically it is resolved within 2-4 weeks (22).
The MPX is characterized by similar symptoms as other poxviruses along with certain distinctive features. The common symptoms include fever, chills, body- and headaches, fatigue, sore throat, and rash that becomes papules and crust later while healing. Because of these similarities, MPX is often misdiagnosed with other poxviral diseases. The main difference between MPX and other poxvirus disease manifestations is that MPXV infection causes enlargement of lymph nodes before the development of rash (57–61). The rash is presented all over the body, usually concentrated on the face and extremities, however, the current multi-country outbreak of human MPX demonstrated the new tendency of atypical presentation. During the 2022 MPXV outbreak the lesions are usually localized in the genitals and/or anus of the infected patients (3, 22–25) and patients experience extreme rectal pain and penile oedema (62, 63). The complications of the MPX disease may be even life-threatening, e.g., encephalitis, sepsis, etc. (22). Besides, MPXV can be vertically transmitted making pregnant women and fetus vulnerable (48). Unfortunately, the lack of surveillance and health care in countries of Africa greatly contributes to the underdiagnoses of MPX and the spread of the virus, meaning that the numbers of daily cases have been more likely much higher than the officially recorded numbers (2). Notably, during the current outbreak, more MPX cases are detected in men who have sex with men (MSM) (62, 64–67). When the MPX cases were identified in Africa before spreading the virus outside the continent, it was notable that more confirmed cases were male. E.g., When 760 cases were detected in DRC during 2005-2007 through the surveillance program, the male patients (62.1%) predominated females (68). Martinez et al. have revealed that in Spain, a region of Madrid, by June 2022, 508 MPX cases were identified out of which 99% were men. 84.1% of the total number of cases had condomless sexual intercourse with multiple partners before the onset of the MPX symptoms. 93% of them were men who had sex with men. The distribution of the rash indicates that this type of close physical contact plays a major role in disease transmission (69). Remarkably, the tendency of smallpox vaccination among MSM is increasing (70). The illustration of MPX symptoms is given in Figure 3.
Reasonings of 2022 MPX outbreak
On the one hand, the global concern has increased as MPXV crossed the African borders and cases have been increased fast, especially, since May 2022. However, it should not be considered an unexpected and unpredicted outbreak as there were many warning signs before MPX spread worldwide. Before the current outbreak took place, there was some favorable basis for MPXV to be disseminated worldwide. Noteworthily, a few months earlier before MPXV turned out into the center of global attention, Bunge et al. systematically reviewed human MPX epidemiology changes. They summarized that MPX cases were escalating, especially in DRC but it was also spreading to other countries, and besides, the median age was growing from young children to young adults (71).
There are several possible reasons that laid the groundwork for spreading MPXV worldwide in an unusual manner since May 2022. Some of the reasonable assumptions are: increased animal trade and making a favorable environment for crossing species barriers (8); increased international travel (3); long-term cryptic dissemination of MPXV in humans and animals in non-indigenous countries (42) along with the lack of the surveillance programs and less funding for health care in endemic countries (8); introduction of the virus in non-endemic countries by the super-spreader events (42); vanishing the global immunity against smallpox due to the eradication of smallpox infection and vaccination cessation since 1980 (5, 8, 72, 73) and acquiring the clinical relevance of MPXV (74); affected immunity due to the COVID-19 pandemic along with the increased adaptability of MPXV via increased mutation rate. The possible reasons for 2022 MPXV multi-country outbreak are illustrated in Figure 4.
As the smallpox vaccine is also effective (up to 85%) (74) in preventing MPXV and today approximately 70% of the world population is unvaccinated to smallpox and lacks vaccine-derived immunity (3), this reason seems to be one of the most rational for the current MPXV outbreak. Moreover, most of the MPX patients are under 50 and have never been vaccinated against smallpox. Interestingly, Adler et al. performed a retrospective observational study and found that there were 7 MPX patients registered in the UK from 2018 to 2021. Four of them acquired the disease outside, while 3 of them were in the UK. Out of these 3 patients, 2 developed the symptoms after arriving from Nigeria while the third patient was a healthcare worker who got MPX symptoms 18 days after contact with one of the other patients while taking care. More importantly, the third patient was vaccinated against smallpox (56). This also underlines the fidelity of long-term cryptic dissemination of the MPXV before the 2022 outbreak. All the abovementioned reasons indicate that the 2022 MPX outbreak was expected to occur.
Prevention and treatment
The genome sequence of currently spread MPXV was reported on May 19, 2022, and was identified as a virus of the West African clade (67). Although this clade is characterized by less mortality and milder forms of the disease (75), it can still cause considerable morbidity and mortality. MPXV is still not a sufficiently studied virus and the treatment and prevention strategies are based on the methods used for the treatment of other Orthopoxviruses such as smallpox. E.g., Matias et al. have demonstrated that a pan-Orthopoxvirus inhibitor tecovirimat (TPOXX) which is approved by food and drug administration (FDA) for treatment of smallpox (76) could treat hospitalized MPX patients (77). Alternatively, there is another FDA-approved drug brincidofovir for the treatment of smallpox which is under investigation and has already shown efficacy against MPX in MPXV prairie dog models (78, 79). Additionally, cidofivir has demonstrated efficacy against Orthopoxviruses in vitro and in vivo studies (80–82). As the VAC immune globulin intravenous (VIGIV) is licensed by FDA for the treatment of complications caused by VAC vaccination, it is also considered one of the reasonable drug targets against MPXV for investigation (82). Currently available vaccines are JYNNEOS (VACV-based) and ACAM2000 (VACV-based) that are originally developed against the smallpox virus (83, 84).
From variolation to nucleic acid vaccines
In 1774, the time when smallpox was spread, in the UK, farmer Benjamin Jesty was the first to immunize his family with material obtained from the cattle infected with cowpox (animal virus) to protect from smallpox (deadly human virus). As a result, all of them stayed healthy despite the numerous exposure to the smallpox virus (85). In 1796, key advancement in vaccinology took place. In the UK, Edward Jenner used a substance taken from the cowpox lesions of young dairymaid to inoculate an 8-year-old boy named James Phipps. Later, Edward Jenner inoculated the boy with the substance obtained from a fresh smallpox lesion and no disease was developed (85). As the word for a cow in Latin is “Vacca” and cowpox was used for the immunization against smallpox, Edward Jenner called this immunization procedure vaccination (85). He was the first person to confer scientific status on this new procedure and pave the way for the following scientific studies. This was the historic origin of immunization even though viruses as the causative agents of diseases were not yet identified (86). Smallpox which killed over 300 million people in the 20th century was finally eradicated thanks to Edward Jenner’s enormous contribution (86). Interestingly, before this milestone, another turning point that had laid the groundwork for the development of immunization was variolation (inoculation)– an ancient Asian technique of infecting people with fluid from the smallpox pustules of a patient with a mild form of the disease. This strategy was also introduced in Europe, particularly, in the UK where Edward Jenner had experienced variolation himself when he was a little boy (87, 88). Several decades after the development of the first vaccine against smallpox (1798), in 1885, Louis Pasteur developed an inactivated virus vaccine against rabies (89). This is when the era of vaccinology and preventive medicine was launched. Anti-rabies vaccine continues saving millions of potential victims globally. Later in 1937, Max Theiler worked on the attenuation of the yellow fever virus and laid the groundwork for using live attenuated viruses for immunization. It was followed by the development of a series of live attenuated vaccines such as measles, rubella, varicella, etc. (86).
World scientists have kept advancing the prophylactic and therapeutic vaccinology all the time and the morbidity and mortality caused by various infectious diseases kept decreasing. Except for the physically or chemically inactivated and live attenuated vaccines, subunit vaccines comprising purified antigens, toxoid vaccines (inactivated bacterial toxins), as well as nucleic acid vaccines have been developed (90). Indeed, since the COVID-19 global pandemic has emerged, the new epoch of next-generation vaccines has begun. Evidently, the mRNA-based approach is advantageous owing to its high efficacy, safety, rapid development, low-cost, and cell-free manufacturing (26, 27, 91). In 1987 a key experiment was done by Robert Malone when he mixed mRNA with the synthetic cationic lipid incorporated into the liposome resulting in the successful transfection into the NIH 3T3 mouse cells and expression (92). Later in 1997 biochemist Katalin Kariko and immunologist Drew Weissman worked on the development of a human immunodeficiency virus (HIV) vaccine based on mRNA technology but as a result, a strong inflammatory response was observed in mice. Hence, they worked on the nucleoside modification and their approach was successful as mRNA was capable to escape innate immune response and increase the translation efficacy (93). Ultimately, the effective mRNA vaccines against COVID-19 that have emergency authorization– BNT162b2 (developed by BioNTech/Pfizer) and mRNA-1273 (developed by Moderna), contain the modified nucleobase N1-methylpseudouridines (m1Ψs) that modulates immune evasion, protein production, and effectiveness (94).
Along with the mRNA, DNA vaccines should not be forgotten as well. Both nucleic acid vaccines carry genetic information of the viral antigen into the host cells and allow them to produce the corresponding protein. This helps the body to develop immunological memory and to fight the real virus in a timely and effective manner in case of exposure (90, 95). mRNA needs to be delivered in the cytoplasm of the host cell to be translated into the target protein while DNA vaccine needs to be transported into the nucleus where it will be transcribed and after translocation of mRNA into the cytoplasm translation into the protein will take place (96). On the other hand, the time of DNA vaccine development from the design to commercialization is shorter (33). DNA vaccines, are considered to be safe (97), and compared to mRNA vaccines they elicit more stability at ambient temperatures (98). There are already approved DNA vaccines for veterinary use against highly pathogenic H5N1 influenza A virus in poultry (98), West Nile virus in horses, etc. (99). Same as in mRNA vaccines, the first human DNA vaccine was also approved for SARS-CoV-2 for emergency use in India (37). Additionally, there are a number of DNA (37, 100) and mRNA (101, 102) vaccines in clinical development.
Need for vaccine development
For many years MPXV was considered to be geographically limited and it was not the center of foci for scientists. It recently re-emerged as the cases increased rapidly outside of Africa. This should be a wake-up call for other viruses as well, such as Crimean-Congo hemorrhagic fever (CCHF) virus, Zika, Ebola, etc. Fortunately, out of two major clades of MPXV, the West African clade is spread which is less severe compared with the Central African clade. Interestingly, the fatality rates for these two clades have a big difference as it is mentioned above (71). Nevertheless, we are not secure that the Central African clade of MPXV will never become an unpredictable deadly pandemic. It is also noteworthy that the MPX outbreak in Nigeria (West Africa) during 2017-2018 reported 122 confirmed cases with 7 deaths out of whom 4 were HIV-positive with poor disease control (103). Gay or bisexual men should be more careful and it is recommended for them to take active preventive measures such as vaccination. Even though it is reported that the smallpox vaccine provides protection against MPXV (104, 105), cases of MPX disease manifestation in smallpox-vaccinated patients are still observed (106). Moreover, rare but serious side effects such as myocarditis and pericarditis are still reported and certain groups of population are vulnerable to vaccination. Besides, the first and the second doses of JYNNEOS are administered 28 days apart. As it is unclear whether the first dose is enough for the sufficient efficacy, in case of post-exposure vaccination, 28 days is too late to protect from the MPX disease. Importantly, the risks of creating new virus strains via exchanging genetic information when infected with MPXV in vaccinated individuals is not understood. Moreover, the rate of recombination of the genes from live or attenuated poxvirus-based vaccines with MPXV is unknown (48). Hence, the specific, truly universal vaccine development for MPXV is essential. Additionally, the case of human-to-dog transmission of MPXV has already been detected which makes the eradication of the outbreak even more difficult (107). Moreover, if we observe the rationale of the tendency of infection outbreaks, we should appreciate the danger of other relatively forgotten viruses such as smallpox that has been already eradicated. If the MPXV emerged because of the wanned immunity against smallpox, smallpox itself with a mortality rate of 10-75% (108) might also re-emerge with a much deadlier outcome. Furthermore, although, MPXV is usually resolved by itself, the current international outbreak showed that it might be life-threatening as well (106). Indeed, MPXV belongs to biosafety level 3 pathogens according to the EU regulations and is categorized as a high-threat virus (109). Alarmingly, the COVID-19 pandemic still exists and infects hundreds of thousands of people daily which makes the world population more vulnerable to MPXV. The high mutation rate of MPXVs isolated during the recent outbreak (42) along with all the abovementioned indicates the urgent need for developing specific, universal MPXV vaccine candidates to ultimately control this emerging virus and be prepared for any sudden outbreak. Considering all the above-stated information along with the extremely advantageous characteristics of nucleic acid-based vaccines, developing a new, next-generation MPXV vaccine certainly makes sense. The efficacy, safety, and simple and rapid production will make the nucleic acid-based MPXV vaccine clinically and socio-economically valuable.
Design of potentially universal MPXV vaccine based on conserved elements
Nucleic acid vaccines do not require a complicated manufacturing process as after the immunization, the body becomes a bioreactor of the viral antigen. Thus, the process of vaccine development is cell-free, simpler, cost and time-effective. Above all, these types of vaccines are favorably safe. In order to design a potentially universal vaccine that will be effective against MPXV, VARV, and VACV, making the multi-epitope vaccine based on the conserved elements of the reasonably selected antigens seems to be the excellent way. First, the antigens should be selected according to their function in viral infection. In case of MPXV, the antigens listed in Table 1 seem to be reasonable targets.
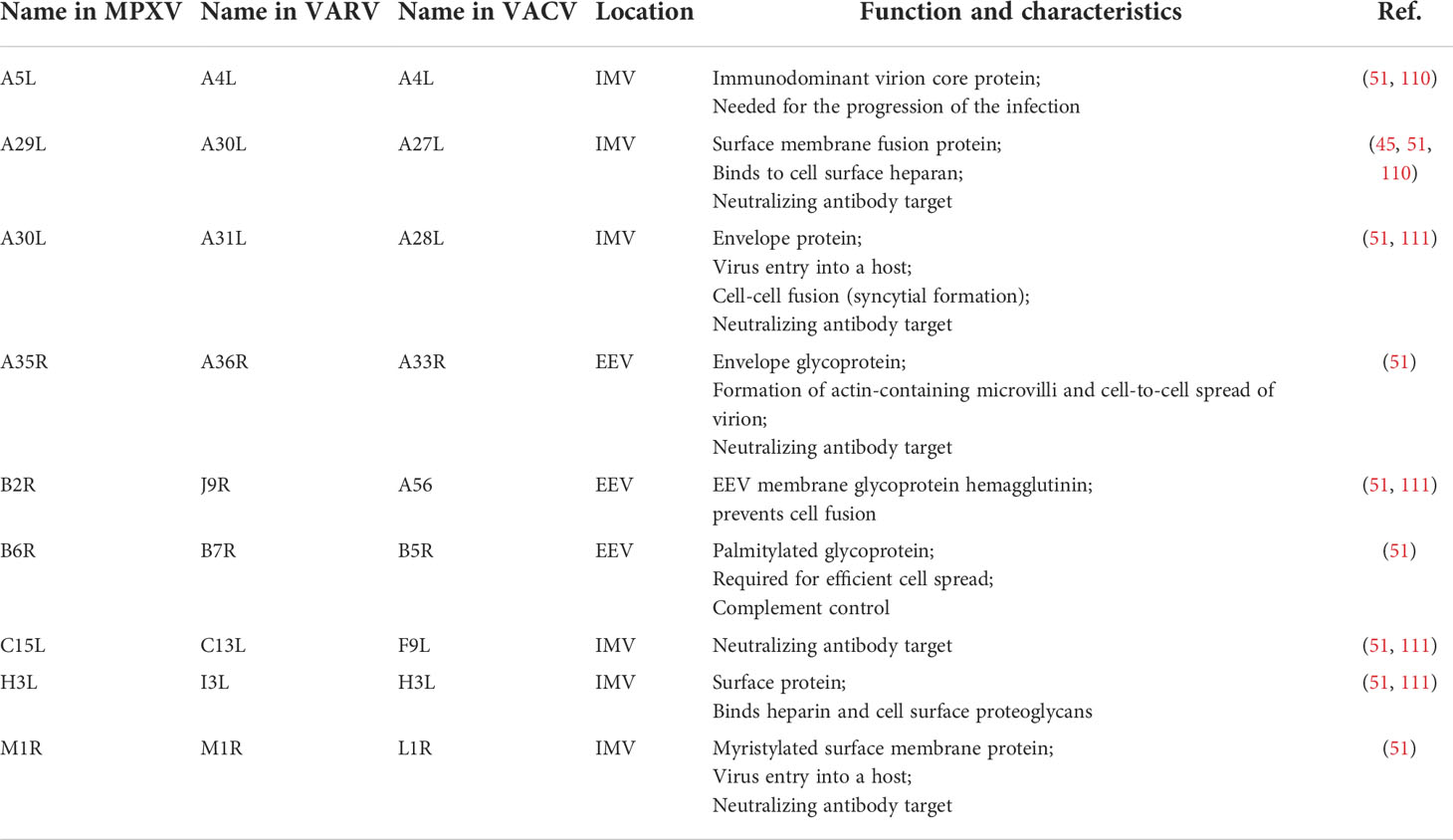
Table 1 List of the potential target antigens of MPXV and their corresponding proteins in VARV and VACV for the nucleic acid vaccine design, their location, function, and characteristics.
After selecting the antigens, the conserved sequences of the selected viral proteins should be determined via immunoinformatics tools (112–114). Luckily, there is a number of immunoinformatics approaches that can be used. The experimentally tested epitopes containing these conserved sequences can be found or predicted on the immune epitope database (IEDB) (115). After the final mRNA or DNA construct is designed using the preferable epitopes and optimized via selecting certain 5’ and 3’ untranslated regions (UTRs), proper linkers, and immune-modulator adjuvants (116), again immunoinformatics analyses should be conducted such as prediction of vaccine structure, immunogenicity, protectiveness, allergenicity, physicochemical properties, receptor-binding capacity, immune response caused via immune simulation, etc. (117–130). This will save time to anticipate the potential outcome of the designed vaccine. Once the results are favorable, the in vitro and in vivo studies will eventually validate the protectiveness of a potentially universal vaccine against MPXV. For the experimental validation, the following steps are proposed to be done: plasmid DNA expressing the gene of interest is synthesized and transformed into DH5α competent E. coli strain for amplification. It is followed by the extraction of plasmids from bacterial cells and purification. When the successful protein expression is confirmed via mammalian cell transfection, the plasmid can be used as a DNA vaccine for further in vivo studies or it should be linearized and in vitro transcribed into mRNA. mRNA is capped (5’-cap) for stability, protection from degradation, and facilitation of ribosomal recruitment (94). After mRNA is purified, its expression levels should be tested via cell transfection that can be followed by encapsulation with the proper delivery system such as lipid nanoparticles (LNPs) (28) and animal immunization experiments can be proceeded. Cellular and humoral immune responses elicited by the mRNA vaccine and the protection after viral challenges should be evaluated with the proper analyses. The outline of the development of a universal nucleic acid vaccine against MPXV is illustrated in Figure 5. In terms of mRNA vaccine side effects, it mainly depends on the dosage. Remarkably, this problem might be solved with self-amplifying (saRNA) or trans-amplifying RNA (taRNA) vaccine development that represents the next-generation nucleic acid vaccines owing to their lower dosage requirements. E.g., Vogel et al. demonstrated that 64-fold less material is needed for inducing the same immunity with saRNA compared to conventional mRNA vaccine against influenza virus (131). Compared with the mRNA molecule (~2000 nt), saRNA is longer (~10,000 nt) as besides the gene of interest, it contains the viral replicase genes which is based on the four non-structural proteins (nsPs) of alphaviruses. The presence of nsPs replicon, allows the molecule to be self-amplified in the host cell, producing the great number of desired antigens (132, 133). The viral replicase replicates the entire RNA as well as the sub-genomic RNA (133). As a result, higher and long-lasting antigen expression takes place. Remarkably, the challenge of saRNA large size can be dealt with using taRNA. The taRNA technology denotes splitting the saRNA molecule into two shorter molecules– encoding replicase and gene of interest separately (133, 134).
Summary
Even though MPXV is not characterized as highly contagious virus as SARS-CoV-2, and smallpox vaccine that is effective against MPXV is available, attention should not be relaxed on developing specific vaccine candidates and seeking treatment ways for this virus. Unequivocally, it is much better to prevent disease in healthy populations than to make an effort to treat disease in already sick patients. The realignment of vaccination strategies as proposed here will work for the common well-being of the human population, particularly for the vulnerable population or those who have close contact with animals such as monkeys or rodents. It is also important that lately MPX was identified in a pet dog which worsens the situation meaning that the virus might circulate in animals and impede its eradication. Here, we provide the rationale for a potentially universal immunization strategy for multi-epitope nucleic acid-based vaccine design. The proposed vaccine construction is based on the conserved epitopes that gives the basis of its potential universality among newly formed mutated strains of MPXVs as well as strengthening the immunity against VARV, and VACV. Thus, the proposed strategy may be one step forward to speeding up overcoming the current outbreak as well as preventing other potential outbreaks.
Author contributions
DP, NR, and YH contributed to the study conceptualization, DP and NR contributed equally to the drafting and editing of the work, CL revised the manuscript, XW contributed to preparing a figure, YH and PGW supervised. All authors contributed to the article and approved the submitted version.
Funding
This work was supported by the Shenzhen Science and Technology Innovation Program (KQTD20200909113758004).
Acknowledgments
Some parts of the figures were created with BioRender.com.
Conflict of interest
The authors declare that the research was conducted in the absence of any commercial or financial relationships that could be construed as a potential conflict of interest.
Publisher’s note
All claims expressed in this article are solely those of the authors and do not necessarily represent those of their affiliated organizations, or those of the publisher, the editors and the reviewers. Any product that may be evaluated in this article, or claim that may be made by its manufacturer, is not guaranteed or endorsed by the publisher.
References
1. Jezek Z, Szczeniowski M, Paluku KM. Human monkeypox: Clinical features of 282 patients. J Infect Dis (1987) 156:293–8. doi: 10.1093/infdis/156.2.293
2. Yang Z. Monkeypox: A potential global threat? J Med Virol (2022) 94:4034–6. doi: 10.1002/jmv.27884
3. Kmiec D, Kirchhoff F. Monkeypox: A new threat? Int J Mol Sci (2022) 23:7866. doi: 10.3390/ijms23147866
4. Kozlov M. Monkeypox outbreaks: 4 key questions researchers have. Nature (2022) 606:238–9. doi: 10.1038/d41586-022-01493-6
5. Xiang Y, White A. Monkeypox virus emerges from the shadow of its more infamous cousin: Family biology matters. Emerg Microbes Infect (2022) 11:1–14. doi: 10.1080/22221751.2022.2095309
6. Gomez-lucia E. Monkeypox: Some keys to understand this emerging disease. Animals (2022) 12:2190. doi: 10.3390/ani12172190
7. Ladnyj D, Ziegler P, Kima E. A human infection caused by monkeypox virus in basankusu territory, democratic republic of the Congo. Bull World Health Organ (1972) 46:593–7.
8. Sklenovská N, Van Ranst M. Emergence of monkeypox as the most important orthopoxvirus infection in humans. Front Public Health (2018) 6:241. doi: 10.3389/fpubh.2018.00241
9. Centers for Disease Control and Prevention (CDC). Past U.S. cases and outbreaks (2022). Available at: https://www.cdc.gov/poxvirus/monkeypox/outbreak/us-outbreaks.html (Accessed September 16, 2022).
10. Gong Q, Wang C, Chuai X, Chiu S. Monkeypox virus: a re-emergent threat to humans. Virol Sin (2022) 37:477–82. doi: 10.1016/j.virs.2022.07.006
11. Rao AK, Schulte J, Chen T-H, Hughes CM, Davidson W, Neff JM, et al. Monkeypox in a traveler returning from Nigeria — Dallas, Texas, July 2021. MMWR Morbid Mortal Weekly Rep (2022) 71:509–16. doi: 10.15585/mmwr.mm7114a1
12. Costello V, Sowash M, Gaur A, Cardis M, Pasieka H, Wortmann G, et al. Imported monkeypox from international traveler, Maryland, USA, 2021. Emerg Infect Dis (2022) 28:1002–5. doi: 10.3201/eid2805.220292
13. Erez N, Achdout H, Milrot E, Schwartz Y, Wiener-Well Y, Paran N, et al. Diagnosis of imported monkeypox, Israel, 2018. Emerg Infect Dis (2019) 25:980–3. doi: 10.3201/eid2505.190076
14. Hobson G, Adamson J, Adler H, Firth R, Gould S, Houlihan C, et al. Family cluster of three cases of monkeypox imported from Nigeria to the united kingdom, may 2021. Eurosurveillance (2021) 26:2100745. doi: 10.2807/1560-7917.ES.2021.26.32.2100745
15. Yong SEF, Ng OT, Ho ZJM, Mak TM, Marimuthu K, Vasoo S, et al. Imported monkeypox, Singapore. Emerg Infect Dis (2020) 26:1826–30. doi: 10.3201/eid2608.191387
16. Vaughan A, Aarons E, Astbury J, Balasegaram S, Beadsworth M, Beck CR, et al. Two cases of monkeypox imported to the united kingdom, september 2018. Eurosurveillance (2018) 23:1800509. doi: 10.2807/1560-7917.ES.2018.23.38.1800509
17. World Health Organization (WHO). WHO director-general declares the ongoing monkeypox outbreak a public health emergency of international concern. (2022). Available at: https://www.who.int/director-general/speeches/detail/who-director-general-s-statement-on-the-press-conference-following-IHR-emergency-committee-regarding-the-multi--country-outbreak-of-monkeypox--23-july-2022
18. Kugelman JR, Johnston SC, Mulembakani PM, Kisalu N, Lee MS, Koroleva G, et al. Genomic variability of monkeypox virus among humans, democratic republic of the Congo. Emerg Infect Dis (2014) 20:232–9. doi: 10.3201/eid2002.130118
19. Moss B. Poxvirus DNA replication. Cold Spring Harbor Perspect Biol (2013) 5:a010199. doi: 10.1101/cshperspect.a010199
20. Thornhill JP, Barkati S, Walmsley S, Rockstroh J, Antinori A, Harrison LB, et al. Monkeypox virus infection in humans across 16 countries — April–June 2022. New Engl J Med (2022) 387:679–91. doi: 10.1056/nejmoa2207323
21. Hutson CL, Olson VA, Carroll DD, Abel JA, Hughes CM, Braden ZH, et al. A prairie dog animal model of systemic orthopoxvirus disease using west African and Congo basin strains of monkeypox virus. J Gen Virol (2009) 90:323–33. doi: 10.1099/vir.0.005108-0
22. World Health Organization (WHO). Monkeypox (2022). Available at: https://www.who.int/news-room/fact-sheets/detail/monkeypox (Accessed September 16, 2022).
23. Moschese D, Giacomelli A, Beltrami M, Pozza G, Mileto D, Reato S, et al. Hospitalisation for monkeypox in Milan, Italy. Travel Med Infect Dis (2022) 49:102417. doi: 10.1016/j.tmaid.2022.102417
24. Mileto D, Riva A, Cutrera M, Moschese D, Mancon A, Meroni L, et al. New challenges in human monkeypox outside Africa: A review and case report from Italy. Travel Med Infect Dis (2022) 49:102386. doi: 10.1016/j.tmaid.2022.102386
25. Sah R, Abdelaal A, Reda A, Katamesh BE, Manirambona E. Monkeypox and its possible sexual transmission: Where are we now with its evidence? Pathogens (2022) 11:924. doi: 10.3390/pathogens11080924
26. Pardi N, Hogan MJ, Porter FW, Weissman D. mRNA vaccines-a new era in vaccinology. Nat Rev Drug Discovery (2018) 17:261–79. doi: 10.1038/nrd.2017.243
27. Kowalzik F, Schreiner D, Jensen C, Teschner D, Gehring S, Zepp F. mRNA-based vaccines. Vaccines (Basel) (2021) 9:390. doi: 10.3390/vaccines9040390
28. Liu C, Rcheulishvili N, Shen Z, Papukashvili D, Xie F, Wang Z, et al. Development of an LNP-encapsulated mRNA-RBD vaccine against SARS-CoV-2 and its variants. Pharmaceutics (2022) 14:1101. doi: 10.3390/pharmaceutics14051101
29. Walsh EE, Frenck RW, Falsey AR, Kitchin N, Absalon J, Gurtman A, et al. Safety and immunogenicity of two RNA-based covid-19 vaccine candidates. New Engl J Med (2020) 383:2439–50. doi: 10.1056/nejmoa2027906
30. Haas EJ, Angulo FJ, McLaughlin JM, Anis E, Singer SR, Khan F, et al. Impact and effectiveness of mRNA BNT162b2 vaccine against SARS-CoV-2 infections and COVID-19 cases, hospitalisations, and deaths following a nationwide vaccination campaign in Israel: an observational study using national surveillance data. Lancet (2021) 397:1819–29. doi: 10.1016/S0140-6736(21)00947-8
31. Corbett KS, Flynn B, Foulds KE, Francica JR, Boyoglu-Barnum S, Werner AP, et al. Evaluation of the mRNA-1273 vaccine against SARS-CoV-2 in nonhuman primates. New Engl J Med (2020) 383:1544–55. doi: 10.1056/nejmoa2024671
32. Flingai S, Czerwonko M, Goodman J, Kudchodkar SB, Muthumani K, Weiner DB. Synthetic DNA vaccines: improved vaccine potency by electroporation and co-delivered genetic adjuvants. Front Immunol (2013) 4:354. doi: 10.3389/fimmu.2013.00354
33. Williams J. Vector design for improved DNA vaccine efficacy, safety and production. Vaccines (2013) 1:225–49. doi: 10.3390/vaccines1030225
34. Xia D, Jin R, Byagathvalli G, Yu H, Ye L, Lu CY, et al. An ultra-low-cost electroporator with microneedle electrodes (ePatch) for SARS-CoV-2 vaccination. Proc Natl Acad Sci United States America (2021) 118 e2110817118. doi: 10.1073/pnas.2110817118
35. Chavda VP, Pandya R, Apostolopoulos V. DNA Vaccines for SARS-CoV-2: toward third-generation vaccination era. Expert Rev Vaccines (2021) 20:1549–60. doi: 10.1080/14760584.2021.1987223
36. Wang X, Rcheulishvili N, Cai J, Liu C, Xie F, Hu X, et al. Development of DNA vaccine candidate against SARS-CoV-2. Viruses (2022) 14:1049. doi: 10.3390/v14051049
37. Sheridan C. First COVID-19 DNA vaccine approved, others in hot pursuit. Nat Biotechnol (2021) 39:1479–82. doi: 10.1038/d41587-021-00023-5
38. Forni D, Molteni C, Cagliani R, Sironi M. Geographic structuring and divergence time frame of monkeypox virus in the endemic region. J Infect Dis (2022), jiac298. doi: 10.1093/infdis/jiac298
39. Kaler J, Hussain A, Flores G, Kheiri S, Desrosiers D. Monkeypox: A comprehensive review of transmission, pathogenesis, and manifestation. Cureus (2022) 14:e26531. doi: 10.7759/cureus.26531
40. Guarner J, Del RC, Malani PN. Monkeypox in 2022 — what clinicians need to know. JAMA - J Am Med Assoc (2022) 328:139–40. doi: 10.1001/jama.2022.10802
41. Tiecco G, Antoni MD, Storti S, Tomasoni LR, Castelli F, Quiros-roldan E. Monkeypox, a literature review: What is new and where does this concerning virus come from? Viruses (2022) 14:1894. doi: 10.3390/v14091894
42. Isidro J, Borges V, Pinto M, Sobral D, Santos JD, Nunes A, et al. Phylogenomic characterization and signs of microevolution in the 2022 multi-country outbreak of monkeypox virus. Nat Med (2022) 28:1569–72. doi: 10.1038/s41591-022-01907-y
43. Luna N, Ramírez AL, Munoz M, Ballesteros N, Patino LH, Castaneda SA, et al. Phylogenomic analysis of the monkeypox virus (MPXV) 2022 outbreak: Emergence of a novel viral lineage? Travel Med Infect Dis (2022) 49:102402. doi: 10.1016/j.tmaid.2022.102402
44. Seet BT, Johnston JB, Brunetti CR, Barrett JW, Everett H, Cameron C, et al. Poxviruses and immune evasion. Annu Rev Immunol (2003) 21:377–423. doi: 10.1146/annurev.immunol.21.120601.141049
45. Pütz MM, Midgley CM, Law M, Smith GL. Quantification of antibody responses against multiple antigens of the two infectious forms of vaccinia virus provides a benchmark for smallpox vaccination. Nat Med (2006) 12:1310–5. doi: 10.1038/nm1457
46. Smith GL, Law M. The exit of vaccinia virus from infected cells. Virus Res (2004) 106:189–97. doi: 10.1016/j.virusres.2004.08.015
48. Lum F-M, Torres-Ruesta A, Tay MZ, Lin RTP, Lye DC, Rénia L, Ng LFP, et al. Monkeypox: disease epidemiology, host immunity and clinical interventions. Nat Rev Immunol (2022), 22:597–613. doi: 10.1038/s41577-022-00775-4
49. Chiu W-L, Lin C-L, Yang M-H, Tzou D-LM, Chang W. Vaccinia virus 4c (A26L) protein on intracellular mature virus binds to the extracellular cellular matrix laminin. J Virol (2007) 81:2149–57. doi: 10.1128/jvi.02302-06
50. Moss B. Poxvirus cell entry: How many proteins does it take? Viruses (2012) 4:688–707. doi: 10.3390/v4050688
51. Shchelkunov SN, Totmenin AV, Safronov PF, Mikheev MV, Gutorov VV, Ryazankina OI, et al. Analysis of the monkeypox virus genome. Virology (2002) 197:172–94. doi: 10.1006/viro.2002.1446
52. Pechenick T, Featherstone RJ, Reynolds DK, Brown HK, James J, Prescott A, et al. RAB1A promotes vaccinia virus replication by facilitating the production of intracellular enveloped virions. Virology (2015) 475:66–73. doi: 10.1016/j.virol.2014.11.007
53. Challberg D, Englund T. Purification and properties of the deoxyribonucleic acid polymerase induced by vaccinia virus. J Biol Chem (1979) 254:7812–9. doi: 10.1016/S0021-9258(18)36019-8
54. Smith GL, Vanderplasschen A, Law M. The formation and function of extracellular enveloped vaccinia virus. J of General Virol (2002) 83:2915–31. doi: 10.1099/0022-1317-83-12-2915
55. Locker JK, Kuehn A, Schleich S, Rutter G, Hohenberg H, Wepf R, et al. Entry of the two infectious forms of vaccinia virus at the plasma membane is signaling-dependent for the IMV but not the EEV. Mol Biol Cell (2000) 11:2497–511. doi: 10.1091/mbc.11.7.2497
56. Adler H, Gould S, Hine P, Snell LB, Wong W, Houlihan CF, et al. Clinical features and management of human monkeypox: a retrospective observational study in the UK. Lancet Infect Dis (2022) 22:1153–62. doi: 10.1016/S1473-3099(22)00228-6
57. Nalca A, Rimoin AW, Bavari S, Whitehouse CA. Reemergence of monkeypox: Prevalence, diagnostics, and countermeasures. Clin Infect Dis (2005) 41:1765–71. doi: 10.1086/498155
58. Sukhdeo SS, Aldhaheri K, Lam PW, Walmsley S. A case of human monkeypox in Canada. CMAJ: Can Med Assoc J = J l’Association Medicale Can (2022) 194:1031–5. doi: 10.1503/cmaj.220886
59. Al-tammemi AB, Albakri R, Alabsi S. The outbreak of human monkeypox in 2022: A changing epidemiology or an impending aftereffect of smallpox eradication? Front Trop Dis (2022) 3:951380. doi: 10.3389/fitd.2022.951380
60. Nicolas-Ruanes B, Vivancos MJ, Azcarraga-Llobet C, Moreno AM, Rodriguez-Dominguez M, Berna-Rico ED, et al. Monkeypox virus case with maculopapular exanthem and proctitis during the Spanish outbreak in 2022. Eur Acad Dermatol Venereol (2022) 36:e658–60. doi: 10.1111/jdv.18300
61. Osadebe L, Hughes CM, Lushima RS, Kabamba J, Nguete B, Malekani J, et al. Enhancing case definitions for surveillance of human monkeypox in the democratic republic of Congo. PloS Negl Trop Dis (2017) 11:e0005857. doi: 10.1371/journal.pntd.0005857
62. Patel A, Bilinska J, Tam JCH, Da D, Fontoura S, Mason CY, et al. Clinical features and novel presentations of human monkeypox in a central London centre during the 2022 outbreak: descriptive case series. Br Med J (2022) 378:e072410. doi: 10.1136/bmj-2022-072410
63. Pfäfflin F, Wendisch D, Scherer R, Jürgens L, Godzick G, Eva N, et al. Monkeypox in-patients with severe anal pain. Infection (2022). doi: 10.1007/s15010-022-01896-7
64. Liu X, Zhu Z, He Y, Lim JW, Lane B, Wang H, et al. Monkeypox claims new victims: the outbreak in men who have sex with men. Infect Dis Poverty (2022) 11:84. doi: 10.1186/s40249-022-01007-6
65. Kupferschmidt K. Why monkeypox is mostly hitting men who have sex with men. Science (2022) 376:1364–5. doi: 10.1126/science.add5966
66. Vallee A, Farfour E, Zucman D. Monkeypox virus: A novel sexually transmitted disease? a case report from France. Travel Med Infect Dis (2022) 49:102394. doi: 10.1016/j.tmaid.2022.102394
67. Otu A, Ebenso B, Walley J, Barceló JM, Ochu CL. Comment global human monkeypox outbreak: atypical presentation demanding urgent public health action. Lancet Microbe (2022) 3:554–5. doi: 10.1016/S2666-5247(22)00153-7
68. Rimoin AW, Mulembakani PM, Johnston SC, Lloyd JO, Kisalu NK, Shongo RL, et al. Major increase in human monkeypox incidence 30 years after smallpox vaccination campaigns cease in the democratic republic of Congo. Proc Natl Acad Sci (PNAS) (2010) 107:16262–7. doi: 10.1073/pnas.1005769107
69. Martínez JI, Montalbán EG, Bueno SJ, Martínez FM, Juliá AN, Díaz S, et al. Monkeypox outbreak predominantly affecting men who have sex with men, Madrid, Spain, 26 April to 16 June 2022. Eurosurveillance (2022) 27:2200471. doi: 10.2807/1560-7917.ES.2022.27.27.2200471
70. World Health Organization (WHO). Perceptions of monkeypox from those most at risk: men who have sex with men having multiple sexual partners (2022). Available at: https://www.who.int/europe/news/item/26-08-2022-perceptions-of-monkeypox-from-those-most-at-risk–men-who-have-sex-with-men-having-multiple-sexual-partners (Accessed September 16, 2022).
71. Bunge EM, Hoet B, Chen L, Lienert F, Weidenthaler H, Baer LR, et al. The changing epidemiology of human monkeypox–a potential threat? a systematic review. PloS Negl Trop Dis (2022) 16:e0010141. doi: 10.1371/journal.pntd.0010141
72. Durski KN, Mccollum AM, Nakazawa Y, Petersen BW, Reynolds MG, Briand S, et al. Emergence of monkeypox — West and central Africa, 1970–2017. MMWR Morbid Mortal Weekly Rep (2018) 67:306–10. doi: 10.15585/mmwr.mm6710a5
73. Weaver JR, Isaacs SN. Monkeypox virus and insights into its immunomodulatory proteins. Immunol Rev (2009) 225:96–113. doi: 10.1111/j.1600-065X.2008.00691.x
74. Nguyen P, Ajisegiri WS, Costantino V, Chughtai AA, Macintyre CR. Reemergence of human monkeypox and declining population immunity in the context of urbanization, Nigeria, 2017–2020. Emerg Infect Dis (2021) 27:1007–14. doi: 10.3201/eid2704.203569
75. Likos AM, Sammons SA, Olson VA, Frace AM, Li Y, Olsen-rasmussen M, et al. A tale of two clades: monkeypox viruses. J Gen Virol (2005) 86:2661–72. doi: 10.1099/vir.0.81215-0
76. Sherwat A, Brooks JT, Birnkrant D, Kim P. Tecovirimat and the treatment of monkeypox — past, present, and future considerations. New Engl J Med (2022) 387:579–81. doi: 10.1056/NEJMp2210125
77. Matias WR, Koshy JM, Nagami EH, Kovac V, Moeng LR, Shenoy ES, et al. Tecovirimat for the treatment of human monkeypox: An initial series from Massachusetts, united states. Open Forum Infect Dis (2022) 9:ofac377. doi: 10.1093/ofid/ofac377
78. Hutson CL, Kondas AV, Mauldin MR, Doty JB, Grossi IM, Morgan CN, et al. Pharmacokinetics and efficacy of a potential smallpox therapeutic, brincidofovir, in a lethal monkeypox virus animal model. mSphere (2021) 6:e00927–20. doi: 10.1128/msphere.00927-20
79. Smith SK, Self J, Weiss S, Carroll D, Braden Z, Regnery RL, et al. Effective antiviral treatment of systemic orthopoxvirus disease: ST-246 treatment of prairie dogs infected with monkeypox virus. J Virol (2011) 85:9176–87. doi: 10.1128/jvi.02173-10
80. De Clercq E. Cidofovir in the therapy and short-term prophylaxis of poxvirus infections. Trends Pharmacol Sci (2002) 23:456–8. doi: 10.1016/S0165-6147(02)02091-6
81. Andrei G, Snoeck R. Cidofovir activity against poxvirus infections. Viruses (2010) 2:2803–30. doi: 10.3390/v2122803
82. Centers for Disease Control and Prevention (CDC). Treatment information for healthcare professionals (2022). Available at: https://www.cdc.gov/poxvirus/monkeypox/clinicians/treatment.html (Accessed September 16, 2022). (also known as Vistide)&text=However%2C it has shown to,including monkeypox) in an outbreak.
83. Rizk JG, Lippi G, Henry BM, Forthal DN, Rizk Y. Prevention and treatment of monkeypox. Drugs (2022) 82:957–63. doi: 10.1007/s40265-022-01742-y
84. Ali A, Khan A, Kaushik AC, Wang Y, Ali SS, Junaid M, et al. Immunoinformatic and systems biology approaches to predict and validate peptide vaccines against Epstein–Barr virus (EBV). Sci Rep (2019) 9:720. doi: 10.1038/s41598-018-37070-z
85. Riedel S. Edward Jenner And the history of smallpox and vaccination. Bristol Medico Chirurgical J (1967) 82:95. doi: 10.1177/000992287201100115
86. Desmond A, Offit PA. On the shoulders of giants — from jenner’s cowpox to mRNA covid vaccines. New Engl J Med (2021) 384:1081–3. doi: 10.1056/NEJMp2034334
87. Stewart AJ, Devlin PM. The history of the smallpox vaccine. J Infect (2006) 52:329–34. doi: 10.1016/j.jinf.2005.07.021
88. Maurer DM, Harrington B, Army D, Hospital C, Hood F, Lane JM. Smallpox vaccine: Contraindications, administration, and adverse reactions. Am Family Physician (2003) 68:889–96.
89. Da M, Gomes M. Louis Pasteur and Dom Pedro II engaged in rabies vaccine development. J Prev Med Hyg (2021) 62:231–6. doi: 10.15167/2421-4248/jpmh2021.62.1.1631
90. Qin F, Xia F, Chen H, Cui B, Feng Y, Zhang P, et al. A guide to nucleic acid vaccines in the prevention and treatment of infectious diseases and cancers: From basic principles to current applications. Front Cell Dev Biol (2021) 9:633776. doi: 10.3389/fcell.2021.633776
91. Damase TR, Sukhovershin R, Boada C, Taraballi F, Pettigrew RI, Cooke JP. The limitless future of RNA therapeutics. Front Bioeng Biotechnol (2021) 9:628137. doi: 10.3389/fbioe.2021.628137
92. Malone RW, Felgner PL, Verma IM. Cationic liposome-mediated RNA transfection. Proc Natl Acad Sci United States America (1989) 86:6077–81. doi: 10.1073/pnas.86.16.6077
93. Karikó K, Muramatsu H, Welsh FA, Ludwig J, Kato H, Akira S, et al. Incorporation of pseudouridine into mRNA yields superior nonimmunogenic vector with increased translational capacity and biological stability. Am Soc Gene Ther (2008) 16:1833–40. doi: 10.1038/mt.2008.200
94. Nance KD, Meier JL. Modifications in an emergency: The role of N1- methylpseudouridine in COVID-19 vaccines. ACS Cent Sci (2021) 7:748–56. doi: 10.1021/acscentsci.1c00197
95. Chavda VP, Hossain K, Beladiya J, Apostolopoulos V. Nucleic acid vaccines for COVID-19: A paradigm shift in the vaccine development arena. Biologics (2021) 1:337–56. doi: 10.3390/biologics1030020
96. Liu MA. A comparison of plasmid DNA and mRNA as vaccine technologies. Vaccines (2019) 7:37. doi: 10.3390/vaccines7020037
97. Hobernik D, Bros M. DNA Vaccines–how far from clinical use? Int J Mol Sci (2018) 19:3605. doi: 10.3390/ijms19113605
98. Lee J, Kumar SA, Jhan YY, Bishop CJ. Engineering DNA vaccines against infectious diseases. Acta Biomaterialia (2018) 15:31–47. doi: 10.1016/j.actbio.2018.08.033
99. Liu MA. DNA Vaccines: an historical perspective and view to the future. Immunol Rev (2011) 239:62–84. doi: 10.1111/j.1600-065X.2010.00980.x
100. Silveira MM, Moreira GMSG, Mendonça M. DNA Vaccines against COVID-19: Perspectives and challenges. Life Sci (2021) 267:118919. doi: 10.1016/j.lfs.2020.118919
101. Bahl K, Senn JJ, Yuzhakov O, Bulychev A, Brito LA, Hassett KJ, et al. Preclinical and clinical demonstration of immunogenicity by mRNA vaccines against H10N8 and H7N9 influenza viruses. Mol Ther (2017) 25:1316–27. doi: 10.1016/j.ymthe.2017.03.035
102. Feldman RA, Fuhr R, Smolenov I, Mick A, Panther L, Watson M, et al. mRNA vaccines against H10N8 and H7N9 influenza viruses of pandemic potential are immunogenic and well tolerated in healthy adults in phase 1 randomized clinical trials. Vaccine (2019) 37:3326–34. doi: 10.1016/j.vaccine.2019.04.074
103. Yinka-ogunleye A, Aruna O, Dalhat M, Ogoina D, Mccollum A, Disu Y, et al. Outbreak of human monkeypox in Nigeria in 2017–18: a clinical and epidemiological report. Lancet Infect Dis (2019) 19:872–9. doi: 10.1016/S1473-3099(19)30294-4
104. Fine PE, Jezek Z, Grab B, Dixon H. The transmission potential of monkeypox virus in human populations. Int J Epidemiol (1988) 17:643–50. doi: 10.1093/ije/17.3.643
105. Burki T. Investigating monkeypox. Lancet (London England) (2022) 399:2254–5. doi: 10.1016/S0140-6736(22)01096-0
106. Meyer H, Perrichot M, Stemmler M, Emmerich P, Schmitz H, Varaine F, et al. Outbreaks of disease suspected of being due to human monkeypox virus infection in the democratic republic of Congo in 2001. J Clin Microbiol (2002) 40:2919–21. doi: 10.1128/JCM.40.8.2919-2921.2002
107. Seang S, Burrel S, Todesco E, Leducq V, Monsel G, Pluart DL, et al. Evidence of human-to-dog transmission of monkeypox virus. Lancet (London England) (2022) 400:658–9. doi: 10.1016/S0140-6736(22)01487-8
108. Javier F, Sánchez M, Martínez-sellés M, Ruiz-galiana J, Ramos PDL. Monkeypox in humans: a new outbreak. Off J Spanish Soc Chemother (2022) 6:2022. doi: 10.37201/req/059.2022
109. Tian D, Zheng T. Comparison and analysis of biological agent category lists based on biosafety and biodefense. PloS One (2014) 9:e101163. doi: 10.1371/journal.pone.0101163
110. Hirao LA, Draghia-akli R, Prigge JT, Yang M, Satishchandran A, Wu L, et al. Multivalent smallpox DNA vaccine delivered by intradermal electroporation drives protective immunity in nonhuman primates against lethal monkeypox challenge. J Infect Dis (2011) 203:95–102. doi: 10.1093/infdis/jiq017
111. Moss B. Smallpox vaccines: Targets of protective immunity. Immunol Rev (2011) 239:8–26. doi: 10.1111/j.1600-065X.2010.00975.x
112. Sievers F, Wilm A, Dineen D, Gibson TJ, Karplus K, Li W, et al. Fast, scalable generation of high-quality protein multiple sequence alignments using clustal omega. Mol Syst Biol (2011) 7:539. doi: 10.1038/msb.2011.75
113. Sievers F, Higgins DG. Clustal omega for making accurate alignments of many protein sequences. Protein Sci (2018) 27:135–45. doi: 10.1002/pro.3290
114. Sievers F, Barton GJ, Higgins DG. Multiple sequence alignments. bioinformatics, 4th ed. Baxevanis AD, Bader GD, Wishart DS, editors (2020). Hoboken, NJ, US: Wiley
115. Vita R, Mahajan S, Overton JA, Dhanda SK, Martini S, Cantrell JR, et al. The immune epitope database (IEDB): 2018 update. Nucleic Acids Res (2019) 47:339–43. doi: 10.1093/nar/gky1006
116. Rybakova Y, Kowalski PS, Huang Y, Gonzalez JT, Heartlein MW, Derosa F, et al. mRNA delivery for therapeutic anti-HER2 antibody expression In vivo. Mol Ther (2019) 27:1415–23. doi: 10.1016/j.ymthe.2019.05.012
117. Saha S, Raghava GPS. AlgPred: Prediction of allergenic proteins and mapping of IgE epitopes. Nucleic Acids Res (2006) 34:202–9. doi: 10.1093/nar/gkl343
118. Dong R, Chu Z, Yu F, Zha Y. Contriving multi-epitope subunit of vaccine for COVID-19: Immunoinformatics approaches. Front Immunol (2020) 11:1784. doi: 10.3389/fimmu.2020.01784
119. Nelson DL, Cox MM, Hoskins AA. Lehninger principles of biochemistry. 8th ed. Austin: Macmillan Learning (2021).
120. Rapin N, Lund O, Castiglione F. Immune system simulation online. Bioinformatics (2011) 27:2013–4. doi: 10.1093/bioinformatics/btr335
121. Kozakov D, Hall DR, Xia B, Porter KA, Padhorny D, Yueh C, et al. The ClusPro web server for protein-protein docking. Nat Protoc (2017) 12:255–78. doi: 10.1038/nprot.2016.169
122. Kozakov D, Brenke R, Comeau SR, Vajda S. PIPER: An FFT-based protein docking program with pairwise potentials. Proteins (2006) 65:392–406. doi: 10.1002/prot.21117
123. Doytchinova IA, Flower DR. Identifying candidate subunit vaccines using an alignment-independent method based on principal amino acid properties. Vaccine (2007) 25:856–66. doi: 10.1016/j.vaccine.2006.09.032
124. Doytchinova IA, Flower DR. VaxiJen: a server for prediction of protective antigens, tumour antigens and subunit vaccines. BMC Bioinf (2007) 8:4. doi: 10.1186/1471-2105-8-4
125. Waterhouse AM, Procter JB, Martin DMA, Clamp M, Barton GJ. Jalview version 2-a multiple sequence alignment editor and analysis workbench. Bioinformatics (2009) 25:1189–91. doi: 10.1093/bioinformatics/btp033
126. Gasteiger E, Hoogland C, Gattiker A, Duvaud S, Wilkins MR, Appel RD, et al. Protein identification and analysis tools on the ExPASy server; the proteomics protocols handbook. Humana Press (2005) 112:531–52. doi: 10.1385/1-59259-890-0:571
127. González-Pech RA, Stephens TG, Chan CX. Commonly misunderstood parameters of NCBI BLAST and important considerations for users. Bioinformatics (2019) 35:2697–8. doi: 10.1093/bioinformatics/bty1018
128. Baek M, Dimaio F, Anishchenko I, Dauparas J, Ovchinnikov S, Lee GR, et al. Accurate prediction of protein structures and interactions using a 3-track neural network. Science (2021) 373:871–6. doi: 10.1126/science.abj8754
129. Zhang J, Liang Y, Zhang Y. Atomic-level protein structure refinement using fragment guided molecular dynamics conformation sampling. Structure (2011) 19:1784–95. doi: 10.1016/j.str.2011.09.022
130. Heo L, Park H, Seok C. GalaxyRefine: protein structure refinement driven by side-chain repacking. Nucleic Acids Res (2013) 41:384–8. doi: 10.1093/nar/gkt458
131. Vogel AB, Lambert L, Kinnear E, Busse D, Erbar S, Reuter KC, et al. Self-amplifying RNA vaccines give equivalent protection against influenza to mRNA vaccines but at much lower doses. Mol Ther (2018) 26:446–55. doi: 10.1016/j.ymthe.2017.11.017
132. Ballesteros-Briones MC, Silva-Pilipich N, Herrador-Canete G, Vanrell L, Smerdou C. A new generation of vaccines based on alphavirus self-amplifying RNA. Curr Opin Virol (2020) 44:145–53. doi: 10.1016/j.coviro.2020.08.003
133. Blakney AK, McKay PF, Shattock RJ. Structural components for amplification of positive and negative strand VEEV splitzicons. Front Mol Biosci (2018) 5:71. doi: 10.3389/fmolb.2018.00071
Keywords: monkeypox virus, MPXV, mRNA vaccine, DNA vaccine, universal vaccine, pandemic
Citation: Papukashvili D, Rcheulishvili N, Liu C, Wang X, He Y and Wang PG (2022) Strategy of developing nucleic acid-based universal monkeypox vaccine candidates. Front. Immunol. 13:1050309. doi: 10.3389/fimmu.2022.1050309
Received: 21 September 2022; Accepted: 12 October 2022;
Published: 27 October 2022.
Edited by:
Mahbuba Rahman, McMaster University, CanadaReviewed by:
Trupti Pandit, Alfred I. duPont Hospital for Children, United StatesRamesh Pandit, Penn Medicine, United States
Copyright © 2022 Papukashvili, Rcheulishvili, Liu, Wang, He and Wang. This is an open-access article distributed under the terms of the Creative Commons Attribution License (CC BY). The use, distribution or reproduction in other forums is permitted, provided the original author(s) and the copyright owner(s) are credited and that the original publication in this journal is cited, in accordance with accepted academic practice. No use, distribution or reproduction is permitted which does not comply with these terms.
*Correspondence: Yunjiao He, aGV5akBzdXN0ZWNoLmVkdS5jbg==; Peng George Wang, d2FuZ3A2QHN1c3RlY2guZWR1LmNu
†These authors have contributed equally to this work