- 1VIVA-University Children’s Cancer Centre, Khoo-Teck Puat-National University Children’s Medical Institute, National University Hospital, Singapore, Singapore
- 2Department of Paediatrics, Yong Loo Lin School of Medicine, National University Singapore, Singapore, Singapore
- 3Clinician Scientist Academy, National University Health System, Singapore, Singapore
- 4Division of Infectious Diseases, Department of Medicine, National University Health System, Singapore, Singapore
- 5Department of Medicine, Yong Loo Lin School of Medicine, National University of Singapore, Singapore, Singapore
- 6Synthetic Biology for Clinical and Technological Innovation (SynCTI), National University of Singapore, Singapore, Singapore
The ideal strategy to fight an infection involves both (i) weakening the invading pathogen through conventional antimicrobial therapy, and (ii) strengthening defense through the augmentation of host immunity. This is even more pertinent in the context of invasive fungal infections whereby the majority of patients have altered immunity and are unable to mount an appropriate host response against the pathogen. Natural killer (NK) cells fit the requirement of an efficient, innate executioner of both tumour cells and pathogens – their unique, targeted cell killing mechanism, combined with other arms of the immune system, make them potent effectors. These characteristics, together with their ready availability (given the various sources of extrinsic NK cells available for harvesting), make NK cells an attractive choice as adoptive cellular therapy against fungi in invasive infections. Improved techniques in ex vivo NK cell activation with expansion, and more importantly, recent advances in genetic engineering including state-of-the-art chimeric antigen receptor platform development, have presented an opportune moment to harness this novel therapeutic as a key component of a multipronged strategy against invasive fungal infections.
Introduction
Despite the availability of treatment options, the risk of mortality from invasive fungal infections (IFIs) remain high, above 50% particularly for mould infections. While this may be attributable in part to the limited range of effective anti-fungal treatments available, the dismal outcomes are primarily accounted for by susceptible, at-risk cohorts such as the severely immunocompromised recipients of chemotherapy, stem cell or organ transplants, as well as those receiving immunosuppressive biologic therapy. These patients may not be competent in mounting an effective host response against the pathogen, even with the aid of an appropriate antimicrobial. On this background, the augmentation of host immunity and antimicrobial responses through the replacement of immune cellular components is a logical strategy. Advances in recent decades have cast a spotlight on T lymphocytes and natural killer (NK) cells which have emerged as promising adoptive cellular therapeutic modalities. This review focuses on capitalizing NK cellular therapy against IFIs, taking reference from parallels in oncology and T cell immunotherapy.
Biology of NK cells
Large lymphocytes with granular cytoplasm by morphology, NK cells are innate lymphoid cells that circulate in the blood, bone marrow and tissue (1). A distinct feature is the expression of CD56 [also known as neural cell adhesion molecule 1 (NCAM1)] and the absence of T cell receptors (2). NK cells are derived from hematopoietic progenitors in the bone marrow where they undergo maturation; NK cell development also occurs in other lymphoid organs (3). However, unlike T cells, trafficking to the thymus is not a requisite for NK cell maturation (4).
NK cells have traditionally been classified as either immunomodulatory (CD56brightCD16dim/negative) or cytotoxic (CD56dimCD16bright), based on CD56 and CD16 expression. More recently, however, advances in multi-parameter cytometry and single cell proteogenomics have led to discoveries of more diverse populations of NK cells. NK cells have been shown to possess memory-like function through higher intensity recall responses following repeated exposures (5, 6). For example, in cytomegalovirus (CMV) infections (7–9), studies have shown that a higher proportion of NK cells demonstrate increased expression of NKG2C, an activating receptor which recognizes and binds to CMV peptide (10).
Peripheral homeostatic maintenance allows NK cells to persist in the blood even when differentiation of progenitor cells fails (11, 12). Human NK cell turnover in the blood occurs every 2 weeks and in vivo doubling time is estimated to be 13.5 days (13, 14). Continuously stimulated NK cells are able to achieve a median of 16 doublings before immune senescence. Reduction of telomere shortening through overexpression of telomerase reverse transcriptase (TERT) increases NK cell lifespan by allowing additional doublings (14).
Role of NK cells in host immunity
The function of NK cells in the immune system is twofold: first, as effector cells in the control of both microbial infections and cancer; second, as regulatory cells that interact with other immune cells such as T cells, B cells and dendritic cells (15).
NK cells play a pivotal role in the control of microbial infections in the immune system. When pathogens reduce the expression of HLA-I on infected cells to evade T cell surveillance, NK cells activate themselves in response to the low HLA-I expression (16, 17). To kill microbe-infected cells, NK cells mediate antibody-dependent cell cytotoxicity (ADCC) on antibody-bound cells (18); in addition, NK cells bind to death receptors such as Fas ligand and tumour necrosis factor-related apoptosis-inducing ligand (TRAIL) to induce apoptosis and cytotoxicity (19). NK cells also augment host anti-microbial responses through the secretion of proinflammatory cytokines including interferon-gamma (IFNγ) (20). NK cells can mount adaptive responses such as the generation of specific NK cells with memory function following repeated exposure, given that different microbes evoke distinct NK cell mediated responses (10, 21, 22).
NK cells further serve as regulators of the immune response through various positive and negative feedback loops, as well as through direct effects on other immune cells (23). NK cells can also interact with dendritic cells by cross presenting antigens of killed targets, triggering a cascade of downstream responses mediated by dendritic cells (24, 25). The secreted cytokines, IFNγ and tumour necrosis factor alpha (TNFα), also support the maturation of dendritic cells which secrete IL-12, which further enhances NK cell activity (26, 27). NK cells can also act directly on T and B cells to moderate overly activated responses against pathogens (15, 28).
Analogously, in cancer, NK cells mediate cytotoxicity against tumour cells through direct interactions based on the presence of NKG2D ligands (which are upregulated in sarcomas) or absence of MHC class I expression (such as in lymphomas) of NK cell receptor ligands on cancer cells (29–31). Cytotoxicity can also be mediated through ADCC as they express constant region (Fc) receptor CD16 which allows recognition and binding to IgG1 and IgG3 antibodies coated on cancer cells. NK cells have also been implicated in tumour immunosurveillance – this is most evident in patients with primary NK cell immunodeficiencies who have been observed to have a higher risk of developing malignancies (32–34). Longitudinal population-based studies suggest a higher relative risk of cancer in those with lower NK cell cytotoxicity (35). Additionally, reduced NK cell function and lower NK cell infiltration in tumours have also been linked to poorer outcomes in cancer patients (36). Conversely, increased NK cell numbers and function were associated with treatment response and disease control in haematological malignancies (37–39).
Licensing and activation
Licensing
Licensing refers to the acquisition of functional competency during NK cell maturation that mediates the capacity of NK cells to respond to activating signals. This is dependent on interactions between killer cell immunoglobulin-like receptors (KIRs) and major histocompatibility complex (MHC) molecules that belong to self (40, 41). MHC class I molecules bind to KIRs which downregulate NK cell function through immunoreceptor tyrosine-based inhibitory motifs (ITIMs) to dampen signals through tyrosine phosphatases (42); these then minimize the destruction of healthy cells (43). NK cell functionality is determined by the amount and type of MHC class I alleles in that interaction with KIRs (42). Once NK cells are functionally competent, mature NK cells are suppressed by ligation of intact self-MHC; suppression is lifted if MHC is altered or reduced (42). The responsiveness of NK cells can be reset or altered in an environment with variant MHC expression, while unlicensed NK cells can still stimulate adaptive immune responses (44, 45). In addition, KIR-mediated inhibition of mature NK cells can also be overcome by stronger activating stimuli (46).
Activation
Activating receptors transmit signals via various pathways. The natural NK cytotoxicity receptors, NKp30 and NKp46, operate through the immunoreceptor tyrosine-based activation motifs (ITAMs) of high-affinity IgE receptor (FcεRIγ) and CD3ζ, while NKp44, CD94-NKG2C, KIR2DS1, KIR2DS2, KIR2DS4 and KIR3DS1 operate via the ITAMs bearing DAP12 adaptor (47). NK cell activation also depends on co-stimulation by molecules such as [1] DNAM1, using a tyrosine and asparagine-based motif (48), [2] 2B4, signalling through an immunoreceptor tyrosine-based switch motif (ITSM) with NK cell receptor 2B4 (49); and, [3] OX40, recruiting tumour necrosis factor-associated factors to initiate signals (50). Signals from activating receptors could be countered by inhibitory receptors (43, 51, 52). The heterodimer CD94-NKG2A engages HLA-E and transduces inhibitory signals via ITIMs (53, 54); this counteracts the activating signals of CD94-NKG2C which then binds to HLA-E with lower affinity (55). The ligands of activating co-stimulatory molecules such as DNAM1 can also bind to inhibitory T cell immunoreceptor with immunoglobulin and ITIM domains (TIGIT) and CD96 (16). NK activation is also mediated by antibodies binding to CD16 Fc receptor, which induces phosphorylation of ITAM domains of FcεRIγ and CD3ζ - launching a cascade that culminates in cytotoxicity of the target cells known as ADCC (56, 57).
Regulatory cytokines
Cytokines are crucial for activation of NK cells, especially in the context of naïve NK cells where binding to individual activating receptors is generally insufficient to trigger a cytotoxic response (58). Interleukin 2 (IL-2) and interleukin 15 (IL-15) are the most well described and commonly used cytokines which can activate NK cells.
Given that the IL-15 receptor (IL15R) shares signalling β and γ subunits with the IL-2 receptor (59), both IL-2 and IL-15 enhance signalling from activating receptors of NK cells (58, 60). In addition, IL-15 also promotes their survival and proliferation (59, 61, 62). Produced primarily by monocytes, macrophages and dendritic cells, IL-15 forms complexes with the α-chain of IL15R on cell surfaces (63, 64). IL-15 demonstrates superior function when presented on the cell surface as membrane-bound IL-15 (mbIL-15) as compared to its soluble form (64). Transduction of mbIL-15 on human NK cells results in increased longer-term survival compared to its secreted form (65). This is due to the paradoxical effect of IL-15 which induces cytokine-inducible SH2-containing protein (CIS) expression, which dampens NK cell activation by promoting the degradation of the tyrosine kinase JAK1 (66).
Other cytokines such as IL-12, IL-18 and IL-21 have also been used in varying combinations to increase the cytotoxicity of NK cells (67, 68). Specifically, human NK cells cultured for 16 hours with IL-12, IL-15 and IL-18, then washed and maintained in IL-15 for 1-2 weeks, exhibited a “memory-like” form upon re-stimulation (69). The complex interplay between the various cytokines may lead to other effects as well. For example, IL-12 can induce expression of inhibitory receptor NKG2A (69, 70) and IL-21 may inhibit IL-15 induced NK cell expansion (71).
Cytotoxic effector capabilities of NK cells
NK cell-mediated cell killing occurs through activation triggered by the formation of a synapse with the target cell. Lytic granules transported on microtubules then concentrate on the synapse, where they fuse with the plasma membrane and are then released (72). Perforin, one of the main components of these granules, forms pores in the cells, leading to osmotic lysis (73). Granzymes, another component of these granules, enter the cells through the pores created by perforin and trigger apoptosis of the cells by activating caspases (72). Lytic granules are very potent as a solitary units in killing target cells (73). NK cells can also proceed to kill other target cells even after degranulation (74); as they can also kill cells through the expression of FAS ligand and TNF-related apoptosis -inducing ligand (TRAIL) (74). In addition to direct cytotoxicity, NK cells also attract and stimulate other immune cells such as dendritic cells (75) through the secretion of various cytokines, chemokines and growth factors (43). An example would be the cytokine IFNγ, which promotes polarization of T helper 1 cells, induces MHC class II expression on antigen presenting cells and, activates macrophages (76).
Specific cytotoxic capabilities of NK cells against fungi
Against fungi, the modus operandi of NK cells is similar: direct killing either via degranulation releasing perforin or granzyme, or secretion of effector cytokines (such as IFNγ and TNFα); where both induce phagocytosis and result in cell death. In vitro, NK cells have exhibited phagocytic-like characteristics, engulfing Candida yeast cells in a contact-dependent manner (77). NK cells execute their main effector role by means of direct cytolytic capacity against fungi, through the release of lytic molecules pre-loaded in granules within the NK cell cytoplasm. Perforin causes direct pore formation in the fungal cell membrane disrupting membrane integrity and facilitating entry of granzymes into the pathogen. Both perforin and granzymes synergize to mediate apoptosis of target cells. Granzymes, classically represented by Granzyme B, induces apoptotic target cell death through caspase-dependent pathways as well as release of inflammatory cytokines such as interleukin 1 alpha and beta (78). The anti-fungal role played by perforins has been well demonstrated against Rhizopus (79), Aspergillus (80) and Candida (77). NK-directed killing is also morphotype-specific, for example, in the case of Aspergillus or Rhizopus, germinating conidia or hyphae are the targets of NK cells.
Host recognition of fungi involves classic pathogen recognition receptors such as toll like receptor (TLR) 2 (81, 82) and C type lectin receptor Dectin-1 (also known as CLEC 7a) (83); which recognize beta-glucan in the fungal cell wall (81). NK-specific receptors, such as Natural Killer Cell Receptors (NCR) subtypes NKp30 and NKp46, have also been implicated. Against Candida and Cryptococcus, NKp30 receptors have been shown to mediate perforin release and fungal cytotoxicity through the PI3K-ERK1/2 pathway (84, 85). Binding between the Candida cell wall adhesins Epa1, Epa6, and Epa7, and NKp46 receptor and its mouse orthologue NCR1, resulted in enhanced NK activation through degranulation marker CD107, resulting in reduced fungal burden (86). Most recently, the Candida adhesin Agglutinin-Like Sequences (ALS) was identified as a ligand for TIGIT, an inhibitory checkpoint inhibitor on NK cells (87). CD56, a classical NK cell marker, has also been shown to be a major pathogen recognition receptor which directly interacts with A. fumigatus. Blockade of CD56 diminished NK activation, with reduced inflammatory cytokine secretion against A. fumigatus (88). ‘Death receptors’ TRAIL-R and Fas by TRAIL and Fas ligand (FasL) (CD95L) have also been observed to be triggered during the degranulation process (18).
IFNγ and proinflammatory TNFα are pivotal mediators of type 1 T helper cell (Th1) host response against fungi (89), while granulocyte-macrophage colony stimulating factor (GMCSF) activates the obligate phagocytes against fungi, macrophages (77). The major source of secreted IFNγ against fungi originates from NK cells. IFNγ is central in orchestrating the host immune response against the pathogen, serving as a bridge between the innate and adaptive immune systems. IFNγ drives the differentiation of CD4 cells towards Th1 subsets on its own (90), or in synergy with TNFα (91, 92) and also mediates NK cross talk with dendritic cells (81). IFNγ also activates tissue macrophages, as shown in the context of Cryptococcus infections (93, 94). Against Candida, IFNγ has also been shown to augment the potency of polymorphonuclear neutrophils (77). Furthermore, NK cells also secrete chemokines such as CXCR2, CCL3/MIP-1α, CCL4/MIP-1β and CCL5/RANTES. The production of these chemokines may be titrated on a dose-dependent response, as seen in Aspergillus infections (95). These chemokines serve to fine-tune NK cell migration, cytotoxicity, and neutrophilic anti-fungal activity (96, 97).
Development and refinement of NK cellular anti-fungal treatment: in-vitro and in-vivo studies
The earliest attempts at utilizing NK cells of murine origin against fungi began more than 30 years ago (Table 1). The process of purification involved passing murine splenocytes through nylon wool, retaining monocytes and B cells. The non-adherent fraction was further differentiated through Percoll gradient fractionation, or selected by panning and rosetting with sheep erythrocytes. The NK-containing cellular fractions demonstrated anti-fungal effects, when tested in vitro with co-incubation against pathogenic fungi of interest, Cryptococcus and Paracoccidioides (98–100). Growth inhibition in excess of 50% could be achieved, albeit with higher effector-to-target (E:T) ratios. The first attempts with human peripheral blood lymphocytes followed on, through density gradient centrifugation and selection for Leu-11b directed against NK surface Fc receptors. In vitro, co-incubation setting against Coccidioides, human NK-enriched cells inhibited C. immitis endospores and spherules effectively. In this setting, interferon was used for immune augmentation and was shown to effectively enhance leucocyte-mediated fungal killing (101). As IL-2 appeared to enhance NK cell activation (102), this was added to CD16/CD56+ cell fractions against Cryptococcus with demonstrable efficacy (103).
The advent of the magnetic-activated cell sorting (MACS) platform has also revolutionized our capacity to sort cellular subtypes via positive or negative selection, enabling us to select for CD56+CD3- NK cells with more than 90% purity. This platform has been widely employed to yield enriched NK cells for further in vitro, and in vivo anti-fungal studies, focusing on more difficult-to-treat moulds such as Aspergillus and Zygomycetes. Interest also remains in the treatment of pathogenic yeasts Candida and Cryptococcus. In tandem, a deeper mechanistic understanding of the anti-fungal properties of NK cells were also gained. For instance, the major roles played by cytokines such as IFNγ; NK-released perforins; the requirement for physical contact between effector-target cells; and, a predilection for specific pathways (e.g. PI3K-ERK1/2 signaling in NK-mediated anti-Cryptococcal activity) (104, 105). The use of human NK cells purified from peripheral blood also regularly involved priming cytokines IL-2, IL-12, IL-15 and IFNα/β concoctions which enhanced NK cellular activity. Against Aspergillus, human NK cells were able to kill A. fumigatus hyphae, but not resting conidia in vitro. Hyphae killing, measured via methoxynitrosulfophenyl-tetrazolium carboxanilide (XTT) assays ranged between 15% to 43% measured hyphae killing of 15-43% was achieved, but required high E:T ratios of 10:1 to 50:1 (80). Similar results were seen against Zygomycetes, whereby R. oryzae hyphae (but not conidia) were susceptible to NK killing (79), as well as in C. albicans (77).
Against the background of the above in-vitro study outcomes saw the development of adoptive transfer of NK cellular therapy against IFI in animals. This was first successfully demonstrated in 2003 with murine splenocytes cultured in IL-12 and IL-18 and purified through negative selection with 95% yield. Two million NK cells injected into immunocompromised mice in a pulmonary aspergillosis mice model in vivo saw significant reduction in lung fungal burden (91, 92). The most recent attempt involved the use of adoptive xeno-transfer of human NK cells to treat mice with pulmonary aspergillosis. Of note, these human NK cells were highly activated, expanded by co-culture with K562 cells genetically modified to express 4-1BB ligand and membrane-bound IL15 (K562-41BBL-mbIL-15). Promisingly, cytotoxicity was observed with a significantly lower E:T ratio of 2:1 where the infusion of 10 million cells led to an approximately 40% reduction in fungal growth, independent of anti-fungal drugs (83).
State of art: Clinical applications and considerations for NK Cell therapy from cancer to IFI
Much of what is currently known about the clinical application of NK cells stems from decades of cancer research in the use of NK cell infusions for cancer treatment (47). NK cells can be obtained from peripheral blood of allogeneic donors, differentiated umbilical cord blood, induced pluripotent stem cells (iPSCs), or irradiated cells of the NK-92 cell line.
The clinical preparation of NK cells for cancer therapy depends on the source of NK cells and it can be broadly divided into the following processes: 1) harvesting from source; 2) ex vivo NK cell preparation which may require differentiation for stem cell sources, and usually involves expansion to generate higher numbers of effector cells, 3) patient lymphodepletion chemotherapy prior to infusion; and, 4) patient IL-2 therapy after infusion. In this section, we will further discuss what is currently known about the clinical application of NK cells in human clinical trials.
Sources of NK cells
Peripheral blood NK cells
The peripheral blood is the most common source of NK cells in clinical trials (Figure 1). Allogeneic donors of peripheral blood NK cells have been preferred over autologous NK sources in the setting of anti-tumour activity for patients with cancer (47). This is primarily due to the self-HLA expression on tumour cells that inhibit the activity of autologous NK cells (47). HLA typing is also required to identify suitable donors. Although allogeneic NK cells generally do not cause graft versus host disease (GVHD) directly (106, 107), adequate T-cell depletion procedures (typical safety limit: 5 x 104 per kg body weight) are still required (108, 109).
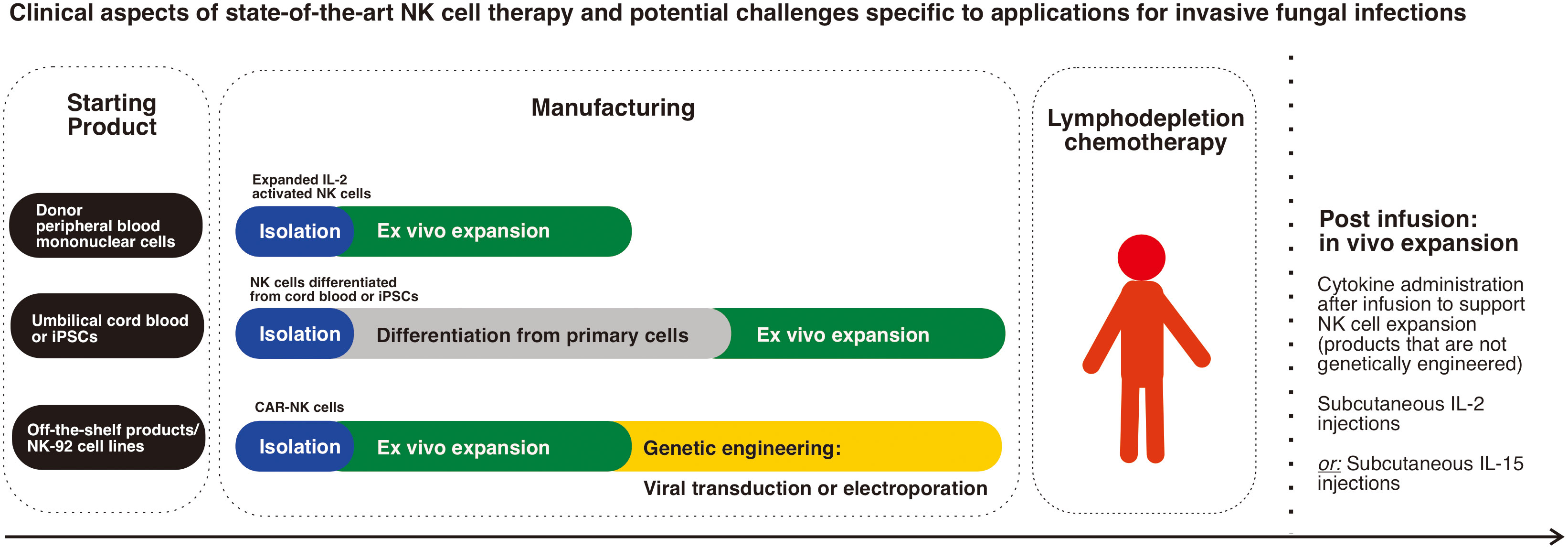
Figure 1 Clinical aspects of state-of-the-art NK cell therapy and potential challenges specific to applications for invasive fungal infections: The main sources of NK cells in clinical trials are donor peripheral blood mononuclear cells which are the most commonly used, while next generation genetically modified products using stem cells (umbilical cord blood cells or iPSCs) are progressively emerging. Schematic diagram representing the main steps in cell manufacturing highlights the complexity and time needed to produce stem cell differentiated products in addition to the need for genetic engineering of CAR-NK cells. The need for lymphodepletion chemotherapy and post infusion cytokine administration, which is the case for most non-genetically engineered products is an important clinical aspect of NK cell therapy.
The process of isolation and enrichment of NK cells from peripheral blood mononuclear cells starts with a leukapheresis procedure (110). In this process, white blood cells are removed from the blood of the donor while the remaining blood components are returned via an apheresis machine (111). Mononuclear cells are further enriched by centrifugation with Ficoll density gradient centrifugation before T cell depletion with CD3 immunomagnetic beads, followed on with CD56 enrichment (112).
Because peripheral blood NK cells of healthy individuals are usually in a resting state, pre-activation is necessary to optimize functionality using IL-2 or IL-15 (113). Short term exposure to high dose IL-2 for 12-16 hours at doses of 1000 international units per millilitre has been shown to adequately activate NK cells in the study by Miller et al. (107). IL-15 has been described to maintain NK cell cytotoxicity for a longer period than IL-2 without repeat exposure (114, 115), however other studies have suggested the possibility of cell exhaustion with other IL-15 dosing regimens (116). Other combinations involving 12-16 hour incubations with IL-12, IL-15 and IL-18 have also been used in clinical trials as pre-activation prior to infusion in patients (117). Recently, inhibitors of glycogen synthase kinase 3 (GSK3) have also been described to induce NK cell maturation and cytotoxicity (118).
Differentiation from stem cells: Umbilical cord blood and iPSCs
NK cells can also be obtained through the differentiation of stem cells such as umbilical cord stem cells and iPSCs. Umbilical cord banks allow the advantage of selection of donors with a specific HLA or NK receptor profile. A higher number of naïve NK cells exist in cord blood (approximately 30%) than in peripheral blood (approximately 10%) (119). While they have lower spontaneous killing of target cells due to lower expression of perforin and granzyme B, they express the same amount of activating receptors (120). With ex vivo activation and expansion, lower cytotoxicity can be overcome to allow broader off-the-shelf applications, including CAR-based therapy (121), given the ubiquity of cord blood banks.
CD34+ hematopoietic cells from umbilical cord blood can be differentiated into NK cells with the use of stem cell factor (SCF), IL-2, IL-7, IL-15, and other growth factors (122, 123). Haematopoietic differentiation of iPSCs can be first induced with SCF, vascular endothelial growth factor (VEGF) and bone morphogenetic protein 4 (BMP4) (124). Thereafter, NK cell differentiation is stimulated using IL-3, IL-7, IL-15, SCF and FLT3L (125). These differentiated NK cells can then be expanded by co-culture with K562 cells with membrane bound IL-21 (125, 126). However, the main limitation of differentiated cells as a source is the significantly longer duration of culture required (about 7 weeks) (127) which may not be practical for clinical use in patients with rapidly progressing conditions.
Off-the-shelf genetically modified NK-92 products
Emerging data suggest the potential for NK-92 cells to be an off-the-shelf source of NK cells for further engineering and clinical development. First established in 1994, NK-92 is a cell line derived from the peripheral blood of a patient with non-Hodgkin’s lymphoma that was first established in 1994 (128); it and has been found to have a high expression of activating receptors (129), granzyme B and perforin (130).
The expansion of NK-92 cells has been adapted to good manufacturing practice (GMP) conditions with a doubling time of 24 to 36 hours with IL-2 support (131, 132). Phase I clinical trials using NK-92 cells in patients with hematologic malignancies and solid tumours have demonstrated safety with some clinical response (133–136). Genetic modification of NK-92 cells through viral transduction with CARs against a variety of cancer targets such as CD19 and CD20 for B-cell malignancies (137–139) have also been developed. The first CAR NK-92 clinical trials were conducted in patients with relapsed or refractory acute myeloid leukemia, using a lentiviral transduced third-generation CAR targeting CD33 (140). While clinical efficacy was not observed, these early studies demonstrated safety as no grade 3-4 adverse events were observed.
While NK-92 cells present an attractive, off-the-shelf option for NK cell-based therapy, a potential major drawback is limited persistence given that the cells are irradiated. Furthermore, most protocols do not include lymphodepleting chemotherapy which limits persistence as the cells are more likely to be rejected very quickly.
Ex-vivo preparation of NK cells: expansion through co-culture with K562 cells
NK cell activation and proliferation can be induced through co-culture with the chronic myelogenous leukaemia derived cell line, K562 (141). Transduction of K562 with both mbIL-15 and 4-1BBL (14, 46, 113) which are then irradiated, further improves NK cell proliferation following co-culture, a method which has also been adapted to clinical use in large-scale, GMP conditions. While irradiation restricts any proliferation of K562 cells, NK cells also effectively kill K562 cells;, ensuring that there will be no viable K562 cells infused to patients (110).
GMP cultures of NK cells isolated from peripheral blood mononuclear cells lasting 10 days can achieve more than 300-fold NK cell expansion (46, 110, 113). This allows sufficient cells for multiple infusions from a single leukapheresis product (110, 142). An even higher amount of NK cells can be generated by prolonging the duration of culture, or through the addition of more irradiated K562-mbIL-15-4-1BBL cells for up to 8-15 weeks, following which senescence may start to set in (14).
Other modifications to K562 cells in an attempt to improve NK cell expansion after co culture have also been studied: 4-1BBL with IL-15 receptor alpha chain (109); 4-1BBL with IL-21 (143); CD64, CD86, CD19 with 4-1BBL; mbIL-15, mbIL-21 or both (111, 126, 144); OX40 ligand with IL-2, IL-15 and IL-21 (145).
Optimizing the host for NK cell therapy: lymphodepletion and cytokine administration
Allogeneic NK cells only persist for 2-3 weeks after infusion. Lymphodepletion is primarily used to improve persistence by overcoming rejection of NK cells by the recipient’s immune system (69, 110, 146). Fludarabine and cyclophosphamide are the most widely used drugs to preferentially deplete recipient lymphoid cells prior to NK cell infusion (107). In the pioneering work by Miller et al. (107), deeper immunosuppression through the addition of cyclophosphamide at 60mg/kg for 2 days to a fludarabine regimen of 25mg/m2 for 5 days led to higher levels of serum IL-15 and resulted in better engraftment. With these lymphodepletion regimens, allogeneic NK cells typically persist for 14-21 days following infusion (69, 110, 146, 147), including that of umbilical cord blood derived NK cells (148).
In addition to lymphodepletion, IL-2 is also commonly administered to improve survival and expansion of donor NK cells. Typically, 6 doses of IL-2 are given subcutaneously over 2 weeks after NK cell infusion (110, 127, 146, 149, 150).
In a study by Miller et al. (107), haploidentical IL-2 activated NK cells were observed to have increased NK cell expansion with higher serum IL-15 levels in patients who received lymphodepletion plus IL-2-diptheria toxin fusion protein, as compared to those who received lymphodepletion chemotherapy alone (127).
IL-15 and its variants have also been used to support NK cell expansion in clinical trials. Cooley et al (151) showed that NK cell expansion was higher than what had been previously described with IL-2; however, subcutaneous IL-15 injections were associated with cytokine release syndrome and associated neurotoxicity. Increased toxicity was hypothesized to be due to IL-15 stimulation of monocytes and T cells in contrast to actual NK cell activation (151).
Adaptation of current NK cellular technology to treatment of IFI: identified challenges and modifications
The perceived attractiveness of NK cellular therapy lies in the capacity of NK cells to directly kill target cells and stimulate the host immune response, without need for prior antigen-specific sensitization, with minimal risk of inducing GVHD (152). While in vitro and animal in vivo NK studies against fungi to date seem promising, these are rudimentary first steps in using NK cells with anti-microbial intent against pathogens compared to the experience accumulated to date with NK infusions for their anti-tumour activity, from which challenges have been recognized. For instance, the requirement for continued IL-2 administration post- NK cell infusion has been pivotal in sustaining viability and expansion but its dosing is limited by the adverse IL-2 cytokine-linked side effects experienced by patients like malaise, nausea, vomiting (153). The potential merits of the use of higher doses of IL-2 (up to 6,000 IU) (113) or with addition of IL-15 and IL-12 have since come under question. IL-15 enhances cytotoxicity, but also increases the risk of immune exhaustion (116). More critical cytokine-related toxicities such as worsening leucopenia, granulocytopenia and thrombocytopenia are likely already inherent in patients susceptible to invasive fungal infections, hypotension, and capillary leak syndrome. The development of IL-15 analogues, such as N-803, primes NK cells to produce much needed IFNγ and TNFα (154) against the fungi. NK cell expansion using irradiated K562 cells with membrane bound IL-15 and 4-1BBL is now being used to produce a good yield of expanded NK cells for cellular therapeutics.
While anti-tumour treatment largely involves single infusions of NK cells, the optimal number of infusions of NK cells, which has important implications on its applicability, remains unknown. The duration of viability of a single infusion of NK cells is estimated to be between 2-3 weeks, subject to rejection by the recipient’s immune system. In order to allow the infused NK cells to dwell and exert their anti-fungal effect, lymphodepletion chemotherapy also acts as a double-edged sword since they are also well recognized to compromise even an immunocompetent host’s immune mechanisms. In the setting of heavily pre-treated patients, or those with existing co-morbidities, fitness to undergo lymphodepletion is a major consideration, given the dose intensity required. In fact, IFI patients who are post stem cell transplant (SCT) or on intensive chemotherapy, are already lymphodepleted by nature of their therapy. Hence, one might view the use of NK adoptive therapy from an allogenic source (or ideally, from the SCT donor) as befitting, under such circumstances.
Historically, the use of autologous NK cells for anti-tumour intent has not yielded significant efficacy. This has been attributed to HLA signals on the tumour cells which may inhibit autologous NK cells from activating and effecting cytotoxicity. However, this is unlikely to be of concern against an invading fungus; instead, it could potentially be an alternative source of NK cells in addition to a conventional allogenic option.
As with other more established forms of personalized cell-based therapy such as CAR-T cell therapy, the issues of manufacturing such as: quality of starting material, turnaround time, schedule availability and requirement for quality control testing prior to product release, need to be addressed when considering the practical aspects of clinical NK cell therapy. Given the clinical urgency, an autologous source may be the most practical. However, if a patient is already in a dire state where the invasive fungal infection is in fact an effect of underlying profound immunosuppression, it may render the harvesting of a suitable starting material impossible. In the same vein, the need for HLA matching and the search for an allogeneic healthy donor starting product may take more time than what a critically ill patient may have.
The role of NK cells as innate lymphocytes effecting non-discriminatory, anti-tumour, and possibly anti-microbial, activity is a double-edged sword. NK cells utilize inhibitory receptors like KIRs which interact with MHC-1 to distinguish between ‘self’ and ‘non-self’, marking out the latter for effector response. While they are not known to display clonotypic receptors, what is apparent is that NK preparation and priming seems to have an effect on fungal morpho-typic activity. NK cells primed by IL-2 by Schmidt et al. displayed specificity for A. fumigatus and R. oryzae hyphae but not conidia (80). Conversely, expanded NK cells expressing K562-41BBL-mbIL-15 by Soe et al. have been observed to be most efficacious against A. fumigatus germinating conidia, and this was attributed to the Dectin-1 receptor (83). As a therapeutic benchmark, voriconazole effectively targets both germinating conidia and active growing hyphae (155). Given the morpho-typic predilection against fungi that has been observed with NK cells from different sources, further development of NK cells with enhanced activity against both conidia and hyphae would be desirable.
The goal ahead: augmenting NK anti-fungal activity through genetic engineering
Efforts in engineering NK cells to augment function have been made via viral transduction or electroporation of mRNA (110, 156). NK cell proliferation is critical in allowing DNA integration of the gene of interest, especially when retroviral transduction is used (46). Gene expression is also generally improved in lentiviral transduction if the cells are proliferating (157). Given concerns regarding oncogenic mutagenesis with viral transductions, mRNA electroporation – a method that does not involve viral vectors – has also been studied. Despite being less costly and faster to prepare, a major limitation is that gene expression is transient and is generally lost within a week (156). While this may be a limitation for cancer-related applications, short-term expression may be still be acceptable for other indications beyond oncology.
To date, genetic engineering efforts have been geared towards 1) enhancing activation and proliferation, 2) decreasing inhibition, and 3) redirecting cells toward specific targets. While most of the studies have been conducted in the context of oncology, significant parallels may also be applicable to an anti-fungal setting.
CAR engineering of NK cells is an emerging field that leverages on the success of CAR-T cell technology in cancer [Figure 2]. The potential major advantage of CAR-NK cells over CAR-T cells is its potential for off-the-shelf use, given its HLA-unrestricted killing (158), which abrogates the need for complex testing and personalised manufacturing, in often dire and urgent clinical scenarios. CAR-NK cells have also demonstrated extended in vivo persistence and a favourable safety profile in ongoing clinical trials (159). Primarily designed to re-direct T cells towards specific targets such as CD19 expressed on B-cell malignancies, second generation CAR-T cells first demonstrated cytotoxicity through the incorporation of the 4-1BB co-stimulatory domain (46). Further observations of a higher basal level of activation that was not dependent on antigen binding have been characterised in a phenomenon known as tonic signalling (160, 161). This suggests that CAR constructs not only redirect T cells to specific targets, they also interact with endogenous receptors leading to more T cell activation (162). While the effects of tonic signalling have not been elucidated in CAR-NK cells, similar cooperative activation in NK cells are plausible (158). With the right target antigen, CAR-NK cells directed against fungi have significant potential for further clinical development.
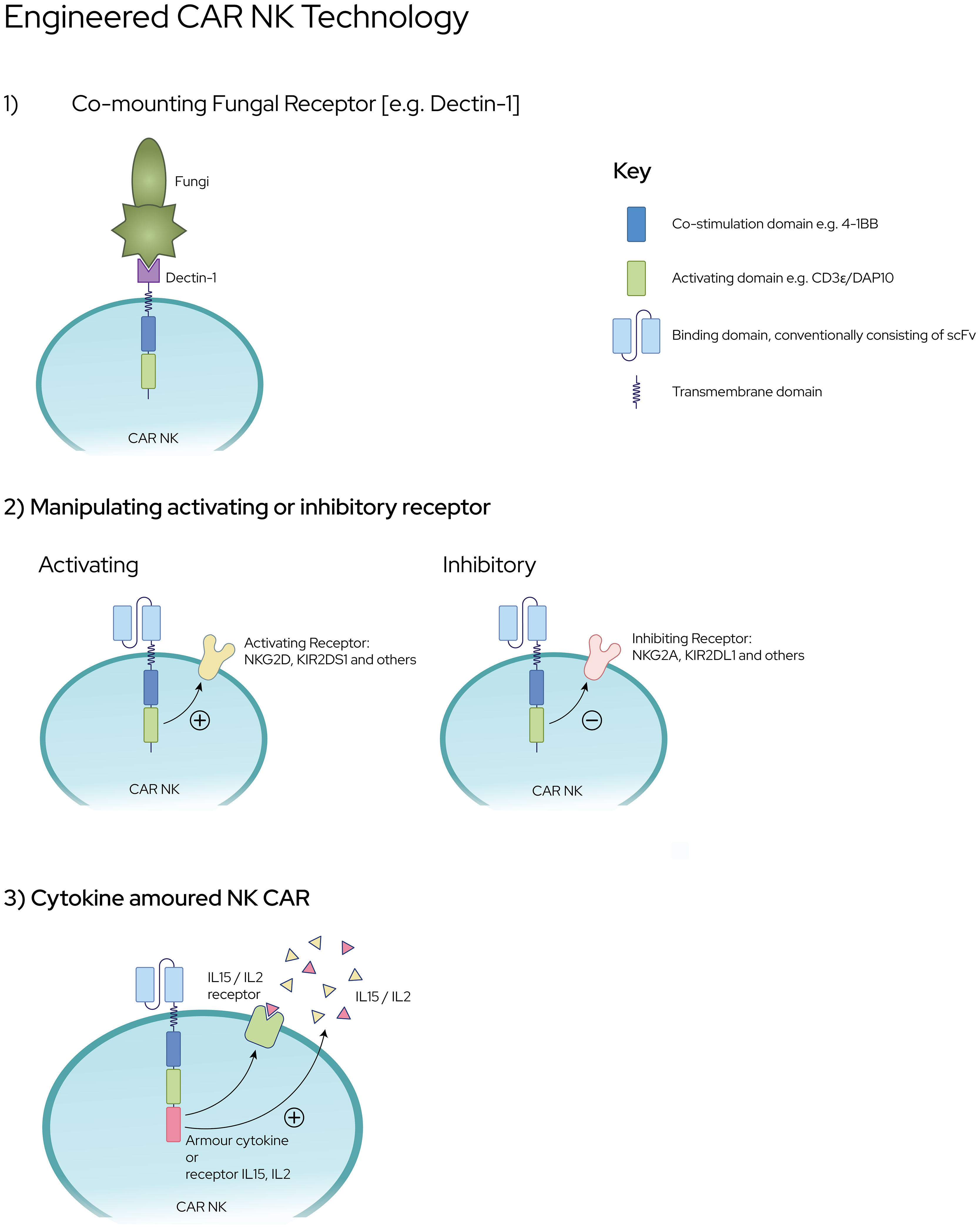
Figure 2 Engineering CAR NK Platforms against Invasive Fungal Infections [1] CAR NK cells co-expressing fungal specific receptors e.g., Dectin-1 fused to co-stimulation and activating domains [2] Enhanced expression of NK-activating receptors or down regulation of NK-inhibitory receptors upon ligation [3] NK cells with ‘cytokine-armouring’ capability for superior persistence and sustenance against fungal pathogens.
Dectin-1, also known as CLEC7A, is a C type lectin receptor which recognizes beta-glucan, a ubiquitous polymer in the cell walls of fungi such as Aspergillus, Candida and Zygomycetes (163, 164). Beta-glucan is most abundantly expressed on actively growing fungal morphotypes such as germinating conidia and hyphal tips (165, 166). Hence, the generation of CAR-NK cells co-expressing Dectin-1 receptor may literally ‘nip the problem in the bud’ given its enhanced specificity over dormant or inactive fungal conidia. In an attempt to study this, Dectin-CAR-T was innovatively modified through the fusion of Dectin-1 receptor to CAR cassette encoding chimeric CD28 and CD3-ζ (167). These Dectin-CAR-T, through their specificity for beta-glucan, were able to induce hyphal damage, thereby functioning as treatment against aspergillosis in mice. This proof-of-concept capability harbours the potential to engineer NK cells with similar intent whereby the likelihood of rejection is lower than T lymphocytes.
Next, is the possibility of manipulating the balance between NK activating receptors (e.g., NKG2D-DAP10) and inhibitory receptors (e.g., NKG2A and KIR) through genetic engineering involving CRISPR-Cas9 gene editing technology. This has now been made possible with the success of CAR-T technology which can also be applied to NK cells as CAR-NK cells with specific targets and increased cytotoxicity. Engineered CAR-NK cells with membrane bound IL-15 (168) (or, with 4-1BBL) and enhanced NKG2D–CD3ζ–DAP10 expression promise to exert greater cytotoxicity with increased cytokine production and degranulation (169). Conversely, down regulation of NK inhibitory receptors is now possible through the use of (i) gene editing techniques; (ii) single-chain variable fragments targeting NKG2A protein expression at the endoplasmic reticulum, and (iii) anti-KIR antibodies (170). Intuitively modifying NK cells to augment expression of IL2R and IL15R also serves to increase the sensitivity of NK cells to enrichment and expansion.
Cytokine “armoured” NK cells are being developed through genetic CAR engineering of NK cells. On the basis that continuous cytokine stimulation of NK cells during ex vivo NK cell preparation renders the cells ‘cytokine-addicted’, these cells are being developed to reduce the effects of “cytokine addiction” such as decreased persistence in vivo (171). Cytokine armouring is being developed in the forms of 1) soluble cytokines that are secreted which also activate other immune cells (172) and, 2) membrane-bound cytokines that are triggered upon cellular interactions (173). When compared with CAR alone, IL-15-armoured CAR NK cells demonstrated superior persistence (168), not only in vivo in preclinical models but also in a clinical study of patients with CD19 CAR-NK cells (159). A similar approach can also be applied in the setting of anti-fungal NK cell therapy to augment NK cell function and overcome the challenges of an immune milieu that is often low in IFNγ and TNFα in the setting of an invasive fungal infection.
The ultimate objectives in optimizing NK cellular therapy against fungi lie in enhancing activation and expansion of NK cells to an ideal E:T ratio. This translates to good cell numbers, viability, and enhanced activity. Beyond the traditional ‘single donor’, use of hematopoietic stem and progenitor cells (HSCs) and iPSC lines (174, 175) provide the advantage of an accessible bank of matched donors. However, inherent obstacles such as the complex cell manufacturing process, need to be overcome through development of off-the-shelf products that do not require HLA matching, given the often-urgent clinical scenarios. Stem cell products can also potentially acquire significant new mutations (176) during the differentiation process which presents an added challenge of regulatory concerns about potential oncogenicity. Other off-the-shelf options such as genetically modified NK-92 cells are promising in terms of time-to-infusion advantage; however, the cytotoxicity and persistence of NK-92 cells that have been lethally irradiated (177) remain to be established until further clinical data from ongoing trials become available (178).
Further studies need to be directed toward an ideal setting where 1) the starting material is readily available; 2) manufacturing can be completed in a reasonable time frame, if not, an off-the-shelf product; 3) lymphodepletion is not required (especially in already immunosuppressed patients); and, 4) NK cells are able to expand in vivo and maintain their own activating signals to mediate effective cytotoxicity.
Conclusion
NK cell technology has improved by leaps and bounds in recent decades, making adoptive cellular transfer a viable therapeutic option that can now be considered against difficult-to-treat fungal infections. However, it is to be highlighted that the use of NK cells as antimicrobial, anti-fungal therapy is unlikely to be implemented independently as a form of monotherapy. A practical strategy would be to administer NK cellular therapy in conjunction with conventional therapeutics such as the new generation azole anti-fungal drugs (i.e., voriconazole, posaconazole, isavuconazole) or even liposomal amphotericin B. The basis for supporting the deployment of a targeted immune- augmenting therapy alongside conventional antimicrobials is strong, given that many patients with invasive fungal infections are immunocompromised.
Author contributions
All authors listed have made a substantial, direct, and intellectual contribution to the work and approved it for publication.
Funding
BO was supported by the Singapore National Medical Research Council Research Training Fellowship NMRC/RTF/MOH/000616. LYAC was supported by the Clinician Scientist Awards (CSA Senior Investigator and Investigator), Individual Research Grant (IRG), Bedside and Bench (B&B) Grants, Centre Grant, and the Training Fellowship Award from the National Medical Research Council (NMRC), Singapore. LYAC also acknowledges the Aspiration Grant and Summit Research Program, iHealthtech Grant, Bench to Bedside Grant, and Seed Fund from the National University Health System, Infectious Diseases and Synthetic Biology Translational Research Program of the National University of Singapore, as well as the Synthetic Biology Research and Development Program of the National Research Foundation, Singapore.
Acknowldgements
We thank Dario Campana and Roger Foo for their advice on the manuscript. We would also like to thank Ms. Sheena Nishanti D/O Ramasamy, medical writer, for her extensive proofreading and editing of the manuscript.
Conflict of interest
The authors declare that the research was conducted in the absence of any commercial or financial relationships that could be construed as a potential conflict of interest.
Publisher’s note
All claims expressed in this article are solely those of the authors and do not necessarily represent those of their affiliated organizations, or those of the publisher, the editors and the reviewers. Any product that may be evaluated in this article, or claim that may be made by its manufacturer, is not guaranteed or endorsed by the publisher.
References
1. Vivier E, Artis D, Colonna M, Diefenbach A, Di Santo JP, Eberl G, et al. Innate lymphoid cells: 10 years on. Cell. (2018) 174(5):1054–66. doi: 10.1016/j.cell.2018.07.017
2. Lanier LL, Testi R, Bindl J, Phillips JH. Identity of leu-19 (CD56) leukocyte differentiation antigen and neural cell adhesion molecule. J Exp Med (1989) 169(6):2233–8. doi: 10.1084/jem.169.6.2233
3. Yu J, Freud AG, Caligiuri MA. Location and cellular stages of natural killer cell development. Trends Immunol (2013) 34(12):573–82. doi: 10.1016/j.it.2013.07.005
4. Lanier LL, Spits H, Phillips JH. The developmental relationship between NK cells and T cells. Immunol Today (1992) 13(10):392–5. doi: 10.1016/0167-5699(92)90087-N
5. O'Sullivan TE, Sun JC, Lanier LL. Natural killer cell memory. Immunity. (2015) 43(4):634–45. doi: 10.1016/j.immuni.2015.09.013
6. Reeves RK, Li H, Jost S, Blass E, Li H, Schafer JL, et al. Antigen-specific NK cell memory in rhesus macaques. Nat Immunol (2015) 16(9):927–32. doi: 10.1038/ni.3227
7. Hammer Q, Ruckert T, Romagnani C. Natural killer cell specificity for viral infections. Nat Immunol (2018) 19(8):800–8. doi: 10.1038/s41590-018-0163-6
8. Kamimura Y, Lanier LL. Homeostatic control of memory cell progenitors in the natural killer cell lineage. Cell Rep (2015) 10(2):280–91. doi: 10.1016/j.celrep.2014.12.025
9. Adams NM, Geary CD, Santosa EK, Lumaquin D, Le Luduec JB, Sottile R, et al. Cytomegalovirus infection drives avidity selection of natural killer cells. Immunity. (2019) 50(6):1381–90 e5. doi: 10.1016/j.immuni.2019.04.009
10. Hammer Q, Ruckert T, Borst EM, Dunst J, Haubner A, Durek P, et al. Peptide-specific recognition of human cytomegalovirus strains controls adaptive natural killer cells. Nat Immunol (2018) 19(5):453–63. doi: 10.1038/s41590-018-0082-6
11. Schlums H, Jung M, Han H, Theorell J, Bigley V, Chiang SC, et al. Adaptive NK cells can persist in patients with GATA2 mutation depleted of stem and progenitor cells. Blood. (2017) 129(14):1927–39. doi: 10.1182/blood-2016-08-734236
12. Corat MA, Schlums H, Wu C, Theorell J, Espinoza DA, Sellers SE, et al. Acquired somatic mutations in PNH reveal long-term maintenance of adaptive NK cells independent of HSPCs. Blood. (2017) 129(14):1940–6. doi: 10.1182/blood-2016-08-734285
13. Lutz CT, Karapetyan A, Al-Attar A, Shelton BJ, Holt KJ, Tucker JH, et al. Human NK cells proliferate and die in vivo more rapidly than T cells in healthy young and elderly adults. J Immunol (2011) 186(8):4590–8. doi: 10.4049/jimmunol.1002732
14. Fujisaki H, Kakuda H, Imai C, Mullighan CG, Campana D. Replicative potential of human natural killer cells. Br J Haematol (2009) 145(5):606–13. doi: 10.1111/j.1365-2141.2009.07667.x
15. Vivier E, Tomasello E, Baratin M, Walzer T, Ugolini S. Functions of natural killer cells. Nat Immunol (2008) 9(5):503–10. doi: 10.1038/ni1582
16. Martinet L, Smyth MJ. Balancing natural killer cell activation through paired receptors. Nat Rev Immunol (2015) 15(4):243–54. doi: 10.1038/nri3799
17. van Erp EA, van Kampen MR, van Kasteren PB, de Wit J. Viral infection of human natural killer cells. Viruses. (2019) 11(3). doi: 10.3390/v11030243
18. Smyth MJ, Cretney E, Kelly JM, Westwood JA, Street SE, Yagita H, et al. Activation of NK cell cytotoxicity. Mol Immunol (2005) 42(4):501–10. doi: 10.1016/j.molimm.2004.07.034
19. Miller JS. Biology of natural killer cells in cancer and infection. Cancer Invest. (2002) 20(3):405–19. doi: 10.1081/CNV-120001185
20. Lanier LL. NK cell recognition. Annu Rev Immunol (2005) 23:225–74. doi: 10.1146/annurev.immunol.23.021704.115526
21. Lee J, Zhang T, Hwang I, Kim A, Nitschke L, Kim M, et al. Epigenetic modification and antibody-dependent expansion of memory-like NK cells in human cytomegalovirus-infected individuals. Immunity. (2015) 42(3):431–42. doi: 10.1016/j.immuni.2015.02.013
22. Soleimanian S, Yaghobi R. Harnessing memory NK cell to protect against COVID-19. Front Pharmacol (2020) 11:1309. doi: 10.3389/fphar.2020.01309
23. Long EO. Ready for prime time: NK cell priming by dendritic cells. Immunity. (2007) 26(4):385–7. doi: 10.1016/j.immuni.2007.04.001
24. Moretta L, Ferlazzo G, Bottino C, Vitale M, Pende D, Mingari MC, et al. Effector and regulatory events during natural killer-dendritic cell interactions. Immunol Rev (2006) 214:219–28. doi: 10.1111/j.1600-065X.2006.00450.x
25. Piccioli D, Sbrana S, Melandri E, Valiante NM. Contact-dependent stimulation and inhibition of dendritic cells by natural killer cells. J Exp Med (2002) 195(3):335–41. doi: 10.1084/jem.20010934
26. Ferlazzo G, Morandi B. Cross-talks between natural killer cells and distinct subsets of dendritic cells. Front Immunol (2014) 5:159. doi: 10.3389/fimmu.2014.00159
27. Holmes TD, Wilson EB, Black EV, Benest AV, Vaz C, Tan B, et al. Licensed human natural killer cells aid dendritic cell maturation via TNFSF14/LIGHT. Proc Natl Acad Sci U S A. (2014) 111(52):E5688–96. doi: 10.1073/pnas.1411072112
28. Schuster IS, Coudert JD, Andoniou CE, Degli-Esposti MA. "Natural regulators": NK cells as modulators of T cell immunity. Front Immunol (2016) 7:235.
29. Karre K, Ljunggren HG, Piontek G, Kiessling R. Selective rejection of h-2-deficient lymphoma variants suggests alternative immune defence strategy. Nature. (1986) 319(6055):675–8. doi: 10.1038/319675a0
30. Diefenbach A, Jensen ER, Jamieson AM, Raulet DH. Rae1 and H60 ligands of the NKG2D receptor stimulate tumour immunity. Nature. (2001) 413(6852):165–71. doi: 10.1038/35093109
31. Cerwenka A, Baron JL, Lanier LL. Ectopic expression of retinoic acid early inducible-1 gene (RAE-1) permits natural killer cell-mediated rejection of a MHC class I-bearing tumor in vivo. Proc Natl Acad Sci U.S.A. (2001) 98(20):11521–6. doi: 10.1073/pnas.201238598
32. Lorenzi L, Tabellini G, Vermi W, Moratto D, Porta F, Notarangelo LD, et al. Occurrence of nodular lymphocyte-predominant hodgkin lymphoma in hermansky-pudlak type 2 syndrome is associated to natural killer and natural killer T cell defects. PloS One (2013) 8(11):e80131. doi: 10.1371/journal.pone.0080131
33. Spinner MA, Sanchez LA, Hsu AP, Shaw PA, Zerbe CS, Calvo KR, et al. GATA2 deficiency: a protean disorder of hematopoiesis, lymphatics, and immunity. Blood. (2014) 123(6):809–21. doi: 10.1182/blood-2013-07-515528
34. Orange JS. Natural killer cell deficiency. J Allergy Clin Immunol (2013) 132(3):515–25. doi: 10.1016/j.jaci.2013.07.020
35. Imai K, Matsuyama S, Miyake S, Suga K, Nakachi K. Natural cytotoxic activity of peripheral-blood lymphocytes and cancer incidence: an 11-year follow-up study of a general population. Lancet (2000) 356(9244):1795–9. doi: 10.1016/S0140-6736(00)03231-1
36. Albertsson PA, Basse PH, Hokland M, Goldfarb RH, Nagelkerke JF, Nannmark U, et al. NK cells and the tumour microenvironment: implications for NK-cell function and anti-tumour activity. Trends Immunol (2003) 24(11):603–9. doi: 10.1016/j.it.2003.09.007
37. Sullivan EM, Jeha S, Kang G, Cheng C, Rooney B, Holladay M, et al. NK cell genotype and phenotype at diagnosis of acute lymphoblastic leukemia correlate with postinduction residual disease. Clin Cancer Res (2014) 20(23):5986–94. doi: 10.1158/1078-0432.CCR-14-0479
38. Palmer S, Hanson CA, Zent CS, Porrata LF, Laplant B, Geyer SM, et al. Prognostic importance of T and NK-cells in a consecutive series of newly diagnosed patients with chronic lymphocytic leukaemia. Br J Haematol (2008) 141(5):607–14. doi: 10.1111/j.1365-2141.2008.07070.x
39. Mizia-Malarz A, Sobol-Milejska G. NK cells as possible prognostic factor in childhood acute lymphoblastic leukemia. Dis Markers. (2019) 2019:3596983. doi: 10.1155/2019/3596983
40. Raulet DH, Vance RE. Self-tolerance of natural killer cells. Nat Rev Immunol (2006) 6(7):520–31. doi: 10.1038/nri1863
41. Elliott JM, Yokoyama WM. Unifying concepts of MHC-dependent natural killer cell education. Trends Immunol (2011) 32(8):364–72. doi: 10.1016/j.it.2011.06.001
42. Brodin P, Lakshmikanth T, Johansson S, Karre K, Hoglund P. The strength of inhibitory input during education quantitatively tunes the functional responsiveness of individual natural killer cells. Blood. (2009) 113(11):2434–41. doi: 10.1182/blood-2008-05-156836
43. Chiossone L, Dumas PY, Vienne M, Vivier E. Natural killer cells and other innate lymphoid cells in cancer. Nat Rev Immunol (2018) 18(11):671–88. doi: 10.1038/s41577-018-0061-z
44. Joncker NT, Shifrin N, Delebecque F, Raulet DH. Mature natural killer cells reset their responsiveness when exposed to an altered MHC environment. J Exp Med (2010) 207(10):2065–72. doi: 10.1084/jem.20100570
45. Zamora AE, Aguilar EG, Sungur CM, Khuat LT, Dunai C, Lochhead GR, et al. Licensing delineates helper and effector NK cell subsets during viral infection. JCI Insight (2017) 2(10). doi: 10.1172/jci.insight.87032
46. Imai C, Iwamoto S, Campana D. Genetic modification of primary natural killer cells overcomes inhibitory signals and induces specific killing of leukemic cells. Blood. (2005) 106(1):376–83. doi: 10.1182/blood-2004-12-4797
47. Shimasaki N, Jain A, Campana D. NK cells for cancer immunotherapy. Nat Rev Drug Discovery (2020) 19(3):200–18. doi: 10.1038/s41573-019-0052-1
48. Zhang Y, Lv G, Lou X, Peng D, Qu X, Yang X, et al. NKG2A expression and impaired function of NK cells in patients with new onset of graves' disease. Int Immunopharmacol. (2015) 24(1):133–9. doi: 10.1016/j.intimp.2014.09.020
49. Eissmann P, Beauchamp L, Wooters J, Tilton JC, Long EO, Watzl C. Molecular basis for positive and negative signaling by the natural killer cell receptor 2B4 (CD244). Blood. (2005) 105(12):4722–9. doi: 10.1182/blood-2004-09-3796
50. Arch RH, Thompson CB. 4-1BB and Ox40 are members of a tumor necrosis factor (TNF)-nerve growth factor receptor subfamily that bind TNF receptor-associated factors and activate nuclear factor kappaB. Mol Cell Biol (1998) 18(1):558–65. doi: 10.1128/MCB.18.1.558
51. Morvan MG, Lanier LL. NK cells and cancer: you can teach innate cells new tricks. Nat Rev Cancer. (2016) 16(1):7–19. doi: 10.1038/nrc.2015.5
52. Guillerey C, Huntington ND, Smyth MJ. Targeting natural killer cells in cancer immunotherapy. Nat Immunol (2016) 17(9):1025–36. doi: 10.1038/ni.3518
53. Houchins JP, Lanier LL, Niemi EC, Phillips JH, Ryan JC. Natural killer cell cytolytic activity is inhibited by NKG2-a and activated by NKG2-c. J Immunol (1997) 158(8):3603–9.
54. Carretero M, Cantoni C, Bellon T, Bottino C, Biassoni R, Rodriguez A, et al. The CD94 and NKG2-a c-type lectins covalently assemble to form a natural killer cell inhibitory receptor for HLA class I molecules. Eur J Immunol (1997) 27(2):563–7. doi: 10.1002/eji.1830270230
55. Kaiser BK, Pizarro JC, Kerns J, Strong RK. Structural basis for NKG2A/CD94 recognition of HLA-e. Proc Natl Acad Sci U S A. (2008) 105(18):6696–701. doi: 10.1073/pnas.0802736105
56. Ferris RL, Jaffee EM, Ferrone S. Tumor antigen-targeted, monoclonal antibody-based immunotherapy: clinical response, cellular immunity, and immunoescape. J Clin Oncol (2010) 28(28):4390–9. doi: 10.1200/JCO.2009.27.6360
57. Bournazos S, Wang TT, Dahan R, Maamary J, Ravetch JV. Signaling by antibodies: Recent progress. Annu Rev Immunol (2017) 35:285–311. doi: 10.1146/annurev-immunol-051116-052433
58. Bryceson YT, March ME, Ljunggren HG, Long EO. Synergy among receptors on resting NK cells for the activation of natural cytotoxicity and cytokine secretion. Blood. (2006) 107(1):159–66. doi: 10.1182/blood-2005-04-1351
59. Fehniger TA, Caligiuri MA. Interleukin 15: biology and relevance to human disease. Blood. (2001) 97(1):14–32. doi: 10.1182/blood.V97.1.14
60. Trinchieri G, Matsumoto-Kobayashi M, Clark SC, Seehra J, London L, Perussia B. Response of resting human peripheral blood natural killer cells to interleukin 2. J Exp Med (1984) 160(4):1147–69. doi: 10.1084/jem.160.4.1147
61. Prlic M, Blazar BR, Farrar MA, Jameson SC. In vivo survival and homeostatic proliferation of natural killer cells. J Exp Med (2003) 197(8):967–76. doi: 10.1084/jem.20021847
62. Miller JS, Morishima C, McNeel DG, Patel MR, Kohrt HEK, Thompson JA, et al. A first-in-Human phase I study of subcutaneous outpatient recombinant human IL15 (rhIL15) in adults with advanced solid tumors. Clin Cancer Res (2018) 24(7):1525–35. doi: 10.1158/1078-0432.CCR-17-2451
63. Dubois S, Mariner J, Waldmann TA, Tagaya Y. IL-15Ralpha recycles and presents IL-15 in trans to neighboring cells. Immunity. (2002) 17(5):537–47. doi: 10.1016/S1074-7613(02)00429-6
64. Kobayashi H, Dubois S, Sato N, Sabzevari H, Sakai Y, Waldmann TA, et al. Role of trans-cellular IL-15 presentation in the activation of NK cell-mediated killing, which leads to enhanced tumor immunosurveillance. Blood. (2005) 105(2):721–7. doi: 10.1182/blood-2003-12-4187
65. Imamura M, Shook D, Kamiya T, Shimasaki N, Chai SM, Coustan-Smith E, et al. Autonomous growth and increased cytotoxicity of natural killer cells expressing membrane-bound interleukin-15. Blood. (2014) 124(7):1081–8. doi: 10.1182/blood-2014-02-556837
66. Delconte RB, Kolesnik TB, Dagley LF, Rautela J, Shi W, Putz EM, et al. CIS is a potent checkpoint in NK cell-mediated tumor immunity. Nat Immunol (2016) 17(7):816–24. doi: 10.1038/ni.3470
67. Parrish-Novak J, Dillon SR, Nelson A, Hammond A, Sprecher C, Gross JA, et al. Interleukin 21 and its receptor are involved in NK cell expansion and regulation of lymphocyte function. Nature. (2000) 408(6808):57–63. doi: 10.1038/35040504
68. Fehniger TA, Shah MH, Turner MJ, VanDeusen JB, Whitman SP, Cooper MA, et al. Differential cytokine and chemokine gene expression by human NK cells following activation with IL-18 or IL-15 in combination with IL-12: implications for the innate immune response. J Immunol (1999) 162(8):4511–20.
69. Romee R, Rosario M, Berrien-Elliott MM, Wagner JA, Jewell BA, Schappe T, et al. Cytokine-induced memory-like natural killer cells exhibit enhanced responses against myeloid leukemia. Sci Transl Med (2016) 8(357):357ra123. doi: 10.1126/scitranslmed.aaf2341
70. Saez-Borderias A, Romo N, Magri G, Guma M, Angulo A, Lopez-Botet M. IL-12-dependent inducible expression of the CD94/NKG2A inhibitory receptor regulates CD94/NKG2C+ NK cell function. J Immunol (2009) 182(2):829–36. doi: 10.4049/jimmunol.182.2.829
71. Kasaian MT, Whitters MJ, Carter LL, Lowe LD, Jussif JM, Deng B, et al. IL-21 limits NK cell responses and promotes antigen-specific T cell activation: a mediator of the transition from innate to adaptive immunity. Immunity. (2002) 16(4):559–69. doi: 10.1016/S1074-7613(02)00295-9
72. Orange JS. Formation and function of the lytic NK-cell immunological synapse. Nat Rev Immunol (2008) 8(9):713–25. doi: 10.1038/nri2381
73. Gwalani LA, Orange JS. Single degranulations in NK cells can mediate target cell killing. J Immunol (2018) 200(9):3231–43. doi: 10.4049/jimmunol.1701500
74. Prager I, Liesche C, van Ooijen H, Urlaub D, Verron Q, Sandstrom N, et al. NK cells switch from granzyme b to death receptor-mediated cytotoxicity during serial killing. J Exp Med (2019) 216(9):2113–27. doi: 10.1084/jem.20181454
75. Bottcher JP, Bonavita E, Chakravarty P, Blees H, Cabeza-Cabrerizo M, Sammicheli S, et al. NK cells stimulate recruitment of cDC1 into the tumor microenvironment promoting cancer immune control. Cell. (2018) 172(5):1022–37 e14. doi: 10.1016/j.cell.2018.01.004
76. Barry KC, Hsu J, Broz ML, Cueto FJ, Binnewies M, Combes AJ, et al. A natural killer-dendritic cell axis defines checkpoint therapy-responsive tumor microenvironments. Nat Med (2018) 24(8):1178–91. doi: 10.1038/s41591-018-0085-8
77. Voigt J, Hunniger K, Bouzani M, Jacobsen ID, Barz D, Hube B, et al. Human natural killer cells acting as phagocytes against candida albicans and mounting an inflammatory response that modulates neutrophil antifungal activity. J Infect Dis (2014) 209(4):616–26. doi: 10.1093/infdis/jit574
78. Voskoboinik I, Whisstock JC, Trapani JA. Perforin and granzymes: function, dysfunction and human pathology. Nat Rev Immunol (2015) 15(6):388–400. doi: 10.1038/nri3839
79. Schmidt S, Tramsen L, Perkhofer S, Lass-Florl C, Hanisch M, Roger F, et al. Rhizopus oryzae hyphae are damaged by human natural killer (NK) cells, but suppress NK cell mediated immunity. Immunobiology. (2013) 218(7):939–44. doi: 10.1016/j.imbio.2012.10.013
80. Schmidt S, Tramsen L, Hanisch M, Latge JP, Huenecke S, Koehl U, et al. Human natural killer cells exhibit direct activity against aspergillus fumigatus hyphae, but not against resting conidia. J Infect Dis (2011) 203(3):430–5. doi: 10.1093/infdis/jiq062
81. Weiss E, Ziegler S, Fliesser M, Schmitt AL, Hunniger K, Kurzai O, et al. First insights in NK-DC cross-talk and the importance of soluble factors during infection with aspergillus fumigatus. Front Cell Infect Microbiol (2018) 8:288. doi: 10.3389/fcimb.2018.00288
82. Azuma M, Sawahata R, Akao Y, Ebihara T, Yamazaki S, Matsumoto M, et al. The peptide sequence of diacyl lipopeptides determines dendritic cell TLR2-mediated NK activation. PloS One (2010) 5(9). doi: 10.1371/journal.pone.0012550
83. Soe WM, Lim JHJ, Williams DL, Goh JG, Tan Z, Sam QH, et al. Using expanded natural killer cells as therapy for invasive aspergillosis. J Fungi (Basel) (2020) 6(4). doi: 10.3390/jof6040231
84. Li SS, Kyei SK, Timm-McCann M, Ogbomo H, Jones GJ, Shi M, et al. The NK receptor NKp30 mediates direct fungal recognition and killing and is diminished in NK cells from HIV-infected patients. Cell Host Microbe (2013) 14(4):387–97. doi: 10.1016/j.chom.2013.09.007
85. Picard LK, Claus M, Fasbender F, Watzl C. Human NK cells responses are enhanced by CD56 engagement. Eur J Immunol (2022) 52(9):1441–51. doi: 10.1002/eji.202249868
86. Vitenshtein A, Charpak-Amikam Y, Yamin R, Bauman Y, Isaacson B, Stein N, et al. NK cell recognition of candida glabrata through binding of NKp46 and NCR1 to fungal ligands Epa1, Epa6, and Epa7. Cell Host Microbe (2016) 20(4):527–34. doi: 10.1016/j.chom.2016.09.008
87. Charpak-Amikam Y, Lapidus T, Isaacson B, Duev-Cohen A, Levinson T, Elbaz A, et al. Candida albicans evades NK cell elimination via binding of agglutinin-like sequence proteins to the checkpoint receptor TIGIT. Nat Commun (2022) 13(1):2463. doi: 10.1038/s41467-022-30087-z
88. Ziegler S, Weiss E, Schmitt AL, Schlegel J, Burgert A, Terpitz U, et al. CD56 is a pathogen recognition receptor on human natural killer cells. Sci Rep (2017) 7(1):6138. doi: 10.1038/s41598-017-06238-4
89. Tripp CS, Wolf SF, Unanue ER. Interleukin 12 and tumor necrosis factor alpha are costimulators of interferon gamma production by natural killer cells in severe combined immunodeficiency mice with listeriosis, and interleukin 10 is a physiologic antagonist. Proc Natl Acad Sci U S A. (1993) 90(8):3725–9. doi: 10.1073/pnas.90.8.3725
90. Scharton TM, Scott P. Natural killer cells are a source of interferon gamma that drives differentiation of CD4+ T cell subsets and induces early resistance to leishmania major in mice. J Exp Med (1993) 178(2):567–77. doi: 10.1084/jem.178.2.567
91. Morrison BE, Park SJ, Mooney JM, Mehrad B. Chemokine-mediated recruitment of NK cells is a critical host defense mechanism in invasive aspergillosis. J Clin Invest. (2003) 112(12):1862–70. doi: 10.1172/JCI18125
92. Park SJ, Hughes MA, Burdick M, Strieter RM, Mehrad B. Early NK cell-derived IFN-{gamma} is essential to host defense in neutropenic invasive aspergillosis. J Immunol (2009) 182(7):4306–12. doi: 10.4049/jimmunol.0803462
93. Kawakami K, Koguchi Y, Qureshi MH, Yara S, Kinjo Y, Uezu K, et al. NK cells eliminate cryptococcus neoformans by potentiating the fungicidal activity of macrophages rather than by directly killing them upon stimulation with IL-12 and IL-18. Microbiol Immunol (2000) 44(12):1043–50. doi: 10.1111/j.1348-0421.2000.tb02601.x
94. Zhang T, Kawakami K, Qureshi MH, Okamura H, Kurimoto M, Saito A. Interleukin-12 (IL-12) and IL-18 synergistically induce the fungicidal activity of murine peritoneal exudate cells against cryptococcus neoformans through production of gamma interferon by natural killer cells. Infect Immun (1997) 65(9):3594–9. doi: 10.1128/iai.65.9.3594-3599.1997
95. Marischen L, Englert A, Schmitt AL, Einsele H, Loeffler J. Human NK cells adapt their immune response towards increasing multiplicities of infection of aspergillus fumigatus. BMC Immunol (2018) 19(1):39. doi: 10.1186/s12865-018-0276-6
96. Robertson MJ. Role of chemokines in the biology of natural killer cells. J Leukoc Biol (2002) 71(2):173–83. doi: 10.1189/jlb.71.2.173
97. Mehrad B, Strieter RM, Moore TA, Tsai WC, Lira SA, Standiford TJ. CXC chemokine receptor-2 ligands are necessary components of neutrophil-mediated host defense in invasive pulmonary aspergillosis. J Immunol (1999) 163(11):6086–94.
98. Jimenez BE, Murphy JW. In vitro effects of natural killer cells against paracoccidioides brasiliensis yeast phase. Infect Immun (1984) 46(2):552–8. doi: 10.1128/iai.46.2.552-558.1984
99. Nabavi N, Murphy JW. In vitro binding of natural killer cells to cryptococcus neoformans targets. Infect Immun (1985) 50(1):50–7. doi: 10.1128/iai.50.1.50-57.1985
100. Hidore MR, Nabavi N, Sonleitner F, Murphy JW. Murine natural killer cells are fungicidal to cryptococcus neoformans. Infect Immun (1991) 59(5):1747–54. doi: 10.1128/iai.59.5.1747-1754.1991
101. Petkus AF, Baum LL. Natural killer cell inhibition of young spherules and endospores of coccidioides immitis. J Immunol (1987) 139(9):3107–11.
102. Lehmann C, Zeis M, Uharek L. Activation of natural killer cells with interleukin 2 (IL-2) and IL-12 increases perforin binding and subsequent lysis of tumour cells. Br J Haematol (2001) 114(3):660–5. doi: 10.1046/j.1365-2141.2001.02995.x
103. Levitz SM, Dupont MP, Smail EH. Direct activity of human T lymphocytes and natural killer cells against cryptococcus neoformans. Infect Immun (1994) 62(1):194–202. doi: 10.1128/iai.62.1.194-202.1994
104. Ma LL, Wang CL, Neely GG, Epelman S, Krensky AM, Mody CH. NK cells use perforin rather than granulysin for anticryptococcal activity. J Immunol (2004) 173(5):3357–65. doi: 10.4049/jimmunol.173.5.3357
105. Wiseman JC, Ma LL, Marr KJ, Jones GJ, Mody CH. Perforin-dependent cryptococcal microbicidal activity in NK cells requires PI3K-dependent ERK1/2 signaling. J Immunol (2007) 178(10):6456–64. doi: 10.4049/jimmunol.178.10.6456
106. Ruggeri L, Capanni M, Urbani E, Perruccio K, Shlomchik WD, Tosti A, et al. Effectiveness of donor natural killer cell alloreactivity in mismatched hematopoietic transplants. Science. (2002) 295(5562):2097–100. doi: 10.1126/science.1068440
107. Miller JS, Soignier Y, Panoskaltsis-Mortari A, McNearney SA, Yun GH, Fautsch SK, et al. Successful adoptive transfer and in vivo expansion of human haploidentical NK cells in patients with cancer. Blood. (2005) 105(8):3051–7. doi: 10.1182/blood-2004-07-2974
108. Muller S, Schulz A, Reiss U, Schwarz K, Schreiner T, Wiesneth M, et al. Definition of a critical T cell threshold for prevention of GVHD after HLA non-identical PBPC transplantation in children. Bone Marrow Transplant. (1999) 24(6):575–81. doi: 10.1038/sj.bmt.1701970
109. Shah NN, Baird K, Delbrook CP, Fleisher TA, Kohler ME, Rampertaap S, et al. Acute GVHD in patients receiving IL-15/4-1BBL activated NK cells following T-cell-depleted stem cell transplantation. Blood. (2015) 125(5):784–92. doi: 10.1182/blood-2014-07-592881
110. Shimasaki N, Coustan-Smith E, Kamiya T, Campana D. Expanded and armed natural killer cells for cancer treatment. Cytotherapy. (2016) 18(11):1422–34. doi: 10.1016/j.jcyt.2016.06.013
111. Lee DA, Verneris MR, Campana D. Acquisition, preparation, and functional assessment of human NK cells for adoptive immunotherapy. Methods Mol Biol (2010) 651:61–77. doi: 10.1007/978-1-60761-786-0_4
112. Williams SM, Sumstad D, Kadidlo D, Curtsinger J, Luo X, Miller JS, et al. Clinical-scale production of cGMP compliant CD3/CD19 cell-depleted NK cells in the evolution of NK cell immunotherapy at a single institution. Transfusion. (2018) 58(6):1458–67. doi: 10.1111/trf.14564
113. Fujisaki H, Kakuda H, Shimasaki N, Imai C, Ma J, Lockey T, et al. Expansion of highly cytotoxic human natural killer cells for cancer cell therapy. Cancer Res (2009) 69(9):4010–7. doi: 10.1158/0008-5472.CAN-08-3712
114. Mao Y, van Hoef V, Zhang X, Wennerberg E, Lorent J, Witt K, et al. IL-15 activates mTOR and primes stress-activated gene expression leading to prolonged antitumor capacity of NK cells. Blood. (2016) 128(11):1475–89. doi: 10.1182/blood-2016-02-698027
115. van Ostaijen-ten Dam MM, Prins HJ, Boerman GH, Vervat C, Pende D, Putter H, et al. Preparation of cytokine-activated NK cells for use in adoptive cell therapy in cancer patients: Protocol optimization and therapeutic potential. J Immunother. (2016) 39(2):90–100. doi: 10.1097/CJI.0000000000000110
116. Felices M, Lenvik AJ, McElmurry R, Chu S, Hinderlie P, Bendzick L, et al. Continuous treatment with IL-15 exhausts human NK cells via a metabolic defect. JCI Insight (2018) 3(3). doi: 10.1172/jci.insight.96219
117. Leong JW, Chase JM, Romee R, Schneider SE, Sullivan RP, Cooper MA, et al. Preactivation with IL-12, IL-15, and IL-18 induces CD25 and a functional high-affinity IL-2 receptor on human cytokine-induced memory-like natural killer cells. Biol Blood Marrow Transplant. (2014) 20(4):463–73. doi: 10.1016/j.bbmt.2014.01.006
118. Cichocki F, Valamehr B, Bjordahl R, Zhang B, Rezner B, Rogers P, et al. GSK3 inhibition drives maturation of NK cells and enhances their antitumor activity. Cancer Res (2017) 77(20):5664–75. doi: 10.1158/0008-5472.CAN-17-0799
119. Kotylo PK, Baenzinger JC, Yoder MC, Engle WA, Bolinger CD. Rapid analysis of lymphocyte subsets in cord blood. Am J Clin Pathol (1990) 93(2):263–6. doi: 10.1093/ajcp/93.2.263
120. Wang Y, Xu H, Zheng X, Wei H, Sun R, Tian Z. High expression of NKG2A/CD94 and low expression of granzyme b are associated with reduced cord blood NK cell activity. Cell Mol Immunol (2007) 4(5):377–82.
121. Herrera L, Santos S, Vesga MA, Anguita J, Martin-Ruiz I, Carrascosa T, et al. Adult peripheral blood and umbilical cord blood NK cells are good sources for effective CAR therapy against CD19 positive leukemic cells. Sci Rep (2019) 9(1):18729. doi: 10.1038/s41598-019-55239-y
122. Spanholtz J, Preijers F, Tordoir M, Trilsbeek C, Paardekooper J, de Witte T, et al. Clinical-grade generation of active NK cells from cord blood hematopoietic progenitor cells for immunotherapy using a closed-system culture process. PloS One (2011) 6(6):e20740. doi: 10.1371/journal.pone.0020740
123. Luevano M, Madrigal A, Saudemont A. Generation of natural killer cells from hematopoietic stem cells in vitro for immunotherapy. Cell Mol Immunol (2012) 9(4):310–20. doi: 10.1038/cmi.2012.17
124. Knorr DA, Ni Z, Hermanson D, Hexum MK, Bendzick L, Cooper LJ, et al. Clinical-scale derivation of natural killer cells from human pluripotent stem cells for cancer therapy. Stem Cells Transl Med (2013) 2(4):274–83. doi: 10.5966/sctm.2012-0084
125. Li Y, Hermanson DL, Moriarity BS, Kaufman DS. Human iPSC-derived natural killer cells engineered with chimeric antigen receptors enhance anti-tumor activity. Cell Stem Cell (2018) 23(2):181–92 e5. doi: 10.1016/j.stem.2018.06.002
126. Denman CJ, Senyukov VV, Somanchi SS, Phatarpekar PV, Kopp LM, Johnson JL, et al. Membrane-bound IL-21 promotes sustained ex vivo proliferation of human natural killer cells. PloS One (2012) 7(1):e30264. doi: 10.1371/journal.pone.0030264
127. Knorr DA, Bachanova V, Verneris MR, Miller JS. Clinical utility of natural killer cells in cancer therapy and transplantation. Semin Immunol (2014) 26(2):161–72. doi: 10.1016/j.smim.2014.02.002
128. Gong JH, Maki G, Klingemann HG. Characterization of a human cell line (NK-92) with phenotypical and functional characteristics of activated natural killer cells. Leukemia. (1994) 8(4):652–8.
129. Maki G, Klingemann HG, Martinson JA, Tam YK. Factors regulating the cytotoxic activity of the human natural killer cell line, NK-92. J Hematother Stem Cell Res (2001) 10(3):369–83. doi: 10.1089/152581601750288975
130. Gong Y, Herberman RR, Reynolds CW. The kinetics of cytoplasmic granule secretion in natural killer cytotoxicity. Chin Med Sci J (1994) 9(3):147–51.
131. Tam YK, Martinson JA, Doligosa K, Klingemann HG. Ex vivo expansion of the highly cytotoxic human natural killer-92 cell-line under current good manufacturing practice conditions for clinical adoptive cellular immunotherapy. Cytotherapy. (2003) 5(3):259–72.
132. Klingemann HG, Wong E, Maki G. A cytotoxic NK-cell line (NK-92) for ex vivo purging of leukemia from blood. Biol Blood Marrow Transplant. (1996) 2(2):68–75.
133. Arai S, Meagher R, Swearingen M, Myint H, Rich E, Martinson J, et al. Infusion of the allogeneic cell line NK-92 in patients with advanced renal cell cancer or melanoma: a phase I trial. Cytotherapy. (2008) 10(6):625–32. doi: 10.1080/14653240802301872
134. Tonn T, Schwabe D, Klingemann HG, Becker S, Esser R, Koehl U, et al. Treatment of patients with advanced cancer with the natural killer cell line NK-92. Cytotherapy. (2013) 15(12):1563–70. doi: 10.1016/j.jcyt.2013.06.017
135. Boyiadzis M, Agha M, Redner RL, Sehgal A, Im A, Hou JZ, et al. Phase 1 clinical trial of adoptive immunotherapy using "off-the-shelf" activated natural killer cells in patients with refractory and relapsed acute myeloid leukemia. Cytotherapy. (2017) 19(10):1225–32. doi: 10.1016/j.jcyt.2017.07.008
136. Williams BA, Law AD, Routy B, denHollander N, Gupta V, Wang XH, et al. A phase I trial of NK-92 cells for refractory hematological malignancies relapsing after autologous hematopoietic cell transplantation shows safety and evidence of efficacy. Oncotarget. (2017) 8(51):89256–68. doi: 10.18632/oncotarget.19204
137. Boissel L, Betancur-Boissel M, Lu W, Krause DS, Van Etten RA, Wels WS, et al. Retargeting NK-92 cells by means of CD19- and CD20-specific chimeric antigen receptors compares favorably with antibody-dependent cellular cytotoxicity. Oncoimmunology. (2013) 2(10):e26527. doi: 10.4161/onci.26527
138. Oelsner S, Friede ME, Zhang C, Wagner J, Badura S, Bader P, et al. Continuously expanding CAR NK-92 cells display selective cytotoxicity against b-cell leukemia and lymphoma. Cytotherapy. (2017) 19(2):235–49. doi: 10.1016/j.jcyt.2016.10.009
139. Liu Q, Xu Y, Mou J, Tang K, Fu X, Li Y, et al. Irradiated chimeric antigen receptor engineered NK-92MI cells show effective cytotoxicity against CD19(+) malignancy in a mouse model. Cytotherapy. (2020) 22(10):552–62. doi: 10.1016/j.jcyt.2020.06.003
140. Tang X, Yang L, Li Z, Nalin AP, Dai H, Xu T, et al. First-in-man clinical trial of CAR NK-92 cells: safety test of CD33-CAR NK-92 cells in patients with relapsed and refractory acute myeloid leukemia. Am J Cancer Res (2018) 8(6):1083–9.
141. Phillips JH, Lanier LL. A model for the differentiation of human natural killer cells. studies on the in vitro activation of leu-11+ granular lymphocytes with a natural killer-sensitive tumor cell, K562. J Exp Med (1985) 161(6):1464–82.
142. Lapteva N, Durett AG, Sun J, Rollins LA, Huye LL, Fang J, et al. Large-Scale ex vivo expansion and characterization of natural killer cells for clinical applications. Cytotherapy. (2012) 14(9):1131–43. doi: 10.3109/14653249.2012.700767
143. Ojo EO, Sharma AA, Liu R, Moreton S, Checkley-Luttge MA, Gupta K, et al. Membrane bound IL-21 based NK cell feeder cells drive robust expansion and metabolic activation of NK cells. Sci Rep (2019) 9(1):14916. doi: 10.1038/s41598-019-51287-6
144. Ciurea SO, Schafer JR, Bassett R, Denman CJ, Cao K, Willis D, et al. Phase 1 clinical trial using mbIL21 ex vivo-expanded donor-derived NK cells after haploidentical transplantation. Blood. (2017) 130(16):1857–68. doi: 10.1182/blood-2017-05-785659
145. Kweon S, Phan MT, Chun S, Yu H, Kim J, Kim S, et al. Expansion of human NK cells using K562 cells expressing OX40 ligand and short exposure to IL-21. Front Immunol (2019) 10:879. doi: 10.3389/fimmu.2019.00879
146. Rubnitz JE, Inaba H, Ribeiro RC, Pounds S, Rooney B, Bell T, et al. NKAML: a pilot study to determine the safety and feasibility of haploidentical natural killer cell transplantation in childhood acute myeloid leukemia. J Clin Oncol (2010) 28(6):955–9. doi: 10.1200/JCO.2009.24.4590
147. Williams RL, Cooley S, Bachanova V, Blazar BR, Weisdorf DJ, Miller JS, et al. Recipient T cell exhaustion and successful adoptive transfer of haploidentical natural killer cells. Biol Blood Marrow Transplant. (2018) 24(3):618–22. doi: 10.1016/j.bbmt.2017.11.022
148. Dolstra H, Roeven MWH, Spanholtz J, Hangalapura BN, Tordoir M, Maas F, et al. Successful transfer of umbilical cord blood CD34(+) hematopoietic stem and progenitor-derived NK cells in older acute myeloid leukemia patients. Clin Cancer Res (2017) 23(15):4107–18. doi: 10.1158/1078-0432.CCR-16-2981
149. Parkhurst MR, Riley JP, Dudley ME, Rosenberg SA. Adoptive transfer of autologous natural killer cells leads to high levels of circulating natural killer cells but does not mediate tumor regression. Clin Cancer Res (2011) 17(19):6287–97. doi: 10.1158/1078-0432.CCR-11-1347
150. Curti A, Ruggeri L, D'Addio A, Bontadini A, Dan E, Motta MR, et al. Successful transfer of alloreactive haploidentical KIR ligand-mismatched natural killer cells after infusion in elderly high risk acute myeloid leukemia patients. Blood. (2011) 118(12):3273–9. doi: 10.1182/blood-2011-01-329508
151. Cooley S, He F, Bachanova V, Vercellotti GM, DeFor TE, Curtsinger JM, et al. First-in-human trial of rhIL-15 and haploidentical natural killer cell therapy for advanced acute myeloid leukemia. Blood Adv (2019) 3(13):1970–80. doi: 10.1182/bloodadvances.2018028332
152. Myers JA, Miller JS. Exploring the NK cell platform for cancer immunotherapy. Nat Rev Clin Oncol (2021) 18(2):85–100. doi: 10.1038/s41571-020-0426-7
153. Pachella LA, Madsen LT, Dains JE. The toxicity and benefit of various dosing strategies for interleukin-2 in metastatic melanoma and renal cell carcinoma. J Adv Pract Oncol (2015) 6(3):212–21.
154. Felices M, Chu S, Kodal B, Bendzick L, Ryan C, Lenvik AJ, et al. IL-15 super-agonist (ALT-803) enhances natural killer (NK) cell function against ovarian cancer. Gynecol Oncol (2017) 145(3):453–61. doi: 10.1016/j.ygyno.2017.02.028
155. Rosowski EE, He J, Huisken J, Keller NP, Huttenlocher A. Efficacy of voriconazole against aspergillus fumigatus infection depends on host immune function. Antimicrob Agents Chemother (2020) 64(2). doi: 10.1128/AAC.00917-19
156. Shimasaki N, Fujisaki H, Cho D, Masselli M, Lockey T, Eldridge P, et al. A clinically adaptable method to enhance the cytotoxicity of natural killer cells against b-cell malignancies. Cytotherapy. (2012) 14(7):830–40. doi: 10.3109/14653249.2012.671519
157. Shimasaki N, Campana D. Natural killer cell reprogramming with chimeric immune receptors. Methods Mol Biol (2013) 969:203–20. doi: 10.1007/978-1-62703-260-5_13
158. Laskowski TJ, Biederstadt A, Rezvani K. Natural killer cells in antitumour adoptive cell immunotherapy. Nat Rev Cancer. (2022). doi: 10.1038/s41568-022-00491-0
159. Liu E, Marin D, Banerjee P, Macapinlac HA, Thompson P, Basar R, et al. Use of CAR-transduced natural killer cells in CD19-positive lymphoid tumors. N Engl J Med (2020) 382(6):545–53. doi: 10.1056/NEJMoa1910607
160. Frigault MJ, Lee J, Basil MC, Carpenito C, Motohashi S, Scholler J, et al. Identification of chimeric antigen receptors that mediate constitutive or inducible proliferation of T cells. Cancer Immunol Res (2015) 3(4):356–67. doi: 10.1158/2326-6066.CIR-14-0186
161. Long AH, Haso WM, Shern JF, Wanhainen KM, Murgai M, Ingaramo M, et al. 4-1BB costimulation ameliorates T cell exhaustion induced by tonic signaling of chimeric antigen receptors. Nat Med (2015) 21(6):581–90. doi: 10.1038/nm.3838
162. Muller YD, Nguyen DP, Ferreira LMR, Ho P, Raffin C, Valencia RVB, et al. The CD28-transmembrane domain mediates chimeric antigen receptor heterodimerization with CD28. Front Immunol (2021) 12:639818. doi: 10.3389/fimmu.2021.639818
163. van de Veerdonk FL, Gresnigt MS, Romani L, Netea MG, Latge JP. Aspergillus fumigatus morphology and dynamic host interactions. Nat Rev Microbiol (2017) 15(11):661–74. doi: 10.1038/nrmicro.2017.90
164. Netea MG, Brown GD, Kullberg BJ, Gow NA. An integrated model of the recognition of candida albicans by the innate immune system. Nat Rev Microbiol (2008) 6(1):67–78. doi: 10.1038/nrmicro1815
165. Hohl TM, Van Epps HL, Rivera A, Morgan LA, Chen PL, Feldmesser M, et al. Aspergillus fumigatus triggers inflammatory responses by stage-specific beta-glucan display. PloS Pathog (2005) 1(3):e30.
166. Lamaris GA, Lewis RE, Chamilos G, May GS, Safdar A, Walsh TJ, et al. Caspofungin-mediated beta-glucan unmasking and enhancement of human polymorphonuclear neutrophil activity against aspergillus and non-aspergillus hyphae. J Infect Dis (2008) 198(2):186–92. doi: 10.1086/589305
167. Kumaresan PR, Manuri PR, Albert ND, Maiti S, Singh H, Mi T, et al. Bioengineering T cells to target carbohydrate to treat opportunistic fungal infection. Proc Natl Acad Sci U S A. (2014) 111(29):10660–5. doi: 10.1073/pnas.1312789111
168. Liu E, Tong Y, Dotti G, Shaim H, Savoldo B, Mukherjee M, et al. Cord blood NK cells engineered to express IL-15 and a CD19-targeted CAR show long-term persistence and potent antitumor activity. Leukemia. (2018) 32(2):520–31. doi: 10.1038/leu.2017.226
169. Chang YH, Connolly J, Shimasaki N, Mimura K, Kono K, Campana D. A chimeric receptor with NKG2D specificity enhances natural killer cell activation and killing of tumor cells. Cancer Res (2013) 73(6):1777–86. doi: 10.1158/0008-5472.CAN-12-3558
170. Kamiya T, Seow SV, Wong D, Robinson M, Campana D. Blocking expression of inhibitory receptor NKG2A overcomes tumor resistance to NK cells. J Clin Invest. (2019) 129(5):2094–106. doi: 10.1172/JCI123955
171. Miller JS. Therapeutic applications: natural killer cells in the clinic. Hematol Am Soc Hematol Educ Program. (2013) 2013:247–53. doi: 10.1182/asheducation-2013.1.247
172. Tarannum M, Romee R. Cytokine-induced memory-like natural killer cells for cancer immunotherapy. Stem Cell Res Ther (2021) 12(1):592. doi: 10.1186/s13287-021-02655-5
173. Anton OM, Peterson ME, Hollander MJ, Dorward DW, Arora G, Traba J, et al. Trans-endocytosis of intact IL-15Ralpha-IL-15 complex from presenting cells into NK cells favors signaling for proliferation. Proc Natl Acad Sci U S A. (2020) 117(1):522–31. doi: 10.1073/pnas.1911678117
174. Moretta F, Petronelli F, Lucarelli B, Pitisci A, Bertaina A, Locatelli F, et al. The generation of human innate lymphoid cells is influenced by the source of hematopoietic stem cells and by the use of G-CSF. Eur J Immunol (2016) 46(5):1271–8. doi: 10.1002/eji.201546079
175. Saetersmoen ML, Hammer Q, Valamehr B, Kaufman DS, Malmberg KJ. Off-the-shelf cell therapy with induced pluripotent stem cell-derived natural killer cells. Semin Immunopathol (2019) 41(1):59–68. doi: 10.1007/s00281-018-0721-x
176. Kuijk E, Jager M, van der Roest B, Locati MD, Van Hoeck A, Korzelius J, et al. The mutational impact of culturing human pluripotent and adult stem cells. Nat Commun (2020) 11(1):2493. doi: 10.1038/s41467-020-16323-4
177. Mitwasi N, Feldmann A, Arndt C, Koristka S, Berndt N, Jureczek J, et al. "UniCAR"-modified off-the-shelf NK-92 cells for targeting of GD2-expressing tumour cells. Sci Rep (2020) 10(1):2141. doi: 10.1038/s41598-020-59082-4
Keywords: augmentation, cytokines, chimeric antigen receptor, adoptive cellular therapy, innate immunity
Citation: Oh BLZ, Chan LWY and Chai LYA (2023) Manipulating NK cellular therapy from cancer to invasive fungal infection: promises and challenges. Front. Immunol. 13:1044946. doi: 10.3389/fimmu.2022.1044946
Received: 16 September 2022; Accepted: 09 December 2022;
Published: 11 January 2023.
Edited by:
Emilie Narni-Mancinelli, INSERM U1104 Centre d’immunologie de Marseille-Luminy (CIML), FranceReviewed by:
Noëlla Lopes, INSERM U1104 Centre d’immunologie de Marseille-Luminy (CIML), FranceCyril Fauriat, INSERM U1068 Centre de Recherche en Cancérologie de Marseille (CRCM), France
Copyright © 2023 Oh, Chan and Chai. This is an open-access article distributed under the terms of the Creative Commons Attribution License (CC BY). The use, distribution or reproduction in other forums is permitted, provided the original author(s) and the copyright owner(s) are credited and that the original publication in this journal is cited, in accordance with accepted academic practice. No use, distribution or reproduction is permitted which does not comply with these terms.
*Correspondence: Louis Yi Ann Chai, chailouis@hotmail.com