- 1State Key Laboratory for Diagnosis and Treatment of Infectious Diseases, National Clinical Research Center for Infectious Diseases, National Medical Center for Infectious Diseases, Collaborative Innovation Center for Diagnosis and Treatment of Infectious Diseases, The First Affiliated Hospital, Zhejiang University School of Medicine, Hangzhou, China
- 2Zhejiang University School of Medicine, Hangzhou, Zhejiang, China
Natural killer T cells (NKTs) are an important part of the immune system. Since their discovery in the 1990s, researchers have gained deeper insights into the physiology and functions of these cells in many liver diseases. NKT cells are divided into two subsets, type I and type II. Type I NKT cells are also named iNKT cells as they express a semi-invariant T cell-receptor (TCR) α chain. As part of the innate immune system, hepatic iNKT cells interact with hepatocytes, macrophages (Kupffer cells), T cells, and dendritic cells through direct cell-to-cell contact and cytokine secretion, bridging the innate and adaptive immune systems. A better understanding of hepatic iNKT cells is necessary for finding new methods of treating liver disease including autoimmune liver diseases, alcoholic liver diseases (ALDs), non-alcoholic fatty liver diseases (NAFLDs), and liver tumors. Here we summarize how iNKT cells are activated, how they interact with other cells, and how they function in the presence of liver disease.
Introduction
Natural killer T (NKT) cells are a group of innate immune cells first recognized in the 1990s (1). These cells feature surface receptors of both T cells and NK cells (e.g., NK1.1 in mice or CD161+/CD56+ in humans). The activation and deactivation of NKT cells are closely tied to our immune activities, such as pathogen elimination, tumor surveillance, and autoimmune responses (2–4). NKT cells can be divided into two subtypes, namely type I and type II. Type I NKT cells, usually referred to as invariant NKT (iNKT) cells, express a semi-invariant mouse Vα14-Jα18/Vβ8 or human Vα24-Jα18/Vβ11 T cell-receptor (TCR) α chain, hence the name. Type I NKT cells are able to recognize lipid antigens (such asglycosphingolipids, glycerophospholipids, lysophospholipids, and cholesterol ester) in the context of CD1d, a non-polymorphic MHC class I-like molecule (5–8). Researchers have found that the injection of α-galactosylceramide(α-GalCer) activates type I NKT cells (9). Type II NKT cells, in contrast, express a relatively diverse range of TCR receptors, and are reactive to a self-glycolipid sulfatide (10). Studies preliminarily suggest contradictory functions for the two types of NKT cells: type I NKT cells are likely pro-inflammatory, while type II are anti-inflammatory (6, 11). Note that type II NKT cells have not been broadly studied due to a lack of distinctive surface characteristics. In this review, we mainly focus on iNKT cells, with also a few contents talking about type II NKT cells, and “NKT cells” will stand for iNKT cells unless otherwise stated.
The liver is a vital part of the human digestive system, and functions as the center of metabolism and detoxification. Though not seen as a primary immune organ, the liver is not to be neglected when we talk about immune reactions. In addition to parenchymal cells (i.e., hepatocytes), the liver also hosts non-parenchymal cells, such as liver sinusoidal endothelial cells, Kupffer cells (macrophages), lymphocytes, and stellate cells. Interestingly, the liver has the highest NKT cell/conventional T cell ratio in the body (12), suggesting that this organ might play an important role in NKT immunology. In this review, we mainly focus on two points: 1) how NKT cells are activated, and 2) how NKT cells interact with other cells (e.g. Kupffer cells, T cells, hepatocytes) in the presence of liver disease.
NKT-cell activators
Briefly, it is known that NKT cells can be activated by lipid antigens (especially α-GalCer) (13–15) cytokines (such as IL-2 (interleukin-2) and IL-18) (16–18) chemokines (including CXCR6) (19), and other substances, but the type and common characteristics of these NKT stimulators remain poorly elucidated. Here we summarize three types of molecules that lead to NKT-cell activation (Figure 1), and briefly discuss their roles in the process of liver diseases.
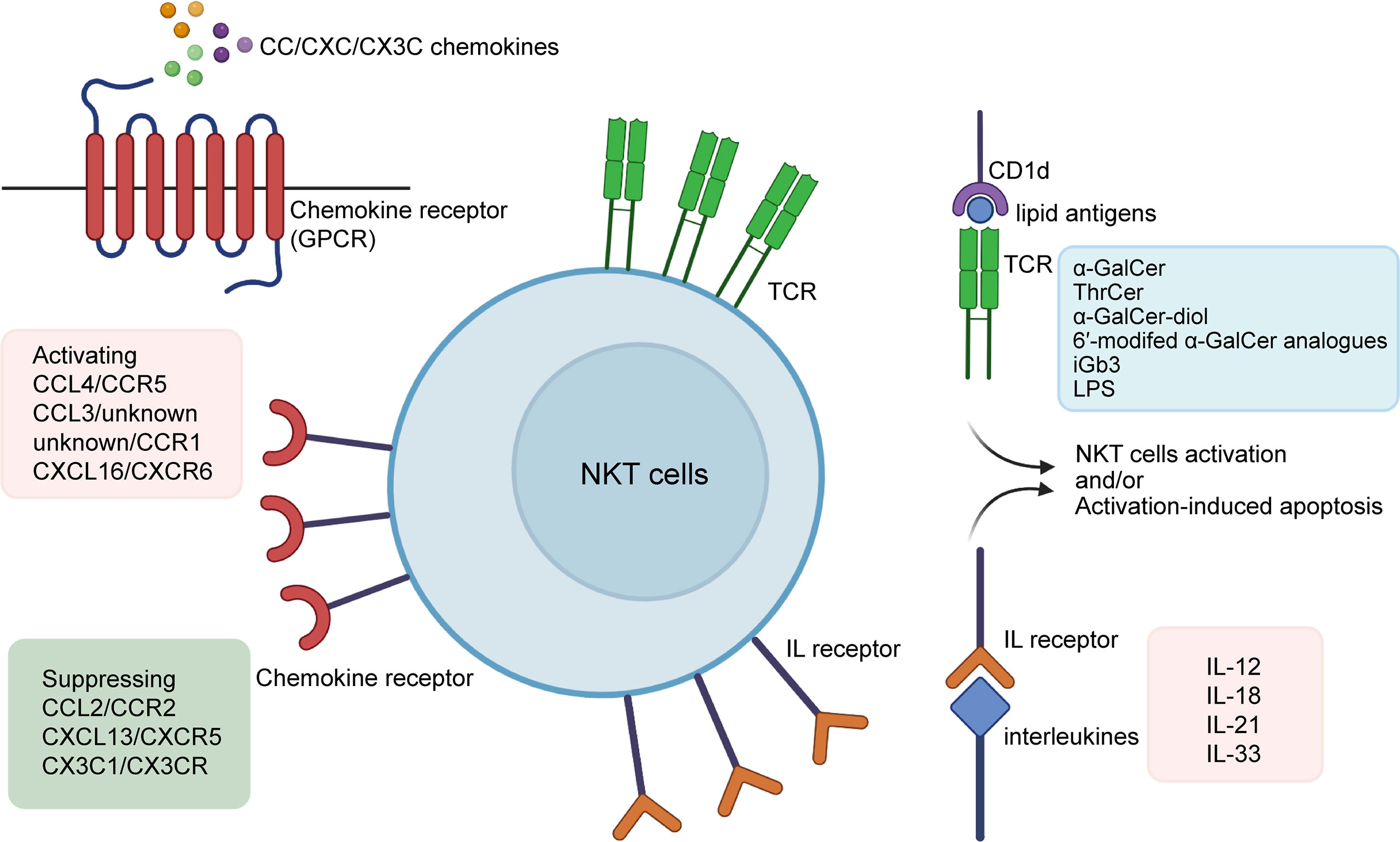
Figure 1 Activators of NKT cells. α-Galcer and some of its analogues (e.g.ThrCer, α-GalCer-diol, 6′-modifed α-GalCer analogues), iGb3, and LPS are among lipid antigens proven to activate NKT cells via the CD1d-dependent pathway; interleukins such as IL-2, IL-12, IL-15, IL-18, IL-21, and IL-33 promote NKT cells by binding directly to their interleukin receptors; CC/CXC/CX3C chemokines are associated with the recruitment and proliferation of NKT cells, with non-exclusive matches with their receptors that come in the form of GPCRs. Also, α-Galcer and some interleukins may also lead to activation-induced apoptosis of NKT cells. α-GalCer, α-galactosylceramide; ThrCer, threitolceramide; iGb3, isoglobotrihexosylceramide; LPS, lipopolysaccharide; IL, interleukin.
α-GalCer and analogues
Previous studies have shown that lipid antigens are presented to NKT cells through CD1d located on the surface of dendritic cells. Among all the lipid antigens, α-GalCer (also known as KRN7000), a synthetic component, is the first to be experimentally confirmed to potentiate NKT cells, both in vitro and in vivo (5, 13, 14).This glycolipid was discovered in an extract of the marine sponge Agelasmauritianus, and its effect on NKT in both in vitro and in vivo activation is widely reported. After α-GalCer administration, there are detectable increases in the number of NKT cells (15)and NKT-derived cytokines (14)(TNF (tumor necrosis factor), IFN-γ (interferon-γ), IL-12, etc.) as well as a degranulation marker (CD107a) (20), and symptoms of experimental animals improve or worsen accordingly (21, 22). Some researchers have also proposed an α-GalCer-based therapy for infection and autoimmune diseases (23, 24), but this proposal has been met with the opposing argument that administration of α-GalCer is also likely to induce hepatocyte damage (15)and NKT-cell anergy (25).
In many types of liver diseases, administration of exogenous α-GalCer changes their pathophysiological process. For example, α-GalCer-induced NKT activation is responsible for exacerbation of ALDs (26). The anti-tumor activity of α-GalCer in the liver is also demonstrated in mice experiments (27). Interestingly, in the mouse model of CCl4-induced acute liver injury, natural activation of NKT cells ameliorates liver damage and inflammation, possibly by suppressing HSC (hepatic stellate cell) activation, while α-GalCer-induced NKT activation accelerated acute liver injury, inflammation and fibrosis (28). Considering its dual effects on liver diseases and hepatic toxicity, further trial of α-GalCer is needed before clinical use.
Furthermore, analogues of α-GalCer are studied for their potential to activate NKT cells. In 2008, Jonathan D. Silk et al. (29)reported that threitolceramide- (ThrCer-) induced activation of NKT cells. In addition, researchers have managed to createα-GalCer analogues artificially, including α-GalCer-diol (with added hydroxyl groups in the acyl chain compared to α-GalCer, Juan Ma et al., 2020) (30)and6′-modified α-GalCer analogues (Matthias Trappeniers et al., 2008) (31). Hopefully, with careful design, these analogues will be applicable as preventative and therapeutic vaccine adjuvants (32, 33).
However, it is worth noting that not allα-GalCer analogues have the potential to activate NKT cells. For instance, in 2005, Jochen Mattner et al. (8)found that injection of α-glucuronosylceramide (PBS 30) or galacturonosylceramide (PBS 59) in mice led to the proliferation of NKT cells, but β-glucuronosylceramide (PBS 50) did not.
Other lipid antigens that activate NKT cells include glycosphingolipid isoglobotrihexosylceramide (iGb3), an endogenous antigen synthesized in the endoplasmic reticulum (ER) and Golgicomplex (34, 35), and bacteria-derived lipopolysaccharide (LPS), a ligand for Toll-like receptor 4 (TLR4) expressed on NKT cells, which corresponds with the roles that NKT cells play during exogenous infection (8, 36, 37).
Interleukins
Interleukins are a group of cytokines that are partially secreted by NKT cells, and some of them have biological effects on NKT cells. In brief, interleukins can activate NKT cells include IL-2, 12, 15, 18, 21, 27, and 33 (Table 1).
Interleukins activate NKT cells by binding to receptors on the cell surface, and many of the working mechanisms of functioning interleukins remain elusive. Activated NKT cells secrete large amounts of Th1/Th2 cytokines, which could be modulated by administration of the interleukins mentioned above, indicating that these interleukins have a profound impact on NKT-cell activation.
IL-2 is found effective for stimulating NKT cells both in vitro and in vivo in many studies focused on mice. Co-administration of IL-2, 12 and 18 results in a stronger ability of NKT cells to secrete IFN-γ (38). Small amounts of IL-2 cDNA (complementary DNA) increases the number of NKT cells in vivo (39), and potentiates the effect of α-GalCer (50). Also, studies found that IL-2 enhances the effect of NKT activation by α-GalCer, but administration of IL-12 alone is not enough to potentiate NKT cells (40). In addition, exogenous IL-2 and/or IL-15 partially overcome the hyporeponsiveness of iNKT cells in chronic HBV patients (51).
Murine models showed that IL-15, partly from Kupffer cells, facilitates the proliferation and maintain the homeostasis of NKT cells (42, 43). IL-15 can potentiate the α-GalCer-stimulated NKT expansion (40). Some researchers have found that IL-15-related NKT activation is associated with the NF-κB signaling pathway, but the exact mechanism remains controversial. Vallabhapurapu S et al. claimed that IL-15-related NKT activation is dependent on the NF-κB signaling pathway, because they found that RelA, a member of Rel/NF-κB family, controls IL-15 signaling by regulating IL-15Rα chain and γC chain, and deficiency of RelA blocks NKT activation by administration of IL-15 (44). However, Locatelli I et al. believed NF-κB deficiency might stimulate NKT recruitment by promoting IL-15 activity (45).Mice experiments showed that the IL-18/IL-18R (IL-18 receptor) axis functions via a rapid NF-κB signaling pathway, directly enhancing IL-4 production by NKT cells. Unlike IL-12,which mainly promotes Th1 response (41), IL-18 stimulates Th1 and Th2 responses simultaneously (16–18); hence the two kinds of cytokines are sometimes co-administered for their combined activation effects (52). Also, we would like to point out that continuous stimulation of IL-18 may result in impaired long-term NKT activation, which is important during clinical practice.
Intriguingly, we noticed an autocrine phenomenon with regard to IL-21, namely that not only does IL-21 enhance the survival of NKT cells, it is also secreted by NKT cells after CD3 and CD28 administration. NKT cells activated by IL-21 exhibit higher granzyme and IL-4 expression (46). Some researchers also report witnessing less IFN-γ and TNF production by NKT cells, indicating that IL-21 leads to “Th1 to Th2” cytokine transformation (47), though this conclusion needs more support. Moreover, α-Galcerhas been found to coordinate with interleukins, including IL-18 and IL-21 (17, 46).
IL-27 and IL-33 also contribute to activation of NKT cells. IL-27 modulates IL-12 secretion of dendritic cells, thus indirectly enhancing maintenance and recruitment of NKT cells (48). IL-33 binds with ST2L (the suppressor of tumorgenicity 2 ligand, and also the receptor of IL-33) on NKT cells to promote IFN-γ secretion as well as FasL expression (49).
Some researchers have studied the functions of interleukins on liver NKT cells and examined them as possible treatment methods. For example, administration of IL-18 potentiates the cytotoxicity of hepatic NKT cells in a perforin-dependent way (53). Co-administration of IL-12 and IL-18 triggers higher IFN-γ release from NKT cells than either administered alone, which demonstrates a higher efficiency for killing liver tumors (54). However, extra work is urgently needed to investigate the effects of interleukins on hepatic NKT cells and their clinical values.
Chemokines
The chemokine superfamily was first discovered in the late 1980s to play a role in inflammation. The protein superfamily consists of four groups, namely XC, CC, CXC, and CX3C, a categorization based on the discrete location of cysteine residues on the initial sequence of the molecules. Chemokine receptors are defined as a group of seven transmembrane-spanning G-protein-coupled receptors (GPCRs), having no one-on-one match with their ligands (55–57). Chemokines are tightly associated with the maturation and localization of NKT cells. The effective ligands and receptors are summarized in Table 2.
Of the four chemokine subgroups listed in Table 2, XC and CX3C have seldom been studied since their discovery; thus, there are very few articles concerning their functions on NKT cells. XC, interestingly, has not been reported so far to potentiate NKT cells. In contrast, CX3C1/CX3CR1 is considered to take part in NKT-cell trafficking within the thymus, but this function may not be of vital importance as CX3CR1-deficient mice do not show NKT-cell developmental disability (67). Also, some researchers hold opposing views on the NKT-cell-activating function of CX3C1/CX3CR1 (68). However, CX3CR1 expression on the cell surface can be utilized to define NKT subtype present in the thymus and peripheral organs (67).
Unlike XC and CX3C, the functions of the other two subgroups are known in more details. In mice models, CCL2, also known as MCP-1, recruits NKT cells to peripheral organs such as the spleen (58) and exerts an anti-inflammatory effect by interacting with CCR2 to prevent IL-4 secretion of NKT cells, which demonstrates a hepatoprotective effect in the liver (59). CCL3 and CCL4, secreted by activated dendritic cells, also attract NKT cells. This effect is accompanied by CXCR3 ligands (CXCL9-11) which derive from the same dendritic cells (61). CCL4 has also been found to induce distinct chemotaxis in different NKT subgroups, attracting CCR5-expressing cells in particular (69). Lack of CCR5 in mice promotes fulminant liver failure because of exacerbated inflammatory responses related to a higher amount of IL-4 from NKT cells that fail to go through apoptosis after activation (62), suggesting a role for CCR5 in NKT-cell regulation. Another CC chemokine receptor on NKT cells surface is CCR1, which together with CCR5 recognizes ligands that come from activated macrophages and dendritic cells (60).
The last type, CXC, is the most comprehensively studied at present, especially CXCR6 and its ligand CXCL16. In short, CXCR6/CXCL16 functions on the distribution rather than maturation of NKT cells. Animal research shows that CXCR6 expression of NKT cells is elevated upon NKT-cell activation, but is not indispensable for NKT-cell development within the thymus, as CXCR6-challenged mice do not show reduced numbers of thymic NKT cells. However, CXCR6 is closely related to localization of NKT cells because of its interaction with CXCL16, which resides on target organs such as the spleen and liver (70). As a result, CXCR6-deficient mice possess fewer NKT cells in their livers, making them more susceptible to infection (63, 70). The CXCR6/CXCL16 reaction boosts IFN-γ and IL-4 release from NKT cells, enhancing inflammatory response (64, 65). In particular, CXCR6/CXCL16 is involved in many liver diseases. For example, CXCR6/CXCL16 expressions greatly increase during liver inflammation (71). Hepatocytes produce CXCL16 in non-alcoholic fatty liver disease (NAFLDs), which ameliorates inflammation and fibrosis (72, 73). In contrast, CXCR5, along with its ligand CXCL13, is reported to reduce NKT-cell activation (66).
The relationship between NKT cells and other cells in liver disease
In the liver, NKT cells have close connections with other cells including hepatocytes (normal liver cells), dendritic cells, macrophages (Kupffer cells), T cells, and B cells, and are able to regulate their functions during innate and acquired immune reactions. This connection is achieved through either direct contact or secretion of cytokines. Evidence shows the significance of this connection because changes in how NKT cells interact with other cells can be found in liver disease and may lead to severe dysfunction of the organ. Here we summarize the ways in which NKT cells coordinate with other liver-resident cells.
NKT cells and hepatocytes
As mentioned above, NKT cells are activated by lipid antigens through CD1d molecules which, in the liver, are expressed on macrophages, dendritic cells, and hepatocytes. CD1d then presents the antigens to the TCR on NKT cells. A decrease in CD1d on hepatocytes results in dysfunction of NKT cells (74). Some studies based on HBV transgenic mice find that during liver diseases such as HBV infection, CD1d expression is elevated on injured hepatocytes, rather than macrophages (75). Meanwhile, hepatocyte-derived IL-7 is also important in the maintenance of NKT cells, which indicates that hepatocytes play a role in the development and maintenance of the immune system (76).
NKT cells attack hepatocytes by expressing FasL, perforin, and granzymes, but their main effects on hepatocytes are achieved by producing Th1 cytokines, especially TNF-α and IFN-γ. Upon activation, NKT cells start to release more TNF-α that directly interacts with TNF receptor 1 (TNFR1)expressed on hepatocytes, on which this molecule has a dual effect, either promoting hepatocyte death or regeneration indifferent contexts (77). Increased level of NKT-derived TNF-α is responsible for exacerbation of α-GalCer-induced liver damage (26). However, in mice that underwent partial hepatectomy, TNF-α promotes regeneration of hepatocytes (78). The interactions between NKT cells and hepatocytes are also tightly associated with a wide range of liver diseases. For instance, in autoimmune liver diseases, NKT cells release death signals to hepatocytes through FasL pathway, and secrete TNF-α, perforin and granzymes in synchronization, promoting the process of autoimmune liver diseases (79). In ALDs, NKT cells also play the role of killing hepatocytes (80). In 2014, Monika Julia Wolf et al. (81)found that TNFSF14 (TNF superfamily 14, also referred to as LIGHT) secreted by NKT cells is responsible for enhanced lipid uptake of hepatocytes as well as liver damage, causing an enhanced possibility of NAFLDs in mice (Figure 2A).
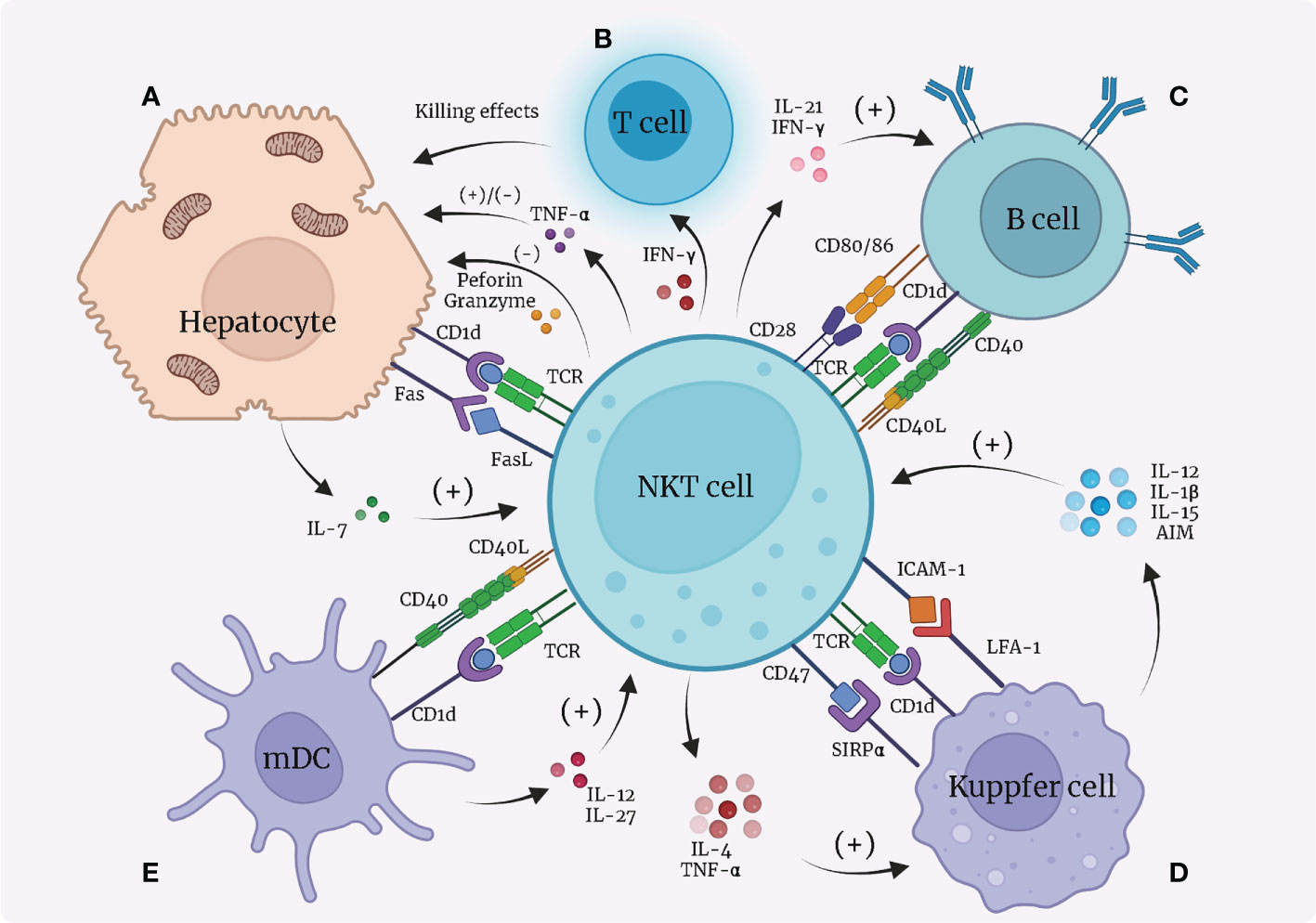
Figure 2 Interactions between NKT cells and hepatocytes, dendritic cells, Kupffer cells, and B cells. (A) Hepatocytes present lipid antigens to TCR on NKT cells via the CD1d-dependent pathway; hepatocytes release IL-7 to activate NKT cells; NKT cells secrete TNF-α that has dual functions on hepatocytes; NKT cells express FasL and induce Fas expression on hepatocytes, leading to apoptosis of hepatocytes. (B) NKT cells secrete IFN-γ to recruit T cells to kill hepatocytes. (C) B cells present lipid antigens to NKT cells through CD1d; NKT cells and B cells contact each other directly through CD40L/CD40 and CD28/CD80, 86; NKT cells secrete IL-21 and IFN-γ to promote B cells. (D) Kupffer cells present lipid antigens to TCR on NKT cells via the CD1d-dependent pathway; Kupffer cells express LFA-1 and SIRPα, which bind with ICAM-1 and CD47, respectively, on NKT cells to activate NKT cells; Kupffer cells secrete IL-12, IL-1β, IL-15, and AIM to recruit and promote NKT cells; NKT cells in return produce pro-inflammatory IL-4 and IFN-γ to function on Kupffer cells. Notably, over-stimulation of NKT cells by Kupffer cells leads to apoptosis of NKT cells. (E) Dendritic cells (especially myeloid dendritic cells, mDC) present lipid antigens via CD1d towards NKT cells to activate NKT cells; dendritic cells secrete IL-27 and IL-12 to activate NKT cells; NKT cells express CD40L to bind with CD40L and reciprocally benefit dendritic cells. mDC, myeloid dendritic cells; IL, interleukin; TNF, tumor necrosis factor; IFN, interferon; SIRP, signal regulatory protein; LFA, lymphocyte function-associated antigen; ICAM, intercellular adhesion molecule; AIM, apoptosis inhibitor expressed by macrophages.
IFN-γ expression is also increased in activated NKT cells. During HCV infection, IFN-γ induces liver sinusoidal endothelial cells to produce CXCL9 and CXCL10 that bind to and recruit CXCR-positive T cells. As a result, more T cells start to locate in the infected liver and negatively affect hepatocytes (82). Moreover, IFN-γ stimulates hepatocytes to express a higher number of Fas, causing liver cell apoptosis after binding with FasL on NKT cells (Figure 2B) (83).
NKT cells and B cells
The interactions between NKT cells and B cells mainly lead to strengthened capacity of B cells. Animal research suggested that CD1d loaded with lipid antigens from B cells surface is a source for NKT-cell activation (84, 85). In return, NKT cells offer helper signals for B cells by expression of CD40L and CD28, which bind to CD40 and CD80/86, respectively, on B cells (86). Secretion of IL-21 (87) and IFN-γ (86) also play a role in B-cell activation. In addition, NKT cells can indirectly enhance B cells by communicating with dendritic and CD4+ T cells (84, 85, 88). Interestingly, stimulation of NKT cells also leads to recruitment of regulatory B cells to the liver that suppress inflammation (Figure 2C) (89). However, up to now, it remains poorly studied how the interactions between NKT and B cells contribute to the pathogenesis of hepatic diseases.
NKT cells and macrophages (Kupffer cells)
The interaction between NKT cells and macrophages is relatively complex as it involves multiple surface and secreted molecules. As one of the main APCs in the liver, macrophages (Kupffer cells) connect with NKT cells in a CD1d-restricted manner. The CD1d molecule located on the surface of Kupffer cells presents exogenous lipid antigens to TCR on NKT cells, leading to NKT-cell activation (90).
Another pivotal means of Kupffer/NKT interaction is through the LFA-1/ICAM-1 pathway. LFA-1 (lymphocyte function-associated antigen 1) and ICAM-1 (intercellular adhesion molecule 1) are resident on the surface of Kupffer cells and NKT cells, respectively, and bind to each other with high affinity. Aside from NKT-cell activation, Kupffer cells are also reported to show quicker iNOS (inducible nitric oxide synthase) and NO synthesis, indicating mutual activating functions between NKT cells and Kupffer cells (91). Notably, over-stimulation of NKT cells by Kupffer cells can result in activation-induced apoptosis and necrosis of NKT cells (90). Signal regulatory protein α (SIRPα) on Kupffer cells binding to CD47 on NKT cells also enhances the function of NKT cells (92).
Kupffer cells secrete many types of cytokines that have biological functions on NKT cells, mostly different interleukins. Kupffer-cell-derived IL-12, IL-1β, and IL-15 are thought to recruit NKT cells, promote NKT-cell activation, and participate in the maintenance of NKT cells (42, 93–95). AIM (“apoptosis inhibitor expressed by macrophages”, also referred to as CD5L), a protein that is normally considered to inhibit apoptosis of CD4+CD8+ double-positive thymocytes, is secreted by Kupffer cells to protect NKT cells from apoptosis (96). On the other hand, NKT cells are capable of producing large quantities of pro-inflammatory IL-4 and IFN-γ, which are associated with granuloma formation around infected Kupffer cells (97).
In conclusion, we believe the relationship between NKT cells and Kupffer cells is reciprocal, enhancing both NKT and Kupffer cells (Figure 2D). In hepatic diseases like inflammation (93, 98), alcoholic liver injury (94) and infection (92), the interactions between Kupffer cells and NKT cells play an indispensable part.
NKT cells and dendritic cells
As a type of APC, dendritic cells participate in lipid antigen (such as α-Galcer) presentation via CD1d towards NKT cells, which leads to NKT-cell activation (99, 100), just like hepatic macrophages do. Dendritic cells also secrete cytokines including IL-27 (48) and IL-12 (101) that both have a positive impact on NKT cells. Notably, only myeloid dendritic cells (mDC), not all dendritic cells, mediate activation of NKT cells, whereas plasmacytoid dendritic cells (pDC) are likely to cause tolerance of NKT cells in a way concerning the activation of type II NKT cells (6). In addition, NKT cells also function as promoters of dendritic cells by expressing CD40L that binds with CD40 on the surface of dendritic cells, forming a reciprocal activating loop (5, 101). It has been observed that dendritic cells respond to TLR stimulation more actively in the presence of NKT cells (Figure 2E) (102, 103).
The positive influence of dendritic cells on NKT cells might provide an insight into treatment of liver diseases. In 2007, Tomohide Tatsumi et al. (104)demonstrated with mice models that α-GalCer-pulsed dendritic cells suppressed liver tumor by activating NK cells, and they proposed that NKT cells might also take a part. Hopefully, future research might provide a more explicit answer.
NKT cells and T cells
T cells consist of a wide range of different cell subgroups including CD8+ T cells, CD4+ T cells, and regulatory T cells (Tregs), each having distinct immune bioactivity.
The interaction between NKT cells and CD8+ T cells seems confusing as researchers have obtained contradictory experimental results. Some people believe NKT cells boost CD8+ T cells just like they do CD4+ T cells, via CD40/CD40L signaling and secretion of cytokines such as IL-4 and IL-13. Activated CD8+ T cells then secrete IFN-γ, a pro-inflammatory cytokine (105, 106). However, other researchers have discovered an inhibitory effect of NKT cells on CD8+ T cells in animal experiments. IFN-γ secreted by activated CD8+ T cells allows NKT cells to produce IL-4 and IL-13, which in turn inhibit CD8+ T cell activity by harassing their chemotaxis to CCL5. Also, NKT cells indirectly suppress CD8+ T cells by potentiating regulatory T cells (Tregs) (107).
The effects of NKT cells on CD4+ T cells are mainly positive, leading to enhanced IFN-γ secretion (105, 108). Normally, CD4+ T cells are the main producers of IL-10, a cytokine that has anti-inflammatory effects, but their IL-10 secretion is profoundly inhibited after activation from NKT cells (105). This indicates that activation of CD4+ T cells by NKT cells may be pro-inflammatory. Dendritic cells are also said to participate in NKT/T crosstalk, enhancing both CD4+ and CD8+ T cells (106). Moreover, NKT-cell-induced Treg activation plays an important role in the depletion of CD4+ T cells (109).
Regulatory T cells (Tregs), on the other hand, are a special subgroup of T cells that can suppress immune reactions. Some studies show a negative feedback relationship between NKT cells and Tregs. Activated NKT cells stimulate activation of Tregs by secreting higher amounts of cytokines such as IL-2, IL-10, and TGF-β. Tregs then suppress the proliferation and functions of NKT cells, reducing their cytotoxic activity. Interestingly, the inhibitory effects of Tregs on NKT cells are achieved in a CD1d-dependent manner that requires direct cell-to-cell contact (110, 111), rather than TGF-β and IL-10, as the suppression of NKT cells continues even after neutralization of the two cytokines (109, 111). This feedback mechanism is likely to prevent over-stimulation of NKT cells and the disastrous cascade immune reactions that could ensue (see Table 3 and Figure 3).
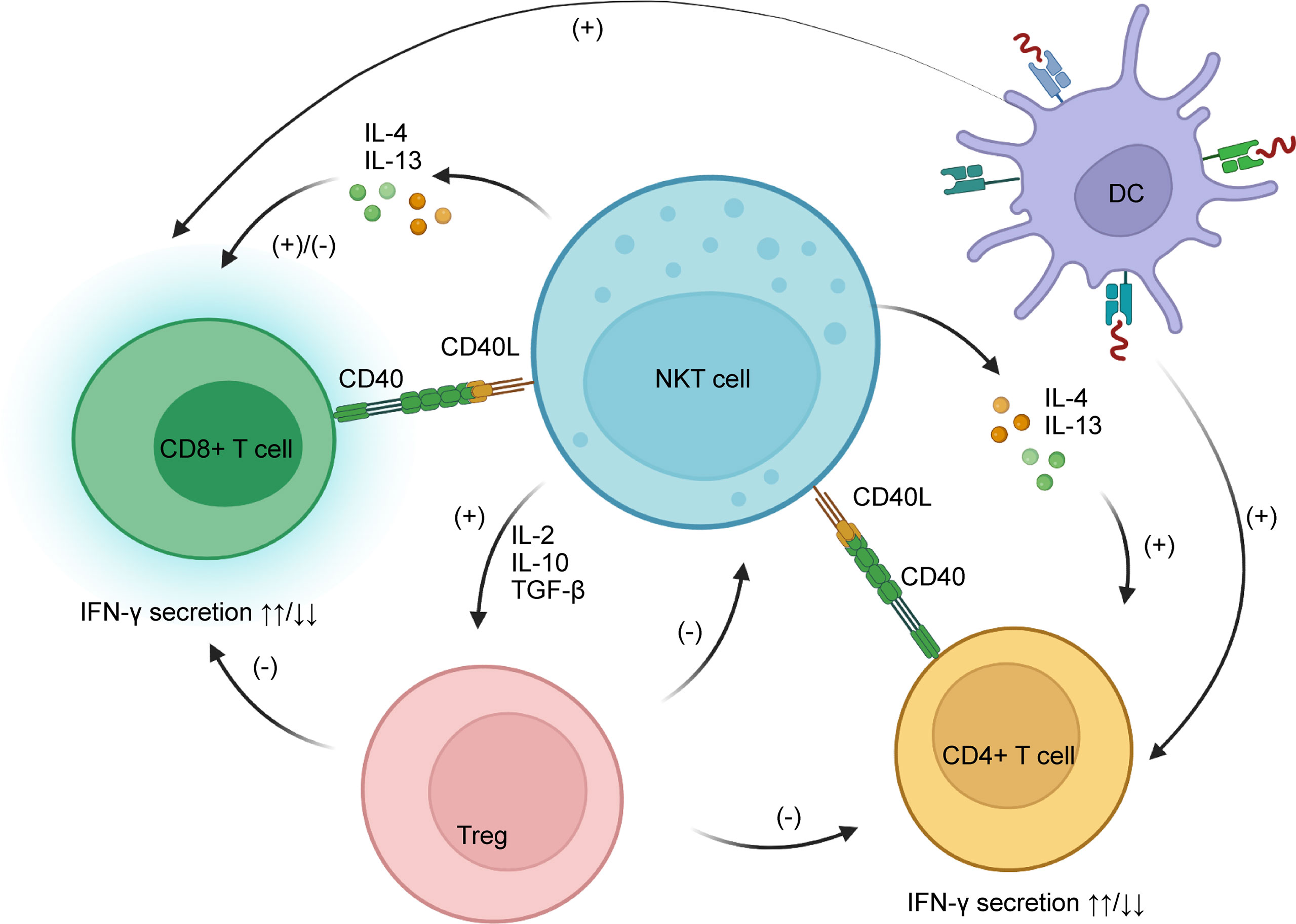
Figure 3 NKT cells interact with CD8+ and CD4+ T cells through CD40/CD40L and secretion of IL-4 and IL-13. The main functions of NKT cells on CD4+ T cells are stimulatory, while on CD8+ T cells they are both stimulatory and inhibitory, as NKT cells can harass their chemotaxis. NKT cells potentiate Tregs by secreting IL-2, IL-10, and TGF-β; Tregs have a negative impact on CD8+ and CD4+ T cells as well as NKT cells. IL, interleukin; TGF, tumor growth factor; Treg, regulatory T cells.
The interactions between NKT and T cells play a pivotal role in liver diseases. For example, during HBV infection in a transgenic mouse model, NKT cells promotes the proliferation of HBV-specific CD8+ T cells (112), and blockade of NKG2D expression prevents hepatitis induced by T cells (113). These results respond with the investigation which found that HCV-specific T cell response comes in accordance of NKG2D expression on NKT cells in healthcare workers who were exposed to small amounts of HCV but showed no obvious liver damage (114). In autoimmune liver disease and NAFLDs, NKT cells contribute to the recruitment of T cells, and potentiate their biological functions (79, 115, 116). As a result, NKT cells are considered as a factor for exacerbation of these two diseases. However, in autoimmune liver diseases, NKT cells promote the activation of Tregs, which ameliorates the killing effect of T cells (117).
NKT cells and other cells
Besides hepatocytes, dendritic cells, macrophages (Kupffer cells), T cells, and B cells, hepatic NKT cells also have close relationship with neutrophils, hepatic stellate cells, and NK cells. These interactions have a significant influence in modulating inflammation as well as immune tolerance, and play a role in the processes of liver cirrhosis and cancerous proliferation. We outline these cell interactions as follows.
NKT cells secrete IL-4 to recruit and promote accumulation of neutrophils, thus enhancing hepatitis and liver fibrosis (115, 118). Additionally, NKT-cell-derived IFN-γ acts as a potent suppressor of neutrophils by inducing apoptosis (118). This may be to prevent over-activation of pro-inflammatory responses.
HSC activation is among the causes of liver fibrosis as it enhances synthesis and accumulation of collagen and extracellular matrix (119). NKT cells can either have a stimulatory or inhibitory effect on HSCs. Normally, NKT cells enhance the growth of HSCs via Hh (Hedgehog) signaling pathway and secreting OPN (osteopontin) (81, 120, 121), but under certain circumstances NKT cells induce HSCs apoptosis via FasL (122). (See 4.3”alcoholic liver diseases (ALDs)” and 4.4”non-alcoholic fatty liver diseases (NAFLDs)”).
Generally, NKT cells are considered to enhance the activation of NK cells. Tomonori Iyoda et al. (123)found activated NKT cells induce NKG2D and DNAM-1 (also known as CD226) expression on NK cells that are necessary for the anti-tumor effects of NK cells. NKT-cell activation also leads to improved cytokine production (e.g IFN-γ) and killing activity of NK cells through the mTOR (mechanistic target of rapamycin) pathway, which brings about enhanced anti-pathogen capacity (124, 125). However, some researchers have also reported an inhibitory effect of NKT cells on IFN-γ secretion of NK cells after alcohol intake (126).
NKT cells in liver diseases
Given all the functions of NKT cells on other cells within the liver, it is easily deduced that NKT cells make an enormous contribution to the pathogenesis and progression of many kinds of liver disease ranging from autoimmune hepatitis to hepatoma. With more insights into how NKT cells work in these diseases, hopefully new methods to treat or cure liver diseases will be discovered. For example, tazarotene, a RAR-γ (retinoic acid receptor-γ) agonist that inhibits NKT-cell proliferation, as well as cytokine release, is tested in mice for treatment of liver steatosis and fibrosis (127). Below, we discuss how NKT cells play their role in autoimmune liver diseases, alcoholic liver diseases (ALDs), non-alcoholic fatty liver diseases (NAFLDs), and liver tumors.
HBV and HCV infection
HBV and HCV infections are two important reasons for liver damage all across the world, affecting approximately 250 million (128) and 80 million (129) people respectively. Thus, research aimed at fighting against viral hepatitis has become a global task. In this chapter, we will review the roles that NKT cells play during the pathophysiological process of HBV and HCV infection.
During HBV infection, hepatocytes that are invaded by virus would present lipid antigens, namely lysophosphatidylethanolamine, to NKT cells via CD1d (130). This leads to NKT activation, causing an elevated amount of IFN-γ, which mediates anti-viral effects (131) but also results in liver damage (132) in mice models. IFN-γ inhibits the proliferation of hepatocytes by inducing apoptosis and negatively regulating cell cycle (75). In addition, NKT cells inhibit HBV by promoting the activation of cytotoxic T lymphocytes (CTL) (112). Blockade of NKG2D is found to ameliorate acute HBV hepatitis both in vitro and in vivo (113). In a retrospective investigation in 2009, India, researchers found that the amount of NKT cells is smaller in fulminant HBV liver failure than acute HBV patients, indicating the role that NKT cells play in limiting HBV infection (133). Notably, any factors that hinder the presentation of lipid antigens of hepatocytes to NKT cells, such as deficiency of NKT cells or CD1d or dysfunction of ER-associated lipid transfer, would result in a delayed anti-viral reaction (130).
However, data collected from clinical patients demonstrated that there is a decreased density (134) and down-regulated function (135) of NKT cells for chronic HBV infection compared to acute HBV infection. Both animal and human studies suggested that NKT cells are associated with over-activation of HSC and excessive healing during HBV infection, which increases the possibility of liver cirrhosis (136, 137). Moreover, the number of NKT cells is positively correlated with the quantity of HBV during chronic HBV infection, and a decrease in NKT number is witnessed after effective anti-viral treatment. This indicates NKT density as a potential marker for evaluating anti-HBV treatments (138).
To this day, plenty of novel NKT-related treating methods of HBV have been experimented on cells or clinical trials. For example, α-GalCer was found to inhibit HBV replication by directly activating NKT cells in mice (131), but clinical trials showed pessimistic results, as administration of α-GalCer alone even decreases NKT density and does not influence density of HBV DNA (139). Also, the function of α-GalCer on NKT activation decreases during chronic HBV infection, but this phenomenon is partly reversible after administration of exogenous IL-2 and/or IL-15 (51). Other ways to activate hepatic NKT cells and inhibit HBV replication include IL-18 (140), thymosin-α1 (141), β-glycosphingolipids (142), CD28/CD80 (143) activation and PD-1/PD-L1 blockade (143), but these methods have not received clinical confirmation yet.
NKT cells also participate in the process of HCV infection. Elevated expression of CD1d on infected biliary cells promotes the activation of NKT cells (144), leading to secretion of cytokines including IL-4 which recruits T cells and perforin and granzyme which mediate liver damage (145). Notably, some researchers claimed that the number of NKT cells in peripheral blood decreases in patients infected with HCV (146, 147), but more researchers did not find significant changes in number of NKT cells (148–150), although hepatic NKT cells showed enhanced activity (147), producing a higher amount of IL-13 that has a pro-Th2 effect (150). The functions of NKT cells during HCV are associated with macrophages and T cells. Macrophages (Kupffer cells) in the liver secrete significantly more IL-15 that boost NKT activation (95). In healthcare workers who were continuously exposed to small amounts of HCV but did not develop symptoms, NKT cells were found to be activated in a way related to specific T cells activation, indicating the protective effect of NKT cells against HCV is partly associated with T cells (114). In patients with chronic HCV, the sustained response to IFN plus ribavirin therapy is associated with elevated dynamism of NK and NKT cells, suggesting NKT cells play a vital role in anti-HCV reaction (151). In addition, in pregnant women infected by HCV, density of NKT cells increase in placenta tissues, which is thought to be responsible for preterm birth (152).
Preclinical studies proposed novel NKT-related anti-HCV therapy including the administration of IFN-α (153) and IL-2/OKT3 (a CD3-specific mAb) (154), which leads to NKT activation and up-regulated IFN-γ expression that inhibit virus replication in mice. Moreover, a clinical trial experimented oral administration of hepatocyte-extracted proteins and HBV or HCV proteins to figure out their anti-viral functions in chronic HBV or HCV patients. Results showed that all patients experienced increased number of NKT cells for at least 2-fold, and histological necro inflammatory score improved in 4/13 (30.7%) and 2/12 (17%) patients of HBV and HCV, respectively (155).
Autoimmune liver diseases
There are three main types of autoimmune liver disease, namely autoimmune hepatitis (AIH), primary biliary cirrhosis (PBC), and primary sclerosing cholangitis (PSC), which are associated with destruction of hepatic parenchyma, small intrahepatic bile ducts, and large bile ducts, respectively. Liver NKT cells primarily reside in liver sinusoids. Numerous studies have found that NKT cells are closely associated with all three types of autoimmune disease (79, 115, 117, 156).
Interestingly, liver NKT cells can either promote or combat autoimmune liver diseases depending on their down-stream target cells (Table 4). Besides, NKT cells promote the development of hepatocyte injury in three ways (1): NKT cells directly kill hepatocytes by expressing FasL and secrete TNF-α, perforin and granzyme B; (2) NKT-derived IFN-γ induces Th0-cell transduction into Th1 cells and CD8+ T-cell transduction into CTLs that bind to the MHC I molecule on the surface of hepatocytes; (3) NKT cells secrete IL-4 that turns Th0 cells into Th2 cells, enhancing B-cell-producing autoimmune antibodies(79). Furthermore, TNF-α and IFN-γ are involved in recruitment of functional T cells, and IL-4 probably promotes neutrophil infiltration within the liver (115). NKT cells are also potent activators of Tregs, which have a negative effect on immune response, thus mitigating autoimmune liver injury (160). Statistics show that simultaneous suppression of NKT cells and promotion of Tregs is helpful for mitigating autoimmune liver injuries in experimental animal models (161).
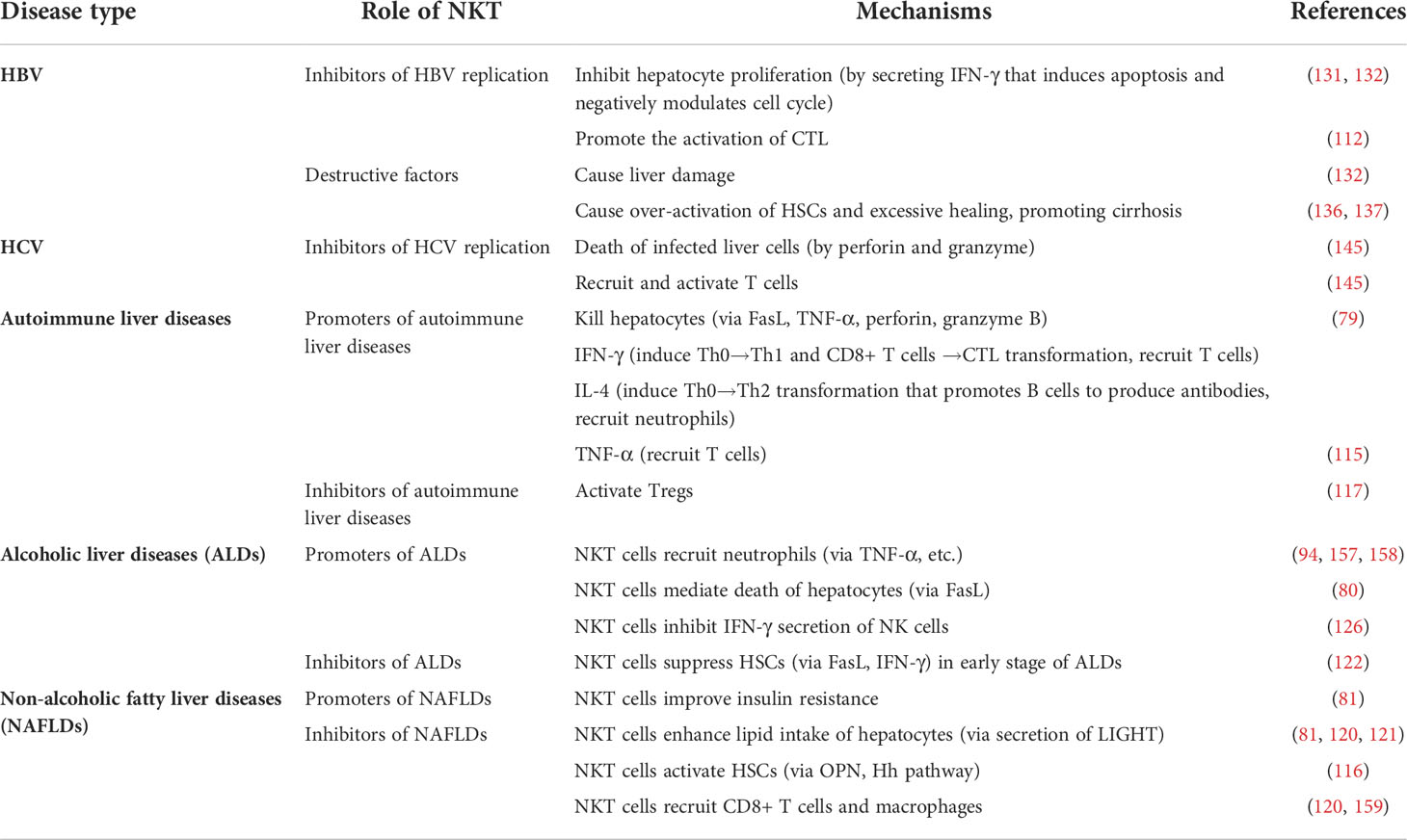
Table 4 Functions of NKT cells in autoimmune liver diseases, alcoholic liver diseases (ALDs) and non-alcoholic fatty liver diseases (NAFLDs).
Apart from inflammation, NKT cells are likely to play a role in liver fibrosis resulting from autoimmune liver diseases. IL-4 and IL-13 from activated NKT cells promote liver fibrosis, suggesting a role for NKT cells in cirrhosis resulting from chronic autoimmune liver injury (77). However, this fibrinogenic effect requires further examination (115).
Given the roles that NKT cells play during autoimmune liver diseases, researchers have been experimenting on modulating NKT cells to find treatments for the disease. In recent years, several substances have been found effective in mice for alleviating concanavalin A (Con A)-induced autoimmune hepatitis partly by inhibiting NKT cells and related production of inflammatory cytokines, including mitochondrial-targeted ubiquinone(MitoQ) (162), diammonium glycyrrhizinate (161) and secoemestrin C (163). Gene modulation such as C6orf120 knockout (164) is also used as a therapeutic target. However, these experiments were only done on mice, and lack of clinical statistics limits extensive application.
Other studies also suggested the role that type II NKT cells plays in autoimmune liver diseases. The number of type II NKT cells was up-regulated in both peripheral blood and liver during autoimmune liver diseases (165). Increased CD1d expression on T cells during autoimmune liver diseases results in activation of type II NKT cells and favors Th1 cytokine production over Th2 within type II NKT cells (166). It is important to understand the physiology of type II NKT cells as they might influence iNKT cells. For example, Ramesh C. Halder et al. reported that activation of type II NKT cells and pDCs are associated with recruitment of anergic iNKT cells (167).
Alcoholic liver diseases
It is widely known that excessive consumption of alcohol ranks high among the risk factors for liver pathogenesis. Alcohol-induced liver diseases include alcoholic hepatitis, steatosis, cirrhosis, and hepatocellular carcinoma (HCC).
ALDs are closely linked to enhanced immune activation (168). Activated inflammatory responses are noticed quickly after alcohol intake with a higher NKT-cell concentration within the liver as well as mesenteric lymph nodes (169–171). Conversely, the absolute number of NKT cells in the whole body is decreased (172), which may be the result of NKT-cell recruitment to the liver and vast consumption of these cells.
Generally, NKT cells are thought to contribute to the development of ALDs, in contrast to type II NKT cells that are considered to attenuate ALDs (157). Binge-feeding with ethanol results in accumulation and activation of NKT cells combined with a higher expression of inflammatory and fibrotic genes in wild-type mice compared to their NKT-deficient counterparts (158). Evidence shows that alcohol potentiates α-Galcer stimulation of NKT cells by facilitating CD1d loading (171). IL-1β from Kupffer cells is also required for hepatic NKT-cell accumulation during ALDs (94).
Activated NKT cells recruit neutrophils by secreting TNF-α and up-regulating expression of neutrophil-attracting MCP-1 (monocyte chemoattractant protein-1, also known as CCL2) (94, 157), ICAM-1 (intercellular adhesion molecule-1), E-selectin, MIP-1α (macrophage inflammatory protein-1α, also known as CCL3), MIP-2, and osteopontin (OPN) (158). Also, NKT cells mediate apoptosis of hepatocytes by expression of FasL (80). Some researchers consider NK cells to be protective against ALDs by secretion of IFN-γ, while NKT cells inhibit this process. However, a subgroup of IL-10-secreting NKT cells (thus called NKT10) facilitates the protective effect of NK cells in ALDs (126).
Controversially, in the early stages of ALDs, NKT cells are likely to play an anti-fibrotic role by suppressing HSCs. The negative influence of NKT cells on HSCs is achieved through direct killing via the Fas/FasL pathway and IFN-γ production (122). Taken together, we conclude that with a few exceptions, NKT cells are mainly promoters of ALDs (Table 4).
Up to now, we have not found any results of NKT-based clinical trial for ALD therapy, but there are experiments done on mice, showing several promising molecules with therapeutic potential, including retinoids and sulfatide (157). These two substances alleviate ALDs by inhibition of NKT cells. Additionally, researchers found that prednisolone, a drug widely used to antagonize inflammation, exacerbates ALDs by inhibiting phagocytosis mediated by macrophages and neutrophils and hepatic regeneration, which provide an insight into management of steroid therapy (173).
Non-alcoholic fatty liver diseases
As the name suggests, the most significant feature of non-alcoholic fatty liver diseases (NAFLDs) is abnormal lipid storage in the liver. NAFLDs include simple stenosis, non-alcoholic steatohepatitis (NASH), cirrhosis, and even liver cancer. In many studies that focus on NAFLDs, a high-fat feeding model is used. Statistics have demonstrated that a high-fat diet, especially one with high concentrations of saturated fatty acids and monounsaturated fatty acids rather than polyunsaturated fatty acids, is associated with liver inflammation, insulin resistance, and NAFLDs (64, 174, 175).
During NAFLDs, the number of NKT cells within the liver decreases. This is because: (1) endothelium stress leads to fewer CD1d’s and impaired lipid presentation (74); (2) Kupffer cells mediate apoptosis and necrosis of over-activated NKT cells and secrete IL-12 to suppress NKT cells (64, 90); (3) normally Kupffer cells-derived IL-15 is stimulating for NKT cells, but in NAFLDs IL-15 secretion is down-regulated (45, 176) and (4) norepinephrine (NE) concentration decreases (176). However, some studies also report an increase in the number of NKT cells in the late stages of NAFLDs, probably because of enhanced activating functions of Kupffer cells via the CD1d-dependent pathway, which is inconsistent with many other study results (120).
Notably, the effects of NKT cells on the development of NAFLDs are rather controversial. While NKT cells ameliorate NAFLDs, probably by improving insulin resistance (120, 159), they are also likely to play a pro-inflammatory role in NAFLDs. Some studies show that NKT cells secrete LIGHT (TNFSF14), which significantly enhances lipid intake of hepatocytes (81). Also, NKT cells lead to activation of HSCs in two ways: (1) they facilitate OPN (osteopontin) secretion; and (2) they promote the Hedgehog (Hh) signaling pathway (note that NKT cells are both inducers and targets of the Hh signaling pathway). HSC activation is associated with exacerbation of liver fibrosis or cirrhosis (81, 120, 121). Activated NKT cells recruit CD8+ T cells and macrophages, too (116). Overall, NKT may have a protective effect in the early stages of NAFLDs but a destructive effect in later stages (Table 4).
In 2017, a published clinical trial said oral administration of β-glucosylceramide improved the hepatic fat content by 14% in NASH patients, which is associated with a decrease in CD4+ and NKT cells, suggesting NKT cells as a possible therapeutic target (177). Mice models also indicate that oral administration of liver-extrated proteins (178), immunoglobulin G-enhanced colostrums (179) and PRX-106 (180) (a recombinant anti-TNF-αfusion protein). The number of hepatic NKT cells was increased in all these mice models.
Liver tumors
Liver tumors are a global health problem that deprives millions of people of their lives. primary hepatic carcinoma (HCC) is the main type of liver tumor. Many studies have shown the tumor-suppressing effect of hepatic NKT cells. Understanding of the roles that NKT cells play in the pathogenesis of both primary and metastatic liver cancer is helpful for finding effective ways of treatment.
NKT cells participate in anti-tumor immune responses mainly by producing IFN-γ. Mice studies found that not only can activated NKT cells secrete IFN-γ, they also stimulate IFN-γ production from NK cells (12, 181). IFN-γ then functions on hepatic T cells and Kupffer cells, enhancing the cytotoxicity and phagocytosis of T cells and Kupffer cells, respectively (182, 183). NKT cells also participate in chemotaxis of T cells as they secrete T cell-recruiting chemokines such as MIP-1α, MIP-1, and IL-8. IFN-γ up-regulates CXCR3 expression of T cells, potentiating T-cell recruitment. Moreover, NKT cells directly mediate the death of tumor cells through FasL, perforin, and granzyme (183). On the other hand, some researchers do not perceive NKT cells as necessary in anti-tumor immunity (184).
Activation of NKT cells in the background of hepatic cancer is closely associated with dendritic cells and Kupffer cells. Dendritic cells communicate with NKT cells in a CD40/CD40L-dependent way. Up-regulated expression of CD40L in NKT cells potentiates DC cells, leading to secretion of IL-12 that in turn activates NKT cells (101). Kupffer cells are also sources of IL-12 (182). Importantly, IL-12-induced NKT activation is linked to reduced primary hepatic tumor and less metastasis to the liver (185) As a result, IL-12-based therapy has been proposed and examined by many researchers (186, 187).
Interestingly, activation of NKT cells is also dependent on CXCR6/CXCL16 interaction, as deficiency of CXCR6 or neutralization of CXCL16 cause hepatic cancer to deteriorate. CXCR6 is expressed on the surface of liver sinusoid epithelium cells, while CXCL16 is a characteristic molecule of NKT cells. However, the deficiency of CXCR6 can be compensated for by systemic NKT-cell activation through other methods (181, 188).
Given the significant functions of NKT cells in development of liver tumors, many novel treatments of hepatic cancer based on NKT cells have been invented in recent years. Mice experiments showed that exogenous IL-12 and α-Galcer (186), direct transfer of ex vivo modulated NKT cells (189), tumor antigens (190, 191), and even antigens of some microorganisms (e.g. LPS from bacteria (182) and some recombinant oncolytic viruses (192)are used to potentiate the anti-tumor effect of NKT cells. Low protein diet (193)and blockade of PD-1/PD-L1 axis (194)are also found useful in suppressing hepatic tumors. Notably, clinical trials have confirmed the effectiveness of some NKT-related treatments. For example, in 2021, Tian-Tian Li et al. (195) reported that stereotactic body radiotherapy had positive effects on peripheral NKT cells in HCC patients, which is associated with a higher overall survival. These results indicate NKT cells as a very promising therapeutic target.
Perspectives and conclusion
In this review, we mainly discussed how NKT cells are activated and the functions of NKT cells during the pathogenesis and development of some liver diseases. It is widely acknowledged that the role NKT cells play in the immunity of the liver and even the whole body is indispensable, and treatment focused on modulating NKT-cell activity is becoming more and more promising. However, considering the dual functions NKT cells have in many liver diseases, the treatment tactics should be studied thoroughly.
In addition, since this review mainly focuses on the physiology of type I NKT cells (iNKT cells), more work needs to be done for a better understanding of type II NKT cells which are more abundant in the human liver than in mice (196). Type II NKT cells are normally considered to be anti-inflammatory, and regulate type I NKT cells (iNKT) and other immune cells and favor tumor growth (101, 197). However, some also report the role that Type II NKT cells play in promoting chronic inflammation (166). Further studies are needed to better demonstrate the functions of Type II NKT cells and how these cells interact with type I NKT cells as well as other participants in our immune system.
Recently, gene analysis has cast new light on NKT researches. Single-cell RNA sequencing indicates distinct populations of functional NKT cell subsets with differences on gene and epigenetic levels (198, 199). This offers a deeper understanding, and is likely to guide future studies within this field.
Author contributions
XG, QC and XM have equal contributions to this study. XG and HZ designed the whole study. JW and CC conducted the statistical analysis. XG, QC, and XM draft the manuscript. JG, YR, and SW made the relevant edits to the manuscript. XG, QC and XM revised the manuscript. All authors read and approved the final manuscript.
Funding
This study was supported by grants awarded by the National Science and Technology Major Project of China (NO 2018ZX10302206), Science and Technology Major Projects of Zhejiang Province (NO 2018C04016), and the Science and Technology Major Projects of Ningbo (NO 2016C51008).
Conflict of interest
The authors declare that the research was conducted in the absence of any commercial or financial relationships that could be construed as a potential conflict of interest.
Publisher’s note
All claims expressed in this article are solely those of the authors and do not necessarily represent those of their affiliated organizations, or those of the publisher, the editors and the reviewers. Any product that may be evaluated in this article, or claim that may be made by its manufacturer, is not guaranteed or endorsed by the publisher.
References
1. Makino Y, Kanno R, Ito T, Higashino K, Taniguchi M. Predominant expression of invariant V alpha 14+ TCR alpha chain in NK1.1+ T cell populations. Int Immunol (1995) 7:1157–61. doi: 10.1093/intimm/7.7.1157
2. Vogt S, Mattner J. NKT cells contribute to the control of microbial infections. Front Cell Infect Microbiol (2021) 11:718350. doi: 10.3389/fcimb.2021.718350
3. Terabe M, Berzofsky JA. Tissue-specific roles of NKT cells in tumor immunity. Front Immunol (2018) 9:1838. doi: 10.3389/fimmu.2018.01838
4. Miyake S, Yamamura T. NKT cells and autoimmune diseases: unraveling the complexity. Curr Top Microbiol Immunol (2007) 314:251–67. doi: 10.1007/978-3-540-69511-0_10
5. Bendelac A, Savage PB, Teyton L. The biology of NKT cells. Annu Rev Immunol (2007) 25:297–336. doi: 10.1146/annurev.immunol.25.022106.141711
6. Kumar V. NKT-cell subsets: promoters and protectors in inflammatory liver disease. J Hepatol (2013) 59:618–20. doi: 10.1016/j.jhep.2013.02.032
7. Wang H, Yin S. Natural killer T cells in liver injury, inflammation and cancer. Expert Rev Gastroenterol Hepatol (2015) 9:1077–85. doi: 10.1586/17474124.2015.1056738
8. Mattner J, Debord KL, Ismail N, Goff RD, Cantu C 3rd, Zhou D, et al. Exogenous and endogenous glycolipid antigens activate NKT cells during microbial infections. Nature (2005) 434:525–9. doi: 10.1038/nature03408
9. Hung JT, Huang JR, Yu AL. Tailored design of NKT-stimulatory glycolipids for polarization of immune responses. J BioMed Sci (2017) 24:22. doi: 10.1186/s12929-017-0325-0
10. Maricic I, Girardi E, Zajonc DM, Kumar V. Recognition of lysophosphatidylcholine by type II NKT cells and protection from an inflammatory liver disease. J Immunol (2014) 193:4580–9. doi: 10.4049/jimmunol.1400699
11. Marrero I, Maricic I, Feldstein AE, Loomba R, Schnabl B, Rivera-Nieves J, et al. Complex network of NKT cell subsets controls immune homeostasis in liver and gut. Front Immunol (2018) 9:2082. doi: 10.3389/fimmu.2018.02082
12. Swain MG. Hepatic NKT cells: Friend or foe? Clin Sci (Lond) (2008) 114:457–66. doi: 10.1042/cs20070328
13. Kobayashi E, Motoki K, Uchida T, Fukushima H, Koezuka Y. KRN7000, a novel immunomodulator, and its antitumor activities. Oncol Res (1995) 7:529–34.
14. Shimizu K, Hidaka M, Bickham K, Moriwaki M, Fujimoto K, Kawano F, et al. Human leukemic cells loaded with alpha-galactosylceramide (alpha-GalCer) activate murine NKT cells in situ. Int J Hematol (2010) 92:152–60. doi: 10.1007/s12185-010-0616-7
15. Osman Y, Kawamura T, Naito T, Takeda K, Van Kaer L, Okumura K, et al. Activation of hepatic NKT cells and subsequent liver injury following administration of alpha-galactosylceramide. Eur J Immunol (2000) 30:1919–28. doi: 10.1002/1521-4141(200007)30:7<1919::Aid-immu1919>3.0.Co;2-3
16. Lind SM, Kuylenstierna C, Moll M, DJ E, Winqvist O, Lundeberg L, et al. IL-18 skews the invariant NKT-cell population via autoreactive activation in atopic eczema. Eur J Immunol (2009) 39:2293–301. doi: 10.1002/eji.200839195
17. Leite-De-Moraes MC, Hameg A, Pacilio M, Koezuka Y, Taniguchi M, Van Kaer L, et al. IL-18 enhances IL-4 production by ligand-activated NKT lymphocytes: A pro-Th2 effect of IL-18 exerted through NKT cells. J Immunol (2001) 166:945–51. doi: 10.4049/jimmunol.166.2.945
18. Uchida T, Kinoshita M, Fukasawa M, Habu Y, Shinomiya N, Seki S. IL-18 time-dependently modulates Th1/Th2 cytokine production by ligand-activated NKT cells. Eur J Immunol (2007) 37:966–77. doi: 10.1002/eji.200636465
19. Mossanen JC, Kohlhepp M, Wehr A, Krenkel O, Liepelt A, Roeth AA, et al. CXCR6 inhibits hepatocarcinogenesis by promoting natural killer T- and CD4(+) T-Cell-Dependent control of senescence. Gastroenterology (2019) 156:1877–89.e4. doi: 10.1053/j.gastro.2019.01.247
20. Fernandez CS, Cameron G, Godfrey DI, Kent SJ. Ex-vivo α-galactosylceramide activation of NKT cells in humans and macaques. J Immunol Methods (2012) 382:150–9. doi: 10.1016/j.jim.2012.05.019
21. Wang Y, Bhave MS, Yagita H, Cardell SL. Natural killer T-cell agonist α-galactosylceramide and PD-1 blockade synergize to reduce tumor development in a preclinical model of colon cancer. Front Immunol (2020) 11:581301. doi: 10.3389/fimmu.2020.581301
22. Chuang YH, Wang TC, Jen HY, Yu AL, Chiang BL. α-galactosylceramide-induced airway eosinophilia is mediated through the activation of NKT cells. J Immunol (2011) 186:4687–92. doi: 10.4049/jimmunol.1003659
23. Sada-Ovalle I, Sköld M, Tian T, Besra GS, Behar SM. Alpha-galactosylceramide as a therapeutic agent for pulmonary mycobacterium tuberculosis infection. Am J Respir Crit Care Med (2010) 182:841–7. doi: 10.1164/rccm.200912-1921OC
24. Van Kaer L. Alpha-galactosylceramide therapy for autoimmune diseases: prospects and obstacles. Nat Rev Immunol (2005) 5:31–42. doi: 10.1038/nri1531
25. Sullivan BA, Kronenberg M. Activation or anergy: NKT cells are stunned by alpha-galactosylceramide. J Clin Invest (2005) 115:2328–9. doi: 10.1172/jci26297
26. Minagawa M, Deng Q, Liu ZX, Tsukamoto H, Dennert G. Activated natural killer T cells induce liver injury by fas and tumor necrosis factor-alpha during alcohol consumption. Gastroenterology (2004) 126:1387–99. doi: 10.1053/j.gastro.2004.01.022
27. Miyagi T, Takehara T, Tatsumi T, Kanto T, Suzuki T, Jinushi M, et al. CD1d-mediated stimulation of natural killer T cells selectively activates hepatic natural killer cells to eliminate experimentally disseminated hepatoma cells in murine liver. Int J Cancer (2003) 106:81–9. doi: 10.1002/ijc.11163
28. Park O, Jeong WI, Wang L, Wang H, Lian ZX, Gershwin ME, et al. Diverse roles of invariant natural killer T cells in liver injury and fibrosis induced by carbon tetrachloride. Hepatology (2009) 49:1683–94. doi: 10.1002/hep.22813
29. Silk JD, Salio M, Reddy BG, Shepherd D, Gileadi U, Brown J, et al. Cutting edge: nonglycosidic CD1d lipid ligands activate human and murine invariant NKT cells. J Immunol (2008) 180:6452–6. doi: 10.4049/jimmunol.180.10.6452
30. Ma J, He P, Zhao C, Ren Q, Dong Z, Qiu J, et al. A designed α-GalCer analog promotes considerable Th1 cytokine response by activating the CD1d-iNKT axis and CD11b-positive Monocytes/Macrophages. Adv Sci (Weinh) (2020) 7:2000609. doi: 10.1002/advs.202000609
31. Trappeniers M, Van Beneden K, Decruy T, Hillaert U, Linclau B, Elewaut D, et al. 6'-derivatised alpha-GalCer analogues capable of inducing strong CD1d-mediated Th1-biased NKT cell responses in mice. J Am Chem Soc (2008) 130:16468–9. doi: 10.1021/ja8064182
32. Li X, Fujio M, Imamura M, Wu D, Vasan S, Wong CH, et al. Design of a potent CD1d-binding NKT cell ligand as a vaccine adjuvant. Proc Natl Acad Sci U.S.A. (2010) 107:13010–5. doi: 10.1073/pnas.1006662107
33. Broecker F, Götze S, Hudon J, Rathwell DCK, Pereira CL, Stallforth P, et al. Synthesis, liposomal formulation, and immunological evaluation of a minimalistic carbohydrate-α-GalCer vaccine candidate. J Med Chem (2018) 61:4918–27. doi: 10.1021/acs.jmedchem.8b00312
34. Yin N, Long X, Goff RD, Zhou D, Cantu C 3rd, Mattner J, et al. Alpha anomers of iGb3 and Gb3 stimulate cytokine production by natural killer T cells. ACS Chem Biol (2009) 4:199–208. doi: 10.1021/cb800277n
35. Li Y, Thapa P, Hawke D, Kondo Y, Furukawa K, Furukawa K, et al. Immunologic glycosphingolipidomics and NKT cell development in mouse thymus. J Proteome Res (2009) 8:2740–51. doi: 10.1021/pr801040h
36. Kawahara K, Moll H, Knirel YA, Seydel U, Zähringer U. Structural analysis of two glycosphingolipids from the lipopolysaccharide-lacking bacterium sphingomonas capsulata. Eur J Biochem (2000) 267:1837–46. doi: 10.1046/j.1432-1327.2000.01189.x
37. Kim JH, Kim HS, Kim HY, Oh SJ, Chung DH. Direct engagement of TLR4 in invariant NKT cells regulates immune diseases by differential IL-4 and IFN-γ production in mice. PloS One (2012) 7:e45348. doi: 10.1371/journal.pone.0045348
38. Shen H, Gu C, Liang T, Liu H, Guo F, Liu X. Unveiling the heterogeneity of NKT cells in the liver through single cell RNA sequencing. Sci Rep (2020) 10:19453. doi: 10.1038/s41598-020-76659-1
39. Ortaldo JR, Winkler-Pickett RT, Bere EW Jr., Watanabe M, Murphy WJ, Wiltrout RH. In vivo hydrodynamic delivery of cDNA encoding IL-2: rapid, sustained redistribution, activation of mouse NK cells, and therapeutic potential in the absence of NKT cells. J Immunol (2005) 175:693–9. doi: 10.4049/jimmunol.175.2.693
40. Ikarashi Y, Iizuka A, Heike Y, Yoshida M, Takaue Y, Wakasugi H. Cytokine production and migration of in vitro-expanded NK1.1(-) invariant Valpha14 natural killer T (Valpha14i NKT) cells using alpha-galactosylceramide and IL-2. Immunol Lett (2005) 101:160–7. doi: 10.1016/j.imlet.2005.05.007
41. Park Y, Kim HS, Ahn JY, Yun D, Cho ML, Hong S, et al. IL-12p35 promotes antibody-induced joint inflammation by activating NKT cells and suppressing TGF-beta. J Immunol (2010) 185:1476–84. doi: 10.4049/jimmunol.1000425
42. Tang ZH, Liang S, Potter J, Jiang X, Mao HQ, Li Z. Tim-3/galectin-9 regulate the homeostasis of hepatic NKT cells in a murine model of nonalcoholic fatty liver disease. J Immunol (2013) 190:1788–96. doi: 10.4049/jimmunol.1202814
43. Ranson T, Vosshenrich CA, Corcuff E, Richard O, Laloux V, Lehuen A, et al. IL-15 availability conditions homeostasis of peripheral natural killer T cells. Proc Natl Acad Sci USA (2003) 100:2663–8. doi: 10.1073/pnas.0535482100
44. Vallabhapurapu S, Powolny-Budnicka I, Riemann M, Schmid RM, Paxian S, Pfeffer K, et al. Rel/NF-kappaB family member RelA regulates NK1.1- to NK1.1+ transition as well as IL-15-induced expansion of NKT cells. Eur J Immunol (2008) 38:3508–19. doi: 10.1002/eji.200737830
45. Locatelli I, Sutti S, Vacchiano M, Bozzola C, Albano E. NF-κB1 deficiency stimulates the progression of non-alcoholic steatohepatitis (NASH) in mice by promoting NKT-cell-mediated responses. Clin Sci (Lond) (2013) 124:279–87. doi: 10.1042/cs20120289
46. Coquet JM, Kyparissoudis K, Pellicci DG, Besra G, Berzins SP, Smyth MJ, et al. IL-21 is produced by NKT cells and modulates NKT cell activation and cytokine production. J Immunol (2007) 178:2827–34. doi: 10.4049/jimmunol.178.5.2827
47. Coquet JM, Skak K, Davis ID, Smyth MJ, Godfrey DI. IL-21 modulates activation of NKT cells in patients with stage IV malignant melanoma. Clin Transl Immunol (2013) 2:e6. doi: 10.1038/cti.2013.7
48. Wei J, Xia S, Sun H, Zhang S, Wang J, Zhao H, et al. Critical role of dendritic cell-derived IL-27 in antitumor immunity through regulating the recruitment and activation of NK and NKT cells. J Immunol (2013) 191:500–8. doi: 10.4049/jimmunol.1300328
49. Chen J, Duan L, Xiong A, Zhang H, Zheng F, Tan Z, et al. Blockade of IL-33 ameliorates con a-induced hepatic injury by reducing NKT cell activation and IFN-γ production in mice. J Mol Med (Berl) (2012) 90:1505–15. doi: 10.1007/s00109-012-0938-4
50. Nishihori Y, Kato K, Tanaka M, Okamoto T, Hagiwara S, Araki N, et al. Interleukin-2 gene transfer potentiates the alpha-galactosylceramide-stimulated antitumor effect by the induction of TRAIL in NKT and NK cells in mouse models of subcutaneous and metastatic carcinoma. Cancer Biol Ther (2009) 8:1763–70. doi: 10.4161/cbt.8.18.9321
51. Tan X, Ding Y, Zhu P, Dou R, Liang Z, Yang D, et al. Elevated hepatic CD1d levels coincide with invariant NKT cell defects in chronic hepatitis b virus infection. J Immunol (2018) 200:3530–8. doi: 10.4049/jimmunol.1701801
52. Baxevanis CN, Gritzapis AD, Papamichail M. In vivo antitumor activity of NKT cells activated by the combination of IL-12 and IL-18. J Immunol (2003) 171:2953–9. doi: 10.4049/jimmunol.171.6.2953
53. Dao T, Mehal WZ, Crispe IN. IL-18 augments perforin-dependent cytotoxicity of liver NK-T cells. J Immunol (1998) 161:2217–22.
54. Subleski JJ, Hall VL, Back TC, Ortaldo JR, Wiltrout RH. Enhanced antitumor response by divergent modulation of natural killer and natural killer T cells in the liver. Cancer Res (2006) 66:11005–12. doi: 10.1158/0008-5472.Can-06-0811
55. Zlotnik A, Yoshie O. The chemokine superfamily revisited. Immunity (2012) 36:705–16. doi: 10.1016/j.immuni.2012.05.008
56. Sokol CL, Luster AD. The chemokine system in innate immunity. Cold Spring Harb Perspect Biol (2015) 7(5):a016303. doi: 10.1101/cshperspect.a016303
57. Charo IF, Ransohoff RM. The many roles of chemokines and chemokine receptors in inflammation. N Engl J Med (2006) 354:610–21. doi: 10.1056/NEJMra052723
58. Faunce DE, Sonoda KH, Stein-Streilein J. MIP-2 recruits NKT cells to the spleen during tolerance induction. J Immunol (2001) 166:313–21. doi: 10.4049/jimmunol.166.1.313
59. Ajuebor MN, Hogaboam CM, Le T, Swain MG. C-c chemokine ligand 2/monocyte chemoattractant protein-1 directly inhibits NKT cell IL-4 production and is hepatoprotective in T cell-mediated hepatitis in the mouse. J Immunol (2003) 170:5252–9. doi: 10.4049/jimmunol.170.10.5252
60. Thomas SY, Hou R, Boyson JE, Means TK, Hess C, Olson DP, et al. CD1d-restricted NKT cells express a chemokine receptor profile indicative of Th1-type inflammatory homing cells. J Immunol (2003) 171:2571–80. doi: 10.4049/jimmunol.171.5.2571
61. Gustafsson K, Junevik K, Werlenius O, Holmgren S, Karlsson-Parra A, Andersson PO. Tumour-loaded α-type 1-polarized dendritic cells from patients with chronic lymphocytic leukaemia produce a superior NK-, NKT- and CD8+ T cell-attracting chemokine profile. Scand J Immunol (2011) 74:318–26. doi: 10.1111/j.1365-3083.2011.02580.x
62. Ajuebor MN, Aspinall AI, Zhou F, Le T, Yang Y, Urbanski SJ, et al. Lack of chemokine receptor CCR5 promotes murine fulminant liver failure by preventing the apoptosis of activated CD1d-restricted NKT cells. J Immunol (2005) 174:8027–37. doi: 10.4049/jimmunol.174.12.8027
63. Geissmann F, Cameron TO, Sidobre S, Manlongat N, Kronenberg M, Briskin MJ, et al. Intravascular immune surveillance by CXCR6+ NKT cells patrolling liver sinusoids. PloS Biol (2005) 3:e113. doi: 10.1371/journal.pbio.0030113
64. Zhu H, Zhang Q, Chen G. CXCR6 deficiency ameliorates ischemia-reperfusion injury by reducing the recruitment and cytokine production of hepatic NKT cells in a mouse model of non-alcoholic fatty liver disease. Int Immunopharmacol (2019) 72:224–34. doi: 10.1016/j.intimp.2019.04.021
65. Shimaoka T, Seino K, Kume N, Minami M, Nishime C, Suematsu M, et al. Critical role for CXC chemokine ligand 16 (SR-PSOX) in Th1 response mediated by NKT cells. J Immunol (2007) 179:8172–9. doi: 10.4049/jimmunol.179.12.8172
66. Jiang J, Karimi O, Ouburg S, Champion CI, Khurana A, Liu G, et al. Interruption of CXCL13-CXCR5 axis increases upper genital tract pathology and activation of NKT cells following chlamydial genital infection. PloS One (2012) 7:e47487. doi: 10.1371/journal.pone.0047487
67. Drennan MB, Govindarajan S, De Wilde K, Schlenner SM, Ware C, Nedospasov S, et al. The thymic microenvironment differentially regulates development and trafficking of invariant NKT cell sublineages. J Immunol (2014) 193:5960–72. doi: 10.4049/jimmunol.1401601
68. Huang D, Shi FD, Jung S, Pien GC, Wang J, Salazar-Mather TP, et al. The neuronal chemokine CX3CL1/fractalkine selectively recruits NK cells that modify experimental autoimmune encephalomyelitis within the central nervous system. FASEB J (2006) 20:896–905. doi: 10.1096/fj.05-5465com
69. Kumari S, Shivam P, Kumar S, Jamal F, Singh MK, Bimal S, et al. Leishmania donovani mediated higher expression of CCL4 induces differential accumulation of CD4(+)CD56(+)NKT and CD8(+)CD56(+)NKT cells at infection site. Cytokine (2018) 110:306–15. doi: 10.1016/j.cyto.2018.03.022
70. Germanov E, Veinotte L, Cullen R, Chamberlain E, Butcher EC, Johnston B. Critical role for the chemokine receptor CXCR6 in homeostasis and activation of CD1d-restricted NKT cells. J Immunol (2008) 181:81–91. doi: 10.4049/jimmunol.181.1.81
71. Ma KL, Wu Y, Zhang Y, Wang GH, Hu ZB, Ruan XZ. Activation of the CXCL16/CXCR6 pathway promotes lipid deposition in fatty livers of apolipoprotein e knockout mice and HepG2 cells. Am J Transl Res (2018) 10:1802–16.
72. Jiang L, Yang M, Li X, Wang Y, Zhou G, Zhao J. CXC motif ligand 16 promotes nonalcoholic fatty liver disease progression via hepatocyte-stellate cell crosstalk. J Clin Endocrinol Metab (2018) 103:3974–85. doi: 10.1210/jc.2018-00762
73. Liepelt A, Wehr A, Kohlhepp M, Mossanen JC, Kreggenwinkel K, Denecke B, et al. CXCR6 protects from inflammation and fibrosis in NEMO(LPC-KO) mice. Biochim Biophys Acta Mol Basis Dis (2019) 1865:391–402. doi: 10.1016/j.bbadis.2018.11.020
74. Yang L, Jhaveri R, Huang J, Qi Y, Diehl AM. Endoplasmic reticulum stress, hepatocyte CD1d and NKT cell abnormalities in murine fatty livers. Lab Invest (2007) 87:927–37. doi: 10.1038/labinvest.3700603
75. Dong Z, Zhang J, Sun R, Wei H, Tian Z. Impairment of liver regeneration correlates with activated hepatic NKT cells in HBV transgenic mice. Hepatology (2007) 45:1400–12. doi: 10.1002/hep.21597
76. Liang B, Hara T, Wagatsuma K, Zhang J, Maki K, Miyachi H, et al. Role of hepatocyte-derived IL-7 in maintenance of intrahepatic NKT cells and T cells and development of b cells in fetal liver. J Immunol (2012) 189:4444–50. doi: 10.4049/jimmunol.1201181
77. Dennert G, Aswad F. The role of NKT cells in animal models of autoimmune hepatitis. Crit Rev Immunol (2006) 26:453–73. doi: 10.1615/critrevimmunol.v26.i5.50
78. Yamada Y, Kirillova I, Peschon JJ, Fausto N. Initiation of liver growth by tumor necrosis factor: deficient liver regeneration in mice lacking type I tumor necrosis factor receptor. Proc Natl Acad Sci USA (1997) 94:1441–6. doi: 10.1073/pnas.94.4.1441
79. Santodomingo-Garzon T, Swain MG. Role of NKT cells in autoimmune liver disease. Autoimmun Rev (2011) 10:793–800. doi: 10.1016/j.autrev.2011.06.003
80. Chelakkot-Govindalayathil AL, Mifuji-Moroka R, D'Alessandro-Gabazza CN, Toda M, Matsuda Y, Gil-Bernabe P, et al. Protein s exacerbates alcoholic hepatitis by stimulating liver natural killer T cells. J Thromb Haemost (2015) 13:142–54. doi: 10.1111/jth.12789
81. Wolf MJ, Adili A, Piotrowitz K, Abdullah Z, Boege Y, Stemmer K, et al. Metabolic activation of intrahepatic CD8+ T cells and NKT cells causes nonalcoholic steatohepatitis and liver cancer via cross-talk with hepatocytes. Cancer Cell (2014) 26:549–64. doi: 10.1016/j.ccell.2014.09.003
82. Ahmad A, Alvarez F. Role of NK and NKT cells in the immunopathogenesis of HCV-induced hepatitis. J Leukoc Biol (2004) 76:743–59. doi: 10.1189/jlb.0304197
83. Santos SA, Andrade DR, Andrade Júnior DR. Rat hepatocyte invasion by listeria monocytogenes and analysis of TNF-alpha role in apoptosis. Rev Inst Med Trop Sao Paulo (2005) 47:73–80. doi: 10.1590/s0036-46652005000200003
84. Bai L, Deng S, Reboulet R, Mathew R, Teyton L, Savage PB, et al. (NKT)-b-cell interactions promote prolonged antibody responses and long-term memory to pneumococcal capsular polysaccharides. Proc Natl Acad Sci USA (2013) 110:16097–102. doi: 10.1073/pnas.1303218110
85. Galli G, Nuti S, Tavarini S, Galli-Stampino L, De Lalla C, Casorati G, et al. Innate immune responses support adaptive immunity: NKT cells induce b cell activation. Vaccine (2003) 21 Suppl 2:S48–54. doi: 10.1016/s0264-410x(03)00200-7
86. Leadbetter EA, Brigl M, Illarionov P, Cohen N, Luteran MC, Pillai S, et al. NK T cells provide lipid antigen-specific cognate help for b cells. Proc Natl Acad Sci USA (2008) 105:8339–44. doi: 10.1073/pnas.0801375105
87. King IL, Fortier A, Tighe M, Dibble J, Watts GF, Veerapen N, et al. Invariant natural killer T cells direct b cell responses to cognate lipid antigen in an IL-21-dependent manner. Nat Immunol (2011) 13:44–50. doi: 10.1038/ni.2172
88. Vomhof-DeKrey EE, Yates J, Leadbetter EA. Invariant NKT cells provide innate and adaptive help for b cells. Curr Opin Immunol (2014) 28:12–7. doi: 10.1016/j.coi.2014.01.007
89. Almishri W, Deans J, Swain MG. Rapid activation and hepatic recruitment of innate-like regulatory b cells after invariant NKT cell stimulation in mice. J Hepatol (2015) 63:943–51. doi: 10.1016/j.jhep.2015.06.007
90. Tang T, Sui Y, Lian M, Li Z, Hua J. Pro-inflammatory activated kupffer cells by lipids induce hepatic NKT cells deficiency through activation-induced cell death. PloS One (2013) 8:e81949. doi: 10.1371/journal.pone.0081949
91. Duwaerts CC, Sun EP, Cheng CW, van Rooijen N, Gregory SH. Cross-activating invariant NKT cells and kupffer cells suppress cholestatic liver injury in a mouse model of biliary obstruction. PloS One (2013) 8:e79702. doi: 10.1371/journal.pone.0079702
92. Beattie L, Svensson M, Bune A, Brown N, Maroof A, Zubairi S, et al. Leishmania donovani-induced expression of signal regulatory protein alpha on kupffer cells enhances hepatic invariant NKT-cell activation. Eur J Immunol (2010) 40:117–23. doi: 10.1002/eji.200939863
93. Hou X, Hao X, Zheng M, Xu C, Wang J, Zhou R, et al. CD205-TLR9-IL-12 axis contributes to CpG-induced oversensitive liver injury in HBsAg transgenic mice by promoting the interaction of NKT cells with kupffer cells. Cell Mol Immunol (2017) 14:675–84. doi: 10.1038/cmi.2015.111
94. Cui K, Yan G, Xu C, Chen Y, Wang J, Zhou R, et al. Invariant NKT cells promote alcohol-induced steatohepatitis through interleukin-1β in mice. J Hepatol (2015) 62:1311–8. doi: 10.1016/j.jhep.2014.12.027
95. Golden-Mason L, Kelly AM, Doherty DG, Traynor O, McEntee G, Kelly J, et al. Hepatic interleuklin 15 (IL-15) expression: implications for local NK/NKT cell homeostasis and development. Clin Exp Immunol (2004) 138:94–101. doi: 10.1111/j.1365-2249.2004.02586.x
96. Kuwata K, Watanabe H, Jiang SY, Yamamoto T, Tomiyama-Miyaji C, Abo T, et al. AIM inhibits apoptosis of T cells and NKT cells in corynebacterium-induced granuloma formation in mice. Am J Pathol (2003) 162:837–47. doi: 10.1016/s0002-9440(10)63880-1
97. Robert-Gangneux F, Drogoul AS, Rostan O, Piquet-Pellorce C, Cayon J, Lisbonne M, et al. Invariant NKT cells drive hepatic cytokinic microenvironment favoring efficient granuloma formation and early control of leishmania donovani infection. PloS One (2012) 7:e33413. doi: 10.1371/journal.pone.0033413
98. Jiang W, Sun R, Zhou R, Wei H, Tian Z. TLR-9 activation aggravates concanavalin a-induced hepatitis via promoting accumulation and activation of liver CD4+ NKT cells. J Immunol (2009) 182:3768–74. doi: 10.4049/jimmunol.0800973
99. Singh AK, Gaur P, Shukla NK, Das SN. Differential dendritic cell-mediated activation and functions of invariant NKT-cell subsets in oral cancer. Oral Dis (2015) 21:e105–13. doi: 10.1111/odi.12238
100. Joshi SK, Lang GA, Devera TS, Johnson AM, Kovats S, Lang ML. Differential contribution of dendritic cell CD1d to NKT cell-enhanced humoral immunity and CD8+ T cell activation. J Leukoc Biol (2012) 91:783–90. doi: 10.1189/jlb.1111559
101. Subleski JJ, Jiang Q, Weiss JM, Wiltrout RH. The split personality of NKT cells in malignancy, autoimmune and allergic disorders. Immunotherapy (2011) 3:1167–84. doi: 10.2217/imt.11.117
102. Osmond TL, Farrand KJ, Painter GF, Ruedl C, Petersen TR, Hermans IF. Activated NKT cells can condition different splenic dendritic cell subsets to respond more effectively to TLR engagement and enhance cross-priming. J Immunol (2015) 195:821–31. doi: 10.4049/jimmunol.1401751
103. Hermans IF, Silk JD, Gileadi U, Masri SH, Shepherd D, Farrand KJ, et al. Dendritic cell function can be modulated through cooperative actions of TLR ligands and invariant NKT cells. J Immunol (2007) 178:2721–9. doi: 10.4049/jimmunol.178.5.2721
104. Tatsumi T, Takehara T, Yamaguchi S, Sasakawa A, Sakamori R, Ohkawa K, et al. Intrahepatic delivery of alpha-galactosylceramide-pulsed dendritic cells suppresses liver tumor. Hepatology (2007) 45:22–30. doi: 10.1002/hep.21447
105. Dondji B, Deak E, Goldsmith-Pestana K, Perez-Jimenez E, Esteban M, Miyake S, et al. Intradermal NKT cell activation during DNA priming in heterologous prime-boost vaccination enhances T cell responses and protection against leishmania. Eur J Immunol (2008) 38:706–19. doi: 10.1002/eji.200737660
106. Hermans IF, Silk JD, Gileadi U, Salio M, Mathew B, Ritter G, et al. NKT cells enhance CD4+ and CD8+ T cell responses to soluble antigen in vivo through direct interaction with dendritic cells. J Immunol (2003) 171:5140–7. doi: 10.4049/jimmunol.171.10.5140
107. Goubier A, Vocanson M, Macari C, Poyet G, Herbelin A, Nicolas JF, et al. Invariant NKT cells suppress CD8(+) T-cell-mediated allergic contact dermatitis independently of regulatory CD4(+) T cells. J Invest Dermatol (2013) 133:980–7. doi: 10.1038/jid.2012.404
108. Shin Y, Hong C, Lee H, Shin JH, Hong S, Park SH. NKT cell-dependent regulation of secondary antigen-specific, conventional CD4+ T cell immune responses. J Immunol (2010) 184:5589–94. doi: 10.4049/jimmunol.0903121
109. Kim HJ, Hwang SJ, Kim BK, Jung KC, Chung DH. NKT cells play critical roles in the induction of oral tolerance by inducing regulatory T cells producing IL-10 and transforming growth factor beta, and by clonally deleting antigen-specific T cells. Immunology (2006) 118:101–11. doi: 10.1111/j.1365-2567.2006.02346.x
110. Hua J, Liang S, Ma X, Webb TJ, Potter JP, Li Z. The interaction between regulatory T cells and NKT cells in the liver: a CD1d bridge links innate and adaptive immunity. PloS One (2011) 6:e27038. doi: 10.1371/journal.pone.0027038
111. La Cava A, Van Kaer L, Fu Dong S. CD4+CD25+ tregs and NKT cells: regulators regulating regulators. Trends Immunol (2006) 27:322–7. doi: 10.1016/j.it.2006.05.003
112. Ito H, Ando K, Ishikawa T, Nakayama T, Taniguchi M, Saito K, et al. Role of Valpha14+ NKT cells in the development of hepatitis b virus-specific CTL: activation of Valpha14+ NKT cells promotes the breakage of CTL tolerance. Int Immunol (2008) 20:869–79. doi: 10.1093/intimm/dxn046
113. Vilarinho S, Ogasawara K, Nishimura S, Lanier LL, Baron JL. Blockade of NKG2D on NKT cells prevents hepatitis and the acute immune response to hepatitis b virus. Proc Natl Acad Sci USA (2007) 104:18187–92. doi: 10.1073/pnas.0708968104
114. Werner JM, Heller T, Gordon AM, Sheets A, Sherker AH, Kessler E, et al. Innate immune responses in hepatitis c virus-exposed healthcare workers who do not develop acute infection. Hepatology (2013) 58:1621–31. doi: 10.1002/hep.26353
115. Mattner J, Natural killer T. (NKT) cells in autoimmune hepatitis. Curr Opin Immunol (2013) 25:697–703. doi: 10.1016/j.coi.2013.09.008
116. Maricic I, Marrero I, Eguchi A, Nakamura R, Johnson CD, Dasgupta S, et al. Differential activation of hepatic invariant NKT cell subsets plays a key role in progression of nonalcoholic steatohepatitis. J Immunol (2018) 201:3017–35. doi: 10.4049/jimmunol.1800614
117. Smyk DS, Mavropoulos A, Mieli-Vergani G, Vergani D, Lenzi M, Bogdanos DP. The role of invariant NKT in autoimmune liver disease: Can vitamin d act as an immunomodulator? Can J Gastroenterol Hepatol (2018) 2018:8197937. doi: 10.1155/2018/8197937
118. Wang H, Feng D, Park O, Yin S, Gao B. Invariant NKT cell activation induces neutrophil accumulation and hepatitis: opposite regulation by IL-4 and IFN-γ. Hepatology (2013) 58:1474–85. doi: 10.1002/hep.26471
119. Mitra A, Satelli A, Yan J, Xueqing X, Gagea M, Hunter CA, et al. IL-30 (IL27p28) attenuates liver fibrosis through inducing NKG2D-rae1 interaction between NKT and activated hepatic stellate cells in mice. Hepatology (2014) 60:2027–39. doi: 10.1002/hep.27392
120. Tajiri K, Shimizu Y. Role of NKT cells in the pathogenesis of NAFLD. Int J Hepatol (2012) 2012:850836. doi: 10.1155/2012/850836
121. Syn WK, Agboola KM, Swiderska M, Michelotti GA, Liaskou E, Pang H, et al. NKT-associated hedgehog and osteopontin drive fibrogenesis in non-alcoholic fatty liver disease. Gut (2012) 61:1323–9. doi: 10.1136/gutjnl-2011-301857
122. Byun JS, Jeong WI. Involvement of hepatic innate immunity in alcoholic liver disease. Immune Netw (2010) 10:181–7. doi: 10.4110/in.2010.10.6.181
123. Iyoda T, Yamasaki S, Hidaka M, Kawano F, Abe Y, Suzuki K, et al. Amelioration of NK cell function driven by Vα24(+) invariant NKT cell activation in multiple myeloma. Clin Immunol (2018) 187:76–84. doi: 10.1016/j.clim.2017.10.007
124. Riese P, Trittel S, May T, Cicin-Sain L, Chambers BJ, Guzmán CA. Activated NKT cells imprint NK-cell differentiation, functionality and education. Eur J Immunol (2015) 45:1794–807. doi: 10.1002/eji.201445209
125. Kim EY, Ner-Gaon H, Varon J, Cullen AM, Guo J, Choi J, et al. Post-sepsis immunosuppression depends on NKT cell regulation of mTOR/IFN-γ in NK cells. J Clin Invest (2020) 130:3238–52. doi: 10.1172/jci128075
126. Cui K, Yan G, Zheng X, Bai L, Wei H, Sun R, et al. Suppression of natural killer cell activity by regulatory NKT10 cells aggravates alcoholic hepatosteatosis. Front Immunol (2017) 8:1414. doi: 10.3389/fimmu.2017.01414
127. Chen Y, Tian Z. Innate lymphocytes: pathogenesis and therapeutic targets of liver diseases and cancer. Cell Mol Immunol (2021) 18:57–72. doi: 10.1038/s41423-020-00561-z
128. Schweitzer A, Horn J, Mikolajczyk RT, Krause G, Ott JJ. Estimations of worldwide prevalence of chronic hepatitis b virus infection: a systematic review of data published between 1965 and 2013. Lancet (2015) 386:1546–55. doi: 10.1016/s0140-6736(15)61412-x
129. Gower E, Estes C, Blach S, Razavi-Shearer K, Razavi H. Global epidemiology and genotype distribution of the hepatitis c virus infection. J Hepatol (2014) 61:S45–57. doi: 10.1016/j.jhep.2014.07.027
130. Zeissig S, Murata K, Sweet L, Publicover J, Hu Z, Kaser A, et al. Hepatitis b virus-induced lipid alterations contribute to natural killer T cell-dependent protective immunity. Nat Med (2012) 18:1060–8. doi: 10.1038/nm.2811
131. Kakimi K, Guidotti LG, Koezuka Y, Chisari FV. Natural killer T cell activation inhibits hepatitis b virus replication. vivo J Exp Med (2000) 192:921–30. doi: 10.1084/jem.192.7.921
132. Baron JL, Gardiner L, Nishimura S, Shinkai K, Locksley R, Ganem D. Activation of a nonclassical NKT cell subset in a transgenic mouse model of hepatitis b virus infection. Immunity (2002) 16:583–94. doi: 10.1016/s1074-7613(02)00305-9
133. Tripathy AS, Das R, Chadha MS, Arankalle VA. Epidemic of hepatitis b with high mortality in India: association of fulminant disease with lack of CCL4 and natural killer T cells. J Viral Hepat (2011) 18:e415–22. doi: 10.1111/j.1365-2893.2011.01457.x
134. Jiang X, Zhang M, Lai Q, Huang X, Li Y, Sun J, et al. Restored circulating invariant NKT cells are associated with viral control in patients with chronic hepatitis b. PloS One (2011) 6:e28871. doi: 10.1371/journal.pone.0028871
135. Yang Z, Lei Y, Chen C, Ren H, Shi T. Roles of the programmed cell death 1, T cell immunoglobulin mucin-3, and cluster of differentiation 288 pathways in the low reactivity of invariant natural killer T cells after chronic hepatitis b virus infection. Arch Virol (2015) 160:2535–45. doi: 10.1007/s00705-015-2539-3
136. Jin Z, Sun R, Wei H, Gao X, Chen Y, Tian Z. Accelerated liver fibrosis in hepatitis b virus transgenic mice: involvement of natural killer T cells. Hepatology (2011) 53:219–29. doi: 10.1002/hep.23983
137. Wei X, Qian J, Yao W, Chen L, Guan H, Chen Y, et al. Hyperactivated peripheral invariant natural killer T cells correlate with the progression of HBV-relative liver cirrhosis. Scand J Immunol (2019) 90:e12775. doi: 10.1111/sji.12775
138. Diao H, He J, Zheng Q, Chen J, Cui G, Wei Y, et al. A possible role for NKT-like cells in patients with chronic hepatitis b during telbivudine treatment. Immunol Lett (2014) 160:65–71. doi: 10.1016/j.imlet.2014.03.013
139. Woltman AM, Ter Borg MJ, Binda RS, Sprengers D, von Blomberg BM, Scheper RJ, et al. Alpha-galactosylceramide in chronic hepatitis b infection: results from a randomized placebo-controlled phase I/II trial. Antivir Ther (2009) 14:809–18. doi: 10.3851/imp1295
140. Kimura K, Kakimi K, Wieland S, Guidotti LG, Chisari FV. Interleukin-18 inhibits hepatitis b virus replication in the livers of transgenic mice. J Virol (2002) 76:10702–7. doi: 10.1128/jvi.76.21.10702-10707.2002
141. Sugahara S, Ichida T, Yamagiwa S, Ishikawa T, Uehara K, Yoshida Y, et al. Thymosin-alpha1 increases intrahepatic NKT cells and CTLs in patients with chronic hepatitis b. Hepatol Res (2002) 24:346–54. doi: 10.1016/s1386-6346(02)00145-6
142. Mizrahi M, Lalazar G, Ben Ya'acov A, Livovsky DM, Horowitz Y, Zolotarov L, et al. Beta-glycoglycosphingolipid-induced augmentation of the anti-HBV immune response is associated with altered CD8 and NKT lymphocyte distribution: a novel adjuvant for HBV vaccination. Vaccine (2008) 26:2589–95. doi: 10.1016/j.vaccine.2008.03.026
143. Wang XF, Lei Y, Chen M, Chen CB, Ren H, Shi TD. PD-1/PDL1 and CD28/CD80 pathways modulate natural killer T cell function to inhibit hepatitis b virus replication. J Viral Hepat (2013) 20 Suppl 1:27–39. doi: 10.1111/jvh.12061
144. Durante-Mangoni E, Wang R, Shaulov A, He Q, Nasser I, Afdhal N, et al. Hepatic CD1d expression in hepatitis c virus infection and recognition by resident proinflammatory CD1d-reactive T cells. J Immunol (2004) 173:2159–66. doi: 10.4049/jimmunol.173.3.2159
145. Kaneko Y, Harada M, Kawano T, Yamashita M, Shibata Y, Gejyo F, et al. Augmentation of Valpha14 NKT cell-mediated cytotoxicity by interleukin 4 in an autocrine mechanism resulting in the development of concanavalin a-induced hepatitis. J Exp Med (2000) 191:105–14. doi: 10.1084/jem.191.1.105
146. Deignan T, Curry MP, Doherty DG, Golden-Mason L, Volkov Y, Norris S, et al. Decrease in hepatic CD56(+) T cells and V alpha 24(+) natural killer T cells in chronic hepatitis c viral infection. J Hepatol (2002) 37:101–8. doi: 10.1016/s0168-8278(02)00072-7
147. Lucas M, Gadola S, Meier U, Young NT, Harcourt G, Karadimitris A, et al. Frequency and phenotype of circulating Valpha24/Vbeta11 double-positive natural killer T cells during hepatitis c virus infection. J Virol (2003) 77:2251–7. doi: 10.1128/jvi.77.3.2251-2257.2003
148. van der Vliet HJ, Molling JW, von Blomberg BM, Kölgen W, Stam AG, de Gruijl TD, et al. Circulating Valpha24+Vbeta11+ NKT cell numbers and dendritic cell CD1d expression in hepatitis c virus infected patients. Clin Immunol (2005) 114:183–9. doi: 10.1016/j.clim.2004.10.001
149. Szereday L, Meggyes M, Halasz M, Szekeres-Bartho J, Par A, Par G. Immunological changes in different patient populations with chronic hepatitis c virus infection. World J Gastroenterol (2016) 22:4848–59. doi: 10.3748/wjg.v22.i20.4848
150. Kanto T, Hayashi N. Innate immunity in hepatitis c virus infection: Interplay among dendritic cells, natural killer cells and natural killer T cells. Hepatol Res (2007) 37 Suppl 3:S319–26. doi: 10.1111/j.1872-034X.2007.00236.x
151. Yamagiwa S, Matsuda Y, Ichida T, Honda Y, Takamura M, Sugahara S, et al. Sustained response to interferon-alpha plus ribavirin therapy for chronic hepatitis c is closely associated with increased dynamism of intrahepatic natural killer and natural killer T cells. Hepatol Res (2008) 38:664–72. doi: 10.1111/j.1872-034X.2008.00317.x
152. Hurtado CW, Golden-Mason L, Brocato M, Krull M, Narkewicz MR, Rosen HR. Innate immune function in placenta and cord blood of hepatitis c–seropositive mother-infant dyads. PloS One (2010) 5:e12232. doi: 10.1371/journal.pone.0012232
153. Miyaki E, Hiraga N, Imamura M, Uchida T, Kan H, Tsuge M, et al. Interferon alpha treatment stimulates interferon gamma expression in type I NKT cells and enhances their antiviral effect against hepatitis c virus. PloS One (2017) 12:e0172412. doi: 10.1371/journal.pone.0172412
154. Ohira M, Ishiyama K, Tanaka Y, Doskali M, Igarashi Y, Tashiro H, et al. Adoptive immunotherapy with liver allograft-derived lymphocytes induces anti-HCV activity after liver transplantation in humans and humanized mice. J Clin Invest (2009) 119:3226–35. doi: 10.1172/jci38374
155. Israeli E, Safadi R, Melhem A, Pappo O, Shibolet O, Klein A, et al. Induction of oral immune regulation towards liver-extracted proteins for treatment of chronic HBV and HCV hepatitis: results of a phase I clinical trial. Liver Int (2004) 24:295–307. doi: 10.1111/j.1478-3231.2004.0935.x
156. Ma WT, Chen DK. Immunological abnormalities in patients with primary biliary cholangitis. Clin Sci (Lond) (2019) 133:741–60. doi: 10.1042/cs20181123
157. Maricic I, Sheng H, Marrero I, Seki E, Kisseleva T, Chaturvedi S, et al. Inhibition of type I natural killer T cells by retinoids or following sulfatide-mediated activation of type II natural killer T cells attenuates alcoholic liver disease in mice. Hepatology (2015) 61:1357–69. doi: 10.1002/hep.27632
158. Mathews S, Feng D, Maricic I, Ju C, Kumar V, Gao B. Invariant natural killer T cells contribute to chronic-plus-binge ethanol-mediated liver injury by promoting hepatic neutrophil infiltration. Cell Mol Immunol (2016) 13:206–16. doi: 10.1038/cmi.2015.06
159. Elinav E, Pappo O, Sklair-Levy M, Margalit M, Shibolet O, Gomori M, et al. Adoptive transfer of regulatory NKT lymphocytes ameliorates non-alcoholic steatohepatitis and glucose intolerance in ob/ob mice and is associated with intrahepatic CD8 trapping. J Pathol (2006) 209:121–8. doi: 10.1002/path.1950
160. Du J, Paz K, Thangavelu G, Schneidawind D, Baker J, Flynn R, et al. Invariant natural killer T cells ameliorate murine chronic GVHD by expanding donor regulatory T cells. Blood (2017) 129:3121–5. doi: 10.1182/blood-2016-11-752444
161. Gao M, Li X, He L, Yang J, Ye X, Xiao F, et al. Diammonium glycyrrhizinate mitigates liver injury Via inhibiting proliferation of NKT cells and promoting proliferation of tregs. Drug Des Devel Ther (2019) 13:3579–89. doi: 10.2147/dddt.S220030
162. Desta YT, Wu M, Bai L, Wu X, Xiong M, Weng X. Mitochondrial-targeted ubiquinone alleviates concanavalin a-induced hepatitis via immune modulation. Int Immunopharmacol (2020) 84:106518. doi: 10.1016/j.intimp.2020.106518
163. Tan X, Sun L, Li Q, Qi C, Fu C, Zhu H, et al. Secoemestrin c inhibits activation of NKT/conventional T cells and protects against concanavalin a-induced autoimmune hepatitis in mice. Am J Transl Res (2020) 12:3389–401.
164. Wu YN, Zhang R, Song XC, Han XX, Zhang J, Li X. C6orf120 gene knockout in rats mitigates concanavalin a−induced autoimmune hepatitis via regulating NKT cells. Cell Immunol (2022) 371:104467. doi: 10.1016/j.cellimm.2021.104467
165. Sebode M, Wigger J, Filpe P, Fischer L, Weidemann S, Krech T, et al. Inflammatory phenotype of intrahepatic sulfatide-reactive type II NKT cells in humans with autoimmune hepatitis. Front Immunol (2019) 10:1065. doi: 10.3389/fimmu.2019.01065
166. Weng X, He Y, Visvabharathy L, Liao CM, Tan X, Balakumar A, et al. Crosstalk between type II NKT cells and T cells leads to spontaneous chronic inflammatory liver disease. J Hepatol (2017) 67:791–800. doi: 10.1016/j.jhep.2017.05.024
167. Halder RC, Aguilera C, Maricic I, Kumar V. Type II NKT cell-mediated anergy induction in type I NKT cells prevents inflammatory liver disease. J Clin Invest (2007) 117:2302–12. doi: 10.1172/jci31602
168. Valenti L, Fracanzani AL, Fargion S. The immunopathogenesis of alcoholic and nonalcoholic steatohepatitis: two triggers for one disease? Semin Immunopathol (2009) 31:359–69. doi: 10.1007/s00281-009-0152-9
169. Haydon G, Lalor PF, Hubscher SG, Adams DH. Lymphocyte recruitment to the liver in alcoholic liver disease. Alcohol (2002) 27:29–36. doi: 10.1016/s0741-8329(02)00208-2
170. Llopis M, Cassard AM, Wrzosek L, Boschat L, Bruneau A, Ferrere G, et al. Intestinal microbiota contributes to individual susceptibility to alcoholic liver disease. Gut (2016) 65:830–9. doi: 10.1136/gutjnl-2015-310585
171. Buschard K, Hansen AK, Jensen K, Lindenbergh-Kortleve DJ, de Ruiter LF, Krohn TC, et al. Alcohol facilitates CD1d loading, subsequent activation of NKT cells, and reduces the incidence of diabetes in NOD mice. PloS One (2011) 6:e17931. doi: 10.1371/journal.pone.0017931
172. Zuluaga P, Teniente-Serra A, Fuster D, Quirant-Sánchez B, Hernandez-Rubio A, Martínez-Cáceres E, et al. Increased natural killer cells are associated with alcohol liver fibrosis and with T cell and cytotoxic subpopulations change. J Clin Med (2022) 11(2):305. doi: 10.3390/jcm11020305
173. Kwon HJ, Won YS, Park O, Feng D, Gao B. Opposing effects of prednisolone treatment on T/NKT cell- and hepatotoxin-mediated hepatitis in mice. Hepatology (2014) 59:1094–106. doi: 10.1002/hep.26748
174. Hua J, Ma X, Webb T, Potter JJ, Oelke M, Li Z. Dietary fatty acids modulate antigen presentation to hepatic NKT cells in nonalcoholic fatty liver disease. J Lipid Res (2010) 51:1696–703. doi: 10.1194/jlr.M003004
175. Ma X, Hua J, Li Z. Probiotics improve high fat diet-induced hepatic steatosis and insulin resistance by increasing hepatic NKT cells. J Hepatol (2008) 49:821–30. doi: 10.1016/j.jhep.2008.05.025
176. Li Z, Oben JA, Yang S, Lin H, Stafford EA, Soloski MJ, et al. Norepinephrine regulates hepatic innate immune system in leptin-deficient mice with nonalcoholic steatohepatitis. Hepatology (2004) 40:434–41. doi: 10.1002/hep.20320
177. Lalazar G, Zigmond E, Weksler-Zangen S, Ya'acov AB, Levy MS, Hemed N, et al. Oral administration of β-glucosylceramide for the treatment of insulin resistance and nonalcoholic steatohepatitis: Results of a double-blind, placebo-controlled trial. J Med Food (2017) 20:458–64. doi: 10.1089/jmf.2016.3753
178. Elinav E, Pappo O, Sklair-Levy M, Margalit M, Shibolet O, Gomori M, et al. Amelioration of non-alcoholic steatohepatitis and glucose intolerance in ob/ob mice by oral immune regulation towards liver-extracted proteins is associated with elevated intrahepatic NKT lymphocytes and serum IL-10 levels. J Pathol (2006) 208:74–81. doi: 10.1002/path.1869
179. Adar T, Ben Ya'acov A, Lalazar G, Lichtenstein Y, Nahman D, Mizrahi M, et al. Oral administration of immunoglobulin G-enhanced colostrum alleviates insulin resistance and liver injury and is associated with alterations in natural killer T cells. Clin Exp Immunol (2012) 167:252–60. doi: 10.1111/j.1365-2249.2011.04511.x
180. Ilan Y, Ben Ya'acov A, Shabbat Y, Gingis-Velitski S, Almon E, Shaaltiel Y. Oral administration of a non-absorbable plant cell-expressed recombinant anti-TNF fusion protein induces immunomodulatory effects and alleviates nonalcoholic steatohepatitis. World J Gastroenterol (2016) 22:8760–9. doi: 10.3748/wjg.v22.i39.8760
181. Ma C, Han M, Heinrich B, Fu Q, Zhang Q, Sandhu M, et al. Gut microbiome-mediated bile acid metabolism regulates liver cancer via NKT cells. Science (2018) 360(6391):eaan5931. doi: 10.1126/science.aan5931
182. Seki S, Nakashima H, Nakashima M, Kinoshita M. Antitumor immunity produced by the liver kupffer cells, NK cells, NKT cells, and CD8 CD122 T cells. Clin Dev Immunol (2011) 2011:868345. doi: 10.1155/2011/868345
183. Subleski JJ, Wiltrout RH, Weiss JM. Application of tissue-specific NK and NKT cell activity for tumor immunotherapy. J Autoimmun (2009) 33:275–81. doi: 10.1016/j.jaut.2009.07.010
184. Park SH, Kyin T, Bendelac A, Carnaud C. The contribution of NKT cells, NK cells, and other gamma-chain-dependent non-T non-b cells to IL-12-mediated rejection of tumors. J Immunol (2003) 170:1197–201. doi: 10.4049/jimmunol.170.3.1197
185. Seki S, Hashimoto W, Ogasawara K, Satoh M, Watanabe H, Habu Y, et al. Antimetastatic effect of NK1+ T cells on experimental haematogenous tumour metastases in the liver and lungs of mice. Immunology (1997) 92:561–6. doi: 10.1046/j.1365-2567.1997.00383.x
186. Smyth MJ, Thia KY, Street SE, Cretney E, Trapani JA, Taniguchi M, et al. Differential tumor surveillance by natural killer (NK) and NKT cells. J Exp Med (2000) 191:661–8. doi: 10.1084/jem.191.4.661
187. Shin T, Nakayama T, Akutsu Y, Motohashi S, Shibata Y, Harada M, et al. Inhibition of tumor metastasis by adoptive transfer of IL-12-activated Valpha14 NKT cells. Int J Cancer (2001) 91:523–8. doi: 10.1002/1097-0215(20010215)91:4<523::aid-ijc1087>3.0.co;2-l
188. Cullen R, Germanov E, Shimaoka T, Johnston B. Enhanced tumor metastasis in response to blockade of the chemokine receptor CXCR6 is overcome by NKT cell activation. J Immunol (2009) 183:5807–15. doi: 10.4049/jimmunol.0803520
189. Margalit M, Shibolet O, Klein A, Elinav E, Alper R, Thalenfeld B, et al. Suppression of hepatocellular carcinoma by transplantation of ex-vivo immune-modulated NKT lymphocytes. Int J Cancer (2005) 115:443–9. doi: 10.1002/ijc.20889
190. Shibolet O, Alper R, Zlotogarov L, Thalenfeld B, Engelhardt D, Rabbani E, et al. NKT and CD8 lymphocytes mediate suppression of hepatocellular carcinoma growth via tumor antigen-pulsed dendritic cells. Int J Cancer (2003) 106:236–43. doi: 10.1002/ijc.11201
191. Shibolet O, Alper R, Zlotogarov L, Thalenfeld B, Engelhardt D, Rabbani E, et al. Suppression of hepatocellular carcinoma growth via oral immune regulation towards tumor-associated antigens is associated with increased NKT and CD8+ lymphocytes. Oncology (2004) 66:323–30. doi: 10.1159/000078334
192. Altomonte J, Wu L, Meseck M, Chen L, Ebert O, Garcia-Sastre A, et al. Enhanced oncolytic potency of vesicular stomatitis virus through vector-mediated inhibition of NK and NKT cells. Cancer Gene Ther (2009) 16:266–78. doi: 10.1038/cgt.2008.74
193. Li C, Bai X, Wang S, Tomiyama-Miyaji C, Nagura T, Kawamura T, et al. Immunopotentiation of NKT cells by low-protein diet and the suppressive effect on tumor metastasis. Cell Immunol (2004) 231:96–102. doi: 10.1016/j.cellimm.2004.12.005
194. Tao L, Wang S, Kang G, Jiang S, Yin W, Zong L, et al. PD-1 blockade improves the anti-tumor potency of exhausted CD3(+)CD56(+) NKT-like cells in patients with primary hepatocellular carcinoma. Oncoimmunology (2021) 10:2002068. doi: 10.1080/2162402x.2021.2002068
195. Li TT, Sun J, Wang Q, Li WG, He WP, Yang RC, et al. The effects of stereotactic body radiotherapy on peripheral natural killer and CD3(+)CD56(+) NKT-like cells in patients with hepatocellular carcinoma. Hepatobiliary Pancreat Dis Int (2021) 20:240–50. doi: 10.1016/j.hbpd.2020.12.015
196. Bandyopadhyay K, Marrero I, Kumar V. NKT cell subsets as key participants in liver physiology and pathology. Cell Mol Immunol (2016) 13:337–46. doi: 10.1038/cmi.2015.115
197. Terabe M, Berzofsky JA. NKT cells in immunoregulation of tumor immunity: a new immunoregulatory axis. Trends Immunol (2007) 28:491–6. doi: 10.1016/j.it.2007.05.008
198. Engel I, Seumois G, Chavez L, Samaniego-Castruita D, White B, Chawla A, et al. Innate-like functions of natural killer T cell subsets result from highly divergent gene programs. Nat Immunol (2016) 17:728–39. doi: 10.1038/ni.3437
Keywords: NKT cells, cytokine, chemokine, liver diseases, immune
Citation: Gu X, Chu Q, Ma X, Wang J, Chen C, Guan J, Ren Y, Wu S and Zhu H (2022) New insights into iNKT cells and their roles in liver diseases. Front. Immunol. 13:1035950. doi: 10.3389/fimmu.2022.1035950
Received: 03 September 2022; Accepted: 14 October 2022;
Published: 26 October 2022.
Edited by:
Xia Liu, ZJU-Hangzhou Global Scientific and Technological Innovation Center, ChinaReviewed by:
Manuel Gomez Del Moral, Universidad Complutense de Madrid, SpainJochen Mattner, University of Erlangen Nuremberg, Germany
Jingbo Pang, University of Illinois at Chicago, United States
Yifang Gao, The First Affiliated Hospital of Sun Yat-sen University, China
Copyright © 2022 Gu, Chu, Ma, Wang, Chen, Guan, Ren, Wu and Zhu. This is an open-access article distributed under the terms of the Creative Commons Attribution License (CC BY). The use, distribution or reproduction in other forums is permitted, provided the original author(s) and the copyright owner(s) are credited and that the original publication in this journal is cited, in accordance with accepted academic practice. No use, distribution or reproduction is permitted which does not comply with these terms.
*Correspondence: Haihong Zhu, zhuhh72@zju.edu.cn
†These authors have contributed equally to this work