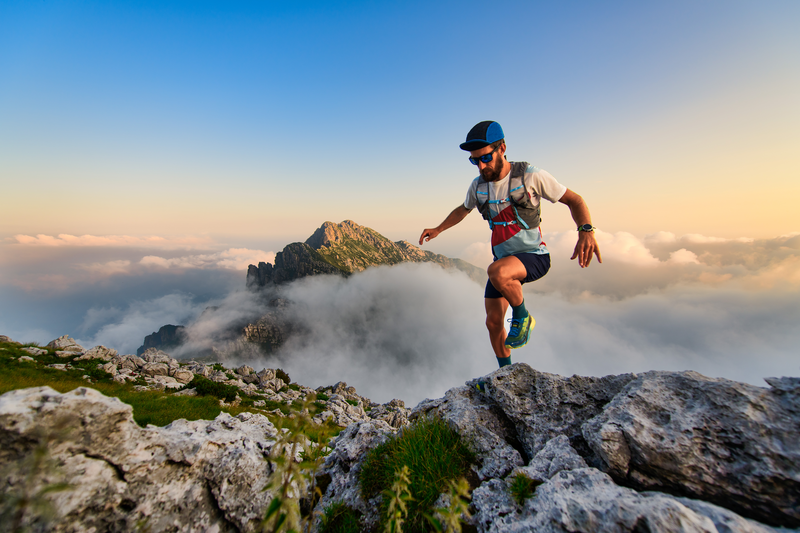
94% of researchers rate our articles as excellent or good
Learn more about the work of our research integrity team to safeguard the quality of each article we publish.
Find out more
SYSTEMATIC REVIEW article
Front. Immunol. , 11 November 2022
Sec. Cancer Immunity and Immunotherapy
Volume 13 - 2022 | https://doi.org/10.3389/fimmu.2022.1027235
CD47-SIRPα interaction acts as a “don’t eat me” signal and is exploited by cancer to downregulate innate and adaptive immune surveillance. There has been intense interest to develop a mechanism of blockade, and we aimed to analyze the emerging data from early clinical trials. We performed a systematic review and meta-analysis of relevant databases and conference abstracts including clinical trials using CD47 and/or SIRPα inhibitors in cancer treatment. Nonlinear mixed models were applied for comparison of response and toxicity. We retrieved 317 articles, 24 of which were eligible. These included 771 response-evaluable patients with hematologic (47.1%) and solid tumors (52.9%). Of these, 6.4% experienced complete response, 10.4% partial response, and 26.1% stable disease for a 16.7% objective response rate (ORR), 42.8% disease control rate, and 4.8-month median duration of response. ORR was significantly higher for hematologic cancers (25.3%) than solid cancers (9.1%, p=0.042). Comparing by mechanism, seven CD47 monoclonal antibodies (mAbs) and six selective SIRPα blockers were given alone or combined with checkpoint inhibitors, targeted therapy, and/or chemotherapy. In solid cancers, selective SIRPα blockade showed a higher ORR (16.2%) than anti-CD47 mAbs (2.8%, p=0.079), which was significant for combination therapies (ORR 28.3% vs 3.0%, respectively, p=0.010). Responses were seen in head and neck, colorectal, endometrial, ovarian, hepatocellular, non-small cell lung, and HER2+gastroesophageal cancers. Dose-limiting toxicity (DLT) was seen in 3.3% of patients (5.4% anti-CD47 mAbs, 1.4% selective SIRPα blockers; p=0.01). The frequency of treatment-related adverse events (TRAEs) ≥grade 3 was 18.0%, similar between the two groups (p=0.082), and mostly laboratory abnormalities. For anti-CD47 mAbs, the most common toxicities included grade 1-2 fatigue (27.2%), headache (21.0%), and anemia (20.5%). For selective SIRPα blockers, these included grade 1-2 infusion reaction (23.1%) and fatigue (15.8%). Anti-CD47 mAbs were significantly more likely than selective SIRPα blockers to cause grade 1-2 fever, chills, nausea/vomiting, headache, and anemia. In conclusion, combination therapies using selective SIRPα blockade had higher response rates in solid tumors than anti-CD47 mAb combinations. Hematologic changes were the main TRAEs, and selective SIRPα blockers seemed to have a better grade 1-2 toxicity profile. Treatment was well-tolerated with minimal DLTs.
Immune checkpoint inhibitors (CPIs) have shown unprecedented clinical activity and revolutionized cancer care. CPIs reinvigorate anti-tumor immune responses by disrupting co-inhibitory T cell signaling, and significant durable responses have been observed. Yet, most patients’ disease fails to respond or initially responds only to give way to progression (1). Factors contributing to the poor immunogenicity of these so-called “cold tumors” include inadequate tumor-associated antigen uptake and impaired cross-priming capability of antigen-presenting cells in the tumor microenvironment, which, in turn, fosters insufficient production of antigen-specific CD8 T cells, functional incompetence of T effectors, and unsustainable antitumor immune memory (2). In light of this limitation, there has been recent interest in therapeutic targeting of the CD47-SIRPα pathway. Inhibition of this interaction, which is heralded as a “myeloid checkpoint,” provides a unique mechanism for targeting the innate immune system.
The CD47-SIRPα axis acts as a “don't eat me” signal and is an essential component of self-tolerance in normal tissue. CD47’s interaction with SIRPα negatively regulates phagocytosis in dendritic cells and macrophages and contributes to homeostasis in T cells and natural killer cells. Both hematologic and solid cancer cells exploit this pathway by overexpressing CD47, which leads to downregulation of immune surveillance and decreased immunogenicity (3, 4). In fact, the level of CD47 overexpression has been directly correlated to poor patient survival outcomes (5). In preclinical studies, inhibition of SIRPα signaling in CD8α+ type I conventional dendritic cells has been shown to enhance sensing of phagocytosed tumor mitochondrial DNA, which triggers the cGAS/STING-mediated type I interferon response that facilitates cross-presentation of tumor antigens to CD8 T cells (6, 7). In vivo, disruption of the CD47-SIRPα interaction enhances antibody-dependent cellular toxicity (8), dendritic cell-mediated cross-priming of T cell response (6), and cytotoxic capacity of NK cells (9). Moreover, when mice previously treated with the SIRPα inhibitor SL-172154 were rechallenged with a second colorectal tumor on the opposite flank, 60% of the mice rejected the tumor, suggesting development of T cell immune memory (10). Given the central role of the CD47-SIRPα pathway in both innate and adaptive immunity against cancer, several agents that block this interaction have entered the clinical space, with numerous others in development.
Inhibitors targeting the CD47-SIRPα axis in clinical development can be categorized by mechanism of action. The CD47 inhibitors that have been investigated thus far are predominantly humanized monoclonal IgG4 antibodies lacking effector function which target CD47 (henceforth, anti-CD47 mAb). Recent interest has focused on specifically blocking the SIRPα interaction with CD47. CD47 is ubiquitously expressed on most cells, especially those of hematopoietic lineage. Thus, anti-CD47 mAb can trigger antibody-dependent cellular cytotoxicity (ADCC) and phagocytosis (ADCP) towards normal cells in an Fc receptor-dependent manner, posing concerns for on-target adverse events such as anemia and thrombocytopenia. In contrast, SIRPα is almost exclusively present on myeloid populations and microglia. Therefore, SIRPα blockade theoretically provides a more targeted inhibition of the CD47-SIRPα axis (11). Further, the preservation of CD47-SIRPγ interaction may benefit anti-tumor immunity (12). Such selective SIRPα blockers in clinical development include SIRPα-IgG or SIRPα-Fc fusion proteins and monoclonal antibodies targeting SIRPα.
As the first wave of clinical trials targeting the CD47-SIRPα pathway are now reporting preliminary results, we conducted a systematic review and meta-analysis to summarize and analyze the emerging clinical data.
We performed a comprehensive systematic search of Ovid MEDLINE, Ovid EMBASE, Cochrane Library, ClinicalTrials.gov, and International Clinical Trials Registry. In addition, we searched “gray literature” resources from conferences, dissertations, reports, and other relevant citations. Searches were restricted to clinical trials and English-language articles. There was no time restriction. Search structures, subject headings, and keywords were tailored to each database by a medical research librarian (KJK) specializing in systematic reviews. MeSH, Emtree, and keywords were searched to identify concepts related to CD47 and/or SIRPα inhibition in human subjects. The full search strings for all databases can be found in the Supplementary Index (S1).
After the initial search, Rayyan was used to screen citations (13). Studies that passed the initial title and abstract review were retrieved for full-text review. Eligible studies included clinical trials of any phase using a CD47 and/or SIRPα inhibitor reporting standardized oncologic response and/or toxicity data. We excluded animal or in vitro studies, multiple reports of the same data, review articles, meta-analyses, and non-peer reviewed literature such as editorials. The PRISMA flow diagram is presented in Supplementary Figure S2 (14). We then classified the CD47 and SIRPα inhibitors by mechanism of action: anti-CD47 mAb targeting CD47 globally, and selective SIRPα blockers targeting the CD47 interaction with SIRPα.
Study characteristics were collected, including type of drug, phase of trial, treatment regimen, and criteria for tumor response and toxicity. Patient and response characteristics collected included types of cancer, number of patients by cancer type, number of prior treatments, number of patients experiencing treatment response, duration of response, and survival data if available. Treatment-related adverse events (TRAEs) were collected according to Common Terminology Criteria for Adverse Events. Dose-limiting toxicities (DLTs), and serious adverse events (SAE) were also collected.
Objective response rate (ORR) was defined as the sum of complete response (CR) rate and partial response (PR) rate, and disease control rate (DCR) was defined as the sum of ORR and the stable disease (SD) rate. Missing data were assumed to be progressive disease for conservative estimation of ORR and DCR. For toxicity calculations, missing data were omitted.
Nonlinear mixed models were applied for comparison of ORR and DCR, with study as a random effect, in the entire cohort as well as subgroups. The subgroups were defined by tumor type (hematologic vs solid) or by regimens (monotherapy vs combinatorial). Patient-level toxicity data were compared using nonlinear mixed models in a similar fashion. Statistical analysis was calculated using SAS version 9.4 (SAS Institute, Cary NC). All p values were two-sided, with 0.05 as the cutoff for level of significance.
We retrieved 317 articles, of which 24 articles met our eligibility criteria. 14 reported on trials of anti-CD47 mAbs and 10 on trials of selective SIRPα blockers. All trials were phase I with the exception of three combined phase I/II trials, and published between 2019-2022. Seven anti-CD47 mAbs were studied, including AK-117, AO-176, CC-90002, Hu5F9-G4, IBI188, SRF231, and TJC4 in monotherapy or in combination with CPI (avelumab), targeted therapy (rituximab, cetuximab), or chemotherapy (azacitidine). Six selective SIRPα blockers were studied, including ALX148, BI 765063, IMM-01, SL-172154, TTI-621, and TTI-622 as monotherapy or combined with CPI (pembrolizumab, ezabenlimab, nivolumab), targeted therapy (rituximab, trastuzumab, ramucirumab), or chemotherapy (platinum-based drugs, paclitaxel, 5-fluorouracil). The selective SIRPα blockers’ mechanism of action included SIRPα-Fc fusion protein with or without inactivated Fc region, SIRPα-Fc-CD40L fusion protein, SIRPα-IgG fusion protein, and monoclonal antibody targeting SIRPα. Cancer types, lines of treatment, sample size, and study-level response in each trial is listed in Table 1.
Table 1 Eligible studies including clinical trials for CD47-SIRPα inhibitors and their study-level efficacy.
Table 2 shows pooled patient-level efficacy data. Eligible studies included a total of 771 response-evaluable patients with hematologic (47.1%) and solid tumors (52.9%). Of these, 6.4% experienced CR, 10.4% experienced PR, and 26.1% experienced SD, for a 16.7% ORR, and 42.8% DCR. The median duration of response was 4.8 months. In all, response in hematologic cancers was higher than in solid cancers (ORR 25.3% vs 9.1%, p=0.042; DCR 56.7% vs 30.4%, p=0.097). Anti-CD47 mAbs and selective SIRPα blockers had similar efficacy in hematologic cancers, with ORRs of 29.8% and 23.0%, respectively (p=0.48, DCR p=0.69). CR or PR was seen in myelodysplastic syndrome, acute myeloid leukemia (AML), non-Hodgkin lymphoma not otherwise specified, mycosis fungoides, Sezary syndrome, diffuse large B cell lymphoma, and peripheral and cutaneous lymphoma. For solid cancers, there was a nonsignificant but notable difference in ORR between patients treated with selective SIRPα blockers (16.2%) versus anti-CD47 mAbs (2.8%, p=0.079); this was also reflected in DCR (41.9% vs 20.3%, respectively; p=0.058). Cancer types that showed CR or PR in anti-CD47 mAb studies included colorectal (n=2), ovarian (n=2), and endometrial (n=1) cancers and adenocarcinoma of the finger (n=1); in selective SIRPα blocker studies, these included HER2+ gastroesophageal (n=13), head and neck (n=9), colorectal (n=1), endometrial (n=1), non-small cell lung (n=1), and hepatocellular (n=1) cancers.
To analyze the contribution of combination regimens on the success of CD47-SIRPα blockade in solid tumors, studies using monotherapy were separated from combination therapies. The ORRs were similar in anti-CD47 mAb (2.6%) and selective SIRPα blockers (1.2%) used as a single agent in solid cancer (p=0.51); DCRs also did not differ significantly (11.2% vs 35.3%, respectively; p=0.081). In contrast, combination therapy with selective SIRPα blockers in solid cancers showed a significantly higher ORR of 28.3% compared to 3.0% in anti-CD47 mAb combinations (p=0.010). Selective SIRPα combination therapy with CPIs (ezabenlimab, pembrolizumab) yielded study-level ORR of 18.8% to 20.0% in patients with recurrent colorectal, endometrial, and head and neck cancers (Table 3) (30, 33, 34). Similar ORR of 21.1% was seen in the study combining a selective SIRPα blocker with trastuzumab in recurrent HER2+ gastroesophageal cancer patients (30). The highest ORR was seen in studies also combining cytotoxic chemotherapy: 38.5% in combination with pembrolizumab, 5-fluorouracil, and platinum drugs in treatment-naïve head and neck cancers (DCR 84.6%), and 72.2% in combination with trastuzumab, ramucirumab, and paclitaxel in recurrent HER2+ gastroesophageal cancers (DCR 88.9%) (31). Combination treatment with pembrolizumab in non-small cell lung cancer was a notable exception to the improved ORR of selective SIRPα combination therapy (ORR 5.0%, DCR 40.0%) (30).
Table 3 Study-level data on the efficacy of selective SIRPα blocker combination therapy in solid cancers.
Table 4 shows pooled patient-level toxicity data reported in the eligible studies. In total, there were 942 patients for whom toxicity could be evaluated. DLTs were seen in 3.3% of patients, with a significant difference between anti-CD47 mAbs and selective SIRPα blockers (5.4% vs 1.4%, respectively; p=0.01). The frequencies of grade 3 or higher TRAEs were not different (19.2% for anti-CD47 mAbs vs 17.0% for selective SIRPα blockers, p=0.082). As expected from the physiology of CD47 expression, hematologic changes were the most common grade 3 or higher TRAEs; neutropenia (6.5%) and thrombocytopenia (8.3%) were the most common in anti-CD47 mAbs and selective SIRPα blockers, respectively. Other grade 3 or higher TRAEs reported in more than 1 patient included infusion-related reaction (IRR), increased bilirubin, increased amylase/lipase, and hypotension (all <2%). For anti-CD47 mAbs, the most common toxicities included grade 1-2 fatigue (27.2%), headache (21.0%), anemia (20.5%), and IRR (17.6%). For selective SIRPα blockers, these included grade 1-2 IRR (23.1%) and fatigue (15.8%). For grade 1-2 toxicities between the groups, anti-CD47 mAbs were significantly more likely than selective SIRPα blockers to cause fever (12.9 vs 5.7%, p=0.042), chills (13.2 vs 8.1%, p=0.002), nausea/vomiting (14.5 vs 5.9%, p=0.005), headache (21.0 vs 4.9%, p=0.002), and anemia (20.5 vs 4.0%, p=0.0002). Other serious adverse events (SAE) included febrile neutropenia (1.4%), infection (1.1%), pneumonia (0.6%), pancreatitis (0.4%), failure to thrive (0.4%), and, in 1 patient each (0.1%) of hemolytic anemia, lactic acidosis, dyspnea, pulmonary embolism, peripheral neuropathy, and death of unknown cause.
The CD47-SIRPα pathway is an emerging target for myeloid checkpoint inhibition. In our analysis, greater response was seen in hematologic cancers, with similar rates for both anti-CD47 mAbs and selective SIRPα blockers. In solid cancers, selective SIRPα blockers yielded higher rates of response than did anti-CD47 mAbs, in large part due to the success of combination therapies. Selective SIRPα blockers seemed to have a better grade 1-2 toxicity profile, but treatment was well tolerated in all groups.
Because CD47 is ubiquitously expressed, particularly in hematopoietic cells (41, 42), consideration of on-target side effects such as anemia and thrombocytopenia have been central to inhibitor development. To mitigate on-target side effects, the majority of anti-CD47 mAb (including AK117, CC-90002, Hu5F9-G4, IBI188, SRF231, and TJC4) are composed of humanized IgG4 which has lower binding affinity for the activating FcγR (43). Nonetheless, earlier studies, including a phase I trial of CC-90002 in AML patients, were closed in part due to concerns about toxicity and the development of anti-drug antibodies (18). In patients receiving escalating doses of Hu5F9-G4, decline in hemoglobin was observed with a median change of -1.0 g/dL, correlating with increased transfusion requirement. In addition, red blood cell agglutination was seen with development of new antibodies and invalid ABO blood grouping (44). However, hemagglutination was not associated with significant clinical toxicity (21). The most advanced anti-CD47 mAb in development is Hu5F9-G4 (magrolimab), which the US Food and Drug Administration granted fast track designation for AML and myelodysplastic syndrome. This was based on results from a phase Ib trial of the drug combined with azacitidine showing an ORR of 64% in AML and 100% in myelodysplastic syndrome (22). Phase III confirmatory trials are in progress. Therapy with Hu5F9-G4 uses a unique priming and maintenance dosing strategy, which mitigates anemia by utilizing the timing of compensatory reticulocytosis since younger red blood cells display lower pro-phagocytic molecules and resistance to phagocytic clearance (11). Blood transfusions were rarely necessary in the population of solid tumor patients, whose rate of grade 3 or higher anemia was 9.7% (21). In early reports of IgG4 isotypes of anti-CD47 mAb, treatment-related anemia for AK117 (16), TJC4 (28), and IBI188 (26) were 40%, 40%, and 15% for grade 1-2 and 0%, 0%, and 5% for grade 3, respectively. For humanized IgG2 anti-CD47 mAb, AO-176 preferentially binds integrin-β1 expressed tumor cells with a lower affinity to red blood cells, and treatment-related anemia of any grade was 22% (17).
Efforts to minimize on-target toxicity led to the development of selective SIRPα blockers. Several different mechanisms of inhibition have been exploited against SIRPα interaction, including monoclonal antibodies and SIRPα-Fc fusion proteins. For instance, ALX148 contains an inactivated Fc domain which prevents Fc-dependent phagocytosis towards the opsonized normal cells while still ensuring an antibody-like pharmacokinetics with a longer half-life (45). TTI-621 is composed of the N-terminal portion of SIRPα, which demonstrates lower binding affinity to human erythrocytes minimizing the risk of anemia (46). In published trial results, the rates of DLT and grade 1-2 anemia were significantly lower for selective SIRPα blockers. However, because of inconsistent reporting of grade 1-2 TRAE, definitive conclusions are limited. Overall, the rate of high grade hematologic toxicity was less than 10% for both groups.
Consistent with preclinical studies, monotherapy with CD47 or SIRPα inhibitors demonstrated unsatisfactory therapeutic efficacy in patients with solid tumors, with ORRs less than 5% (17, 21, 27, 30, 35, 37). Combination therapy significantly improved response. Because CD8 T cells are upregulated in response to CD47-SIRPα blockade, PD-L1/PD-1 inhibitors have been proposed to synergistically augment both innate and adaptive antitumor response (47). SIRPα combination therapy with CPI (ezabenlimab, pembrolizumab) yielded a study-level ORR of 18.8% to 20.0% in colorectal, endometrial, and head and neck cancer patients. The three partial responses shown in a study using BI 765063 are noteworthy in that all patients were microsatellite stable (33, 34). Given the limited efficacy of CPI alone for microsatellite stable cancers with ORR ranging from 0-7% (48–50), these promising findings suggest that dual blockade of SIRPα and PD-1 may augment phagocytosis and play a role in overcoming CPI resistance.
Furthermore, physiologic initiation of phagocytosis requires expression of pro-phagocytic molecules, such as damage-associated molecular patterns, on tumor cells in addition to the absence of inhibitory signals. Emerging evidence indicates that αCD47 or αSIRPα monotherapy exerts limited therapeutic efficacy due to lack of endogenous pro-phagocytic “eat me” triggers on tumor cells (11, 51). Hence, combining a cytotoxic therapy to induce the display of activating signals has shown to magnify the therapeutic advantages of phagocytosis checkpoint blockade against poorly immunogenic tumors. Targeted therapies such as cetuximab or trastuzumab have shown success by inducing both ADCC and ADCP through Fc receptor interaction (5, 52), which translated clinically (Table 3). Moreover, several successful combinations with chemotherapy have been presented. These include the combination of ALX148 with trastuzumab and chemotherapy in HER2+ gastroesophageal cancer, which has shown a remarkable ORR of up to 72% (53, 54). While difficult to generalize due to patient selection and lack of a comparison arm, this favorable outcome relative to monotherapy implies that CD47-SIRPα inhibition is more effective when combined with cytotoxic therapy.
Intriguingly, various preclinical studies suggested that selective SIRPα blockade is more efficacious than CD47 inhibition in solid tumors (51, 55). The lower therapeutic effect of anti-CD47 mAbs may result from antibody sequestration by the healthy tissue “sink”, which thereby limits the blockade efficiency against the tumor cells. More importantly, CD47 also engages SIRPγ, which is pivotal for T cell endothelial transmigration, cell-cell adhesion, and costimulation between T cells and dendritic cells (56, 57). Global inhibition of CD47 compromises SIRPγ-mediated T cell activation, proliferation, and migration, resulting in a dampened antitumor T cell response. In contrast, selective SIRPα blockade may preserve these essential T cell functions, allowing higher T cell infiltration and pro-inflammatory cytokine production (12). The role of binding affinity as it relates to clinical efficacy, especially in the more newly developed fusion proteins and bispecific antibodies, is complex (52, 58), and will become apparent as pharmacokinetic and pharmacodynamic data from the ongoing trials become available. In all, due to the relatively limited array of combination therapies studied in anti-CD47 mAbs for solid cancers, differing patient enrollment criteria, and heterogeneous drug designs, conclusions regarding the inherent superiority of selective SIRPα blockers in this setting cannot be drawn.
Finally, patient selection seems to be an important component of response and may therefore direct future research. In general, most cancer types with CD47 alteration/amplification, such as ovarian, esophageal, endometrial, and head and neck cancers (59), seemed to respond to CD47-SIRPα blockade. However, non-small cell lung cancer was a notable exception with a 5.0% ORR in combination therapy (30). Results from ongoing pharmacodynamic studies may shed light on predictive biomarkers. For example, patient selection based on expression of SIRPα V1 allele was a novel approach (33). Furthermore, future drug design should focus on the specificity of SIRPα inhibition so as to minimize on-target side effects (52). While not yet observed, theoretical toxicity due to the minor expression of SIRPα in the central and peripheral nervous system should be considered (46). Scientifically rational combination therapies to further augment the activating “eat me” signal should be explored.
Our study has some limitations. The chief limitation is that the protocols of the included studies varied considerably. This was necessary because published data in their final form are scarce for this topic. Similarly, our inclusion of gray literature, which enabled us to include the most recent data, also led to missing variables and incomplete protocol information. In particular, there was a paucity of patient-level toxicity data. Because the studies of anti-CD47 mAbs tend to predate those of selective SIRPα blockers, the former had more complete toxicity data, which may have overrepresented this effect in our analysis. We attempted to account for this by omitting missing toxicity data in statistical comparisons. In addition, the array of combination therapies seemed relatively limited in anti-CD47 mAbs, particularly combinations with cytotoxic therapy. Along this vein, the efficacy comparison of combination therapy was largely driven by one selective SIRPα blocker, ALX148. While data presented herein was statistically significant and thought-provoking, our conclusion should be considered merely hypothesis-generating. Survival and duration of response were often not available because the studies are ongoing. Similarly, pharmacokinetic and pharmacodynamic data from the source studies are much anticipated. Despite these limitations, this represents the first comprehensive review and analysis of patient-level data on CD47-SIRPα inhibitors in clinical trials.
CD47-SIRPα inhibition shows promise in cancer therapy; selective SIRPα blockade in combination with cytotoxic therapies in particular seem to maximize anti-tumoral benefit. While there was no difference in higher grade TRAEs, selective SIRPα blockers appear to have a milder grade 1-2 toxicity profile and lower DLT thus far. Treatment was well tolerated for both groups. Many clinical trials - including those in Table 1 and others that have yet to report data, such as TQB2928, ZL-1201, STI-6643, IMC-002 among many others - are ongoing.
The original contributions presented in the study are included in the article/Supplementary Material. Further inquiries can be directed to the corresponding author.
JS performed the study design, data collection, formal data analysis, and manuscript writing. RC-EH performed manuscript writing and editing and formal data analysis. HL performed the formal statistical analysis and manuscript editing. KK is the research librarian who performed the initial systematic search of the relevant databases. AB, YY, and JW performed manuscript review and editing. MC and DH are the senior authors who contributed conceptualization, study design, formal data analysis, and manuscript editing. All authors contributed to the article and approved the submitted version.
The authors declare no funding directly related to this study. We acknowledge support through the MD Anderson Cancer Center Support Grant from the National Cancer Institute of the National Institutes of Health (NIH/NCI P30 CA016672, CA217685), the T32 training grant CA101642 (JS), Cancer Prevention and Research Institute of Texas (CPRIT) Research Training Grant RP170067 (RCH).
We thank Bryan Tutt in the Research Medical Library at The University of Texas MD Anderson Cancer Center for editing this article.
The authors declare no conflict of interest directly relating to this study. Possible competing interests outside of this work include: JS received institutional research or grant funding from NIH/NCI P30CA016672, CA217685 and the T32 training grant CA101642. RC-EH formal data analysis received institutional research or grant funding from Cancer Prevention and Research Institute of Texas CPRIT research training grant RP170067. YY has served as a statistical consultant to AbbVie, Amgen, Bexion Pharmaceuticals, BeyondSpring Pharmaceuticals, Boehringer Ingelheim Pharmaceuticals, Bristol Myers Squibb, Century Therapeutics, Enliven Therapeutics, NGM Biopharmaceuticals, Repare Therapeutics, Servier Pharmaceuticals, Starpax Pharmaceuticals, and Vertex Pharmaceuticals. JW has received institutional research or grant funding from GlaxoSmithKline, Bristol Meyers Squibb, Merck, Nanobiotix, RefleXion, Alkermes, Artidis, Mavu Pharma, Takeda, Varian, Checkmate Pharmaceuticals, HotSpot Therapeutics, Inc., Gilead and Kiromic. JW serves/served on the scientific advisory board for Legion Healthcare Partners, RefleXion Medical, MolecularMatch, Merck, AstraZeneca, Aileron Therapeutics, OncoResponse, Checkmate Pharmaceuticals, Mavu Pharma, Alpine Immune Sciences, Ventana Medical Systems, Nanobiotix, China Medical Tribune, GI Innovation, Genentech and Nanorobotix. JW serves as consultant for Lifescience Dynamics Limited. JW has/had Speaking Engagements for Ventana Medical Systems, US Oncology, Alkermes, Boehringer Ingelheim, Accuray and RSS. JW holds/held stock or ownership in Alpine Immune Sciences, Checkmate Pharmaceuticals, Healios, Mavu Pharma, Legion Healthcare Partners, MolecularMatch, Nanorobotix, OncoResponse, and RefleXion. JW has accepted honoraria in the form of travel costs from Nanobiotix, RefleXion, Varian, Shandong University, The Korea Society of Radiology, Aileron Therapeutics and Ventana. JW has the following patents; MP470 amuvatinib, MRX34 regulation of PDL1, XRT technique to overcome immune resistance. MD Anderson Cancer Center has a trademark for RadScopal™. MC has received institutional research or grant funding from ImmunoGenesis, Inc., ImmunoMet, Inc.; received consulting fees from ImmunoGenesis, Inc., Alligator Bioscience, Inc., from ImmunOS, Inc., ImmunoMet, Inc., Oncoresponse, Inc., from Pieris, Inc., from Nurix, Inc., Aptevo, Inc., Servier, Inc., Kineta, Inc., Salarius, Inc., Xencor, Inc., Agenus, Inc., Mereo, Inc., Amunix, Inc., Adagene, Inc. MC has a patent Methods and Composition for Localized Secretion of Anti-CTLA-4 Antibodies with royalties paid to multiple licensees, a patent Dual specificity antibodies which bind both PD-L1 and PD-L2 and prevent their binding to PD-1 with royalties paid to ImmunoGenesis, Inc., and a patent Cyclic Dinucleotides as Agonists of Stimulator of Interferon Gene Dependent Signaling licensed to ImmunoGenesis, Inc. DH has received institutional research or grant funding from AbbVie, Adaptimmune, Adlai-Nortye, Amgen, Astra-Zeneca, Bayer, Bristol-Myers Squibb, Daiichi-Sankyo, Deciphera, Eisai, Eli Lilly, Endeavor, Erasca, F. Hoffmann-LaRoche, Fate Therapeutics, Genentech, Genmab, Ignyta, Infinity, Kite, Kyowa Kirin, LOXO, Merck, Medimmune, Mirati, Mologen, Navier, NCI-CTEP, Novartis, Numab, Pfizer, Pyramid Bio, SeaGen, Takeda, TCR2, Teckro, Turning Point Therapeutics, and VM Oncology; held a consulting, speaker, or advisory role for Abbvie, Adaptimmune, Alpha Insights, Acuta, Alkermes, Amgen, Aumbiosciences, Axiom, Baxter, Bayer, Boxer Capital, BridgeBio, COR2ed, COG, Cowen, Ecor1, Gennao Bio, Genentech, Gilead, GLG, Group H, Guidepoint, HCW Precision, Immunogen, Infinity, Janssen, Liberium, MedaCorp, Medscape, Numab, Oncologia Brasil, Pfizer, Pharma Intelligence, POET Congress, Prime Oncology, RAIN, SeaGen, ST Cube, Takeda, Tavistock, Trieza Therapeutics, Turning Point Therapeutics, WebMD, YingLing Pharma, and Ziopharm; travel, accommodation, or expense support from AACR, ASCO, Bayer, Genmab, Gilead, SITC, and Telperian; and has other ownership interests in Molecular Match advisor, OncoResponse founder, advisor, and Telperian founder, advisor.
The remaining authors declare that the research was conducted in the absence of any commercial or financial relationships that could be construed as a potential conflict of interest.
All claims expressed in this article are solely those of the authors and do not necessarily represent those of their affiliated organizations, or those of the publisher, the editors and the reviewers. Any product that may be evaluated in this article, or claim that may be made by its manufacturer, is not guaranteed or endorsed by the publisher.
The Supplementary Material for this article can be found online at: https://www.frontiersin.org/articles/10.3389/fimmu.2022.1027235/full#supplementary-material
1. Jenkins RW, Barbie DA, Flaherty KT. Mechanisms of resistance to immune checkpoint inhibitors. Br J Cancer (2018) 118(1):9–16. doi: 10.1038/bjc.2017.434
2. Jhunjhunwala S, Hammer C, Delamarre L. Antigen presentation in cancer: insights into tumour immunogenicity and immune evasion. Nat Rev Cancer (2021) 21(5):298–312. doi: 10.1038/s41568-021-00339-z
3. Jaiswal S, Jamieson CHM, Pang WW, Park CY, Chao MP, Majeti R, et al. CD47 is upregulated on circulating hematopoietic stem cells and leukemia cells to avoid phagocytosis. Cell (2009) 138(2):271–85. doi: 10.1016/j.cell.2009.05.046
4. Betancur PA, Abraham BJ, Yiu YY, Willingham SB, Khameneh F, Zarnegar M, et al. A CD47-associated super-enhancer links pro-inflammatory signalling to CD47 upregulation in breast cancer. Nat Commun (2017) 8(1):14802. doi: 10.1038/ncomms14802
5. Willingham SB, Volkmer J-P, Gentles AJ, Sahoo D, Dalerba P, Mitra SS, et al. The CD47-signal regulatory protein alpha (SIRPa) interaction is a therapeutic target for human solid tumors. Proc Natl Acad Sci (2012) 109(17):6662–7. doi: 10.1073/pnas.1121623109
6. Liu X, Pu Y, Cron K, Deng L, Kline J, Frazier WA, et al. CD47 blockade triggers T cell-mediated destruction of immunogenic tumors. Nat Med (2015) 21(10):1209–15. doi: 10.1038/nm.3931
7. Xu MM, Pu Y, Han D, Shi Y, Cao X, Liang H, et al. Dendritic cells but not macrophages sense tumor mitochondrial DNA for cross-priming through signal regulatory protein α signaling. Immunity (2017) 47(2):363–373.e5. doi: 10.1016/j.immuni.2017.07.016
8. Zhao XW, van Beek EM, Schornagel K, Van der Maaden H, Van Houdt M, Otten MA, et al. CD47-signal regulatory protein- (SIRP ) interactions form a barrier for antibody-mediated tumor cell destruction. Proc Natl Acad Sci (2011) 108(45):18342–7. doi: 10.1073/pnas.1106550108
9. Deuse T, Hu X, Agbor-Enoh S, Jang MK, Alawi M, Saygi C, et al. The SIRPα-CD47 immune checkpoint in NK cells. J Exp Med (2021) 218(3):e20200839. doi: 10.1084/jem.20200839
10. de Silva S, Fromm G, Shuptrine CW, Johannes K, Patel A, Yoo KJ, et al. CD40 enhances type I interferon responses downstream of CD47 blockade, bridging innate and adaptive immunity. Cancer Immunol Res (2020) 8(2):230–45. doi: 10.1158/2326-6066.CIR-19-0493
11. Logtenberg MEW, Scheeren FA, Schumacher TN. The CD47-SIRPα immune checkpoint. Immunity (2020) 52(5):742–52. doi: 10.1016/j.immuni.2020.04.011
12. Gauttier V, Pengam S, Durand J, Biteau K, Mary C, Morello A, et al. Selective SIRPα blockade reverses tumor T cell exclusion and overcomes cancer immunotherapy resistance. J Clin Invest (2020) 130(11):6109–23. doi: 10.1172/JCI135528
13. Ouzzani M, Hammady H, Fedorowicz Z, Elmagarmid A. Rayyan–a web and mobile app for systematic reviews. Syst Rev (2016) 5(1):210. doi: 10.1186/s13643-016-0384-4
14. Page MJ, McKenzie JE, Bossuyt PM, Boutron I, Hoffmann TC, Mulrow CD, et al. The PRISMA 2020 statement: an updated guideline for reporting systematic reviews. BMJ (2021) n71. doi: 10.1136/bmj.n71
15. Kaur S, Cicalese KV, Banerjee R, Roberts DD. Preclinical and clinical development of therapeutic antibodies targeting functions of CD47 in the tumor microenvironment. Antib Ther (2020) 3(3):179–92. doi: 10.1093/abt/tbaa017
16. Gan HK, Coward J, Rachelle A, Mislang A, Cosman R, Nagrial A, et al. Safety of AK117, an anti-CD47 monoclonal antibody, in patients with advanced or metastatic solid tumors in a phase I study. J Clin Oncol Conf Annu Meet Am Soc Clin Oncol ASCO (2021) 39(15):2630. doi: 10.1200/JCO.2021.39.15_suppl.2630
17. Burris HA III, Spira AI, Taylor MH, Yeku OO, Liu JF, Munster PN, et al. A first-in-human study of AO-176, a highly differentiated anti-CD47 antibody, in patients with advanced solid tumors. J Clin Oncol (2021) 39(15_suppl):2516–6. doi: 10.1200/JCO.2021.39.15_suppl.2516
18. Zeidan AM, DeAngelo DJ, Palmer JM, Seet CS, Tallman MS, Wei X, et al. A phase I study of CC-90002, a monoclonal antibody targeting CD47, in patients with relapsed and/or refractory (R/R) acute myeloid leukemia (AML) and high-risk myelodysplastic syndromes (MDS): final results. Blood (2019) 134:1320. doi: 10.1182/blood-2019-125363
19. Abrisqueta P, Sancho JM, Cordoba R, Persky DO, Andreadis C, Huntington SF, et al. Anti-CD47 antibody, CC-90002, in combination with rituximab in subjects with relapsed and/or refractory non-Hodgkin lymphoma (R/R NHL). Blood (2019) 134(supplement 1):4089. doi: 10.1182/blood-2019-125310
20. Vyas P, Knapper S, Kelly R, Salim R, Lubowiecki M, Royston D, et al. Initial phase 1 results of the first-in-class anti-CD47 antibody HU5F9-G4 in relapsed/refractory acute myeloid leukemia patients. In: European Hematology Association Annual Meeting. Abstract PF232. Stockholm: HemaSphere (2018) 2.
21. Sikic BI, Lakhani N, Patnaik A, Shah SA, Chandana SR, Rasco D, et al. First-in-human, first-in-class phase I trial of the anti-CD47 antibody Hu5F9-G4 in patients with advanced cancers. J Clin Oncol (2019) 37(12):946–53. doi: 10.1200/JCO.18.02018
22. Sallman D, Asch A, Kambhampati S, Malki MA, Zeidner J, Donnellan W, et al. AML-196: the first-in-class anti-CD47 antibody magrolimab in combination with azacitidine is well tolerated and effective in AML patients: phase 1b results. Clin Lymphoma Myeloma Leuk (2021) 21(supplement 1):S290. doi: 10.1016/S2152-2650(21)01694-3
23. Advani R, Flinn I, Popplewell L, Forero A, Bartlett NL, Ghosh N, et al. CD47 blockade by Hu5F9-G4 and rituximab in non-hodgkin’s lymphoma. N Engl J Med (2018) 379(18):1711–21. doi: 10.1056/NEJMoa1807315
24. Lakhani NJ, Patnaik A, Liao JB, Moroney JW, Miller DS, Fleming GF, et al. A phase ib study of the anti-CD47 antibody magrolimab with the PDL1 inhibitor avelumab (A) in solid tumor (ST) and ovarian cancer (OC) patients. J Clin Oncol Conf (2020) 38(5):18. doi: 10.1200/JCO.2020.38.5_suppl.18
25. Fisher GA, Lakhani NJ, Eng C, Hecht JR, Bendell JC, Philip PA, et al. A phase Ib/II study of the anti- CD47 antibody magrolimab with cetuximab in solid tumor and colorectal cancer patients. J Clin Oncol Conf (2020) 38(4):114. doi: 10.1200/JCO.2020.38.4_suppl.114
26. Lakhani N, Orloff M, Fu S, Liu Y, Wang Y, Zhou H, et al. First-in-human phase i trial of IBI188, an antiCD47 targeting monoclonal antibody, in patients with advanced solid tumors and lymphomas. J Immunother Cancer (2020) 8(3):A180. doi: 10.1136/jitc-2020-SITC2020.0295
27. Patnaik A, Spreafico A, Paterson AM, Peluso M, Chung JK, Bowers B, et al. Results of a first-in-human phase I study of SRF231, a fully human, high-affinity anti-CD47 antibody. J Clin Oncol Conf (2020) 38(15):3064. doi: 10.1200/JCO.2020.38.15_suppl.3064
28. Qi J, Li J, Jiang B, Cao X, Zhang M, Meng Y, et al. A phase I/IIa study of lemzoparlimab, a monoclonal antibody targeting CD47, in patients with relapsed and/or refractory acute myeloid leukemia (AML) and myelodysplastic syndrome (MDS): Initial phase I results. Blood (2020) 136(supplement 1):30–31. doi: 10.1182/blood-2020-134391
29. Berlin J, Harb W, Adjei A, Xing Y, Swiecicki P, Seetharam MN, et al. A first-in-human study of lemzoparlimab, a differentiated anti-CD47 antibody, in subjects with relapsed/refractory malignancy: initial monotherapy results. J Immunother Cancer (2020) 8:A233–4. doi: 10.1136/jitc-2020-SITC2020.0385
30. Lakhani NJ, Chow LQM, Gainor JF, LoRusso P, Lee K.-W, Chung HC, et al. Evorpacept alone and in combination with pembrolizumab or trastuzumab in patients with advanced solid tumours (ASPEN-01): A first-in-human, open-label, multicentre, phase 1 dose-escalation and dose-expansion study. Lancet Oncol (2021) 22(12):1740–51. doi: 10.1016/S1470-2045(21)00584-2
31. Lee K-W, Chung H, Kim TM, Lakhani N, Messersmith W, Santana-Davila R, et al. 498 evorpacept (ALX148), a CD47 myeloid checkpoint inhibitor, in patients with head and neck squamous cell carcinoma (HNSCC) and with gastric/gastroesophageal cancer (GC); ASPEN-01. J Immunother Cancer (2021) 9(Suppl 2):A530–0. doi: 10.1136/jitc-2021-SITC2021.498
32. Kim TM, Lakhani N, Gainor J, Kamdar M, Fanning P, Squifflet P, et al. ALX148, a CD47 blocker, in combination with rituximab in patients with non-Hodgkin lymphoma. Blood (2020) 136(supplement 1):13–14. doi: 10.1182/blood-2020-135941
33. Kotecki N, Champiat S, Delord JP, Vinceneux A, Jungels C, Marabelle A, et al. Phase I dose escalation study in patients (pts) with advanced solid tumours receiving first-in-class BI 765063, a selective signal-regulatory protein alpha (SIRPalpha) inhibitor, in combination with ezabenlimab (BI 754091), a programmed cell death protein 1 (PD-1) inhibitor. Ann Oncol (2021) 32:S841–2. doi: 10.1016/j.annonc.2021.08.1367
34. Kotecki N. (2021). Phase I dose escalation study in patients with advanced solid tumours receiving first-in-class BI 765063, a selective signal-regulatory protein α (SIRPα) inhibitor, in combination with ezabenlimab (BI 754091), a programmed cell death protein-1 (PD-1) inhibitor, in: Proceedings of ESMO,. Luganom, Switzerland.
35. Champiat S, Cassier PA, Kotecki N, Korakis I, Vinceneux A, Jungels C, et al. Safety, pharmacokinetics, efficacy, and preliminary biomarker data of first-in-class BI 765063, a selective SIRPalpha inhibitor: Results of monotherapy dose escalation in phase 1 study in patients with advanced solid tumors. J Clin Oncol Conf Annu Meet Am Soc Clin Oncol ASCO (2021) 39(15):2623. doi: 10.1200/JCO.2021.39.15_suppl.2623
36. Sun M, Qi J, Zheng W, Song L, Jiang B, Wang Z, et al. Preliminary results of a first-inhuman phase I dtudy of IMM01, SIRPalpha fc protein in patients with relapsed or refractory lymphoma. J Clin Oncol Conf Annu Meet Am Soc Clin Oncol ASCO (2021) 39(15):2550. doi: 10.1200/JCO.2021.39.15_suppl.2550
37. Lakhani N, Richardson D, Kristedja T, Rangwala F, McKay H, Gonzalez L, et al. 429 phase 1 dose escalation study of the agonist redirected checkpoint, SL-172154 (SIRPα-Fc-CD40L) in subjects with platinum-resistant ovarian cancer. J Immunother Cancer (2021) 9(Suppl 2):A459. doi: 10.1136/jitc-2021-SITC2021.429
38. Patel K, Ramchandren R, et al. Investigational CD47-blocker TTI-622 shows single-agent activity in patients with advanced relapsed or refractory lymphoma: Update from the ongoing first-in-human dose escalation study. Blood (2020) 136:46–7. doi: 10.1182/blood-2020-136607
39. Querfeld C, Thompson J, Taylor MH, Pillai R, Johnson LDS, Catalano T, et al. Intralesional administration of the CD47 antagonist TTI-621 (SIRPalphaFc) induces responses in both injected and non-injected lesions in patients with relapsed/refractory mycosis fungoides and sezary syndrome: Interim results of a multicenter phase I trial. Blood Conf 60th Annu Meet Am Soc Hematol ASH (2018) 132(supplement 1):1653. doi: 10.1182/blood-2018-99-116915
40. Ansell SM, Maris MB, Lesokhin AM, Chen RW, Flinn IW, Sawas A, et al. Phase I study of the CD47 blocker TTI-621 in patients with relapsed or refractory hematologic malignancies. Clin Cancer Res Off J Am Assoc Cancer Res (2021) 27(8):2190–9. doi: 10.1158/1078-0432.CCR-20-3706
41. Oldenborg P-A, Zheleznyak A, Fang Y-F, Lagenaur CF, Gresham HD, Lindberg FP. Role of CD47 as a marker of self on red blood cells. Science (2000) 288(5473):2051–4. doi: 10.1126/science.288.5473.2051
42. Olsson M, Bruhns P, Frazier WA, Ravetch JV, Oldenborg P-A. Platelet homeostasis is regulated by platelet expression of CD47 under normal conditions and in passive immune thrombocytopenia. Blood (2005) 105(9):3577–82. doi: 10.1182/blood-2004-08-2980
43. Jiang Z, Sun H, Yu J, Tian W, Song Y. Targeting CD47 for cancer immunotherapy. J Hematol OncolJ Hematol Oncol (2021) 14(1):180. doi: 10.1186/s13045-021-01197-w
44. Brierley CK, Staves J, Roberts C, Johnson H, Vyas P, Goodnough LT, et al. The effects of monoclonal anti-CD47 on RBCs, compatibility testing, and transfusion requirements in refractory acute myeloid leukemia. Transfusion (Paris) (2019) 59(7):2248–2254. doi: 10.1111/trf.15397
45. Evorpacept mechnism of action ALX oncol. Available at: https://alxoncology.com/science/#evorpacept
46. Zhang W, Huang Q, Xiao W, Zhao Y, Pi J, Xu H, et al. Advances in anti-tumor treatments targeting the CD47/SIRPα axis. Front Immunol (2020) 11:18. doi: 10.3389/fimmu.2020.00018
47. Sockolosky JT, Dougan M, Ingram JR, Ho CCM, Kauke MJ, Almo SC, et al. Durable antitumor responses to CD47 blockade require adaptive immune stimulation. Proc Natl Acad Sci (2016) 113(19):E2646–54. doi: 10.1073/pnas.1604268113
48. Le DT, Uram JN, Wang H, Bartlett BR, Kemberling H, Eyring AD, et al. PD-1 blockade in tumors with mismatch-repair deficiency. N Engl J Med (2015) 372(26):2509–20. doi: 10.1056/NEJMoa1500596
49. Brahmer JR, Drake CG, Wollner I, Powderly JD, Picus J, Sharfman WH, et al. Phase I study of single-agent anti-programmed death-1 (MDX-1106) in refractory solid tumors: safety, clinical activity, pharmacodynamics, and immunologic correlates. J Clin Oncol Off J Am Soc Clin Oncol (2010) 28(19):3167–75. doi: 10.1200/JCO.2009.26.7609
50. Topalian SL, Hodi FS, Brahmer JR, Gettinger SN, Smith DC, McDermott DF, et al. Safety, activity, and immune correlates of anti–PD-1 antibody in cancer. N Engl J Med (2012) 366(26):2443–54. doi: 10.1056/NEJMoa1200690
51. Hsieh RC-E, Krishnan S, Wu R-C, Boda AR, Liu A, Winkler M, et al. ATR-mediated CD47 and PD-L1 up-regulation restricts radiotherapy-induced immune priming and abscopal responses in colorectal cancer. Sci Immunol (2022) 7(72):eabl9330. doi: 10.1126/sciimmunol.abl9330
52. Weiskopf K, Ring AM, Ho CCM, Ho CCM, Volkmer J.-P, Levin AM, et al. Engineered SIRPα variants as immunotherapeutic adjuvants to anticancer antibodies. Science (2013) 341(6141):88–91. doi: 10.1126/science.1238856
53. Wan H, Chow L, Gainor J, Lakhani N, Chung H, Lee KW, et al. Pharmacodynamic biomarker characterization of ALX148, a CD47 blocker, in combination with established anticancer antibodies in patients with advanced malignancy. J Immunother Cancer Conf 34th Annu Meet Pre Conf Programs Soc Immunother Cancer Part (2019) 7. doi: 10.1200/JCO.2019.37.15_suppl.2514
54. Chung H, Lee K, Kim W, Gainor J, Lakhani N, Chow L, et al. ASPEN-01: A phase 1 study of ALX148, a CD47 blocker, in combination with trastuzumab, ramucirumab and paclitaxel in patients with second-line HER2-positive advanced gastric or gastroesophageal junction cancer. Ann Oncol (2021) 32(S3):S215. doi: 10.1016/j.annonc.2021.05.055
55. Abe T, Tanaka Y, Piao J, Tanimine N, Oue N, Hinoi T, et al. Signal regulatory protein alpha blockade potentiates tumoricidal effects of macrophages on gastroenterological neoplastic cells in syngeneic immunocompetent mice. Ann Gastroenterol Surg (2018) 2(6):451–62. doi: 10.1002/ags3.12205
56. Dehmani S, Nerrière-Daguin V, Néel M, Elain-Duret N, Heslan J.-M, Belarif L, et al. SIRPγ-CD47 interaction positively regulates the activation of human T cells in situation of chronic stimulation. Front Immunol (2021) 12:732530. doi: 10.3389/fimmu.2021.732530
57. Stefanidakis M, Newton G, Lee WY, Parkos CA, Luscinskas FW. Endothelial CD47 interaction with SIRPgamma is required for human T-cell transendothelial migration under shear flow conditions in vitro. Blood (2008) 112(4):1280–9. doi: 10.1182/blood-2008-01-134429
58. Jalil AR, Andrechak JC, Discher DE. Macrophage checkpoint blockade: results from initial clinical trials, binding analyses, and CD47-SIRPα structure–function. Antib Ther (2020) 3(2):80–94. doi: 10.1093/abt/tbaa006
Keywords: CD47 inhibitor, SIRPα inhibitor, next generation checkpoint inhibition, checkpoint inhibitor resistance, clinical trial, novel therapeutics
Citation: Son J, Hsieh RC-E, Lin HY, Krause KJ, Yuan Y, Biter AB, Welsh J, Curran MA and Hong DS (2022) Inhibition of the CD47-SIRPα axis for cancer therapy: A systematic review and meta-analysis of emerging clinical data. Front. Immunol. 13:1027235. doi: 10.3389/fimmu.2022.1027235
Received: 24 August 2022; Accepted: 30 September 2022;
Published: 11 November 2022.
Edited by:
Lekh N. Dahal, University of liverpool, United KingdomReviewed by:
Sukhbir Kaur, National Institutes of Health (NIH), United StatesCopyright © 2022 Son, Hsieh, Lin, Krause, Yuan, Biter, Welsh, Curran and Hong. This is an open-access article distributed under the terms of the Creative Commons Attribution License (CC BY). The use, distribution or reproduction in other forums is permitted, provided the original author(s) and the copyright owner(s) are credited and that the original publication in this journal is cited, in accordance with accepted academic practice. No use, distribution or reproduction is permitted which does not comply with these terms.
*Correspondence: David S. Hong, ZHNob25nQG1kYW5kZXJzb24ub3Jn
†These authors share first authorship
Disclaimer: All claims expressed in this article are solely those of the authors and do not necessarily represent those of their affiliated organizations, or those of the publisher, the editors and the reviewers. Any product that may be evaluated in this article or claim that may be made by its manufacturer is not guaranteed or endorsed by the publisher.
Research integrity at Frontiers
Learn more about the work of our research integrity team to safeguard the quality of each article we publish.