- 1Department of Oral and Maxillofacial Surgery, Tianjin Medical University Stomatology Hospital, Tianjin, Tianjin, China
- 2School of Stomatology, Tianjin Medical University, Tianjin, China
- 3Department of Endodontics & Laboratory for Dental Stem Cells and Endocrine Immunology, Tianjin Medical University School of Stomatology, Tianjin, China
The oral mucosa is a membranous structure comprising epithelial and connective tissue that covers the oral cavity. The oral mucosa is the first immune barrier to protect the body against pathogens for systemic protection. It is frequently exposed to mechanical abrasion, chemical erosion, and pathogenic invasion, resulting in oral mucosal lesions, particularly inflammatory diseases. Epithelial-mesenchymal transition (EMT) is a crucial biological process in the pathogenesis of oral mucosal disorders, which are classified into three types (types 1, 2, and 3) based on their physiological consequences. Among these, type-2 EMT is crucial in wound repair, organ fibrosis, and tissue regeneration. It causes infectious and dis-infectious immunological diseases, such as oral lichen planus (OLP), oral leukoplakia, oral submucosal fibrosis, and other precancerous lesions. However, the mechanism and cognition between type-2 EMT and oral mucosal inflammatory disorders remain unknown. This review first provides a comprehensive evaluation of type-2 EMT in chronically inflammatory oral mucosal disorders. The aim is to lay a foundation for future research and suggest potential treatments.
Introduction
In the 1980s, epithelial-mesenchymal transition (EMT) was identified as a feature of embryogenesis (1). Under different stimuli, epithelial cells lose polarity and cell-cell junctions and thus gain the ability to migrate, transforming into spindle-like mesenchymal cells. The reverse process of EMT is known as a mesenchymal-epithelial transition (MET). Both are crucial biological processes in embryonic development and tissue genesis in the dynamic balance of alteration (2). (Figure 1) Whether EMT or MET describes a process, quasi-mesenchymal cells are a type of transitional cell with characteristics of both epithelial and mesenchymal cells. Cancer metastasis and invasion have been linked to hybrid cells. They undergo partial EMT and have unique properties such as collective cell migration (3).
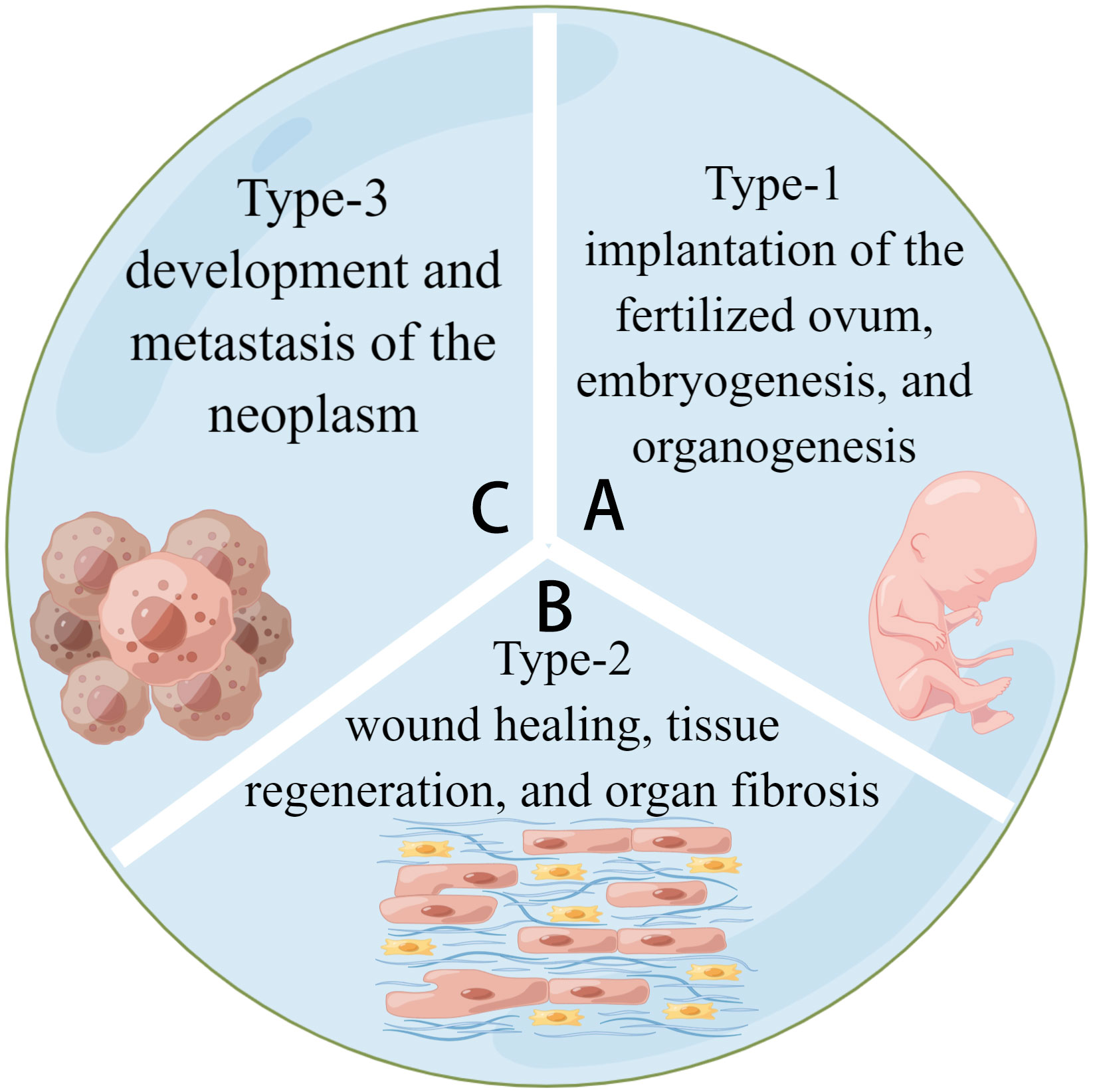
Figure 1 Three types of EMT. (A) Type-1 EMT is related to the embryogenesis of tissues and organs. (B) Type-2 EMT is related to tissue regeneration and fibrosis. (C) Type-3 EMT is related to tumor development and metastasis. They share a common model of cell activities.
EMT is classified into three types based on the biological environment in which it occurs: types 1, 2, and 3. Types 1 and 2 EMTs are associated with embryonic, regenerative, and pathological processes, respectively, with no abnormal cell proliferation (4, 5). Type-3 EMT is critical for tumor development and metastasis because it allows cancerous cells to generate, develop, and spread (6). (See Figure 2) Most current research focuses on Type-3 EMT, which is responsible for tumor genesis. However, types 1 and 2 EMTs have received little attention. Types 1 and 2 EMTs conclude the biological development of craniofacial tissues and organs from birth and the pathological processes of abnormal transition after the individual fully develops.
Fibrosis of the oral mucosa occurs in the inflammatory microenvironment of type-2 EMT. The immune microenvironment regulates cytokines and molecules in some signaling pathways, and the mechanisms are systematically generalized. Scientists have worked on EMT to treat degenerative diseases, repair injuries, rebuild tissue and organs, and delay senescence (7).
This review summarized the function and mechanism of type-1 and type-2 EMTs in oral mucosal nonneoplastic diseases. We proposed potential therapies for EMT-related diseases to lay a foundation for future clinical use in stomatology.
Type-2 EMT in oral mucosa
The oral cavity is protected by oral mucosa, primarily squamous epithelium, an immune system component. This review highlighted the oral mucosa as the human body’s immune barrier to defend against pathogens for systematic protection. Innate and adaptive immunity combine to form oral mucosal immunity. The former includes the physical barrier of mucosal epithelium, which excretes defensin, interleukin (IL)-8, and tumor necrosis factor-α (TNF-α); the normal flora, which alters the surrounding environment to inhibit the growth of potential pathogens; and the innate immune cells such as dendritic, Langerhans, and mast cells. The latter is known as the Mucosal Immune System (MIS). It produces secretory IgA in the Waldeyer’s ring and connects the inducer and effector sites via cell homing. MIS is involved in the local adaptive immune response and can cause mucosal cytotoxicity.
Type-2 EMT occurs in wound healing, tissue regeneration, and organ fibrosis and may result in keloids in the repair of the human epithelium in the inflammatory microenvironment. In the oral mucosa, it has been reported that simple wound healing causes no scar, which differs from the skin (8). However, certain microbiomes and tobacco and alcohol use can cause the pathological formation of fibrosis and keloids in the oral mucosa via EMT, which may delay the ultimate healing of the oral mucosa. There is ample space for further investigation in this area, the details of which remain unknown.
EMT in oral mucosal wound repair and regeneration
When a wound occurs, the skin and mucosa go through hemostasis, inflammation, proliferation, and remodeling. Granulation tissue forms in the inflammatory microenvironment and then progresses to the proliferation stage, where keratinocytes and fibroblasts migrate to the wound bed. The former is in charge of barrier reconstruction, while the latter is in charge of secreting extracellular matrix and remodeling granulation tissue (9).
Oral health has long been closely linked to systemic health but has received little attention. The oral cavity, located at the beginning of the human digestive tract, is critical for mastication, digestion, pronunciation, and aesthetics. Even though the oral mucosa is often exposed to mechanical abrasion and tension, it heals much faster with less scarring than the skin (10). The reason might be that oral mucosal fibroblasts and dermal fibroblasts have different cell behaviors and responses to growth factors. When exposed to transforming growth factor-β (TGF-β1), oral mucosal fibroblasts have a higher average proliferation rate, a lower shrinkage capacity, and synthesize more collagen (11).
Type-2 EMT is an after-birth reactivation recognized as a way to control inflammation and tissue regeneration. In recent decades, scientists have committed to identifying the factors initiating EMT. One explanation for the phenomenon is that in acute and mild trauma, wounded epithelial cells differentiate into fibroblast-like cells to reproduce tissues and organs, which is a reparative biological process (6). However, in the case of long-term continuing inflammation, the keloid is considered pathological fibrosis. It ceases once the repair is completed (12). The interaction between TGF-β1 and pro-inflammatory cytokines can generate a microenvironment for autoregulatory loops to strengthen the EMT. Polyriboinosinic: polyribocytidylic acid (Poly (I: C)) has been shown to accelerate collective HaCaT cell migration via autocrine/paracrine IL-8 secretions and EMT (13). It promotes leukocyte accumulation and improves chemokine expression during wound healing (14). Poly I: C induced IL-8 production by keratinocytes by stimulating Toll-like receptor 3 (TLR3), and TLR3 is a component of wound healing in regulating inflammation, during which NF-κB is activated (15). Poly I: C can stimulate EMT and improve wound healing (16). Interestingly, it has also been found that excessive poly I: C stimulation contributes to delayed wound healing (17). The complicated mechanisms of the double effect of Poly I: C remain to be investigated further. Another explanation for activating EMT during a wound in the mucosa is that the loss of apical-basal polarity can initiate the transition. It has been demonstrated that normal apical-basal cell polarity inhibits EMT via SNAI1 degradation mediated by the PAR complex (18). This points to the potential role of the cell-cell junction in regulating EMT.
EMT with oral mucosal dis-infectious diseases
Pathological processes associated with type 2 EMT include abnormal metastasis, keloid formation, and fibrosis. Scientists have reported the formation of keloids related to TGF-β1, EGF, and fibroblast growth factor (FGF) signaling pathways (19). Keratinocytes and fibroblasts influence the keloid and fibrosis of abnormal tissue, causing oral leukoplakia (OLK) or oral submucous fibrosis (20). Fibrosis has also been linked to inflammation.
Oral leukoplakia (OLK) is the most common underlying precancerous lesion and potentially malignant disorder (21). EMT can cause OLK to progress into oral squamous cell carcinoma (OSCC), linked to smoking (22) and chewing tobacco (23). It has been observed that in non-smokers, OLK occurs in conjunction with an immunosuppressive microenvironment established by activation of the PD-1/PD-L1 pathway and recruitment of CD163+ tumor-associated macrophages (TAMs), which may function in the early and transforming stages of oral tumorigenesis. The findings demonstrate that EGFR and WNT pathway proteins are overexpressed in all OLK samples, triggered by chewing tobacco, and may be a risk factor for the type of proliferation. Remarkably, the lncRNA oral leukoplakia progressed associated 1(LOLA1) has been found to promote oral mucosa epithelial migration, invasion, and EMT via the AKT/GSK‐3β pathway, thereby accelerating the progression of OLK (24). Elevated levels of some novel biomarkers, such as Snail and Axin2, with a high correlation to OLK malignant transformation, can predict oral tumorigenesis (25).
Oral submucous fibrosis (OSF) develops in a constant pro-inflammatory environment and has the characteristics of tissue fibrosis and degeneration diseases in various tissues and organs (26). Patients with OSF have difficulty opening their mouths and have stiff oral mucosa. It is caused by EMT, which causes oral submucous fibrosis (27). Arecoline is known as the pathogenic factor of OSF and has been shown to increase Twist expression (28).Chewing areca causes microtrauma and activates a protective inflammatory response, releasing many growth factors such as TGF-β, platelet-derived growth factor, basic FGF, and cytokines such as IL-6 and TNF-α, which promote fibrosis (29). Hinokitiol has been shown to downregulate Snail, lowering a-SMA expression and myofibroblast properties as an anti-fibrosis agent (30).
The World Health Organization classifies OLP as a premalignant chronic inflammatory disease mediated by T-lymphocytes. Smad 3 expression in OLP is higher and statistically significant than in normal oral mucosa, consistent with apoptosis, inflammation, and EMT functions (31, 32). It has also been reported that in OLP, claudin‐1, claudin‐4, and E‐cadherin are downregulated, disrupting the epithelial barrier and causing T‐lymphocytes to migrate into epithelial cells (33). Furthermore, OLP liquefaction degeneration is an EMT result primarily induced by IFN-γ, which can improve the malignant transition (34). The current studies also illustrated that the submucosal infiltration of T and B lymphocytes is more distinct in OLP than in OLK, and the immunological response is also stronger in OLP (35).
EMT with oral mucosal infectious diseases
The oral cavity is a huge reservoir for microorganisms to grow, develop and manipulate. Millions of viruses, bacteria, and fungi colonize the mucosa epithelium forming a balanced biofilm. Once the equilibrium is disrupted, opportunistic pathogens take over and cause continuous inflammatory reactions. Oral microbiota has been found to manipulate cell migration by modulating the EMT process in such an inflammatory microenvironment. Microbiota degrades epithelial tight junction proteins, improves mesenchymal properties, and induces partial or complete EMT (36). It is frequently associated with oral mucosa infectious diseases such as gingivitis, oral candidiasis, herpes, and others. Pathogens in the oral cavity cause disease via different regulatory mechanisms of the EMT.
Porphyromonas gingivalis (P. gingivalis) degrades E-cadherin to regulate the epithelial function of the barrier (37). NNMT, CCAT1, and GAS6 genes are involved in cell migration and invasion. These gene’s messenger RNA (mRNA) levels are high in P. gingivalis-infected oral epithelial cells (38). It also modulates the β-catenin pathway and uncouples the β-catenin destruction complex in gingival epithelial cells, facilitating nuclear translocation to activate TCF/LEF promoter elements in the following step (39).
Streptococcus gordonii suppresses FOXO1 and activates the TAK1-NLK negative regulatory pathway for ZEB2 induction resistance (40). Upregulation of partial EMT genes has been observed in Fusobacterium nucleatum-infected OSCC cells (41). The signal transducer and activator of the transcription-3 signaling pathway is activated, increasing the expression of EMT-associated genes such as E-cadherin, Snail, and Twist. The EMT has been widely debated over the years, particularly its role in cancer progression. However, the significance of EMT in embryogenesis, tissue regeneration, and fibrosis is rarely discussed. This review discusses the types 1 and 2 EMTs in craniofacial tissues and organs. Related disorders such as palatal cleft, dental defect, OLK, and OSF are also evaluated. There remains a long way to go to reduce the negative effects of EMT, such as the formation of keloid and fibrosis and the facilitation of neoplasm to provide theoretical support for the following research and applications of types 1 and 2 EMTs so that experimental trials of EMT can be used in the clinic and theoretical knowledge can transform from bench to bedside. Certain bacteria, lower PH, signaling molecules, loss of apical-basal polarity, and other approaches have been used to activate EMT. The EMT process, particularly type-2 EMT, strongly correlates with inflammation regulated by the immunological microenvironment. However, there remains a long way to go before determining the complete blueprint of the crosstalk among various cytokines and signaling pathways. As previously stated, we have concluded complicated mechanisms of types 1 and 2 EMTs. Many details of regulation and alteration remain unknown. It may be important for researchers to investigate the differential expression of cytokines and signaling pathways during both biological and pathological processes of EMT activities (Table 1).
EMT with immune regulation of the oral mucosa
Oral mucosal disease, particularly oral mucosal precancerous lesions, has been linked to changes in the immune microenvironment. The infiltration of high-grade CD8+ lymphocytes within the epithelium was linked to increased in remission rates (43). Intraepithelial CD8+ lymphocytes are likely to serve as a biomarker of remission and a potential area of biomedical research regarding OLP’s etiology and premalignant potential. The host immune system may bypass PD-L1-expressing dysplastic epithelial and recruited subepithelial cells in oral precancerous lesions. Furthermore, by inhibiting the PD-1/PD-L1 pathways, oral precancerous lesions can be prevented from transforming into cancer, and advanced cancer can be treated (44). According to previous research, OLP lesions are caused by IL-4, which is produced by several factors. It also affects various cells, resulting in OLP lesions (45).
Immune mediators are not only directly linked to precancerous lesions, but they are also indirectly mediated by EMT. It has been reported that activated oncogenic Ras post-transcriptionally enhances premalignant cell mutations, intensifying malignancy and cell invasion. There is a significant change in mRNA levels, which correlates with protein abundance and is consistent with EMT. These proteins also changed following Ras transformation, suggesting that premalignant cells were primed to become malignant. Therefore, Ras-induced EMT-associated invasion in primed premalignant cells via post-transcriptional mechanisms (46). SCC-4 cells synthesize and release IL-6 independently, a process aided by TLR2/TLR6 agonists. In contrast to precancerous human tongue DOK cells, cancerous tongue SCC-4 cells exhibit a classic EMT profile (47). Beyond their immune function, CD4+ T cells are abundant in the dense stroma surrounding ductal epithelium in CP tissues associated with EMT. CD4+ T cells can induce EMT in premalignant cells (48). EMT is also facilitated by abnormal immune mediator expression in precancerous lesions. T cell dysfunction (49) or genetic changes (50) also contributes to developing immunosuppressive microenvironments during the malignant transformation of the oral mucosa by inducing EMT. The TGF-β (51) and PI3K-AKT (19) signaling pathways are critical in this transformation.
Prospect
EMT is a common physiological process during embryogenesis, wound healing, fibrosis, tumorigenesis, and cancer metastasis. It has been artificially divided into three types based on different biological backgrounds, but the boundaries are not always clear. It is recommended to command the differential expressions of EMT in various situations to regulate the microenvironment to maintain equilibrium. EMT has applications in tissue regeneration and fibrosis inhibition, and we propose prospects. Concerning tooth tissue regeneration, two types of cells are indispensable: epithelial stem cells and mesenchymal stem cells (MSCs). They interact with and transform into one another under certain conditions. In dental tissue engineering, epithelial stem cells are primarily derived from embryonic tooth epithelium, while MSCs are derived from tooth germ and bone marrow stem cells.
EMT and traditional approaches to tissue engineering
The interaction between seed cells, scaffold materials, and the microenvironment is central to current tissue engineering. Wang et al. invented the new concept of bio-root and saw it through to completion (52). Fruitful research on tooth regeneration has been published in the last few decades (53), and it is attractive to realize the clinical transformation of bio-root. However, common cell culture approaches are somewhat complicated for incorporating both epithelial stem cells and MSCs into the regenerative system, and increasing the workload. As a result, an EMT-induced culture system might be advantageous. Only one type of seed cell is added, and another type can be transferred from the original one via the regulation of cytokines and other signaling pathways. This method simplifies the operation and may provide a solution to the cell source shortage.
Recent studies on EMT have demonstrated that three-dimensional models can better simulate the extracellular matrix microenvironment, improve cell vitality, and reduce mortality than two-dimensional models, particularly for cartilage formation (54). The hydrogel is a porous, jelly-like structure that provides a biocompatible and non-toxic environment for cell growth, differentiation, and proliferation. It creates an environment for EMT/MET to occur by containing specific biomarkers and nutrient materials. The biocompatibility and histocompatibility of the ECM-derived hydrogel are higher (55). TGF-β1 induces EMT within the three-dimensional system for the decreased epithelial markers (E-cadherin), increased mesenchymal markers (Vimentin and α-SMA), and enhanced migratory and invasion capacity (56). (Figure 3) Furthermore, the treated dentin matrix, hyaluronic acid, PCL, and ceramics are widely used; they can be chosen during various tissue engineering study processes involving EMT (57–61).
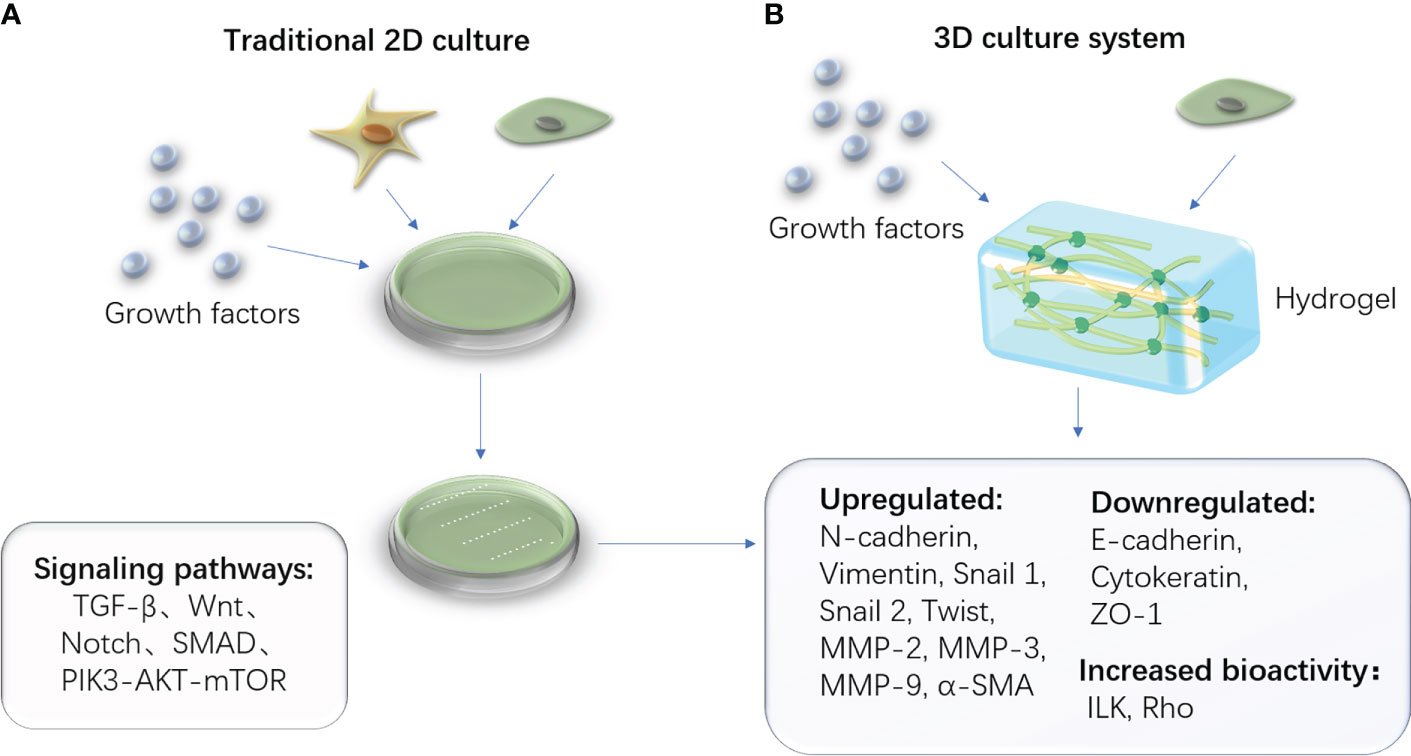
Figure 3 Traditional 2D cell culture system and advanced 3D cell culture system. (A) The schematic diagram of the traditional 2D culturing method, which contains stem cells and necessary cytokines in a culture medium. (B) The schematic diagram of a novel 3D culturing method in which the culture medium is replaced by the biomaterial hydrogel.
However, the use of EMT in traditional tissue engineering is restricted by a lack of experimental conditions, unknown mechanisms, and operating techniques. Moreover, the condition of unclear regulation can contribute to tumor genesis and make the process uncontrollable. There remains much work to be done before we can put these considerations into practice.
EMT in developmental biological regeneration
Unlike traditional tissue engineering, biological regeneration eliminates exogenous scaffolds and stimulates the organism’s regrowth. It is safer, easier, and has fewer side effects (62). On the other hand, the high demands for immunological microenvironments pose challenges. Bacterial pathogens, acid microenvironment, growth factors, proteins of primary signaling pathways, loss of apical-basal polarity, hypoxia, and other factors have all been implicated in the activation of EMT (18, 63–65). For instance, during long-term infection with the opportunistic pathogen P. gingivalis, human primary epithelial cells develop an EMT phenotype (66). Anaerobic periodontal pathogens have been shown to induce EMT in primary oral keratinocytes, destroying the periodontal barrier and contributing to periodontitis (63). EMT-associated transcription factors such as Slug, Snail, and Zeb1 showed significant increases in response to pathogen exposure. The treatment of EMT-related oral diseases may benefit from focusing on critical factors. The key to successful regeneration is determining how to precisely control these variables.
Coffee and EMT research has also received widespread attention. Coffee components can reverse EMT transitions or even rescue the functions of EMT inducers. For instance, Trigonelline extracted from natural coffee beans reduces renal fibrosis by inhibiting EMT (67). Chlorogenic acid derived from coffee has antitumor and anti-metastatic properties by interfering with the NF-κB/EMT signaling pathway (68). They exert pharmacological functions as EMT inhibitors, but more studies for clinical transformation are needed. Furthermore, because low PH promotes EMT, an alkaline diet and anti-acid drugs may effectively prevent EMT in the craniofacial tissues and organs (64).
Different signaling pathways and molecules regulate EMT in various biological or pathological processes. As a result, additional research into the individual mechanisms of each process is required to achieve precise control. Otherwise, it is considering how to find a balance between promoting practical functions and maintaining cell vitality. We are now at tipping in combining EMT and tissue engineering. They mutually aid in the advancement of regenerative medicine. The multi-discipline study is now the mainstream of research and has a promising future (69).
Methods of preventing dysfibrosis
It is challenging to reduce the negative effects of dysfibrosis and keloids. As previously elucidated, oral mucosa has less keloid and fibrosis and heals wounds faster than the skin. The mechanisms of improved mucosal quality are primarily concluded as follows (9, 10): 1. Fewer pro-inflammatory factors and less inflammatory response; 2. Reduced recruitment of neutrophils, macrophages, and T cells after injury; 3. Certain microorganisms activate the immune system for wound healing cascade; 4. the suitable environment of saliva, which provides a biomimetic idea of hydrogel, applies to skin healing to accelerate the process (70). By inhibiting EMT, we may be able to design a type of epithelium with high regenerative capacity and self-repair without many keloids in the future.
The anti-EMT mainstream of reducing fibrosis during tissue regeneration or OSF (71). One solution is to improve EMT inhibitors such as phosphatase and tensin homolog, which inhibits the PI3K/AKT pathway to reduce hypertrophic scar fibroblast proliferation and eliminate keloid and fibrotic scars (72).
Another option is to use MSCs, which have anti-fibrotic, anti-oxidative, and angiogenesis properties, indicating that the cell is an ideal anti-fibrotic target.26 The functioning mechanism is attributed to inhibiting the TGF-β1 pathway via N-cadherin and vimentin downregulation (73, 74). MSCs are produced from epithelial cells through the continuous process of EMT. They have significantly higher levels of expression of several biomarkers, including CD105, CD73, and CD90 (75). Specific induction causes MSCs to differentiate into osteoblasts, adipocytes, and chondrocytes, differentiating into dental pulp stem cells (DPSCs), dental follicle stem cells (DFSCs), periodontal ligament stem cells (PDLSCs), and others. As a result, EMT serves as a unique source of seed cells for tissue regeneration.
MSCs are also critical in halting the process of OSF for immunomodulatory, anti-fibrotic, anti-oxidative, and angiogenic functions. Areca chewing can increase pro-inflammatory cytokines such as TNF-α and IL-6 in response to the microtrauma it causes, thereby promoting fibrosis progression (29). MSCs also suppress TNF-α expression via IL-10 secretion and downregulate TNF- α and IL-6 by inhibiting IFN-γ expression (76). MSCs also suppress the TGF-β pathway by secreting hepatocyte growth factor and TNF-stimulated gene 6 protein, which restores the TGF-β1/TGF-β3 balance for anti-fibrotic microenvironment production (77).
In this review, we present our expectations that in the future, we can apply EMT to regenerative medicine with or without scaffolding materials for profound progress in the following research. We owe the huge leap in the basic study of EMT to the progress made in the past few years. EMT significantly impact on scientific research and clinical transformation once the functional mechanisms are identified. We anticipate developing novel medicines for the treatment of EMT-related diseases in stomatology such as developmental malformation, wound repair, keloid and fibrosis, and other oral mucosa pathological alterations in the future.
Author contributions
ZM, and TY wrote this manuscript. DL revised this manuscript. All authors contributed to the article and approved the submitted version.
Funding
This research was funded by Tianjin Municipal Science and Technology Foundation grant number 21JCQNJC01190 and Tianjin Health Science and Technology Project grant number KJ20081. This research was also supported by the National Natural Science Foundation of China (82071079 to Dayong Liu)
Acknowledgments
Authors thank the Bullet Edits for revising the English language. Authors also thank Figdraw of Home for Researchers, which supported Figures drawing.
Conflict of interest
The authors declare that the research was conducted in the absence of any commercial or financial relationships that could be construed as a potential conflict of interest.
Publisher’s note
All claims expressed in this article are solely those of the authors and do not necessarily represent those of their affiliated organizations, or those of the publisher, the editors and the reviewers. Any product that may be evaluated in this article, or claim that may be made by its manufacturer, is not guaranteed or endorsed by the publisher.
References
1. Greenburg G, Hay ED. Epithelia suspended in collagen gels can lose polarity and express characteristics of migrating mesenchymal cells. J Cell Biol (1982) 95(1):333–9. doi: 10.1083/jcb.95.1.333
2. Haensel D, Dai X. Epithelial-to-mesenchymal transition in cutaneous wound healing: Where we are and where we are heading. Dev dynamics: an Off Publ Am Assoc Anatom (2018) 247(3):473–80. doi: 10.1002/dvdy.24561
3. Liao T-T, Yang M-H. Hybrid Epithelial/Mesenchymal state in cancer metastasis: Clinical significance and regulatory mechanisms. Cells (2020) 9(3):623. doi: 10.3390/cells9030623
4. Zavadil J, Haley J, Kalluri R, Muthuswamy SK, Thompson E. Epithelial-mesenchymal transition. Cancer Res (2008) 68(23):9574–7. doi: 10.1158/0008-5472.CAN-08-2316
5. Kalluri R, Weinberg RA. The basics of epithelial-mesenchymal transition. J Clin Invest (2009) 119(6):1420–8. doi: 10.1172/JCI39104
6. Marconi GD, Fonticoli L, Rajan TS, Pierdomenico SD, Trubiani O, Pizzicannella J, et al. Epithelial-mesenchymal transition (EMT): The type-2 EMT in wound healing, tissue regeneration and organ fibrosis. Cells (2021) 10(7):1587. doi: 10.3390/cells10071587
7. Thiery JP, Acloque H, Huang RYJ, Nieto MA. Epithelial-mesenchymal transitions in development and disease. Cell (2009) 139(5):871–90. doi: 10.1016/j.cell.2009.11.007
8. Leavitt T, Hu MS, Marshall CD, Barnes LA, Lorenz HP, Longaker MT. Scarless wound healing: finding the right cells and signals. Cell Tissue Res (2016) 365(3):483–93. doi: 10.1007/s00441-016-2424-8
9. Nikoloudaki G, Creber K, Hamilton DW. Wound healing and fibrosis: a contrasting role for periostin in skin and the oral mucosa. Am J Physiol Cell Physiol (2020) 318(6):C1065–C77. doi: 10.1152/ajpcell.00035.2020
10. Waasdorp M, Krom BP, Bikker FJ, van Zuijlen PPM, Niessen FB, Gibbs S. The bigger picture: Why oral mucosa heals better than skin. Biomolecules (2021) 11(8):1161. doi: 10.3390/biom11081165
11. Lee HG, Eun HC. Differences between fibroblasts cultured from oral mucosa and normal skin: implication to wound healing. J Dermatol Sci (1999) 21(3):176–82. doi: 10.1016/S0923-1811(99)00037-7
12. López-Novoa JM, Nieto MA. Inflammation and EMT: An alliance towards organ fibrosis and cancer progression. EMBO Mol Med (2009) 1(6-7):303–14. doi: 10.1002/emmm.200900043
13. Takada K, Komine-Aizawa S, Hirohata N, Trinh QD, Nishina A, Kimura H, et al. Poly I:C induces collective migration of HaCaT keratinocytes via IL-8. BMC Immunol (2017) 18(1):19. doi: 10.1186/s12865-017-0202-3
14. Lin Q, Wang L, Lin Y, Liu X, Ren X, Wen S, et al. Toll-like receptor 3 ligand polyinosinic:polycytidylic acid promotes wound healing in human and murine skin. J Invest Dermatol (2012) 132(8):2085–92. doi: 10.1038/jid.2012.120
15. Kawai T, Akira S. Toll-like receptor downstream signaling. Arthritis Res Ther (2005) 7(1):12–9. doi: 10.1186/ar1469
16. Harada K, Sato Y, Ikeda H, Isse K, Ozaki S, Enomae M, et al. Epithelial-mesenchymal transition induced by biliary innate immunity contributes to the sclerosing cholangiopathy of biliary atresia. J pathol (2009) 217(5):654–64. doi: 10.1002/path.2488
17. Grimstad O, Husebye H, Espevik T. TLR3 mediates release of IL-1beta and cell death in keratinocytes in a caspase-4 dependent manner. J Dermatol Sci (2013) 72(1):45–53. doi: 10.1016/j.jdermsci.2013.05.006
18. Jung HY FL, Tsai JH, Kajimoto T, Chang Q, Newton AC, Yang J. Apical-basal polarity inhibits epithelial-mesenchymal transition and tumour metastasis by PAR-complex-mediated SNAI1 degradation. Nat Cell Biol (2019) 21(3):359–71. doi: 10.1038/s41556-019-0291-8
19. Shetty SS, Sharma M, Fonseca FP, Jayaram P, Tanwar AS, Kabekkodu SP, et al. Signaling pathways promoting epithelial mesenchymal transition in oral submucous fibrosis and oral squamous cell carcinoma. Jpn Dent Sci Rev (2020) 56(1):97–108. doi: 10.1016/j.jdsr.2020.07.002
20. Hahn JM, Glaser K, McFarland KL, Aronow BJ, Boyce ST, Supp DM. Keloid-derived keratinocytes exhibit an abnormal gene expression profile consistent with a distinct causal role in keloid pathology. Wound Repair Regen (2013) 21(4):530–44. doi: 10.1111/wrr.12060
21. Lv Z, Cong R, Li J, Cao K, Bao Q, Li L, et al. PTHLH predicts the prognosis of patients with oral leukoplakia. Onco Targets Ther (2020) 13:10013–23. doi: 10.2147/OTT.S261124
22. Teh M-T, Yagyuu T, Funayama N, Imada M, Kirita T. Effect of smoking status and programmed death-ligand 1 expression on the microenvironment and malignant transformation of oral leukoplakia: A retrospective cohort study. PloS One (2021) 16(4):e0250359. doi: 10.1371/journal.pone.0250359
23. Bhattacharyya S, Ray S, Saha D, Mustafi SM, Alam N, Sarkar A, et al. Chewing tobacco may act as a risk factor for dysplastic transformation of squamous cells in oral leukoplakia- a cytochemistry based approach. Pathol - Res Pract (2021) 218:153287. doi: 10.1016/j.prp.2020.153287
24. Liu W, Yao Y, Shi L, Tang G, Wu L. A novel lncRNA LOLA1 may predict malignant progression and promote migration, invasion, and EMT of oral leukoplakia via the AKT/GSK-3beta pathway. J Cell Biochem (2021) 122(10):1302–12. doi: 10.1002/jcb.29951
25. Zhang X, Kim KY, Zheng Z, Kim HS, Cha IH, Yook JI. Snail and Axin2 expression predict the malignant transformation of oral leukoplakia. Oral Oncol (2017) 73:48–55. doi: 10.1016/j.oraloncology.2017.08.004
26. Kheur S, Sanap A, Kharat A, Gupta AA, Raj AT, Kheur M, et al. Hypothesizing the therapeutic potential of mesenchymal stem cells in oral submucous fibrosis. Med Hypotheses (2020) 144:110204. doi: 10.1016/j.mehy.2020.110204
27. Lien K, Mayer W, Herrera R, Rosbe K, Tugizov SM. HIV-1 proteins gp120 and tat induce the epithelial-mesenchymal transition in oral and genital mucosal epithelial cells. PloS One (2019) 14(12):e0226343. doi: 10.1371/journal.pone.0226343
28. Lee Y-H, Yang L-C, Hu F-W, Peng C-Y, Yu C-H, Yu C-C. Elevation of twist expression by arecoline contributes to the pathogenesis of oral submucous fibrosis. J Formosan Med Assoc (2016) 115(5):311–7. doi: 10.1016/j.jfma.2015.05.009
29. Rajalalitha P, Vali S. Molecular pathogenesis of oral submucous fibrosis–a collagen metabolic disorder. J Oral Pathol Med (2005) 34(6):321–8. doi: 10.1111/j.1600-0714.2005.00325.x
30. Yang HW, Lu MY, Chiu YW, Liao YW, Huang YF, Ju Chueh P, et al. Hinokitiol ablates myofibroblast activation in precancerous oral submucous fibrosis by targeting snail. Environ Toxicol (2018) 33(4):454–62. doi: 10.1002/tox.22531
31. Karatsaidis A, Schreurs O, Axéll T, Helgeland K, Schenck K. Inhibition of the transforming growth factor-beta/Smad signaling pathway in the epithelium of oral lichen. J Invest Dermatol (2003) 121(6):1283–90. doi: 10.1046/j.1523-1747.2003.12633.x
32. Danielsson K, Wahlin YB, Coates PJ, Nylander K. Increased expression of smad proteins, and in particular Smad3, in oral lichen planus compared to normal oral mucosa. J Oral Pathol Med (2010) 39(8):639–44. doi: 10.1111/j.1600-0714.2010.00902.x
33. Hamalainen L, Soini Y, Pasonen-Seppanen S, Siponen M. Alterations in the expression of EMT-related proteins claudin-1, claudin-4 and claudin-7, e-cadherin, TWIST1 and ZEB1 in oral lichen planus. J Oral Pathol Med (2019) 48(8):735–44. doi: 10.1111/jop.12917
34. Liu Y, Liu G, Liu Q, Tan J, Hu X, Wang J, et al. The cellular character of liquefaction degeneration in oral lichen planus and the role of interferon gamma. J Oral Pathol Med (2017) 46(10):1015–22. doi: 10.1111/jop.12595
35. Dafar A, Siarov A, Mostaghimi Y, Robledo-Sierra J, De Lara S, Giglio D, et al. Langerhans cells, T cells, and b cells in oral lichen planus and oral leukoplakia. Int J Dent (2022) 2022:5430309. doi: 10.1155/2022/5430309
36. Bai H, Yang J, Meng S, Liu C. Oral microbiota-driven cell migration in carcinogenesis and metastasis. Front Cell Infect Microbiol (2022) 12:864479. doi: 10.3389/fcimb.2022.864479
37. Abdulkareem AA, Shelton RM, Landini G, Cooper PR, Milward MR. Periodontal pathogens promote epithelial-mesenchymal transition in oral squamous carcinoma cells in vitro. Cell Adh Migr (2018) 12(2):127–37. doi: 10.1080/19336918.2017.1322253
38. Geng F, Liu J, Guo Y, Li C, Wang H, Wang H, et al. Persistent exposure to porphyromonas gingivalis promotes proliferative and invasion capabilities, and tumorigenic properties of human immortalized oral epithelial cells. Front Cell Infect Microbiol (2017) 7:57. doi: 10.3389/fcimb.2017.00057
39. Zhou Y, Sztukowska M, Wang Q, Inaba H, Potempa J, Scott DA, et al. Noncanonical activation of beta-catenin by porphyromonas gingivalis. Infect Immun (2015) 83(8):3195–203. doi: 10.1128/IAI.00302-15
40. Ohshima J, Wang Q, Fitzsimonds ZR, Miller DP, Sztukowska MN, Jung YJ, et al. Streptococcus gordonii programs epithelial cells to resist ZEB2 induction by porphyromonas gingivalis. Proc Natl Acad Sci United States America (2019) 116(17):8544–53. doi: 10.1073/pnas.1900101116
41. Shao W, Fujiwara N, Mouri Y, Kisoda S, Yoshida K, Yoshida K, et al. Conversion from epithelial to partial-EMT phenotype by fusobacterium nucleatum infection promotes invasion of oral cancer cells. Sci Rep (2021) 11(1):14943. doi: 10.1038/s41598-021-94384-1
42. Yin X, Li J, Salmon B, Huang L, Lim WH, Liu B, et al. Wnt Signaling and Its Contribution to Craniofacial Tissue Homeostasis. J Dent Res (2015) 94(11):1487–94. doi: 10.1177/0022034515599772
43. Enomoto A, Sato E, Yasuda T, Isomura T, Nagao T, Chikazu D. Intraepithelial CD8+ lymphocytes as a predictive diagnostic biomarker for the remission of oral lichen planus. Hum pathol (2018) 74:43–53. doi: 10.1016/j.humpath.2017.12.008
44. Yagyuu T, Hatakeyama K, Imada M, Kurihara M, Matsusue Y, Yamamoto K, et al. Programmed death ligand 1 (PD-L1) expression and tumor microenvironment: Implications for patients with oral precancerous lesions. Oral Oncol (2017) 68:36–43. doi: 10.1016/j.oraloncology.2017.03.006
45. Mehrbani SP, Motahari P, Azar FP, Ahari MA. Role of interleukin-4 in pathogenesis of oral lichen planus: A systematic review. Med Oral Patol Oral Cir Bucal (2020) 25(3):e410–e5. doi: 10.4317/medoral.23460
46. Bisogno LS, Friedersdorf MB, Keene JD. Ras post-transcriptionally enhances a pre-malignantly primed EMT to promote invasion. iScience (2018) 4:97–108. doi: 10.1016/j.isci.2018.05.011
47. Karavyraki M, Porter RK. Evidence of a role for interleukin-6 in anoikis resistance in oral squamous cell carcinoma. Med Oncol (2022) 39(5):60. doi: 10.1007/s12032-022-01664-5
48. Goebel L, Grage-Griebenow E, Gorys A, Helm O, Genrich G, Lenk L, et al. CD4(+) T cells potently induce epithelial-mesenchymal-transition in premalignant and malignant pancreatic ductal epithelial cells-novel implications of CD4(+) T cells in pancreatic cancer development. Oncoimmunology (2015) 4(4):e1000083. doi: 10.1080/2162402X.2014.1000083
49. Wen L, Lu H, Li Q, Li Q, Wen S, Wang D, et al. Contributions of T cell dysfunction to the resistance against anti-PD-1 therapy in oral carcinogenesis. J Exp Clin Cancer Res (2019) 38(1):299. doi: 10.1186/s13046-019-1185-0
50. Rangel R, Pickering CR, Sikora AG, Spiotto MT. Genetic changes driving immunosuppressive microenvironments in oral premalignancy. Front Immunol (2022) 13:840923. doi: 10.3389/fimmu.2022.840923
51. Hao Y, Baker D, Ten Dijke P. TGF-beta-Mediated epithelial-mesenchymal transition and cancer metastasis. Int J Mol Sci (2019) 20(11):E2767. doi: 10.3390/ijms20112767
52. Wang S, Liu Y, Fang D, Shi S. The miniature pig: a useful large animal model for dental and orofacial research. Oral Dis (2007) 13(6):530–7. doi: 10.1111/j.1601-0825.2006.01337.x
53. Yang X, Ma Y, Guo W, Yang B, Tian W. Stem cells from human exfoliated deciduous teeth as an alternative cell source in bio-root regeneration. Theranostics (2019) 9(9):2694–711. doi: 10.7150/thno.31801
54. Pal M, Chen H, Lee BH, Lee JYH, Yip YS, Tan NS, et al. Epithelial-mesenchymal transition of cancer cells using bioengineered hybrid scaffold composed of hydrogel/3D-fibrous framework. Sci Rep (2019) 9(1):8997. doi: 10.1038/s41598-019-45384-9
55. Zhou J, Wu P, Sun H, Zhou H, Zhang Y, Xiao Z. Lung tissue extracellular matrix-derived hydrogels protect against radiation-induced lung injury by suppressing epithelial-mesenchymal transition. J Cell Physiol (2020) 235(3):2377–88. doi: 10.1002/jcp.29143
56. Yao Y, Chen R, Wang G, Zhang Y, Liu F. Exosomes derived from mesenchymal stem cells reverse EMT via TGF-beta1/Smad pathway and promote repair of damaged endometrium. Stem Cell Res Ther (2019) 10(1):225. doi: 10.1186/s13287-019-1332-8
57. Li R, Guo W, Yang B, Guo L, Sheng L, Chen G, et al. Human treated dentin matrix as a natural scaffold for complete human dentin tissue regeneration. Biomaterials (2011) 32(20):4525–38. doi: 10.1016/j.biomaterials.2011.03.008
58. Yang B, Chen G, Li J, Zou Q, Xie D, Chen Y, et al. Tooth root regeneration using dental follicle cell sheets in combination with a dentin matrix - based scaffold. Biomaterials (2012) 33(8):2449–61. doi: 10.1016/j.biomaterials.2011.11.074
59. Ferroni L, Gardin C, Sivolella S, Brunello G, Berengo M, Piattelli A, et al. A hyaluronan-based scaffold for the in vitro construction of dental pulp-like tissue. Int J Mol Sci (2015) 16(3):4666–81. doi: 10.3390/ijms16034666
60. Park CH, Rios HF, Jin Q, Bland ME, Flanagan CL, Hollister SJ, et al. Biomimetic hybrid scaffolds for engineering human tooth-ligament interfaces. Biomaterials (2010) 31(23):5945–52. doi: 10.1016/j.biomaterials.2010.04.027
61. Nam S, Won JE, Kim CH, Kim HW. Odontogenic differentiation of human dental pulp stem cells stimulated by the calcium phosphate porous granules. J Tissue Eng (2011) 2011:812547. doi: 10.4061/2011/812547
62. Park HM, Kim SH, Choi BH, Park SH. Effects of induction culture on osteogenesis of scaffold-free engineered tissue for bone regeneration applications. Tissue Eng Regener Med (2022) 19(2):417–29. doi: 10.1007/s13770-021-00418-0
63. Abdulkareem AA, Shelton RM, Landini G, Cooper PR, Milward MR. Potential role of periodontal pathogens in compromising epithelial barrier function by inducing epithelial-mesenchymal transition. J Periodontal Res (2018) 53(4):565–74. doi: 10.1111/jre.12546
64. Suzuki A MT, Baba Y, Shimamura K, Kato Y. Acidic extracellular pH promotes epithelial mesenchymal transition in Lewis lung carcinoma model. Cancer Cell Int (2014) 14(1):129. doi: 10.1186/s12935-014-0129-1
65. Jiao M, Nan KJ. Activation of PI3 kinase/Akt/HIF-1alpha pathway contributes to hypoxia-induced epithelial-mesenchymal transition and chemoresistance in hepatocellular carcinoma. Int J Oncol (2012) 40(2):461–8. doi: 10.3892/ijo.2011.1197
66. Lee J, Roberts JS, Atanasova KR, Chowdhury N, Han K, Yilmaz O. Human primary epithelial cells acquire an epithelial-Mesenchymal-Transition phenotype during long-term infection by the oral opportunistic pathogen, porphyromonas gingivalis. Front Cell Infect Microbiol (2017) 7:493. doi: 10.3389/fcimb.2017.00493
67. Peerapen P, Thongboonkerd V. Protective roles of trigonelline against oxalate-induced epithelial-to-mesenchymal transition in renal tubular epithelial cells: An in vitro study. Food Chem Toxicol (2020) 135:110915. doi: 10.1016/j.fct.2019.110915
68. Zeng A, Liang X, Zhu S, Liu C, Wang S, Zhang Q, et al. Chlorogenic acid induces apoptosis, inhibits metastasis and improves antitumor immunity in breast cancer via the NFkappaB signaling pathway. Oncol Rep (2021) 45(2):717–27. doi: 10.3892/or.2020.7891
69. Abdallah BM, Khattab HM. Recent approaches to isolating and culturing mouse bone marrow-derived mesenchymal stromal stem cells. Curr Stem Cell Res Ther (2021) 16(5):599–607. doi: 10.2174/1574888X15666200708134337
70. Kong X, Fu J, Shao K, Wang L, Lan X, Shi J. Biomimetic hydrogel for rapid and scar-free healing of skin wounds inspired by the healing process of oral mucosa. Acta Biomater (2019) 100:255–69. doi: 10.1016/j.actbio.2019.10.011
71. Li M, Luan F, Zhao Y, Hao H, Zhou Y, Han W, et al. Epithelial-mesenchymal transition: An emerging target in tissue fibrosis. Exp Biol Med (2015) 241(1):1–13. doi: 10.1177/1535370215597194
72. Guo L, Chen L, Bi S, Chai L, Wang Z, Cao C, et al. PTEN inhibits proliferation and functions of hypertrophic scar fibroblasts. Mol Cell Biochem (2012) 361(1-2):161–8. doi: 10.1007/s11010-011-1100-2
73. Tsuji K, Kitamura S, Wada J. Immunomodulatory and regenerative effects of mesenchymal stem cell-derived extracellular vesicles in renal diseases. Int J Mol Sci (2020) 21(3):E756. doi: 10.3390/ijms21030756
74. Usunier B, Benderitter M, Tamarat R, Chapel A. Management of fibrosis: The mesenchymal stromal cells breakthrough. Stem Cells Int (2014) 2014:1–26. doi: 10.1155/2014/340257
75. Harrell CR, Djonov V, Volarevic V. The cross-talk between mesenchymal stem cells and immune cells in tissue repair and regeneration. Int J Mol Sci (2021) 22(5):2472. doi: 10.3390/ijms22052472
76. Sotiropoulou PA, Perez SA, Gritzapis AD, Baxevanis CN, Papamichail M. Interactions between human mesenchymal stem cells and natural killer cells. Stem Cells (2006) 24(1):74–85. doi: 10.1634/stemcells.2004-0359
Keywords: epithelial-mesenchymal transition, craniofacial embryogenesis, oral mucosa alterations, keloid, fibrosis, immunological microenvironment
Citation: Meng Z, Yang T and Liu D (2022) Type-2 epithelial-mesenchymal transition in oral mucosal nonneoplastic diseases. Front. Immunol. 13:1020768. doi: 10.3389/fimmu.2022.1020768
Received: 16 August 2022; Accepted: 13 October 2022;
Published: 31 October 2022.
Edited by:
Junji Xu, Capital Medical University, ChinaReviewed by:
Debora Heller, Universidade Cruzeiro do Sul, BrazilLei Hu, Beijing Stomatological Hospital, Capital Medical University, China
Copyright © 2022 Meng, Yang and Liu. This is an open-access article distributed under the terms of the Creative Commons Attribution License (CC BY). The use, distribution or reproduction in other forums is permitted, provided the original author(s) and the copyright owner(s) are credited and that the original publication in this journal is cited, in accordance with accepted academic practice. No use, distribution or reproduction is permitted which does not comply with these terms.
*Correspondence: Dayong Liu, ZHlsaXVwZXJpb0B0bXUuZWR1LmNu
†These authors have contributed equally to this work