- 1College of Stomatology, Chongqing Medical University, Chongqing, China
- 2Chongqing Key Laboratory of Oral Diseases and Biomedical Sciences, Chongqing, China
- 3Chongqing Municipal Key Laboratory of Oral Biomedical Engineering of Higher Education, Chongqing, China
Purpose: There is a bidirectional relationship between periodontitis and type 2 diabetes mellitus (T2DM). The aim of this study was to further explore the pathogenesis of this comorbidity, screen out ferroptosis-related genes involved in the pathological process, and predict potential drug targets to develop new therapeutic strategies.
Methods: Common cross-talk genes were identified from periodontitis datasets (GSE16134, GSE10334 and GSE106090) and T2DM databases (DisGeNET and GeneCard). Then, GO and KEGG enrichment analyses, PPI network analysis and hub gene identification were performed. The association between ferroptosis and periodontitis with T2DM was investigated by Pearson correlation analysis. Core ferroptosis-related cross-talk genes were identified and verified by qRT-PCR. Potential drugs targeting these core genes were predicted via DGIDB.
Results: In total, 67 cross-talk genes and two main signalling pathways (immuno-inflammatory pathway and AGE-RAGE signalling pathway) were identified. Pearson correlation analysis indicated that ferroptosis served as a crucial target in the pathological mechanism and treatment of periodontitis with T2DM. IL-1β, IL-6, NFE2L2 and ALOX5 were identified as core ferroptosis-related genes and the qRT-PCR detection results were statistically different. In total, 13 potential drugs were screened out, among which, Echinacea and Ibudilast should be developed first.
Conclusions: This study contributes to a deeper understanding of the common pathogenesis of periodontitis and T2DM and provides new insights into the role of ferroptosis in this comorbidity. In addition, two drugs with potential clinical application value were identified. The potential utility of these drugs requires further experimental investigation.
Introduction
Periodontitis, triggered by microbiota dysbiosis, is an immuno-inflammatory disease of the periodontal tissue (1, 2). It affects approximately 61.9% of the worldwide population, making it one of the most significant lifestyle disorders (3). Type 2 diabetes mellitus (T2DM) is a metabolic disease characterised by hyperglycaemia, insulin resistance and insufficient insulin secretion (4, 5). In 2005, the International Diabetes Federation (IDF) reported that, worldwide, there were about 415 million people aged 20–79 years old living with T2DM, and it was estimated that this number will increase to more than 640 million by 2040 (6, 7). Both periodontitis and T2DM pose serious global public health and financial burdens, especially in developing economies (8–10).
In 2008, the American Diabetes Association (ADA) defined periodontitis as a complication of diabetes. Many epidemiological studies, clinical trials and scientific experiments have been performed in an attempt to elucidate the biological relevance of this relationship (11–13). Several epidemiological studies have provided strong evidence for a direct correlation between periodontitis and T2DM (14, 15). The risk of periodontitis in diabetics is 3-4 times that of patients without T2DM (16–18). Studies have also found increased expression of inflammatory factors in the gingival tissue of diabetic rats, including IL-1β, IL-6, TNF-α and IL-17A, which may be mediated by advanced glycation end products (AGEs) and their ligands (19, 20). On the other hand, periodontitis is not only a complication of diabetes but also a risk factor for it (11, 14). Several reports suggest that periodontitis can have an adverse effect on glycemic control in diabetes (12, 15). It is hypothesised that bacteria and their products in the periodontal pocket can enter the circulatory system through the damaged epithelial barrier, increasing the risk of insulin resistance and diabetic complications (11). Thus, today, scientists and doctors are increasingly using the term “comorbidity” to portray the bidirectional relationship between periodontitis and T2DM. Therefore, it is of great significance to study the interaction mechanism between periodontitis and T2DM to further understand the pathogenesis and treatment of these disorders.
Previous studies have provided preliminary evidence suggesting that the biological link between periodontitis and T2DM overlaps with the immuno-inflammatory response, microbiota dysbiosis and oxidative stress (11, 21, 22). There is growing literature indicating that an overabundance of reactive oxygen species (ROS) plays a role in the establishment of oxidative stress microenvironments that underlie the pathogenesis of many chronic inflammatory diseases, such as cardiovascular diseases, inflammatory bowel disease, periodontitis and T2DM (23–25). ROS can substantially increase the expression levels of proinflammatory factors, resulting in periodontal tissue destruction (26). As one of the main pathogenic factors in T2DM and its complications, the generation of AGEs is also related to ROS (27). Under normal conditions, ROS are beneficial for antimicrobial defence, signal transduction and gene regulation (28, 29). However, excessive ROS cause a series of pathological changes, mainly via lipid peroxidation; these changes include biological macromolecule and cell membrane damage, which is cytotoxic to the host cell and can even lead to cell death (30). Indeed, a variety of programmed cell death processes, such as apoptosis, pyroptosis, necroptosis and ferroptosis, are all related to the development of chronic inflammation (31, 32). Among them, ferroptosis is characterised by a dramatic increase in iron-dependent ROS, lipid peroxidation, a decrease in mitochondria and decreased or inactivated glutathione peroxidase-4 (GPX-4) activity; together, these lead to oxidative stress damage in vivo (33, 34). In the ferroptosis process, the cytoplasm may release a large number of danger signals, such as proinflammatory factors and iron, to the extracellular environment due to the rupture of the plasma membrane (23). Meng et al. reported that HMOX1 upregulation promotes ferroptosis in diabetic atherosclerosis (35). Thus, ferroptosis has become a target for study of the aetiology and treatment of various inflammatory diseases (33–35). Since ferroptosis and periodontitis with T2DM overlap biochemical pathways including ROS overload and lipid peroxidation, it is necessary to explore the role of ferroptosis in the pathogenesis of periodontitis with T2DM. However, to date, there is no published research on the role of ferroptosis in periodontitis with T2DM.
With the development of modern sequencing technology, bioinformatic analysis has allowed for the exploration of interrelationships and pathogenesis connections between diseases based on the use of human samples, rather than animal or cell models (36). This means that more convincing conclusions can be reached. Thus, using bioinformatics analysis and qRT-PCR validation, this study explored essential genes and signalling pathways in periodontitis and T2DM, provided new insights into the biological mechanisms of ferroptosis in periodontitis with T2DM and identified potential new therapeutic target drugs.
Methods and materials
Data collection
The GSE16134, GSE10334 and GSE106090 datasets were extracted from the Gene Expression Omnibus (GEO, https://www.ncbi.nlm.nih.gov/geo/) database. GEO is a public online database that is available to researchers. Each dataset contained both periodontitis samples and control samples. Text mining was performed on the DisGeNET and GeneCard databases to investigate genes related to T2DM.
Identification of differentially expressed genes and cross-talk genes
After normalization and removal of the batch effect using R package inSilicoMerging, GEO2R (http://www.ncbi.nlm.nih.gov/geo/geo2r) was employed to identify DEGs between periodontitis and control samples. DEGs were identified based on P < 0.05 and |log fold change (FC)|≥ 0.5. The search term ‘type 2 diabetes mellitus’ was employed to identify and download all reported genes related to T2DM. To add authenticity, the genes overlapping in the two databases were identified as DEGs for T2DM. The common DEGs were identified as cross-talk genes of periodontitis and T2DM.
Functional and pathway enrichment analysis of cross-talk genes
To comprehensively explore the functional information of these genes, Gene Ontology (GO) and Kyoto Encyclopedia of Genes and Genomes (KEGG) pathway enrichment analyses of the cross-talk genes were performed using DAVID (version 6.8, https://david.ncifcrf.gov/). GO enrichment analysis includes three parts: biological processes (BP), molecular functions (MF) and cellular components (CC). P < 0.05 and a false discovery rate (FDR) < 5% were the cut-off criteria.
Protein-protein interaction network construction, hub gene identification and module analysis
To obtain a PPI network, the cross-talk genes were imported into STRING (http://www.string-db.org/), an online database used for predicting direct and indirect protein-protein functional interactions through correlation analyses. The combined score ≥ 0.4 was chosen for the PPI network construction. Then, the result was visualized using Cytoscape software (version 3.9.1). CytoHubba, a plugin in Cytoscape, was used to identify hub genes. Another plugin in Cytoscape, MCODE, was used to investigate the most significant module in the PPI network.
Correlation analysis between cross-talk genes and ferroptosis-related genes
To explore the specific role of ferroptosis in periodontitis with T2DM, 388 FRGs were downloaded from the FerrDb website (http://www.zhounan.org/ferrdb/), including 186 drivers, 132 suppressors and 113 markers (Supplementary Table S1). The FerrDb website is an online database for identifying the newest genes related to ferroptosis. Then, the correlations between the cross-talk genes and FRGs were assessed using Pearson correlation analysis. The overlapping cross-talk genes and FRGs were defined as ferroptosis-related cross-talk genes (FR-cross-talk genes) for subsequent investigation. Functional and pathway enrichment analysis of FR-cross-talk genes was performed using the method described above.
Receiver operating characteristic curve analysis
To evaluate the sensitivity and specificity of the FR-cross-talk genes for diagnosis, receiver operating characteristic (ROC) curve analysis was conducted using the R package pROC (version 1.17.0.1). The confidence interval was set as 95%. Genes with an area under the ROC curve (AUC) of more than 0.7 were considered significant and were defined as core genes (37, 38).
Experimental validation of core genes by clinical samples
To experimentally validate the core genes, healthy gingival samples were harvested from six individuals without periodontitis or T2DM during crown lengthening surgery, and diseased periodontal samples in deep periodontal pocket were harvested from six individuals with periodontitis and four individuals with periodontitis and T2DM during periodontal flap surgery. Samples were immediately transferred to liquid nitrogen for storage. The diagnostic criteria for T2DM were those established by the ADA in 2021 (39), i.e., random plasma glucose ≥11.1 mmol/L or 2-h plasma glucose ≥11.1 mmol/L during Oral glucose tolerance tests (OGTT) or fasting plasma glucose ≥7.0 mmol/L or random plasma glucose ≥11.1 mmol/L or glycosylated hemoglobin A1C ≥6.5% (39). Periodontitis was diagnosed according to the 2017 World Workshop on the Classification of Periodontal and Peri-Implant Diseases and Conditions criteria (40). The exclusion criteria included the use of immunosuppressive drugs or antibiotics (for more than seven days within the past 30 days) or non-steroidal anti-inflammatory drugs (except daily use of aspirin 75-325 mg); the presence of systemic disease; and excessive alcohol use. All individuals were selected from the Affiliated Hospital of Stomatology, Chongqing Medical University and they had not had periodontal surgery before. Periodontitis or T2DM was diagnosed by qualified doctors. The study was approved by the Ethics Committee of the Affiliated Hospital of Stomatology, Chongqing Medical University (2022059) and complied with the 1964 Helsinki declaration and later amendments and ethics standards.
Quantitative real-time polymerase chain reaction and statistical analysis
After sufficient grinding, total RNA was extracted from the gingiva using RNAiso Plus (Takara, Japan). Next, 5 × PrimeScript RT Master Mix (Takara, Japan) was used for reverse transcription and a TB Green PCR Core Kit (TaKaRa, Japan) was used for PCR amplification. The reaction and detection were conducted on a CFX96TM system (Bio-Rad, USA). Beta-actin was used as the standardized reference gene. The relative mRNA expression levels of each target gene were calculated using the 2-ΔΔCT method. The primer sequences of genes used in the study are listed in Table 1. The qRT-PCR data were analysed by ANOVA using GraphPad Prism software (version 6.0, USA). A p-value <0.05 was considered statistically significant. Radar chart was used to visualize different genes expression levels between groups.
Potential drugs prediction
The drug-gene interaction database (DGIDB, http://www.dgidb.org) is a free database that provides interaction information about drugs and genes of interest. In this study, DGIDB was used to screen potential drugs targeting core genes in order to identify new therapeutic targets. A query score >1 was set as the cut-off criterion (41, 42).
Results
Flowchart of the study and identification of cross-talk genes
A detailed flowchart of the study is presented in Figure 1A. In brief, a total of 1117 DEGs for periodontitis, 733 DEGs for T2DM and 67 cross-talk genes were identified through data mining and processing (Figures 1B-E). The genes were listed in Supplementary Table S2.
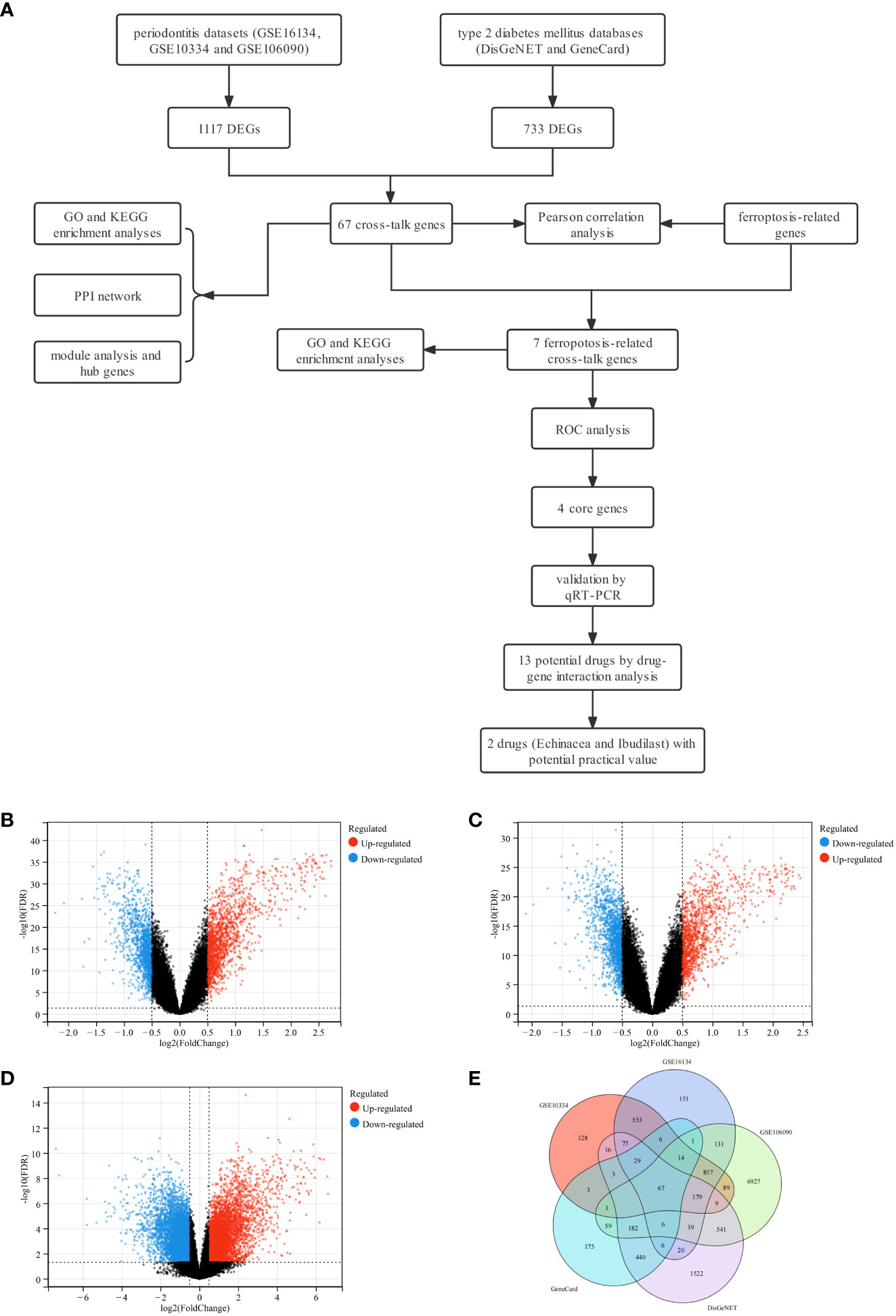
Figure 1 Study design flowchart, volcano diagram and venn diagram. (A) The detailed flowchart of the study. (B) The volcano map of GSE16134. (C) The volcano map of GSE10334. (D) The volcano map of GSE106090. Upregulated genes are marked in red; downregulated genes are marked in blue. (E) Venn diagram of cross-talk genes from three datasets and two databases.
Functional and pathway enrichment analysis of cross-talk genes
For GO analysis, changes in BP included significant enrichment of several immuno-inflammatory processes, such as the cytokine-mediated signalling pathway, inflammatory response, signal transduction and immune response (Figure 2A). Changes in CC included significant enrichment of the basic structure of the cell, such as extracellular space, extracellular region, plasma membrane and cytoplasm (Figure 2B). Changes in MF included significant enrichment of binding-related functions, such as protein binding, identical protein binding, zinc ion binding and integrin binding (Figure 2C). For KEGG analysis, as expected, the cross-talk genes were enriched in several ferroptosis-related signalling pathways, such as the PI3K-Akt signalling pathway and MAPK signalling pathway; these are also two of the most prominent signalling pathways implicated in human inflammatory diseases (31–34). The cross-talk genes were also enriched in other pathways, including the AGE-RAGE signalling pathway in diabetic complications, TNF signalling pathway and NOD-like receptor signalling pathway (Figure 2D).
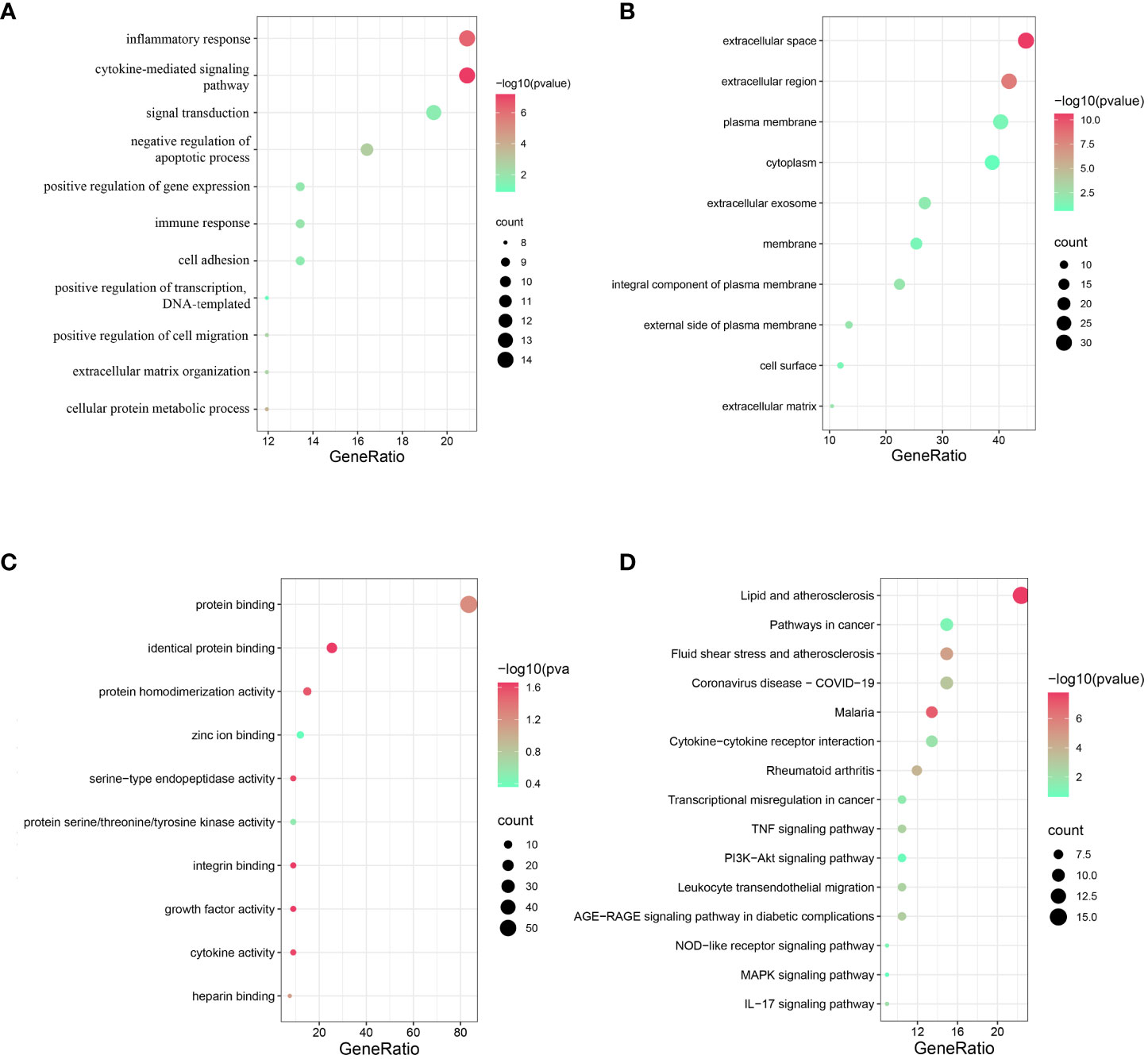
Figure 2 Functional and pathway enrichment analysis of cross-talk genes. (A) Top 10 BP terms. (B) Top 10 CC terms. (C) Top 10 MF terms. (D) Top 15 KEGG terms. P < 0.05 was considered significant.
PPI network, hub gene identification and module analysis
The 67 cross-talk genes from the above analysis were all uploaded to the STRING database to build a PPI network. The network contained 62 nodes and 336 edges (Figure 3A). Based on the MCODE scores, 10 hub genes (IL6, IL1B, MMP9, APOE, CXCL8, VCAM1, CCL5, CXCL12, PECAM1 and TIMP1) were screened out (Figure 3B). Next, the most significant modules were extracted, of which, module 1 contained 19 nodes and 151 edges (Figure 3C), and module 2 contained 4 nodes and 5 edges (Figure 3D). Both network and significant modules indicated that periodontitis and T2DM share some common potential molecular mechanisms.
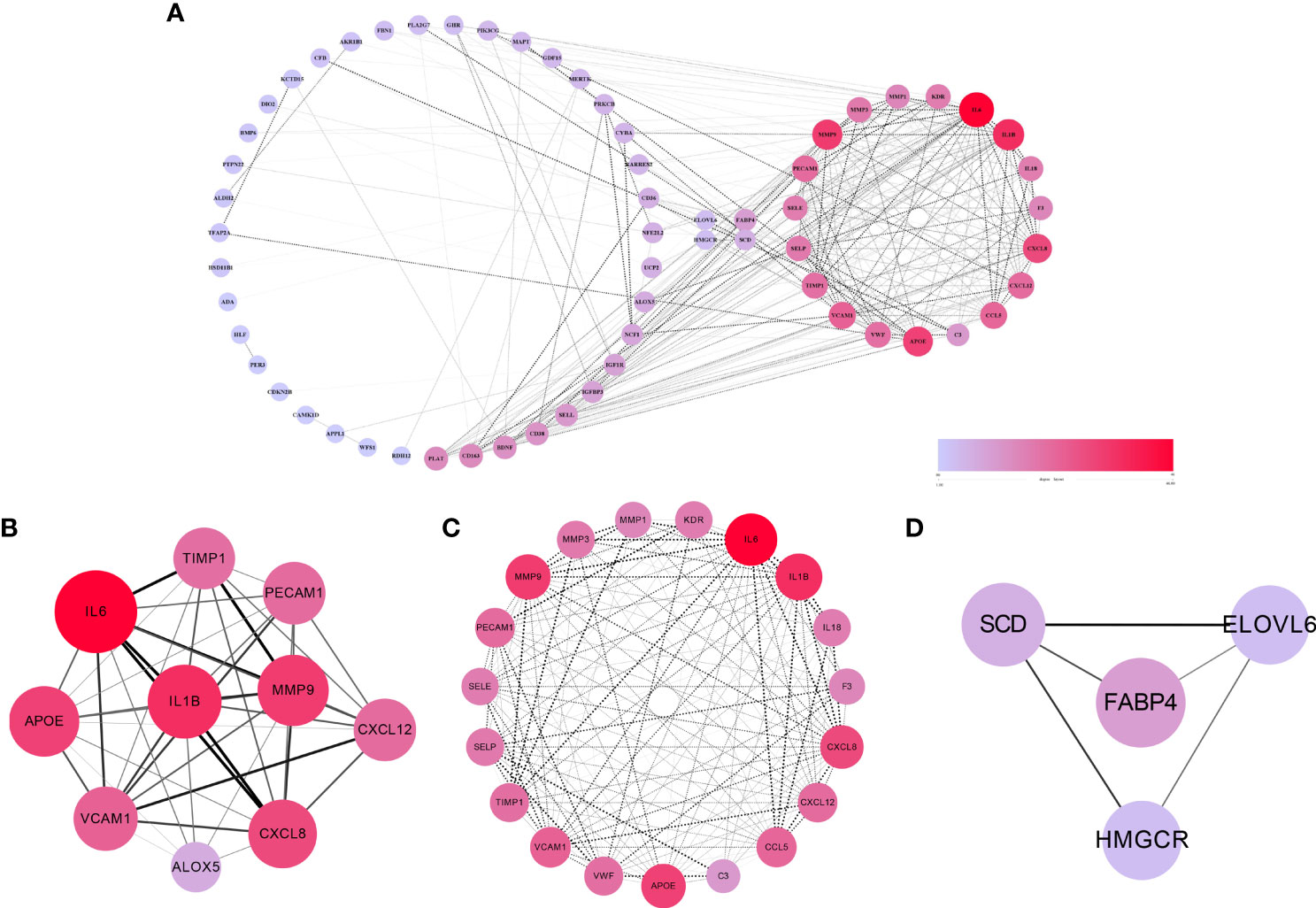
Figure 3 The results of PPI network, hub genes and significant modules. (A) PPI network of cross-talk genes. (B) Top 10 hub genes. (C) Significant module 1 (contained 19 nodes and 151 edges). (D) Significant module 2 (contained 4 nodes and 5 edges).
Correlation analysis between cross-talk genes and FRGs
After batch correction and normalization, a periodontitis dataset was obtained and correlation analysis between cross-talk genes and FRGs was carried out. The Pearson analysis results showed that all the correlation coefficients were statistically significant (P < 0.05) and most of the cross-talk genes had large correlation coefficient sums (Figure 4; Supplementary Table S3), indicating that ferroptosis may play a role in the pathological process of periodontitis with T2DM and may be a therapeutic target for future research. Then, a Venn diagram (Figure 5A) was used to screen out seven FR-cross-talk genes (IL-1β, IL-6, ALOX5, NFE2L2, GDF15, SCD and TFAP2A); the sums of their correlation coefficients were 29.71, 19.87, 30.09, 29.52, 8.77, 13.18 and 16.41, respectively (Supplementary Table S3).
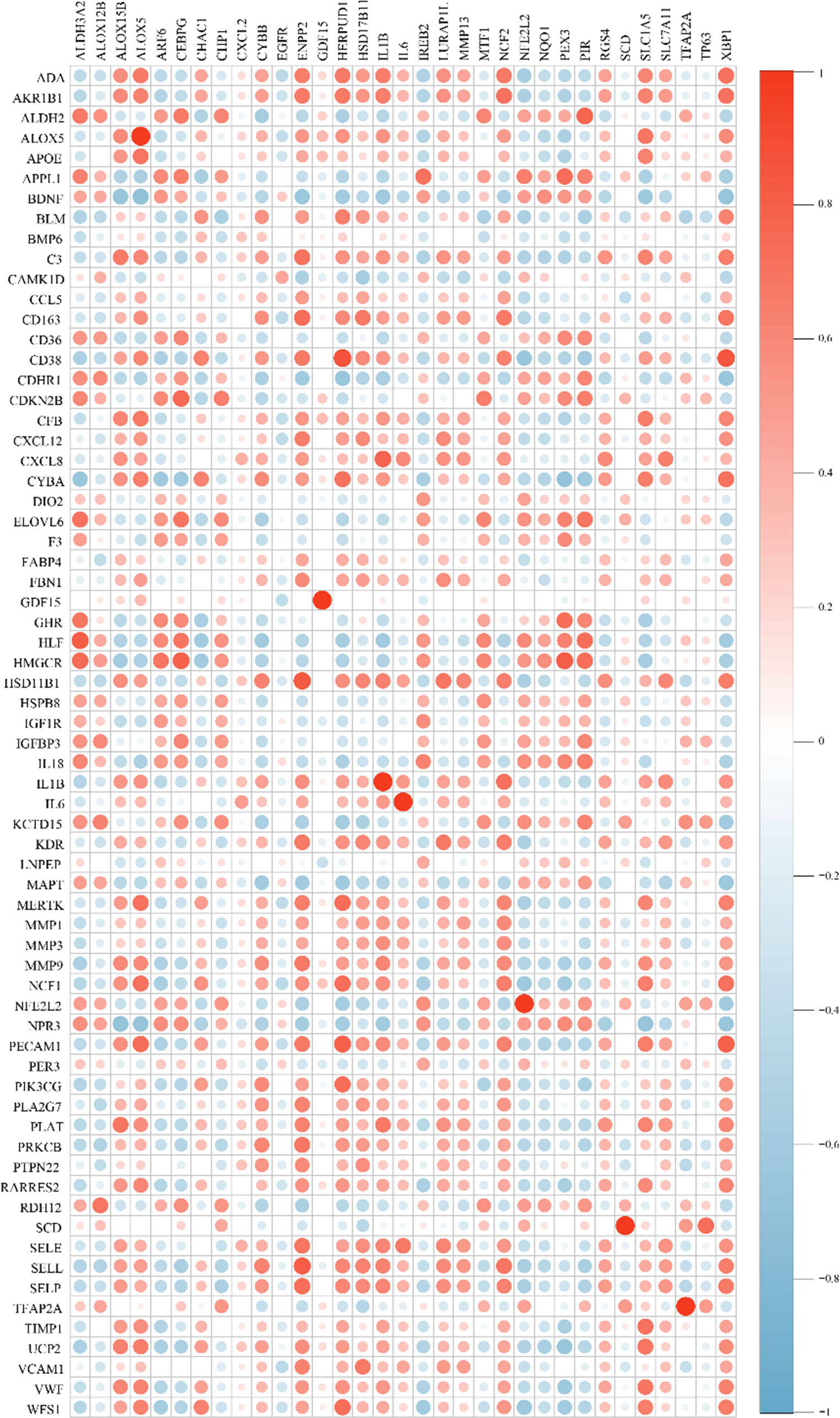
Figure 4 Correlation analysis between cross-talk genes and ferroptosis-related genes. Genes in the vertical line are cross-talk genes and genes in in the horizontal line are FRGs. Red indicates a positive correlation, blue indicates a negative correlation. The size of circle designates the correlation coefficient between cross-talk genes and ferroptosis-related genes.
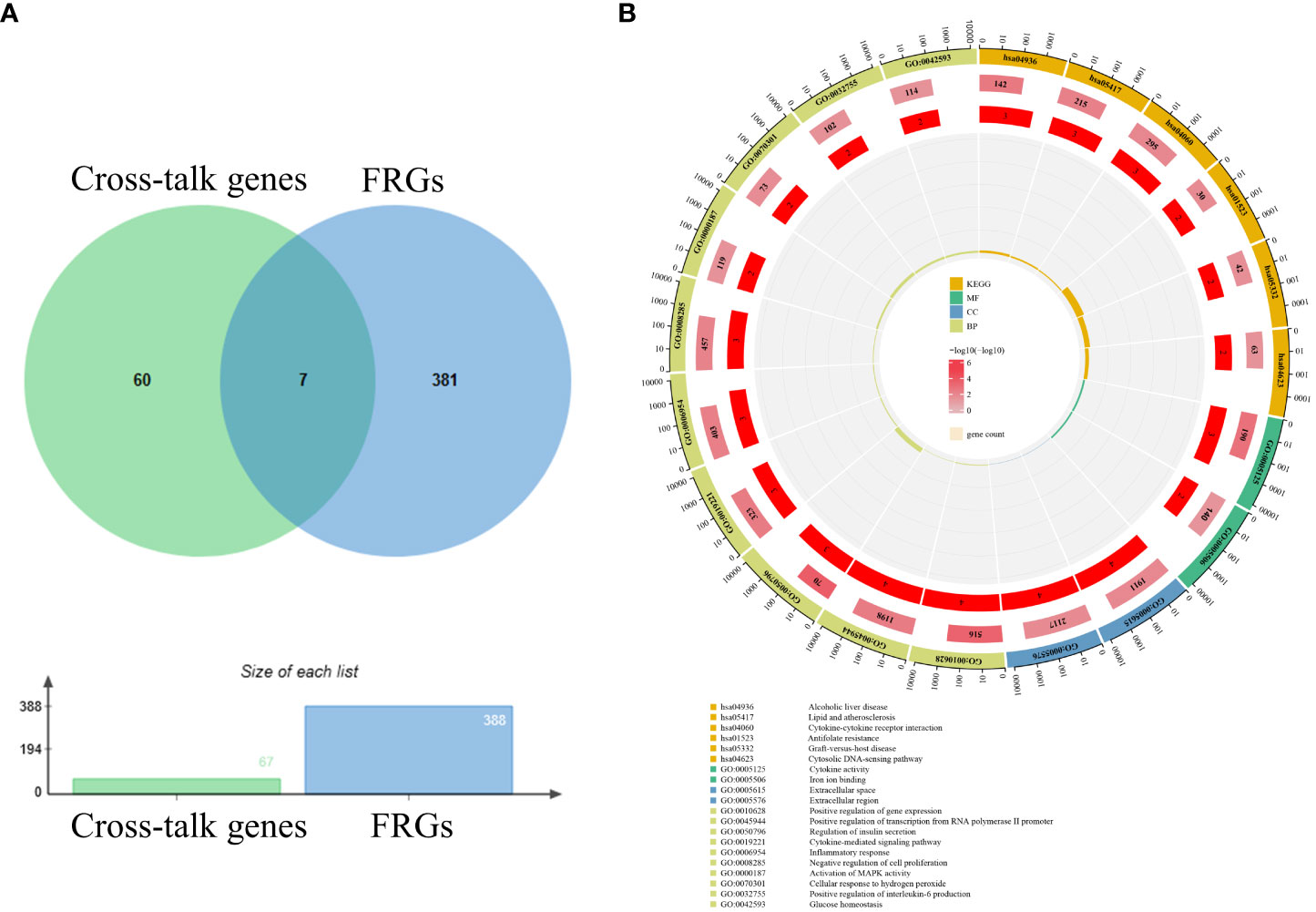
Figure 5 Venn diagram and enrichment analysis of FR-cross-talk genes. (A) Seven FR-cross-talk genes were identified by venn diagram. (B)The GO and KEGG enrichment analysis of FR-cross-talk genes.
Functional and pathway enrichment analysis of FR-cross-talk genes
To explore the biological functions and pathways related to the FR-cross-talk genes, GO and KEGG analyses were performed (Figure 5B). The GO analysis results revealed that the FR-cross-talk genes were significantly enriched in i) regulation of insulin secretion, cytokine-mediated signalling pathway, inflammatory response, cellular response to hydrogen peroxide, positive regulation of interleukin-6 production and glucose homeostasis (BP); ii) extracellular space and extracellular region (CC); and iii) iron ion binding and cytokine activity (MF). The KEGG analysis results revealed that cytokine-cytokine receptor interaction, antifolate resistance and the cytosolic DNA-sensing pathway were significantly enriched. The above terms are related to iron metabolism, glucose metabolism, inflammation and oxidative stress, and some are consistent with the enrichment analysis results of the cross-talk genes.
ROC curve analysis
As illustrated in Figure 6. the AUCs of IL-1β, IL-6, ALOX5 and NFE2L2 were 0.82, 0.73, 0.80 and 0.83, respectively. The four genes were considered to be of high accuracy in diagnosing periodontitis with T2DM and were defined as core genes (Supplementary Table S4). On the contrary, GDF15, SCD and TFAP2A had lower sensitivity in predicting periodontitis with T2DM, with AUCs of 0.54, 0.60 and 0.66, respectively.
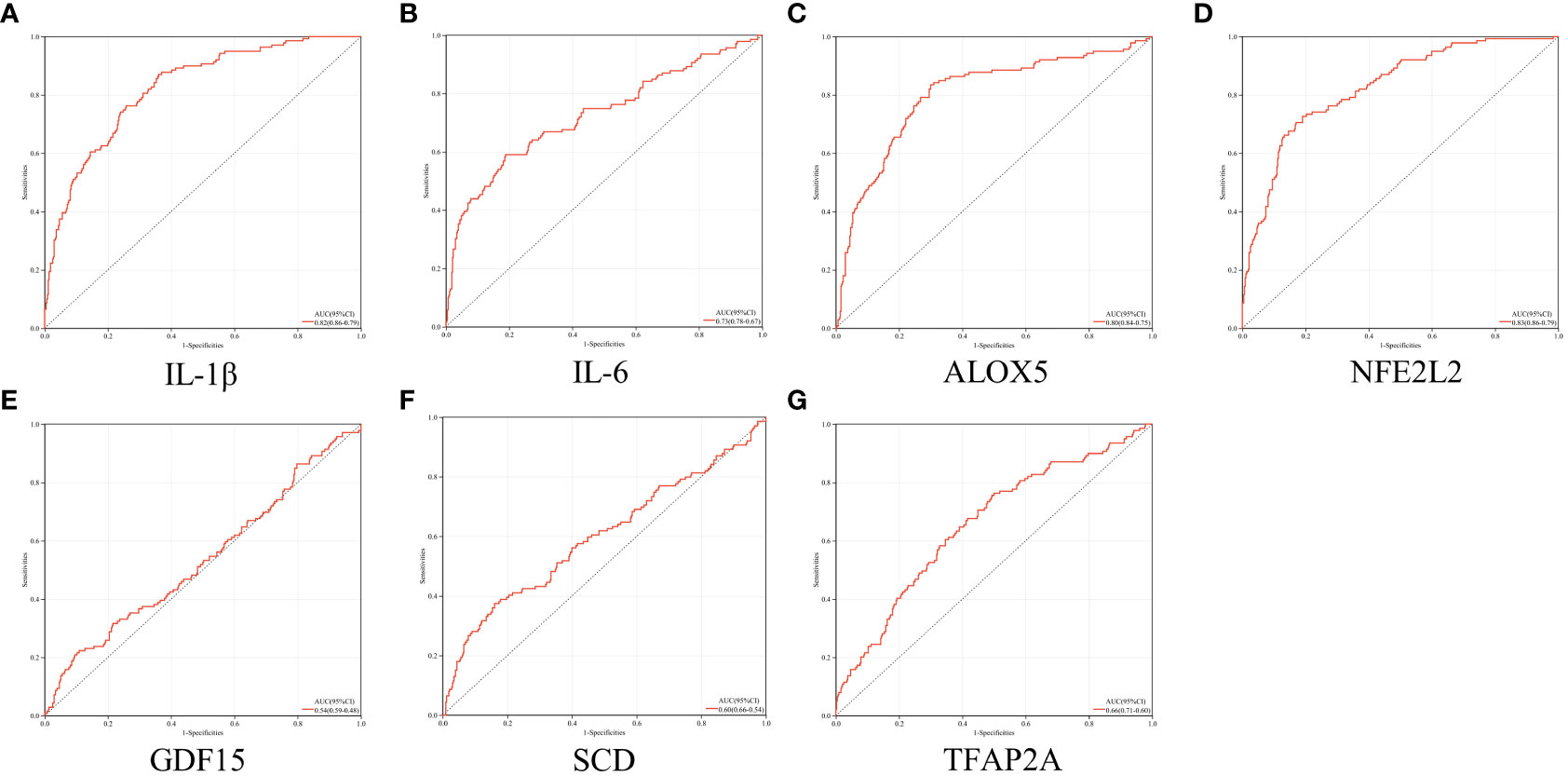
Figure 6 ROC analysis. (A–G) ROC analysis results of IL-1β, IL-6, ALOX5, NFE2L2, GDF15, SCD and TFAP2A, respectively.
Validation of core gene expression by qRT-PCR
To further increase our confidence in the findings, qRT-PCR was used to detect the expression of the core genes in the different sample groups (Figure 7; Supplementary Table S5). Compared with the control group, the mRNA expression levels of IL-1β, IL-6 and ALOX5 were increased in the periodontitis group and further increased in the periodontitis with T2DM group. The expression levels of IL-1β and IL-6 were higher than the other two genes in the periodontitis group and periodontitis with T2DM group. The mRNA expression level of NFE2L2 was decreased in the periodontitis group and further decreased in the periodontitis with T2DM group. The results indicate that periodontitis can lead to inflammatory and oxidative stress damage, and that T2DM can play a synergistic role.
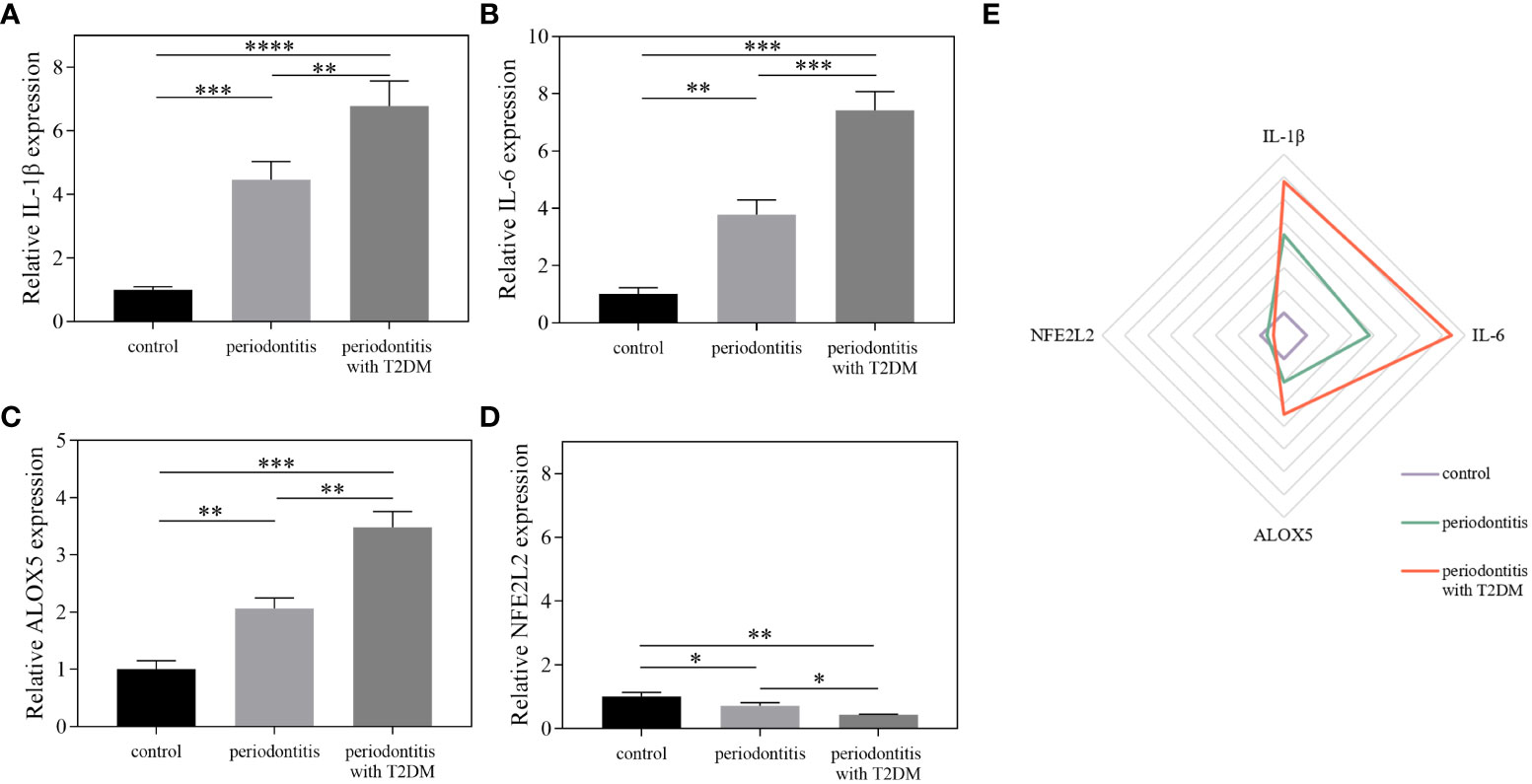
Figure 7 The results of qRT-PCR. (A–D) The relative expression levels of IL-1β, IL-6, ALOX5 and NFE2L2 between different groups using qRT-PCR. (E) Differentially expressed factors were shown as a Radar Chart. The qRT-PCR data were analysed by ANOVA. A p-value < 0.05 was considered statistically significant. All data were presented as the mean ± SD. *P < 0.05, **P < 0.01, ***P < 0.001, ****P < 0.0001.
Drug-gene interaction analysis
To predict drugs with potential therapeutic effects for periodontitis with T2DM, the five core genes were introduced into DGIDB. In total, 37, 25, 821 and 25 target drugs were obtained for IL-1β, IL-6, NFE2L2 and ALOX5, respectively (Supplementary Table S6). Most of the drugs were anticancer, inhibitor, antibody or antagonist drugs. Drugs that target more than one gene were considered more credible and effective (Table 2). Some of them, including Methotrexate, Melatonin, Resveratrol, Diacerein, Lithium and Infliximab, have been used to treat periodontitis or T2DM in animal experiments (43–47, 49), while the remaining drugs (Hydroquinone, Echinacea, Hydrocortisone, Cisplatin, Omeprazole, Ibudilast, Lansoprazole) have not been found to have therapeutic effects in periodontitis with T2DM. Notably, Cisplatin, a metal-based anticancer agent, has been reported to aggravate periodontitis (48).
Discussion
Periodontitis and T2DM are global epidemic diseases that involve multiple genes and signalling pathways (1, 5). Clinically, periodontitis and T2DM are risk factors for each other, showing a bidirectional link, but the underlying mechanisms responsible for the link are not fully understood. Oxidative stress plays an important role in the pathogenesis and therapeutic targets of periodontitis and T2DM (22, 50, 51). Moreover, ferroptosis can cause oxidative damage and oxidative stress imbalance in vivo. At present, no study has examined the role of ferroptosis in periodontitis with T2DM. Thus, in this study, we attempted to investigate the association and mechanism between the two diseases, explore the role of ferroptosis-related genes in periodontitis with T2DM, and search for potential effective therapeutic target drugs using bioinformatics analysis combined with experimental validation.
In the present study, 67 cross-talk genes that were overlapping in periodontitis and T2DM were identified, including 10 hub genes (IL-6, IL-1B, MMP9, APOE, CXCL8, VCAM1, CCL5, CXCL12, PECAM1 and TIMP1). Functional and pathway enrichment analyses demonstrated that these cross-talk genes were mainly enriched in immuno-inflammatory pathways, such as cytokine-cytokine receptor interaction, TNF signalling pathway, PI3K-Akt signalling pathway, leukocyte transendothelial migration, IL-17 signalling pathway, immune response and cytokine activity. At present, periodontitis and T2DM are considered to be chronic inflammatory diseases characterized by the production of a large number of proinflammatory factors (11, 20, 22). In periodontitis, increased expression levels of proinflammatory factors stimulate osteoclast activity by altering the RANKL/osteoproteinin (OPG) ratio, leading to progressive alveolar bone resorption (52). At the same time, periodontitis contributes to low-grade systemic inflammation by remote transfer of local bacteria, bacterial products and proinflammatory mediators (21), which can worsen insulin resistance and dysglycemia, fuelling the development of T2DM (11). On the other hand, T2DM upregulates the expression of proinflammatory factors in local periodontal tissues and the circulatory system (22, 51). Higher levels of IL-17, PGE2, IL-23 and interferon-γ have been found in the gingival crevicular fluid (GCF) of patients with periodontitis with T2DM, compared to systematically healthy patients with periodontitis (19, 20). Importantly, the above processes interact and form a vicious cycle (11, 53, 54). According to the KEGG results of this study, the AGE-RAGE signalling pathway is also closely associated with both periodontitis and T2DM. AGEs are formed by irreversible glycosylation and oxidation of proteins, nucleic acids and lipids caused by hyperglycemia (55). The combination of AGEs and receptor of AGEs (RAGEs) increases the expression of proinflammatory cytokines (such as IL-6, TNF-α, IL-1β) to promote periodontal inflammation (55, 56). With the activation of RAGEs and other receptors, nuclear factor-Kappa B is also activated, which leads to an increase in ROS and oxidative stress damage (56). The accumulation of AGEs in alveolar bone interferes with bone remodelling and regeneration by disrupting the normal physiological function of the RANKL/OPG axis (57).
It is well-accepted that oxidative stress plays a critical role in the pathophysiological process of periodontitis and T2DM, whereas ferroptosis is closely related to the establishment of an oxidative stress microenvironment characterized by ROS production and lipid peroxidation in inflammatory diseases (23–25). FRGs may play a driving, suppressing and/or marking role in ferroptosis (58). Cross-talk genes are common DEGs in periodontitis and T2DM. The GO and KEGG enrichment analyses illustrated that FRGs and cross-talk genes jointly regulate the biological processes related to oxidative stress, iron metabolism, glucose metabolism and inflammation, suggesting a potential association between ferroptosis and periodontitis with T2DM. Additionally, we further investigated the link between ferroptosis and comorbid periodontitis and T2DM by performing Pearson correlation analysis between the FRGs and cross-talk genes. The results showed that most of the cross-talk genes were highly correlated with the FRGs, indicating that ferroptosis is an important target for studying the aetiology and treatment of periodontitis with T2DM. Then, we identified four core genes (IL-1β, IL-6, NFE2L2 and ALOX5) from the ferroptosis-related cross-talk genes by ROC curve analysis. The AUCs of these four genes were all greater than 0.7, suggesting that they have clinical value for diagnosing and monitoring periodontitis with T2DM. Furthermore, we collected clinical samples from different patient populations (control group, periodontitis group and periodontitis with T2DM group) and conducted qRT-PCR to verify the above results. The qRT-PCR results revealed upregulation of IL-1β, IL-6 and ALOX5 in the periodontitis group and further upregulation in the periodontitis with T2DM group, while the expression of NFE2L2 was the opposite. This is consistent with the ROC analysis results. Both IL-1β and IL-6 are crucial mediators of the inflammatory response, and the expression levels of IL-1β and IL-6 can be downregulated by inhibiting ferroptosis (59). Nuclear factor erythroid-derived 2-like 2 (NFE2L2), also known as Nrf2, encodes a transcription factor that binds to protective genes with antioxidant elements in the promoter region to rescue oxidative damage (58). Cui et al. reported that RSL3 induces Nrf2 expression to protect cells from ferroptosis in lipopolysaccharide-induced inflammation (60). Arachidonate 5-lipoxygenase (ALOX5) encodes a protein that is a member of the lipid oxidase family and catalyses the oxidation of arachidonic acid to produce leukotriene (61). According to previous studies, ALOX5 plays a crucial role in cell death, including apoptosis, pyroptosis, necroptosis and ferroptosis. Upregulation of ALOX5 induces ferroptosis through the accumulation of leukotriene and lipid peroxidation, which exacerbates the inflammatory response (61, 62).
Based on the results, 13 drugs with effective therapeutic effects or priority for development were screened out. Aside from Cisplatin, which aggravates periodontitis, Methotrexate, Melatonin, Resveratrol, Diacerein, Lithium and Infliximab have been reported to have therapeutic effects on periodontitis or T2DM via different pathways in animal models (43–49). The remaining six drugs (Hydroquinone, Echinacea, Hydrocortisone, Omeprazole, Ibudilast and Lansoprazole) have not been reported in the treatment of periodontitis with T2DM. Among them, Echinacea and Ibudilast deserve our attention. Echinacea, a herb belonging to the family Asteraceae, is a traditional medicine with antibacterial, antioxidant and immunomodulatory activities (63). Its main chemical components are polysaccharides, volatile terpenes, caffeic acid derivatives, alkylamides, polyphenols and alkaloids, and these components may regulate the MAPK signalling pathway, JNK signalling pathway and Nrf2/HO-1 signalling pathway (64). Because of its excellent pharmacological activities, Echinacea has been used in the clinical treatment of the common cold, respiratory infections, conjunctivitis and some cancers, and has been shown to be safe (63, 64). Ibudilast is a safe orally-available phosphodiesterase 4 inhibitor that has been used in clinical practice for over 20 years. It inhibits the production of proinflammatory factors and the Th17 response in vivo to exert anti-inflammatory effects (65, 66). Ibudilast can be used to treat acute kidney injury and rheumatoid arthritis in animal models (67). These drugs are potential new target drugs for the treatment of periodontitis with T2DM; however, more animal model and clinical cohort studies will be needed to verify their use.
Although previous studies have separately explored the role of ferroptosis in periodontitis and T2DM through bioinformatics analysis (68–70), few studies have reported the potential relationship between ferroptosis and periodontitis with T2DM. This study might provide new insights into the biological mechanisms of ferroptosis in periodontitis with T2DM. Moreover, potential targeted drugs were predicted. However, there are some limitations to the present study. Firstly, since this study is based on existing public datasets, the results are largely exploratory and should be treated with caution. Secondly, the FRGs are derived from FerrDb, a continuously updated website, and more related genes are yet to be discovered. Moreover, the four ferroptosis-related core genes (IL-1β, IL-6, NFE2L2 and ALOX5) identified in this study are also involved in other pathways. Therefore, future studies are needed to explore the specific role of these four genes in ferroptosis.
Conclusion
In conclusion, through bioinformatics analysis and qRT-PCR validation, we identified common hub genes and signalling pathways involved in periodontitis and T2DM and demonstrated a strong association between ferroptosis and this comorbidity. In addition, four ferroptosis-related core genes (IL-1β, IL-6, NFE2L2 and ALOX5) were identified and verified by qRT-PCR. These may serve as potential biomarkers for the clinical diagnosis and treatment of periodontitis with T2DM. Meanwhile, 13 drugs targeting these core genes were screened, among which, Echinacea and Ibudilast appear to be of potential practical value in the treatment of periodontitis with T2DM. This study provides novel insights into the pathogenesis and treatment of periodontitis with T2DM. The findings of this study should be validated in future clinical and experimental studies.
Data availability statement
The original contributions presented in the study are included in the article/Supplementary Material. Further inquiries can be directed to the corresponding authors.
Ethics statement
The studies involving human participants were reviewed and approved by Ethics Committee of the College of Stomatology, Chongqing Medical University (2022059). The patients/participants provided their written informed consent to participate in this study.
Author contributions
JSong and SP developed and designed the major study plan. SP and BH analyzed the data, interpreted the data and draw charts. JSun and ZY collected the literature and reference. ZH and WY helped checked the data. SP wrote the first draft of the paper. JSong and XG supervised the study. All authors contributed to the article and approved the submitted version.
Funding
This study was supported by the National Natural Science Foundation of China (31600788), the Chongqing Research Program of Basic Research and Frontier Technology (cstc2015jcyjA10035), the Postgraduate Students’ Innovative Research and Development Projects of Chongqing (CYB19158), 2019 Chongqing Graduate Tutor Team Construction Project (dstd201903).
Conflict of interest
The authors declare that the research was conducted in the absence of any commercial or financial relationships that could be construed as a potential conflict of interest.
Publisher’s note
All claims expressed in this article are solely those of the authors and do not necessarily represent those of their affiliated organizations, or those of the publisher, the editors and the reviewers. Any product that may be evaluated in this article, or claim that may be made by its manufacturer, is not guaranteed or endorsed by the publisher.
Supplementary material
The Supplementary Material for this article can be found online at: https://www.frontiersin.org/articles/10.3389/fimmu.2022.1015491/full#supplementary-material
References
1. Kinane DF, Stathopoulou PG, Papapanou PN. Periodontal diseases. Nat Rev Dis Primers (2017) 3:17038. doi: 10.1038/nrdp.2017.38
2. Pihlstrom BL, Michalowicz BS, Johnson NW. Periodontal diseases. Lancet (2005) 366(9499):1809–20. doi: 10.1016/s0140-6736(05)67728-8
3. Ortigara GB, Mário Ferreira TG, Tatsch KF, Romito GA, Ardenghi TM, Sfreddo CS, et al. The 2018 EFP/AAP periodontitis case classification demonstrates high agreement with the 2012 CDC/AAP criteria. J Clin Periodontol (2021) 48(7):886–95. doi: 10.1111/jcpe.13462
4. Zheng Y, Ley SH, Hu FB. Global aetiology and epidemiology of type 2 diabetes mellitus and its complications. Nat Rev Endocrinol (2018) 14(2):88–98. doi: 10.1038/nrendo.2017.151
5. Zimmet PZ, Magliano DJ, Herman WH, Shaw JE. Diabetes: a 21st century challenge. Lancet Diabetes Endocrinol (2014) 2(1):56–64. doi: 10.1016/s2213-8587(13)70112-8
6. Ogurtsova K, da Rocha Fernandes JD, Huang Y, Linnenkamp U, Guariguata L, Cho NH, et al. IDF diabetes atlas: Global estimates for the prevalence of diabetes for 2015 and 2040. Diabetes Res Clin Pract (2017) 128:40–50. doi: 10.1016/j.diabres.2017.03.024
7. Chen L, Magliano DJ, Zimmet PZ. The worldwide epidemiology of type 2 diabetes mellitus–present and future perspectives. Nat Rev Endocrinol (2011) 8(4):228–36. doi: 10.1038/nrendo.2011.183
8. Lovic D, Piperidou A, Zografou I, Grassos H, Pittaras A, Manolis A. The growing epidemic of diabetes mellitus. Curr Vasc Pharmacol (2020) 18(2):104–9. doi: 10.2174/1570161117666190405165911
9. Kassebaum NJ, Bernabé E, Dahiya M, Bhandari B, Murray CJ, Marcenes W. Global burden of severe periodontitis in 1990-2010: A systematic review and meta-regression. J Dent Res (2014) 93(11):1045–53. doi: 10.1177/0022034514552491
10. Jin LJ, Lamster IB, Greenspan JS, Pitts NB, Scully C, Warnakulasuriya S. Global burden of oral diseases: emerging concepts, management and interplay with systemic health. Oral Dis (2016) 22(7):609–19. doi: 10.1111/odi.12428
11. Lalla E, Papapanou PN. Diabetes mellitus and periodontitis: a tale of two common interrelated diseases. Nat Rev Endocrinol (2011) 7(12):738–48. doi: 10.1038/nrendo.2011.106
12. Taylor GW, Burt BA, Becker MP, Genco RJ, Shlossman M, Knowler WC, et al. Severe periodontitis and risk for poor glycemic control in patients with non-insulin-dependent diabetes mellitus. J Periodontol (1996) 67(10 Suppl):1085–93. doi: 10.1902/jop.1996.67.10s.1085
13. Genco RJ, Borgnakke WS. Diabetes as a potential risk for periodontitis: association studies. Periodontol 2000 (2020) 83(1):40–5. doi: 10.1111/prd.12270
14. Borgnakke WS, Ylöstalo PV, Taylor GW, Genco RJ. Effect of periodontal disease on diabetes: systematic review of epidemiologic observational evidence. J Clin Periodontol (2013) 40 Suppl 14:S135–52. doi: 10.1111/jcpe.12080
15. Demmer RT, Jacobs DR Jr., Desvarieux M. Periodontal disease and incident type 2 diabetes: Results from the first national health and nutrition examination survey and its epidemiologic follow-up study. Diabetes Care (2008) 31(7):1373–9. doi: 10.2337/dc08-0026
16. Watanabe K. Periodontitis in diabetics: is collaboration between physicians and dentists needed? Dis Mon (2011) 57(4):206–13. doi: 10.1016/j.disamonth.2011.03.007
17. Borgnakke WS, Ylöstalo PV, Taylor GW, Genco RJ. Effect of periodontal disease on diabetes: Systematic review of epidemiologic observational evidence. J Periodontol (2013) 84(4 Suppl):S135–52. doi: 10.1902/jop.2013.1340013
18. Artese HP, Foz AM, Rabelo Mde S, Gomes GH, Orlandi M, Suvan J, et al. Periodontal therapy and systemic inflammation in type 2 diabetes mellitus: a meta-analysis. PloS One (2015) 10(5):e0128344. doi: 10.1371/journal.pone.0128344
19. Salvi GE, Beck JD, Offenbacher S. PGE2, IL-1 beta, and TNF-alpha responses in diabetics as modifiers of periodontal disease expression. Ann Periodontol (1998) 3(1):40–50. doi: 10.1902/annals.1998.3.1.40
20. Salvi GE, Yalda B, Collins JG, Jones BH, Smith FW, Arnold RR, et al. Inflammatory mediator response as a potential risk marker for periodontal diseases in insulin-dependent diabetes mellitus patients. J Periodontol (1997) 68(2):127–35. doi: 10.1902/jop.1997.68.2.127
21. Kumar M, Mishra L, Mohanty R, Nayak R. "Diabetes and gum disease: the diabolic duo". Diabetes Metab Syndr (2014) 8(4):255–8. doi: 10.1016/j.dsx.2014.09.022
22. Polak D, Sanui T, Nishimura F, Shapira L. Diabetes as a risk factor for periodontal disease-plausible mechanisms. Periodontol 2000 (2020) 83(1):46–58. doi: 10.1111/prd.12298
23. Mao H, Zhao Y, Li H, Lei L. Ferroptosis as an emerging target in inflammatory diseases. Prog Biophys Mol Biol (2020) 155:20–8. doi: 10.1016/j.pbiomolbio.2020.04.001
24. Li D, Jiang C, Mei G, Zhao Y, Chen L, Liu J, et al. Quercetin alleviates ferroptosis of pancreatic β cells in type 2 diabetes. Nutrients (2020) 12(10):2954. doi: 10.3390/nu12102954
25. Yu Y, Yan Y, Niu F, Wang Y, Chen X, Su G, et al. Ferroptosis: A cell death connecting oxidative stress, inflammation and cardiovascular diseases. Cell Death Discovery (2021) 7(1):193. doi: 10.1038/s41420-021-00579-w
26. Sczepanik FSC, Grossi ML, Casati M, Goldberg M, Glogauer M, Fine N, et al. Periodontitis is an inflammatory disease of oxidative stress: We should treat it that way. Periodontol 2000 (2020) 84(1):45–68. doi: 10.1111/prd.12342
27. Giacco F, Brownlee M. Oxidative stress and diabetic complications. Circ Res (2010) 107(9):1058–70. doi: 10.1161/circresaha.110.223545
28. Valko M, Leibfritz D, Moncol J, Cronin MT, Mazur M, Telser J. Free radicals and antioxidants in normal physiological functions and human disease. Int J Biochem Cell Biol (2007) 39(1):44–84. doi: 10.1016/j.biocel.2006.07.001
29. Yang B, Chen Y, Shi J. Reactive oxygen species (ROS)-based nanomedicine. Chem Rev (2019) 119(8):4881–985. doi: 10.1021/acs.chemrev.8b00626
30. Zhang J, Wang X, Vikash V, Ye Q, Wu D, Liu Y, et al. ROS and ROS-mediated cellular signaling. Oxid Med Cell Longev (2016) 2016:4350965. doi: 10.1155/2016/4350965
31. Khan I, Yousif A, Chesnokov M, Hong L, Chefetz I. A decade of cell death studies: Breathing new life into necroptosis. Pharmacol Ther (2021) 220:107717. doi: 10.1016/j.pharmthera.2020.107717
32. Christgen S, Tweedell RE, Kanneganti TD. Programming inflammatory cell death for therapy. Pharmacol Ther (2022) 232:108010. doi: 10.1016/j.pharmthera.2021.108010
33. Tang D, Chen X, Kang R, Kroemer G. Ferroptosis: Molecular mechanisms and health implications. Cell Res (2021) 31(2):107–25. doi: 10.1038/s41422-020-00441-1
34. Chen X, Kang R, Kroemer G, Tang D. Ferroptosis in infection, inflammation, and immunity. J Exp Med (2021) 218(6):e20210518. doi: 10.1084/jem.20210518
35. Meng Z, Liang H, Zhao J, Gao J, Liu C, Ma X, et al. HMOX1 upregulation promotes ferroptosis in diabetic atherosclerosis. Life Sci (2021) 284:119935. doi: 10.1016/j.lfs.2021.119935
36. Chen C, Hou J, Tanner JJ, Cheng J. Bioinformatics methods for mass spectrometry-based proteomics data analysis. Int J Mol Sci (2020) 21(8):2873. doi: 10.3390/ijms21082873
37. Li Z, Huang B, Yi W, Wang F, Wei S, Yan H, et al. Identification of potential early diagnostic biomarkers of sepsis. J Inflammation Res (2021) 14:621–31. doi: 10.2147/jir.S298604
38. Nordqvist SF, Boesen VB, Rasmussen >ÅK, Feldt-Rasmussen U, Hegedüs L, Bonnema SJ, et al. Determining minimal important change for the thyroid-related quality of life questionnaire ThyPRO. Endocr Connect (2021) 10(3):316–24. doi: 10.1530/ec-21-0026
39. American Diabetes Association. 2. Classification and diagnosis of diabetes: Standards of medical care in diabetes-2021. Diabetes care 44(Suppl 1):S15–33. doi: 10.2337/dc21-S002
40. Tonetti MS, Greenwell H, Kornman KS. Staging and grading of periodontitis: Framework and proposal of a new classification and case definition. J Periodontol (2018) 89 Suppl 1:S159–s172. doi: 10.1002/jper.18-0006
41. Freshour SL, Kiwala S, Cotto KC, Coffman AC, McMichael JF, Song JJ, et al. Integration of the drug-gene interaction database (DGIdb 4.0) with open crowdsource efforts. Nucleic Acids Res (2021) 49(D1):D1144–d1151. doi: 10.1093/nar/gkaa1084
42. Chen Z, Zhong Z, Zhang W, Su G, Yang P. Integrated analysis of key pathways and drug targets associated with vogt-Koyanagi-Harada disease. Front Immunol (2020) 11:587443. doi: 10.3389/fimmu.2020.587443
43. Lübcke PM, Ebbers MNB, Volzke J, Bull J, Kneitz S, Engelmann R, et al. Periodontal treatment prevents arthritis in mice and methotrexate ameliorates periodontal bone loss. Sci Rep (2019) 9(1):8128. doi: 10.1038/s41598-019-44512-9
44. Kose O, Kurt Bayrakdar S, Unver B, Altin A, Akyildiz K, Mercantepe T, et al. Melatonin improves periodontitis-induced kidney damage by decreasing inflammatory stress and apoptosis in rats. J Periodontol (2021) 92(6):22–34. doi: 10.1002/jper.20-0434
45. Bhattarai G, Poudel SB, Kook SH, Lee JC. Resveratrol prevents alveolar bone loss in an experimental rat model of periodontitis. Acta Biomater (2016) 29:398–408. doi: 10.1016/j.actbio.2015.10.031
46. Silva RCL, Sasso-Cerri E, Cerri PS. Diacerein-induced interleukin-1β deficiency reduces the inflammatory infiltrate and immunoexpression of matrix metalloproteinase-8 in periodontitis in rat molars. J Periodontol (2022). doi: 10.1002/jper.21-0375
47. de Souza Malta F, Napimoga MH, Marins LM, Miranda TS, de Oliveira FB, Posch AT, et al. Lithium chloride assuages bone loss in experimental periodontitis in estrogen-deficient rats. Clin Oral Investig (2020) 24(6):2025–36. doi: 10.1007/s00784-019-03067-9
48. Gusman DJR, Ervolino E, Theodoro LH, Garcia VG, Nagata MJH, Alves BES, et al. Antineoplastic agents exacerbate periodontal inflammation and aggravate experimental periodontitis. J Clin Periodontol (2019) 46(4):457–69. doi: 10.1111/jcpe.13101
49. Kim JH, Kim AR, Choi YH, Jang S, Woo GH, Cha JH, et al. Tumor necrosis factor-α antagonist diminishes osteocytic RANKL and sclerostin expression in diabetes rats with periodontitis. PloS One (2017) 12(12):e0189702. doi: 10.1371/journal.pone.0189702
50. Allen EM, Matthews J.B. DJOH, Griffiths HR, Chapple IL. Oxidative and inflammatory status in type 2 diabetes patients with periodontitis. J Clin Periodontol (2011) 38(10):894–901. doi: 10.1111/j.1600-051X.2011.01764.x
51. Sanz M, Ceriello A, Buysschaert M, Chapple I, Demmer RT, Graziani F, et al. Scientific evidence on the links between periodontal diseases and diabetes: Consensus report and guidelines of the joint workshop on periodontal diseases and diabetes by the international diabetes federation and the European federation of periodontology. J Clin Periodontol (2018) 45(2):138–49. doi: 10.1111/jcpe.12808
52. Santos VR, Lima JA, Gonçalves TE, Bastos MF, Figueiredo LC, Shibli JA, et al. Receptor activator of nuclear factor-kappa b ligand/osteoprotegerin ratio in sites of chronic periodontitis of subjects with poorly and well-controlled type 2 diabetes. J Periodontol (2010) 81(10):1455–65. doi: 10.1902/jop.2010.100125
53. Xiao E, Mattos M, Vieira GHA, Chen S, Corrêa JD, Wu Y, et al. Diabetes enhances IL-17 expression and alters the oral microbiome to increase its pathogenicity. Cell Host Microbe (2017) 22(1):120–128.e4. doi: 10.1016/j.chom.2017.06.014
54. Polak D, Shapira L. An update on the evidence for pathogenic mechanisms that may link periodontitis and diabetes. J Clin Periodontol (2018) 45(2):150–66. doi: 10.1111/jcpe.12803
55. Byun K, Yoo Y, Son M, Lee J, Jeong GB, Park YM, et al. Advanced glycation end-products produced systemically and by macrophages: A common contributor to inflammation and degenerative diseases. Pharmacol Ther (2017) 177:44–55. doi: 10.1016/j.pharmthera.2017.02.030
56. Nonaka K, Kajiura Y, Bando M, Sakamoto E, Inagaki Y, Lew JH, et al. Advanced glycation end-products increase IL-6 and ICAM-1 expression via RAGE, MAPK and NF-κB pathways in human gingival fibroblasts. J Periodontal Res (2018) 53(3):334–44. doi: 10.1111/jre.12518
57. Alikhani M, Alikhani Z, Boyd C, MacLellan CM, Raptis M, Liu R, et al. Advanced glycation end products stimulate osteoblast apoptosis via the MAP kinase and cytosolic apoptotic pathways. Bone (2007) 40(2):345–53. doi: 10.1016/j.bone.2006.09.011
58. Liu H, Gao L, Xie T, Li J, Zhai TS, Xu Y. Identification and validation of a prognostic signature for prostate cancer based on ferroptosis-related genes. Front Oncol (2021) 11:623313. doi: 10.3389/fonc.2021.623313
59. Shou Y, Yang L, Yang Y, Xu J. Inhibition of keratinocyte ferroptosis suppresses psoriatic inflammation. Cell Death Dis (2021) 12(11):1009. doi: 10.1038/s41419-021-04284-5
60. Cui Y, Zhang Z, Zhou X, Zhao Z, Zhao R, Xu X, et al. Microglia and macrophage exhibit attenuated inflammatory response and ferroptosis resistance after RSL3 stimulation via increasing Nrf2 expression. J Neuroinflamm (2021) 18(1):249. doi: 10.1186/s12974-021-02231-x
61. Sun QY, Zhou HH, Mao XY. Emerging roles of 5-lipoxygenase phosphorylation in inflammation and cell death. Oxid Med Cell Longev (2019) 2019:2749173. doi: 10.1155/2019/2749173
62. Günes Günsel G, Conlon TM, Jeridi A, Kim R, Ertüz Z, Lang NJ, et al. The arginine methyltransferase PRMT7 promotes extravasation of monocytes resulting in tissue injury in COPD. Nat Commun (2022) 13(1):1303. doi: 10.1038/s41467-022-28809-4
63. Sharifi-Rad M, Mnayer D, Morais-Braga MFB, Carneiro JNP, Bezerra CF, Coutinho HDM, et al. Echinacea plants as antioxidant and antibacterial agents: From traditional medicine to biotechnological applications. Phytother Res (2018) 32(9):1653–63. doi: 10.1002/ptr.6101
64. Xu W, Zhu H, Hu B, Cheng Y, Guo Y, Yao W, et al. Echinacea in hepatopathy: A review of its phytochemistry, pharmacology, and safety. Phytomedicine (2021) 87:153572. doi: 10.1016/j.phymed.2021.153572
65. Li X, Zou Y, Fu YY, Xing J, Wang KY, Wan PZ, et al. Ibudilast attenuates folic acid-induced acute kidney injury by blocking pyroptosis through TLR4-mediated NF-κB and MAPK signaling pathways. Front Pharmacol (2021) 12:650283. doi: 10.3389/fphar.2021.650283
66. Oliveros G, Wallace CH, Chaudry O, Liu Q, Qiu Y, Xie L, et al. Repurposing ibudilast to mitigate alzheimer's disease by targeting inflammation. Brain (2022) awac136 doi: 10.1093/brain/awac136
67. Clanchy FIL, Williams RO. Ibudilast inhibits chemokine expression in rheumatoid arthritis synovial fibroblasts and exhibits immunomodulatory activity in experimental arthritis. Arthritis Rheumatol (2019) 71(5):703–11. doi: 10.1002/art.40787
68. Zhang C, Xue P, Ke J, Cai Q. Development of ferroptosis-associated ceRNA network in periodontitis. Int Dent J (2022). doi: 10.1016/j.identj.2022.05.004
69. Zhang S, Jin H, Da J, Zhang K, Liu L, Guo Y, et al. Role of ferroptosis-related genes in periodontitis based on integrated bioinformatics analysis. PloS One (2022) 17(7):e0271202. doi: 10.1371/journal.pone.0271202
Keywords: ferroptosis, drug prediction, periodontitis, type 2 diabetes mellitus, pathway
Citation: Pan S, Hu B, Sun J, Yang Z, Yu W, He Z, Gao X and Song J (2022) Identification of cross-talk pathways and ferroptosis-related genes in periodontitis and type 2 diabetes mellitus by bioinformatics analysis and experimental validation. Front. Immunol. 13:1015491. doi: 10.3389/fimmu.2022.1015491
Received: 09 August 2022; Accepted: 13 September 2022;
Published: 29 September 2022.
Edited by:
Pedro Paulo Chaves Souza, Universidade Federal de Goiás, BrazilReviewed by:
Dogukan Yilmaz, University of Turku, FinlandRaquel Mantuaneli Scarel-Caminaga, Universidade Estadual Paulista, Brazil
Jeffrey Ebersole, University of Nevada, Las Vegas, United States
Copyright © 2022 Pan, Hu, Sun, Yang, Yu, He, Gao and Song. This is an open-access article distributed under the terms of the Creative Commons Attribution License (CC BY). The use, distribution or reproduction in other forums is permitted, provided the original author(s) and the copyright owner(s) are credited and that the original publication in this journal is cited, in accordance with accepted academic practice. No use, distribution or reproduction is permitted which does not comply with these terms.
*Correspondence: Jinlin Song, songjinlin@hospital.cqmu.edu.cn; Xiang Gao, xiangg@hospital.cqmu.edu.cn
†These authors have contributed equally to this work and share first authorship