- 1Department of Medicine, College of Medicine, State University of New York, Upstate Medical University, Syracuse, NY, United States
- 2Department of Biochemistry and Molecular Biology, College of Medicine, State University of New York, Upstate Medical University, Syracuse, NY, United States
- 3Department of Microbiology and Immunology, College of Medicine, State University of New York, Upstate Medical University, Syracuse, NY, United States
- 4The Zabludowicz Center for Autoimmune Diseases, Sheba Medical Center, Ramat Gan, Israel
- 5Department of Immunology and Rheumatology, Instituto Nacional de Ciencias Médicas y Nutrición Salvador Zubirán, Mexico City, Mexico
- 6Tecnologico de Monterrey, Escuela de Medicina y Ciencias de la Salud, Monterrey, Mexico
- 7Department of Inflammation & Immunity, Lerner Research Institute, Cleveland Clinic, Cleveland, OH, United States
Editorial on the Research Topic
New biomarkers for the diagnosis and treatment of systemic lupus erythematosus
Systemic lupus erythematosus is an autoimmune disease of unknown etiology that primarily affects females of child-bearing age with various morbidities (1). Mortality of SLE still exceeds 10% over 5 years (2, 3). While current treatments are partially effective, they carry significant side effects (4), with infections due to toxicity of immunosuppressant medications being a major cause of death (5, 6). This includes belimumab, the 1st drug approved by the FDA for SLE treatment in 56 years (7), and more recently anifrolumab, both of which also predispose to infections (8). Therefore, a significant unmet need exists to identify biomarkers that can be targeted for safe and effective therapeutic intervention in SLE. A Research Topic centered around new biomarkers for the Diagnosis and Treatment of SLE included 15 publications with a wide range of focus and experimental design. This Editorial addresses the challenges of integrating a series of newly reported single biomarkers, composite biomarkers based multi-omics approaches, and biomarkers based on machine learning with the complex systems biology of SLE. These newly reported biomarkers are shown in Table 1.
S100 calcium-binding protein A8 protein (S100A8) levels as biomarkers for systemic lupus erythematosus (SLE) were quantified in serum, urine, and saliva samples from 249 patients with SLE and 52 age- and sex-matched healthy controls (HCs) and a receiver operating characteristic curve was used to analyze whether they may be used as biomarkers for diagnosis and prediction of flares (17). For SLE diagnosis, the area under the receiver operating characteristic curve (AUC) was 0.831 for serum S100A8 (95% CI, 0.765–0.897), 0.751 for urine S100A8 (95% CI, 0.648–0.854), and 0.729 for salivary S100A8 (95% CI, 0.646–0.812). Pearson’s correlation analysis showed that S100A8 in serum, urine, and saliva was significantly associated with the SLEDAI (r = 0.267, p < 0.001; r = 0.274, p < 0.001; and r = 0.629, p < 0.001, respectively). Among the clinical manifestations, nephritis was the only organ involvement that was associated with increased concentration of S100A8 in serum, urine, and saliva in comparison to SLE patients without LN (17). An independent study demonstrated that enhanced glomerular S100A8 staining in class IV LN patients over controls (16). S100A8 has been identified as a differentially expressed gene (DEG) with overexpression in kidneys of LN patients (16).
Mass spectroscopy of circulating immune complexes identified 300 proteins in the serum of SLE patients, several of which were found to be highly associated with LN in two independent patient-control cohorts (14). Prolyl 3-hydroxylase 1 (P3H1), phosphatase and actin regulator 4 (PHACTR4), and regulator of G-protein signaling 12 (RGS12) discriminated LN AUC values of 0.82, 0.99, and 0.90, respectively.
Serial kidney biopsies for initial diagnosis and subsequent monitoring of lupus nephritis (LN) remain challenging, thus non-invasive biomarkers are needed. Urinary ALCAM, PF4, and VCAM-1 were identified as potential biomarkers for predicting kidney disease activity in childhood-onset SLE with ALCAM (AUC 0.83) being the single most predictive (15). Herpes virus entry mediator (HVEM) demonstrated comparable diagnostic ability to creatinine normalization when distinguishing active lupus nephritis from inactive SLE patients using the candidate biomarker ALCAM (20). In a 3-stage study including a total of 321 LN patients, a combination of four biomarkers, adiponectin, MCP-1, sVCAM-1 and PF4, were found to have the greatest predictive value for the detection of proliferative, active LN (18). Patients with LN exhibit a profound depletion of atypical age-associated B-cell (ABC) like CD11c+T-bet+CD21hi B cells in comparison with healthy individuals and SLE patients without LN (19). Selected from 284 DEGs identified in two independent SLE cohorts in the Gene Expression Omnibus (GEO) database, machine learning validated ABCB1, IFI27, and PLSCR1 as top predictors of SLE in a Chinese validation cohort of patients over ethnically matched controls (13). Expression of each these genes was correlated with the expansion of pro-inflammatory lineages of the adaptive and innate immune systems (13). Separately, a comprehensive analysis of gut microbiome genome databases newly identified Bacilli and Lactobacillales as promoters of SLE and Bacillales, Coprobacter and Lachnospira as protectors from SLE (9).
Among plasma sphingolipids, lactoceramide has been identified as a potential predictor of cardiovascular disease (CVD) in African-American patients with SLE (11). Tan et al. provide an extensive review of biomarker development for SLE (21). The biomarkers are divided by their molecular nature: i) autoantibodies; ii) serum proteins (cytokines, chemokines, complement components, soluble receptors and transporters); iii) microRNAs and long non-coding RNA (LncRNAs); and relevance for organ involvement, such as nephritis, neuropsychiatric lupus, and cutaneous lupus. The review does not discuss cellular biomarkers, such as Tregs, memory B or T cells, or metabolites. Indeed, a comprehensive review of all biomarkers implicated in lupus pathogenesis and patients care remains daunting. In contrast, Ole Petter Rekvig focuses on the role of DNA structure in triggering anti-DNA antibodies and its relationship to lupus nephritis (12). Mitochondrial N-formyl methionine (fMet) is newly implicated in promoting neutrophil-mediated inflammation in systemic sclerosis (22).
Neuropsychiatric SLE (NPSLE) can be diagnosed in the majority of patients with appropriate screening instruments (23). NPSLE and particularly depression has been associated with elevated levels of type 1 interferons, TNFs, and IL-6 in the cerebrospinal fluid (CSF) of SLE patients (24). Accumulation of senescent cells in the hippocampus has been linked to major depression (25). Apparently, depression in lupus-prone MRL/lpr mice is associated with the accumulation of senescent cells in the cornu ammonis 3 (CA3) region of hippocampus (10). Importantly, oral fisetin, a senolytic drug, reduced the number of senescent neural cells and IL-6 mRNA in the hippocampus and improved depressive behavior in the MRL/lpr mice (10).
Given the diversity of hypotheses, methodologies, experimental models and study design, integration of the newly reported biomarkers with the systems biology of SLE present multiple challenges. Such challenges can be easily attributed to a general lack of understanding of lupus pathogenesis. However, several key facts need to be considered when integrating such interesting but diverse outcome. A hallmark of SLE is the production of antinuclear autoantibodies (ANA) (26). Although ANAs are directed to an ever growing number of nucleic acid and nucleoprotein targets and their titers can greatly vary due to the course of disease and impact of therapies, their detection remains a key criterion of diagnostic workup (27). Thus, biomarkers may be mechanistically connected, either upstream or downstream, to the generation or handling of autoantibodies and cell-mediated autoreactivity. T (28) and B cells of the adaptive immune system (29, 30) and IFN-producing dendritic cells are essential for lupus pathogenesis (31). Therefore, integrating biomarkers into the signaling networks that connect the adaptive and innate arms of a dysfunctional immune system is critical for appreciating their significance for controlling pathogenesis, predicting flares or serving as target for treatment in SLE. Notably, only few of these studies involved cellular biomarkers (13, 19) that can be connected to central pathways of lupus pathogenesis. Nevertheless, certain easily detectable biomarkers may also serve other purposes, such as sensing organ damage and obviating the need for invasive procedures, i.e., renal biopsy in LN (15, 17–19). Fisetin was found to control depression by preventing the senescence of neuronal cells in the hippocampus of MRl/lpr mice (10). Fisetin is known to exert its antiaging effect by blocking the mechanistic target of rapamycin (mTOR) (32, 33) (Figure 1), which serves a sensor of cellular stress and central regulator of pro-inflammatory lineage development in the immune system (36). Importantly, T cells of SLE patients (37–41) and mice exhibit activation of the mechanistic target of rapamycin (mTOR) (42, 43). Th17 and IL-4 and IL-17-producing DN T cells are expanded, while CD8 EMT cells (44, 45) and Tregs are deficient in SLE patients due to cell type-specific skewing of autophagy that can be corrected with therapeutic efficacy by mTOR blockade (45, 46). Rapamycin blocks nephritis in SLE (47–49). Rapamycin also blocks the production of vascular cell adhesion molecule-1 (VCAM-1) by vascular endothelial cells (50). Of note, VCAM1 was identified as a sensitive biomarker of active LN by two independent studies published under this Research Topic (15, 18). Therefore, it’s possible that mTOR blockade with sirolimus or fisetin would block LN via reducing the expression and urinary excretion of VCAM1 and other adhesion molecules.
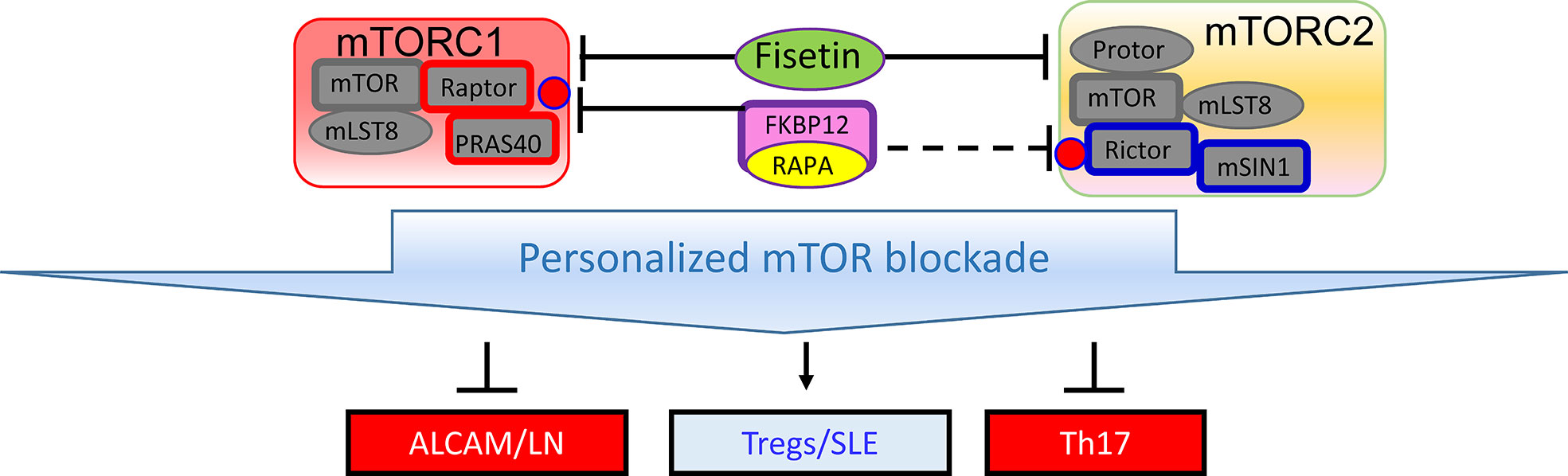
Figure 1 Schematic diagram of mTOR complexes 1 (mTORC1) and 2 (mTORC2) in SLE. Similar to rapamycin, fisetin inhibits both mTOR complexes (34), which may be involved in expanding Tregs (35) and thus improving depression and other organ involvement in SLE (10, 35).
In conclusion, while a single marker may not adequately capture all the finesses of LN, SLE-CVD, NPSLE or other subcategories of SLE, it is conceivable that the creation of easily accessible screening assays measuring one or more factors will provide fast and reliable information about the development of organ-specific symptoms, the onset of flares, and the prediction of different therapeutic intervention among diverse SLE patients. Before we can reach such goal, it is however important that new markers are tested across different patient groups. For example, it will be of interest to know if S100A8, PF4 and (s)VCAM-1 are similarly upregulated in SLE patients with NPSLE or CVD, or if this phenotype is specific for LN. Future studies are clearly warranted to substantiate the importance of these biomarkers for the diagnosis and treatment of SLE.
Author contributions
AP conceived and wrote the paper. All authors contributed to the article and approved the submitted version.
Funding
This work was supported in part by grants AI072648 (AP), AI122176 (AP), and AR076092 (AP), and R01 AI118774 (TJ) from the National Institutes of Health, the Phillips Lupus and Autoimmunity Center of Excellence (AP), and the Central New York Community Foundation (AP).
Conflict of interest
The authors declare that the research was conducted in the absence of any commercial or financial relationships that could be construed as a potential conflict of interest.
Publisher’s note
All claims expressed in this article are solely those of the authors and do not necessarily represent those of their affiliated organizations, or those of the publisher, the editors and the reviewers. Any product that may be evaluated in this article, or claim that may be made by its manufacturer, is not guaranteed or endorsed by the publisher.
References
1. Tsokos GC. Systemic lupus erythematosus. N Engl J Med (2011) 365:2110. doi: 10.1056/NEJMra1100359
2. Trager J. And ward, m. m. 2001. mortality and causes of death in systemic lupus erythematosus. Curr Opin Rheumatol (2001) 13:345. doi: 10.1097/00002281-200109000-00002
3. Pons-Estel GJ, Alarcon GS, Scofield L, Reinlib L, Cooper GS. Understanding the epidemiology and progression of systemic lupus erythematosus. Semin Arth Rheumatol (2010) 39:257. doi: 10.1016/j.semarthrit.2008.10.007
4. Francis L, Perl A. Pharmacotherapy of systemic lupus erythematosus. Expert Opin Pharmacother (2009) 10:1481. doi: 10.1517/14656560902971003
5. Bernatsky S, Boivin JF, Joseph L, Manzi S, Ginzler E, Gladman DD, et al. Mortality in systemic lupus erythematosus. Arthritis Rheumatol (2006) 54:2550. doi: 10.1002/art.21955
6. Yurkovich M, Vostretsova K, Chen W, Avina-Zubieta JA. Overall and cause-specific mortality in patients with systemic lupus erythematosus: A meta-analysis of observational studies. Arthritis Care Res (2014) 66:608. doi: 10.1002/acr.22173
7. Navarra SV, Guzman RM, Gallacher AE, Hall S, Levy RA, Jimenez RE, et al. Efficacy and safety of belimumab in patients with active systemic lupus erythematosus: a randomised, placebo-controlled, phase 3 trial. Lancet (2011) 377:721. doi: 10.1016/S0140-6736(10)61354-2
8. Bruce IN, Urowitz M, van Vollenhoven R, Aranow C, Fettiplace J, Oldham M, et al. Long-term organ damage accrual and safety in patients with SLE treated with belimumab plus standard of care. Lupus (2016) 25:699. doi: 10.1177/0961203315625119
9. Xiang K, Wang P, Xu Z, Hu YQ, He YS, Chen Y, et al. Causal effects of gut microbiome on systemic lupus erythematosus: A two-sample mendelian randomization study. Front Immunol (2021) 12:667097. doi: 10.3389/fimmu.2021.667097
10. Saito Y, Miyajima M, Yamamoto S, Sato T, Miura N, Fujimiya M, et al. Accumulation of senescent neural cells in murine lupus with depression-like behavior. Front Immunol (2021) 12:692321. doi: 10.3389/fimmu.2021.692321
11. Hammad SM, Harden OC, Wilson DA, Twal WO, Nietert PJ, Oates JC. Plasma sphingolipid profile associated with subclinical atherosclerosis and clinical disease markers of systemic lupus erythematosus: Potential predictive value. Front Immunol (2021) 12:694318. doi: 10.3389/fimmu.2021.694318
12. Rekvig OP. The anti-DNA antibodies: Their specificities for unique DNA structures and their unresolved clinical ImpactGÇöA system criticism and a hypothesis. Front Immunol (2022) 12:808008. doi: 10.3389/fimmu.2021.808008
13. Zhong Y, Zhang W, Hong X, Zeng Z, Chen Y, Liao S, et al. Screening biomarkers for systemic lupus erythematosus based on machine learning and exploring their expression correlations with the ratios of various immune cells. Front Immunol (2022) 13:873787. doi: 10.3389/fimmu.2022.873787
14. Tang C, Fang M, Tan G, Zhang S, Yang B, Li Y, et al. Discovery of novel circulating immune complexes in lupus nephritis using immunoproteomics. Front Immunol (2022) 13:850015. doi: 10.3389/fimmu.2022.850015
15. Soliman SA, Haque A, Vanarsa K, Zhang T, Ismail F, Lee KH, et al. PF4 and VCAM-1 surpass conventional metrics in identifying nephritis disease activity in childhood-onset systemic lupus erythematosus. Front Immunol (2022) 13:885307. doi: 10.3389/fimmu.2022.885307
16. Qijiao W, Zhihan C, Makota P, Qing Y, Fei G, Zhihong W, et al. Glomerular expression of S100A8 in lupus nephritis: An integrated bioinformatics analysis. Front Immunol (2022) 13:843576. doi: 10.3389/fimmu.2022.843576
17. Kim JW, Jung JY, Lee SW, Baek WY, Kim HA, Suh CH. S100A8 in serum, urine, and saliva as a potential biomarker for systemic lupus erythematosus. Front Immunol (2022) 13:886209. doi: 10.3389/fimmu.2022.886209
18. Whittall-Garcia L, Goliad K, Kim M, Bonilla D, Gladman D, Urowitz M, et al. Identification and validation of a urinary biomarker panel to accurately diagnose and predict response to therapy in lupus nephritis. Front Immunol (2022) 13:889931. doi: 10.3389/fimmu.2022.889931
19. Sosa-Hernandez VA, Romero-Ramirez S, Cervantes-Diaz R, Carrillo-Vazquez DA, Navarro-Hernandez IC, Whittall-Garcia LP, et al. CD11c+ T-bet+ CD21hi b cells are negatively associated with renal impairment in systemic lupus erythematosus and act as a marker for nephritis remission. Front Immunol (2022) 13:892241. doi: 10.3389/fimmu.2022.892241
20. Soomro S, Stanley S, Lei R, Saxena R, Petri M, Mohan C. Comprehensive urinomic identification of protein alternatives to creatinine normalization for diagnostic assessment of lupus nephritis. Front Immunol (2022) 13:853778. doi: 10.3389/fimmu.2022.853778
21. Tan G, Baby B, Zhou Y, Wu T. Emerging molecular markers towards potential diagnostic panels for lupus. Front Immunol (2022) 12:808839. doi: 10.3389/fimmu.2021.808839
22. Kuley R, Stultz RD, Duvvuri B, Wang T, Fritzler MJ, Hesselstrand R, et al. N-formyl methionine peptide-mediated neutrophil activation in systemic sclerosis. Front Immunol (2022) 12:785275. doi: 10.3389/fimmu.2021.785275
23. Meszaros-Szombathyne Z, Perl A, Faraone SV. Psychiatric symptoms in systemic lupus erythematosus: A systematic review. J Clin Psychiatry (2012) 73:993. doi: 10.4088/JCP.11r07425
24. Schwartz N, Stock AD, Putterman C. Neuropsychiatric lupus: new mechanistic insights and future treatment directions. Nat Rev Rheumatol (2019) 15:137. doi: 10.1038/s41584-018-0156-8
25. MacQueen G, Frodl T. The hippocampus in major depression: evidence for the convergence of the bench and bedside in psychiatric research? Mol Psychiatry (2011) 16:252. doi: 10.1038/mp.2010.80
26. Perl A. Systems biology of lupus: Mapping the impact of genomic and environmental factors on gene expression signatures, cellular signaling, metabolic pathways, hormonal and cytokine imbalance, and selecting targets for treatment. Autoimmunity (2010) 43:32. doi: 10.3109/08916930903374774
27. Pisetsky DS, Lipsky PE. New insights into the role of antinuclear antibodies in systemic lupus erythematosus. Nat Rev Rheumatol (2020) 16:565. doi: 10.1038/s41584-020-0480-7
28. Mihara M, Ohsugi Y, Saito K, Miyai T, Togashi M, Ono S, et al. Immunologic abnormality in NZB/NZW F1 mice. thymus-independent occurrence of b cell abnormality and requirement for T cells in the development of autoimmune disease, as evidenced by an analysis of the athymic nude individuals. J Immunol (1988) 141:85.
29. Shlomchik MJ, Madaio MP, Ni D, Trounstein M, Huszar D. The role of b cells in lpr/lpr-induced autoimmunity. J Exp Med (1994) 180:1295. doi: 10.1084/jem.180.4.1295
30. Jacob N, Guo S, Mathian A, Koss MN, Gindea S, Putterman C, et al. And BAFF dependence of IFN-a-Exaggerated disease in systemic lupus erythematosus-prone NZM 2328 mice. J Immunol (2011) 186:4984. doi: 10.4049/jimmunol.1000466
31. Peschke K, Achleitner M, Frenzel K, Gerbaulet A, Ada SR, Zeller N, et al. And XXXR. behrendt. 2016. loss of Trex1 in dendritic cells is sufficient to trigger systemic autoimmunity. J Immunol (2016) 197:2157. doi: 10.4049/jimmunol.1600722
32. Deeba N, Vaqar M, Imran M, Mukhtar H. Inhibition of Akt/mTOR signaling by the dietary flavonoid fisetin. Anti-Cancer Agents Med Chem (2013) 13:995. doi: 10.2174/18715206113139990129
33. Xiao Y, Liu Y, Gao Z, Li X, Weng M, Shi C, et al. Fisetin inhibits the proliferation, migration and invasion of pancreatic cancer by targeting PI3K/AKT/mTOR signaling. Aging (Albany NY) (2001) 13:24753. doi: 10.18632/aging.203713
34. Suh Y, Afaq F, Khan N, Johnson JJ, Khusro FH, Mukhtar H. Fisetin induces autophagic cell death through suppression of mTOR signaling pathway in prostate cancer cells. Carcinogenesis (2010) 31:1424. doi: 10.1093/carcin/bgq115
35. Xu S-P, Li Y-S. Fisetin inhibits pristine-induced systemic lupus erythematosus in a murine model through CXCLs regulation. Int J Mol Med (2018) 42:3220. doi: 10.3892/ijmm.2018.3903
36. Perl A. Mechanistic target of rapamycin pathway activation in rheumatic diseases. Nat Rev Rheumatol (2016) 12:169. doi: 10.1038/nrrheum.2015.172
37. Fernandez D, Bonilla E, Mirza N, Niland B, Perl A. Rapamycin reduces disease activity and normalizes T-cell activation-induced calcium fluxing in patients with systemic lupus erythematosus. Arthritis Rheumatol (2006) 54:2983. doi: 10.1002/art.22085
38. Fernandez DR, Telarico T, Bonilla E, Li Q, Banerjee S, Middleton FA, et al. Activation of mTOR controls the loss of TCRζ in lupus T cells through HRES-1/Rab4-regulated lysosomal degradation. J Immunol (2009) 182:2063. doi: 10.4049/jimmunol.0803600
39. Lai Z-W, Borsuk R, Shadakshari A, Yu J, Dawood M, Garcia R, et al. mTOR activation triggers IL-4 production and necrotic death of double-negative T cells in patients with systemic lupus erythematosus. J Immunol (2013) 191:2236. doi: 10.4049/jimmunol.1301005
40. Kato H, Perl A. MTORC1 expands Th17 and IL-4+ DN T cells and contracts tregs in SLE. J Immunol (2014) 192:4134. doi: 10.4049/jimmunol.1301859
41. Kato H, Perl A. The IL-21-mTOR axis blocks treg differentiation and function by suppression of autophagy in patients with systemic lupus erythematosus. Arthritis Rheumatol (2018) 70:427. doi: 10.1002/art.40380
42. Caza TN, Fernandez D, Talaber G, Oaks Z, Haas M, Madaio MP, et al. HRES-1/RAB4-Mediated depletion of DRP1 impairs mitochondrial homeostasis and represents a target for treatment in SLE. Ann Rheumatol Dis (2014) 73:1887. doi: 10.1136/annrheumdis-2013-203794
43. Oaks Z, Winans T, Caza T, Fernandez D, Liu Y, Landas SK, et al. Mitochondrial dysfunction in the liver and antiphospholipid antibody production precede disease onset and respond to rapamycin in lupus-prone mice. Arthritis Rheumatol (2016) 68:2728. doi: 10.1002/art.39791
44. Comte D, Karampetsou MP, Yoshida N, Kis-Toth K, Kyttaris VC, Tsokos GC. Signaling lymphocytic activation molecule family member 7 engagement restores defective effector CD8+ T cell function in systemic lupus erythematosus. Arthritis Rheumatol (2017) 69:1035. doi: 10.1002/art.40038
45. Lai Z, Kelly R, Winans T, Marchena I, Shadakshari A, Yu J, et al. Sirolimus in patients with clinically active systemic lupus erythematosus resistant to, or intolerant of, conventional medications: a single-arm, open-label, phase 1/2 trial. Lancet (2018) 391:1186. doi: 10.1016/S0140-6736(18)30485-9
46. Lai Z-W, Hanczko R, Bonilla E, Caza TN, Clair B, Bartos A, et al. N-acetylcysteine reduces disease activity by blocking mTOR in T cells of lupus patients. Arthritis Rheumatol (2012) 64:2937. doi: 10.1002/art.34502
47. Yap DYH, Tang C, Chan GCW, Kwan LPY, Ma MKM, Mok MMY, et al. Longterm data on sirolimus treatment in patients with lupus nephritis. J Rheumatol (2018) 45:1663. doi: 10.3899/jrheum.180507
48. Piranavan P, Perl A. Improvement of renal and non-renal SLE outcome measures on sirolimus therapy - a 21-year follow-up study of 73 patients. Clin Immunol (2021) 229:108781. doi: 10.1016/j.clim.2021.108781
49. Mao Z, Tan Y, Tao J, Li L, Wang H, Yu F, et al. Renal mTORC1 activation is associated with disease activity and prognosis in lupus nephritis. Rheumatology (2022) 61:3830–40. doi: 10.1093/rheumatology/keac037
Keywords: lupus (SLE), biomarker, multi-omics analyses, machine learning, systems biology
Citation: Perl A, Agmon-Levin N, Crispín JC and Jorgensen TN (2022) Editorial: New biomarkers for the diagnosis and treatment of systemic lupus erythematosus. Front. Immunol. 13:1009038. doi: 10.3389/fimmu.2022.1009038
Received: 01 August 2022; Accepted: 20 September 2022;
Published: 12 October 2022.
Edited by:
Chris Wincup, King’s College Hospital NHS Foundation Trust, United KingdomReviewed by:
Elizabeth C. Jury, University College London, United KingdomCopyright © 2022 Perl, Agmon-Levin, Crispín and Jorgensen. This is an open-access article distributed under the terms of the Creative Commons Attribution License (CC BY). The use, distribution or reproduction in other forums is permitted, provided the original author(s) and the copyright owner(s) are credited and that the original publication in this journal is cited, in accordance with accepted academic practice. No use, distribution or reproduction is permitted which does not comply with these terms.
*Correspondence: Andras Perl, cGVybGFAdXBzdGF0ZS5lZHU=