- 1Department of Gastroenterology, Digestive Disease Hospital, Affiliated Hospital of Zunyi Medical University, Zunyi, China
- 2Division of Gastroenterology & Hepatology, Graduate School of Medical and Dental Sciences, Niigata University, Niigata, Japan
- 3Department of General Surgery, Affiliated Hospital of Zunyi Medical University, Zunyi, China
Alarmins exist outside cells and are early warning signals to the immune system; as such, alarmin receptors are widely distributed on various immune cells. Alarmins, proinflammatory molecular patterns associated with tissue damage, are usually released into the extracellular space, where they induce immune responses and participate in the damage and repair processes of mucosal diseases.In the stomach, gastric alarmin release has been shown to be involved in gastric mucosal inflammation, antibacterial defense, adaptive immunity, and wound healing; moreover, this release causes damage and results in the development of gastric mucosal diseases, including various types of gastritis, ulcers, and gastric cancer. Therefore, it is necessary to understand the role of alarmins in gastric mucosal diseases. This review focuses on the contribution of alarmins, including IL33, HMGB1, defensins and cathelicidins, to the gastric mucosal barrier and their role in gastric mucosal diseases. Here, we offer a new perspective on the prevention and treatment of gastric mucosal diseases.
Introduction
Alarmins are endogenous, constitutive molecules that are rapidly released from cells in response to infection or tissue damage; thus, alarmins act as early warning signals to the immune system by promoting the chemoattraction of antigen-presenting cells and activating innate and adaptive immunity (1). Alarmins are distinct from microbial pathogen-associated molecular patterns (PAMPs); rather, alarmins are derived from host cell damage-associated molecular patterns (DAMPs) generated upon host cell injury and bind to pattern recognition receptors (PRRs) of the innate immune system, thereby initiating downstream inflammatory responses (2, 3). Under normal circumstances, alarmins are present in intracellular granules, the nucleus or the cytoplasm and are involved in protein regulation (4, 5). After alarmins are rapidly released into the extracellular space in response to stimuli such as degranulation, cell death, and induction, receptor-expressing immune cells are recruited and activated, which can help restore immune homeostasis and promote epithelial repair (6); however, alarmin release can also lead to persistent tissue damage (7, 8). All alarmins have the ability to promote inflammation and immunity, but these factors play different roles after recognition by different receptors (1, 9–12). Alarmins are involved in processes such as inflammation, antibacterial defense, adaptive immunity, and wound healing and result in tissue damage and repair, highlighting the potential value of alarmins (13–19).
Alarmins are diverse and can be generally classified as antimicrobial peptides (AMPs), nuclear binding proteins, heat shock proteins (HSPs), ionic binders, nucleotides/metabolites (adenosine triphosphate and uric acid) and extracellular matrix degradation products (20–24). However, some alarmin studies revealed that nuclear binding proteins, including IL-33 (25–27) and high mobility group B1 (HMGB1) (28), and AMPs, including defensins (29, 30) and cathelicidin (31, 32), were mainly involved in the development of gastric mucosal diseases.
Gastric mucosal diseases involve mucosal tissue damage and include various types of gastritis, gastric ulcers (GUs) and gastric tumors (33–35). Recent studies have shown that alarmins are highly expressed in gastric mucosal diseases and initiate the close cooperation between innate and adaptive immunity to regulate host damage and repair (36). Therefore, this review mainly focuses on the pathophysiological roles of alarmins in gastric mucosal injury and disease (Figure 1; Table 1) to provide new insights into gastric mucosal diseases.
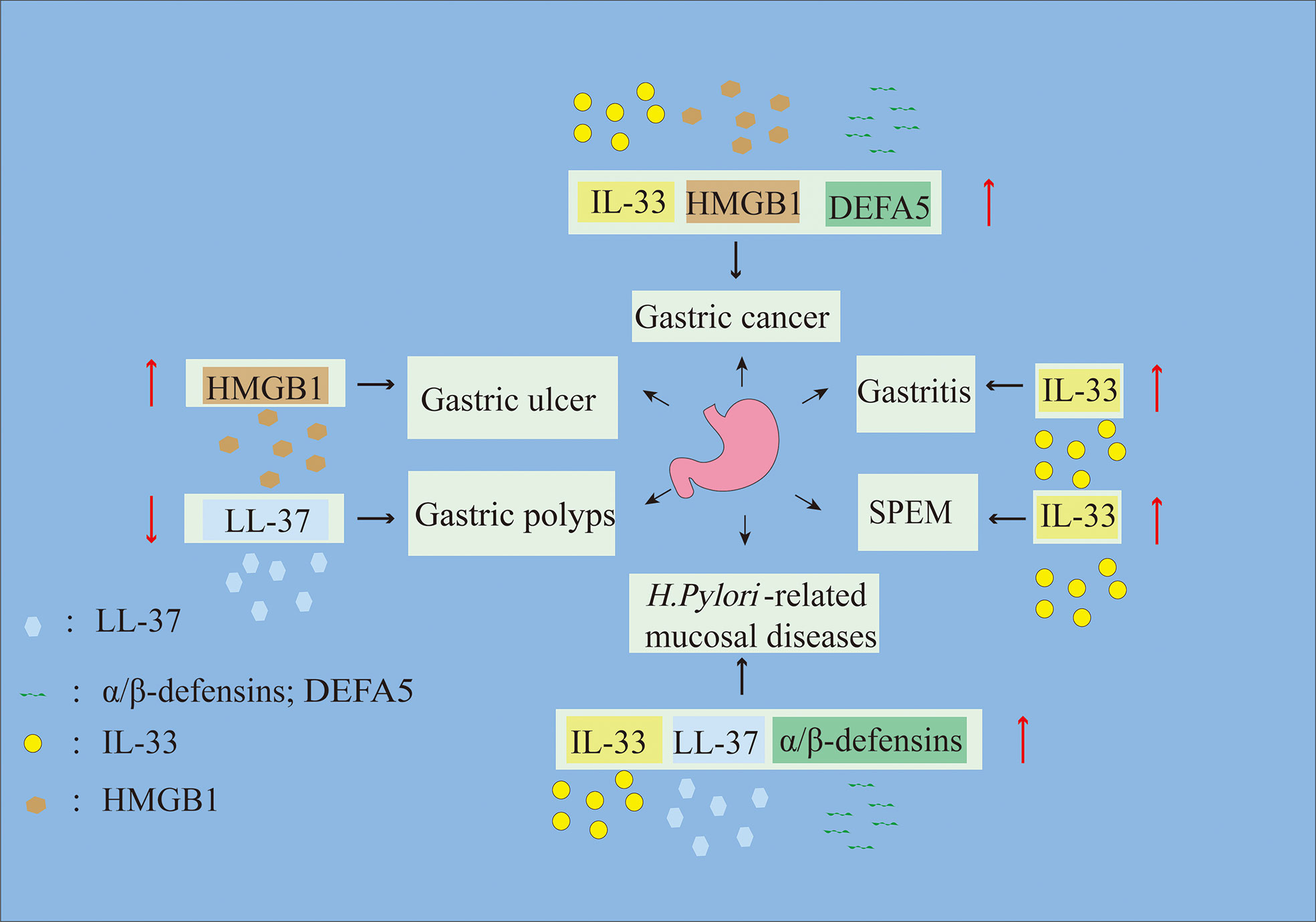
Figure 1 Alarmins are involved in the progression of gastric mucosal diseases. Up arrows represent alarmin upregulation, and down arrows represent alarmin downregulation.
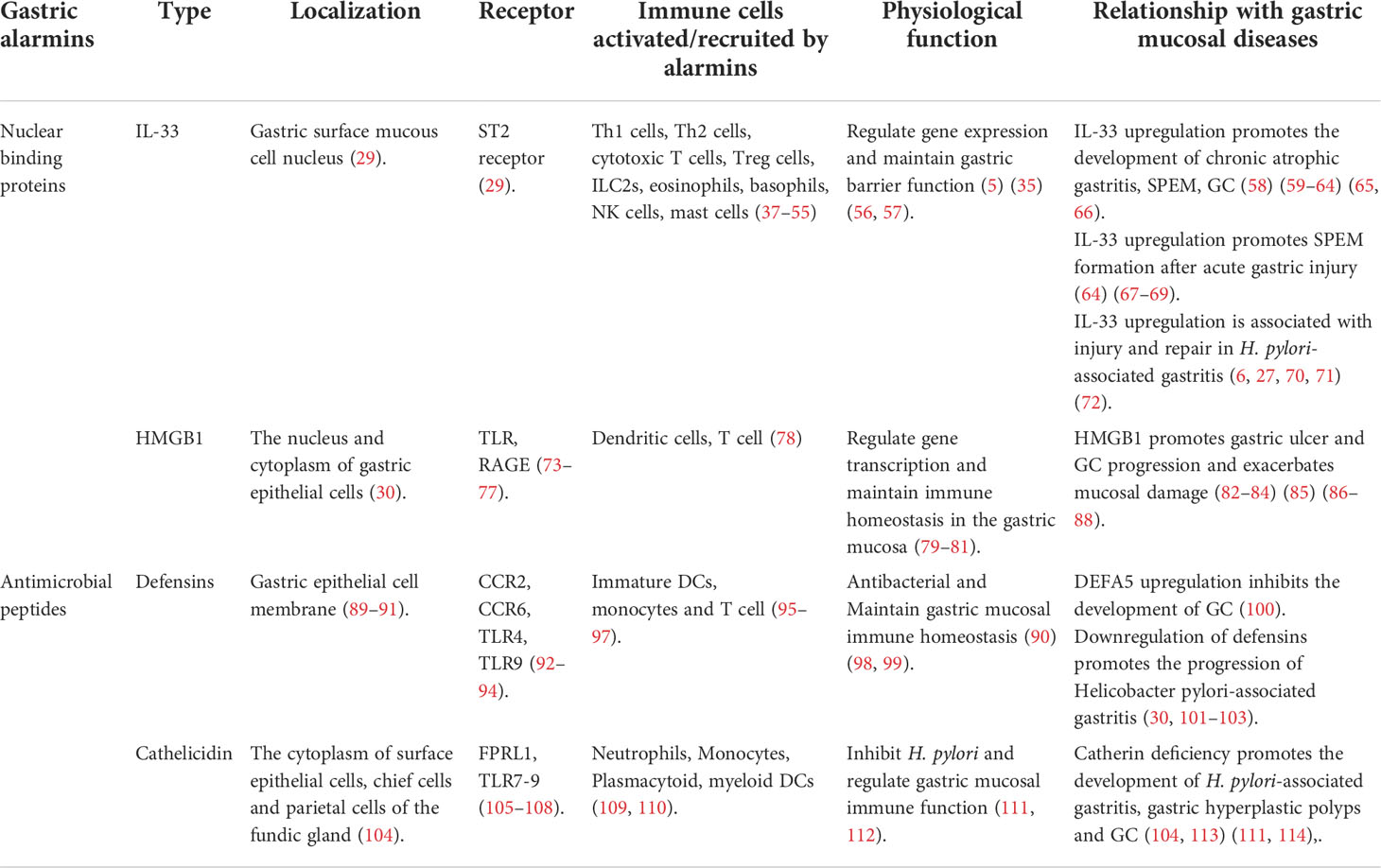
Table 1 Multiple gastric alarmins involved in the development of gastric mucosal diseases are mentioned in this review.
Gastric alarmin nuclear binding proteins: IL-33 and HMGB1
Nuclear binding proteins are a class of DNA-binding proteins that are involved in the regulation of transcription, replication and repair (37). Alarmins classified nuclear binding proteins in the stomach include IL-33 (38) and HMGB1 (39, 40), which are released extracellularly, causing immune changes and participating in the development of gastric mucosal diseases.
The expression pattern and functional role of IL-33 in the stomach
IL-33 is a nuclear binding protein in the IL-1 cytokine family (6, 41). Under physiological conditions, IL-33 translocates to the nucleus and is involved in regulating gene expression (5) and the maintenance of barrier function (42). The alarmin IL-33 is released in response to epithelial barrier damage or cell necrosis (43, 65) and signals through the IL-33 receptor complex, which consists of interleukin-1 receptor-like 1 (ST2) and interleukin-1 receptor accessory protein (IL1RAcP) (38). These receptors are widely expressed on various immune cells, including T helper type 2 (Th2) cells (44–46), activated T helper type 1 (Th1) cells (47), cytotoxic T cells (48, 49), type 2 innate lymphocytes (ILC2s) (50–54), eosinophils (55, 115), basophils (56, 116), natural killer (NK) cells, NKT cells (57), mast cells (MCs) (58, 117–119) and Treg cells (120). Therefore, these factors are involved in coordinating immune defense and repair mechanisms (121) and trigger adaptive immune responses (122).
In the stomach, IL-33 is highly expressed and localized to the nuclei of a subset of foveolar cells (also known as surface mucous cells (SMCs) or pit cells) (38), but a small fraction of IL-33 positive cells colocalize with Ki-67. This finding suggests that IL-33 expression changes as progenitors differentiate into pre-SMCs; specifically, surface IL-33 expression is inhibited as SMCs mature and migrate (65). Recent studies have shown that IL-33, which is released extracellularly, is involved in gastric injury and repair (36). In addition, recent studies have identified IL-33 as a DAMP that signals innate immune cells in response to stress or cell membrane disruption (123, 124) and that participates in the development of gastric mucosal diseases (65).
IL-33 release is involved in the development of gastric mucosal diseases
IL-33 upregulation promotes the development of chronic atrophic gastritis, gastric metaplasia and gastric cancer
Chronic atrophic gastritis, spasmolytic polypeptide-expressing metaplasia (SPEM) and GC are states along the continuous developmental trajectory of gastric mucosal disease, and IL-33 is a potential promoter of this process. IL33 is mainly induced by chronic phosphorylation of signal transducer and activator of transcription 3 (STAT3), and previous studies have shown that overactivated STAT3 is involved in gastric inflammation (59) and can lead to Th2-mediated gastric metaplasia (65). Gastric metaplasia includes SPEM (60, 61) and intestinal metaplasia. In SPEM, chief cells transdifferentiate into cells with a mucinous metaplastic phenotype, usually in the presence of parietal cell atrophy, epithelial barrier impairment, and ulceration (62–64, 125–127), and express Muc6 and TFF2 at the base of the gland (128). These findings suggest that SPEM develops as a physiological healing response after injury through the transdifferentiation of zymogen-secreting cells into mucinous cell metaplasia. Furthermore, studies in a long-term adrenalectomy SPEM model (129) have shown that SPEM progression is delayed in only IL-33 KO mice and that treatment of mice with recombinant IL-33 induces gastritis with a potential SPEM phenotype (65). Thus, IL-33 may be an important initiator of SPEM development. However, another study reported (66) that IL-13 KO mice are resistant to SPEM but still exhibit immune cell infiltration, possibly due to limitations of the L635-induced acute SPEM model. However, IL-33 is critical for promoting signaling through the downstream factor IL-13 to drive the induction of metaplasia (66); notably, the IL-33 and IL-13 cytokine signaling networks promote SPEM by driving M2 macrophage polarization (130) and affect mitochondrial metabolism (131). These findings were validated in mouse models and in human metaplastic tissues; one study confirmed that M2 macrophages promote SPEM expansion and intestinal metaplasia in the presence of inflammation and parietal cell shrinkage (130), and other studies validated that M2 macrophages promote SPEM progression (66, 130). In addition, IL-33 can affect many immune cell types to regulate SPEM development. ILC2s play an important role in this process, as gastric immune cell infiltration and SPEM are largely blocked by ILC2 depletion (65, 67), suggesting that these cells may be the main responders to gastric IL-33 release. However, some researchers have suggested that MCs may drive SPEM development through IL-13 (68), and IL-33 is upstream of IL-13 (66). Therefore, the IL-13-mediated activation of ILC2s or MCs may be a complementary mechanism by which immune cells induce SPEM development. In addition, it has been suggested that eosinophils contribute to SPEM (25), although some researchers believe these cells are dispensable (69). However, the depletion of eosinophils significantly reduces local IL-33-producing M2 macrophages and SPEM (25). Thus, IL-33 is a key mediator of chronic gastritis (CG) and intestinal metaplasia that promotes metaplasia and M2 macrophage-dependent eosinophilic infiltration, leading to SPEM progression (25). These data suggest that IL-33 is a potential therapeutic target for precancerous lesions of the gastrointestinal tract.
Furthermore, the metaplastic program can be perpetuated by persistent injury and chronic inflammatory stimulation, leading to the transformation to GC (36). Some researchers have shown that IL-33 can promote continuous GC cell growth by inducing the MC-dependent production and release of macrophage-attracting factors (27) or by activating the mitogen-activated protein kinase (MAPK) pathway, which includes extracellular signal-regulated kinases (ERKs) such as ERK1/2, JNK and p38 (70, 71), thereby promoting GC proliferation, differentiation, migration and apoptosis (132). Interestingly, a recent study showed that IL-33 can inhibit cyclin C (CCNC, G0/G1 transition) and cyclin B1 (CCNB1, G2/M transition) and that cysteine aspartase-3 (CASP3) activation decreases tumor cell proliferation and thus may be involved in cell proliferation in an environment- and cell type-dependent manner (72). Therefore, IL-33 may be an immunotherapy target for preventing the progression of CG to early stage GC.
Moreover, it is worth mentioning that IL-33 promotes the development of SPEM upon acute gastric mucosal injury (67). The occurrence of SPEM at the ulcer margins of regenerating gastric glands (63, 133) represents a repair process after acute mucosal injury (63, 134). Moreover, recent studies have shown that IL-33 mRNA is rapidly upregulated in acute gastric injury through TFF2 and phosphorylated ERK1/2, while the activation of ERK1/2 and the expression of the innate immunity cytokines IL1α, IL1β, and IL6 are increased (65), suggesting that IL-33 can mediate acute inflammatory injury in the stomach. IL-33 is released into the extracellular environment, and the IL-33 receptor engages a signaling pathway as a key regulator of SPEM development, thereby promoting cytokine and immune regulation in response to acute gastric epithelial injury (66). Mice lacking IL-33 or subunits of the IL-33 receptor complex fail to develop SPEM following acute parietal cell loss (66). Therefore, the gastric alarmin IL-33 plays an important role in gastric focal and diffuse injury, and alterations in IL-33 result in the development of gastric mucosal diseases.
IL-33 is involved in the occurrence of Helicobacter pylori (H. pylori)-related mucosal diseases
Recent studies have suggested that IL-33 may be an alarmin in H. pylori-positive patients (135). IL-33 is highly expressed in the mucosa of patients with H. pylori-infected gastritis (136), and ST2 is recruited into membrane rafts in response to IL-33 release by H. pylori-infected gastric epithelial cells (137), which promotes the production of tumor necrosis factor-α (TNF-α) by MCs and inhibits the proliferation of gastric epithelial cells, leading to the progression of H. pylori-associated gastritis and bacterial colonization (138). Moreover, one study found that IL-33 can regulate the phenotype and activity of MCs. IL-33 stimulates the expression of the Dectin-1, Dectin-2, RIG-I and nucleotide-binding oligomerization domain-containing protein 1 (NOD1) receptors in mature MCs to enhance and modulate the inflammatory response, perhaps stimulating MCs to release numerous proinflammatory and immunomodulatory factors and induce migratory responses during H. pylori infection (139). However, another study showed that IL-33 expression increases during acute H. pylori infection and may promote gastric mucosal regenerative activity through collagen I (140). This finding suggests that IL-33 not only represents damage in H. pylori-related gastric mucosal disease but also may be involved in tissue repair processes. IL-33 is released into the extracellular environment, where it has been shown to recruit immune cells to enhance mucosal immune defense and repair mechanisms. IL-33 attracts circulating innate immune cells by activating resident IL-1R4+ (ST2) MCs and dendritic cells (DCs) and releasing cytokines and chemoattractants (141, 142), essential processes for damage repair. Proteases released by activated MCs convert IL-33 to a more active form (143), thereby amplifying the initial effect of IL-33 and attracting more immune cells to migrate to the site of injury. Moreover, activated MCs and DCs produce IL-33 (144, 145), activating specific immune cells to release mediators that stimulate fibroblasts to initiate wound healing (146) and close the gap in the barrier (147–149). Furthermore, IL-33 production is promoted by NOD1 signaling in chronic H. pylori-infected gastritis, which prevents excessive inflammation (150). Therefore, IL-33 has a dichotomous role in H. pylori infection-related gastric mucosal disease, acting as both an accelerator of disease progression and a key factor in reversing disease exacerbation.
Furthermore, it is interesting that IL33 induces a Th2-biased response. However, the downregulation of IL33 mRNA or IL33 knockout in H. pylori-positive human gastric samples and mice with a chronic H. pylori infection may lead to Th1/Th17 immune dysregulation during subsequent pathology (65), and both conditions have been shown to evoke precancerous changes (78, 151, 152). These data suggest that chronic H. pylori-mediated inhibition of gastric IL33 may be a key event in GC progression, preventing the induction of Th2 immunity and dysregulating the local immune response to Th1/Th17 cells, thereby exacerbating carcinogenesis. Therefore, IL-33 is critical in H. pylori-associated gastritis and GC.
The expression pattern and functional role of HMGB1 in the stomach
The HMG superfamily of nucleosome-binding proteins can be divided into three subfamilies: HMGA, HMGB, and HMGN (73). HMGB is the most common gastric HMG protein, and HMGB1 has been widely studied in gastric diseases (39, 40). Initially, HMGB1 was shown to be a DAMP. Therefore, as an alarmin, HMGB1 can be actively secreted by various inflammatory cells (74), passively released by necrotic and apoptotic cells (75–77, 153, 154), and selectively released through tumor cell autophagy (75, 79). Extracellular HMGB1 activates DCs to promote their functional maturation and stimulates them to secrete HMGB1, thereby maintaining antigen-specific T-cell proliferation, preventing T-cell activation-dependent apoptosis and promoting Th1-skewed differentiation (80). In addition, HMGB1 usually binds to Toll-like receptors (TLRs) or receptors for advanced glycation end products (RAGEs) (81, 82, 155–157) to activate innate immunity (83, 84), thereby participating in tissue repair.
In the stomach under physiological conditions, HMGB1 is mainly localized in the nucleus and cytoplasm of gastric epithelial cells (39) and is involved in transcriptional regulation as a chromatin-binding factor associated with specific DNA-binding proteins (158, 159). HMGB1 is significantly upregulated in gastric mucosal diseases, and upon upregulation, HMGB1 acts as a potent chemokine, triggering the infiltration of inflammatory immune cells and increasing the progression of gastric mucosal diseases (40, 160). Therefore, HMGB1 is a key inflammatory signal in controlling gastric mucosal diseases (160, 161).
HMGB1 promotes the development of gastric mucosal diseases
HMGB1 enhances GC proliferation and metastasis
Early studies showed that HMGB1 levels are highly correlated with the depth of invasion, lymph node metastasis, tumor size and poor prognosis of GC (85). Therefore, a great deal of research has been conducted to understand the relationship between HMGB1 and GC. Initially, some researchers suggested that inhibiting HMGB1 could upregulate Mcl-1 transcription, thereby increasing autophagy and promoting GC cell apoptosis (28), which paved the way for later studies on the role of HMGB1 in GC. Since, researchers have proposed many mechanisms to explain the involvement of HMGB1 in GC proliferation and metastasis, such as the HMGB1-mediated PI3K/Akt/HIF-1α signaling pathway (162) and activation of the MEK/ERK or NF-κB signaling pathway to induce GC cell proliferation through interactions with RAGE (163, 164). Moreover, HMGB1 can also enhance the expression of cyclins, thereby inducing epithelial–mesenchymal transition and matrix metalloproteinase (MMP) expression and promoting the upregulation of RAGE, which activates the Akt/mTOR/P70S6K and ERK/P90RSK/CREB signaling pathways to regulate GC cell proliferation and migration (165). In addition, another study showed that the HMGB1/TLR4/MyD88 signaling pathway promotes GC progression and that silencing HMGB1/TLR4/MyD88 signaling in GC cells with HMGB1 siRNA significantly inhibits GC cell proliferation, migration and invasion and induces apoptosis via the NF-κB pathway (86). Moreover, recent studies have shown that exosomes released by GC cells carry HMGB1, which can induce N2 neutrophil polarization through the HMGB1/TLR4/NF-κB signaling pathway, resulting in GC cell proliferation and migration (87). These data suggest that HMGB1 may represent a new therapeutic target in GC.
HMGB1 is involved in the progression of GUs
HMGB1 can be activated and released into the extracellular environment through inflammatory stimulation, oxidative stress and other injuries (88). HMGB1 is released into the extracellular environment and stimulates cytokine production through RAGE or TLR4, which triggers inflammation and recruits leukocytes to the site of tissue damage (166). Neutrophils are the most common cell type recruited, and excess neutrophil infiltration is a negative regulator of GU healing (167). Neutrophil extravasation to the injury site increases ROS levels (168), and high ROS levels can damage the gastric mucosal barrier by oxidizing cellular proteins and lipids (169), thereby increasing permeability and leading to inflammation. Moreover, ROS production can stimulate the release of inflammatory cytokines such as TNF-α and NF-κB from macrophages (169). TNF-α blocks gastric microcirculation around the mucosa of the ulcer, further delaying ulcer healing (170).
Moreover, HMGB1 binds to RAGE or TLR4, which can inhibit the phosphorylation and proteasomal degradation of IκBα, releasing NF-κBp65 for transport to the nucleus and thereby activating the proinflammatory NF-κB pathway [6,7] and triggering the transcription of proinflammatory cytokines such as IL-1β and TNF-α (171) to exacerbate gastric ulceration (172, 173). Moreover, reducing gastric oxidative stress can interfere with NF-κBp65 binding to the promoter region of target proinflammatory cytokines and thereby inhibit the redox-sensitive NF-κB pathway (174). Therefore, inhibiting the HMGB1/RAGE pathway may protect against GU injury.
Furthermore, GU induction experiments showed that HMGB1 expression increases in response to activation of the nucleotide-binding domain and leucine-rich repeat protein 3 (NLRP3) inflammasome and NF-κBp65 (175). Previous studies have demonstrated that HMGB1 activates the NLRP3 inflammasome (176) and is involved in a variety of inflammatory diseases (177–179), which may create a positive feedback effect on GU. The NLRP3 inflammasome, an important proinflammatory mediator that is involved in ulcer pathogenesis, is activated by binding to PRRs, which increases the expression of pro-IL-1β and pro-TNF-α (95), thereby damaging the gastric mucosa. Therefore, high HMGB1 expression is one reason for delayed GU healing. When the HMGB1/NLRP3/NF-κB pathway is inhibited, the expression of IL-1β and TNF-α is downregulated, thereby promoting GU healing. However, some researchers hold the opposite view because extracellular HMGB1 and RAGE induce the migration and proliferation of vascular-associated stem cells (angioblasts), which may promote tissue regeneration (96). Moreover, studies have demonstrated that HMGB1 can reduce tissue damage in inflammatory bowel disease and other complex inflammatory diseases by regulating autophagy and apoptosis (97). Therefore, HMGB1 may have dual roles in GUs, both damaging tissue and promoting tissue repair and resisting damage.
Gastric alarmin AMPs: Defensins and cathelicidin
AMPs are an original immune mechanism, and this class of peptides and small proteins has microbicidal activity. Initially, AMPs were extensively studied in insects and other invertebrates. However, there is growing evidence that AMPs also play a crucial role in human immunity. There are two types of AMPs in human tissues and cells called defensins and cathelicidins, which are mainly produced by epithelial cells and neutrophils (92). Because of the immune effect of defensin and cathelicidin in gastric diseases, they have received increasing attention. AMPs have antimicrobial activity in the stomach, acting as a mucosal defense mechanism at key locations in the mucus layer (93).
Expression patterns and functional roles of defensins in the stomach
Defensins, including α-, β-, and θ-defensins, are cationic antimicrobial host defense peptides consisting of a characteristic β-sheet and six disulfide-linked cysteines (89, 94). In humans, the defensin subfamily mainly includes α- and β-defensins (18). Of the six human α-defensins, human neutrophil peptides 1-4 (HNP-1, HNP-2, HNP-3 and HNP-4) are mainly produced by neutrophils (90), whereas the best-studied β-defensins (HBDs, HBD1–HBD4) (91) are produced by various epithelial and mucosal cells (98). Human defensins bind to receptors (99, 180, 181), including CC chemokine receptor 2 (CCR2) (182), CCR6 (100), TLR4 (183) and TLR9, to induce chemokine-mediated immune cell migration to sites of tissue damage and thus participate in tissue damage and repair.
Defensins are localized at the cell membranes in the gastric surface epithelium (32, 184), where they participate in host antibacterial defense (29) and coordinate innate and adaptive immunity to maintain gastric mucosal homeostasis (101, 102). Defensin expression is increased in gastric mucosal diseases, including CG, GU, benign gastric polyps (BGPs) and GC (30, 103, 185), and defensins are involved in the progression of gastric mucosal diseases.
Defensins promote the development of gastric mucosal diseases
Defensins are involved in the development of GC
Recent studies have shown that α-defensin 5 (DEFA5) overexpression inhibits the development of GC (186). Mechanistically, DEFA5 induces cell cycle arrest by binding to BMI1, reducing its binding to the CDKN2a locus and upregulating the expression of the cyclin-dependent kinase inhibitors p16 and p19, therefore significantly increasing the number of cells in the G1 phase and inhibiting tumor growth (186). Therefore, DEFA5 may act as a tumor suppressor in GC. Overall, defensins play key regulatory roles in the occurrence of GC.
Defensin release caused by H. pylori-associated gastric mucosal diseases
Recent studies have shown that defensins, including HNP1-3 and HBD1-4 (30, 187–189), are highly expressed in H. pylori-associated gastritis, and this increased expression may represent a defensive response of the gastric mucosal barrier to limit infection (190). However, as bacteria and immunity begin to compete, H. pylori virulence factors activate the NLRC4 inflammasome and the NF-κB pathway, resulting in the downregulation of defensin expression (191). Moreover, H. pylori-infected cells block interferon β (IFNβ), IL-6 and IL-22 signaling to suppress defensin production (192), which results in increased colonization of the stomach by antibiotic-resistant bacteria (193). These events promote the persistence of bacteria in the gastric niche, leading to gastritis, ulcers and even cancer (188). However, another study reported unexpected results; specifically, the marked increase in defensin HNP1-3 in H. pylori-infected patients (30) formed a positive feedback cycle with neutrophils (194). This finding may relate to IL-8 release by H. pylori-infected gastric epithelial cells, which stimulates massive neutrophil infiltration (195) and the release of α-defensins (105, 109, 110), with direct toxic effects on tissue cells. Moreover, α-defensins stimulate epithelial cells to secrete IL-8 (106), which further increases gastric inflammation and exacerbates injury (107, 108). Thus, defensins have dual roles in H. pylori-associated gastritis: they are involved in protecting the gastric mucosa and can deleteriously promote the recruitment and accumulation of inflammatory cells that mediate the progression of gastritis.
In addition, there is some evidence that defensins are involved in the progression of GC. H. pylori infection induces HBD-2 and HBD-3 mRNA expression in human gastric adenocarcinoma cell lines (104, 113), but HBD-2 protein was not detected in specimens from H. pylori-negative patients (196). In conclusion, defensins may be novel targets for the treatment of H. pylori-associated gastritis and GC.
Expression pattern and functional role of cathelicidin in the stomach
Cathelicidins are a conserved family of host defense peptides that inhibit microorganisms (110) and modulate immune responses. Typically, cathelicidins are expressed in epithelial cells, lymphocytes and monocytes (110, 111). Cathelicidins use human formyl peptide receptor-like-1 (FPRL1) to induce neutrophil and monocyte migration (112, 114) or activate plasmacytoid and myeloid DCs via TLR7, 8 or 9, all of which have been shown to contribute to autoimmune disease and wound healing (197–200). In the cathelicidin family, LL-37/human cationic AMP 18 (hCAP18) is the only member expressed in humans. LL-37 is expressed in the cytoplasm of normal gastric fundic gland surface epithelial cells, chief cells and parietal cells (nuclear or membrane); however, when the gastric mucosa is damaged, hCAP18 activates the innate immune system in the stomach to participate in disease progression (31).
Cathelicidins promote the development of gastric mucosal diseases
Inhibitory effect of cathelicidins on gastric hyperplastic polyps and GC
As the only cathelicidin in humans, LL-37 is absent or expressed at very low levels in gastric hyperplastic polyps and gastric tumors (31, 201). Moreover, exogenous LL-37 can block GC cells in the G0/G1 phase and inhibit cell proliferation, and the deletion of endogenous LL-37 stimulates DNA synthesis in GC cells, indicating its antiproliferative effect. LL-37 reduces the production of cytokines such as TNF-α and IL-6 by activating p44/42 MAPK and controlling IL-32 (202), which may inhibit tumor growth. In addition, LL-37 may inhibit mitosis through a proteasome-dependent mechanism that activates bone morphogenetic protein (BMP) signaling (201). Therefore, LL-37 may function as a tumor suppressor peptide in gastric carcinogenesis. Moreover, studies have demonstrated that inhibiting LL-33 N-formyl peptide receptors (FPR1, FPR2, and FPR3) leads to the inhibition of tumor angiogenesis (31). Therefore, LL-37 is a protective factor in gastric hyperplastic polyps and GC, bringing new hope for anticancer therapeutics.
Cathelicidin deficiency promotes the development of H. pylori-associated gastric mucosal diseases
Cathelicidin is an important promoter of gastric mucosal repair and mucosal barrier protection (203). Increased production of LL-37/hCAP18 by gastric epithelial cells contributes to host mucosal defense in H. pylori-associated gastritis (204). Moreover, cathelicidin can inhibit the growth of H. pylori, destroy the bacterial biofilm, and induce morphological changes in the H. pylori membrane, thereby resisting damage (203). In addition, studies have shown that cathelicidin-knockout mice exhibit greater H. pylori colonization; increased production of the proinflammatory cytokines IL-6, IL-1β and ICAM1; and lower expression of the anti-inflammatory cytokine IL-10, which causes tissue damage (203). In addition, rat studies have shown that the host defense peptide rCRAMP promotes GU healing by directly stimulating the transforming growth factor alpha (TGF-α)-dependent transactivation of epidermal growth factor receptor (EGFR) and the downstream signaling mediators ERK1/2 in epithelial cells of the stomach (205). Therefore, cathelicidin plays an important regulatory role in H. pylori-associated gastritis and GU and may be a new target for the treatment of H. pylori-associated gastric mucosal diseases.
Alarmins function as diagnostic markers or therapeutic factors in other immune diseases
Recent studies have revealed that alarmins may be diagnostic markers and therapeutic targets (206–209). For example, the release of the active 18/21 kDa fragments of IL-33 may promote the expansion of proinflammatory signaling, a potential indicator of inflammation in response to damage (206). In addition, studies have shown that the progression of intestinal inflammation can be significantly inhibited by anti-HMGB1 neutralizing antibodies (210, 211), suggesting that host defense peptides may have a role in the development of antibacterial, anti-inflammatory and immunomodulatory therapeutics (212). Alarmins have also been used in studies of other diseases as an inflammatory factor assay (213), prognostic assessment (214), and drug therapy in patients with asthma (215, 216) or atopic dermatitis (217).
Conclusions
Previous studies have revealed the role of certain alarmins in gastric mucosal repair and have provided convincing evidence that these alarmins promote the proliferation and migration of neighboring cells, recruit various immune cells for antibacterial responses and tissue damage repair, and protect the mucosal barrier. Based on these functions, alarmins are potential therapeutic targets in inflammation and even cancer. However, continued alarmin stimulation can also lead to tissue damage and even cancer. This review provides a basic, systematic summary of gastric alarmins, which will prompt researchers to focus on the functional diversity of alarmins in gastric mucosal diseases and provide new perspectives for both treatment and prevention.
Author contributions
XL and TL conceived and designed the review. EW drafted the manuscript. ZM participated in the data investigation and analysis. JZ assisted in the preparation of the charts. BT, TL and XL edited and revised the manuscript. ST and KM revised and checked this paper. All authors contributed to the article and approved the submitted version.
Funding
This research was supported by the National Natural Science Foundation of China (81860103 and 82070536 to X.L., 82160505 and 81660098 to T.L., and 82073087 to B.T.) and the Guizhou Province International Science and Technology Cooperation (Gastroenterology) Base [Qian Ke He Platform Talents-HZJD (2021) 001 to X.L.].
Acknowledgments
We are grateful to GRW, HJ and JXA, who provided suggestions for the article and supported the daily experiments. Simultaneously, I would like to thank the Collaborative Innovation Center of Chinese Ministry of Education (2020-39) for providing funding.
Conflict of interest
The authors declare that the research was conducted in the absence of any commercial or financial relationships that could be construed as a potential conflict of interest.
Publisher’s note
All claims expressed in this article are solely those of the authors and do not necessarily represent those of their affiliated organizations, or those of the publisher, the editors and the reviewers. Any product that may be evaluated in this article, or claim that may be made by its manufacturer, is not guaranteed or endorsed by the publisher.
References
1. Oppenheim J, Yang D. Alarmins: Chemotactic Activators Immune Responses. Curr Opin Immunol (2005) 17(4):359–65. doi: 10.1016/j.coi.2005.06.002
2. Zindel J, Kubes P. Damps, pamps, and lamps in immunity and sterile inflammation. Annu Rev Pathol (2020) 15:493–518. doi: 10.1146/annurev-pathmechdis-012419-032847
3. Tang D, Kang R, Coyne CB, Zeh HJ, Lotze MT. Pamps and damps: Signal 0s that spur autophagy and immunity. Immunol Rev (2012) 249(1):158–75. doi: 10.1111/j.1600-065X.2012.01146.x
4. Agresti A, Bianchi M. Hmgb proteins and gene expression. Curr Opin Genet Dev (2003) 13(2):170–8. doi: 10.1016/s0959-437x(03)00023-6
5. Carriere V, Roussel L, Ortega N, Lacorre D, Americh L, Aguilar L, et al. Il-33, the il-1-Like cytokine ligand for St2 receptor, is a chromatin-associated nuclear factor in vivo. Proc Natl Acad Sci USA (2007) 104(1):282–7. doi: 10.1073/pnas.0606854104
6. Yang D, Han Z, Oppenheim JJ. Alarmins and immunity. Immunol Rev (2017) 280(1):41–56. doi: 10.1111/imr.12577
7. Alvarez F, Fritz J, Piccirillo C. Pleiotropic effects of il-33 on Cd4 T cell differentiation and effector functions. Front Immunol (2019) 10:522. doi: 10.3389/fimmu.2019.00522
8. Yang D, Oppenheim JJ. Antimicrobial proteins act as "Alarmins" in joint immune defense. Arthritis rheumatism (2004) 50(11):3401–3. doi: 10.1002/art.20604
9. Bianchi M. Damps, pamps and alarmins: All we need to know about danger. J Leukoc Biol (2007) 81(1):1–5. doi: 10.1189/jlb.0306164
10. Yang D, Chen Q, Su S, Zhang P, Kurosaka K, Caspi R, et al. Eosinophil-derived neurotoxin acts as an alarmin to activate the Tlr2-Myd88 signal pathway in dendritic cells and enhances Th2 immune responses. J Exp Med. (2008) 205(1):79–90. doi: 10.1084/jem.20062027
11. Yang D, de la Rosa G, Tewary P, Oppenheim JJ. Alarmins link neutrophils and dendritic cells. Trends Immunol (2009) 30(11):531–7. doi: 10.1016/j.it.2009.07.004
12. Yang D, Oppenheim JJ. Alarmins and antimicrobial immunity. Med Mycol (2009), 47(Suppl 1):S146–53. doi: 10.1080/13693780902721416
13. Wei F, Yang D, Tewary P, Li Y, Li S, Chen X, et al. The alarmin Hmgn1 contributes to antitumor immunity and is a potent immunoadjuvant. Cancer Res. (2014) 74(21):5989–98. doi: 10.1158/0008-5472.Can-13-2042
14. Saito T, Liu M, Binnie M, Sato M, Hwang D, Azad S, et al. Distinct expression patterns of alveolar "Alarmins" in subtypes of chronic lung allograft dysfunction. Am J Transplant. (2014) 14(6):1425–32. doi: 10.1111/ajt.12718
15. Vogl T, Eisenblätter M, Völler T, Zenker S, Hermann S, van Lent P, et al. Alarmin S100a8/S100a9 as a biomarker for molecular imaging of local inflammatory activity. Nat Commun (2014) 5:4593. doi: 10.1038/ncomms5593
16. Alt J, Qin X, Pulsipher A, Orb Q, Orlandi R, Zhang J, et al. Topical cathelicidin (Ll-37) an innate immune peptide induces acute olfactory epithelium inflammation in a mouse model. Int Forum Allergy Rhinol (2015) 5(12):1141–50. doi: 10.1002/alr.21634
17. van den Bosch M, Blom A, Schelbergen R, Vogl T, Roth J, Slöetjes A, et al. Induction of canonical wnt signaling by the alarmins S100a8/A9 in murine knee joints: Implications for osteoarthritis. Arthritis Rheumatol (2016) 68(1):152–63. doi: 10.1002/art.39420
18. Ganz T. Defensins: Antimicrobial peptides of innate immunity. Nat Rev Immunol (2003) 3(9):710–20. doi: 10.1038/nri1180
19. Chan J, Roth J, Oppenheim J, Tracey K, Vogl T, Feldmann M, et al. Alarmins: Awaiting a clinical response. J Clin Invest (2012) 122(8):2711–9. doi: 10.1172/jci62423
20. Kol A, Lichtman A, Finberg R, Libby P, Kurt-Jones EJ. Cutting edge: Heat shock protein (Hsp) 60 activates the innate immune response: Cd14 is an essential receptor for Hsp60 activation of mononuclear cells. J Immunol (2000) 164(1):13–7. doi: 10.4049/jimmunol.164.1.13
21. Ogawa K, Takamori Y, Suzuki K, Nagasawa M, Takano S, Kasahara Y, et al. Granulysin in human serum as a marker of cell-mediated immunity. Eur J Immunol (2003) 33(7):1925–33. doi: 10.1002/eji.200323977
22. Wargnier A, Sasportes M, Lagrange P. Granulysin: Antimicrobial molecule of innate and acquired immunity in human tuberculosis. Pathol Biol (Paris) (2005) 53:516–21. doi: 10.1016/j.patbio.2005.07.003
23. Austermann J, Friesenhagen J, Fassl S, Petersen B, Ortkras T, Burgmann J, et al. Alarmins Mrp8 and Mrp14 induce stress tolerance in phagocytes under sterile inflammatory conditions. Cell Rep (2014) 9(6):2112–23. doi: 10.1016/j.celrep.2014.11.020
24. Yang D, Tewary P, de la Rosa G, Wei F, Oppenheim J. The alarmin functions of high-mobility group proteins. Biochim Biophys Acta (2010) 1799:157–63. doi: 10.1016/j.bbagrm.2009.11.002
25. De Salvo C, Pastorelli L, Petersen CP, Buttò LF, Buela K-A, Omenetti S, et al. Interleukin 33 triggers early eosinophil-dependent events leading to metaplasia in a chronic model of gastritis-prone mice. Gastroenterology (2021) 160(1):302–16.e7. doi: 10.1053/j.gastro.2020.09.040
26. Jeong H, Lee B, Kim KH, Cho SY, Cho Y, Park J, et al. Wfdc2 promotes spasmolytic polypeptide-expressing metaplasia through the up-regulation of Il33 in response to injury. Gastroenterology (2021) 161(3):953–67.e15. doi: 10.1053/j.gastro.2021.05.058
27. Eissmann MF, Dijkstra C, Jarnicki A, Phesse T, Brunnberg J, Poh AR, et al. Il-33-Mediated mast cell activation promotes gastric cancer through macrophage mobilization. Nat Commun (2019) 10(1):2735. doi: 10.1038/s41467-019-10676-1
28. Zhan Z, Li Q, Wu P, Ye Y, Tseng H-Y, Zhang L, et al. Autophagy-mediated Hmgb1 release antagonizes apoptosis of gastric cancer cells induced by vincristine via transcriptional regulation of mcl-1. Autophagy (2012) 8(1):109–21. doi: 10.4161/auto.8.1.18319
29. Bajaj-Elliott M, Fedeli P, Smith GV, Domizio P, Maher L, Ali RS, et al. Modulation of host antimicrobial peptide (Beta-defensins 1 and 2) expression during gastritis. Gut (2002) 51(3):356–61. doi: 10.1136/gut.51.3.356
30. Isomoto H, Mukae H, Ishimoto H, Date Y, Nishi Y, Inoue K, et al. Elevated concentrations of alpha-defensins in gastric juice of patients with helicobacter pylori infection. Am J Gastroenterol (2004) 99(10):1916–23. doi: 10.1111/j.1572-0241.2004.40334.x
31. Hase K, Murakami M, Iimura M, Cole S, Horibe Y, Ohtake T, et al. Expression of ll-37 by human gastric epithelial cells as a potential host defense mechanism against helicobacter pylori. Gastroenterology (2003) 125(6):1613–25. doi: 10.1053/j.gastro.2003.08.028
32. Hamanaka Y, Nakashima M, Wada A, Ito M, Kurazono H, Hojo H, et al. Expression of human beta-defensin 2 (Hbd-2) in helicobacter pylori induced gastritis: Antibacterial effect of hbd-2 against helicobacter pylori. Gut (2001) 49(4):481–7. doi: 10.1136/gut.49.4.481
33. Schubert M, Peura DJG. Control of gastric acid secretion in health and disease. Gastroenterology (2008) 134(7):1842–60. doi: 10.1053/j.gastro.2008.05.021
34. Conti L, Annibale B, Lahner EJM. Autoimmune gastritis and gastric microbiota. Microorganisms (2020) 8(11):1827. doi: 10.3390/microorganisms8111827
35. Wang F, Meng W, Wang B, Qiao L. Helicobacter pylori-induced gastric inflammation and gastric cancer. Cancer Lett (2014) 345(2):196–202. doi: 10.1016/j.canlet.2013.08.016
36. Meyer AR, Goldenring JR. Injury, repair, inflammation and metaplasia in the stomach. J Physiol (2018) 596(17):3861–7. doi: 10.1113/JP275512
37. Kang R, Chen R, Zhang Q, Hou W, Wu S, Cao L, et al. Hmgb1 in health and disease. Mol Aspects Med (2014) 40:1–116. doi: 10.1016/j.mam.2014.05.001
38. Schmitz J, Owyang A, Oldham E, Song Y, Murphy E, McClanahan T, et al. Il-33, an interleukin-1-Like cytokine that signals via the il-1 receptor-related protein St2 and induces T helper type 2-associated cytokines. Immunity (2005) 23(5):479–90. doi: 10.1016/j.immuni.2005.09.015
39. Lin H, Hsu F, Chen W, Lee C, Lin Y, Chen Y, et al. Helicobacter pylori activates Hmgb1 expression and recruits rage into lipid rafts to promote inflammation in gastric epithelial cells. Front Immunol (2016) 7:341. doi: 10.3389/fimmu.2016.00341
40. Nadatani Y, Watanabe T, Tanigawa T, Ohkawa F, Takeda S, Higashimori A, et al. High-mobility group box 1 inhibits gastric ulcer healing through toll-like receptor 4 and receptor for advanced glycation end products. PLoS One. (2013) 8(11):e80130. doi: 10.1371/journal.pone.0080130
41. Cayrol C, Girard J. Interleukin-33 (Il-33): A nuclear cytokine from the il-1 family. Immunol Rev (2018) 281(1):154–68. doi: 10.1111/imr.12619
42. Martin N, Martin M. Interleukin 33 is a guardian of barriers and a local alarmin. Nat Immunol. (2016) 17(2):122–31. doi: 10.1038/ni.3370
43. Schwartz C, O'Grady K, Lavelle E, Fallon P. Interleukin 33: An innate alarm for adaptive responses beyond Th2 immunity-emerging roles in obesity, intestinal inflammation, and cancer. Eur J Immunol (2016) 46(5):1091–100. doi: 10.1002/eji.201545780
44. Löhning M, Stroehmann A, Coyle A, Grogan J, Lin S, Gutierrez-Ramos J, et al. T1/St2 is preferentially expressed on murine Th2 cells, independent of interleukin 4, interleukin 5, and interleukin 10, and important for Th2 effector function. Proc Natl Acad Sci USA (1998) 95(12):6930–5. doi: 10.1073/pnas.95.12.6930
45. Xu D, Chan W, Leung B, Huang F, Wheeler R, Piedrafita D, et al. Selective expression of a stable cell surface molecule on type 2 but not type 1 helper T cells. J Exp Med (1998) 187(5):787–94. doi: 10.1084/jem.187.5.787
46. Löhning M, Grogan J, Coyle A, Yazdanbakhsh M, Meisel C, Gutierrez-Ramos J, et al. T1/St2 expression is enhanced on Cd4+ T cells from schistosome egg-induced granulomas: Analysis of Th cell cytokine coexpression ex vivo. J Immunol (1999) 162(7):3882–9.
47. Baumann C, Bonilla W, Fröhlich A, Helmstetter C, Peine M, Hegazy A, et al. T-Bet- and Stat4-dependent il-33 receptor expression directly promotes antiviral Th1 cell responses. Proc Natl Acad Sci USA. (2015) 112(13):4056–61. doi: 10.1073/pnas.1418549112
48. Bonilla W, Fröhlich A, Senn K, Kallert S, Fernandez M, Johnson S, et al. The alarmin interleukin-33 drives protective antiviral Cd8+ T cell responses. Science (2012) 335(6071):984–9. doi: 10.1126/science.1215418
49. Yang Q, Li G, Zhu Y, Liu L, Chen E, Turnquist H, et al. Il-33 synergizes with tcr and il-12 signaling to promote the effector function of Cd8+ T cells. Eur J Immunol (2011) 41(11):3351–60. doi: 10.1002/eji.201141629
50. Monticelli L, Sonnenberg G, Abt M, Alenghat T, Ziegler C, Doering T, et al. Innate lymphoid cells promote lung-tissue homeostasis after infection with influenza virus. Nat Immunol (2011) 12(11):1045–54. doi: 10.1031/ni.2131
51. Mjösberg J, Trifari S, Crellin N, Peters C, van Drunen C, Piet B, et al. Human il-25- and il-33-Responsive type 2 innate lymphoid cells are defined by expression of Crth2 and Cd161. Nat Immunol (2011) 12(11):1055–62. doi: 10.1038/ni.2104
52. Neill D, Wong S, Bellosi A, Flynn R, Daly M, Langford T, et al. Nuocytes represent a new innate effector leukocyte that mediates type-2 immunity. Nature (2010) 464(7293):1367–70. doi: 10.1038/nature08900
53. Moro K, Yamada T, Tanabe M, Takeuchi T, Ikawa T, Kawamoto H, et al. Innate production of T(H)2 cytokines by adipose tissue-associated c-Kit(+)Sca-1(+) lymphoid cells. Nature (2010) 463(7280):540–4. doi: 10.1038/nature08636
54. Saenz S, Siracusa M, Perrigoue J, Spencer S, Urban J, Tocker J, et al. Il25 elicits a multipotent progenitor cell population that promotes T(H)2 cytokine responses. Nature (2010) 464(7293):1362–6. doi: 10.1038/nature08901
55. Pecaric-Petkovic T, Didichenko S, Kaempfer S, Spiegl N, Dahinden CJB. Human basophils and eosinophils are the direct target leukocytes of the novel il-1 family member il-33. Blood (2009) 113(7):1526–34. doi: 10.1182/blood-2008-05-157818
56. Suzukawa M, Iikura M, Koketsu R, Nagase H, Tamura C, Komiya A, et al. An il-1 cytokine member, il-33, induces human basophil activation Via its St2 receptor. J Immunol (2008) 181(9):5981–9. doi: 10.4049/jimmunol.181.9.5981
57. Bourgeois E, Van L, Samson M, Diem S, Barra A, Roga S, et al. The pro-Th2 cytokine il-33 directly interacts with invariant nkt and nk cells to induce ifn-gamma production. Eur J Immunol (2009) 39(4):1046–55. doi: 10.1002/eji.200838575
58. Moritz D, Rodewald H, Gheyselinck J, Klemenz R. The il-1 receptor-related T1 antigen is expressed on immature and mature mast cells and on fetal blood mast cell progenitors. J Immunol (1998) 161(9):4866–74.
59. Meeroff J, Paulsen G, Guth P. Parenteral aspirin produces and enhances gastric mucosal lesions and bleeding in rats. Am J Dig Dis (1975) 20(9):847–52. doi: 10.1007/bf01070953
60. Caldwell B, Meyer A, Weis J, Engevik A, Choi E. Chief Cell plasticity is the origin of metaplasia following acute injury in the stomach mucosa. Gut (2021) 71(6):1068–77. doi: 10.1136/gutjnl-2021-325310
61. Schmidt P, Lee J, Joshi V, Playford R, Poulsom R, Wright N, et al. Identification of a metaplastic cell lineage associated with human gastric adenocarcinoma. Lab Invest (1999) 79(6):639–46.
62. Choi E, Hendley A, Bailey J, Leach S, Goldenring J. Expression of activated ras in gastric Chief cells of mice leads to the full spectrum of metaplastic lineage transitions. Gastroenterology (2016) 150(4):918–30.e13. doi: 10.1053/j.gastro.2015.11.049
63. Engevik A, Feng R, Choi E, White S, Bertaux-Skeirik N, Li J, et al. The development of spasmolytic Polypeptide/Tff2-expressing metaplasia (Spem) during gastric repair is absent in the aged stomach. Cell Mol Gastroenterol Hepatol (2016) 2(5):605–24. doi: 10.1016/j.jcmgh.2016.05.004
64. Leushacke M, Tan S, Wong A, Swathi Y, Hajamohideen A, Tan L, et al. Lgr5-expressing Chief cells drive epithelial regeneration and cancer in the oxyntic stomach. Nat Cell Biol (2017) 19(7):774–86. doi: 10.1038/ncb3541
65. Buzzelli JN, Chalinor HV, Pavlic DI, Sutton P, Menheniott TR, Giraud AS, et al. Il33 is a stomach alarmin that initiates a skewed Th2 response to injury and infection. Cell Mol Gastroenterol Hepatol (2015) 1(2):203–21.e3. doi: 10.1016/j.jcmgh.2014.12.003
66. Petersen CP, Meyer AR, De Salvo C, Choi E, Schlegel C, Petersen A, et al. A signalling cascade of il-33 to il-13 regulates metaplasia in the mouse stomach. Gut (2018) 67(5):805–17. doi: 10.1136/gutjnl-2016-312779
67. Meyer AR, Engevik AC, Madorsky T, Belmont E, Stier MT, Norlander AE, et al. Group 2 innate lymphoid cells coordinate damage response in the stomach. Gastroenterology (2020) 159(6):2077–91.e8. doi: 10.1053/j.gastro.2020.08.051
68. Noto CN, Hoft SG, Bockerstett KA, Jackson NM, Ford EL, Vest LS, et al. Il13 acts directly on gastric epithelial cells to promote metaplasia development during chronic gastritis. Cell Mol Gastroenterol Hepatol (2022) 13(2):623–42. doi: 10.1016/j.jcmgh.2021.09.012
69. Busada JT, Ramamoorthy S, Cain DW, Xu X, Cook DN, Cidlowski JA. Endogenous glucocorticoids prevent gastric metaplasia by suppressing spontaneous inflammation. J Clin Invest (2019) 129(3):1345–58. doi: 10.1172/JCI123233
70. Sui X, Kong N, Ye L, Han W, Zhou J, Zhang Q, et al. P38 and jnk mapk pathways control the balance of apoptosis and autophagy in response to chemotherapeutic agents. Cancer Lett (2014) 344(2):174–9. doi: 10.1016/j.canlet.2013.11.019
71. Ye X, Zhao Y, Weng G, Chen Y, Wei X, Shao J, et al. Il-33-Induced jnk pathway activation confers gastric cancer chemotherapy resistance. Oncol Rep (2015) 33(6):2746–52. doi: 10.3892/or.2015.3898
72. Pisani L, Tontini G, Gentile C, Marinoni B, Teani I, Nandi N, et al. Proinflammatory interleukin-33 induces dichotomic effects on cell proliferation in normal gastric epithelium and gastric cancer. Int J Mol Sci (2021) 22(11):5792. doi: 10.3390/ijms22115792
73. Hock R, Furusawa T, Ueda T, Bustin M. Hmg chromosomal proteins in development and disease. Trends Cell Biol (2007) 17(2):72–9. doi: 10.1016/j.tcb.2006.12.001
74. Qin S, Wang H, Yuan R, Li H, Ochani M, Ochani K, et al. Role of Hmgb1 in apoptosis-mediated sepsis lethality. J Exp Med. (2006) 203(7):1637–42. doi: 10.1084/jem.20052203
75. Scaffidi P, Misteli T, Bianchi MJN. Release of chromatin protein Hmgb1 by necrotic cells triggers inflammation. Nature (2002) 418(6894):191–5. doi: 10.1038/nature00858
76. Bell C, Jiang W, Reich C, Pisetsky D. The extracellular release of Hmgb1 during apoptotic cell death. Am J Physiol Cell Physiol (2006) 291(6):C1318–25. doi: 10.1152/ajpcell.00616.2005
77. Charoonpatrapong K, Shah R, Robling A, Alvarez M, Clapp D, Chen S, et al. Hmgb1 expression and release by bone cells. J Cell Physiol. (2006) 207(2):480–90. doi: 10.1002/jcp.20577
78. Rubenstein J, Inadomi J, Scheiman J, Schoenfeld P, Appelman H, Zhang M, et al. Association between helicobacter pylori and barrett's esophagus, erosive esophagitis, and gastroesophageal reflux symptoms. Clin Gastroenterol Hepatol (2014) 12(2):239–45. doi: 10.1016/j.cgh.2013.08.029
79. Thorburn J, Horita H, Redzic J, Hansen K, Frankel A, Thorburn A, et al. Autophagy regulates selective Hmgb1 release in tumor cells that are destined to die. Cell Death Differ (2009) 16(1):175–83. doi: 10.1038/cdd.2008.143
80. Bianchi M, Manfredi A. High-mobility group box 1 (Hmgb1) protein at the crossroads between innate and adaptive immunity. Immunol Rev (2007) 220:35–46. doi: 10.1111/j.1600-065X.2007.00574.x
81. Dumitriu I, Baruah P, Bianchi M, Manfredi A, Rovere-Querini P. Requirement of Hmgb1 and rage for the maturation of human plasmacytoid dendritic cells. Eur J Immunol (2005) 35(7):2184–90. doi: 10.1002/eji.200526066
82. Sims G, Rowe D, Rietdijk S, Herbst R, Coyle A. Hmgb1 and rage in inflammation and cancer. Annu Rev Immunol (2010) 28:367–88. doi: 10.1146/annurev.immunol.021908.132603
83. Lotze M, Tracey K. High-mobility group box 1 protein (Hmgb1): Nuclear weapon in the immune arsenal. Nat Rev Immunol (2005) 5(4):331–42. doi: 10.1038/nri1594
84. Andersson U, Tracey K. Hmgb1 is a therapeutic target for sterile inflammation and infection. Annu Rev Immunol (2011) 29:139–62. doi: 10.1146/annurev-immunol-030409-101323
85. Chung H, Lee S, Kim H, Hong D, Chung J, Stroncek D, et al. Serum high mobility group box-1 (Hmgb1) is closely associated with the clinical and pathologic features of gastric cancer. J Transl Med. (2009) 7:38. doi: 10.1186/1479-5876-7-38
86. Yue Y, Zhou T, Gao Y, Zhang Z, Li L, Liu L, et al. High mobility group box 1/Toll-like receptor 4/Myeloid differentiation factor 88 signaling promotes progression of gastric cancer. Tumour Biol (2017) 39(3):1010428317694312. doi: 10.1177/1010428317694312
87. Zhang X, Shi H, Yuan X, Jiang P, Qian H, Xu W. Tumor-derived exosomes induce N2 polarization of neutrophils to promote gastric cancer cell migration. Mol Cancer (2018) 17(1):146. doi: 10.1186/s12943-018-0898-6
88. Yu Y, Tang D, Kang R. Oxidative stress-mediated Hmgb1 biology. Front Physiol. (2015) 6:93. doi: 10.3389/fphys.2015.00093
89. Selsted M, Harwig S, Ganz T, Schilling J, Lehrer R. Primary structures of three human neutrophil defensins. J Clin Invest (1985) 76(4):1436–9. doi: 10.1172/jci112121
90. Doss M, White M, Tecle T, Hartshorn K. Human defensins and ll-37 in mucosal immunity. J Leukoc Biol (2010) 87(1):79–92. doi: 10.1189/jlb.0609382
91. Suarez-Carmona M, Hubert P, Delvenne P, Herfs M. Defensins: "Simple" antimicrobial peptides or broad-spectrum molecules? Cytokine Growth Factor Rev (2015) 26(3):361–70. doi: 10.1016/j.cytogfr.2014.12.005
92. Wiesner J, Vilcinskas A. Antimicrobial peptides: The ancient arm of the human immune system. Virulence (2010) 1(5):440–64. doi: 10.4161/viru.1.5.12983
93. Padra M, Benktander J, Robinson K, Lindén S. Carbohydrate-dependent and antimicrobial peptide defence mechanisms against helicobacter pylori infections. (2019) 421:179–207. doi: 10.1007/978-3-030-15138-6_8
94. Ganz T, Selsted M, Szklarek D, Harwig S, Daher K, Bainton D, et al. Defensins. natural peptide antibiotics of human neutrophils. Curr Top Microbiol (1985) 76(4):1427–35. doi: 10.1172/jci112120
95. Ma C, Liu S, Zhang S, Xu T, Yu X, Gao Y, et al. Evidence and perspective for the role of the Nlrp3 inflammasome signaling pathway in ischemic stroke and its therapeutic potential (Review). Int J Mol Med (2018) 42(6):2979–90. doi: 10.3892/ijmm.2018.3911
96. Palumbo R, Sampaolesi M, De Marchis F, Tonlorenzi R, Colombetti S, Mondino A, et al. Extracellular Hmgb1, a signal of tissue damage, induces mesoangioblast migration and proliferation. J Cell Biol (2004) 164(3):441–9. doi: 10.1083/jcb.200304135
97. Zhu X, Messer J, Wang Y, Lin F, Cham C, Chang J, et al. Cytosolic Hmgb1 controls the cellular Autophagy/Apoptosis checkpoint during inflammation. J Clin Invest (2015) 125(3):1098–110. doi: 10.1172/jci76344
98. Wehkamp J, Schauber J, Stange E. Defensins and cathelicidins in gastrointestinal infections. Curr Opin Gastroenterol. (2007) 23(1):32–8. doi: 10.1097/MOG.0b013e32801182c2
99. Yang D, Chen Q, Chertov O, Oppenheim J. Human neutrophil defensins selectively chemoattract naive T and immature dendritic cells. J Leukoc Biol (2000) 68(1):9–14.
100. Presicce P, Giannelli S, Taddeo A, Villa M, Della Bella S. Human defensins activate monocyte-derived dendritic cells, promote the production of proinflammatory cytokines, and up-regulate the surface expression of Cd91. J Leukoc Biol (2009) 86(4):941–8. doi: 10.1189/jlb.0708412
101. Dhople V, Krukemeyer A, Ramamoorthy A. The human beta-Defensin-3, an antibacterial peptide with multiple biological functions. Biochim Biophys Acta (2006) 1758(9):1499–512. doi: 10.1016/j.bbamem.2006.07.007
102. Froy O, Chapnik N, Nussinovitch A. Defensin carriers for better mucosal immunity in the digestive system. Int J Pharm (2010) 393:263–7. doi: 10.1016/j.ijpharm.2010.04.038
103. Tanabe H, Sato T, Watari J, Maemoto A, Fujiya M, Fijiya M, et al. Functional role of metaplastic paneth cell defensins in helicobacter pylori-infected stomach. Helicobacter (2008) 13(5):370–9. doi: 10.1111/j.1523-5378.2008.00621.x
104. Bauer B, Wex T, Kuester D, Meyer T, Malfertheiner P. Differential expression of human beta defensin 2 and 3 in gastric mucosa of helicobacter pylori-infected individuals. Helicobacter (2013) 18(1):6–12. doi: 10.1111/hel.12000
105. Rodríguez-García M, Oliva H, Climent N, García F, Gatell J, Gallart T. Human immature monocyte-derived dendritic cells produce and secrete alpha-defensins 1-3. J Leukoc Biol (2007) 82(5):1143–6. doi: 10.1189/jlb.0507295
106. Van Wetering S, Mannesse-Lazeroms S, Van Sterkenburg M, Daha M, Dijkman J, Hiemstra P. Effect of defensins on interleukin-8 synthesis in airway epithelial cells. Am J Physiol (1997) 272:L888–96. doi: 10.1152/ajplung.1997.272.5.L888
107. Nozawa Y, Nishihara K, Peek R, Nakano M, Uji T, Ajioka H, et al. Identification of a signaling cascade for interleukin-8 production by helicobacter pylori in human gastric epithelial cells. Biochem Pharmacol (2002) 64(1):21–30. doi: 10.1016/s0006-2952(02)01030-4
108. Crabtree J, Wyatt J, Trejdosiewicz L, Peichl P, Nichols P, Ramsay N, et al. Interleukin-8 expression in helicobacter pylori infected, normal, and neoplastic gastroduodenal mucosa. J Clin Pathol (1994) 47(1):61–6. doi: 10.1136/jcp.47.1.61
109. Chertov O, Yang D, Howard O, Oppenheim J. Leukocyte granule proteins mobilize innate host defenses and adaptive immune responses. Immunol Rev (2000) 177:68–78. doi: 10.1034/j.1600-065x.2000.17702.x
110. Agerberth B, Charo J, Werr J, Olsson B, Idali F, Lindbom L, et al. The human antimicrobial and chemotactic peptides ll-37 and alpha-defensins are expressed by specific lymphocyte and monocyte populations. Blood (2000) 96(9):3086–93.
111. Bals R, Wang X, Zasloff M, Wilson J. The peptide antibiotic ll-37/Hcap-18 is expressed in epithelia of the human lung where it has broad antimicrobial activity at the airway surface. Proc Natl Acad Sci USA (1998) 95(16):9541–6. doi: 10.1073/pnas.95.16.9541
112. Wantha S, Alard J, Megens R, van der Does A, Döring Y, Drechsler M, et al. Neutrophil-derived cathelicidin promotes adhesion of classical monocytes. Circ Res (2013) 112(5):792–801. doi: 10.1161/circresaha.112.300666
113. Kawauchi K, Yagihashi A, Tsuji N, Uehara N, Furuya D, Kobayashi D, et al. Human beta-Defensin-3 induction in h. pylori-infected gastric mucosal tissues. World J Gastroenterol (2006) 12(36):5793–7. doi: 10.3748/wjg.v12.i36.5793
114. De Yang, Chen Q, Schmidt A, Anderson G, Wang J, Wooters J, et al. Ll-37, the neutrophil granule- and epithelial cell-derived cathelicidin, utilizes formyl peptide receptor-like 1 (Fprl1) as a receptor to chemoattract human peripheral blood neutrophils, monocytes, and T cells. J Exp Med (2000) 192(7):1069–74. doi: 10.1084/jem.192.7.1069
115. Cherry W, Yoon J, Bartemes K, Iijima K, Kita H. A novel il-1 family cytokine, il-33, potently activates human eosinophils. J Allergy Clin Immunol (2008) 121(6):1484–90. doi: 10.1016/j.jaci.2008.04.005
116. Smithgall M, Comeau M, Yoon B, Kaufman D, Armitage R, Smith D. Il-33 amplifies both Th1- and Th2-type responses through its activity on human basophils, allergen-reactive Th2 cells, inkt and nk cells. Int Immunol (2008) 20(8):1019–30. doi: 10.1093/intimm/dxn060
117. Gächter T, Moritz D, Gheyselinck J, Klemenz R. Gata-dependent expression of the interleukin-1 receptor-related T1 gene in mast cells. Mol Cell Biol (1998) 18(9):5320–31. doi: 10.1128/mcb.18.9.5320
118. Gächter T, Werenskiold A, Klemenz R. Transcription of the interleukin-1 receptor-related T1 gene is initiated at different promoters in mast cells and fibroblasts. J Biol Chem (1996) 271(1):124–9. doi: 10.1074/jbc.271.1.124
119. Moritz D, Gheyselinck J, Klemenz R. Expression analysis of the soluble and membrane-associated forms of the interleukin-1 receptor-related T1 protein in primary mast cells and fibroblasts. Hybridoma (1998) 17(2):107–16. doi: 10.1089/hyb.1998.17.107
120. Schiering C, Krausgruber T, Chomka A, Fröhlich A, Adelmann K, Wohlfert E, et al. The alarmin il-33 promotes regulatory T-cell function in the intestine. Nature (2014) 513(7519):564–8. doi: 10.1038/nature13577
121. Millar N, O'Donnell C, McInnes I, Brint E. Wounds that heal and wounds that don't - the role of the il-33/St2 pathway in tissue repair and tumorigenesis. Semin Cell Dev Biol (2017) 61:41–50. doi: 10.1016/j.semcdb.2016.08.007
122. Lott J, Sumpter T, Turnquist H. New dog and new tricks: Evolving roles for il-33 in type 2 immunity. J Leukoc Biol (2015) 97(6):1037–48. doi: 10.1189/jlb.3RI1214-595R
123. Shi Y, Evans J, Rock K. Molecular identification of a danger signal that alerts the immune system to dying cells. Nature (2003) 425(6957):516–21. doi: 10.1038/nature01991
124. Kono H, Rock K. How dying cells alert the immune system to danger. Nat Rev Immunol (2008) 8(4):279–89. doi: 10.1038/nri2215
125. Matsuo J, Kimura S, Yamamura A, Koh C, Hossain M, Heng D, et al. Identification of stem cells in the epithelium of the stomach corpus and antrum of mice. Gastroenterology (2017) 152(1):218–31.e14. doi: 10.1053/j.gastro.2016.09.018
126. Nam K, Lee H, Sousa J, Weis V, O'Neal R, Finke P, et al. Mature Chief cells are cryptic progenitors for metaplasia in the stomach. Gastroenterology (2010) 139(6):2028–37.e9. doi: 10.1053/j.gastro.2010.09.005
127. Radyk M, Burclaff J, Willet S, Mills J. Metaplastic cells in the stomach arise, independently of stem cells, Via dedifferentiation or transdifferentiation of Chief cells. Gastroenterology (2018) 154(4):839–43.e2. doi: 10.1053/j.gastro.2017.11.278
128. Weis V, Sousa J, LaFleur B, Nam K, Weis J, Finke P, et al. Heterogeneity in mouse spasmolytic polypeptide-expressing metaplasia lineages identifies markers of metaplastic progression. Gut (2013) 62(9):1270–9. doi: 10.1136/gutjnl-2012-302401
129. Busada JT, Peterson KN, Khadka S, Xu X, Oakley RH, Cook DN, et al. Glucocorticoids and androgens protect from gastric metaplasia by suppressing group 2 innate lymphoid cell activation. Gastroenterology (2021) 161(2):637–52.e4. doi: 10.1053/j.gastro.2021.04.075
130. Petersen C, Weis V, Nam K, Sousa J, Fingleton B, Goldenring J. Macrophages promote progression of spasmolytic polypeptide-expressing metaplasia after acute loss of parietal cells. Gastroenterology (2014) 146(7):1727–38.e8. doi: 10.1053/j.gastro.2014.02.007
131. Xu H, Sun L, He Y, Yuan X, Niu J, Su J, et al. Deficiency in il-33/St2 axis reshapes mitochondrial metabolism in lipopolysaccharide-stimulated macrophages. Front Immunol (2019) 10:127. doi: 10.3389/fimmu.2019.00127
132. Huang N, Cui X, Li W, Zhang C, Liu L, Li J. Il−33/St2 promotes the malignant progression of gastric cancer Via the mapk pathway. Mol Med Rep (2021) 23(5):361. doi: 10.3892/mmr.2021.12000
133. Bertaux-Skeirik N, Wunderlich M, Teal E, Chakrabarti J, Biesiada J, Mahe M, et al. Cd44 variant isoform 9 emerges in response to injury and contributes to the regeneration of the gastric epithelium. J Pathol (2017) 242(4):463–75. doi: 10.1002/path.4918
134. Farrell J, Taupin D, Koh T, Chen D, Zhao C, Podolsky D, et al. Tff2/Sp-deficient mice show decreased gastric proliferation, increased acid secretion, and increased susceptibility to nsaid injury. J Clin Invest (2002) 109(2):193–204. doi: 10.1172/jci12529
135. da Silva E, da Silva N, Rodrigues R, Adad S, de Lima Pereira S, Ribeiro B, et al. Il-33arginase-1 and treg profile appear to modulate inflammatory process in patients with chronic gastritis: May be the alarm cytokine in -positive patients. Mediators Inflamm (2019) 2019:2536781. doi: 10.1155/2019/2536781
136. Shahi H, Reiisi S, Bahreini R, Bagheri N, Salimzadeh L, Shirzad H, et al. Association between helicobacter pylori caga, Baba2 virulence factors and gastric mucosal interleukin-33 mrna expression and clinical outcomes in dyspeptic patients. Int J Mol Cell Med (2015) 4(4):227–34.
137. Kuo C, Chen C, Lo H, Feng C, Wu H, Huang M, et al. Helicobacter pylori induces il-33 production and recruits St-2 to lipid rafts to exacerbate inflammation. Cells (2019) 8(10):1290. doi: 10.3390/cells8101290
138. Lv Y, Teng Y, Mao F, Peng L, Zhang J, Cheng P, et al. Helicobacter pylori-induced il-33 modulates mast cell responses, benefits bacterial growth, and contributes to gastritis. Cell Death Dis (2018) 9(5):457. doi: 10.1038/s41419-018-0493-1
139. Agier J, Brzezińska-Błaszczyk E, Różalska S, Wiktorska M, Kozłowska E, Żelechowska P. Mast cell phenotypic plasticity and their activity under the influence of cathelicidin-related antimicrobial peptide (Cramp) and il-33 alarmins. Cell Immunol (2021) 369:104424. doi: 10.1016/j.cellimm.2021.104424
140. Gonciarz W, Krupa A, Chmiela M. Helicobacter pyloriproregenerative activity of il-33 in gastric tissue cells undergoing -induced apoptosis. Int J Mol Sci(2020) 21(5):1801. doi: 10.3390/ijms21051801
141. Hammad H, Lambrecht BJI. Barrier epithelial cells and the control of type 2 immunity. Immunity (2015) 43(1):29–40. doi: 10.1016/j.immuni.2015.07.007
142. Divekar R, Kita H. Recent advances in epithelium-derived cytokines (Il-33, il-25, and thymic stromal lymphopoietin) and allergic inflammation. Curr Opin Allergy Clin Immunol (2015) 15(1):98–103. doi: 10.1097/aci.0000000000000133
143. Lefrançais E, Duval A, Mirey E, Roga S, Espinosa E, Cayrol C, et al. Central domain of il-33 is cleaved by mast cell proteases for potent activation of group-2 innate lymphoid cells. Proc Natl Acad Sci USA (2014) 111(43):15502–7. doi: 10.1073/pnas.1410700111
144. Williams J, Tjota M, Clay B, Vander Lugt B, Bandukwala H, Hrusch C, et al. Transcription factor Irf4 drives dendritic cells to promote Th2 differentiation. Nat Commun (2013) 4:2990. doi: 10.1038/ncomms3990
145. Tjota M, Hrusch C, Blaine K, Williams J, Barrett N, Sperling A, et al. Signaling through fcrγ-associated receptors on dendritic cells drives il-33-Dependent Th2-type responses. J Allergy Clin Immunol. (2014) 134(3):706–13.e8. doi: 10.1016/j.jaci.2014.06.013
146. Hayashi H, Kawakita A, Okazaki S, Murai H, Yasutomi M, Ohshima Y. Il-33 enhanced the proliferation and constitutive production of il-13 and il-5 by fibrocytes. Biomed Res Int (2014) 2014:738625. doi: 10.1155/2014/738625
147. Komai-Koma M, Gilchrist D, McKenzie A, Goodyear C, Xu D, Liew F. Il-33 activates B1 cells and exacerbates contact sensitivity. J Immunol (2011) 186(4):2584–91. doi: 10.4049/jimmunol.1002103
148. Ahmed A, Koma M. Interleukin-33 triggers B1 cell expansion and its release of Monocyte/Macrophage chemoattractants and growth factors. Scand J Immunol (2015) 82(2):118–24. doi: 10.1111/sji.12312
149. Sattler S, Ling G, Xu D, Hussaarts L, Romaine A, Zhao H, et al. Il-10-Producing regulatory b cells induced by il-33 (Breg(Il-33)) effectively attenuate mucosal inflammatory responses in the gut. J Autoimmun (2014) 50:107–22. doi: 10.1016/j.jaut.2014.01.032
150. Tran L, Tran D, De Paoli A, D'Costa K, Creed S, Ng G, et al. Nod1 is required for helicobacter pylori induction of il-33 responses in gastric epithelial cells. Cell Microbiol (2018) 20(5):e12826. doi: 10.1111/cmi.12826
151. Csendes A, Smok G, Cerda G, Burdiles P, Mazza D, Csendes P. Prevalence of helicobacter pylori infection in 190 control subjects and in 236 patients with gastroesophageal reflux, erosive esophagitis or barrett's esophagus. Dis Esophagus (1997) 10(1):38–42. doi: 10.1093/dote/10.1.38
152. Kao S, Chen W, Hsu P, Chuah S, Lu C, Lai K, et al. The frequencies of gastroesophageal and extragastroesophageal symptoms in patients with mild erosive esophagitis, severe erosive esophagitis, and barrett's esophagus in Taiwan. Gastroenterol Res Pract (2013) 2013:480325. doi: 10.1155/2013/480325
153. Ito N, DeMarco R, Mailliard R, Han J, Rabinowich H, Kalinski P, et al. Cytolytic cells induce Hmgb1 release from melanoma cell lines. J Leukoc Biol (2007) 81(1):75–83. doi: 10.1189/jlb.0306169
154. Vande Walle L, Kanneganti T, Lamkanfi M. Hmgb1 release by inflammasomes. Virulence. (2011) 2(2):162–5. doi: 10.4161/viru.2.2.15480
155. Rauvala H, Rouhiainen A. Rage as a receptor of Hmgb1 (Amphoterin): Roles in health and disease. Curr Mol Med (2007) 7(8):725–34. doi: 10.2174/156652407783220750
156. Kokkola R, Andersson A, Mullins G, Ostberg T, Treutiger C, Arnold B, et al. Rage is the major receptor for the proinflammatory activity of Hmgb1 in rodent macrophages. Scand J Immunol (2005) 61(1):1–9. doi: 10.1111/j.0300-9475.2005.01534.x
157. Ellerman J, Brown C, de Vera M, Zeh H, Billiar T, Rubartelli A, et al. Masquerader: High mobility group box-1 and cancer. Clin Cancer Res (2007) 13(10):2836–48. doi: 10.1158/1078-0432.Ccr-06-1953
158. Takaki W, Konishi H, Matsubara D, Shoda K, Arita T, Kataoka S, et al. Role of extracellular high-mobility group box-1 as a therapeutic target of gastric cancer. Int J Mol Sci (2022) 23(6):3264. doi: 10.3390/ijms23063264
159. Müller S, Scaffidi P, Degryse B, Bonaldi T, Ronfani L, Agresti A, et al. New embo members' review: The double life of Hmgb1 chromatin protein: Architectural factor and extracellular signal. EMBO J (2001) 20(16):4337–40. doi: 10.1093/emboj/20.16.4337
160. Badr A, El-Orabi N, Ali R. The implication of the crosstalk of Nrf2 with noxs, and Hmgb1 in ethanol-induced gastric ulcer: Potential protective effect is afforded by raspberry ketone. PLoS One (2019) 14(8):e0220548. doi: 10.1371/journal.pone.0220548
161. Arab H, Salama S, Eid A, Kabel A, Shahin N. Targeting mapks, nf-Kb, and Pi3k/Akt pathways by methyl palmitate ameliorates ethanol-induced gastric mucosal injury in rats. J Cell Physiol (2019) 234(12):22424–38. doi: 10.1002/jcp.28807
162. Zhou P, Zheng Z, Wan T, Wu J, Liao C, Sun X. Vitexin inhibits gastric cancer growth and metastasis through Hmgb1-mediated inactivation of the Pi3k/Akt/Mtor/Hif-1α signaling pathway. J Gastric Cancer. (2021) 21(4):439–56. doi: 10.5230/jgc.2021.21.e40
163. Zhang Q, Wu L, Zhang T, Han Y, Lin X. Autophagy-mediated Hmgb1 release promotes gastric cancer cell survival via rage activation of extracellular signal-regulated kinases 1/2. Oncol Rep (2015) 33(4):1630–8. doi: 10.3892/or.2015.3782
164. Zhang J, Kou Y, Zhu J, Chen W, Li S. Knockdown of Hmgb1 inhibits growth and invasion of gastric cancer cells through the nf-Kb pathway in vitro and in vivo. Int J Oncol (2014) 44(4):1268–76. doi: 10.3892/ijo.2014.2285
165. Tang T, Wang S, Cai T, Cheng Z, Meng Y, Qi S, et al. High mobility group box 1 regulates gastric cancer cell proliferation and migration via rage-Mtor/Erk feedback loop. J Cancer (2021) 12(2):518–29. doi: 10.7150/jca.51049
166. He S, Cheng J, Feng X, Yu Y, Tian L, Huang Q. The dual role and therapeutic potential of high-mobility group box 1 in cancer. Oncotarget (2017) 8(38):64534–50. doi: 10.18632/oncotarget.17885
167. Shimizu N, Watanabe T, Arakawa T, Fujiwara Y, Higuchi K, Kuroki T. Pentoxifylline accelerates gastric ulcer healing in rats: Roles of tumor necrosis factor alpha and neutrophils during the early phase of ulcer healing. Digestion (2000) 61(3):157–64. doi: 10.1159/000007752
168. Lee M, Shin I, Jeon W, Seo C, Ha H, Huh J, et al. Protective effect of bojungikki-tang, a traditional herbal formula, against alcohol-induced gastric injury in rats. J Ethnopharmacol (2012) 142(2):346–53. doi: 10.1016/j.jep.2012.04.043
169. Bhattacharyya A, Chattopadhyay R, Mitra S, Crowe SE. Oxidative stress: An essential factor in the pathogenesis of gastrointestinal mucosal diseases. Physiol Rev (2014) 94(2):329–54. doi: 10.1152/physrev.00040.2012
170. Konturek P, Brzozowski T, Burnat G, Szlachcic A, Koziel J, Kwiecien S, et al. Gastric ulcer healing and stress-lesion preventive properties of pioglitazone are attenuated in diabetic rats. J Physiol Pharmacol (2010) 61(4):429–36.
171. Suryavanshi S, Kulkarni Y. Nf-Kβ: A potential target in the management of vascular complications of diabetes. Front Pharmacol (2017) 8:798. doi: 10.3389/fphar.2017.00798
172. Aziz R, Siddiqua A, Shahzad M, Shabbir A, Naseem N. Biomedecine p, et al. oxyresveratrol ameliorates ethanol-induced gastric ulcer via downregulation of il-6, tnf-A, nf-ĸb, and cox-2 levels, and upregulation of tff-2 levels. Biomed Pharmacother (2019) 110:554–60. doi: 10.1016/j.biopha.2018.12.002
173. Arab H, Salama S, Omar H, Arafa E-S, Maghrabi I. Diosmin protects against ethanol-induced gastric injury in rats: Novel anti-ulcer actions. PLoS One (2015) 10(3):e0122417. doi: 10.1371/journal.pone.0122417
174. Tse H, Milton M, Piganelli J. Mechanistic analysis of the immunomodulatory effects of a catalytic antioxidant on antigen-presenting cells: Implication for their use in targeting oxidation-reduction reactions in innate immunity. Free Radic Biol Med (2004) 36(2):233–47. doi: 10.1016/j.freeradbiomed.2003.10.029
175. Alzokaky A, Abdelkader E, El-Dessouki A, Khaleel S, Raslan N. C-phycocyanin protects against ethanol-induced gastric ulcers in rats: Role of Hmgb1/Nlrp3/Nf-Kb pathway. Basic Clin Pharmacol Toxicol (2020) 127(4):265–77. doi: 10.1111/bcpt.13415
176. Chi W, Chen H, Li F, Zhu Y, Yin W, Zhuo Y. Hmgb1 promotes the activation of Nlrp3 and caspase-8 inflammasomes Via nf-Kb pathway in acute glaucoma. J Neuroinflamm (2015) 12:137. doi: 10.1186/s12974-015-0360-2
177. Duewell P, Kono H, Rayner K, Sirois C, Vladimer G, Bauernfeind F, et al. Nlrp3 inflammasomes are required for atherogenesis and activated by cholesterol crystals. Nature (2010) 464(7293):1357–61. doi: 10.1038/nature08938
178. Villani A, Lemire M, Fortin G, Louis E, Silverberg M, Collette C, et al. Common variants in the Nlrp3 region contribute to crohn's disease susceptibility. Nat Genet (2009) 41(1):71–6. doi: 10.1038/ng.285
179. Lee H, Kim J, Kim H, Shong M, Ku B, Jo E. Upregulated Nlrp3 inflammasome activation in patients with type 2 diabetes. Diabetes (2013) 62(1):194–204. doi: 10.2337/db12-0420
180. Hubert P, Herman L, Maillard C, Caberg J, Nikkels A, Pierard G, et al. Defensins induce the recruitment of dendritic cells in cervical human papillomavirus-associated (Pre)Neoplastic lesions formed in vitro and transplanted in vivo. FASEB J (2007) 21(11):2765–75. doi: 10.1096/fj.06-7646com
181. Cappelletti M, Presicce P, Calcaterra F, Mavilio D, Della Bella S. Bright expression of Cd91 identifies highly activated human dendritic cells that can be expanded by defensins. Immunology (2015) 144(4):661–7. doi: 10.1111/imm.12418
182. Yang D, Chertov O, Bykovskaia S, Chen Q, Buffo M, Shogan J, et al. Beta-defensins: Linking innate and adaptive immunity through dendritic and T cell Ccr6. Science (1999) 286(5439):525–8. doi: 10.1126/science.286.5439.525
183. Yang Y, Jiang Y, Yin Q, Liang H, She R. Chicken intestine defensins activated murine peripheral blood mononuclear cells through the Tlr4-Nf-Kappab pathway. Vet Immunol Immunopathol (2010) 133(1):59–65. doi: 10.1016/j.vetimm.2009.07.008
184. Cunliffe R. Alpha-defensins in the gastrointestinal tract. Mol Immunol (2003) 40(7):463–7. doi: 10.1016/s0161-5890(03)00157-3
185. Nishi Y, Isomoto H, Mukae H, Ishimoto H, Wen C, Wada A, et al. Concentrations of alpha- and beta-defensins in gastric juice of patients with various gastroduodenal diseases. World J Gastroenterol (2005) 11(1):99–103. doi: 10.3748/wjg.v11.i1.99
186. Wu Z, Ding Z, Cheng B, Cui Z. The inhibitory effect of human Defa5 in growth of gastric cancer by targeting Bmi1. Cancer Sci (2021) 112(3):1075–83. doi: 10.1111/cas.14827
187. Boughan PK, Argent RH, Body-Malapel M, Park J-H, Ewings KE, Bowie AG, et al. Nucleotide-binding oligomerization domain-1 and epidermal growth factor receptor: Critical regulators of beta-defensins during helicobacter pylori infection. J Biol Chem (2006) 281(17):11637–48. doi: 10.1074/jbc.M510275200
188. Patel SR, Smith K, Letley DP, Cook KW, Memon AA, Ingram RJM, et al. Helicobacter pylori downregulates expression of human B-defensin 1 in the gastric mucosa in a type iv secretion-dependent fashion. Cell Microbiol (2013) 15(12):2080–92. doi: 10.1111/cmi.12174
189. Otte JM, Neumann HM, Brand S, Schrader H, Schmidt WE, Schmitz F. Expression of beta-defensin 4 is increased in human gastritis. Eur J Clin Invest (2009) 39(2):126–38. doi: 10.1111/j.1365-2362.2008.02071.x
190. Taha AS, Faccenda E, Angerson WJ, Balsitis M, Kelly RW. Gastric epithelial anti-microbial peptides–histological correlation and influence of anatomical site and peptic ulcer disease. Dig Liver Dis (2005) 37(1):51–6. doi: 10.1016/j.dld.2004.07.019
191. Semper R, Vieth M, Gerhard M, Mejías-Luque R. Helicobacter pylori exploits the Nlrc4 inflammasome to dampen host defenses. J Immunol (2019) 203(8):2183–93. doi: 10.4049/jimmunol.1900351
192. Morey P, Pfannkuch L, Pang E, Boccellato F, Sigal M, Imai-Matsushima A, et al. Helicobacter pylori depletes cholesterol in gastric glands to prevent interferon gamma signaling and escape the inflammatory response. Gastroenterology (2018) 154(5):1391–404.e9. doi: 10.1053/j.gastro.2017.12.008
193. Zhang X, Jiang A, Qi B, Yu H, Xiong Y, Zhou G, et al. Secretion expression of human neutrophil peptide 1 (Hnp1) in pichia pastoris and its functional analysis against antibiotic-resistant helicobacter pylori. Appl Microbiol Biotechnol (2018) 102(11):4817–27. doi: 10.1007/s00253-018-8982-9
194. Soylu O, Ozturk Y, Ozer E. Alpha-defensin expression in the gastric tissue of children with helicobacter pylori-associated chronic gastritis: An immunohistochemical study. J Pediatr Gastroenterol Nutr (2008) 46(4):474–7. doi: 10.1097/MPG.0b013e31815a9923
195. Okrent D, Lichtenstein A, Ganz T. Direct cytotoxicity of polymorphonuclear leukocyte granule proteins to human lung-derived cells and endothelial cells. Am Rev Respir Dis (1990) 141(1):179–85. doi: 10.1164/ajrccm/141.1.179
196. Uehara N, Yagihashi A, Kondoh K, Tsuji N, Fujita T, Hamada H, et al. Human beta-Defensin-2 induction in helicobacter pylori-infected gastric mucosal tissues: Antimicrobial effect of overexpression. J Med Microbiol (2003) 52(Pt 1):41–5. doi: 10.1099/jmm.0.04985-0
197. Chen K, Liu M, Liu Y, Wang C, Yoshimura T, Gong W, et al. Signal relay by cc chemokine receptor 2 (Ccr2) and formylpeptide receptor 2 (Fpr2) in the recruitment of monocyte-derived dendritic cells in allergic airway inflammation. J Biol Chem (2013) 288(23):16262–73. doi: 10.1074/jbc.M113.450635
198. Kin N, Chen Y, Stefanov E, Gallo R, Kearney J. Cathelin-related antimicrobial peptide differentially regulates T- and b-cell function. Eur J Immunol (2011) 41(10):3006–16. doi: 10.1002/eji.201141606
199. Gregorio J, Meller S, Conrad C, Di Nardo A, Homey B, Lauerma A, et al. Plasmacytoid dendritic cells sense skin injury and promote wound healing through type I interferons. J Exp Med (2010) 207(13):2921–30. doi: 10.1084/jem.20101102
200. Ganguly D, Chamilos G, Lande R, Gregorio J, Meller S, Facchinetti V, et al. Self-Rna-Antimicrobial peptide complexes activate human dendritic cells through Tlr7 and Tlr8. J Exp Med (2009) 206(9):1983–94. doi: 10.1084/jem.20090480
201. Wu W, Sung J, To K, Yu L, Li H, Li Z, et al. The host defense peptide ll-37 activates the tumor-suppressing bone morphogenetic protein signaling Via inhibition of proteasome in gastric cancer cells. J Cell Physiol (2010) 223(1):178–86. doi: 10.1002/jcp.22026
202. Choi K, Napper S, Mookherjee N. Human cathelicidin ll-37 and its derivative ig-19 regulate interleukin-32-Induced inflammation. Immunology (2014) 143(1):68–80. doi: 10.1111/imm.12291
203. Zhang L, Wu W, Gallo R, Fang E, Hu W, Ling T, et al. Critical role of antimicrobial peptide cathelicidin for controlling helicobacter pylori survival and infection. J Immunol (2016) 196(4):1799–809. doi: 10.4049/jimmunol.1500021
204. Wu W, Wang G, Coffelt S, Betancourt A, Lee C, Fan D, et al. Emerging roles of the host defense peptide ll-37 in human cancer and its potential therapeutic applications. Int J Cancer (2010) 127(8):1741–7. doi: 10.1002/ijc.25489
205. Yang Y, Wu W, Tai E, Wong H, Lam E, So W, et al. The cationic host defense peptide rcramp promotes gastric ulcer healing in rats. J Pharmacol Exp Ther (2006) 318(2):547–54. doi: 10.1124/jpet.106.102467
206. Perez F, Ruera CN, Miculan E, Carasi P, Dubois-Camacho K, Garbi L, et al. Il-33 alarmin and its active proinflammatory fragments are released in small intestine in celiac disease. Front Immunol (2020) 11:581445. doi: 10.3389/fimmu.2020.581445
207. Du J, Guan W, Shen X. Il-33 in gastric metaplasia-implications for therapeutic targets. Gastroenterology (2021) 160(7):2629–30. doi: 10.1053/j.gastro.2021.01.231
208. Ngo Thi Phuong N, Palmieri V, Adamczyk A, Klopfleisch R, Langhorst J, Hansen W, et al. Il-33 drives expansion of type 2 innate lymphoid cells and regulatory T cells and protects mice from severe, acute colitis. Front Immunol (2021) 12:669787. doi: 10.3389/fimmu.2021.669787
209. Singh A, Gupta N, Khandakar H, Kaushal S, Seth A, Pandey RM, et al. Autophagy-associated hmgb-1 as a novel potential circulating non-invasive diagnostic marker for detection of urothelial carcinoma of bladder. Mol Cell Biochem (2022) 477(2):493–505. doi: 10.1007/s11010-021-04299-8
210. Chen L, Li J, Ye Z, Sun B, Wang L, Chen Y, et al. Anti-high mobility group box 1 neutralizing-antibody ameliorates dextran sodium sulfate colitis in mice. Front Immunol (2020) 11:585094. doi: 10.3389/fimmu.2020.585094
211. Yang H, Wang H, Andersson U. Targeting inflammation driven by Hmgb1. Front Immunol (2020) 11:484. doi: 10.3389/fimmu.2020.00484
212. Zhang L, Wei X, Zhang R, Petitte JN, Si D, Li Z, et al. Design and development of a novel peptide for treating intestinal inflammation. Front Immunol (2019) 10:1841. doi: 10.3389/fimmu.2019.01841
213. Gruen DS, Brown JB, Guyette FX, Vodovotz Y, Johansson PI, Stensballe J, et al. Prehospital plasma is associated with distinct biomarker expression following injury. JCI Insight (2020) 5(8):e135350. doi: 10.1172/jci.insight.135350
214. Krychtiuk KA, Stojkovic S, Lenz M, Brekalo M, Huber K, Wojta J, et al. Predictive value of low interleukin-33 in critically ill patients. Cytokine (2018) 103:109–13. doi: 10.1016/j.cyto.2017.09.017
215. Kelsen SG, Agache IO, Soong W, Israel E, Chupp GL, Cheung DS, et al. Astegolimab (Anti-St2) efficacy and safety in adults with severe asthma: A randomized clinical trial. J Allergy Clin Immunol (2021) 148(3):790–8. doi: 10.1016/j.jaci.2021.03.044
216. Wechsler ME, Ruddy MK, Pavord ID, Israel E, Rabe KF, Ford LB, et al. Efficacy and safety of itepekimab in patients with moderate-to-Severe asthma. N Engl J Med (2021) 385(18):1656–68. doi: 10.1056/NEJMoa2024257
Keywords: gastric alarmin, IL-33, high mobility group (HMG) family, antimicrobial peptides (AMPs), defensin, cathelicidin, gastric mucosal diseases
Citation: Wu E, Zhu J, Ma Z, Tuo B, Terai S, Mizuno K, Li T and Liu X (2022) Gastric alarmin release: A warning signal in the development of gastric mucosal diseases. Front. Immunol. 13:1008047. doi: 10.3389/fimmu.2022.1008047
Received: 31 July 2022; Accepted: 14 September 2022;
Published: 06 October 2022.
Edited by:
Jaime Hook, Icahn School of Medicine at Mount Sinai, United StatesReviewed by:
Justyna Agier, Medical University of Lodz, PolandJonathan Busada, West Virginia University, United States
Copyright © 2022 Wu, Zhu, Ma, Tuo, Terai, Mizuno, Li and Liu. This is an open-access article distributed under the terms of the Creative Commons Attribution License (CC BY). The use, distribution or reproduction in other forums is permitted, provided the original author(s) and the copyright owner(s) are credited and that the original publication in this journal is cited, in accordance with accepted academic practice. No use, distribution or reproduction is permitted which does not comply with these terms.
*Correspondence: Xuemei Liu, b25seW9uZWxpdXh1ZW1laUAxNjMuY29t; Taolang Li, MDA3ODAyOUBzaW5hLmNvbQ==