- 1Wesfarmers Centre of Vaccines and Infectious Diseases, Telethon Kids Institute, Perth, WA, Australia
- 2Menzies School of Health Research Charles, Darwin University, Darwin, NT, Australia
- 3School of Medicine, University of Western Australia, Perth, WA, Australia
- 4Research School of Population Health, Australian National University, Canberra, ACT, Australia
- 5Centre for Child Health Research, University of Western Australia, Perth, WA, Australia
Introduction: Children in low-mid income countries, and First Nations children in high-income countries, experience disproportionately high rates of Streptococcus pneumoniae and Haemophilus influenzae infections and diseases including pneumonia and otitis media. We previously observed that infants from Papua New Guinea had no evidence of waning maternal immunity for H. influenzae-specific antibodies. In this study, we assessed S. pneumoniae and H. influenzae antibody titres in Australian First Nation mothers and infants to determine antigen-specific antibody ontogenies and whether H. influenzae antibody titres in infants were due to low maternal antibody titres or lack of placental transfer.
Methods: Breast milk, infant nasopharyngeal swabs and ear assessment data were collected 1-, 2-, 7-months post-birth as well as maternal, cord and 7-month-old infant sera, from 85 Australian Aboriginal and Torres Strait Islander mother-infant pairs. Serum IgG and breast milk IgG and IgA antibody titres to S. pneumoniae antigens (PspA1, PspA2, CbpA, Ply) and H. influenzae antigens (PD, ChimV4, OMP26, rsPilA) were measured.
Results: IgG titres in maternal and cord sera were similar for all antigens, except Ply (higher in cord; p=0.004). Sera IgG titres at 7-months of age were lower than cord sera IgG titres for all S. pneumoniae antigens (p<0.001). Infant sera IgG titres were higher than cord sera for H. influenzae PD (p=0.029), similar for OMP26 (p=0.817) and rsPilA (p=0.290), and lower for ChimV4 (p=0.004). Breast milk titres were similar for all antigens at 1, 2 and 7-months except OMP26 IgA (lower at 7-months than 1-month; p=0.035), PspA2 IgG (p=0.012) and Ply IgG that increased by 7-months (p=0.032). One third of infants carried nontypeable Haemophilus influenzae (NTHi), 45% carried S. pneumoniae and 52% had otitis media (OM) observed at least once over the 7-months. 73% of infants who carried either S. pneumoniae or NTHi, also had otitis media observed.
Conclusions: Similarities between maternal and cord IgG titres, and absence of waning, support a lack of maternal H. influenzae IgG antibodies available for cross-placental transfer. Increased maternal anti-PD IgG could offer some protection from early carriage with NTHi, and maternal immunisation strategies should be considered for passive-active immunisation of infants to protect against S. pneumoniae and H. influenzae diseases.
Trial registration: ClinicalTrials.gov NCT00714064 and NCT00310349.
Introduction
Early life protection from infection is generally provided by the trans-placental transfer of IgG antibodies from mother to infant. These antibodies circulate in the infant’s bloodstream and decline over the first 6- to 12-months of life (1, 2). Passive transfer of maternal IgA antibodies through colostrum and breast milk also occurs when the child is breastfed. These antibodies are supplied for the duration of breastfeeding and protect against infections of the gastrointestinal and respiratory tracts (3, 4). The provision of maternal antibodies offers valuable protection to infants too young to be vaccinated or where vaccines are not yet available. Several maternal vaccines are available or in development, offering protection for infants against respiratory pathogens including influenza (5), pertussis (6), respiratory syncytial virus (RSV) (7), and SARS-CoV-2 (8).
Streptococcus pneumoniae and Haemophilus influenzae infections cause otitis media (OM), pneumonia and bacteraemia, which are major contributors to childhood mortality and morbidity, despite the widespread use of pneumococcal conjugate vaccines (PCVs) and the H influenzae type b (Hib) vaccine (9). The burden of S. pneumoniae and H. influenzae disease is particularly high in infants (10), the elderly (11, 12), in populations of low-middle-income countries, and for First Nation people in high-income countries (13). Unfortunately, current vaccines offer protection against a limited number of S. pneumoniae serotypes (14), and there is no licensed vaccine for nontypeable H. influenzae (NTHi), which is now the leading cause of H. influenzae infections (15). Thus, serotype-independent vaccines are being developed including whole-cell and protein-based subunit-vaccines for S. pneumoniae (16) and H. influenzae (17, 18, 19, 20) in attempt to offer broader protection against these bacterial pathogens.
Measuring the concentrations of circulating antibodies against putative vaccine candidate antigens following natural pathogen exposure provides a valuable indication of the antigen immunogenicity and in terms of maternal vaccines, the transplacental antigen-specific antibody transfer efficiency. We previously observed the natural waning of sera IgG to S. pneumoniae, but not H. influenzae, protein antigens between birth and 9 months of age among Papua New Guinean (PNG) infants (21). However, it was unclear due to lack of appropriately matched samples whether the lack of observed waning simply related to low baseline levels due to either (i) low maternal levels or (ii) poor transplacental transfer.
Antibody waning to S. pneumoniae antigens has also been shown in Finnish, Filipino, and North American children (22, 23, 24). Efficient cross-placental transfer of S. pneumoniae anti-Ply and anti-PspA antibodies has been observed in a PNG study (25). Maternal transfer of H. influenzae specific antibodies, to our knowledge, has not been investigated before. This is critical to understanding the waning dynamics. A cross-sectional study in China revealed that antibody titres to H. influenzae protein antigens Protein D (PD) and Protein 6 are at their lowest in adults of childbearing age (26), suggesting that the lack of H. influenzae specific antibody waning in infants may be due to low maternal titres available for placental transfer.
Understanding maternal-infant antibody titres to vaccine candidate antigens will help provide information for vaccine strategies for the development of future S. pneumoniae and H. influenzae vaccines. For example, increasing maternal titres of protective antibody through maternal immunisation may help provide enhanced protection against S. pneumoniae and H. influenzae infections from birth. While there is insufficient evidence on the efficacy of maternal pneumococcal polysaccharide vaccines providing protection against S. pneumoniae carriage and disease in infants (27, 28), protein-based vaccines such as pertussis are now routinely administered during pregnancy to protect infants in the first months of life (6). Thus, it is possible that future maternal immunisation strategies for protein-based S. pneumoniae and H. influenzae vaccines are viable options.
The level of maternal antibody waning in an infant is dependent on the initial maternal transfer titre. It is important to understand maternal transfer and subsequent waning of maternal antibodies when considering maternal vaccination strategies (29). To investigate further the maternal transfer of naturally induced antibody to S. pneumoniae antigens and H. influenzae antigens, antigen-specific IgG titres were compared between maternal and cord blood sera collected at delivery, and infant sera collected at 7-months of age, from Australian Aboriginal and Torres Strait Islander families. Antigen-specific IgA and IgG titres were also measured in breast milk samples collected from the mothers at 1-, 2- and 7-months post-delivery. Here we aimed to understand the potential protection afforded by systemic and mucosal protein specific antibody against colonisation and disease, the relationships between antibody titres, and nasopharyngeal colonisation, and the development of OM in the first 7-months. While these vaccine candidates are still in early clinical development, it is important to understand whether antibody to these candidate antigens can be passed from mother to infant.
Methods
Study cohort
This was a retrospective analysis of samples and clinical data collected from Aboriginal and Torres Strait Islander women and infants in the Northern Territory during the PneuMum randomized controlled trial (2006-2011) (30). Briefly, women in the PneuMum study were randomized to receive the 23-valent pneumococcal polysaccharide vaccine (23vPPV) 1) either while pregnant, 2) within 72 hours of delivering their baby, or 3) at the end of the study (offered only). All infants were offered their routine PCV as recommended at 2-, 4- and 6-months of age (7vPCV from June 2001 or 10vPHiD-CV from October 2009) (30). By the 7-month visit, 72% of infants had received 2 PCV doses and 52% had received 3 doses. Two infants providing samples for this antibody study received 10vPHiD-CV, which contains PD and were thus excluded from the sera IgG PD analysis.
Ethics approval and community involvement
Approval was received from the Human Research Ethics Committee of the Northern Territory Department of Health and Menzies School of Health Research HREC and the Aboriginal Ethics Sub-Committee (HREC 2020-3800). Extensive community consultation with the PneuMum Indigenous Reference Group in the Northern Territory, the Menzies Child Health Division First Nations Reference group, the Kulunga Aboriginal Unit at Telethon Kids Institute, and the Ear Health Aboriginal Community Advisory Group in Western Australia ensured culturally appropriate processes were maintained for the additional analysis of collected specimens.
Sample collection and processing
Maternal venous blood and cord blood samples were collected at birth, and infant blood samples were collected at 7-months of age (30). Sera was separated from blood and stored at -70°C. Manually expressed breast milk was collected at 1-, 2- and 7-months post-birth and transported at 4°C to the laboratory and centrifuged at 10,000 g for 30 minutes at 4°C to remove fat, with supernatant stored at -70°C until required. Nasopharyngeal swabs (NPS) were collected from the mother on the day of birth, and from the infant at 1-, 2- and 7-months of age. Swabs were placed in skim milk, tryptone, glucose, and glycerol (STGG) transport medium, vortexed and frozen at -70°C until used for standard microbiological culture (31). All H. influenzae isolated from these swabs were NTHi (30). Aliquots of maternal, cord and infant sera and breast milk were shipped on dry ice from the Menzies School of Health Research in Darwin, Northern Territory to the laboratories at the Telethon Kids Institute in Perth, Western Australia for analysis of antibody titres.
Ear assessments
Infant’s ears were assessed by trained research nurses (and reviewed by an independent assessor) using tympanometry and otoscopy at 1-, 2- and 7-months of age and scored for presence or absence of middle ear infection (OM) in the infants’ worst ear according to recommended guidelines for clinical practice in Aboriginal and Torres Strait Islander children (30, 32). Outcomes of clinical phenotypes of OM e.g. acute OM or OM with effusion are not presented. Based on these diagnoses, sub-analyses were conducted comparing infants with OM observed at any study visit over the 7-months and those without.
S. pneumoniae and H. influenzae antigens used in this study
S. pneumoniae antigens PspA1, PspA2, CbpA and Ply were prepared as previously described (33). The sequence for PD was selected based on H. influenzae strain 772 (34). Pure, stable and endotoxin free recombinant PD was produced by the Protein Expression Facility (The University of Queensland) according to optimised protocols and adapted from methods used previously (33). H. influenzae antigens OMP26, rsPilA and ChimV4 were prepared as described previously (21).
Measurement of IgG and IgA against S. pneumoniae antigens PspA1, PspA2, CbpA, Ply and H. influenzae antigens PD, OMP26, rsPilA and ChimV4
Sera were assessed for specific IgG antibodies against S. pneumoniae antigens PspA1, PspA2, CbpA, Ply, and H. influenzae antigens PD, OMP26, rsPilA and ChimV4 using multiplex bead-based immunoassays that have been described previously (21). The final assays consisted of a 5-plex immunoassay containing PspA1, PD, ScpA (Streptococcus pyogenes antigen, data not shown here), OMP26 and rsPilA, and a 4-plex immunoassay containing PspA2, CbpA, Ply and ChimV4. Test sera were diluted at 1:500 in sample diluent (phosphate-buffered saline with 2% newborn bovine sera and 0.05% Tween 20 (Sigma-Aldrich)). A 3-fold dilution of standards was prepared at a starting ratio of 1:20. Diluted sera (25µL) and standards (25µL) were added to the plate and mixed with prepared beads (25µL containing 4000 beads per region) and incubated in the dark at room temperature for 30 minutes, followed by standard bioplex protocol (35).
Breast milk was assessed for antigen-specific IgA antibodies against S. pneumoniae antigens, PspA1, PspA2 and Ply, and H. influenzae specific antigens PD, OMP26, rsPilA and ChimV4 using multiplexed bead-based immunoassays as for sera. A breast milk reference sample was prepared from pooled breast milk samples from 8 donors from a maternal S. pneumoniae vaccination study (Princess Margaret Hospital for Children Human Ethics Committee 721EP) and diluted 2-fold starting at 1:2.5. Antigen-specific IgG titres were also measured in breast milk samples, except for rsPilA as antibody titres to this antigen in the reference sample were below the limit of detection and a standard curve could not be established for analysis. Test samples were diluted 1:20 in sample diluent and immunoassays were conducted as above for sera. Antibody titres to CbpA could not be measured in breast milk as CbpA binds to the secretory component of secretory IgA in breast milk (36).
The BioPlex® 200 System (BioRad) was used to measure the fluorescence of 100 beads per specific bead region. The mean fluorescence intensity (MFI) values were generated with the Bio-plex Manager 6.0 software into arbitrary units (AU/mL). Inter-assay variability was assessed using the percentage of coefficient of variation (%CV) of MFI of the standard curve and remained within 10-15% between plates. Out of range readings were repeated at an appropriate higher or lower dilution (down to 1:20 for sera and 1:5 for breast milk) to fit within the standard curve.
Statistical analyses
All antibody concentration data were non-parametric and therefore log-transformed to normalise prior to analysis. Cord, maternal and infant sera, and breast milk samples were then analysed using parametric paired t-tests. Unpaired t-tests were used to compare carriage and non-carriage groups, OM and no OM groups, and between 23vPPV vaccine groups. Fold changes were calculated by dividing paired cord/infant titres and maternal transfer ratios were calculated by dividing paired cord/maternal titres. A paired mixed-effects model was used to compare breast milk antigen-specific IgA and IgG tires over time. Geometric mean titres (GMT) were calculated with 95% confidence intervals (CI). All data were analysed using SPSS version 25 (IBM, Armonk, New York, United States of America) and graphs prepared with GraphPad Prism 8 (GraphPad, La Jolla, California, United States of America).
Results
Study cohort
The PneuMum study enrolled 227 mothers, of which 85 gave consent for use of their samples in future studies including this study (Figure 1). Of those 85 mothers, 25 (29.4%) received 23vPPV between the 30th-36th week of pregnancy, 29 (34.0%) received 23vPPV within 4 hours of delivery and 31 (36.5%) received 23vPPV upon exit from the study (when the infant was 7-months old). The GMT of maternal, cord and infant sera IgG and breast milk IgA and IgG (Supplementary Table S1) for all 8 antigens studied here, were compared between the maternal vaccination groups, with no differences as expected (p≥ 0.05) (except breast milk Ply IgG at 1- and 2-months; p= 0.001). Therefore, timing of 23vPPV vaccination was not considered a confounder and groups were combined for further study analyses. Slightly more male infants were represented (56%). All babies were delivered at term, with a mean of 39.2 weeks [95% CI: 39.0 - 39.5 weeks] (Table 1). The average birth weight of study infants was 3452 g [95% CI: 2060 g – 4425 g] and the mean maternal age was 25.1 years [95% CI: 17 - 37 years of age] (Table 1).
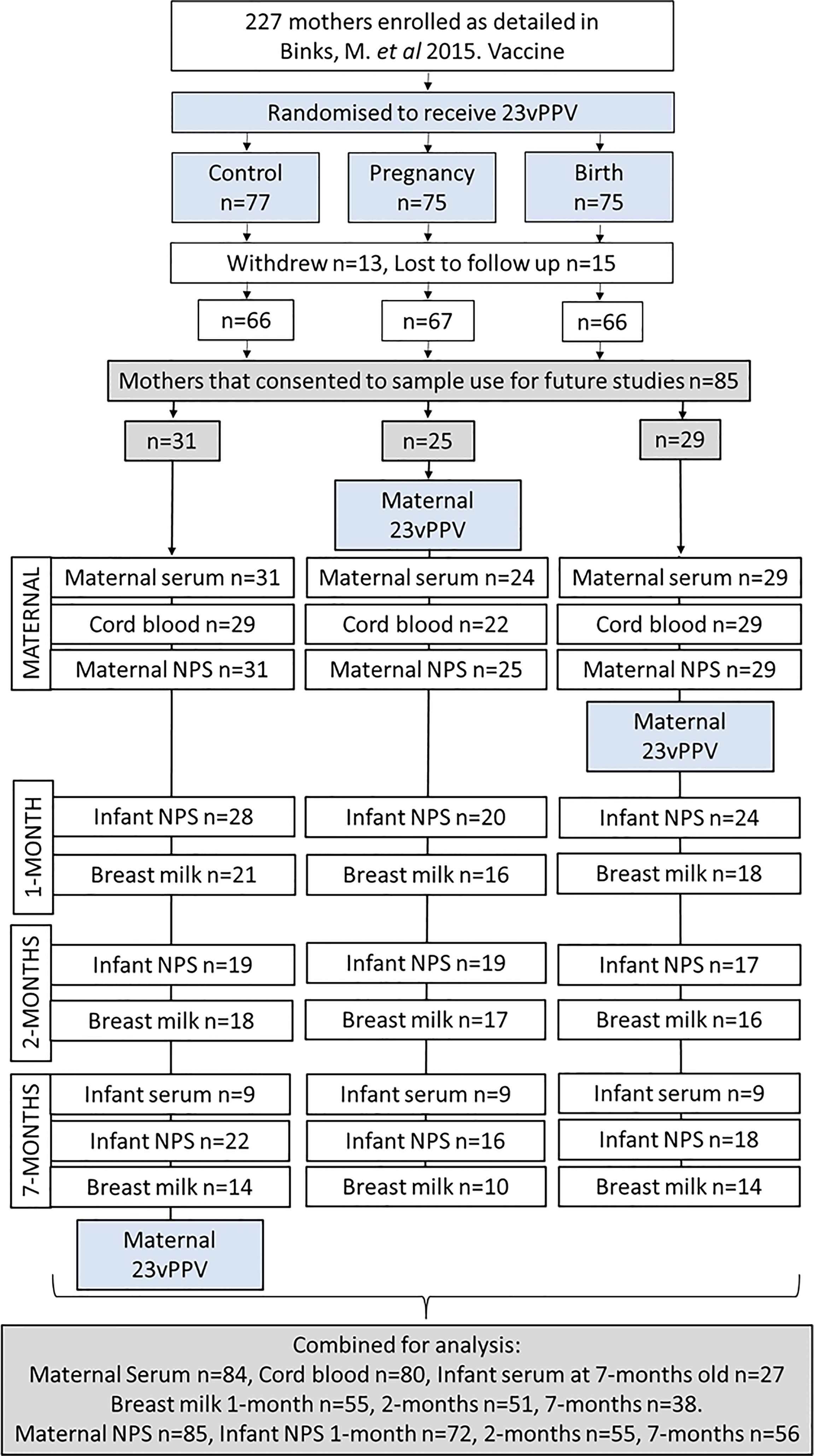
Figure 1 Participant flow chart and sample numbers. Of the 85 mother:infant pairs in this study 1 maternal serum sample was not collected (n=84); 5 mothers had no cord blood sera collected (n=80), and infant sera was not collected from 58 of the 85 infants (n=27).
Eighty-four maternal sera were collected (1 not collected), 53 cord sera (32 not collected) and 27 infant sera at 7-months old (58 not collected) (Figure 1). A total of 144 breast milk samples were collected. Of the 65/85 mothers who were breastfeeding at 1-month, 55 provided a breast milk sample, at 2-months, 52/85 mothers were breastfeeding with 51 providing a breast milk sample. At 7-months, 40/85 mothers were breastfeeding with 38 provided a breast milk sample. Infant study visits were scheduled at 1-, 2- and 7-months of age. There were 13 out of 85 infants who missed the 1-month study visit (no NPS collected, and no ear assessment done), 30 missed at 2-months and 29 missed at 7-months. 23 infants attended only 1 study visit, 26 infants attended 2 study visits and 36 infants attended all three study visits and had their ears assessed and NPS collected (Figure 1).
By 7-months of age nearly half of the infants carried either S. pneumoniae or NTHi and this was associated with an OM diagnosis
Nasopharyngeal swabs were collected from all 85 mothers at the time of delivery (Figure 1) with maternal carriage uncommon (Table 1). Nasopharyngeal swabs were collected from 72 infants at 1-month, 55 infants at 2-months, and 56 infants at 7-months (Figure 1). At 1-month of age, 8/72 infants (11%) were colonised with NTHi, and 6/72 infants (8%) were colonised with S. pneumoniae (Table 1). At 2-months of age, 5/55 infants (9%) were carrying NTHi and 14/55 infants (26%) were colonised with S. pneumoniae. At 7-months of age 25/56 infants (45%) carried NTHi, and 26/56 infants (46%) carried S. pneumoniae. Of those infants with all 3 NPS collected, 2/36 (6%) had S. pneumoniae detected at all 3 study visits and 2/36 (6%) had NTHi detected at all 3 study visits, with one of those infants being positive for both pathogens at all 3 study visits.
At the 1-month study visit, 12/71 (17%) infants had OM observed, increasing to 19/53 (36%) infants at the 2-months study visit. At the 7-month study visit, 33/54 (61%) infants had OM observed. Over the three study visits, 29 (34%) children had 1 OM episode observed, 10 (12%) had 2 OM episodes observed and 5 (6%) had OM observed at all 3 visits. Over the whole 7-months of the study, 44/85 (52%) children had OM observed at 1 or more study visit.
Overall, 29/44 (66%) infants who were colonised with S. pneumoniae and 26/44 (59%) infants who were colonised with NTHi on at least one of their three study visits also had OM observed during at least one of their three study visits.
Antibody titres were similar in maternal and cord sera for all antigens measured
IgG GMT in cord and maternal sera were similar for S. pneumoniae antigens PspA1, PspA2 and CbpA (p≥0.05; Figures 2A–C). For the S. pneumoniae antigen Ply, IgG was higher in cord sera than in maternal sera (Figure 2D, p=0.004). IgG titres in cord and maternal sera were similar for all H. influenzae antigens (p≥0.05; Figures 2E–H). All GMT and 95% CI are shown in Supplementary Table S2. The geometric mean cord/maternal IgG antibody ratios were all above 1 (anti-PspA1: 1.21 [range 1.11-1.31], anti-PspA2: 1.18 [range 1.10-1.26], anti-CbpA: 1.19 [range 1.09-1.29], anti-Ply: 1.99 [range 1.81-2.20], anti-PD: 1.27 [range 1.19-1.35], anti-ChimV4: 1.01 [range 0.93-1.10], anti-rsPilA: 1.07 [range 1.00-1.14] and anti-OMP26: 1.09 [range 1.01-1.18]).
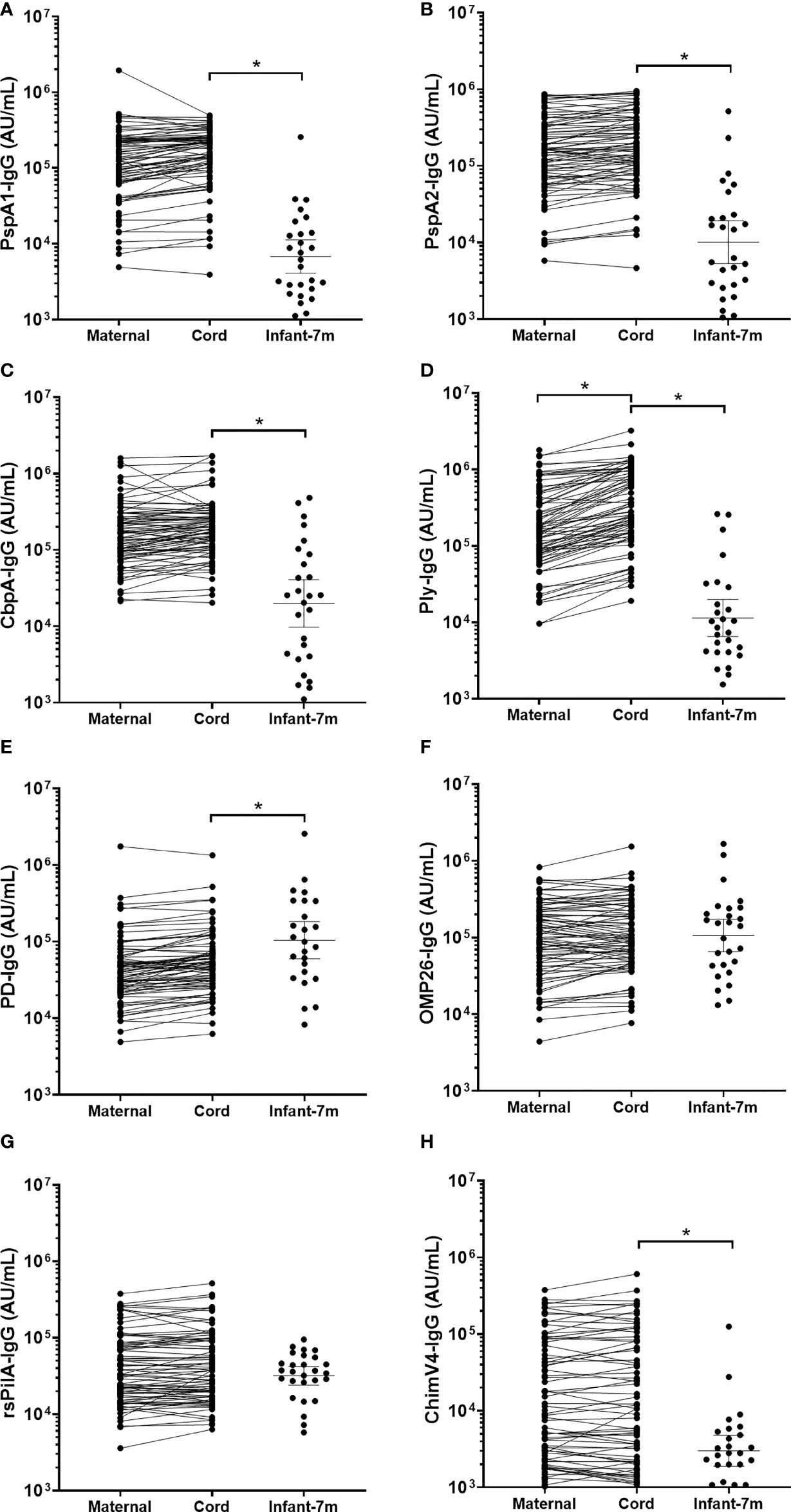
Figure 2 IgG titres against Streptococcus pneumoniae and Haemophilus influenzae antigens in maternal, cord and infant sera. Each point represents sera IgG titres against S. pneumoniae antigens (A) PspA1, (B) PspA2, (C) CbpA, (D) Ply and H. influenzae antigens (E) PD, (F) OMP26, (G) rsPilA and (H) ChimV4. PspA1, pneumococcal surface protein A family 1; PspA2, pneumococcal surface protein A family 2; CbpA, choline-binding protein A; Ply, pneumolysin. PD, Protein D; OMP26, outer membrane protein 26; rsPilA, recombinant soluble pilus A protein; ChimV4, chimeric vaccine antigen 4 (rsPilA and P5). * p-value <0.05, Paired t-tests were conducted on the logarithmically transformed data. All samples had measurable antibody titres.
S. pneumoniae-specific IgG titres in cord sera were up to 20-fold higher than infant serum at 7-months of age
Cord blood IgG titres were higher than those in infant sera at 7-months of age for all 4 S. pneumoniae antigens (p<0.001). Geometric mean cord/infant IgG fold changes were as follows, 19.7-fold for anti-PspA1 [range 180 to 1.26], 14.8-fold for anti-PspA2 [range 98.1 to 0.5], 9.61-fold for anti-CbpA [range 110 to 0.25] and 21.7-fold for anti-Ply [range 216 to 0.17].
H. influenzae-specific IgG titres were similar between cord and infant sera
Antigen-specific cord sera IgG GMT were lower compared to infant IgG at 7-months of age for PD (p=0.029), but higher for ChimV4 (p=0.001) (Figures 2E, H). There were no differences in cord versus infant IgG GMT for OMP26 (p=0.999) or rsPilA (p=0.207) (Figures 2F, G). All GMT and 95% CI are shown in Supplementary Table S2. The geometric mean cord/infant IgG fold changes 0.46-fold for anti-PD [range 10.6 to 0.02], 1.0-fold for anti-OMP26 [range 75.4 to 0.02], 4.2-fold for anti-ChimV4 [range 59.3 to 0.06] and 1.4-fold for anti-rsPilA [range 21.1 to 0.14].
S. pneumoniae antigen-specific sera IgG titres were higher in infants colonised with S. pneumoniae in the first 7-months of life
Overall, 35/85 (41%) infants had at least one NPS positive for S. pneumoniae over the 7-months (Table 1). Infant sera IgG titres at 7-months of age against S. pneumoniae specific PspA2 (p=0.045), CbpA (p=0.001) and Ply (p=0.025) were all higher in infants that carried S. pneumoniae at any study visit over the 7-months Figure 3). Titres of anti-CbpA IgG in cord sera (p=0.009) and CbpA IgG in maternal sera (p=0.006) were higher in those mothers whose infant had any positive NPS for S. pneumoniae carriage over the 7-months (Figure 3C).
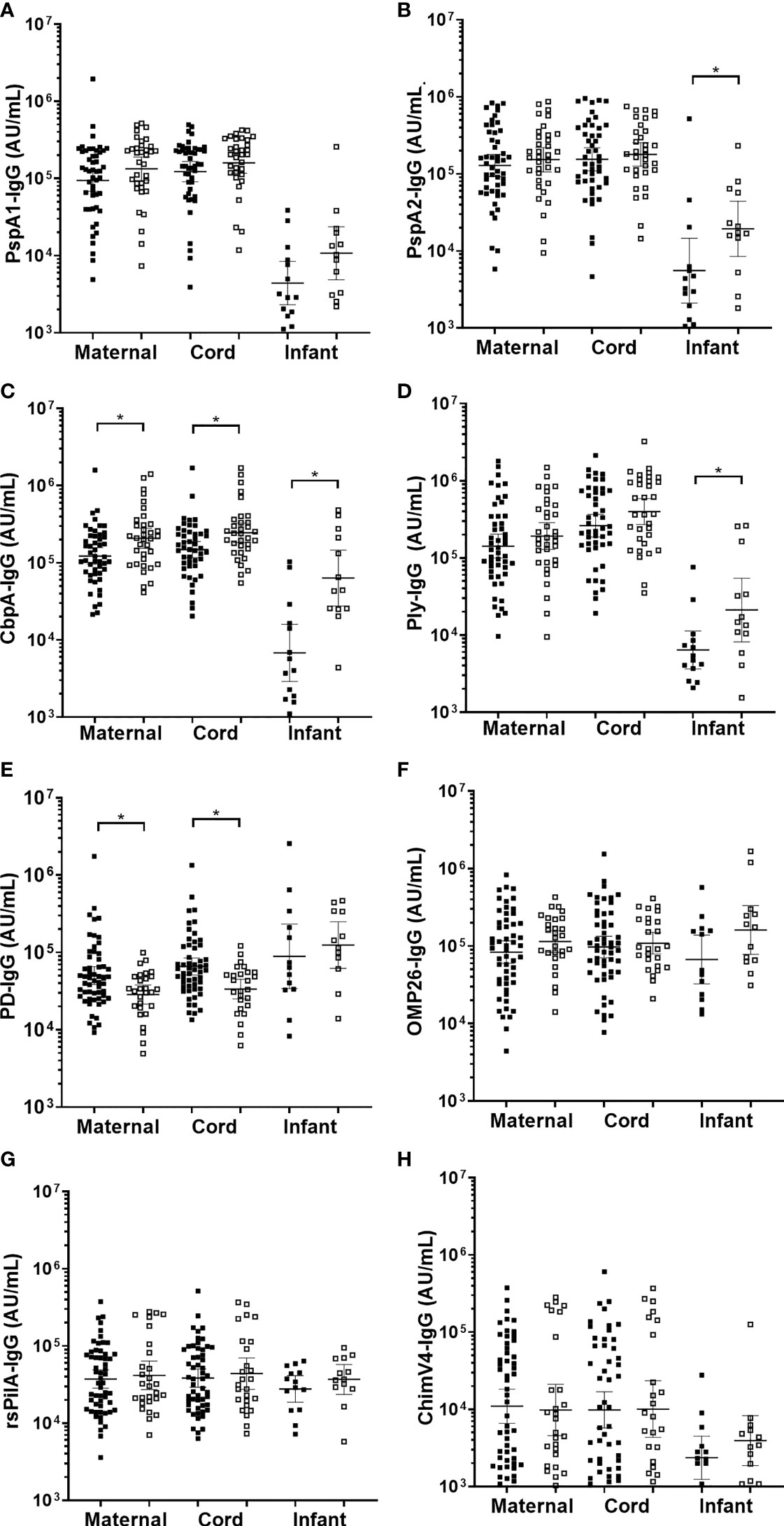
Figure 3 Sera IgG titres against Streptococcus pneumoniae or Haemophilus influenzae separated based on any NPS positive to S. pneumoniae or NTHi in the first 7-months of life, in maternal venous sera, cord sera and infant venous sera from matched mother-infant pairs. Each point represents the sera IgG titres against S. pneumoniae antigens (A) PspA1, (B) PspA2, (C) CbpA, (D) Ply and H. influenzae antigens (E) PD, (F) OMP26, (G) rsPilA and (H) ChimV4. PspA1, pneumococcal surface protein A family 1; PspA2, pneumococcal surface protein A family 2; CbpA, choline-binding protein A; Ply, pneumolysin. PD, Protein D; OMP26, outer membrane protein 26; rsPilA, recombinant soluble pilus A protein; ChimV4, chimeric vaccine antigen 4 (rsPilA and P5). *p-value <0.05, Unpaired t-tests were conducted on logarithmically transformed data. Positive NPS = open squares.
H. influenzae antigen titres were similar between infants with and without NTHi carriage, except higher anti-PD IgG titres in cord and maternal sera were associated with lower prevalence of infant NTHi carriage
Overall, 28/85 (33%) infants had at least one NPS positive for NTHi over the 7-months. There were no differences in sera IgG titres against H. influenzae antigens in infants carrying NTHi at any study visit over the 7-months. Infants that were not colonised with NTHi at 1-, 2- or 7-months of age had higher anti-PD IgG titres in their cord serum (p=0.002) (and their mother's serum; p=0.009) (Figure 3E) with no differences in OMP26, rsPilA and ChimV4 (Figures 3F–H).
Antigen specific maternal, cord and infant sera IgG titres did not protect against all cause OM by 7-months of age
There were no differences in infant sera for GMT for anti-PspA1, anti-PspA2, anti-CbpA, or anti-Ply between those children with OM and without OM (Figures 4A–D). Maternal sera from mothers of infants with any OM observed by 7-months of age had higher GMT than those without OM for anti-PspA1 IgG (p=0.009) (Figure 4A) and CbpA (p=0.006) (Figure 4C). There were no differences for anti-PspA2 or anti-Ply antibody titres in maternal sera between those mothers of children with OM and without OM (Figures 4B, D). H. influenzae antigen IgG tires against PD, OMP26, rsPilA and ChimV4 in maternal, cord or infant sera were similar between children with and without an OM diagnosis (Figures 4E–H).
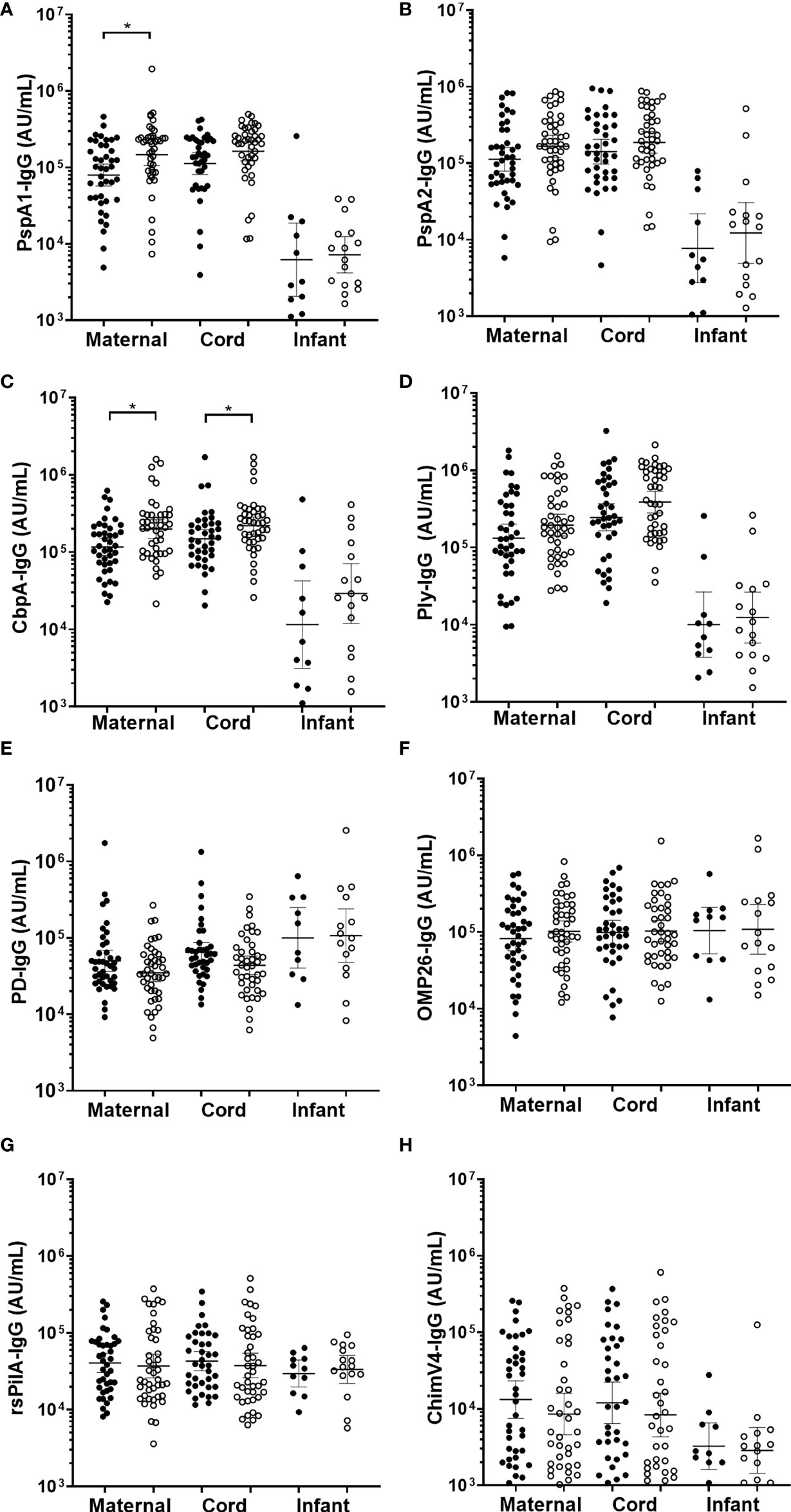
Figure 4 IgG antibodies against Streptococcus pneumoniae and Haemophilus influenzae antigens in maternal, cord and infant sera from matched mother-infant pairs, separated based on otitis media diagnosis in the first 7-months of life or not. Each point represents sera IgG titres against S. pneumoniae antigens (A) PspA1, (B) PspA2, (C) CbpA, (D) Ply and H. influenzae antigens (E) PD, (F) OMP26, (G) rsPilA and (H) ChimV4. PspA1, pneumococcal surface protein A family 1; PspA2, pneumococcal surface protein A family 2; CbpA, choline-binding protein A; Ply, pneumolysin. Protein D; OMP26, outer membrane protein 26; rsPilA, recombinant soluble pilus A protein; ChimV4, chimeric vaccine antigen 4 (rsPilA and P5). *p-value <0.05, Unpaired t-tests were conducted on the logarithmically transformed data. With otitis media diagnosis = open circles.
IgA and IgG to S. pneumoniae and H. influenzae antigens were detected in breast milk, with levels sustained for 7-months post-birth
In breast milk, the IgA GMT were similar at 1-, 2- and 7-months post-birth for all S. pneumoniae antigens tested (PspA1, PspA2 and Ply) and for 3 of the 4 H. influenzae antigens (PD, rsPilA and ChimV4), (Figure 5). The IgA GMT against OMP26 were lower in breast milk at 7-months post-birth compared to 1-month (p=0.035) (Figure 5E). All samples had measurable IgA titres except for rsPilA, with 6 samples below the limit of detection. All GMT and 95% CI are shown in Supplementary Table S3.
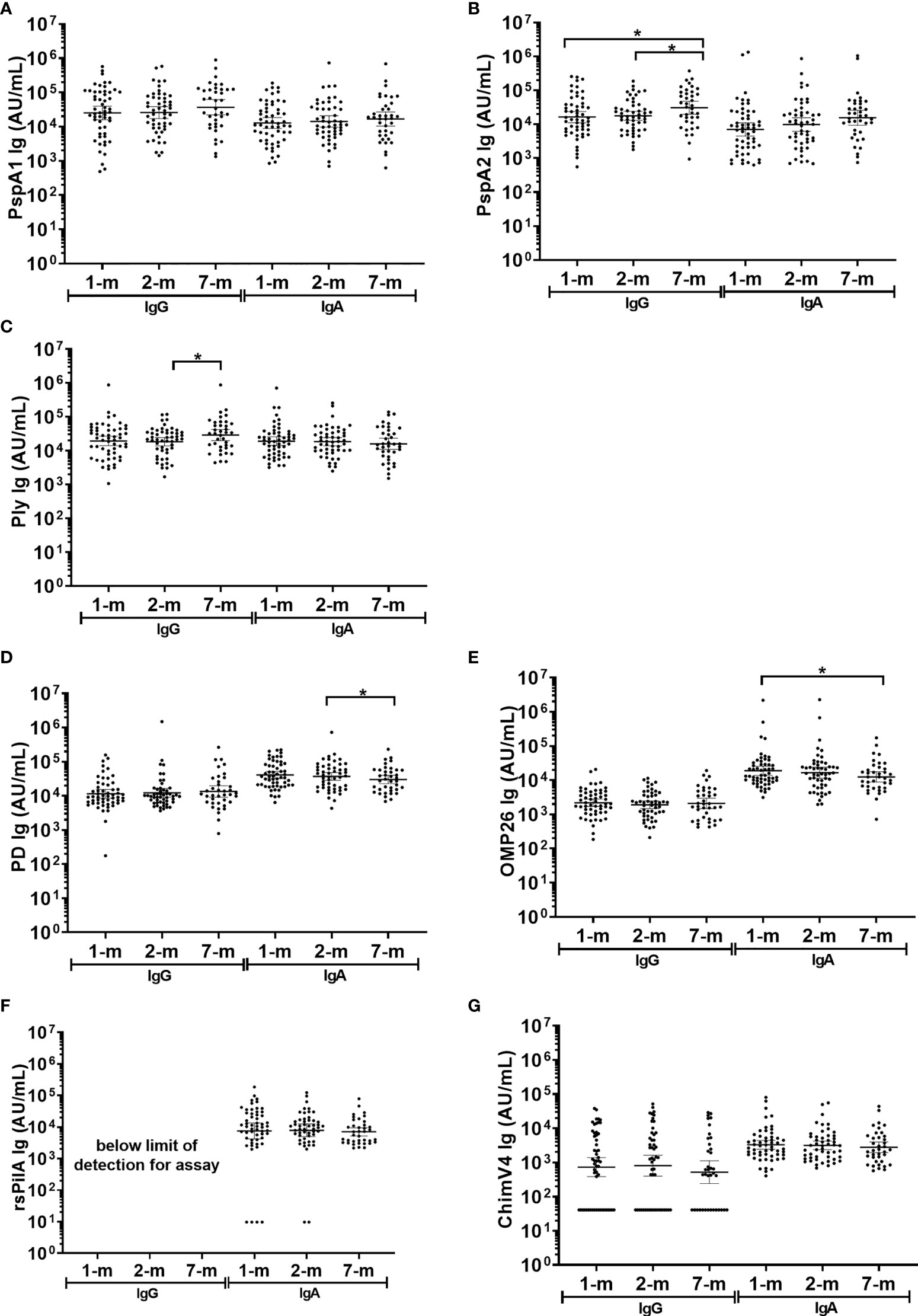
Figure 5 Breast milk IgG and IgA titres against Streptococcus pneumoniae and Haemophilus influenzae antigens. Each point represents the breast milk IgG and IgA titres against S. pneumoniae antigens (A) PspA1, (B) PspA2, (C) Ply and H. influenzae antigens (D) PD, (E) OMP26, (F) rsPilA and (G) ChimV4. PspA1, pneumococcal surface protein A family 1; PspA2, pneumococcal surface protein A family 2; Ply, pneumolysin. PD, Protein D; OMP26, outer membrane protein 26; rsPilA, recombinant soluble pilus A protein; ChimV4, chimeric vaccine antigen 4 (rsPilA and P5). * p-value <0.05, paired t-tested were conducted on the logarithmically transformed data. 1-month n=55, 2-months n=51, 7-months n=38. All samples had measurable antibody titres except for rsPilA, for which 6 samples were below the limit of detection for anti-rsPilA IgA. The assay reference breast milk had minimal rsPilA IgG therefore anti-rsPilA IgG titres could not be quantified.
Fifty-one breast milk samples (35%) had no detectable anti-ChimV4 IgG and were assigned half the limit of detection (41 AU/mL). Anti-rsPilA IgG was below the detection limit in standards required for assay establishment and therefore could not be measured here, IgG to all other antigens were detectable. Anti-PspA1, anti-PD, anti-OMP26 and anti-ChimV4 IgG GMT did not change over the 7-months (p≥0.05) (Figures 5A, D, E, G). Anti-PspA2 IgG titres increased by 7-months of age: 1-month versus 7-months, p=0.012; 2-months versus 7-months, p=0.020, (Supplementary Table S3 and Figure 5B). Anti-Ply IgG titres in breast milk also increased between 2- and 7-months of age (p=0.032) (Figure 5C).
Higher anti-PspA IgG and IgA titres in breast milk were observed from mothers of infants with S. pneumoniae carriage, while higher anti-PD IgG titres in breast milk at 1-month were observed in infants that were not colonised with NTHi at any study visit
In general, antigen-specific IgG and IgA titres in breast milk were similar between infants that were colonised with either S. pneumoniae or NTHi (on at least one of the 1-, 2-, or 7-month visit) versus infants that were not colonised at a study visit (Figure 6). Of note was the PspA2 antibody titres in breast milk, where PspA2 IgG and IgA titres were higher in 1-month (IgA only p=0.001), 2-month (IgG p=0.006; IgA p=0.018) and 7-month (IgG p=0.006; IgA p=0.010) breast milk samples for infants colonised with S. pneumoniae compared with infants that were not colonised at a study visit (Figure 6B). Anti-PspA1 IgA titres were also higher in the 7-month breast milk sample when children were colonised with S. pneumoniae compared with those that were not colonised (p=0.038) (Figure 6A). Higher anti-PD IgG titres in breast milk at 1-month post-birth were associated with decreased prevalence of infant NTHi carriage at any study visit (p=0.041) (Figure 6D).
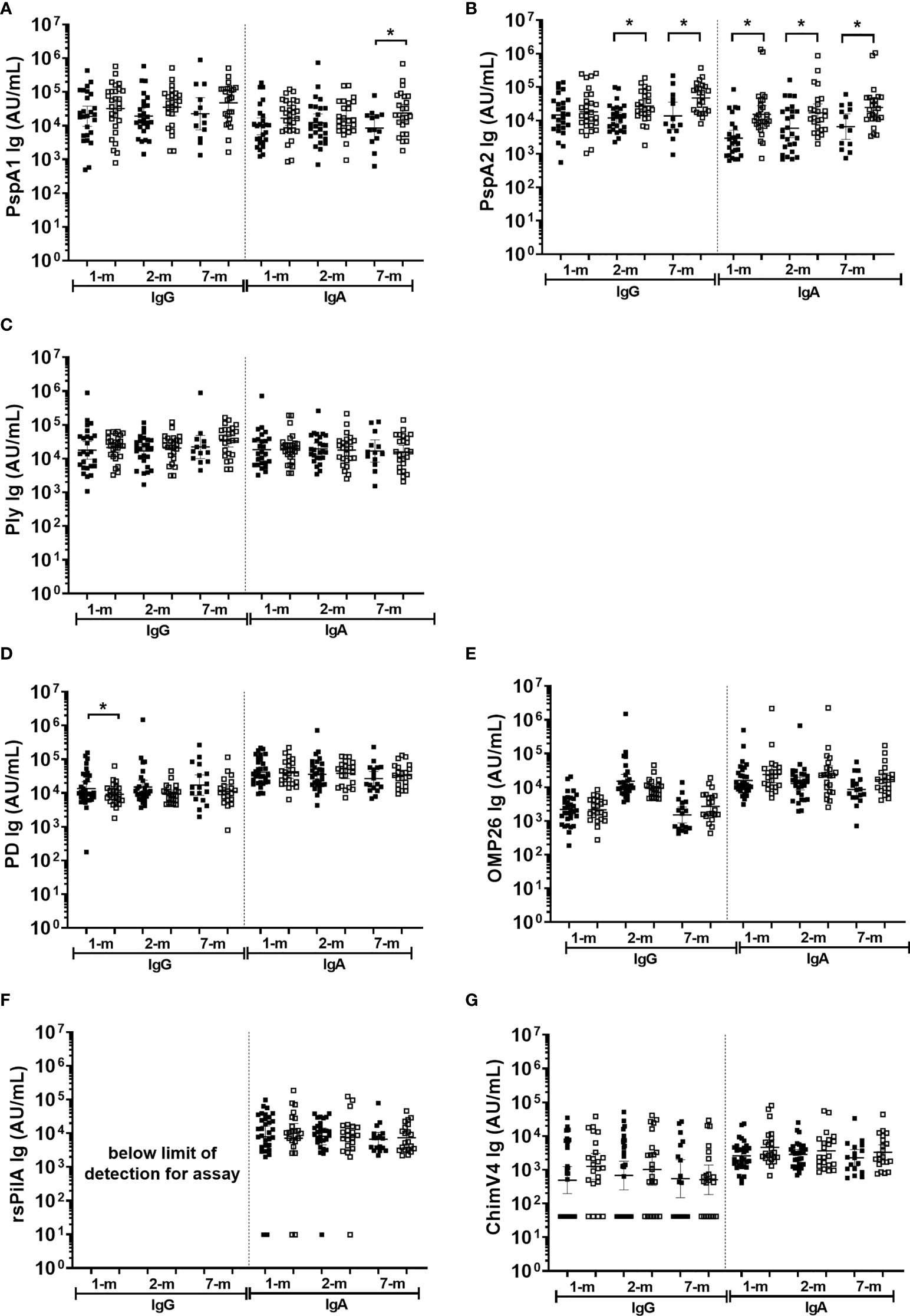
Figure 6 Breast milk IgG and IgA titres against Streptococcus pneumoniae and Haemophilus influenzae separated based on any NPS positive to S. pneumoniae or NTHi in the first 7-months of life. Each point represents the breast milk IgG or IgA titres against S. pneumoniae antigens (A) PspA1, (B) PspA2, (C) Ply and H. influenzae antigens (D) PD, (E) OMP26, (F) rsPilA, (G) ChimV4. PspA1, pneumococcal surface protein A family 1; PspA2, pneumococcal surface protein A family 2; Ply, pneumolysin. PD, Protein D; OMP26, outer membrane protein 26; rsPilA, recombinant soluble pilus A protein; ChimV4, chimeric vaccine antigen 4 (rsPilA and P5). Unpaired t-tests were conducted on logarithmically transformed data with * p<0.05. For the 1-month visit n=55, 2-months visit n=51, 7-months visit n=38. Positive NPS = open squares.
Antigen specific antibody in breast milk did not protect against all cause OM by 7-months of age
Antigen-specific IgA titres against S. pneumoniae or H. influenzae antigens in breast milk were similar between infants with and without an OM diagnosis at a study visit (p≥0.05; Figure 7). The exception was anti-PspA1 IgG and PspA2 IgA titres, where higher titres were observed in breast milk at 1-month post-birth from mothers of infants with an OM diagnosis at one or more study visit (Figures 7A, B) (p=0.017) and anti-PspA2 IgA GMT in 1-month breast milk sample of mother with infant OM diagnosis (p=0.048). Anti-PspA1 IgG titres were higher in the 2-month breast milk sample for mothers of infants with an OM diagnosis (p=0.001). At 2-months, anti-PspA2 IgG titres were also increased in breast milk from mothers whose infant developed OM (p=0.016).
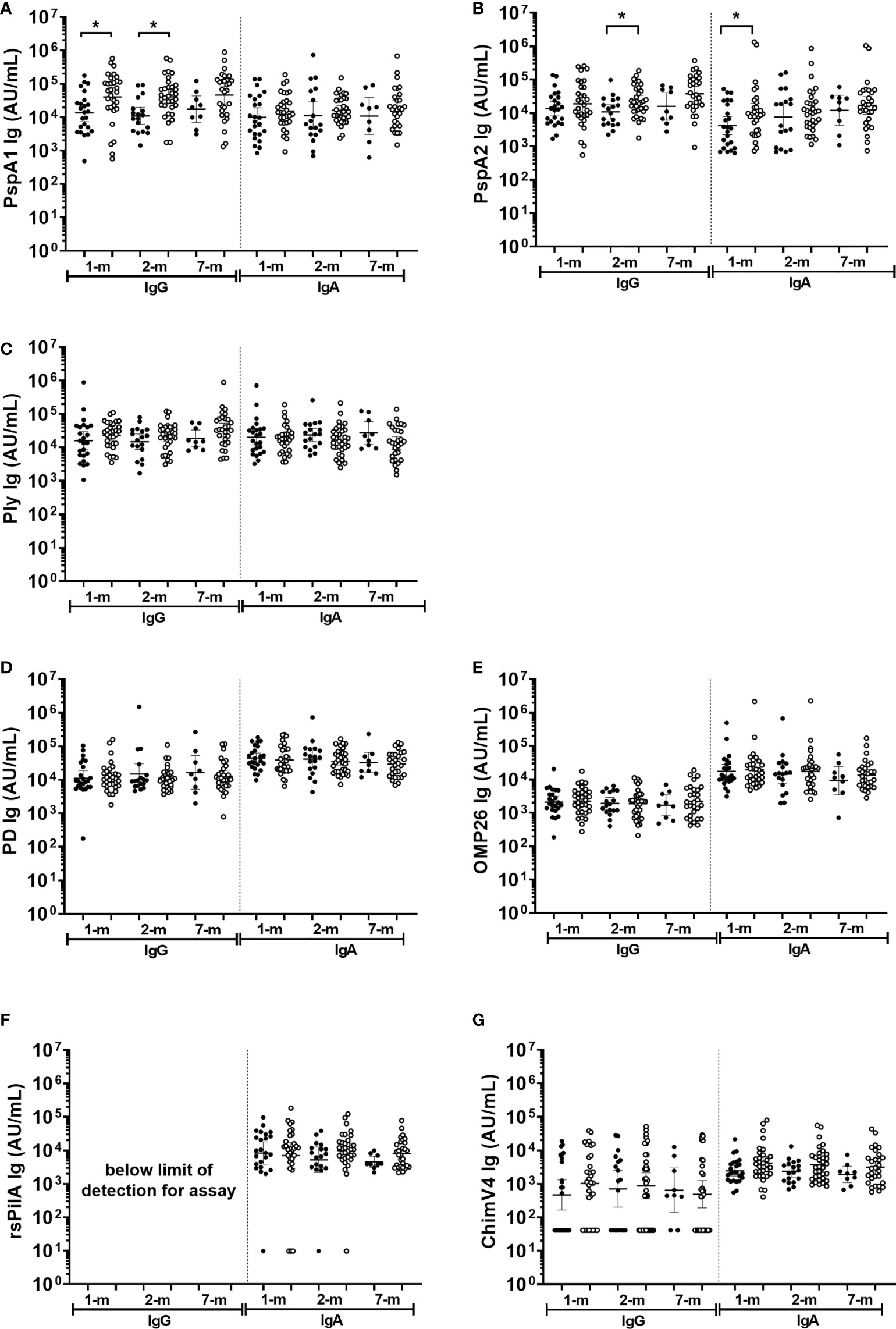
Figure 7 Breast milk IgG and IgA antibodies against Streptococcus pneumoniae and Haemophilus influenzae antigens separated based on otitis media diagnosis in the first 7-months of life or not. Each point represents breast milk IgG or IgA titres against S. pneumoniae antigens (A) PspA1, (B) PspA2, (C) Ply and H. influenzae antigens (D) PD, (E) OMP26, (F) rsPilA, (G) ChimV4. PspA1, pneumococcal surface protein A family 1; PspA2, pneumococcal surface protein A family 2; Ply, pneumolysin. PD, Protein D; OMP26, outer membrane protein 26; rsPilA, recombinant soluble pilus A protein; ChimV4, chimeric vaccine antigen 4 (rsPilA and P5). Unpaired t-tests were conducted on logarithmically transformed data with * p<0.05. 1-month n=55, 2-months n=51, 7-months n=38. With otitis media= open circles.
Discussion
Insight into maternal transfer of antibodies and early life protection against S. pneumoniae and H. influenzae is important to inform vaccine development and immunisation strategies, especially for infants at high risk of disease from these pathogens. In this study, we observed that antigen-specific natural antibody to S. pneumoniae and H. influenzae vaccine candidate antigens is present in serum and breast milk of Australian Aboriginal and Torres Strait Islander mothers. We demonstrated that cord antigen-specific serum IgG titres reflected maternal titres, indicating efficient placental transfer of antibody for every antigen tested. For the S. pneumoniae antibodies, we observed a 20- to 50-fold reduction in S. pneumoniae-specific IgG titres in infant sera at 7-months of age in comparison to cord sera titres, indicative of waning maternal antibodies in the first months of life. This has been reported in other studies of mother-infant pairs from Papua New Guinea (25), Burmese refugees at the Thai border (37), and the Philippines (23). In contrast, there was no change in H. influenzae-specific IgG titres between cord and infant sera at 7-months of age (i.e. no evidence of waning maternal antibodies). To our knowledge, this is the first investigation into maternal transfer of antibody to H. influenzae protein vaccine antigen candidates. We previously reported high titres of serum IgG against S. pneumoniae antigens in 1-month-old Papua New Guinean infants, which wane between 4- to 10-months of age, while H. influenzae antibody titres gradually increased from 1-month of age with no evidence of waning (21). This current study provides evidence that the maternal H. influenzae antigen-specific IgG that is available is transferred to infants (maternal:cord ratio = 1 for all H. influenzae antigens tested). It is therefore likely that mothers simply do not have much H. influenzae-specific antibody to transfer, and the infant must rely on making their own. Further support of low maternal H. influenzae protein antigen IgG titres available for transfer comes from a study in China, where antibody titres to H. influenzae PD and P6 steadily increased from 1-month of age, peaking at 7-months of age, with the lowest antibody titres observed in adults aged 21-40 years (i.e. child-bearing age) (26).
Understanding natural antibody ontogeny in mothers and infants is important when considering maternal and infant vaccination strategies. Demonstration of the ability for placental transfer of S. pneumoniae and H. influenzae-specific IgG holds promise for future immunisation strategies to confer early in life protection from these major pathogens. Our specific observation that higher H. influenzae PD IgG titres in maternal and cord blood were associated with protection of infants from NTHi carriage warrants further investigation. This is particularly important as this finding also applied to breast milk, where infants of mothers with higher breast milk PD IgG titres at 1-month post-birth were less likely to be colonised with NTHi by 7-month of age. This highlights the potential for maternal immunisation studies with a PD-containing vaccine (e.g. PCV10 or newer PD containing formulations in development) to delay NTHi carriage and thus H. influenzae-associated infections in young infants. While no correlates of protection currently exist for these antigens, placental transfer of antibodies to bacterial proteins in vaccines has been shown to be more effective than antibodies to bacterial polysaccharides in vaccines (38), further supporting the potential for maternal immunisation with PD-containing vaccines. This is of particular importance for populations such as Australian Aboriginal and Torres Strait Islanders who have high rates of NTHi carriage and NTHi OM (39, 40).
Our observation that breast milk IgA antibody titres remained constant over the first 7-months post-birth, for both S. pneumoniae and H. influenzae antigens, suggests that breast milk is a conduit for maternal antibody transfer long after birth. While breast-feeding is known to reduce the risk of S. pneumoniae and H. influenzae diseases such as OM (41, 42), the specific protective role of antigen-specific antibodies in breast milk has not been specifically investigated. A recent study using protein microarray to measure antibodies to multiple pathogens in breast milk of mothers from low- and high-income countries identified high variability in antigen-specific IgG and IgA repertoires for S. pneumoniae depending on geography/socioeconomic status (43). The use of breast milk to impart immunity to the surrounding environment is yet to be harnessed for prevention of S. pneumoniae and H. influenzae infections i.e. through maternal immunisation.
Limitations for this study include a single sampling point for infant sera at 7-months of age that does not enable kinetic assessment of maternal antibody waning. As middle ear samples are not able to be collected at the time of OM in Australia, the aetiology remains unknown. Some data and sample collection points were absent in this longitudinal clinical cohort, and as such the prevalence of carriage and OM could be higher than that reported. Despite these samples being collected between 2006 and 2011, serum antibody titres remain stable when samples are correctly stored and the major OM pathogens are still the same. Additionally, similar antibody waning patterns are observed in other cohorts such as the PNG study that was collected between 2014-2016 (21).
Conclusion
Antibody to all S. pneumoniae and H. influenzae vaccine candidate antigens assessed could be measured in maternal, cord and infant sera from Aboriginal and Torres Strait Islander mother-infant pairs. The similarities between maternal and cord IgG titres confirm placental transfer of these antibodies. The absence of waning antibody to H. influenzae antigens between cord and infant sera supports a lack of maternal H. influenzae IgG antibodies available for cross-placental transfer. The observation that increased maternal anti-PD IgG (and anti-PD IgA in breast milk) may offer protection from infant NTHi carriage points to the potential value of considering PD-containing vaccines in maternal immunisation strategies. Maternal PCV10 vaccination is currently being investigated in the PneuMatters study for prevention of acute lower respiratory infection (ACTRN12618000150246). Sustained titres of antigen-specific antibody in breast milk over the first 7-months post-birth also highlights the great potential for passive-active immunisation of infants to protect against S. pneumoniae and H. influenzae disease.
Data availability statement
The raw data supporting the conclusions of this article will be made available by the authors, without undue reservation.
Ethics statement
The studies involving human participants were reviewed and approved by Human Research Ethics Committee of the Northern Territory Department of Health and the Menzies School of Health Research HREC and the Aboriginal Ethics Sub-Committee (HREC 2020-3800). Extensive community consultation with the PneuMum Indigenous Reference Group, Menzies Child Health Division First Nations Reference group in the Northern Territory, the Kulunga Aboriginal Unit at the Telethon Kids Institute, and the Ear Health Aboriginal Community Advisory Group in Western Australia ensured culturally appropriate processes were maintained for the additional analysis of collected specimens. Written informed consent to participate in this study was provided by the participants’ legal guardian/next of kin.
Author contributions
ES, MB, AB, RT, PR, RA and L-AK contributed to the conceptualization of this study. KM and SC performed laboratory assays. KM analyzed the data and wrote the original draft manuscript under the supervision of L-AK. All authors have contributed to the article and have read and agreed to the published version of the article.
Funding
This study was funded by a seed grant from the Wesfarmers Centre of Vaccines and Infectious Diseases at the Telethon Kids Institute awarded to ES, MB, RT, PR, RA and L-AK. RT is funded by a fellowship from the Passe and Williams Foundation. L-AK is funded by a fellowship from the Perron Foundation. MB is funded by Menzies School of Health fellowship.
Acknowledgments
ChimV4 and rsPilA antigens were generously provided by Professor Lauren Bakaletz. OMP26 antigen was generously provided by Professor Allan Cripps. Ply antigen was generously provided by Professor Tim Mitchell. We also acknowledge and thank the study participants, clinical staff and the Telethon Kids Institute Kulunga team members and the Menzies Child Health Division First Nations Reference group.
Conflict of interest
The authors declare that the research was conducted in the absence of any commercial or financial relationships that could be construed as a potential conflict of interest.
Publisher’s note
All claims expressed in this article are solely those of the authors and do not necessarily represent those of their affiliated organizations, or those of the publisher, the editors and the reviewers. Any product that may be evaluated in this article, or claim that may be made by its manufacturer, is not guaranteed or endorsed by the publisher.
Supplementary material
The Supplementary Material for this article can be found online at: https://www.frontiersin.org/articles/10.3389/fimmu.2022.1005344/full#supplementary-material
References
1. Watanaveeradej V, Endy TP, Samakoses R, Kerdpanich A, Simasathien S, Polprasert N, et al. Transplacentally transferred maternal-infant antibodies to dengue virus. Am J Trop Med Hyg (2003) 69(2):123–8. doi: 10.4269/ajtmh.2003.69.123
2. Kiliç A, Altinkaynak S, Ertekin V, Inandi T. The duration of maternal measles antibodies in children. J Trop Pediatr (2003) 49(5):302–5. doi: 10.1093/tropej/49.5.302
3. Rogier EW, Frantz AL, Bruno MEC, Wedlund L, Cohen DA, Stromberg AJ, et al. Secretory antibodies in breast milk promote long-term intestinal homeostasis by regulating the gut microbiota and host gene expression. Proc Natl Acad Sci (2014) 111(8):3074–9. doi: 10.1073/pnas.1315792111
4. Tromp I, Jong Kiefte-de J, Raat H, Jaddoe V, Franco O, Hofman A, et al. Breastfeeding and the risk of respiratory tract infections after infancy: The generation r study. PloS One (2017) 12(2):e0172763. doi: 10.1371/journal.pone.0172763
5. Benowitz I, Esposito DB, Gracey KD, Shapiro ED, Vázquez M. Influenza vaccine given to pregnant women reduces hospitalization due to influenza in their infants. Clin Infect Dis (2010) 51(12):1355–61. doi: 10.1086/657309
6. Switzer C, D’Heilly C, Macina D. Immunological and clinical benefits of maternal immunization against pertussis: A systematic review. Infect Dis Ther (2019) 8(4):499–541. doi: 10.1007/s40121-019-00264-7
7. Ochola R, Sande C, Fegan G, Scott PD, Medley GF, Cane PA, et al. The level and duration of RSV-specific maternal IgG in infants in kilifi Kenya. PloS One (2009) 4(12):e8088. doi: 10.1371/journal.pone.0008088
8. Dagan N, Barda N, Biron-Shental T, Makov-Assif M, Key C, Kohane IS, et al. Effectiveness of the BNT162b2 mRNA COVID-19 vaccine in pregnancy. Nat Med (2021) 27(10):1693–5. doi: 10.1038/s41591-021-01490-8
9. Wahl B, K L, Greenbaum A, Majumder A, Liu L, Chu Y, et al. Burden of streptococcus pneumoniae and haemophilus influenzae type b disease in children in the era of conjugate vaccines: global, regional, and national estimates for 2000-15. Lancet Global Health (2018) 6(7):e744–57. doi: 10.1016/S2214-109X(18)30247-X
10. O’Brien KL, Wolfson LJ, Watt JP, Henkle E, Deloria-Knoll M, McCall N, et al. Burden of disease caused by streptococcus pneumoniae in children younger than 5 years: global estimates. Lancet (2009) 374(9693):893–902. doi: 10.1016/S0140-6736(09)61204-6
11. Earle K, Williams S. Burden of pneumococcal disease in adults aged 65 years and older: an Australian perspective. Pneumonia (2016) 8(1):9. doi: 10.1186/s41479-016-0008-8
12. Blain A, MacNeil J, Wang X, Bennett N, Farley MM, Harrison LH, et al. Invasive haemophilus influenzae disease in adults ≥65 years, united states, 2011. Open Forum Infect Dis (2014) 1(2):ofu044–4. doi: 10.1093/ofid/ofu044
13. Bryce J, Boschi-Pinto C, Shibuya K, Black RE. WHO estimates of the causes of death in children. Lancet (2005) 365(9465):1147–52. doi: 10.1016/S0140-6736(05)71877-8
14. Daniels CC, Rogers PD, Shelton CM. A review of pneumococcal vaccines: Current polysaccharide vaccine recommendations and future protein antigens. J Pediatr Pharmacol therapeut: JPPT: Off J PPAG (2016) 21(1):27–35. doi: 10.5863/1551-6776-21.1.27
15. Slack MPE. The evidence for non-typeable haemophilus influenzae as a causative agent of childhood pneumonia. Pneumonia (2017) 9(1):9. doi: 10.1186/s41479-017-0033-2
16. Briles DE, Paton JC, Mukerji R, Swiatlo E, Crain MJ. Pneumococcal vaccines. Microbiol Spectr (2019) 7(6):GPP3-0028-2018. doi: 10.1128/microbiolspec.GPP3-0028-2018
17. Prymula R, Pazdiora P, Traskine M, Rüggeberg JU, Borys D. Safety and immunogenicity of an investigational vaccine containing two common pneumococcal proteins in toddlers: a phase II randomized clinical trial. Vaccine (2014) 32(25):3025–34. doi: 10.1016/j.vaccine.2014.03.066
18. Leroux-Roels G, Maes C, Boever De F, Traskine M, Rüggeberg JU, Borys D. Safety, reactogenicity and immunogenicity of a novel pneumococcal protein-based vaccine in adults: a phase I/II randomized clinical study. Vaccine (2014) 32(50):6838–46. doi: 10.1016/j.vaccine.2014.02.052
19. Novotny LA, Adams LD, Kang DR, Wiet GJ, Cai X, Sethi S, et al. Epitope mapping immunodominant regions of the PilA protein of nontypeable haemophilus influenzae (NTHI) to facilitate the design of two novel chimeric vaccine candidates. Vaccine (2009) 28(1):279–89. doi: 10.1016/j.vaccine.2009.08.017
20. Ysebaert C, Denoël P, Weynants V, Bakaletz LO, Novotny LA, Godfroid F, et al. A protein e-PilA fusion protein shows vaccine potential against nontypeable haemophilus influenzae in mice and chinchillas. Infect Immun (2019) 87(8):e00345–19. doi: 10.1128/IAI.00345-19
21. Martinovich KM, Rahman T, Gier C, Seppanen EJ, Orami T, Granland CM, et al. Differences in pneumococcal and haemophilus influenzae natural antibody development in Papua new guinean children in the first year of life. Front Immunol (2021) 12(3249). doi: 10.3389/fimmu.2021.725244
22. Simell B, Ahokas P, Lahdenkari M, Poolman J, Henckaerts I, Kilpi TM, et al. Pneumococcal carriage and acute otitis media induce serum antibodies to pneumococcal surface proteins CbpA and PhtD in children. Vaccine (2009) 27(34):4615–21. doi: 10.1016/j.vaccine.2009.05.071
23. Holmlund E, Quiambao B, Ollgren J, Jaakkola T, Neyt C, Poolman J, et al. Antibodies to pneumococcal proteins PhtD, CbpA, and LytC in Filipino pregnant women and their infants in relation to pneumococcal carriage. Clin Vaccine immunol: CVI (2009) 16(6):916–23. doi: 10.1128/CVI.00050-09
24. Kaur R, Casey JR, Pichichero ME. Serum antibody response to three non-typeable haemophilus influenzae outer membrane proteins during acute otitis media and nasopharyngeal colonization in otitis prone and non-otitis prone children. Vaccine (2011) 29(5):1023–8. doi: 10.1016/j.vaccine.2010.11.055
25. Francis JP, Richmond PC, Pomat WS, Michael A, Keno H, Phuanukoonnon S, et al. Maternal antibodies to pneumolysin but not to pneumococcal surface protein a delay early pneumococcal carriage in high-risk Papua new guinean infants. Clin Vaccine immunol: CVI (2009) 16(11):1633–8. doi: 10.1128/CVI.00247-09
26. Hua CZ, Hu WL, Shang SQ, Li JP, Hong LQ, Yan J. Serum concentrations of antibodies against outer membrane protein P6, protein d, and T- and b-cell combined antigenic epitopes of nontypeable haemophilus influenzae in children and adults of different ages. Clin Vaccine Immunol (2016) 23(2):155–61. doi: 10.1128/CVI.00506-15
27. Clarke E, Kampmann B, Goldblatt D. Maternal and neonatal pneumococcal vaccination - where are we now? Expert Rev Vaccines (2016) 15(10):1305–17. doi: 10.1586/14760584.2016.1167602
28. Chaithongwongwatthana S, Yamasmit W, Limpongsanurak S, Lumbiganon P, Tolosa JE. Pneumococcal vaccination during pregnancy for preventing infant infection. Cochrane Database System Rev (2015) 2015(1):CD004903. doi: 10.1002/14651858.CD004903.pub4
29. Niewiesk S. Maternal antibodies: clinical significance, mechanism of interference with immune responses, and possible vaccination strategies. Front Immunol (2014) 5:446–6. doi: 10.3389/fimmu.2014.00446
30. Binks MJ, Moberley SA, Balloch A, Leach AJ, Nelson S, Hare KM, et al. PneuMum: Impact from a randomised controlled trial of maternal 23-valent pneumococcal polysaccharide vaccination on middle ear disease amongst indigenous infants, northern territory, Australia. Vaccine (2015) 33(48):6579–87. doi: 10.1016/j.vaccine.2015.10.101
31. O’Brien KL, Nohynek H. Report from a WHO working group: standard method for detecting upper respiratory carriage of streptococcus pneumoniae. Pediatr Infect Dis J (2003) 22(2):e1–11. doi: 10.1097/01.inf.0000049347.42983.77
32. Morris P, Ballinger D, Leach A, Koops H, Hayhurst B, Stubbs L, et al. Recommendations for clinical care guidelines on the management of otitis media in aboriginal and Torres strait islander populations. Canberra: Office of Aboriginal and Torres Strait Islander Health (2001).
33. Wiertsema SP, Corscadden KJ, Mowe EN, Zhang G, Vijayasekaran S, Coates HL, et al. IgG responses to pneumococcal and haemophilus influenzae protein antigens are not impaired in children with a history of recurrent acute otitis media. PloS One (2012) 7(11):e49061. doi: 10.1371/journal.pone.0049061
34. Forsgren A, Riesbeck K, Janson H. Protein d of haemophilus influenzae: a protective nontypeable h. influenzae antigen and a carrier for pneumococcal conjugate vaccines. Clin Infect Dis (2008) 46(5):726–31. doi: 10.1086/527396
35. Thornton RB, Kirkham LS, Corscadden KJ, Wiertsema S.P, Fuery A, Jones BJ, et al. Australian Aboriginal children with otitis media have reduced antibody titers to specific nontypeable haemophilus influenzae vaccine antigens. Clin Vaccine Immunol (2017) 24(4):E551–8. doi: 10.1128/CVI.00556-16
36. Brock SC, McGraw PA, Wright PF, Crowe JE Jr. The human polymeric immunoglobulin receptor facilitates invasion of epithelial cells by streptococcus pneumoniae in a strain-specific and cell type-specific manner. Infect Immun (2002) 70(9):5091–5. doi: 10.1128/IAI.70.9.5091-5095.2002
37. Turner P, Turner C, Green N, Ashton L, Lwe E, Jankhot A, et al. Serum antibody responses to pneumococcal colonization in the first 2 years of life: results from an SE Asian longitudinal cohort study. Clin Microbiol Infect (2013) 19(12):E551–8. doi: 10.1111/1469-0691.12286
38. de Voer RM, Klis der van FR, Nooitgedagt JE, Versteegh FG, Huisseling van JC, Rooijen van DM, et al. Seroprevalence and placental transportation of maternal antibodies specific for neisseria meningitidis serogroup c, haemophilus influenzae type b, diphtheria, tetanus, and pertussis. Clin Infect Dis (2009) 49(1):58–64. doi: 10.1086/599347
39. Sun W, Jacoby P, Riley TV, Bowman J, Leach AJ, Coates H, et al. Association between early bacterial carriage and otitis media in aboriginal and non-aboriginal children in a semi-arid area of Western Australia: a cohort study. BMC Infect Dis (2012) 12:366. doi: 10.1186/1471-2334-12-366
40. Leach AJ, Wigger C, Beissbarth J, Woltring D, rews R, Chatfield MD, et al. General health, otitis media, nasopharyngeal carriage and middle ear microbiology in northern territory aboriginal children vaccinated during consecutive periods of 10-valent or 13-valent pneumococcal conjugate vaccines. Int J Pediatr Otorhinol (2016) 86:224–32. doi: 10.1016/j.ijporl.2016.05.011
41. Kørvel-Hanquist A, Djurhuus BD, Homøe P. The effect of breastfeeding on childhood otitis media. Curr Allergy Asthma Rep (2017) 17(7):45. doi: 10.1007/s11882-017-0712-3
42. Frank NM, Lynch KF, Uusitalo U, Yang J, Lönnrot M, Virtanen SM, et al. The relationship between breastfeeding and reported respiratory and gastrointestinal infection rates in young children. BMC Pediatr (2019) 19(1):339. doi: 10.1186/s12887-019-1693-2
43. McGuire MK, Randall AZ, Seppo AE, Järvinen KM, Meehan CL, Gindola D, et al. Multipathogen analysis of IgA and IgG antigen specificity for selected pathogens in milk produced by women from diverse geographical regions: The INSPIRE study. Front Immunol (2021) 11:614372–2. doi: 10.3389/fimmu.2020.614372
Keywords: Haemophilus influenzae, Streptococcus pneumoniae, maternal, breast milk, IgG, IgA
Citation: Martinovich KM, Seppanen EJ, Bleakley AS, Clark SL, Andrews RM, Richmond PC, Binks MJ, Thornton RB and Kirkham L-AS (2022) Evidence of maternal transfer of antigen-specific antibodies in serum and breast milk to infants at high-risk of S. pneumoniae and H. influenzae disease. Front. Immunol. 13:1005344. doi: 10.3389/fimmu.2022.1005344
Received: 28 July 2022; Accepted: 24 August 2022;
Published: 21 September 2022.
Edited by:
Ahmed Sherif Attia, Cairo University, EgyptReviewed by:
Lovro Lamot, University of Zagreb, CroatiaDalia ElGhoneimy, Ain Shams University, Egypt
Rajlakshmi Viswanathan, National Institute of Virology, (ICMR), India
Copyright © 2022 Martinovich, Seppanen, Bleakley, Clark, Andrews, Richmond, Binks, Thornton and Kirkham. This is an open-access article distributed under the terms of the Creative Commons Attribution License (CC BY). The use, distribution or reproduction in other forums is permitted, provided the original author(s) and the copyright owner(s) are credited and that the original publication in this journal is cited, in accordance with accepted academic practice. No use, distribution or reproduction is permitted which does not comply with these terms.
*Correspondence: Lea-Ann S. Kirkham, lea-ann.kirkham@telethonkids.org.au