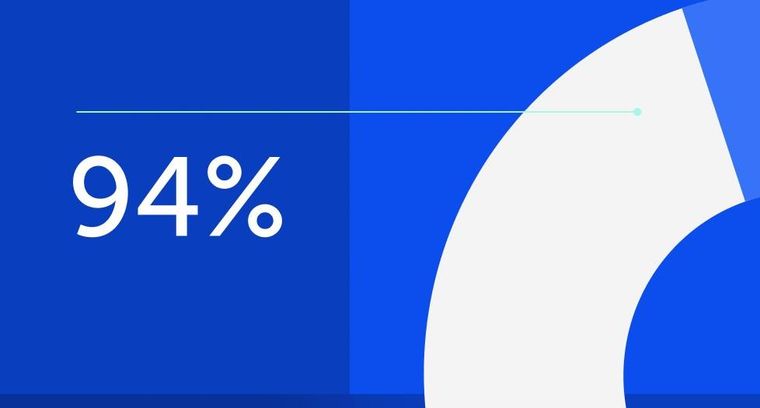
94% of researchers rate our articles as excellent or good
Learn more about the work of our research integrity team to safeguard the quality of each article we publish.
Find out more
REVIEW article
Front. Immunol., 14 February 2023
Sec. Autoimmune and Autoinflammatory Disorders : Autoimmune Disorders
Volume 13 - 2022 | https://doi.org/10.3389/fimmu.2022.1000951
This article is part of the Research TopicAutoimmune Pre-DiseaseView all 26 articles
Shift work is associated with systemic chronic inflammation, impaired host and tumor defense and dysregulated immune responses to harmless antigens such as allergens or auto-antigens. Thus, shift workers are at higher risk to develop a systemic autoimmune disease and circadian disruption with sleep impairment seem to be the key underlying mechanisms. Presumably, disturbances of the sleep-wake cycle also drive skin-specific autoimmune diseases, but epidemiological and experimental evidence so far is scarce. This review summarizes the effects of shift work, circadian misalignment, poor sleep, and the effect of potential hormonal mediators such as stress mediators or melatonin on skin barrier functions and on innate and adaptive skin immunity. Human studies as well as animal models were considered. We will also address advantages and potential pitfalls in animal models of shift work, and possible confounders that could drive skin autoimmune diseases in shift workers such as adverse lifestyle habits and psychosocial influences. Finally, we will outline feasible countermeasures that may reduce the risk of systemic and skin autoimmunity in shift workers, as well as treatment options and highlight outstanding questions that should be addressed in future studies.
Our 24/7 society leads to an increase in shift work with work schedules that fall outside the standard work hours from 7 AM to 6 PM. Shift work includes early morning, evening, or night shifts, as well as fixed or rotating shifts. About 15-25% of the world-wide population is working in shifts (1), in particular health care workers. In this latter population epidemiological studies provide alarming data on shift workers showing increased rates of longstanding illnesses (2). In addition, the Nurses’ Health Study demonstrated enhanced mortality due to cardiovascular diseases or lung cancer (3). Shift workers are forced to be active and to sleep at time periods that are out of sync with their endogenous time-keeping system. This internal clock, the circadian system, is comprised of clock genes in many, if not all cells of the body. The molecular machinery of cellular clocks involves -among others- the core clock genes Brain and Muscle ARNT-Like1 (in humans BMAL1, in mice Bmal1) and Circadian Locomotor Output Cycles Kaput (CLOCK, Clock), which form dimers upon translation and initialize the transcription of Period (PER, Per) genes. These form a dimer with Cryptochrome (CRY, Cry) genes. This in turn inhibits the transcription of BMAL1. Moreover, the BMAL1-CLOCK-dimer is binding together with the dimer of NFIL3 and DBP to the E box of REVERB/Reverb genes, initiating their transcription. In turn, REVERB/Reverb genes inhibit the transcription of NFIL3/Nfil3, forming another feedback-loop (4). With the interaction of other genes and proteins, a network of interconnected loops is formed, taking approximately 24 hours (h) to be executed. The master clock in the hypothalamic suprachiasmatic nuclei (SCN) is synchronized to external time cues like 24 h light-dark changes, a process that is called entrainment (4). In turn, the SCN entrain peripheral clocks by systemic signals such as core body temperature, mediators of the stress systems (such as the sympathetic nervous system (SNS) and the hypothalamus-pituitary-adrenal (HPA)-axis, as well as melatonin, the pineal hormone of darkness (5). The circadian system controls virtually all body functions like sleep and wakefulness, behavioral changes in physical activity and food intake, thermoregulation, cell proliferation and metabolism, the cardiovascular, the endo-crine, the digestive, the reproductive and the immune system (6–16). Apart from light, several non-photic external time cues have been described that were summarized in a review by Mistlberger and Skene (17). Exemplarily, food is a non-photic external time cue that can feedback to the SCN to further entrain 24 h rhythms
When shift workers experience a mismatch of their internal clock with environmental cues and obligations this circadian misalignment can result in circadian disruption of body functions from the molecular to the behavioral level. Potential outcomes are poor sleep, chronic stress (18), burn out syndrome (19), social isolation (20) and adverse lifestyle habits like physical inactivity (18), unhealthy diet (21, 22), or substance abuse (23). All these processes can trigger systemic chronic inflammation (SCI) (24, 25) and a dysregulation of innate and adaptive immune responses. That alone or in combination may foster infectious, cardiovascular, metabolic and cancer diseases in shift workers (22, 26, 27). In addition, the STRESSJEM Study, which was conducted in France, analyzed mortality and cause-specific mortality due to night and shift work. Niedhammer et al. described a sex-specific association between night and shift work and cerebrovascular diseases, ischemic heart diseases, respiratory cancers and breast cancer as causes of mortality (27). Thus, a life against the internal clock can impair host defense against pathogens (28, 29) and tumors (30) and also seems to promote unwanted immune responses to harmless antigens, like allergens (31) or auto-antigens (32). While shift workers are at higher risk to develop systemic autoimmune diseases as shown for rheumatoid arthritis (RA) (33), data on associated skin manifestations or skin-specific autoimmune diseases is only limited. In the following, we will outline that shift work likely impairs skin physiology and immunity and thus could promote skin autoimmune diseases, a causality that, however, needs to be experimentally clarified in future studies.
In detail, we will first describe 24 h in the life of a physician during a night shift in a narrative in section 2 and outline (Table 1) experimental approaches to delineate the effects of shift work on the skin and the immune system in section 3. In section 4, we will then summarize epidemiological and experimental evidence in humans and animals indicating that shift work could promote skin autoimmune diseases, before we give more detailed reports on the cellular effects of shift work on skin physiology, skin innate immunity and skin adaptive immunity in section 5. In section 6, we will discuss candidate neuroendocrine mediators linking shift work with skin autoimmune diseases and in section 7 we will highlight potential countermeasures and therapeutic approaches to prevent, ameliorate or treat skin autoimmune diseases in shift workers. In the last part of our review, in section 8, we will summarize the outlined findings and give an outlook on outstanding questions that should be addressed in future experiments
In a narrative describing 24 h in the life of an intensive care unit physician who we called Dr. S.W. on night shift, we would like to outline shift work-induced changes in behavior, neuroendocrine mediators, thermoregulation, skin physiology and immune functions in comparison to regular 24 h rhythms. We hypothesize that fine-tuned physiological rhythms in neuroendocrine-immune interactions foster skin barrier functions and that disturbances thereof could promote patho-physiological processes of skin autoimmune diseases. We chose a tabular form with 4 h intervals (Table 1) (34–55).
In this chapter we aim to describe the different types of studies in humans as well as in animals, which can be used to assess the effects of shift work on the skin and the immune system.
The circadian system controls skin physiology (56) as well as leukocyte ontogeny, differentiation, traffic, and function and thus various aspects of innate and adaptive immunity (57, 58).
Skin physiology in humans can be assessed in vivo non-invasively by inspection, by photo documentation, by imaging techniques like optical coherence tomography (59), as well as by measurements of skin temperature, skin pH, skin conductance, or transepidermal water loss (60). Skin physiology can also be measured invasively by harvesting suction blister fluid, by skin biopsies, or by injecting substances that induce an observable skin reaction.
Delayed type hypersensitivity (DTH) describes the cell mediated allergic immune reaction to a certain substance. This takes several days to develop as it involves antigen presenting cells as well as T helper 1 cells (Th1) and T helper 17 (Th17) cells. These recognize the antigen and release cytokines, attracting cytotoxic T cells, which kill the target cells (61, 62). A DTH-reaction also occurs in several autoimmune diseases such as RA where collagen is attacked as well as thyroiditis with the thyroglobulin antigen as a target (63, 64).
Immune parameters in humans are mainly assessed in blood (e.g., numbers of certain leukocyte subsets, levels of cytokines) and immune functions can be tested in vitro by using leukocyte cell lines, ex vivo by culturing freshly sampled blood leukocytes or in vivo by administering immunomodulatory substances and measuring the emerging immune response (e.g., antibody response to vaccination). The assessment of innate and adaptive immunity of the skin requires the invasive methods described above that allow the measurement of immune cells or mediators in fluids or tissues (e.g., histology, immunohistochemistry, immunofluorescence, fluorescent activated cell sorting, enzyme linked immunosorbant assay (ELISA), Western blotting).
The circadian system is closely linked to sleep that itself has manifold effects on skin health and skin ageing (65) and the immune system (47). 24 h rhythms of human behavior including sleep-wake behavior are monitored by questionnaires or wearables like actigraphy watches (66, 67). The latter can also track ambient light and temperature, heart rate, skin temperature and skin conductance. The gold standard to measure sleep with its different stages from light sleep to deep sleep (slow wave sleep (SWS)) and rapid eye movement (REM) sleep is polysomnography, encompassing electroencephalography for brain activity, electrooculography for eye movements and electromyography for muscle activity. It can be recorded with ambulatory devices in the home setting or in the sleep laboratory, where it can be combined with videotaping, monitoring of core body temperature and repeated blood sampling. The two-process model of sleep regulation describes the control of the onset, duration and quality of sleep, as well as increases in alertness and performance during wakefulness. The homeostatic “process S” involves sleep regulatory substances such as adenosine, tumor necrosis factor (TNF) and interleukin (IL)-1. This is combined with the circadian “process C”, which regulates wakefulness by wake-promoting neurotransmitters such as catecholamines (68, 69). Interestingly, the likelihood to fall asleep is highest, when temperature of the distal skin regions (e.g., fingers and toes) is rising in the evening (44). Further, sleep can be deepened by passive warming of the skin (70). Apart from the circadian regulation of sleep by process C, sleep can feedback to the circadian system and impact body rhythms on the level of the SCN (71–73) and the periphery (74–78). Consequently, 24 h rhythms in skin physiology or a given immune parameter, could stem from the effects of the circadian system, from sleep, or both. Moreover, experimental manipulation of the circadian system likely changes sleep and vice versa, experimental manipulation of sleep can impact 24 h rhythms of skin and immune parameters.
Along this line, it has been shown in cross-sectional and longitudinal epidemiological studies that shift work induces both circadian disruption and sleep disturbances (79, 80). About 20-30% of shift workers even suffer from shift work disorder, a primary circadian rhythm sleep disorder with debilitating sleep disturbances and/or excessive sleepiness (81). Notably, also other primary sleep disorders (e.g., obstructive sleep apnea) and secondary sleep complaints due to comorbidities (e.g., depression) or medication (e.g., steroids) should be ruled out when studying shift work-autoimmune relationships (82, 83). To mimic shift work, circadian disruption can be induced in healthy volunteers experimentally by changes of the light-dark cycle, mistimed food intake, or mistimed sleep by delaying, depriving or fragmenting sleep (84, 85). Comparable experimental procedures in animals to directly manipulate the circadian system or sleep on the cellular level will be summarized in the next section.
Most animal experiments in biomedical research are performed in mice that are active at night and sleep during the day (86). Wild mice are orientating on zeitgebers such as the light/dark cycle, ambient temperature and seasonal dynamics (87). During the day, the mice are asleep and when the sun is downing and the temperature is lowering, this is the signal to get awake and be active (Figure 1). These wild mice are exposed to a completely different life and stressors (87) than their counterparts in the laboratories. They have many more options to explore, more space to run around and also larger territories than in a standard cage. The social groups form dynamically and are not gender specific (88). Wild mice are rarely disturbed during the rest phase; however, they have to cope with the stressors of predators, pathogens and limited access to food (87, 89, 90). Unfortunately, these natural conditions cannot be mimicked in the laboratory setting (91) (Figure 2 and Box 1) (92–98).
Figure 1 24 hours in the life of Ferry the forest mouse. In nature, mice follow their natural rhythm guided by abiotic zeitgebers such as light and temperature. They sleep during the day, cuddling in their warm nest in the soil (1). When the sun is downing, they wake up and leave the nest (2). The mice use the night and their highest alertness to seek for food and watch out for predators (3). A lot of running, climbing, and collecting of food during the night (4), releases them happily tired into the day and their sleep (5).
Figure 2 24 hours in the life of Blacky the lab mouse. Mice are nocturnal animals, being active during the night, which is opposite to humans. This leads to various interruptions of their rest period (1, 2), accompanied by stress and changes in behavior. Grooming and eating alleviates some stress caused by sleep deprivation (3). The cage-environment is highly restricted in space, movement, and explorative options, resulting in coping behavior (4). Moreover, mice might catch up some sleep that was missed in the rest period (5). More information can be found in the Box 1.
BOX 1 Blacky, the lab mouse (Figure 2).
Blacky, a C57BL/6 mouse, is living with his brothers in a ventilated cage with 501 cm2. Their home is comfortable with a fluffy nest. During the day, when the light is turned on, all brothers want to sleep and cuddle in their nest to stay warm and get some rest (1). It is not as cozy as it sounds because there is constantly noise from humans around them and the ambient temperature is below the brothers’ thermoneutral zone of 30°C. The temperature of 22°C is constant for 24 hours, which makes it even more difficult to decide whether it is time to be awake or asleep, it is just always cold. From time to time, their entire home is picked up while they are asleep and put somewhere else or the nest is exchanged for a new nest. Somedays even all brothers are woken up and placed in a new home (2). These days are truly horrible and to compensate the stress, the brothers groom each other, even plucking off the whiskers or entire patches of fur. Some of the brothers also get hungry or feel the urge to chew to get rid of the stress (3) (92, 93). So, a little meal in between is very common and especially Black-Jack, the biggest brother who leads the group, starts accumulating excessive fat. When the light is turned off, the brothers’ activity period starts. It is getting quiet around them, they do not hear the humans anymore, just other mice from other homes nearby. This is a good time to take a meal and luckily, they do not need to search long for their food, there is enough for everyone. Apart from food, their cage is boring because there is nothing to explore and barely anything to play with or space to run around. This is why Blacky and his brothers groom each other a lot (4) (94) causing small bold patches, sometimes even wounds and infections (95, 96). The grooming follows a strict hierarchy, and Black-Jack gives all the calls. If another brother has a different opinion, Black-Jack is showing him who is the boss by biting him. These fights and dominance behaviors alter neuroendocrine mediators such as corticosterone and tyrosine hydroxylase (97). After feeding, grooming and fighting, there is nothing much to do and since the brothers were woken up several times during their rest period, this is a good time to sleep again (5) (98). Unfortunately, this sleep is never as good as when the lights are on but who knows when to be active and when to sleep anyways?
Laboratory mice do not have to scare predatory animals, but care takers and scientists are fulfilling this aspect sufficiently. It was shown in several experimental studies that handling and exchange or cleaning of cages is inducing stress in mice (92, 93), especially when male care takers and scientists are involved (99) (Figure 2 and Box 1). The laboratory mice cannot entirely follow their circadian rhythm as most experimental interventions take place during the work hours of the scientists and animal caretakers, thus in the rest period of these animals during the day. It therefore cannot be excluded that laboratory mice are constantly sleep deprived and suffer somehow from “shift work disorder”. Keeping a mouse below its thermoneutral zone of 30°C ambient temperature may further activate the stress systems and interfere with circadian regulation (100), sleep and immunity (101). In most cases, studies in mice on the circadian system or sleep address these issues. In their experimental designs the light-dark cycle is changed on purpose (i.e., the dark period for the animals is during the daytime working hours of experimenters). Manipulations are in this way mainly scheduled to the active period of the animal and experimenters work in dim or red light that does not impact the SCN. As shown in Figure 1, mice in nature are exposed to circadian changes in ambient temperature, which are not present in laboratories, also leading to differences in sleep and circadian alignment (100, 102). However, the fact that warmer ambient temperature, the availability of nesting material and group housing support sleep in mice is taken into account in most laboratories (45).
Shift work can be mimicked experimentally in mice by changes in the light-dark schedule. As food intake is an important external time cue, time restricted feeding can be seen as another method to mimic shift work, in particular, when food is offered during the light phase, thus during the rest period of mice (103, 104). In ad libitum feeding protocols, food intake is often not controlled for, although it has a major impact on skin clocks (105) and on immune outcomes in response to circadian or sleep manipulations (106). Apart from changes in external time cues to mimic shift work, in animals the circadian system can be directly targeted by SCN lesions or genetic manipulations of clock genes in the germline or on a cell-specific level (e.g., Clock knock-out in particular immune cells, or knock-downs by adeno-associated viruses) (10, 107–110). Notably, these interventions might also induce sleep changes that should be controlled for (111). On the other hand, sleep can be manipulated by various more or less stressful techniques of sleep deprivation or fragmentation, or by optogenetics (112). However, it should be kept in mind, that mice cannot follow a constant routine to avoid confounding influences of physical activity during induced wakefulness. Mice are still the most common animal model although they are nocturnal animals with a complementary sleep-wake rhythm compared to humans. However, not all cellular or endocrine factors have complementary rhythms. Melatonin for example has a similar pattern in mice and humans, underlining its role as a dark-signal, which is downstream activating different pathways. Noteworthy, it is under debate for many mouse strains whether they are able to synthesize melatonin (113, 114). Despite this, melatonin was shown to affect mice regarding depressive-like and stress behavior and also circadian alignment (115–117).
On the other hand, mice offer plenty of possibilities to study sleep-wake behavior, e.g. by implanting electrodes or by assessing circadian locomotor activity patterns with electronic running wheels (even in group housed mice) (118). It is also possible to implant radiotelemetry transmitters to constantly measure the heart rate and blood pressure (119, 120) or using photobeam, and electroencephalograms. Metabolic feeding cages using indirect calorimetry are also a great option to follow the circadian metabolic patterns of mice (121). Genetic knock-outs, knock-downs and knock-ins make these animals a valuable tool to examine the effect of specific genes. It should be taken into account, however, that extended breeding, husbandry and genetic manipulation of lab mice resulted in profound changes in gene expression distancing them further from wild animals (89).
The murine immune system is only partly comparable to that of humans with various cellular and molecular differences (122, 123). Neutrophils display about 10-25% of the cells in the peripheral blood in mice whereas these are 50-70% in humans. Lymphocytes are the most abundant cell type in peripheral blood in mice with 75-90%, compared to only 30-50% in humans (122). Mice are also commonly used to assess skin biology. This is an interesting option to induce certain diseases such as epidermolysis bullosa acquisita (EBA) (124–126), and allows to take skin and organ biopsies in a degree that is not possible to gain from human patients. Human and murine skin are composed of the same layers. However, the thickness of human skin is much higher and more adherent to underlying tissues. Accordingly, mice have decreased barrier function and enhanced percutaneous absorption, which should be considered when using murine models for topic drug delivery. Moreover, mice have fur and therefore more hair follicles, which leads to differences in wound healing (127, 128). In contrast to humans, mice also show a subcutaneous layer called panniculus carnosus, which is a muscle layer, enabling skin contraction. This is of interest since large wounds require muscle contraction for healing, whereas in humans wound healing is achieved by formation of granulation tissue and reepithelization (129).
Even though rodents have the above-mentioned limitations, they are nevertheless a valuable and hitherto irreplaceable tool for studying effects of sleep and the circadian machinery on the immune system. Murine studies allow to explore the relations and connections between different organs and influences of the lifestyle, which cannot be shown in other models. This is either due to limitations in genetic manipulations in non-rodents or because the model is not a full organism such as cell- or organ-cultures. Hopefully, future techniques are overcoming the need for murine studies and thereby offer new models without the above-mentioned drawbacks of mice. It is important to address difficulties with certain models to be able to overcome these. Nevertheless, there are various well-working mouse models, which delivered valuable results. Just to mention two out of many studies, we would like to outline the work of Toth et al. who studied the effects of shift work in a lupus mouse model, which resembled also typical human outcomes (130) as well as the work of Sadeghi et al. who used an EBA mouse model with an unbiased genetic approach to investigate inflammatory processes and discovered the role of the clock gene retinoic acid-related orphan receptor-alpha (Rora) in this disease (126).
Apart from invertebrates that cannot be used to assess skin diseases, other animal models are fish to study melanoma (131, 132) or mammals such as pigs to study skin and circadian regulation of the immune system (133, 134). However, porcine models are difficult to establish as the animals need a lot of space and require comparably long breeding spans, although some genetical modifications such as CRISPR/Cas are already well-established (135). Regarding non-human primates, pigs are ethically more accepted for experimental purposes and show higher numbers of offspring, allowing to gain sufficient animals for statistical analysis of the experiments.
This section outlines the connection between shift work and skin autoimmune diseases. Firstly, described by shift works’ general effect on the immune system and then depicting more detailed effects on certain skin autoimmune diseases.
Shift work drives SCI (25, 136, 137) that is associated with endothelial dysfunction, atherosclerosis, cardiovascular diseases, impaired glucose tolerance, metabolic syndrome, diabetes mellitus, obesity, mood disorders and neurodegenerative diseases (22, 24, 136, 138). Likewise, experimental circadian disruption as well as sleep deprivation in humans and animal models can induce an inflammatory response (40, 139, 140) and dysfunctions of cardiovascular processes (75, 141, 142), metabolism (143, 144), mood and cognition (145). Presumably, SCI in the periphery and a parallel neuroinflammatory response in the brain are the consequence of innate immune cells responding to sterile immune stimuli (e.g., reactive oxygen species, metabolites, danger/damage associated molecular patterns) (37, 146–148) and failures in counter-regulatory, anti-inflammatory mechanisms that normally regulate and resolve inflammation (e.g., IL-10, resolvins, M2 macrophages, regulatory T cells (Tregs)) (47, 149–151). While the innate immune system fights this unnecessary battle against sterile stimuli, targeted and protective immune responses are compromised. Thus, shift work or experimental circadian disruption, as well as insufficient sleep are associated with failures in innate and adaptive immunity against pathogens (152) and tumors (153–156) and with reduced vaccination-driven T cell and antibody responses (157–160), both in humans and in mice. On the other hand, shift work seems to boost unwanted adaptive immune responses to harmless antigens such as allergens and auto-antigens. In detail, shift work, circadian misalignment, clock gene polymorphisms or poor sleep are associated with a higher risk to develop allergic or autoimmune diseases (31, 161). The latter include (i) connective tissue diseases such as systemic lupus erythematosus (SLE) (162), systemic sclerosis (SSc) (161), and Sjögren’s syndrome (163, 164), (ii) different forms of arthritis such as rheumatoid arthritis (RA) (165), and spondyloarthritis (SpA) (161, 166) including psoriasis arthritis (163), (iii) inflammatory bowel disease (IBD) (167–173), (iv) autoimmune thyroiditis (32, 174–176), and (v) multiple sclerosis (177). Undoubtedly, sleep is impaired in patients with systemic autoimmune diseases (178). Moreover, they show disturbances in 24 h rhythms of their stress systems (179, 180), cardiovascular functions (181), and melatonin (182–185). These changes may be the consequence rather than the cause of the autoimmune disease, as disease symptoms such as pain, itch, respiratory or gastrointestinal dysfunctions can heavily interfere with a regular sleep-wake behavior (186). However, lupus-prone mice show disturbed 24 h rhythms of corticosterone and melatonin already in asymptomatic phases, thus before manifestation of the disease (187). Moreover, experimental circadian disruption and/or sleep deprivation in animal models can promote autoimmune diseases like lupus (130, 188). It can also worsen colonic inflammation in murine models of IBD (189) or attenuate others such as experimental auto-immune encephalomyelitis (190). Thus, autoimmune processes and wake-sleep-disturbances most likely show bidirectional relationships that could feed into a vicious circle.
Many systemic autoimmune diseases affect the barrier organs, thus the mucosa of the respiratory, gastrointestinal, or urogenital tract or the epidermis, dermis and subcutis of the skin (Table 2) (191–260). Cutaneous manifestations are leading symptoms in SSc (e.g., puffy fingers, skin fibrosis and calcinosis cutis), or characteristic clinical presentations of SLE (e.g., malar ‘butterfly’ rash), of Sjögren’s syndrome [e.g., dry skin called xerosis cutis or xeroderma (232, 233)], of RA [e.g., rheumatoid nodules (241)], of SpA, celiac disease or IBD (e.g., pyoderma gangrenosum) (242, 252), or of thyroiditis [e.g., myxedema (259)]. Connective tissue diseases, but also any other systemic autoimmune disease can lead to secondary Raynaud’s phenomenon with an exaggerated cold-induced vasoconstriction of arteriovenous anastomoses in distal skin regions (e.g., fingers and toes). Currently, there is only limited data on the impact of the sleep-wake cycle on cutaneous symptoms of systemic autoimmune diseases or thyroiditis. The circadian system may be involved in photosensitivity in SLE (218). Moreover, poor sleep correlates with enhanced skin thickness in SSc (225, 227) and with genital ulcers in the vasculitis Behçet’s disease (235, 236, 261). However, although shift work has been shown to impair skin health with respect to allergic and cancerous conditions (262, 263), to our knowledge epidemiological or experimental studies on skin manifestations of systemic autoimmune diseases are currently lacking. Even for Raynaud’s phenomenon that involves a pathophysiology closely linked to circadian and sleep-dependent thermoregulation (which could be easily monitored by wearables), we did not find a single study that investigated this condition in shift workers.
Likewise, studies on the association between shift work, circadian disruption or poor sleep on skin-specific autoimmune diseases are rare and mainly focus on psoriasis. Psoriasis typically presents with cutaneous erythematosquamous plaques and approximately 50% of patients develop typical nail changes. Rarely, pustular changes occur, which can affect the palms and soles, but also the entire body. One aspect of psoriasis research that has recently been investigated is the time-of-day variability in disease symptoms and severity with a peak of itch and psoriasis flares in the evening and at night. However, the reasons for this observation remain unclear (194). Related diseases such as SpA show similar peaks of symptoms at night (249).
Early circadian research on psoriasis investigated time-of-day-dependent changes in the epidermis. One study revealed no diurnal differences in mitotic index (264), whereas another one showed increased cell proliferation at 6 AM compared to healthy controls (265). A further study investigating circadian cell kinetics revealed a stable epidermal and dermal infiltrate cell proliferation over the day both in uninvolved and involved psoriatic skin, but with a circadian rhythm in epidermal DNA synthesis (266). However, in 1985, a 24 h rhythm of neutrophil migration in psoriatic skin with a peak at around 10 PM was detected that could not be shown in the skin of healthy controls (193). Further reports suggest a systemic circadian perturbation, with disruption of circadian rhythms of urinary and haema-tological parameters (192), of blood pressure and heart rate (237, 267) and of plasma melatonin levels (202) in psoriasis (and vasculitis) patients. In addition, lower urinary 24 h cortisol levels, lower serum cortisol at 8 AM and 5 PM were found in patients with psoriasis and IBD (251) compared to healthy controls suggesting an altered function of the HPA-axis (268).
To unravel the link between the circadian clock and psoriasis, a transcriptomic study showed a downregulation of CRY1/2, REVERBA, CLOCK, BMAL1 and RORA/C in keratinocytes from psoriatic lesions (269). A recent study found changes in core clock genes and clock proteins in non-lesional and lesional human skin samples in psoriasis (270). In a mouse model, imiquimod-induced psoriasis-like dermatitis was ameliorated in mice with a loss of function mutation in the Clock gene compared to wild-type mice. Accordingly, in mice with a loss of function mutation in the Per2 gene imiquimod-induced psoriasis-like dermatitis was exaggerated because PER2 inhibits CLOCK activity (271).
Based on the described investigations, few groups studied the application of chronotherapy in psoriasis. Balneotherapy (bathing therapy) has the highest efficiency when it is applied in the morning (272). Topical corticosteroid application in the evening has a higher efficiency than application in the morning after two days of treatment. However, this difference evened out after five treatment days (273). Discussed reasons for the higher effectiveness are an improved corticosteroid absorption in the evening due to a higher cutaneous perfusion and a higher skin barrier permeability or a higher therapeutic potential due to a rise in inflammation and cell proliferation in the skin during evening hours (273, 274). Recently, chronotherapy of maxa-calcitol, a vitamin D analogue, was investigated in a mouse model of psoriasis in which the skin inflammation was induced by topical 12-O-tetradecanoylphorbol-13-acetate (TPA). In the skin of mice, expression of the nuclear vitamin D receptor exhibits a distinct daily variation with a peak in the middle of the active period. Accordingly, in TPA-mice application of maxacalcitol during early to middle of the active period had the highest therapeutic efficacy (275).
Various studies describe the occurrence of sleep disturbances and fatigue in patients with SLE, Sjögren’s syndrome, vasculitis, psoriasis, and psoriatic arthritis (197, 198, 219, 238, 276–279). In both conditions, patients rate sleep disturbances as a factor severely impairing quality of life (280–283). Sleep disturbances in patients with psoriasis and psoriatic arthritis are caused not only by disease manifestations such as nocturnal itching or pain (20, 284), but also by gastroesophageal reflux disease, anxiety or depression (285, 286). In addition, an association between psoriasis and prevalence of sleep disorders, such as obstructive sleep apnea or restless legs syndrome, has been described. Data on the prevalence of sleep disorders in psoriasis and potential effects of psoriasis treatment with biologics on sleep and sleep disorders are presented in a recently published review (287). Until now, the effect of immunomodulatory and immuno-suppressive therapeutics on sleep disturbances remains elusive (288–290). In addition, studies investigating the relationship between sleep quality and disease activity in psoriasis and psoriatic arthritis give contradictory results (276, 291–298). So far, studies on lifestyle interventions that affect sleep and investigate respective effects on disease activity are lacking (299). Impairment of sleep in patients with psoriasis, psoriatic arthritis and axial SpA increases the risk for psychiatric diseases, which themselves might impair sleep, resulting in a vicious circle (300–302). Moreover, patients with psoriasis and sleep disturbances have a higher risk of stroke and ischemic heart disease compared to psoriatic patients without sleep disturbances (303). Finally, jet-lag in patients with psoriasis experiencing a flight crossing at least two time-zones increases self-reported disease severity (304).
Circadian and diurnal variations as well as sleep dis-turbances are well investigated in psoriasis and psoriatic arthritis but only a single study investigated the influence of shift work on the risk of psoriasis. Li and colleagues published a study in 2013 showing that enhanced duration of rotating night shift work increases the risk of psoriasis independently of important behavioral risk factors for psoriasis, namely body mass index and smoking (191). Moreover, night shift work is associated with an increased risk of psoriasis comorbidities, e.g. myocardial infarction (305).
Apart from psoriasis further skin-specific autoimmune diseases are vitiligo, pemphigus, EBA, and bullous pemphigoid (BP). Poor sleepers show a higher risk of vitiligo (306, 307). There are also reports on sleep disturbances in patients with BP (67) or vitiligo (207, 208). For EBA, the clock gene Rora was found to be a genetic risk locus in the murine passive anti-collagen type VII transfer model (126). The core-loop of the circadian clock consists of Bmal1/Clock and Per/Cry. However, more genes interact and form additional loops. The transcription of this clock gene Rora is initiated by Bmal1/Clock, which itself fosters the transcription of Bmal1 (308). Sadeghi and colleagues found that a knockout of Rora in mice diminished the skin lesions upon anti-COL7 challenge. They could further show that even a Rora blockade was able to reduce skin inflammation and blistering in this model (126). Patients with BP (mostly elderly) are often also diagnosed with neuro-psychiatric comorbidities years before skin manifestations of BP appear (309). It turned out that anti-BP230 (one of the autoantibodies emerging in BP), is an independent predictor of neuro-psychiatric illnesses in BP patients (310). In addition to the dermis, BP230 is also expressed in the central nervous system and an immunologic cross-reaction of the autoantibodies causing neuroinflammation might explain the BP-associated neuropsychiatric disorders (311). Circadian disruption (e.g by aging or sleep disturbance) increases neuroinflammation in rats (312, 313) and humans (24, 314) and might amplify neuropsychiatric symptoms in BP patients. If this scenario is translatable to shift work remains to be elucidated. Symptoms of autoimmune skin diseases peak at night, as shown by actigraphy also in BP patients (66, 67). This could further disrupt sleep and circadian rhythms thus feeding into a vicious cycle.
Apart from autoimmune skin diseases, disturbances of the wake-sleep cycle seem to promote also infectious skin diseases such as bacterial invasions (315), allergic skin diseases such as atopic dermatitis (316), contact hypersensitivity (317), or skin cancers (318).
In general, skin diseases show distinct 24 h rhythms in symptoms (163), with a nocturnal peak in pain, pruritus and scratching that heavily interferes with sleep (195, 244, 319–321). The dermatology life quality index (DLQI) questionnaire is not considering sleep disturbances (322), whereas the bullous pemphigoid disease area index (BPDAI) explicitly queries sleep impairments to quantify the extend of pruritus (217, 323). These questionnaires are not standardized to span different (autoimmune) skin diseases, which renders it difficult to compare them with each other. The Pittsburg Sleep Quality Index (PSQI) covers various aspects of sleep, its duration and disruptions but does not include any aspects of autoimmunity besides unspecified pain at night (324). Sleep disturbances due to nocturnal symptoms should therefore more often be rated in clinical scores of skin diseases or by objective assessments using, e.g., wearables (66, 67).
So far, we described the manifold influences of the circadian system on sleep, behavior, thermoregulation, neuroendocrine mediators, the immune system, skin manifestations of systemic autoimmune diseases and skin-specific autoimmune diseases. In the following, we will outline how the circadian system and the sleep-wake cycle physiologically regulate skin and immune cells and how disturbances of this regulation, e.g. due to shift work, could drive the pathophysiology of these disorders.
It is hypothesized that the circadian system evolved to protect proliferating cells from DNA damage due to UV light (10, 325, 326). Such a rhythmic adaptation to light or to other environmental stimuli like ambient temperature, moisture, or pathogens conceivably is most relevant in the skin, the major barrier between the outer and the inner world (Figure 3). Thus, multiple epidermal, dermal and hair follicle clocks tick in stem cells, keratinocytes, fibroblasts, and melanocytes and jointly seem to serve protection against environmental challenges during the activity period (49, 109, 327–332). Notably, clock genes also regulate cellular functions in human keratinocyte and melanoma cell lines (327). The subcutis with its fat depots and its dense sympathetic innervation and vascularization also seems to have a circadian regulation that might serve in particular metabolic and thermoregulatory functions (333). Presumably, this skin clockwork maintains skin physiology and skin integrity (39, 331). Thus, skin blood flow, temperature, pH, transepidermal water loss, and sebum excretion show 24 h rhythms in humans (334–336) and animals (337). The circadian system also impacts wound healing in mice (338) and hamsters (339). Experimental disruptions of this finetuned rhythmic skin regulation by circadian misalignment or sleep deprivation impairs skin integrity (65, 340), regeneration (341, 342), and wound healing (339, 343, 344), accelerates skin aging (345), enhances the activity of skin proteases (196), and leads to skin ulcers and hyperkeratosis (346). These outcomes prompt symptoms such as itching and pain. Interestingly, the pheno-menon that pruritus and scratching peak at night, is presumably driven by the described rhythmic changes in skin barrier functions and by enhanced nocturnal skin temperature (214). Although pain perception shows conflicting results with respect to circadian regulation in humans (347, 348), it clearly increases upon circadian or sleep disturbances (349). Vice versa, as outlined above itching and pain at night are likely explanations of sleep disturbances in patients with skin diseases (277, 319). Thus, circadian disruption, sleep loss, enhanced scratching, and exaggerated pain perception likely feed into a vicious circle that further fosters skin barrier damage. Shift workers show disrupted rhythms in hair follicle cells and interfollicular epidermal cells (341, 350) and changes in pain perception (351). However, it is presently unknown, whether shift workers suffer from impairments of skin physiology or integrity. Itching leads to scratching, causing wounds that need to heal. In mice, wounds occurring during the rest phase healed less quickly than wounds that occurred during the active phase. Responsible for this is the rhythmic mobilization of fibroblasts by dynamic actin (338). Similarly, it was found in mice that sleep fragmentation delays wound healing (352). Likewise, difficulties in wound healing are commonly observed in patients with autoimmune diseases and sleep abnormalities (353).
Figure 3 Potential consequences of shift work. Circadian misalignment and/or sleep impairments as a result of shift work presumably lead to changes in the skin barrier function. An intact barrier (left part) is able to block physical challenges as well as pathogens and prevents transepidermal water loss, whereas a disturbed barrier (middle part) is not able to do so. Noxi and intruders then can reach the epidermis, induce damage and activate immune cells and thus local inflammation can occur. A severely disturbed barrier (right part) shows breaches, through which bacteria, viruses and fungi enter the skin and cause inflammation with the attraction of various innate and adaptive immune cells. Likely, consequences are itching, scratching, and pain. Molecular clocks tick in skin cells, in innate and adaptive immune cells, as well as in endothelial cells and could be entrained by light, temperature, and neuroendocrine mediators such as cortisol, catecholamines and melatonin. We hypothesize, that this circadian system of the skin strengthens barrier functions during daytime and that shift work-induced changes favor the development of autoreactive T cells and autoantibodies resulting in autoimmune diseases.
The skin is populated by transient and resident innate immune cells. Previous studies showed a sleep-wake cycle-dependent hematopoietic release of granulocytes and monocytes and subsequent traffic of these cells to various tissues (110, 354–356). Although rhythmic leukocyte homing to skin seems to be neglectable in the steady state (355), indirect evidence indicates that there is a circadian regulation of immune cell traffic to the skin upon wounding and upon microbial or antigenic challenges (357–362). Likewise, tissue-resident innate immune cells like dendritic cells (363, 364), mast cells (317, 365, 366) or macrophages (367–369) show circadian regulation that impacts cutaneous responses to antigens and allergens, respectively. Several genes controlling immune functions are rhythmically regulated in murine skin (331) and the expression of cytokines (e.g. TNF) and chemokines (e.g. IL-8 or C-X-C-motif ligand 1) in human skin are under clock control as well (370). Overall, disturbances of these fine-tuned rhythms in leukocyte traffic and function seem to result in unchecked innate immunity. This is a mechanism that could also contribute to systemic inflammatory responses with increases in blood leukocytes, neutrophils, monocytes, and C-reactive protein in shift workers (75, 136, 138, 371–376). Along this line, both experimental circadian disruption and sleep deprivation enhance the responsiveness of the innate immune system to inflammatory stimuli and trigger inflammation (75, 106, 139, 354, 356). The outlined interactions between the sleep-wake cycle and innate immunity may be relevant for skin diseases, as sleep deprivation induces systemic increases in pro-inflammatory cytokines also in a mouse model of psoriasis (196), and as neutrophil traffic into the skin of psoriatic patients shows rhythmic regulation (193). Apart from granulocytes, monocytes, dendritic cells, and mast cells, also natural killer (NK) cells show 24 h rhythms of their numbers and their activity in human blood (377–379) and rodent spleen (154, 380). Circadian and sleep manipulations alter these parameters (154, 340, 377, 378, 380–382). Likewise, experimentally simulated shift work in healthy individuals changed gene transcripts of NK cell-mediated immune responses (77) and shift workers show impaired NK cell-function (383, 384). Also, in patients with systemic autoimmune diseases (385) or with vitiligo (204, 205), altered rhythms of NK cell-activity in peripheral blood were described. Time-of-day dependent changes in NK cell numbers or functions in healthy or diseased skin, however, were not tested so far.
T cells play key roles in a variety of autoimmune diseases (239, 240, 247, 250, 258, 260) and can enter and reside in the epidermis and dermis. Their recirculation between blood, lymphoid organs and other tissues is regulated by the circadian system (190, 382, 386) and by sleep (387, 388). This is presumably mediated by sleep-wake cycle dependent changes in T cell selectins, integrins, and chemokine receptors, and in corresponding ligands on endothelial cells and surrounding tissues (190, 386, 389, 390). Moreover, T cell functions like proliferation (151, 391), Th1-, Th2-, and Th17-differentiation or cytokine production (392–398), and the activity of Tregs (151, 399, 400) are linked to the sleep-wake cycle. Rhythmic changes in T cell traffic and functions seem to be regulated by T cell intrinsic clocks such as REVERBA (398, 400, 401) and by effects of the SCN or sleep on neuroendocrine mediators (see next section) (387, 390, 402). In shift workers, increases in T cell numbers (384, 403) and impairments in T cell proliferation (28) were reported.
Joint effects of the circadian system and sleep on T cell immunity could also contribute to sleep-wake cycle dependent changes in cutaneous T cell responses and disturbances thereof in shift workers. Indeed, the T cell driven induction or recall of cutaneous DTH reactions show rhythmic modulation in humans (404, 405) and rats (406, 407). Primary DTH responses were impaired in stroke patients showing sleep rhythm disturbances (408) or in hamsters upon experimental circadian disruption (363) or light at night (409). In contrast, Clock mutant mice showed enhanced T cell driven contact hypersensitivity to allergens upon challenge (317). Likewise, constant light in mice enhanced allergic skin responses, while the development of immune tolerance and subsequent Treg infiltration in the challenged skin was impaired (410). Another mouse experiment demonstrated reductions in skin allograft rejection and in T cell infiltration of the graft by sleep deprivation (411). Overall, the picture suggests, that dis-turbances of the circadian system and/or sleep could impair developing but exaggerate established T cell responses in the skin. With respect to skin autoimmune diseases, one study reported disrupted rhythms in blood CD4 T cell numbers in patients with vitiligo (206). In mouse models of psoriasis, clock gene mutations changed skin inflammation by modulating IL-23 receptor expression in gamma delta T cells and subsequent IL-17 and IL-22 production (271) and treatment with a REVERB agonist suppressed IL-17 production in gamma-delta T cells and improved dermatitis (199). In humans, clock genes might likewise impact cutaneous T cell responses, although this was so far only elaborated in the context of skin cancer (412).
Clocks also tick in B cells of mice (413) and humans. There are 24 h rhythms in human B cell numbers in blood (414) and murine B cell numbers in spleens and lymph nodes (415), and in systemic levels of antibodies, so called immunoglobulins (Ig) of the three subtypes IgG, IgA, and IgM (416–418). In line with exaggerated allergic DTH responses that are driven by T cells, Clock mutant mice also show enhanced IgE reactions to allergens (317). There is evidence that day-night-shift rotations attenuate the release of the anti-inflammatory cytokine IL-10 by B cells (419). This lack of immunologic regulation could be deleterious as the IL-10 releasing B cells of shift workers were unable to inhibit the proliferation of T cells (419). It could also be shown that CLOCK expression in peripheral B cells of shift workers was higher, which leads to a reduced expression of transforming growth factor beta (a cytokine mainly released by Tregs) (420). Cry 1/2 deficient mice showed an autoimmune phenotype with elevated levels of serum IgG, antinuclear antibodies (ANAs), and immune complexes, as Cry presumably regulates B cell development and B cell receptor signaling (421). On the other hand, the distribution of B cell subsets in spleen, lymph nodes and peritoneal cavity in wild type and Bmal1 knockout mice did not differ. The maturation of B cells was also not influenced by the knockout of Bmal1. Moreover, there was minor circadian regulation of Per2, which was detected by a reporter mouse model. It seemed as if cell intrinsic circadian clocks did not affect the B cells. They were probably gated by cell-extrinsic circadian variations (422).
B cells generate long-lasting immunologic memory by becoming (auto-)antibody-producing plasma cells and being able to survive decades in niches in the bone marrow (423). Therefore, plasma cells can be key in autoimmunity. In several cutaneous autoimmune diseases, auto-antibodies are a common diagnostic criterion and B cells are also one of the most often targeted cell types in the treatment of autoimmune diseases (424). In accordance, disease severity of pemphigus, BP, and SSc correlated with the number of B cells infiltrating the skin (210, 212, 215, 216, 229, 425). Auto-antibodies can be developed against all kinds of self-molecules. For connective tissue diseases such as SLE and SSc, for example, ANAs are formed against contents of the cell nucleus (220). This content is presumably presented for extended time to the immune system due to insufficient clearance after cell death (426). Apoptosis is a natural process but can also be triggered by UV light, explaining the photosensitivity in SLE (218).
Plasma cells cannot only develop in primary and secondary lymphoid organs by the help of T cells but also in a T cell-independent manner in the skin. This phenomenon was observed in several autoimmune and inflammatory diseases and the effect of local auto-antibody secretion is believed to play a role in chronic inflammation (424). Unfortunately, the influence of the circadian system or sleep on these skin-resident B cells is currently unknown.
This section concentrates on central and peripheral hormonal agents that connect circadian, neuronal, and immunologic mechanisms.
The exact contributions of the SCN (109) with their systemic signals (e.g., stress mediators or core body temperature), of external time cues that directly affect the skin (e.g., extraretinal photoreception by keratinocytes, changes in ambient temperature) (427, 428) or of sleep and associated changes in behavior (e.g., darkness, supine position, reduced physical activity, fasting) (333, 390) in the entrainment of skin clocks and in the circadian regulation of the skin remain to be elucidated (429) (Figure 4). Whatever the case, the stress systems and melatonin seem to be key, either as SCN outputs to the periphery, as signals in cutaneous light perception or thermoregulation or as mediators that change during sleep (22). In humans, blood levels of stress mediators and melatonin oscillate in anti-phase with peak levels during daytime activity for stress mediators and nocturnal sleep for melatonin, respectively (430, 431). These changes induce an increase in skin temperature in the evening that in turn essentially contributes to sleep onset (44). Sleep and its associated behavioral changes further reduce nocturnal levels of stress mediators and enhance nocturnal levels of melatonin. The peripheral hormone of the HPA-axis is cortisol that regulates human keratinocyte clock functions and suppresses proliferation of these epidermal cells (49). The mediators of the SNS are the catecholamines epinephrine and norepinephrine that together with melatonin are key in thermo- and vasoregulation. Thus, the supporting effects of melatonin intake on sleep initiation is assumed to be mediated by vasodilatatory effects of this hormone (432). Cortisol, catecholamines, and melatonin also impact immune cell clocks, traffic and functions in blood and various tissues (154, 381, 390), including the skin (363). They are not only released systemically, but also produced locally in the epidermis and dermis (433, 434). In mice and rats, the peripheral hormone of the HPA-axis is corticosterone that likewise increases sharply at the transition from the rest to the activity period (435, 436). For blood and tissue levels of catecholamines in rodents, rhythms and peak times were rather inconsistent (437–439). In contrast to humans, mice show highest melatonin levels during their activity period, thus in phase with corticosterone (114). As melatonin has many effects on skin physiology and immunity (440), it therefore seems not very straightforward to study the effects of the circadian system and sleep on skin immunity in mice. A counter argument could be that many laboratory mouse strains like C57BL/6 and Balb/C seem to be genetically incapable to synthesize sufficient amounts of systemic melatonin (441) or to express melatonin receptors. C57BL/6 mice assume to lack both melatonin receptors, yet scientists bred a strain of melatonin receptor (MT)1 and MT2 knockout mice and backcrossed them into a melatonin proficient strain to study the effects of each receptor separately (442). Recently, a melatonin proficient C57BL/6 strain was also developed (443). In sum, these different mouse strains could serve to study the effects of melatonin administrations on skin immunity, but experimental design needs to be chosen with caution.
Figure 4 Layers of systems in the human body that are affected by shift work. Circadian disruptions due to shift work can affect the suprachiasmatic nuclei (SCN) and the major outputs of this master clock such as core body temperature and neuroendocrine mediators. These systemic influences as well as direct effects of ambient temperature and light can affect the circadian system in the skin and the cellular clocks of skin leukocytes of the innate (top) and adaptive (bottom) immune system.
Stress mediators and melatonin may play a role in SLE (221–224), SSc (230, 231, 444), psoriasis (196, 200–202, 268, 445), Sjögren’s syndrome (234), and vitiligo (203, 209). A failure in the HPA-axis to control inflammation is discussed in autoimmune diseases (180, 248) and glucocorticoids are widely used therapeutically as immunosuppressants.
Vasodilatatory, anti-inflammatory, and melatonin-releasing effects of catecholamines are mediated by beta-adrenoceptors (389, 446). Beta-blockers therefore can have manifold unwanted effects on the circadian system, sleep, and the immune system and in this way may contribute to disease flares in psoriasis (447). Also other hormones and mediators such as growth hormone, prolactin, aldosterone, thyroid hormones, sex hormones, ghrelin, leptin, prostaglandins, serotonin, histamine, adenosine, endorphins, αMSH, neuropeptides, and vitamin D are regulated by the wake-sleep cycle (22, 35, 47, 226, 389, 390, 448), are involved in autoimmune diseases (180, 449), can impact skin physiology, and immunity (205, 209, 450) and may therefore be of relevance in the etiopathology of skin autoimmune diseases in shift workers.
As itch and pain are key, interrelated symptoms in skin autoimmune diseases, underlying mediators could be of particular interest in studying effects of shift work on dermal cells and leukocytes. Neuronal pathways of itch and pain involve A- and C-fibers in the epidermis, which are activated by histamine, neuropeptides, and cytokines, as well as forwarding of the signals via the dorsal root ganglion to the brain. Beta-endorphins are able to act as analgesics by binding to opiod receptors, starting a cascade of interactions, which finally results in the inhibition of pain signalling (451). Histamine is often released at the site of inflammation in the skin (452) and plasma levels of beta-endorphin are enhanced in children with atopic dermatitis (453). In healthy humans, plasma levels of beta-endorphins (but not of histamine (454)) show rhythmic regulation being highest in the morning and reduced in the night (455–457). Ligand binding to histamine receptors on leukocytes likewise changes from night to day with complex patterns in healthy and atopic individuals (458). Moreover, in healthy individuals blood levels of cortisol and beta-endorphins are coupled, meaning that cortisol follows beta-endorphin with a lag-phase of ten minutes (459). Glucocorticoids are potent antipruritic drugs, but are also known to inhibit pain pathways (460). Therefore, not only low beta-endorphin levels but also low cortisol levels could explain why symptoms of itch and pain are most pronounced at night, as it was demonstrated for itch in patients with psoriasis (211, 461).
The effect of sex hormones is expected to explain the sexual dimorphism in autoimmune diseases that predominantly affect women (462). The effect of estrogen on sleep and circadian rhythms becomes also visible in women, when they reach the menopause and suffer from sleep disorders due to a decline or imbalance of this hormone (463).
Shift workers show enhanced average cortisol levels (25) and disrupted melatonin rhythms (464). However, until yet there is no data on catecholamines, histamines, endorphins, sex hormones, or other neuroendocrine mediators in shift workers.
Until now, this review focused on potential circadian drivers of autoimmunity. However, there are also some factors that might improve and stabilize the circadian system and thereby might be able to alleviate symptoms and negative effects of shift work.
Shift work is indispensable in healthcare, public protection and transportation. Some industries use 24 h schedules due to difficulties in stopping machines and production chains. However, shift work may also serve to maximize profit. From a health perspective, night shifts should only be demanded from workers when absolutely required. To minimize health issues, employers should use sophisticated shift work schedules (i) favoring a forward rotating system instead of a backwards rotating one, (ii) allowing rest periods of at least 11 h between two shifts, days off after night shifts and free weekends, (iii) avoiding early morning starts and long night-time working hours, and (iv) limiting the number of (consecutive) evening and night shifts (465, 466). Ideally, the chronotype of the workers should be assessed (i.e., being a morning or evening person) and whenever possible, this should be taken into account when scheduling shifts (e.g., avoiding night shifts in morning persons) (467). To facilitate alignment to night shifts and re-alignment to the regular sleep-wake cycle, bright light can help to suppress melatonin secretion and sleepiness during wake periods, whereas melatonin supplementation, as well as a cool and dark bedroom with a bedding that facilitates a suitable skin temperature might help to catch up on sleep (465, 468, 469).
Melatonin acts as dark signal, which is only to some degree able to facilitate sleep. Nevertheless, the idea of melatonin supplementation is to re-gain the circadian rhythm if it was disturbed. Even though the available data on this topic is limited, there are some studies on melatonin supplementation in shift workers and also as treatment for SLE. Nabatian-Asl and colleagues were able to show that 10 mg/day melatonin supplementation for 12 weeks is reducing serum malondialdehyde, which is a marker for oxidative stress. Oxidative stress levels are known to correlate with SLE-activity (221). A recent systematic review by Carriedo-Diez and colleagues investigated melatonin supplementation in shift workers. The investigated studies used between 1 and 10 mg melatonin and recognized improvements such as reduced day-time sleepiness and increased total sleep period (470). However, the studies varied in their design and group sizes and ages. More future work will hopefully shed light on this topic, also investigating other autoimmune diseases and effects as well as side-effects of melatonin.
As food intake entrains rhythms, there are some recommendations for meal timing and composition during shifts (465, 471). Caffeine and physical activity promote wakefulness and the latter seems to protect from shift work disorder (34, 81). Pharmacological interventions in shift workers with sleeping aids such as zopiclone or wake-promoting substances such as modafinil did not lead to clear improvement of sleep or alertness, respectively (472).
The psychosocial and socioeconomic situation of the workers cannot be neglected. Apart from physical conse-quences, shift workers may also suffer from emotional and mental health issues. The socioeconomic status differs among shift workers and many report increased job stress (473) or social isolation (20). As social stress and isolation were shown to be associated with the conserved transcriptional response to adversity as an indicator of SCI (474, 475), they may mediate or influence the effects of shift work on skin autoimmune diseases. Older age, female gender, being married, or having children is increasing the risk of suffering from sleep related impairment in response to shift work (81). Medical surveillance, in particular in employees with these kind of risk factors, should also cover skin health and care. Considering working time preferences and giving employees shift schedules one month in advance can help them to plan activities with family and friends and thus to improve the work-life balance and social contacts (18, 465, 466). Further countermeasures to avoid negative health outcomes in shift workers are financial compensation, individual counselling, health education (e.g. dietary habits, physical activity, avoidance of substance abuse), and information about non-pharma-cological interventions to reduce stress (e.g., mindfulness based stress reduction) and to ease sleep (e.g. sleep hygiene, napping) (466, 476).
In case that a systemic or skin-specific autoimmune disease has already occurred, physicians might recommend to avoid night shifts, as stress and stressful events could worsen symptoms and cause further disease flares (213). If this is not feasible, wise timing of topic and/or systemic drug treatments, thus during work hours or before bedtime and not at standard clock times might be advantageous (477).
Available evidence indicates that shift work by disrupting the circadian system and sleep impairs skin physiology and immunity and presumably contributes to skin autoimmune diseases. Circadian and sleep aspects should be considered in basic research on immunity in particular in experiments on nocturnal animals. Moreover, other animal models such as pigs should be considered in immunologic and chronobiologic studies.
The influences of shift work and disrupted circadian clocks are manifold, ranging from fatigue and metabolic disturbances over SCI to the development of autoimmune diseases. Innate and adaptive immune cells (as well as all other cells) show rhythmic regulation and may react adversely to different stimuli such as pathogens, allergens or (auto-)antigens if the rhythm is disrupted. The signaling of the SCN downwards to peripheral organs and cells is mediated by hormones like melatonin and cortisol. These hormones should be monitored in shift workers, to delineate their role in the development of autoimmune diseases. The skin as the largest human organ is in contact with the outer world and therefore an important barrier, which seems to be weakened by circadian disruption, sleep loss, or shift work. In our society, shift work is unavoidable, however, this review offers several opportunities to improve the health of shift workers.
All authors contributed in writing and revising the manuscript. TL and JH developed the concept, structure and both tables, SS prepared the figures and wrote paragraphs about animal studies and B cells, HG provided her clinical insight and knowledge especially regarding psoriasis, arthritis and inflammatory bowel disease and wrote these sections. All authors contributed to the article and approved the submitted version.
This study was supported by grants dedicated to Jennifer Hundt and Tanja Lange of the Research Training Group “Defining and Targeting Autoimmune Pre-Disease” (GRK 2633/1 – 2021, 429175970) and the Excellence Cluster “Precision Medicine in Chronic Inflammation” (EXC 2167), both from the Deutsche Forschungsgemeinschaft.
We thank our research groups for their wonderful scientific work and the colleagues from the intensive care unit 11a/12a and A.311 of the UKSH Campus Luebeck for enjoyable night shifts. All figures were prepared using BioRender.com.
The authors declare that the research was conducted in the absence of any commercial or financial relationships that could be construed as a potential conflict of interest.
All claims expressed in this article are solely those of the authors and do not necessarily represent those of their affiliated organizations, or those of the publisher, the editors and the reviewers. Any product that may be evaluated in this article, or claim that may be made by its manufacturer, is not guaranteed or endorsed by the publisher.
ANA, Antinuclear antibody; BMAL1, Brain and muscle ARNT-Like1; BP, Bullous pemphigoid; BPDAI, Bullous pemphigoid disease area index; CLOCK, Circadian locomotor output cycles kaput; CRY, Cryptochrome; Dbp, D-site of albumin promoter binding protein; DLQI, Daily quality of life index; DTH, Delayed type hypersensitivity; EBA, Epidermolysis bullosa acquisita; ELISA, Enzyme-linked immunosorbent assay; h, hours; HPA-axis, Hypothalamus-pituitary-adrenal axis; IBD, Inflammatory bowel disease; Ig, Immunoglobulin; IL, Interleukin; NFIL3, Nuclear factor interleukin 3 regulated protein; NK, Natural killer cell; PER, Period; PSQI, Pittsburgh sleep quality index; RA, Rheumatoid arthritis; REM, Rapid eye movement; RORa, Retinoic acid-related orphan receptor-alpha; SCI, Systemic chronic inflammation; SCN, Suprachiasmatic nuclei; SLE, Systemic lupus erythematosus; SNS, Sympathetic nervous system; SpA, Spondyloarthritis; SSc, Systemic sclerosis; SWS, Slow wave sleep; TNF, Tumor necrosis factor; TPA, 12-O-tetradecanoylphorbol-13-acetate; Tregs, Regulatory T cells.
1. Almeida CMO, Malheiro A. Sleep, immunity and shift workers: A review. Sleep Sci September (2016) 9(3):164–8. doi: 10.1016/j.slsci.2016.10.007
2. Kecklund G, Axelsson J. Health consequences of shift work and insufficient sleep. BMJ (2016) 355:i5210. doi: 10.1136/bmj.i5210
3. Gu F, Han J, Laden F, Pan A, Caporaso NE, Stampfer MJ. Total and cause-specific mortality of U.S. nurses working rotating night shifts. Am J Prev Med (2015) 48(3):241–52.
4. Cox KH, Takahashi JS. Circadian clock genes and the transcriptional architecture of the clock mechanism. J Mol Endocrinol (2019) 63(4):R93–102. doi: 10.1530/JME-19-0153
5. Kotronoulas G, Stamatakis A, Stylianopoulou F. Hormones, hormonal agents, and neuropeptides involved in the neuroendocrine regulation of sleep in humans. Hormones (Athens) (2009) 8(4):232–48. doi: 10.14310/horm.2002.1239
6. Smith RE. Circadian variations in human thermoregulatory responses. J Appl Physiol (1969) 26(5):554–60. doi: 10.1152/jappl.1969.26.5.554
7. Aschoff J. Circadian control of body temperature. J Thermal Biol (1983) 8(1):143–7. doi: 10.1016/0306-4565(83)90094-3
8. Beersma DGM, Gordijn MCM. Circadian control of the sleep-wake cycle. Physiol Behav (2007) 90(2–3):190–5. doi: 10.1016/j.physbeh.2006.09.010
9. Haus E. Chronobiology in the endocrine system. Advanced Drug Delivery Rev (2007) 59(9):985–1014. doi: 10.1016/j.addr.2007.01.001
10. Geyfman M, Kumar V, Liu Q, Ruiz R, Gordon W, Espitia F. Brain and muscle arnt-like protein-1 (BMAL1) controls circadian cell proliferation and susceptibility to UVB-induced DNA damage in the epidermis. . Proc Natl Acad Sci U S A. (2012) 109(29):11758–63. doi: 10.1073/pnas.1209592109
11. Scheiermann C, Kunisaki Y, Frenette PS. Circadian control of the immune system. Nat Rev Immunol März (2013) 13(3):190–8. doi: 10.1038/nri3386
12. Miller B, Takahashi J. Central circadian control of female reproductive function. Front Endocrinol (2014) 4:195. doi: 10.3389/fendo.2013.00195
13. Thosar SS, Shea SA. Circadian control of human cardiovascular function. Curr Opin Pharmacol (2021) 57:89–97. doi: 10.1016/j.coph.2021.01.002
14. Challet E. The circadian regulation of food intake. Nat Rev Endocrinol Juli (2019) 15(7):393–405. doi: 10.1038/s41574-019-0210-x
15. Kalsbeek A, la Fleur S, Fliers E. Circadian control of glucose metabolism. Mol Metab (2014) 3(4):372–83. doi: 10.1016/j.molmet.2014.03.002
16. Segers A, Depoortere I. Circadian clocks in the digestive system. Nat Rev Gastroenterol Hepatol (2021) 18(4):239–51. doi: 10.1038/s41575-020-00401-5
17. Mistlberger RE, Skene DJ. Nonphotic entrainment in humans? J biol rhythms. August (2005) 20(4):339–52.
18. Awosoga O, Steinke C, Nord C, Doan J, Varsanyi S, Meadows J. Exploring the role of shift work in the self-reported health and wellbeing of long-term and assisted-living professional caregivers in Alberta, Canada. Hum Resour Health (2020) 18(1):70. doi: 10.1186/s12960-020-00515-6
19. Wisetborisut A, Angkurawaranon C, Jiraporncharoen W, Uaphanthasath R, Wiwatanadate P. Shift work and burnout among health care workers. Occup Med (Lond) (2014) 64(4):279–86. doi: 10.1093/occmed/kqu009
20. Jensen HI, Larsen JW, Thomsen TD. The impact of shift work on intensive care nurses’ lives outside work: A cross-sectional study. J Clin Nurs (2018) 27(3–4):e703–9.
21. Buss J. Associations between obesity and stress and shift work among nurses. Workplace Health Saf (2012) 60(10):453–8. doi: 10.1177/216507991206001007
22. Potter GDM, Skene DJ, Arendt J, Cade JE, Grant PJ, Hardie LJ. Circadian rhythm and sleep disruption: Causes, metabolic consequences, and countermeasures. Endocr Rev (2016) 37(6):584–608. doi: 10.1210/er.2016-1083
23. Trinkoff AM, Storr CL. Work schedule characteristics and substance use in nurses. Am J Ind Med (1998) 34(3):266–71. doi: 10.1002/(SICI)1097-0274(199809)34:3<266::AID-AJIM9>3.0.CO;2-T
24. Furman D, Campisi J, Verdin E, Carrera-Bastos P, Targ S, Franceschi C. Chronic inflammation in the etiology of disease across the life span. Nat Med Dezember (2019) 25(12):1822–32. doi: 10.1038/s41591-019-0675-0
25. Atwater AQ, Immergluck LC, Davidson AJ, Castanon-Cervantes O. Shift work predicts increases in lipopolysaccharide-binding protein, interleukin-10, and leukocyte counts in a cross-sectional study of healthy volunteers carrying low-grade systemic inflammation. Int J Environ Res Public Health (2021) 18(24). doi: 10.3390/ijerph182413158
26. Prather AA, Carroll JE. Associations between sleep duration, shift work, and infectious illness in the united states: Data from the national health interview survey. Sleep Health (2021) 7(5):638–43. doi: 10.1016/j.sleh.2021.05.004
27. Niedhammer I, Coutrot T, Geoffroy-Perez B, Chastang JF. Shift and night work and all-cause and cause-specific mortality: Prospective results from the STRESSJEM study. J Biol Rhythms (2022) 37(3):249–59. doi: 10.1177/07487304221092103
28. Nakano Y, Miura T, Hara I, Aono H, Miyano N, Miyajima K. The effect of shift work on cellular immune function. J Hum Ergol (Tokyo) (1982) 11(Suppl):131–7.
29. Abo SMC, Layton AT. Modeling the circadian regulation of the immune system: Sexually dimorphic effects of shift work. PloS Comput Biol März (2021) 17(3):e1008514. doi: 10.1371/journal.pcbi.1008514
30. Hansen J. Risk of breast cancer after night- and shift work: current evidence and ongoing studies in Denmark. Cancer Causes Control. Mai (2006) 17(4):531–7. doi: 10.1007/s10552-005-9006-5
31. Nakao A. Circadian regulation of the biology of allergic disease: Clock disruption can promote allergy. Front Immunol (2020) 11:1237. doi: 10.3389/fimmu.2020.01237
32. Magrini A, Pietroiusti A, Coppeta L, Babbucci A, Barnaba E, Papadia C. Shift work and autoimmune thyroid disorders. Int J Immunopathol Pharmacol (2006) 19(4 Suppl):31–6.
33. Hedström AK, Åkerstedt T, Klareskog L, Alfredsson L. Relationship between shift work and the onset of rheumatoid arthritis. RMD Open (2017) 3(2):e000475.
34. McHill AW, Smith BJ, Wright KPJ. Effects of caffeine on skin and core temperatures, alertness, and recovery sleep during circadian misalignment. J Biol Rhythms (2014) 29(2):131–43. doi: 10.1177/0748730414523078
35. Morris CJ, Aeschbach D, Scheer FAJL. Circadian system, sleep and endocrinology. Mol Cell Endocrinol (2012) 349(1):91–104. doi: 10.1016/j.mce.2011.09.003
36. Xie L, Kang H, Xu Q, Chen MJ, Liao Y, Thiyagarajan M. Sleep drives metabolite clearance from the adult brain. Science (2013) 342(6156):373–7. doi: 10.1126/science.1241224
37. Krueger JM. The role of cytokines in sleep regulation. Curr Pharm Des (2008) 14(32):3408–16. doi: 10.2174/138161208786549281
38. Axelsson J, Sundelin T, Ingre M, Van Someren EJW, Olsson A, Lekander M. Beauty sleep: experimental study on the perceived health and attractiveness of sleep deprived people. BMJ (2010) 341:c6614. doi: 10.1136/bmj.c6614
39. Kahan V, Andersen ML, Tomimori J, Tufik S. Can poor sleep affect skin integrity? med hypotheses. Dezember (2010) 75(6):535–7.
40. Irwin MR. Why sleep is important for health: a psychoneuroimmunology perspective. Annu Rev Psychol (2015) 66:143–72. doi: 10.1146/annurev-psych-010213-115205
41. Ribeiro JA, Sebastião AM. Caffeine and adenosine. J Alzheimers Dis (2010) 20(Suppl 1):S3–15. doi: 10.3233/JAD-2010-1379
42. Bhattacharya M. Could a coffee a day keep the inflammasome away? Sci Transl Med (2017) 9(376):eaam6057. doi: 10.1126/scitranslmed.aam6057
43. Chen J, Tang Z, Slominski AT, Li W, Żmijewski MA, Liu Y. Vitamin d and its analogs as anticancer and anti-inflammatory agents. Eur J Med Chem (2020) 207:112738. doi: 10.1016/j.ejmech.2020.112738
44. Kräuchi K, Cajochen C, Werth E, Wirz-Justice A. Warm feet promote the rapid onset of sleep. Nature (1999) 401(6748):36–7. doi: 10.1038/43366
45. Harding EC, Franks NP, Wisden W. The temperature dependence of sleep. Front Neurosci (2019) 13:336. doi: 10.3389/fnins.2019.00336
46. Tan X, van Egmond L, Partinen M, Lange T, Benedict C. A narrative review of interventions for improving sleep and reducing circadian disruption in medical inpatients. Sleep Med Juli (2019) 59:42–50. doi: 10.1016/j.sleep.2018.08.007
47. Besedovsky L, Lange T, Haack M. The sleep-immune crosstalk in health and disease. Physiol Rev (2019) 99(3):1325–80. doi: 10.1152/physrev.00010.2018
48. Charloux A, Gronfier C, Chapotot F, Ehrhart J, Piquard F, Brandenberger G. Sleep deprivation blunts the night time increase in aldosterone release in humans. J Sleep Res (2001) 10(1):27–33. doi: 10.1046/j.1365-2869.2001.00235.x
49. Spörl F, Korge S, Jürchott K, Wunderskirchner M, Schellenberg K, Heins S. Krüppel-like factor 9 is a circadian transcription factor in human epidermis that controls proliferation of keratinocytes. Proc Natl Acad Sci U.S.A. (2012) 109(27):10903–8. doi: 10.1073/pnas.1118641109
50. Yarrington A, Mehta P. Does sleep promote recovery after bone marrow transplantation?–a hypothesis. Pediatr Transplant (1998) 2(1):51–5.
51. Rasch B, Dodt C, Mölle M, Born J. Sleep-stage-specific regulation of plasma catecholamine concentration. Psychoneuroendocrinology (2007) 32(8–10):884–91. doi: 10.1016/j.psyneuen.2007.06.007
52. Wirth MD, Burch J, Shivappa N, Steck SE, Hurley TG, Vena JE. Dietary inflammatory index scores differ by shift work status: NHANES 2005 to 2010. J Occup Environ Med (2014) 56(2):145–8. doi: 10.1097/JOM.0000000000000088
53. Thomas GN, Wang MP, Ho SY, Mak KH, Cheng KK, Lam TH. Adverse lifestyle leads to an annual excess of 2 million deaths in China. PloS One (2014) 9(2):e89650. doi: 10.1371/journal.pone.0089650
54. Dawson D, Reid K. Fatigue, alcohol and performance impairment. Nature. (1997) 388(6639):235. doi: 10.1038/40775
55. Rahman SA, Sullivan JP, Barger LK, St Hilaire MA, O’Brien CS, Stone KL. Extended work shifts and neurobehavioral performance in resident-physicians. Pediatrics (2021) 147(3):e2020009936. doi: 10.1542/peds.2020-009936
56. Lubov JE, Cvammen W, Kemp MG. The impact of the circadian clock on skin physiology and cancer development. Int J Mol Sci (2021) 22(11). doi: 10.3390/ijms22116112
57. Haus E, Smolensky MH. Biologic rhythms in the immune system. Chronobiol Int (1999) 16(5):581–622. doi: 10.3109/07420529908998730
58. Labrecque N, Cermakian N. Circadian clocks in the immune system. J Biol Rhythms (2015) 30(4):277–90. doi: 10.1177/0748730415577723
59. Ha L, Hundt JE. Optical coherence tomography for fast bedside imaging, assessment and monitoring of autoimmune inflammatory skin diseases? J Dtsch Dermatol Ges (2020) 18(9):937–42. doi: 10.1111/ddg.14266
60. Mayrovitz HN, Berthin T. Assessing potential circadian, diurnal, and ultradian variations in skin biophysical properties. Cureus. September (2021) 13(9):e17665. doi: 10.7759/cureus.17665
61. Kobayashi K, Kaneda K, Kasama T. Immunopathogenesis of delayed-type hypersensitivity. Microscopy Res Technique. (2001) 53(4):241–5. doi: 10.1002/jemt.1090
62. Gan PY, Steinmetz OM, Tan DSY, O’Sullivan KM, Ooi JD, Iwakura Y. Th17 cells promote autoimmune anti-myeloperoxidase glomerulonephritis. JASN (2010) 21(6):925–31. doi: 10.1681/ASN.2009070763
63. Atkinson SM, Usher PA, Kvist PH, Markholst H, Haase C, Nansen A. Establishment and characterization of a sustained delayed-type hypersensitivity model with arthritic manifestations in C57BL/6J mice. Arthritis Res Ther (2012) 14(3):R134. doi: 10.1186/ar3867
64. Doniach D, Hudson RV, Roitt IM. Human auto-immune thyroiditis: Clinical studies. Br Med J (1960) 1(5170):365–73. doi: 10.1136/bmj.1.5170.365
65. Oyetakin-White P, Suggs A, Koo B, Matsui MS, Yarosh D, Cooper KD. Does poor sleep quality affect skin ageing? Clin Exp Dermatol (2015) 40(1):17–22. doi: 10.1111/ced.12455
66. Hon K lun E, Chiu L shan M, Lam M chin A, Choi C lung P, Chan S, Luk N-M. Measurement of pruritus in a Chinese woman with pemphigoid gestationis using a wrist movement detector. Int J Dermatol (2008) 47(1):64–7.
67. Kalinska-Bienias A, Piotrowski T, Kowalczyk E, Lesniewska A, Kaminska M, Jagielski P. Actigraphy-measured nocturnal wrist movements and assessment of sleep quality in patients with bullous pemphigoid: a pilot case-control study. Clin Exp Dermatol Oktober (2019) 44(7):759–65. doi: 10.1111/ced.13902
69. Pandi-Perumal SR, Cardinali DP, Zaki NFW, Karthikeyan R, Spence DW, Reiter RJ. Timing is everything: Circadian rhythms and their role in the control of sleep. Front Neuroendocrinol (2022) 66:100978. doi: 10.1016/j.yfrne.2022.100978
70. Raymann RJEM, Swaab DF, Van Someren EJW. Skin deep: enhanced sleep depth by cutaneous temperature manipulation. Brain (2008) 131(Pt 2):500–13. doi: 10.1093/brain/awm315
71. Deboer T, Vansteensel MJ, Détári L, Meijer JH. Sleep states alter activity of suprachiasmatic nucleus neurons. Nat Neurosci Oktober (2003) 6(10):1086–90. doi: 10.1038/nn1122
72. Fuller PM, Gooley JJ, Saper CB. Neurobiology of the sleep-wake cycle: sleep architecture, circadian regulation, and regulatory feedback. J Biol Rhythms (2006) 21(6):482–93. doi: 10.1177/0748730406294627
73. Scammell TE, Arrigoni E, Lipton JO. Neural circuitry of wakefulness and sleep. Neuron (2017) 93(4):747–65. doi: 10.1016/j.neuron.2017.01.014
74. Archer SN, Laing EE, Möller-Levet CS, van der Veen DR, Bucca G, Lazar AS. Mistimed sleep disrupts circadian regulation of the human transcriptome. Proc Natl Acad Sci U.S.A. (2014) 111(6):E682–691. doi: 10.1073/pnas.1316335111
75. Qin B, Deng Y. Overexpression of circadian clock protein cryptochrome (CRY) 1 alleviates sleep deprivation-induced vascular inflammation in a mouse model. Immunol Lett Januar (2015) 163(1):76–83. doi: 10.1016/j.imlet.2014.11.014
76. Husse J, Kiehn JT, Barclay JL, Naujokat N, Meyer-Kovac J, Lehnert H. Tissue-specific dissociation of diurnal transcriptome rhythms during sleep restriction in mice. Sleep (2017) 40(6). doi: 10.1093/sleep/zsx068
77. Kervezee L, Cuesta M, Cermakian N, Boivin DB. Simulated night shift work induces circadian misalignment of the human peripheral blood mononuclear cell transcriptome. Proc Natl Acad Sci U.S.A. (2018) 115(21):5540–5. doi: 10.1073/pnas.1720719115
78. Hoekstra MM, Jan M, Katsioudi G, Emmenegger Y, Franken P. The sleep-wake distribution contributes to the peripheral rhythms in PERIOD-2. Elife (2021) 10:e69773.
79. Lajoie P, Aronson KJ, Day A, Tranmer J. A cross-sectional study of shift work, sleep quality and cardiometabolic risk in female hospital employees. BMJ Open (2015) 5(3):e007327. doi: 10.1136/bmjopen-2014-007327
80. Zhang Y, Shen J, Zhou Z, Sang L, Zhuang X, Chu M. Relationships among shift work, hair cortisol concentration and sleep disorders: a cross-sectional study in China. BMJ Open (2020) 10(11):e038786. doi: 10.1136/bmjopen-2020-038786
81. Booker LA, Magee M, Rajaratnam SMW, Sletten TL, Howard ME. Individual vulnerability to insomnia, excessive sleepiness and shift work disorder amongst healthcare shift workers. A systematic review. Sleep Med Rev (2018) 41:220–33. doi: 10.1016/j.smrv.2018.03.005
82. Nokes BT, Raza HA, Cartin-Ceba R, Lyng PJ, Krahn LE, Wesselius L. Individuals with scleroderma may have increased risk of sleep-disordered breathing. J Clin Sleep Med (2019) 15(11):1665–9. doi: 10.5664/jcsm.8036
83. Simon RD. Shift work disorder: clinical assessment and treatment strategies. J Clin Psychiatry (2012) 73(6):e20.
84. Dumont M, Blais H, Roy J, Paquet J. Controlled patterns of daytime light exposure improve circadian adjustment in simulated night work. J Biol Rhythms (2009) 24(5):427–37. doi: 10.1177/0748730409343795
85. Santos HO, Genario R, Tinsley GM, Ribeiro P, Carteri RB, Coelho-Ravagnani C de F. A scoping review of intermittent fasting, chronobiology, and metabolism. Am J Clin Nutr (2022) 115(4):991–1004. doi: 10.1093/ajcn/nqab433
86. Hickman DL, Johnson J, Vemulapalli TH, Crisler JR, Shepherd R. Commonly used animal models. Principles Anim Res Graduate Undergraduate Students (2017), 117–75. doi: 10.1016/B978-0-12-802151-4.00007-4
87. Singleton GR, Krebs CJ. Chapter 3 - the secret world of wild mice. In: Fox JG, Davisson MT, Quimby FW, Barthold SW, Newcomer CE, Smith AL, Herausgeber, editors. The mouse in biomedical research, 2nd ed.. Burlington: Academic Press (2007). p. 25–51. Available at: https://www.sciencedirect.com/science/article/pii/B9780123694546500157.
88. Raulo A, Allen BE, Troitsky T, Husby A, Firth JA, Coulson T. Social networks strongly predict the gut microbiota of wild mice. ISME J (2021) 15(9):2601–13. doi: 10.1038/s41396-021-00949-3
89. Guénet JL, Bonhomme F. Wild mice: an ever-increasing contribution to a popular mammalian model. Trends Genet (2003) 19(1):24–31. doi: 10.1016/S0168-9525(02)00007-0
90. Abolins SR, Pocock MJO, Hafalla JCR, Riley EM, Viney ME. Measures of immune function of wild mice, mus musculus. Mol Ecology. (2011) 20(5):881–92. doi: 10.1111/j.1365-294X.2010.04910.x
91. Abolins S, King EC, Lazarou L, Weldon L, Hughes L, Drescher P. The comparative immunology of wild and laboratory mice, mus musculus domesticus. Nat Commun (2017) 8(1):14811. doi: 10.1038/ncomms14811
92. Gray S, Hurst JL. The effects of cage cleaning on aggression within groups of male laboratory mice. Anim Behaviour. (1995) 49(3):821–6. doi: 10.1016/0003-3472(95)80213-4
93. Longordo F, Fan J, Steimer T, Kopp C, Lüthi A. Do mice habituate to “gentle handling?” a comparison of resting behavior, corticosterone levels and synaptic function in handled and undisturbed C57BL/6J mice. Sleep (2011) 34(5):679–81. doi: 10.1093/sleep/34.5.679
94. Harri M, Lindblom J, Malinen H, Hyttinen M, Lapveteläinen T, Eskola S. Effect of access to a running wheel on behavior of C57BL/6J mice. Lab Anim Sci (1999) 49(4):401–5.
95. Smolinsky AN, Bergner CL, LaPorte JL, Kalueff AV. Analysis of grooming behavior and its utility in studying animal stress, anxiety, and depression. In: Gould TD, Herausgeber, editors. Mood and anxiety related phenotypes in mice: Characterization using behavioral tests. Totowa, NJ: Humana Press (2009). p. 21–36. doi: 10.1007/978-1-60761-303-9_2
96. Foltz CJ, Ullman-Cullere M. Guidelines for assessing the health and condition of mice. Lab Animal. (1999) 28(4):5.
97. Ely DL, Henry JP. Neuroendocrine response patterns in dominant and subordinate mice. Horm Behav April (1978) 10(2):156–69. doi: 10.1016/0018-506X(78)90005-3
98. Olini N, Rothfuchs I, Azzinnari D, Pryce CR, Kurth S, Huber R. Chronic social stress leads to altered sleep homeostasis in mice. Behav Brain Res 1. Juni (2017) 327:167–73.
99. Sorge RE, Martin LJ, Isbester KA, Sotocinal SG, Rosen S, Tuttle AH. Olfactory exposure to males, including men, causes stress and related analgesia in rodents. Nat Methods Juni (2014) 11(6):629–32. doi: 10.1038/nmeth.2935
100. van der Vinne V, Riede SJ, Gorter JA, Eijer WG, Sellix MT, Menaker M. Cold and hunger induce diurnality in a nocturnal mammal. Proc Natl Acad Sci U S A. 21. Oktober (2014) 111(42):15256–60. doi: 10.1073/pnas.1413135111
101. Hylander BL, Gordon CJ, Repasky EA. Manipulation of ambient housing temperature to study the impact of chronic stress on immunity and cancer in mice. J Immunol 1. Februar (2019) 202(3):631–6. doi: 10.4049/jimmunol.1800621
102. Gaskill B, Rohr S, Pajor E, Lucas J, Garner J. Some like it hot: Mouse temperature preferences in laboratory housing. Appl Anim Behav Sci - Appl Anim Behav Sci (2009) 116:279–85. doi: 10.1016/j.applanim.2008.10.002
103. Hatori M, Panda S. Response of peripheral rhythms to the timing of food intake. Methods Enzymol (2015) 552:145–61. doi: 10.1016/bs.mie.2014.10.027
104. Schroder EA, Delisle BP. Time restricted feeding to the light cycle dissociates canonical circadian clocks and physiological rhythms in heart rate. Front Pharmacol (2022) 13:910195. doi: 10.3389/fphar.2022.910195
105. Wang H, van Spyk E, Liu Q, Geyfman M, Salmans ML, Kumar V. Time-restricted feeding shifts the skin circadian clock and alters UVB-induced DNA damage. Cell Rep (2017) 20(5):1061–72. doi: 10.1016/j.celrep.2017.07.022
106. Guerrero-Vargas NN, Guzmán-Ruiz M, Fuentes R, García J, Salgado-Delgado R, Basualdo M del C. Shift work in rats results in increased inflammatory response after lipopolysaccharide administration: A role for food consumption. J Biol Rhythms (2015) 30(4):318–30. doi: 10.1177/0748730415586482
107. Oishi K, Fukui H, Ishida N. Rhythmic expression of BMAL1 mRNA is altered in clock mutant mice: differential regulation in the suprachiasmatic nucleus and peripheral tissues. Biochem Biophys Res Commun (2000) 268(1):164–71. doi: 10.1006/bbrc.1999.2054
108. Duguay D, Cermakian N. The crosstalk between physiology and circadian clock proteins. Chronobiology Int (2009) 26(8):1479–513. doi: 10.3109/07420520903497575
109. Tanioka M, Yamada H, Doi M, Bando H, Yamaguchi Y, Nishigori C. Molecular clocks in mouse skin. J Invest Dermatol (2009) 129(5):1225–31. doi: 10.1038/jid.2008.345
110. Gibbs J, Ince L, Matthews L, Mei J, Bell T, Yang N. An epithelial circadian clock controls pulmonary inflammation and glucocorticoid action. Nat Med (2014) 20(8):919–26. doi: 10.1038/nm.3599
111. Ibuka N, Nihonmatsu I, Sekiguchi S. Sleep-wakefulness rhythms in mice after suprachiasmatic nucleus lesions. Waking Sleeping (1980) 4(2):167–73.
112. Vanini G, Torterolo P. Sleep-wake neurobiology. Adv Exp Med Biol (2021) 1297:65–82. doi: 10.1007/978-3-030-61663-2_5
113. Conti A, Maestroni GJ. Melatonin rhythms in mice: role in autoimmune and lymphoproliferative diseases. Ann N Y Acad Sci (1998) 840:395–410. doi: 10.1111/j.1749-6632.1998.tb09578.x
114. Kennaway DJ. Melatonin research in mice: a review. Chronobiol Int (2019) 36(9):1167–83. doi: 10.1080/07420528.2019.1624373
115. Onaolapo AY, Adebayo AN, Onaolapo OJ. Exogenous daytime melatonin modulates response of adolescent mice in a repeated unpredictable stress paradigm. Naunyn Schmiedebergs Arch Pharmacol (2017) 390(2):149–61. doi: 10.1007/s00210-016-1314-7
116. Yin J, Li Y, Han H, Ma J, Liu G, Wu X. Administration of exogenous melatonin improves the diurnal rhythms of the gut microbiota in mice fed a high-fat diet. mSystems (2020) 5(3). doi: 10.1128/mSystems.00002-20
117. Yang Y, Jiang W, Feng Y, Liu J, Chen H, Wang D. Melatonin alleviates hippocampal GR inhibition and depression-like behavior induced by constant light exposure in mice. Ecotoxicol Environ Saf. (2021) 228:112979. doi: 10.1016/j.ecoenv.2021.112979
118. Mayr KA, Young L, Molina LA, Tran MA, Whelan PJ. An economical solution to record and control wheel-running for group-housed mice. J Neurosci Methods (2020) 331:108482. doi: 10.1016/j.jneumeth.2019.108482
119. Mills PA, Huetteman DA, Brockway BP, Zwiers LM, Gelsema AJ, Schwartz RS. A new method for measurement of blood pressure, heart rate, and activity in the mouse by radiotelemetry. J Appl Physiol (1985) 88(5):1537–44.
120. Tang X, Sanford LD. Telemetric recording of sleep and home cage activity in mice. Sleep (2002) 25(6):691–9. doi: 10.1093/sleep/25.6.677
121. Tschöp MH, Speakman JR, Arch JRS, Auwerx J, Brüning JC, Chan L. A guide to analysis of mouse energy metabolism. Nat Methods (2011) 9(1):57–63.
122. Mestas J, Hughes CCW. Of mice and not men: differences between mouse and human immunology. J Immunol (2004) 172(5):2731–8. doi: 10.4049/jimmunol.172.5.2731
123. Zschaler J, Schlorke D, Arnhold J. Differences in innate immune response between man and mouse. Crit Rev Immunol (2014) 34(5):433–54. doi: 10.1615/CritRevImmunol.2014011600
124. Kasperkiewicz M, Sadik CD, Bieber K, Ibrahim SM, Manz RA, Schmidt E. Epidermolysis bullosa acquisita: From pathophysiology to novel therapeutic options. J Invest Dermatol 136(1):24–33. doi: 10.1038/JID.2015.356
125. Kasprick A, Bieber K, Ludwig RJ. Drug discovery for pemphigoid diseases. Curr Protoc Pharmacol (2019) 84(1):e55. doi: 10.1002/cpph.55
126. Sadeghi H, Gupta Y, Möller S, Samavedam U, Behnen M, Muller S. The retinoid-related orphan receptor alpha is essential for the end-stage effector phase of experimental epidermolysis bullosa acquisita. Bd. 237 J Pathol (2015). doi: 10.1002/path.4556
127. Ansell DM, Kloepper JE, Thomason HA, Paus R, Hardman MJ. Exploring the “hair growth-wound healing connection”: anagen phase promotes wound re-epithelialization. J Invest Dermatol (2011) 131(2):518–28. doi: 10.1038/jid.2010.291
128. Jimenez F, Poblet E, Izeta A. Reflections on how wound healing-promoting effects of the hair follicle can be translated into clinical practice. Exp Dermatol (2015) 24(2):91–4. doi: 10.1111/exd.12521
129. Zomer HD, Trentin AG. Skin wound healing in humans and mice: Challenges in translational research. J Dermatol Sci (2018) 90(1):3–12. doi: 10.1016/j.jdermsci.2017.12.009
130. Toth LA, Trammell RA, Liberati T, Verhulst S, Hart ML, Moskowitz JE. Influence of chronic exposure to simulated shift work on disease and longevity in disease-prone inbred mice. Comp Med (2017) 67(2):116–26.
131. Frantz WT, Ceol CJ. From tank to treatment: Modeling melanoma in zebrafish. Cells 22 (2020) 9(5):E1289. doi: 10.3390/cells9051289
132. Kaufman CK. Zebrafish melanoma. Adv Exp Med Biol (2016) 916:439–50. doi: 10.1007/978-3-319-30654-4_19
133. Schneider MR, Wolf E. Genetically engineered pigs as investigative and translational models in dermatology. Br J Dermatol (2016) 174(1):237–9. doi: 10.1111/bjd.14092
134. Engert LC, Weiler U, Pfaffinger B, Stefanski V, Schmucker SS. Photoperiodic effects on diurnal rhythms in cell numbers of peripheral leukocytes in domestic pigs. Front Immunol (2019) 10:393. doi: 10.3389/fimmu.2019.00393
135. Fan N, Lai L. Genetically modified pig models for human diseases. J Genet Genomics (2013) 40(2):67–73. doi: 10.1016/j.jgg.2012.07.014
136. Sookoian S, Gemma C, Fernández Gianotti T, Burgueño A, Alvarez A, González CD. Effects of rotating shift work on biomarkers of metabolic syndrome and inflammation. J Intern Med (2007) 261(3):285–92. doi: 10.1111/j.1365-2796.2007.01766.x
137. Puttonen S, Viitasalo K, Härmä M. Effect of shiftwork on systemic markers of inflammation. Chronobiol Int (2011) 28(6):528–35. doi: 10.3109/07420528.2011.580869
138. Pavanello S, Stendardo M, Mastrangelo G, Bonci M, Bottazzi B, Campisi M. Inflammatory long pentraxin 3 is associated with leukocyte telomere length in night-shift workers. Front Immunol (2017) 8:516. doi: 10.3389/fimmu.2017.00516
139. Castanon-Cervantes O, Wu M, Ehlen JC, Paul K, Gamble KL, Johnson RL. Dysregulation of inflammatory responses by chronic circadian disruption. J Immunol (2010) 185(10):5796–805. doi: 10.4049/jimmunol.1001026
140. Inokawa H, Umemura Y, Shimba A, Kawakami E, Koike N, Tsuchiya Y. Chronic circadian misalignment accelerates immune senescence and abbreviates lifespan in mice. Sci Rep (2020) 10(1):2569. doi: 10.1038/s41598-020-59541-y
141. Sauvet F, Leftheriotis G, Gomez-Merino D, Langrume C, Drogou C, Van Beers P. Effect of acute sleep deprivation on vascular function in healthy subjects. J Appl Physiol (1985) 108(1):68–75.
142. Carreras A, Zhang SX, Peris E, Qiao Z, Gileles-Hillel A, Li RC. Chronic sleep fragmentation induces endothelial dysfunction and structural vascular changes in mice. Sleep (2014) 37(11):1817–24. doi: 10.5665/sleep.4178
143. Spiegel K, Leproult R, Van Cauter E. Impact of sleep debt on metabolic and endocrine function. Lancet (1999) 354(9188):1435–9. doi: 10.1016/S0140-6736(99)01376-8
144. Hu S, Li P, Zhang R, Liu X, Wei S. Integrated metabolomics and proteomics analysis reveals energy metabolism disorders in the livers of sleep-deprived mice. J Proteomics (2021) 245:104290. doi: 10.1016/j.jprot.2021.104290
145. Ju YES, Lucey BP, Holtzman DM. Sleep and Alzheimer disease pathology–a bidirectional relationship. Nat Rev Neurol (2014) 10(2):115–9. doi: 10.1038/nrneurol.2013.269
146. Chen GY, Nuñez G. Sterile inflammation: sensing and reacting to damage. Nat Rev Immunol (2010) 10(12):826–37. doi: 10.1038/nri2873
147. Rock KL, Latz E, Ontiveros F, Kono H. The sterile inflammatory response. Annu Rev Immunol (2010) 28:321–42. doi: 10.1146/annurev-immunol-030409-101311
148. Cunningham C, Hennessy E. Co-Morbidity and systemic inflammation as drivers of cognitive decline: new experimental models adopting a broader paradigm in dementia research. Alzheimers Res Ther (2015) 7(1):33. doi: 10.1186/s13195-015-0117-2
149. Lange T, Dimitrov S, Fehm HL, Westermann J, Born J. Shift of monocyte function toward cellular immunity during sleep. Arch Intern Med (2006) 166(16):1695–700. doi: 10.1001/archinte.166.16.1695
150. Lawrence T, Gilroy DW. Chronic inflammation: a failure of resolution? Int J Exp Pathol (2007) 88(2):85–94. doi: 10.1111/j.1365-2613.2006.00507.x
151. Bollinger T, Bollinger A, Skrum L, Dimitrov S, Lange T, Solbach W. Sleep-dependent activity of T cells and regulatory T cells. Clin Exp Immunol Februar (2009) 155(2):231–8. doi: 10.1111/j.1365-2249.2008.03822.x
152. Waggoner SN. Circadian rhythms in immunity. Curr Allergy Asthma Rep (2020) 20(1):2. doi: 10.1007/s11882-020-0896-9
153. Filipski E, King VM, Li X, Granda TG, Mormont MC, Claustrat B. Disruption of circadian coordination accelerates malignant growth in mice. Pathol Biol (Paris) (2003) 51(4):216–9. doi: 10.1016/S0369-8114(03)00034-8
154. Logan RW, Zhang C, Murugan S, O’Connell S, Levitt D, Rosenwasser AM. Chronic shift-lag alters the circadian clock of NK cells and promotes lung cancer growth in rats. J Immunol (2012) 188(6):2583–91. doi: 10.4049/jimmunol.1102715
155. Hakim F, Wang Y, Zhang SXL, Zheng J, Yolcu ES, Carreras A. Fragmented sleep accelerates tumor growth and progression through recruitment of tumor-associated macrophages and TLR4 signaling. Cancer Res (2014) 74(5):1329–37. doi: 10.1158/0008-5472.CAN-13-3014
156. Salamanca-Fernández E, Rodríguez-Barranco M, Guevara M, Ardanaz E, Olry de Labry Lima A, Sánchez MJ. Night-shift work and breast and prostate cancer risk: updating the evidence from epidemiological studies. Sist Sanit Navar (2018) 41(2):211–26.
157. Brown R, Pang G, Husband AJ, King MG. Suppression of immunity to influenza virus infection in the respiratory tract following sleep disturbance. Reg Immunol (1989) 2(5):321–5.
158. Spiegel K, Sheridan JF, Van Cauter E. Effect of sleep deprivation on response to immunization. JAMA (2002) 288(12):1471–2. doi: 10.1001/jama.288.12.1469
159. Lange T, Dimitrov S, Bollinger T, Diekelmann S, Born J. Sleep after vaccination boosts immunological memory. J Immunol (2011) 187(1):283–90. doi: 10.4049/jimmunol.1100015
160. Ruiz FS, Rosa DS, Zimberg IZ, Dos Santos Quaresma MV, Nunes JO, Apostolico JS. Night shift work and immune response to the meningococcal conjugate vaccine in healthy workers: a proof of concept study. Sleep Med (2020) 75:263–75. doi: 10.1016/j.sleep.2020.05.032
161. Hsiao YH, Chen YT, Tseng CM, Wu LA, Lin WC, Su VYF. Sleep disorders and increased risk of autoimmune diseases in individuals without sleep apnea. Sleep (2015) 38(4):581–6. doi: 10.5665/sleep.4574
162. Cooper GS, Parks CG, Treadwell EL, St Clair EW, Gilkeson GS, Dooley MA. Occupational risk factors for the development of systemic lupus erythematosus. J Rheumatol (2004) 31(10):1928–33.
163. Smolensky MH, Portaluppi F, Manfredini R, Hermida RC, Tiseo R, Sackett-Lundeen LL. Diurnal and twenty-four hour patterning of human diseases: acute and chronic common and uncommon medical conditions. Sleep Med Rev (2015) 21:12–22. doi: 10.1016/j.smrv.2014.06.005
164. Adeola HA, Papagerakis S, Papagerakis P. Systems biology approaches and precision oral health: A circadian clock perspective. Front Physiol (2019) 10:399. doi: 10.3389/fphys.2019.00399
165. Gao RC, Sang N, Jia CZ, Zhang MY, Li BH, Wei M. Association between sleep traits and rheumatoid arthritis: A mendelian randomization study. Front Public Health (2022) 10:940161. doi: 10.3389/fpubh.2022.940161
166. Focan C, Alvin M, Mazy V, Focan-Henrard D, Levi F, Franchimont P. Chronobiological aspects of spondylarthritis. Annu Rev Chronopharmacology. (1988) 5:17–20.
167. Keefer L, Stepanski EJ, Ranjbaran Z, Benson LM, Keshavarzian A. An initial report of sleep disturbance in inactive inflammatory bowel disease. J Clin Sleep Med (2006) 2(4):409–16. doi: 10.5664/jcsm.26656
168. Ranjbaran Z, Keefer L, Farhadi A, Stepanski E, Sedghi S, Keshavarzian A. Impact of sleep disturbances in inflammatory bowel disease. J Gastroenterol Hepatol (2007) 22(11):1748–53. doi: 10.1111/j.1440-1746.2006.04820.x
169. Ananthakrishnan AN, Khalili H, Konijeti GG, Higuchi LM, de Silva P, Fuchs CS. Sleep duration affects risk for ulcerative colitis: a prospective cohort study. Clin Gastroenterol Hepatol (2014) 12(11):1879–86. doi: 10.1016/j.cgh.2014.04.021
170. Gombert M, Carrasco-Luna J, Pin-Arboledas G, Codoñer-Franch P. The connection of circadian rhythm to inflammatory bowel disease. Transl Res April (2019) 206:107–18. doi: 10.1016/j.trsl.2018.12.001
171. Hasosah M, Alhashmi W, Abualsaud R, Alamoudi A, Aljawad A, Tunkar M. Environmental risk factors for childhood inflammatory bowel diseases: A multicenter case-control study. Children (Basel) (2022) 9(3):438. doi: 10.3390/children9030438
172. van der Sloot KWJ, Geertsema P, Rijkmans HC, Voskuil MD, van Dullemen HM, Visschedijk MC. Environmental factors associated with biological use and surgery in inflammatory bowel disease. J Gastroenterol Hepatol April (2021) 36(4):1022–34. doi: 10.1111/jgh.15223
173. Sonnenberg A. Occupational distribution of inflammatory bowel disease among German employees. Gut. September (1990) 31(9):1037–40. doi: 10.1136/gut.31.9.1037
174. Helvaci N, Oguz SH, Kabacam S, Karabulut E, Akbiyik F, Alikasifoglu M. Clock gene PERIOD3 polymorphism is associated with susceptibility to graves’ disease but not to hashimoto’s thyroiditis. Chronobiol Int (2019) 36(10):1343–50. doi: 10.1080/07420528.2019.1642909
175. Shaker SA, Meghad MA, Mahfouz M, Hassanien TE, Mahmoud BF, Salem TM. Thyroid –pineal axis: Melatonin, circadian clock genes expression and vitamin d in hashimoto’s thyroiditis patients [Internet]. in review (2022). Available at: https://www.researchsquare.com/article/rs-1498029/v1.
176. Xerfan EMS, Facina AS, Andersen ML, Tufik S, Tomimori J. Hashimoto thyroiditis as a cause or consequence of obstructive sleep apnea. J Clin Sleep Med (2019) 15(11):1703–3. doi: 10.5664/jcsm.8054
177. Olsson T, Barcellos LF, Alfredsson L. Interactions between genetic, lifestyle and environmental risk factors for multiple sclerosis. Nat Rev Neurol (2017) 13(1):25–36. doi: 10.1038/nrneurol.2016.187
178. Abad VC, Sarinas PSA, Guilleminault C. Sleep and rheumatologic disorders. Sleep Med Rev (2008) 12(3):211–28. doi: 10.1016/j.smrv.2007.09.001
179. Shoenfeld Y, Zandman-Goddard G, Stojanovich L, Cutolo M, Amital H, Levy Y. The mosaic of autoimmunity: hormonal and environmental factors involved in autoimmune diseases–2008. Isr Med Assoc J Januar (2008) 10(1):8–12.
180. Straub RH, Bijlsma JWJ, Masi A, Cutolo M. Role of neuroendocrine and neuroimmune mechanisms in chronic inflammatory rheumatic diseases–the 10-year update. Semin Arthritis Rheum (2013) 43(3):392–404. doi: 10.1016/j.semarthrit.2013.04.008
181. Ferri C, Emdin M, Giuggioli D, Carpeggiani C, Maielli M, Varga A. Autonomic dysfunction in systemic sclerosis: time and frequency domain 24 hour heart rate variability analysis. Br J Rheumatol (1997) 36(6):669–76. doi: 10.1093/rheumatology/36.6.669
182. Kotulska A, Brzezinska-Wcislo L, Kucharz EJ. Circadian profile of serum melatonin in patients with systemic sclerosis. Clin Exp Rheumatol (2005) 23(5):728–9.
183. Cutolo M, Straub RH, Buttgereit F. Circadian rhythms of nocturnal hormones in rheumatoid arthritis: translation from bench to bedside. Ann Rheum Dis (2008) 67(7):905–8. doi: 10.1136/ard.2008.088955
184. Chen M, Mei Q, Xu J, Lu C, Fang H, Liu X. Detection of melatonin and homocysteine simultaneously in ulcerative colitis. Clin Chim Acta (2012) 413(1–2):30–3. doi: 10.1016/j.cca.2011.06.036
185. Zheng Y, Huang S, Zhang J, Hou J, Wu F, Wang W. Melatonin alleviates vascular endothelial cell damage by regulating an autophagy-apoptosis axis in Kawasaki disease. Cell Proliferation. (2022) 55(6):e13251. doi: 10.1111/cpr.13251
186. Parekh PJ, Oldfield Iv EC, Challapallisri V, Ware JC, Johnson DA. Sleep disorders and inflammatory disease activity: chicken or the egg? Am J Gastroenterol (2015) 110(4):484–8. doi: 10.1038/ajg.2014.247
187. Lechner O, Dietrich H, Oliveira dos Santos A, Wiegers GJ, Schwarz S, Harbutz M. Altered circadian rhythms of the stress hormone and melatonin response in lupus-prone MRL/MP-fas(Ipr) mice. J Autoimmun (2000) 14(4):325–33. doi: 10.1006/jaut.2000.0375
188. Palma BD, Gabriel A, Colugnati FAB, Tufik S. Effects of sleep deprivation on the development of autoimmune disease in an experimental model of systemic lupus erythematosus. Am J Physiol Regul Integr Comp Physiol (2006) 291(5):R1527–1532. doi: 10.1152/ajpregu.00186.2006
189. Tang Y, Preuss F, Turek FW, Jakate S, Keshavarzian A. Sleep deprivation worsens inflammation and delays recovery in a mouse model of colitis. Sleep Med (2009) 10(6):597–603. doi: 10.1016/j.sleep.2008.12.009
190. Druzd D, Matveeva O, Ince L, Harrison U, He W, Schmal C. Lymphocyte circadian clocks control lymph node trafficking and adaptive immune responses. Immunity (2017) 46(1):120–32. doi: 10.1016/j.immuni.2016.12.011
191. Li WQ, Qureshi AA, Schernhammer ES, Han J. Rotating night-shift work and risk of psoriasis in US women. J Invest Dermatol (2013) 133(2):565–7. doi: 10.1038/jid.2012.285
192. Radaelli A, Carandente F, Tadini G, Ronchi M, Zoccoli A, Caccialanza P. Circadian temporal structure in psoriasis. Chronobiologia (1982) 9(2):203–9.
193. Pigatto PD, Radaelli A, Tadini G, Polenghi MM, Brambilla L, Altomare G. Circadian rhythm of the in vivo migration of neutrophils in psoriatic patients. Arch Dermatol Res (1985) 277(3):185–9. doi: 10.1007/BF00404314
194. Ferguson FJ, Lada G, Hunter HJA, Bundy C, Henry AL, Griffiths CEM. Diurnal and seasonal variation in psoriasis symptoms. J Eur Acad Dermatol Venereol (2021) 35(1):e45–7. doi: 10.1111/jdv.16791
195. Yosipovitch G, Goon A, Wee J, Chan YH, Goh CL. The prevalence and clinical characteristics of pruritus among patients with extensive psoriasis. Br J Dermatol (2000) 143(5):969–73. doi: 10.1046/j.1365-2133.2000.03829.x
196. Hirotsu C, Rydlewski M, Araújo MS, Tufik S, Andersen ML. Sleep loss and cytokines levels in an experimental model of psoriasis. PloS One (2012) 7(11):e51183. doi: 10.1371/journal.pone.0051183
197. Haugeberg G, Lund Nilsen TI, Kavanaugh A, Thomsen RS, Gulati AM, Hoff M. Physical and psychosocial burden of psoriatic arthritis: Longitudinal data from a population-based study in Norway. Arthritis Care Res (Hoboken) (2021) 73(1):138–45. doi: 10.1002/acr.24412
198. Skoie IM, Ternowitz T, Jonsson G, Norheim K, Omdal R. Fatigue in psoriasis: a phenomenon to be explored. Br J Dermatol (2015) 172(5):1196–203. doi: 10.1111/bjd.13647
199. Wang S, Kozai M, Mita H, Cai Z, Masum MA, Ichii O. REV-ERB agonist suppresses IL-17 production in γδT cells and improves psoriatic dermatitis in a mouse model. BioMed Pharmacother (2021) 144:112283. doi: 10.1016/j.biopha.2021.112283
200. Keen MA, Hassan I. Serum prolactin levels in psoriasis and its association with disease activity: a case-control study. Indian J Dermatol (2014) 59(6):562–6. doi: 10.4103/0019-5154.143512
201. Khatri G, Mahajan VK, Mehta KS, Sharma KK, Bhushan S, Chauhan PS. Serum prolactin levels in patients with psoriasis: Results of a pilot study. Indian J Med Res (2017) 145(2):250–2.
202. Mozzanica N, Tadini G, Radaelli A, Negri M, Pigatto P, Morelli M. Plasma melatonin levels in psoriasis. Acta Derm Venereol. (1988) 68(4):312–6.
203. Ghafourian E, Ghafourian S, Sadeghifard N, Mohebi R, Shokoohini Y, Nezamoleslami S. Vitiligo: Symptoms, pathogenesis and treatment. Int J Immunopathol Pharmacol (2014) 27(4):485–9. doi: 10.1177/039463201402700403
204. Mozzanica N, Frigerio U, Negri M, Tadini G, Villa ML, Mantovani M. Circadian rhythm of natural killer cell activity in vitiligo. J Am Acad Dermatol April (1989) 20(4):591–6. doi: 10.1016/S0190-9622(89)70069-4
205. Mozzanica N, Villa ML, Foppa S, Vignati G, Cattaneo A, Diotti R. Plasma alpha-melanocyte-stimulating hormone, beta-endorphin, met-enkephalin, and natural killer cell activity in vitiligo. J Am Acad Dermatol (1992) 26(5 Pt 1):693–700. doi: 10.1016/0190-9622(92)70094-V
206. Mozzanica N, Frigerio U, Finzi AF, Cattaneo A, Negri M, Scaglione F. T Cell subpopulations in vitiligo: a chronobiologic study. J Am Acad Dermatol (1990) 22(2 Pt 1):223–30. doi: 10.1016/0190-9622(90)70029-H
207. Sharma N, Koranne RV, Singh RK. Psychiatric morbidity in psoriasis and vitiligo: a comparative study. J Dermatol (2001) 28(8):419–23. doi: 10.1111/j.1346-8138.2001.tb00003.x
208. Khudhair OS, Gorial FI, Hameed AF. Fibromyalgia syndrome and vitiligo: A novel association. Arch Rheumatol (2018) 33(2):174–80. doi: 10.5606/ArchRheumatol.2018.6524
209. Kotb El-Sayed MI, Abd El-Ghany AA, Mohamed RR. Neural and endocrinal pathobiochemistry of vitiligo: Comparative study for a hypothesized mechanism. Front Endocrinol (Lausanne) (2018) 9:197. doi: 10.3389/fendo.2018.00197
211. Pedroni MN, Hirotsu C, Porro AM, Tufik S, Andersen ML. The role of sleep in pemphigus: a review of mechanisms and perspectives. Arch Dermatol Res (2017) 309(8):659–64. doi: 10.1007/s00403-017-1765-9
212. Yuan H, Zhou S, Liu Z, Cong W, Fei X, Zeng W. Pivotal role of lesional and perilesional T/B lymphocytes in pemphigus pathogenesis. J Invest Dermatol (2017) 137(11):2362–70. doi: 10.1016/j.jid.2017.05.032
213. Morell-Dubois S, Carpentier O, Cottencin O, Queyrel V, Hachulla E, Hatron PY. Stressful life events and pemphigus. DRM. (2008) 216(2):104–8. doi: 10.1159/000111506
214. Patel T, Ishiuji Y, Yosipovitch G. Nocturnal itch: why do we itch at night? Acta Derm Venereol (2007) 87(4):295–8. doi: 10.2340/00015555-0280
215. Kasperkiewicz M, Zillikens D. The pathophysiology of bullous pemphigoid. Clin Rev Allergy Immunol (2007) 33(1–2):67–77. doi: 10.1007/s12016-007-0030-y
216. Ludwig RJ. Model systems duplicating epidermolysis bullosa acquisita: a methodological review. Autoimmunity. Februar (2012) 45(1):102–10. doi: 10.3109/08916934.2011.606451
217. Miyamoto D, Santi CG, Aoki V, Maruta CW. Bullous pemphigoid. Bras Dermatol (2019) 94:133–46. doi: 10.1590/abd1806-4841.20199007
218. Wolf SJ, Estadt SN, Gudjonsson JE, Kahlenberg JM. Human and murine evidence for mechanisms driving autoimmune photosensitivity. Front Immunol (2018) 9:2430. doi: 10.3389/fimmu.2018.02430
219. Van Oers ML, Bossema ER, Thoolen BJ, Hartkamp A, Dekkers JC, Godaert GLR. Variability of fatigue during the day in patients with primary sjögren’s syndrome, systemic lupus erythematosus, and rheumatoid arthritis. Clin Exp Rheumatol (2010) 28(5):715–21.
220. Habibagahi M, Habibagahi Z, Jaberipour M, Aghdashi A. Quantification of regulatory T cells in peripheral blood of patients with systemic lupus erythematosus. Rheumatol Int (2011) 31(9):1219–25. doi: 10.1007/s00296-010-1427-0
221. Nabatian-Asl M, Ghorbanihaghjo A, Malek Mahdavi A, Khabbazi A, Hajialilo M, Ghojazadeh M. Effects of melatonin supplementation on serum oxidative stress markers and disease activity in systemic lupus erythematosus patients: A randomised, double-blind, placebo-controlled trial. Int J Clin Pract (2021) 75(7):e14246. doi: 10.1111/ijcp.14246
222. Gutiérrez MA, Garcia ME, Rodriguez JA, Rivero S, Jacobelli S. Hypothalamic-pituitary-adrenal axis function and prolactin secretion in systemic lupus erythematosus. Lupus. (1998) 7(6):404–8. doi: 10.1191/096120398678920343
223. Morand EF. Systemic lupus erythematosus: Stress and the onset of SLE. Nat Rev Rheumatol (2018) 14(3):127–8. doi: 10.1038/nrrheum.2018.2
224. Lee KH, Lee H, Lee CH, Kim JY, Kim JM, Kim SS. Adrenal insufficiency in systematic lupus erythematosus (SLE) and antiphospholipid syndrome (APS): A systematic review. Autoimmun Rev (2019) 18(1):1–8. doi: 10.1016/j.autrev.2018.06.014
225. Bassel M, Hudson M, Taillefer SS, Schieir O, Baron M, Thombs BD. Frequency and impact of symptoms experienced by patients with systemic sclerosis: results from a Canadian national survey. Rheumatology (2011) 50(4):762–7. doi: 10.1093/rheumatology/keq310
226. Hilty C, Brühlmann P, Sprott H, Gay RE, Michel BA, Gay S. Altered diurnal rhythm of prolactin in systemic sclerosis. J Rheumatol (2000) 27(9):2160–5.
227. Bagnato GL, Fiorenza A, Cordova F, Roberts WN, Moore C, Greco D. Clinical, autoimmune, and psychiatric parameters correlate with sleep disturbance in patients with systemic sclerosis and rheumatoid arthritis. Clin Exp Rheumatol (2016) 34 Suppl 100(5):49–55.
228. Emmrich R, Petzold H. Medicinal sleep therapy in the treatment of scleroderma and related diseases. Dtsch Med Wochenschr (1954) 79(25):1003–7. doi: 10.1055/s-0028-1115598
229. Sakkas LI, Chikanza IC, Platsoucas CD. Mechanisms of disease: the role of immune cells in the pathogenesis of systemic sclerosis. Nat Clin Pract Rheumatol (2006) 2(12):679–85. doi: 10.1038/ncprheum0346
230. Bagnato G, Cordova F, Sciortino D, Miceli G, Bruno A, Ferrera A. Association between cortisol levels and pain threshold in systemic sclerosis and major depression. Rheumatol Int (2018) 38(3):433–41. doi: 10.1007/s00296-017-3866-3
231. Todisco M. Effectiveness of a treatment based on melatonin in five patients with systemic sclerosis. Am J Ther (2006) 13(1):84–7. doi: 10.1097/01.mjt.0000162012.58990.4f
232. Kittridge A, Routhouska SB, Korman NJ. Dermatologic manifestations of sjögren syndrome. J Cutan Med Surg (2011) 15(1):8–14. doi: 10.2310/7750.2010.09033
233. Nocturne G, Mariette X. B cells in the pathogenesis of primary sjögren syndrome. Nat Rev Rheumatol (2018) 14(3):133–45. doi: 10.1038/nrrheum.2018.1
234. Johnson EO, Kostandi M, Moutsopoulos HM. Hypothalamic-pituitary-adrenal axis function in sjögren’s syndrome: mechanisms of neuroendocrine and immune system homeostasis. Ann N Y Acad Sci (2006) 1088:41–51. doi: 10.1196/annals.1366.018
235. Chen KR, Carlson JA. Clinical approach to cutaneous vasculitis. Am J Clin Dermatol (2008) 9(2):71–92. doi: 10.2165/00128071-200809020-00001
236. Micheletti RG, Pagnoux C. Management of cutaneous vasculitis. La Presse Médicale. 1. Oktober (2020) 49(3):104033. doi: 10.1016/j.lpm.2020.104033
237. Shilkina NP, Петровна ШН, Yunonin IE, Евгеньевич ЮИ, Stolyarova SA, Анатольевна СС. Arterial hypertension in patients with systemic connective tissue diseases and hemorrhagic vasculitis. Terapevticheskii arkhiv (2010) 82(5):40–5.
238. Basu N, McClean A, Harper L, Amft EN, Dhaun N, Luqmani RA. Explaining fatigue in ANCA-associated vasculitis. Rheumatology (2013) 52(9):1680–5. doi: 10.1093/rheumatology/ket191
239. Weyand CM, Goronzy JJ. Immune mechanisms in medium and large-vessel vasculitis. Nat Rev Rheumatol (2013) 9(12):731–40. doi: 10.1038/nrrheum.2013.161
240. Żabińska M, Kościelska-Kasprzak K, Krajewska J, Bartoszek D, Augustyniak-Bartosik H, Krajewska M. Immune cells profiling in ANCA-associated vasculitis patients–relation to disease activity. Cells. (2021) 10(7):1773.
241. Lora V, Cerroni L, Cota C. Skin manifestations of rheumatoid arthritis. G Ital Dermatol Venereol (2018) 153(2):243–55. doi: 10.23736/S0392-0488.18.05872-8
242. Meier K, Schloegl A, Poddubnyy D, Ghoreschi K. Skin manifestations in spondyloarthritis. Ther Adv Musculoskeletal. (2020) 12:1759720X20975915. doi: 10.1177/1759720X20975915
243. Puttonen S, Oksanen T, Vahtera J, Pentti J, Virtanen M, Salo P. Is shift work a risk factor for rheumatoid arthritis? the Finnish public sector study. Ann Rheum Dis (2010) 69(4):779–80. doi: 10.1136/ard.2008.099184
244. Harkness JA, Richter MB, Panayi GS, de Pette KV, Unger A, Pownall R. Circadian variation in disease activity in rheumatoid arthritis. Br Med J (Clin Res Ed) (1982) 284(6315):551–4. doi: 10.1136/bmj.284.6315.551
245. Ursini F, De Giorgi A, D’Onghia M, De Giorgio R, Fabbian F, Manfredini R. Chronobiology and chronotherapy in inflammatory joint diseases. Pharmaceutics (2021) 13(11):1832. doi: 10.3390/pharmaceutics13111832
246. Yap HY, Tee SZY, Wong MMT, Chow SK, Peh SC, Teow SY. Pathogenic role of immune cells in rheumatoid arthritis: Implications in clinical treatment and biomarker development. Cells (2018) 7(10):E161. doi: 10.3390/cells7100161
247. Lories RJ. Advances in understanding the pathophysiology of spondyloarthritis. Best Pract Res Clin Rheumatol (2018) 32(3):331–41. doi: 10.1016/j.berh.2018.12.001
248. Spies CM, Straub RH, Cutolo M, Buttgereit F. Circadian rhythms in rheumatology–a glucocorticoid perspective. Arthritis Res Ther (2014) 16(Suppl 2):S3. doi: 10.1186/ar4687
249. Karacaer C, Bes C, Seri̇n E, Soy M. Serum melatonin levels related with night pain and high disease activity in seronegative spondyloarthritis patients. J Hum Rhythm (2020) 6(4):177–87.
250. Baumgart DC, Carding SR. Inflammatory bowel disease: cause and immunobiology. Lancet (2007) 369(9573):1627–40. doi: 10.1016/S0140-6736(07)60750-8
251. Payer J, Huorka M, Duris I, Mikulecký M, Kratochvíl’ová H, Ondrejka P. Circadian rhythmicity and cross correlation of plasma gastrin, cortisol and somatostatin levels in ulcerative colitis patients and healthy subjects. Hepatogastroenterology (1993) 40(3):272–5.
252. Therrien A, Kelly CP, Silvester JA. Celiac disease: Extraintestinal manifestations and associated conditions. J Clin Gastroenterol (2020) 54(1):8–21. doi: 10.1097/MCG.0000000000001267
253. Zingone F, Siniscalchi M, Capone P, Tortora R, Andreozzi P, Capone E. The quality of sleep in patients with coeliac disease. Aliment Pharmacol Ther (2010) 32(8):1031–6. doi: 10.1111/j.1365-2036.2010.04432.x
254. Mårild K, Morgenthaler TI, Somers VK, Kotagal S, Murray JA, Ludvigsson JF. Increased use of hypnotics in individuals with celiac disease: a nationwide case-control study. BMC Gastroenterol (2015) 15:10. doi: 10.1186/s12876-015-0236-z
255. Zylberberg HM, Demmer RT, Murray JA, Green PHR, Lebwohl B. Depression and insomnia among individuals with celiac disease or on a gluten-free diet in the USA: results from a national survey. Eur J Gastroenterol Hepatol (2017) 29(9):1091–6. doi: 10.1097/MEG.0000000000000932
256. Yerushalmy-Feler A, Tauman R, Derowe A, Averbuch E, Ben-Tov A, Weintraub Y. Gluten-free diet may improve obstructive sleep apnea-related symptoms in children with celiac disease. BMC Pediatr (2018) 18(1):35. doi: 10.1186/s12887-018-1039-5
257. Suzer Gamli I, Keceli Basaran M. The effect of a gluten-free diet on sleep disturbances in children with celiac disease. Nat Sci Sleep. (2022) 14:449–56. doi: 10.2147/NSS.S354811
258. De Re V, Magris R, Cannizzaro R. New insights into the pathogenesis of celiac disease. Front Med (Lausanne). (2017) 4:137. doi: 10.3389/fmed.2017.00137
259. Bastenie PA, Bonnyns M, Vanhaelst L. Natural history of primary myxedema. Am J Med (1985) 79(1):91–100. doi: 10.1016/0002-9343(85)90551-0
260. Ragusa F, Fallahi P, Elia G, Gonnella D, Paparo SR, Giusti C. Hashimotos’ thyroiditis: Epidemiology, pathogenesis, clinic and therapy. Best Pract Res Clin Endocrinol Metab (2019) 33(6):101367.
261. Yazmalar L, Batmaz İ, Sarıyıldız MA, Yıldız M, Uçmak D, Türkçü F. Sleep quality in patients with behçet’s disease. Int J Rheum Dis (2017) 20(12):2062–9. doi: 10.1111/1756-185X.12459
262. Gehlert S, Clanton M. Shift work and breast cancer. Int J Environ Res Public Health (2020) 17(24):E9544. doi: 10.3390/ijerph17249544
263. Coppeta L, De Zordo LM, Papa F, Pietroiusti A, Magrini A. Skin sensitization among night shift and daytime healthcare workers: a cross sectional study. Cent Eur J Public Health (2021) 29(3):191–4. doi: 10.21101/cejph.a6260
264. Milstein HG, Cornell RC. Diurnal mitotic studies of psoriatic epidermis. J Invest Dermatol (1973) 61(3):180–2. doi: 10.1111/1523-1747.ep12676228
265. Pullmann H, Lennartz KJ, Steigleder GK. In vitro examination of cell proliferation in normal and psoriatic epidermis, with special regard to diurnal variations. Arch Dermatol Forsch. (1974) 250(3):177–84. doi: 10.1007/BF00561186
266. Gelfant S, Ozawa A, Chalker DK, Smith JG. Circadian rhythms and differences in epidermal and in dermal cel proliferation in uninvolved and involved psoriatic skin in vivo. J Invest Dermatol (1982) 78(1):58–62. doi: 10.1111/1523-1747.ep12497933
267. Bacaksiz A, Akif Vatankulu M, Sonmez O, Erdogan E, Tasal A, Turfan M. Non-dipping nocturnal blood pressure in psoriasis vulgaris. Wien Klin Wochenschr (2012) 124(23–24):822–9. doi: 10.1007/s00508-012-0294-y
268. Thaller V, Vrkljan M, Hotujac L, Thakore J. The potential role of hypocortisolism in the pathophysiology of PTSD and psoriasis. Coll Antropol (1999) 23(2):611–9.
269. Pasquali L, Srivastava A, Meisgen F, Das Mahapatra K, Xia P, Xu Landén N. The keratinocyte transcriptome in psoriasis: Pathways related to immune responses, cell cycle and keratinization. Acta Derm Venereol (2019) 99(2):196–205. doi: 10.2340/00015555-3066
270. Németh V, Horváth S, Kinyó Á, Gyulai R, Lengyel Z. Expression patterns of clock gene mRNAs and clock proteins in human psoriatic skin samples. Int J Mol Sci (2021) 23(1):121.
271. Ando N, Nakamura Y, Aoki R, Ishimaru K, Ogawa H, Okumura K. Circadian gene clock regulates psoriasis-like skin inflammation in mice. J Invest Dermatol (2015) 135(12):3001–8. doi: 10.1038/jid.2015.316
272. Militenko SA, Mar’iasis ED, Sokolovski SR, Fadeeva MI. [An improvement in the therapy of psoriasis at the pyatigorsk health resort taking into account circadian biorhythms]. Vopr Kurortol Fizioter Lech Fiz Kult Dezember (1989) 6):38–40.
273. Nguyen S, Bahakeem H, Alkhalifah A, Cavalié M, Boukari F, Montaudié H. Topical corticosteroids application in the evening is more effective than in the morning in psoriasis: results of a prospective comparative study. J Eur Acad Dermatol Venereol (2017) 31(5):e263–4. doi: 10.1111/jdv.14027
274. Gu WJ, Mi N. Topical corticosteroids application in the evening is more effective than in the morning in psoriasis: comment. J Eur Acad Dermatol Venereol. September (2017) 31(9):e405. doi: 10.1111/jdv.14214
275. Yoshioka D, Ando H, Ushijima K, Kumazaki M, Fujimura A. Chronotherapy of maxacalcitol on skin inflammation induced by topical 12-O-tetradecanoylphorbol-13-acetate in mice. Chronobiol Int (2018) 35(9):1269–80. doi: 10.1080/07420528.2018.1474890
276. Melikoglu M. Sleep quality and its association with disease severity in psoriasis. Eurasian J Med (2017) 49(2):124–7. doi: 10.5152/eurasianjmed.2017.17132
277. Kaaz K, Szepietowski JC, Matusiak Ł.. Sleep quality among adult patients with chronic dermatoses. Postepy Dermatol Alergol (2019) 36(6):659–66. doi: 10.5114/ada.2019.84007
278. Saçmacı H, Gürel G. Sleep disorders in patients with psoriasis: a cross-sectional study using non-polysomnographical methods. Sleep Breath (2019) 23(3):893–8.
279. Luca M, Musumeci ML, D’Agata E, Micali G. Depression and sleep quality in psoriatic patients: impact of psoriasis severity. Int J Psychiatry Clin Pract (2020) 24(1):102–4. doi: 10.1080/13651501.2019.1659372
280. Nyunt WWT, Low WY, Ismail R, Sockalingam S, Min AKK. Determinants of health-related quality of life in psoriasis patients in Malaysia. Asia Pac J Public Health (2015) 27(2):NP662–673. doi: 10.1177/1010539513492561
281. Henry AL, Bundy C, Kyle SD, Griffiths CEM, Chisholm A. Understanding the experience of sleep disturbance in psoriasis: a qualitative exploration using the common-sense model of self-regulation. Br J Dermatol (2019) 180(6):1397–404. doi: 10.1111/bjd.17685
282. Haugeberg G, Michelsen B, Kavanaugh A. Impact of skin, musculoskeletal and psychosocial aspects on quality of life in psoriatic arthritis patients: A cross-sectional study of outpatient clinic patients in the biologic treatment era. RMD Open (2020) 6(1):e001223. doi: 10.1136/rmdopen-2020-001223
283. Ogdie A, Michaud K, Nowak M, Bruce R, Cantor S, Hintzen C. Patient’s experience of psoriatic arthritis: a conceptual model based on qualitative interviews. RMD Open (2020) 6(3):e001321.
284. Hawro T, Hawro M, Zalewska-Janowska A, Weller K, Metz M, Maurer M. Pruritus and sleep disturbances in patients with psoriasis. Arch Dermatol Res März (2020) 312(2):103–11. doi: 10.1007/s00403-019-01998-7
285. Palominos PE, Coates L, Kohem CL, Orbai AM, Smolen J, de Wit M. Determinants of sleep impairment in psoriatic arthritis: An observational study with 396 patients from 14 countries. Joint Bone Spine (2020) 87(5):449–54. doi: 10.1016/j.jbspin.2020.03.014
286. Sahin E, Hawro M, Weller K, Sabat R, Philipp S, Kokolakis G. Prevalence and factors associated with sleep disturbance in adult patients with psoriasis. J Eur Acad Dermatol Venereol (2022) 36(5):688–97. doi: 10.1111/jdv.17917
287. Halioua B, Chelli C, Misery L, Taieb J, Taieb C. Sleep disorders and psoriasis: An update. Acta Derm Venereol (2022) 102:adv00699. doi: 10.2340/actadv.v102.1991
288. Strober BE, Sobell JM, Duffin KC, Bao Y, Guérin A, Yang H. Sleep quality and other patient-reported outcomes improve after patients with psoriasis with suboptimal response to other systemic therapies are switched to adalimumab: results from PROGRESS, an open-label phase IIIB trial. Br J Dermatol (2012) 167(6):1374–81. doi: 10.1111/bjd.12000
289. Stinco G, Trevisan G, Piccirillo F, Di Meo N, Nan K, Deroma L. Psoriasis vulgaris does not adversely influence the quality of sleep. G Ital Dermatol Venereol (2013) 148(6):655–9.
290. Thaçi D, Galimberti R, Amaya-Guerra M, Rosenbach T, Robertson D, Pedersen R. Improvement in aspects of sleep with etanercept and optional adjunctive topical therapy in patients with moderate-to-severe psoriasis: results from the PRISTINE trial. J Eur Acad Dermatol Venereol. (2014) 28(7):900–6.
291. Gezer O, Batmaz İ, Sariyildiz MA, Sula B, Ucmak D, Bozkurt M. Sleep quality in patients with psoriatic arthritis. Int J Rheum Dis (2017) 20(9):1212–8. doi: 10.1111/1756-185X.12505
292. Henry AL, Kyle SD, Chisholm A, Griffiths CEM, Bundy C. A cross-sectional survey of the nature and correlates of sleep disturbance in people with psoriasis. Br J Dermatol (2017) 177(4):1052–9. doi: 10.1111/bjd.15469
293. Henry AL, Chisholm A, Carter LA, Bundy C, Griffiths CEM, Kyle SD. The relationship between sleep disturbance, symptoms and daytime functioning in psoriasis: a prospective study integrating actigraphy and experience sampling methodology. Sleep Med (2020) 72:144–9. doi: 10.1016/j.sleep.2020.03.013
294. Wong ITY, Chandran V, Li S, Gladman DD. Sleep disturbance in psoriatic disease: Prevalence and associated factors. J Rheumatol (2017) 44(9):1369–74. doi: 10.3899/jrheum.161330
295. Smith MP, Ly K, Thibodeaux Q, Weerasinghe T, Beck K, Shankle L. Factors influencing sleep difficulty and sleep quantity in the citizen pscientist psoriatic cohort. Dermatol Ther (Heidelb) (2019) 9(3):511–23. doi: 10.1007/s13555-019-0306-1
296. Haugeberg G, Hoff M, Kavanaugh A, Michelsen B. Psoriatic arthritis: exploring the occurrence of sleep disturbances, fatigue, and depression and their correlates. Arthritis Res Ther (2020) 22(1):198. doi: 10.1186/s13075-020-02294-w
297. Tas B, Kabeloglu V, Soysal A, Atakli D. Sleep quality in psoriasis patients and its relations with possible affecting factors. Sisli Etfal Hastan Tip Bul. (2020) 54(2):181–7.
298. Skougaard M, Stisen ZR, Jørgensen TS, Egeberg A, Hansen RL, Perez-Chada LM. Increased prevalence of sleep disturbance in psoriatic arthritis is associated with inflammatory and non-inflammatory measures. Scand J Rheumatol (2022), 1–9. doi: 10.1080/03009742.2022.2044116
299. van Acht MR, van den Reek JMPA, de Jong EMGJ, Seyger MMB. The effect of lifestyle changes on disease severity and quality of life in patients with plaque psoriasis: A narrative review. Psoriasis (Auckl). (2022) 12:35–51.
300. Cano-García L, Mena-Vázquez N, Manrique Arija S, Hernández-Sánchez MD, Segura-Ruiz R, Domínguez-Quesada C. Psychological factors associated with sleep disorders in patients with axial spondyloarthritis or psoriatic arthritis: A multicenter cross-sectional observational study. J Clin Nurs. (2021) 30(1–2):266–75. doi: 10.1111/jocn.15546
301. Nowowiejska J, Baran A, Flisiak I. Mutual relationship between sleep disorders, quality of life and psychosocial aspects in patients with psoriasis. Front Psychiatry (2021) 12:674460. doi: 10.3389/fpsyt.2021.674460
302. Tas B, Kabeloglu V. Prevalence of metabolic syndrome and its parameters and their correlations with psoriasis duration, severity, and sleep quality in psoriasis patients: A cross-sectional study. Dermatol Pract Concept (2021) 11(3):e2021049. doi: 10.5826/dpc.1103a49
303. Chiu HY, Hsieh CF, Chiang YT, Tsai YW, Huang WF, Li CY, et al. concomitant sleep disorders significantly increase the risk of cardiovascular disease in patients with psoriasis. PloS One (2016) 11(1):e0146462. doi: 10.1371/journal.pone.0146462
304. Damiani G, Bragazzi NL, Garbarino S, Chattu VK, Shapiro CM, Pacifico A, et al. Psoriatic and psoriatic arthritis patients with and without jet-lag: does it matter for disease severity scores? insights and implications from a pilot, prospective study. Chronobiol Int (2019) 36(12):1733–40. doi: 10.1080/07420528.2019.1678629
305. Gelfand JM, Neimann AL, Shin DB, Wang X, Margolis DJ, Troxel AB. Risk of myocardial infarction in patients with psoriasis. JAMA (2006) 296(14):1735–41. doi: 10.1001/jama.296.14.1735
306. Mouzas O, Angelopoulos N, Papaliagka M, Tsogas P. Increased frequency of self-reported parasomnias in patients suffering from vitiligo. Eur J Dermatol (2008) 18(2):165–8.
307. Seo HM, Kim TL, Kim JS. The risk of alopecia areata and other related autoimmune diseases in patients with sleep disorders: a Korean population-based retrospective cohort study. Sleep (2018) 41(9). doi: 10.1093/sleep/zsy111
308. Akashi M, Takumi T. The orphan nuclear receptor RORalpha regulates circadian transcription of the mammalian core-clock Bmal1. Nat Struct Mol Biol (2005) 12(5):441–8. doi: 10.1038/nsmb925
309. Kridin K, Hübner F, Recke A, Linder R, Schmidt E. The burden of neurological comorbidities in six autoimmune bullous diseases: a population-based study. J Eur Acad Dermatol Venereology. (2021) 35(10):2074–8. doi: 10.1111/jdv.17465
310. Ständer S, Hammers CM, Vorobyev A, Schmidt E, Hundt JE, Sadik CD, et al. coexistence of bullous pemphigoid with neuropsychiatric comorbidities is associated with anti-BP230 seropositivity. J Eur Acad Dermatol Venereol. (2021) 35(10):2067–73. doi: 10.1111/jdv.17304
311. Försti AK, Huilaja L, Schmidt E, Tasanen K. Neurological and psychiatric associations in bullous pemphigoid-more than skin deep? Exp Dermatol (2017) 26(12):1228–34. doi: 10.1111/exd.13401
312. Fonken LK, Kitt MM, Gaudet AD, Barrientos RM, Watkins LR, Maier SF. Diminished circadian rhythms in hippocampal microglia may contribute to age-related neuroinflammatory sensitization. Neurobiol Aging (2016) 47:102–12. doi: 10.1016/j.neurobiolaging.2016.07.019
313. Xing C, Zhou Y, Xu H, Ding M, Zhang Y, Zhang M, et al. Sleep disturbance induces depressive behaviors and neuroinflammation by altering the circadian oscillations of clock genes in rats. Neurosci Res (2021) 171:124–32. doi: 10.1016/j.neures.2021.03.006
314. Lananna BV, Musiek ES. The wrinkling of time: Aging, inflammation, oxidative stress, and the circadian clock in neurodegeneration. Neurobiol Dis (2020) 139:104832. doi: 10.1016/j.nbd.2020.104832
315. Everson CA, Toth LA. Systemic bacterial invasion induced by sleep deprivation. Am J Physiol Regul Integr Comp Physiol (2000) 278(4):R905–916. doi: 10.1152/ajpregu.2000.278.4.R905
316. Chang YS, Chiang BL. Sleep disorders and atopic dermatitis: A 2-way street? J Allergy Clin Immunol (2018) 142(4):1033–40. doi: 10.1016/j.jaci.2018.08.005
317. Takita E, Yokota S, Tahara Y, Hirao A, Aoki N, Nakamura Y, et al. Biological clock dysfunction exacerbates contact hypersensitivity in mice. Br J Dermatol (2013) 168(1):39–46. doi: 10.1111/j.1365-2133.2012.11176.x
318. Yousef E, Mitwally N, Noufal N, Tahir MR. Shift work and risk of skin cancer: A systematic review and meta-analysis. Sci Rep (2020) 10(1):2012. doi: 10.1038/s41598-020-59035-x
319. Milette K, Hudson M, Körner A, Baron M, Thombs BD. Canadian Scleroderma research group. Sleep disturbances systemic sclerosis: evidence role gastrointestinal symptoms Pain pruritus. Rheumatol (Oxford) (2013) 52(9):1715–20.
320. Vachiramon V, Onprasert W, Harnchoowong S, Chanprapaph K. Prevalence and clinical characteristics of itch in vitiligo and its clinical significance. BioMed Res Int (2017) 2017:5617838. doi: 10.1155/2017/5617838
321. Heckman CJ, Riley M, Valdes-Rodriguez R, Yosipovitch G. Development and initial psychometric properties of two itch-related measures: Scratch intensity and impact, sleep-related itch and scratch. J Invest Dermatol (2020) 140(11):2138–2145.e1. doi: 10.1016/j.jid.2020.03.961
322. de Ruiter CC, Rustemeyer T. Biologics can significantly improve dermatology life quality index (DLQI) in psoriatic patients: A systematic review. Psoriasis (Auckl). (2022) 12:99–112. doi: 10.2147/PTT.S356568
323. Masmoudi W, Vaillant M, Vassileva S, Patsatsi A, Quereux G, Moltrasio C, et al. International validation of the bullous pemphigoid disease area index severity score and calculation of cut-off values for defining mild, moderate and severe types of bullous pemphigoid. Br J Dermatol (2021) 184(6):1106–12. doi: 10.1111/bjd.19611
324. Buysse DJ, Reynolds CF, Monk TH, Berman SR, Kupfer DJ. The Pittsburgh sleep quality index: a new instrument for psychiatric practice and research. Psychiatry Res (1989) 28(2):193–213. doi: 10.1016/0165-1781(89)90047-4
325. Rosbash M. The implications of multiple circadian clock origins. PloS Biol (2009) 7(3):e62. doi: 10.1371/journal.pbio.1000062
326. Khapre RV, Samsa WE, Kondratov RV. Circadian regulation of cell cycle: Molecular connections between aging and the circadian clock. Ann Med (2010) 42(6):404–15. doi: 10.3109/07853890.2010.499134
327. Zanello SB, Jackson DM, Holick MF. Expression of the circadian clock genes clock and period1 in human skin. J Invest Dermatol (2000) 115(4):757–60. doi: 10.1046/j.1523-1747.2000.00121.x
328. Sandu C, Dumas M, Malan A, Sambakhe D, Marteau C, Nizard C, et al. Human skin keratinocytes, melanocytes, and fibroblasts contain distinct circadian clock machineries. Cell Mol Life Sci (2012) 69(19):3329–39. doi: 10.1007/s00018-012-1026-1
329. Watanabe M, Hida A, Kitamura S, Enomoto M, Ohsawa Y, Katayose Y, et al. Rhythmic expression of circadian clock genes in human leukocytes and beard hair follicle cells. Biochem Biophys Res Commun (2012) 425(4):902–7. doi: 10.1016/j.bbrc.2012.08.008
330. Janich P, Toufighi K, Solanas G, Luis NM, Minkwitz S, Serrano L, et al. Human epidermal stem cell function is regulated by circadian oscillations. Cell Stem Cell (2013) 13(6):745–53. doi: 10.1016/j.stem.2013.09.004
331. Plikus MV, Van Spyk EN, Pham K, Geyfman M, Kumar V, Takahashi JS, et al. The circadian clock in skin: implications for adult stem cells, tissue regeneration, cancer, aging, and immunity. J Biol Rhythms (2015) 30(3):163–82. doi: 10.1177/0748730414563537
332. Solanas G, Peixoto FO, Perdiguero E, Jardí M, Ruiz-Bonilla V, Datta D, et al. Aged stem cells reprogram their daily rhythmic functions to adapt to stress. Cell (2017) 170(4):678–692.e20. doi: 10.1016/j.cell.2017.07.035
333. Sindrup JH, Kastrup J, Jørgensen B, Bülow J, Lassen NA. Nocturnal variations in subcutaneous blood flow rate in lower leg of normal human subjects. Am J Physiol (1991) 260(2 Pt 2):H480–485. doi: 10.1152/ajpheart.1991.260.2.H480
334. Reinberg AE, Touitou Y, Soudant E, Bernard D, Bazin R, Mechkouri M. Oral contraceptives alter circadian rhythm parameters of cortisol, melatonin, blood pressure, heart rate, skin blood flow, transepidermal water loss, and skin amino acids of healthy young women. Chronobiol Int (1996) 13(3):199–211. doi: 10.3109/07420529609012653
335. Yosipovitch G, Xiong GL, Haus E, Sackett-Lundeen L, Ashkenazi I, Maibach HI. Time-dependent variations of the skin barrier function in humans: transepidermal water loss, stratum corneum hydration, skin surface pH, and skin temperature. J Invest Dermatol (1998) 110(1):20–3. doi: 10.1046/j.1523-1747.1998.00069.x
336. Le Fur I, Reinberg A, Lopez S, Morizot F, Mechkouri M, Tschachler E. Analysis of circadian and ultradian rhythms of skin surface properties of face and forearm of healthy women. J Invest Dermatol (2001) 117(3):718–24. doi: 10.1046/j.0022-202x.2001.01433.x
337. Ohmori K, Minamide K, Goto S, Nagai M, Shirai J, Oku K. Time-of-day-dependent variations of scratching behavior and transepidermal water loss in mice that developed atopic dermatitis. J Vet Med Sci (2014) 76(11):1501–4. doi: 10.1292/jvms.14-0173
338. Hoyle NP, Seinkmane E, Putker M, Feeney KA, Krogager TP, Chesham JE, et al. circadian actin dynamics drive rhythmic fibroblast mobilization during wound healing. Sci Transl Med (2017) 9(415). doi: 10.1126/scitranslmed.aal2774
339. Cable EJ, Onishi KG, Prendergast BJ. Circadian rhythms accelerate wound healing in female Siberian hamsters. Physiol Behav (2017) 171:165–74. doi: 10.1016/j.physbeh.2016.12.019
340. Altemus M, Rao B, Dhabhar FS, Ding W, Granstein RD. Stress-induced changes in skin barrier function in healthy women. J Invest Dermatol (2001) 117(2):309–17. doi: 10.1046/j.1523-1747.2001.01373.x
341. Deshayes N, Genty G, Berthelot F, Paris M. Human long-term deregulated circadian rhythm alters regenerative properties of skin and hair precursor cells. Eur J Dermatol (2018) 28(4):467–75. doi: 10.1684/ejd.2018.3358
342. Elkhenany H, AlOkda A, El-Badawy A, El-Badri N. Tissue regeneration: Impact of sleep on stem cell regenerative capacity. Life Sci (2018) 214:51–61. doi: 10.1016/j.lfs.2018.10.057
343. Kowalska E, Ripperger JA, Hoegger DC, Bruegger P, Buch T, Birchler T, et al. NONO couples the circadian clock to the cell cycle. Proc Natl Acad Sci U.S.A. (2013) 110(5):1592–9. doi: 10.1073/pnas.1213317110
344. Smith TJ, Wilson MA, Karl JP, Orr J, Smith CD, Cooper AD, et al. Impact of sleep restriction on local immune response and skin barrier restoration with and without “multinutrient” nutrition intervention. J Appl Physiol (1985) 124(1):190–200.
345. Kondratov RV, Kondratova AA, Gorbacheva VY, Vykhovanets OV, Antoch MP. Early aging and age-related pathologies in mice deficient in BMAL1, the core componentof the circadian clock. Genes Dev (2006) 20(14):1868–73. doi: 10.1101/gad.1432206
346. Kushida CA, Everson CA, Suthipinittharm P, Sloan J, Soltani K, Bartnicke B, et al. Sleep deprivation in the rat: VI. skin changes. Sleep (1989) 12(1):42–6.
347. Labrecque G, Vanier MC. Biological rhythms in pain and in the effects of opioid analgesics. Pharmacol Ther (1995) 68(1):129–47. doi: 10.1016/0163-7258(95)02003-9
348. Bachmann CG, Nitsche MA, Pfingsten M, Gersdorff N, Harder C, Baier PC, et al. Diurnal time course of heat pain perception in healthy humans. Neurosci Lett (2011) 489(2):122–5. doi: 10.1016/j.neulet.2010.11.080
349. Alexandre C, Latremoliere A, Ferreira A, Miracca G, Yamamoto M, Scammell TE, et al. Decreased alertness due to sleep loss increases pain sensitivity in mice. Nat Med (2017) 23(6):768–74. doi: 10.1038/nm.4329
350. Akashi M, Soma H, Yamamoto T, Tsugitomi A, Yamashita S, Yamamoto T, et al. Noninvasive method for assessing the human circadian clock using hair follicle cells. Proc Natl Acad Sci U.S.A. (2010) 107(35):15643–8. doi: 10.1073/pnas.1003878107
351. Schestatsky P, Dall-Agnol L, Gheller L, Stefani LC, Sanches PRS, de Souza IC, et al. Pain-autonomic interaction after work-induced sleep restriction. Eur J Neurol (2013) 20(4):638–46. doi: 10.1111/ene.12011
352. McLain JM, Alami WH, Glovak ZT, Cooley CR, Burke SJ, Collier JJ, et al. Sleep fragmentation delays wound healing in a mouse model of type 2 diabetes. Sleep (2018) 41(11). doi: 10.1093/sleep/zsy156
353. Zhou K, Ma Y, Brogan MS. Chronic and non-healing wounds: The story of vascular endothelial growth factor. Med Hypotheses (2015) 85(4):399–404. doi: 10.1016/j.mehy.2015.06.017
354. Nguyen KD, Fentress SJ, Qiu Y, Yun K, Cox JS, Chawla A. Circadian gene Bmal1 regulates diurnal oscillations of Ly6C(hi) inflammatory monocytes. Science (2013) 341(6153):1483–8. doi: 10.1126/science.1240636
355. He W, Holtkamp S, Hergenhan SM, Kraus K, de Juan A, Weber J, et al. circadian expression of migratory factors establishes lineage-specific signatures that guide the homing of leukocyte subsets to tissues. Immunity (2018) 49(6):1175–1190.e7. doi: 10.1016/j.immuni.2018.10.007
356. McAlpine CS, Kiss MG, Rattik S, He S, Vassalli A, Valet C, et al. Sleep modulates haematopoiesis and protects against atherosclerosis. Nature (2019) 566(7744):383–7. doi: 10.1038/s41586-019-0948-2
357. Herrscher RF, Kasper C, Sullivan TJ. Endogenous cortisol regulates immunoglobulin e-dependent late phase reactions. J Clin Invest (1992) 90(2):596–603. doi: 10.1172/JCI115898
358. Dhabhar FS, Miller AH, Stein M, McEwen BS, Spencer RL. Diurnal and acute stress-induced changes in distribution of peripheral blood leukocyte subpopulations. Brain Behav Immun (1994) 8(1):66–79. doi: 10.1006/brbi.1994.1006
359. Dhabhar FS. Psychological stress and immunoprotection versus immunopathology in the skin. Clin Dermatol (2013) 31(1):18–30. doi: 10.1016/j.clindermatol.2011.11.003
360. Kiessling S, Dubeau-Laramée G, Ohm H, Labrecque N, Olivier M, Cermakian N. The circadian clock in immune cells controls the magnitude of leishmania parasite infection. Sci Rep (2017) 7(1):10892. doi: 10.1038/s41598-017-11297-8
361. Fawcett S, Al Kassas R M, Dykes I, Hughes AT, Ghali F, Ross K. A time to heal: microRNA and circadian dynamics in cutaneous wound repair. Clin Sci (2022) 136(8):579–97. doi: 10.1042/CS20220011
362. Kinoshita C, Okamoto Y, Aoyama K, Nakaki T. MicroRNA: A key player for the interplay of circadian rhythm abnormalities, sleep disorders and neurodegenerative diseases. Clocks Sleep. September (2020) 2(3):282–307. doi: 10.3390/clockssleep2030022
363. Prendergast KA, Kirman JR. Dendritic cell subsets in mycobacterial infection: control of bacterial growth and T cell responses. Tuberculosis (Edinb) (2013) 93(2):115–22. doi: 10.1016/j.tube.2012.10.008
364. Holtkamp SJ, Ince LM, Barnoud C, Schmitt MT, Sinturel F, Pilorz V, et al. circadian clocks guide dendritic cells into skin lymphatics. Nat Immunol (2021) 22(11):1375–81. doi: 10.1038/s41590-021-01040-x
365. Christ P, Sowa AS, Froy O, Lorentz A. The circadian clock drives mast cell functions in allergic reactions. Front Immunol (2018) 9:1526. doi: 10.3389/fimmu.2018.01526
366. Nakao A. Clockwork allergy: How the circadian clock underpins allergic reactions. J Allergy Clin Immunol (2018) 142(4):1021–31. doi: 10.1016/j.jaci.2018.08.007
367. Sato S, Sakurai T, Ogasawara J, Takahashi M, Izawa T, Imaizumi K, et al. A circadian clock gene, rev-erbα, modulates the inflammatory function of macrophages through the negative regulation of Ccl2 expression. J Immunol (2014) 192(1):407–17. doi: 10.4049/jimmunol.1301982
368. Oishi Y, Hayashi S, Isagawa T, Oshima M, Iwama A, Shimba S, et al. Bmal1 regulates inflammatory responses in macrophages by modulating enhancer RNA transcription. Sci Rep (2017) 7(1):7086. doi: 10.1038/s41598-017-07100-3
369. Curtis AM, Fagundes CT, Yang G, Palsson-McDermott EM, Wochal P, McGettrick AF, et al. circadian control of innate immunity in macrophages by miR-155 targeting Bmal1. Proc Natl Acad Sci U.S.A. (2015) 112(23):7231–6. doi: 10.1073/pnas.1501327112
370. Park S, Kim K, Bae IH, Lee SH, Jung J, Lee TR, et al. TIMP3 is a CLOCK-dependent diurnal gene that inhibits the expression of UVB-induced inflammatory cytokines in human keratinocytes. FASEB J (2018) 32(3):1510–23. doi: 10.1096/fj.201700693R
371. Khosro S, Alireza S, Omid A, Forough S. Night work and inflammatory markers. Indian J Occup Environ Med (2011) 15(1):38–41.
372. Kim SW, Jang EC, Kwon SC, Han W, Kang MS, Nam YH, et al. Night shift work and inflammatory markers in male workers aged 20-39 in a display manufacturing company. Ann Occup Environ Med (2016) 28:48. doi: 10.1186/s40557-016-0135-y
373. Wirth MD, Shivappa N, Burch JB, Hurley TG, Hébert JR. The dietary inflammatory index, shift work, and depression: Results from NHANES. Health Psychol (2017) 36(8):760–9. doi: 10.1037/hea0000514
374. Lu LF, Wang CP, Tsai IT, Hung WC, Yu TH, Wu CC, et al. Relationship between shift work and peripheral total and differential leukocyte counts in Chinese steel workers. J Occup Health (2016) 58(1):81–8. doi: 10.1539/joh.15-0137-OA
375. Loef B, van Baarle D, van der Beek AJ, Sanders EAM, Bruijning-Verhagen P, Proper KI. Shift work and respiratory infections in health-care workers. Am J Epidemiol (2019) 188(3):509–17. doi: 10.1093/aje/kwy258
376. Streng AA, Loef B, Dollé MET, van der Horst GTJ, Chaves I, Proper KI, et al. Night shift work characteristics are associated with several elevated metabolic risk factors and immune cell counts in a cross-sectional study. Sci Rep (2022) 12(1):2022. doi: 10.1038/s41598-022-06122-w
377. Matsumoto Y, Mishima K, Satoh K, Tozawa T, Mishima Y, Shimizu T, et al. Total sleep deprivation induces an acute and transient increase in NK cell activity in healthy young volunteers. Sleep (2001) 24(7):804–9.
378. Dimitrov S, Lange T, Nohroudi K, Born J. Number and function of circulating human antigen presenting cells regulated by sleep. Sleep (2007) 30(4):401–11. doi: 10.1093/sleep/30.4.401
379. Fondell E, Axelsson J, Franck K, Ploner A, Lekander M, Bälter K, et al. Short natural sleep is associated with higher T cell and lower NK cell activities. Brain Behav Immun (2011) 25(7):1367–75. doi: 10.1016/j.bbi.2011.04.004
380. Logan RW, Wynne O, Levitt D, Price D, Sarkar DK. Altered circadian expression of cytokines and cytolytic factors in splenic natural killer cells of Per1(-/-) mutant mice. J Interferon Cytokine Res (2013) 33(3):108–14. doi: 10.1089/jir.2012.0092
381. De Lorenzo BHP, de Oliveira Marchioro L, Greco CR, Suchecki D. Sleep-deprivation reduces NK cell number and function mediated by β-adrenergic signalling. Psychoneuroendocrinology (2015) 57:134–43. doi: 10.1016/j.psyneuen.2015.04.006
382. Beam CA, Wasserfall C, Woodwyk A, Akers M, Rauch H, Blok T, et al. Synchronization of the normal human peripheral immune system: A comprehensive circadian systems immunology analysis. Sci Rep (2020) 10(1):672. doi: 10.1038/s41598-019-56951-5
383. Okamoto H, Tsunoda T, Teruya K, Takeda N, Uemura T, Matsui T, et al. An occupational health study of emergency physicians in Japan: health assessment by immune variables (CD4, CD8, CD56, and NK cell activity) at the beginning of work. J Occup Health (2008) 50(2):136–46. doi: 10.1539/joh.L6084
384. Nagai M, Morikawa Y, Kitaoka K, Nakamura K, Sakurai M, Nishijo M, et al. Effects of fatigue on immune function in nurses performing shift work. J Occup Health (2011) 53(5):312–9. doi: 10.1539/joh.10-0072-OA
385. Masera RG, Carignola R, Staurenghi AH, Sartori ML, Lazzero A, Griot G, et al. Altered circadian rhythms of natural killer (NK) cell activity in patients with autoimmune rheumatic diseases. Chronobiologia (1994) 21(1–2):127–32.
386. Dimitrov S, Benedict C, Heutling D, Westermann J, Born J, Lange T. Cortisol and epinephrine control opposing circadian rhythms in T cell subsets. Blood (2009) 113(21):5134–43. doi: 10.1182/blood-2008-11-190769
387. Besedovsky L, Ngo HVV, Dimitrov S, Gassenmaier C, Lehmann R, Born J. Auditory closed-loop stimulation of EEG slow oscillations strengthens sleep and signs of its immune-supportive function. Nat Commun (2017) 8(1):1984. doi: 10.1038/s41467-017-02170-3
388. Besedovsky L, Dimitrov S, Born J, Lange T. Nocturnal sleep uniformly reduces numbers of different T-cell subsets in the blood of healthy men. Am J Physiol Regul Integr Comp Physiol (2016) 311(4):R637–42. doi: 10.1152/ajpregu.00149.2016
389. Dimitrov S, Lange T, Gouttefangeas C, Jensen ATR, Szczepanski M, Lehnnolz J, et al. Gαs-coupled receptor signaling and sleep regulate integrin activation of human antigen-specific T cells. J Exp Med (2019) 216(3):517–26. doi: 10.1084/jem.20181169
390. Lange T, Luebber F, Grasshoff H, Besedovsky L. The contribution of sleep to the neuroendocrine regulation of rhythms in human leukocyte traffic. Semin Immunopathol (2022) 44(2):239–54. doi: 10.1007/s00281-021-00904-6
391. Fortier EE, Rooney J, Dardente H, Hardy MP, Labrecque N, Cermakian N. Circadian variation of the response of T cells to antigen. J Immunol (2011) 187(12):6291–300. doi: 10.4049/jimmunol.1004030
392. Petrovsky N. Towards a unified model of neuroendocrine-immune interaction. Immunol Cell Biol (2001) 79(4):350–7. doi: 10.1046/j.1440-1711.2001.01029.x
393. Dimitrov S, Lange T, Tieken S, Fehm HL, Born J. Sleep associated regulation of T helper 1/T helper 2 cytokine balance in humans. Brain Behav Immun (2004) 18(4):341–8. doi: 10.1016/j.bbi.2003.08.004
394. Bollinger T, Bollinger A, Naujoks J, Lange T, Solbach W. The influence of regulatory T cells and diurnal hormone rhythms on T helper cell activity. Immunology (2010) 131(4):488–500. doi: 10.1111/j.1365-2567.2010.03320.x
395. Axelsson J, Rehman J, Akerstedt T, Ekman R, Miller GE, Höglund CO, et al. Effects of sustained sleep restriction on mitogen-stimulated cytokines, chemokines and T helper 1/ T helper 2 balance in humans. PloS One (2013) 8(12):e82291. doi: 10.1371/journal.pone.0082291
396. Yu X, Rollins D, Ruhn KA, Stubblefield JJ, Green CB, Kashiwada M, et al. TH17 cell differentiation is regulated by the circadian clock. Science (2013) 342(6159):727–30. doi: 10.1126/science.1243884
397. Cuesta M, Boudreau P, Dubeau-Laramée G, Cermakian N, Boivin DB. Simulated night shift disrupts circadian rhythms of immune functions in humans. J Immunol (2016) 196(6):2466–75. doi: 10.4049/jimmunol.1502422
398. Mosure SA, Wilson AN, Solt LA. Targeting nuclear receptors for TH17-mediated inflammation: REV-ERBerations of circadian rhythm and metabolism. Immunometabolism (2022) 4(2):e220006. doi: 10.20900/immunometab20220006
399. Khalyfa A, Poroyko VA, Qiao Z, Gileles-Hillel A, Khalyfa AA, Akbarpour M, et al. Exosomes and metabolic function in mice exposed to alternating dark-light cycles mimicking night shift work schedules. Front Physiol (2017) 8:882. doi: 10.3389/fphys.2017.00882
400. Hand LE, Gray KJ, Dickson SH, Simpkins DA, Ray DW, Konkel JE, et al. Regulatory T cells confer a circadian signature on inflammatory arthritis. Nat Commun (2020) 11(1):1658. doi: 10.1038/s41467-020-15525-0
401. Bollinger T, Leutz A, Leliavski A, Skrum L, Kovac J, Bonacina L, et al. circadian clocks in mouse and human CD4+ T cells. PloS One (2011) 6(12):e29801. doi: 10.1371/journal.pone.0029801
402. Filipski E, King VM, Li X, Granda TG, Mormont MC, Liu X, et al. Host circadian clock as a control point in tumor progression. J Natl Cancer Inst (2002) 94(9):690–7. doi: 10.1093/jnci/94.9.690
403. Loef B, Nanlohy NM, Jacobi RHJ, van de Ven C, Mariman R, van der Beek AJ, et al. Immunological effects of shift work in healthcare workers. Sci Rep (2019) 9(1):18220. doi: 10.1038/s41598-019-54816-5
404. Cove-Smith JR, Kabler P, Pownall R, Knapp MS. Circadian variation in an immune response in man. Br Med J (1978) 2(6132):253–4. doi: 10.1136/bmj.2.6132.253
405. Poellman L, Poellmann B. Tagesrhythmische unterschiede bei der antikörperbildung nach hepatitis-B-Schutzimpfung [Circadian differences of antibody response to hepatitis b vaccination]. Gentner Verlag: Arbeitsmedizin im Gesundheitsdienst [Occupational medicine in medical service] Stuttgart (1988) p. 83–7.
406. Pownall R, Knapp MS. Circadian rhythmicity of delayed hypersensitivity to oxazolone in the rat. Clin Sci Mol Med (1978) 54(4):447–9. doi: 10.1042/cs0540447
407. Pownall R, Knapp MS. A circadian study of corticosteroid suppression of delayed hypersensitivity. Int J Immunopharmacol. (1979) 1(4):293–8. doi: 10.1016/0192-0561(79)90005-5
408. Fiorina P, Lattuada G, Silvestrini C, Ponari O, Dall’Aglio P. Disruption of nocturnal melatonin rhythm and immunological involvement in ischaemic stroke patients. Scand J Immunol (1999) 50(2):228–31. doi: 10.1046/j.1365-3083.1999.00579.x
409. Bedrosian TA, Fonken LK, Walton JC, Nelson RJ. Chronic exposure to dim light at night suppresses immune responses in Siberian hamsters. Biol Lett (2011) 7(3):468–71. doi: 10.1098/rsbl.2010.1108
410. Mizutani H, Tamagawa-Mineoka R, Minami Y, Yagita K, Katoh N. Constant light exposure impairs immune tolerance development in mice. J Dermatol Sci (2017) 86(1):63–70. doi: 10.1016/j.jdermsci.2016.12.016
411. Ruiz FS, Andersen ML, Guindalini C, Araujo LP, Lopes JD, Tufik S. Sleep influences the immune response and the rejection process alters sleep pattern: Evidence from a skin allograft model in mice. Brain Behav Immun (2017) 61:274–88. doi: 10.1016/j.bbi.2016.12.027
412. de Assis LVM, Kinker GS, Moraes MN, Markus RP, Fernandes PA, Castrucci AM de L. Expression of the circadian clock gene BMAL1 positively correlates with antitumor immunity and patient survival in metastatic melanoma. Front Oncol (2018) 8:185. doi: 10.3389/fonc.2018.00185
413. Silver AC, Arjona A, Walker WE, Fikrig E. The circadian clock controls toll-like receptor 9-mediated innate and adaptive immunity. Immunity (2012) 36(2):251–61. doi: 10.1016/j.immuni.2011.12.017
414. Born J, Lange T, Hansen K, Mölle M, Fehm HL. Effects of sleep and circadian rhythm on human circulating immune cells. J Immunol (1997) 158(9):4454–64. doi: 10.4049/jimmunol.158.9.4454
415. Downton P, Early JO, Gibbs JE. Circadian rhythms in adaptive immunity. Immunology (2020) 161(4):268–77. doi: 10.1111/imm.13167
416. Cajochen C, Weber J, Estrada AF, Kobayashi K, Gabel V. Circadian and homeostatic sleep-wake regulation of secretory immunoglobulin a (sIgA): Effects of environmental light and recovery sleep. Brain Behav Immun Health (2022) 19:100394. doi: 10.1016/j.bbih.2021.100394
417. Wada M, Orihara K, Kamagata M, Hama K, Sasaki H, Haraguchi A, et al. circadian clock-dependent increase in salivary IgA secretion modulated by sympathetic receptor activation in mice. Sci Rep (2017) 7(1):8802. doi: 10.1038/s41598-017-09438-0
418. Casale G, Marinoni GL, d’Angelo R, de Nicola P. Circadian rhythm of immunoglobulins in aged persons. Age Ageing (1983) 12(1):81–5. doi: 10.1093/ageing/12.1.81
419. Wang Q, Li L, Li C, Cao H, Chen Y, Zhou W, et al. circadian protein CLOCK modulates regulatory b cell functions of nurses engaging day-night shift rotation. Cell Signal (2022) 96:110362. doi: 10.1016/j.cellsig.2022.110362
420. Yang H, Yang LT, Liu J, Tang S, Zhao X, Wang Q, et al. circadian protein CLK suppresses transforming growth factor-β expression in peripheral b cells of nurses with day-night shift rotation. Am J Transl Res (2018) 10(12):4331–7.
421. Cao Q, Zhao X, Bai J, Gery S, Sun H, Lin DC, et al. circadian clock cryptochrome proteins regulate autoimmunity. Proc Natl Acad Sci U.S.A. (2017) 114(47):12548–53. doi: 10.1073/pnas.1619119114
422. Hemmers S, Rudensky AY. The cell-intrinsic circadian clock is dispensable for lymphocyte differentiation and function. Cell Rep (2015) 11(9):1339–49. doi: 10.1016/j.celrep.2015.04.058
423. Winter O, Dame C, Jundt F, Hiepe F. Pathogenic long-lived plasma cells and their survival niches in autoimmunity, malignancy, and allergy. J Immunol (2012) 189(11):5105–11. doi: 10.4049/jimmunol.1202317
424. Fetter T, Niebel D, Braegelmann C, Wenzel J. Skin-associated b cells in the pathogenesis of cutaneous autoimmune diseases-implications for therapeutic approaches. Cells (2020) 9(12). doi: 10.3390/cells9122627
425. Bosello S, Angelucci C, Lama G, Alivernini S, Proietti G, Tolusso B, et al. characterization of inflammatory cell infiltrate of scleroderma skin: B cells and skin score progression. Arthritis Res Ther (2018) 20(1):75. doi: 10.1186/s13075-018-1569-0
426. Elkon K, Casali P. Nature and functions of autoantibodies. Nat Rev Rheumatol (2008) 4(9):491–8. doi: 10.1038/ncprheum0895
427. Campbell SS, Murphy PJ. Extraocular circadian phototransduction in humans. Science (1998) 279(5349):396–9. doi: 10.1126/science.279.5349.396
428. Kawara S, Mydlarski R, Mamelak AJ, Freed I, Wang B, Watanabe H, et al. Low-dose ultraviolet b rays alter the mRNA expression of the circadian clock genes in cultured human keratinocytes. J Invest Dermatol (2002) 119(6):1220–3. doi: 10.1046/j.1523-1747.2002.19619.x
429. Xiang K, Xu Z, Hu YQ, He YS, Wu GC, Li TY, et al. circadian clock genes as promising therapeutic targets for autoimmune diseases. Autoimmun Rev (2021) 20(8):102866. doi: 10.1016/j.autrev.2021.102866
430. Reis ES, Lange T, Köhl G, Herrmann A, Tschulakow AV, Naujoks J, et al. Sleep and circadian rhythm regulate circulating complement factors and immunoregulatory properties of C5a. Brain Behav Immun (2011) 25(7):1416–26. doi: 10.1016/j.bbi.2011.04.011
431. Dimitrov S, Besedovsky L, Born J, Lange T. Differential acute effects of sleep on spontaneous and stimulated production of tumor necrosis factor in men. Brain Behav Immun (2015) 47:201–10. doi: 10.1016/j.bbi.2014.11.017
432. Kräuchi K, Cajochen C, Pache M, Flammer J, Wirz-Justice A. Thermoregulatory effects of melatonin in relation to sleepiness. Chronobiol Int (2006) 23(1–2):475–84. doi: 10.1080/07420520500545854
433. Arck PC, Slominski A, Theoharides TC, Peters EMJ, Paus R. Neuroimmunology of stress: skin takes center stage. J Invest Dermatol (2006) 126(8):1697–704. doi: 10.1038/sj.jid.5700104
434. Fischer TW, Slominski A, Zmijewski MA, Reiter RJ, Paus R. Melatonin as a major skin protectant: from free radical scavenging to DNA damage repair. Exp Dermatol (2008) 17(9):713–30. doi: 10.1111/j.1600-0625.2008.00767.x
435. Kalsbeek A, Yi CX, Cailotto C, la Fleur SE, Fliers E, Buijs RM. Mammalian clock output mechanisms. Essays Biochem (2011) 49(1):137–51.
436. Sollars PJ, Weiser MJ, Kudwa AE, Bramley JR, Ogilvie MD, Spencer RL, et al. Altered entrainment to the day/night cycle attenuates the daily rise in circulating corticosterone in the mouse. PloS One (2014) 9(11):e111944. doi: 10.1371/journal.pone.0111944
437. Logan RW, Arjona A, Sarkar DK. Role of sympathetic nervous system in the entrainment of circadian natural-killer cell function. Brain Behav Immun (2011) 25(1):101–9. doi: 10.1016/j.bbi.2010.08.007
438. Wang C, Lutes LK, Barnoud C, Scheiermann C. The circadian immune system. Sci Immunol (2022) 7(72):eabm2465. doi: 10.1126/sciimmunol.abm2465
439. Maestroni GJ, Cosentino M, Marino F, Togni M, Conti A, Lecchini S, et al. Neural and endogenous catecholamines in the bone marrow. circadian association of norepinephrine with hematopoiesis? Exp Hematol (1998) 26(12):1172–7.
440. Claustrat B, Leston J. Melatonin: Physiological effects in humans. Neurochirurgie (2015) 61(2–3):77–84. doi: 10.1016/j.neuchi.2015.03.002
441. Tosini G, Owino S, Guillaume JL, Jockers R. Understanding melatonin receptor pharmacology: latest insights from mouse models, and their relevance to human disease. Bioessays (2014) 36(8):778–87. doi: 10.1002/bies.201400017
442. Jin X, von Gall C, Pieschl RL, Gribkoff VK, Stehle JH, Reppert SM, et al. Targeted disruption of the mouse Mel(1b) melatonin receptor. Mol Cell Biol (2003) 23(3):1054–60. doi: 10.1128/MCB.23.3.1054-1060.2003
443. Zhang Z, Silveyra E, Jin N, Ribelayga CP. A congenic line of the C57BL/6J mouse strain that is proficient in melatonin synthesis. J Pineal Res (2018) 65(3):e12509. doi: 10.1111/jpi.12509
444. Thoreau B, Chaigne B, Renaud A, Mouthon L. Pathophysiology of systemic sclerosis. Presse Med (2021) 50(1):104087. doi: 10.1016/j.lpm.2021.104087
445. Buske-Kirschbaum A, Ebrecht M, Kern S, Hellhammer DH. Endocrine stress responses in TH1-mediated chronic inflammatory skin disease (psoriasis vulgaris)–do they parallel stress-induced endocrine changes in TH2-mediated inflammatory dermatoses (atopic dermatitis)? Psychoneuroendocrinology (2006) 31(4):439–46. doi: 10.1016/j.psyneuen.2005.10.006
446. Dimitrov S, Lange T, Born J. Selective mobilization of cytotoxic leukocytes by epinephrine. J Immunol (2010) 184(1):503–11. doi: 10.4049/jimmunol.0902189
447. Abel EA, DiCicco LM, Orenberg EK, Fraki JE, Farber EM. Drugs in exacerbation of psoriasis. J Am Acad Dermatol (1986) 15(5 Pt 1):1007–22. doi: 10.1016/S0190-9622(86)70265-X
448. Nakamura Y, Ishimaru K, Shibata S, Nakao A. Regulation of plasma histamine levels by the mast cell clock and its modulation by stress. Sci Rep (2017) 7:39934. doi: 10.1038/srep39934
449. Zielinski MR, Systrom DM, Rose NR. Fatigue, sleep, and autoimmune and related disorders. Front Immunol (2019) 10:1827. doi: 10.3389/fimmu.2019.01827
450. Venier A, De Simone C, Forni L, Ghirlanda G, Uccioli L, Serri F, et al. Treatment of severe psoriasis with somatostatin: four years of experience. Arch Dermatol Res (1988) 280 Suppl:S51–54.
451. Sutaria N, Adawi W, Goldberg R, Roh YS, Choi J, Kwatra SG. Itch: Pathogenesis and treatment. J Am Acad Dermatol (2022) 86(1):17–34. doi: 10.1016/j.jaad.2021.07.078
452. Ikoma A, Steinhoff M, Ständer S, Yosipovitch G, Schmelz M. The neurobiology of itch. Nat Rev Neurosci (2006) 7(7):535–47. doi: 10.1038/nrn1950
453. Georgala S, Schulpis KH, Papaconstantinou ED, Stratigos J. Raised β-endorphin serum levels in children with atopic dermatitis and pruritus. J Dermatol Sci (1994) 8(2):125–8. doi: 10.1016/0923-1811(94)90006-X
454. Bates ME, Clayton M, Calhoun W, Jarjour N, Schrader L, Geiger K, et al. Relationship of plasma epinephrine and circulating eosinophils to nocturnal asthma. Am J Respir Crit Care Med (1994) 149(3 Pt 1):667–72. doi: 10.1164/ajrccm.149.3.8118634
455. Petraglia F, Facchinetti F, Parrini D, Micieli G, Luca SD, Genazzani AR. Simultaneous circadian variations of plasma ACTH, beta-lipotropin, beta-endorphin and cortisol. HRM (1983) 17(3):147–52.
456. Segal JP, Tresidder KA, Bhatt C, Gilron I, Ghasemlou N. Circadian control of pain and neuroinflammation. J Neurosci Res (2018) 96(6):1002–20. doi: 10.1002/jnr.24150
457. Straub RH, Cutolo M. Circadian rhythms in rheumatoid arthritis: Implications for pathophysiology and therapeutic management. Arthritis Rheumatism. (2007) 56(2):399–408. doi: 10.1002/art.22368
458. Zak-Nejmark T, Nowak IA, Kraus-Filarska M. Circadian variations of histamine binding to lymphocytes and neutrophils and skin reactivity to histamine in atopic and healthy subjects. Arch Immunol Ther Exp (Warsz) (2006) 54(4):283–7. doi: 10.1007/s00005-006-0032-0
459. Iranmanesh A, Lizarralde G, Johnson ML, Veldhuis JD. Circadian, ultradian, and episodic release of beta-endorphin in men, and its temporal coupling with cortisol. J Clin Endocrinol Metab (1989) 68(6):1019–26. doi: 10.1210/jcem-68-6-1019
460. McLain RF, Kapural L, Mekhail NA. Epidural steroid therapy for back and leg pain: mechanisms of action and efficacy. Spine J (2005) 5(2):191–201. doi: 10.1016/j.spinee.2004.10.046
461. Luengas-Martinez A, Paus R, Iqbal M, Bailey L, Ray DW, Young HS. Circadian rhythms in psoriasis and the potential of chronotherapy in psoriasis management. Exp Dermatol (2022). doi: 10.1111/exd.14649
462. Moulton VR. Sex hormones in acquired immunity and autoimmune disease. Front Immunol (2018). doi: 10.3389/fimmu.2018.02279
463. Moe KE. Reproductive hormones, aging, and sleep. Semin Reprod Med (1999) 17(4):339–48. doi: 10.1055/s-2007-1016243
464. Samanta S. Melatonin: A potential antineoplastic agent in breast cancer. JEP(T) (2022) 41(4). https://www.dl.begellhouse.com/journals/0ff459a57a4c08d0,6b57eefe5f7fdc1a,3ef3eeb570d21560.html.
465. Knauth P, Hornberger S. Preventive and compensatory measures for shift workers. Occup Med (Lond) (2003) 53(2):109–16. doi: 10.1093/occmed/kqg049
466. d’Ettorre G, Pellicani V. Preventing shift work disorder in shift health-care workers. Saf Health Work (2020) 11(2):244–7. doi: 10.1016/j.shaw.2020.03.007
467. Vetter C, Fischer D, Matera JL, Roenneberg T. Aligning work and circadian time in shift workers improves sleep and reduces circadian disruption. Curr Biol (2015) 25(7):907–11. doi: 10.1016/j.cub.2015.01.064
468. Santhi N, Duffy JF, Horowitz TS, Czeisler CA. Scheduling of sleep/darkness affects the circadian phase of night shift workers. Neurosci Lett (2005) 384(3):316–20. doi: 10.1016/j.neulet.2005.04.094
469. Sadeghniiat-Haghighi K, Aminian O, Pouryaghoub G, Yazdi Z. Efficacy and hypnotic effects of melatonin in shift-work nurses: double-blind, placebo-controlled crossover trial. J Circadian Rhythms (2008) 6:10. doi: 10.1186/1740-3391-6-10
470. Carriedo-Diez B, Tosoratto-Venturi JL, Cantón-Manzano C, Wanden-Berghe C, Sanz-Valero J. The effects of the exogenous melatonin on shift work sleep disorder in health personnel: A systematic review. Int J Environ Res Public Health (2022) 19(16):10199. doi: 10.3390/ijerph191610199
471. Nogueira LFR, Pellegrino P, Cipolla-Neto J, Moreno CRC, Marqueze EC. Timing and composition of last meal before bedtime affect sleep parameters of night workers. Clocks Sleep (2021) 3(4):536–46. doi: 10.3390/clockssleep3040038
472. Liira J, Verbeek J, Ruotsalainen J. Pharmacological interventions for sleepiness and sleep disturbances caused by shift work. JAMA (2015) 313(9):961–2. doi: 10.1001/jama.2014.18422
473. Golding H, Ritonja JA, Day AG, Aronson KJ, Tranmer J. Modeling the relationship between shift work and cardiometabolic risk through circadian disruption, sleep and stress pathways. Chronobiol Int (2022) 39(5):704–13. doi: 10.1080/07420528.2022.2032124
474. Powell ND, Sloan EK, Bailey MT, Arevalo JMG, Miller GE, Chen E, et al. Social stress up-regulates inflammatory gene expression in the leukocyte transcriptome via β-adrenergic induction of myelopoiesis. Proc Natl Acad Sci U.S.A. (2013) 110(41):16574–9. doi: 10.1073/pnas.1310655110
475. Mascaro JS, Wallace A, Hyman B, Haack C, Hill CC, Moore MA, et al. Flourishing in healthcare trainees: Psychological well-being and the conserved transcriptional response to adversity. Int J Environ Res Public Health (2022) 19(4):2255. doi: 10.3390/ijerph19042255
476. Redeker NS, Caruso CC, Hashmi SD, Mullington JM, Grandner M, Morgenthaler TI. Workplace interventions to promote sleep health and an alert, healthy workforce. J Clin Sleep Med (2019) 15(4):649–57. doi: 10.5664/jcsm.7734
Keywords: shift work, skin, sleep, circadian, autoimmune, cortisol, melatonin, inflammation
Citation: Stenger S, Grasshoff H, Hundt JE and Lange T (2023) Potential effects of shift work on skin autoimmune diseases. Front. Immunol. 13:1000951. doi: 10.3389/fimmu.2022.1000951
Received: 22 July 2022; Accepted: 29 November 2022;
Published: 14 February 2023.
Edited by:
Marco Emilio Bianchi, Vita-Salute San Raffaele University, ItalyReviewed by:
José Jiram Torres-Ruiz, Instituto Nacional de Ciencias Médicas y Nutrición Salvador Zubirán (INCMNSZ), MexicoCopyright © 2023 Stenger, Grasshoff, Hundt and Lange. This is an open-access article distributed under the terms of the Creative Commons Attribution License (CC BY). The use, distribution or reproduction in other forums is permitted, provided the original author(s) and the copyright owner(s) are credited and that the original publication in this journal is cited, in accordance with accepted academic practice. No use, distribution or reproduction is permitted which does not comply with these terms.
*Correspondence: Sarah Stenger, c2FyYWguc3RlbmdlckBzdHVkZW50LnVuaS1sdWViZWNrLmRl; Tanja Lange, VGFuamEubGFuZ2VAdWtzaC5kZQ==
†ORCID: Sarah Stenger, orcid.org/0000-0003-3860-622X
Hanna Grasshoff, orcid.org/0000-0003-1395-3268
Jennifer E. Hundt, orcid.org/0000-0002-9644-556X
Tanja Lange, orcid.org/000-0001-8309-2112
Disclaimer: All claims expressed in this article are solely those of the authors and do not necessarily represent those of their affiliated organizations, or those of the publisher, the editors and the reviewers. Any product that may be evaluated in this article or claim that may be made by its manufacturer is not guaranteed or endorsed by the publisher.
Research integrity at Frontiers
Learn more about the work of our research integrity team to safeguard the quality of each article we publish.