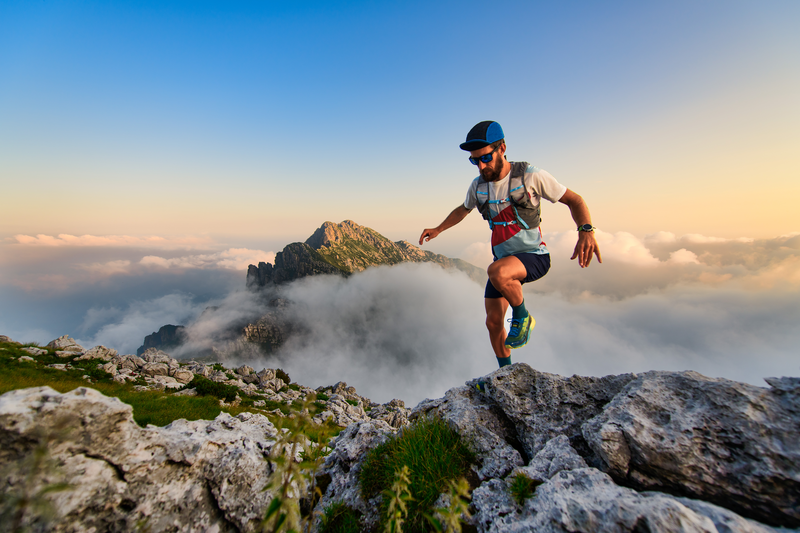
94% of researchers rate our articles as excellent or good
Learn more about the work of our research integrity team to safeguard the quality of each article we publish.
Find out more
ORIGINAL RESEARCH article
Front. Immunol. , 06 October 2022
Sec. Inflammation
Volume 13 - 2022 | https://doi.org/10.3389/fimmu.2022.1000491
Peritoneal adhesions commonly occur after abdominal or pelvic surgery. These scars join internal organs to each other or to the cavity wall and can present with abdominal or pelvic pain, and bowel obstruction or female infertility. The mechanisms underlying adhesion formation remain unclear and thus, effective treatments are not forthcoming. Peritoneal macrophages accumulate after surgery and previous studies have attributed either pro- or anti-scarring properties to these cells. We propose that there are complex and nuanced responses after surgery with respect to both resident and also monocyte-derived peritoneal macrophage subpopulations. Moreover, we contend that differences in responses of specific macrophage subpopulations in part explain the risk of developing peritoneal scars. We characterized alterations in peritoneal macrophage subpopulations after surgery-induced injury using two strains of mice, BALB/c and C57BL/6, with known differences in macrophage response post-infection. At 14 days post-surgery, BALB/c mice displayed more adhesions compared with C57BL/6 mice. This increase in scarring correlated with a lower influx of monocyte-derived macrophages at day 3 post-surgery. Moreover, BALB/c mice showed distinct macrophage repopulation dynamics after surgery. To confirm a role for monocyte-derived macrophages, we used Ccr2-deficient mice as well as antibody-mediated depletion of CCR2 expressing cells during initial stages of adhesion formation. Both Ccr2-deficient and CCR2-depleted mice showed a significant increase in adhesion formation associated with the loss of peritoneal monocyte influx. These findings revealed an important protective role for monocyte-derived cells in reducing adhesion formation after surgery.
Peritoneal adhesions commonly occur after abdominal or pelvic surgery and abnormally conjoin internal organs to each other or to the adjacent cavity wall. Adhesions may cause a range of complications, including severe chronic pelvic pain, female infertility, intestinal obstruction, and are a contraindication for future abdominal surgery (1). The peritoneum lines the cavity wall and majority of internal organs, and consists of a surface monolayer of mesothelial cells over a layer of submesothelial connective tissue (2). During surgery, this gliding interface is disrupted and adhesions may develop from fibrin-rich clots that span between injured tissues (3). Several key events, including reduced fibrinolysis (4) and mesothelial-to-mesenchymal transition (MMT) (5, 6), have been shown to be pivotal in adhesion formation. However, cellular and molecular pathways underlying their development are not well defined. Consequently, attempts to prevent adhesions occurring, such as atraumatic surgical technique and use of barrier material, show mixed success and hence are not consistently applied (1). An improved understanding of surgical adhesion formation will lead to better prevention strategies.
Peritoneal macrophages, although known to provide immune surveillance of the abdominal cavity (7), are also involved in the formation of post-surgical adhesions (8–10). Critically, however, there is a lack of consensus as to their exact contribution, with studies attributing both protective as well as pathological roles to macrophages. Experimental expansion of peritoneal macrophages has been shown to have protective effects (9, 11), while somewhat in contradiction, depletion of peritoneal macrophages also reduces adhesion formation (12). The peritoneal cavity contains at least three macrophage subpopulations: a long-lived, self-renewing resident population (F4/80high) also known as large peritoneal macrophages, a population derived from monocytes recruited from the blood (F4/80low) (13) also known as small peritoneal macrophages, and an intermediate population (F4/80int), which consists of monocyte-derived macrophages in the process of differentiating into a cavity resident phenotype (14). Functional differences between these subsets (14–16) and time of analysis may explain the contradictory outcome of studies that have manipulated macrophage numbers. However, which specific subsets are responsible for protective or pathologic properties remains unclear.
Notably, fundamental differences exist between common laboratory mouse strains, BALB/c and C57BL/6, in terms of the response of serous cavity macrophages to infection (17, 18). Furthermore, BALB/c mice have been found to be more susceptible to peritoneal adhesion formation compared with C57BL/6 mice (19). Thus, we hypothesized that the difference in propensity to form adhesions is related to the different profiles of peritoneal cavity macrophages in the different mouse strains. Here, we investigated the dynamics of peritoneal macrophage subsets in BALB/c and C57BL/6 mice after performing surgery to induce adhesions. We found that BALB/c mice were more susceptible to mature peritoneal adhesion formation compared with C57BL/6 mice, but this difference was not apparent until day 14 post-surgery. Surprisingly, adhesion susceptibility in BALB/c mice was associated with a reduced influx of monocyte-derived cells at day 3 after surgery compared with C57BL/6 mice. To confirm a protective role for monocyte-derived macrophages, we analyzed surgical adhesion formation in Ccr2-deficient mice as well as in monocyte-depleted C57BL/6 mice. These findings revealed an important protective role for monocyte-derived macrophages in reducing mature adhesion formation after surgery.
Female BALB/c and C57BL/6 mice, 10 to 12 weeks of age, and approximate weight of 20 g, were purchased from a commercial vendor (Envigo, Hillcrest, UK). The Ccr2-knock out (KO) colony [originally from The Jackson Laboratory, USA (20)] was backcrossed to a C57BL/6 background for at least 10 generations, bred in-house and shared by Dr. John Grainger (The University of Manchester, UK). Ten-to twelve-week-old male and female KO mice and their wild-type (WT) littermates were used. All animals were maintained in individually ventilated cages under specific-pathogen-free conditions in the Biological Services Facilities (BSF) of the University of Manchester and provided with food and water ad libitum. Animal experiments were performed according to the UK Animals (Scientific Procedures) Act (1986) under a Project License (P1208AD89) granted by the UK Home Office and approved by the University of Manchester Ethical Review Committee.
Abdominal surgery was performed following a previously described protocol with slight modification (21, 22). Briefly, mice were anesthetized with a mixture of inhaled isoflurane and oxygen, received s.c. injection of buprenorphine analgesia (50-100 μl/kg), were shaved and the surgery site cleaned with sterile wipes. All surgical procedures were performed in a dedicated surgical theatre. A midline incision was made through the abdominal wall and peritoneum. Circular wounding, approximately 7 mm2 of the peritoneal cavity wall (approximately 1 cm caudal to the xiphoid cartilage and 1 cm from the midline incision) was created using a trauma instrument developed by Dr. M. Eastwood (Department of Biomedical Sciences, University of Westminster, London, UK). The instrument comprised a clamping device that allowed the trauma to be size- and site-specific, and an abrading rod with collar that restricted the depth of insertion and hence pressure applied (Supplementary Figure 1). The inserted rod was rotated three times to produce a consistent lesion on the peritoneal cavity wall. Thereafter, approximately 1 cm length of caecum serosa was abraded with a No 15 surgical blade thirty times and then closely opposed to the wounded peritoneal wall by placing two simple interrupted sutures (8-0 Polyamide, non-absorbable, ETHILON W8170, Ethicon, New Jersey, US) horizontally 3-5 mm either side from the peritoneal wound. Linea alba and skin were closed separately using 6-0 non-absorbable monofilament polyamide sutures (ETHICON W1600T, Ethicon, New Jersey, US). A graphical illustration of the experimental approach and a photo of the trauma instrument are provided in Supplementary Figure 1. The mice were necropsied by CO2 asphyxiation in a rising concentration at day 3, day 7, and day 14 post-surgery.
To assess the importance of monocyte-derived peritoneal macrophages during surgery-induced peritoneal adhesion formation, female C57BL/6 mice were treated with 20 μg of α-CCR2 mAb (clone: MC21, RRID : AB_2314128 (23); or isotype control antibody (Rat IgG2b, Biolegend) in 200 μl of PBS via daily intra-peritoneal (i.p.) injection at day -1, 0, and day 1 post-surgery.
Collection of peritoneal lavage fluid and peritoneal exudate cells (PECs) was performed by flushing the peritoneal cavity with 3 ml ice cold RPMI 1640 media (Sigma-Aldrich, Dorset, UK) containing 1% Penicillin/Streptomycin (Sigma-Aldrich, Dorset, UK) 3 times. The first 3 ml of the wash was centrifuged, and the supernatant used for the detection of soluble mediators using ELISA. The cell pellet left was mixed with the remaining washes and PECs collected by centrifugation for flow cytometry analysis. To remove erythrocytes, cells were incubated with red blood cell lysis buffer (Sigma-Aldrich) following the manufacturer’s instructions. Cell number was determined using a Countess II FL automated cell counter (Invitrogen). Adhesion profile was assessed by two independent observers to determine the total number of adhesions within the peritoneal cavity after surgery and also an arbitrary adhesion score. The adhesion score was measured based on the type of adhesion (Supplementary Table 1). The more distal the adhesion from the injury site, the higher the adhesion score. The observers were blinded to the treatment groups during the adhesion score assessment but not to the strain of mouse. Adhesion tissue was collected and fixed in 4% buffered paraformaldehyde overnight and then stored in 70% EtOH before being processed and embedded in paraffin wax for histology analysis.
An aliquot (1x106) of total PECs were stained using a viability assay (Zombie UV, Biolegend) and blocked with 5 μg/mL anti-CD16/32 (clone 93; Biolegend) and heat inactivated normal mouse serum (1:10, Sigma-Aldrich) in flow cytometry buffer (0.5% BSA and 2 mM EDTA in Dulbecco’s PBS) before surface staining on ice with antibodies listed in Supplementary Tables 2, 3. For intracellular staining, samples were fixed and permeabilized using eBioscience Foxp3 Staining Buffer kit (Fisher Scientific) following the manufacturer’s instructions before adding antibodies in 1x eBioscience™ Permeabilization Buffer (Thermo Fisher Scientific). All antibodies were purchased from Biolegend unless stated otherwise. PECs were analyzed on a BD FACSymphony machine using BD FACSDiva software (BD Biosciences) and post-acquisition analysis performed using FlowJo v10 software (BD Biosciences). The number of cells per cavity for each population (e.g. F4/80high resident macrophages) was calculated by determining the percentage of single live cells for each population using FlowJo multiplied by the total number of PECs divided by 100. The gating strategy for flow cytometry analysis is shown in Supplementary Figures 2, 3.
Serial sections (5-μm-thick) of peritoneal adhesion tissue were collected, de-waxed and rehydrated using serial dilutions of alcohol prior to Masson’s trichrome staining following manufacturer’s instruction (Sigma-Aldrich). Images were acquired using a [20×/0.80 Plan Apo] objective using the Pannoramic 250 Flash II slide scanner (3D Histech Ltd., Hungary). Collagen profile was analyzed by quantifying the selected site-specific adhesion area (Supplementary Figure 4) in µm2 using RGB thresholding by QuantCentre and HistoQuant plugin on SlideViewer software (version 2.5).
Interleukin (IL)-6, IL-10, IL-12, IL-13, TNF-α, IFN-γ, Relmα and Ym1 in peritoneal lavage fluid were detected by enzyme linked immunosorbant assay (ELISA). Ninety-six-well immunoGrade plates (BrandTech Scientific, Inc) were coated with primary antibodies diluted in appropriate buffer at 4°C overnight. After washing and non-specific binding blocking, the wash supernatant and standard were added, and the samples were incubated at 4°C overnight. Plates were washed and biotinylated detection antibody was added before the plates were incubated with Streptavidin peroxidase (1:1,000, Sigma) for 60 min. Plates were washed and then the TMB substrate solution (Biolegend) was added. After adding stop solution, plates were read at 450 nm on a VersaMax Microplate reader (Molecular Devices). List of coating and detection antibodies is shown in Supplementary Table 4.
Statistical analysis was performed using Prism 9 (Graph-Pad software Inc., La Jolla, CA). Data were tested for normal distribution using a Shapiro-Wilk test prior to analysis of significant differences between groups. Data deemed to follow Gaussian distribution were subsequently analyzed using t-test for analysis of two groups, or ANOVA followed by Tukey or Sidak posthoc test for experiments with more than 2 groups. Data with non-Gaussian distribution were analyzed using Mann Whitney test (2 groups) or Kruskal-Wallis ANOVA followed by Dunn’s multiple comparison test for experiments with more than 2 groups. Differences with a p-value of less than 0.05 were considered statistically significant.
To determine whether BALB/c and C57BL/6 mice showed differences in susceptibility to peritoneal adhesions and if this related to variations in macrophage subset responses, mice of each strain were subjected to surgical injury. Three, 7 and 14 days after surgery, animals were analyzed for adhesion formation between the injured sites on the caecum and the peritoneal cavity wall (site-specific) as well as to other organs or the midline incision (non-site-specific) (see scoring system, Supplementary Table 1). Similar to previously published data (19), we found that BALB/c mice were more likely to develop an increased number of peritoneal adhesions as well as involving other organs compared with C57BL/6 mice. However, in our model this enhanced susceptibility did not become apparent until day 14 after surgery (Figures 1A, B). Collagen profile in site-specific adhesion tissue, as assessed by Masson’s trichrome staining, was similar between the two strains at day 3 and 7 post-surgery (Supplementary Figure 5). However, BALB/c mice showed significantly higher collagen deposition in the site-specific adhesion tissue at day 14 (Figures 1C, D).
Figure 1 BALB/c mice showed more peritoneal adhesions than C57BL/6 mice at day 14 post-surgery (p.s.), and fewer monocytes and macrophages at day 3. Number of adhesions after surgery at different time points (A) and adhesion score at day 14 post-surgery (B). Representative image and corresponding histological appearance (Masson’s trichrome staining) of adhesions between mouse strains at day 14 after surgery (C). Collagen analysis in adhesion histological sections at day 14 post-surgery (D). Total cell number of total peritoneal cavity macrophages (E), peritoneal resident macrophages (F), monocytes (G), peritoneal F4/80low macrophages (H) and peritoneal F4/80int intermediate macrophages (I). Data shows mean ± SEM, pooled from 6 separate experiments for naïve or 2 separate experiments for day 3, 7, and 14 post-surgery., n= 6-20 mice/group; * P <0.05, ** P <0.01, *** P <0.001, **** P <0.0001. (A) ANOVA with Sidak’s multiple comparison test; (B, C) Mann Whitney test and (E–I) ANOVA with Tukey’s multiple comparison test. Scale bar: 40μm.
To assess whether this difference in adhesion formation was associated with variation in macrophage profiles, we analyzed PECs by flow cytometry using panels (Supplementary Tables 2, 3) that allow us to characterize different subsets of peritoneal macrophages. Although there was no significant difference in the total number of PECs between BALB/c and C57BL/6 prior to and after surgery (Supplementary Figure 6), BALB/c mice showed significantly lower number of total peritoneal macrophages (CD11b+Lin-Ly6C-) under steady state conditions as well as on day 3 post-surgery (Figure 1E). This difference was largely due to reduced numbers of cavity resident macrophages (F4/80high), which however reached similar numbers in both strains from day 7 (Figure 1F). Moreover, although both strains of mice showed recruitment of monocytes (CD11b+Lin-MHCII-F4/80-Ly6Chigh) from day 3 post-surgery with the number staying elevated up to day 14 post-surgery, the early influx of monocytes (day 3 post-surgery) was significantly more pronounced in C57BL/6 compared with BALB/c mice (Figure 1G). Infiltrating monocytes have been shown to differentiate into mature macrophages (F4/80low) within the cavity (24). Moreover, recruited monocytes can also give rise to resident macrophages (F4/80high) via an intermediate population (F4/80int) (14). In line with the enhanced early influx of monocytes, C57BL/6 mice also showed an enhanced total cell number of F4/80low (Figure 1H) and F4/80int macrophages (Figure 1I) at day 3 post-surgery. In summary, all peritoneal macrophage subsets (F4/80low, F4/80int, F4/80high) were significantly less abundant in BALB/c mice compared with C57BL/6, particularly at day 3 post-surgery and this was associated with enhanced adhesions with increased collagen deposition in BALB/c mice at day 14 after surgery.
Changes in macrophage dynamics, in particular the loss of F4/80high cavity resident macrophages after surgery, has previously been reported with C57BL/6 mice (25). However, specific differences between BALB/c and C57BL/6 mice have not been investigated; hence we performed a more in-depth characterization of the dynamic changes in peritoneal macrophage subsets following surgery in the two strains. CD102, and the transcription factor Gata6 are known as defining markers of F4/80high cavity resident macrophages, upregulated at different stages of the differentiation process (26). Both markers were expressed at similar levels by F4/80high macrophages present in both BALB/c and C57BL/6 mice (Figures 2A, B), indicating that although surgery induced considerable changes in the number of these cells with reduced number in BALB/c at day 3 post-surgery (Figure 1F), they retained their peritoneal cavity resident identity. However, at day 3 after surgery F4/80high macrophages of both strains showed a dramatic loss in the proportion of cells expressing Tim4, another defining marker of F4/80high cavity resident macrophages (Figure 2C). Of note, F4/80high macrophages in BALB/c mice regained Tim4 expression over the course of the study with expression at day 14 post-surgery similar to naïve mice (Figure 2C). In contrast, this recovery of Tim4+ F4/80high cells did not occur in C57BL/6 mice (Figure 2C). Expression of Tim4 by F4/80high macrophages has been implicated as a marker of an embryonic origin i.e. established resident peritoneal population, whereas monocyte-derived resident macrophages largely fail to express Tim4 (14). Thus, the persistent loss of Tim4 expression in F4/80high cavity macrophages of C57BL/6 mice might indicate an enhanced integration of monocyte-derived cells into the resident macrophage pool.
Figure 2 Peritoneal macrophage markers and cytokine profiles post-surgery in BALB/c mice were altered compared with C57BL/6 mice. Relative % of CD102+ (A), Gata6+ (B), and Tim4+ (C) of F4/80high population. Relative % and total cell number of CD226+ F4/80low (D, E, respectively). Relative % of Sema4a+ (F) and Lyve1+ (G) of F4/80low macrophage population. Total cell number of Sca1+ (H), Relmα+ (I), and Ym1+ (J) in myeloid cells (CD11b+lin-Ly6C- cells) by flow cytometry. Peritoneal lavage levels for Relmα (K) and Ym1 (L) by ELISA. Data shows mean ± SEM, pooled from 6 separate experiments for naïve or 2 separate experiments for day 3, 7, and 14 p.s., n= 6-20 mice/group; * P <0.05, ** P <0.01, **** P< 0.0001, ANOVA with Tukey’s multiple comparison test.
Abdominal surgery in C57BL/6 mice has previously been shown to induce the macrophage disappearance reaction of tissue resident F4/80high macrophages (27). We also observed reduced numbers of F4/80high macrophages in the peritoneal cavity 3 days after surgery in both C57BL/6 as well as BALB/c mice (Figure 1F). In addition, CD226 (aka DNAM-1) is highly expressed by F4/80low macrophages present in the peritoneal cavity under steady state conditions (28) and these homeostatic CD226+ cells are displaced from the peritoneal cavity under inflammatory conditions (29) similar to the disappearance reaction described for F4/80high resident macrophages (30). In line with this, the proportion of CD226+ cells within F4/80low macrophages as well as the absolute number were reduced at day 3 after surgery in C57BL/6 and BALB/c mice compared with naïve animals (Figures 2D, E). Of note, C57BL/6 mice harbored significantly more CD226+F4/80low as well as F4/80high macrophages at day 3 after surgery as compared with BALB/c mice (Figures 2E, 1F). These data suggest that both mouse strains undergo the macrophage disappearance reaction but differences exist in the re-population dynamics. Semaphorin 4a (Sema4a) is expressed by circulating inflammatory monocytes and is upregulated on peritoneal macrophages during inflammation (31). Furthermore, it is retained during resolution of inflammation on F4/80int and F4/80high cells of monocytic origin (14). In line with a lower monocyte-derived macrophage influx on day 3 post-surgery, BALB/c mice showed fewer Sema4a+F4/80low macrophages, whereas Sema4a expression was maintained on F4/80low macrophages in C57BL/6 mice (Figure 2F). Similarly, Lymphatic Vessel Endothelial Receptor-1 (Lyve-1) expression has been associated with differentiation of monocyte-derived cells to F4/80int macrophages (18). Interestingly, we found a significant decrease in Lyve-1 expression by F4/80low macrophages in C57BL/6 mice compared with BALB/c mice on day 14 post-surgery (Figure 2G), but we found no difference in the proportion of F4/80int or F4/80high macrophages expressing Lyve-1 between the strains (Supplementary Figure 7). This may indicate that following surgery, there is an enhanced progression of monocyte-derived cells to F4/80int cells in C57BL/6 but not BALB/c mice. Of note, we found that none of the markers previously associated with specific macrophage subsets (e.g. CD102, Tim4, Lyve-1) were exclusively expressed by only one peritoneal macrophage subset (F4/80low, F4/80int, F4/80high) but were shared between several subsets (Supplementary Figure 8). This indicates a certain degree of phenotypic plasticity between the described macrophage subsets rather than exclusive expression patterns.
With regard to cellular activation, we did not detect a significant induction of either M1 (Sca1, TNFα) or M2 (Relmα, Ym1, CD206) activation markers in response to surgery in either BALB/c or C57BL/6 mice (Figures 2G–I; Supplementary Figure 7). However, BALB/c mice showed a significantly lower number of Sca-1+ myeloid cells (CD11b+lin-Ly6C- cells) prior and at day 3 post-surgery (Figure 2H), as well as a lower number of Relmα+ cells at day 3 post-surgery (Figure 2I) and lower numbers of Ym1+ cells at day 14 after surgery compared with C57BL/6 (Figure 2J). We also analyzed cytokine levels in the peritoneal lavage of BALB/c and C57BL/6 mice prior and after surgery (Figures 2K, L; Supplementary Figure 9). Interestingly, the level of Relmα in the peritoneal lavage of BALB/c mice was significantly lower compared with C57BL/6 at day 7 post-surgery (Figure 2K), a slight delay compared with the Relmα+ cell reduction found at day 3 post-surgery with flow cytometry (Figure 2I). Similar to the flow cytometry data (Figure 2J), a significantly lower concentration of Ym-1 in the peritoneal lavage of BALB/c mice was detectable at day 14 post-surgery compared with C57BL/6 (Figure 2L). The remaining cytokines did not show a significant difference in levels between BALB/c and C57BL/6 mice prior and post-surgery (Supplementary Figure 9). These data indicate that in addition to a reduced early influx of monocyte-derived macrophages in BALB/c mice, these cells also failed to fully integrate into the resident macrophage pool during the later resolution phase of repair following surgery.
Our findings indicated that BALB/c mice were more likely to have increased peritoneal adhesions at day 14 after surgery, compared with C57BL/6 mice, potentially due to reduced monocyte infiltration early following surgery. Therefore, adhesion-inducing surgery was performed in Ccr2- deficient mice (Ccr2KO on a C57BL/6 background). Due to the targeted disruption of the Ccr2 gene, Ccr2KO mice fail to recruit monocytes to sites of inflammation/injury, in part because of defective egress from the bone marrow (32). As expected, following surgery, Ccr2KO mice showed a significantly higher number of peritoneal adhesions as well as adhesion score at day 7 compared with WT littermates (Figures 3A–C). Ccr2KO mice also showed a significantly higher relative percentage of F4/80high macrophages after surgery (Figure 3D), but there was no significant difference in the total number of F4/80high resident macrophages between Ccr2KO and WT littermates prior and post-surgery (Figure 3E). Thus, it seems unlikely that the difference in adhesion formation was due to changes in the dynamics of F4/80high resident macrophages. In contrast, and in line with reduced monocyte infiltration, the relative percentages and total numbers of infiltrating monocytes (Figures 3F, G), F4/80low monocyte-derived macrophages (Figures 3H, I), and F4/80int converting cells (Figures 3J, K) were significantly lower in Ccr2KO mice compared with WT littermates after surgery. The data thus supports the hypothesis that infiltrating monocyte-derived cells play a protective role in surgery-induced peritoneal adhesion formation.
Figure 3 Ccr2-deficient mice were more susceptible to peritoneal adhesion formation post-surgery compared with their WT littermates. Number of adhesions (A), adhesion scores (B) and representative adhesion image at day 7 after surgery (C). Relative percentage and total cell number of peritoneal F4/80high resident macrophages (D, E, respectively), monocytes (F, G, respectively), peritoneal F4/80low monocyte-derived macrophages (H, I, respectively), and peritoneal F4/80int macrophages (J, K, respectively). Data shows mean ± SEM, pooled from 5 separate experiments for naïve or 4 separate experiments for day 7, n= 6-9 mice/group; mixed male and female; * P <0.05, *** P <0.001, **** P <0.0001; (A, B) Mann Whitney test, (D–K) ANOVA with Tukey’s multiple comparison test.
As expected, the lack of Ccr2 expression in Ccr2KO mice reduced monocyte influx into the peritoneal cavity compared with WT littermates. Consequently, similar to our data with BALB/c mice (Figure 2C), the relative percentage of Tim4+ cells within F4/80high macrophages at day 7 after surgery in Ccr2KO mice was significantly higher compared with WT littermates (Figure 4A). The lack of monocyte influx in Ccr2KO mice also caused significantly lower relative percentage of Sema4a+ cells within F4/80low (Figure 4B), F4/80int (Figure 4C), and F4/80high macrophage subsets (Figure 4D) compared with WT littermates. In addition, the relative percentage of Lyve-1+ cells within F4/80low (Figure 4E), F4/80int (Figure 4F), and F4/80high (Figure 4G) macrophage subsets in Ccr2KO mice was also significantly lower compared with WT littermates. The relative percentage of CD226 within F4/80low macrophage population did not show any significant difference between the groups (Figure 4H), but Ccr2KO mice had significantly lower total number of CD226+ cells compared with WT littermates (Figure 4I). We also analyzed the activation of all macrophage populations and found that Ccr2KO peritoneal macrophages had significantly lower CD206 (Figure 4J) and Relmα expression (Figure 4K) prior and post-surgery compared with WT littermates. Similar to our flow cytometry data, the level of Relmα in the peritoneal lavage were also significantly lower in Ccr2KO at day 7 post-surgery compared with WT littermates (Figure 4L). The levels of other cytokines, including Ym1, IFN-γ, IL-6, IL-10, IL-12, and IL-13 remained the same between the groups (Supplementary Figure 10).
Figure 4 Peritoneal macrophage markers associated with monocyte-derived and intermediate subsets were altered in Ccr2-deficient mice. Relative % of Tim+ (A) within F4/80high population, Relative % of Sema4a+ within F4/80low (B), F4/80int (C), and F4/80high (D). Relative % of Lyve1+ within F4/80low (E), F4/80int (F), and F4/80high (G). Relative % of CD226+ within F4/80low (H). Total number of CD226+ cells within F4/80low subset (I) Total number of CD206+ (J) and Relmα+ (K) cells within total peritoneal macrophages. Cytokine level of Relmα in peritoneal lavage (L) using ELISA. Data shows mean ± SEM, pooled from 4-5 separate experiments for naïve or 4 separate experiments for day 7, n= 4-6 mice/group; mixed male and female, * P <0.05, ** P <0.01, *** P <0.001, **** P <0.0001, ANOVA with Tukey’s multiple comparison test.
As Ccr2KO mice do not express the Ccr2 gene, monocyte influx is defective throughout their life course, thus, it is not possible to determine whether early influx of monocytes, as observed in C57BL/6 mice on day 3 post-surgery (Figure 1G), was contributing to the reduction in adhesions or whether influx of monocytes at a later stage (day 7 or 14 post-surgery) was also of importance. In addition, due to effects on their replenishment, phenotypically distinct resident macrophages accumulate in Ccr2KO animals with age (26). Therefore, the observed susceptibility to adhesion formation may have been due to altered functional responses of cavity resident macrophages in Ccr2KO mice rather than defective monocyte recruitment. To address this issue, C57BL/6 mice were treated with an anti-CCR2 monoclonal antibody (mAb; clone MC21 (23); injected i.p. at day -1, 0, and 1 after surgery) to specifically deplete monocytes during the early phases of repair. Rat IgG2b was used as isotype control, and mice were analyzed at day 14 post-surgery (Figure 5A). MC21 mAb has been shown to effectively deplete CCR2+ (CD11b+Ly6Chigh) monocytes (23). Similar to our findings with BALB/c and Ccr2KO mice, early depletion of infiltrating monocytes enhanced the susceptibility of C57BL/6 mice to surgery-induced adhesions at day 14 post-surgery compared with isotype control-antibody treated mice (Figures 5B–D). Moreover, the site-specific adhesion tissue in anti-CCR2 mAb treated mice also showed significantly higher collagen deposition at day 14 post-surgery compared with isotype control treated mice (Figures 5D, E). Both at day 3 as well as day 14 post-surgery, no significant difference in the total number of PECs (Figure 5F), F4/80high macrophages (Figure 5G) or monocytes (Figure 5H) could be detected between the groups. However, anti-CCR2 mAb treated mice regained significantly higher Tim4 expression within F4/80high macrophage subset at day 14 post-surgery compared with isotype control-treated mice (Figure 5I), indicative of reduced monocyte-integration in anti-CCR2 Ab treated mice. Of note, unlike BALB/c (Figure 2C) and Ccr2KO mice (Figure 4A), anti-CCR2 mAb treated mice only partially regained Tim4 expression (~ 44%) and did not reach the same level as detected in naïve C57BL/6 mice (~ 92%, Figure 2C), suggesting a delayed integration of monocyte-derived cells. In line with this hypothesis, no significant difference in any of the other peritoneal macrophage markers (e.g. Sema4a, Lyve-1) was found between anti-CCR2 mAb treated and isotype control-treated mice after surgery (Supplementary Figure 11 and 12). Interestingly, the level of Relmα in the peritoneal lavage of anti-CCR2 mAb-treated mice at day 14 post-surgery was significantly higher compared with isotype control-treated mice (Figure 5J), which may indicate a delay in the induction of surgery-induced responses (Figure 2K) or over-compensatory mechanisms. All other cytokines remained similar between the groups (Supplementary Figure 13). Together, the treatment of C57BL/6 mice with a monocyte-depleting antibody thus provided direct evidence that monocytes contribute to protection against adhesion formation.
Figure 5 Depletion of monocyte-derived peritoneal macrophages enhanced susceptibility of C57BL/6 mice to peritoneal adhesions post-surgery. Schematic of experiment approach (A). Number of adhesions (B) and adhesion score at day 14 post-surgery (C). Representative image and corresponding histological appearance (Masson’s trichrome staining) of adhesions at day 14 in mice treated with CCR2-mAb or control IgG2b (D). Collagen analysis in site-specific adhesion histological sections at day 14 after surgery (E). Total peritoneal exudate cells (F). Total cell number of peritoneal F4/80high resident macrophages (G) and monocytes (H) at day 3 and 14 post-surgery. Relative % of Tim4+ cells within F4/80high population (I). Level of Relmα in peritoneal lavage at day 14 (J). Data shows mean ± SEM, pooled from 2 separate experiments, n= 6-8 mice/group; * P <0.05, ** P <0.01; (B, C, E, J) Mann Whitney test, (F–I) ANOVA with Tukey’s multiple comparison test. Scale bar 40μm.
The contribution of peritoneal macrophages to adhesion formation after surgery remains ill-defined, with both a protective and pathological role reported (9, 11, 12). Furthermore, mouse strain differences in susceptibility to surgical adhesion formation following pneumoperitoneum/laparoscopy and uterine horn/side wall trauma was demonstrated in a previous study. (19), but the mechanisms driving these differences remain largely unexplored. Here, we found that C57BL/6 mice showed a significantly lower peritoneal adhesion score after surgery compared with BALB/c mice in agreement with the previous study (19). However, unlike that previous study (19), we found that the susceptibility of BALB/c mice to peritoneal adhesions did not become apparent until day 14 post-surgery. Moreover, site-specific adhesions that did form displayed a relatively lower collagen deposition suggesting the adhesions maybe less mature and more readily degraded. Importantly, C57BL/6 mice showed an enhanced influx of monocytes and monocyte-derived macrophages at day 3 post-surgery. Blocking the recruitment of circulating monocytes in C57BL/6 mice, either by using Ccr2KO mice or antibody-mediated CCR2 depletion, resulted in an enhanced susceptibility to peritoneal adhesions after surgery. Together, these data provide evidence that circulating monocytes or monocyte-derived macrophages help prevent peritoneal adhesions following surgery (Figure 6).
Figure 6 Monocytes and/or monocyte-derived peritoneal macrophages are essential in preventing peritoneal adhesion formation after surgery. BALB/c mice showed a significantly lower influx of monocytes and monocyte-derived macrophages at day 3 post-surgery, leading to greater peritoneal adhesion formation at day 14 post-surgery (A) compared with C57BL/6 mice (B). Blocking the recruitment of circulating monocytes in C57BL/6 mice resulted in an enhanced susceptibility to peritoneal adhesions after surgery (C). The illustration was created using Biorender.com.
Our data complements and advances two previous studies of surgery-induced adhesion formation, in which experimental enhancement of monocyte recruitment showed a protective effect. In a rabbit study, recruitment of inflammatory cells, including monocytes, by intraperitoneal injection of protease peptone prior to surgery reduced the number of peritoneal adhesions formed (9). In a mouse model of ischemic button- induced adhesion formation, thioglycolate or monocyte chemoattractant protein-1 (MCP-1) injection increased inflammatory monocyte influx and reduced adhesion formation (11). In this latter study, transient neutrophil depletion was also proposed as a complementary mechanism for reducing adhesions (11). In this context, it is interesting to note, that although BALB/c mice were more susceptible to peritoneal adhesions in our model, an equivalent increase in neutrophils in the peritoneal cavity at day 3 after surgery was found compared with C57BL/6 mice (Supplementary Figure 14). The relative percentage and total cell number of other populations, including eosinophils, B cells, and T cells are also shown in Supplementary Figure 14. Moreover, Ccr2KO mice have been shown to recruit equivalent numbers of neutrophils in a model of bacteria-induced inflammation (33). Thus, the protective effect of monocytes or monocyte-derived cells in our model seems unlikely to be dependent on an altered neutrophil response.
Similarly, two recent studies suggested that rapid recruitment of F4/80high peritoneal resident macrophages to the site of mesothelial injury may result in the creation of a barrier preventing excessive fibrin attachment and consequently preventing the formation of adhesions after surgery (25, 34). We found that C57BL/6 mice showed a significantly higher number of peritoneal F4/80high resident macrophages prior and at day 3 post-surgery compared with BALB/c mice. Thus, C57BL/6 mice may be inherently protected from peritoneal adhesion formation by forming a macrophage-rich barrier. However, the number and dynamics of the resident macrophage population in both Ccr2KO and antibody-depleted mice were not altered compared with their respective controls, while these mice were still more susceptible to peritoneal adhesion formation. Our data from Ccr2KO and anti-CCR2 mAb depleted animals, both on a C57BL/6 background, therefore argue for an additional protective role for monocytes and monocyte-derived cells as described, independent of a possible protective barrier function of F4/80high resident macrophages.
Of note, we found that the difference in adhesion score between BALB/c and C57BL/6 mice occurred at a later stage of adhesion formation (day 14 post-surgery). Whilst the adhesion score in BALB/c mice remained the same between day 7 and day 14 after surgery, C57BL/6 mice showed a reduced adhesion score at day 14 suggesting that some adhesions that initially formed in C57BL/6 may have been immature and cleared possibly due to lower collagen deposition to stabilize development. Clearance of early fibrin-rich adhesions has been associated with increased fibrinolysis in the initial stages post-surgery (4) and so the protective effect of monocytes or monocyte-derived macrophages may be due to promotion of proteolytic activity and hence degradation of collagen-poor unstable adhesions between days 7 and 14 post-surgery. This hypothesis is consistent with the finding that monocyte-derived macrophages degrade fibrin-rich aggregates that form in response to intraperitoneal bacterial infection (35). Interestingly, the response of resident F4/80high Gata6 dependent macrophages was found to depend on the severity of the insult (34). Minor injury (laser injury) induced cavity resident macrophages to aggregate on the injured site to form a temporary wound covering or barrier. However, with more severe injury (ischemic button), these aggregates expanded to form superaggregates spanning adjacent tissues and became a nidus for subsequent adhesion formation (34). Thus, the protective effects of monocytes or monocyte-derived macrophages in our model may be explained by active degradation of immature adhesions through fibrinolytic activity or by disrupting F4/80high resident macrophage-derived superaggregates.
Importantly, we cannot distinguish whether the protective effect is mediated via monocytes themselves or via macrophages that have differentiated from monocytes in the cavity (24). Differences in the recruitment of monocytes to the peritoneal cavity between BALB/c and C57BL/6 mice were largely restricted to the early phases of the repair process (day 3 post-surgery, Figure 1F) and early but transient depletion of monocytes significantly affected adhesion formation (Figure 5B). Changes in monocyte-derived macrophage populations (F4/80low and F4/80int) were slightly prolonged, but similarly temporary (Figures 1G, H). Thus, it is unclear if these cells act early during the adhesion formation process, but the outcome of these actions was not visible until later (day 14 post-surgery), or whether the cells acquire an activation phenotype at later stages dependent on their earlier influx. Interestingly, we found a marked change in the phenotype of F4/80high resident macrophages at later stages as indicated by the loss of Tim4 expression by these cells (Figure 2C). Tim4 has been linked to the embryonic origin of resident macrophages, while a loss of Tim4 expression indicates integration of monocyte-derived macrophages into the resident pool (24). Thus, the maintained expression of Tim4 by F4/80high macrophages in BALB/c, Ccr2KO, and partially in anti-CCR2 mAb-treated mice indicates a failure to integrate monocyte-derived cells into the resident pool. Importantly, these monocyte-derived resident cells are functionally different to their embryonic-derived counterparts (14). Therefore, it is possible that the protective effect of monocytes is exerted at the level of monocyte-derived, F4/80high Tim4- resident cells. In this case, the reason why we do not observe notable differences during the early stages of the adhesion process may be due to the delay necessitated by the differentiation process of these cells.
Overall, our data suggest that several macrophage populations possess the capacity to prevent adhesion formation, with each acting at different phases of the resolution process (e.g. resident cells early versus. monocyte-derived cells later) and utilizing different, non-redundant mechanisms (e.g. barrier-function versus fibrinolysis). Importantly, the effectiveness of these mechanisms is likely to be further influenced by other factors like severity of the injury (34), the presence of inflammatory stimuli (36) or neutrophil infiltration (11) as well as a possible genetic differences as shown here. Our findings reveal an important role of monocyte-derived cells to prevent adhesion formation during the recovery phase after surgery. The results of our current study may inform the idea that manipulation of monocytes/macrophages may in future be used to ameliorate surgical adhesions. More work, however, is needed in experimental models before this can be translated to humans. It has previously been suggested that injection of an artificial CCR2 ligand (recombinant MCP-1) to attract monocytes to the peritoneal cavity at the time of surgery can reduce adhesion formation in mice, however the effect did not reach statistical significance (11) and MCP-1/CCL2 is not specific for monocytes but also attracts memory T lymphocytes and natural killer (NK) cells (37). Thus, such a treatment may yield unwanted side-effects. Other chemokines acting via CCR2 (e.g. PC3-secreted microprotein (PSPM)) have been suggested to more specifically target monocytes, but their effect on adhesion formation is not known (38). In addition, chemokines or any CCR-2 based treatment may be hampered by genetic polymorphisms in the CCR-2 gene (39). As an alternative systemic CSF-1 delivery has been shown to increase intestinal monocyte and MHC-IIlow macrophage numbers overcoming CCR2 deficiency and promoting the resolution of gut inflammation (40) and, thus, could be adapted for the peritoneal cavity. However, caution is needed as bacterial contamination after surgery can enhance adhesion formation in an EGFR-dependent manner and, importantly, bone marrow-/monocyte-derived macrophages are the main source of EGFR-ligands under these circumstances (36). Therefore, in contrast to our relatively sterile conditions, monocytes/monocyte-derived cells may actually enhance adhesion formation in the presence of bacterial contaminants. Thus, further research is required to identify conclusively the specific phenotype of monocyte-derived cells harboring this protective capacity as well as to decipher their pivotal mechanism of action. Such data will allow the development of an innovative approach in preventing peritoneal adhesion maturation by modulating monocyte-derived macrophages following surgery.
All data sets supporting this study are available on request.
All animal experiments were reviewed and approved by the University of Manchester Animal Welfare and Ethical Review Board and performed under the regulation of the Home Office Scientific Procedures Act (1986) and the Home Office approved licence (P1208AD89).
RS: designed and performed experiments, analyzed data, and wrote the manuscript. KD: perfomed the surgery and edited the manuscript. DR and SH: designed experiments, analyzed data, wrote the manuscript and obtained funding. AW and JA: designed experiments, provided input for interpretation, edited the manuscript and obtained funding. MM: provided input for interpretation and edited the manuscript. All authors contributed to the article and approved the submitted version.
This study was funded by Medical Research Council (MRC) UK grant (MR/S02560X/1) awarded to AW, JA, DR, and SH and MRC UK grant (MR/P02615X/1) awarded to DR.
We would like to thank Dr. John Grainger and Dr. Kara Filbey for sharing the Ccr2-deficient mice. We would also like to thank Dr. Gareth Howell from the flow cytometry facility, staffs in the Bioimaging facility and BSF facility at the University of Manchester, and Stella Pearson for technical assistence sectioning histology samples. Some aspects of this manuscript have previously been released as a preprint (41).
The authors declare that the research was conducted in the absence of any commercial or financial relationships that could be construed as a potential conflict of interest.
All claims expressed in this article are solely those of the authors and do not necessarily represent those of their affiliated organizations, or those of the publisher, the editors and the reviewers. Any product that may be evaluated in this article, or claim that may be made by its manufacturer, is not guaranteed or endorsed by the publisher.
The Supplementary Material for this article can be found online at: https://www.frontiersin.org/articles/10.3389/fimmu.2022.1000491/full#supplementary-material
1. Tang J, Xiang Z, Bernards MT, Chen S. Peritoneal adhesions: Occurrence, prevention and experimental models. Acta biomaterialia (2020) 116:84–104. doi: 10.1016/j.actbio.2020.08.036
2. Herrick SE, Allen JE. Surgical adhesions: A sticky macrophage problem. Science (2021) 371(6533):993–4. doi: 10.1126/science.abg5416
3. Ryan GB, Grobéty J, Majno G. Postoperative peritoneal adhesions: A study of the mechanisms. Am J pathology (1971) 65(1):117.
4. Brokelman WJ, Holmdahl L, Janssen IM, Falk P, Bergström M, Klinkenbijl JH, et al. Decreased peritoneal tissue plasminogen activator during prolonged laparoscopic surgery. J Surg Res (2009) 151(1):89–93. doi: 10.1016/j.jss.2008.01.005
5. Tsai JM, Sinha R, Seita J, Fernhoff N, Christ S, Koopmans T, et al. Surgical adhesions in mice are derived from mesothelial cells and can be targeted by antibodies against mesothelial markers. Sci Trans Med (2018) 10(469):eaan6735. doi: 10.1126/scitranslmed.aan6735
6. Sandoval P, Jiménez-Heffernan JA, Guerra-Azcona G, Pérez-Lozano ML, Rynne-Vidal Á, Albar-Vizcaíno P, et al. Mesothelial-to-mesenchymal transition in the pathogenesis of post-surgical peritoneal adhesions. J pathology (2016) 239(1):48–59. doi: 10.1002/path.4695
7. Kubicka U, Olszewski W, Tarnowski W, Bielecki K, Ziółkowska A, Wierzbicki Z. Normal human immune peritoneal cells: subpopulations and functional characteristics. Scandinavian J Immunol (1996) 44(2):157–63. doi: 10.1046/j.1365-3083.1996.d01-297.x
8. Burnett SH, Beus BJ, Avdiushko R, Qualls J, Kaplan AM, Cohen DA. Development of peritoneal adhesions in macrophage depleted mice. J Surg Res (2006) 131(2):296–301. doi: 10.1016/j.jss.2005.08.026
9. Ar'Rajab A, Dawidson I, Sentementes J, Sikes P, Harris R, Mileski W. Enhancement of peritoneal macrophages reduces postoperative peritoneal adhesion formation. J Surg Res (1995) 58(3):307–12. doi: 10.1006/jsre.1995.1048
10. Hoshino A, Kawamura YI, Yasuhara M, Toyama-Sorimachi N, Yamamoto K, Matsukawa A, et al. Inhibition of CCL1-CCR8 interaction prevents aggregation of macrophages and development of peritoneal adhesions. J Immunol (2007) 178(8):5296–304. doi: 10.4049/jimmunol.178.8.5296
11. Tsai JM, Shoham M, Fernhoff NB, George BM, Marjon KD, McCracken MN, et al. Neutrophil and monocyte kinetics play critical roles in mouse peritoneal adhesion formation. Blood advances (2019) 3(18):2713–21. doi: 10.1182/bloodadvances.2018024026
12. Honjo K, Munakata S, Tashiro Y, Salama Y, Shimazu H, Eiamboonsert S, et al. Plasminogen activator inhibitor-1 regulates macrophage-dependent postoperative adhesion by enhancing EGF-HER1 signaling in mice. FASEB J (2017) 31(6):2625–37. doi: 10.1096/fj.201600871RR
13. Cassado A, D’Império Lima MR, Bortoluci KR. Revisiting mouse peritoneal macrophages: heterogeneity, development, and function. Front Immunol (2015) 6:225. doi: 10.3389/fimmu.2015.00225
14. Louwe P, Badiola Gomez L, Webster H, Perona-Wright G, Bain C, Forbes S, et al. Recruited macrophages that colonize the post-inflammatory peritoneal niche convert into functionally divergent resident cells. Nat Commun (2021) 12(1):1–15. doi: 10.1038/s41467-021-21778-0
15. Ghosn EEB, Cassado AA, Govoni GR, Fukuhara T, Yang Y, Monack DM, et al. Two physically, functionally, and developmentally distinct peritoneal macrophage subsets. Proc Natl Acad Sci (2010) 107(6):2568–73. doi: 10.1073/pnas.0915000107
16. Bain CC, Jenkins SJ. The biology of serous cavity macrophages. Cell Immunol (2018) 330:126–35. doi: 10.1016/j.cellimm.2018.01.003
17. Campbell SM, Knipper JA, Ruckerl D, Finlay CM, Logan N, Minutti CM, et al. Myeloid cell recruitment versus local proliferation differentiates susceptibility from resistance to filarial infection. Elife (2018) 7:e30947. doi: 10.7554/eLife.30947
18. Finlay CM, Parkinson JE, Chan BH, Ajendra J, Chenery A, Morrison A, et al. Genotype and Th2 cells control monocyte to tissue resident macrophage differentiation during nematode infection of the pleural cavity. bioRxiv. (2021) 2021:12.17.472661. doi: 10.2139/ssrn.3992680
19. Molinas CR, Binda MM, Campo R, Koninckx PR. Adhesion formation and interanimal variability in a laparoscopic mouse model varies with strains. Fertility sterility (2005) 83(6):1871–4. doi: 10.1016/j.fertnstert.2004.11.084
20. Boring L, Gosling J, Chensue SW, Kunkel SL, Farese R, Broxmeyer HE, et al. Impaired monocyte migration and reduced type 1 (Th1) cytokine responses in CC chemokine receptor 2 knockout mice. J Clin Invest (1997) 100(10):2552–61. doi: 10.1172/JCI119798
21. Sulaiman H, Gabella G, Davis C, Mutsaers SE, Boulos P, Laurent GJ, et al. Growth of nerve fibres into murine peritoneal adhesions. J pathology. (2000) 192(3):396–403. doi: 10.1002/1096-9896(2000)9999:9999<::AID-PATH710>3.0.CO;2-4
22. Gorvy DA, Herrick SE, Shah M, Ferguson MW. Experimental manipulation of transforming growth factor-β isoforms significantly affects adhesion formation in a murine surgical model. Am J pathol (2005) 167(4):1005–19. doi: 10.1016/S0002-9440(10)61190-X
23. Mack M, Cihak J, Simonis C, Luckow B, Proudfoot AE, Plachý Jí, et al. Expression and characterization of the chemokine receptors CCR2 and CCR5 in mice. J Immunol (2001) 166(7):4697–704. doi: 10.4049/jimmunol.166.7.4697
24. Bain CC, Hawley CA, Garner H, Scott CL, Schridde A, Steers NJ, et al. Long-lived self-renewing bone marrow-derived macrophages displace embryo-derived cells to inhabit adult serous cavities. Nat Commun (2016) 7(1):1–14. doi: 10.1038/ncomms11852
25. Ito T, Shintani Y, Fields L, Shiraishi M, Podaru MN, Kainuma S, et al. Cell barrier function of resident peritoneal macrophages in post-operative adhesions. Nat Commun (2021) 12(1):1–12. doi: 10.1038/s41467-021-22536-y
26. Bain C, Gibson D, Steers N, Boufea K, Louwe P, Doherty C, et al. Rate of replenishment and microenvironment contribute to the sexually dimorphic phenotype and function of peritoneal macrophages. Sci Immunol (2020) 5(48):eabc4466. doi: 10.1126/sciimmunol.abc4466
27. Goswami DG, Rubio AJ, Mata J, Munoz S, Gallegos A, Walker WE. Large Peritoneal macrophages and transitional premonocytes promote survival during abdominal sepsis. Immunohorizons (2021) 5(12):994–1007. doi: 10.4049/immunohorizons.2100086
28. Takenaka E, Van Vo A, Yamashita-Kanemaru Y, Shibuya A, Shibuya K. Selective DNAM-1 expression on small peritoneal macrophages contributes to CD4+ T cell costimulation. Sci Rep (2018) 8(1):1–8. doi: 10.1038/s41598-018-33437-4
29. Liao CT, Rosas M, Davies LC, Giles PJ, Tyrrell VJ, O'Donnell VB, et al. IL-10 differentially controls the infiltration of inflammatory macrophages and antigen-presenting cells during inflammation. Eur J Immunol (2016) 46(9):2222–32. doi: 10.1002/eji.201646528
30. Zwicky SN, Stroka D, Zindel J. Sterile injury repair and adhesion formation at serosal surfaces. Front Immunol (2021) 12, 1692. doi: 10.3389/fimmu.2021.684967
31. Meda C, Molla F, De Pizzol M, Regano D, Maione F, Capano S, et al. Semaphorin 4A exerts a proangiogenic effect by enhancing vascular endothelial growth factor-a expression in macrophages. J Immunol (2012) 188(8):4081–92. doi: 10.4049/jimmunol.1101435
32. Tsou C-L, Peters W, Si Y, Slaymaker S, Aslanian AM, Weisberg SP, et al. Critical roles for CCR2 and MCP-3 in monocyte mobilization from bone marrow and recruitment to inflammatory sites. J Clin Invest (2007) 117(4):902–9. doi: 10.1172/JCI29919
33. Rückerl D, Campbell SM, Duncan S, Sutherland TE, Jenkins SJ, Hewitson JP, et al. Macrophage origin limits functional plasticity in helminth-bacterial co-infection. PloS pathogens. (2017) 13(3):e1006233.
34. Zindel J, Peiseler M, Hossain M, Deppermann C, Lee W, Haenni B, et al. Primordial GATA6 macrophages function as extravascular platelets in sterile injury. Science (2021) 371(6533):eabe0595. doi: 10.1126/science.abe0595
35. Vega-Pérez A, Villarrubia LH, Godio C, Gutiérrez-González A, Feo-Lucas L, Ferriz M, et al. Resident macrophage-dependent immune cell scaffolds drive anti-bacterial defense in the peritoneal cavity. Immunity (2021) 54(11):2578-94.e5. doi: 10.1016/j.immuni.2021.10.007
36. Zindel J, Mittner J, Bayer J, April-Monn SL, Kohler A, Nusse Y, et al. Intraperitoneal microbial contamination drives post-surgical peritoneal adhesions by mesothelial EGFR-signaling. Nat Commun (2021) 12(1):1–17. doi: 10.1038/s41467-021-27612-x
37. Deshmane SL, Kremlev S, Amini S, Sawaya BE. Monocyte chemoattractant protein-1 (MCP-1): an overview. J Interferon Cytokine Res (2009) 29(6):313–26. doi: 10.1089/jir.2008.0027
38. Pei X, Zheng D, She S, Ma J, Guo C, Mo X, et al. The PSMP-CCR2 interactions trigger monocyte/macrophage-dependent colitis. Sci Rep (2017) 7(1):1–14. doi: 10.1038/s41598-017-05255-7
39. Fantuzzi L, Tagliamonte M, Gauzzi MC, Lopalco L. Dual CCR5/CCR2 targeting: opportunities for the cure of complex disorders. Cell Mol Life Sci (2019) 76(24):4869–86. doi: 10.1007/s00018-019-03255-6
40. Farro G, Stakenborg M, Gomez-Pinilla PJ, Labeeuw E, Goverse G, Di Giovangiulio M, et al. CCR2-dependent monocyte-derived macrophages resolve inflammation and restore gut motility in postoperative ileus. Gut (2017) 66(12):2098–109. doi: 10.1136/gutjnl-2016-313144
Keywords: peritoneum, macrophages, adhesion formation, monocytes, post-surgery
Citation: Sahputra R, Dejyong K, Woolf AS, Mack M, Allen JE, Rückerl D and Herrick SE (2022) Monocyte-derived peritoneal macrophages protect C57BL/6 mice against surgery-induced adhesions. Front. Immunol. 13:1000491. doi: 10.3389/fimmu.2022.1000491
Received: 22 July 2022; Accepted: 13 September 2022;
Published: 06 October 2022.
Edited by:
Kari Ann Shirey, University of Maryland, United StatesReviewed by:
Daniel Prantner, University of Maryland, Baltimore, United StatesCopyright © 2022 Sahputra, Dejyong, Woolf, Mack, Allen, Rückerl and Herrick. This is an open-access article distributed under the terms of the Creative Commons Attribution License (CC BY). The use, distribution or reproduction in other forums is permitted, provided the original author(s) and the copyright owner(s) are credited and that the original publication in this journal is cited, in accordance with accepted academic practice. No use, distribution or reproduction is permitted which does not comply with these terms.
*Correspondence: Rinal Sahputra, cmluYWwuc2FocHV0cmFAbWFuY2hlc3Rlci5hYy51aw==; Dominik Rückerl, ZG9taW5pay5ydWNrZXJsQG1hbmNoZXN0ZXIuYWMudWs=; Sarah E. Herrick, c2FyYWguaGVycmlja0BtYW5jaGVzdGVyLmFjLnVr
†These authors share last authorship
Disclaimer: All claims expressed in this article are solely those of the authors and do not necessarily represent those of their affiliated organizations, or those of the publisher, the editors and the reviewers. Any product that may be evaluated in this article or claim that may be made by its manufacturer is not guaranteed or endorsed by the publisher.
Research integrity at Frontiers
Learn more about the work of our research integrity team to safeguard the quality of each article we publish.