- Department of Microbiology and Immunology, University of Oklahoma Health Sciences Center, Oklahoma City, OK, United States
Adjuvant combinations may enhance or broaden the expression of immune responses to vaccine antigens. Information on whether established Alum type adjuvants can be combined with experimental CD1d ligand adjuvants is currently lacking. In this study, we used a murine Clostridioides difficile immunization and challenge model to evaluate Alum (Alhydrogel™), α-galactosylceramide (α-GC), and one of its analogs 7DW8-5 singly and in combination as vaccine adjuvants. We observed that the Alum/α-GC combination caused modest enhancement of vaccine antigen-specific IgG1 and IgG2b responses, and a broadening to include IgG2c that did not significantly impact overall protection. Similar observations were made using the Alum/7DW8-5 combination. Examination of the impact of adjuvants on NKT cells revealed expansion of invariant NKT (iNKT) cells with modest expansion of their iNKTfh subset and little effect on diverse NKT (dNKT) cells. Side effects of the adjuvants was determined and revealed transient hepatotoxicity when Alum/α-GC was used in combination but not singly. In summary these results showed that the Alum/α-GC or the Alum/7DW8-5 combination could exert distinct effects on the NKT cell compartment and on isotype switch to produce Th1-driven IgG subclasses in addition to Alum/Th2-driven subclasses. While Alum alone was efficacious in stimulating IgG-mediated protection, and α-GC offered no apparent additional benefit in the C. difficile challenge model, the work herein reveals immune response features that could be optimized and harnessed in other vaccine contexts.
Introduction
The range of vaccine adjuvants currently deployed in the clinical setting is limited. Aluminum-based ‘Alum’ formulations are and have been for decades, those most commonly used. Commensurate with development of prototype adjuvants that operate through diverse mechanisms, there is interest in development of combination adjuvant platforms (1–3). Such adjuvant combinations may enhance or broaden the expression of immune responses to vaccine efficacy or bring other benefits such as dose sparing.
Alum has a positive track record for efficacy when a Th2-driven humoral immune response is desired, but it does not stimulate good Th1-driven humoral (IgG2c) or cellular (CD8+ CTL) immunity [reviewed in (4, 5)]. Alum-enhanced humoral responses require damage-associated molecular patterns (DAMPs) such as uric acid to be released from Alum-damaged cells (6). Uric acid in turn acts in an inflammasome-dependent manner to boost antigen presentation by APCs such as dendritic cells (DCs) and Th cell priming (7, 8). Alum also leads to recruitment of numerous cell types including monocytes, eosinophils, neutrophils, Natural Killer (NK) cells, and Natural Killer T (NKT) cells to immunization sites (9).
Murine invariant NKT (iNKT) cells express a semi-invariant T cell receptor (TCR) combining a Vα14-Jα18 rearrangement with Vβ chains 8.2, 7, or 2 in mice (10–12). Human iNKT cells express a semi-invariant Vα24,Jα18,Vβ11 TCR (13). The TCR on murine and human iNKT cells recognizes the cell surface CD1d loaded with glycolipid ligands such as α-galactosylceramide (α-GC) on APCs (14). CD1d/glycolipid and TCR interactions facilitate activation of NKT cells leading to regulation of anti-microbial and tumor immunity, autoimmunity and self-tolerance (15). In studies by our group and others, it has been observed that immunization with iNKT-activating ligands enhance humoral and cellular immune responses to co-administered antigens (Ags), pathogens, or tumors (16–28). The α-GC glycolipid enhances adaptive immune responses to malaria, cancer, influenza, and bacteria (and their toxins) (15, 19, 20, 26–36). Although α-GC and its derivatives can induce a strong Th1 response in vivo (32, 34), in the context of humoral immunity, a more balanced Th1/Th2 response is also observed (16, 18, 19, 36). The α-GC adjuvant has a shorter track record than Alum, but is well tolerated in human subjects, and has shown some promise in boosting tumor-specific Cytotoxic T Lymphocyte (CTL) responses (37–39). Following intraperitoneal (i.p.) or intravenous (i.v.) delivery using a polysorbate vehicle, α-GC can over-stimulate iNKT cells and cause functional anergy (40, 41). However, subcutaneous delivery in the absence of polysorbate can avoid iNKT anergy (17). The 7DW8-5 molecule, an analog of α-GC also has potent adjuvant effects on adaptive immune responses (42, 43). 7DW8-5 has a higher affinity for CD1d than α-GC and consequently when delivered by the intramuscular route (i.m.) is retained locally unlike α-GC which disperses and exerts systemic effects (44).
Follicular helper iNKT (iNKTfh) cells represent a subset of iNKT cells that arise through proliferation and activation of a Bcl6 transcription factor-driven differentiation program (45–47). The iNKTfh subset is characterized as TCRβ+, CD1dtetramer+,CD44hi, CXCR5hi, PD-1hi and secrete IL-4 and IL-21 (47, 48). Although a role in B cell help is evident for B cell responses, their precise contribution to T-dependent versus T-independent and primary versus recall/memory remains unclear (31, 46, 49).
Also related to iNKT cells, a group of CD1d-restricted diverse NKT (dNKT) cells has been described which are non-reactive with α-GC and express a variable TCR (50). The dNKT subset is protective in autoimmune diabetes, experimental autoimmune encephalomyelitis and Concanavalin A-induced hepatitis (51–53) and can suppress tumor immuno-surveillance (52). In contrast to iNKT cells that are known to mediate enhanced antibody (Ab) responses against foreign Ag, little is known about dNKT contributions to humoral immunity other than one report by our group documenting reduced responsiveness to Imject™ Alum adjuvant in mice lacking dNKT cells (54).
C. difficile is a Gram positive bacterium that is the leading cause of hospital derived infections, antibiotic-associated diarrhea and pseudomembranous colitis. C. difficile can also cause systemic disease that includes cardiotoxicity and multiple organ dysfunction (55, 56). One of the defense mechanisms and correlates of protection against C. difficile infection (CDI) includes a serum IgG response against C. difficile toxins A and B (TcdA and TcdB) (57–59). We have previously shown that both Alum (Imject™ and Alhydrogel™ (AL)) and the α-GC adjuvant can boost TcdB-specific Ab responses (36). IgG-dependent protection against a toxin challenge and a live pathogen challenge in a mouse infection model has been demonstrated following immunization against TcdB when Alhydrogel™ was used as an adjuvant (36, 60). Evidence is also accumulating that targeting of oligo- or polysaccharide antigens on C. difficile is a viable vaccine strategy (61, 62) and recent studies from our group showed that α-GC could enhance protection following vaccination with C. difficile polysaccharides (63). Although a combination toxin- and carbohydrate-based vaccine may be necessary for success in the clinic, toxin-based approaches allow a clear examination of the contribution of the humoral immune response to protection in challenge models.
In this study we evaluated Alum and α-GC adjuvants singly and in combination for their ability to enhance immunity and protection against C. difficile as compared to either adjuvant alone. We measured TcdB-specific humoral immunity, systemic toxicity, iNKT and dNKT expansion and differentiation into iNKTfh cells, as well as protection against a live C. difficile challenge. We show that a combination of α-GC and Alum can broaden the humoral immune responses and that 7DW8-5 can differentially stimulate iNKT and the Alum / {alpha}-GC combination iNKTfh expansion. However, there was little additional benefit with regard to protection against C. difficile disease and the Alum / {alpha}-GC combination was associated with transient hepatotoxicity. In summary the Alum/α-GC combination may be useful for broadening humoral immunity to some pathogens but may have limited utility for application to C. difficile toxin-based vaccines.
Materials And Methods
Ethics
This study was carried out in accordance with the recommendations in the Guide for the Care and Use of Laboratory Animals of the National Institutes of Health. All animal procedures were approved by the OUHSC Institutional Animal Care and Use Committee.
Reagents
Key reagents were purchased as follows: HRP-conjugated anti-mouse IgM, IgG1, IgG2b, IgG2c, and IgG3 (Southern Biotech, Birmingham, AL); Biotin-conjugated anti-CXCR5 (2G8), and FITC-conjugated anti-CD4 (GK1.5) mAbs and APC-conjugated streptavidin were from BD Biosciences (San Jose, CA). The PE-Cy7-conjugated anti-PD-1 (RPMI-30), PE-conjugated anti-IgD (11-26c.2a), and BV421-conjugated anti-CD44 (IM7) mAbs were purchased from Biolegend (San Diego, CA). FcR-blocking mAb 2.4G2 was from BioXCell (Lebanon, NH). Alhydrogel™ (In vivogen, San Diego, CA); α-GC (Axorra, Farmingdale, NY); 7DW8-5 (Diagnocine, Hackensack, NJ); 2,2’-azino-bis(3-ethylbenzothiazoline-6-sulphonic acid), ABTS (KPL, Gaithersburg, MD); Cefoperazone (Sigma, St. Louis, MO). C. difficile was cultured and TcdB and CTD purified as previously described [28]. The CTD-encoding region of tcdb gene (YP_001087135.1: nucleotides 4961–7111) from C. difficile strain VPI-10463 was codon optimized and cloned into pET15b (Genscript). The CTD gene was amplified using primers 5′-GATCATATGCTGTATGTGGGTAACCG-3′ and 5′-AACGGATCCTTATTCGCTAATAACCA-3′ containing BamHI and Nde1 sites for cloning into pET15b. CTD (representing VPI-10463 TcdB1651–2366) was expressed in Escherichia coli BL21 star DE3 (Invitrogen) and purified by Ni2+ affinity chromatography (HisTrap, GE Life Sciences).
Mice
Female C57Bl/6 mice were purchased from Charles River (Bethesda, MD, USA). Before experiments, all mice were housed under the same specific-pathogen free conditions. Mice were 6-8 week-old mice at the time of immunization and 10-12 weeks old at the time of antibiotic treatment and C. difficile infection.
Adjuvant Dose and Immunizations
Previous work by our group documented that 1 to 4 µg α-GC when administered s.c. led to iNKT expansion and activation without over-stimulation (17), and with the higher dose boosting T-dependent humoral immune responses (16, 17). Similarly 100 µl of a 2% suspension of Alum (Alhydrogel™, In vivogen, San Diego, CA) exerted a strong adjuvant effect on CTD-specific IgG responses in mice (36). Work by the Tsuji laboratory compared effects of 1 µg and 10 µg 7DW8-5 adjuvant on anti-hemagglutinin IgG responses and protection against influenza challenge in mice, showing good protection at the higher dose and more modest effects at the lower dose (42). For this study, Alum, α-GC, and 7DW8-5 doses were reduced to determine if suboptimal protection when adjuvants were administered singly could be enhanced by their combination. Mice were anesthetized with a vaporized 4% isoflurane/96% medical air mix and immunized subcutaneously (s.c). Unless indicated otherwise, mice received the following formulations: 10 µg of CTD in sterile phosphate-buffered saline (PBS), adsorbed to Alum (25 µl of the 2% suspension) (36); CTD mixed with 1 µg α-GC or 2 µg 7DW8-5 (49); CTD adsorbed to Alum and mixed with α-GC or 7DW8-5. For prime boost experiments, mice received NP-KLH adsorbed to Alum then NP-KLH mixed with 7DW8-5 14 days later (or vice versa). Where indicated, mice received adjuvants but not antigens, including in toxicity determinations. The timelines and vaccination schemes for this study are outlined in Figure S1).
Flow Cytometry
Inguinal, and axillary lymph node cells and spleens were isolated by mechanical disruption and red blood cells were removed by hypotonic lysis with Tris-buffered Ammonium Chloride. The cells were suspended in RPMI media with 1% FBS. The cells were incubated with anti-FcR–blocking antibody (2.4G2, 20 µg/ml) for 5 min and stained with fluorochrome conjugated mAbs for 30 min at room temperature. Cells were then washed with ice-cold PBS three times (290 RCF, 5 min, 22°C) and fixed with 2% w/v paraformaldehyde in PBS. The cells were analyzed on a Stratedigm S1200Ex flow cytometer (Stratedigm, San Jose, CA). Data were analyzed with FlowJo software (Tree Star, Ashland, OR).
Toxicity Assay
Mice were immunized s.c. with Alum, α-GC, 7DW8-5, Alum/α-GC, or Alum/7D5-8W in the amounts used for immunization and protection experiments. Heparinized blood samples were collected on days 1, 2 and 7. Blood samples were pooled (3 per group to obtain 150 µl for analysis) and tested within 1 hr using an Abaxa VetScan VS2 veterinary blood analyzer (Union City, CA) in conjunction with a Comprehensive Diagnostic test cartridge which measures the following: Albumin (ALB); Alkaline Phosphate (ALP); Alanine Transaminase (ALT); Amylase (AMY); Bilirubin (TBIL); Blood Urea Nitrogen (BUN); Calcium (CA); Phosphate (PHOS); Creatinine (CRE); Glucose (GLU); Sodium (NA+); Potassium (K+); Total Protein (TP); Globulin (GLOB).
Bleeds
Blood samples were obtained by the retro-orbital route using heparinized capillary tubes. Erythrocytes were removed by centrifugation producing plasma samples that were stored at 4°C or -20°C as required.
ELISA
To measure antigen-specific antibodies, ELISA Max™ enzyme-linked immunosorbent assay (ELISA) 96-well plates (Thermo Fisher Scientific, Rochester, NY). were coated with 10 µg/ml of antigen in Phosphate coating buffer (0.1 M Na2HPO4 in deionized water, pH=9.0) overnight at 4°C. Wells were blocked with 1% Bovine Serum Albumin in PBS-T (PBS 1X, 0.05% tween) for 2 hours at room temperature, and incubated overnight at 4°C with serially –diluted mouse sera. Wells were washed with PBS-T and then incubated for 1h with Horse-Radish Peroxidase (HRP)-conjugated IgM (1:5000), IgG1 (1:8,000), IgG2b (1:5000), IgG2c (1:5000), or IgG3 (1:5000). Wells were washed and developed for 5 min at room temperature with ABTS substrate (KPL, Gaithersburg, MD). A 10% w/v SDS solution was used to stop the reaction. Endpoint Ab titers were determined by measuring the O.D at 405 nm.
Preparation of C. difficile Spores
In this study, the VPI 10463 strain of C. difficile was used for infection of mice (64). C. difficile VPI 10463 spores were prepared and isolated as previously described and all steps were performed anaerobically at 37°C. Briefly, pre-reduced Columbia Broth (BD) was inoculated with a single colony of bacteria. The culture was then transferred and grown in Clospore media, a liquid media that allow production of high titers of C. difficile spores (65). Spores were harvested and stored in sterile water.
C. difficile Infection
C. difficile infection was induced in mice as previously described (60). Briefly, mice were treated with Cefoperazone sodium salt (Sigma-Aldrich, St. Louis, MO) at a final concentration of 0.5 mg/ml in distilled drinking water for five days followed by a two-day sterile water period. Mice were then orally gavaged with 105 CFU of C. difficile VPI 10463 spores. The weights of the mice were monitored once per day for up to 14 days. Fecal samples were collected, and C. difficile bacteria were quantified on day 3 post gavage to assess bacterial burden.
Fecal Bacteria Enumeration
Numbers of shed bacteria were quantified on day 3 post-gavage, unless otherwise indicated. Fecal pellets were homogenized with 1X PBS, serially diluted, plated on TCCFA and cultured under anaerobic conditions at 37°C. CFUs were counted within 24 and 48 hours (66).
Statistics
Data were analyzed using GraphPad Prism 8.1 (La Jolla, CA, USA). A two tailed T-test or a Mann-Whitney test, and One-way ANOVA with Dunnett’s multiple comparison test were used for statistical analysis between two and multiple experimental groups respectively. A Two-way repeated measures ANOVA with Dunnett’s multiple comparisons test was used to determine statistical significance in weight loss over time.
Results
A Single Dose of Alum-Adsorbed CTD Affords Sufficient Protection Against C. difficile but Inclusion of CD1d Ligand Broadens the Humoral Immune Response to TcdB
As expected, naïve mice exhibited severe weight loss over 3 days following C. difficile spore challenge before recovery over a further 7 day period (Figure 1A). Mice immunized with the Alum and α-GC adjuvants in the absence of antigen (CTD) had a similar disease course to naïve mice showing that the adjuvants afforded no protection in the absence of antigen. Immunization with the CTD antigen in the absence of adjuvant resulted in partial protection, blunting overall weight loss, but did not alter the course of disease. Immunization with CTD plus α-GC had a modest effect on weight loss and delayed maximum weight loss by 1-2 days. Immunization with the Alum-adsorbed CTD vaccine was strongly protective with minimal weight loss. Immunization with Alum-adsorbed CTD plus α-GC (referred to hereafter as the combination vaccine), had no further discernable effect on weight loss. The CTD-specific Ab response was dominated by IgG1 (Figure 1B). Alum-adsorbed CTD induced a strong IgG1 response whereas adjuvant alone, CTD alone, and CTD plus α-GC did not. The combination vaccine modestly increased IgG1 titers and the effects were statistically significant. Although the CTD-specific IgG2b responses were lower than that observed for IgG1, effects of the adjuvants singly and in combination were similar. In contrast, all vaccine modalities failed to induce an IgG2c response with the exception of the combination vaccine, which therefore broadened the humoral response. As expected, none of the immunization modalities affected bacterial burden (Figure 1C). This is because the vaccine targets secreted toxins which largely account for pathology associated with C. difficile infection. In a follow up experiment, CTD was tested in combination with a higher 4 µg α-GC dose and followed with a booster vaccine consisting of CTD only. In that experiment, our prior observations that α-GC could enhance the IgG response were observed (36, 49), confirming that α-GC was functional in our study (data not shown). These data demonstrate that inclusion of α-GC in a vaccine platform containing Alum has little effect on protection against C. difficile but does broaden the humoral immune response to include IgG2c.
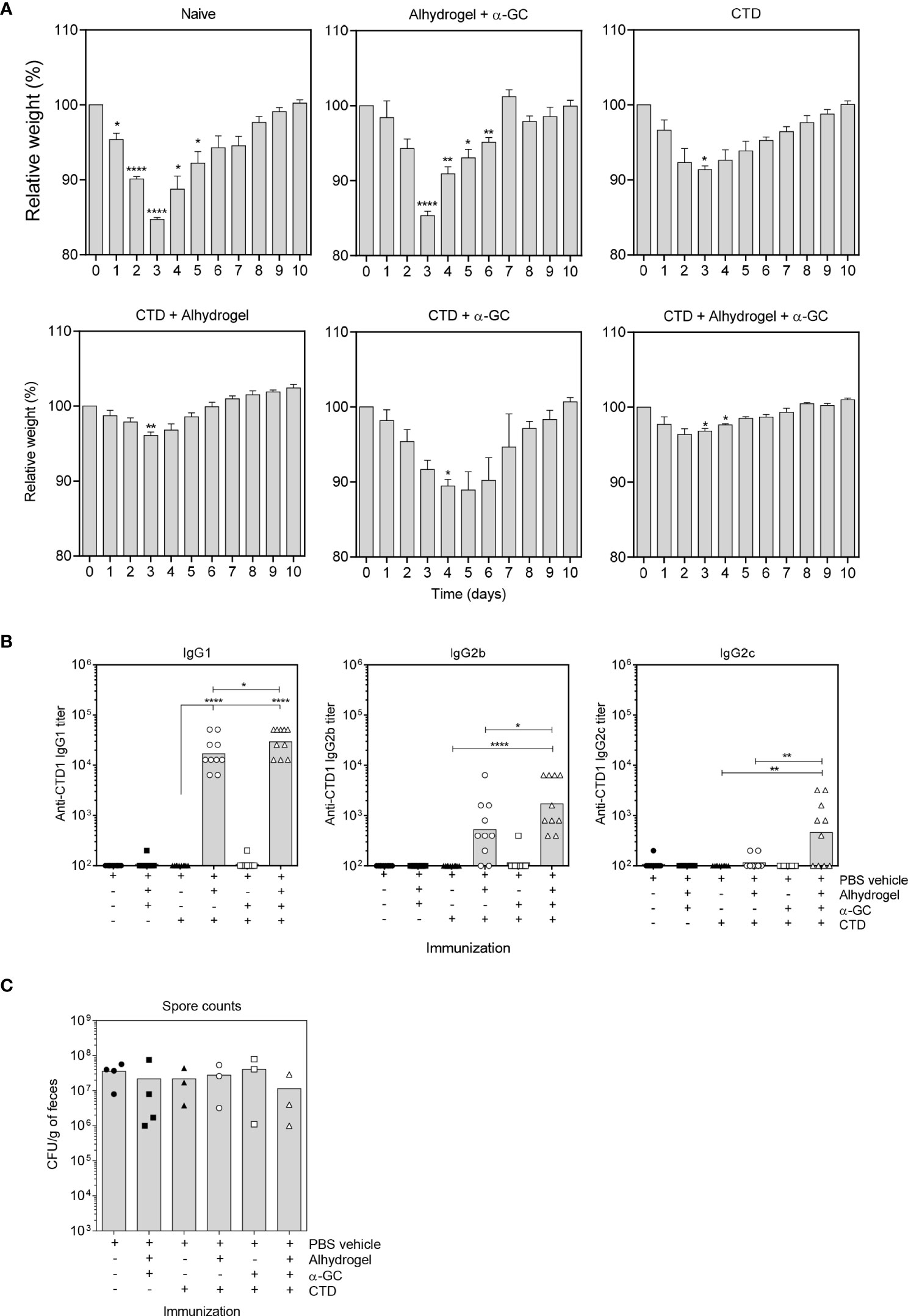
Figure 1 A single dose of Alum-adsorbed CTD affords sufficient protection against C. difficile but inclusion of α-GC broadens the humoral immune response to TcdB. (A) B6 mice were immunized s.c. as indicated with a single dose of each vaccine. After 28 days, mice were bled and treated with Cefoperazone for 5 days. After antibiotic withdrawal and provision of regular drinking water for 2 days, mice were orally gavaged with 5 x 104 live C. difficile spores. Weights were then monitored daily. Graphs show mean ± SEM percentages of pre-infection weights for 5 mice per group. A Two-way repeated measures ANOVA with Dunnett’s multiple comparisons test was used to detect statistically significant differences in weight. Data are representative of two similar experiments. (B) Anti-CTD IgG1 (left), IgG2b (middle), and IgG2c (right) were detected by ELISA. Endpoint titers are shown, and each data point represents an individual mouse. Data from two pooled experiments is shown. Statistical significance was detected by ANOVA with Dunnett’s multiple comparison test. (*P < 0.05; **P < 0.01; ****P < 0.0001). (C) C. difficile spore counts in day 3 fecal pellets were determined as described in materials and methods. Pellets could not be collected from every mouse which was attributed to dehydration.
We also tested a combination vaccine consisting of Alum-adsorbed CTD plus the functional α-GC analog 7DW8-5 to determine if there were discernable features from the response to the α-GC-containing vaccine (Figure 2). As expected, naïve mice lost a significant amount of body weight then mounted a partial recovery showing a more prolonged course of infection than in the previous experiment (Figure 2A). In contrast, immunized mice were protected from weight loss. The Ab titers were similar to that observed when using α-GC as the adjuvant with a high IgG1 titer, moderate IgG2b titer and low IgG2c titer (Figure 2B). C. difficile spore counts in the fecal pellets were unaffected by vaccination as expected (Figure 2C). These data show that 7DW8-5 did not result in changes to Ab profiles or protection beyond that induced by α-GC.
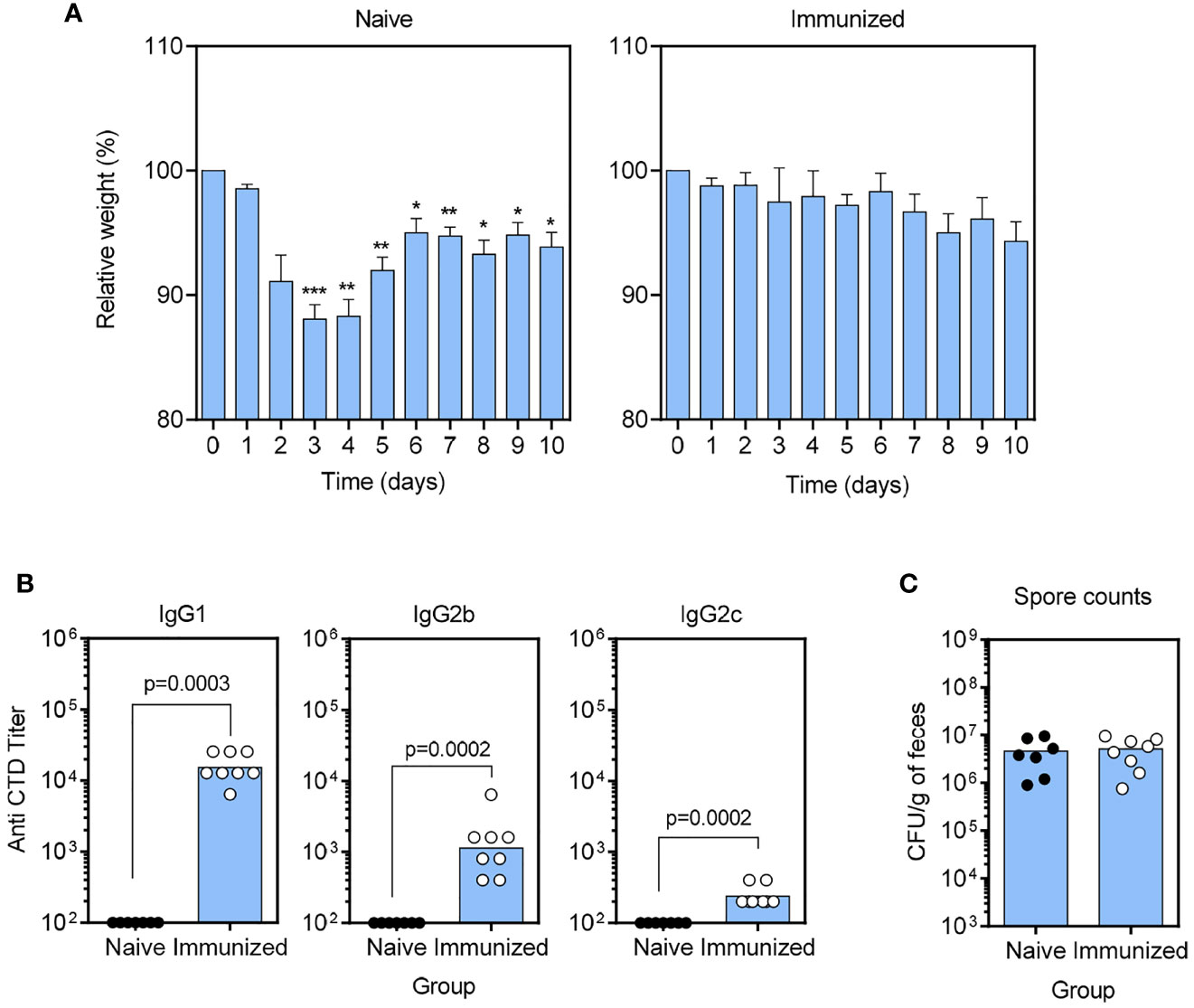
Figure 2 Inclusion of the α-GC analog 7DW8-5 in the CTD/Alum vaccine does not confer changes to IgG subclass or protection beyond that provided by α-GC. (A) B6 mice were immunized s.c. with PBS vehicle (naïve) a single dose of the CTD/Alum plus 7DW8-5 vaccine before antibiotic treatment and C. difficile spore challenge. Weights were then monitored daily. Graphs show mean ± SEM percentages of pre-infection weights (Naïve, n=7, Immunized, n=8). A Two-way repeated measures ANOVA with Dunnett’s multiple comparisons test was used to detect statistically significant differences in weight. (*P < 0.05; **P < 0.01; ***P < 0.001). (B) Anti-CTD IgG1 (left), IgG2b (middle), and IgG2c (right) were detected by ELISA. Endpoint titers are shown, and each data point represents an individual mouse. Statistical significance was detected by 2-tailed Mann-Whitney U test. (C) C. difficile spore counts in day 3 fecal pellets were determined as described in Materials and Methods.
NKT Cell Expansion With Single and Combination Adjuvants
Since α-GC and 7DW8-5 are well established to activate iNKT cells and humoral immune responses to Alum Imject adjuvant have a partial dependence on dNKT cells, we examined these populations by flow cytometry 8 days after adjuvant administration (Figure 3 and Figure S2). Splenocytes were examined for TCRβ+, NK1.1+ lymphocytes which are consistent with iNKT and dNKT cells collectively. Using CD1d tetramers loaded with the PBS57 artificial α-GC ligand allows differentiation between the majority iNKT (TCRβ+, NK1.1+, tetramer+) and minority dNKT (TCRβ+, NK1.1+, tetramer-) populations. When Alum and α-GC were administered singly significant increases in the frequency of iNKT cells and dNKT cells were not observed. The Alum/α-GC combination led to an increase in iNKT cell frequency (Figure 3A and Figure S3A). Neither Alum and α-GC administered singly or combined led to significant increases in absolute numbers of iNKT cells and dNKT cells. However, α-GC and the Alum/α-GC combination led to a significant increase in the iNKT/dNKT cell ratio. 7DW8-5 alone or in combination with Alum had no significant effect on iNKT or dNKT numbers (Figure 3B).
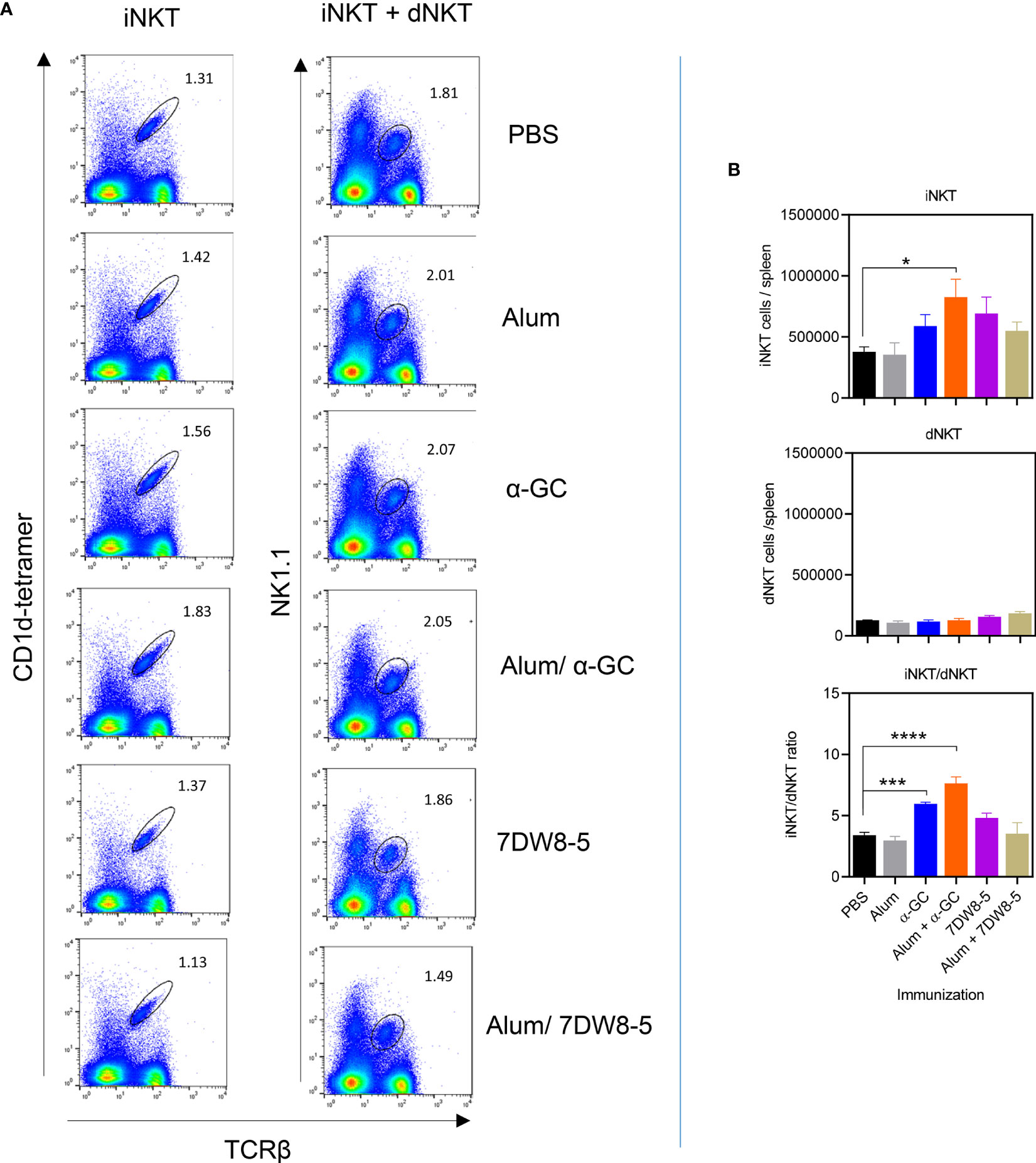
Figure 3 The Alum/α-GC combination adjuvant increases iNKT cell numbers and the iNKT/dNKT ratio. Mice were immunized as indicated and after 8 days, splenocytes prepared and examined by flow cytometry. (A) Shows representative flow cytometry plots of total NKT cells (iNKT and dNKT, left column) and of iNKT cells (right column). (B) Shows mean ± SEM absolute numbers of iNKT cells (upper panel) and dNKT cells (middle panel). dNKT numbers are calculated by subtracting the TcRβ+/tetramer+ population from the TCRβ+/NK1.1+ population. Also shown is the iNKT/dNKT ratio for absolute numbers (lower panel). Data show 3 pooled experiments with similar results (PBS, Alum n=12, α-GC, Alum/α-GC n=11, 7DW8-5, Alum/7DW8-5 n=3). One way ANOVA with Dunnett’s post-test was used to detect significant differences (*P < 0.05; ***P < 0.001; ****P < 0.0001).
Lymph node cells were also analyzed for the presence of iNKTfh cells (Figure 4). The iNKTfh population can be defined as those expressing high levels of PD-1 and CXCR5 within the PD-1+/CXCR5+ population (47). Indeed we previously demonstrated that ablation of the Bcl6 transcription factor in the CD4+ lineage resulted in a selective loss of the PD-1hi/CXCR5hi population (49). Alum did not cause detectable expansion of PD-1+/CXCR+ or PD-1hi/CXCR5hi iNKT cells. The α-GC adjuvant and the Alum/α-GC combination did not cause statistically significant increases in iNKT frequencies or that of the PD-1+/CXCR5+ or PD-1hi/CXCR5hi populations (Figure 4A and Figure S3B). However, α-GC and the Alum/α-GC combination caused statistically significant increases in the absolute numbers of iNKT cells, while Alum/α-GC significantly increased the PD-1+/CXCR5+ population (Figure 4B). The Alum/α-GC combination caused a variable increase in the PD-1hi/CXCR5hi population which was not statistically significant (Figure 4B). The 7DW8-5 adjuvant and the Alum/7DW8-5 combination did not significantly increase frequencies or total numbers of iNKT cells, PD-1lo/CXCRlo or PD-1hi/CXCR5hi iNKT cells. These data show that α-GC and the Alum/α-GC combination in the amounts given expand PD-1+/CXCR5+ iNKT cells but poorly stimulate expansion and differentiation of functional follicular helper PD-1hi/CXCR5hi iNKT cells.
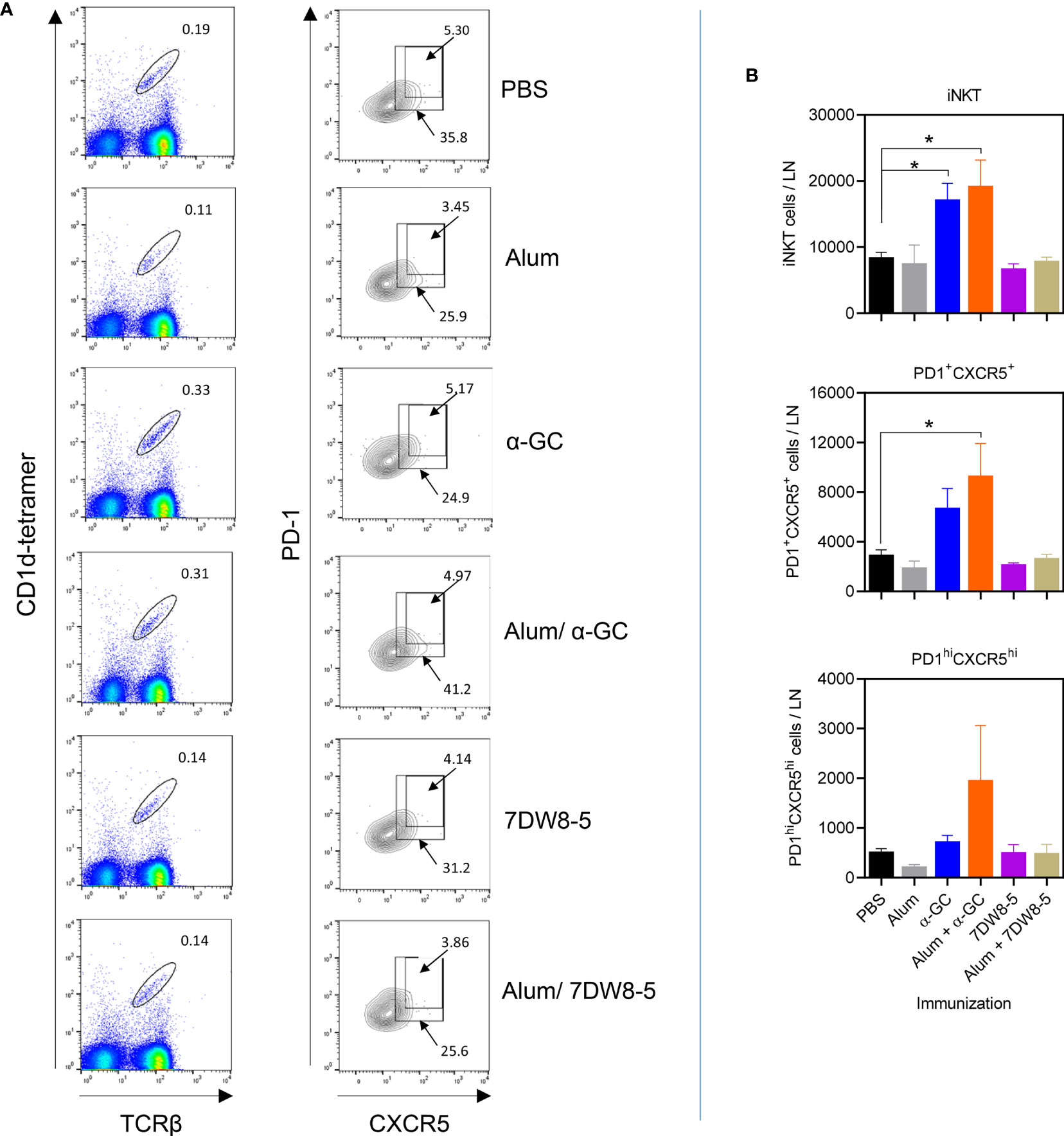
Figure 4 Expansion of iNKTfh cells following administration of single and combination adjuvants. Lymph nodes from mice described in this figure were analyzed by flow cytometry for iNKTfh cells. (A) Shows representative flow cytometry plots of CXCR5+/PD-1+ and CXCR5hi/PD-1hi events after gating on TCRβ+/tetramer+ events. (B) Graphs show mean ± SEM absolute numbers of TCRβ+/tetramer+, CXCR5+/PD-1+ and CXCR5hi/PD-1hi events. Data shown 2 pooled experiments with similar results (PBS, Alum n=6, α-GC, Alum/α-GC n=6, 7DW8-5, Alum/7DW8-5 n=3). One way ANOVA with Dunnett’s post-test was used to detect significant differences (*p < 0.05).
Transient Toxicity on Combining Alum and CD1d-Binding Adjuvants
Following immunization with Alum, or α-GC, significant hepatic toxicity could not be detected in response to either agent and was evidenced by maintenance of baseline levels of Alanine Transferase (ALT) on days 1, 2 and 7 following immunization (Figure 5A). In contrast, 7DW8-5 alone, the combination of Alum/α-GC and of Alum/7DW8-5 led to a transient increase in serum ALT concentration. The ALT concentrations were elevated on days 1 and returned to baseline by day 2 or between day 2 and 7 (Figure 5A). A comparison of ALT concentrations at their peak on day 1, revealed that the Alum/α-GC combination caused more ALT release than 7DW8-5 alone or the Alum/7DW8-5 combination (Figure 5B).
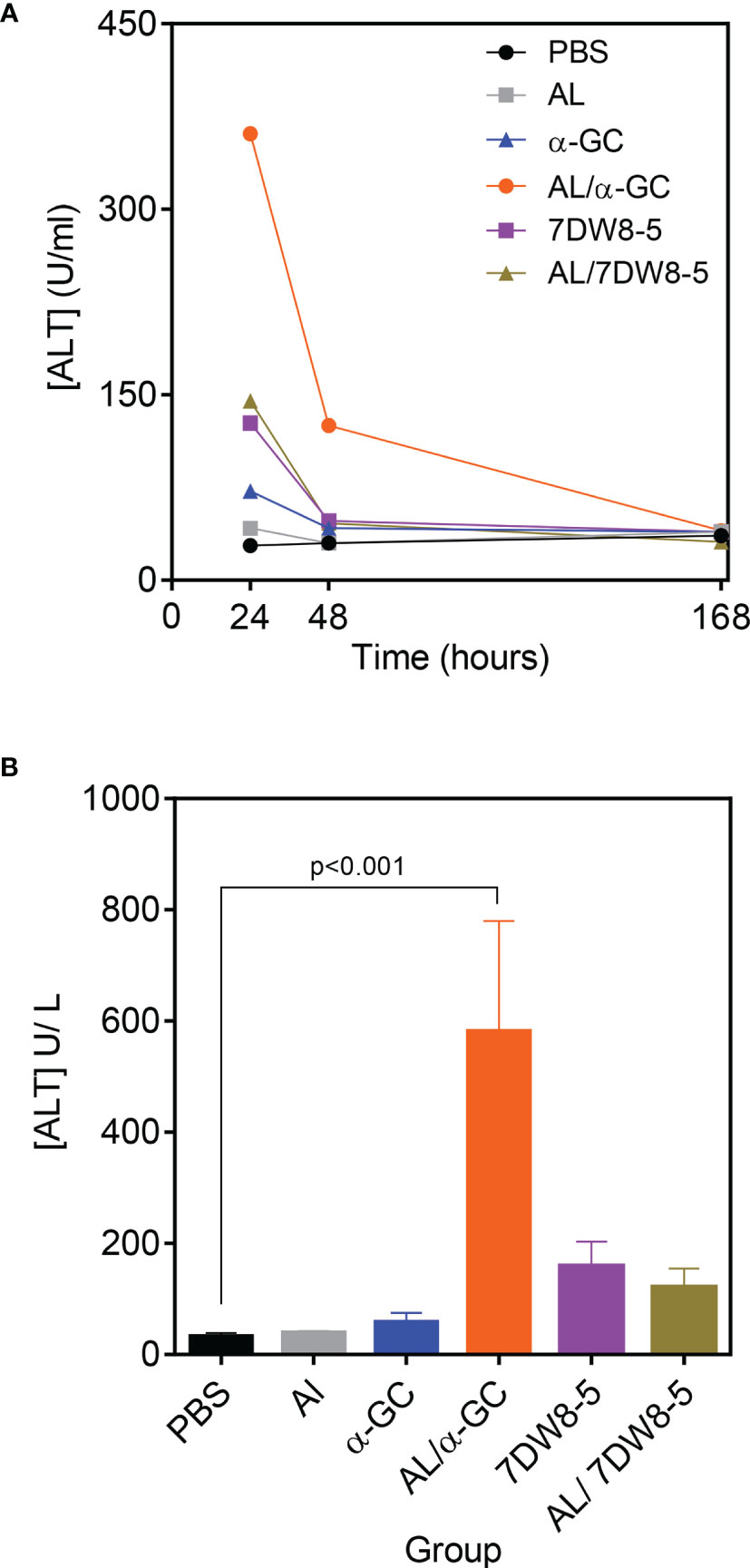
Figure 5 Transient hepatotoxicity on combining Alum and CD1d-binding adjuvants. B6 mice were immunized s.c. with adjuvants indicated singly or in combination and in doses used for other experiments in the study. Heparinized blood samples were collected and pooled and analyzed as described in materials and methods. (A) Graph shows ALT concentration over time and is representative of four independent experiments. Each data point represents pooled blood samples from 3 individual mice. (B) Shows the mean ± SD ALT concentration for 4 experiments at the 24 hr time point. Data were analyzed by ANOVA followed by Dunnett’s post-test.
Several other factors were not significantly affected by the Alum/α-GC or the Alum/7DW8-5 combinations (Table 1). 7DW8-5 alone and both adjuvant combinations caused a temporary drop in Alkaline Phosphatase concentrations for reasons that are unclear. All adjuvants singly and in combination caused a temporary drop in blood glucose concentration which only reached significance with the Alum/α-GC combination. 7DW8-5 alone and the Alum/7DW8-5 combination caused elevations in globulin concentration, but responses were variable and non-significant. This may indicate inflammation following adjuvant administration. These data show that the combination of Alum and CD1d-binding adjuvants resulted in transient hepatotoxicity, and which resolved quickly after immunization. Other factors were not altered to a significant degree, indicating that the adjuvant combination appeared to be generally well-tolerated.
Humoral Immunity Using Alum and 7DW8-5 in a Prime Boost Strategy
Since additive or synergistic effects of administering Alum and α-GC or 7DW8-5 together were not observed, mice were subject to a prime-boost strategy in which they received NP-KLH/Alum in an initial vaccine then NP-KLH/7DW8-5 in a booster vaccine (Figure S4). Controls included omission of the adjuvant from the prime and from the booster vaccine. IgM and IgG2c titers were observed to be uniform across all experimental groups. Clear adjuvant effects of Alum in the priming dose were observed for IgG1, IgG2b, and IgG3. However, 7DW8-5 exerted no additional effect on titers when administered in the booster vaccine. Absence of adjuvant in the priming dose followed by inclusion of 7DW8-5 in the booster failed to exert a significant adjuvant effect on titers. Therefore, as expected, Alum exerted a strong adjuvant effect on Th2 responses (IgG1) and 7DW8-5 when included in a booster vaccine did not alter the IgG subclass profile.
Another group of mice was immunized with NP-KLH/7DW8-5 in the priming dose with NP-KLH/Alum being administered as the booster dose, reversing the order from the previous experiment. Comparison of these two groups revealed no difference in IgM or IgG3 titers in primary or secondary bleeds (Figure S5). In contrast, 7DW8-5 was less effective at stimulating production of IgG1, IgG2b, and IgG2c than Alum as evidenced by primary bleed titers. Upon completion of the immunization schedule, secondary bleed titers revealed that the order in which the adjuvants was administered had no effect on IgM, IgG1, or IgG3 production. However, administration of 7DW8-5 first led to lower IgG2b and IgG2c titers than administration of Alum first. This data therefore suggests that the order in which adjuvants are administered affected Ig class switch and thus the overall production of Th1-driven subclasses (IgG2c).
Discussion
We have shown that the combination of Alum (Alhydrogel™) with α-GC or 7DW8-5 did not confer substantial advantage with regards to protection against C. difficile. This was the case using relatively low amounts of the CTD antigen and Alum which proved to be quite effective. Examination of Ab subclasses revealed that inclusion of α-GC led to increased production of IgG1, IgG2b, and IgG2c, perhaps altering the Th2/Th1 balance induced by Alum. Although this did not translate to advantages in protection against C. difficile, this broadening of the Ab subclasses could be useful in other infectious disease contexts where a Th1-driven Ab response can be beneficial.
Inclusion of α-GC or 7DW8-5 in an Alum/CTD vaccine conferred the same protection against C. difficile and was associated with similar IgG1, IgG2b, and IgG2c profiles. However, α-GC was more efficient at stimulating iNKT cell expansion than 7DW8-5. Interestingly, 7DW8-5 and Alum/7DW8-5 did not stimulate increases in PD-1+/CXCR5+ or PD-1hi/CXCR5hi (iNKTfh) cells. In a previous report, we demonstrated that iNKT cell expansion following α-GC treatment was a product of proliferation and differentiation (46), which contrasts with 7DW8-5. The localized versus systemic distribution of 7DW8-5 and α-GC respectively (44) may be responsible for differential iNKTfh expansion. This is because different CD1d-expressing APCs could present the ligand to distinct iNKT populations in different environments and exert distinct effects on their expansion and differentiation into iNKT cells. This suggests that different CD1d ligands could be engineered to expand iNKTfh cells or to avoid this effect dependent on the response required.
There are several reports that the iNKT cell population can influence memory to protein antigens (17, 21, 27). Thus far it remains unclear whether iNKTfh cells are associated with enhanced memory B cell responses against protein antigens. Some information is available regarding iNKTfh cells and polysaccharide antigens. The Bendelac group demonstrated iNKT/iNKTfh cell-driven anti-polysaccharide responses (31). They reported that immunization with capsular pneumococcal polysaccharides and α-GC resulted in class-switch recombination, affinity maturation and B cell memory but with a limited expansion of iNKTfh cells (31). We were unable to observe convincing Ab recall responses to T-independent carbohydrate Ags co-administered with α-GC although increased iNKTfh expansion, primary Ab responses and class switch were observed (67). Taken together, these studies suggests that iNKTfh cells provide additional B cell help for anti-polysaccharide responses but do not induce B cell memory.
Administration of Alum, α-GC, and 7DW8-5 singly did not result in overt signs of toxicity as evidenced by stable concentrations of serum ALT and several other metabolites and serum proteins. In contrast, the Alum/α-GC combination resulted in a transient elevation of ALT. Approximately 30% of murine hepatic lymphocytes are iNKT cells (68). In humans, the frequency of iNKT cells in liver is much lower at around 0.5% (68) and α-GC has been well-tolerated in the clinic in experimental cancer immunotherapies (39). The Alum/α-GC combination may not be of concern in terms of future administration to humans, but our results do suggest that the systemic distribution of α-GC in combination with Alum can result in subsequent NKT-dependent damage to hepatocytes. Crucially, toxicity can be minimized using 7DW8-5 which remains more localized than α-GC (44).
The data herein, suggest that inclusion of α-GC or its analogs in Alum-based vaccines could be optimized and applied to vaccination against C. difficile or other pathogens. We recently reported that α-GC is a good adjuvant for stimulating protection against C. difficile when surface polysaccharide II (PSII), a T-independent antigen is used for immunization (63). In that study, anti-PSII IgG1 was observed using a PSII/α-GC vaccine and crucially lowered the bacterial burden. Arguably, an Alum/CTD prime followed by a PSII/α-GC booster could be a useful strategy, although as our initial data show, the order of events in a prime boost strategy may be important.
Our findings therefore suggest that α-GC could be optimized for inclusion in existing adjuvant platforms but toxicity, desired Ab subclass, and the order of events in a prime boost strategy will need to be examined. The physicochemical properties of Alum formulations including Alhydrogel such as size, shape, charge, hydration, antigen adsorption, and aggregation can all be manipulated [reviewed in (69)]. Arguably, the interaction of Alum with glycolipid adjuvants warrants investigation and optimization.
Data Availability Statement
The raw data supporting the conclusions of this article will be made available by the authors, without undue reservation.
Ethics Statement
The animal study was reviewed and approved by OUHSC Institutional Animal Care and Use Committee.
Author Contributions
GL designed and performed experiments, analyzed data, and wrote the manuscript. KB-N performed experiments, analyzed data, and edited the manuscript. SA performed experiments. TS performed experiments. JB provided critical resources and input on experiment method and edited the manuscript. ML conceived and designed the project, designed and assisted with experiments, analyzed data, and wrote the manuscript. All authors contributed to the article and approved the submitted version.
Funding
This work was supported by NIH grants AI134719 (ML) and AI119048 (JB).
Conflict of Interest
The authors declare that the research was conducted in the absence of any commercial or financial relationships that could be construed as a potential conflict of interest.
Publisher’s Note
All claims expressed in this article are solely those of the authors and do not necessarily represent those of their affiliated organizations, or those of the publisher, the editors and the reviewers. Any product that may be evaluated in this article, or claim that may be made by its manufacturer, is not guaranteed or endorsed by the publisher.
Acknowledgments
We thank Nicole Reuter, Comparative Medicine, University of Oklahoma Health Sciences for assistance with whole blood analyses. We thank the Laboratory for Molecular Biology and Cytometry Research at OUHSC for the use of the Flow Cytometry service.
Supplementary Material
The Supplementary Material for this article can be found online at: https://www.frontiersin.org/articles/10.3389/fimmu.2021.818734/full#supplementary-material.
References
1. Mount A, Koernig S, Silva A, Drane D, Maraskovsky E, Morelli AB. Combination of Adjuvants: The Future of Vaccine Design. Expert Rev Vaccines (2013) 12:733–46. doi: 10.1586/14760584.2013.811185
2. Mutwiri G, Gerdts V, van Drunen Littel-van den Hurk S, Auray G, Eng N, Garlapati S, et al. Combination Adjuvants: The Next Generation of Adjuvants? Expert Rev Vaccines (2011) 10:95–107. doi: 10.1586/erv.10.154
3. Del Giudice G, Rappuoli R, Didierlaurent AM. Correlates of Adjuvanticity: A Review on Adjuvants in Licensed Vaccines. Semin Immunol (2018) 39:14–21. doi: 10.1016/j.smim.2018.05.001
4. Wen Y, Shi Y. Alum: An Old Dog With New Tricks. Emerg Microbes Infect (2016) 5:e25. doi: 10.1038/emi.2016.40
5. Kool M, Fierens K, Lambrecht BN. Alum Adjuvant: Some of the Tricks of the Oldest Adjuvant. J Med Microbiol (2012) 61:927–34. doi: 10.1099/jmm.0.038943-0
6. Kono H, Rock KL. How Dying Cells Alert the Immune System to Danger. Nat Rev Immunol (2008) 8:279–89. doi: 10.1038/nri2215
7. Marichal T, Ohata K, Bedoret D, Mesnil C, Sabatel C, Kobiyama K, et al. DNA Released From Dying Host Cells Mediates Aluminum Adjuvant Activity. Nat Med (2011) 17:996–1002. doi: 10.1038/nm.2403
8. Kool M, Willart MA, van Nimwegen M, Bergen I, Pouliot P, Virchow JC, et al. An Unexpected Role for Uric Acid as an Inducer of T Helper 2 Cell Immunity to Inhaled Antigens and Inflammatory Mediator of Allergic Asthma. Immunity (2011) 34:527–40. doi: 10.1016/j.immuni.2011.03.015
9. McKee AS, Munks MW, MacLeod MK, Fleenor CJ, Van Rooijen N, Kappler JW, et al. Alum Induces Innate Immune Responses Through Macrophage and Mast Cell Sensors, But These Sensors Are Not Required for Alum to Act as an Adjuvant for Specific Immunity. J Immunol (2009) 183:4403–14. doi: 10.4049/jimmunol.0900164
10. Lantz O, Bendelac A. An Invariant T Cell Receptor Alpha Chain is Used by a Unique Subset of Major Histocompatibility Complex Class I-Specific CD4+ and CD4-8- T Cells in Mice and Humans. J Exp Med (1994) 180:1097–106. doi: 10.1084/jem.180.3.1097
11. Arase H, Arase N, Ogasawara K, Good RA, Onoe K. An NK1.1+ CD4+8- Single-Positive Thymocyte Subpopulation That Expresses a Highly Skewed T-Cell Antigen Receptor V Beta Family. Proc Natl Acad Sci USA (1992) 89:6506–10. doi: 10.1073/pnas.89.14.6506
12. Hayakawa K, Lin BT, Hardy RR. Murine Thymic CD4+ T Cell Subsets: A Subset (Thy0) That Secretes Diverse Cytokines and Overexpresses the V Beta 8 T Cell Receptor Gene Family. J Exp Med (1992) 176:269–74. doi: 10.1084/jem.176.1.269
13. Zeng SG, Ghnewa YG, O'Reilly VP, Lyons VG, Atzberger A, Hogan AE, et al. Human Invariant NKT Cell Subsets Differentially Promote Differentiation, Antibody Production, and T Cell Stimulation by B Cells In Vitro. J Immunol (2013) 191:1666–76. doi: 10.4049/jimmunol.1202223
14. Hayakawa Y, Godfrey DI, Smyth MJ. Alpha-Galactosylceramide: Potential Immunomodulatory Activity and Future Application. Curr med Chem (2004) 11:241–52. doi: 10.2174/0929867043456115
15. Taniguchi M, Harada M, Kojo S, Nakayama T, Wakao H. The Regulatory Role of Valpha14 NKT Cells in Innate and Acquired Immune Response. Annu Rev Immunol (2003) 21:483–513. doi: 10.1146/annurev.immunol.21.120601.141057
16. Lang GA, Exley MA, Lang ML. The CD1d-Binding Glycolipid Alpha-Galactosylceramide Enhances Humoral Immunity to T-Dependent and T-Independent Antigen in a CD1d-Dependent Manner. Immunology (2006) 119:116–25. doi: 10.1111/j.1365-2567.2006.02413.x
17. Devera TS, Shah HB, Lang GA, Lang ML. Glycolipid-Activated NKT Cells Support the Induction of Persistent Plasma Cell Responses and Antibody Titers. Eur J Immunol (2008) 38:1001–11. doi: 10.1002/eji.200738000
18. Lang GA, Devera TS, Lang ML. Requirement for CD1d Expression by B Cells to Stimulate NKT Cell-Enhanced Antibody Production. Blood (2008) 111:2158–62. doi: 10.1182/blood-2007-10-117309
19. Devera TS, Aye LM, Lang GA, Joshi SK, Ballard JD, Lang ML. CD1d-Dependent B-Cell Help by NK-Like T Cells Leads to Enhanced and Sustained Production of Bacillus Anthracis Lethal Toxin-Neutralizing Antibodies. Infect Immun (2010) 78:1610–7. doi: 10.1128/IAI.00002-10
20. Devera TS, Joshi SK, Aye LM, Lang GA, Ballard JD, Lang ML. Regulation of Anthrax Toxin-Specific Antibody Titers by Natural Killer T Cell-Derived IL-4 and IFNgamma. PloS One (2011) 6:e23817. doi: 10.1371/journal.pone.0023817
21. Lang GA, Johnson AM, Devera TS, Joshi SK, Lang ML. Reduction of CD1d Expression In Vivo Minimally Affects NKT-Enhanced Antibody Production But Boosts B-Cell Memory. Int Immunol (2011) 23:251–60. doi: 10.1093/intimm/dxq477
22. Shah HB, Joshi SK, Lang ML. CD40L-Null NKT Cells Provide B Cell Help for Specific Antibody Responses. Vaccine (2011) 29:9132–6. doi: 10.1016/j.vaccine.2011.09.060
23. Shah HB, Joshi SK, Rampuria P, Devera TS, Lang GA, Stohl W, et al. BAFF- and APRIL-Dependent Maintenance of Antibody Titers After Immunization With T-Dependent Antigen and CD1d-Binding Ligand. J Immunol (2013) 191:1154–63. doi: 10.4049/jimmunol.1300263
24. Lang ML. The Importance of B Cell CD1d Expression for Humoral Immunity. Expert Rev Vaccines (2014) 13:1275–8. doi: 10.1586/14760584.2014.932693
25. Fujii S, Shimizu K, Hemmi H, Steinman RM. Innate Valpha14(+) Natural Killer T Cells Mature Dendritic Cells, Leading to Strong Adaptive Immunity. Immunol Rev (2007) 220:183–98. doi: 10.1111/j.1600-065X.2007.00561.x
26. Fujii S, Shimizu K, Kronenberg M, Steinman RM. Prolonged IFN-Gamma-Producing NKT Response Induced With Alpha-Galactosylceramide-Loaded DCs. Nat Immunol (2002) 3:867–74. doi: 10.1038/ni827
27. Galli G, Pittoni P, Tonti E, Malzone C, Uematsu Y, Tortoli M, et al. Invariant NKT Cells Sustain Specific B Cell Responses and Memory. Proc Natl Acad Sci USA (2007) 104:3984–9. doi: 10.1073/pnas.0700191104
28. Ko SY, Ko HJ, Chang WS, Park SH, Kweon MN, Kang CY. Alpha-Galactosylceramide can Act as a Nasal Vaccine Adjuvant Inducing Protective Immune Responses Against Viral Infection and Tumor. J Immunol (2005) 175:3309–17. doi: 10.4049/jimmunol.175.5.3309
29. Bendelac A, Savage PB, Teyton L. The Biology of NKT Cells. Annu Rev Immunol (2007) 25:297–336. doi: 10.1146/annurev.immunol.25.022106.141711
30. Carreno LJ, Kharkwal SS, Porcelli SA. Optimizing NKT Cell Ligands as Vaccine Adjuvants. Immunotherapy (2014) 6:309–20. doi: 10.2217/imt.13.175
31. Bai L, Deng S, Reboulet R, Mathew R, Teyton L, Savage PB, et al. Natural Killer T (NKT)-B-Cell Interactions Promote Prolonged Antibody Responses and Long-Term Memory to Pneumococcal Capsular Polysaccharides. Proc Natl Acad Sci USA (2013) 110:16097–102. doi: 10.1073/pnas.1303218110
32. Hermans IF, Silk JD, Gileadi U, Salio M, Mathew B, Ritter G, et al. NKT Cells Enhance CD4+ and CD8+ T Cell Responses to Soluble Antigen In Vivo Through Direct Interaction With Dendritic Cells. J Immunol (2003) 171:5140–7. doi: 10.4049/jimmunol.171.10.5140
33. Kobrynski LJ, Sousa AO, Nahmias AJ, Lee FK. Cutting Edge: Antibody Production to Pneumococcal Polysaccharides Requires CD1 Molecules and CD8+ T Cells. J Immunol (2005) 174:1787–90. doi: 10.4049/jimmunol.174.4.1787
34. Schmieg J, Yang G, Franck RW, Tsuji M. Superior Protection Against Malaria and Melanoma Metastases by a C-Glycoside Analogue of the Natural Killer T Cell Ligand Alpha-Galactosylceramide. J Exp Med (2003) 198:1631–41. doi: 10.1084/jem.20031192
35. Belperron AA, Dailey CM, Bockenstedt LK. Infection-Induced Marginal Zone B Cell Production of Borrelia Hermsii-Specific Antibody is Impaired in the Absence of CD1d. J Immunol (2005) 174:5681–6. doi: 10.4049/jimmunol.174.9.5681
36. Devera TS, Lang GA, Lanis JM, Rampuria P, Gilmore CL, James JA, et al. Memory B Cells Encode Neutralizing Antibody Specific for Toxin B From the Clostridium Difficile Strains VPI 10463 and NAP1/BI/027 But With Superior Neutralization of VPI 10463 Toxin B. Infect Immun (2016) 84:194–204. doi: 10.1128/IAI.00011-15
37. Chang DH, Osman K, Connolly J, Kukreja A, Krasovsky J, Pack M, et al. Sustained Expansion of NKT Cells and Antigen-Specific T Cells After Injection of Alpha-Galactosyl-Ceramide Loaded Mature Dendritic Cells in Cancer Patients. J Exp Med (2005) 201:1503–17. doi: 10.1084/jem.20042592
38. Nieda M, Okai M, Tazbirkova A, Lin H, Yamaura A, Ide K, et al. Therapeutic Activation of Valpha24+Vbeta11+ NKT Cells in Human Subjects Results in Highly Coordinated Secondary Activation of Acquired and Innate Immunity. Blood (2004) 103:383–9. doi: 10.1182/blood-2003-04-1155
39. Fujii S, Shimizu K, Okamoto Y, Kunii N, Nakayama T, Motohashi S, et al. NKT Cells as an Ideal Anti-Tumor Immunotherapeutic. Front Immunol (2013) 4:409. doi: 10.3389/fimmu.2013.00409
40. Parekh VV, Wilson MT, Olivares-Villagomez D, Singh AK, Wu L, Wang CR, et al. Glycolipid Antigen Induces Long-Term Natural Killer T Cell Anergy in Mice. J Clin Invest (2005) 115:2572–83. doi: 10.1172/JCI24762
41. Sullivan BA, Kronenberg M. Activation or Anergy: NKT Cells Are Stunned by Alpha-Galactosylceramide. J Clin Invest (2005) 115:2328–9. doi: 10.1172/JCI26297
42. Feng H, Nakajima N, Wu L, Yamashita M, Lopes TJS, Tsuji M, et al. A Glycolipid Adjuvant, 7dw8-5, Enhances the Protective Immune Response to the Current Split Influenza Vaccine in Mice. Front Microbiol (2019) 10:2157. doi: 10.3389/fmicb.2019.02157
43. Padte NN, Boente-Carrera M, Andrews CD, McManus J, Grasperge BF, Gettie A, et al. A Glycolipid Adjuvant, 7DW8-5, Enhances CD8+ T Cell Responses Induced by an Adenovirus-Vectored Malaria Vaccine in Non-Human Primates. PloS One (2013) 8:e78407. doi: 10.1371/journal.pone.0078407
44. Li X, Kawamura A, Andrews CD, Miller JL, Wu D, Tsao T, et al. Colocalization of a CD1d-Binding Glycolipid With a Radiation-Attenuated Sporozoite Vaccine in Lymph Node-Resident Dendritic Cells for a Robust Adjuvant Effect. J Immunol (2015) 195:2710–21. doi: 10.4049/jimmunol.1403017
45. Tonti E, Fedeli M, Napolitano A, Iannacone M, von Andrian UH, Guidotti LG, et al. Follicular Helper NKT Cells Induce Limited B Cell Responses and Germinal Center Formation in the Absence of CD4+ T Cell Help. J Immunol (2012) 188:3217–22. doi: 10.4049/jimmunol.1103501
46. Rampuria P, Lang ML. CD1d-Dependent Expansion of NKT Follicular Helper Cells In Vivo and In Vitro is a Product of Cellular Proliferation and Differentiation. Int Immunol (2015) 27:253–63. doi: 10.1093/intimm/dxv007
47. Chang PP, Barral P, Fitch J, Pratama A, Ma CS, Kallies A, et al. Identification of Bcl-6-Dependent Follicular Helper NKT Cells That Provide Cognate Help for B Cell Responses. Nat Immunol (2012) 13:35–43. doi: 10.1038/ni.2166
48. King IL, Fortier A, Tighe M, Dibble J, Watts GF, Veerapen N, et al. Invariant Natural Killer T Cells Direct B Cell Responses to Cognate Lipid Antigen in an IL-21-Dependent Manner. Nat Immunol (2012) 13:44–50. doi: 10.1038/ni.2172
49. Rampuria P, Lang GA, Devera TS, Gilmore C, Ballard JD, Lang ML. Coordination Between T Helper Cells, iNKT Cells, and Their Follicular Helper Subsets in the Humoral Immune Response Against Clostridium Difficile Toxin B. J leukocyte Biol (2017) 101:567–76. doi: 10.1189/jlb.4A0616-271R
50. Arrenberg P, Halder R, Dai Y, Maricic I, Kumar V. Oligoclonality and Innate-Like Features in the TCR Repertoire of Type II NKT Cells Reactive to a Beta-Linked Self-Glycolipid. Proc Natl Acad Sci USA (2010) 107:10984–9. doi: 10.1073/pnas.1000576107
51. Duarte N, Stenstrom M, Campino S, Bergman ML, Lundholm M, Holmberg D, et al. Prevention of Diabetes in Nonobese Diabetic Mice Mediated by CD1d-Restricted Nonclassical NKT Cells. J Immunol (2004) 173:3112–8. doi: 10.4049/jimmunol.173.5.3112
52. Ambrosino E, Terabe M, Halder RC, Peng J, Takaku S, Miyake S, et al. Cross-Regulation Between Type I and Type II NKT Cells in Regulating Tumor Immunity: A New Immunoregulatory Axis. J Immunol (2007) 179:5126–36. doi: 10.4049/jimmunol.179.8.5126
53. Jahng A, Maricic I, Aguilera C, Cardell S, Halder RC, Kumar V. Prevention of Autoimmunity by Targeting a Distinct, Noninvariant CD1d-Reactive T Cell Population Reactive to Sulfatide. J Exp Med (2004) 199:947–57. doi: 10.1084/jem.20031389
54. Shah HB, Devera TS, Rampuria P, Lang GA, Lang ML. Type II NKT Cells Facilitate Alum-Sensing and Humoral Immunity. J leukocyte Biol (2012) 92:883–93. doi: 10.1189/jlb.0412177
55. Steele J, Chen K, Sun X, Zhang Y, Wang H, Tzipori S, et al. Systemic Dissemination of Clostridium Difficile Toxins A and B is Associated With Severe, Fatal Disease in Animal Models. J Infect Dis (2012) 205:384–91. doi: 10.1093/infdis/jir748
56. Hamm EE, Voth DE, Ballard JD. Identification of Clostridium Difficile Toxin B Cardiotoxicity Using a Zebrafish Embryo Model of Intoxication. Proc Natl Acad Sci U S A (2006) 103:14176–81. doi: 10.1073/pnas.0604725103
57. Kelly CP, Kyne L. The Host Immune Response to Clostridium Difficile. J Med Microbiol (2011) 60:1070–9. doi: 10.1099/jmm.0.030015-0
58. Steele J, Mukherjee J, Parry N, Tzipori S. Antibody Against TcdB, But Not TcdA, Prevents Development of Gastrointestinal and Systemic Clostridium Difficile Disease. J Infect Dis (2013) 207:323–30. doi: 10.1093/infdis/jis669
59. Leav BA, Blair B, Leney M, Knauber M, Reilly C, Lowy I, et al. Serum Anti-Toxin B Antibody Correlates With Protection From Recurrent Clostridium Difficile Infection (CDI). Vaccine (2010) 28:965–9. doi: 10.1016/j.vaccine.2009.10.144
60. Amadou Amani S, Shadid T, Ballard JD, Lang ML. Clostridioides Difficile Infection Induces an Inferior IgG Response to That Induced by Immunization and Is Associated With a Lack of T Follicular Helper Cell and Memory B Cell Expansion. Infect Immun (2020) 88(3):e00829–19. doi: 10.1128/IAI.00829-19
61. Monteiro MA. The Design of a Clostridium Difficile Carbohydrate-Based Vaccine. Methods Mol Biol (2016) 1403:397–408. doi: 10.1007/978-1-4939-3387-7_21
62. Broecker F, Wegner E, Seco BMS, Kaplonek P, Brautigam M, Ensser A, et al. Synthetic Oligosaccharide-Based Vaccines Protect Mice From Clostridioides Difficile Infections. ACS Chem Biol (2019) 14:2720–8. doi: 10.1021/acschembio.9b00642
63. Lang GA, Shrestha B, Amadou Amani S, Shadid TM, Ballard JD, Lang ML. Alpha-Galactosylceramide-Reactive NKT Cells Increase IgG1 Class Switch Against a Clostridioides Difficile Polysaccharide Antigen and Enhance Immunity Against a Live Pathogen Challenge. Infect Immun (2021) 89(11):e0043821. doi: 10.1128/IAI.00438-21
64. Theriot CM, Koumpouras CC, Carlson PE, Bergin II, Aronoff DM, Young VB. Cefoperazone-Treated Mice as an Experimental Platform to Assess Differential Virulence of Clostridium Difficile Strains. Gut Microbes (2011) 2:326–34. doi: 10.4161/gmic.19142
65. Perez J, Springthorpe VS, Sattar SA. Clospore: A Liquid Medium for Producing High Titers of Semi-Purified Spores of Clostridium Difficile. J AOAC Int (2011) 94:618–26. doi: 10.1093/jaoac/94.2.618
66. Winston JA, Thanissery R, Montgomery SA, Theriot CM. Cefoperazone-Treated Mouse Model of Clinically-Relevant Clostridium Difficile Strain R20291. JoVE (Journal Visualized Experiments) (2016) 118:e54850. doi: 10.3791/54850
67. Lang GA, Amadou Amani S, Quinn JL, Axtell RC, Lang ML. Immunization-Expanded NKT Follicular Helper Cells Drive IgG1 Isotype Switch Against an Exogenous T-Independent Polysaccharide But Do Not Promote Recall Responses. Immunohorizons (2019) 3:88–93. doi: 10.4049/immunohorizons.1800081
68. Kenna T, Golden-Mason L, Porcelli SA, Koezuka Y, Hegarty JE, O'Farrelly C, et al. NKT Cells From Normal and Tumor-Bearing Human Livers Are Phenotypically and Functionally Distinct From Murine NKT Cells. J Immunol (2003) 171:1775–9. doi: 10.4049/jimmunol.171.4.1775
Keywords: adjuvant, Alhydrogel™, α-galactosylceramide, CD1d, immunization, Alum
Citation: Lang GA, Norman K, Amadou Amani S, Shadid TM, Ballard JD and Lang ML (2022) Use of a Clostridioides difficile Murine Immunization and Challenge Model to Evaluate Single and Combination Vaccine Adjuvants Consisting of Alum and NKT Cell-Activating Ligands. Front. Immunol. 12:818734. doi: 10.3389/fimmu.2021.818734
Received: 19 November 2021; Accepted: 27 December 2021;
Published: 14 January 2022.
Edited by:
Jochen Mattner, University of Erlangen Nuremberg, GermanyReviewed by:
Luc Van Kaer, Vanderbilt University, United StatesDapeng Zhou, Tongji University, China
Paul Savage, Brigham Young University, United States
Copyright © 2022 Lang, Norman, Amadou Amani, Shadid, Ballard and Lang. This is an open-access article distributed under the terms of the Creative Commons Attribution License (CC BY). The use, distribution or reproduction in other forums is permitted, provided the original author(s) and the copyright owner(s) are credited and that the original publication in this journal is cited, in accordance with accepted academic practice. No use, distribution or reproduction is permitted which does not comply with these terms.
*Correspondence: Mark L. Lang, mark-lang@ouhsc.edu